Abstract
The bioelectrical properties of Purkinje cells were analysed in sagittal slices of adult rat cerebellum by the use of intracellular recordings performed at a somatic level in current or in voltage clamp. The passive electrical constants of Purkinje cells were determined by measuring the time course and the amplitude of the voltage responses induced by hyperpolarizing current pulses. The mean value of input resistance was 21 +/- 1 M omega. Mean values of the membrane time constant and of the total electrotonic length of Purkinje cells were 19.5 +/- 1.7 ms and 0.59 +/- 0.01 ms respectively. A time dependent inward rectification was present in all cells. In current-clamp experiments it appeared as a sag in hyperpolarizing voltage responses which were followed by well developed anodal breaks. In voltage-clamped cells, the inward relaxation induced by hyperpolarizing commands fitted to a single exponential. It was already present near resting potential and could reach an amplitude of up to 4 nA for jumps near to -120 mV. This relaxation was provisionally termed Ih. Tail current relaxations also fitted to a single exponential when they were recorded in the presence of tetrodotoxin (TTX) and of Co. The inward relaxation induced by hyperpolarizing commands was readily blocked by Cs, whereas it was unaffected when Ba replaced Ca in the bath, except near rest where it was strongly reduced. The Ca channel blockers Cd, Co and D600 also markedly depressed or even suppressed the inward rectification near resting