Abstract
86Rb efflux from pinched-off rat brain presynaptic nerve terminals (synaptosomes) was used to measure the K permeability of the terminals. Synaptosomes were pre-loaded with 86Rb and the suspensions were then filtered on glass fibre filters. The terminals trapped on the filters were superfused with 'efflux solutions', and the effluent and filters were then counted. 86Rb efflux into physiological saline (PSS) containing 5 mM-K and 145 mM-Na was about 0.4% of the 86Rb load per second (component 'R'). Increasing extracellular K concentration [( K]o), or adding veratridine and sea anemone toxin, stimulated efflux; presumably by depolarizing the nerve terminals. The K-stimulated 86Rb efflux was a graded function of [K]o. High [K]o evoked at least three components of efflux: a 'fast phase' (T) that apparently inactivated in less than 1 s, a 'slower phase' (S) that was linear for 3-5 s, and a Ca-dependent phase (C). Some, but not all, of the slow phase 86Rb efflux (component S) may be attributable to increased efflux mediated by the 'resting' K permeability mechanism when the driving force is increased by depolarization. K efflux was also studied and was found to be qualitatively similar to 86Rb efflux. 86Rb: 42K permeability ratios were 0.6-0.8 for most components of the efflux. Raising the Mg concentration in the efflux solution shifted the 86Rb efflux versus [K]o curve in the direction of increased [K]o. This