Abstract
The forelimb motor cortex was stimulated via chronically implanted microelectrodes whilst electromyographic (e.m.g.) responses were recorded from muscles in the contralateral forelimb in cats walking steadily at 0.5 m/s. The stimuli were brief trains of 0.2 ms pulses (11 pulses at 330 Hz), intensity 5-20 microA and e.m.g.s were recorded from the following muscles: biceps brachii, brachialis, long and lateral heads of triceps brachii, latissimus dorsi, cleidobrachialis, extensor digitorum communis, palmaris longus and flexor and extensor carpi ulnaris. During locomotion, stimulation at 20 microA readily elicited brief, short-latency changes in the normal locomotor patterns of activity in all muscles studied. The changes included production of e.m.g. at times in the step cycle when the muscles are normally inactive and brief augmentations or diminution of the normal locomotor e.m.g.s. Individual electrodes usually influenced several muscles, and muscles acting antagonistically about the same joint were sometimes co-contracted. The first effect on locomotor flexor muscles (i.e. muscles active in relation to the swing phase of the step cycle) was almost always excitatory and such effects were often phase-dependent, usually occurring when the muscle was normally active or about to become active. Extensor muscles were excited from some cortical loci but inhibited from others (inhibitions were necessarily detectable only when the muscles exhibited locomotor-related e.m.g.s). Some micro-electrodes elicited excitation during swing (when the extensors are inactive) but elicited inhibition during stance. In several muscles the latencies of the excitatory e.m.g. changes could be as short as 6 ms measured from the first pulse in the stimulus train. In flexors, but