Abstract
Water movements have been studied in sheets of isolated rabbit ileum using a method which measures net volume flows across the mucosal and serosal surfaces of the tissue continuously with high resolution. At 35 degrees C, with the tissues incubated in isotonic Ringer solution containing D-glucose (25 mM) on both sides, there is a steady net inflow of fluid at the rate of 24 +/- 2 microliter cm-2 h-1 across the mucosal surface (Jm) and an outflow of 8 +/- 1 microliter cm-2 h-1 across the serosal surface (Js) (n = 16). The stable transepithelial p.d. across these tissues is 2.7 +/- 0.2 mV, serosa positive. Jm can be reversibly inhibited by anoxia. Ouabain (0.1 mM) added to the serosal solution inhibits inflow across the mucosal and serosal surfaces by 75% (n = 7) within 30 min. If phlorizin (0.1 mM) is added to the mucosal Ringer solution containing glucose (20 mM) within 30 min of the commencement of in vitro absorption, Jm is reduced from 37 +/- 3 to 28 +/- 2 microliter cm-2 h-1 (n = 3). Dilution of the mucosal Ringer solution by 50 mosmol kg-1 (with the serosal solution kept isosmolar) results in a rapid transient increase in mucosal inflow. An increase of 50 mosmol kg-1 in the mucosal Ringer solution with NaCl, sucrose or mannitol causes a transient reversal of mucosal flow, followed by a return of inflow at a reduced level. Rabbit ileum can transport water against gradients of approximately 75 mosmol kg-1 of sucrose, NaCl, or mannitol. Addition of polyethylene glycol (mol. wt. 20000; 3 mosmol kg-1) causes a sustained reversal of mucosal inflow; inflow can be restored only by removing polyethylene glycol from the mucosal Ringer solution. The tissue can absorb water against an osmotic gradient of 200 mM-glycerol. The above data have been incorporated into a new model to explain isotonic flow of fluid by this epithelium. The main features are that the hydraulic conductivity (Lp) of the mucosal boundary of the lateral intercellular space is approximately 1 X 10(-8) cm s-1 cmH2O-1. This Lp is too low to sustain isotonicity of the