Abstract
1. The ion-selective and ion transport properties of glycine receptor (GlyR) and gamma-aminobutyric acid receptor (GABAR) channels in the soma membrane of mouse spinal cord neurones were investigated using the whole-cell, cell-attached and outside-out patch versions of the patch-clamp technique. 2. Current-voltage (I-V) relations of transmitter-activated currents obtained from whole-cell measurements with 145 mM-Cl- intracellularly and extracellularly, showed outward rectification. In voltage-jump experiments, the instantaneous I-V relations were linear, and the steady-state I-V relations were rectifying outwardly indicating that the gating of GlyR and GABAR channels is voltage sensitive. 3. The reversal potential of whole-cell currents shifted 56 mV per tenfold change in internal Cl- activity indicating activation of Cl(-)-selective channels. The permeability ratio of K+ to Cl- (PK/PCl) was smaller than 0.05 for both channels. 4. The permeability sequence for large polyatomic anions was formate greater than bicarbonate greater than acetate greater than phosphate greater than propionate for GABAR channels; phosphate and propionate were not measurably permeant in GlyR channels. This indicates that open GlyR and GABAR channels have effective pore diameters of 5.2 and 5.6 A, respectively. The sequence of relative permeabilities for small anions was SCN- greater than I- greater than Br- greater than Cl- greater than F- for both channels. 5. GlyR and GABAR channels are multi-conductance-state channels. In cell-attached patches the single-channel slope conductances close to 0 mV membrane potential were 29, 18 and 10 pS for glycine, and 28, 17 and 10 pS for GABA-activated channels. The most frequently observed (main) conductance states were 29 and 17 pS for the GlyR and GABAR channel, respectively. 