Abstract
86Rb efflux was examined in isolated presynaptic nerve terminals (synaptosomes) from rat brain in a study designed to assess K permeability (PK) changes sensitive to alterations in internal Ca activity. Rb efflux from 86Rb-loaded synaptosomes into nominally Ca-free physiological saline (PSS) containing 5 mM-K was about 0.3-0.4%/s. Raising extracellular K concentration [( K]o), to depolarize the synaptosomes, stimulated the 86Rb efflux. Addition of Ca to the 5 mM-K PSS had no effect, but Ca did further stimulate 86Rb efflux into K-rich solutions. The effect of Ca was graded, with apparent half-maximal activation, KA approximately equal to 0.5 mM-Ca. These data fit the view that, during depolarization, Ca enters the terminals through voltage-regulated Ca channels, and that the rise in intracellular Ca concentration opens certain (Ca-activated) K channels. The Ca-dependent stimulation of 86Rb efflux was greatest during the initial seconds of incubation (component CT), and then declined to a much lower rate (component CS). Much of this change in rate could be attributed to inactivation of voltage-regulated Ca channels and reduced entry of Ca. The Ca-dependent increase in 86Rb efflux was completely inhibited by 100 microM-La. In the presence of Ca, but not in its absence, the Ca ionophore A23187 stimulated 86Rb efflux both in 5 and 100 mM-K PSS. The effect in 100 mM-K was quantitatively greater, perhaps because of the