Abstract
1. The mechanisms of action of acetylcholine (ACh) in the medial (m.g.n.) and dorsal lateral geniculate (l.g.n.d.) nuclei were investigated using intracellular recordings techniques in guinea-pig and cat in vitro thalamic slices. 2. Application of ACh to neurones in guinea-pig geniculate nuclei resulted in a hyperpolarization in all neurones followed by a slow depolarization in 52% of l.g.n.d. and 46% of m.g.n. neurones. Neither the hyperpolarization nor the slow depolarization were eliminated by blockade of synaptic transmission and both were activated by acetyl-beta-methylcholine and DL-muscarine and blocked by scopolamine, indicating that these responses are mediated by direct activation of muscarinic receptors on the cells studied. 3. The ACh-induced hyperpolarization was associated with an increase in apparent input conductance (Gi) of 4-13 nS. The reversal potential of the ACh-induced hyperpolarization varied in a Nernstian manner with changes in extracellular [K+] and was greatly reduced by bath application of the K+ antagonist Ba2+ or intracellular injection of Cs+. These findings show that the muscarinic hyperpolarization is mediated by an increase in K+ conductance. 4. The ACh-induced slow depolarization was associated with a decrease in Gi of 2-15 nS, had an extrapolated reversal potential near EK, and was sensitive to [K+]o, indicating that this response is due to a decrease in K+ conductance. 5. In contrast to effects on guinea-pig geniculate neurones, applications of ACh to cat l.g.n.d. and m.g.n. cells resulted in a rapid depolarization in nearly all cells, followed in some neurones by a hyperpolarization and/or a slow depolarization. The rapid excitatory response was associated with an increase in membrane conductance, had an estimated reversal potential of -49 to -4 mV and may be mediated by nicotinic receptors. The hyperpolarization and slow depolarization were similar to those of the guinea-pig in that they were associated with an increase and decrease, respectively, of Gi, and were mediated by muscarinic receptors. 