Abstract
1. The Vaseline-gap technique was used to record slow calcium currents and asymmetric charge movement in single fibres of fast-twitch muscles (extensor digitorum longus (e.d.l.) and sternomastoid) and slow-twitch muscles (soleus) from rat and rabbit, at a holding potential of -90 mV. 2. The slow calcium current in soleus fibres was about one-third of the size of the current in e.d.l. fibres, but was very similar otherwise. In both e.d.l. and soleus fibres, the dihydropyridine (DHP), nifedipine, suppressed the calcium current entirely. 3. In these normally polarized fibres, nifedipine suppressed only part (qns) of the asymmetric charge movement. The proportion of qns suppressed by various concentrations of nifedipine was linearly related to the associated reduction of the calcium current. Half-maximal suppression of both parameters was obtained with about 0.5 microM-nifedipine. The calcium current and the qns component of the charge movement also were suppressed over the same time course by nifedipine. Another DHP calcium antagonist, (+)PN200/110, was indistinguishable from nifedipine in its effects of suppressing calcium currents and qns. 4. In all muscle types, the total amount of qns in each fibre was linearly related to the size of the calcium current (in the absence of DHP). On average, qns was 3.3 times larger in e.d.l. fibres than in soleus fibres. 5. In contrast to the other dihydropyridines, (-)bay K8644, a calcium channel agonist, did not suppress any asymmetric charge movement. 6. The potential dependence of the slow calcium current implied a minimum gating charge of about five or six electronic charges. The movement of qns occurred over a more negative potential range than the change in calcium conductance. 7. Experiments on the binding of (+)PN200/110 indicated that e.d.l. muscles had between about 2 and 3 times more specific DHP binding sites than did soleus muscle. 