Abstract
1. When the Na+-K+ pump of cultured embryonic chick heart cells was inhibited by addition of ouabain with or without removal of external K+, the membrane potential rapidly depolarized to -40 mV and the Na+ content approximately doubled within 3 min. 2. After this, exposure to an [Na+]o of 27 mM caused a fall in Na+ content, a gain in Ca2+ content and a hyperpolarization. The hyperpolarization was approximately 25 mV in a [K+]o of 0 or 5.4 mM after 3 min of pump inhibition. After approximately 10 min of pump inhibition, the same hyperpolarization was observed in a [K+]o of 5.4 mM but in K+-free solution the hyperpolarization increased to approximately 44 mV. 3. Varying [K+]o during the 10 min period of Na+-K+ pump inhibition showed that the increase in hyperpolarization was associated with the period of exposure to K+-free solution rather than the [K+]o at the time of lowering [Na+]o. 4. Changes in Na+ and Ca2+ content induced by exposure to an [Na+]o of 27 mM in K+-free solution were similar at 3 and 10 min. This and the above observations suggest that the increased hyperpolarization was due to an increased membrane resistance. 5. 10 mM-Cs+ reduced the low-[Na+]o hyperpolarization by 26% but did not significantly affect the movements of Na+ and Ca2+. 1 mM-La3+ reduced the low-[Na+]o hyperpolarization by 15%: it also totally blocked the rise in Ca2+ content and partially blocked the fall in Na+ content. 1 mM-Ba2+ reduced the low-[Na+]o hyperpolarization by 20%. 