Abstract
1. The repolarization of the action potential, and a fast after-hyperpolarization (a.h.p.) were studied in CA1 pyramidal cells (n = 76) in rat hippocampal slices (28-37 degrees C). Single spikes were elicited by brief (1-3 ms) current pulses, at membrane potentials close to rest (-60 to -70 mV). 2. Each action potential was followed by four after-potentials: (a) the fast a.h.p., lasting 2-5 ms; (b) an after-depolarization; (c) a medium a.h.p., (50-100 ms); and (d) a slow a.h.p. (1-2 s). Both the fast a.h.p. and the slow a.h.p. (but not the medium a.h.p.) were inhibited by Ca2+-free medium or Ca2+-channel blockers (Co2+, Mn2+ or Cd2+); but tetraethylammonium (TEA; 0.5-2 nM) blocked only the fast a.h.p., and noradrenaline (2-5 microM) only the slow a.h.p. This suggests that two Ca2+-activated K+ currents were involved: a fast, TEA-sensitive one (IC) underlying the fast a.h.p., and a slow noradrenaline-sensitive one (IAHP) underlying the slow a.h.p. 3. Like the fast a.h.p., spike repolarization seems to depend on a Ca2+-dependent K+ current of the fast, TEA-sensitive kind (IC). The repolarization was slowed by Ca2+-free medium, Co2+, Mn2+, Cd2+, or TEA, but not by noradrenaline. Charybdotoxin (CTX; 30 nM), a scorpion toxin which blocks the large-conductance Ca2+-activated K+ channel in muscle, had a similar effect to TEA. The effects of TEA and Cd2+ (or Mn2+) showed mutual occlusion. Raising the external K+ concentration reduced the fast a.h.p. and slowed the spike repolarization, whereas Cl- loading of the cell was ineffective. 4. The transient K+ current, IA, seems also to contribute to spike repolarization, because: (a) 4-aminopyridine (4-AP; 0.1 mM), which blocks IA, slowed the spike repolarization; (b) depolarizing pre-pulses, which inactivate IA, had a similar effect; (c) hyperpolarizing pre-pulses speeded up the spike repolarization; (d) the effects of 4-AP and pre-pulses persisted during Ca2+ blockade (like IA); and (e) depolarizing pre-pulses reduced the effect of 4-AP. 5. Pre-pulses or 4-AP broadened the spike less, and in a different manner, than Ca2+-free medium, Cd2+, Co2+, Mn2+, TEA or CTX. The former broadening was uniform, with little effect on the fast a.h.p., whereas the latter affected mostly the last two-thirds of the spike repolarization and abolished the fast a.h.p.(ABSTRACT TRUNCATED AT 400 WORDS)