6. The muscarinic hyperpolarization interacted with the intrinsic properties of the thalamic neurones to inhibit single-spike activity while promoting the occurrence of burst discharges. The muscarinic slow depolarization had the opposite effect; it brought the membrane potential into the range where burst firing was blocked and single-spike firing predominated. Depending upon the membrane potential, the rapid excitatory response of cat geniculate neurones could activate either a burst or a train of action potentials.(ABSTRACT TRUNCATED AT 400 WORDS)
Full text
PDF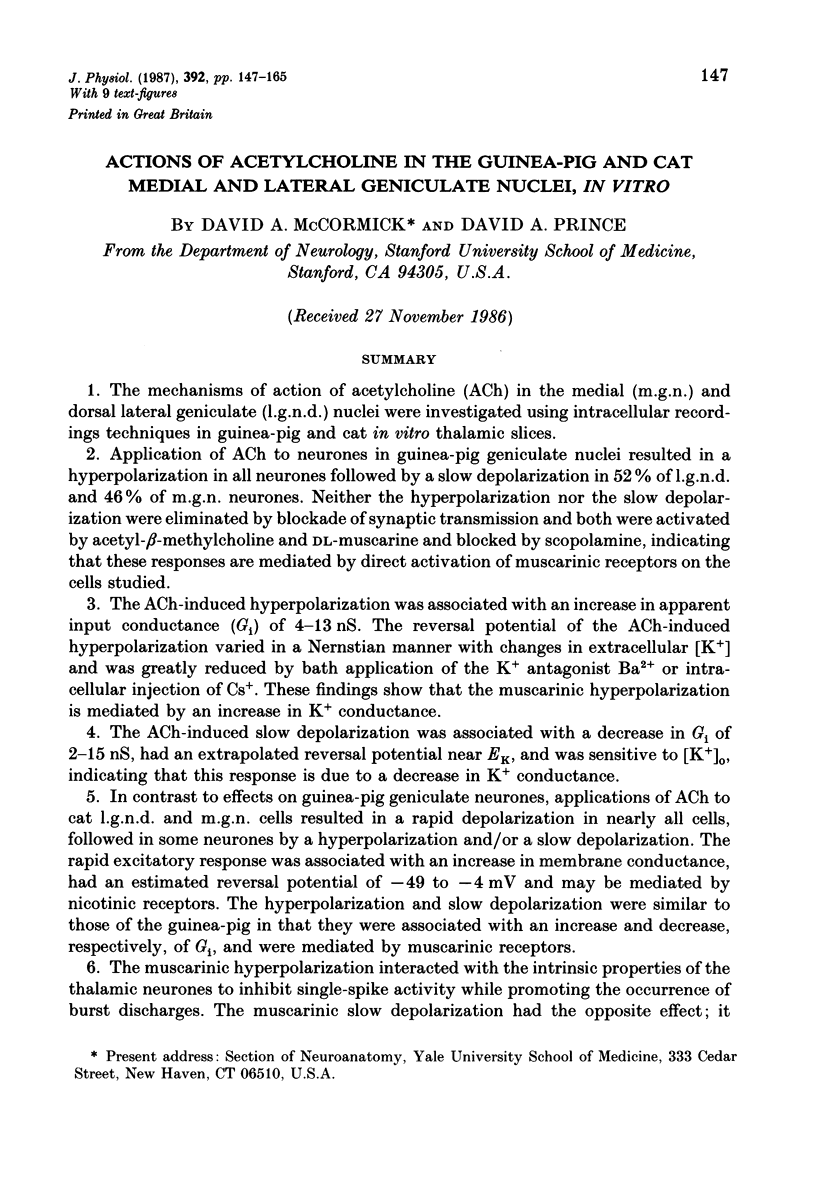
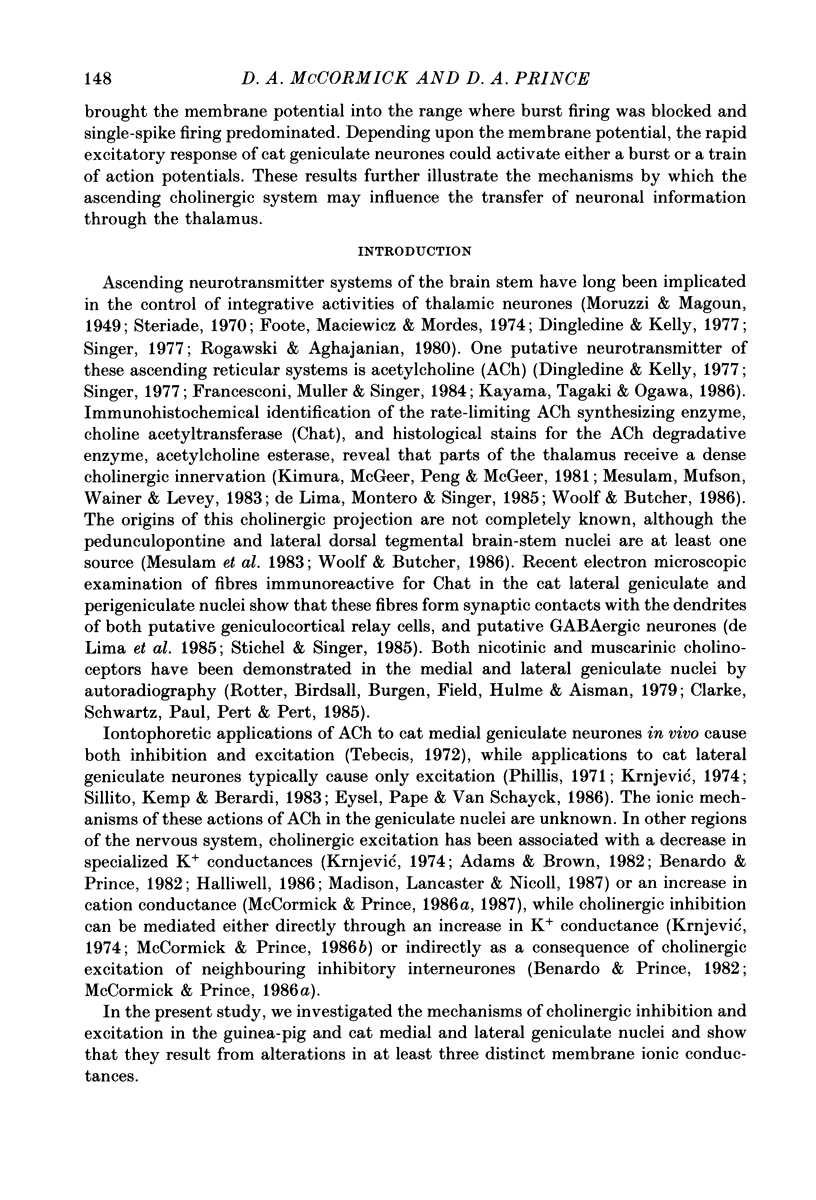
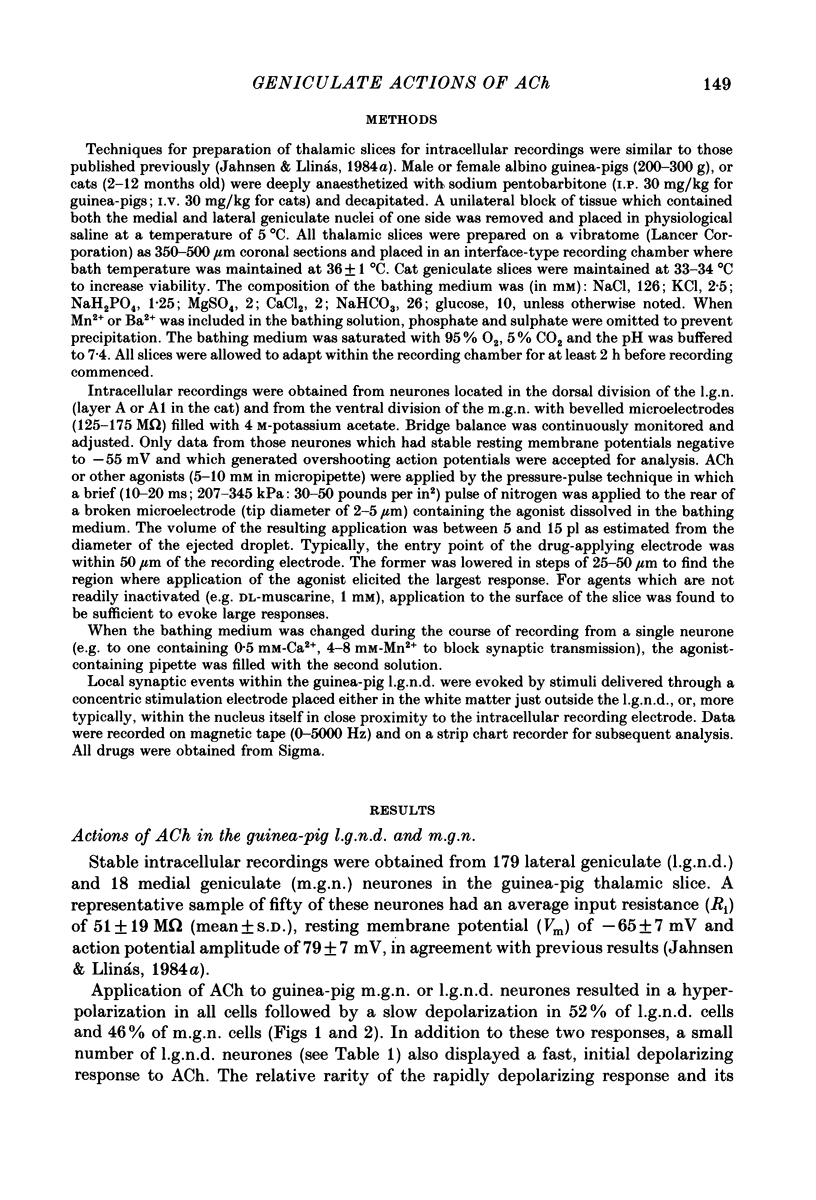
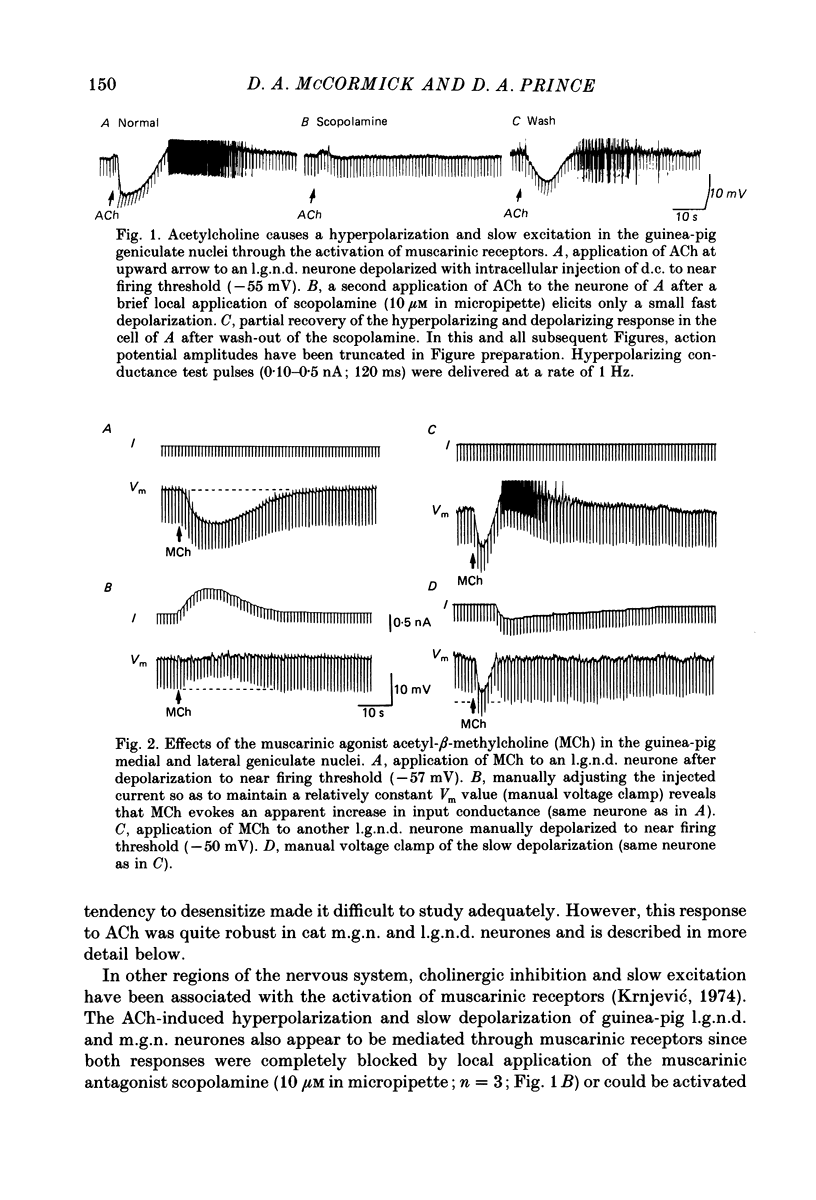
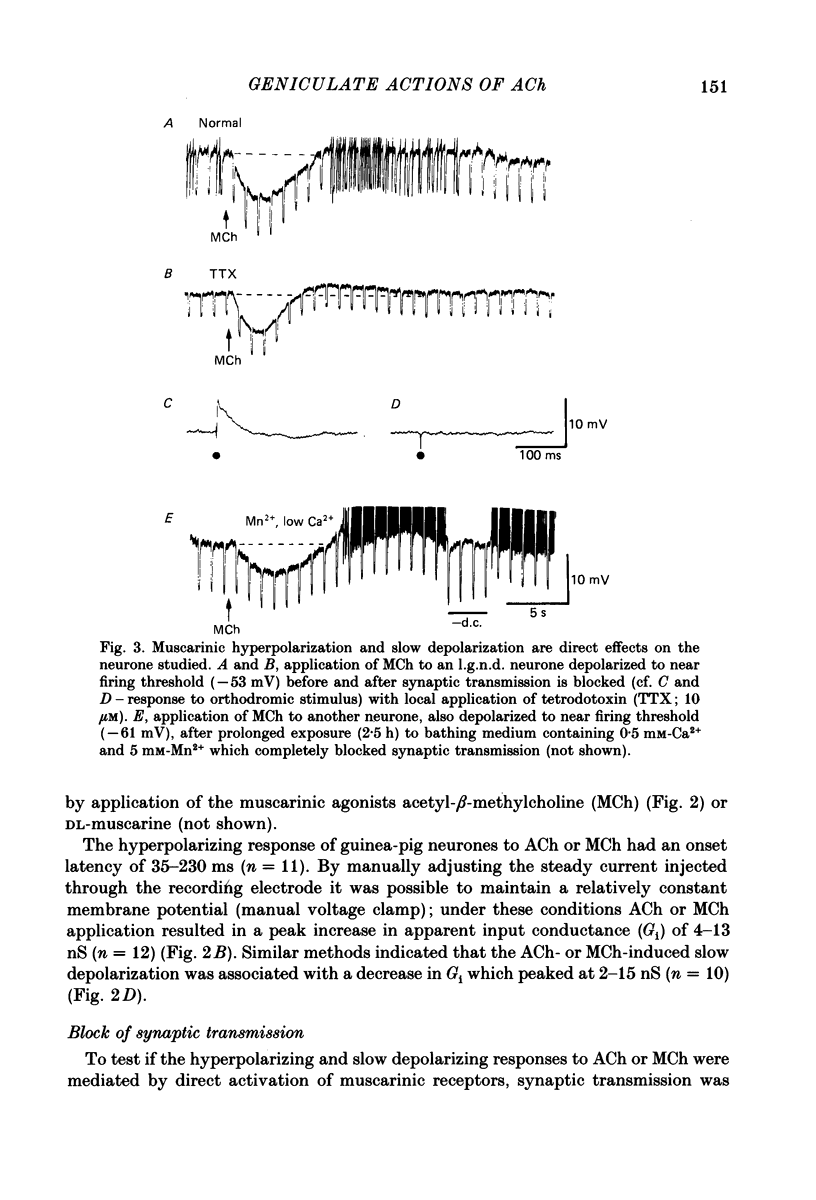
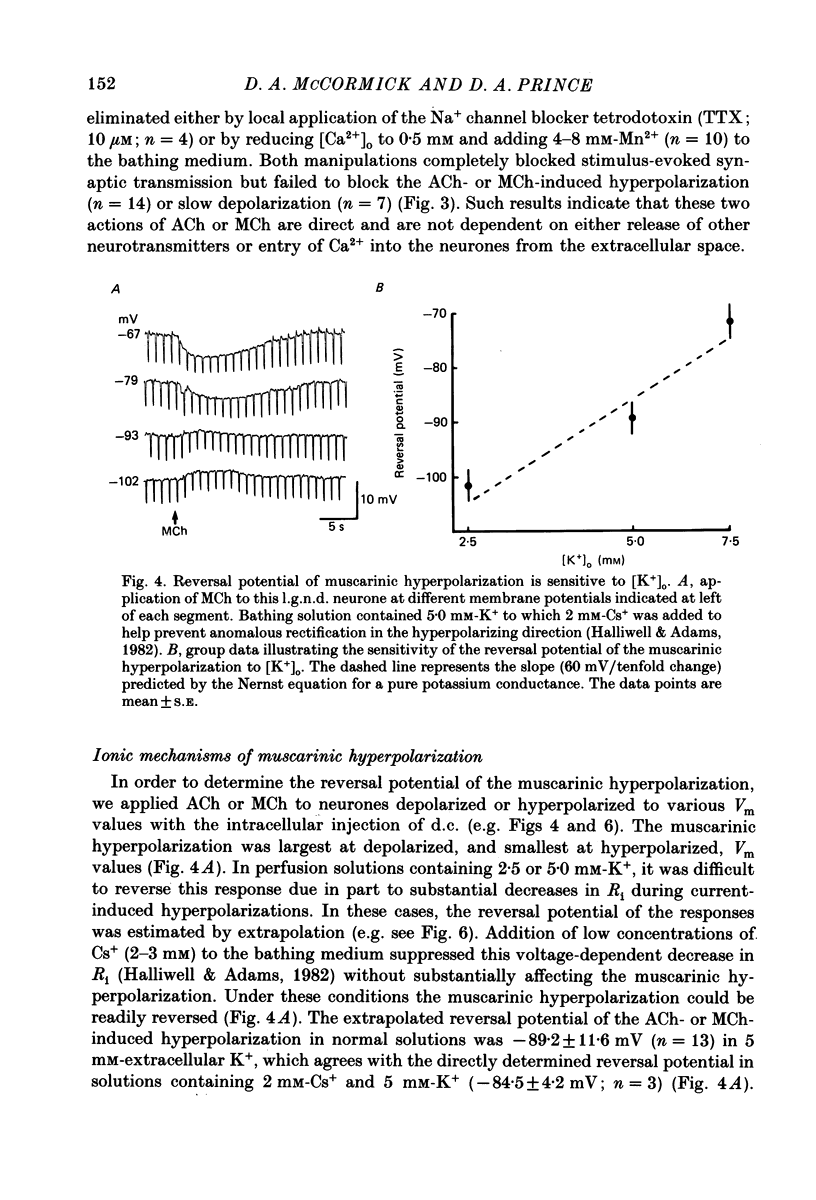
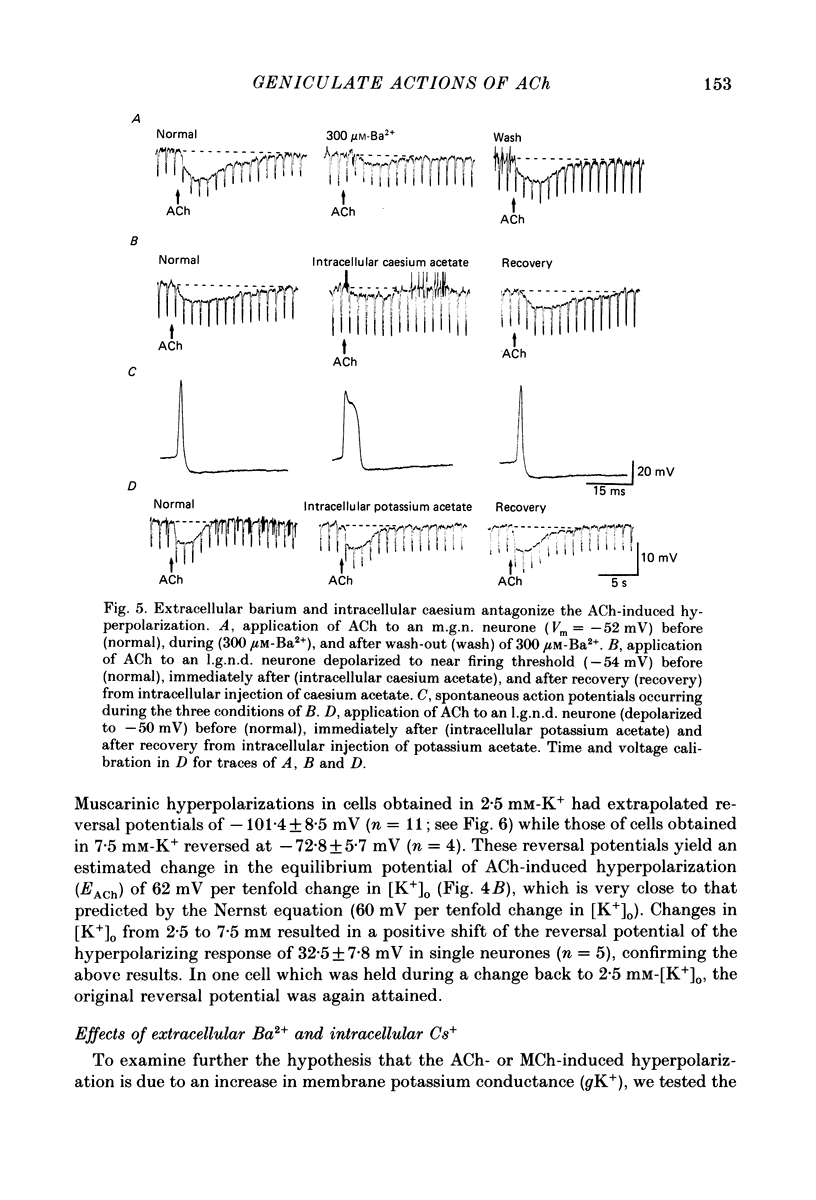
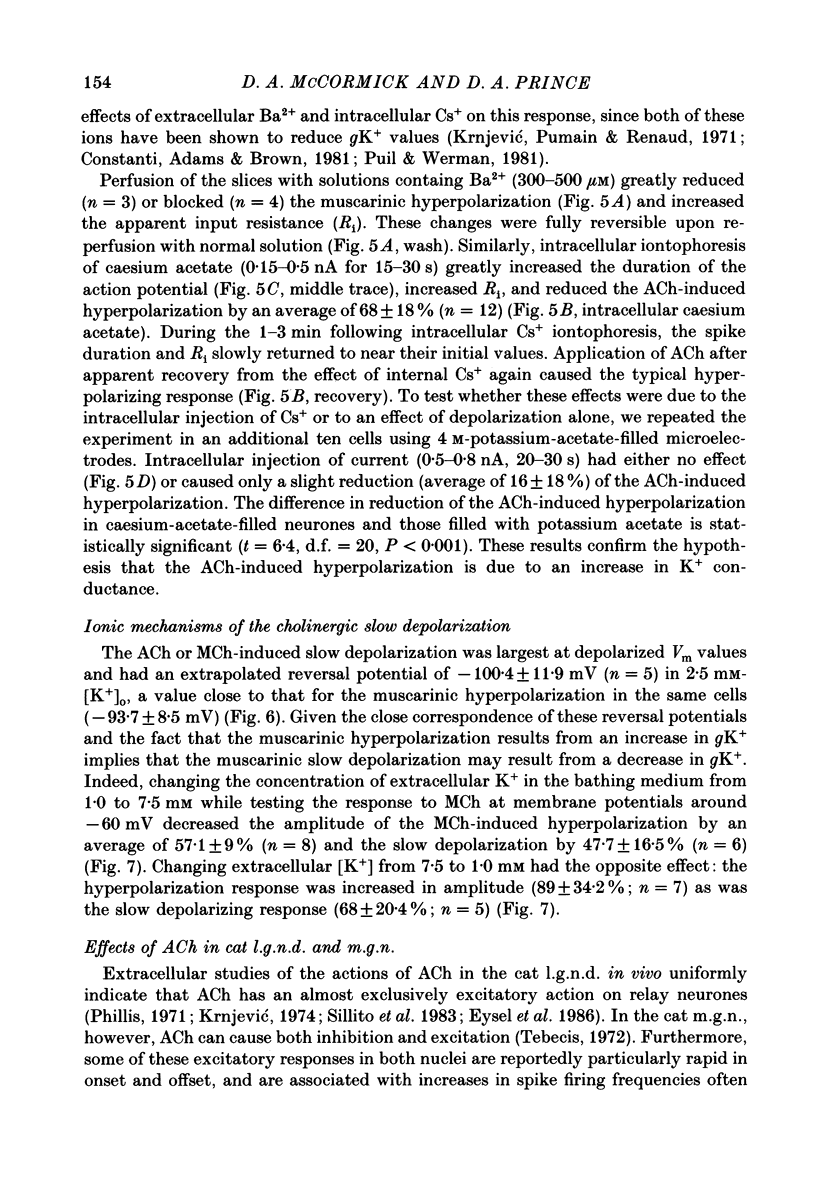
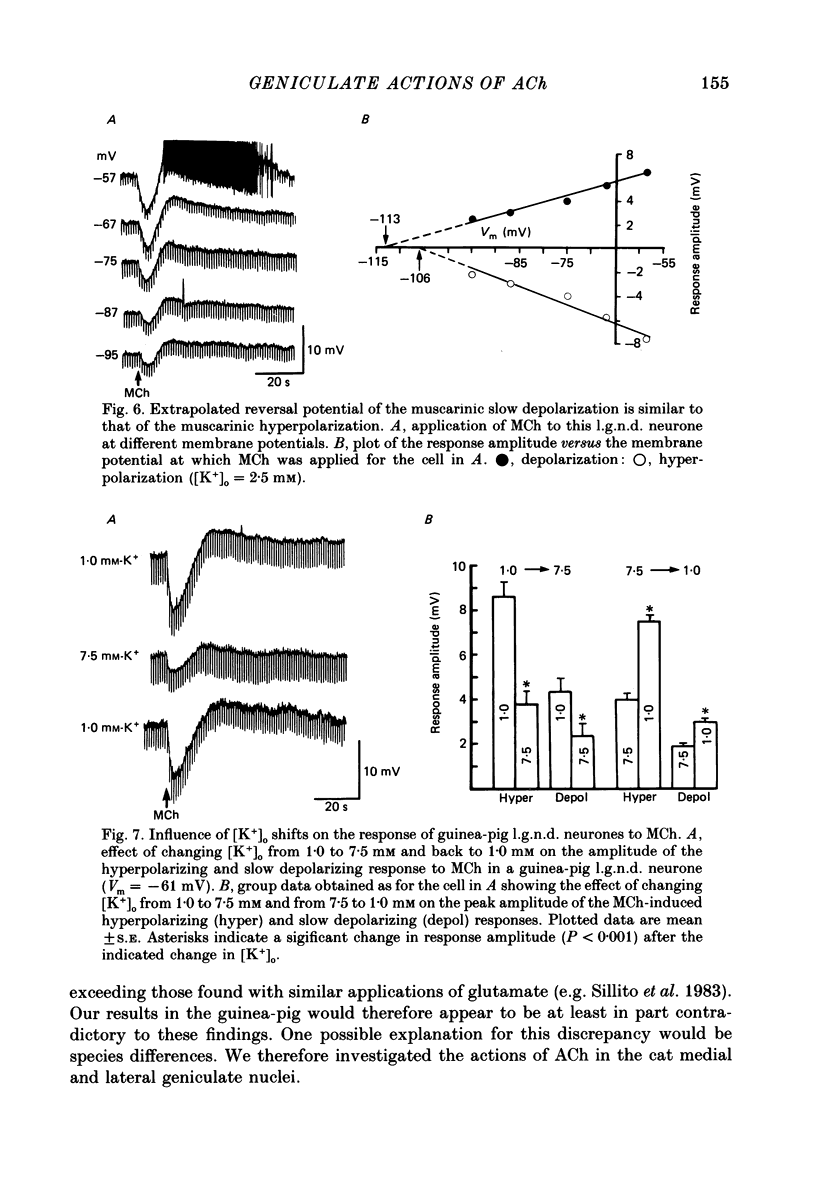
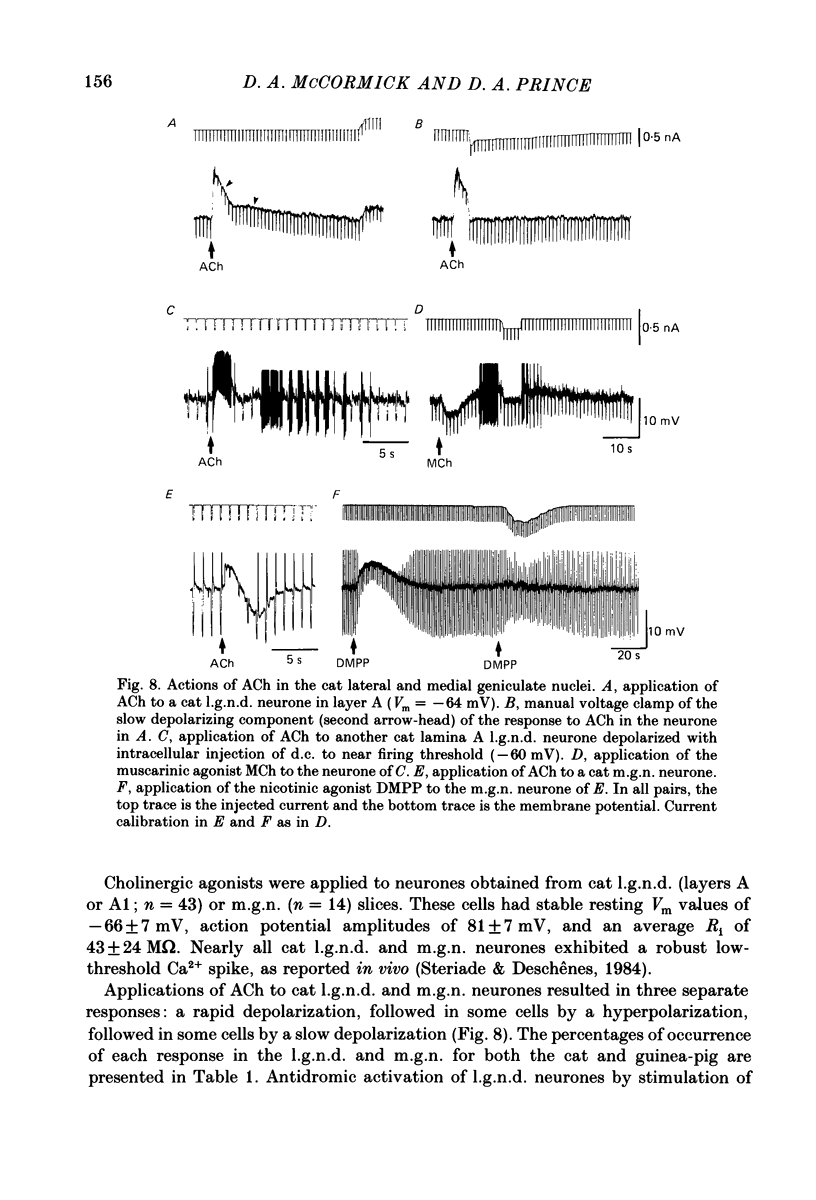
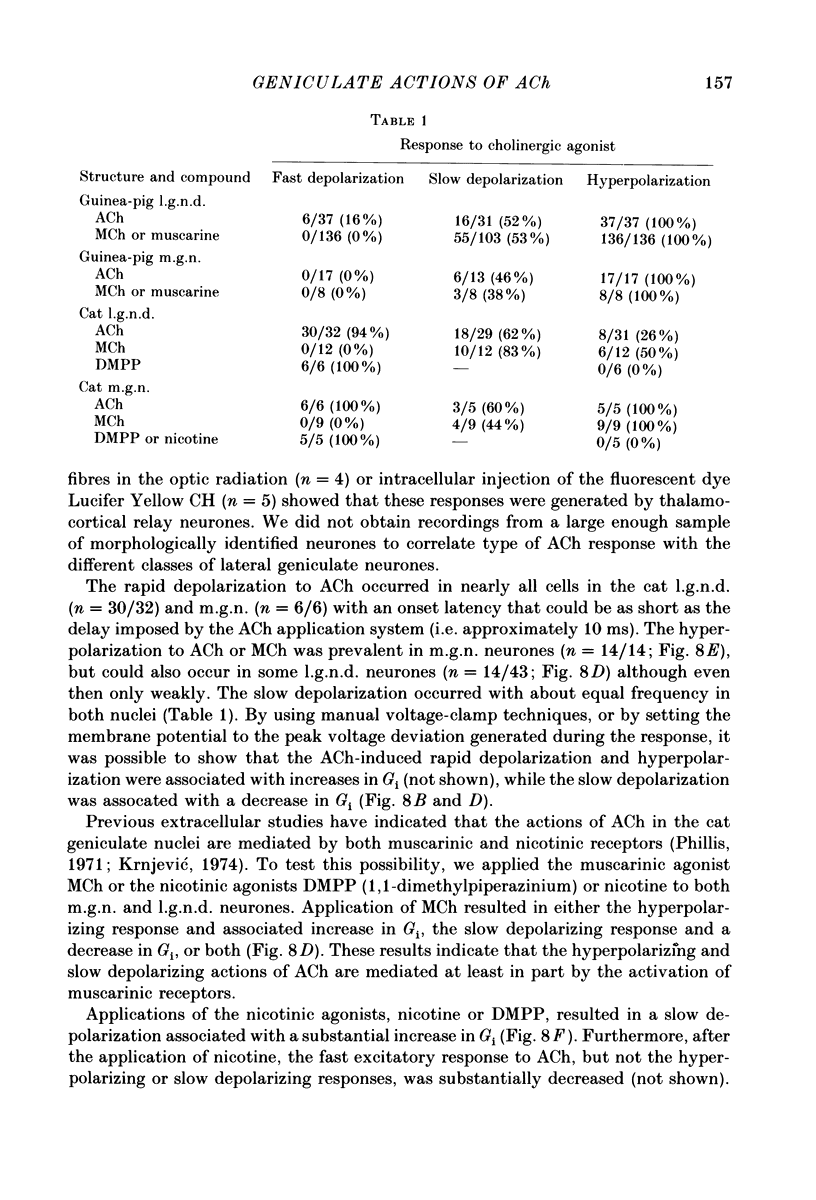
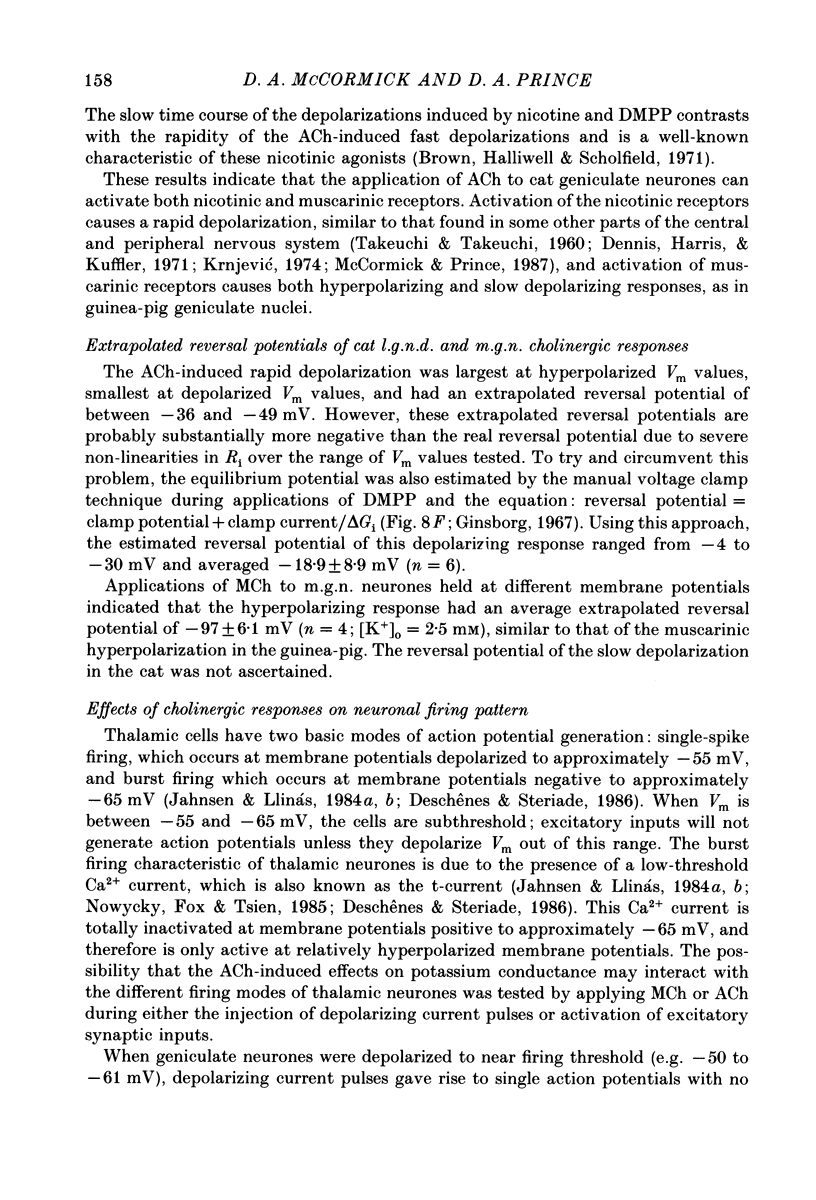
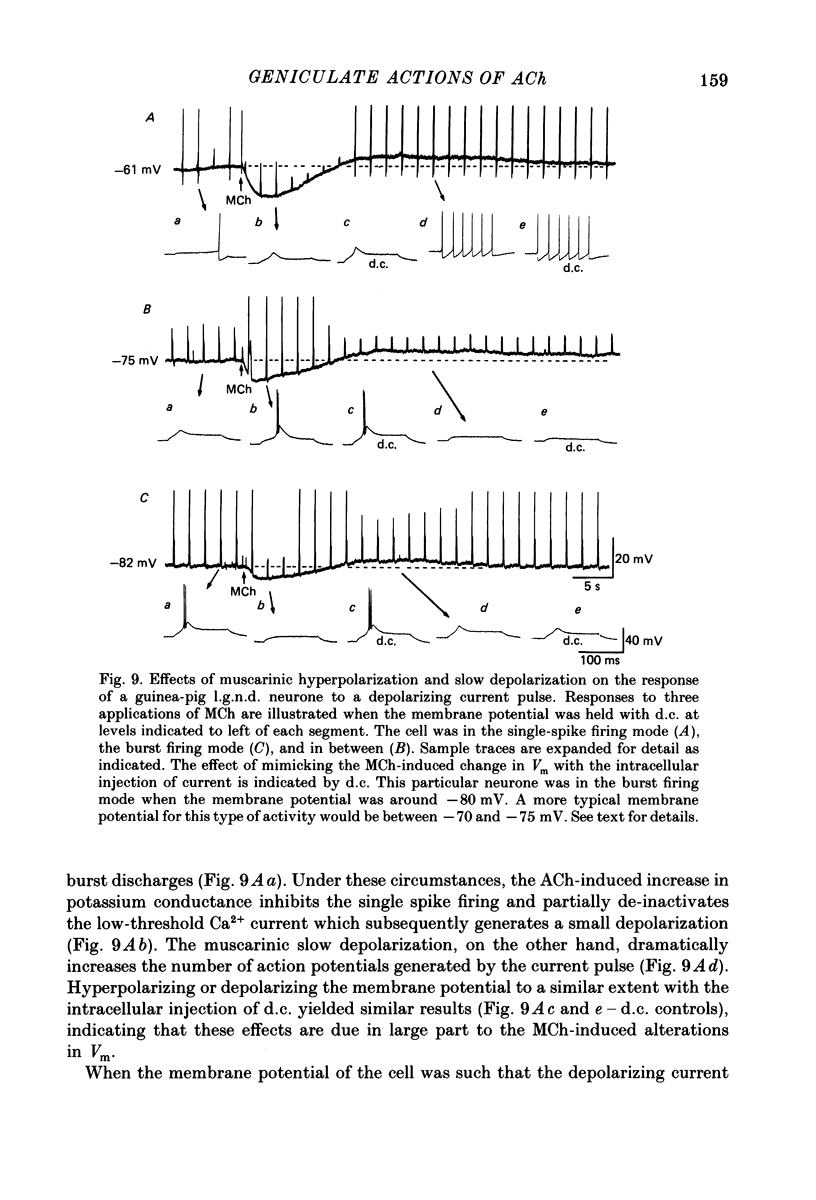
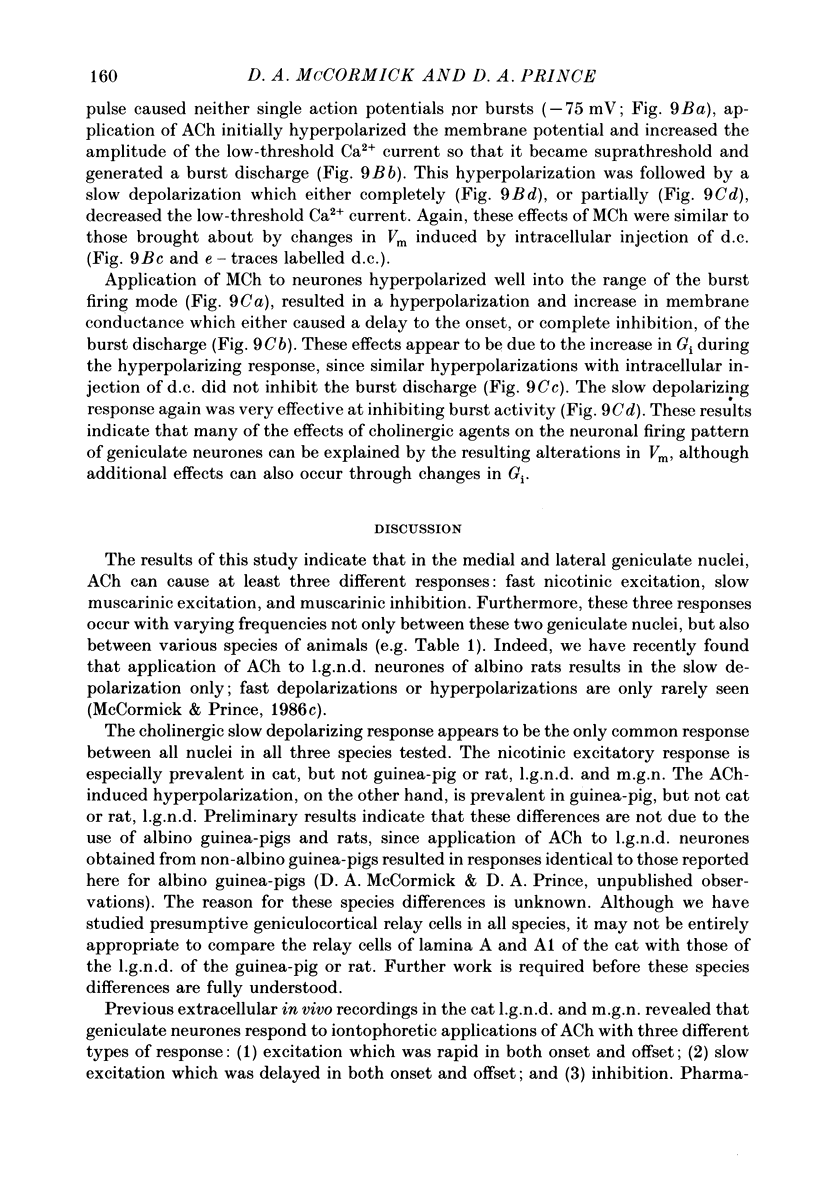
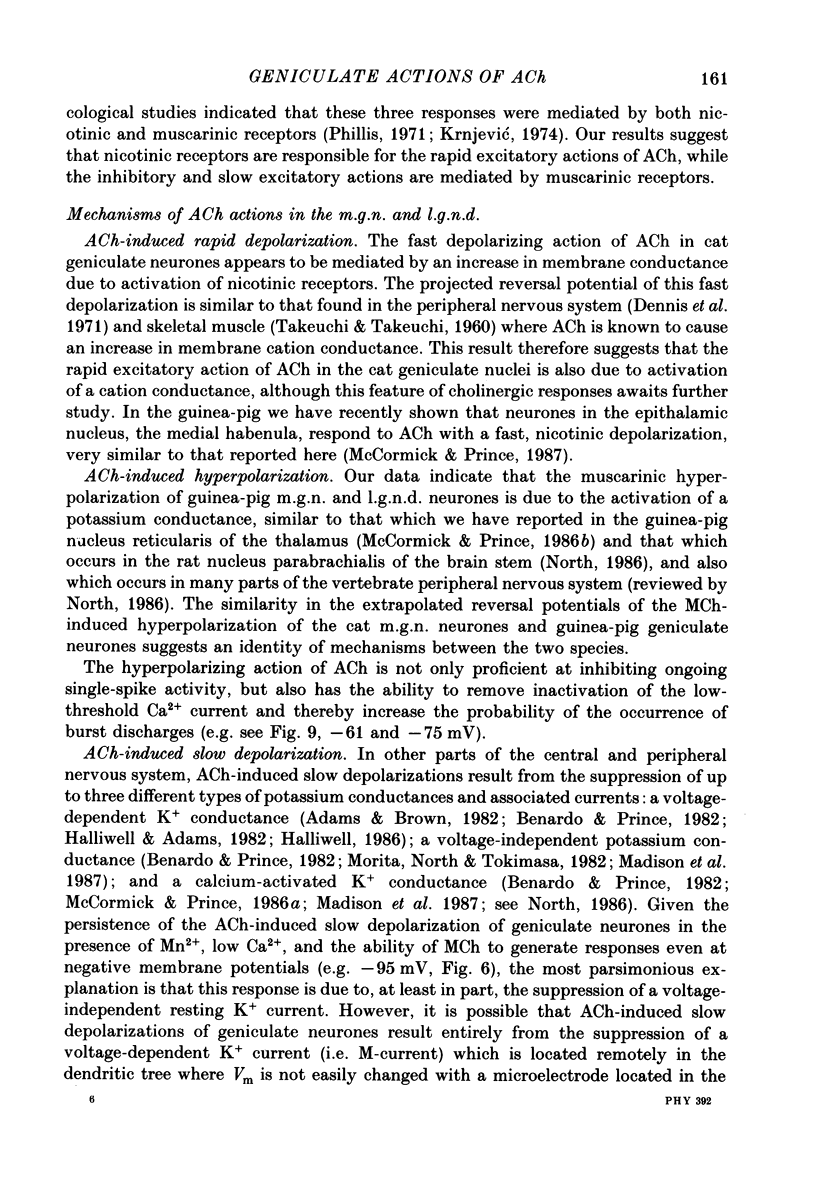
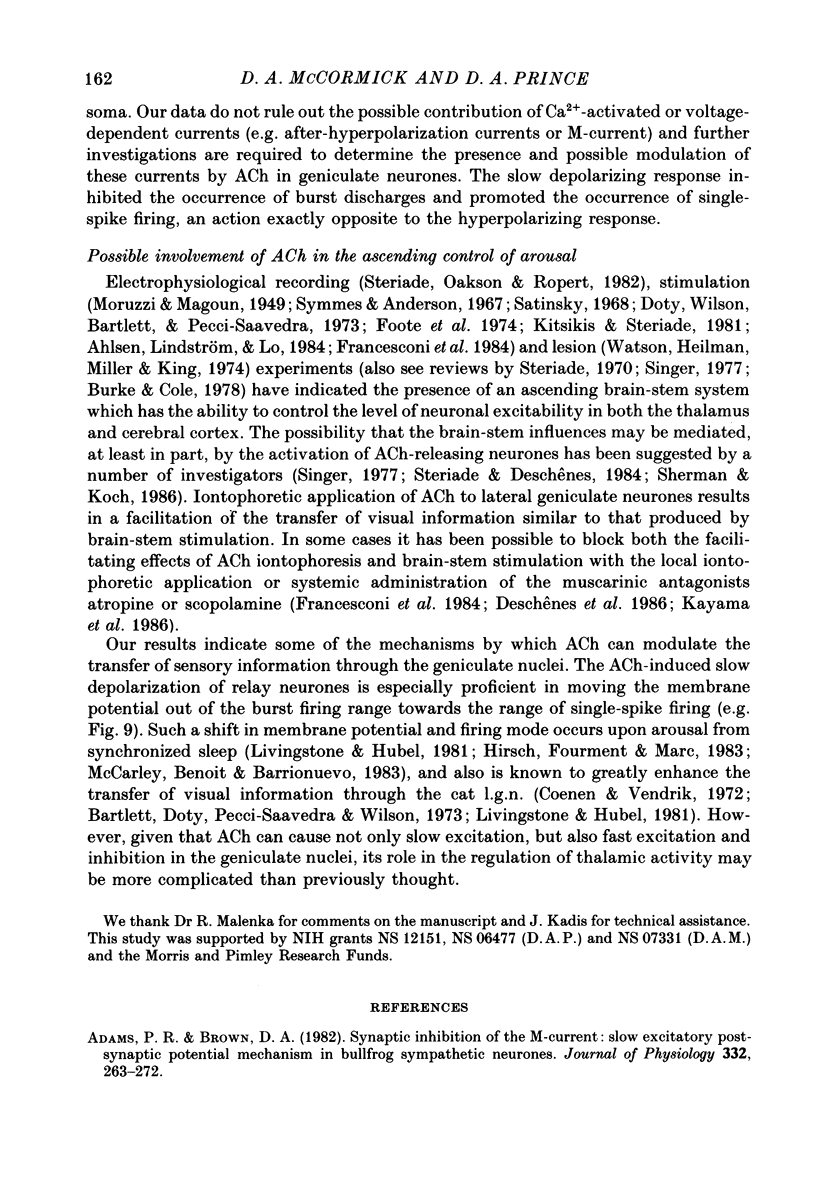
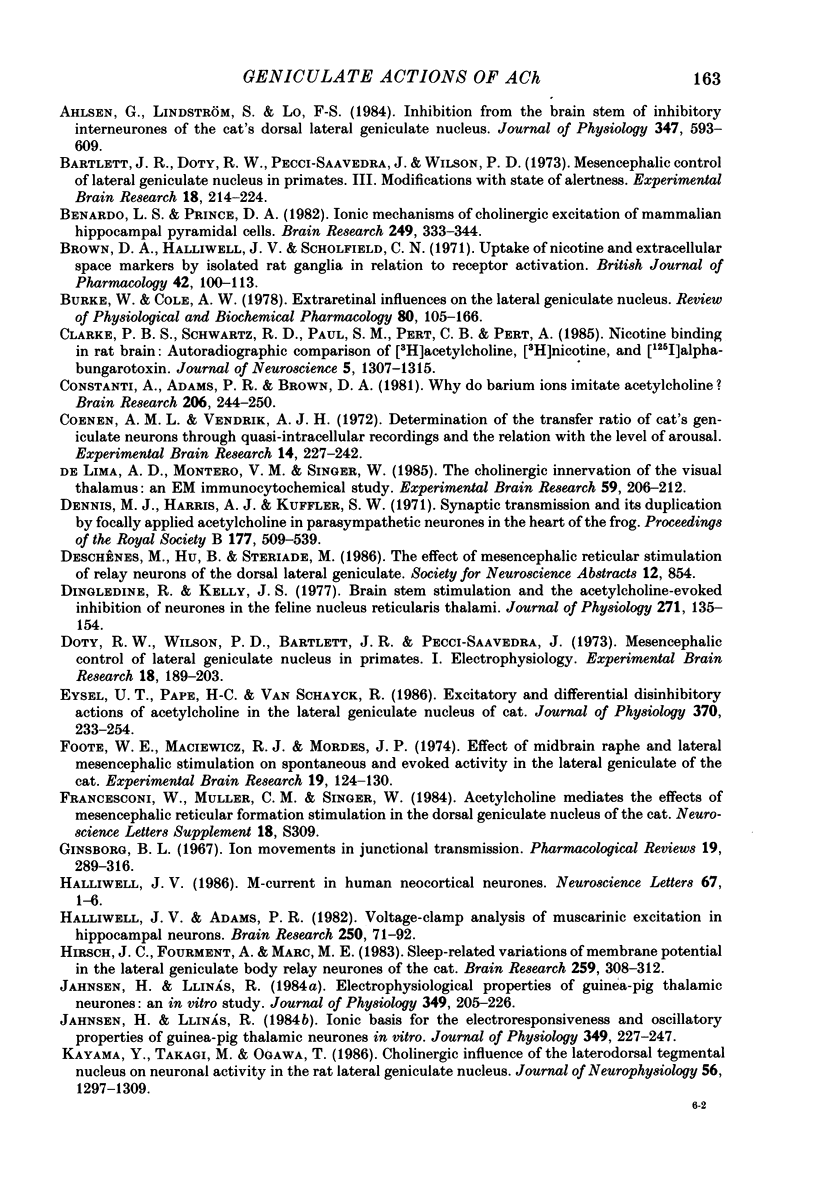
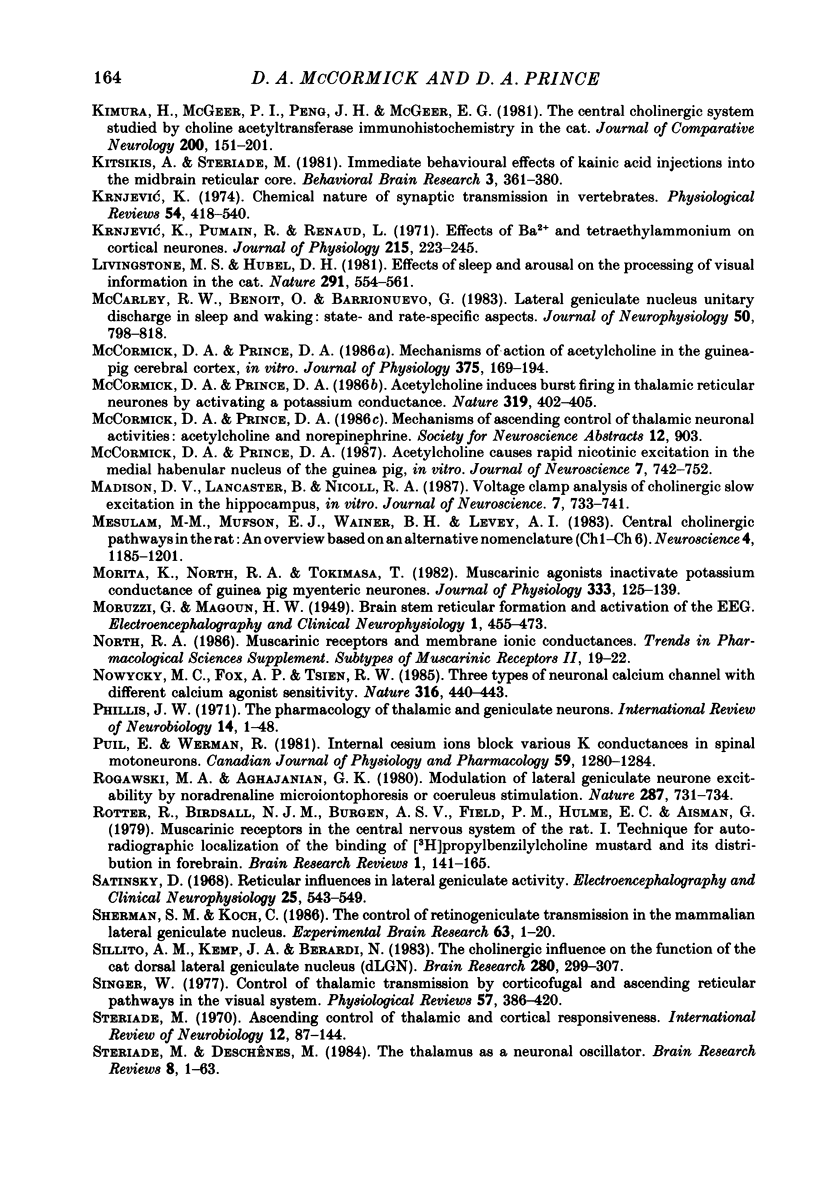
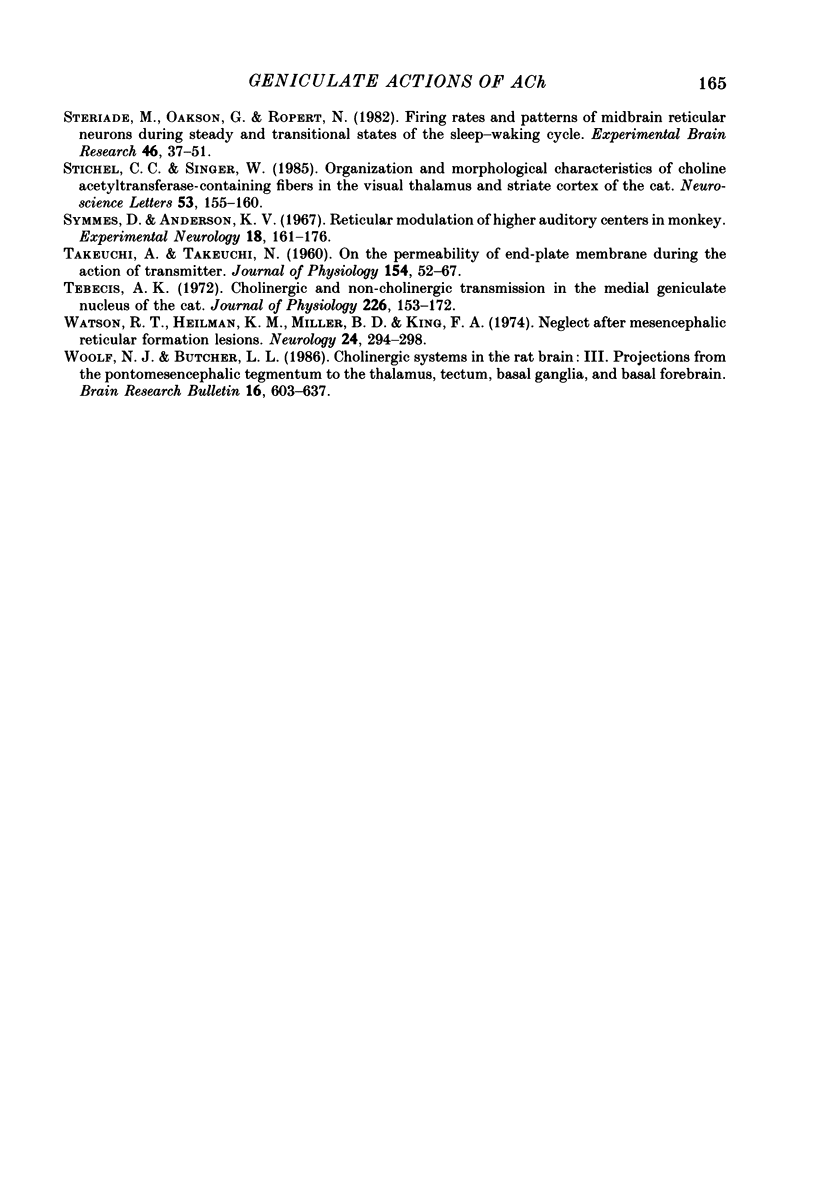
Selected References
These references are in PubMed. This may not be the complete list of references from this article.
- Adams P. R., Brown D. A. Synaptic inhibition of the M-current: slow excitatory post-synaptic potential mechanism in bullfrog sympathetic neurones. J Physiol. 1982 Nov;332:263–272. doi: 10.1113/jphysiol.1982.sp014412. [DOI] [PMC free article] [PubMed] [Google Scholar]
