Abstract
1. Right ventricular rabbit papillary muscles were arterially perfused with a mixture of Tyrode solution, bovine erythrocytes, dextran and albumin. In the recording chamber, they were surrounded by a H2O-saturated atmosphere of O2 and CO2 which served as an electrical insulator. 2. Conduction velocity and passive electrical properties were determined from intra- and extracellular potentials measured during excitation and during flow of subthreshold current. 3. The propagation of the action potential was linear along the muscle at a velocity of 55.6 cm/s. The extracellular wave-front voltage was 51.5 mV. 4. The following values for passive cable properties were obtained: (i) a ratio of extra- to intracellular longitudinal resistance of 1.2; (ii) an extracellular specific resistance (Ro) of 63 omega cm; (iii) an intracellular specific resistance (Ri) of 166 omega cm; (iv) a space constant lambda of 0.357 mm; (v) a membrane time constant tau of 2.57 ms. The space constant lambda* recalculated for zero extracellular resistance was 0.528 mm. 5. Arresting perfusion with drop of perfusion pressure was associated with an immediate increase of the extracellular longitudinal resistance by 35% and a decrease of conduction velocity by 13%. 6. The present results demonstrate the important contribution of the extracellular resistance to electrotonic interaction and propagation in densely packed myocardial tissue. Moreover, changes in perfusion pressure are associated with changes in extracellular resistance, probably as a consequence of changes in intravascular volume.
Full text
PDF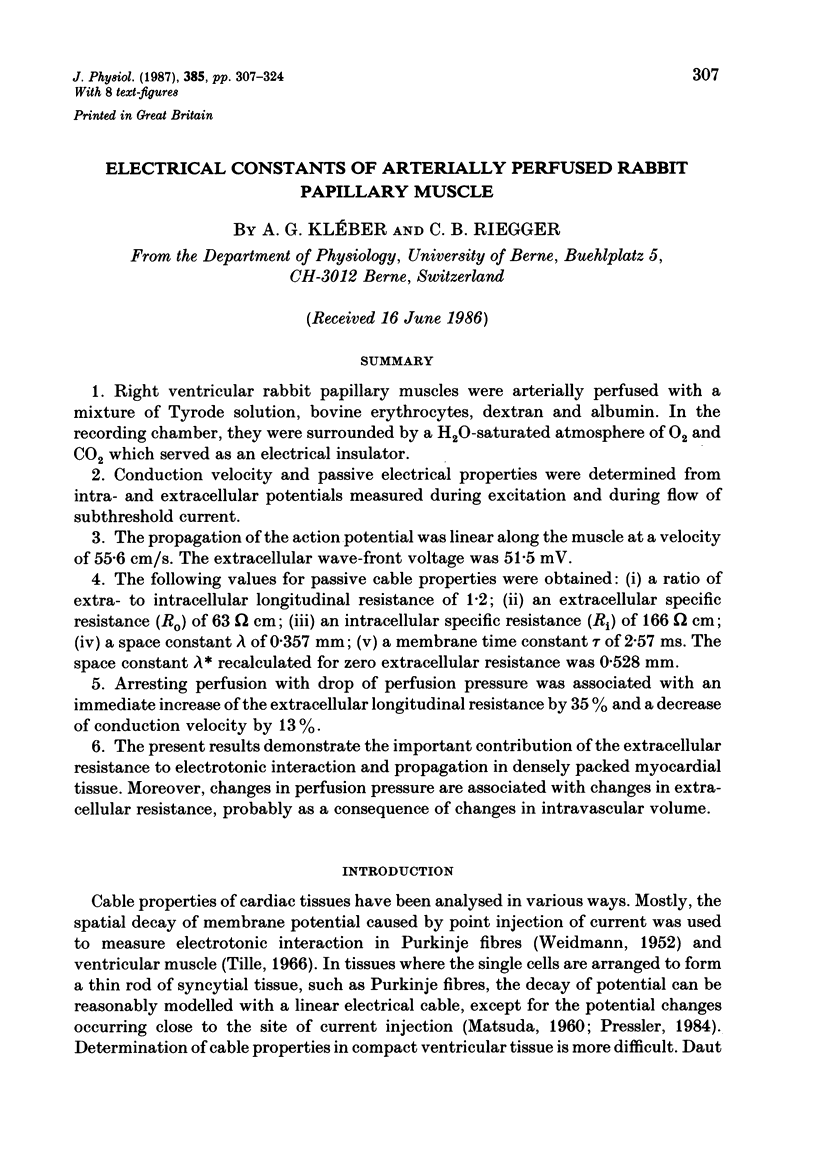
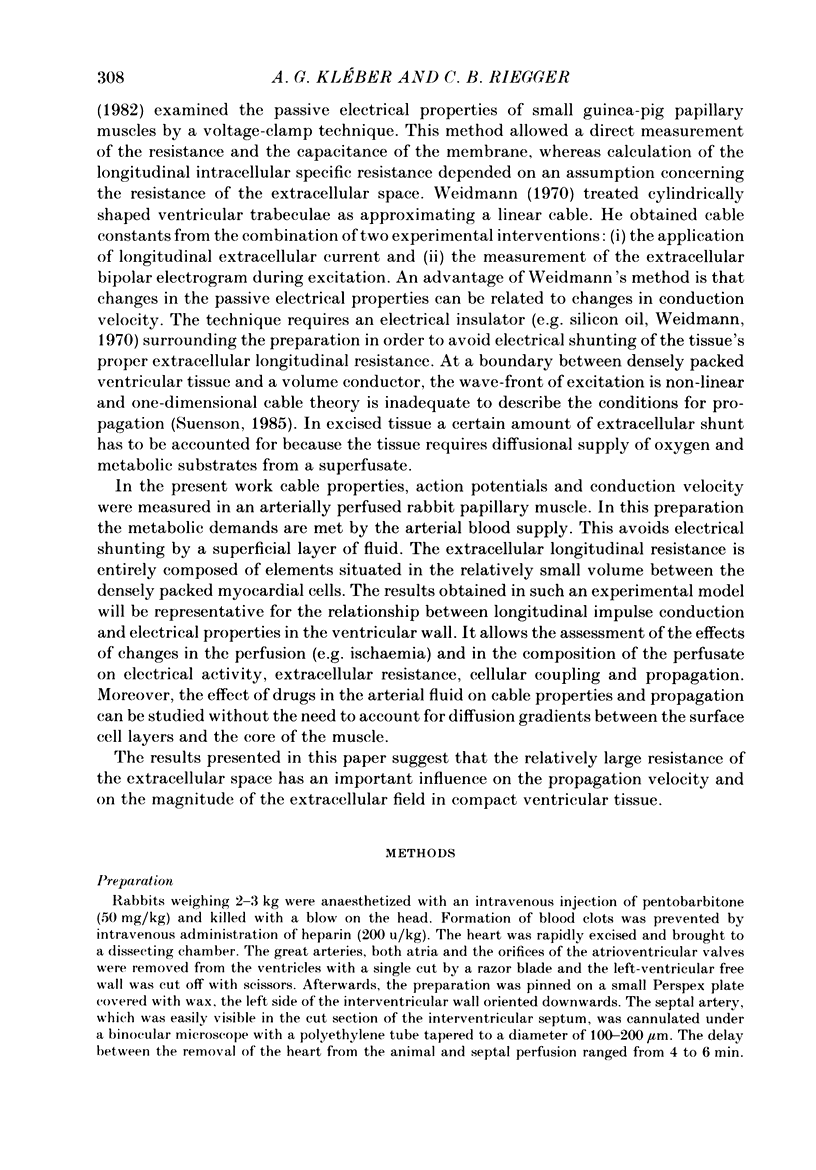
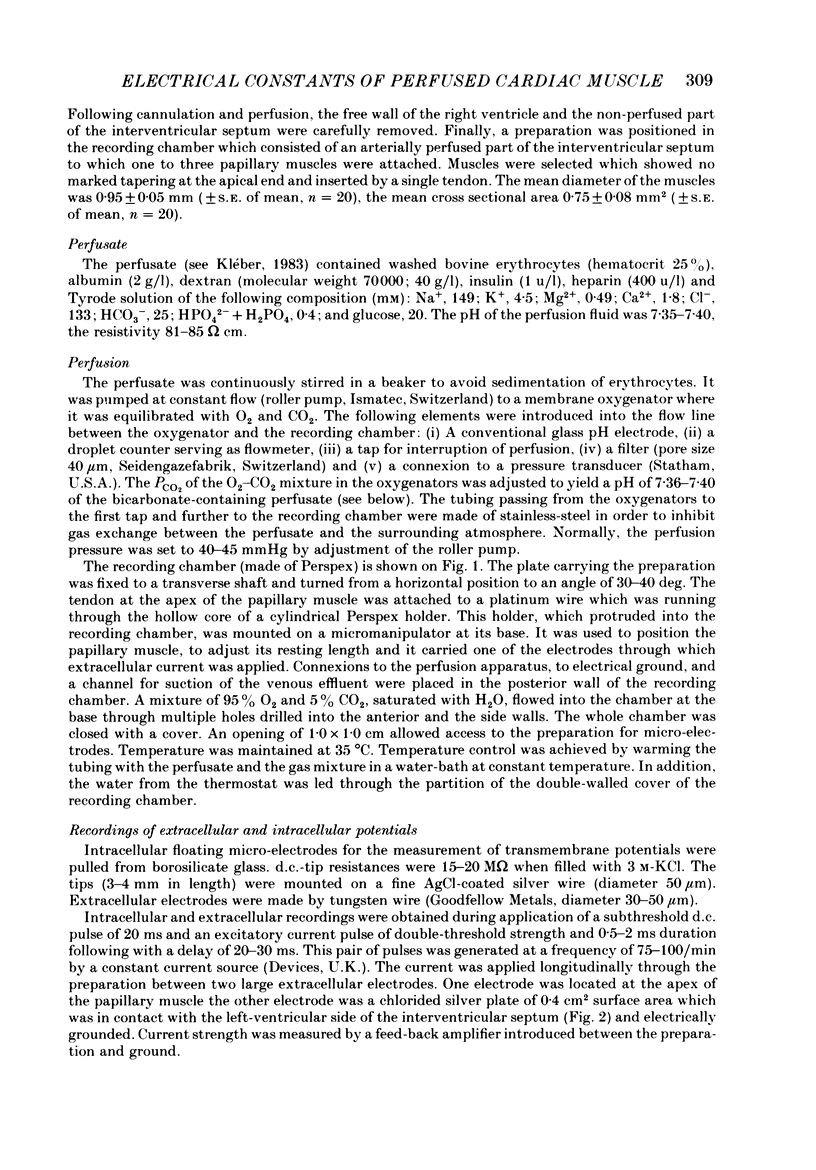
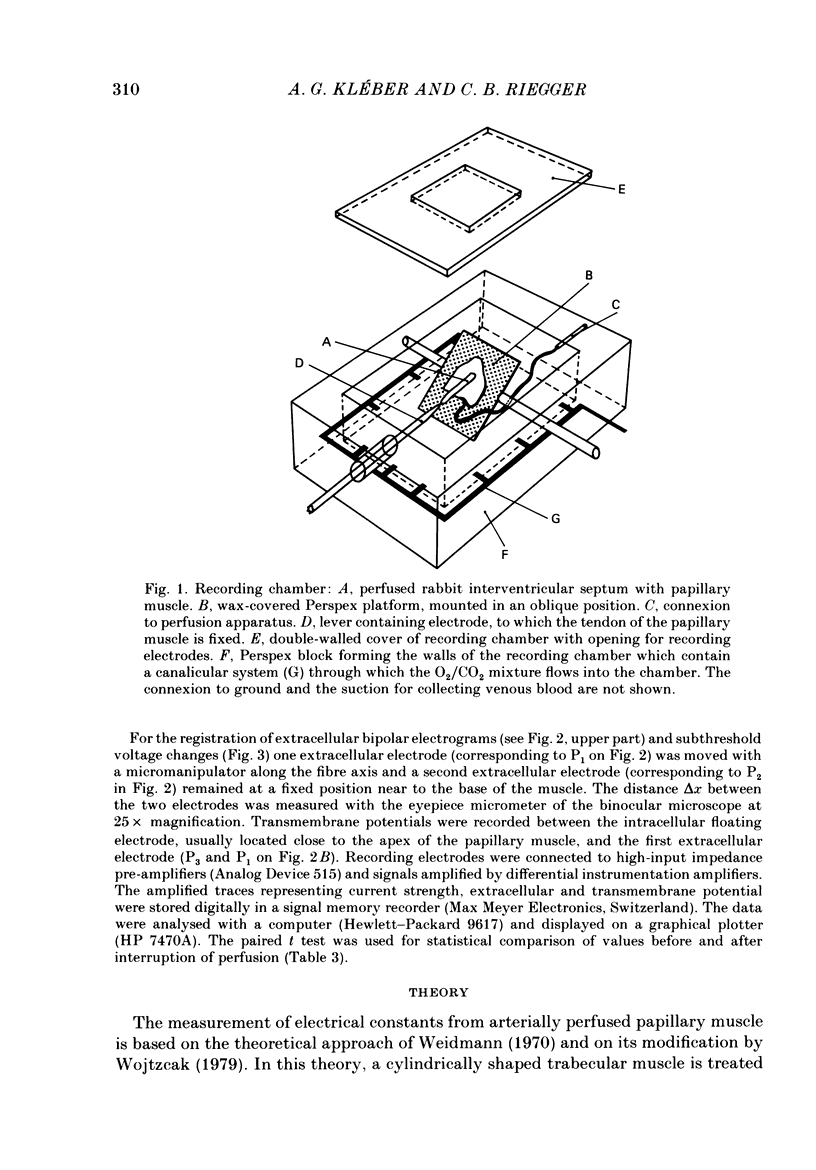
