Abstract
The fine structure of ion-channel activations by junctional nicotinic receptors in adult frog muscle fibres has been investigated. The agonists used were acetylcholine (ACh), carbachol (CCh), suberyldicholine (SubCh) and decan-1,10-dicarboxylic acid dicholine ester (DecCh). Individual activations (bursts) were interrupted by short closed periods; the distribution of their durations showed a major fast component ('short gaps') and a minor slower component ('intermediate gaps'). The mean duration of both short and intermediate gaps was dependent on the nature of the agonist. For short gaps the mean durations (microseconds) were: ACh, 20; SubCh, 43; DecCh, 71; CCh, 13. The mean number of short gaps per burst were: ACh, 1.9; SubCh, 4.1; DecCh, 2.0. The mean number of short gaps per burst, and the mean number per unit open time, were dependent on the nature of the agonist, but showed little dependence on agonist concentration or membrane potential for ACh, SubCh and DecCh. The short gaps in CCh increased in frequency with agonist concentration and were mainly produced by channel blockages by CCh itself. Partially open channels (subconductance states) were clearly resolved rarely (0.4% of gaps within bursts) but regularly. Conductances of 18% (most commonly) and 71% of the main value were found. However, most short gaps were probably full closures. The distribution of burst lengths had two components. The faster component represented mainly isolated short openings that were much more common at low agonist concentrations. The slower component represented bursts of longer openings. Except at very low concentrations more than 85% of activations were of this type, which corresponds to the 'channel lifetime' found by noise analysis. The frequency of channel openings increased slightly with hyperpolarization. The short gaps during activations were little affected when (a) the [H+]o or [Ca2+]o were reduced to 1/10th of normal, (b) when extracellular Ca2+ was replaced by Mg2+, (c) when the [Cl-]i was raised or (d) when, in one experiment on an isolated inside-out patch, the normal intracellular constituents were replaced by KCl. Reduction of [Ca2+]O to 1/10 of normal increased the single-channel conductance by 50%, and considerably increased the number of intermediate gaps. No temporal asymmetry was detectable in the bursts of openings. Positive correlations were found between the lengths of successive apparent open times at low SubCh concentrations, but no correlations between burst lengths were detectable. The component of brief openings behaves, at low concentrations, as though it originates from openings of singly occupied channels.(ABSTRACT TRUNCATED AT 400 WORDS)