Full text
PDF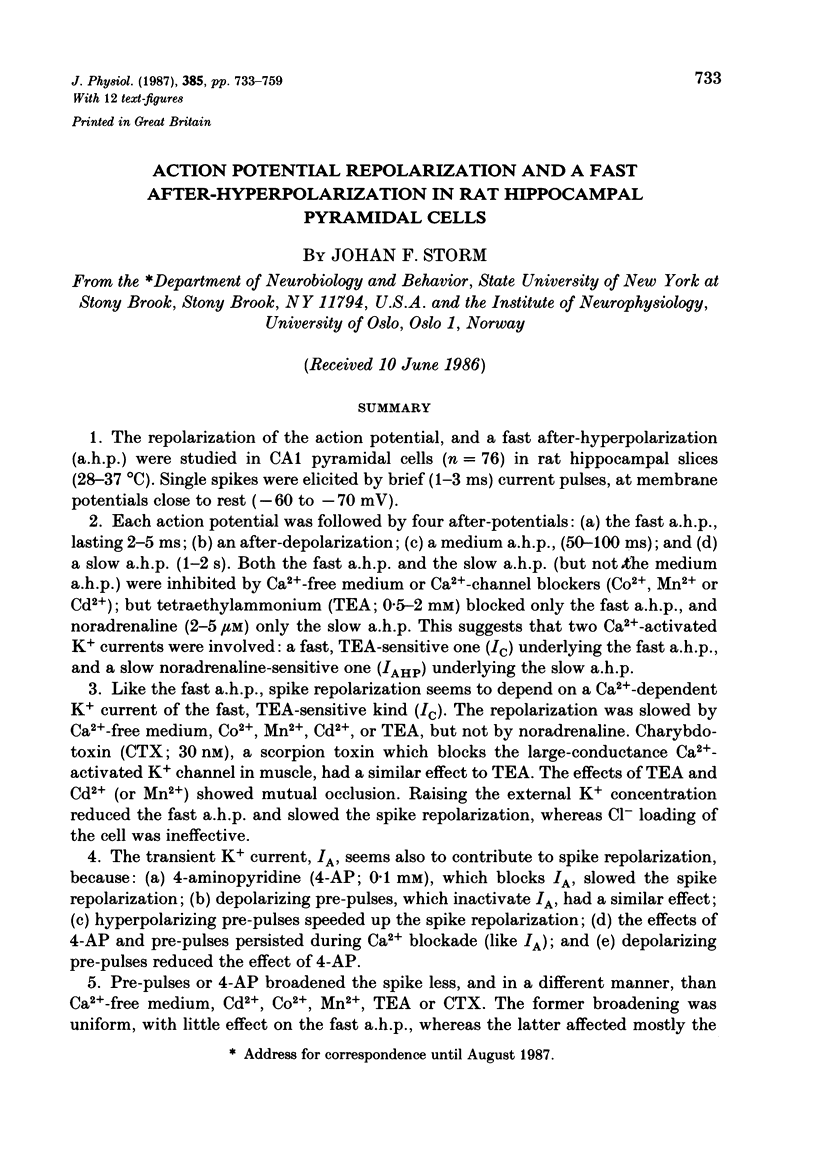
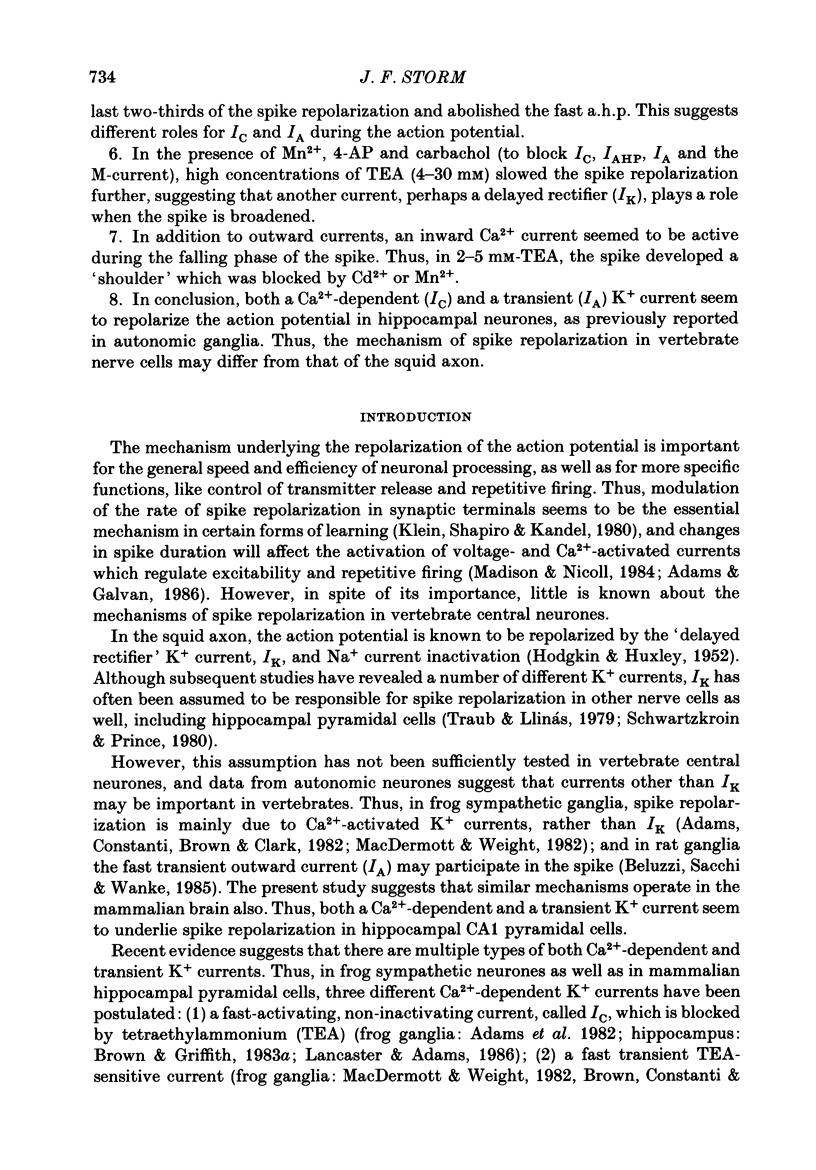
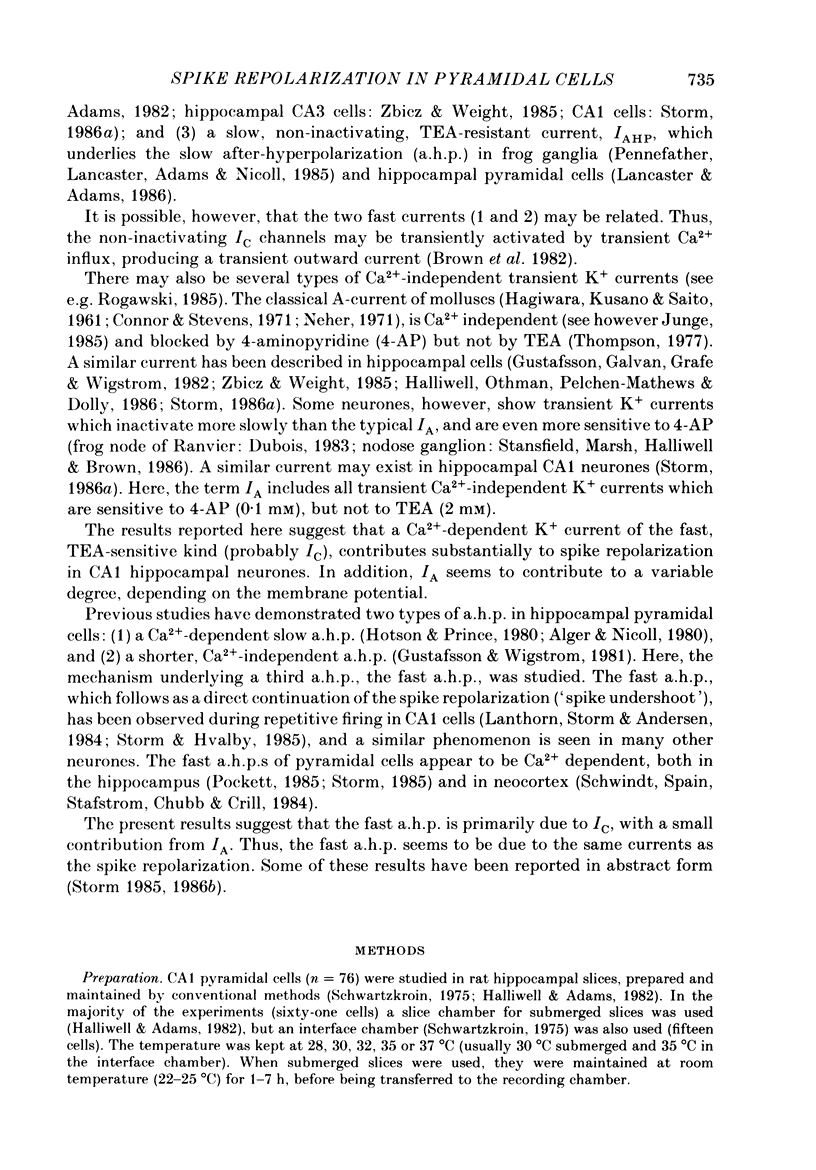
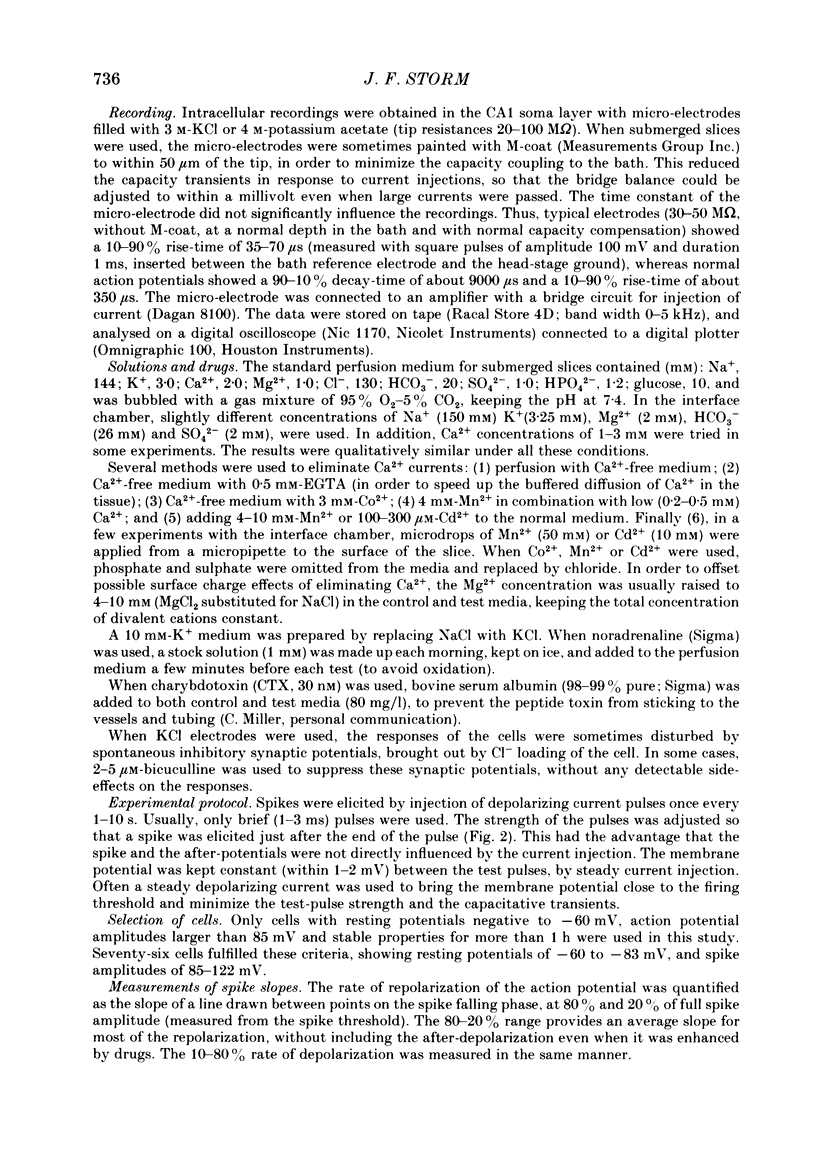
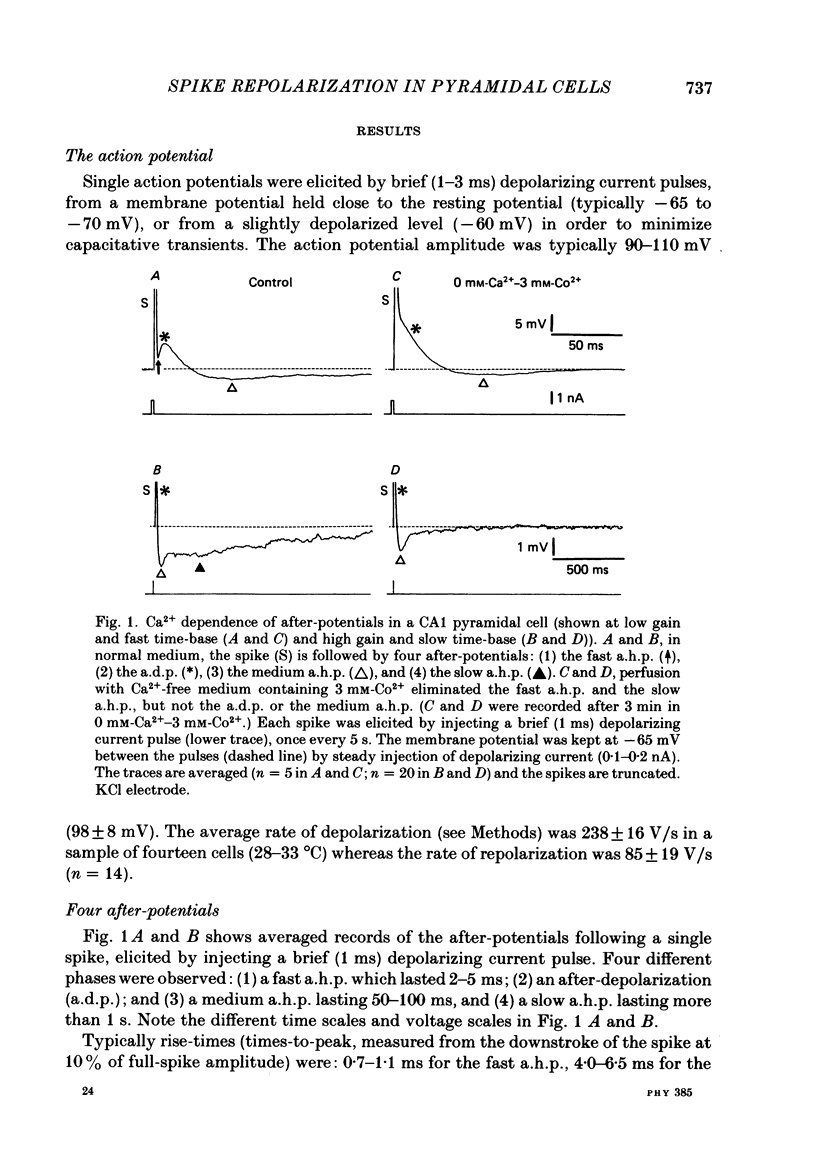
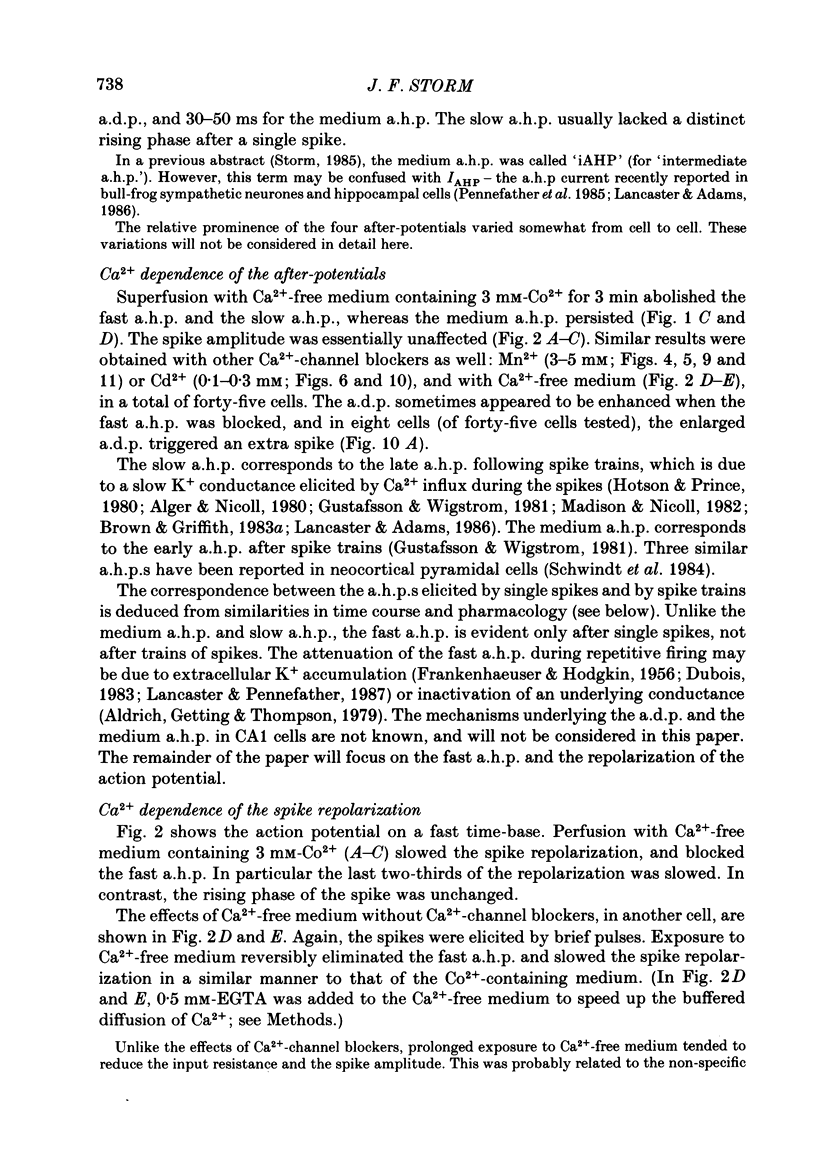
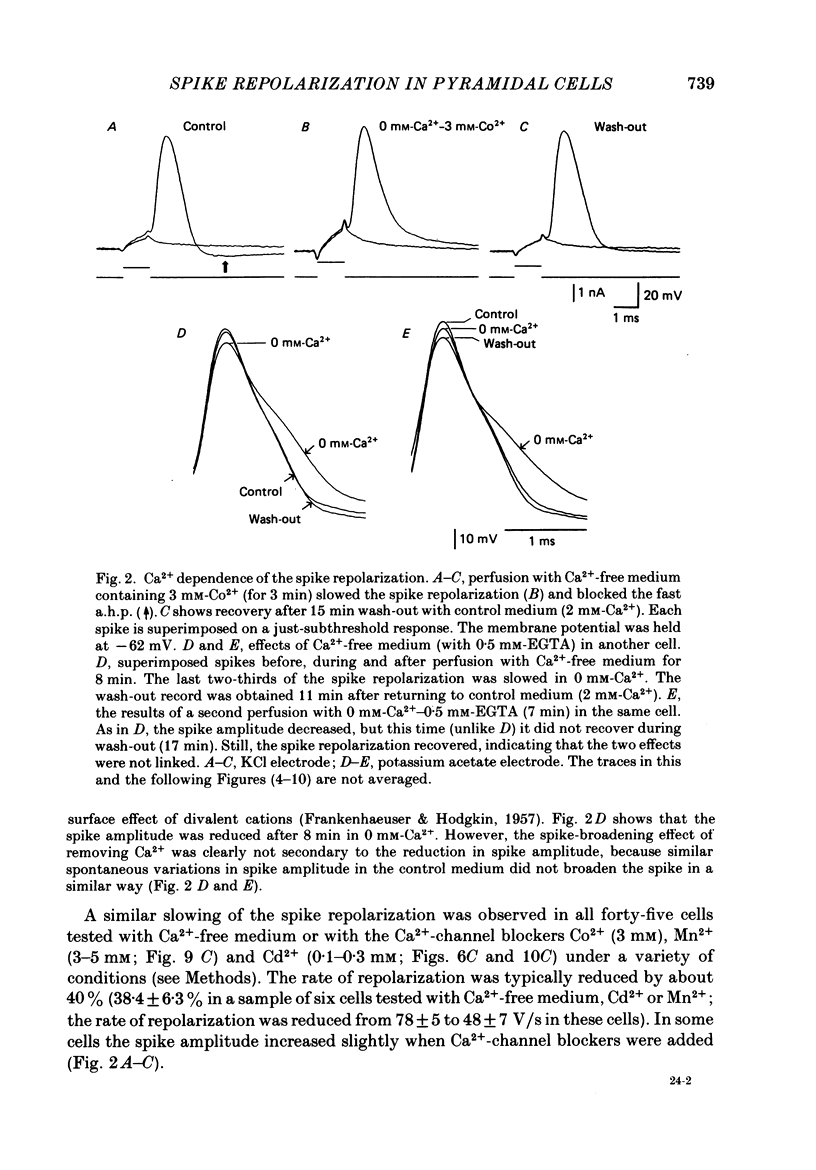
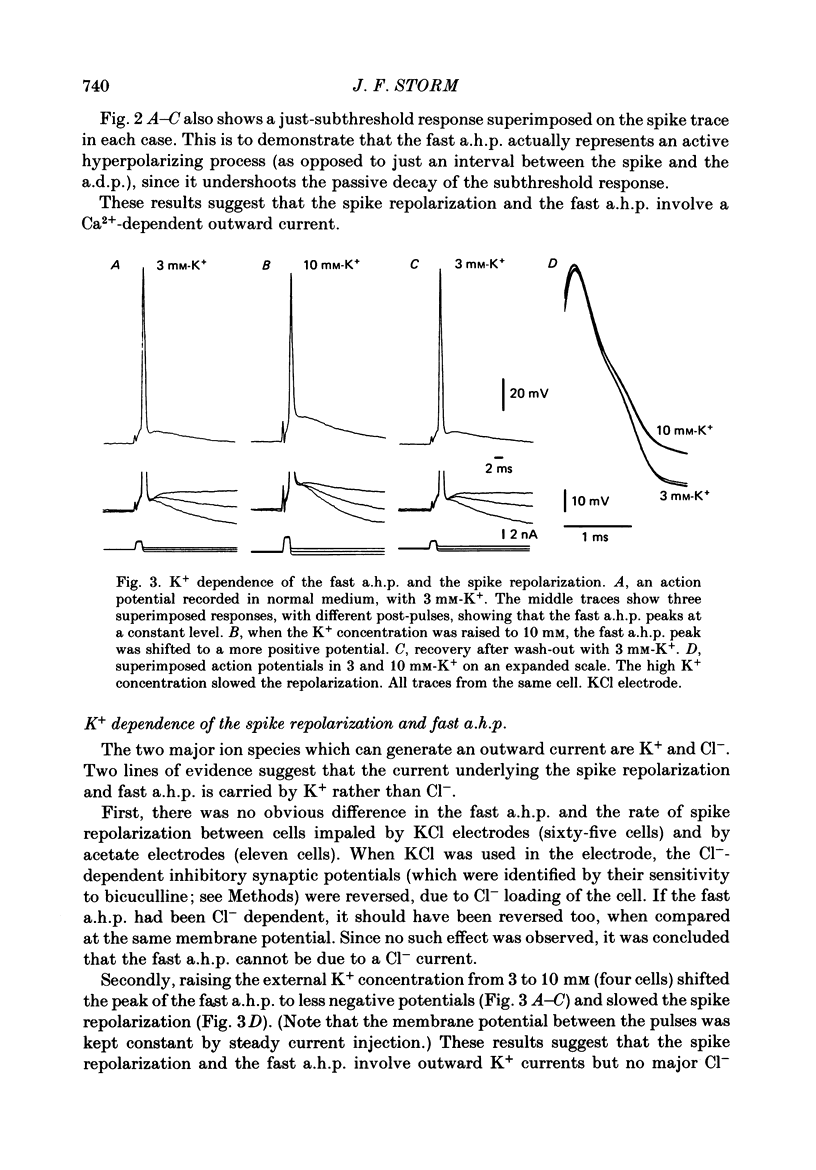
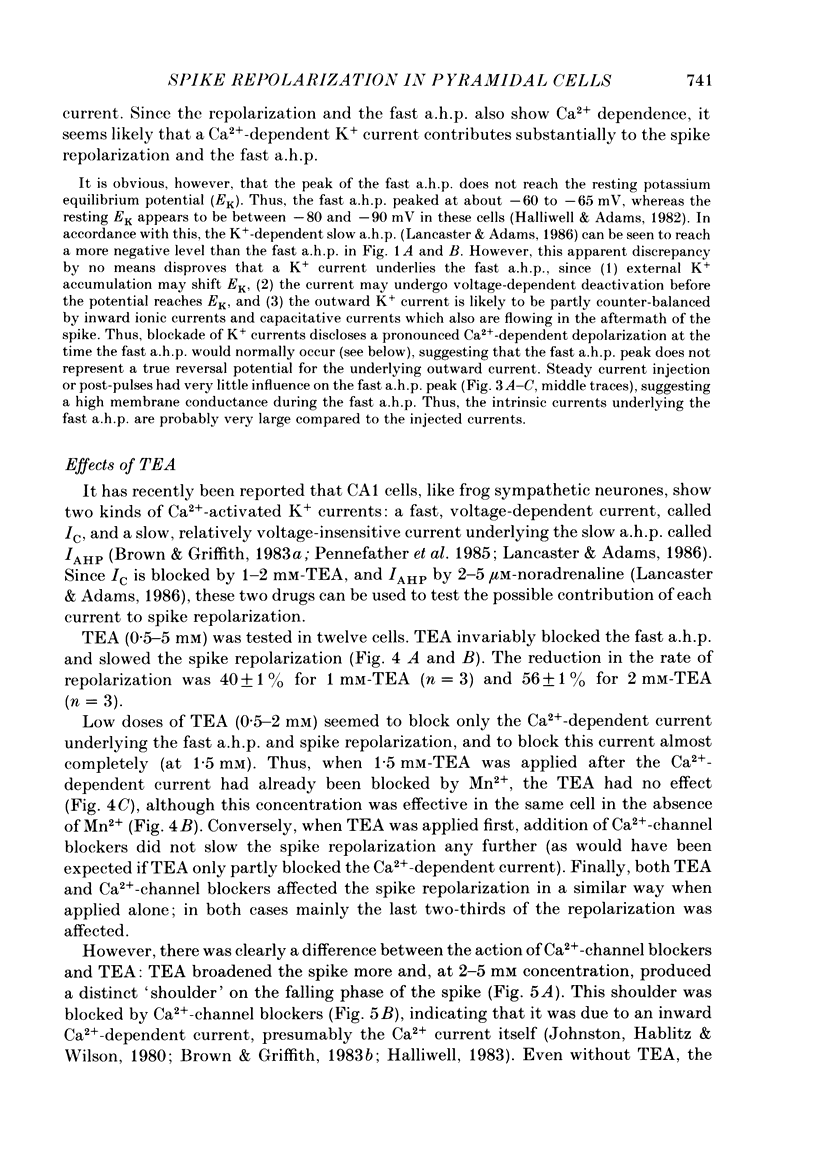
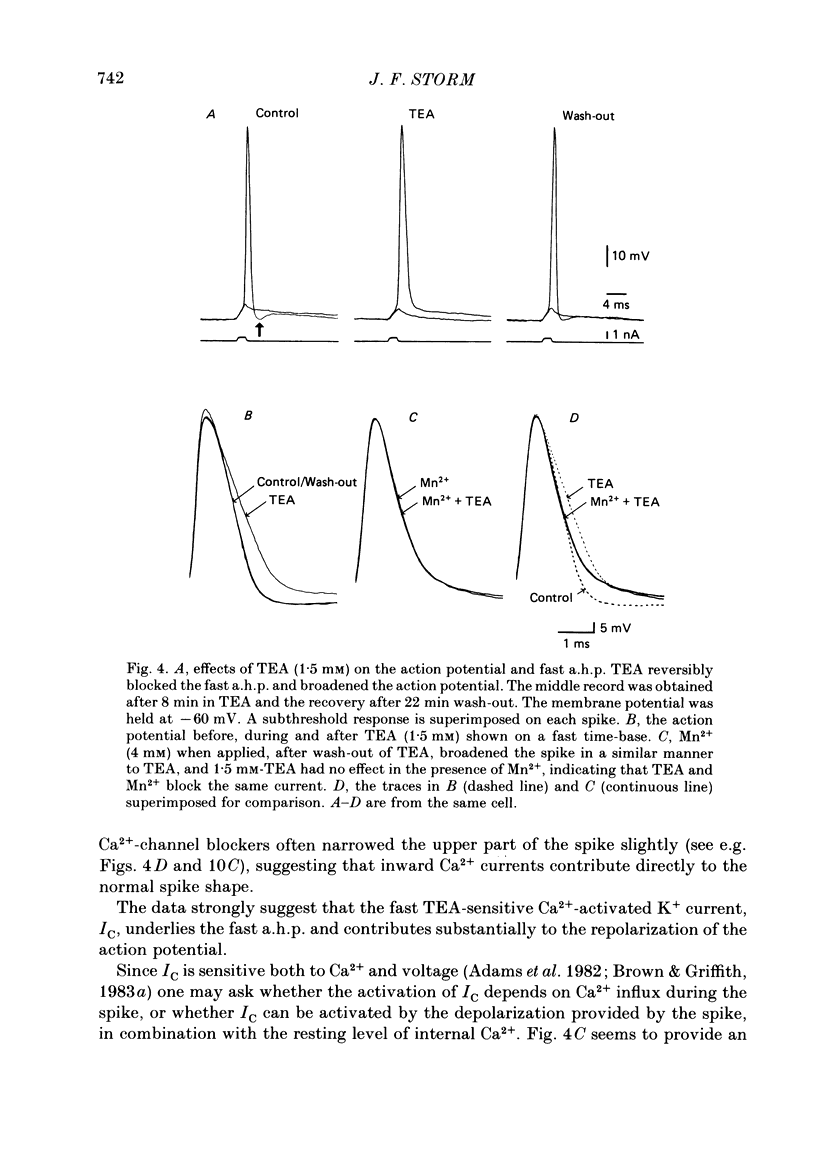
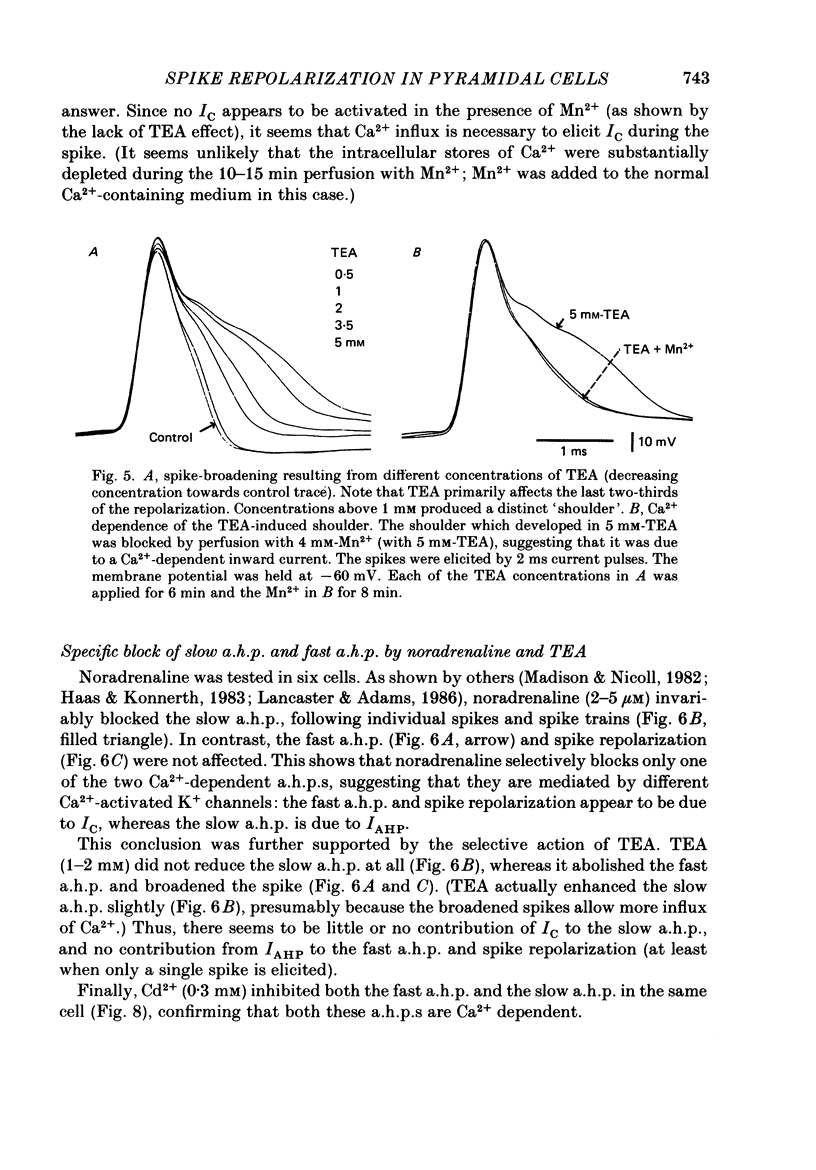
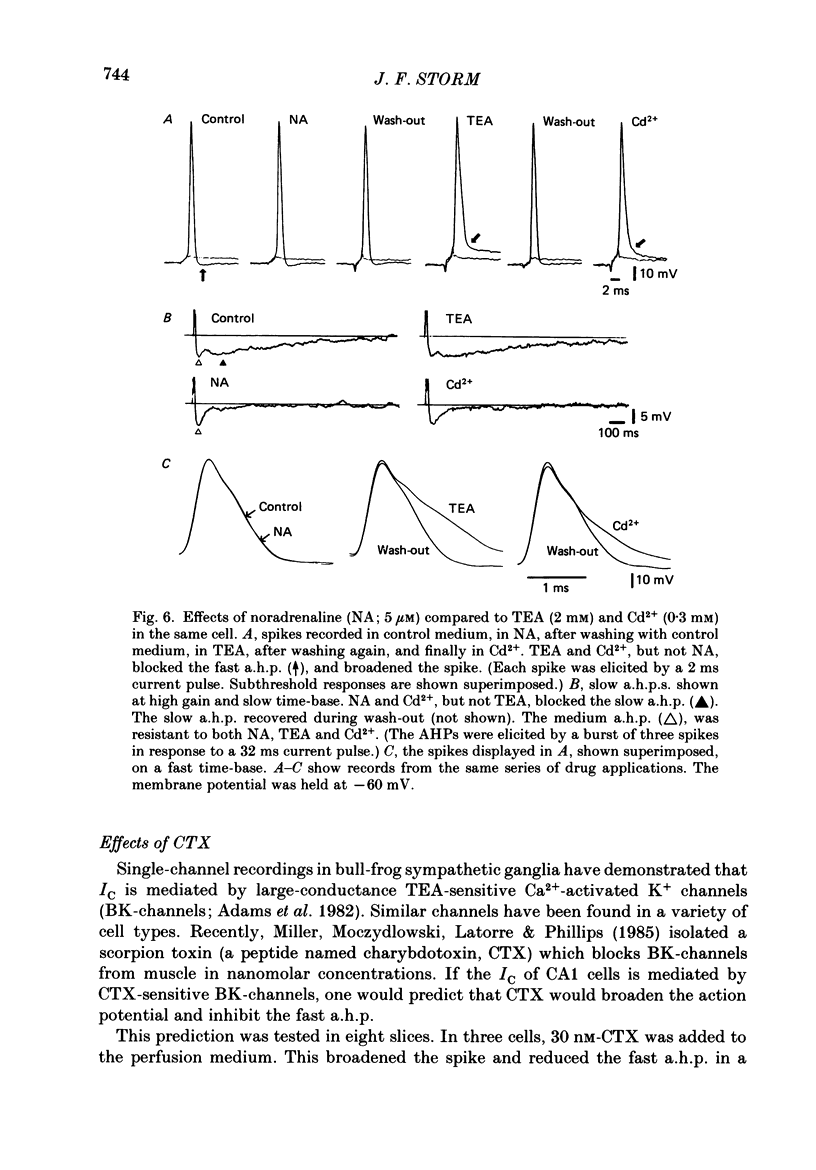
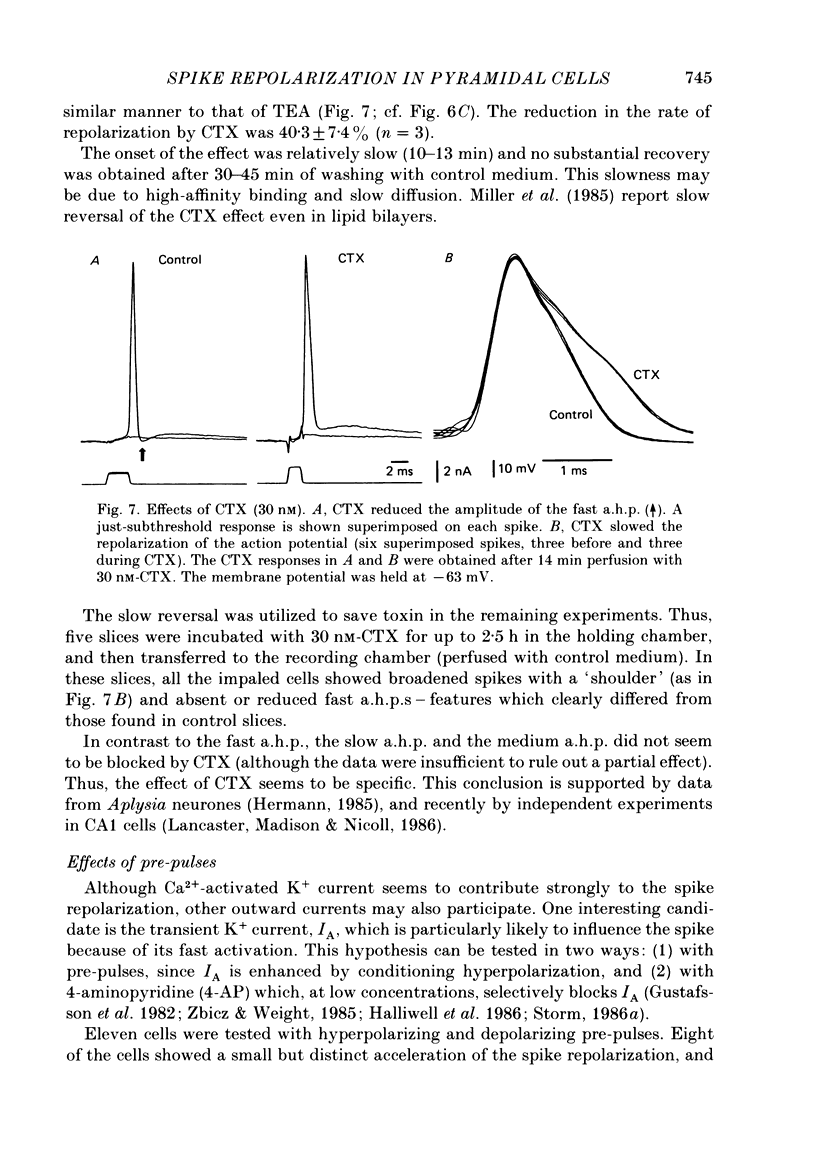
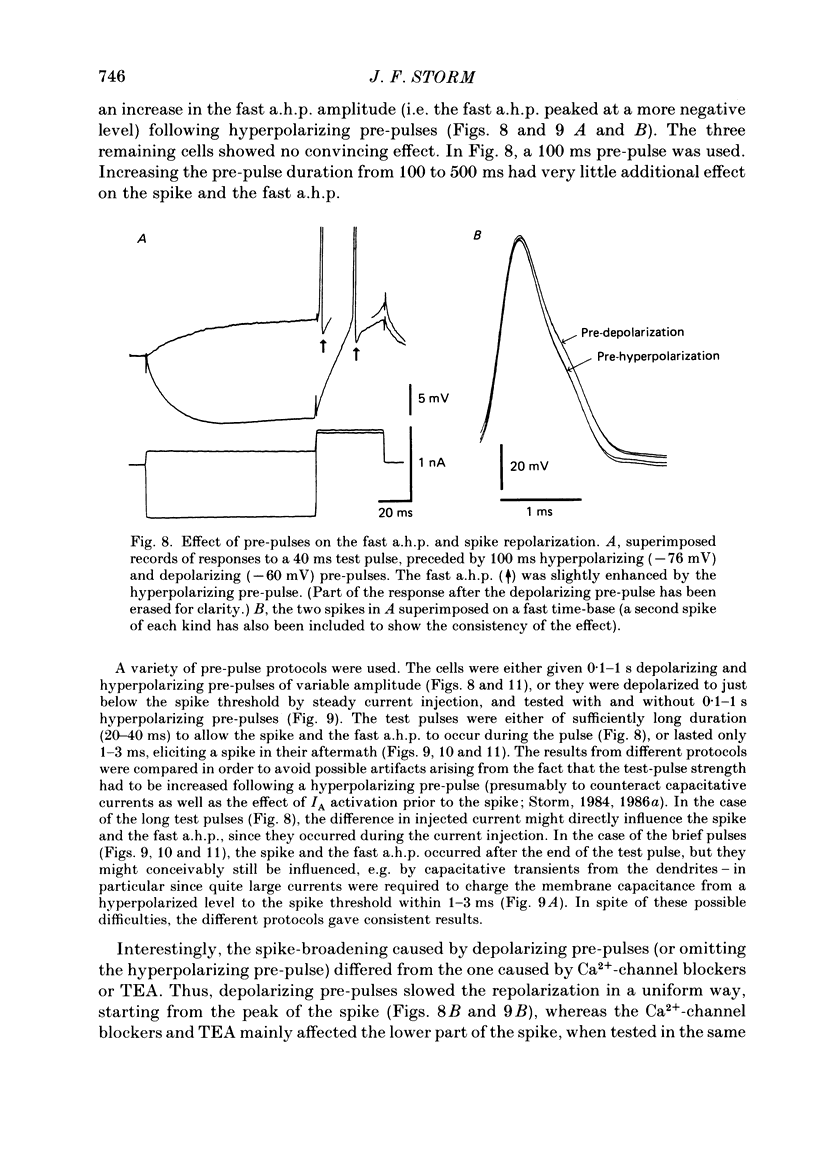
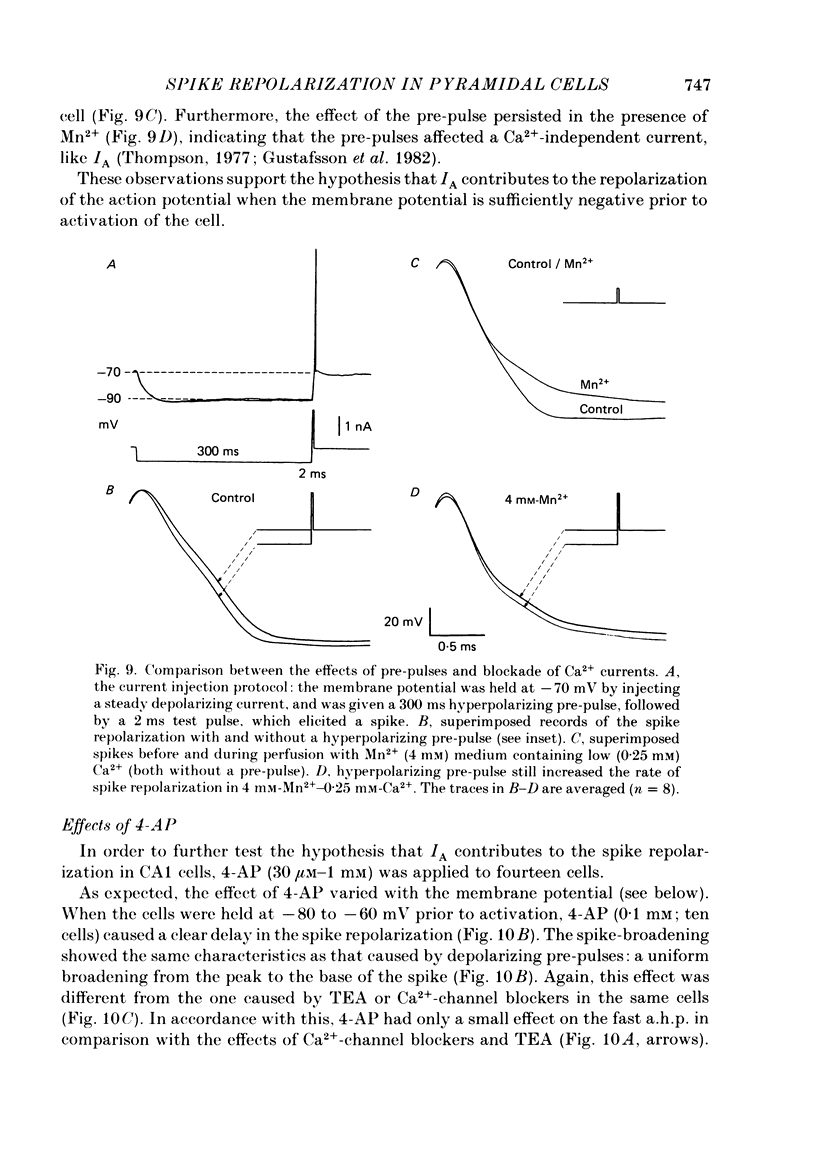
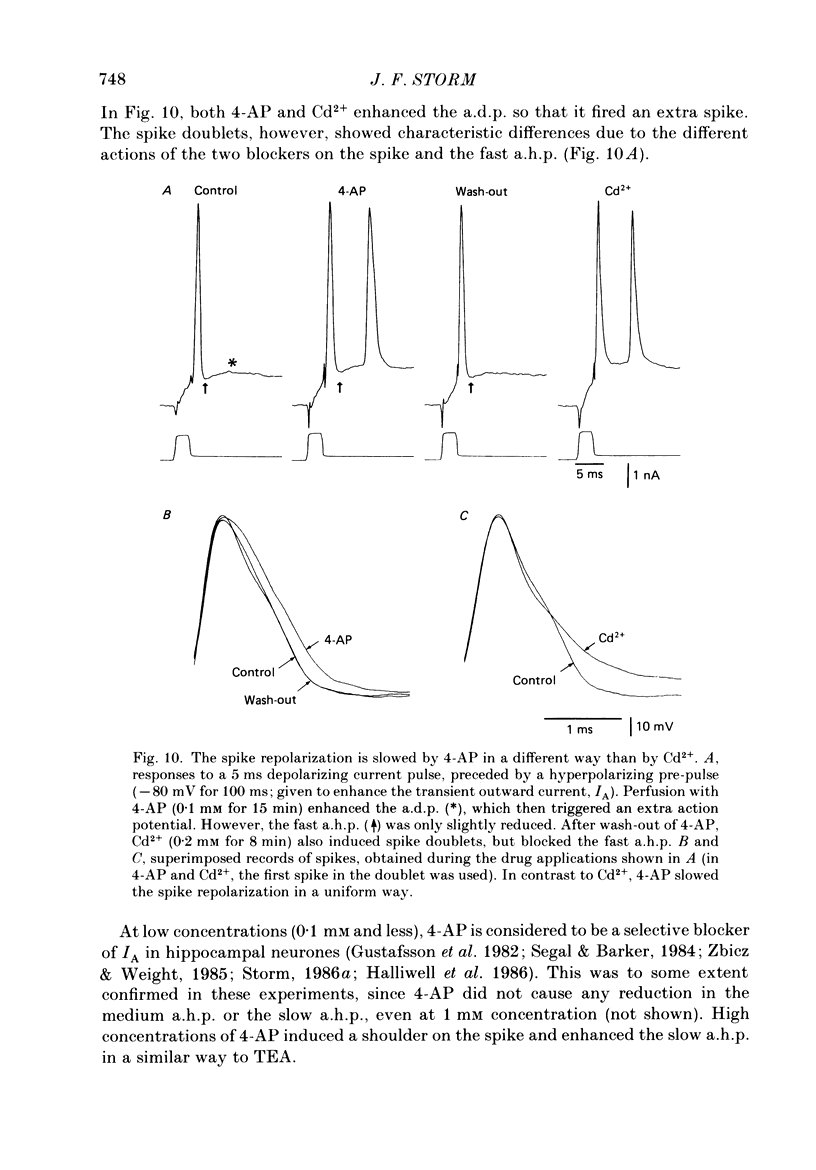
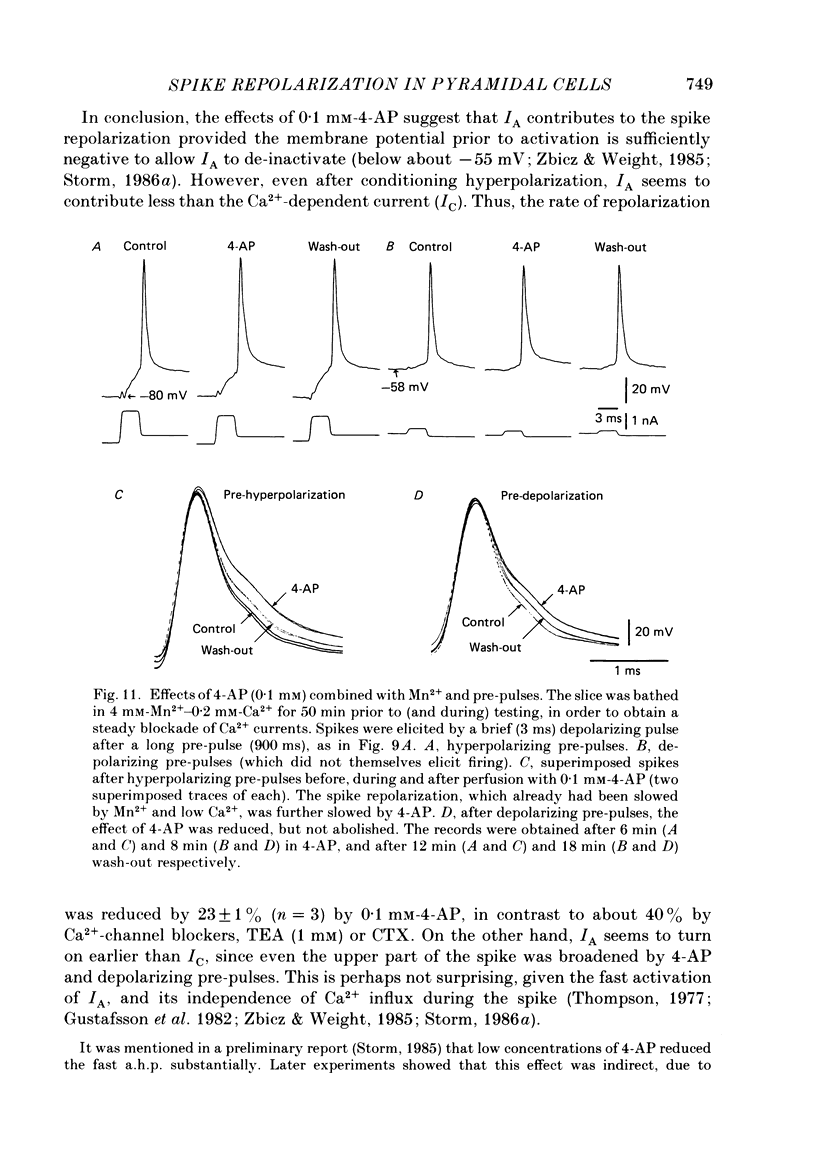
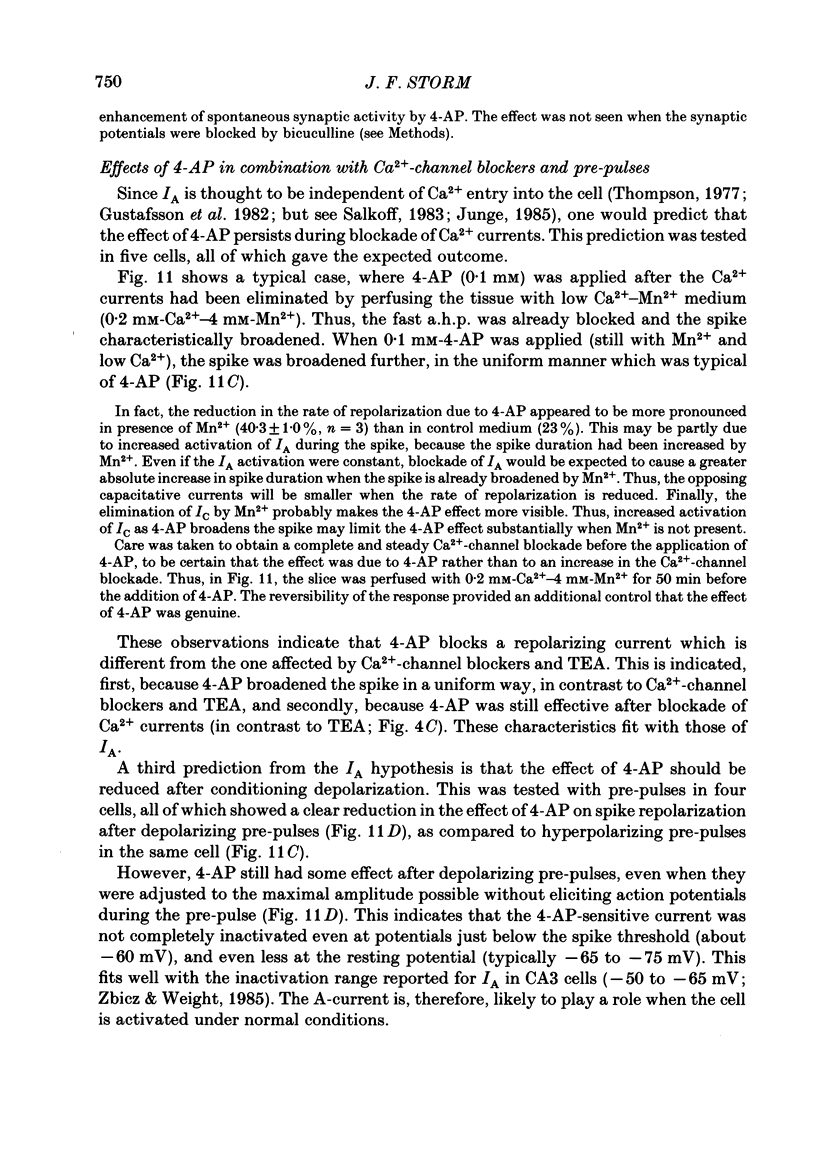
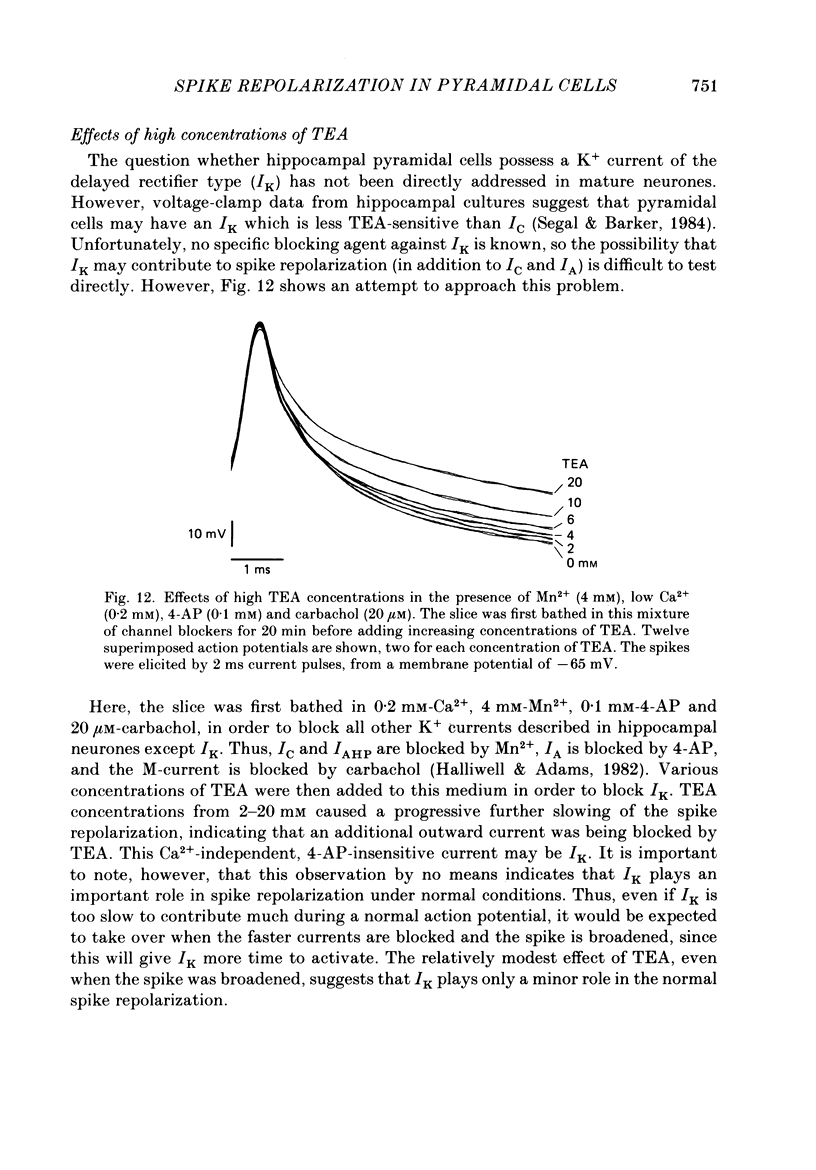
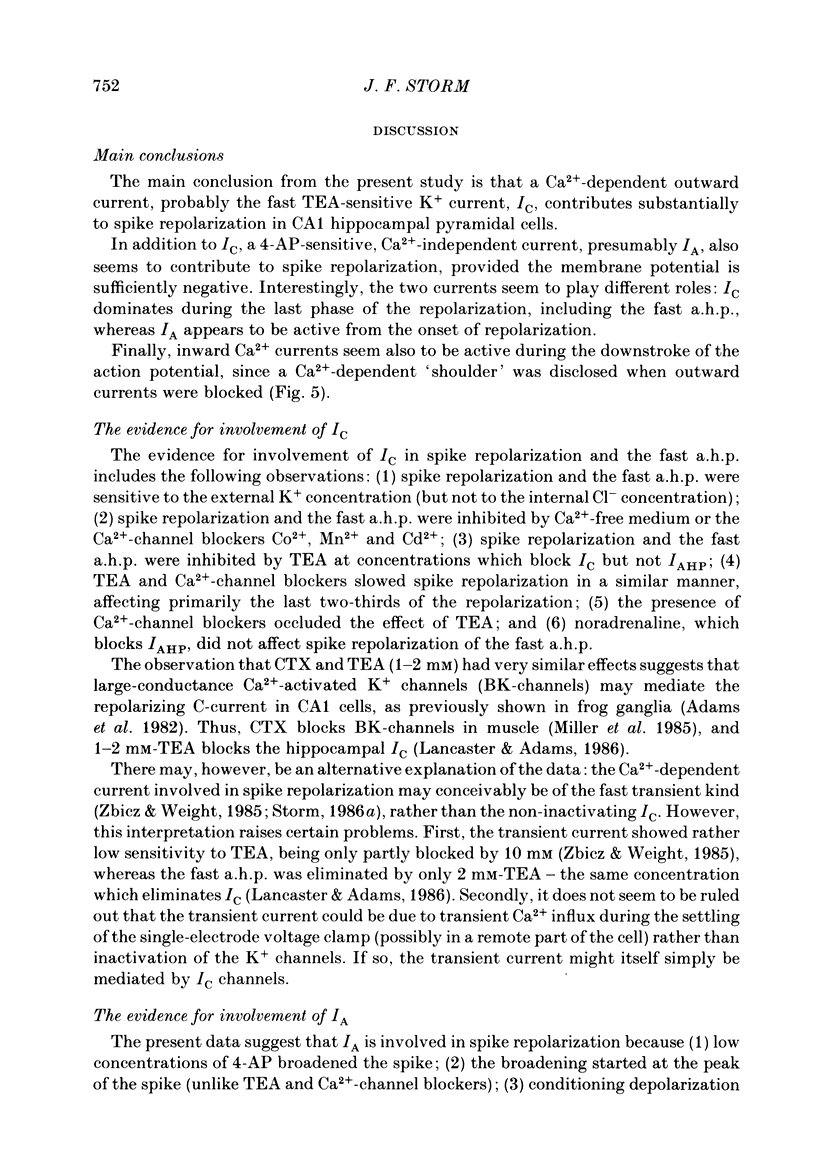
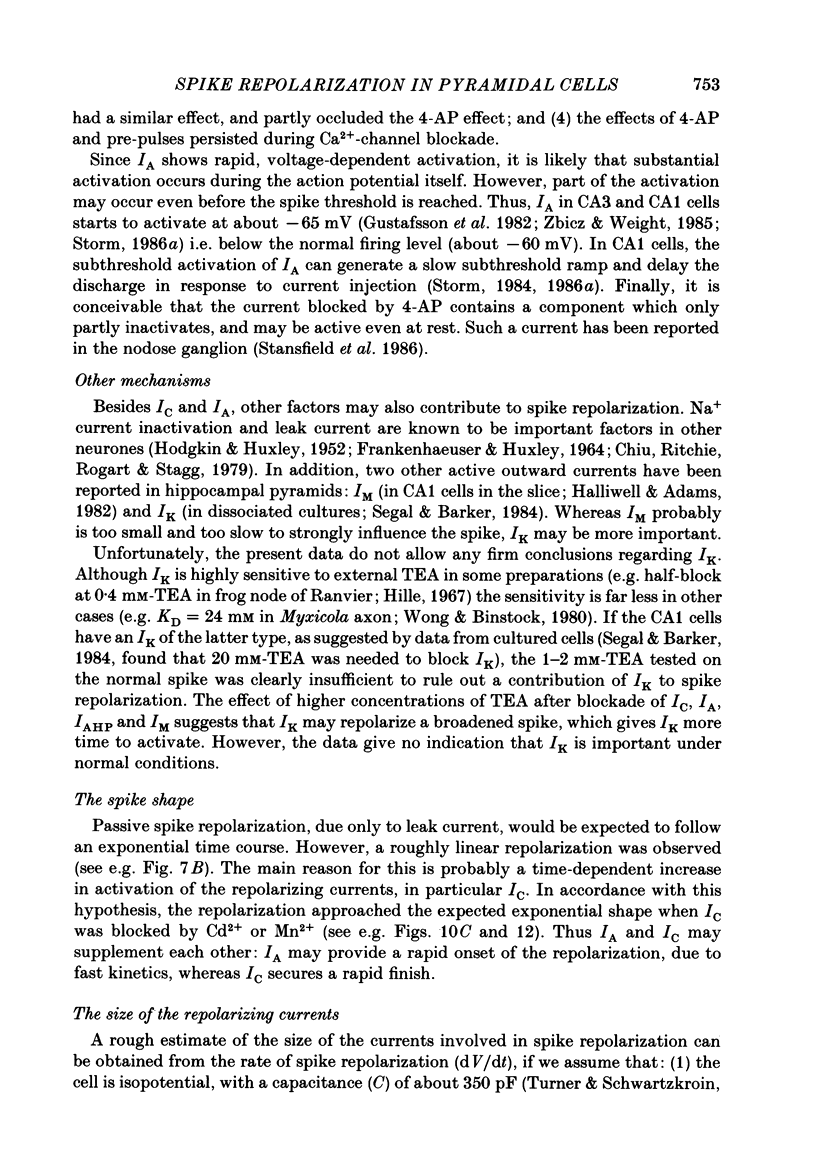
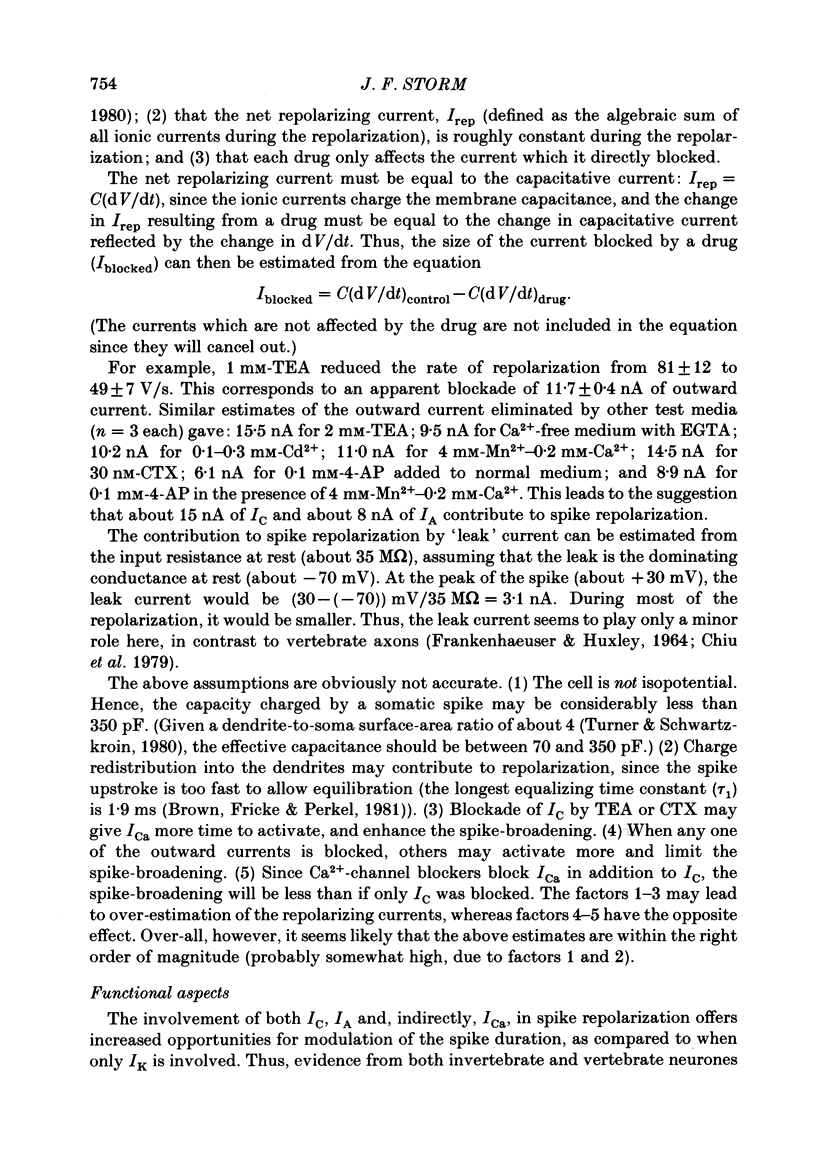
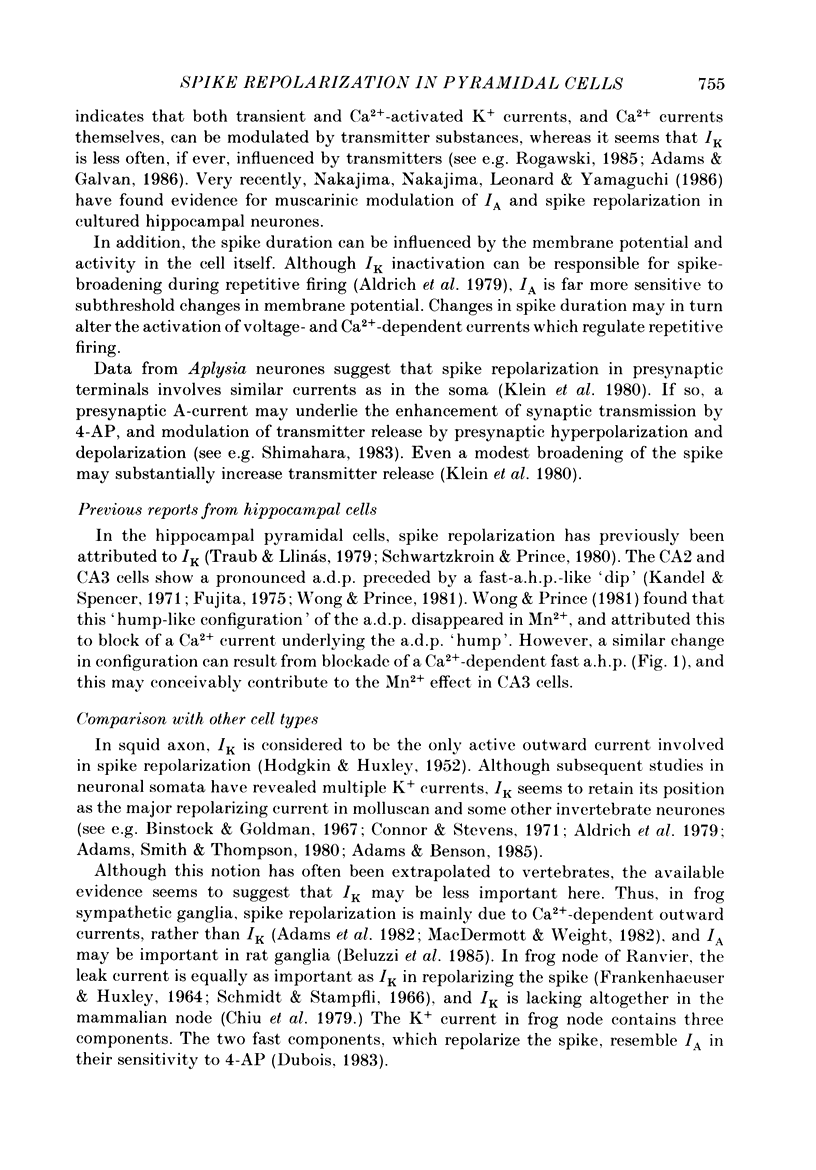
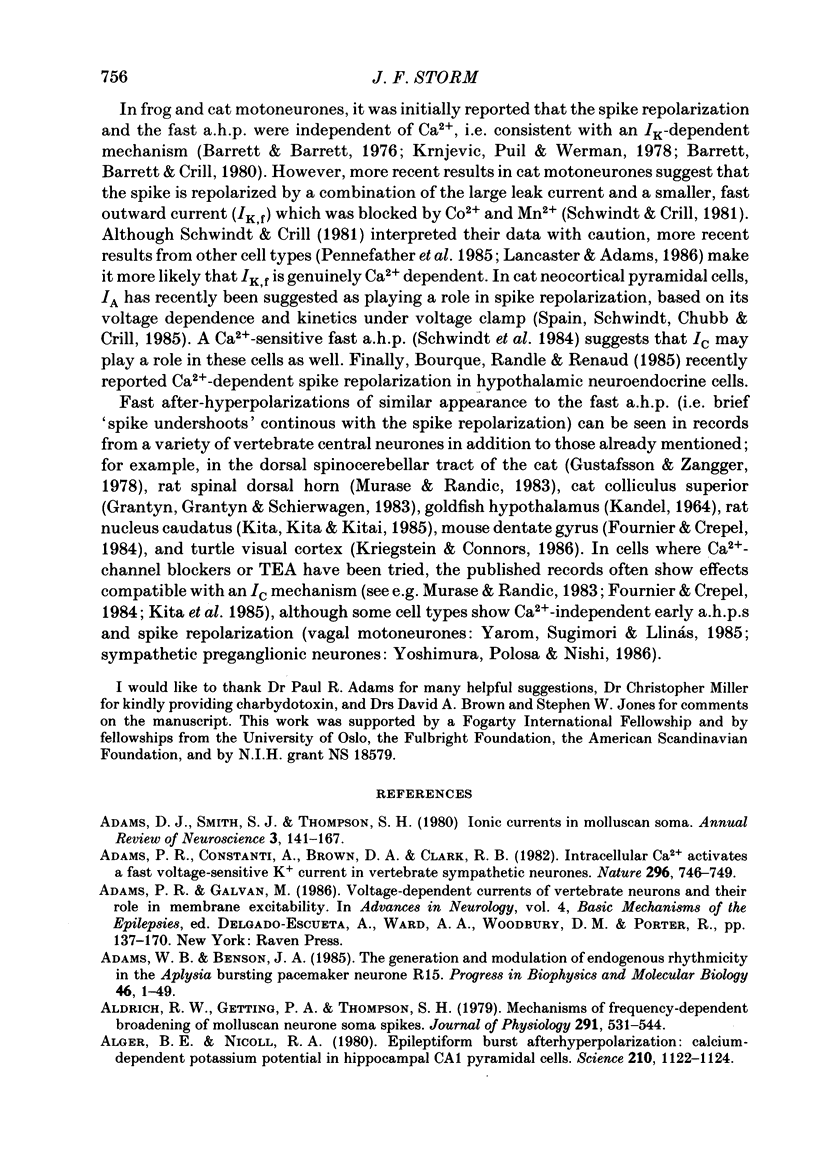
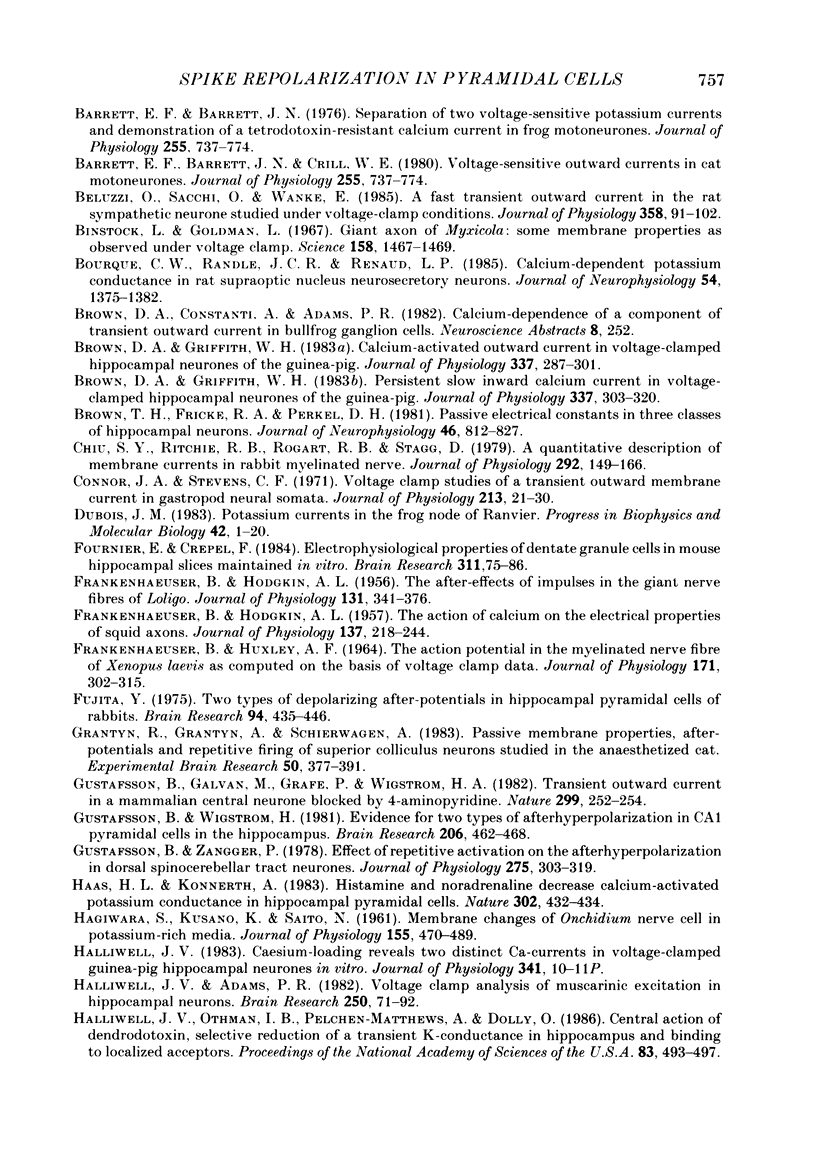
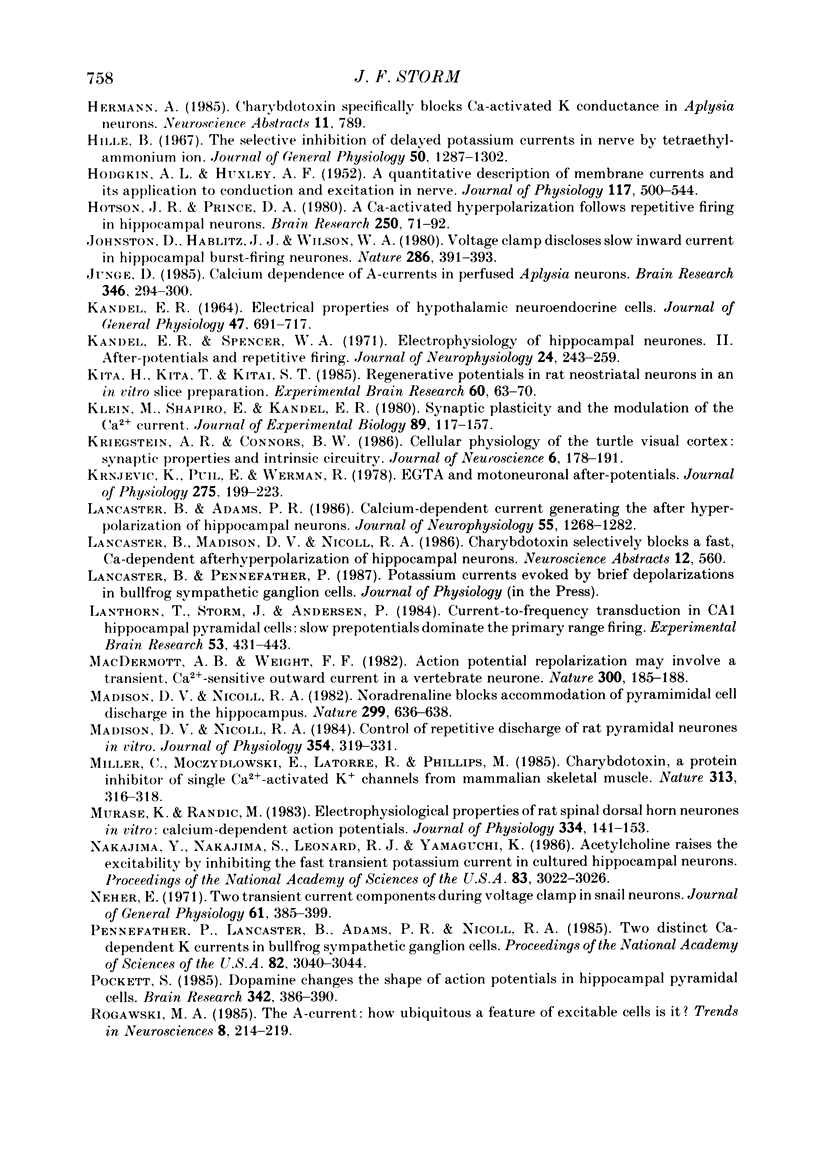
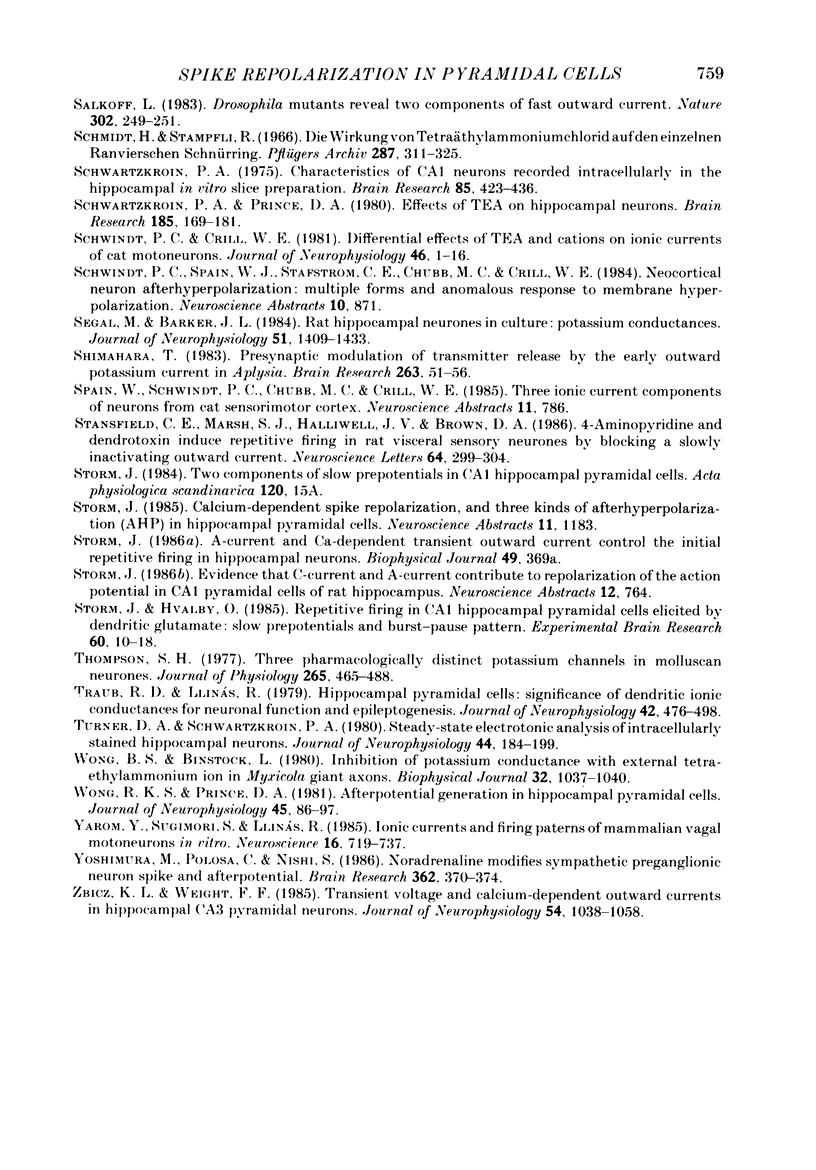
Selected References
These references are in PubMed. This may not be the complete list of references from this article.
- Adams D. J., Smith S. J., Thompson S. H. Ionic currents in molluscan soma. Annu Rev Neurosci. 1980;3:141–167. doi: 10.1146/annurev.ne.03.030180.001041. [DOI] [PubMed] [Google Scholar]
- Adams P. R., Constanti A., Brown D. A., Clark R. B. Intracellular Ca2+ activates a fast voltage-sensitive K+ current in vertebrate sympathetic neurones. Nature. 1982 Apr 22;296(5859):746–749. doi: 10.1038/296746a0. [DOI] [PubMed] [Google Scholar]
- Adams P. R., Galvan M. Voltage-dependent currents of vertebrate neurons and their role in membrane excitability. Adv Neurol. 1986;44:137–170. [PubMed] [Google Scholar]
- Adams W. B., Benson J. A. The generation and modulation of endogenous rhythmicity in the Aplysia bursting pacemaker neurone R15. Prog Biophys Mol Biol. 1985;46(1):1–49. doi: 10.1016/0079-6107(85)90011-2. [DOI] [PubMed] [Google Scholar]
- Aldrich R. W., Jr, Getting P. A., Thompson S. H. Mechanism of frequency-dependent broadening of molluscan neurone soma spikes. J Physiol. 1979 Jun;291:531–544. doi: 10.1113/jphysiol.1979.sp012829. [DOI] [PMC free article] [PubMed] [Google Scholar]
