Abstract
1. Ganglion cells were dissociated from the enzyme-treated rat retina, identified with specific fluorescent labels, and maintained in vitro. Electrophysiological properties of solitary retinal ganglion cells were investigated with both conventional intracellular and patch-clamp recordings. Although comparable results were obtained for most measurements some important differences were noted. 2. The input resistance of solitary retinal ganglion cells was considerably higher when measured with 'giga-seal' suction pipettes than with conventional intracellular electrodes. Under current-clamp conditions with both intracellular and patch pipettes, these central mammalian neurones maintained resting potentials of about -60 mV and displayed action potentials followed by an after-hyperpolarization in response to small depolarizations. The membrane currents during this activity, analysed under voltage clamp with patch pipettes, consisted of five components: Na+ current (INa), Ca2+ current (ICa), and currents with properties similar to the delayed outward, the transient (A-type), and the Ca2+-activated K+ currents (IK, IA and IK(Ca), respectively). 3. Ionic substitution, pharmacological agents, and voltage-clamp experiments revealed that the regenerative currents were carried by both Na+ and Ca2+. 100 nM-1 microM-tetradotoxin (TTX) reversibly blocked the fast spikes carried by the presumptive INa, which under voltage-clamp analysis had classical Hodgkin-Huxley-type activation and inactivation. 4. Single-channel recordings of the Na+ current (iNa) permitted comparison of these 'microscopic' events with the 'macroscopic' whole-cell current (INa). The inactivation time constant (tau h) fitted to the averaged single-channel recordings of iNa in outside-out patches was slower than the tau h obtained during whole-cell recordings of INa. 5. In the presence of 1-40 microM-TTX and 20 mM-TEA, slow action potentials appeared in intracellular recordings and were probably mediated by Ca2+. The potentials were abrogated by 3 mM-Co2+ or 200 microM-Cd2+; conversely, increasing the extracellular Ca2+ concentration from 2.5 to 10-25 mM or substitution of 1 mM-Ba2+ for 2.5 mM-Ca2+ enhanced their amplitude. ICa was measured directly in whole-cell recordings with patch pipettes after blocking INa with extracellular 1 microM-TTX and K+ currents with intracellular 120-mM Cs+ and 20 mM-TEA. 6. During whole-cell recordings with patch electrodes, extracellular 20 mM-TEA suppressed IK and, to a lesser extent, IA. Extracellular 5 mM-4-AP or a pre-pulse of the membrane potential to -40 mV prior to stronger depolarization completely blocked IA.(ABSTRACT TRUNCATED AT 400 WORDS)
