Abstract
1. We have studied effects of electrical diffusion potentials on active Na+-K+ exchange in phospholipid vesicles reconstituted with pig kidney Na+, K+-ATPase. 2. Diffusion potentials, negative inside, were established using outwardly directed K+ gradients plus valinomycin or Li+ gradients plus a Li+ ionophore, AS701. Measurement of fluorescence changes of the carbocyanine dye DiS-C3-(5) showed that the ionophores generated potentials of the expected orientation and of sufficient stability for their effects on active transport to be assessed. Measurement of rates of passive 22Na+ fluxes, over a wide range of diffusion potentials, were consistent with the quantitative predictions of the constant-field flux equation. This result demonstrates that values of diffusion potentials calculated from the Nernst or constant-field equation are accurate. 3. In some conditions, the inside-negative potential (-130 to -180 mV) accelerated the rate of ATP-dependent Na+-K+ exchange on inside-out-oriented pumps, compared to 'control' without the ionophores. Reduction in the size of the diffusion potentials by addition to the medium of Li+ with AS701 or Cs+ with the valinomycin progressively annulled the acceleratory effects, consistent with these being true effects of a change in membrane potentials. 4. At saturating cytoplasmic Na+ and ATP concentrations, the diffusion potential accelerated ATP-dependent Na+-K+ exchange by up to about 30% compared to control but this effect disappeared at rate-limiting ATP concentrations (approximately 1 microM). 5. Using prior knowledge of rate-limiting steps, we interpret this finding to mean that the conformational transition E2(2K)----E12K associated with transport of two K+ ions is voltage insensitive while E1P(3Na)----E2P3Na associated with transport of three Na+ ions is voltage sensitive. The simplest explanation is that the net charge in the transport domain of the protein when no ions, 2K+ or 3Na+ are bound is -2, 0 and +1 respectively. 6. The accelerating effect of the negative-inside diffusion potential on Na+-K+ exchange is greater at limitingly low cytoplasmic Na+ concentrations than at saturating cytoplasmic Na+ concentrations. Cytoplasmic Na+ activation curves show that the diffusion potential increases the apparent cytoplasmic Na+ affinity and reduces the sigmoidicity of cytoplasmic Na+ activation. 