8. These results point to a close relationship between slow calcium channels, the qns component of the charge movement and DHP binding sites, in both fast- and slow-twitch mammalian muscle. qns appears to be part of the gating current of the T-system calcium channels.
Full text
PDF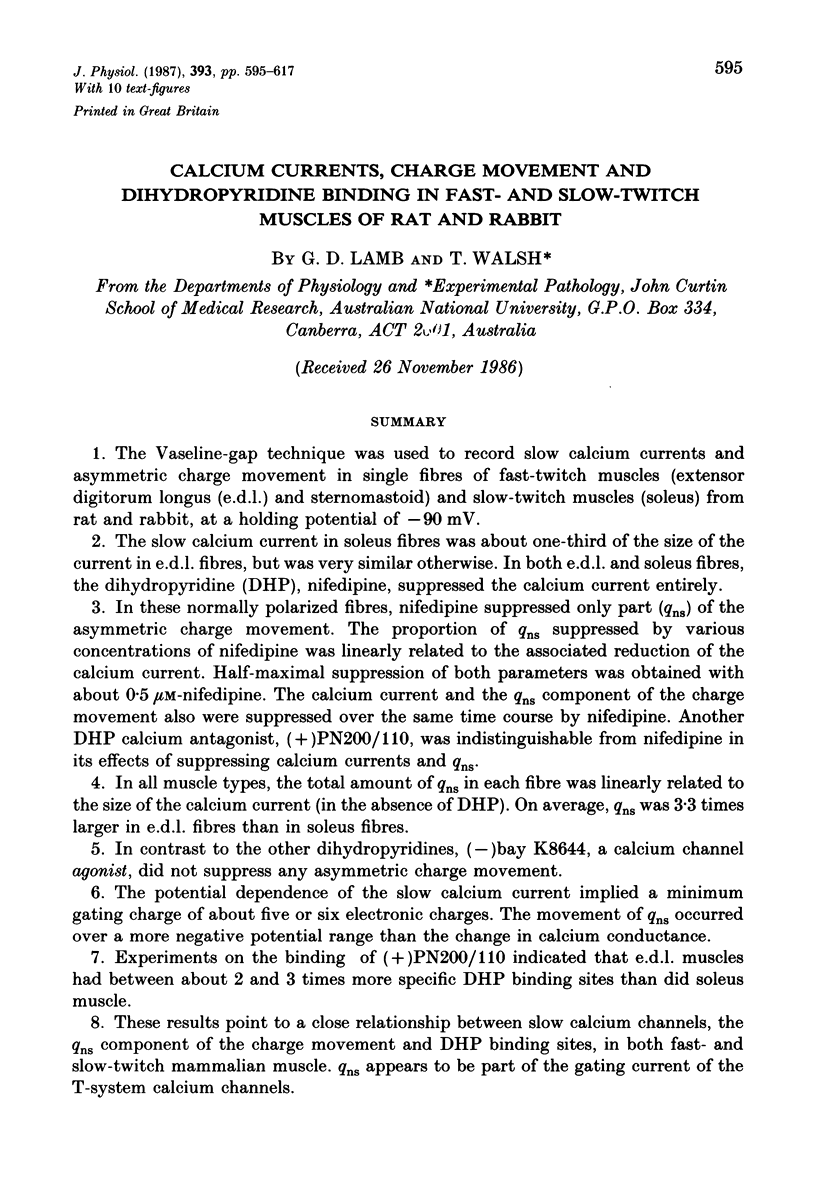
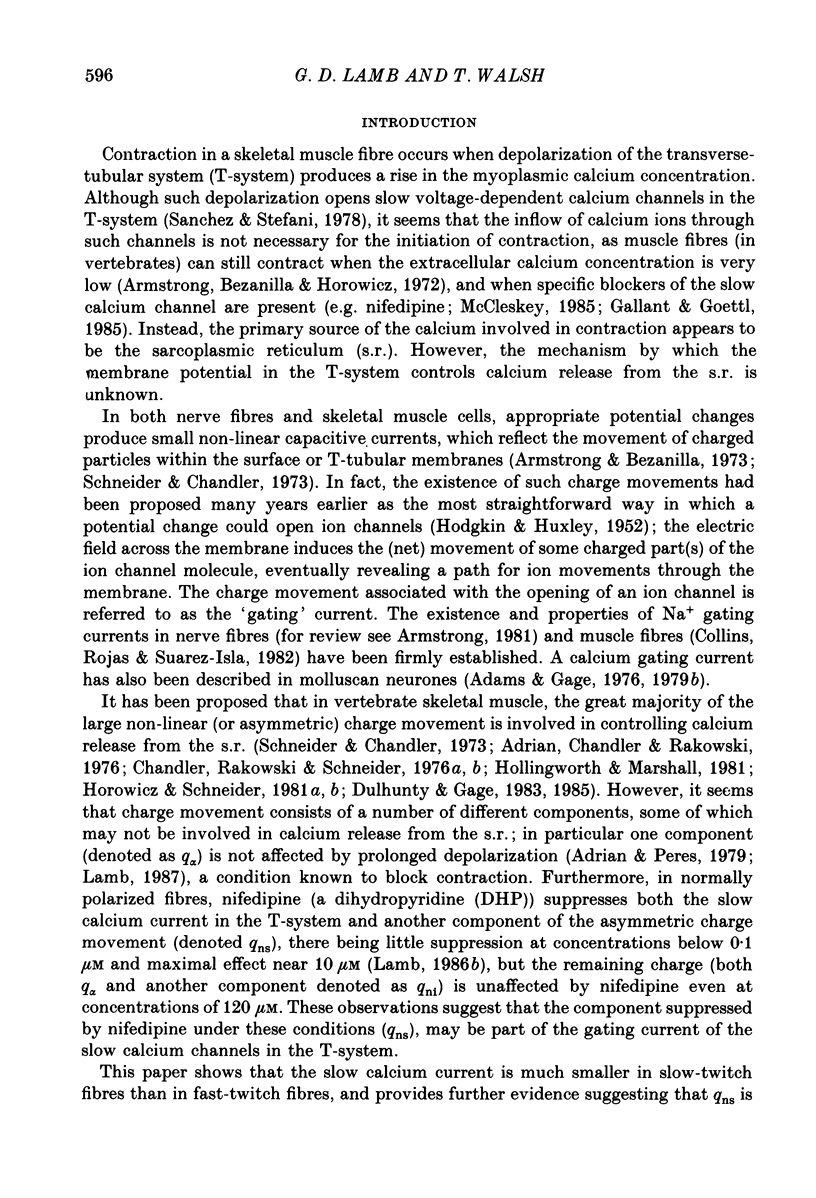
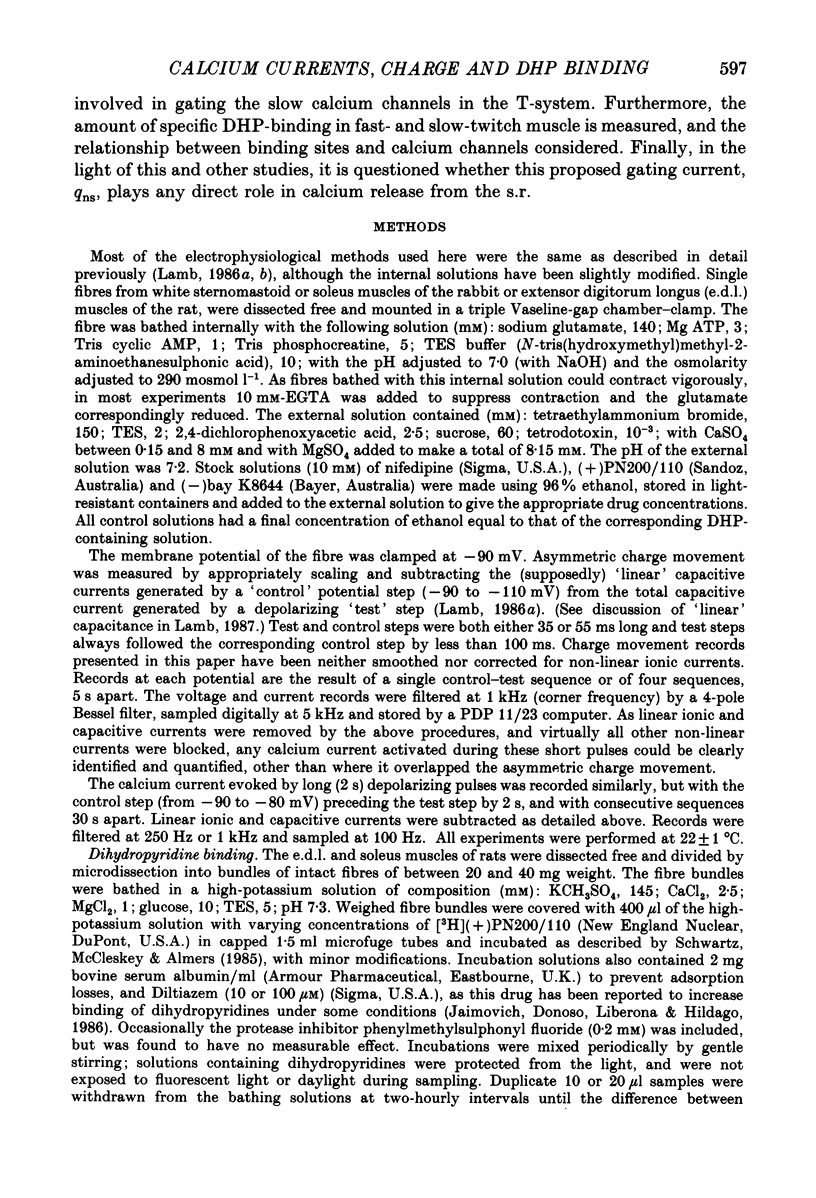