- Alger B. E., Nicoll R. A. Epileptiform burst afterhyperolarization: calcium-dependent potassium potential in hippocampal CA1 pyramidal cells. Science. 1980 Dec 5;210(4474):1122–1124. doi: 10.1126/science.7444438. [DOI] [PubMed] [Google Scholar]
- Barrett E. F., Barret J. N. Separation of two voltage-sensitive potassium currents, and demonstration of a tetrodotoxin-resistant calcium current in frog motoneurones. J Physiol. 1976 Mar;255(3):737–774. doi: 10.1113/jphysiol.1976.sp011306. [DOI] [PMC free article] [PubMed] [Google Scholar]
- Barrett E. F., Barret J. N. Separation of two voltage-sensitive potassium currents, and demonstration of a tetrodotoxin-resistant calcium current in frog motoneurones. J Physiol. 1976 Mar;255(3):737–774. doi: 10.1113/jphysiol.1976.sp011306. [DOI] [PMC free article] [PubMed] [Google Scholar]
- Belluzzi O., Sacchi O., Wanke E. A fast transient outward current in the rat sympathetic neurone studied under voltage-clamp conditions. J Physiol. 1985 Jan;358:91–108. doi: 10.1113/jphysiol.1985.sp015542. [DOI] [PMC free article] [PubMed] [Google Scholar]
- Binstock L., Goldman L. Giant axon of Myxicola: some membrane properties as observed under voltage clamp. Science. 1967 Dec 15;158(3807):1467–1469. doi: 10.1126/science.158.3807.1467. [DOI] [PubMed] [Google Scholar]
- Bourque C. W., Randle J. C., Renaud L. P. Calcium-dependent potassium conductance in rat supraoptic nucleus neurosecretory neurons. J Neurophysiol. 1985 Dec;54(6):1375–1382. doi: 10.1152/jn.1985.54.6.1375. [DOI] [PubMed] [Google Scholar]
- Brown D. A., Griffith W. H. Calcium-activated outward current in voltage-clamped hippocampal neurones of the guinea-pig. J Physiol. 1983 Apr;337:287–301. doi: 10.1113/jphysiol.1983.sp014624. [DOI] [PMC free article] [PubMed] [Google Scholar]
- Brown D. A., Griffith W. H. Persistent slow inward calcium current in voltage-clamped hippocampal neurones of the guinea-pig. J Physiol. 1983 Apr;337:303–320. doi: 10.1113/jphysiol.1983.sp014625. [DOI] [PMC free article] [PubMed] [Google Scholar]
- Brown T. H., Fricke R. A., Perkel D. H. Passive electrical constants in three classes of hippocampal neurons. J Neurophysiol. 1981 Oct;46(4):812–827. doi: 10.1152/jn.1981.46.4.812. [DOI] [PubMed] [Google Scholar]
