Abstract
Quasi steady-state electric fields were applied across the isolated turtle cerebellum to study the relationship between applied field, neuronal morphology and the modulation of the neuronal spike firing pattern. Spiking elements were identified electrophysiologically using extracellular recording methods and by subsequent horseradish peroxidase injection, which revealed their dendritic morphology and orientation. The electric field was precisely defined by measuring the voltage gradients induced in the cerebellum by 40 s constant-current pulses. The field was constant in the vertical (dorso-ventral) axis and zero in the horizontal plane, in agreement with theory. Neurones were modulated by applying a sinusoidal field at frequencies between 0.05 and 1.0 Hz. Modulated cells exhibited an increase in firing frequency and fell into one of four classes, depending on the direction of the field that produced the modulation. Thus neurones were excited by: ventricle-directed fields (V modulation), pia-directed fields (P modulation), both of the above (V/P modulation) or showed no consistent modulation (non-modulation). Most Purkinje somata and primary dendrites (nineteen out of twenty-eight) and most Purkinje dendrites (eighteen out of thirty), were V modulated with maximum rate proportional to the peak field intensity. The dendrites of these cells were consistently oriented toward the pia. Among the stellate cells, the lower molecular layer stellates, with dendrites extending predominantly towards the pia, were mostly (nineteen out of thirty-two) V modulated. The mid-molecular layer stellates, which showed much variability in dendritic orientation, were distributed among all four of the modulation classes. The upper molecular layer stellates, with a mostly horizontal dendritic alignment, were mainly (nine out of sixteen) non-modulated. All groups of spiking elements showed a correlation between patterns of modulation by applied fields and dendritic orientation, which suggests the degree of differential polarization of the