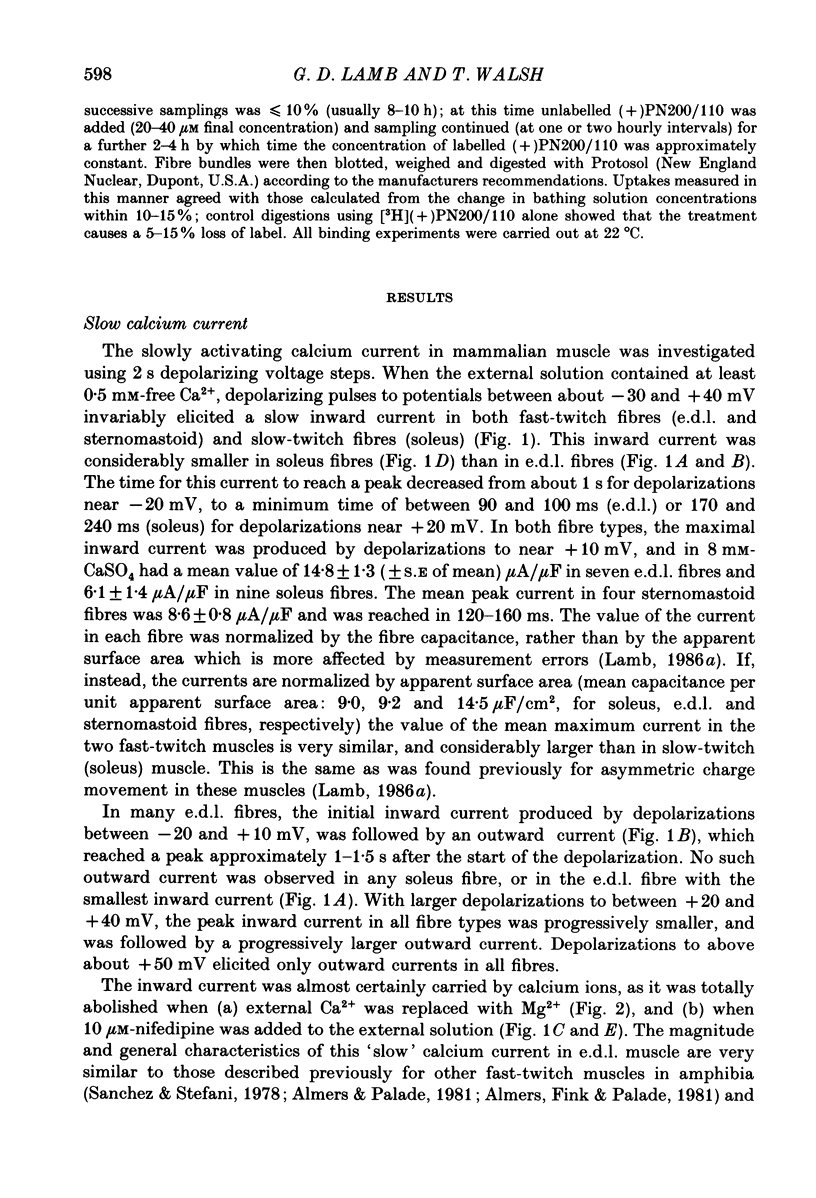
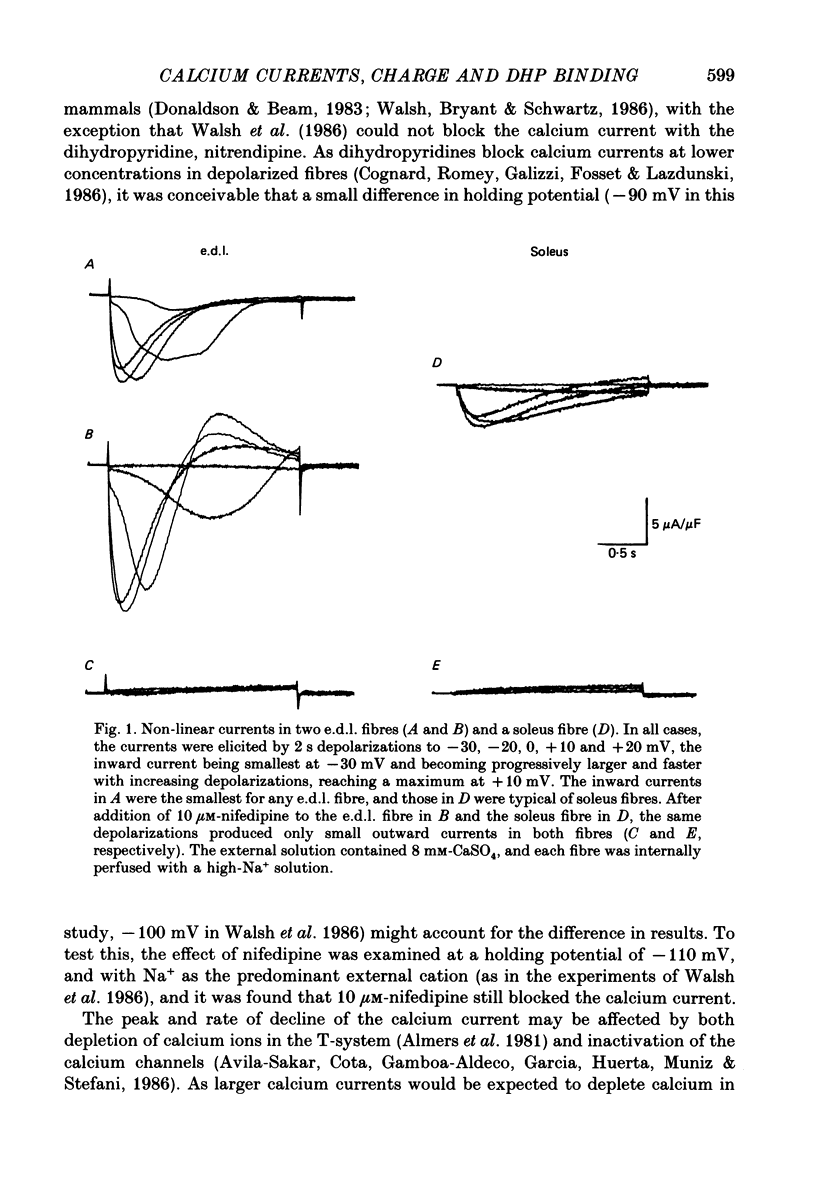
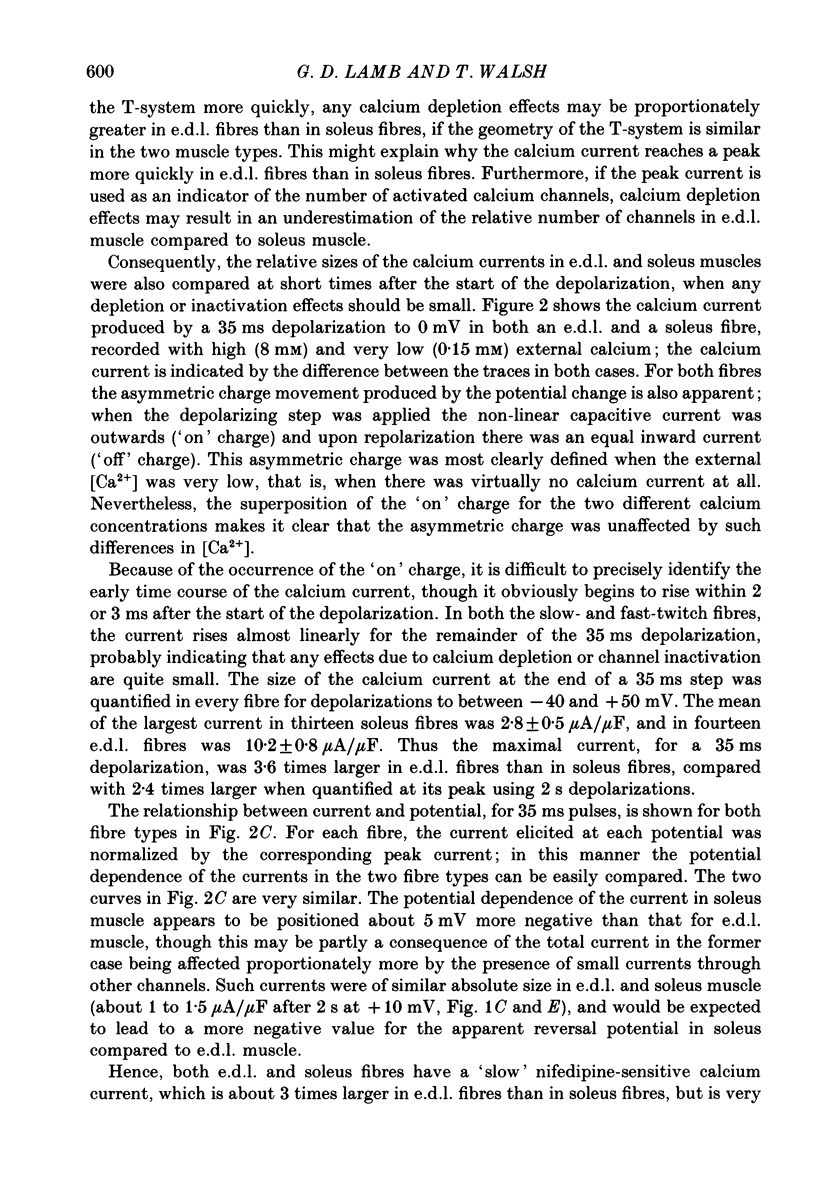
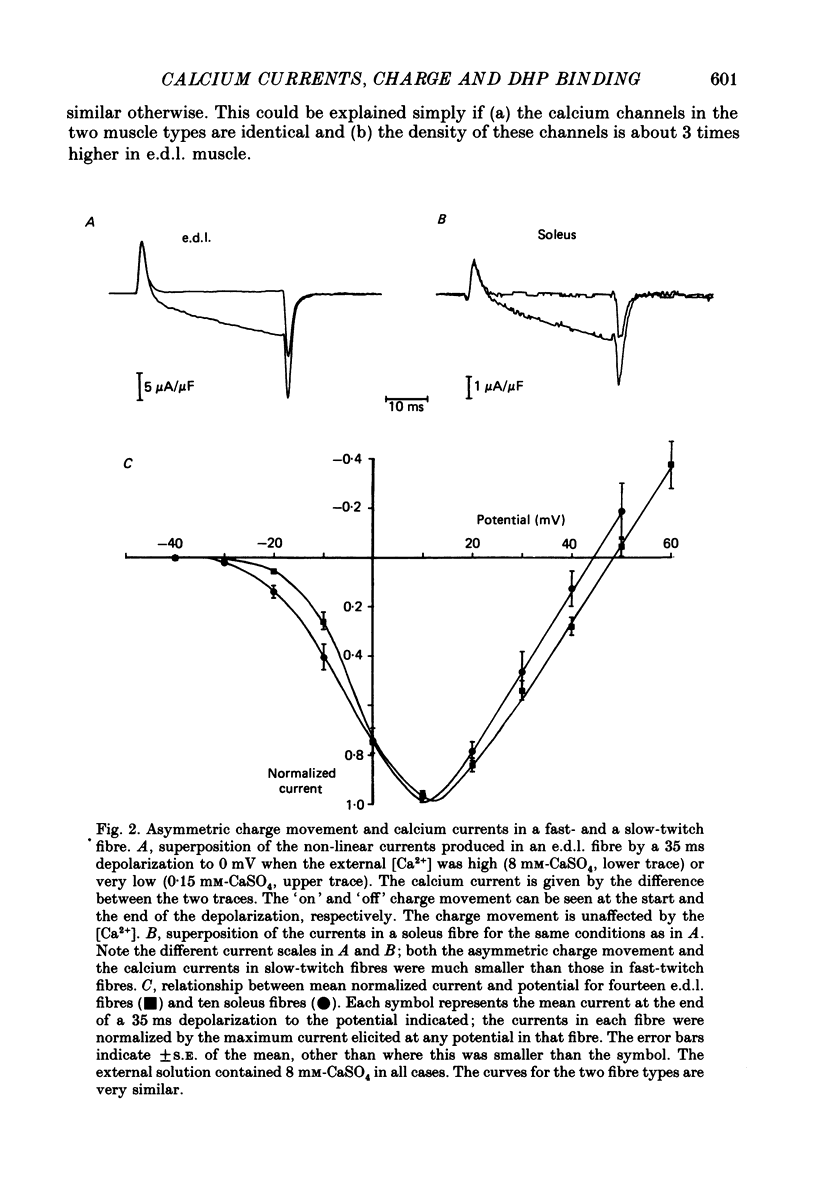
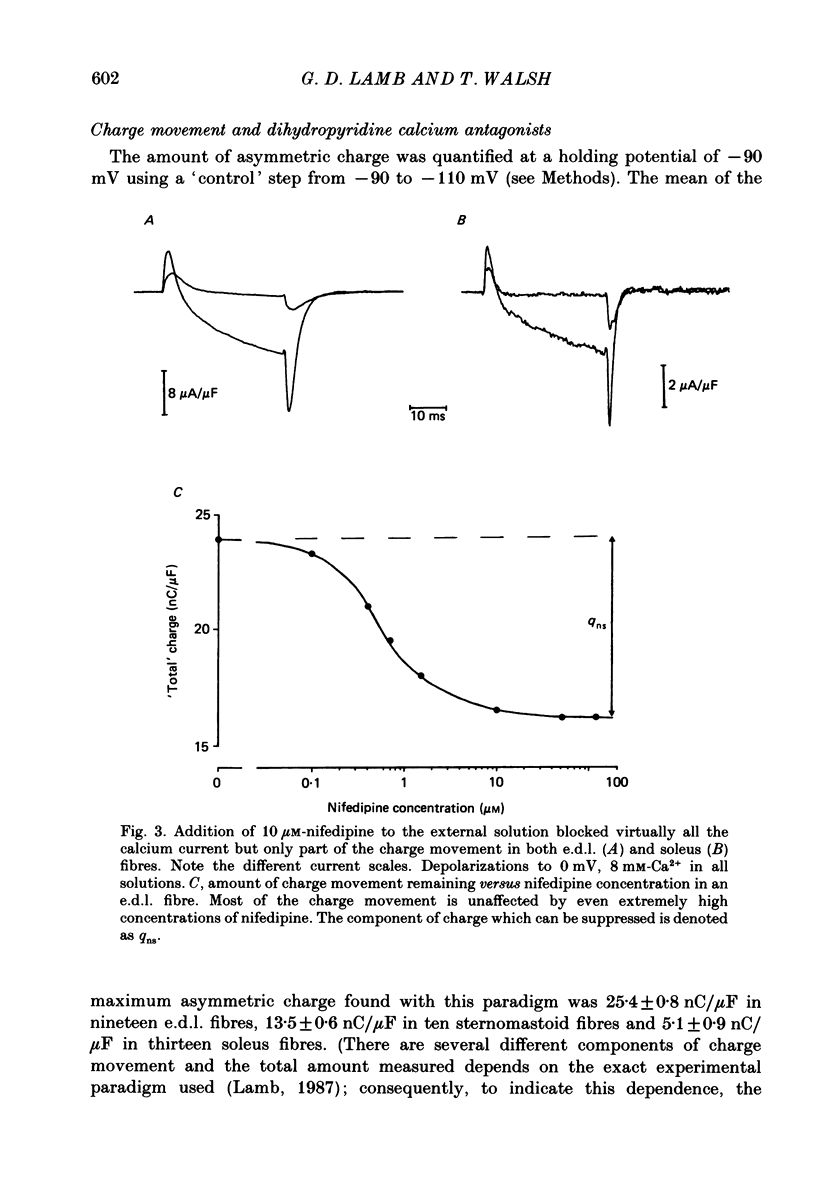
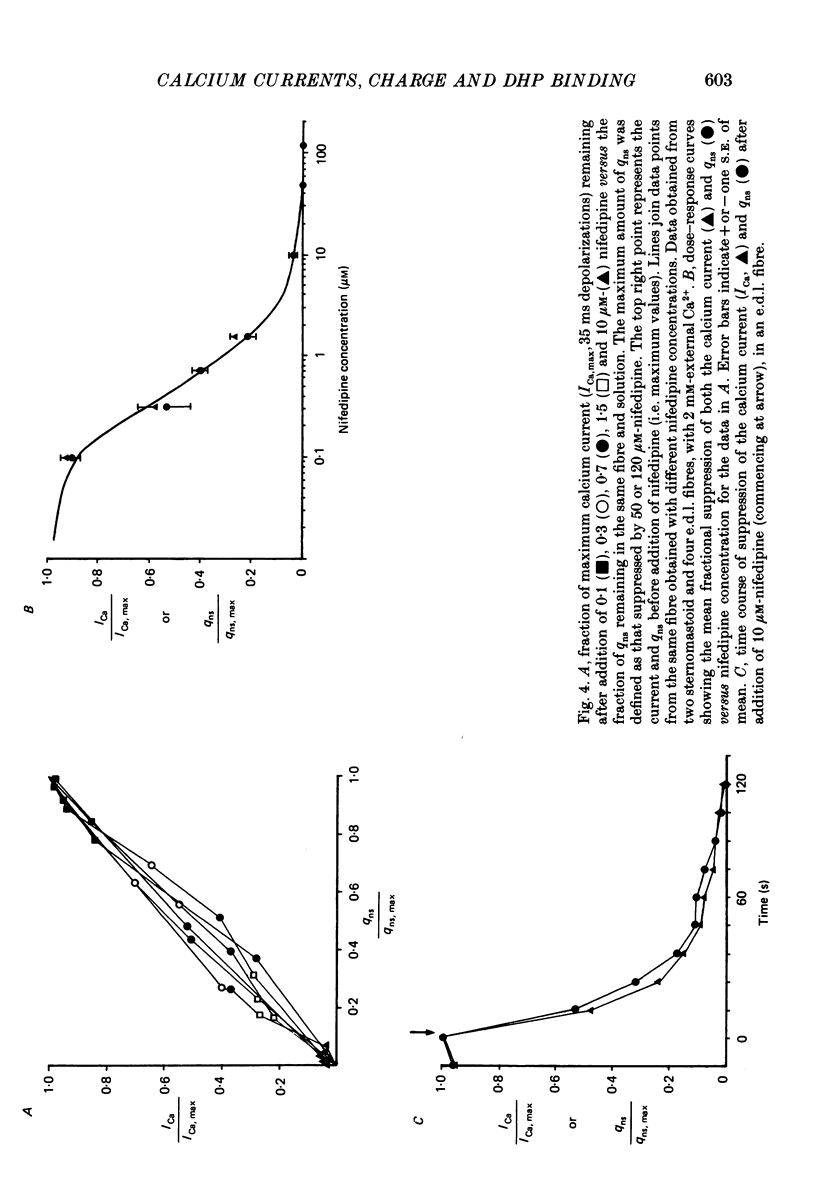
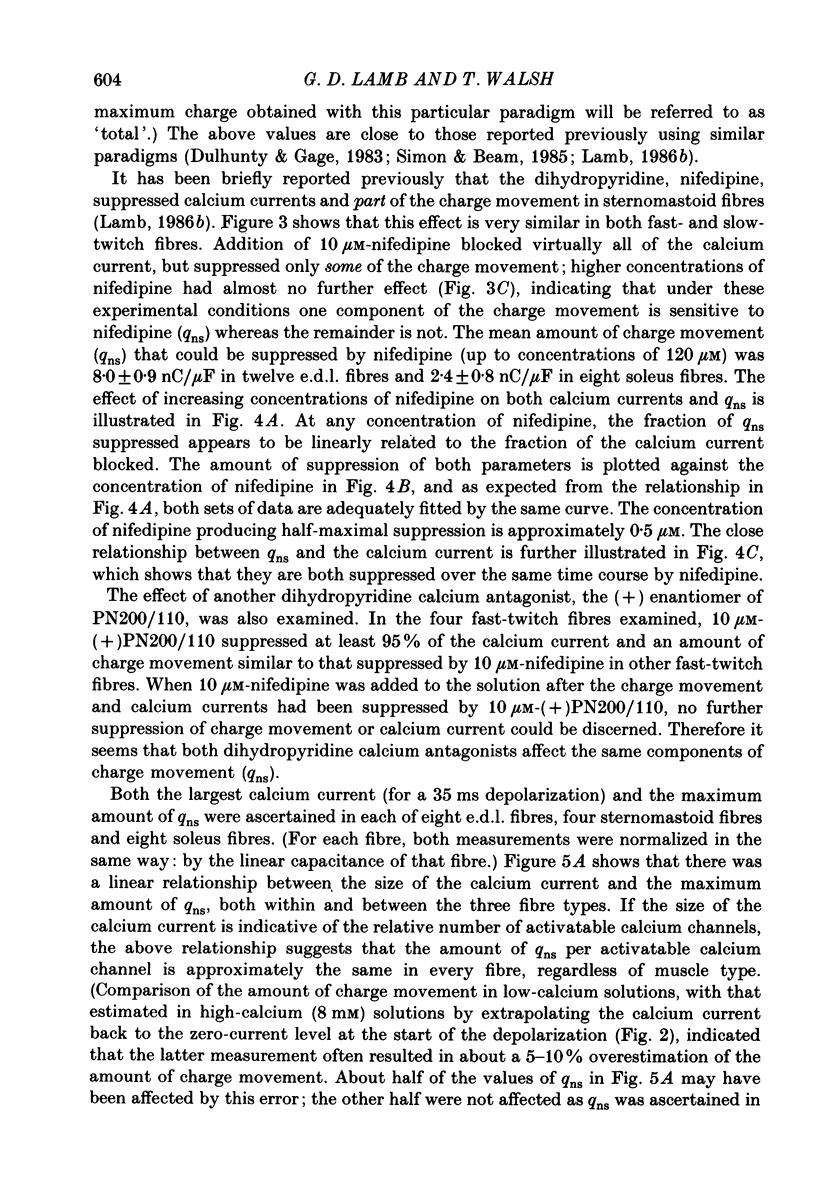
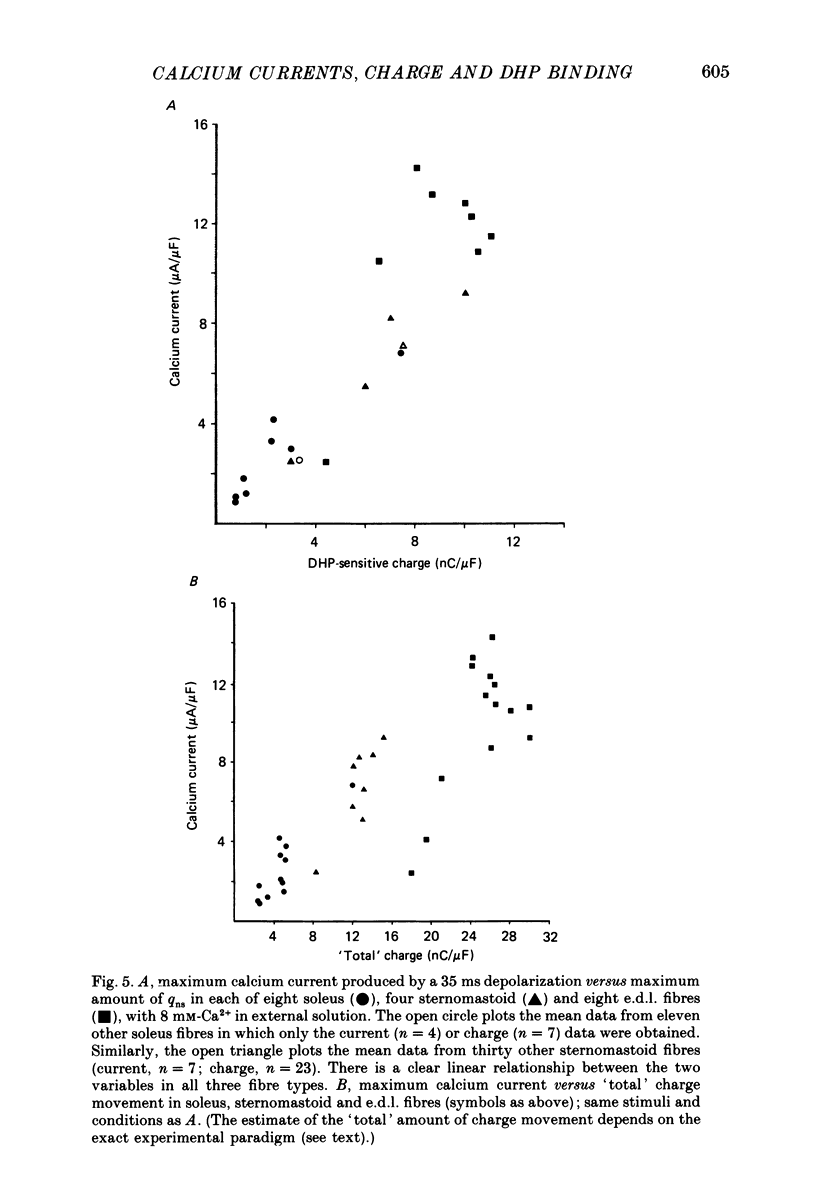
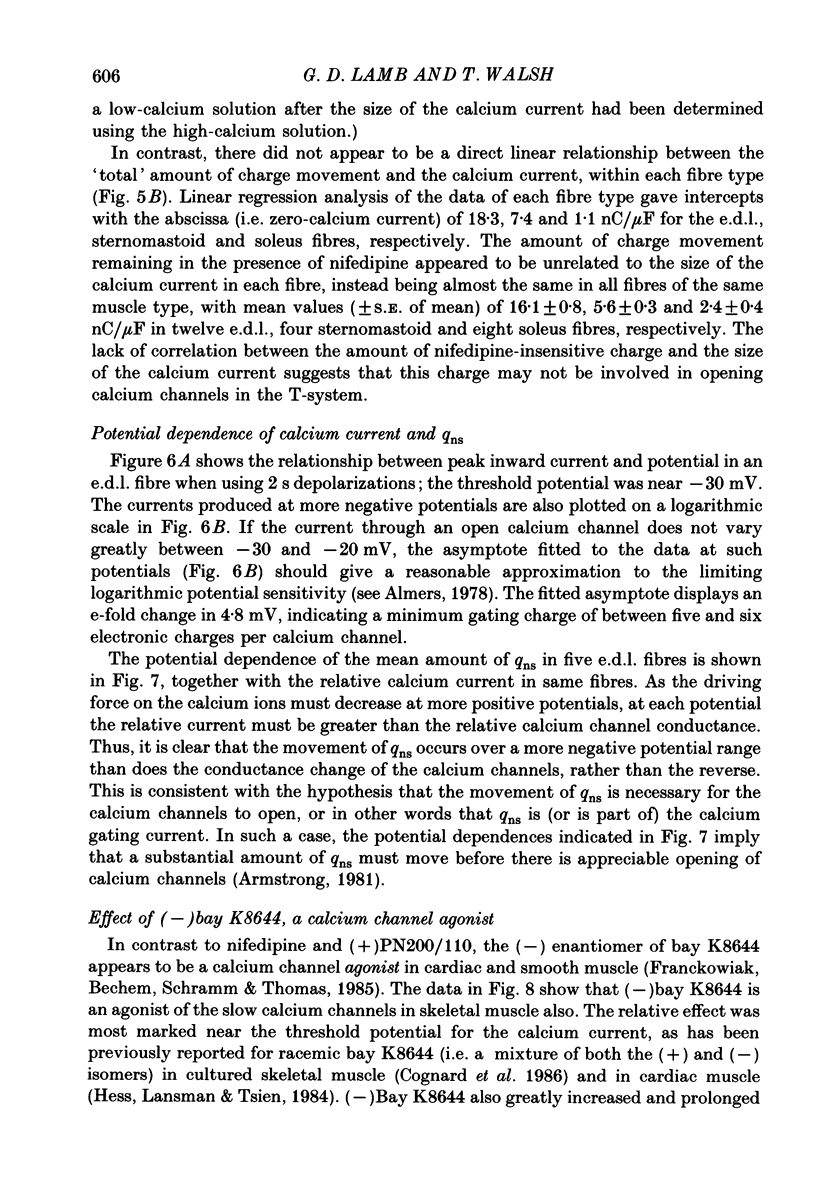
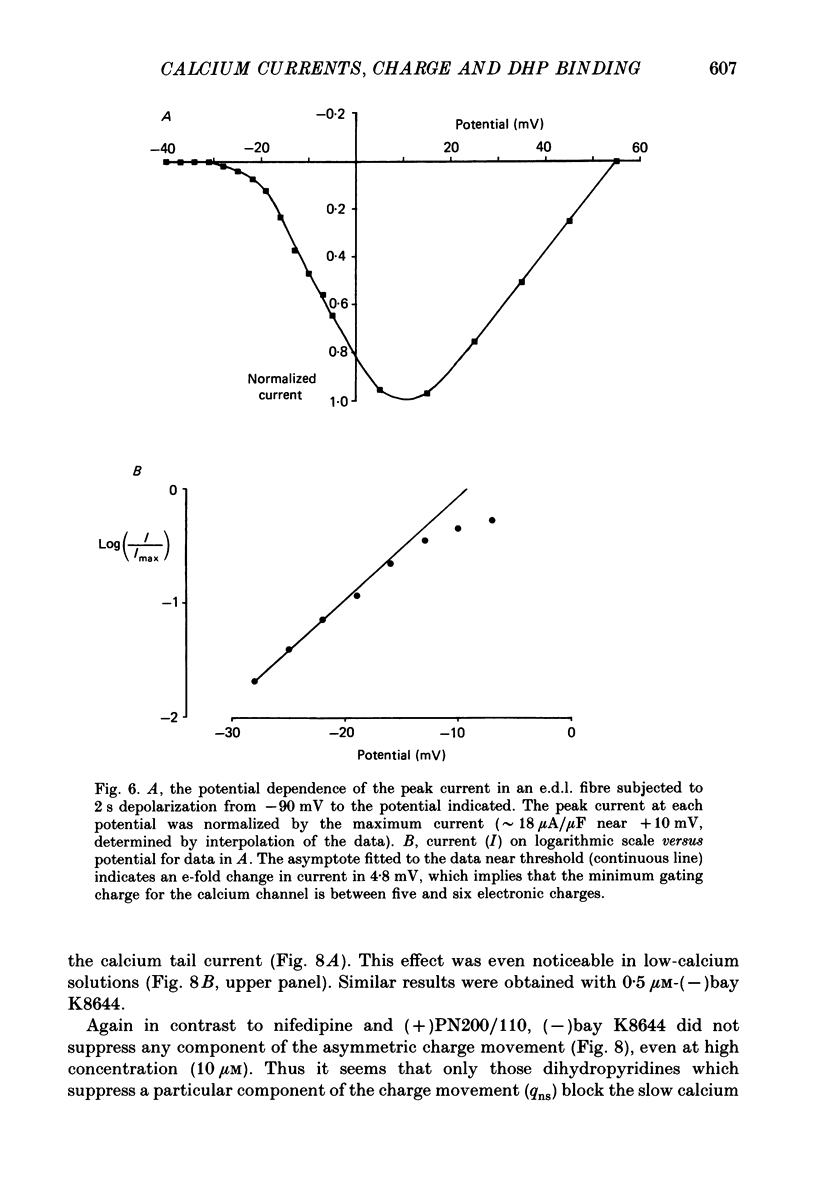
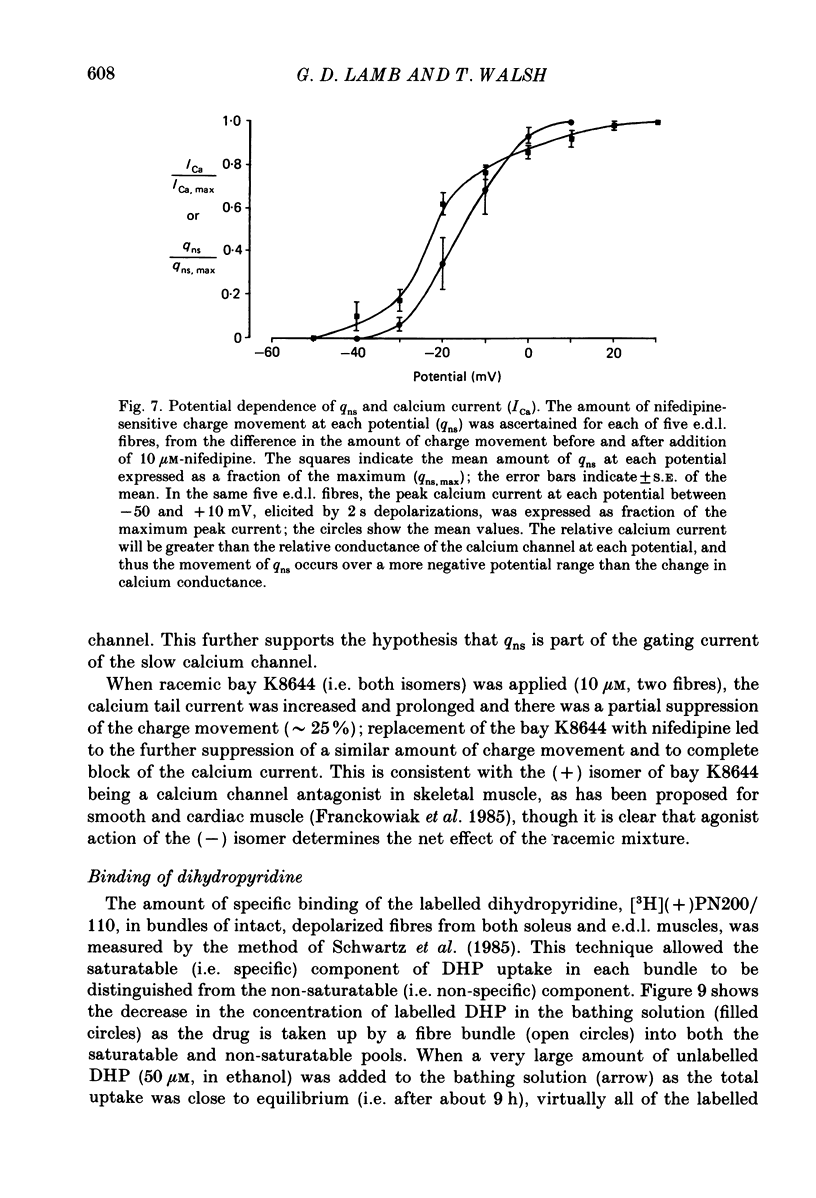
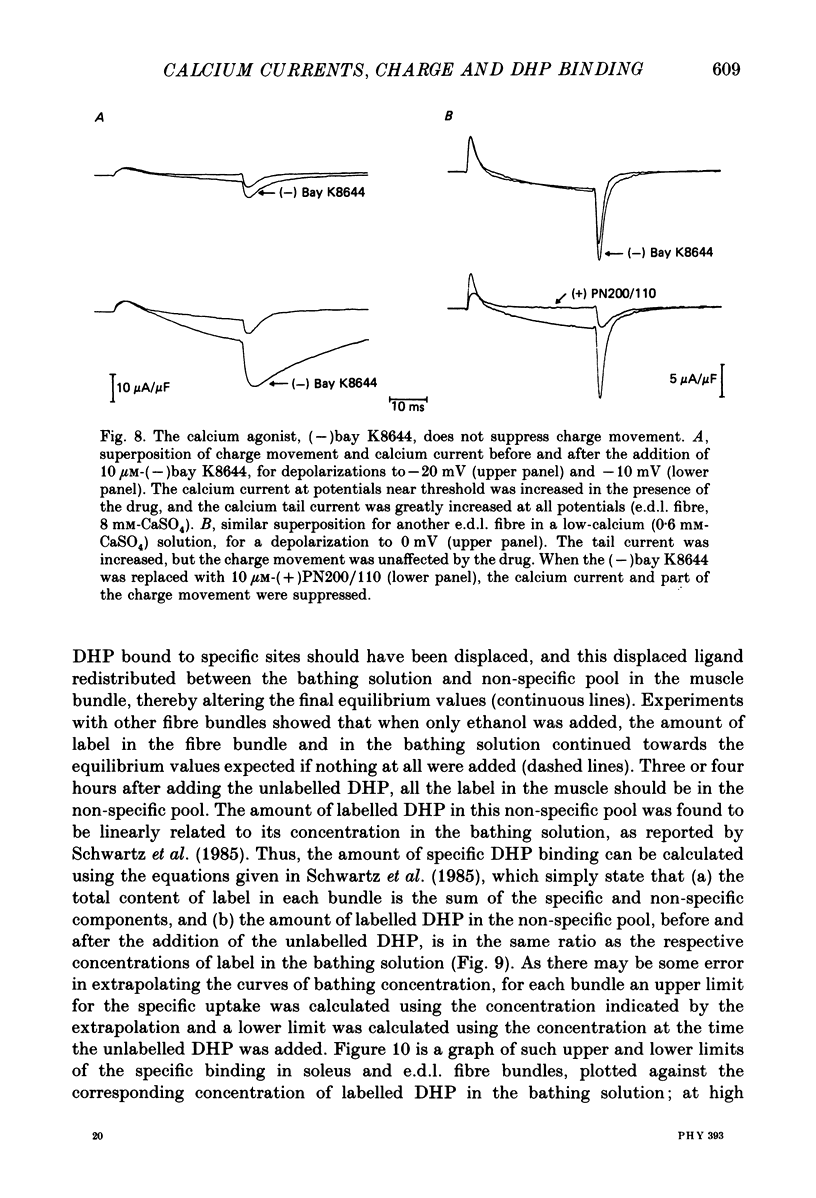