Full text
PDF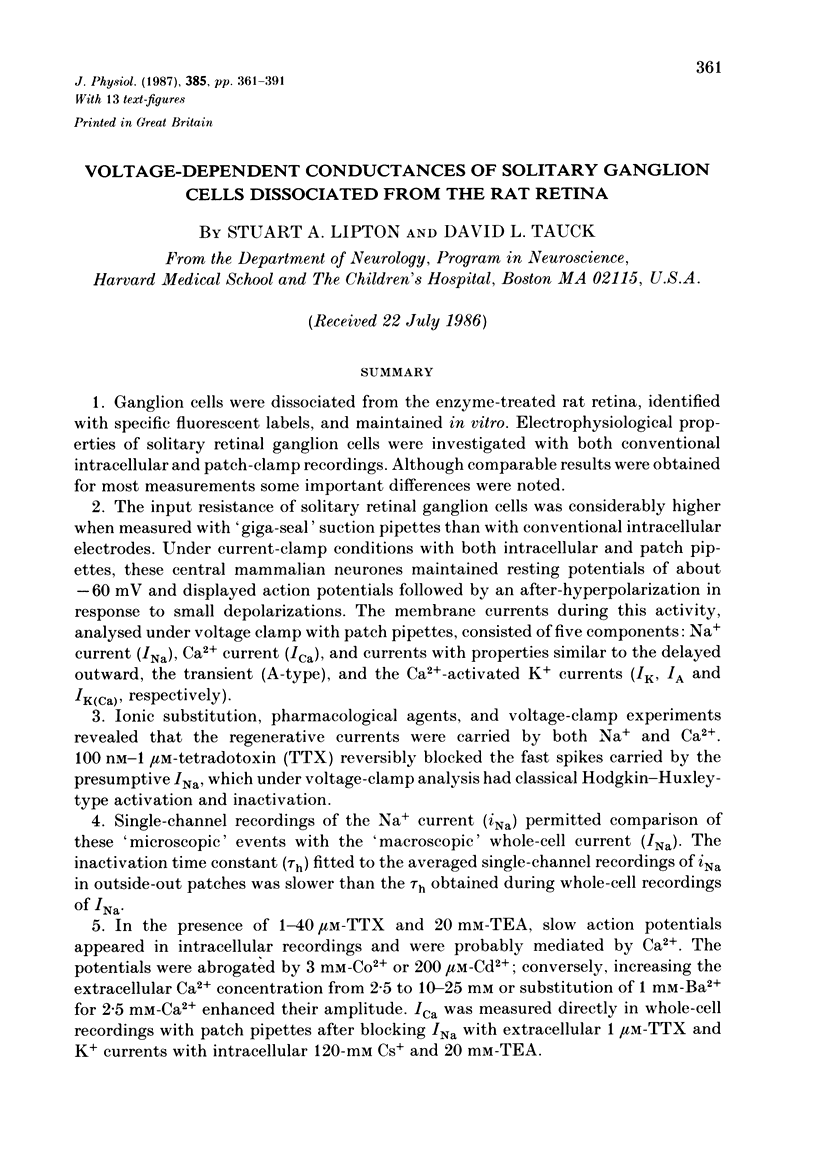
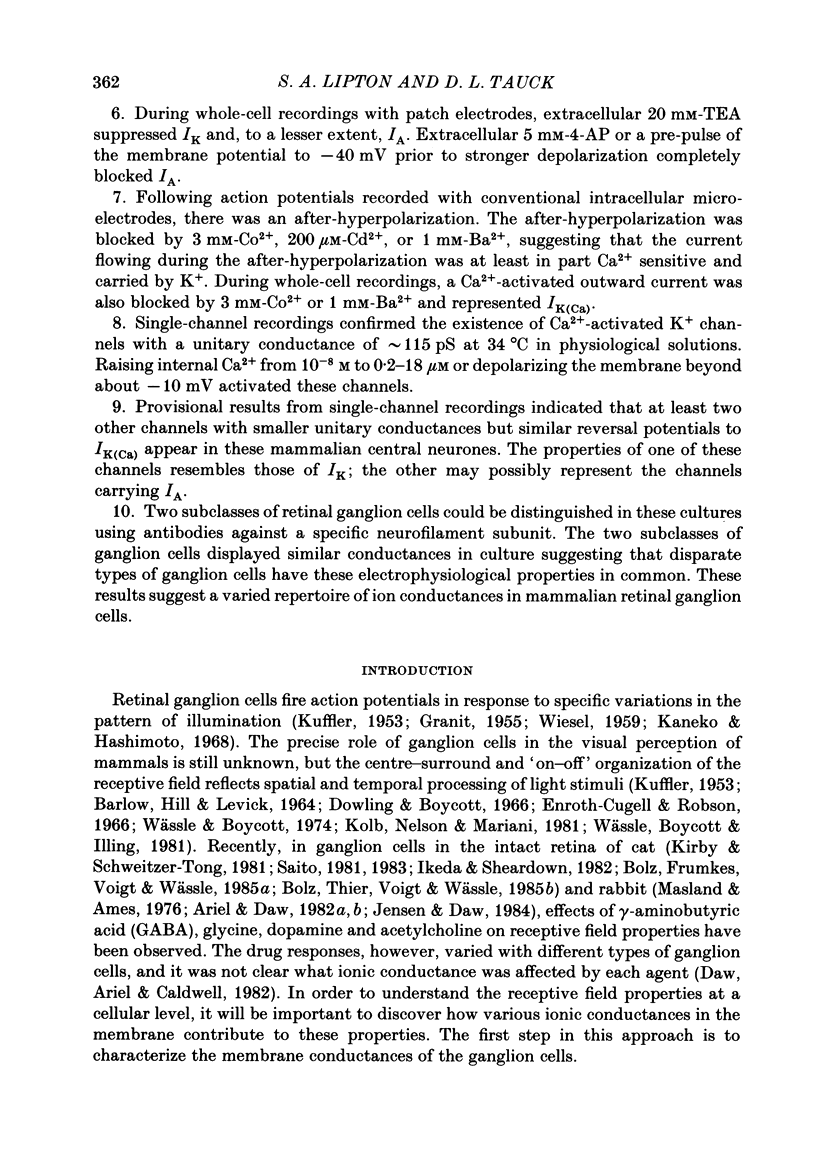
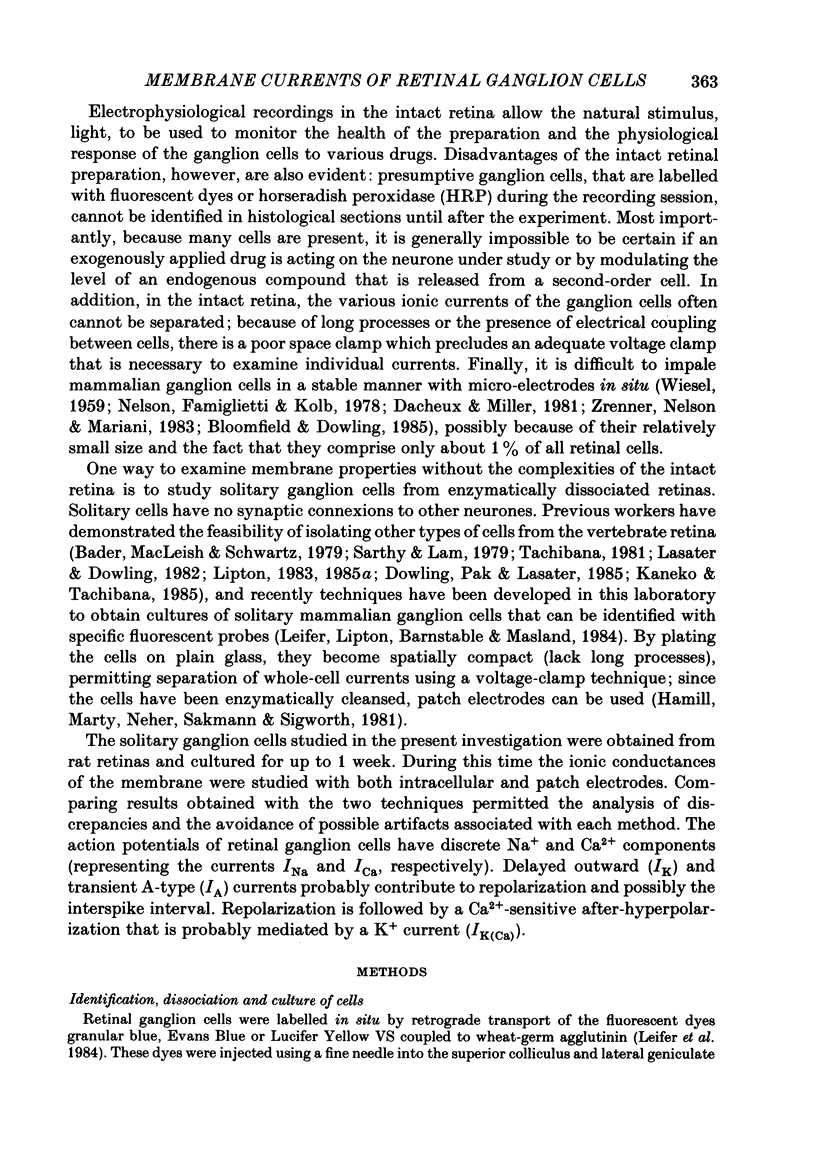
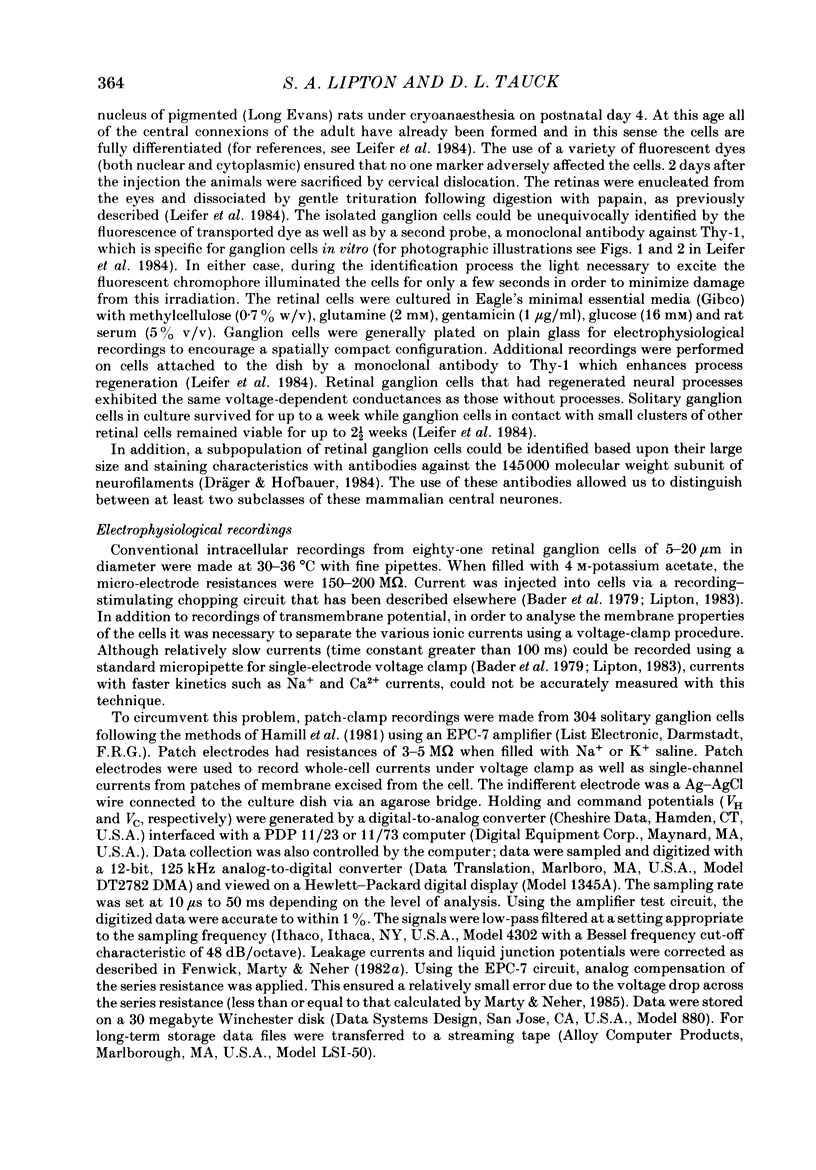
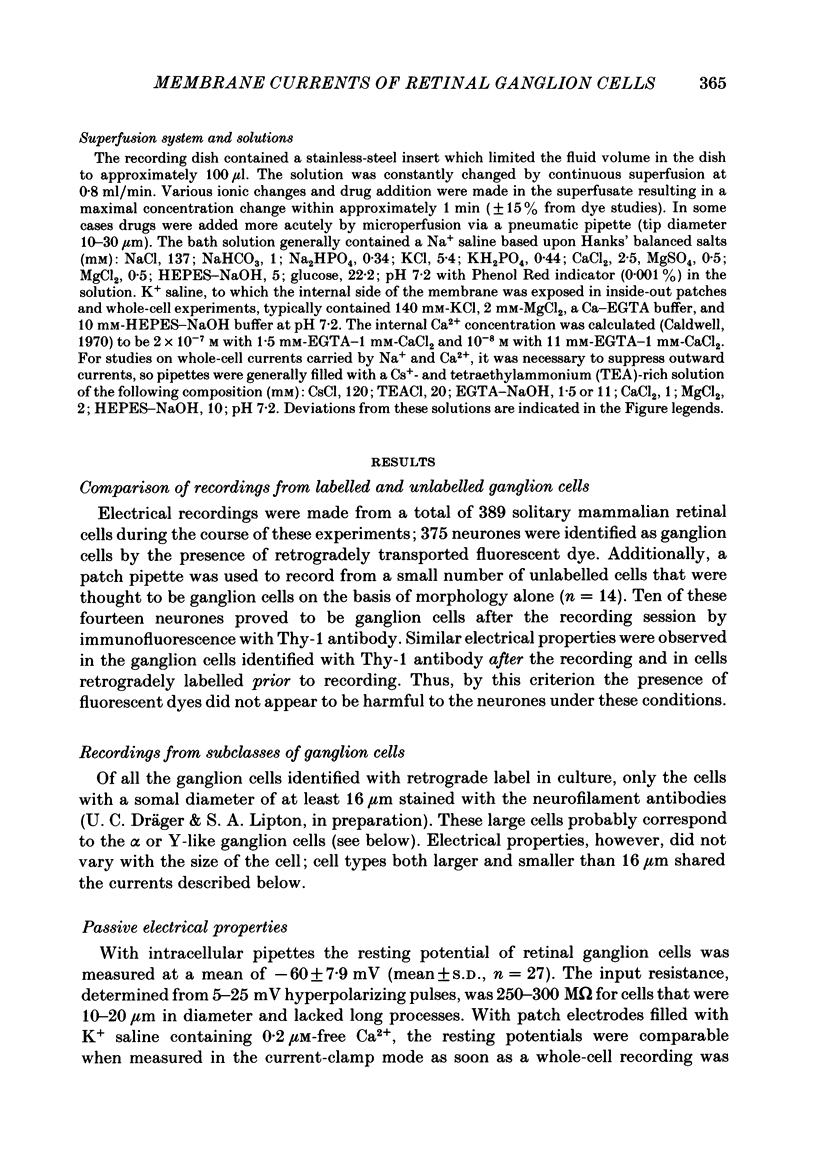
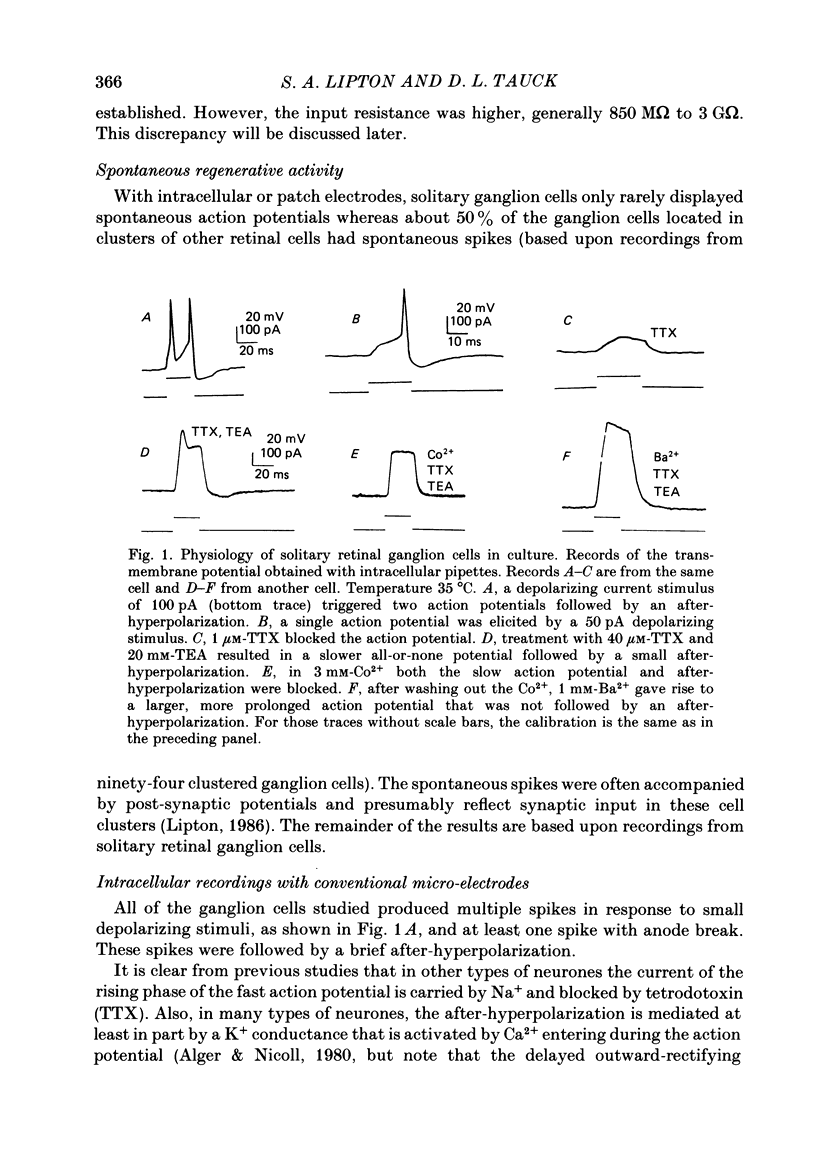
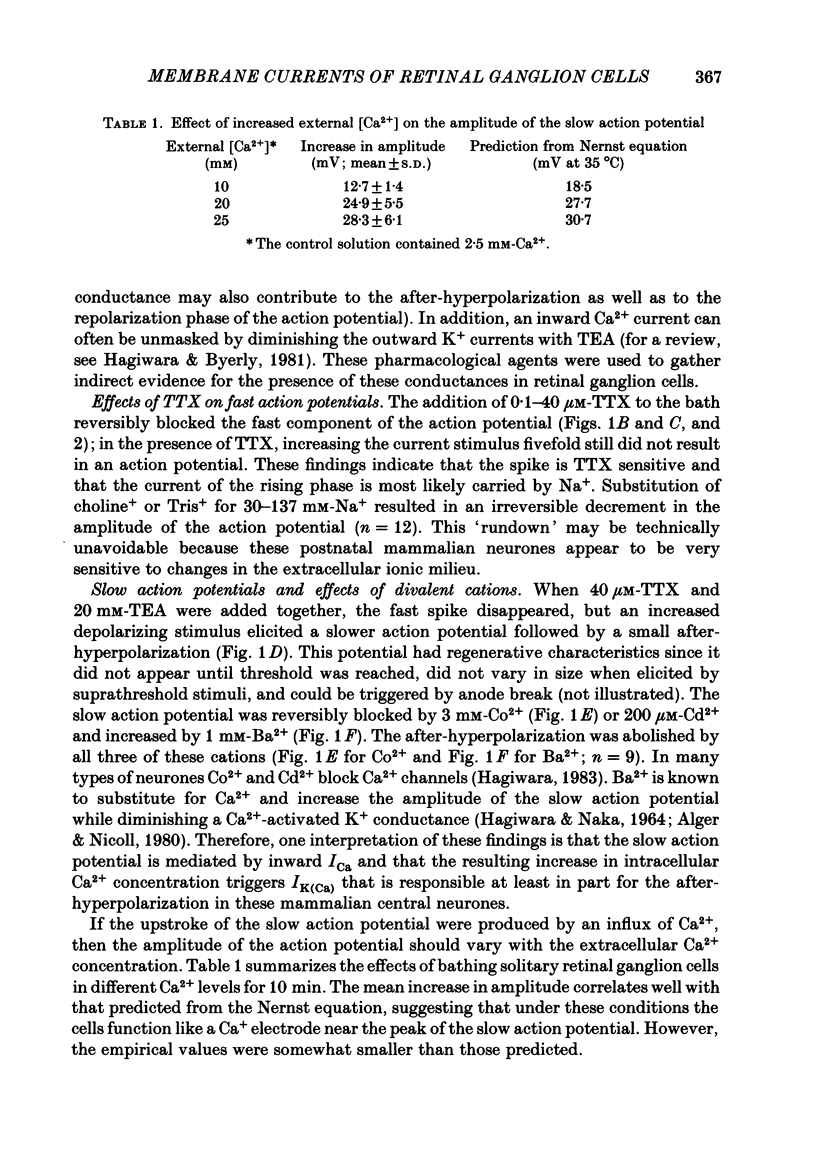
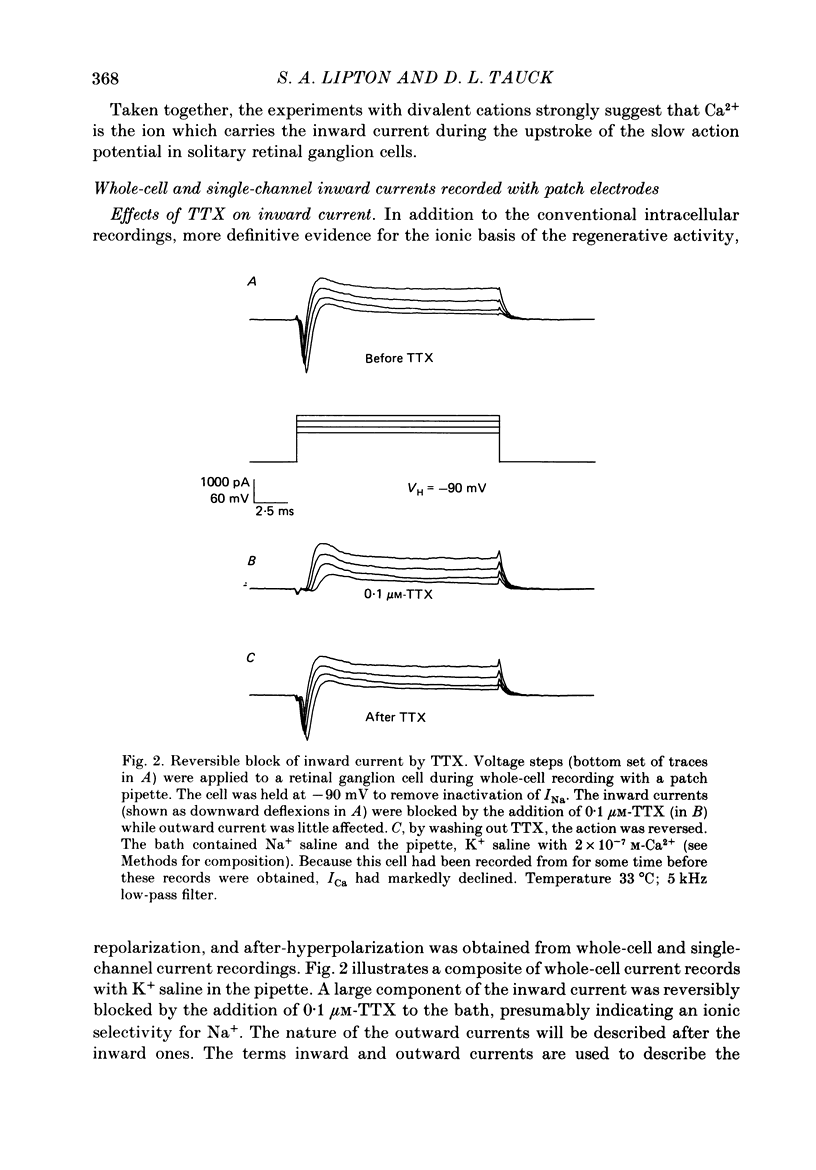
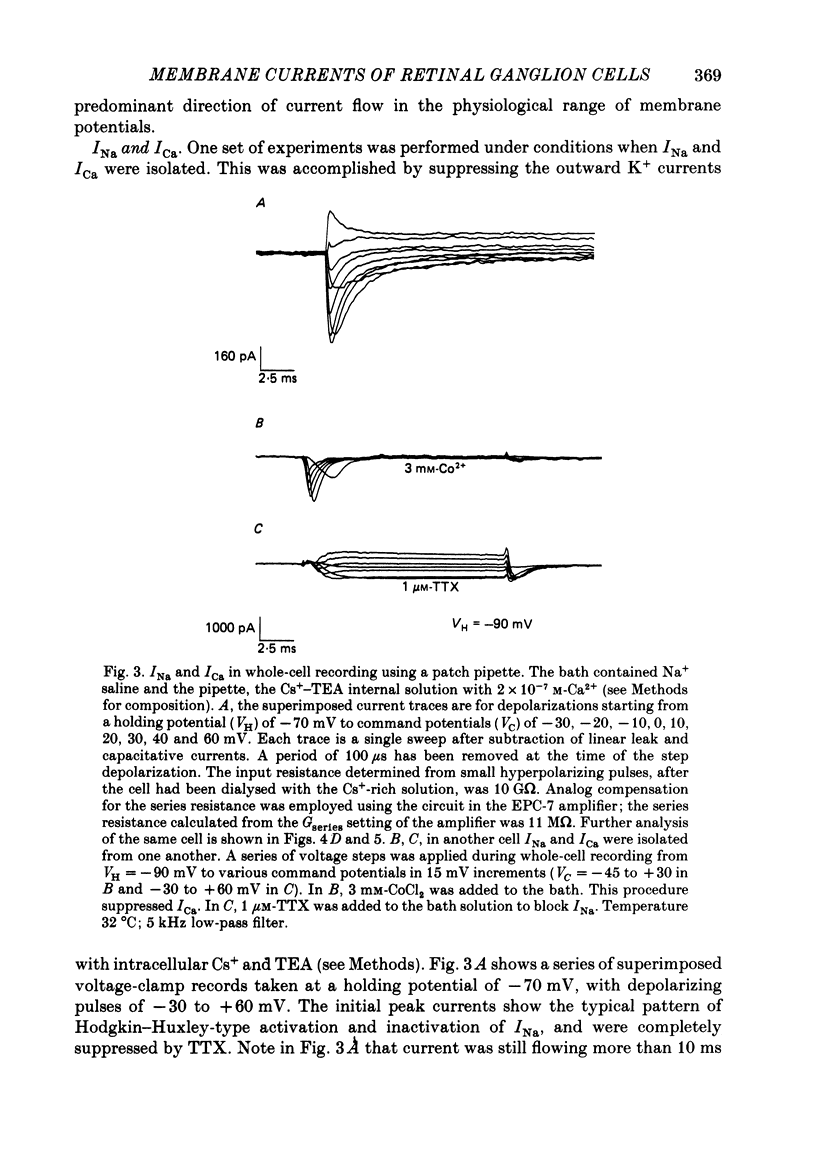
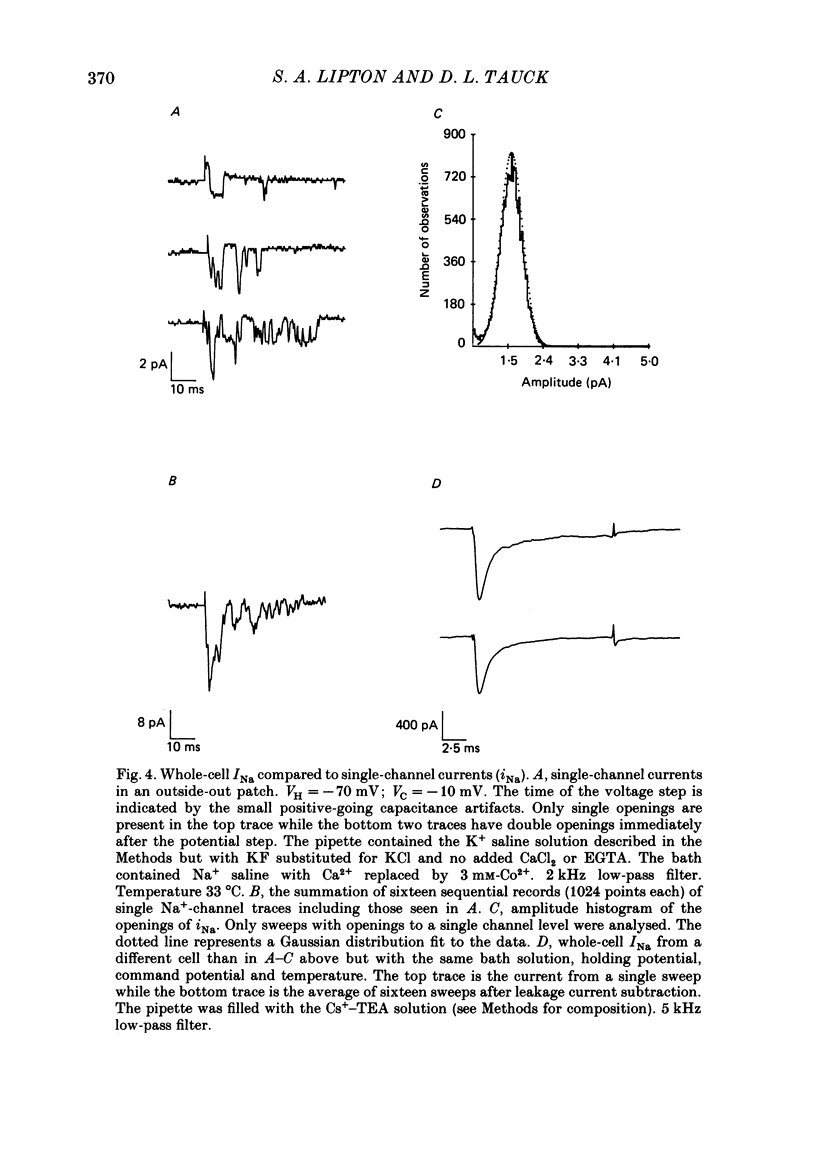
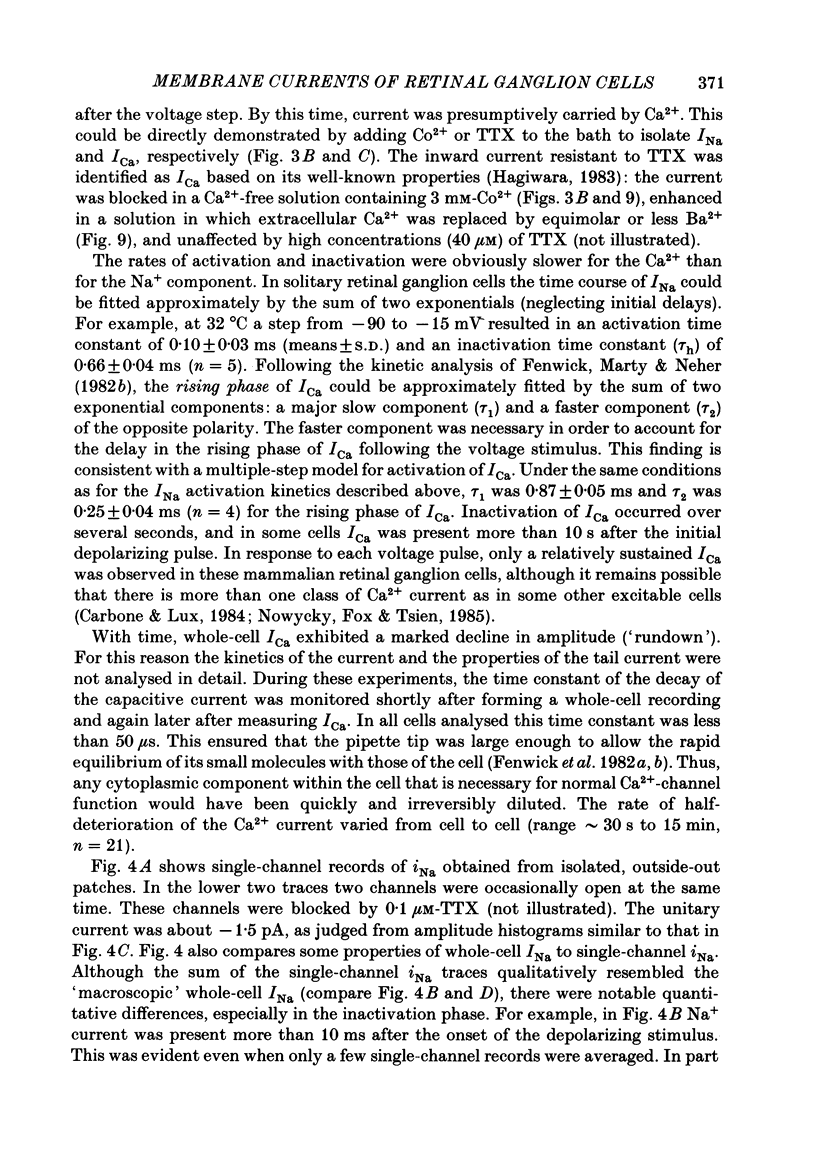
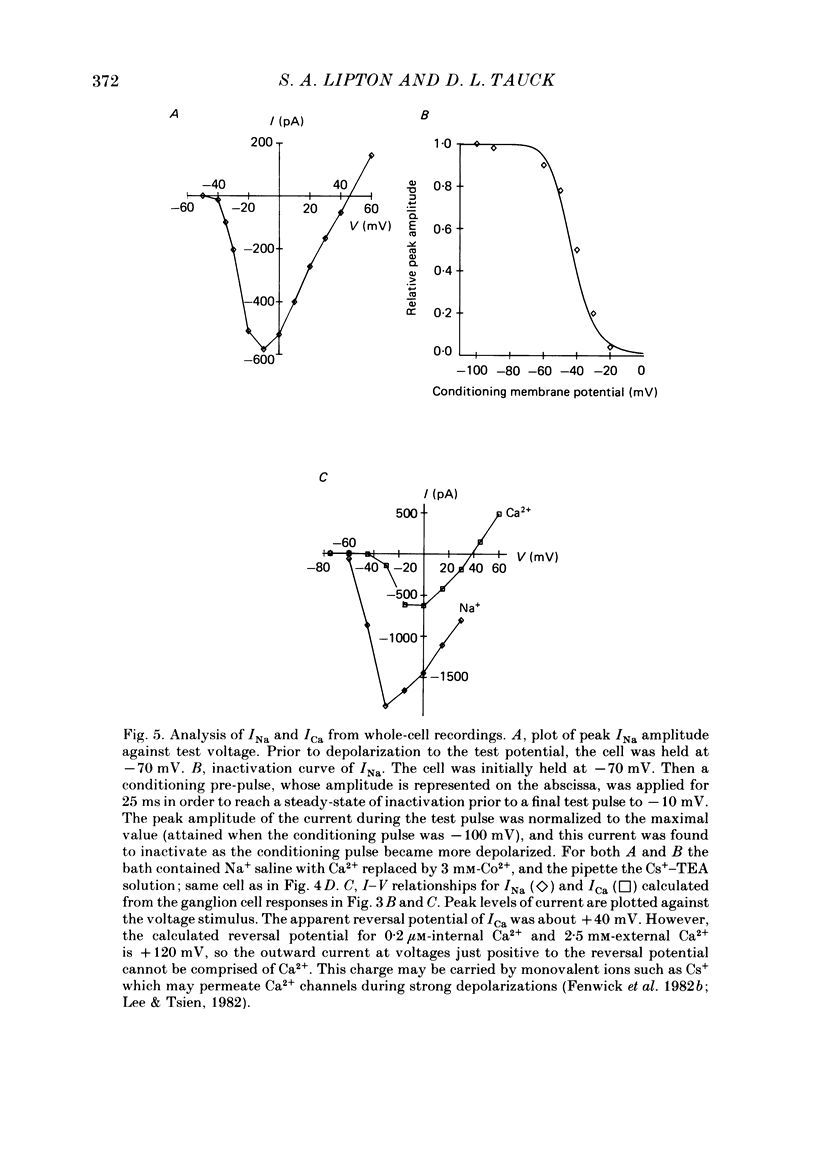
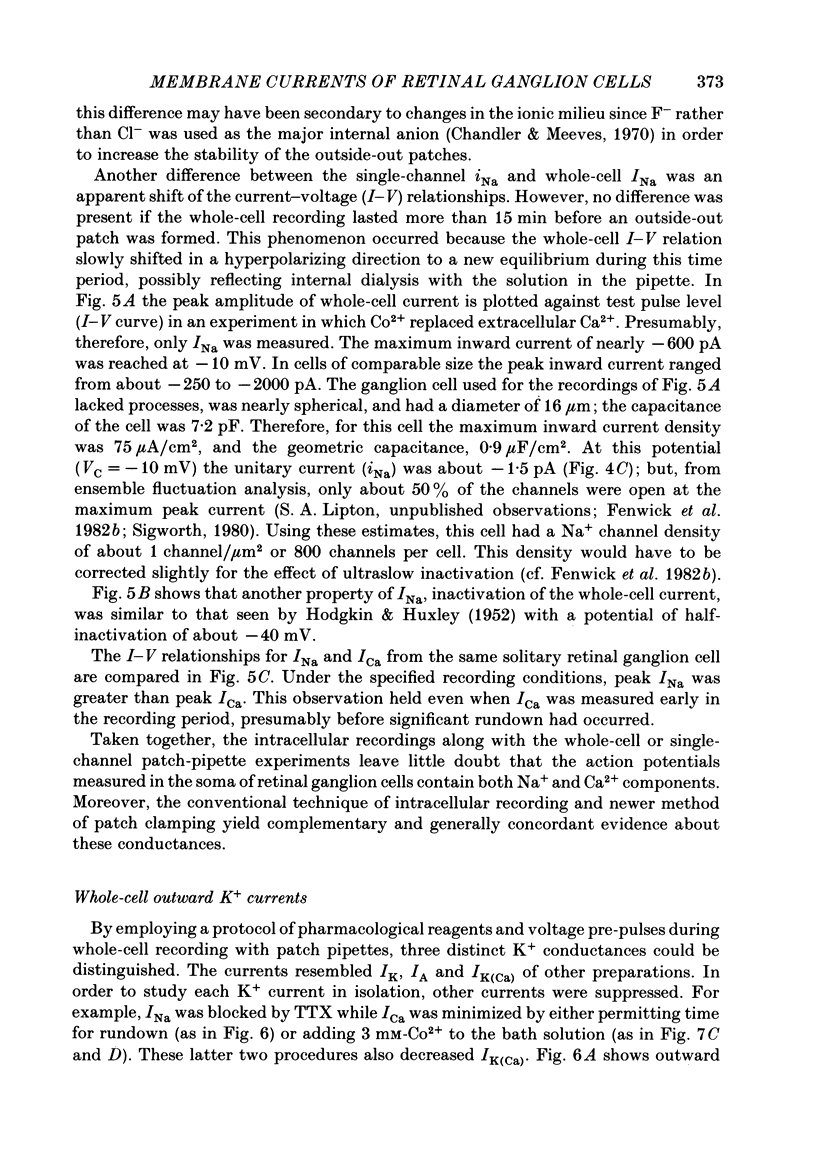
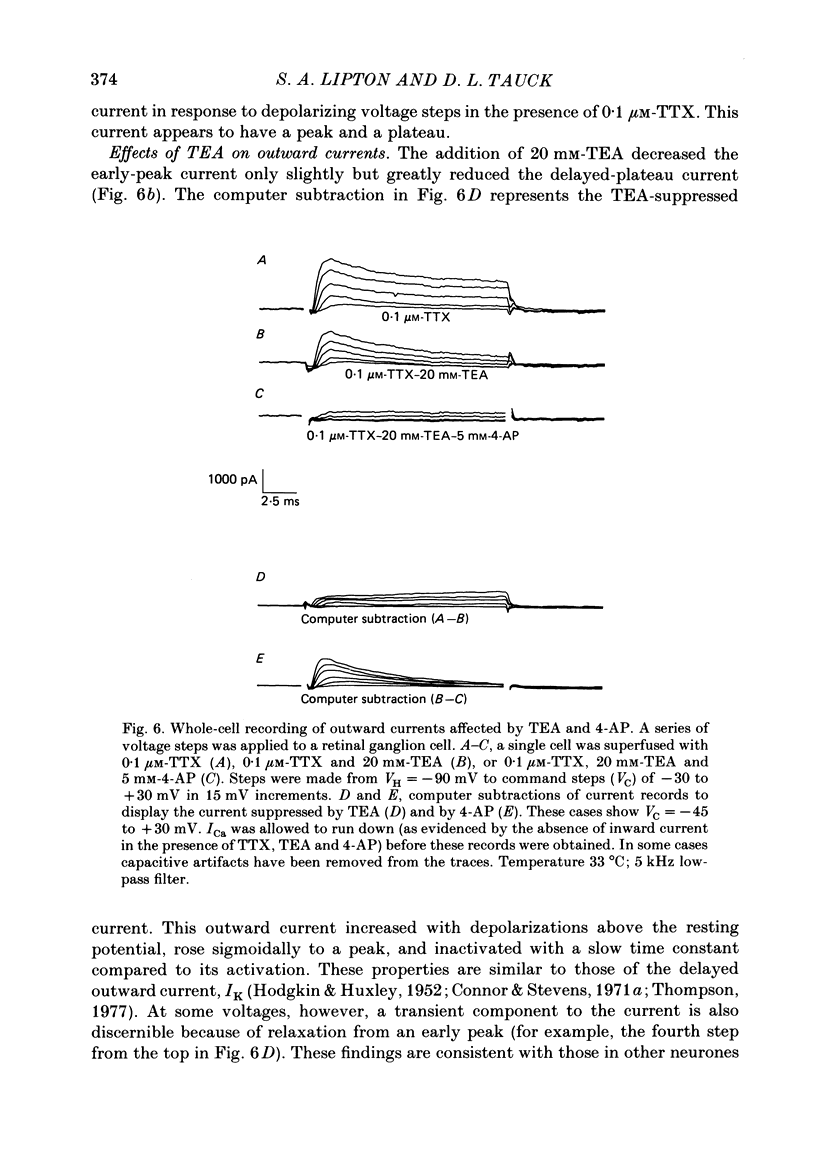
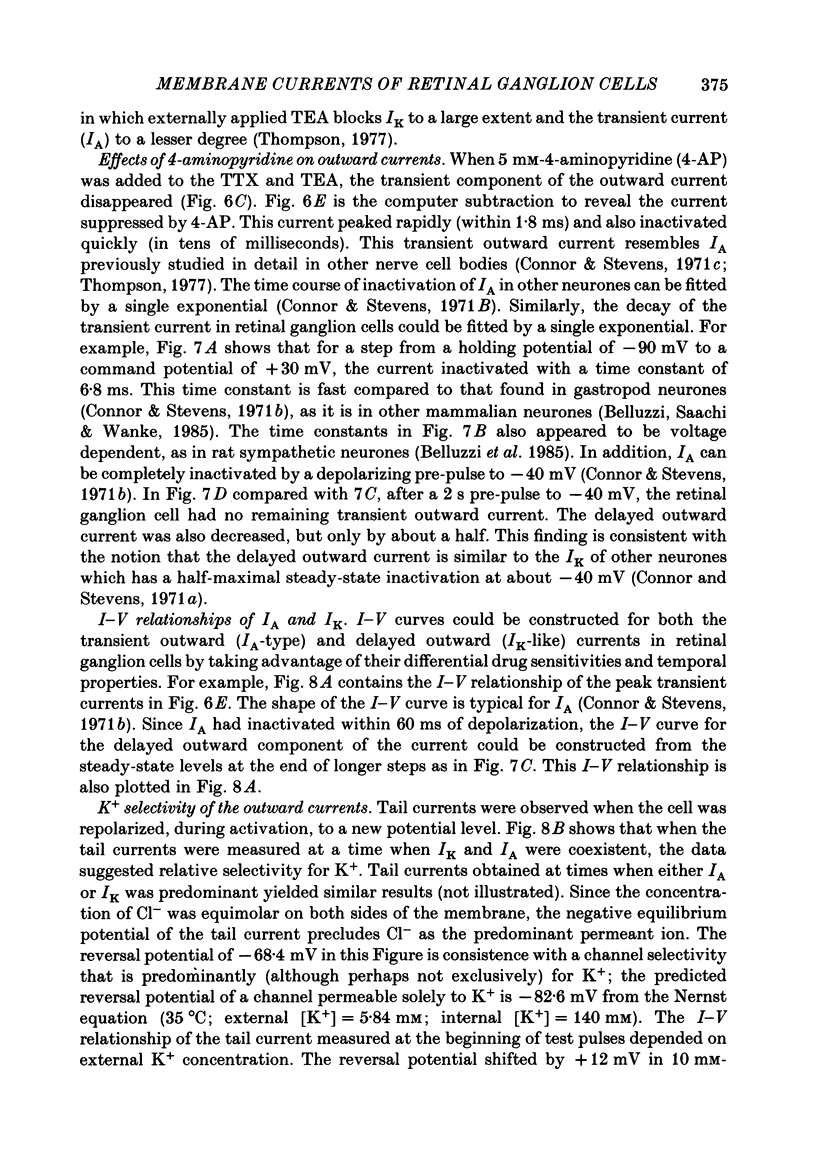
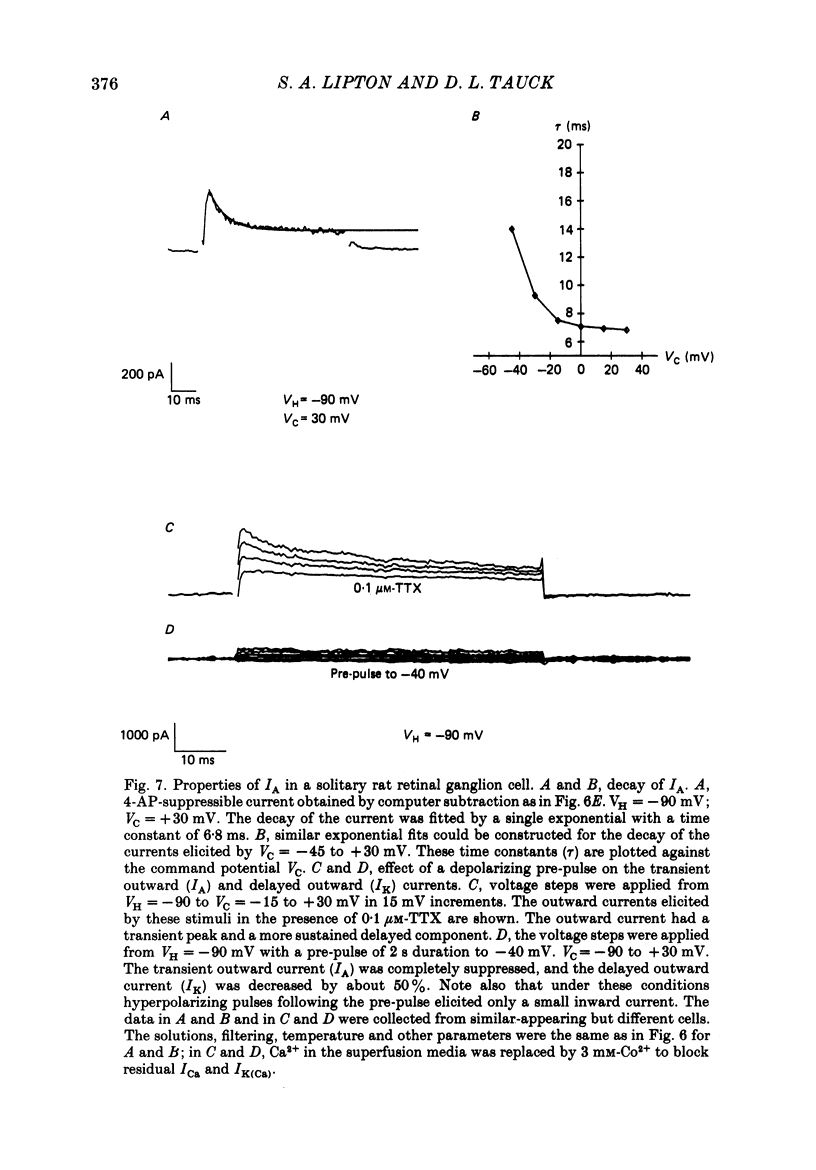
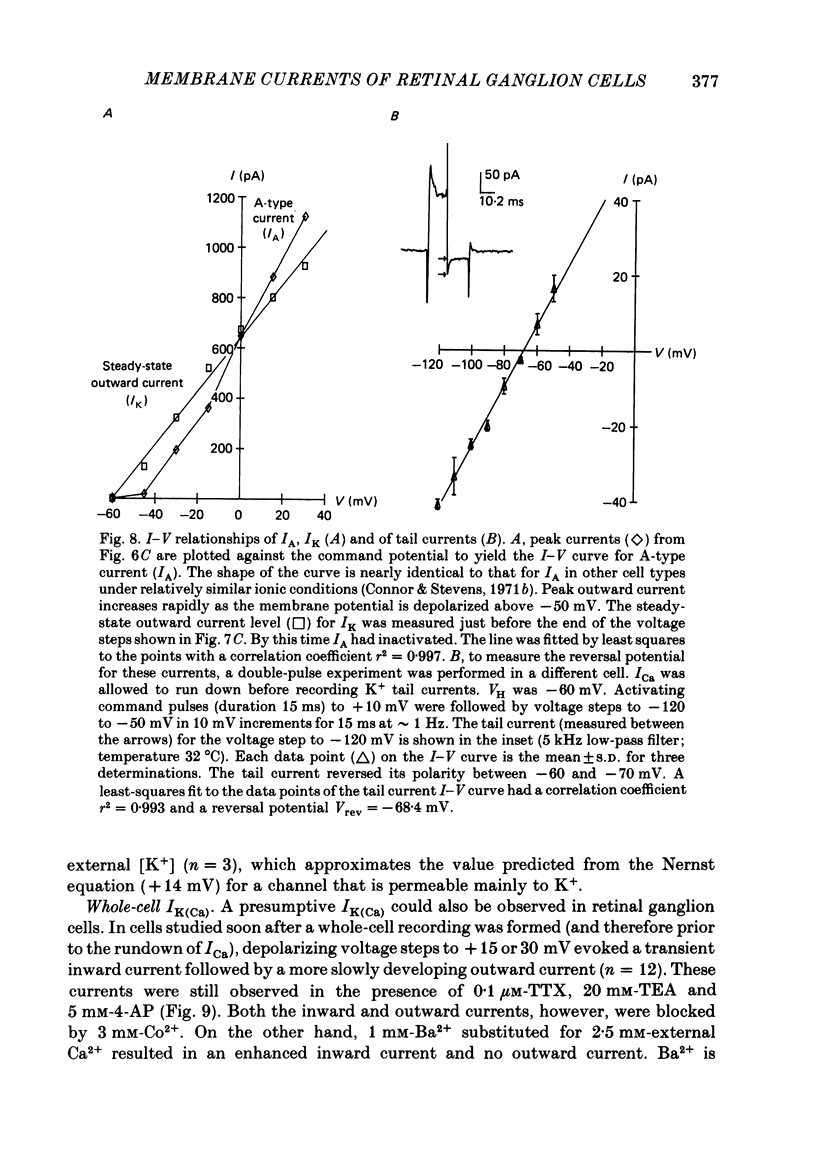
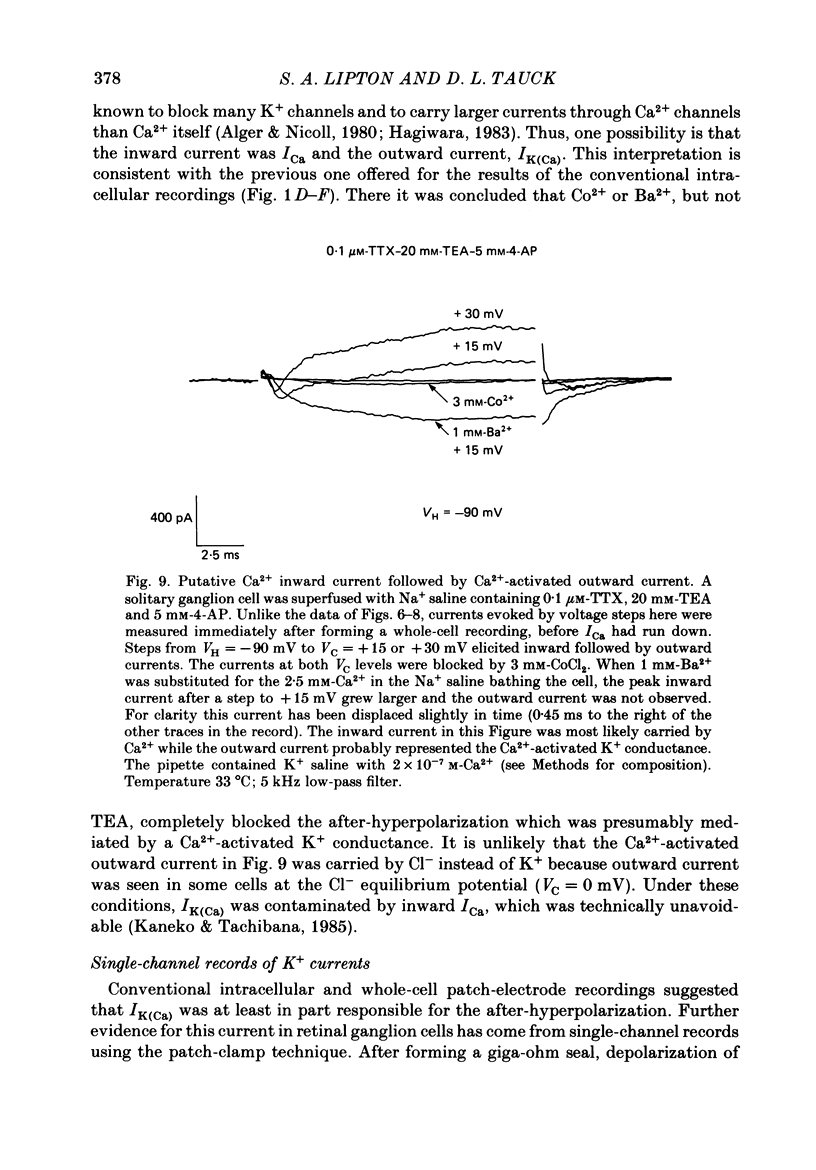
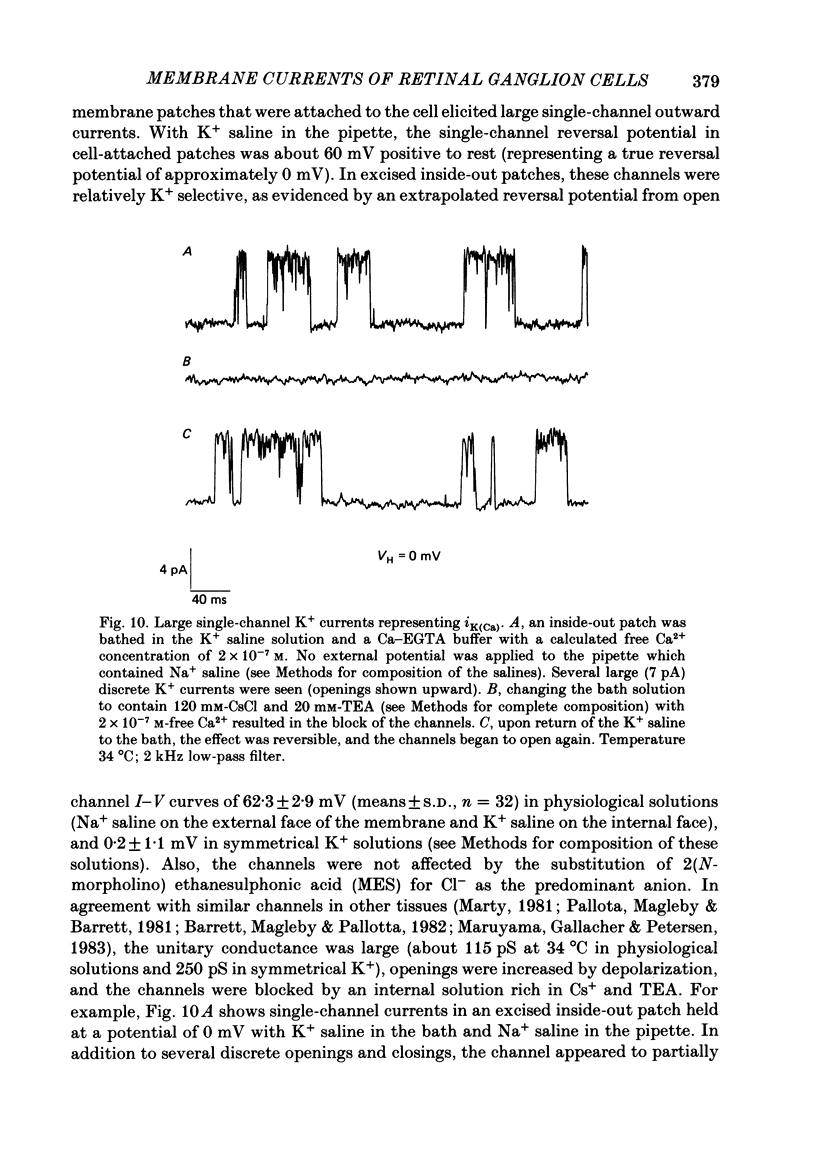
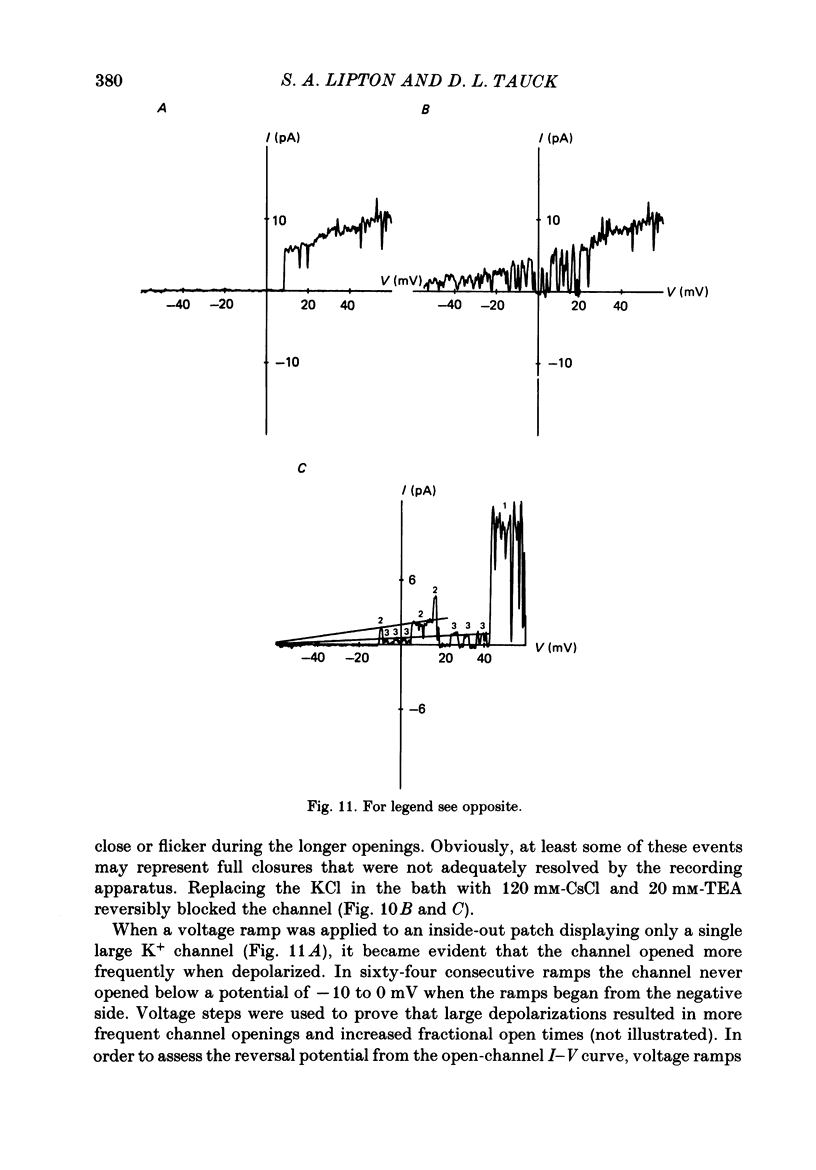
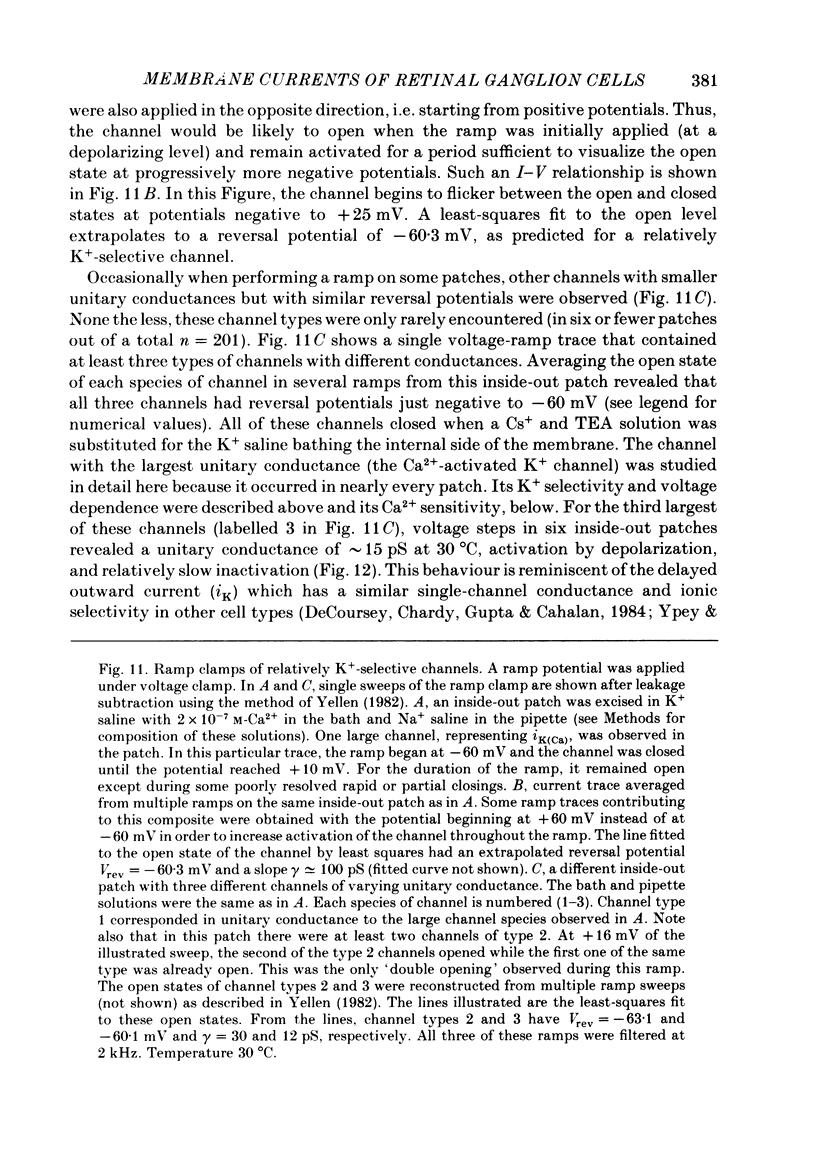
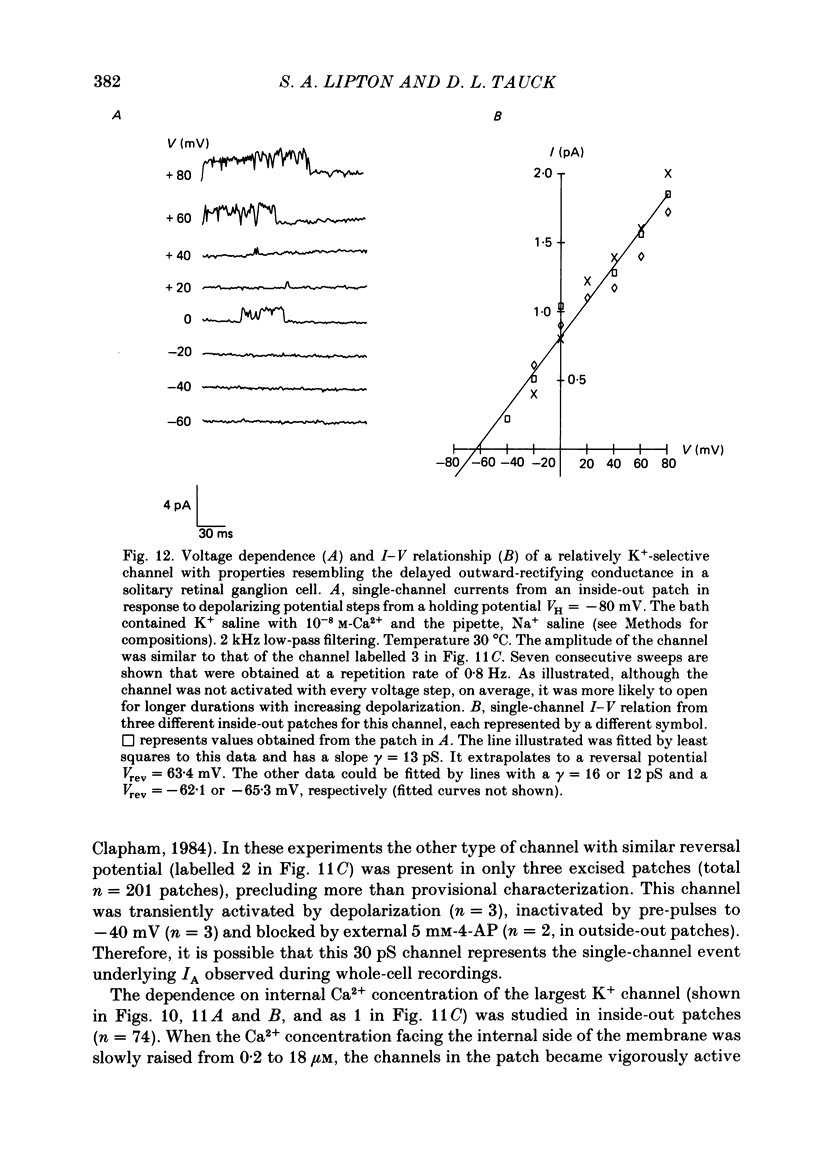
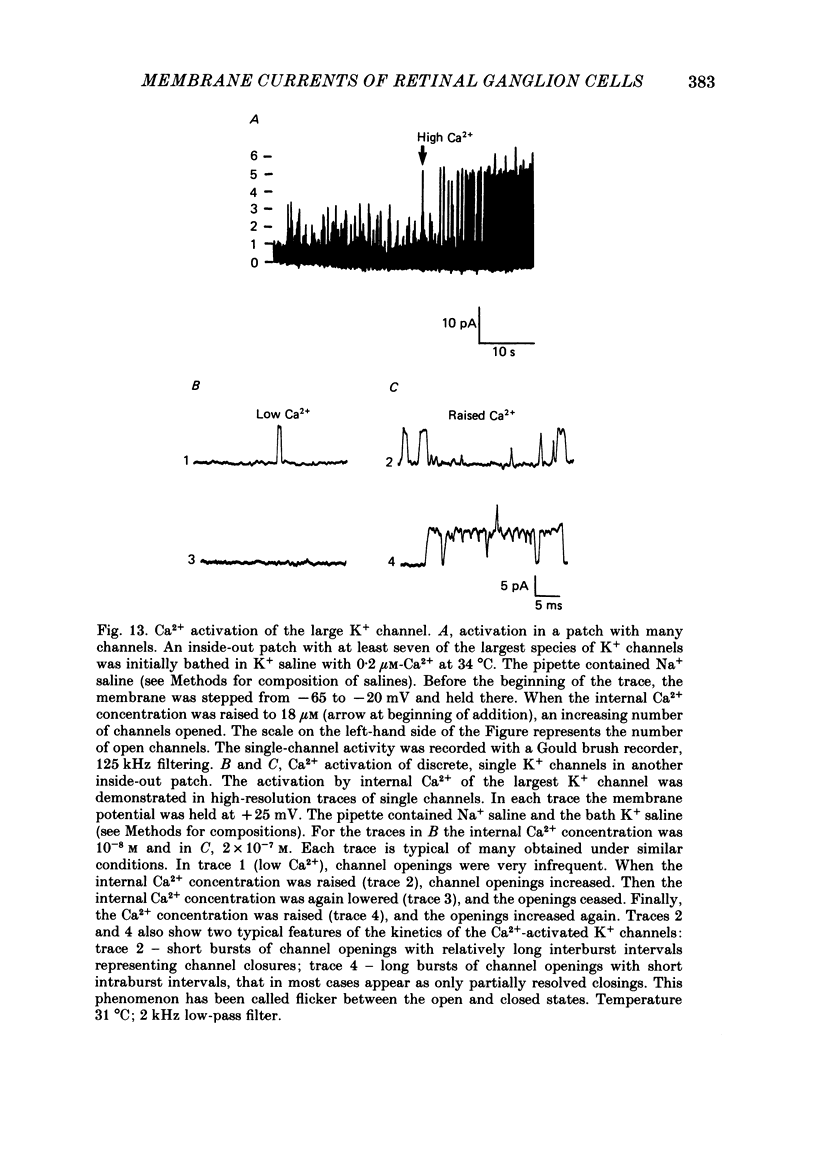
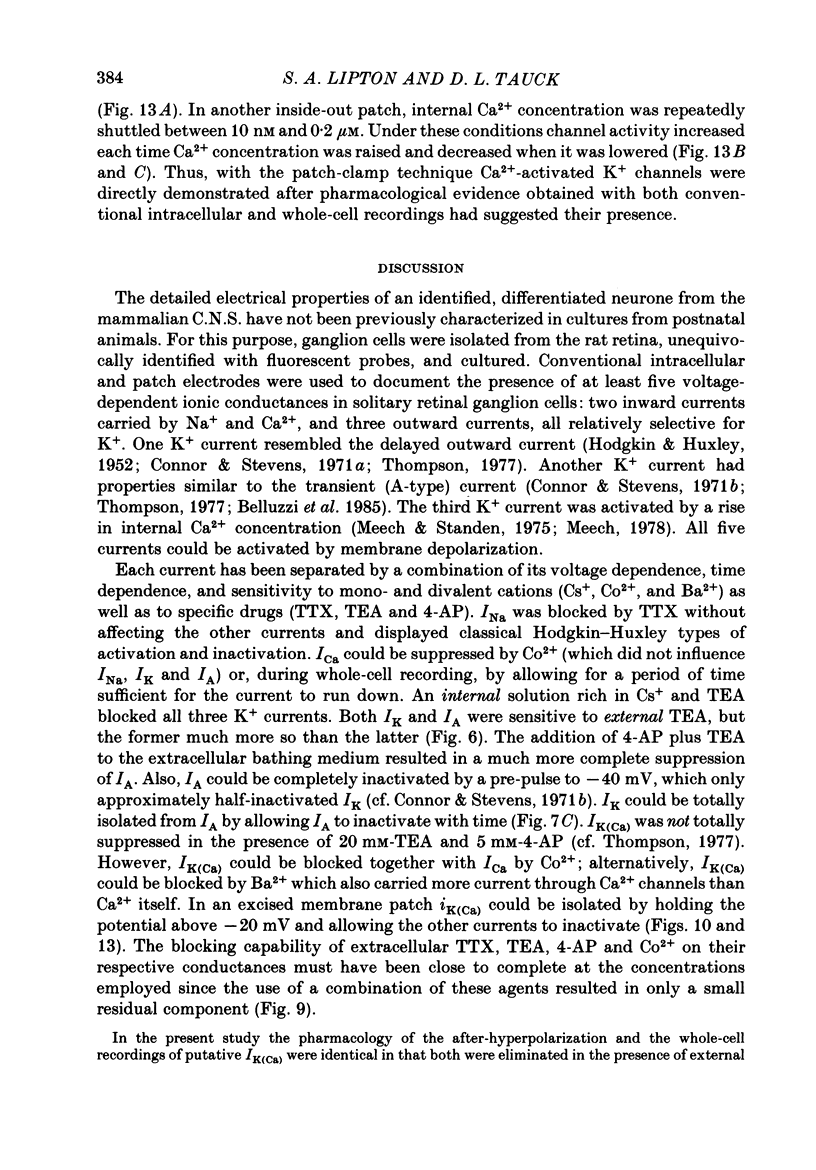
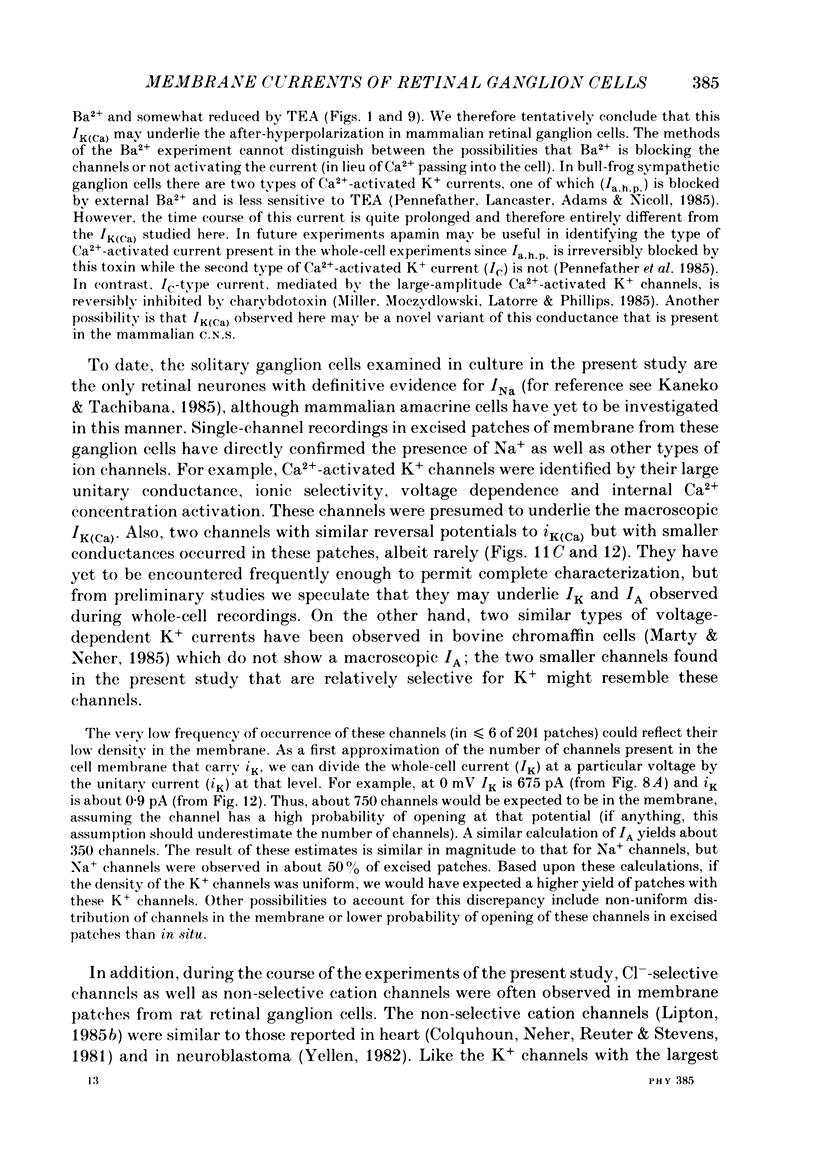
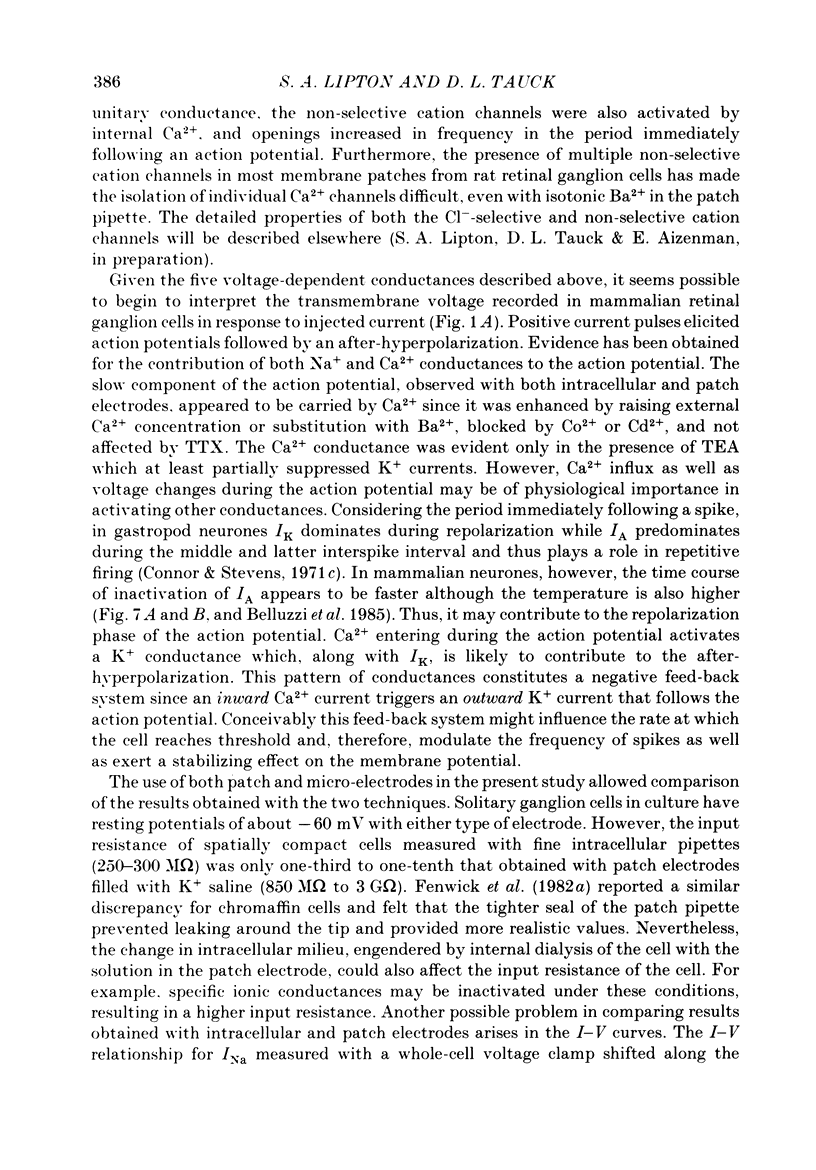
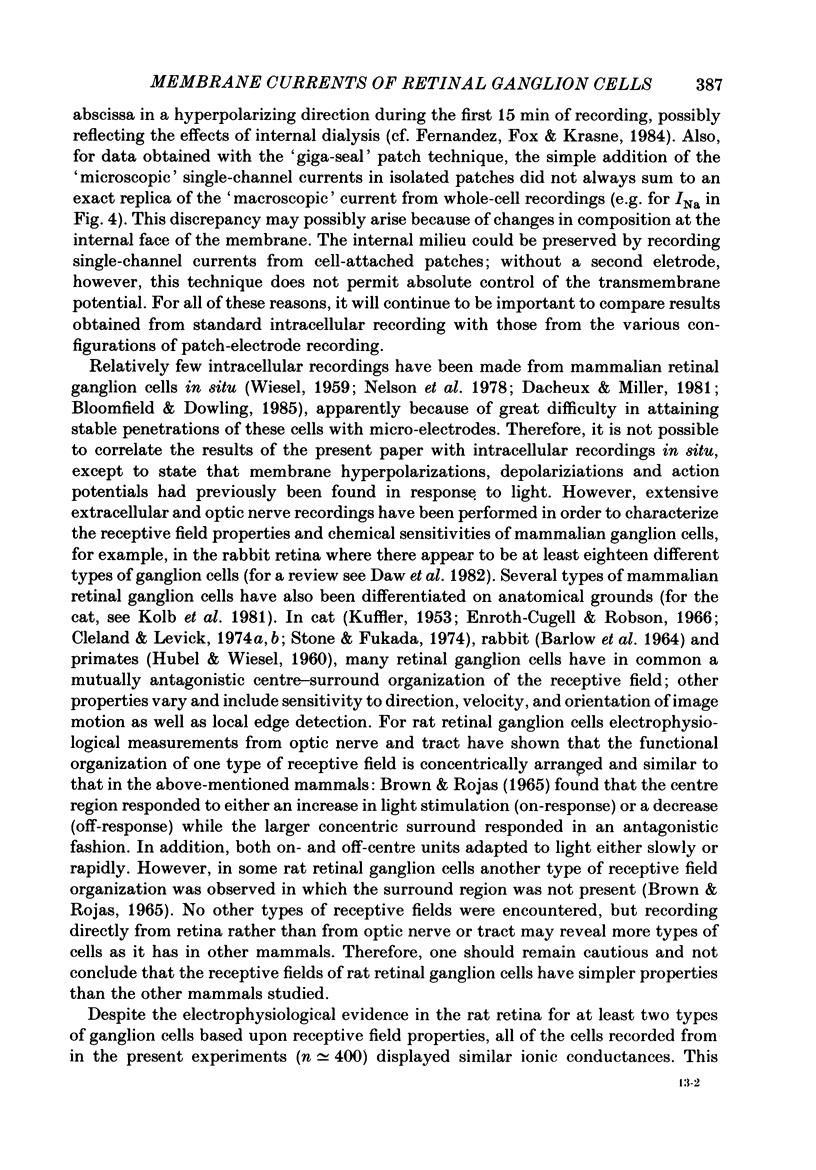
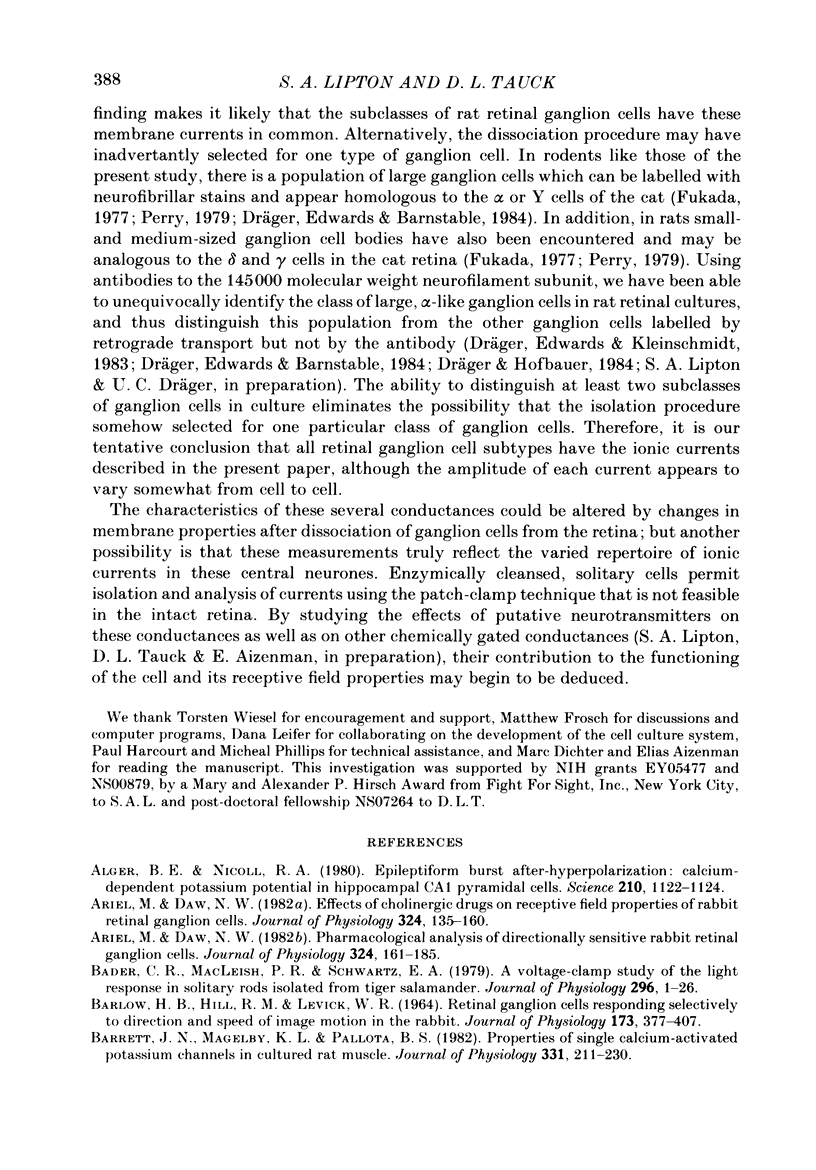
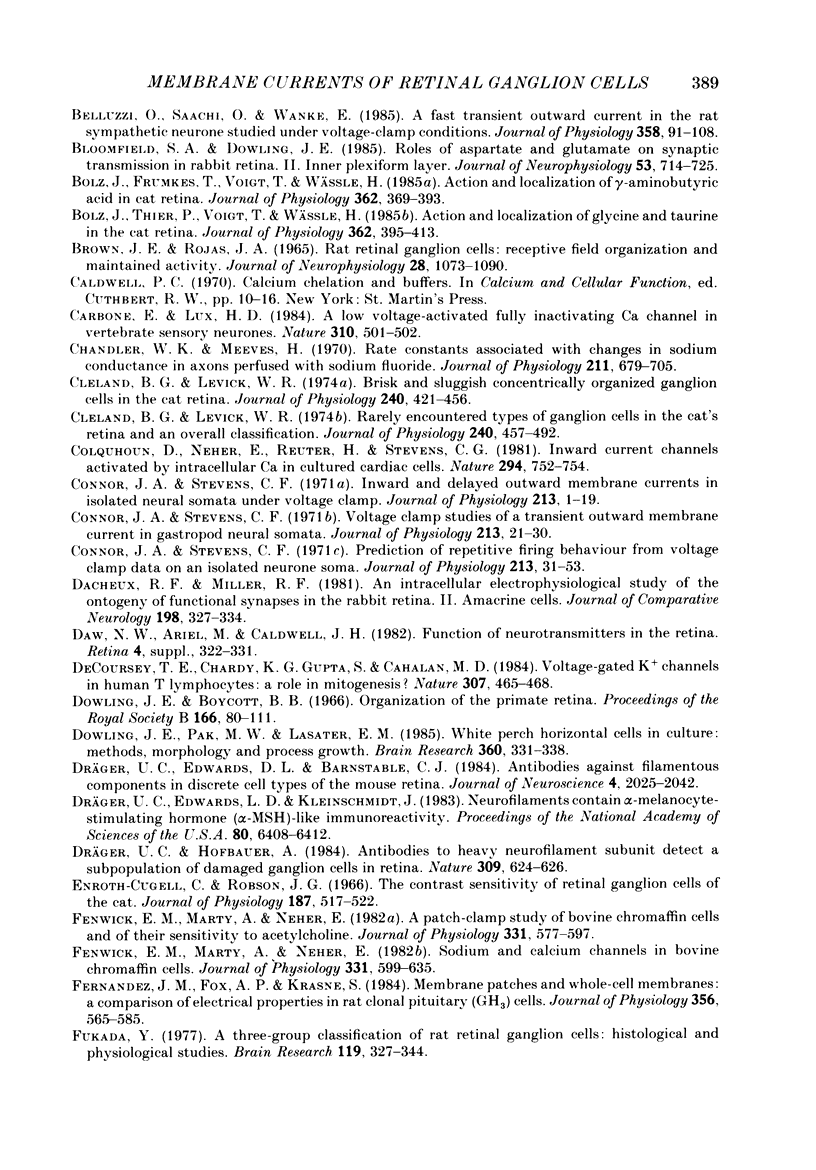
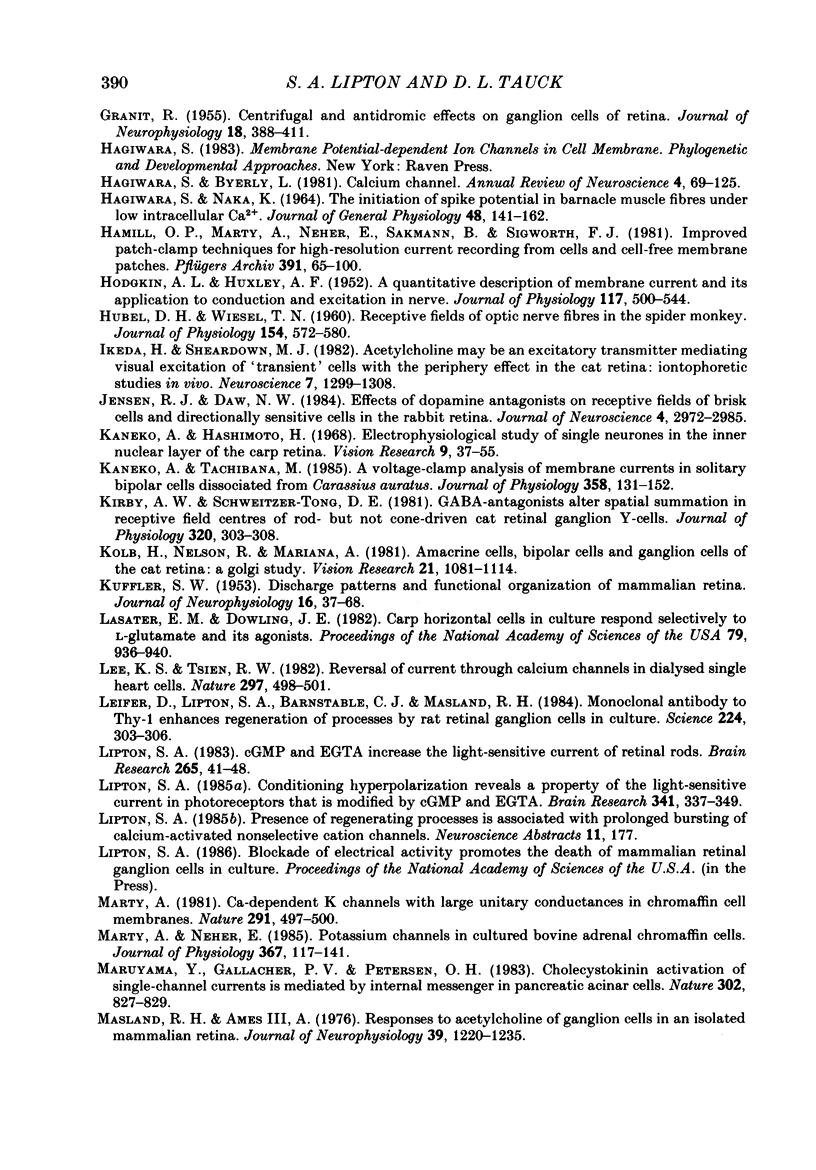
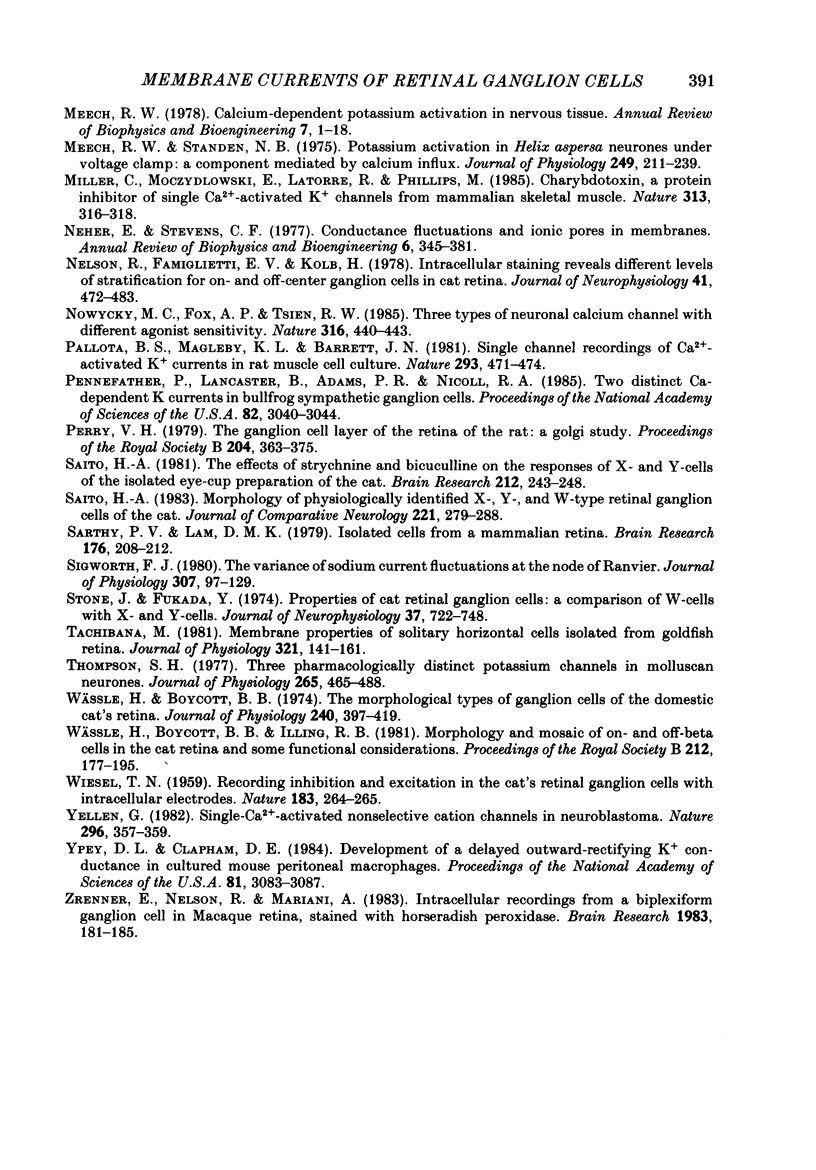
Selected References
These references are in PubMed. This may not be the complete list of references from this article.
- Alger B. E., Nicoll R. A. Epileptiform burst afterhyperolarization: calcium-dependent potassium potential in hippocampal CA1 pyramidal cells. Science. 1980 Dec 5;210(4474):1122–1124. doi: 10.1126/science.7444438. [DOI] [PubMed] [Google Scholar]
- Ariel M., Daw N. W. Effects of cholinergic drugs on receptive field properties of rabbit retinal ganglion cells. J Physiol. 1982 Mar;324:135–160. doi: 10.1113/jphysiol.1982.sp014104. [DOI] [PMC free article] [PubMed] [Google Scholar]
- Ariel M., Daw N. W. Pharmacological analysis of directionally sensitive rabbit retinal ganglion cells. J Physiol. 1982 Mar;324:161–185. doi: 10.1113/jphysiol.1982.sp014105. [DOI] [PMC free article] [PubMed] [Google Scholar]
- BARLOW H. B., HILL R. M., LEVICK W. R. RETINAL GANGLION CELLS RESPONDING SELECTIVELY TO DIRECTION AND SPEED OF IMAGE MOTION IN THE RABBIT. J Physiol. 1964 Oct;173:377–407. doi: 10.1113/jphysiol.1964.sp007463. [DOI] [PMC free article] [PubMed] [Google Scholar]
- Bader C. R., Macleish P. R., Schwartz E. A. A voltage-clamp study of the light response in solitary rods of the tiger salamander. J Physiol. 1979 Nov;296:1–26. doi: 10.1113/jphysiol.1979.sp012988. [DOI] [PMC free article] [PubMed] [Google Scholar]