6. In outside-out patches with equal extracellular and intracellular concentrations of 145 mM-Cl-, the conductance states were 46, 30, 20 and 12 pS for GlyR channels and 44, 30, 19 and 12 pS for GABAR channels. The most frequently occurring main state was 46 pS for the GlyR and 30 pS for the GABAR channel. 7. Single-channel conductances measured in equal 140 mM concentrations of small anions on both membrane faces revealed a conductance sequence of Cl- greater than Br- greater than I- greater than SCN- greater than F- for both channels. This is nearly the inverse sequence of that found for the permeability of these ions indicating the presence of binding sites for ions in the channel.(ABSTRACT TRUNCATED AT 400 WORDS)
Full text
PDF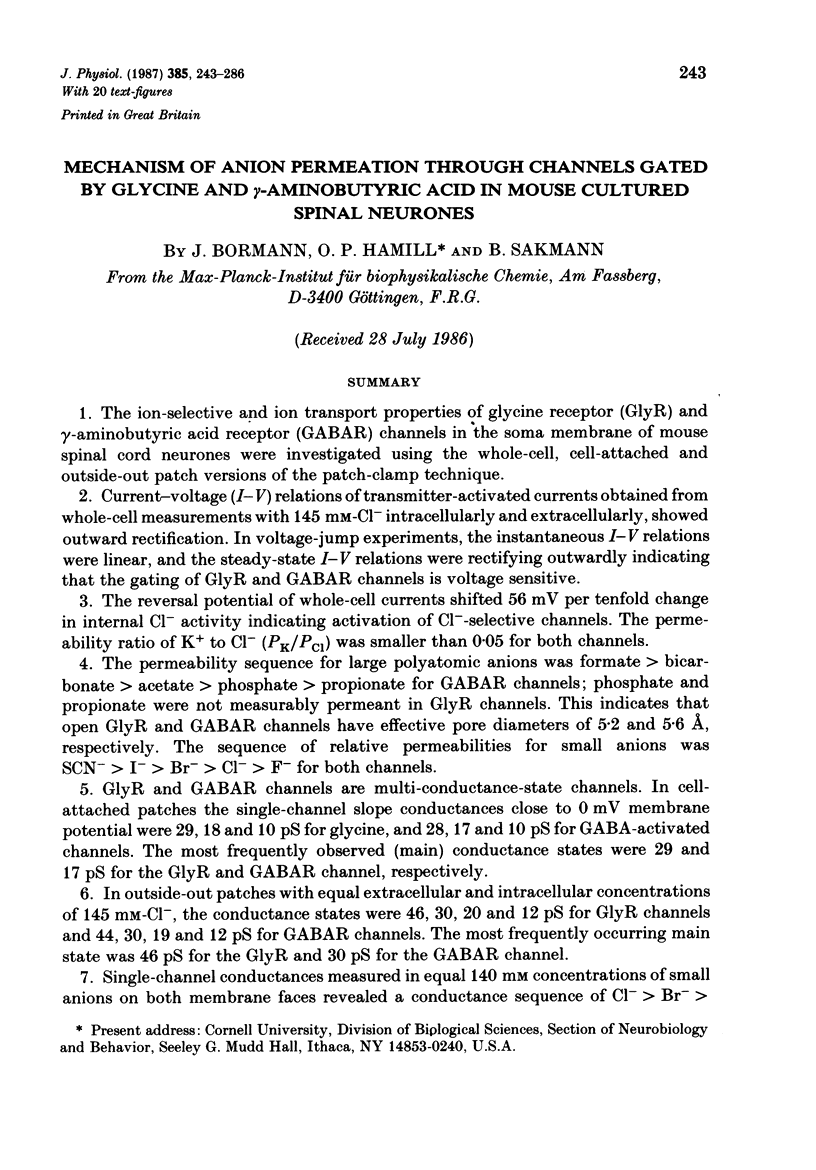
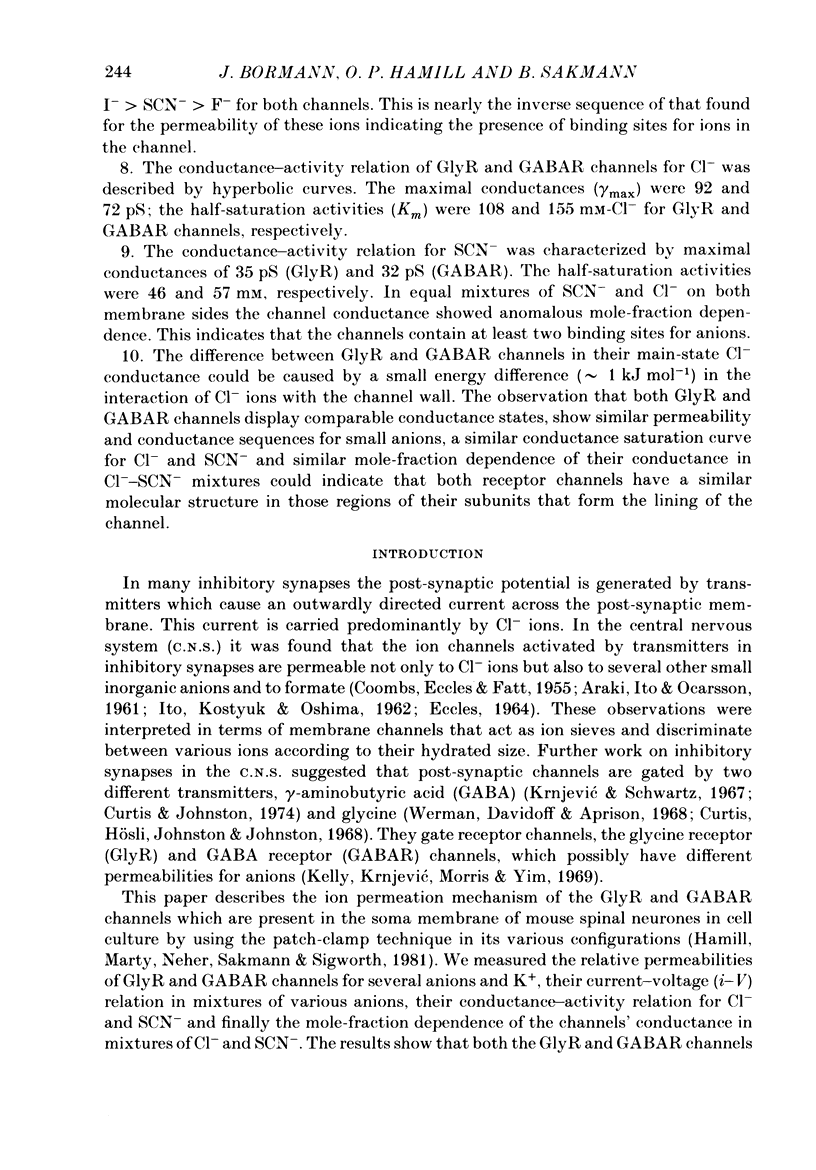
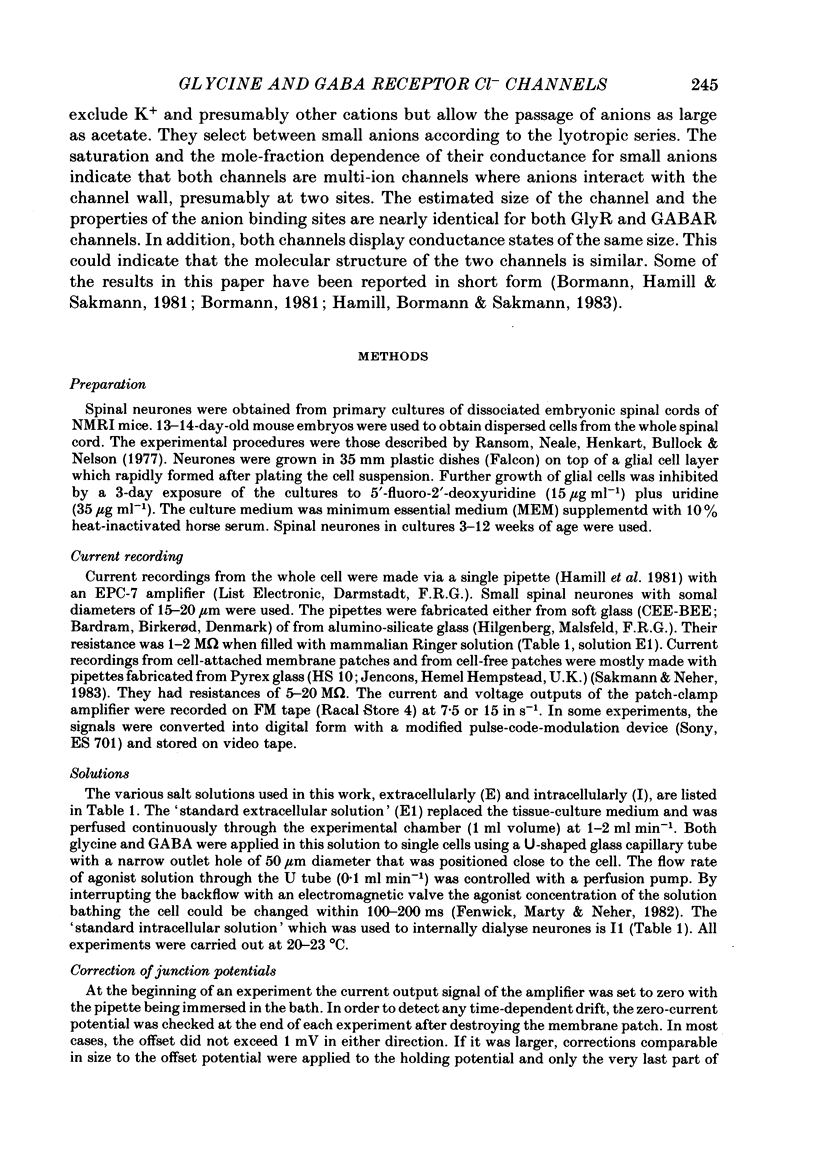
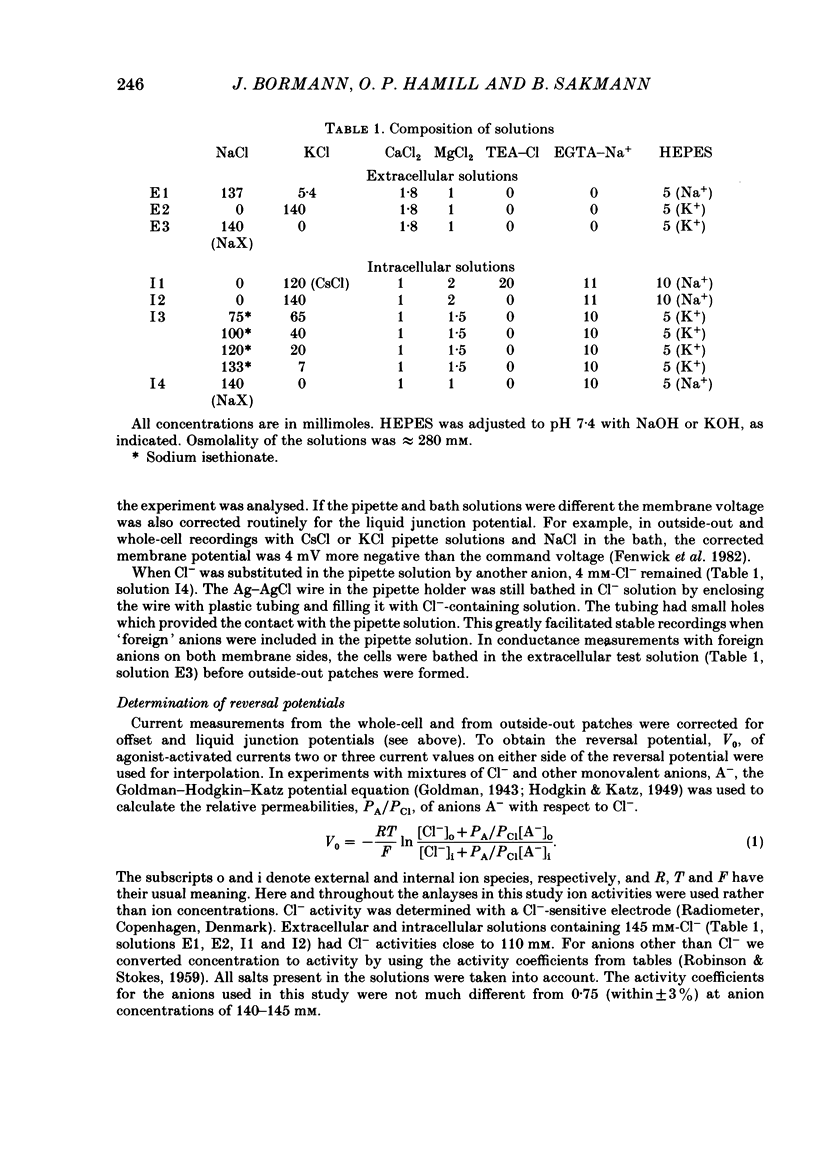
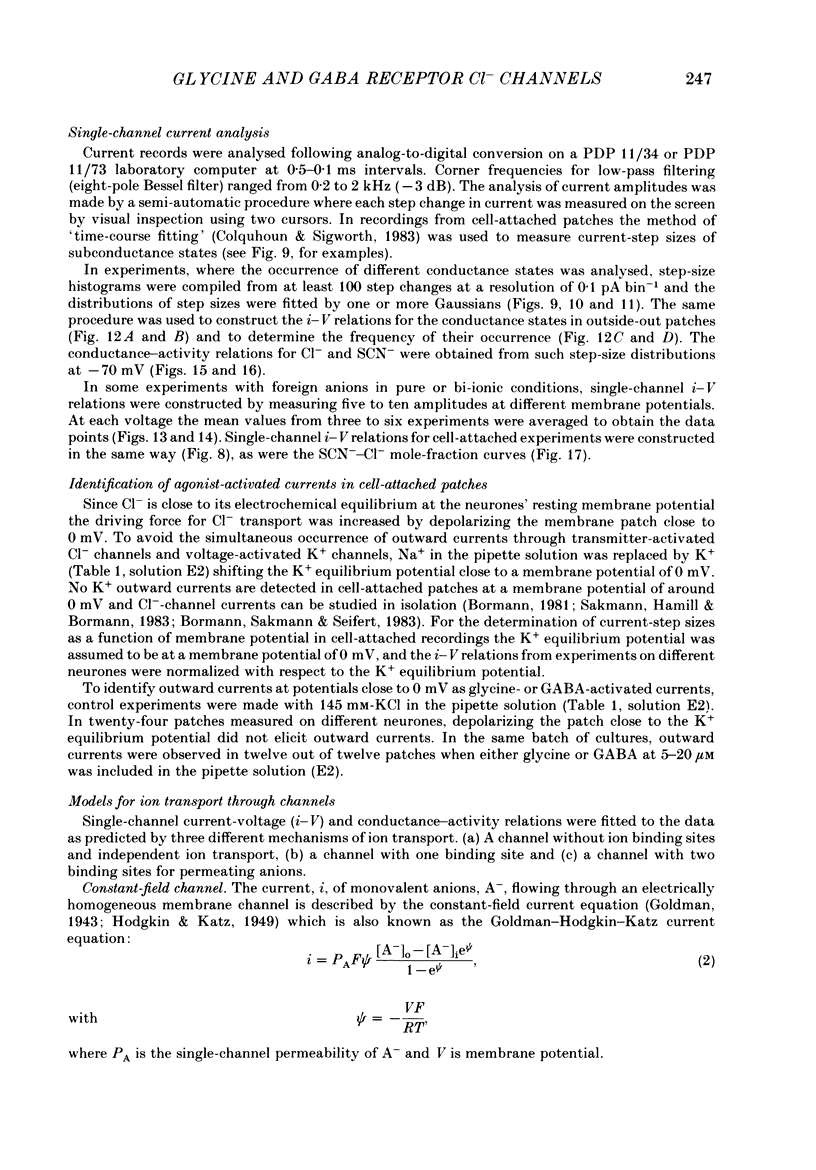
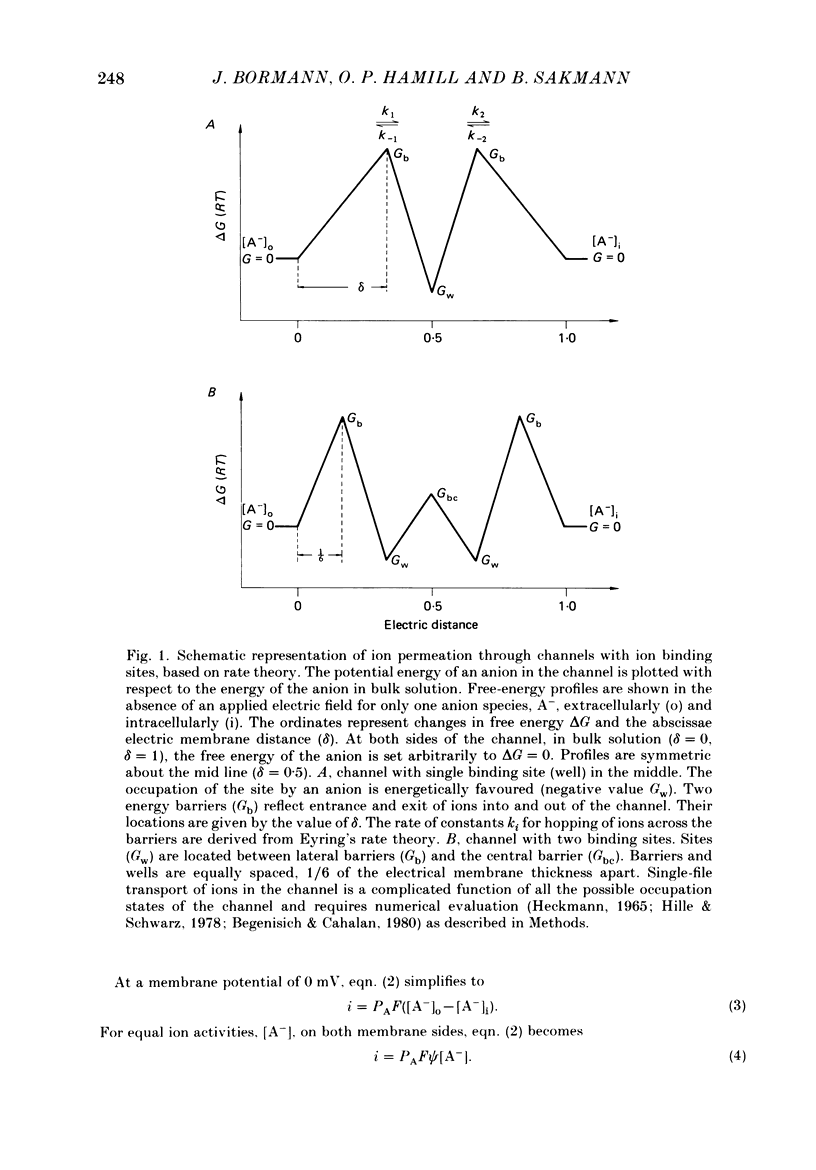
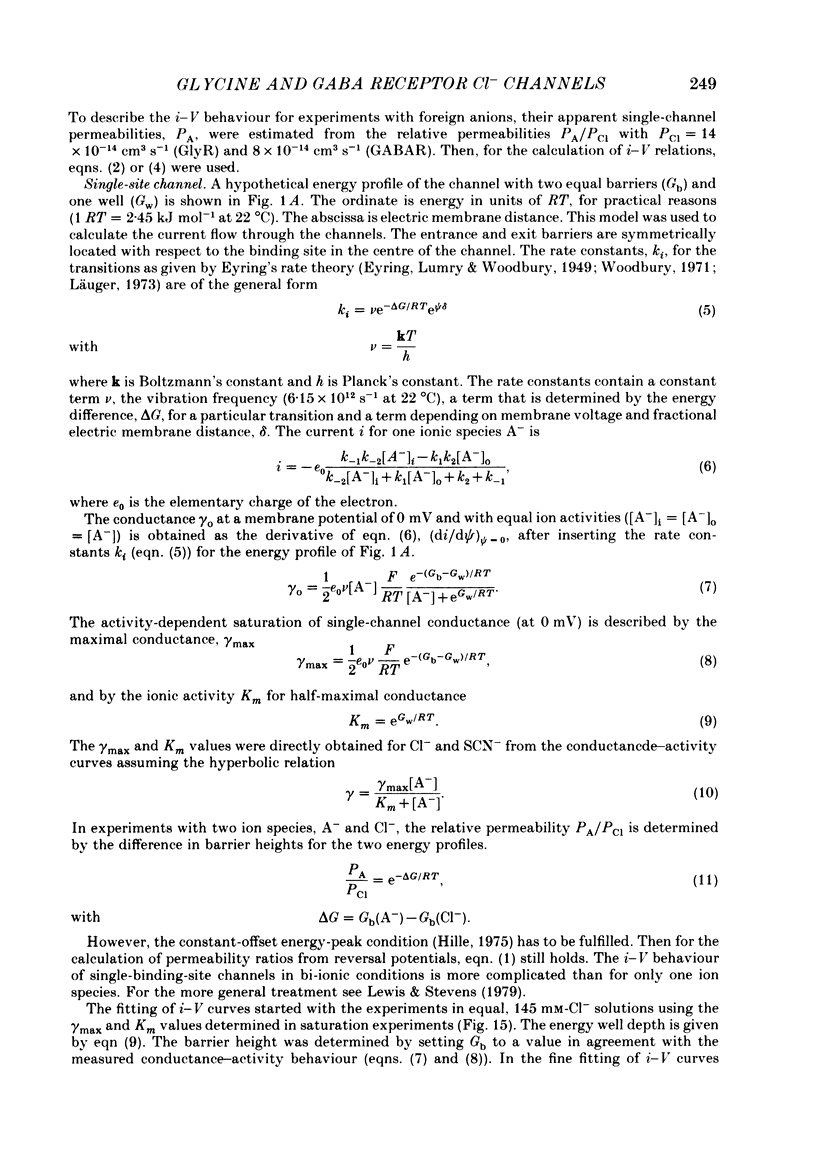
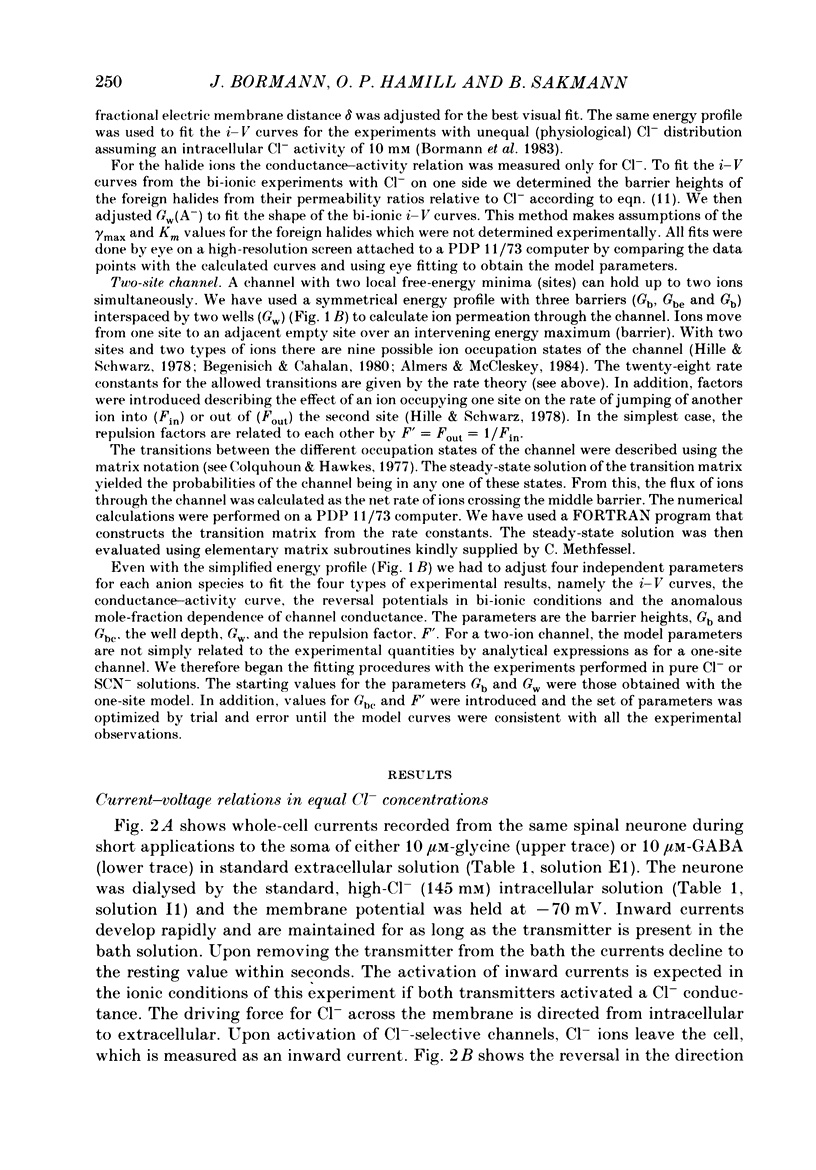
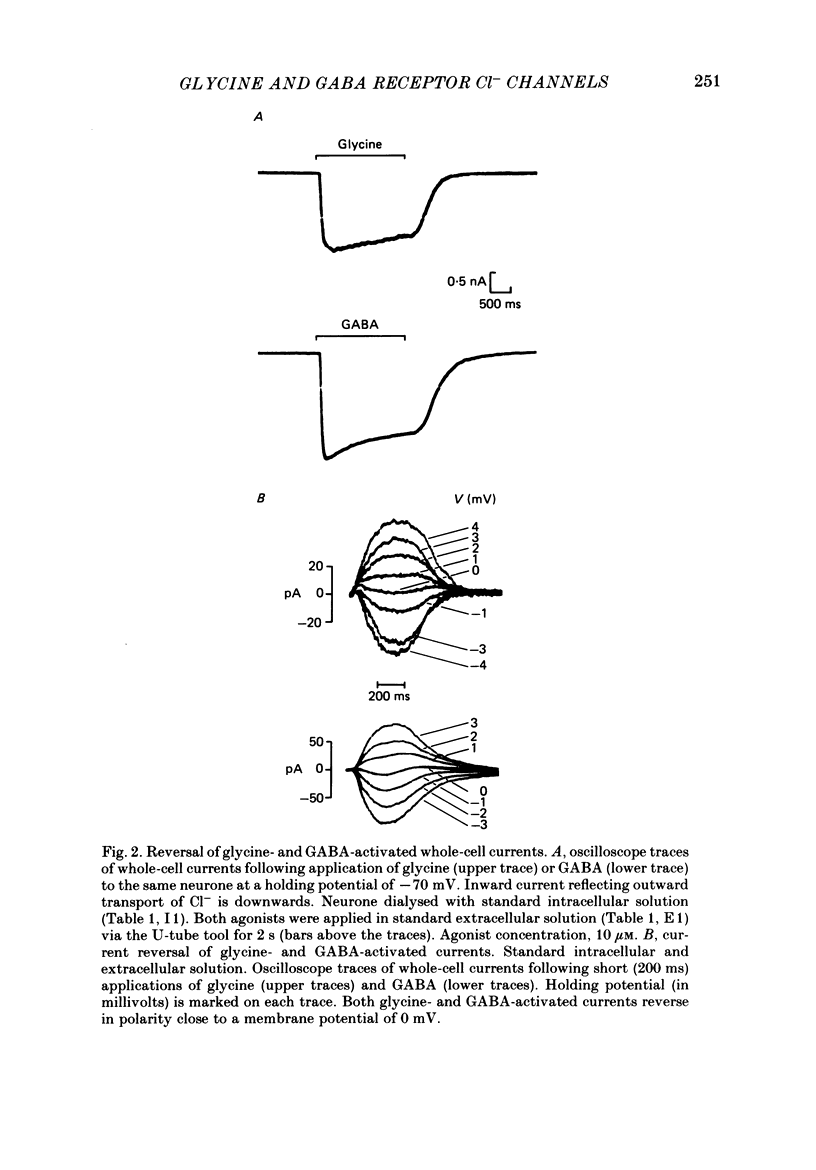
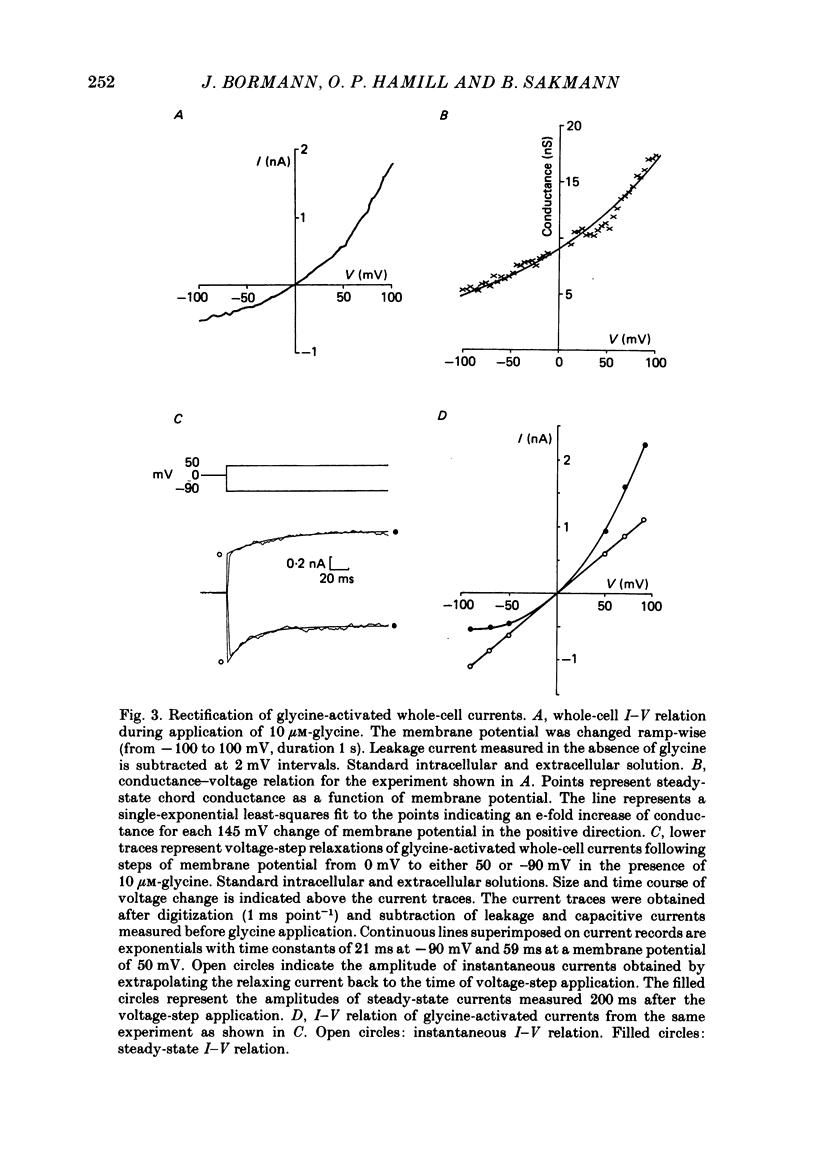
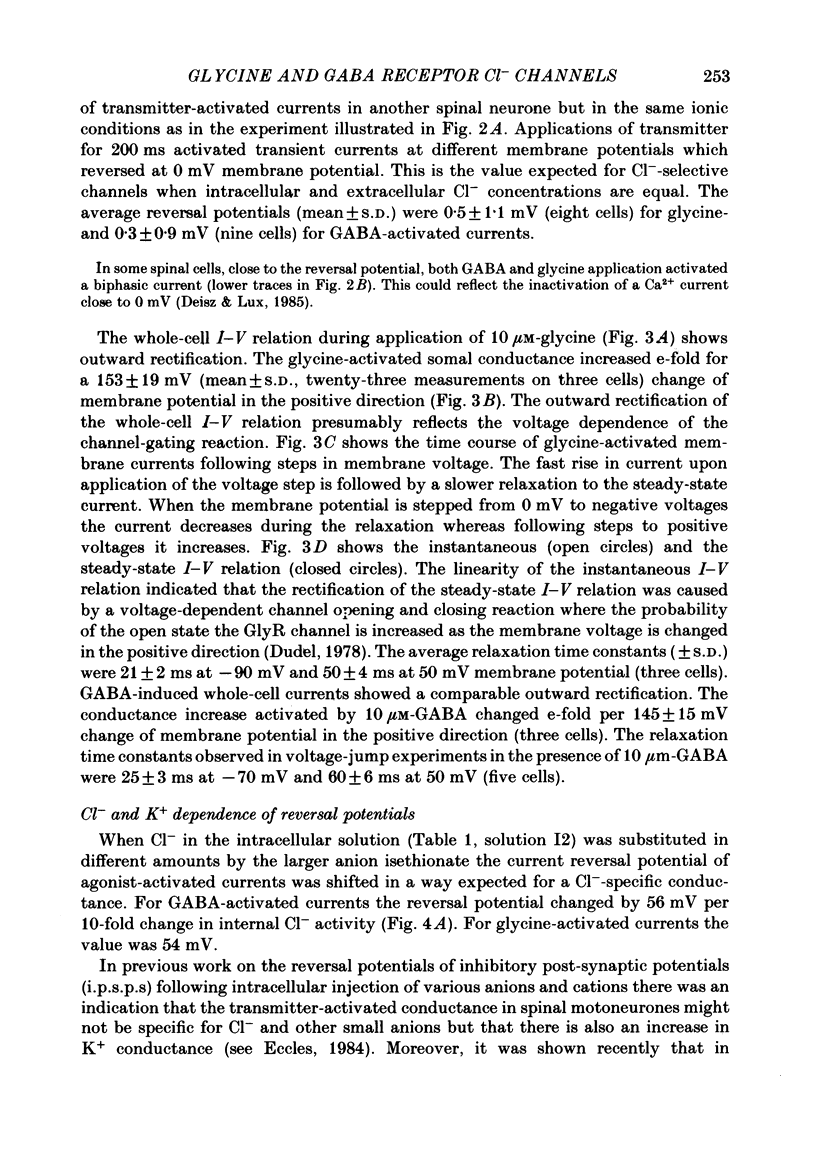
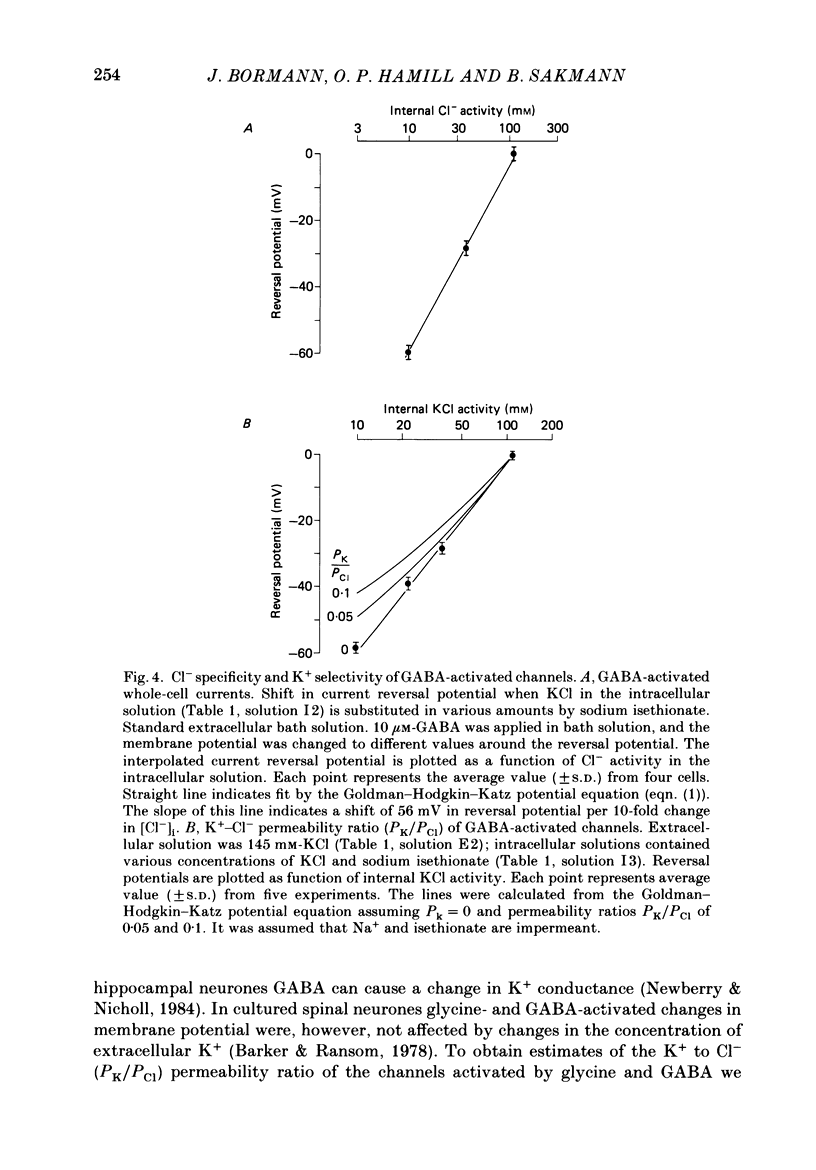
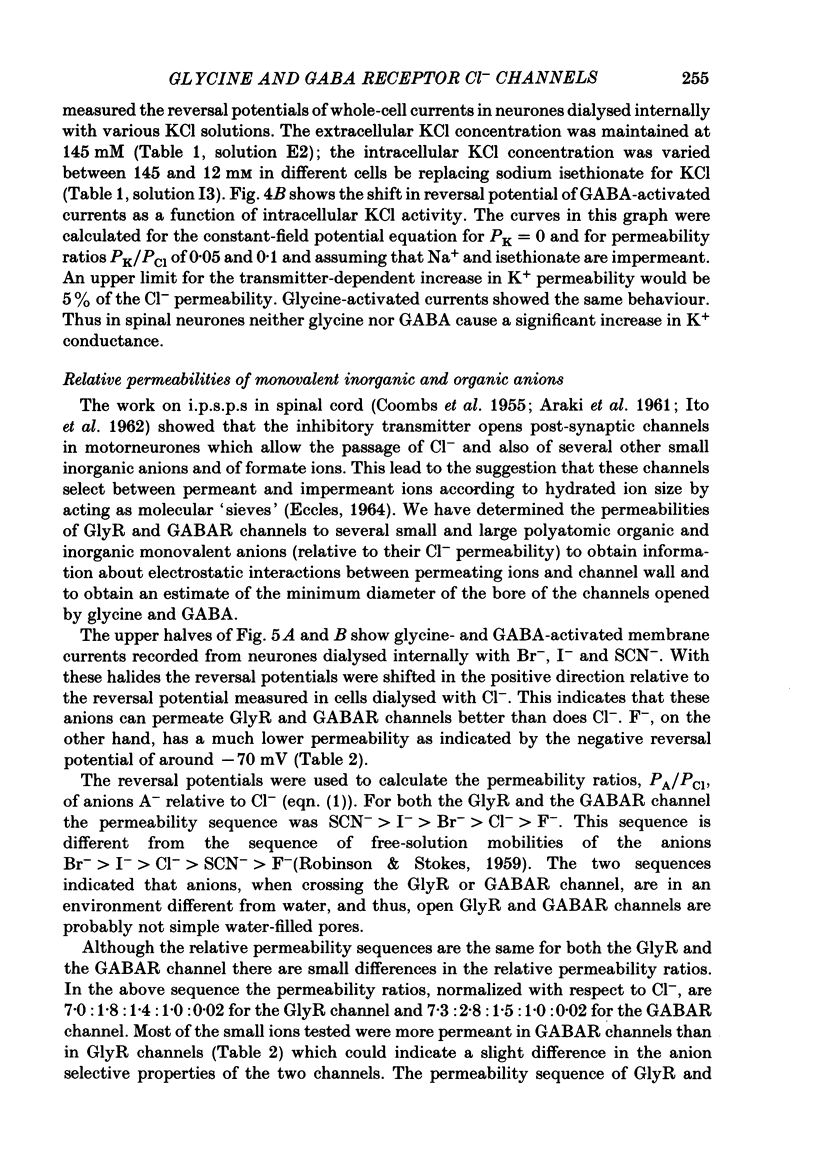
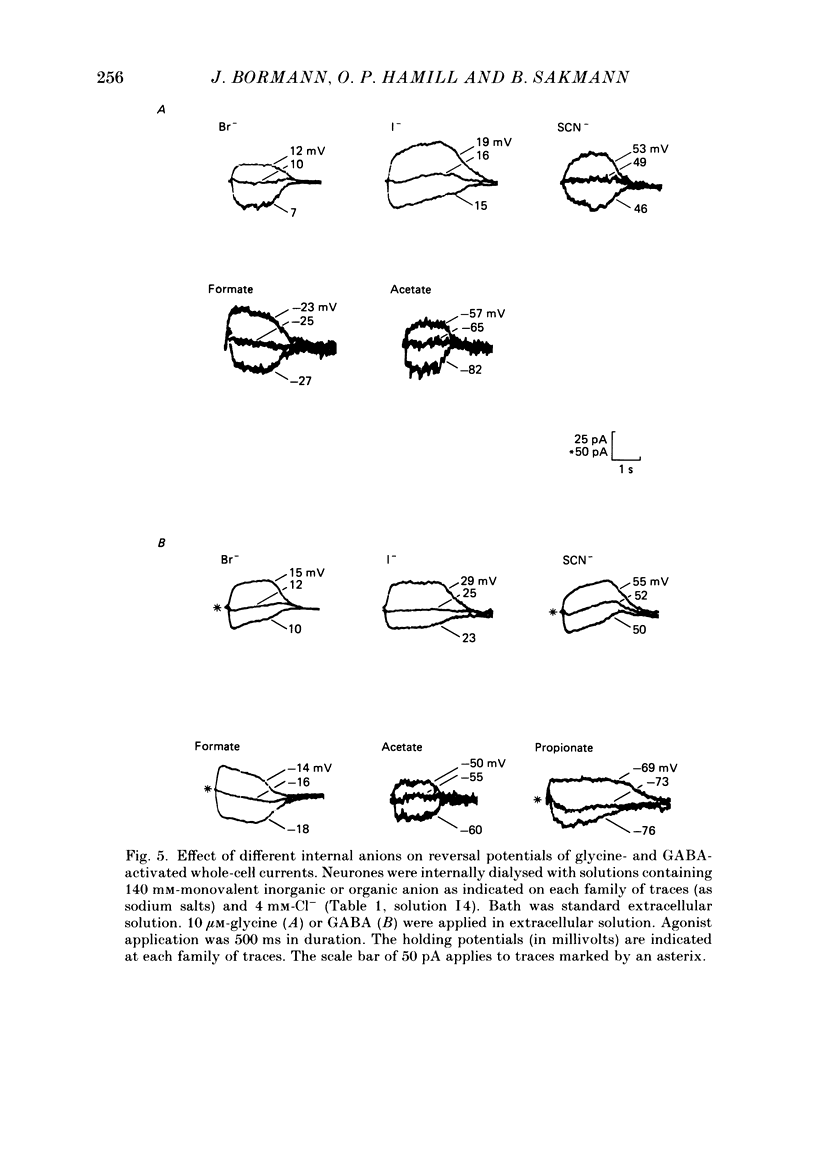
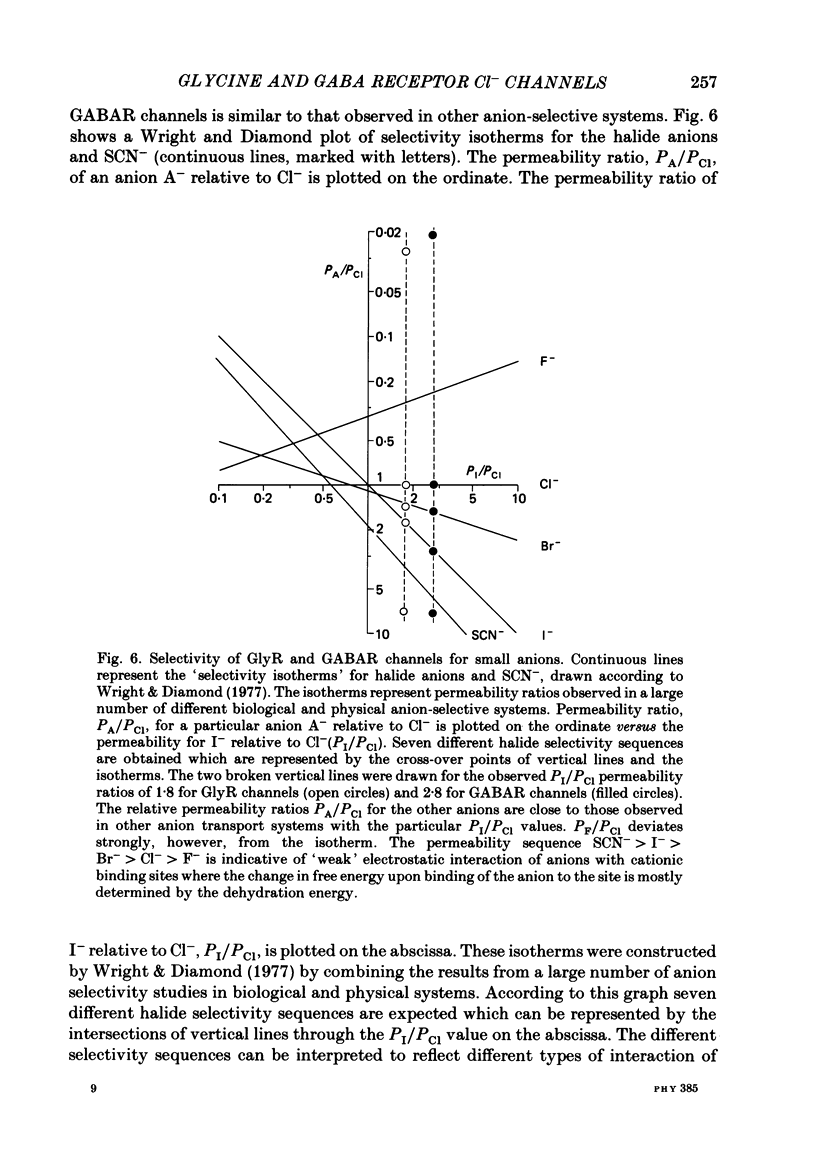
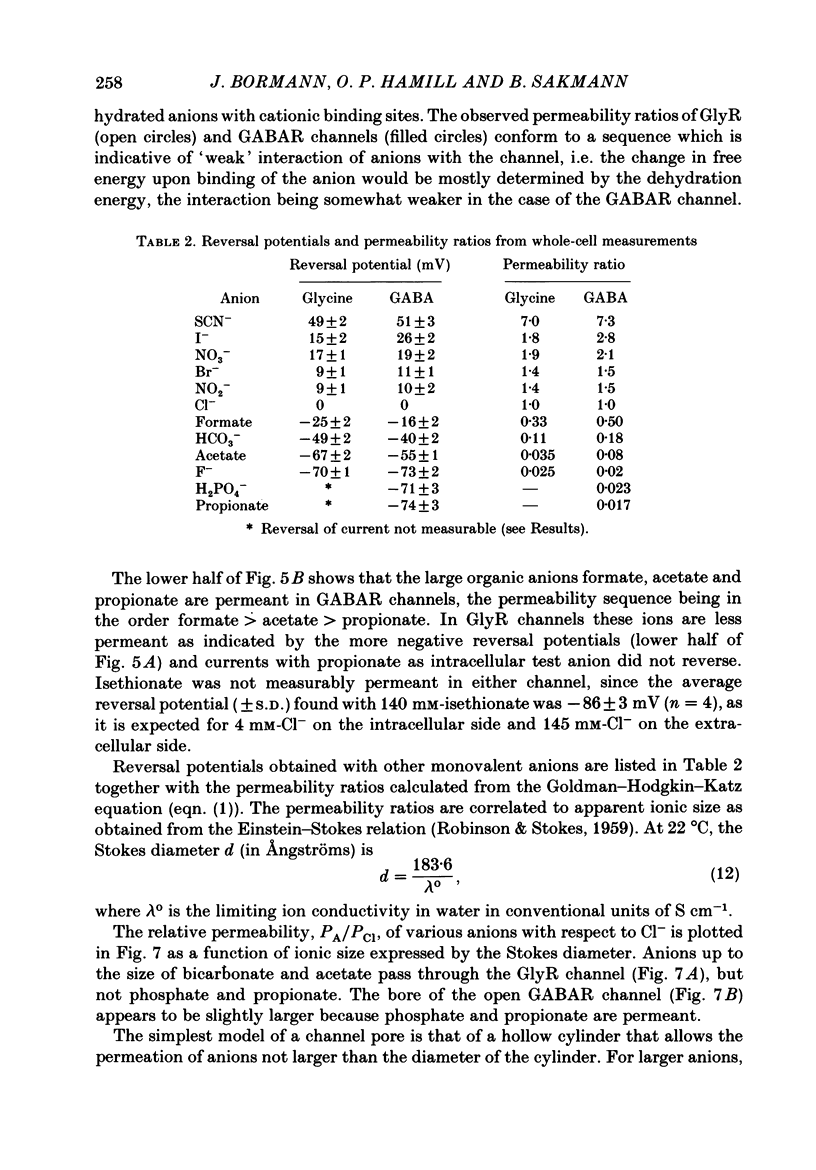
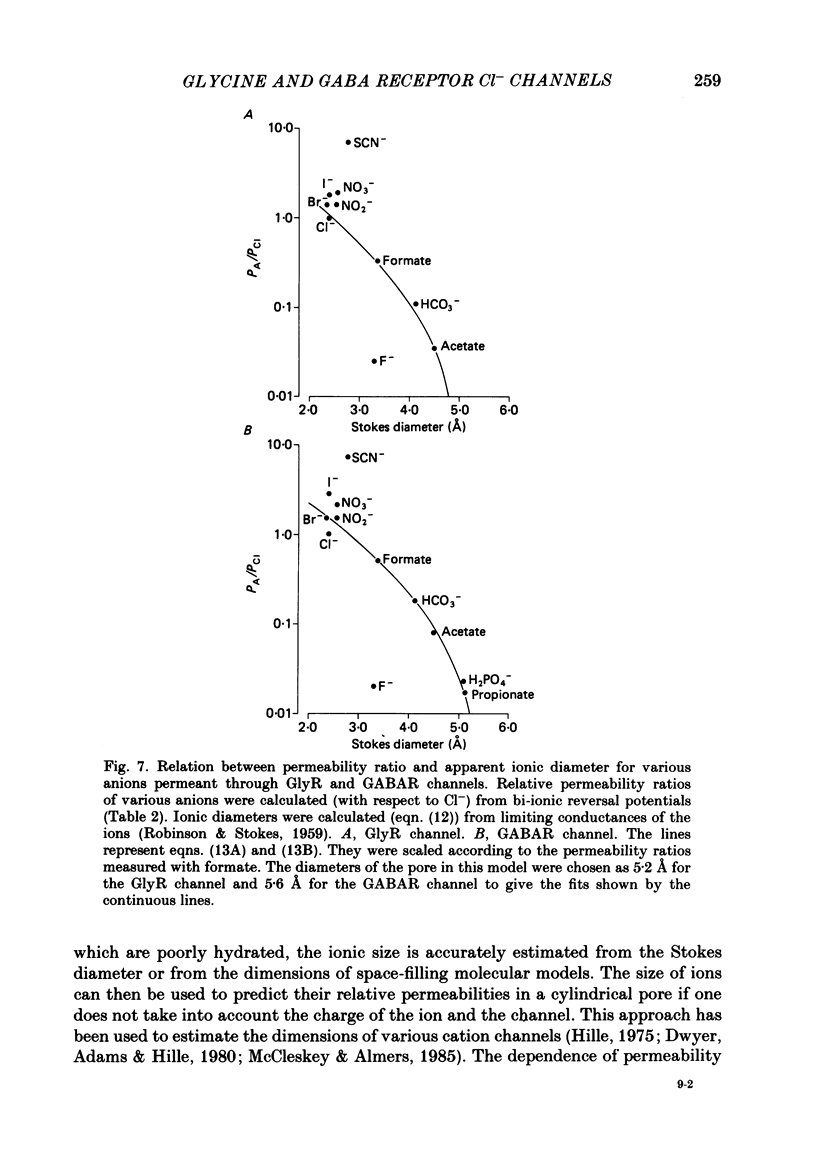
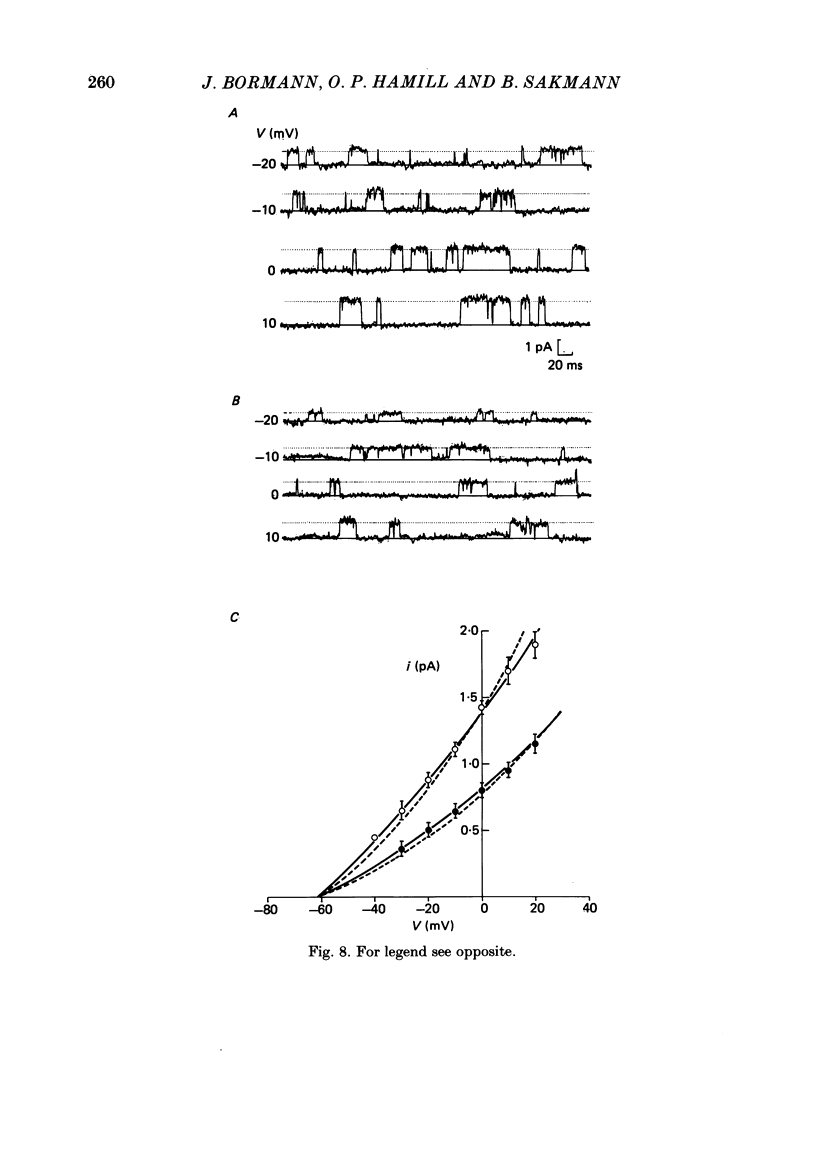
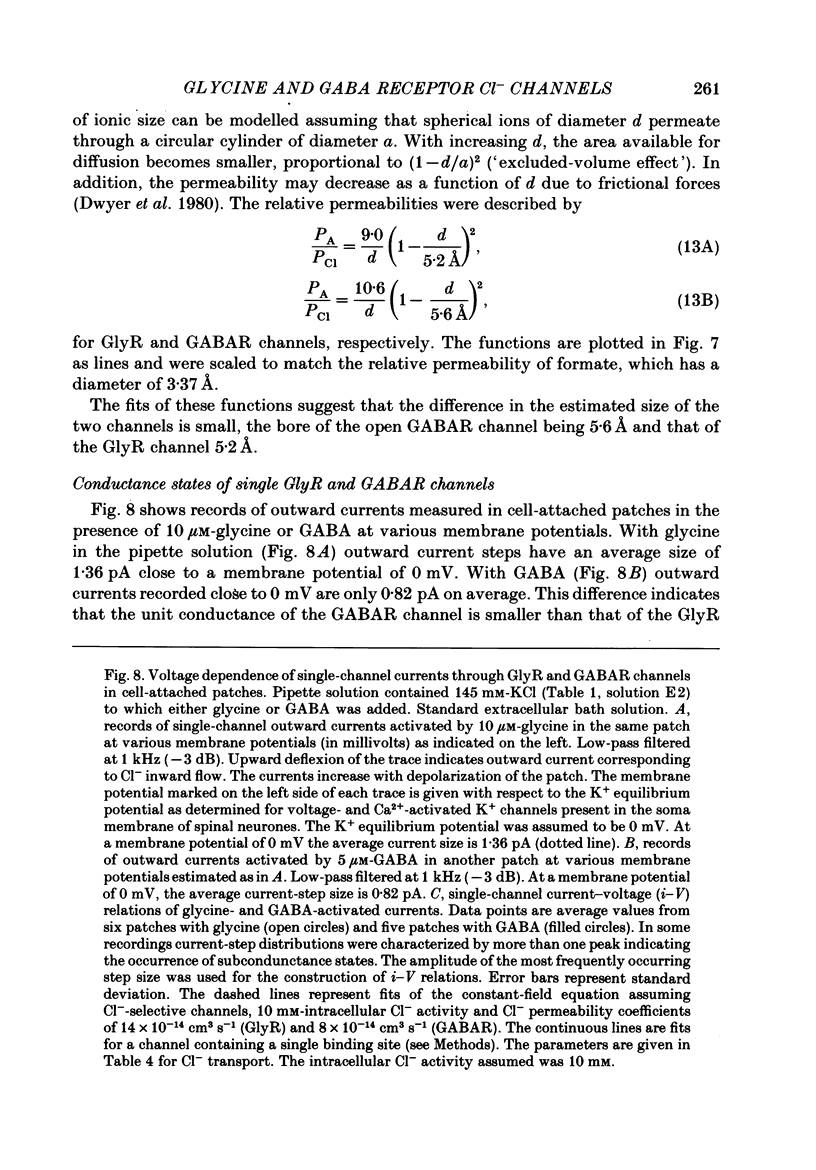
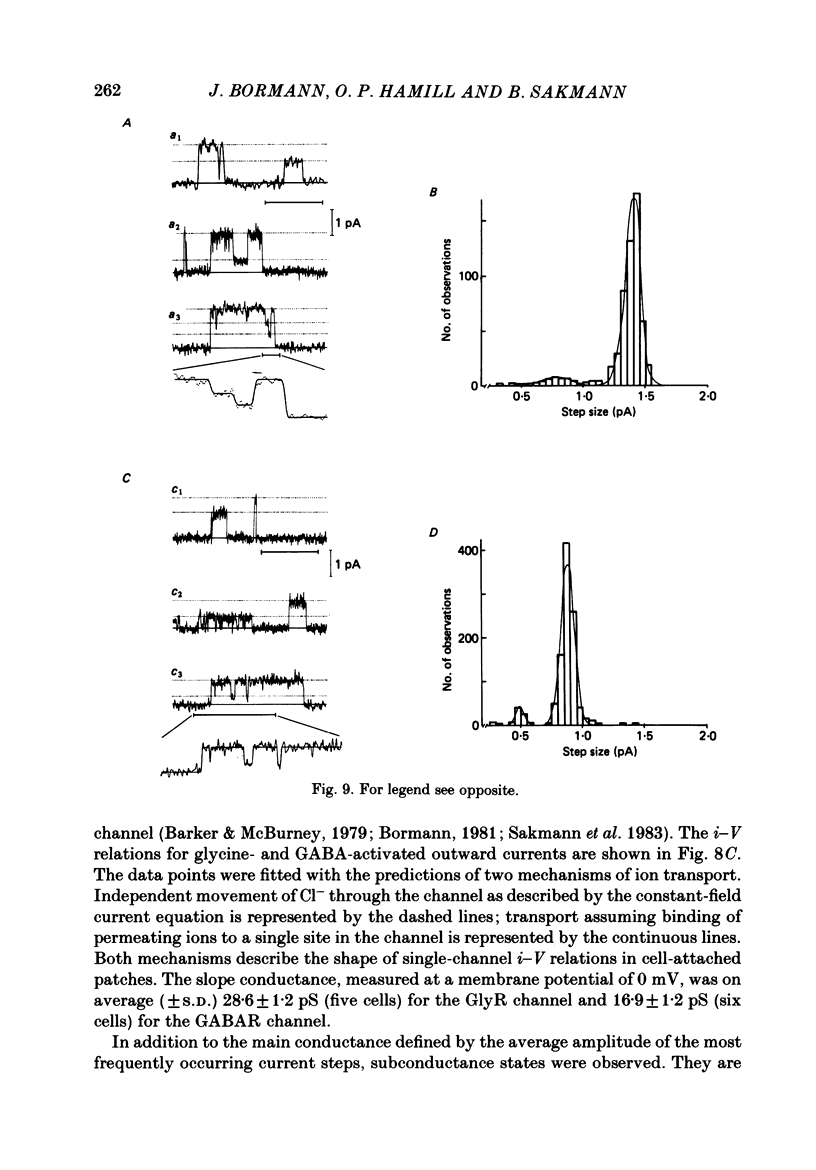
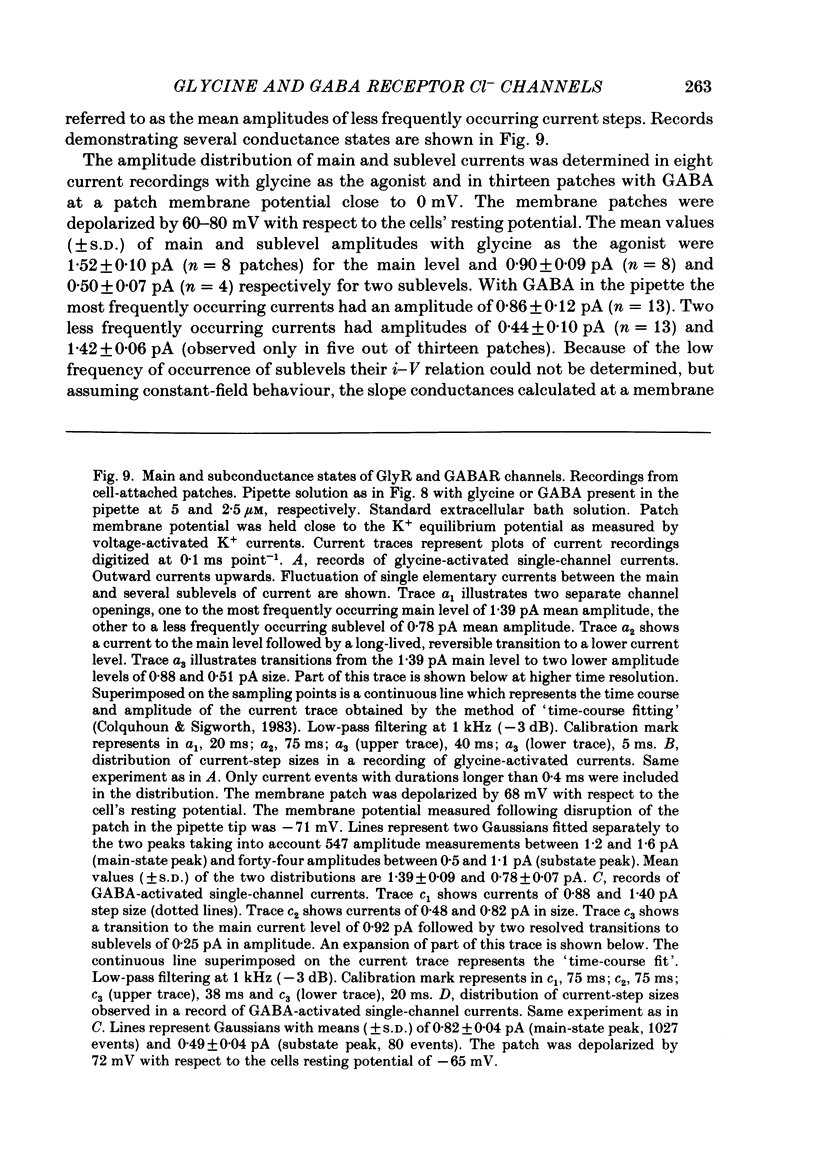
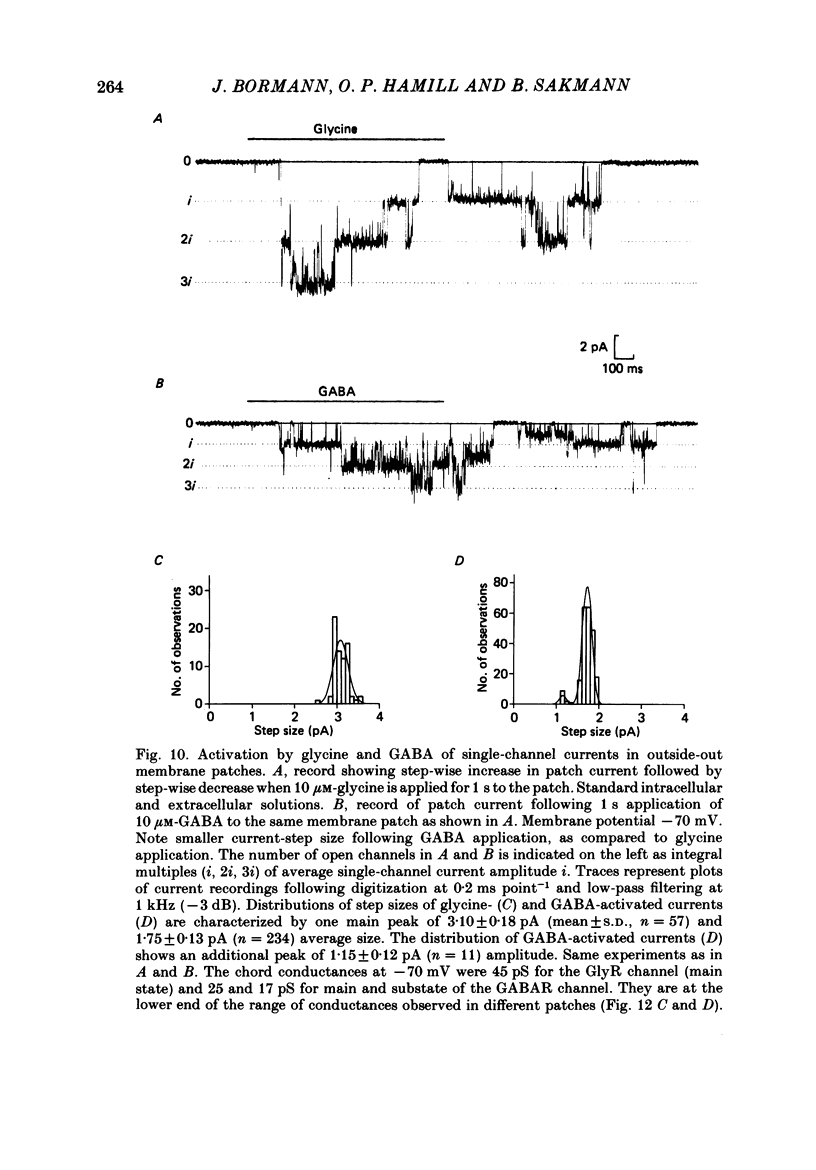
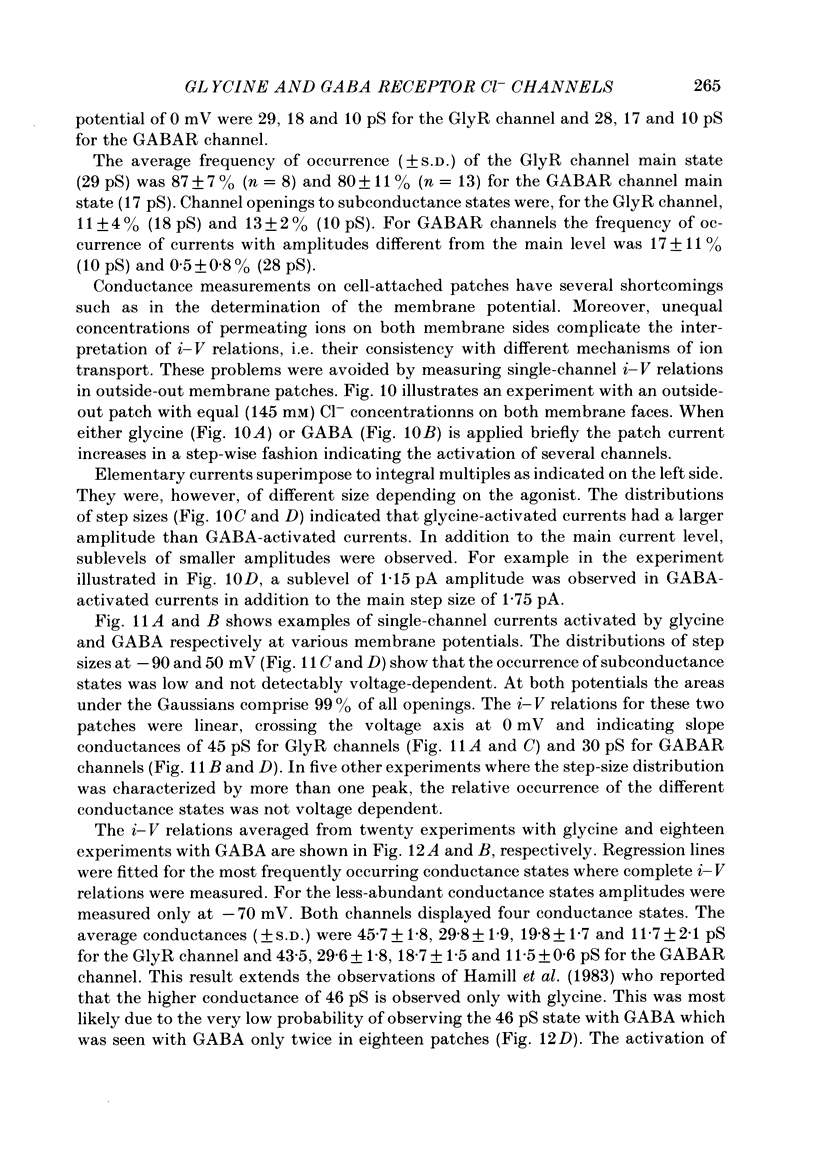
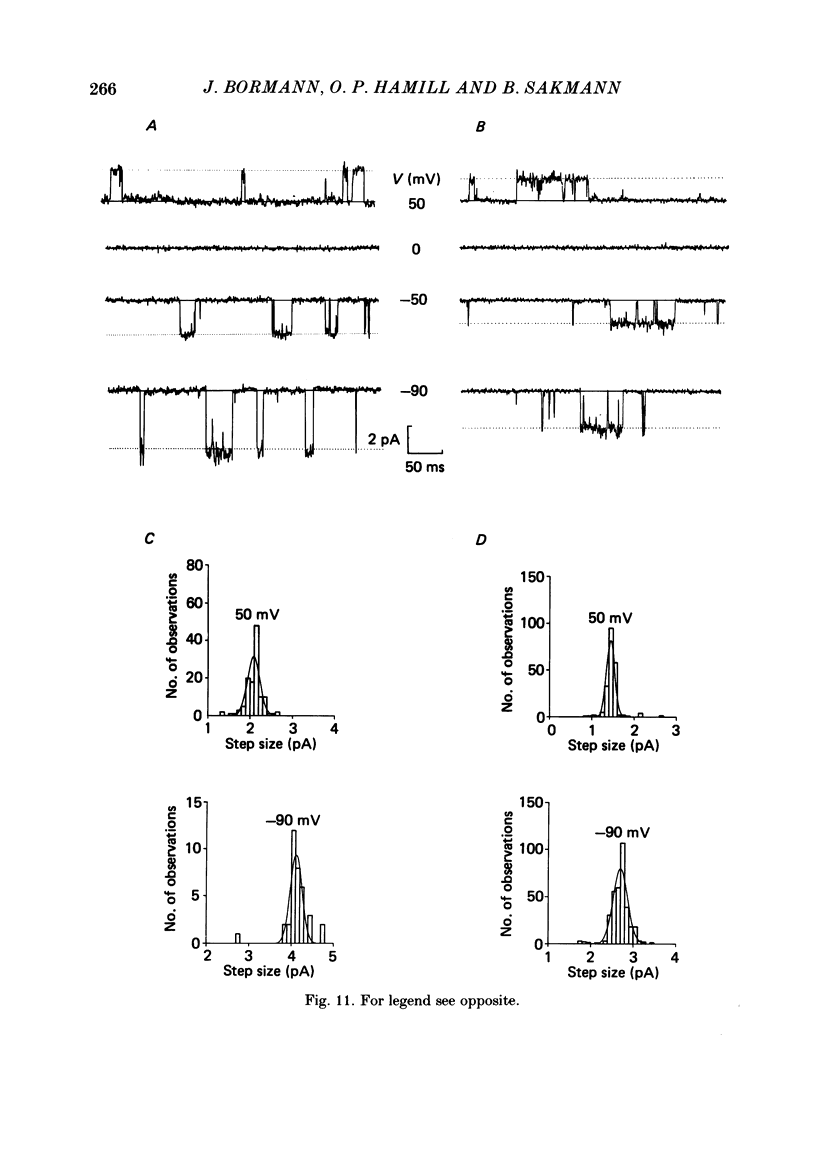
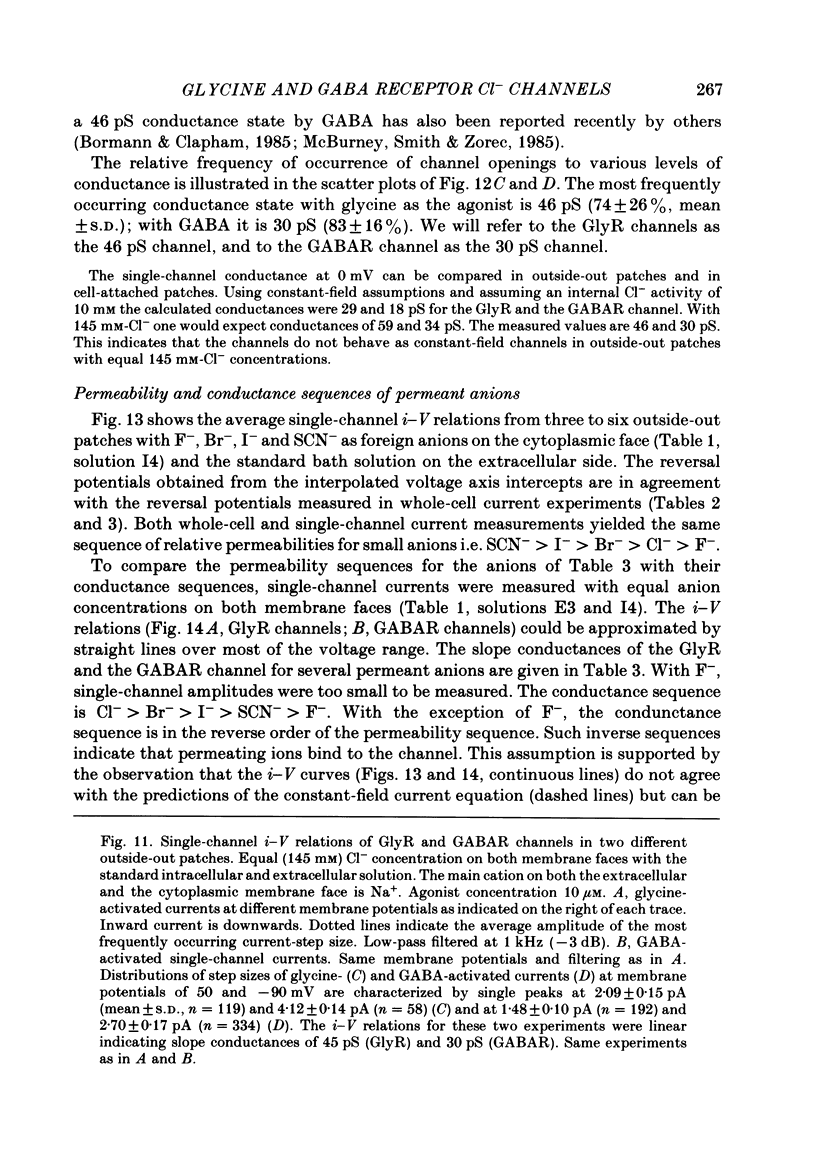
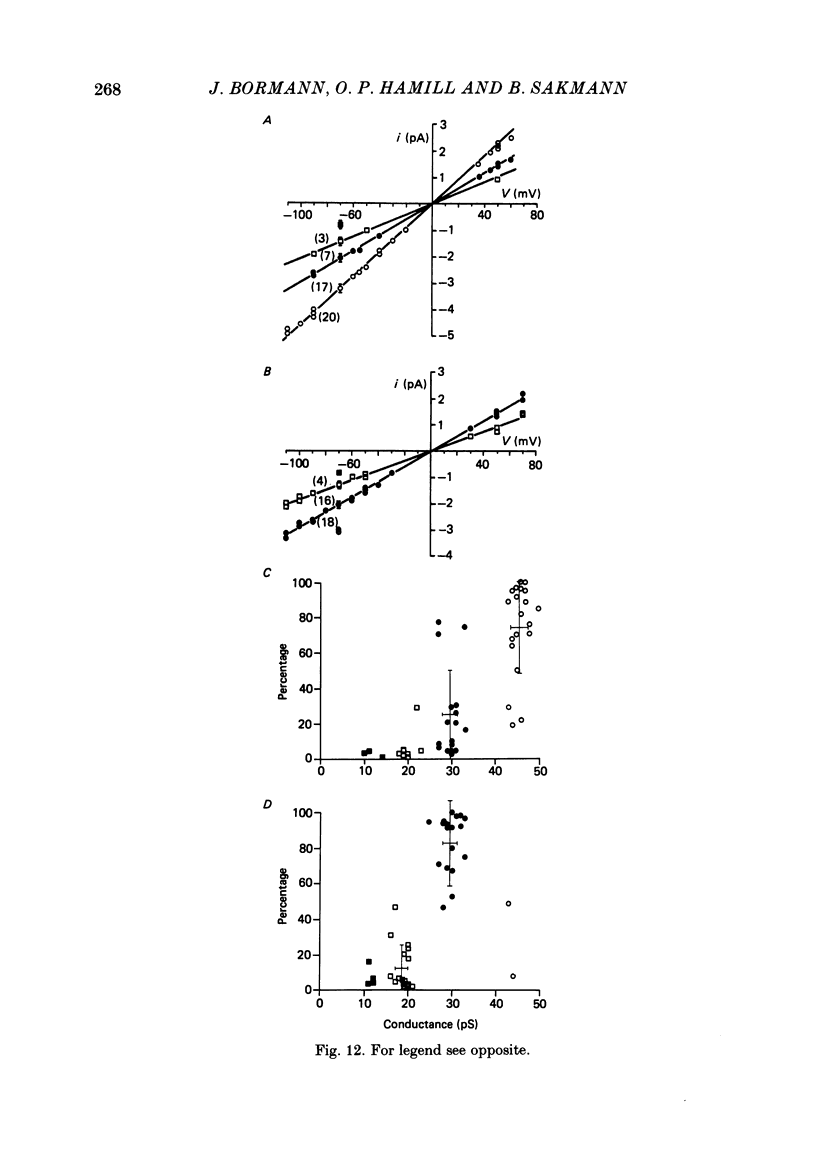
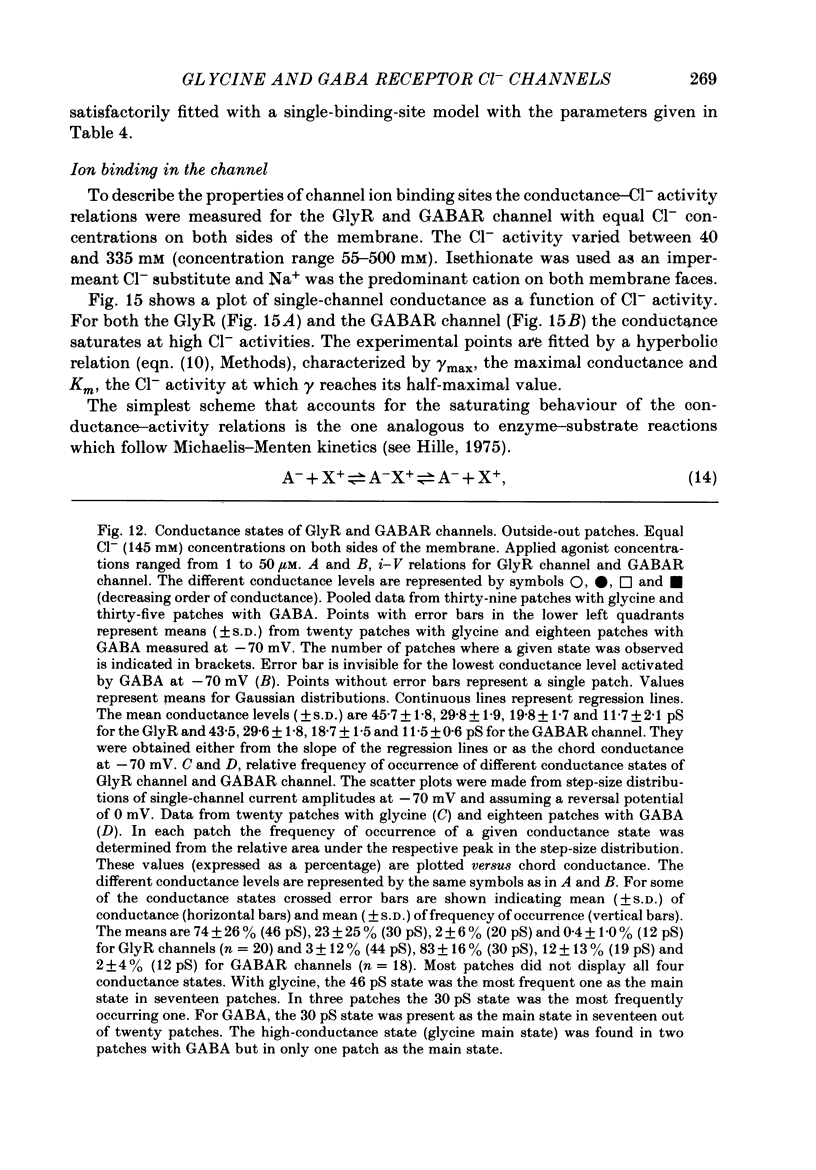