- Chiu S. Y., Ritchie J. M., Rogart R. B., Stagg D. A quantitative description of membrane currents in rabbit myelinated nerve. J Physiol. 1979 Jul;292:149–166. doi: 10.1113/jphysiol.1979.sp012843. [DOI] [PMC free article] [PubMed] [Google Scholar]
- Connor J. A., Stevens C. F. Voltage clamp studies of a transient outward membrane current in gastropod neural somata. J Physiol. 1971 Feb;213(1):21–30. doi: 10.1113/jphysiol.1971.sp009365. [DOI] [PMC free article] [PubMed] [Google Scholar]
- Dubois J. M. Potassium currents in the frog node of Ranvier. Prog Biophys Mol Biol. 1983;42(1):1–20. doi: 10.1016/0079-6107(83)90002-0. [DOI] [PubMed] [Google Scholar]
- FRANKENHAEUSER B., HODGKIN A. L. The action of calcium on the electrical properties of squid axons. J Physiol. 1957 Jul 11;137(2):218–244. doi: 10.1113/jphysiol.1957.sp005808. [DOI] [PMC free article] [PubMed] [Google Scholar]
- FRANKENHAEUSER B., HODGKIN A. L. The after-effects of impulses in the giant nerve fibres of Loligo. J Physiol. 1956 Feb 28;131(2):341–376. doi: 10.1113/jphysiol.1956.sp005467. [DOI] [PMC free article] [PubMed] [Google Scholar]
- FRANKENHAEUSER B., HUXLEY A. F. THE ACTION POTENTIAL IN THE MYELINATED NERVE FIBER OF XENOPUS LAEVIS AS COMPUTED ON THE BASIS OF VOLTAGE CLAMP DATA. J Physiol. 1964 Jun;171:302–315. doi: 10.1113/jphysiol.1964.sp007378. [DOI] [PMC free article] [PubMed] [Google Scholar]
- Fournier E., Crepel F. Electrophysiological properties of dentate granule cells in mouse hippocampal slices maintained in vitro. Brain Res. 1984 Oct 8;311(1):75–86. doi: 10.1016/0006-8993(84)91400-8. [DOI] [PubMed] [Google Scholar]
- Fujita Y. Two types of depolarizing after-potentials in hippocampal pyramidal cells of rabbits. Brain Res. 1975 Sep 5;94(3):435–446. doi: 10.1016/0006-8993(75)90227-9. [DOI] [PubMed] [Google Scholar]
- Grantyn R., Grantyn A., Schierwagen A. Passive membrane properties, afterpotentials and repetitive firing of superior colliculus neurons studied in the anesthetized cat. Exp Brain Res. 1983;50(2-3):377–391. doi: 10.1007/BF00239204. [DOI] [PubMed] [Google Scholar]
- Gustafsson B., Galvan M., Grafe P., Wigström H. A transient outward current in a mammalian central neurone blocked by 4-aminopyridine. Nature. 1982 Sep 16;299(5880):252–254. doi: 10.1038/299252a0. [DOI] [PubMed] [Google Scholar]