- Barrett J. N., Magleby K. L., Pallotta B. S. Properties of single calcium-activated potassium channels in cultured rat muscle. J Physiol. 1982 Oct;331:211–230. doi: 10.1113/jphysiol.1982.sp014370. [DOI] [PMC free article] [PubMed] [Google Scholar]
- Belluzzi O., Sacchi O., Wanke E. A fast transient outward current in the rat sympathetic neurone studied under voltage-clamp conditions. J Physiol. 1985 Jan;358:91–108. doi: 10.1113/jphysiol.1985.sp015542. [DOI] [PMC free article] [PubMed] [Google Scholar]
- Bloomfield S. A., Dowling J. E. Roles of aspartate and glutamate in synaptic transmission in rabbit retina. II. Inner plexiform layer. J Neurophysiol. 1985 Mar;53(3):714–725. doi: 10.1152/jn.1985.53.3.714. [DOI] [PubMed] [Google Scholar]
- Bolz J., Frumkes T., Voigt T., Wässle H. Action and localization of gamma-aminobutyric acid in the cat retina. J Physiol. 1985 May;362:369–393. doi: 10.1113/jphysiol.1985.sp015684. [DOI] [PMC free article] [PubMed] [Google Scholar]
- Bolz J., Thier P., Voigt T., Wässle H. Action and localization of glycine and taurine in the cat retina. J Physiol. 1985 May;362:395–413. doi: 10.1113/jphysiol.1985.sp015685. [DOI] [PMC free article] [PubMed] [Google Scholar]
- Boycott B. B., Wässle H. The morphological types of ganglion cells of the domestic cat's retina. J Physiol. 1974 Jul;240(2):397–419. doi: 10.1113/jphysiol.1974.sp010616. [DOI] [PMC free article] [PubMed] [Google Scholar]
- Brown J. E., Rojas J. A. Rat retinal ganglion cells: receptive field organization and maintained activity. J Neurophysiol. 1965 Nov;28(6):1073–1090. doi: 10.1152/jn.1965.28.6.1073. [DOI] [PubMed] [Google Scholar]
- Carbone E., Lux H. D. A low voltage-activated, fully inactivating Ca channel in vertebrate sensory neurones. Nature. 1984 Aug 9;310(5977):501–502. doi: 10.1038/310501a0. [DOI] [PubMed] [Google Scholar]
- Chandler W. K., Meves H. Rate constants associated with changes in sodium conductance in axons perfused with sodium fluoride. J Physiol. 1970 Dec;211(3):679–705. doi: 10.1113/jphysiol.1970.sp009299. [DOI] [PMC free article] [PubMed] [Google Scholar]
- Cleland B. G., Levick W. R. Brisk and sluggish concentrically organized ganglion cells in the cat's retina. J Physiol. 1974 Jul;240(2):421–456. doi: 10.1113/jphysiol.1974.sp010617. [DOI] [PMC free article] [PubMed] [Google Scholar]
- Cleland B. G., Levick W. R. Properties of rarely encountered types of ganglion cells in the cat's retina and an overall classification. J Physiol. 1974 Jul;240(2):457–492. doi: 10.1113/jphysiol.1974.sp010618. [DOI] [PMC free article] [PubMed] [Google Scholar]
