Abstract
1. A technique was developed to generate 2-8 degrees C step temperature perturbations (T-jumps) in single muscle fibres to study the thermodynamics of muscle contraction. A solid-state pulsed holmium laser emitting at 2.065 microns heated the fibre and surrounding solution in approximately 150 mus. The signal from a 100 microns thermocouple fed back to a heating wire maintained the elevated temperature after the laser pulse. 2. Tension of glycerol-extracted muscle fibres from rabbit psoas muscle did not change significantly following T-jumps when the fibre was relaxed. 3. In rigor, tension decreased abruptly on heating indicating normal (not rubber-like) thermoelasticity. The thermoelastic coefficient (negative ratio of relative length change to relative temperature change) of the fibre was estimated to be -0.021 at sarcomere lengths of 2.5-2.8 microns. Rigor tension was constant after the temperature step and returned to the original value on recooling. 4. In maximal Ca2+ activation, tension transients initiated by T-jumps had several phases. An immediate tension decrease suggests that thermoelasticity during contraction is similar to that in rigor. Active tension then recovered to the value before the T-jump with an apparent rate constant of approximately 400 s-1 (at 10-20 degrees C). This rate constant did not have an appreciable dependence on the final temperature. Finally, tension increased exponentially to a new higher level with a rate constant of approximately 20 s-1 at 20 degrees C. This rate constant increased with temperature with a Q10 of 1.4. 