extended cable elements of the neurone by the applied field as the basic mechanism for field-induced excitation or inhibition. The threshold for modulation among all neurones was 15-20 mV/mm, which is similar to the fields that modulate other nervous tissues. This suggests that many neurones can be modulated by fields of the order of 10-20 mV/mm.
Full text
PDF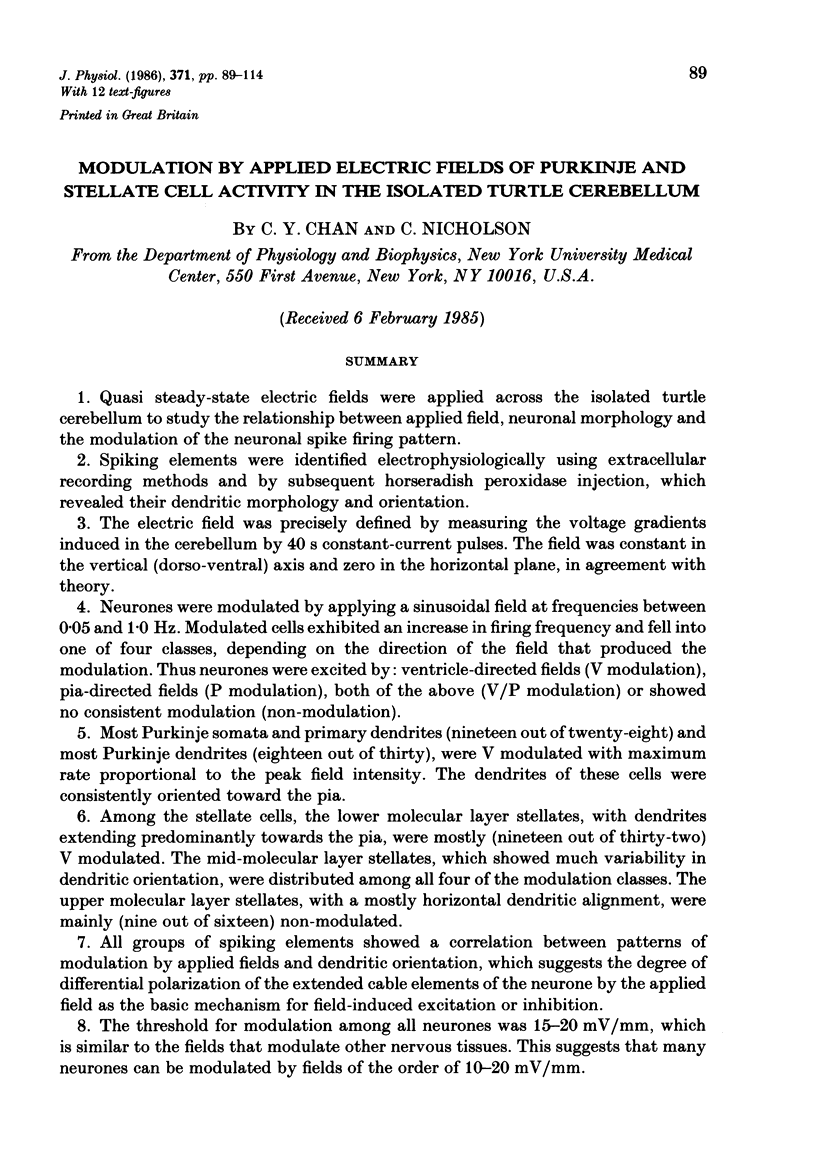
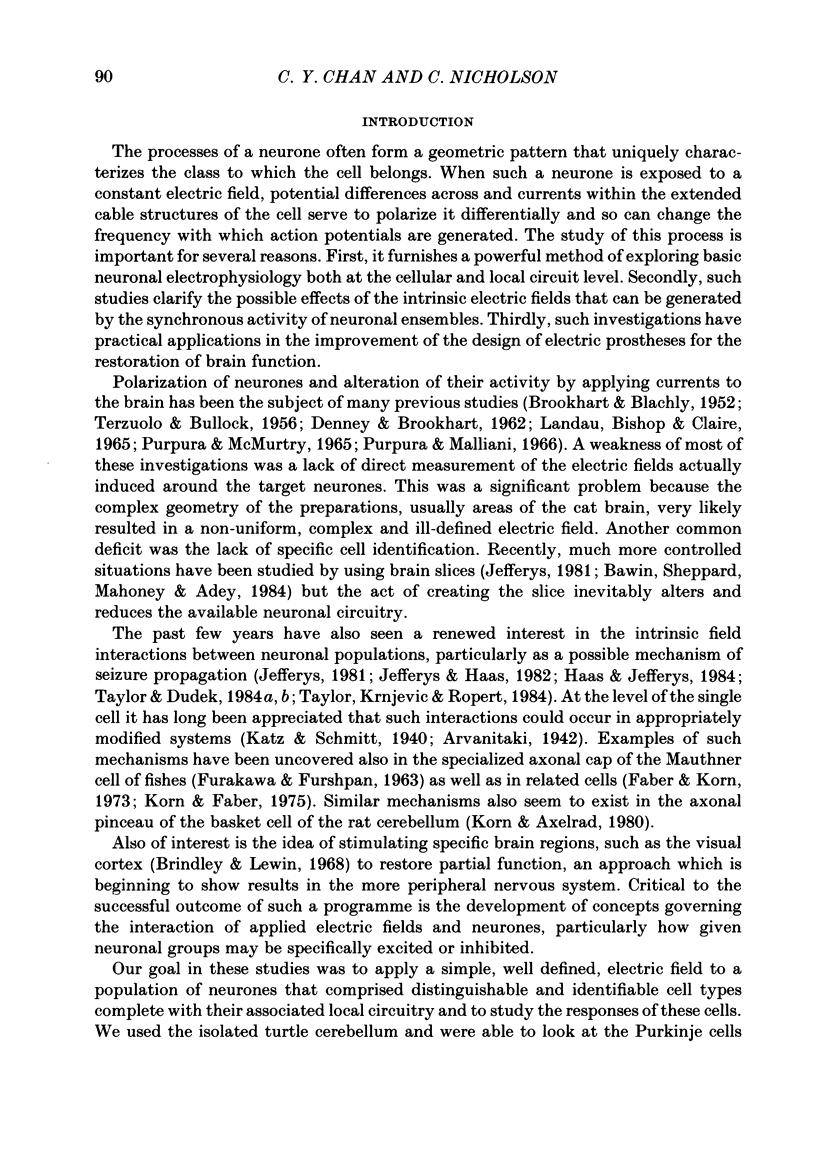
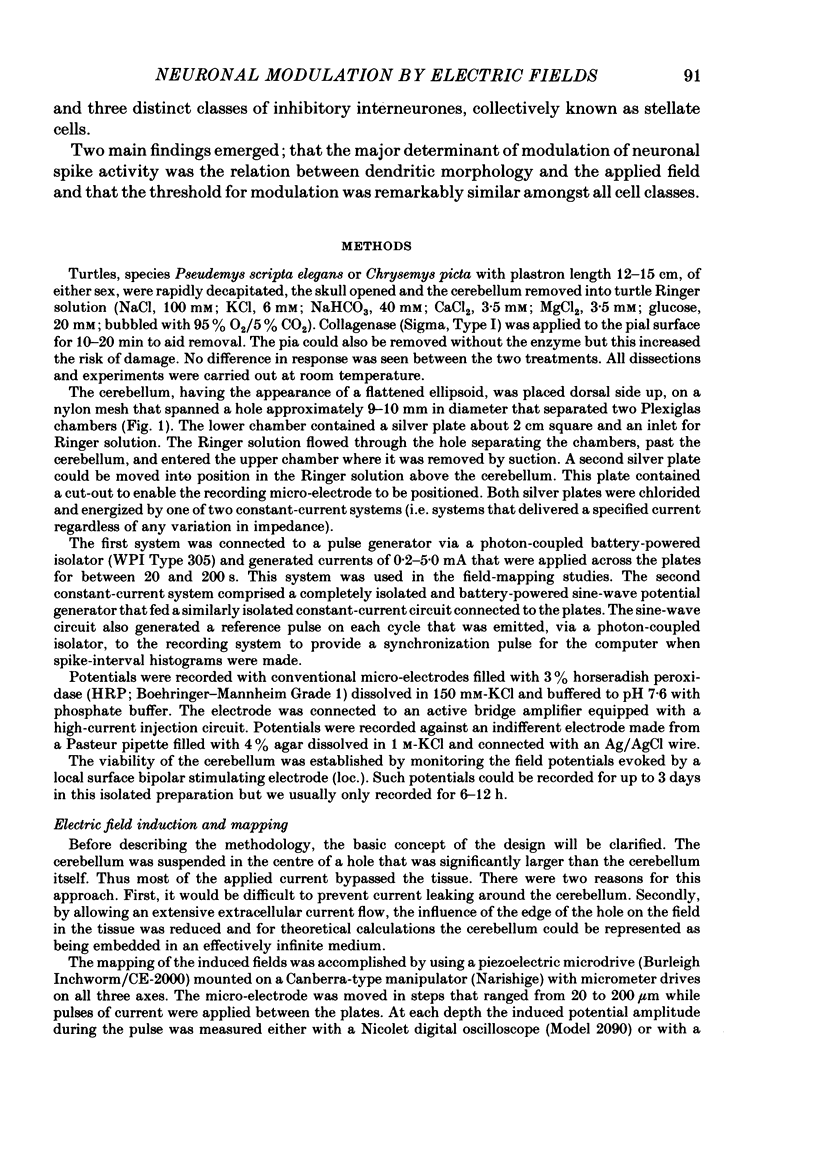
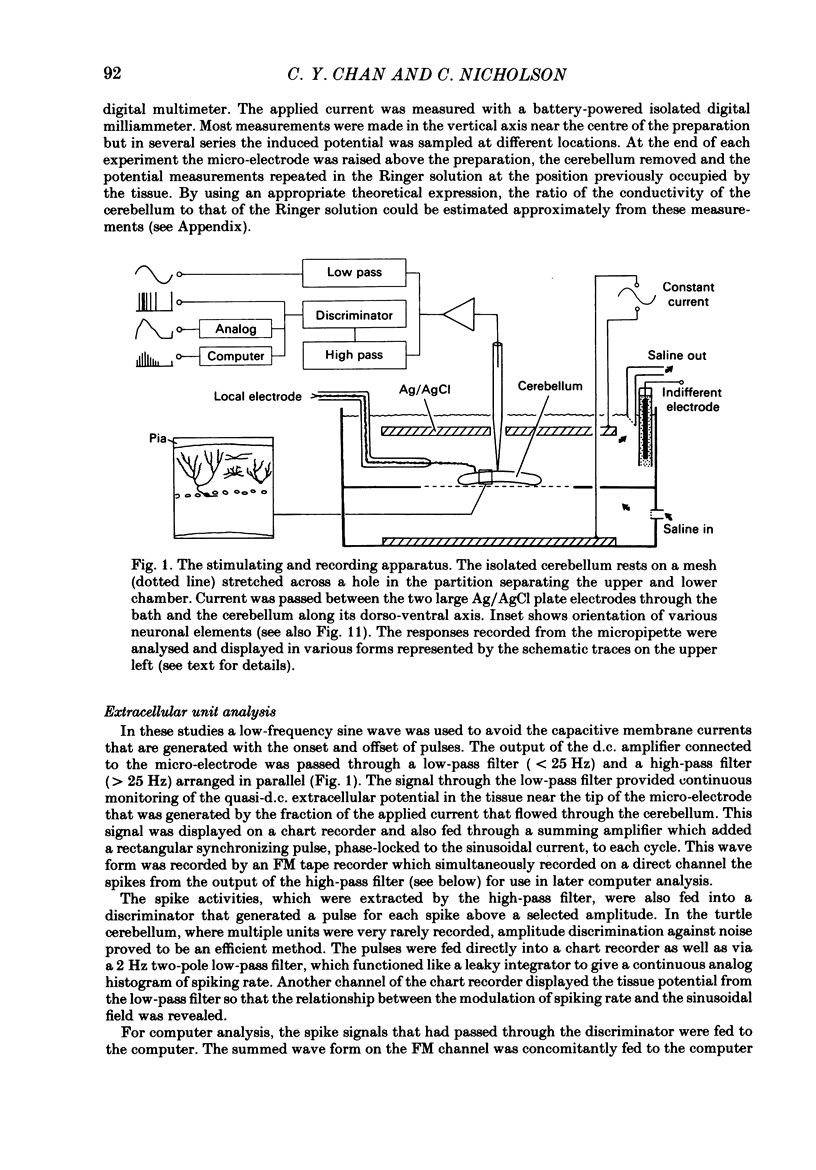
