Abstract
The whole-cell patch-electrode technique of Fenwick, Marty & Neher (1982) has been applied to single suspension-cultured mouse fibroblasts. Seals in the range of 10-50 G omega were obtained without special cleaning of the cell membranes. Rupture of the membrane patch inside the electrode was accompanied by a shift of measured potential into the range -10 to -25 mV, but in most cases with little change in the recorded resistance. The latter fact implied that the absolute resistance of the cell membrane must be in the same range as the seal resistance and the recorded potential is a poor measure of actual cell membrane potential. Steady-state current-voltage curves (range -160 mV to +80 mV) were generated before and after rupture of the membrane patch, and the difference between these gave (zero-current) membrane potentials of -50 to -75 mV, which represents a leak-corrected estimate of the true cell-membrane potential. The associated slope conductivity of the cell membrane was 5-15 microS/cm2 (assumed smooth-sphere geometry, cells 13-15 microns in diameter) and was K+-dominated. With 0.1 mM (or more) free Ca2+ filling the patch electrode, membrane potentials in the range -60 to -85 mV were observed following patch rupture, with associated slope conductivities of 200-400 microS/cm2, also K+-dominated. Similar voltages and conductivities were observed at the peak of pulse-induced 'hyperpolarizing activation' (Nelson, Peacock, & Minna, 1972), and the two phenomena probably reflect the behaviour of Ca2+-activated K+ channels. Both the pulse-induced conductance and the Ca2+-activated conductance spontaneously decayed, the latter over periods of 5-15 min following patch rupture. Sr2+, Ba2+, and Co2+ could also activate the putative K+ channels, but only Sr2+ really mimicked Ca2+. Co2+ and Ba2+ activated