- Ahlsén G., Lindström S., Lo F. S. Inhibition from the brain stem of inhibitory interneurones of the cat's dorsal lateral geniculate nucleus. J Physiol. 1984 Feb;347:593–609. doi: 10.1113/jphysiol.1984.sp015085. [DOI] [PMC free article] [PubMed] [Google Scholar]
- Atinsky D. Reticular influences on lateral geniculate neuron activity. Electroencephalogr Clin Neurophysiol. 1968 Dec;25(6):543–549. doi: 10.1016/0013-4694(68)90233-2. [DOI] [PubMed] [Google Scholar]
- Bartlett J. R., Doty R. W., Pecci-Saavedra J., Wilson P. D. Mesencephalic control of lateral geniculate nucleus in primates. 3. Modifications with state of alertness. Exp Brain Res. 1973 Sep 29;18(2):214–224. doi: 10.1007/BF00234725. [DOI] [PubMed] [Google Scholar]
- Benardo L. S., Prince D. A. Ionic mechanisms of cholinergic excitation in mammalian hippocampal pyramidal cells. Brain Res. 1982 Oct 14;249(2):333–344. doi: 10.1016/0006-8993(82)90067-1. [DOI] [PubMed] [Google Scholar]
- Brown D. A., Halliwell J. V., Scholfield C. N. Uptake of nicotine and extracellular space markers by isolated rat ganglia in relation to receptor activation. Br J Pharmacol. 1971 May;42(1):100–113. doi: 10.1111/j.1476-5381.1971.tb07090.x. [DOI] [PMC free article] [PubMed] [Google Scholar]
- Burke W., Cole A. M. Extraretinal influences on the lateral geniculate nucleus. Rev Physiol Biochem Pharmacol. 1978;80:105–166. doi: 10.1007/3540084665_3. [DOI] [PubMed] [Google Scholar]
- Clarke P. B., Schwartz R. D., Paul S. M., Pert C. B., Pert A. Nicotinic binding in rat brain: autoradiographic comparison of [3H]acetylcholine, [3H]nicotine, and [125I]-alpha-bungarotoxin. J Neurosci. 1985 May;5(5):1307–1315. doi: 10.1523/JNEUROSCI.05-05-01307.1985. [DOI] [PMC free article] [PubMed] [Google Scholar]
- Coenen A. M., Vendrik A. J. Determination of the transfer ratio of cat's geniculate neurons through quasi-intracellular recordings and the relation with the level of alertness. Exp Brain Res. 1972;14(3):227–242. doi: 10.1007/BF00816160. [DOI] [PubMed] [Google Scholar]
