Abstract
1. The electrophysiological properties of testosterone-secreting cells (i.e. Leydig cells) in the mouse were studied using patch electrodes. The cells appeared solitarily or in clusters after mechanical dissociation from testes. They were confirmed to be Leydig cells on the basis of 3 beta-hydroxysteroid dehydrogenase staining. 2. Under current-clamp conditions in the whole-cell configuration, Leydig cells immersed in standard saline were able to generate action potential-like responses. The active responses occurred after cessation of membrane hyperpolarization or when cells were held in a hyperpolarized condition and stimulated with depolarizing current pulses. 3. In Leydig cells under voltage clamp, depolarizations more positive than -50 mV evoked transient inward currents which decayed completely during the duration of depolarization (130 ms). No obvious outward currents were evoked by pulses less positive than 30 mV. 4. The inward currents were identified as Ca2+ current, since replacement of external Ca2+ with Mn2+ reversibly diminished the current whereas Ba2+ or Sr2+ substituted for Ca2+. 5. With voltage pulses more positive than 40 mV, outward currents were evoked. The currents were dependent on K+ concentration and were blocked by quinine or tetraethylammonium. The amplitudes of outward currents were increased with raised internal Ca2+ concentration. 6. Single-channel recordings of the outward currents revealed that the unitary conductance was 130 pS when internal K+ was 131-143 mM and external K+ was 5 mM. The open probability of the channel showed marked dependence on the membrane potential and the internal Ca2+ concentration. Thus, the current was identified as being Ca2+- and membrane potential-dependent K+ current. 7. Leydig cells within a cluster possessed distinct intercellular couplings. The mean coupling ratio obtained by applying two patch electrodes to a pair of cells was 0.84. Transfer of injected dye (Lucifer Yellow) to adjacent cells was also confirmed. 