5. At submaximal Ca2+ activation the tension rise was followed by a decay to below the value before the T-jump. This decline was expected from the temperature dependence of steady pCa-tension curves. The final tension decline occurred on the 1-5 s time scale. 6. The value and amplitude dependence of the rate constant for the quick recovery following T-jumps were similar to those of the quick recovery following length steps during active contractions. The enthalpy change associated with the quick tension recovery following temperature-step perturbations was estimated to be positive suggesting that the recovery process is an endothermic reaction. Slower reaction steps on the 10-30 ms timescale, as well as reactions corresponding to the quick recovery, may contribute to the cross-bridge power stroke.
Full text
PDF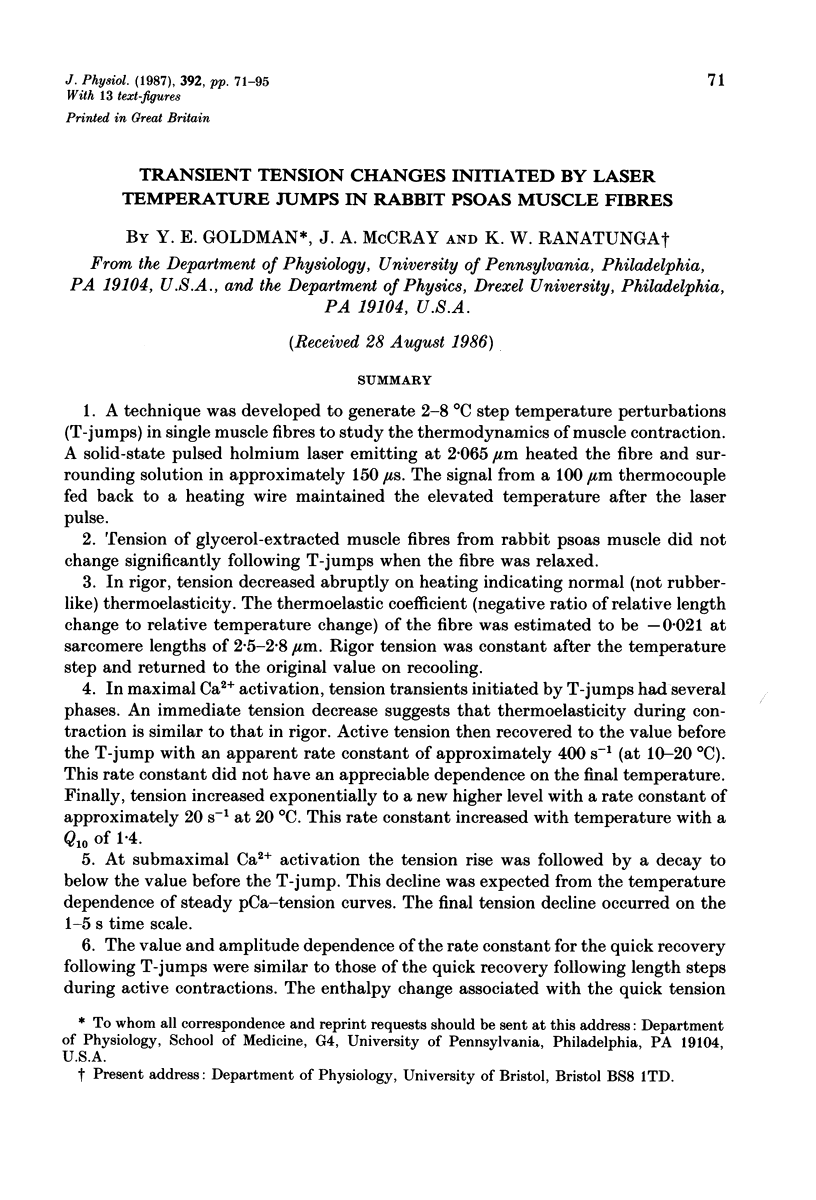
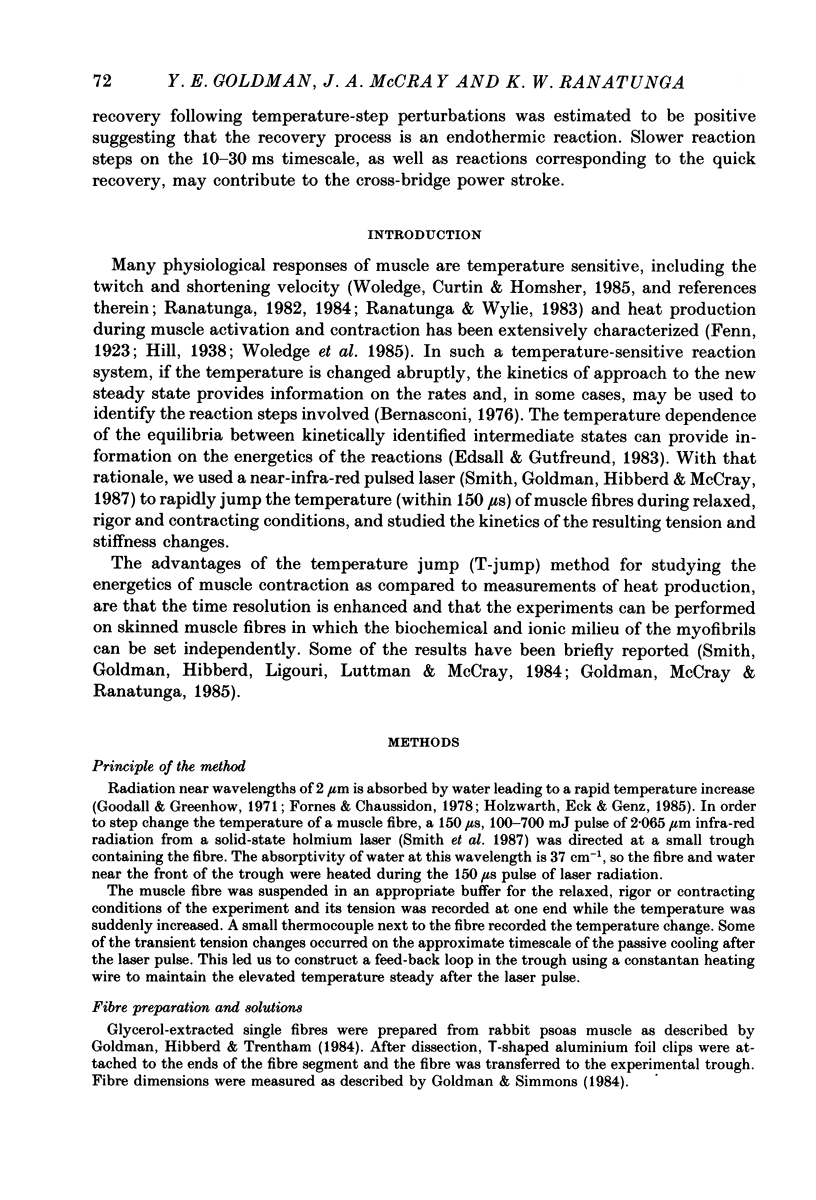
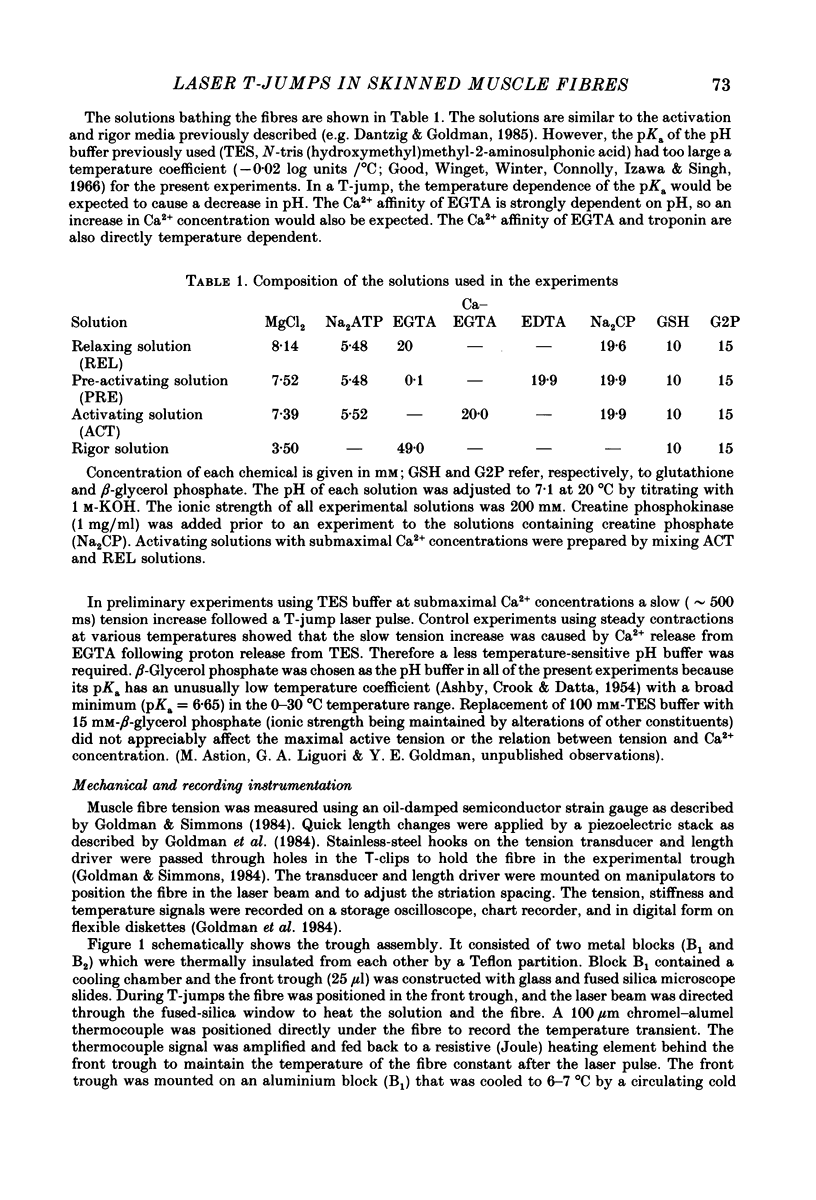
