Abstract
1. The post-natal development of the electrophysiological properties of cortical layer V pyramidal neurons was investigated with intracellular recordings from rat sensorimotor cortical slices, in vitro. 2. At all ages post-natally (post-natal day 1 to day 36; P1-P36) neurons were capable of generating a train of Na+-dependent action potentials in response to intracellular injection of sufficient depolarizing current. During the second and third week post-natally, these action potentials changed substantially, becoming faster in both their rising and falling phases, shorter in duration, and larger in amplitude. 3. Both mature (greater than P21) and immature (P2-P4) cortical neurones could generate Ca2+-dependent action potentials only if a substantial portion of K+ conductances were blocked. The maximum rate of rise of Ca2+ spikes also increased with age. 4. The apparent input resistance, specific membrane resistance, and membrane time constant all decreased with age from P1 to P30. Immature neurones had I-V relationships that were substantially more linear than those of adult cells, although rectification was often present in both the hyperpolarizing and depolarizing range. Inward rectification in the depolarizing range was Na+ dependent and was substantially larger in mature versus immature neurones. 5. Single, or trains of, action potentials in immature neurones were followed by short duration (10-50 ms) and long duration (1-5 s) after-hyperpolarizations (a.h.p.s) respectively. The duration of the latter appeared to decrease with age. The presence of large a.h.p.s indicates that Ca2+ entry occurs during the action potential of immature, as well as mature, neurones. 6. Responses to intracellular injection of depolarizing current pulses indicated that immature neurones have frequency versus injected current (f-I) relationships which are in general less steep than those for adult neurones and more limited in terms of the range of firing frequencies. 