Abstract
Cephalopod molluscs have complex brains and behaviour, yet little is known about the permeability of their blood-brain interface. The accompanying paper characterized the fluid compartments of the brain and presented evidence for restricted permeability of the blood-brain interface to albumin. The present paper investigates the permeability of the interface to small non-electrolytes. [14C]Polyethylene glycol (PEG, mol. wt. 4000), and [51Cr]EDTA (mol. wt. 342) were injected intravenously or intramuscularly, and their penetration into brain and muscle studied up to 48 h. Tracers equilibrated with muscle interstitial fluid (ISF) at relatively short times, but in brain ISF reached only 0.5-0.65 X their plasma concentration. This is qualitative evidence for the presence in brain of a barrier to these molecules and an efficient drainage mechanism for ISF. Quantitative treatment of the uptake data allows calculation of the permeability X surface area product (PS) and the permeability coefficient (P). For the brain PS and P are in the range 1-3 X 10(-4) ml g-1 min-1 and 1-3 X 10(-8) cm s-1 respectively, (PEG), and 3 X 10(-4) ml g-1 min-1 and 3-4 X 10(-8) cm s-1 respectively (Cr-EDTA). The P values are close to those reported for mammalian brain. Assuming that the lack of equilibration in brain is due to ISF flow, the rate of flow can be calculated. Values for vertical and optic lobe are approximately 0.2 microliter g-1 min-1, again close to those