8. It was concluded that Leydig cells have at least two kinds of voltage-dependent channels in the membrane. The Ca2+ channel may be activated by physiological changes in membrane potential, leading to an influx of Ca2+. The Ca2+-dependent K+ channel hardly seems to be activated unless the internal Ca2+ concentration increases remarkably. It is presumed that intercellular coupling may play a role in synchronizing or intensifying the endocrine activities of Leydig cells located within a cluster.
Full text
PDF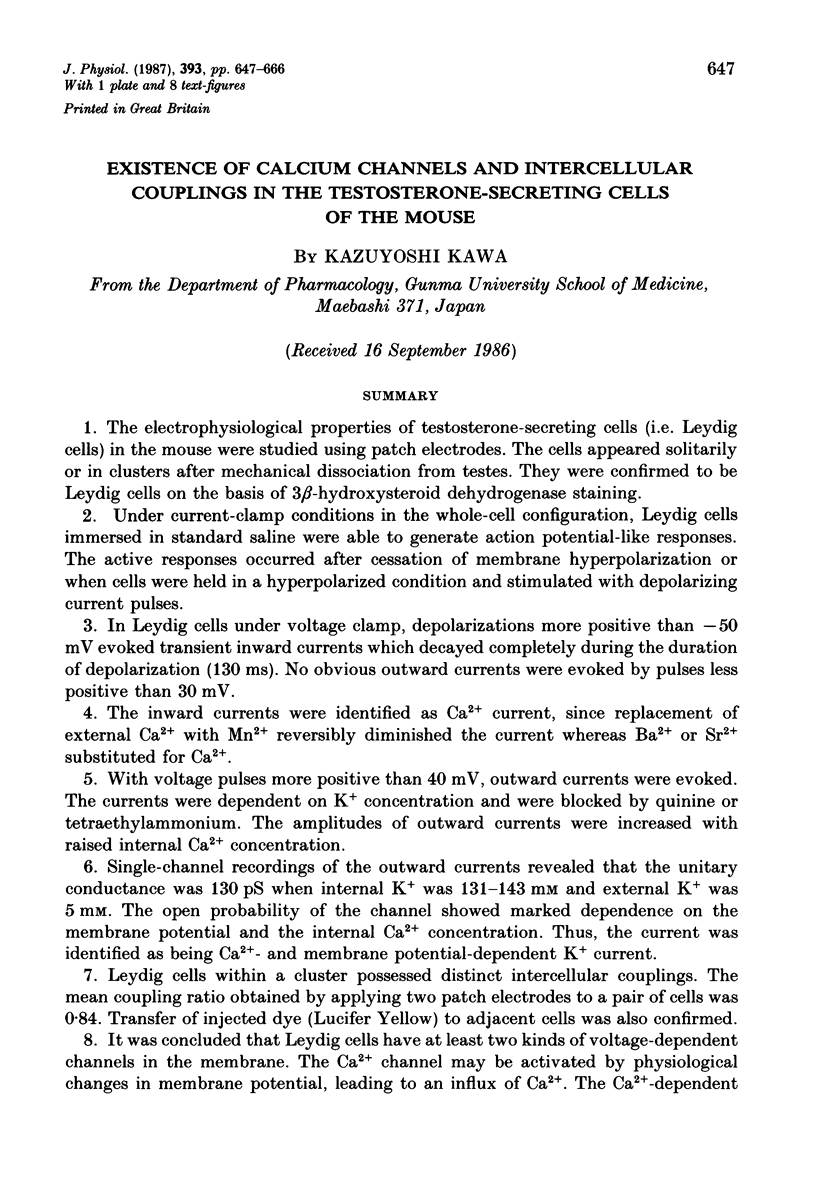
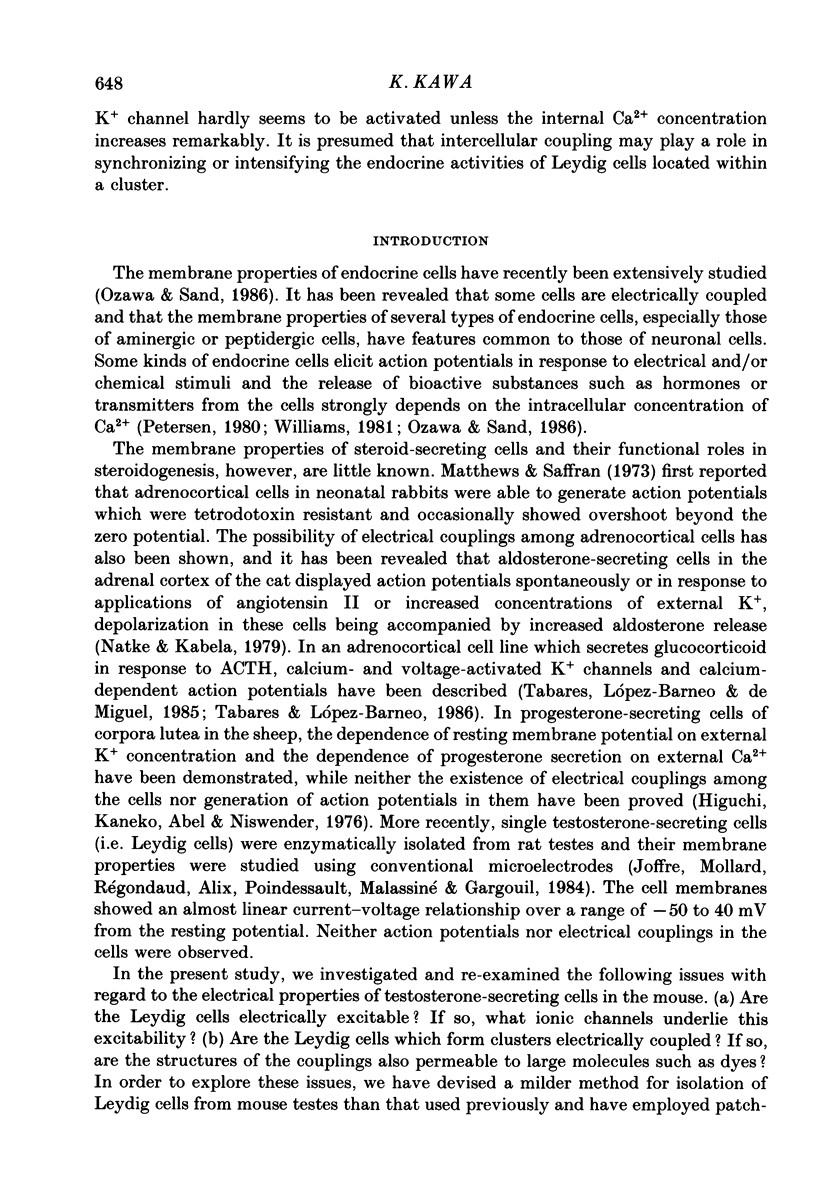
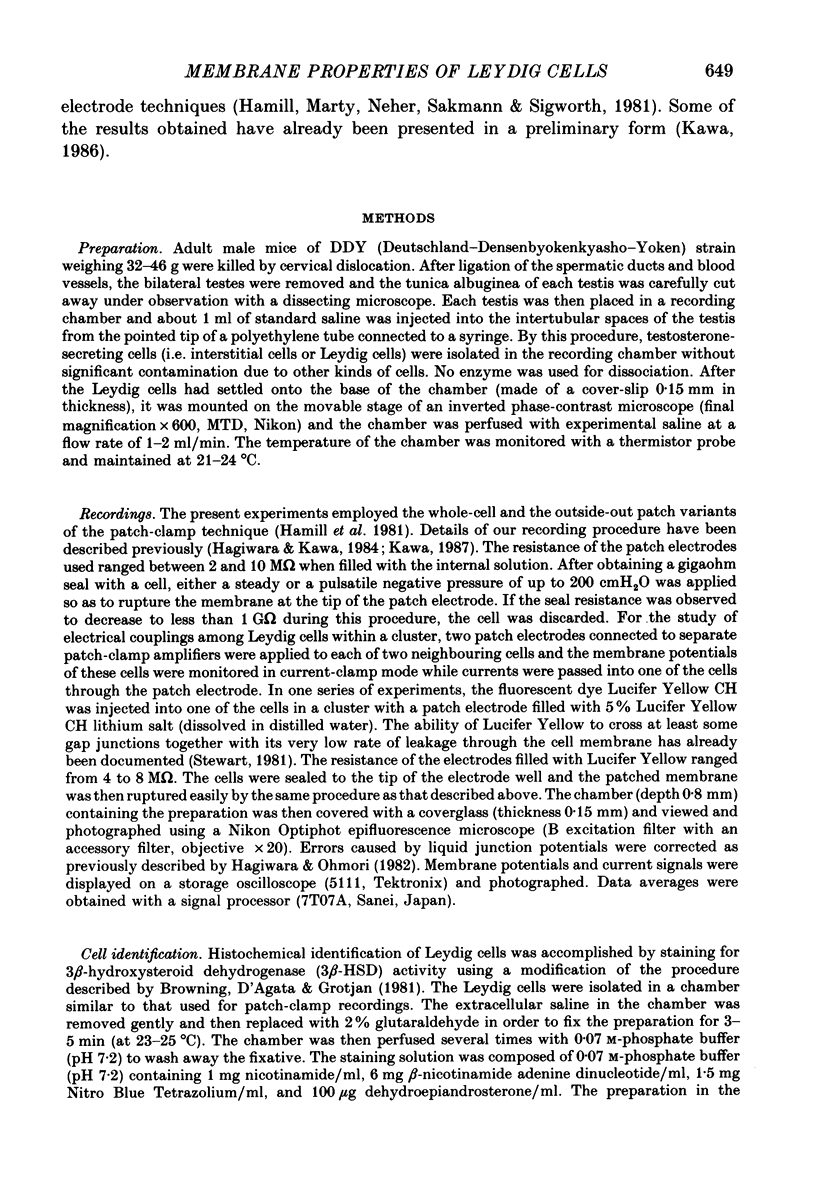
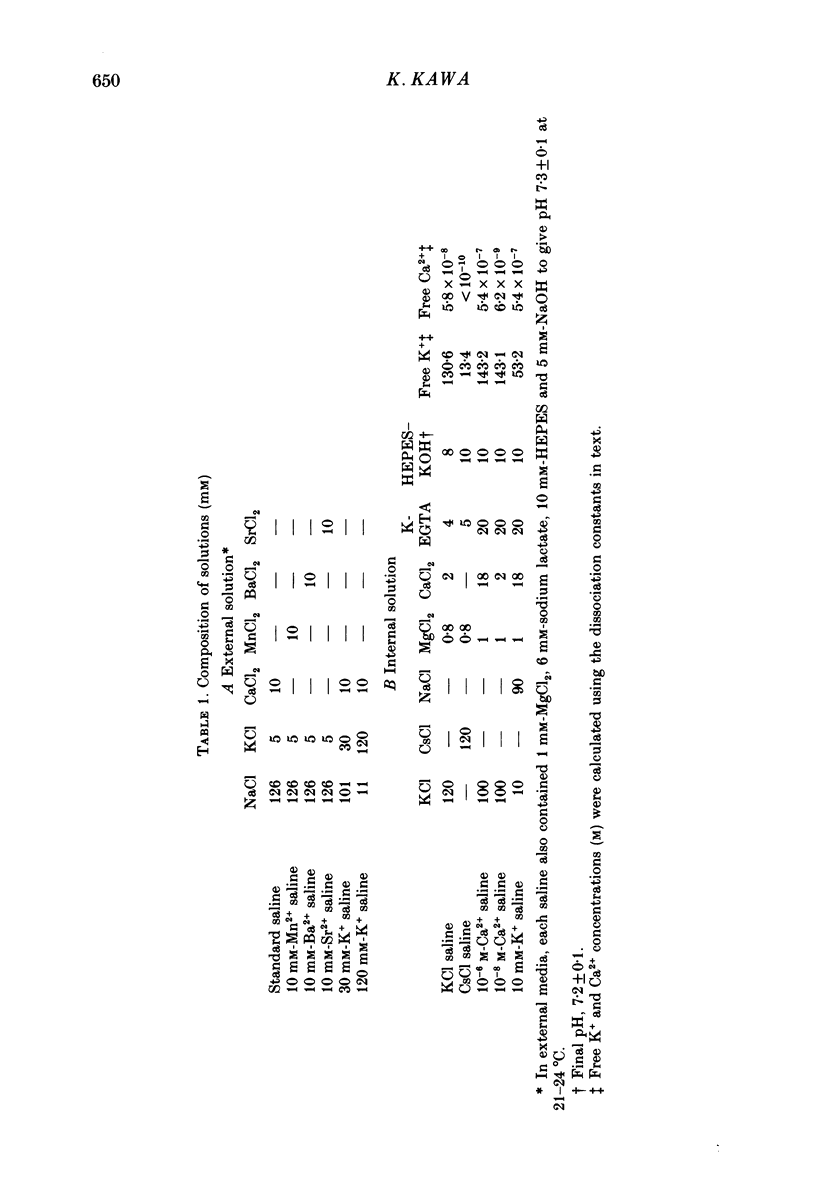
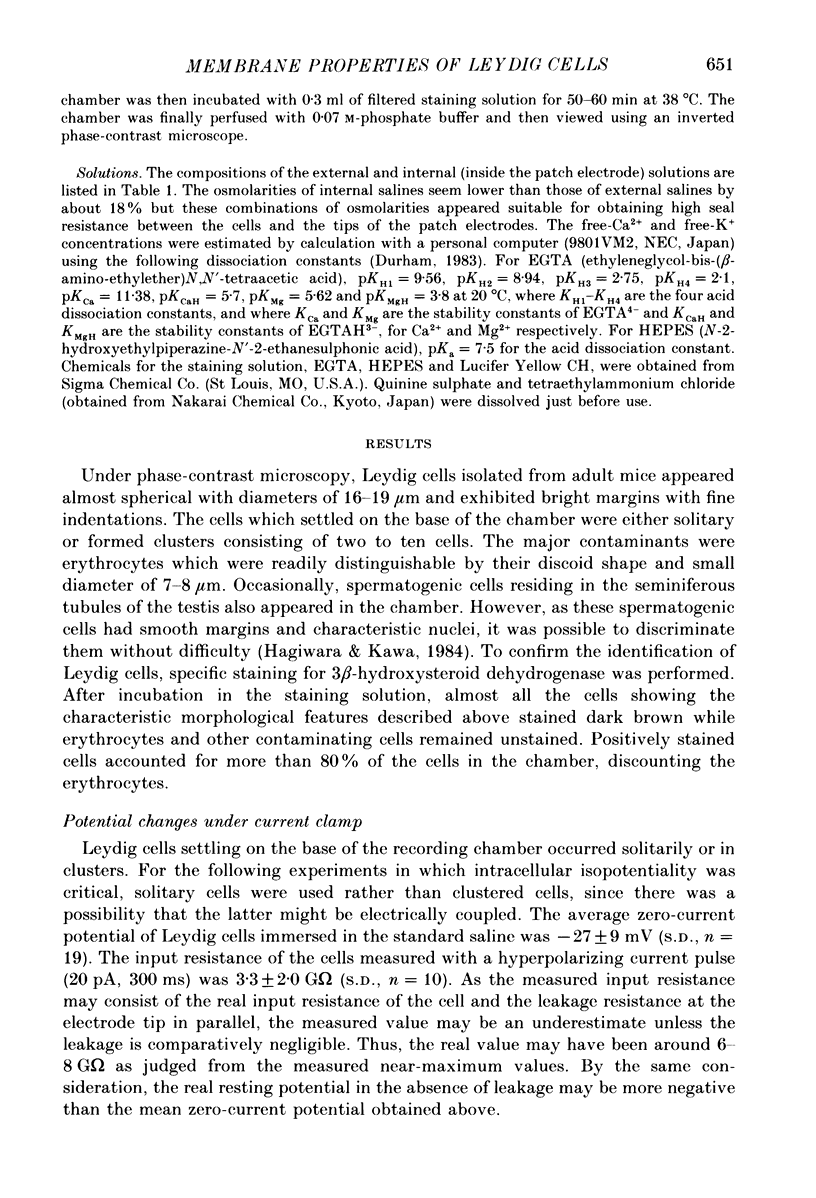
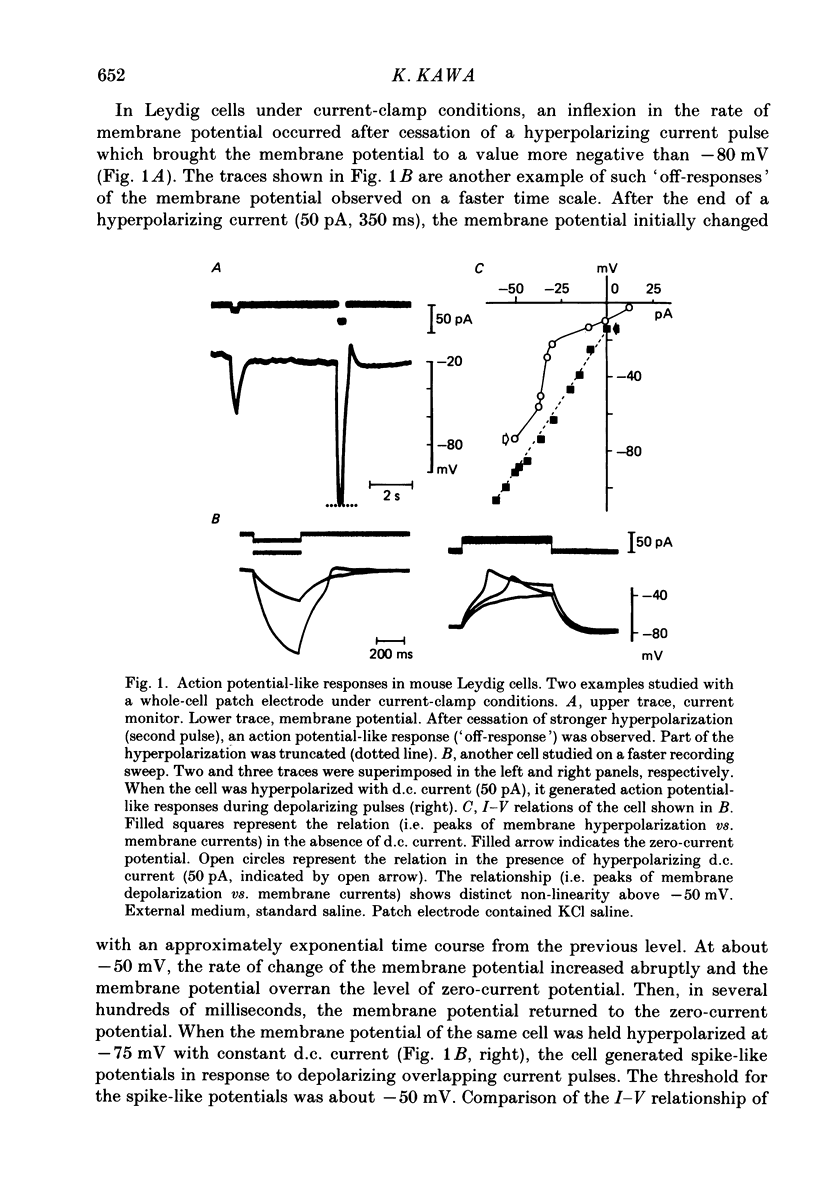
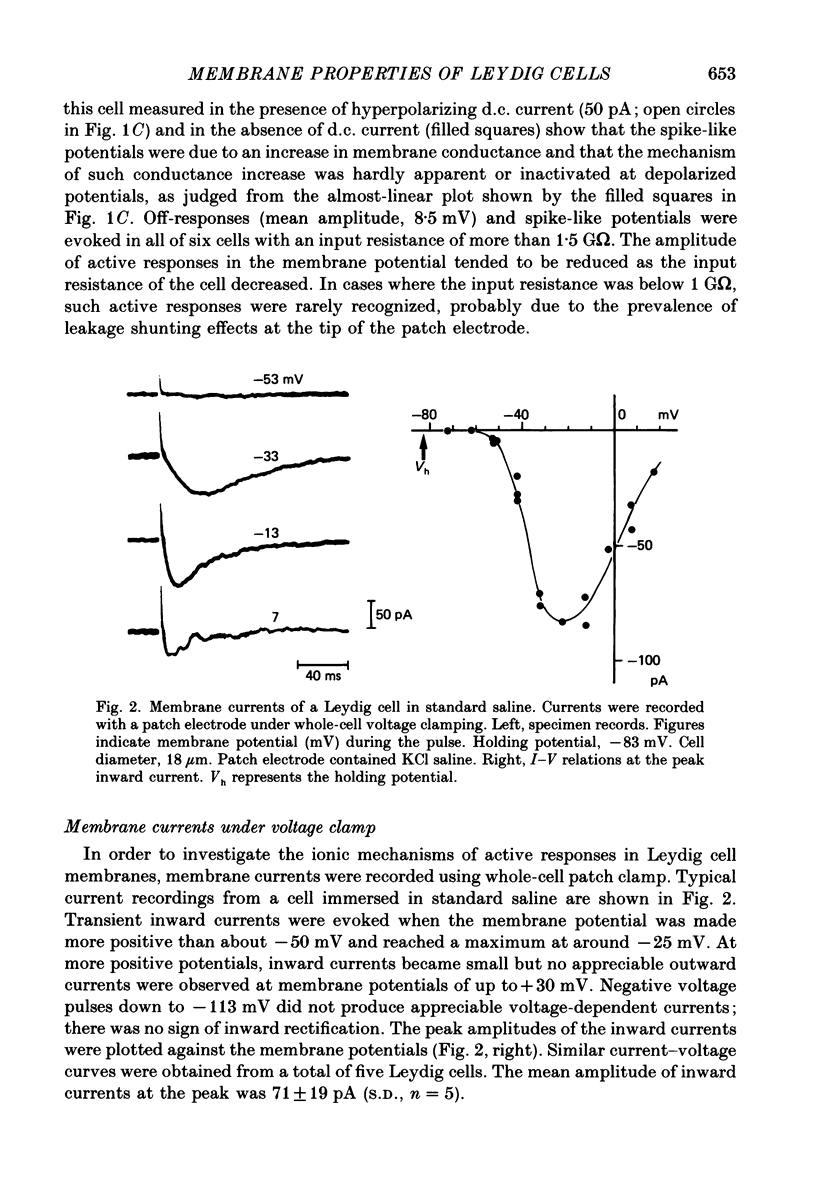
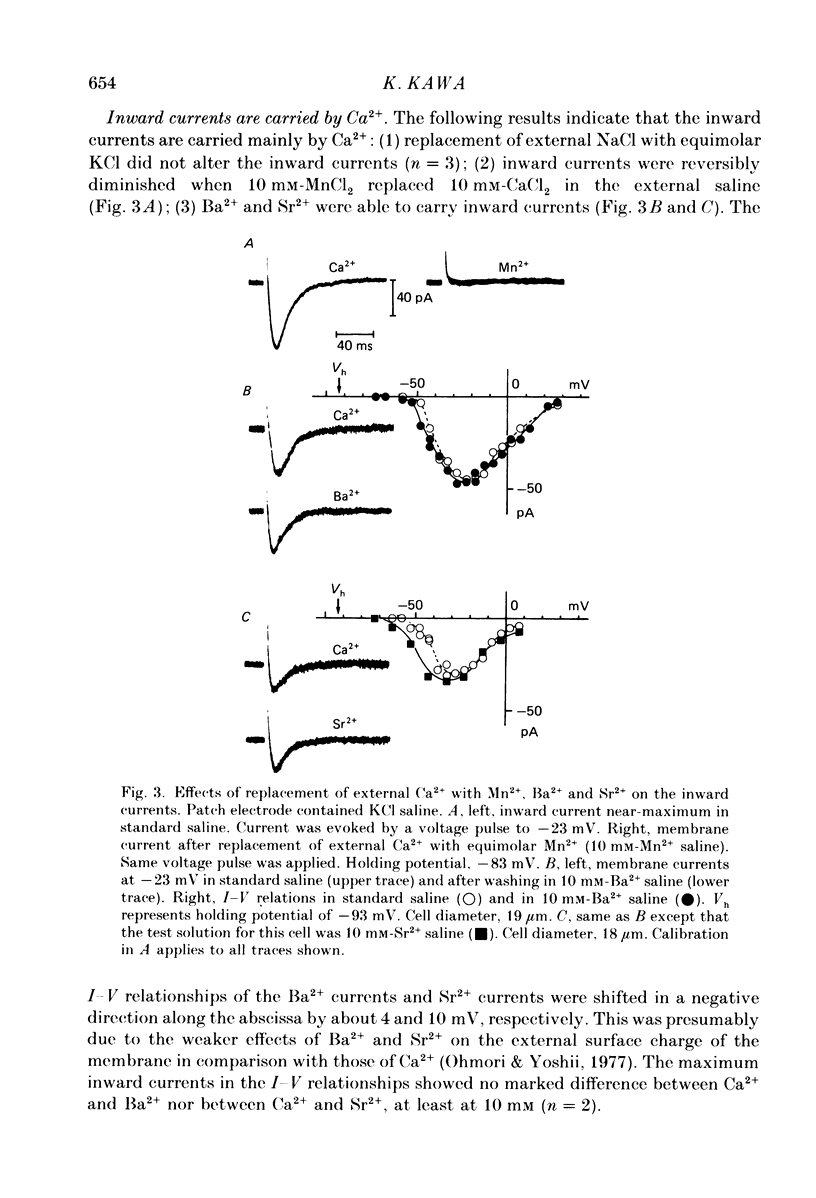
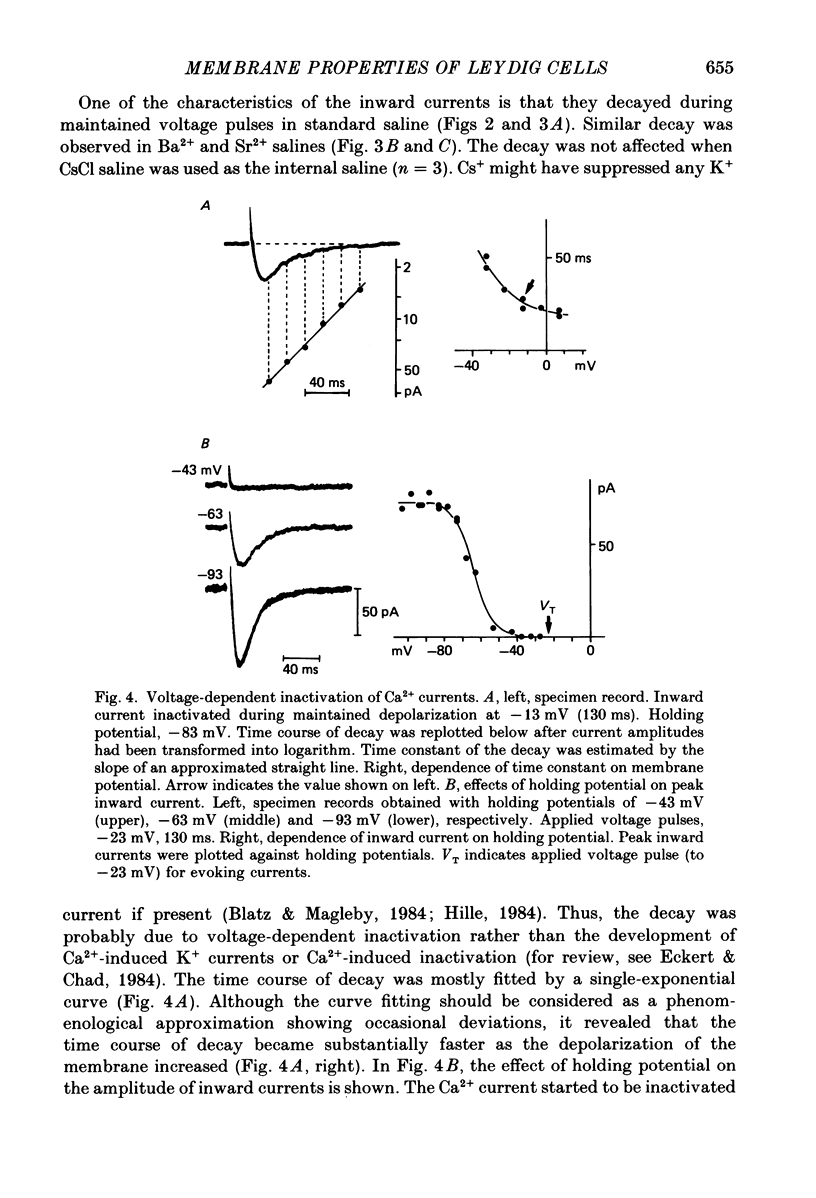
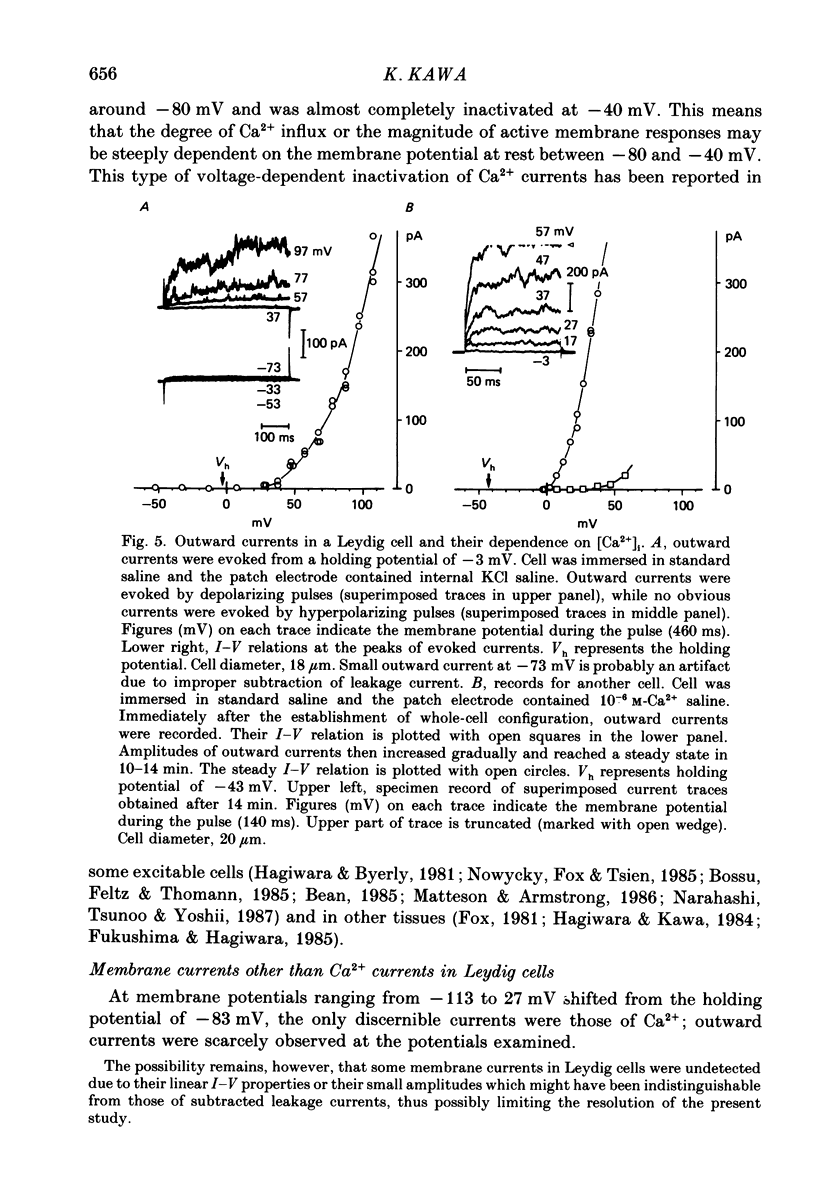
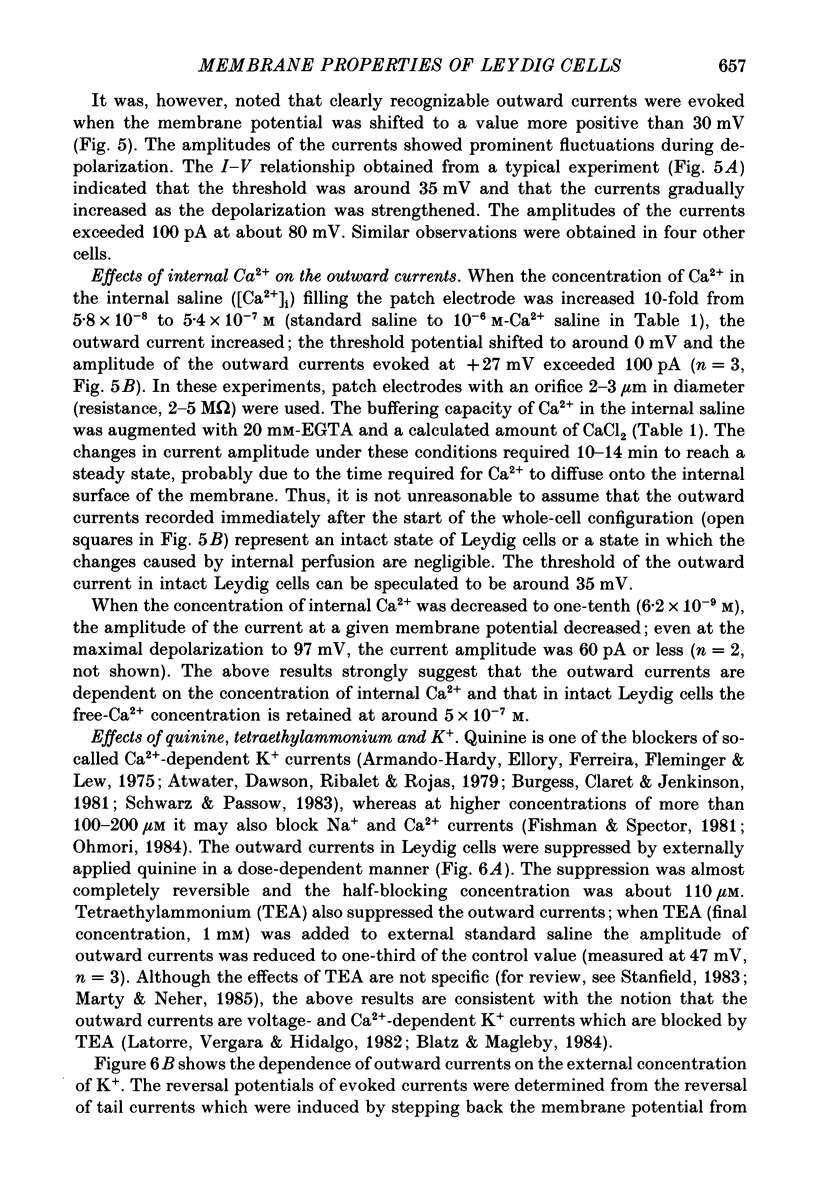
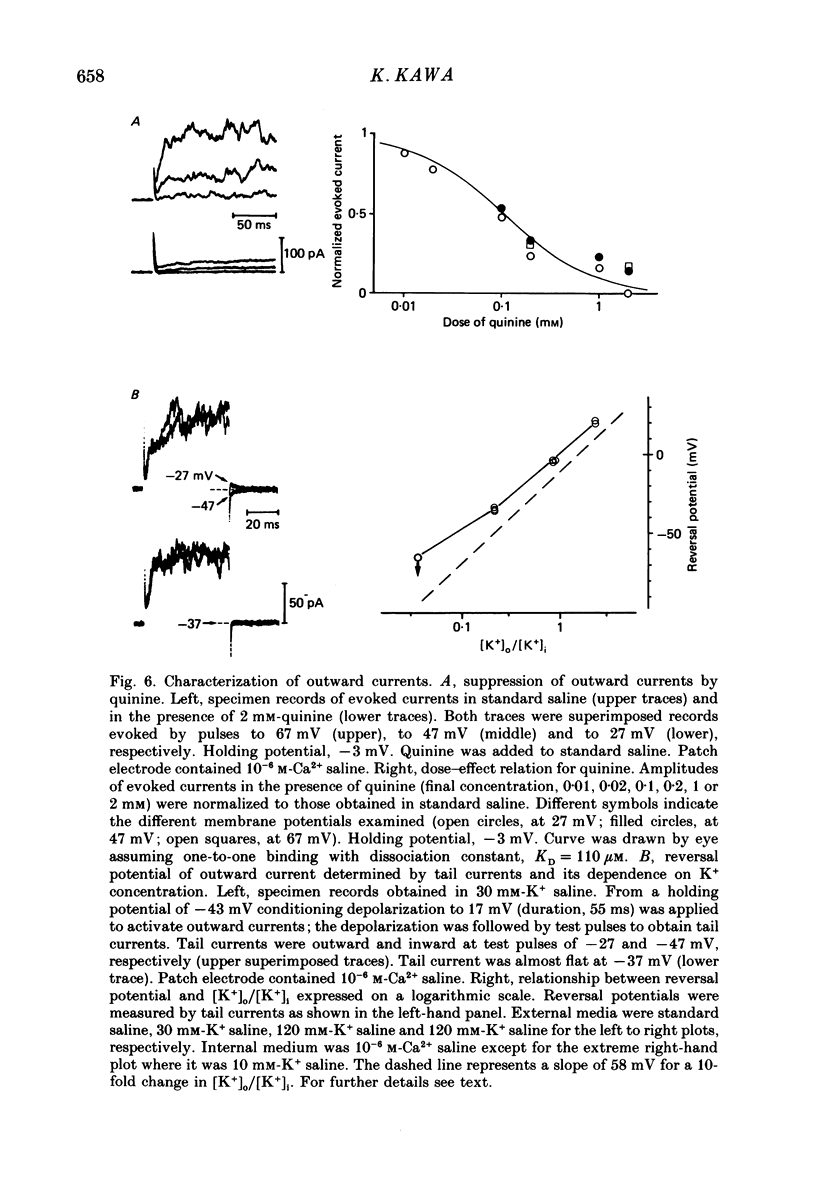
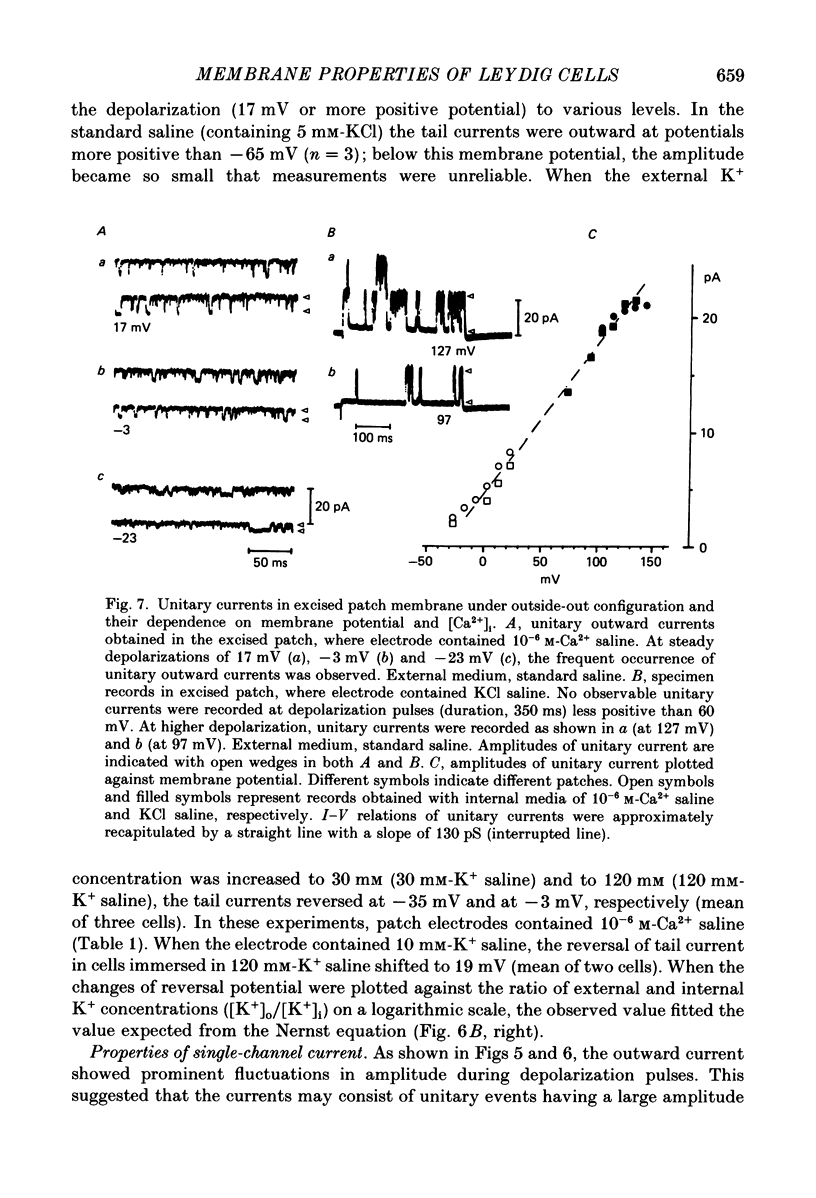
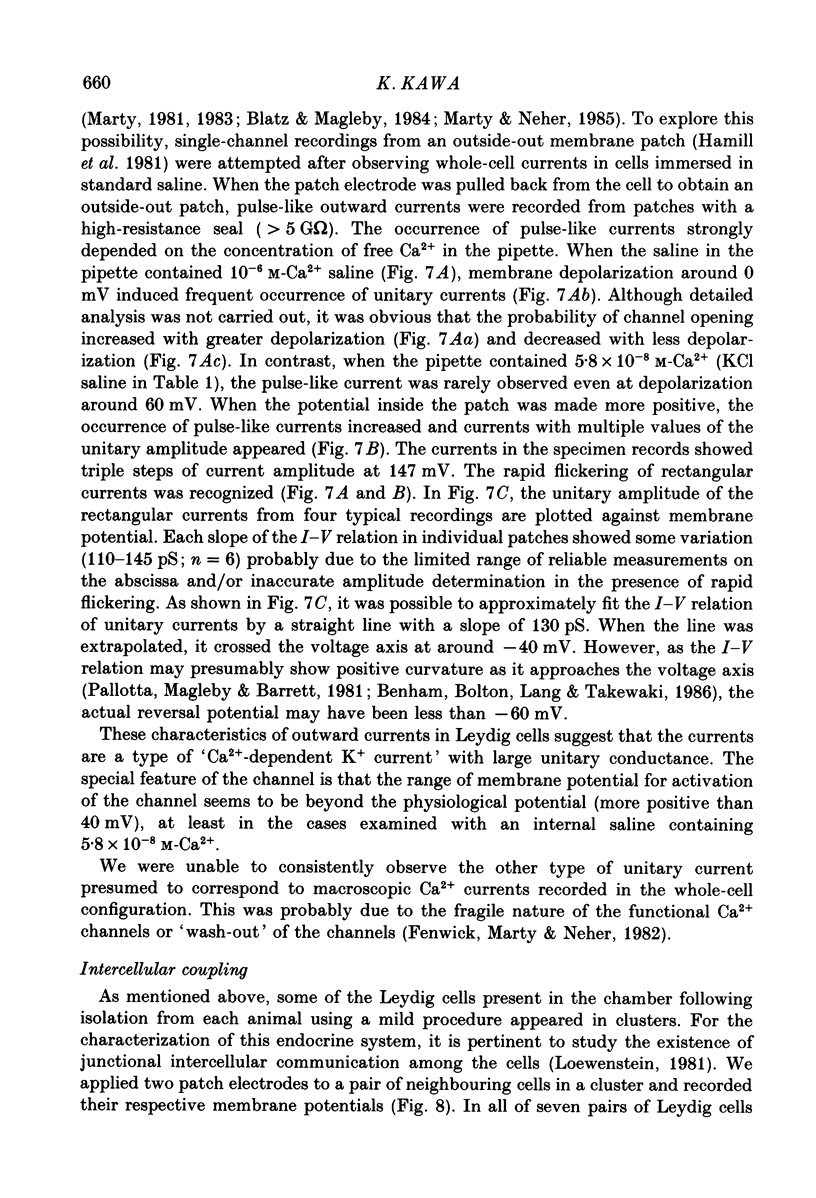
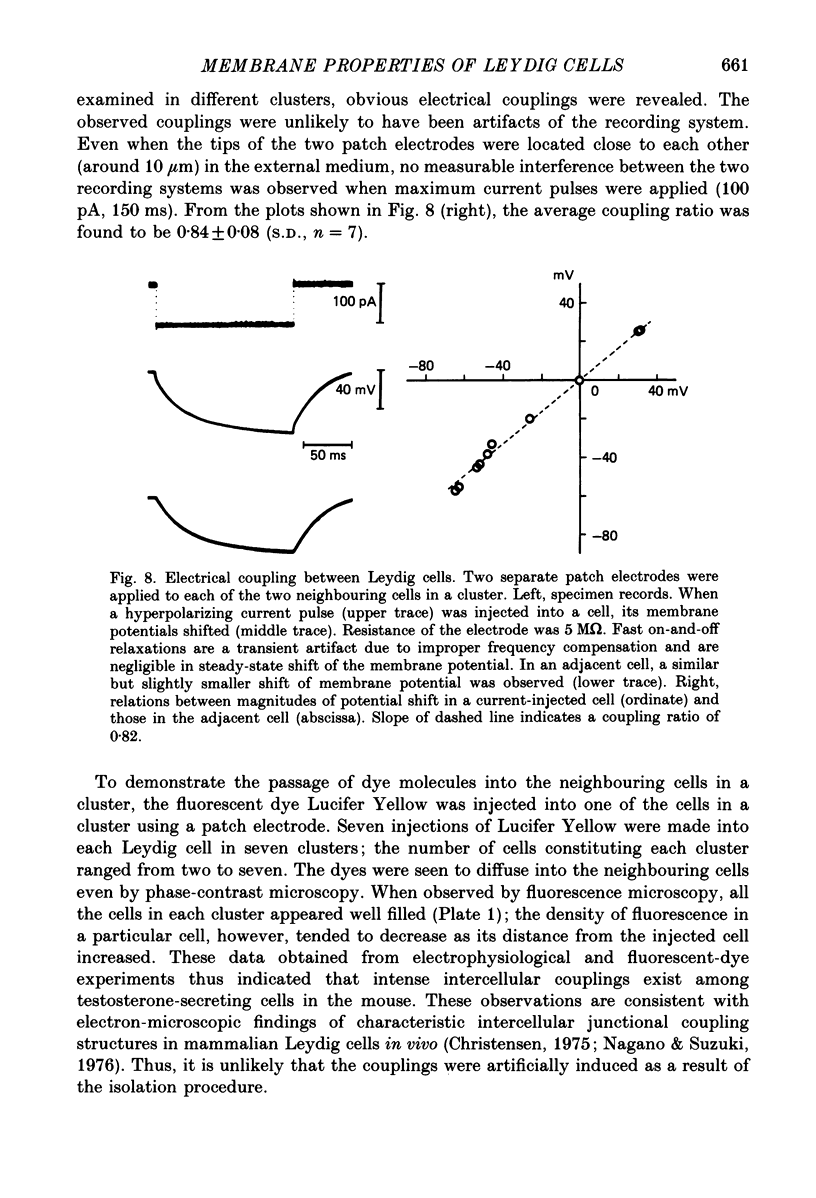
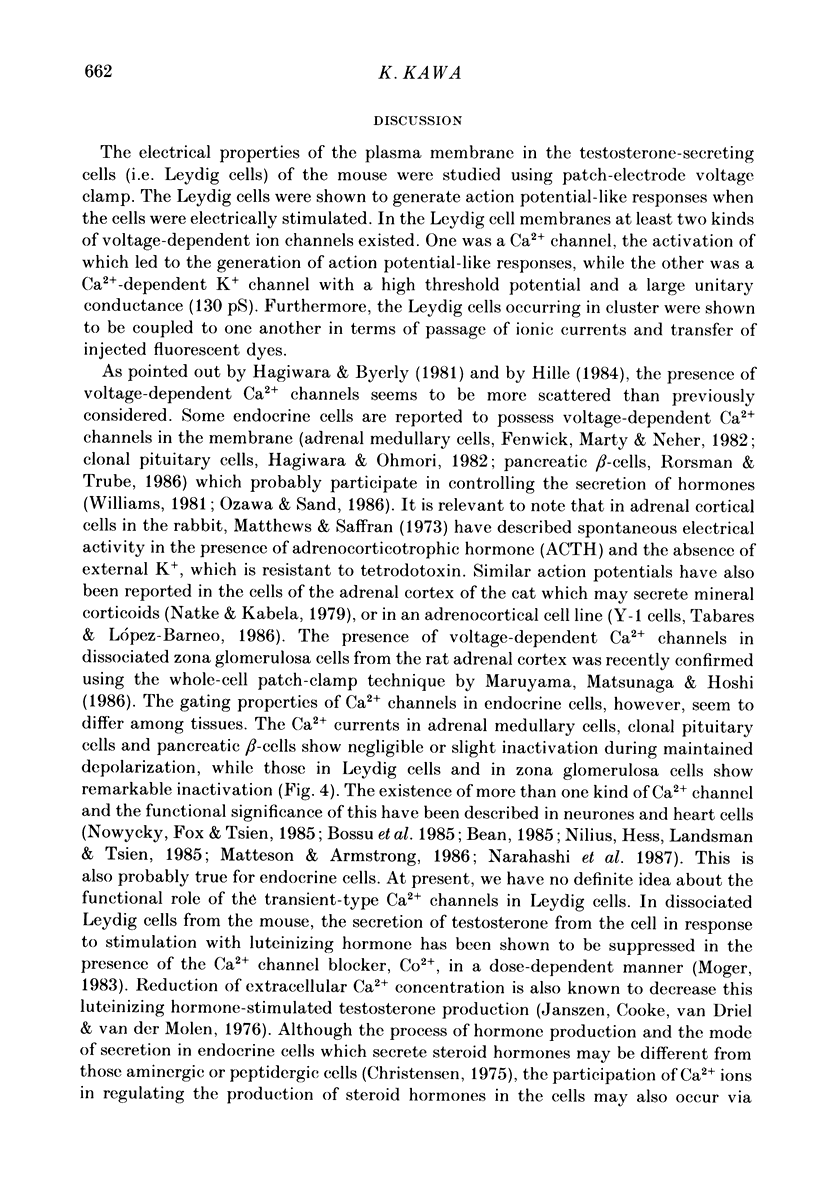
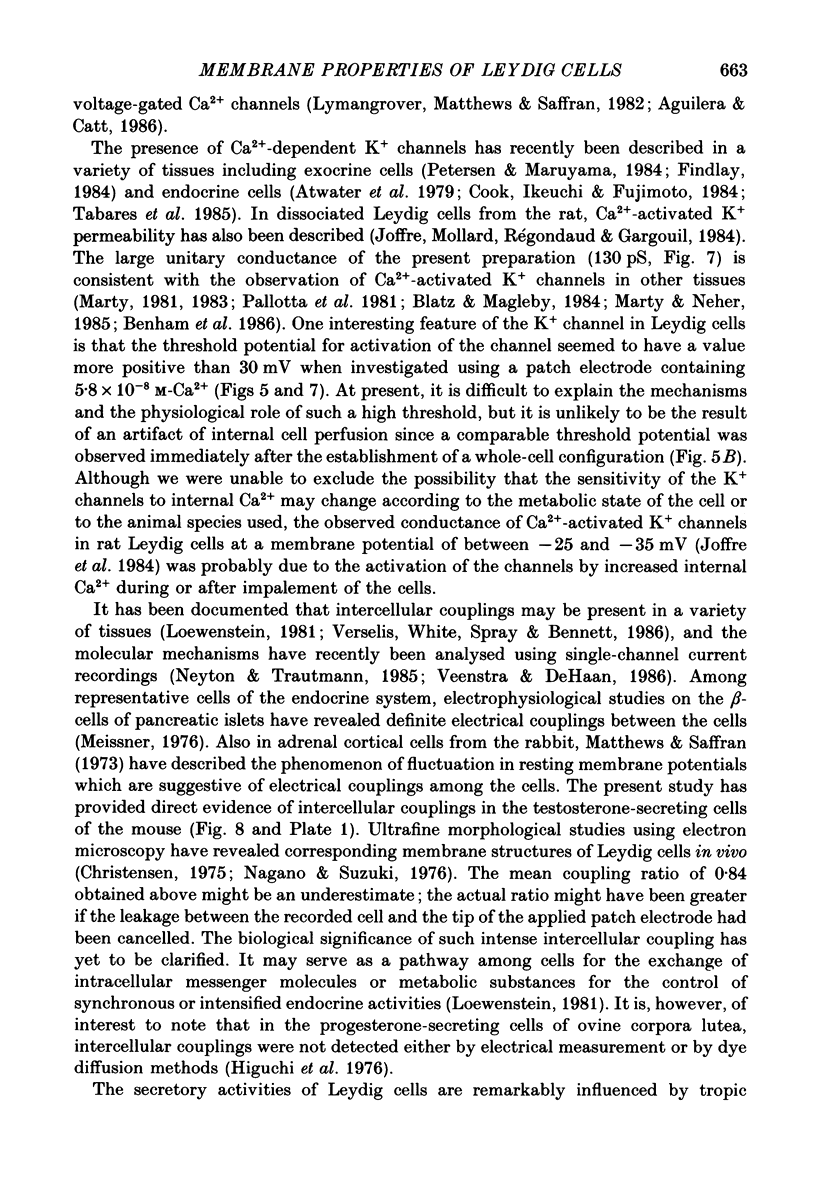
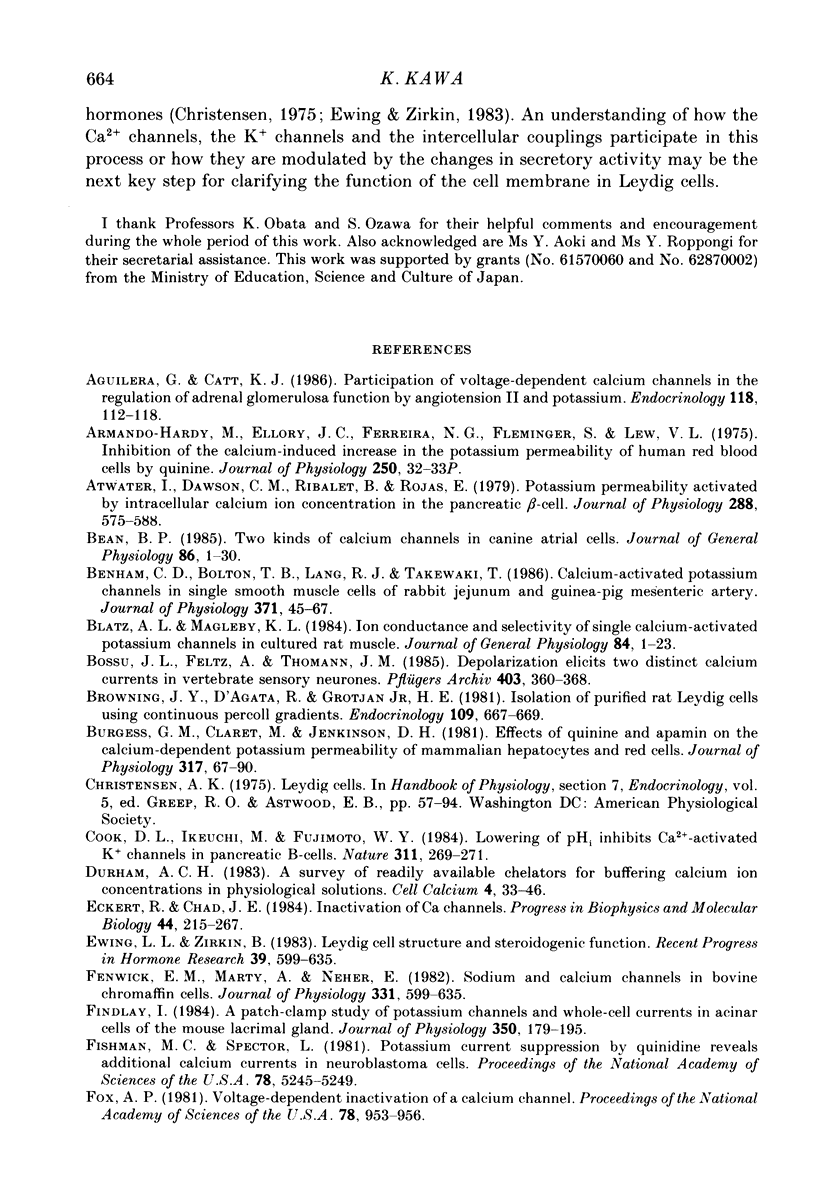
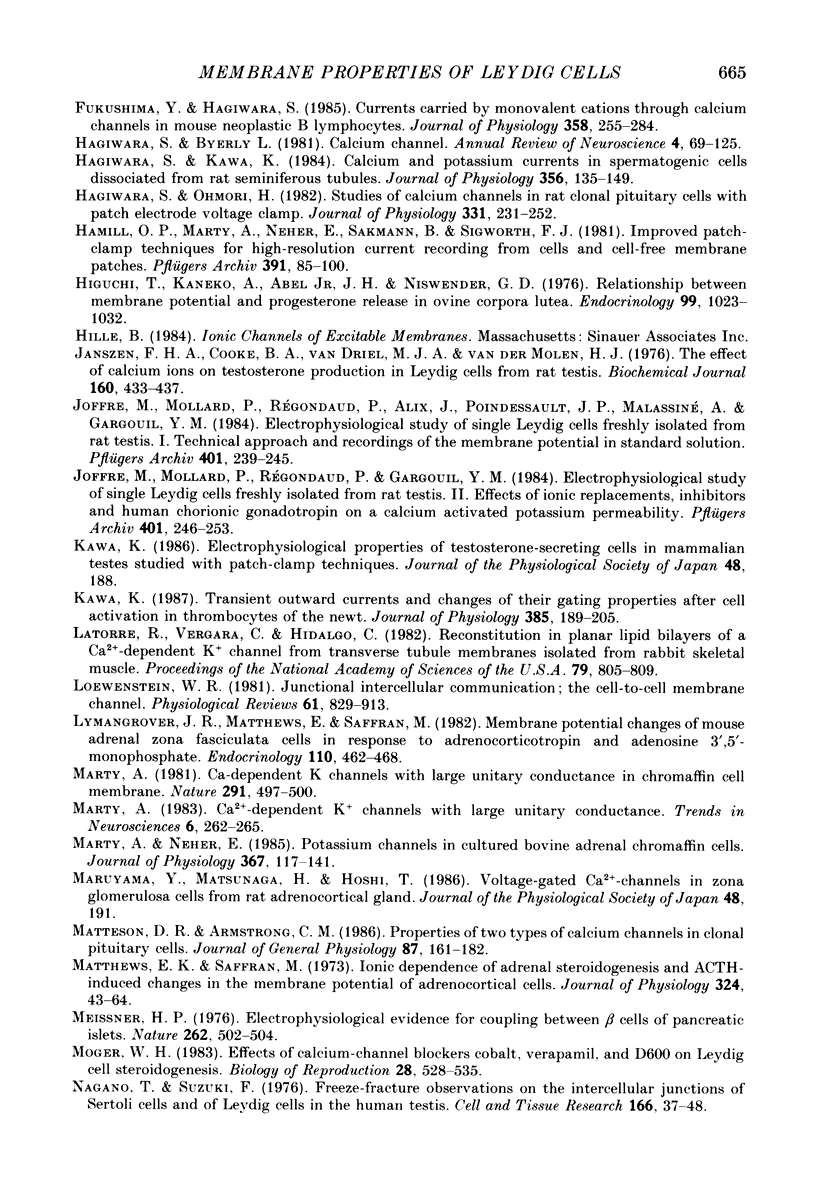
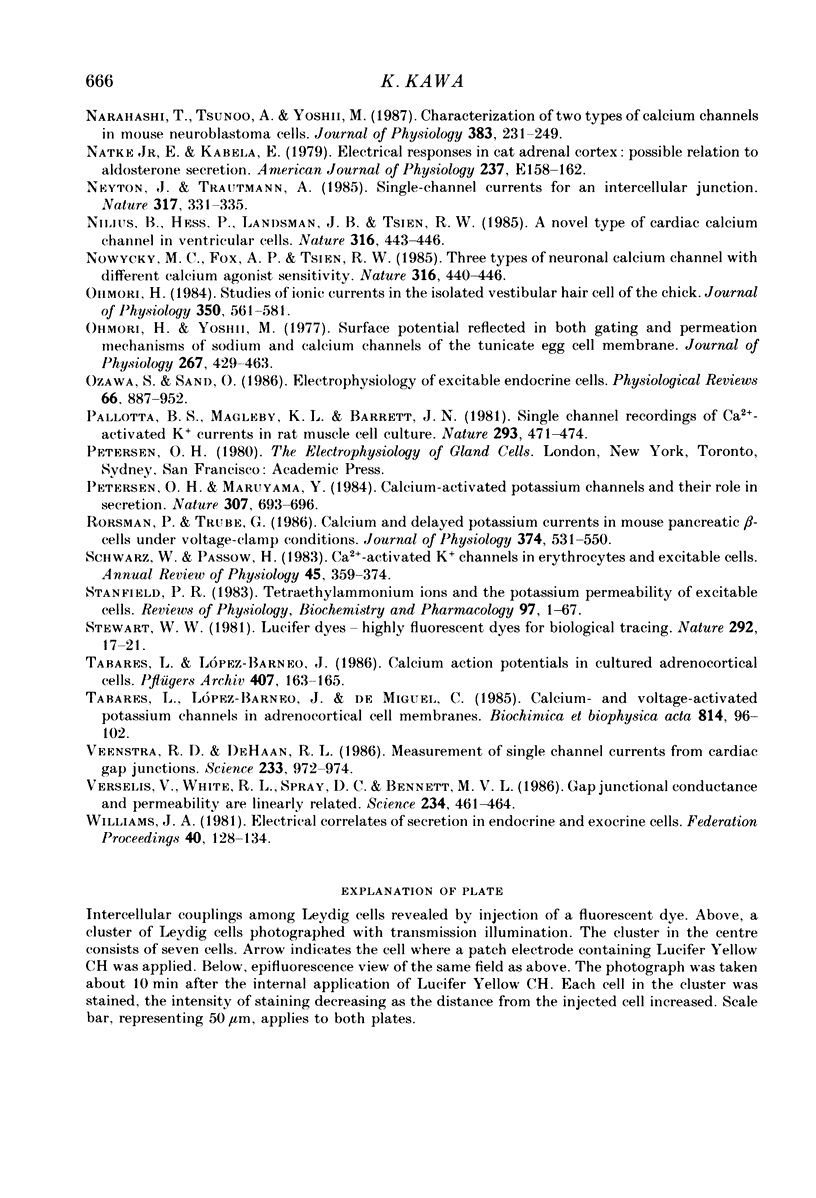
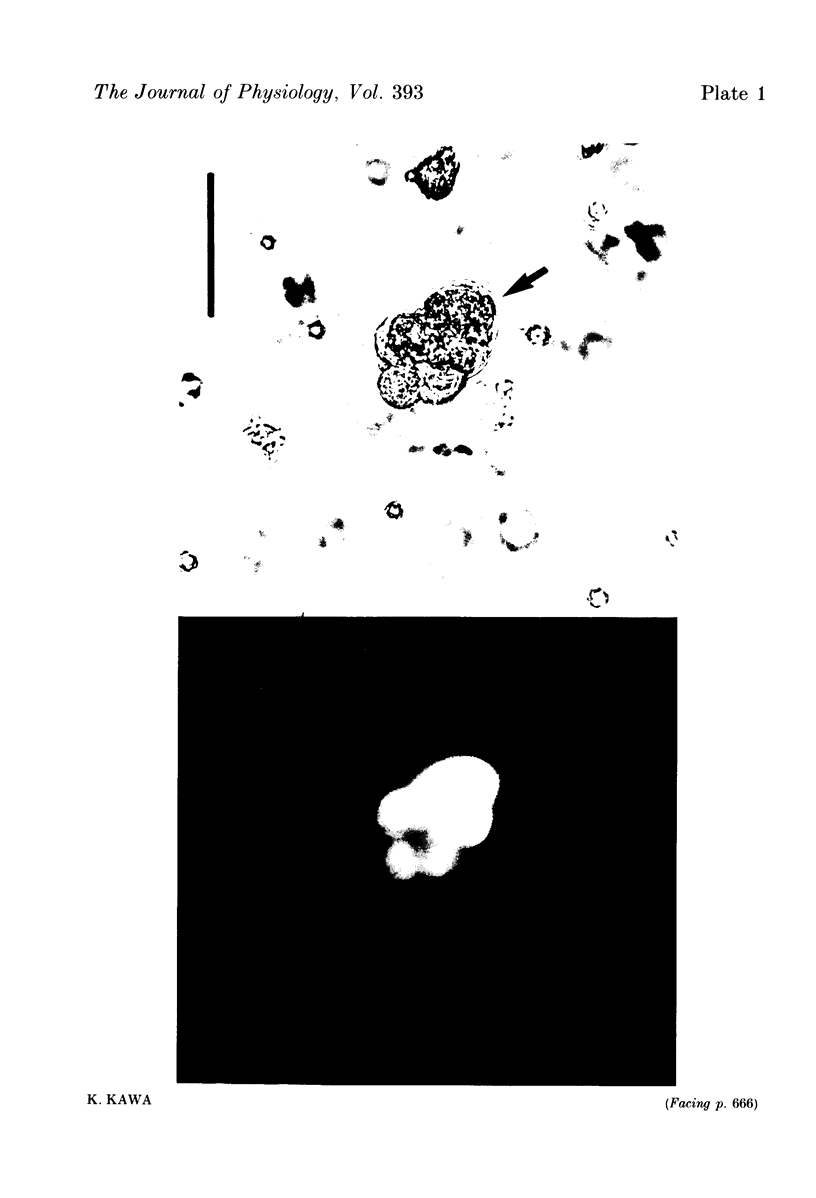
Images in this article
Selected References
These references are in PubMed. This may not be the complete list of references from this article.
- Aguilera G., Catt K. J. Participation of voltage-dependent calcium channels in the regulation of adrenal glomerulosa function by angiotensin II and potassium. Endocrinology. 1986 Jan;118(1):112–118. doi: 10.1210/endo-118-1-112. [DOI] [PubMed] [Google Scholar]
- Armando-Hardy M., Ellory J. C., Ferreira H. G., Fleminger S., Lew V. L. Inhibition of the calcium-induced increase in the potassium permeability of human red blood cells by quinine. J Physiol. 1975 Aug;250(1):32P–33P. [PubMed] [Google Scholar]