- Colquhoun D., Neher E., Reuter H., Stevens C. F. Inward current channels activated by intracellular Ca in cultured cardiac cells. Nature. 1981 Dec 24;294(5843):752–754. doi: 10.1038/294752a0. [DOI] [PubMed] [Google Scholar]
- Connor J. A., Stevens C. F. Inward and delayed outward membrane currents in isolated neural somata under voltage clamp. J Physiol. 1971 Feb;213(1):1–19. doi: 10.1113/jphysiol.1971.sp009364. [DOI] [PMC free article] [PubMed] [Google Scholar]
- Connor J. A., Stevens C. F. Prediction of repetitive firing behaviour from voltage clamp data on an isolated neurone soma. J Physiol. 1971 Feb;213(1):31–53. doi: 10.1113/jphysiol.1971.sp009366. [DOI] [PMC free article] [PubMed] [Google Scholar]
- Connor J. A., Stevens C. F. Voltage clamp studies of a transient outward membrane current in gastropod neural somata. J Physiol. 1971 Feb;213(1):21–30. doi: 10.1113/jphysiol.1971.sp009365. [DOI] [PMC free article] [PubMed] [Google Scholar]
- Dacheux R. F., Miller R. F. An intracellular electrophysiological study of the ontogeny of functional synapses in the rabbit retina. II. Amacrine cells. J Comp Neurol. 1981 May 10;198(2):327–334. doi: 10.1002/cne.901980210. [DOI] [PubMed] [Google Scholar]
- Daw N. W., Ariel M., Caldwell J. H. Function of neurotransmitters in the retina. Retina. 1982;2(4):322–331. [PubMed] [Google Scholar]
- DeCoursey T. E., Chandy K. G., Gupta S., Cahalan M. D. Voltage-gated K+ channels in human T lymphocytes: a role in mitogenesis? Nature. 1984 Feb 2;307(5950):465–468. doi: 10.1038/307465a0. [DOI] [PubMed] [Google Scholar]
- Dowling J. E., Boycott B. B. Organization of the primate retina: electron microscopy. Proc R Soc Lond B Biol Sci. 1966 Nov 15;166(1002):80–111. doi: 10.1098/rspb.1966.0086. [DOI] [PubMed] [Google Scholar]
- Dowling J. E., Pak M. W., Lasater E. M. White perch horizontal cells in culture: methods, morphology and process growth. Brain Res. 1985 Dec 23;360(1-2):331–338. doi: 10.1016/0006-8993(85)91250-8. [DOI] [PubMed] [Google Scholar]
- Dräger U. C., Edwards D. L., Barnstable C. J. Antibodies against filamentous components in discrete cell types of the mouse retina. J Neurosci. 1984 Aug;4(8):2025–2042. doi: 10.1523/JNEUROSCI.04-08-02025.1984. [DOI] [PMC free article] [PubMed] [Google Scholar]
- Dräger U. C., Edwards D. L., Kleinschmidt J. Neurofilaments contain alpha-melanocyte-stimulating hormone (alpha-MSH)-like immunoreactivity. Proc Natl Acad Sci U S A. 1983 Oct;80(20):6408–6412. doi: 10.1073/pnas.80.20.6408. [DOI] [PMC free article] [PubMed] [Google Scholar]