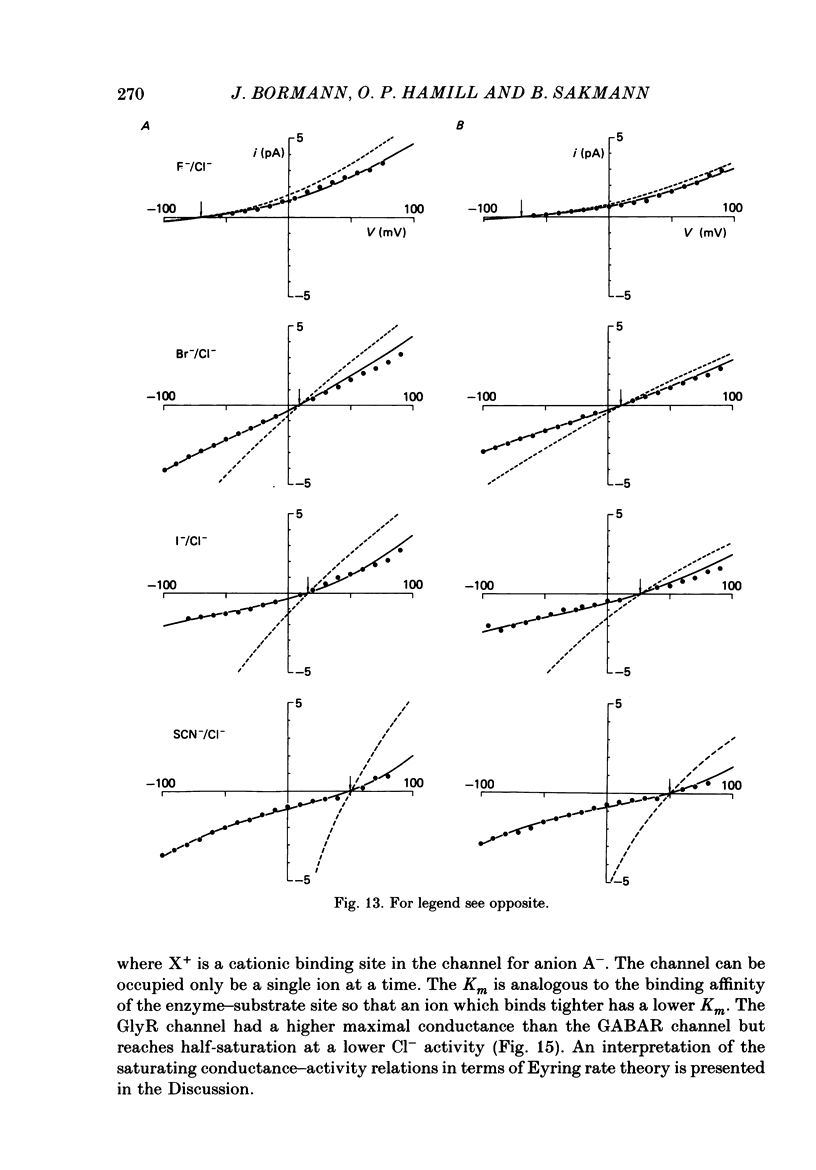
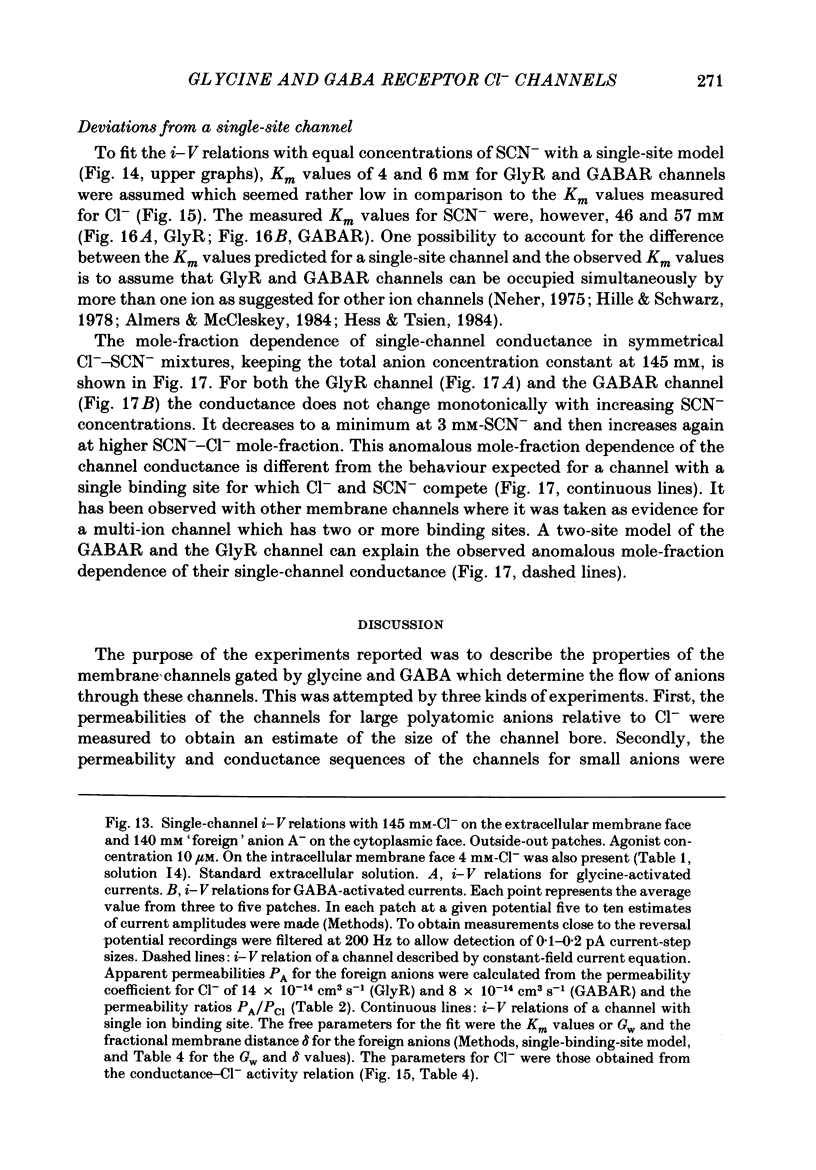
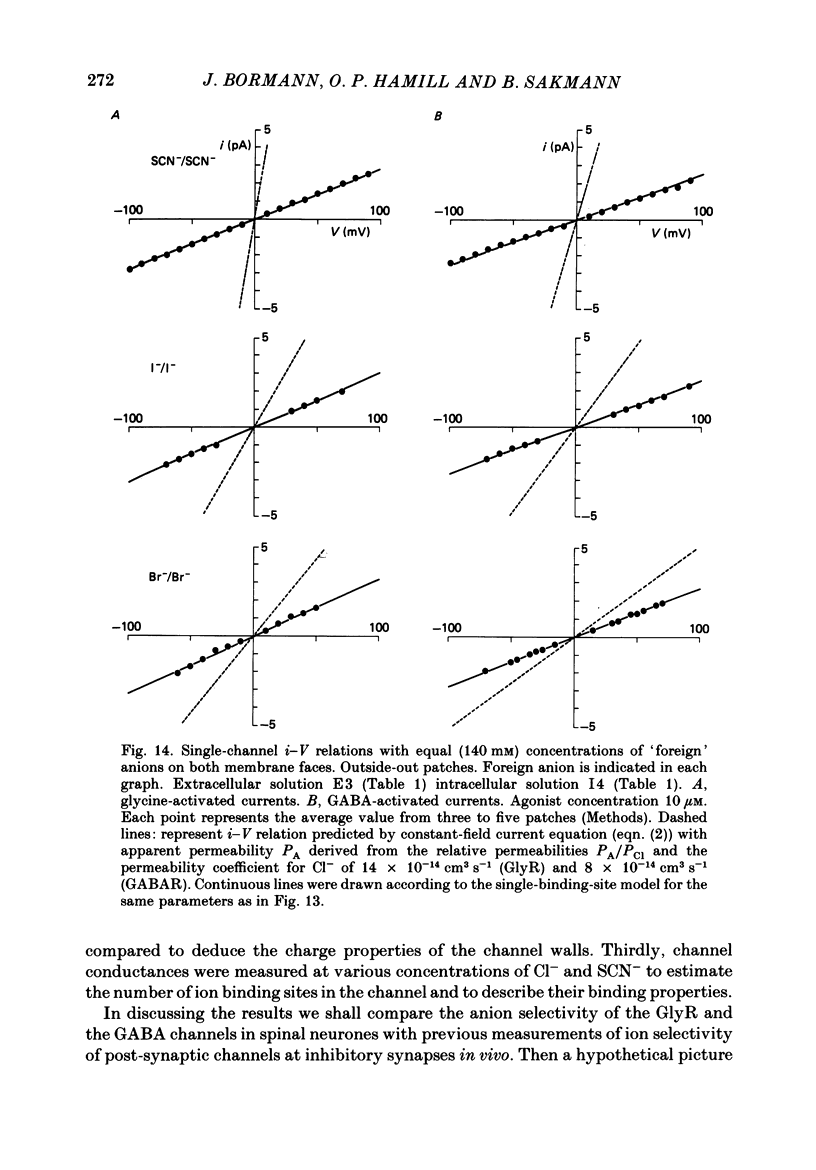
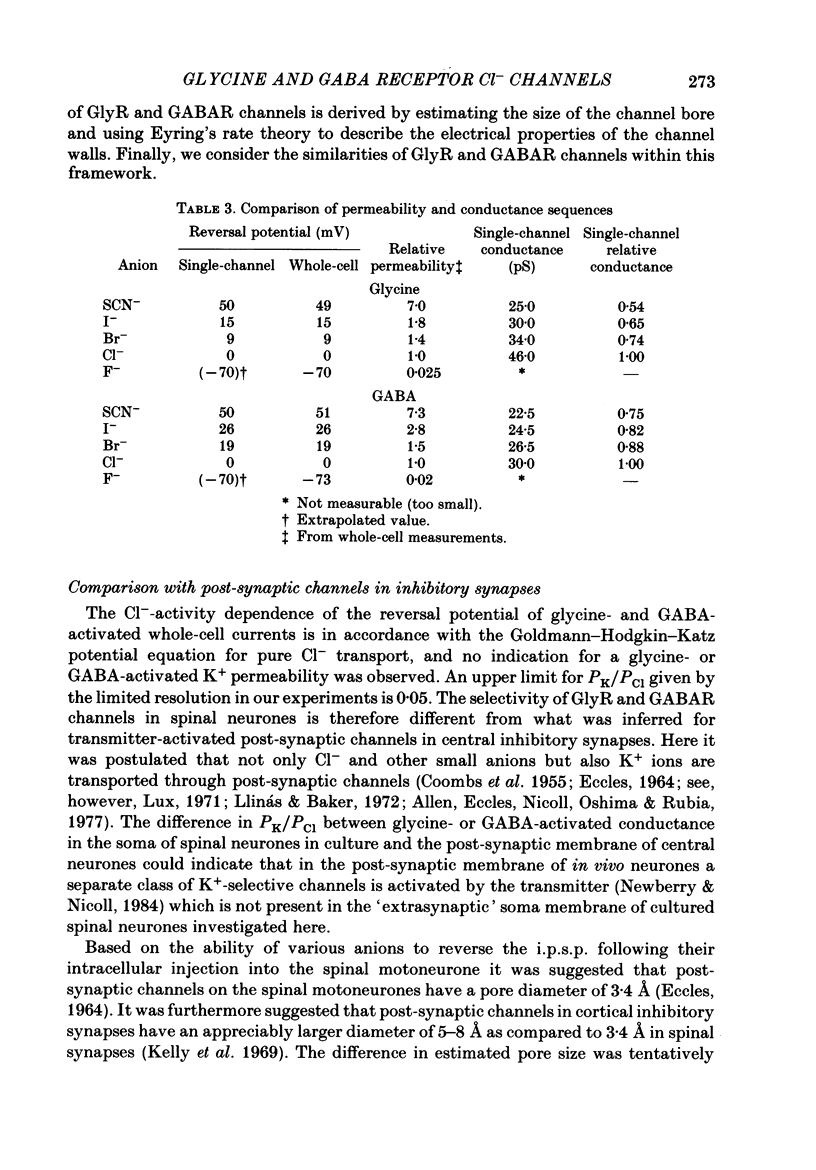
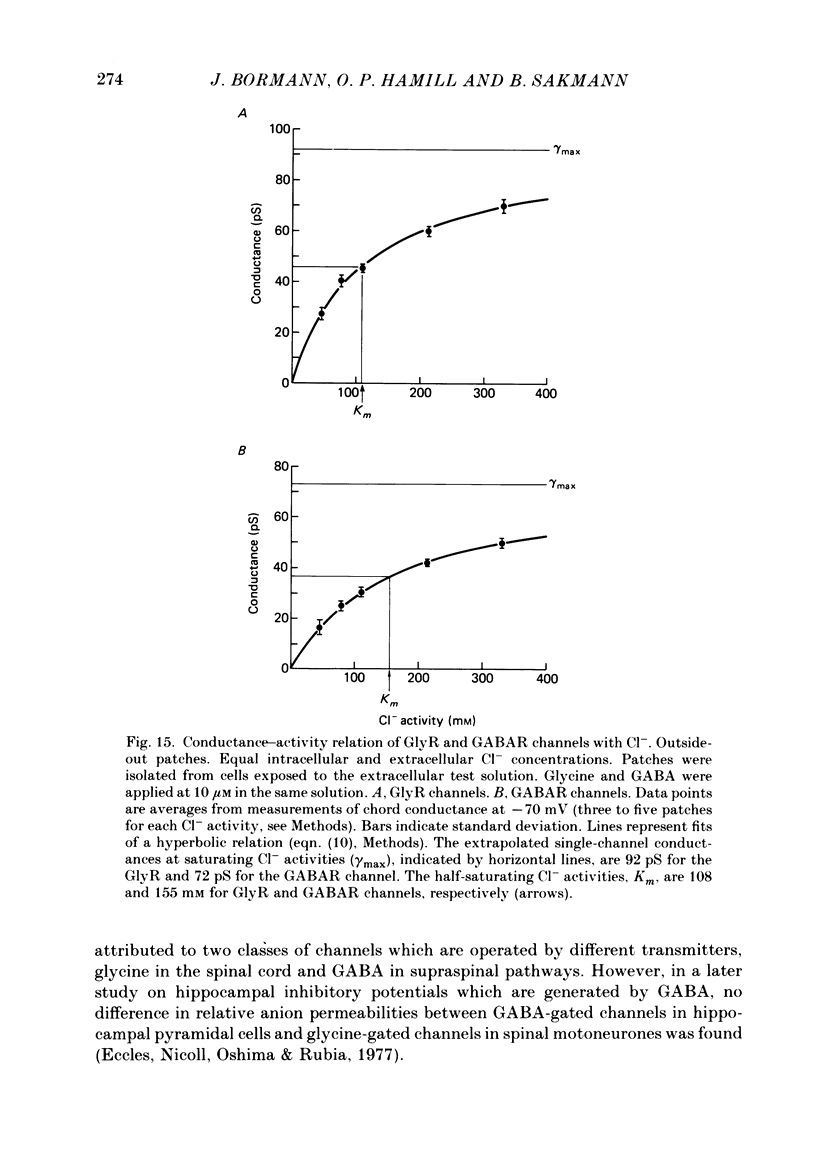
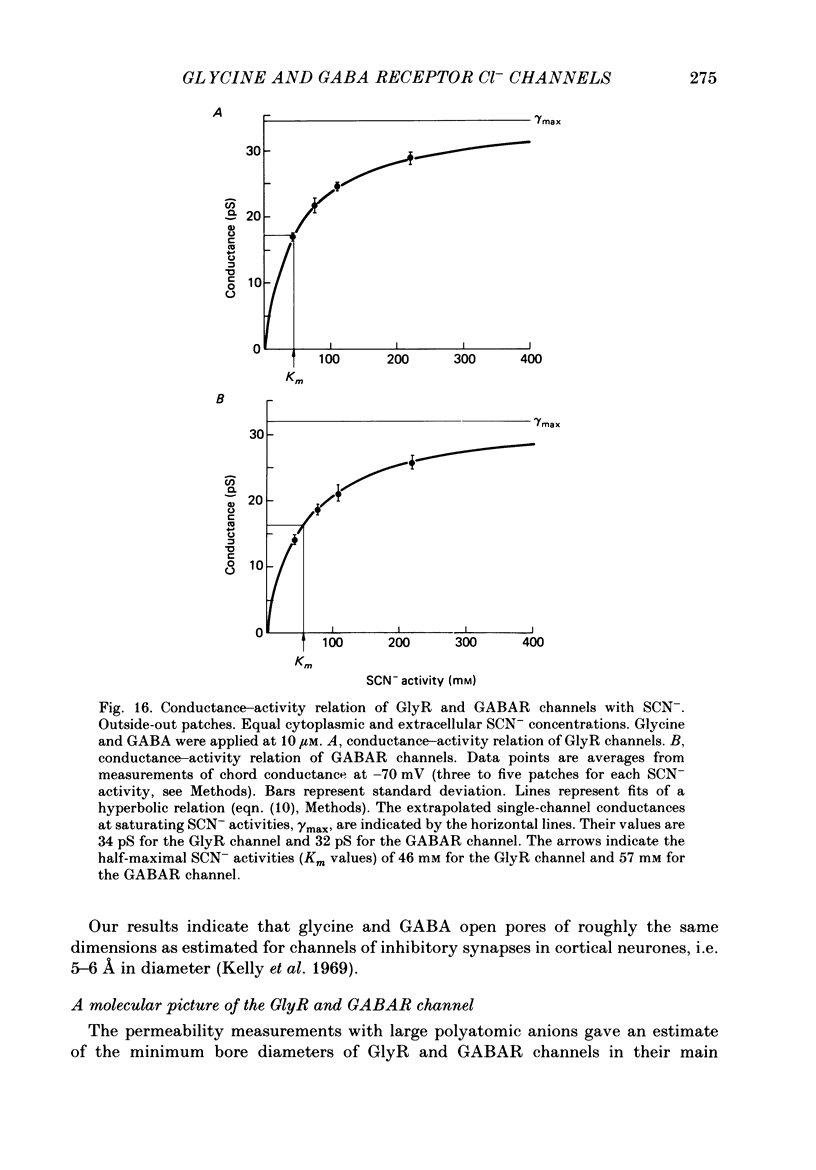
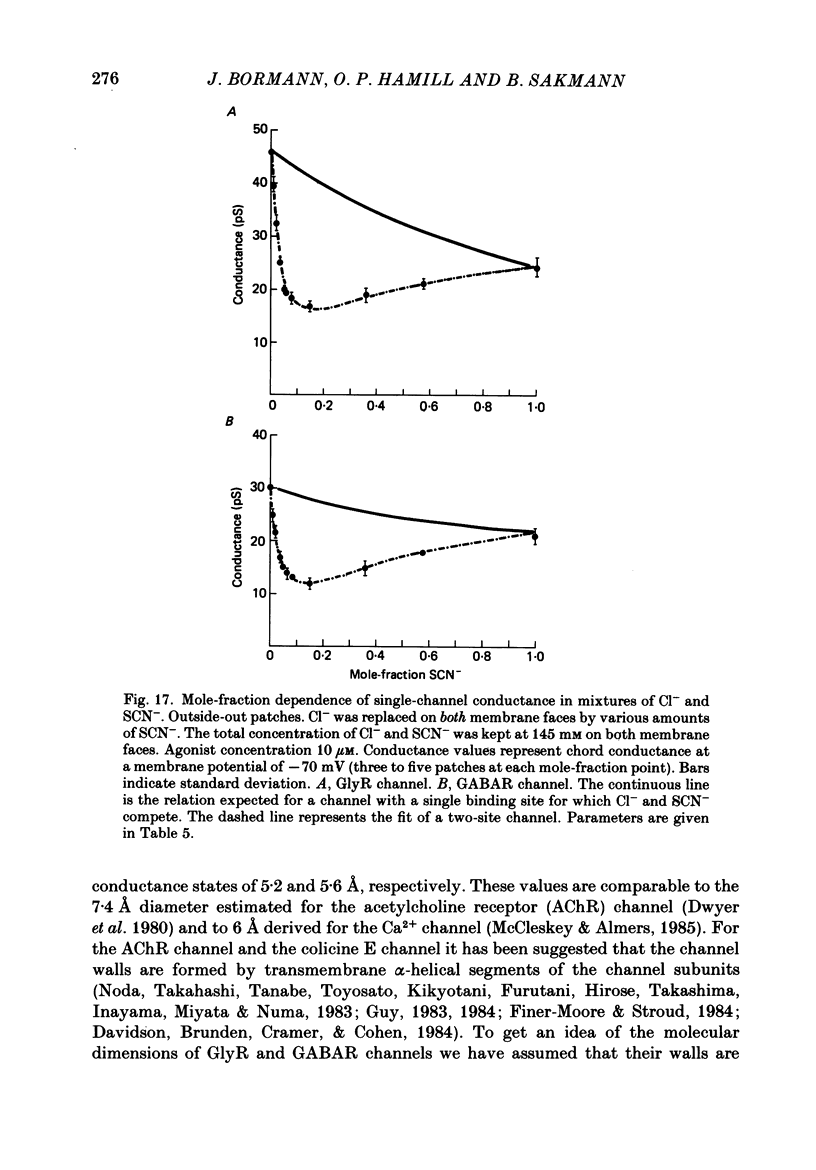
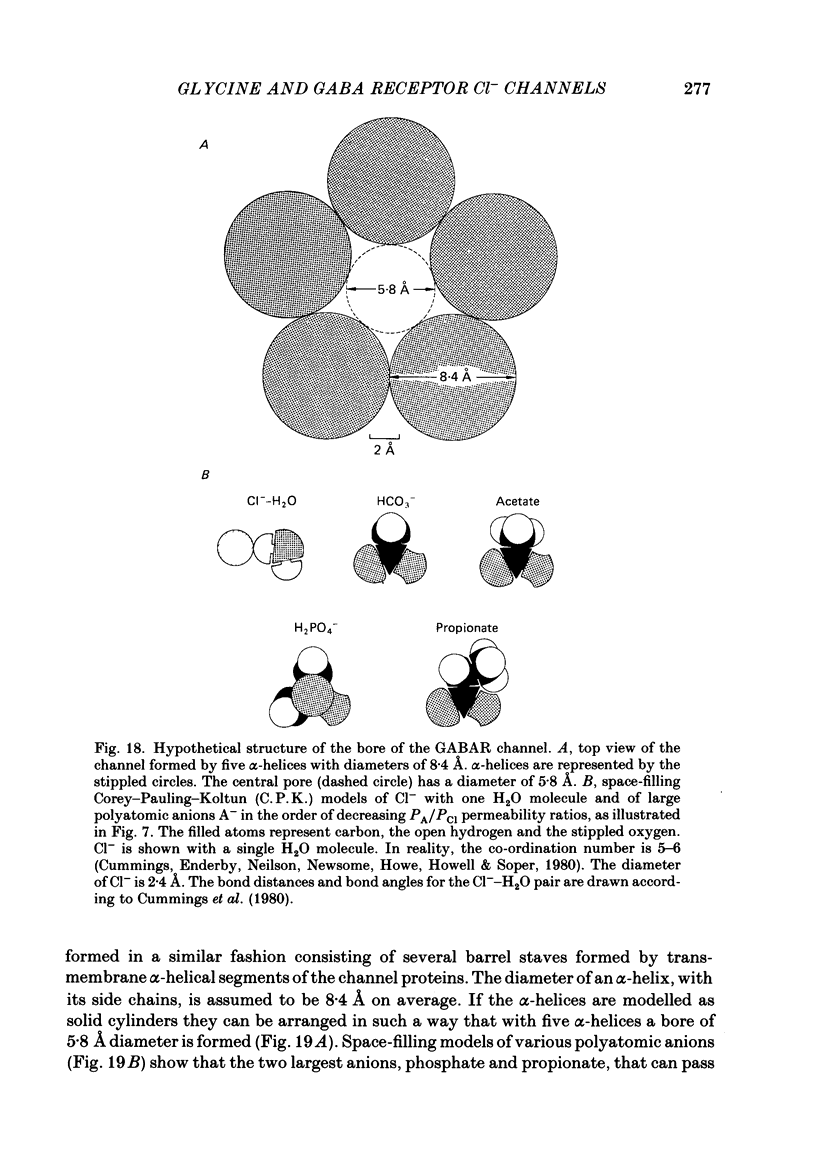
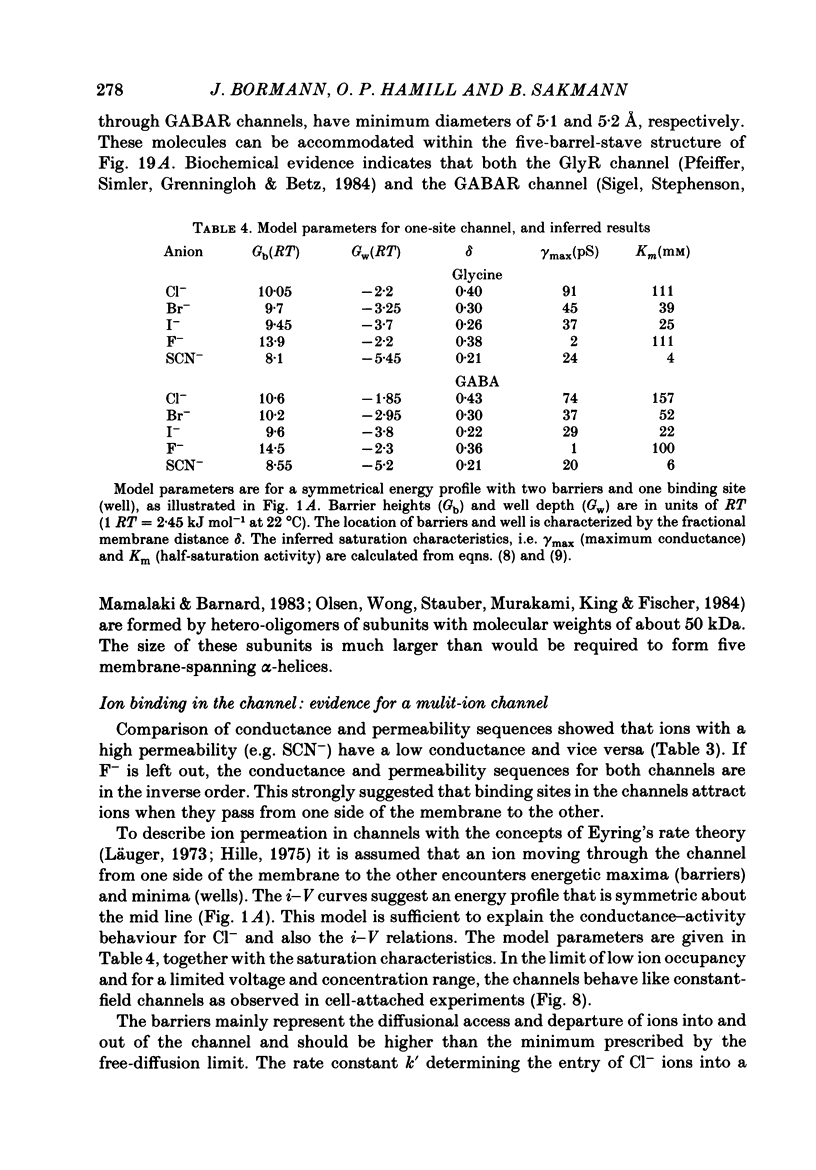
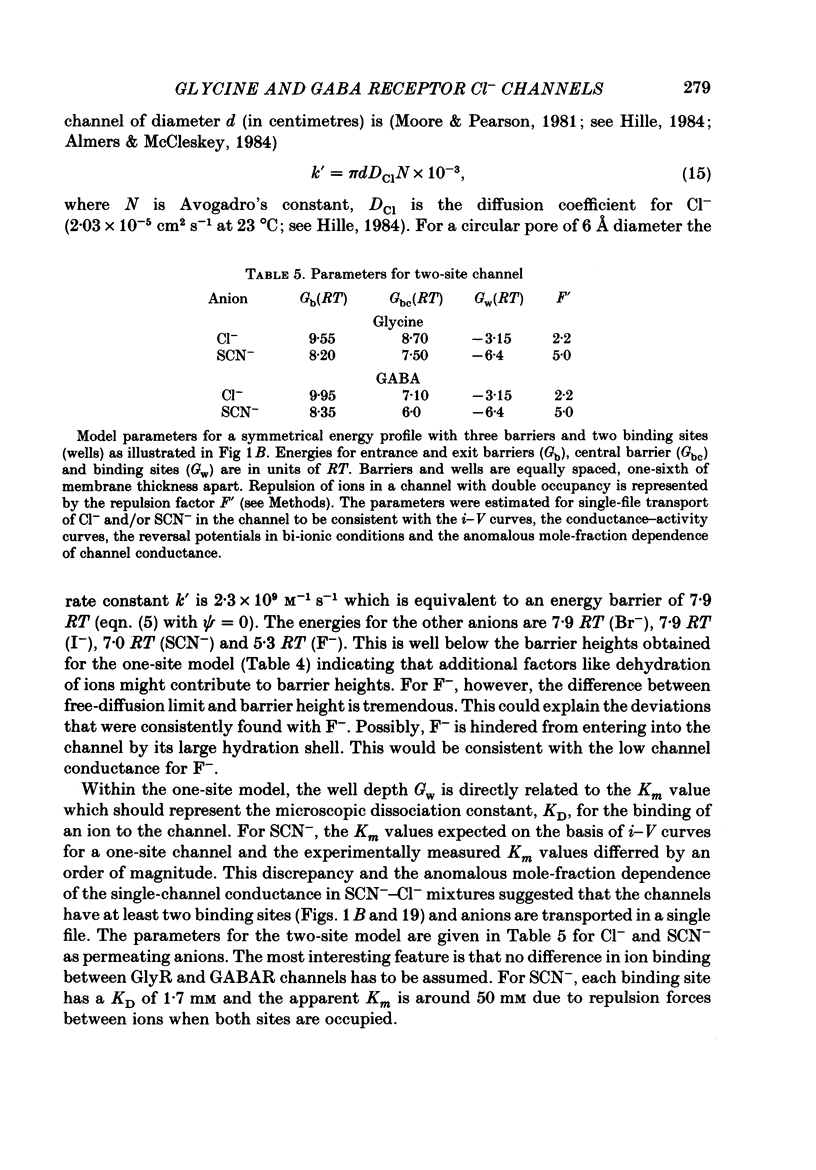
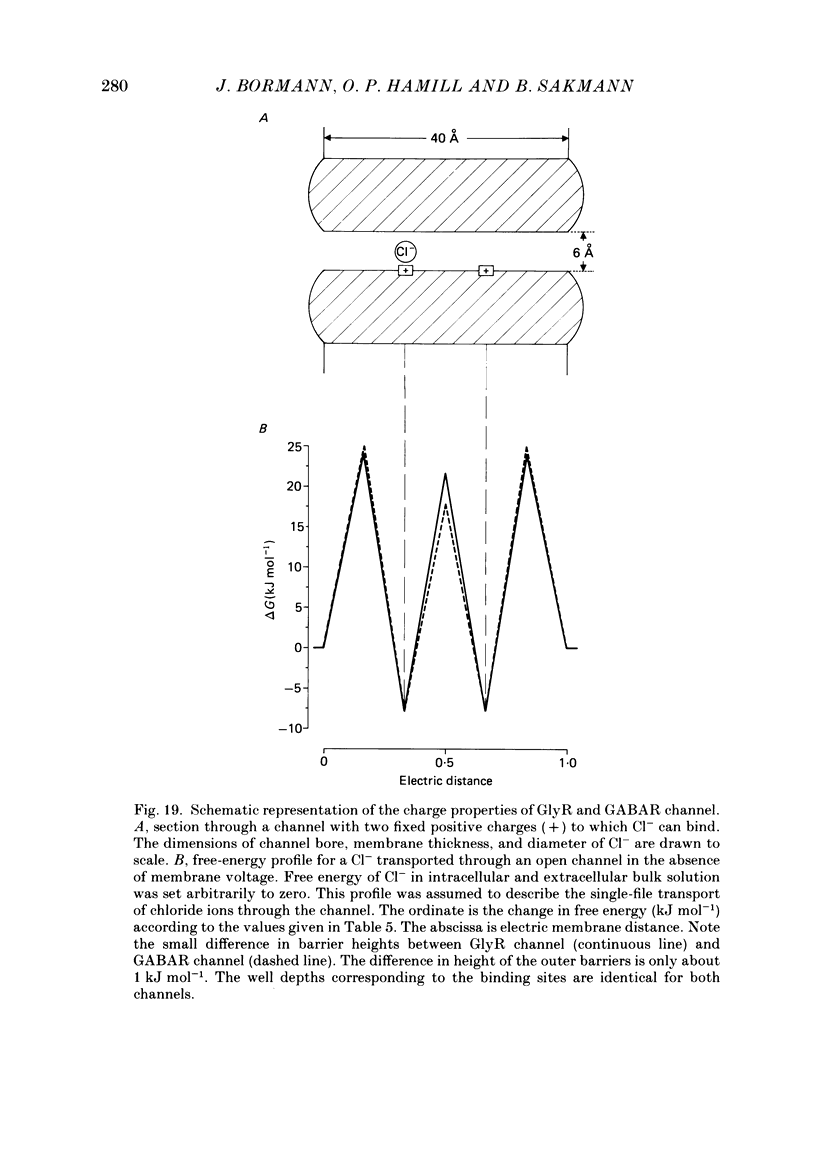
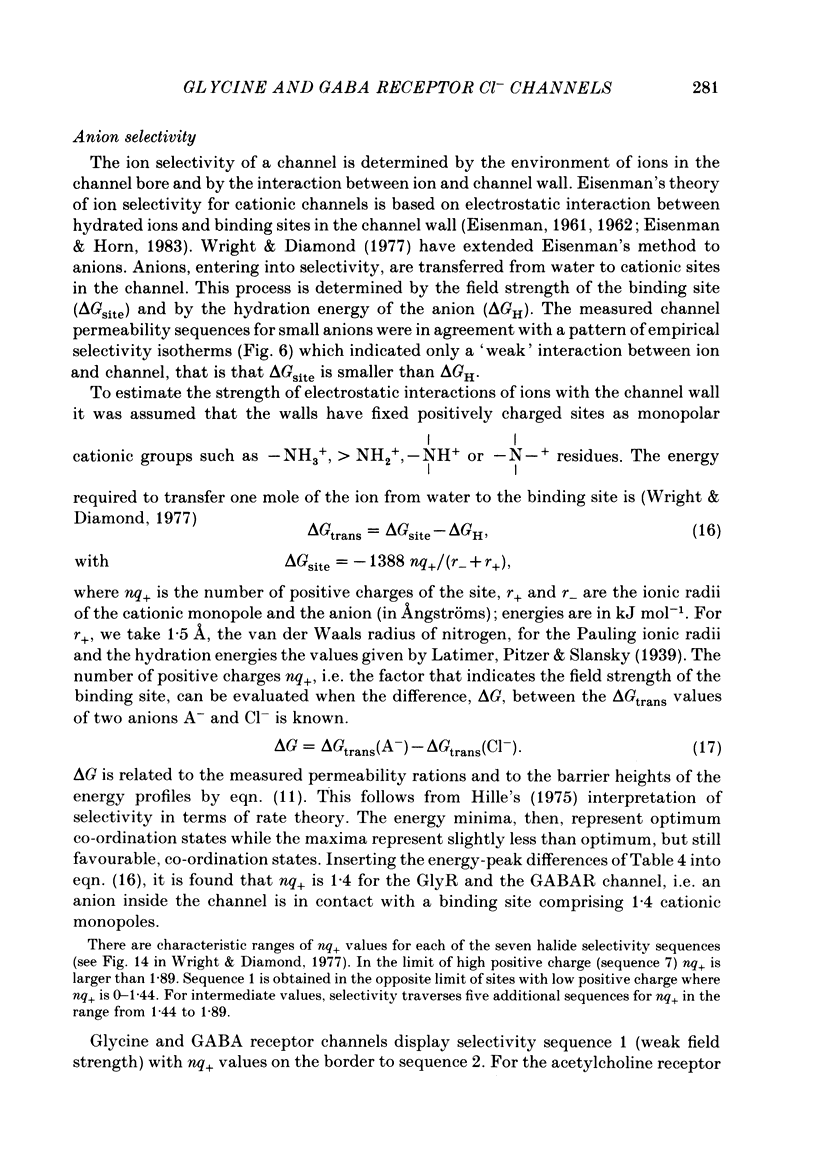
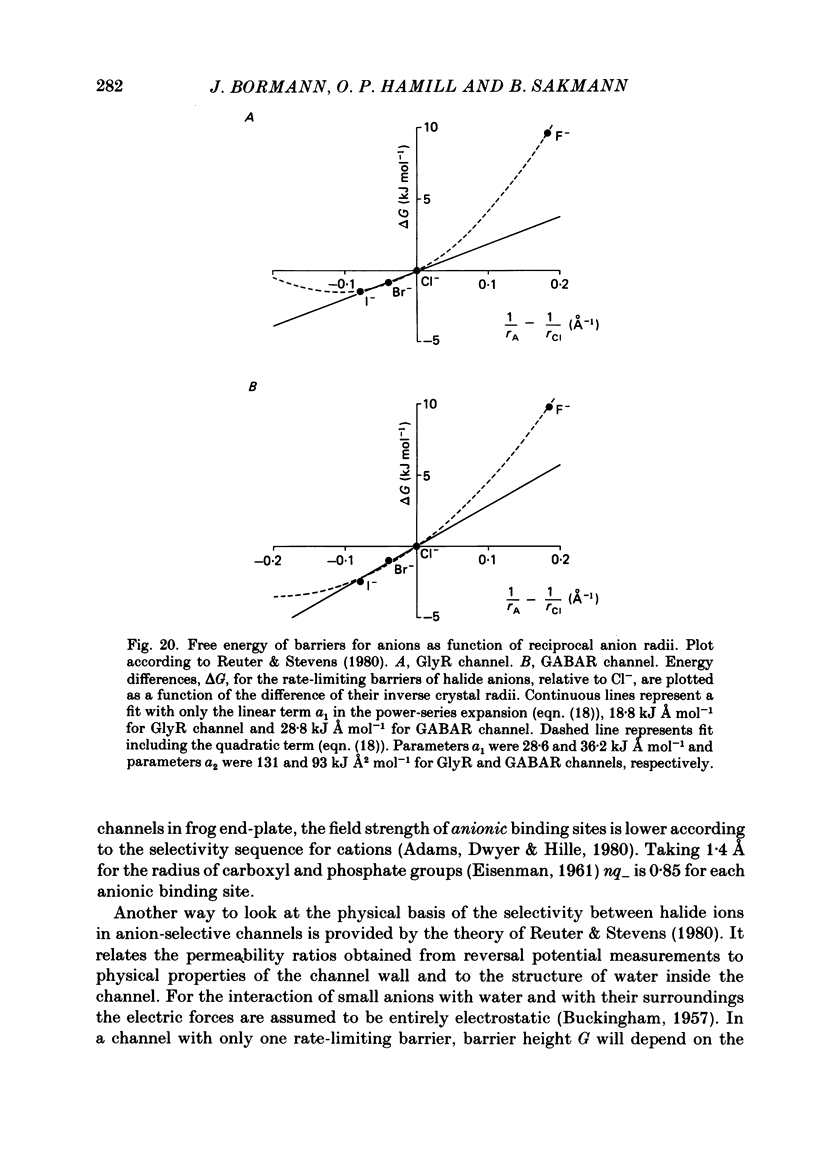
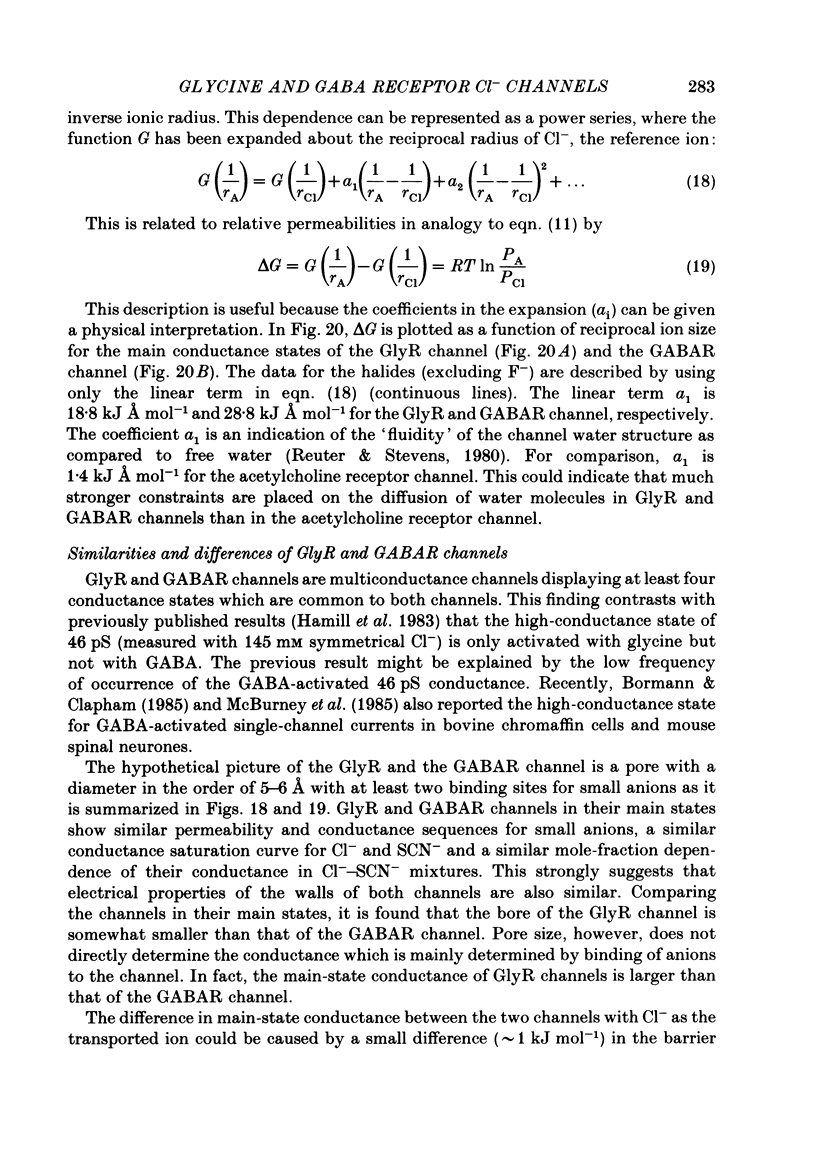
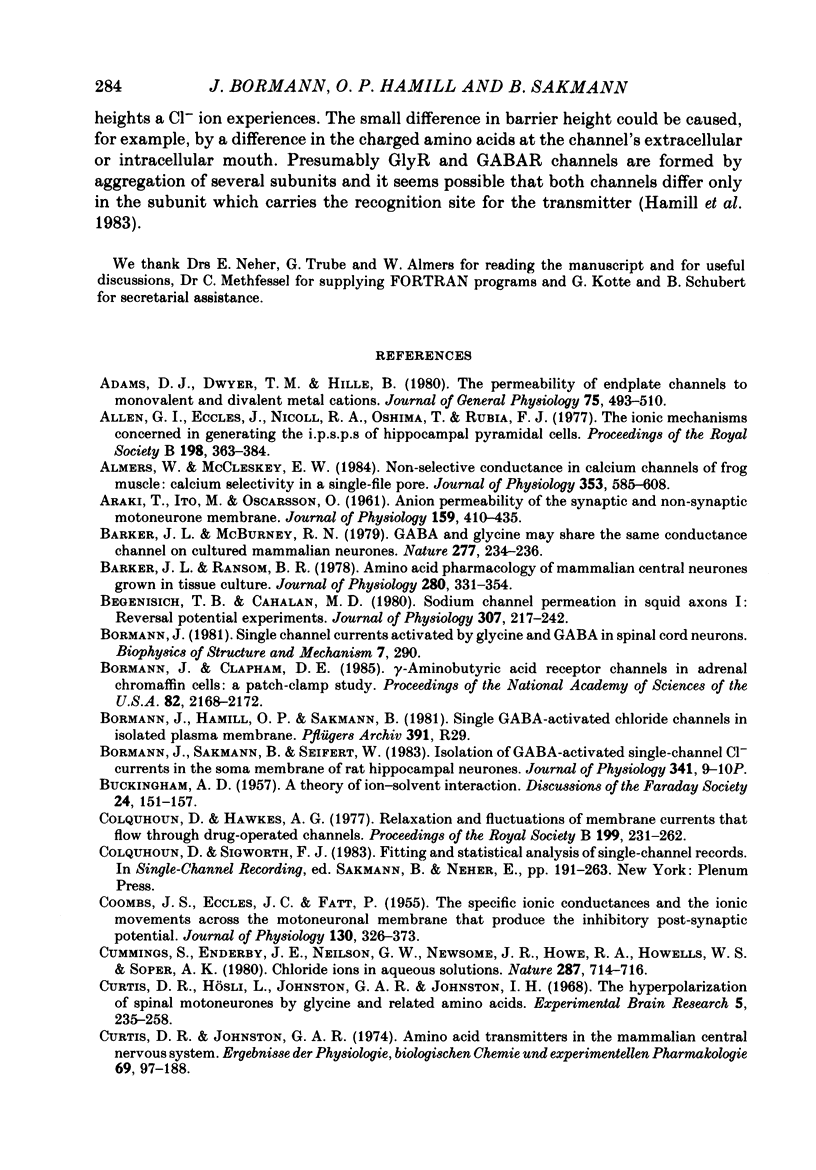
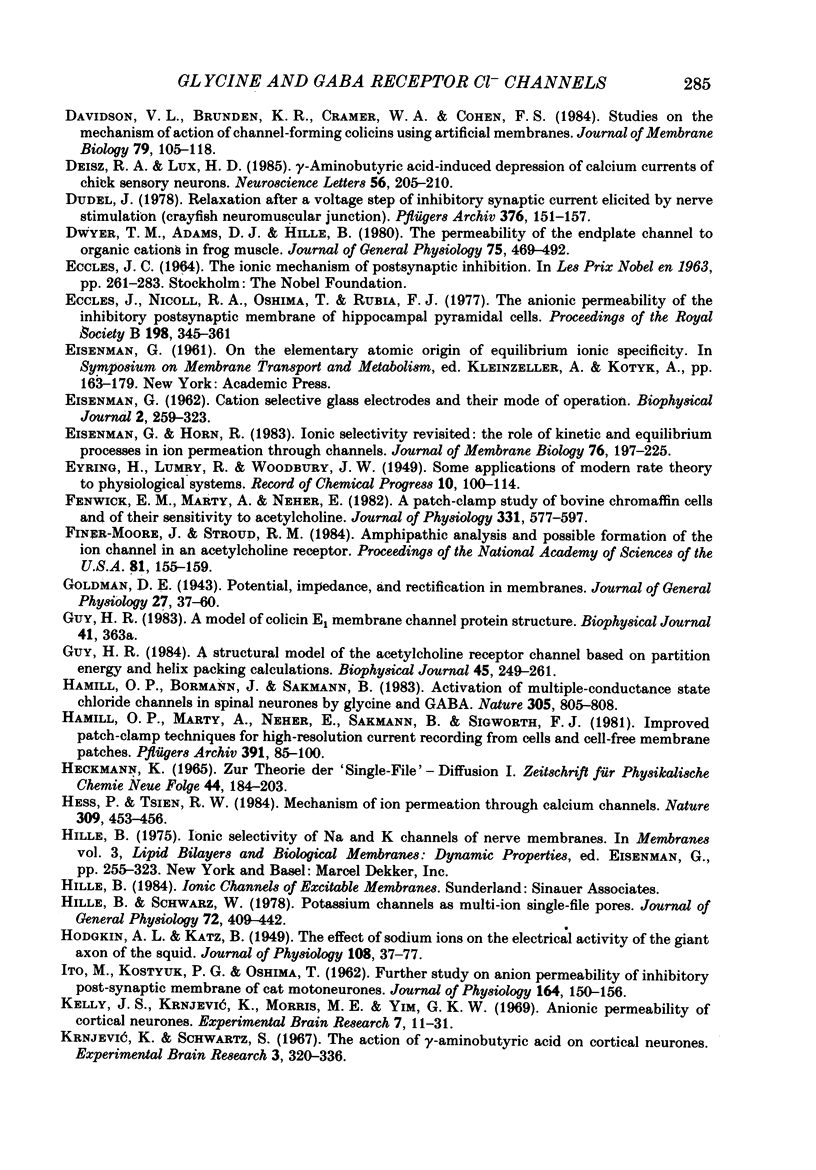
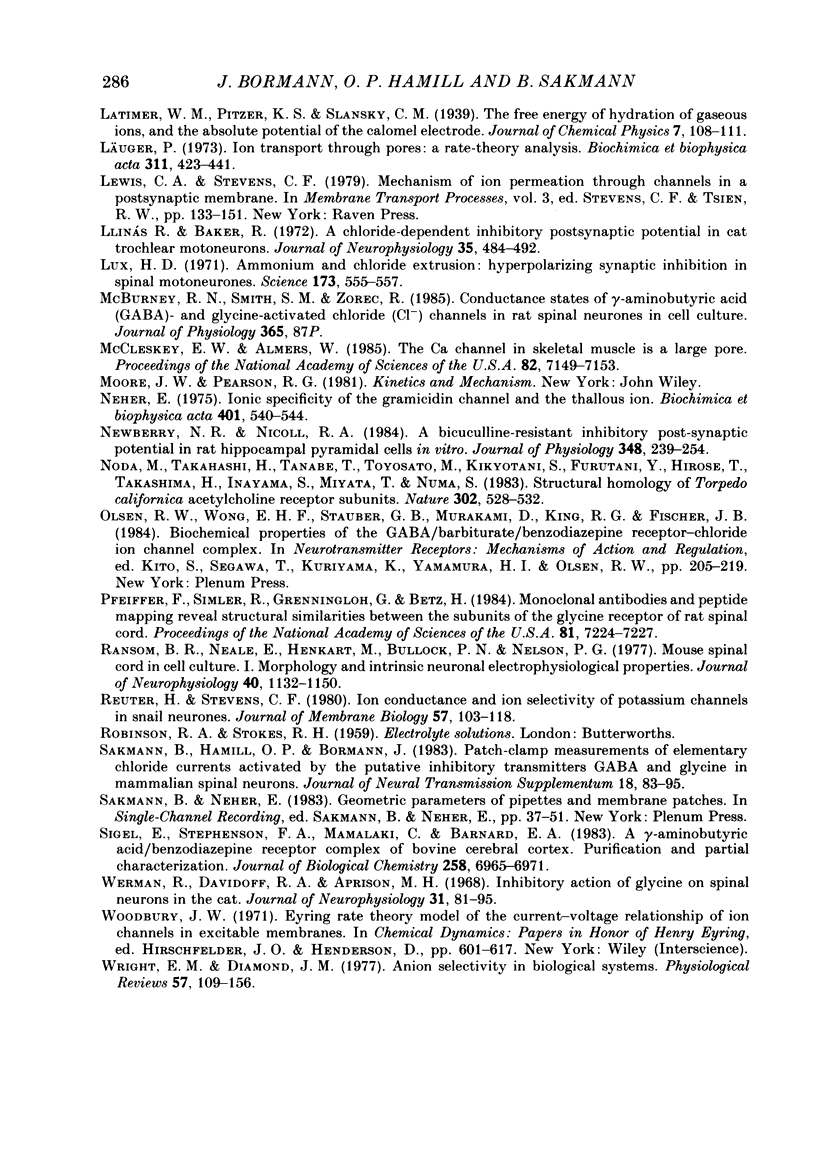
Selected References
These references are in PubMed. This may not be the complete list of references from this article.
- ARAKI T., ITO M., OSCARSSON O. Anion permeability of the synaptic and non-synaptic motoneurone membrane. J Physiol. 1961 Dec;159:410–435. doi: 10.1113/jphysiol.1961.sp006818. [DOI] [PMC free article] [PubMed] [Google Scholar]
- Adams D. J., Dwyer T. M., Hille B. The permeability of endplate channels to monovalent and divalent metal cations. J Gen Physiol. 1980 May;75(5):493–510. doi: 10.1085/jgp.75.5.493. [DOI] [PMC free article] [PubMed] [Google Scholar]
- Allen G. I., Eccles J., Nicoll R. A., Oshima T., Rubia F. J. The ionic mechanisms concerned in generating the i.p.s.ps of hippocampal pyramidal cells. Proc R Soc Lond B Biol Sci. 1977 Sep 19;198(1133):363–384. doi: 10.1098/rspb.1977.0103. [DOI] [PubMed] [Google Scholar]
- Almers W., McCleskey E. W. Non-selective conductance in calcium channels of frog muscle: calcium selectivity in a single-file pore. J Physiol. 1984 Aug;353:585–608. doi: 10.1113/jphysiol.1984.sp015352. [DOI] [PMC free article] [PubMed] [Google Scholar]