7. Our results are consistent with the hypothesis that there is a considerable increase in the density of voltage-dependent ionic channels underlying the electro-responsiveness of cortical pyramidal neurones during post-natal development.
Full text
PDF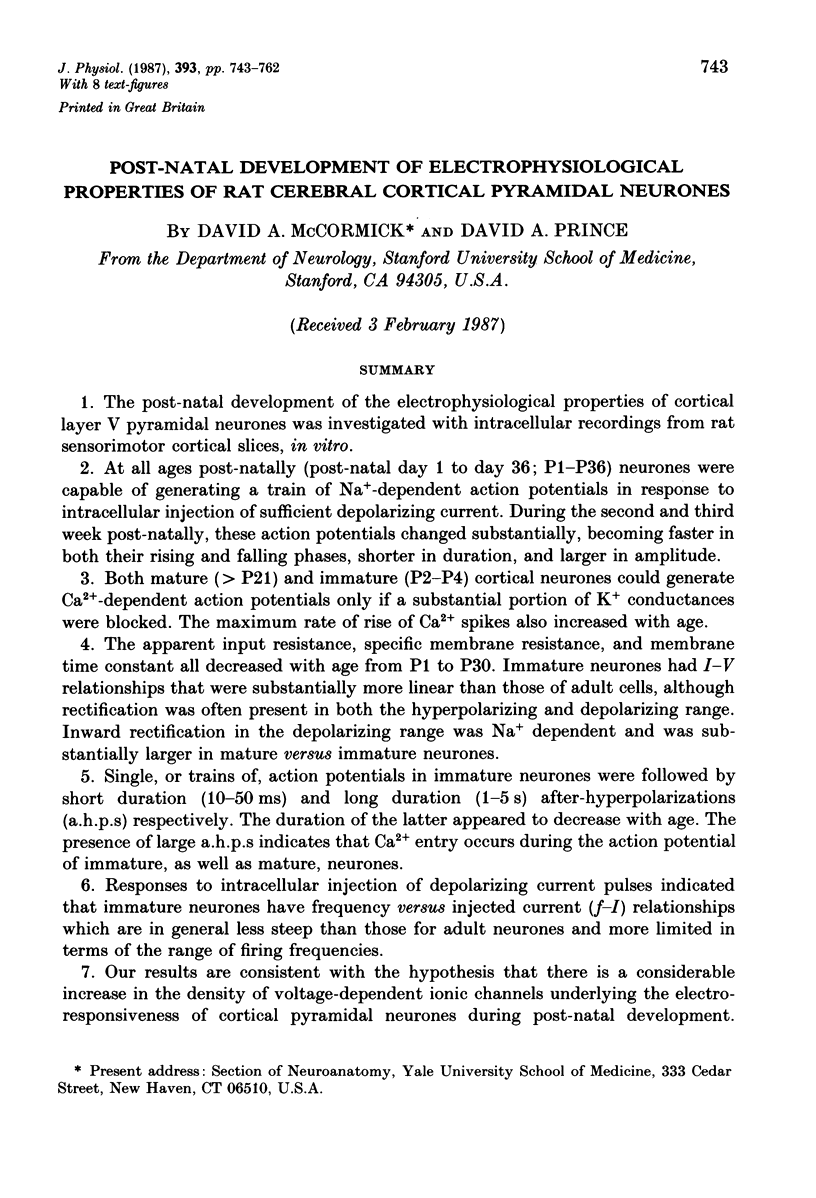
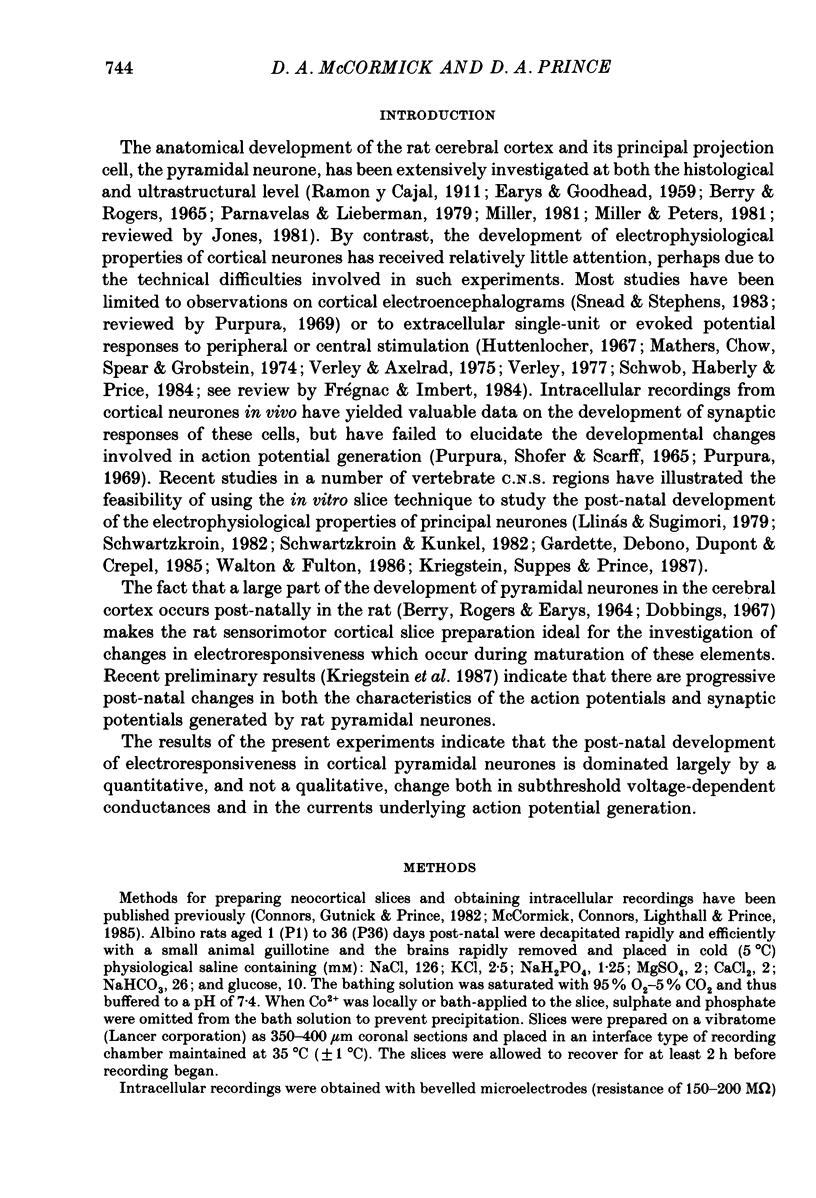
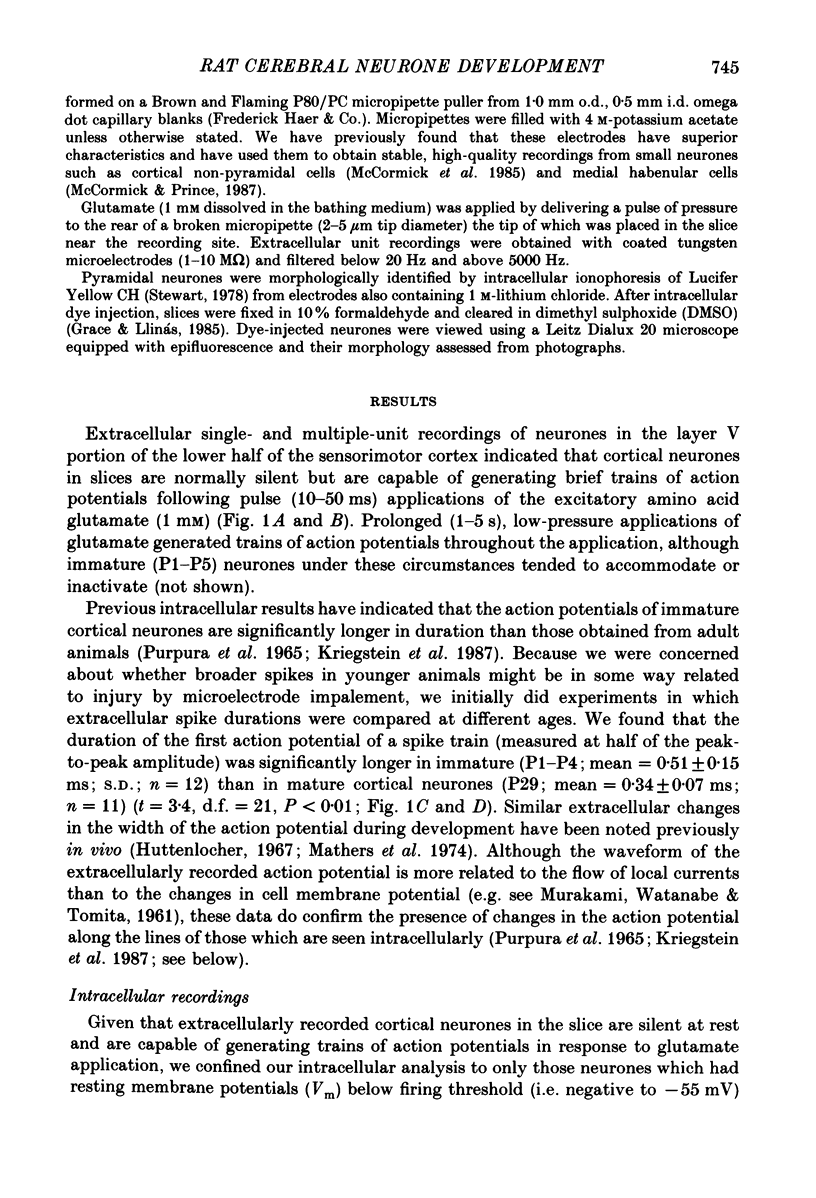
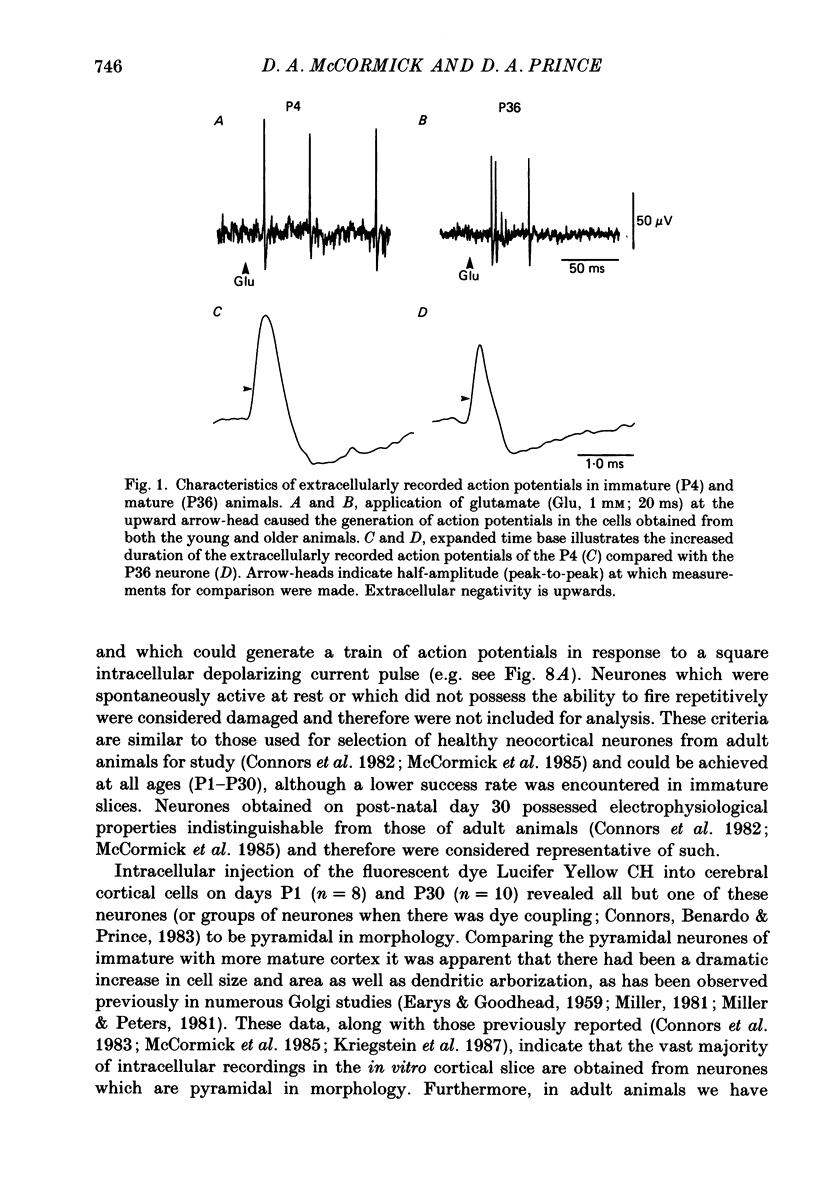
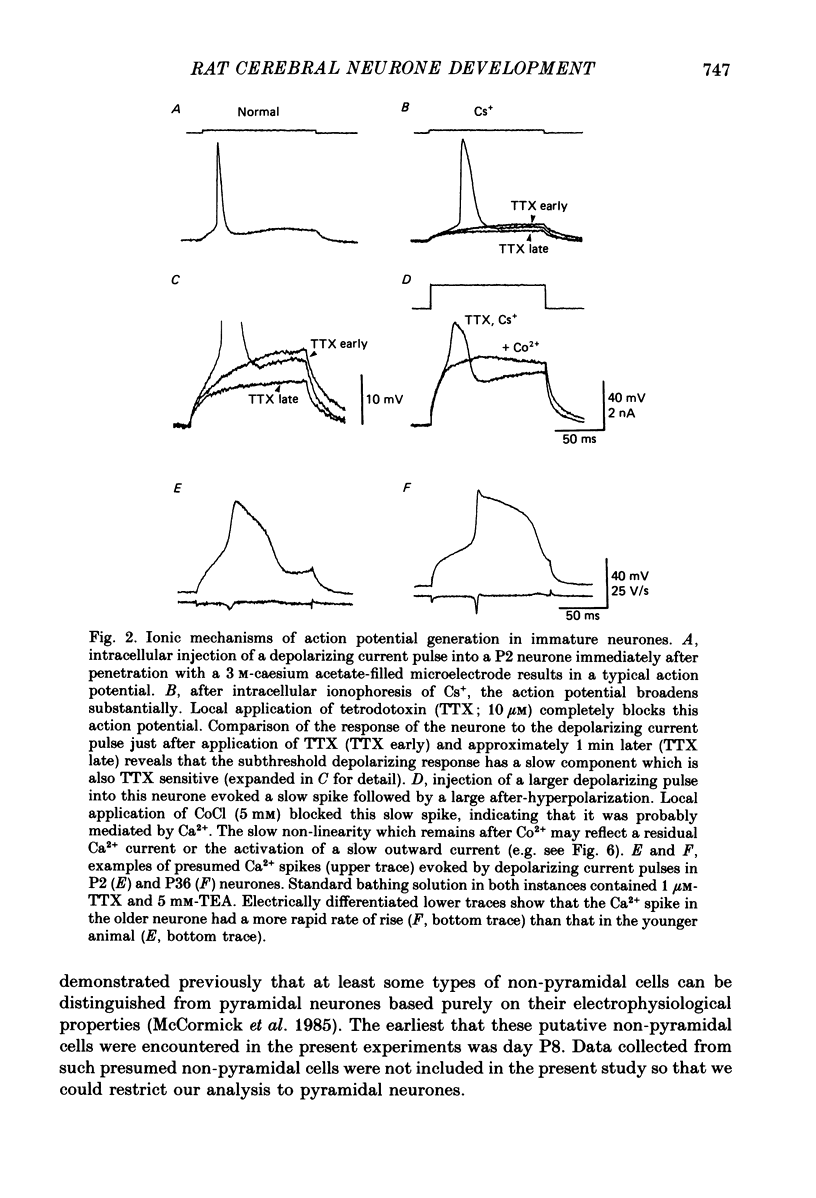
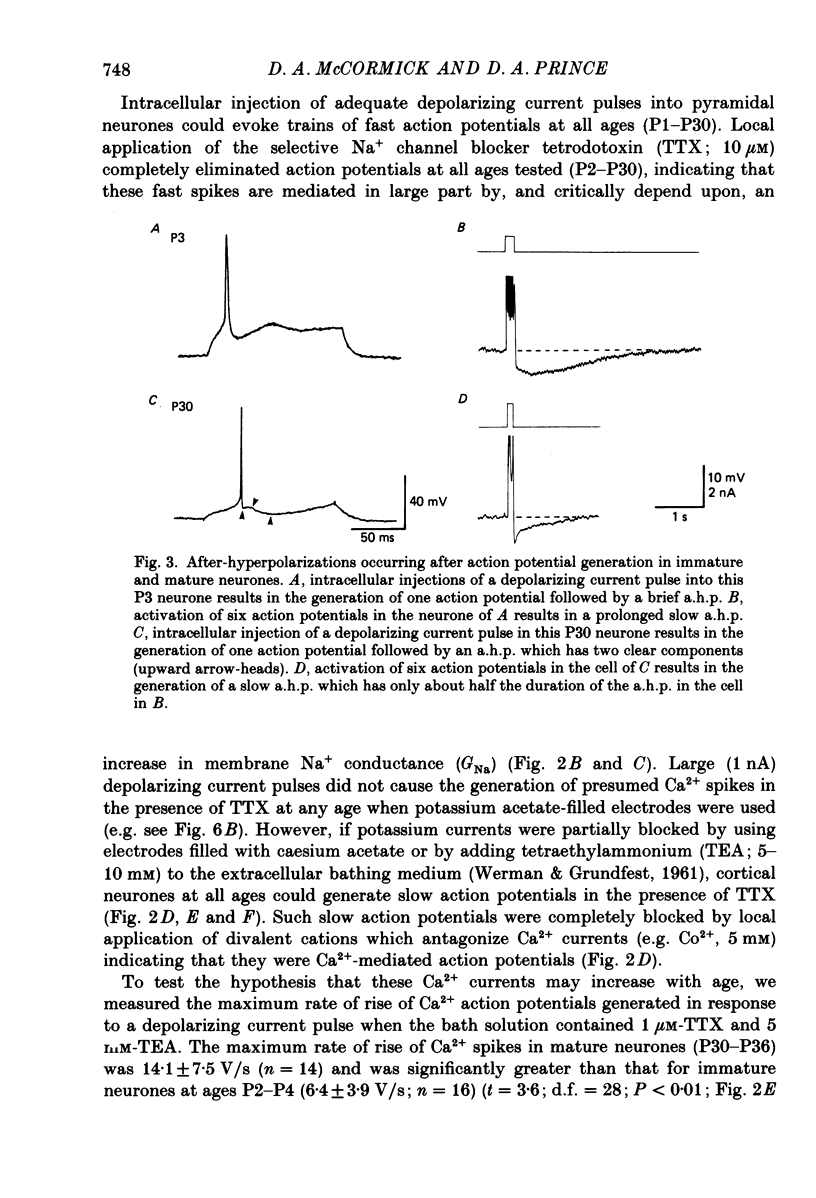
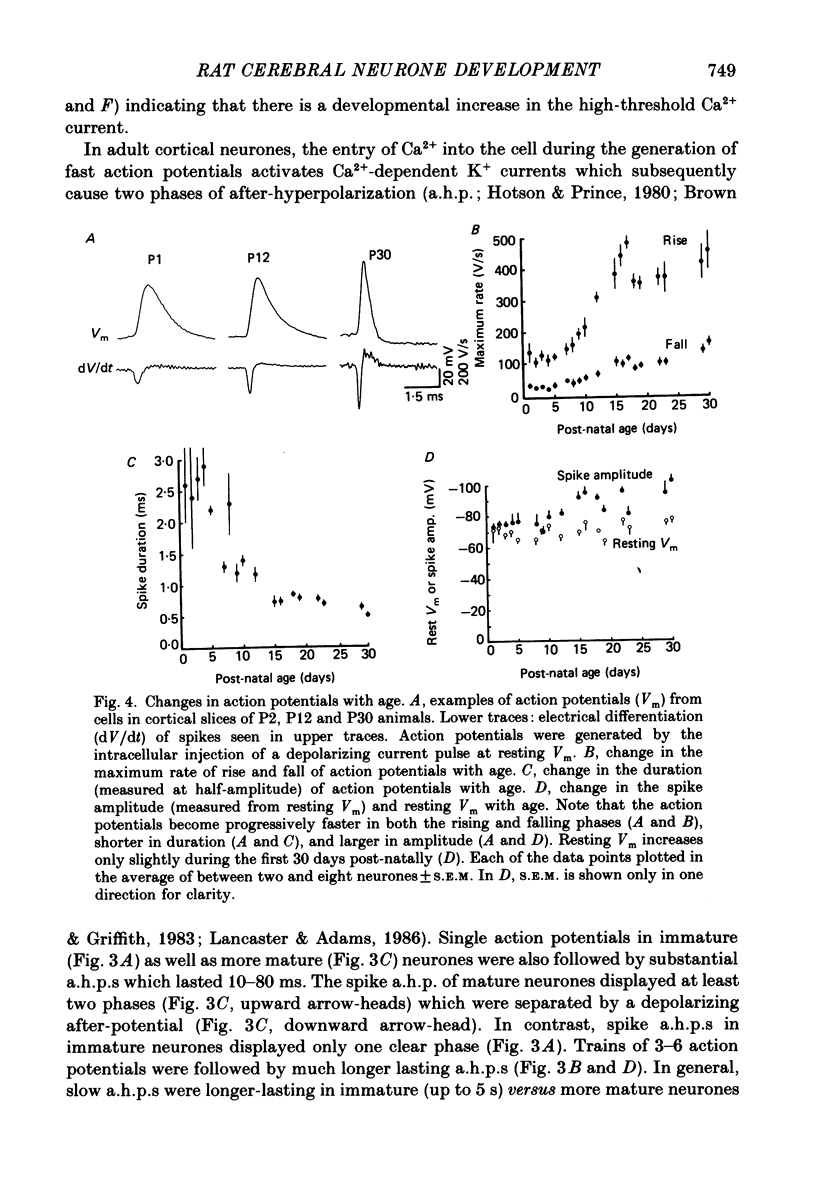
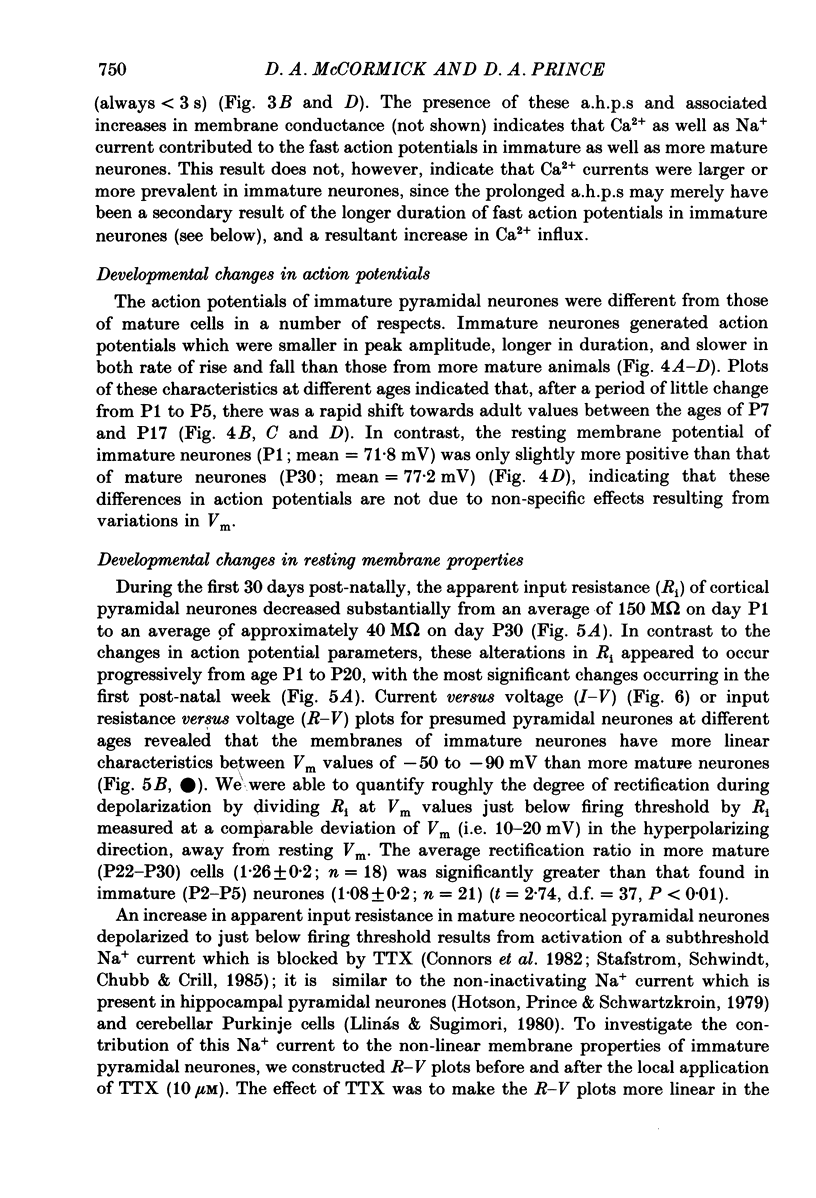
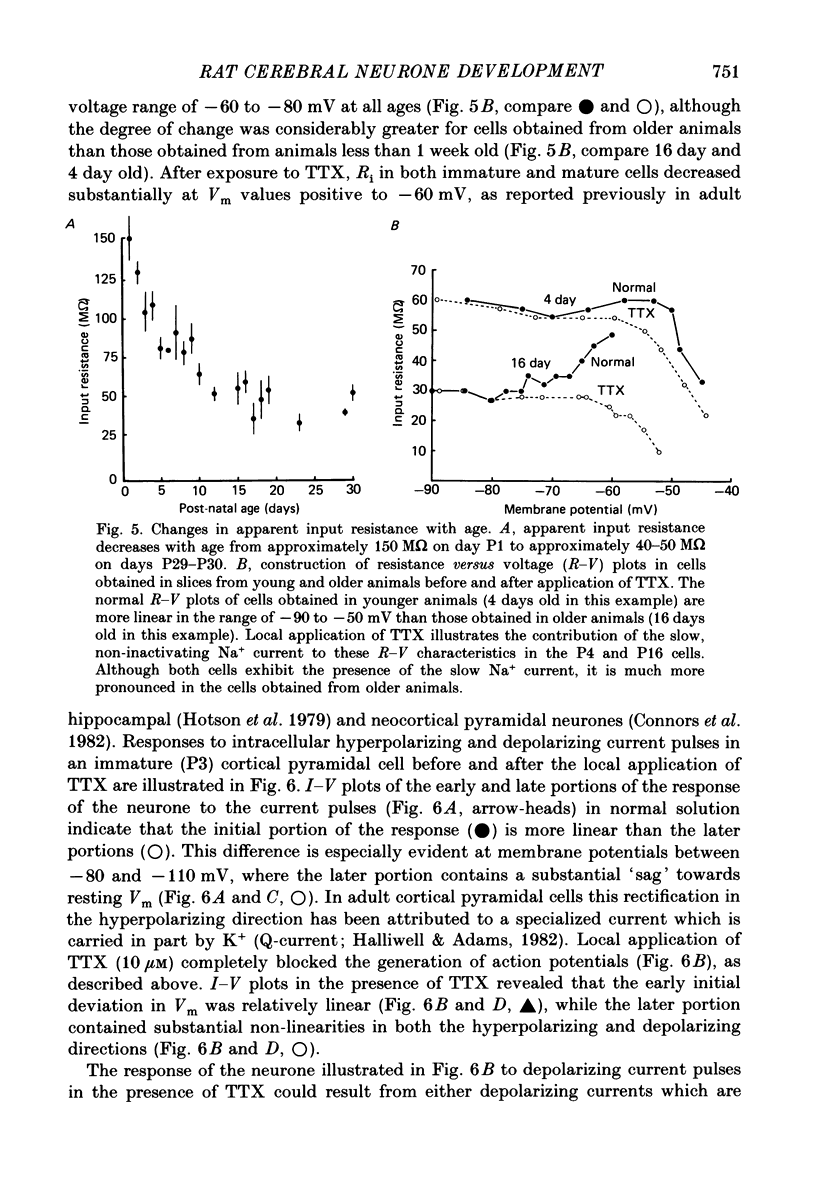
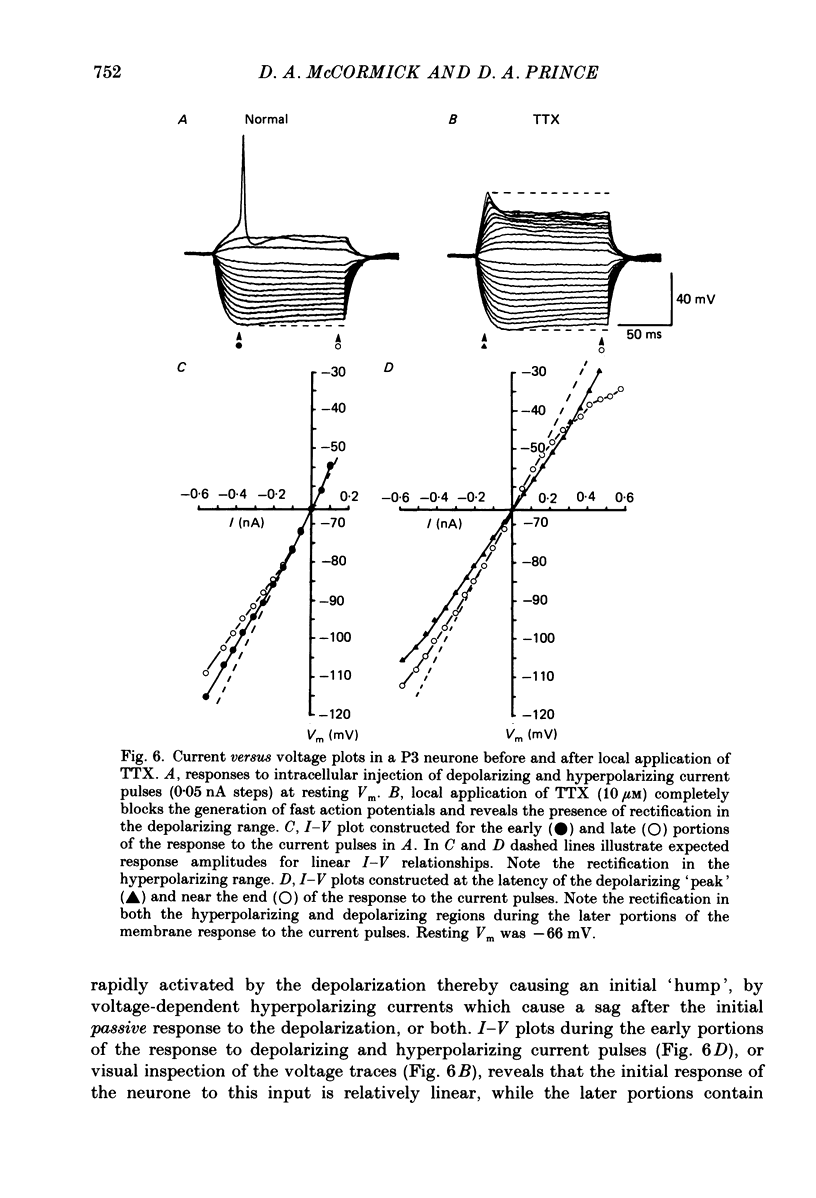
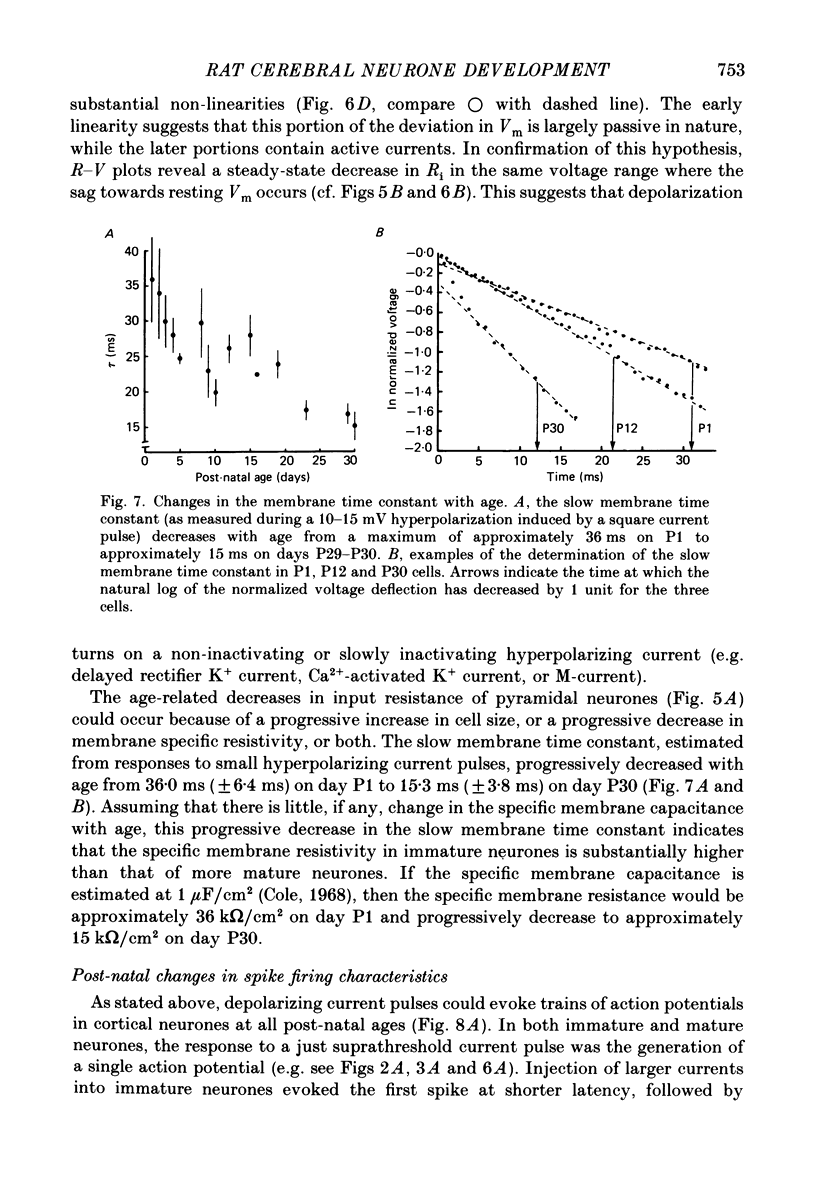
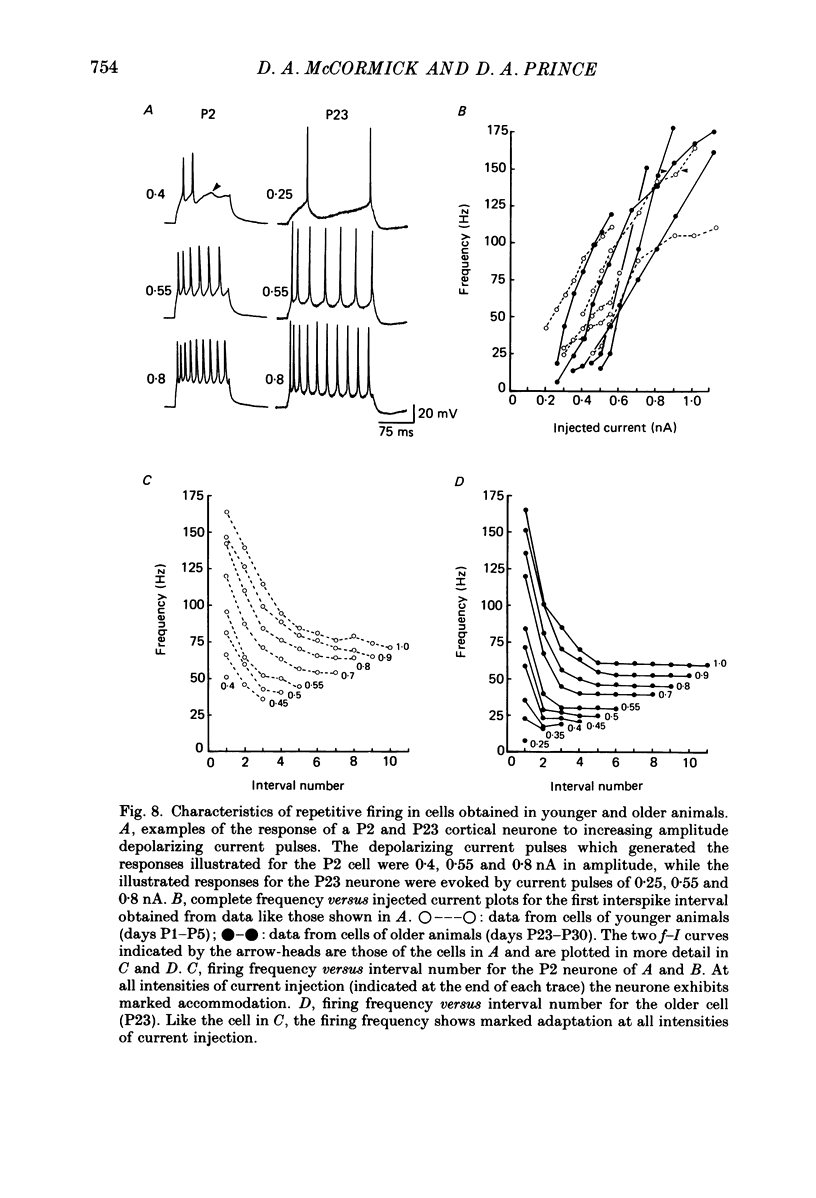
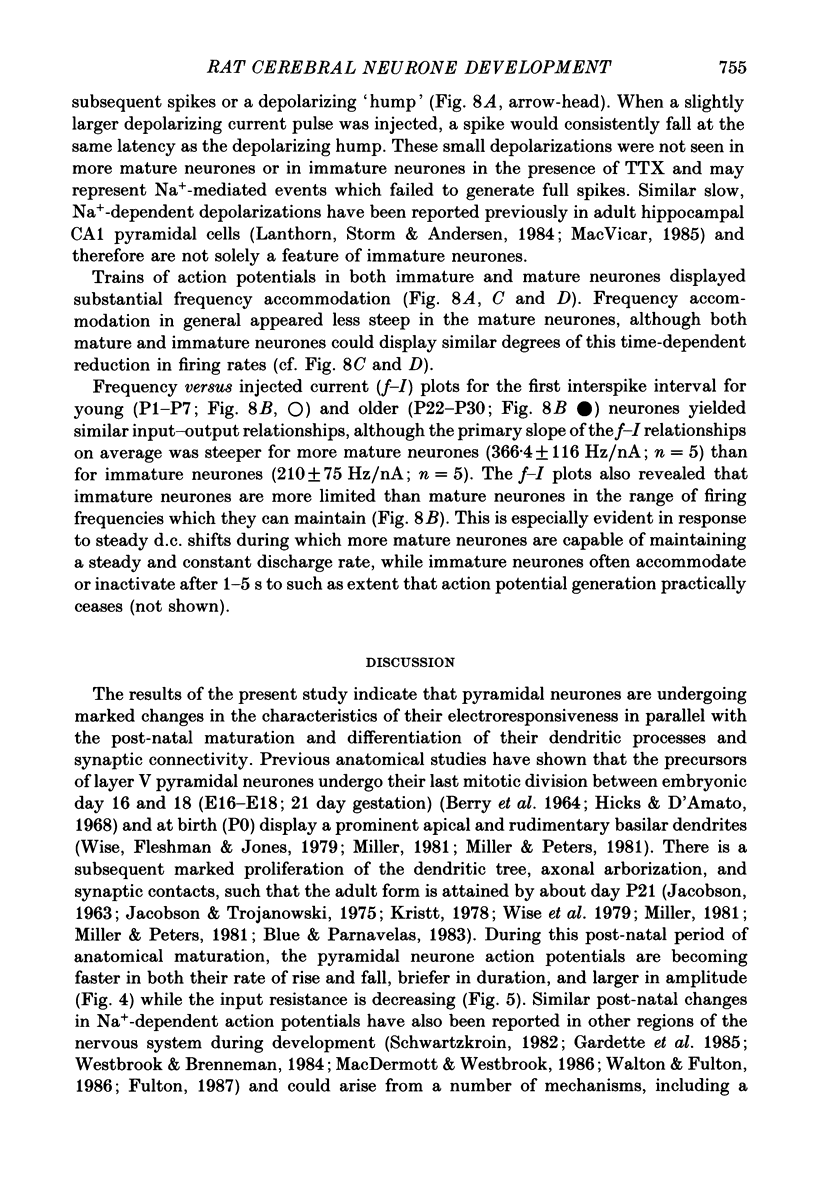
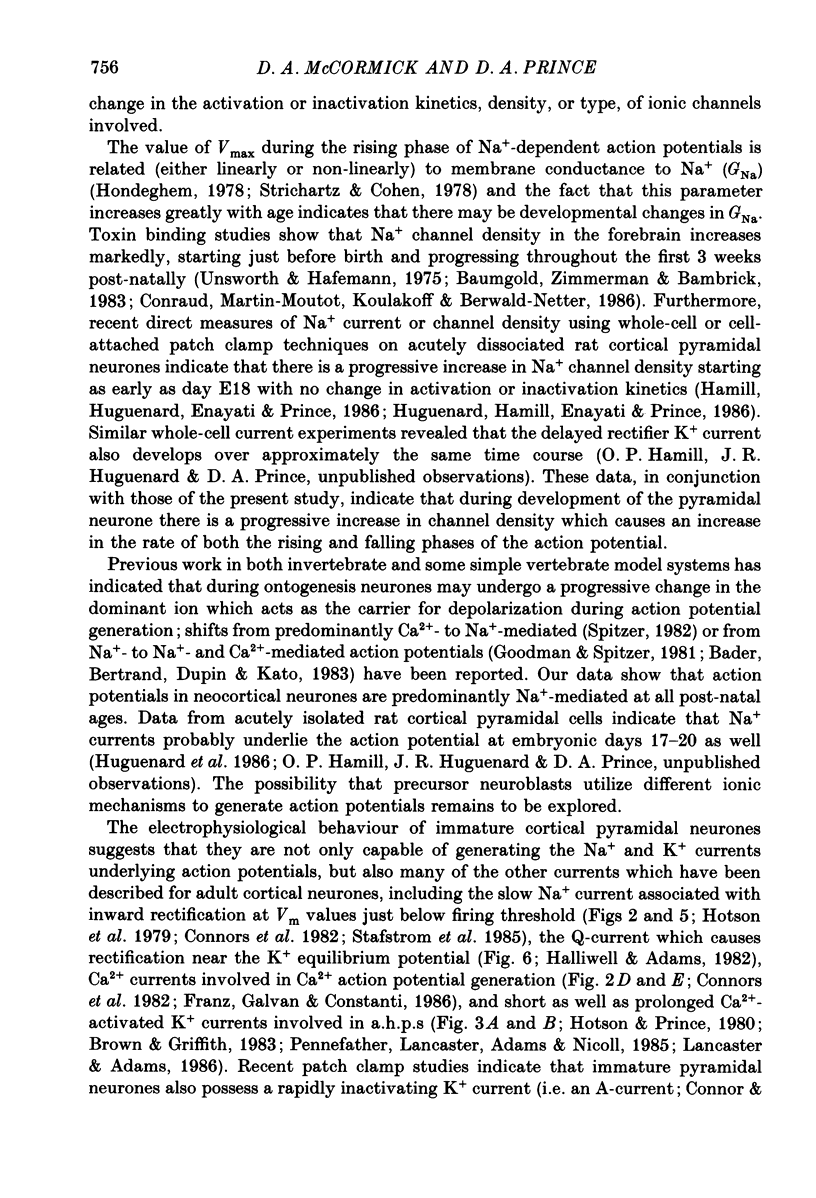
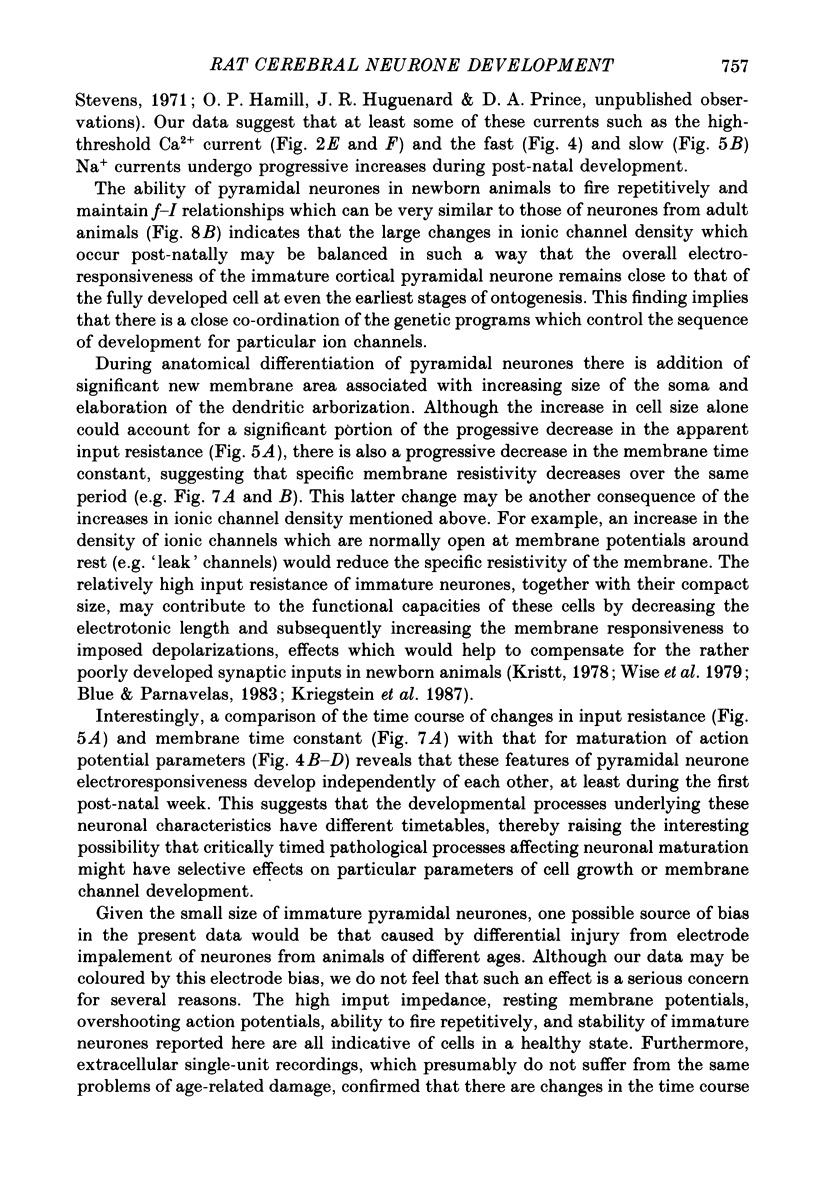
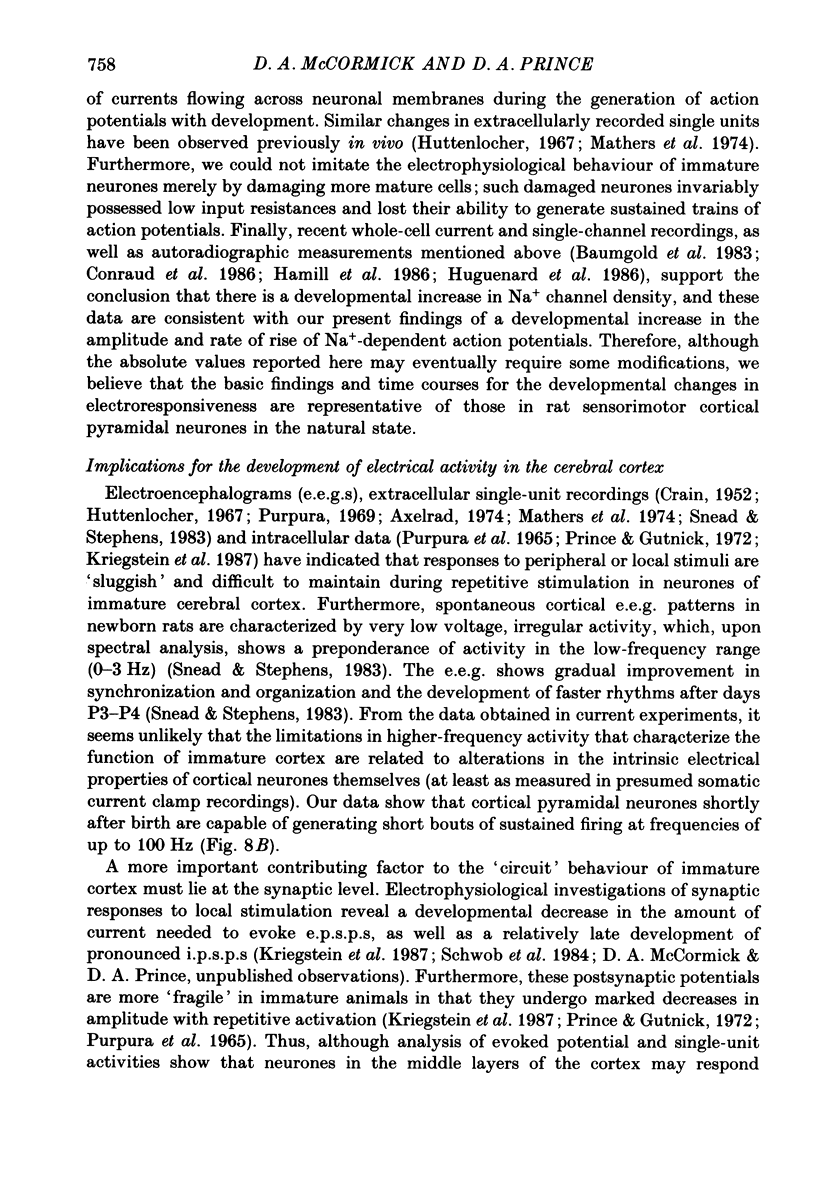
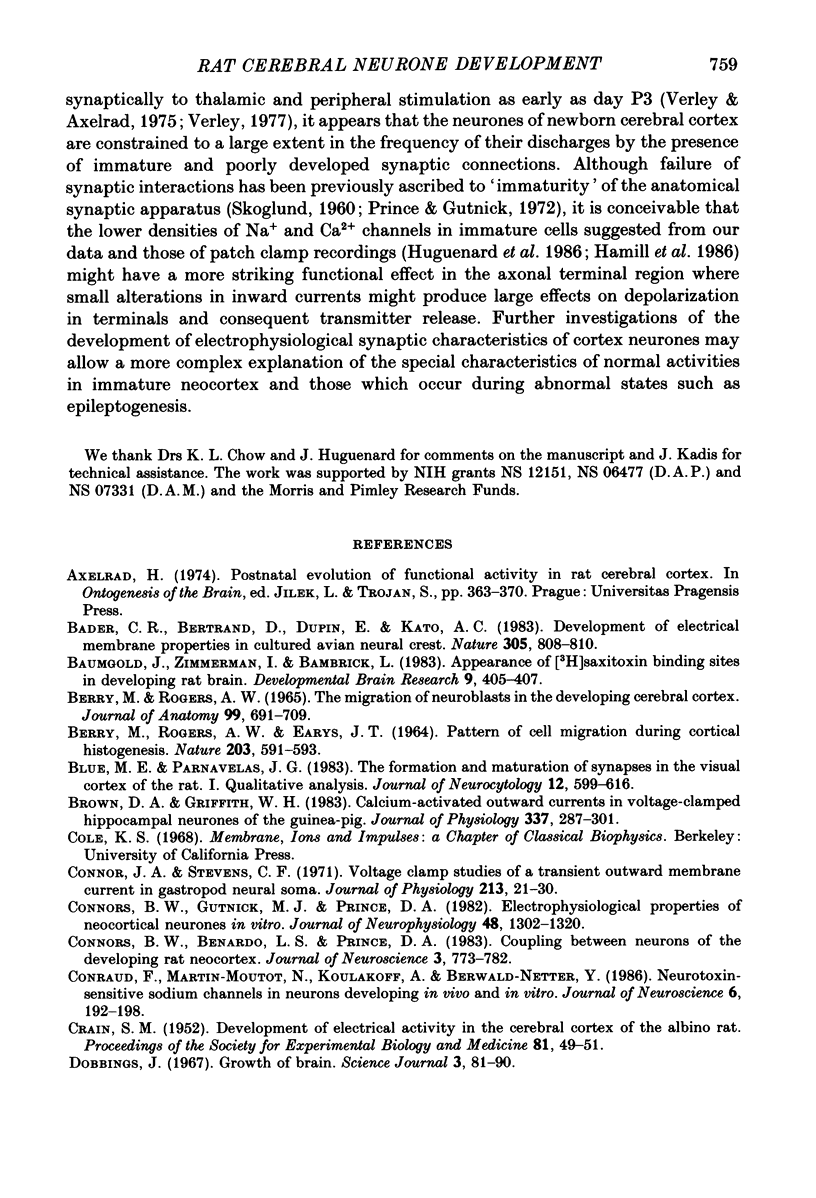
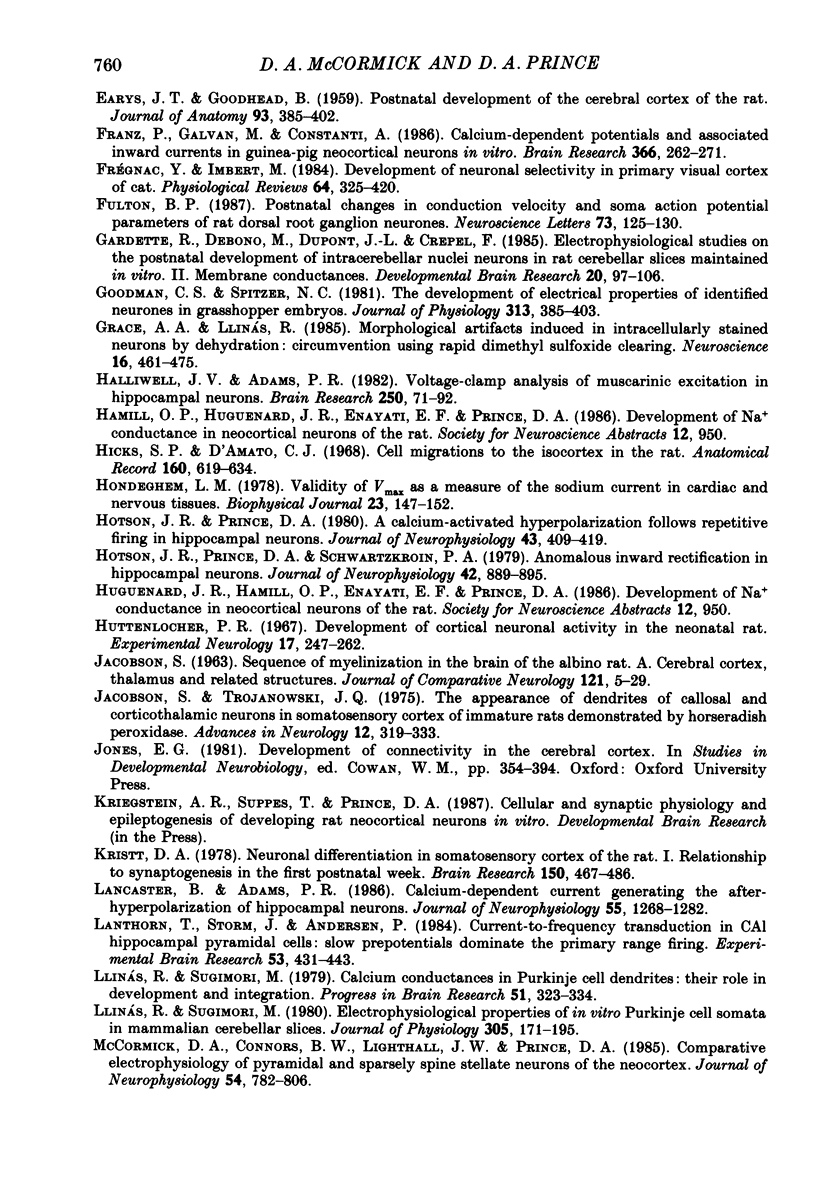
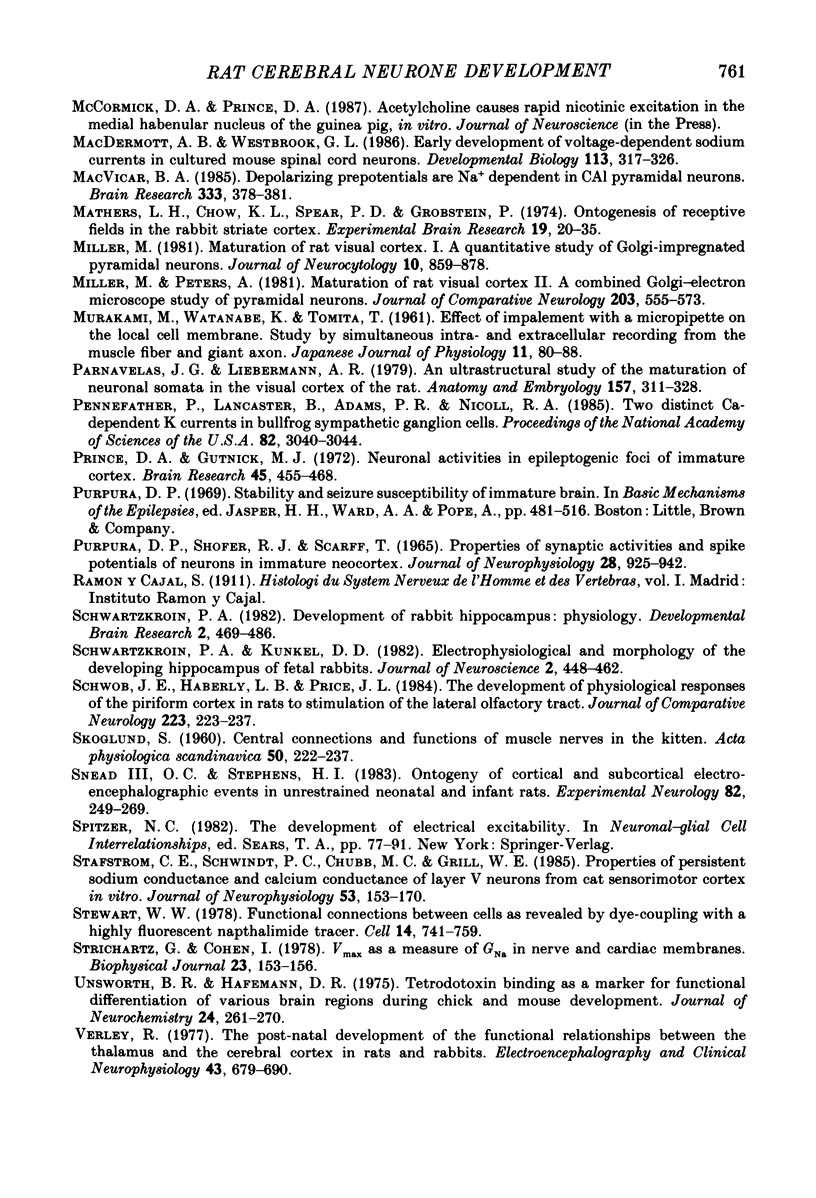
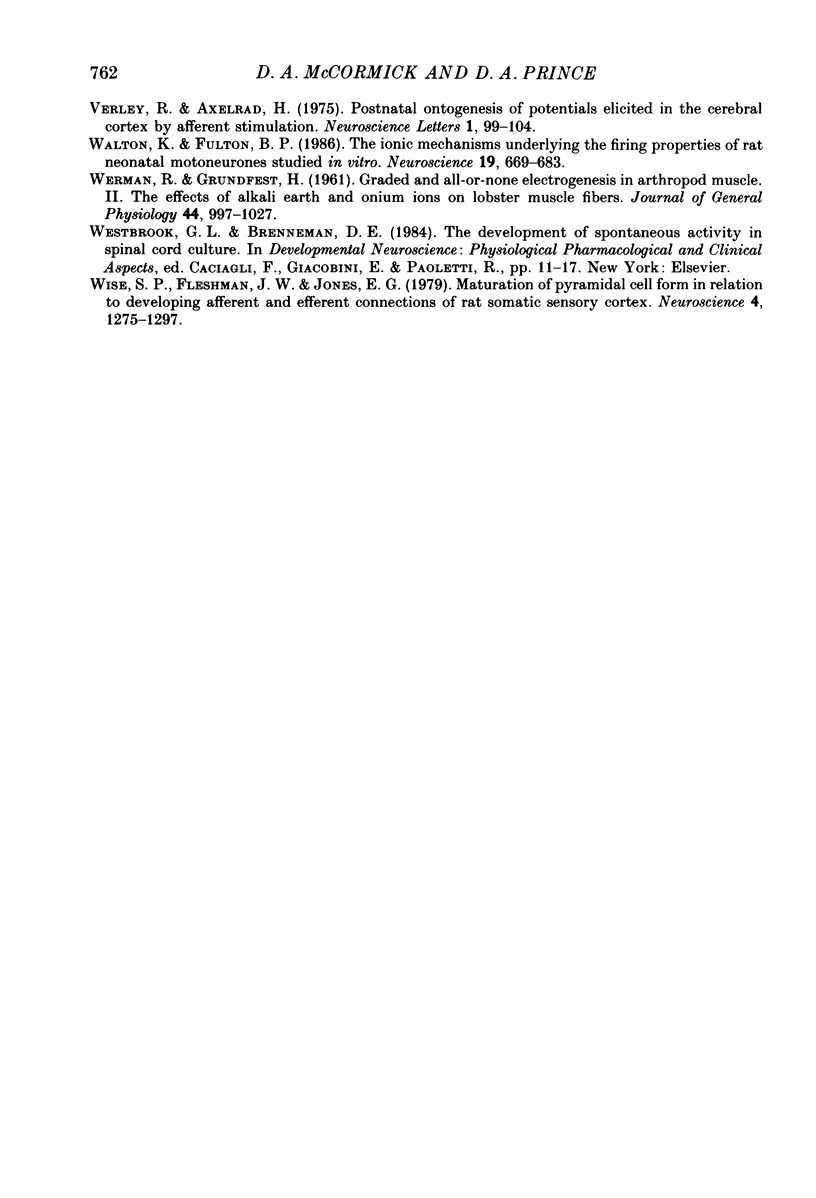
Selected References
These references are in PubMed. This may not be the complete list of references from this article.
- BERRY M., ROGERS A. W., EAYRS J. T. PATTERN OF CELL MIGRATION DURING CORTICAL HISTOGENESIS. Nature. 1964 Aug 8;203:591–593. doi: 10.1038/203591b0. [DOI] [PubMed] [Google Scholar]
- Bader C. R., Bertrand D., Dupin E., Kato A. C. Development of electrical membrane properties in cultured avian neural crest. 1983 Oct 27-Nov 2Nature. 305(5937):808–810. doi: 10.1038/305808a0. [DOI] [PubMed] [Google Scholar]
- Baumgold J., Zimmerman I., Bambrick L. Appearance of [3H]saxitoxin binding sites in developing rat brain. Brain Res. 1983 Sep;285(3):405–407. doi: 10.1016/0165-3806(83)90040-8. [DOI] [PubMed] [Google Scholar]