reported for mammalian brain. It is concluded that the tightness of the Sepia blood-brain barrier approaches that of mammals, and a flowing ISF system is present. An association between a tight barrier and higher central nervous system integrative function is suggested. The significance of these findings for the evolution of control of the brain microenvironment is discussed.
Full text
PDF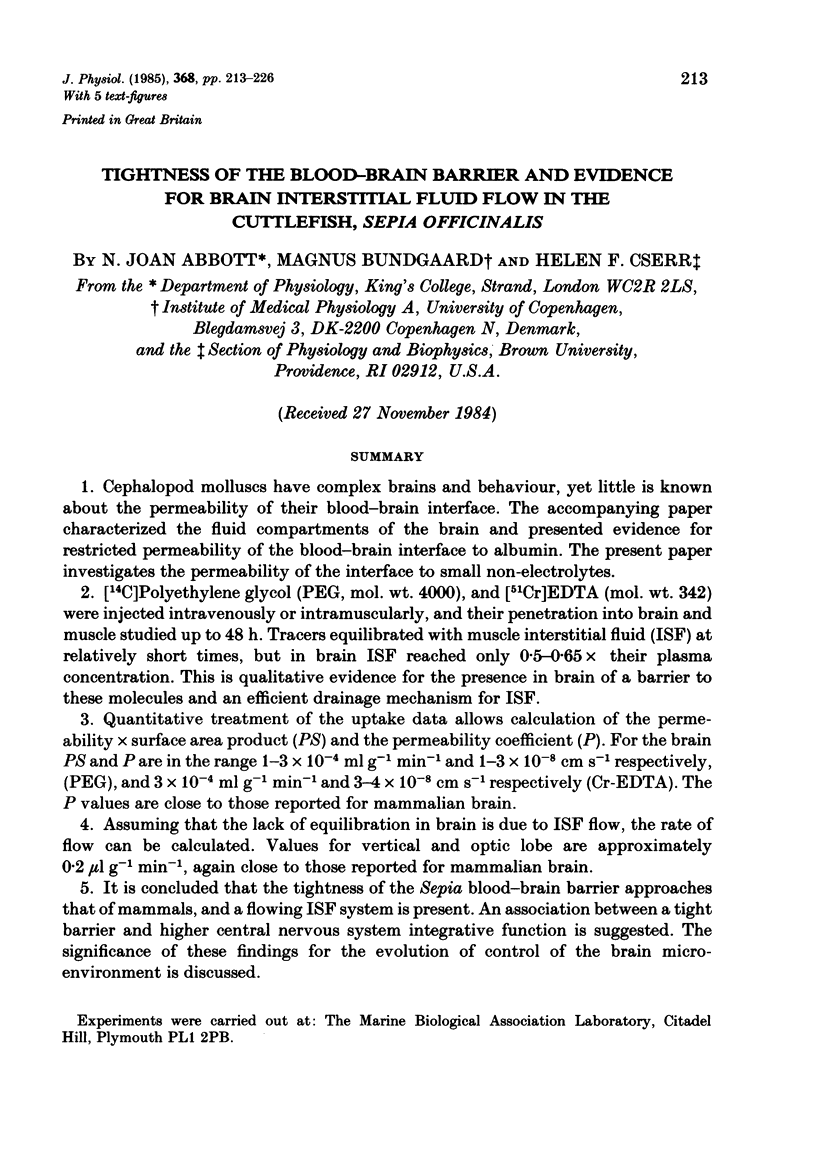
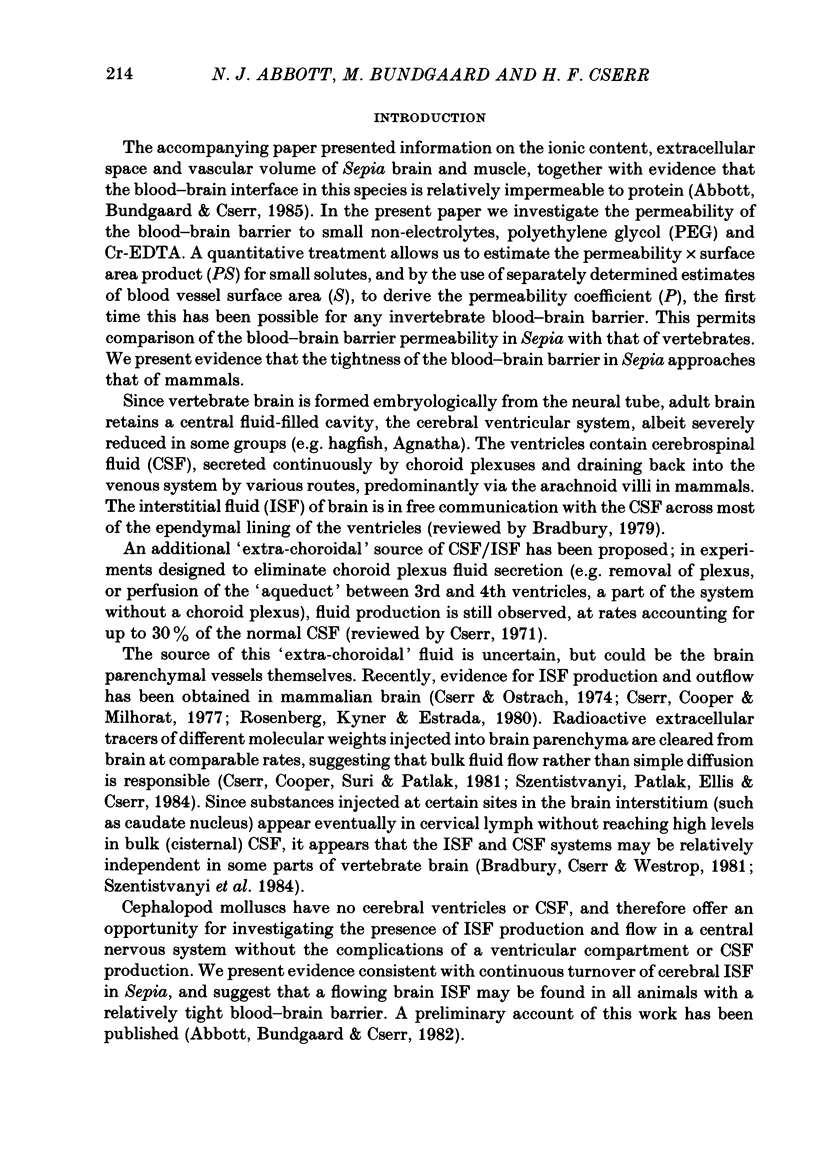
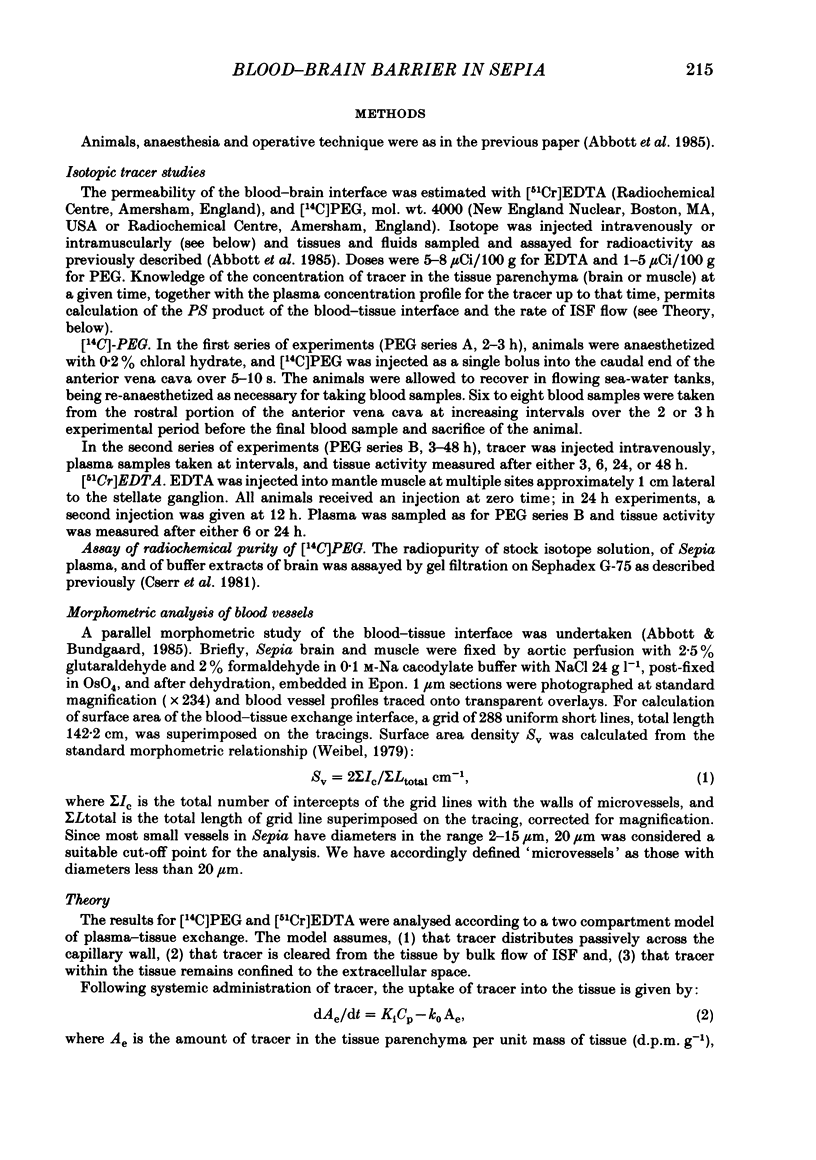
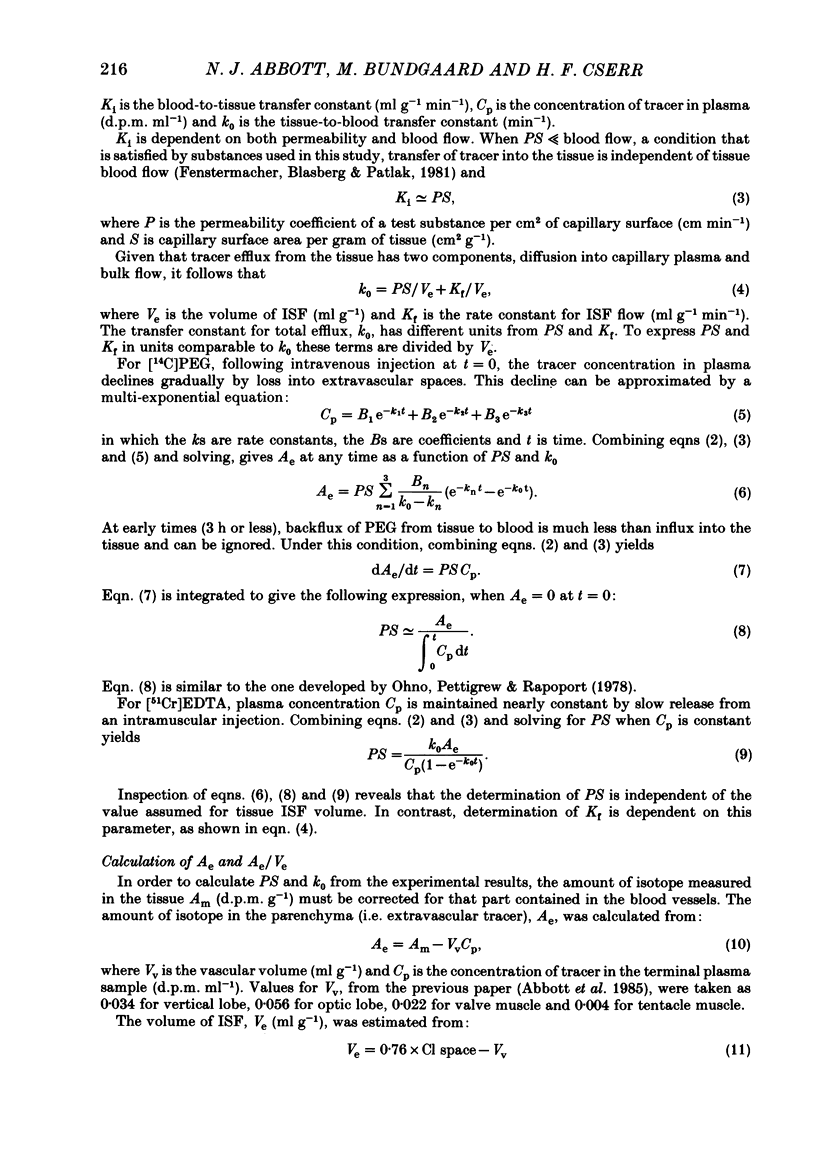
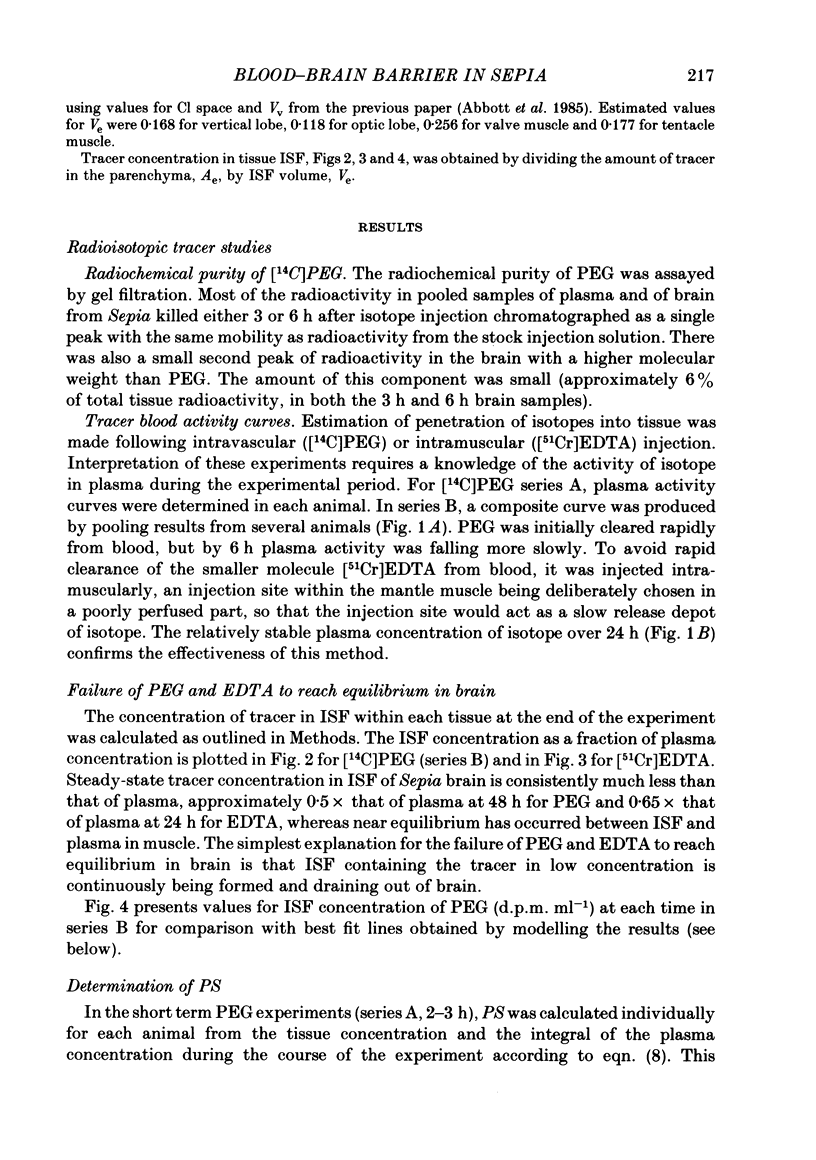
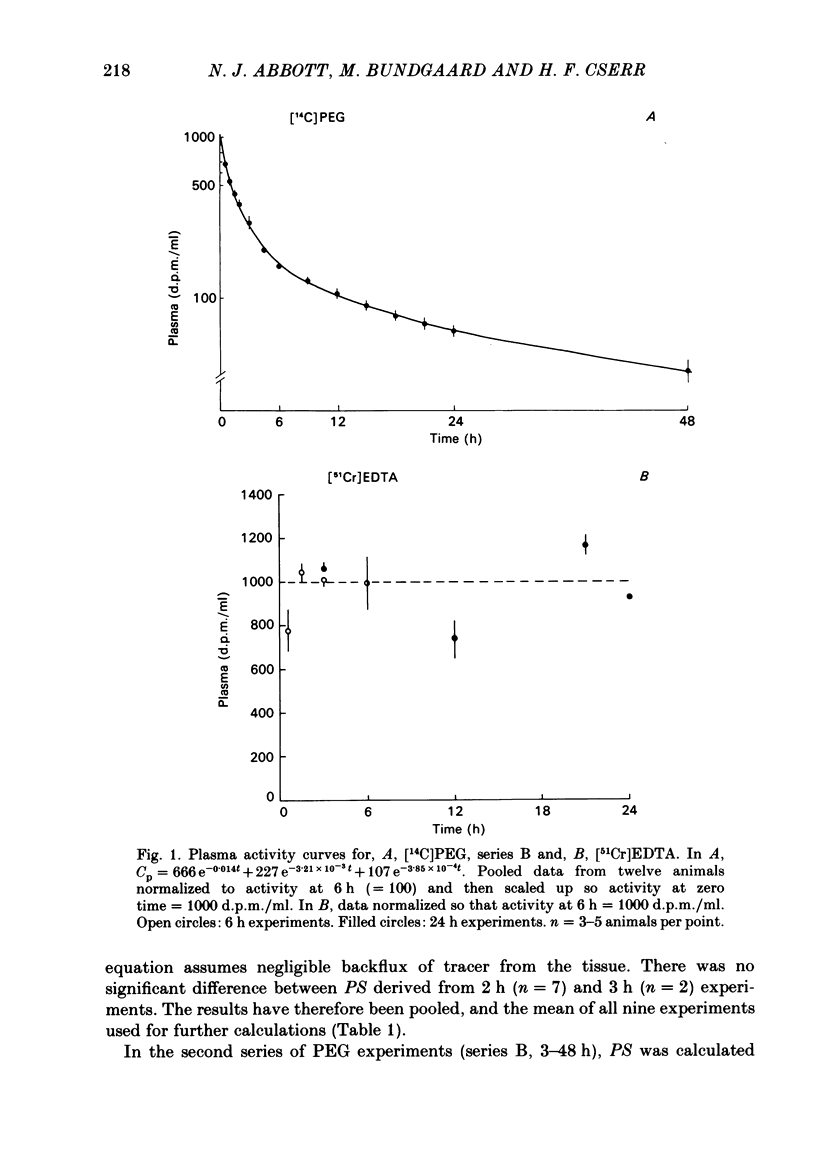
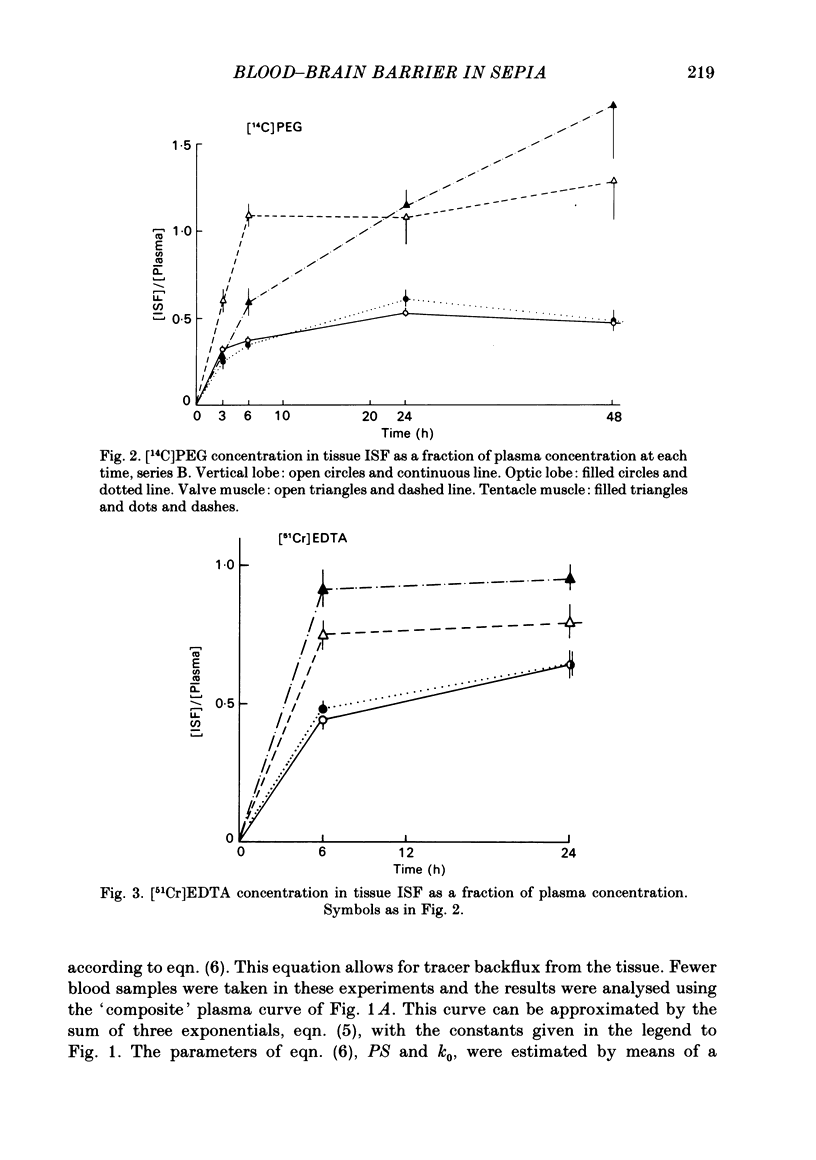
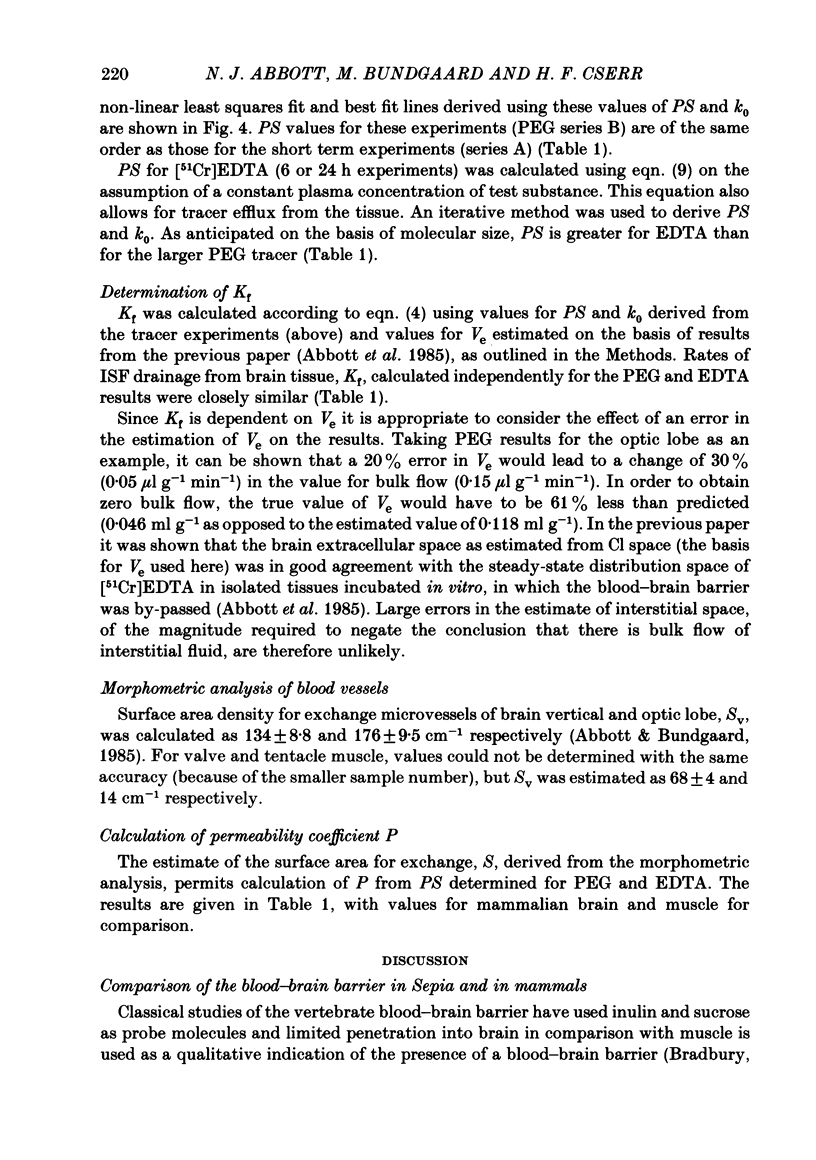