- Barker J. L., McBurney R. N. GABA and glycine may share the same conductance channel on cultured mammalian neurones. Nature. 1979 Jan 18;277(5693):234–236. doi: 10.1038/277234a0. [DOI] [PubMed] [Google Scholar]
- Barker J. L., Ransom B. R. Amino acid pharmacology of mammalian central neurones grown in tissue culture. J Physiol. 1978 Jul;280:331–354. doi: 10.1113/jphysiol.1978.sp012387. [DOI] [PMC free article] [PubMed] [Google Scholar]
- Begenisich T. B., Cahalan M. D. Sodium channel permeation in squid axons. I: Reversal potential experiments. J Physiol. 1980 Oct;307:217–242. doi: 10.1113/jphysiol.1980.sp013432. [DOI] [PMC free article] [PubMed] [Google Scholar]
- Bormann J., Clapham D. E. gamma-Aminobutyric acid receptor channels in adrenal chromaffin cells: a patch-clamp study. Proc Natl Acad Sci U S A. 1985 Apr;82(7):2168–2172. doi: 10.1073/pnas.82.7.2168. [DOI] [PMC free article] [PubMed] [Google Scholar]
- COOMBS J. S., ECCLES J. C., FATT P. The specific ionic conductances and the ionic movements across the motoneuronal membrane that produce the inhibitory post-synaptic potential. J Physiol. 1955 Nov 28;130(2):326–374. doi: 10.1113/jphysiol.1955.sp005412. [DOI] [PMC free article] [PubMed] [Google Scholar]
- Colquhoun D., Hawkes A. G. Relaxation and fluctuations of membrane currents that flow through drug-operated channels. Proc R Soc Lond B Biol Sci. 1977 Nov 14;199(1135):231–262. doi: 10.1098/rspb.1977.0137. [DOI] [PubMed] [Google Scholar]
- Curtis D. R., Hösli L., Johnston G. A., Johnston I. H. The hyperpolarization of spinal motoneurones by glycine and related amino acids. Exp Brain Res. 1968;5(3):235–258. doi: 10.1007/BF00238666. [DOI] [PubMed] [Google Scholar]
- Curtis D. R., Johnston G. A. Amino acid transmitters in the mammalian central nervous system. Ergeb Physiol. 1974;69(0):97–188. doi: 10.1007/3-540-06498-2_3. [DOI] [PubMed] [Google Scholar]