with a delay of several minutes following patch rupture, and deactivated quickly with a small decrease of conductance and a large decrease of membrane potential. Evidently, Co2+ and Ba2+ affect channel specificity as well as channel opening and closing kinetics.
Full text
PDF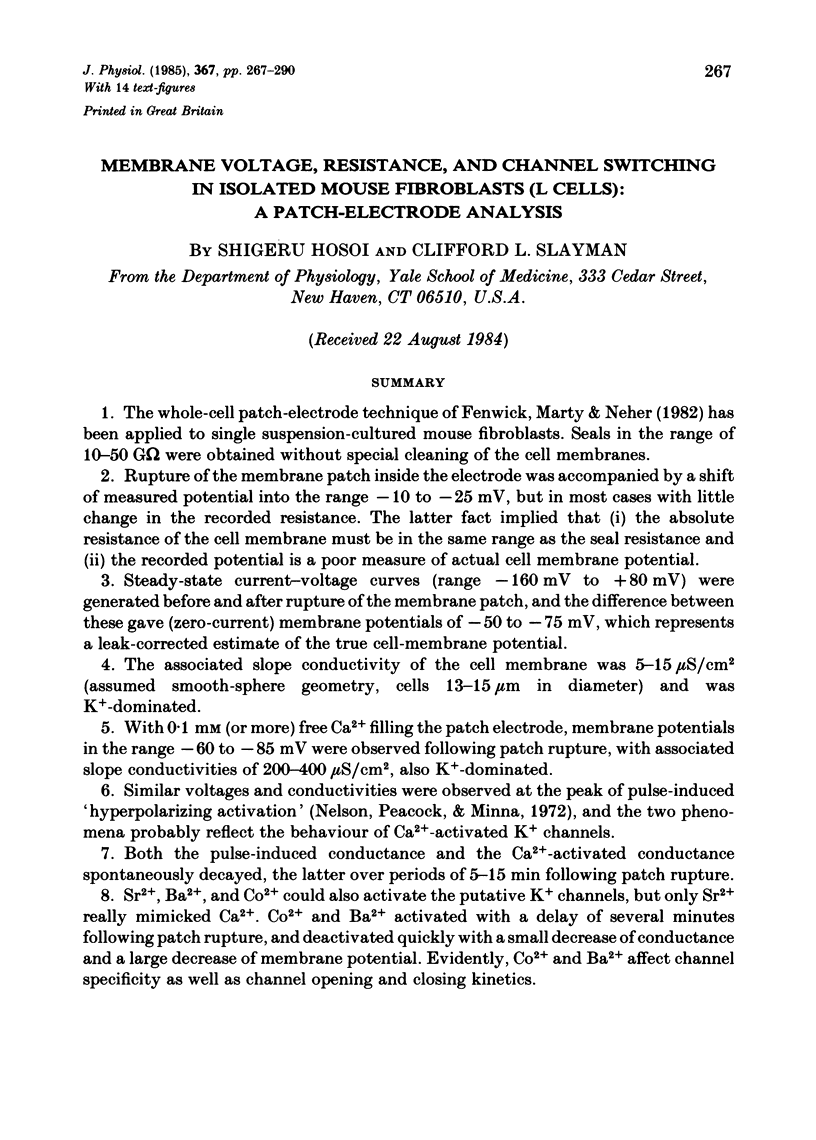
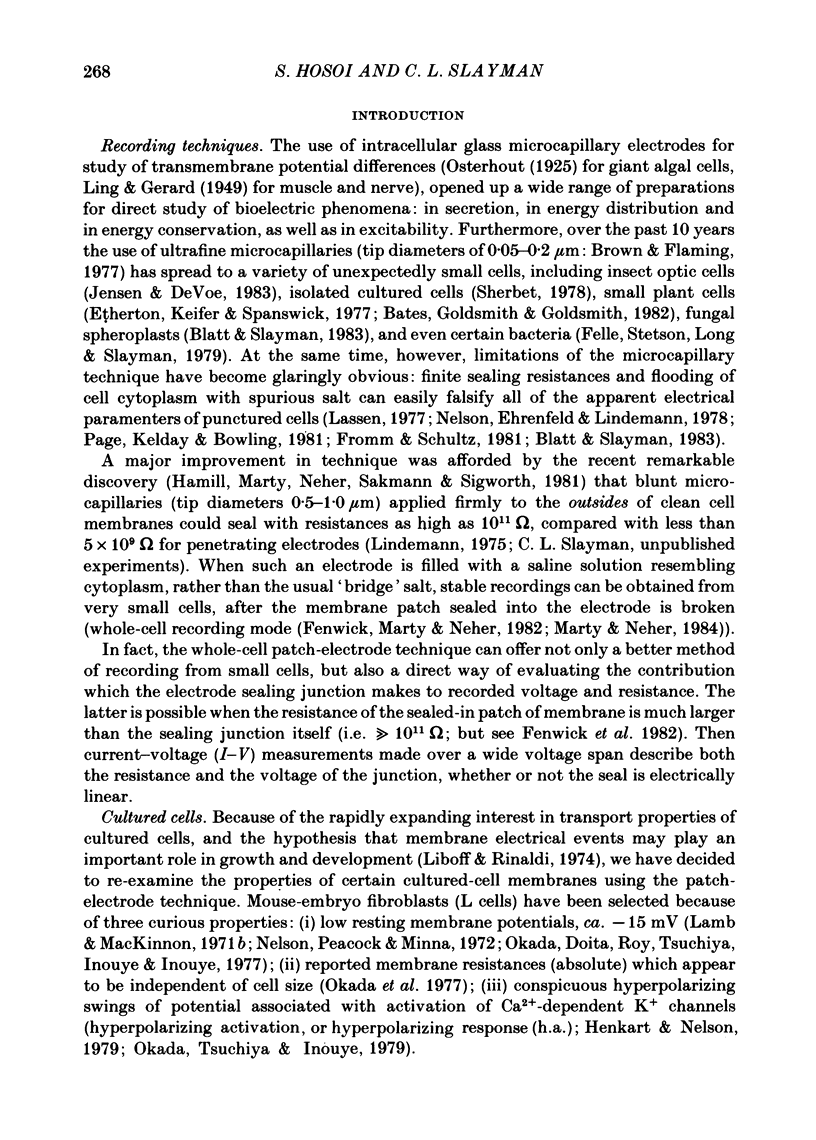
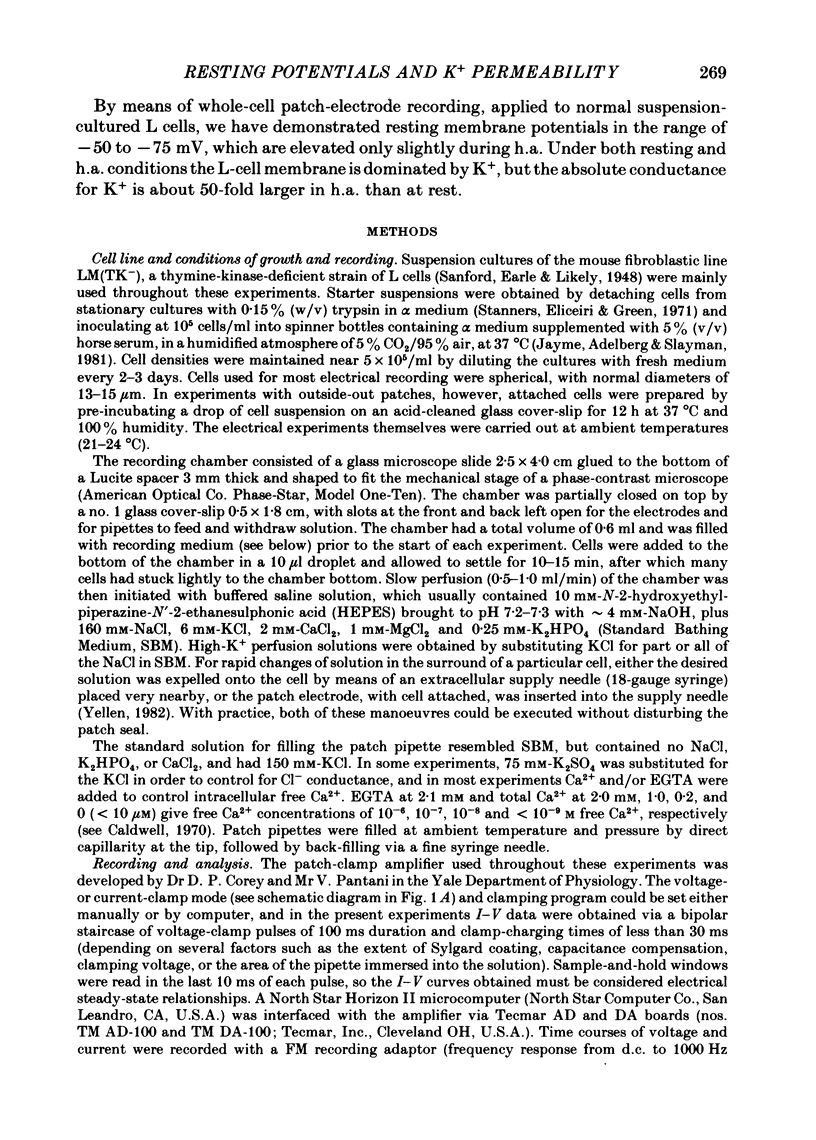
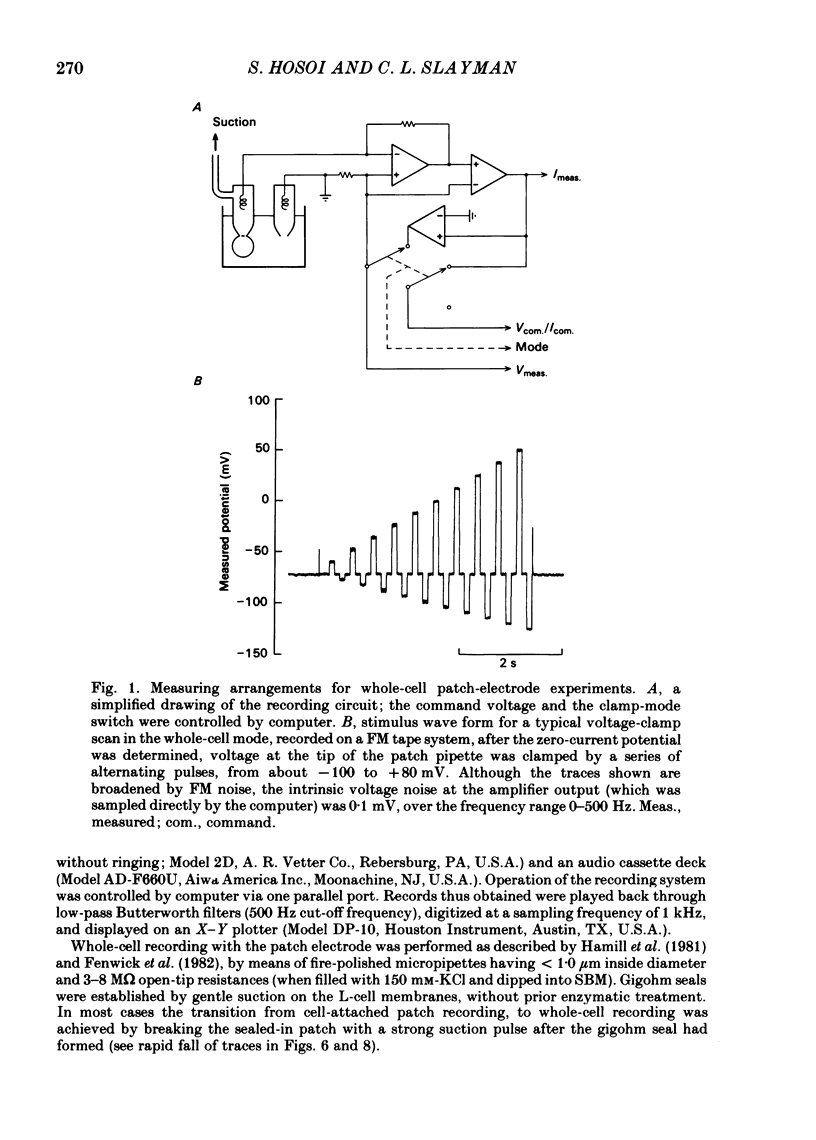
