Abstract
1. Motor-unit size was measured by tension recording in neonatal (3-5 day) rat skeletal muscle (fourth deep lumbrical muscle). Each unit was then depleted of glycogen and its fibres studied in mid-belly frozen sections, by staining for glycogen (periodic acid-Schiff reagent and antibody labelling for slow myosin. The contralateral muscle acted as control, and further controls for the method are described. 2. All the motor units contained both slow-myosin-containing (S; antibody-positive) and slow-myosin-free (F; antibody-negative) fibres. 3. The proportion of each unit that was made up of S fibres was compared with the whole muscle. Of the twelve units studied seven were not selectively innervated, four may have been selectively innervated in favour of F fibres, and one was selectively innervated in favour of S fibres. The last unit was much smaller than the others. 4. Fibre cross-sectional areas were measured in units and in the whole muscles. Mean cross-sectional areas for individual F fibres in all the motor units were smaller than in the corresponding whole muscles (ratio 0.71), implying that small fibres have higher levels of polyneuronal innervation than larger ones (each small fibre occurring in more overlapping units than each larger fibre). There was no such difference in S fibres (ratio 0.96). 5. Motor-unit sizes (as a percentage of whole muscle) were smaller when obtained from summed fibre cross-sectional areas than from fibre counts (this follows from 4, above). Comparisons with unit sizes from tension recording are discussed. 6. Controls show that there is little, if any, non-specific fatigue of muscle fibres that are not part of the unit subjected to glycogen depletion. 