Full text
PDF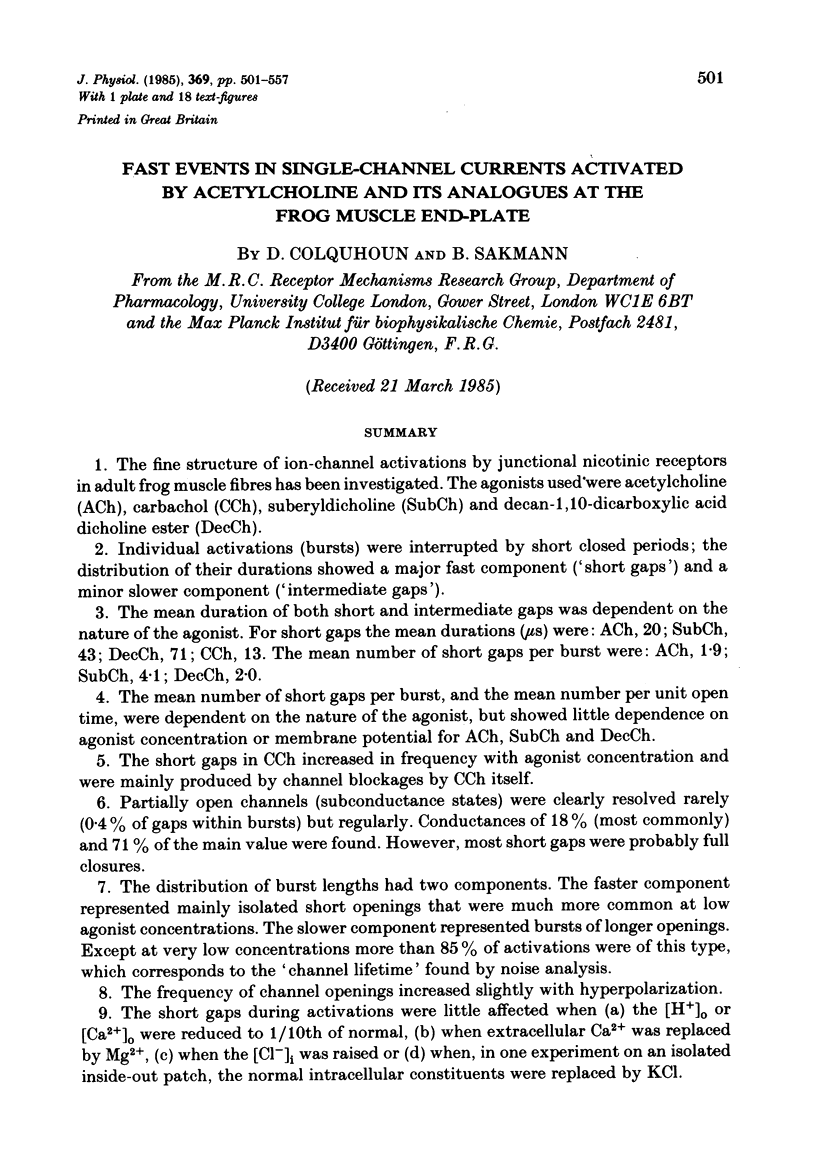
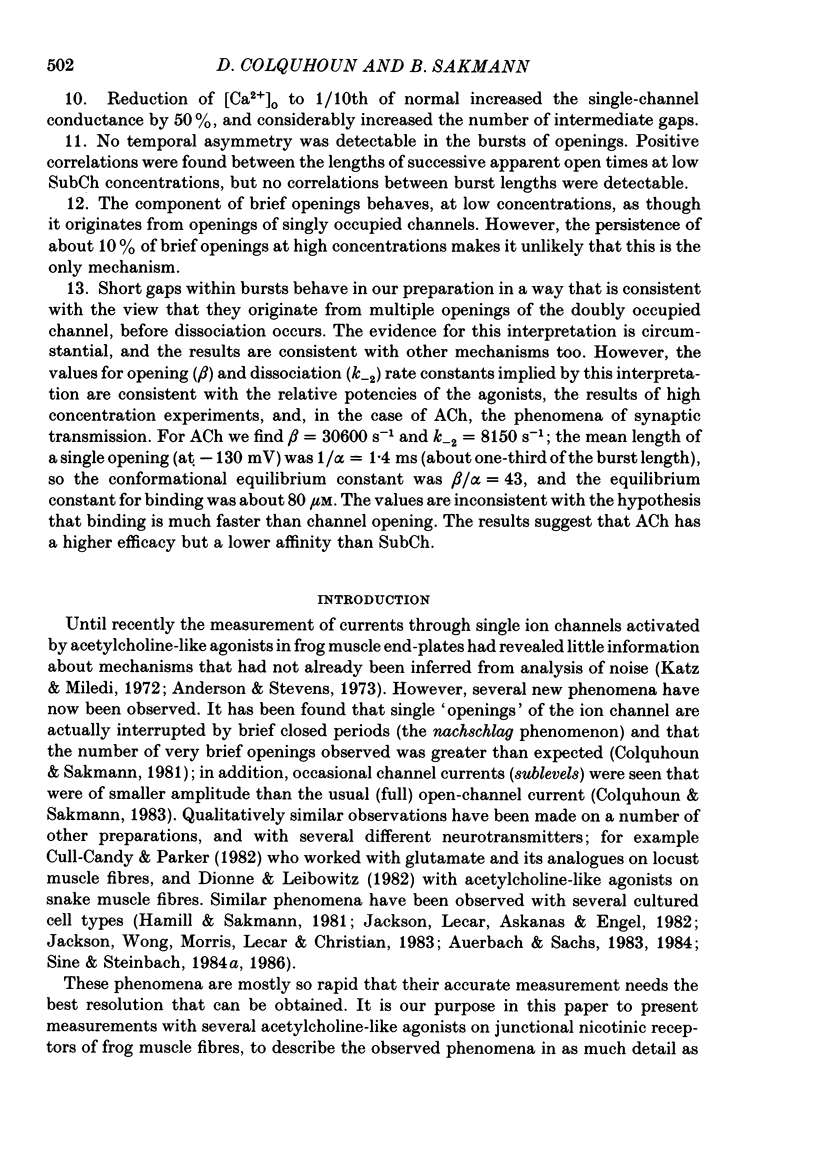
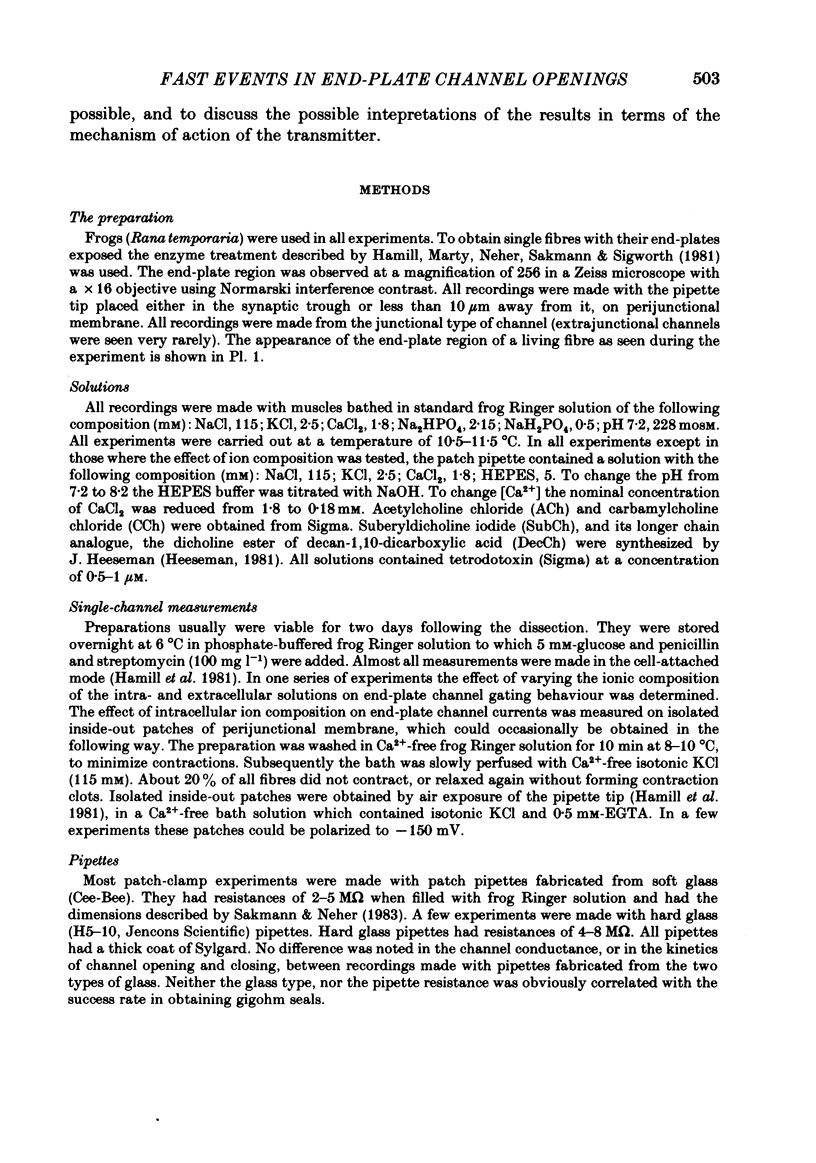
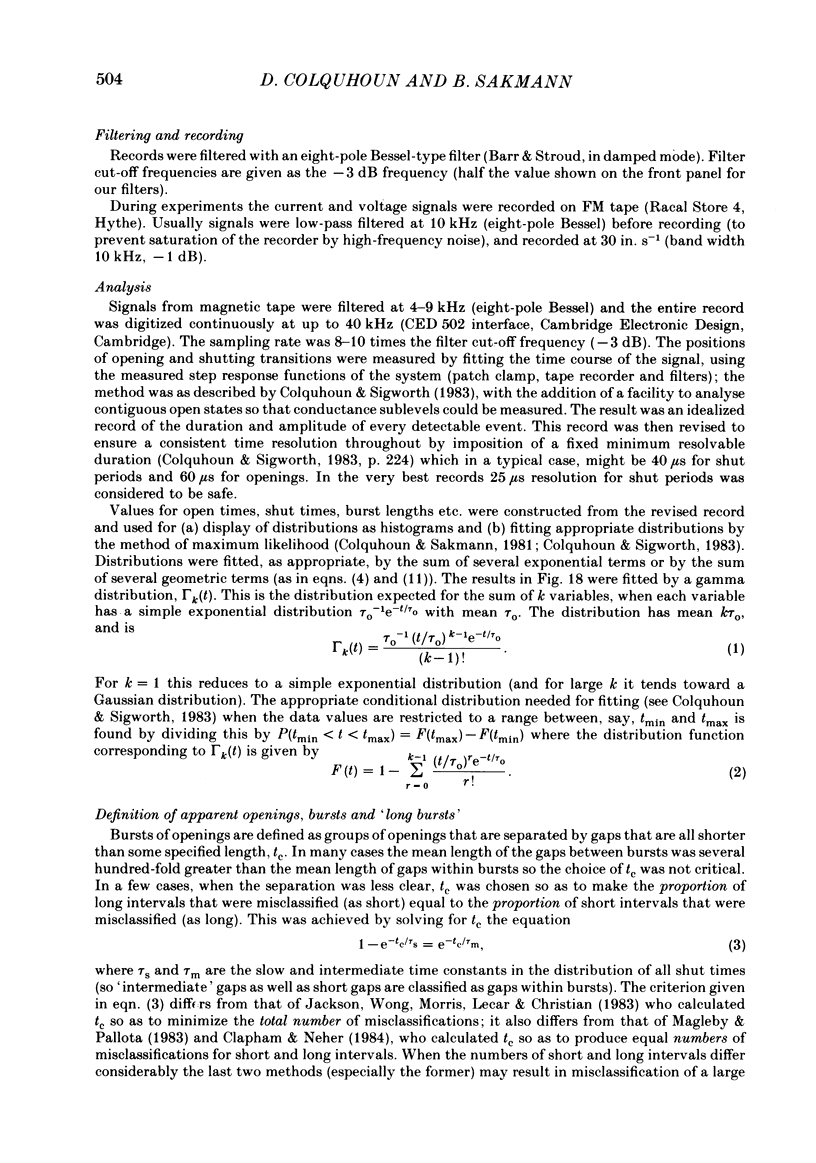
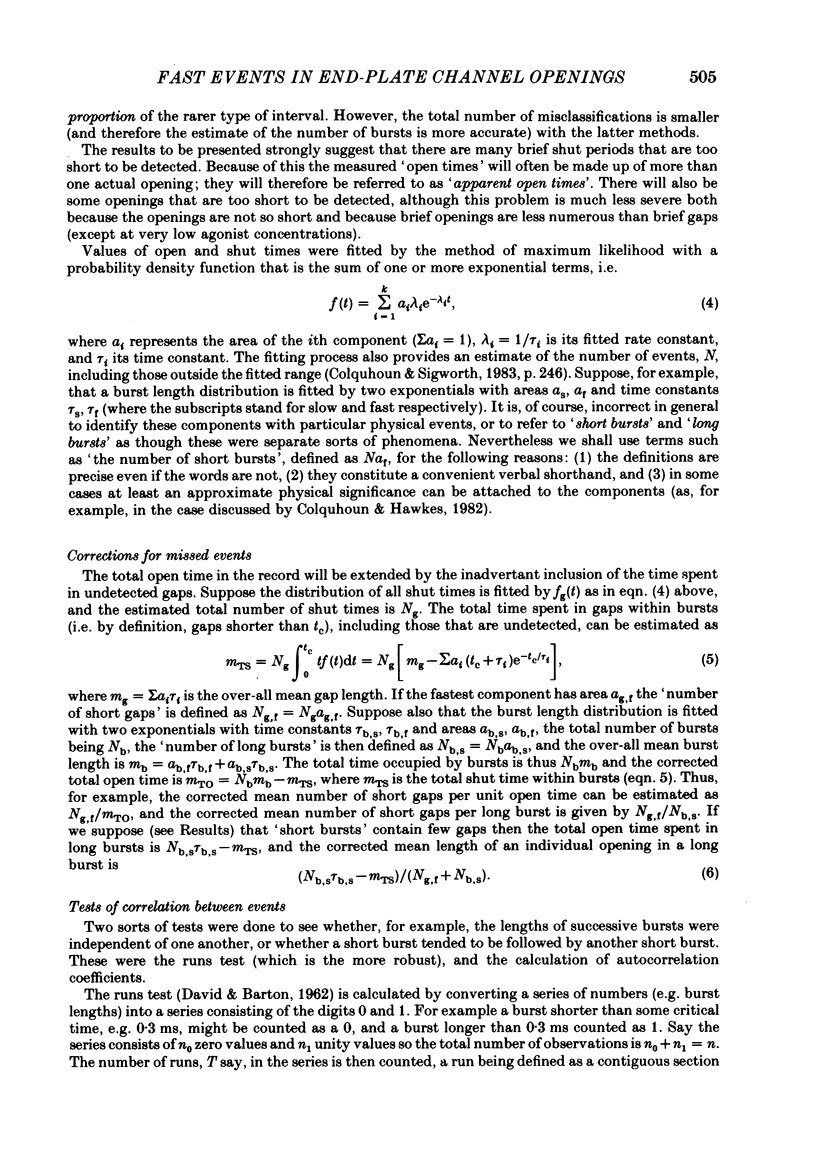
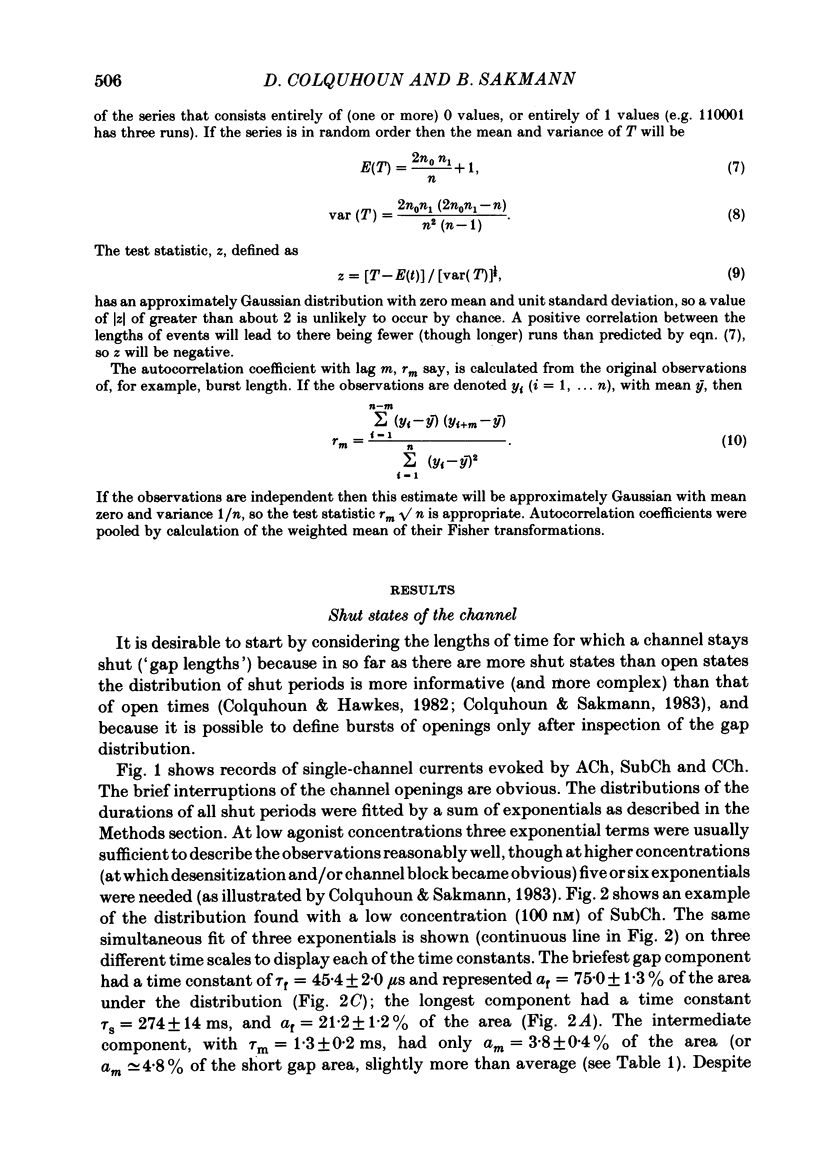
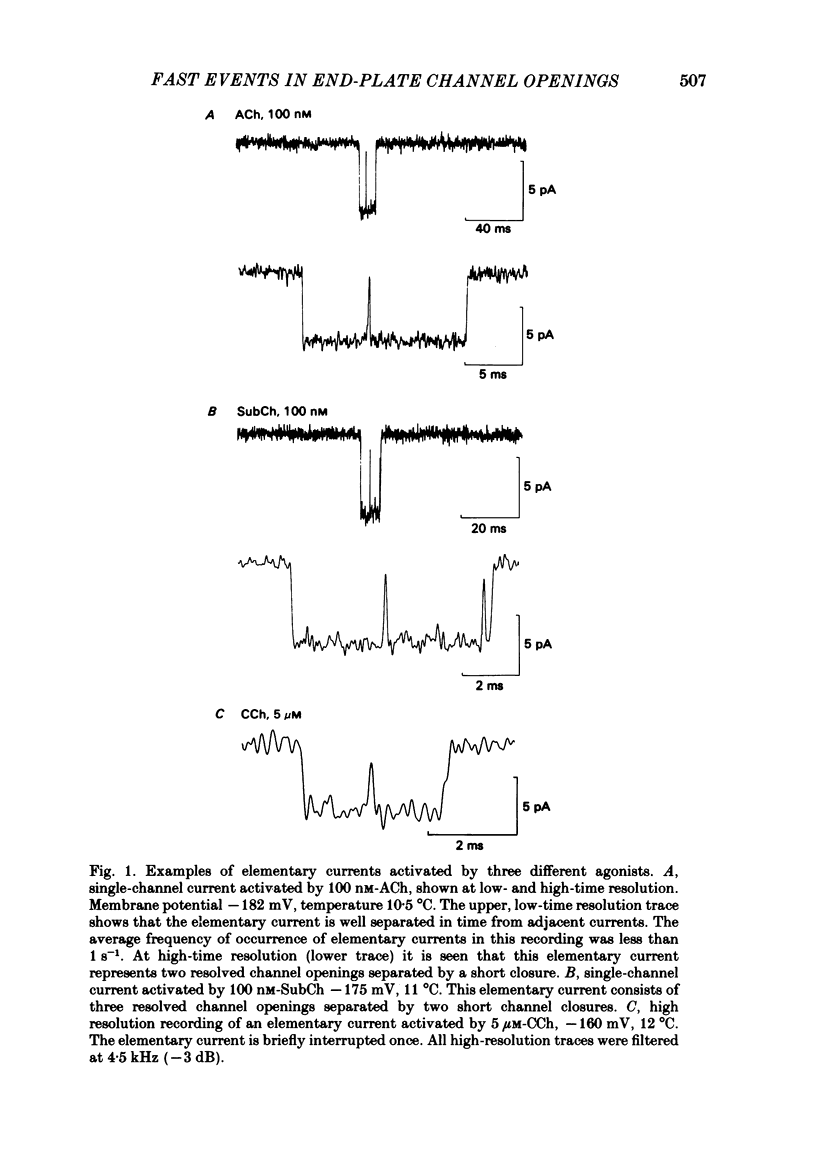
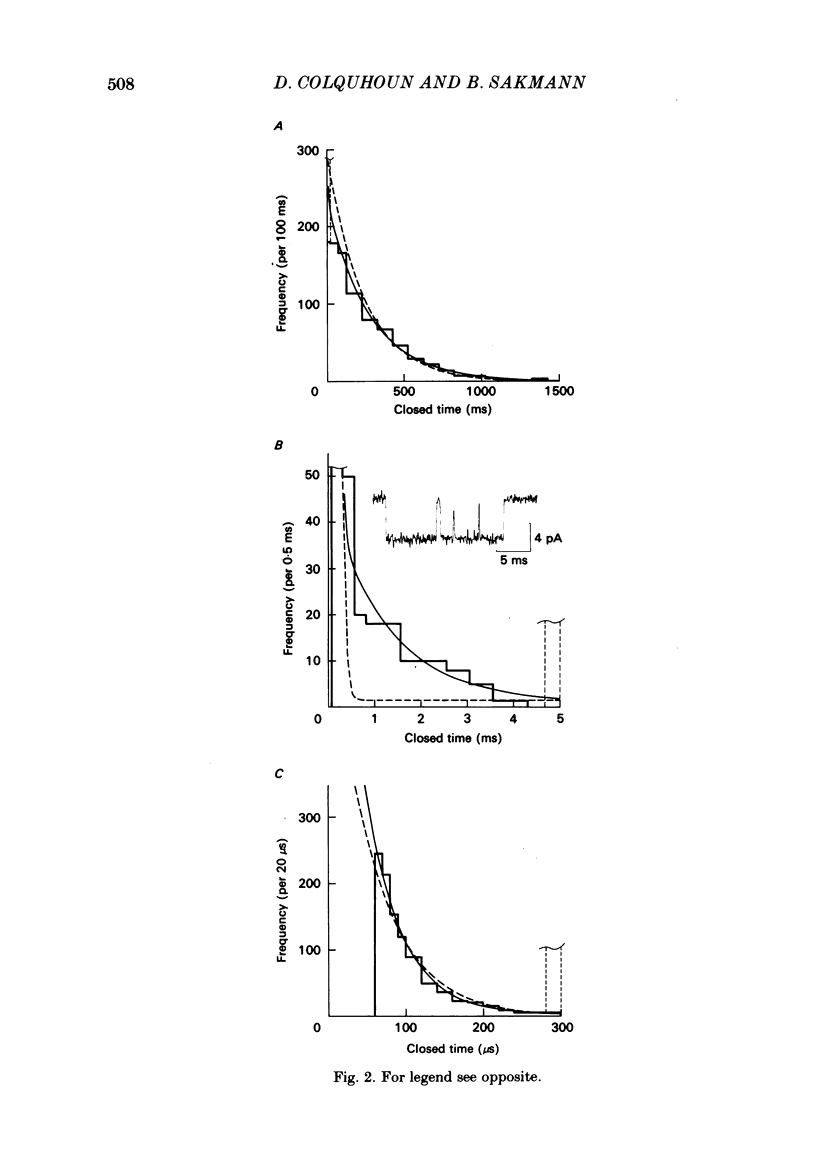
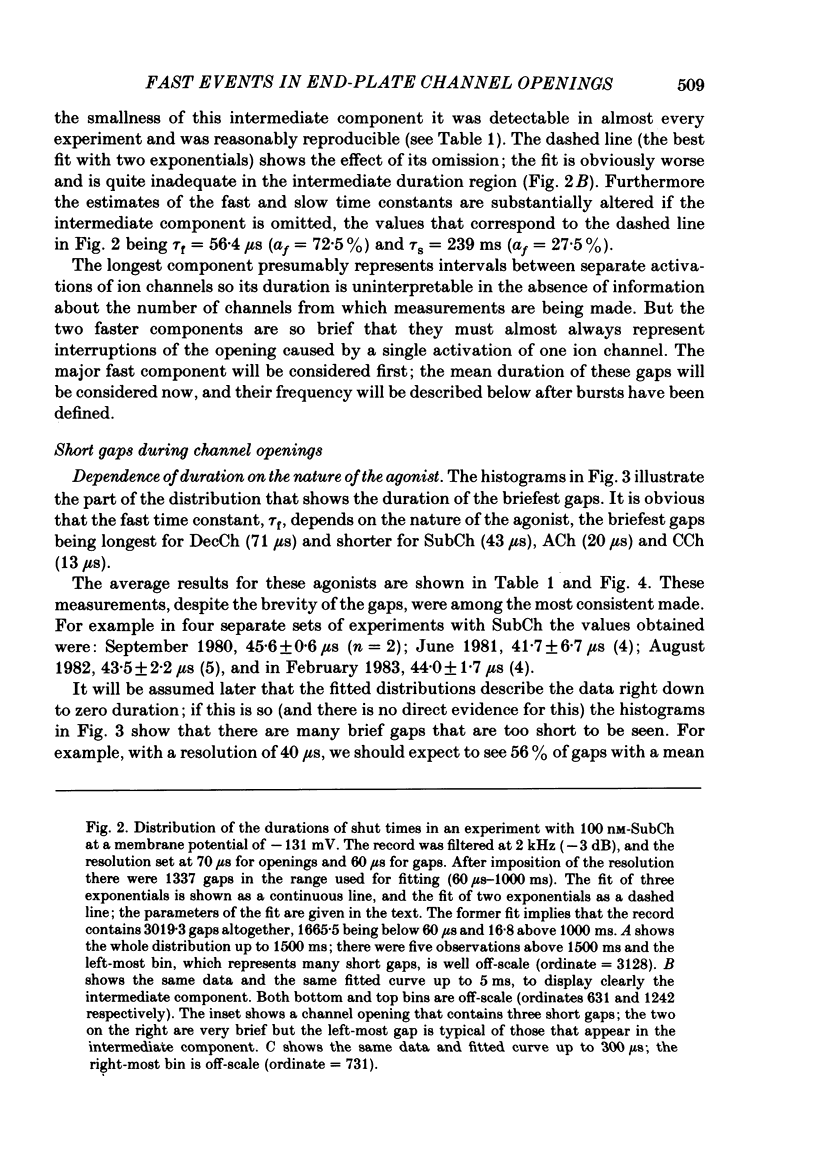
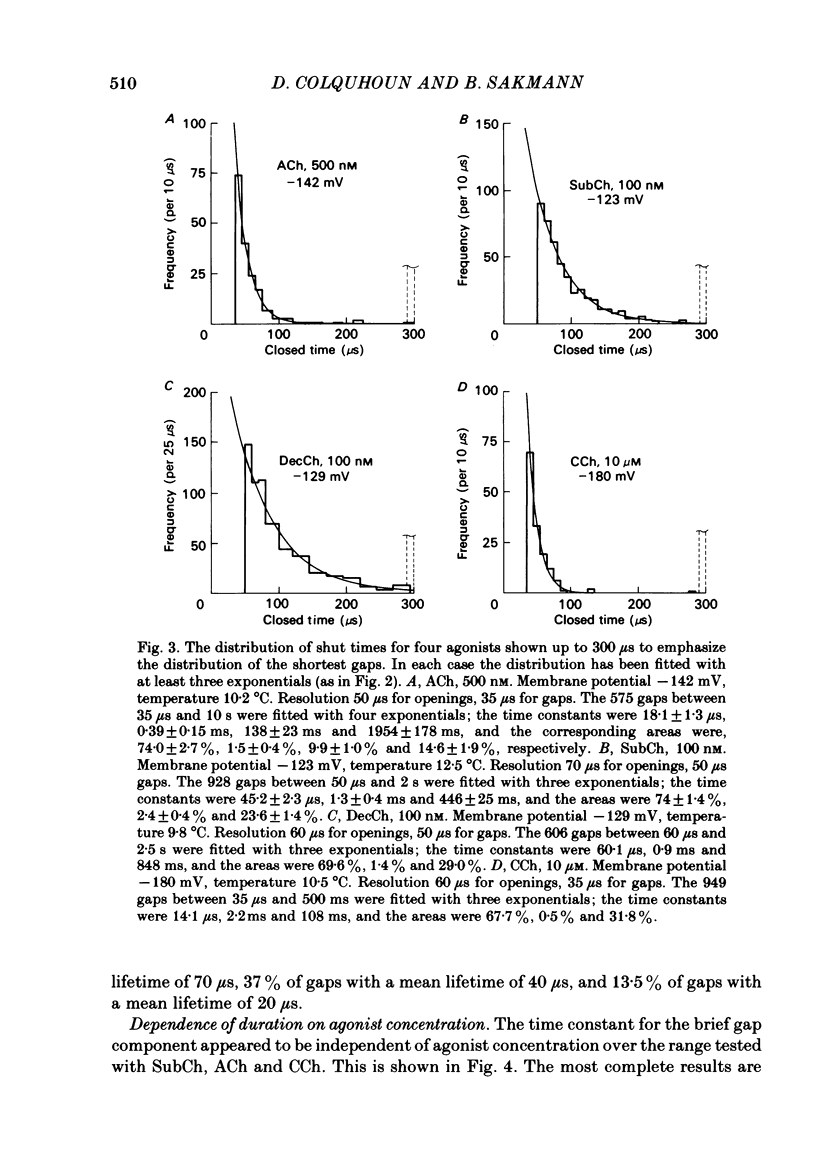
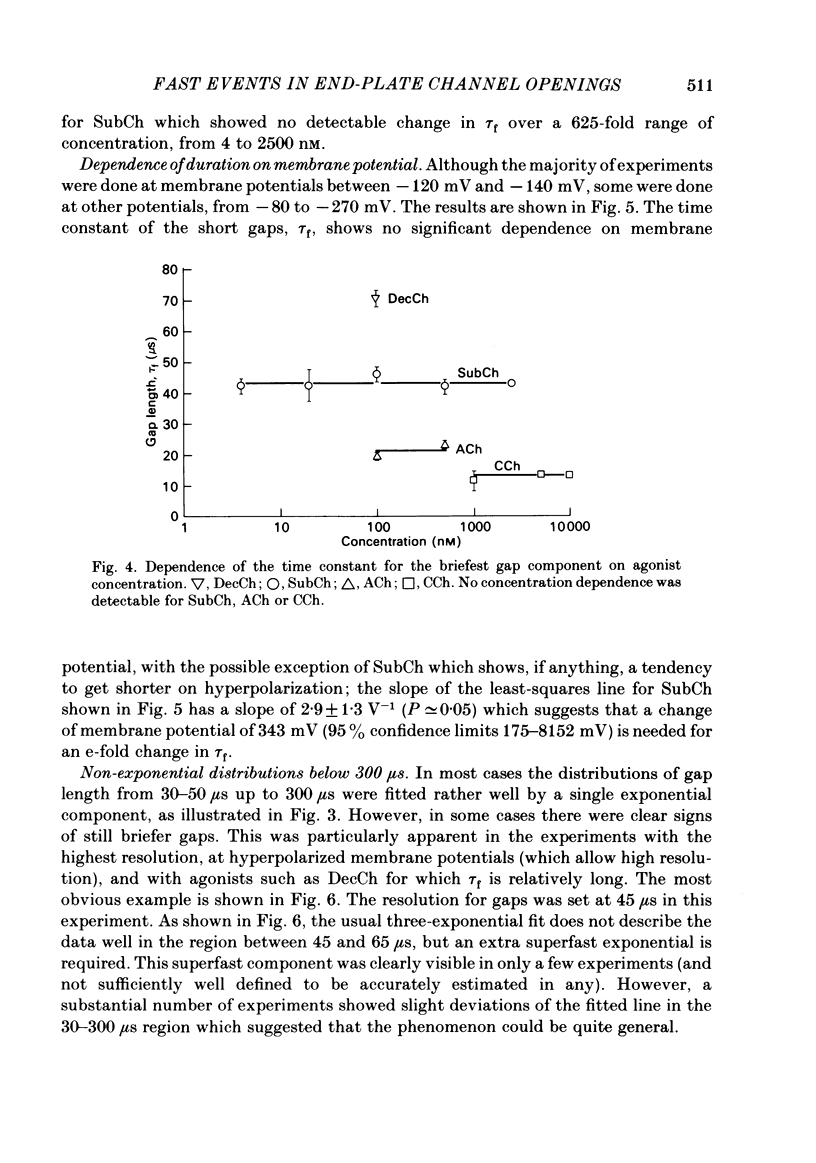
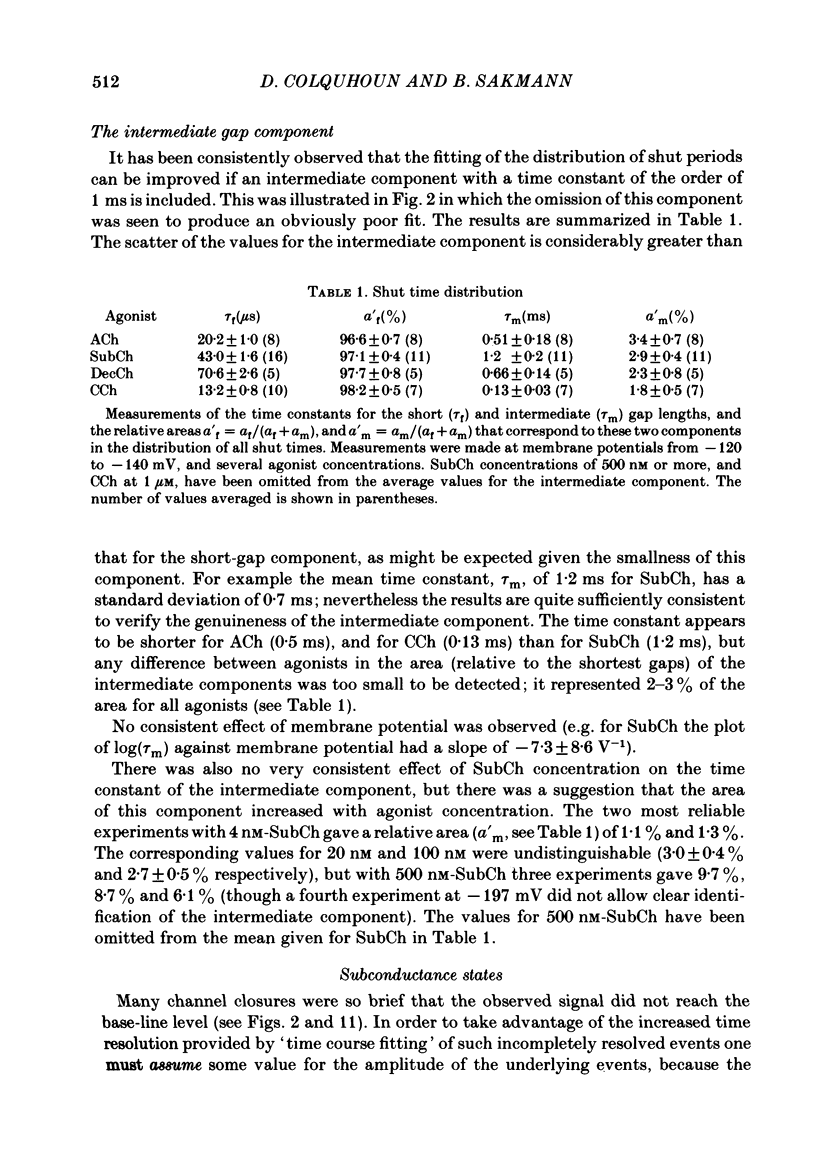
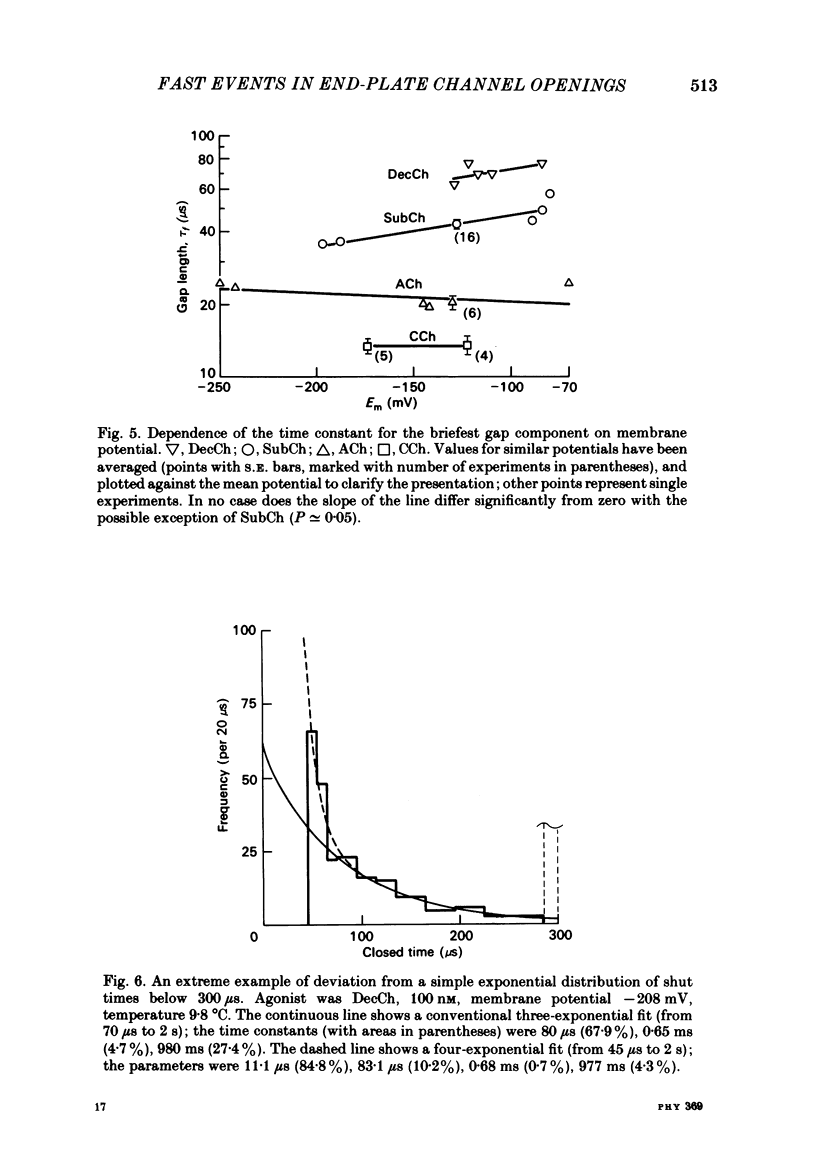
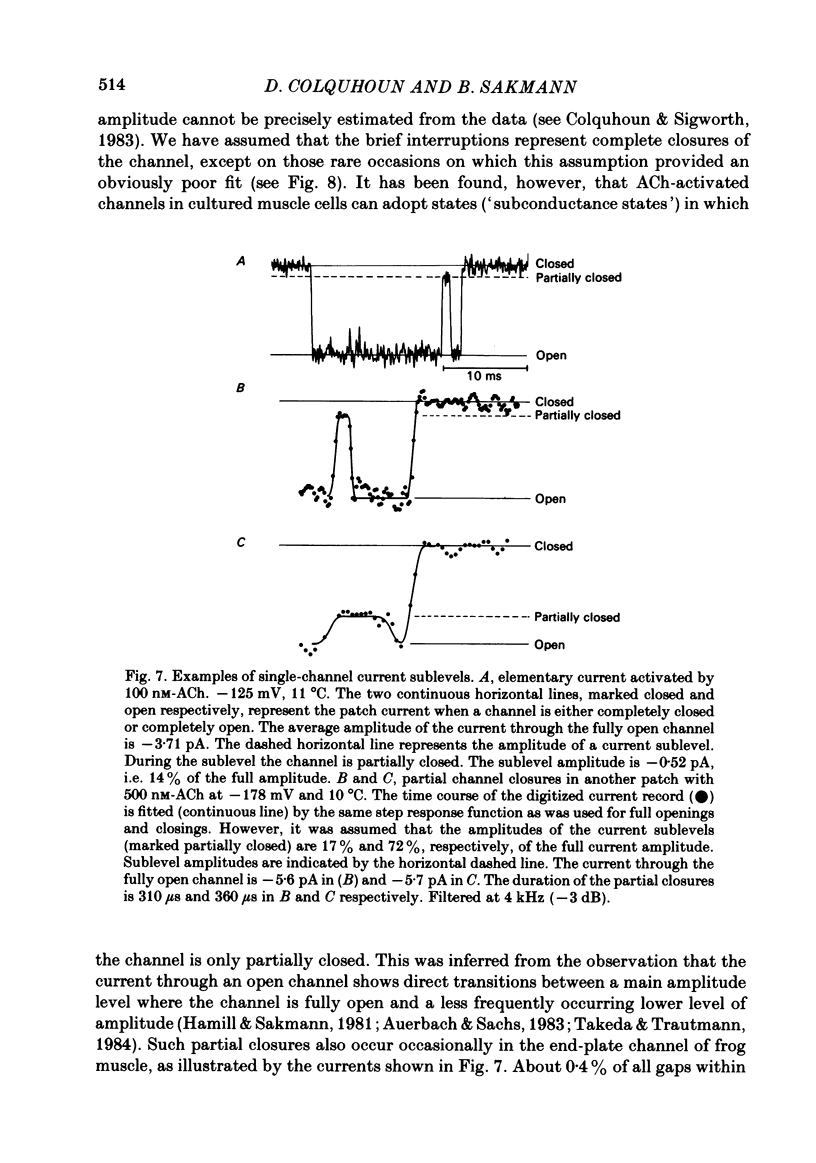
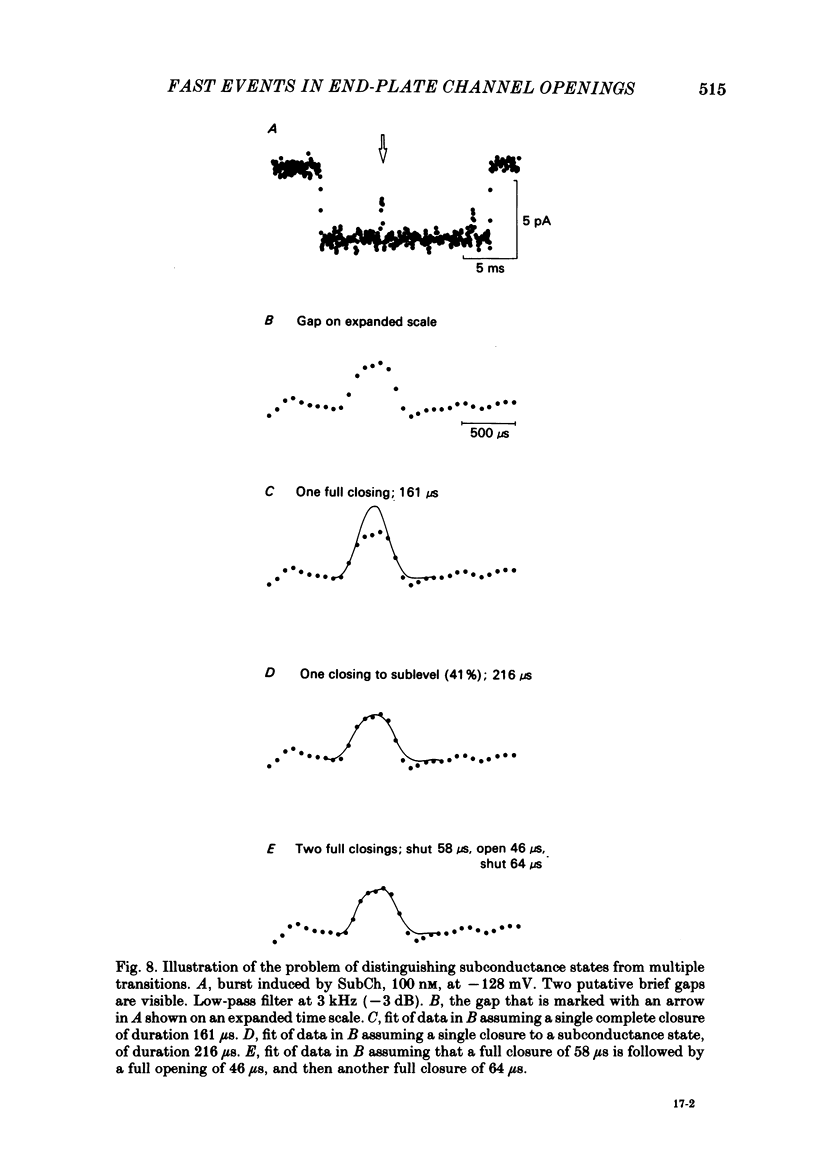
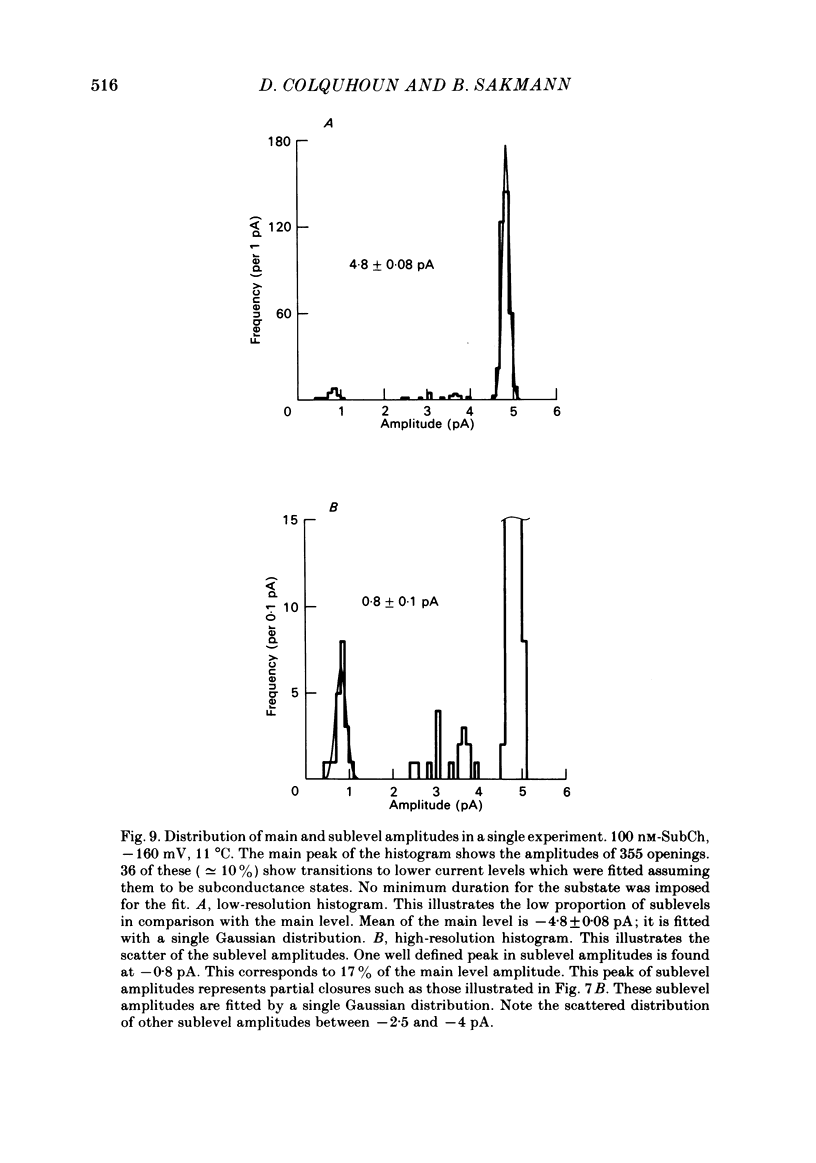
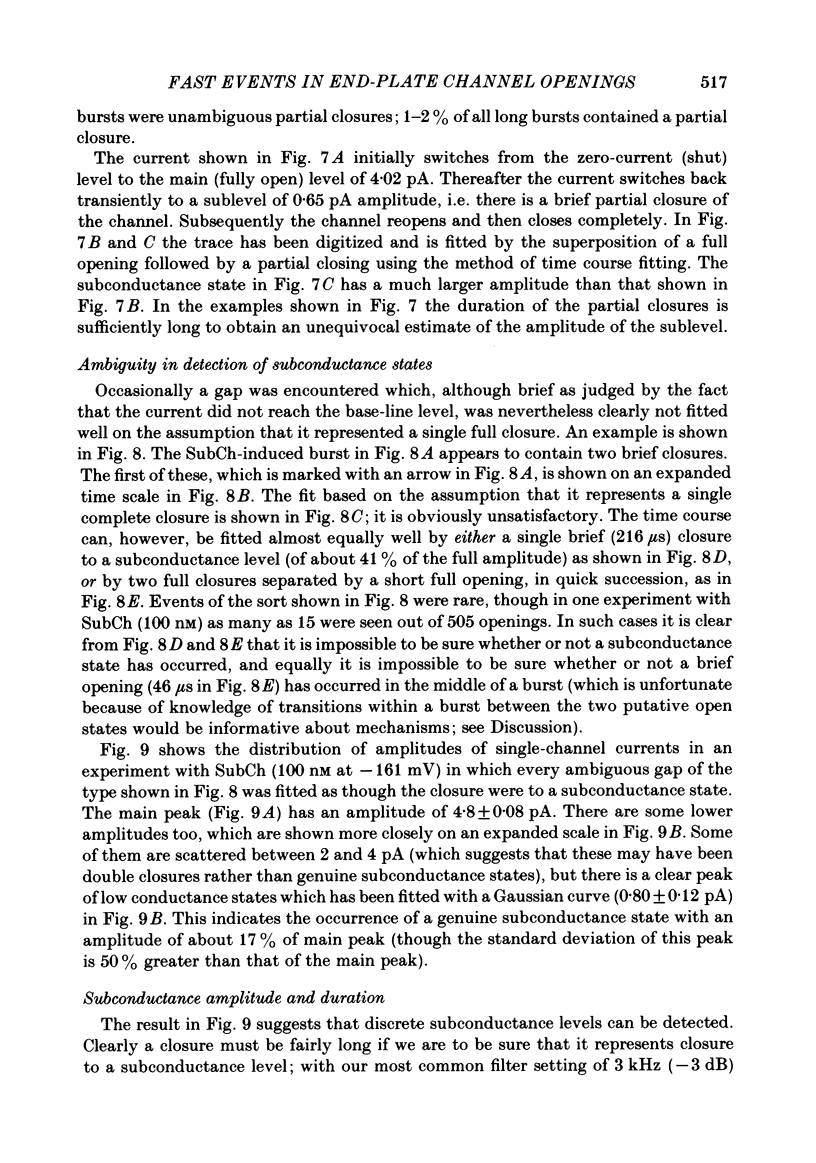
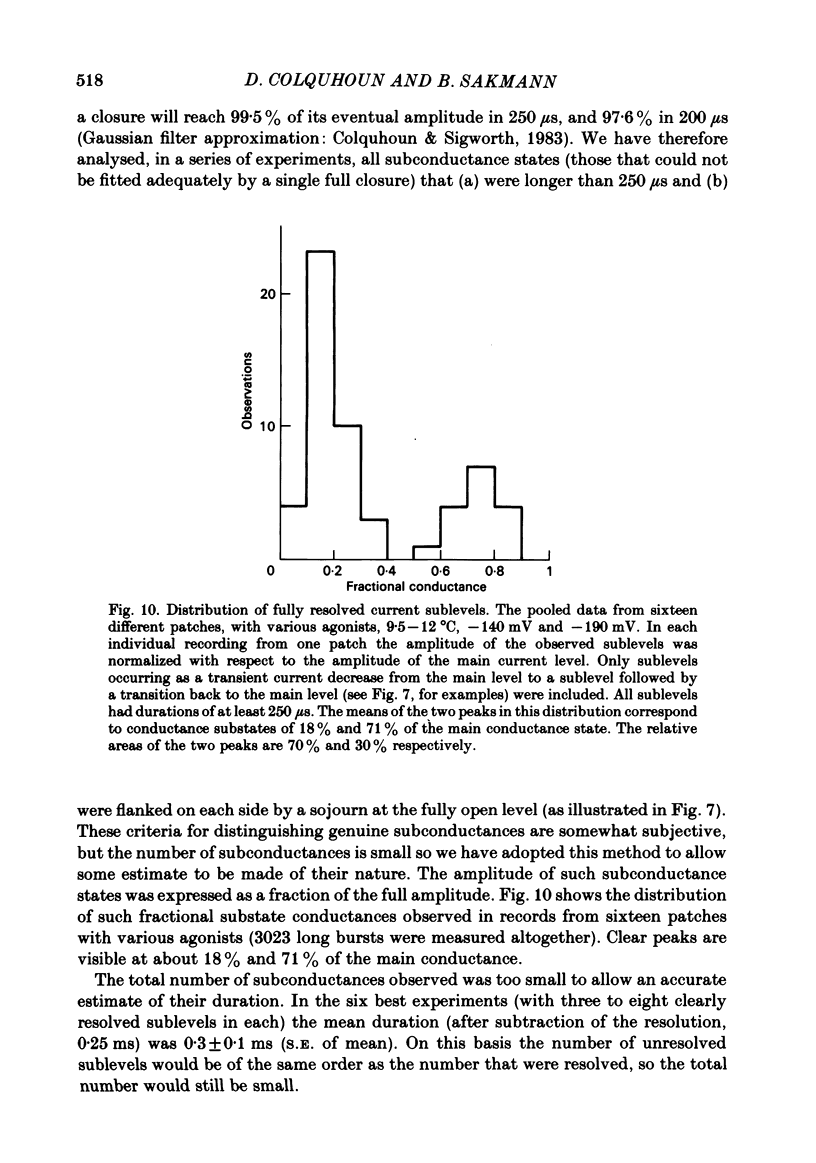
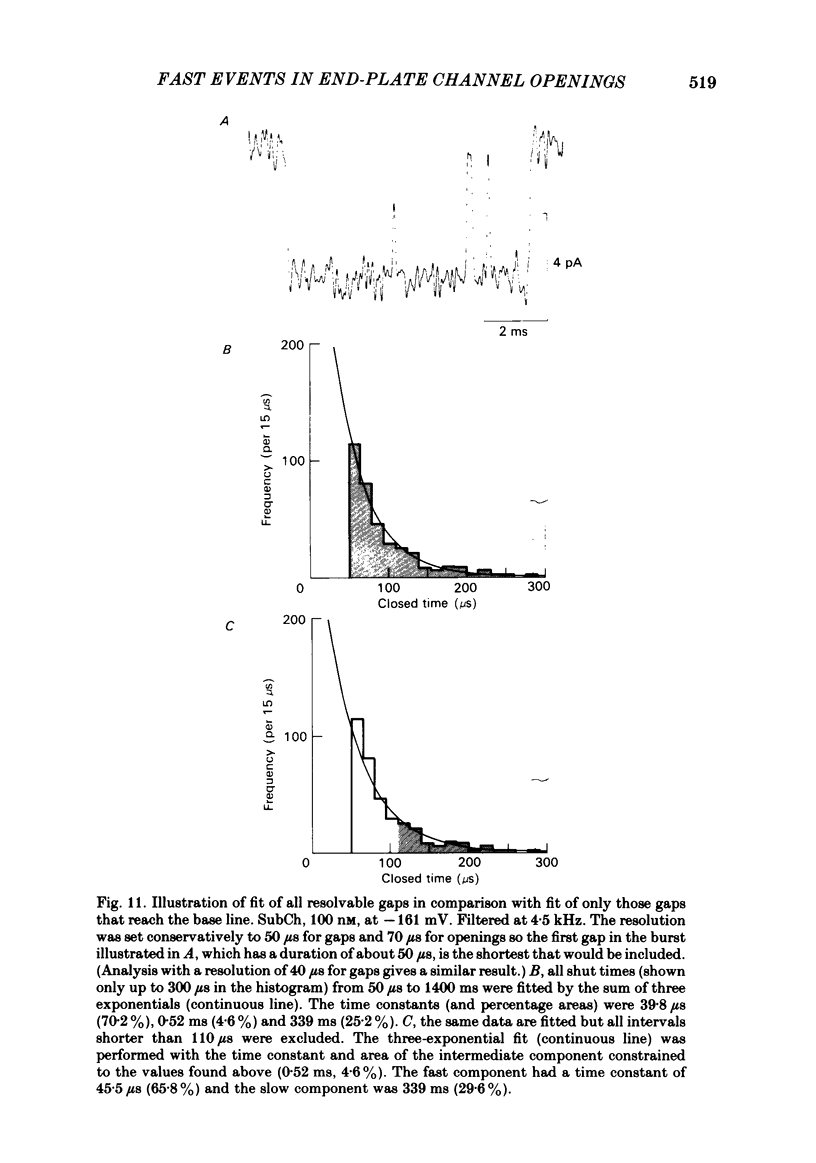
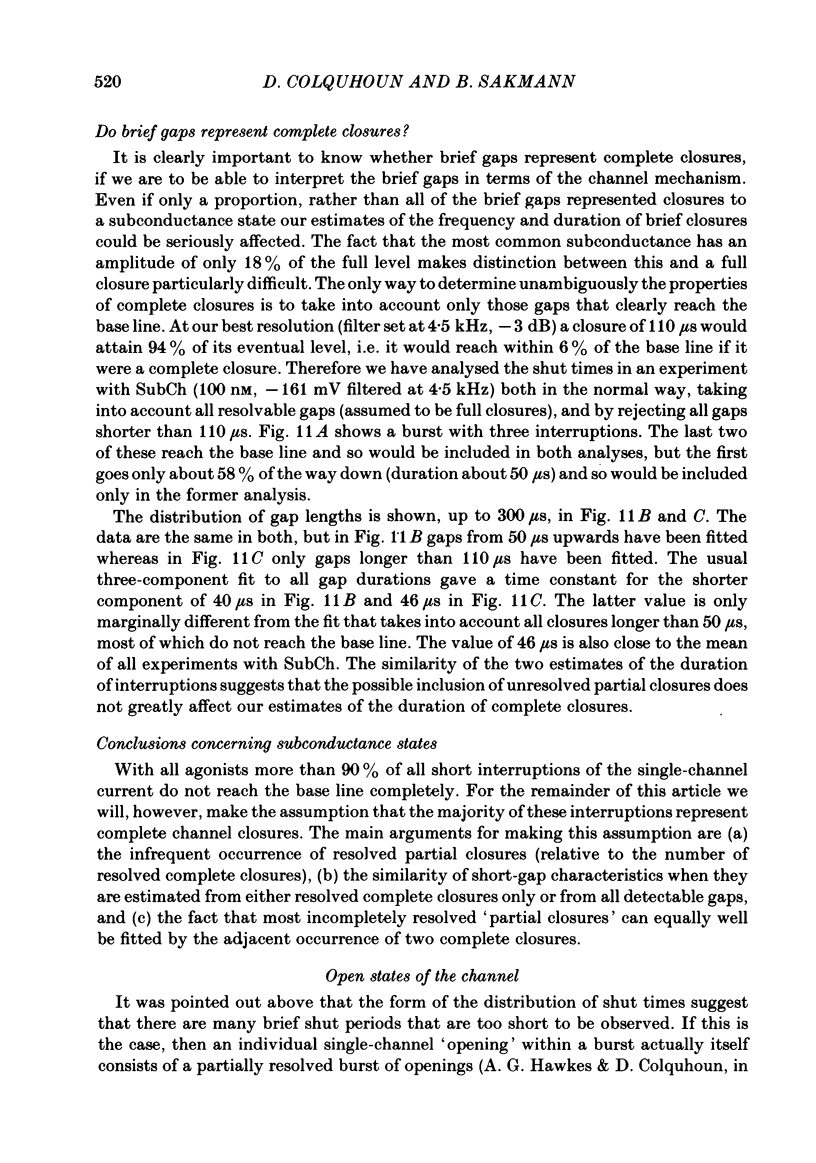
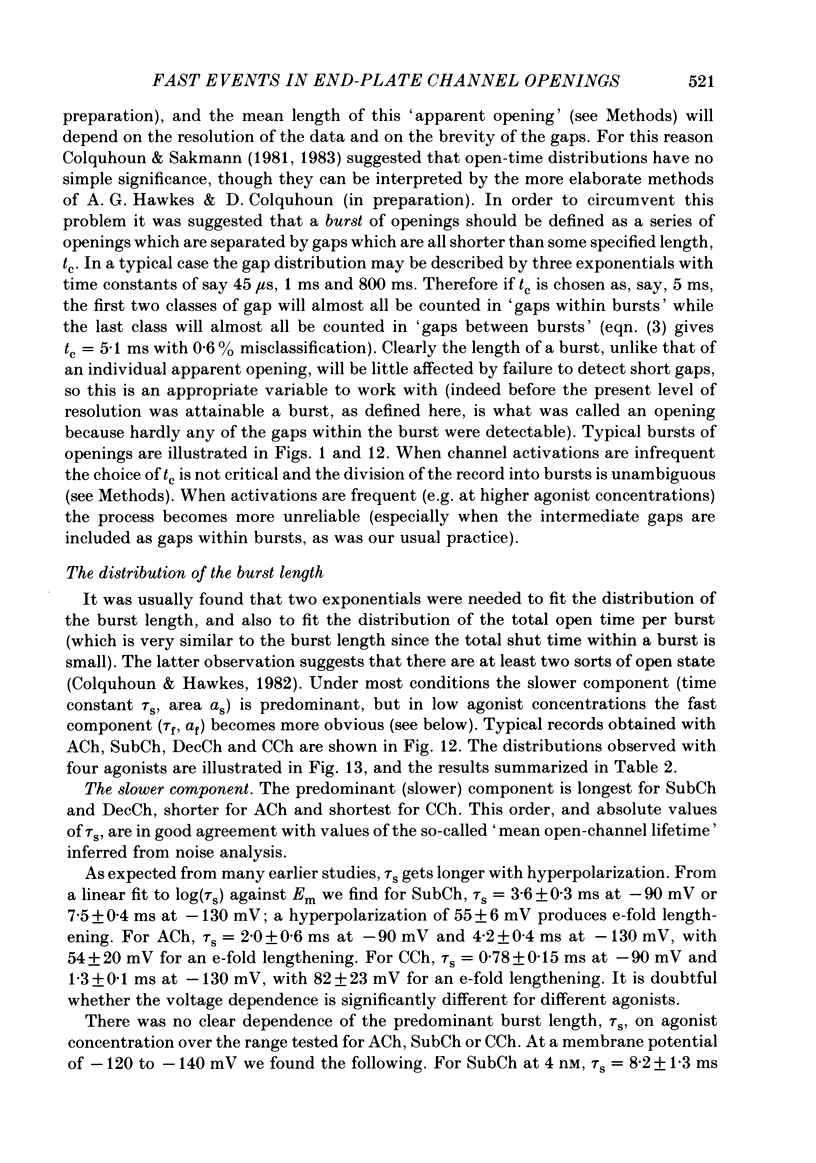
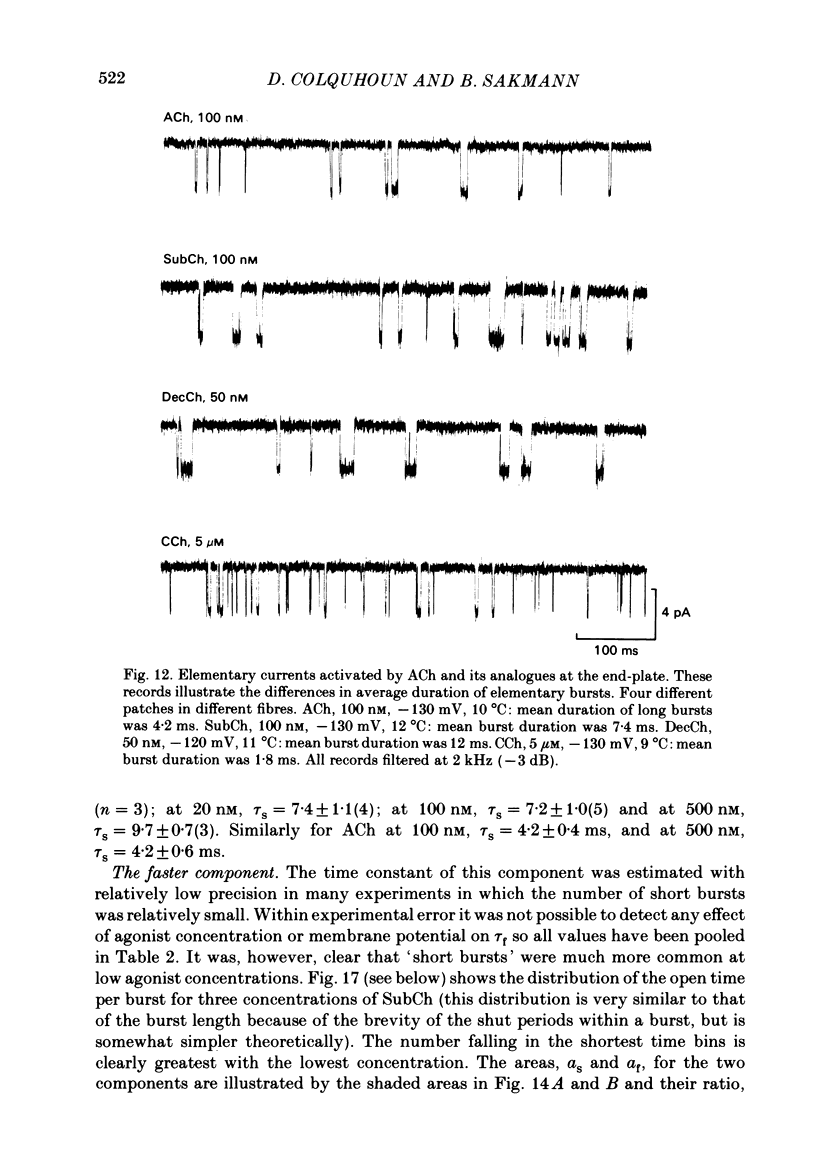
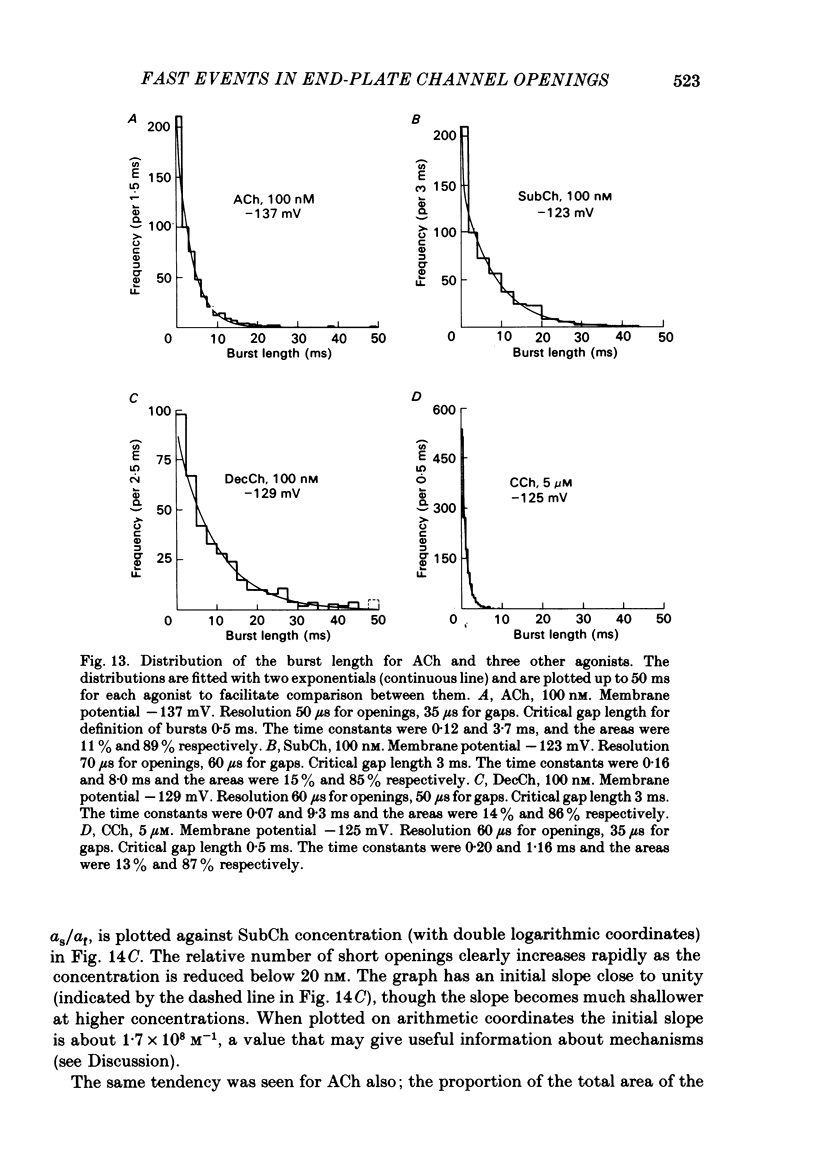
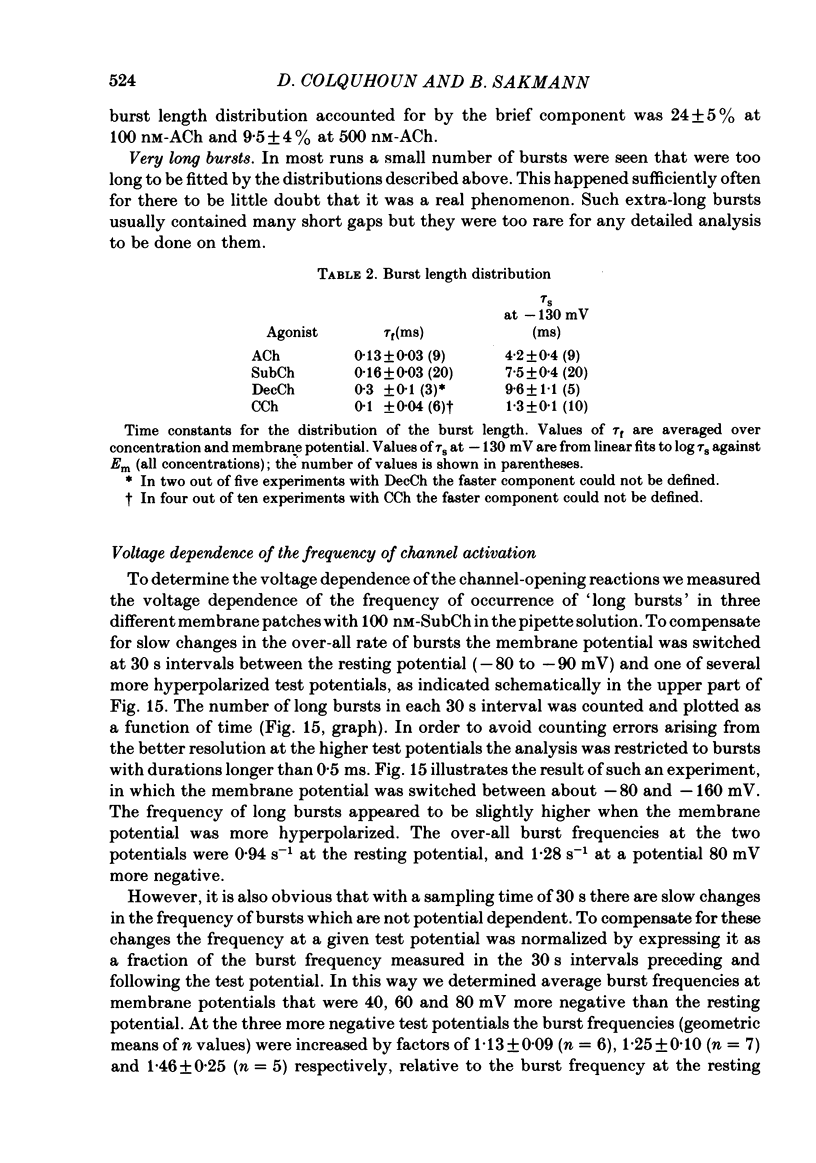
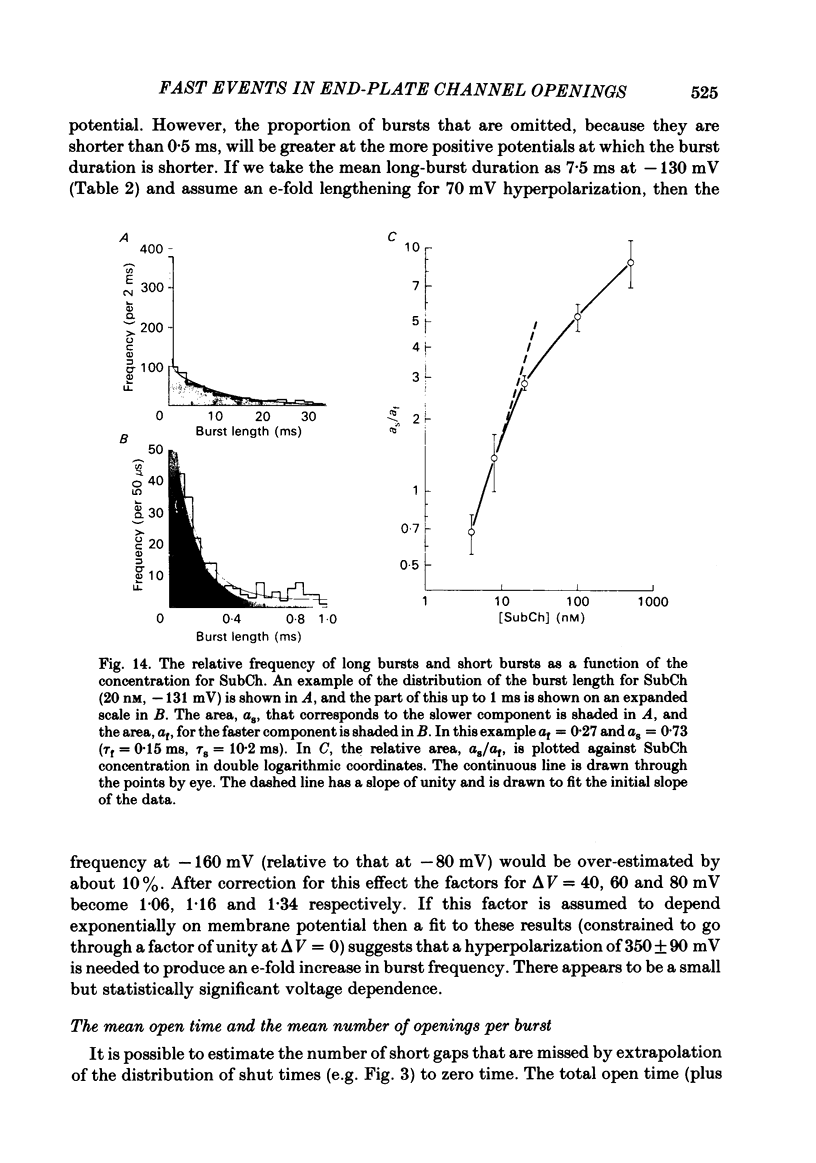
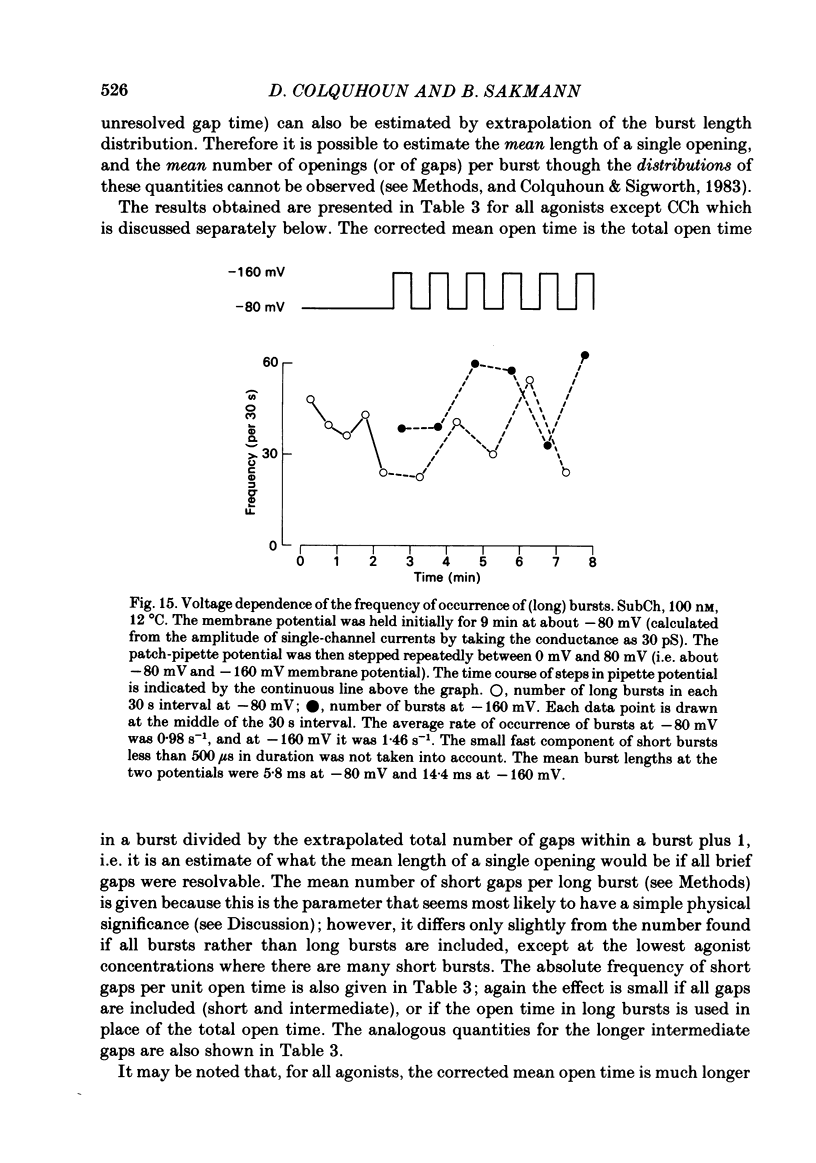
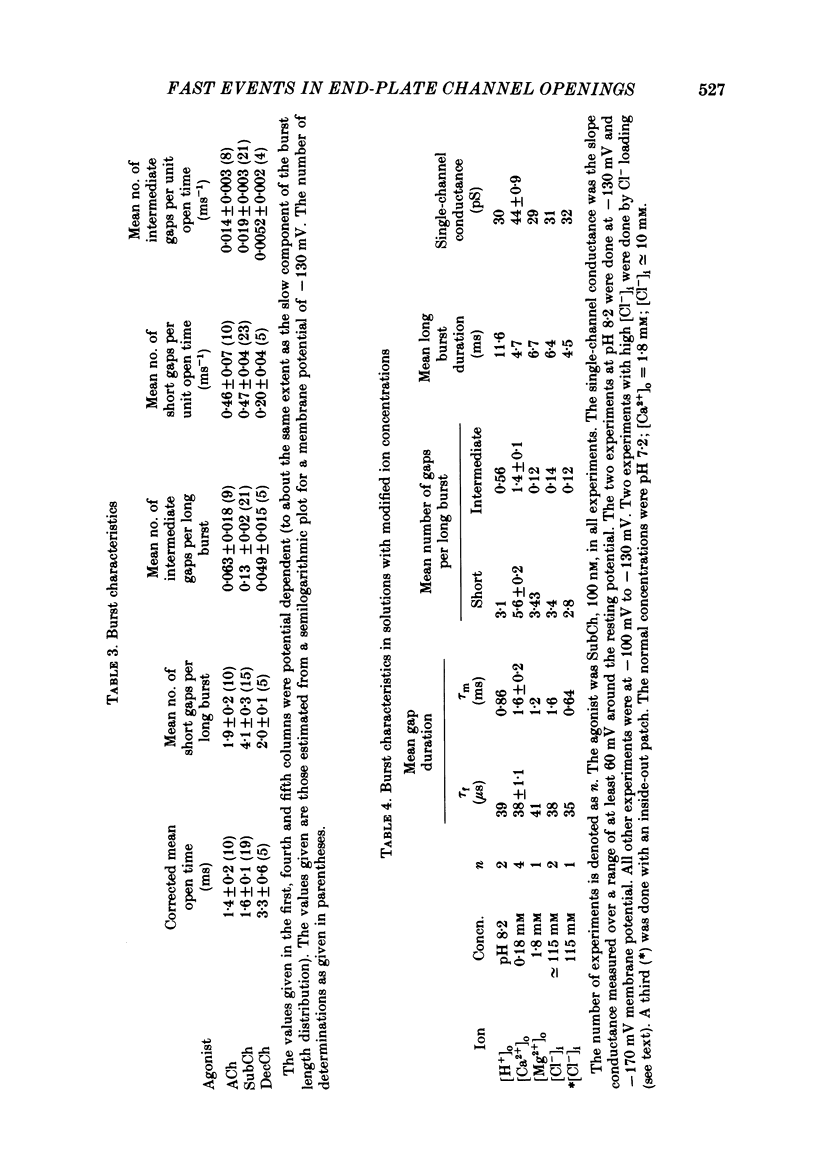
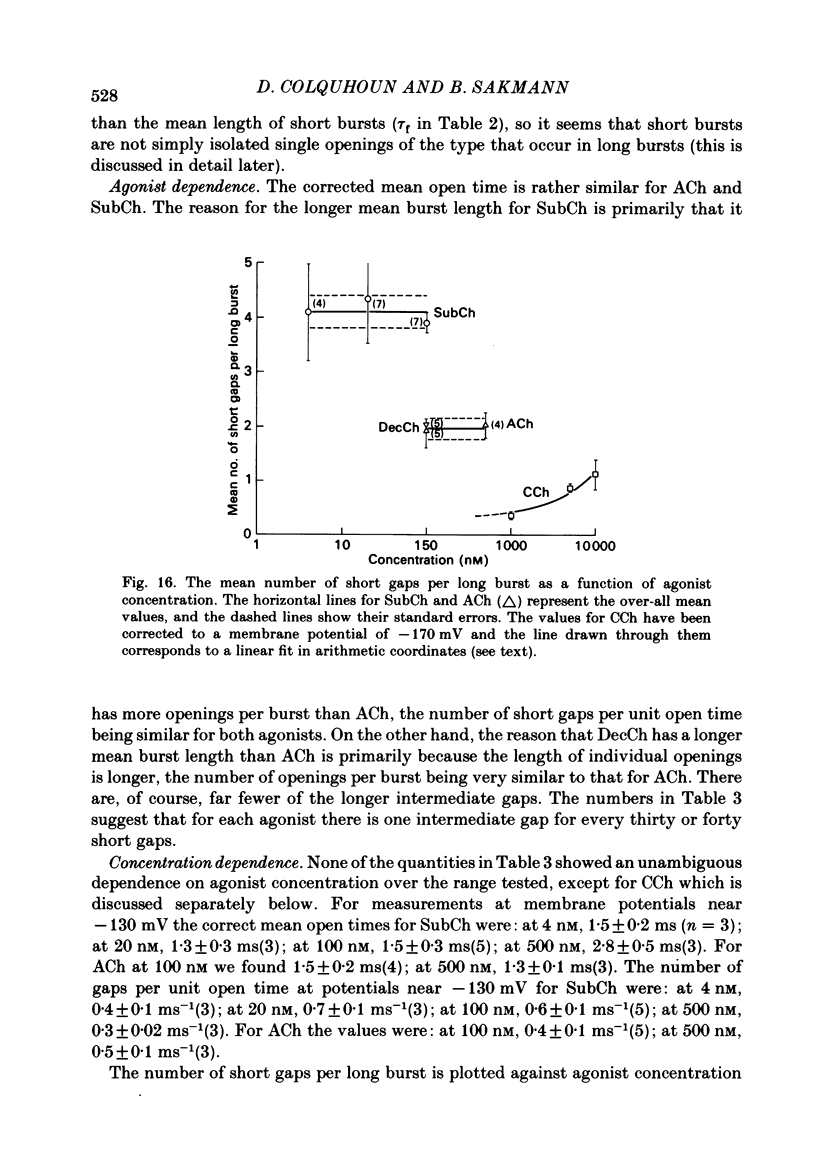
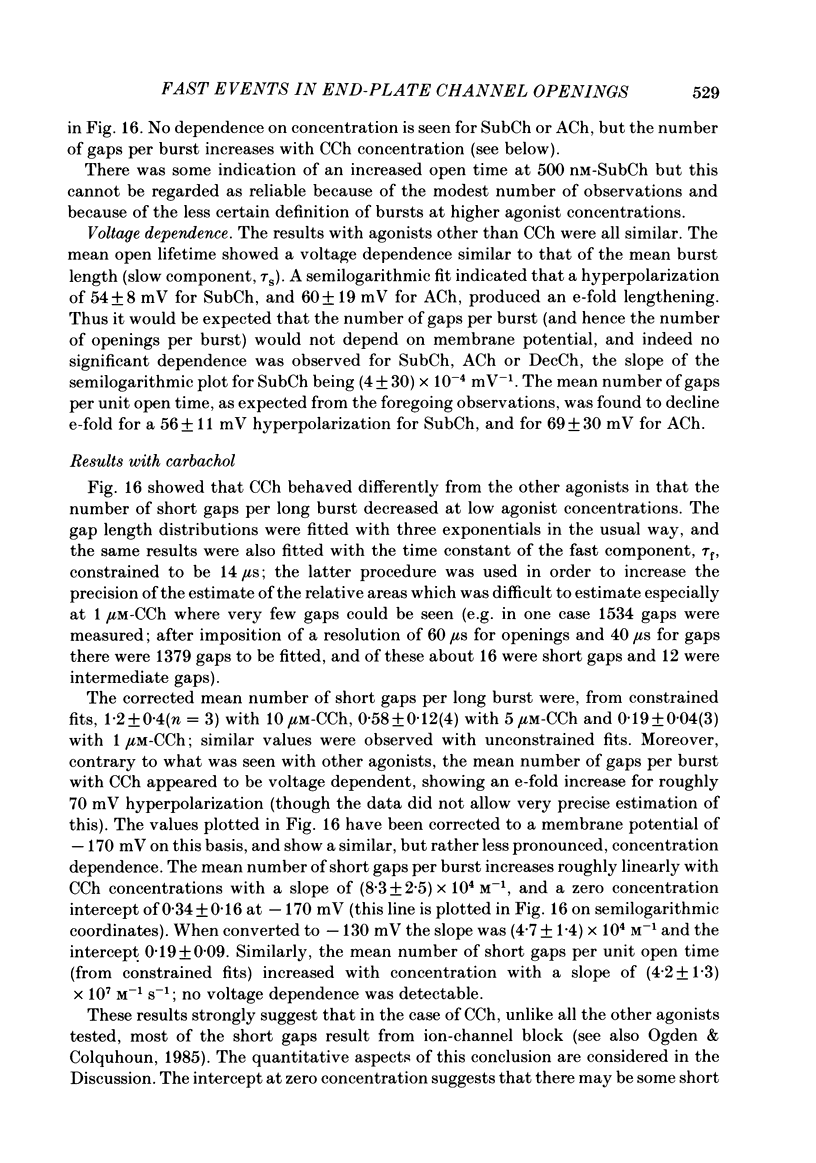
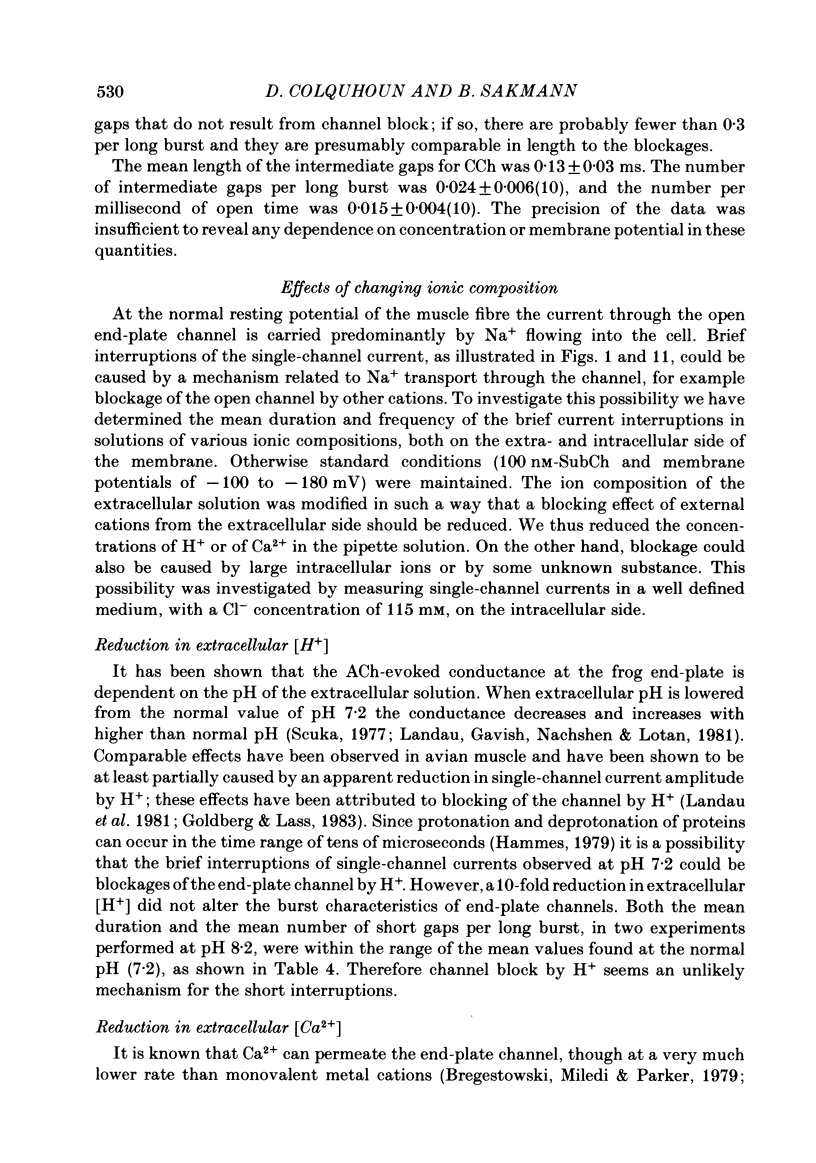
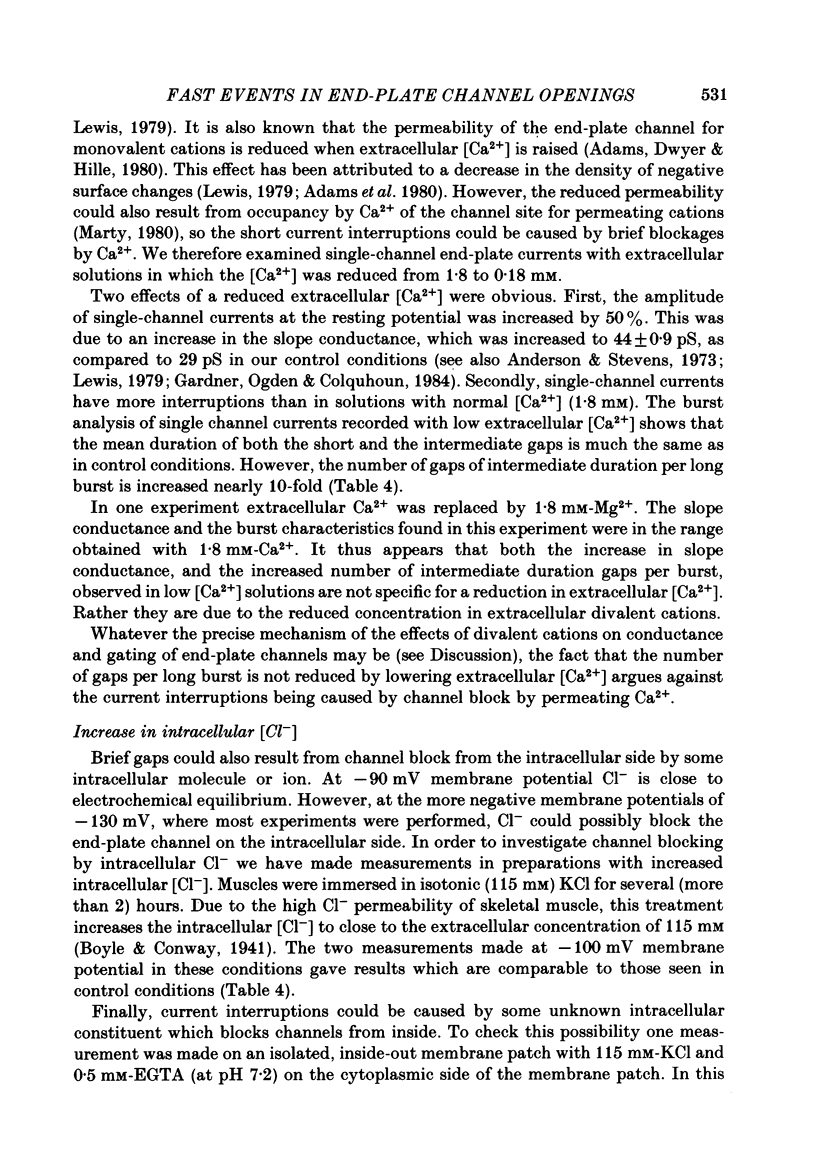
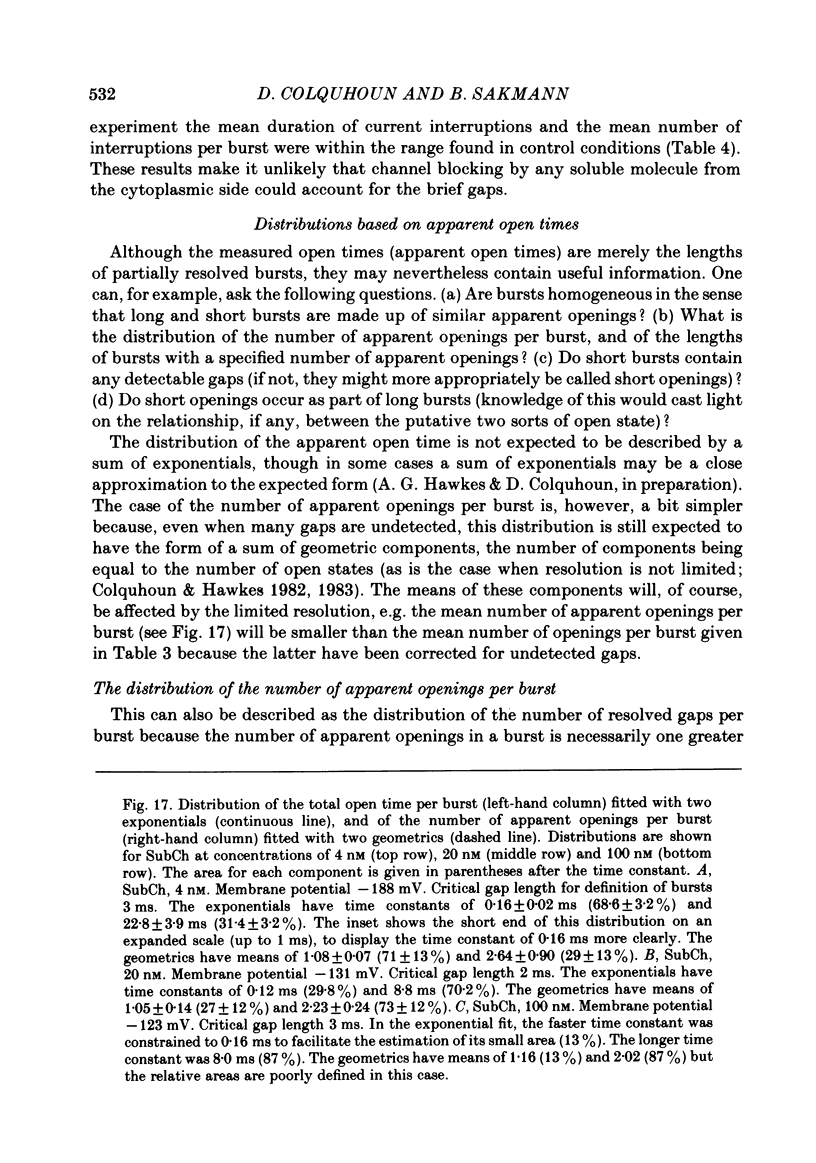
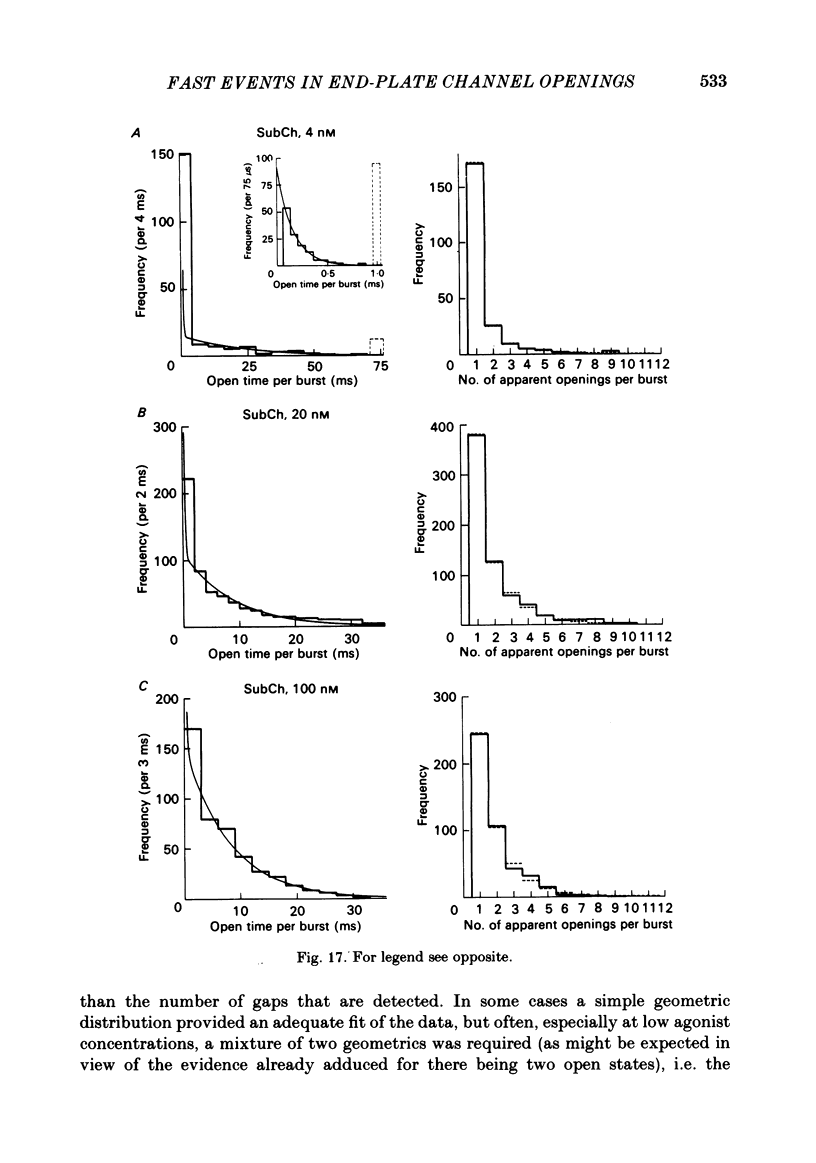
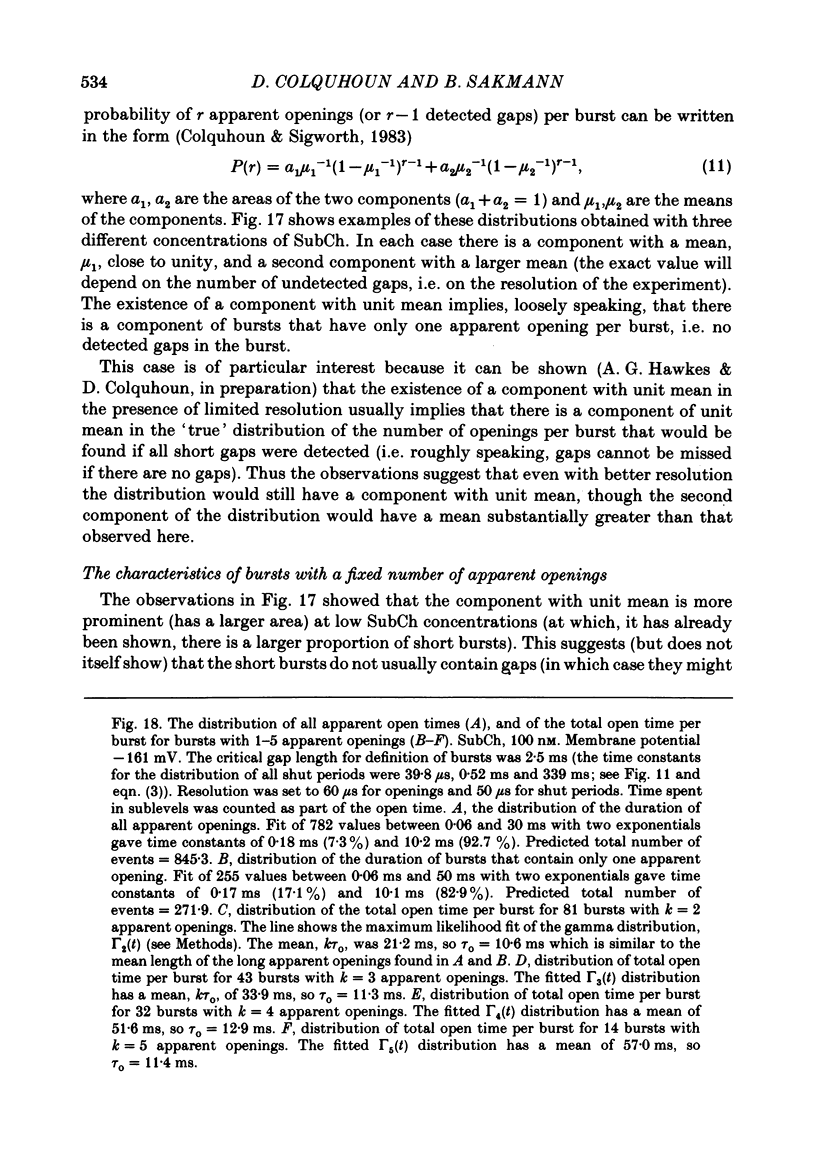
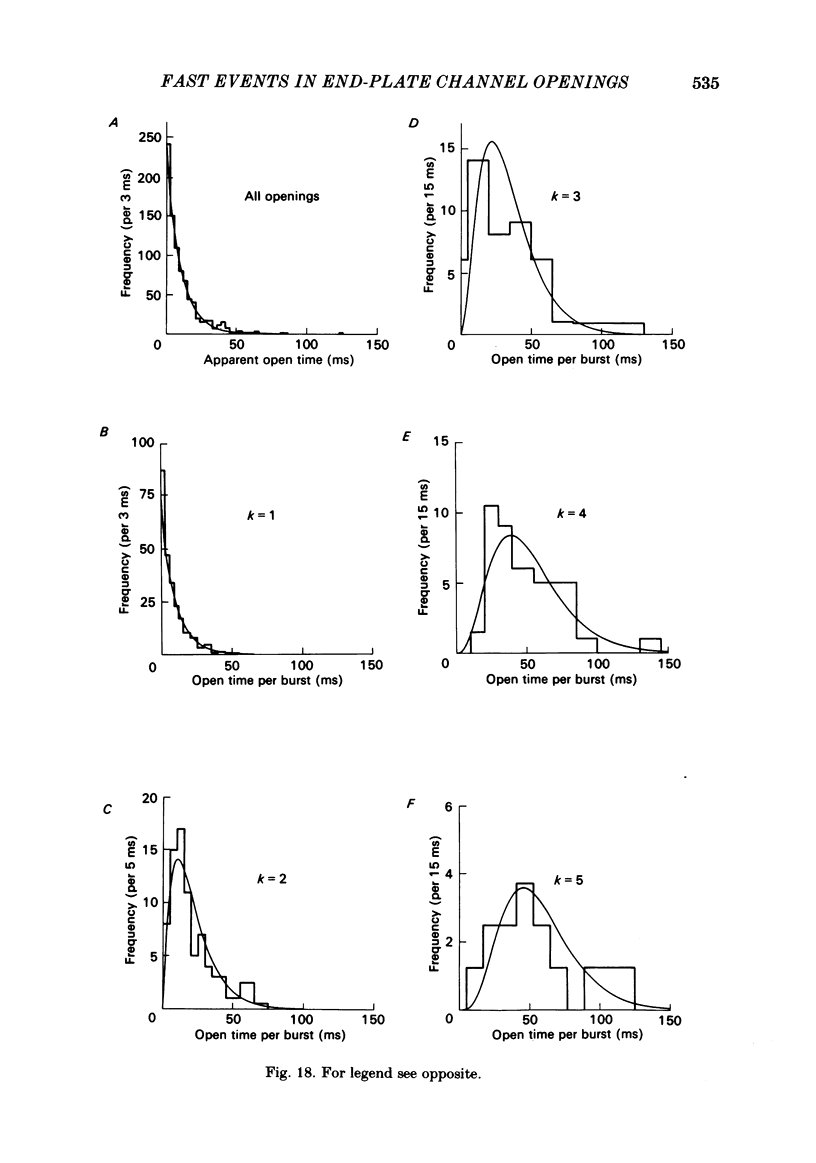
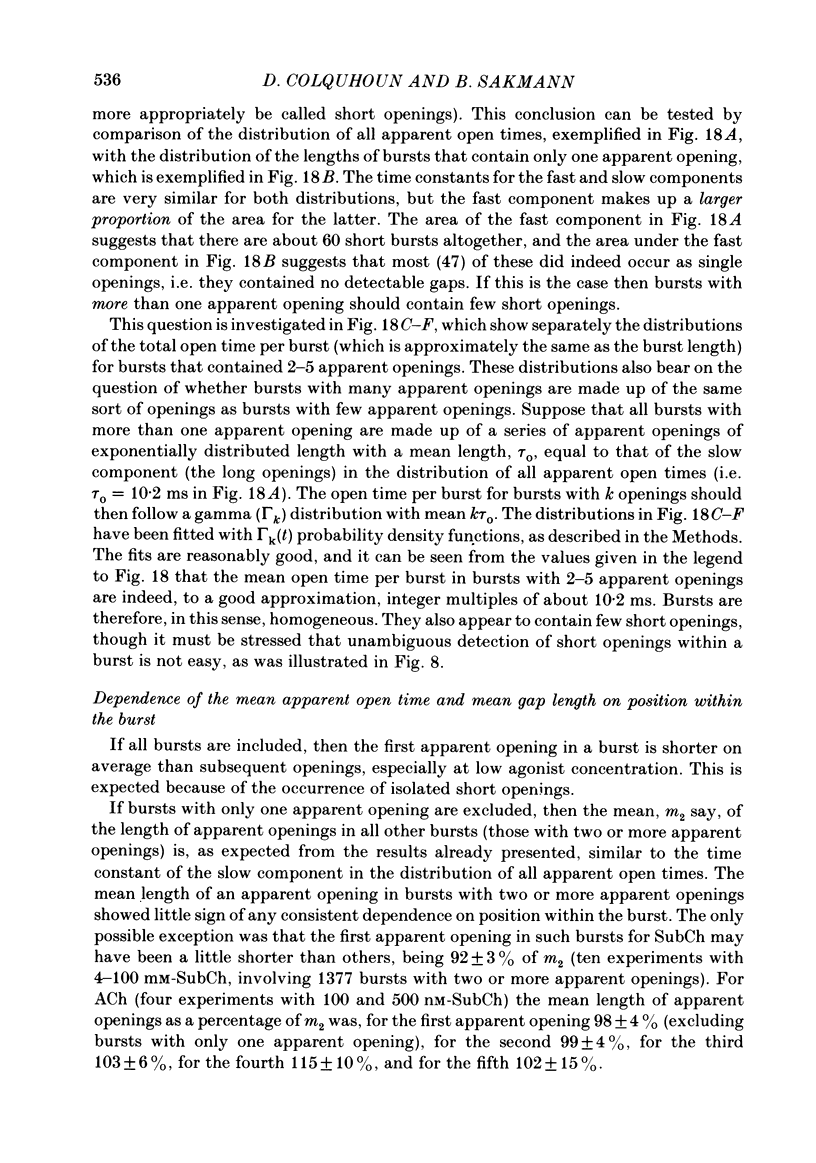
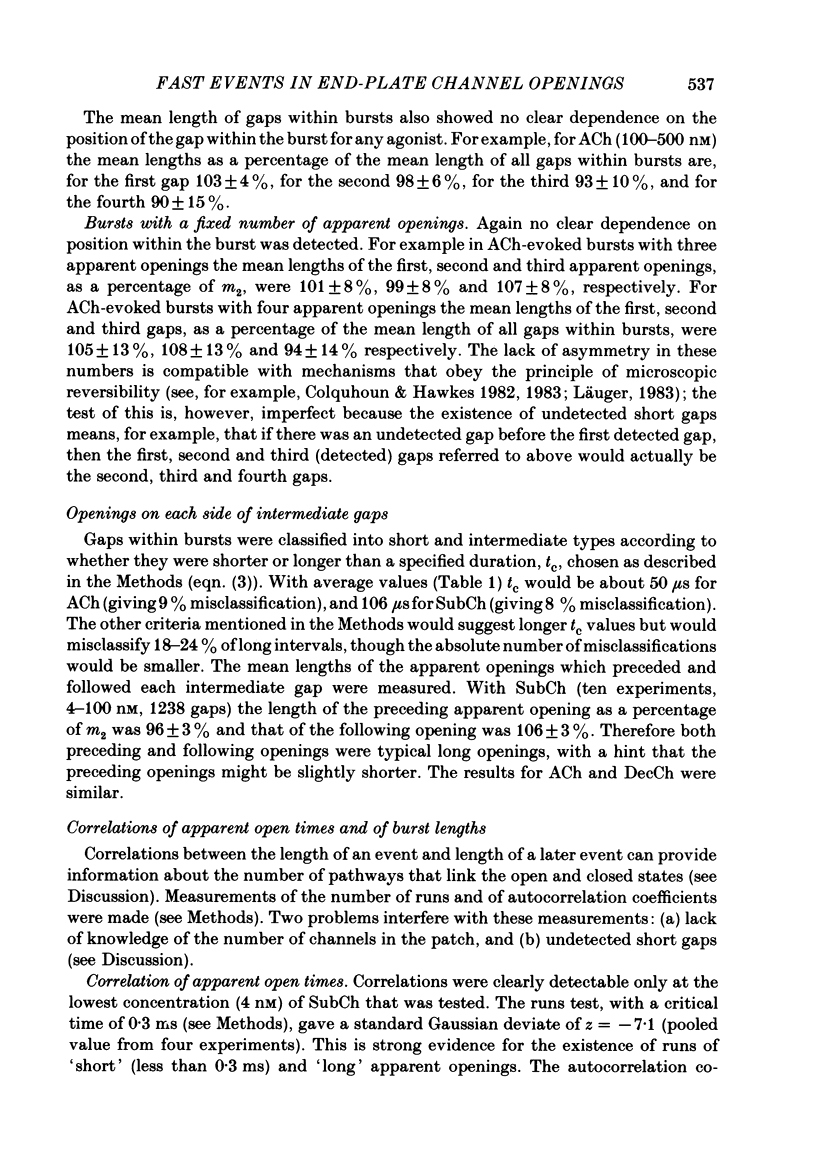
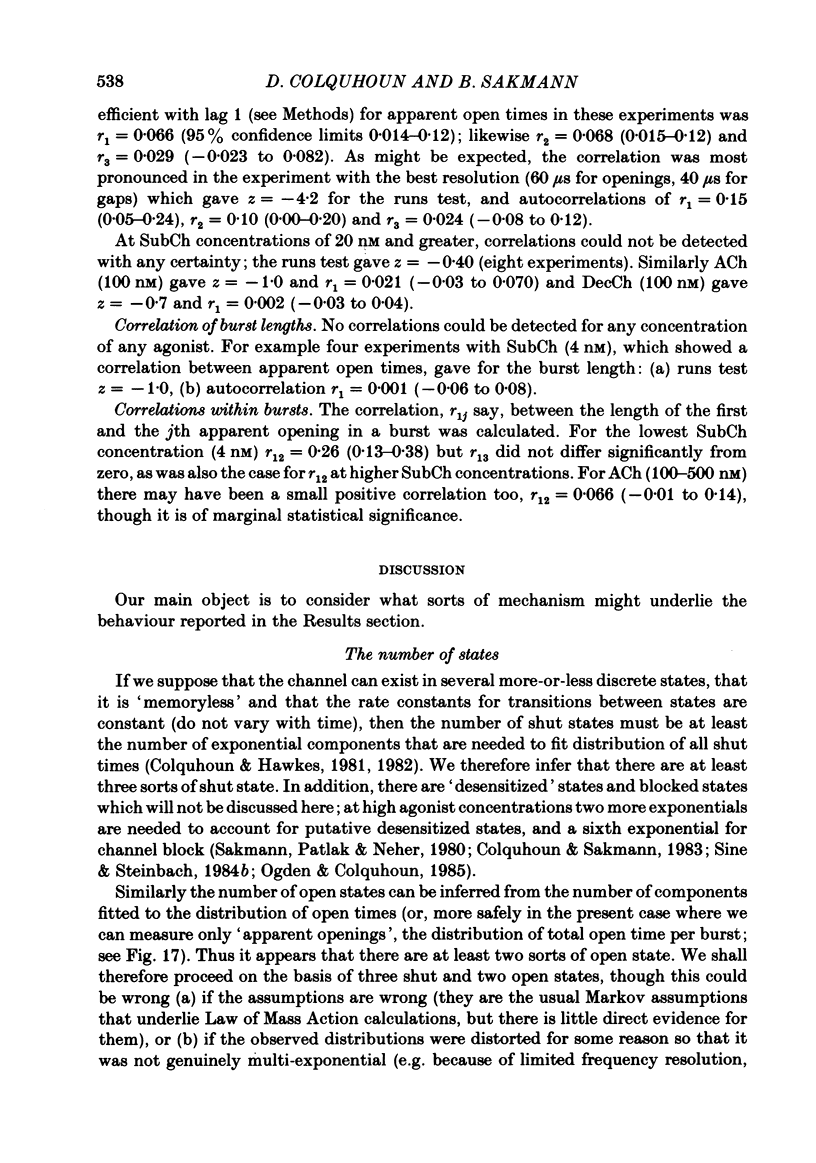
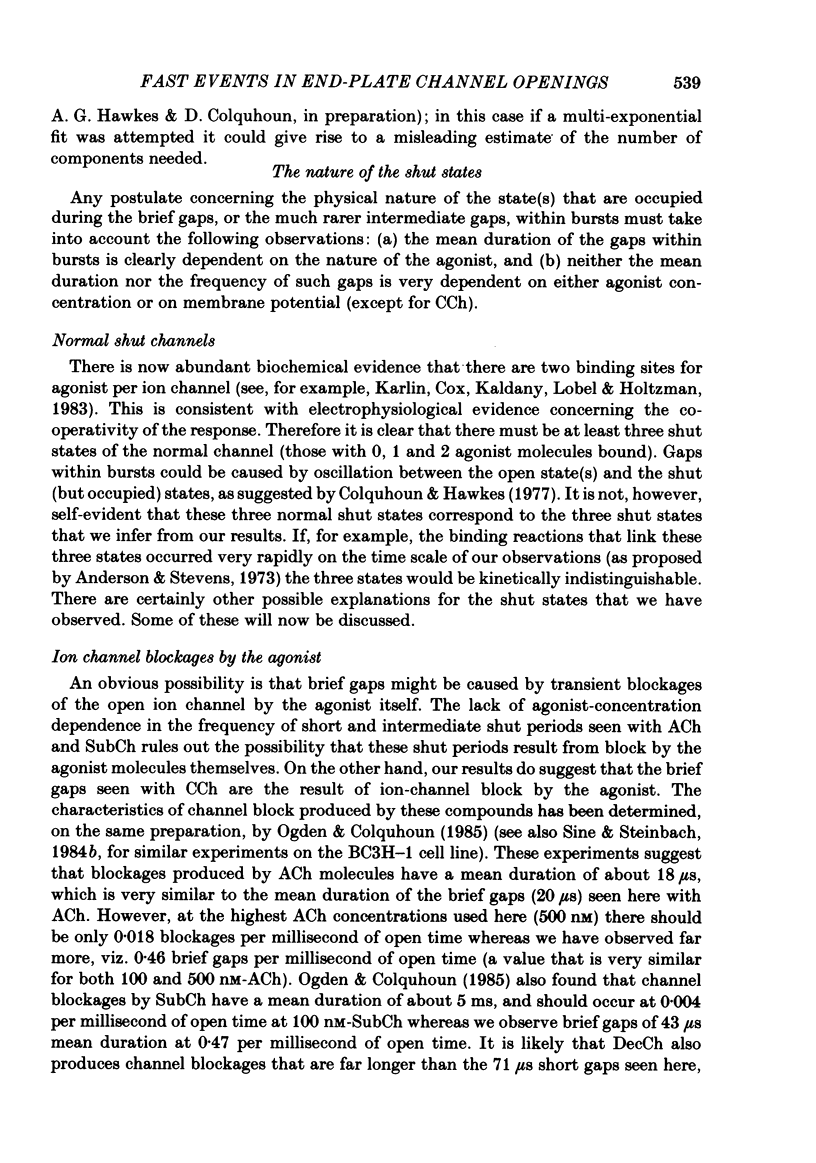
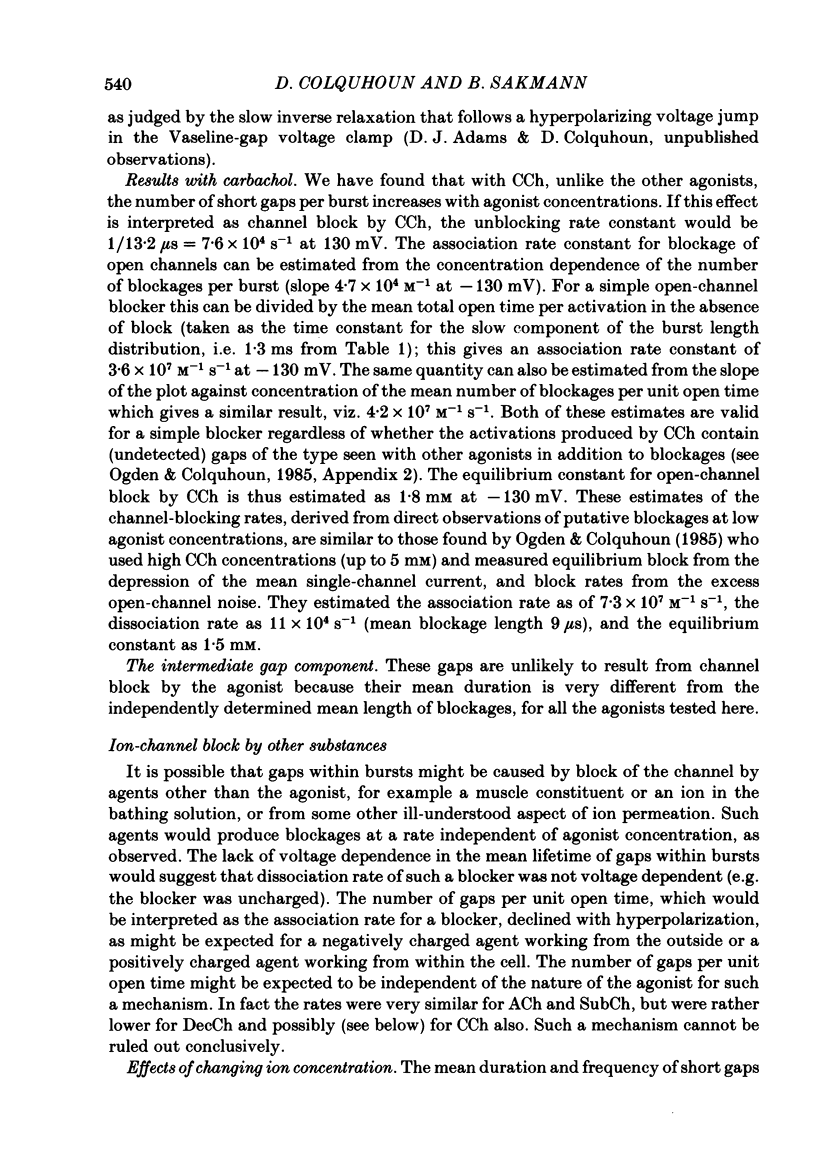
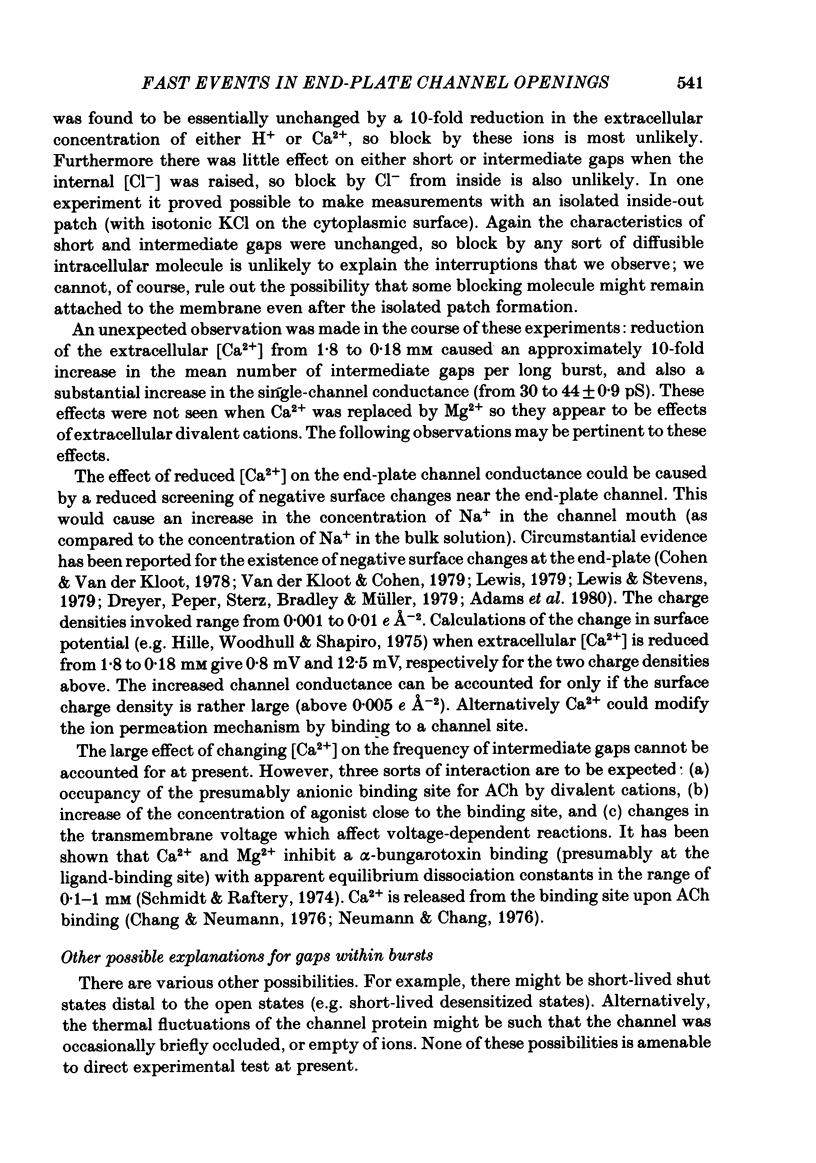
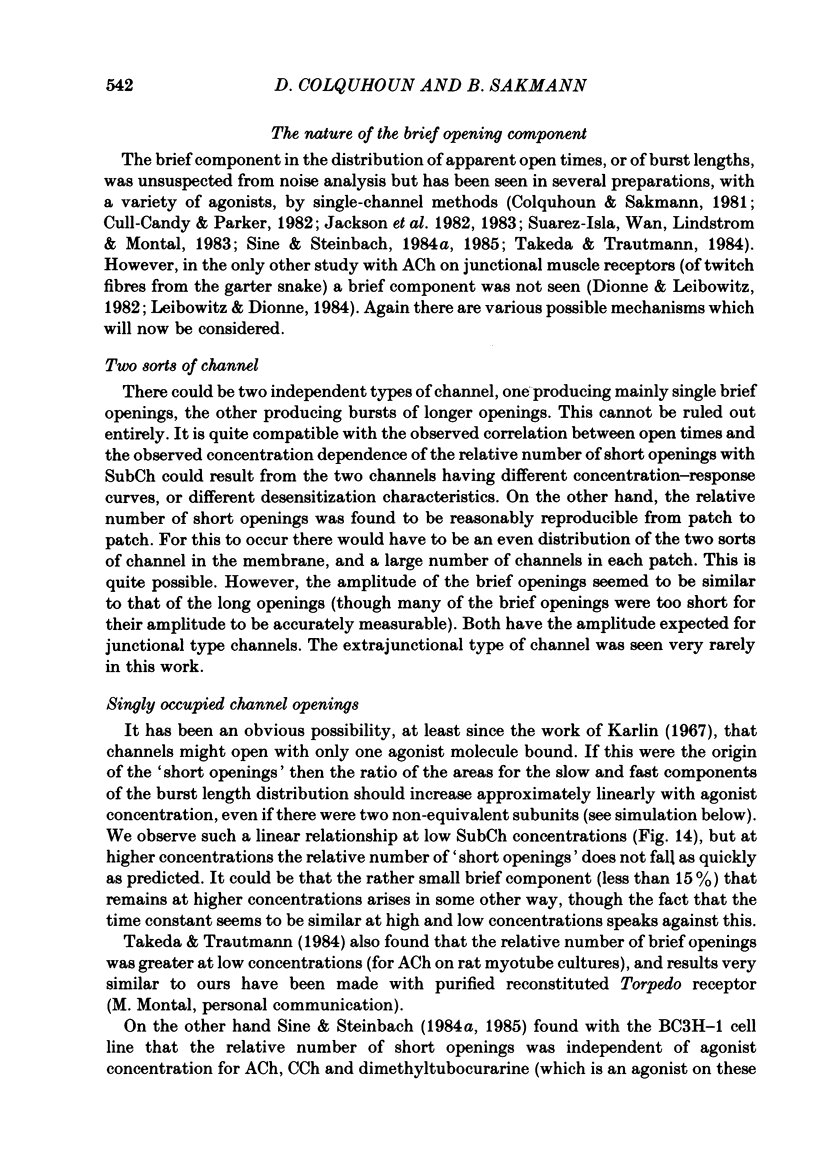
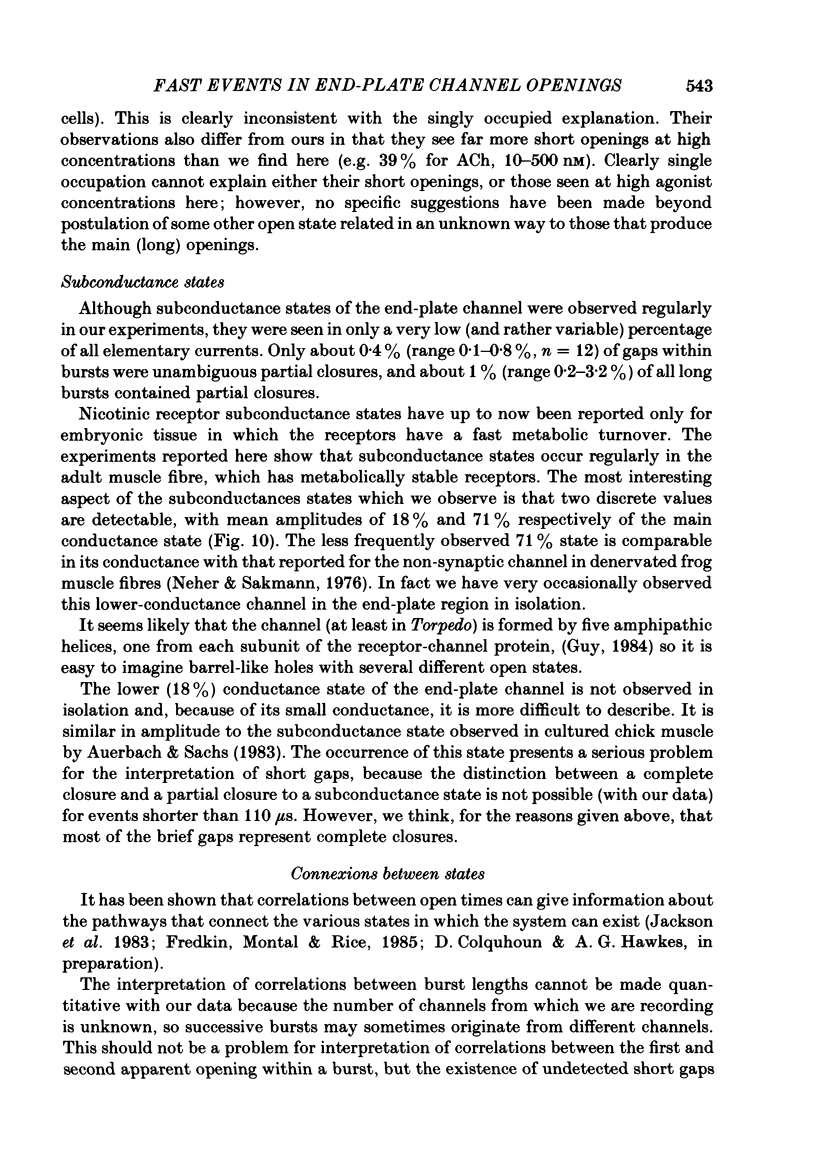
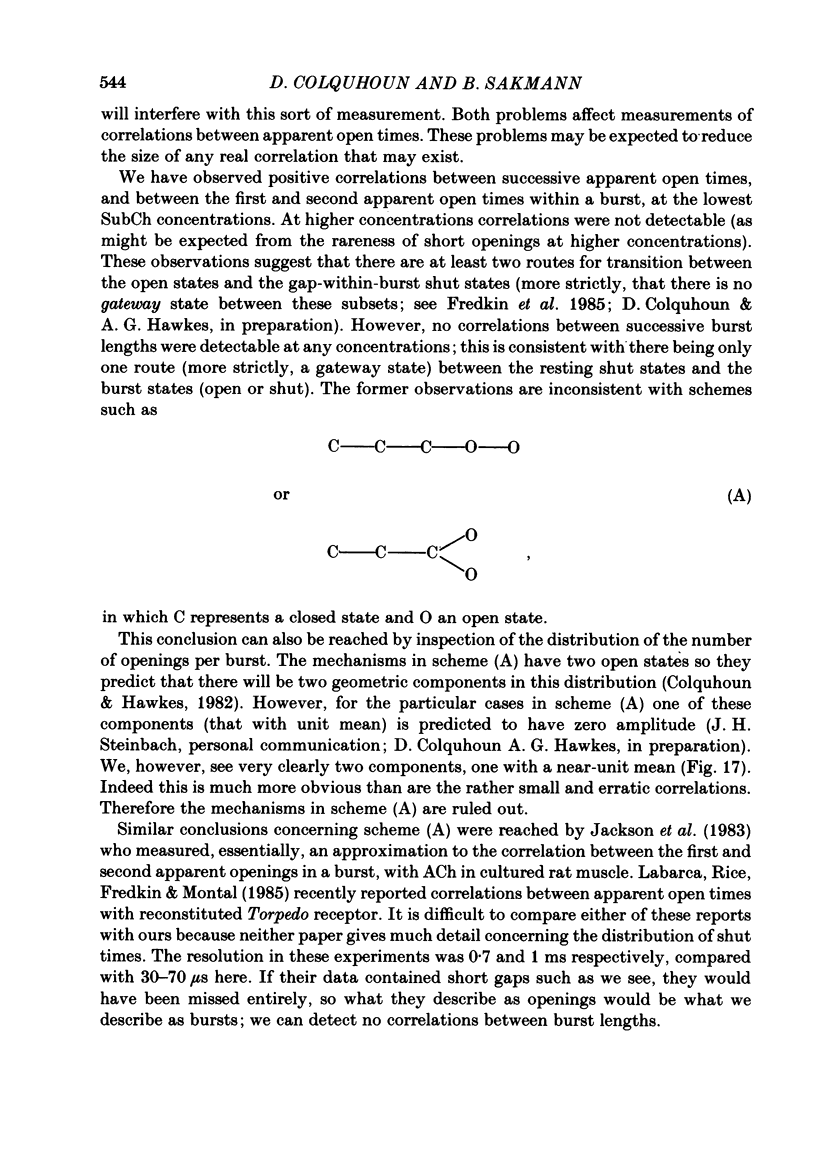
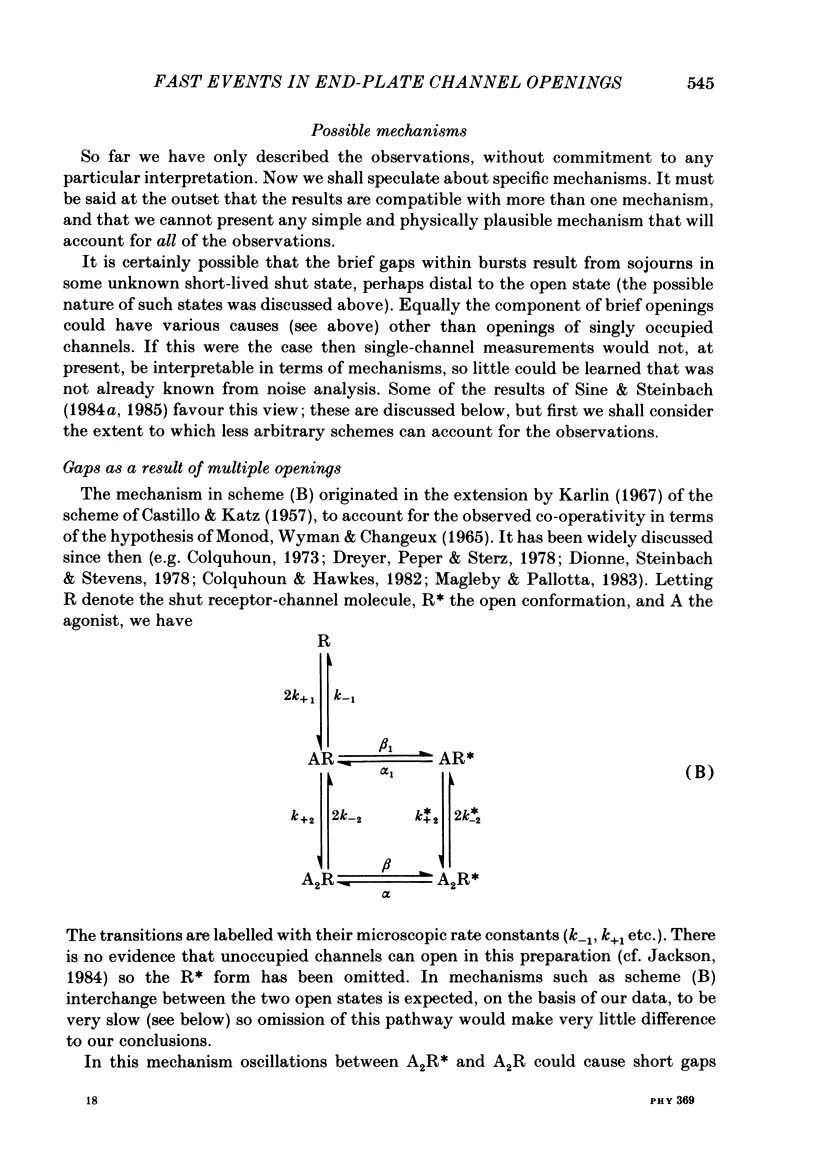
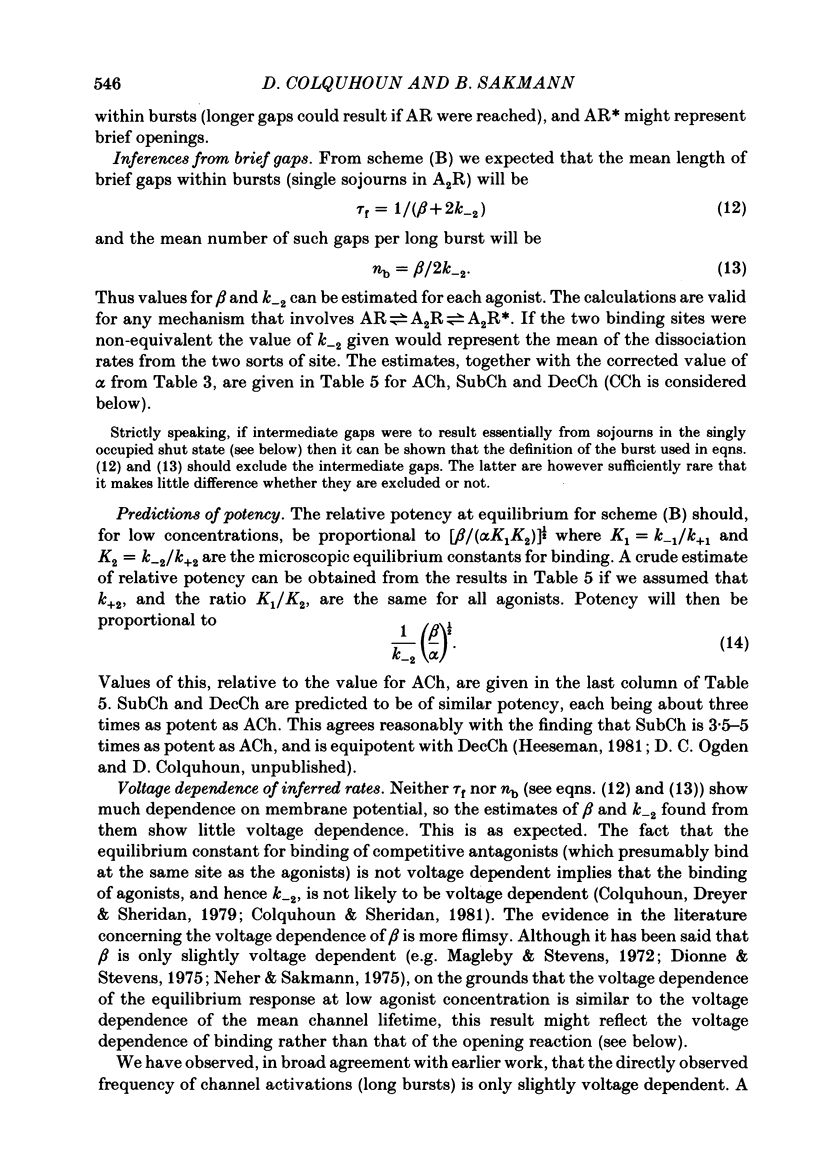
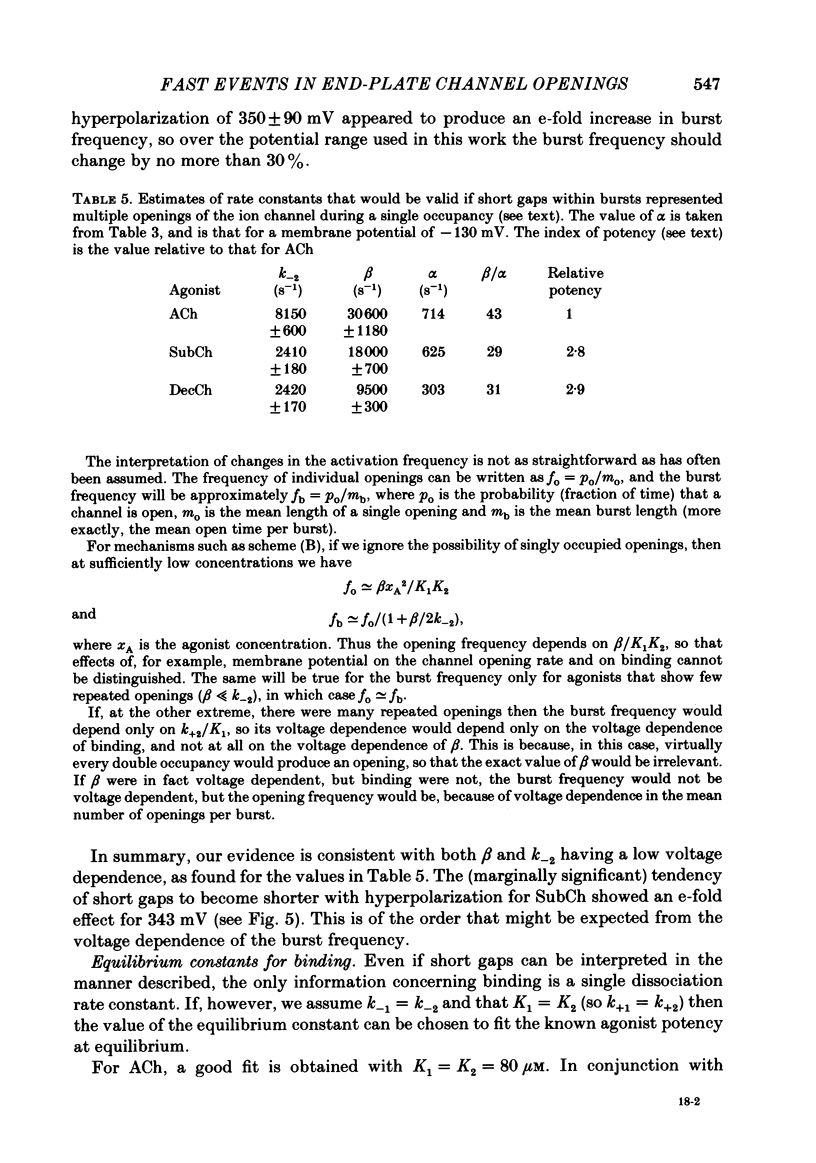
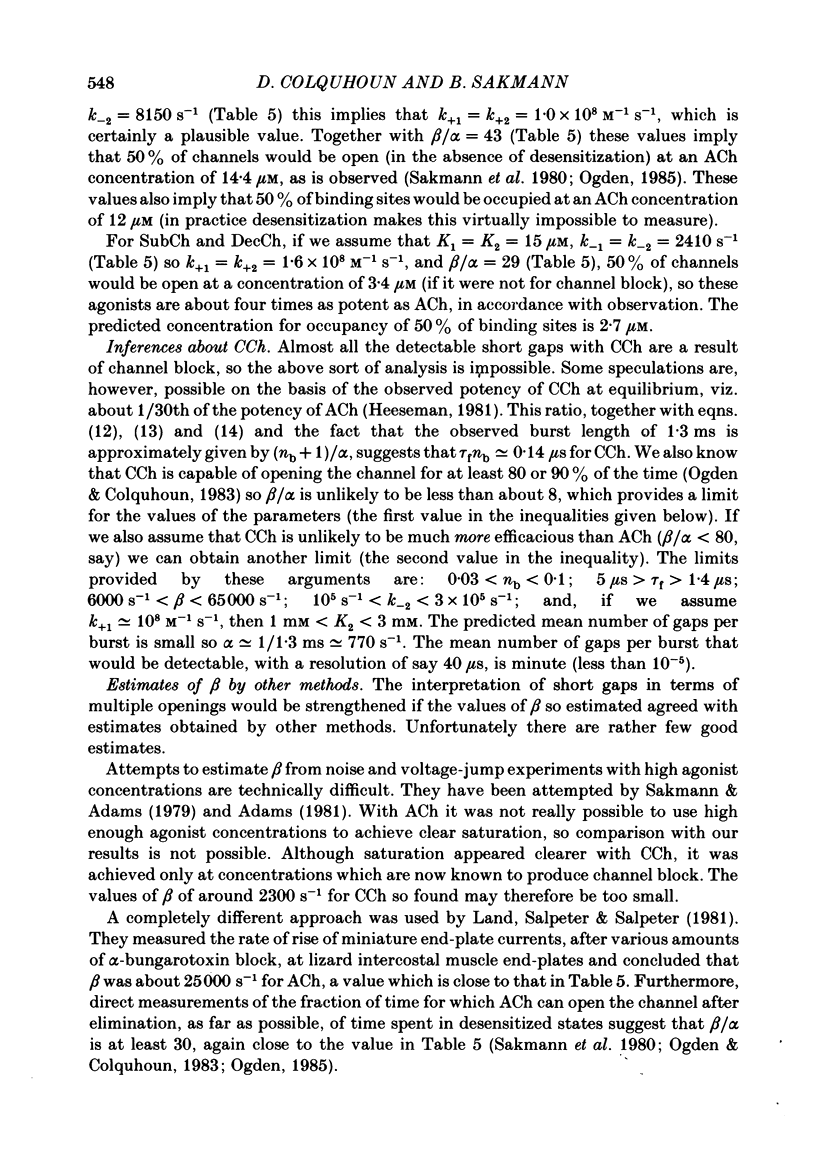
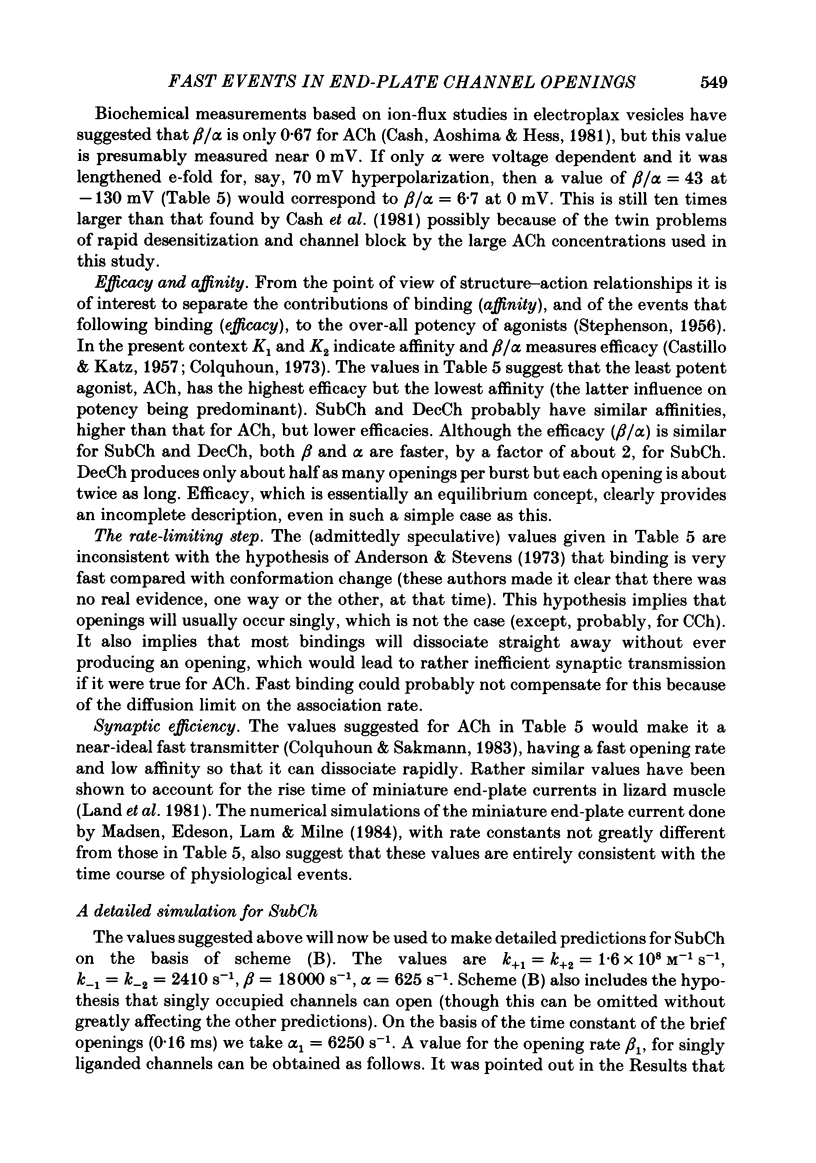
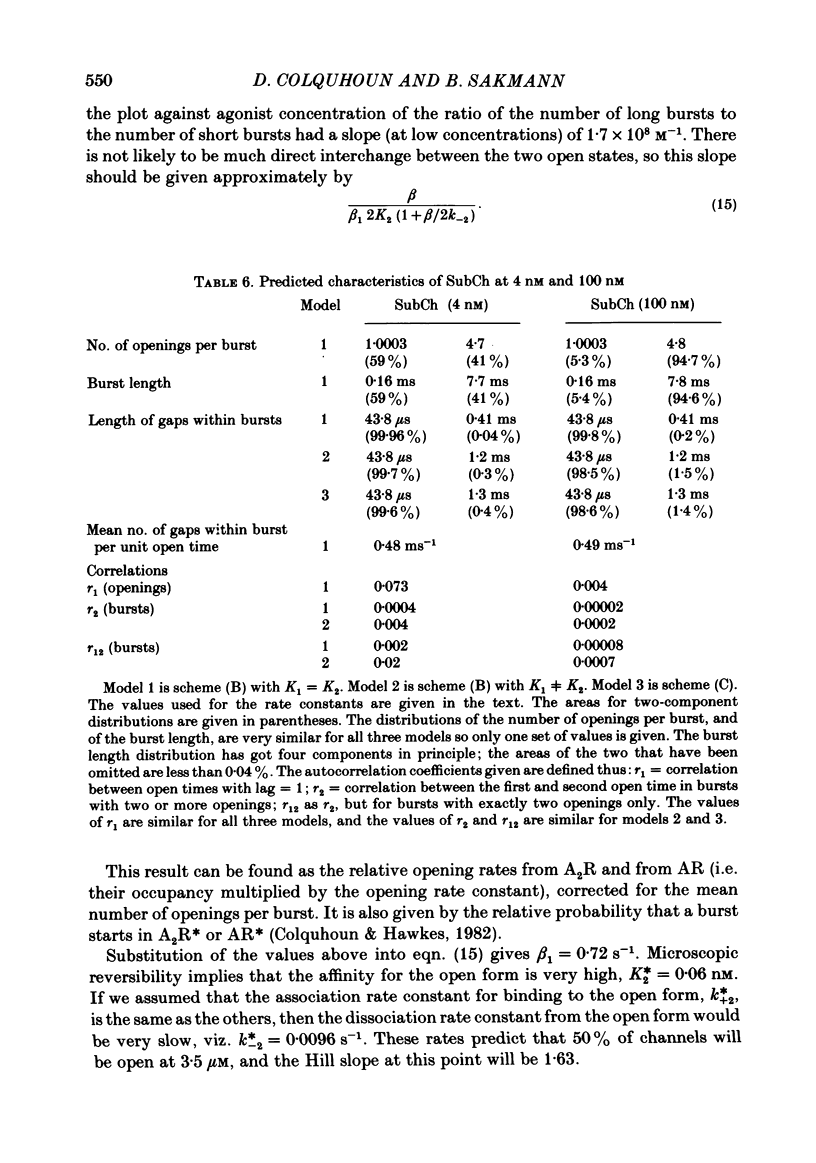
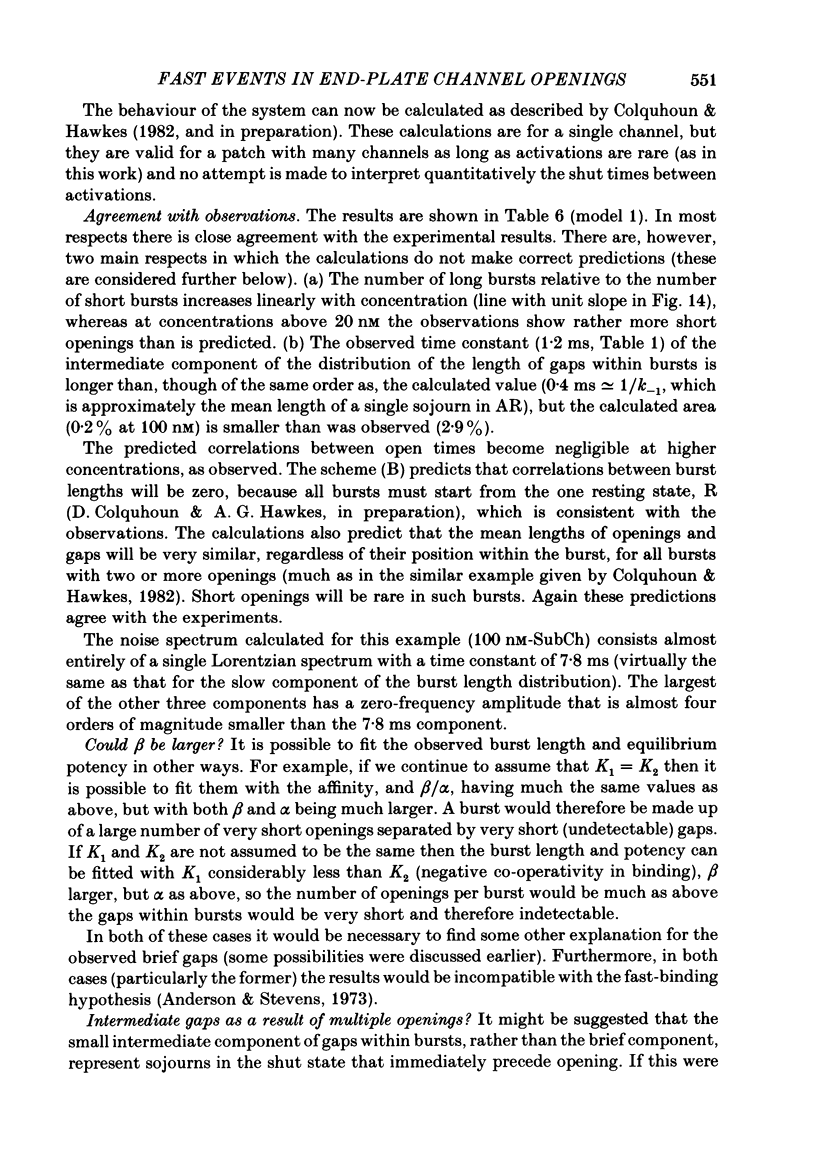