7. A kinetic analysis reveals that this effect on apparent affinity is due to an increase in intrinsic Na+ binding and occurs in addition to the effect on a transport rate constant.(ABSTRACT TRUNCATED AT 400 WORDS)
Full text
PDF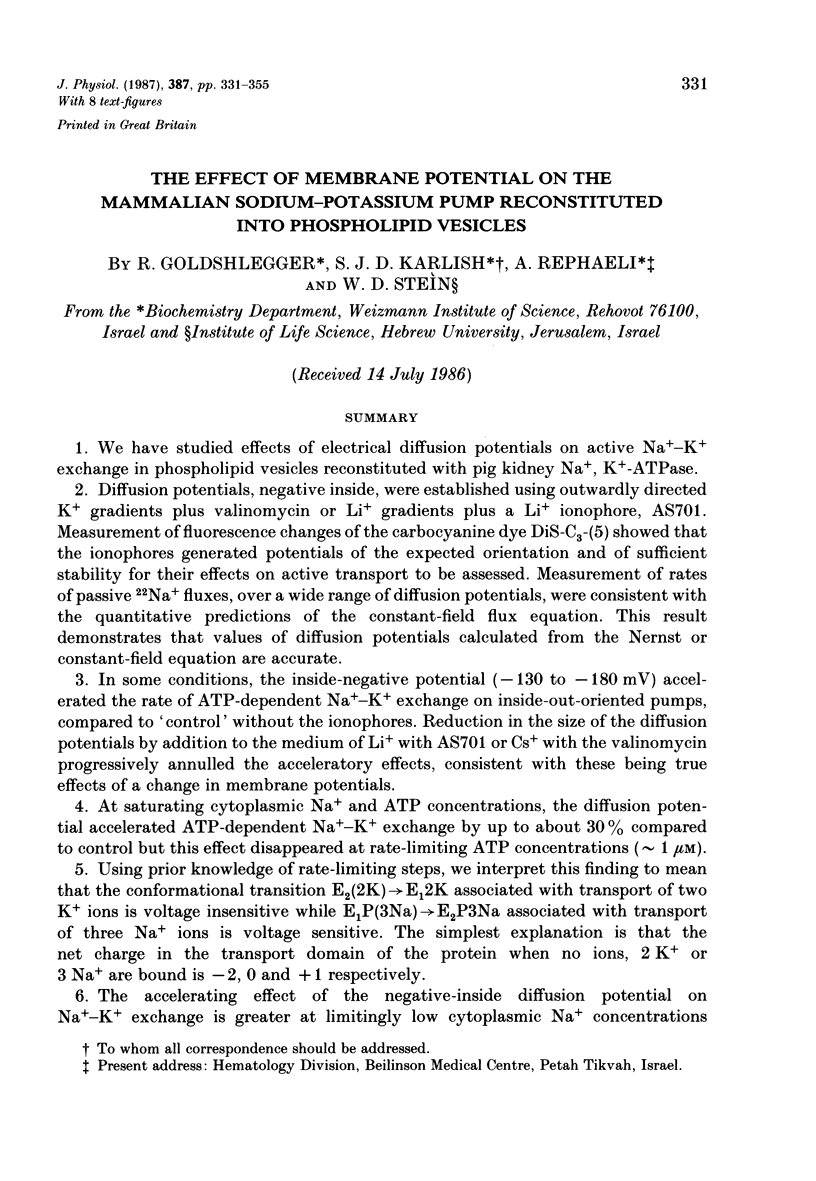
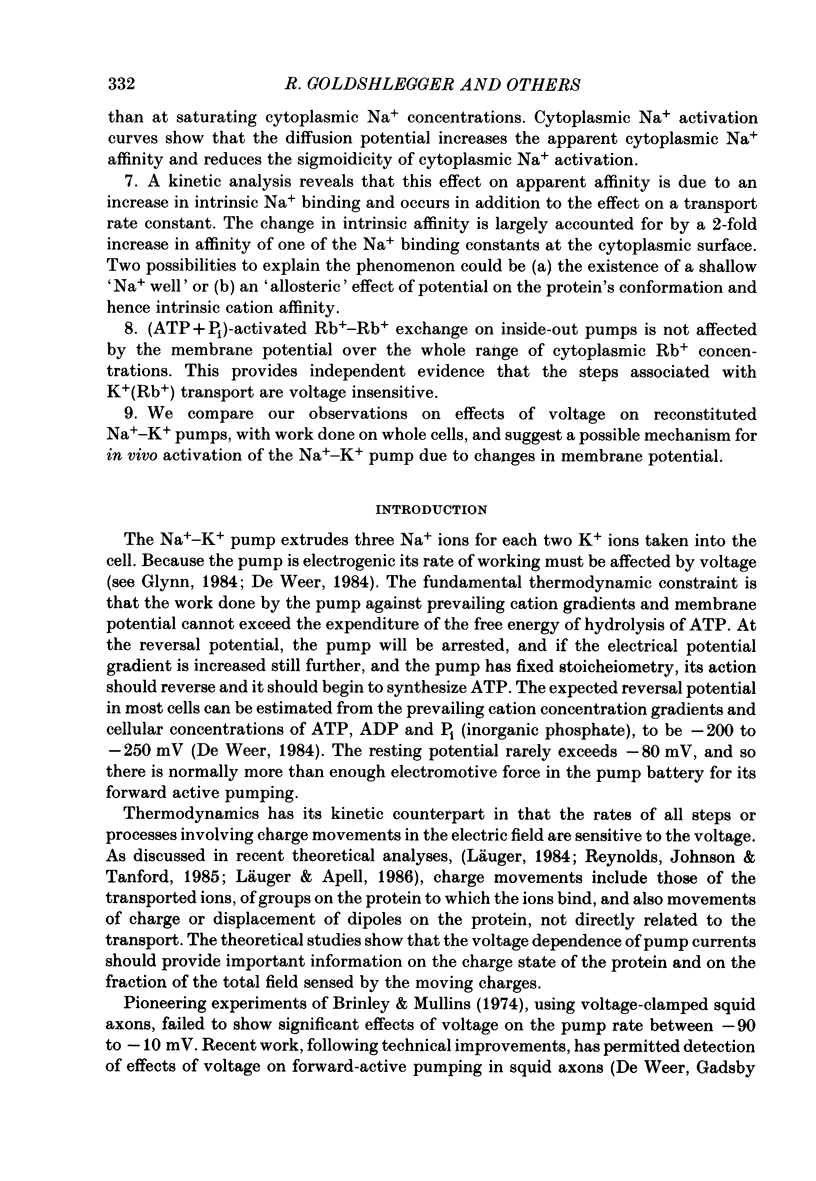
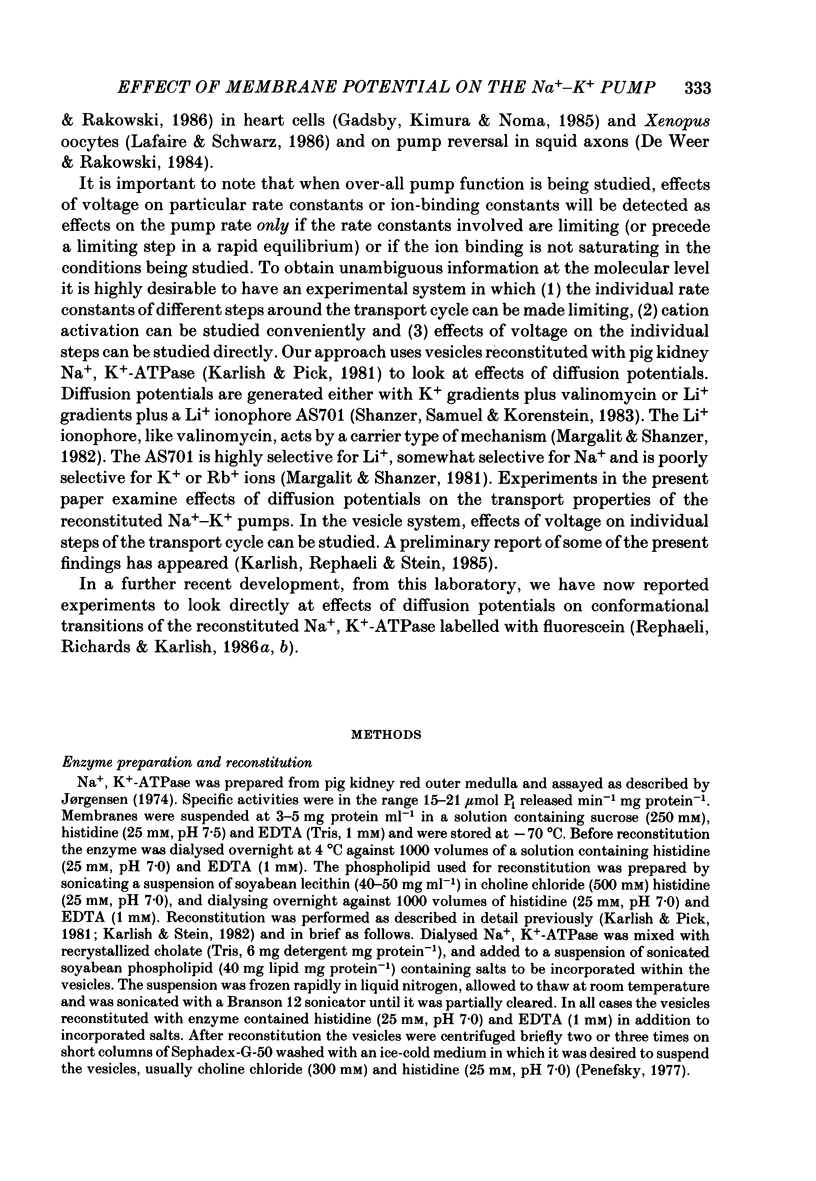
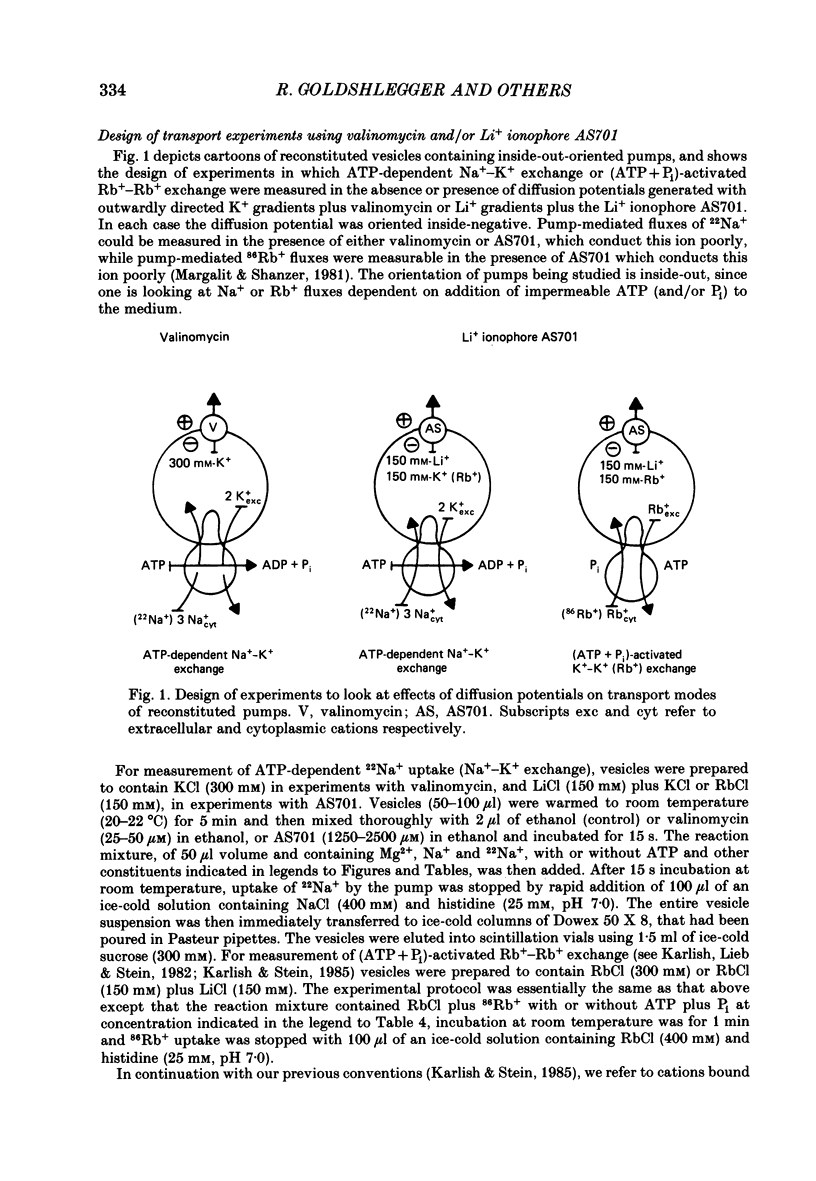
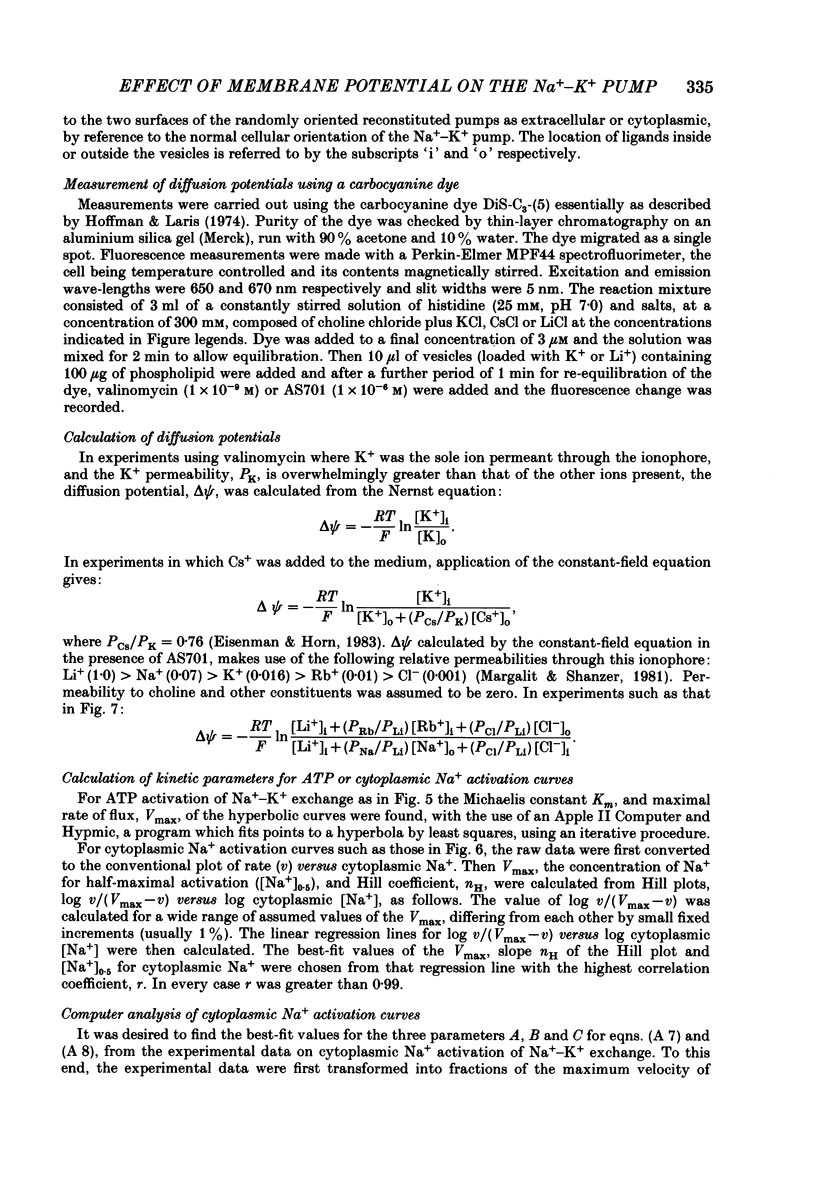
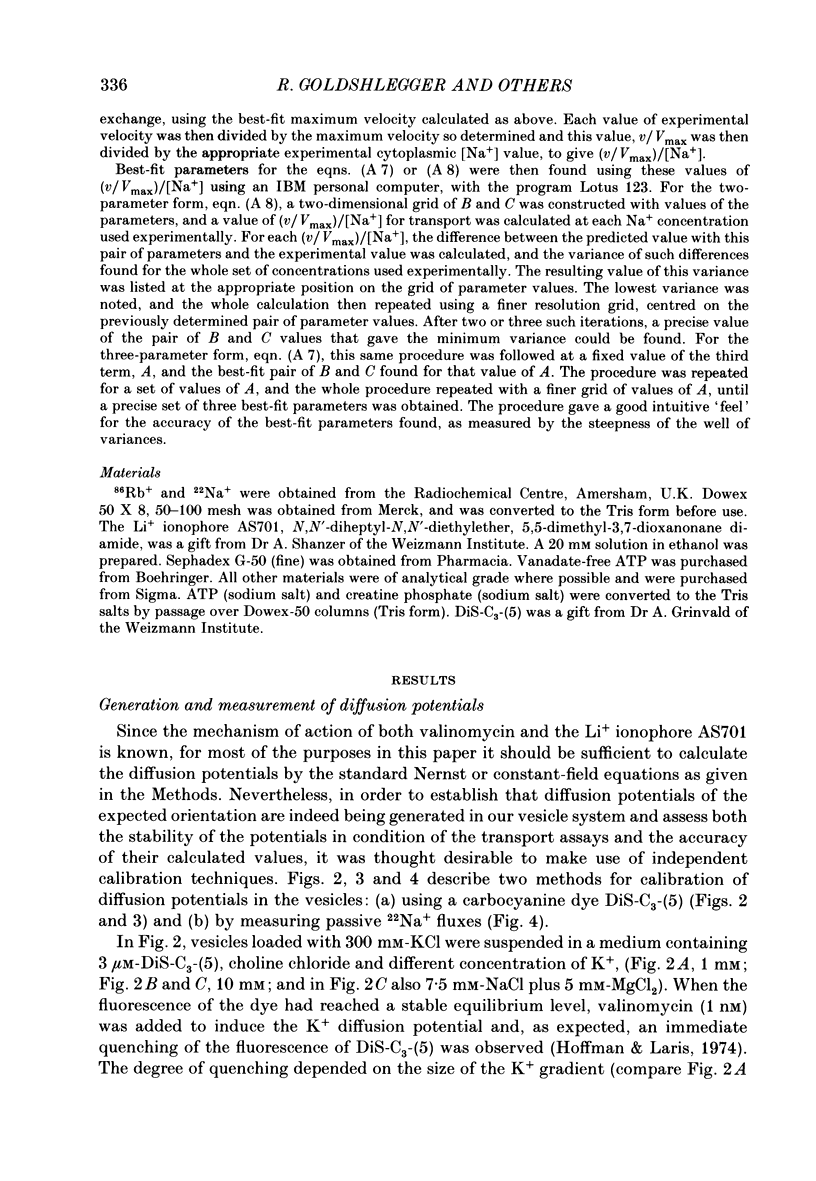
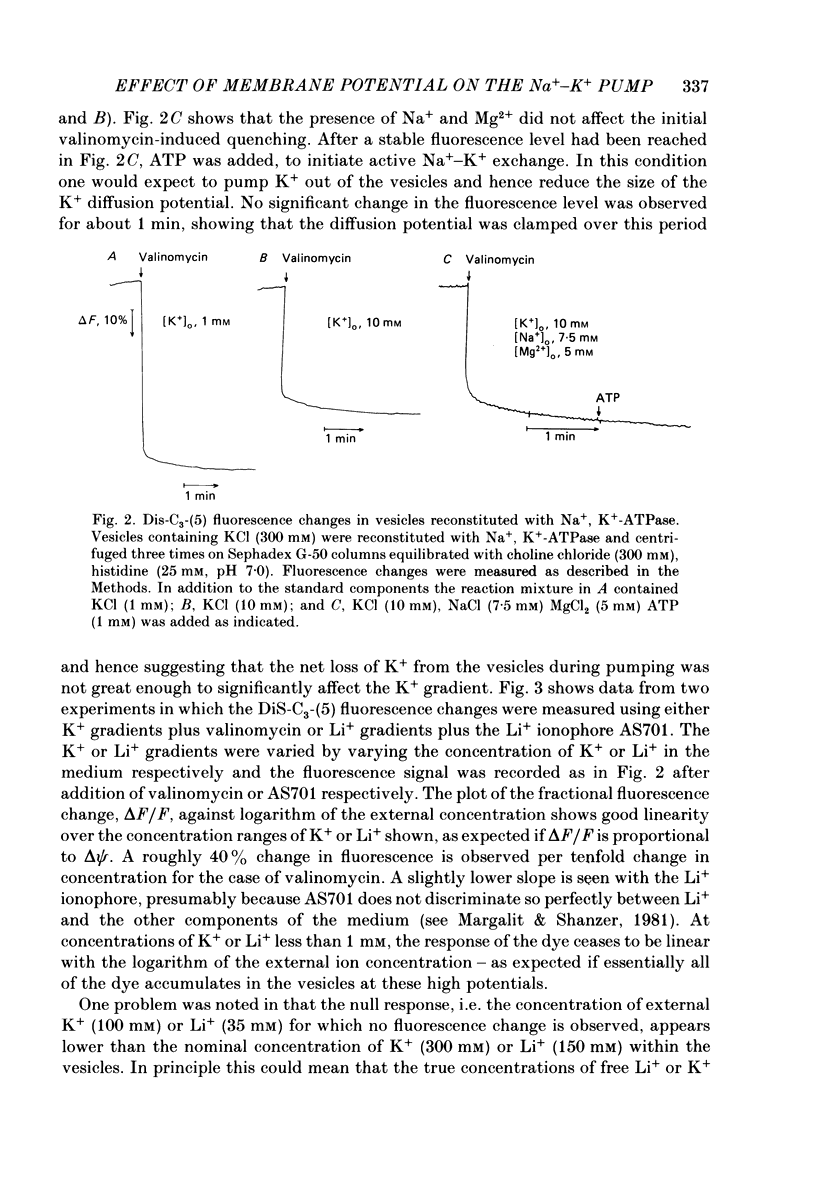
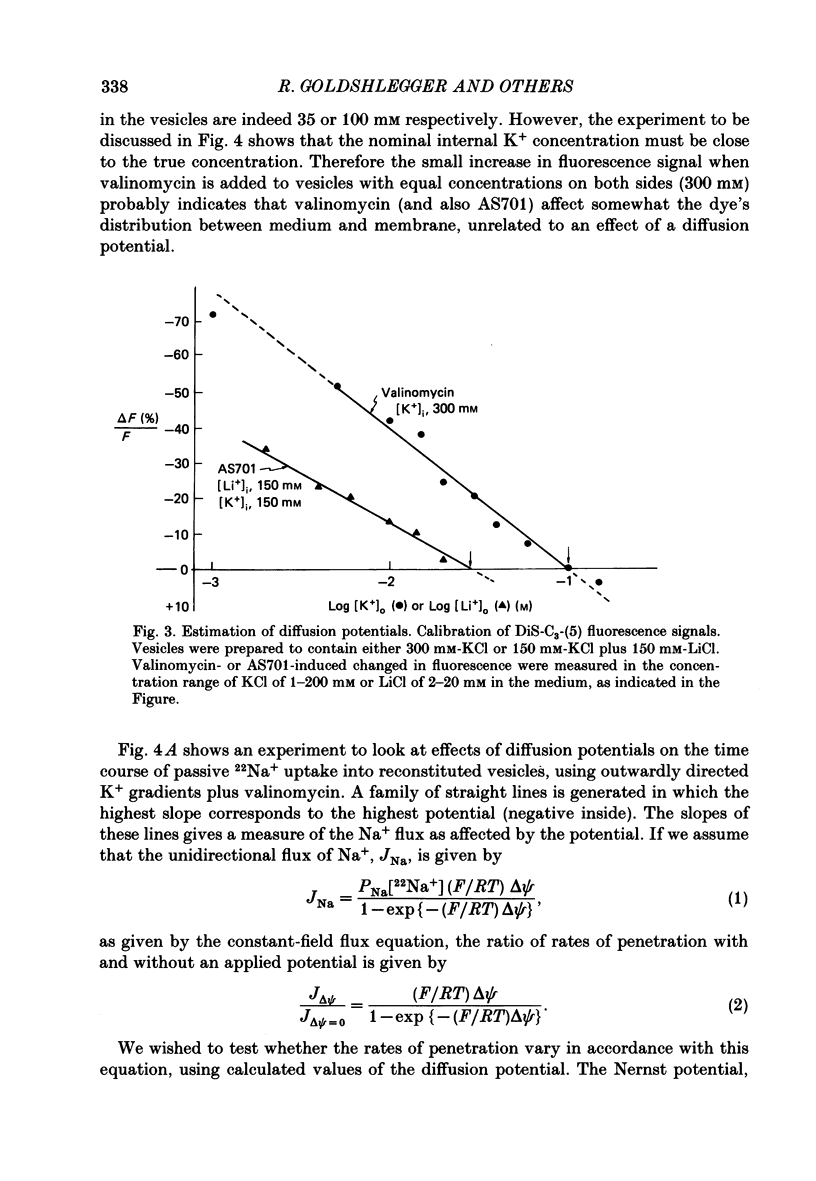
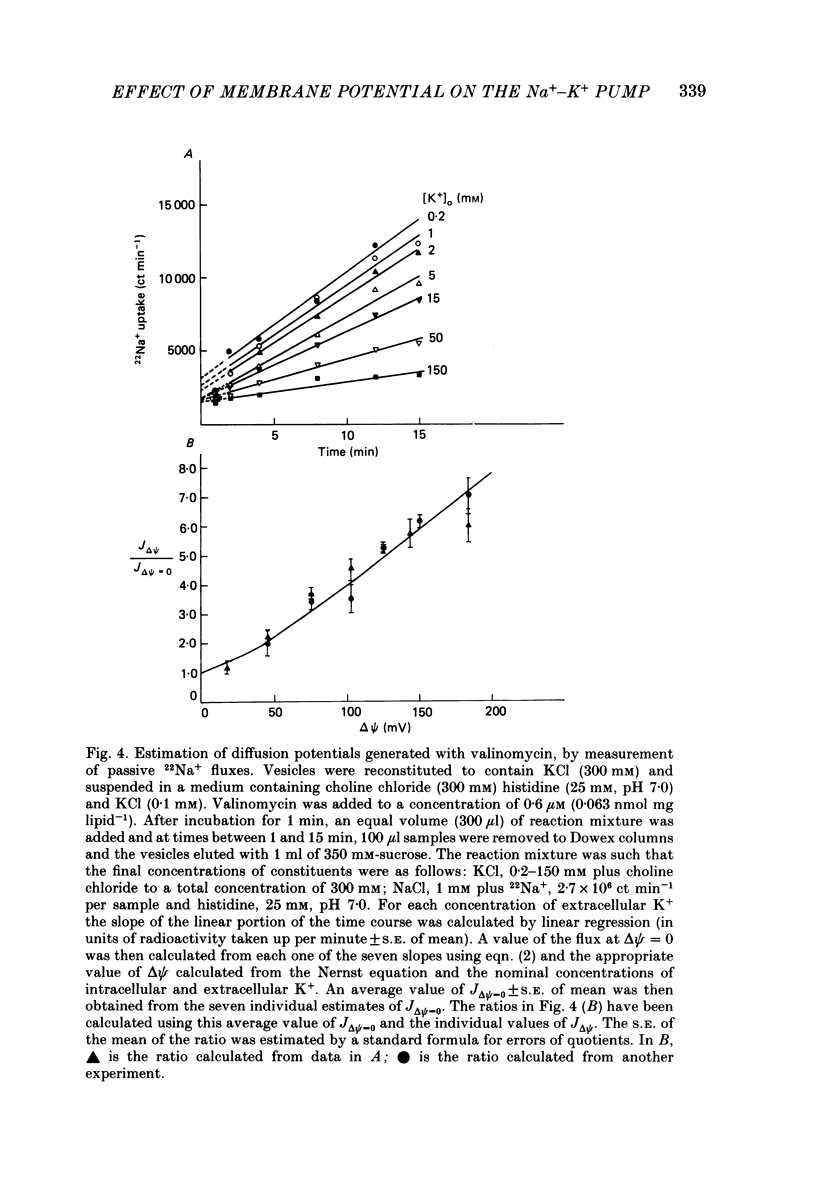
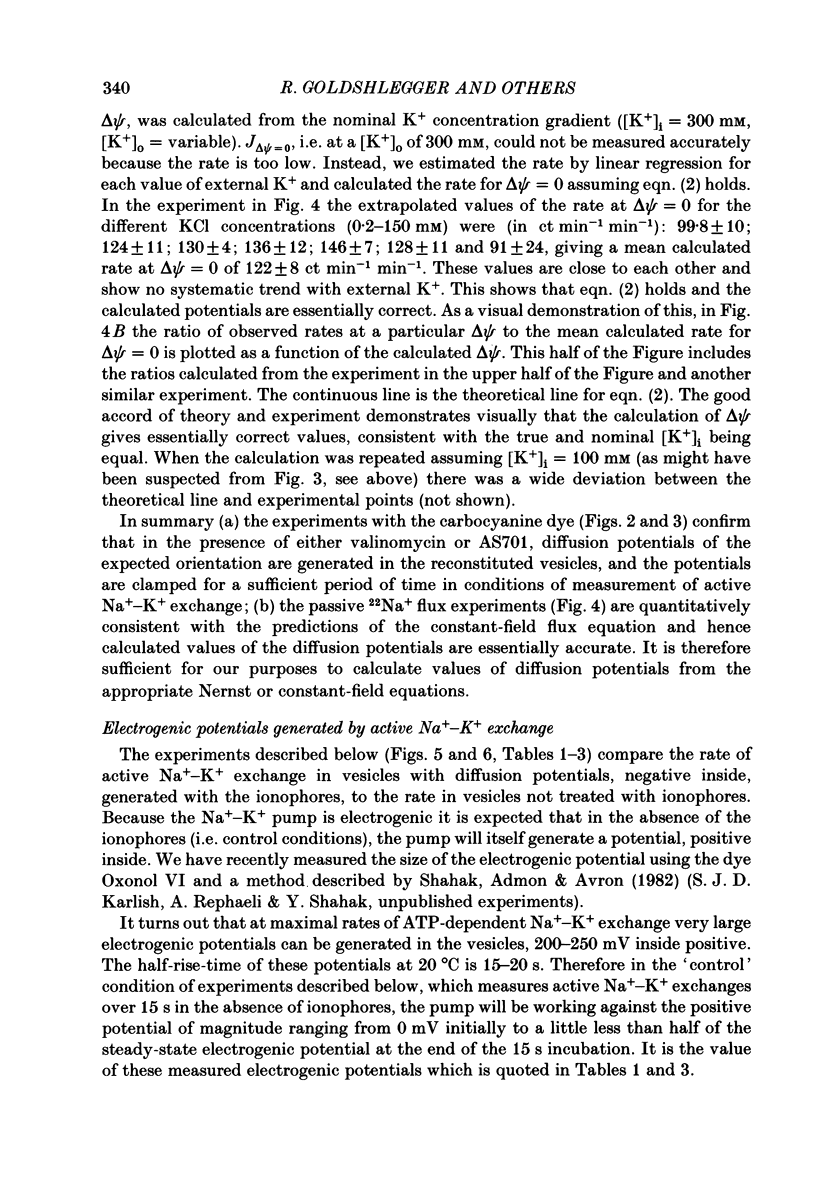
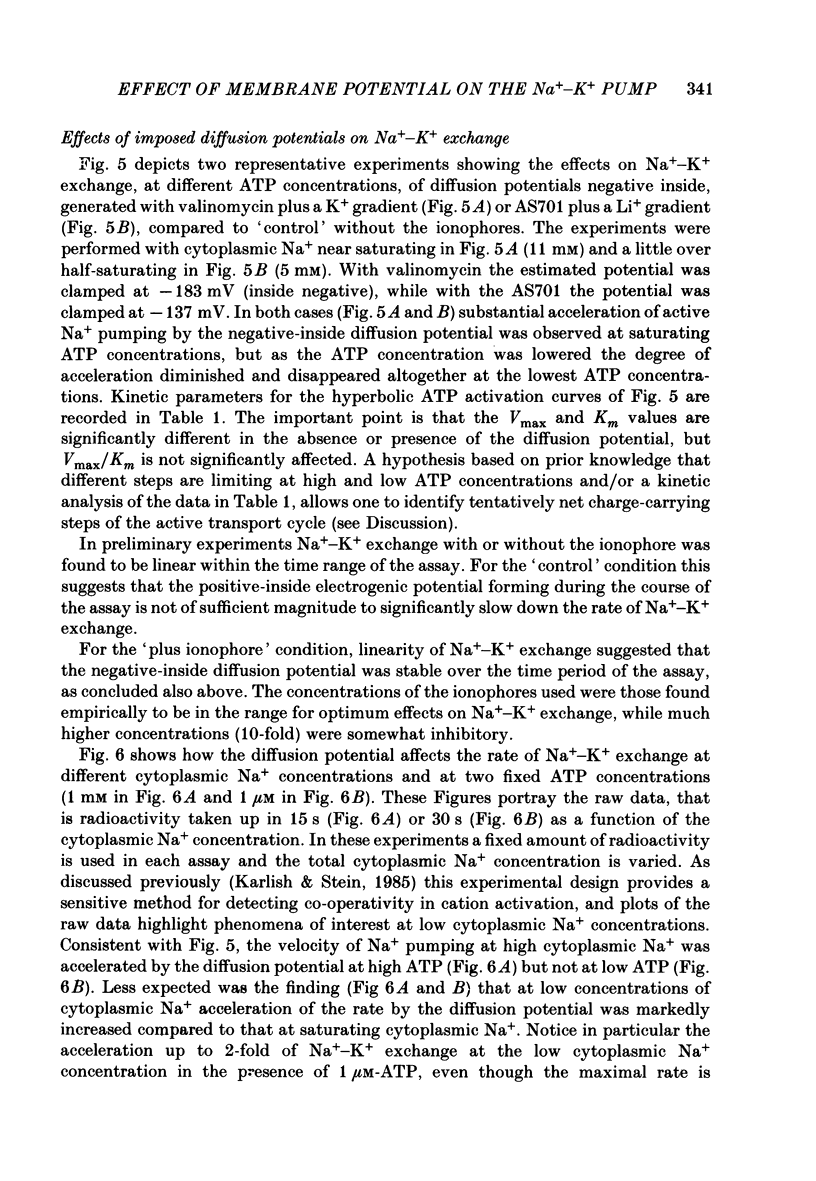
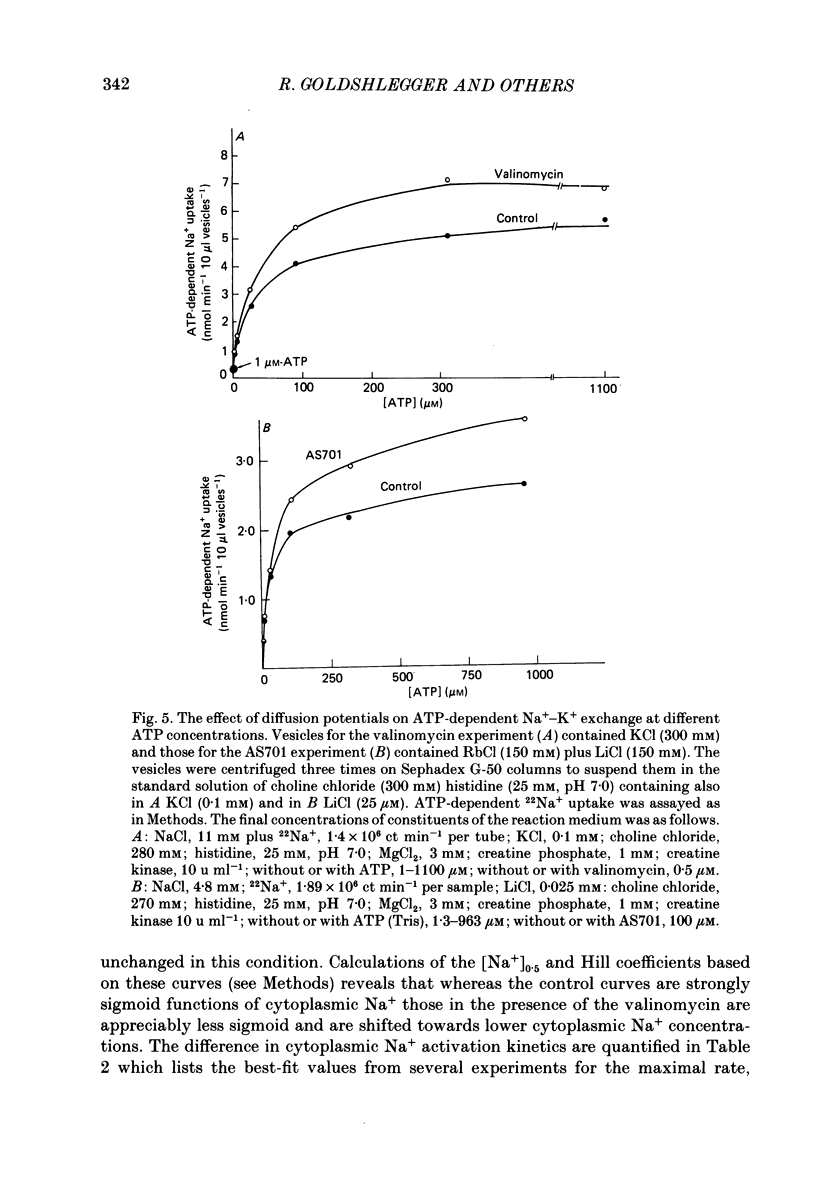
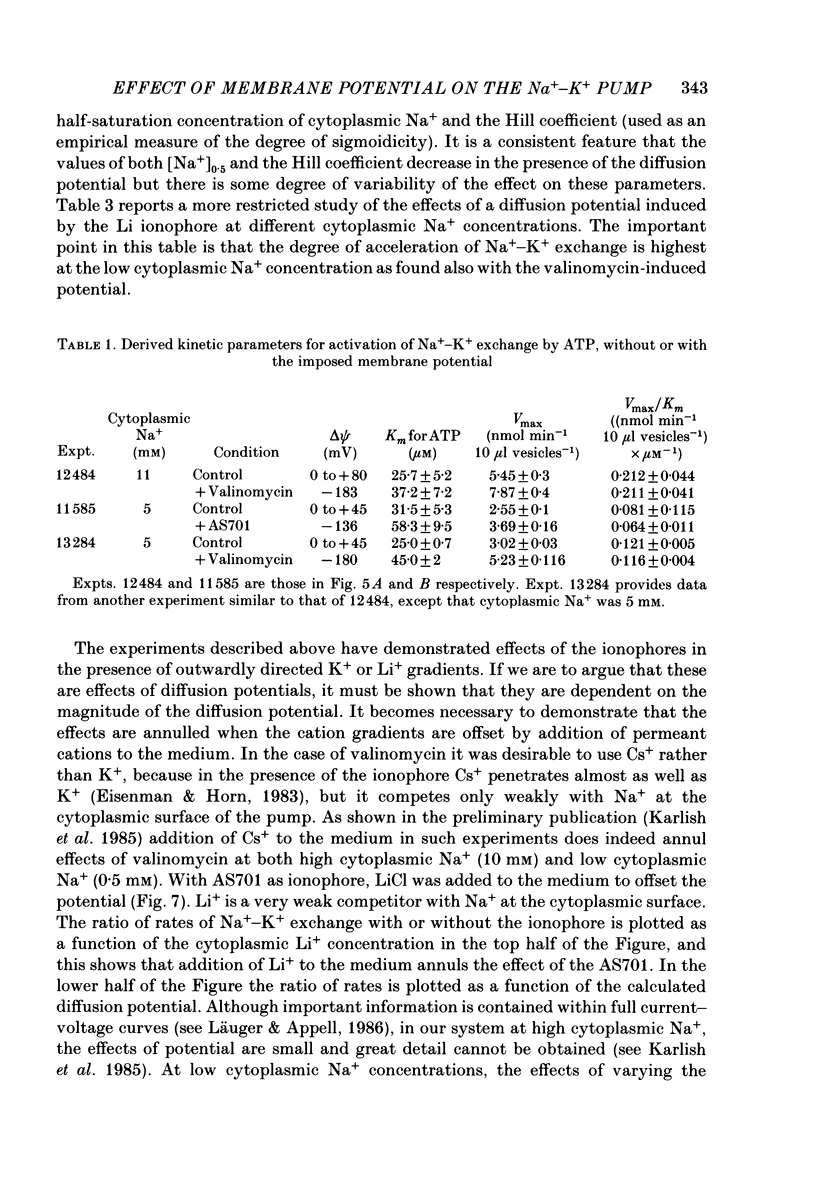
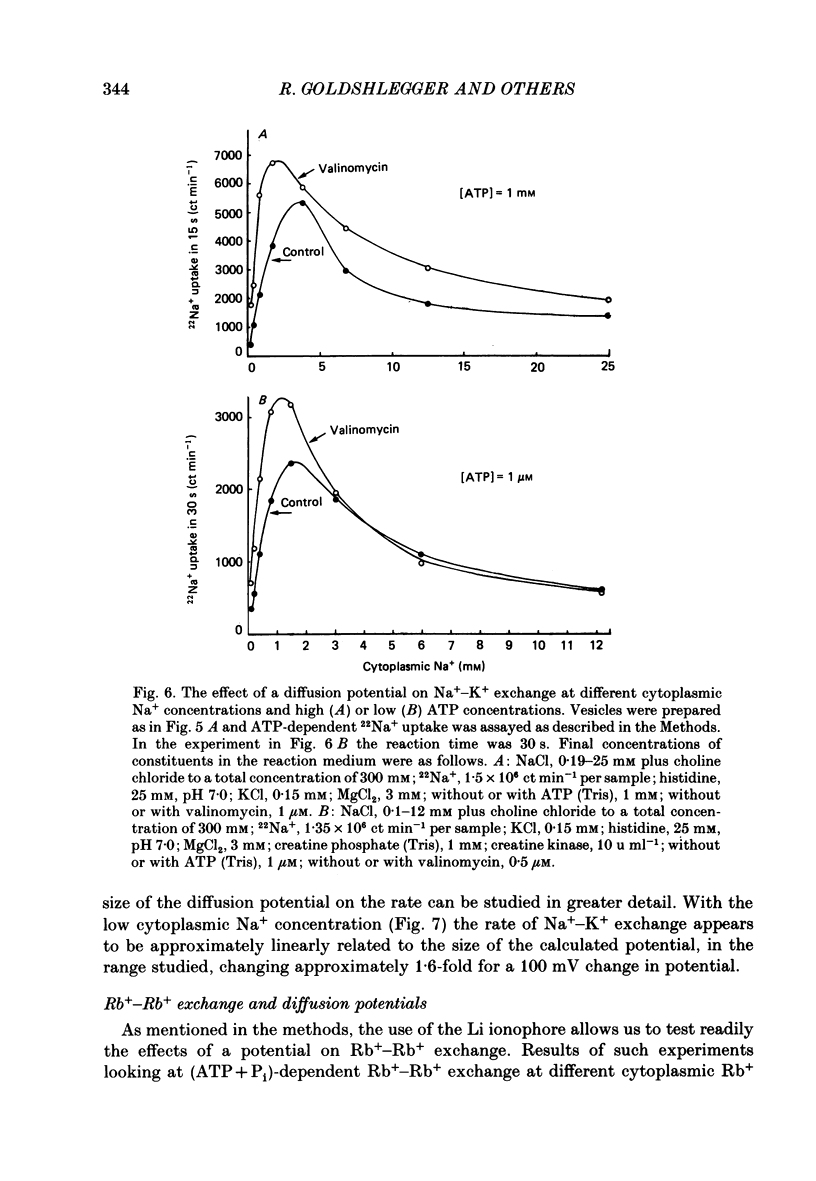
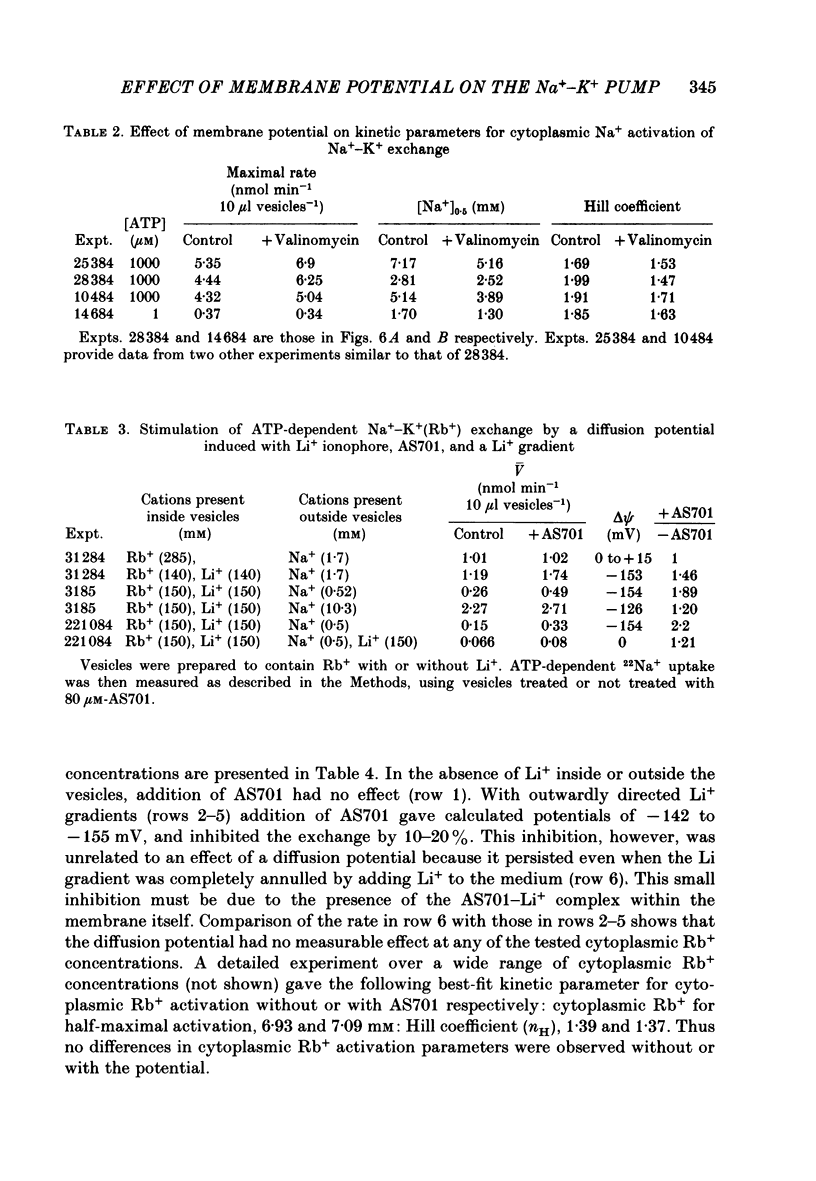
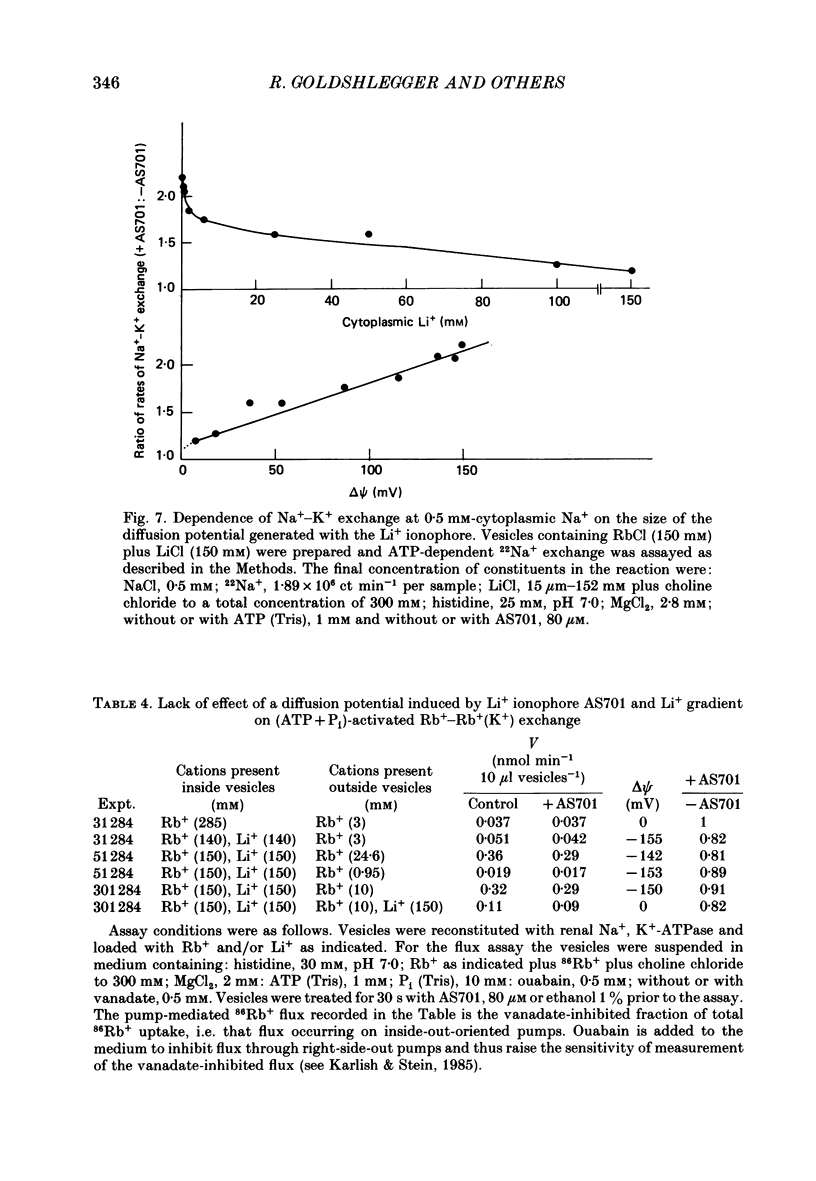
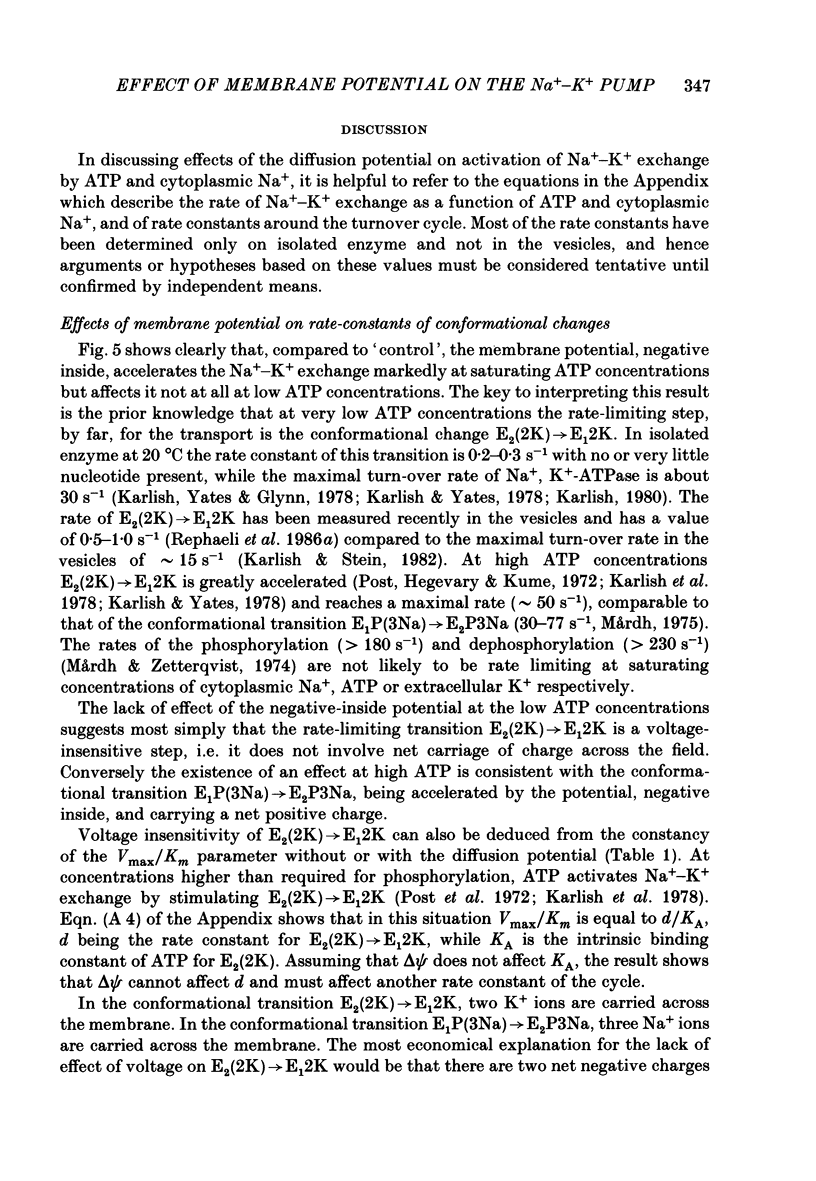
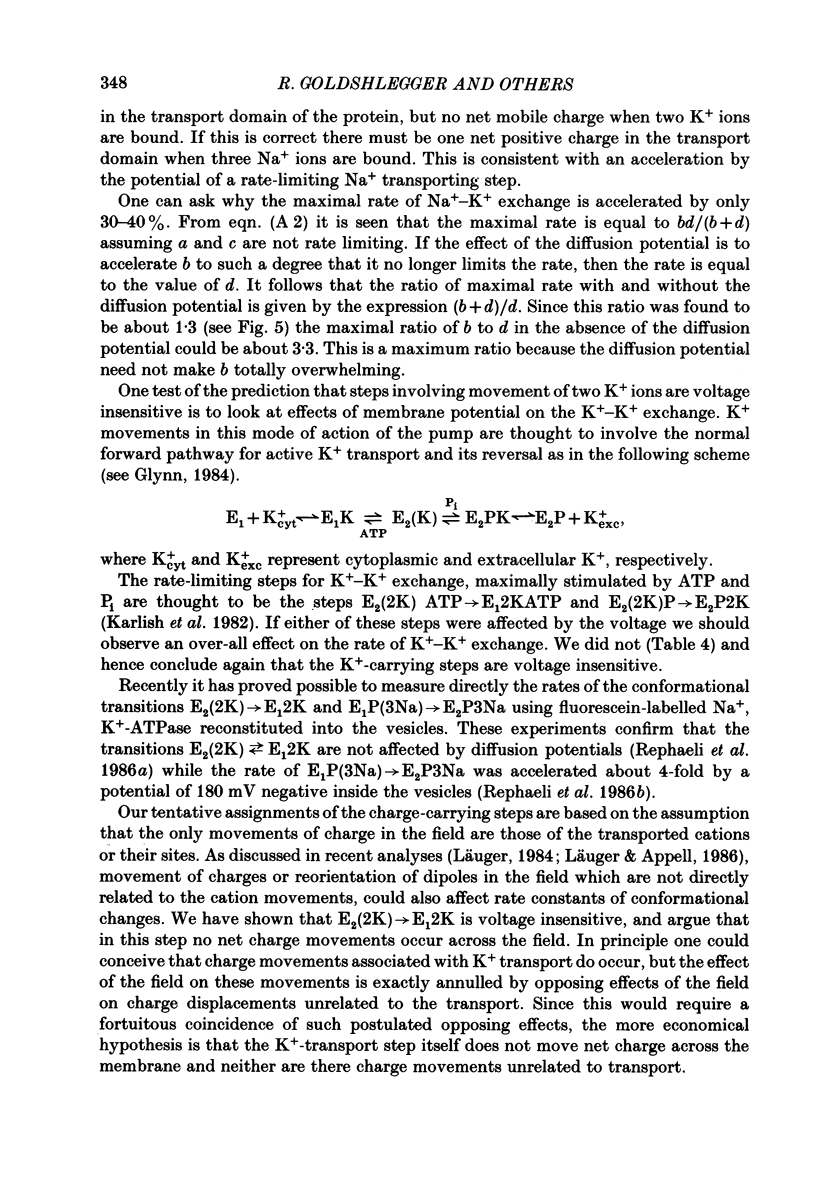
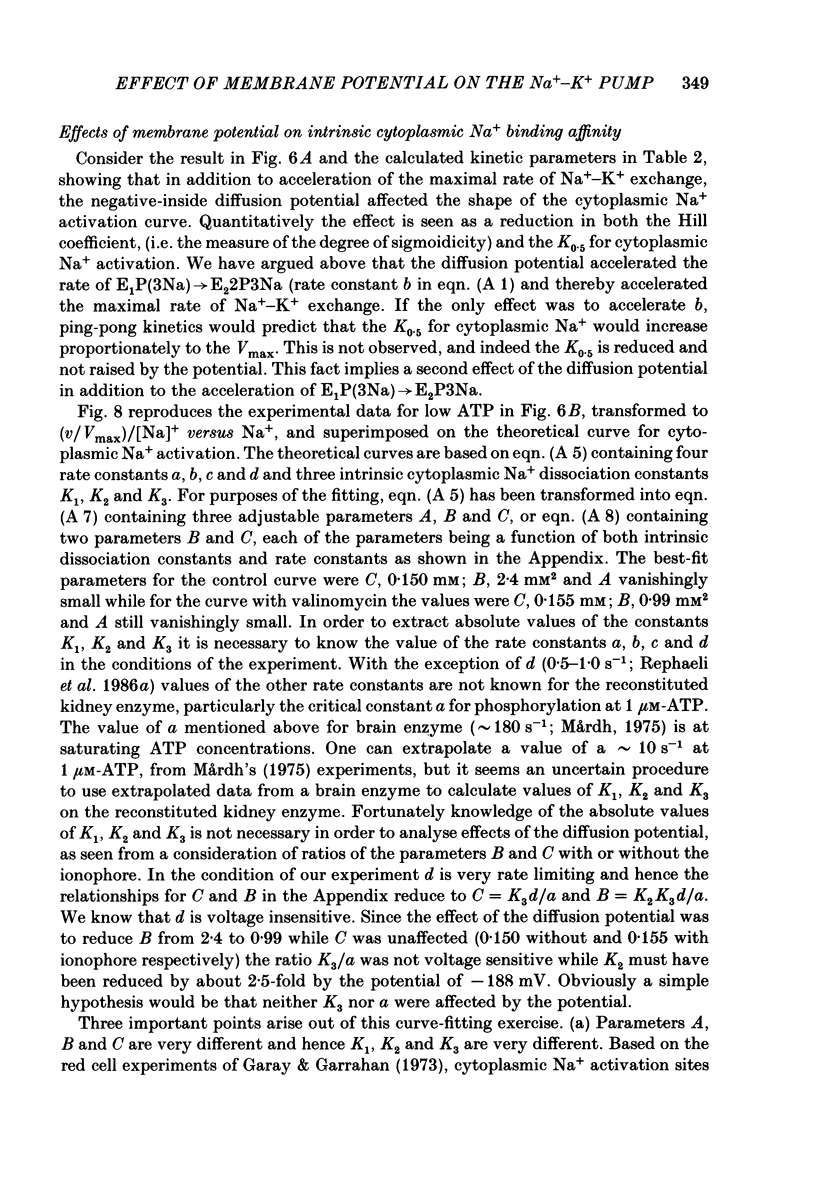
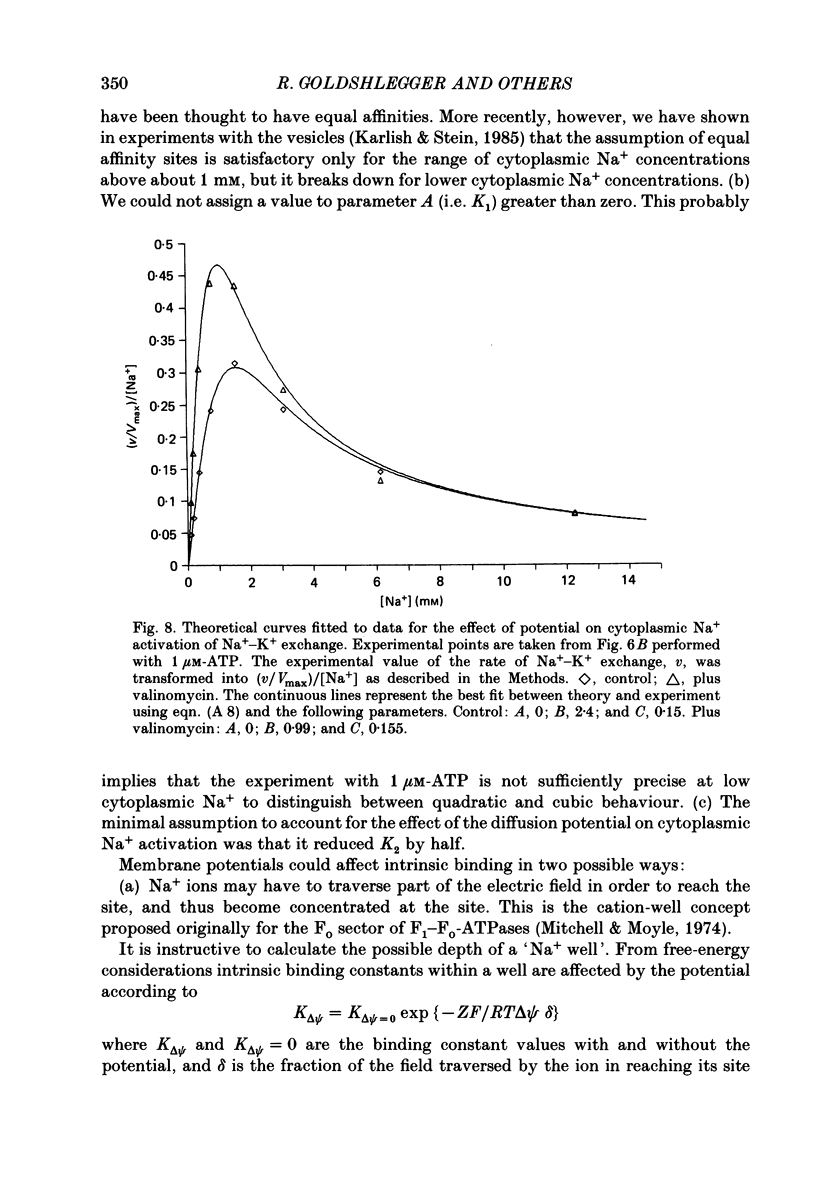