- Constanti A., Adams P. R., Brown D. A. Who do barium ions imitate acetylcholine? Brain Res. 1981 Feb 9;206(1):244–250. doi: 10.1016/0006-8993(81)90125-6. [DOI] [PubMed] [Google Scholar]
- Dennis M. J., Harris A. J., Kuffler S. W. Synaptic transmission and its duplication by focally applied acetylcholine in parasympathetic neurons in the heart of the frog. Proc R Soc Lond B Biol Sci. 1971 Apr 27;177(1049):509–539. doi: 10.1098/rspb.1971.0045. [DOI] [PubMed] [Google Scholar]
- Dingledine R., Kelly J. S. Brain stem stimulation and the acetylcholine-evoked inhibition of neurones in the feline nucleus reticularis thalami. J Physiol. 1977 Sep;271(1):135–154. doi: 10.1113/jphysiol.1977.sp011994. [DOI] [PMC free article] [PubMed] [Google Scholar]
- Doty R. W., Wilson P. D., Bartlett J. R., Pecci-Saavedra J. Mesencephalic control of lateral geniculate nucleus in primates. I. Electrophysiology. Exp Brain Res. 1973 Sep 29;18(2):189–203. doi: 10.1007/BF00234723. [DOI] [PubMed] [Google Scholar]
- Eysel U. T., Pape H. C., Van Schayck R. Excitatory and differential disinhibitory actions of acetylcholine in the lateral geniculate nucleus of the cat. J Physiol. 1986 Jan;370:233–254. doi: 10.1113/jphysiol.1986.sp015932. [DOI] [PMC free article] [PubMed] [Google Scholar]
- Foote W. E., Maciewicz R. J., Mordes J. P. Effect of midbrain raphe and lateral mesencephalic stimulation on spontaneous and evoked activity in the lateral geniculate of the cat. Exp Brain Res. 1974 Jan 31;19(2):124–130. doi: 10.1007/BF00238529. [DOI] [PubMed] [Google Scholar]
- Ginsborg B. L. Ion movements in junctional transmission. Pharmacol Rev. 1967 Sep;19(3):289–316. [PubMed] [Google Scholar]
- Halliwell J. V., Adams P. R. Voltage-clamp analysis of muscarinic excitation in hippocampal neurons. Brain Res. 1982 Oct 28;250(1):71–92. doi: 10.1016/0006-8993(82)90954-4. [DOI] [PubMed] [Google Scholar]
- Halliwell J. V. M-current in human neocortical neurones. Neurosci Lett. 1986 Jun 6;67(1):1–6. doi: 10.1016/0304-3940(86)90198-9. [DOI] [PubMed] [Google Scholar]