- Davidson V. L., Brunden K. R., Cramer W. A., Cohen F. S. Studies on the mechanism of action of channel-forming colicins using artificial membranes. J Membr Biol. 1984;79(2):105–118. doi: 10.1007/BF01872115. [DOI] [PubMed] [Google Scholar]
- Deisz R. A., Lux H. D. gamma-Aminobutyric acid-induced depression of calcium currents of chick sensory neurons. Neurosci Lett. 1985 May 14;56(2):205–210. doi: 10.1016/0304-3940(85)90130-2. [DOI] [PubMed] [Google Scholar]
- Dudel J. Relaxation after a voltage step of inhibitory synaptic current elicited by nerve stimulation (crayfish neuromuscular junction). Pflugers Arch. 1978 Sep 6;376(2):151–157. doi: 10.1007/BF00581578. [DOI] [PubMed] [Google Scholar]
- Dwyer T. M., Adams D. J., Hille B. The permeability of the endplate channel to organic cations in frog muscle. J Gen Physiol. 1980 May;75(5):469–492. doi: 10.1085/jgp.75.5.469. [DOI] [PMC free article] [PubMed] [Google Scholar]
- EISENMAN G. Cation selective glass electrodes and their mode of operation. Biophys J. 1962 Mar;2(2 Pt 2):259–323. doi: 10.1016/s0006-3495(62)86959-8. [DOI] [PMC free article] [PubMed] [Google Scholar]
- Eccles J., Nicoll R. A., Oshima T., Rubia F. J. The anionic permeability of the inhibitory postsynaptic membrane of hippocampal pyramidal cells. Proc R Soc Lond B Biol Sci. 1977 Sep 19;198(1133):345–361. doi: 10.1098/rspb.1977.0102. [DOI] [PubMed] [Google Scholar]
- Eisenman G., Horn R. Ionic selectivity revisited: the role of kinetic and equilibrium processes in ion permeation through channels. J Membr Biol. 1983;76(3):197–225. doi: 10.1007/BF01870364. [DOI] [PubMed] [Google Scholar]
- Fenwick E. M., Marty A., Neher E. A patch-clamp study of bovine chromaffin cells and of their sensitivity to acetylcholine. J Physiol. 1982 Oct;331:577–597. doi: 10.1113/jphysiol.1982.sp014393. [DOI] [PMC free article] [PubMed] [Google Scholar]
