Abstract
1. A reliable and simple fish brain slice preparation was obtained from the cerebellum of the skate, and its properties were described. 2. A potentiometric oxonol dye, RH-482, and multiple site optical recording of transmembrane voltage (MSORTV) were used to reveal the electrophysiological properties of the parallel fibre action potential and to measure its conduction (0.13 m/s). The parallel fibre action potential was blocked in the presence of tetrodotoxin (TTX) and prolonged by tetraethylammonium (TEA), suggesting that the upstroke depends upon sodium entry and the repolarization upon potassium efflux. An after-hyperpolarization results from a calcium-dependent potassium conductance. 3. A second potentiometric dye, RH-155, differing only slightly from RH-482, exhibited a high affinity for glial cell membrane, and could be used to monitor changes in extracellular potassium concentration by detecting changes in glial membrane potential. 4. Calcium channel blockers such as cadmium ions blocked the optical signal that reflected the extracellular accumulation of potassium. 5. Interventions that modified the extracellular volume, and thereby affected the accumulation of potassium, produced large changes in the optical signal that monitored glial depolarization. Hypertonic and hypotonic bathing solutions resulted in decreases and increases, respectively, in the magnitude of the extrinsic absorption change that tracked potassium accumulation. 6. Blocking sodium-potassium pump activity by means of ouabain prolonged the time course of the optical signal that was related to potassium accumulation in the extracellular space. 