flow emerging from the lateral intercellular space at the observed rates. Hypertonic fluid emerging from the lateral intercellular space is diluted by transcellular water flow generated by the hypertonicity of the submucosa and back-diffusion of solute via mucosal shunt channels.(ABSTRACT TRUNCATED AT 400 WORDS)
Full text
PDF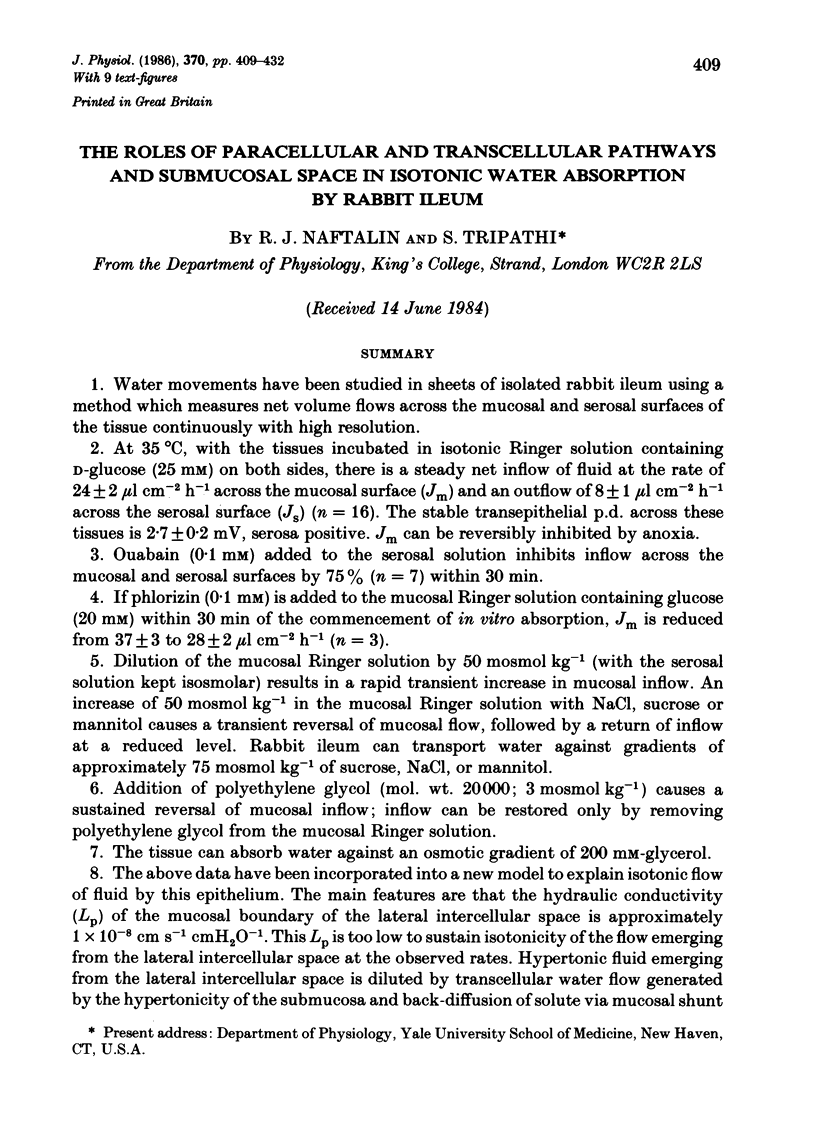
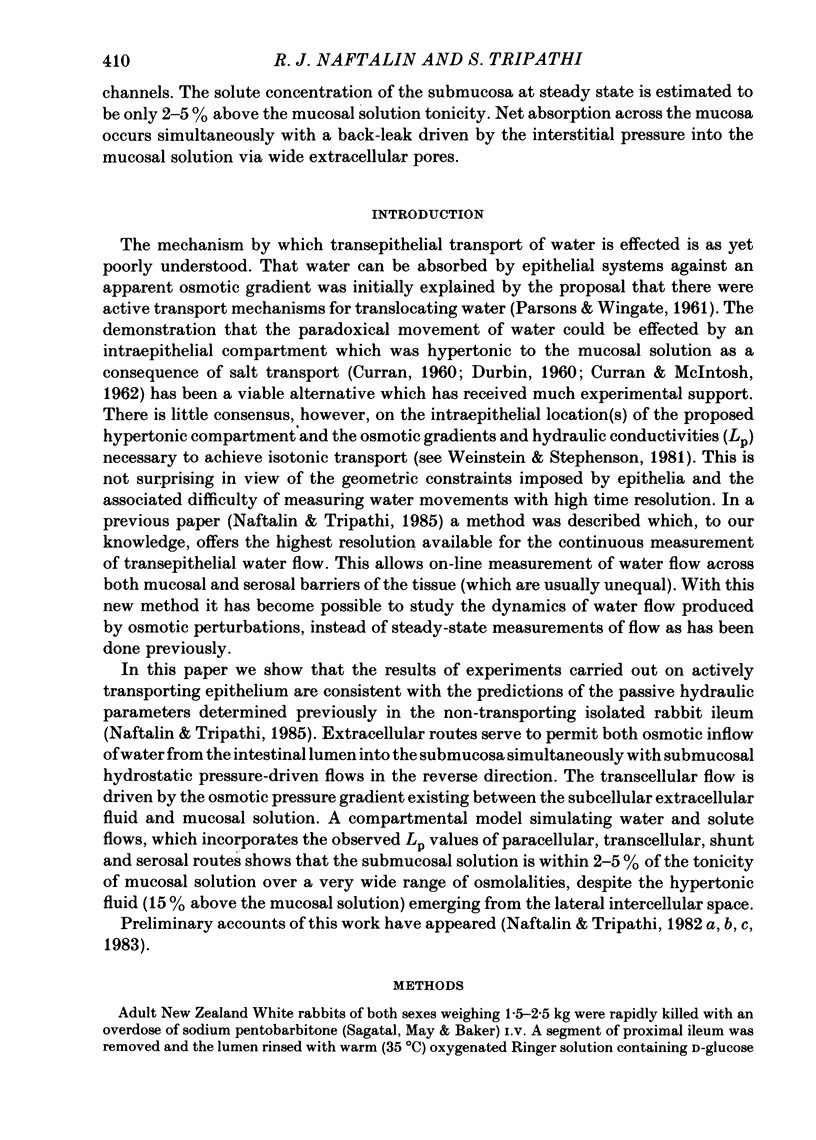
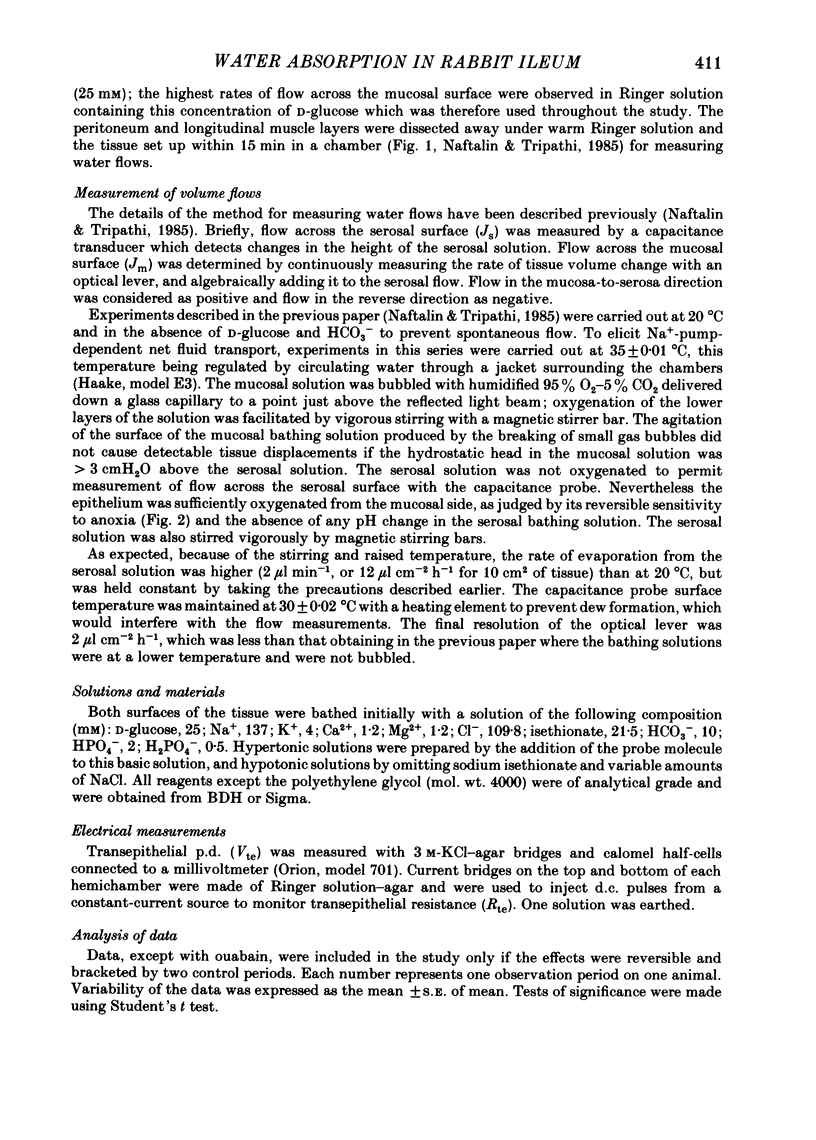
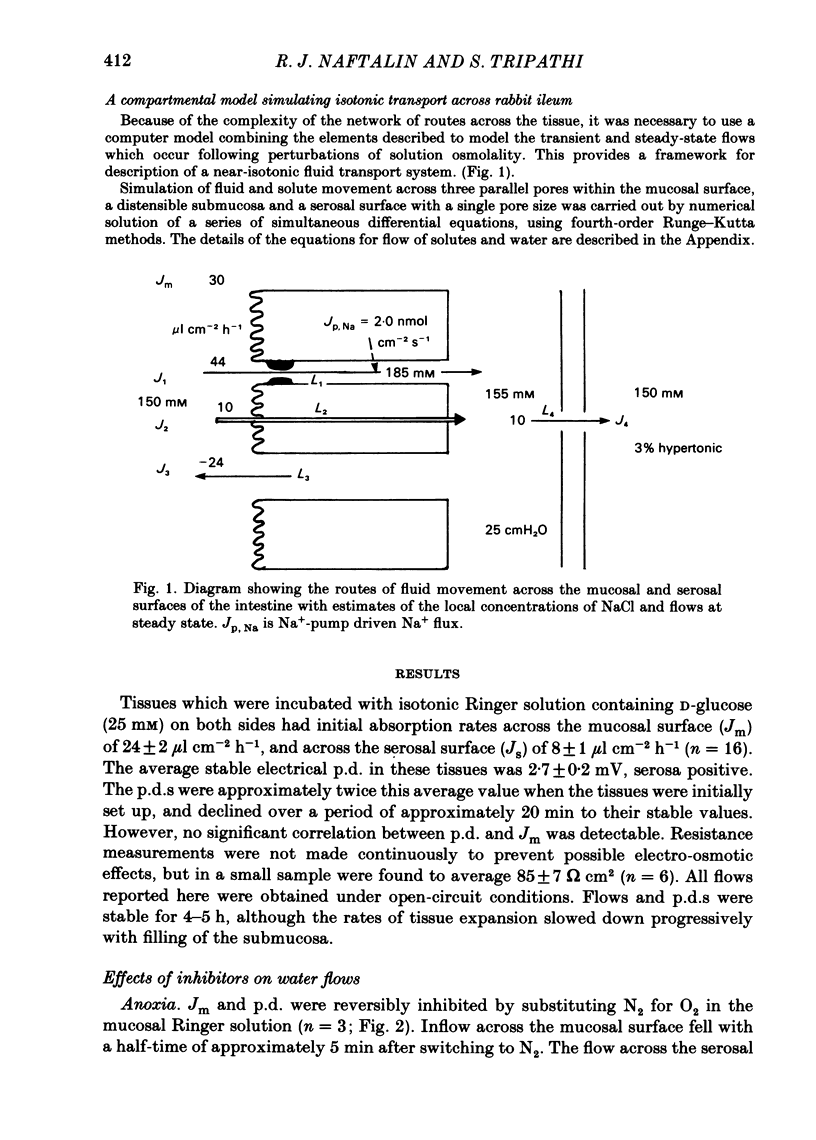
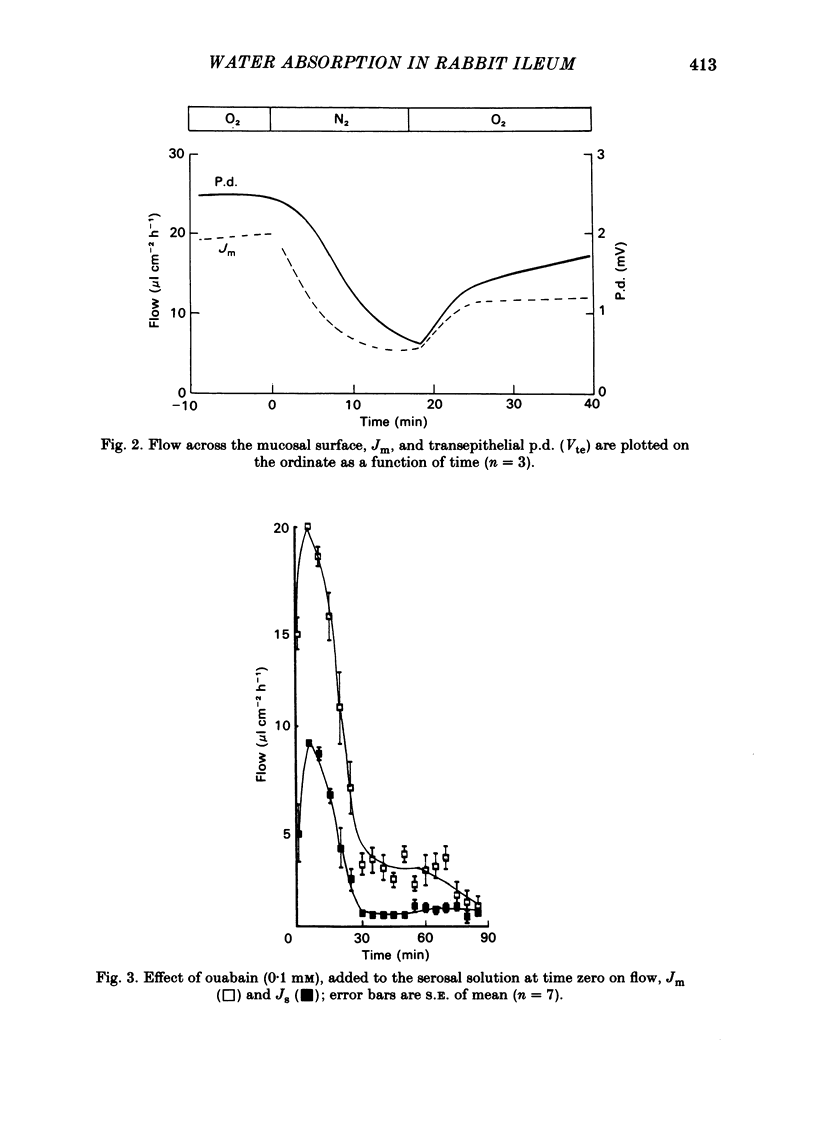
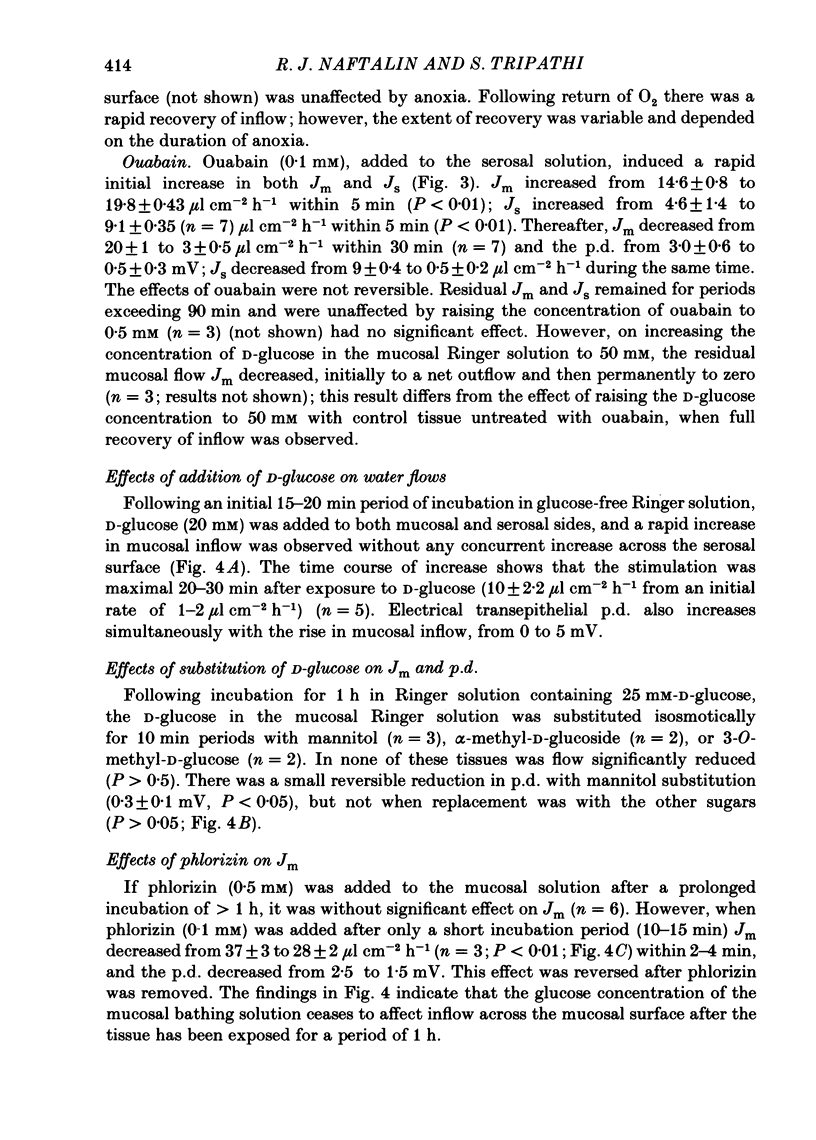
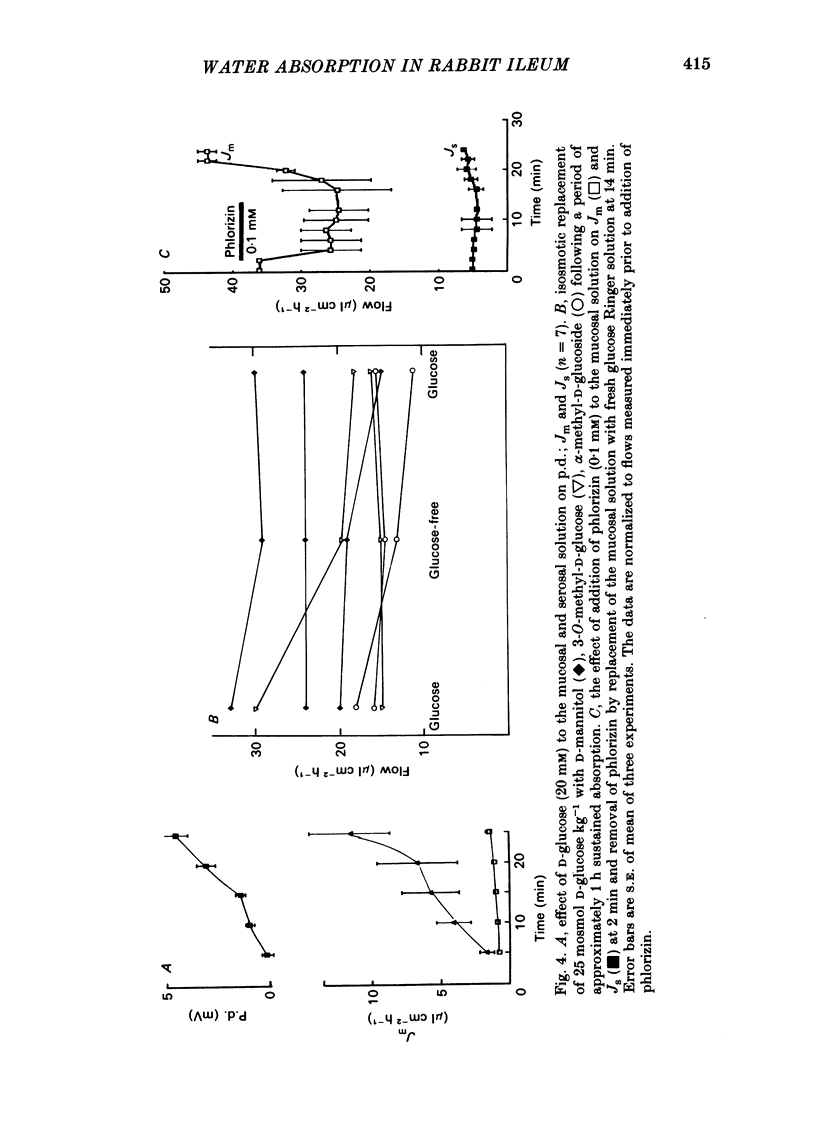
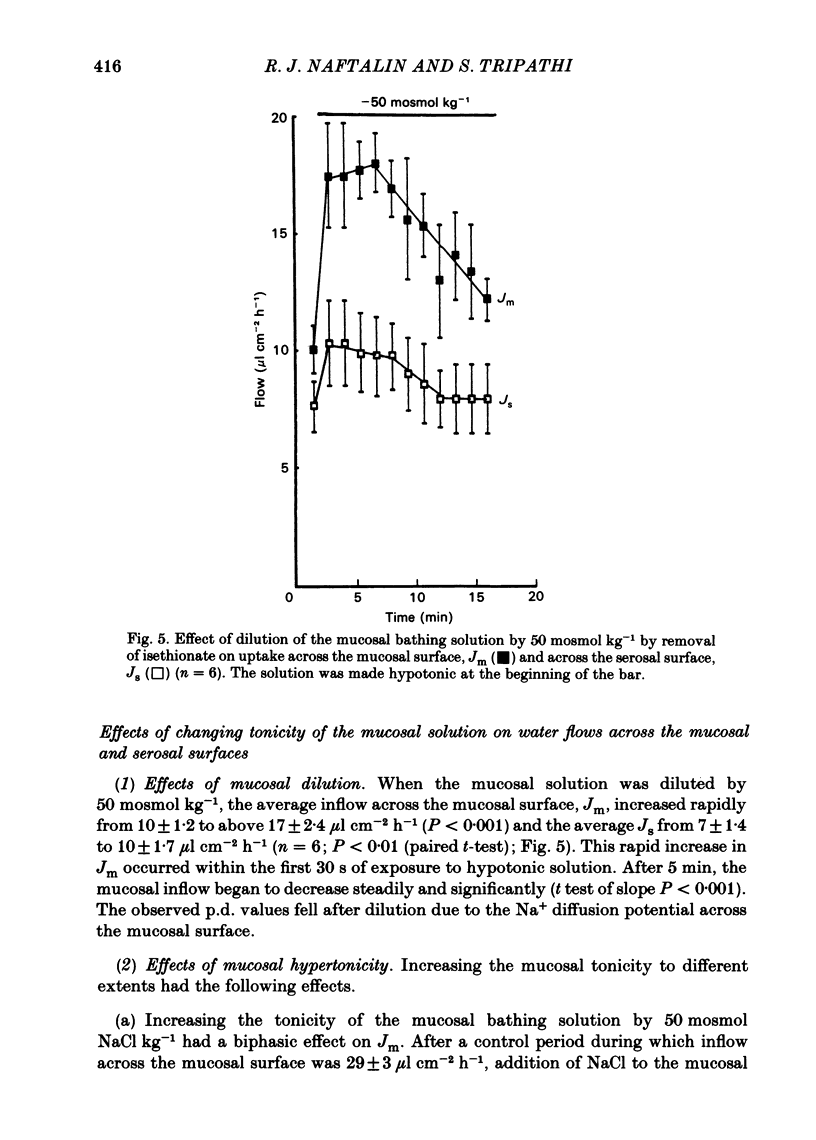
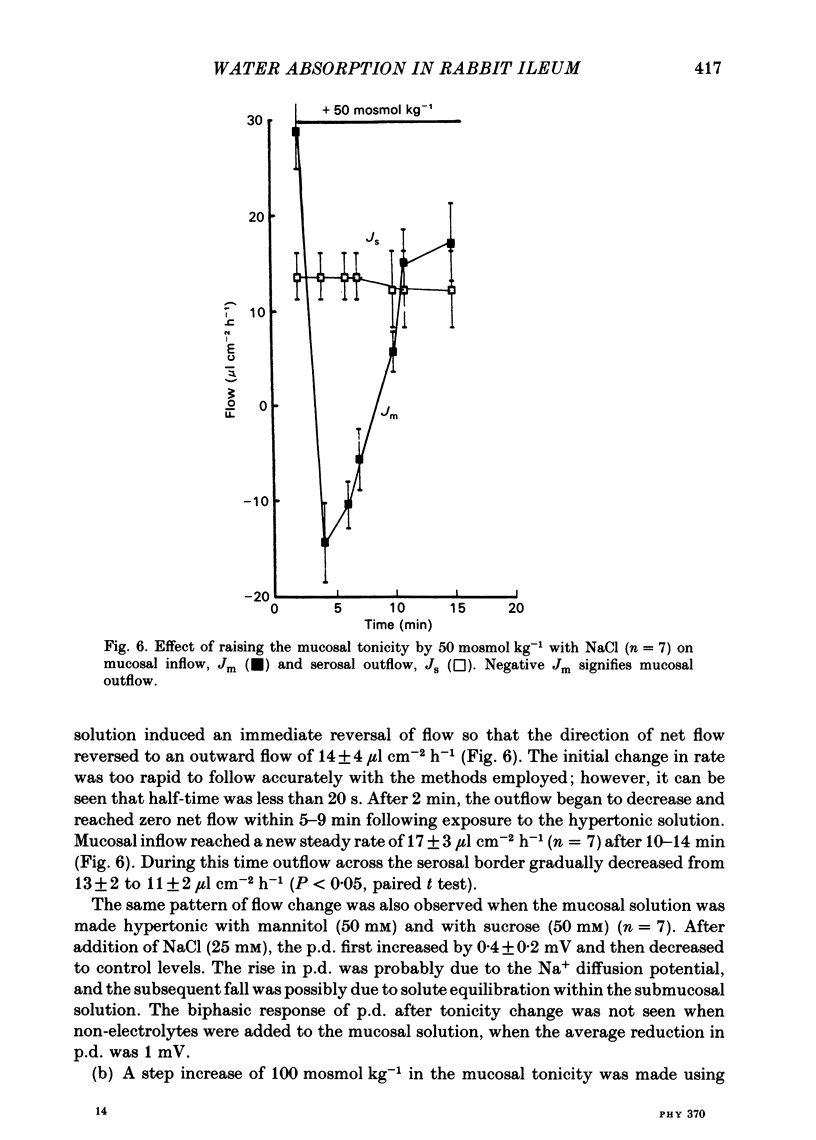
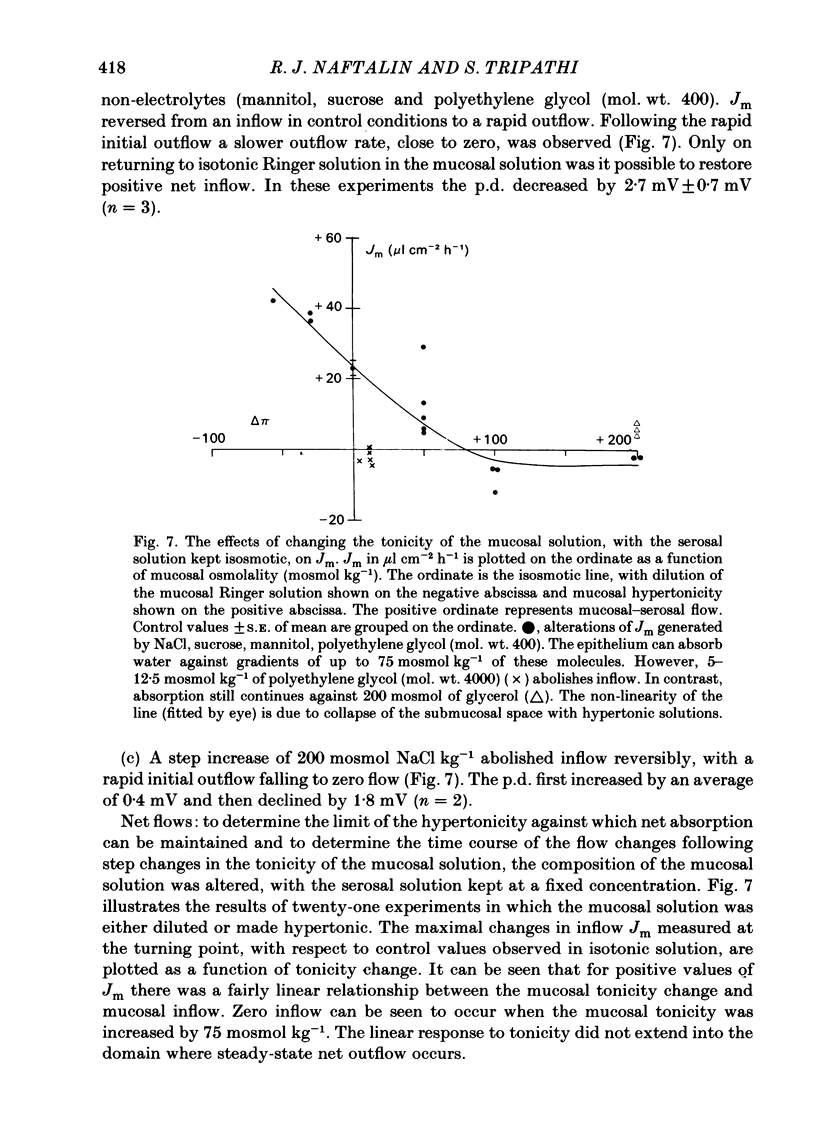
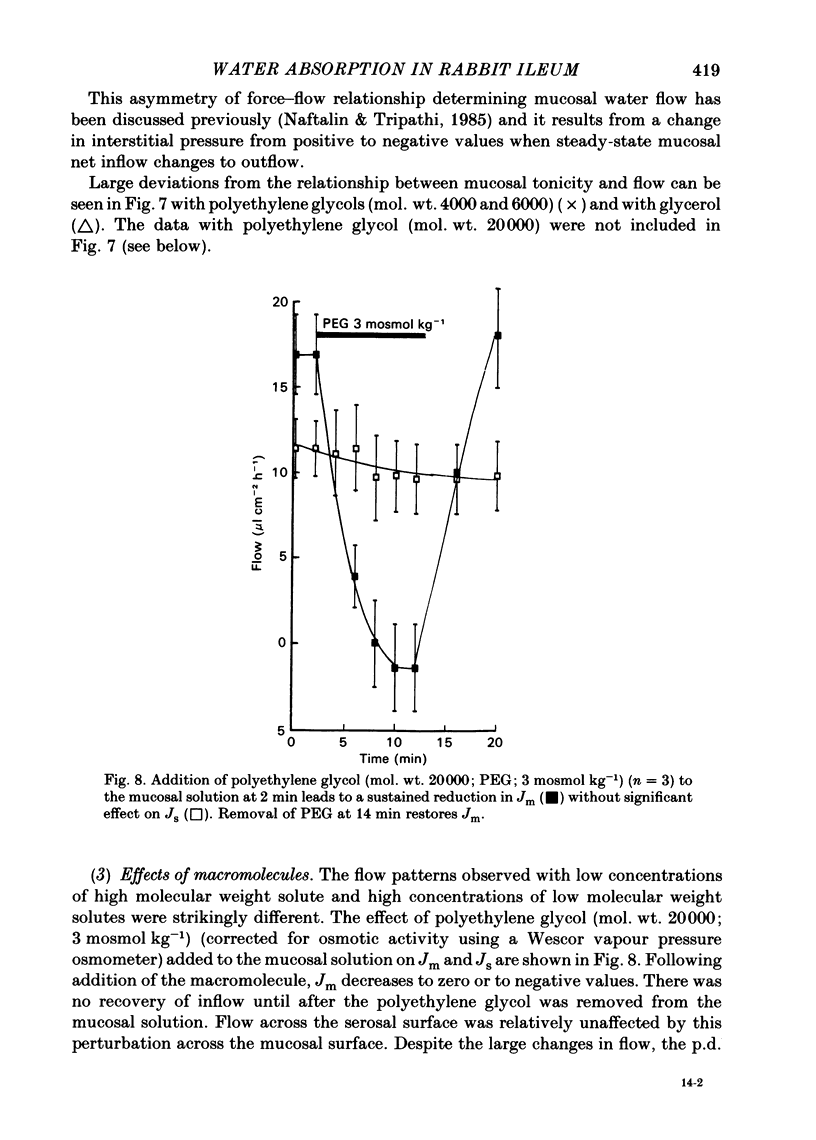
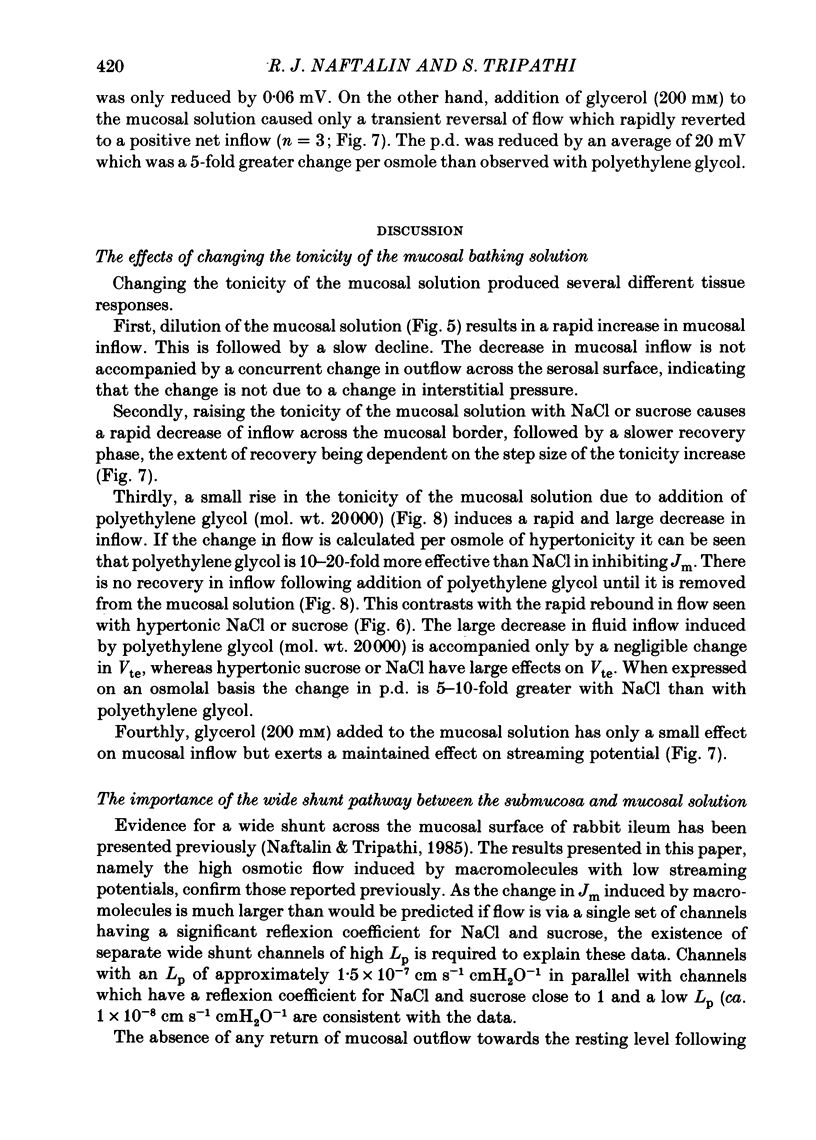
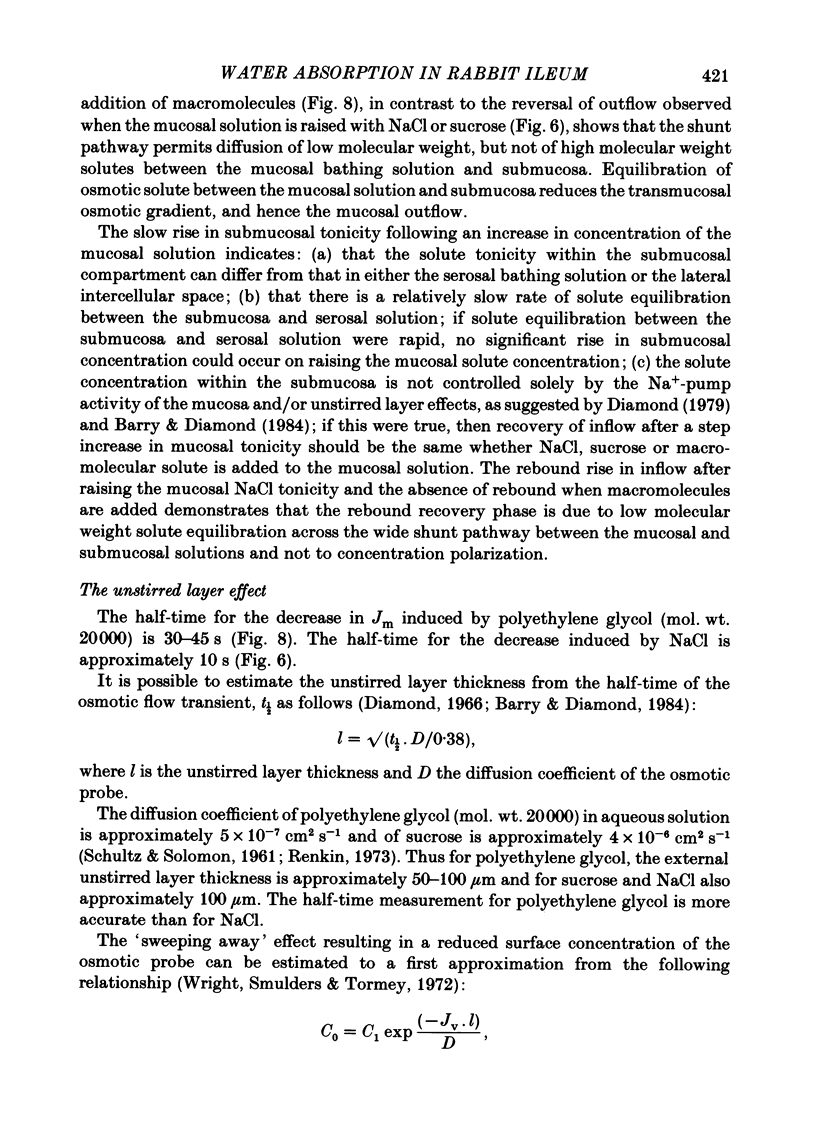
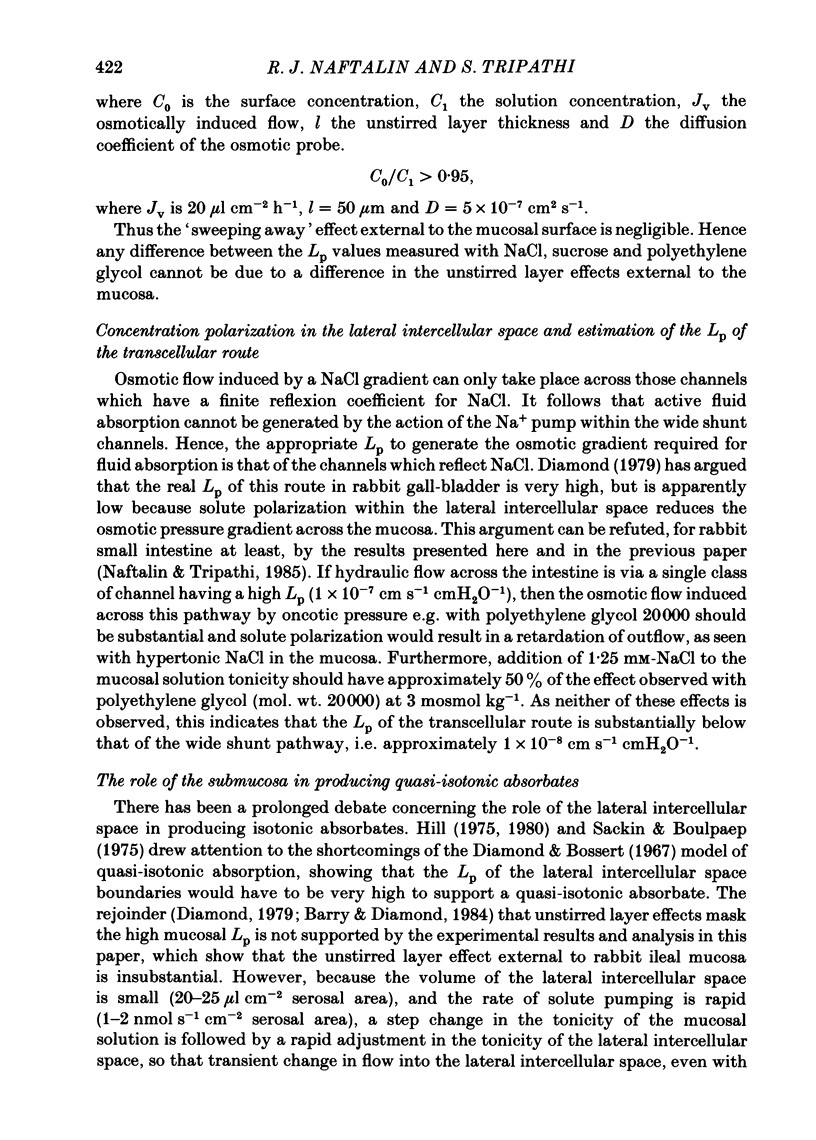
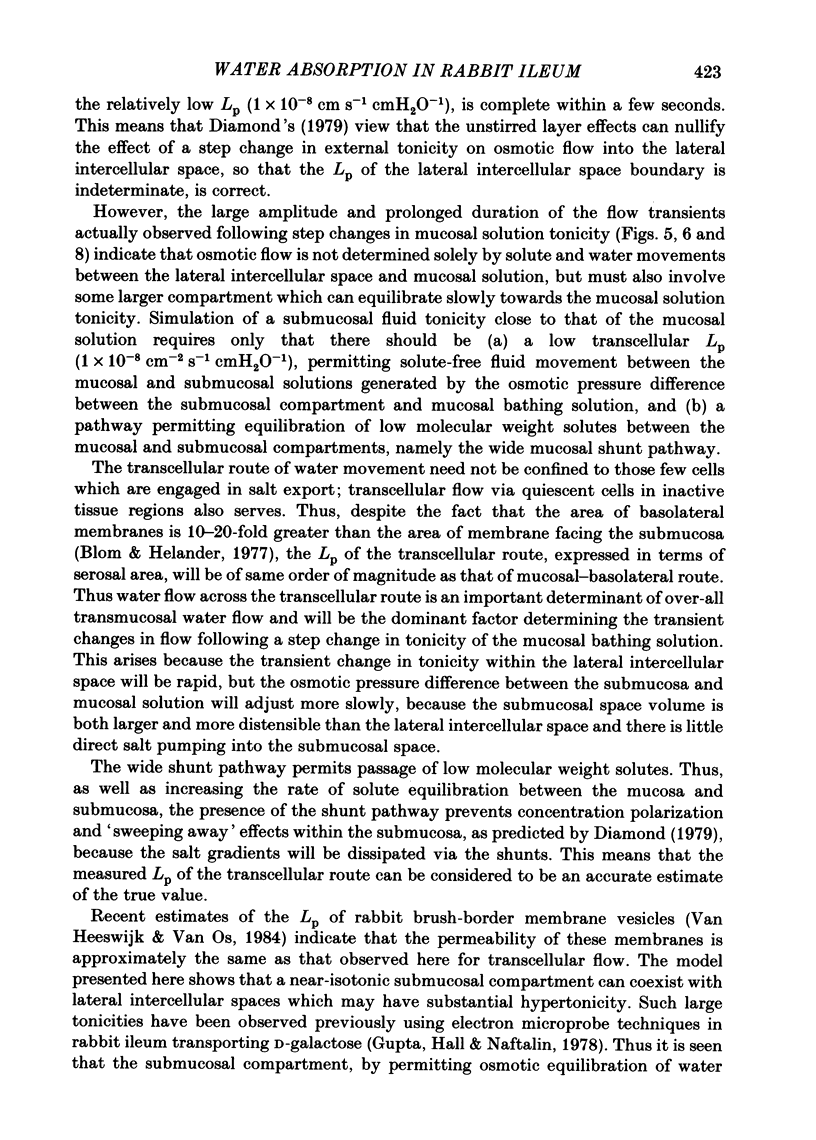
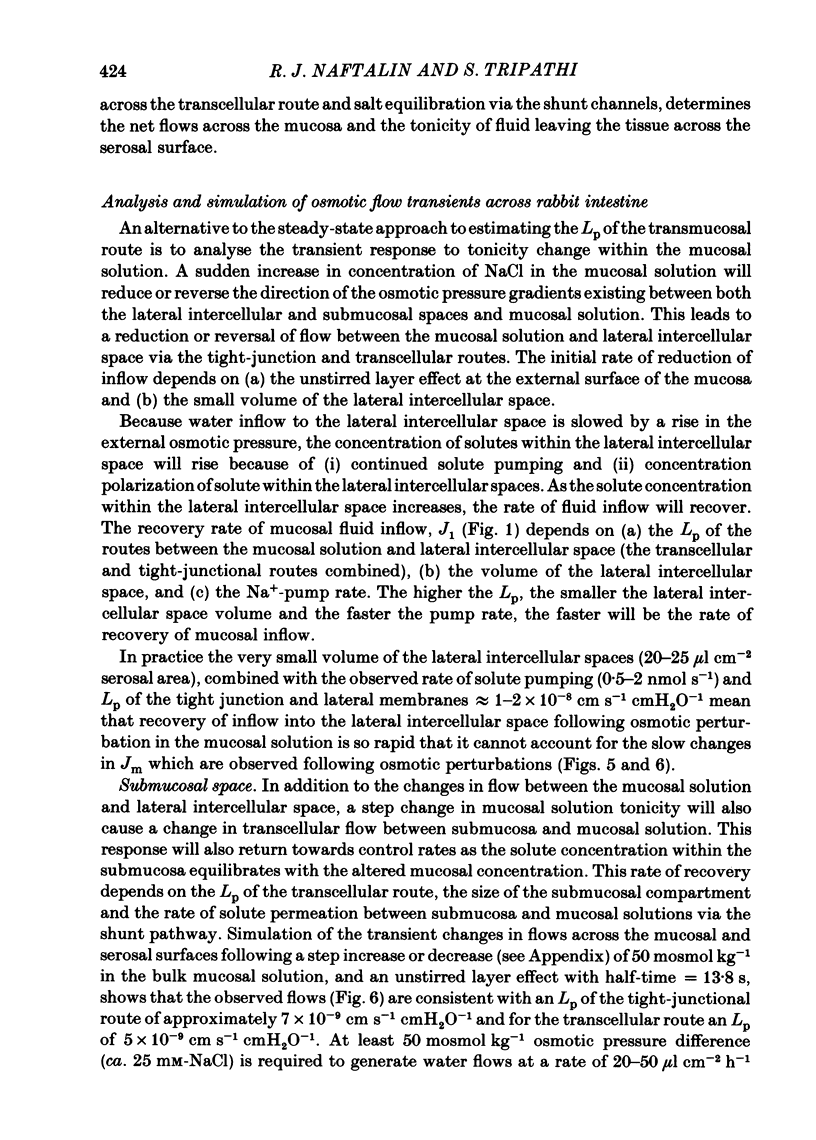
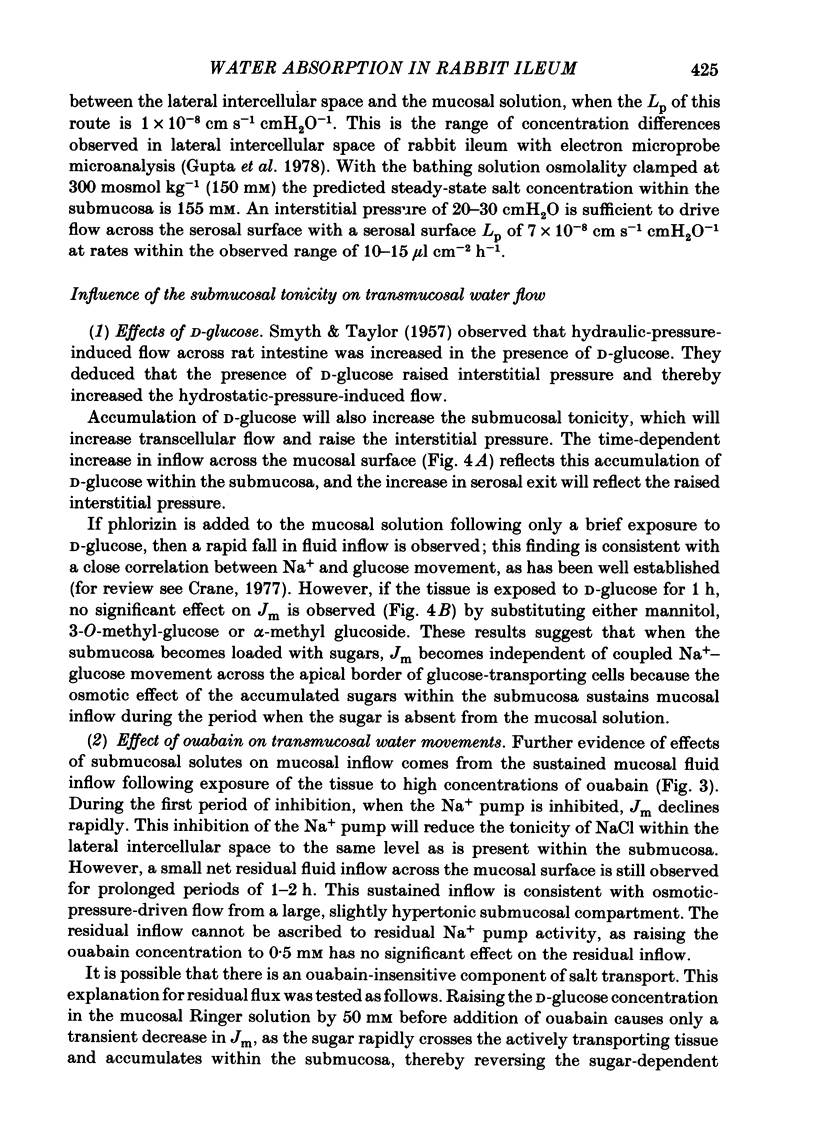