increased outward driving force on Rb in depolarized synaptosomes. When synaptosomes were suspended in media containing the voltage-sensitive fluorescent dye, DiS-C3-(5) (1,1'-dipentyl-2,2'-thiocarbocyanine), the addition of Ca+ A23187 decreased the fluorescence intensity (= synaptosome hyperpolarization) when the media contained 5 mM-K but not 100 mM-K. This implies that in the presence of Ca + A23187, PK was increased, and the membrane potential moved closer to the K equilibrium potential, EK. Quinine sulphate, a blocker of Ca-activated K channels, reduced the Ca-stimulated 86Rb efflux with high affinity (apparent half-maximal inhibition, KI approximately equal to 1 microM). Tetraethylammonium chloride, another agent known to block Ca-activated K channels, was also a relatively potent inhibitor of Ca-stimulated 86Rb efflux (KI approximately equal to 0.2 mM). The K-channel blocker, 4-aminopyridine, partially inhibited Ca-stimulated 86Rb efflux at concentrations below 0.5 mM, but stimulated this efflux at concentrations greater than or equal to 1 mM.(ABSTRACT TRUNCATED AT 400 WORDS)
Full text
PDF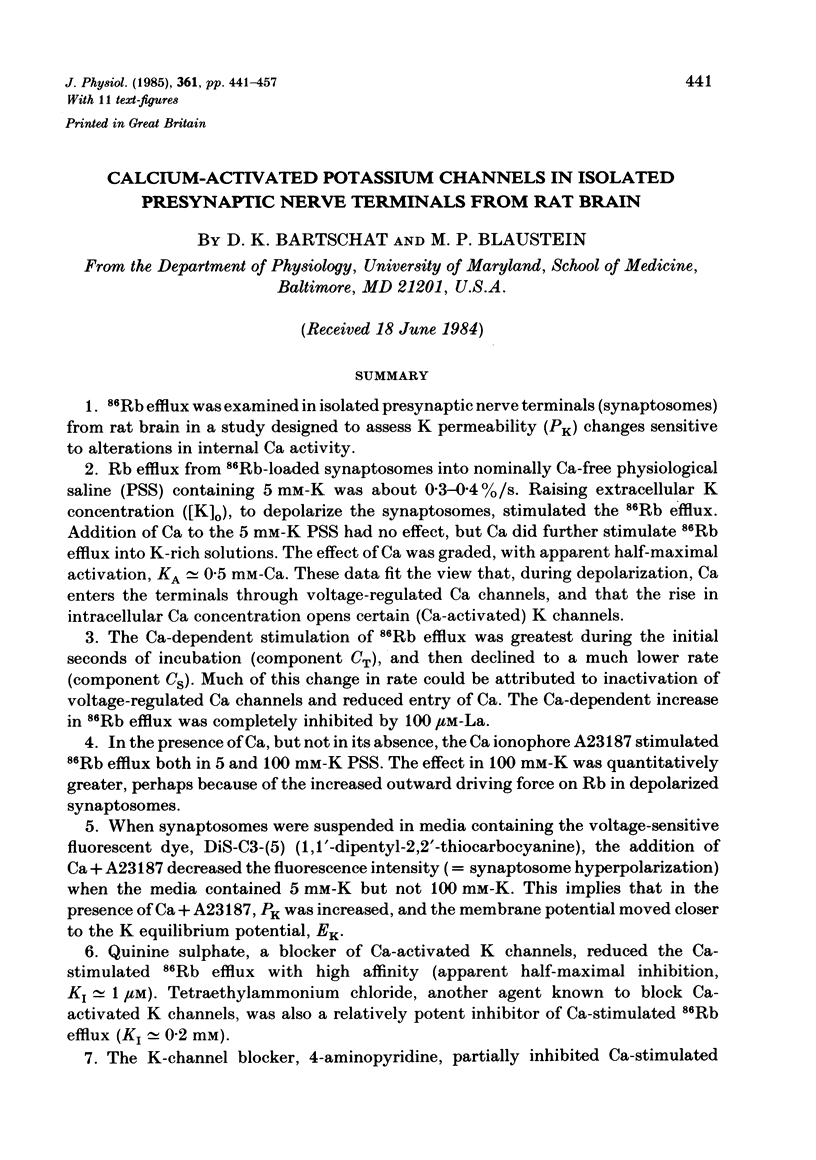
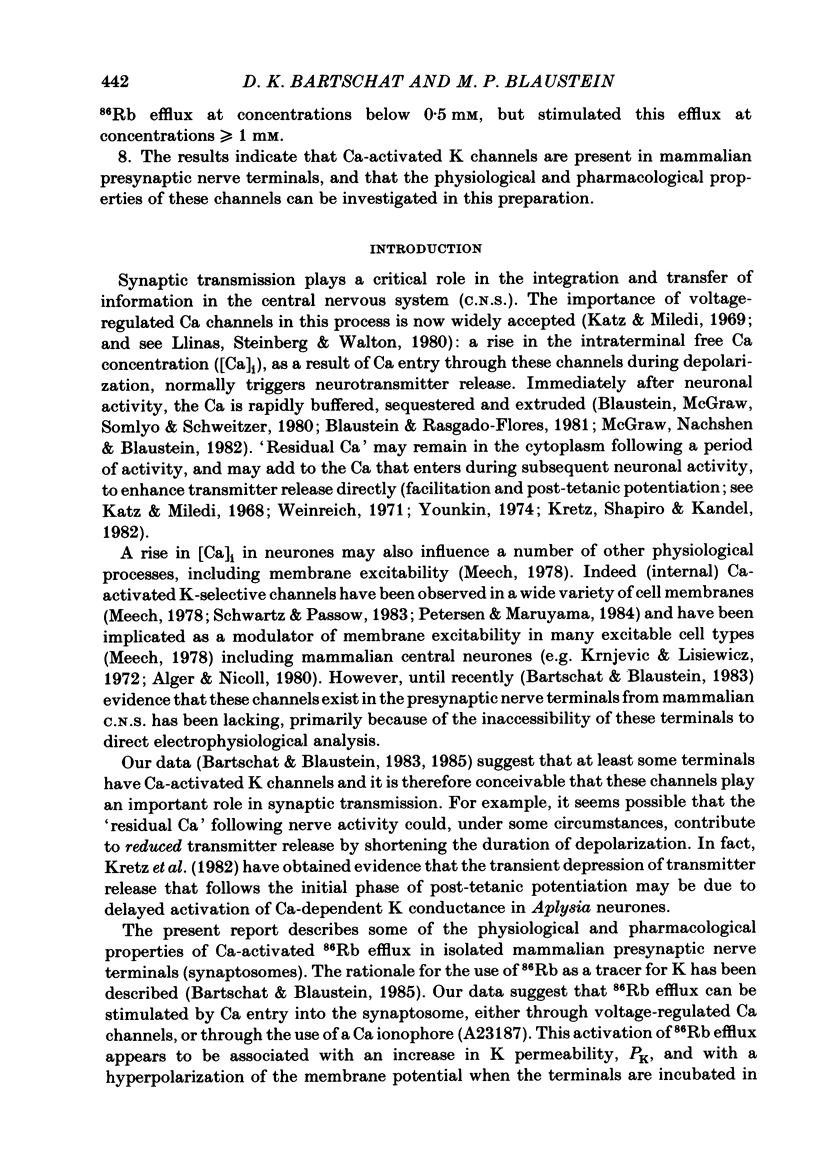
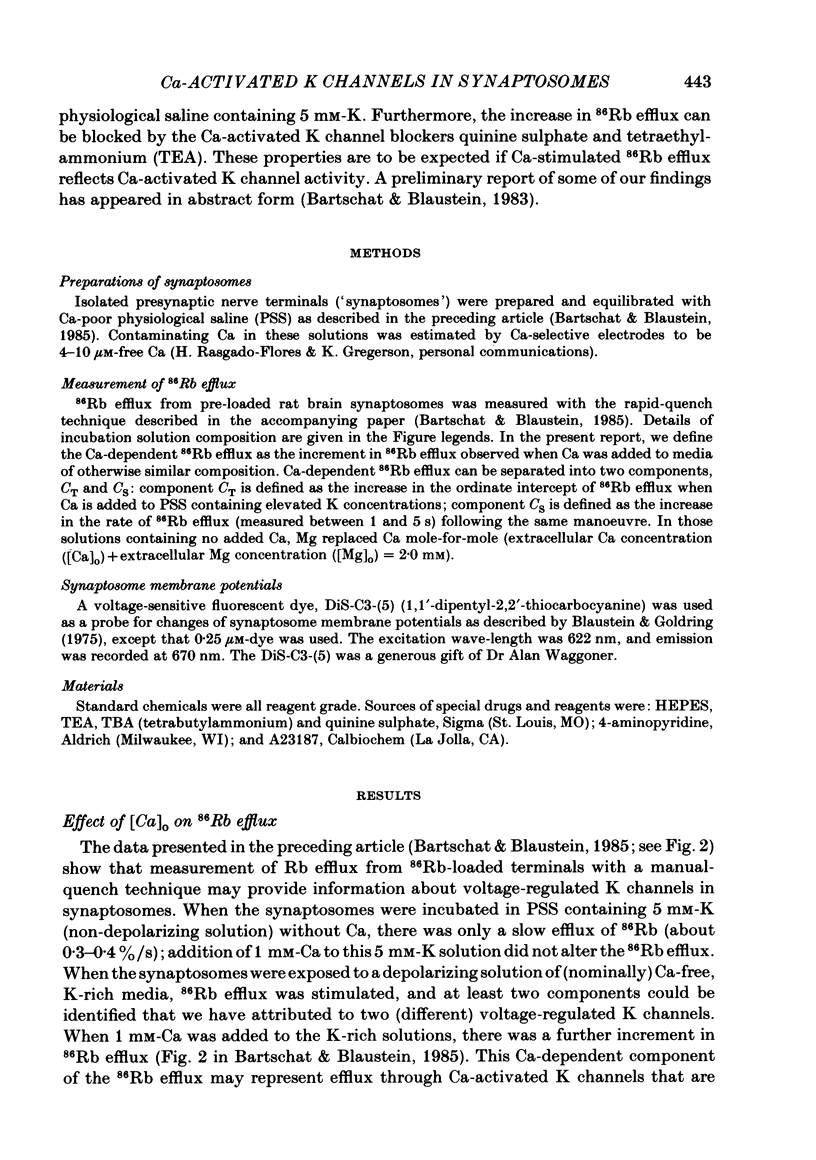
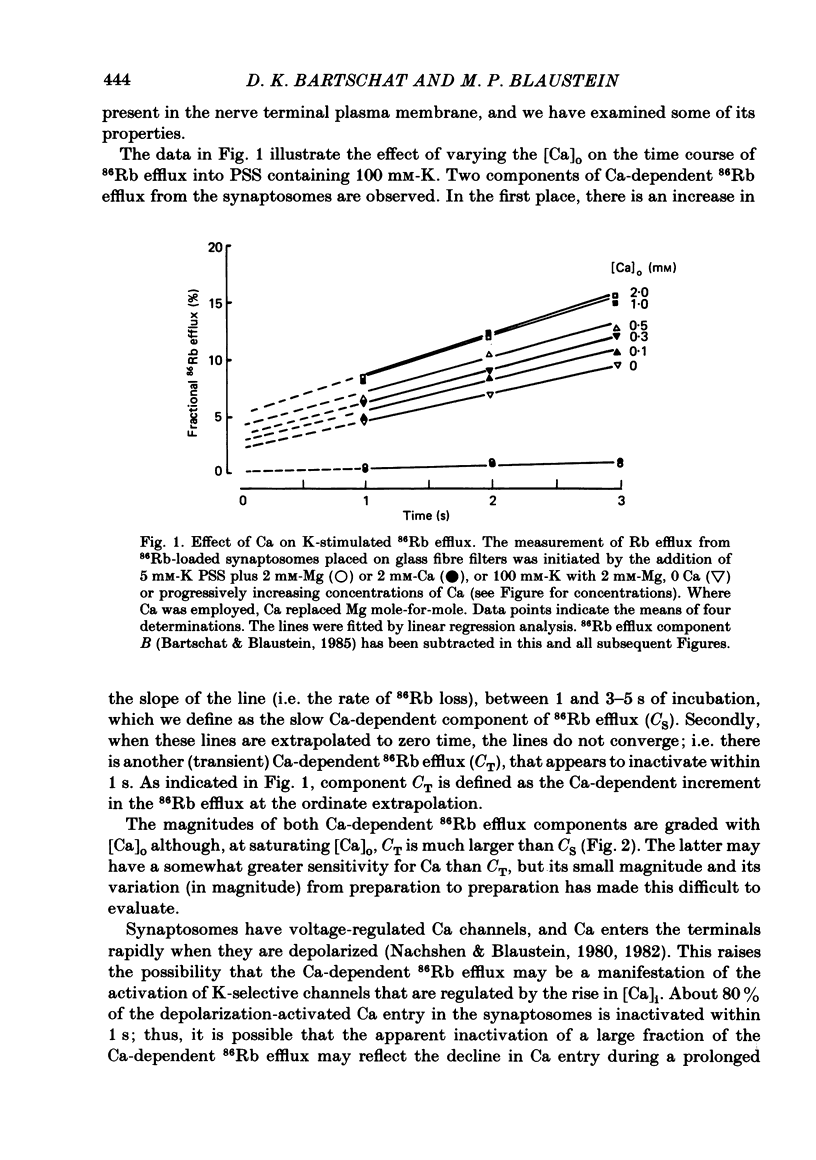
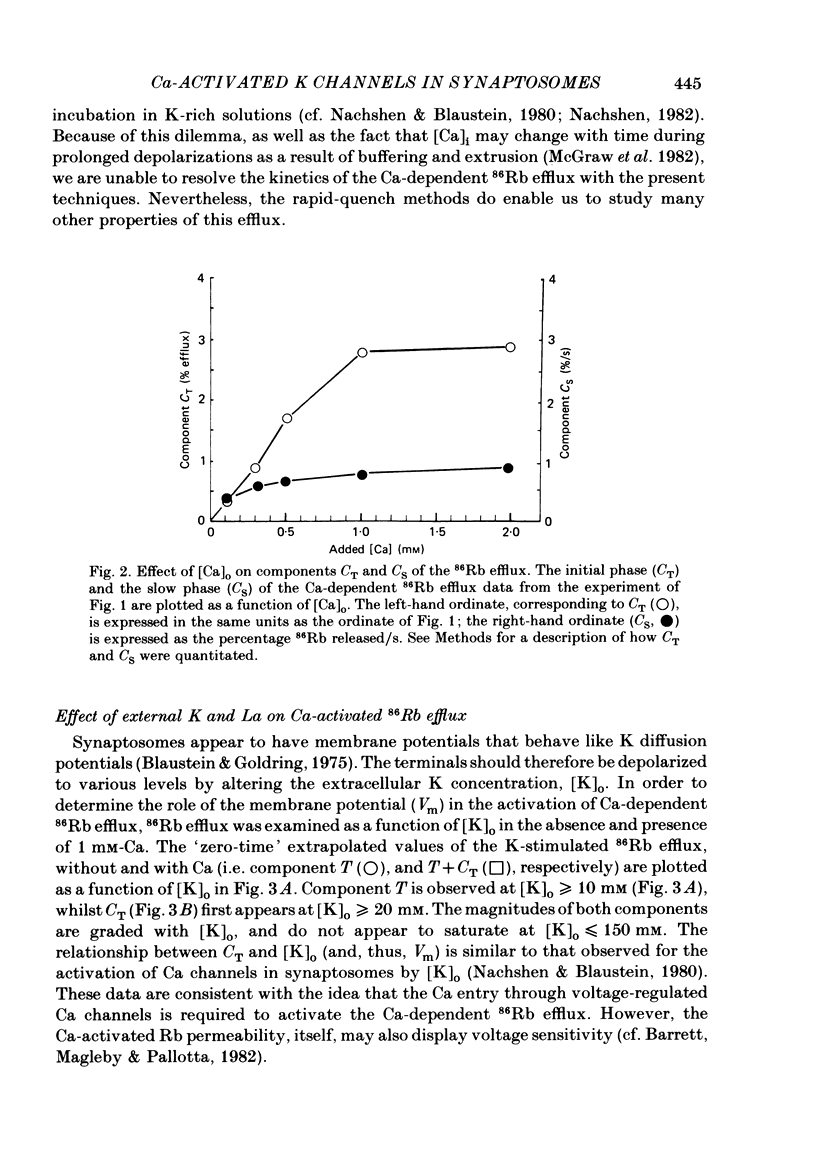
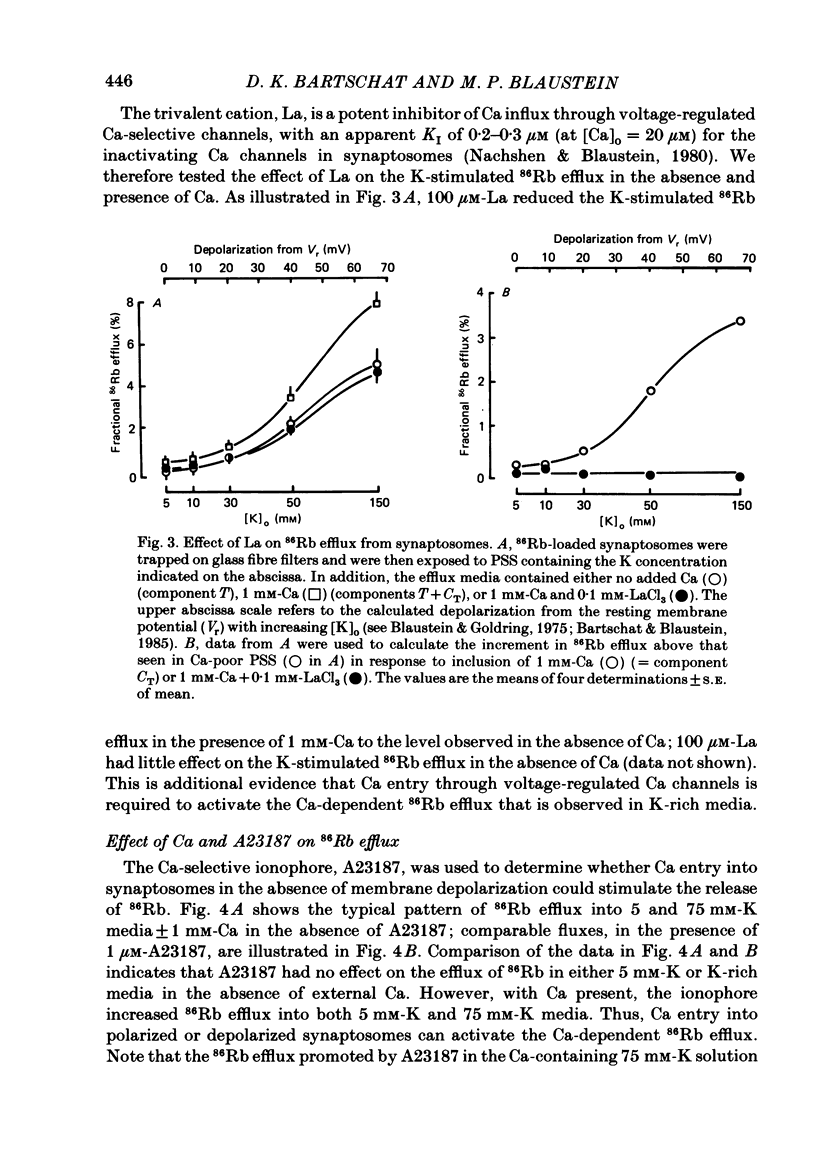
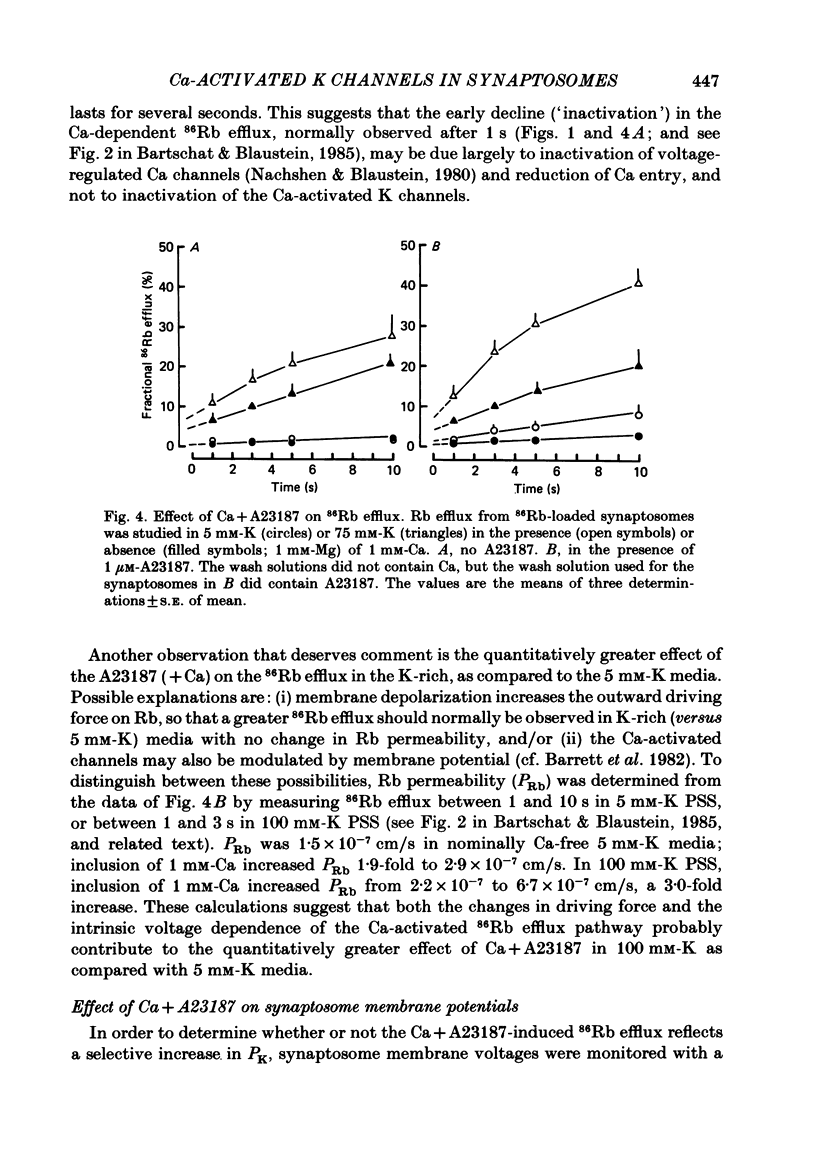
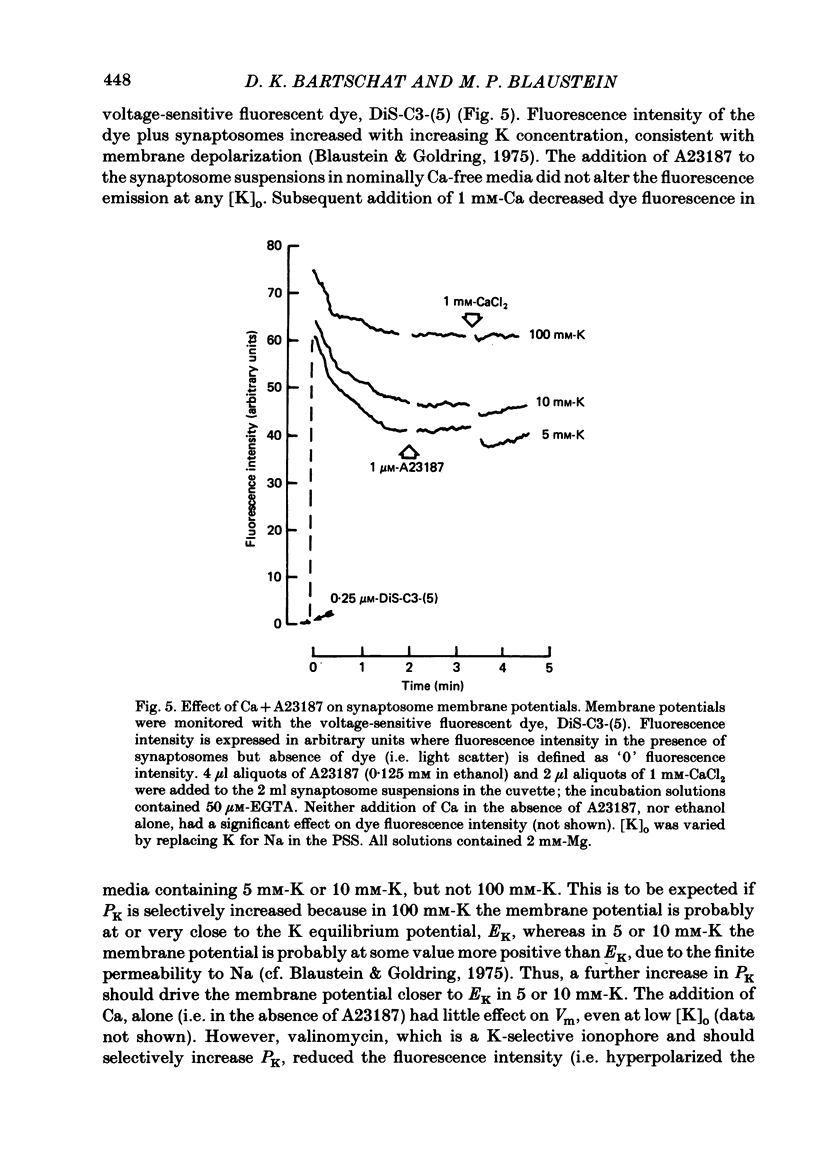