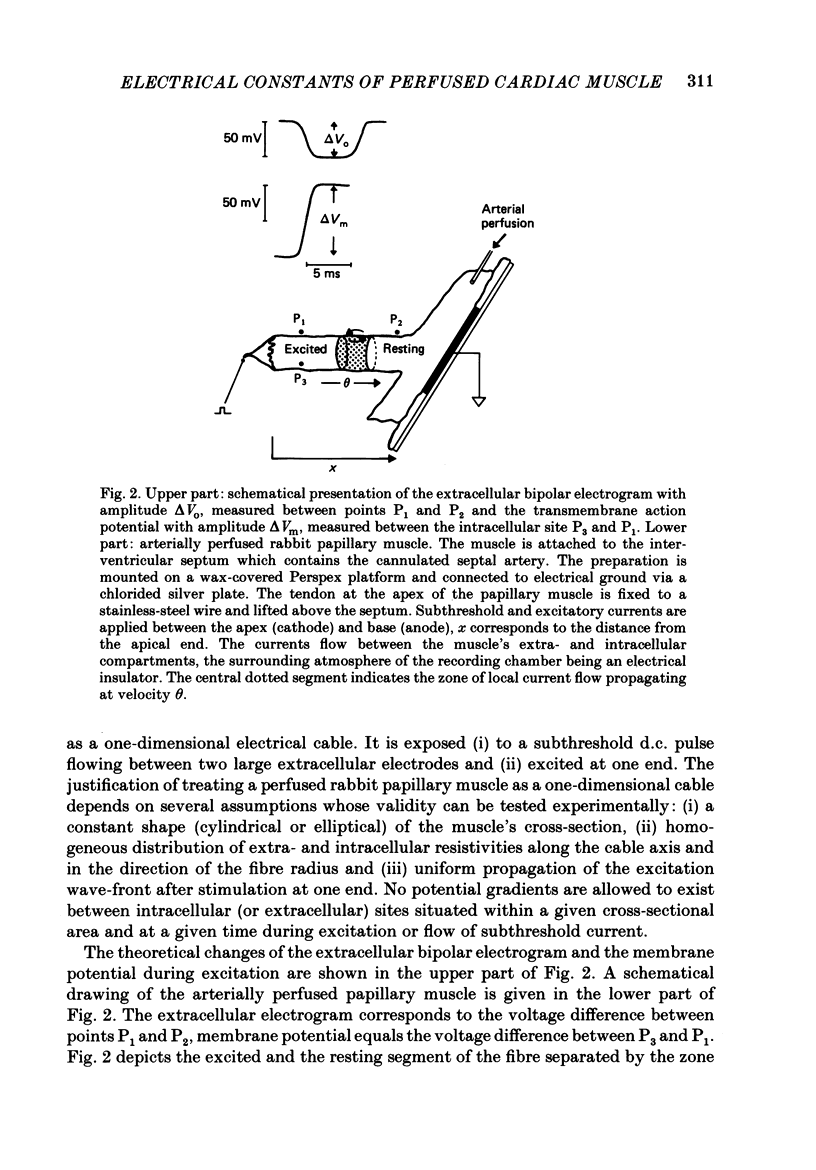
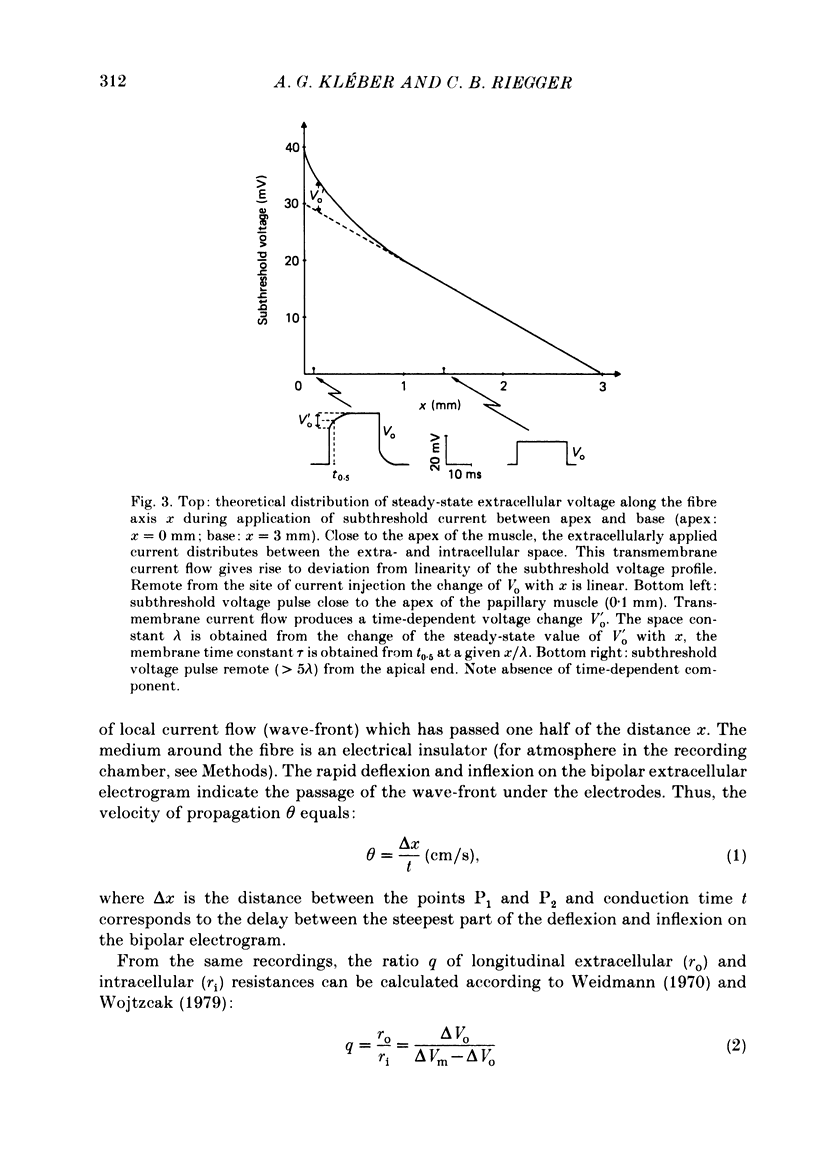
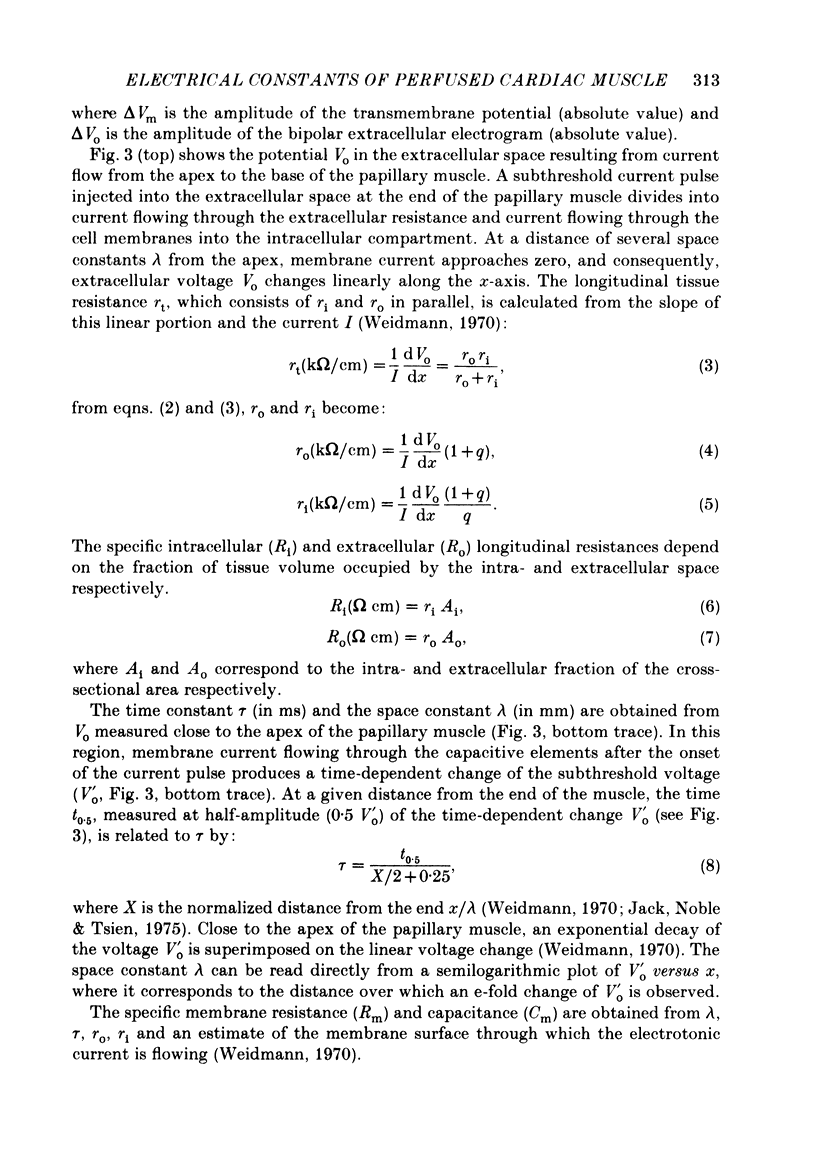
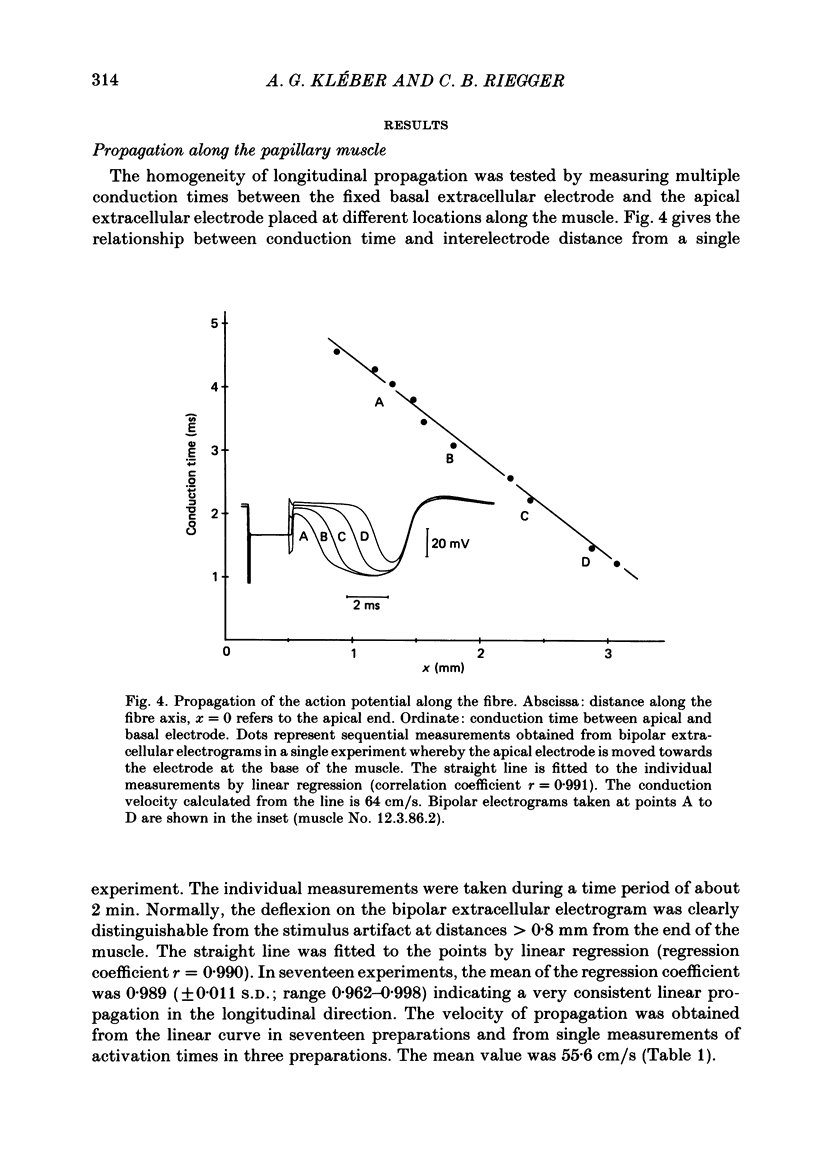
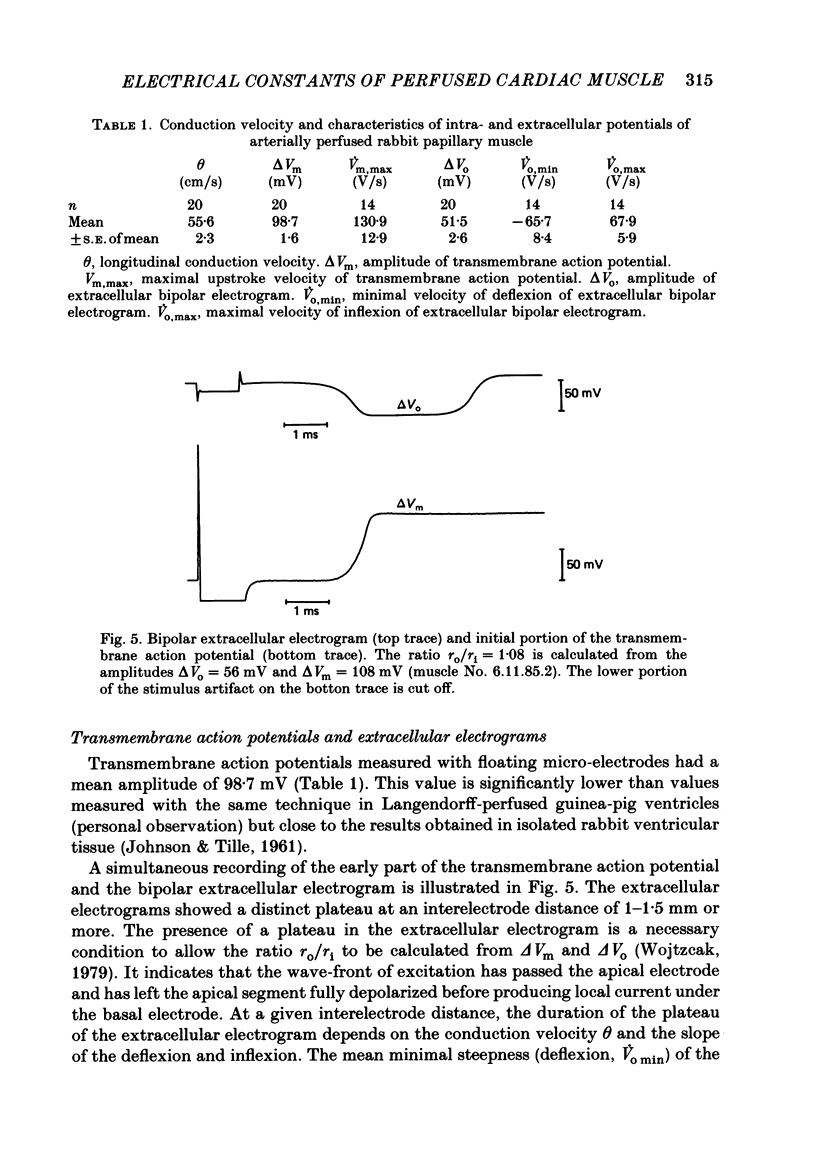
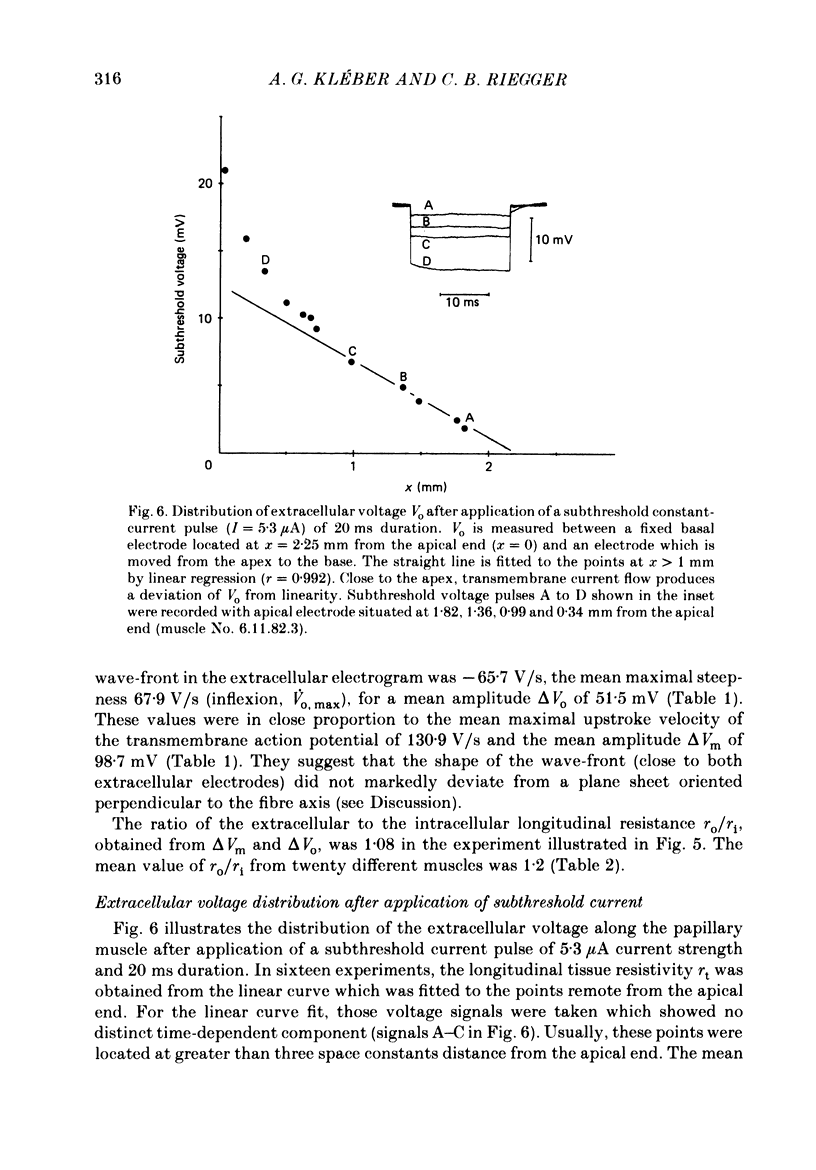
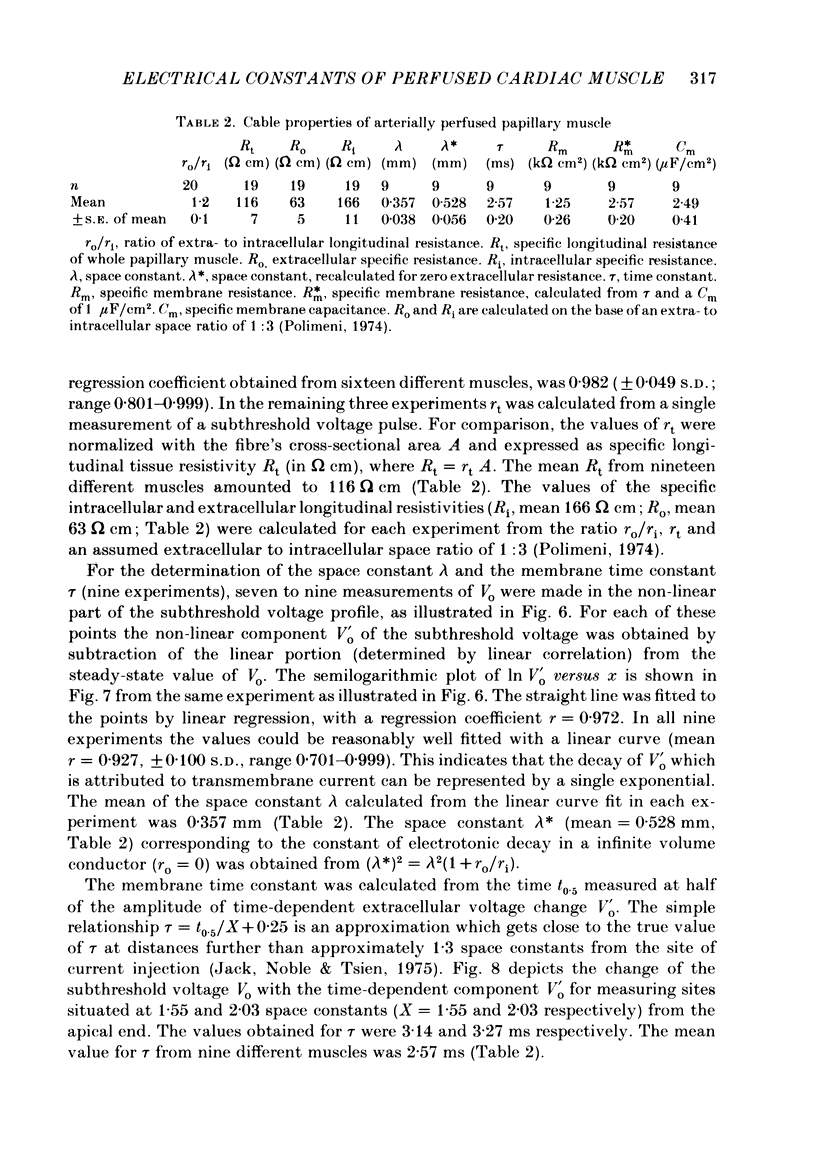
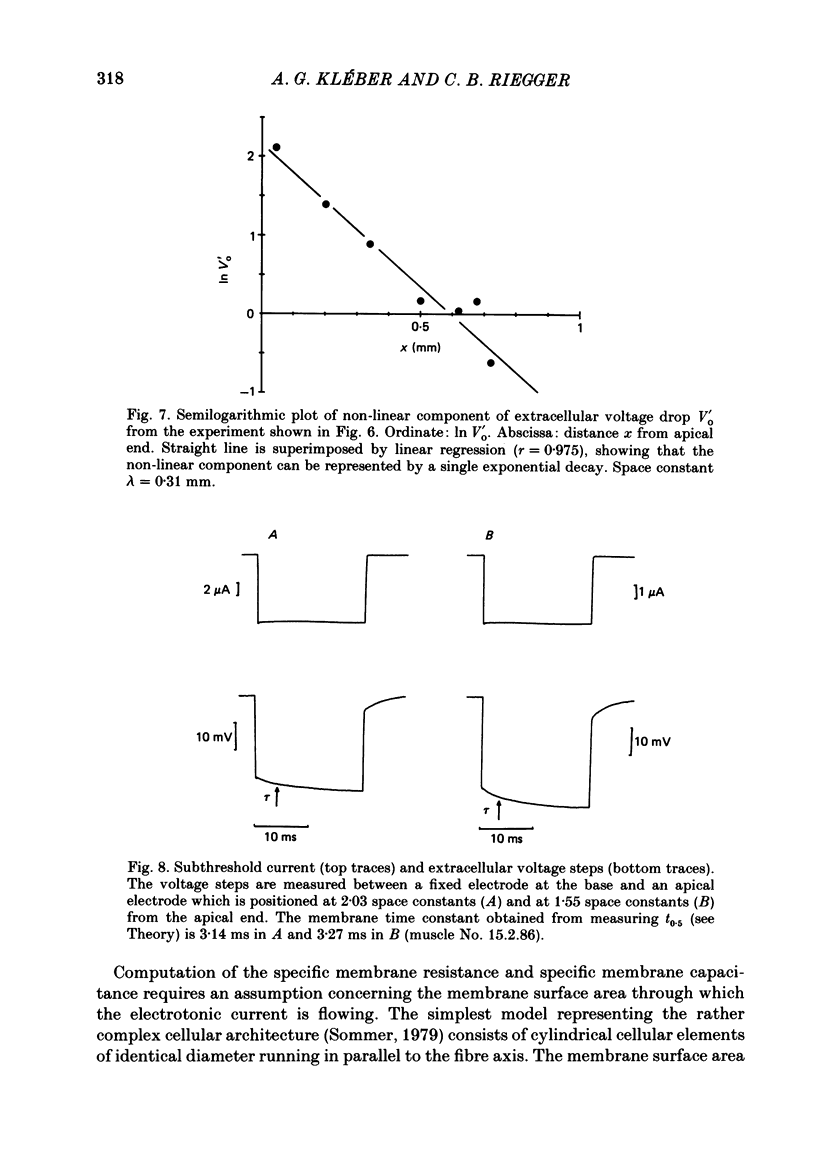