potential, and up to about -85 mV, whereas this blocking effect was much less apparent or even absent at more negative potentials. Ih was clearly enhanced when the external K concentration was raised up to 20 mM. In the presence of TTX and Co in the bath, inward relaxations induced by hyperpolarizing jumps were unaffected in Na-free solution, whereas the amplitude of tail currents was reduced. Furthermore, the reversal potential of Ih which ranged between -45 and -56 mV in the Co plus TTX containing solution, shifted toward more negative values in the Na-free medium. In contrast, Ih remained unchanged in low Cl solution. From these experiments, it is likely that K and Na are the main charge carriers of Ih. Furthermore, this current seems to be contaminated near resting potential by a Ca-dependent K current. Anodal breaks following hyperpolarizing commands were slightly attenuated when Cd or TTX were added to the bath.(ABSTRACT TRUNCATED AT 400 WORDS)
Full text
PDF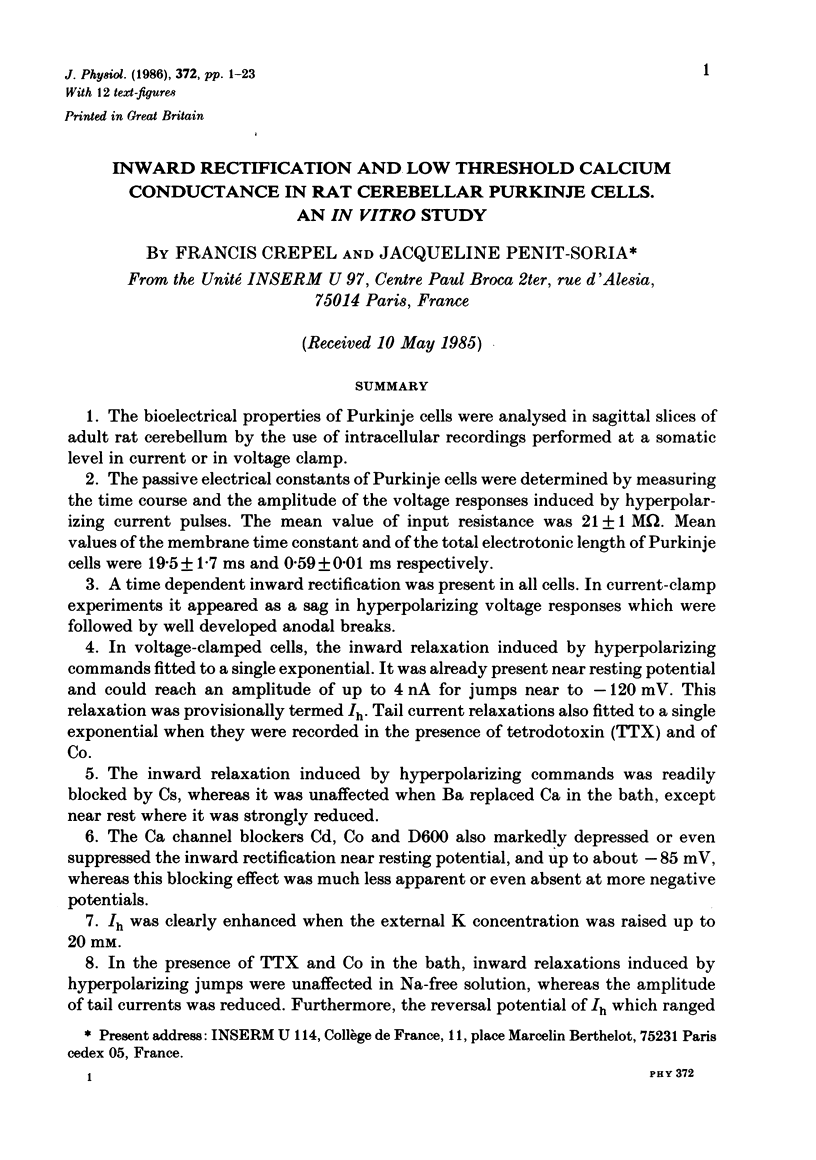