- Dräger U. C., Hofbauer A. Antibodies to heavy neurofilament subunit detect a subpopulation of damaged ganglion cells in retina. Nature. 1984 Jun 14;309(5969):624–626. doi: 10.1038/309624a0. [DOI] [PubMed] [Google Scholar]
- Enroth-Cugell C., Robson J. G. The contrast sensitivity of retinal ganglion cells of the cat. J Physiol. 1966 Dec;187(3):517–552. doi: 10.1113/jphysiol.1966.sp008107. [DOI] [PMC free article] [PubMed] [Google Scholar]
- Fenwick E. M., Marty A., Neher E. A patch-clamp study of bovine chromaffin cells and of their sensitivity to acetylcholine. J Physiol. 1982 Oct;331:577–597. doi: 10.1113/jphysiol.1982.sp014393. [DOI] [PMC free article] [PubMed] [Google Scholar]
- Fenwick E. M., Marty A., Neher E. Sodium and calcium channels in bovine chromaffin cells. J Physiol. 1982 Oct;331:599–635. doi: 10.1113/jphysiol.1982.sp014394. [DOI] [PMC free article] [PubMed] [Google Scholar]
- GRANIT R. Centrifugal and antidromic effects on ganglion cells of retina. J Neurophysiol. 1955 Jul;18(4):388–411. doi: 10.1152/jn.1955.18.4.388. [DOI] [PubMed] [Google Scholar]
- HAGIWARA S., NAKA K. I. THE INITIATION OF SPIKE POTENTIAL IN BARNACLE MUSCLE FIBERS UNDER LOW INTRACELLULAR CA++. J Gen Physiol. 1964 Sep;48:141–162. doi: 10.1085/jgp.48.1.141. [DOI] [PMC free article] [PubMed] [Google Scholar]
- HODGKIN A. L., HUXLEY A. F. A quantitative description of membrane current and its application to conduction and excitation in nerve. J Physiol. 1952 Aug;117(4):500–544. doi: 10.1113/jphysiol.1952.sp004764. [DOI] [PMC free article] [PubMed] [Google Scholar]
- HUBEL D. H., WIESEL T. N. Receptive fields of optic nerve fibres in the spider monkey. J Physiol. 1960 Dec;154:572–580. doi: 10.1113/jphysiol.1960.sp006596. [DOI] [PMC free article] [PubMed] [Google Scholar]
- Hagiwara S., Byerly L. Calcium channel. Annu Rev Neurosci. 1981;4:69–125. doi: 10.1146/annurev.ne.04.030181.000441. [DOI] [PubMed] [Google Scholar]
- Hamill O. P., Marty A., Neher E., Sakmann B., Sigworth F. J. Improved patch-clamp techniques for high-resolution current recording from cells and cell-free membrane patches. Pflugers Arch. 1981 Aug;391(2):85–100. doi: 10.1007/BF00656997. [DOI] [PubMed] [Google Scholar]
- Ikeda H., Sheardown M. J. Acetylcholine may be an excitatory transmitter mediating visual excitation of 'transient' cells with the periphery effect in the cat retina: iontophoretic studies in vivo. Neuroscience. 1982 May;7(5):1299–1308. doi: 10.1016/0306-4522(82)91135-6. [DOI] [PubMed] [Google Scholar]