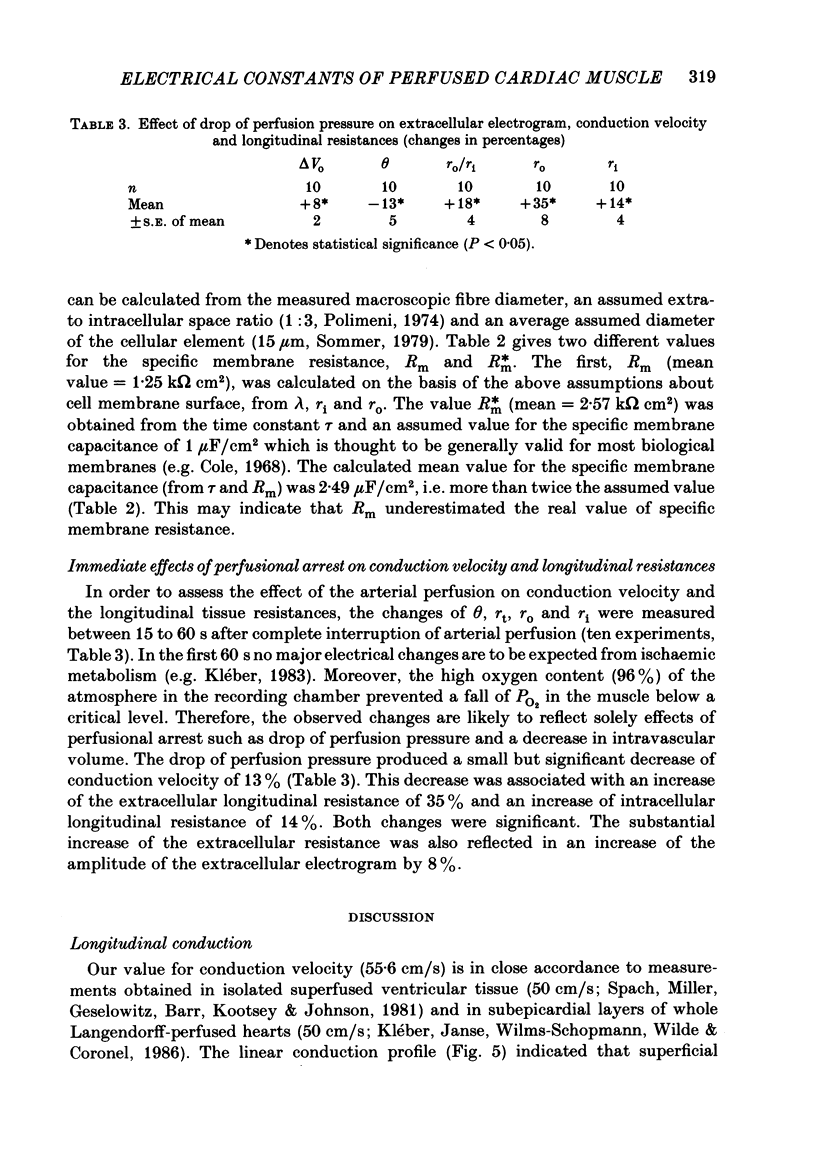
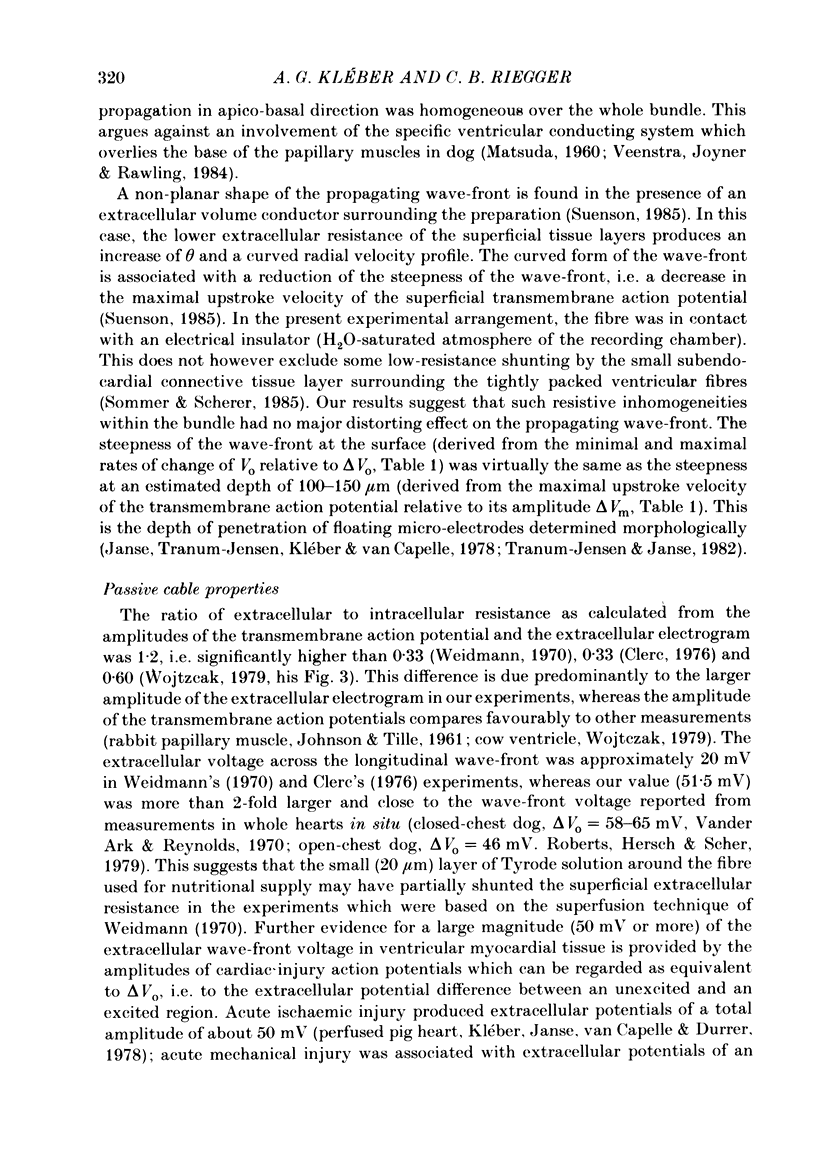
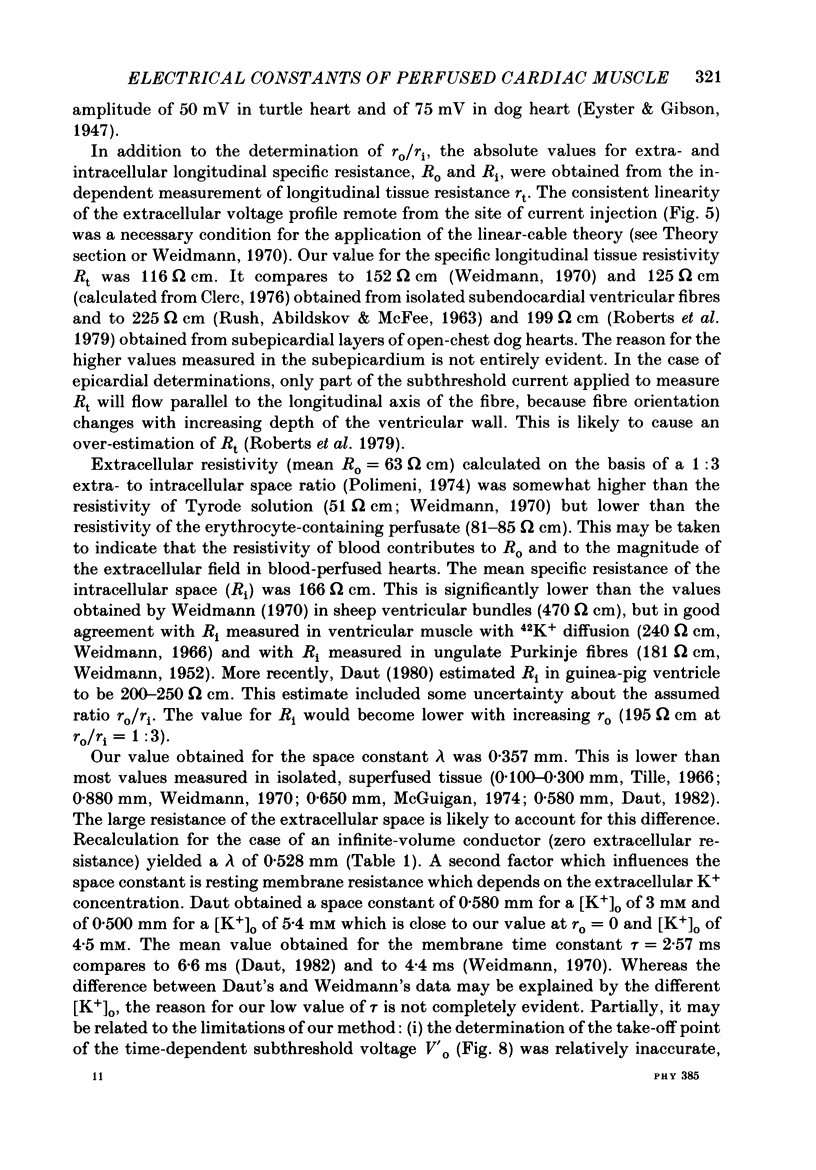
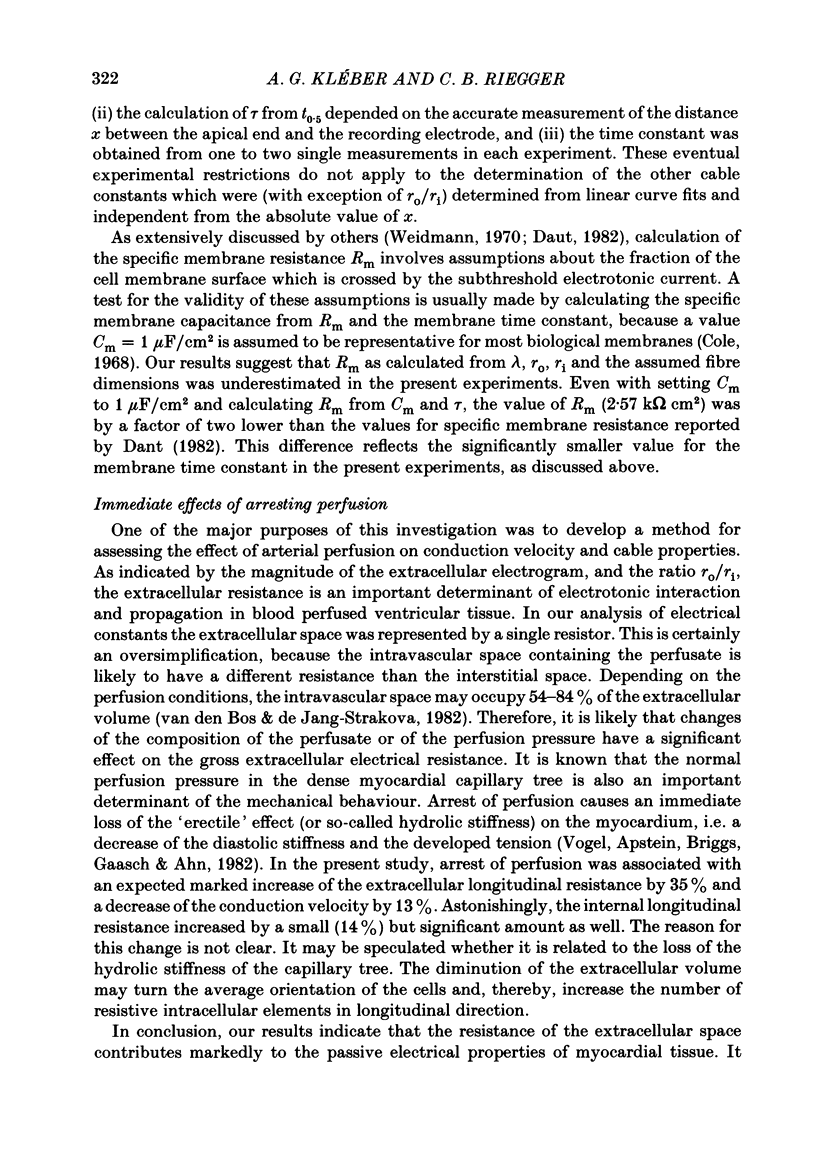
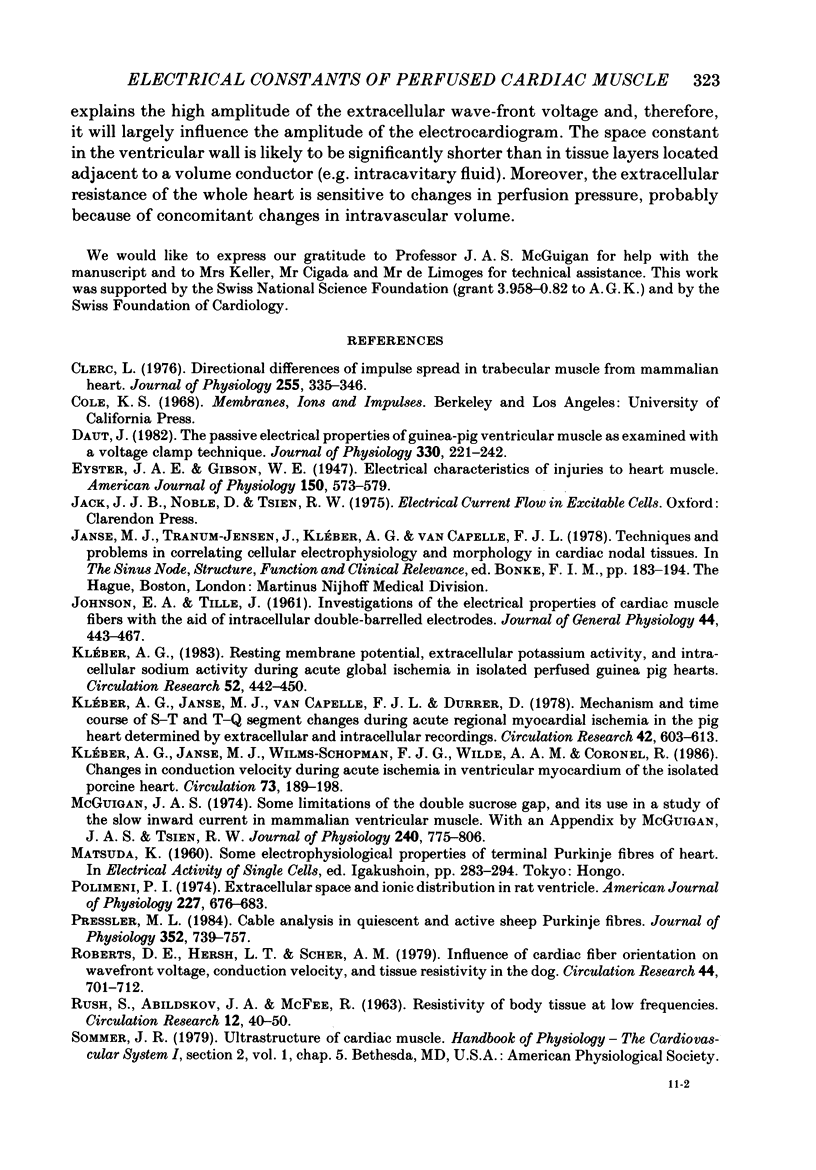
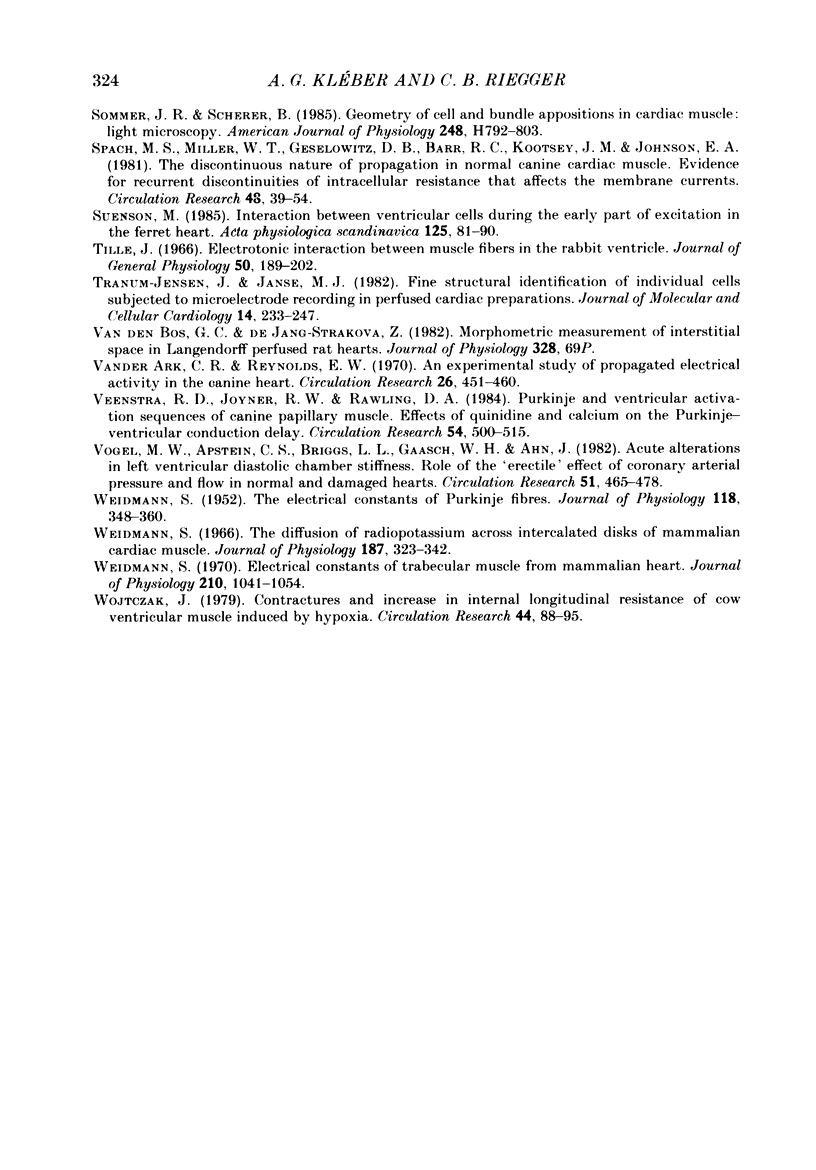
Selected References
These references are in PubMed. This may not be the complete list of references from this article.