shift may be the result of screening of surface charges by Mg. Several agents that block various K channels in other preparations inhibited K-stimulated 86Rb efflux in synaptosomes: tetraethylammonium (TEA), tetrabutylammonium (TBA), and 4-aminopyridine (4-AP). The fast component (T) of high [K]o-stimulated 86Rb efflux was selectively blocked by low concentrations of 4-AP (apparent half-maximal inhibition, KI = 0.1-0.2 mM); it was also blocked by TEA (KI = 0.6 mM) and TBA (KI = 0.8-1.0 mM). Dose-response curves for inhibition of component T by all three agents were monophasic. the slow component (S) of the K-stimulated 86Rb efflux was much less sensitive to all three agents, than was component T; the broad dose-response curves were consistent with the view that two (or more) different K conductances may contribute to component S.(ABSTRACT TRUNCATED AT 400 WORDS)
Full text
PDF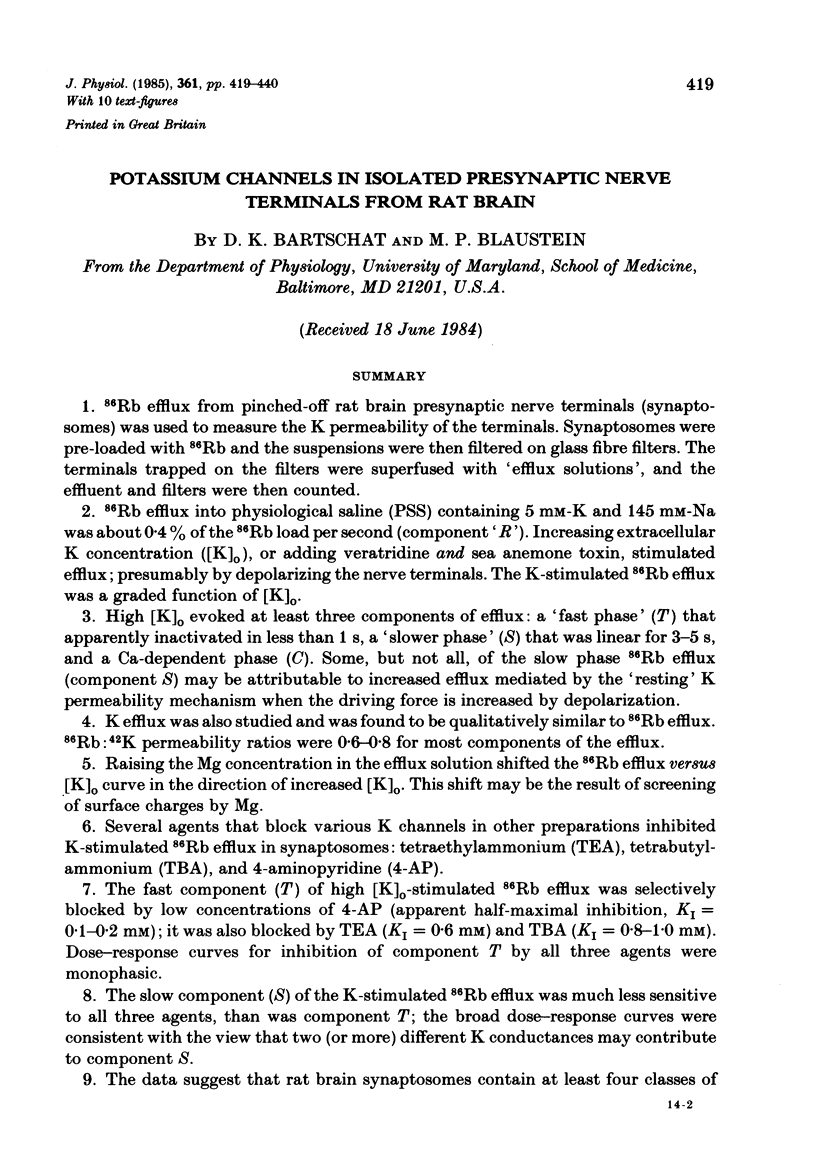
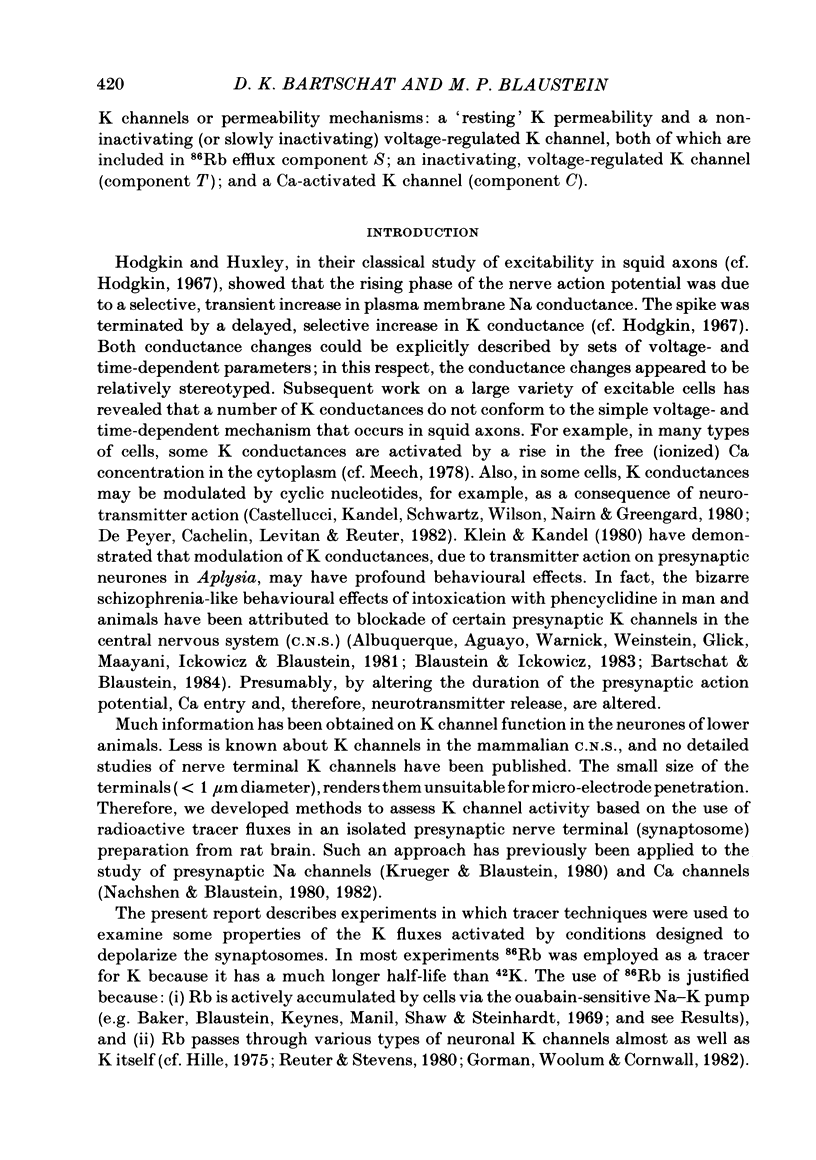