6. Raising [Ca2+]o from 2.7 to 13.5 mM produced similar but smaller hyperpolarizations (approximately 6 mV after 3 min pump inhibition). High [Ca2+]o caused a rise in Ca2+ content but no significant drop in Na+ content. The hyperpolarization in high [Ca2+]o was insensitive to verapamil (20 microM) and 10 mM-Cs+. 7. We conclude from the disparities between the magnitudes of the hyperpolarizations and the changes in ion contents that Na+-Ca2+ exchange cannot be unequivocally identified as electrogenic solely from the low-[Na+]o hyperpolarizations.
Full text
PDF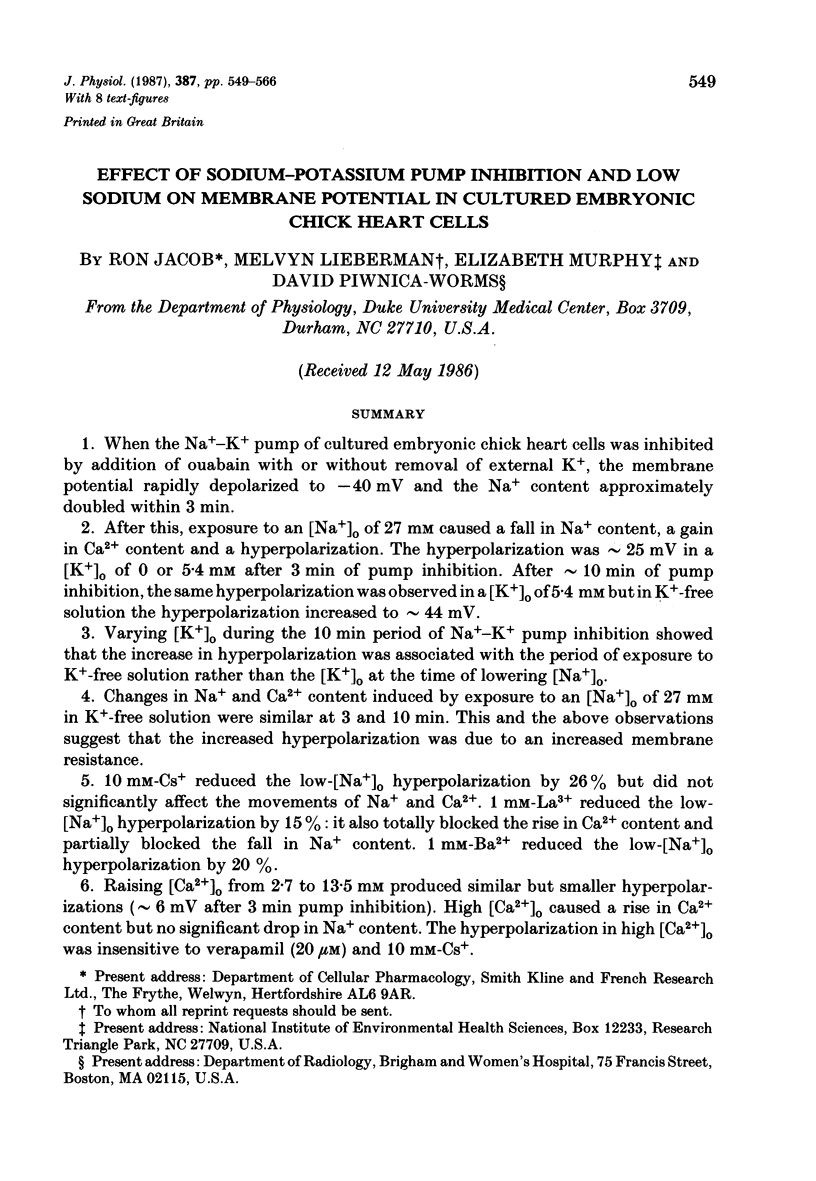
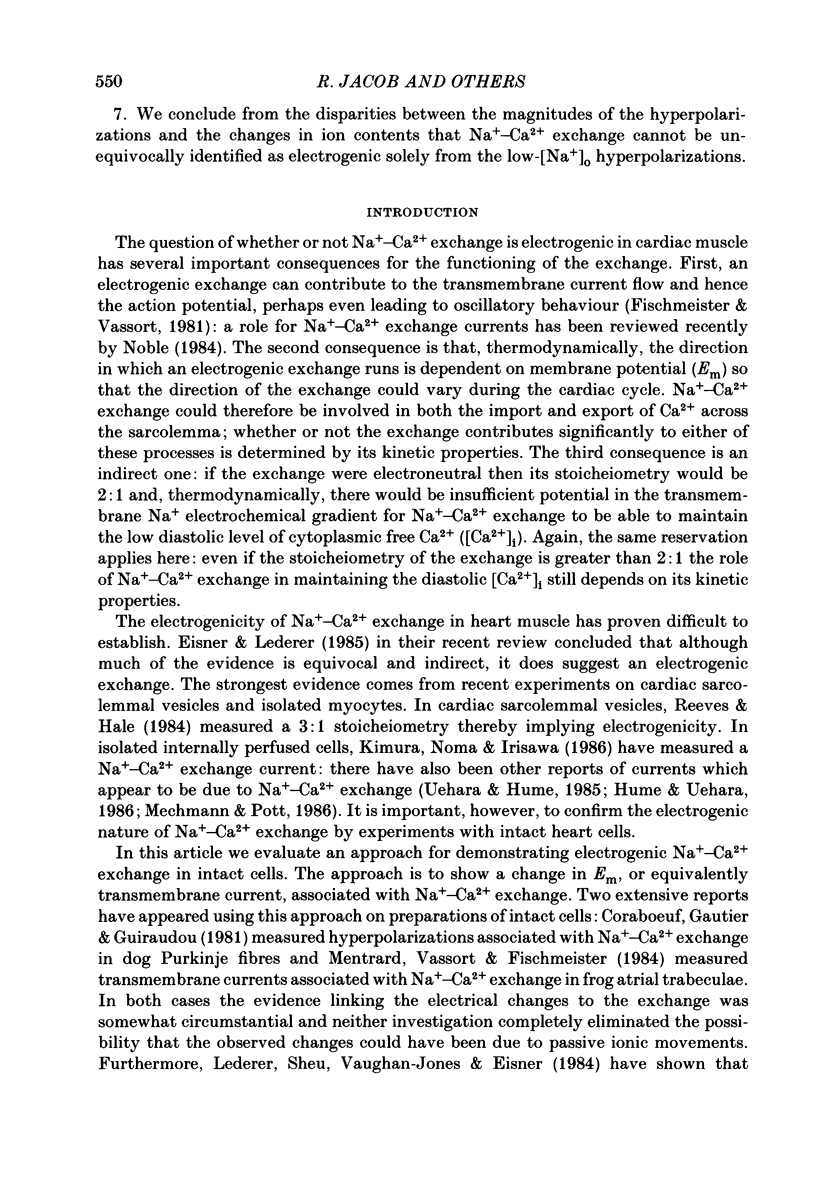
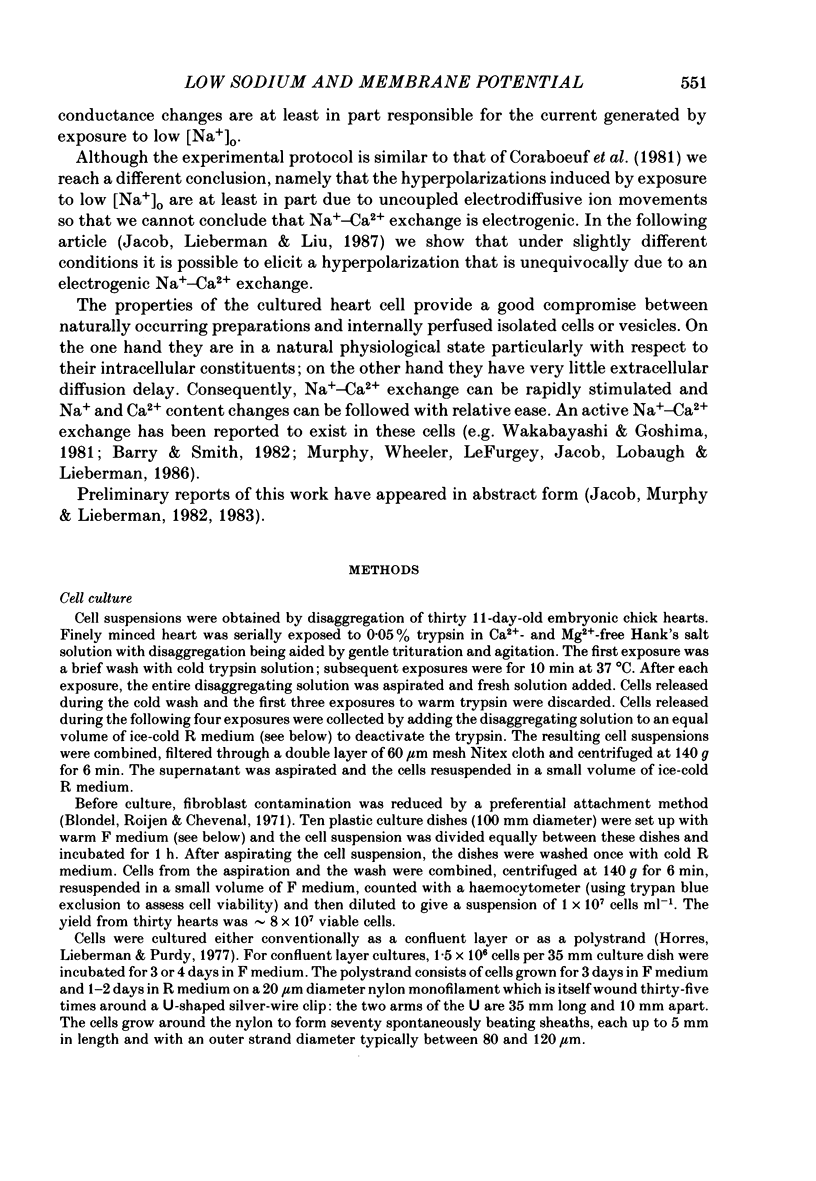
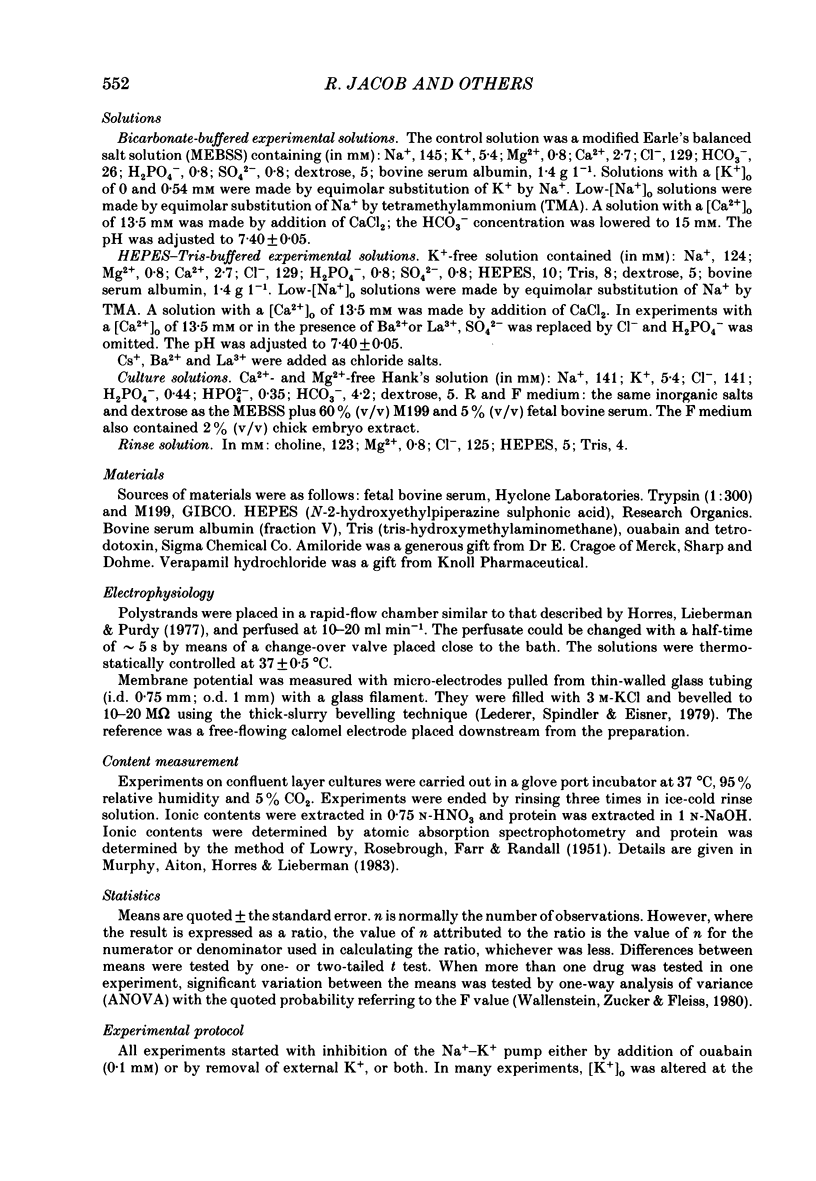
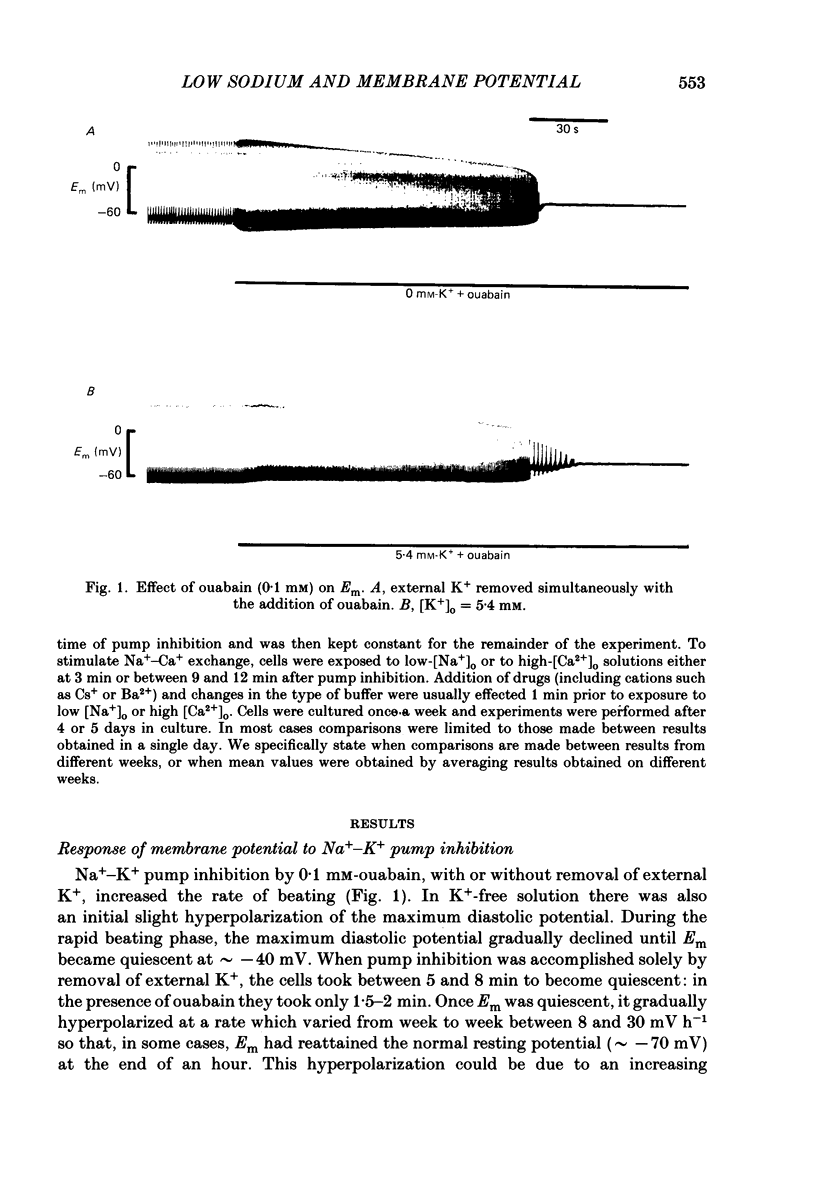
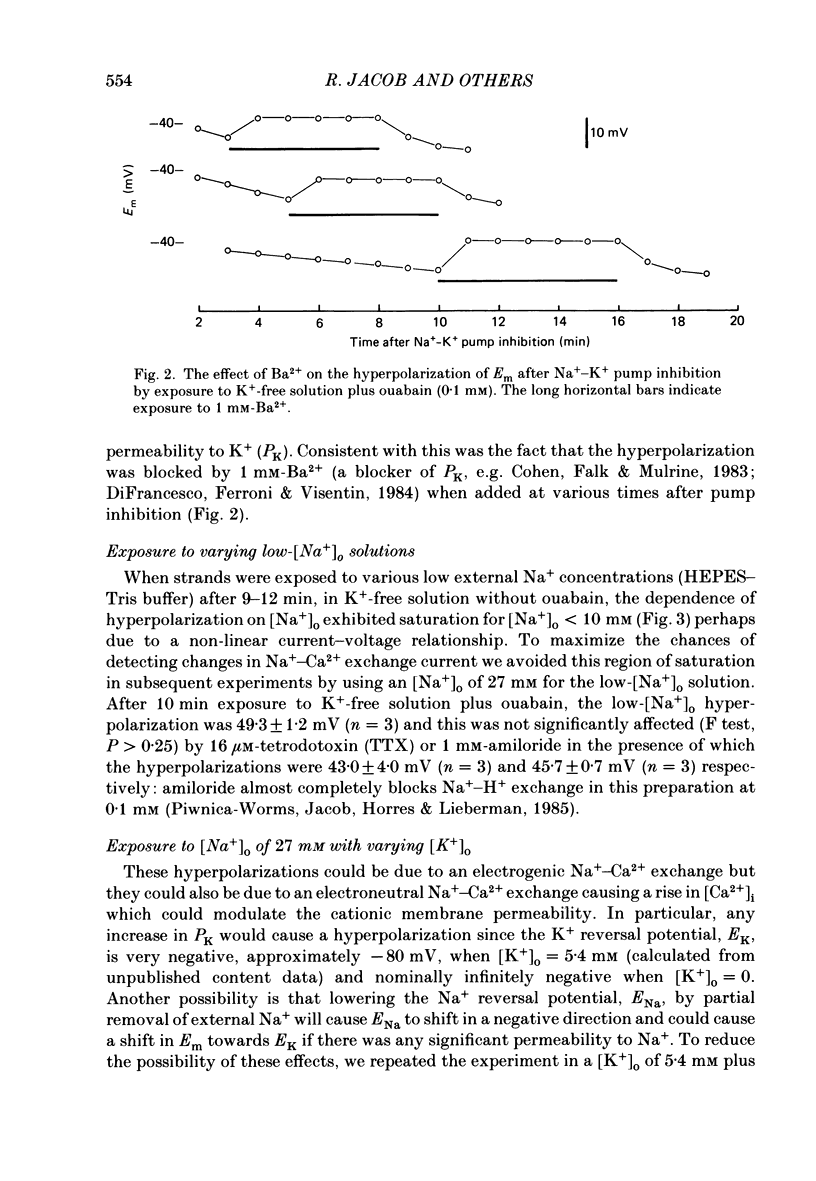
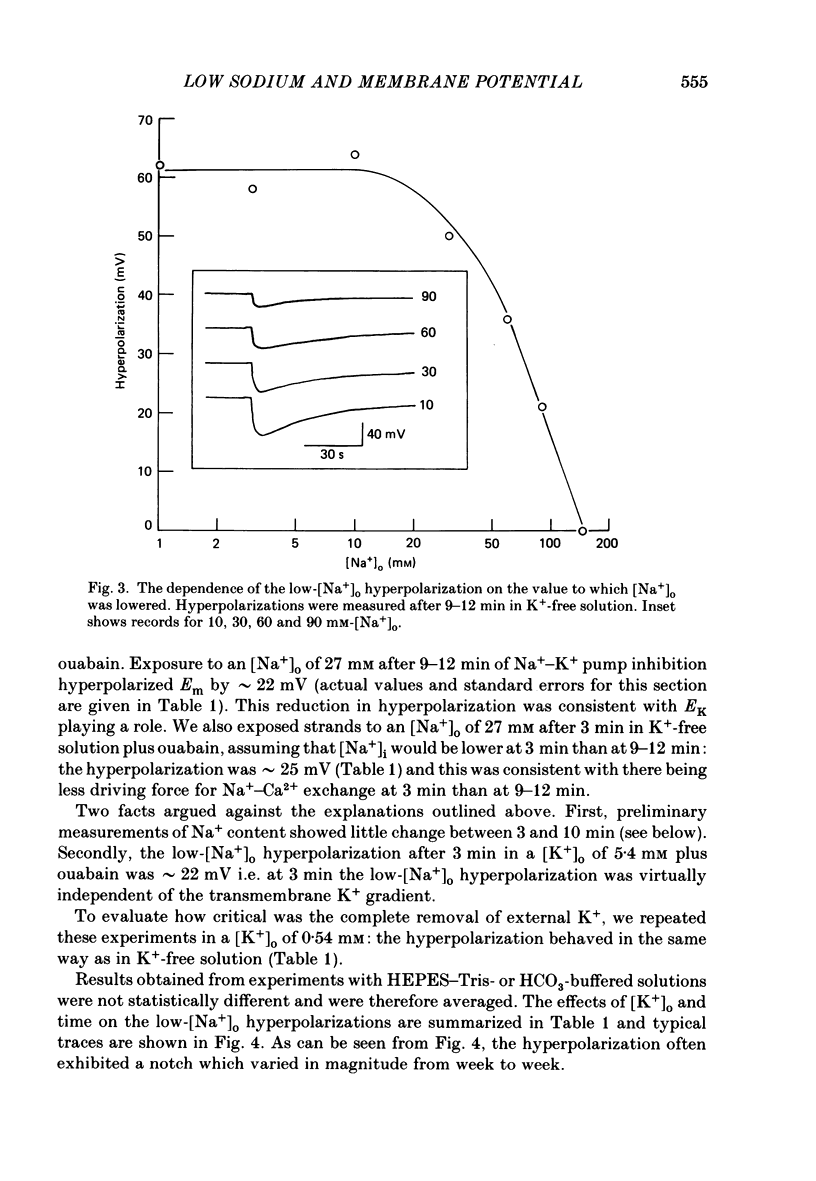
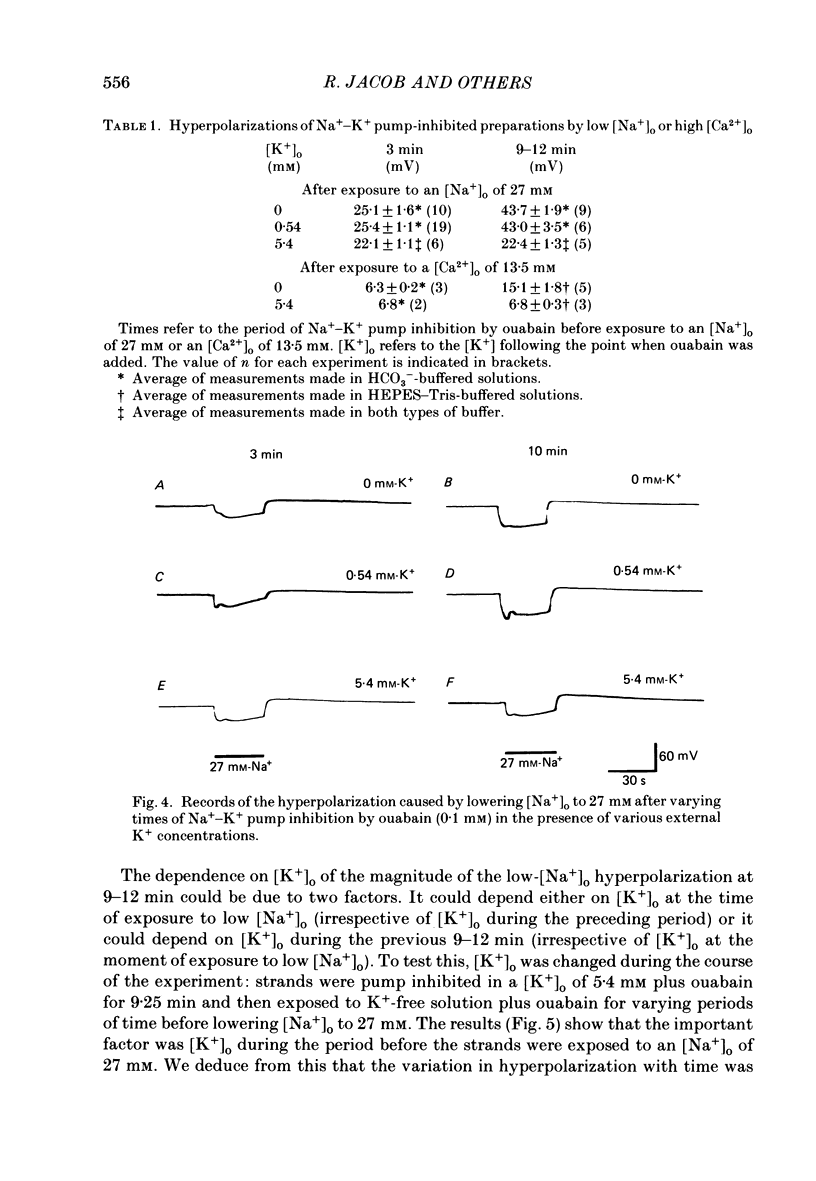
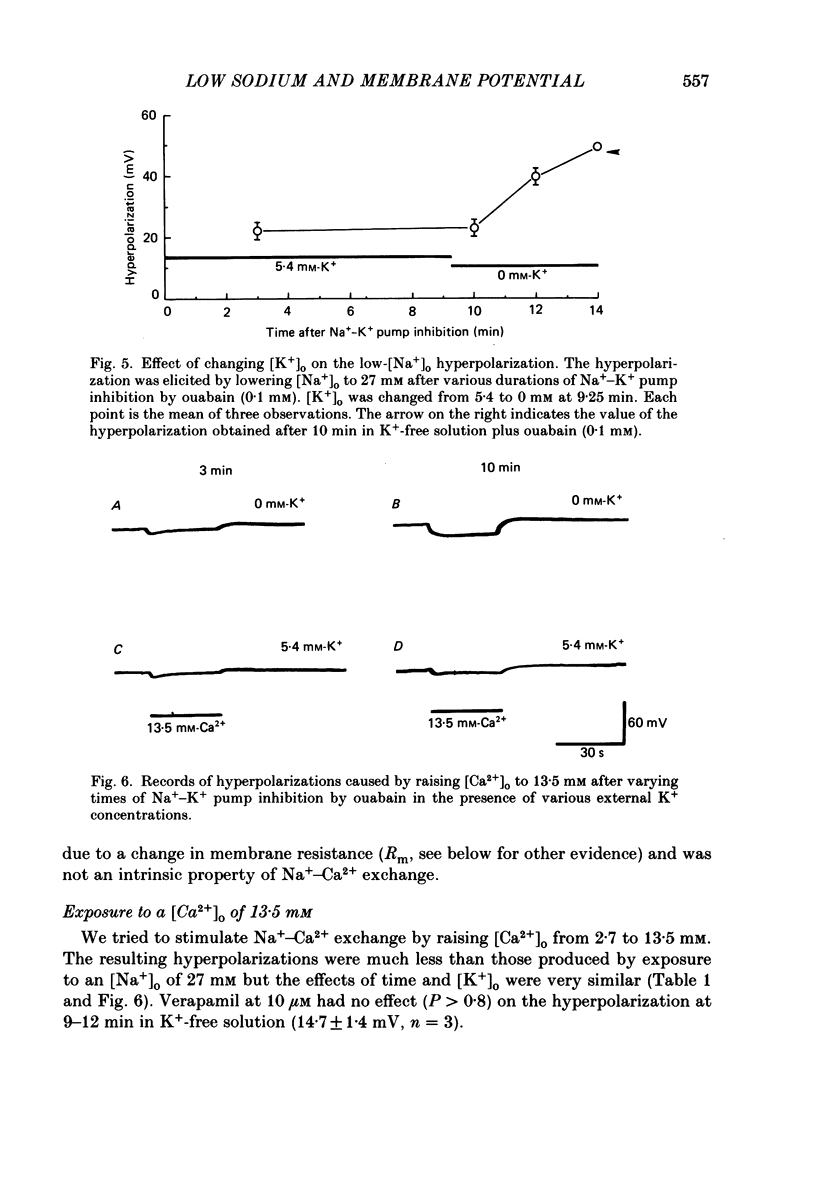
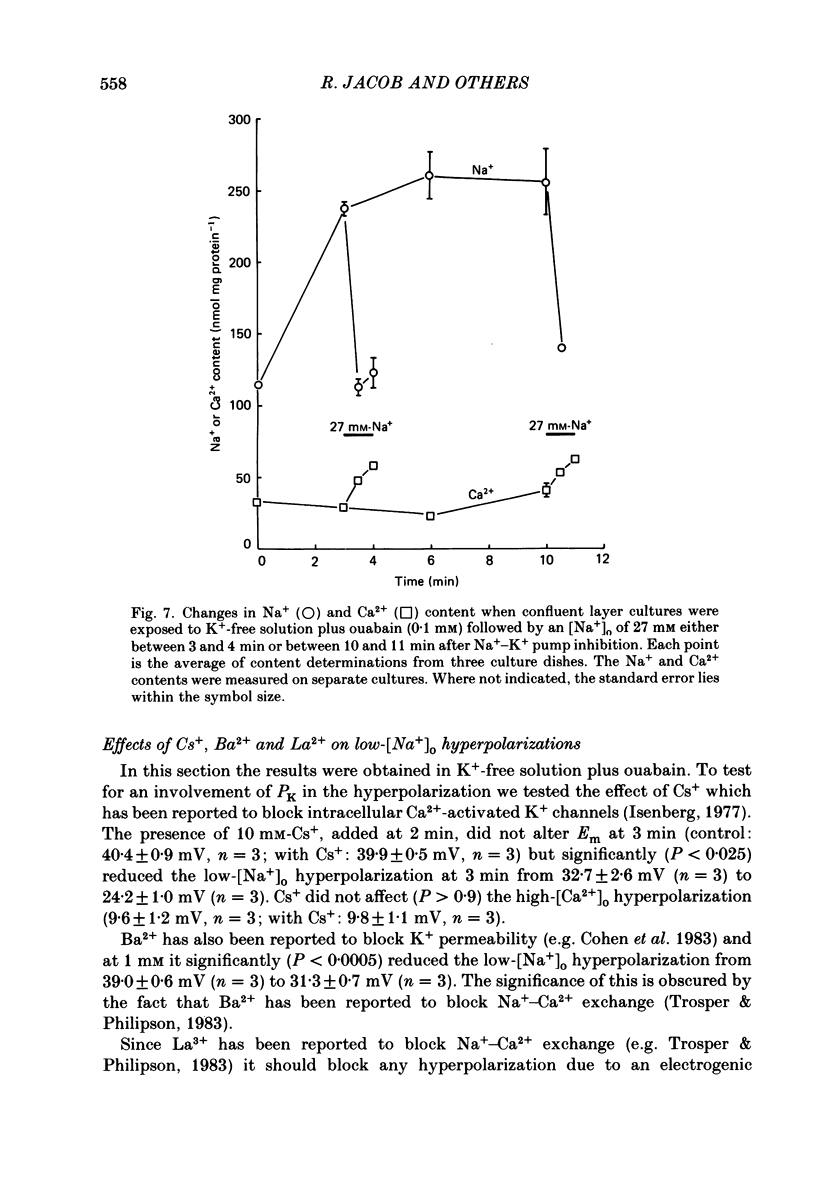
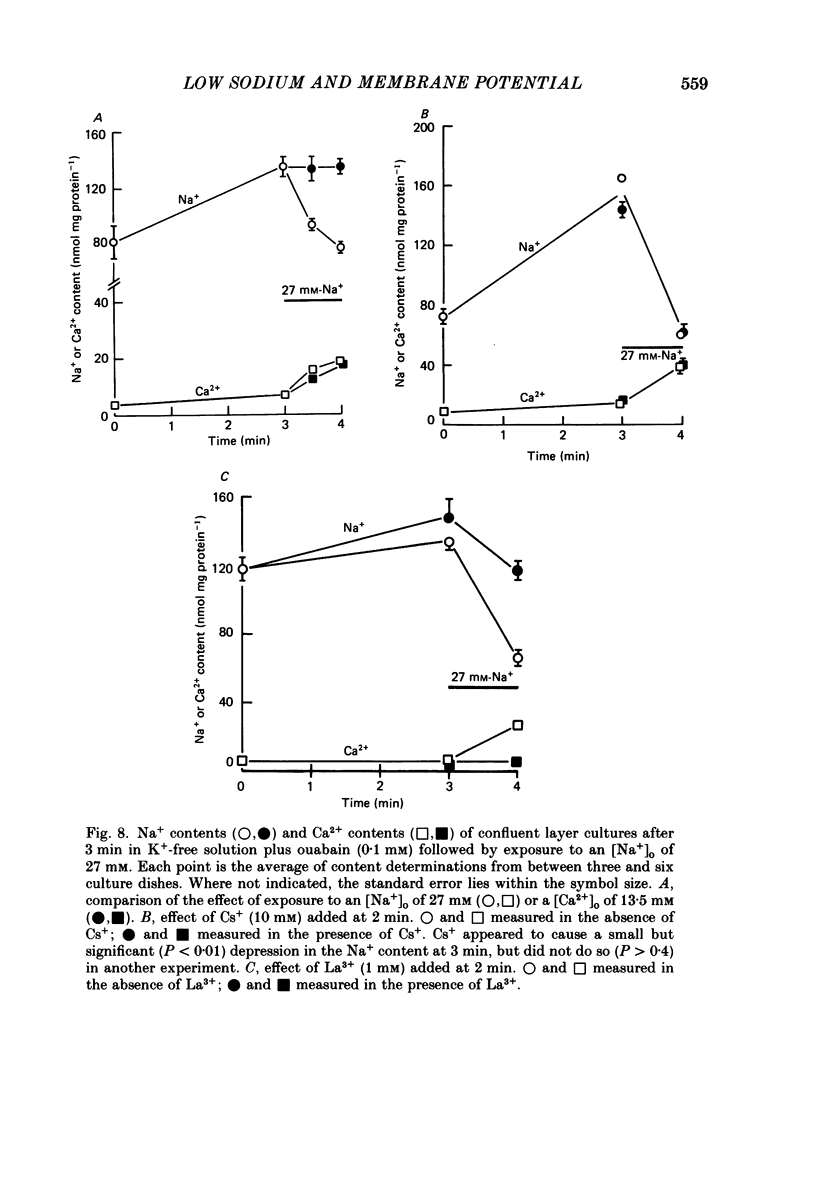
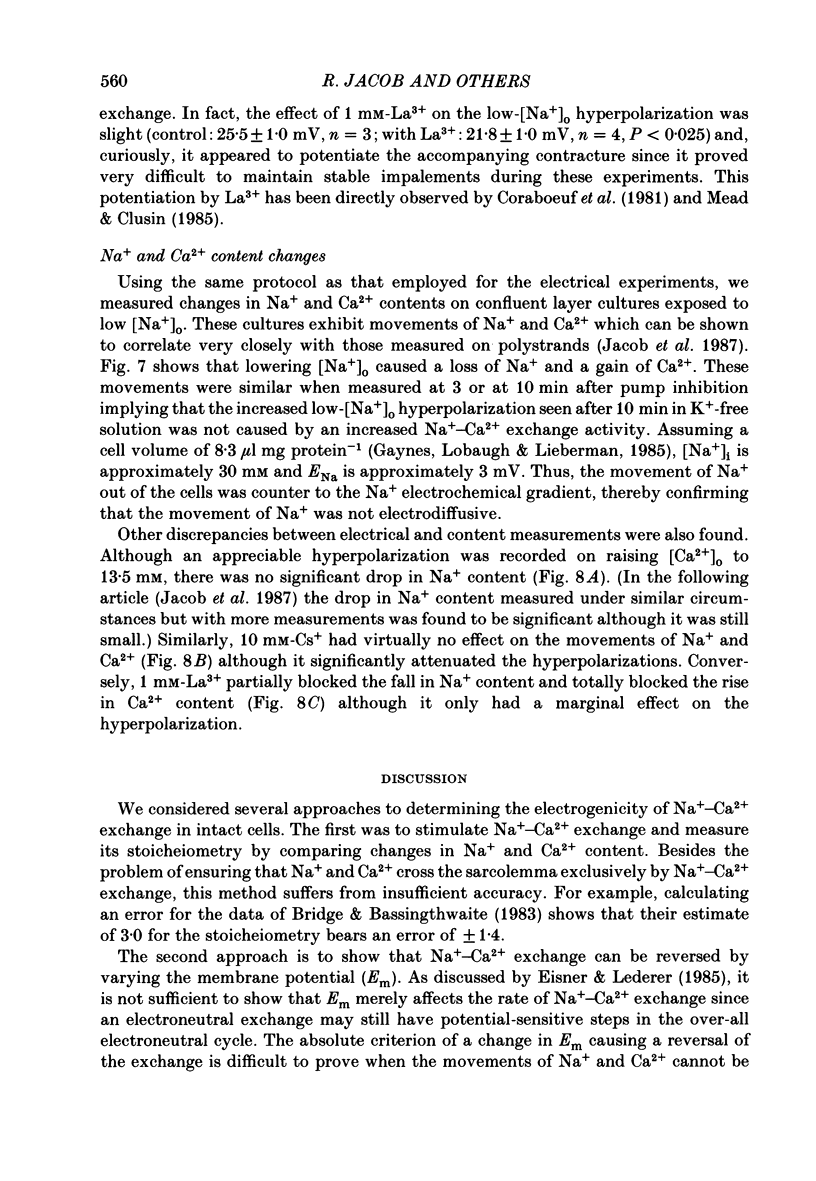
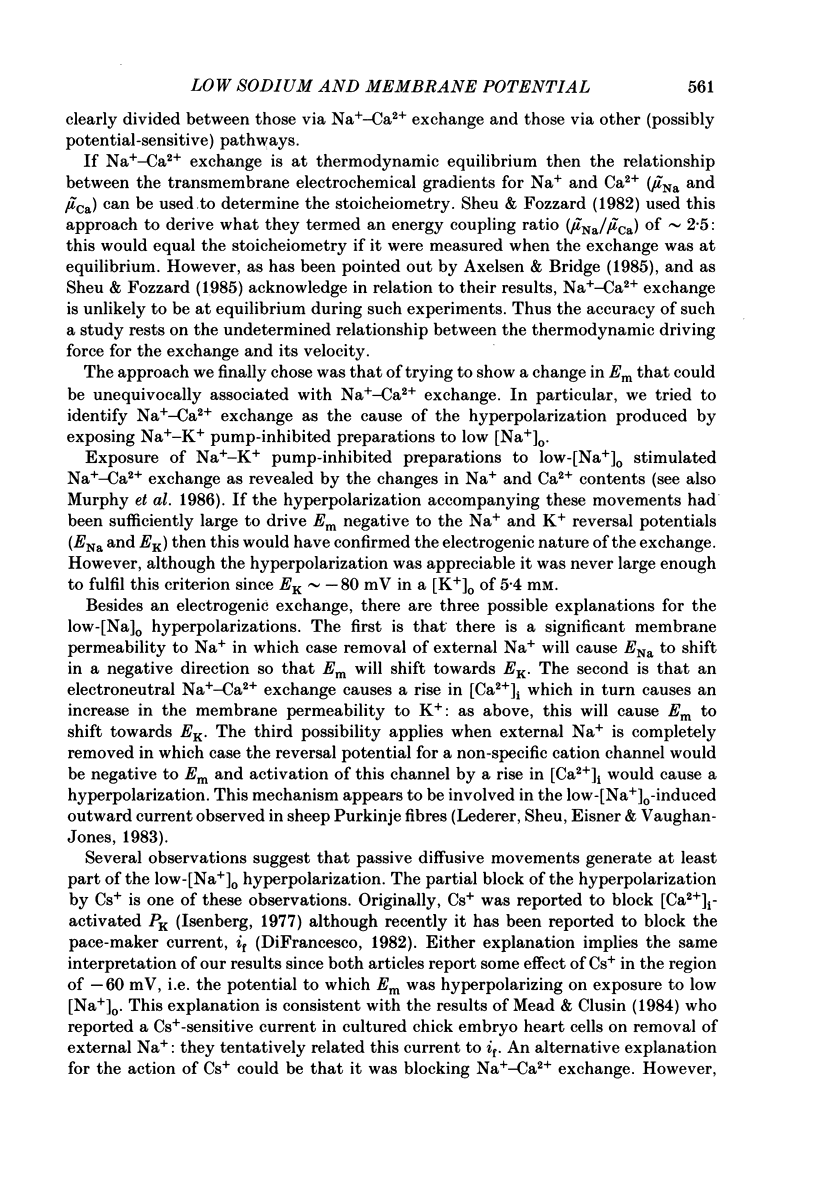
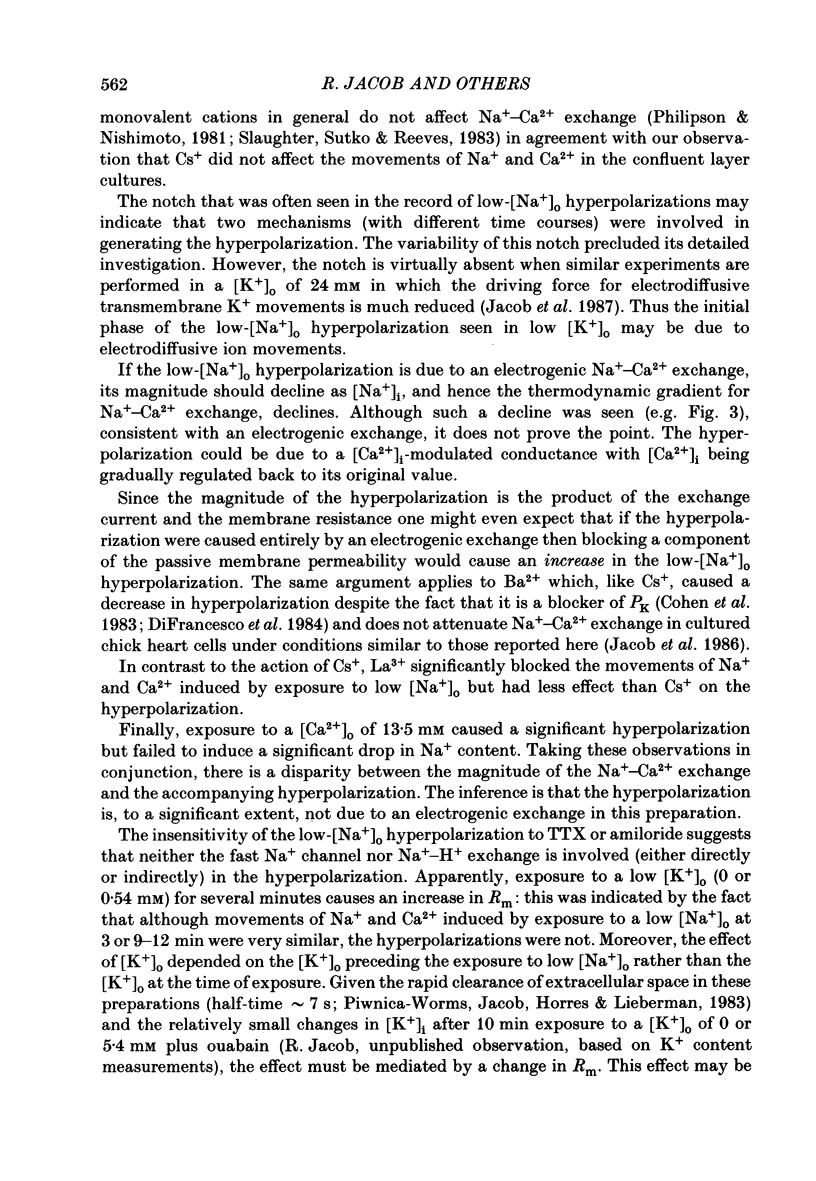
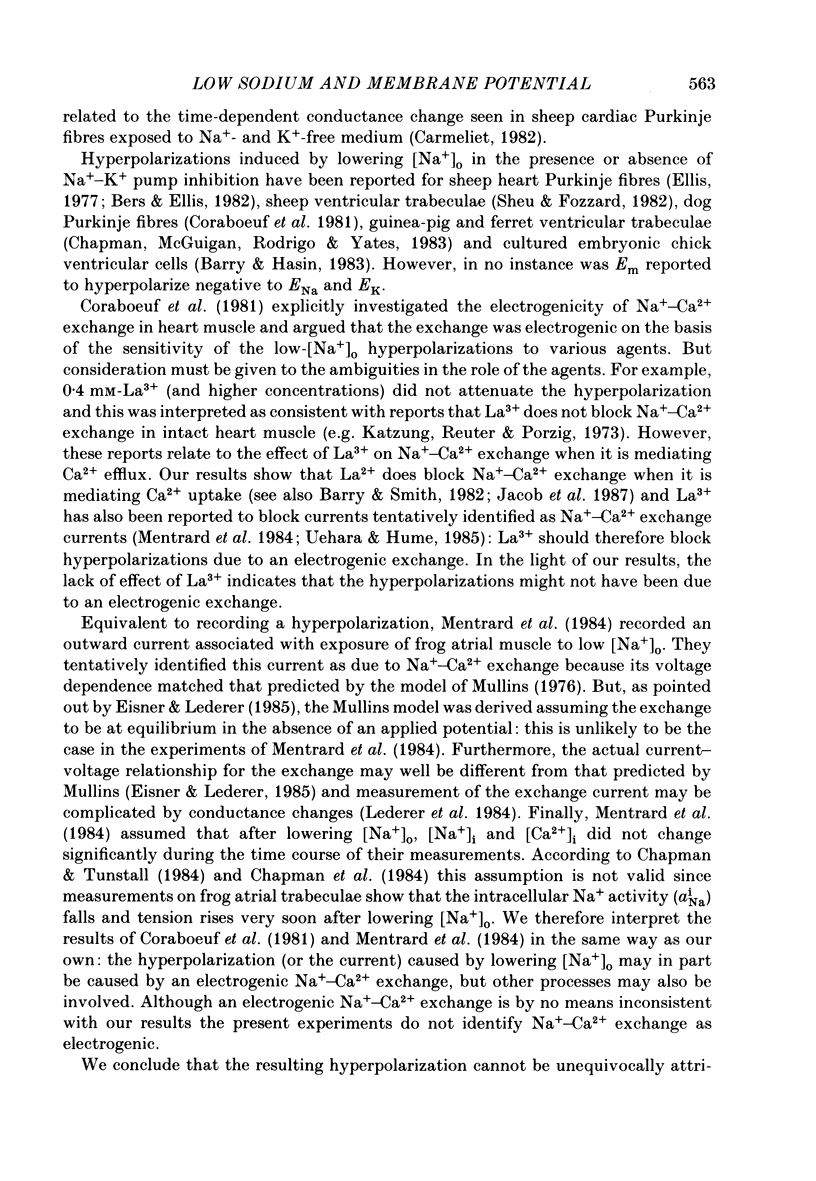
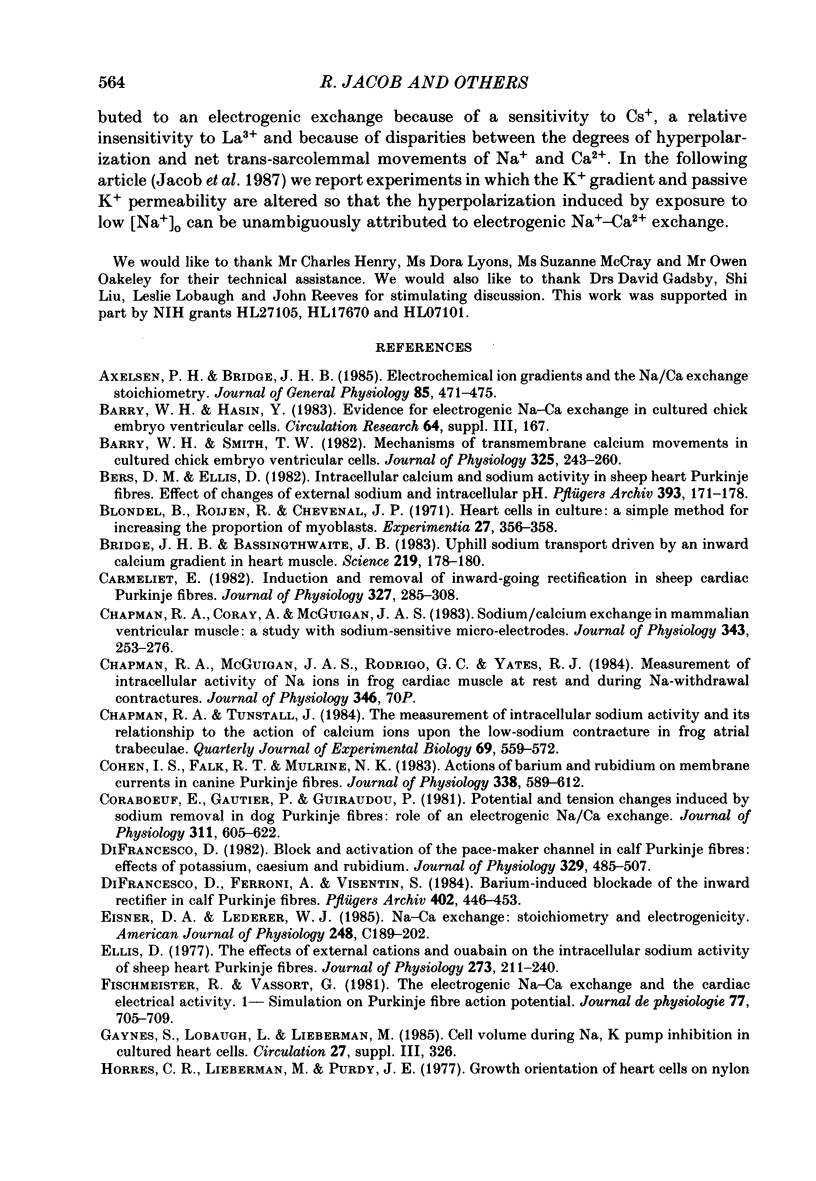
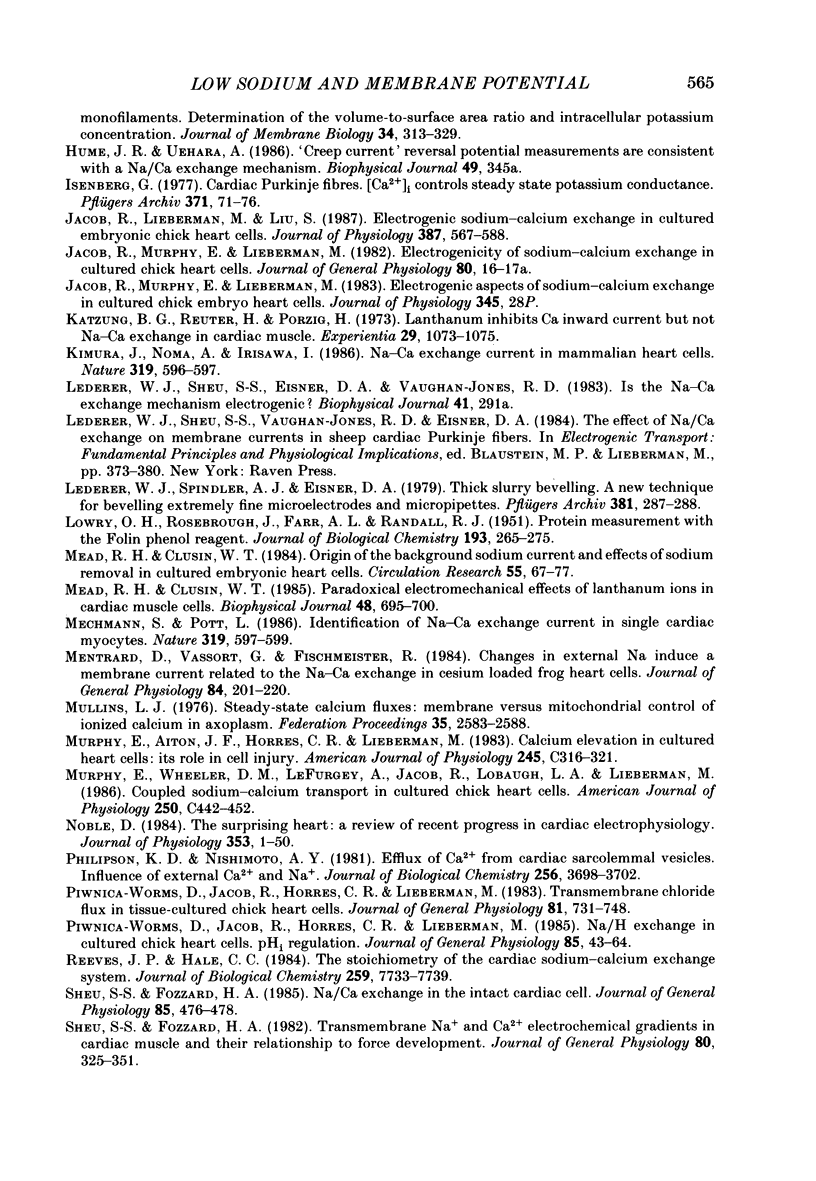
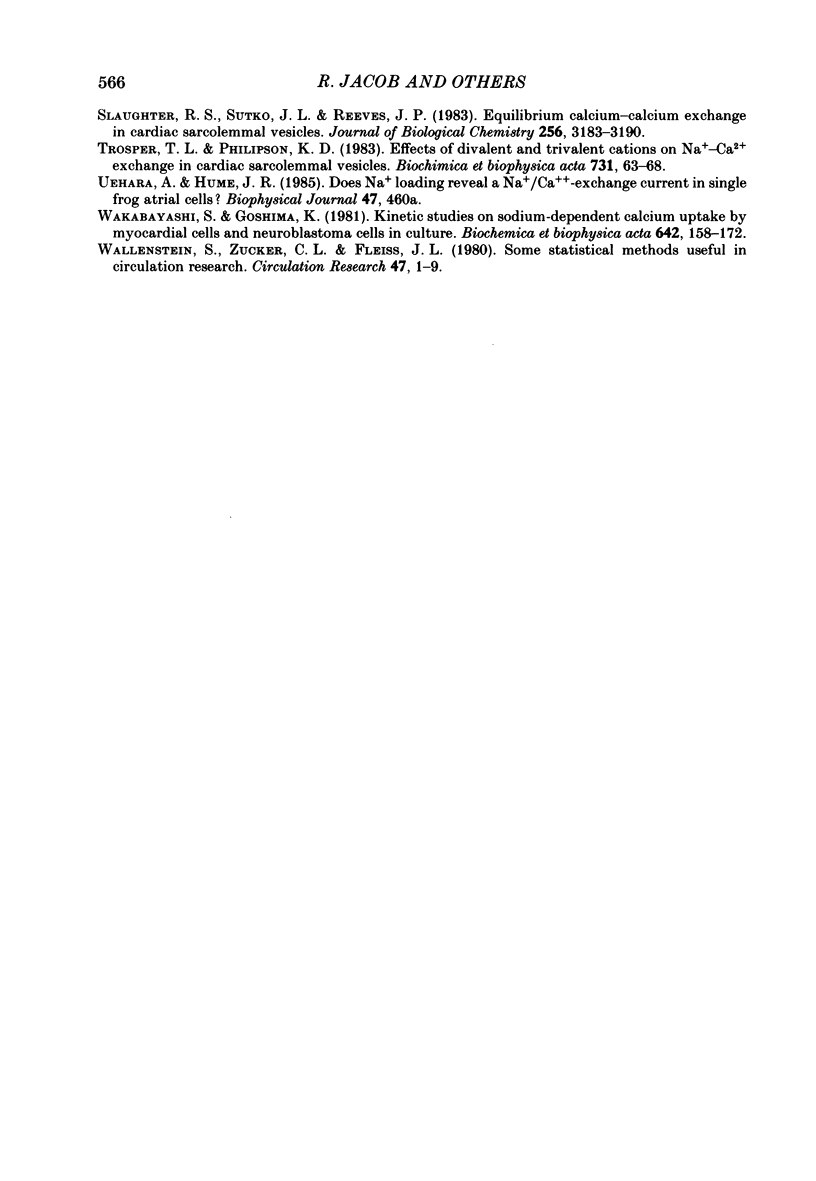
Selected References
These references are in PubMed. This may not be the complete list of references from this article.