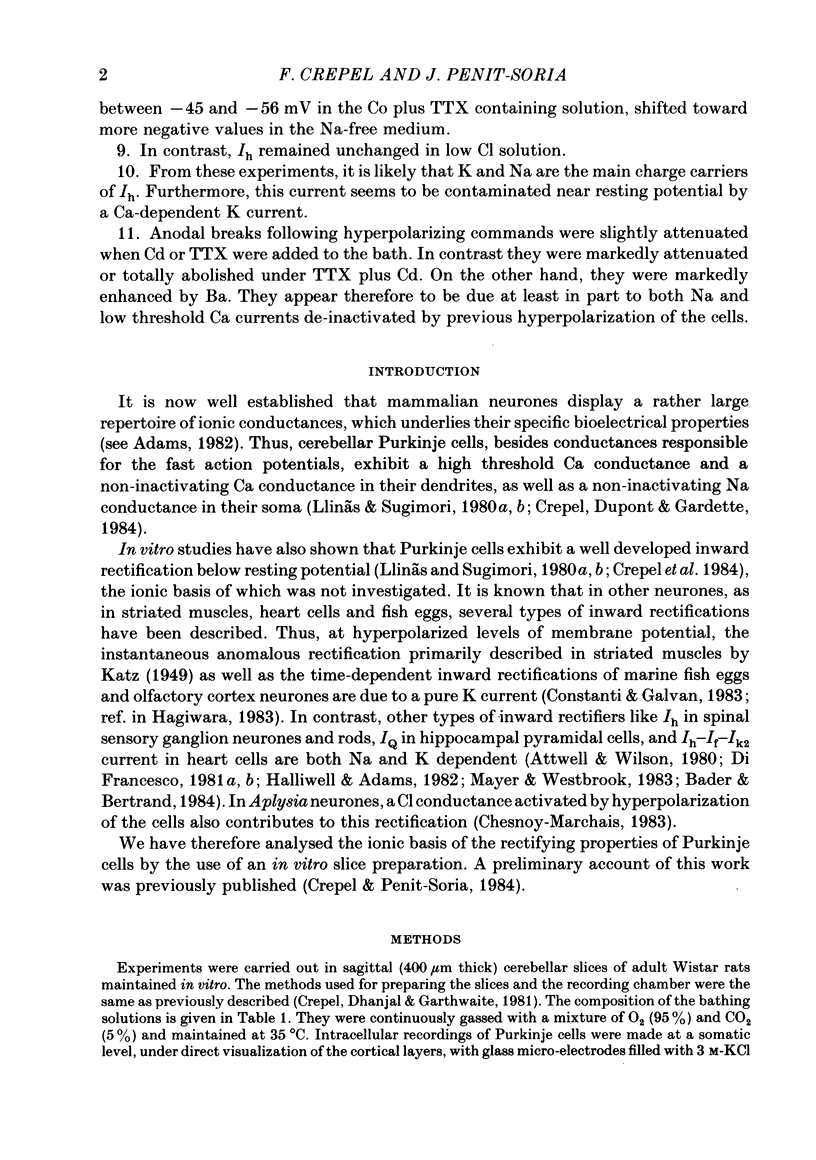
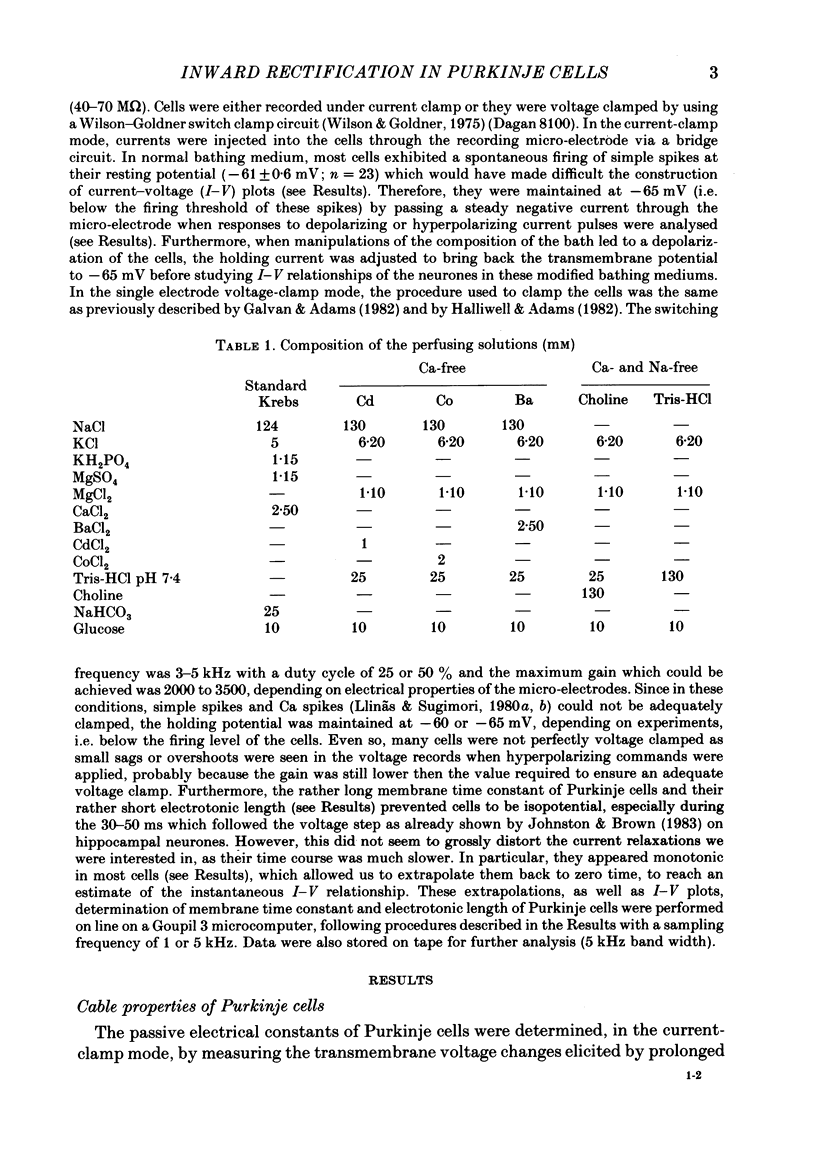
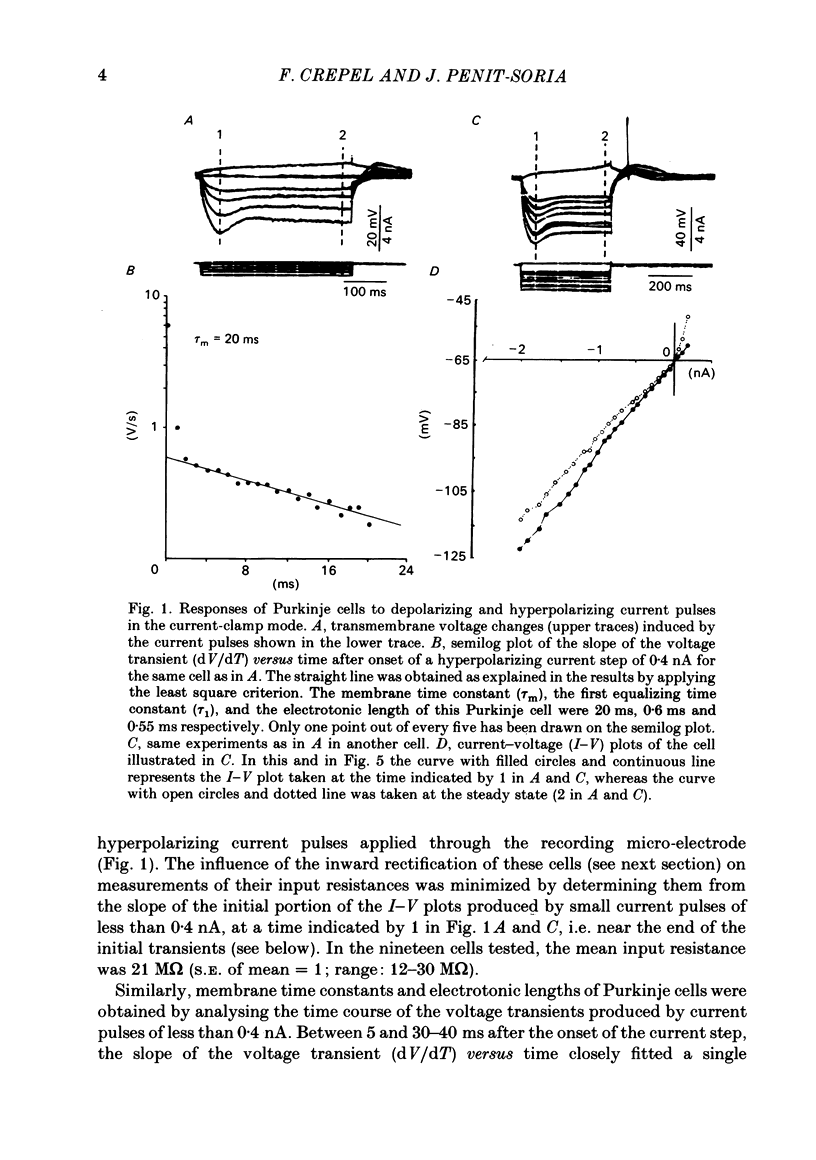
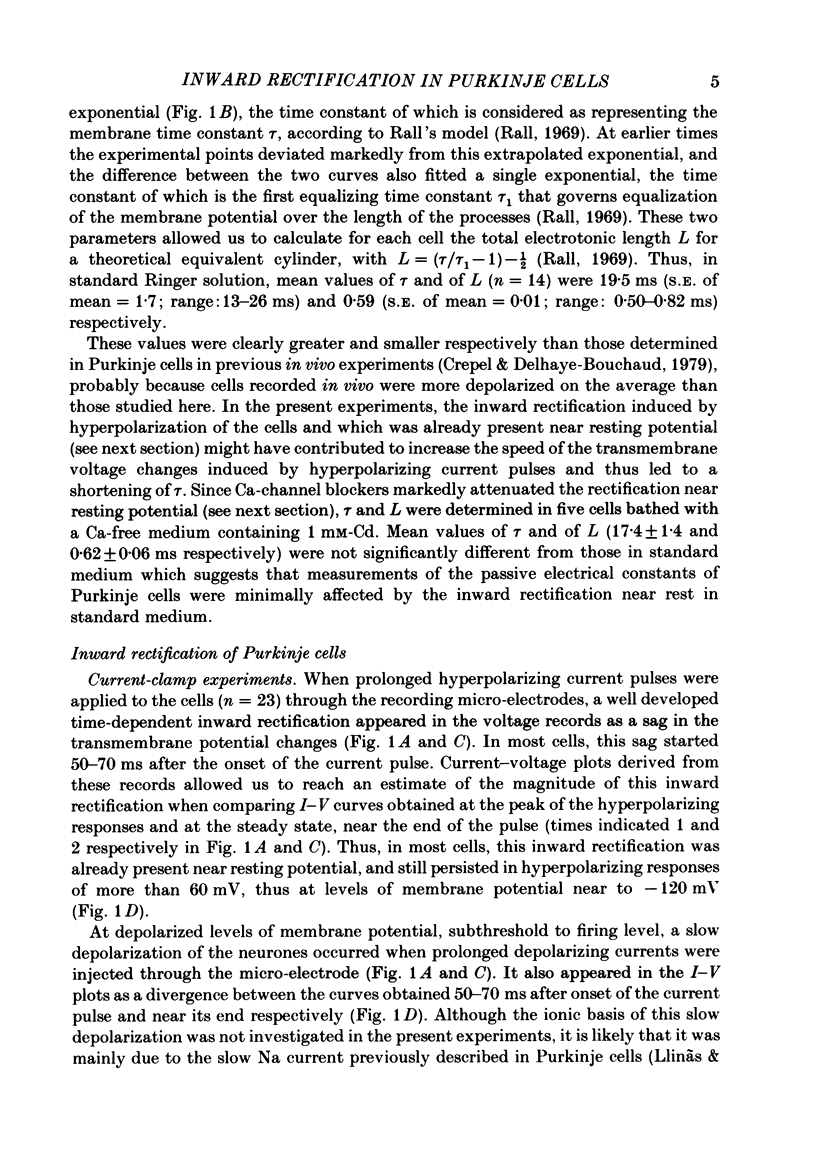
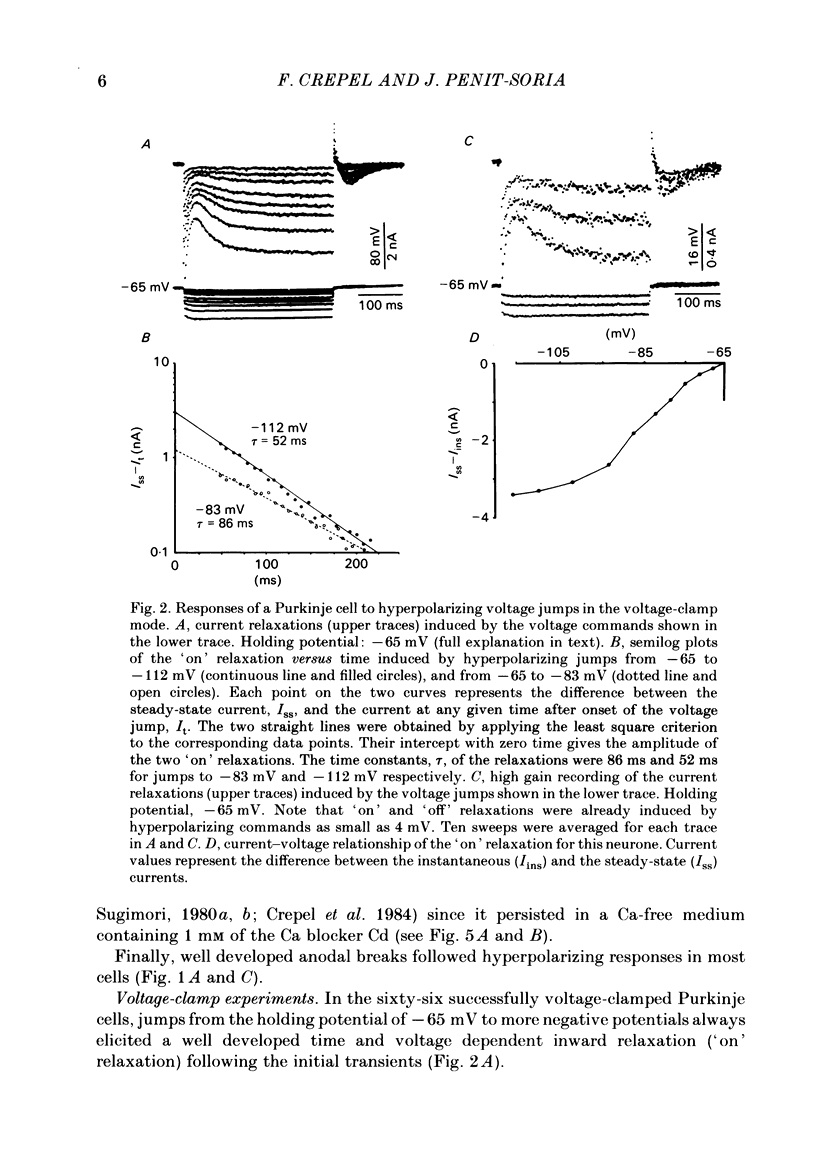
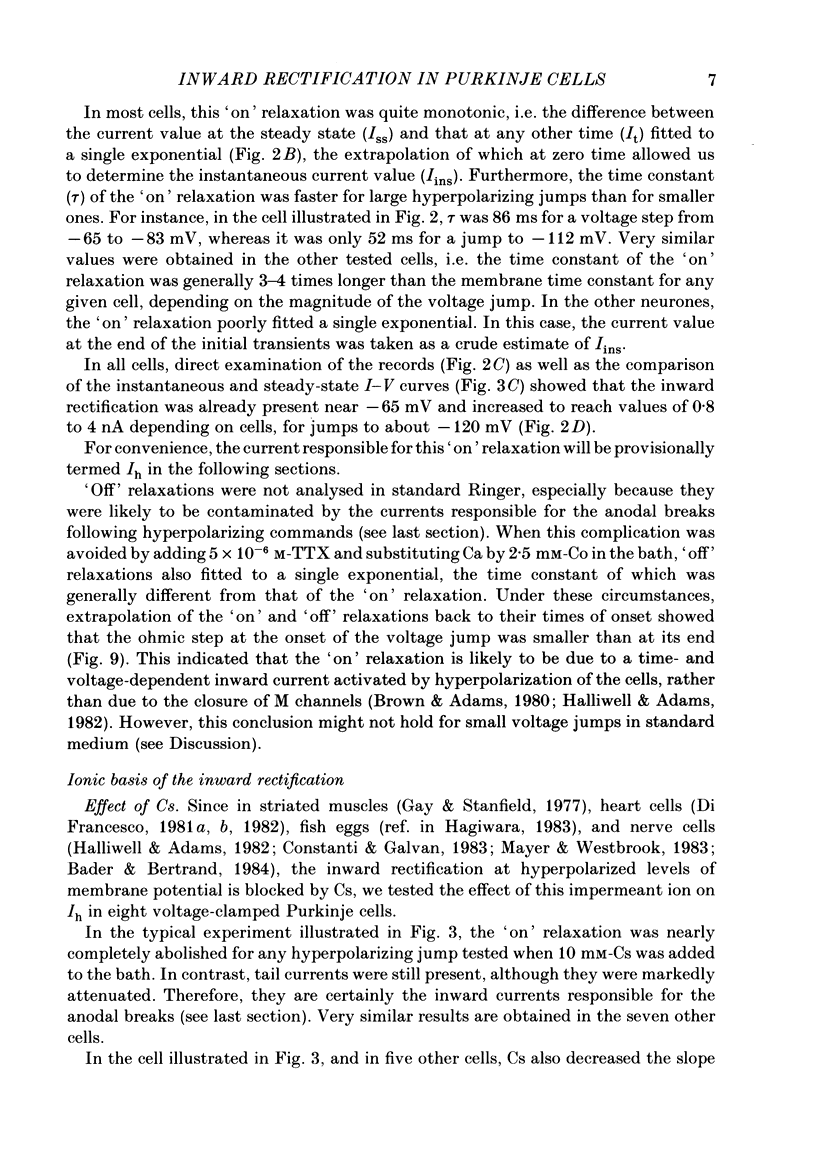
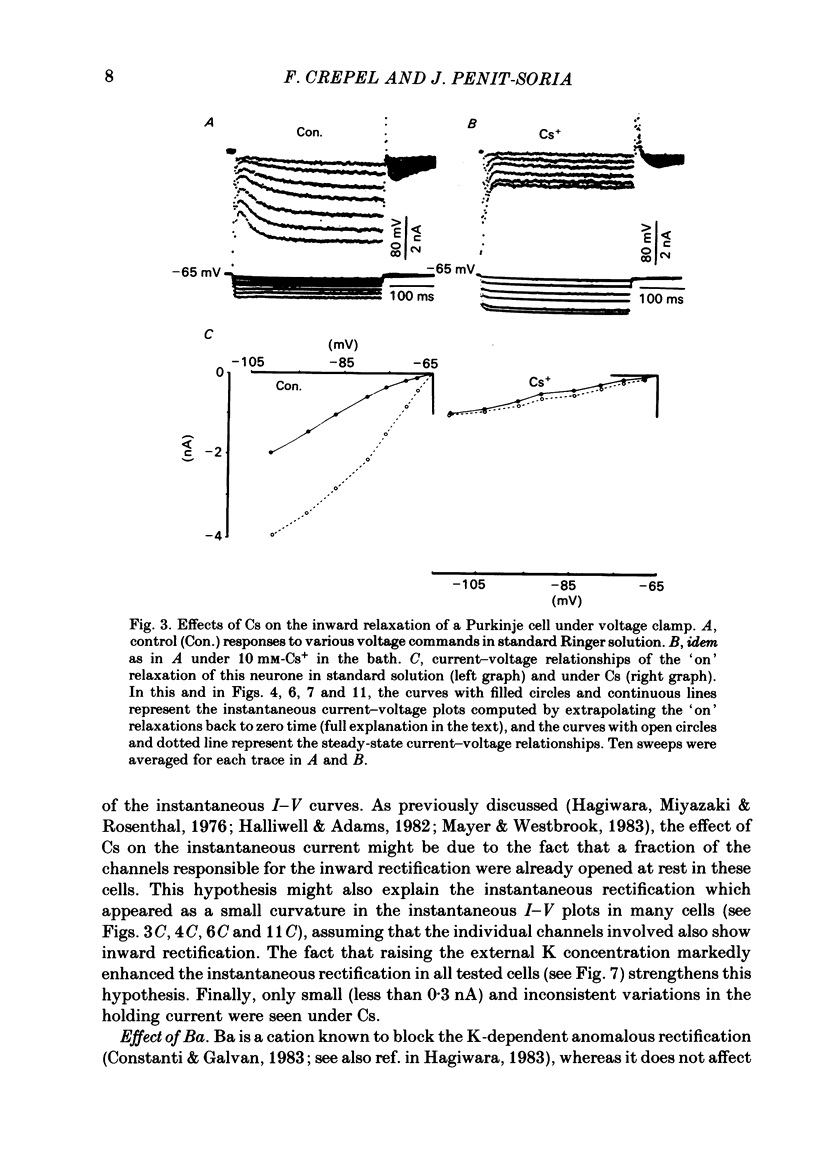
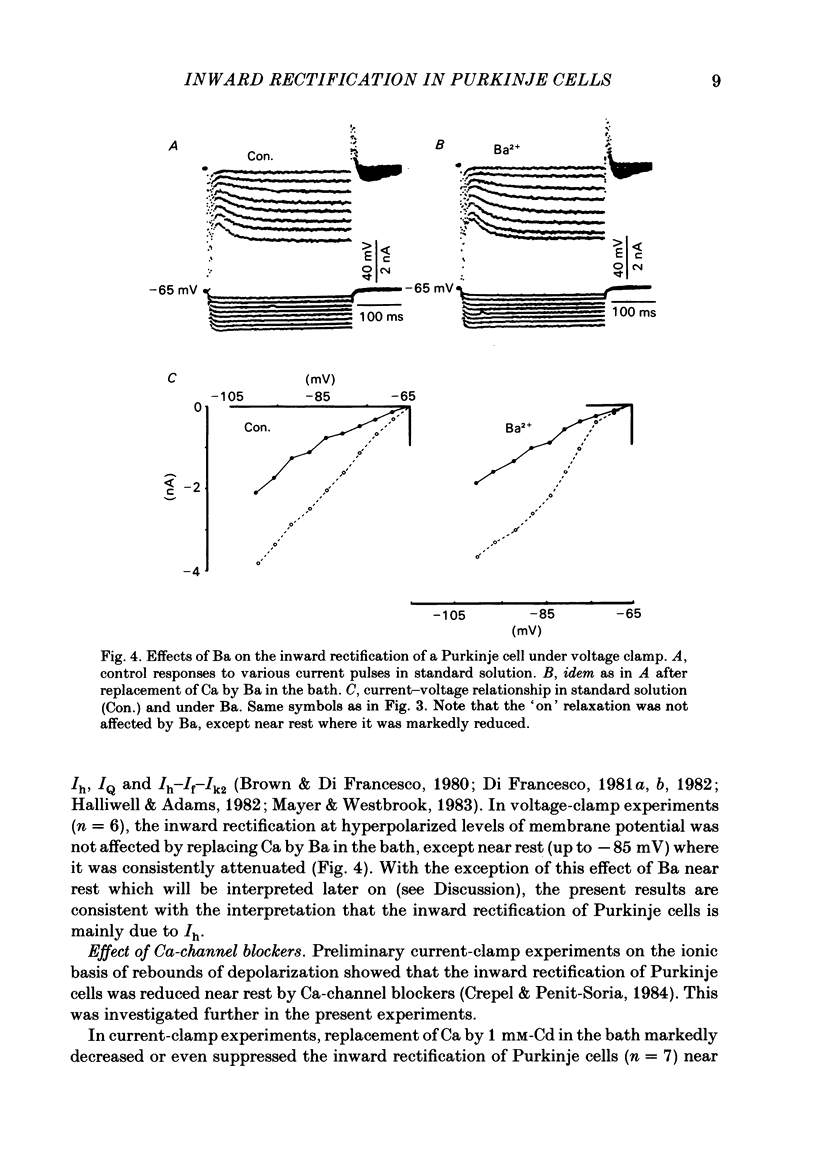
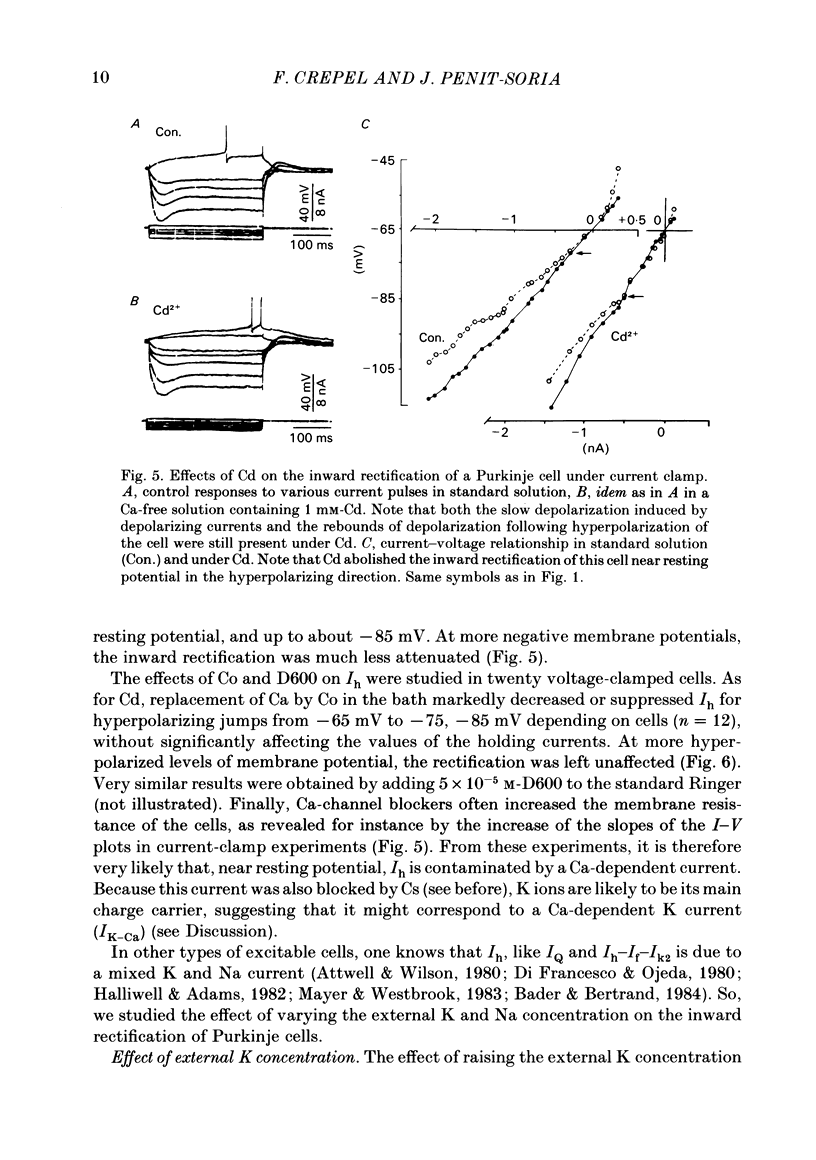
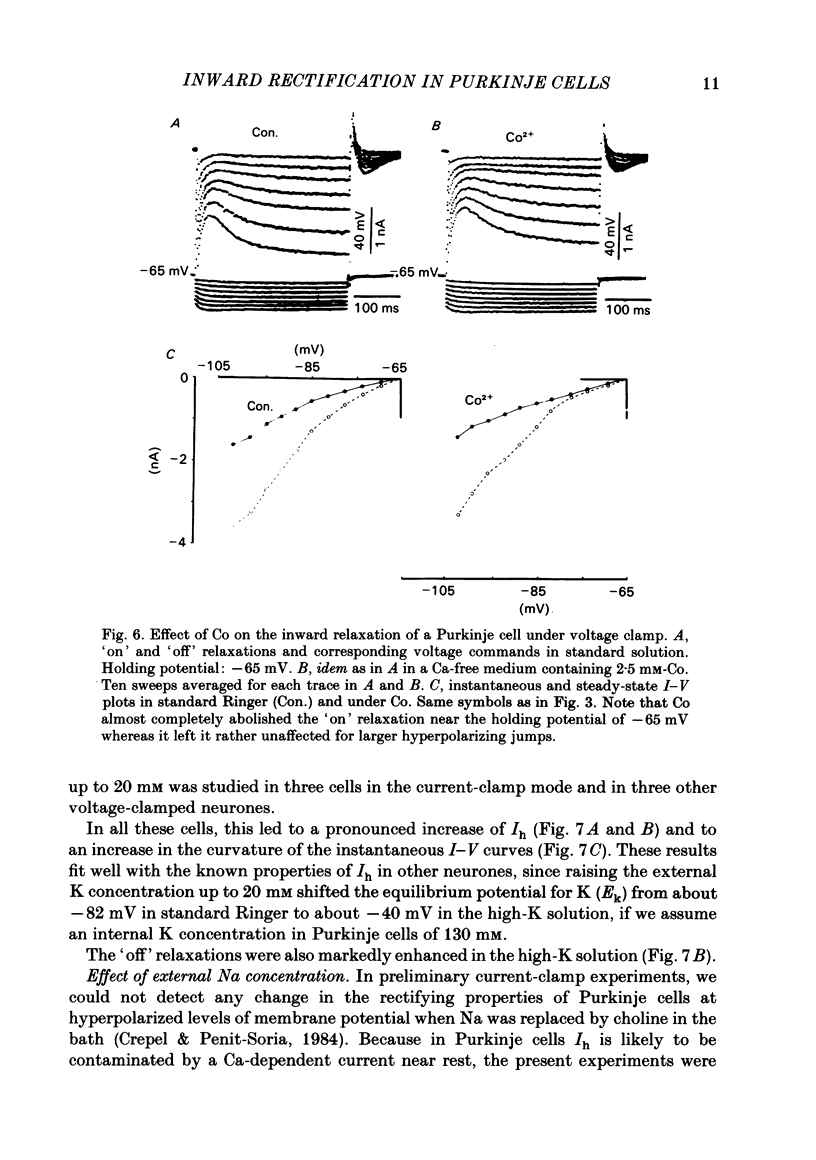
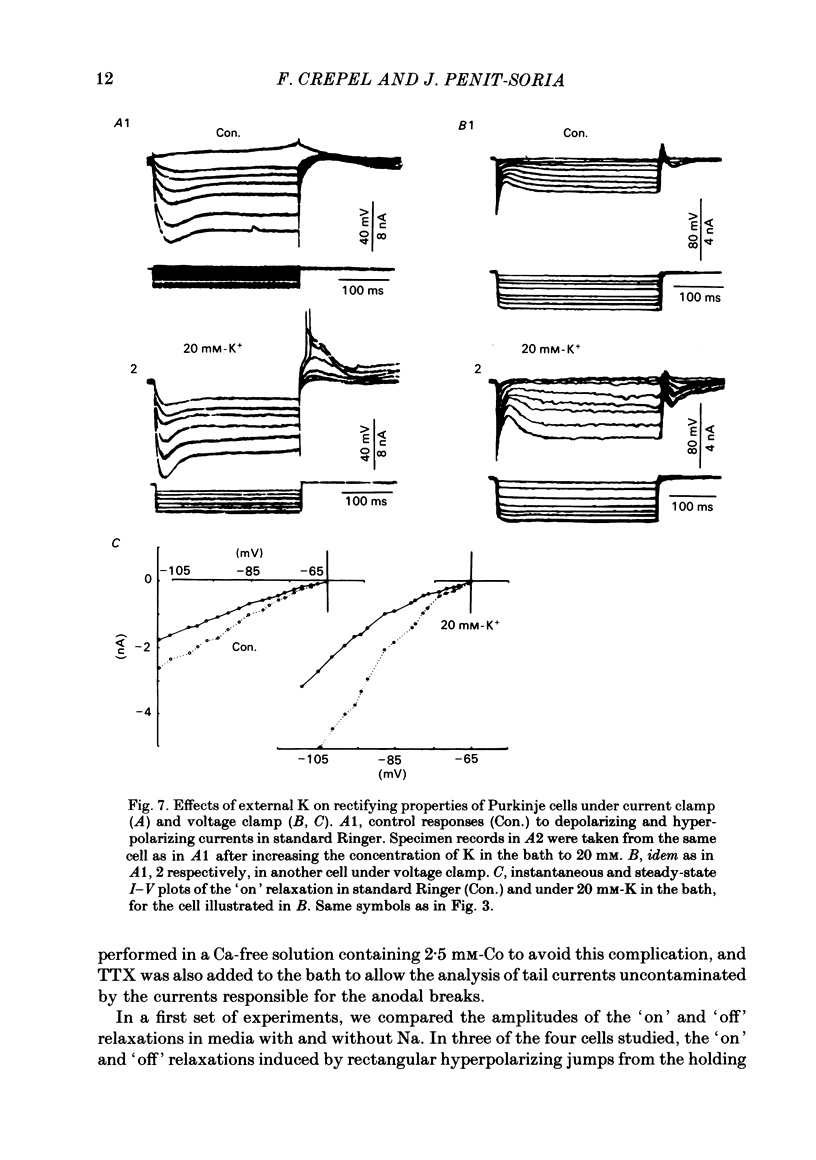
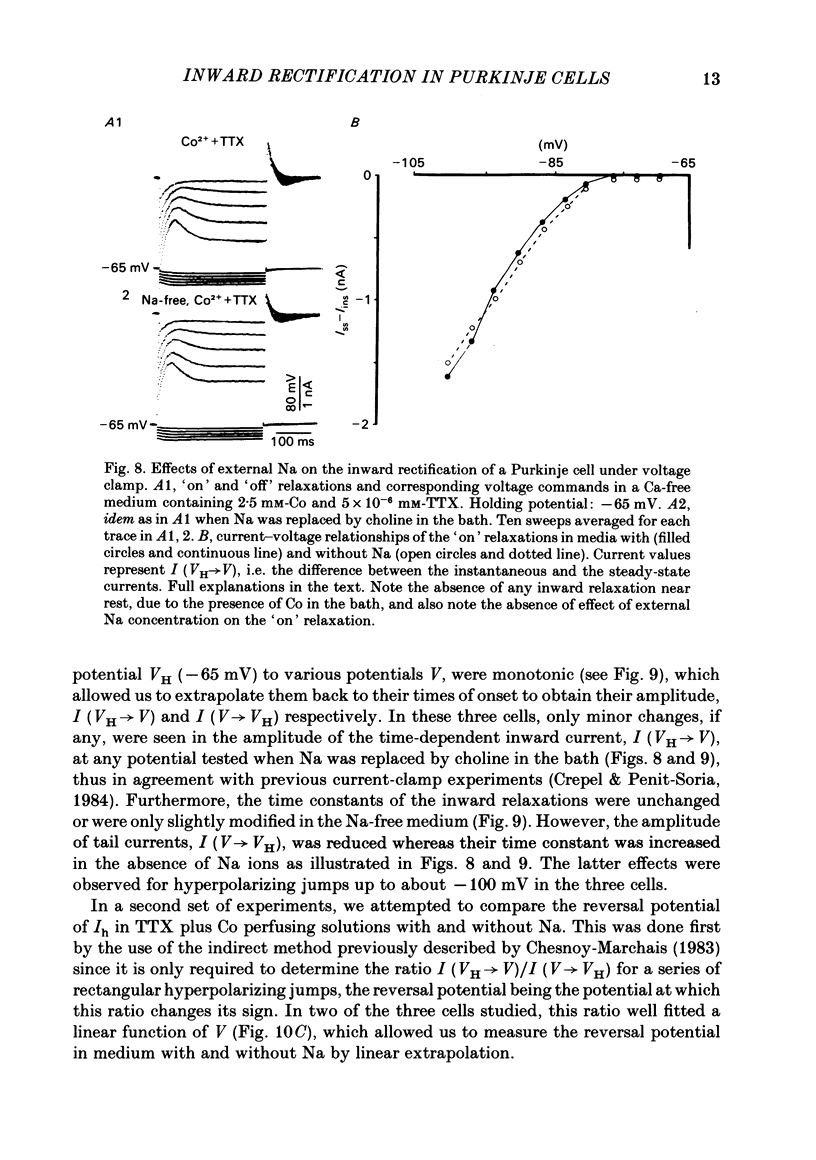