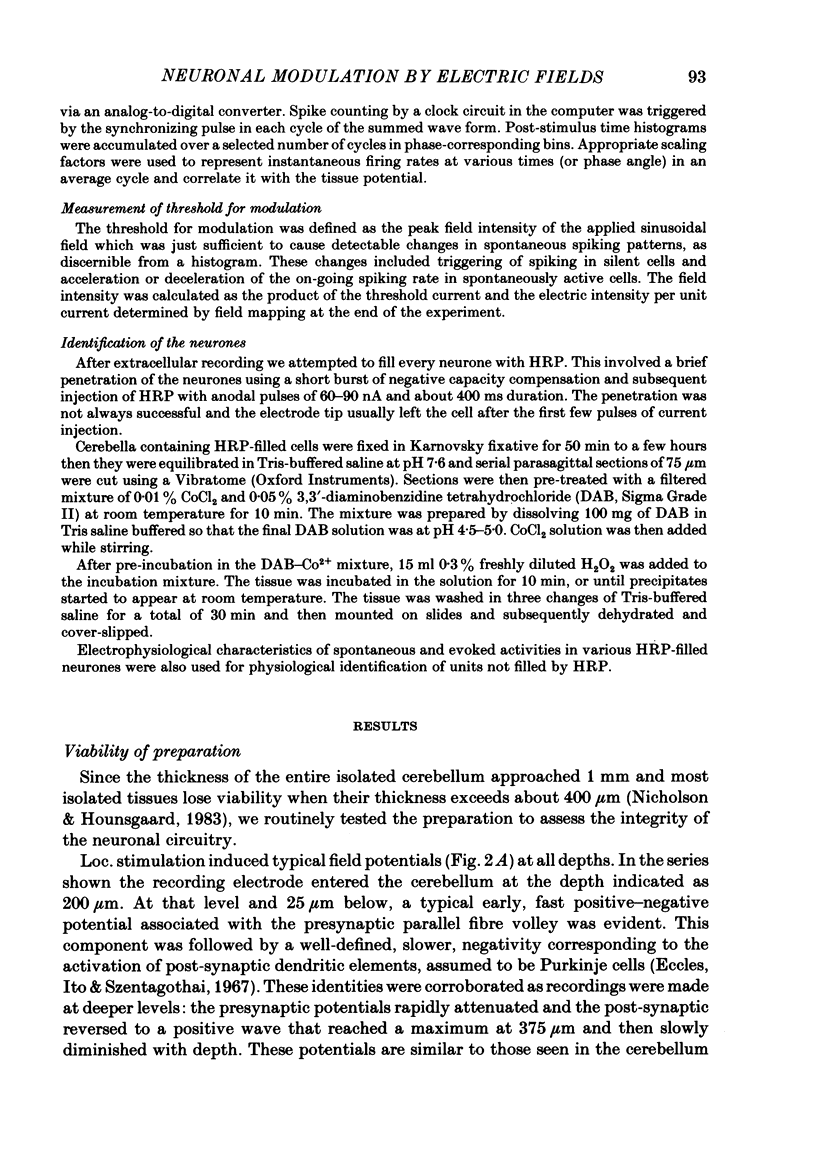
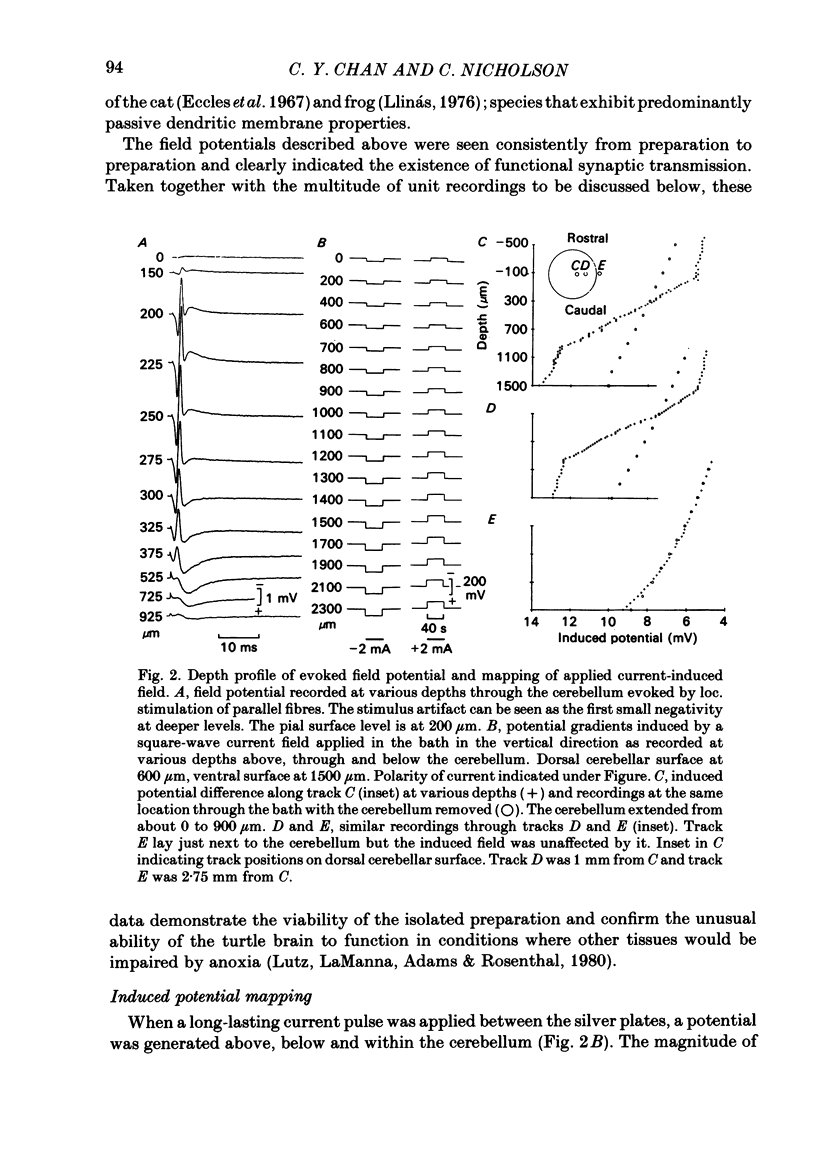
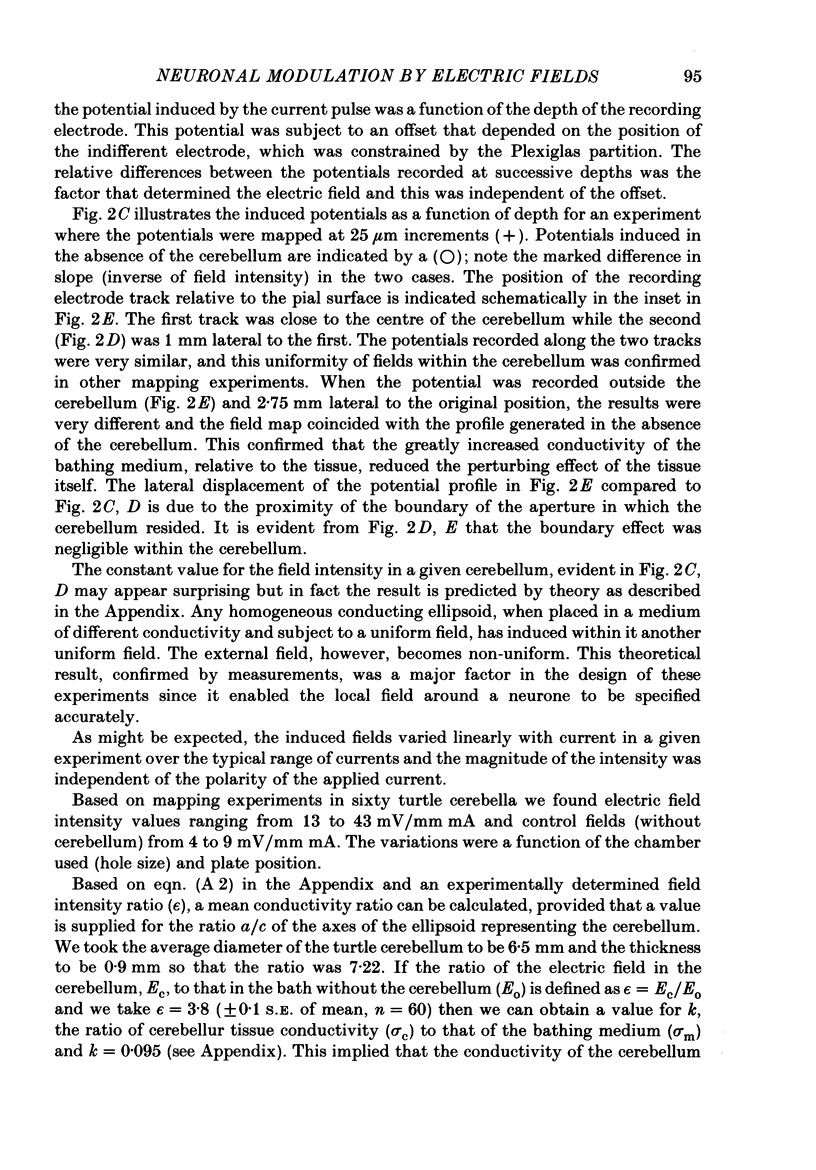
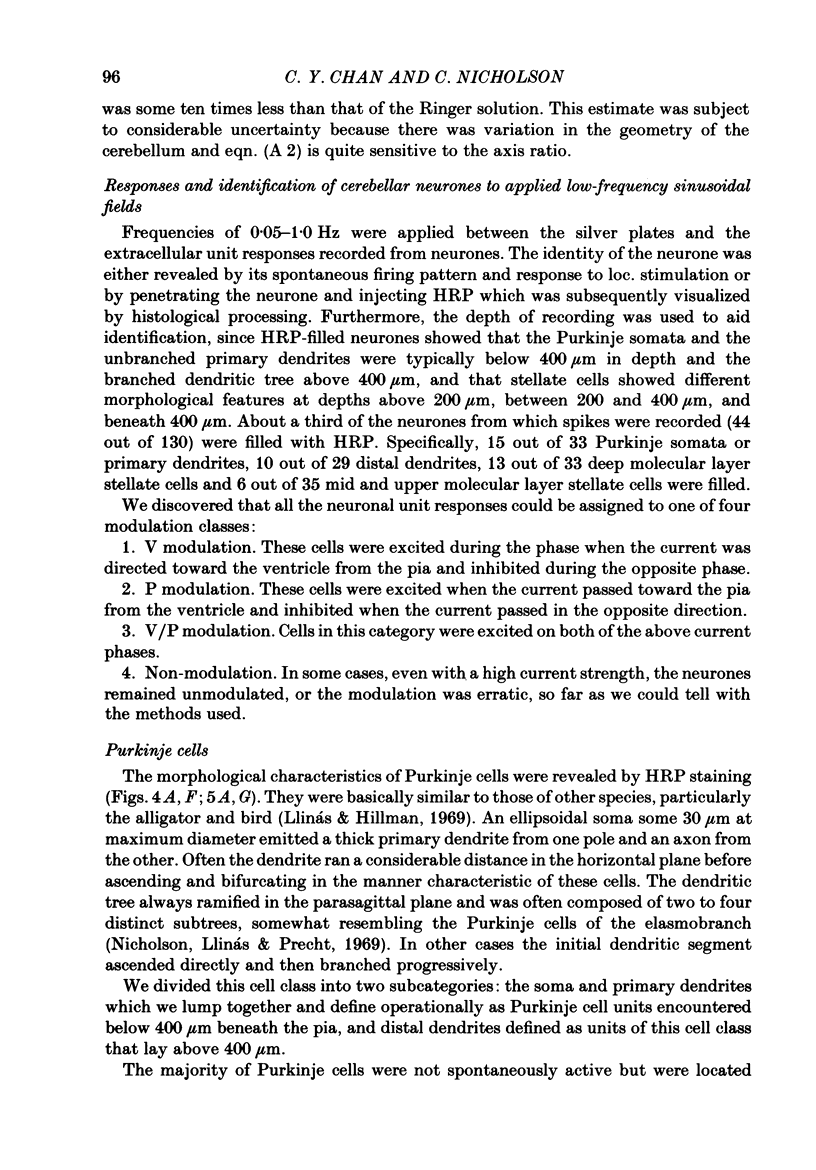
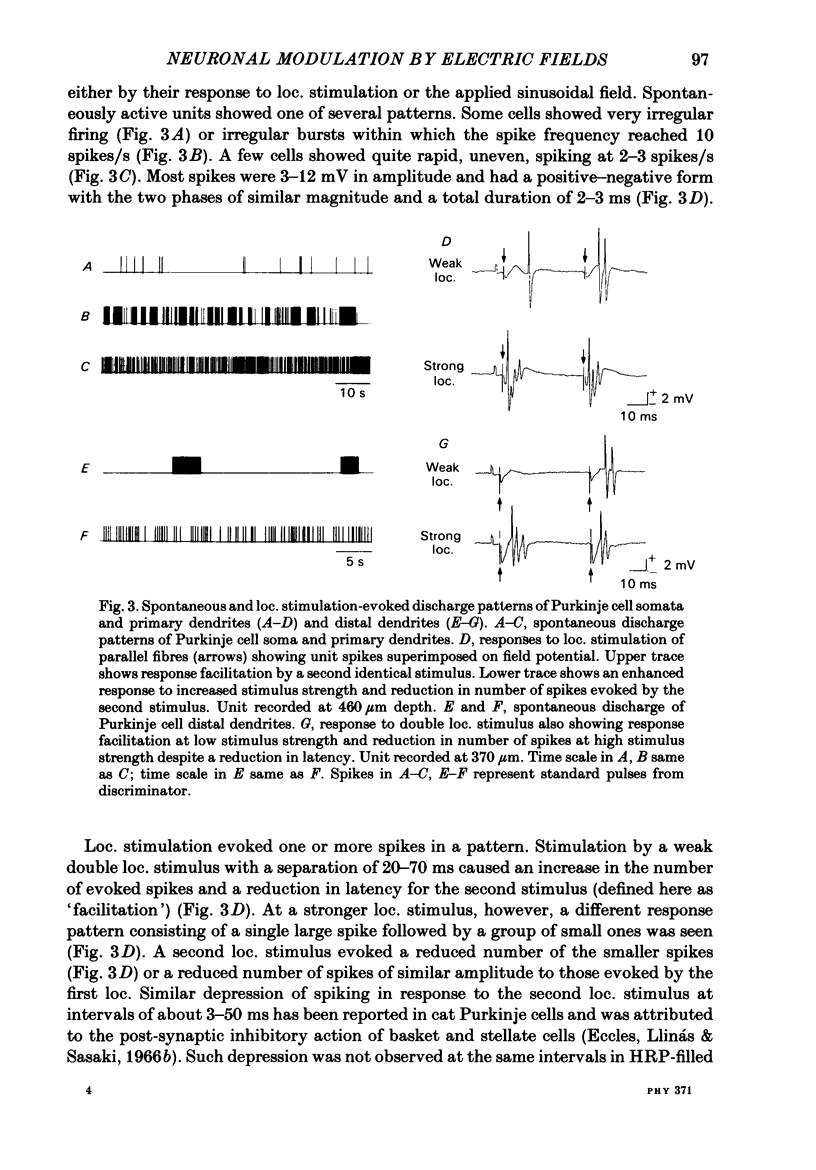
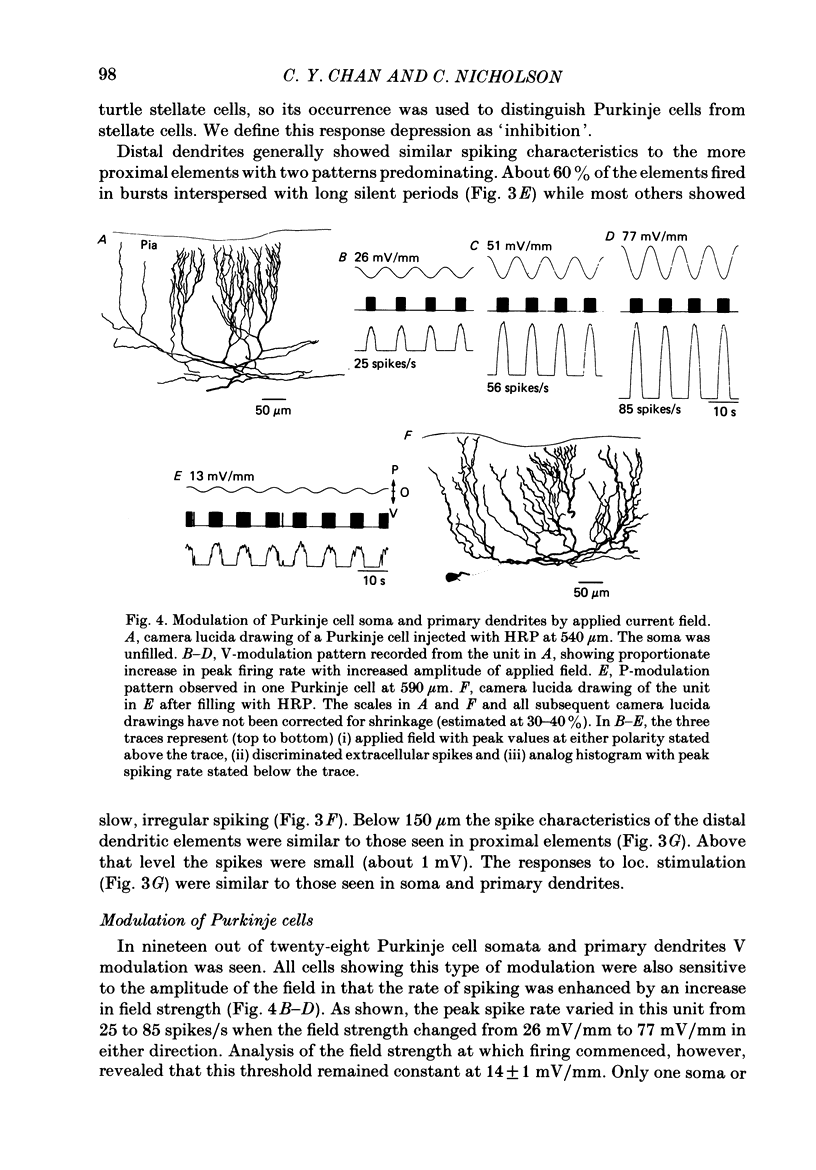
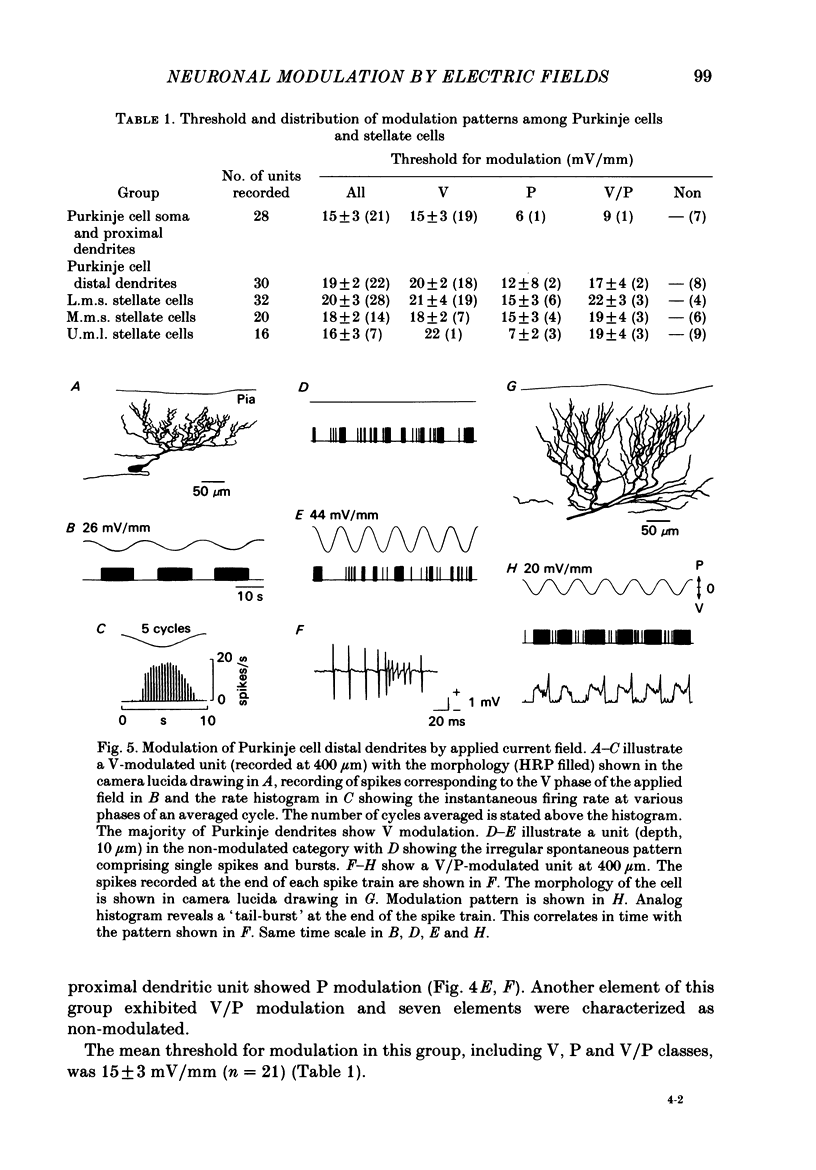
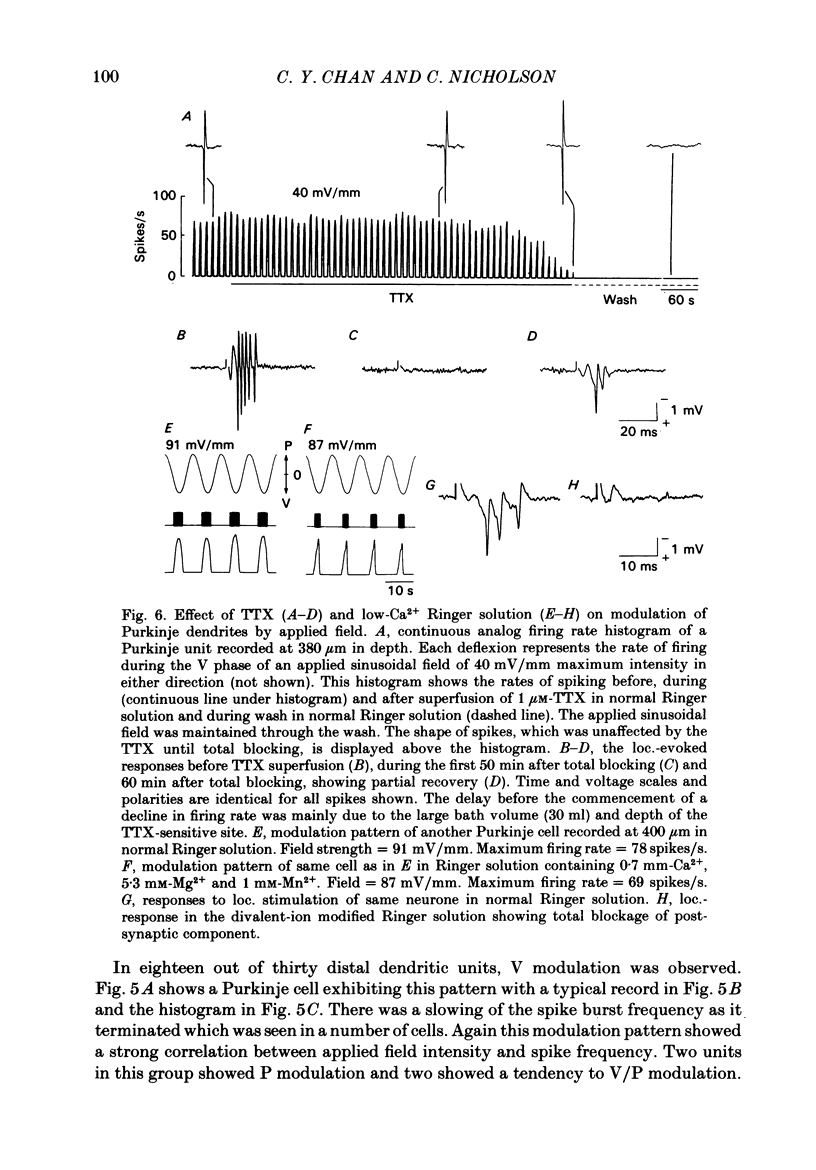
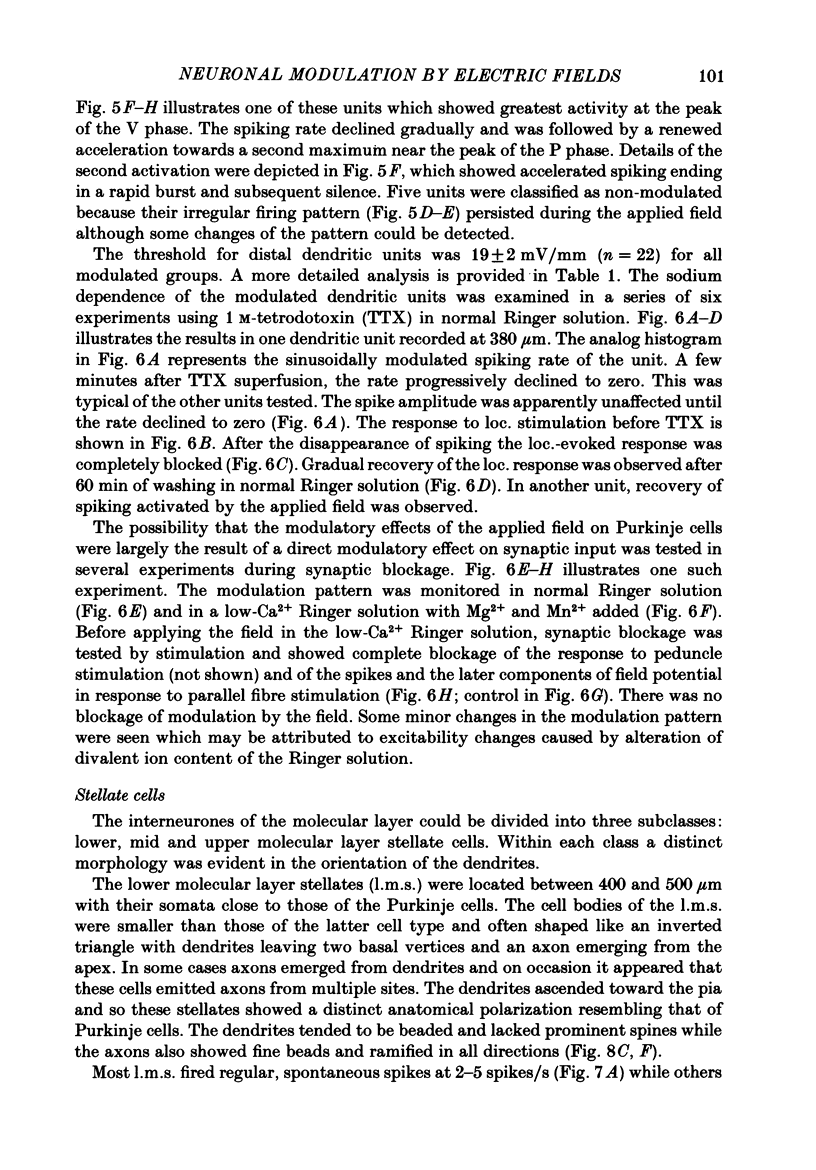
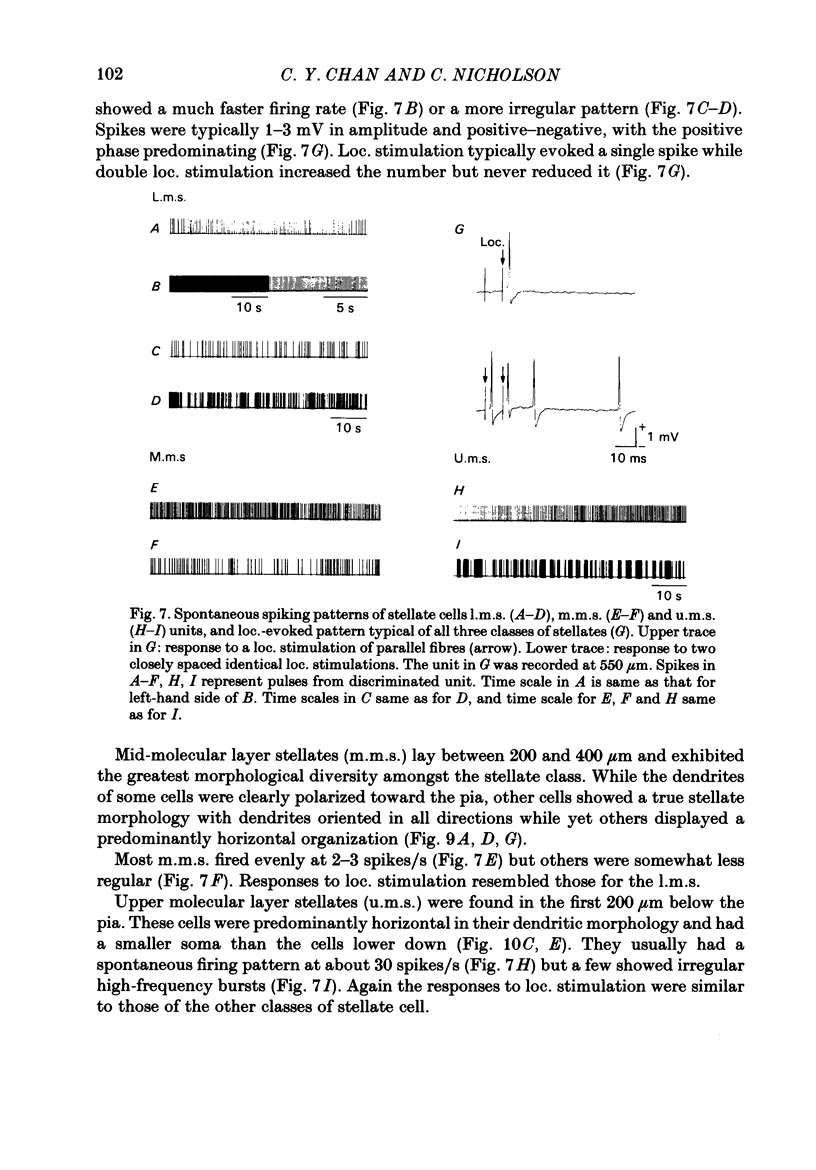
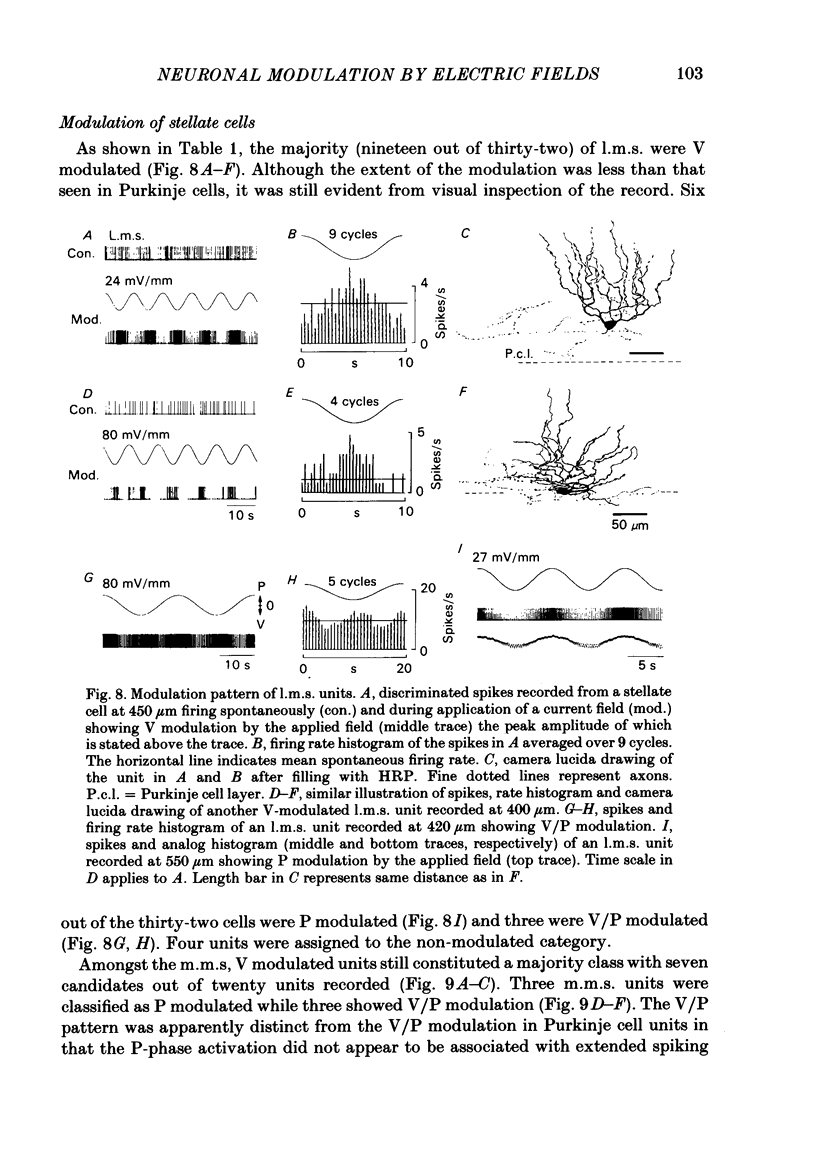
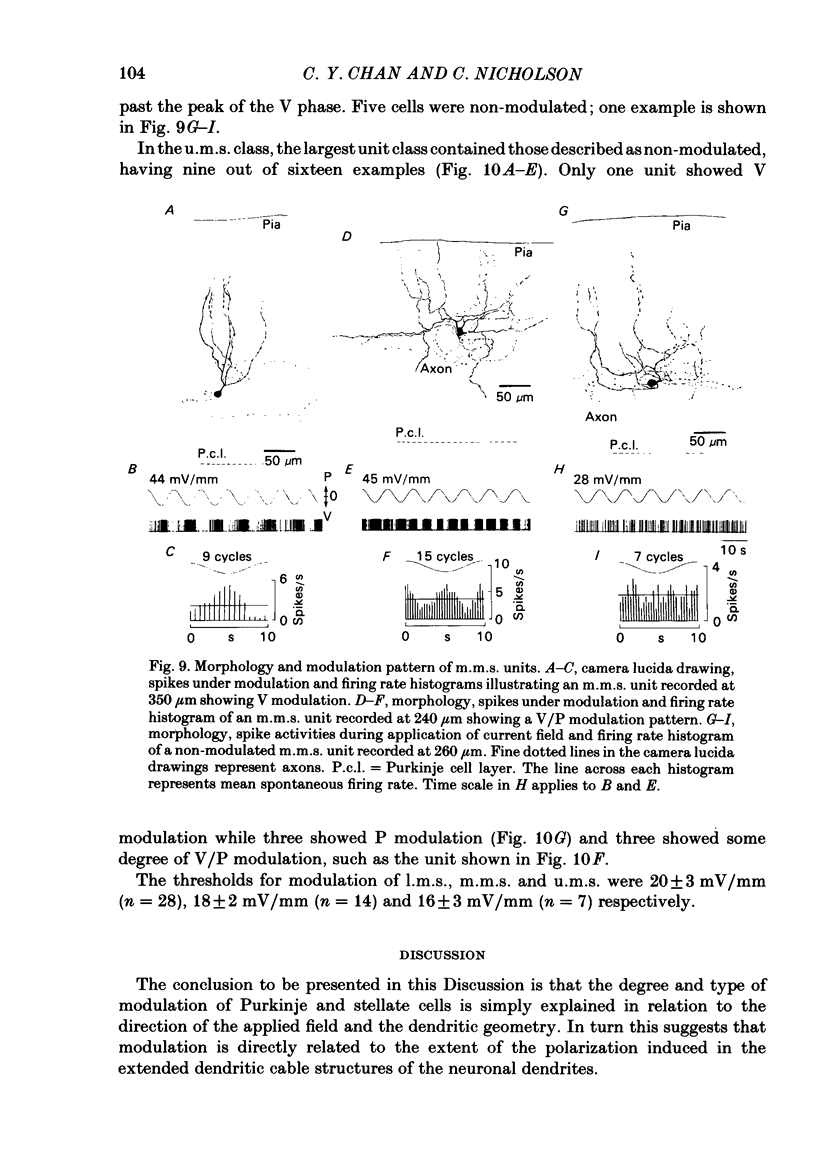