- Finer-Moore J., Stroud R. M. Amphipathic analysis and possible formation of the ion channel in an acetylcholine receptor. Proc Natl Acad Sci U S A. 1984 Jan;81(1):155–159. doi: 10.1073/pnas.81.1.155. [DOI] [PMC free article] [PubMed] [Google Scholar]
- Goldman D. E. POTENTIAL, IMPEDANCE, AND RECTIFICATION IN MEMBRANES. J Gen Physiol. 1943 Sep 20;27(1):37–60. doi: 10.1085/jgp.27.1.37. [DOI] [PMC free article] [PubMed] [Google Scholar]
- Guy H. R. A structural model of the acetylcholine receptor channel based on partition energy and helix packing calculations. Biophys J. 1984 Jan;45(1):249–261. doi: 10.1016/S0006-3495(84)84152-1. [DOI] [PMC free article] [PubMed] [Google Scholar]
- HODGKIN A. L., KATZ B. The effect of sodium ions on the electrical activity of giant axon of the squid. J Physiol. 1949 Mar 1;108(1):37–77. doi: 10.1113/jphysiol.1949.sp004310. [DOI] [PMC free article] [PubMed] [Google Scholar]
- Hamill O. P., Bormann J., Sakmann B. Activation of multiple-conductance state chloride channels in spinal neurones by glycine and GABA. 1983 Oct 27-Nov 2Nature. 305(5937):805–808. doi: 10.1038/305805a0. [DOI] [PubMed] [Google Scholar]
- Hamill O. P., Marty A., Neher E., Sakmann B., Sigworth F. J. Improved patch-clamp techniques for high-resolution current recording from cells and cell-free membrane patches. Pflugers Arch. 1981 Aug;391(2):85–100. doi: 10.1007/BF00656997. [DOI] [PubMed] [Google Scholar]
- Hess P., Tsien R. W. Mechanism of ion permeation through calcium channels. 1984 May 31-Jun 6Nature. 309(5967):453–456. doi: 10.1038/309453a0. [DOI] [PubMed] [Google Scholar]
- Hille B., Schwarz W. Potassium channels as multi-ion single-file pores. J Gen Physiol. 1978 Oct;72(4):409–442. doi: 10.1085/jgp.72.4.409. [DOI] [PMC free article] [PubMed] [Google Scholar]