7. Extracellular potassium accumulation was revealed to be critically dependent upon intracellular calcium ions.
Full text
PDF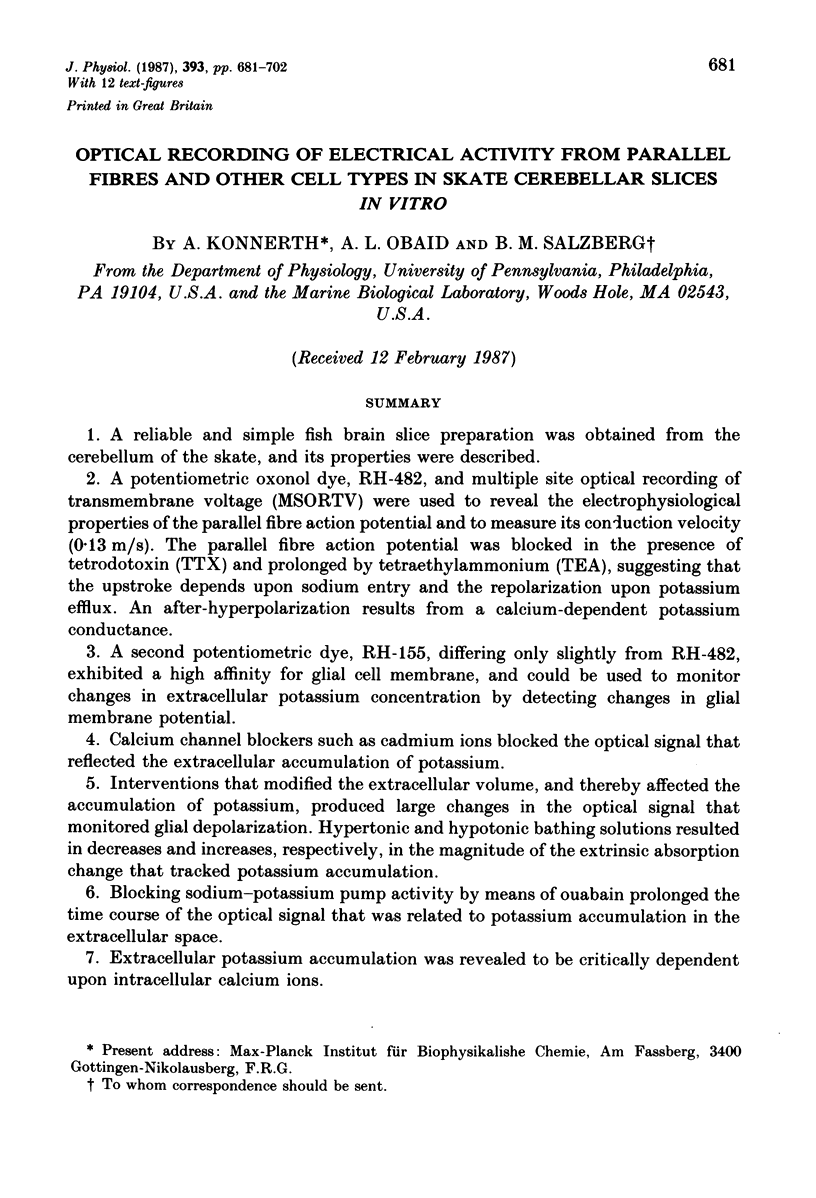
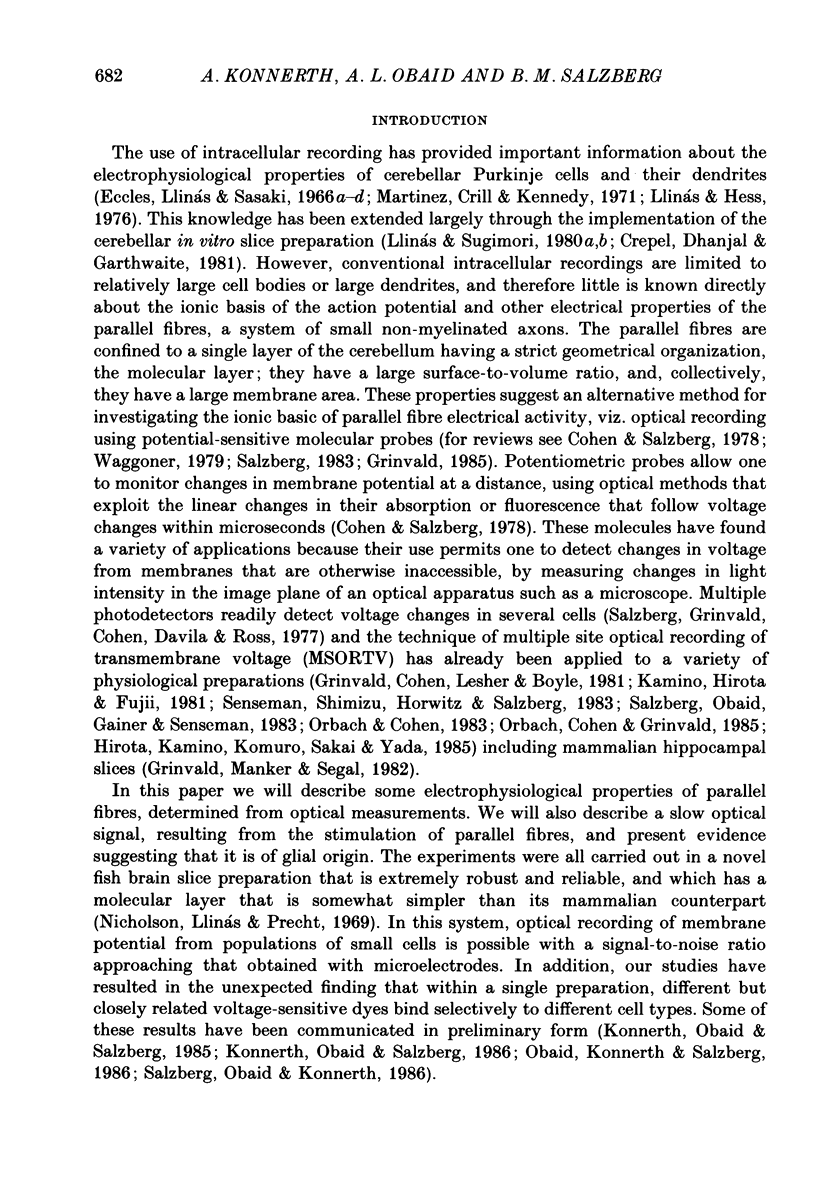
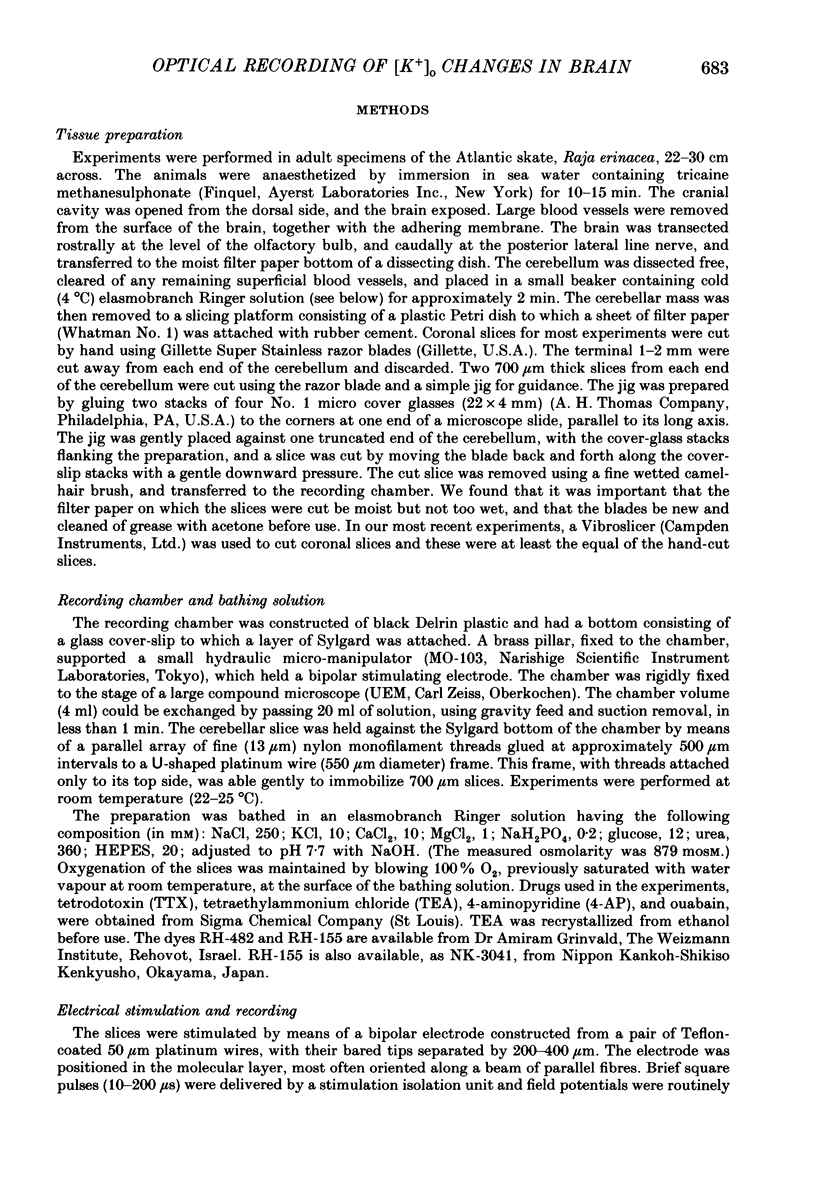
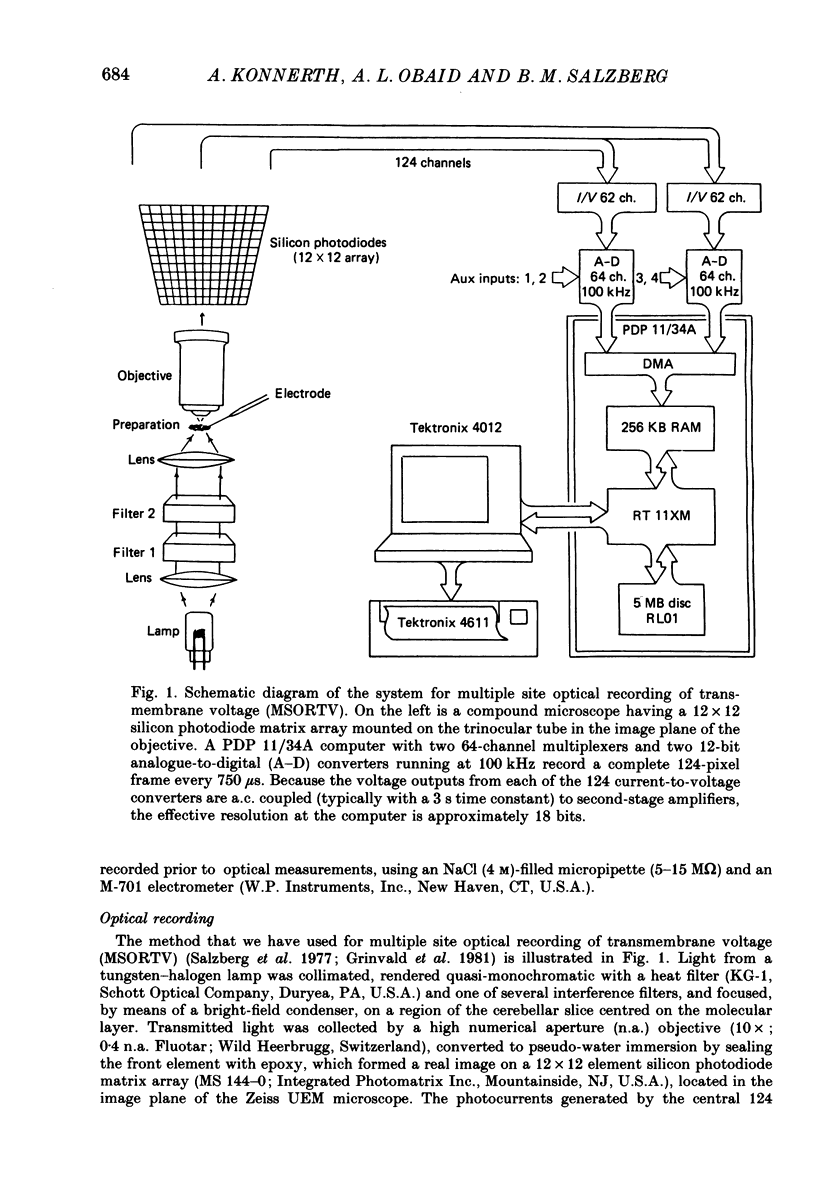
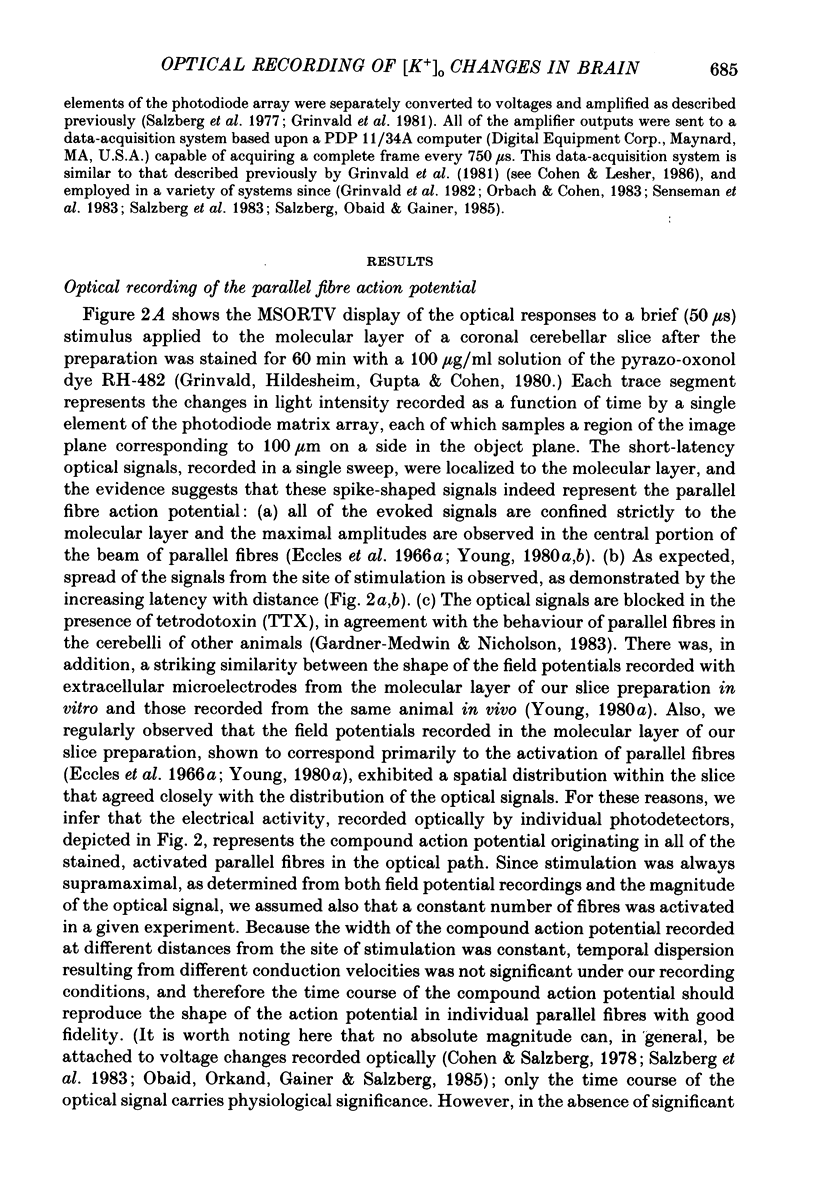
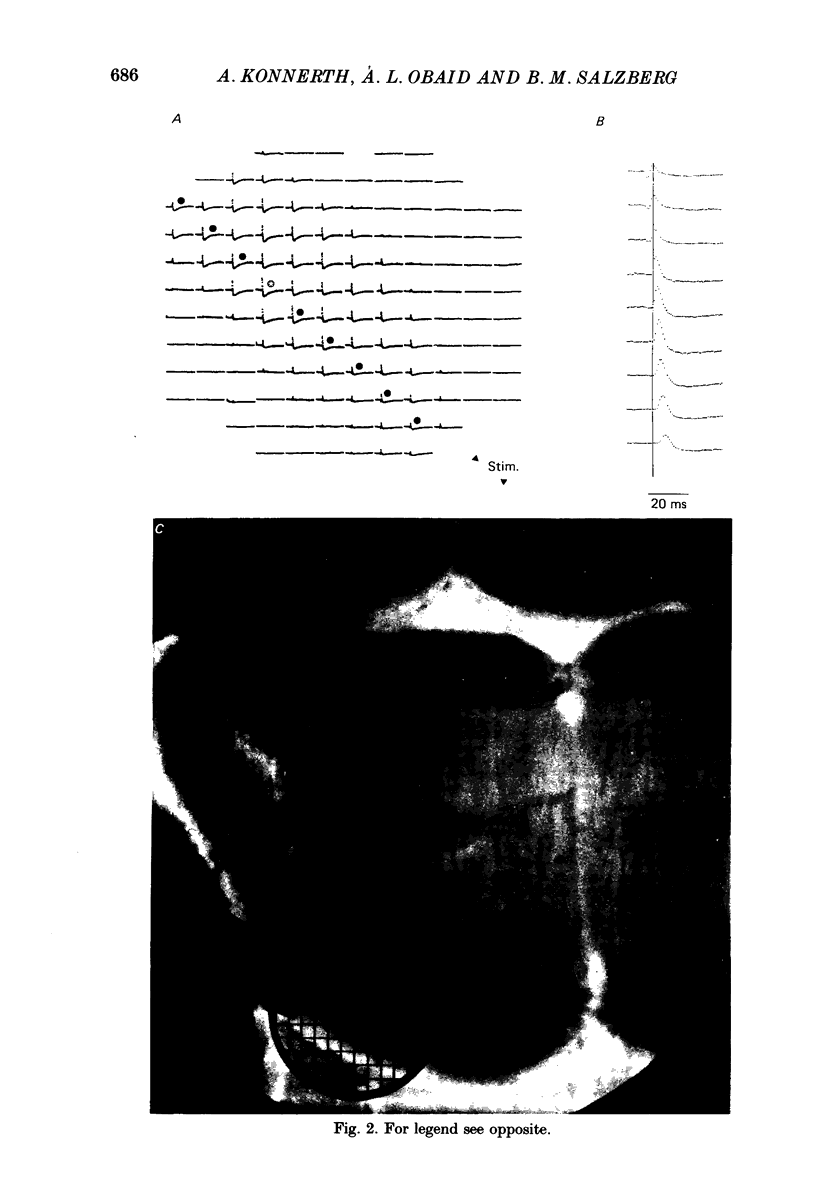