- Jensen R. J., Daw N. W. Effects of dopamine antagonists on receptive fields of brisk cells and directionally selective cells in the rabbit retina. J Neurosci. 1984 Dec;4(12):2972–2985. doi: 10.1523/JNEUROSCI.04-12-02972.1984. [DOI] [PMC free article] [PubMed] [Google Scholar]
- Kaneko A., Hashimoto H. Electrophysiological study of single neurons in the inner nuclear layer of the carp retina. Vision Res. 1969 Jan;9(1):37–55. doi: 10.1016/0042-6989(69)90030-3. [DOI] [PubMed] [Google Scholar]
- Kaneko A., Tachibana M. A voltage-clamp analysis of membrane currents in solitary bipolar cells dissociated from Carassius auratus. J Physiol. 1985 Jan;358:131–152. doi: 10.1113/jphysiol.1985.sp015544. [DOI] [PMC free article] [PubMed] [Google Scholar]
- Kirby A. W., Schweitzer-Tong D. E. Gaba-antagonists alter spatial summation in receptive field centres of rod- but not cone-drive cat retinal ganglion Y-cells. J Physiol. 1981 Nov;320:303–308. doi: 10.1113/jphysiol.1981.sp013950. [DOI] [PMC free article] [PubMed] [Google Scholar]
- Kolb H., Nelson R., Mariani A. Amacrine cells, bipolar cells and ganglion cells of the cat retina: a Golgi study. Vision Res. 1981;21(7):1081–1114. doi: 10.1016/0042-6989(81)90013-4. [DOI] [PubMed] [Google Scholar]
- Lasater E. M., Dowling J. E. Carp horizontal cells in culture respond selectively to L-glutamate and its agonists. Proc Natl Acad Sci U S A. 1982 Feb;79(3):936–940. doi: 10.1073/pnas.79.3.936. [DOI] [PMC free article] [PubMed] [Google Scholar]
- Lee K. S., Tsien R. W. Reversal of current through calcium channels in dialysed single heart cells. Nature. 1982 Jun 10;297(5866):498–501. doi: 10.1038/297498a0. [DOI] [PubMed] [Google Scholar]
- Leifer D., Lipton S. A., Barnstable C. J., Masland R. H. Monoclonal antibody to Thy-1 enhances regeneration of processes by rat retinal ganglion cells in culture. Science. 1984 Apr 20;224(4646):303–306. doi: 10.1126/science.6143400. [DOI] [PubMed] [Google Scholar]
- Lipton S. A. Conditioning hyperpolarization reveals a property of the light-sensitive current in photoreceptors that is modified by cGMP and EGTA. Brain Res. 1985 Aug 26;341(2):337–349. doi: 10.1016/0006-8993(85)91073-x. [DOI] [PubMed] [Google Scholar]
- Lipton S. A. cGMP and EGTA increase the light-sensitive current of retinal rods. Brain Res. 1983 Apr 11;265(1):41–48. doi: 10.1016/0006-8993(83)91331-8. [DOI] [PubMed] [Google Scholar]
- Marty A. Ca-dependent K channels with large unitary conductance in chromaffin cell membranes. Nature. 1981 Jun 11;291(5815):497–500. doi: 10.1038/291497a0. [DOI] [PubMed] [Google Scholar]
- Marty A., Neher E. Potassium channels in cultured bovine adrenal chromaffin cells. J Physiol. 1985 Oct;367:117–141. doi: 10.1113/jphysiol.1985.sp015817. [DOI] [PMC free article] [PubMed] [Google Scholar]