- Gustafsson B., Wigström H. Evidence for two types of afterhyperpolarization in CA1 pyramidal cells in the hippocampus. Brain Res. 1981 Feb 16;206(2):462–468. doi: 10.1016/0006-8993(81)90548-5. [DOI] [PubMed] [Google Scholar]
- Gustafsson B., Zangger P. Effect of repetitive activation on the afterhyperpolarization in dorsal spinocerebellar tract neurones. J Physiol. 1978 Feb;275:303–319. doi: 10.1113/jphysiol.1978.sp012191. [DOI] [PMC free article] [PubMed] [Google Scholar]
- HAGIWARA S., KUSANO K., SAITO N. Membrane changes of Onchidium nerve cell in potassium-rich media. J Physiol. 1961 Mar;155:470–489. doi: 10.1113/jphysiol.1961.sp006640. [DOI] [PMC free article] [PubMed] [Google Scholar]
- HODGKIN A. L., HUXLEY A. F. A quantitative description of membrane current and its application to conduction and excitation in nerve. J Physiol. 1952 Aug;117(4):500–544. doi: 10.1113/jphysiol.1952.sp004764. [DOI] [PMC free article] [PubMed] [Google Scholar]
- Haas H. L., Konnerth A. Histamine and noradrenaline decrease calcium-activated potassium conductance in hippocampal pyramidal cells. 1983 Mar 31-Apr 6Nature. 302(5907):432–434. doi: 10.1038/302432a0. [DOI] [PubMed] [Google Scholar]
- Halliwell J. V., Adams P. R. Voltage-clamp analysis of muscarinic excitation in hippocampal neurons. Brain Res. 1982 Oct 28;250(1):71–92. doi: 10.1016/0006-8993(82)90954-4. [DOI] [PubMed] [Google Scholar]
- Halliwell J. V., Othman I. B., Pelchen-Matthews A., Dolly J. O. Central action of dendrotoxin: selective reduction of a transient K conductance in hippocampus and binding to localized acceptors. Proc Natl Acad Sci U S A. 1986 Jan;83(2):493–497. doi: 10.1073/pnas.83.2.493. [DOI] [PMC free article] [PubMed] [Google Scholar]
- Hille B. The selective inhibition of delayed potassium currents in nerve by tetraethylammonium ion. J Gen Physiol. 1967 May;50(5):1287–1302. doi: 10.1085/jgp.50.5.1287. [DOI] [PMC free article] [PubMed] [Google Scholar]
- Junge D. Calcium dependence of A-currents in perfused Aplysia neurons. Brain Res. 1985 Nov 4;346(2):294–300. doi: 10.1016/0006-8993(85)90863-7. [DOI] [PubMed] [Google Scholar]
- KANDEL E. R. ELECTRICAL PROPERTIES OF HYPOTHALAMIC NEUROENDOCRINE CELLS. J Gen Physiol. 1964 Mar;47:691–717. doi: 10.1085/jgp.47.4.691. [DOI] [PMC free article] [PubMed] [Google Scholar]