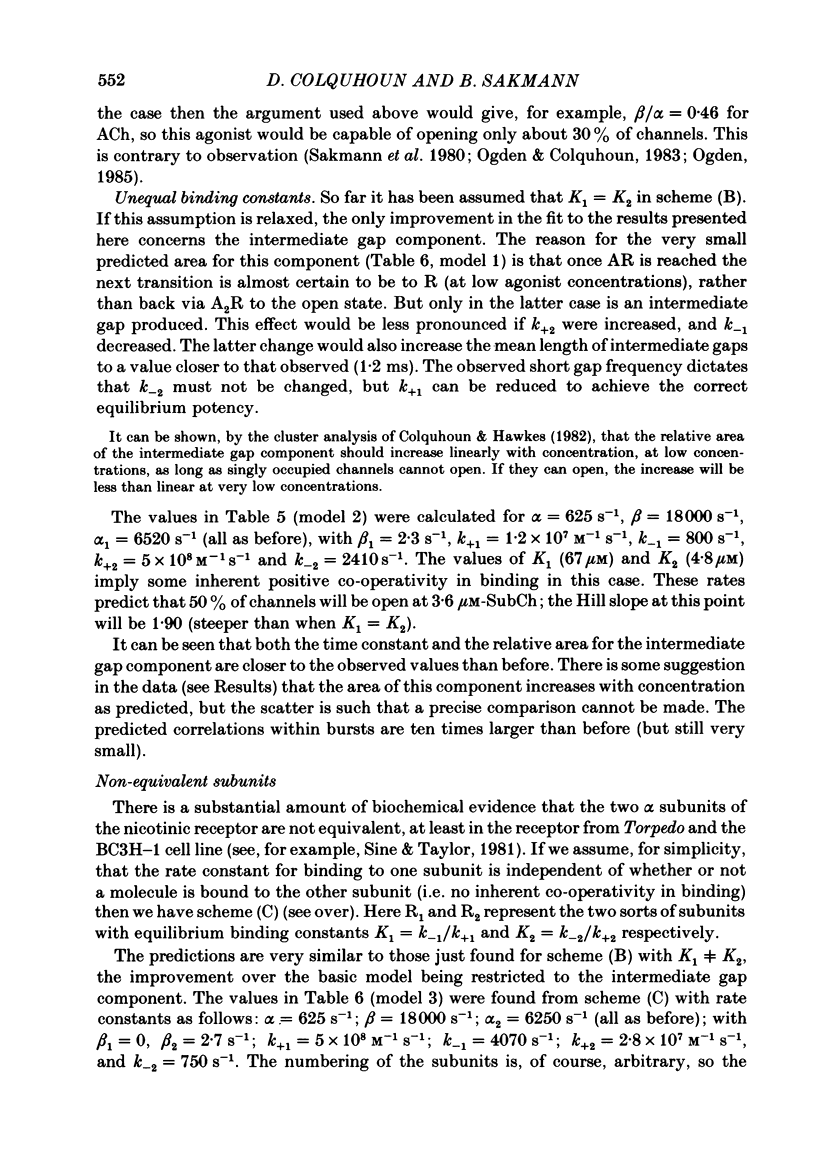
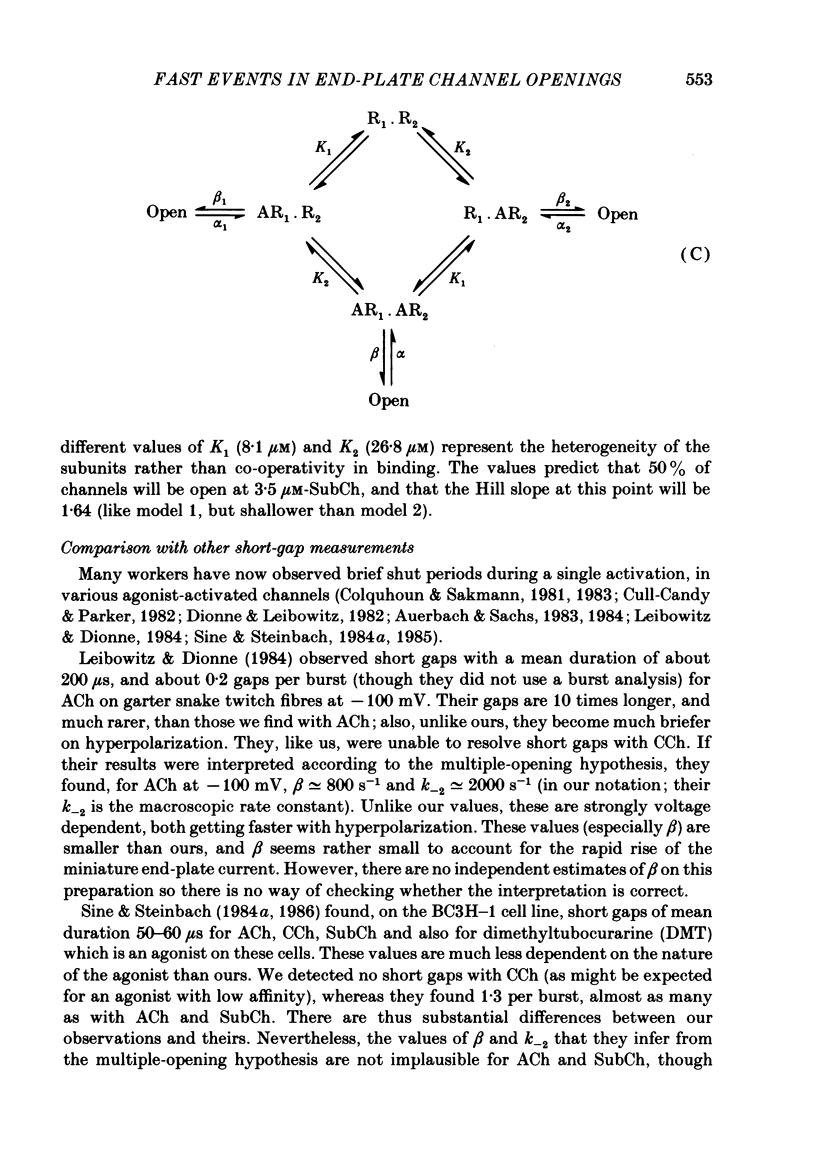
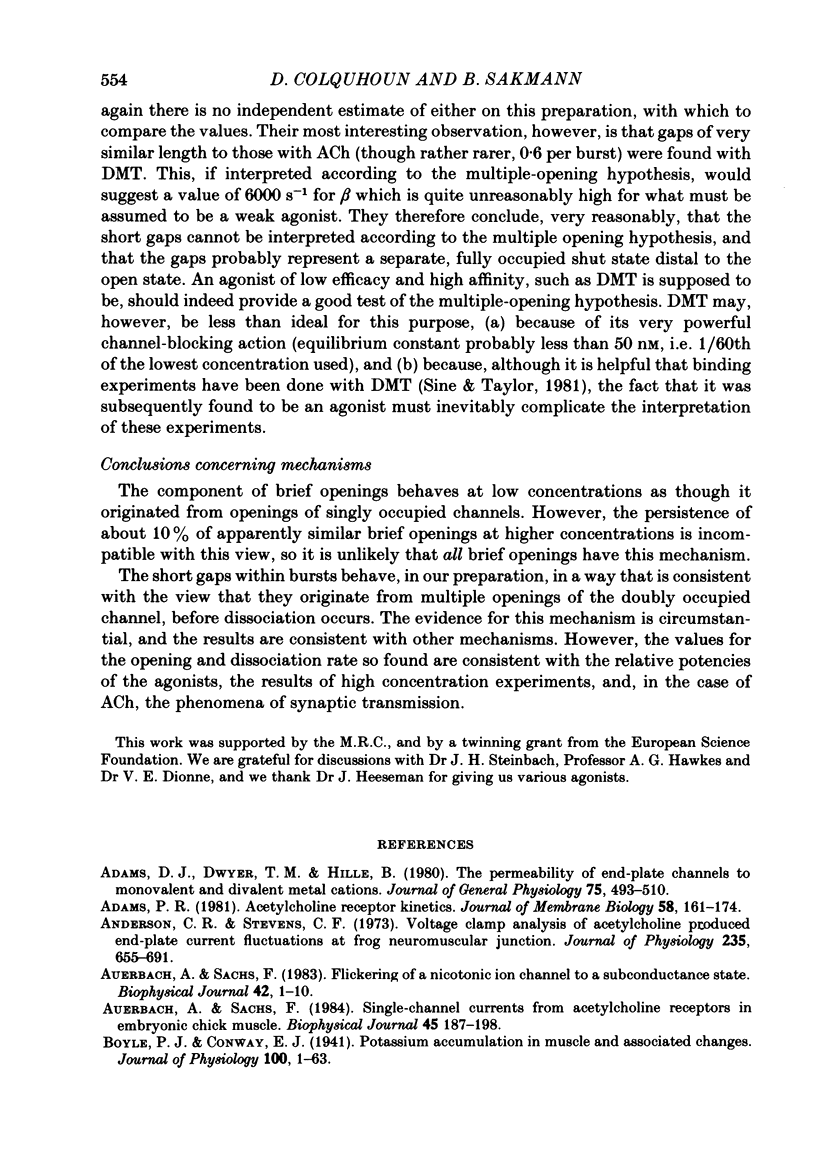
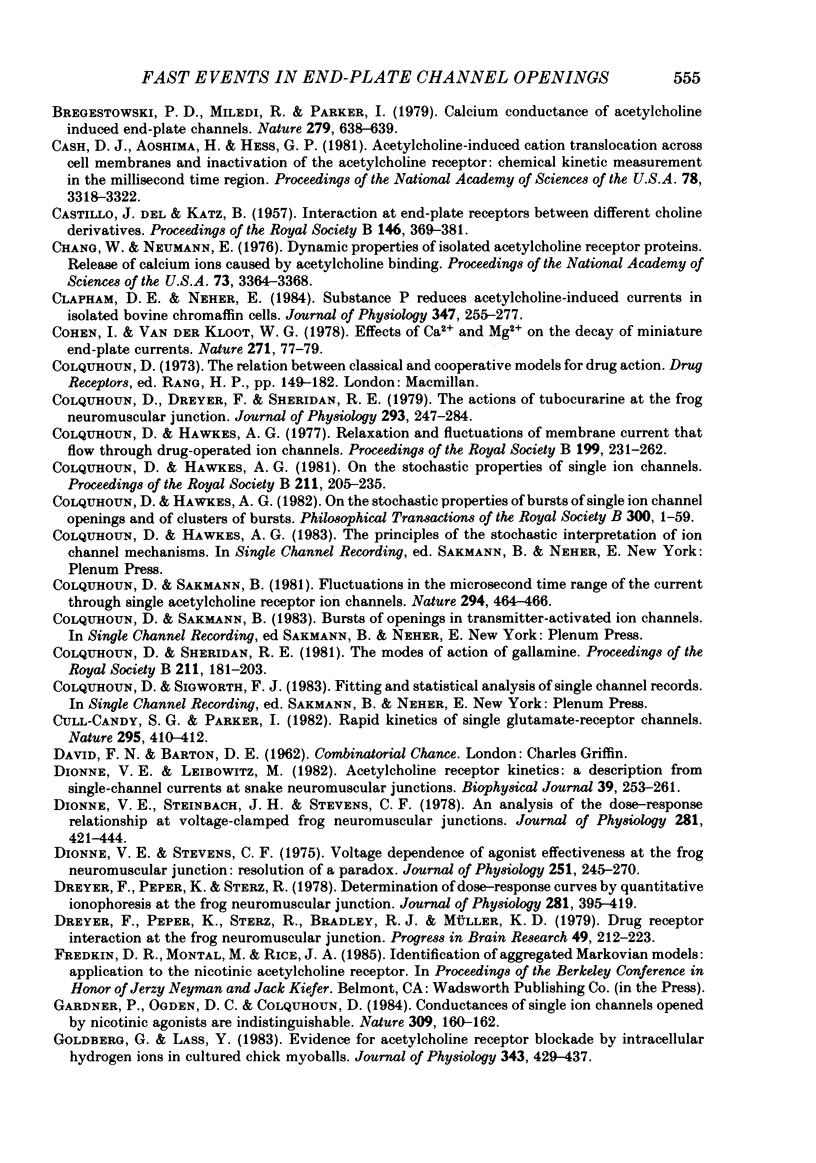
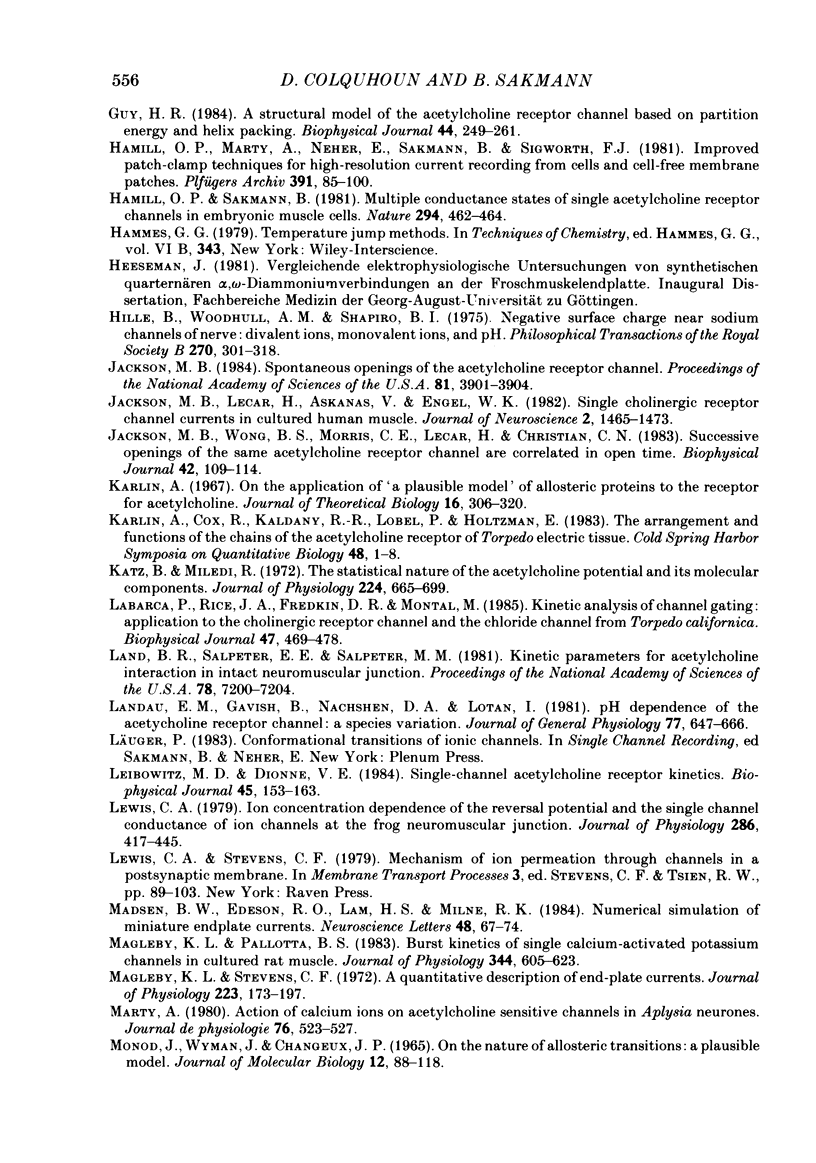
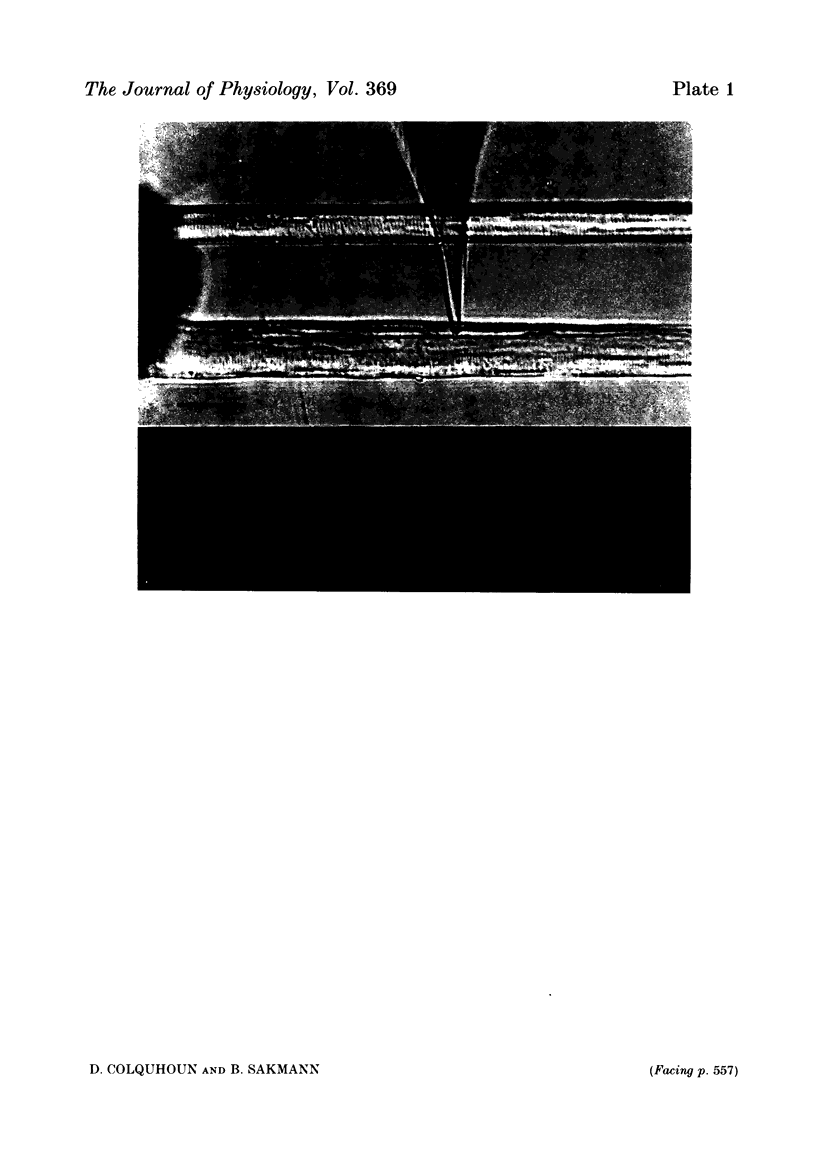
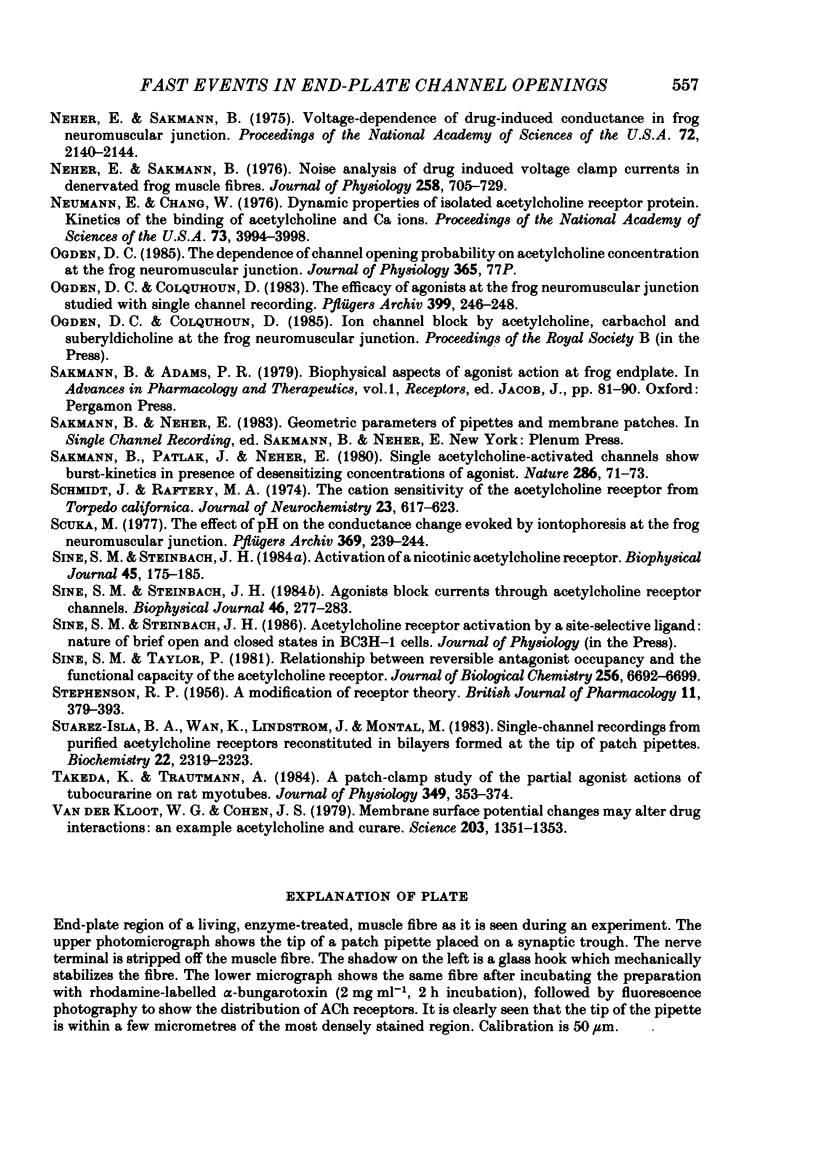
Images in this article
Selected References
These references are in PubMed. This may not be the complete list of references from this article.
- Adams D. J., Dwyer T. M., Hille B. The permeability of endplate channels to monovalent and divalent metal cations. J Gen Physiol. 1980 May;75(5):493–510. doi: 10.1085/jgp.75.5.493. [DOI] [PMC free article] [PubMed] [Google Scholar]
- Adams P. R. Acetylcholine receptor kinetics. J Membr Biol. 1981 Feb 28;58(3):161–174. doi: 10.1007/BF01870902. [DOI] [PubMed] [Google Scholar]
- Anderson C. R., Stevens C. F. Voltage clamp analysis of acetylcholine produced end-plate current fluctuations at frog neuromuscular junction. J Physiol. 1973 Dec;235(3):655–691. doi: 10.1113/jphysiol.1973.sp010410. [DOI] [PMC free article] [PubMed] [Google Scholar]
- Auerbach A., Sachs F. Flickering of a nicotinic ion channel to a subconductance state. Biophys J. 1983 Apr;42(1):1–10. doi: 10.1016/S0006-3495(83)84362-8. [DOI] [PMC free article] [PubMed] [Google Scholar]
- Auerbach A., Sachs F. Single-channel currents from acetylcholine receptors in embryonic chick muscle. Kinetic and conductance properties of gaps within bursts. Biophys J. 1984 Jan;45(1):187–198. doi: 10.1016/S0006-3495(84)84147-8. [DOI] [PMC free article] [PubMed] [Google Scholar]
- Boyle P. J., Conway E. J. Potassium accumulation in muscle and associated changes. J Physiol. 1941 Aug 11;100(1):1–63. doi: 10.1113/jphysiol.1941.sp003922. [DOI] [PMC free article] [PubMed] [Google Scholar]
- Bregestovski P. D., Miledi R., Parker I. Calcium conductance of acetylcholine-induced endplate channels. Nature. 1979 Jun 14;279(5714):638–639. doi: 10.1038/279638a0. [DOI] [PubMed] [Google Scholar]
- Cash D. J., Aoshima H., Hess G. P. Acetylcholine-induced cation translocation across cell membranes and inactivation of the acetylcholine receptor: chemical kinetic measurements in the millisecond time region. Proc Natl Acad Sci U S A. 1981 Jun;78(6):3318–3322. doi: 10.1073/pnas.78.6.3318. [DOI] [PMC free article] [PubMed] [Google Scholar]