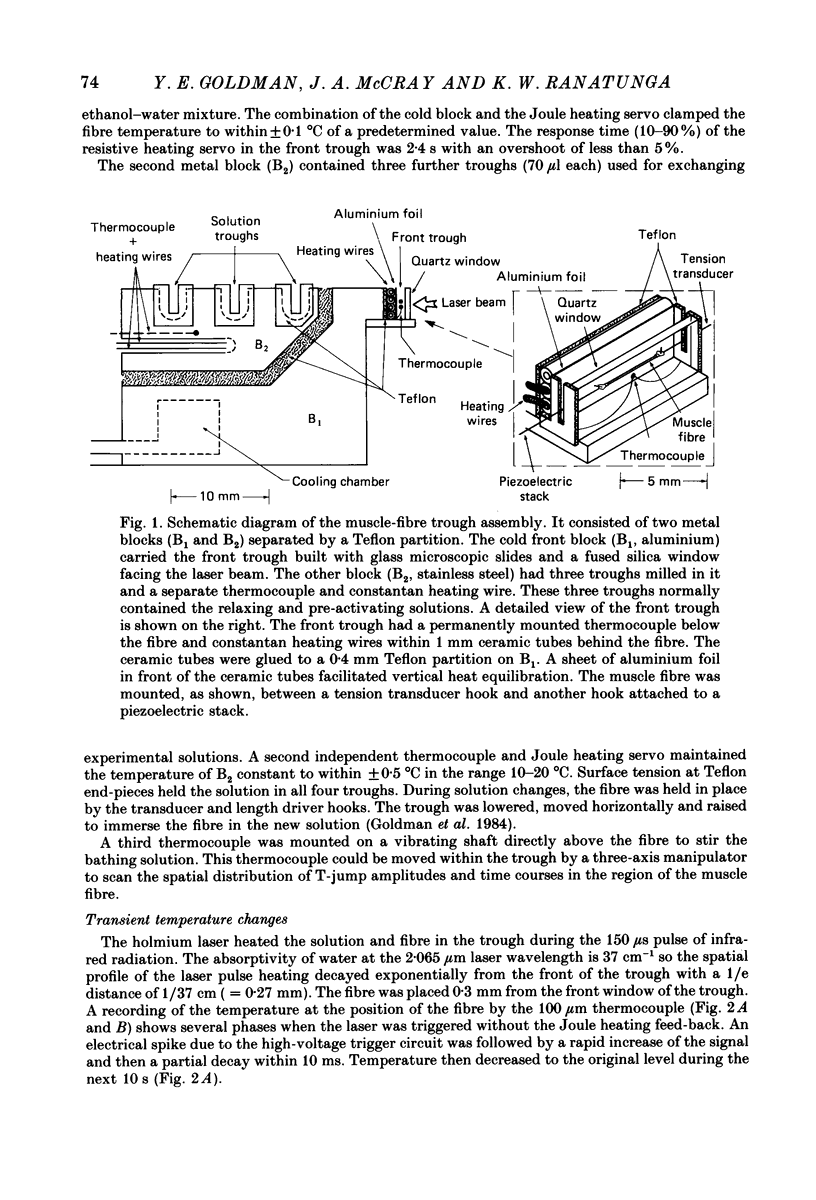
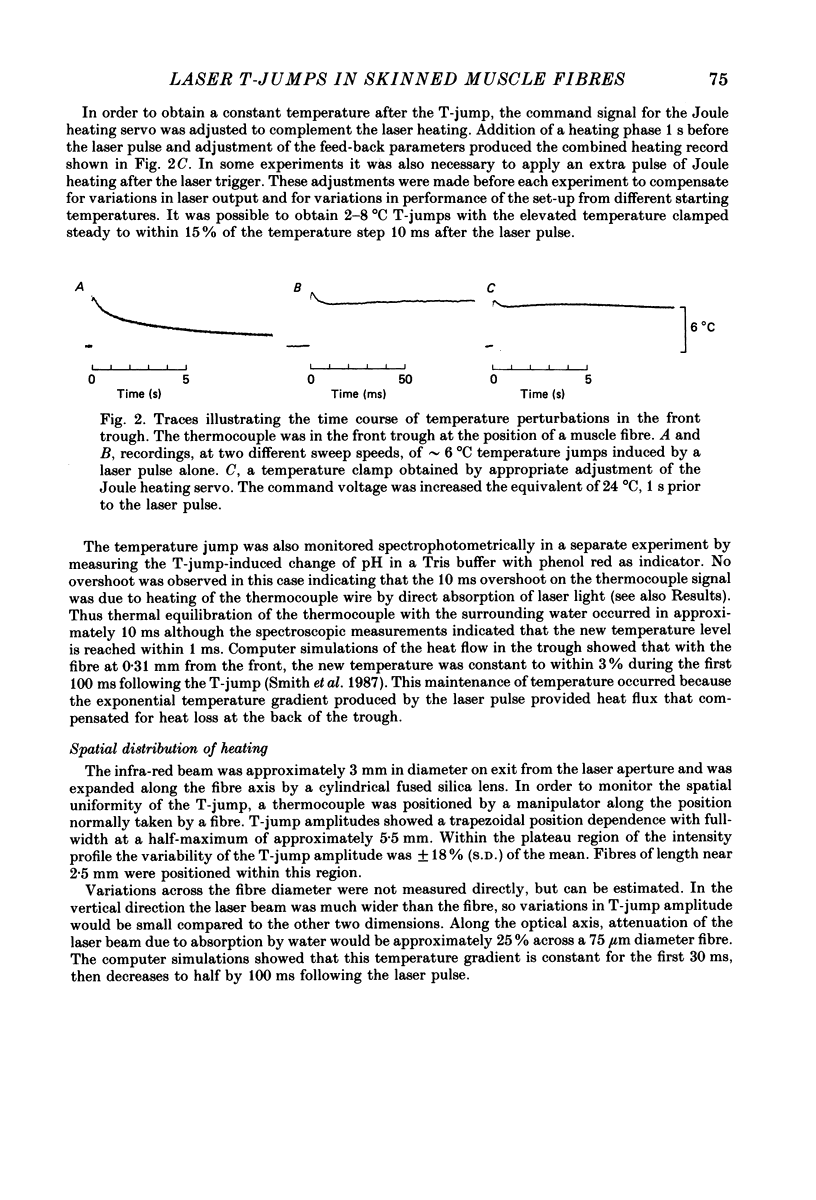
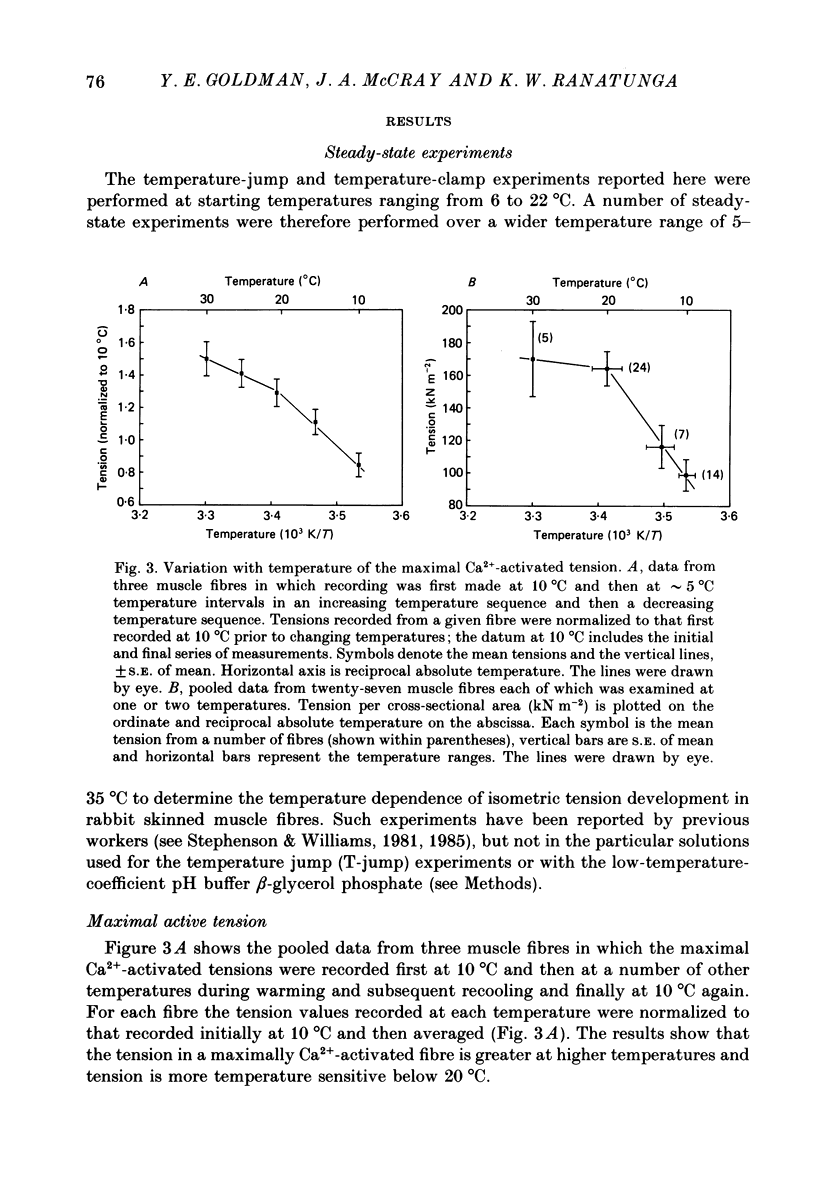
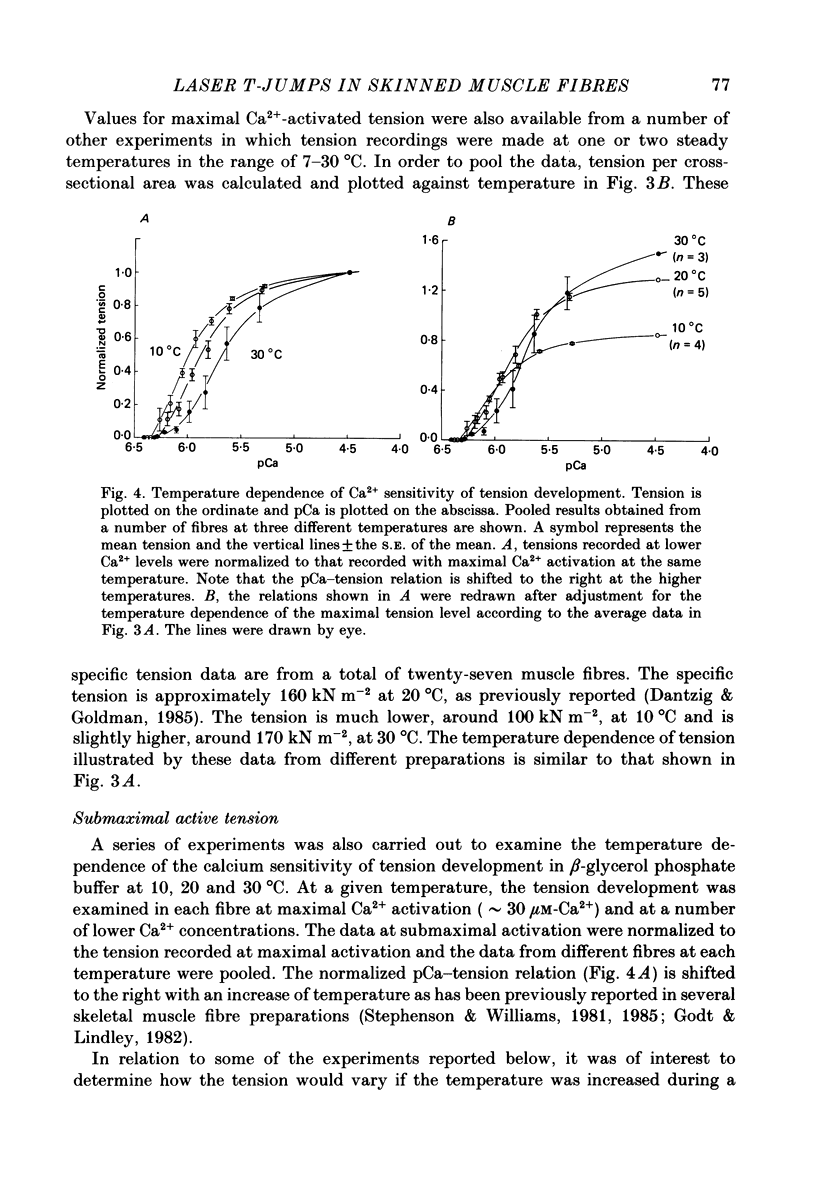
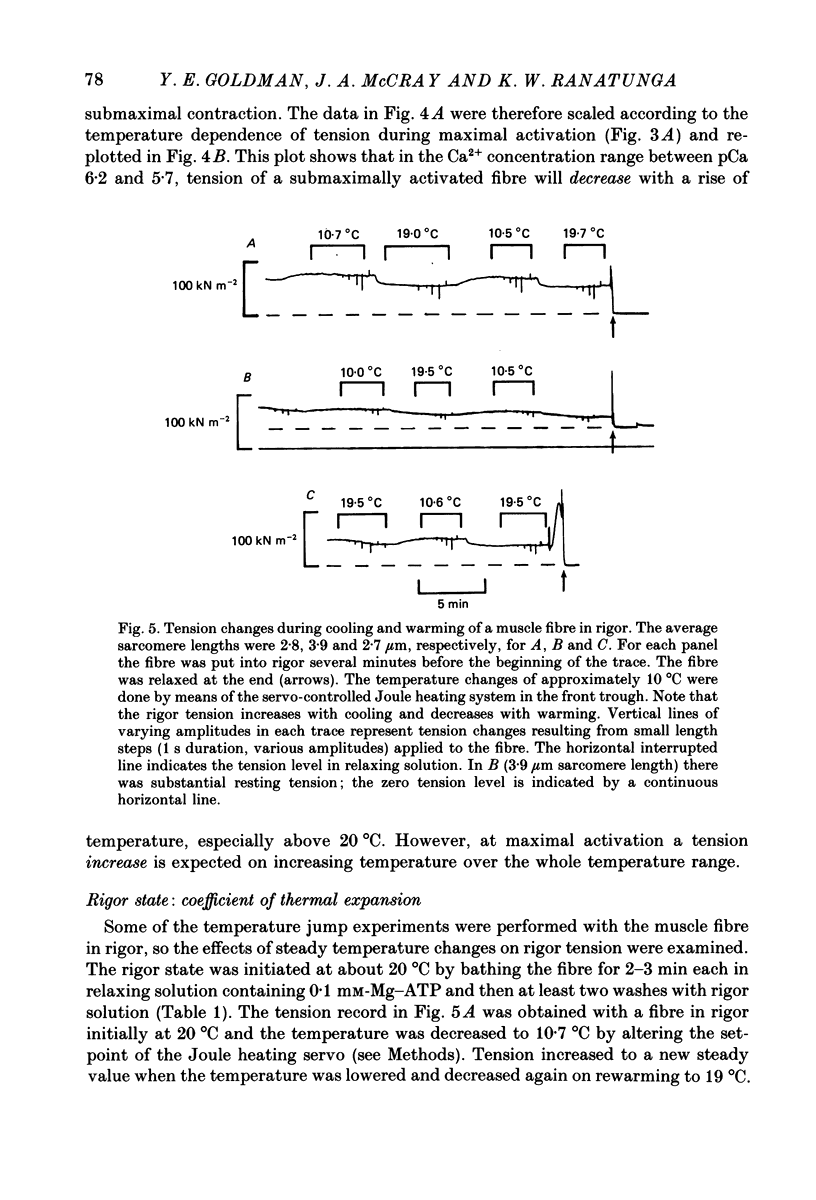
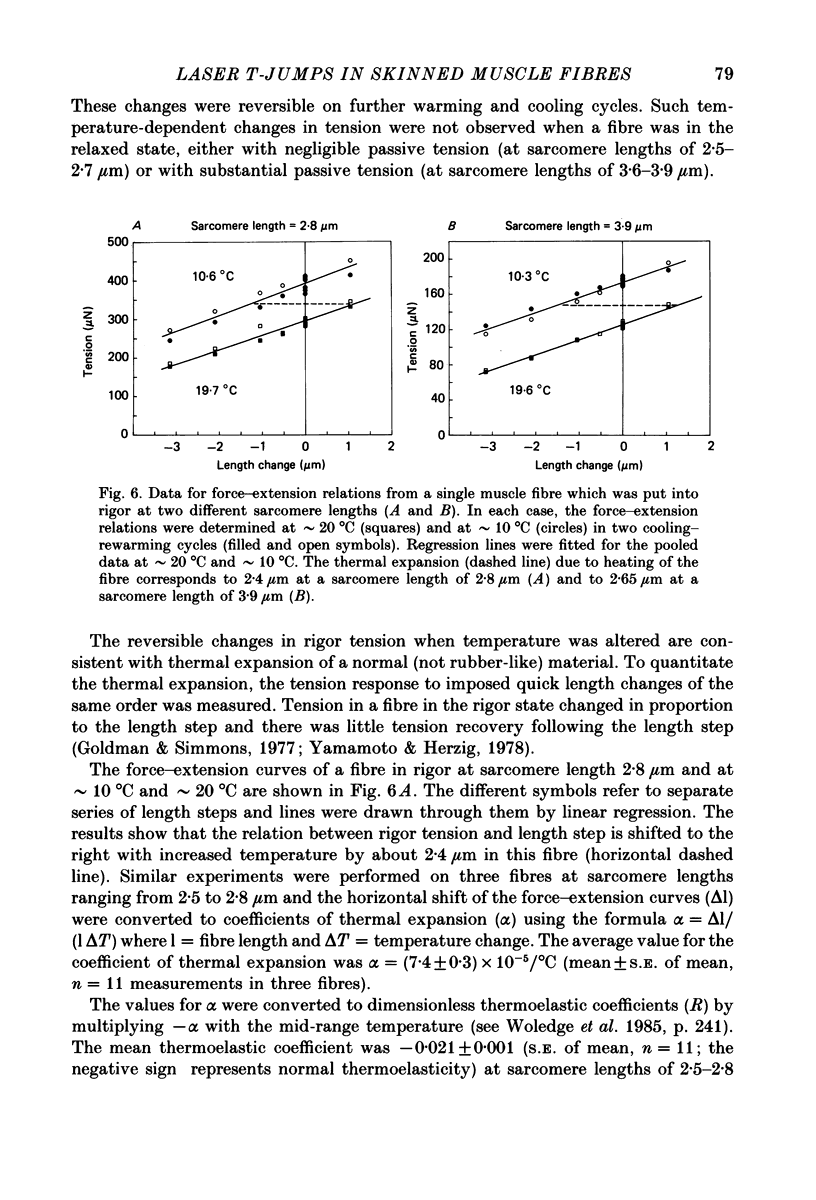
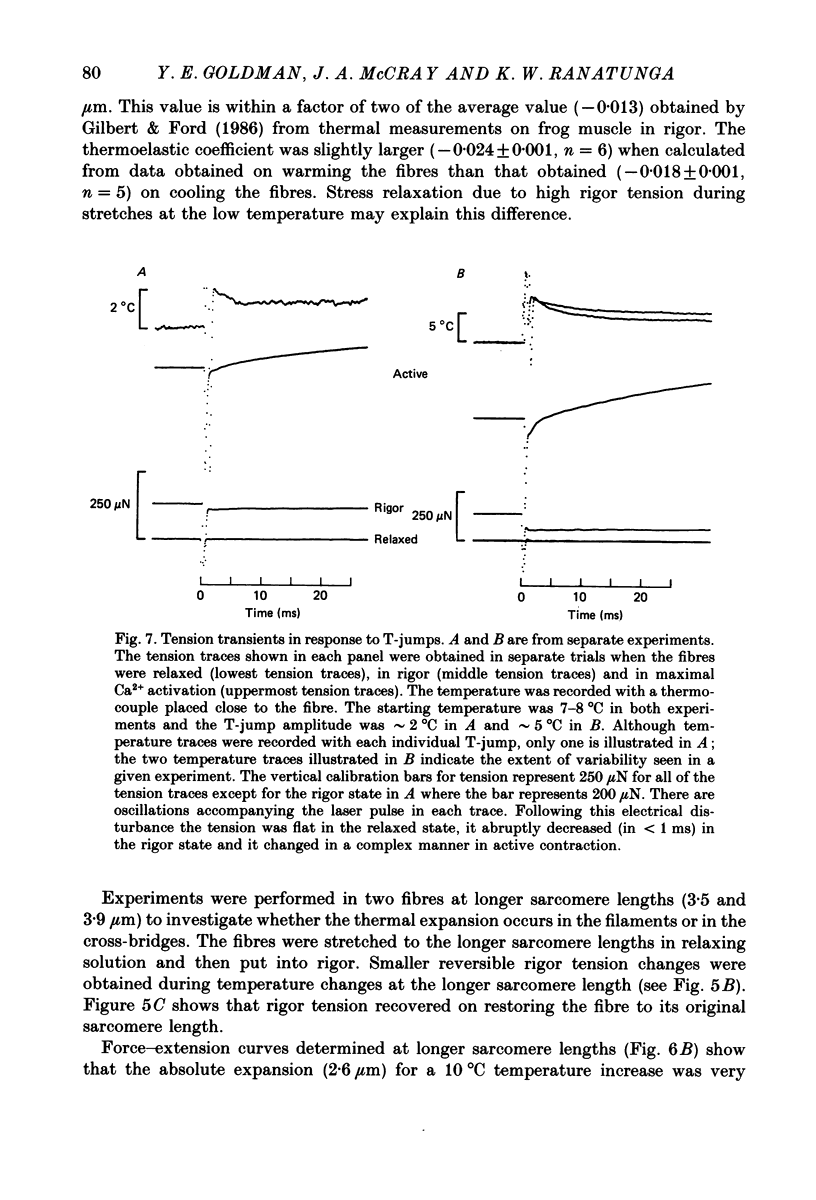
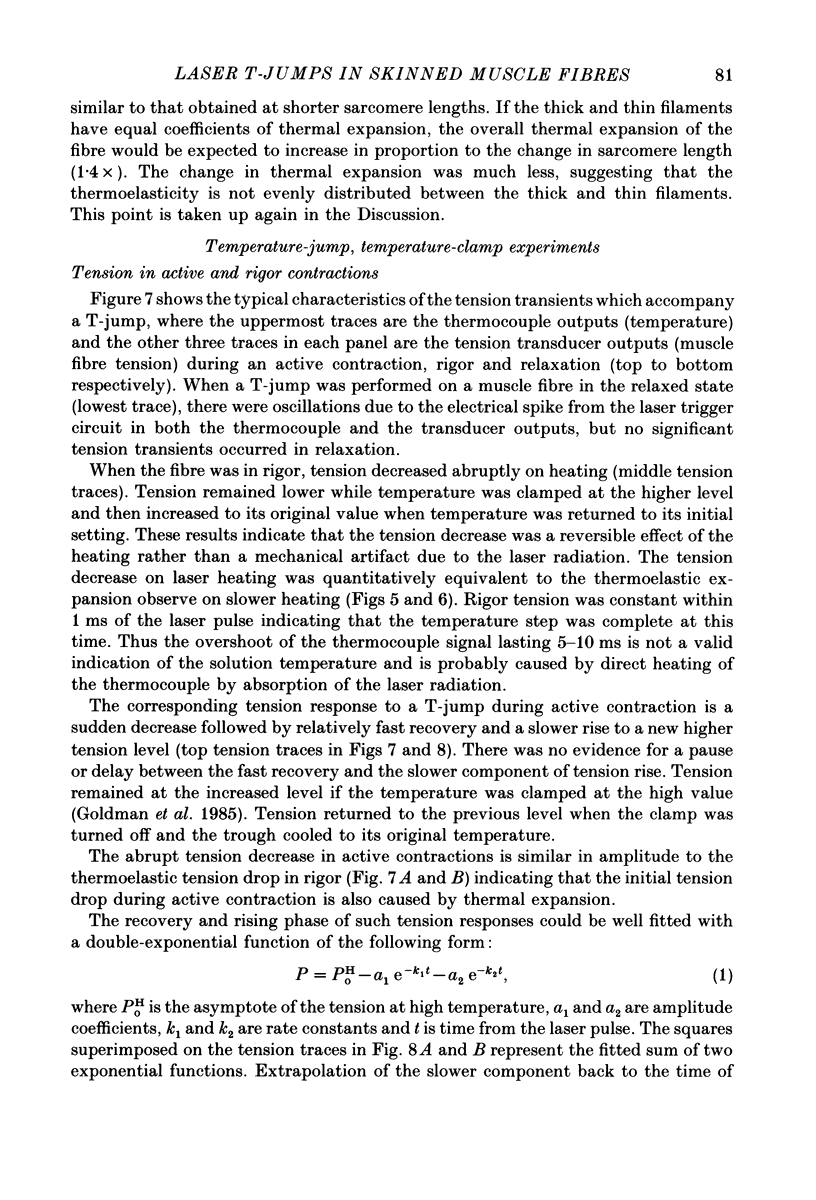
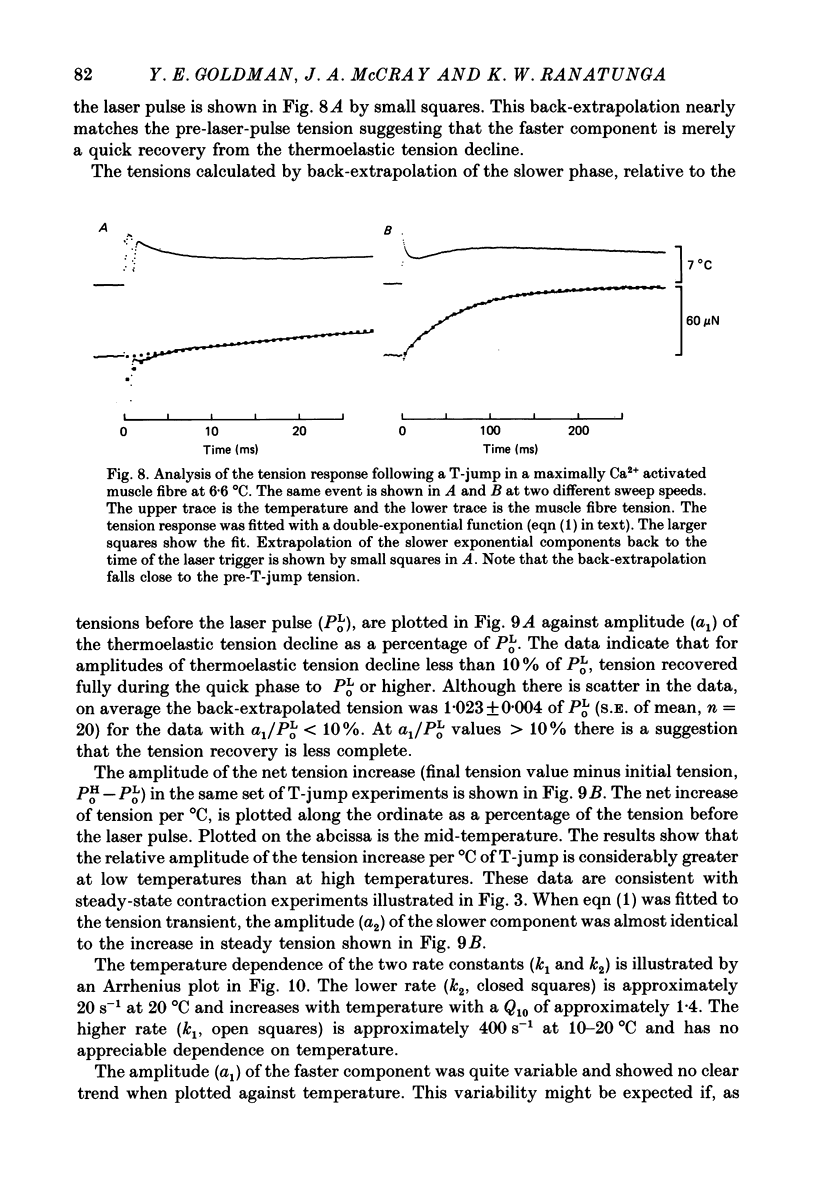
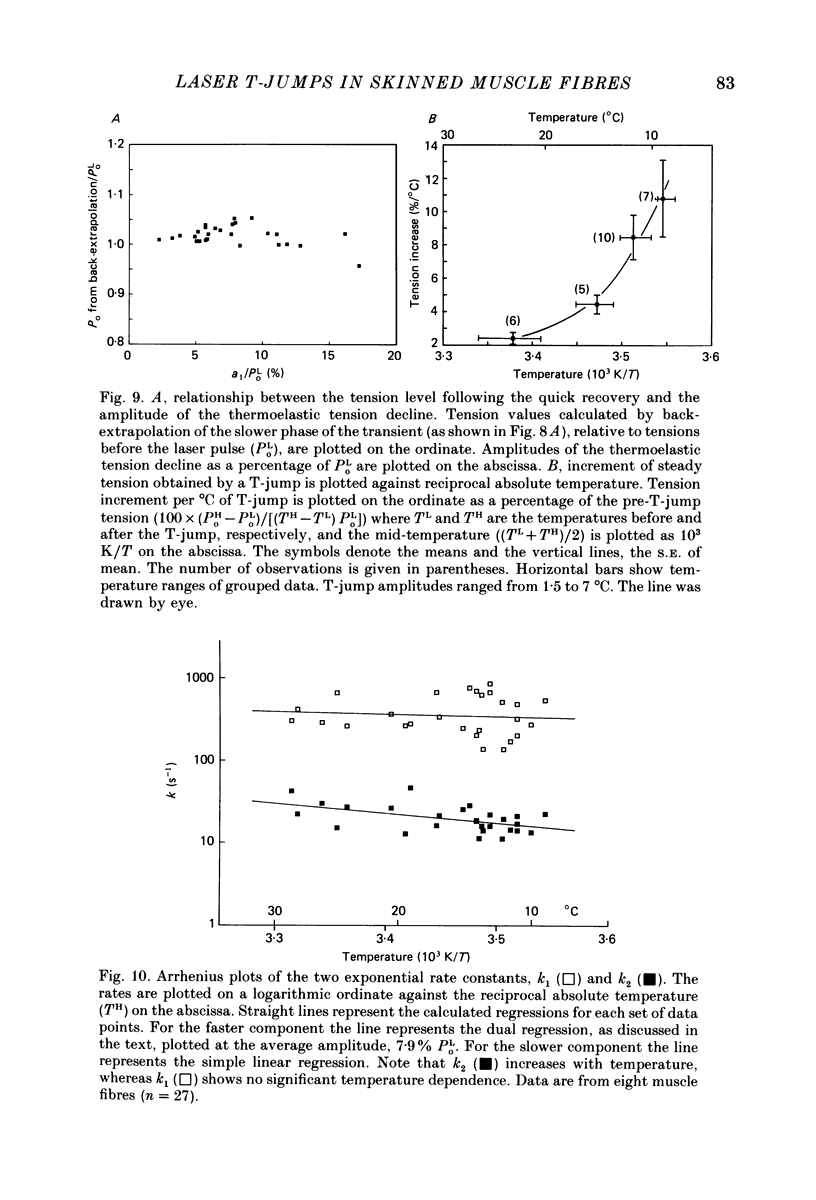
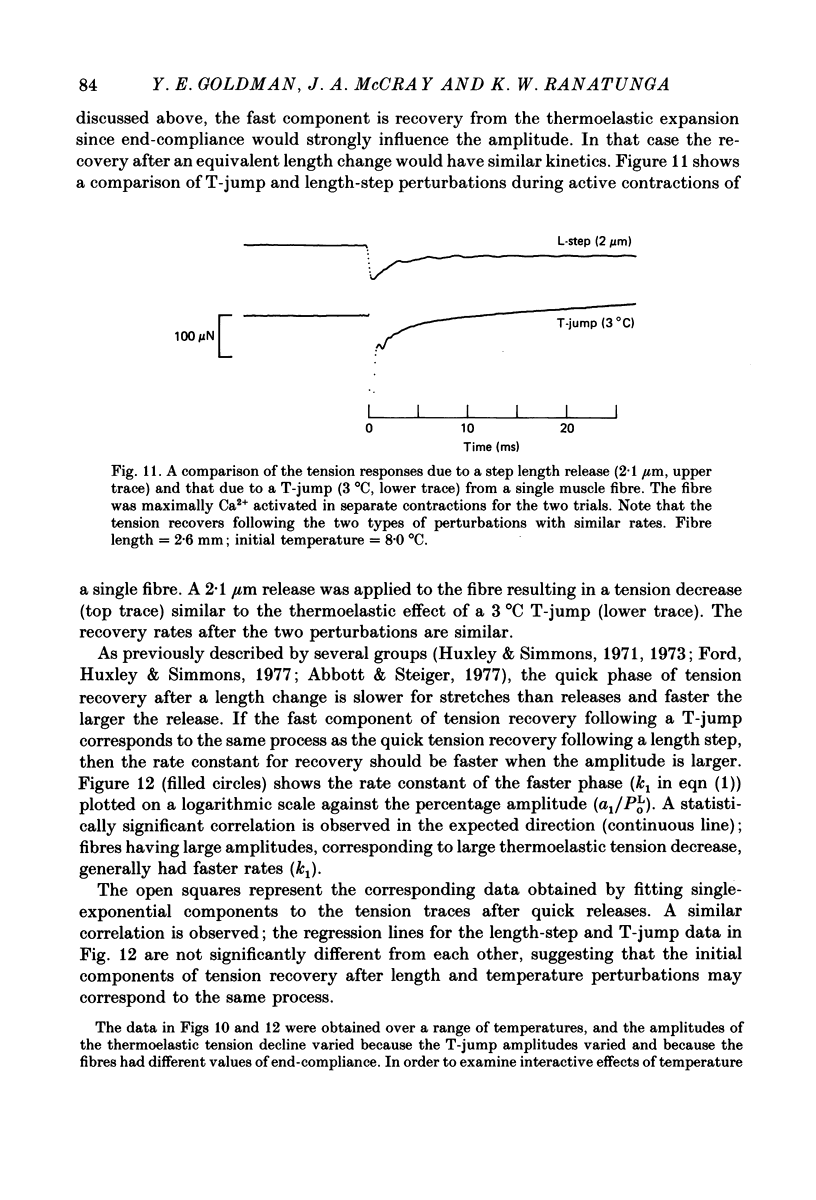
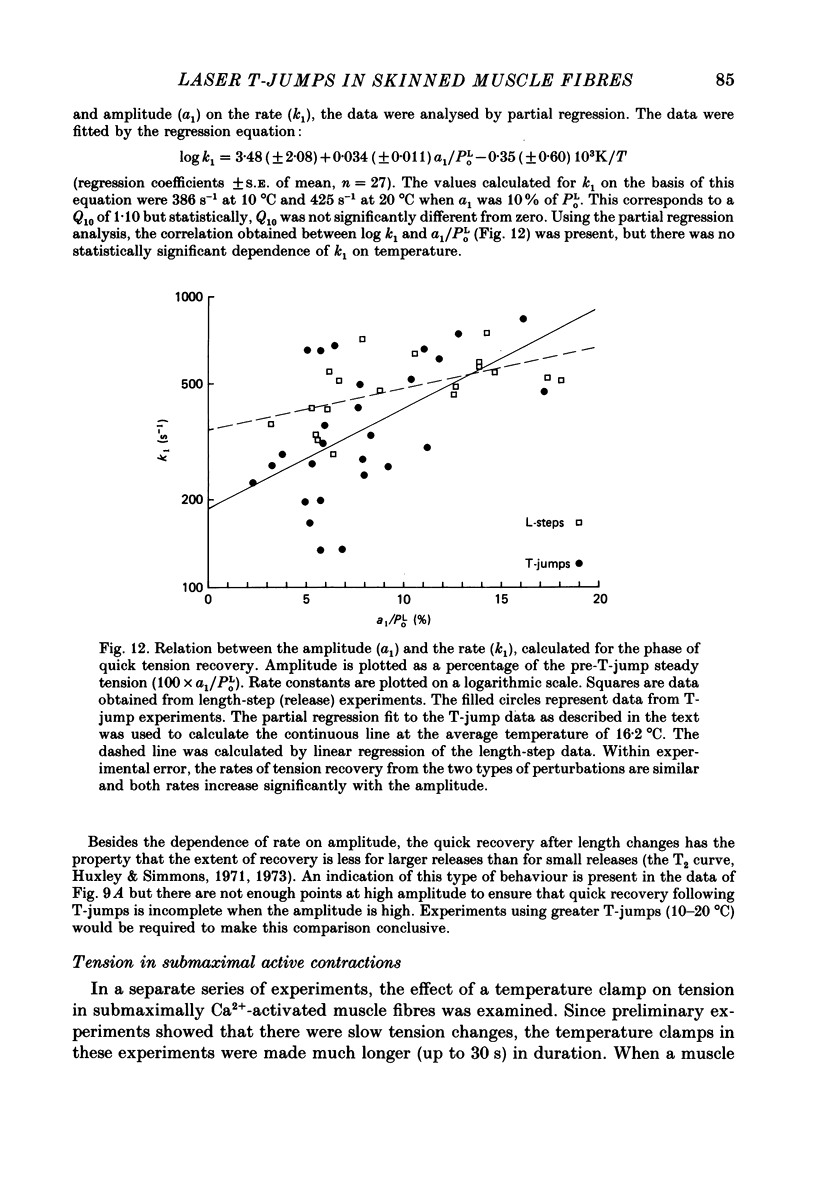
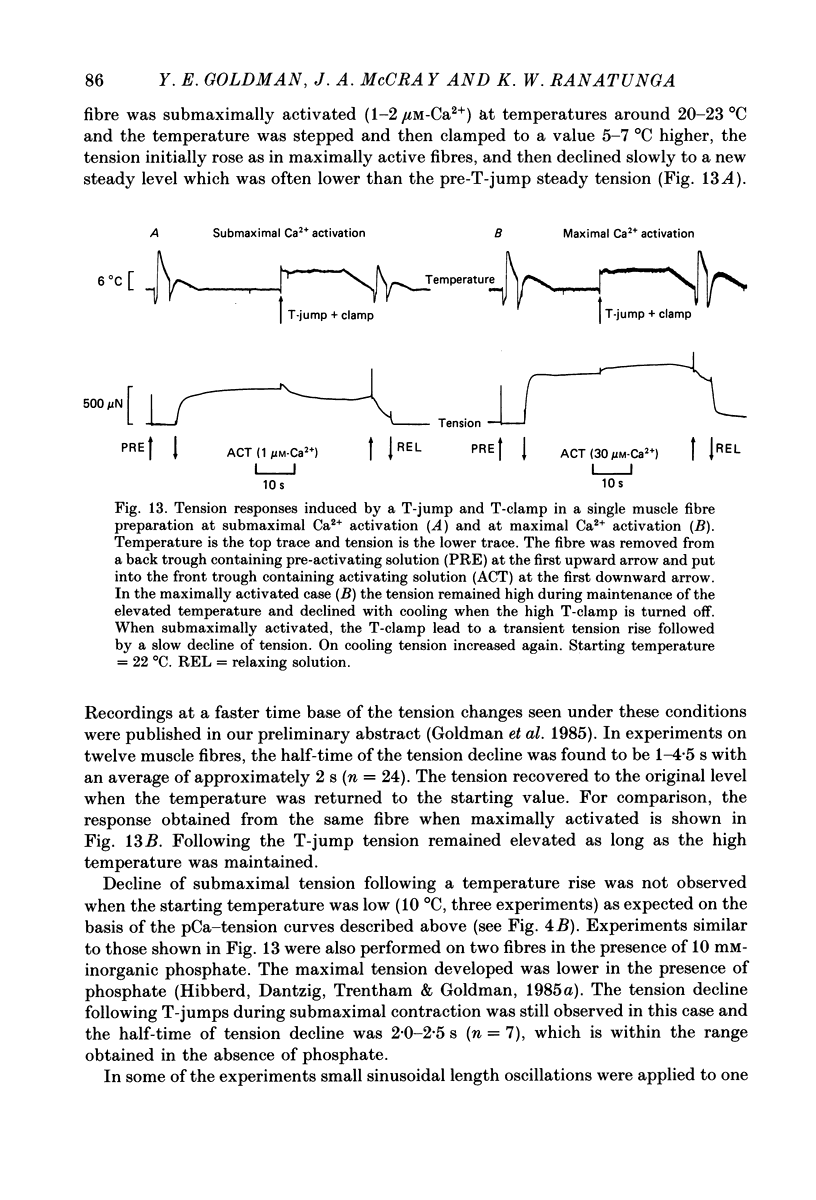