- Atwater I., Dawson C. M., Ribalet B., Rojas E. Potassium permeability activated by intracellular calcium ion concentration in the pancreatic beta-cell. J Physiol. 1979 Mar;288:575–588. [PMC free article] [PubMed] [Google Scholar]
- Bean B. P. Two kinds of calcium channels in canine atrial cells. Differences in kinetics, selectivity, and pharmacology. J Gen Physiol. 1985 Jul;86(1):1–30. doi: 10.1085/jgp.86.1.1. [DOI] [PMC free article] [PubMed] [Google Scholar]
- Benham C. D., Bolton T. B., Lang R. J., Takewaki T. Calcium-activated potassium channels in single smooth muscle cells of rabbit jejunum and guinea-pig mesenteric artery. J Physiol. 1986 Feb;371:45–67. doi: 10.1113/jphysiol.1986.sp015961. [DOI] [PMC free article] [PubMed] [Google Scholar]
- Blatz A. L., Magleby K. L. Ion conductance and selectivity of single calcium-activated potassium channels in cultured rat muscle. J Gen Physiol. 1984 Jul;84(1):1–23. doi: 10.1085/jgp.84.1.1. [DOI] [PMC free article] [PubMed] [Google Scholar]
- Bossu J. L., Feltz A., Thomann J. M. Depolarization elicits two distinct calcium currents in vertebrate sensory neurones. Pflugers Arch. 1985 Apr;403(4):360–368. doi: 10.1007/BF00589247. [DOI] [PubMed] [Google Scholar]
- Browning J. Y., D'Agata R., Grotjan H. E., Jr Isolation of purified rat Leydig cells using continuous Percoll gradients. Endocrinology. 1981 Aug;109(2):667–669. doi: 10.1210/endo-109-2-667. [DOI] [PubMed] [Google Scholar]
- Burgess G. M., Claret M., Jenkinson D. H. Effects of quinine and apamin on the calcium-dependent potassium permeability of mammalian hepatocytes and red cells. J Physiol. 1981 Aug;317:67–90. doi: 10.1113/jphysiol.1981.sp013814. [DOI] [PMC free article] [PubMed] [Google Scholar]
- Cook D. L., Ikeuchi M., Fujimoto W. Y. Lowering of pHi inhibits Ca2+-activated K+ channels in pancreatic B-cells. Nature. 1984 Sep 20;311(5983):269–271. doi: 10.1038/311269a0. [DOI] [PubMed] [Google Scholar]
- Durham A. C. A survey of readily available chelators for buffering calcium ion concentrations in physiological solutions. Cell Calcium. 1983 Feb;4(1):33–46. doi: 10.1016/0143-4160(83)90047-7. [DOI] [PubMed] [Google Scholar]