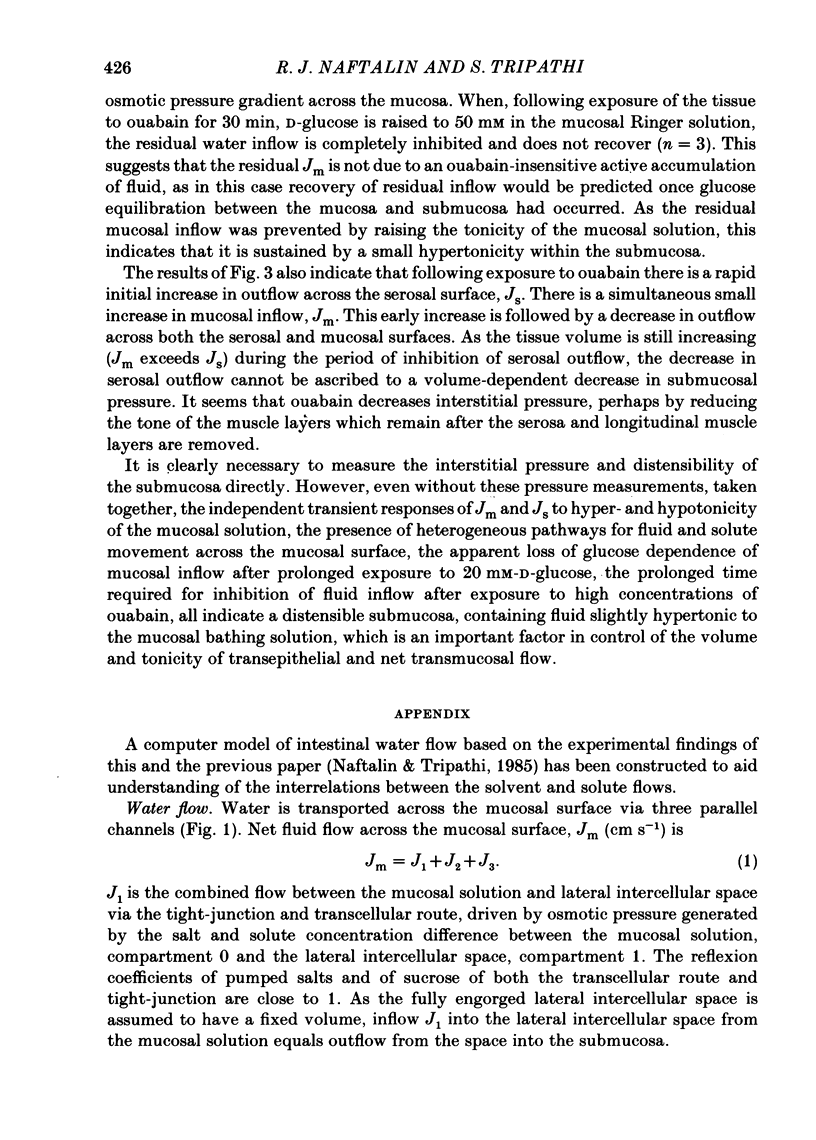
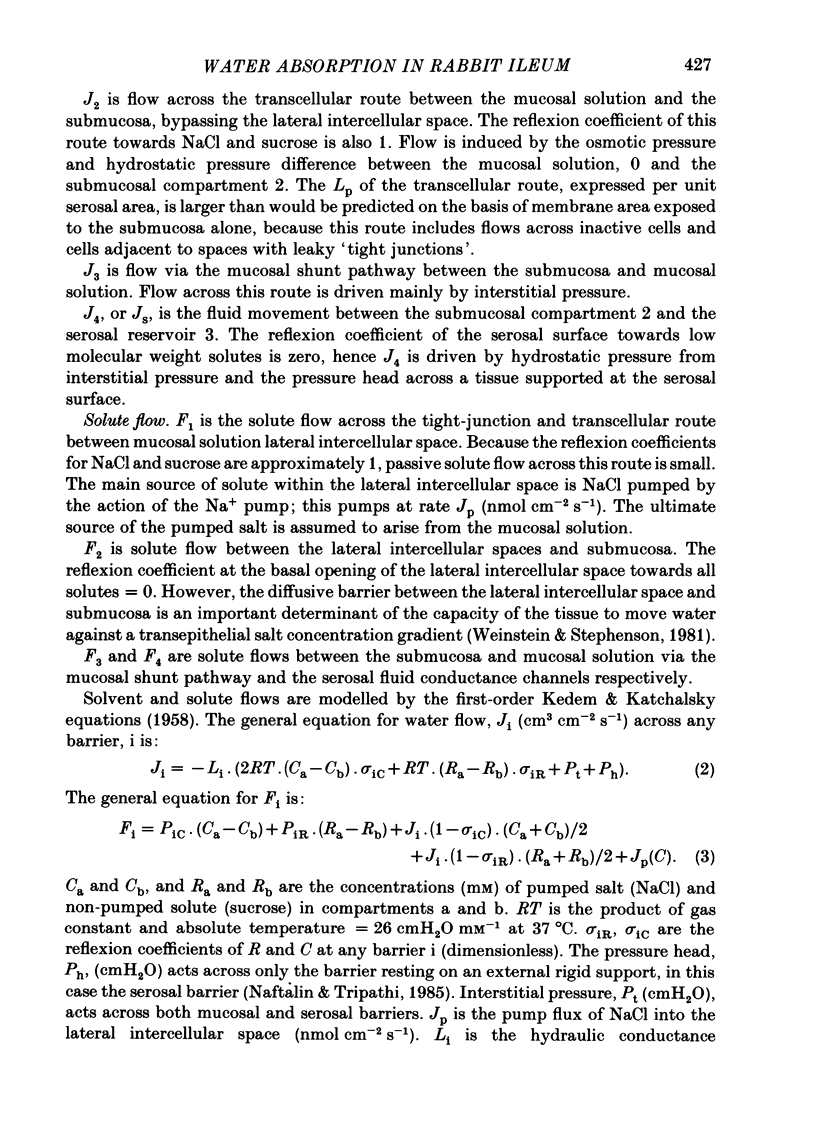
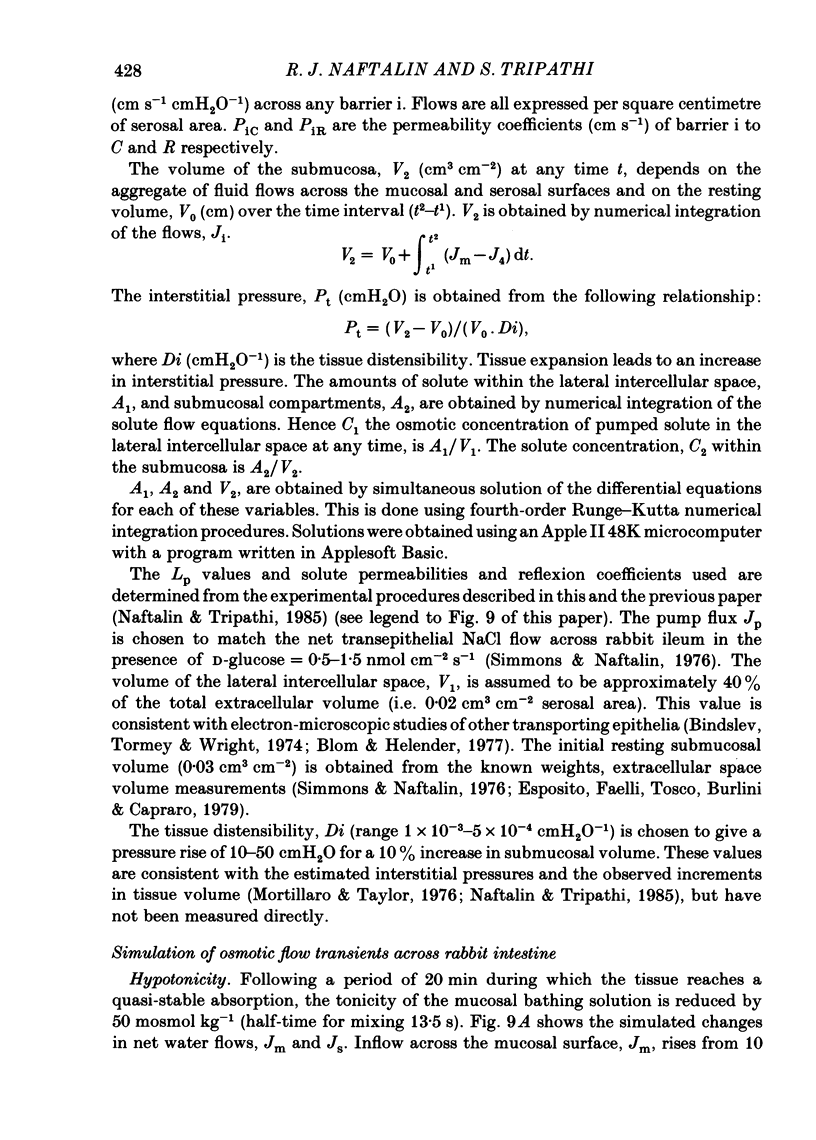
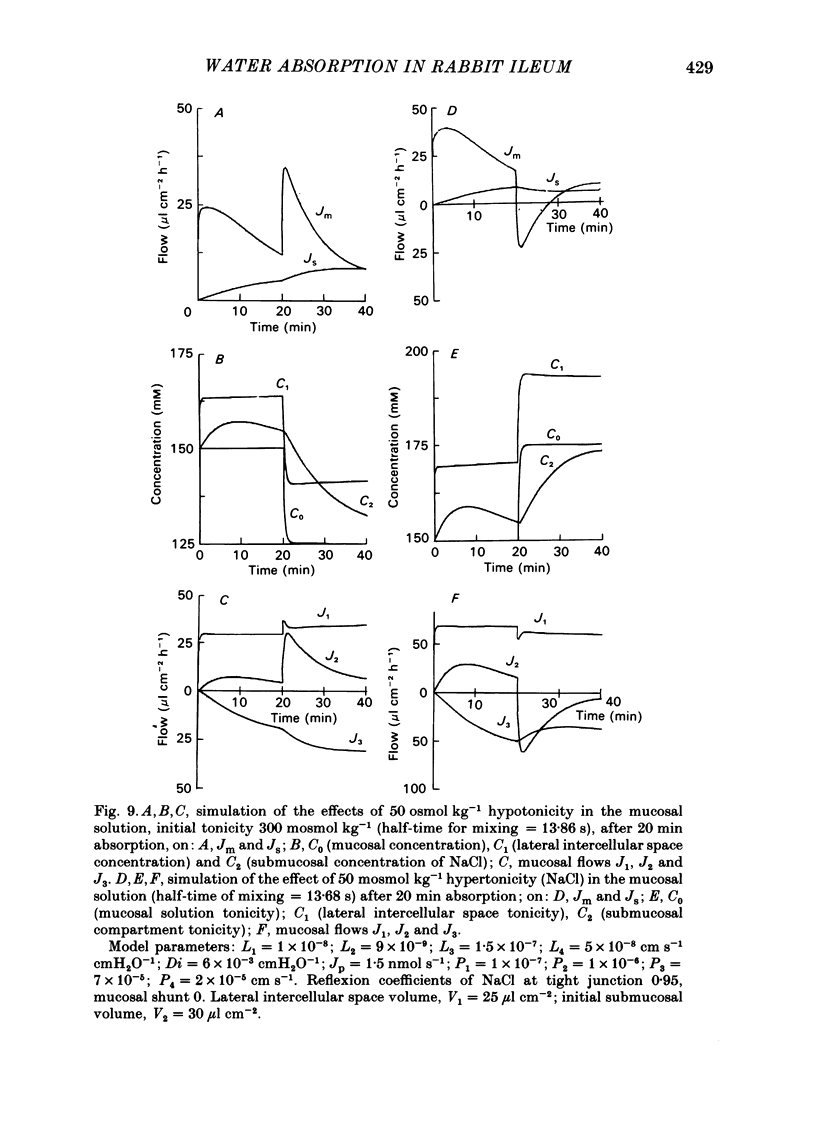
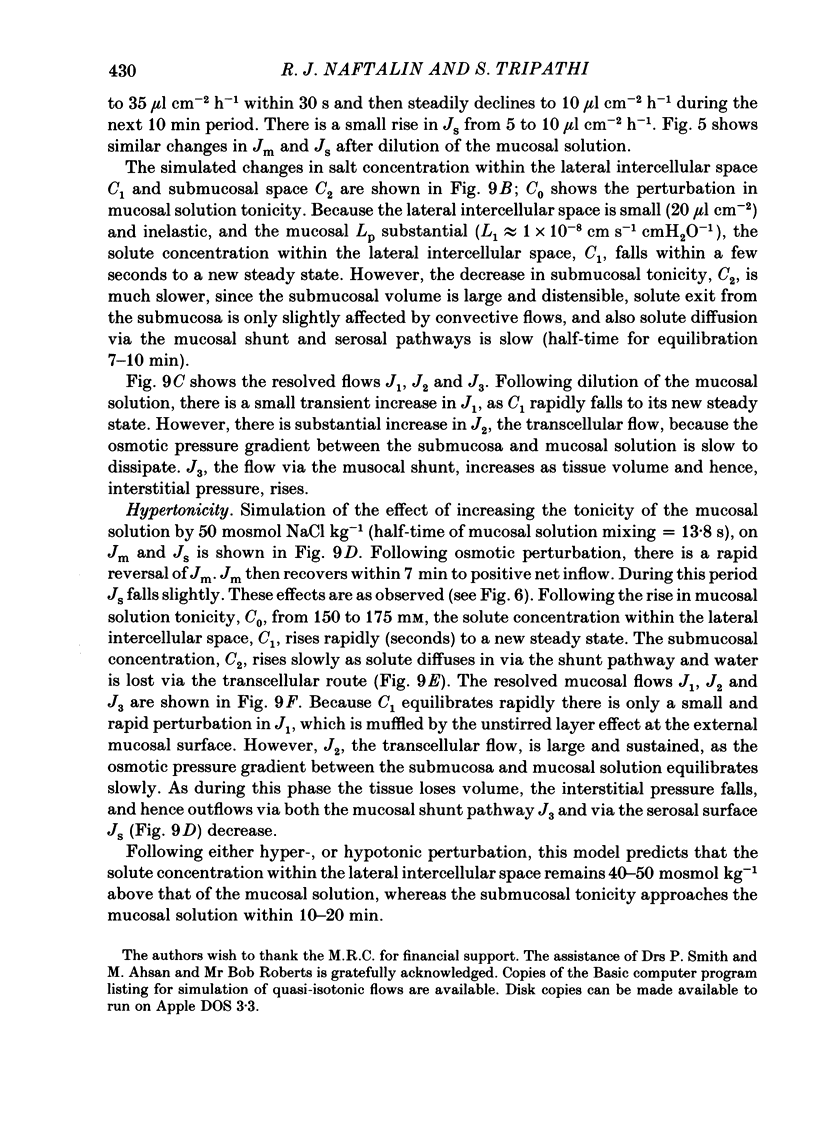
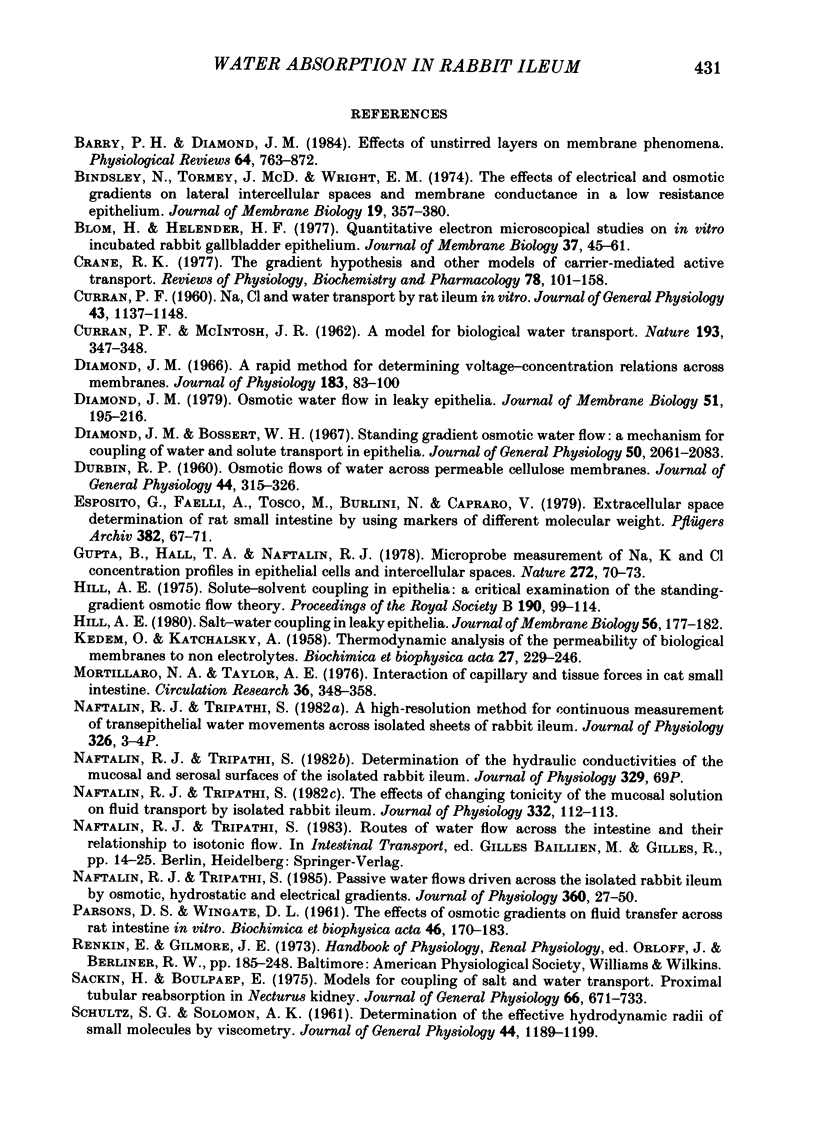
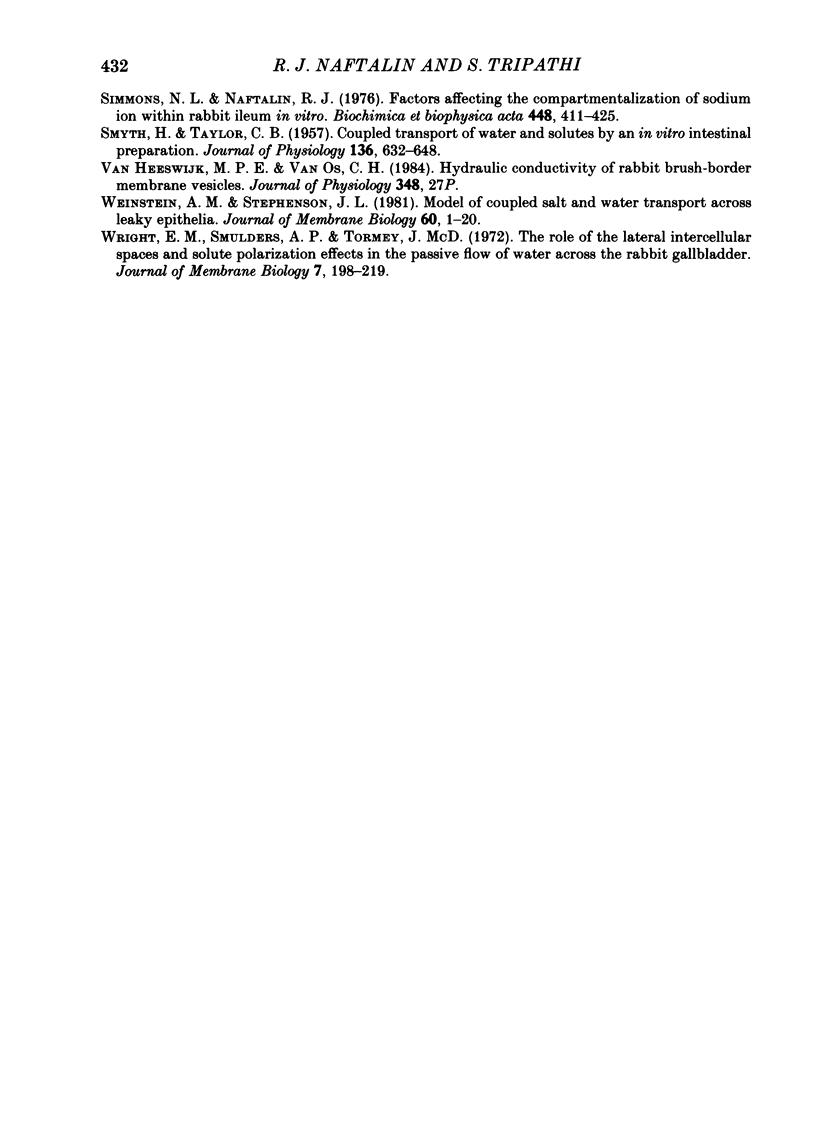
Selected References
These references are in PubMed. This may not be the complete list of references from this article.
- Barry P. H., Diamond J. M. Effects of unstirred layers on membrane phenomena. Physiol Rev. 1984 Jul;64(3):763–872. doi: 10.1152/physrev.1984.64.3.763. [DOI] [PubMed] [Google Scholar]
- Bindslev N., Tormey J. M., Wright E. M. The effects of electrical and osmotic gradients on lateral intercellular spaces and membrane conductance in a low resistance epithelium. J Membr Biol. 1974;19(4):357–380. doi: 10.1007/BF01869986. [DOI] [PubMed] [Google Scholar]
- Blom H., Helander H. F. Quantitative electron microscopical studies on in vitro incubated rabbit gallbladder epithelium. J Membr Biol. 1977 Oct 3;37(1):45–61. doi: 10.1007/BF01940923. [DOI] [PubMed] [Google Scholar]