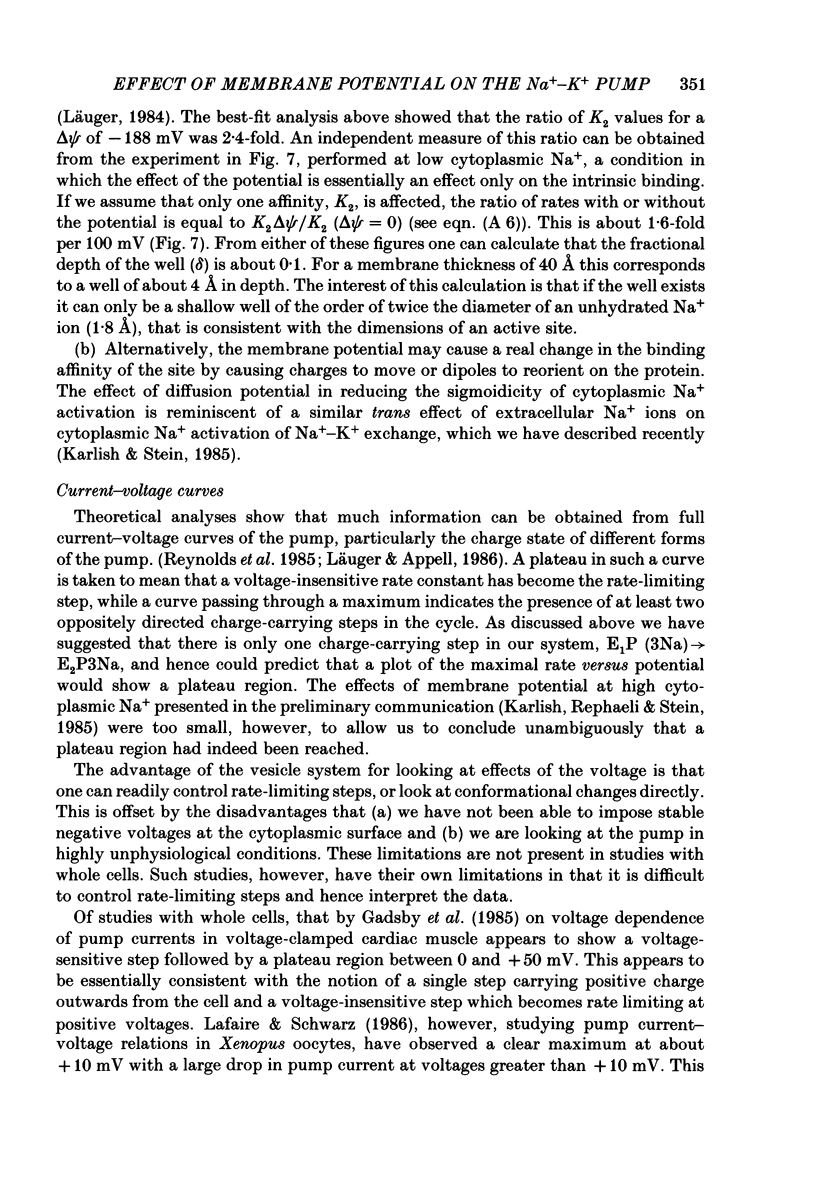
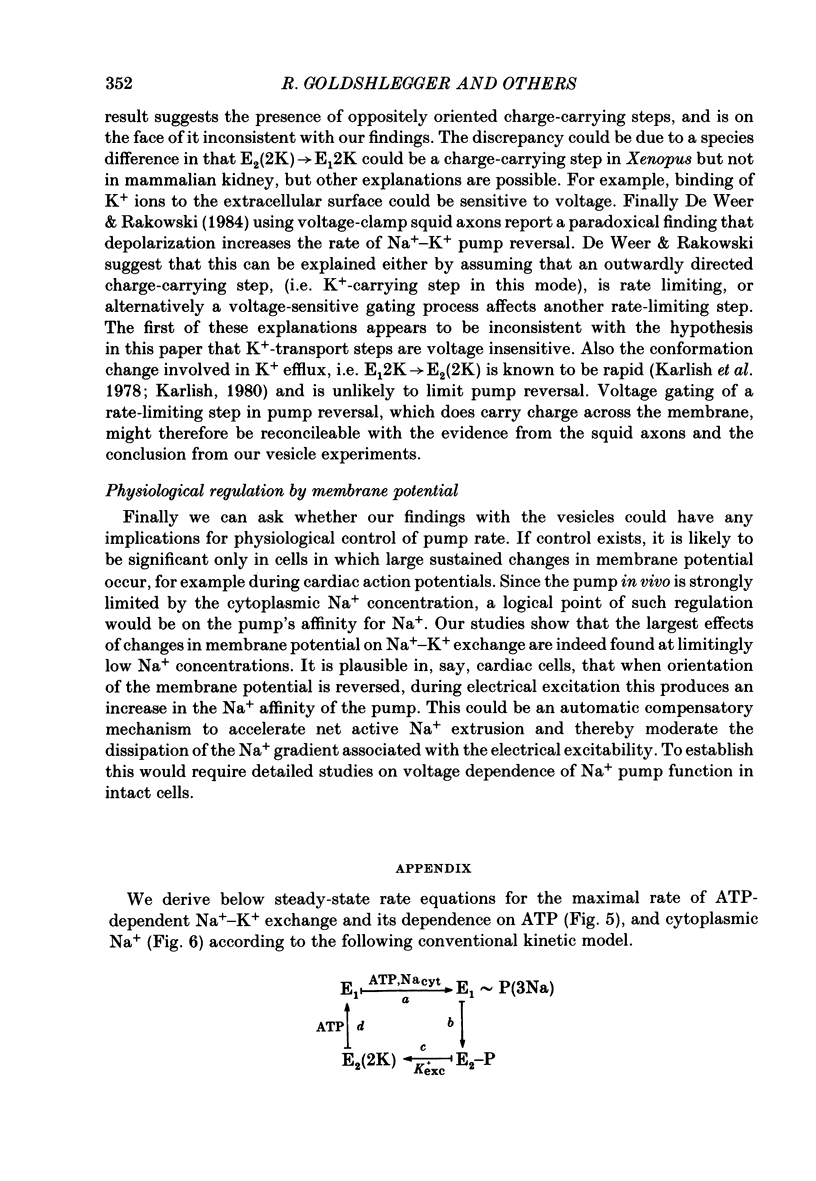
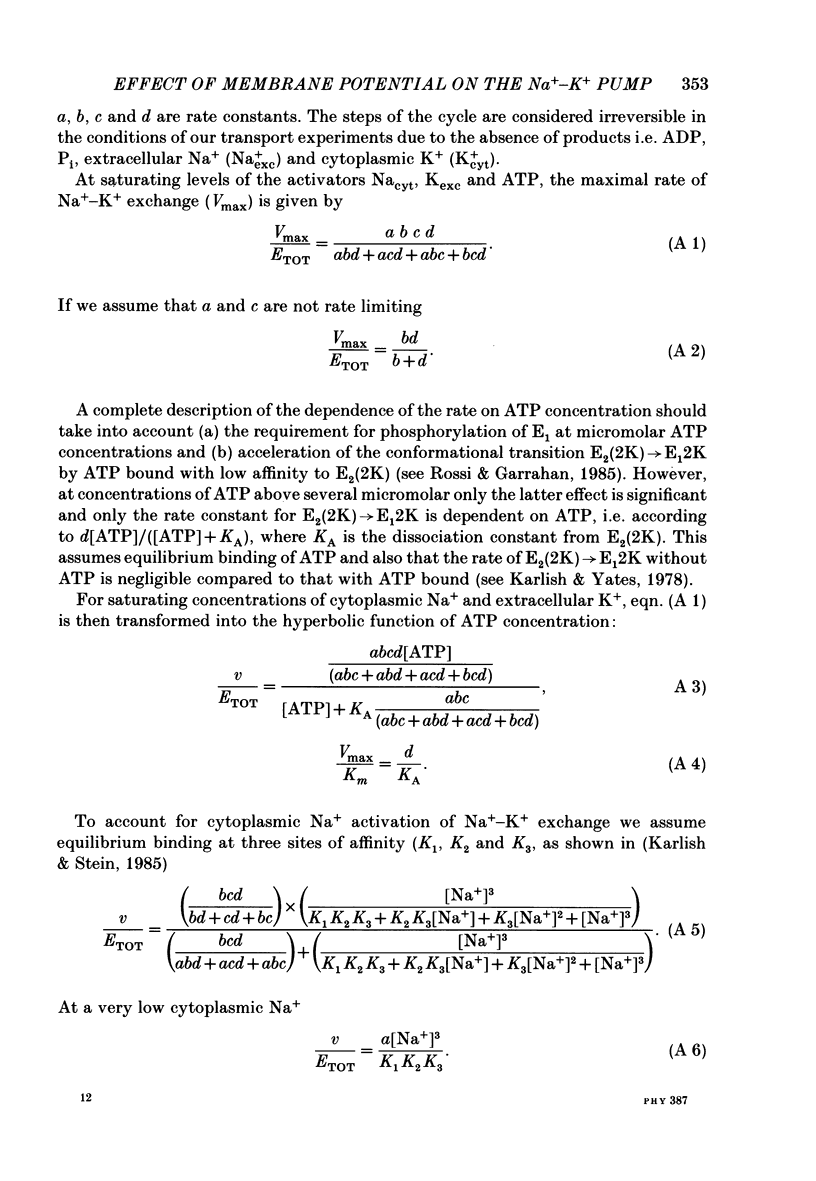
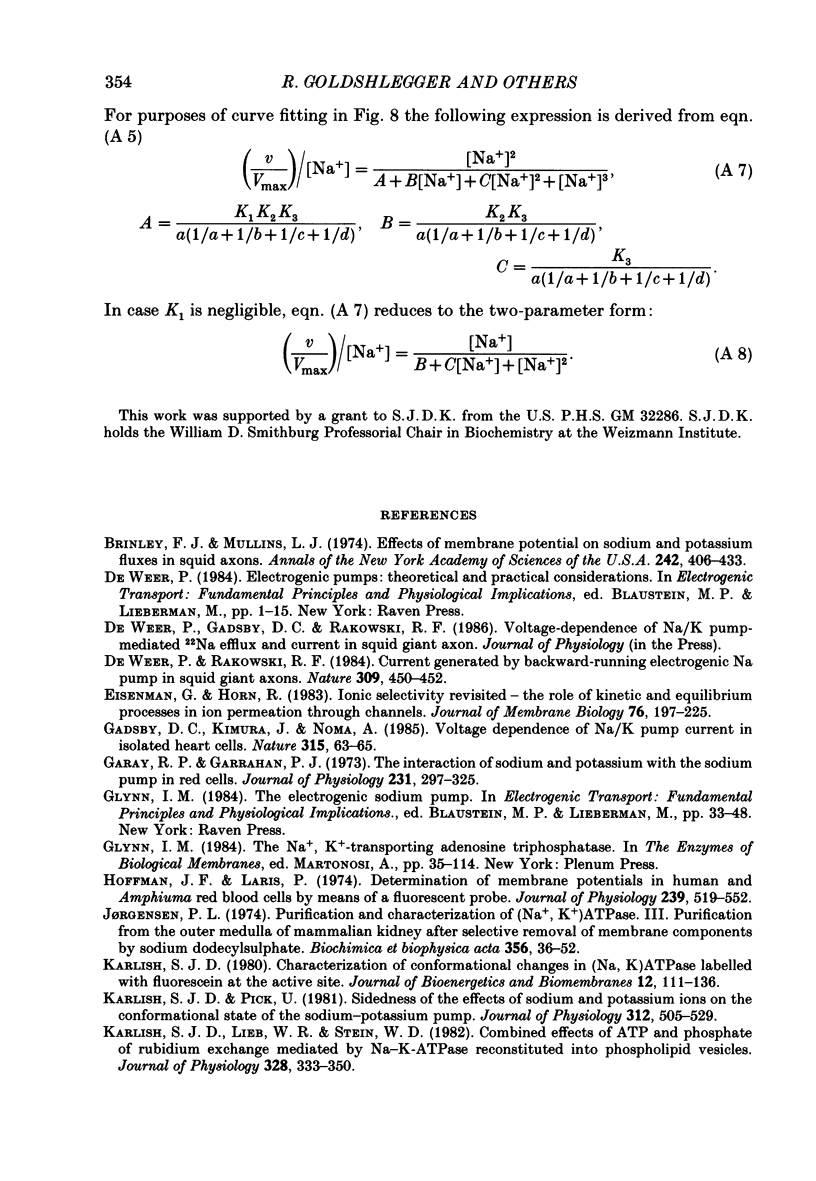
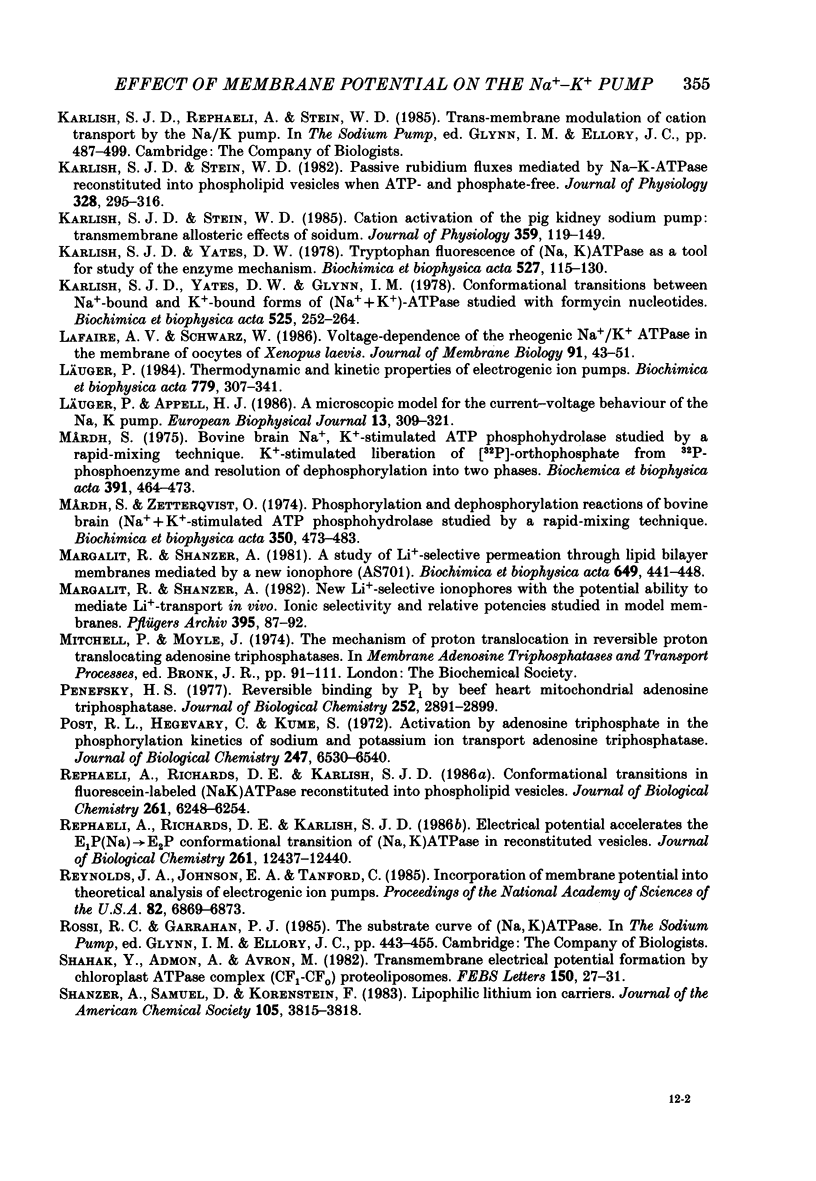
Selected References
These references are in PubMed. This may not be the complete list of references from this article.
- Brinley F. J., Jr, Mullins L. J. Effects of membrane potential on sodium and potassium fluxes in squid axons. Ann N Y Acad Sci. 1974;242(0):406–433. doi: 10.1111/j.1749-6632.1974.tb19106.x. [DOI] [PubMed] [Google Scholar]
- De Weer P., Rakowski R. F. Current generated by backward-running electrogenic Na pump in squid giant axons. 1984 May 31-Jun 6Nature. 309(5967):450–452. doi: 10.1038/309450a0. [DOI] [PubMed] [Google Scholar]
- Eisenman G., Horn R. Ionic selectivity revisited: the role of kinetic and equilibrium processes in ion permeation through channels. J Membr Biol. 1983;76(3):197–225. doi: 10.1007/BF01870364. [DOI] [PubMed] [Google Scholar]
- Gadsby D. C., Kimura J., Noma A. Voltage dependence of Na/K pump current in isolated heart cells. Nature. 1985 May 2;315(6014):63–65. doi: 10.1038/315063a0. [DOI] [PubMed] [Google Scholar]
