Abstract
Single fast myotomal fibres and small bundles of slow fibres (from the adductor pectoralis profundus muscle) were isolated from the Antarctic teleost Notothenia neglecta. Fibres were skinned by a brief detergent treatment. The effects of phosphate on the mechanical properties and ATPase activity of fast and slow fibres were studied. 20 mM-phosphate inhibited maximum isometric tension in slow fibres by 34%, but by only 11% in fast fibres. A half-maximal response was obtained at approximately 5 mM-phosphate. These concentrations are within the range measured in muscle, and the effect is probably of physiological significance. This species is of particular interest, since there is evidence that the energy supply to the fast muscle is largely based on phosphocreatine breakdown, which would result in large changes in intracellular phosphate concentration during exercise. The maximum contraction velocity of both fast and slow fibres was not affected by 10 mM-phosphate, nor was the ATPase activity of the slow fibres during isometric contraction. The phosphate-induced depression in tension in slow fibres was associated with a proportional decrease in stiffness. The rate of force recovery after rapid, small amplitude stretches and releases was increased by phosphate, as was the rate of rise of force during stretch activation. The results are discussed with reference to the different patterns of energy supply for contraction in muscle, and an attempt is made at explaining the data in terms of changes in cross-bridge kinetics.
Full text
PDF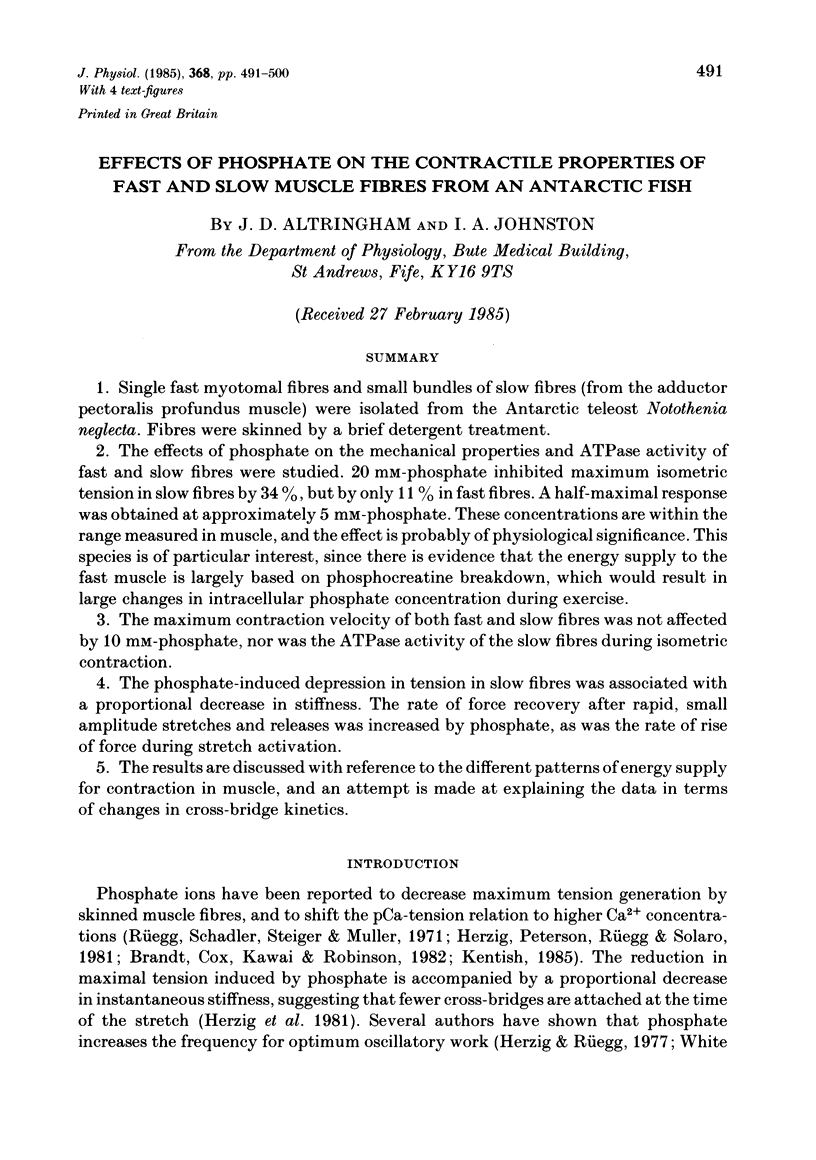
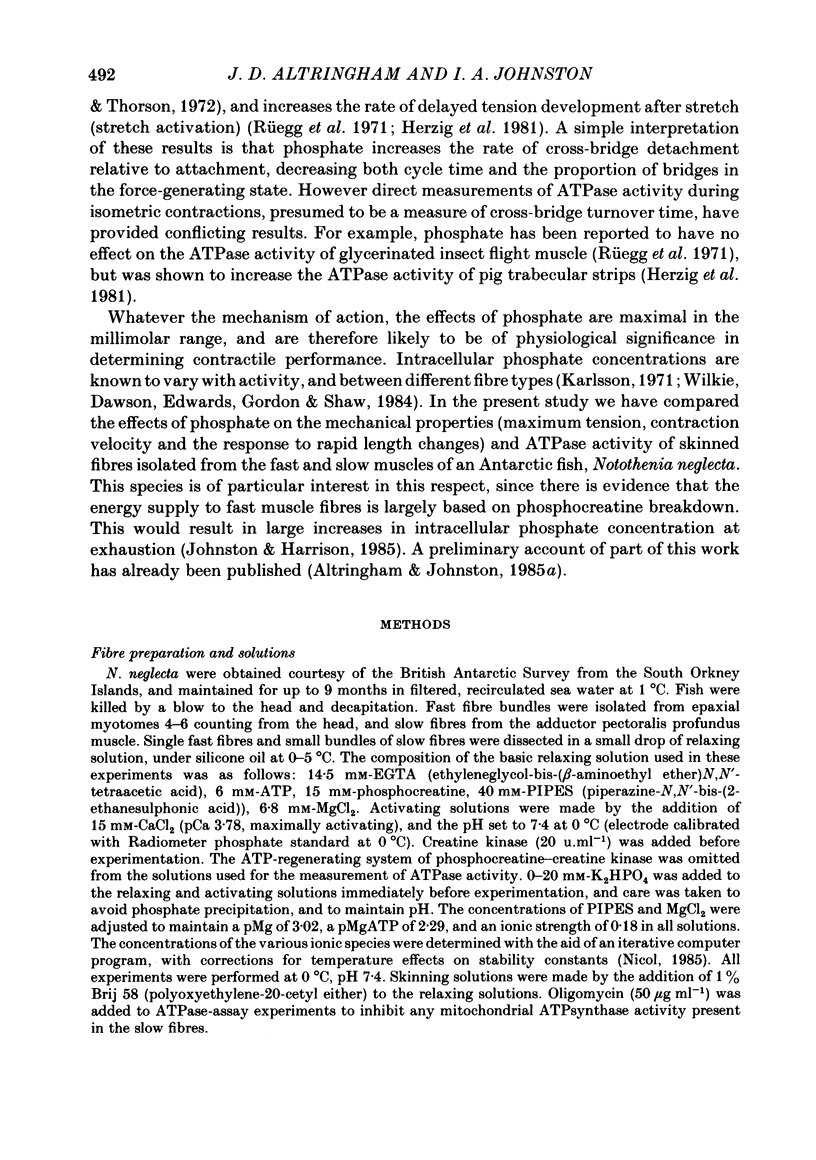
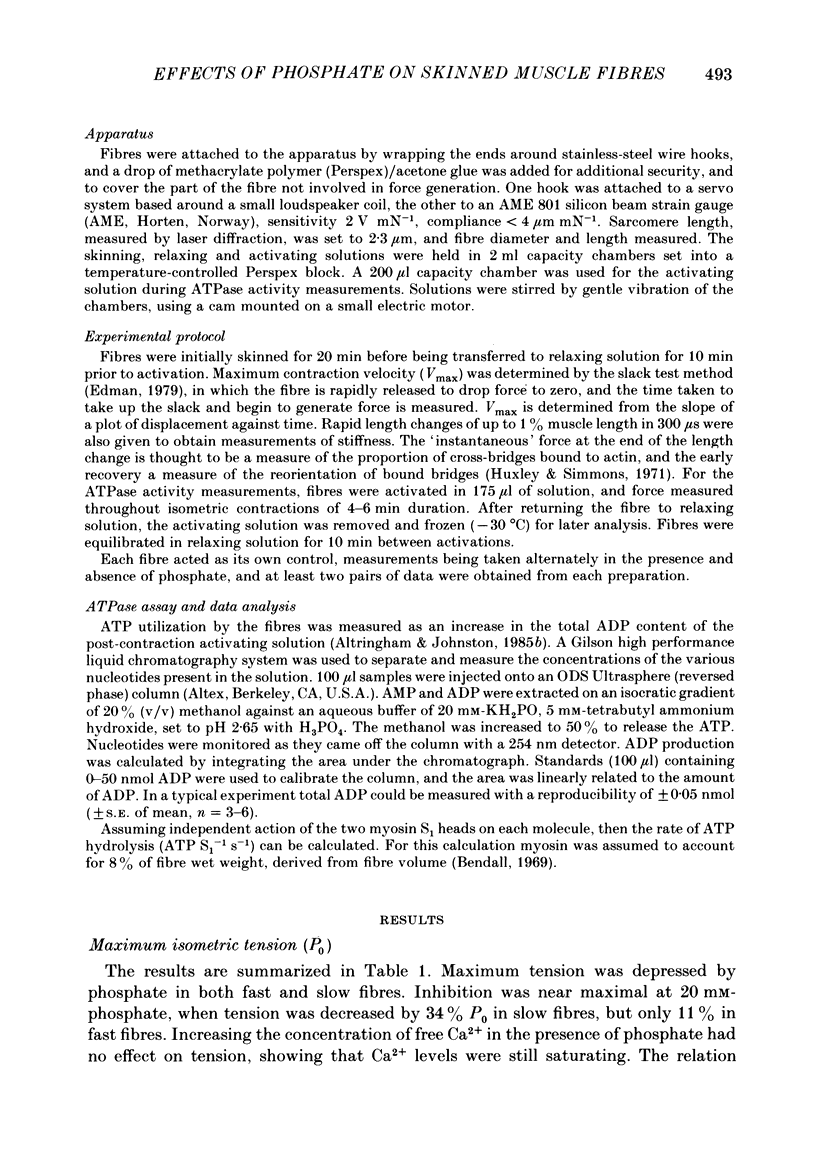
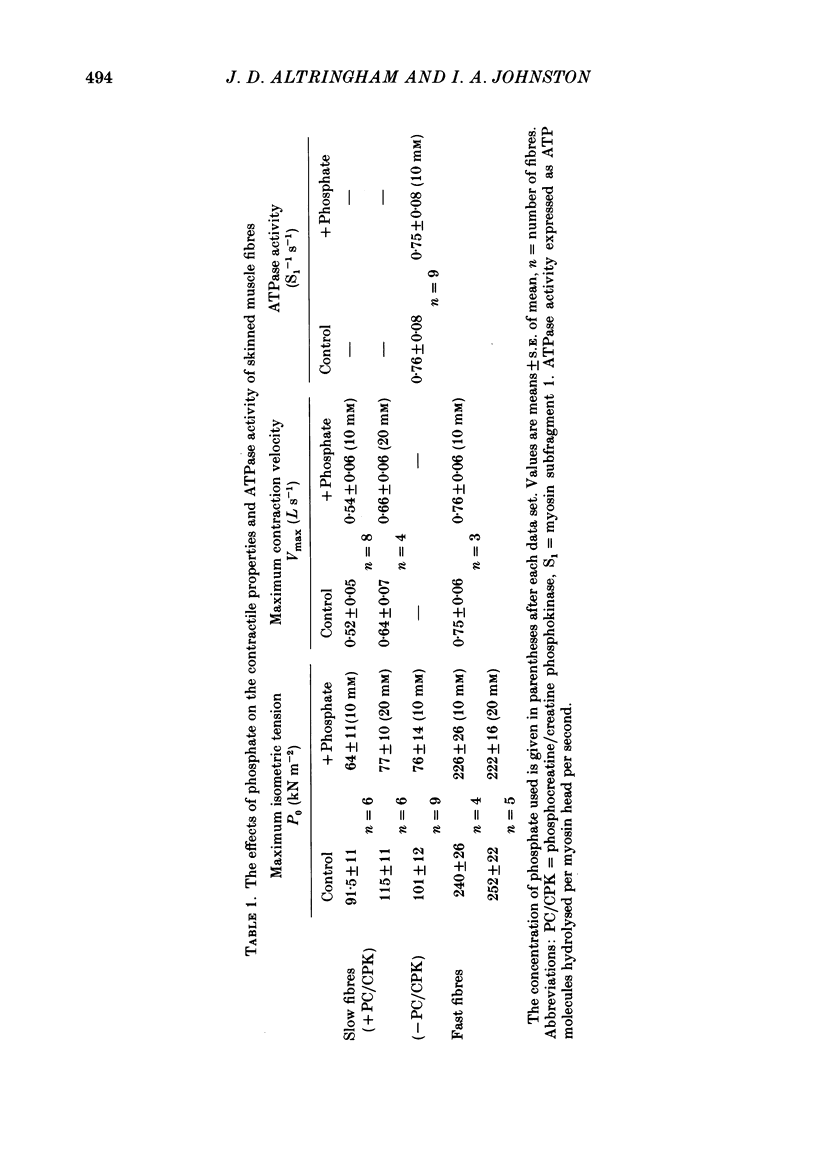
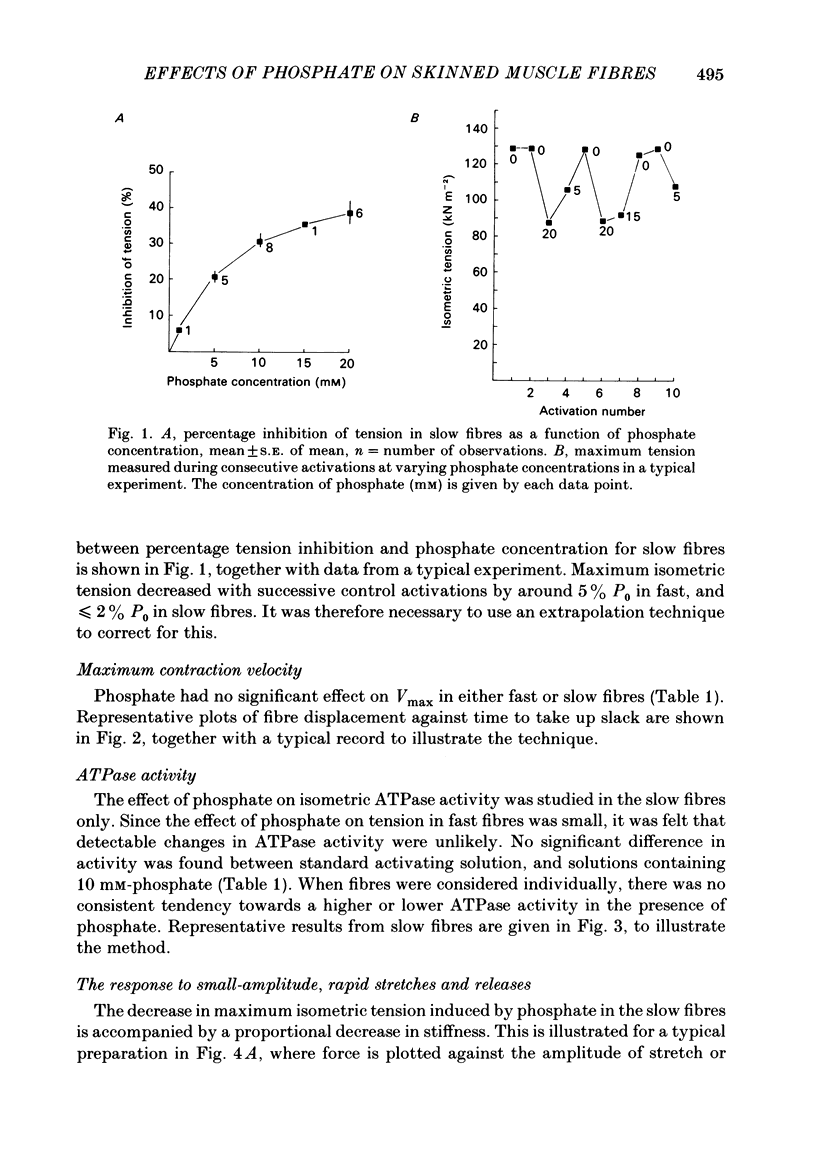
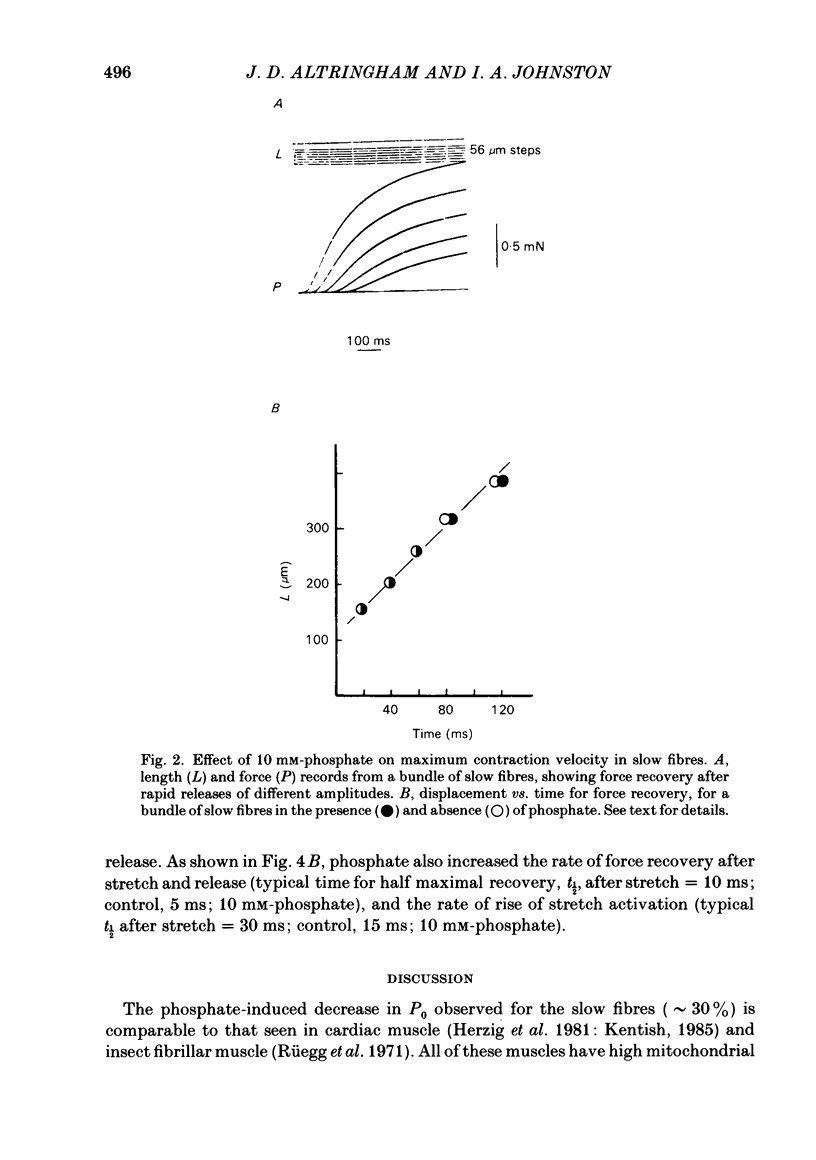