not in extensors, latencies fluctuated according to the timing of the stimuli relative to the step cycle. Reduction in stimulus intensity reduced the amplitude of the e.m.g. changes, the number of muscles influenced and often increased the latency. However, both excitations and inhibitions were sometimes evident at 5 microA and thresholds for excitatory responses were, over-all, substantially lower than in the resting animal. Longer trains of stimuli were capable of resetting the step cycle. Response thresholds were greatly increased after pyramidectomy. These findings support the view that the natural bursts of impulses discharged by pyramidal tract neurones during steady locomotion are likely to contribute to regulating forelimb muscle activity on a step-by-step basis.
Full text
PDF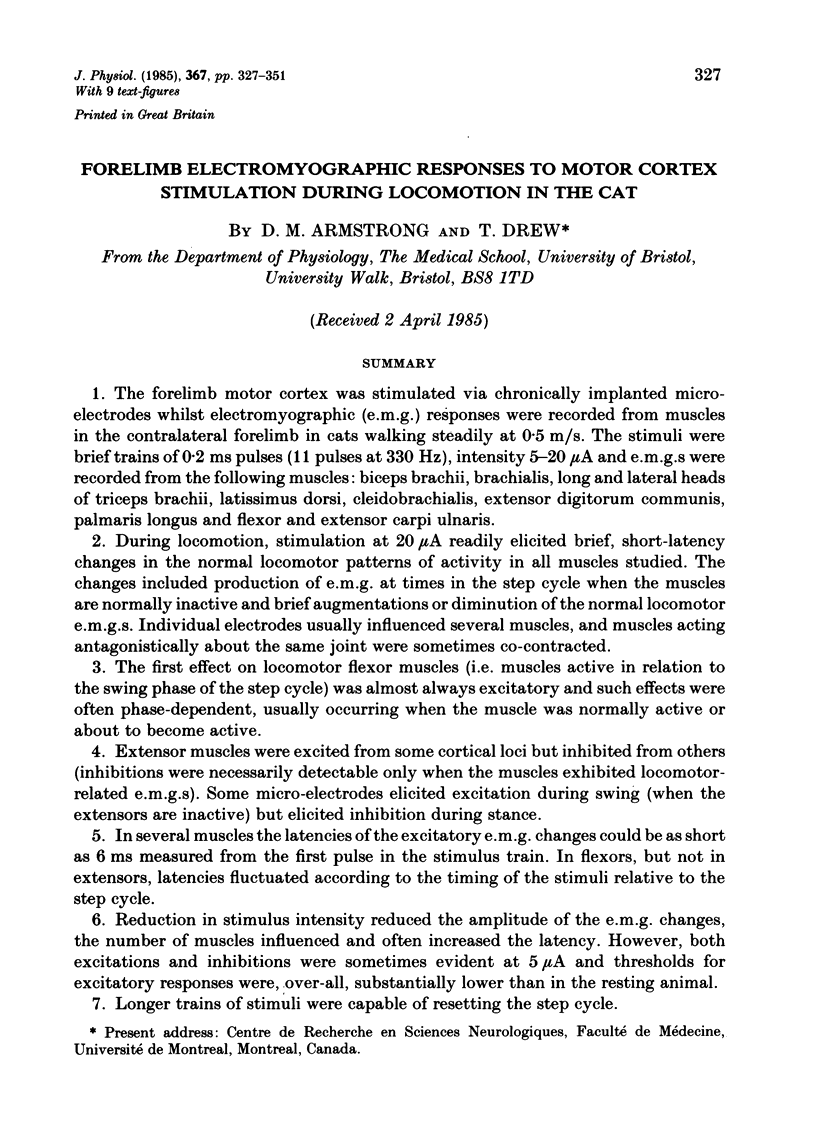
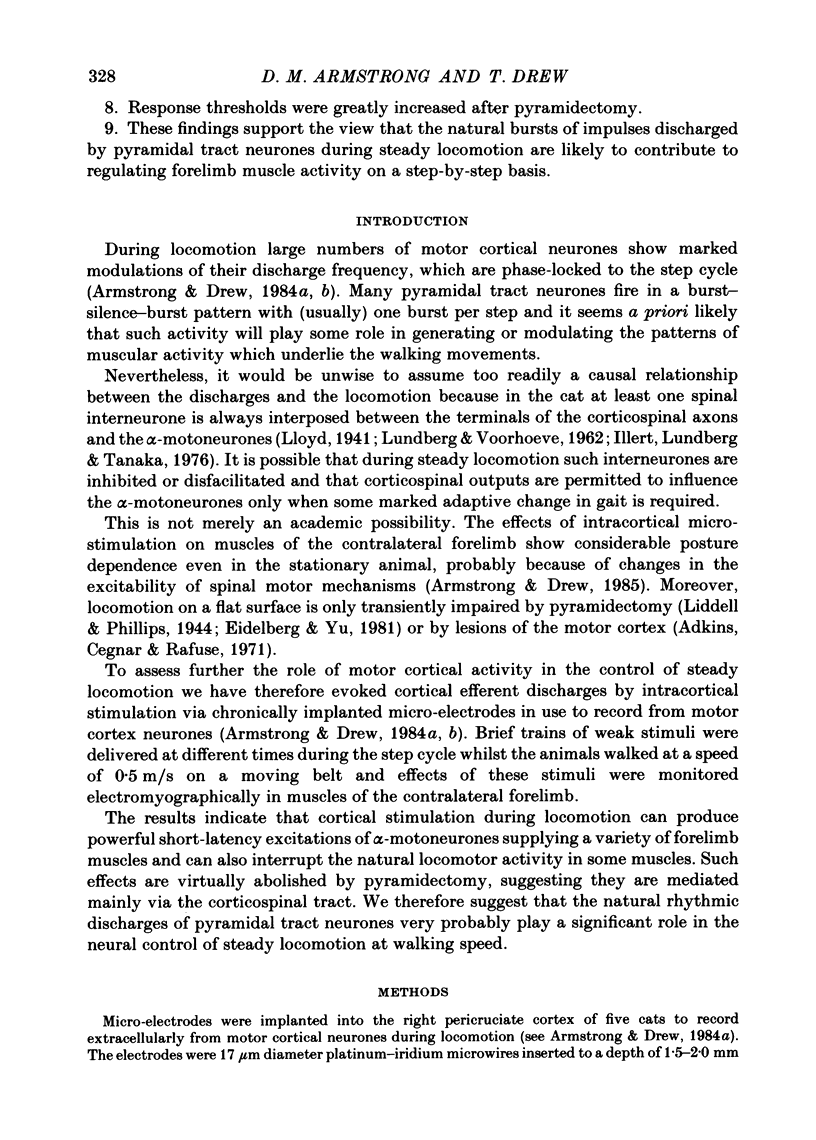
