Abstract
1. Tension and heat production were measured at 0 degree C in sartorius muscles from Rana temporaria in response to two extents of shortening at five velocities. Shortening was from approximately 2.4 to 2.2 microns, 2.4 to 2.3 microns and 2.3 to 2.2 micron at velocities per half-sarcomere from 0.2 to 1.56 micron s-1. 2. Tension became approximately constant at all velocities. Records of heat rate obtained by differentiating traces from which thermoelastic heat had been subtracted became negative early in shortening and then rose. Heat rate became constant during shortening only at the lowest velocity and was still rising at the end of shortening at higher velocities. The highest heat rate occurred at the end of shortening at the two highest velocities. At the end of shortening at all velocities heat rate gradually approached the isometric level measured at the short length, the half-time for decline being largest following the slowest larger shortening. 3. Heat produced as a consequence of shortening but not associated with tension recovery was determined by subtracting shortening heat measured in response to two extents of shortening to the same muscle length. The differences in shortening heat continued to increase after shortening ended, and more of the extra heat produced in response to shortening appeared after the end of rapid shortening than during shortening itself. 4. Shortening heat coefficients calculated in different ways were similar to coefficients determined in previous studies. Coefficients calculated from measurements that excluded heat produced by tension recovery and allowed for continued production of heat by processes initiated by shortening were found to increase linearly with the force maintained during shortening. 