- Axelsen P. H., Bridge J. H. Electrochemical ion gradients and the Na/Ca exchange stoichiometry. Measurements of these gradients are thermodynamically consistent with a stoichiometric coefficient greater than or equal to 3. J Gen Physiol. 1985 Mar;85(3):471–475. doi: 10.1085/jgp.85.3.471. [DOI] [PMC free article] [PubMed] [Google Scholar]
- Barry W. H., Smith T. W. Mechanisms of transmembrane calcium movement in cultured chick embryo ventricular cells. J Physiol. 1982 Apr;325:243–260. doi: 10.1113/jphysiol.1982.sp014148. [DOI] [PMC free article] [PubMed] [Google Scholar]
- Bers D. M., Ellis D. Intracellular calcium and sodium activity in sheep heart Purkinje fibres. Effect of changes of external sodium and intracellular pH. Pflugers Arch. 1982 Apr;393(2):171–178. doi: 10.1007/BF00582941. [DOI] [PubMed] [Google Scholar]
- Blondel B., Roijen I., Cheneval J. P. Heart cells in culture: a simple method for increasing the proportion of myoblasts. Experientia. 1971 Mar 15;27(3):356–358. doi: 10.1007/BF02138197. [DOI] [PubMed] [Google Scholar]
- Bridge J. H., Bassingthwaighte J. B. Uphill sodium transport driven by an inward calcium gradient in heart muscle. Science. 1983 Jan 14;219(4581):178–180. doi: 10.1126/science.6849128. [DOI] [PMC free article] [PubMed] [Google Scholar]
- Carmeliet E. Induction and removal of inward-going rectification in sheep cardiac Purkinje fibres. J Physiol. 1982 Jun;327:285–308. doi: 10.1113/jphysiol.1982.sp014232. [DOI] [PMC free article] [PubMed] [Google Scholar]
- Chapman R. A., Coray A., McGuigan J. A. Sodium/calcium exchange in mammalian ventricular muscle: a study with sodium-sensitive micro-electrodes. J Physiol. 1983 Oct;343:253–276. doi: 10.1113/jphysiol.1983.sp014891. [DOI] [PMC free article] [PubMed] [Google Scholar]