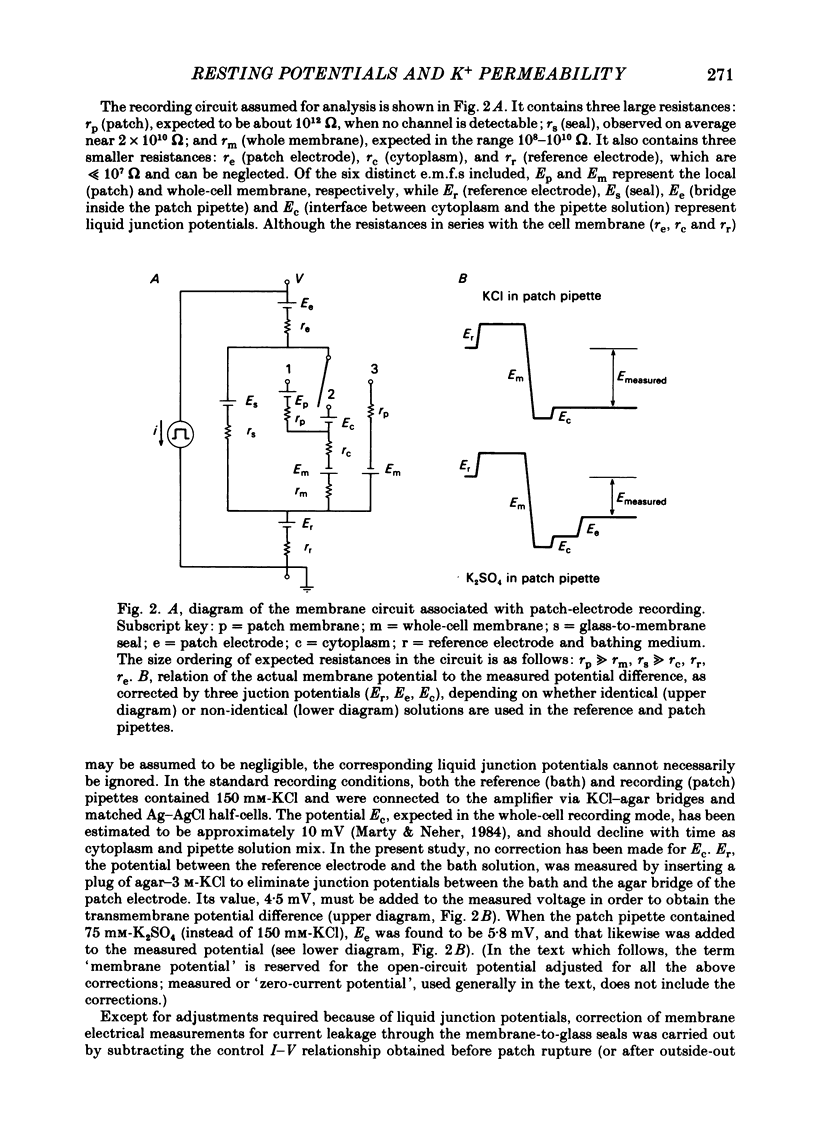
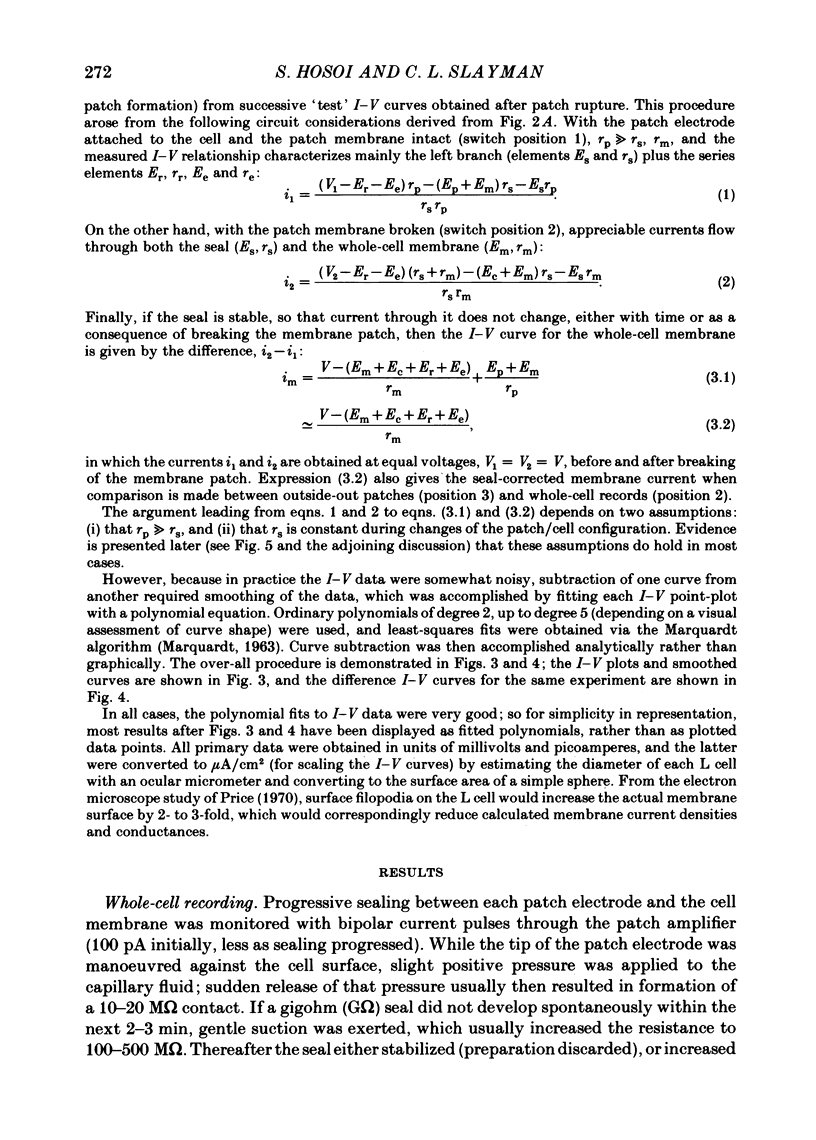
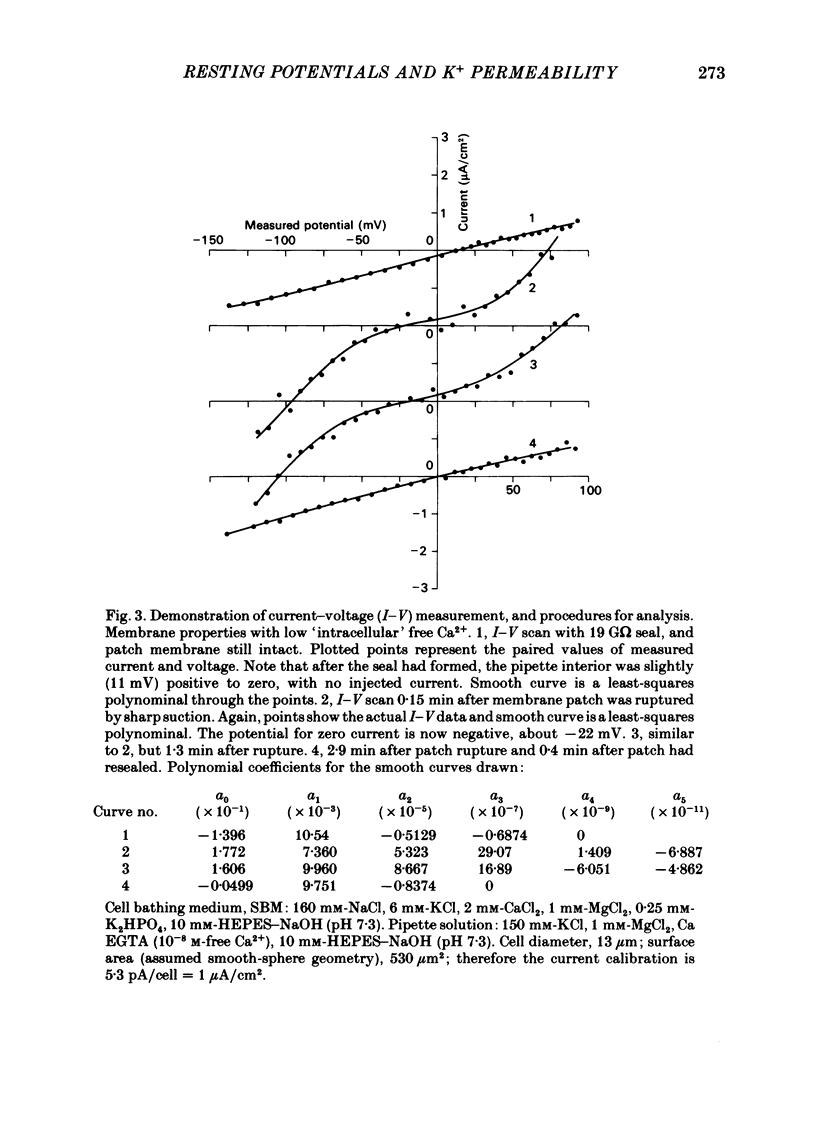
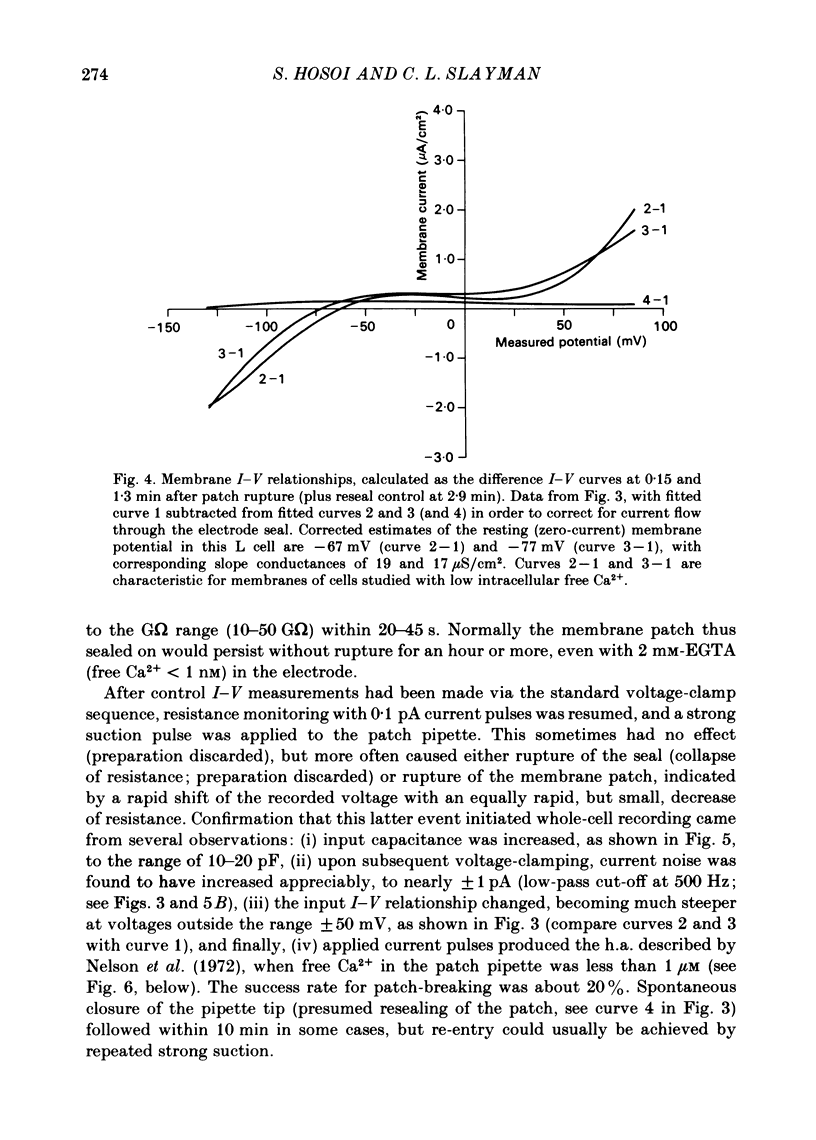
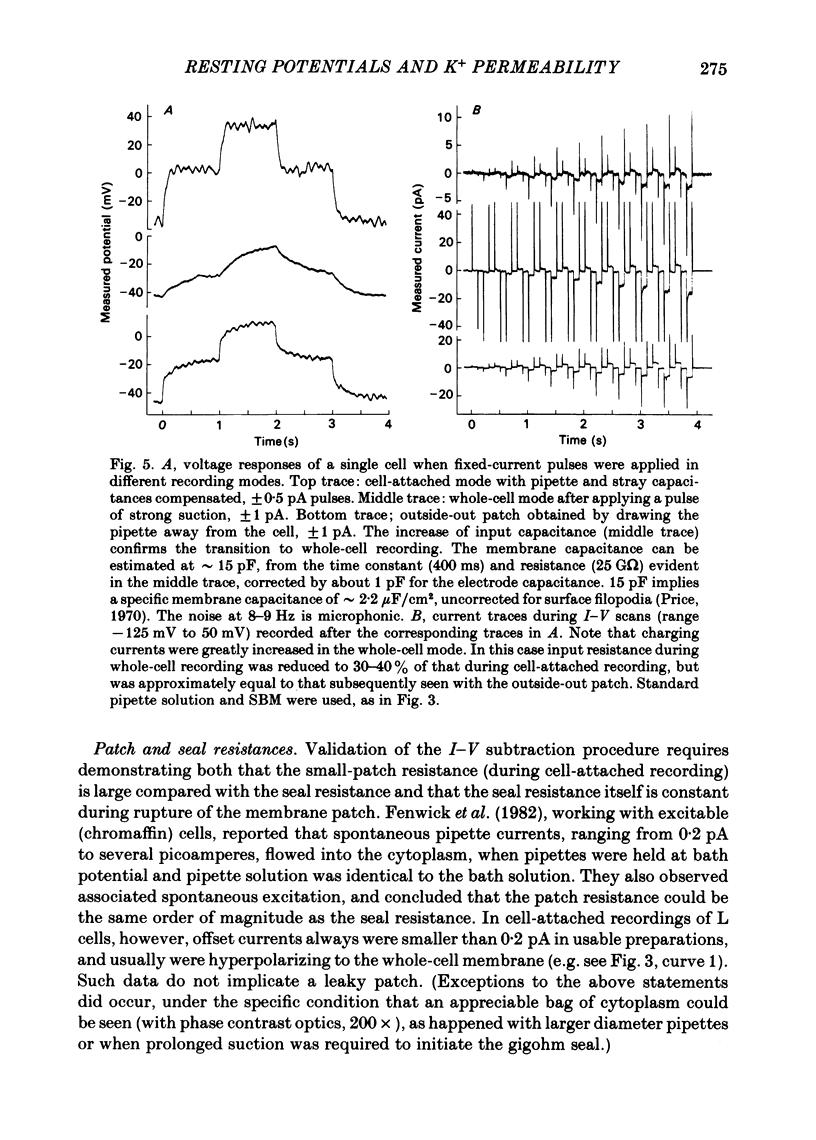
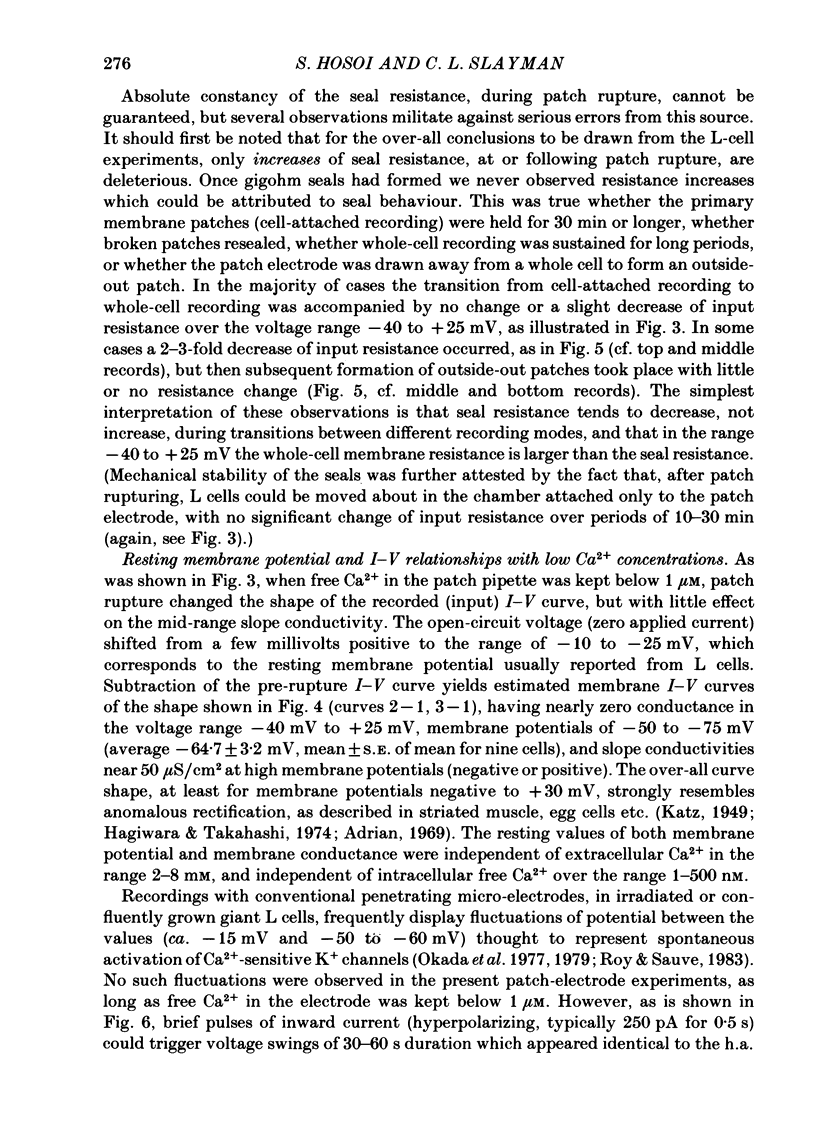
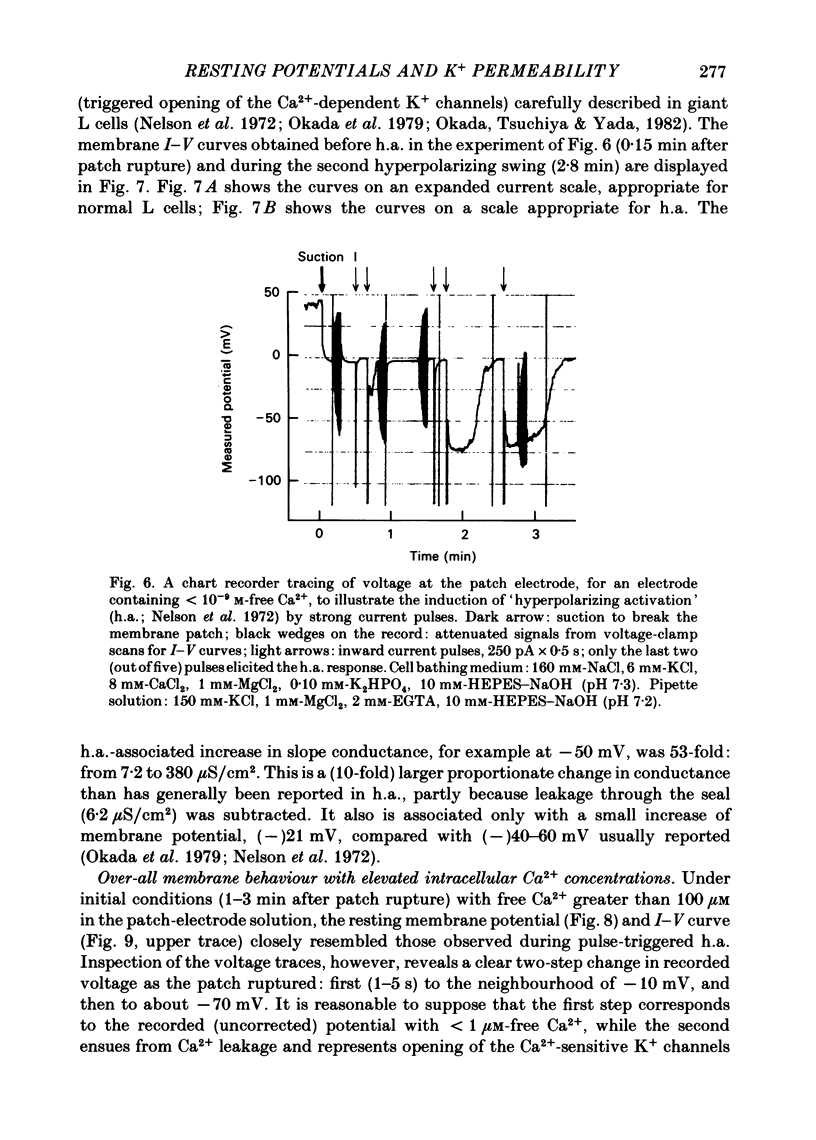
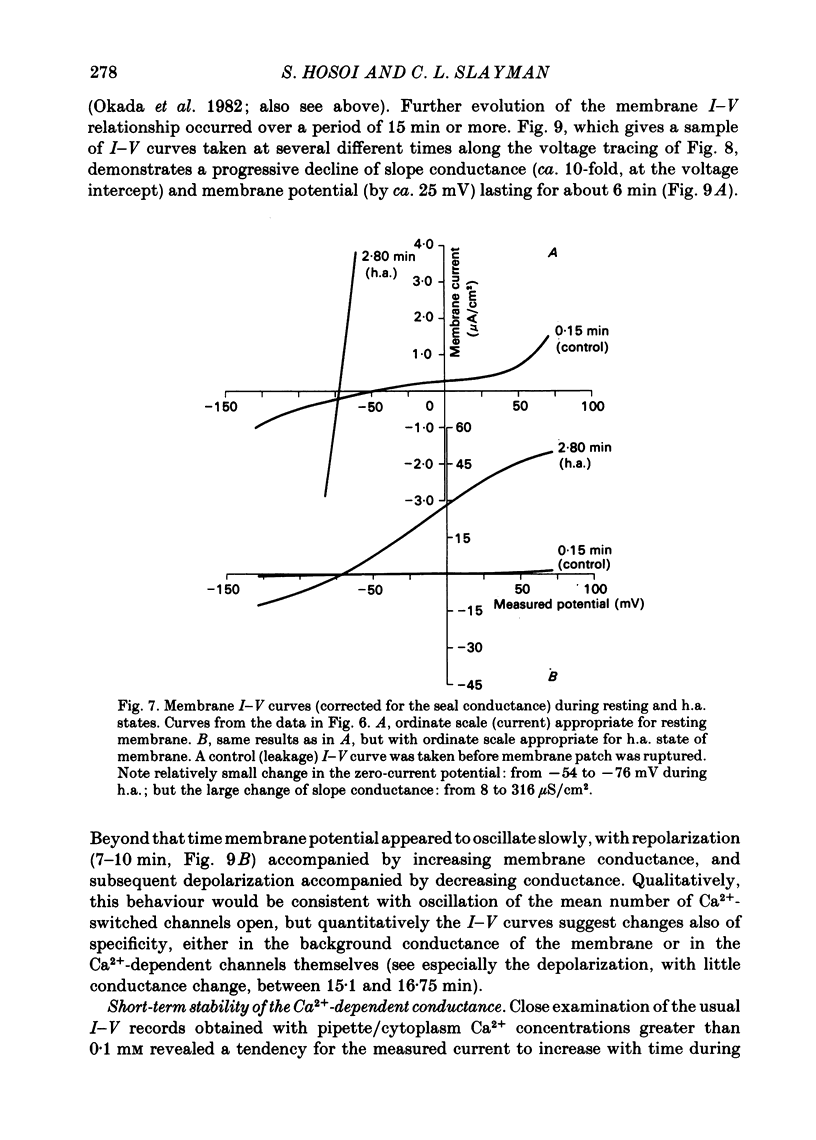
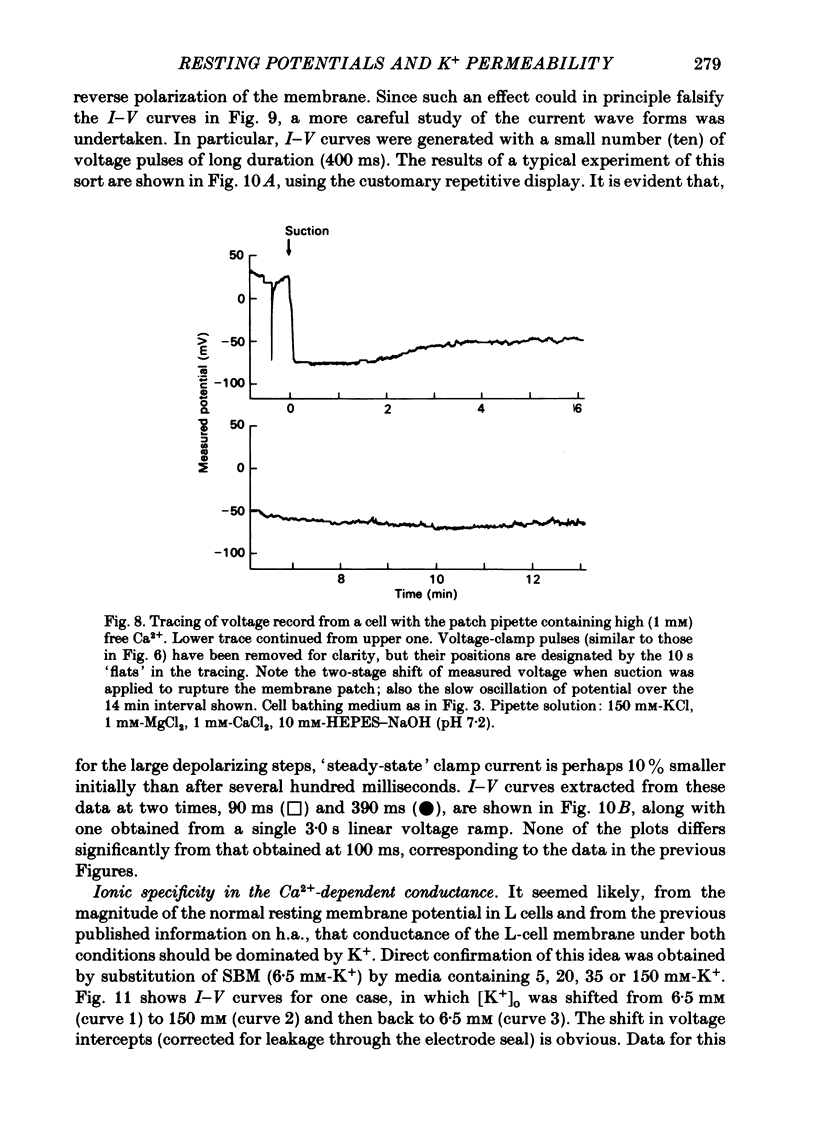
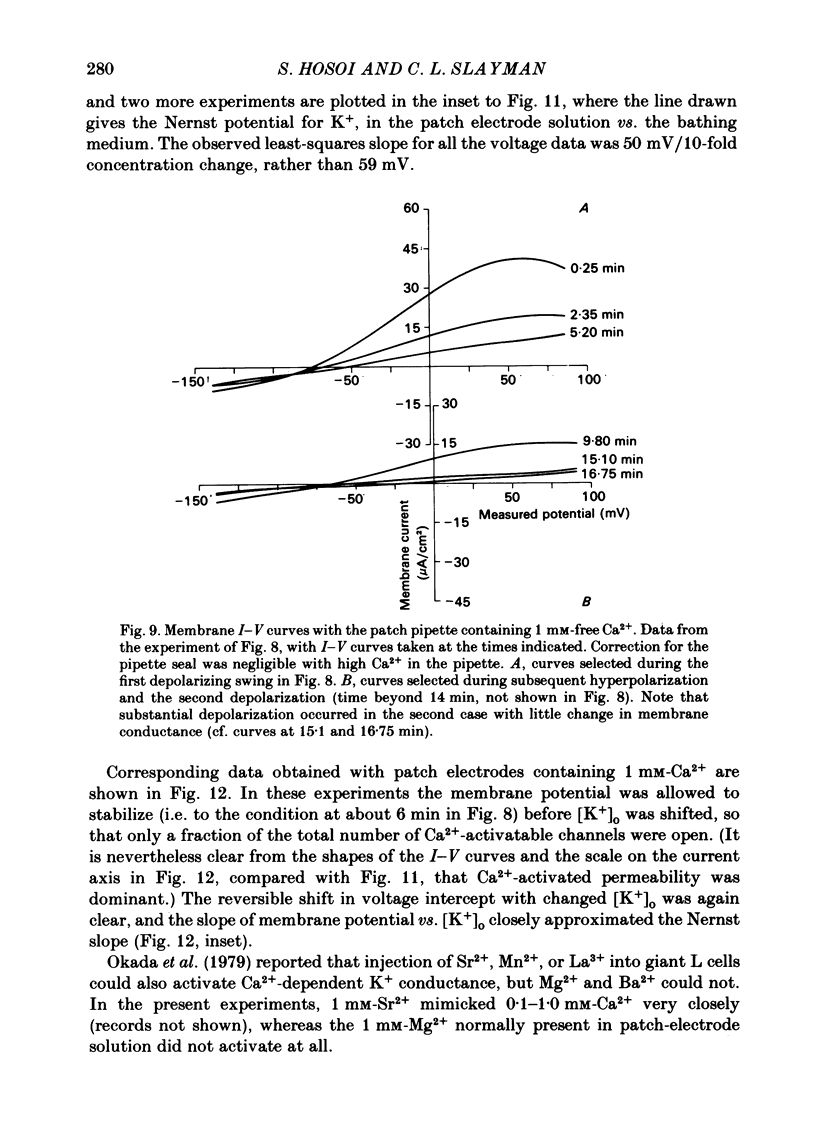
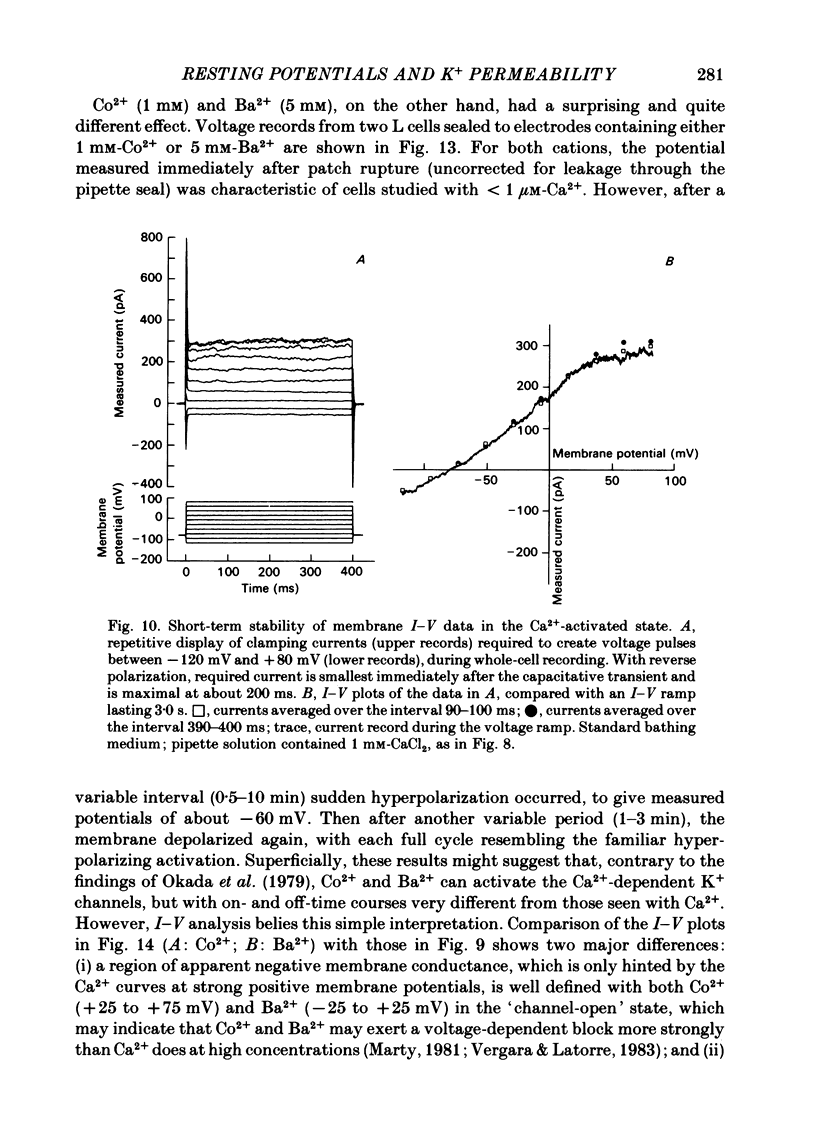
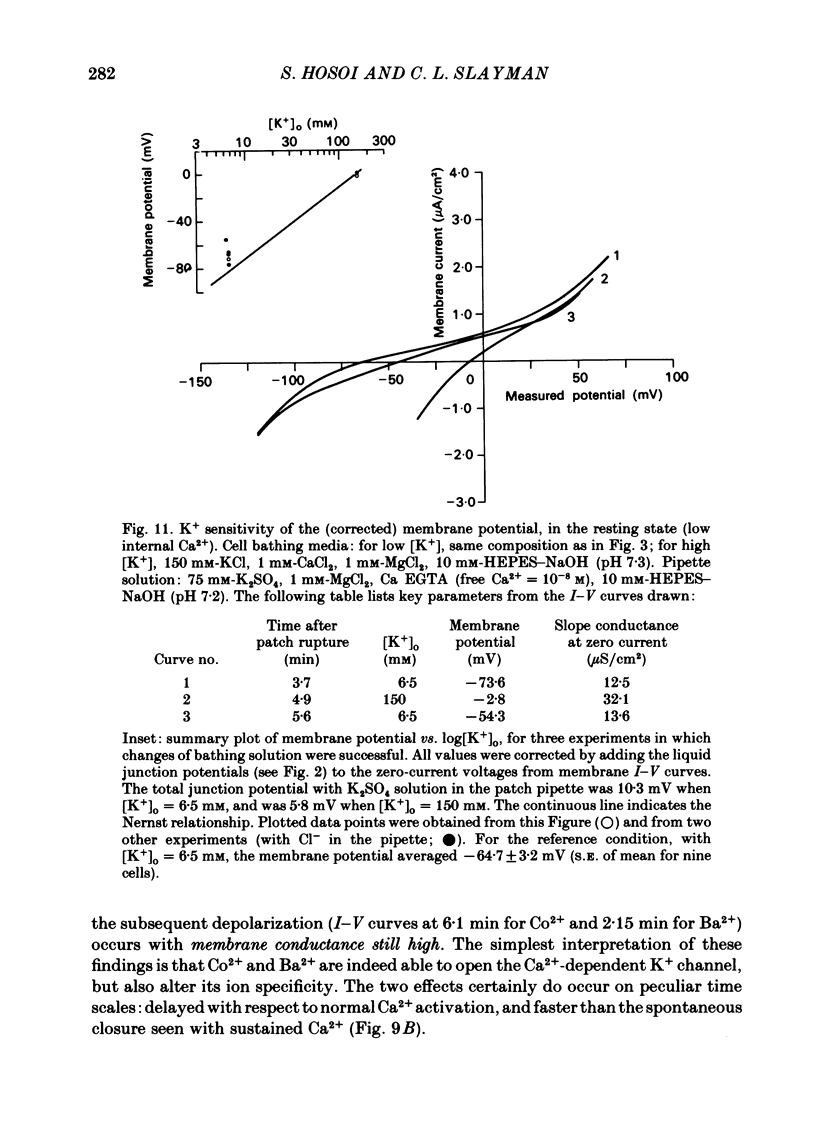