5. The results show that the kinetics of heat production during and after shortening are very sensitive to the speed of shortening and that steady rates of energy liberation are not attained during shortening of less than or equal to 10% of muscle length at velocities greater than or equal to 12% of maximum velocity.
Full text
PDF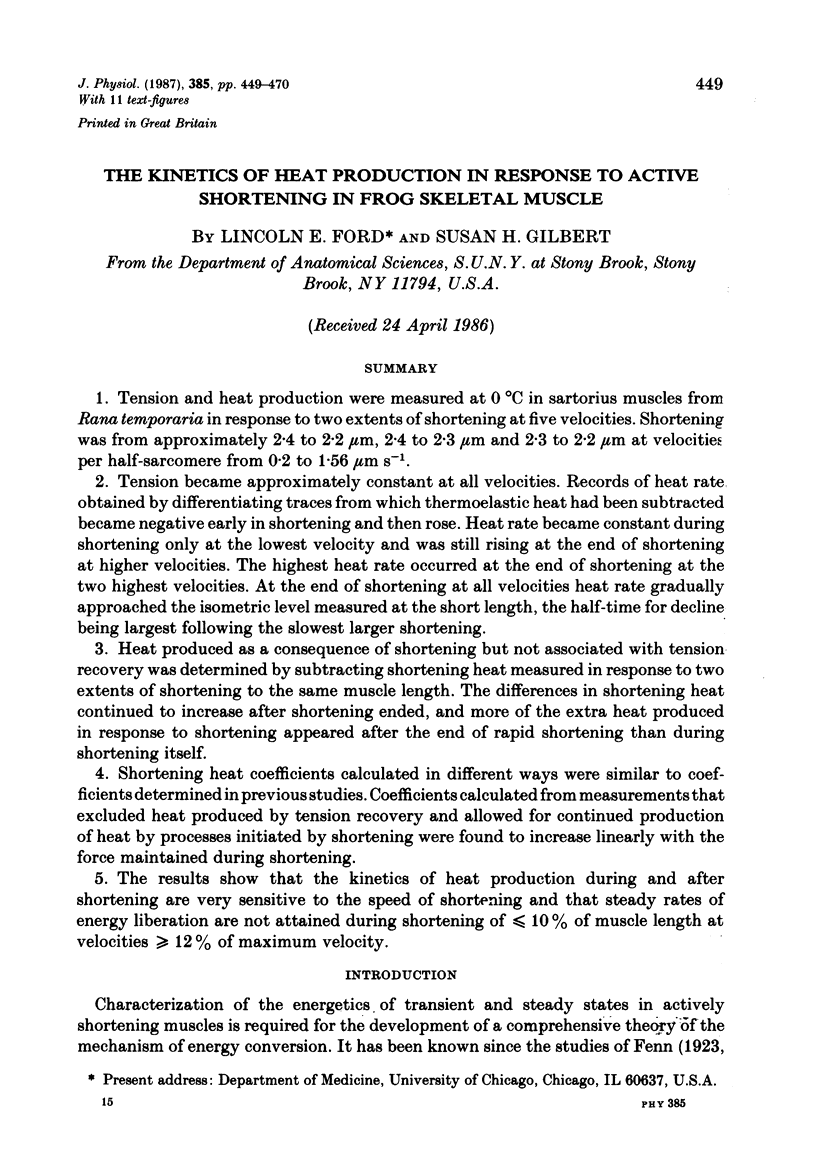

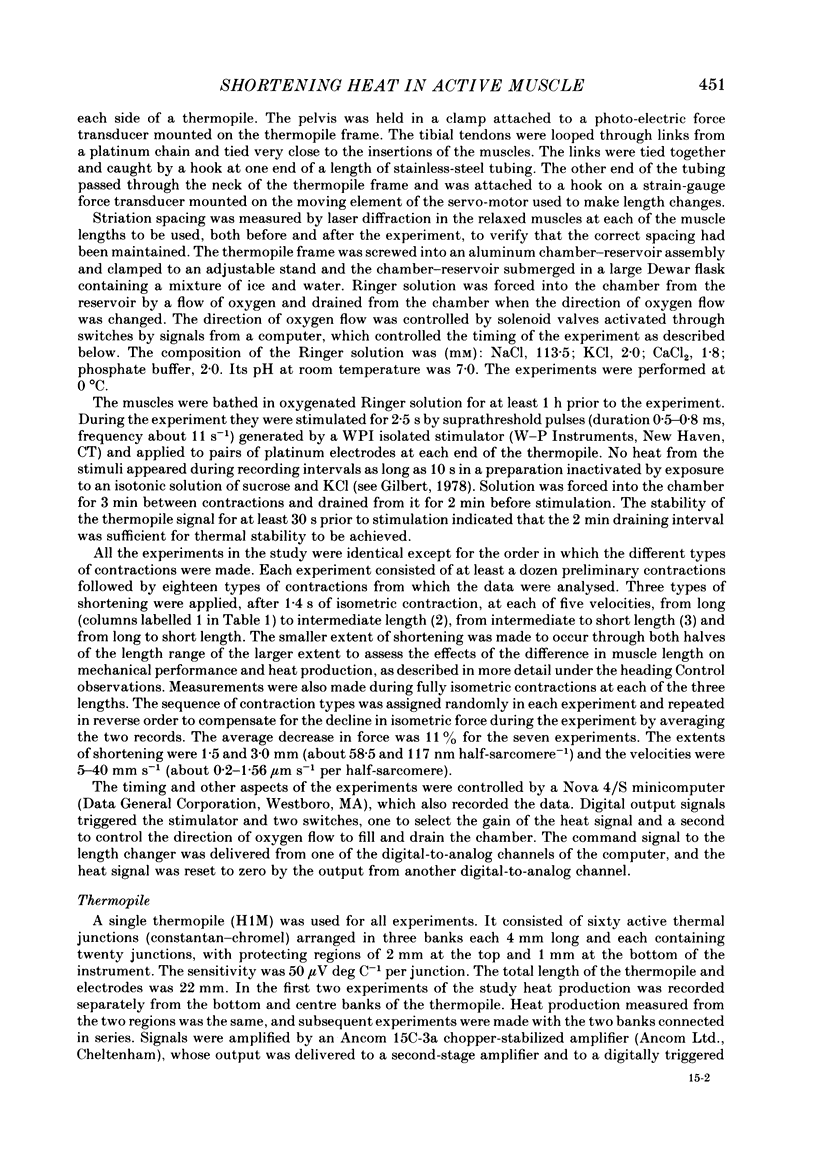
