Abstract
1. The magnesium dependence of net and isotopic (using 86Rb as tracer) potassium transport was measured in fed ferret red cells. Bumetanide (0.1 mM) was used to dissect total flux into two components: bumetanide sensitive and bumetanide resistant. 2. Increasing the external magnesium concentration from zero (added) to 2 mM stimulated bumetanide-sensitive uptake by 16% but inhibited the bumetanide-resistant component by about 20%. 3. Ionophore A23187 was used to control internal magnesium concentration. A23187 was usually present in the cells during measurement of isotopic fluxes but was washed away before measurement of net fluxes. The magnesium-buffering characteristics of fed ferret red cells were assessed during these experiments. The cytoplasm acts as a high-capacity, low-affinity magnesium buffer over most of the range. Some high-affinity binding was seen in the presence of A23187 and 2 mM-EDTA. 4. A23187 itself slightly inhibits bumetanide-sensitive potassium transport. 5. Bumetanide-sensitive potassium transport is strongly dependent on the concentration of internal ionized magnesium. Transport is 35% maximal at 10(-7) M and increases up to the maximal rate at 1.3 mM. Further increase in ionized magnesium concentration to 3.5 mM has no additional effect. The curve relating activity to magnesium concentration is steepest at the physiological magnesium concentration. The effects of changing magnesium concentration are fully reversible. 6. Reduction of internal ionized magnesium concentration to 10(-7) M with A23187 and EDTA