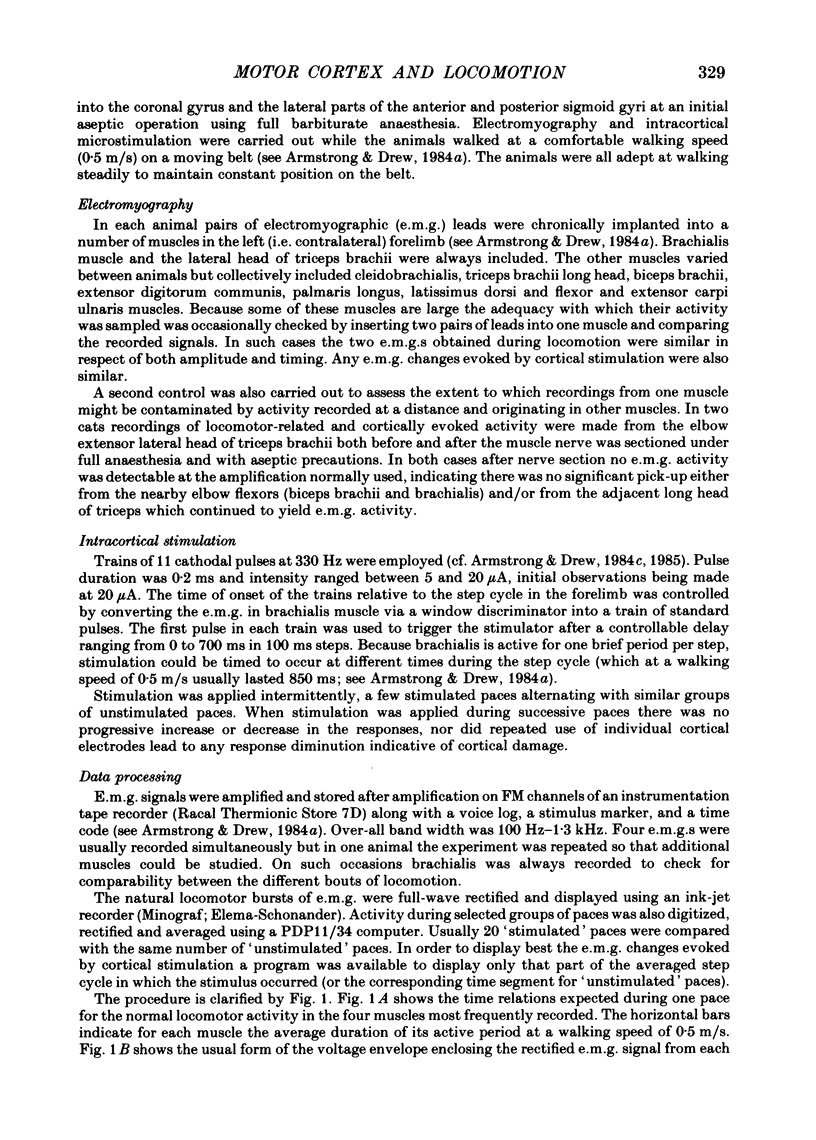
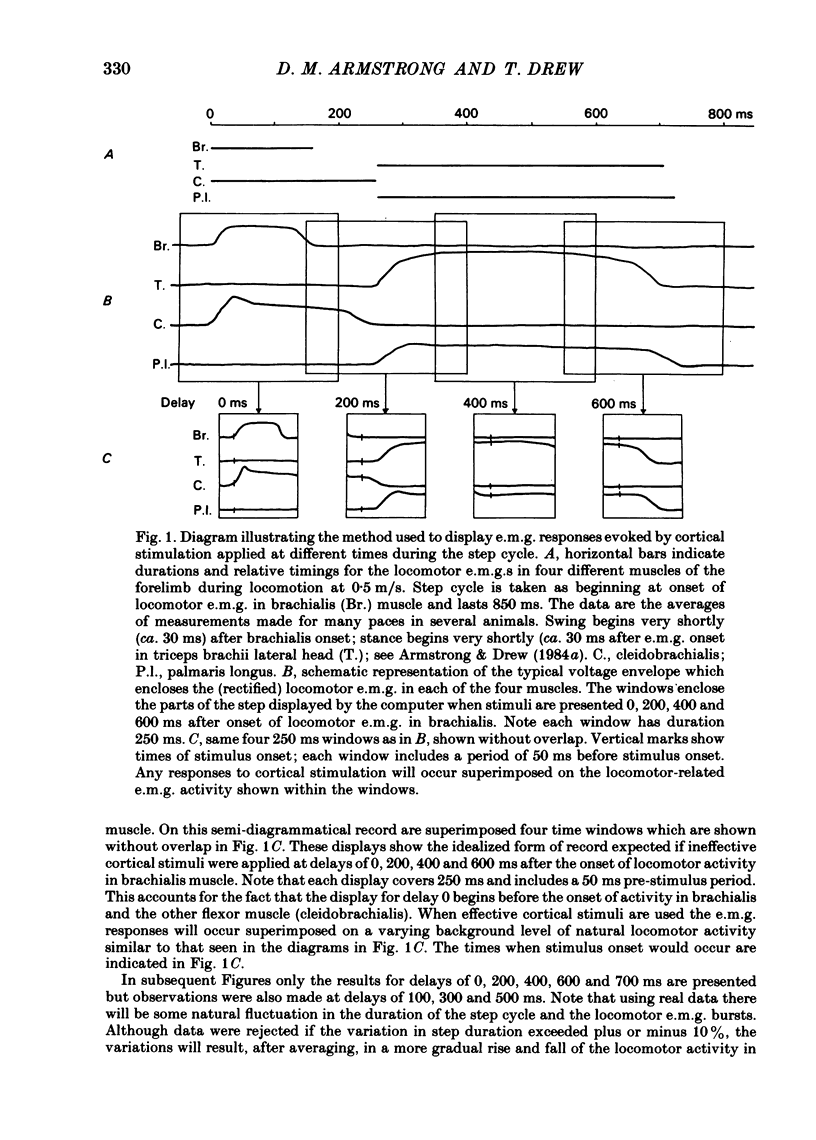
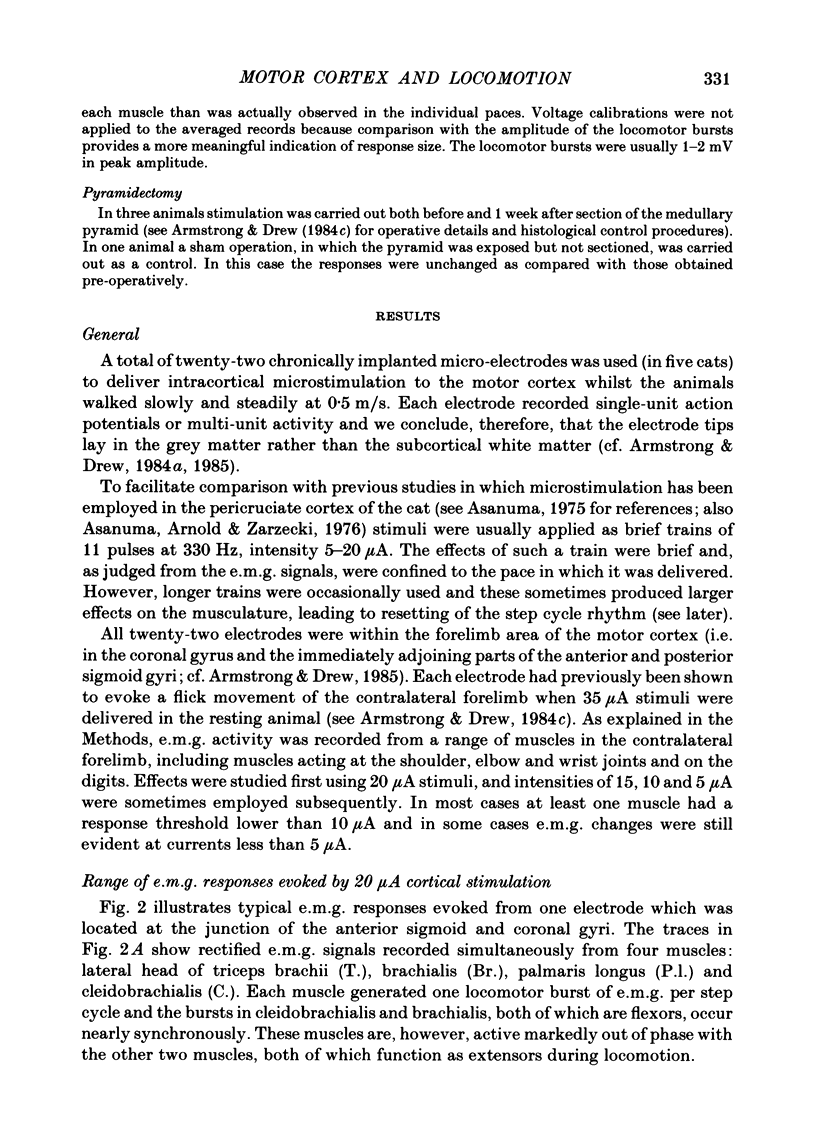
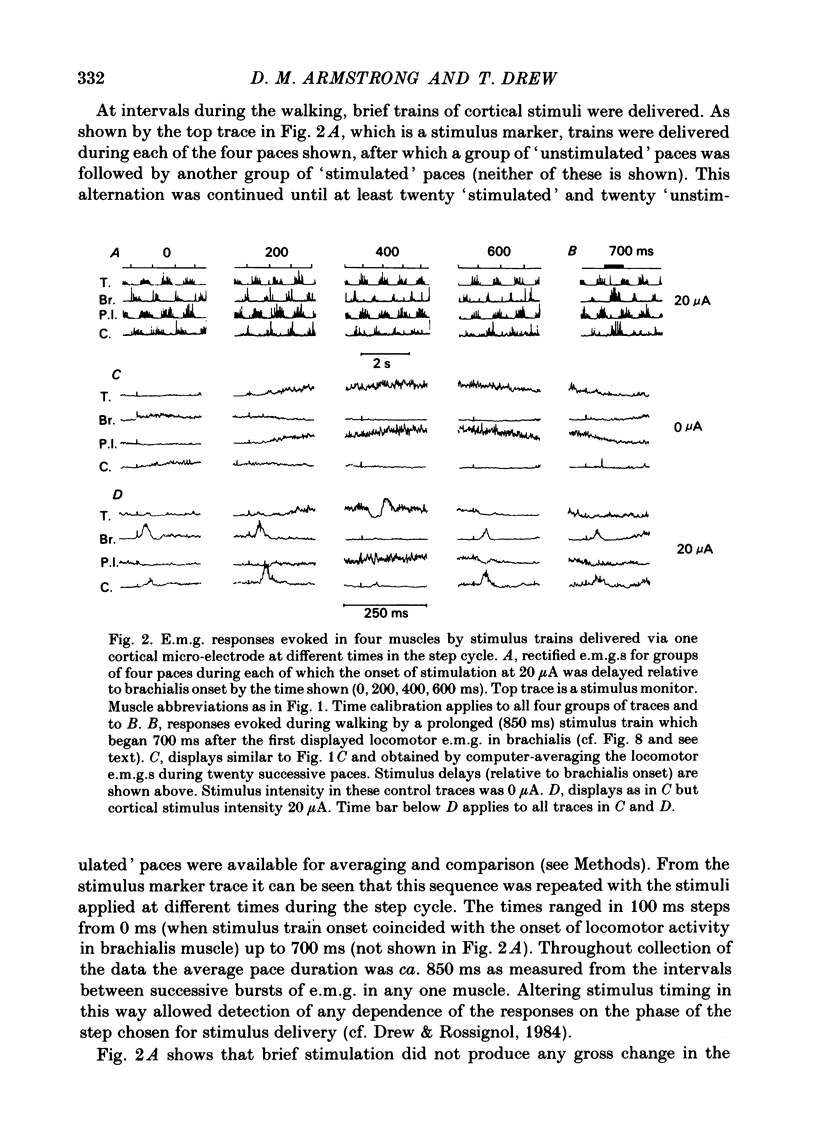
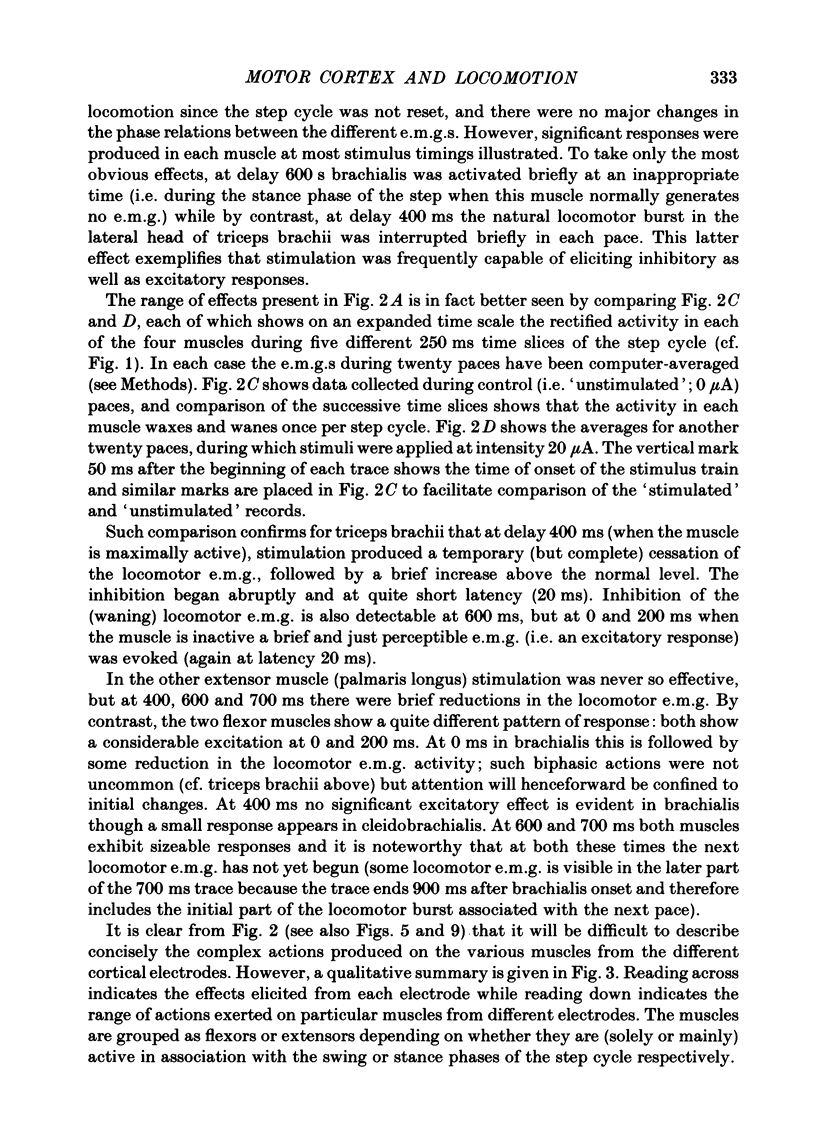
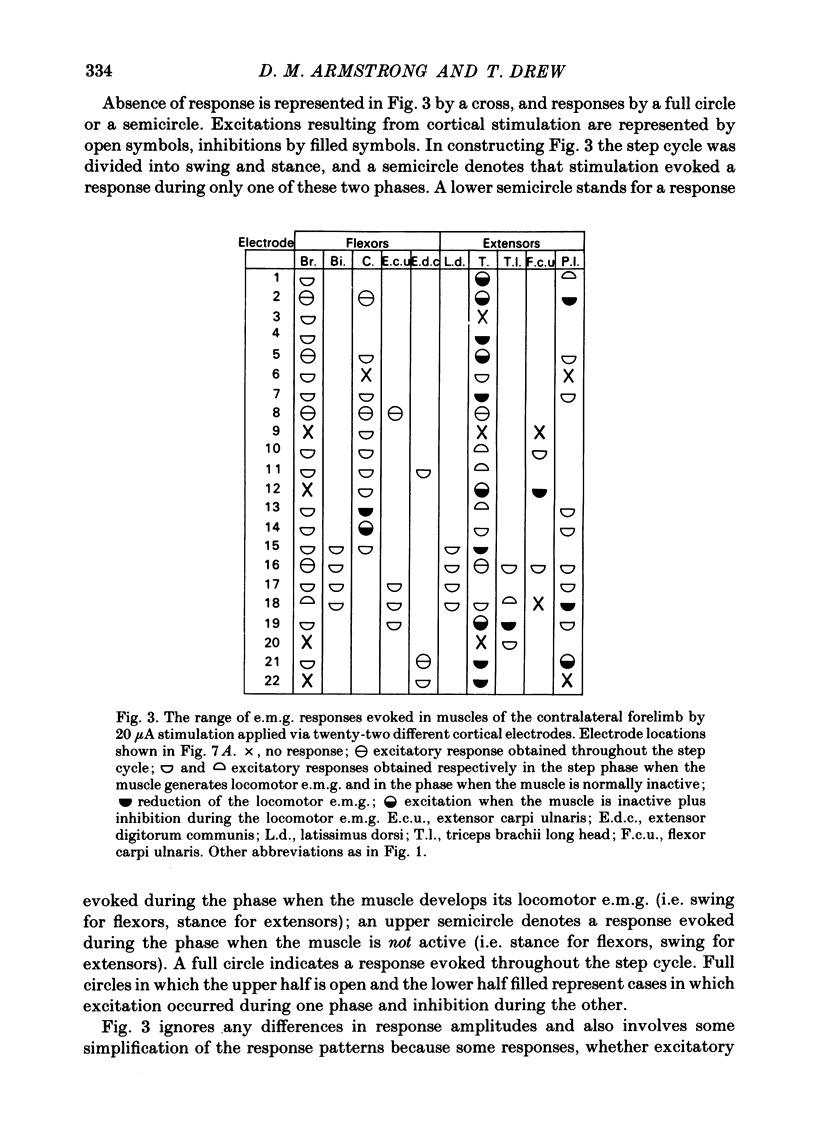
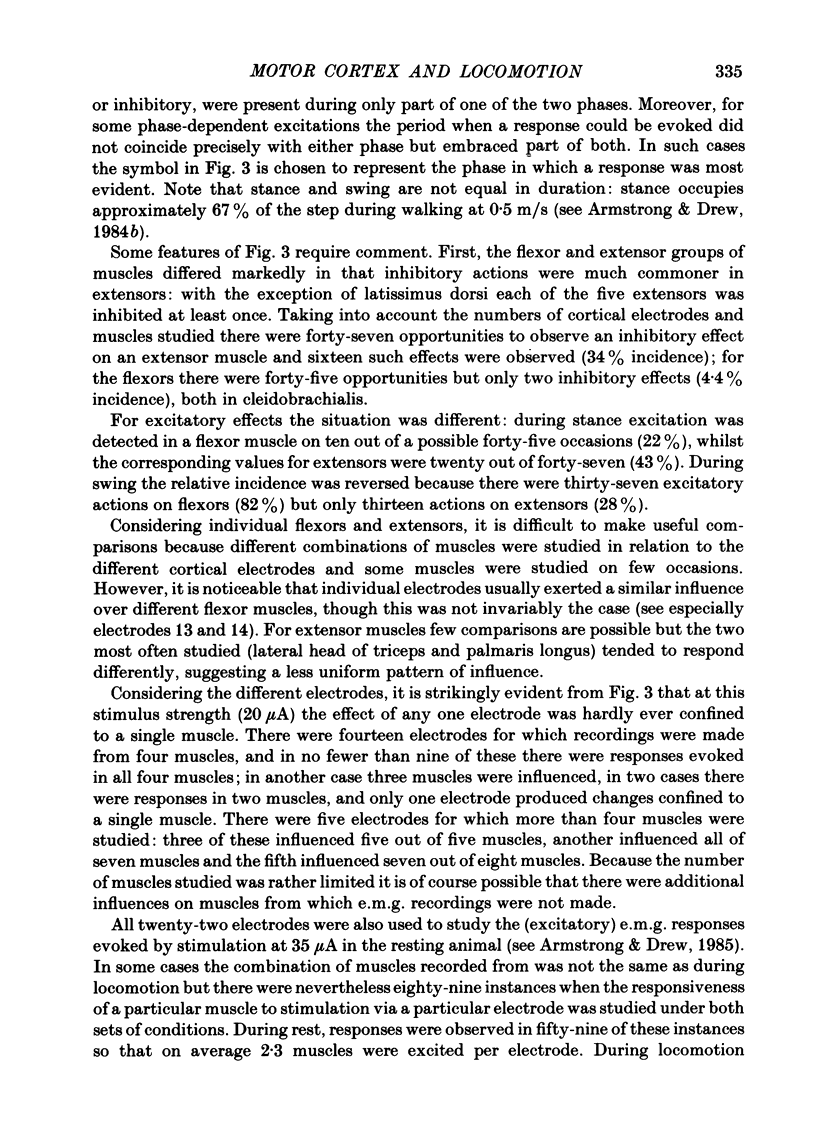
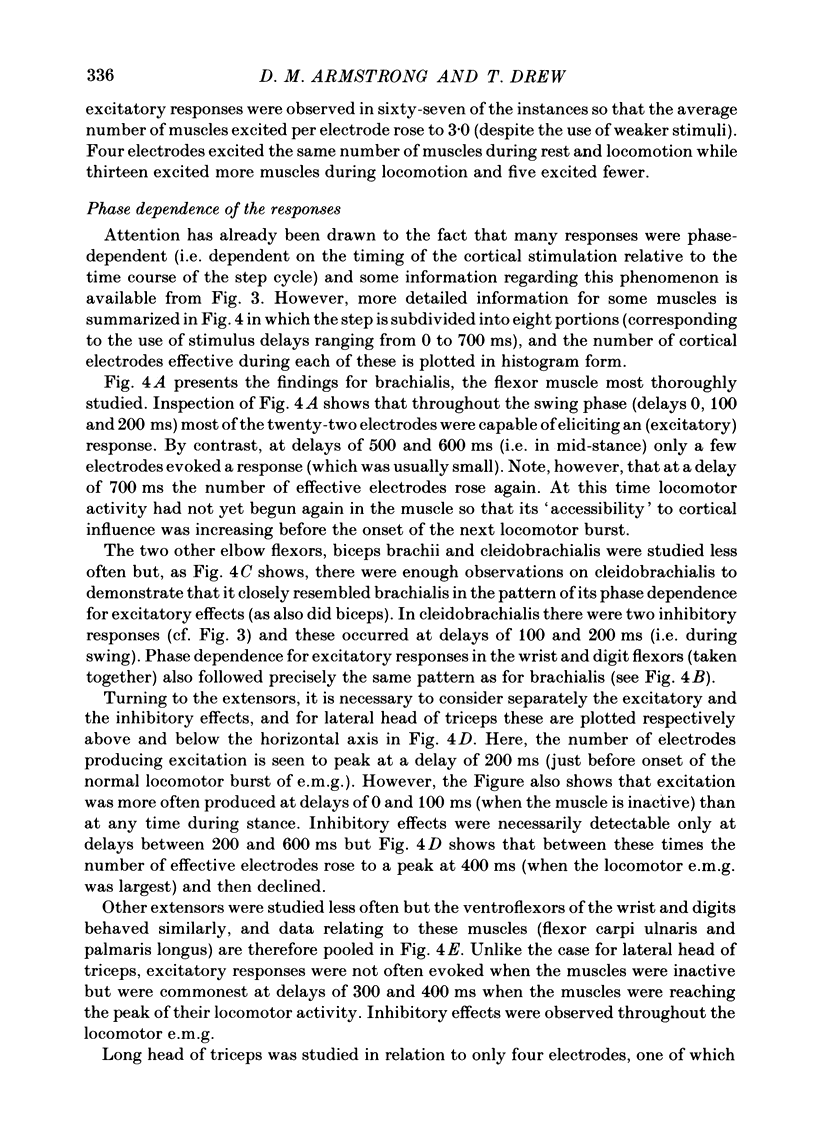
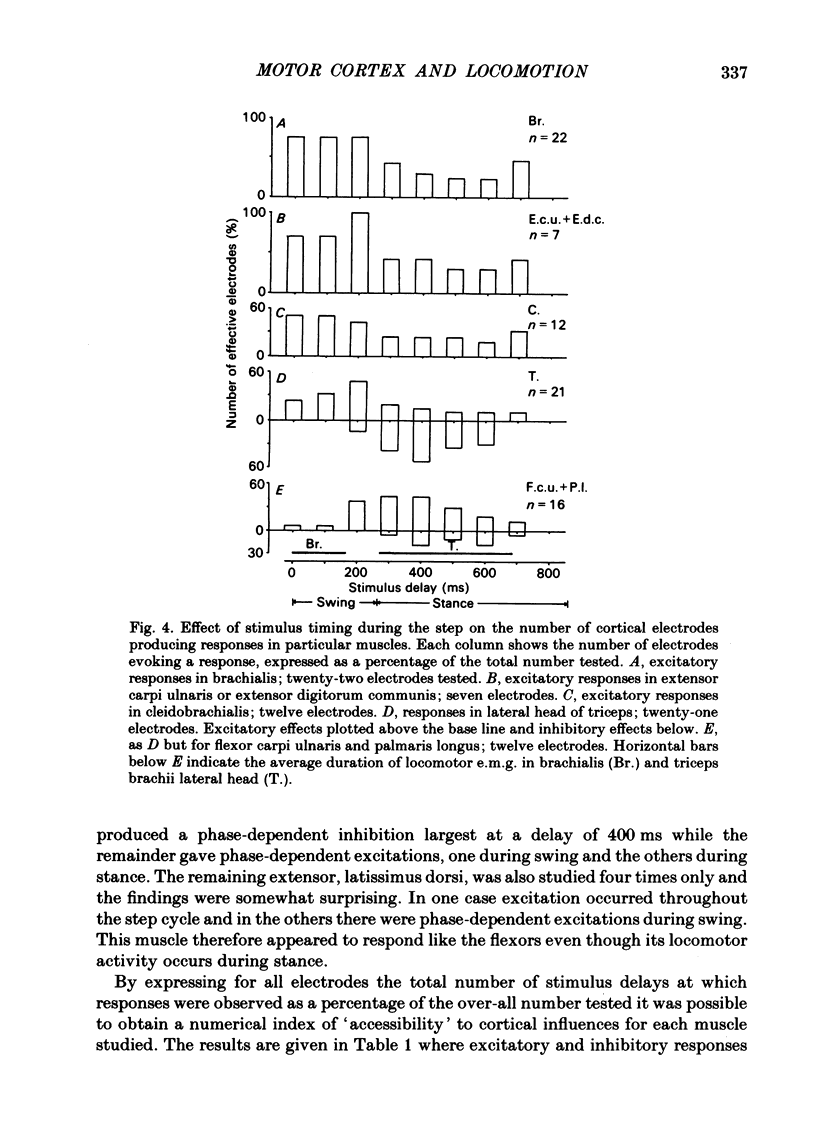
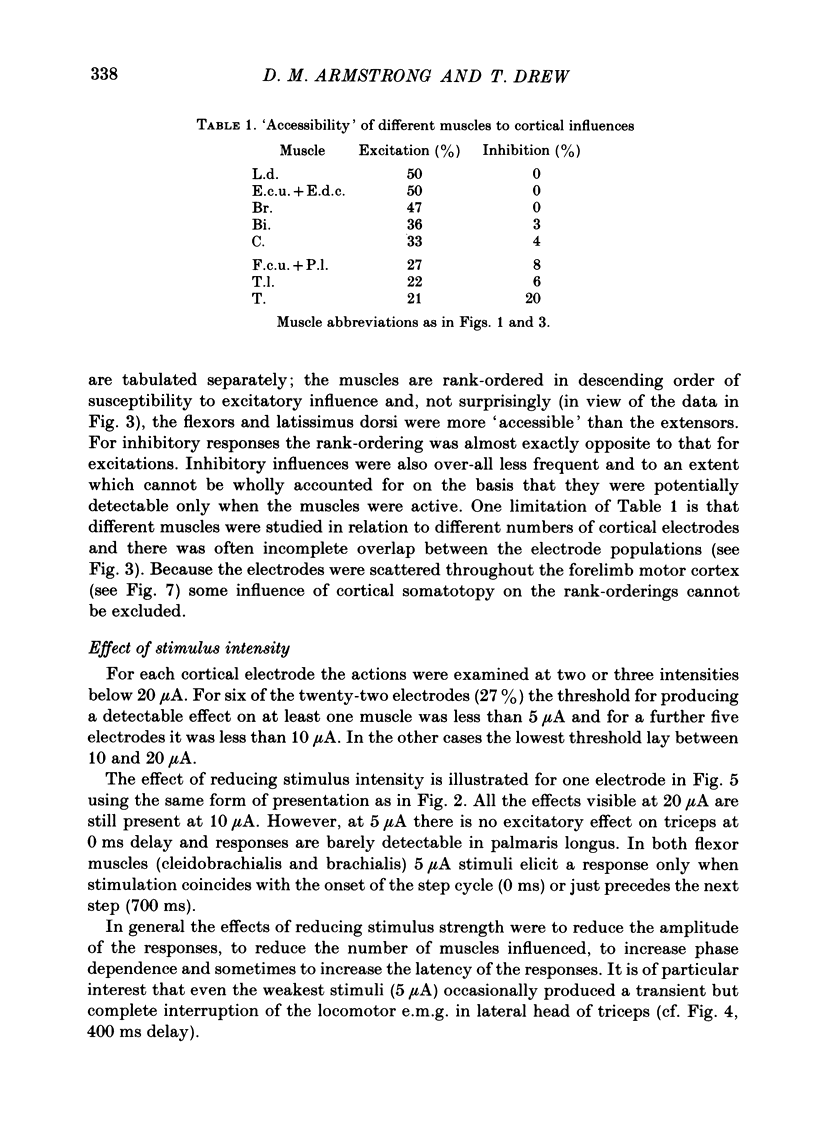
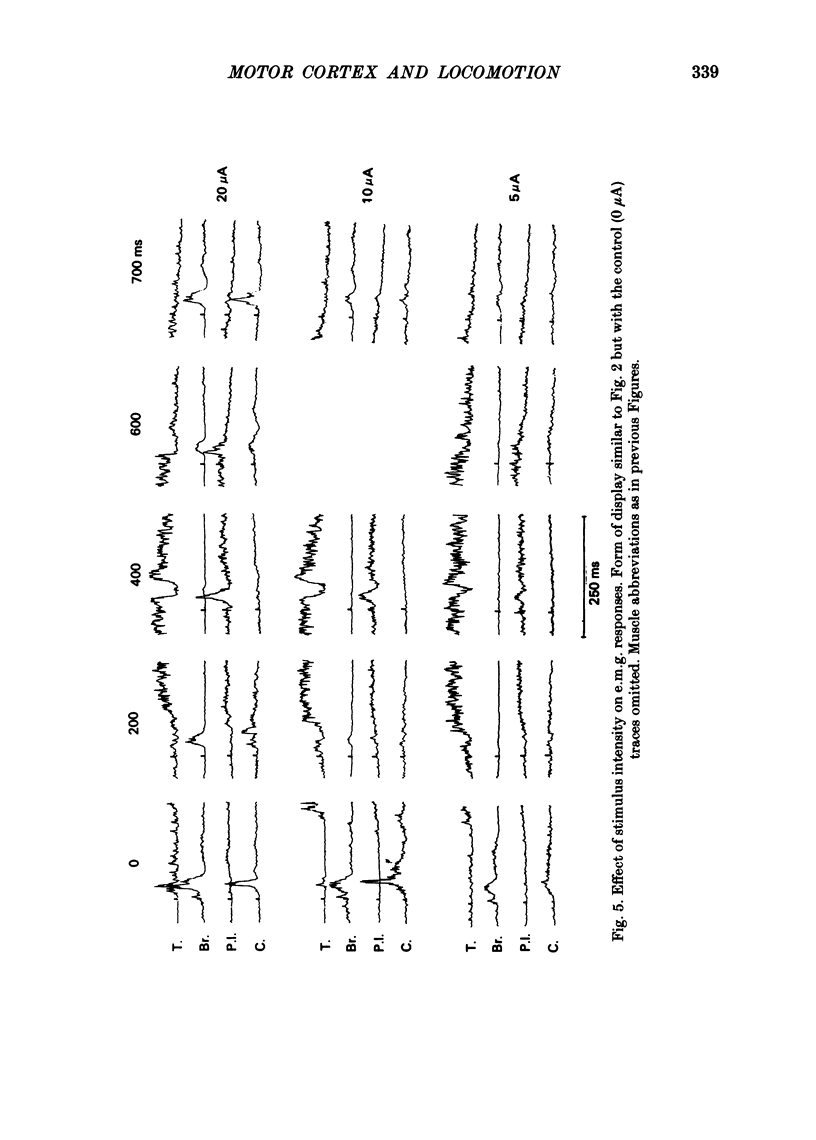