- Chapman R. A., Tunstall J. The measurement of intracellular sodium activity and its relationship to the action of calcium ions upon the low-sodium contracture in frog atrial trabeculae. Q J Exp Physiol. 1984 Jul;69(3):559–572. doi: 10.1113/expphysiol.1984.sp002842. [DOI] [PubMed] [Google Scholar]
- Cohen I. S., Falk R. T., Mulrine N. K. Actions of barium and rubidium on membrane currents in canine Purkinje fibres. J Physiol. 1983 May;338:589–612. doi: 10.1113/jphysiol.1983.sp014691. [DOI] [PMC free article] [PubMed] [Google Scholar]
- Croaboeuf E., Gautier P., Giuraudou P. Potential and tension changes induced by sodium removal in dog Purkinje fibres: role of an electrogenic sodium-calcium exchange. J Physiol. 1981 Feb;311:605–622. doi: 10.1113/jphysiol.1981.sp013607. [DOI] [PMC free article] [PubMed] [Google Scholar]
- DiFrancesco D. Block and activation of the pace-maker channel in calf purkinje fibres: effects of potassium, caesium and rubidium. J Physiol. 1982 Aug;329:485–507. doi: 10.1113/jphysiol.1982.sp014315. [DOI] [PMC free article] [PubMed] [Google Scholar]
- DiFrancesco D., Ferroni A., Visentin S. Barium-induced blockade of the inward rectifier in calf Purkinje fibres. Pflugers Arch. 1984 Dec;402(4):446–453. doi: 10.1007/BF00583946. [DOI] [PubMed] [Google Scholar]
- Eisner D. A., Lederer W. J. Na-Ca exchange: stoichiometry and electrogenicity. Am J Physiol. 1985 Mar;248(3 Pt 1):C189–C202. doi: 10.1152/ajpcell.1985.248.3.C189. [DOI] [PubMed] [Google Scholar]