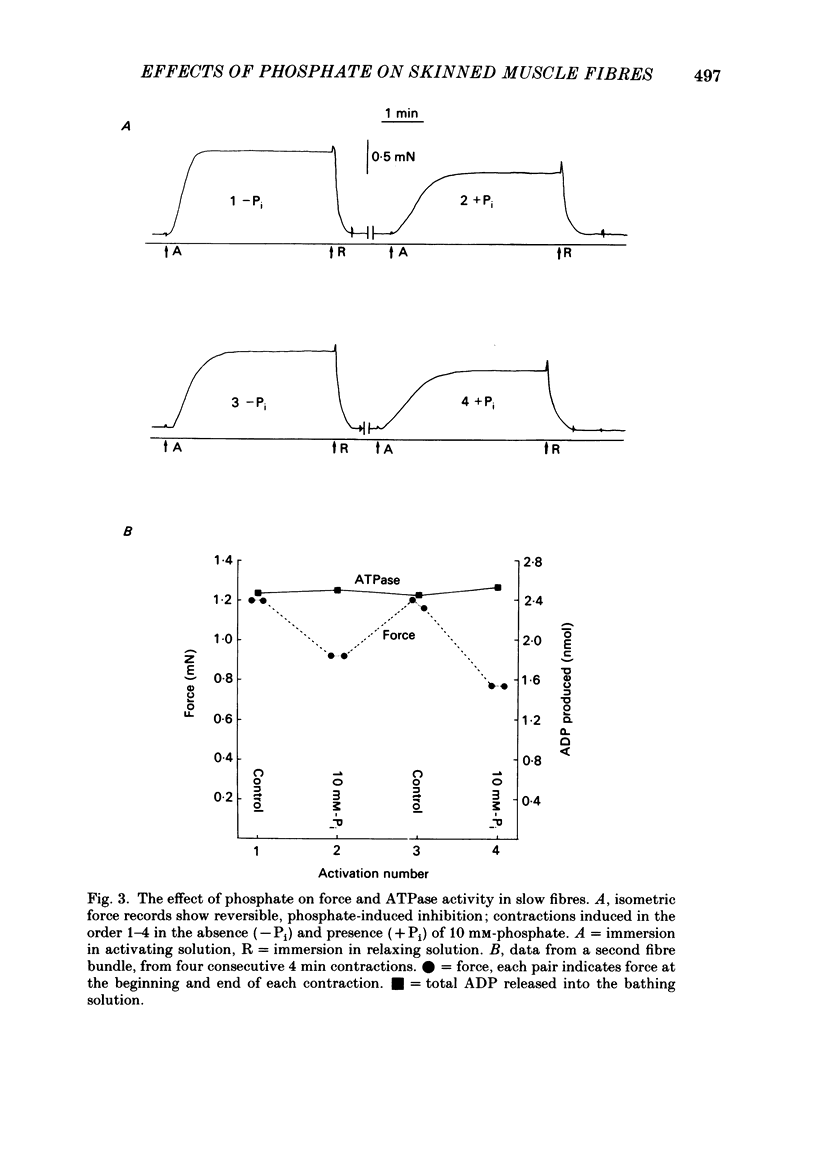
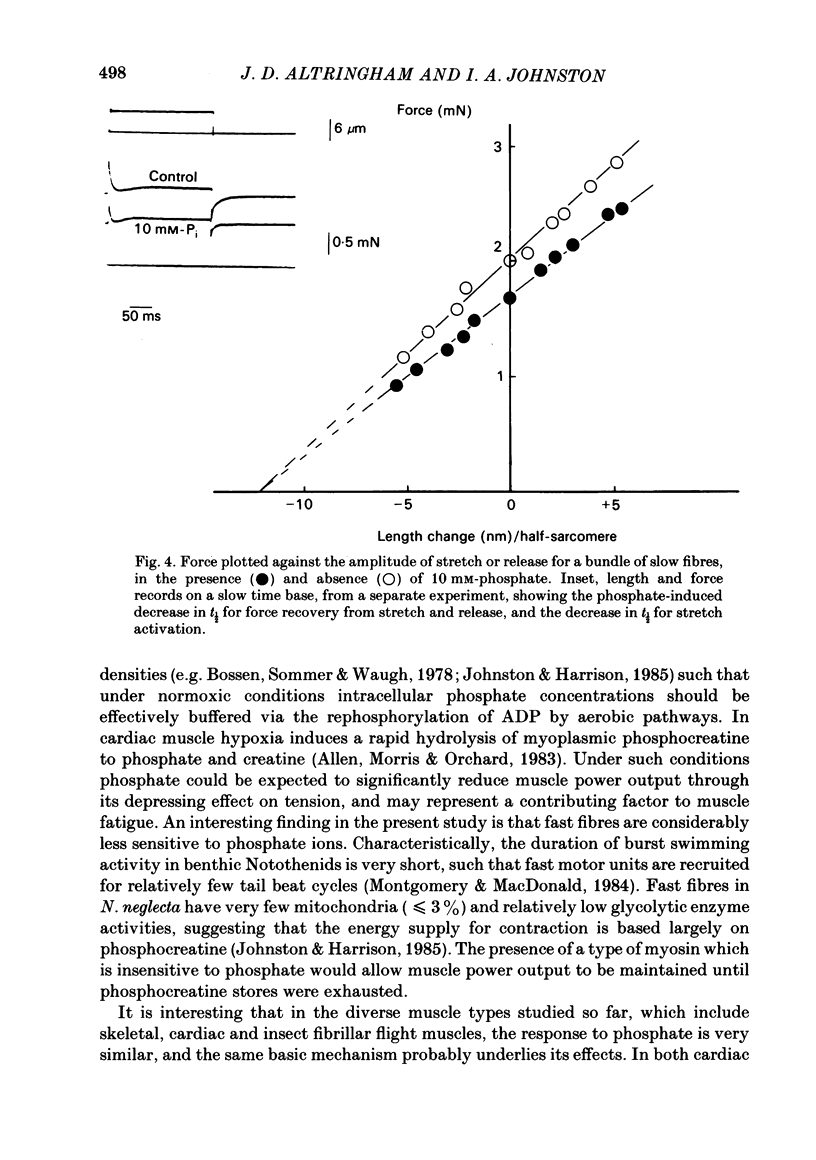
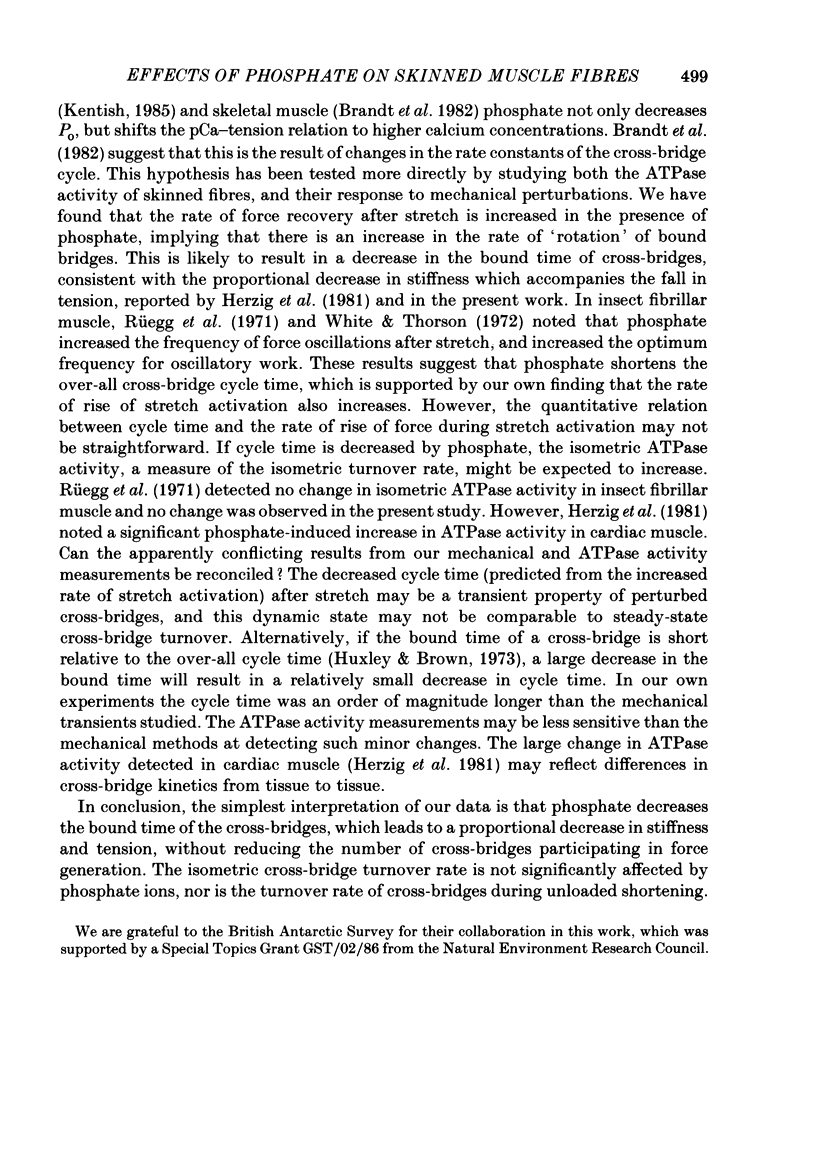
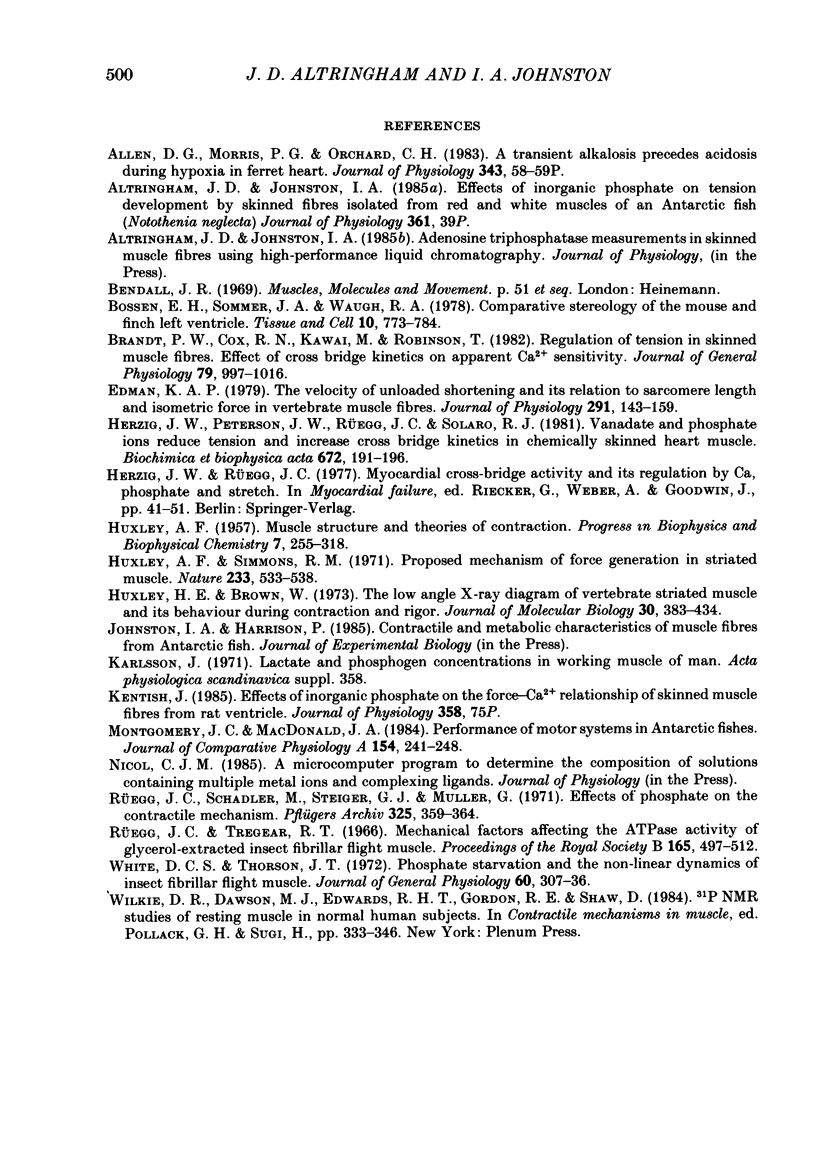
Selected References
These references are in PubMed. This may not be the complete list of references from this article.
