Abstract
The post-natal development of pyramidal tract neurones (p.t.n.s) was investigated in twenty-one barbiturate-anaesthetized kittens from birth to 28 days of age using a combination of electrophysiological and anatomical techniques. P.t.n. responses were recorded intracellularly as well as extracellularly with glass micropipettes filled with horseradish peroxidase (HRP) on stimulation of the medullary pyramid and cerebellar nuclei. Latency histograms of antidromic responses of p.t.n.s were compared at various ages. In the neonate, p.t.n.s were divided into two groups which were presumed to be analogous with fast and slow p.t.n.s in adult animals. During the first post-natal week, latency shortening was not conspicuous, but by the end of the second post-natal week, the faster group showed a marked decrease of latencies (up to around 10 ms at 14 days of age), while those of the slower group did not change so much. The slower group increased their conduction velocity during the third post-natal week (latencies up to around 18 ms). At the end of the fourth post-natal week, the distribution of antidromic latencies was in a narrower range, but the values were still longer than those reported in adult animals. Intracellular HRP staining revealed that apical dendrites of p.t.n.s spread fully to the pial surface even at birth. The somata of these neurones were characteristically covered with somatic appendages and development of the basal dendritic tree was immature in 0-1-day-old kittens. Basal dendrites developed nearly completely by 7 days, but somata were still covered with appendages. During the fourth post-natal week, these appendages disappeared almost completely. The sizes of the dendritic field, especially of apical dendrites, became larger in parallel with the development of cortical layers. From the morphological point of view, differentiation of fast and slow p.t.n.s was not clear until 28 days of age except in somatic volumes, which were already