Abstract
1. The dynamics of the centre mechanism of individual cat X retinal ganglion cells is investigated. The visual stimuli consist of temporal contrast modulation of stationary patterns. In order to study the response of the centre mechanism, patterns were either sine gratings of high spatial frequency or small circular spots positioned over the receptive-field centre. 2. Responses to contrast reversal are approximately linear. However, as the modulation depth of the stimulus increases, responses become more transient. Ganglion cell responses show this phenomenon at moderate contrasts (e.g. 0.1), which do not elicit discharges that approach the maximum firing rate of the ganglion cell. 3. A sequence of dynamical models are constructed from responses elicited by sum-of-sinusoids modulation of the spatial pattern. The first model is strictly linear. It consists of a series of low-pass filters and a single high-pass filter. The linear model predicts the approximate shape of the step response, but does not account for the change in shape of the response as a function of modulation depth. 4. The second model, a quasi-linear model, allows the 'linear' dynamics to vary slowly with a neural measure of contrast. The main effect of high contrast is a shorter time constant in the high-pass filter. This model accounts qualitatively for the increased transience of the response, but fails to predict the magnitude of the effect at higher modulation depths. 5. In the third model, the transfer characteristics of the centre response adjust rapidly as contrast changes. This intrinsically non-linear model provides excellent agreement with observed response to steps and more complex modulation patterns. 