- Eckert R., Chad J. E. Inactivation of Ca channels. Prog Biophys Mol Biol. 1984;44(3):215–267. doi: 10.1016/0079-6107(84)90009-9. [DOI] [PubMed] [Google Scholar]
- Ewing L. L., Zirkin B. Leydig cell structure and steroidogenic function. Recent Prog Horm Res. 1983;39:599–635. doi: 10.1016/b978-0-12-571139-5.50019-7. [DOI] [PubMed] [Google Scholar]
- Fenwick E. M., Marty A., Neher E. Sodium and calcium channels in bovine chromaffin cells. J Physiol. 1982 Oct;331:599–635. doi: 10.1113/jphysiol.1982.sp014394. [DOI] [PMC free article] [PubMed] [Google Scholar]
- Findlay I. A patch-clamp study of potassium channels and whole-cell currents in acinar cells of the mouse lacrimal gland. J Physiol. 1984 May;350:179–195. doi: 10.1113/jphysiol.1984.sp015195. [DOI] [PMC free article] [PubMed] [Google Scholar]
- Fishman M. C., Spector I. Potassium current suppression by quinidine reveals additional calcium currents in neuroblastoma cells. Proc Natl Acad Sci U S A. 1981 Aug;78(8):5245–5249. doi: 10.1073/pnas.78.8.5245. [DOI] [PMC free article] [PubMed] [Google Scholar]
- Fox A. P. Voltage-dependent inactivation of a calcium channel. Proc Natl Acad Sci U S A. 1981 Feb;78(2):953–956. doi: 10.1073/pnas.78.2.953. [DOI] [PMC free article] [PubMed] [Google Scholar]
- Fukushima Y., Hagiwara S. Currents carried by monovalent cations through calcium channels in mouse neoplastic B lymphocytes. J Physiol. 1985 Jan;358:255–284. doi: 10.1113/jphysiol.1985.sp015550. [DOI] [PMC free article] [PubMed] [Google Scholar]
- Hagiwara S., Byerly L. Calcium channel. Annu Rev Neurosci. 1981;4:69–125. doi: 10.1146/annurev.ne.04.030181.000441. [DOI] [PubMed] [Google Scholar]
- Hagiwara S., Kawa K. Calcium and potassium currents in spermatogenic cells dissociated from rat seminiferous tubules. J Physiol. 1984 Nov;356:135–149. doi: 10.1113/jphysiol.1984.sp015457. [DOI] [PMC free article] [PubMed] [Google Scholar]