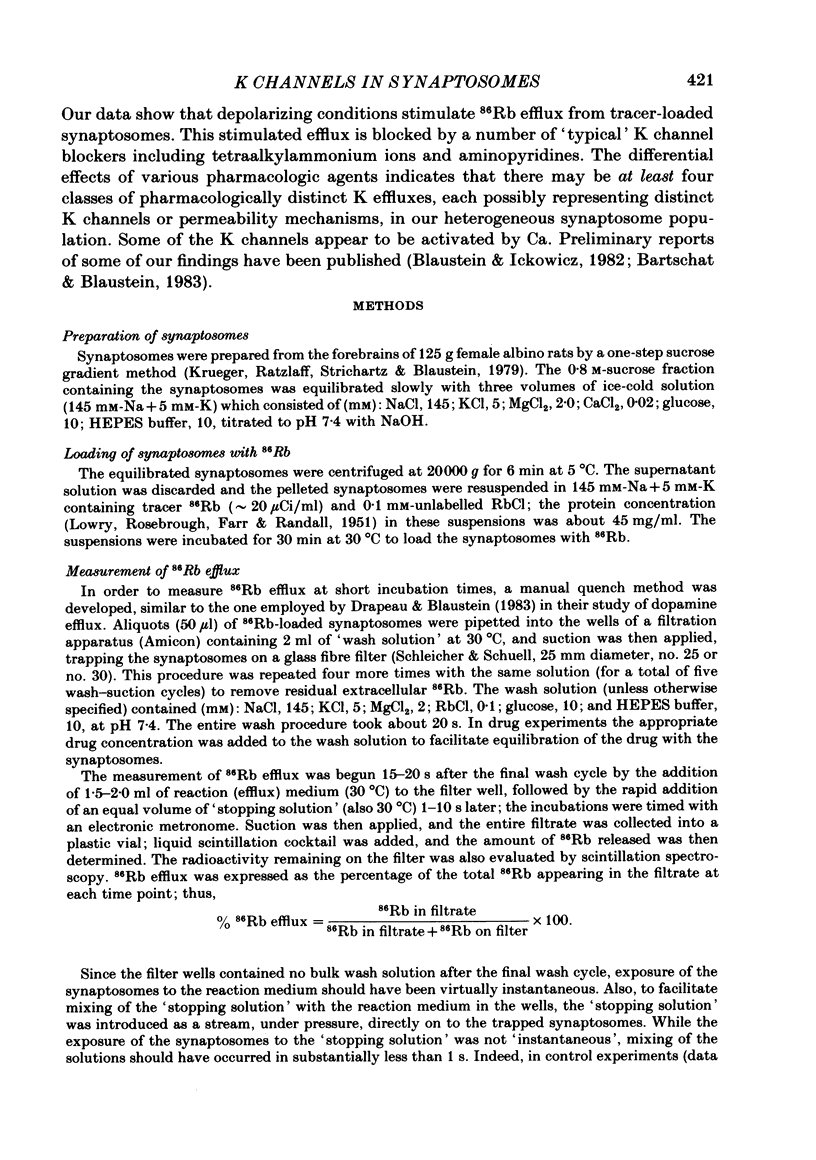
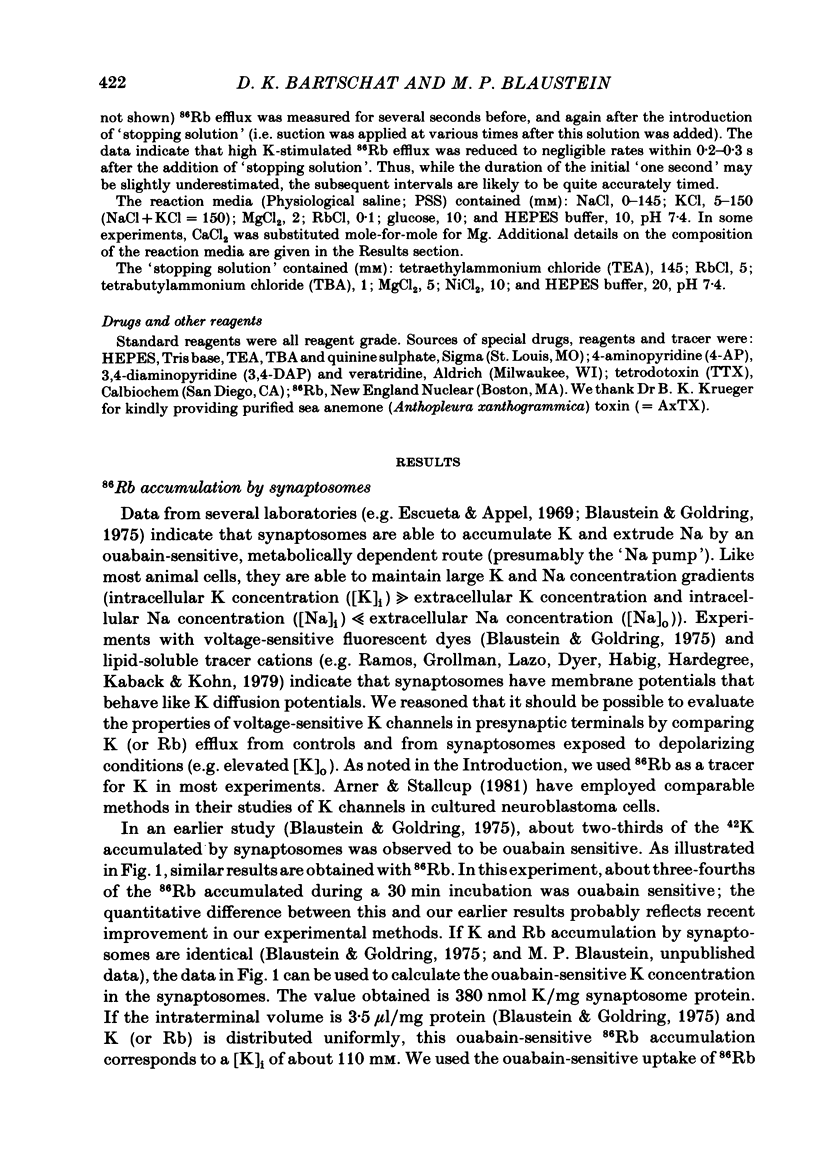
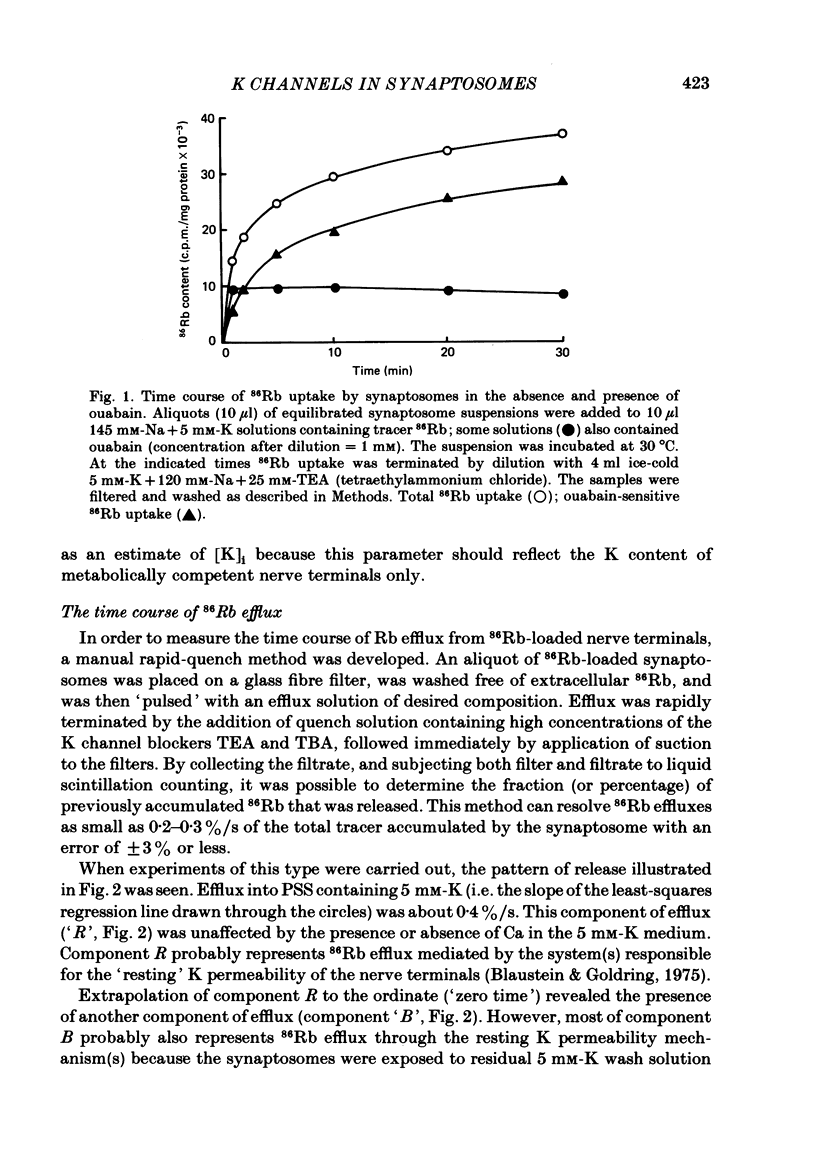
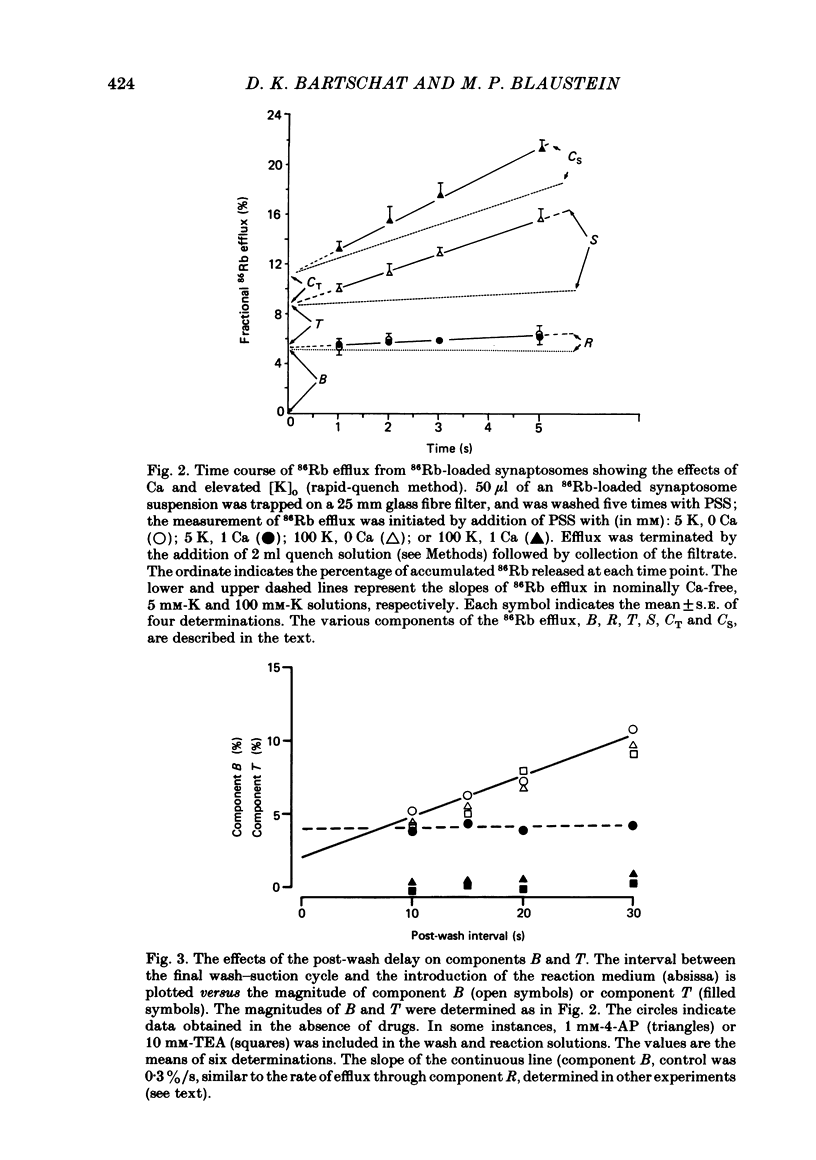
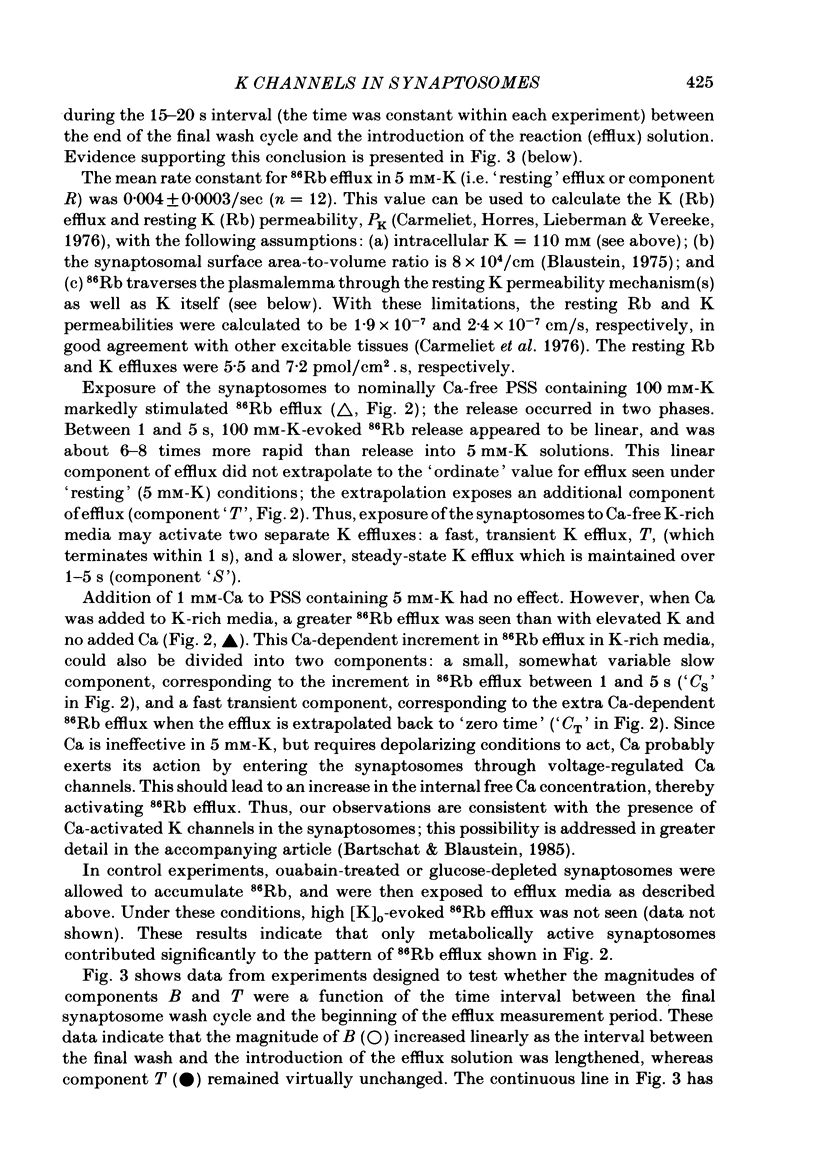
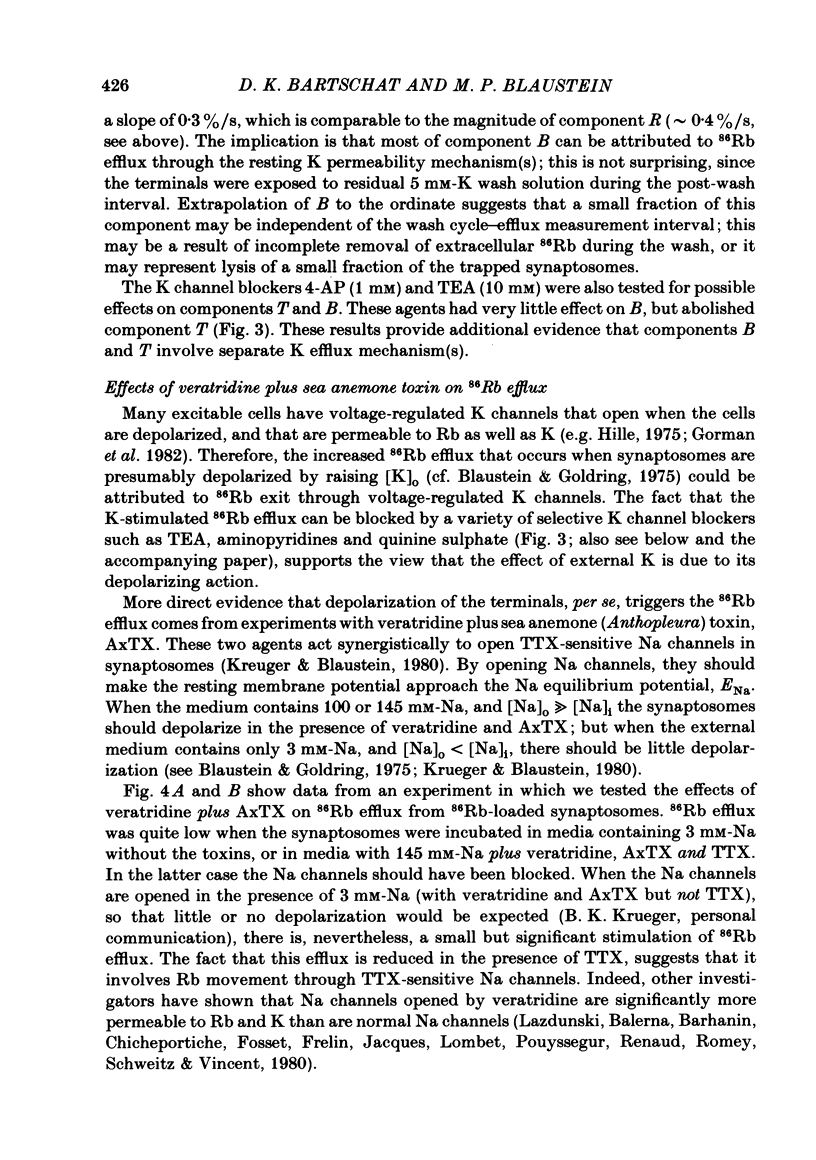
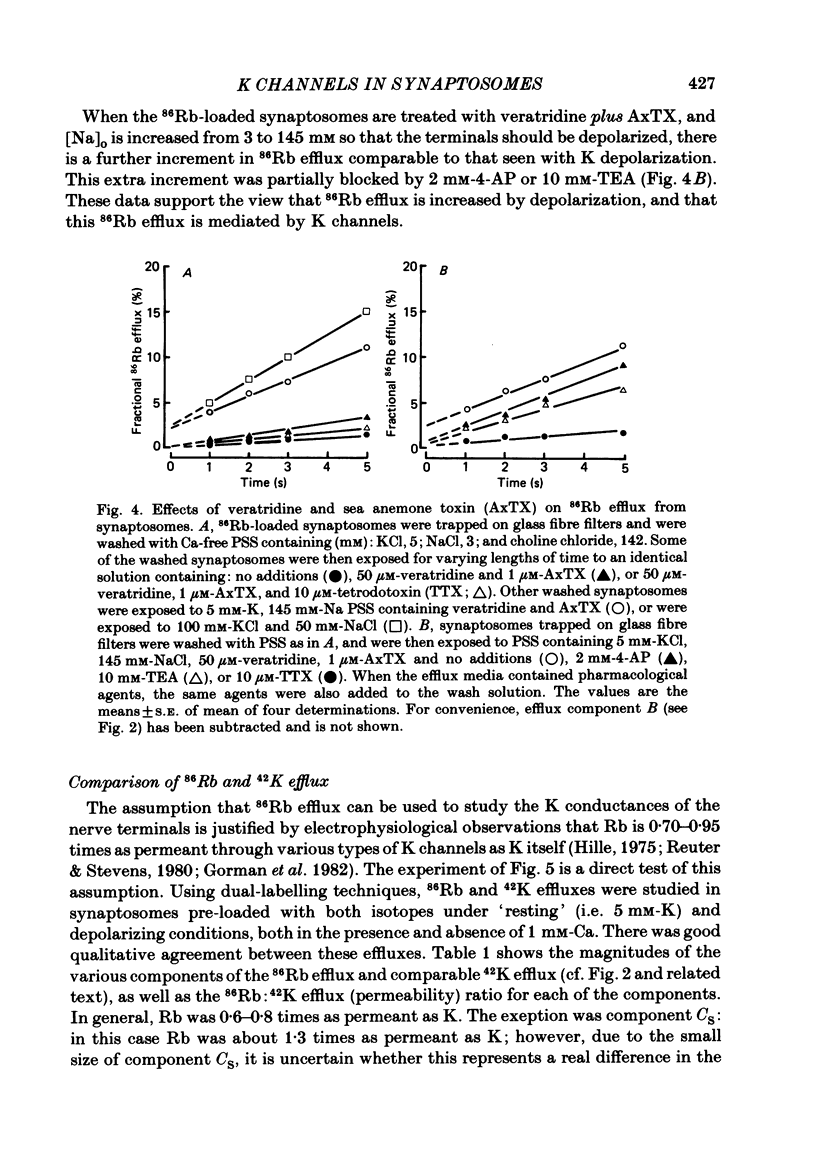
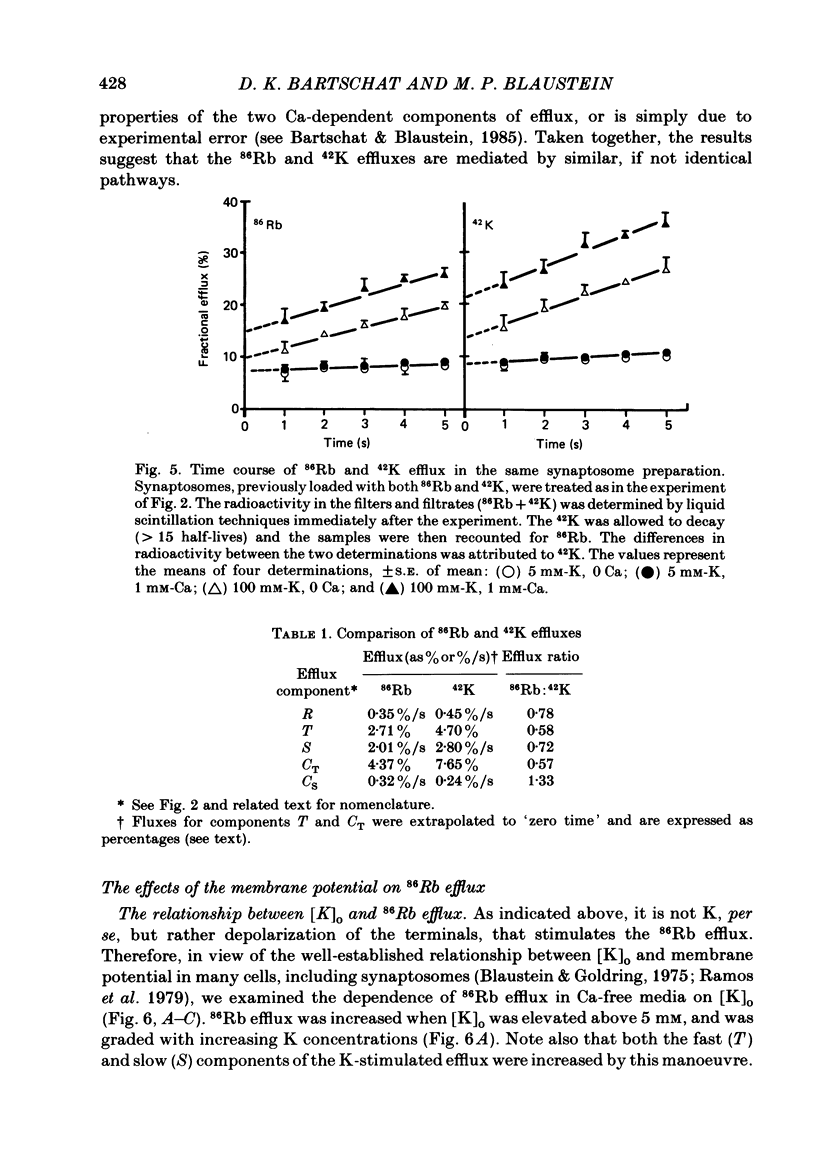
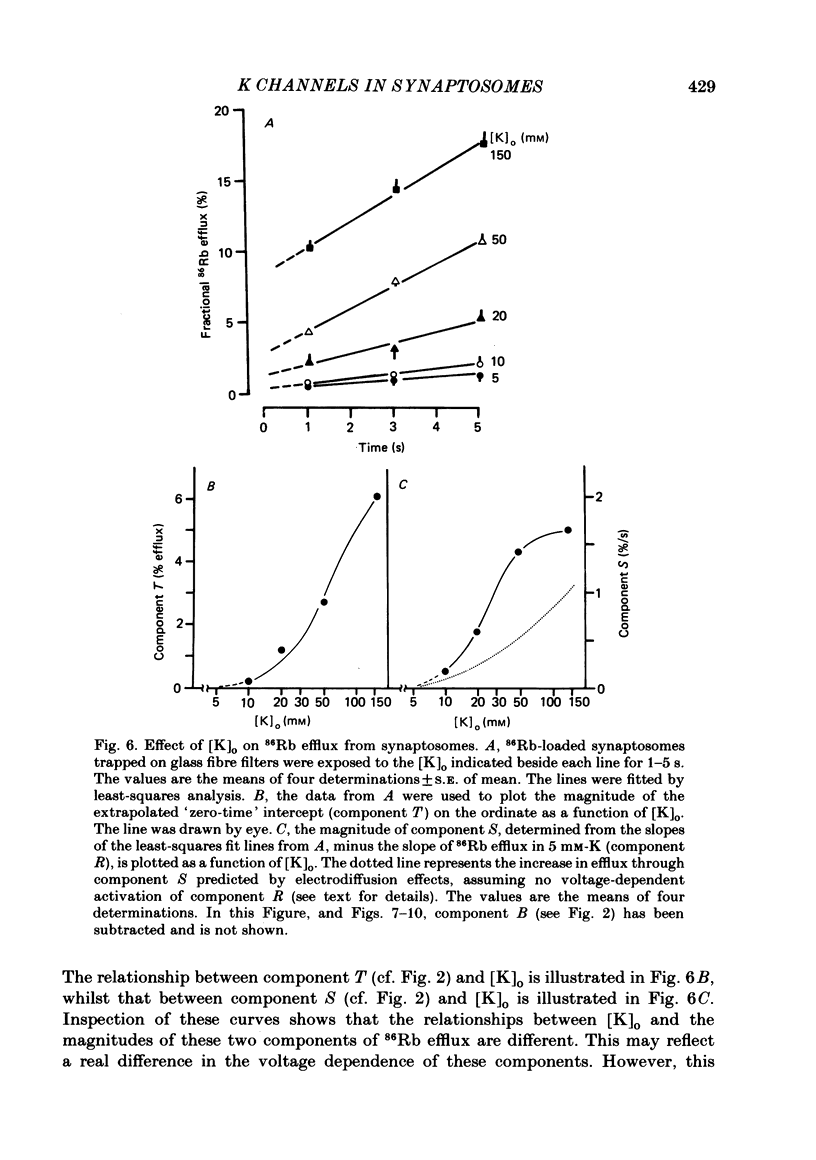
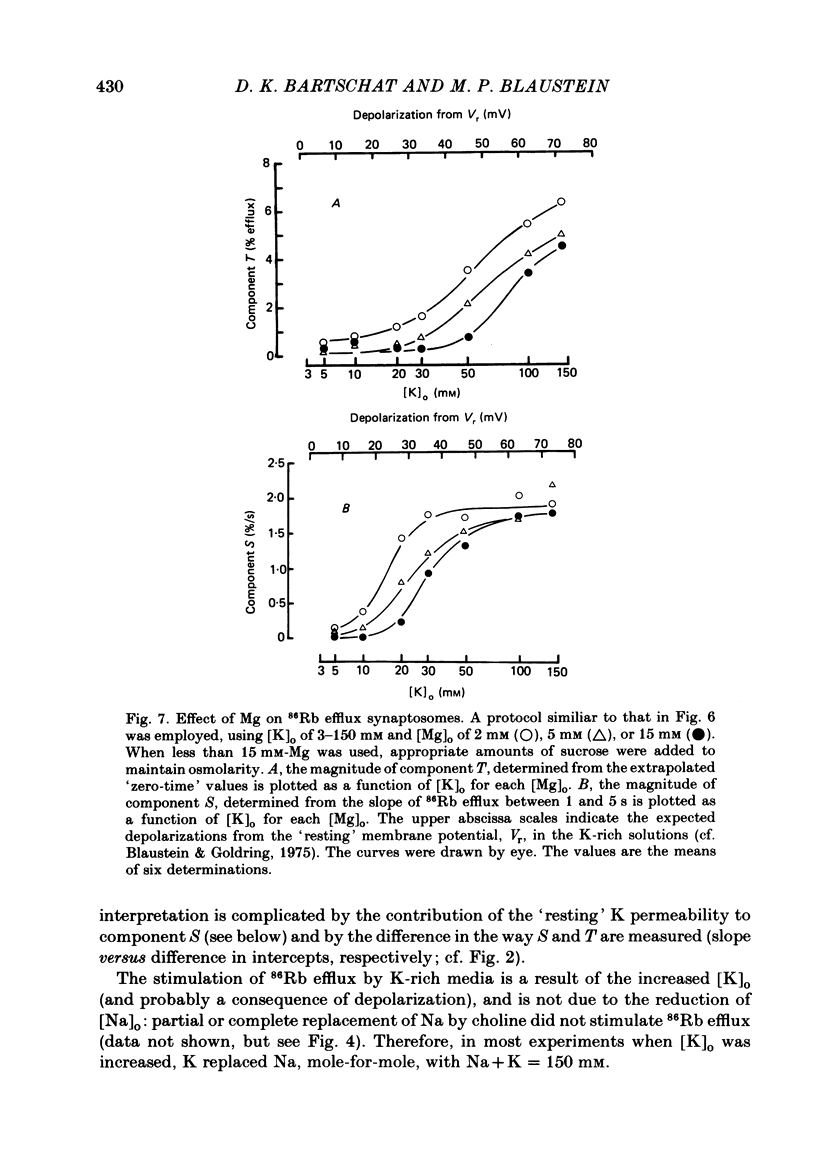
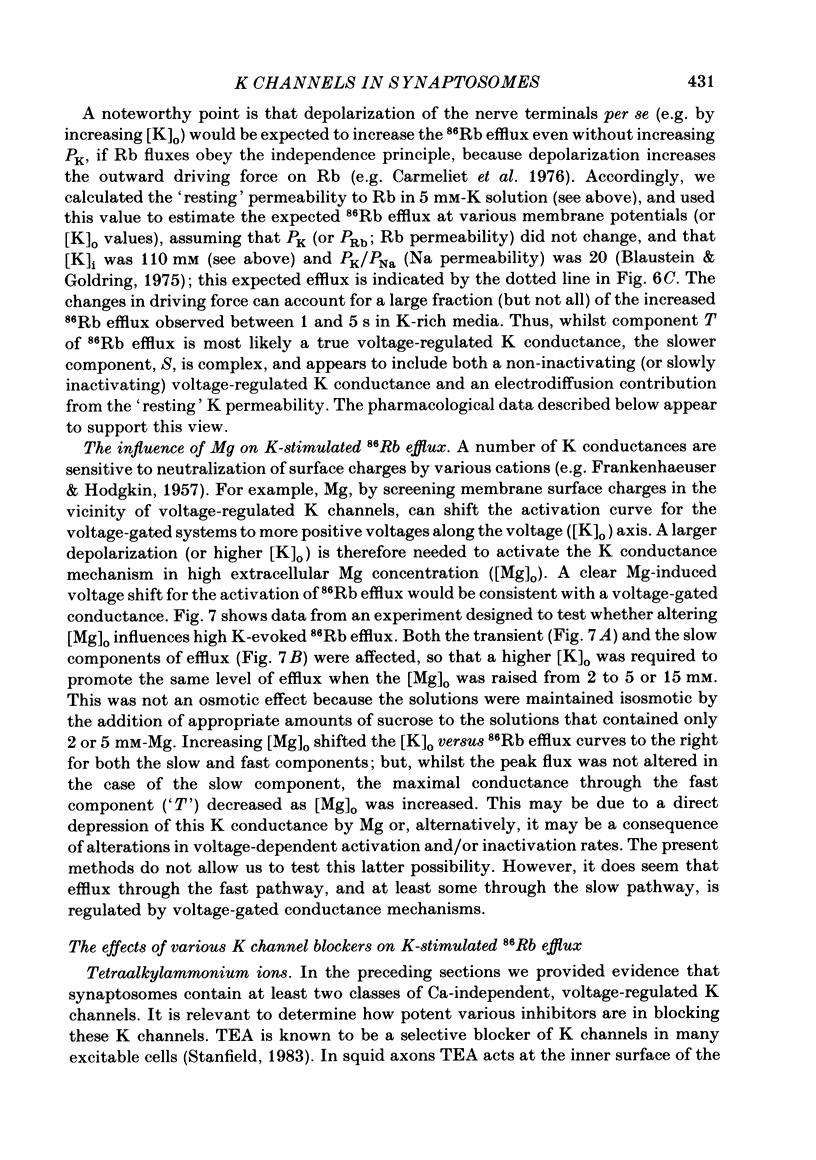