6. The non-linearity necessitated by a voltage-to-spikes transduction is analysed quantitatively. In most ganglion cells, a simple truncation at 0 impulses/s (and no saturation) explains the changes in apparent gain and mean firing rate that occur as modulation depth is increased. A non-linear voltage-to-spike transduction per se cannot account for the observed effect of contrast on dynamics. 7. The parameters of the dynamical model are measured for a population of twenty-seven X ganglion cells (nineteen on-centre and eight off-centre). The low-pass stage and the strength of the high-pass stage are relatively uniform across the population. The over-all gain and the dynamics of the high-pass stage vary substantially across the population, but show no consistent dependence on the on-off distinction or on retinal location. Some implications of this variability for retinal function are discussed.
Full text
PDF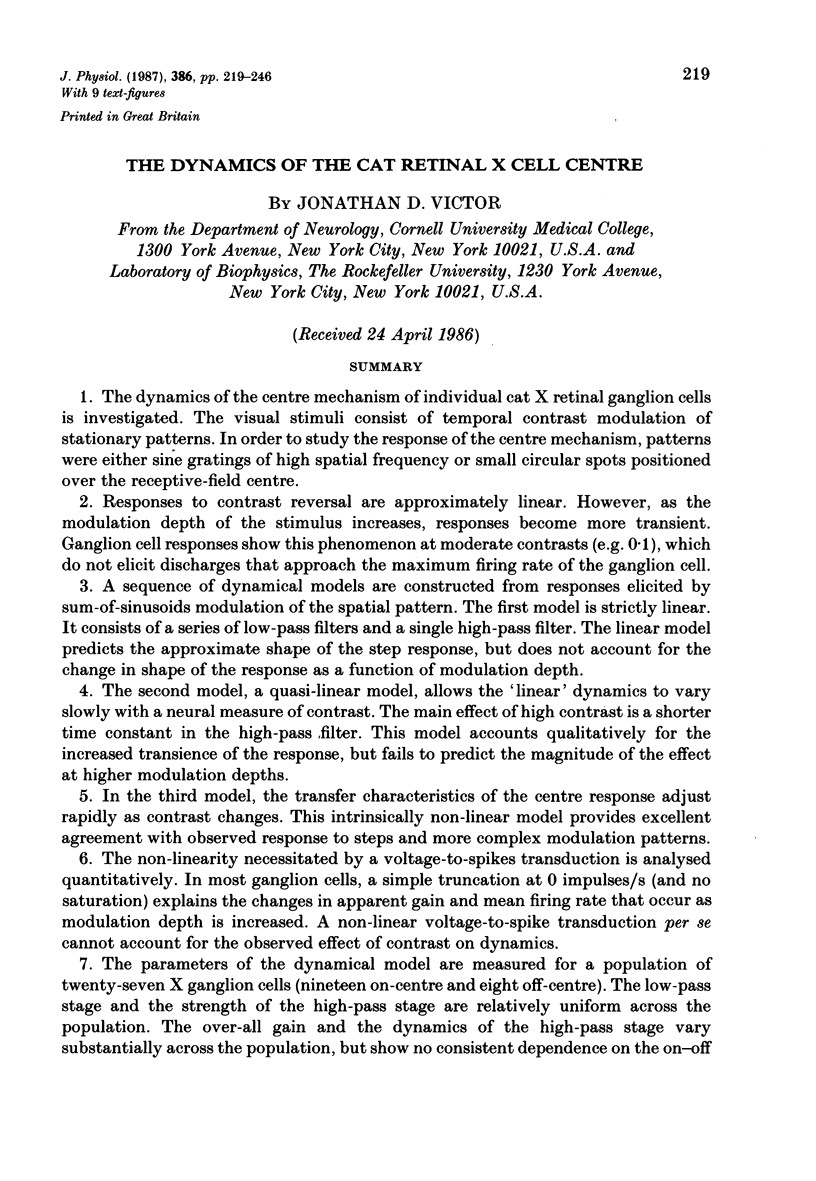
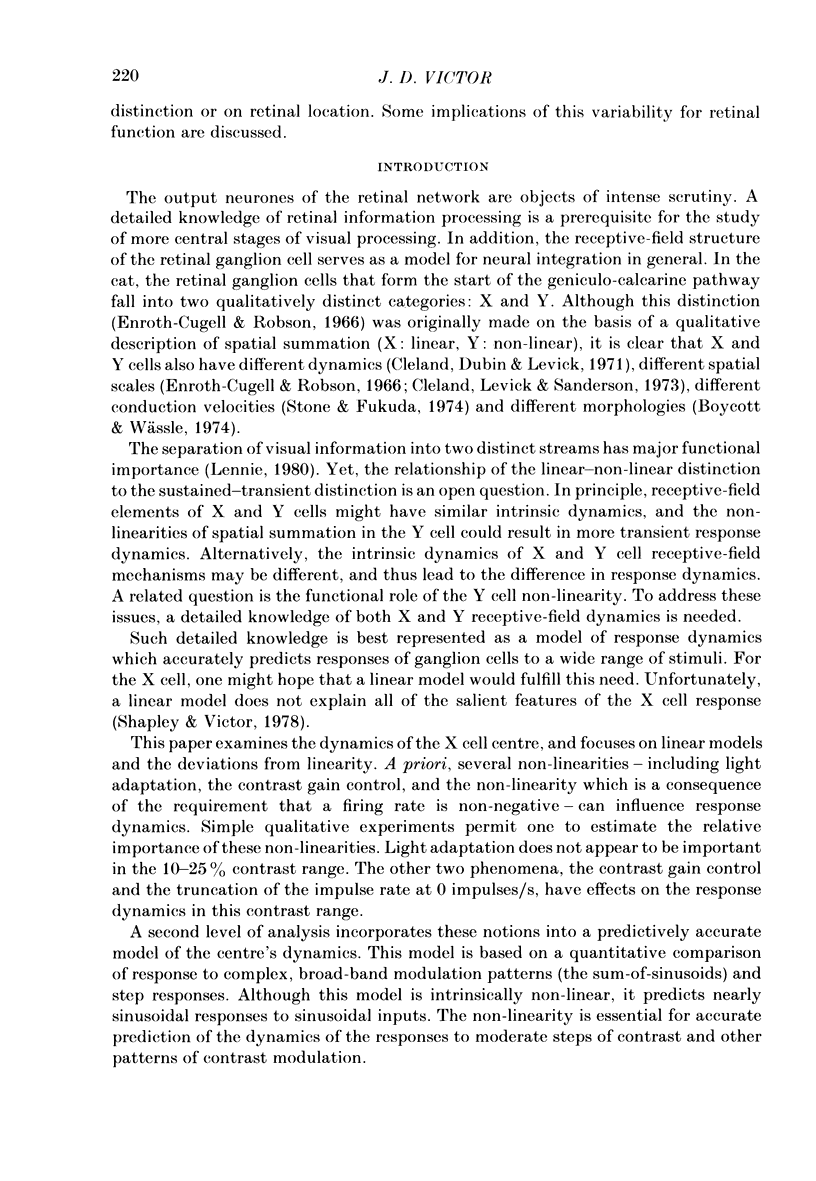
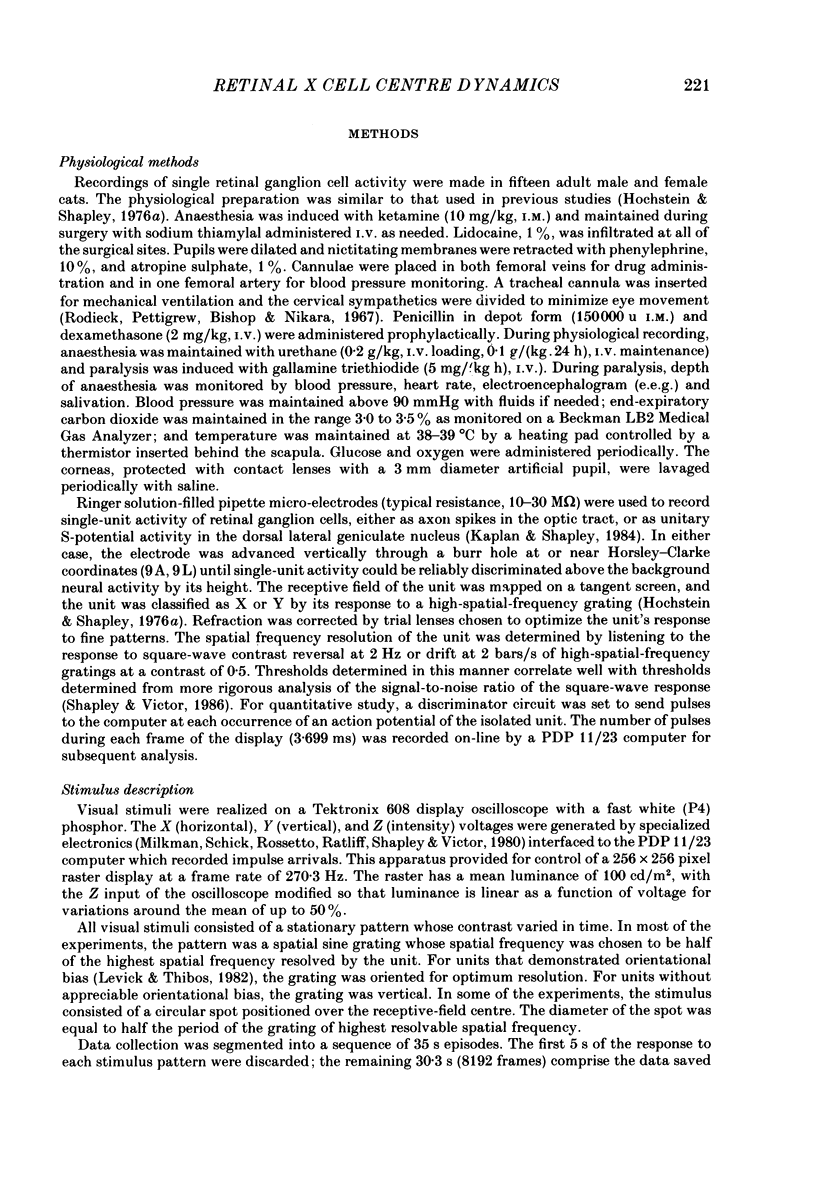
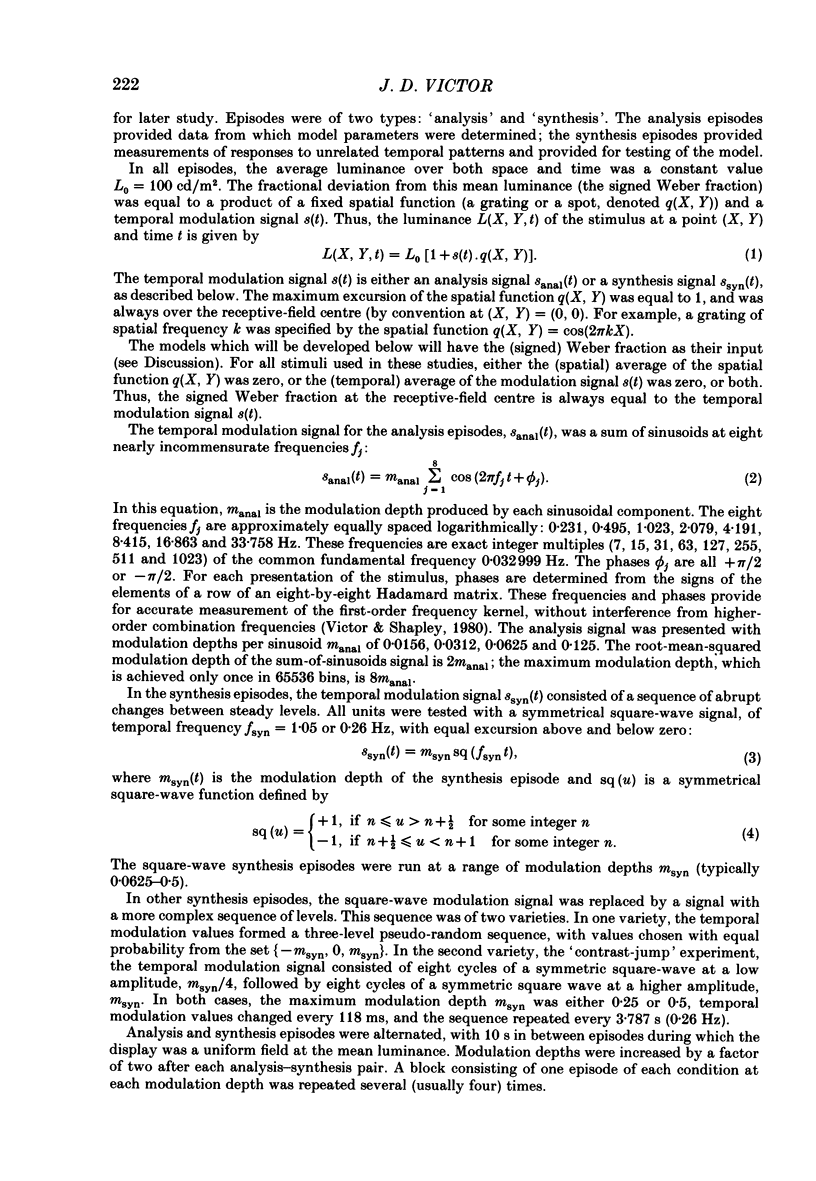