- Berry M., Rogers A. W. The migration of neuroblasts in the developing cerebral cortex. J Anat. 1965 Oct;99(Pt 4):691–709. [PMC free article] [PubMed] [Google Scholar]
- Blue M. E., Parnavelas J. G. The formation and maturation of synapses in the visual cortex of the rat. I. Qualitative analysis. J Neurocytol. 1983 Aug;12(4):599–616. doi: 10.1007/BF01181526. [DOI] [PubMed] [Google Scholar]
- Brown D. A., Griffith W. H. Calcium-activated outward current in voltage-clamped hippocampal neurones of the guinea-pig. J Physiol. 1983 Apr;337:287–301. doi: 10.1113/jphysiol.1983.sp014624. [DOI] [PMC free article] [PubMed] [Google Scholar]
- CRAIN S. M. Development of electrical activity in the cerebral cortex of the albino rat. Proc Soc Exp Biol Med. 1952 Oct;81(1):49–51. [PubMed] [Google Scholar]
- Connor J. A., Stevens C. F. Voltage clamp studies of a transient outward membrane current in gastropod neural somata. J Physiol. 1971 Feb;213(1):21–30. doi: 10.1113/jphysiol.1971.sp009365. [DOI] [PMC free article] [PubMed] [Google Scholar]
- Connors B. W., Benardo L. S., Prince D. A. Coupling between neurons of the developing rat neocortex. J Neurosci. 1983 Apr;3(4):773–782. doi: 10.1523/JNEUROSCI.03-04-00773.1983. [DOI] [PMC free article] [PubMed] [Google Scholar]
- Connors B. W., Gutnick M. J., Prince D. A. Electrophysiological properties of neocortical neurons in vitro. J Neurophysiol. 1982 Dec;48(6):1302–1320. doi: 10.1152/jn.1982.48.6.1302. [DOI] [PubMed] [Google Scholar]
- Couraud F., Martin-Moutot N., Koulakoff A., Berwald-Netter Y. Neurotoxin-sensitive sodium channels in neurons developing in vivo and in vitro. J Neurosci. 1986 Jan;6(1):192–198. doi: 10.1523/JNEUROSCI.06-01-00192.1986. [DOI] [PMC free article] [PubMed] [Google Scholar]
- EAYRS J. T., GOODHEAD B. Postnatal development of the cerebral cortex in the rat. J Anat. 1959 Oct;93:385–402. [PMC free article] [PubMed] [Google Scholar]