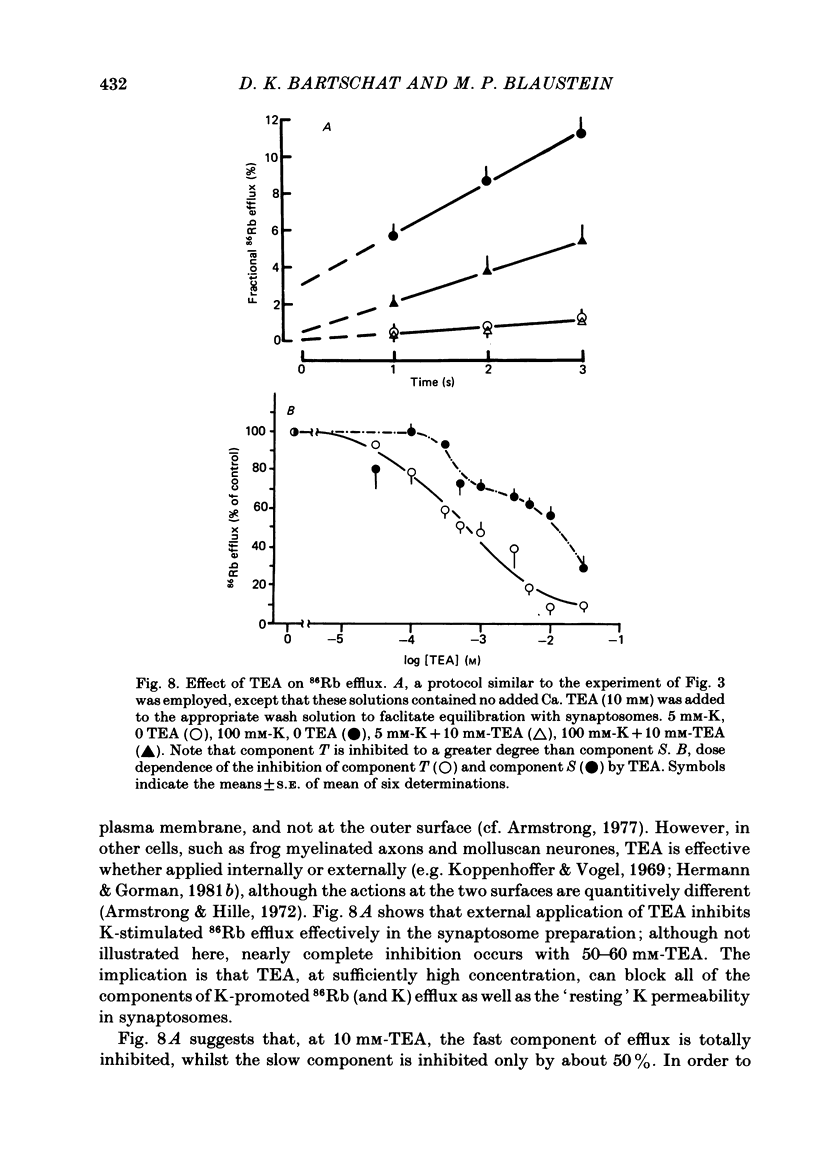
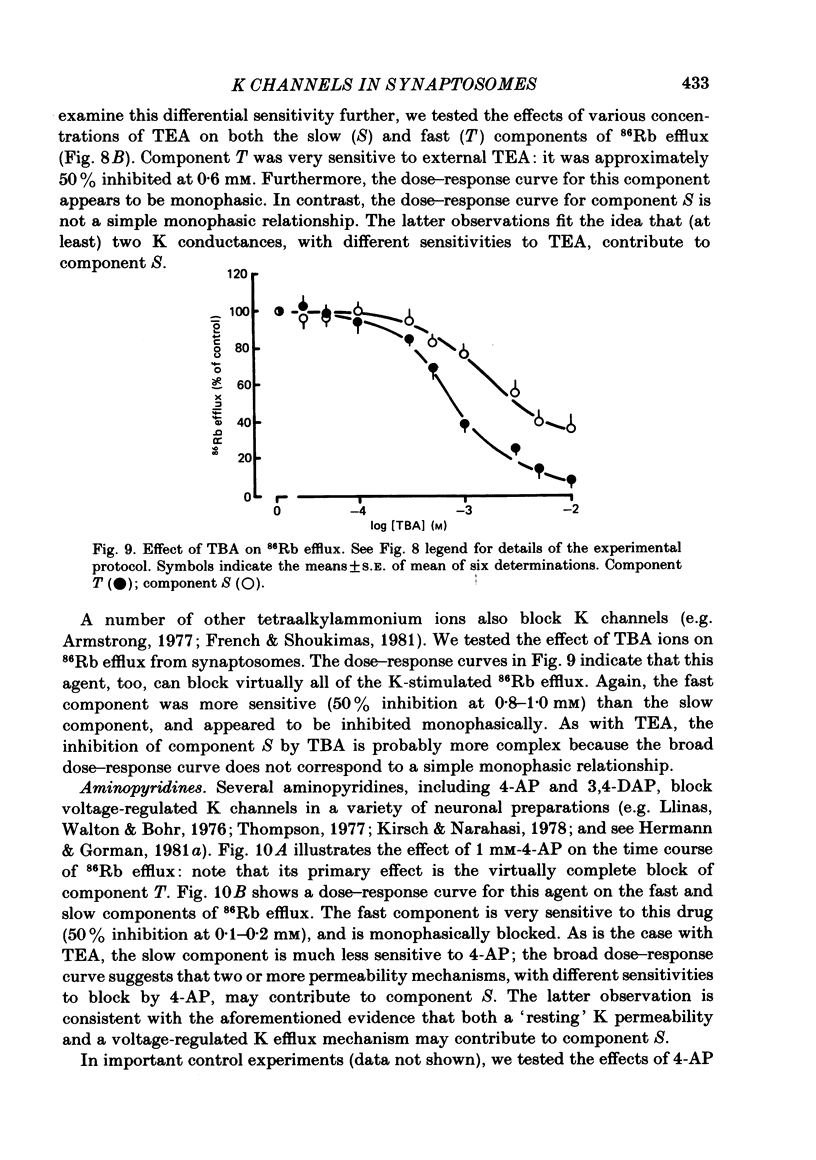
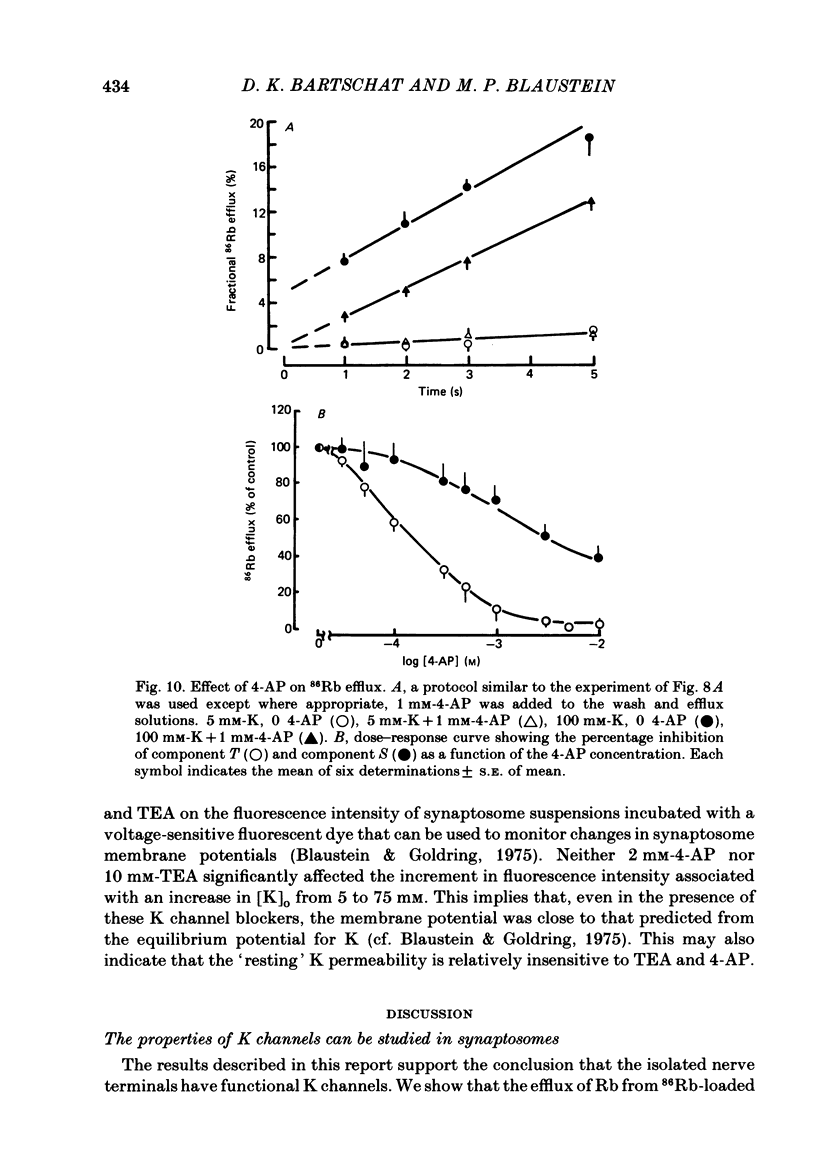
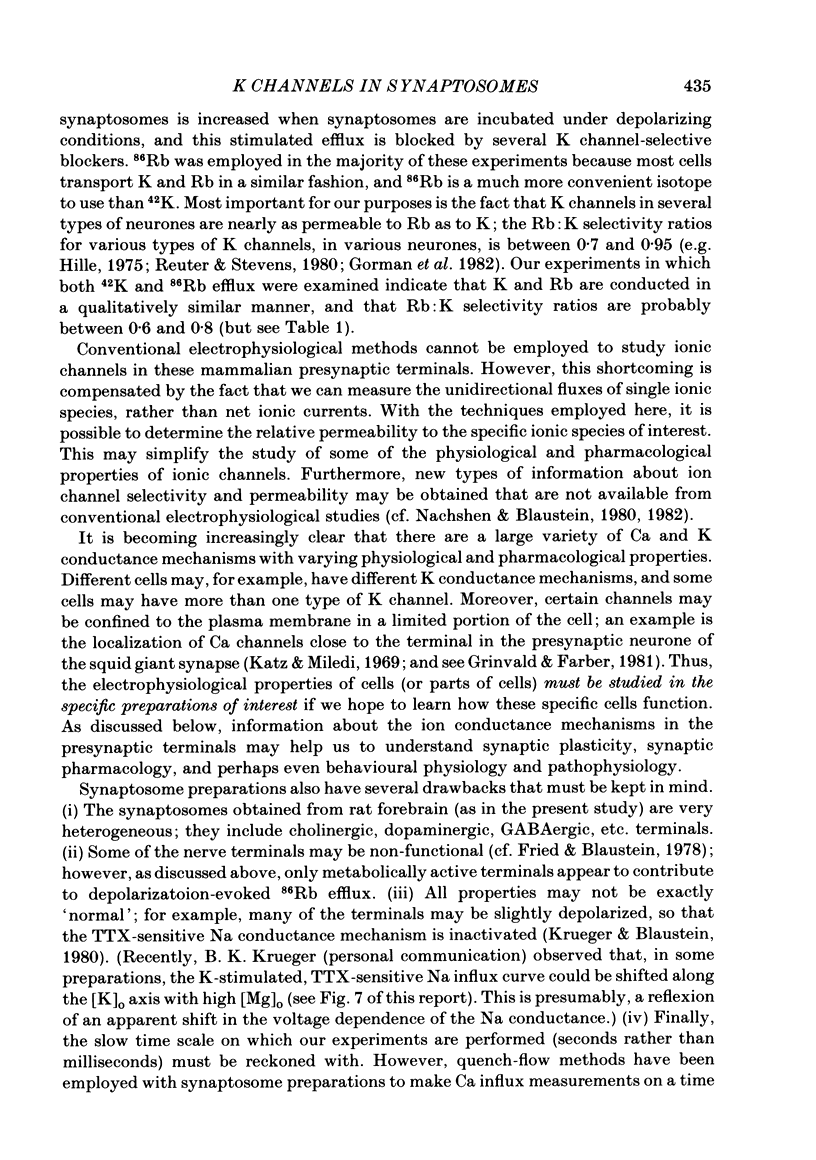
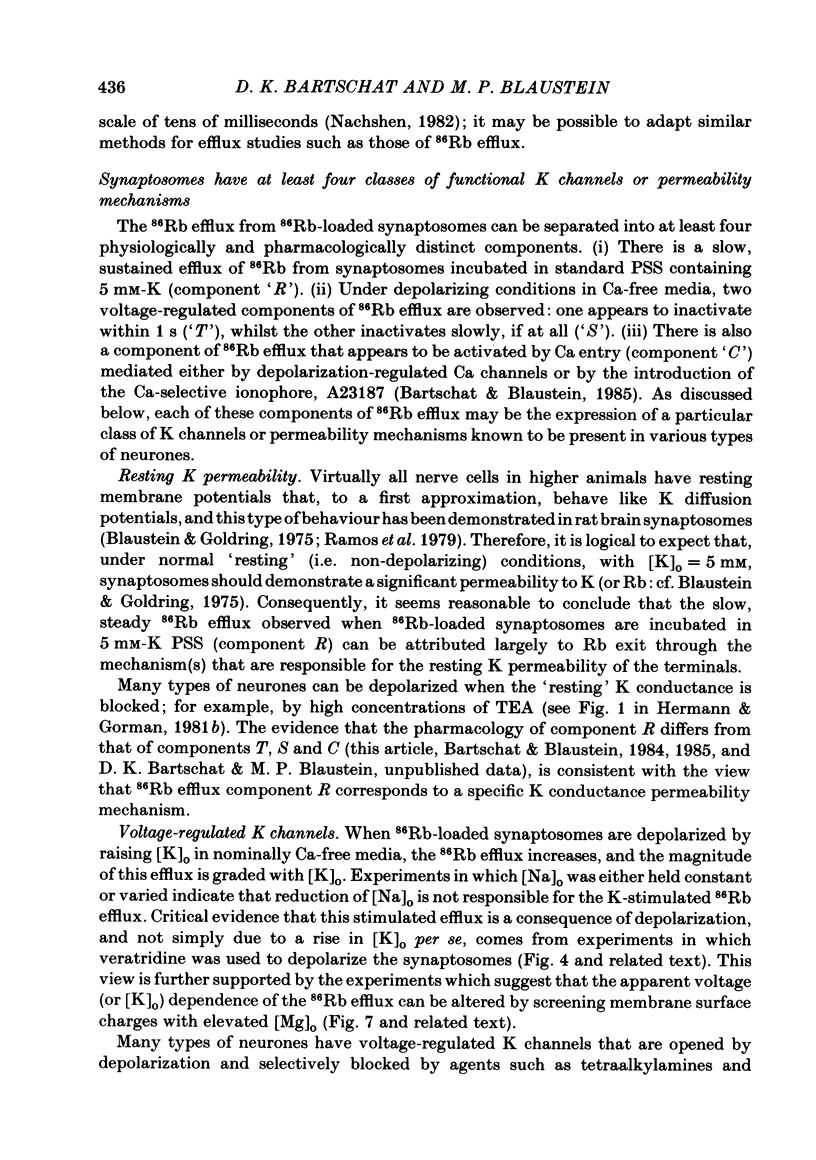
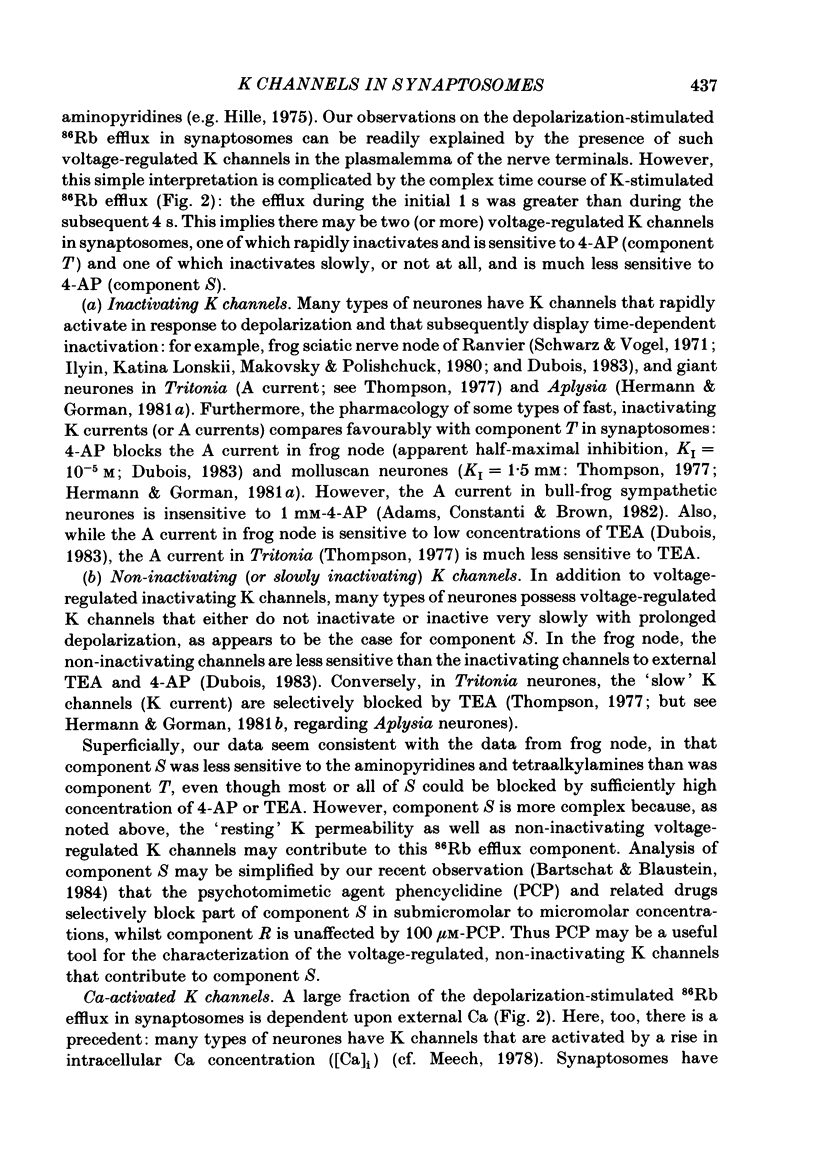
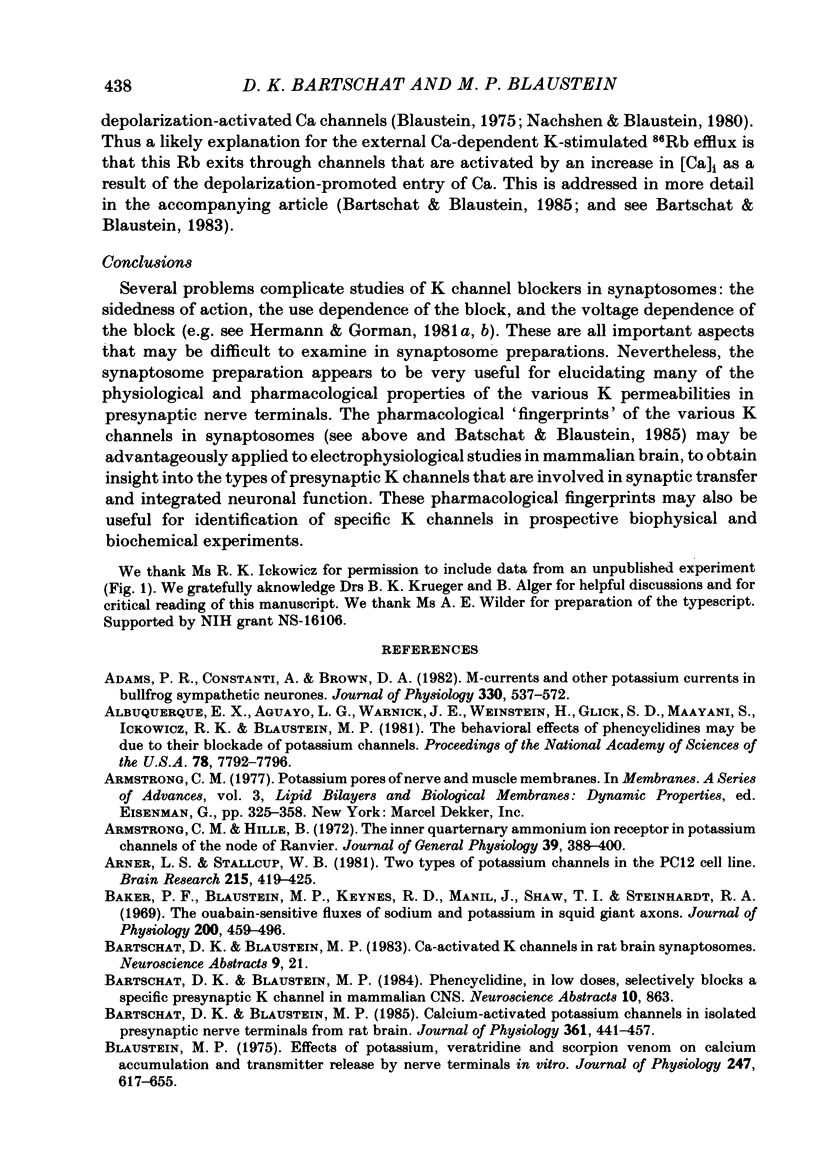
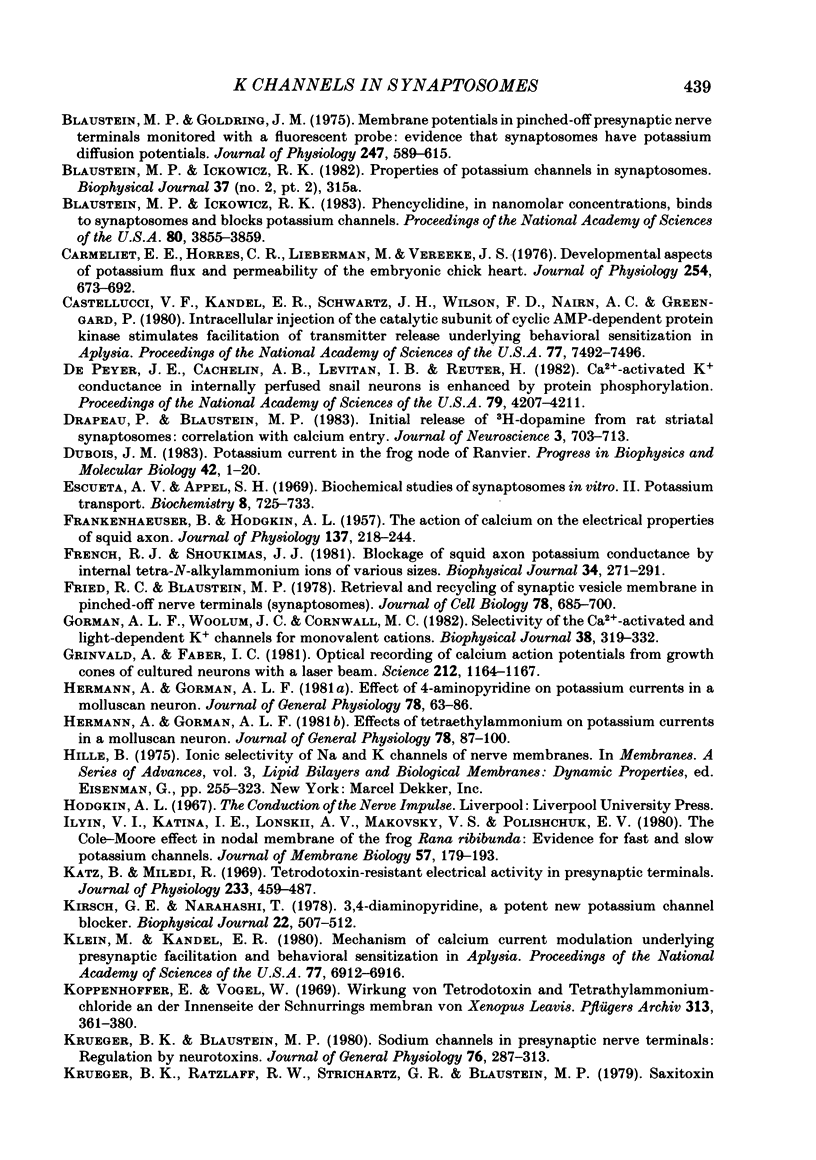
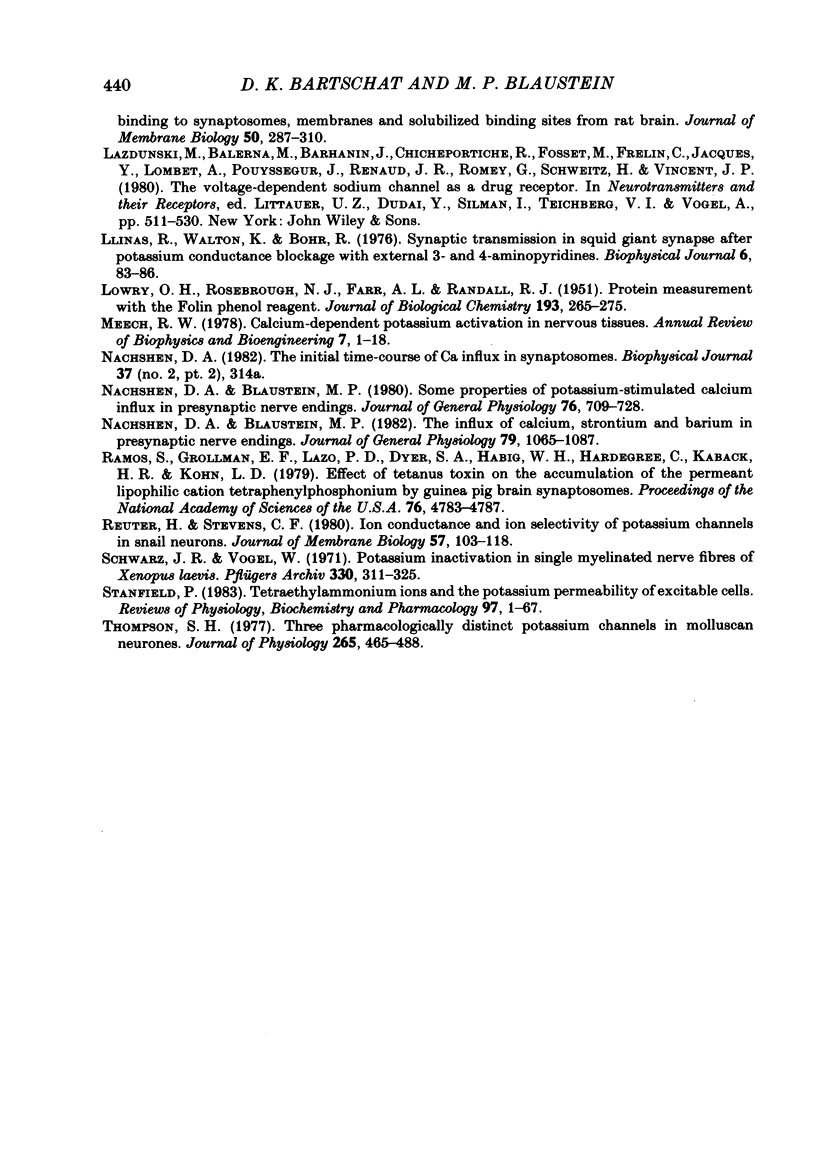
Selected References
These references are in PubMed. This may not be the complete list of references from this article.