- Garay R. P., Garrahan P. J. The interaction of sodium and potassium with the sodium pump in red cells. J Physiol. 1973 Jun;231(2):297–325. doi: 10.1113/jphysiol.1973.sp010234. [DOI] [PMC free article] [PubMed] [Google Scholar]
- Glynn I. M. The electrogenic sodium pump. Soc Gen Physiol Ser. 1984;38:33–48. [PubMed] [Google Scholar]
- Hoffman J. F., Laris P. C. Determination of membrane potentials in human and Amphiuma red blood cells by means of fluorescent probe. J Physiol. 1974 Jun;239(3):519–552. doi: 10.1113/jphysiol.1974.sp010581. [DOI] [PMC free article] [PubMed] [Google Scholar]
- Karlish S. J. Characterization of conformational changes in (Na,K) ATPase labeled with fluorescein at the active site. J Bioenerg Biomembr. 1980 Aug;12(3-4):111–136. doi: 10.1007/BF00744678. [DOI] [PubMed] [Google Scholar]
- Karlish S. J., Lieb W. R., Stein W. D. Combined effects of ATP and phosphate on rubidium exchange mediated by Na-K-ATPase reconstituted into phospholipid vesicles. J Physiol. 1982 Jul;328:333–350. doi: 10.1113/jphysiol.1982.sp014267. [DOI] [PMC free article] [PubMed] [Google Scholar]
