Abstract
Single intact fibres from frog muscle at 0-1 degrees C were stimulated to produce isometric tetani at a sarcomere length of about 2.25 micron, using a spot-follower apparatus to control the length of the central part of a fibre. When the plateau of the tetanus was reached the fibre was forced to shorten by applying a step and ramp length change in an approximation to an isotonic release. When tension had reached a steady level, Ti, during shortening, tension transients were elicited by applying step changes of length, complete within 0.2 ms, ranging from a stretch of 1.5 nm per half-sarcomere to a release of 6 nm per half-sarcomere. The tension transients recorded during shortening were qualitatively similar to those previously recorded in isometric tetani. There were four phases: phase 1, the change of tension during the step; phase 2, a rapid partial recovery of tension; phase 3, a delay or reversal of recovery; phase 4, a slower recovery of tension to the level before the step was applied. Measurements were made of the extreme tension, T1, attained during a step, and the level, T2, to which tension recovers in phase 2. The excursion of tension, [T1-Ti], during a small step of given size, fell with increase of shortening velocity, reaching about 40% of the isometric value near the maximum velocity of shortening. T2 fell as shortening velocity was increased and the fraction of steady tension recovered, T2/Ti, also decreased, so that the proportion of tension recovery in phase 4 increased. All the recovery phases became progressively more rapid with increase of shortening velocity. The early tension response was matched with a delay-line simulator so as to estimate the value of the instantaneous stiffness. Stiffness during shortening was found to decrease approximately linearly with tension, reaching about 35% of the isometric value as tension approached zero. It was impossible to match the early tension response in a rapidly shortening fibre without assuming decreased stiffness. The decline of stiffness is interpreted as due largely to reduced number of attached cross-bridges, but quantitative estimates would be affected by possible filament compliance and non-linearity of cross-bridge stiffness. The decrease in T2 also suggests fewer cross-bridges