- Hirsch J. C., Fourment A., Marc M. E. Sleep-related variations of membrane potential in the lateral geniculate body relay neurons of the cat. Brain Res. 1983 Jan 24;259(2):308–312. doi: 10.1016/0006-8993(83)91264-7. [DOI] [PubMed] [Google Scholar]
- Jahnsen H., Llinás R. Electrophysiological properties of guinea-pig thalamic neurones: an in vitro study. J Physiol. 1984 Apr;349:205–226. doi: 10.1113/jphysiol.1984.sp015153. [DOI] [PMC free article] [PubMed] [Google Scholar]
- Jahnsen H., Llinás R. Ionic basis for the electro-responsiveness and oscillatory properties of guinea-pig thalamic neurones in vitro. J Physiol. 1984 Apr;349:227–247. doi: 10.1113/jphysiol.1984.sp015154. [DOI] [PMC free article] [PubMed] [Google Scholar]
- Kayama Y., Takagi M., Ogawa T. Cholinergic influence of the laterodorsal tegmental nucleus on neuronal activity in the rat lateral geniculate nucleus. J Neurophysiol. 1986 Nov;56(5):1297–1309. doi: 10.1152/jn.1986.56.5.1297. [DOI] [PubMed] [Google Scholar]
- Kimura H., McGeer P. L., Peng J. H., McGeer E. G. The central cholinergic system studied by choline acetyltransferase immunohistochemistry in the cat. J Comp Neurol. 1981 Aug 1;200(2):151–201. doi: 10.1002/cne.902000202. [DOI] [PubMed] [Google Scholar]
- Kitsikis A., Steriade M. Immediate behavioral effects of kainic acid injections into the midbrain reticular core. Behav Brain Res. 1981 Nov;3(3):361–380. doi: 10.1016/0166-4328(81)90005-x. [DOI] [PubMed] [Google Scholar]
- Krnjević K., Pumain R., Renaud L. Effects of Ba2+ and tetraethylammonium on cortical neurones. J Physiol. 1971 May;215(1):223–245. doi: 10.1113/jphysiol.1971.sp009466. [DOI] [PMC free article] [PubMed] [Google Scholar]
- Livingstone M. S., Hubel D. H. Effects of sleep and arousal on the processing of visual information in the cat. Nature. 1981 Jun 18;291(5816):554–561. doi: 10.1038/291554a0. [DOI] [PubMed] [Google Scholar]