- Hagiwara S., Ohmori H. Studies of calcium channels in rat clonal pituitary cells with patch electrode voltage clamp. J Physiol. 1982 Oct;331:231–252. doi: 10.1113/jphysiol.1982.sp014371. [DOI] [PMC free article] [PubMed] [Google Scholar]
- Hamill O. P., Marty A., Neher E., Sakmann B., Sigworth F. J. Improved patch-clamp techniques for high-resolution current recording from cells and cell-free membrane patches. Pflugers Arch. 1981 Aug;391(2):85–100. doi: 10.1007/BF00656997. [DOI] [PubMed] [Google Scholar]
- Higuchi T., Kaneko A., Abel J. H., Jr, Niswender G. D. Relationship between membrane potential and progesterone release in ovine corpora lutea. Endocrinology. 1976 Oct;99(4):1023–1032. doi: 10.1210/endo-99-4-1023. [DOI] [PubMed] [Google Scholar]
- Janszen F. H., Cooke B. A., Van Driel M. J., Van Der Molen H. J. The effect of calcium ions on testosterone production in Leydig cells from rat testis. Biochem J. 1976 Dec 15;160(3):433–437. doi: 10.1042/bj1600433. [DOI] [PMC free article] [PubMed] [Google Scholar]
- Joffre M., Mollard P., Régondaud P., Alix J., Poindessault J. P., Malassiné A., Gargouïl Y. M. Electrophysiological study of single Leydig cells freshly isolated from rat testis. I. Technical approach and recordings of the membrane potential in standard solution. Pflugers Arch. 1984 Jul;401(3):239–245. doi: 10.1007/BF00582590. [DOI] [PubMed] [Google Scholar]
- Joffre M., Mollard P., Régondaud P., Gargouïl Y. M. Electrophysiological study of single Leydig cells freshly isolated from rat testis. II. Effects of ionic replacements, inhibitors and human chorionic gonadotropin on a calcium activated potassium permeability. Pflugers Arch. 1984 Jul;401(3):246–253. doi: 10.1007/BF00582591. [DOI] [PubMed] [Google Scholar]
- Kawa K. Transient outward currents and changes of their gating properties after cell activation in thrombocytes of the newt. J Physiol. 1987 Apr;385:189–205. doi: 10.1113/jphysiol.1987.sp016491. [DOI] [PMC free article] [PubMed] [Google Scholar]