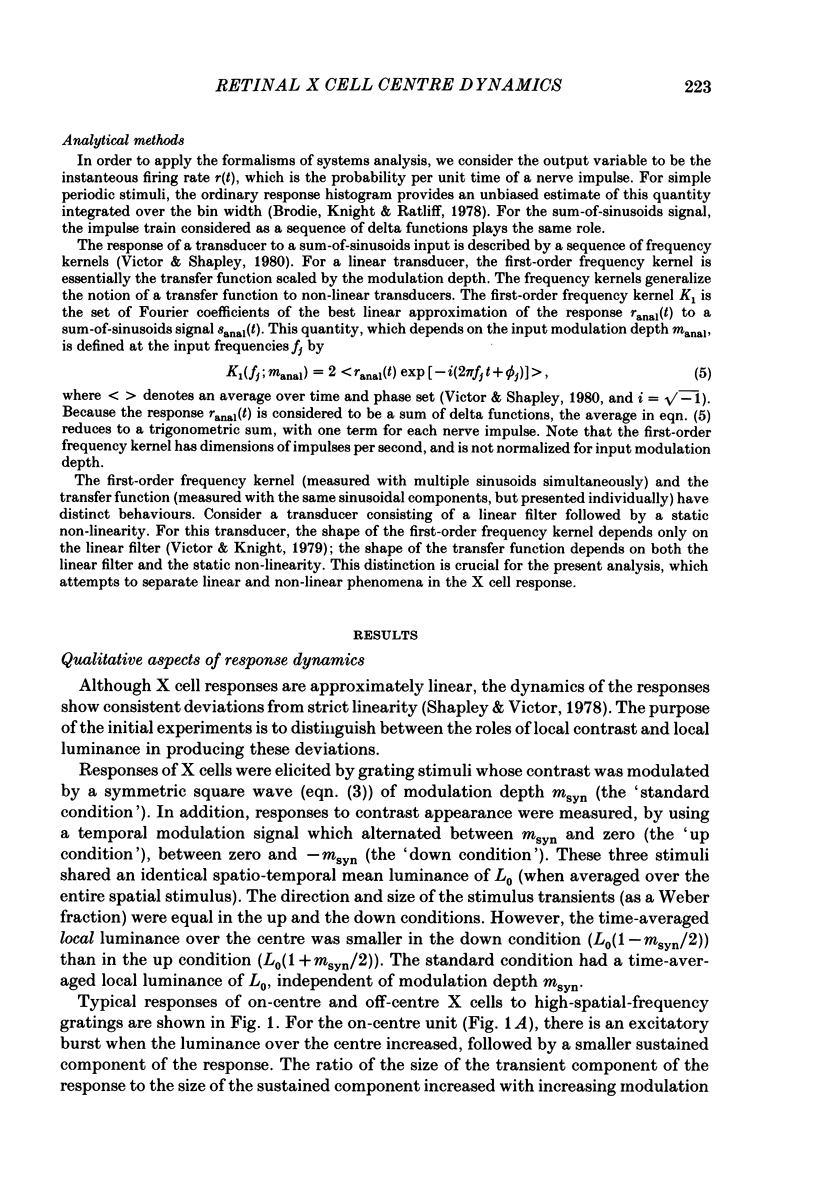
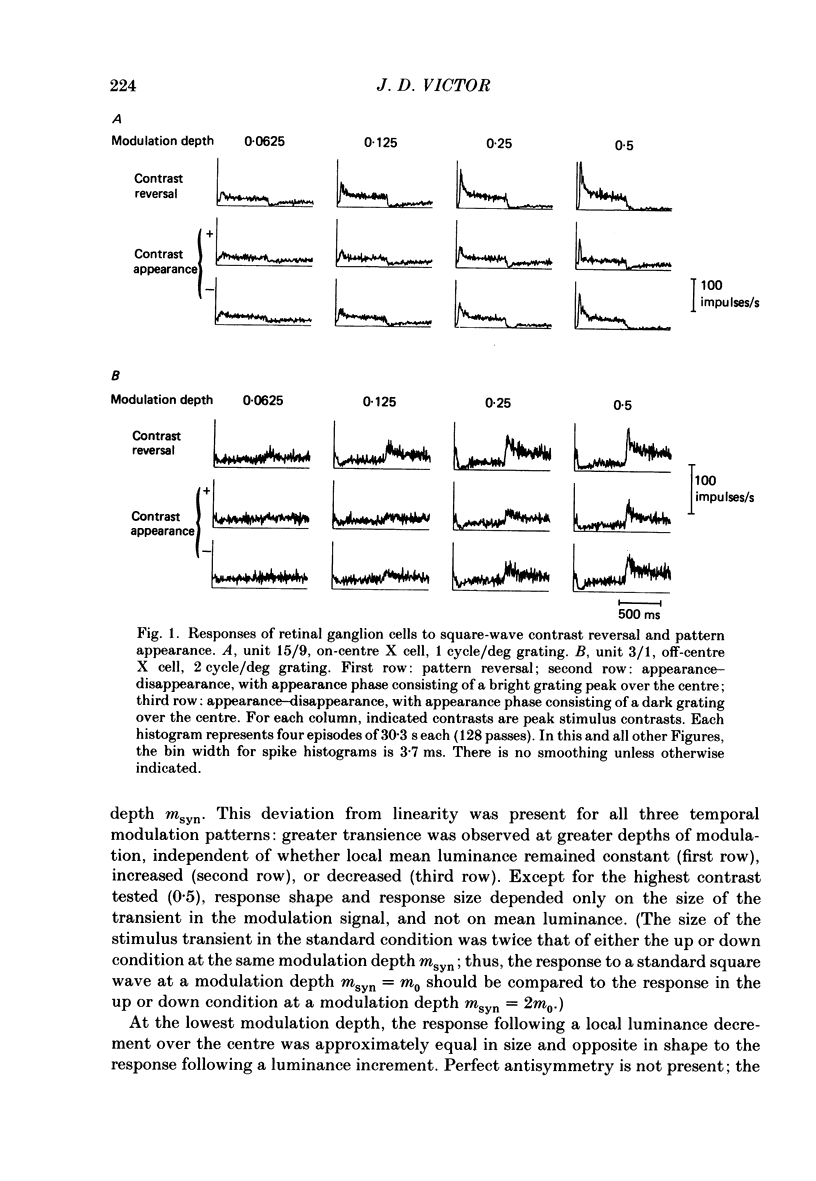
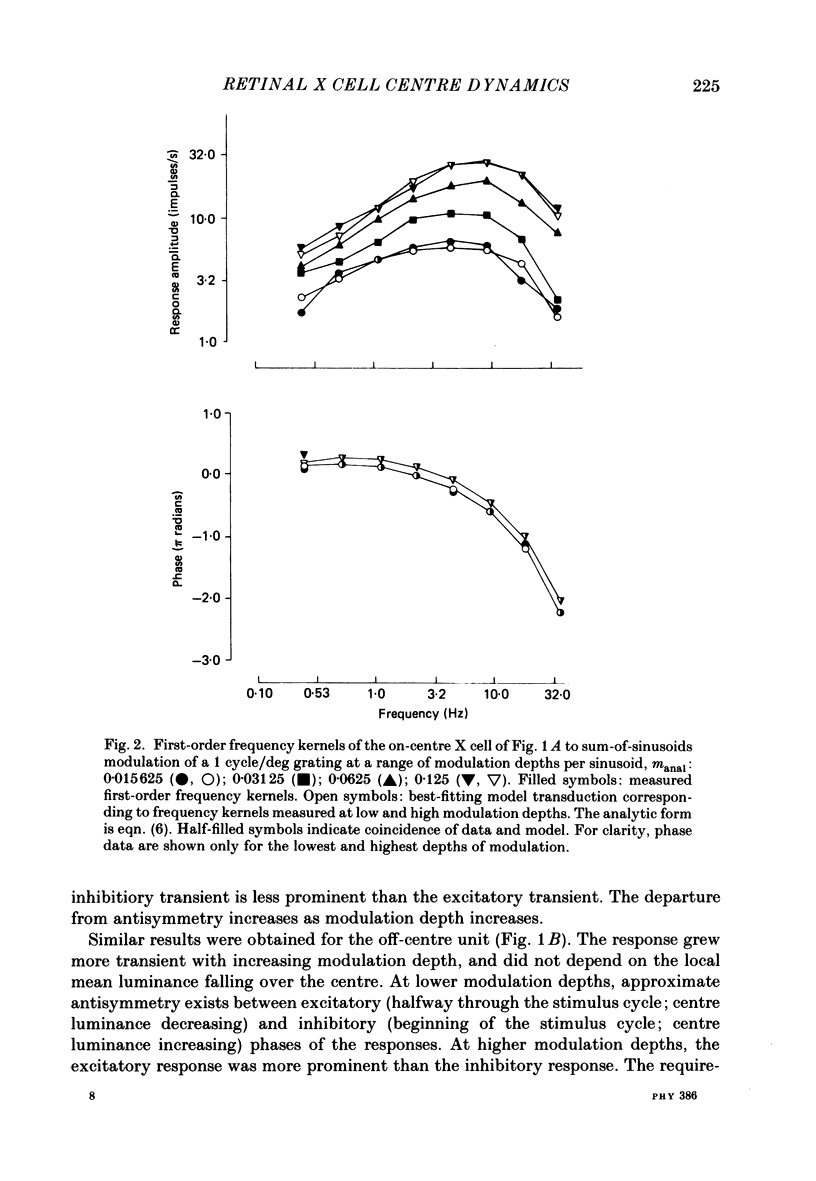
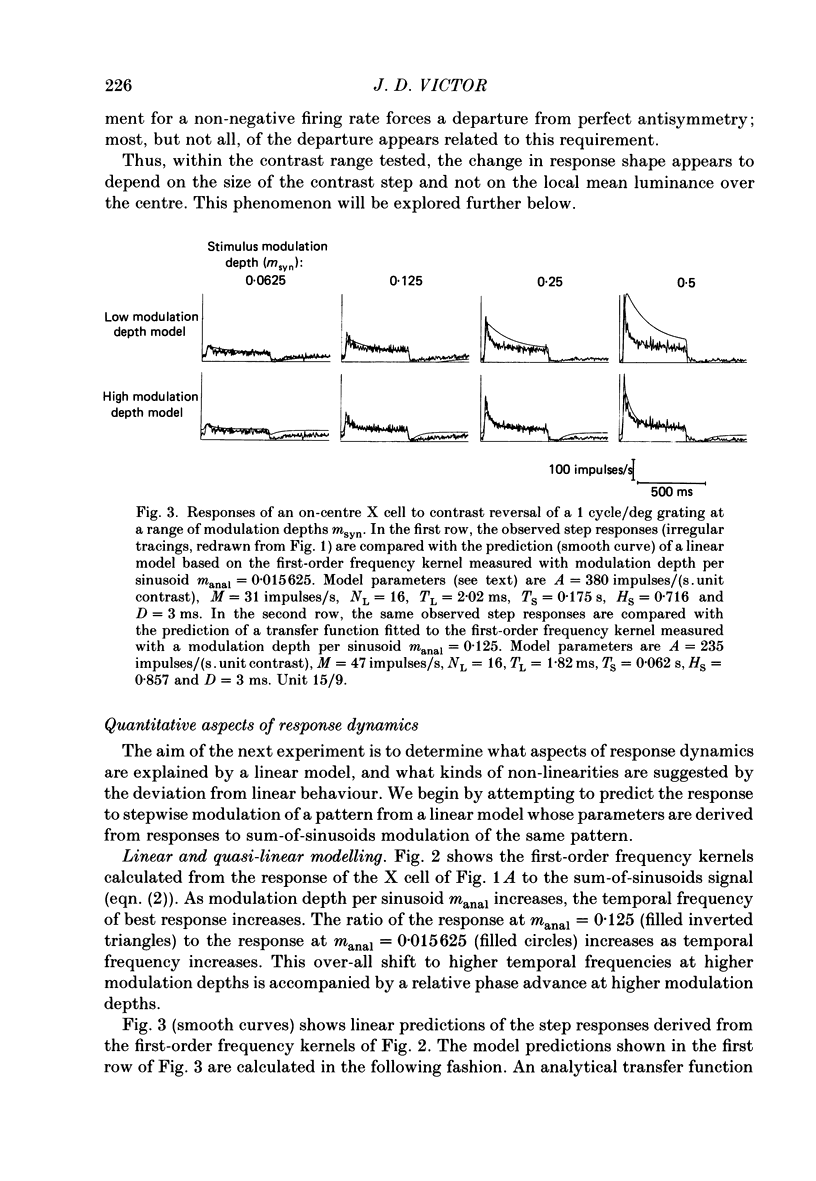
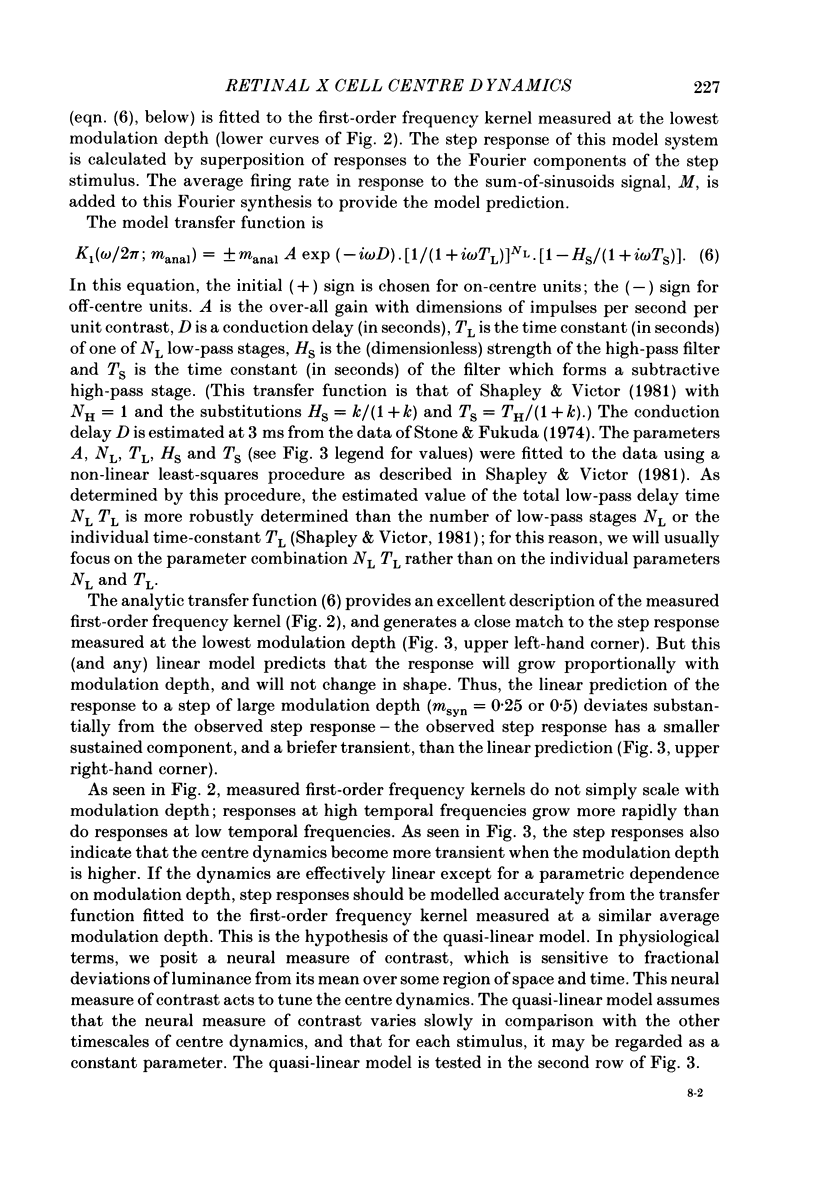
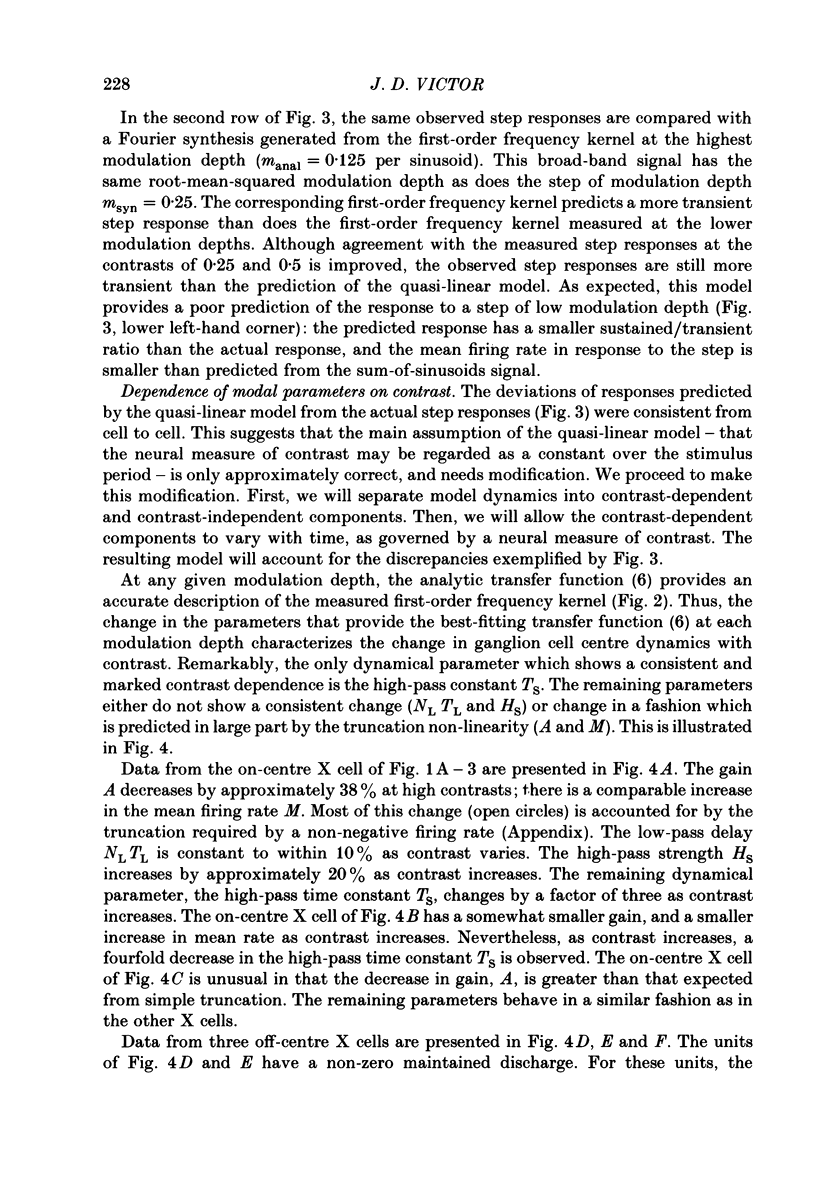
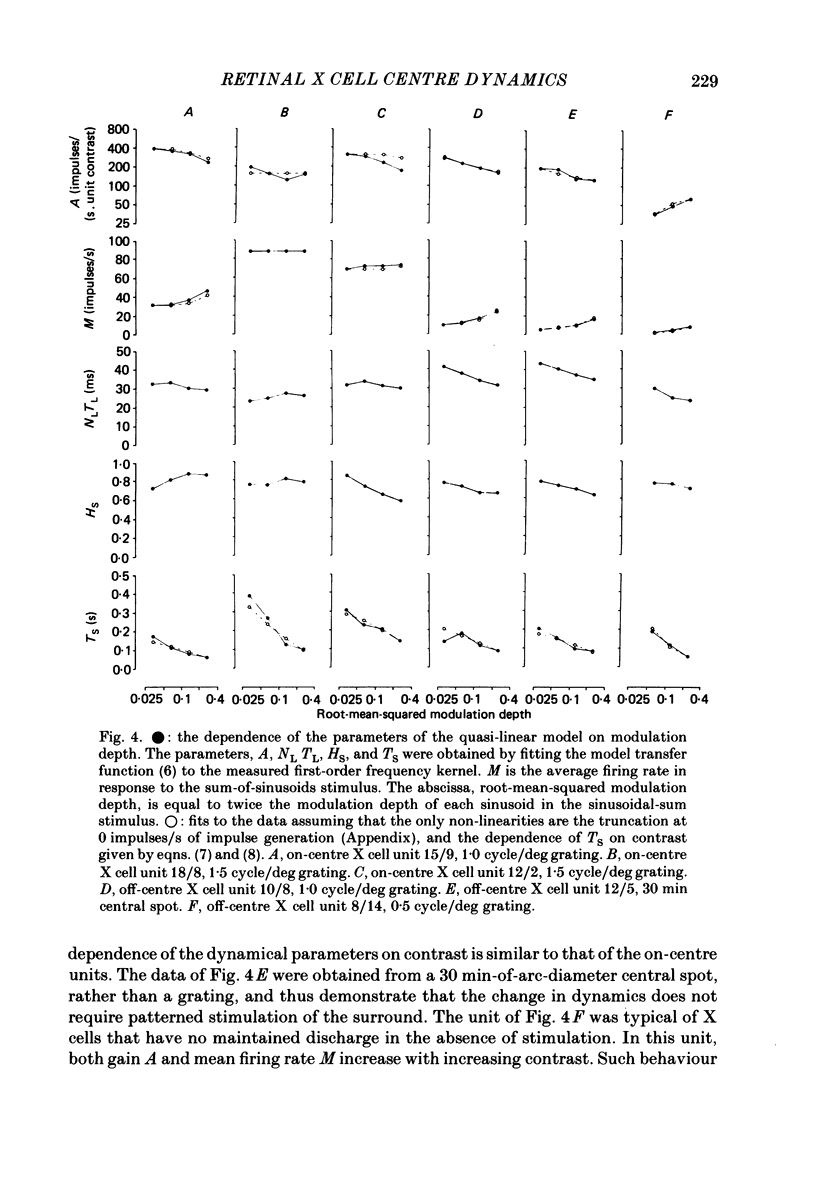
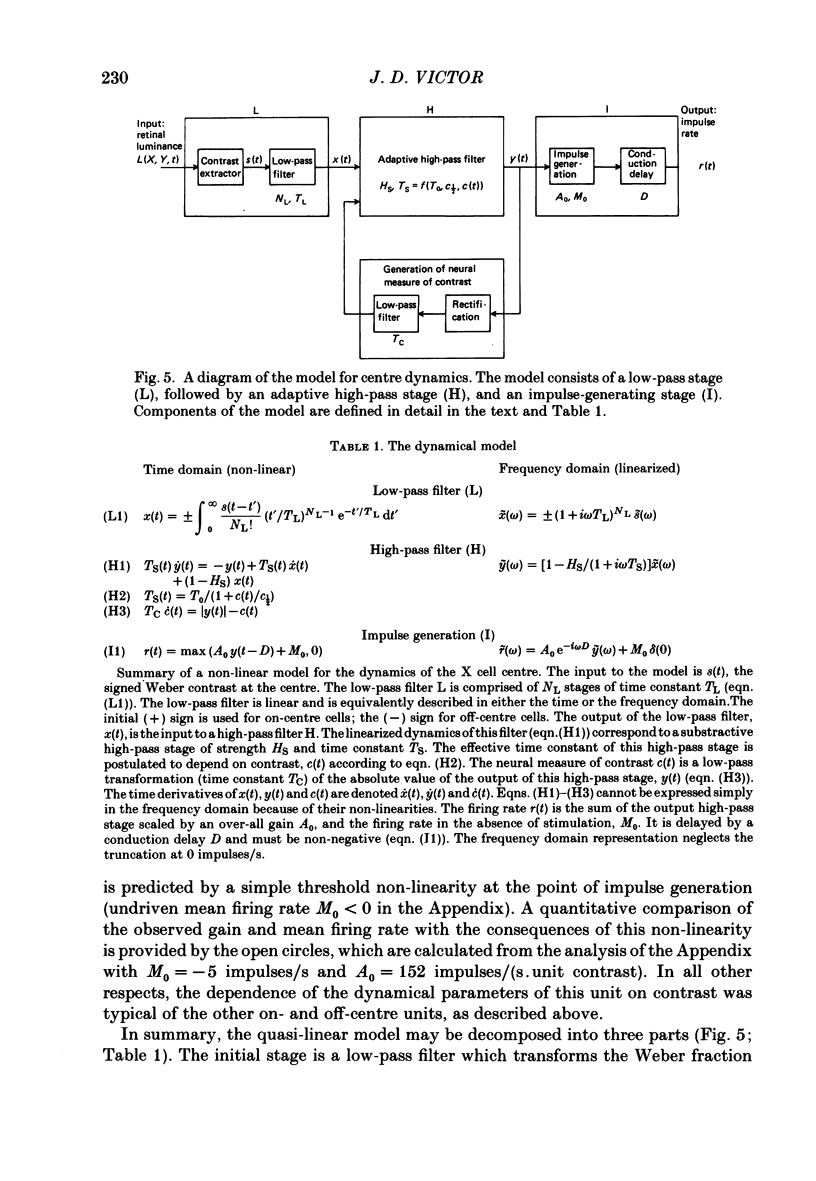
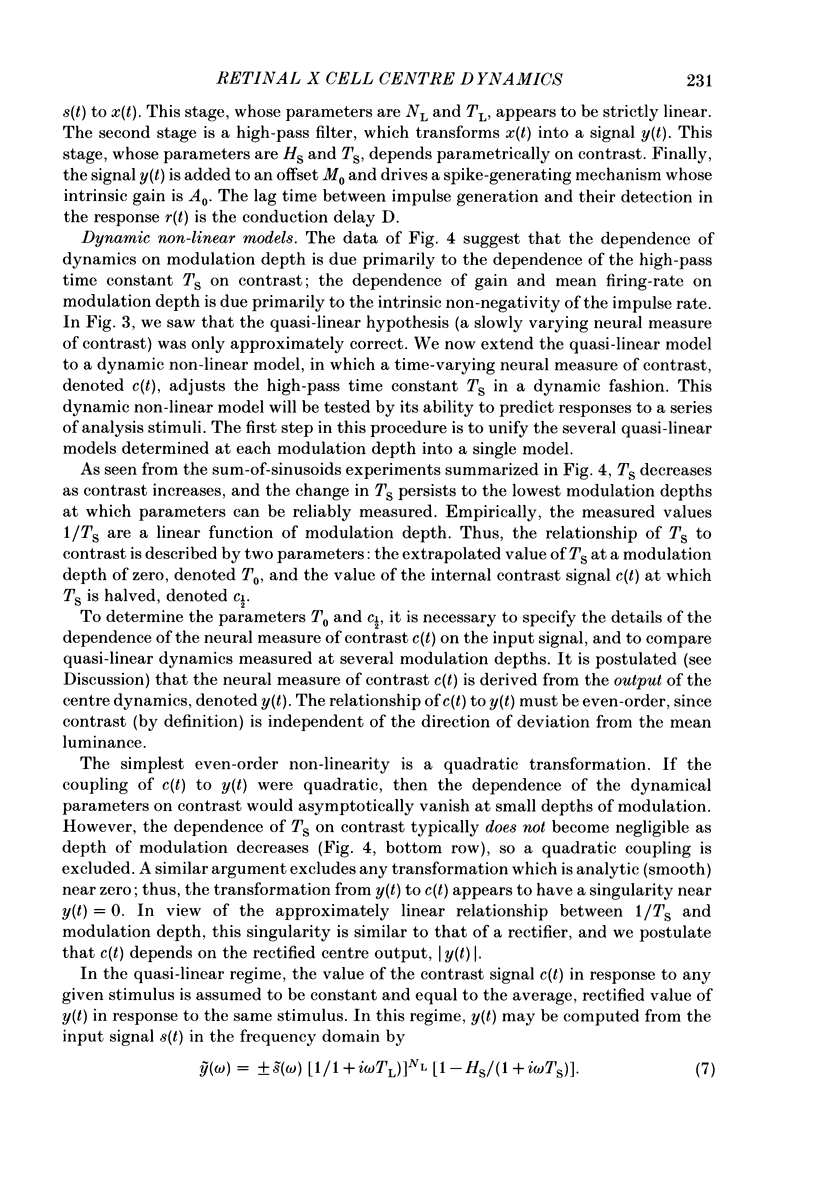
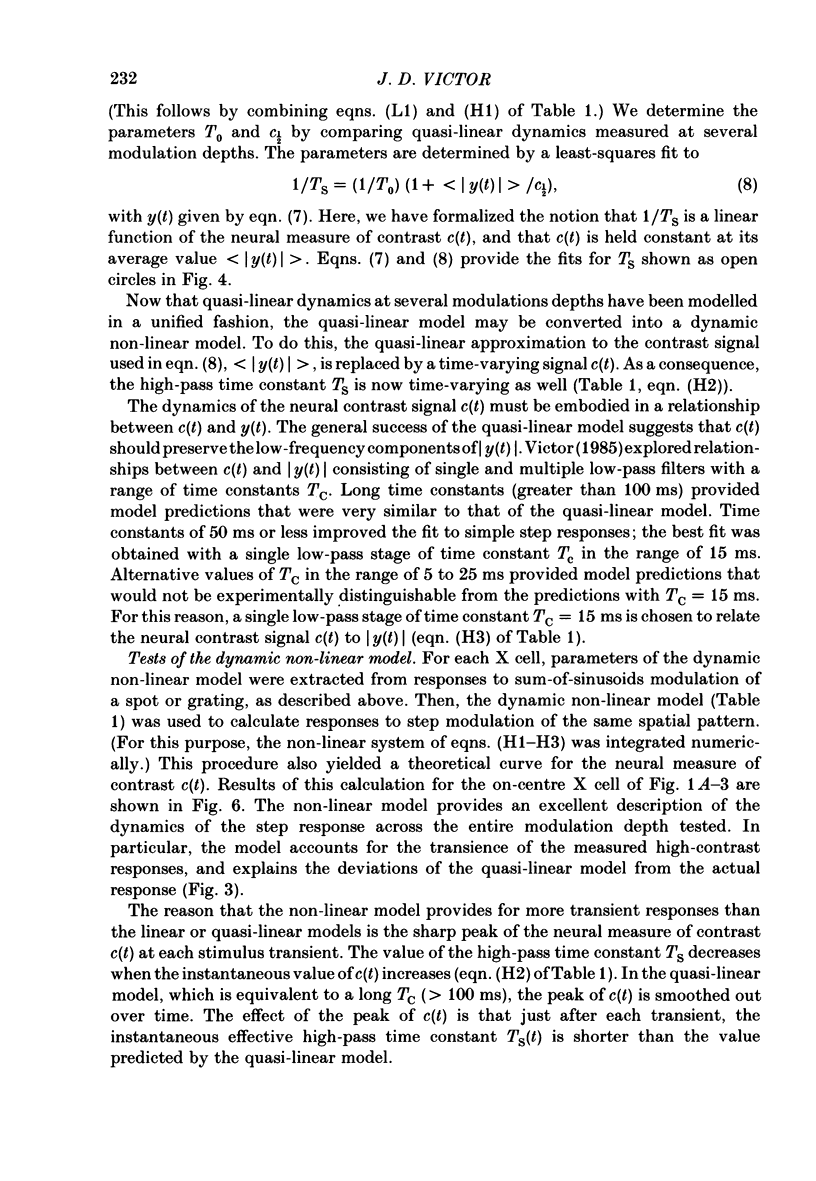
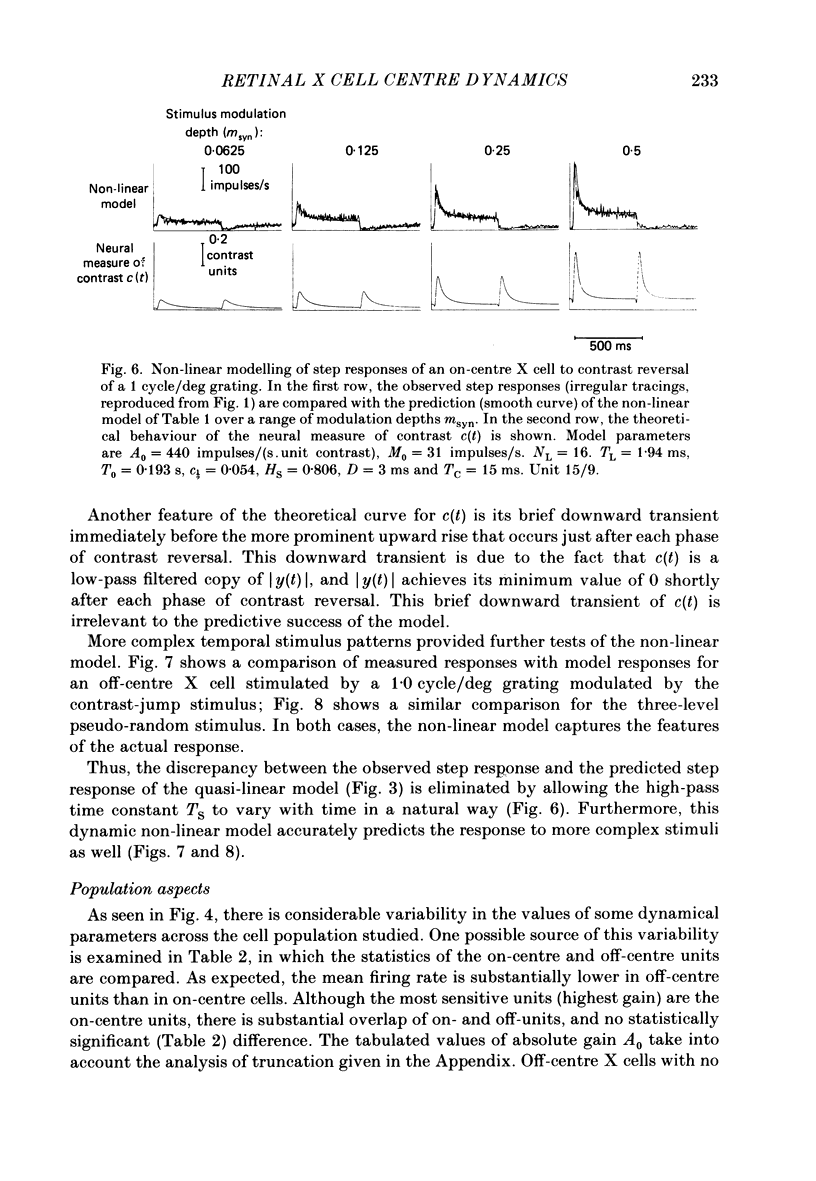
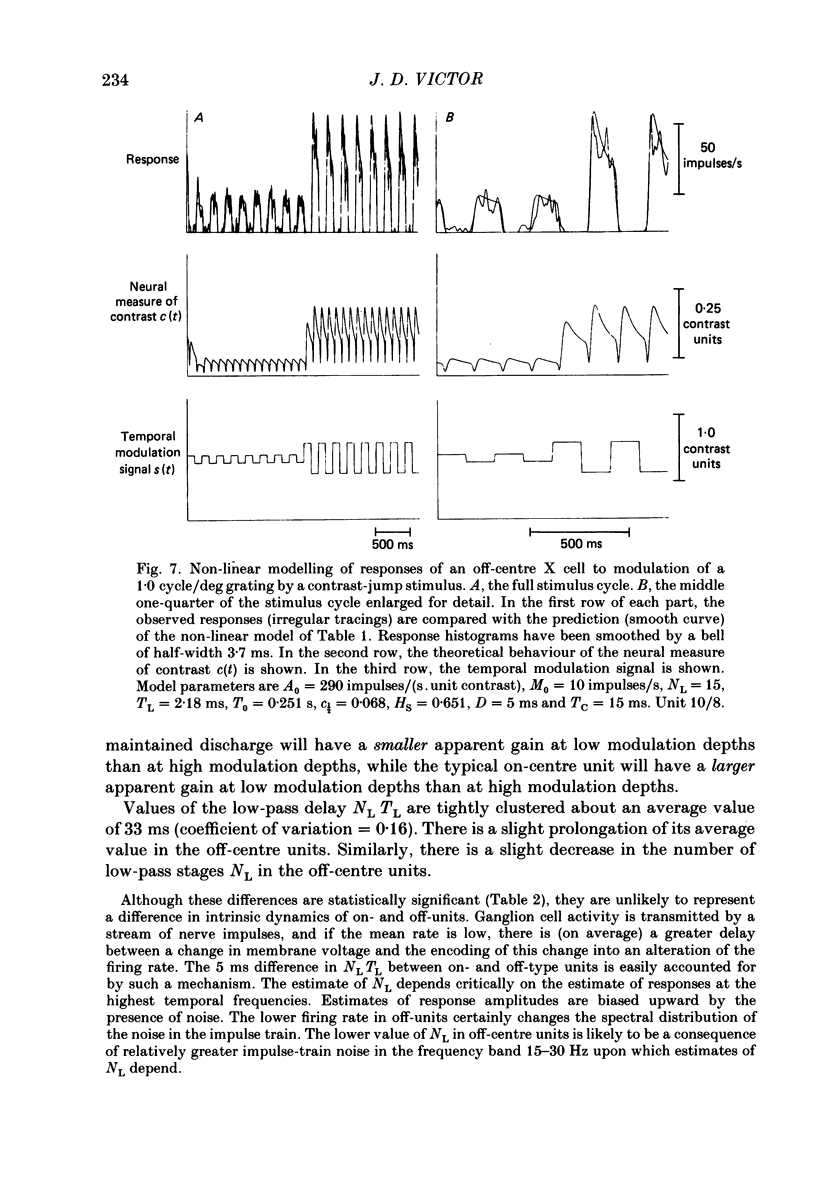
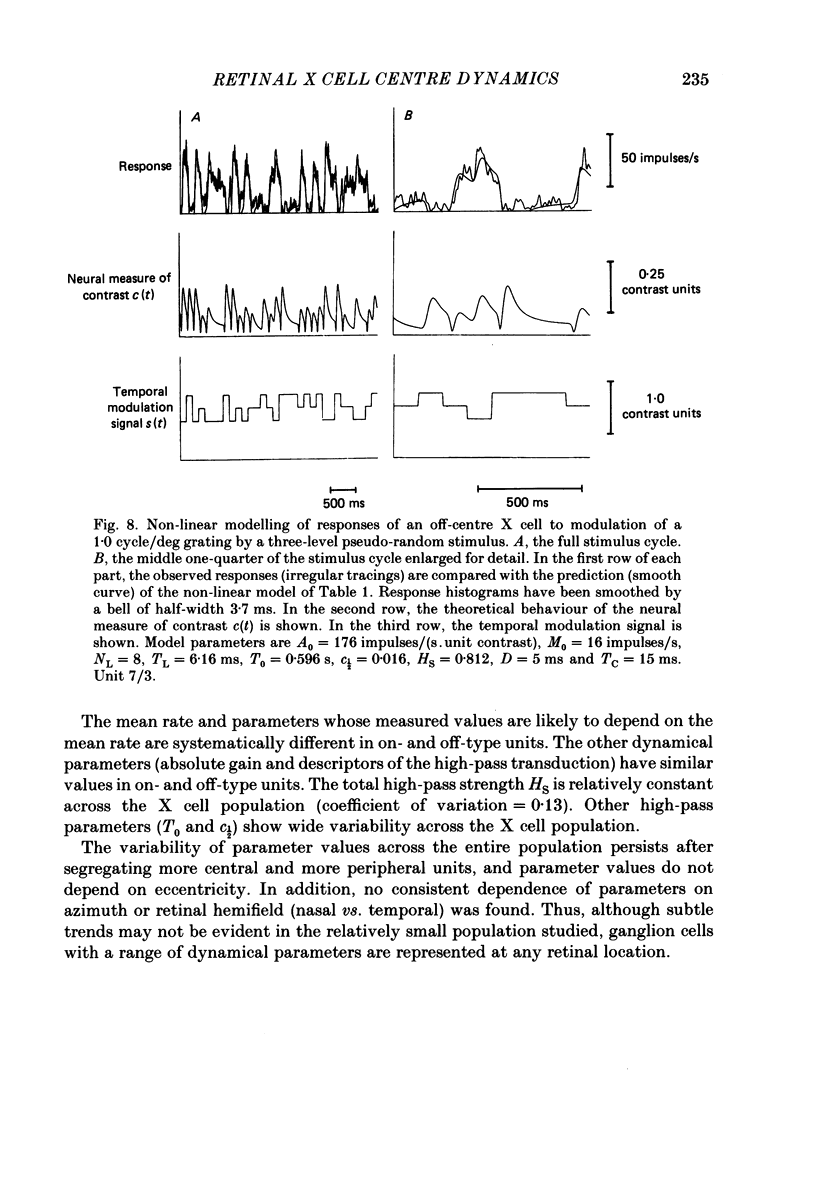