- Adams P. R., Brown D. A., Constanti A. M-currents and other potassium currents in bullfrog sympathetic neurones. J Physiol. 1982 Sep;330:537–572. doi: 10.1113/jphysiol.1982.sp014357. [DOI] [PMC free article] [PubMed] [Google Scholar]
- Albuquerque E. X., Aguayo L. G., Warnick J. E., Weinstein H., Glick S. D., Maayani S., Ickowicz R. K., Blaustein M. P. The behavioral effects of phencyclidines may be due to their blockade of potassium channels. Proc Natl Acad Sci U S A. 1981 Dec;78(12):7792–7796. doi: 10.1073/pnas.78.12.7792. [DOI] [PMC free article] [PubMed] [Google Scholar]
- Armstrong C. M., Hille B. The inner quaternary ammonium ion receptor in potassium channels of the node of Ranvier. J Gen Physiol. 1972 Apr;59(4):388–400. doi: 10.1085/jgp.59.4.388. [DOI] [PMC free article] [PubMed] [Google Scholar]
- Arner L. S., Stallcup W. B. Two types of potassium channels in the PC12 cell line. Brain Res. 1981 Jun 29;215(1-2):419–425. doi: 10.1016/0006-8993(81)90528-x. [DOI] [PubMed] [Google Scholar]
- Baker P. F., Blaustein M. P., Keynes R. D., Manil J., Shaw T. I., Steinhardt R. A. The ouabain-sensitive fluxes of sodium and potassium in squid giant axons. J Physiol. 1969 Feb;200(2):459–496. doi: 10.1113/jphysiol.1969.sp008703. [DOI] [PMC free article] [PubMed] [Google Scholar]
- Bartschat D. K., Blaustein M. P. Calcium-activated potassium channels in isolated presynaptic nerve terminals from rat brain. J Physiol. 1985 Apr;361:441–457. doi: 10.1113/jphysiol.1985.sp015654. [DOI] [PMC free article] [PubMed] [Google Scholar]
- Blaustein M. P. Effects of potassium, veratridine, and scorpion venom on calcium accumulation and transmitter release by nerve terminals in vitro. J Physiol. 1975 Jun;247(3):617–655. doi: 10.1113/jphysiol.1975.sp010950. [DOI] [PMC free article] [PubMed] [Google Scholar]
- Blaustein M. P., Goldring J. M. Membrane potentials in pinched-off presynaptic nerve ternimals monitored with a fluorescent probe: evidence that synaptosomes have potassium diffusion potentials. J Physiol. 1975 Jun;247(3):589–615. doi: 10.1113/jphysiol.1975.sp010949. [DOI] [PMC free article] [PubMed] [Google Scholar]
- Blaustein M. P., Ickowicz R. K. Phencyclidine in nanomolar concentrations binds to synaptosomes and blocks certain potassium channels. Proc Natl Acad Sci U S A. 1983 Jun;80(12):3855–3859. doi: 10.1073/pnas.80.12.3855. [DOI] [PMC free article] [PubMed] [Google Scholar]
- Carmeliet E. E., Horres C. R., Lieberman M., Vereecke J. S. Developmental aspects of potassium flux and permeability of the embryonic chick heart. J Physiol. 1976 Jan;254(3):673–692. doi: 10.1113/jphysiol.1976.sp011252. [DOI] [PMC free article] [PubMed] [Google Scholar]
- Castellucci V. F., Kandel E. R., Schwartz J. H., Wilson F. D., Nairn A. C., Greengard P. Intracellular injection of t he catalytic subunit of cyclic AMP-dependent protein kinase simulates facilitation of transmitter release underlying behavioral sensitization in Aplysia. Proc Natl Acad Sci U S A. 1980 Dec;77(12):7492–7496. doi: 10.1073/pnas.77.12.7492. [DOI] [PMC free article] [PubMed] [Google Scholar]
- Drapeau P., Blaustein M. P. Initial release of [3H]dopamine from rat striatal synaptosomes: correlation with calcium entry. J Neurosci. 1983 Apr;3(4):703–713. doi: 10.1523/JNEUROSCI.03-04-00703.1983. [DOI] [PMC free article] [PubMed] [Google Scholar]
- Dubois J. M. Potassium currents in the frog node of Ranvier. Prog Biophys Mol Biol. 1983;42(1):1–20. doi: 10.1016/0079-6107(83)90002-0. [DOI] [PubMed] [Google Scholar]
- Escueta A. V., Appel S. H. Biochemical studies of synapses in vitro. II. Potassium transport. Biochemistry. 1969 Feb;8(2):725–733. doi: 10.1021/bi00830a038. [DOI] [PubMed] [Google Scholar]
- FRANKENHAEUSER B., HODGKIN A. L. The action of calcium on the electrical properties of squid axons. J Physiol. 1957 Jul 11;137(2):218–244. doi: 10.1113/jphysiol.1957.sp005808. [DOI] [PMC free article] [PubMed] [Google Scholar]
- French R. J., Shoukimas J. J. Blockage of squid axon potassium conductance by internal tetra-N-alkylammonium ions of various sizes. Biophys J. 1981 May;34(2):271–291. doi: 10.1016/S0006-3495(81)84849-7. [DOI] [PMC free article] [PubMed] [Google Scholar]
- Fried R. C., Blaustein M. P. Retrieval and recycling of synaptic vesicle membrane in pinched-off nerve terminals (synaptosomes). J Cell Biol. 1978 Sep;78(3):685–700. doi: 10.1083/jcb.78.3.685. [DOI] [PMC free article] [PubMed] [Google Scholar]
- Gorman A. L., Woolum J. C., Cornwall M. C. Selectivity of the Ca2+-activated and light-dependent K+ channels for monovalent cations. Biophys J. 1982 Jun;38(3):319–322. doi: 10.1016/S0006-3495(82)84565-7. [DOI] [PMC free article] [PubMed] [Google Scholar]
- Grinvald A., Farber I. C. Optical recording of calcium action potentials from growth cones of cultured neurons with a laser microbeam. Science. 1981 Jun 5;212(4499):1164–1167. doi: 10.1126/science.7233210. [DOI] [PubMed] [Google Scholar]
- Hermann A., Gorman A. L. Effects of 4-aminopyridine on potassium currents in a molluscan neuron. J Gen Physiol. 1981 Jul;78(1):63–86. doi: 10.1085/jgp.78.1.63. [DOI] [PMC free article] [PubMed] [Google Scholar]
- Ilyin V. I., Katina I. E., Lonskii A. V., Makovsky V. S., Polishchuk E. V. The Cole-Moore effect in nodal membrane of the frog Rana ridibunda: evidence for fast and slow potassium channels. J Membr Biol. 1980 Dec 30;57(3):179–193. doi: 10.1007/BF01869586. [DOI] [PubMed] [Google Scholar]
- Katz B., Miledi R. Tetrodotoxin-resistant electric activity in presynaptic terminals. J Physiol. 1969 Aug;203(2):459–487. doi: 10.1113/jphysiol.1969.sp008875. [DOI] [PMC free article] [PubMed] [Google Scholar]
- Kirsch G. E., Narahashi T. 3,4-diaminopyridine. A potent new potassium channel blocker. Biophys J. 1978 Jun;22(3):507–512. doi: 10.1016/S0006-3495(78)85503-9. [DOI] [PMC free article] [PubMed] [Google Scholar]
- Klein M., Kandel E. R. Mechanism of calcium current modulation underlying presynaptic facilitation and behavioral sensitization in Aplysia. Proc Natl Acad Sci U S A. 1980 Nov;77(11):6912–6916. doi: 10.1073/pnas.77.11.6912. [DOI] [PMC free article] [PubMed] [Google Scholar]
- Koppenhöfer E., Vogel W. Wirkung von Tetrodotoxin und Tetraäthylammoniumchlorid an der Innenseite der Schnürringsmembran von Xenopus laevis. Pflugers Arch. 1969;313(4):361–380. doi: 10.1007/BF00593959. [DOI] [PubMed] [Google Scholar]
- Krueger B. K., Blaustein M. P., Ratzlaff R. W. Sodium channels in presynaptic nerve terminals. Regulation by neurotoxins. J Gen Physiol. 1980 Sep;76(3):287–313. doi: 10.1085/jgp.76.3.287. [DOI] [PMC free article] [PubMed] [Google Scholar]
- Krueger B. K., Ratzlaff R. W., Strichartz G. R., Blaustein M. P. Saxitoxin binding to synaptosomes, membranes, and solubilized binding sites from rat brain. J Membr Biol. 1979 Nov 30;50(3-4):287–310. doi: 10.1007/BF01868894. [DOI] [PubMed] [Google Scholar]
- LOWRY O. H., ROSEBROUGH N. J., FARR A. L., RANDALL R. J. Protein measurement with the Folin phenol reagent. J Biol Chem. 1951 Nov;193(1):265–275. [PubMed] [Google Scholar]
- Llinás R., Walton K., Bohr V. Synaptic transmission in squid giant synapse after potassium conductance blockage with external 3- and 4-aminopyridine. Biophys J. 1976 Jan;16(1):83–86. doi: 10.1016/S0006-3495(76)85664-0. [DOI] [PMC free article] [PubMed] [Google Scholar]
- Meech R. W. Calcium-dependent potassium activation in nervous tissues. Annu Rev Biophys Bioeng. 1978;7:1–18. doi: 10.1146/annurev.bb.07.060178.000245. [DOI] [PubMed] [Google Scholar]
- Nachshen D. A., Blaustein M. P. Influx of calcium, strontium, and barium in presynaptic nerve endings. J Gen Physiol. 1982 Jun;79(6):1065–1087. doi: 10.1085/jgp.79.6.1065. [DOI] [PMC free article] [PubMed] [Google Scholar]
- Nachshen D. A., Blaustein M. P. Some properties of potassium-stimulated calcium influx in presynaptic nerve endings. J Gen Physiol. 1980 Dec;76(6):709–728. doi: 10.1085/jgp.76.6.709. [DOI] [PMC free article] [PubMed] [Google Scholar]
- Ramos S., Grollman E. F., Lazo P. S., Dyer S. A., Habig W. H., Hardegree M. C., Kaback H. R., Kohn L. D. Effect of tetanus toxin on the accumulation of the permeant lipophilic cation tetraphenylphosphonium by guinea pig brain synaptosomes. Proc Natl Acad Sci U S A. 1979 Oct;76(10):4783–4787. doi: 10.1073/pnas.76.10.4783. [DOI] [PMC free article] [PubMed] [Google Scholar]
- Reuter H., Stevens C. F. Ion conductance and ion selectivity of potassium channels in snail neurones. J Membr Biol. 1980 Dec 15;57(2):103–118. doi: 10.1007/BF01868997. [DOI] [PubMed] [Google Scholar]
- Stanfield P. R. Tetraethylammonium ions and the potassium permeability of excitable cells. Rev Physiol Biochem Pharmacol. 1983;97:1–67. doi: 10.1007/BFb0035345. [DOI] [PubMed] [Google Scholar]
- Thompson S. H. Three pharmacologically distinct potassium channels in molluscan neurones. J Physiol. 1977 Feb;265(2):465–488. doi: 10.1113/jphysiol.1977.sp011725. [DOI] [PMC free article] [PubMed] [Google Scholar]
- de Peyer J. E., Cachelin A. B., Levitan I. B., Reuter H. Ca2+ -activated K+ conductance in internally perfused snail neurons is enhanced by protein phosphorylation. Proc Natl Acad Sci U S A. 1982 Jul;79(13):4207–4211. doi: 10.1073/pnas.79.13.4207. [DOI] [PMC free article] [PubMed] [Google Scholar]