- Latorre R., Vergara C., Hidalgo C. Reconstitution in planar lipid bilayers of a Ca2+-dependent K+ channel from transverse tubule membranes isolated from rabbit skeletal muscle. Proc Natl Acad Sci U S A. 1982 Feb;79(3):805–809. doi: 10.1073/pnas.79.3.805. [DOI] [PMC free article] [PubMed] [Google Scholar]
- Loewenstein W. R. Junctional intercellular communication: the cell-to-cell membrane channel. Physiol Rev. 1981 Oct;61(4):829–913. doi: 10.1152/physrev.1981.61.4.829. [DOI] [PubMed] [Google Scholar]
- Lymangrover J. R., Matthews E. K., Saffran M. Membrane potential changes of mouse adrenal zona fasciculata cells in response to adrenocorticotropin and adenosine 3',5'-monophosphate. Endocrinology. 1982 Feb;110(2):462–468. doi: 10.1210/endo-110-2-462. [DOI] [PubMed] [Google Scholar]
- Marty A. Ca-dependent K channels with large unitary conductance in chromaffin cell membranes. Nature. 1981 Jun 11;291(5815):497–500. doi: 10.1038/291497a0. [DOI] [PubMed] [Google Scholar]
- Marty A., Neher E. Potassium channels in cultured bovine adrenal chromaffin cells. J Physiol. 1985 Oct;367:117–141. doi: 10.1113/jphysiol.1985.sp015817. [DOI] [PMC free article] [PubMed] [Google Scholar]
- Matteson D. R., Armstrong C. M. Properties of two types of calcium channels in clonal pituitary cells. J Gen Physiol. 1986 Jan;87(1):161–182. doi: 10.1085/jgp.87.1.161. [DOI] [PMC free article] [PubMed] [Google Scholar]
- Matthews E. K., Saffran M. Ionic dependence of adrenal steroidogenesis and ACTH-induced changes in the membrane potential of adrenocortical cells. J Physiol. 1973 Oct;234(1):43–64. doi: 10.1113/jphysiol.1973.sp010333. [DOI] [PMC free article] [PubMed] [Google Scholar]
- Meissner H. P. Electrophysiological evidence for coupling between beta cells of pancreatic islets. Nature. 1976 Aug 5;262(5568):502–504. doi: 10.1038/262502a0. [DOI] [PubMed] [Google Scholar]
- Moger W. H. Effects of the calcium-channel blockers cobalt, verapamil, and D600 on Leydig cell steroidogenesis. Biol Reprod. 1983 Apr;28(3):528–535. doi: 10.1095/biolreprod28.3.528. [DOI] [PubMed] [Google Scholar]