- Ellis D. The effects of external cations and ouabain on the intracellular sodium activity of sheep heart Purkinje fibres. J Physiol. 1977 Dec;273(1):211–240. doi: 10.1113/jphysiol.1977.sp012090. [DOI] [PMC free article] [PubMed] [Google Scholar]
- Fischmeister R., Vassort G. The electrogenic Na-Ca exchange and the cardiac electrical activity. I--Simulation on Purkinje fibre action potential. J Physiol (Paris) 1981 Sep;77(6-7):705–709. [PubMed] [Google Scholar]
- Horres C. R., Lieberman M., Purdy J. E. Growth orientation of heart cells on nylon monofilament. Determination of the volume-to-surface area ratio and intracellular potassium concentration. J Membr Biol. 1977 Jun 15;34(4):313–329. doi: 10.1007/BF01870306. [DOI] [PubMed] [Google Scholar]
- Isenberg G. Cardiac Purkinje fibres: [Ca2+]i controls steady state potassium conductance. Pflugers Arch. 1977 Oct 19;371(1-2):71–76. doi: 10.1007/BF00580774. [DOI] [PubMed] [Google Scholar]
- Jacob R., Lieberman M., Liu S. Electrogenic sodium-calcium exchange in cultured embryonic chick heart cells. J Physiol. 1987 Jun;387:567–588. doi: 10.1113/jphysiol.1987.sp016589. [DOI] [PMC free article] [PubMed] [Google Scholar]
- Katzung B. G., Reuter H., Porzig H. Lanthanum inhibits Ca inward current but not Na-Ca exchange in cardiac muscle. Experientia. 1973 Sep 15;29(9):1073–1075. doi: 10.1007/BF01946727. [DOI] [PubMed] [Google Scholar]
- Kimura J., Noma A., Irisawa H. Na-Ca exchange current in mammalian heart cells. Nature. 1986 Feb 13;319(6054):596–597. doi: 10.1038/319596a0. [DOI] [PubMed] [Google Scholar]