- Franz P., Galvan M., Constanti A. Calcium-dependent action potentials and associated inward currents in guinea-pig neocortical neurons in vitro. Brain Res. 1986 Feb 26;366(1-2):262–271. doi: 10.1016/0006-8993(86)91303-x. [DOI] [PubMed] [Google Scholar]
- Frégnac Y., Imbert M. Development of neuronal selectivity in primary visual cortex of cat. Physiol Rev. 1984 Jan;64(1):325–434. doi: 10.1152/physrev.1984.64.1.325. [DOI] [PubMed] [Google Scholar]
- Fulton B. P. Postnatal changes in conduction velocity and soma action potential parameters of rat dorsal root ganglion neurones. Neurosci Lett. 1987 Jan 14;73(2):125–130. doi: 10.1016/0304-3940(87)90005-x. [DOI] [PubMed] [Google Scholar]
- Gardette R., Debono M., Dupont J. L., Crepel F. Electrophysiological studies on the postnatal development of intracerebellar nuclei neurons in rat cerebellar slices maintained in vitro. II. Membrane conductances. Brain Res. 1985 May;352(1):97–106. doi: 10.1016/0165-3806(85)90091-4. [DOI] [PubMed] [Google Scholar]
- Goodman C. S., Spitzer N. C. The development of electrical properties of identified neurones in grasshopper embryos. J Physiol. 1981;313:385–403. doi: 10.1113/jphysiol.1981.sp013672. [DOI] [PMC free article] [PubMed] [Google Scholar]
- Grace A. A., Llinás R. Morphological artifacts induced in intracellularly stained neurons by dehydration: circumvention using rapid dimethyl sulfoxide clearing. Neuroscience. 1985 Oct;16(2):461–475. doi: 10.1016/0306-4522(85)90018-1. [DOI] [PubMed] [Google Scholar]
- Halliwell J. V., Adams P. R. Voltage-clamp analysis of muscarinic excitation in hippocampal neurons. Brain Res. 1982 Oct 28;250(1):71–92. doi: 10.1016/0006-8993(82)90954-4. [DOI] [PubMed] [Google Scholar]
- Hicks S. P., D'Amato C. J. Cell migrations to the isocortex in the rat. Anat Rec. 1968 Mar;160(3):619–634. doi: 10.1002/ar.1091600311. [DOI] [PubMed] [Google Scholar]