- CURRAN P. F., MACINTOSH J. R. A model system for biological water transport. Nature. 1962 Jan 27;193:347–348. doi: 10.1038/193347a0. [DOI] [PubMed] [Google Scholar]
- CURRAN P. F. Na, Cl, and water transport by rat ileum in vitro. J Gen Physiol. 1960 Jul;43:1137–1148. doi: 10.1085/jgp.43.6.1137. [DOI] [PMC free article] [PubMed] [Google Scholar]
- DURBIN R. P. Osmotic flow of water across permeable cellulose membranes. J Gen Physiol. 1960 Nov;44:315–326. doi: 10.1085/jgp.44.2.315. [DOI] [PMC free article] [PubMed] [Google Scholar]
- Diamond J. M. A rapid method for determining voltage-concentration relations across membranes. J Physiol. 1966 Mar;183(1):83–100. doi: 10.1113/jphysiol.1966.sp007852. [DOI] [PMC free article] [PubMed] [Google Scholar]
- Diamond J. M., Bossert W. H. Standing-gradient osmotic flow. A mechanism for coupling of water and solute transport in epithelia. J Gen Physiol. 1967 Sep;50(8):2061–2083. doi: 10.1085/jgp.50.8.2061. [DOI] [PMC free article] [PubMed] [Google Scholar]