7. Evidence is given that muscle fibre conduction block occurs during the depletion regime, leading to less glycogen depletion towards the ends of the muscle fibres than in the end-plate zone.
Full text
PDF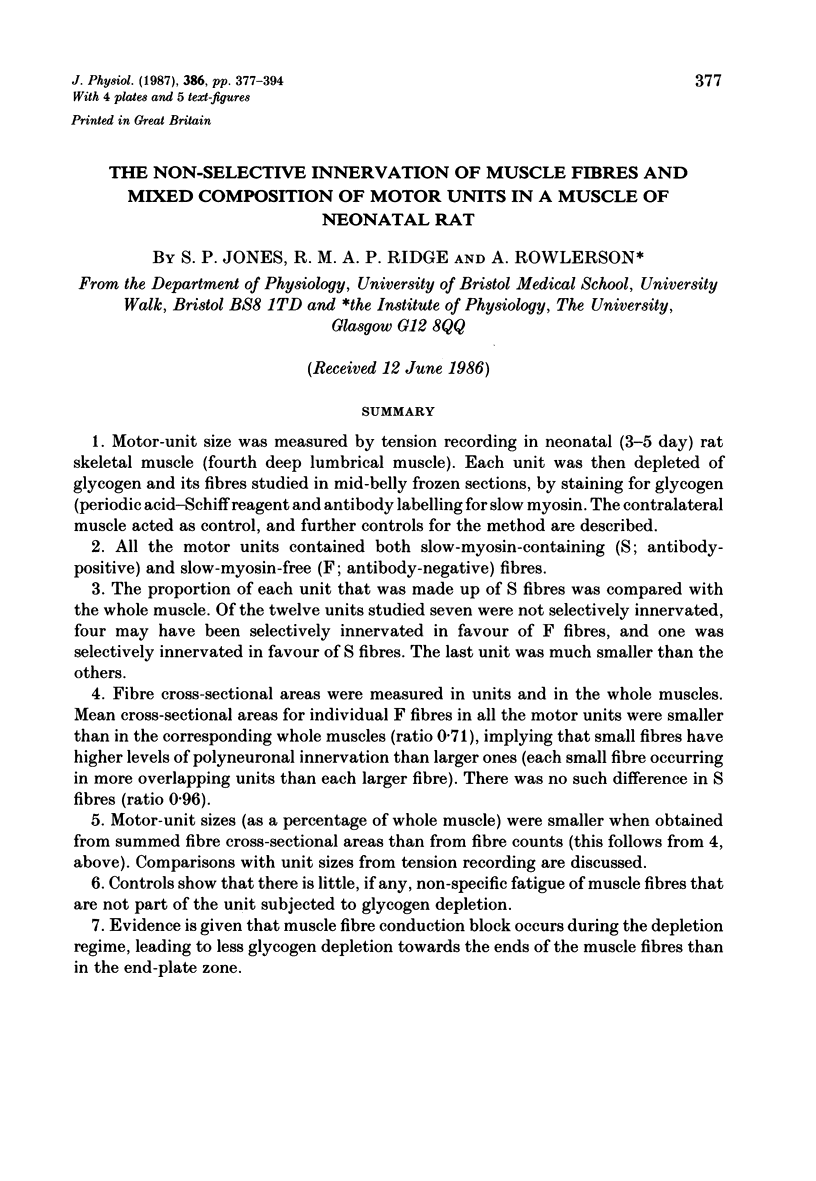
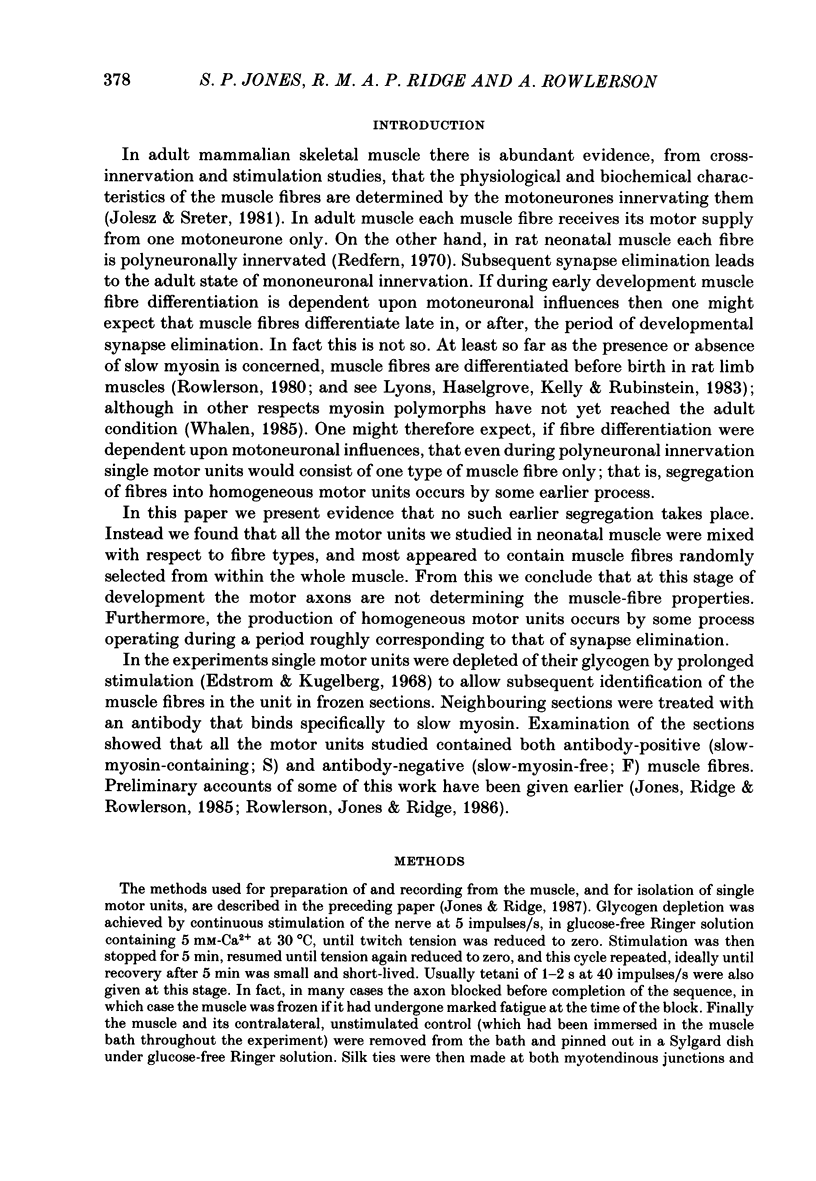
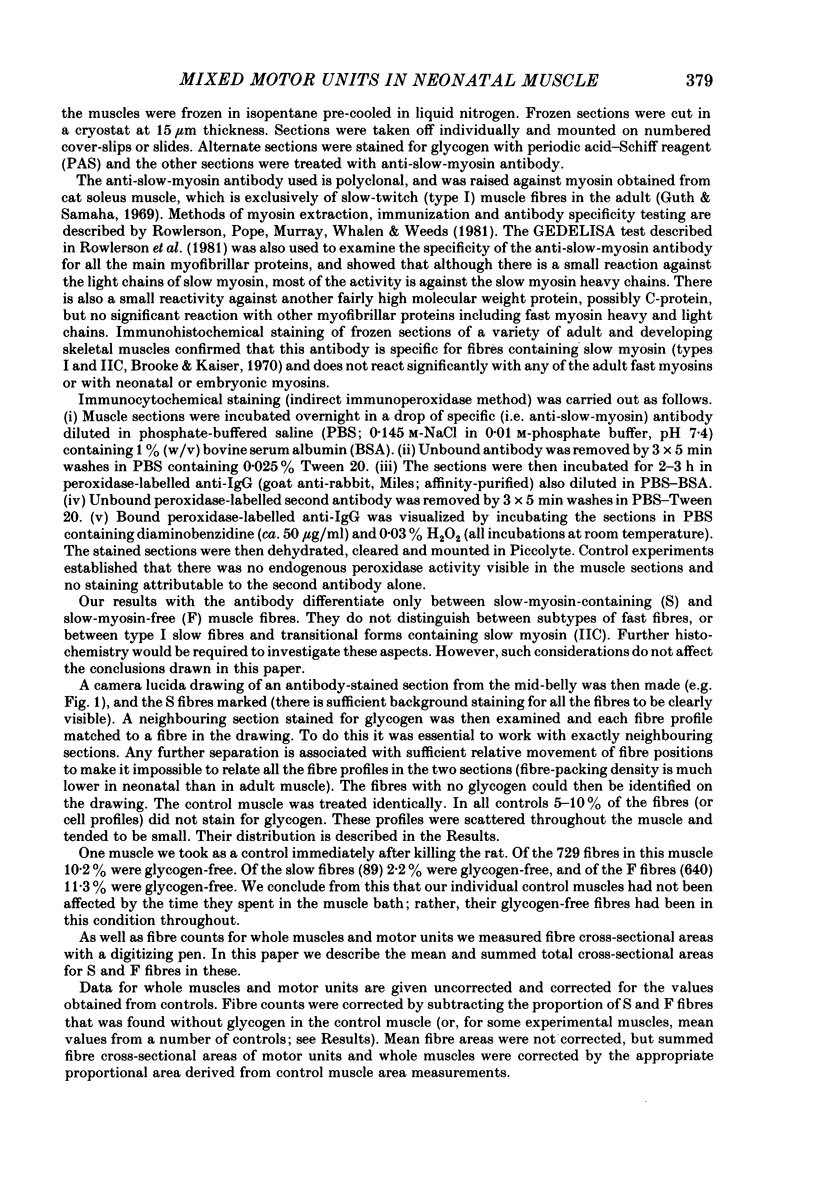
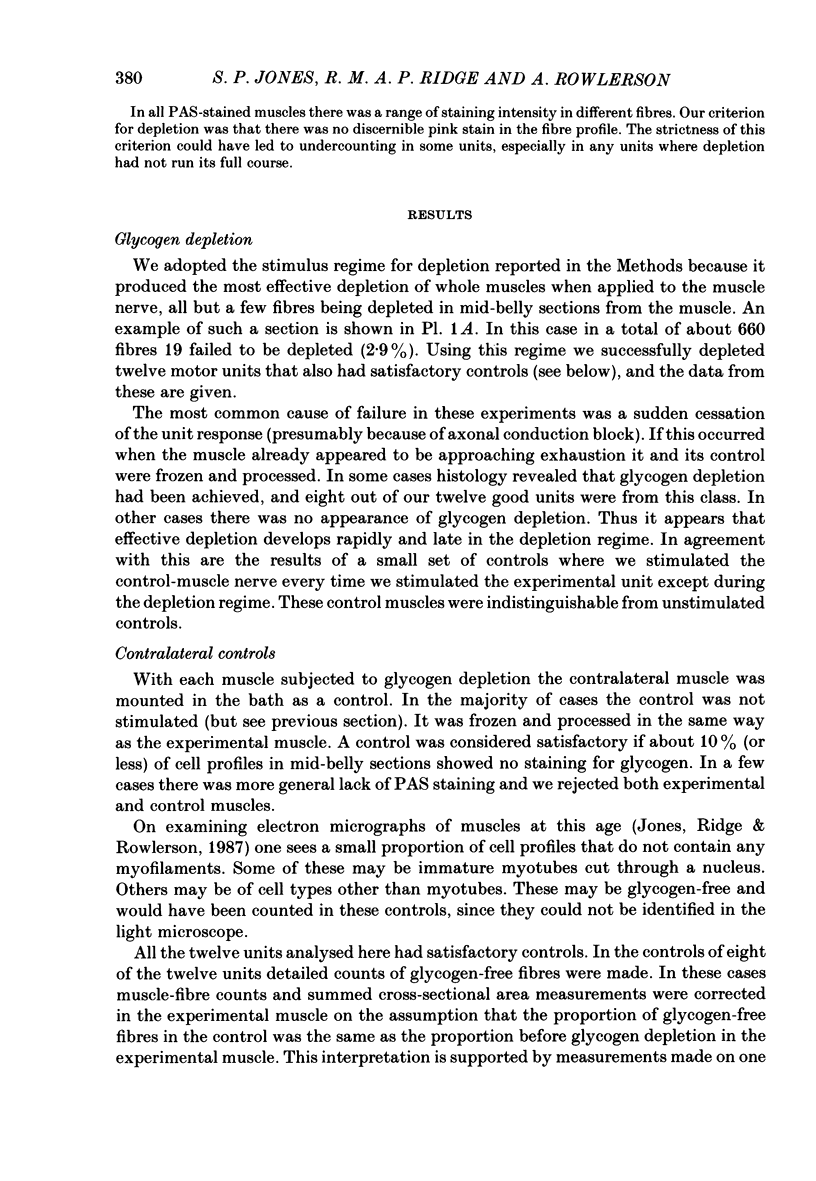
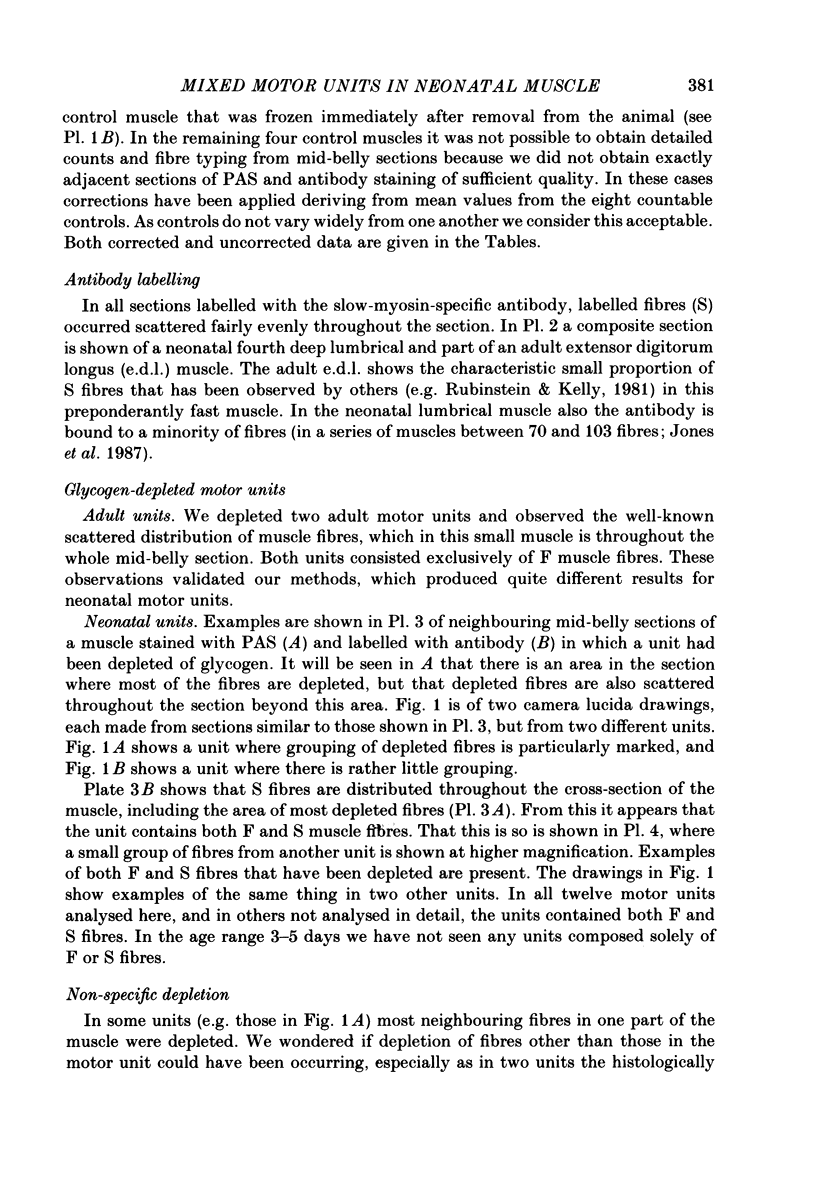
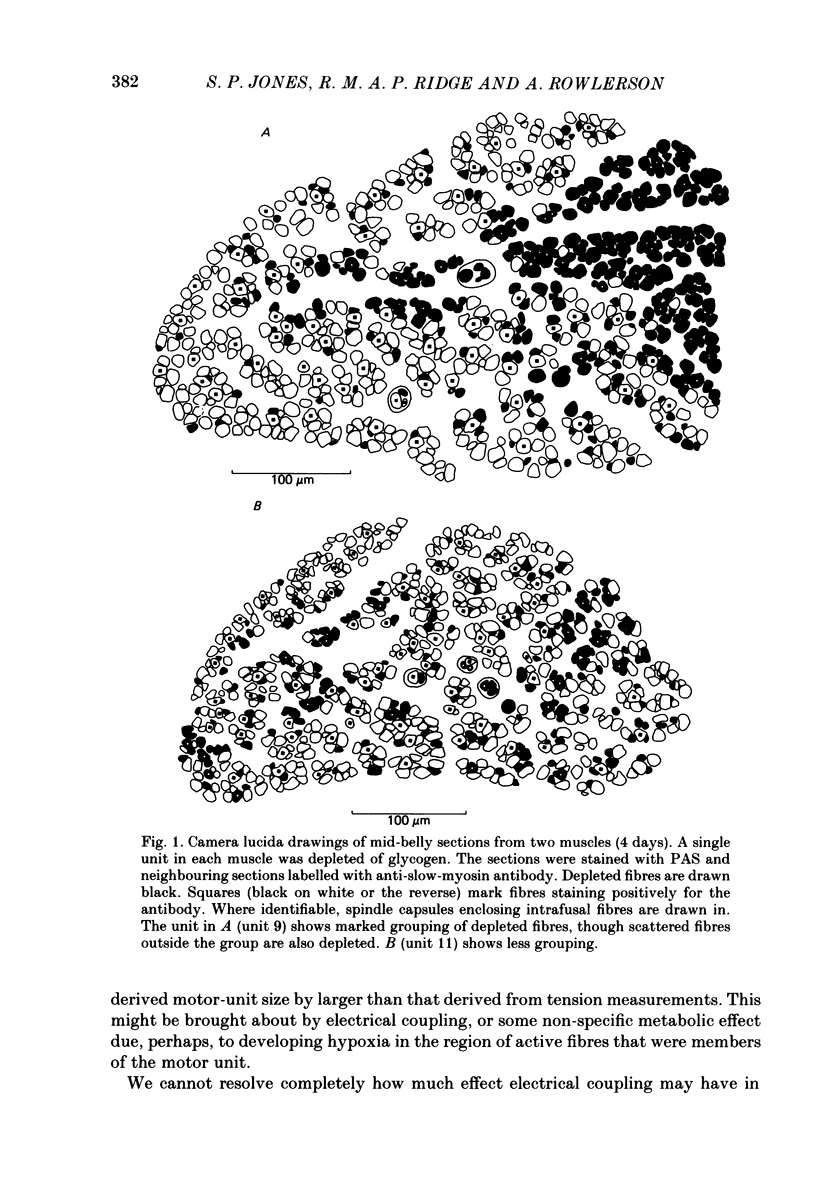
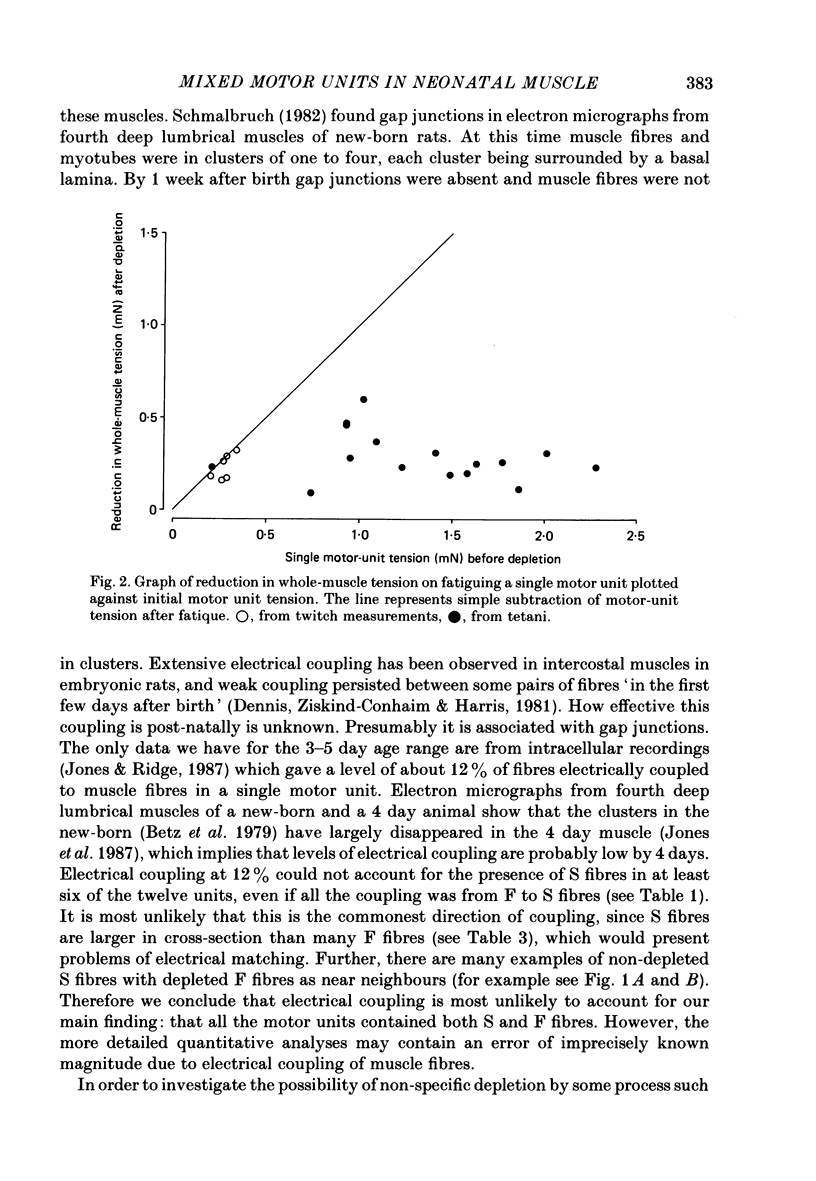
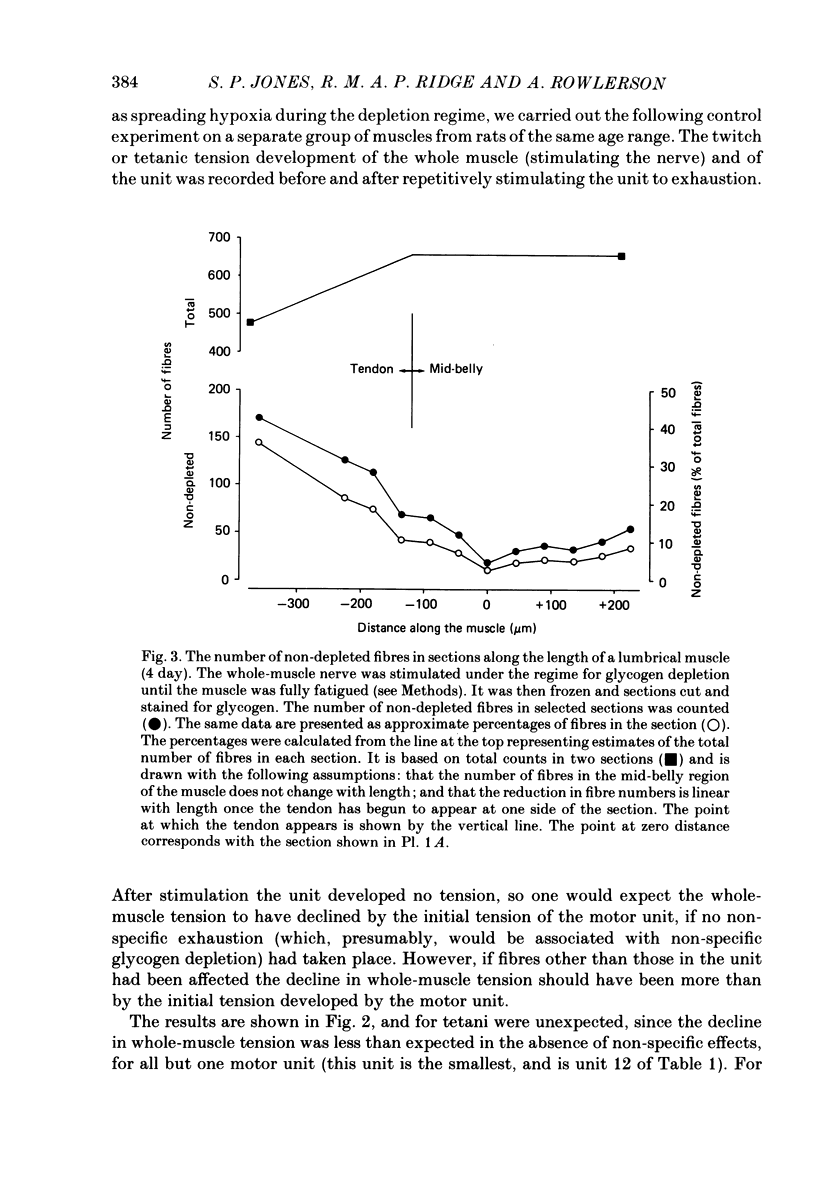
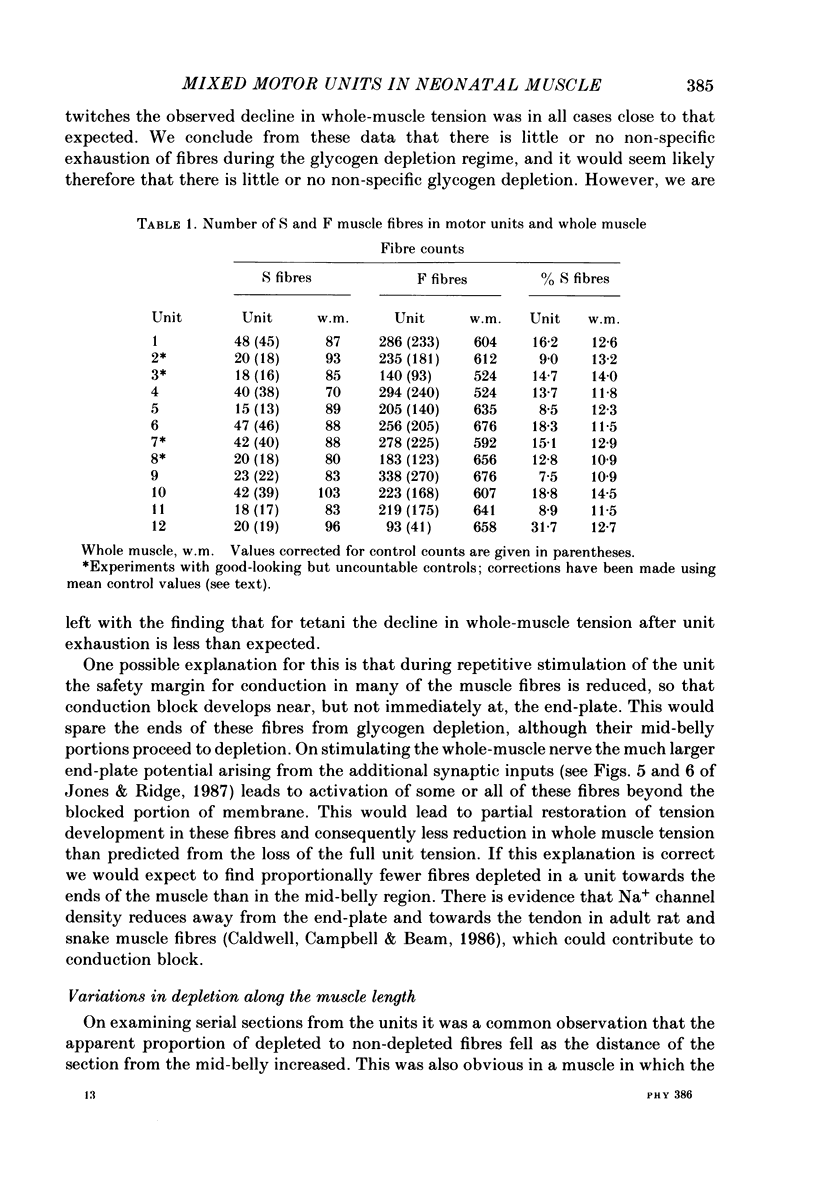
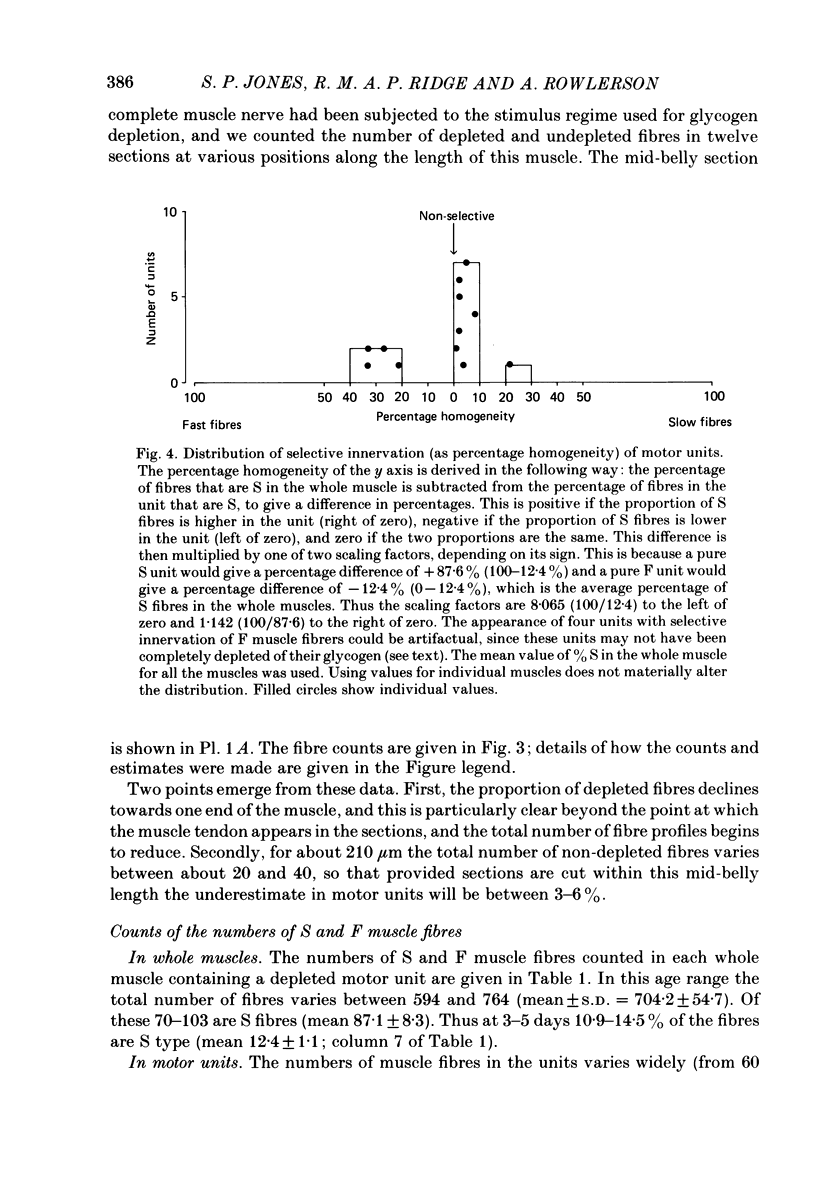
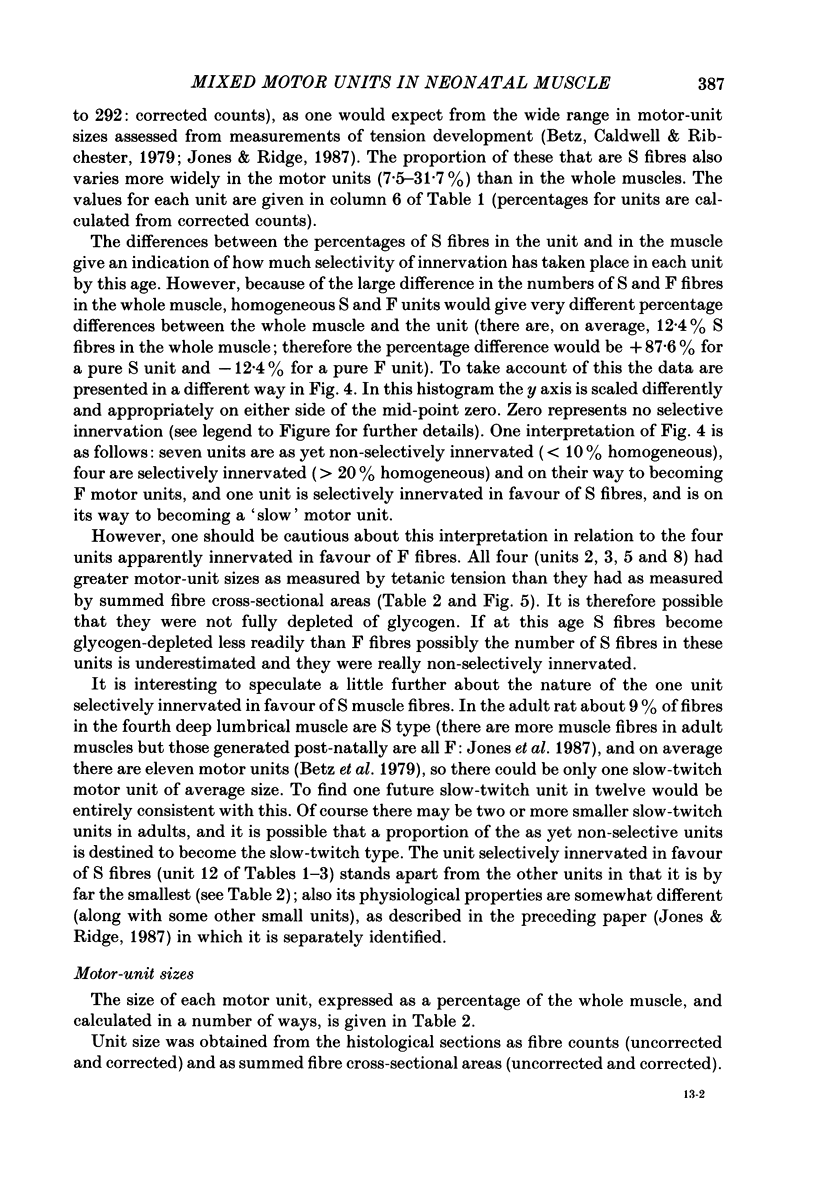
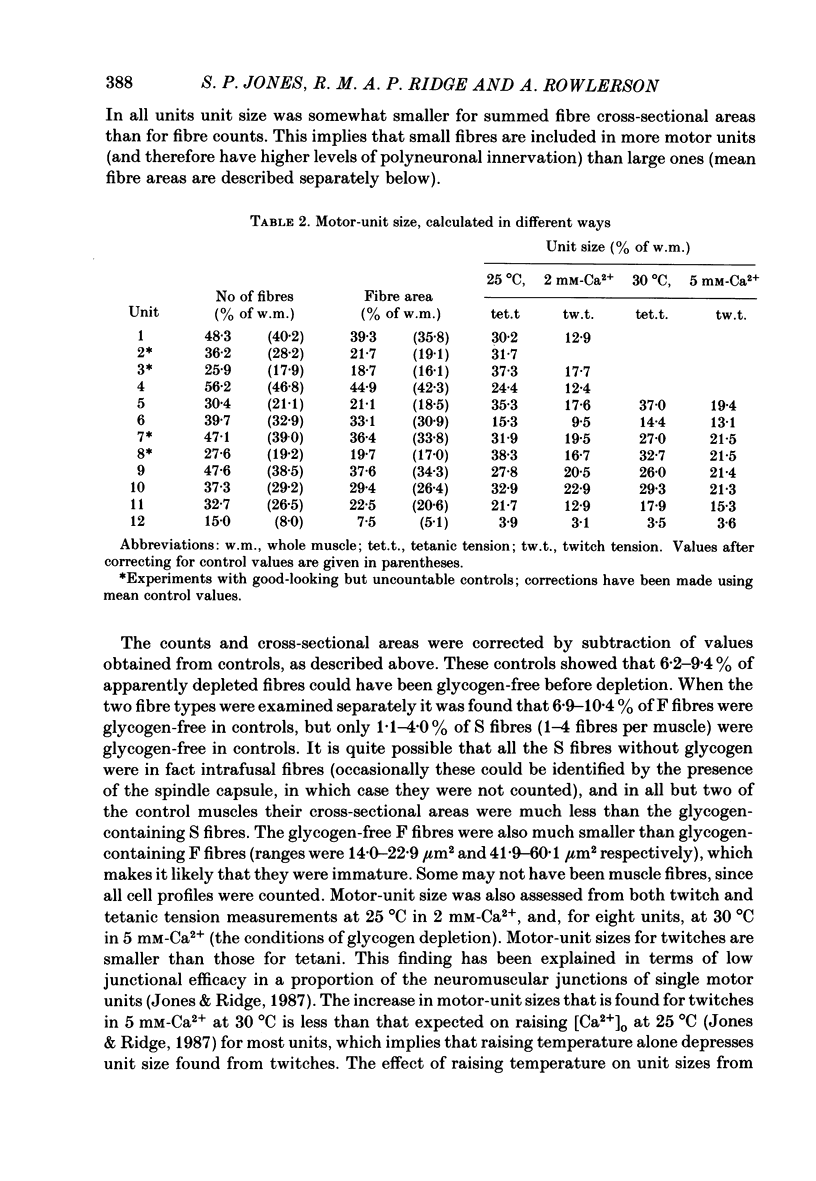
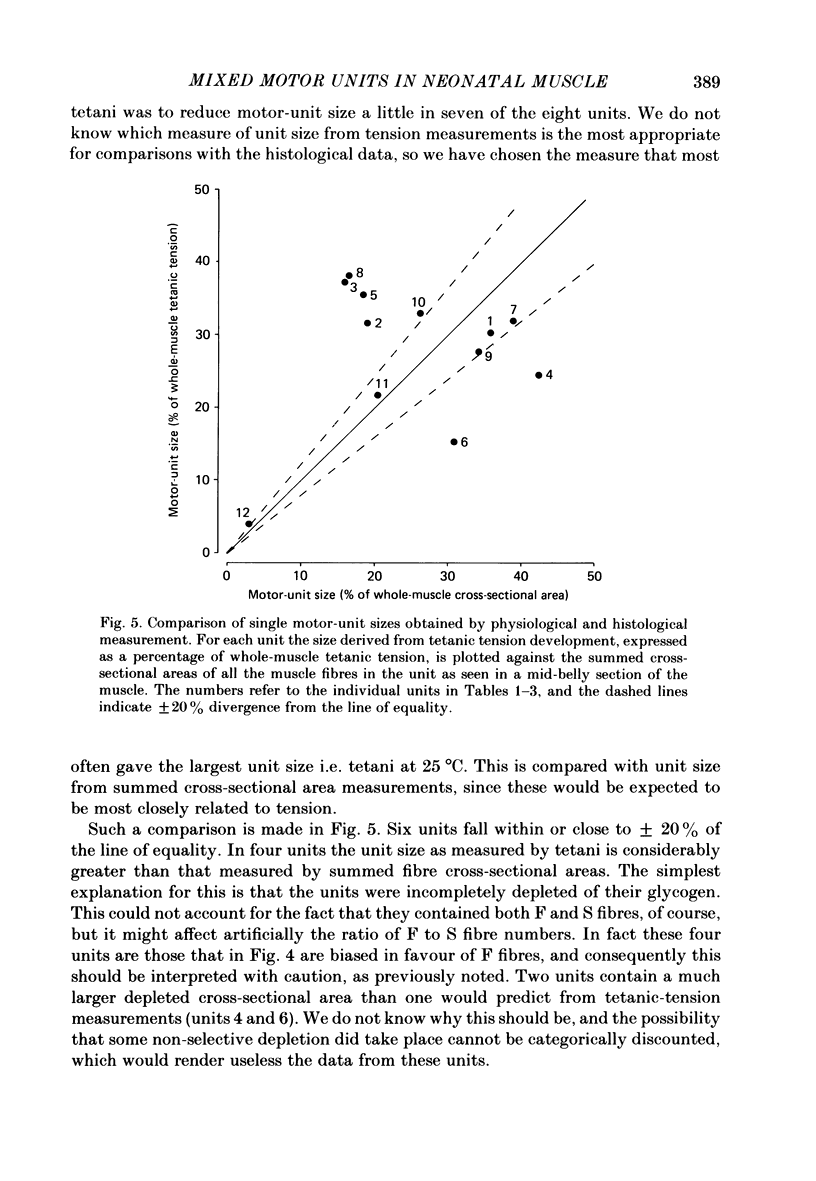
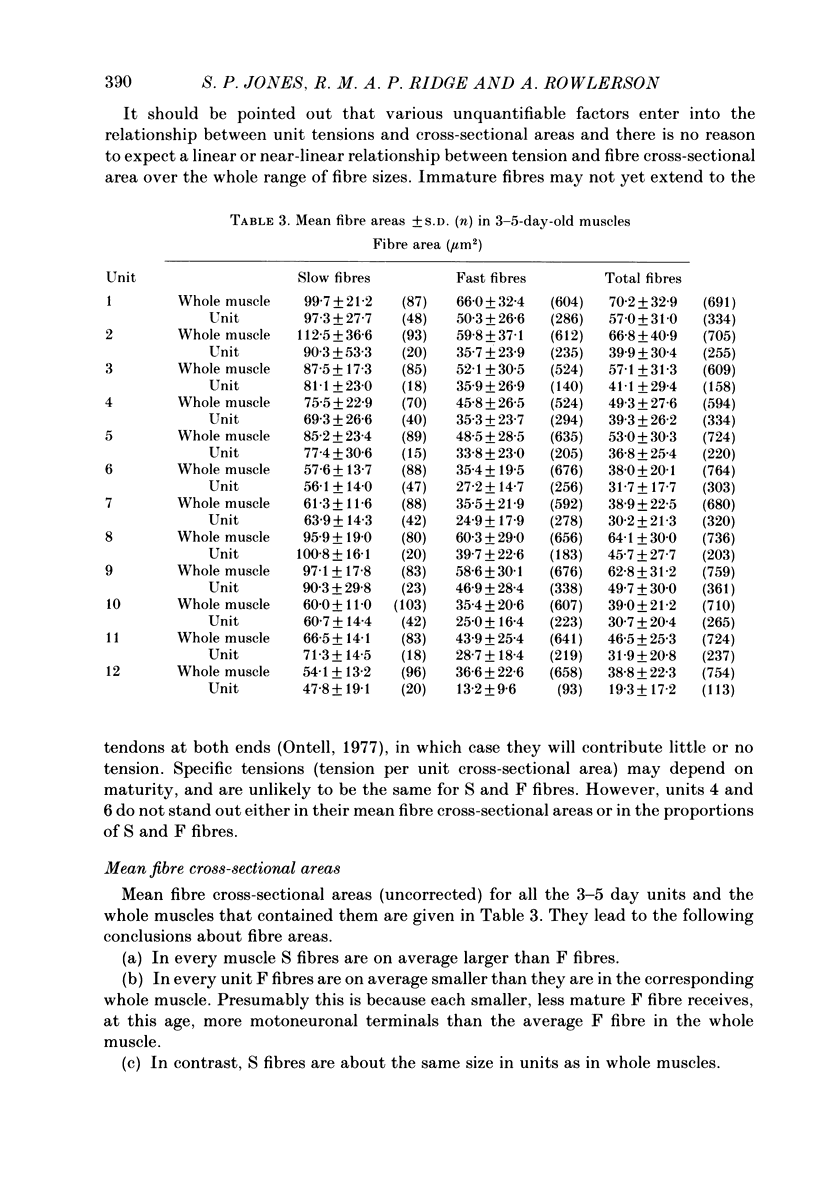
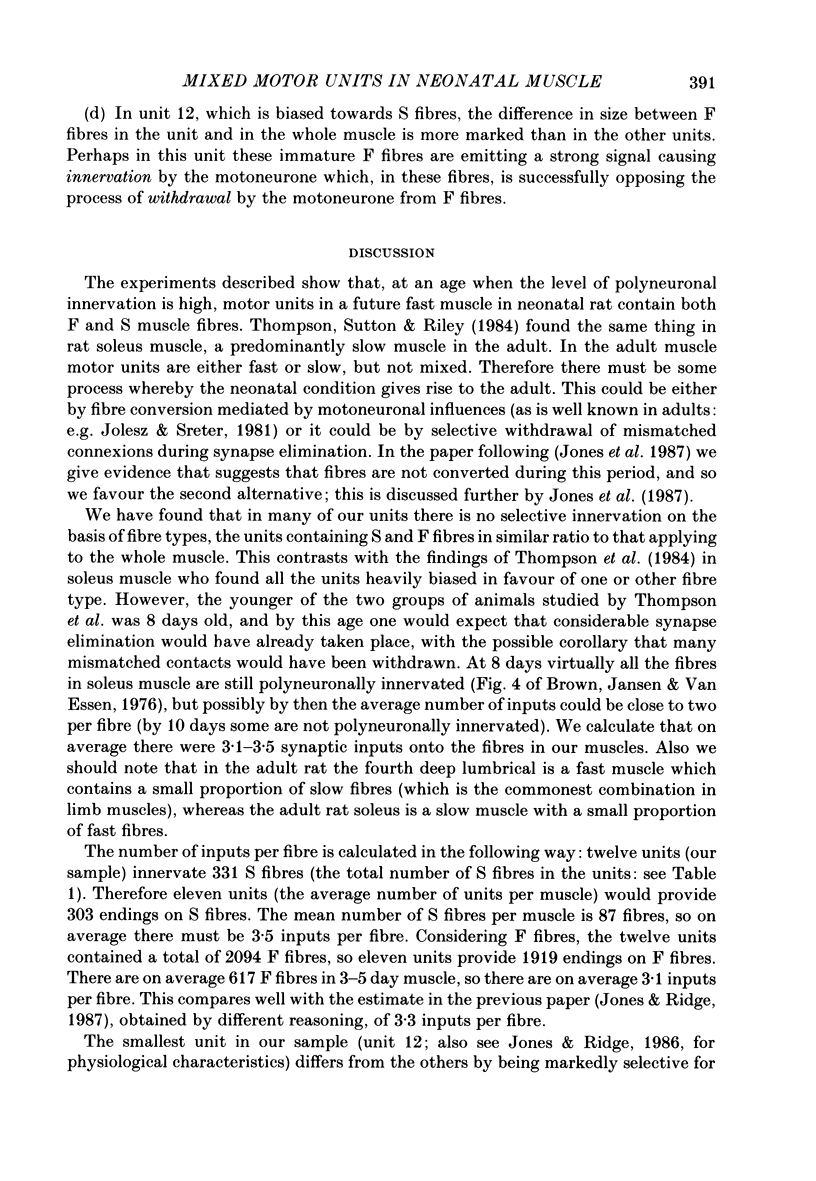
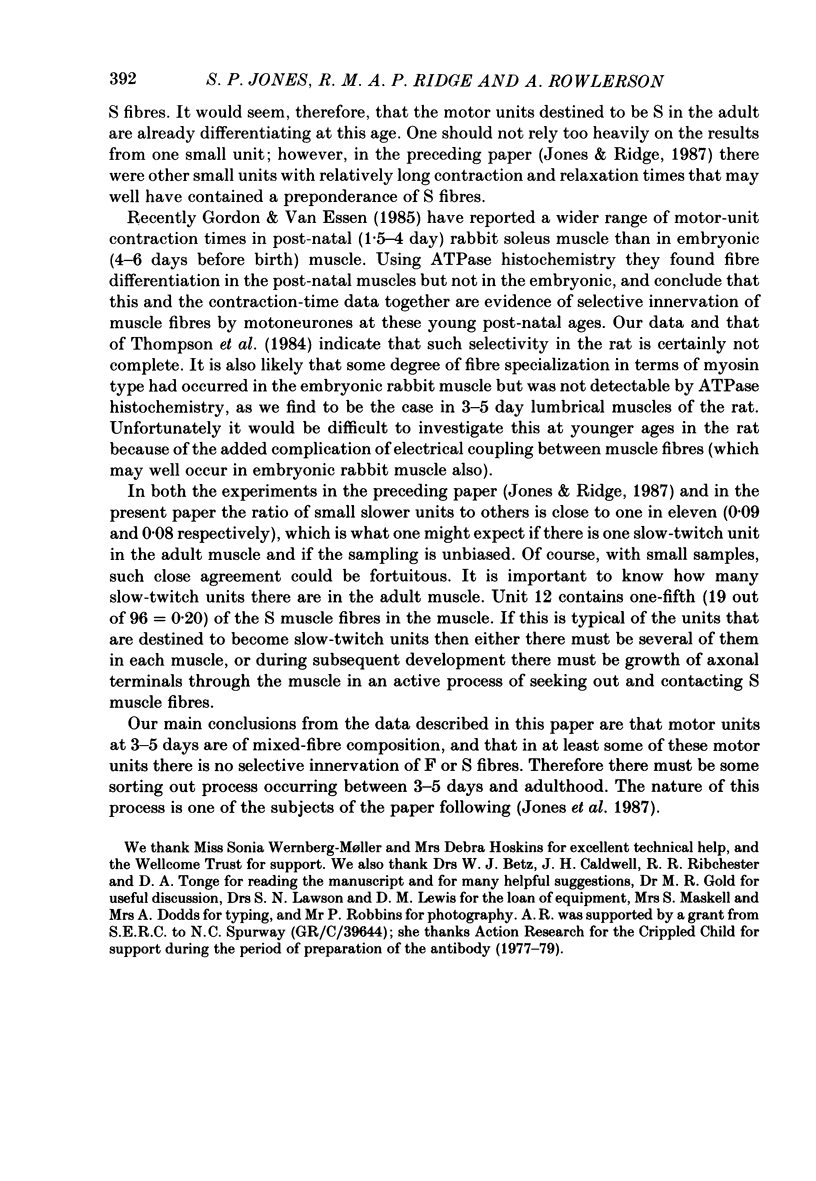
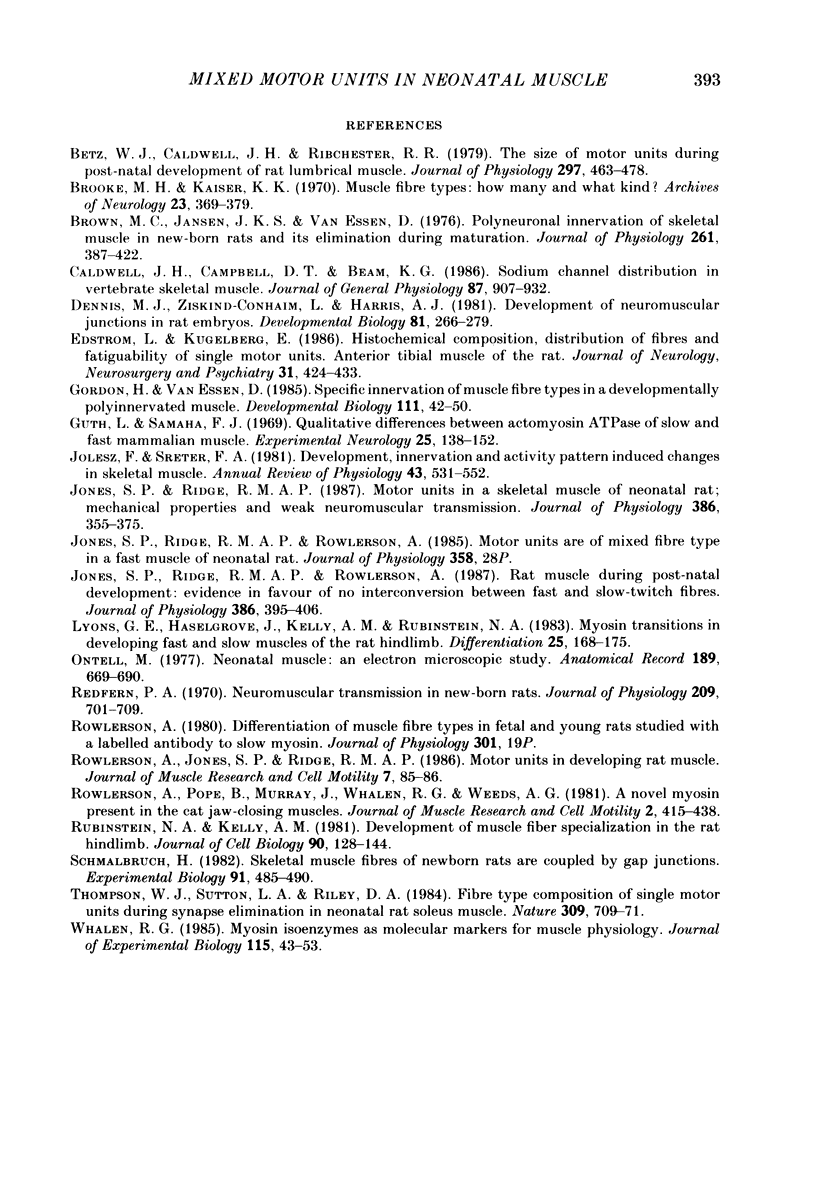