- Madison D. V., Lancaster B., Nicoll R. A. Voltage clamp analysis of cholinergic action in the hippocampus. J Neurosci. 1987 Mar;7(3):733–741. doi: 10.1523/JNEUROSCI.07-03-00733.1987. [DOI] [PMC free article] [PubMed] [Google Scholar]
- McCarley R. W., Benoit O., Barrionuevo G. Lateral geniculate nucleus unitary discharge in sleep and waking: state- and rate-specific aspects. J Neurophysiol. 1983 Oct;50(4):798–818. doi: 10.1152/jn.1983.50.4.798. [DOI] [PubMed] [Google Scholar]
- McCormick D. A., Prince D. A. Acetylcholine causes rapid nicotinic excitation in the medial habenular nucleus of guinea pig, in vitro. J Neurosci. 1987 Mar;7(3):742–752. doi: 10.1523/JNEUROSCI.07-03-00742.1987. [DOI] [PMC free article] [PubMed] [Google Scholar]
- McCormick D. A., Prince D. A. Acetylcholine induces burst firing in thalamic reticular neurones by activating a potassium conductance. 1986 Jan 30-Feb 5Nature. 319(6052):402–405. doi: 10.1038/319402a0. [DOI] [PubMed] [Google Scholar]
- McCormick D. A., Prince D. A. Mechanisms of action of acetylcholine in the guinea-pig cerebral cortex in vitro. J Physiol. 1986 Jun;375:169–194. doi: 10.1113/jphysiol.1986.sp016112. [DOI] [PMC free article] [PubMed] [Google Scholar]
- Mesulam M. M., Mufson E. J., Wainer B. H., Levey A. I. Central cholinergic pathways in the rat: an overview based on an alternative nomenclature (Ch1-Ch6). Neuroscience. 1983 Dec;10(4):1185–1201. doi: 10.1016/0306-4522(83)90108-2. [DOI] [PubMed] [Google Scholar]
- Morita K., North R. A., Tokimasa T. Muscarinic agonists inactivate potassium conductance of guinea-pig myenteric neurones. J Physiol. 1982 Dec;333:125–139. doi: 10.1113/jphysiol.1982.sp014443. [DOI] [PMC free article] [PubMed] [Google Scholar]
- Nowycky M. C., Fox A. P., Tsien R. W. Three types of neuronal calcium channel with different calcium agonist sensitivity. Nature. 1985 Aug 1;316(6027):440–443. doi: 10.1038/316440a0. [DOI] [PubMed] [Google Scholar]