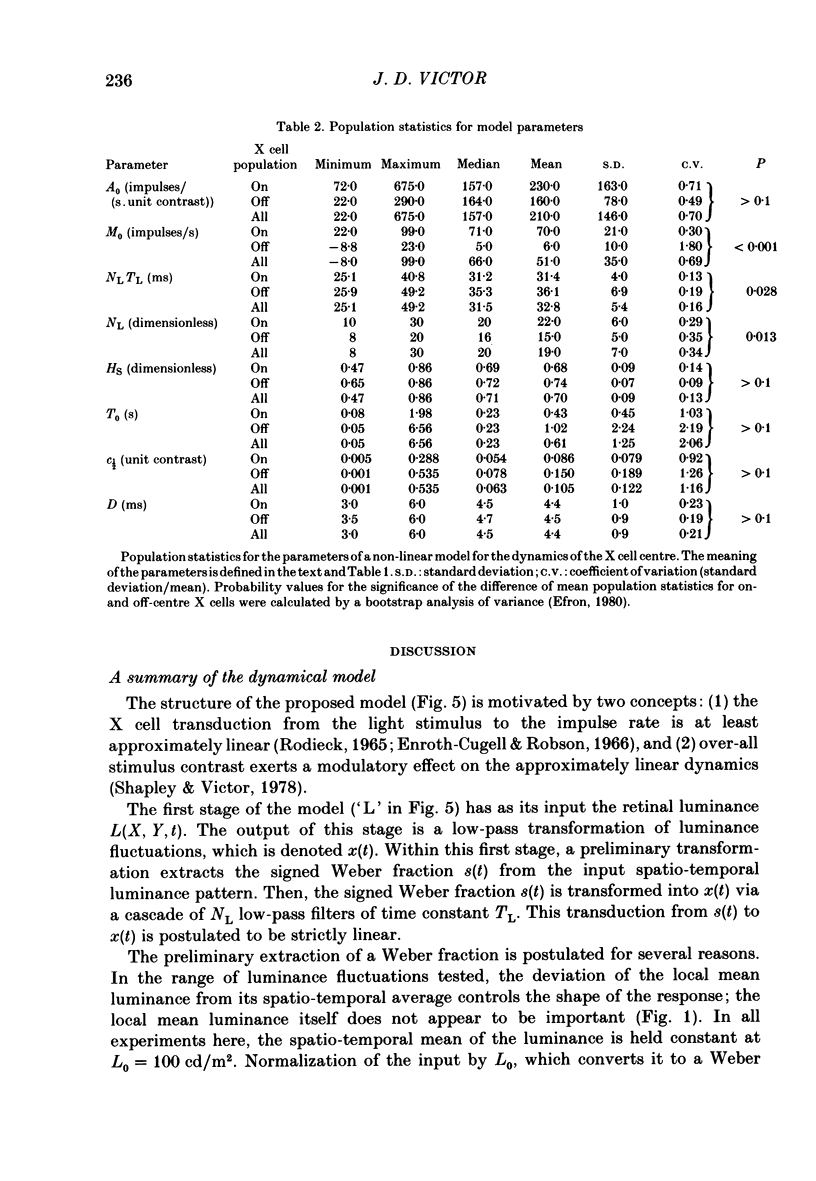
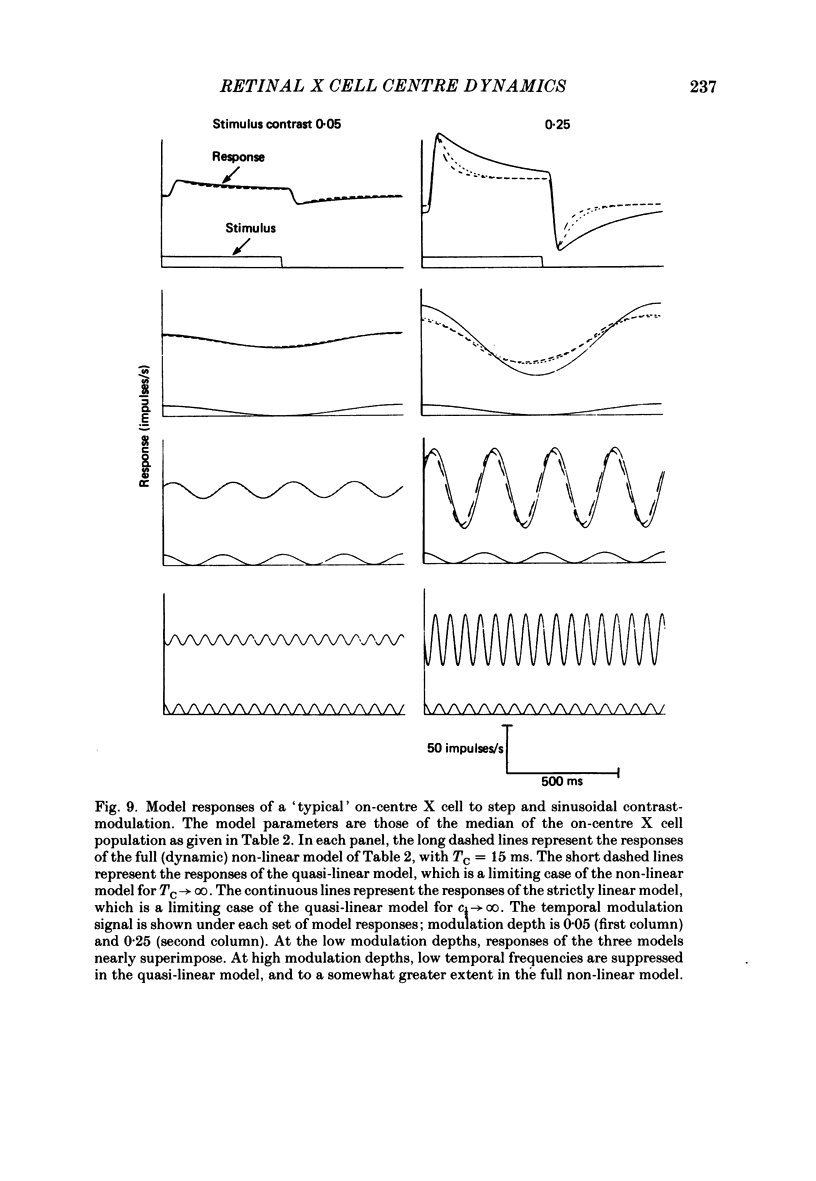
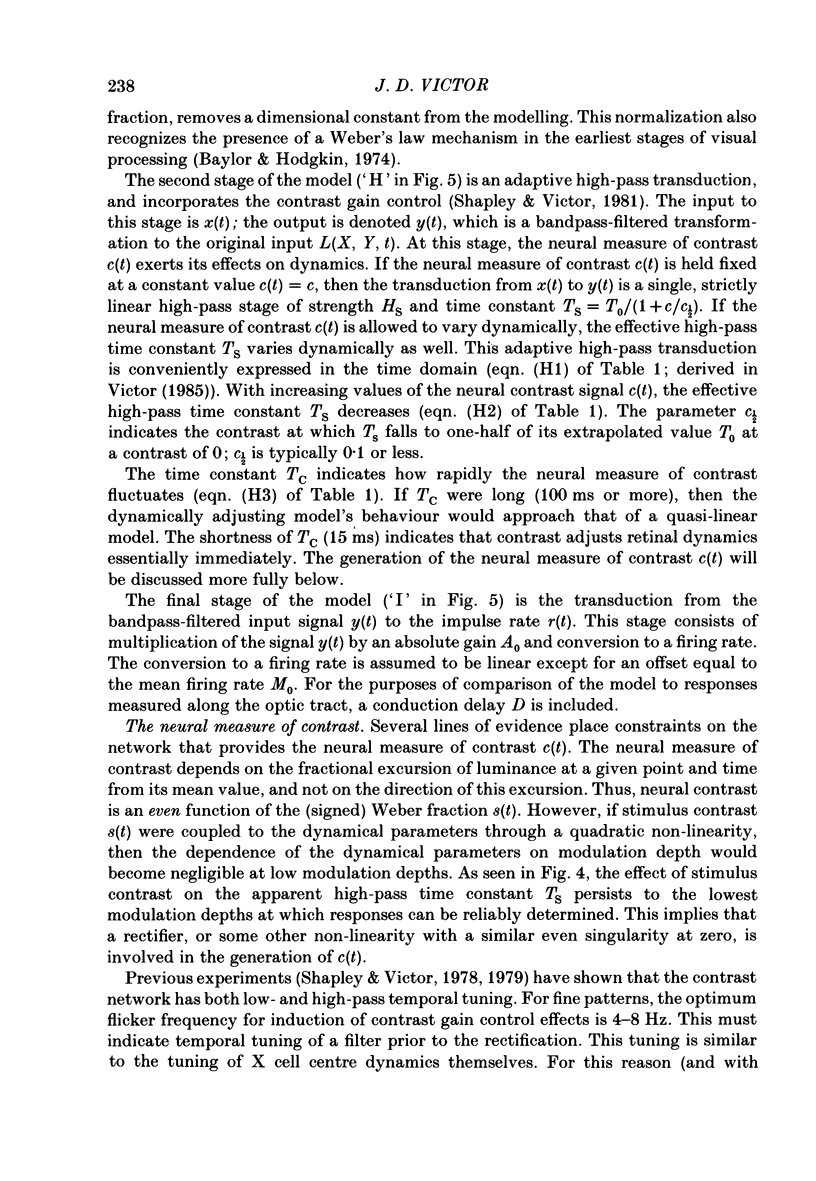
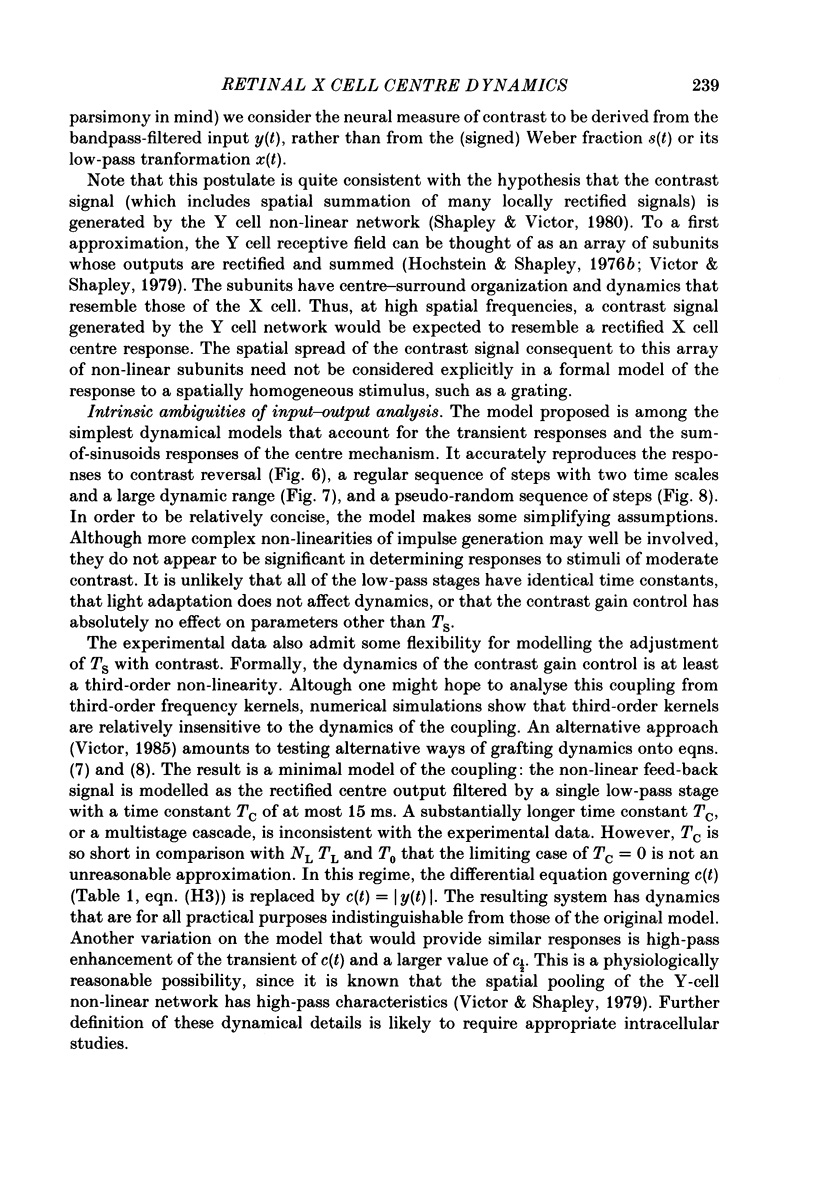
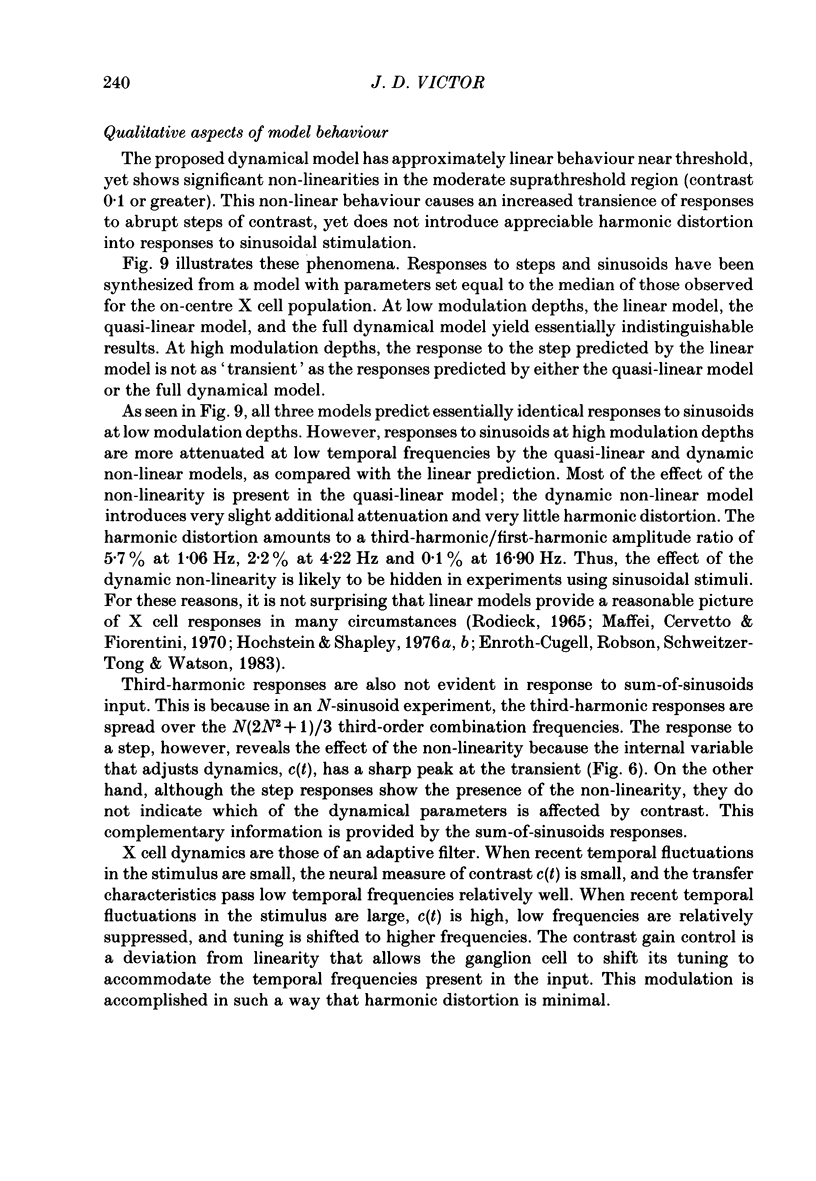
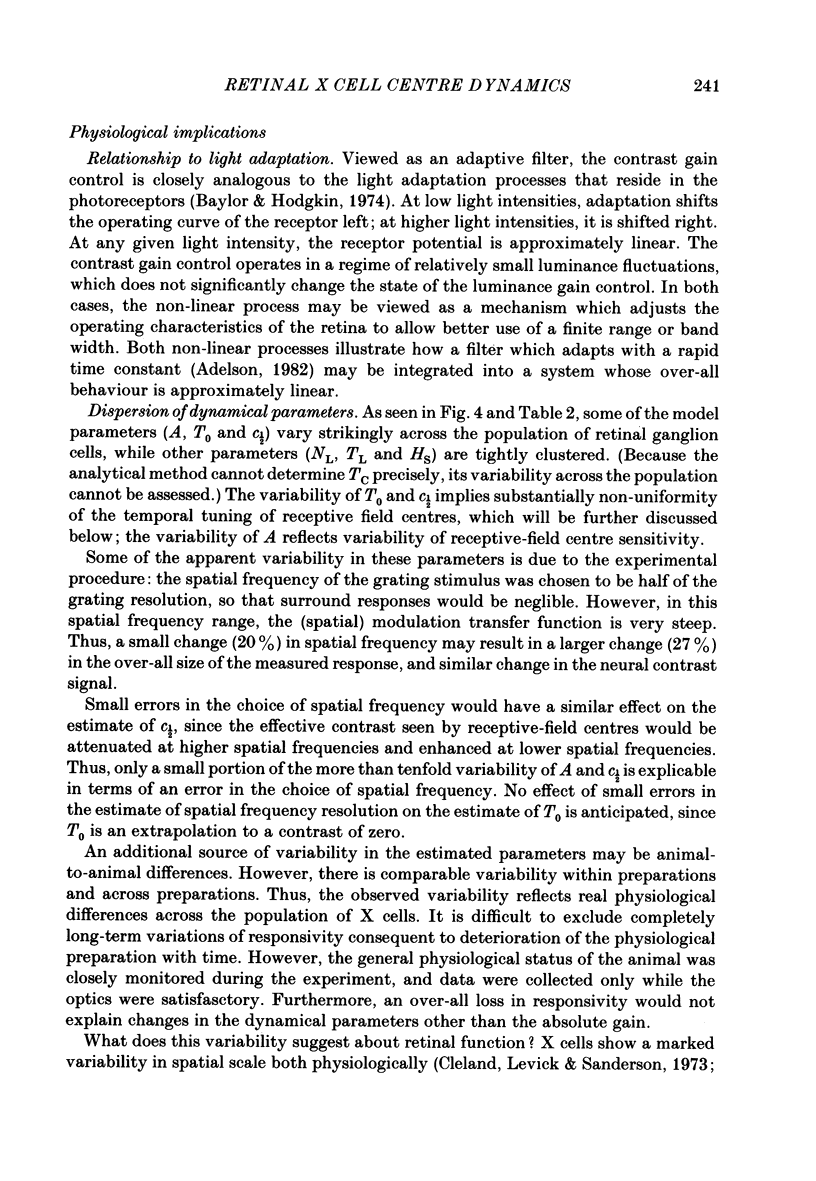
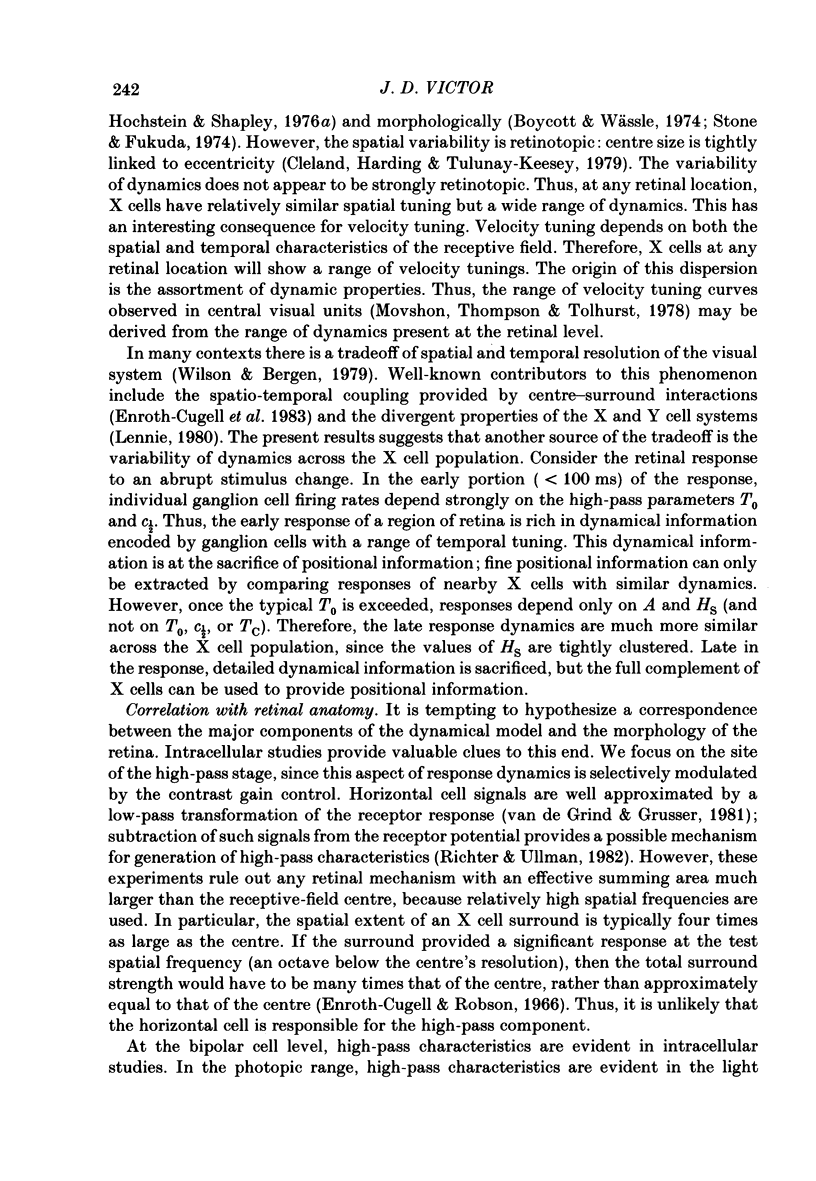
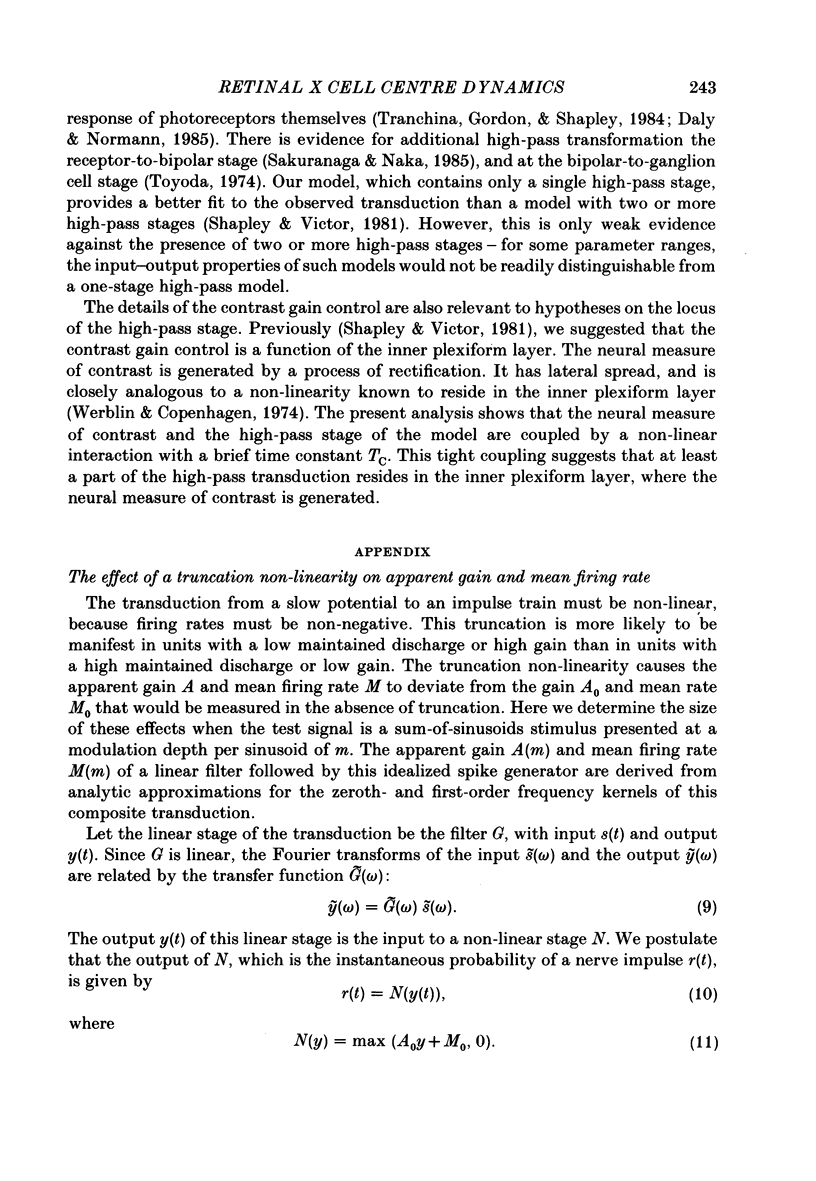
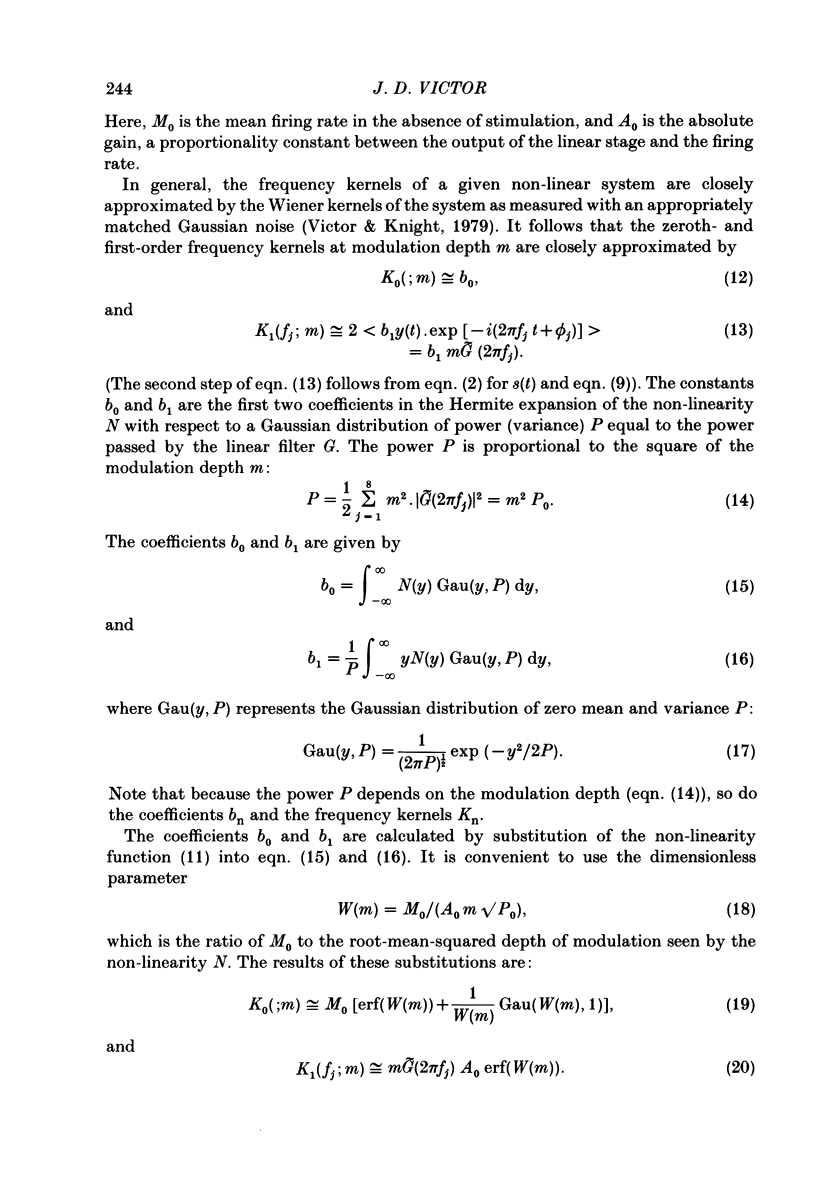
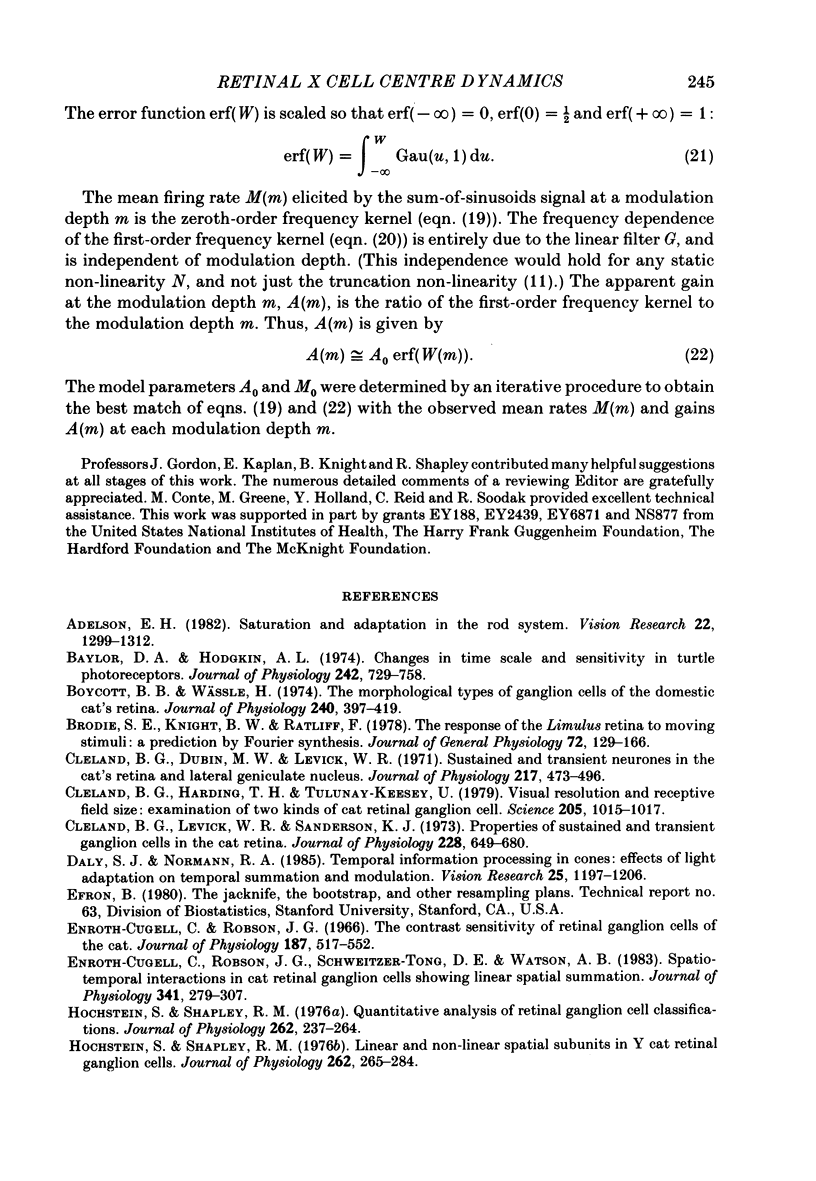
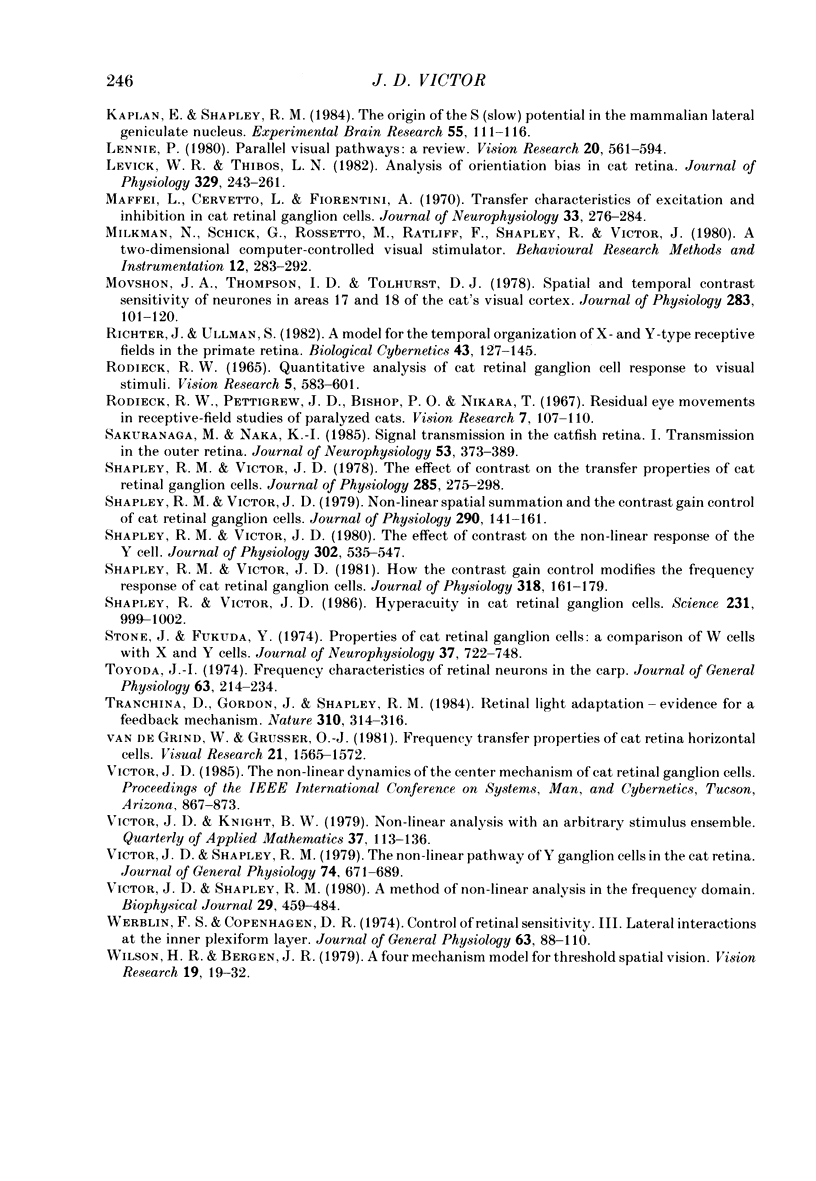
Selected References
These references are in PubMed. This may not be the complete list of references from this article.