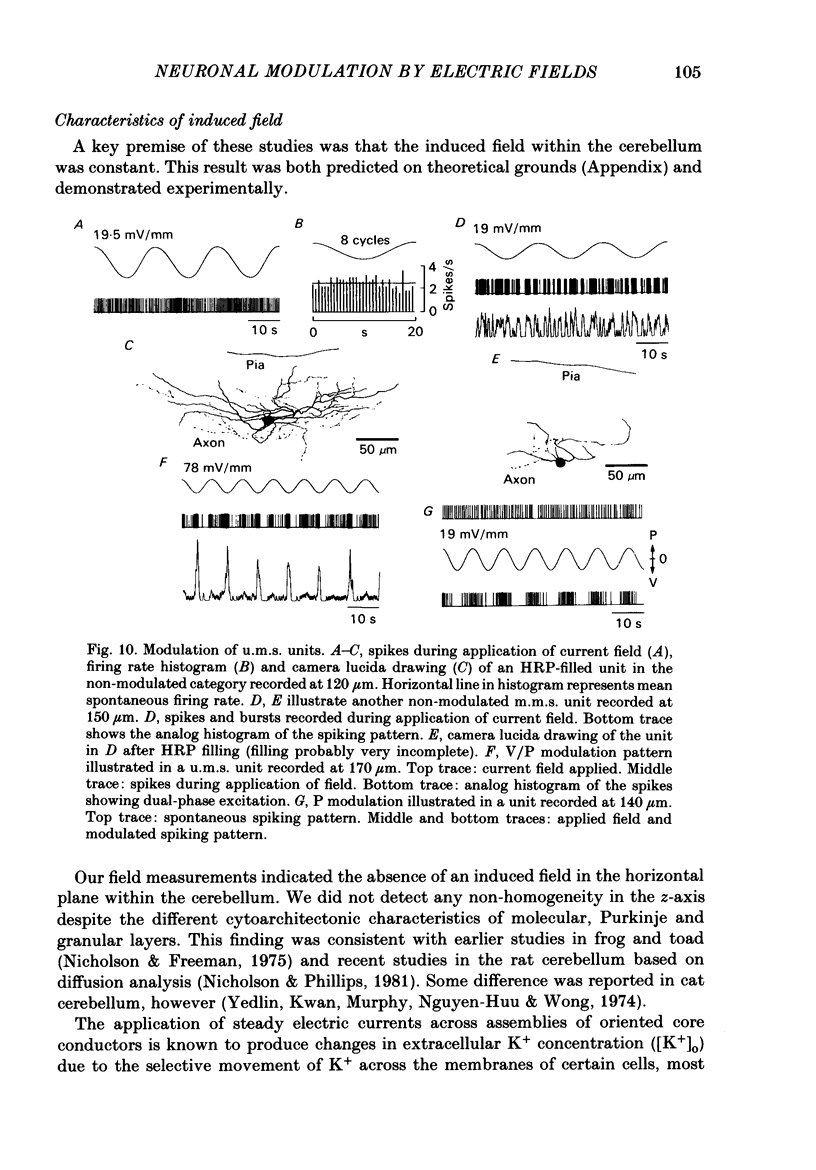
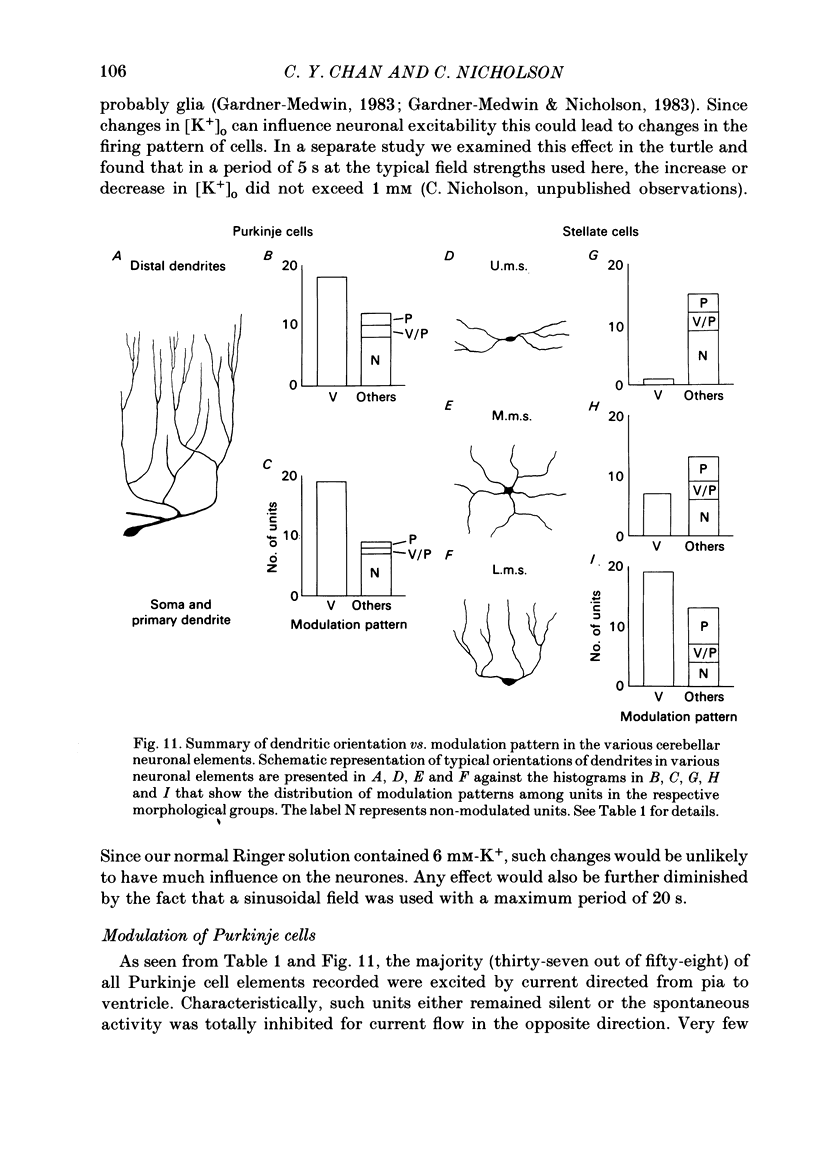
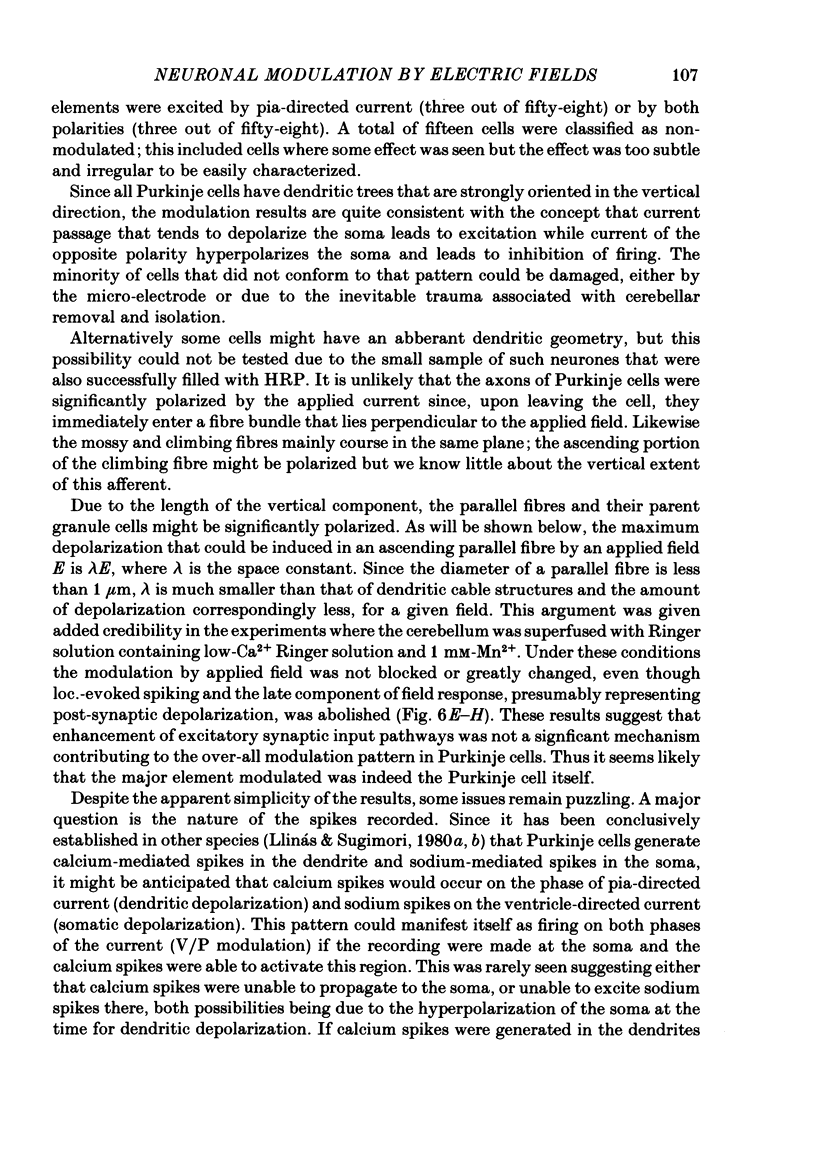
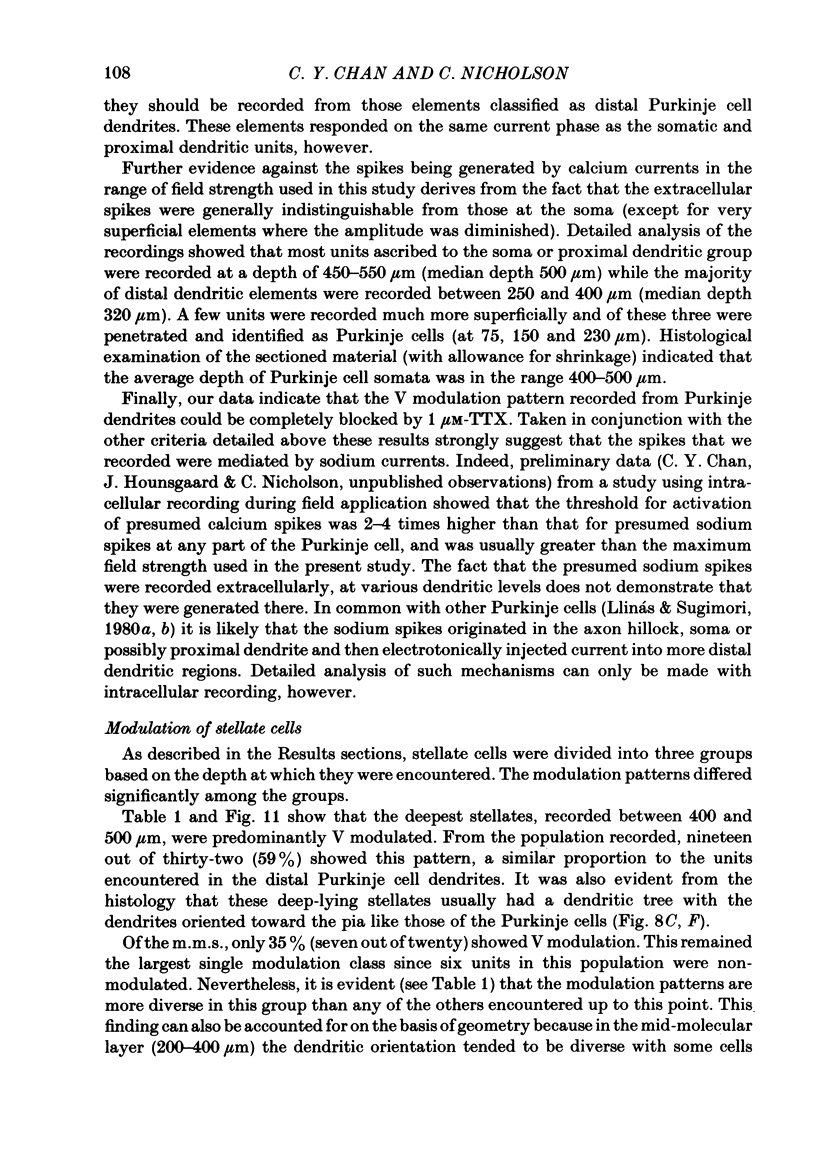
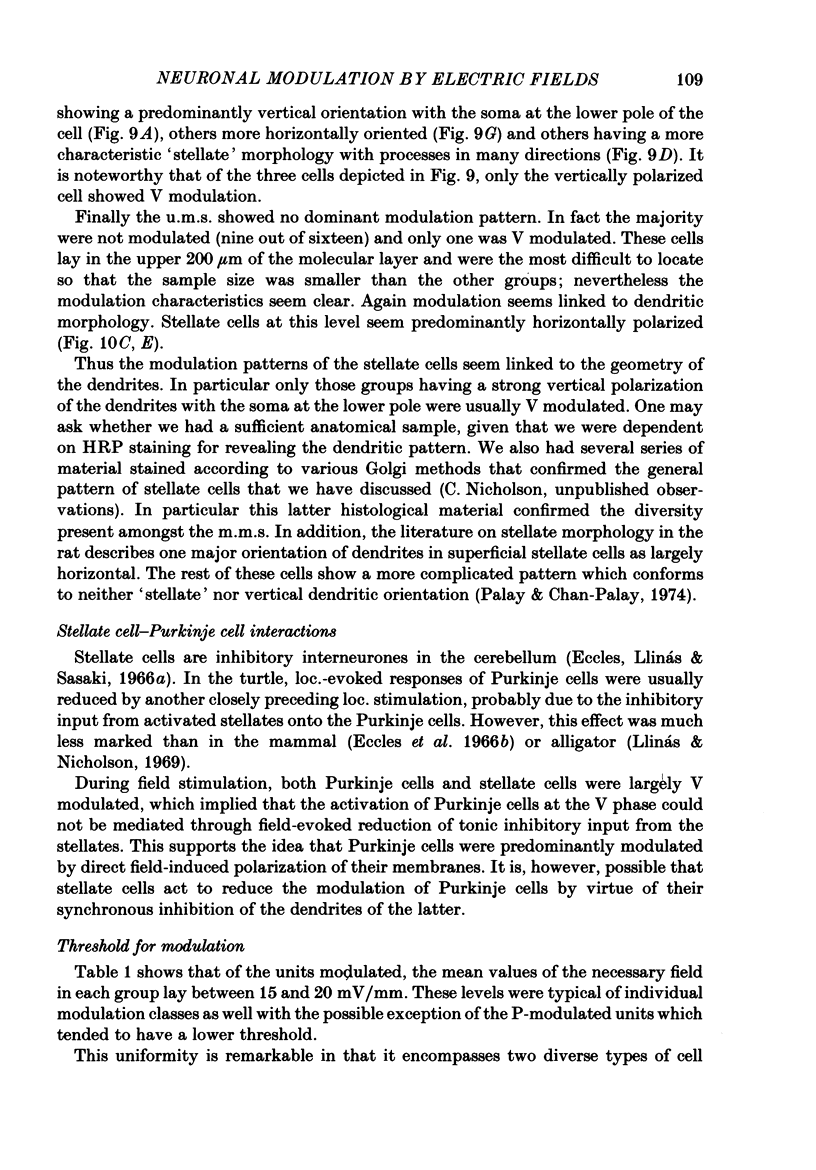
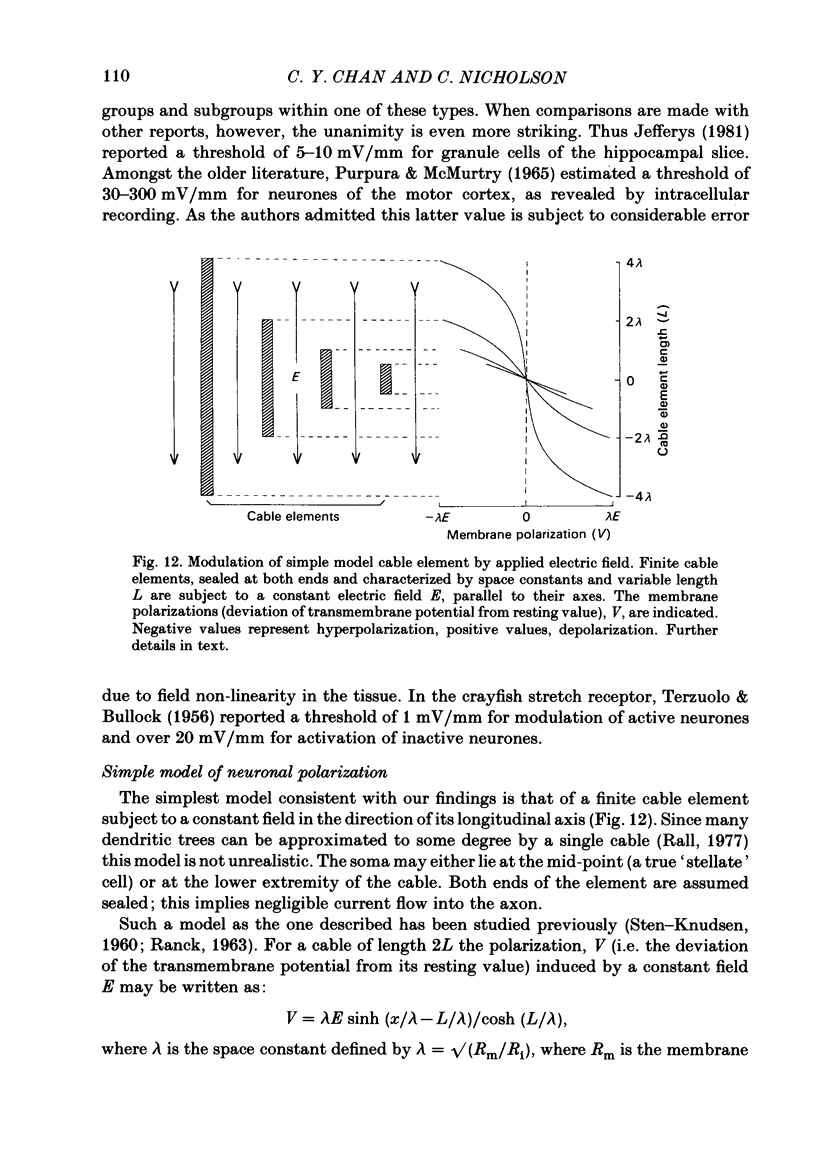
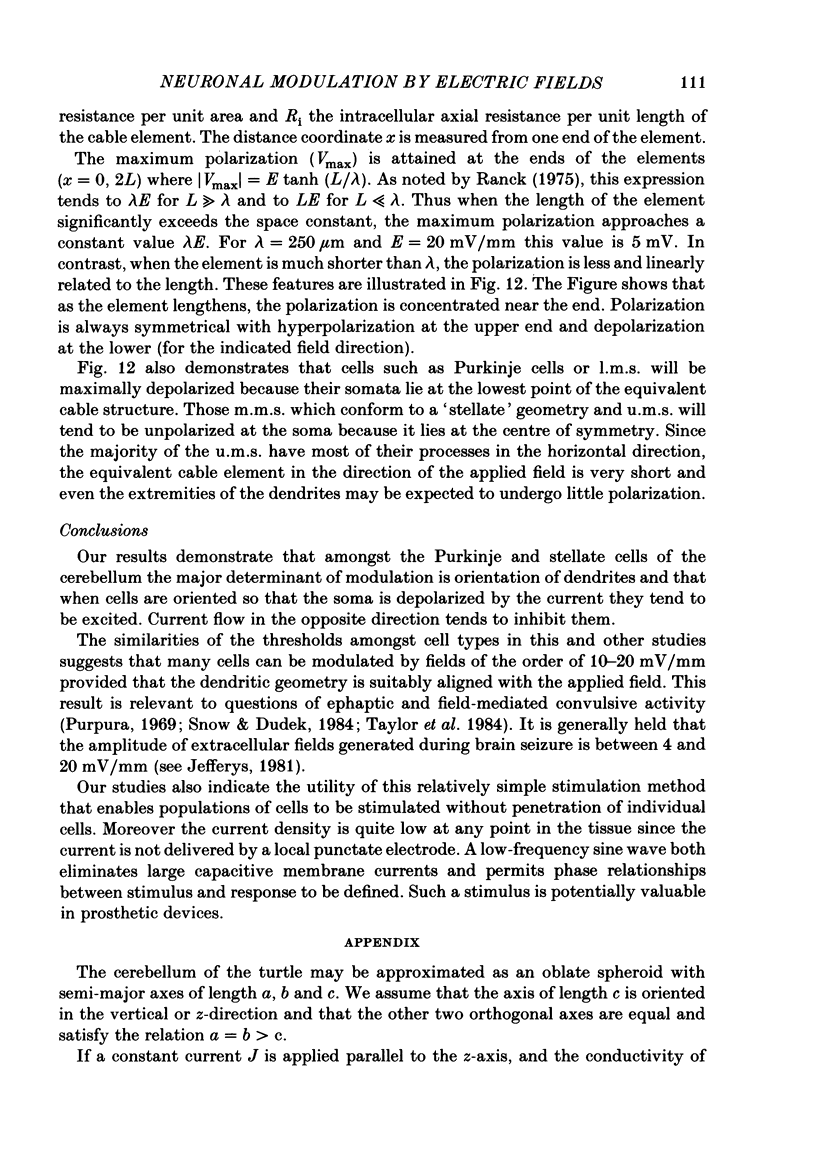
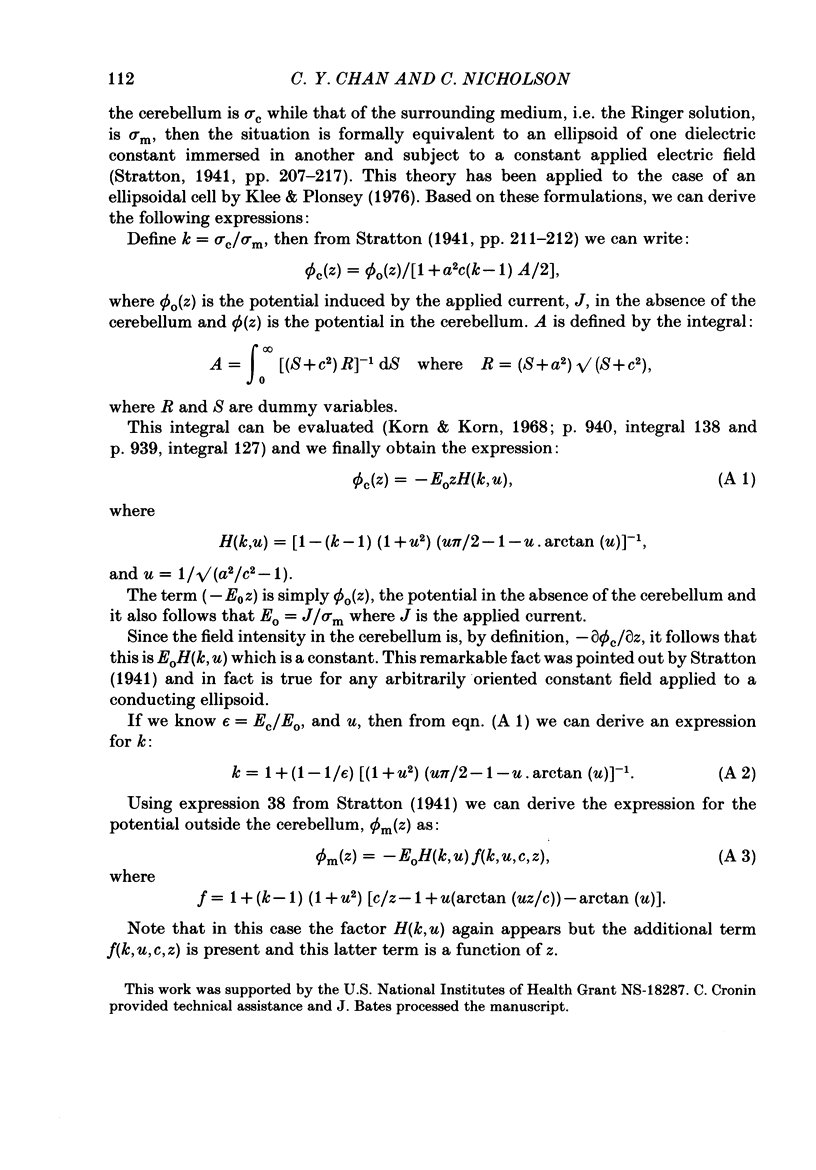
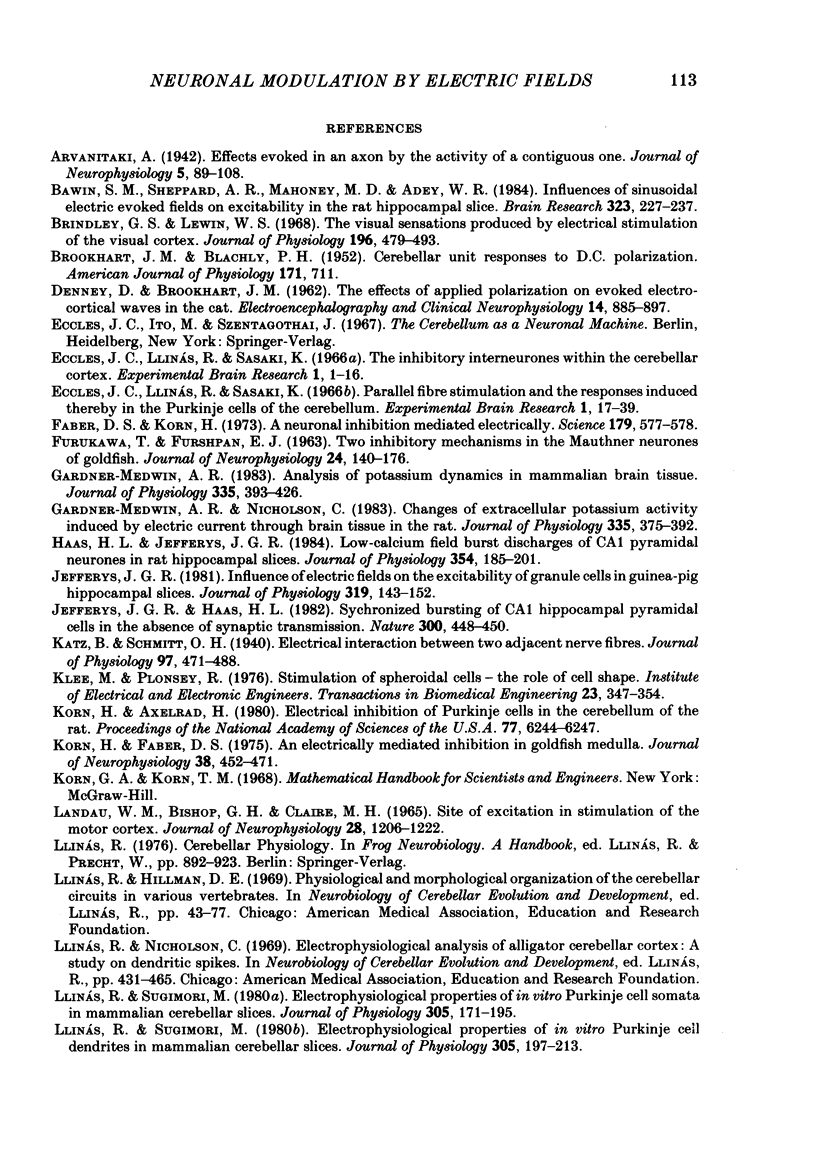
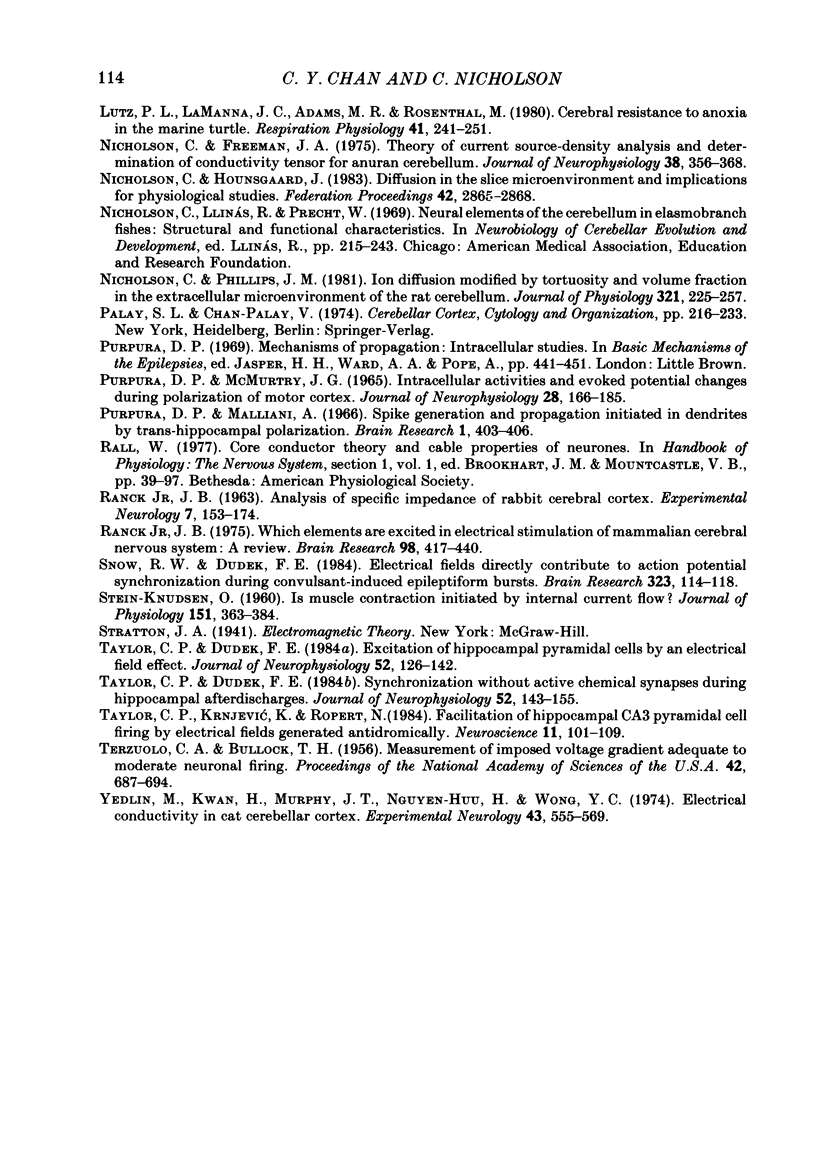
Selected References
These references are in PubMed. This may not be the complete list of references from this article.