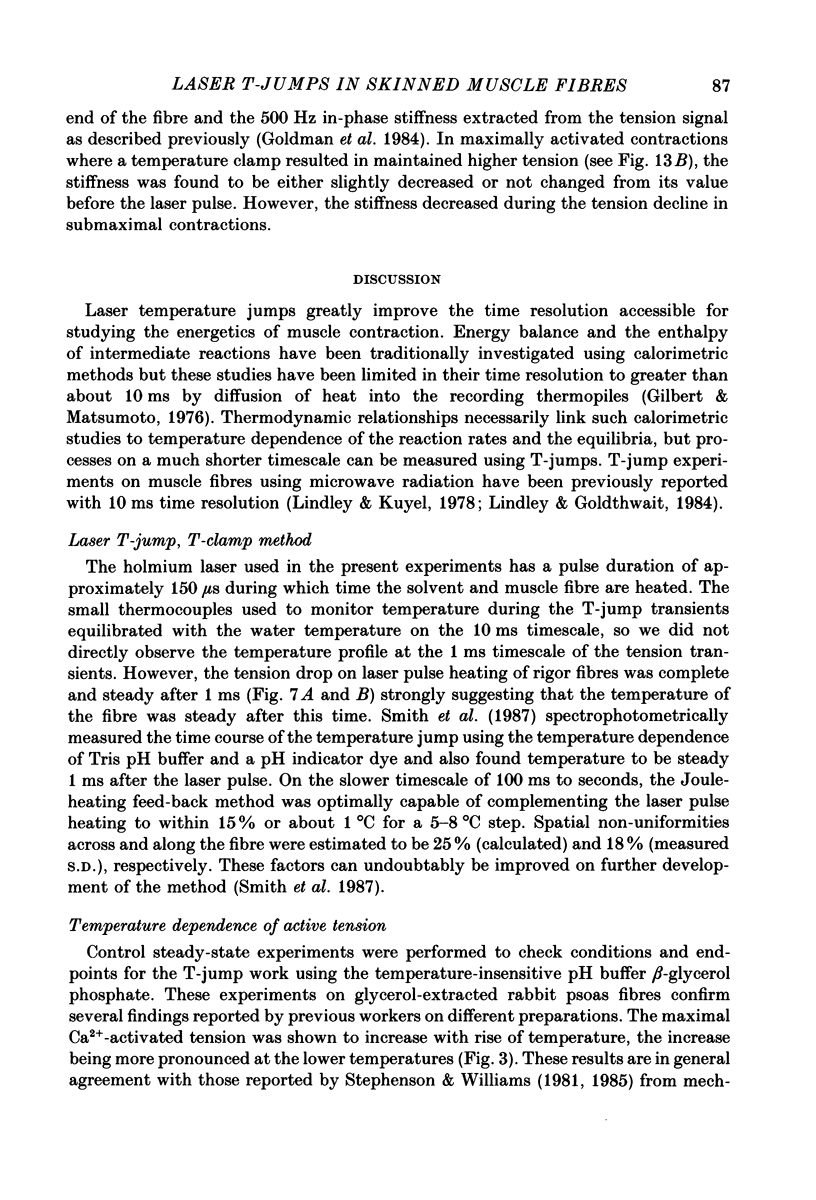
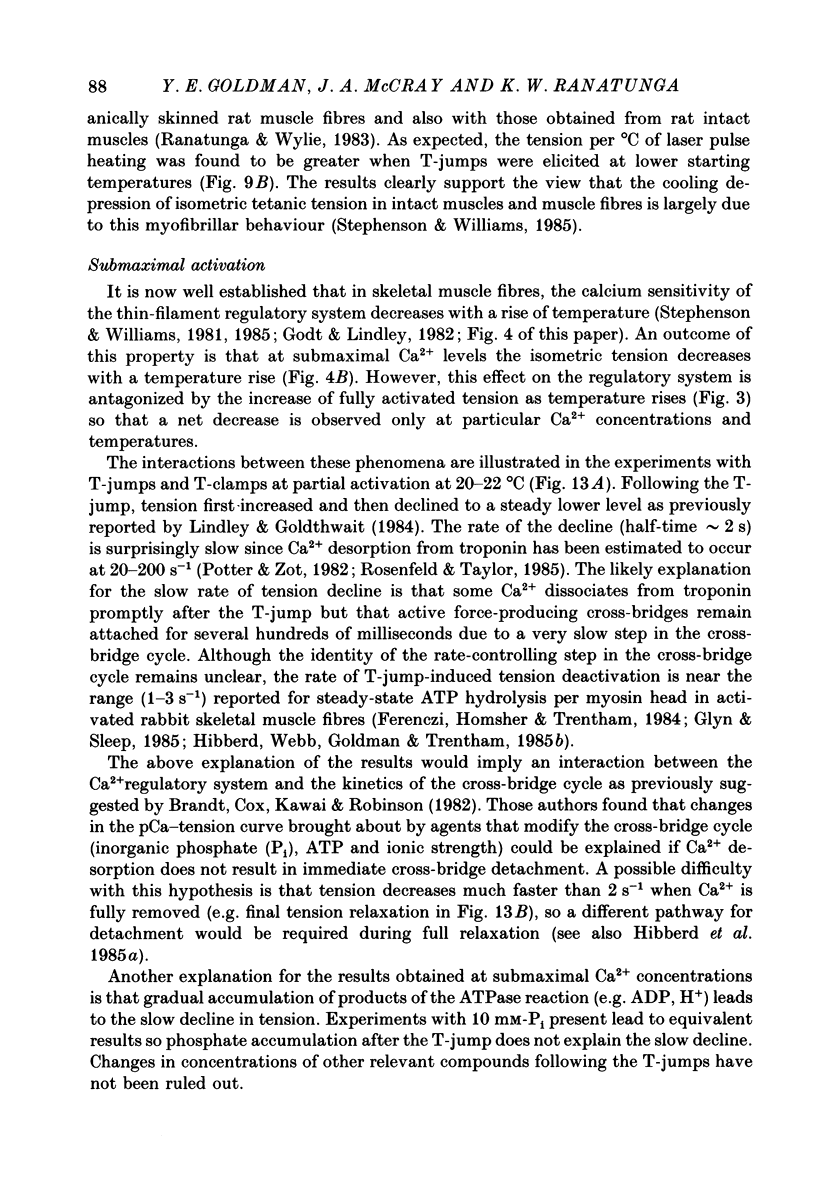
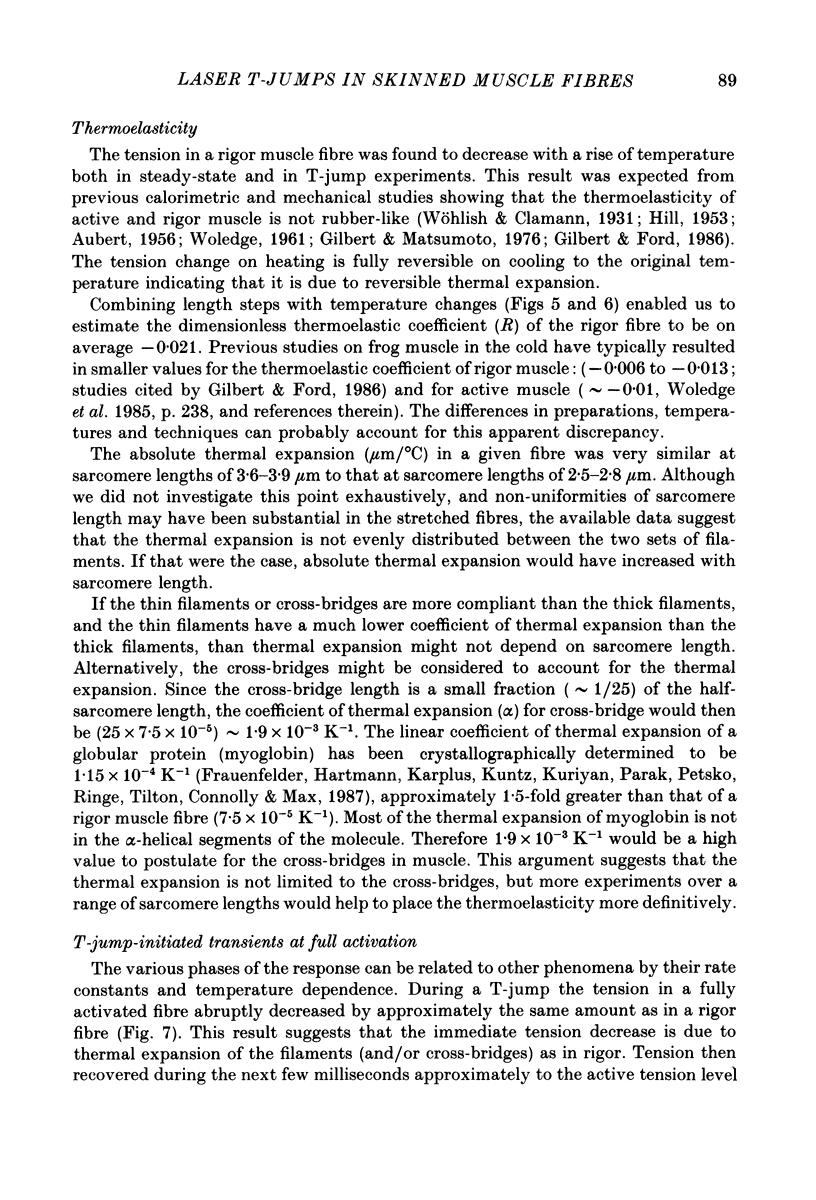
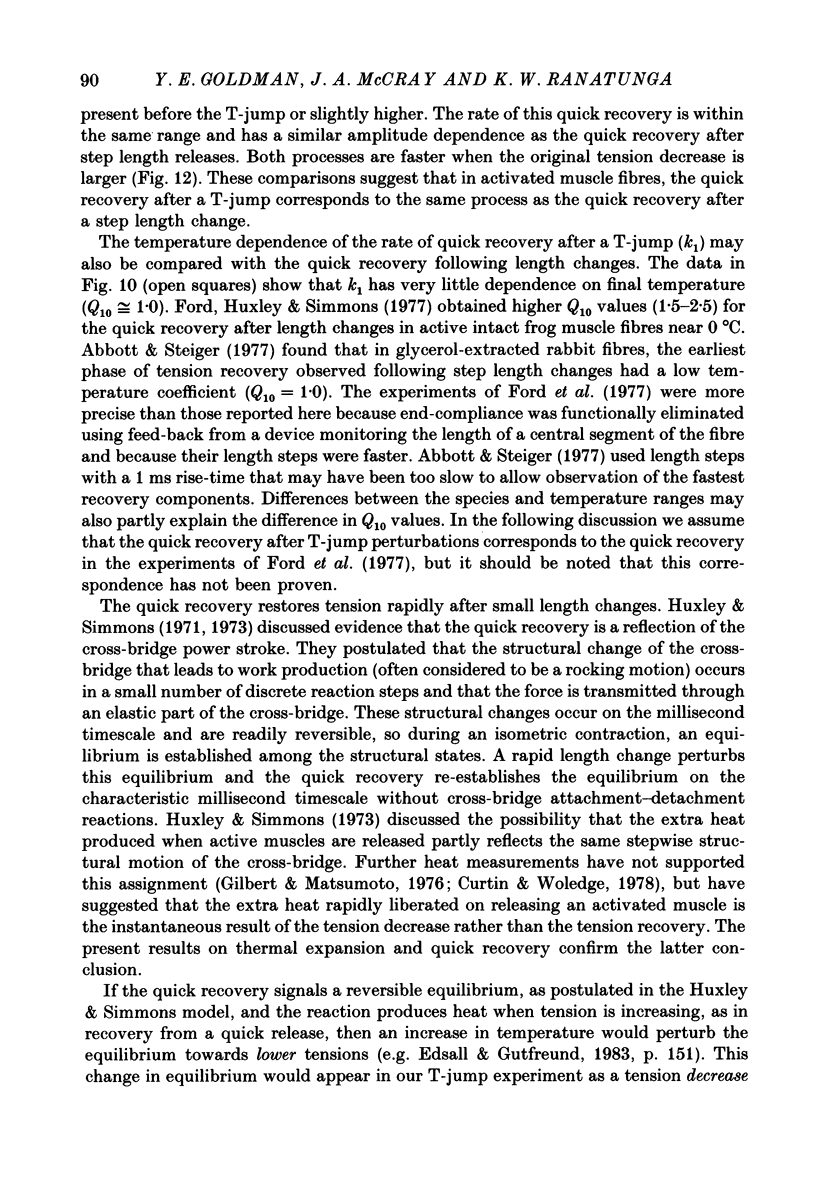
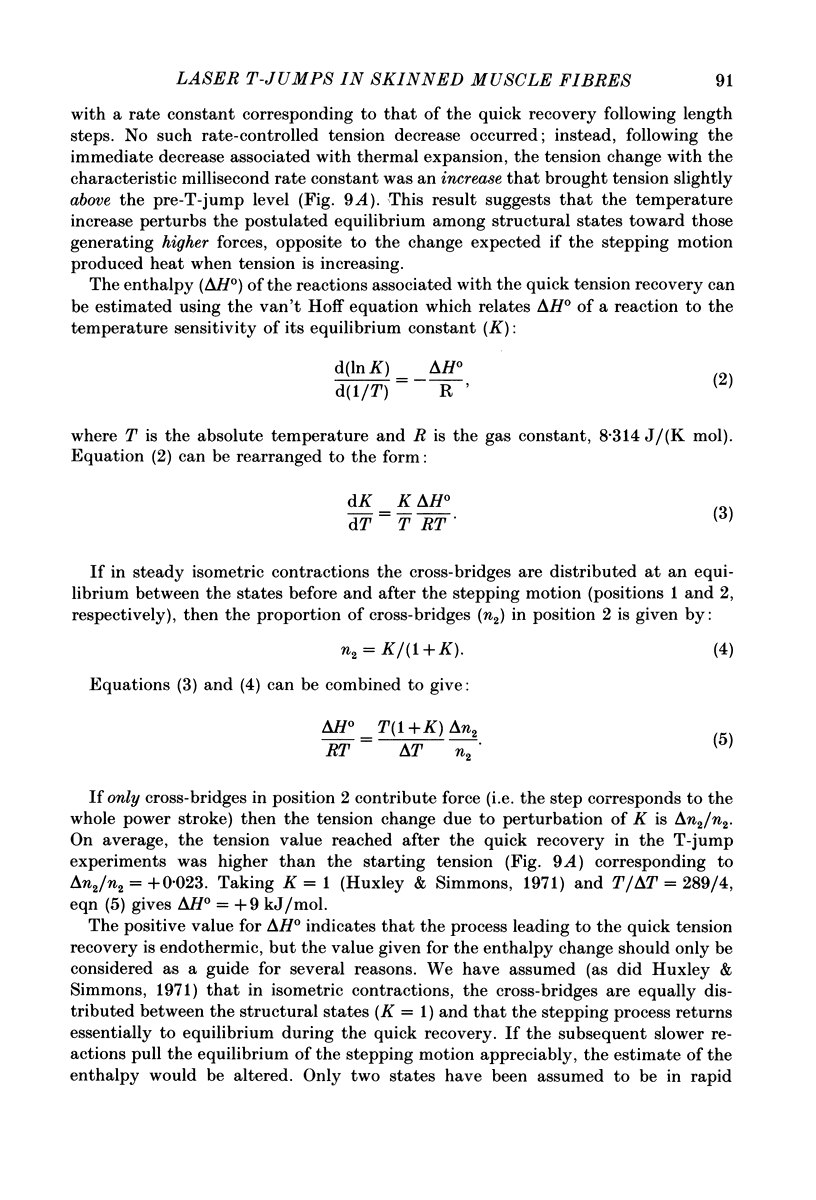
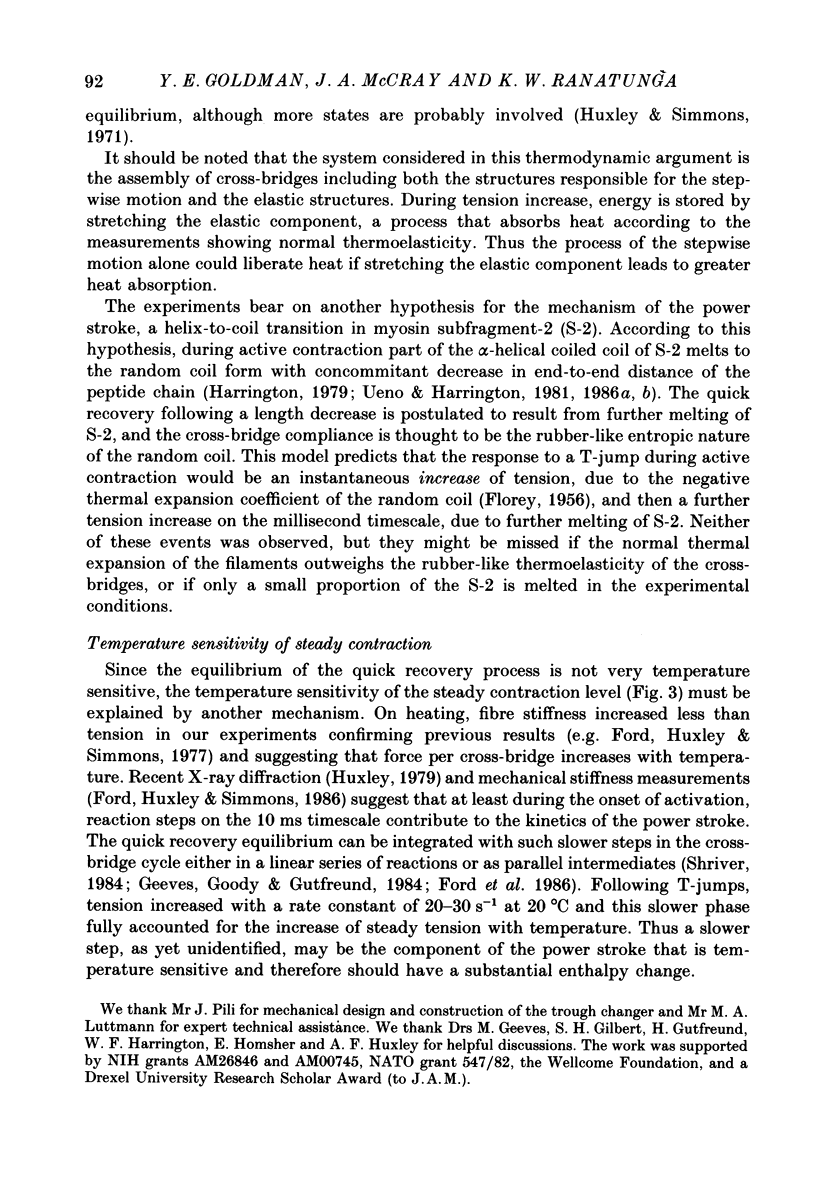
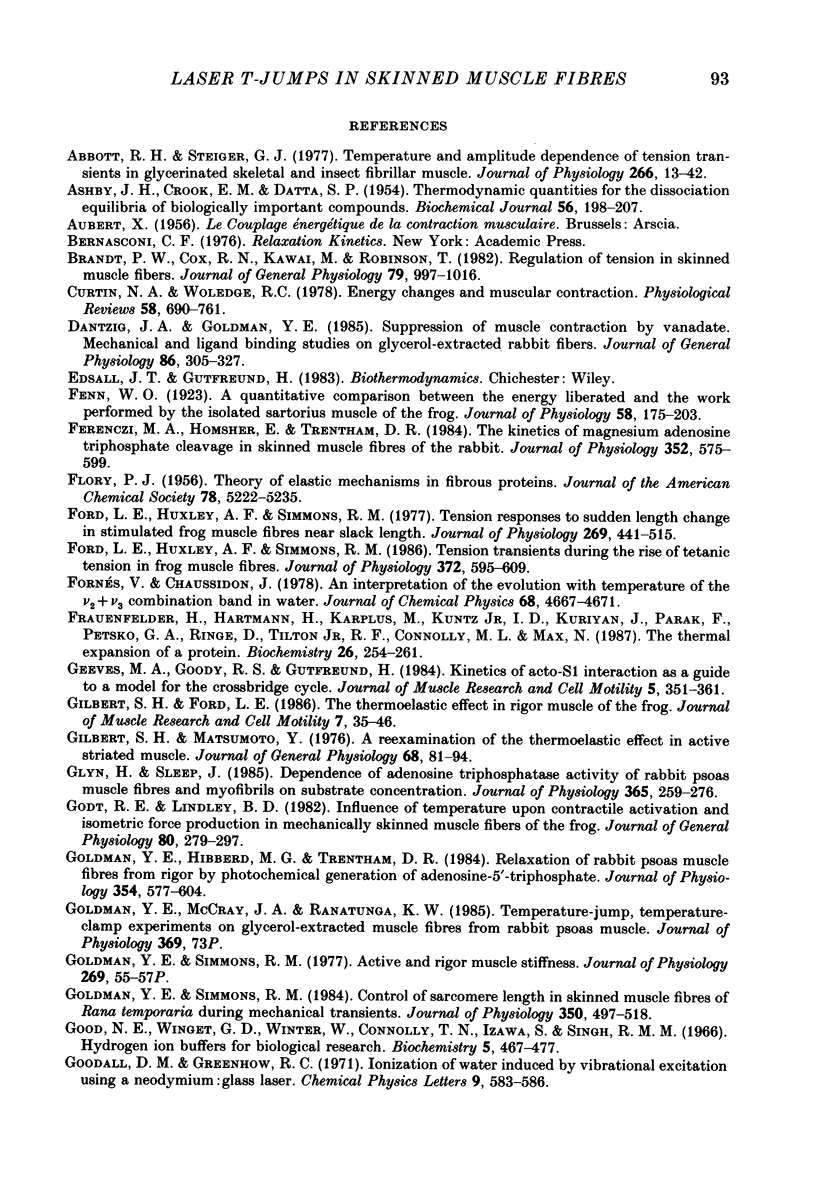
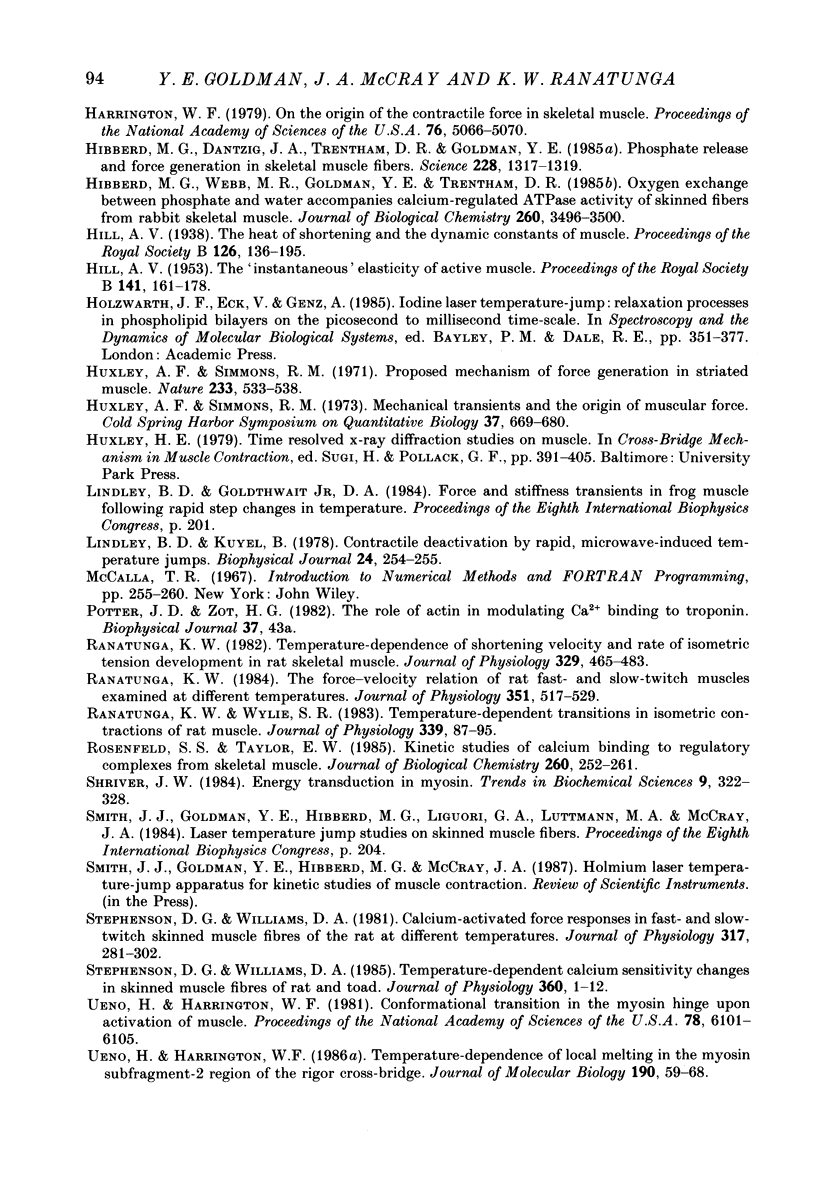
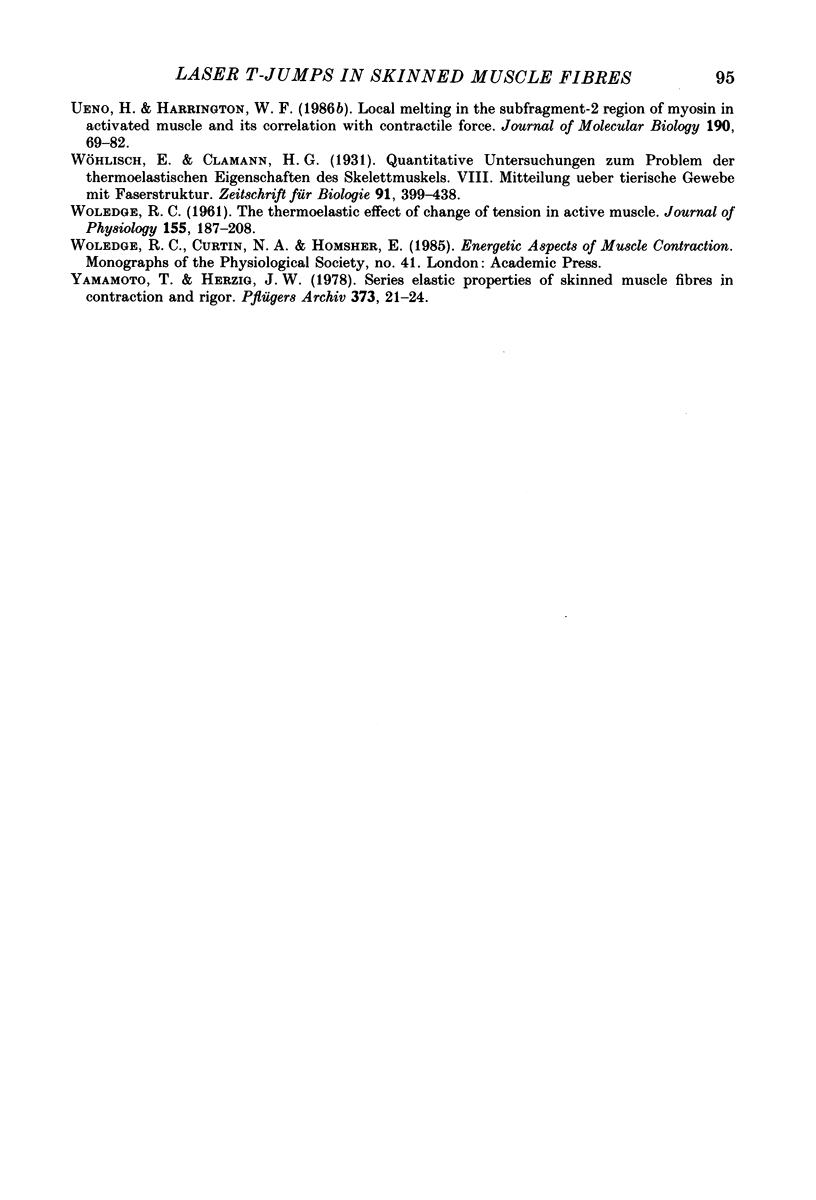
Selected References
These references are in PubMed. This may not be the complete list of references from this article.