- Adelson E. H. Saturation and adaptation in the rod system. Vision Res. 1982;22(10):1299–1312. doi: 10.1016/0042-6989(82)90143-2. [DOI] [PubMed] [Google Scholar]
- Baylor D. A., Hodgkin A. L. Changes in time scale and sensitivity in turtle photoreceptors. J Physiol. 1974 Nov;242(3):729–758. doi: 10.1113/jphysiol.1974.sp010732. [DOI] [PMC free article] [PubMed] [Google Scholar]
- Boycott B. B., Wässle H. The morphological types of ganglion cells of the domestic cat's retina. J Physiol. 1974 Jul;240(2):397–419. doi: 10.1113/jphysiol.1974.sp010616. [DOI] [PMC free article] [PubMed] [Google Scholar]
- Brodie S. E., Knight B. W., Ratliff F. The response of the Limulus retina to moving stimuli: a prediction by Fourier synthesis. J Gen Physiol. 1978 Aug;72(2):129–166. doi: 10.1085/jgp.72.2.129. [DOI] [PMC free article] [PubMed] [Google Scholar]
- Cleland B. G., Dubin M. W., Levick W. R. Sustained and transient neurones in the cat's retina and lateral geniculate nucleus. J Physiol. 1971 Sep;217(2):473–496. doi: 10.1113/jphysiol.1971.sp009581. [DOI] [PMC free article] [PubMed] [Google Scholar]
- Cleland B. G., Harding T. H., Tulunay-Keesey U. Visual resolution and receptive field size: examination of two kinds of cat retinal ganglion cell. Science. 1979 Sep 7;205(4410):1015–1017. doi: 10.1126/science.472720. [DOI] [PubMed] [Google Scholar]
- Cleland B. G., Levick W. R., Sanderson K. J. Properties of sustained and transient ganglion cells in the cat retina. J Physiol. 1973 Feb;228(3):649–680. doi: 10.1113/jphysiol.1973.sp010105. [DOI] [PMC free article] [PubMed] [Google Scholar]
- Daly S. J., Normann R. A. Temporal information processing in cones: effects of light adaptation on temporal summation and modulation. Vision Res. 1985;25(9):1197–1206. doi: 10.1016/0042-6989(85)90034-3. [DOI] [PubMed] [Google Scholar]
- Enroth-Cugell C., Robson J. G., Schweitzer-Tong D. E., Watson A. B. Spatio-temporal interactions in cat retinal ganglion cells showing linear spatial summation. J Physiol. 1983 Aug;341:279–307. doi: 10.1113/jphysiol.1983.sp014806. [DOI] [PMC free article] [PubMed] [Google Scholar]
- Enroth-Cugell C., Robson J. G. The contrast sensitivity of retinal ganglion cells of the cat. J Physiol. 1966 Dec;187(3):517–552. doi: 10.1113/jphysiol.1966.sp008107. [DOI] [PMC free article] [PubMed] [Google Scholar]
- Hochstein S., Shapley R. M. Linear and nonlinear spatial subunits in Y cat retinal ganglion cells. J Physiol. 1976 Nov;262(2):265–284. doi: 10.1113/jphysiol.1976.sp011595. [DOI] [PMC free article] [PubMed] [Google Scholar]
- Hochstein S., Shapley R. M. Quantitative analysis of retinal ganglion cell classifications. J Physiol. 1976 Nov;262(2):237–264. doi: 10.1113/jphysiol.1976.sp011594. [DOI] [PMC free article] [PubMed] [Google Scholar]
- Kaplan E., Shapley R. The origin of the S (slow) potential in the mammalian lateral geniculate nucleus. Exp Brain Res. 1984;55(1):111–116. doi: 10.1007/BF00240504. [DOI] [PubMed] [Google Scholar]
- Lennie P. Parallel visual pathways: a review. Vision Res. 1980;20(7):561–594. doi: 10.1016/0042-6989(80)90115-7. [DOI] [PubMed] [Google Scholar]
- Levick W. R., Thibos L. N. Analysis of orientation bias in cat retina. J Physiol. 1982 Aug;329:243–261. doi: 10.1113/jphysiol.1982.sp014301. [DOI] [PMC free article] [PubMed] [Google Scholar]
- Maffei L., Cervetto L., Fiorentini A. Transfer characteristics of excitation and inhibition in cat retinal ganglion cells. J Neurophysiol. 1970 Mar;33(2):276–284. doi: 10.1152/jn.1970.33.2.276. [DOI] [PubMed] [Google Scholar]
- Movshon J. A., Thompson I. D., Tolhurst D. J. Spatial and temporal contrast sensitivity of neurones in areas 17 and 18 of the cat's visual cortex. J Physiol. 1978 Oct;283:101–120. doi: 10.1113/jphysiol.1978.sp012490. [DOI] [PMC free article] [PubMed] [Google Scholar]
- Richter J., Ullman S. A model for the temporal organization of X- and Y-type receptive fields in the primate retina. Biol Cybern. 1982;43(2):127–145. doi: 10.1007/BF00336975. [DOI] [PubMed] [Google Scholar]
- Rodieck R. W., Pettigrew J. D., Bishop P. O., Nikara T. Residual eye movements in receptive-field studies of paralyzed cats. Vision Res. 1967 Jan;7(1):107–110. doi: 10.1016/0042-6989(67)90031-4. [DOI] [PubMed] [Google Scholar]
- Rodieck R. W. Quantitative analysis of cat retinal ganglion cell response to visual stimuli. Vision Res. 1965 Dec;5(11):583–601. doi: 10.1016/0042-6989(65)90033-7. [DOI] [PubMed] [Google Scholar]
- Sakuranaga M., Naka K. Signal transmission in the catfish retina. I. Transmission in the outer retina. J Neurophysiol. 1985 Feb;53(2):373–389. doi: 10.1152/jn.1985.53.2.373. [DOI] [PubMed] [Google Scholar]
- Shapley R. M., Victor J. D. How the contrast gain control modifies the frequency responses of cat retinal ganglion cells. J Physiol. 1981 Sep;318:161–179. doi: 10.1113/jphysiol.1981.sp013856. [DOI] [PMC free article] [PubMed] [Google Scholar]
- Shapley R. M., Victor J. D. Nonlinear spatial summation and the contrast gain control of cat retinal ganglion cells. J Physiol. 1979 May;290(2):141–161. doi: 10.1113/jphysiol.1979.sp012765. [DOI] [PMC free article] [PubMed] [Google Scholar]
- Shapley R. M., Victor J. D. The effect of contrast on the non-linear response of the Y cell. J Physiol. 1980 May;302:535–547. doi: 10.1113/jphysiol.1980.sp013259. [DOI] [PMC free article] [PubMed] [Google Scholar]
- Shapley R. M., Victor J. D. The effect of contrast on the transfer properties of cat retinal ganglion cells. J Physiol. 1978 Dec;285:275–298. doi: 10.1113/jphysiol.1978.sp012571. [DOI] [PMC free article] [PubMed] [Google Scholar]
- Shapley R., Victor J. Hyperacuity in cat retinal ganglion cells. Science. 1986 Feb 28;231(4741):999–1002. doi: 10.1126/science.3945816. [DOI] [PubMed] [Google Scholar]
- Stone J., Fukuda Y. Properties of cat retinal ganglion cells: a comparison of W-cells with X- and Y-cells. J Neurophysiol. 1974 Jul;37(4):722–748. doi: 10.1152/jn.1974.37.4.722. [DOI] [PubMed] [Google Scholar]
- Toyoda J. Frequency characteristics of retinal neurons in the carp. J Gen Physiol. 1974 Feb;63(2):214–234. doi: 10.1085/jgp.63.2.214. [DOI] [PMC free article] [PubMed] [Google Scholar]
- Tranchina D., Gordon J., Shapley R. M. Retinal light adaptation--evidence for a feedback mechanism. 1984 Jul 26-Aug 1Nature. 310(5975):314–316. doi: 10.1038/310314a0. [DOI] [PubMed] [Google Scholar]
- Victor J. D., Shapley R. M. The nonlinear pathway of Y ganglion cells in the cat retina. J Gen Physiol. 1979 Dec;74(6):671–689. doi: 10.1085/jgp.74.6.671. [DOI] [PMC free article] [PubMed] [Google Scholar]
- Victor J., Shapley R. A method of nonlinear analysis in the frequency domain. Biophys J. 1980 Mar;29(3):459–483. doi: 10.1016/S0006-3495(80)85146-0. [DOI] [PMC free article] [PubMed] [Google Scholar]
- Werblin F. S., Copenhagen D. R. Control of retinal sensitivity. 3. Lateral interactions at the inner plexiform layer. J Gen Physiol. 1974 Jan;63(1):88–110. doi: 10.1085/jgp.63.1.88. [DOI] [PMC free article] [PubMed] [Google Scholar]
- Wilson H. R., Bergen J. R. A four mechanism model for threshold spatial vision. Vision Res. 1979;19(1):19–32. doi: 10.1016/0042-6989(79)90117-2. [DOI] [PubMed] [Google Scholar]
- van de Grind W., Grüsser O. J. Frequency transfer properties of cat retina horizontal cells. Vision Res. 1981;21(11):1565–1572. doi: 10.1016/0042-6989(81)90033-x. [DOI] [PubMed] [Google Scholar]