are attached as shortening velocity increases, but uncertainties about the processes determining phase 2 during shortening do not permit a precise estimate of stiffness to be made.(ABSTRACT TRUNCATED AT 400 WORDS)
Full text
PDF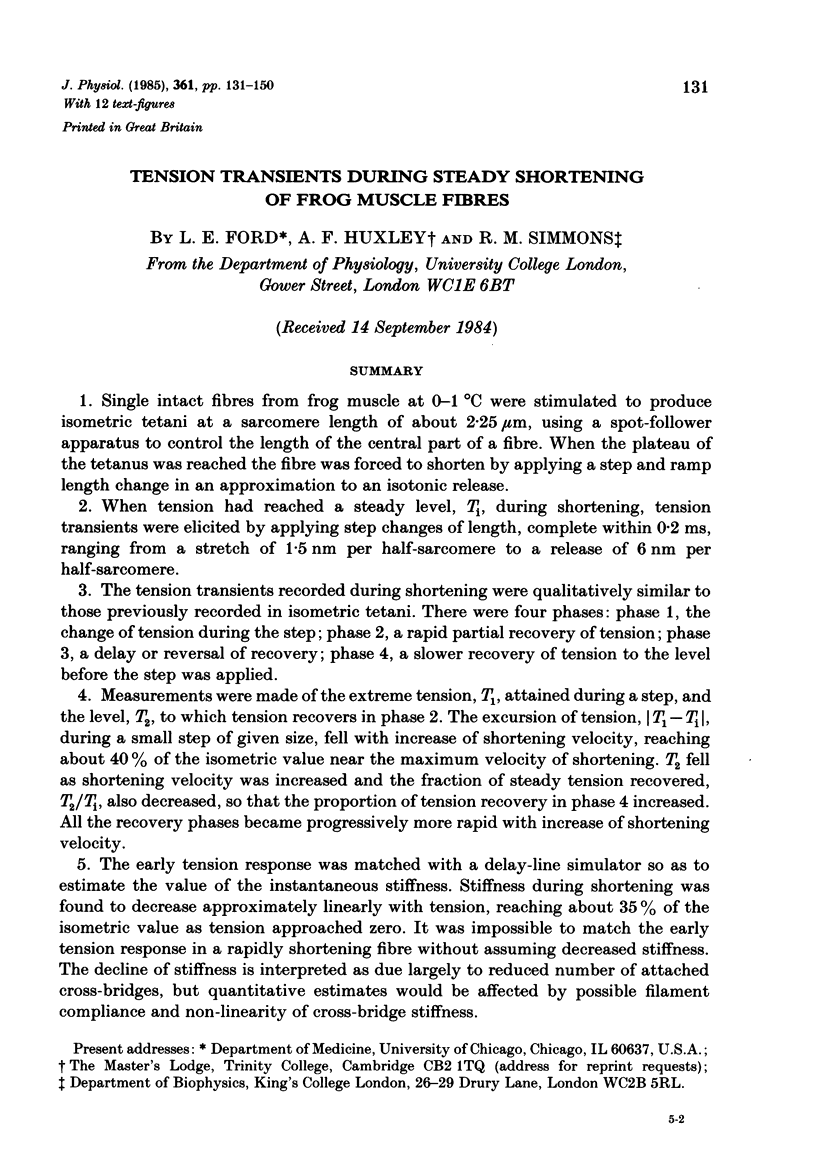
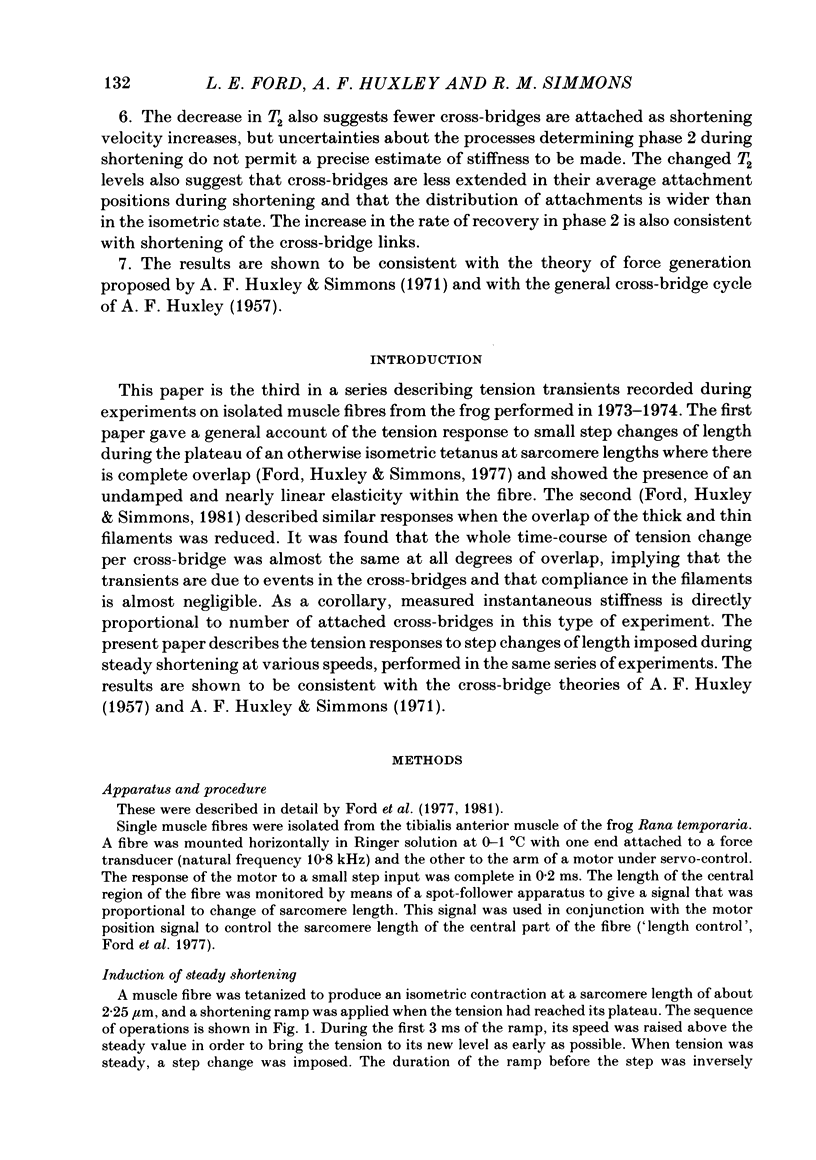
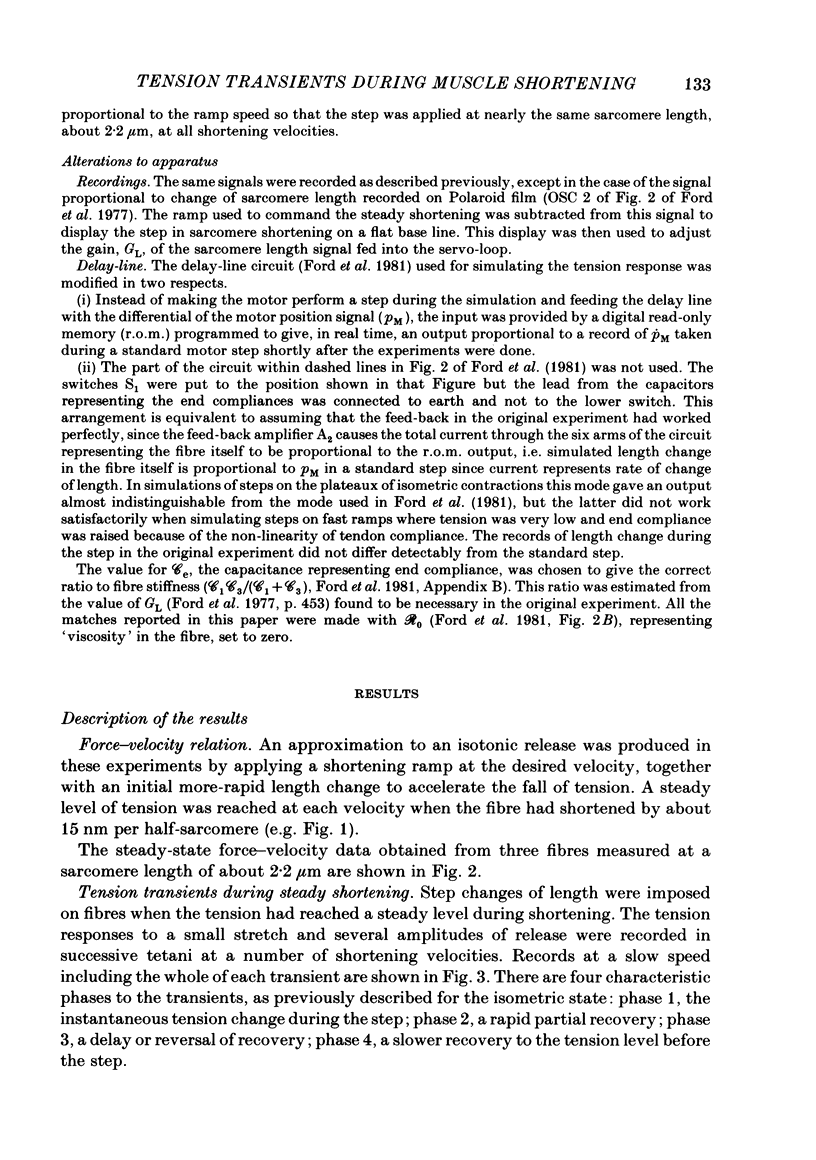
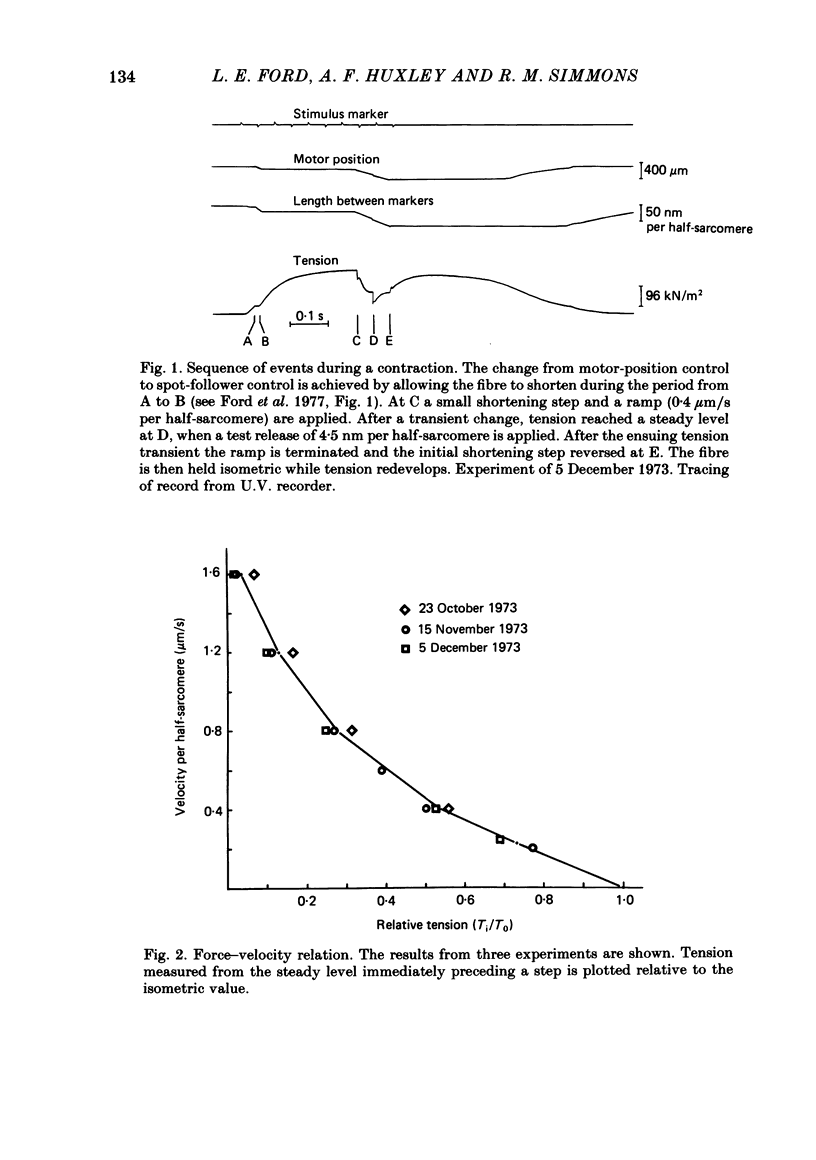
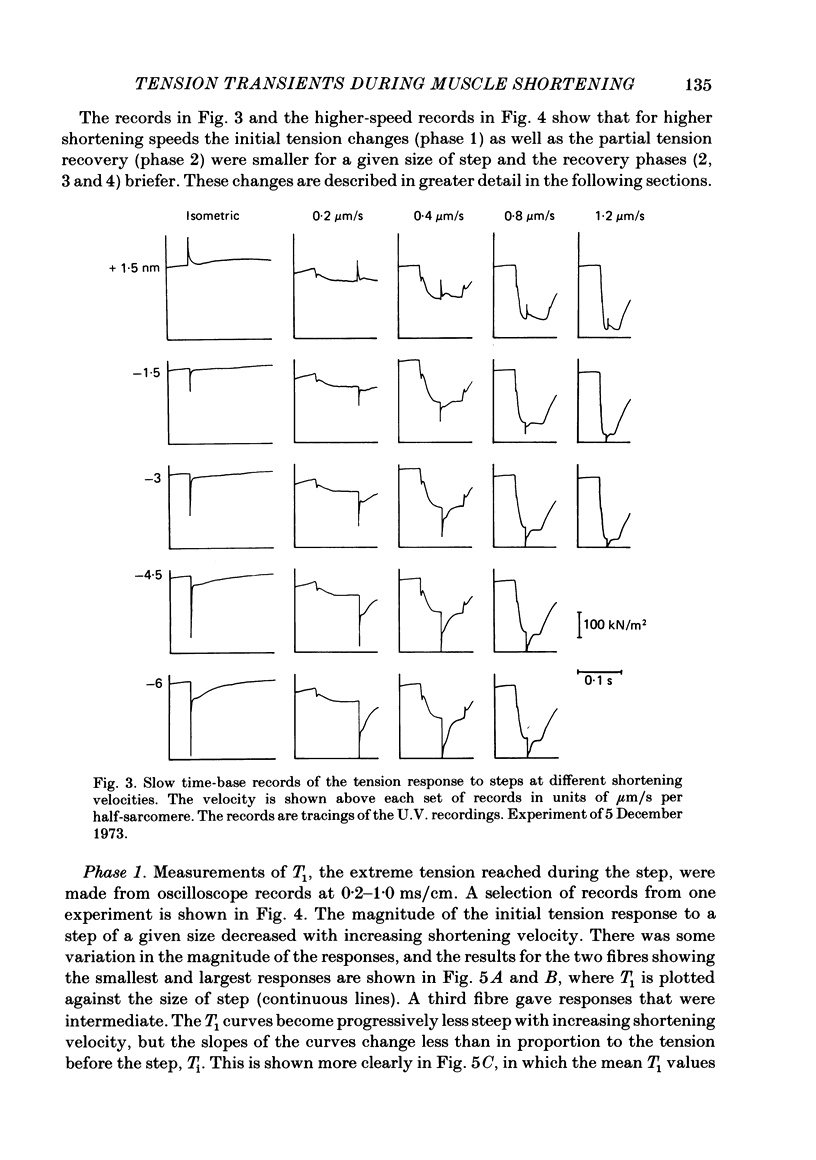
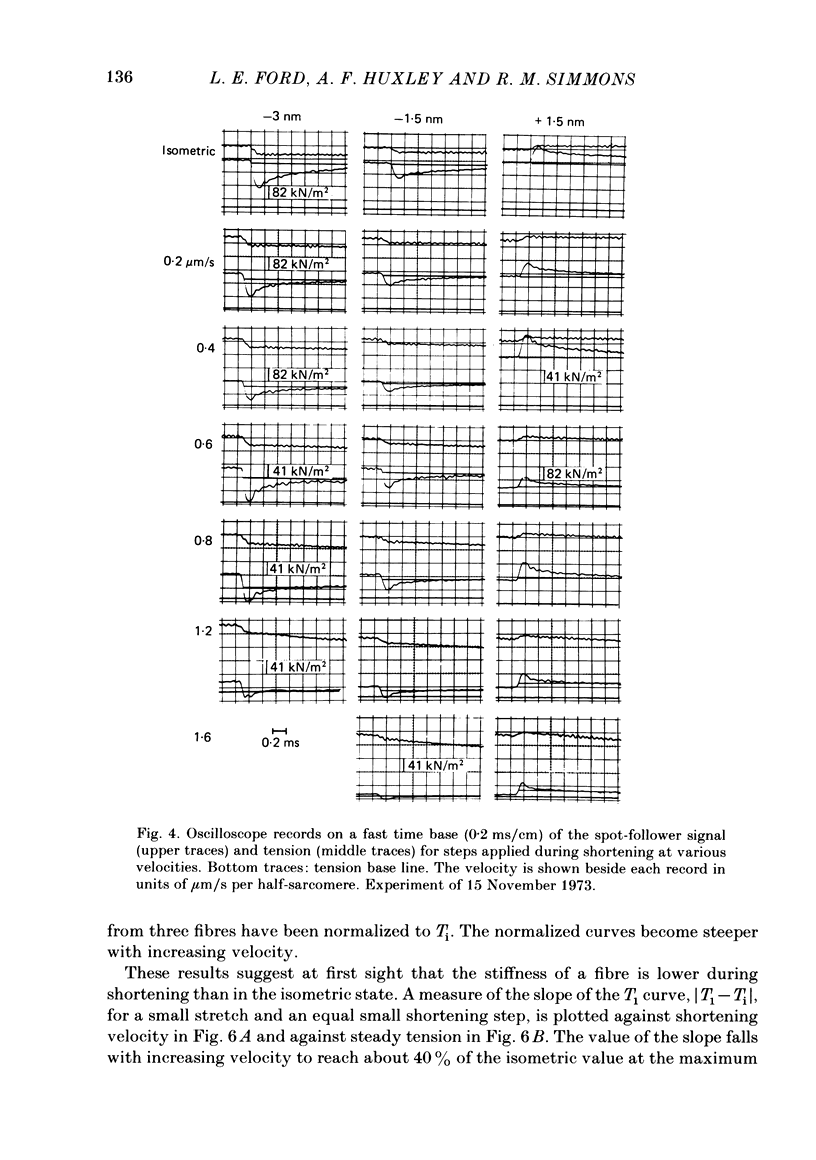
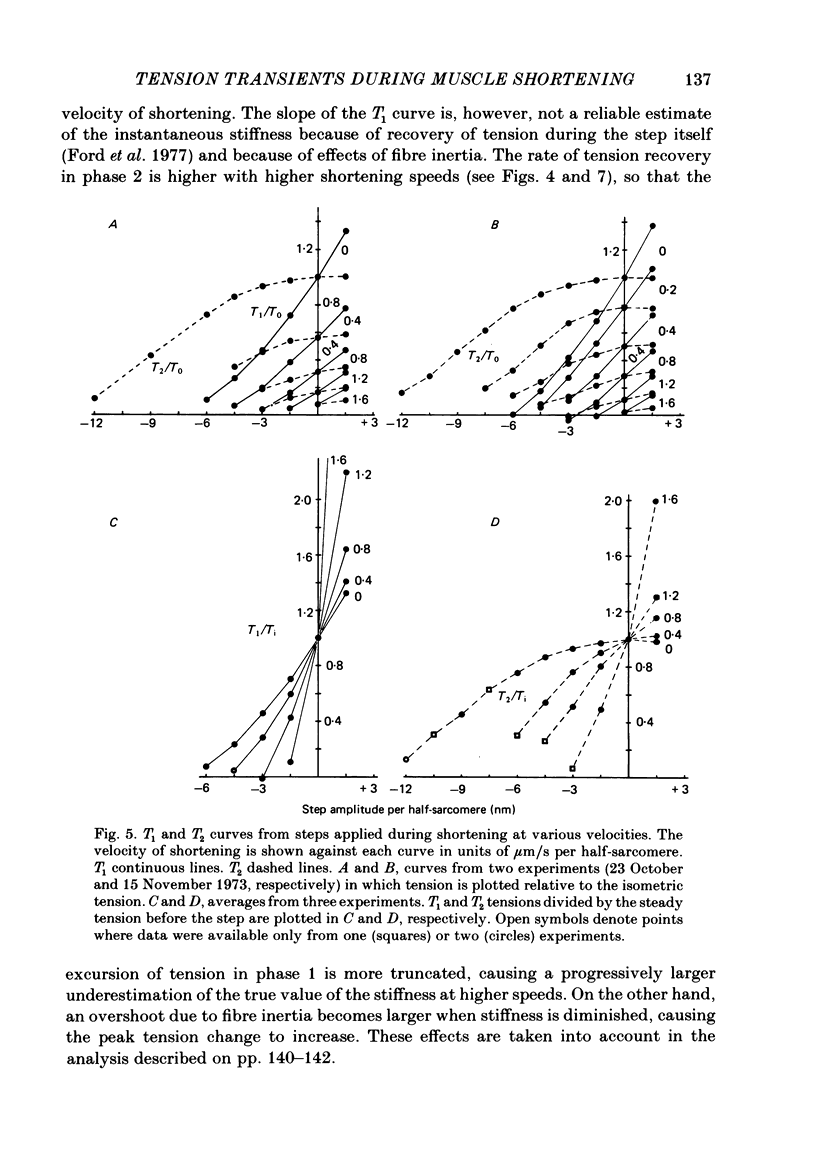
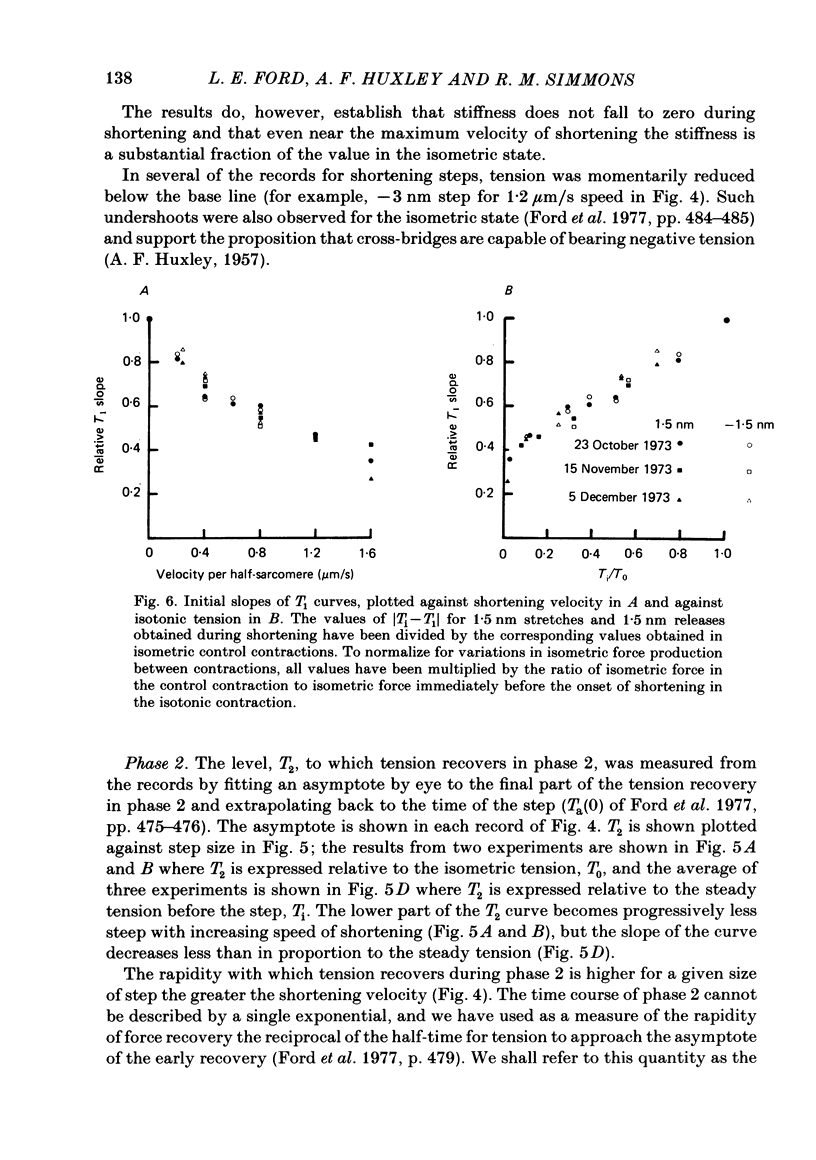
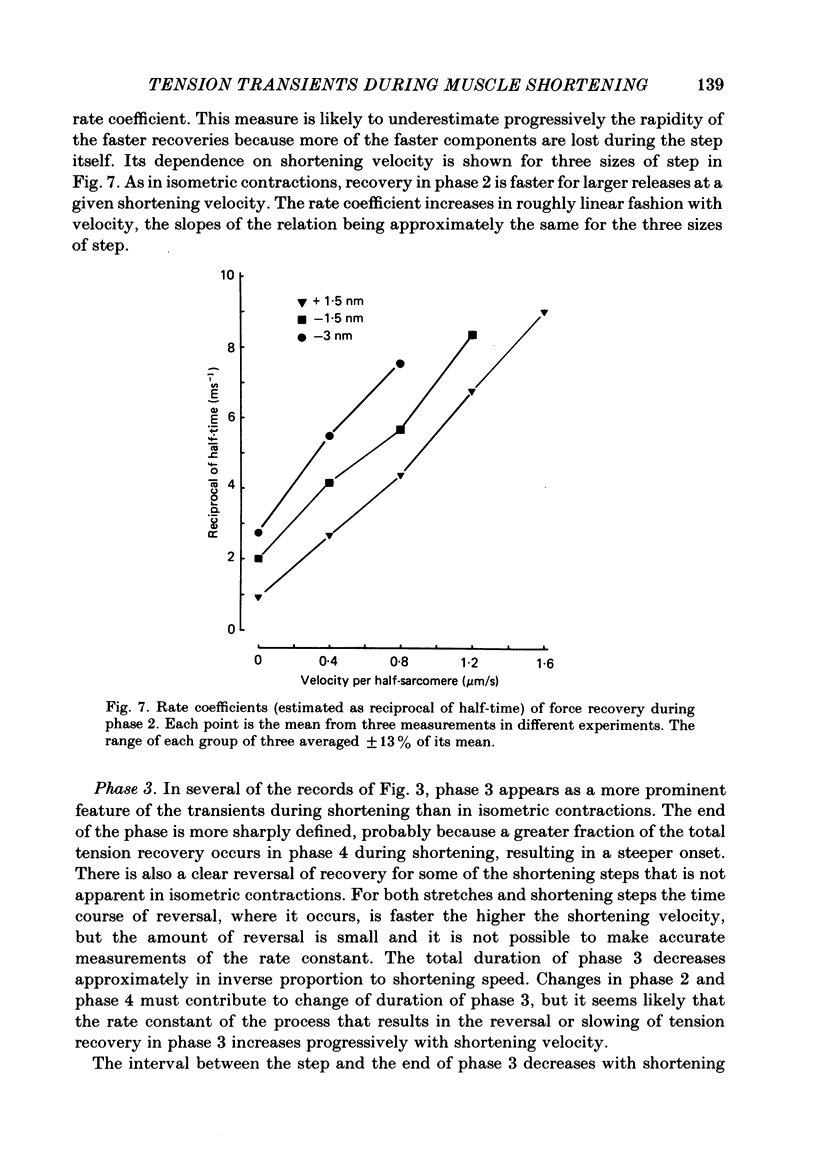
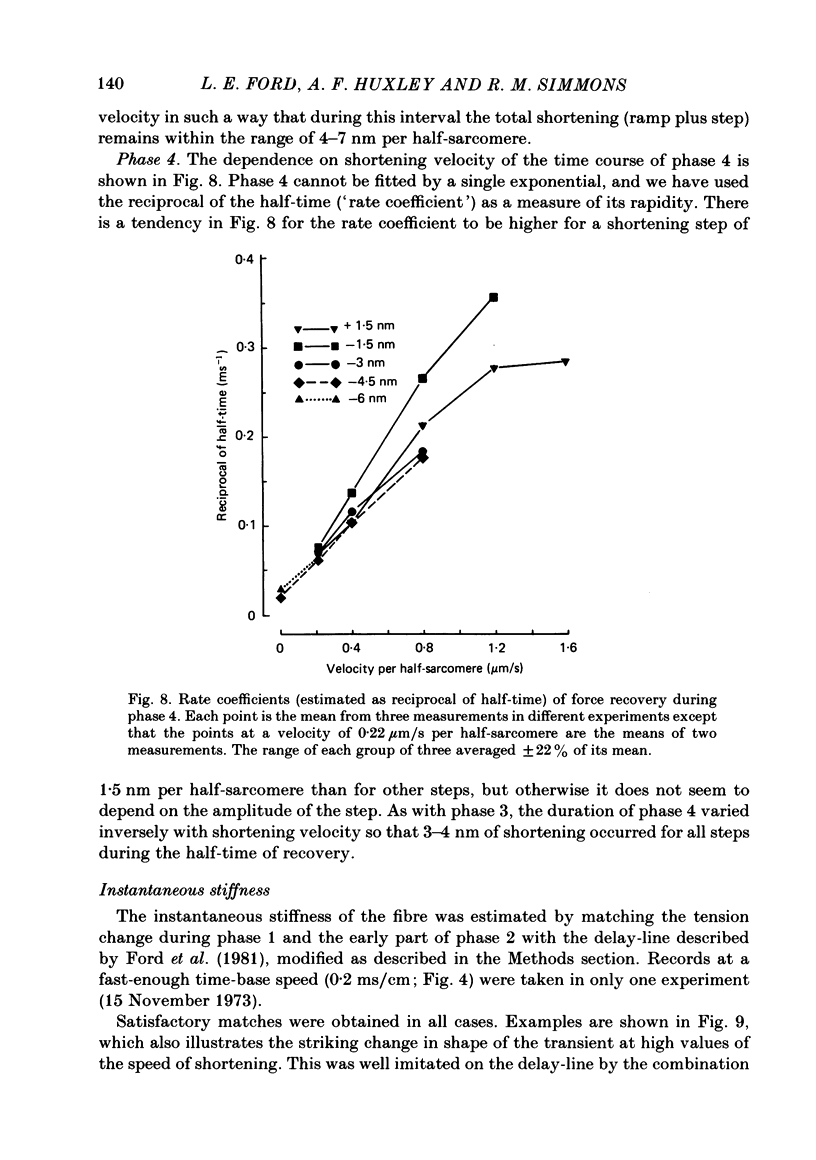
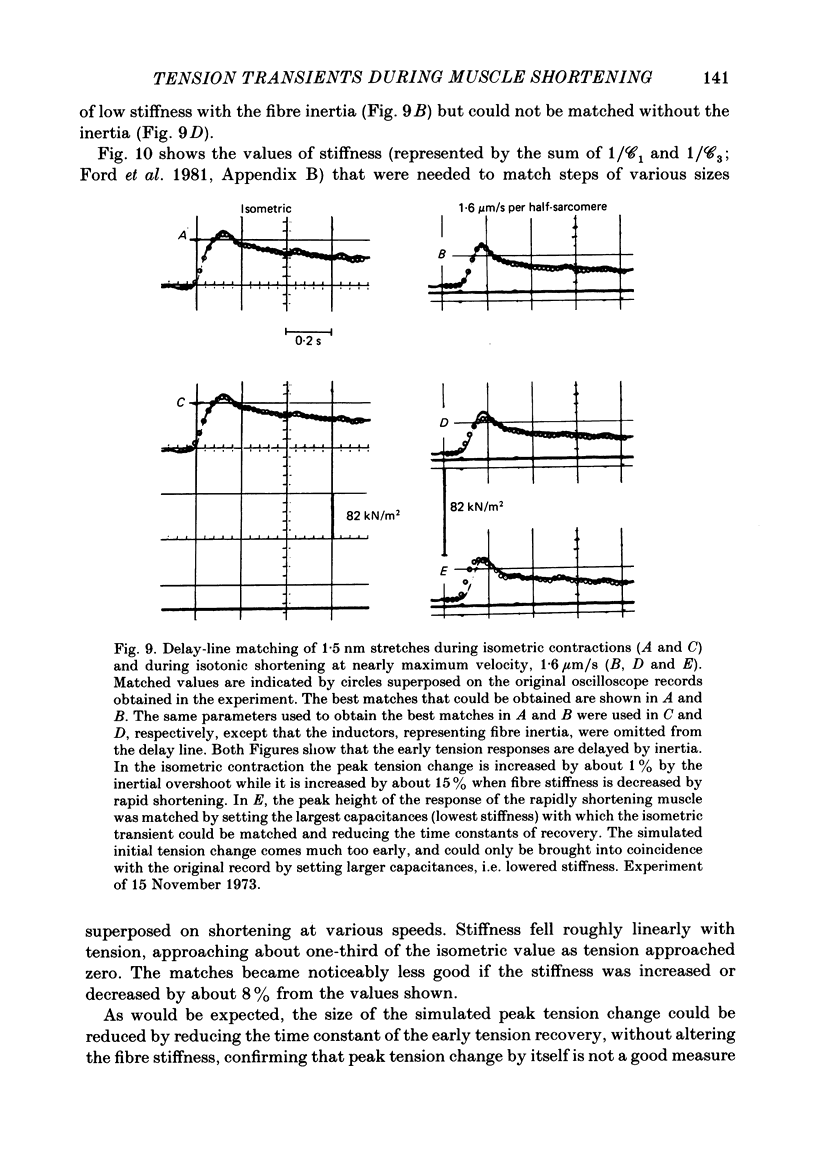
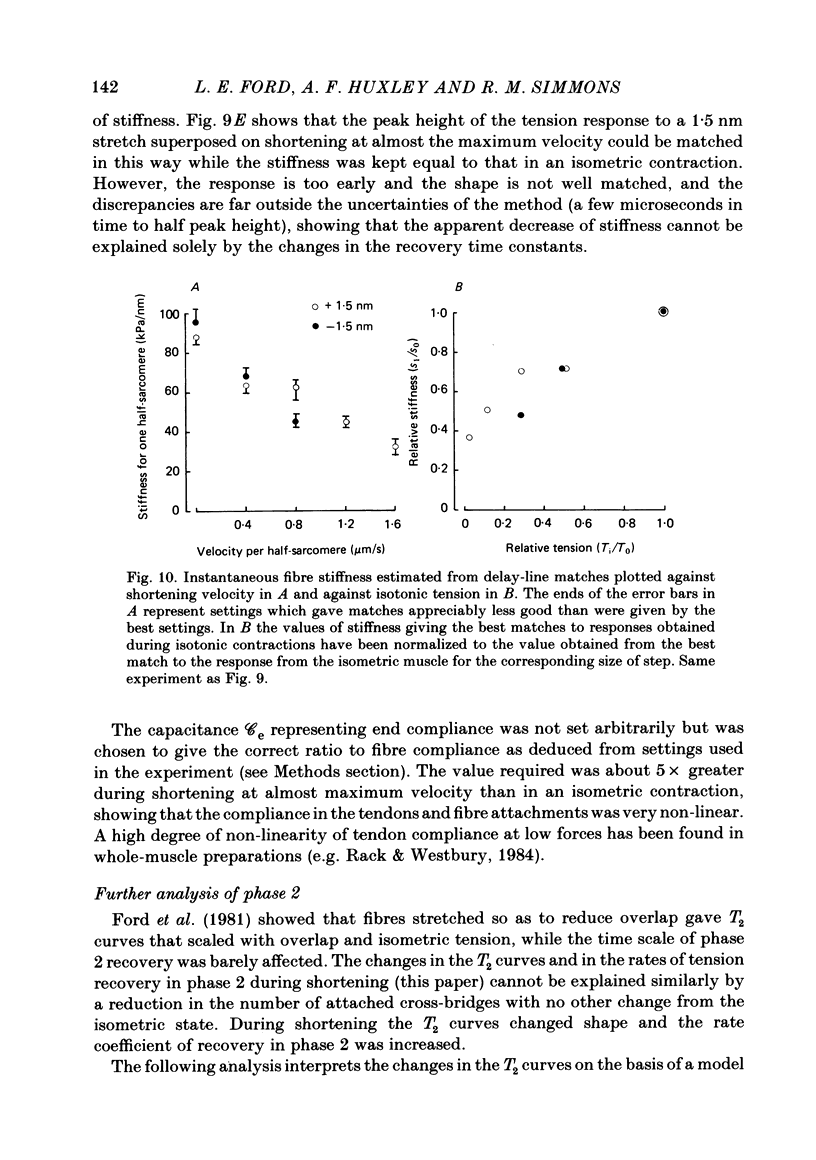
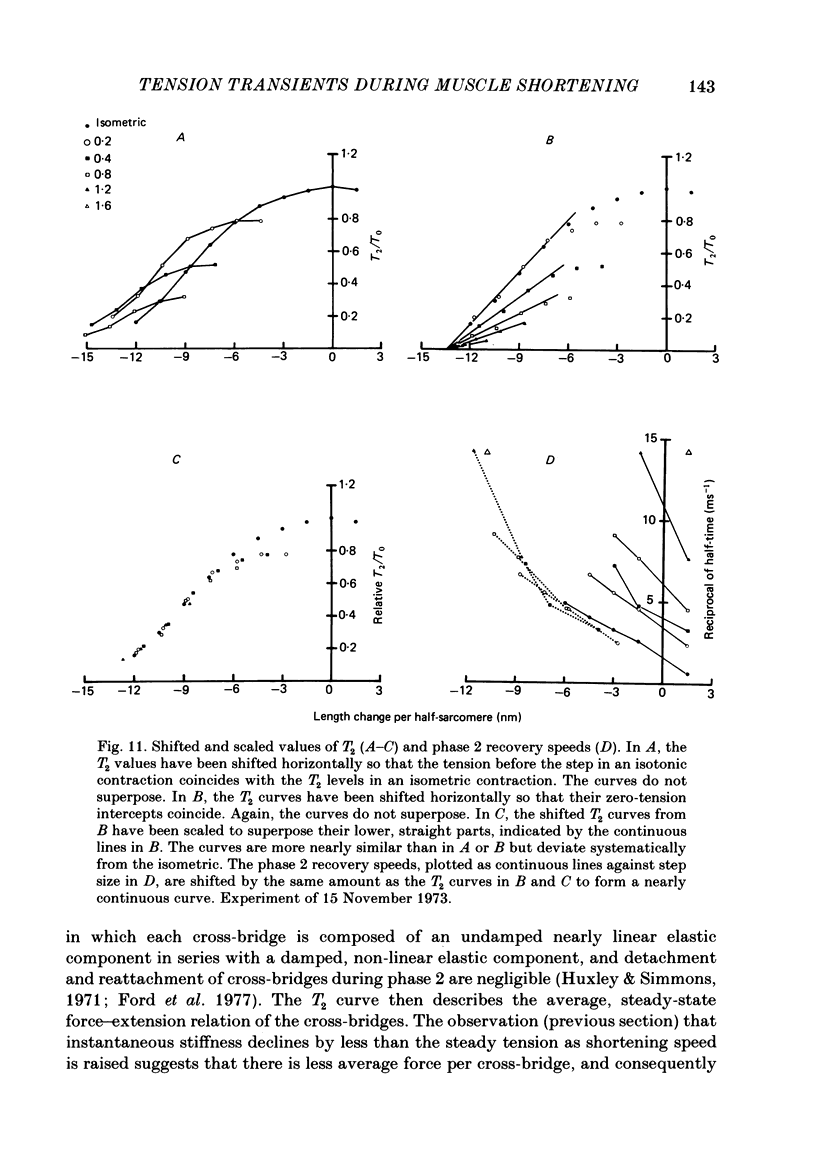