- KANDEL E. R., SPENCER W. A. Electrophysiology of hippocampal neurons. II. After-potentials and repetitive firing. J Neurophysiol. 1961 May;24:243–259. doi: 10.1152/jn.1961.24.3.243. [DOI] [PubMed] [Google Scholar]
- Kita H., Kita T., Kitai S. T. Regenerative potentials in rat neostriatal neurons in an in vitro slice preparation. Exp Brain Res. 1985;60(1):63–70. doi: 10.1007/BF00237019. [DOI] [PubMed] [Google Scholar]
- Klein M., Shapiro E., Kandel E. R. Synaptic plasticity and the modulation of the Ca2+ current. J Exp Biol. 1980 Dec;89:117–157. doi: 10.1242/jeb.89.1.117. [DOI] [PubMed] [Google Scholar]
- Kriegstein A. R., Connors B. W. Cellular physiology of the turtle visual cortex: synaptic properties and intrinsic circuitry. J Neurosci. 1986 Jan;6(1):178–191. doi: 10.1523/JNEUROSCI.06-01-00178.1986. [DOI] [PMC free article] [PubMed] [Google Scholar]
- Krnjević K., Puil E., Werman R. EGTA and motoneuronal after-potentials. J Physiol. 1978 Feb;275:199–223. doi: 10.1113/jphysiol.1978.sp012186. [DOI] [PMC free article] [PubMed] [Google Scholar]
- Lancaster B., Adams P. R. Calcium-dependent current generating the afterhyperpolarization of hippocampal neurons. J Neurophysiol. 1986 Jun;55(6):1268–1282. doi: 10.1152/jn.1986.55.6.1268. [DOI] [PubMed] [Google Scholar]
- Lanthorn T., Storm J., Andersen P. Current-to-frequency transduction in CA1 hippocampal pyramidal cells: slow prepotentials dominate the primary range firing. Exp Brain Res. 1984;53(2):431–443. doi: 10.1007/BF00238173. [DOI] [PubMed] [Google Scholar]
- MacDermott A. B., Weight F. F. Action potential repolarization may involve a transient, Ca2+-sensitive outward current in a vertebrate neurone. Nature. 1982 Nov 11;300(5888):185–188. doi: 10.1038/300185a0. [DOI] [PubMed] [Google Scholar]
- Madison D. V., Nicoll R. A. Noradrenaline blocks accommodation of pyramidal cell discharge in the hippocampus. Nature. 1982 Oct 14;299(5884):636–638. doi: 10.1038/299636a0. [DOI] [PubMed] [Google Scholar]
- Miller C., Moczydlowski E., Latorre R., Phillips M. Charybdotoxin, a protein inhibitor of single Ca2+-activated K+ channels from mammalian skeletal muscle. Nature. 1985 Jan 24;313(6000):316–318. doi: 10.1038/313316a0. [DOI] [PubMed] [Google Scholar]