- Karlish S. J., Pick U. Sidedness of the effects of sodium and potassium ions on the conformational state of the sodium-potassium pump. J Physiol. 1981 Mar;312:505–529. doi: 10.1113/jphysiol.1981.sp013641. [DOI] [PMC free article] [PubMed] [Google Scholar]
- Karlish S. J., Stein W. D. Cation activation of the pig kidney sodium pump: transmembrane allosteric effects of sodium. J Physiol. 1985 Feb;359:119–149. doi: 10.1113/jphysiol.1985.sp015578. [DOI] [PMC free article] [PubMed] [Google Scholar]
- Karlish S. J., Stein W. D. Passive rubidium fluxes mediated by Na-K-ATPase reconstituted into phospholipid vesicles when ATP- and phosphate-free. J Physiol. 1982 Jul;328:295–316. doi: 10.1113/jphysiol.1982.sp014265. [DOI] [PMC free article] [PubMed] [Google Scholar]
- Karlish S. J., Yates D. W., Glynn I. M. Conformational transitions between Na+-bound and K+-bound forms of (Na+ + K+)-ATPase, studied with formycin nucleotides. Biochim Biophys Acta. 1978 Jul 7;525(1):252–264. doi: 10.1016/0005-2744(78)90219-x. [DOI] [PubMed] [Google Scholar]