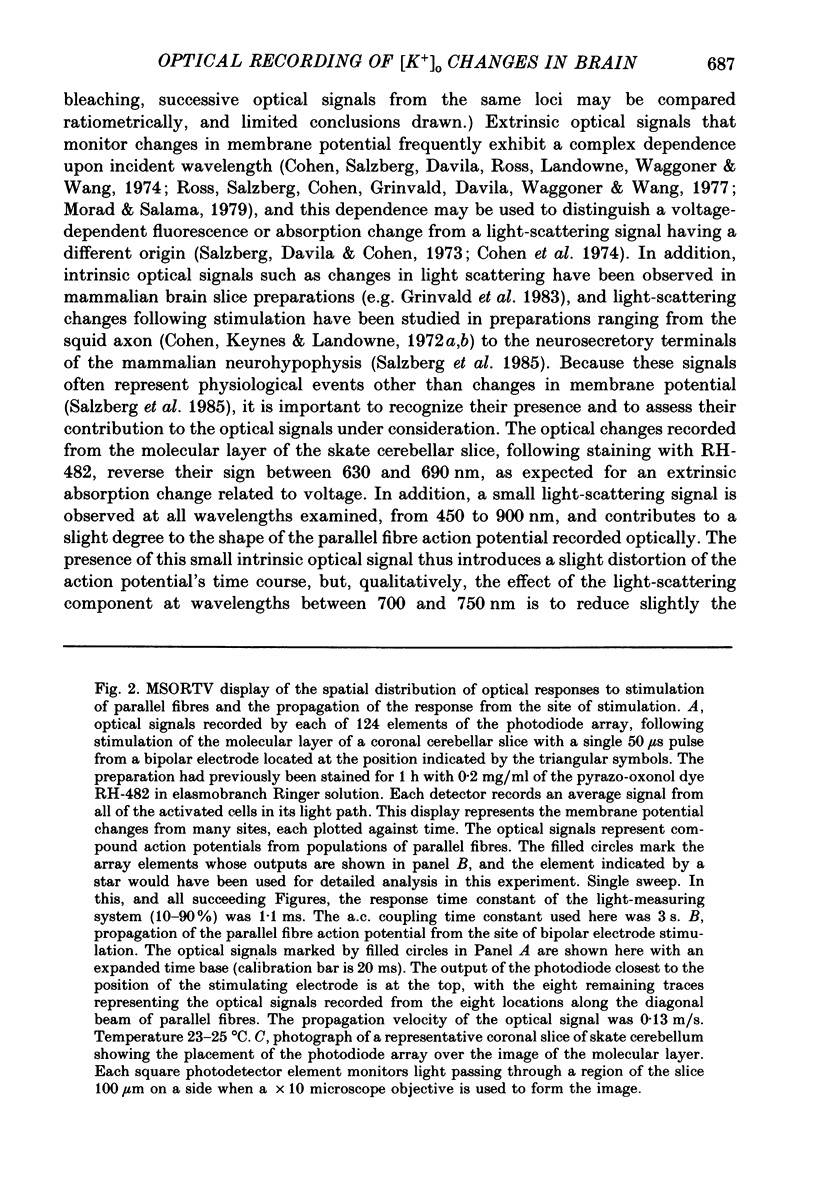
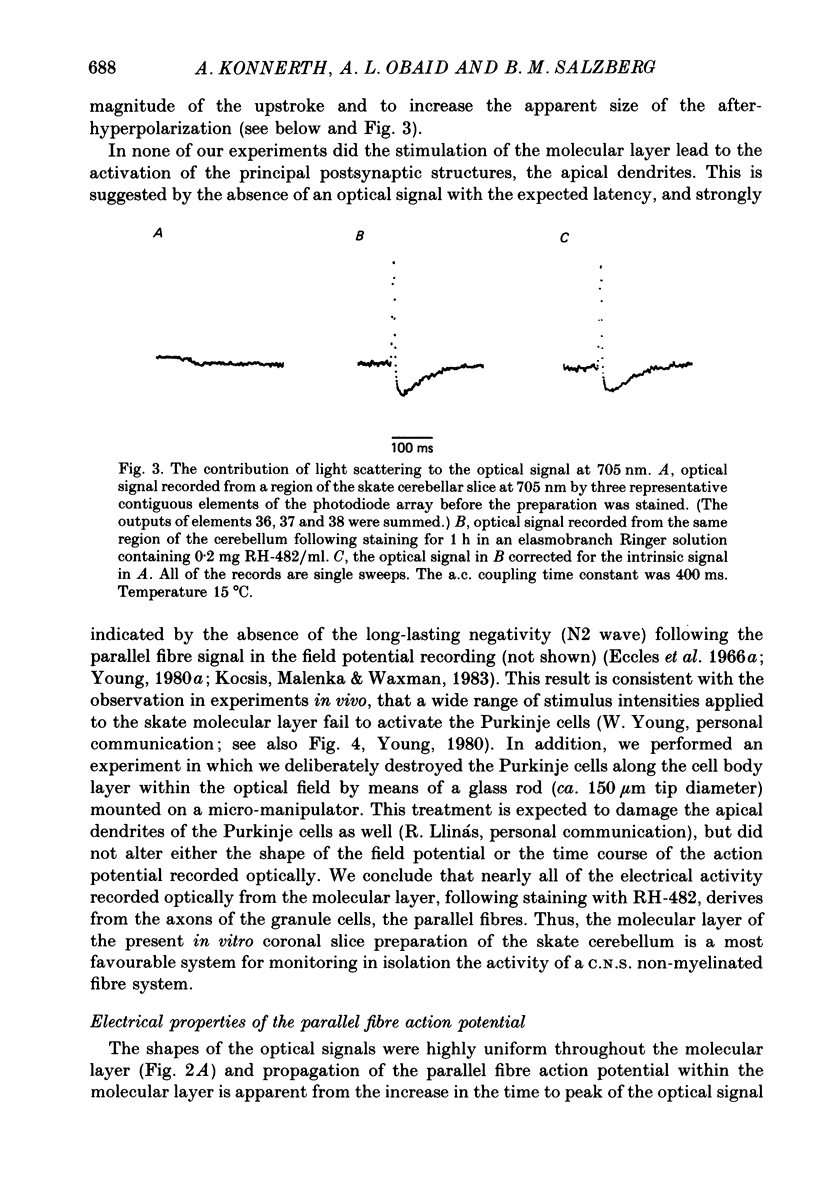
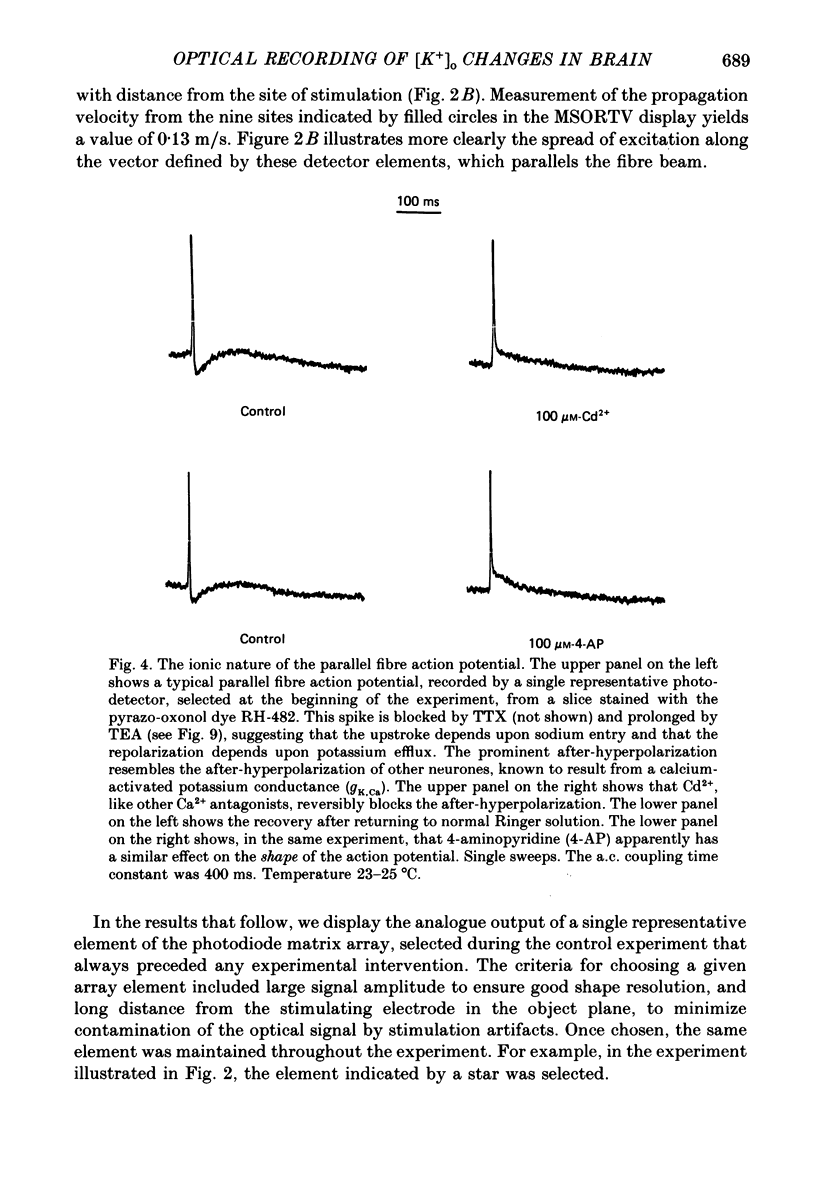
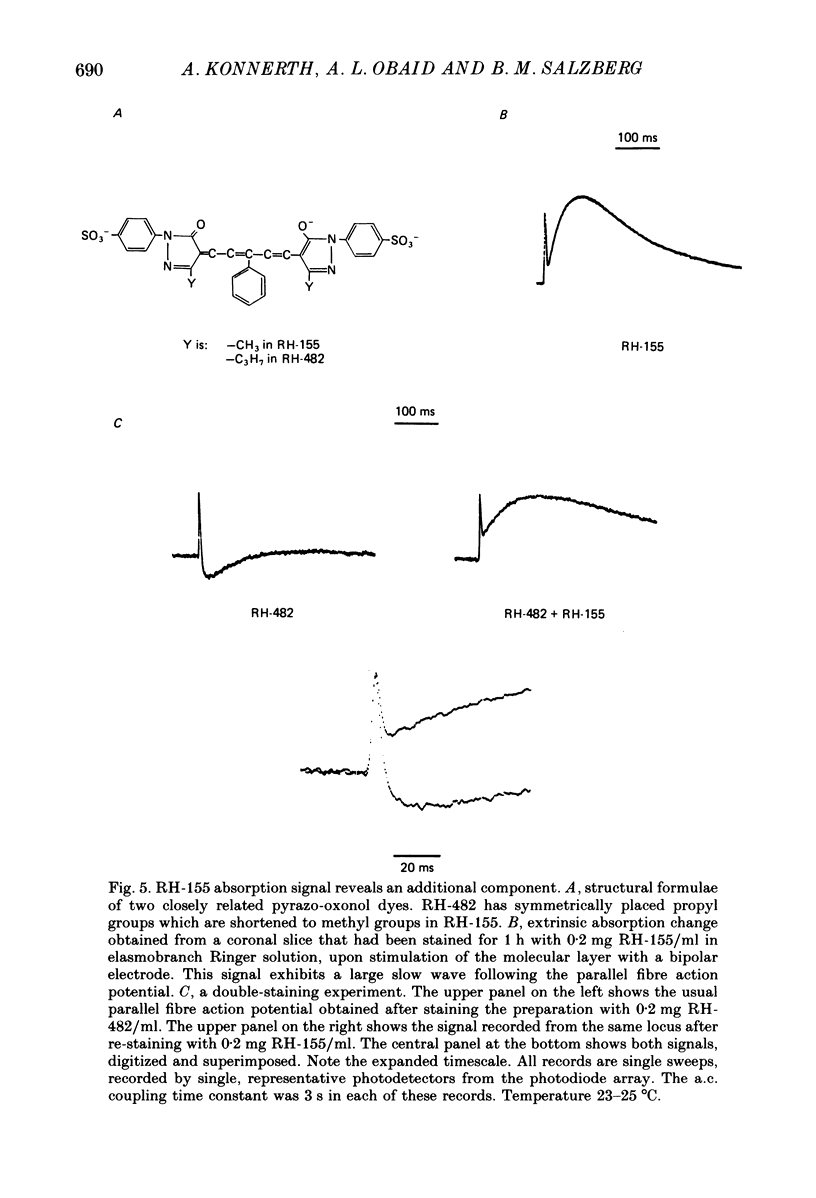
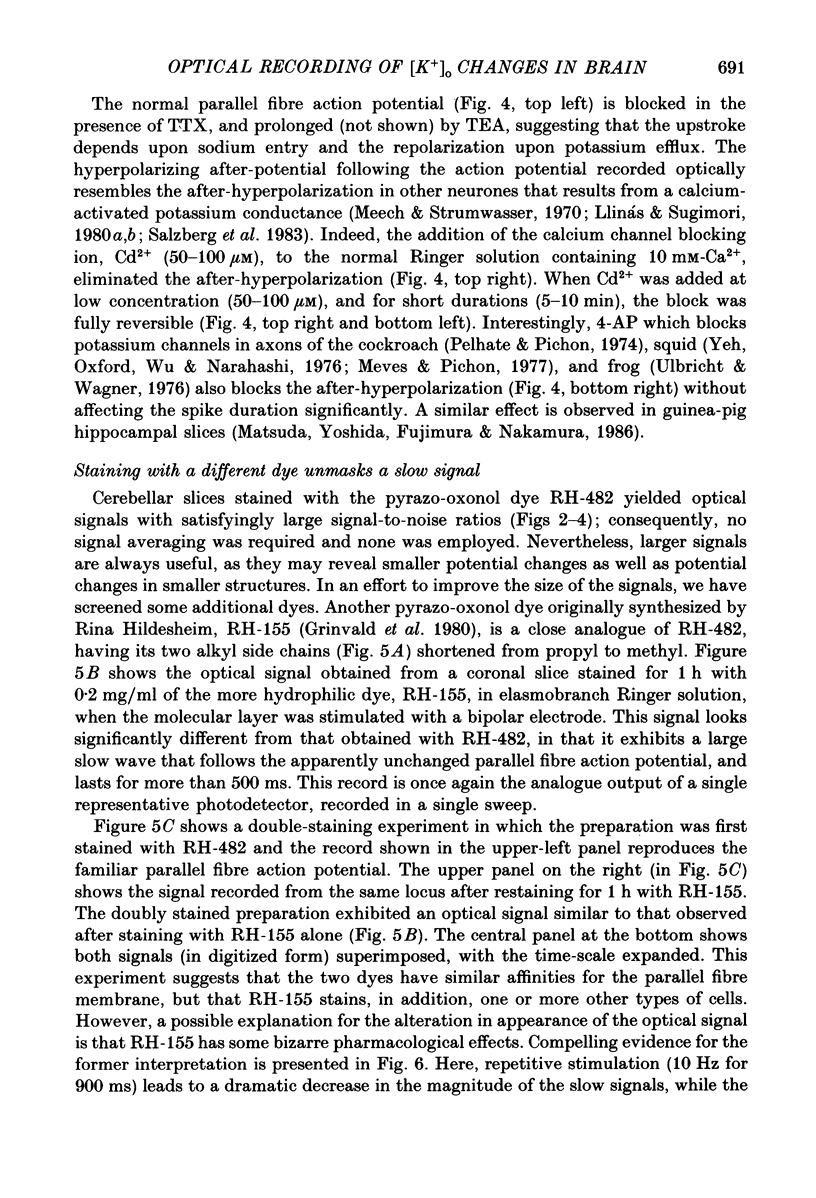
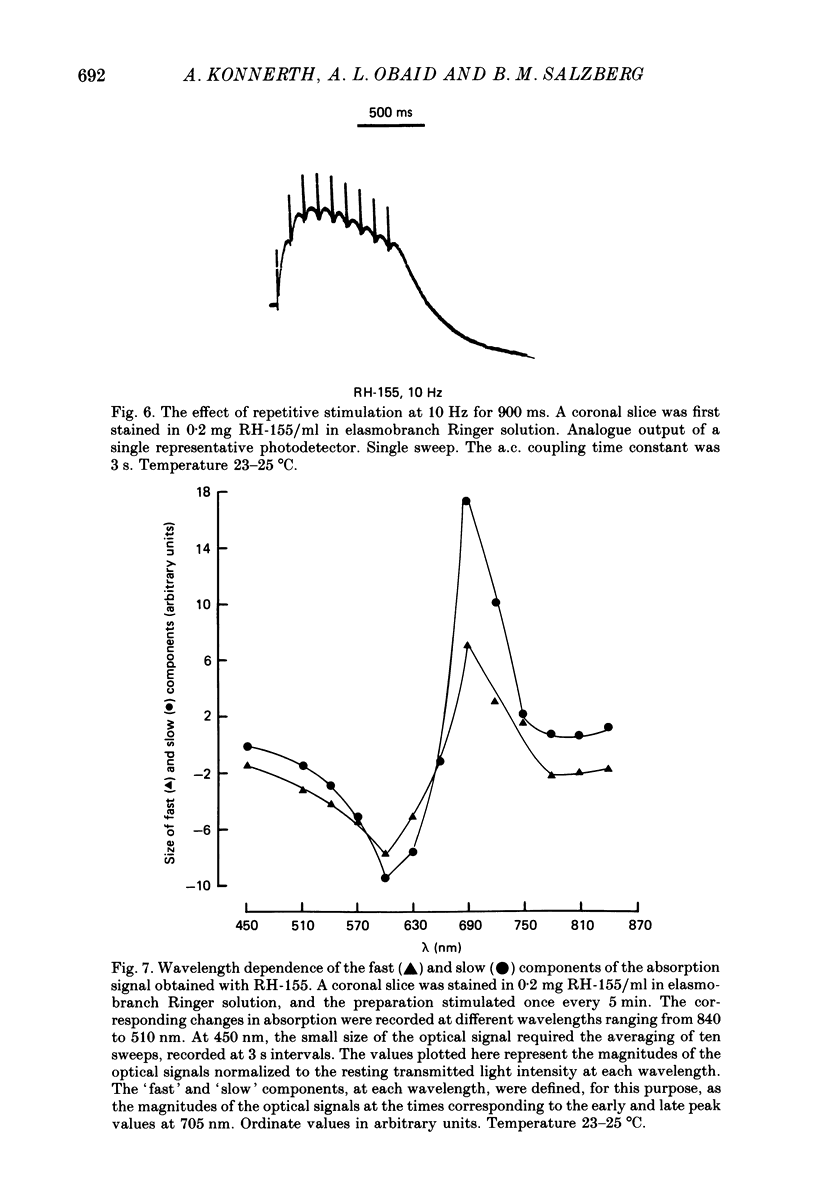
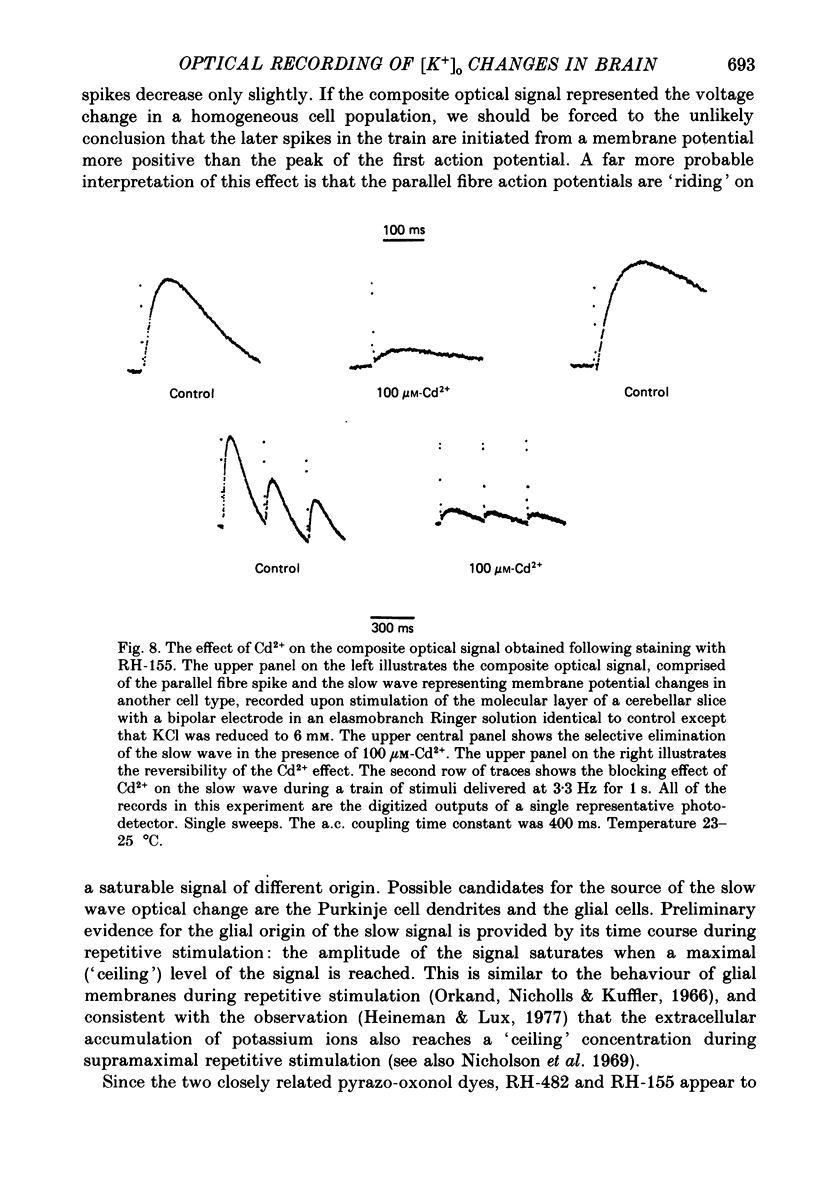
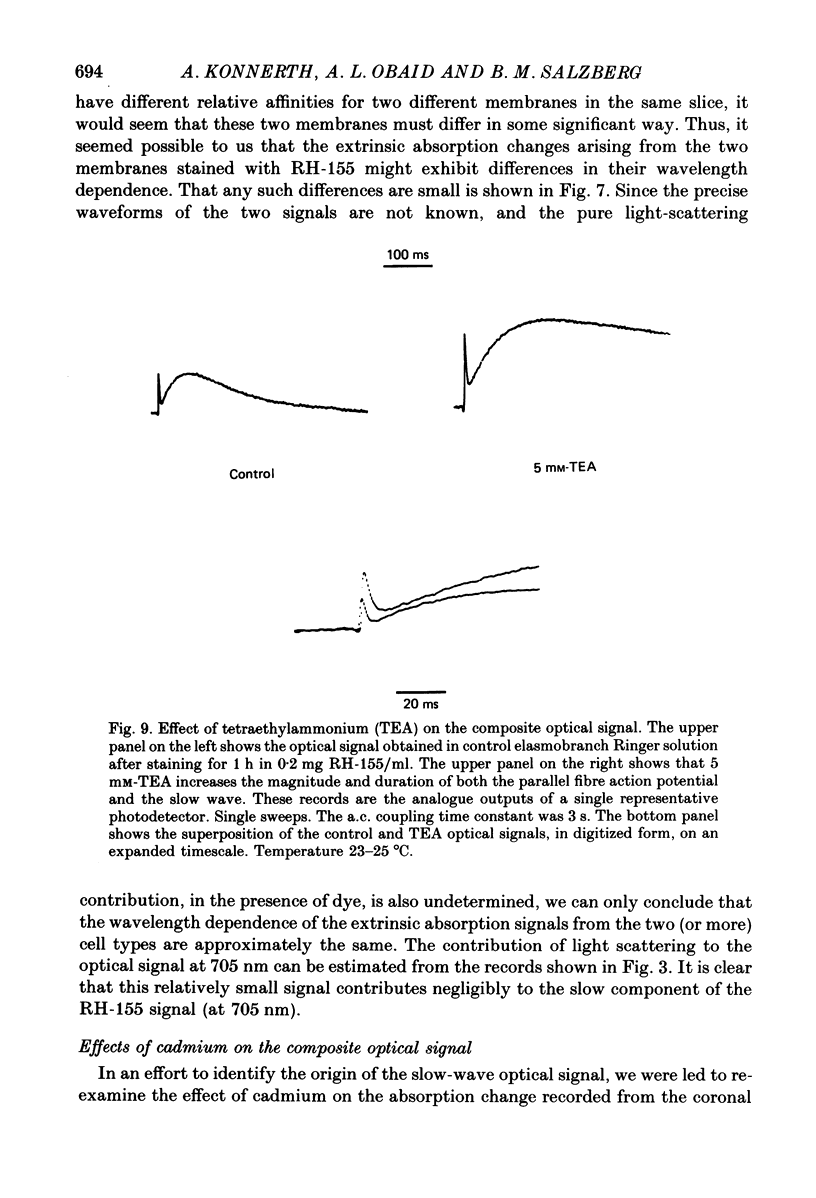
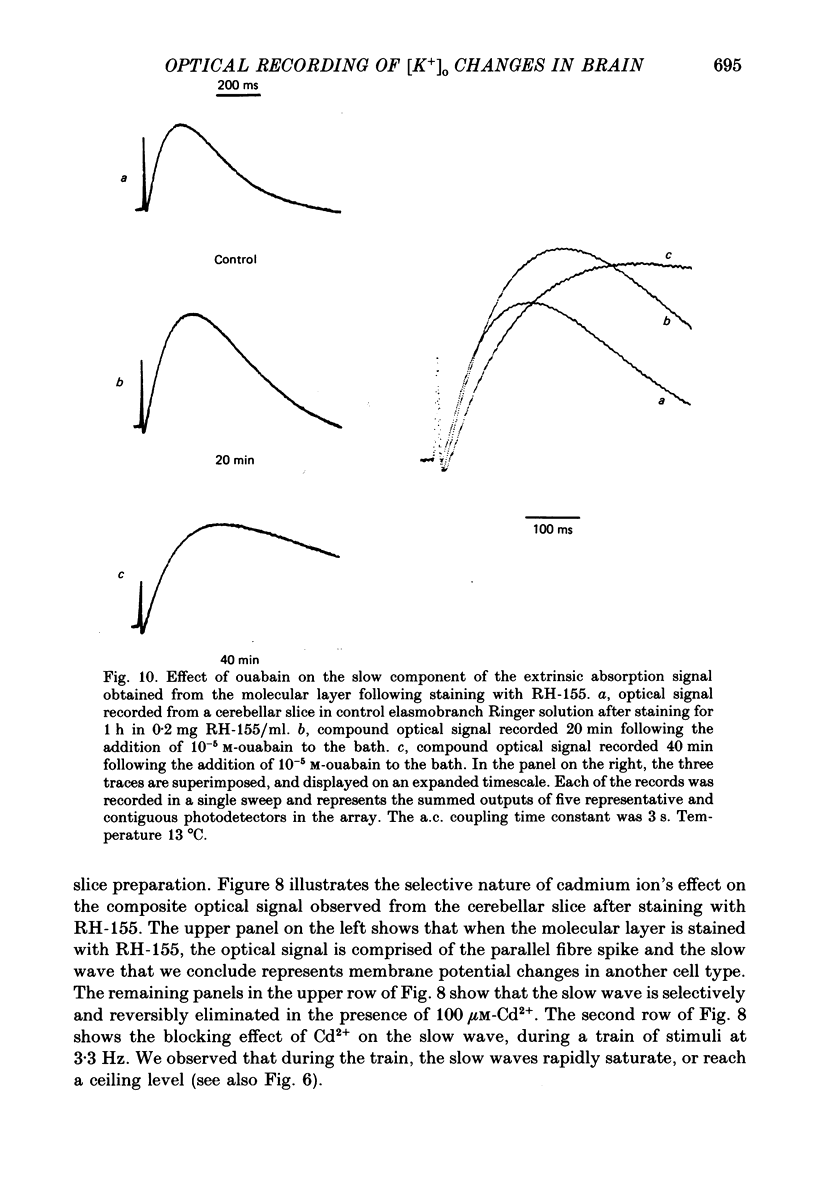
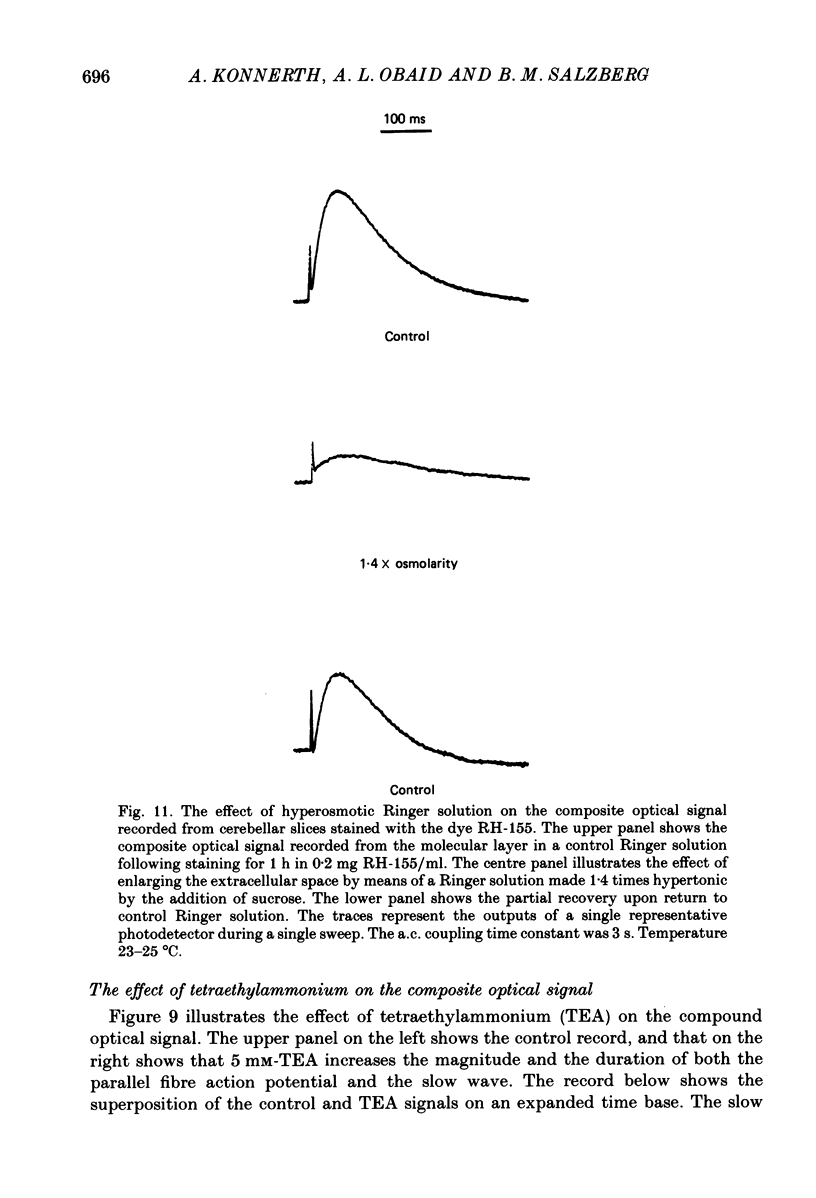