- Chang H. W., Neumann E. Dynamic properties of isolated acetylcholine receptor proteins: release of calcium ions caused by acetylcholine binding. Proc Natl Acad Sci U S A. 1976 Oct;73(10):3364–3368. doi: 10.1073/pnas.73.10.3364. [DOI] [PMC free article] [PubMed] [Google Scholar]
- Clapham D. E., Neher E. Substance P reduces acetylcholine-induced currents in isolated bovine chromaffin cells. J Physiol. 1984 Feb;347:255–277. doi: 10.1113/jphysiol.1984.sp015065. [DOI] [PMC free article] [PubMed] [Google Scholar]
- Cohen I., Van der Kloot W. Effects of [Ca2+] and [Mg2+] on the decay of miniature endplate currents. Nature. 1978 Jan 5;271(5640):77–79. doi: 10.1038/271077a0. [DOI] [PubMed] [Google Scholar]
- Colquhoun D., Dreyer F., Sheridan R. E. The actions of tubocurarine at the frog neuromuscular junction. J Physiol. 1979 Aug;293:247–284. doi: 10.1113/jphysiol.1979.sp012888. [DOI] [PMC free article] [PubMed] [Google Scholar]
- Colquhoun D., Hawkes A. G. On the stochastic properties of bursts of single ion channel openings and of clusters of bursts. Philos Trans R Soc Lond B Biol Sci. 1982 Dec 24;300(1098):1–59. doi: 10.1098/rstb.1982.0156. [DOI] [PubMed] [Google Scholar]
- Colquhoun D., Hawkes A. G. On the stochastic properties of single ion channels. Proc R Soc Lond B Biol Sci. 1981 Mar 6;211(1183):205–235. doi: 10.1098/rspb.1981.0003. [DOI] [PubMed] [Google Scholar]
- Colquhoun D., Hawkes A. G. Relaxation and fluctuations of membrane currents that flow through drug-operated channels. Proc R Soc Lond B Biol Sci. 1977 Nov 14;199(1135):231–262. doi: 10.1098/rspb.1977.0137. [DOI] [PubMed] [Google Scholar]
- Colquhoun D., Sakmann B. Fluctuations in the microsecond time range of the current through single acetylcholine receptor ion channels. Nature. 1981 Dec 3;294(5840):464–466. doi: 10.1038/294464a0. [DOI] [PubMed] [Google Scholar]
- Colquhoun D., Sheridan R. E. The modes of action of gallamine. Proc R Soc Lond B Biol Sci. 1981 Mar 6;211(1183):181–203. doi: 10.1098/rspb.1981.0002. [DOI] [PubMed] [Google Scholar]
- Cull-Candy S. G., Parker I. Rapid kinetics of single glutamate-receptor channels. Nature. 1982 Feb 4;295(5848):410–412. doi: 10.1038/295410a0. [DOI] [PubMed] [Google Scholar]
- DEL CASTILLO J., KATZ B. Interaction at end-plate receptors between different choline derivatives. Proc R Soc Lond B Biol Sci. 1957 May 7;146(924):369–381. doi: 10.1098/rspb.1957.0018. [DOI] [PubMed] [Google Scholar]