- Karlish S. J., Yates D. W. Tryptophan fluorescence of (Na+ + K+)-ATPase as a tool for study of the enzyme mechanism. Biochim Biophys Acta. 1978 Nov 10;527(1):115–130. doi: 10.1016/0005-2744(78)90261-9. [DOI] [PubMed] [Google Scholar]
- Lafaire A. V., Schwarz W. Voltage dependence of the rheogenic Na+/K+ ATPase in the membrane of oocytes of Xenopus laevis. J Membr Biol. 1986;91(1):43–51. doi: 10.1007/BF01870213. [DOI] [PubMed] [Google Scholar]
- Margalit R., Shanzar A. New Li+-selective ionophores with the potential ability to mediate Li+-transport in vivo. Ionic selectivity and relative potencies, studied in model membranes. Pflugers Arch. 1982 Nov 1;395(2):87–92. doi: 10.1007/BF00584719. [DOI] [PubMed] [Google Scholar]
- Margalit R., Shanzer A. A study of Li+-selective permeation through lipid bilayer membranes mediated by a new ionophore (AS701). Biochim Biophys Acta. 1981 Dec 7;649(2):441–448. doi: 10.1016/0005-2736(81)90434-x. [DOI] [PubMed] [Google Scholar]
- Mårdh S., Zetterqvist O. Phosphorylation and dephosphorylation reactions of bovine brain (Na+-K+)-stimulated ATP phosphohydrolase studied by a rapid mixing technique. Biochim Biophys Acta. 1974 Jun 18;350(2):473–483. doi: 10.1016/0005-2744(74)90523-3. [DOI] [PubMed] [Google Scholar]