- Phillis J. W. The pharmacology of thalamic and geniculate neurons. Int Rev Neurobiol. 1971;14:1–48. doi: 10.1016/s0074-7742(08)60182-8. [DOI] [PubMed] [Google Scholar]
- Puil E., Werman R. Internal cesium ions block various K conductances in spinal motoneurons. Can J Physiol Pharmacol. 1981 Dec;59(12):1280–1284. doi: 10.1139/y81-201. [DOI] [PubMed] [Google Scholar]
- Rogawski M. A., Aghajanian G. K. Modulation of lateral geniculate neurone excitability by noradrenaline microiontophoresis or locus coeruleus stimulation. Nature. 1980 Oct 23;287(5784):731–734. doi: 10.1038/287731a0. [DOI] [PubMed] [Google Scholar]
- Rotter A., Birdsall N. J., Burgen A. S., Field P. M., Hulme E. C., Raisman G. Muscarinic receptors in the central nervous system of the rat. I. Technique for autoradiographic localization of the binding of [3H]propylbenzilylcholine mustard and its distribution in the forebrain. Brain Res. 1979;180(2):141–165. doi: 10.1016/0165-0173(79)90002-x. [DOI] [PubMed] [Google Scholar]
- Sherman S. M., Koch C. The control of retinogeniculate transmission in the mammalian lateral geniculate nucleus. Exp Brain Res. 1986;63(1):1–20. doi: 10.1007/BF00235642. [DOI] [PubMed] [Google Scholar]
- Sillito A. M., Kemp J. A., Berardi N. The cholinergic influence on the function of the cat dorsal lateral geniculate nucleus (dLGN). Brain Res. 1983 Dec 5;280(2):299–307. doi: 10.1016/0006-8993(83)90059-8. [DOI] [PubMed] [Google Scholar]
- Singer W. Control of thalamic transmission by corticofugal and ascending reticular pathways in the visual system. Physiol Rev. 1977 Jul;57(3):386–420. doi: 10.1152/physrev.1977.57.3.386. [DOI] [PubMed] [Google Scholar]
- Steriade M. Ascending control of thalamic and cortical responsiveness. Int Rev Neurobiol. 1970;12:87–144. doi: 10.1016/s0074-7742(08)60059-8. [DOI] [PubMed] [Google Scholar]
- Steriade M., Deschenes M. The thalamus as a neuronal oscillator. Brain Res. 1984 Nov;320(1):1–63. doi: 10.1016/0165-0173(84)90017-1. [DOI] [PubMed] [Google Scholar]