- Diamond J. M. Osmotic water flow in leaky epithelia. J Membr Biol. 1979 Dec 31;51(3-4):195–216. doi: 10.1007/BF01869084. [DOI] [PubMed] [Google Scholar]
- Esposito G., Faelli A., Tosco M., Burlini N., Capraro V. Extracellular space determination in rat small intestine by using markers of different molecular weights. Pflugers Arch. 1979 Oct;382(1):67–71. doi: 10.1007/BF00585906. [DOI] [PubMed] [Google Scholar]
- Gupta B. L., Hall T. A., Naftalin R. J. Microprobe measurement of Na, K and Cl concentration profiles in epithelial cells and intercellular spaces of rabbit ileum. Nature. 1978 Mar 2;272(5648):70–73. doi: 10.1038/272070a0. [DOI] [PubMed] [Google Scholar]
- Hill A. E. Solute-solvent coupling in epithelia: a critical examination of the standing-gradient osmotic flow theory. Proc R Soc Lond B Biol Sci. 1975 Jun 20;190(1098):99–114. doi: 10.1098/rspb.1975.0081. [DOI] [PubMed] [Google Scholar]
- Hill A. Salt-water coupling in leaky epithelia. J Membr Biol. 1980 Oct 31;56(3):177–182. doi: 10.1007/BF01869474. [DOI] [PubMed] [Google Scholar]