approximately doubles bumetanide-resistant potassium transport. 7. Bumetanide-sensitive fluxes occur via the sodium-potassium-chloride co-transport system under the conditions used. Results described in this paper thus suggest that internal magnesium may be an important physiological controller of sodium-potassium-chloride co-transport activity.
Full text
PDF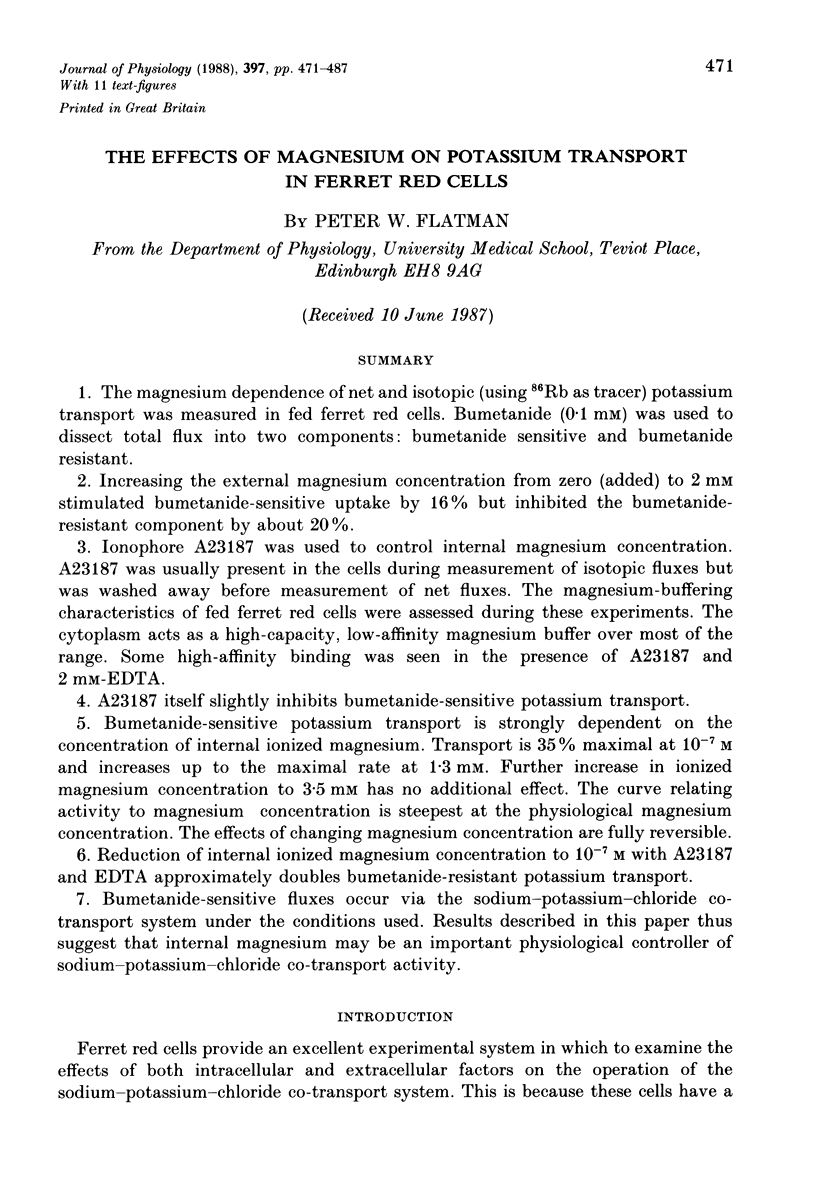