different in the first post-natal week. At the end of the fourth post-natal week, p.t.n.s with short antidromic latencies had a tendency to bear spines more sparsely over the secondary and tertiary dendritic surface in comparison with p.t.n.s with longer latencies. Intracortical axonal trajectories developed fairly well in the immature cerebral cortex, and the general pattern of ramification changed little during the first month after birth.(ABSTRACT TRUNCATED AT 400 WORDS)
Full text
PDF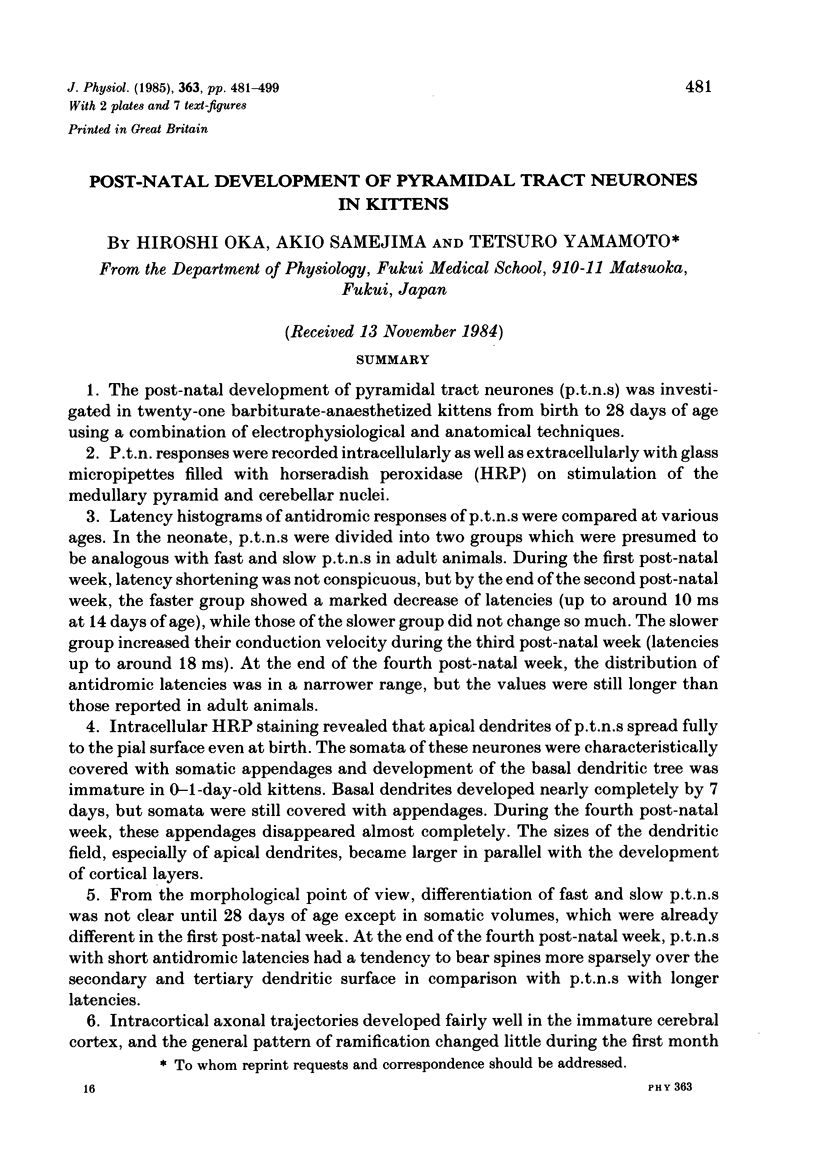
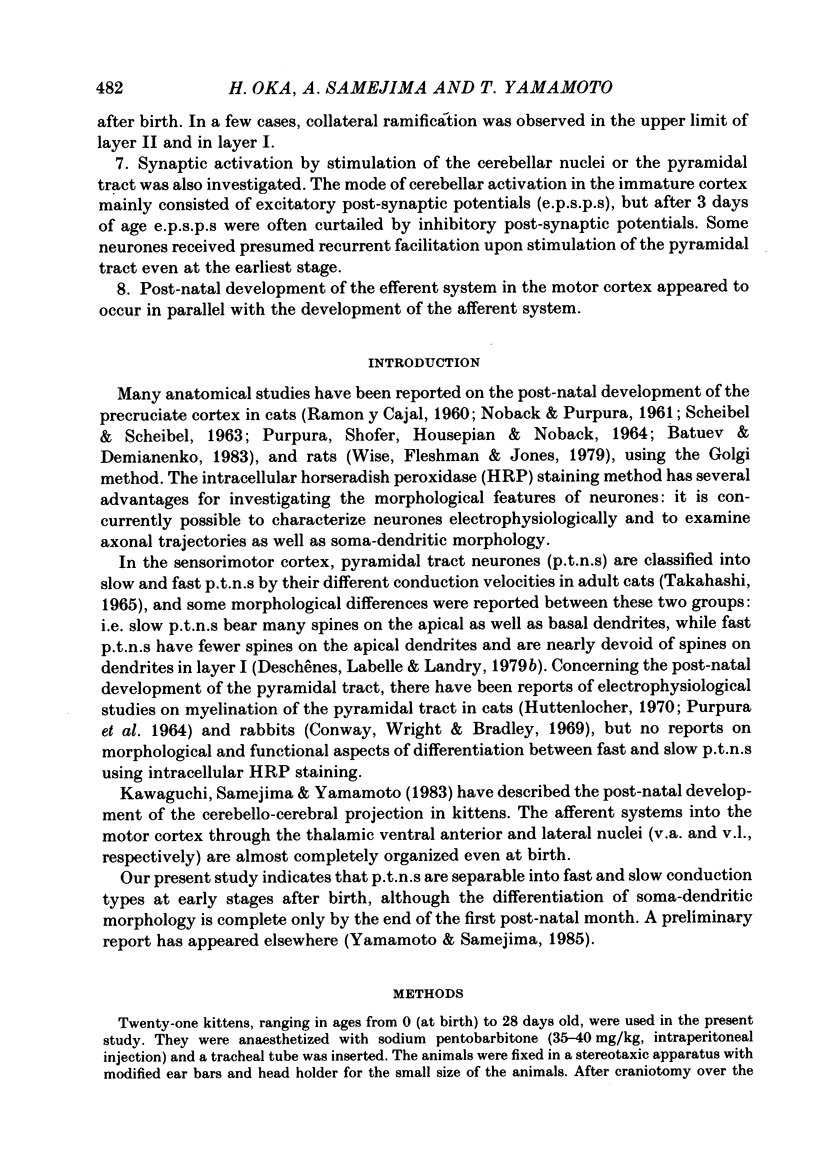
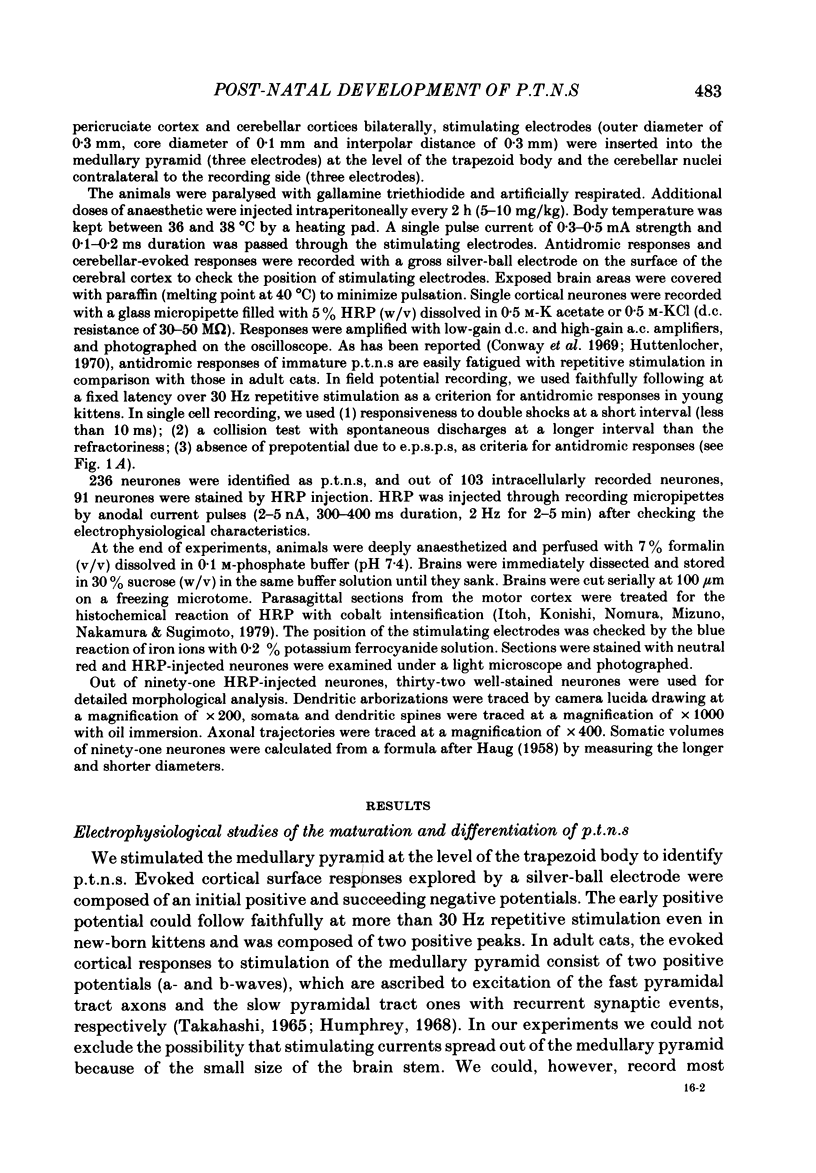
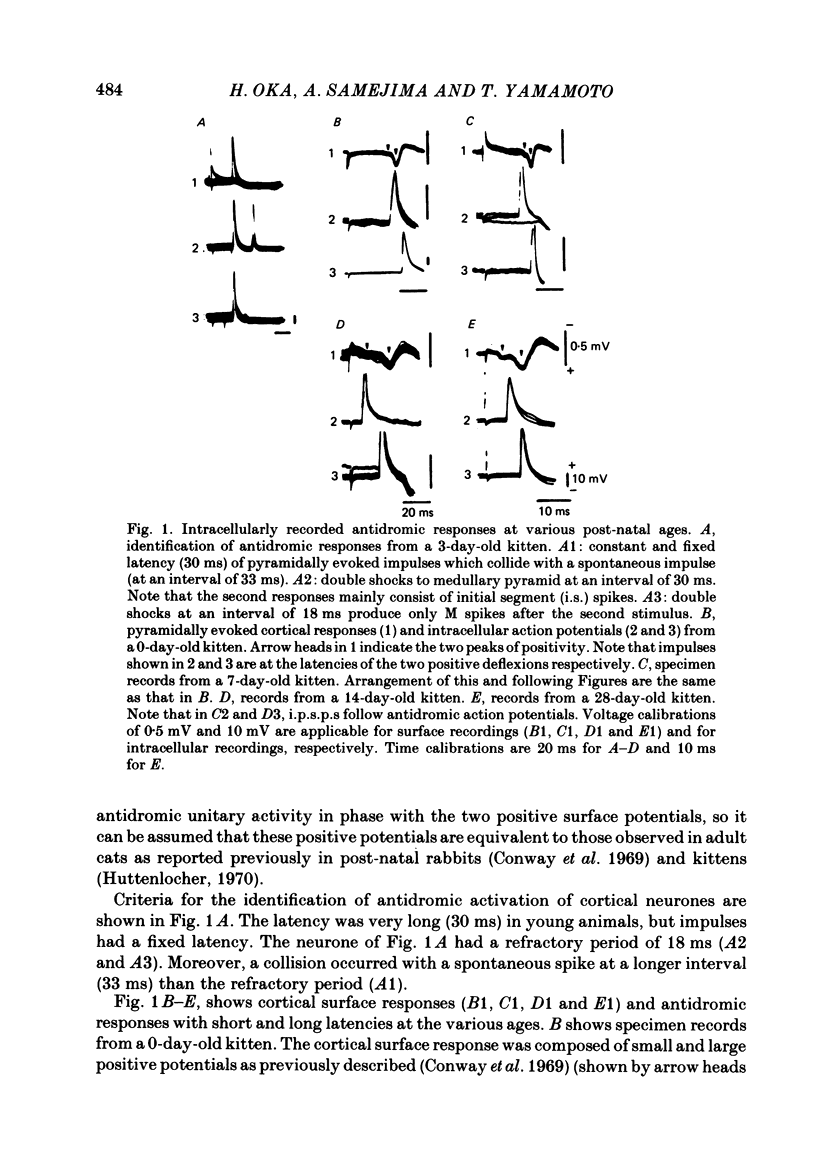
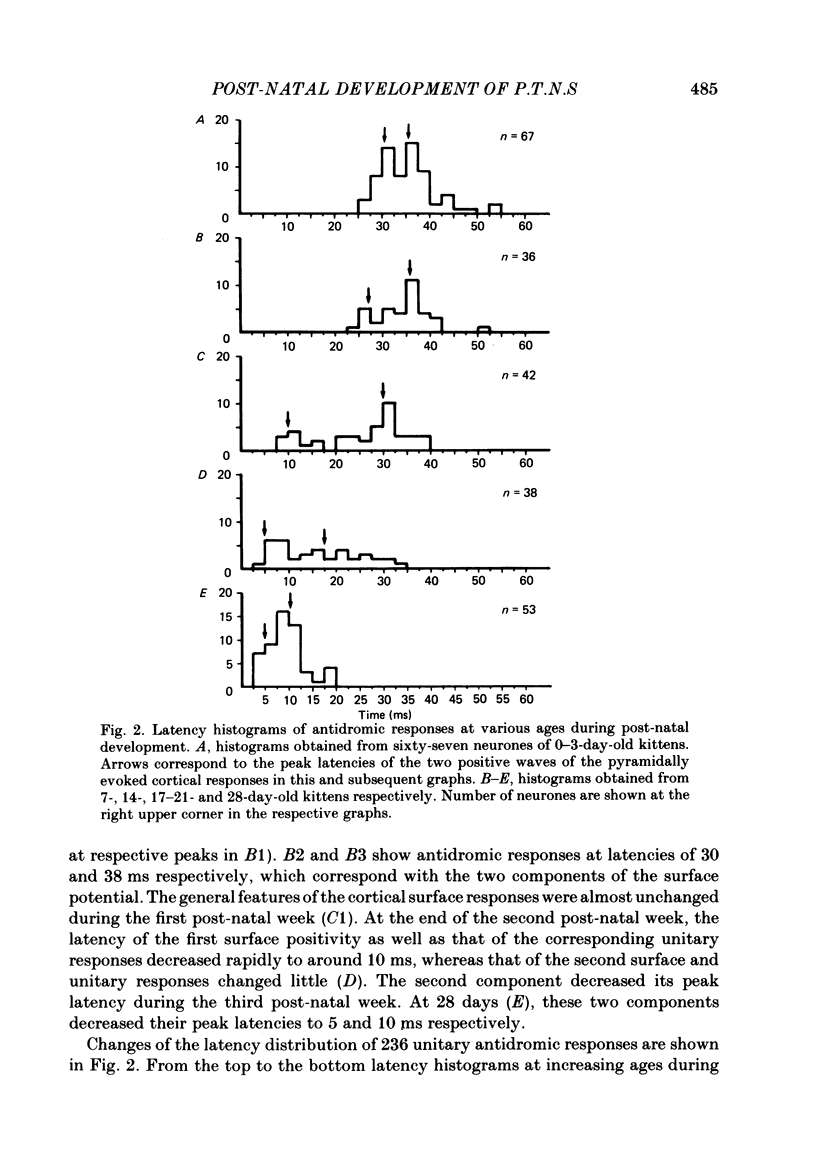
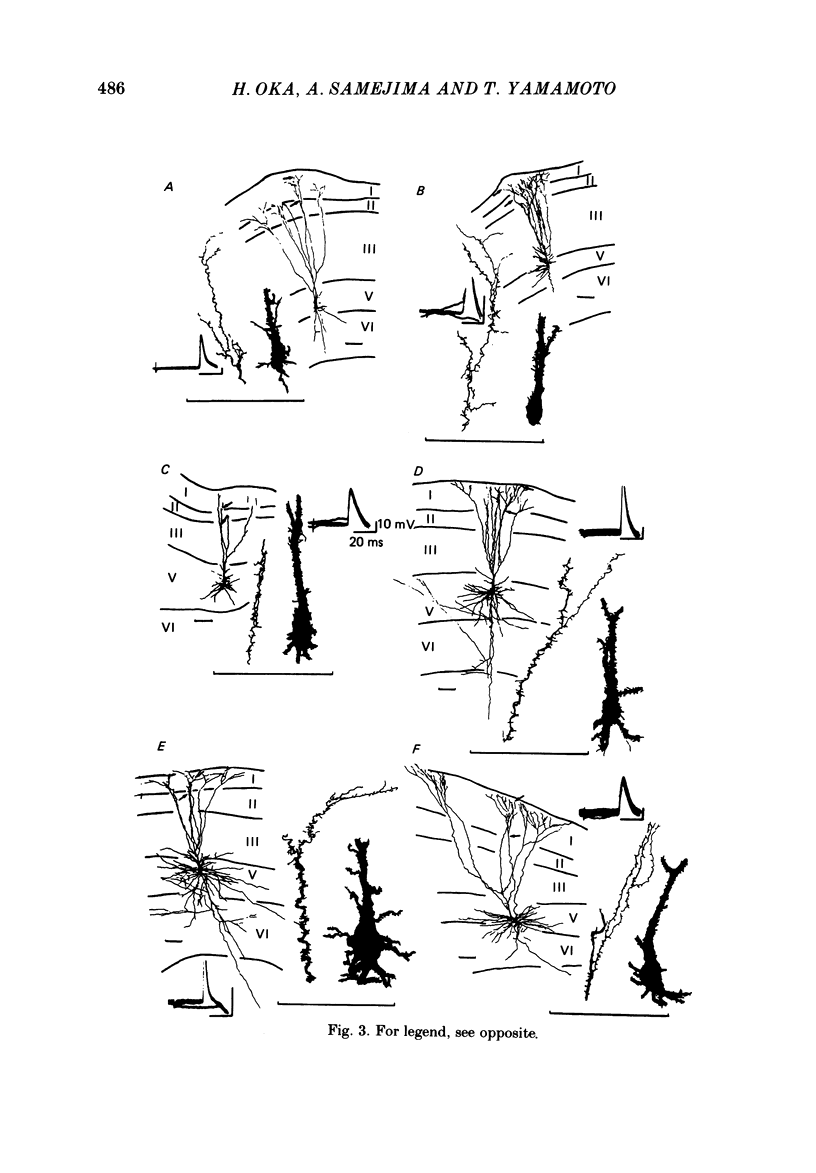
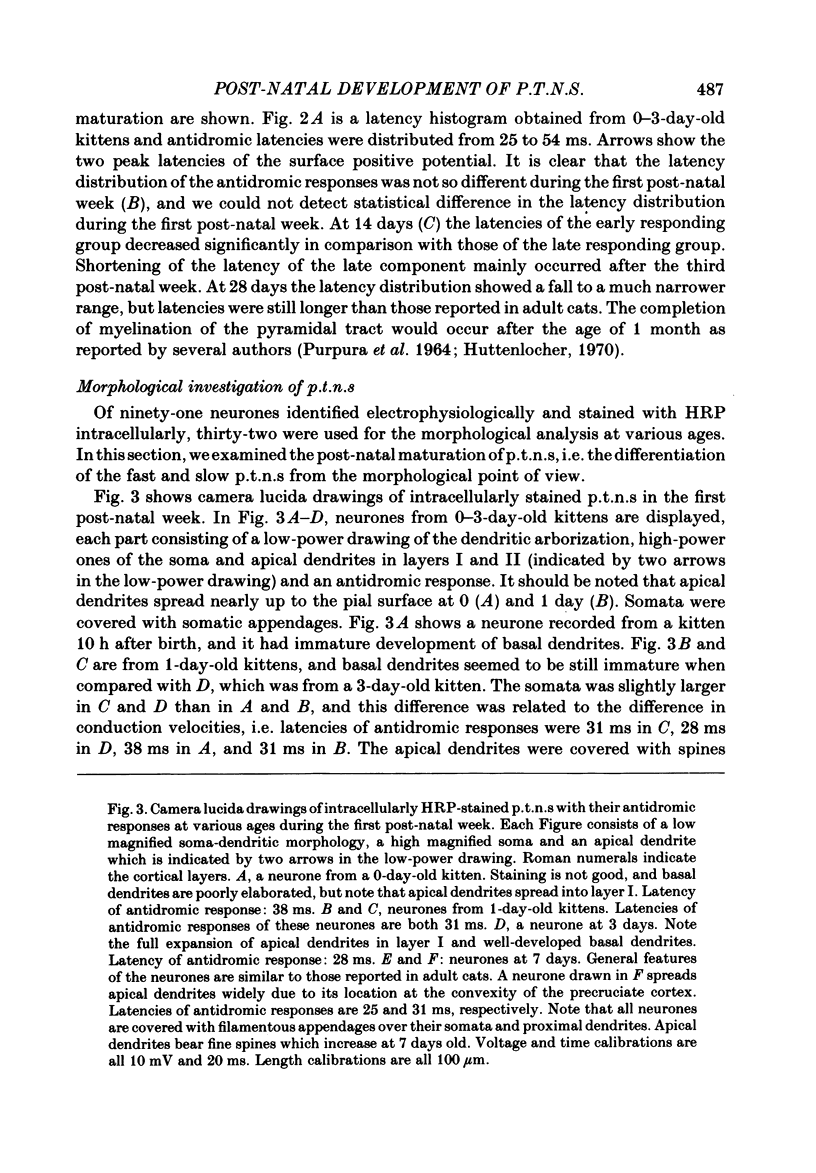
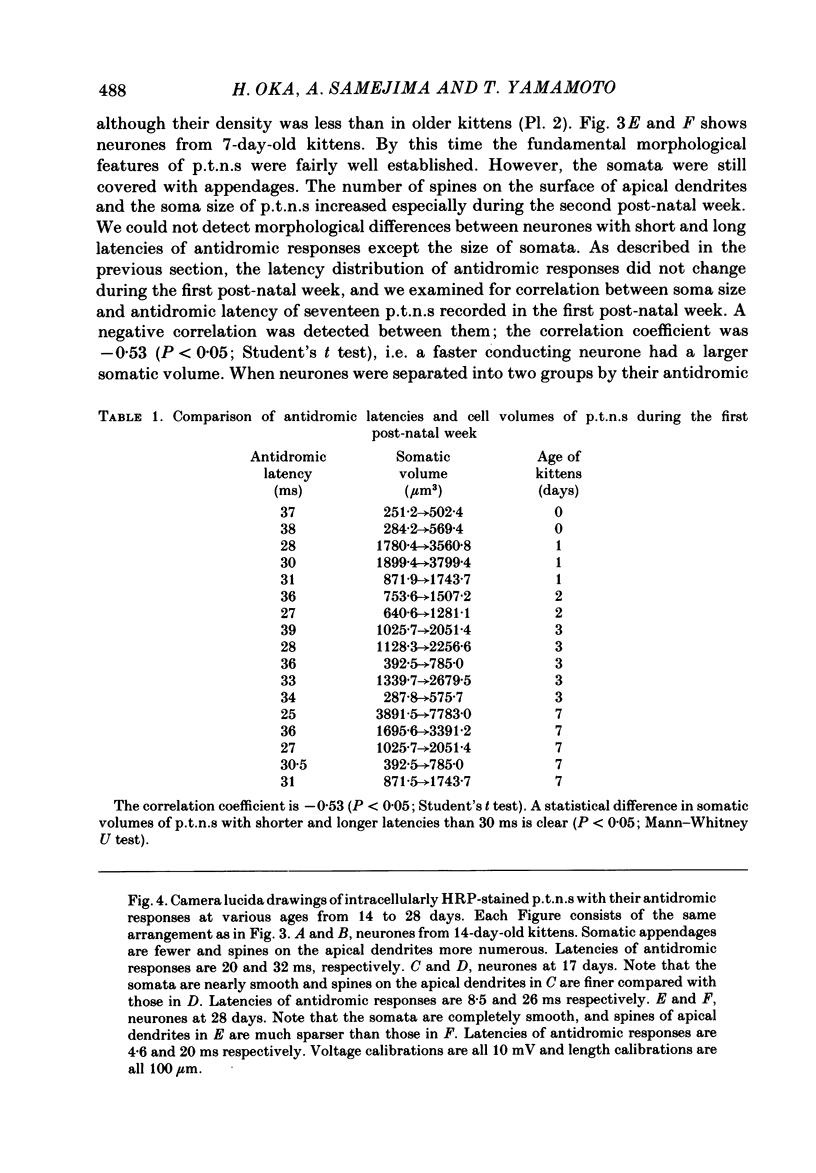
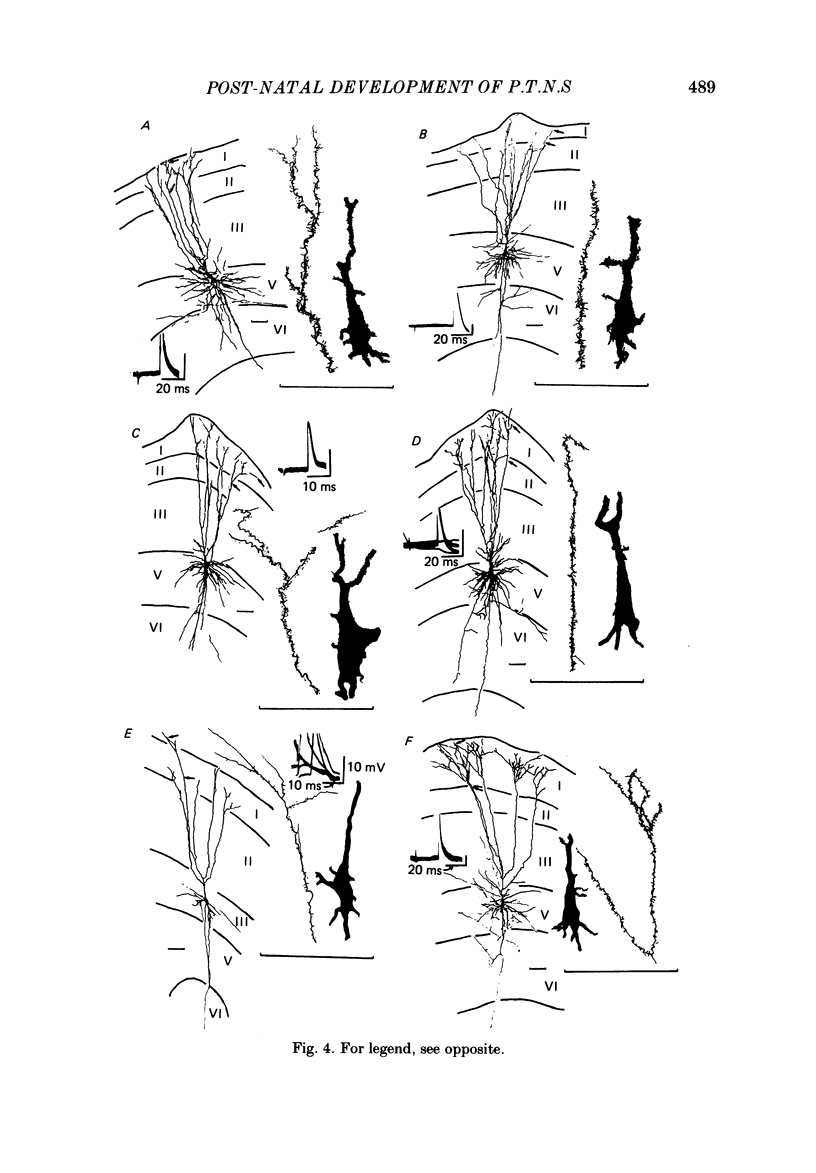
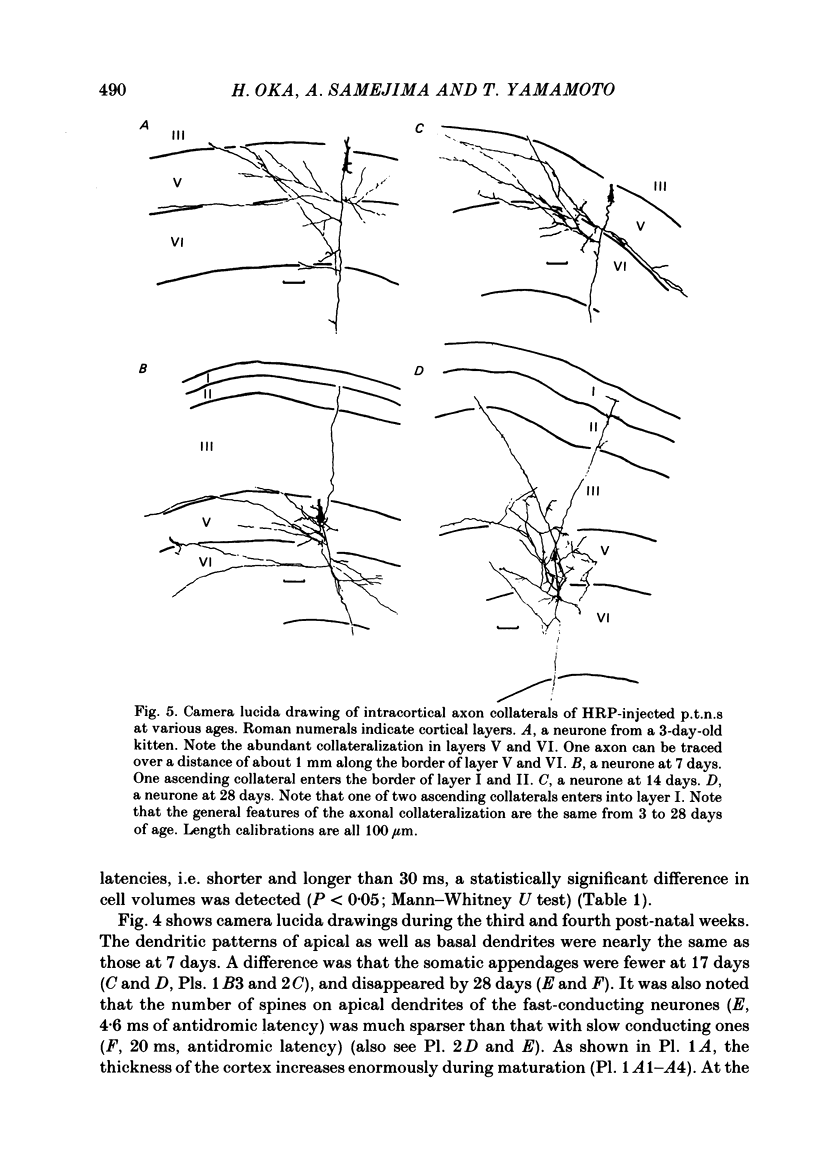
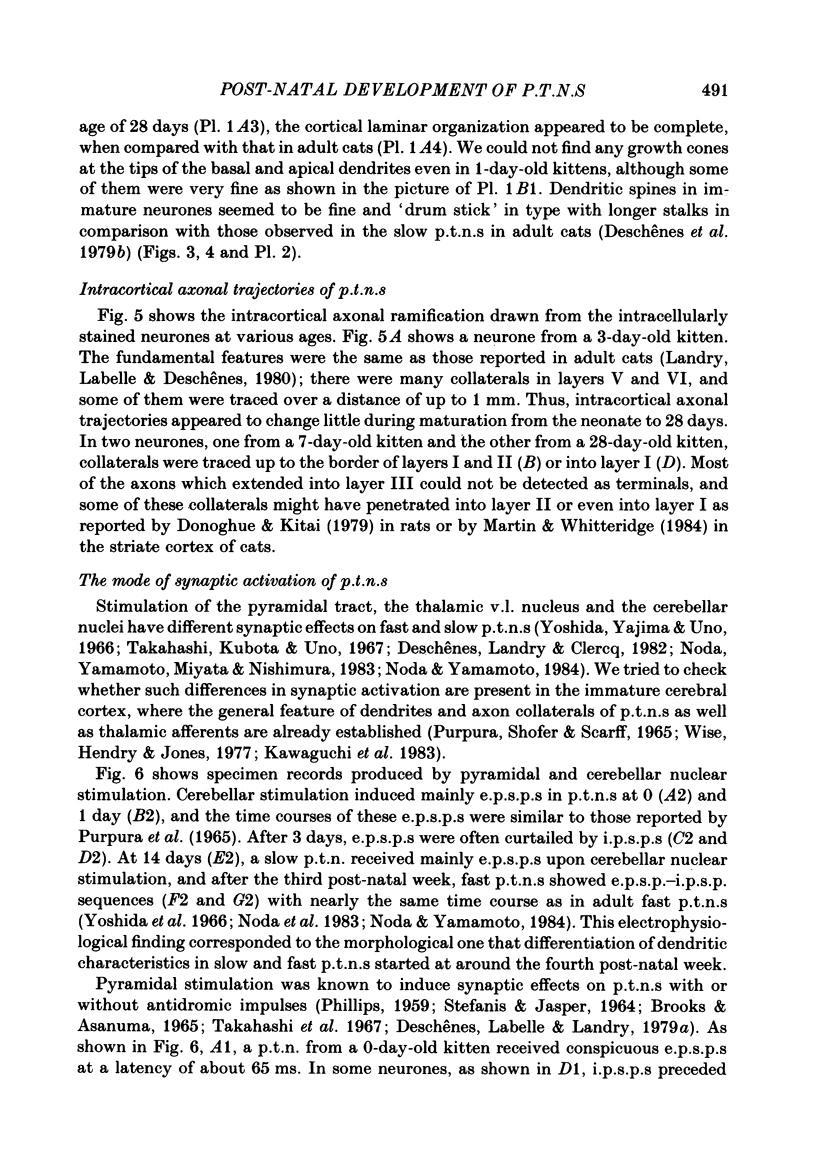
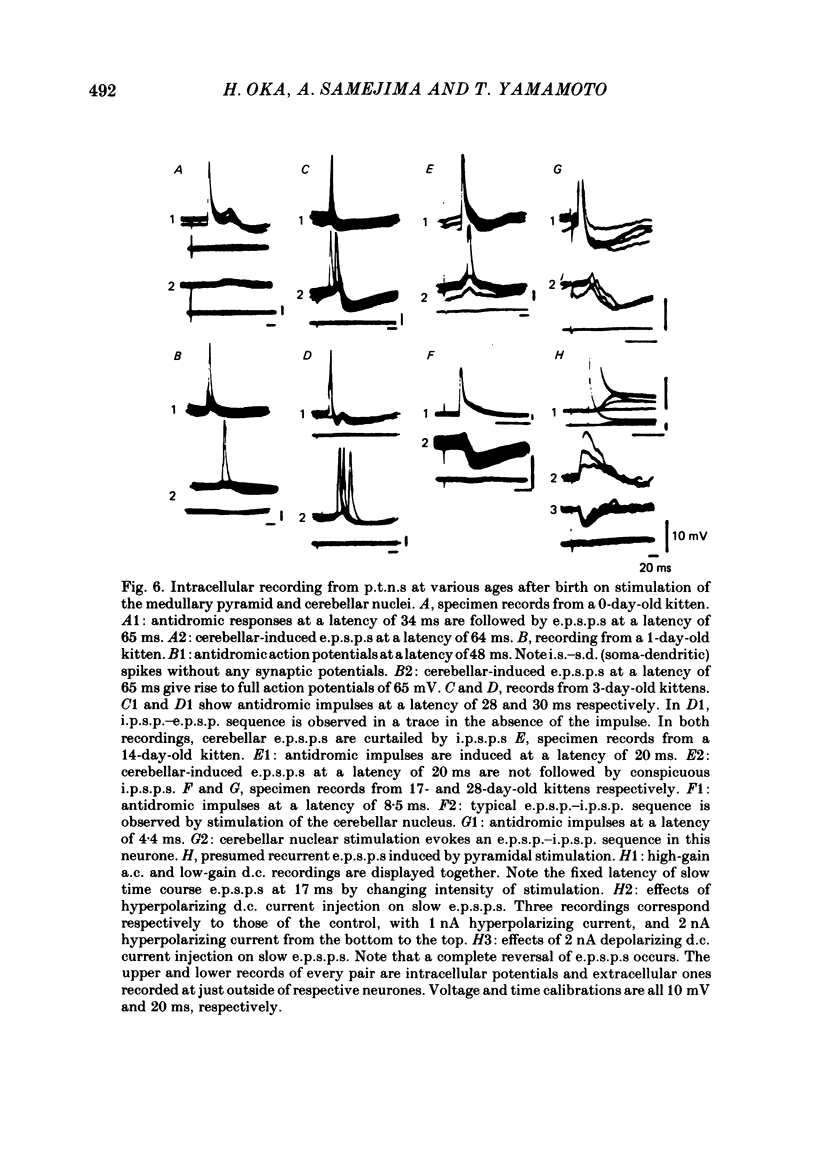