- Dionne V. E., Leibowitz M. D. Acetylcholine receptor kinetics. A description from single-channel currents at snake neuromuscular junctions. Biophys J. 1982 Sep;39(3):253–261. doi: 10.1016/S0006-3495(82)84515-3. [DOI] [PMC free article] [PubMed] [Google Scholar]
- Dionne V. E., Steinbach J. H., Stevens C. F. An analysis of the dose-response relationship at voltage-clamped frog neuromuscular junctions. J Physiol. 1978 Aug;281:421–444. doi: 10.1113/jphysiol.1978.sp012431. [DOI] [PMC free article] [PubMed] [Google Scholar]
- Dionne V. E., Stevens C. F. Voltage dependence of agonist effectiveness at the frog neuromuscular junction: resolution of a paradox. J Physiol. 1975 Oct;251(2):245–270. doi: 10.1113/jphysiol.1975.sp011090. [DOI] [PMC free article] [PubMed] [Google Scholar]
- Dreyer F., Peper K., Sterz R., Bradley R. J., Müller K. D. Drug-receptor interaction at the frog neuromuscular junction. Prog Brain Res. 1979;49:213–223. doi: 10.1016/S0079-6123(08)64635-X. [DOI] [PubMed] [Google Scholar]
- Dreyer F., Peper K., Sterz R. Determination of dose-response curves by quantitative ionophoresis at the frog neuromuscular junction. J Physiol. 1978 Aug;281:395–419. doi: 10.1113/jphysiol.1978.sp012430. [DOI] [PMC free article] [PubMed] [Google Scholar]
- Gardner P., Ogden D. C., Colquhoun D. Conductances of single ion channels opened by nicotinic agonists are indistinguishable. Nature. 1984 May 10;309(5964):160–162. doi: 10.1038/309160a0. [DOI] [PubMed] [Google Scholar]
- Goldberg G., Lass Y. Evidence for acetylcholine receptor blockade by intracellular hydrogen ions in cultured chick myoballs. J Physiol. 1983 Oct;343:429–437. doi: 10.1113/jphysiol.1983.sp014901. [DOI] [PMC free article] [PubMed] [Google Scholar]
- Guy H. R. A structural model of the acetylcholine receptor channel based on partition energy and helix packing calculations. Biophys J. 1984 Jan;45(1):249–261. doi: 10.1016/S0006-3495(84)84152-1. [DOI] [PMC free article] [PubMed] [Google Scholar]
- Hamill O. P., Marty A., Neher E., Sakmann B., Sigworth F. J. Improved patch-clamp techniques for high-resolution current recording from cells and cell-free membrane patches. Pflugers Arch. 1981 Aug;391(2):85–100. doi: 10.1007/BF00656997. [DOI] [PubMed] [Google Scholar]
- Hamill O. P., Sakmann B. Multiple conductance states of single acetylcholine receptor channels in embryonic muscle cells. Nature. 1981 Dec 3;294(5840):462–464. doi: 10.1038/294462a0. [DOI] [PubMed] [Google Scholar]