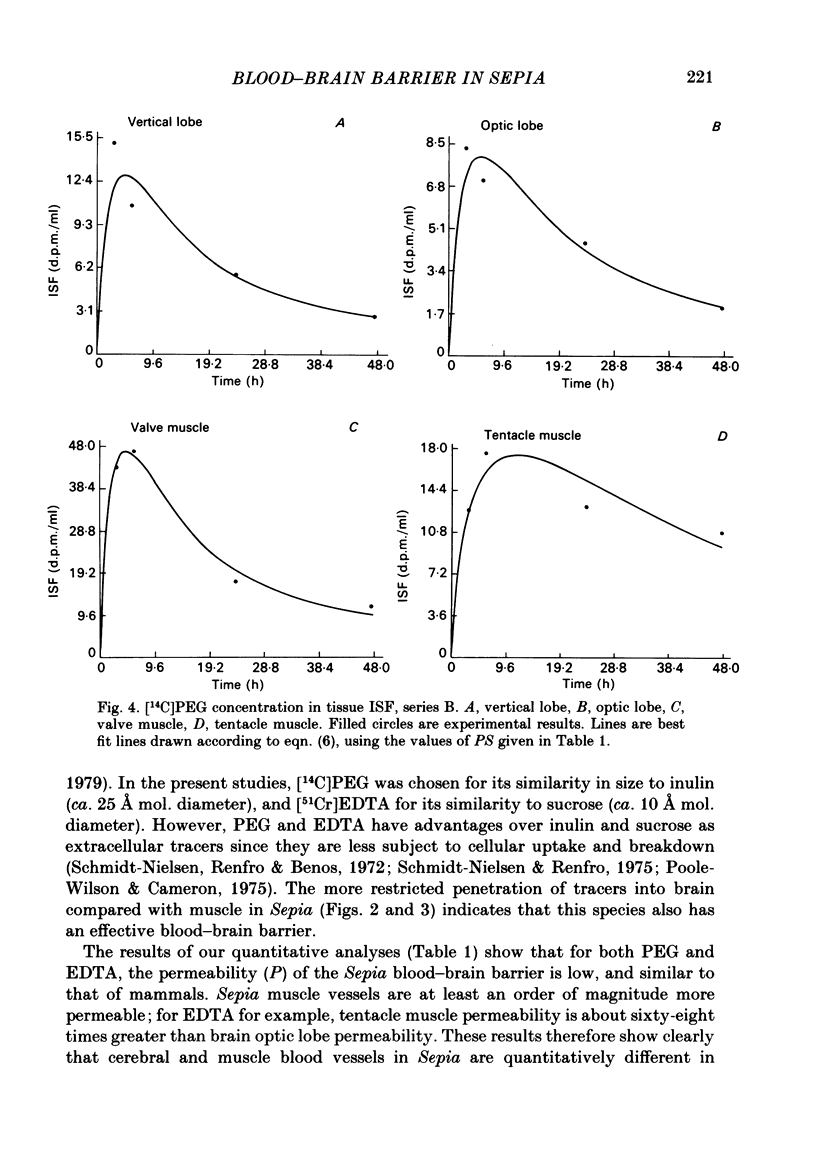
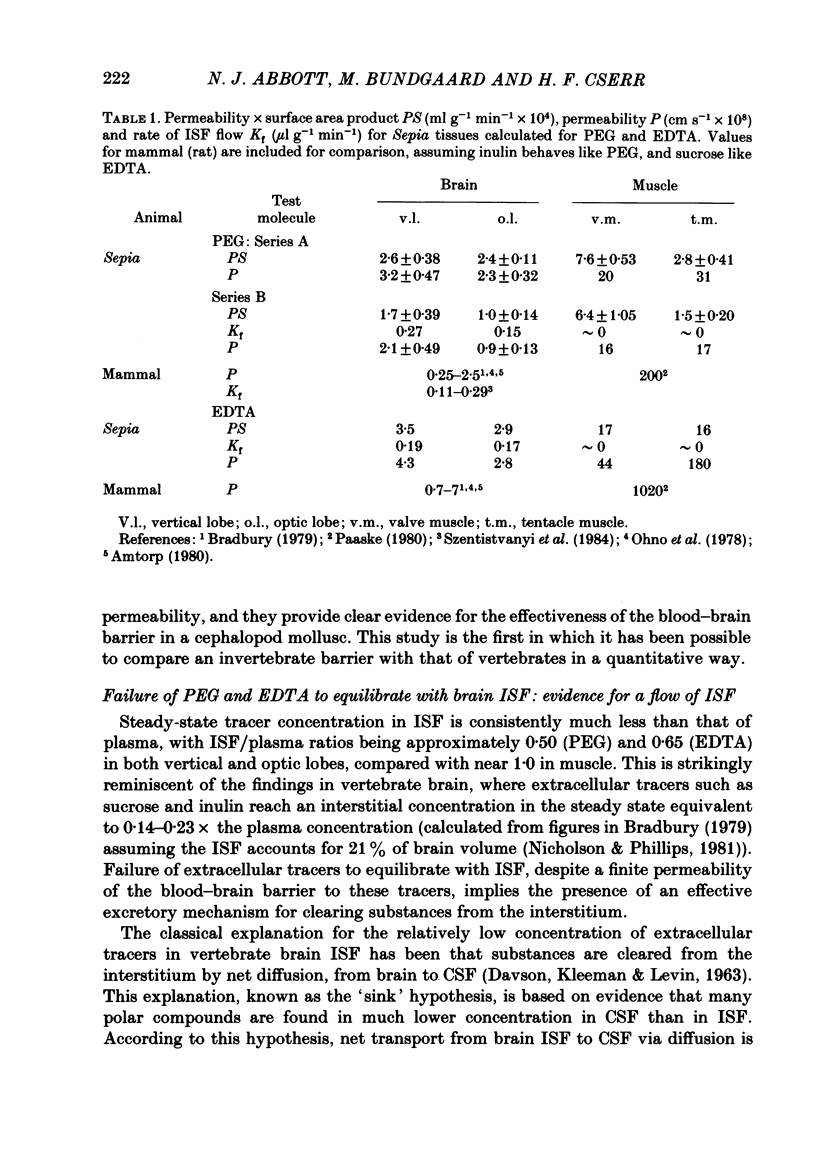
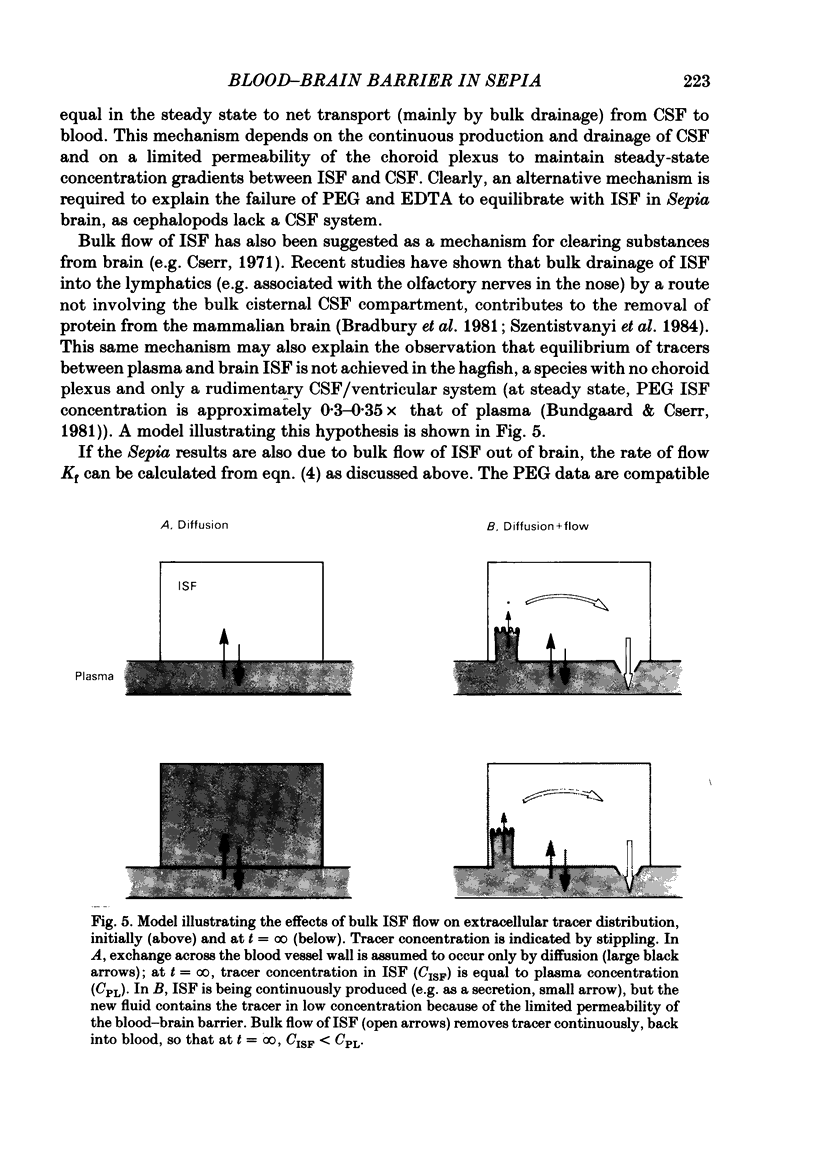
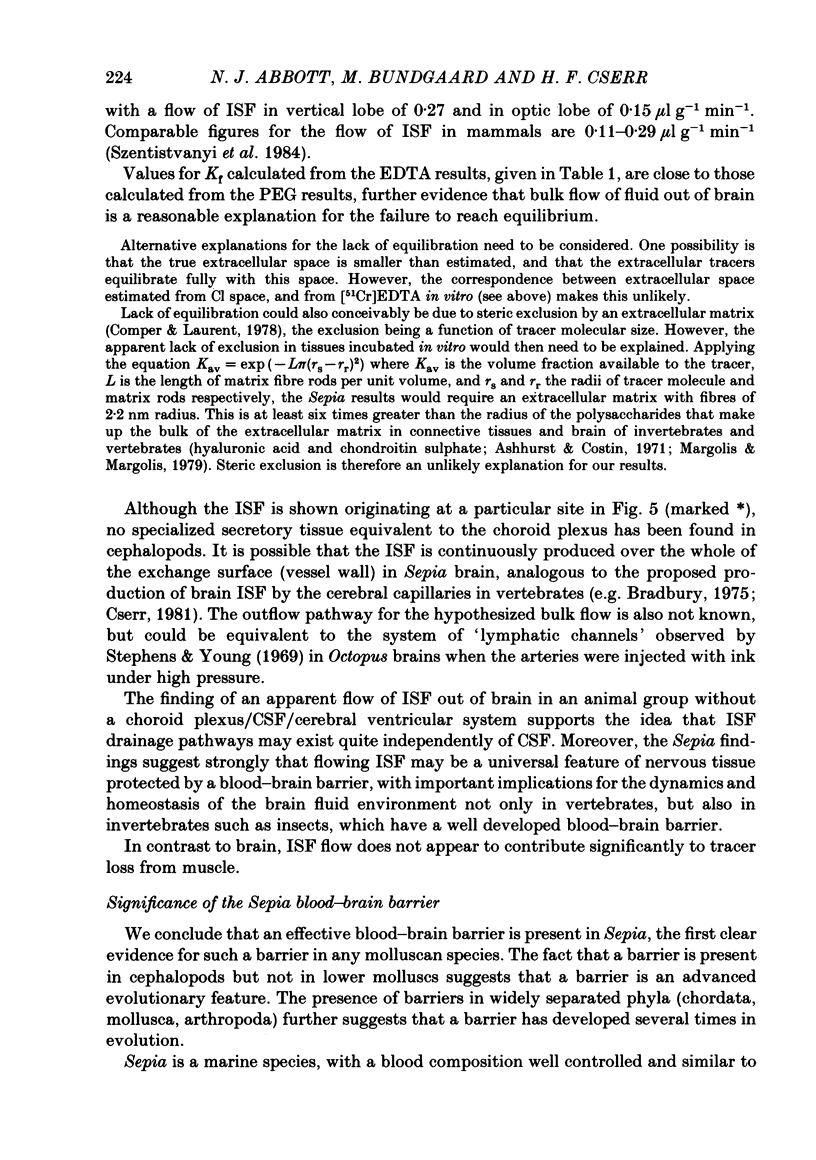
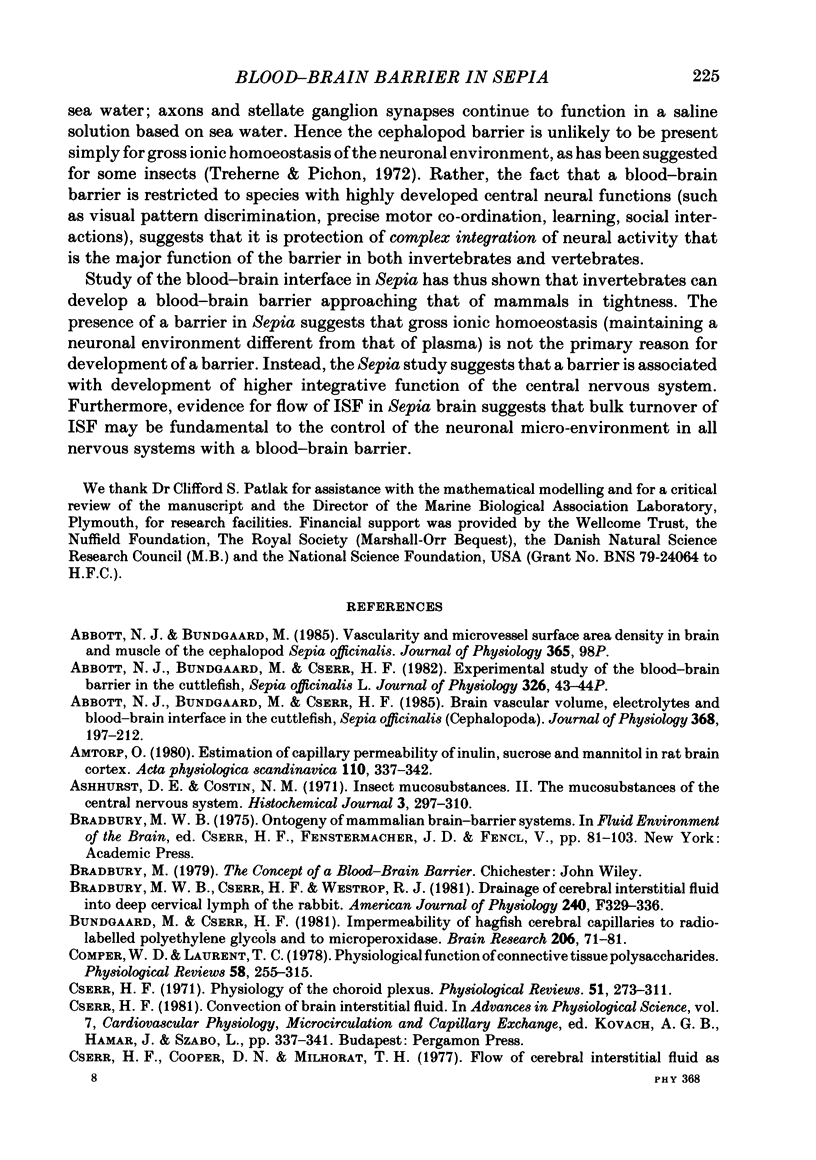
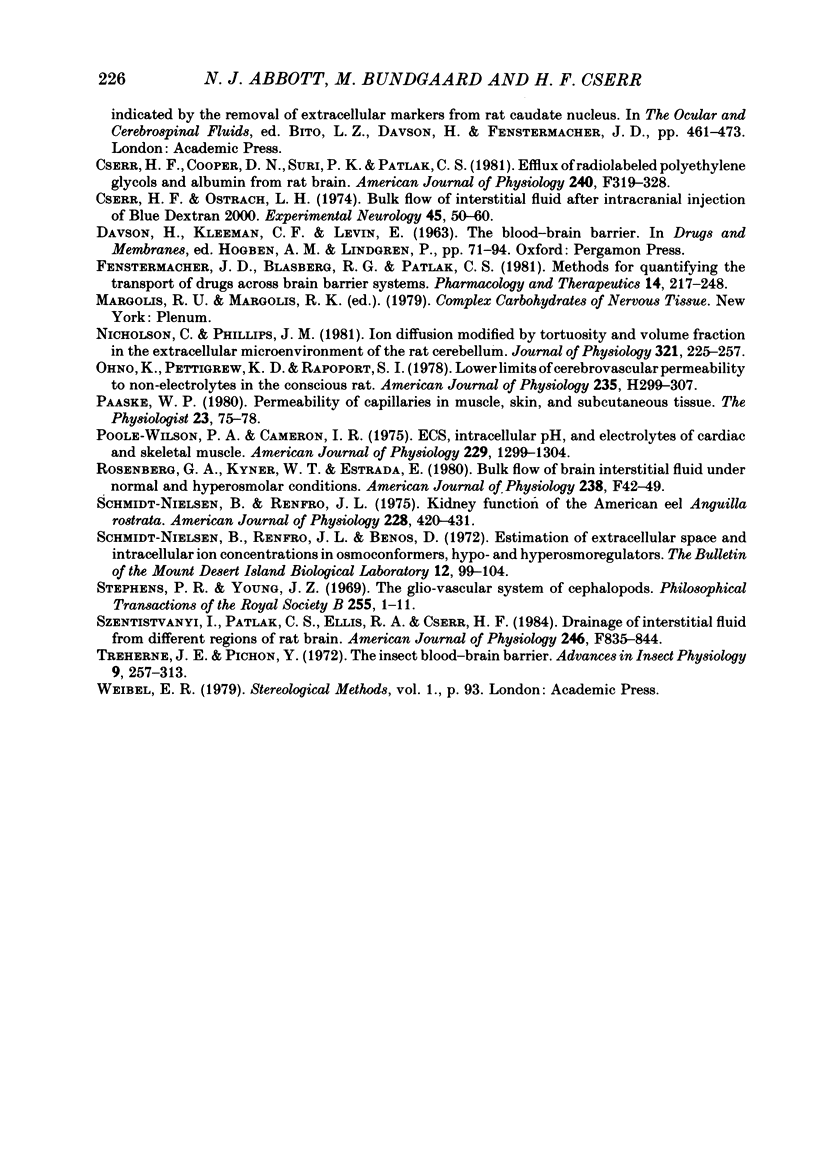
Images in this article
Selected References
These references are in PubMed. This may not be the complete list of references from this article.