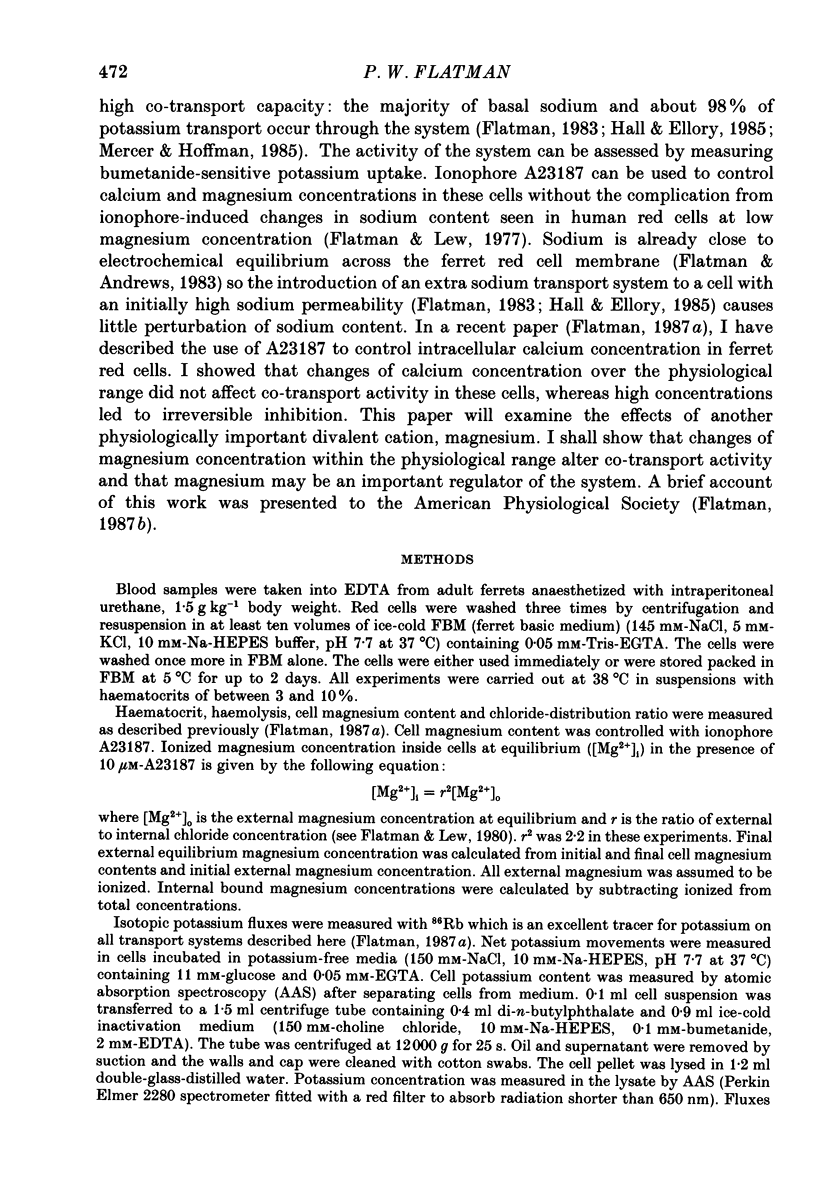
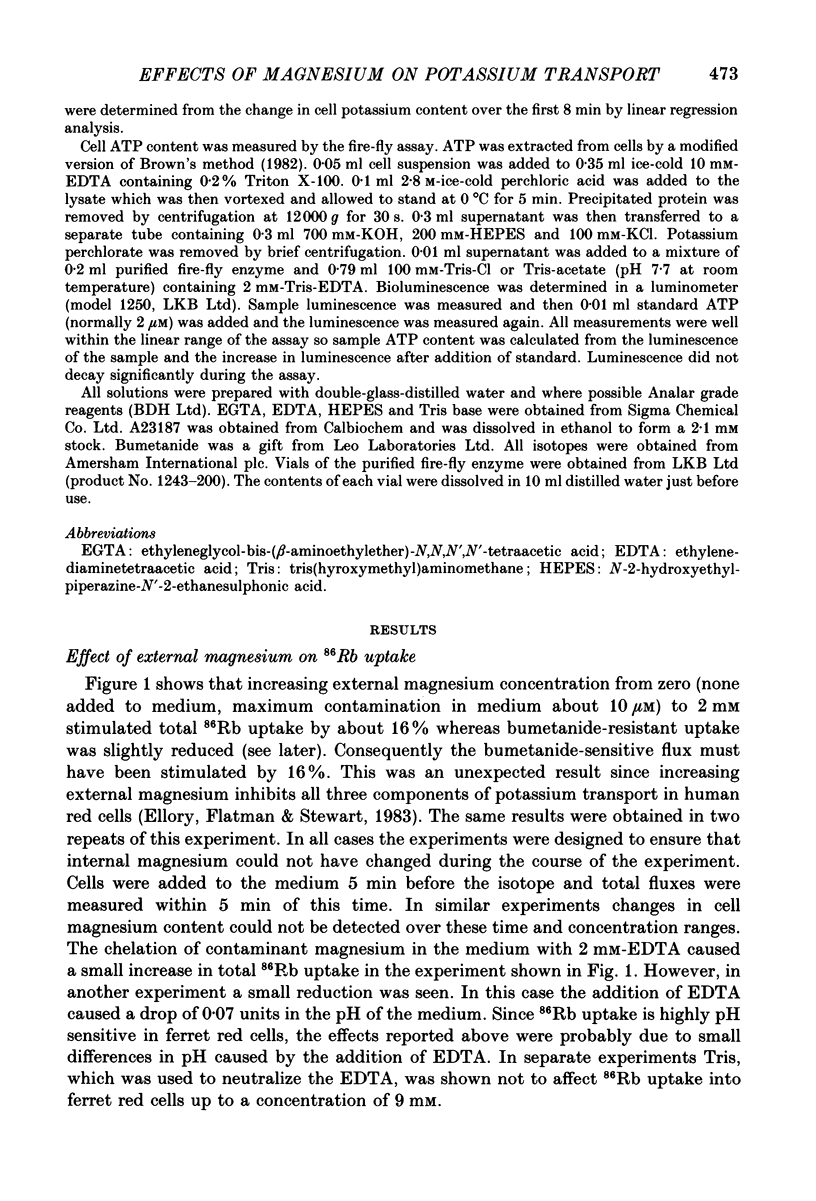
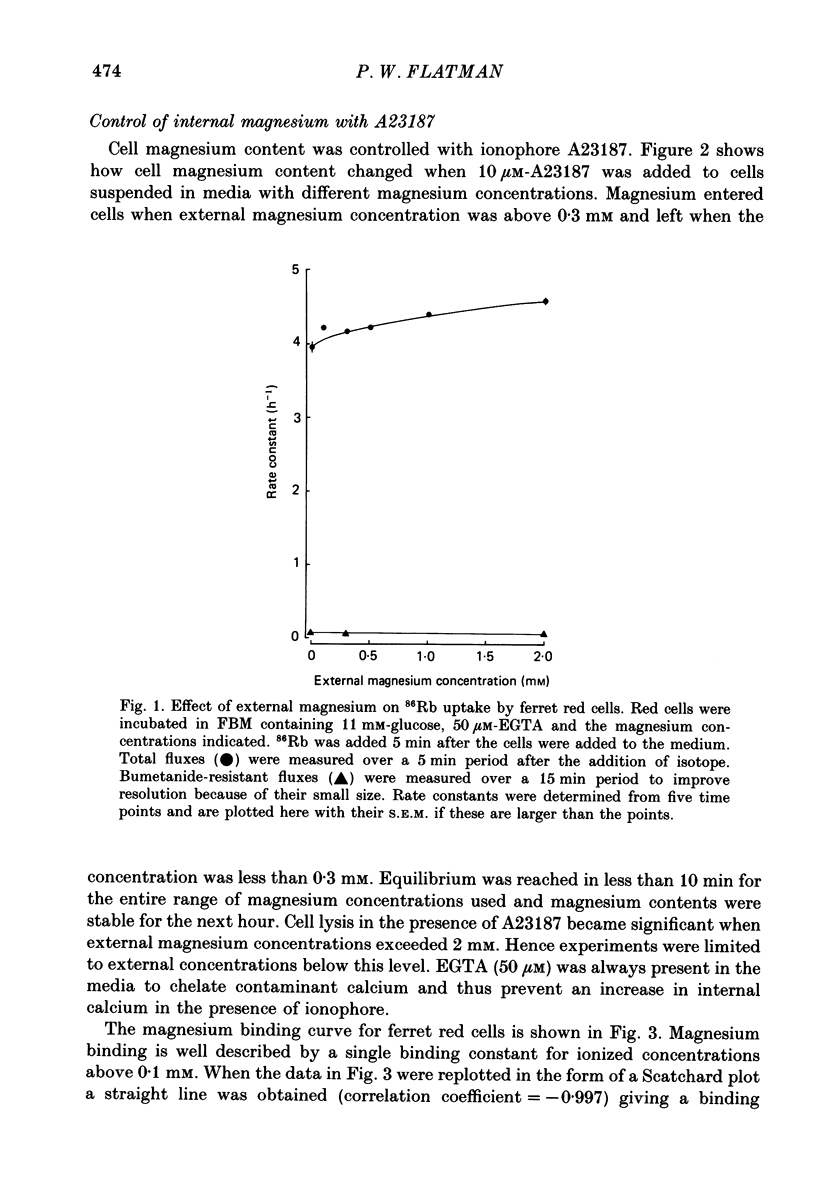
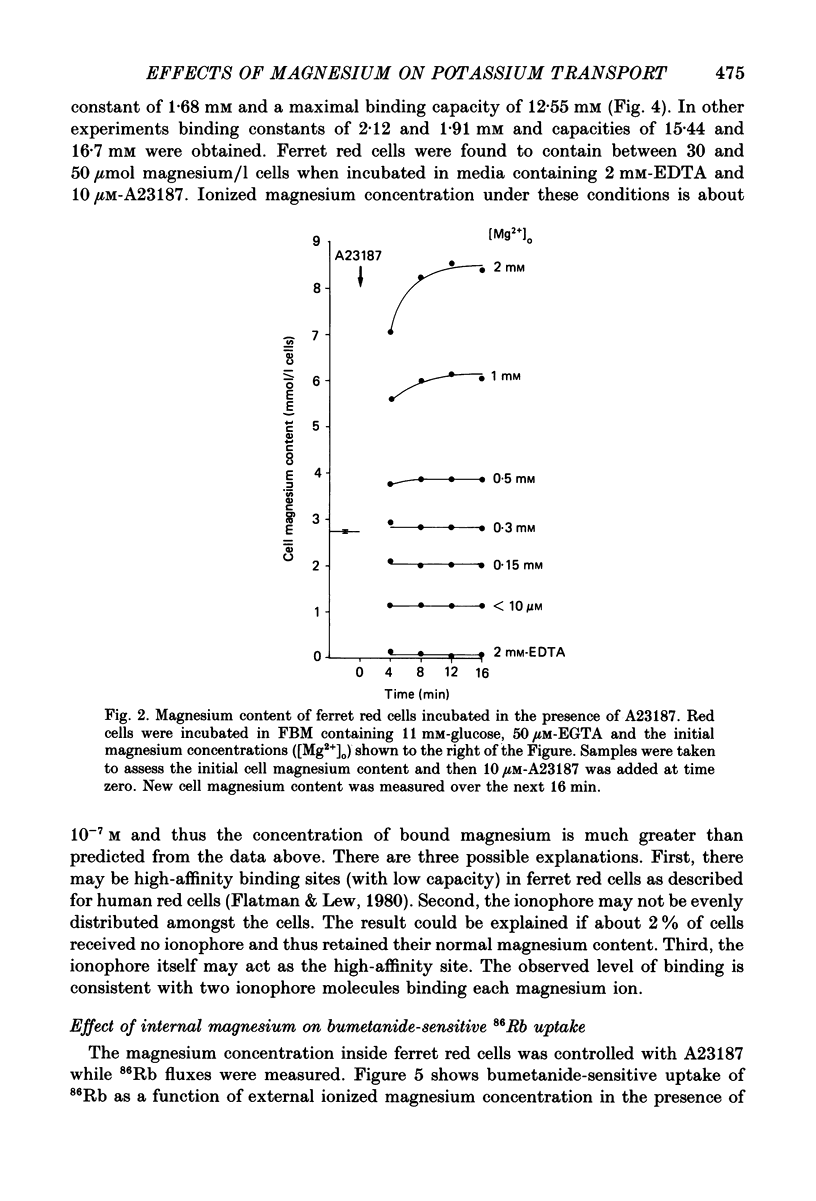
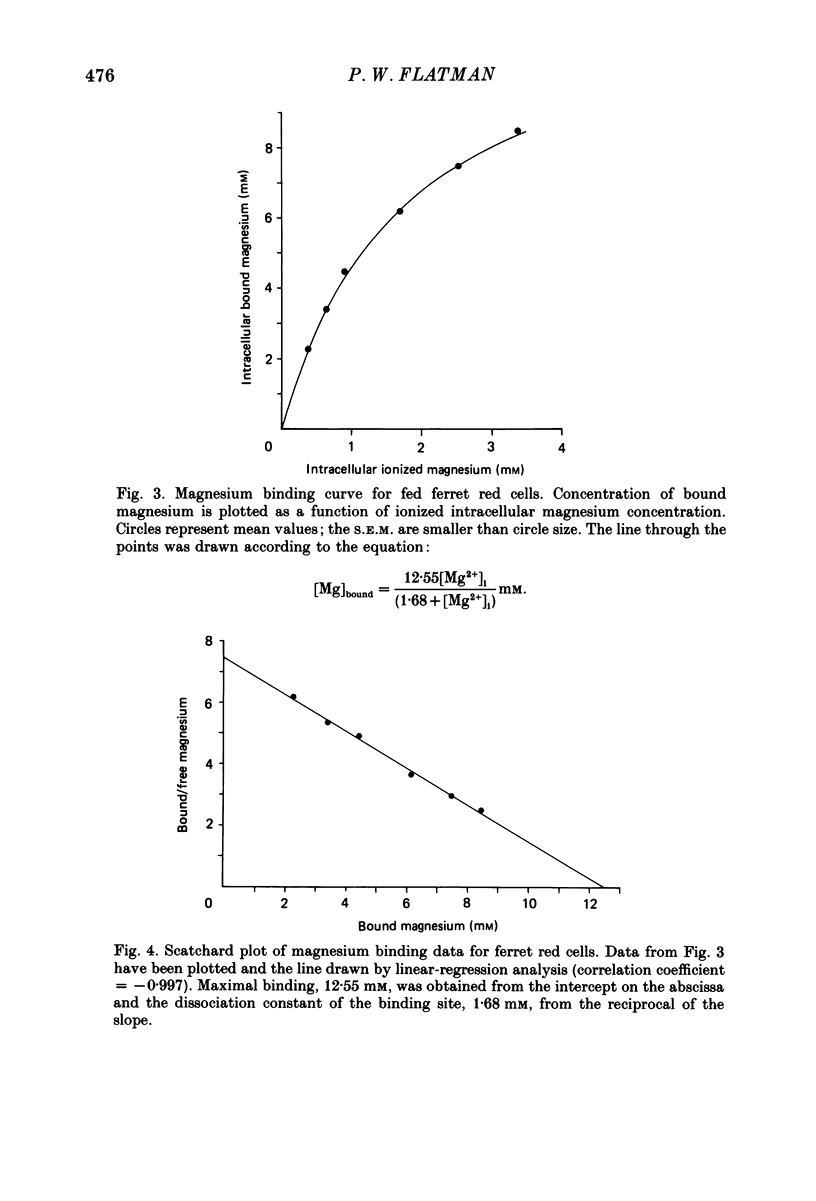
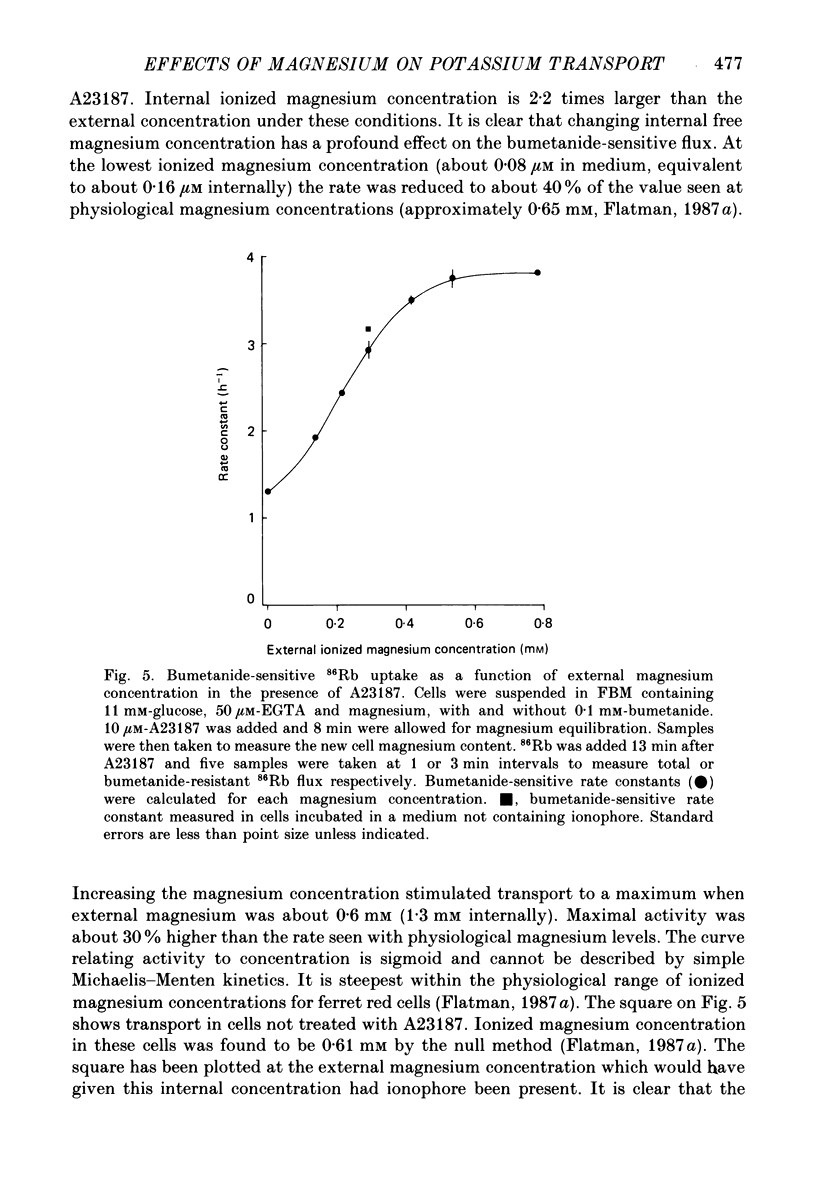
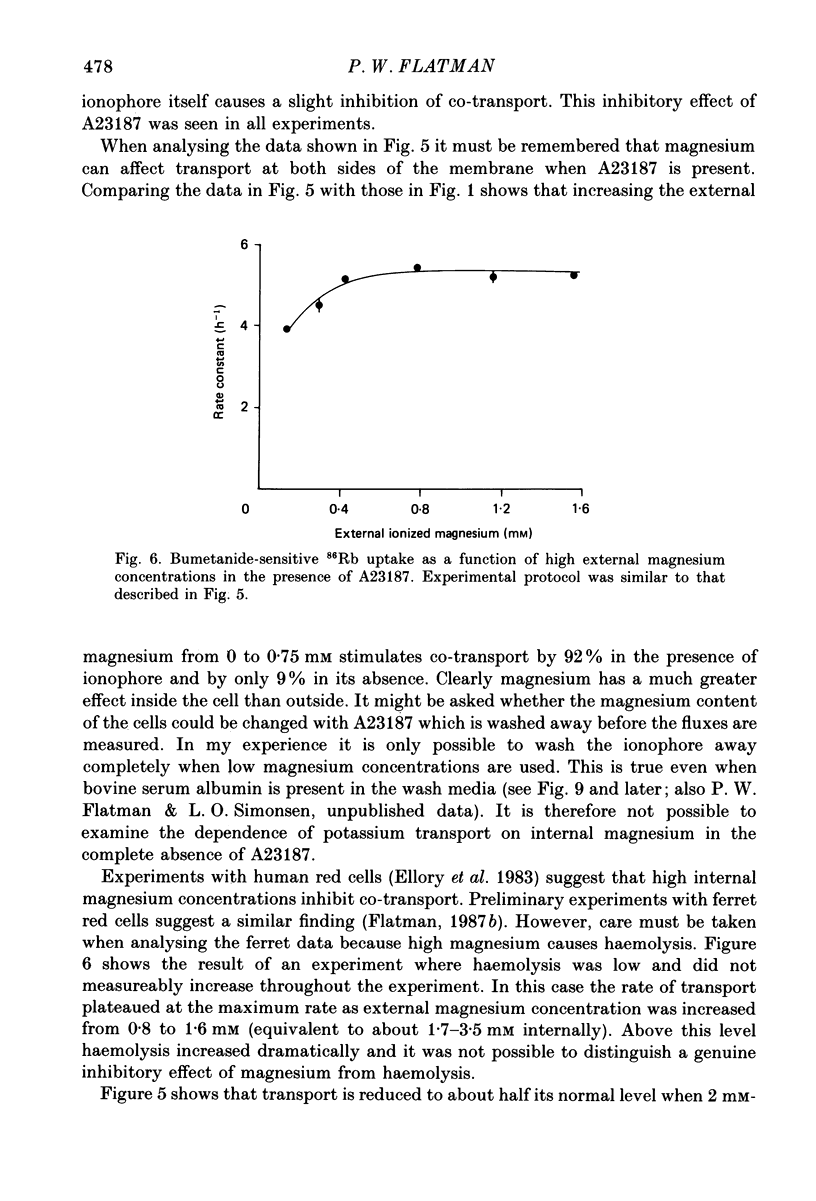
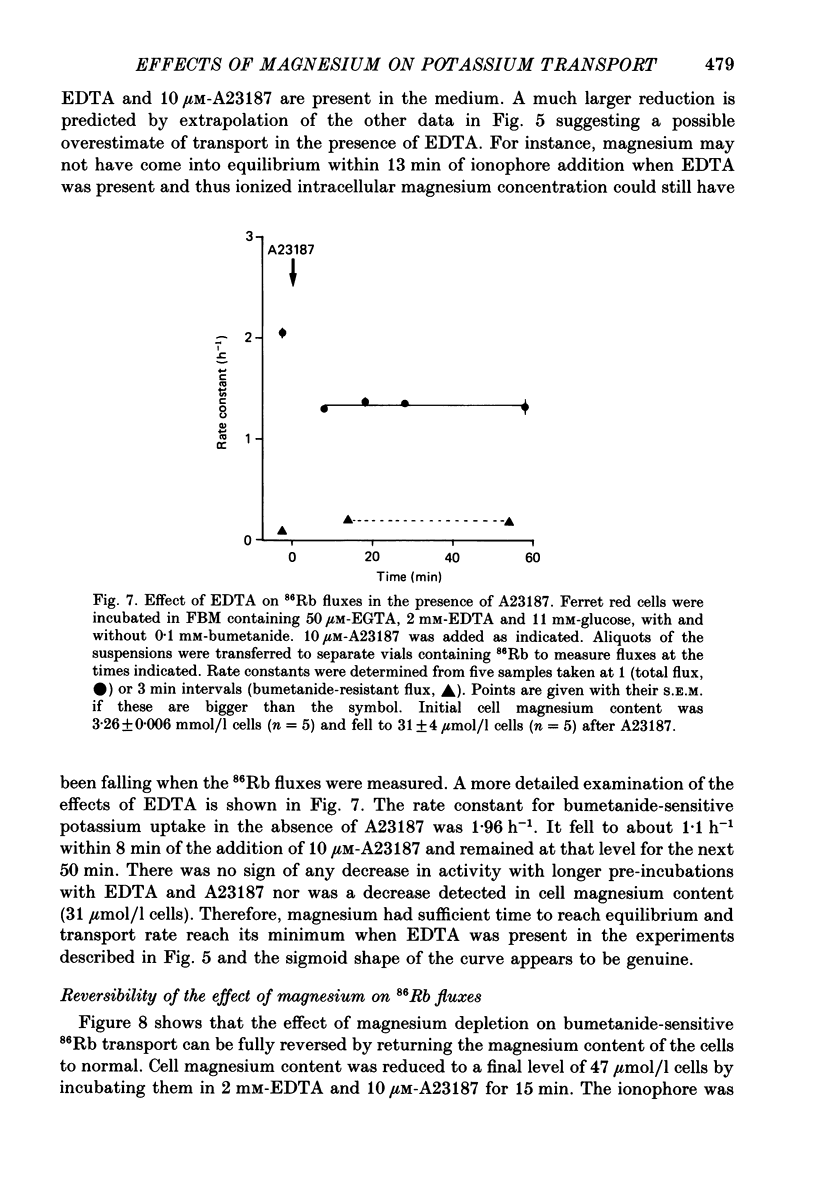
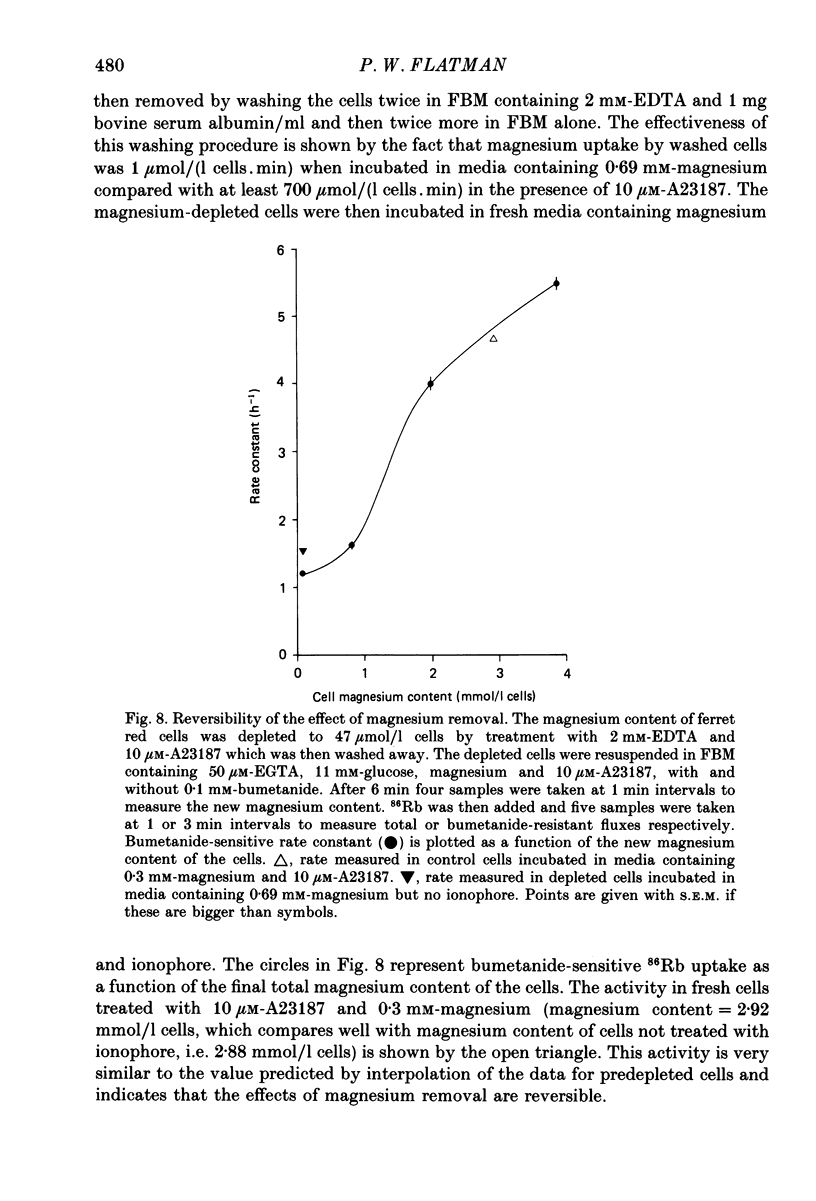