- KEDEM O., KATCHALSKY A. Thermodynamic analysis of the permeability of biological membranes to non-electrolytes. Biochim Biophys Acta. 1958 Feb;27(2):229–246. doi: 10.1016/0006-3002(58)90330-5. [DOI] [PubMed] [Google Scholar]
- Mortillaro N. A., Taylor A. E. Interaction of capillary and tissue forces in the cat small intestine. Circ Res. 1976 Sep;39(3):348–358. doi: 10.1161/01.res.39.3.348. [DOI] [PubMed] [Google Scholar]
- Naftalin R. J., Tripathi S. Passive water flows driven across the isolated rabbit ileum by osmotic, hydrostatic and electrical gradients. J Physiol. 1985 Mar;360:27–50. doi: 10.1113/jphysiol.1985.sp015602. [DOI] [PMC free article] [PubMed] [Google Scholar]
- PARSONS D. S., WINGATE D. L. The effect of osmotic gradients on fluid transfer across rat intestine in vitro. Biochim Biophys Acta. 1961 Jan 1;46:170–183. doi: 10.1016/0006-3002(61)90660-6. [DOI] [PubMed] [Google Scholar]
- SCHULTZ S. G., SOLOMON A. K. Determination of the effective hydrodynamic radii of small molecules by viscometry. J Gen Physiol. 1961 Jul;44:1189–1199. doi: 10.1085/jgp.44.6.1189. [DOI] [PMC free article] [PubMed] [Google Scholar]
- SMYTH D. H., TAYLOR C. B. Transfer of water and solutes by an in vitro intestinal preparation. J Physiol. 1957 May 23;136(3):632–648. doi: 10.1113/jphysiol.1957.sp005788. [DOI] [PMC free article] [PubMed] [Google Scholar]
- Sackin H., Boulpaep E. L. Models for coupling of salt and water transport; Proximal tubular reabsorption in Necturus kidney. J Gen Physiol. 1975 Dec;66(6):671–733. doi: 10.1085/jgp.66.6.671. [DOI] [PMC free article] [PubMed] [Google Scholar]
- Simmons N. L., Naftalin R. J. Factors affecting the compartmentalization of sodium ion within rabbit ileum in vitro. Biochim Biophys Acta. 1976 Oct 19;448(3):411–425. doi: 10.1016/0005-2736(76)90297-2. [DOI] [PubMed] [Google Scholar]
- Weinstein A. M., Stephenson J. L. Models of coupled salt and water transport across leaky epithelia. J Membr Biol. 1981 May 15;60(1):1–20. doi: 10.1007/BF01870828. [DOI] [PubMed] [Google Scholar]