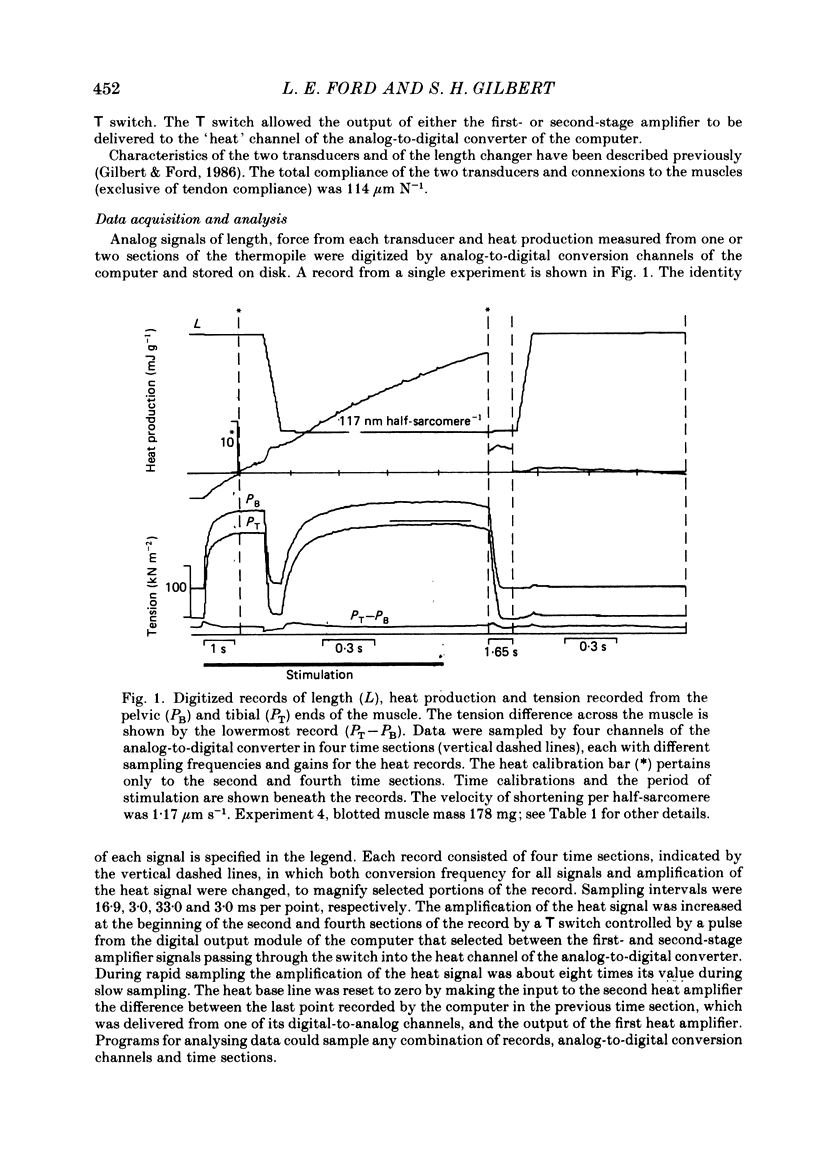
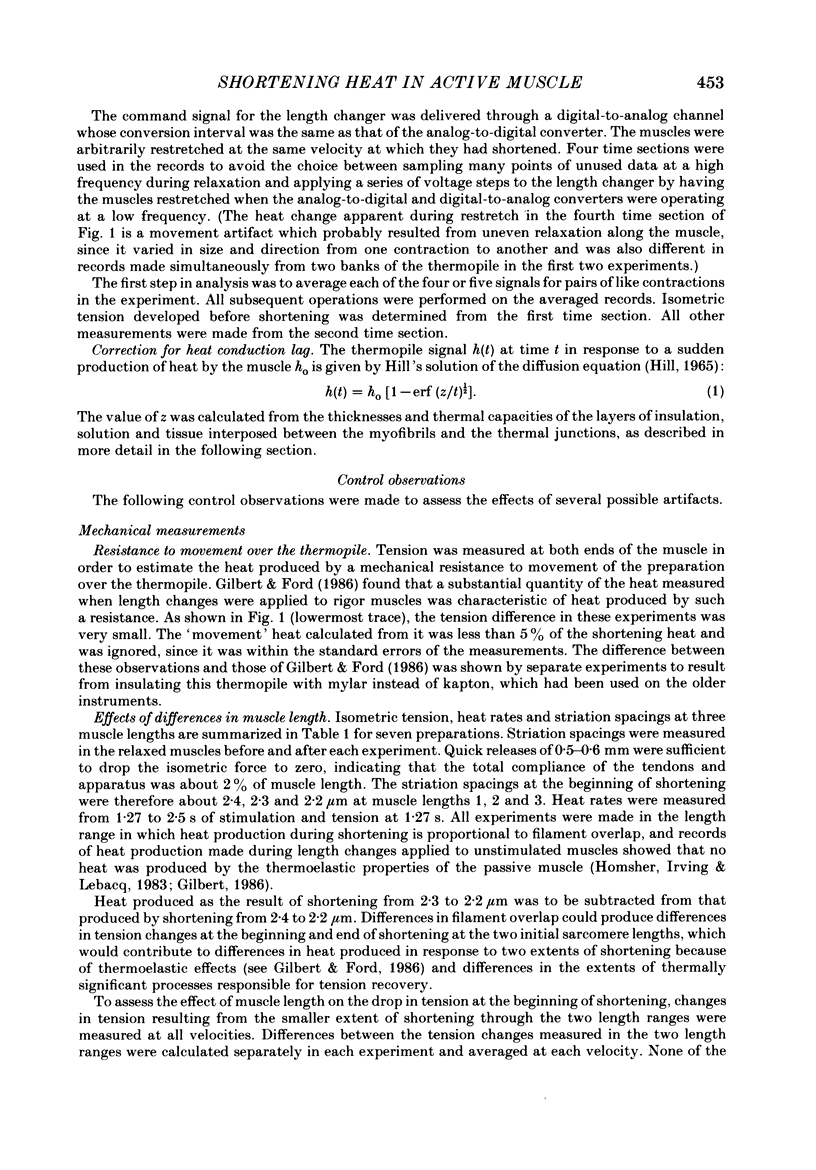
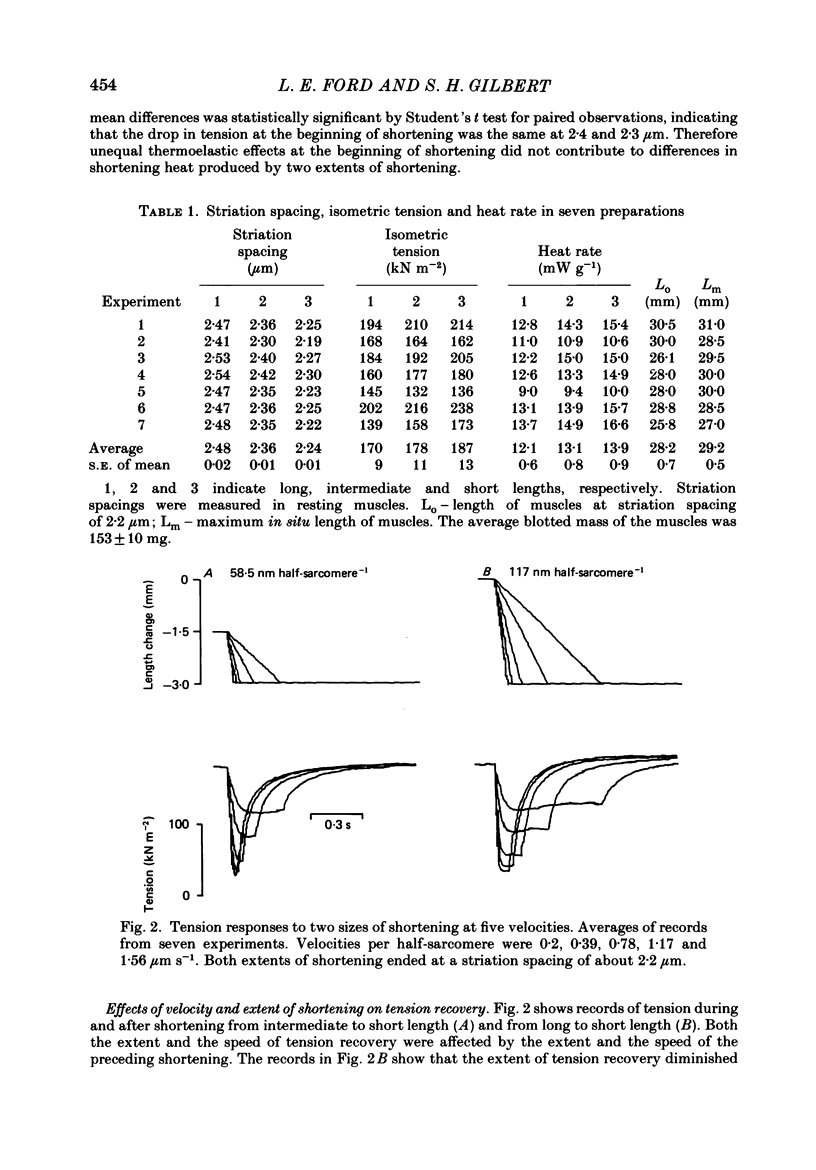
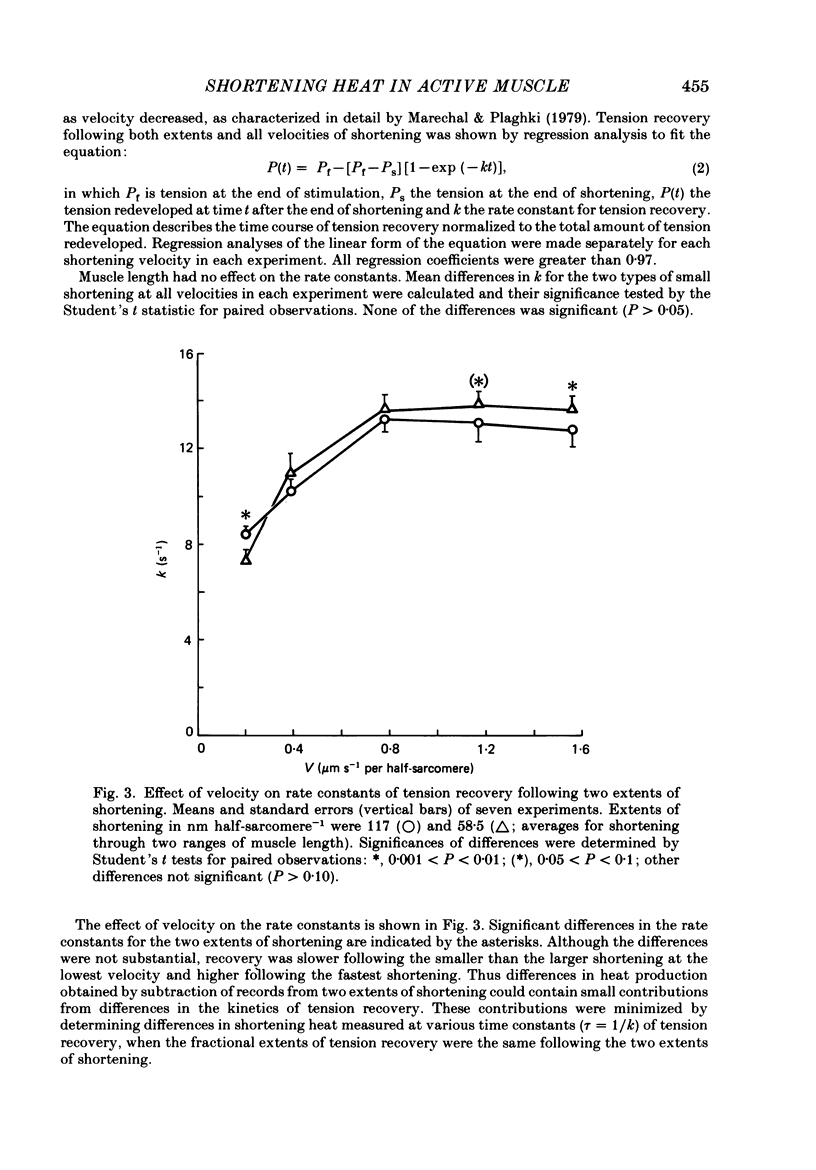
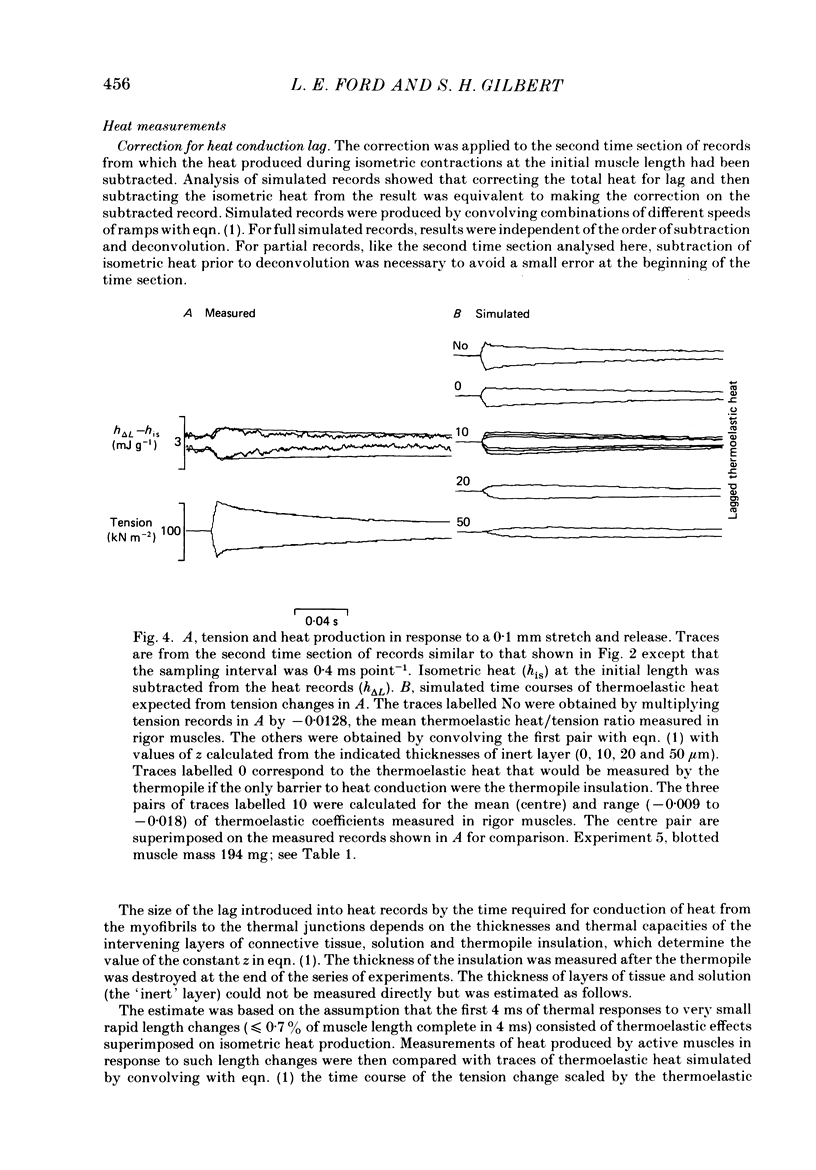
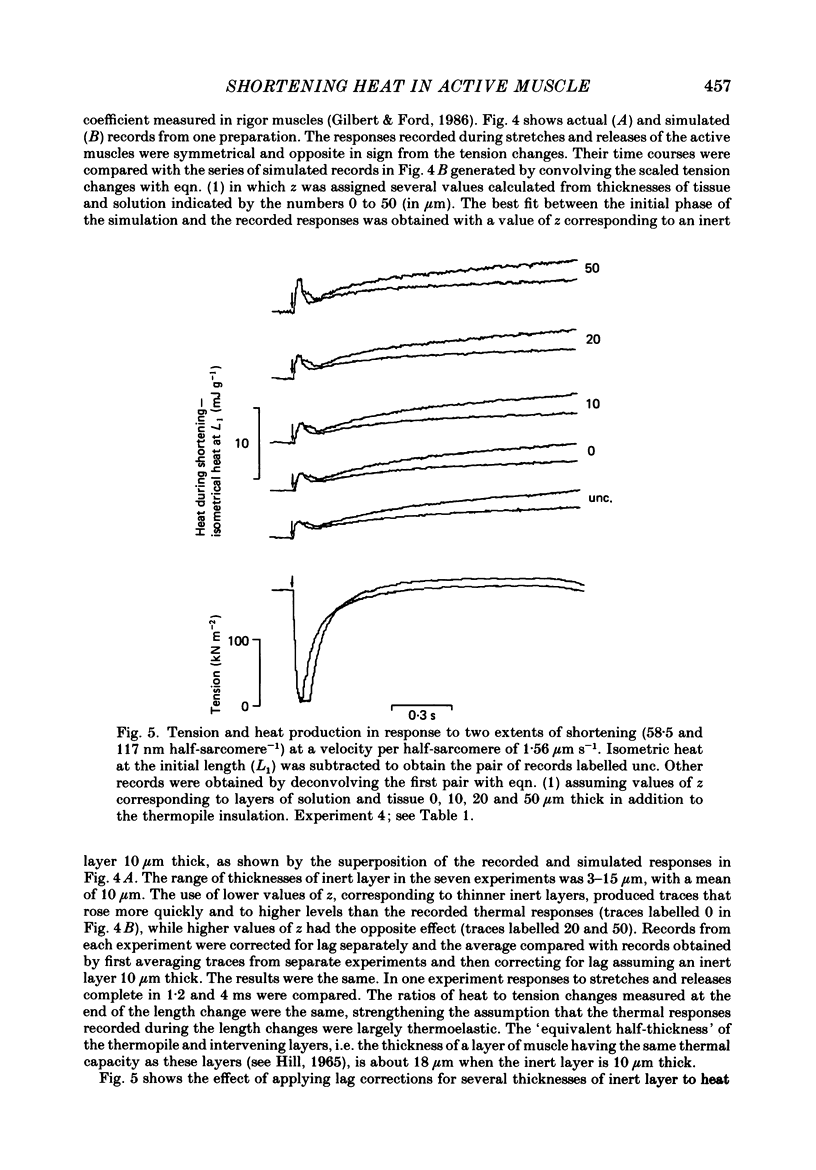

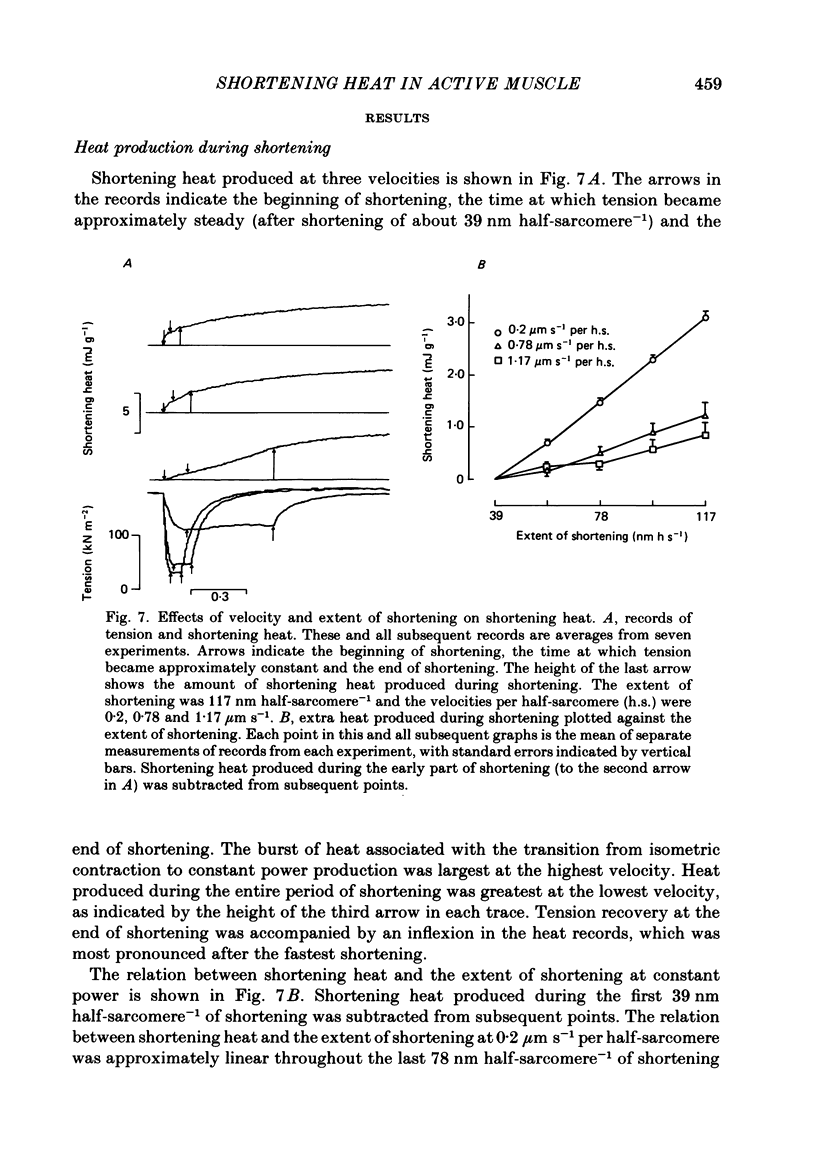
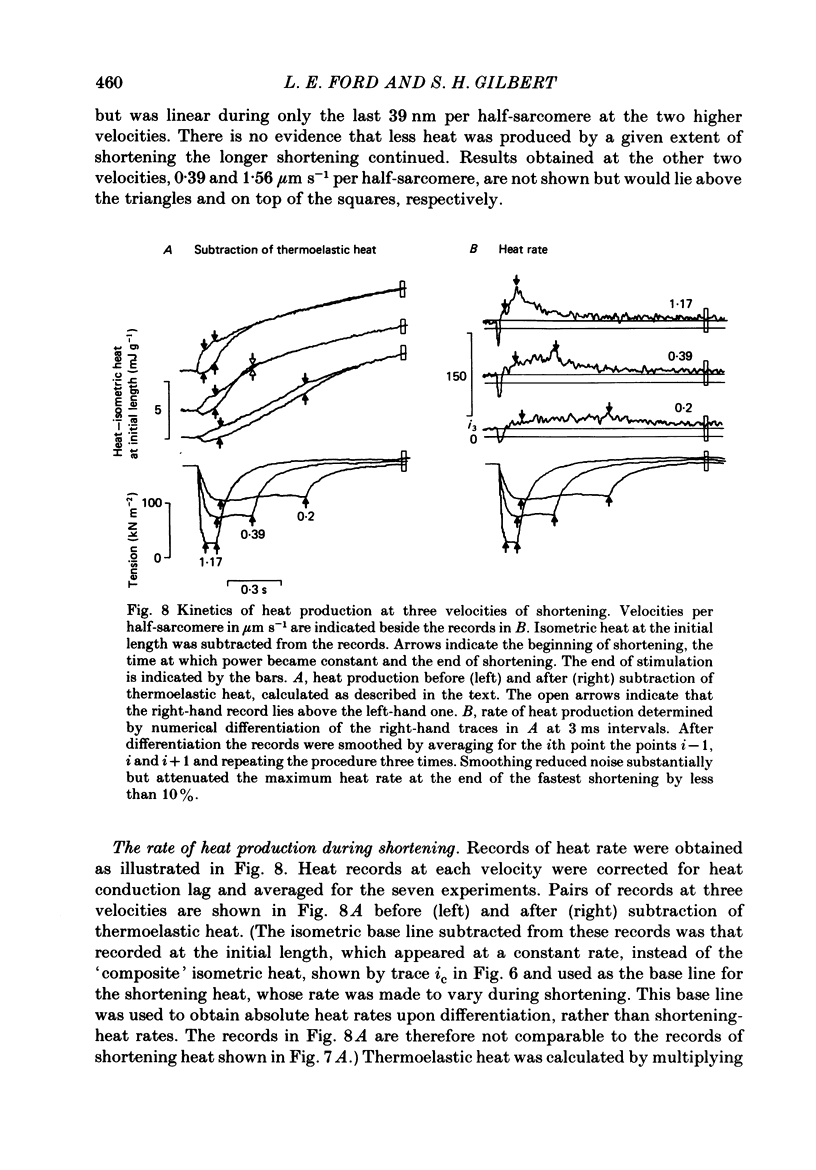
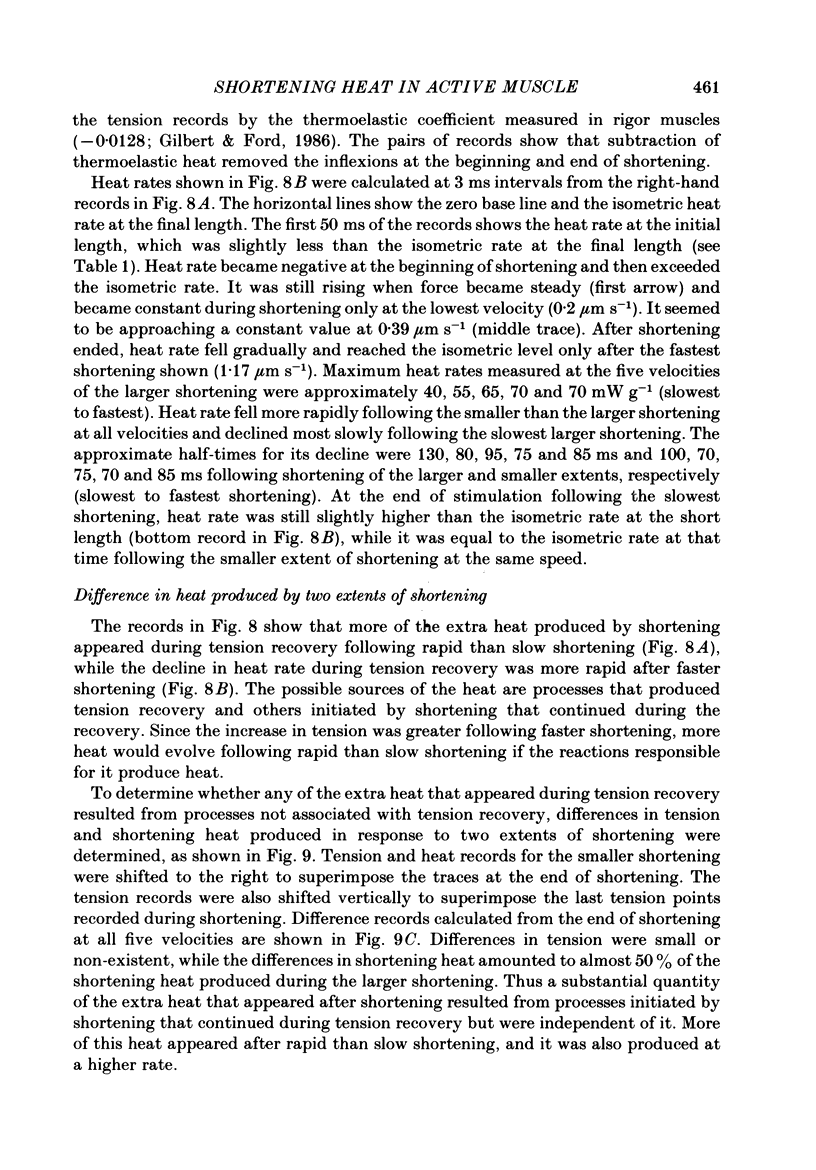
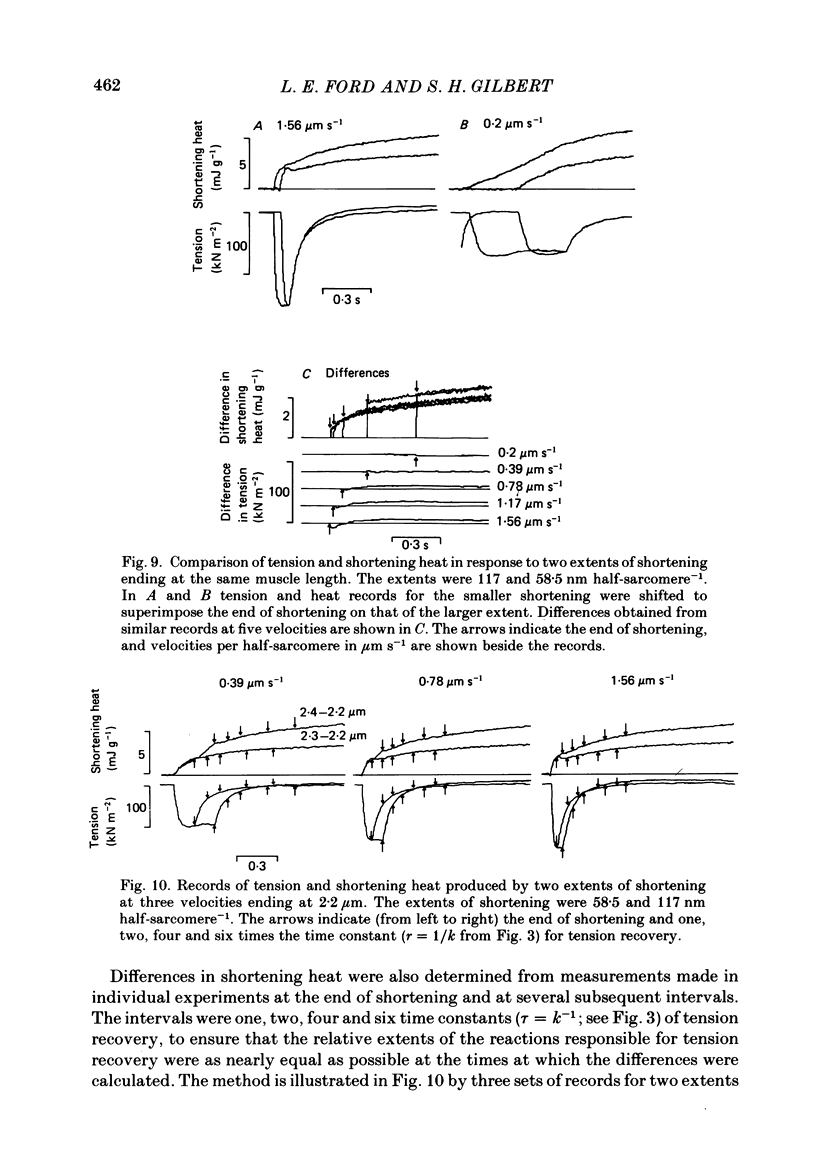