- Maruyama Y., Gallacher D. V., Petersen O. H. Voltage and Ca2+-activated K+ channel in baso-lateral acinar cell membranes of mammalian salivary glands. Nature. 1983 Apr 28;302(5911):827–829. doi: 10.1038/302827a0. [DOI] [PubMed] [Google Scholar]
- Masland R. H., Ames A., 3rd Responses to acetylcholine of ganglion cells in an isolated mammalian retina. J Neurophysiol. 1976 Nov;39(6):1220–1235. doi: 10.1152/jn.1976.39.6.1220. [DOI] [PubMed] [Google Scholar]
- Meech R. W. Calcium-dependent potassium activation in nervous tissues. Annu Rev Biophys Bioeng. 1978;7:1–18. doi: 10.1146/annurev.bb.07.060178.000245. [DOI] [PubMed] [Google Scholar]
- Meech R. W., Standen N. B. Potassium activation in Helix aspersa neurones under voltage clamp: a component mediated by calcium influx. J Physiol. 1975 Jul;249(2):211–239. doi: 10.1113/jphysiol.1975.sp011012. [DOI] [PMC free article] [PubMed] [Google Scholar]
- Miller C., Moczydlowski E., Latorre R., Phillips M. Charybdotoxin, a protein inhibitor of single Ca2+-activated K+ channels from mammalian skeletal muscle. Nature. 1985 Jan 24;313(6000):316–318. doi: 10.1038/313316a0. [DOI] [PubMed] [Google Scholar]
- Neher E., Stevens C. F. Conductance fluctuations and ionic pores in membranes. Annu Rev Biophys Bioeng. 1977;6:345–381. doi: 10.1146/annurev.bb.06.060177.002021. [DOI] [PubMed] [Google Scholar]
- Nelson R., Famiglietti E. V., Jr, Kolb H. Intracellular staining reveals different levels of stratification for on- and off-center ganglion cells in cat retina. J Neurophysiol. 1978 Mar;41(2):472–483. doi: 10.1152/jn.1978.41.2.472. [DOI] [PubMed] [Google Scholar]
- Nowycky M. C., Fox A. P., Tsien R. W. Three types of neuronal calcium channel with different calcium agonist sensitivity. Nature. 1985 Aug 1;316(6027):440–443. doi: 10.1038/316440a0. [DOI] [PubMed] [Google Scholar]
- Pallotta B. S., Magleby K. L., Barrett J. N. Single channel recordings of Ca2+-activated K+ currents in rat muscle cell culture. Nature. 1981 Oct 8;293(5832):471–474. doi: 10.1038/293471a0. [DOI] [PubMed] [Google Scholar]
- Pennefather P., Lancaster B., Adams P. R., Nicoll R. A. Two distinct Ca-dependent K currents in bullfrog sympathetic ganglion cells. Proc Natl Acad Sci U S A. 1985 May;82(9):3040–3044. doi: 10.1073/pnas.82.9.3040. [DOI] [PMC free article] [PubMed] [Google Scholar]
- Perry V. H. The ganglion cell layer of the retina of the rat: a Golgi study. Proc R Soc Lond B Biol Sci. 1979 May 23;204(1156):363–375. doi: 10.1098/rspb.1979.0033. [DOI] [PubMed] [Google Scholar]