- Hondeghem L. M. Validity of Vmax as a measure of the sodium current in cardiac and nervous tissues. Biophys J. 1978 Jul;23(1):147–152. doi: 10.1016/S0006-3495(78)85439-3. [DOI] [PMC free article] [PubMed] [Google Scholar]
- Hotson J. R., Prince D. A. A calcium-activated hyperpolarization follows repetitive firing in hippocampal neurons. J Neurophysiol. 1980 Feb;43(2):409–419. doi: 10.1152/jn.1980.43.2.409. [DOI] [PubMed] [Google Scholar]
- Hotson J. R., Prince D. A., Schwartzkroin P. A. Anomalous inward rectification in hippocampal neurons. J Neurophysiol. 1979 May;42(3):889–895. doi: 10.1152/jn.1979.42.3.889. [DOI] [PubMed] [Google Scholar]
- Huttenlocher P. R. Development of cortical neuronal activity in the neonatal cat. Exp Neurol. 1967 Mar;17(3):247–262. doi: 10.1016/0014-4886(67)90104-5. [DOI] [PubMed] [Google Scholar]
- JACOBSON S. SEQUENCE OF MYELINIZATION IN THE BRAIN OF THE ALBINO RAT. A. CEREBRAL CORTEX, THALAMUS AND RELATED STRUCTURES. J Comp Neurol. 1963 Aug;121:5–29. doi: 10.1002/cne.901210103. [DOI] [PubMed] [Google Scholar]
- Jacobson S., Trojanowski J. Q. The appearance of dendrites of callosal and corticothalamic neurons in somatosensory cortex of immature rats demonstrated by horseradish peroxidase. Adv Neurol. 1975;12:319–333. [PubMed] [Google Scholar]
- Kristt D. A. Neuronal differentiation in somatosensory cortex of the rat. I. Relationship to synaptogenesis in the first postnatal week. Brain Res. 1978 Jul 21;150(3):467–486. doi: 10.1016/0006-8993(78)90814-4. [DOI] [PubMed] [Google Scholar]
- Lancaster B., Adams P. R. Calcium-dependent current generating the afterhyperpolarization of hippocampal neurons. J Neurophysiol. 1986 Jun;55(6):1268–1282. doi: 10.1152/jn.1986.55.6.1268. [DOI] [PubMed] [Google Scholar]
- Lanthorn T., Storm J., Andersen P. Current-to-frequency transduction in CA1 hippocampal pyramidal cells: slow prepotentials dominate the primary range firing. Exp Brain Res. 1984;53(2):431–443. doi: 10.1007/BF00238173. [DOI] [PubMed] [Google Scholar]