- Hamm T. M., Reinking R. M., Roscoe D. D., Stuart D. G. Synchronous afferent discharge from a passive muscle of the cat: significance for interpreting spike-triggered averages. J Physiol. 1985 Aug;365:77–102. doi: 10.1113/jphysiol.1985.sp015760. [DOI] [PMC free article] [PubMed] [Google Scholar]
- Hille B., Woodhull A. M., Shapiro B. I. Negative surface charge near sodium channels of nerve: divalent ions, monovalent ions, and pH. Philos Trans R Soc Lond B Biol Sci. 1975 Jun 10;270(908):301–318. doi: 10.1098/rstb.1975.0011. [DOI] [PubMed] [Google Scholar]
- Jackson M. B., Lecar H., Askanas V., Engel W. K. Single cholinergic receptor channel currents in cultured human muscle. J Neurosci. 1982 Oct;2(10):1465–1473. doi: 10.1523/JNEUROSCI.02-10-01465.1982. [DOI] [PMC free article] [PubMed] [Google Scholar]
- Jackson M. B. Spontaneous openings of the acetylcholine receptor channel. Proc Natl Acad Sci U S A. 1984 Jun;81(12):3901–3904. doi: 10.1073/pnas.81.12.3901. [DOI] [PMC free article] [PubMed] [Google Scholar]
- Jackson M. B., Wong B. S., Morris C. E., Lecar H., Christian C. N. Successive openings of the same acetylcholine receptor channel are correlated in open time. Biophys J. 1983 Apr;42(1):109–114. doi: 10.1016/S0006-3495(83)84375-6. [DOI] [PMC free article] [PubMed] [Google Scholar]
- Karlin A. On the application of "a plausible model" of allosteric proteins to the receptor for acetylcholine. J Theor Biol. 1967 Aug;16(2):306–320. doi: 10.1016/0022-5193(67)90011-2. [DOI] [PubMed] [Google Scholar]
- Katz B., Miledi R. The statistical nature of the acetycholine potential and its molecular components. J Physiol. 1972 Aug;224(3):665–699. doi: 10.1113/jphysiol.1972.sp009918. [DOI] [PMC free article] [PubMed] [Google Scholar]
- Labarca P., Rice J. A., Fredkin D. R., Montal M. Kinetic analysis of channel gating. Application to the cholinergic receptor channel and the chloride channel from Torpedo californica. Biophys J. 1985 Apr;47(4):469–478. doi: 10.1016/S0006-3495(85)83939-4. [DOI] [PMC free article] [PubMed] [Google Scholar]
- Land B. R., Salpeter E. E., Salpeter M. M. Kinetic parameters for acetylcholine interaction in intact neuromuscular junction. Proc Natl Acad Sci U S A. 1981 Nov;78(11):7200–7204. doi: 10.1073/pnas.78.11.7200. [DOI] [PMC free article] [PubMed] [Google Scholar]