- LOWRY O. H., ROSEBROUGH N. J., FARR A. L., RANDALL R. J. Protein measurement with the Folin phenol reagent. J Biol Chem. 1951 Nov;193(1):265–275. [PubMed] [Google Scholar]
- Lederer W. J., Sheu S. S., Vaughan-Jones R. D., Eisner D. A. The effects of Na-Ca exchange on membrane currents in sheep cardiac Purkinje fibers. Soc Gen Physiol Ser. 1984;38:373–380. [PubMed] [Google Scholar]
- Lederer W. J., Spindler A. J., Eisner D. A. Thick slurry bevelling: a new technique for bevelling extremely fine microelectrodes and micropipettes. Pflugers Arch. 1979 Sep;381(3):287–288. doi: 10.1007/BF00583261. [DOI] [PubMed] [Google Scholar]
- Mead R. H., Clusin W. T. Origin of the background sodium current and effects of sodium removal in cultured embryonic cardiac cells. Circ Res. 1984 Jul;55(1):67–77. doi: 10.1161/01.res.55.1.67. [DOI] [PubMed] [Google Scholar]
- Mead R. H., Clusin W. T. Paradoxical electromechanical effect of lanthanum ions in cardiac muscle cells. Biophys J. 1985 Nov;48(5):695–700. doi: 10.1016/S0006-3495(85)83827-3. [DOI] [PMC free article] [PubMed] [Google Scholar]
- Mechmann S., Pott L. Identification of Na-Ca exchange current in single cardiac myocytes. Nature. 1986 Feb 13;319(6054):597–599. doi: 10.1038/319597a0. [DOI] [PubMed] [Google Scholar]
- Mentrard D., Vassort G., Fischmeister R. Changes in external Na induce a membrane current related to the Na-Ca exchange in cesium-loaded frog heart cells. J Gen Physiol. 1984 Aug;84(2):201–220. doi: 10.1085/jgp.84.2.201. [DOI] [PMC free article] [PubMed] [Google Scholar]