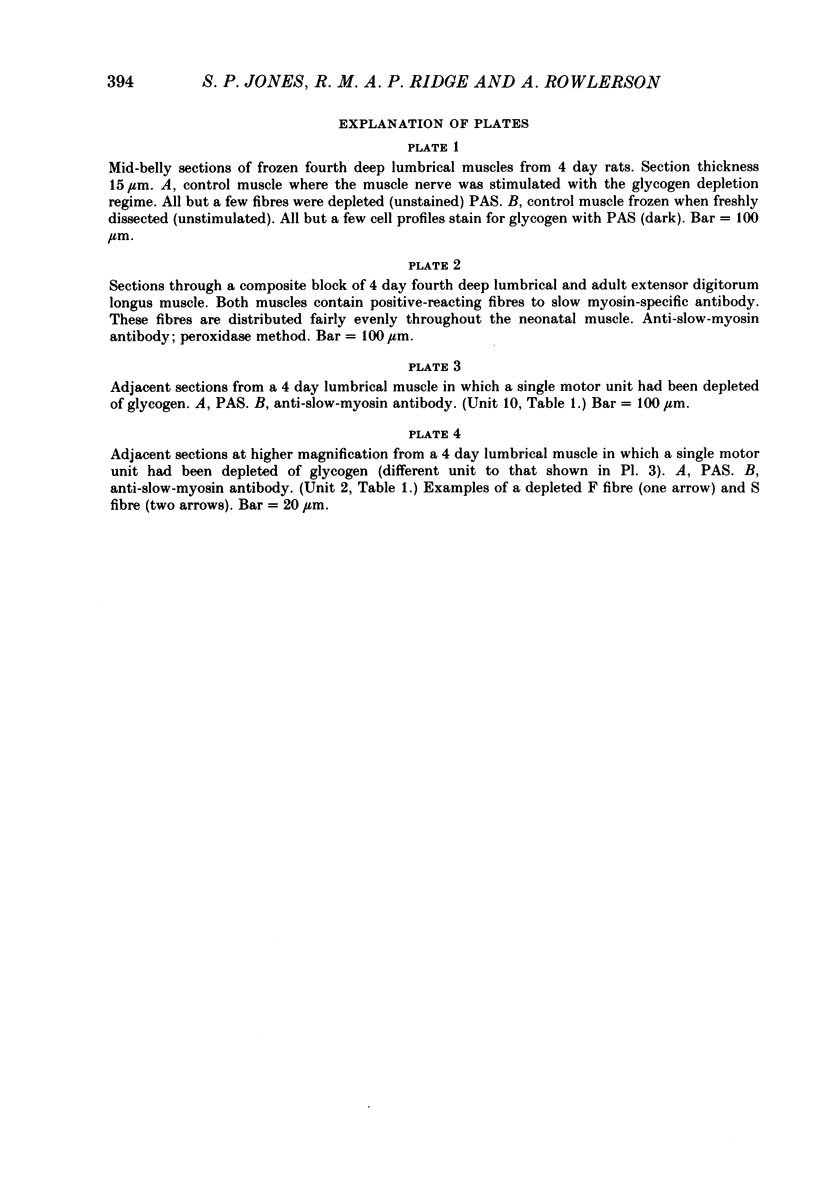
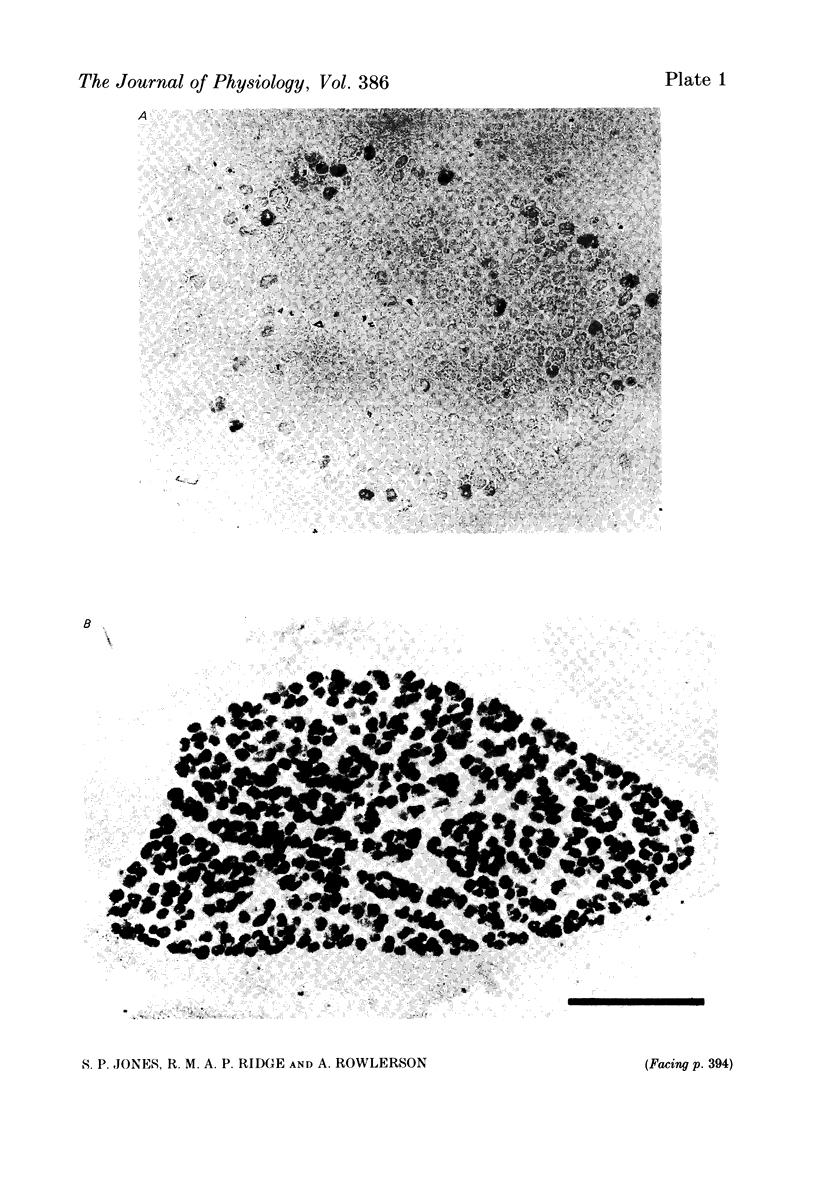
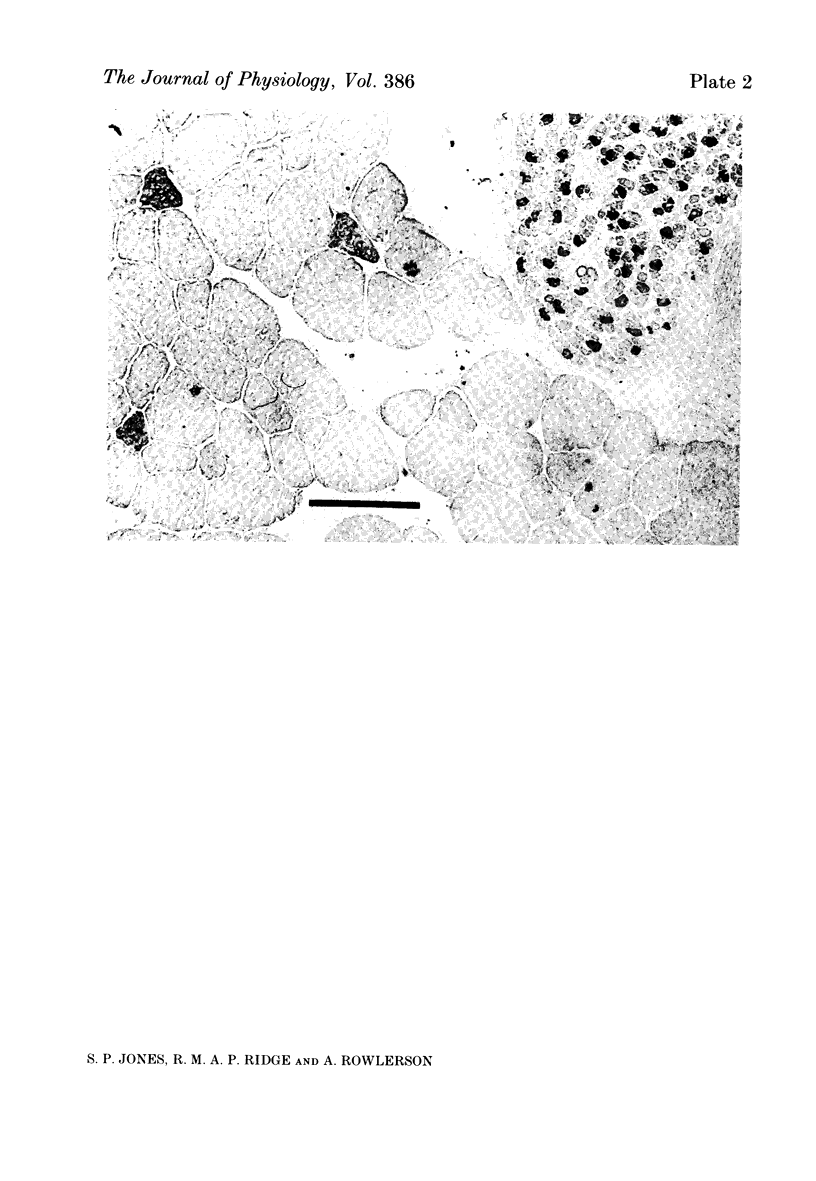
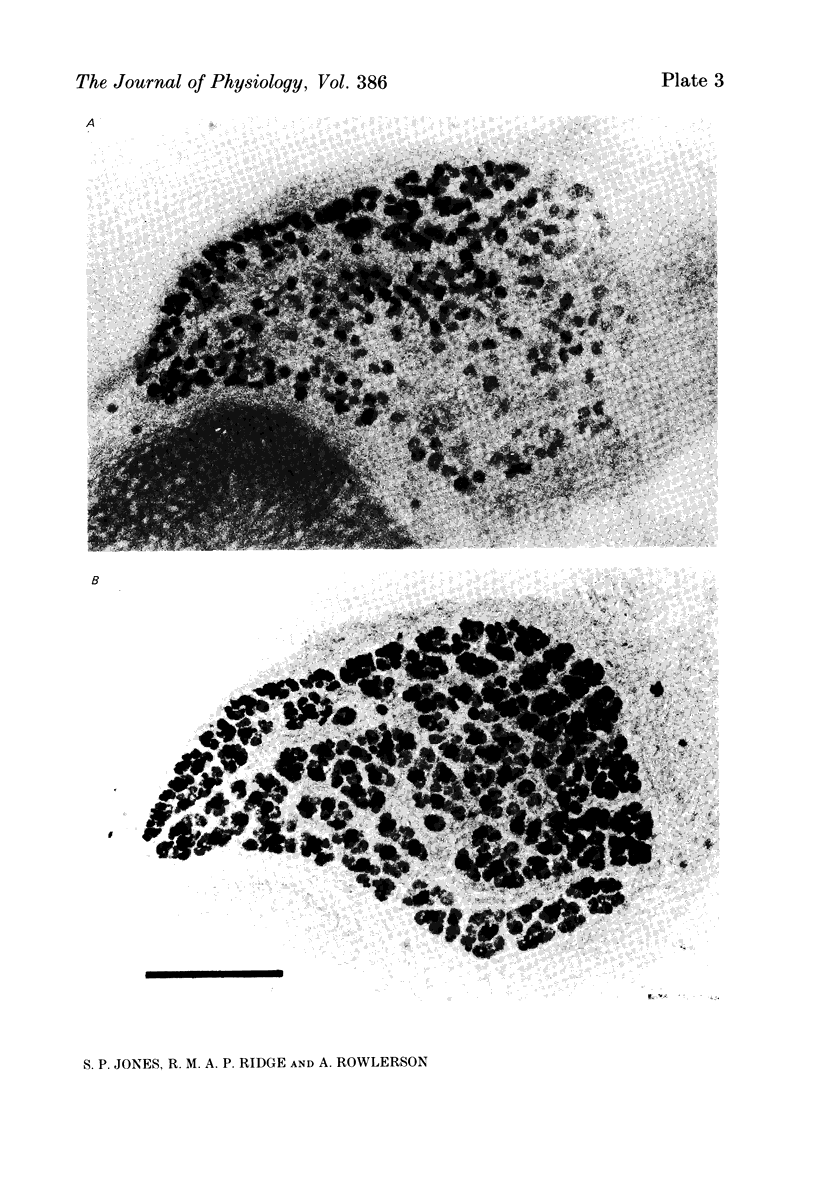
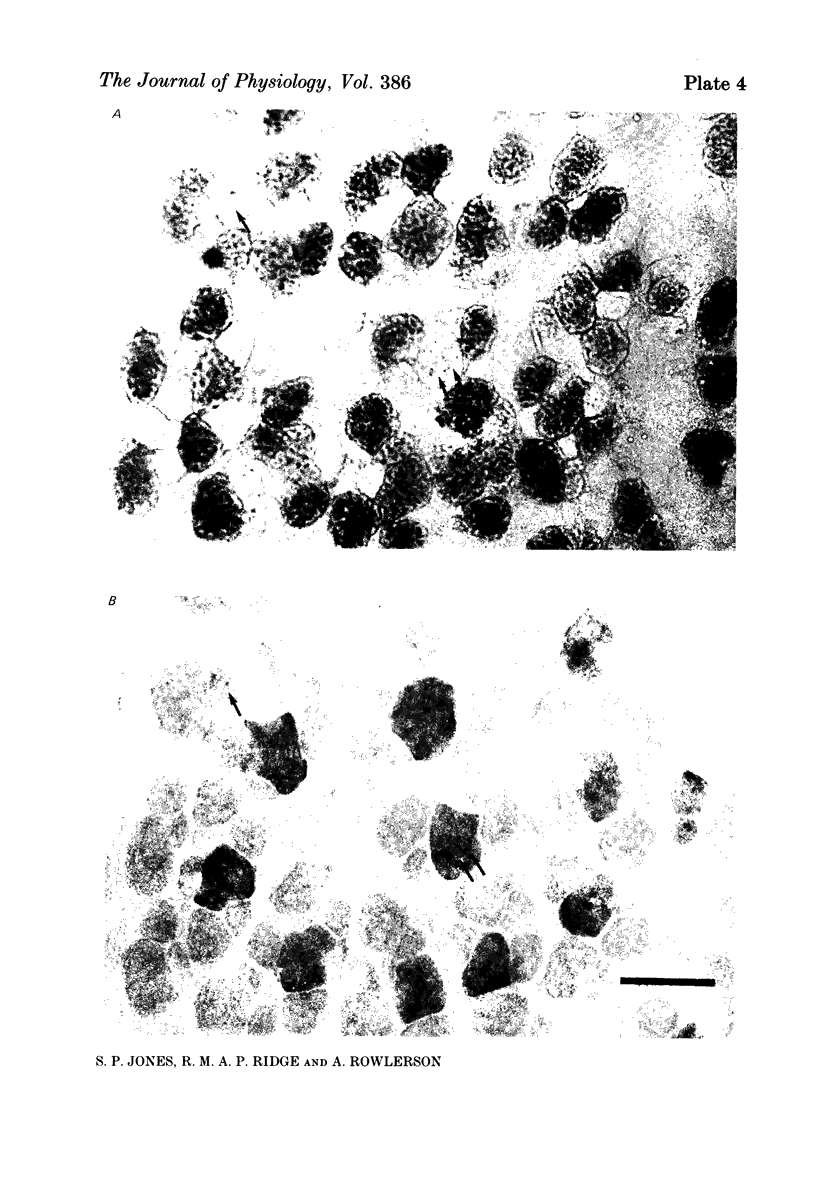
Images in this article
Selected References
These references are in PubMed. This may not be the complete list of references from this article.
- Betz W. J., Caldwell J. H., Ribchester R. R. The size of motor units during post-natal development of rat lumbrical muscle. J Physiol. 1979 Dec;297(0):463–478. doi: 10.1113/jphysiol.1979.sp013051. [DOI] [PMC free article] [PubMed] [Google Scholar]
- Brooke M. H., Kaiser K. K. Muscle fiber types: how many and what kind? Arch Neurol. 1970 Oct;23(4):369–379. doi: 10.1001/archneur.1970.00480280083010. [DOI] [PubMed] [Google Scholar]