- Landau E. M., Gavish B., Nachshen D. A., Lotan I. pH dependence of the acetylcholine receptor channel: a species variation. J Gen Physiol. 1981 Jun;77(6):647–666. doi: 10.1085/jgp.77.6.647. [DOI] [PMC free article] [PubMed] [Google Scholar]
- Leibowitz M. D., Dionne V. E. Single-channel acetylcholine receptor kinetics. Biophys J. 1984 Jan;45(1):153–163. doi: 10.1016/S0006-3495(84)84144-2. [DOI] [PMC free article] [PubMed] [Google Scholar]
- Lewis C. A. Ion-concentration dependence of the reversal potential and the single channel conductance of ion channels at the frog neuromuscular junction. J Physiol. 1979 Jan;286:417–445. doi: 10.1113/jphysiol.1979.sp012629. [DOI] [PMC free article] [PubMed] [Google Scholar]
- MONOD J., WYMAN J., CHANGEUX J. P. ON THE NATURE OF ALLOSTERIC TRANSITIONS: A PLAUSIBLE MODEL. J Mol Biol. 1965 May;12:88–118. doi: 10.1016/s0022-2836(65)80285-6. [DOI] [PubMed] [Google Scholar]
- Madsen B. W., Edeson R. O., Lam H. S., Milne R. K. Numerical simulation of miniature endplate currents. Neurosci Lett. 1984 Jul 13;48(1):67–74. doi: 10.1016/0304-3940(84)90290-8. [DOI] [PubMed] [Google Scholar]
- Magleby K. L., Pallotta B. S. Burst kinetics of single calcium-activated potassium channels in cultured rat muscle. J Physiol. 1983 Nov;344:605–623. doi: 10.1113/jphysiol.1983.sp014958. [DOI] [PMC free article] [PubMed] [Google Scholar]
- Magleby K. L., Stevens C. F. A quantitative description of end-plate currents. J Physiol. 1972 May;223(1):173–197. doi: 10.1113/jphysiol.1972.sp009840. [DOI] [PMC free article] [PubMed] [Google Scholar]
- Marty A. Action of calcium ions on acetylcholine-sensitive channels in Aplysia neurones. J Physiol (Paris) 1980 Sep;76(5):523–527. [PubMed] [Google Scholar]
- Neher E., Sakmann B. Noise analysis of drug induced voltage clamp currents in denervated frog muscle fibres. J Physiol. 1976 Jul;258(3):705–729. doi: 10.1113/jphysiol.1976.sp011442. [DOI] [PMC free article] [PubMed] [Google Scholar]
- Neher E., Sakmann B. Voltage-dependence of drug-induced conductance in frog neuromuscular junction. Proc Natl Acad Sci U S A. 1975 Jun;72(6):2140–2144. doi: 10.1073/pnas.72.6.2140. [DOI] [PMC free article] [PubMed] [Google Scholar]
- Neumann E., Chang H. W. Dynamic properties of isolated acetylcholine receptor protein: kinetics of the binding of acetylcholine and Ca ions. Proc Natl Acad Sci U S A. 1976 Nov;73(11):3994–3998. doi: 10.1073/pnas.73.11.3994. [DOI] [PMC free article] [PubMed] [Google Scholar]