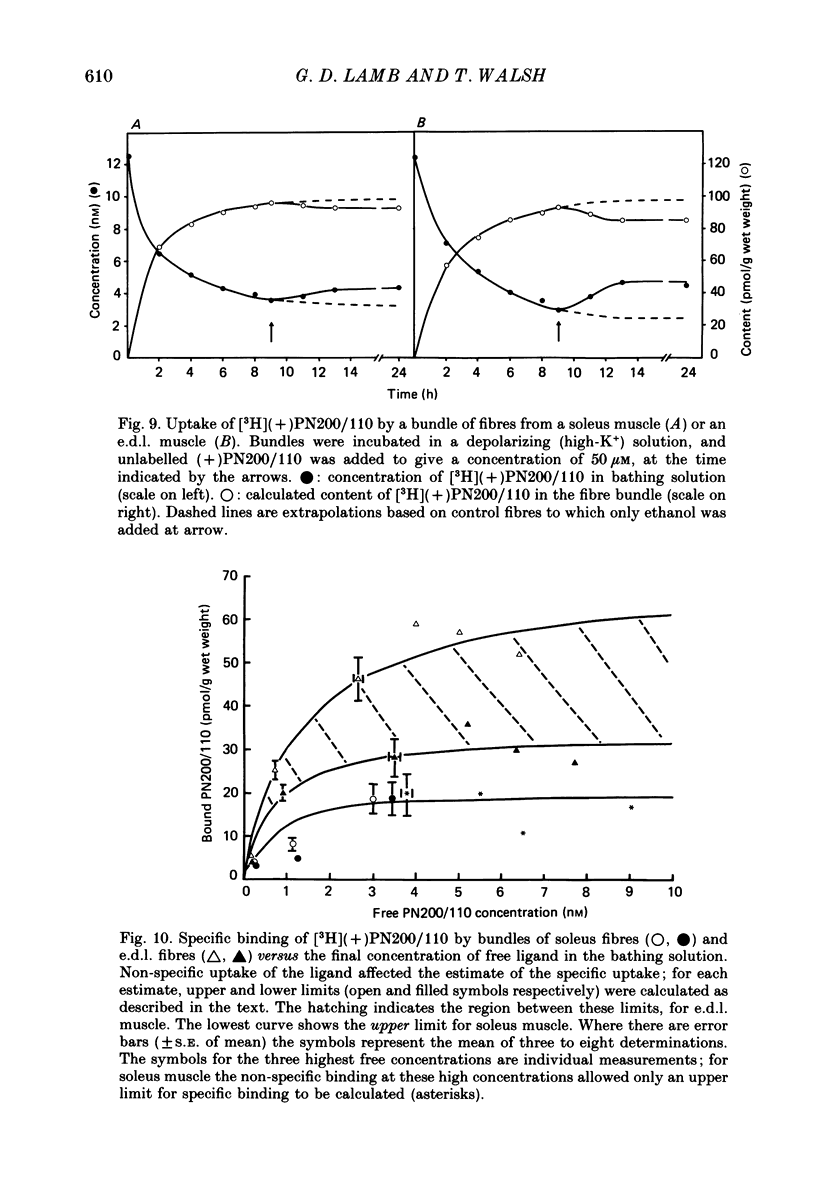
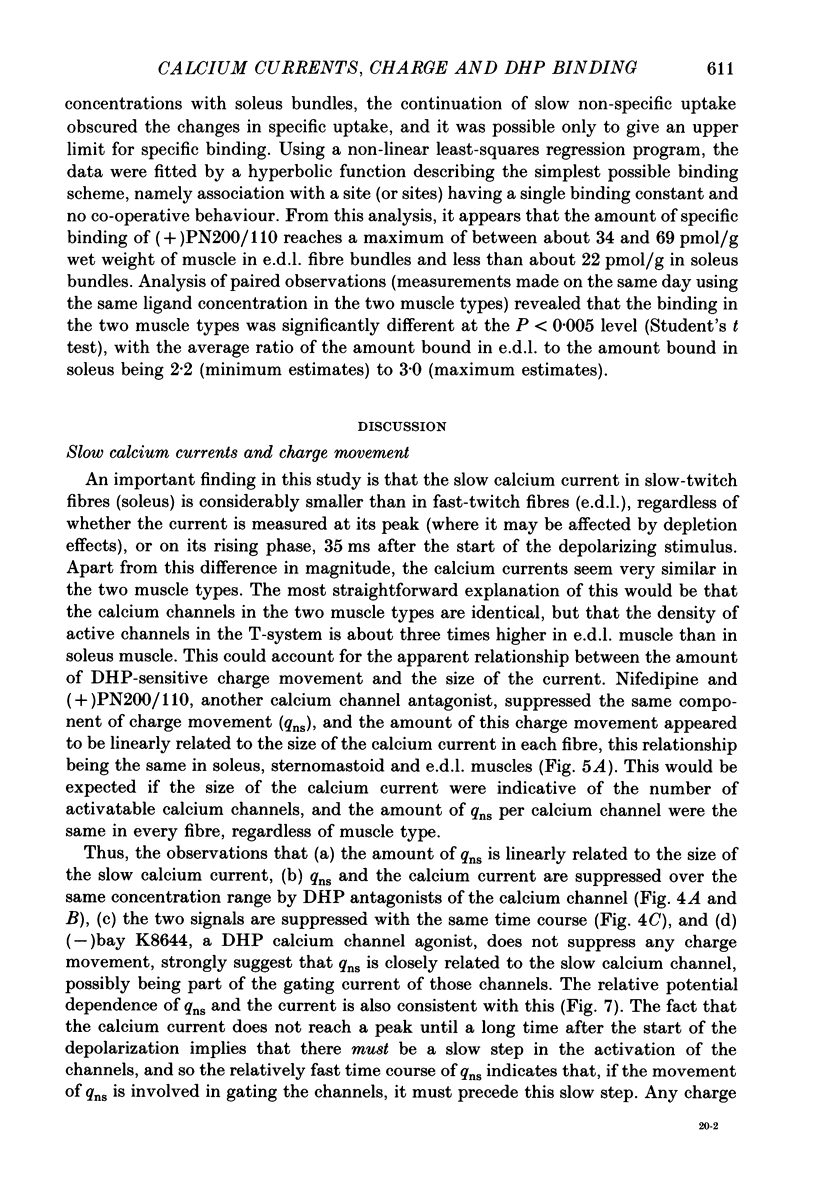
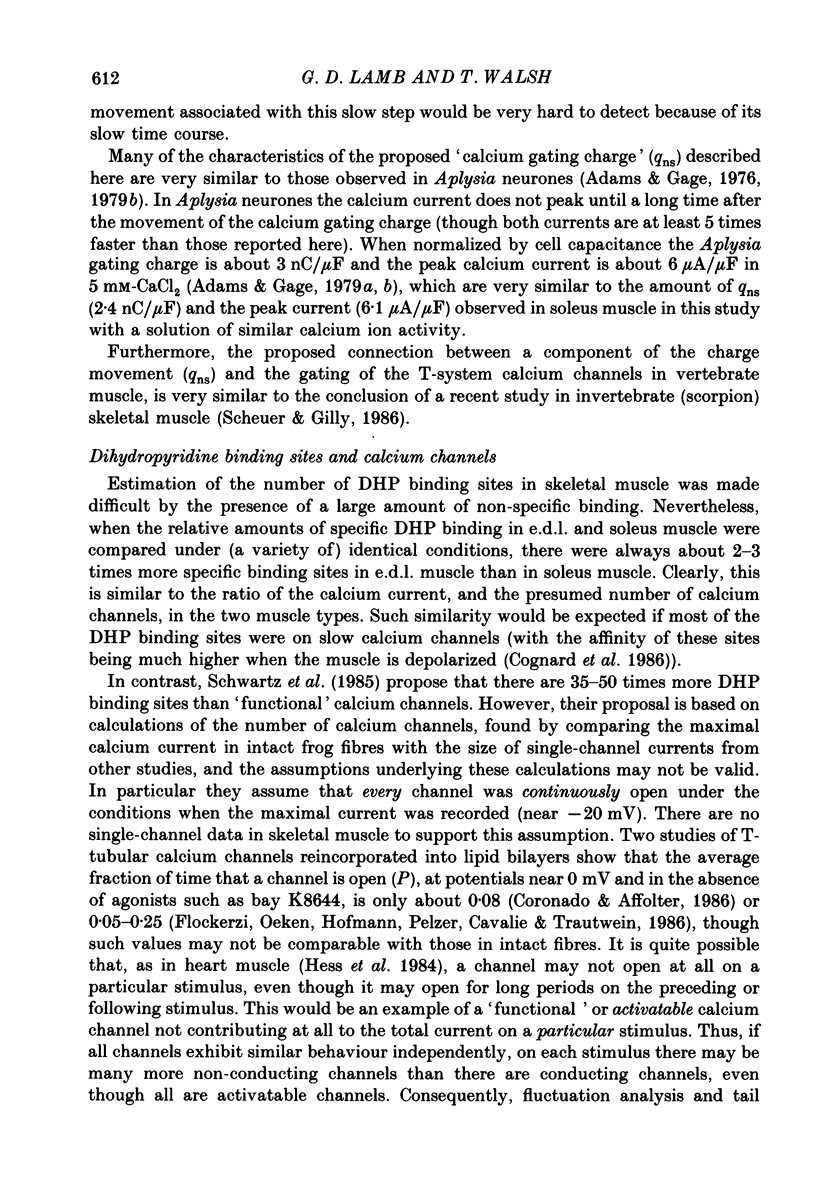
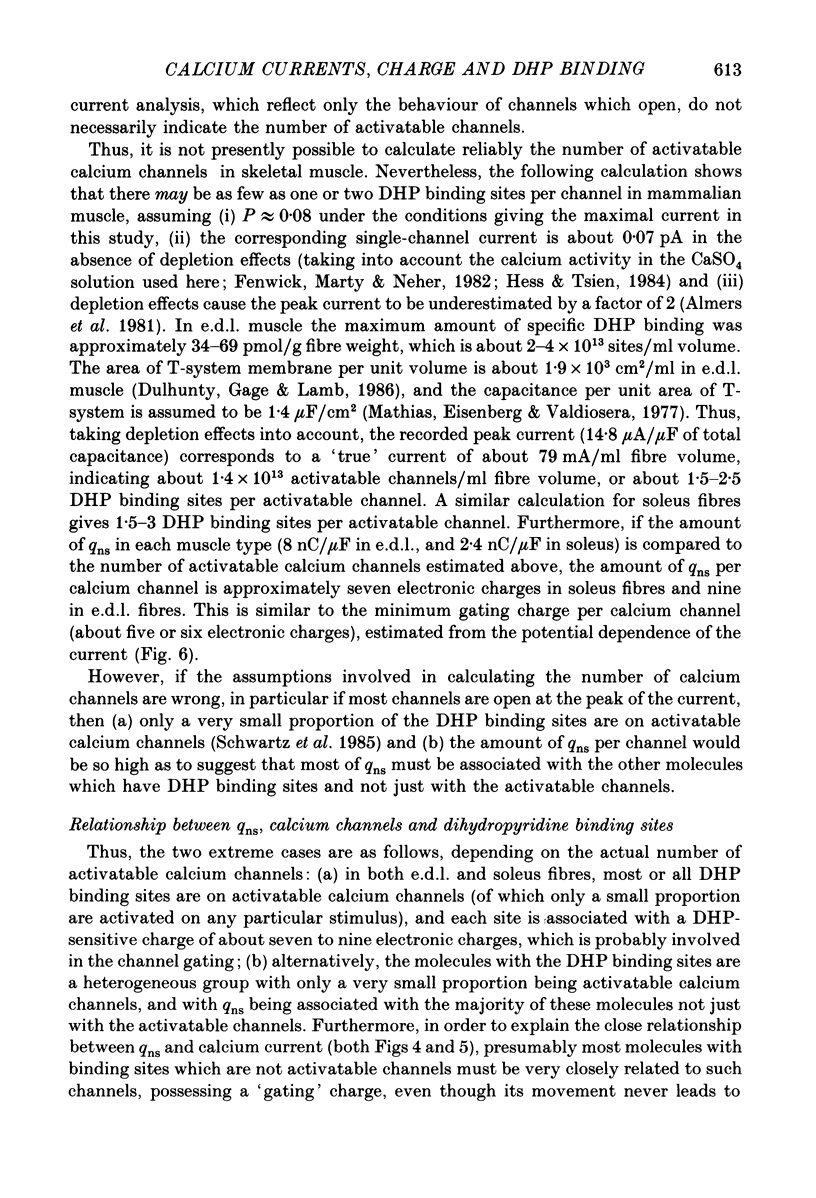
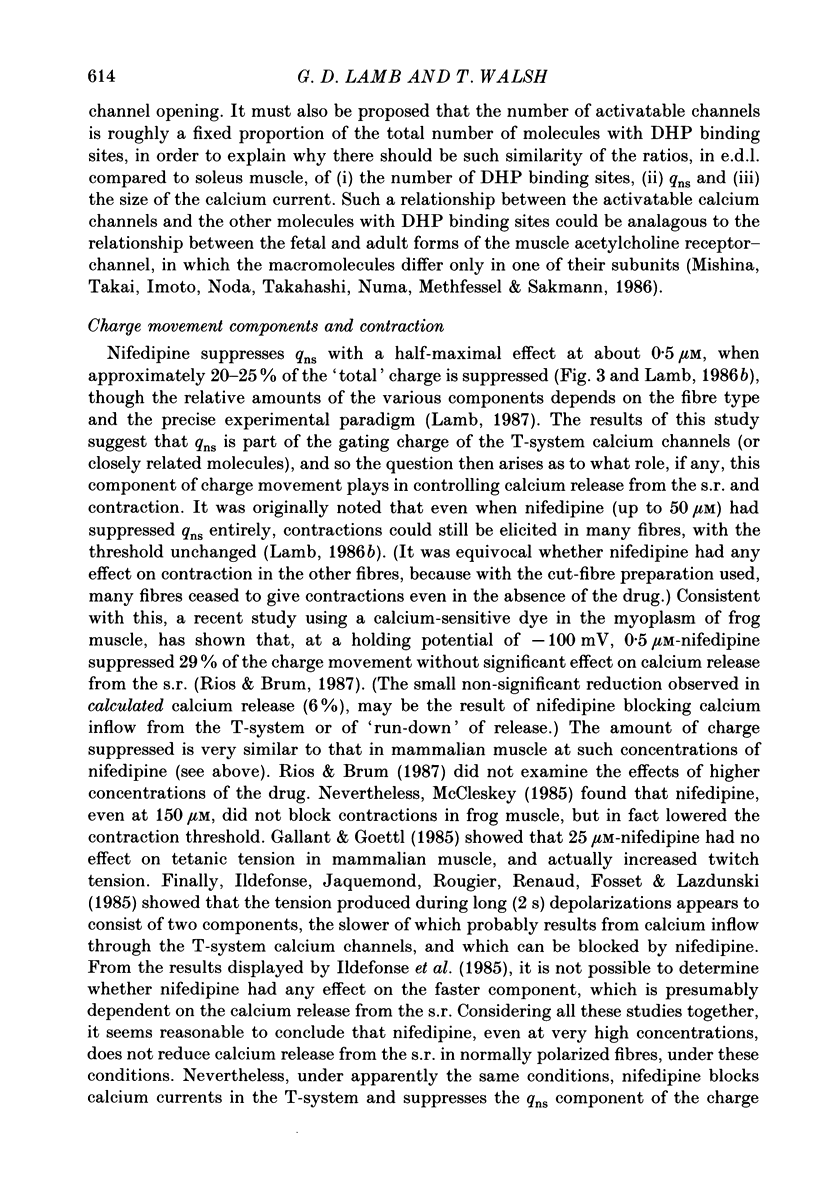
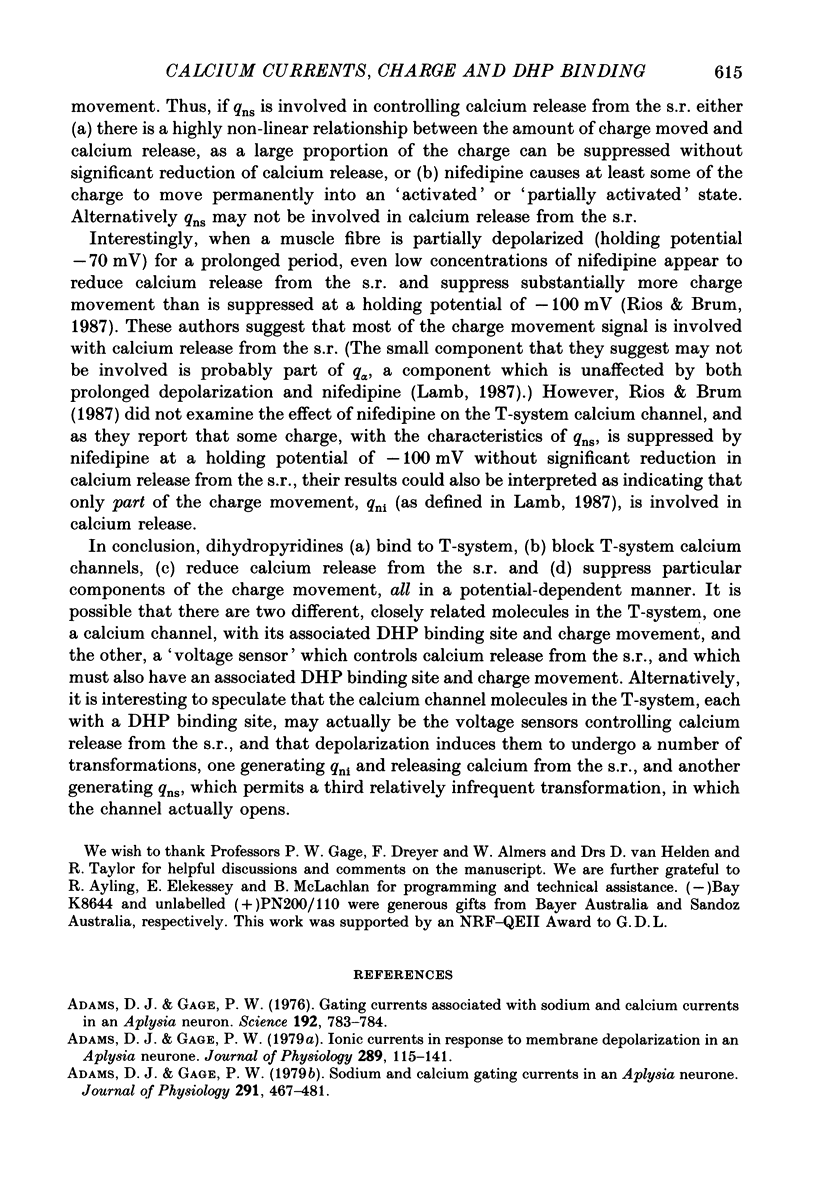
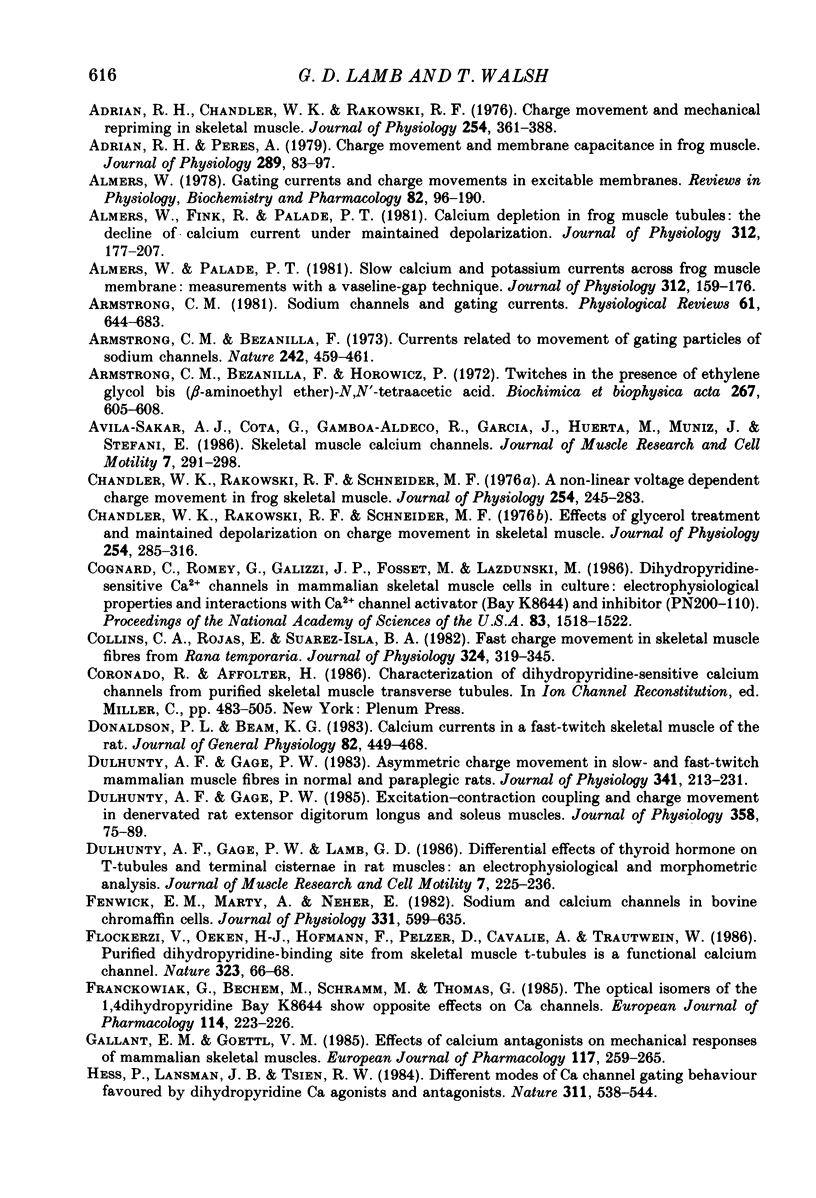
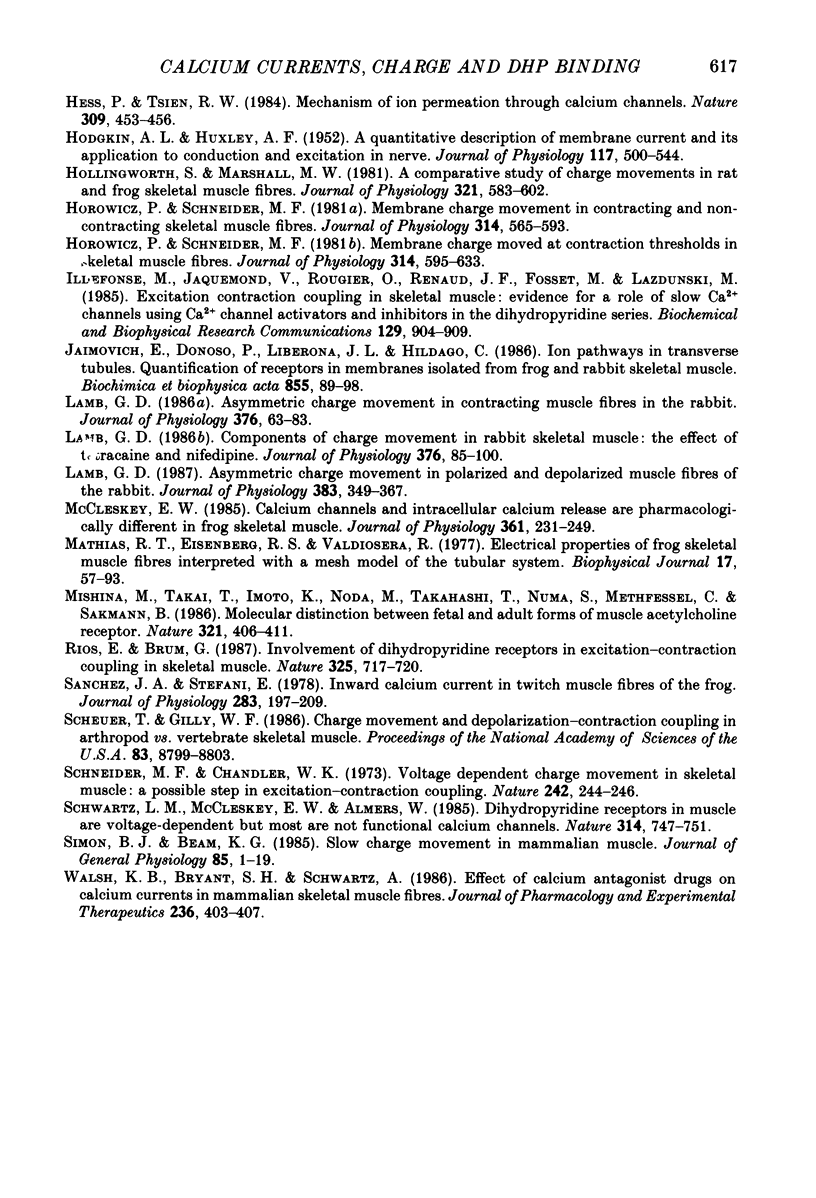
Selected References
These references are in PubMed. This may not be the complete list of references from this article.