- Brown M. C., Jansen J. K., Van Essen D. Polyneuronal innervation of skeletal muscle in new-born rats and its elimination during maturation. J Physiol. 1976 Oct;261(2):387–422. doi: 10.1113/jphysiol.1976.sp011565. [DOI] [PMC free article] [PubMed] [Google Scholar]
- Caldwell J. H., Campbell D. T., Beam K. G. Na channel distribution in vertebrate skeletal muscle. J Gen Physiol. 1986 Jun;87(6):907–932. doi: 10.1085/jgp.87.6.907. [DOI] [PMC free article] [PubMed] [Google Scholar]
- Dennis M. J., Ziskind-Conhaim L., Harris A. J. Development of neuromuscular junctions in rat embryos. Dev Biol. 1981 Jan 30;81(2):266–279. doi: 10.1016/0012-1606(81)90290-6. [DOI] [PubMed] [Google Scholar]
- Edström L., Kugelberg E. Histochemical composition, distribution of fibres and fatiguability of single motor units. Anterior tibial muscle of the rat. J Neurol Neurosurg Psychiatry. 1968 Oct;31(5):424–433. doi: 10.1136/jnnp.31.5.424. [DOI] [PMC free article] [PubMed] [Google Scholar]
- Gordon H., Van Essen D. C. Specific innervation of muscle fiber types in a developmentally polyinnervated muscle. Dev Biol. 1985 Sep;111(1):42–50. doi: 10.1016/0012-1606(85)90433-6. [DOI] [PubMed] [Google Scholar]
- Guth L., Samaha F. J. Qualitative differences between actomyosin ATPase of slow and fast mammalian muscle. Exp Neurol. 1969 Sep;25(1):138–152. doi: 10.1016/0014-4886(69)90077-6. [DOI] [PubMed] [Google Scholar]
- Jolesz F., Sreter F. A. Development, innervation, and activity-pattern induced changes in skeletal muscle. Annu Rev Physiol. 1981;43:531–552. doi: 10.1146/annurev.ph.43.030181.002531. [DOI] [PubMed] [Google Scholar]
- Jones S. P., Ridge R. M. Motor units in a skeletal muscle of neonatal rat: mechanical properties and weak neuromuscular transmission. J Physiol. 1987 May;386:355–375. doi: 10.1113/jphysiol.1987.sp016538. [DOI] [PMC free article] [PubMed] [Google Scholar]