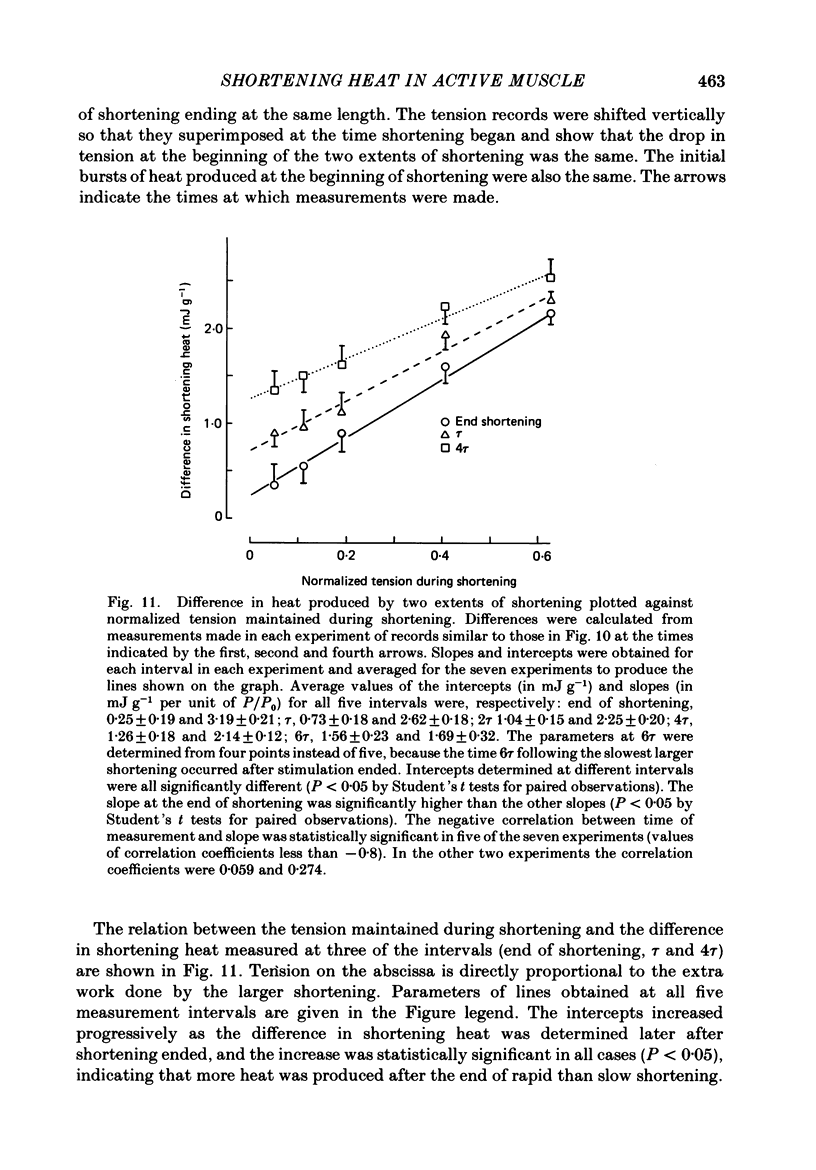
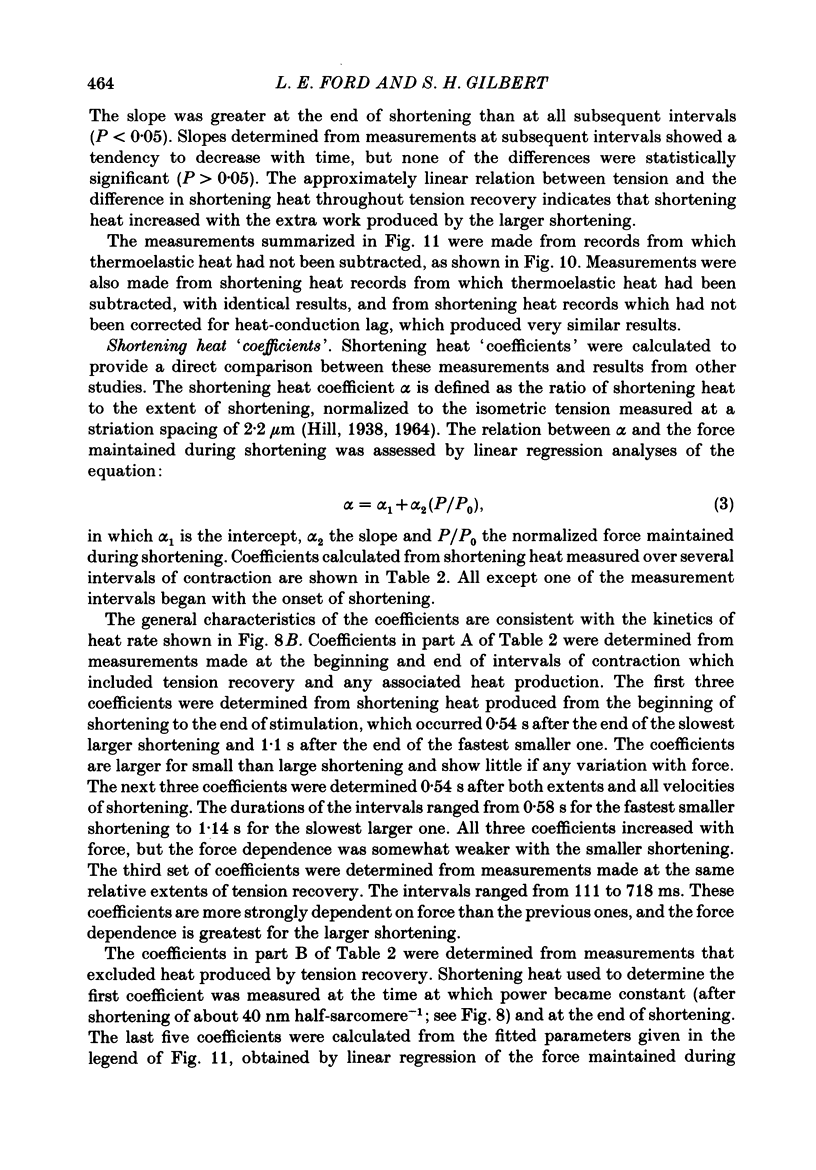
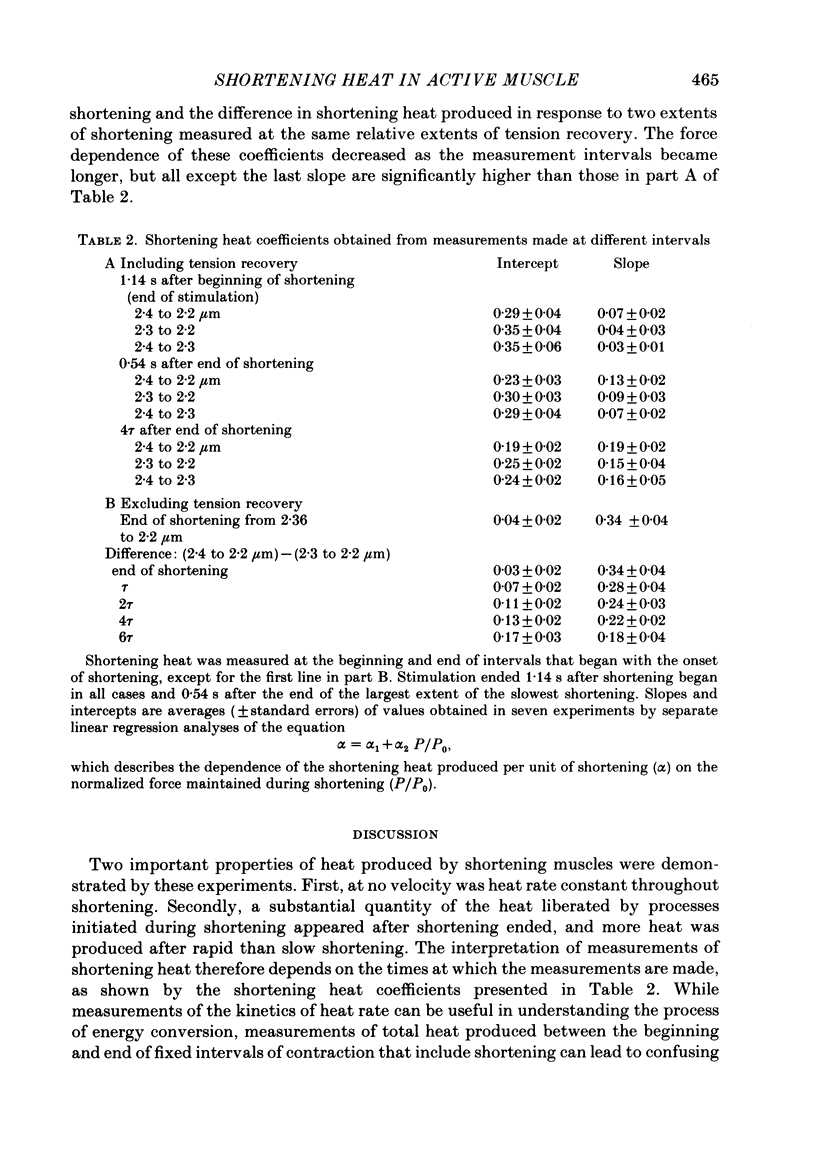
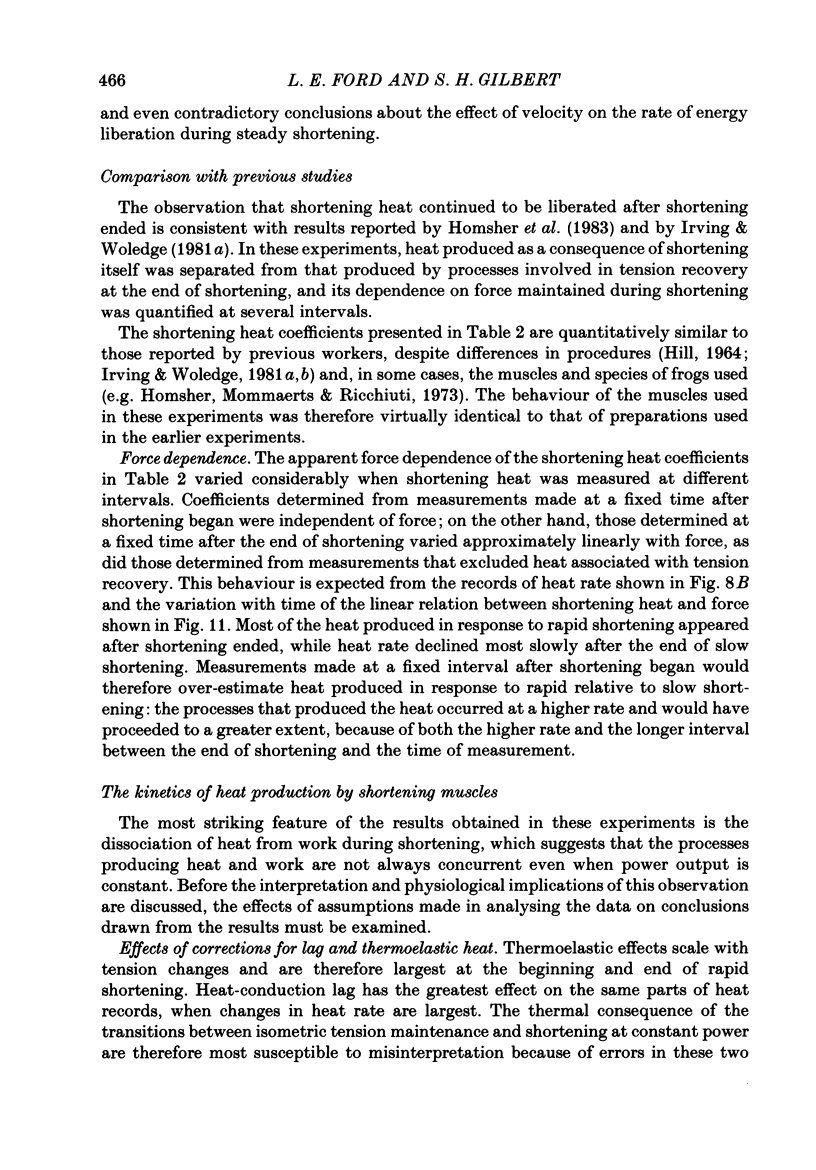
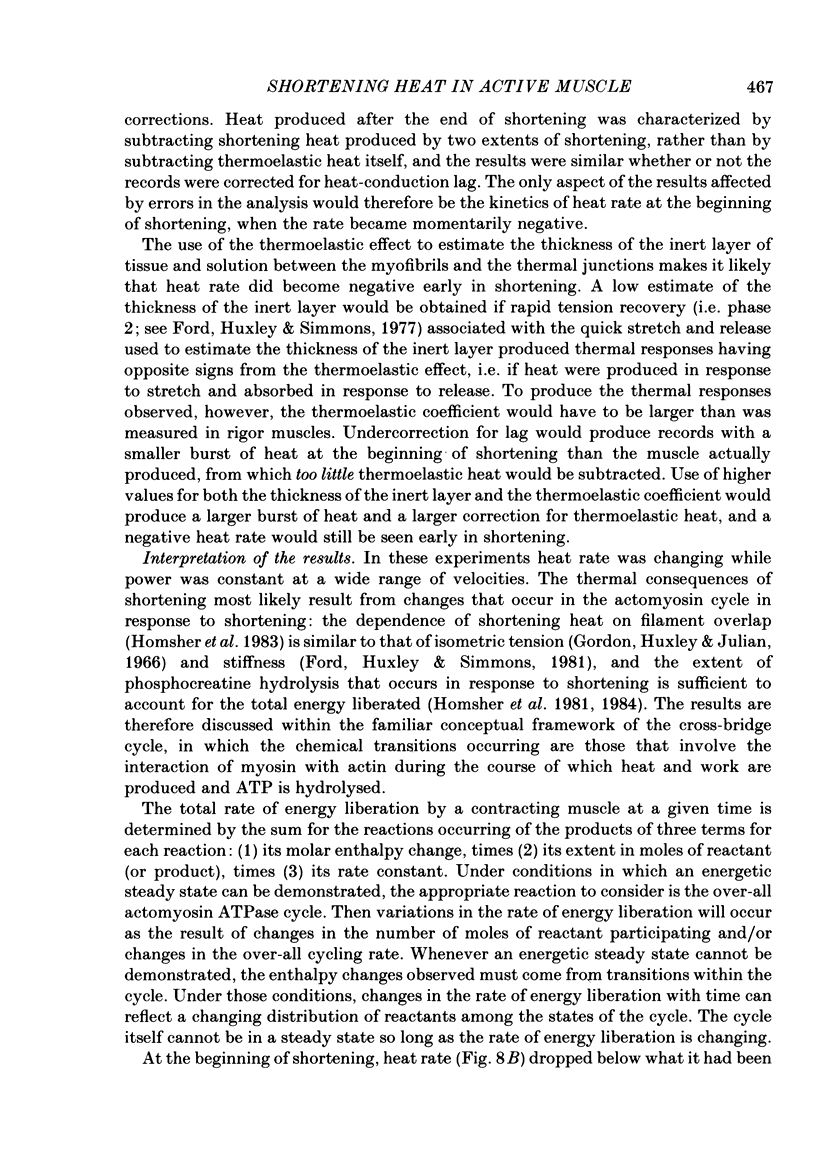
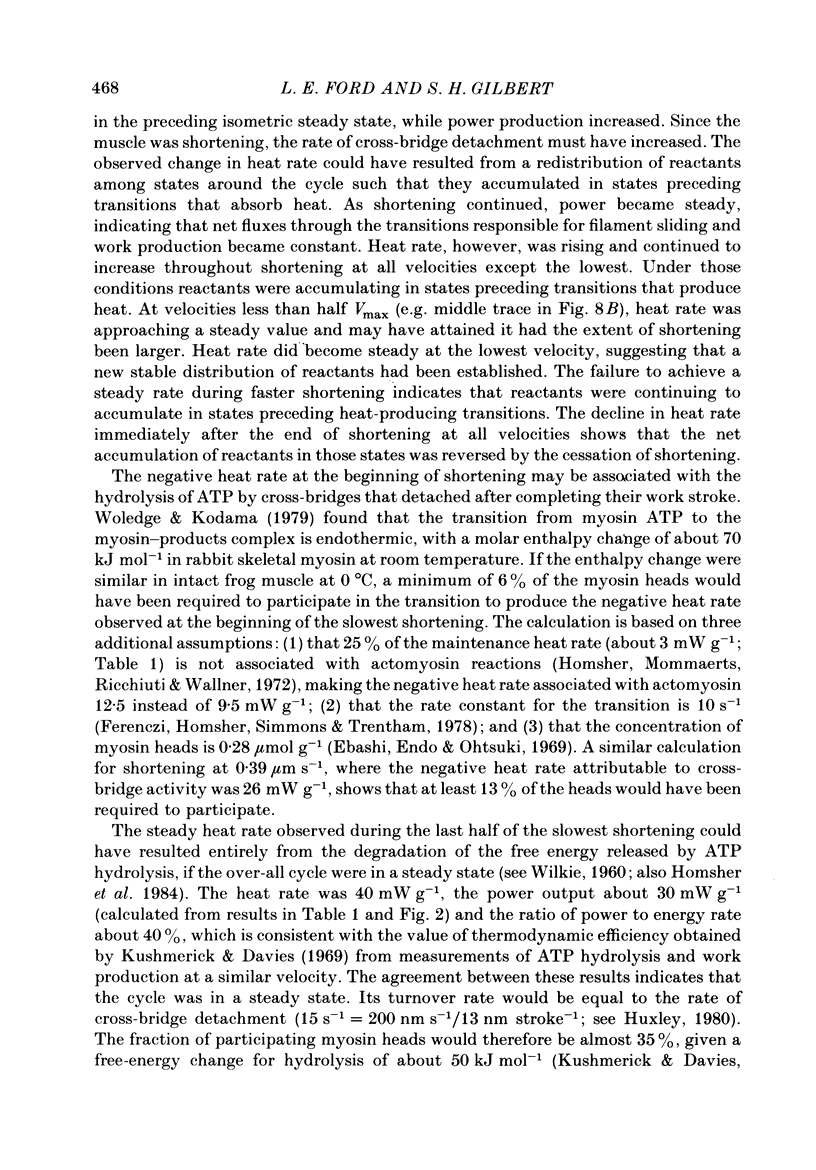
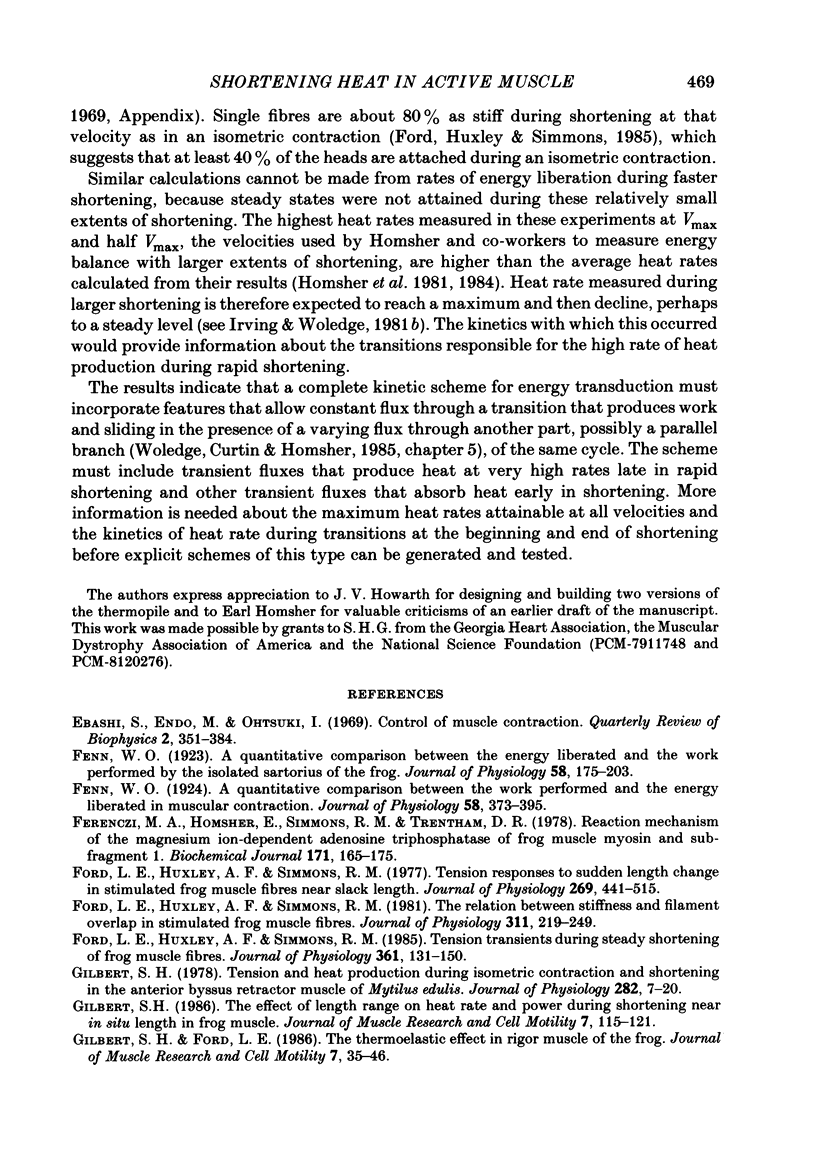
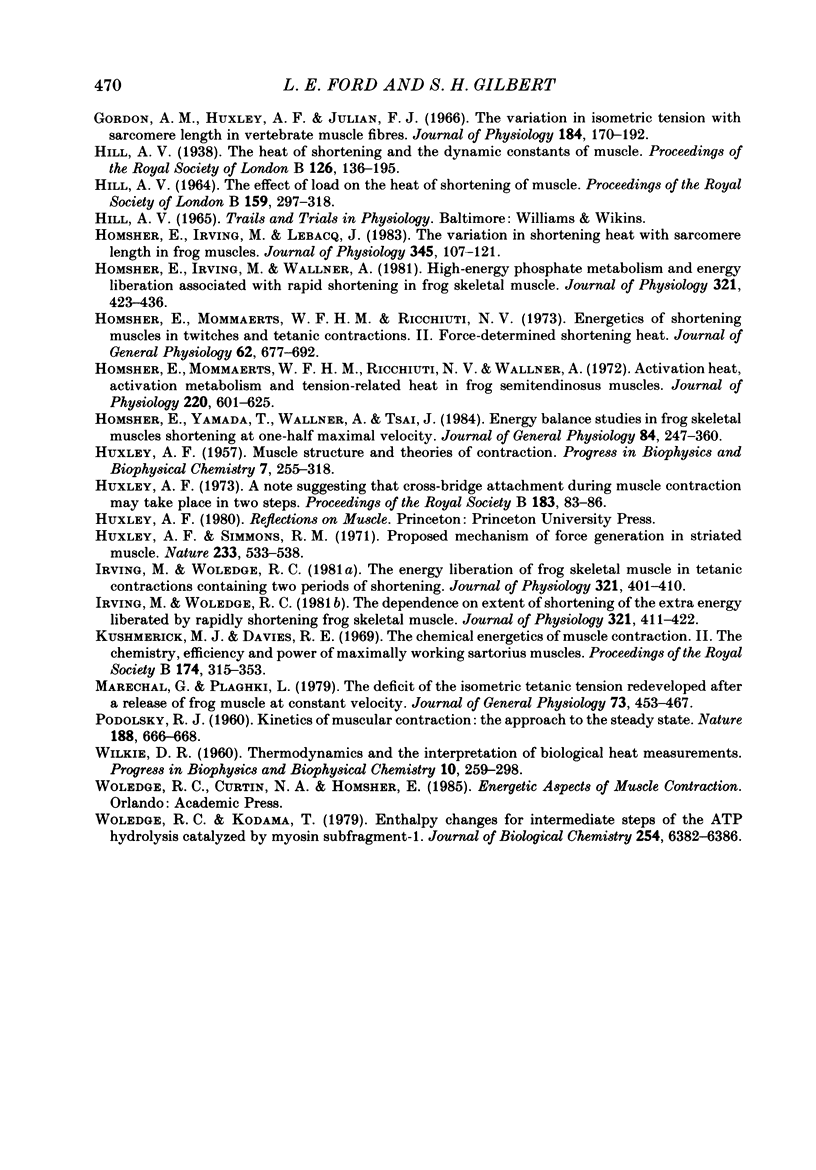
Selected References
These references are in PubMed. This may not be the complete list of references from this article.