- Saito H. A. Morphology of physiologically identified X-, Y-, and W-type retinal ganglion cells of the cat. J Comp Neurol. 1983 Dec 10;221(3):279–288. doi: 10.1002/cne.902210304. [DOI] [PubMed] [Google Scholar]
- Saito H. The effects of strychnine and bicuculline on the responses of X- and Y-cells of the isolated eye-cut preparation of the cat. Brain Res. 1981 May 11;212(1):243–248. doi: 10.1016/0006-8993(81)90061-5. [DOI] [PubMed] [Google Scholar]
- Sarthy P. V., Lam D. M. Isolated cells from a mammalian retina. Brain Res. 1979 Oct 26;176(1):208–212. doi: 10.1016/0006-8993(79)90889-8. [DOI] [PubMed] [Google Scholar]
- Sigworth F. J. The variance of sodium current fluctuations at the node of Ranvier. J Physiol. 1980 Oct;307:97–129. doi: 10.1113/jphysiol.1980.sp013426. [DOI] [PMC free article] [PubMed] [Google Scholar]
- Stone J., Fukuda Y. Properties of cat retinal ganglion cells: a comparison of W-cells with X- and Y-cells. J Neurophysiol. 1974 Jul;37(4):722–748. doi: 10.1152/jn.1974.37.4.722. [DOI] [PubMed] [Google Scholar]
- Tachibana M. Membrane properties of solitary horizontal cells isolated from goldfish retina. J Physiol. 1981 Dec;321:141–161. doi: 10.1113/jphysiol.1981.sp013976. [DOI] [PMC free article] [PubMed] [Google Scholar]
- Thompson S. H. Three pharmacologically distinct potassium channels in molluscan neurones. J Physiol. 1977 Feb;265(2):465–488. doi: 10.1113/jphysiol.1977.sp011725. [DOI] [PMC free article] [PubMed] [Google Scholar]
- WIESEL T. N. Recording inhibition and excitation in the cat's retinal ganglion cells with intracellular electrodes. Nature. 1959 Jan 24;183(4656):264–265. doi: 10.1038/183264a0. [DOI] [PubMed] [Google Scholar]
- Wässle H., Boycott B. B., Illing R. B. Morphology and mosaic of on- and off-beta cells in the cat retina and some functional considerations. Proc R Soc Lond B Biol Sci. 1981 May 22;212(1187):177–195. doi: 10.1098/rspb.1981.0033. [DOI] [PubMed] [Google Scholar]
- Yellen G. Single Ca2+-activated nonselective cation channels in neuroblastoma. Nature. 1982 Mar 25;296(5855):357–359. doi: 10.1038/296357a0. [DOI] [PubMed] [Google Scholar]
- Ypey D. L., Clapham D. E. Development of a delayed outward-rectifying K+ conductance in cultured mouse peritoneal macrophages. Proc Natl Acad Sci U S A. 1984 May;81(10):3083–3087. doi: 10.1073/pnas.81.10.3083. [DOI] [PMC free article] [PubMed] [Google Scholar]
- Zrenner E., Nelson R., Mariani A. Intracellular recordings from a biplexiform ganglion cell in macaque retina, stained with horseradish peroxidase. Brain Res. 1983 Mar 7;262(2):181–185. doi: 10.1016/0006-8993(83)91007-7. [DOI] [PubMed] [Google Scholar]