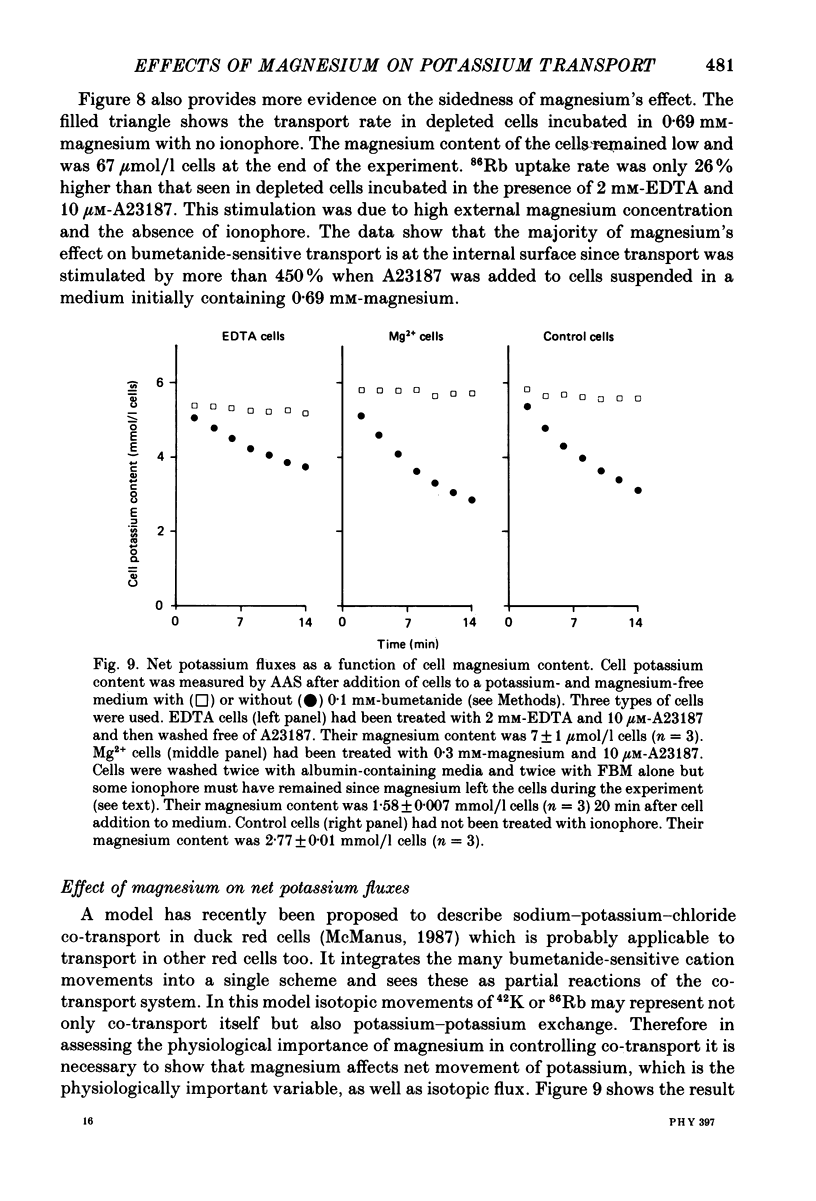
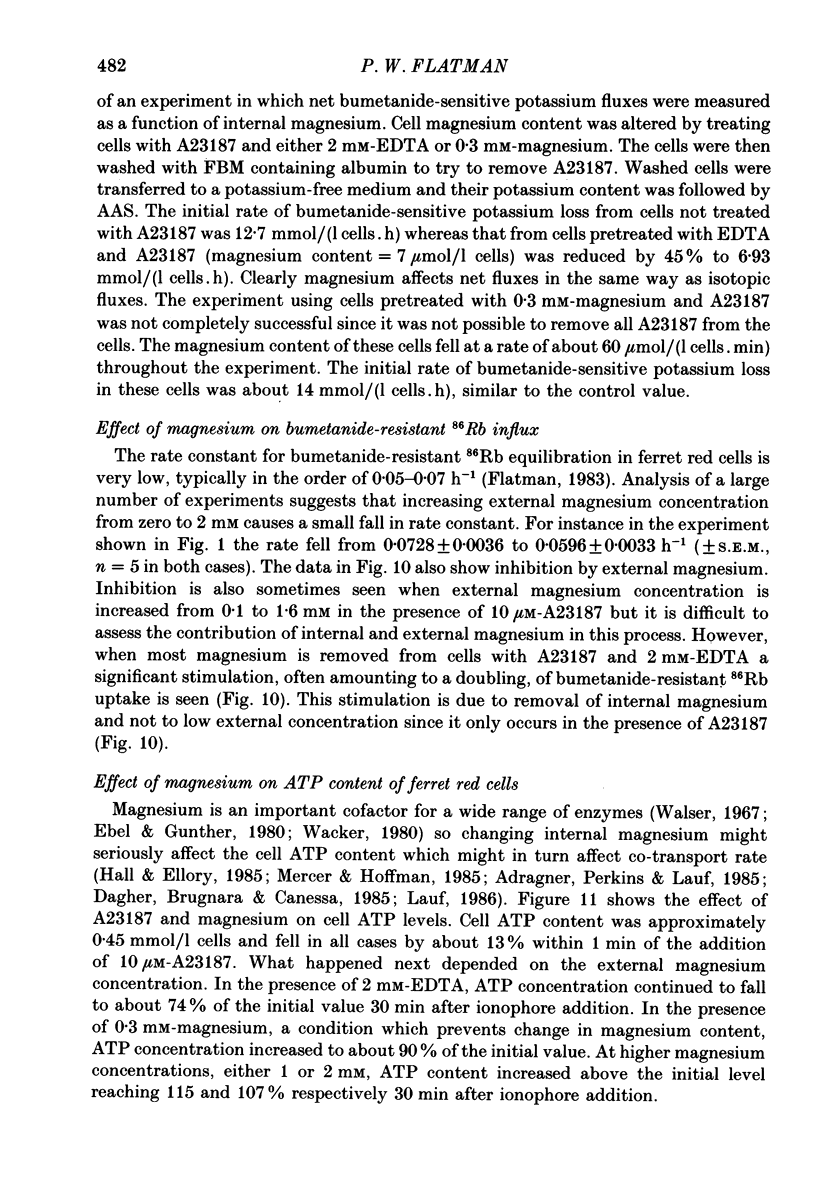
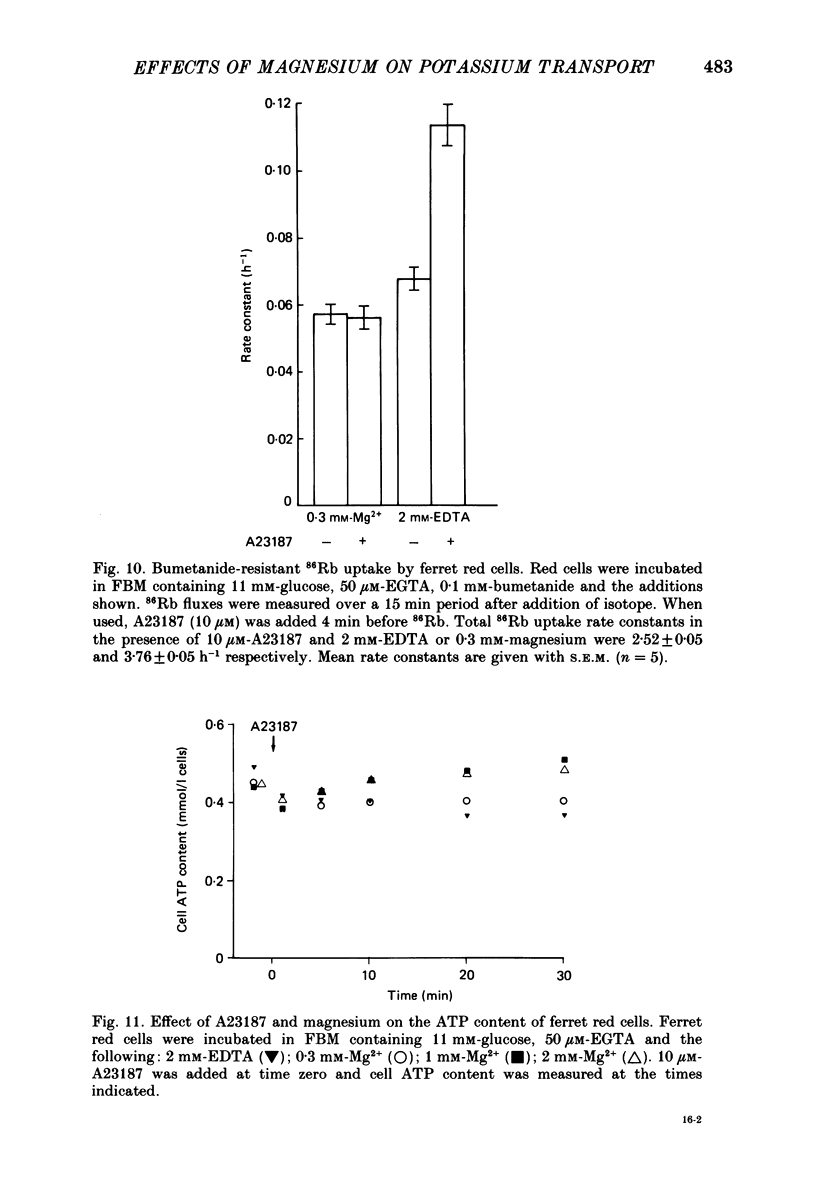
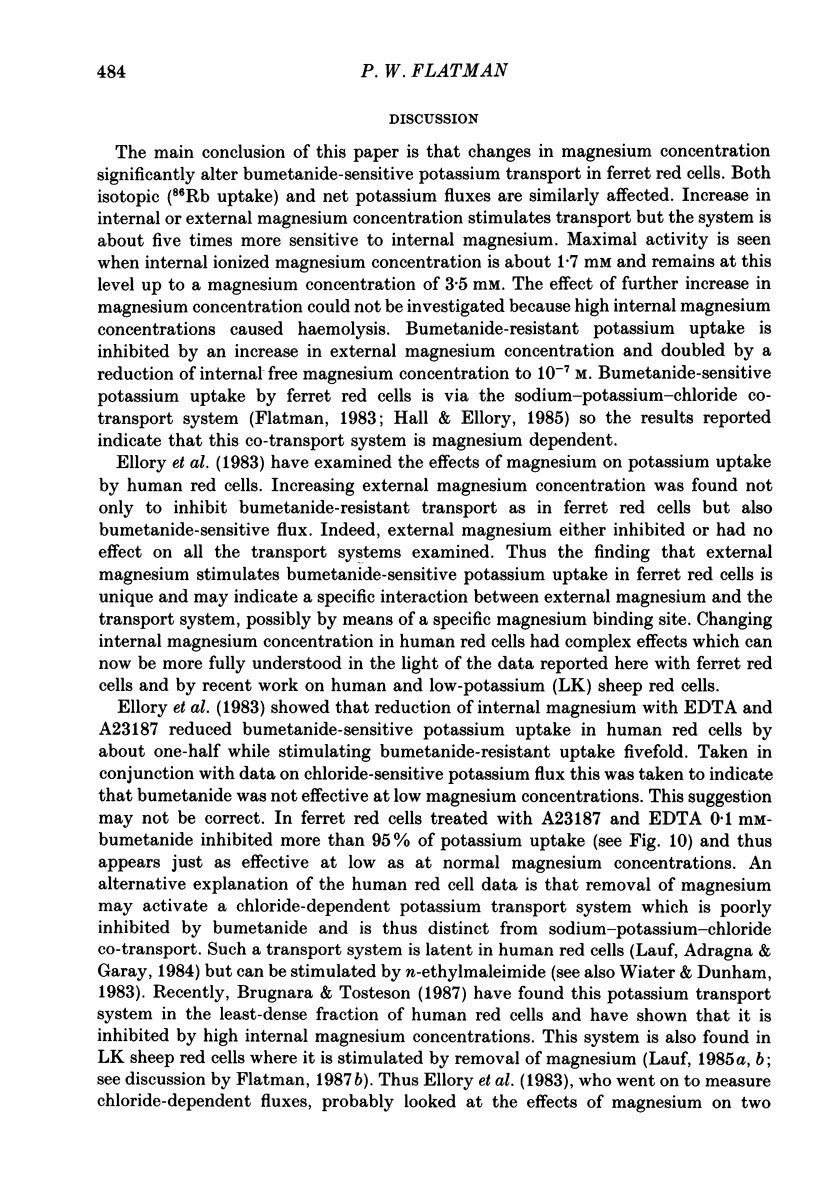
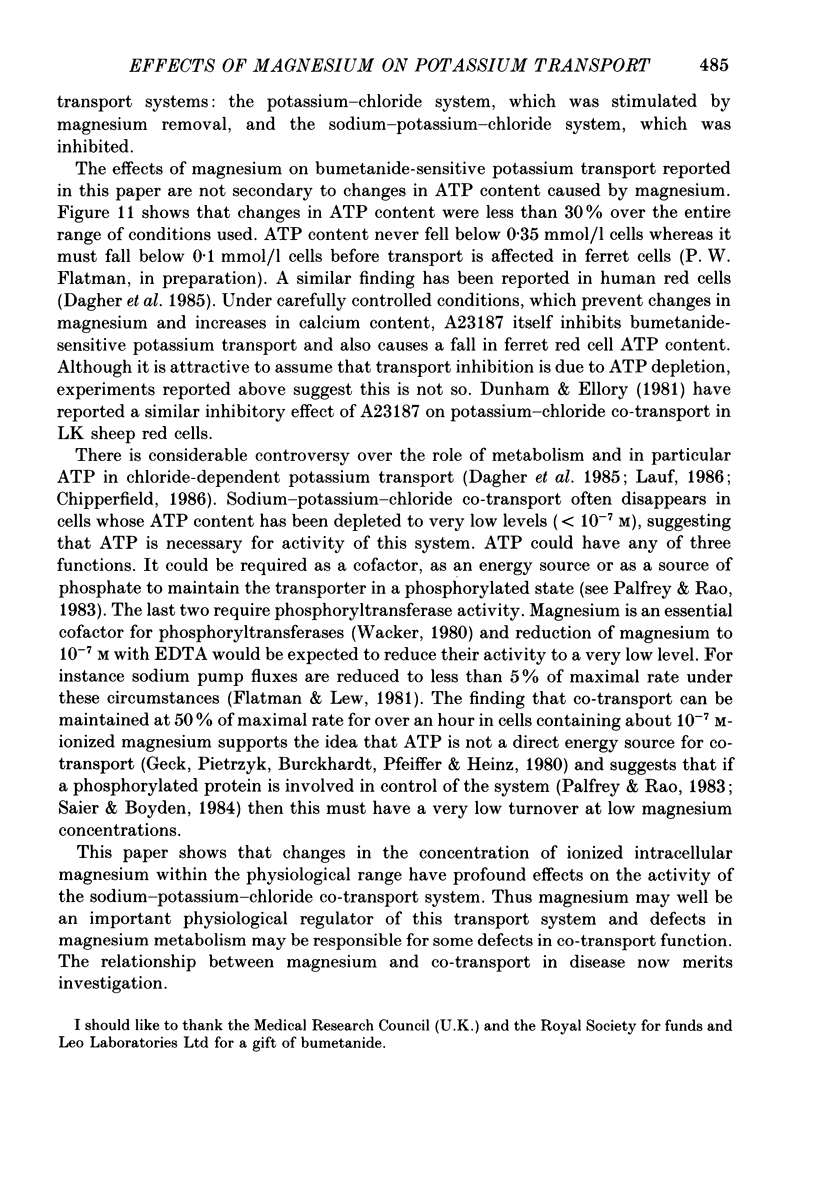
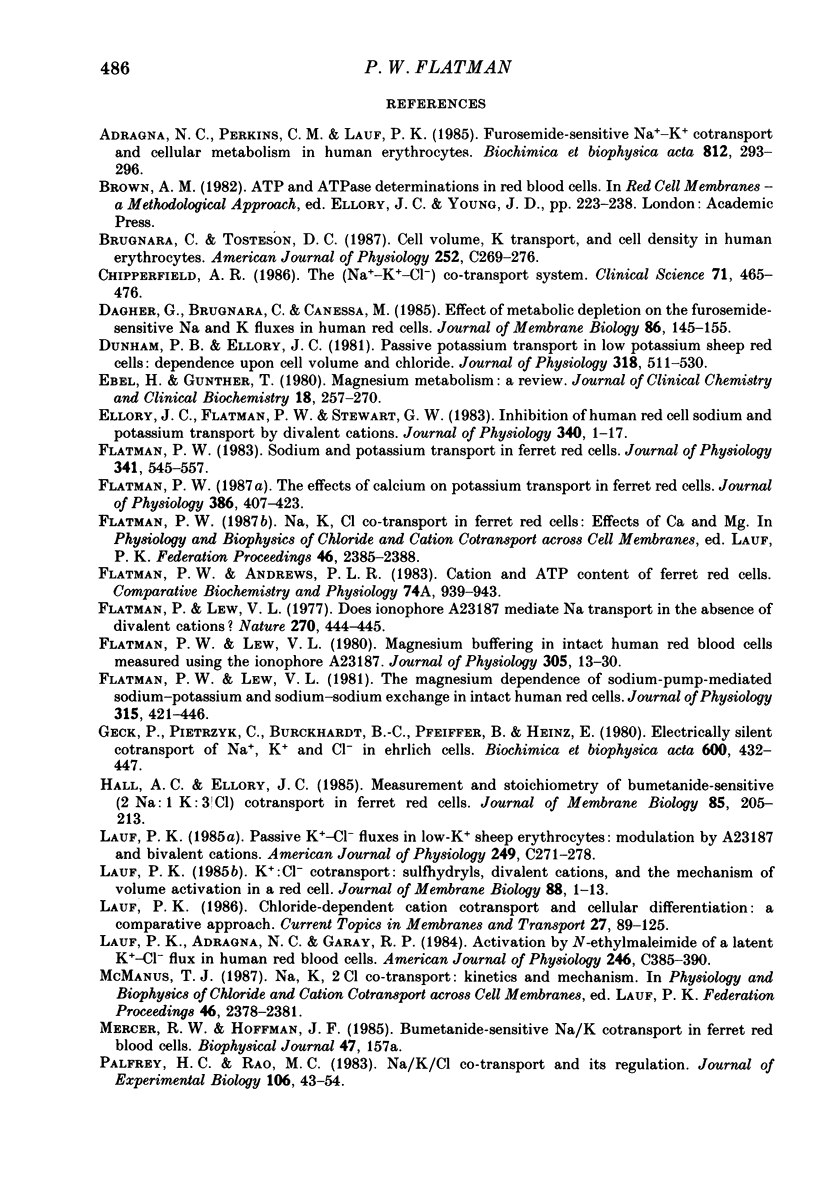
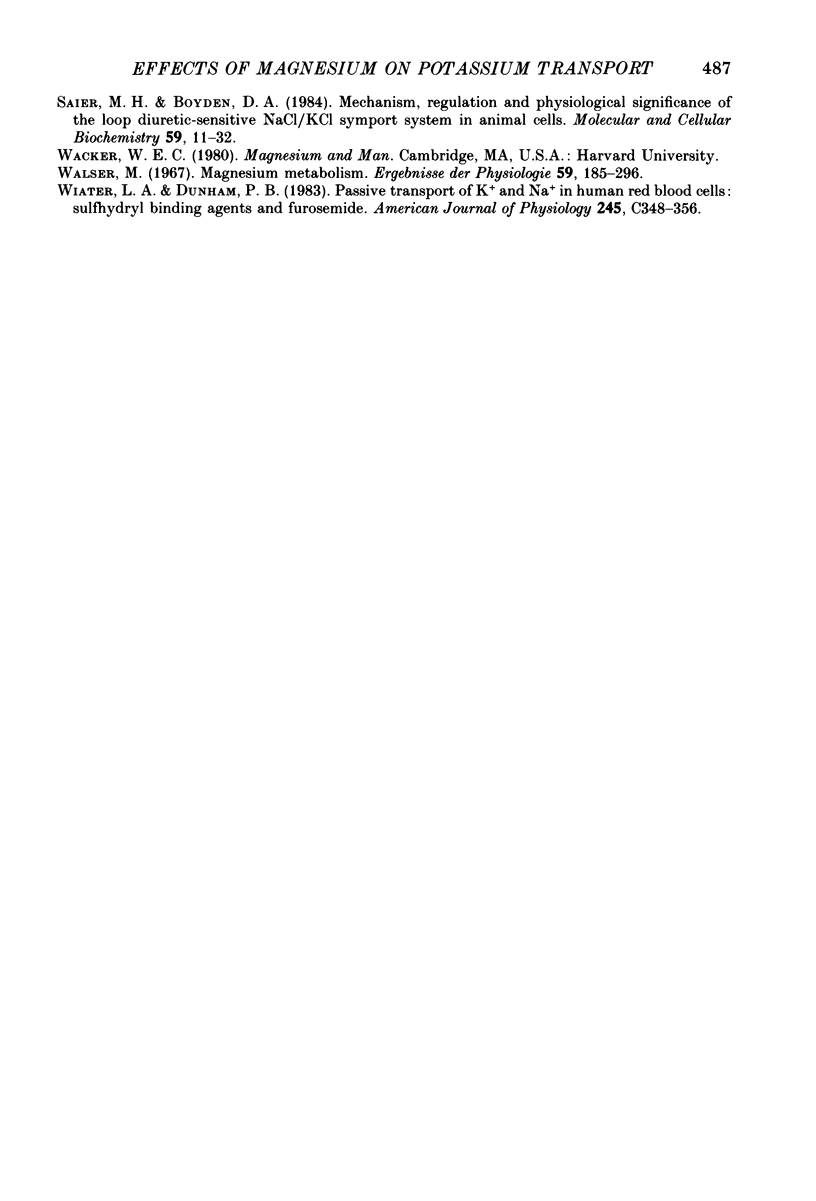
Selected References
These references are in PubMed. This may not be the complete list of references from this article.