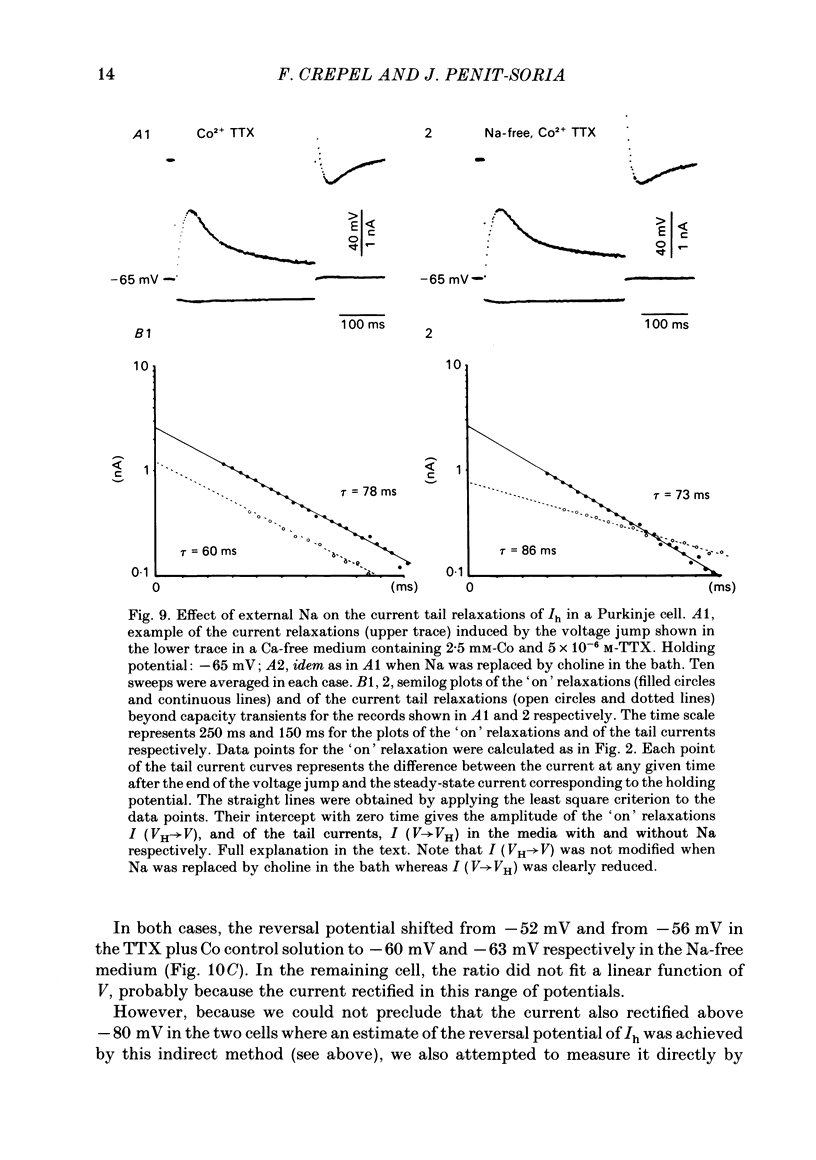
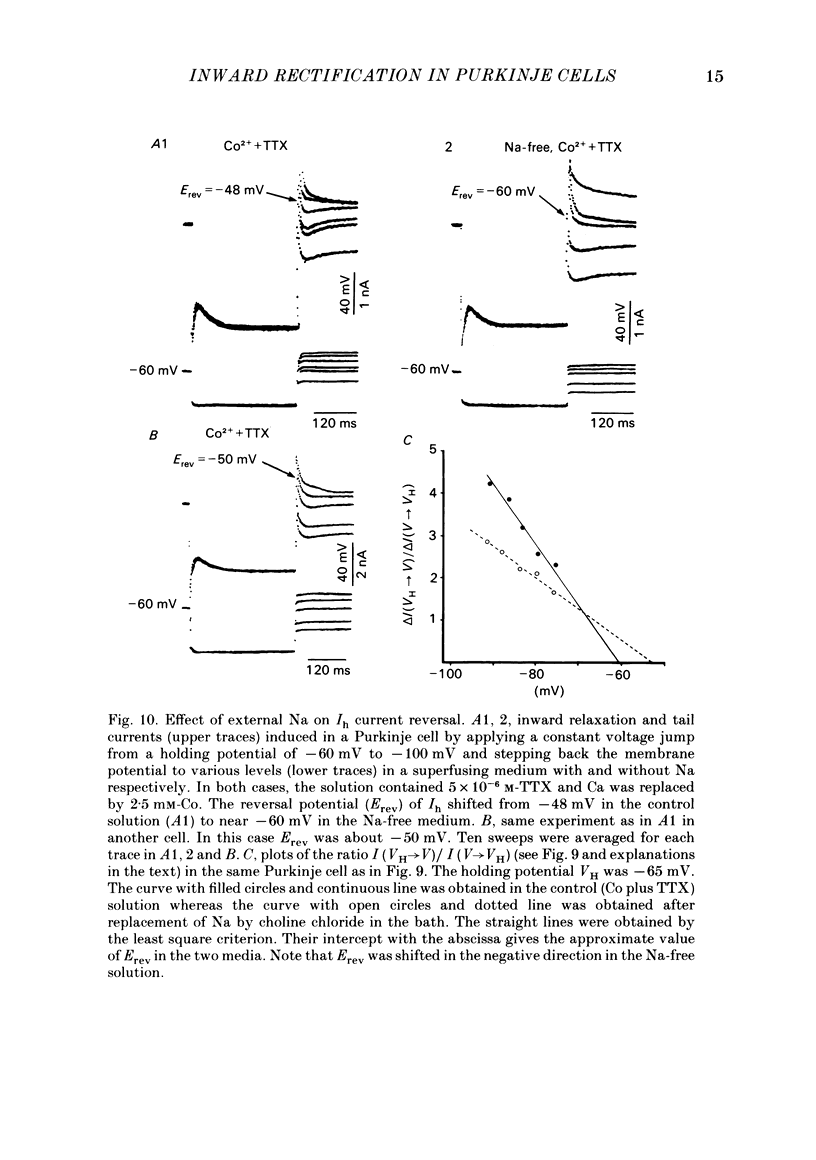
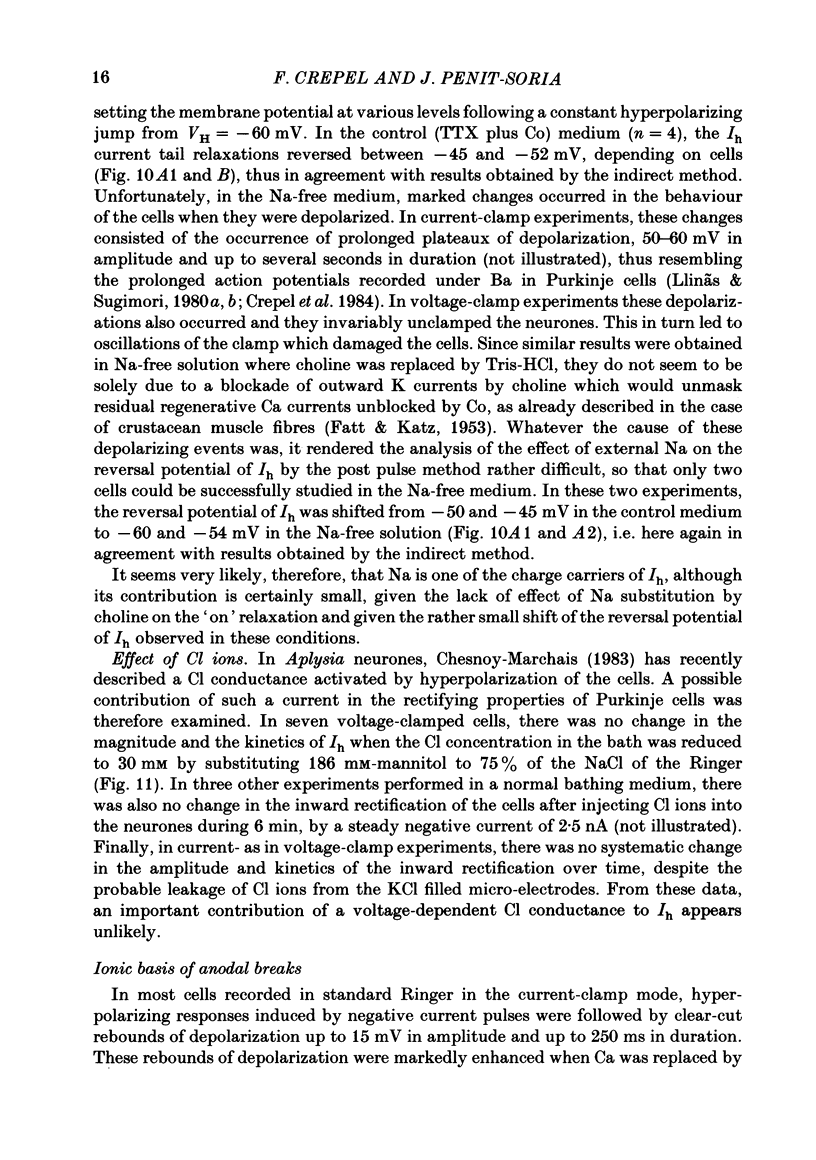
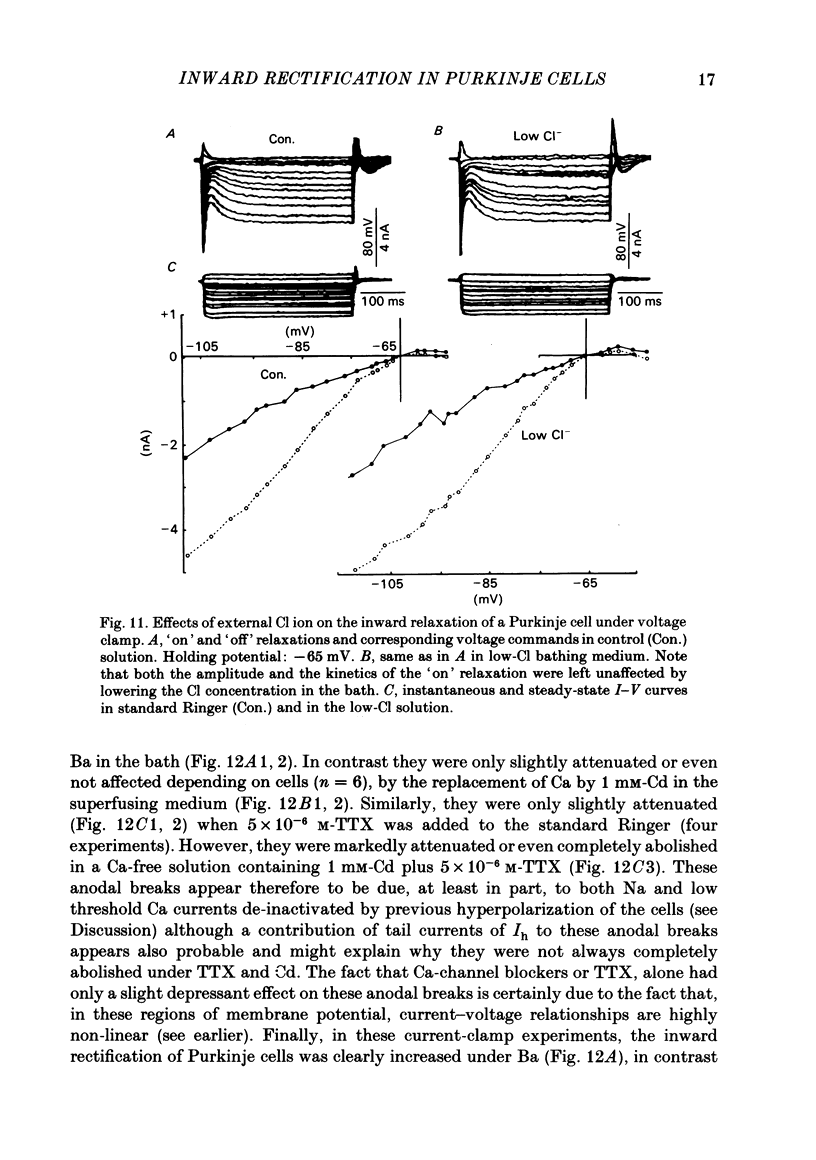
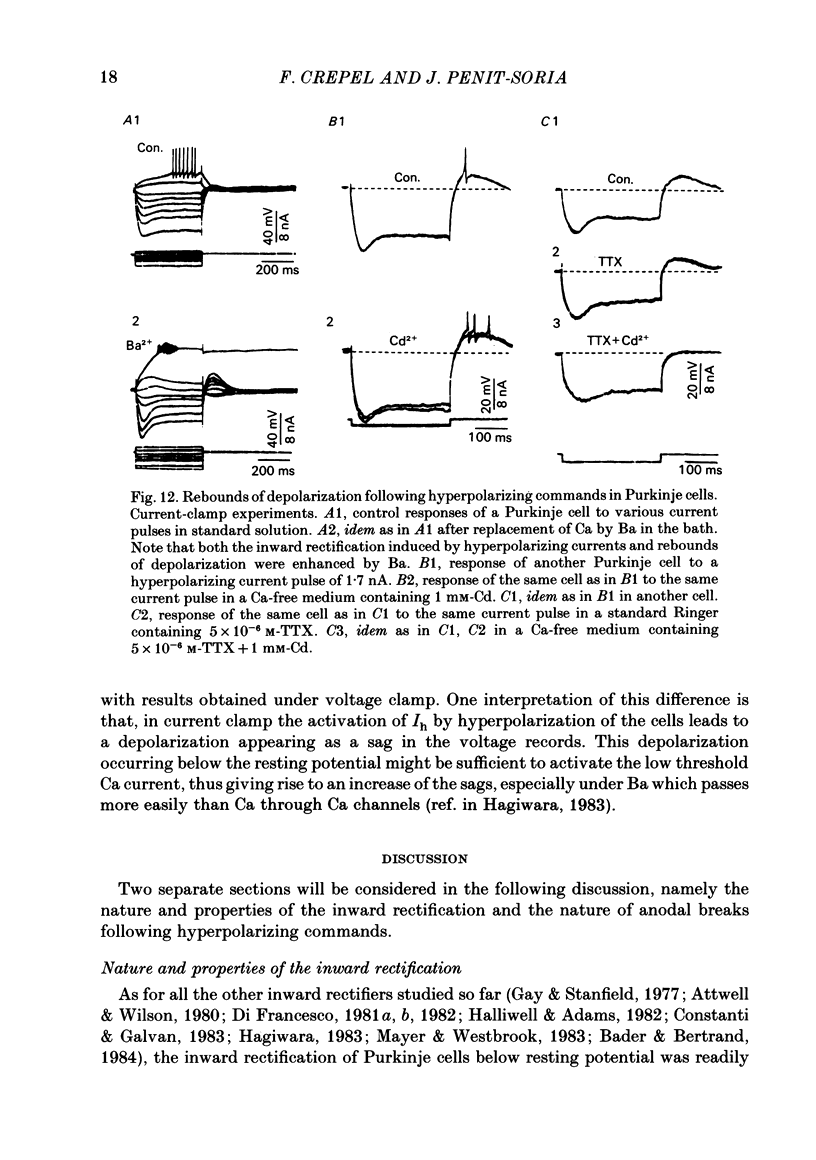
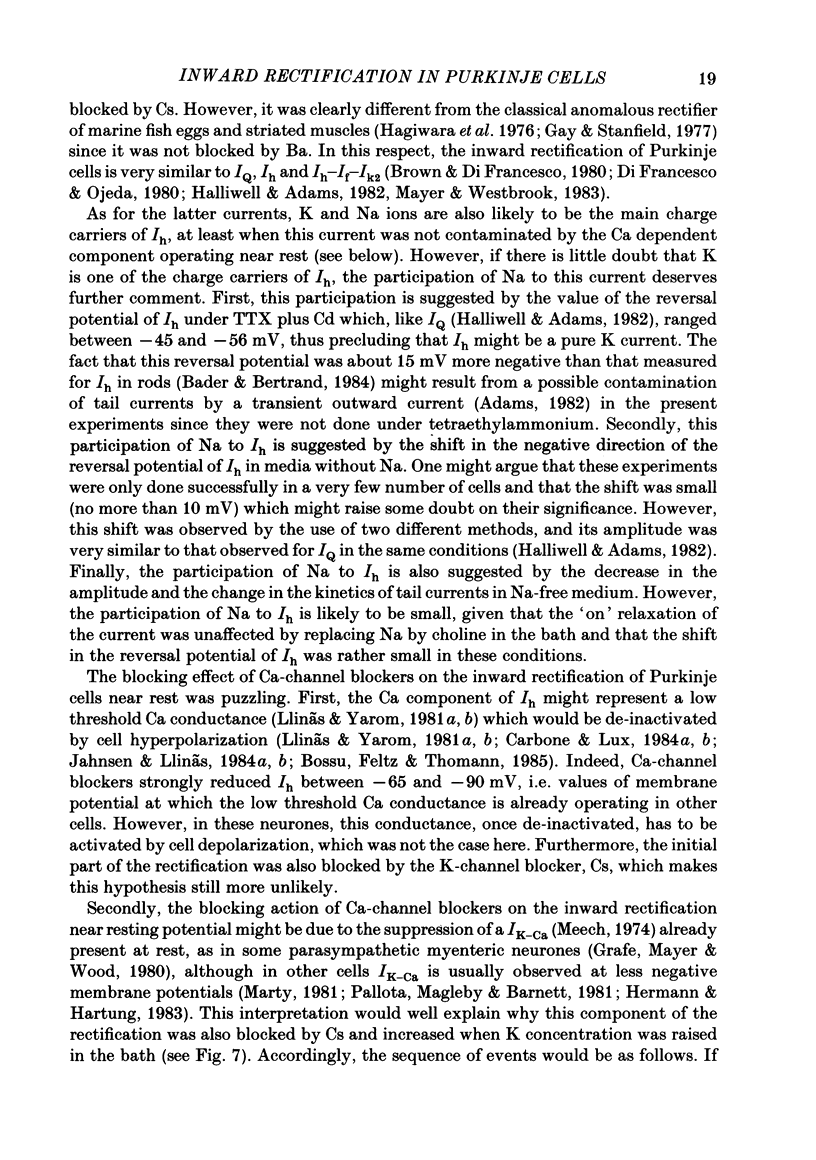
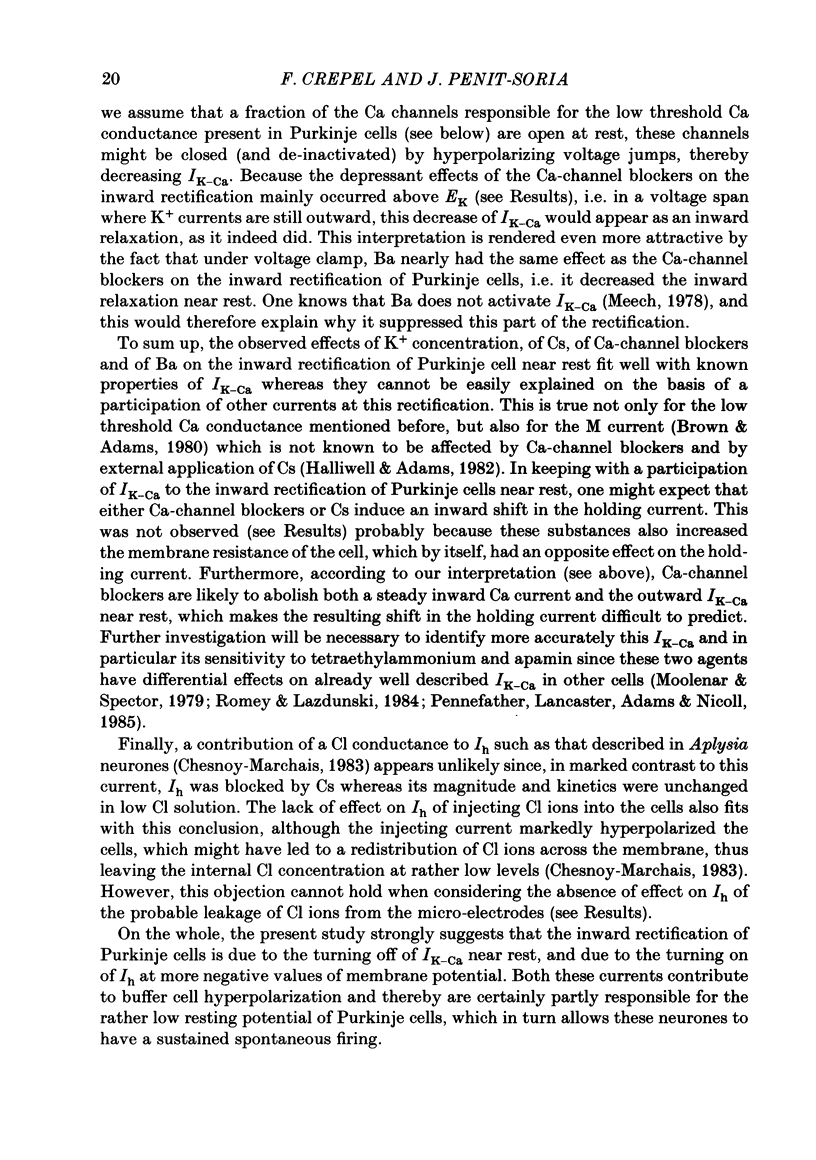
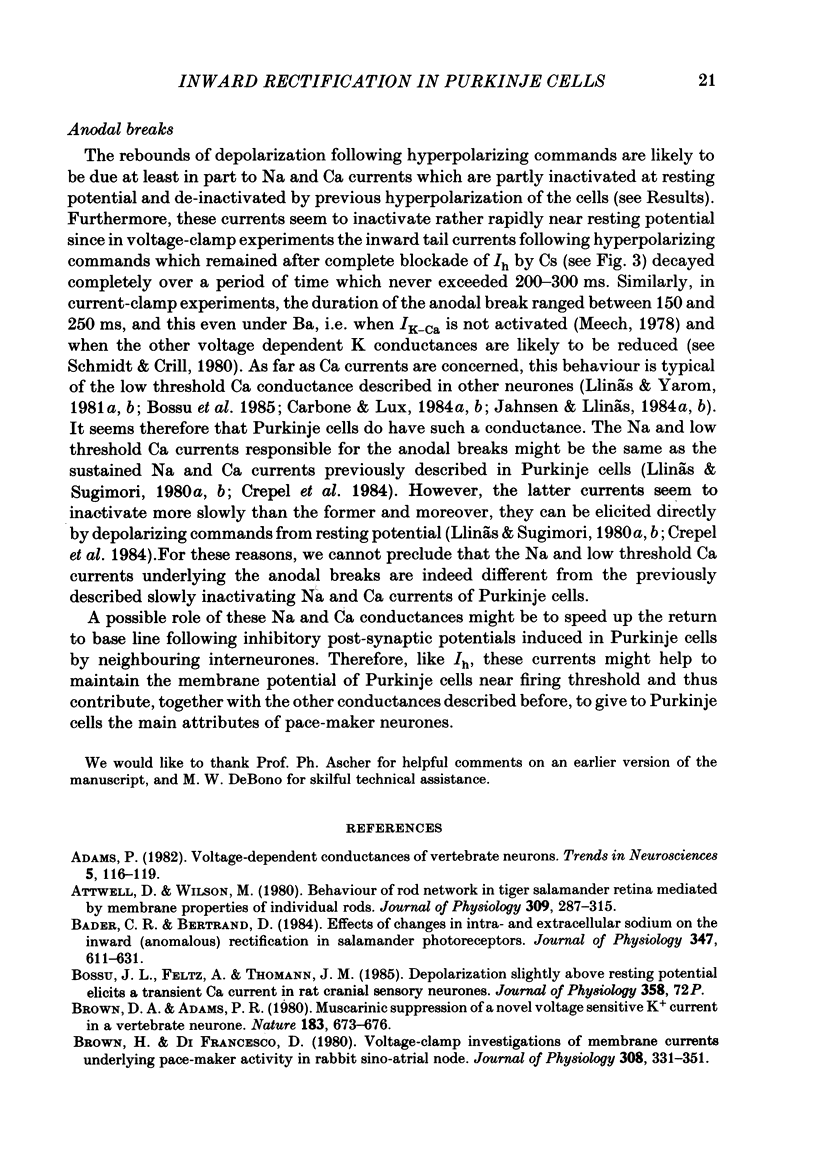
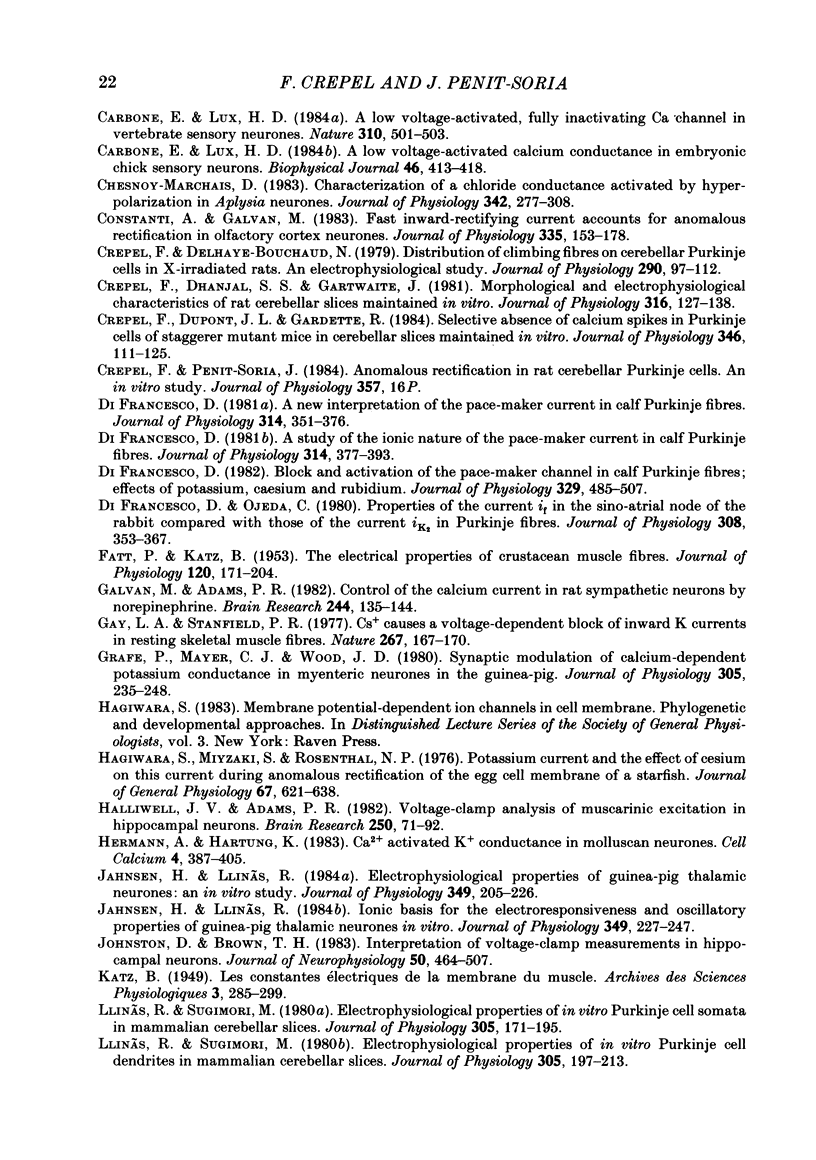
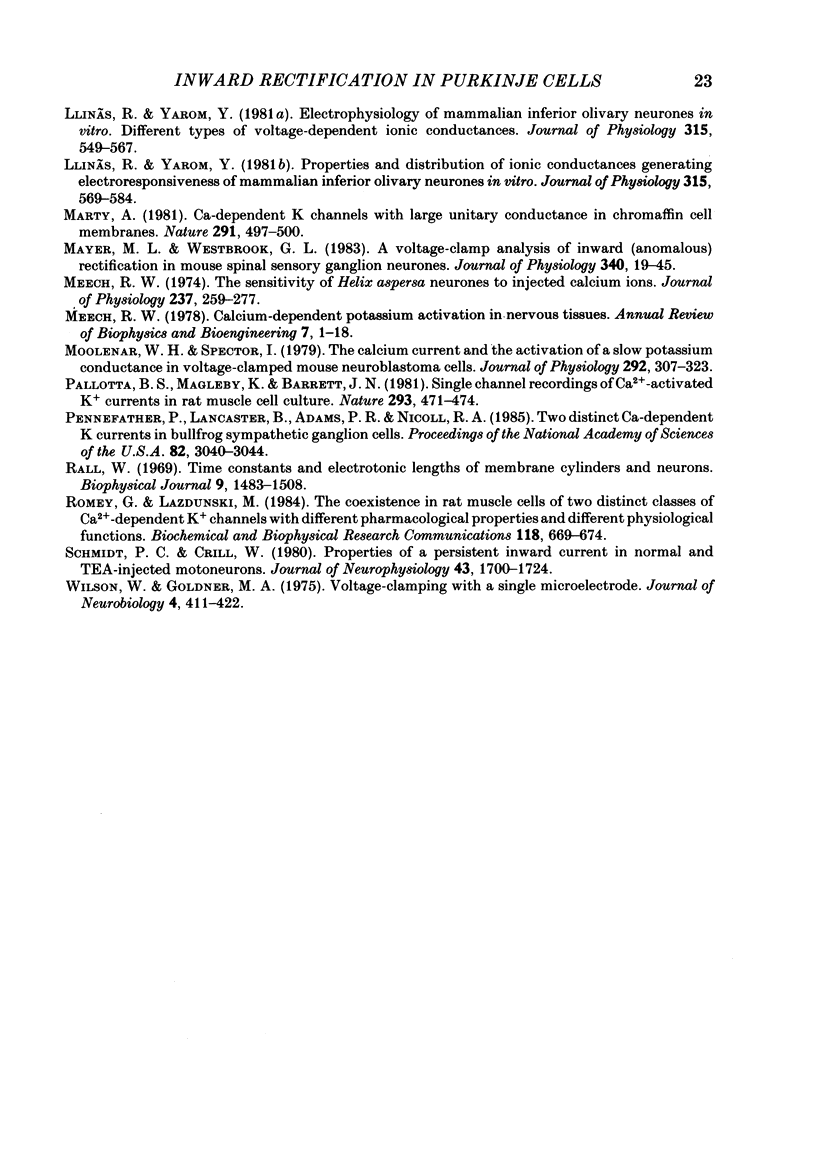
Selected References
These references are in PubMed. This may not be the complete list of references from this article.