- ASHBY J. H., CROOK E. M., DATTA S. P. Thermodynamic quantities for the dissociation equilibria of biologically important compounds. 2. The acid dissociations of glycerol 2-phosphoric acid. Biochem J. 1954 Feb;56(2):198–207. doi: 10.1042/bj0560198. [DOI] [PMC free article] [PubMed] [Google Scholar]
- Abbott R. H., Steiger G. J. Temperature and amplitude dependence of tension transients in glycerinated skeletal and insect fibrillar muscle. J Physiol. 1977 Mar;266(1):13–42. doi: 10.1113/jphysiol.1977.sp011754. [DOI] [PMC free article] [PubMed] [Google Scholar]
- Brandt P. W., Cox R. N., Kawai M., Robinson T. Effect of cross-bridge kinetics on apparent Ca2+ sensitivity. J Gen Physiol. 1982 Jun;79(6):997–1016. doi: 10.1085/jgp.79.6.997. [DOI] [PMC free article] [PubMed] [Google Scholar]
- Curtin N. A., Woledge R. C. Energy changes and muscular contraction. Physiol Rev. 1978 Jul;58(3):690–761. doi: 10.1152/physrev.1978.58.3.690. [DOI] [PubMed] [Google Scholar]
- Dantzig J. A., Goldman Y. E. Suppression of muscle contraction by vanadate. Mechanical and ligand binding studies on glycerol-extracted rabbit fibers. J Gen Physiol. 1985 Sep;86(3):305–327. doi: 10.1085/jgp.86.3.305. [DOI] [PMC free article] [PubMed] [Google Scholar]
- Fenn W. O. A quantitative comparison between the energy liberated and the work performed by the isolated sartorius muscle of the frog. J Physiol. 1923 Dec 28;58(2-3):175–203. doi: 10.1113/jphysiol.1923.sp002115. [DOI] [PMC free article] [PubMed] [Google Scholar]
- Ferenczi M. A., Homsher E., Trentham D. R. The kinetics of magnesium adenosine triphosphate cleavage in skinned muscle fibres of the rabbit. J Physiol. 1984 Jul;352:575–599. doi: 10.1113/jphysiol.1984.sp015311. [DOI] [PMC free article] [PubMed] [Google Scholar]
- Ford L. E., Huxley A. F., Simmons R. M. Tension responses to sudden length change in stimulated frog muscle fibres near slack length. J Physiol. 1977 Jul;269(2):441–515. doi: 10.1113/jphysiol.1977.sp011911. [DOI] [PMC free article] [PubMed] [Google Scholar]
- Ford L. E., Huxley A. F., Simmons R. M. Tension transients during the rise of tetanic tension in frog muscle fibres. J Physiol. 1986 Mar;372:595–609. doi: 10.1113/jphysiol.1986.sp016027. [DOI] [PMC free article] [PubMed] [Google Scholar]
- Frauenfelder H., Hartmann H., Karplus M., Kuntz I. D., Jr, Kuriyan J., Parak F., Petsko G. A., Ringe D., Tilton R. F., Jr, Connolly M. L. Thermal expansion of a protein. Biochemistry. 1987 Jan 13;26(1):254–261. doi: 10.1021/bi00375a035. [DOI] [PubMed] [Google Scholar]
- Geeves M. A., Goody R. S., Gutfreund H. Kinetics of acto-S1 interaction as a guide to a model for the crossbridge cycle. J Muscle Res Cell Motil. 1984 Aug;5(4):351–361. doi: 10.1007/BF00818255. [DOI] [PubMed] [Google Scholar]
- Gilbert S. H., Ford L. E. The thermoelastic effect in rigor muscle of the frog. J Muscle Res Cell Motil. 1986 Feb;7(1):35–46. doi: 10.1007/BF01756200. [DOI] [PubMed] [Google Scholar]
- Gilbert S. H., Matsumoto Y. A reexamination of the thermoelastic effect in active striated muscle. J Gen Physiol. 1976 Jul;68(1):81–94. doi: 10.1085/jgp.68.1.81. [DOI] [PMC free article] [PubMed] [Google Scholar]
- Glyn H., Sleep J. Dependence of adenosine triphosphatase activity of rabbit psoas muscle fibres and myofibrils on substrate concentration. J Physiol. 1985 Aug;365:259–276. doi: 10.1113/jphysiol.1985.sp015770. [DOI] [PMC free article] [PubMed] [Google Scholar]
- Godt R. E., Lindley B. D. Influence of temperature upon contractile activation and isometric force production in mechanically skinned muscle fibers of the frog. J Gen Physiol. 1982 Aug;80(2):279–297. doi: 10.1085/jgp.80.2.279. [DOI] [PMC free article] [PubMed] [Google Scholar]
- Goldman Y. E., Hibberd M. G., Trentham D. R. Relaxation of rabbit psoas muscle fibres from rigor by photochemical generation of adenosine-5'-triphosphate. J Physiol. 1984 Sep;354:577–604. doi: 10.1113/jphysiol.1984.sp015394. [DOI] [PMC free article] [PubMed] [Google Scholar]
- Goldman Y. E., Simmons R. M. Active and rigor muscle stiffness [proceedings]. J Physiol. 1977 Jul;269(1):55P–57P. [PubMed] [Google Scholar]
- Goldman Y. E., Simmons R. M. Control of sarcomere length in skinned muscle fibres of Rana temporaria during mechanical transients. J Physiol. 1984 May;350:497–518. doi: 10.1113/jphysiol.1984.sp015215. [DOI] [PMC free article] [PubMed] [Google Scholar]
- Good N. E., Winget G. D., Winter W., Connolly T. N., Izawa S., Singh R. M. Hydrogen ion buffers for biological research. Biochemistry. 1966 Feb;5(2):467–477. doi: 10.1021/bi00866a011. [DOI] [PubMed] [Google Scholar]
- HILL A. V. The instantaneous elasticity of active muscle. Proc R Soc Lond B Biol Sci. 1953 Apr 17;141(903):161–178. doi: 10.1098/rspb.1953.0033. [DOI] [PubMed] [Google Scholar]
- Harrington W. F. On the origin of the contractile force in skeletal muscle. Proc Natl Acad Sci U S A. 1979 Oct;76(10):5066–5070. doi: 10.1073/pnas.76.10.5066. [DOI] [PMC free article] [PubMed] [Google Scholar]
- Hibberd M. G., Dantzig J. A., Trentham D. R., Goldman Y. E. Phosphate release and force generation in skeletal muscle fibers. Science. 1985 Jun 14;228(4705):1317–1319. doi: 10.1126/science.3159090. [DOI] [PubMed] [Google Scholar]
- Hibberd M. G., Webb M. R., Goldman Y. E., Trentham D. R. Oxygen exchange between phosphate and water accompanies calcium-regulated ATPase activity of skinned fibers from rabbit skeletal muscle. J Biol Chem. 1985 Mar 25;260(6):3496–3500. [PubMed] [Google Scholar]
- Huxley A. F., Simmons R. M. Proposed mechanism of force generation in striated muscle. Nature. 1971 Oct 22;233(5321):533–538. doi: 10.1038/233533a0. [DOI] [PubMed] [Google Scholar]
- Lindley B. D., Kuyel B. Contractile deactivation by rapid, microwave-induced temperature jumps. Biophys J. 1978 Oct;24(1):254–255. doi: 10.1016/S0006-3495(78)85367-3. [DOI] [PMC free article] [PubMed] [Google Scholar]
- Ranatunga K. W. Temperature-dependence of shortening velocity and rate of isometric tension development in rat skeletal muscle. J Physiol. 1982 Aug;329:465–483. doi: 10.1113/jphysiol.1982.sp014314. [DOI] [PMC free article] [PubMed] [Google Scholar]
- Ranatunga K. W. The force-velocity relation of rat fast- and slow-twitch muscles examined at different temperatures. J Physiol. 1984 Jun;351:517–529. doi: 10.1113/jphysiol.1984.sp015260. [DOI] [PMC free article] [PubMed] [Google Scholar]
- Ranatunga K. W., Wylie S. R. Temperature-dependent transitions in isometric contractions of rat muscle. J Physiol. 1983 Jun;339:87–95. doi: 10.1113/jphysiol.1983.sp014704. [DOI] [PMC free article] [PubMed] [Google Scholar]
- Rosenfeld S. S., Taylor E. W. Kinetic studies of calcium binding to regulatory complexes from skeletal muscle. J Biol Chem. 1985 Jan 10;260(1):252–261. [PubMed] [Google Scholar]
- Stephenson D. G., Williams D. A. Calcium-activated force responses in fast- and slow-twitch skinned muscle fibres of the rat at different temperatures. J Physiol. 1981 Aug;317:281–302. doi: 10.1113/jphysiol.1981.sp013825. [DOI] [PMC free article] [PubMed] [Google Scholar]
- Stephenson D. G., Williams D. A. Temperature-dependent calcium sensitivity changes in skinned muscle fibres of rat and toad. J Physiol. 1985 Mar;360:1–12. doi: 10.1113/jphysiol.1985.sp015600. [DOI] [PMC free article] [PubMed] [Google Scholar]
- Ueno H., Harrington W. F. Conformational transition in the myosin hinge upon activation of muscle. Proc Natl Acad Sci U S A. 1981 Oct;78(10):6101–6105. doi: 10.1073/pnas.78.10.6101. [DOI] [PMC free article] [PubMed] [Google Scholar]
- Ueno H., Harrington W. F. Local melting in the subfragment-2 region of myosin in activated muscle and its correlation with contractile force. J Mol Biol. 1986 Jul 5;190(1):69–82. doi: 10.1016/0022-2836(86)90076-8. [DOI] [PubMed] [Google Scholar]
- Ueno H., Harrington W. F. Temperature-dependence of local melting in the myosin subfragment-2 region of the rigor cross-bridge. J Mol Biol. 1986 Jul 5;190(1):59–68. doi: 10.1016/0022-2836(86)90075-6. [DOI] [PubMed] [Google Scholar]
- WOLEDGE R. C. The thermoelastic effect of change of tension in active muscle. J Physiol. 1961 Jan;155:187–208. doi: 10.1113/jphysiol.1961.sp006622. [DOI] [PMC free article] [PubMed] [Google Scholar]
- Yamamoto T., Herzig J. W. Series elastic properties of skinned muscle fibres in contraction and rigor. Pflugers Arch. 1978 Jan 31;373(1):21–24. doi: 10.1007/BF00581144. [DOI] [PubMed] [Google Scholar]