- Llinás R., Sugimori M. Calcium conductances in Purkinje cell dendrites: their role in development and integration. Prog Brain Res. 1979;51:323–334. doi: 10.1016/S0079-6123(08)61312-6. [DOI] [PubMed] [Google Scholar]
- Llinás R., Sugimori M. Electrophysiological properties of in vitro Purkinje cell somata in mammalian cerebellar slices. J Physiol. 1980 Aug;305:171–195. doi: 10.1113/jphysiol.1980.sp013357. [DOI] [PMC free article] [PubMed] [Google Scholar]
- MURAKAMI M., WATANABE K., TOMITA T. Effect of impalement with a micropipette on the local cell membrane. Study by simultaneous intra- and extracellular recording from the muscle fiber and giant axon. Jpn J Physiol. 1961 Feb 15;11:80–88. doi: 10.2170/jjphysiol.11.80. [DOI] [PubMed] [Google Scholar]
- MacDermott A. B., Westbrook G. L. Early development of voltage-dependent sodium currents in cultured mouse spinal cord neurons. Dev Biol. 1986 Feb;113(2):317–326. doi: 10.1016/0012-1606(86)90167-3. [DOI] [PubMed] [Google Scholar]
- Macvicar B. A. Depolarizing prepotentials are Na+ dependent in CA1 pyramidal neurons. Brain Res. 1985 May 6;333(2):378–381. doi: 10.1016/0006-8993(85)91597-5. [DOI] [PubMed] [Google Scholar]
- Mathers L. H., Chow K. L., Spear P. D., Grobstein P. Ontogenesis of receptive fields in the rabbit striate cortex. Exp Brain Res. 1974 Jan 22;19(1):20–35. doi: 10.1007/BF00233393. [DOI] [PubMed] [Google Scholar]
- McCormick D. A., Connors B. W., Lighthall J. W., Prince D. A. Comparative electrophysiology of pyramidal and sparsely spiny stellate neurons of the neocortex. J Neurophysiol. 1985 Oct;54(4):782–806. doi: 10.1152/jn.1985.54.4.782. [DOI] [PubMed] [Google Scholar]
- Miller M. Maturation of rat visual cortex. I. A quantitative study of Golgi-impregnated pyramidal neurons. J Neurocytol. 1981 Oct;10(5):859–878. doi: 10.1007/BF01262658. [DOI] [PubMed] [Google Scholar]