- Mullins L. J. Steady-state calcium fluxes: membrane versus mitochondrial control of ionized calcium in axoplasm. Fed Proc. 1976 Dec;35(14):2583–2588. [PubMed] [Google Scholar]
- Murphy E., Aiton J. F., Horres C. R., Lieberman M. Calcium elevation in cultured heart cells: its role in cell injury. Am J Physiol. 1983 Nov;245(5 Pt 1):C316–C321. doi: 10.1152/ajpcell.1983.245.5.C316. [DOI] [PubMed] [Google Scholar]
- Murphy E., Wheeler D. M., LeFurgey A., Jacob R., Lobaugh L. A., Lieberman M. Coupled sodium-calcium transport in cultured chick heart cells. Am J Physiol. 1986 Mar;250(3 Pt 1):C442–C452. doi: 10.1152/ajpcell.1986.250.3.C442. [DOI] [PubMed] [Google Scholar]
- Noble D. The surprising heart: a review of recent progress in cardiac electrophysiology. J Physiol. 1984 Aug;353:1–50. doi: 10.1113/jphysiol.1984.sp015320. [DOI] [PMC free article] [PubMed] [Google Scholar]
- Philipson K. D., Nishimoto A. Y. Efflux of Ca2+ from cardiac sarcolemmal vesicles. Influence of external Ca2+ and Na+. J Biol Chem. 1981 Apr 25;256(8):3698–3702. [PubMed] [Google Scholar]
- Piwnica-Worms D., Jacob R., Horres C. R., Lieberman M. Na/H exchange in cultured chick heart cells. pHi regulation. J Gen Physiol. 1985 Jan;85(1):43–64. doi: 10.1085/jgp.85.1.43. [DOI] [PMC free article] [PubMed] [Google Scholar]
- Piwnica-Worms D., Jacob R., Horres C. R., Lieberman M. Transmembrane chloride flux in tissue-cultured chick heart cells. J Gen Physiol. 1983 May;81(5):731–748. doi: 10.1085/jgp.81.5.731. [DOI] [PMC free article] [PubMed] [Google Scholar]