- Jones S. P., Ridge R. M., Rowlerson A. Rat muscle during post-natal development: evidence in favour of no interconversion between fast- and slow-twitch fibres. J Physiol. 1987 May;386:395–406. doi: 10.1113/jphysiol.1987.sp016540. [DOI] [PMC free article] [PubMed] [Google Scholar]
- Lyons G. E., Haselgrove J., Kelly A. M., Rubinstein N. A. Myosin transitions in developing fast and slow muscles of the rat hindlimb. Differentiation. 1983;25(2):168–175. doi: 10.1111/j.1432-0436.1984.tb01352.x. [DOI] [PubMed] [Google Scholar]
- Ontell M. Neonatal muscle: an electron microscopic study. Anat Rec. 1977 Dec;189(4):669–690. doi: 10.1002/ar.1091890410. [DOI] [PubMed] [Google Scholar]
- Redfern P. A. Neuromuscular transmission in new-born rats. J Physiol. 1970 Aug;209(3):701–709. doi: 10.1113/jphysiol.1970.sp009187. [DOI] [PMC free article] [PubMed] [Google Scholar]
- Rubinstein N. A., Kelly A. M. Development of muscle fiber specialization in the rat hindlimb. J Cell Biol. 1981 Jul;90(1):128–144. doi: 10.1083/jcb.90.1.128. [DOI] [PMC free article] [PubMed] [Google Scholar]
- Schmalbruch H. Skeletal muscle fibers of newborn rats are coupled by gap junctions. Dev Biol. 1982 Jun;91(2):485–490. doi: 10.1016/0012-1606(82)90056-2. [DOI] [PubMed] [Google Scholar]
- Thompson W. J., Sutton L. A., Riley D. A. Fibre type composition of single motor units during synapse elimination in neonatal rat soleus muscle. Nature. 1984 Jun 21;309(5970):709–711. doi: 10.1038/309709a0. [DOI] [PubMed] [Google Scholar]
- Whalen R. G. Myosin isoenzymes as molecular markers for muscle physiology. J Exp Biol. 1985 Mar;115:43–53. doi: 10.1242/jeb.115.1.43. [DOI] [PubMed] [Google Scholar]