- Nagano T., Suzuki F. Freeze-fracture observations on the intercellular junctions of Sertoli cells and of Leydig cells in the human testis. Cell Tissue Res. 1976 Feb 6;166(1):37–48. doi: 10.1007/BF00215123. [DOI] [PubMed] [Google Scholar]
- Narahashi T., Tsunoo A., Yoshii M. Characterization of two types of calcium channels in mouse neuroblastoma cells. J Physiol. 1987 Feb;383:231–249. doi: 10.1113/jphysiol.1987.sp016406. [DOI] [PMC free article] [PubMed] [Google Scholar]
- Natke E., Jr, Kabela E. Electrical responses in cat adrenal cortex: possible relation to aldosterone secretion. Am J Physiol. 1979 Aug;237(2):E158–E162. doi: 10.1152/ajpendo.1979.237.2.E158. [DOI] [PubMed] [Google Scholar]
- Neyton J., Trautmann A. Single-channel currents of an intercellular junction. 1985 Sep 26-Oct 2Nature. 317(6035):331–335. doi: 10.1038/317331a0. [DOI] [PubMed] [Google Scholar]
- Nilius B., Hess P., Lansman J. B., Tsien R. W. A novel type of cardiac calcium channel in ventricular cells. Nature. 1985 Aug 1;316(6027):443–446. doi: 10.1038/316443a0. [DOI] [PubMed] [Google Scholar]
- Nowycky M. C., Fox A. P., Tsien R. W. Three types of neuronal calcium channel with different calcium agonist sensitivity. Nature. 1985 Aug 1;316(6027):440–443. doi: 10.1038/316440a0. [DOI] [PubMed] [Google Scholar]
- Ohmori H. Studies of ionic currents in the isolated vestibular hair cell of the chick. J Physiol. 1984 May;350:561–581. doi: 10.1113/jphysiol.1984.sp015218. [DOI] [PMC free article] [PubMed] [Google Scholar]
- Ohmori H., Yoshii M. Surface potential reflected in both gating and permeation mechanisms of sodium and calcium channels of the tunicate egg cell membrane. J Physiol. 1977 May;267(2):429–463. doi: 10.1113/jphysiol.1977.sp011821. [DOI] [PMC free article] [PubMed] [Google Scholar]
- Ozawa S., Sand O. Electrophysiology of excitable endocrine cells. Physiol Rev. 1986 Oct;66(4):887–952. doi: 10.1152/physrev.1986.66.4.887. [DOI] [PubMed] [Google Scholar]
- Pallotta B. S., Magleby K. L., Barrett J. N. Single channel recordings of Ca2+-activated K+ currents in rat muscle cell culture. Nature. 1981 Oct 8;293(5832):471–474. doi: 10.1038/293471a0. [DOI] [PubMed] [Google Scholar]