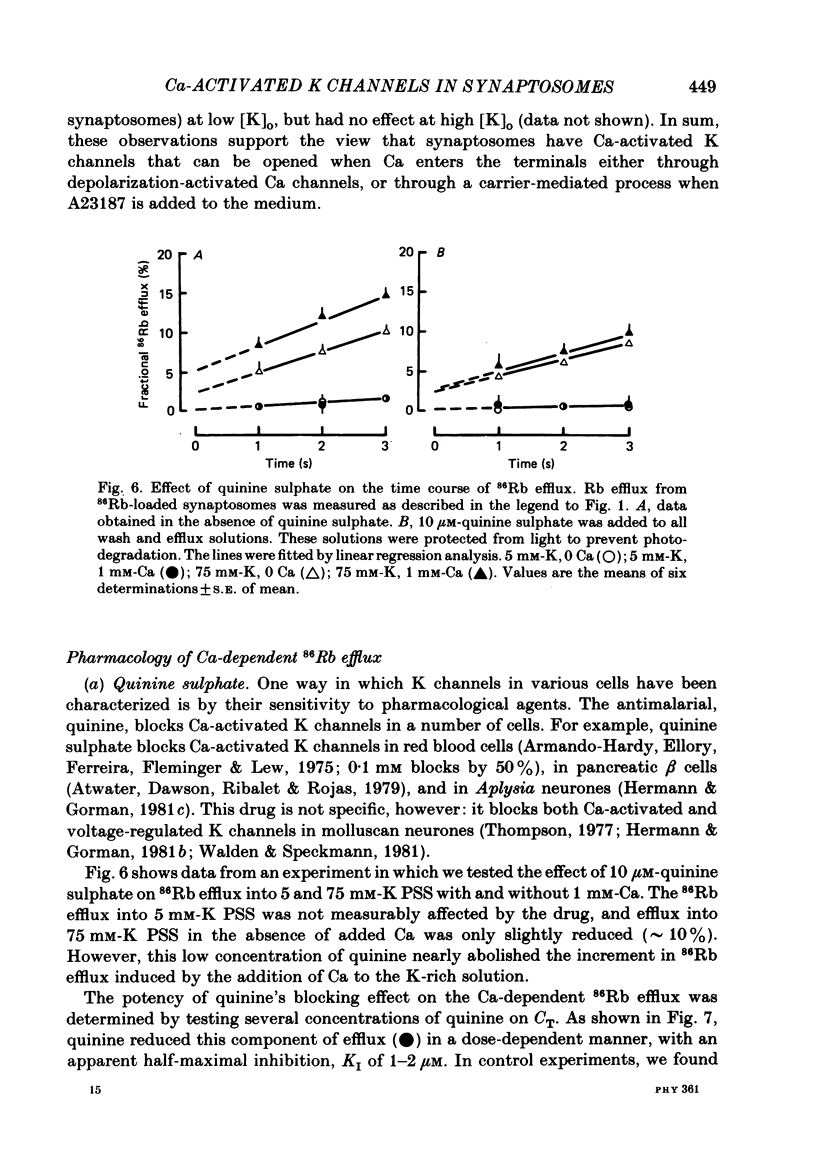
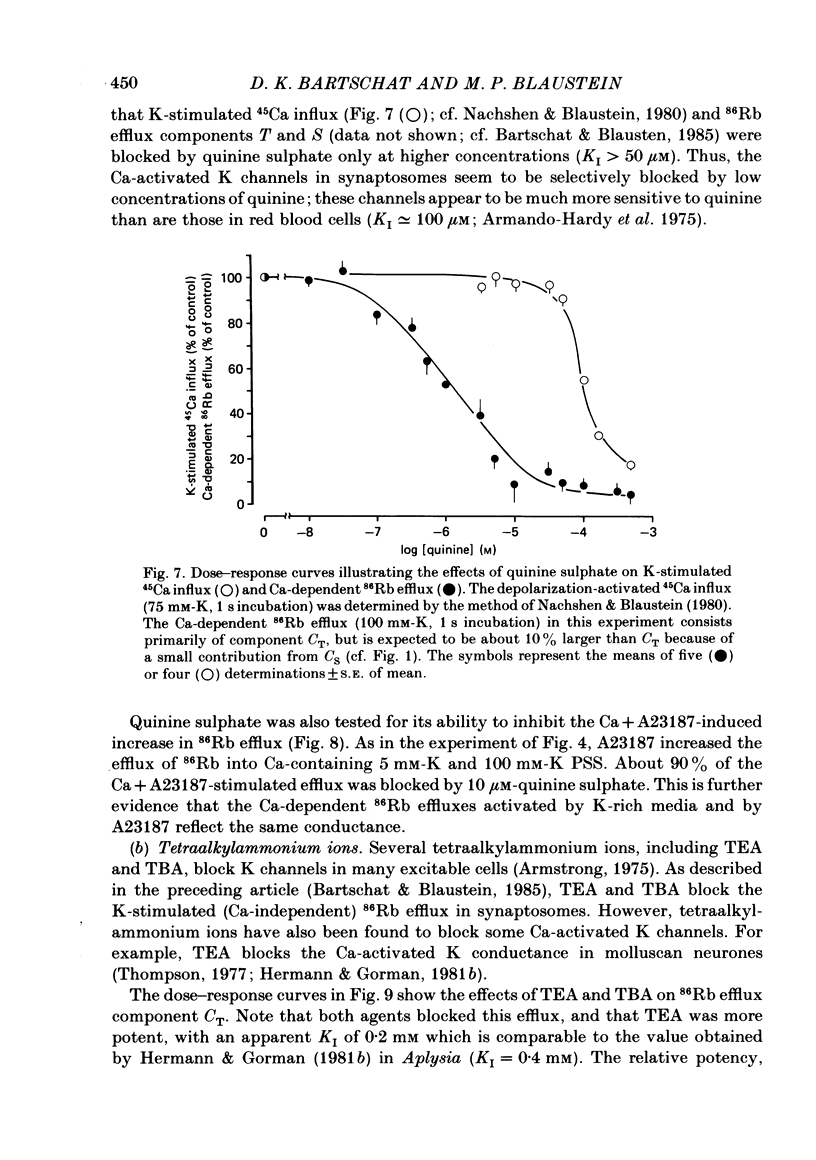
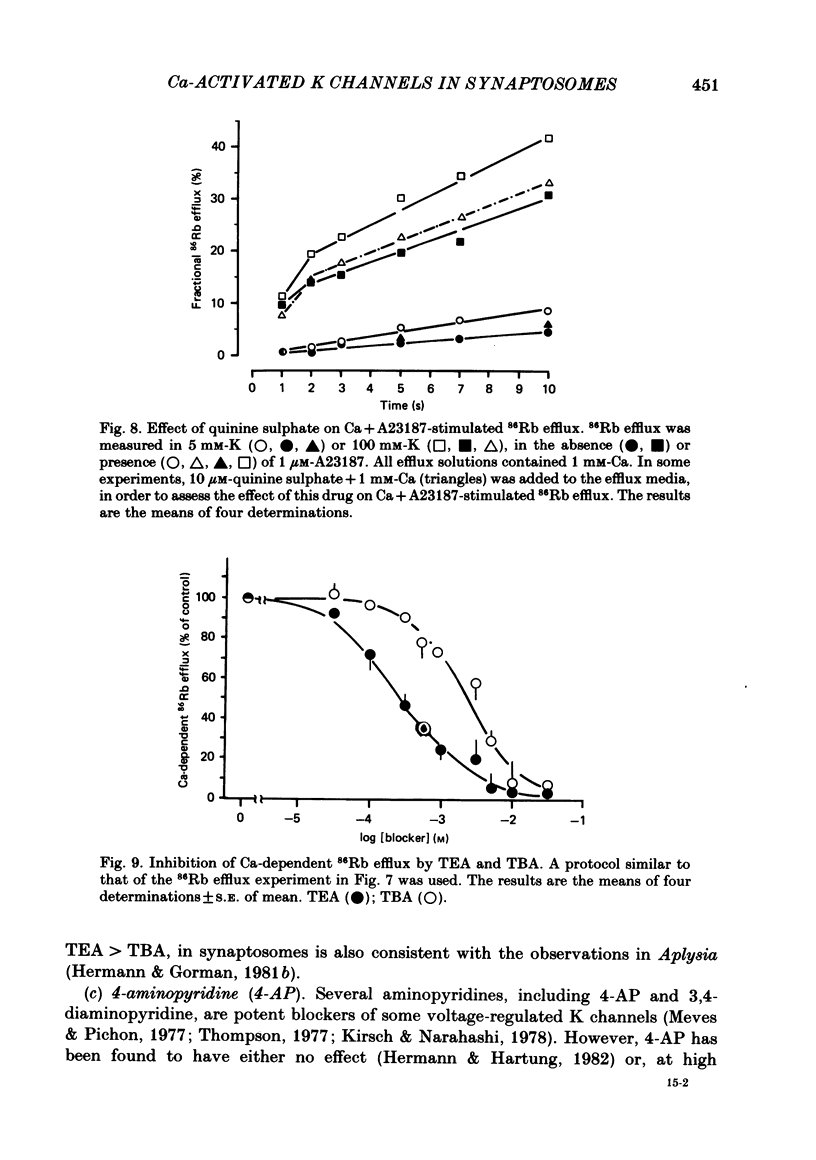
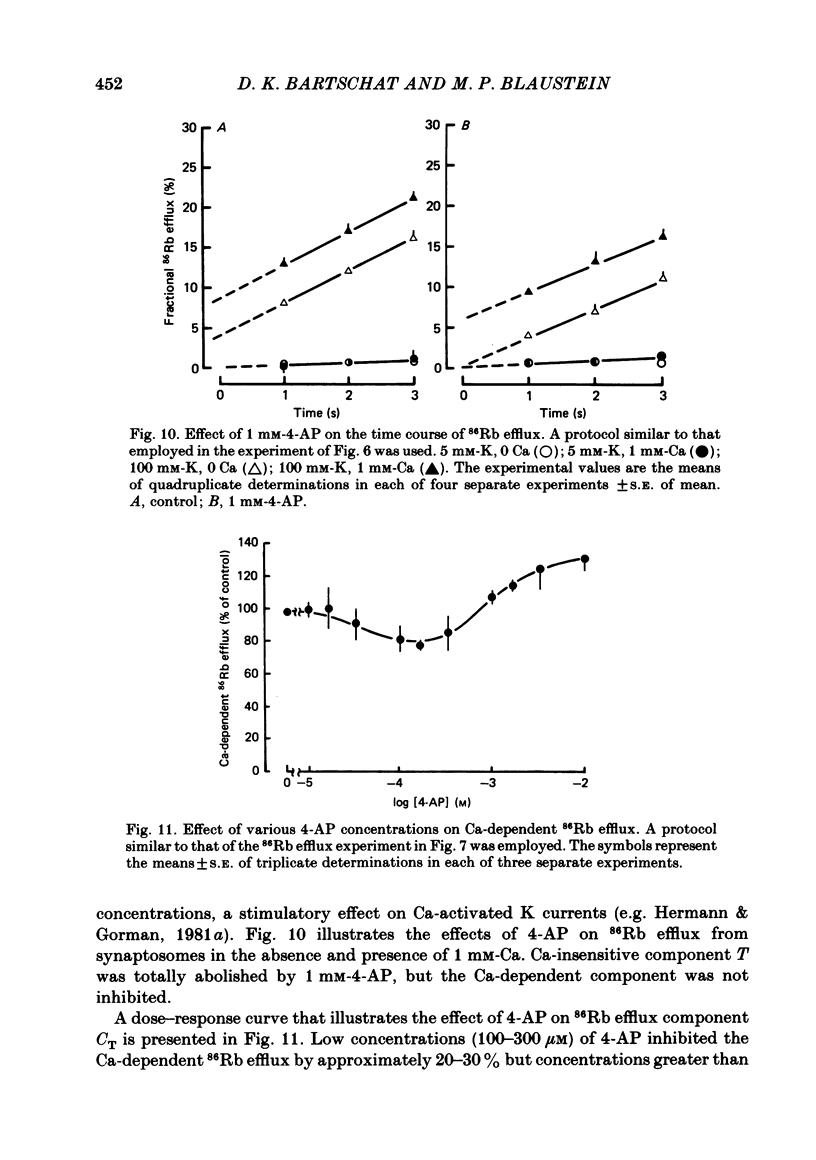
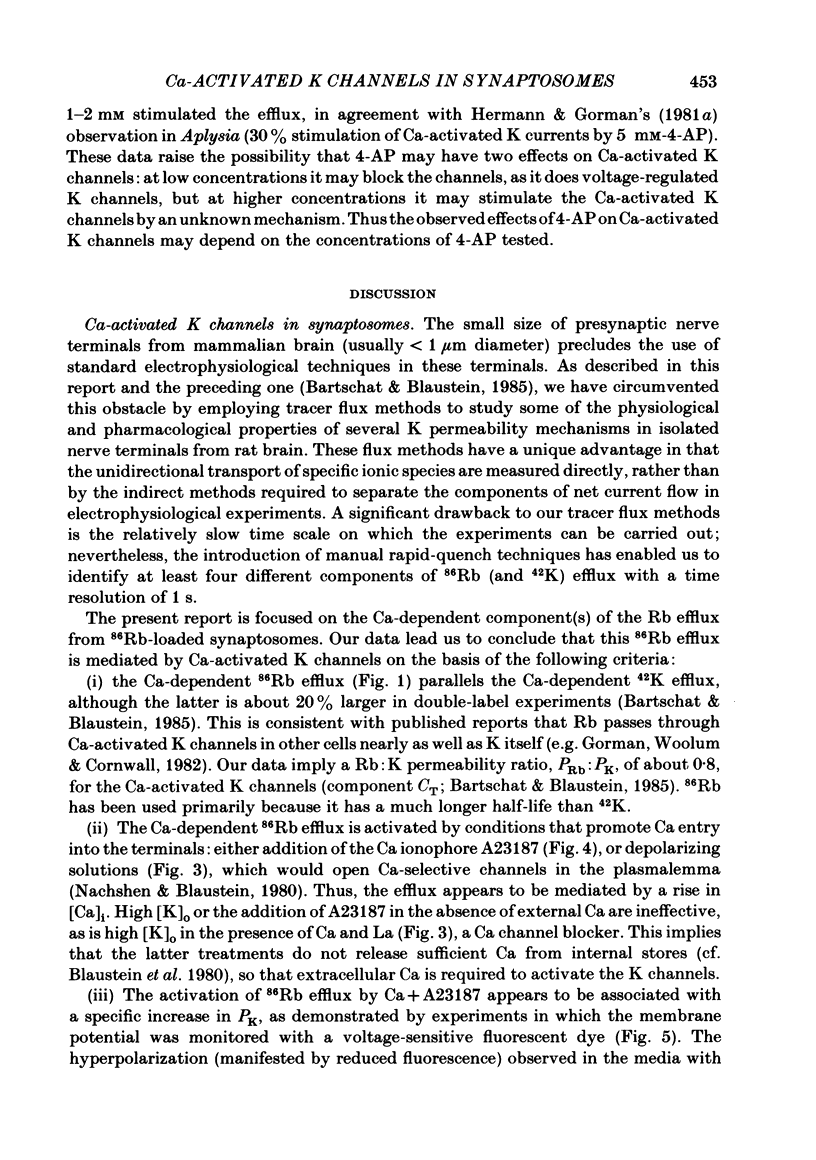
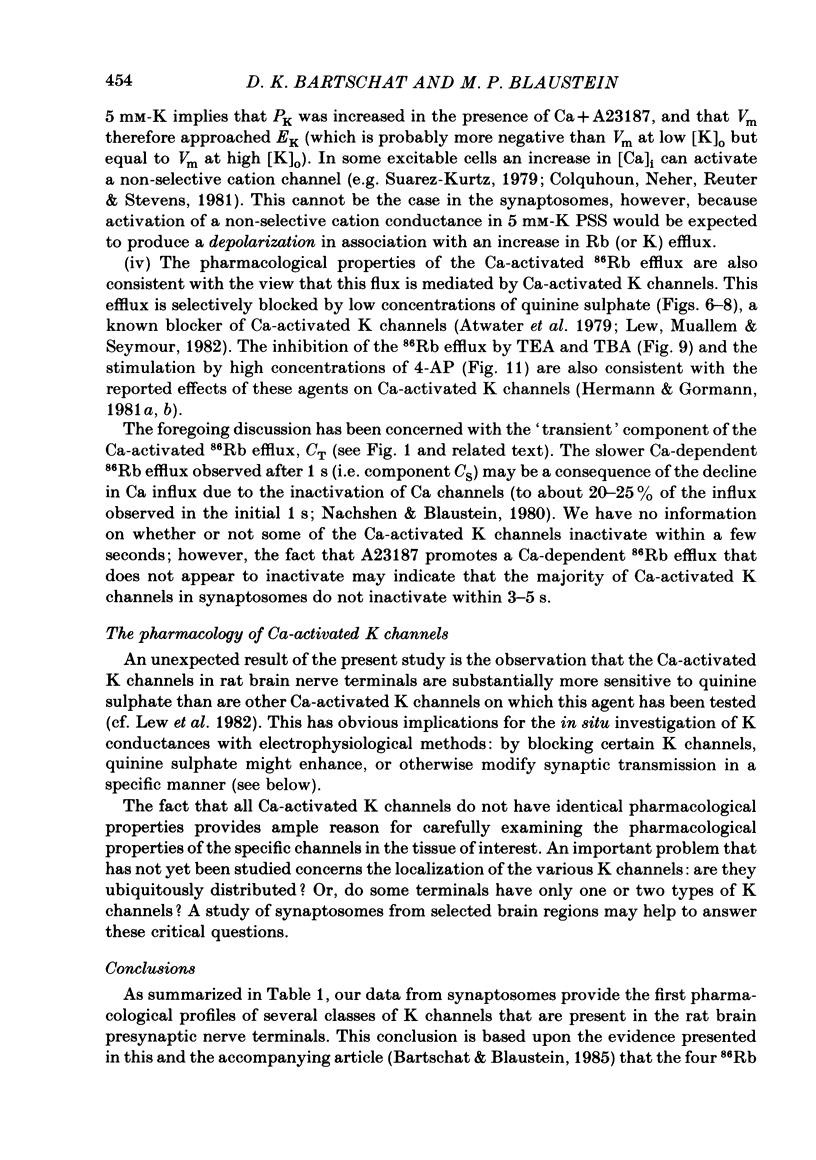
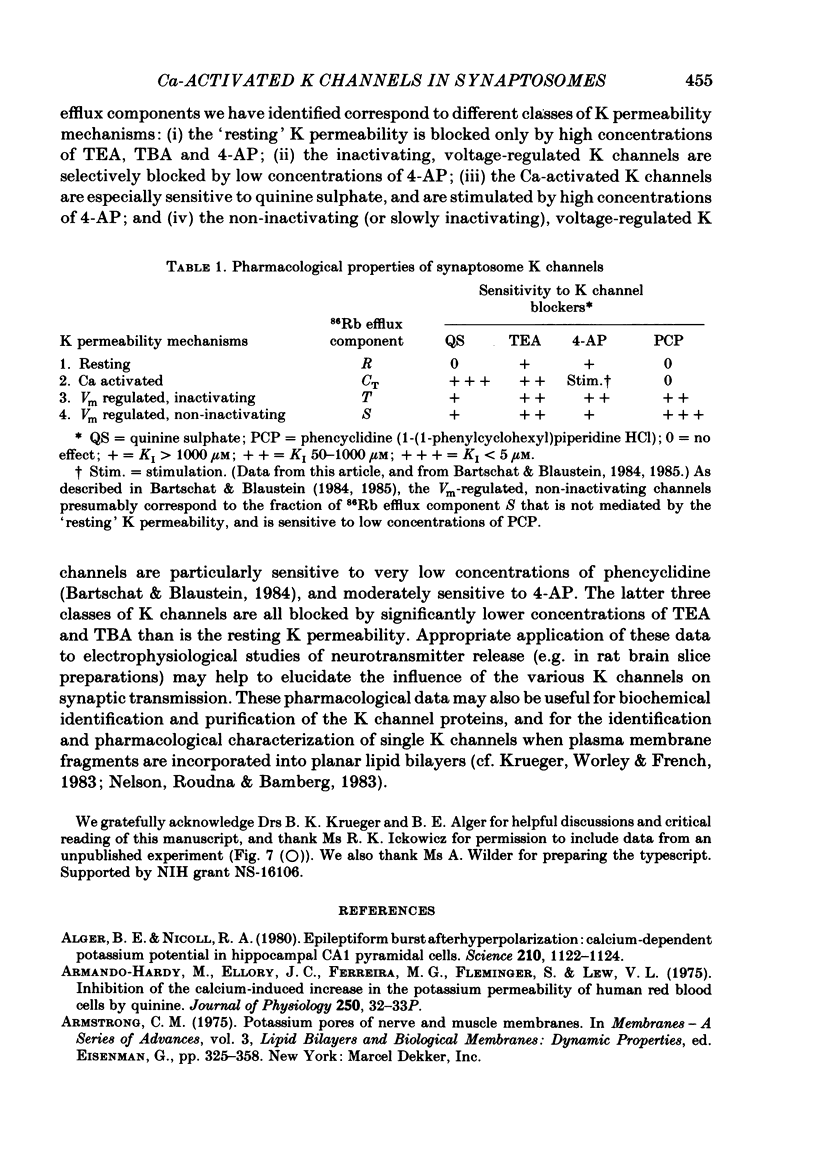
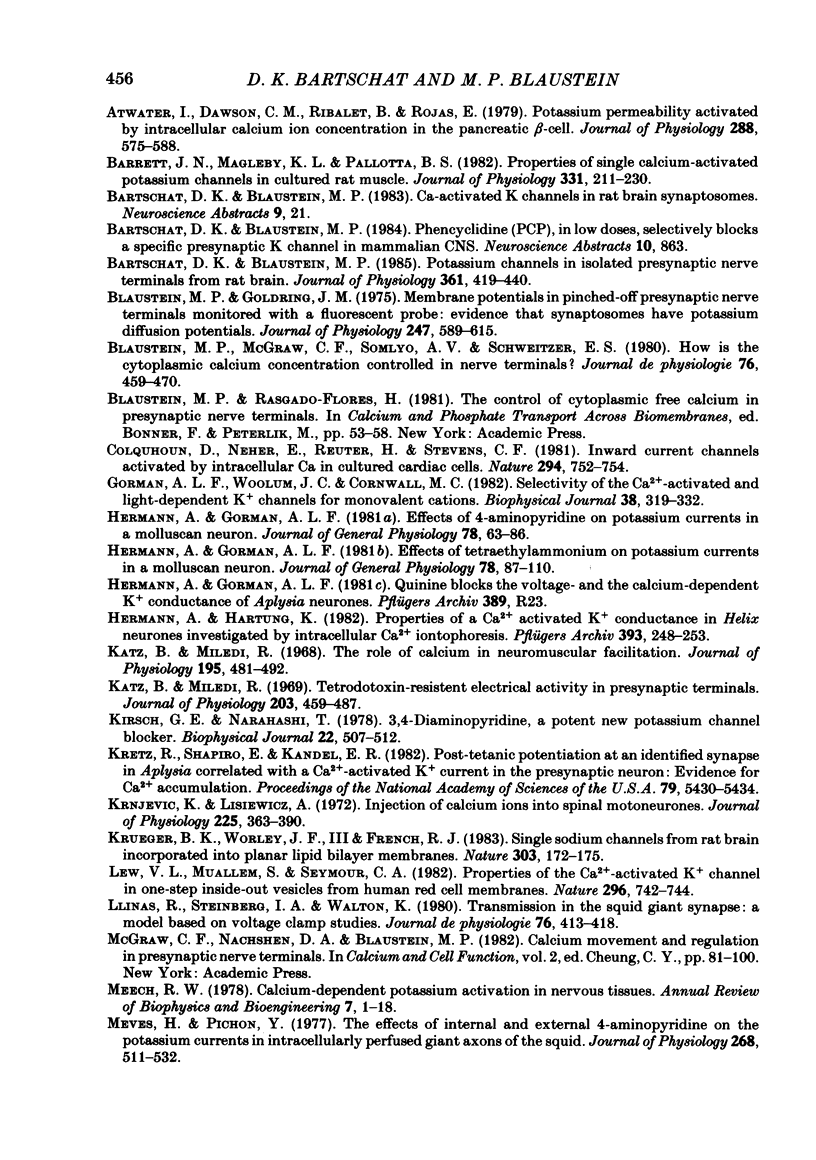
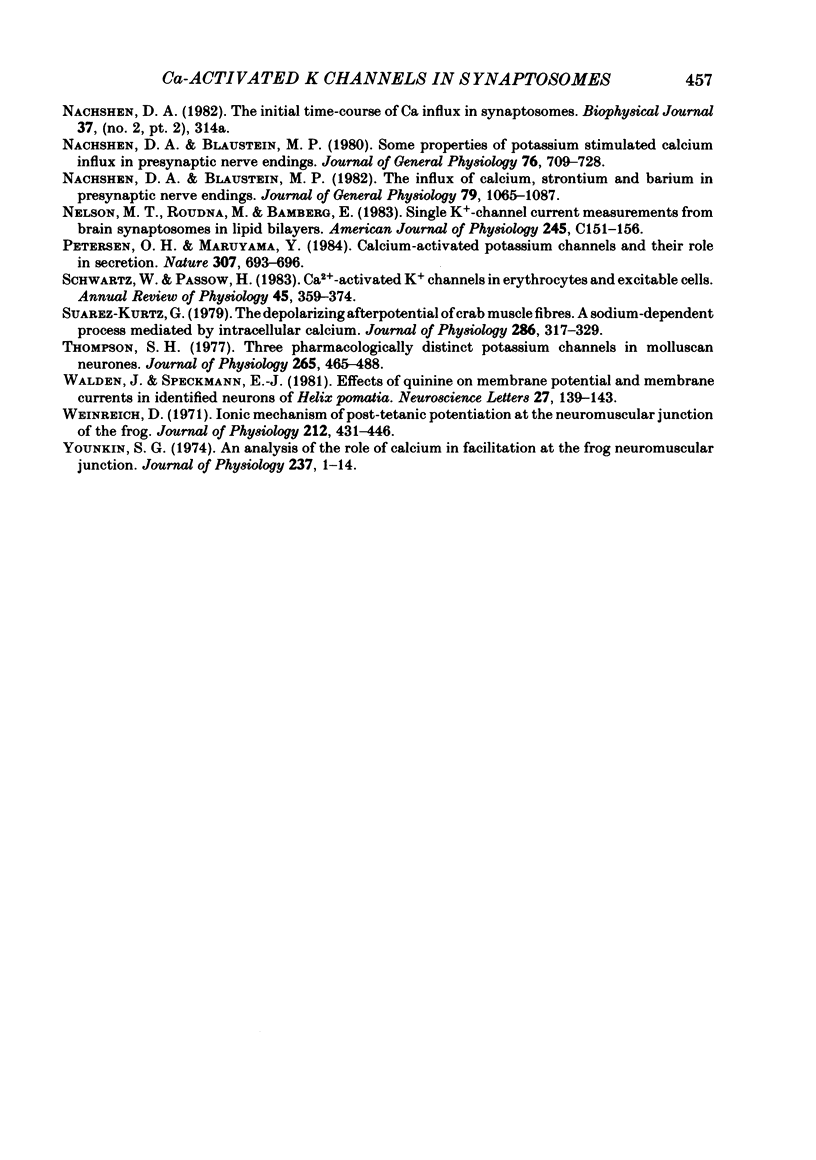
Selected References
These references are in PubMed. This may not be the complete list of references from this article.