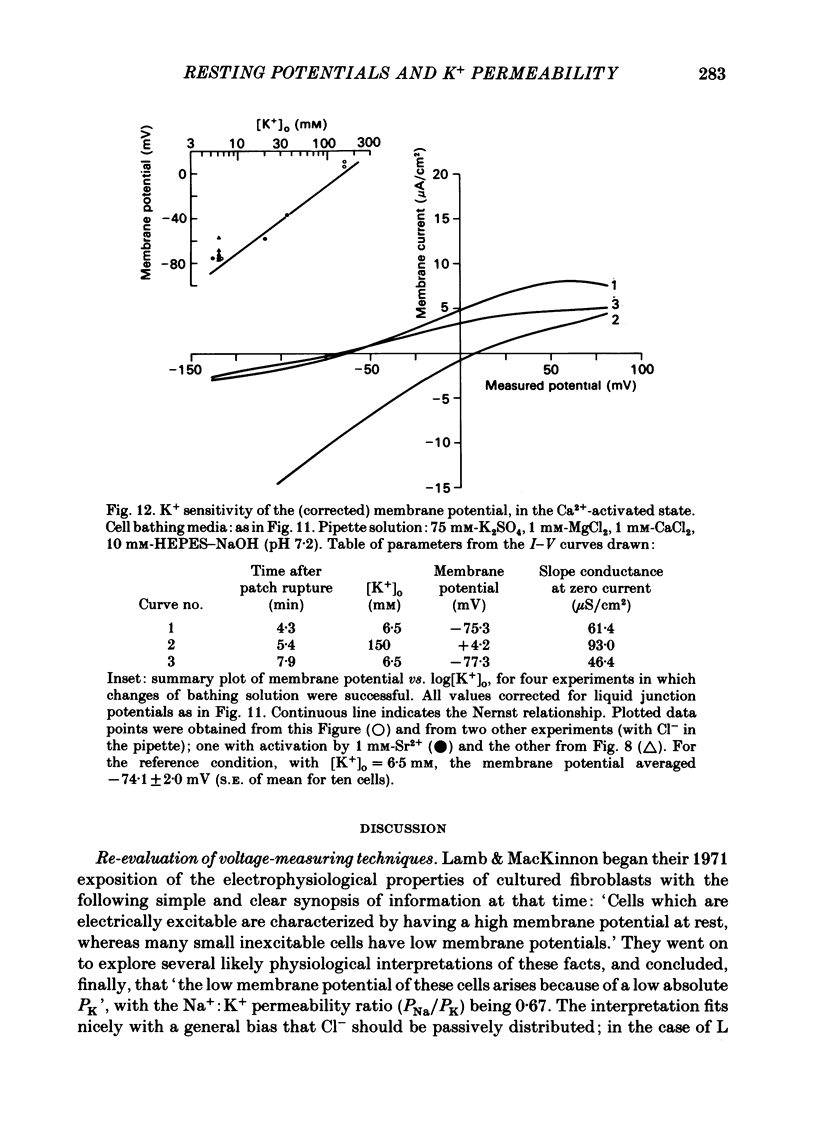
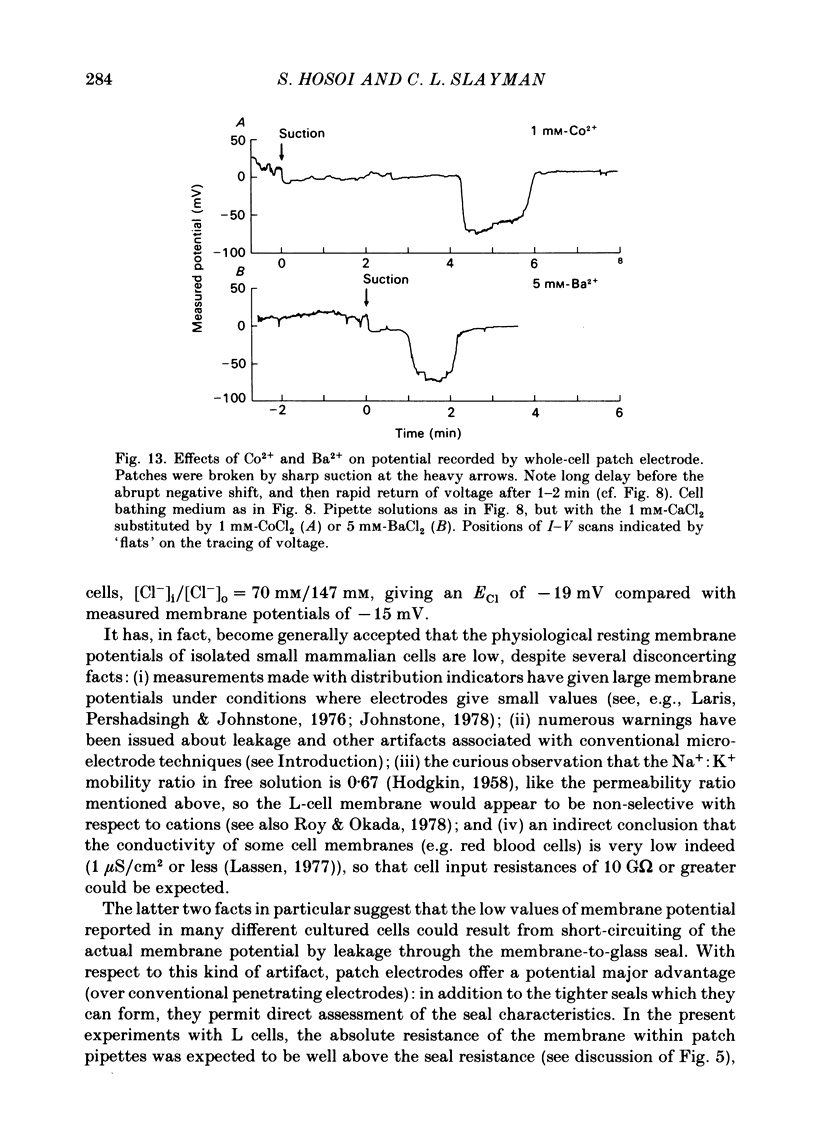
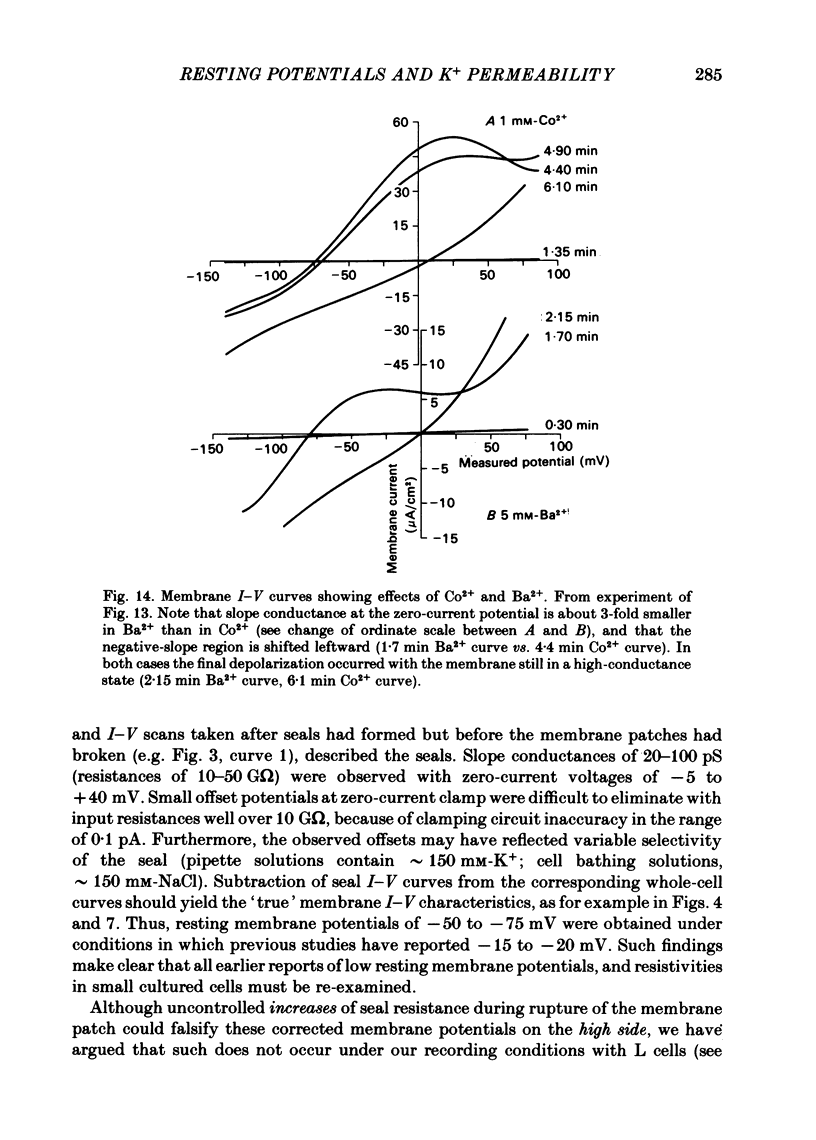
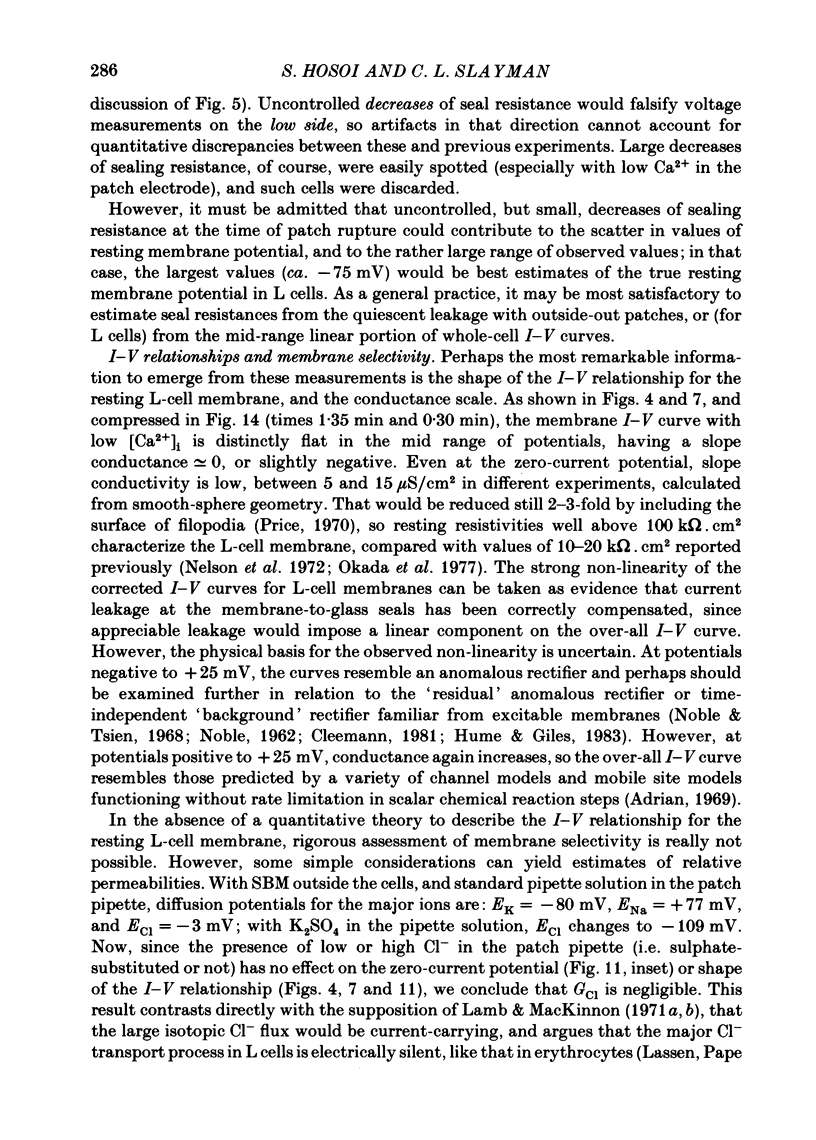
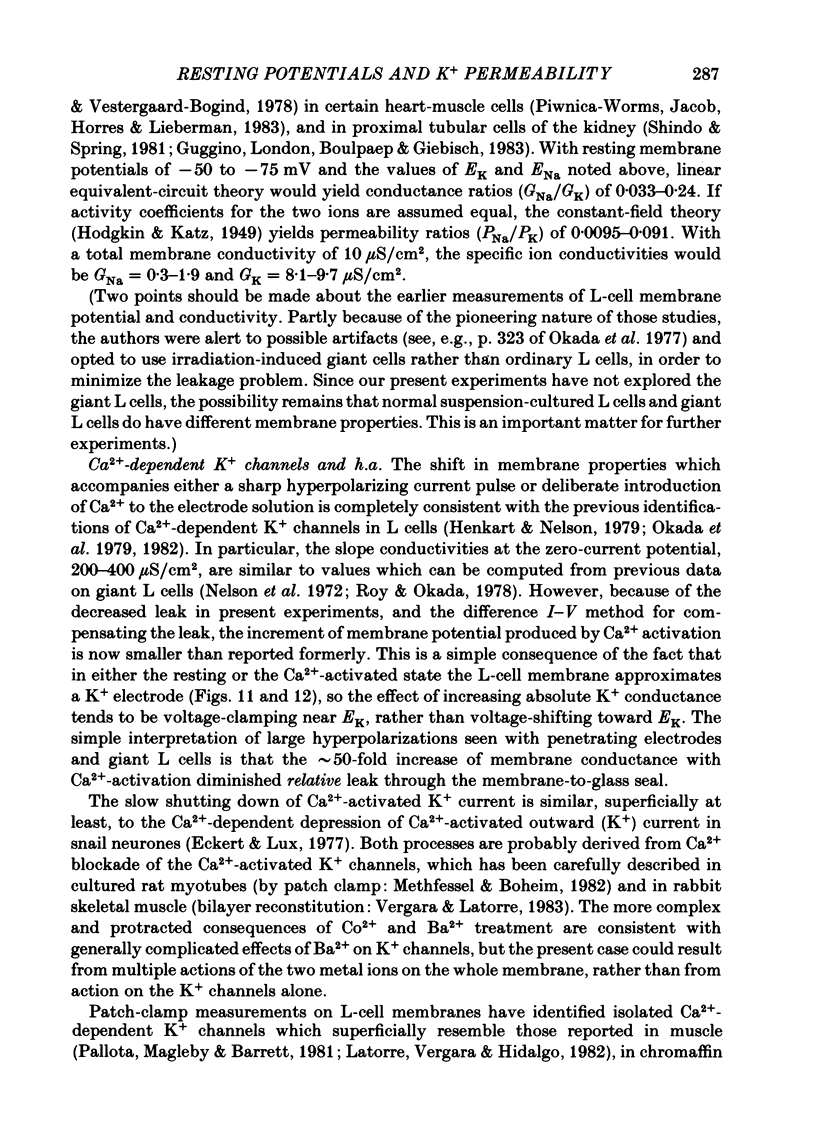
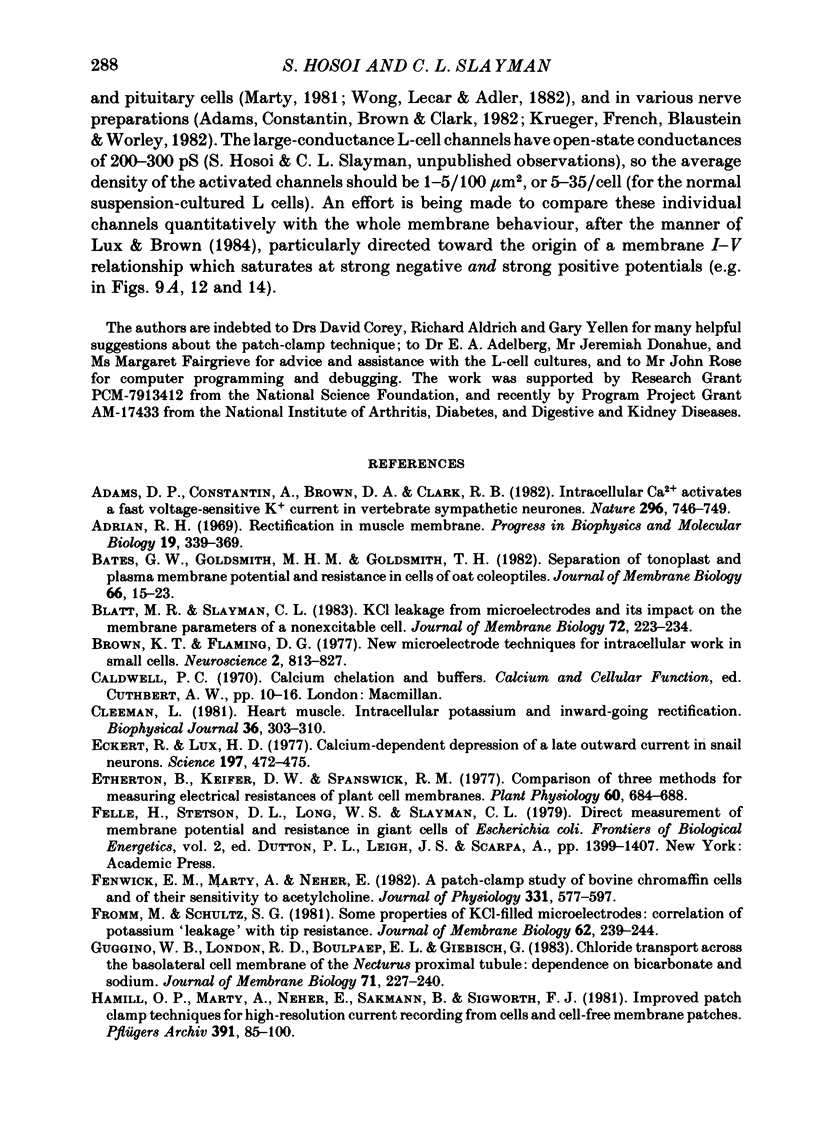
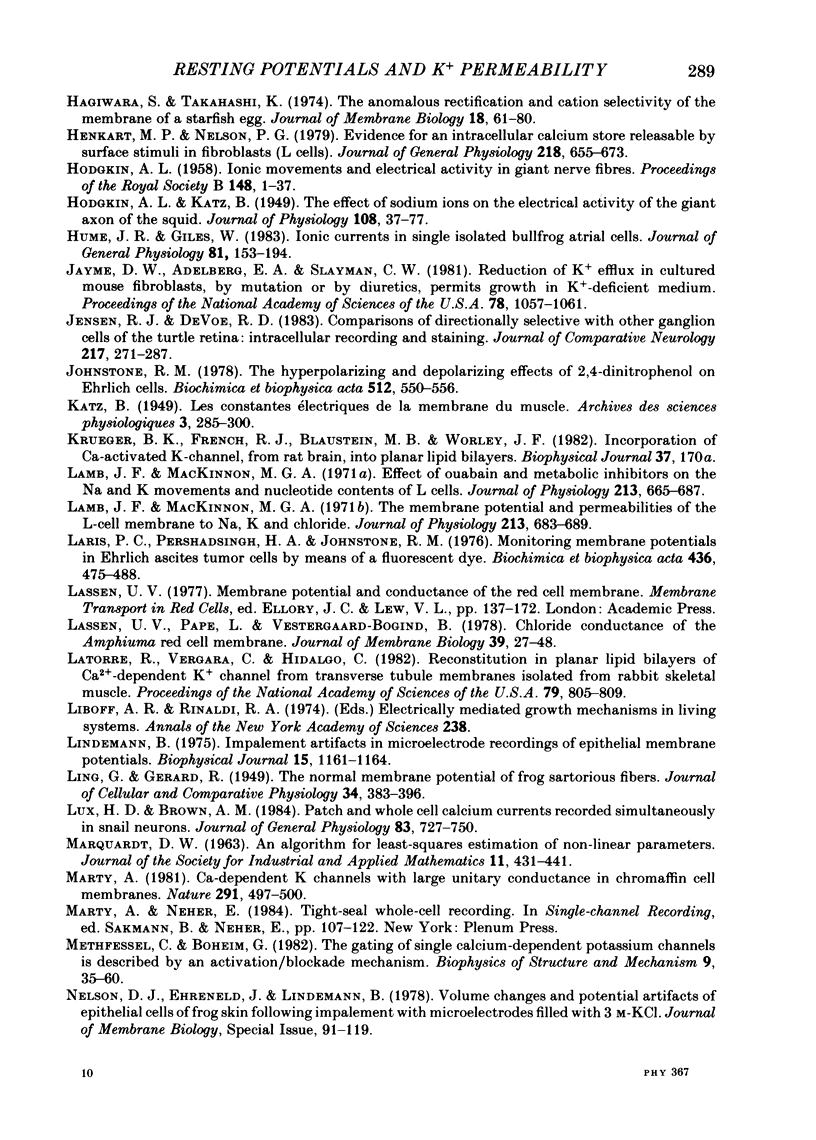
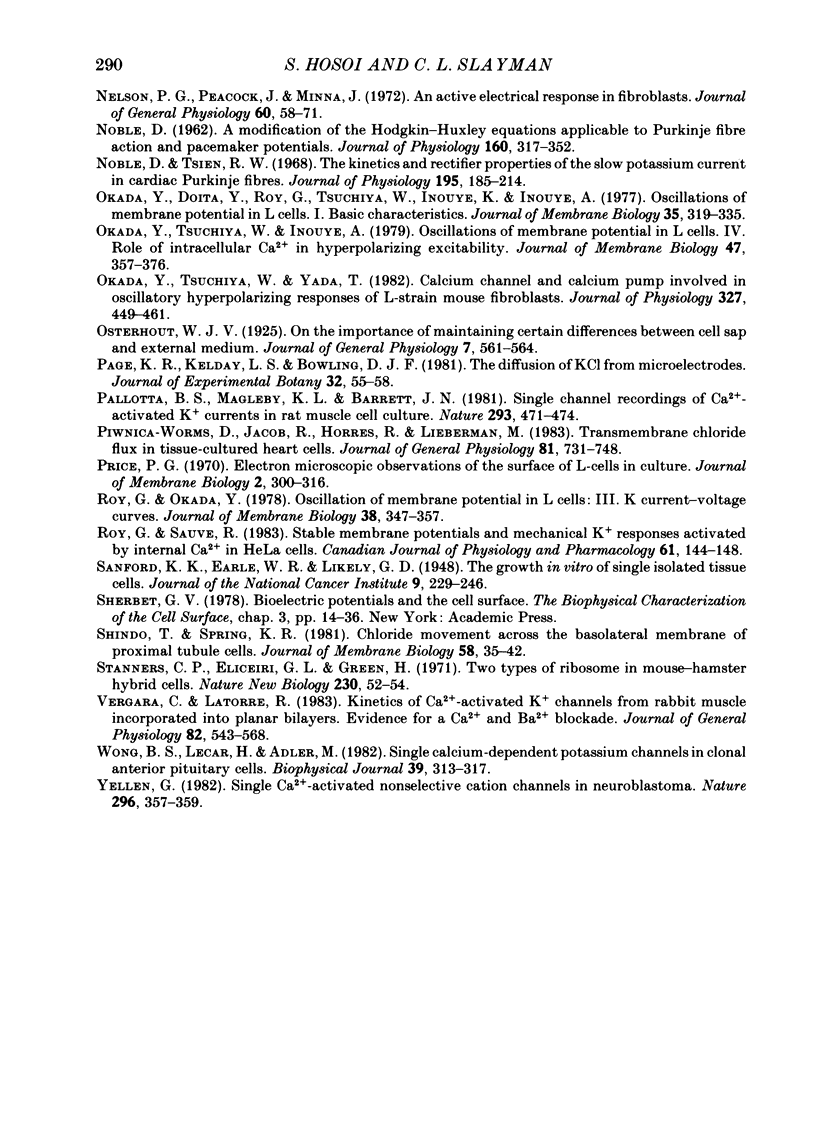
Selected References
These references are in PubMed. This may not be the complete list of references from this article.