- ITO M., KOSTYUK P. G., OSHIMA T. Further study on anion permeability of inhibitory post-synaptic membrane of cat motoneurones. J Physiol. 1962 Oct;164:150–156. doi: 10.1113/jphysiol.1962.sp007009. [DOI] [PMC free article] [PubMed] [Google Scholar]
- Kelly J. S., Krnjević K., Morris M. E., Yim G. K. Anionic permeability of cortical neurones. Exp Brain Res. 1969;7(1):11–31. doi: 10.1007/BF00236105. [DOI] [PubMed] [Google Scholar]
- Krnjević K., Schwartz S. The action of gamma-aminobutyric acid on cortical neurones. Exp Brain Res. 1967;3(4):320–336. doi: 10.1007/BF00237558. [DOI] [PubMed] [Google Scholar]
- Llinas R., Baker R. A chloride-dependent inhibitory postsynaptic potential in cat trochlear motoneurons. J Neurophysiol. 1972 Jul;35(4):484–492. doi: 10.1152/jn.1972.35.4.484. [DOI] [PubMed] [Google Scholar]
- Lux H. D. Ammonium and chloride extrusion: hyperpolarizing synaptic inhibition in spinal motoneurons. Science. 1971 Aug 6;173(3996):555–557. doi: 10.1126/science.173.3996.555. [DOI] [PubMed] [Google Scholar]
- McCleskey E. W., Almers W. The Ca channel in skeletal muscle is a large pore. Proc Natl Acad Sci U S A. 1985 Oct;82(20):7149–7153. doi: 10.1073/pnas.82.20.7149. [DOI] [PMC free article] [PubMed] [Google Scholar]
- Newberry N. R., Nicoll R. A. A bicuculline-resistant inhibitory post-synaptic potential in rat hippocampal pyramidal cells in vitro. J Physiol. 1984 Mar;348:239–254. doi: 10.1113/jphysiol.1984.sp015107. [DOI] [PMC free article] [PubMed] [Google Scholar]
- Noda M., Takahashi H., Tanabe T., Toyosato M., Kikyotani S., Furutani Y., Hirose T., Takashima H., Inayama S., Miyata T. Structural homology of Torpedo californica acetylcholine receptor subunits. Nature. 1983 Apr 7;302(5908):528–532. doi: 10.1038/302528a0. [DOI] [PubMed] [Google Scholar]