- Penefsky H. S. Reversible binding of Pi by beef heart mitochondrial adenosine triphosphatase. J Biol Chem. 1977 May 10;252(9):2891–2899. [PubMed] [Google Scholar]
- Post R. L., Hegyvary C., Kume S. Activation by adenosine triphosphate in the phosphorylation kinetics of sodium and potassium ion transport adenosine triphosphatase. J Biol Chem. 1972 Oct 25;247(20):6530–6540. [PubMed] [Google Scholar]
- Rephaeli A., Richards D. E., Karlish S. J. Electrical potential accelerates the E1P(Na)----E2P conformational transition of (Na,K)-ATPase in reconstituted vesicles. J Biol Chem. 1986 Sep 25;261(27):12437–12440. [PubMed] [Google Scholar]
- Rephaeli A., Richards D., Karlish S. J. Conformational transitions in fluorescein-labeled (Na,K)ATPase reconstituted into phospholipid vesicles. J Biol Chem. 1986 May 15;261(14):6248–6254. [PubMed] [Google Scholar]
- Reynolds J. A., Johnson E. A., Tanford C. Incorporation of membrane potential into theoretical analysis of electrogenic ion pumps. Proc Natl Acad Sci U S A. 1985 Oct;82(20):6869–6873. doi: 10.1073/pnas.82.20.6869. [DOI] [PMC free article] [PubMed] [Google Scholar]