- Ebashi S., Endo M., Otsuki I. Control of muscle contraction. Q Rev Biophys. 1969 Nov;2(4):351–384. doi: 10.1017/s0033583500001190. [DOI] [PubMed] [Google Scholar]
- Fenn W. O. A quantitative comparison between the energy liberated and the work performed by the isolated sartorius muscle of the frog. J Physiol. 1923 Dec 28;58(2-3):175–203. doi: 10.1113/jphysiol.1923.sp002115. [DOI] [PMC free article] [PubMed] [Google Scholar]
- Fenn W. O. The relation between the work performed and the energy liberated in muscular contraction. J Physiol. 1924 May 23;58(6):373–395. doi: 10.1113/jphysiol.1924.sp002141. [DOI] [PMC free article] [PubMed] [Google Scholar]
- Ferenczi M. A., Homsher E., Simmons R. M., Trentham D. R. Reaction mechanism of the magnesium ion-dependent adenosine triphosphatase of frog muscle myosin and subfragment 1. Biochem J. 1978 Apr 1;171(1):165–175. doi: 10.1042/bj1710165. [DOI] [PMC free article] [PubMed] [Google Scholar]
- Ford L. E., Huxley A. F., Simmons R. M. Tension responses to sudden length change in stimulated frog muscle fibres near slack length. J Physiol. 1977 Jul;269(2):441–515. doi: 10.1113/jphysiol.1977.sp011911. [DOI] [PMC free article] [PubMed] [Google Scholar]
- Ford L. E., Huxley A. F., Simmons R. M. Tension transients during steady shortening of frog muscle fibres. J Physiol. 1985 Apr;361:131–150. doi: 10.1113/jphysiol.1985.sp015637. [DOI] [PMC free article] [PubMed] [Google Scholar]
- Ford L. E., Huxley A. F., Simmons R. M. The relation between stiffness and filament overlap in stimulated frog muscle fibres. J Physiol. 1981 Feb;311:219–249. doi: 10.1113/jphysiol.1981.sp013582. [DOI] [PMC free article] [PubMed] [Google Scholar]
- Gilbert S. H., Ford L. E. The thermoelastic effect in rigor muscle of the frog. J Muscle Res Cell Motil. 1986 Feb;7(1):35–46. doi: 10.1007/BF01756200. [DOI] [PubMed] [Google Scholar]
- Gilbert S. H. Tension and heat production during isometric contractions and shortening in the anterior byssus retractor muscle of Mytilus edulis. J Physiol. 1978 Sep;282:7–20. doi: 10.1113/jphysiol.1978.sp012444. [DOI] [PMC free article] [PubMed] [Google Scholar]
- Gilbert S. H. The effect of length range on heat rate and power during shortening near in situ length in frog muscle. J Muscle Res Cell Motil. 1986 Apr;7(2):115–121. doi: 10.1007/BF01753412. [DOI] [PubMed] [Google Scholar]
- Gordon A. M., Huxley A. F., Julian F. J. The variation in isometric tension with sarcomere length in vertebrate muscle fibres. J Physiol. 1966 May;184(1):170–192. doi: 10.1113/jphysiol.1966.sp007909. [DOI] [PMC free article] [PubMed] [Google Scholar]
- HILL A. V. THE EFFECT OF LOAD ON THE HEAT OF SHORTENING OF MUSCLE. Proc R Soc Lond B Biol Sci. 1964 Jan 14;159:297–318. doi: 10.1098/rspb.1964.0004. [DOI] [PubMed] [Google Scholar]
- HUXLEY A. F. Muscle structure and theories of contraction. Prog Biophys Biophys Chem. 1957;7:255–318. [PubMed] [Google Scholar]
- Homsher E., Irving M., Lebacq J. The variation in shortening heat with sarcomere length in frog muscle. J Physiol. 1983 Dec;345:107–121. doi: 10.1113/jphysiol.1983.sp014968. [DOI] [PMC free article] [PubMed] [Google Scholar]
- Homsher E., Irving M., Wallner A. High-energy phosphate metabolism and energy liberation associated with rapid shortening in frog skeletal muscle. J Physiol. 1981 Dec;321:423–436. doi: 10.1113/jphysiol.1981.sp013994. [DOI] [PMC free article] [PubMed] [Google Scholar]
- Homsher E., Mommaerts W. F., Ricchiuti N. V. Energetics of shortening muscles in twitches and tetanic contractions. II. Force-determined shortening heat. J Gen Physiol. 1973 Dec;62(6):677–692. doi: 10.1085/jgp.62.6.677. [DOI] [PMC free article] [PubMed] [Google Scholar]
- Homsher E., Mommaerts W. F., Ricchiuti N. V., Wallner A. Activation heat, activation metabolism and tension-related heat in frog semitendinosus muscles. J Physiol. 1972 Feb;220(3):601–625. doi: 10.1113/jphysiol.1972.sp009725. [DOI] [PMC free article] [PubMed] [Google Scholar]
- Huxley A. F. A note suggesting that the cross-bridge attachment during muscle contraction may take place in two stages. Proc R Soc Lond B Biol Sci. 1973 Feb 27;183(1070):83–86. doi: 10.1098/rspb.1973.0006. [DOI] [PubMed] [Google Scholar]
- Huxley A. F., Simmons R. M. Proposed mechanism of force generation in striated muscle. Nature. 1971 Oct 22;233(5321):533–538. doi: 10.1038/233533a0. [DOI] [PubMed] [Google Scholar]
- Irving M., Woledge R. C. The dependence on extent of shortening of the extra energy liberated by rapidly shortening frog skeletal muscle. J Physiol. 1981 Dec;321:411–422. doi: 10.1113/jphysiol.1981.sp013993. [DOI] [PMC free article] [PubMed] [Google Scholar]
- Irving M., Woledge R. C. The energy liberation of frog skeletal muscle in tetanic contractions containing two periods of shortening. J Physiol. 1981 Dec;321:401–410. doi: 10.1113/jphysiol.1981.sp013992. [DOI] [PMC free article] [PubMed] [Google Scholar]
- Kodama T., Woledge R. C. Enthalpy changes for intermediate steps of the ATP hydrolysis catalyzed by myosin subfragment-1. J Biol Chem. 1979 Jul 25;254(14):6382–6386. [PubMed] [Google Scholar]
- Kushmerick M. J., Davies R. E. The chemical energetics of muscle contraction. II. The chemistry, efficiency and power of maximally working sartorius muscles. Appendix. Free energy and enthalpy of atp hydrolysis in the sarcoplasm. Proc R Soc Lond B Biol Sci. 1969 Dec 23;174(1036):315–353. doi: 10.1098/rspb.1969.0096. [DOI] [PubMed] [Google Scholar]
- Maréchal G., Plaghki L. The deficit of the isometric tetanic tension redeveloped after a release of frog muscle at a constant velocity. J Gen Physiol. 1979 Apr;73(4):453–467. doi: 10.1085/jgp.73.4.453. [DOI] [PMC free article] [PubMed] [Google Scholar]
- PODOLSKY R. J. Kinetics of muscular contraction: the approach to the steady state. Nature. 1960 Nov 19;188:666–668. doi: 10.1038/188666a0. [DOI] [PubMed] [Google Scholar]
- WILKIE D. R. Thermodynamics and the interpretation of biological heat measurements. Prog Biophys Mol Biol. 1960;10:259–298. [PubMed] [Google Scholar]