- Abbott N. J., Bundgaard M., Cserr H. F. Brain vascular volume, electrolytes and blood-brain interface in the cuttlefish Sepia officinalis (Cephalopoda). J Physiol. 1985 Nov;368:197–212. doi: 10.1113/jphysiol.1985.sp015853. [DOI] [PMC free article] [PubMed] [Google Scholar]
- Amtorp O. Estimation of capillary permeability of inulin, sucrose and mannitol in rat brain cortex. Acta Physiol Scand. 1980 Dec;110(4):337–342. doi: 10.1111/j.1748-1716.1980.tb06678.x. [DOI] [PubMed] [Google Scholar]
- Ashhurst D. E., Costin N. M. Insect mucosubstances. II. The mucosubstances of the central nervous system. Histochem J. 1971 Jul;3(4):297–310. doi: 10.1007/BF01005228. [DOI] [PubMed] [Google Scholar]
- Bradbury M. W., Cserr H. F., Westrop R. J. Drainage of cerebral interstitial fluid into deep cervical lymph of the rabbit. Am J Physiol. 1981 Apr;240(4):F329–F336. doi: 10.1152/ajprenal.1981.240.4.F329. [DOI] [PubMed] [Google Scholar]
- Bundgaard M., Cserr H. F. Impermeability of hagfish cerebral capillaries to radio-labelled polyethylene glycols and to microperoxidase. Brain Res. 1981 Feb 9;206(1):71–81. doi: 10.1016/0006-8993(81)90101-3. [DOI] [PubMed] [Google Scholar]
- Comper W. D., Laurent T. C. Physiological function of connective tissue polysaccharides. Physiol Rev. 1978 Jan;58(1):255–315. doi: 10.1152/physrev.1978.58.1.255. [DOI] [PubMed] [Google Scholar]
- Cserr H. F., Ostrach L. H. Bulk flow of interstitial fluid after intracranial injection of blue dextran 2000. Exp Neurol. 1974 Oct;45(1):50–60. doi: 10.1016/0014-4886(74)90099-5. [DOI] [PubMed] [Google Scholar]
- Cserr H. F. Physiology of the choroid plexus. Physiol Rev. 1971 Apr;51(2):273–311. doi: 10.1152/physrev.1971.51.2.273. [DOI] [PubMed] [Google Scholar]
- Fenstermacher J. D., Blasberg R. G., Patlak C. S. Methods for Quantifying the transport of drugs across brain barrier systems. Pharmacol Ther. 1981;14(2):217–248. doi: 10.1016/0163-7258(81)90062-0. [DOI] [PubMed] [Google Scholar]
- Nicholson C., Phillips J. M. Ion diffusion modified by tortuosity and volume fraction in the extracellular microenvironment of the rat cerebellum. J Physiol. 1981 Dec;321:225–257. doi: 10.1113/jphysiol.1981.sp013981. [DOI] [PMC free article] [PubMed] [Google Scholar]
- Ohno K., Pettigrew K. D., Rapoport S. I. Lower limits of cerebrovascular permeability to nonelectrolytes in the conscious rat. Am J Physiol. 1978 Sep;235(3):H299–H307. doi: 10.1152/ajpheart.1978.235.3.H299. [DOI] [PubMed] [Google Scholar]
- Paaske W. P. Permeability of capillaries in muscle, skin, and subcutaneous tissue. Physiologist. 1980 Feb;23(1):75–78. [PubMed] [Google Scholar]
- Poole-Wilson P. A., Cameron I. R. ECS, intracellular pH, and electrolytes of cardiac and skeletal muscle. Am J Physiol. 1975 Nov;229(5):1299–1304. doi: 10.1152/ajplegacy.1975.229.5.1299. [DOI] [PubMed] [Google Scholar]
- Rosenberg G. A., Kyner W. T., Estrada E. Bulk flow of brain interstitial fluid under normal and hyperosmolar conditions. Am J Physiol. 1980 Jan;238(1):F42–F49. doi: 10.1152/ajprenal.1980.238.1.F42. [DOI] [PubMed] [Google Scholar]
- Schmidt-Nielsen B., Renfro J. L. Kidney function of the American eel Anguilla rostrata. Am J Physiol. 1975 Feb;228(2):420–431. doi: 10.1152/ajplegacy.1975.228.2.420. [DOI] [PubMed] [Google Scholar]
- Szentistványi I., Patlak C. S., Ellis R. A., Cserr H. F. Drainage of interstitial fluid from different regions of rat brain. Am J Physiol. 1984 Jun;246(6 Pt 2):F835–F844. doi: 10.1152/ajprenal.1984.246.6.F835. [DOI] [PubMed] [Google Scholar]