- Miller M., Peters A. Maturation of rat visual cortex. II. A combined Golgi-electron microscope study of pyramidal neurons. J Comp Neurol. 1981 Dec 20;203(4):555–573. doi: 10.1002/cne.902030402. [DOI] [PubMed] [Google Scholar]
- Parnavelas J. G., Lieberman A. R. An ultrastructural study of the maturation of neuronal somata in the visual cortex of the rat. Anat Embryol (Berl) 1979;157(3):311–328. doi: 10.1007/BF00304996. [DOI] [PubMed] [Google Scholar]
- Pennefather P., Lancaster B., Adams P. R., Nicoll R. A. Two distinct Ca-dependent K currents in bullfrog sympathetic ganglion cells. Proc Natl Acad Sci U S A. 1985 May;82(9):3040–3044. doi: 10.1073/pnas.82.9.3040. [DOI] [PMC free article] [PubMed] [Google Scholar]
- Prince D. A., Gutnick M. J. Neuronal activities in epileptogenic foci of immature cortex. Brain Res. 1972 Oct 27;45(2):455–468. doi: 10.1016/0006-8993(72)90474-x. [DOI] [PubMed] [Google Scholar]
- Purpura D. P., Shofer R. J., Scarff T. Properties of synaptic activities and spike potentials of neurons in immature neocortex. J Neurophysiol. 1965 Sep;28(5):925–942. doi: 10.1152/jn.1965.28.5.925. [DOI] [PubMed] [Google Scholar]
- Schwartzkroin P. A., Kunkel D. D. Electrophysiology and morphology of the developing hippocampus of fetal rabbits. J Neurosci. 1982 Apr;2(4):448–462. doi: 10.1523/JNEUROSCI.02-04-00448.1982. [DOI] [PMC free article] [PubMed] [Google Scholar]
- Schwob J. E., Haberly L. B., Price J. L. The development of physiological responses of the piriform cortex in rats to stimulation of the lateral olfactory tract. J Comp Neurol. 1984 Feb 20;223(2):223–237. doi: 10.1002/cne.902230206. [DOI] [PubMed] [Google Scholar]
- Snead O. C., 3rd, Stephens H. I. Ontogeny of cortical and subcortical electroencephalographic events in unrestrained neonatal and infant rats. Exp Neurol. 1983 Nov;82(2):249–269. doi: 10.1016/0014-4886(83)90400-4. [DOI] [PubMed] [Google Scholar]