- Petersen O. H., Maruyama Y. Calcium-activated potassium channels and their role in secretion. Nature. 1984 Feb 23;307(5953):693–696. doi: 10.1038/307693a0. [DOI] [PubMed] [Google Scholar]
- Rorsman P., Trube G. Calcium and delayed potassium currents in mouse pancreatic beta-cells under voltage-clamp conditions. J Physiol. 1986 May;374:531–550. doi: 10.1113/jphysiol.1986.sp016096. [DOI] [PMC free article] [PubMed] [Google Scholar]
- Schwarz W., Passow H. Ca2+-activated K+ channels in erythrocytes and excitable cells. Annu Rev Physiol. 1983;45:359–374. doi: 10.1146/annurev.ph.45.030183.002043. [DOI] [PubMed] [Google Scholar]
- Stanfield P. R. Tetraethylammonium ions and the potassium permeability of excitable cells. Rev Physiol Biochem Pharmacol. 1983;97:1–67. doi: 10.1007/BFb0035345. [DOI] [PubMed] [Google Scholar]
- Stewart W. W. Lucifer dyes--highly fluorescent dyes for biological tracing. Nature. 1981 Jul 2;292(5818):17–21. doi: 10.1038/292017a0. [DOI] [PubMed] [Google Scholar]
- Tabares L., López-Barneo J. Calcium action potentials in cultured adrenocortical cells. Pflugers Arch. 1986 Aug;407(2):163–165. doi: 10.1007/BF00580669. [DOI] [PubMed] [Google Scholar]
- Tabares L., López-Barneo J., de Miguel C. Calcium- and voltage-activated potassium channels in adrenocortical cell membranes. Biochim Biophys Acta. 1985 Mar 28;814(1):96–102. doi: 10.1016/0005-2736(85)90423-7. [DOI] [PubMed] [Google Scholar]
- Veenstra R. D., DeHaan R. L. Measurement of single channel currents from cardiac gap junctions. Science. 1986 Aug 29;233(4767):972–974. doi: 10.1126/science.2426781. [DOI] [PubMed] [Google Scholar]
- Verselis V., White R. L., Spray D. C., Bennett M. V. Gap junctional conductance and permeability are linearly related. Science. 1986 Oct 24;234(4775):461–464. doi: 10.1126/science.3489990. [DOI] [PubMed] [Google Scholar]
- Williams J. A. Electrical correlates of secretion in endocrine and exocrine cells. Fed Proc. 1981 Feb;40(2):128–134. [PubMed] [Google Scholar]