- Steriade M., Oakson G., Ropert N. Firing rates and patterns of midbrain reticular neurons during steady and transitional states of the sleep-waking cycle. Exp Brain Res. 1982;46(1):37–51. doi: 10.1007/BF00238096. [DOI] [PubMed] [Google Scholar]
- Stichel C. C., Singer W. Organization and morphological characteristics of choline acetyltransferase-containing fibers in the visual thalamus and striate cortex of the cat. Neurosci Lett. 1985 Jan 21;53(2):155–160. doi: 10.1016/0304-3940(85)90178-8. [DOI] [PubMed] [Google Scholar]
- Symmes D., Anderson K. V. Reticular modulation of higher auditory centers in monkey. Exp Neurol. 1967 Jun;18(2):161–176. doi: 10.1016/0014-4886(67)90038-6. [DOI] [PubMed] [Google Scholar]
- TAKEUCHI A., TAKEUCHI N. On the permeability of end-plate membrane during the action of transmitter. J Physiol. 1960 Nov;154:52–67. doi: 10.1113/jphysiol.1960.sp006564. [DOI] [PMC free article] [PubMed] [Google Scholar]
- Tebecis A. K. Cholinergic and non-cholinergic transmission in the medial geniculate nucleus of the cat. J Physiol. 1972 Oct;226(1):153–172. doi: 10.1113/jphysiol.1972.sp009978. [DOI] [PMC free article] [PubMed] [Google Scholar]
- Watson R. T., Heilman K. M., Miller B. D., King F. A. Neglect after mesencephalic reticular formation lesions. Neurology. 1974 Mar;24(3):294–298. doi: 10.1212/wnl.24.3.294. [DOI] [PubMed] [Google Scholar]
- Woolf N. J., Butcher L. L. Cholinergic systems in the rat brain: III. Projections from the pontomesencephalic tegmentum to the thalamus, tectum, basal ganglia, and basal forebrain. Brain Res Bull. 1986 May;16(5):603–637. doi: 10.1016/0361-9230(86)90134-6. [DOI] [PubMed] [Google Scholar]
- de Lima A. D., Montero V. M., Singer W. The cholinergic innervation of the visual thalamus: an EM immunocytochemical study. Exp Brain Res. 1985;59(1):206–212. doi: 10.1007/BF00237681. [DOI] [PubMed] [Google Scholar]