- Stafstrom C. E., Schwindt P. C., Chubb M. C., Crill W. E. Properties of persistent sodium conductance and calcium conductance of layer V neurons from cat sensorimotor cortex in vitro. J Neurophysiol. 1985 Jan;53(1):153–170. doi: 10.1152/jn.1985.53.1.153. [DOI] [PubMed] [Google Scholar]
- Stewart W. W. Functional connections between cells as revealed by dye-coupling with a highly fluorescent naphthalimide tracer. Cell. 1978 Jul;14(3):741–759. doi: 10.1016/0092-8674(78)90256-8. [DOI] [PubMed] [Google Scholar]
- Strichartz G., Cohen I. Vmax as a measure of GNa in nerve and cardiac membranes. Biophys J. 1978 Jul;23(1):153–156. doi: 10.1016/S0006-3495(78)85440-X. [DOI] [PMC free article] [PubMed] [Google Scholar]
- Unsworth B. R., Hafemann D. R. Tetrodotoxin binding as a marker for functional differentiation of various brain regions during chick and mouse development. J Neurochem. 1975 Feb;24(2):261–270. doi: 10.1111/j.1471-4159.1975.tb11874.x. [DOI] [PubMed] [Google Scholar]
- Verley R. The post-natal development of the functional relationships between the thalamus and the cerebral cortex in rats and rabbits. Electroencephalogr Clin Neurophysiol. 1977 Nov;43(5):679–690. doi: 10.1016/0013-4694(77)90083-9. [DOI] [PubMed] [Google Scholar]
- WERMAN R., GRUNDFEST H. Graded and all-or-none electrogenesis in arthropod muscle. II. The effects of alkali-earth and onium ions on lobster muscle fibers. J Gen Physiol. 1961 May;44:997–1027. doi: 10.1085/jgp.44.5.997. [DOI] [PMC free article] [PubMed] [Google Scholar]
- Walton K., Fulton B. P. Ionic mechanisms underlying the firing properties of rat neonatal motoneurons studied in vitro. Neuroscience. 1986 Nov;19(3):669–683. doi: 10.1016/0306-4522(86)90291-5. [DOI] [PubMed] [Google Scholar]
- Wise S. P., Fleshman J. W., Jr, Jones E. G. Maturation of pyramidal cell form in relation to developing afferent and efferent connections of rat somatic sensory cortex. Neuroscience. 1979;4(9):1275–1297. doi: 10.1016/0306-4522(79)90157-x. [DOI] [PubMed] [Google Scholar]