- Bossen E. H., Sommer J. R., Waugh R. A. Comparative stereology of the mouse and finch left ventricle. Tissue Cell. 1978;10(4):773–784. doi: 10.1016/0040-8166(78)90062-9. [DOI] [PubMed] [Google Scholar]
- Brandt P. W., Cox R. N., Kawai M., Robinson T. Effect of cross-bridge kinetics on apparent Ca2+ sensitivity. J Gen Physiol. 1982 Jun;79(6):997–1016. doi: 10.1085/jgp.79.6.997. [DOI] [PMC free article] [PubMed] [Google Scholar]
- Dulhunty A. F., Gage P. W. Excitation-contraction coupling and charge movement in denervated rat extensor digitorum longus and soleus muscles. J Physiol. 1985 Jan;358:75–89. doi: 10.1113/jphysiol.1985.sp015541. [DOI] [PMC free article] [PubMed] [Google Scholar]
- Edman K. A. The velocity of unloaded shortening and its relation to sarcomere length and isometric force in vertebrate muscle fibres. J Physiol. 1979 Jun;291:143–159. doi: 10.1113/jphysiol.1979.sp012804. [DOI] [PMC free article] [PubMed] [Google Scholar]
- HUXLEY A. F. Muscle structure and theories of contraction. Prog Biophys Biophys Chem. 1957;7:255–318. [PubMed] [Google Scholar]
- Herzig J. W., Peterson J. W., Rüegg J. C., Solaro R. J. Vanadate and phosphate ions reduce tension and increase cross-bridge kinetics in chemically skinned heart muscle. Biochim Biophys Acta. 1981 Jan 21;672(2):191–196. doi: 10.1016/0304-4165(81)90392-5. [DOI] [PubMed] [Google Scholar]
- Huxley A. F., Simmons R. M. Proposed mechanism of force generation in striated muscle. Nature. 1971 Oct 22;233(5321):533–538. doi: 10.1038/233533a0. [DOI] [PubMed] [Google Scholar]
- Huxley H. E., Brown W. The low-angle x-ray diagram of vertebrate striated muscle and its behaviour during contraction and rigor. J Mol Biol. 1967 Dec 14;30(2):383–434. doi: 10.1016/s0022-2836(67)80046-9. [DOI] [PubMed] [Google Scholar]
- Rüegg J. C., Schädler M., Steiger G. J., Müller G. Effects of inorganic phosphate on the contractile mechanism. Pflugers Arch. 1971;325(4):359–364. doi: 10.1007/BF00592176. [DOI] [PubMed] [Google Scholar]
- Rüegg J. C., Tregear R. T. Mechanical factors affecting the ATPase activity of glycerol-extracted insect fibrillar flight muscle. Proc R Soc Lond B Biol Sci. 1966 Oct 11;165(1001):497–512. doi: 10.1098/rspb.1966.0080. [DOI] [PubMed] [Google Scholar]
- White D. C., Thorson J. Phosphate starvation and the nonlinear dynamics of insect fibrillar flight muscle. J Gen Physiol. 1972 Sep;60(3):307–336. doi: 10.1085/jgp.60.3.307. [DOI] [PMC free article] [PubMed] [Google Scholar]
- Wilkie D. R., Dawson M. J., Edwards R. H., Gordon R. E., Shaw D. 31P NMR studies of resting muscle in normal human subjects. Adv Exp Med Biol. 1984;170:333–347. doi: 10.1007/978-1-4684-4703-3_28. [DOI] [PubMed] [Google Scholar]