- Alger B. E., Nicoll R. A. Epileptiform burst afterhyperolarization: calcium-dependent potassium potential in hippocampal CA1 pyramidal cells. Science. 1980 Dec 5;210(4474):1122–1124. doi: 10.1126/science.7444438. [DOI] [PubMed] [Google Scholar]
- Armando-Hardy M., Ellory J. C., Ferreira H. G., Fleminger S., Lew V. L. Inhibition of the calcium-induced increase in the potassium permeability of human red blood cells by quinine. J Physiol. 1975 Aug;250(1):32P–33P. [PubMed] [Google Scholar]
- Atwater I., Dawson C. M., Ribalet B., Rojas E. Potassium permeability activated by intracellular calcium ion concentration in the pancreatic beta-cell. J Physiol. 1979 Mar;288:575–588. [PMC free article] [PubMed] [Google Scholar]
- Barrett J. N., Magleby K. L., Pallotta B. S. Properties of single calcium-activated potassium channels in cultured rat muscle. J Physiol. 1982 Oct;331:211–230. doi: 10.1113/jphysiol.1982.sp014370. [DOI] [PMC free article] [PubMed] [Google Scholar]
- Bartschat D. K., Blaustein M. P. Potassium channels in isolated presynaptic nerve terminals from rat brain. J Physiol. 1985 Apr;361:419–440. doi: 10.1113/jphysiol.1985.sp015653. [DOI] [PMC free article] [PubMed] [Google Scholar]
- Blaustein M. P., Goldring J. M. Membrane potentials in pinched-off presynaptic nerve ternimals monitored with a fluorescent probe: evidence that synaptosomes have potassium diffusion potentials. J Physiol. 1975 Jun;247(3):589–615. doi: 10.1113/jphysiol.1975.sp010949. [DOI] [PMC free article] [PubMed] [Google Scholar]
- Blaustein M. P., McGraw C. F., Somlyo A. V., Schweitzer E. S. How is the cytoplasmic calcium concentration controlled in nerve terminals? J Physiol (Paris) 1980 Sep;76(5):459–470. [PubMed] [Google Scholar]
- Colquhoun D., Neher E., Reuter H., Stevens C. F. Inward current channels activated by intracellular Ca in cultured cardiac cells. Nature. 1981 Dec 24;294(5843):752–754. doi: 10.1038/294752a0. [DOI] [PubMed] [Google Scholar]
- Gorman A. L., Woolum J. C., Cornwall M. C. Selectivity of the Ca2+-activated and light-dependent K+ channels for monovalent cations. Biophys J. 1982 Jun;38(3):319–322. doi: 10.1016/S0006-3495(82)84565-7. [DOI] [PMC free article] [PubMed] [Google Scholar]
- Hermann A., Gorman A. L. Effects of 4-aminopyridine on potassium currents in a molluscan neuron. J Gen Physiol. 1981 Jul;78(1):63–86. doi: 10.1085/jgp.78.1.63. [DOI] [PMC free article] [PubMed] [Google Scholar]
- Hermann A., Hartung K. Properties of a Ca2+ activated K+ conductance in Helix neurones investigated by intracellular Ca2+ ionophoresis. Pflugers Arch. 1982 May;393(3):248–253. doi: 10.1007/BF00584078. [DOI] [PubMed] [Google Scholar]
- Katz B., Miledi R. Tetrodotoxin-resistant electric activity in presynaptic terminals. J Physiol. 1969 Aug;203(2):459–487. doi: 10.1113/jphysiol.1969.sp008875. [DOI] [PMC free article] [PubMed] [Google Scholar]
- Katz B., Miledi R. The role of calcium in neuromuscular facilitation. J Physiol. 1968 Mar;195(2):481–492. doi: 10.1113/jphysiol.1968.sp008469. [DOI] [PMC free article] [PubMed] [Google Scholar]
- Kirsch G. E., Narahashi T. 3,4-diaminopyridine. A potent new potassium channel blocker. Biophys J. 1978 Jun;22(3):507–512. doi: 10.1016/S0006-3495(78)85503-9. [DOI] [PMC free article] [PubMed] [Google Scholar]
- Kretz R., Shapiro E., Kandel E. R. Post-tetanic potentiation at an identified synapse in Aplysia is correlated with a Ca2+-activated K+ current in the presynaptic neuron: evidence for Ca2+ accumulation. Proc Natl Acad Sci U S A. 1982 Sep;79(17):5430–5434. doi: 10.1073/pnas.79.17.5430. [DOI] [PMC free article] [PubMed] [Google Scholar]
- Krnjević K., Lisiewicz A. Injections of calcium ions into spinal motoneurones. J Physiol. 1972 Sep;225(2):363–390. doi: 10.1113/jphysiol.1972.sp009945. [DOI] [PMC free article] [PubMed] [Google Scholar]
- Krueger B. K., Worley J. F., 3rd, French R. J. Single sodium channels from rat brain incorporated into planar lipid bilayer membranes. Nature. 1983 May 12;303(5913):172–175. doi: 10.1038/303172a0. [DOI] [PubMed] [Google Scholar]
- Lew V. L., Muallem S., Seymour C. A. Properties of the Ca2+-activated K+ channel in one-step inside-out vesicles from human red cell membranes. Nature. 1982 Apr 22;296(5859):742–744. doi: 10.1038/296742a0. [DOI] [PubMed] [Google Scholar]
- Llinás R., Steinberg I. Z., Walton K. Transmission in the squid giant synapse: a model based on voltage clamp studies. J Physiol (Paris) 1980 Sep;76(5):413–418. [PubMed] [Google Scholar]
- Meech R. W. Calcium-dependent potassium activation in nervous tissues. Annu Rev Biophys Bioeng. 1978;7:1–18. doi: 10.1146/annurev.bb.07.060178.000245. [DOI] [PubMed] [Google Scholar]
- Meves H., Pichon Y. The effect of internal and external 4-aminopyridine on the potassium currents in intracellularly perfused squid giant axons. J Physiol. 1977 Jun;268(2):511–532. doi: 10.1113/jphysiol.1977.sp011869. [DOI] [PMC free article] [PubMed] [Google Scholar]
- Nachshen D. A., Blaustein M. P. Influx of calcium, strontium, and barium in presynaptic nerve endings. J Gen Physiol. 1982 Jun;79(6):1065–1087. doi: 10.1085/jgp.79.6.1065. [DOI] [PMC free article] [PubMed] [Google Scholar]
- Nachshen D. A., Blaustein M. P. Some properties of potassium-stimulated calcium influx in presynaptic nerve endings. J Gen Physiol. 1980 Dec;76(6):709–728. doi: 10.1085/jgp.76.6.709. [DOI] [PMC free article] [PubMed] [Google Scholar]
- Nelson M. T., Roudna M., Bamberg E. Single K+-channel current measurements from brain synaptosomes in lipid bilayers. Am J Physiol. 1983 Jul;245(1):C151–C156. doi: 10.1152/ajpcell.1983.245.1.C151. [DOI] [PubMed] [Google Scholar]
- Petersen O. H., Maruyama Y. Calcium-activated potassium channels and their role in secretion. Nature. 1984 Feb 23;307(5953):693–696. doi: 10.1038/307693a0. [DOI] [PubMed] [Google Scholar]
- Schwarz W., Passow H. Ca2+-activated K+ channels in erythrocytes and excitable cells. Annu Rev Physiol. 1983;45:359–374. doi: 10.1146/annurev.ph.45.030183.002043. [DOI] [PubMed] [Google Scholar]
- Suarez-Kurtz G. The depolarizing afterpotential of crab muscle fibres. A sodium-dependent process mediated by intracellular calcium. J Physiol. 1979 Jan;286:317–329. doi: 10.1113/jphysiol.1979.sp012621. [DOI] [PMC free article] [PubMed] [Google Scholar]
- Thompson S. H. Three pharmacologically distinct potassium channels in molluscan neurones. J Physiol. 1977 Feb;265(2):465–488. doi: 10.1113/jphysiol.1977.sp011725. [DOI] [PMC free article] [PubMed] [Google Scholar]
- Walden J., Speckmann E. J. Effects of quinine on membrane potential and membrane currents in identified neurons of Helix pomatia. Neurosci Lett. 1981 Dec 11;27(2):139–143. doi: 10.1016/0304-3940(81)90258-5. [DOI] [PubMed] [Google Scholar]
- Weinreich D. Ionic mechanism of post-tetanic potentiation at the neuromuscular junction of the frog. J Physiol. 1971 Jan;212(2):431–446. doi: 10.1113/jphysiol.1971.sp009333. [DOI] [PMC free article] [PubMed] [Google Scholar]
- Younkin S. G. An analysis of the role of calcium in facilitation at the frog neuromuscular junction. J Physiol. 1974 Feb;237(1):1–14. doi: 10.1113/jphysiol.1974.sp010466. [DOI] [PMC free article] [PubMed] [Google Scholar]