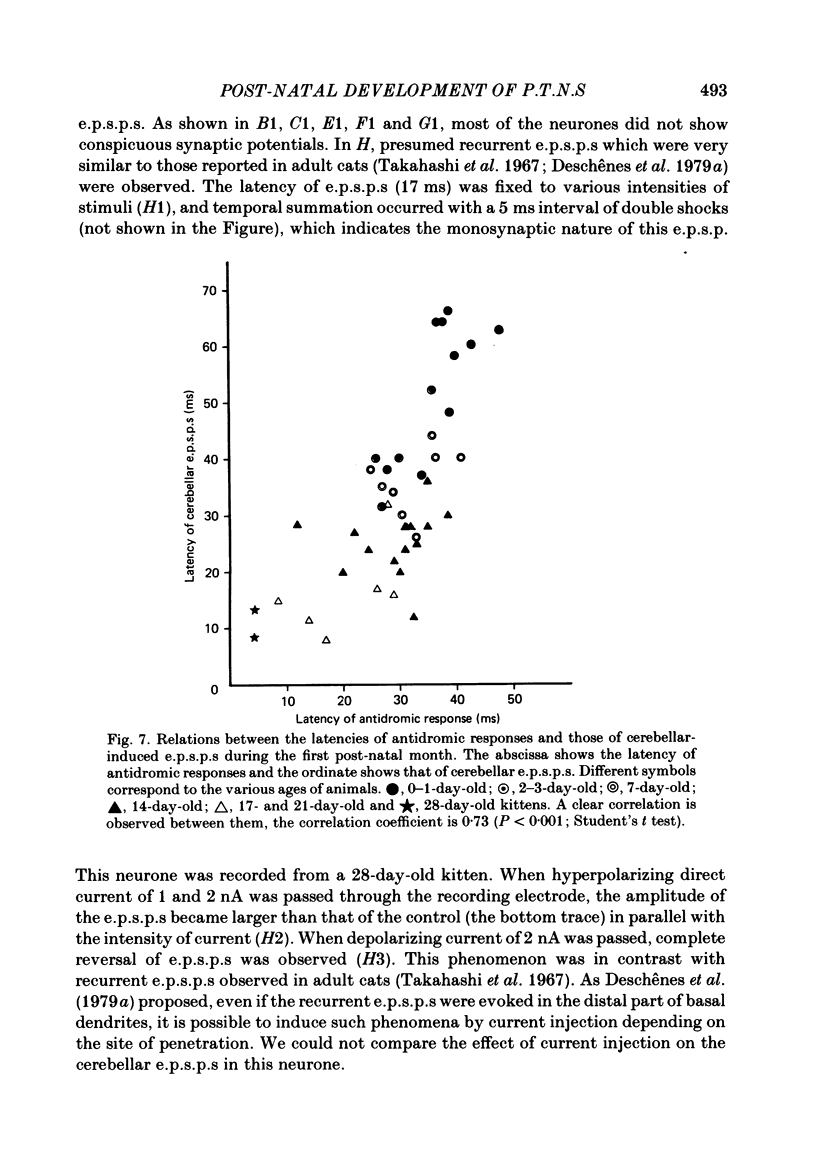
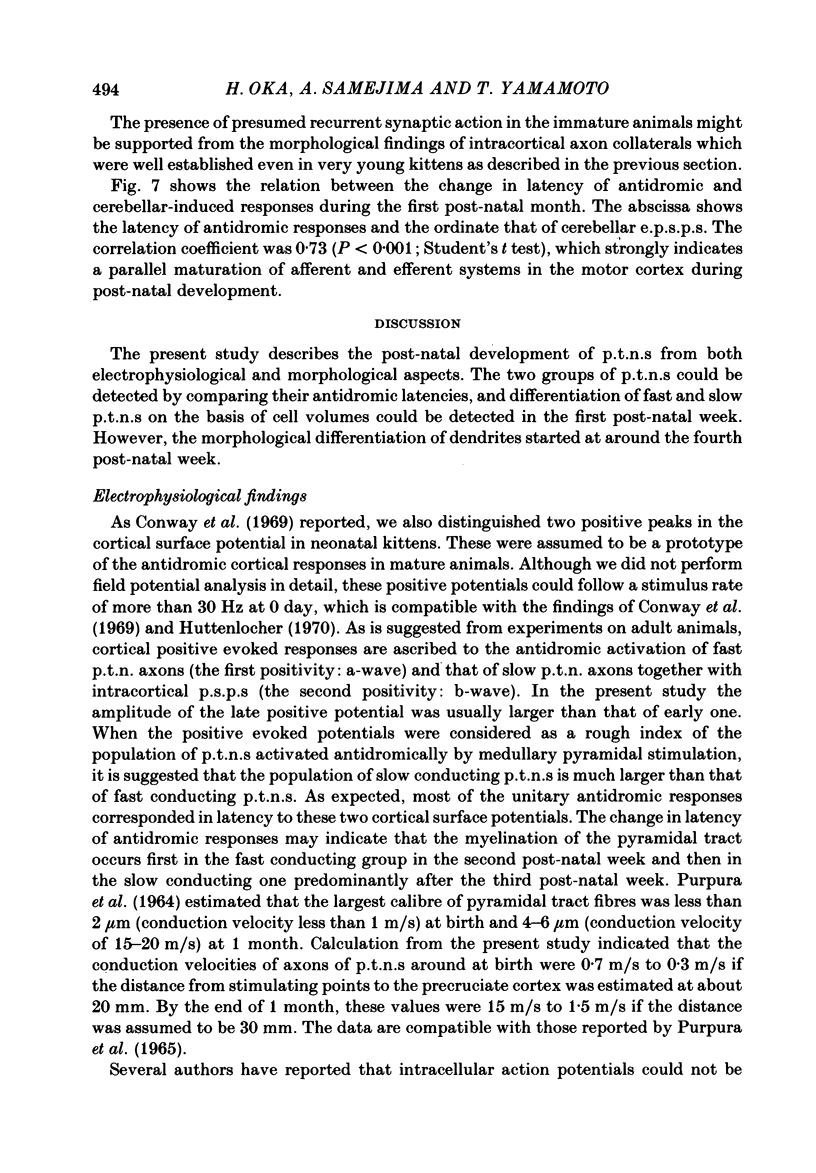
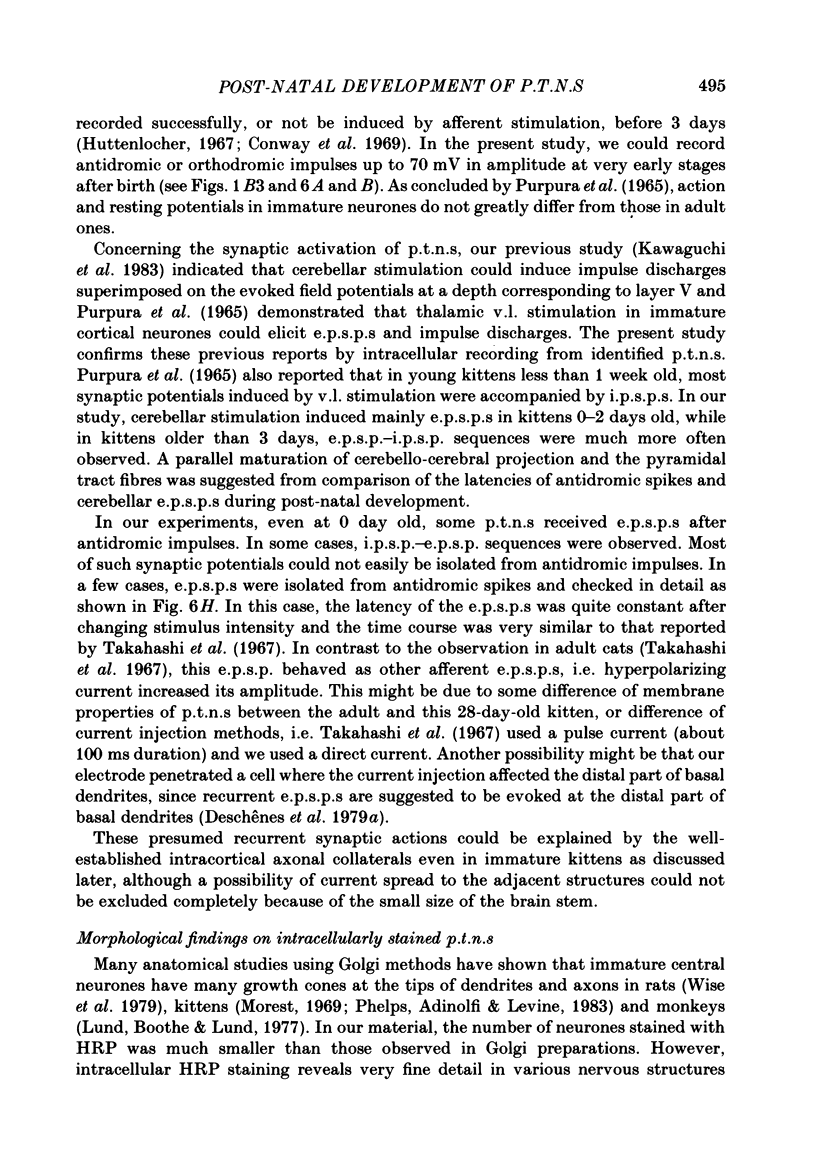
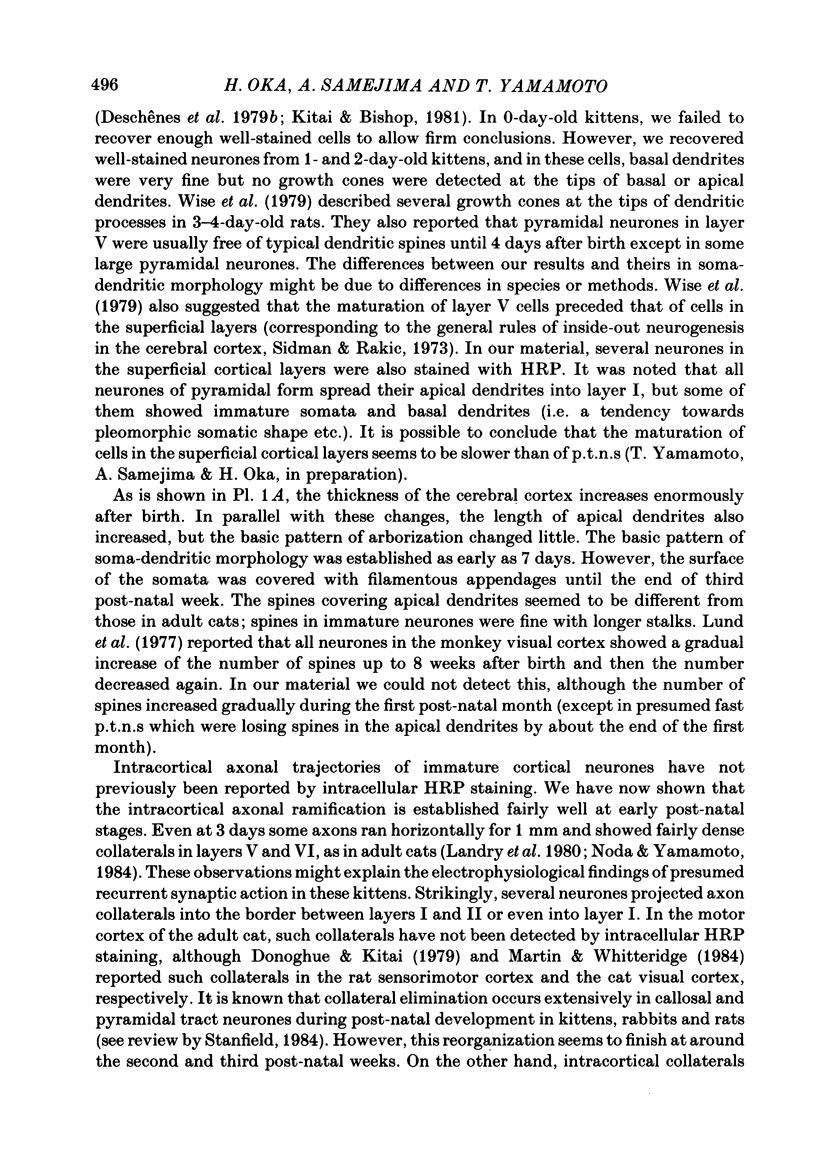
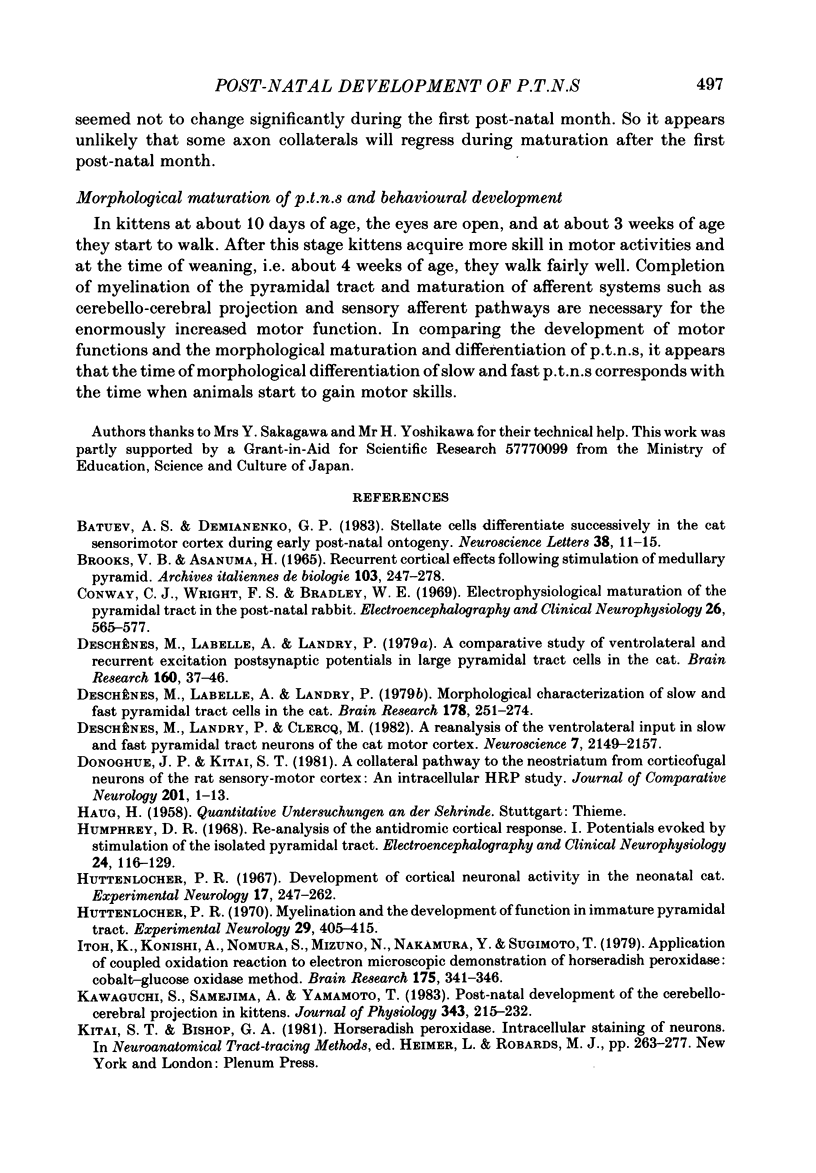
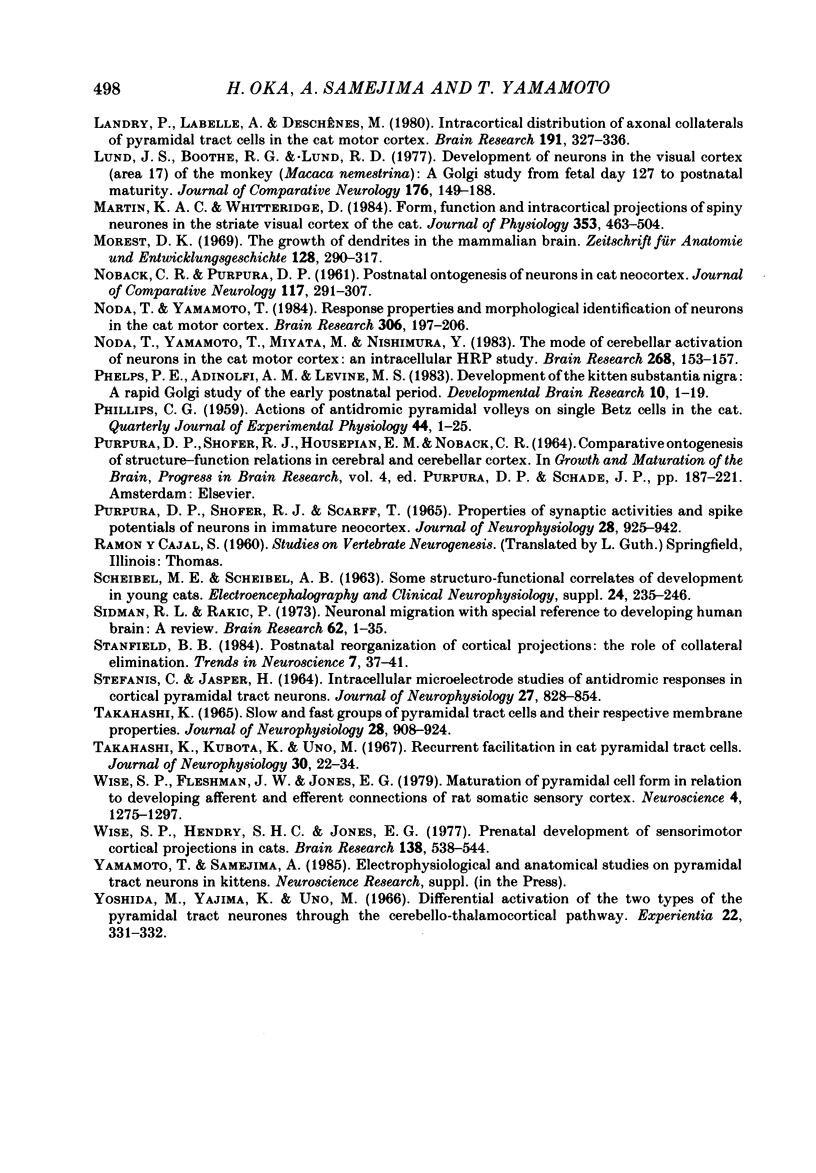
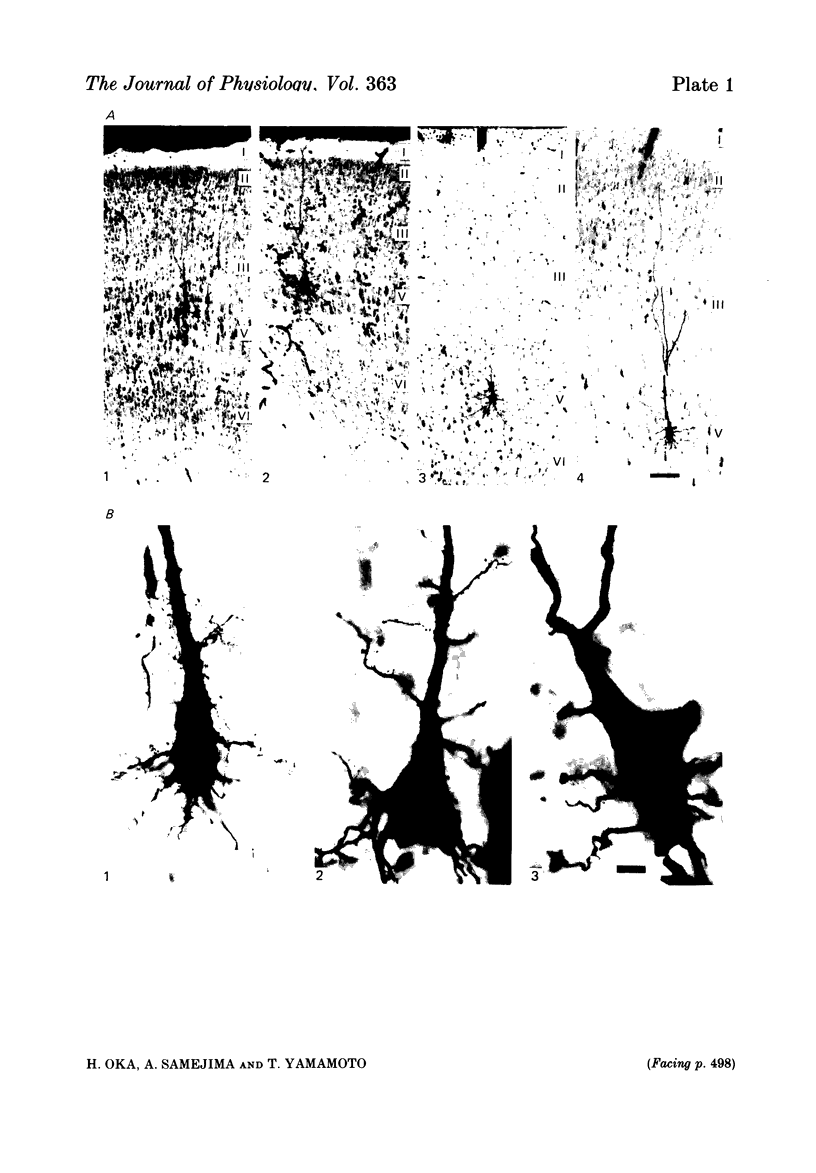
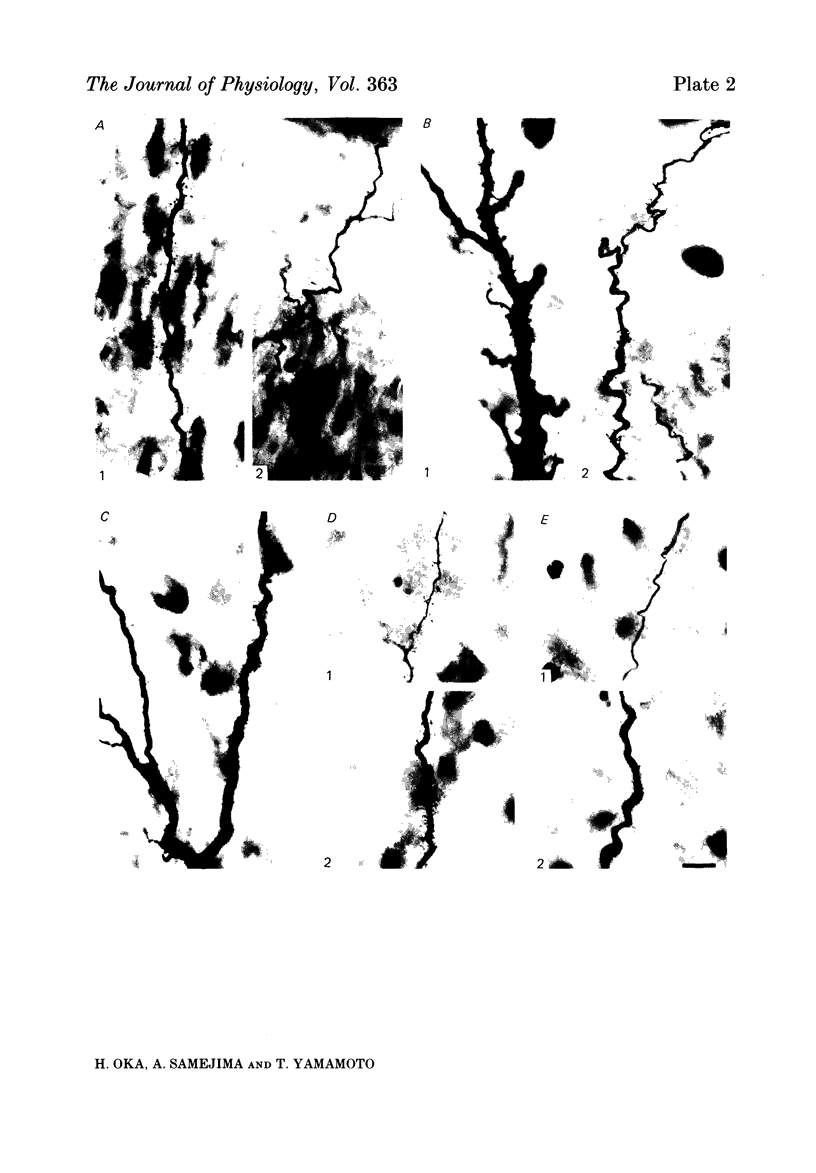
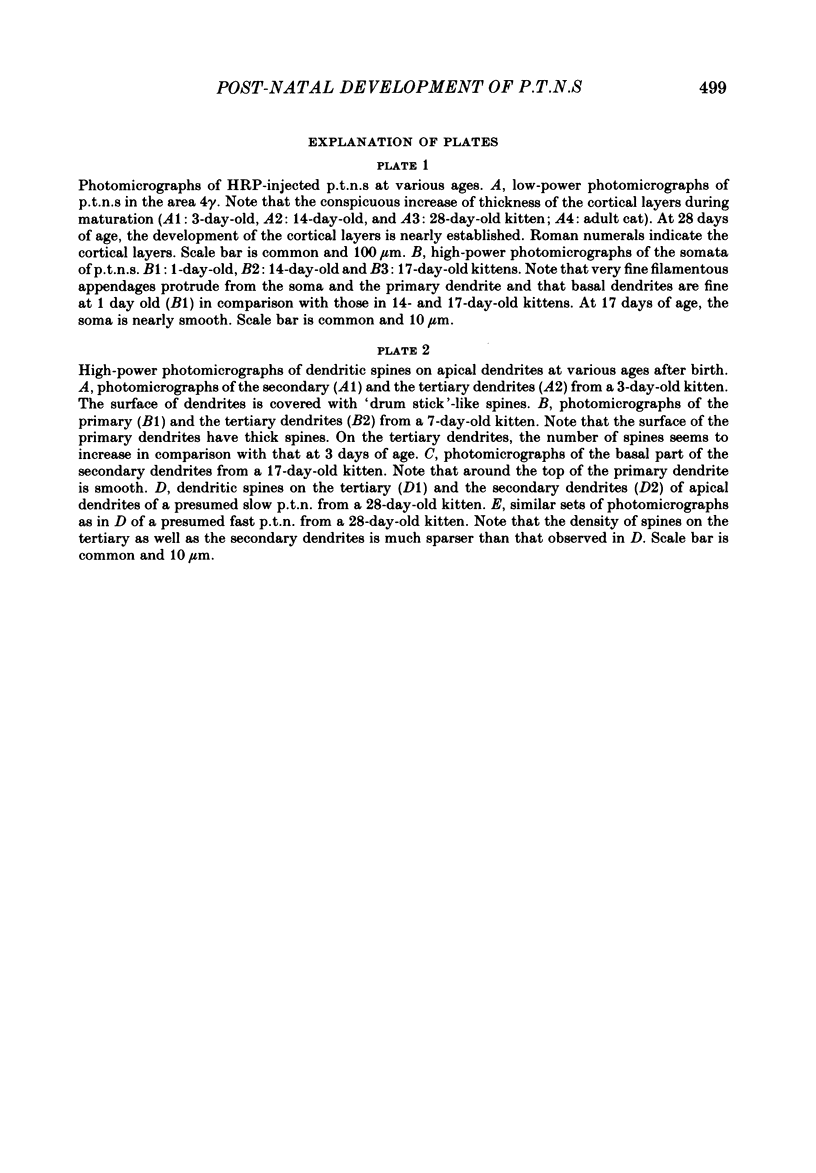
Images in this article
Selected References
These references are in PubMed. This may not be the complete list of references from this article.