- Adragna N. C., Perkins C. M., Lauf P. K. Furosemide-sensitive Na+-K+ cotransport and cellular metabolism in human erythrocytes. Biochim Biophys Acta. 1985 Jan 10;812(1):293–296. doi: 10.1016/0005-2736(85)90551-6. [DOI] [PubMed] [Google Scholar]
- Brugnara C., Tosteson D. C. Cell volume, K transport, and cell density in human erythrocytes. Am J Physiol. 1987 Mar;252(3 Pt 1):C269–C276. doi: 10.1152/ajpcell.1987.252.3.C269. [DOI] [PubMed] [Google Scholar]
- Chipperfield A. R. The (Na+-K+-Cl-) co-transport system. Clin Sci (Lond) 1986 Nov;71(5):465–476. doi: 10.1042/cs0710465. [DOI] [PubMed] [Google Scholar]
- Dagher G., Brugnara C., Canessa M. Effect of metabolic depletion on the furosemide-sensitive Na and K fluxes in human red cells. J Membr Biol. 1985;86(2):145–155. doi: 10.1007/BF01870781. [DOI] [PubMed] [Google Scholar]
- Dunham P. B., Ellory J. C. Passive potassium transport in low potassium sheep red cells: dependence upon cell volume and chloride. J Physiol. 1981 Sep;318:511–530. doi: 10.1113/jphysiol.1981.sp013881. [DOI] [PMC free article] [PubMed] [Google Scholar]
- Ebel H., Günther T. Magnesium metabolism: a review. J Clin Chem Clin Biochem. 1980 May;18(5):257–270. doi: 10.1515/cclm.1980.18.5.257. [DOI] [PubMed] [Google Scholar]
- Ellory J. C., Flatman P. W., Stewart G. W. Inhibition of human red cell sodium and potassium transport by divalent cations. J Physiol. 1983 Jul;340:1–17. doi: 10.1113/jphysiol.1983.sp014746. [DOI] [PMC free article] [PubMed] [Google Scholar]
- Flatman P. W., Andrews P. L. Cation and ATP content of ferret red cells. Comp Biochem Physiol A Comp Physiol. 1983;74(4):939–943. doi: 10.1016/0300-9629(83)90373-0. [DOI] [PubMed] [Google Scholar]
- Flatman P. W., Lew V. L. Magnesium buffering in intact human red blood cells measured using the ionophore A23187. J Physiol. 1980 Aug;305:13–30. doi: 10.1113/jphysiol.1980.sp013346. [DOI] [PMC free article] [PubMed] [Google Scholar]
- Flatman P. W., Lew V. L. The magnesium dependence of sodium-pump-mediated sodium-potassium and sodium-sodium exchange in intact human red cells. J Physiol. 1981 Jun;315:421–446. doi: 10.1113/jphysiol.1981.sp013756. [DOI] [PMC free article] [PubMed] [Google Scholar]
- Flatman P. W. Sodium and potassium transport in ferret red cells. J Physiol. 1983 Aug;341:545–557. doi: 10.1113/jphysiol.1983.sp014823. [DOI] [PMC free article] [PubMed] [Google Scholar]
- Flatman P. W. The effects of calcium on potassium transport in ferret red cells. J Physiol. 1987 May;386:407–423. doi: 10.1113/jphysiol.1987.sp016541. [DOI] [PMC free article] [PubMed] [Google Scholar]
- Flatman P., Lew V. L. Does ionophore A23187 mediate Na transport in the absence of divalent cations? Nature. 1977 Dec 1;270(5636):444–445. doi: 10.1038/270444a0. [DOI] [PubMed] [Google Scholar]
- Geck P., Pietrzyk C., Burckhardt B. C., Pfeiffer B., Heinz E. Electrically silent cotransport on Na+, K+ and Cl- in Ehrlich cells. Biochim Biophys Acta. 1980 Aug 4;600(2):432–447. doi: 10.1016/0005-2736(80)90446-0. [DOI] [PubMed] [Google Scholar]
- Hall A. C., Ellory J. C. Measurement and stoichiometry of bumetanide-sensitive (2Na:1K:3Cl) cotransport in ferret red cells. J Membr Biol. 1985;85(3):205–213. doi: 10.1007/BF01871515. [DOI] [PubMed] [Google Scholar]
- Lauf P. K., Adragna N. C., Garay R. P. Activation by N-ethylmaleimide of a latent K+-Cl- flux in human red blood cells. Am J Physiol. 1984 May;246(5 Pt 1):C385–C390. doi: 10.1152/ajpcell.1984.246.5.C385. [DOI] [PubMed] [Google Scholar]
- Lauf P. K. K+:Cl- cotransport: sulfhydryls, divalent cations, and the mechanism of volume activation in a red cell. J Membr Biol. 1985;88(1):1–13. doi: 10.1007/BF01871208. [DOI] [PubMed] [Google Scholar]
- Lauf P. K. Passive K+-Cl- fluxes in low-K+ sheep erythrocytes: modulation by A23187 and bivalent cations. Am J Physiol. 1985 Sep;249(3 Pt 1):C271–C278. doi: 10.1152/ajpcell.1985.249.3.C271. [DOI] [PubMed] [Google Scholar]
- Palfrey H. C., Rao M. C. Na/K/Cl co-transport and its regulation. J Exp Biol. 1983 Sep;106:43–54. doi: 10.1242/jeb.106.1.43. [DOI] [PubMed] [Google Scholar]
- Saier M. H., Jr, Boyden D. A. Mechanism, regulation and physiological significance of the loop diuretic-sensitive NaCl/KCl symport system in animal cells. Mol Cell Biochem. 1984;59(1-2):11–32. doi: 10.1007/BF00231303. [DOI] [PubMed] [Google Scholar]
- Walser M. Magnesium metabolism. Ergeb Physiol. 1967;59:185–296. doi: 10.1007/BF02269144. [DOI] [PubMed] [Google Scholar]
- Wiater L. A., Dunham P. B. Passive transport of K+ and Na+ in human red blood cells: sulfhydryl binding agents and furosemide. Am J Physiol. 1983 Nov;245(5 Pt 1):C348–C356. doi: 10.1152/ajpcell.1983.245.5.C348. [DOI] [PubMed] [Google Scholar]