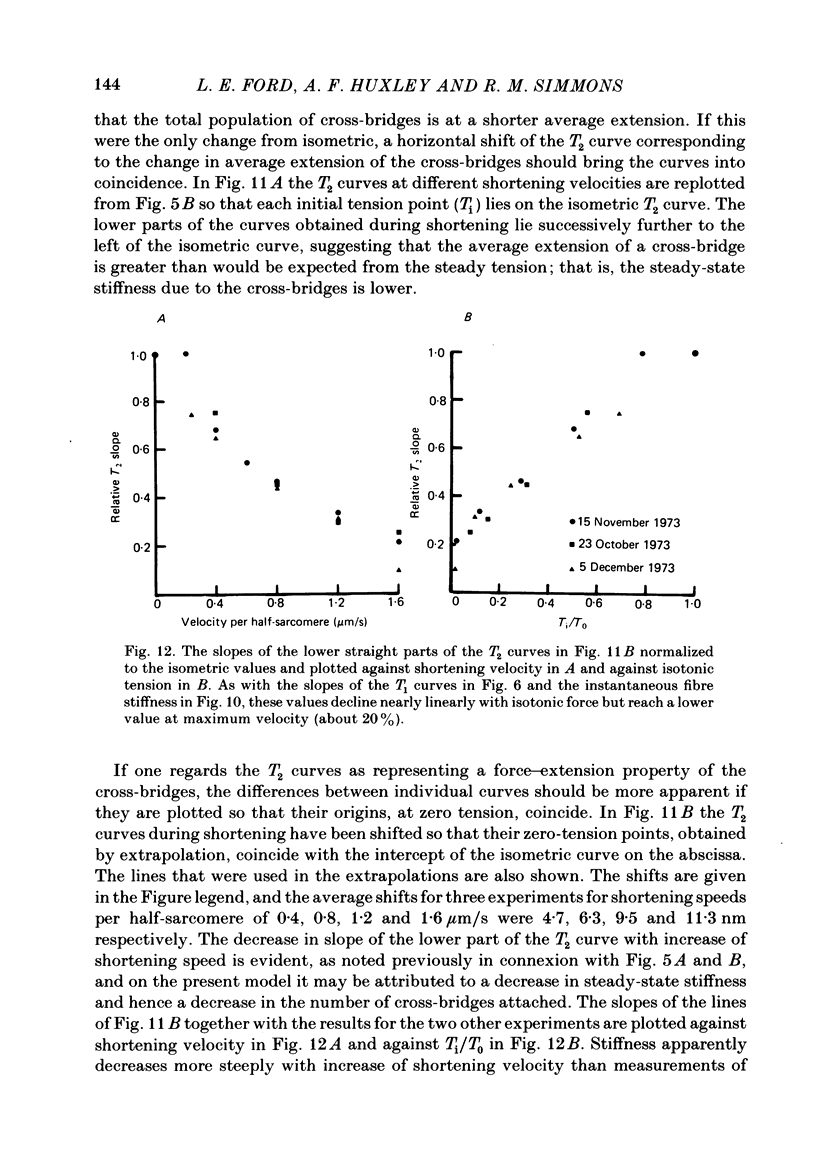
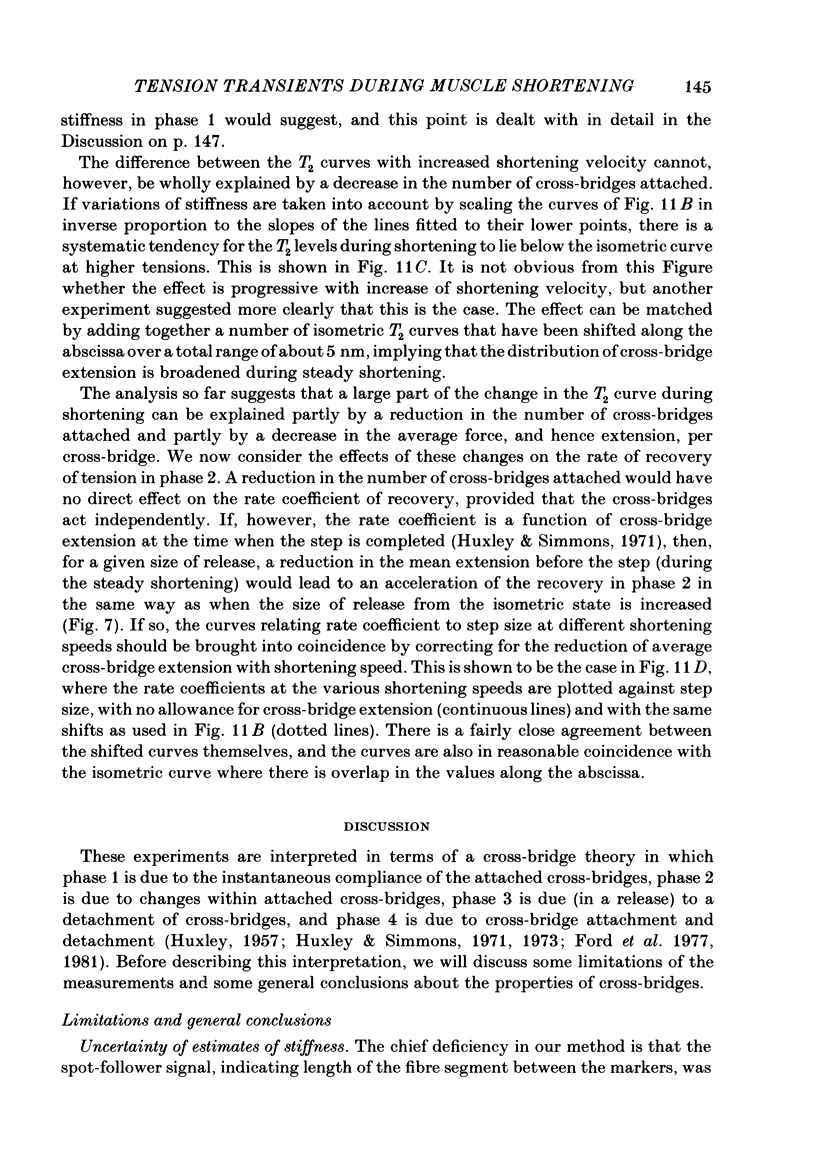
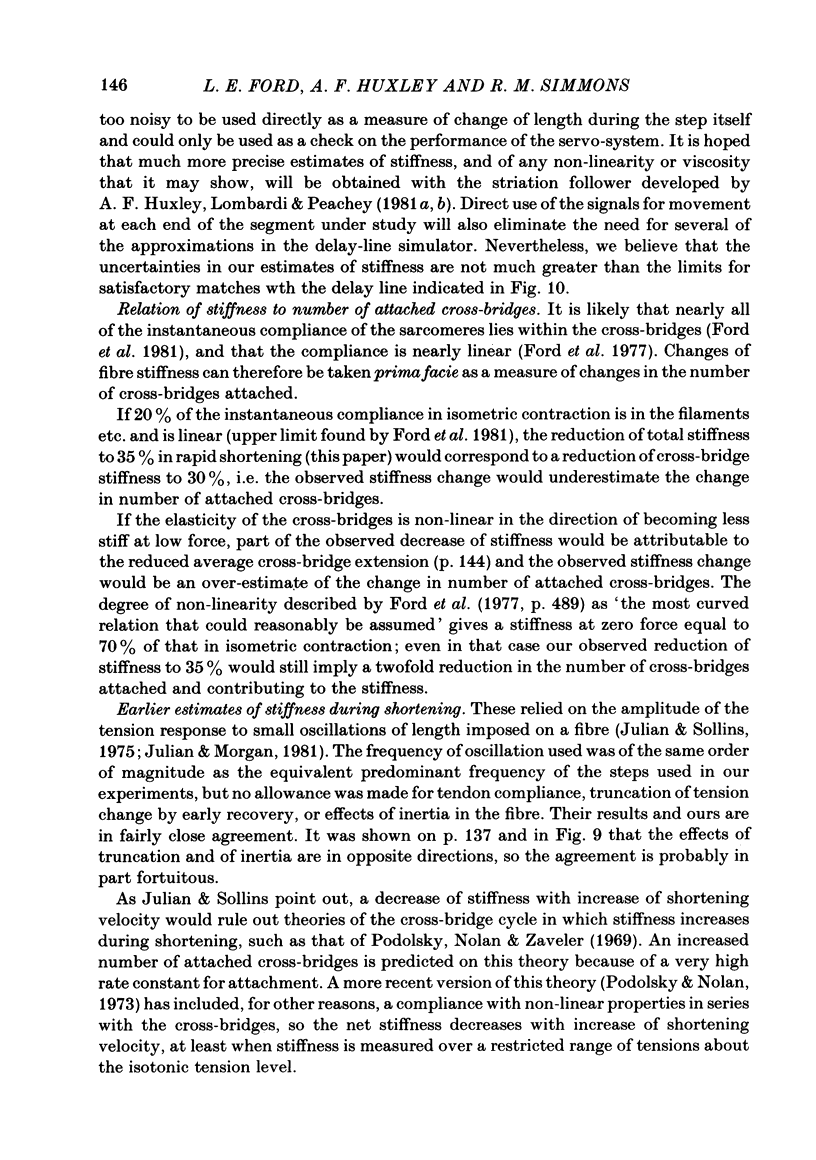
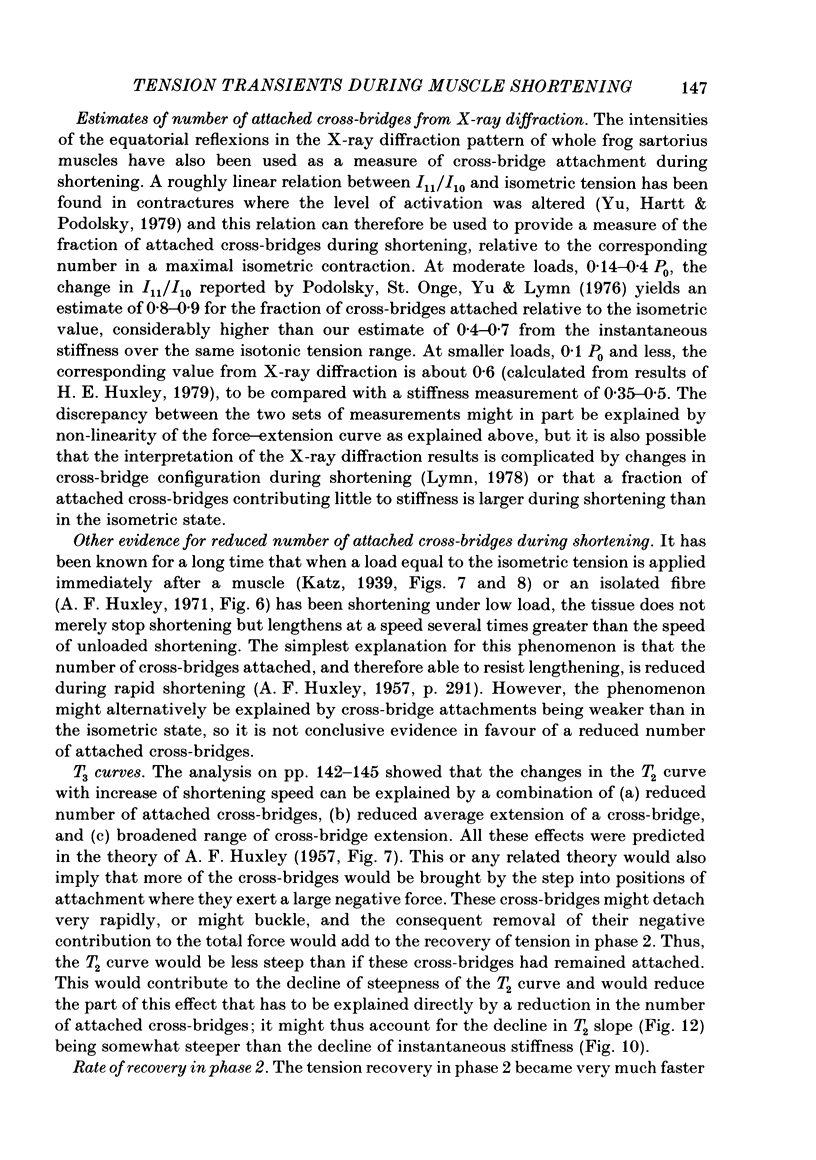
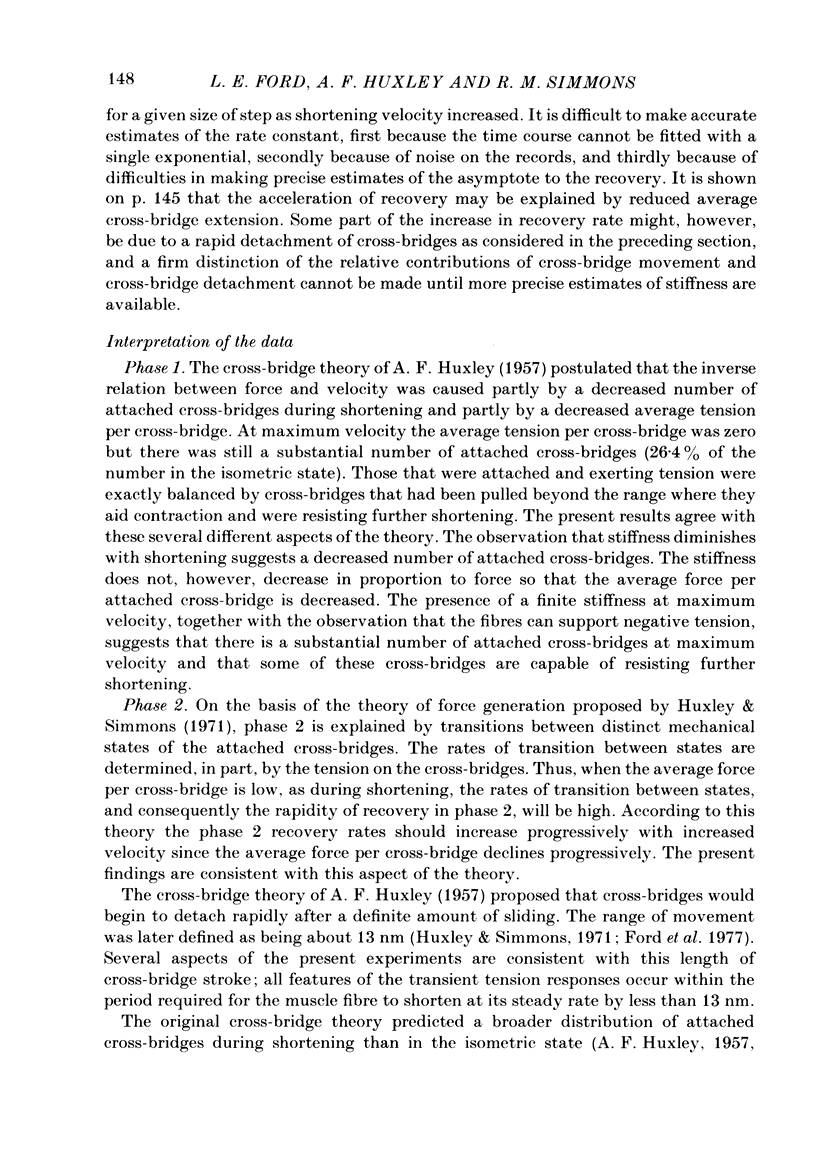
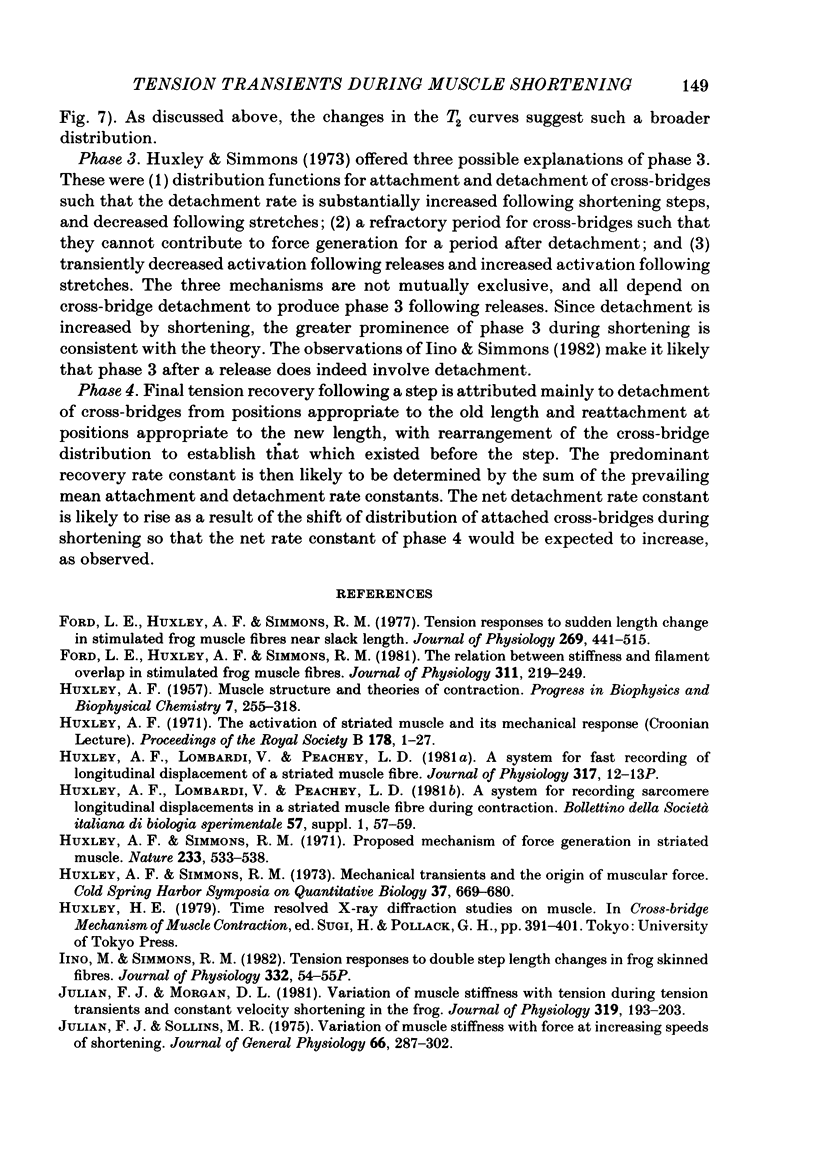
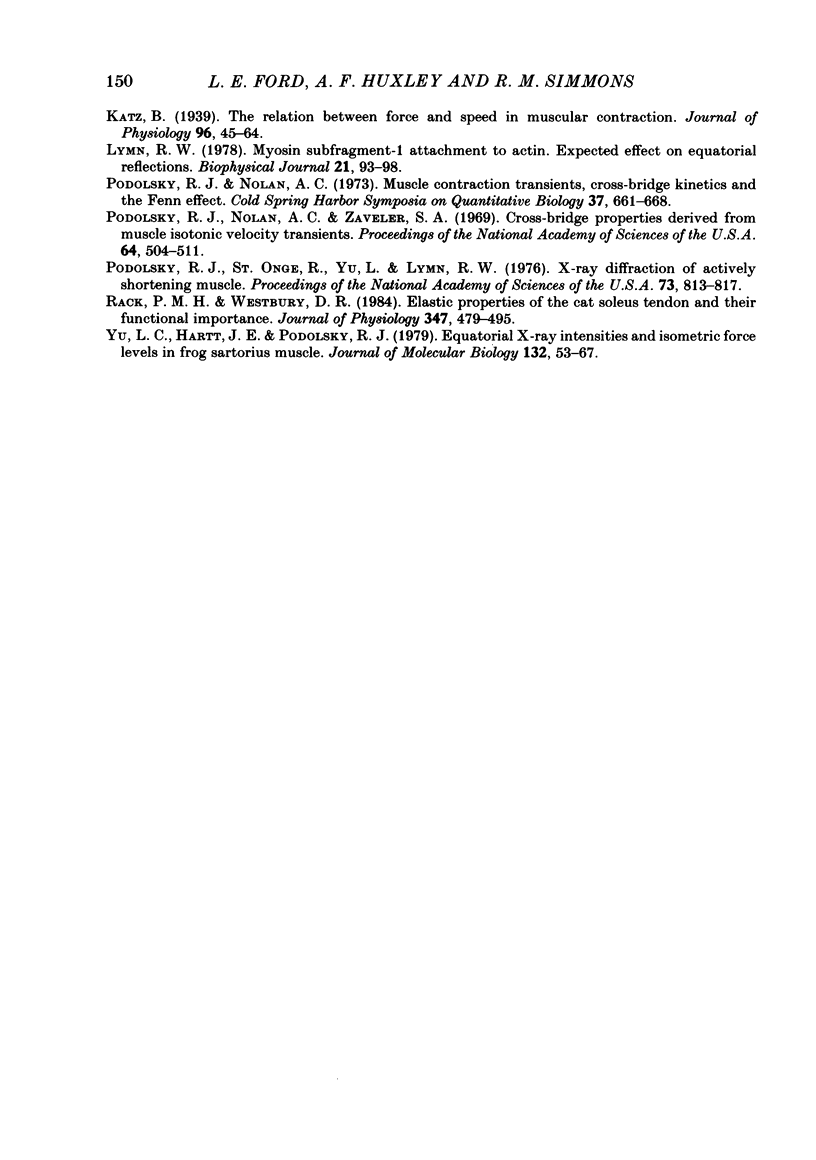
Selected References
These references are in PubMed. This may not be the complete list of references from this article.
- Ford L. E., Huxley A. F., Simmons R. M. Tension responses to sudden length change in stimulated frog muscle fibres near slack length. J Physiol. 1977 Jul;269(2):441–515. doi: 10.1113/jphysiol.1977.sp011911. [DOI] [PMC free article] [PubMed] [Google Scholar]