- BROOKS V. B., ASANUMA H. RECURRENT CORTICAL EFFECTS FOLLOWING STIMULATION OF MEDULLARY PYRAMID. Arch Ital Biol. 1965 Jun 10;103:247–278. [PubMed] [Google Scholar]
- Batuev A. S., Demianenko G. P. Stellate cells differentiate successively in the cat sensorimotor cortex during early postnatal ontogeny. Neurosci Lett. 1983 Jul 15;38(1):11–15. doi: 10.1016/0304-3940(83)90102-7. [DOI] [PubMed] [Google Scholar]
- Conway C. J., Wright F. S., Bradley W. E. Electrophysiological maturation of the pyramidal tract in the post-natal rabbit. Electroencephalogr Clin Neurophysiol. 1969 Jun;26(6):565–577. doi: 10.1016/0013-4694(69)90002-9. [DOI] [PubMed] [Google Scholar]
- Deschênes M., Labelle A., Landry P. A comparative study of ventrolateral and recurrent excitatory postsynaptic potentials in large pyramidal tract cells in the cat. Brain Res. 1979 Jan 5;160(1):37–46. doi: 10.1016/0006-8993(79)90598-5. [DOI] [PubMed] [Google Scholar]
- Deschênes M., Labelle A., Landry P. Morphological characterization of slow and fast pyramidal tract cells in the cat. Brain Res. 1979 Dec 14;178(2-3):251–274. doi: 10.1016/0006-8993(79)90693-0. [DOI] [PubMed] [Google Scholar]
- Deschênes M., Landry P., Clercq M. A reanalysis of the ventrolateral input in slow and fast pyramidal tract neurons of the cat motor cortex. Neuroscience. 1982;7(9):2149–2157. doi: 10.1016/0306-4522(82)90126-9. [DOI] [PubMed] [Google Scholar]
- Donoghue J. P., Kitai S. T. A collateral pathway to the neostriatum from corticofugal neurons of the rat sensory-motor cortex: an intracellular HRP study. J Comp Neurol. 1981 Sep 1;201(1):1–13. doi: 10.1002/cne.902010102. [DOI] [PubMed] [Google Scholar]
- Humphrey D. R. Re-analysis of the antidromic cortical response. I. Potentials evoked by stimulation of the isolated pyramidal tract. Electroencephalogr Clin Neurophysiol. 1968 Feb;24(2):116–129. doi: 10.1016/0013-4694(68)90118-1. [DOI] [PubMed] [Google Scholar]
- Huttenlocher P. R. Development of cortical neuronal activity in the neonatal cat. Exp Neurol. 1967 Mar;17(3):247–262. doi: 10.1016/0014-4886(67)90104-5. [DOI] [PubMed] [Google Scholar]
- Huttenlocher P. R. Myelination and the development of function in immature pyramidal tract. Exp Neurol. 1970 Dec;29(3):405–415. doi: 10.1016/0014-4886(70)90068-3. [DOI] [PubMed] [Google Scholar]
- Itoh K., Konishi A., Nomura S., Mizuno N., Nakamura Y., Sugimoto T. Application of coupled oxidation reaction to electron microscopic demonstration of horseradish peroxidase: cobalt-glucose oxidase method. Brain Res. 1979 Oct 19;175(2):341–346. doi: 10.1016/0006-8993(79)91013-8. [DOI] [PubMed] [Google Scholar]
- Kawaguchi S., Samejima A., Yamamoto T. Post-natal development of the cerebello-cerebral projection in kittens. J Physiol. 1983 Oct;343:215–232. doi: 10.1113/jphysiol.1983.sp014889. [DOI] [PMC free article] [PubMed] [Google Scholar]
- Landry P., Labelle A., Deschênes M. Intracortical distribution of axonal collaterals of pyramidal tract cells in the cat motor cortex. Brain Res. 1980 Jun 9;191(2):327–336. doi: 10.1016/0006-8993(80)91284-6. [DOI] [PubMed] [Google Scholar]
- Lund J. S., Boothe R. G., Lund R. D. Development of neurons in the visual cortex (area 17) of the monkey (Macaca nemestrina): a Golgi study from fetal day 127 to postnatal maturity. J Comp Neurol. 1977 Nov 15;176(2):149–188. doi: 10.1002/cne.901760203. [DOI] [PubMed] [Google Scholar]
- Martin K. A., Whitteridge D. Form, function and intracortical projections of spiny neurones in the striate visual cortex of the cat. J Physiol. 1984 Aug;353:463–504. doi: 10.1113/jphysiol.1984.sp015347. [DOI] [PMC free article] [PubMed] [Google Scholar]
- Morest D. K. The growth of dendrites in the mammalian brain. Z Anat Entwicklungsgesch. 1969;128(4):290–317. doi: 10.1007/BF00522529. [DOI] [PubMed] [Google Scholar]
- NOBACK C. R., PURPURA D. P. Postnatal ontogenesis of neurons in cat neocortex. J Comp Neurol. 1961 Dec;117:291–307. doi: 10.1002/cne.901170303. [DOI] [PubMed] [Google Scholar]
- Noda T., Yamamoto T., Miyata M., Nishimura Y. The mode of cerebellar activation of neurons in the cat motor cortex: an intracellular HRP study. Brain Res. 1983 May 23;268(1):153–157. doi: 10.1016/0006-8993(83)90400-6. [DOI] [PubMed] [Google Scholar]
- Noda T., Yamamoto T. Response properties and morphological identification of neurons in the cat motor cortex. Brain Res. 1984 Jul 23;306(1-2):197–206. doi: 10.1016/0006-8993(84)90369-x. [DOI] [PubMed] [Google Scholar]
- PHILLIPS C. G. Actions of antidromic pyramidal volleys on single Betz cells in the cat. Q J Exp Physiol Cogn Med Sci. 1959 Jan;44(1):1–25. doi: 10.1113/expphysiol.1959.sp001364. [DOI] [PubMed] [Google Scholar]
- Phelps P. E., Adinolfi A. M., Levine M. S. Development of the kitten substantia nigra: a rapid Golgi study of the early postnatal period. Brain Res. 1983 Oct;312(1):1–19. doi: 10.1016/0165-3806(83)90116-5. [DOI] [PubMed] [Google Scholar]
- Purpura D. P., Shofer R. J., Scarff T. Properties of synaptic activities and spike potentials of neurons in immature neocortex. J Neurophysiol. 1965 Sep;28(5):925–942. doi: 10.1152/jn.1965.28.5.925. [DOI] [PubMed] [Google Scholar]
- STEFANIS C., JASPER H. INTRACELLULAR MICROELECTRODE STUDIES OF ANTIDROMIC RESPONSES IN CORTICAL PYRAMIDAL TRACT NEURONS. J Neurophysiol. 1964 Sep;27:828–854. doi: 10.1152/jn.1964.27.5.828. [DOI] [PubMed] [Google Scholar]
- Scheibel M. E., Scheibel A. B. Some structuro-functional correlates of development in young cats. Electroencephalogr Clin Neurophysiol. 1963;(Suppl):235+–235+. [PubMed] [Google Scholar]
- Sidman R. L., Rakic P. Neuronal migration, with special reference to developing human brain: a review. Brain Res. 1973 Nov 9;62(1):1–35. doi: 10.1016/0006-8993(73)90617-3. [DOI] [PubMed] [Google Scholar]
- Takahashi K. Slow and fast groups of pyramidal tract cells and their respective membrane properties. J Neurophysiol. 1965 Sep;28(5):908–924. doi: 10.1152/jn.1965.28.5.908. [DOI] [PubMed] [Google Scholar]
- Wise S. P., Fleshman J. W., Jr, Jones E. G. Maturation of pyramidal cell form in relation to developing afferent and efferent connections of rat somatic sensory cortex. Neuroscience. 1979;4(9):1275–1297. doi: 10.1016/0306-4522(79)90157-x. [DOI] [PubMed] [Google Scholar]
- Wise S. P., Hendry S. H., Jones E. G. Prenatal development of sensorimotor cortical projections in cats. Brain Res. 1977 Dec 23;138(3):538–544. doi: 10.1016/0006-8993(77)90690-4. [DOI] [PubMed] [Google Scholar]
- Yoshida M., Yajima K., Uno M. Different activation of the 2 types of the pyramidal tract neurones through the cerebello-thalamocortical pathway. Experientia. 1966 May 15;22(5):331–332. doi: 10.1007/BF01900484. [DOI] [PubMed] [Google Scholar]