- Adams P. R., Constanti A., Brown D. A., Clark R. B. Intracellular Ca2+ activates a fast voltage-sensitive K+ current in vertebrate sympathetic neurones. Nature. 1982 Apr 22;296(5859):746–749. doi: 10.1038/296746a0. [DOI] [PubMed] [Google Scholar]
- Adrian R. H. Rectification in muscle membrane. Prog Biophys Mol Biol. 1969;19(2):339–369. [PubMed] [Google Scholar]
- Blatt M. R., Slayman C. L. KCl leakage from microelectrodes and its impact on the membrane parameters of a nonexcitable cell. J Membr Biol. 1983;72(3):223–234. doi: 10.1007/BF01870589. [DOI] [PubMed] [Google Scholar]
- Cleemann L. Heart muscle. Intracellular potassium and inward-going rectification. Biophys J. 1981 Oct;36(1):303–310. doi: 10.1016/S0006-3495(81)84730-3. [DOI] [PMC free article] [PubMed] [Google Scholar]
- Eckert R., Lux H. D. Calcium-dependent depression of a late outward current in snail neurons. Science. 1977 Jul 29;197(4302):472–475. doi: 10.1126/science.17921. [DOI] [PubMed] [Google Scholar]
- Etherton B. Comparison of three methods for measuring electrical resistances of plant cell membranes. Plant Physiol. 1977 Nov;60(5):684–688. doi: 10.1104/pp.60.5.684. [DOI] [PMC free article] [PubMed] [Google Scholar]
- Fenwick E. M., Marty A., Neher E. A patch-clamp study of bovine chromaffin cells and of their sensitivity to acetylcholine. J Physiol. 1982 Oct;331:577–597. doi: 10.1113/jphysiol.1982.sp014393. [DOI] [PMC free article] [PubMed] [Google Scholar]
- Fromm M., Schultz S. G. Some properties of KCl-filled microelectrodes: correlation of potassium "leakage" with tip resistance. J Membr Biol. 1981;62(3):239–244. doi: 10.1007/BF01998169. [DOI] [PubMed] [Google Scholar]
- Guggino W. B., London R., Boulpaep E. L., Giebisch G. Chloride transport across the basolateral cell membrane of the Necturus proximal tubule: dependence on bicarbonate and sodium. J Membr Biol. 1983;71(3):227–240. doi: 10.1007/BF01875464. [DOI] [PubMed] [Google Scholar]
- HODGKIN A. L. Ionic movements and electrical activity in giant nerve fibres. Proc R Soc Lond B Biol Sci. 1958 Jan 1;148(930):1–37. doi: 10.1098/rspb.1958.0001. [DOI] [PubMed] [Google Scholar]
- HODGKIN A. L., KATZ B. The effect of sodium ions on the electrical activity of giant axon of the squid. J Physiol. 1949 Mar 1;108(1):37–77. doi: 10.1113/jphysiol.1949.sp004310. [DOI] [PMC free article] [PubMed] [Google Scholar]
- Hagiwara S., Takahashi K. The anomalous rectification and cation selectivity of the membrane of a starfish egg cell. J Membr Biol. 1974;18(1):61–80. doi: 10.1007/BF01870103. [DOI] [PubMed] [Google Scholar]
- Hamill O. P., Marty A., Neher E., Sakmann B., Sigworth F. J. Improved patch-clamp techniques for high-resolution current recording from cells and cell-free membrane patches. Pflugers Arch. 1981 Aug;391(2):85–100. doi: 10.1007/BF00656997. [DOI] [PubMed] [Google Scholar]
- Henkart M. P., Nelson P. G. Evidence for an intracellular calcium store releasable by surface stimuli ifibroblasts (L cells). J Gen Physiol. 1979 May;73(5):655–673. doi: 10.1085/jgp.73.5.655. [DOI] [PMC free article] [PubMed] [Google Scholar]
- Hume J. R., Giles W. Ionic currents in single isolated bullfrog atrial cells. J Gen Physiol. 1983 Feb;81(2):153–194. doi: 10.1085/jgp.81.2.153. [DOI] [PMC free article] [PubMed] [Google Scholar]
- Jayme D. W., Adelberg E. A., Slayman C. W. Reduction of K+ efflux in cultured mouse fibroblasts, by mutation or by diuretics, permits growth in K+-deficient medium. Proc Natl Acad Sci U S A. 1981 Feb;78(2):1057–1061. doi: 10.1073/pnas.78.2.1057. [DOI] [PMC free article] [PubMed] [Google Scholar]
- Jensen R. J., DeVoe R. D. Comparisons of directionally selective with other ganglion cells of the turtle retina: intracellular recording and staining. J Comp Neurol. 1983 Jul 1;217(3):271–287. doi: 10.1002/cne.902170305. [DOI] [PubMed] [Google Scholar]
- Johnstone R. M. The hyperpolarizing and depolarizing effects of 2,4-dinitrophenol on Ehrlich cells. Biochim Biophys Acta. 1978 Oct 4;512(3):550–556. doi: 10.1016/0005-2736(78)90164-5. [DOI] [PubMed] [Google Scholar]
- LING G., GERARD R. W. The normal membrane potential of frog sartorius fibers. J Cell Physiol. 1949 Dec;34(3):383–396. doi: 10.1002/jcp.1030340304. [DOI] [PubMed] [Google Scholar]
- Lamb J. F., MacKinnon M. G. Effect of ouabain and metabolic inhibitors on the Na and K movements and nucleotide contents of L cells. J Physiol. 1971 Mar;213(3):665–682. doi: 10.1113/jphysiol.1971.sp009407. [DOI] [PMC free article] [PubMed] [Google Scholar]
- Lamb J. F., MacKinnon M. G. The membrane potential and permeabilities of the L cell membrane to Na, K and chloride. J Physiol. 1971 Mar;213(3):683–689. doi: 10.1113/jphysiol.1971.sp009408. [DOI] [PMC free article] [PubMed] [Google Scholar]
- Laris P. C., Pershadsingh H. A., Johnstone R. M. Monitoring membrane potentials in Ehrlich ascites tumor cells by means of a fluorescent dye. Biochim Biophys Acta. 1976 Jun 17;436(2):475–488. doi: 10.1016/0005-2736(76)90209-1. [DOI] [PubMed] [Google Scholar]
- Lassen U. V., Pape L., Vestergaard-Bogind B. Chloride conductance of the amphiuma red cell membrane. J Membr Biol. 1978 Feb 6;39(1):27–48. doi: 10.1007/BF01872753. [DOI] [PubMed] [Google Scholar]
- Latorre R., Vergara C., Hidalgo C. Reconstitution in planar lipid bilayers of a Ca2+-dependent K+ channel from transverse tubule membranes isolated from rabbit skeletal muscle. Proc Natl Acad Sci U S A. 1982 Feb;79(3):805–809. doi: 10.1073/pnas.79.3.805. [DOI] [PMC free article] [PubMed] [Google Scholar]
- Lindemann B. Letter: Impalement artifacts in microelectrode recordings of epithelial membrane potentials. Biophys J. 1975 Nov;15(11):1161–1164. doi: 10.1016/S0006-3495(75)85892-9. [DOI] [PMC free article] [PubMed] [Google Scholar]
- Lux H. D., Brown A. M. Patch and whole cell calcium currents recorded simultaneously in snail neurons. J Gen Physiol. 1984 May;83(5):727–750. doi: 10.1085/jgp.83.5.727. [DOI] [PMC free article] [PubMed] [Google Scholar]
- Marty A. Ca-dependent K channels with large unitary conductance in chromaffin cell membranes. Nature. 1981 Jun 11;291(5815):497–500. doi: 10.1038/291497a0. [DOI] [PubMed] [Google Scholar]
- Methfessel C., Boheim G. The gating of single calcium-dependent potassium channels is described by an activation/blockade mechanism. Biophys Struct Mech. 1982;9(1):35–60. doi: 10.1007/BF00536014. [DOI] [PubMed] [Google Scholar]
- NOBLE D. A modification of the Hodgkin--Huxley equations applicable to Purkinje fibre action and pace-maker potentials. J Physiol. 1962 Feb;160:317–352. doi: 10.1113/jphysiol.1962.sp006849. [DOI] [PMC free article] [PubMed] [Google Scholar]
- Nelson D. J., Ehrenfeld J., Lindemann B. Volume changes and potential artifacts of epithelial cells of frog skin following impalement with microelectrodes filled with 3 m KCl. J Membr Biol. 1978;40(Spec No):91–119. doi: 10.1007/BF02026000. [DOI] [PubMed] [Google Scholar]
- Nelson P. G., Peacock J., Minna J. An active electrical response in fibroblasts. J Gen Physiol. 1972 Jul;60(1):58–71. doi: 10.1085/jgp.60.1.58. [DOI] [PMC free article] [PubMed] [Google Scholar]
- Noble D., Tsien R. W. The kinetics and rectifier properties of the slow potassium current in cardiac Purkinje fibres. J Physiol. 1968 Mar;195(1):185–214. doi: 10.1113/jphysiol.1968.sp008454. [DOI] [PMC free article] [PubMed] [Google Scholar]
- Okada Y., Doida Y., Roy G., Tsuchiya W., Inouye K., Inouye A. Oscillations of membrane potential in L cells. I. Basic characteristics. J Membr Biol. 1977 Aug 4;35(4):319–335. doi: 10.1007/BF01869957. [DOI] [PubMed] [Google Scholar]
- Okada Y., Tsuchiya W., Inouye A. Oscillations of membrane potential in L cells. IV. Role of intracellular Ca2+ in hyperpolarizing excitability. J Membr Biol. 1979 Jun 7;47(4):357–376. doi: 10.1007/BF01869744. [DOI] [PubMed] [Google Scholar]
- Okada Y., Tsuchiya W., Yada T. Calcium channel and calcium pump involved in oscillatory hyperpolarizing responses of L-strain mouse fibroblasts. J Physiol. 1982 Jun;327:449–461. doi: 10.1113/jphysiol.1982.sp014242. [DOI] [PMC free article] [PubMed] [Google Scholar]
- Osterhout W. J. ON THE IMPORTANCE OF MAINTAINING CERTAIN DIFFERENCES BETWEEN CELL SAP AND EXTERNAL MEDIUM. J Gen Physiol. 1925 Mar 20;7(4):561–564. doi: 10.1085/jgp.7.4.561. [DOI] [PMC free article] [PubMed] [Google Scholar]
- Pallotta B. S., Magleby K. L., Barrett J. N. Single channel recordings of Ca2+-activated K+ currents in rat muscle cell culture. Nature. 1981 Oct 8;293(5832):471–474. doi: 10.1038/293471a0. [DOI] [PubMed] [Google Scholar]
- Piwnica-Worms D., Jacob R., Horres C. R., Lieberman M. Transmembrane chloride flux in tissue-cultured chick heart cells. J Gen Physiol. 1983 May;81(5):731–748. doi: 10.1085/jgp.81.5.731. [DOI] [PMC free article] [PubMed] [Google Scholar]
- Roy G., Okada Y. Oscillation of membrane potential in L cells: III K + current-voltage curves. J Membr Biol. 1978 Feb 3;38(4):347–357. doi: 10.1007/BF01870151. [DOI] [PubMed] [Google Scholar]
- Roy G., Sauvé R. Stable membrane potentials and mechanical K+ responses activated by internal Ca2+ in HeLa cells. Can J Physiol Pharmacol. 1983 Feb;61(2):144–148. doi: 10.1139/y83-021. [DOI] [PubMed] [Google Scholar]
- SANFORD K. K., EARLE W. R., LIKELY G. D. The growth in vitro of single isolated tissue cells. J Natl Cancer Inst. 1948 Dec;9(3):229–246. [PubMed] [Google Scholar]
- Shindo T., Spring K. R. Chloride movement across the basolateral membrane of proximal tubule cells. J Membr Biol. 1981 Jan 30;58(1):35–42. doi: 10.1007/BF01871032. [DOI] [PubMed] [Google Scholar]
- Stanners C. P., Eliceiri G. L., Green H. Two types of ribosome in mouse-hamster hybrid cells. Nat New Biol. 1971 Mar 10;230(10):52–54. doi: 10.1038/newbio230052a0. [DOI] [PubMed] [Google Scholar]
- Vergara C., Latorre R. Kinetics of Ca2+-activated K+ channels from rabbit muscle incorporated into planar bilayers. Evidence for a Ca2+ and Ba2+ blockade. J Gen Physiol. 1983 Oct;82(4):543–568. doi: 10.1085/jgp.82.4.543. [DOI] [PMC free article] [PubMed] [Google Scholar]
- Wong B. S., Lecar H., Adler M. Single calcium-dependent potassium channels in clonal anterior pituitary cells. Biophys J. 1982 Sep;39(3):313–317. doi: 10.1016/S0006-3495(82)84522-0. [DOI] [PMC free article] [PubMed] [Google Scholar]
- Yellen G. Single Ca2+-activated nonselective cation channels in neuroblastoma. Nature. 1982 Mar 25;296(5855):357–359. doi: 10.1038/296357a0. [DOI] [PubMed] [Google Scholar]