- Adams D. J., Gage P. W. Gating currents associated with sodium and calcium currents in an Aplysia neuron. Science. 1976 May 21;192(4241):783–784. doi: 10.1126/science.1265479. [DOI] [PubMed] [Google Scholar]
- Adams D. J., Gage P. W. Ionic currents in response to membrane depolarization in an Aplysia neurone. J Physiol. 1979 Apr;289:115–141. doi: 10.1113/jphysiol.1979.sp012728. [DOI] [PMC free article] [PubMed] [Google Scholar]
- Adams D. J., Gage P. W. Sodium and calcium gating currents in an Aplysia neurone. J Physiol. 1979 Jun;291:467–481. doi: 10.1113/jphysiol.1979.sp012826. [DOI] [PMC free article] [PubMed] [Google Scholar]
- Adrian R. H., Chandler W. K., Rakowski R. F. Charge movement and mechanical repriming in skeletal muscle. J Physiol. 1976 Jan;254(2):361–388. doi: 10.1113/jphysiol.1976.sp011236. [DOI] [PMC free article] [PubMed] [Google Scholar]
- Adrian R. H., Peres A. Charge movement and membrane capacity in frog muscle. J Physiol. 1979 Apr;289:83–97. doi: 10.1113/jphysiol.1979.sp012726. [DOI] [PMC free article] [PubMed] [Google Scholar]
- Almers W., Fink R., Palade P. T. Calcium depletion in frog muscle tubules: the decline of calcium current under maintained depolarization. J Physiol. 1981 Mar;312:177–207. doi: 10.1113/jphysiol.1981.sp013623. [DOI] [PMC free article] [PubMed] [Google Scholar]
- Almers W. Gating currents and charge movements in excitable membranes. Rev Physiol Biochem Pharmacol. 1978;82:96–190. doi: 10.1007/BFb0030498. [DOI] [PubMed] [Google Scholar]
- Almers W., Palade P. T. Slow calcium and potassium currents across frog muscle membrane: measurements with a vaseline-gap technique. J Physiol. 1981 Mar;312:159–176. doi: 10.1113/jphysiol.1981.sp013622. [DOI] [PMC free article] [PubMed] [Google Scholar]
- Armstrong C. M., Bezanilla F. M., Horowicz P. Twitches in the presence of ethylene glycol bis( -aminoethyl ether)-N,N'-tetracetic acid. Biochim Biophys Acta. 1972 Jun 23;267(3):605–608. doi: 10.1016/0005-2728(72)90194-6. [DOI] [PubMed] [Google Scholar]
- Armstrong C. M., Bezanilla F. Currents related to movement of the gating particles of the sodium channels. Nature. 1973 Apr 13;242(5398):459–461. doi: 10.1038/242459a0. [DOI] [PubMed] [Google Scholar]
- Armstrong C. M. Sodium channels and gating currents. Physiol Rev. 1981 Jul;61(3):644–683. doi: 10.1152/physrev.1981.61.3.644. [DOI] [PubMed] [Google Scholar]
- Avila-Sakar A. J., Cota G., Gamboa-Aldeco R., Garcia J., Huerta M., Muñiz J., Stefani E. Skeletal muscle Ca2+ channels. J Muscle Res Cell Motil. 1986 Aug;7(4):291–298. doi: 10.1007/BF01753649. [DOI] [PubMed] [Google Scholar]
- Chandler W. K., Rakowski R. F., Schneider M. F. A non-linear voltage dependent charge movement in frog skeletal muscle. J Physiol. 1976 Jan;254(2):245–283. doi: 10.1113/jphysiol.1976.sp011232. [DOI] [PMC free article] [PubMed] [Google Scholar]
- Chandler W. K., Rakowski R. F., Schneider M. F. Effects of glycerol treatment and maintained depolarization on charge movement in skeletal muscle. J Physiol. 1976 Jan;254(2):285–316. doi: 10.1113/jphysiol.1976.sp011233. [DOI] [PMC free article] [PubMed] [Google Scholar]
- Cognard C., Romey G., Galizzi J. P., Fosset M., Lazdunski M. Dihydropyridine-sensitive Ca2+ channels in mammalian skeletal muscle cells in culture: electrophysiological properties and interactions with Ca2+ channel activator (Bay K8644) and inhibitor (PN 200-110). Proc Natl Acad Sci U S A. 1986 Mar;83(5):1518–1522. doi: 10.1073/pnas.83.5.1518. [DOI] [PMC free article] [PubMed] [Google Scholar]
- Collins C. A., Rojas E., Suarez-Isla B. A. Fast charge movements in skeletal muscle fibres from Rana temporaria. J Physiol. 1982 Mar;324:319–345. doi: 10.1113/jphysiol.1982.sp014115. [DOI] [PMC free article] [PubMed] [Google Scholar]
- Donaldson P. L., Beam K. G. Calcium currents in a fast-twitch skeletal muscle of the rat. J Gen Physiol. 1983 Oct;82(4):449–468. doi: 10.1085/jgp.82.4.449. [DOI] [PMC free article] [PubMed] [Google Scholar]
- Dulhunty A. F., Gage P. W. Asymmetrical charge movement in slow- and fast-twitch mammalian muscle fibres in normal and paraplegic rats. J Physiol. 1983 Aug;341:213–231. doi: 10.1113/jphysiol.1983.sp014802. [DOI] [PMC free article] [PubMed] [Google Scholar]
- Dulhunty A. F., Gage P. W. Excitation-contraction coupling and charge movement in denervated rat extensor digitorum longus and soleus muscles. J Physiol. 1985 Jan;358:75–89. doi: 10.1113/jphysiol.1985.sp015541. [DOI] [PMC free article] [PubMed] [Google Scholar]
- Dulhunty A. F., Gage P. W., Lamb G. D. Differential effects of thyroid hormone on T-tubules and terminal cisternae in rat muscles: an electrophysiological and morphometric analysis. J Muscle Res Cell Motil. 1986 Jun;7(3):225–236. doi: 10.1007/BF01753555. [DOI] [PubMed] [Google Scholar]
- Fenwick E. M., Marty A., Neher E. Sodium and calcium channels in bovine chromaffin cells. J Physiol. 1982 Oct;331:599–635. doi: 10.1113/jphysiol.1982.sp014394. [DOI] [PMC free article] [PubMed] [Google Scholar]
- Flockerzi V., Oeken H. J., Hofmann F., Pelzer D., Cavalié A., Trautwein W. Purified dihydropyridine-binding site from skeletal muscle t-tubules is a functional calcium channel. Nature. 1986 Sep 4;323(6083):66–68. doi: 10.1038/323066a0. [DOI] [PubMed] [Google Scholar]
- Franckowiak G., Bechem M., Schramm M., Thomas G. The optical isomers of the 1,4-dihydropyridine BAY K 8644 show opposite effects on Ca channels. Eur J Pharmacol. 1985 Aug 15;114(2):223–226. doi: 10.1016/0014-2999(85)90631-4. [DOI] [PubMed] [Google Scholar]
- Gallant E. M., Goettl V. M. Effects of calcium antagonists on mechanical responses of mammalian skeletal muscles. Eur J Pharmacol. 1985 Nov 5;117(2):259–265. doi: 10.1016/0014-2999(85)90611-9. [DOI] [PubMed] [Google Scholar]
- HODGKIN A. L., HUXLEY A. F. A quantitative description of membrane current and its application to conduction and excitation in nerve. J Physiol. 1952 Aug;117(4):500–544. doi: 10.1113/jphysiol.1952.sp004764. [DOI] [PMC free article] [PubMed] [Google Scholar]
- Hess P., Lansman J. B., Tsien R. W. Different modes of Ca channel gating behaviour favoured by dihydropyridine Ca agonists and antagonists. Nature. 1984 Oct 11;311(5986):538–544. doi: 10.1038/311538a0. [DOI] [PubMed] [Google Scholar]
- Hess P., Tsien R. W. Mechanism of ion permeation through calcium channels. 1984 May 31-Jun 6Nature. 309(5967):453–456. doi: 10.1038/309453a0. [DOI] [PubMed] [Google Scholar]
- Hollingworth S., Marshall M. W. A comparative study of charge movement in rat and frog skeletal muscle fibres. J Physiol. 1981 Dec;321:583–602. doi: 10.1113/jphysiol.1981.sp014004. [DOI] [PMC free article] [PubMed] [Google Scholar]
- Horowicz P., Schneider M. F. Membrane charge moved at contraction thresholds in skeletal muscle fibres. J Physiol. 1981 May;314:595–633. doi: 10.1113/jphysiol.1981.sp013726. [DOI] [PMC free article] [PubMed] [Google Scholar]
- Horowicz P., Schneider M. F. Membrane charge movement in contracting and non-contracting skeletal muscle fibres. J Physiol. 1981 May;314:565–593. doi: 10.1113/jphysiol.1981.sp013725. [DOI] [PMC free article] [PubMed] [Google Scholar]
- Ildefonse M., Jacquemond V., Rougier O., Renaud J. F., Fosset M., Lazdunski M. Excitation contraction coupling in skeletal muscle: evidence for a role of slow Ca2+ channels using Ca2+ channel activators and inhibitors in the dihydropyridine series. Biochem Biophys Res Commun. 1985 Jun 28;129(3):904–909. doi: 10.1016/0006-291x(85)91977-1. [DOI] [PubMed] [Google Scholar]
- Jaimovich E., Donoso P., Liberona J. L., Hidalgo C. Ion pathways in transverse tubules. Quantification of receptors in membranes isolated from frog and rabbit skeletal muscle. Biochim Biophys Acta. 1986 Feb 13;855(1):89–98. doi: 10.1016/0005-2736(86)90192-6. [DOI] [PubMed] [Google Scholar]
- Lamb G. D. Asymmetric charge movement in contracting muscle fibres in the rabbit. J Physiol. 1986 Jul;376:63–83. doi: 10.1113/jphysiol.1986.sp016142. [DOI] [PMC free article] [PubMed] [Google Scholar]
- Lamb G. D. Asymmetric charge movement in polarized and depolarized muscle fibres of the rabbit. J Physiol. 1987 Feb;383:349–367. doi: 10.1113/jphysiol.1987.sp016413. [DOI] [PMC free article] [PubMed] [Google Scholar]
- Lamb G. D. Components of charge movement in rabbit skeletal muscle: the effect of tetracaine and nifedipine. J Physiol. 1986 Jul;376:85–100. doi: 10.1113/jphysiol.1986.sp016143. [DOI] [PMC free article] [PubMed] [Google Scholar]
- Mathias R. T., Eisenberg R. S., Valdiosera R. Electrical properties of frog skeletal muscle fibers interpreted with a mesh model of the tubular system. Biophys J. 1977 Jan;17(1):57–93. doi: 10.1016/S0006-3495(77)85627-0. [DOI] [PMC free article] [PubMed] [Google Scholar]
- McCleskey E. W. Calcium channels and intracellular calcium release are pharmacologically different in frog skeletal muscle. J Physiol. 1985 Apr;361:231–249. doi: 10.1113/jphysiol.1985.sp015643. [DOI] [PMC free article] [PubMed] [Google Scholar]
- Mishina M., Takai T., Imoto K., Noda M., Takahashi T., Numa S., Methfessel C., Sakmann B. Molecular distinction between fetal and adult forms of muscle acetylcholine receptor. Nature. 1986 May 22;321(6068):406–411. doi: 10.1038/321406a0. [DOI] [PubMed] [Google Scholar]
- Rios E., Brum G. Involvement of dihydropyridine receptors in excitation-contraction coupling in skeletal muscle. Nature. 1987 Feb 19;325(6106):717–720. doi: 10.1038/325717a0. [DOI] [PubMed] [Google Scholar]
- Sanchez J. A., Stefani E. Inward calcium current in twitch muscle fibres of the frog. J Physiol. 1978 Oct;283:197–209. doi: 10.1113/jphysiol.1978.sp012496. [DOI] [PMC free article] [PubMed] [Google Scholar]
- Scheuer T., Gilly W. F. Charge movement and depolarization-contraction coupling in arthropod vs. vertebrate skeletal muscle. Proc Natl Acad Sci U S A. 1986 Nov;83(22):8799–8803. doi: 10.1073/pnas.83.22.8799. [DOI] [PMC free article] [PubMed] [Google Scholar]
- Schneider M. F., Chandler W. K. Voltage dependent charge movement of skeletal muscle: a possible step in excitation-contraction coupling. Nature. 1973 Mar 23;242(5395):244–246. doi: 10.1038/242244a0. [DOI] [PubMed] [Google Scholar]
- Schwartz L. M., McCleskey E. W., Almers W. Dihydropyridine receptors in muscle are voltage-dependent but most are not functional calcium channels. 1985 Apr 25-May 1Nature. 314(6013):747–751. doi: 10.1038/314747a0. [DOI] [PubMed] [Google Scholar]
- Simon B. J., Beam K. G. Slow charge movement in mammalian skeletal muscle. J Gen Physiol. 1985 Jan;85(1):1–19. doi: 10.1085/jgp.85.1.1. [DOI] [PMC free article] [PubMed] [Google Scholar]
- Walsh K. B., Bryant S. H., Schwartz A. Effect of calcium antagonist drugs on calcium currents in mammalian skeletal muscle fibers. J Pharmacol Exp Ther. 1986 Feb;236(2):403–407. [PubMed] [Google Scholar]