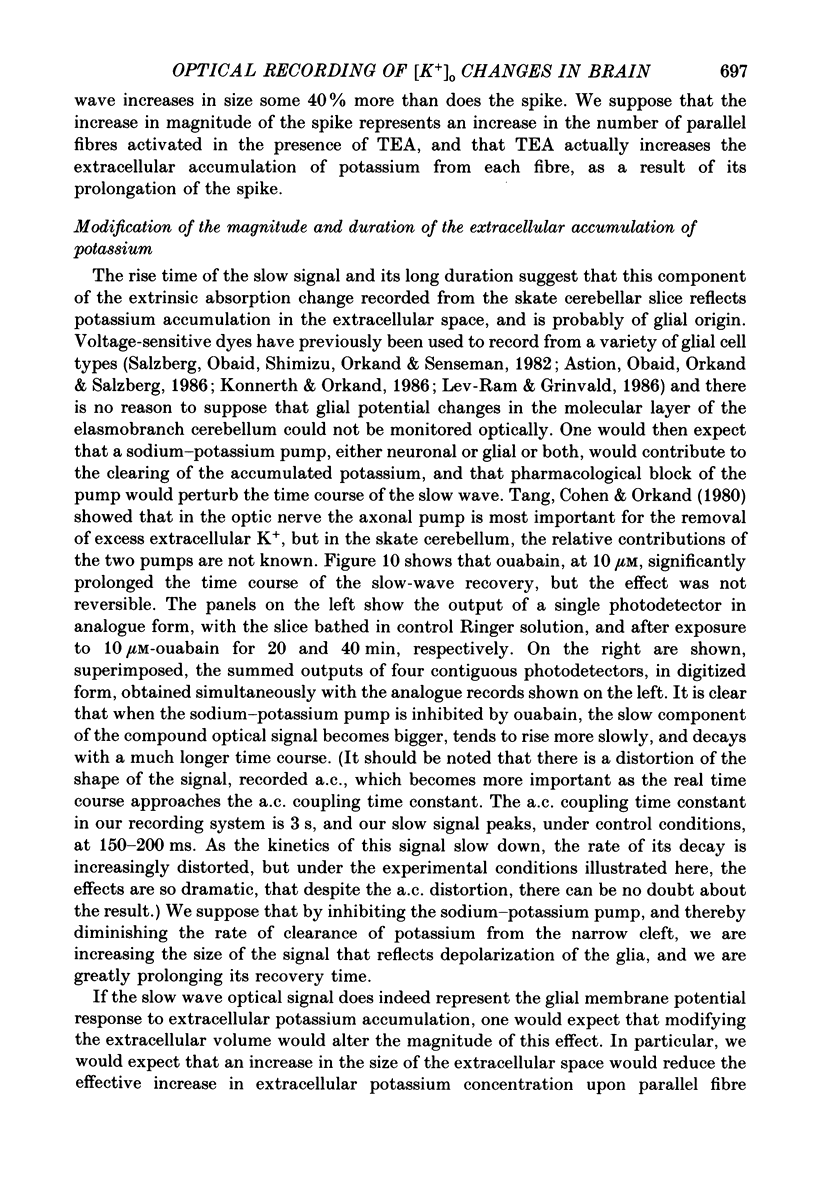
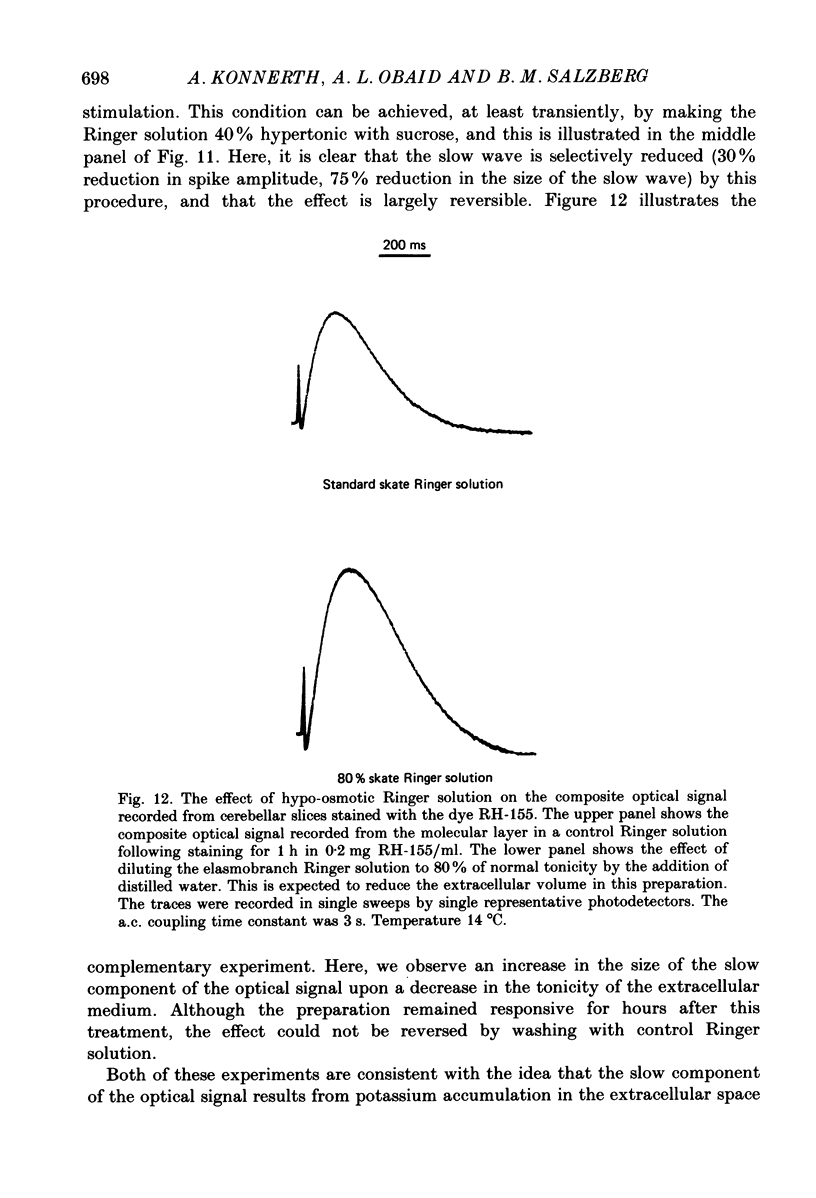
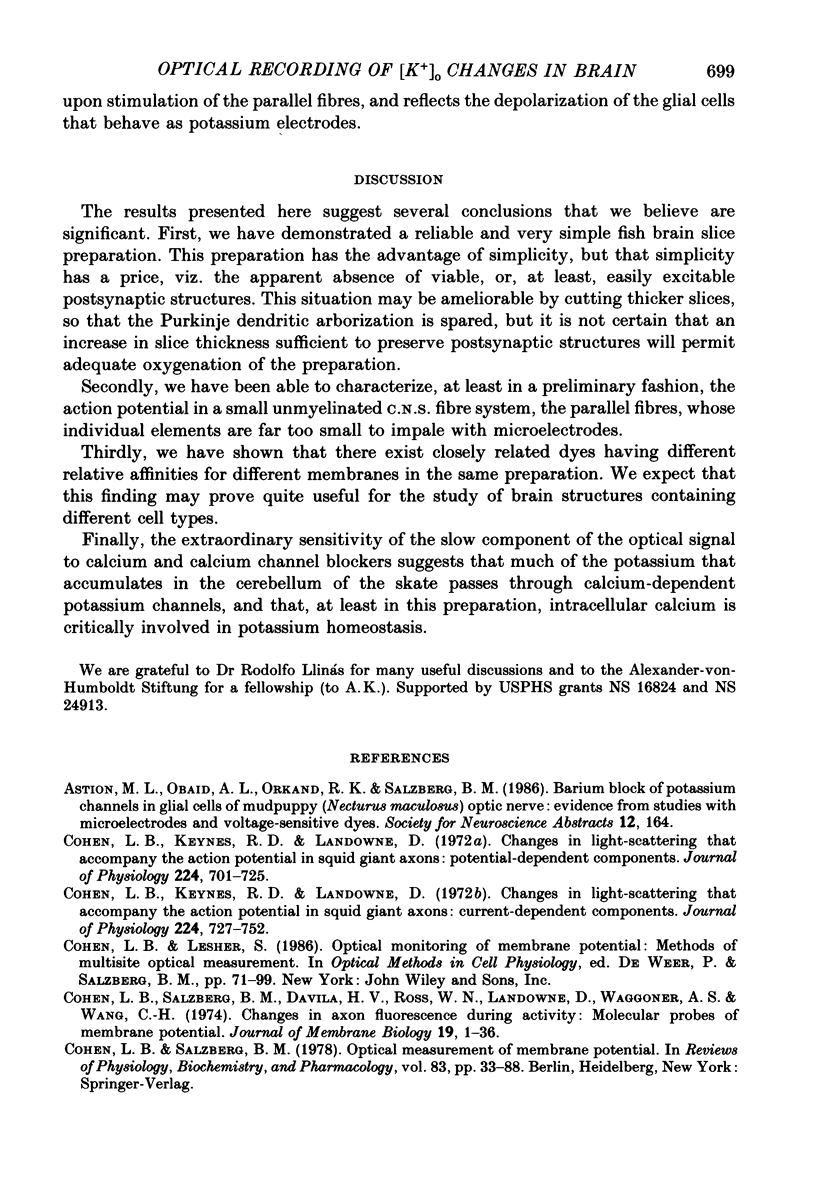
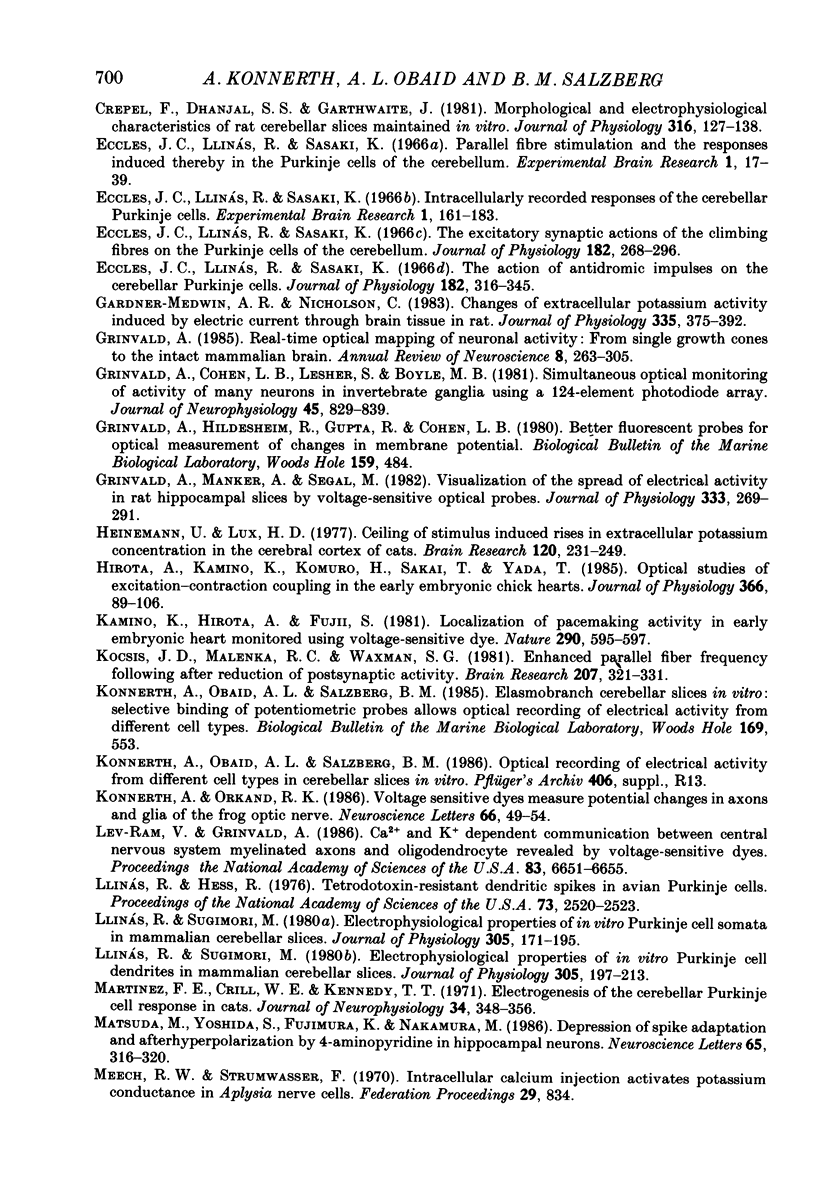
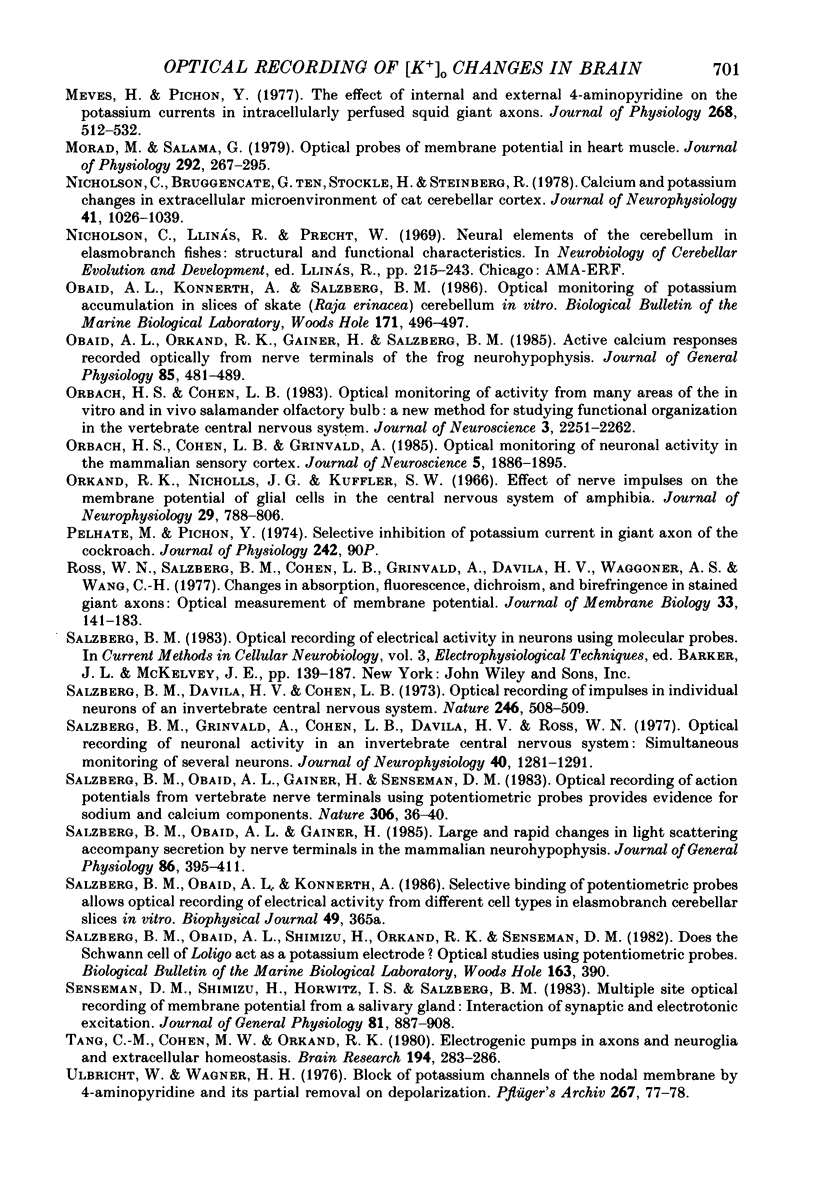
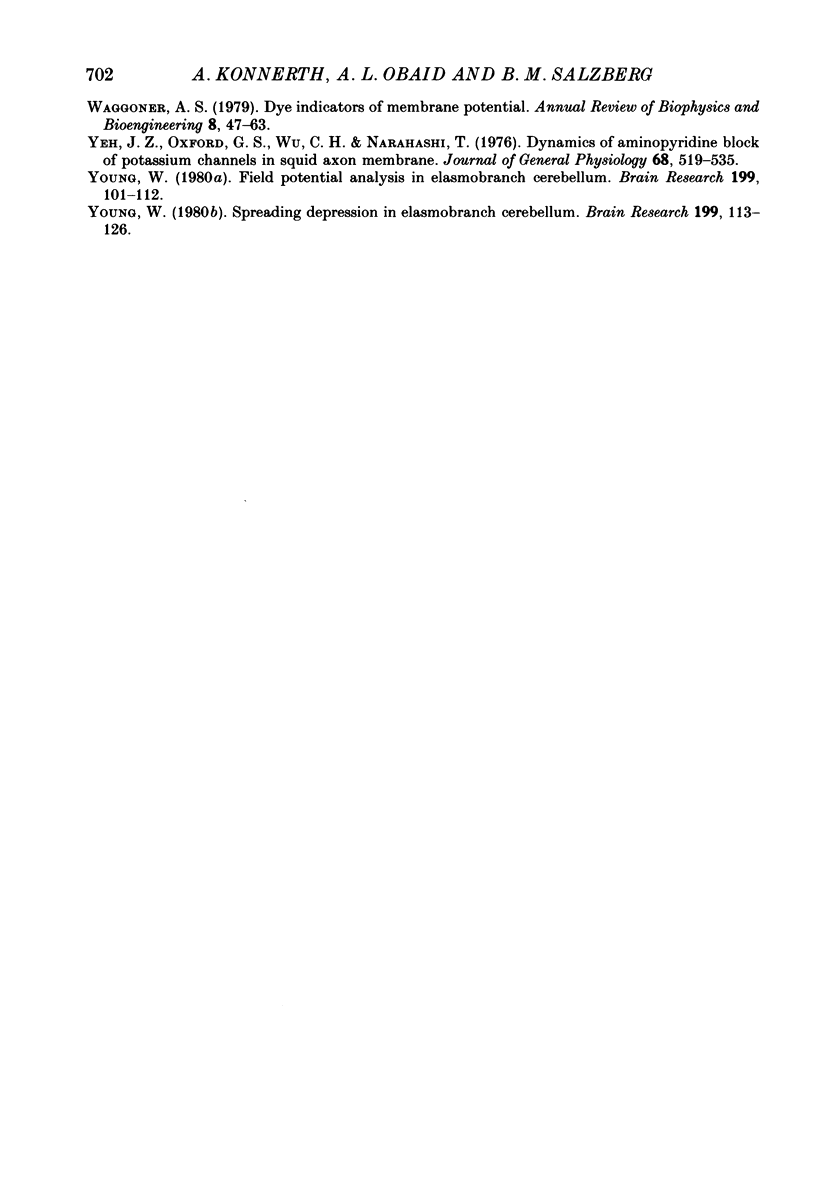
Images in this article
Selected References
These references are in PubMed. This may not be the complete list of references from this article.