- Ford L. E., Huxley A. F., Simmons R. M. The relation between stiffness and filament overlap in stimulated frog muscle fibres. J Physiol. 1981 Feb;311:219–249. doi: 10.1113/jphysiol.1981.sp013582. [DOI] [PMC free article] [PubMed] [Google Scholar]
- HUXLEY A. F. Muscle structure and theories of contraction. Prog Biophys Biophys Chem. 1957;7:255–318. [PubMed] [Google Scholar]
- Huxley A. F., Simmons R. M. Proposed mechanism of force generation in striated muscle. Nature. 1971 Oct 22;233(5321):533–538. doi: 10.1038/233533a0. [DOI] [PubMed] [Google Scholar]
- Julian F. J., Morgan D. L. Variation of muscle stiffness with tension during tension transients and constant velocity shortening in the frog. J Physiol. 1981;319:193–203. doi: 10.1113/jphysiol.1981.sp013901. [DOI] [PMC free article] [PubMed] [Google Scholar]
- Julian F. J., Sollins M. R. Variation of muscle stiffness with force at increasing speeds of shortening. J Gen Physiol. 1975 Sep;66(3):287–302. doi: 10.1085/jgp.66.3.287. [DOI] [PMC free article] [PubMed] [Google Scholar]
- Katz B. The relation between force and speed in muscular contraction. J Physiol. 1939 Jun 14;96(1):45–64. doi: 10.1113/jphysiol.1939.sp003756. [DOI] [PMC free article] [PubMed] [Google Scholar]
- Lymn R. W. Myosin subfragment-1 attachment to actin. Expected effect on equatorial reflections. Biophys J. 1978 Jan;21(1):93–98. doi: 10.1016/S0006-3495(78)85510-6. [DOI] [PMC free article] [PubMed] [Google Scholar]
- Podolsky R. J., Nolan A. C., Zaveler S. A. Cross-bridge properties derived from muscle isotonic velocity transients. Proc Natl Acad Sci U S A. 1969 Oct;64(2):504–511. doi: 10.1073/pnas.64.2.504. [DOI] [PMC free article] [PubMed] [Google Scholar]
- Podolsky R. J., St Onge H., Yu L., Lymn R. W. X-ray diffraction of actively shortening muscle. Proc Natl Acad Sci U S A. 1976 Mar;73(3):813–817. doi: 10.1073/pnas.73.3.813. [DOI] [PMC free article] [PubMed] [Google Scholar]
- Rack P. M., Westbury D. R. Elastic properties of the cat soleus tendon and their functional importance. J Physiol. 1984 Feb;347:479–495. doi: 10.1113/jphysiol.1984.sp015077. [DOI] [PMC free article] [PubMed] [Google Scholar]
- Yu L. P., Hartt J. E., Podolsky R. J. Equatorial x-ray intensities and isometric force levels in frog sartorius muscle. J Mol Biol. 1979 Jul 25;132(1):53–67. doi: 10.1016/0022-2836(79)90495-9. [DOI] [PubMed] [Google Scholar]