- Clerc L. Directional differences of impulse spread in trabecular muscle from mammalian heart. J Physiol. 1976 Feb;255(2):335–346. doi: 10.1113/jphysiol.1976.sp011283. [DOI] [PMC free article] [PubMed] [Google Scholar]
- Daut J. The passive electrical properties of guinea-pig ventricular muscle as examined with a voltage-clamp technique. J Physiol. 1982 Sep;330:221–242. doi: 10.1113/jphysiol.1982.sp014338. [DOI] [PMC free article] [PubMed] [Google Scholar]
- JOHNSON E. A., TILLE J. Investigations of the electrical properties of cardiac muscle fibres with the aid of intracellular double-barrelled electrodes. J Gen Physiol. 1961 Jan;44:443–467. doi: 10.1085/jgp.44.3.443. [DOI] [PMC free article] [PubMed] [Google Scholar]
- Kléber A. G., Janse M. J., Wilms-Schopmann F. J., Wilde A. A., Coronel R. Changes in conduction velocity during acute ischemia in ventricular myocardium of the isolated porcine heart. Circulation. 1986 Jan;73(1):189–198. doi: 10.1161/01.cir.73.1.189. [DOI] [PubMed] [Google Scholar]
- Kléber A. G., Janse M. J., van Capelle F. J., Durrer D. Mechanism and time course of S-T and T-Q segment changes during acute regional myocardial ischemia in the pig heart determined by extracellular and intracellular recordings. Circ Res. 1978 May;42(5):603–613. doi: 10.1161/01.res.42.5.603. [DOI] [PubMed] [Google Scholar]
- Kléber A. G. Resting membrane potential, extracellular potassium activity, and intracellular sodium activity during acute global ischemia in isolated perfused guinea pig hearts. Circ Res. 1983 Apr;52(4):442–450. doi: 10.1161/01.res.52.4.442. [DOI] [PubMed] [Google Scholar]
- McGuigan J. A. Some limitations of the double sucrose gap, and its use in a study of the slow outward current in mammalian ventricular muscle. J Physiol. 1974 Aug;240(3):775–806. doi: 10.1113/jphysiol.1974.sp010634. [DOI] [PMC free article] [PubMed] [Google Scholar]
- Polimeni P. I. Extracellular space and ionic distribution in rat ventricle. Am J Physiol. 1974 Sep;227(3):676–683. doi: 10.1152/ajplegacy.1974.227.3.676. [DOI] [PubMed] [Google Scholar]
- Pressler M. L. Cable analysis in quiescent and active sheep Purkinje fibres. J Physiol. 1984 Jul;352:739–757. doi: 10.1113/jphysiol.1984.sp015319. [DOI] [PMC free article] [PubMed] [Google Scholar]
- RUSH S., ABILDSKOV J. A., McFEER Resistivity of body tissues at low frequencies. Circ Res. 1963 Jan;12:40–50. doi: 10.1161/01.res.12.1.40. [DOI] [PubMed] [Google Scholar]
- Roberts D. E., Hersh L. T., Scher A. M. Influence of cardiac fiber orientation on wavefront voltage, conduction velocity, and tissue resistivity in the dog. Circ Res. 1979 May;44(5):701–712. doi: 10.1161/01.res.44.5.701. [DOI] [PubMed] [Google Scholar]
- Sommer J. R., Scherer B. Geometry of cell and bundle appositions in cardiac muscle: light microscopy. Am J Physiol. 1985 Jun;248(6 Pt 2):H792–H803. doi: 10.1152/ajpheart.1985.248.6.H792. [DOI] [PubMed] [Google Scholar]
- Spach M. S., Miller W. T., 3rd, Geselowitz D. B., Barr R. C., Kootsey J. M., Johnson E. A. The discontinuous nature of propagation in normal canine cardiac muscle. Evidence for recurrent discontinuities of intracellular resistance that affect the membrane currents. Circ Res. 1981 Jan;48(1):39–54. doi: 10.1161/01.res.48.1.39. [DOI] [PubMed] [Google Scholar]
- Suenson M. Interaction between ventricular cells during the early part of excitation in the ferret heart. Acta Physiol Scand. 1985 Sep;125(1):81–90. doi: 10.1111/j.1748-1716.1985.tb07694.x. [DOI] [PubMed] [Google Scholar]
- Tille J. Electrotonic interaction between muscle fibers in the rabbit ventricle. J Gen Physiol. 1966 Sep;50(1):189–202. doi: 10.1085/jgp.50.1.189. [DOI] [PMC free article] [PubMed] [Google Scholar]
- Tranum-Jensen J., Janse M. J. Fine structural identification of individual cells subjected to microelectrode recording in perfused cardiac preparations. J Mol Cell Cardiol. 1982 Apr;14(4):233–247. doi: 10.1016/0022-2828(82)90192-4. [DOI] [PubMed] [Google Scholar]
- Vander Ark C. R., Reynolds E. W., Jr An experimental study of propagated electrical activity in the canine heart. Circ Res. 1970 Apr;26(4):451–460. doi: 10.1161/01.res.26.4.451. [DOI] [PubMed] [Google Scholar]
- Veenstra R. D., Joyner R. W., Rawling D. A. Purkinje and ventricular activation sequences of canine papillary muscle. Effects of quinidine and calcium on the Purkinje-ventricular conduction delay. Circ Res. 1984 May;54(5):500–515. doi: 10.1161/01.res.54.5.500. [DOI] [PubMed] [Google Scholar]
- Vogel W. M., Apstein C. S., Briggs L. L., Gaasch W. H., Ahn J. Acute alterations in left ventricular diastolic chamber stiffness. Role of the "erectile" effect of coronary arterial pressure and flow in normal and damaged hearts. Circ Res. 1982 Oct;51(4):465–478. doi: 10.1161/01.res.51.4.465. [DOI] [PubMed] [Google Scholar]
- WEIDMANN S. The electrical constants of Purkinje fibres. J Physiol. 1952 Nov;118(3):348–360. doi: 10.1113/jphysiol.1952.sp004799. [DOI] [PMC free article] [PubMed] [Google Scholar]
- Weidmann S. Electrical constants of trabecular muscle from mammalian heart. J Physiol. 1970 Nov;210(4):1041–1054. doi: 10.1113/jphysiol.1970.sp009256. [DOI] [PMC free article] [PubMed] [Google Scholar]
- Weidmann S. The diffusion of radiopotassium across intercalated disks of mammalian cardiac muscle. J Physiol. 1966 Nov;187(2):323–342. doi: 10.1113/jphysiol.1966.sp008092. [DOI] [PMC free article] [PubMed] [Google Scholar]
- Wojtczak J. Contractures and increase in internal longitudianl resistance of cow ventricular muscle induced by hypoxia. Circ Res. 1979 Jan;44(1):88–95. doi: 10.1161/01.res.44.1.88. [DOI] [PubMed] [Google Scholar]