- Attwell D., Wilson M. Behaviour of the rod network in the tiger salamander retina mediated by membrane properties of individual rods. J Physiol. 1980 Dec;309:287–315. doi: 10.1113/jphysiol.1980.sp013509. [DOI] [PMC free article] [PubMed] [Google Scholar]
- Bader C. R., Bertrand D. Effect of changes in intra- and extracellular sodium on the inward (anomalous) rectification in salamander photoreceptors. J Physiol. 1984 Feb;347:611–631. doi: 10.1113/jphysiol.1984.sp015086. [DOI] [PMC free article] [PubMed] [Google Scholar]
- Brown D. A., Adams P. R. Muscarinic suppression of a novel voltage-sensitive K+ current in a vertebrate neurone. Nature. 1980 Feb 14;283(5748):673–676. doi: 10.1038/283673a0. [DOI] [PubMed] [Google Scholar]
- Brown H., Difrancesco D. Voltage-clamp investigations of membrane currents underlying pace-maker activity in rabbit sino-atrial node. J Physiol. 1980 Nov;308:331–351. doi: 10.1113/jphysiol.1980.sp013474. [DOI] [PMC free article] [PubMed] [Google Scholar]
- Carbone E., Lux H. D. A low voltage-activated calcium conductance in embryonic chick sensory neurons. Biophys J. 1984 Sep;46(3):413–418. doi: 10.1016/S0006-3495(84)84037-0. [DOI] [PMC free article] [PubMed] [Google Scholar]
- Carbone E., Lux H. D. A low voltage-activated, fully inactivating Ca channel in vertebrate sensory neurones. Nature. 1984 Aug 9;310(5977):501–502. doi: 10.1038/310501a0. [DOI] [PubMed] [Google Scholar]
- Chesnoy-Marchais D. Characterization of a chloride conductance activated by hyperpolarization in Aplysia neurones. J Physiol. 1983 Sep;342:277–308. doi: 10.1113/jphysiol.1983.sp014851. [DOI] [PMC free article] [PubMed] [Google Scholar]
- Constanti A., Galvan M. Fast inward-rectifying current accounts for anomalous rectification in olfactory cortex neurones. J Physiol. 1983 Feb;335:153–178. doi: 10.1113/jphysiol.1983.sp014526. [DOI] [PMC free article] [PubMed] [Google Scholar]
- Crepel F., Delhaye-Bouchaud N. Distribution of climbing fibres on cerebellar Purkinje cells in X-irradiated rats. An electrophysiological study. J Physiol. 1979 May;290(2):97–112. doi: 10.1113/jphysiol.1979.sp012762. [DOI] [PMC free article] [PubMed] [Google Scholar]
- Crepel F., Dhanjal S. S., Garthwaite J. Morphological and electrophysiological characteristics of rat cerebellar slices maintained in vitro. J Physiol. 1981 Jul;316:127–138. doi: 10.1113/jphysiol.1981.sp013777. [DOI] [PMC free article] [PubMed] [Google Scholar]
- Crepel F., Dupont J. L., Gardette R. Selective absence of calcium spikes in Purkinje cells of staggerer mutant mice in cerebellar slices maintained in vitro. J Physiol. 1984 Jan;346:111–125. doi: 10.1113/jphysiol.1984.sp015010. [DOI] [PMC free article] [PubMed] [Google Scholar]
- DiFrancesco D. Block and activation of the pace-maker channel in calf purkinje fibres: effects of potassium, caesium and rubidium. J Physiol. 1982 Aug;329:485–507. doi: 10.1113/jphysiol.1982.sp014315. [DOI] [PMC free article] [PubMed] [Google Scholar]
- DiFrancesco D., Ojeda C. Properties of the current if in the sino-atrial node of the rabbit compared with those of the current iK, in Purkinje fibres. J Physiol. 1980 Nov;308:353–367. doi: 10.1113/jphysiol.1980.sp013475. [DOI] [PMC free article] [PubMed] [Google Scholar]
- FATT P., KATZ B. The electrical properties of crustacean muscle fibres. J Physiol. 1953 Apr 28;120(1-2):171–204. doi: 10.1113/jphysiol.1953.sp004884. [DOI] [PMC free article] [PubMed] [Google Scholar]
- Galvan M., Adams P. R. Control of calcium current in rat sympathetic neurons by norepinephrine. Brain Res. 1982 Jul 22;244(1):135–144. doi: 10.1016/0006-8993(82)90911-8. [DOI] [PubMed] [Google Scholar]
- Gay L. A., Stanfield P. R. Cs(+) causes a voltage-dependent block of inward K currents in resting skeletal muscle fibres. Nature. 1977 May 12;267(5607):169–170. doi: 10.1038/267169a0. [DOI] [PubMed] [Google Scholar]
- Grafe P., Mayer C. J., Wood J. D. Synaptic modulation of calcium-dependent potassium conductance in myenteric neurones in the guinea-pig. J Physiol. 1980 Aug;305:235–248. doi: 10.1113/jphysiol.1980.sp013360. [DOI] [PMC free article] [PubMed] [Google Scholar]
- Hagiwara S., Miyazaki S., Rosenthal N. P. Potassium current and the effect of cesium on this current during anomalous rectification of the egg cell membrane of a starfish. J Gen Physiol. 1976 Jun;67(6):621–638. doi: 10.1085/jgp.67.6.621. [DOI] [PMC free article] [PubMed] [Google Scholar]
- Halliwell J. V., Adams P. R. Voltage-clamp analysis of muscarinic excitation in hippocampal neurons. Brain Res. 1982 Oct 28;250(1):71–92. doi: 10.1016/0006-8993(82)90954-4. [DOI] [PubMed] [Google Scholar]
- Hermann A., Hartung K. Ca2+ activated K+ conductance in molluscan neurones. Cell Calcium. 1983 Dec;4(5-6):387–405. doi: 10.1016/0143-4160(83)90016-7. [DOI] [PubMed] [Google Scholar]
- Jahnsen H., Llinás R. Electrophysiological properties of guinea-pig thalamic neurones: an in vitro study. J Physiol. 1984 Apr;349:205–226. doi: 10.1113/jphysiol.1984.sp015153. [DOI] [PMC free article] [PubMed] [Google Scholar]
- Jahnsen H., Llinás R. Ionic basis for the electro-responsiveness and oscillatory properties of guinea-pig thalamic neurones in vitro. J Physiol. 1984 Apr;349:227–247. doi: 10.1113/jphysiol.1984.sp015154. [DOI] [PMC free article] [PubMed] [Google Scholar]
- Johnston D., Brown T. H. Interpretation of voltage-clamp measurements in hippocampal neurons. J Neurophysiol. 1983 Aug;50(2):464–486. doi: 10.1152/jn.1983.50.2.464. [DOI] [PubMed] [Google Scholar]
- Llinás R., Sugimori M. Electrophysiological properties of in vitro Purkinje cell dendrites in mammalian cerebellar slices. J Physiol. 1980 Aug;305:197–213. doi: 10.1113/jphysiol.1980.sp013358. [DOI] [PMC free article] [PubMed] [Google Scholar]
- Llinás R., Sugimori M. Electrophysiological properties of in vitro Purkinje cell somata in mammalian cerebellar slices. J Physiol. 1980 Aug;305:171–195. doi: 10.1113/jphysiol.1980.sp013357. [DOI] [PMC free article] [PubMed] [Google Scholar]
- Llinás R., Yarom Y. Electrophysiology of mammalian inferior olivary neurones in vitro. Different types of voltage-dependent ionic conductances. J Physiol. 1981 Jun;315:549–567. doi: 10.1113/jphysiol.1981.sp013763. [DOI] [PMC free article] [PubMed] [Google Scholar]
- Llinás R., Yarom Y. Properties and distribution of ionic conductances generating electroresponsiveness of mammalian inferior olivary neurones in vitro. J Physiol. 1981 Jun;315:569–584. doi: 10.1113/jphysiol.1981.sp013764. [DOI] [PMC free article] [PubMed] [Google Scholar]
- Marty A. Ca-dependent K channels with large unitary conductance in chromaffin cell membranes. Nature. 1981 Jun 11;291(5815):497–500. doi: 10.1038/291497a0. [DOI] [PubMed] [Google Scholar]
- Mayer M. L., Westbrook G. L. A voltage-clamp analysis of inward (anomalous) rectification in mouse spinal sensory ganglion neurones. J Physiol. 1983 Jul;340:19–45. doi: 10.1113/jphysiol.1983.sp014747. [DOI] [PMC free article] [PubMed] [Google Scholar]
- Meech R. W. Calcium-dependent potassium activation in nervous tissues. Annu Rev Biophys Bioeng. 1978;7:1–18. doi: 10.1146/annurev.bb.07.060178.000245. [DOI] [PubMed] [Google Scholar]
- Meech R. W. The sensitivity of Helix aspersa neurones to injected calcium ions. J Physiol. 1974 Mar;237(2):259–277. doi: 10.1113/jphysiol.1974.sp010481. [DOI] [PMC free article] [PubMed] [Google Scholar]
- Moolenaar W. H., Spector I. The calcium current and the activation of a slow potassium conductance in voltage-clamped mouse neuroblastoma cells. J Physiol. 1979 Jul;292:307–323. doi: 10.1113/jphysiol.1979.sp012852. [DOI] [PMC free article] [PubMed] [Google Scholar]
- Pallotta B. S., Magleby K. L., Barrett J. N. Single channel recordings of Ca2+-activated K+ currents in rat muscle cell culture. Nature. 1981 Oct 8;293(5832):471–474. doi: 10.1038/293471a0. [DOI] [PubMed] [Google Scholar]
- Pennefather P., Lancaster B., Adams P. R., Nicoll R. A. Two distinct Ca-dependent K currents in bullfrog sympathetic ganglion cells. Proc Natl Acad Sci U S A. 1985 May;82(9):3040–3044. doi: 10.1073/pnas.82.9.3040. [DOI] [PMC free article] [PubMed] [Google Scholar]
- Rall W. Time constants and electrotonic length of membrane cylinders and neurons. Biophys J. 1969 Dec;9(12):1483–1508. doi: 10.1016/S0006-3495(69)86467-2. [DOI] [PMC free article] [PubMed] [Google Scholar]
- Romey G., Lazdunski M. The coexistence in rat muscle cells of two distinct classes of Ca2+-dependent K+ channels with different pharmacological properties and different physiological functions. Biochem Biophys Res Commun. 1984 Jan 30;118(2):669–674. doi: 10.1016/0006-291x(84)91355-x. [DOI] [PubMed] [Google Scholar]
- Schwindt P. C., Crill W. E. Properties of a persistent inward current in normal and TEA-injected motoneurons. J Neurophysiol. 1980 Jun;43(6):1700–1724. doi: 10.1152/jn.1980.43.6.1700. [DOI] [PubMed] [Google Scholar]
- Wilson W. A., Goldner M. M. Voltage clamping with a single microelectrode. J Neurobiol. 1975 Jul;6(4):411–422. doi: 10.1002/neu.480060406. [DOI] [PubMed] [Google Scholar]