- Olsen R. W., Wong E. H., Stauber G. B., Murakami D., King R. G., Fischer J. B. Biochemical properties of the GABA/barbiturate/benzodiazepine receptor-chloride ion channel complex. Adv Exp Med Biol. 1984;175:205–219. doi: 10.1007/978-1-4684-4805-4_17. [DOI] [PubMed] [Google Scholar]
- Pfeiffer F., Simler R., Grenningloh G., Betz H. Monoclonal antibodies and peptide mapping reveal structural similarities between the subunits of the glycine receptor of rat spinal cord. Proc Natl Acad Sci U S A. 1984 Nov;81(22):7224–7227. doi: 10.1073/pnas.81.22.7224. [DOI] [PMC free article] [PubMed] [Google Scholar]
- Ransom B. R., Neale E., Henkart M., Bullock P. N., Nelson P. G. Mouse spinal cord in cell culture. I. Morphology and intrinsic neuronal electrophysiologic properties. J Neurophysiol. 1977 Sep;40(5):1132–1150. doi: 10.1152/jn.1977.40.5.1132. [DOI] [PubMed] [Google Scholar]
- Reuter H., Stevens C. F. Ion conductance and ion selectivity of potassium channels in snail neurones. J Membr Biol. 1980 Dec 15;57(2):103–118. doi: 10.1007/BF01868997. [DOI] [PubMed] [Google Scholar]
- Sakmann B., Hamill O. P., Bormann J. Patch-clamp measurements of elementary chloride currents activated by the putative inhibitory transmitter GABA and glycine in mammalian spinal neurons. J Neural Transm Suppl. 1983;18:83–95. [PubMed] [Google Scholar]
- Sigel E., Stephenson F. A., Mamalaki C., Barnard E. A. A gamma-aminobutyric acid/benzodiazepine receptor complex of bovine cerebral cortex. J Biol Chem. 1983 Jun 10;258(11):6965–6971. [PubMed] [Google Scholar]
- Werman R., Davidoff R. A., Aprison M. H. Inhibitory of glycine on spinal neurons in the cat. J Neurophysiol. 1968 Jan;31(1):81–95. doi: 10.1152/jn.1968.31.1.81. [DOI] [PubMed] [Google Scholar]
- Wright E. M., Diamond J. M. Anion selectivity in biological systems. Physiol Rev. 1977 Jan;57(1):109–156. doi: 10.1152/physrev.1977.57.1.109. [DOI] [PubMed] [Google Scholar]