- Murase K., Randić M. Electrophysiological properties of rat spinal dorsal horn neurones in vitro: calcium-dependent action potentials. J Physiol. 1983 Jan;334:141–153. doi: 10.1113/jphysiol.1983.sp014485. [DOI] [PMC free article] [PubMed] [Google Scholar]
- Nakajima Y., Nakajima S., Leonard R. J., Yamaguchi K. Acetylcholine raises excitability by inhibiting the fast transient potassium current in cultured hippocampal neurons. Proc Natl Acad Sci U S A. 1986 May;83(9):3022–3026. doi: 10.1073/pnas.83.9.3022. [DOI] [PMC free article] [PubMed] [Google Scholar]
- Pennefather P., Lancaster B., Adams P. R., Nicoll R. A. Two distinct Ca-dependent K currents in bullfrog sympathetic ganglion cells. Proc Natl Acad Sci U S A. 1985 May;82(9):3040–3044. doi: 10.1073/pnas.82.9.3040. [DOI] [PMC free article] [PubMed] [Google Scholar]
- Pockett S. Dopamine changes the shape of action potentials in hippocampal pyramidal cells. Brain Res. 1985 Sep 9;342(2):386–390. doi: 10.1016/0006-8993(85)91143-6. [DOI] [PubMed] [Google Scholar]
- Salkoff L. Drosophila mutants reveal two components of fast outward current. Nature. 1983 Mar 17;302(5905):249–251. doi: 10.1038/302249a0. [DOI] [PubMed] [Google Scholar]
- Schmidt H., Stämpfli R. Die Wirkung von Tetraäthylammoniumchlorid auf den einzelnen Ranvierschen Schnürring. Pflugers Arch Gesamte Physiol Menschen Tiere. 1966;287(4):311–325. [PubMed] [Google Scholar]
- Schwartzkroin P. A. Characteristics of CA1 neurons recorded intracellularly in the hippocampal in vitro slice preparation. Brain Res. 1975 Mar 7;85(3):423–436. doi: 10.1016/0006-8993(75)90817-3. [DOI] [PubMed] [Google Scholar]
- Schwartzkroin P. A., Prince D. A. Effects of TEA on hippocampal neurons. Brain Res. 1980 Mar 3;185(1):169–181. doi: 10.1016/0006-8993(80)90680-0. [DOI] [PubMed] [Google Scholar]
- Schwindt P. C., Crill W. E. Differential effects of TEA and cations on outward ionic currents of cat motoneurons. J Neurophysiol. 1981 Jul;46(1):1–16. doi: 10.1152/jn.1981.46.1.1. [DOI] [PubMed] [Google Scholar]
- Segal M., Barker J. L. Rat hippocampal neurons in culture: potassium conductances. J Neurophysiol. 1984 Jun;51(6):1409–1433. doi: 10.1152/jn.1984.51.6.1409. [DOI] [PubMed] [Google Scholar]
- Shimahara T. Presynaptic modulation of transmitter release by the early outward potassium current in Aplysia. Brain Res. 1983 Mar 14;263(1):51–56. doi: 10.1016/0006-8993(83)91199-x. [DOI] [PubMed] [Google Scholar]