- Cohen L. B., Keynes R. D., Landowne D. Changes in axon light scattering that accompany the action potential: current-dependent components. J Physiol. 1972 Aug;224(3):727–752. doi: 10.1113/jphysiol.1972.sp009920. [DOI] [PMC free article] [PubMed] [Google Scholar]
- Cohen L. B., Keynes R. D., Landowne D. Changes in light scattering that accompany the action potential in squid giant axons: potential-dependent components. J Physiol. 1972 Aug;224(3):701–725. doi: 10.1113/jphysiol.1972.sp009919. [DOI] [PMC free article] [PubMed] [Google Scholar]
- Cohen L. B., Lesher S. Optical monitoring of membrane potential: methods of multisite optical measurement. Soc Gen Physiol Ser. 1986;40:71–99. [PubMed] [Google Scholar]
- Cohen L. B., Salzberg B. M., Davila H. V., Ross W. N., Landowne D., Waggoner A. S., Wang C. H. Changes in axon fluorescence during activity: molecular probes of membrane potential. J Membr Biol. 1974;19(1):1–36. doi: 10.1007/BF01869968. [DOI] [PubMed] [Google Scholar]
- Crepel F., Dhanjal S. S., Garthwaite J. Morphological and electrophysiological characteristics of rat cerebellar slices maintained in vitro. J Physiol. 1981 Jul;316:127–138. doi: 10.1113/jphysiol.1981.sp013777. [DOI] [PMC free article] [PubMed] [Google Scholar]
- Eccles J. C., Llinás R., Sasaki K. Intracellularly recorded responses of the cerebellar Purkinje cells. Exp Brain Res. 1966;1(2):161–183. doi: 10.1007/BF00236869. [DOI] [PubMed] [Google Scholar]
- Eccles J. C., Llinás R., Sasaki K. Parallel fibre stimulation and the responses induced thereby in the Purkinje cells of the cerebellum. Exp Brain Res. 1966;1(1):17–39. doi: 10.1007/BF00235207. [DOI] [PubMed] [Google Scholar]
- Eccles J. C., Llinás R., Sasaki K. The excitatory synaptic action of climbing fibres on the Purkinje cells of the cerebellum. J Physiol. 1966 Jan;182(2):268–296. doi: 10.1113/jphysiol.1966.sp007824. [DOI] [PMC free article] [PubMed] [Google Scholar]
- Gardner-Medwin A. R., Nicholson C. Changes of extracellular potassium activity induced by electric current through brain tissue in the rat. J Physiol. 1983 Feb;335:375–392. doi: 10.1113/jphysiol.1983.sp014540. [DOI] [PMC free article] [PubMed] [Google Scholar]
- Grinvald A., Cohen L. B., Lesher S., Boyle M. B. Simultaneous optical monitoring of activity of many neurons in invertebrate ganglia using a 124-element photodiode array. J Neurophysiol. 1981 May;45(5):829–840. doi: 10.1152/jn.1981.45.5.829. [DOI] [PubMed] [Google Scholar]
- Grinvald A., Manker A., Segal M. Visualization of the spread of electrical activity in rat hippocampal slices by voltage-sensitive optical probes. J Physiol. 1982 Dec;333:269–291. doi: 10.1113/jphysiol.1982.sp014453. [DOI] [PMC free article] [PubMed] [Google Scholar]
- Grinvald A. Real-time optical mapping of neuronal activity: from single growth cones to the intact mammalian brain. Annu Rev Neurosci. 1985;8:263–305. doi: 10.1146/annurev.ne.08.030185.001403. [DOI] [PubMed] [Google Scholar]
- Heinemann U., Lux H. D. Ceiling of stimulus induced rises in extracellular potassium concentration in the cerebral cortex of cat. Brain Res. 1977 Jan 21;120(2):231–249. doi: 10.1016/0006-8993(77)90903-9. [DOI] [PubMed] [Google Scholar]
- Hirota A., Kamino K., Komuro H., Sakai T., Yada T. Optical studies of excitation-contraction coupling in the early embryonic chick heart. J Physiol. 1985 Sep;366:89–106. doi: 10.1113/jphysiol.1985.sp015786. [DOI] [PMC free article] [PubMed] [Google Scholar]
- Kamino K., Hirota A., Fujii S. Localization of pacemaking activity in early embryonic heart monitored using voltage-sensitive dye. Nature. 1981 Apr 16;290(5807):595–597. doi: 10.1038/290595a0. [DOI] [PubMed] [Google Scholar]
- Kocsis J. D., Malenka R. C., Waxman S. G. Enhanced parallel fiber frequency-following after reduction of postsynaptic activity. Brain Res. 1981 Mar 2;207(2):321–331. doi: 10.1016/0006-8993(81)90367-x. [DOI] [PubMed] [Google Scholar]
- Konnerth A., Orkand R. K. Voltage-sensitive dyes measure potential changes in axons and glia of the frog optic nerve. Neurosci Lett. 1986 May 6;66(1):49–54. doi: 10.1016/0304-3940(86)90164-3. [DOI] [PubMed] [Google Scholar]
- Lev-Ram V., Grinvald A. Ca2+- and K+-dependent communication between central nervous system myelinated axons and oligodendrocytes revealed by voltage-sensitive dyes. Proc Natl Acad Sci U S A. 1986 Sep;83(17):6651–6655. doi: 10.1073/pnas.83.17.6651. [DOI] [PMC free article] [PubMed] [Google Scholar]
- Llinás R., Hess R. Tetrodotoxin-resistant dendritic spikes in avian Purkinje cells. Proc Natl Acad Sci U S A. 1976 Jul;73(7):2520–2523. doi: 10.1073/pnas.73.7.2520. [DOI] [PMC free article] [PubMed] [Google Scholar]
- Llinás R., Sugimori M. Electrophysiological properties of in vitro Purkinje cell dendrites in mammalian cerebellar slices. J Physiol. 1980 Aug;305:197–213. doi: 10.1113/jphysiol.1980.sp013358. [DOI] [PMC free article] [PubMed] [Google Scholar]
- Llinás R., Sugimori M. Electrophysiological properties of in vitro Purkinje cell somata in mammalian cerebellar slices. J Physiol. 1980 Aug;305:171–195. doi: 10.1113/jphysiol.1980.sp013357. [DOI] [PMC free article] [PubMed] [Google Scholar]
- Martinez F. E., Crill W. E., Kennedy T. T. Electrogenesis of cerebellar Purkinje cell responses in cats. J Neurophysiol. 1971 May;34(3):348–356. doi: 10.1152/jn.1971.34.3.348. [DOI] [PubMed] [Google Scholar]
- Matsuda Y., Yoshida S., Fujimura K., Nakamura M. Depression of spike adaptation and afterhyperpolarization by 4-aminopyridine in hippocampal neurons. Neurosci Lett. 1986 Apr 24;65(3):316–320. doi: 10.1016/0304-3940(86)90281-8. [DOI] [PubMed] [Google Scholar]
- Meves H., Pichon Y. The effect of internal and external 4-aminopyridine on the potassium currents in intracellularly perfused squid giant axons. J Physiol. 1977 Jun;268(2):511–532. doi: 10.1113/jphysiol.1977.sp011869. [DOI] [PMC free article] [PubMed] [Google Scholar]
- Morad M., Salama G. Optical probes of membrane potential in heart muscle. J Physiol. 1979 Jul;292:267–295. doi: 10.1113/jphysiol.1979.sp012850. [DOI] [PMC free article] [PubMed] [Google Scholar]
- Nicholson C., ten Bruggencate G., Stöckle H., Steinberg R. Calcium and potassium changes in extracellular microenvironment of cat cerebellar cortex. J Neurophysiol. 1978 Jul;41(4):1026–1039. doi: 10.1152/jn.1978.41.4.1026. [DOI] [PubMed] [Google Scholar]
- Obaid A. L., Orkand R. K., Gainer H., Salzberg B. M. Active calcium responses recorded optically from nerve terminals of the frog neurohypophysis. J Gen Physiol. 1985 Apr;85(4):481–489. doi: 10.1085/jgp.85.4.481. [DOI] [PMC free article] [PubMed] [Google Scholar]
- Orbach H. S., Cohen L. B., Grinvald A. Optical mapping of electrical activity in rat somatosensory and visual cortex. J Neurosci. 1985 Jul;5(7):1886–1895. doi: 10.1523/JNEUROSCI.05-07-01886.1985. [DOI] [PMC free article] [PubMed] [Google Scholar]
- Orbach H. S., Cohen L. B. Optical monitoring of activity from many areas of the in vitro and in vivo salamander olfactory bulb: a new method for studying functional organization in the vertebrate central nervous system. J Neurosci. 1983 Nov;3(11):2251–2262. doi: 10.1523/JNEUROSCI.03-11-02251.1983. [DOI] [PMC free article] [PubMed] [Google Scholar]
- Orkand R. K., Nicholls J. G., Kuffler S. W. Effect of nerve impulses on the membrane potential of glial cells in the central nervous system of amphibia. J Neurophysiol. 1966 Jul;29(4):788–806. doi: 10.1152/jn.1966.29.4.788. [DOI] [PubMed] [Google Scholar]
- Pelhate M., Pichon Y. Proceedings: Selective inhibition of potassium current in the giant axon of the cockroach. J Physiol. 1974 Oct;242(2):90P–91P. [PubMed] [Google Scholar]
- Ross W. N., Salzberg B. M., Cohen L. B., Grinvald A., Davila H. V., Waggoner A. S., Wang C. H. Changes in absorption, fluorescence, dichroism, and Birefringence in stained giant axons: : optical measurement of membrane potential. J Membr Biol. 1977 May 6;33(1-2):141–183. doi: 10.1007/BF01869514. [DOI] [PubMed] [Google Scholar]
- Salzberg B. M., Davila H. V., Cohen L. B. Optical recording of impulses in individual neurones of an invertebrate central nervous system. Nature. 1973 Dec 21;246(5434):508–509. doi: 10.1038/246508a0. [DOI] [PubMed] [Google Scholar]
- Salzberg B. M., Grinvald A., Cohen L. B., Davila H. V., Ross W. N. Optical recording of neuronal activity in an invertebrate central nervous system: simultaneous monitoring of several neurons. J Neurophysiol. 1977 Nov;40(6):1281–1291. doi: 10.1152/jn.1977.40.6.1281. [DOI] [PubMed] [Google Scholar]
- Salzberg B. M., Obaid A. L., Gainer H. Large and rapid changes in light scattering accompany secretion by nerve terminals in the mammalian neurohypophysis. J Gen Physiol. 1985 Sep;86(3):395–411. doi: 10.1085/jgp.86.3.395. [DOI] [PMC free article] [PubMed] [Google Scholar]
- Salzberg B. M., Obaid A. L., Senseman D. M., Gainer H. Optical recording of action potentials from vertebrate nerve terminals using potentiometric probes provides evidence for sodium and calcium components. Nature. 1983 Nov 3;306(5938):36–40. doi: 10.1038/306036a0. [DOI] [PubMed] [Google Scholar]
- Senseman D. M., Shimizu H., Horwitz I. S., Salzberg B. M. Multiple-site optical recording of membrane potential from a salivary gland. Interaction of synaptic and electrotonic excitation. J Gen Physiol. 1983 Jun;81(6):887–908. doi: 10.1085/jgp.81.6.887. [DOI] [PMC free article] [PubMed] [Google Scholar]
- Tang C. M., Cohen M. W., Orkand R. K. Electrogenic pumps in axons and neuroglia and extracellular potassium homeostasis. Brain Res. 1980 Jul 21;194(1):283–286. doi: 10.1016/0006-8993(80)91345-1. [DOI] [PubMed] [Google Scholar]
- Yeh J. Z., Oxford G. S., Wu C. H., Narahashi T. Dynamics of aminopyridine block of potassium channels in squid axon membrane. J Gen Physiol. 1976 Nov;68(5):519–535. doi: 10.1085/jgp.68.5.519. [DOI] [PMC free article] [PubMed] [Google Scholar]
- Young W. Field potential analysis in elasmobranch cerebellum. Brain Res. 1980 Oct 13;199(1):101–112. doi: 10.1016/0006-8993(80)90233-4. [DOI] [PubMed] [Google Scholar]
- Young W. Spreading depression in elasmobranch cerebellum. Brain Res. 1980 Oct 13;199(1):113–126. doi: 10.1016/0006-8993(80)90234-6. [DOI] [PubMed] [Google Scholar]