- Reeves J. P., Hale C. C. The stoichiometry of the cardiac sodium-calcium exchange system. J Biol Chem. 1984 Jun 25;259(12):7733–7739. [PubMed] [Google Scholar]
- Sheu S. S., Fozzard H. A. Na/Ca exchange in the intact cardiac cell. J Gen Physiol. 1985 Mar;85(3):476–478. [PMC free article] [PubMed] [Google Scholar]
- Sheu S. S., Fozzard H. A. Transmembrane Na+ and Ca2+ electrochemical gradients in cardiac muscle and their relationship to force development. J Gen Physiol. 1982 Sep;80(3):325–351. doi: 10.1085/jgp.80.3.325. [DOI] [PMC free article] [PubMed] [Google Scholar]
- Slaughter R. S., Sutko J. L., Reeves J. P. Equilibrium calcium-calcium exchange in cardiac sarcolemmal vesicles. J Biol Chem. 1983 Mar 10;258(5):3183–3190. [PubMed] [Google Scholar]
- Trosper T. L., Philipson K. D. Effects of divalent and trivalent cations on Na+-Ca2+ exchange in cardiac sarcolemmal vesicles. Biochim Biophys Acta. 1983 May 26;731(1):63–68. doi: 10.1016/0005-2736(83)90398-x. [DOI] [PubMed] [Google Scholar]
- Wakabayashi S., Goshima K. Kinetic studies on sodium-dependent calcium uptake by myocardial cells and neuroblastoma cells in culture. Biochim Biophys Acta. 1981 Mar 20;642(1):158–172. doi: 10.1016/0005-2736(81)90146-2. [DOI] [PubMed] [Google Scholar]
- Wallenstein S., Zucker C. L., Fleiss J. L. Some statistical methods useful in circulation research. Circ Res. 1980 Jul;47(1):1–9. doi: 10.1161/01.res.47.1.1. [DOI] [PubMed] [Google Scholar]