- Stansfeld C. E., Marsh S. J., Halliwell J. V., Brown D. A. 4-Aminopyridine and dendrotoxin induce repetitive firing in rat visceral sensory neurones by blocking a slowly inactivating outward current. Neurosci Lett. 1986 Mar 14;64(3):299–304. doi: 10.1016/0304-3940(86)90345-9. [DOI] [PubMed] [Google Scholar]
- Storm J., Hvalby O. Repetitive firing of CA1 hippocampal pyramidal cells elicited by dendritic glutamate: slow prepotentials and burst-pause pattern. Exp Brain Res. 1985;60(1):10–18. doi: 10.1007/BF00237013. [DOI] [PubMed] [Google Scholar]
- Thompson S. H. Three pharmacologically distinct potassium channels in molluscan neurones. J Physiol. 1977 Feb;265(2):465–488. doi: 10.1113/jphysiol.1977.sp011725. [DOI] [PMC free article] [PubMed] [Google Scholar]
- Traub R. D., Llinás R. Hippocampal pyramidal cells: significance of dendritic ionic conductances for neuronal function and epileptogenesis. J Neurophysiol. 1979 Mar;42(2):476–496. doi: 10.1152/jn.1979.42.2.476. [DOI] [PubMed] [Google Scholar]
- Turner D. A., Schwartzkroin P. A. Steady-state electrotonic analysis of intracellularly stained hippocampal neurons. J Neurophysiol. 1980 Jul;44(1):184–199. doi: 10.1152/jn.1980.44.1.184. [DOI] [PubMed] [Google Scholar]
- Wong B. S., Binstock L. Inhibition of potassium conductance with external tetraethylammonium ion in Myxicola giant axons. Biophys J. 1980 Dec;32(3):1037–1042. doi: 10.1016/S0006-3495(80)85034-X. [DOI] [PMC free article] [PubMed] [Google Scholar]
- Wong R. K., Prince D. A. Afterpotential generation in hippocampal pyramidal cells. J Neurophysiol. 1981 Jan;45(1):86–97. doi: 10.1152/jn.1981.45.1.86. [DOI] [PubMed] [Google Scholar]
- Yarom Y., Sugimori M., Llinás R. Ionic currents and firing patterns of mammalian vagal motoneurons in vitro. Neuroscience. 1985 Dec;16(4):719–737. doi: 10.1016/0306-4522(85)90090-9. [DOI] [PubMed] [Google Scholar]
- Yoshimura M., Polosa C., Nishi S. Noradrenaline modifies sympathetic preganglionic neuron spike and afterpotential. Brain Res. 1986 Jan 8;362(2):370–374. doi: 10.1016/0006-8993(86)90466-x. [DOI] [PubMed] [Google Scholar]
- Zbicz K. L., Weight F. F. Transient voltage and calcium-dependent outward currents in hippocampal CA3 pyramidal neurons. J Neurophysiol. 1985 Apr;53(4):1038–1058. doi: 10.1152/jn.1985.53.4.1038. [DOI] [PubMed] [Google Scholar]