- Bawin S. M., Sheppard A. R., Mahoney M. D., Adey W. R. Influences of sinusoidal electric fields on excitability in the rat hippocampal slice. Brain Res. 1984 Dec 10;323(2):227–237. doi: 10.1016/0006-8993(84)90293-2. [DOI] [PubMed] [Google Scholar]
- Brindley G. S., Lewin W. S. The sensations produced by electrical stimulation of the visual cortex. J Physiol. 1968 May;196(2):479–493. doi: 10.1113/jphysiol.1968.sp008519. [DOI] [PMC free article] [PubMed] [Google Scholar]
- DENNEY D., BROOKHART J. M. The effects of applied polarization on evoked electro-cortical waves in the cat. Electroencephalogr Clin Neurophysiol. 1962 Dec;14:885–897. doi: 10.1016/0013-4694(62)90139-6. [DOI] [PubMed] [Google Scholar]
- Eccles J. C., Llinás R., Sasaki K. The inhibitory interneurones within the cerebellar cortex. Exp Brain Res. 1966;1(1):1–16. doi: 10.1007/BF00235206. [DOI] [PubMed] [Google Scholar]
- FURUKAWA T., FURSHPAN E. J. Two inhibitory mechanisms in the Mauthner neurons of goldfish. J Neurophysiol. 1963 Jan;26:140–176. doi: 10.1152/jn.1963.26.1.140. [DOI] [PubMed] [Google Scholar]
- Faber D. S., Korn H. A neuronal inhibition mediated electrically. Science. 1973 Feb 9;179(4073):577–578. doi: 10.1126/science.179.4073.577. [DOI] [PubMed] [Google Scholar]
- Gardner-Medwin A. R. Analysis of potassium dynamics in mammalian brain tissue. J Physiol. 1983 Feb;335:393–426. doi: 10.1113/jphysiol.1983.sp014541. [DOI] [PMC free article] [PubMed] [Google Scholar]
- Gardner-Medwin A. R., Nicholson C. Changes of extracellular potassium activity induced by electric current through brain tissue in the rat. J Physiol. 1983 Feb;335:375–392. doi: 10.1113/jphysiol.1983.sp014540. [DOI] [PMC free article] [PubMed] [Google Scholar]
- Haas H. L., Jefferys J. G. Low-calcium field burst discharges of CA1 pyramidal neurones in rat hippocampal slices. J Physiol. 1984 Sep;354:185–201. doi: 10.1113/jphysiol.1984.sp015371. [DOI] [PMC free article] [PubMed] [Google Scholar]
- Jefferys J. G., Haas H. L. Synchronized bursting of CA1 hippocampal pyramidal cells in the absence of synaptic transmission. Nature. 1982 Dec 2;300(5891):448–450. doi: 10.1038/300448a0. [DOI] [PubMed] [Google Scholar]
- Jefferys J. G. Influence of electric fields on the excitability of granule cells in guinea-pig hippocampal slices. J Physiol. 1981;319:143–152. doi: 10.1113/jphysiol.1981.sp013897. [DOI] [PMC free article] [PubMed] [Google Scholar]
- Katz B., Schmitt O. H. Electric interaction between two adjacent nerve fibres. J Physiol. 1940 Feb 14;97(4):471–488. doi: 10.1113/jphysiol.1940.sp003823. [DOI] [PMC free article] [PubMed] [Google Scholar]
- Klee M., Plonsey R. Stimulation of spheroidal cells--the role of cell shape. IEEE Trans Biomed Eng. 1976 Jul;23(4):347–354. doi: 10.1109/tbme.1976.324597. [DOI] [PubMed] [Google Scholar]
- Korn H., Axelrad H. Electrical inhibition of Purkinje cells in the cerebellum of the rat. Proc Natl Acad Sci U S A. 1980 Oct;77(10):6244–6247. doi: 10.1073/pnas.77.10.6244. [DOI] [PMC free article] [PubMed] [Google Scholar]
- Korn H., Faber D. S. An electrically mediated inhibition in goldfish medulla. J Neurophysiol. 1975 Mar;38(2):452–471. doi: 10.1152/jn.1975.38.2.452. [DOI] [PubMed] [Google Scholar]
- Landau W. M., Bishop G. H., Clare M. H. Site of excitation in stimulation of the motor cortex. J Neurophysiol. 1965 Nov;28(6):1206–1222. doi: 10.1152/jn.1965.28.6.1206. [DOI] [PubMed] [Google Scholar]
- Llinás R., Sugimori M. Electrophysiological properties of in vitro Purkinje cell dendrites in mammalian cerebellar slices. J Physiol. 1980 Aug;305:197–213. doi: 10.1113/jphysiol.1980.sp013358. [DOI] [PMC free article] [PubMed] [Google Scholar]
- Llinás R., Sugimori M. Electrophysiological properties of in vitro Purkinje cell somata in mammalian cerebellar slices. J Physiol. 1980 Aug;305:171–195. doi: 10.1113/jphysiol.1980.sp013357. [DOI] [PMC free article] [PubMed] [Google Scholar]
- Lutz P. L., LaManna J. C., Adams M. R., Rosenthal M. Cerebral resistance to anoxia in the marine turtle. Respir Physiol. 1980 Sep;41(3):241–251. doi: 10.1016/0034-5687(80)90074-2. [DOI] [PubMed] [Google Scholar]
- Nicholson C., Freeman J. A. Theory of current source-density analysis and determination of conductivity tensor for anuran cerebellum. J Neurophysiol. 1975 Mar;38(2):356–368. doi: 10.1152/jn.1975.38.2.356. [DOI] [PubMed] [Google Scholar]
- Nicholson C., Hounsgaard J. Diffusion in the slice microenvironment and implications for physiological studies. Fed Proc. 1983 Sep;42(12):2865–2868. [PubMed] [Google Scholar]
- Nicholson C., Phillips J. M. Ion diffusion modified by tortuosity and volume fraction in the extracellular microenvironment of the rat cerebellum. J Physiol. 1981 Dec;321:225–257. doi: 10.1113/jphysiol.1981.sp013981. [DOI] [PMC free article] [PubMed] [Google Scholar]
- PURPURA D. P., MCMURTRY J. G. INTRACELLULAR ACTIVITIES AND EVOKED POTENTIAL CHANGES DURING POLARIZATION OF MOTOR CORTEX. J Neurophysiol. 1965 Jan;28:166–185. doi: 10.1152/jn.1965.28.1.166. [DOI] [PubMed] [Google Scholar]
- Purpura D. P., Malliani A. Spike generation and propagation initiated in dendrites by transhippocampal polarization. Brain Res. 1966 May-Jun;1(4):403–406. doi: 10.1016/0006-8993(66)90132-6. [DOI] [PubMed] [Google Scholar]
- RANCK J. B., Jr Analysis of specific impedance of rabbit cerebral cortex. Exp Neurol. 1963 Feb;7:153–174. doi: 10.1016/s0014-4886(63)80006-0. [DOI] [PubMed] [Google Scholar]
- Ranck J. B., Jr Which elements are excited in electrical stimulation of mammalian central nervous system: a review. Brain Res. 1975 Nov 21;98(3):417–440. doi: 10.1016/0006-8993(75)90364-9. [DOI] [PubMed] [Google Scholar]
- STEN-KNUDSEN O. Is muscle contraction initiated by internal current flow? J Physiol. 1960 May;151:363–384. doi: 10.1113/jphysiol.1960.sp006444. [DOI] [PMC free article] [PubMed] [Google Scholar]
- Snow R. W., Dudek F. E. Electrical fields directly contribute to action potential synchronization during convulsant-induced epileptiform bursts. Brain Res. 1984 Dec 3;323(1):114–118. doi: 10.1016/0006-8993(84)90271-3. [DOI] [PubMed] [Google Scholar]
- Taylor C. P., Dudek F. E. Excitation of hippocampal pyramidal cells by an electrical field effect. J Neurophysiol. 1984 Jul;52(1):126–142. doi: 10.1152/jn.1984.52.1.126. [DOI] [PubMed] [Google Scholar]
- Taylor C. P., Dudek F. E. Synchronization without active chemical synapses during hippocampal afterdischarges. J Neurophysiol. 1984 Jul;52(1):143–155. doi: 10.1152/jn.1984.52.1.143. [DOI] [PubMed] [Google Scholar]
- Taylor C. P., Krnjević K., Ropert N. Facilitation of hippocampal CA3 pyramidal cell firing by electrical fields generated antidromically. Neuroscience. 1984 Jan;11(1):101–109. doi: 10.1016/0306-4522(84)90216-1. [DOI] [PubMed] [Google Scholar]
- Terzuolo C. A., Bullock T. H. MEASUREMENT OF IMPOSED VOLTAGE GRADIENT ADEQUATE TO MODULATE NEURONAL FIRING. Proc Natl Acad Sci U S A. 1956 Sep;42(9):687–694. doi: 10.1073/pnas.42.9.687. [DOI] [PMC free article] [PubMed] [Google Scholar]
- Yedlin M., Kwan H., Murphy J. T., Nguyen-Huu H., Wong Y. C. Electrical conductivity in cat cerebellar cortex. Exp Neurol. 1974 Jun;43(3):555–569. doi: 10.1016/0014-4886(74)90195-2. [DOI] [PubMed] [Google Scholar]