- Ogden D. C., Colquhoun D. The efficacy of agonists at the frog neuromuscular junction studied with single channel recording. Pflugers Arch. 1983 Nov;399(3):246–248. doi: 10.1007/BF00656725. [DOI] [PubMed] [Google Scholar]
- STEPHENSON R. P. A modification of receptor theory. Br J Pharmacol Chemother. 1956 Dec;11(4):379–393. doi: 10.1111/j.1476-5381.1956.tb00006.x. [DOI] [PMC free article] [PubMed] [Google Scholar]
- Sakmann B., Patlak J., Neher E. Single acetylcholine-activated channels show burst-kinetics in presence of desensitizing concentrations of agonist. Nature. 1980 Jul 3;286(5768):71–73. doi: 10.1038/286071a0. [DOI] [PubMed] [Google Scholar]
- Schmidt J., Raftery M. A. The cation sensitivity of the acetylcholine receptor from Torpedo californica. J Neurochem. 1974 Oct;23(4):617–623. doi: 10.1111/j.1471-4159.1974.tb04383.x. [DOI] [PubMed] [Google Scholar]
- Scuka M. The effects of pH on the conductance change evoked by iontophoresis in the frog neuromuscular junction. Pflugers Arch. 1977 Jul 19;369(3):239–244. doi: 10.1007/BF00582190. [DOI] [PubMed] [Google Scholar]
- Sine S. M., Steinbach J. H. Activation of a nicotinic acetylcholine receptor. Biophys J. 1984 Jan;45(1):175–185. doi: 10.1016/S0006-3495(84)84146-6. [DOI] [PMC free article] [PubMed] [Google Scholar]
- Sine S. M., Steinbach J. H. Agonists block currents through acetylcholine receptor channels. Biophys J. 1984 Aug;46(2):277–283. doi: 10.1016/S0006-3495(84)84022-9. [DOI] [PMC free article] [PubMed] [Google Scholar]
- Sine S. M., Taylor P. Relationship between reversible antagonist occupancy and the functional capacity of the acetylcholine receptor. J Biol Chem. 1981 Jul 10;256(13):6692–6699. [PubMed] [Google Scholar]
- Suarez-Isla B. A., Wan K., Lindstrom J., Montal M. Single-channel recordings from purified acetylcholine receptors reconstituted in bilayers formed at the tip of patch pipets. Biochemistry. 1983 May 10;22(10):2319–2323. doi: 10.1021/bi00279a003. [DOI] [PubMed] [Google Scholar]
- Takeda K., Trautmann A. A patch-clamp study of the partial agonist actions of tubocurarine on rat myotubes. J Physiol. 1984 Apr;349:353–374. doi: 10.1113/jphysiol.1984.sp015160. [DOI] [PMC free article] [PubMed] [Google Scholar]
- Van der Kloot W. G., Cohen I. Membrane surface potential changes may alter drug interactions: an example, acetylcholine and curare. Science. 1979 Mar 30;203(4387):1351–1352. doi: 10.1126/science.424757. [DOI] [PubMed] [Google Scholar]