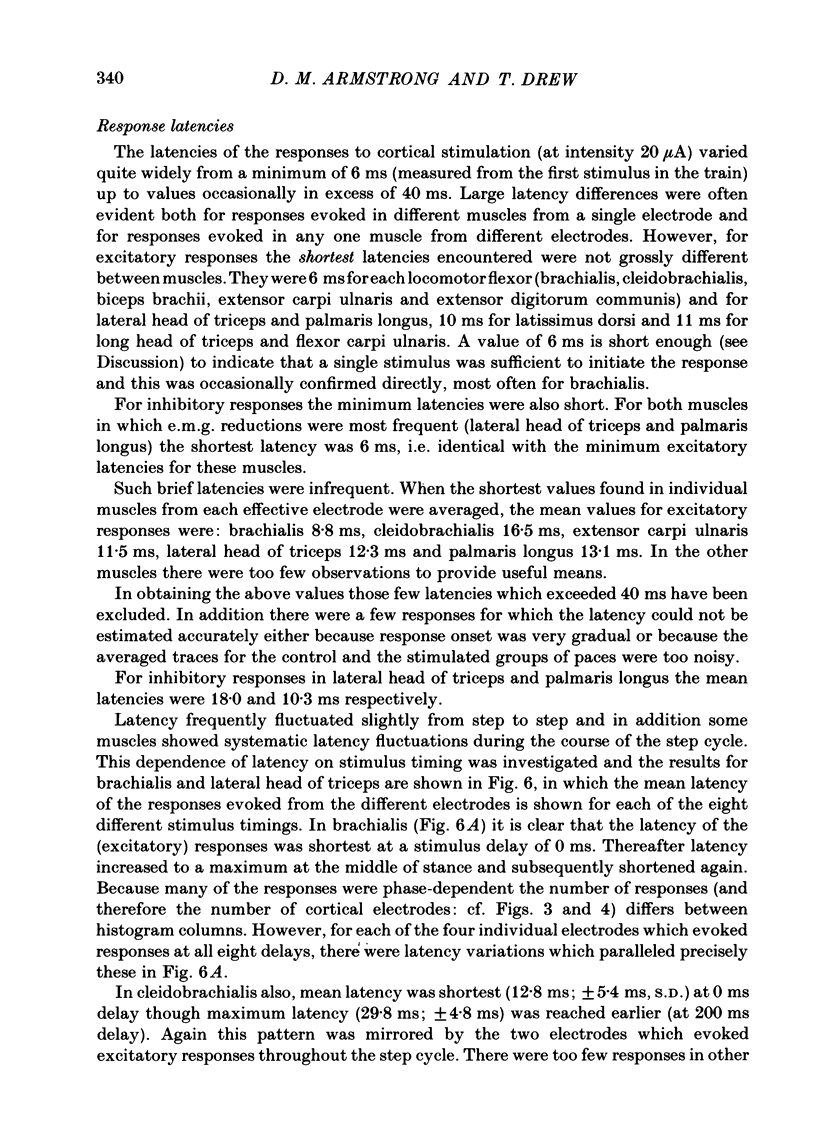
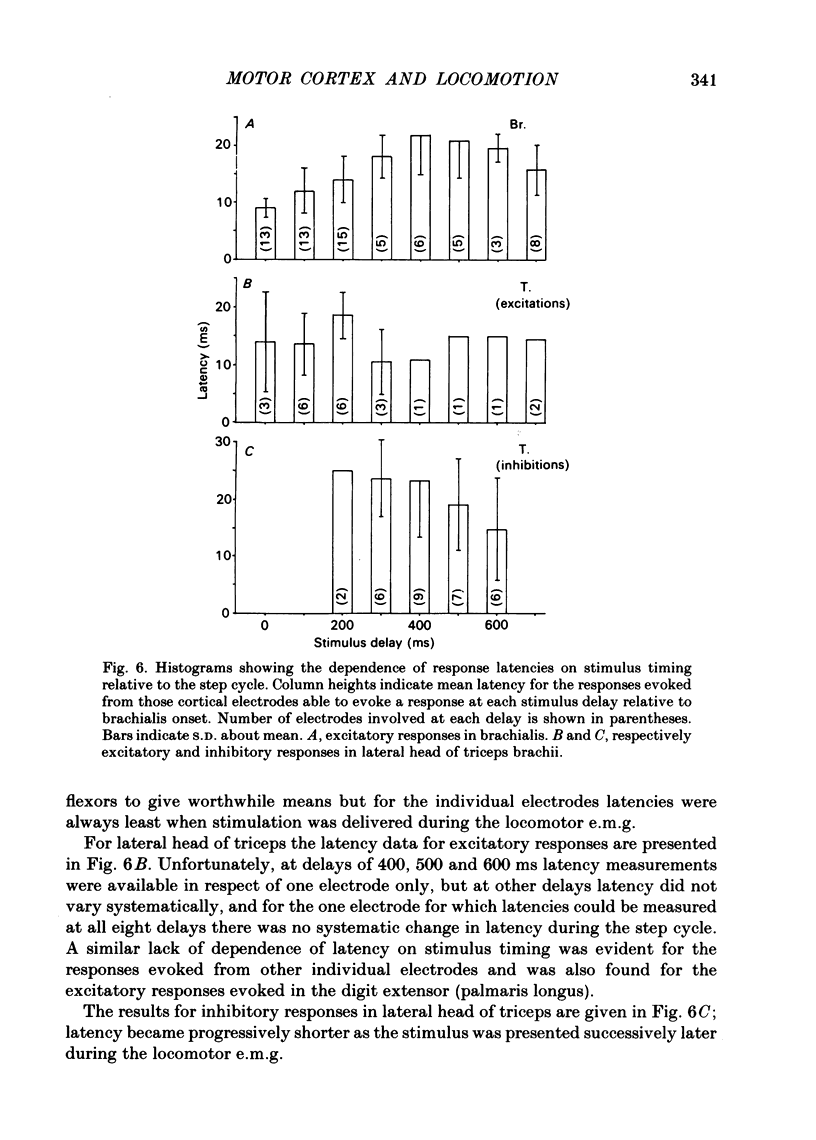
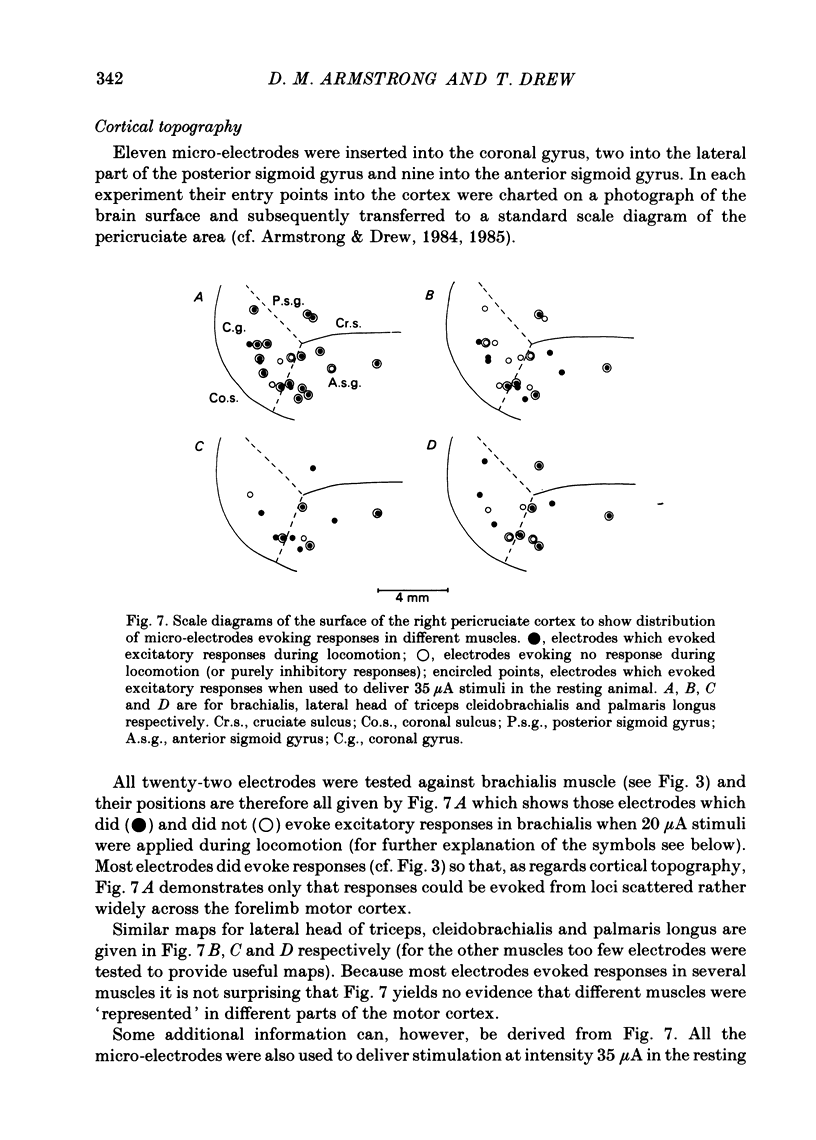
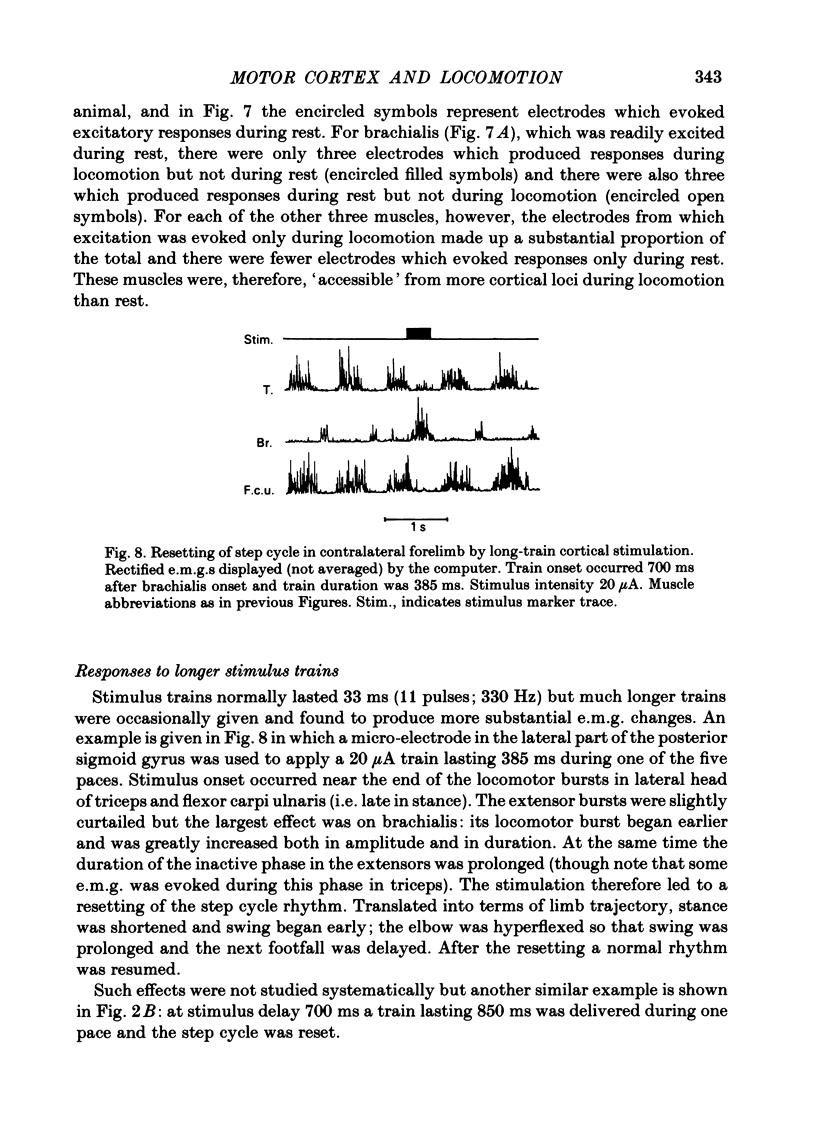
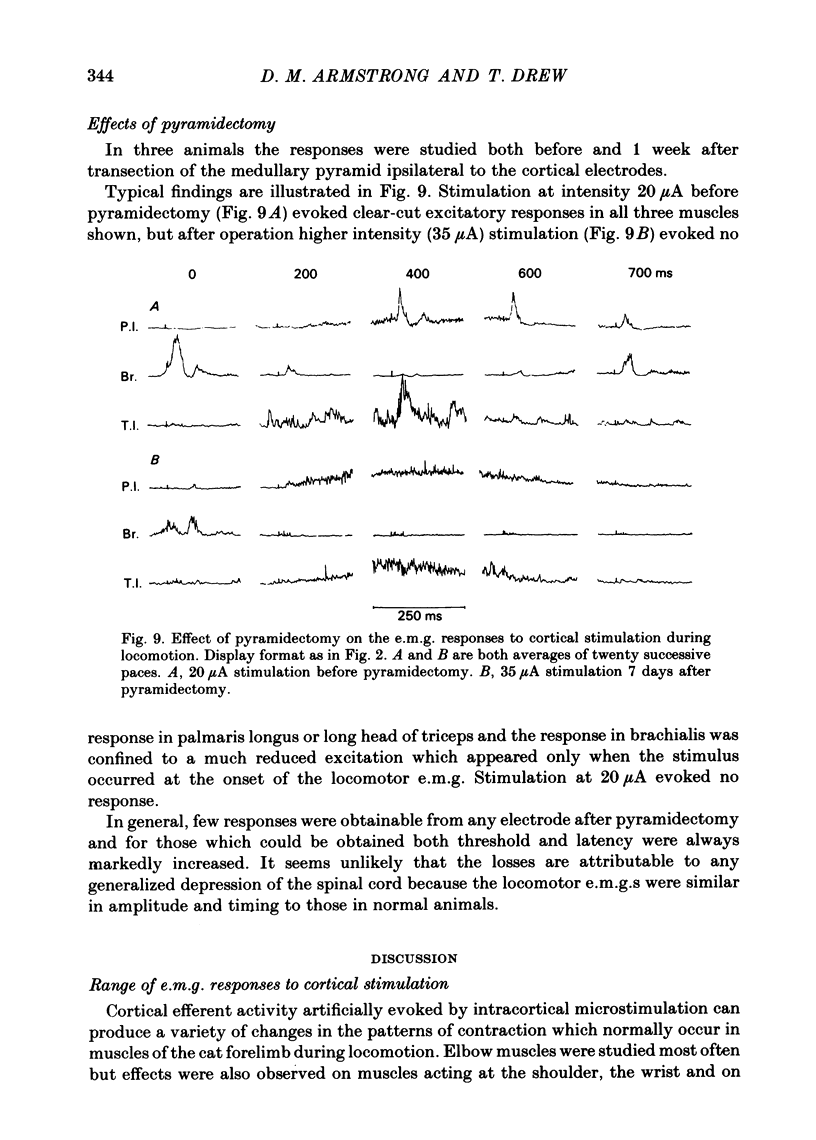
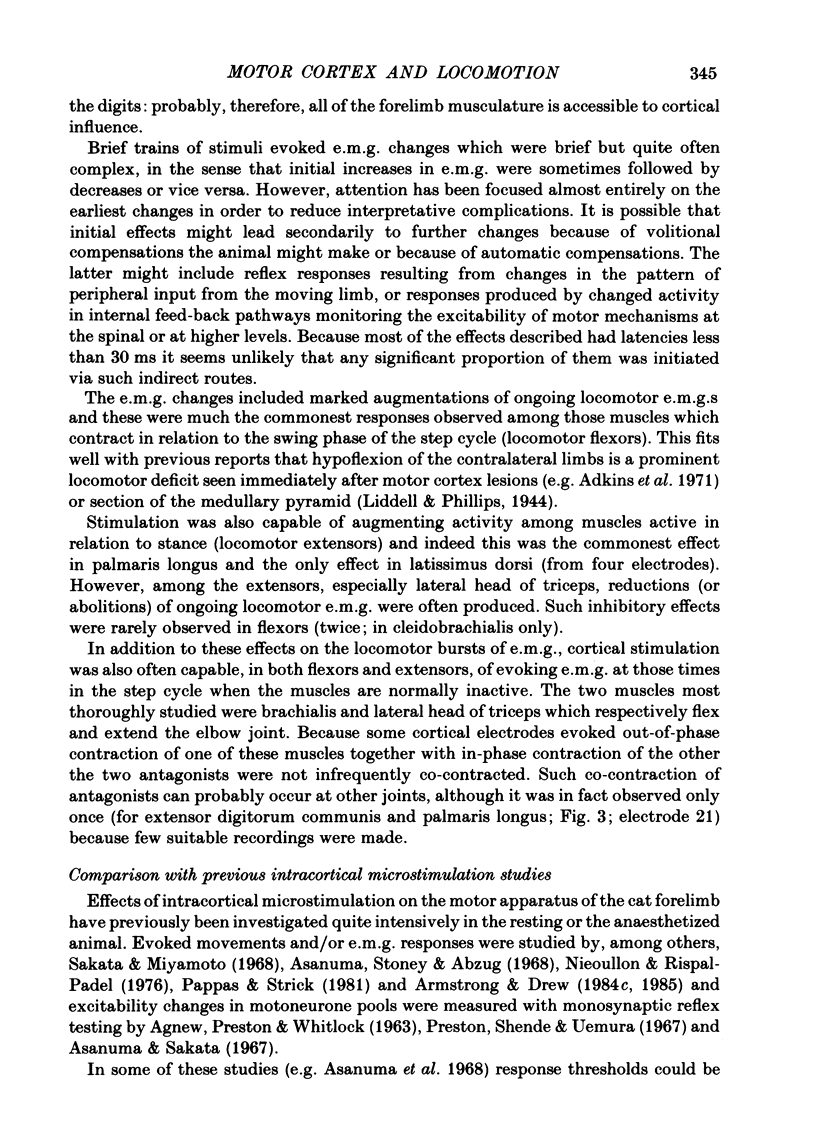
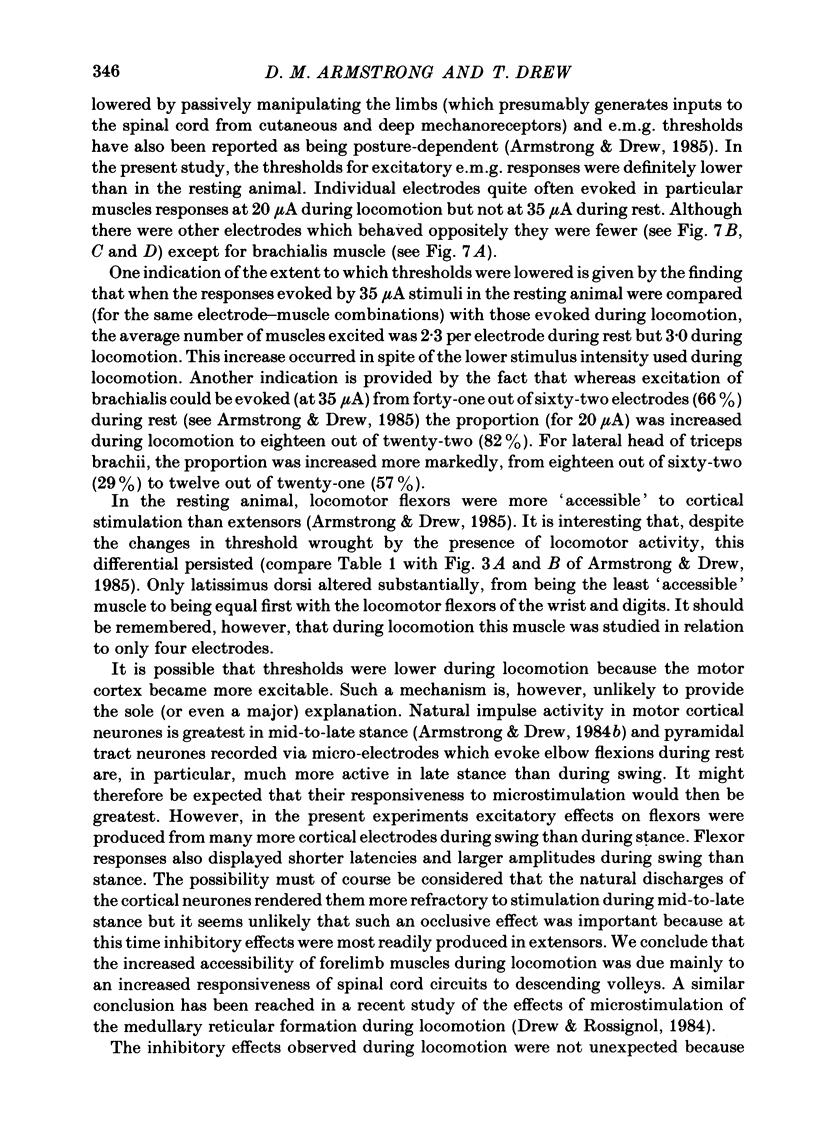
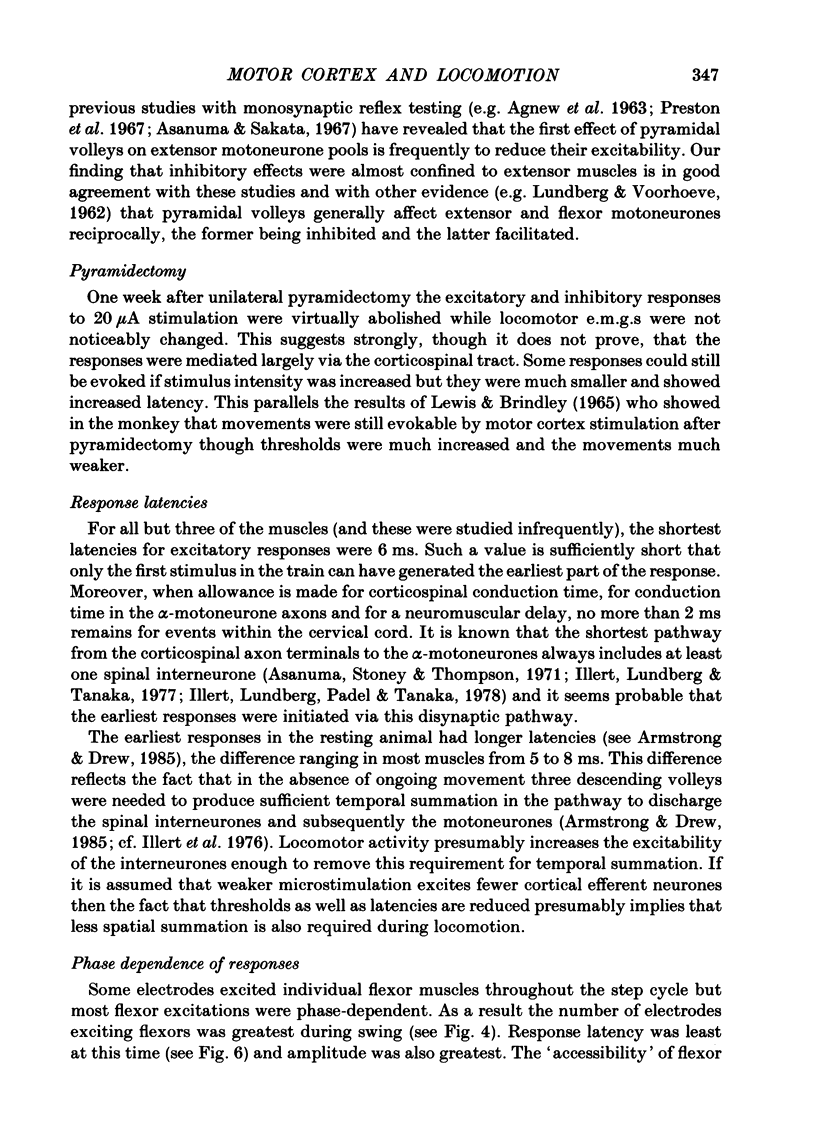
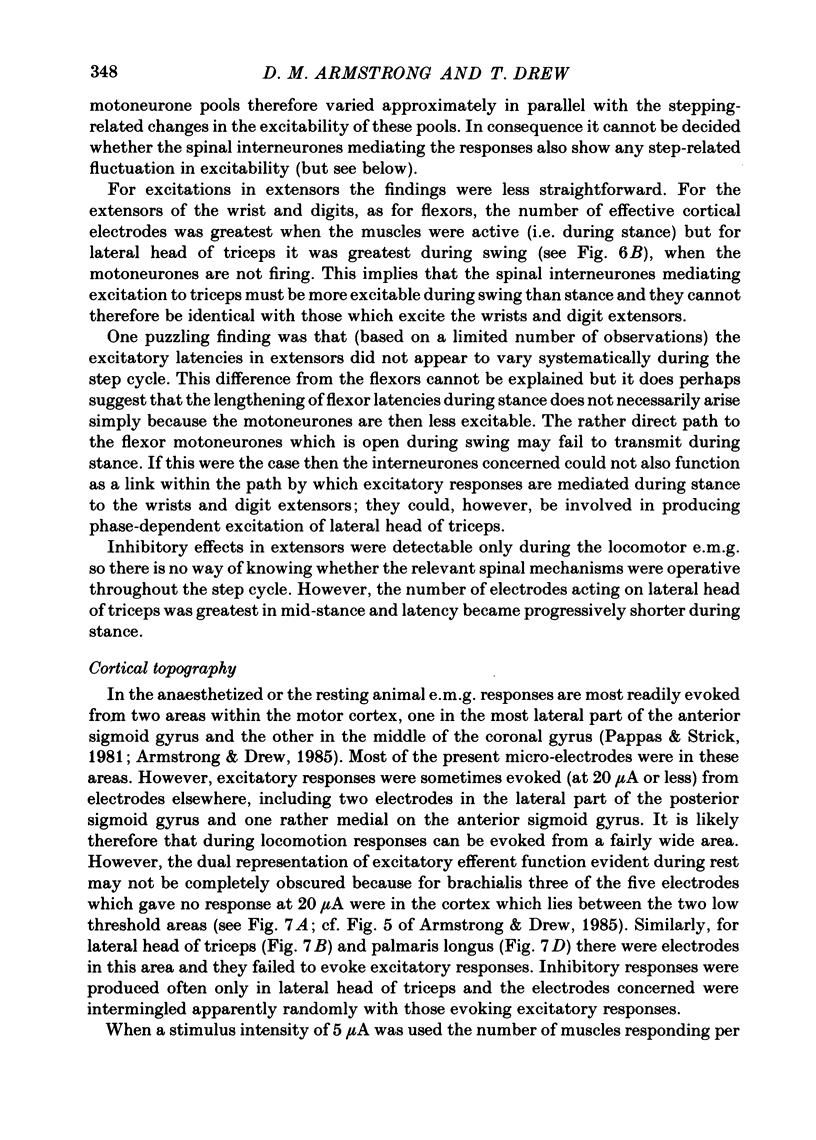
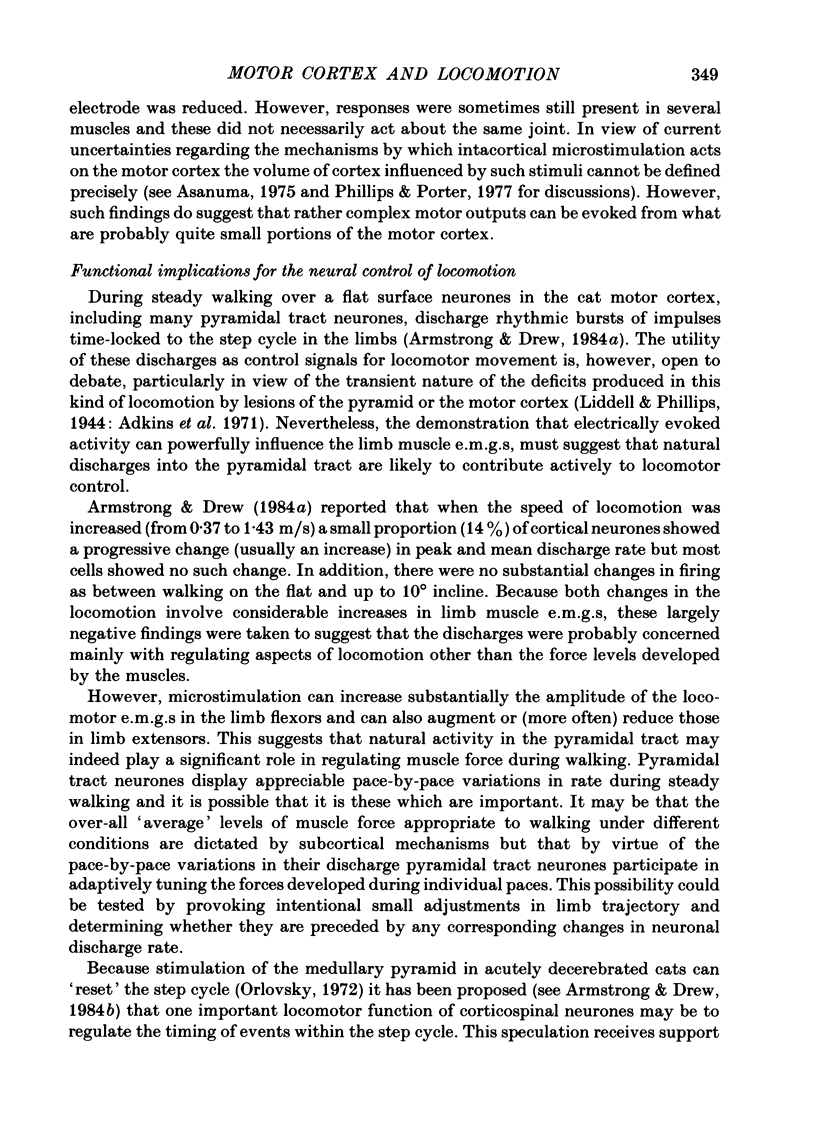
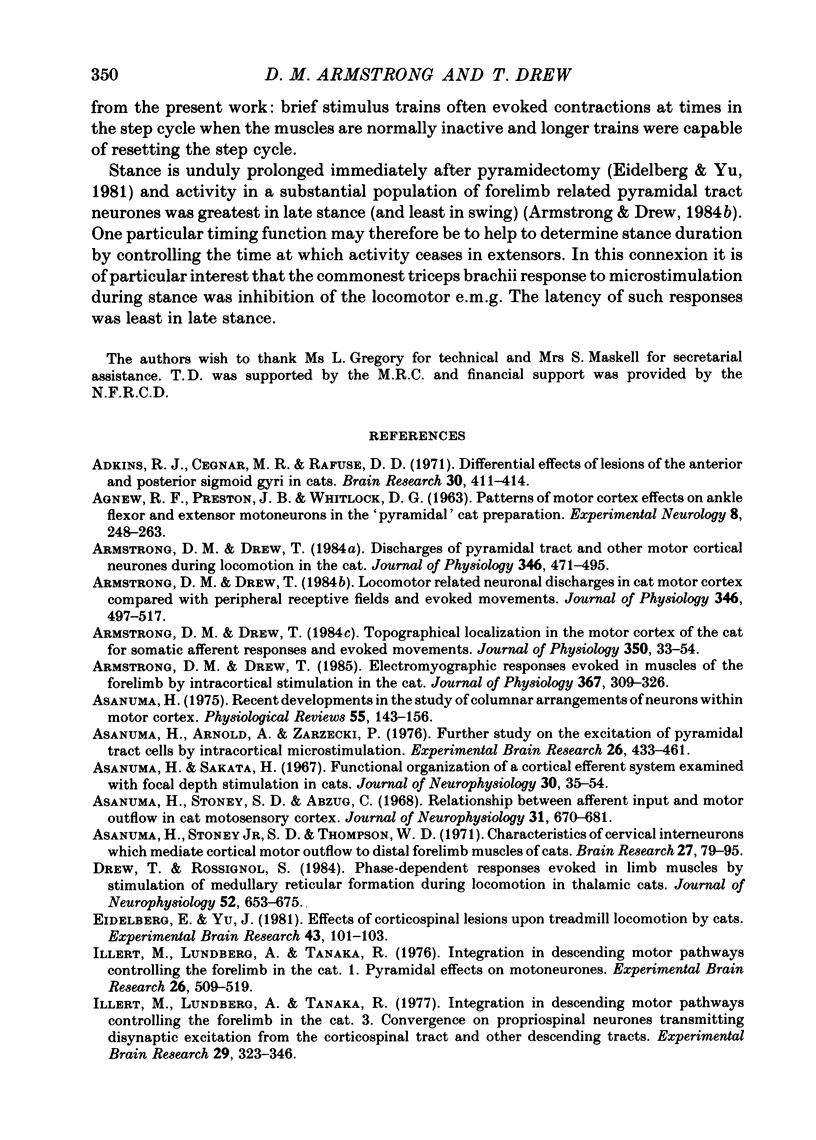
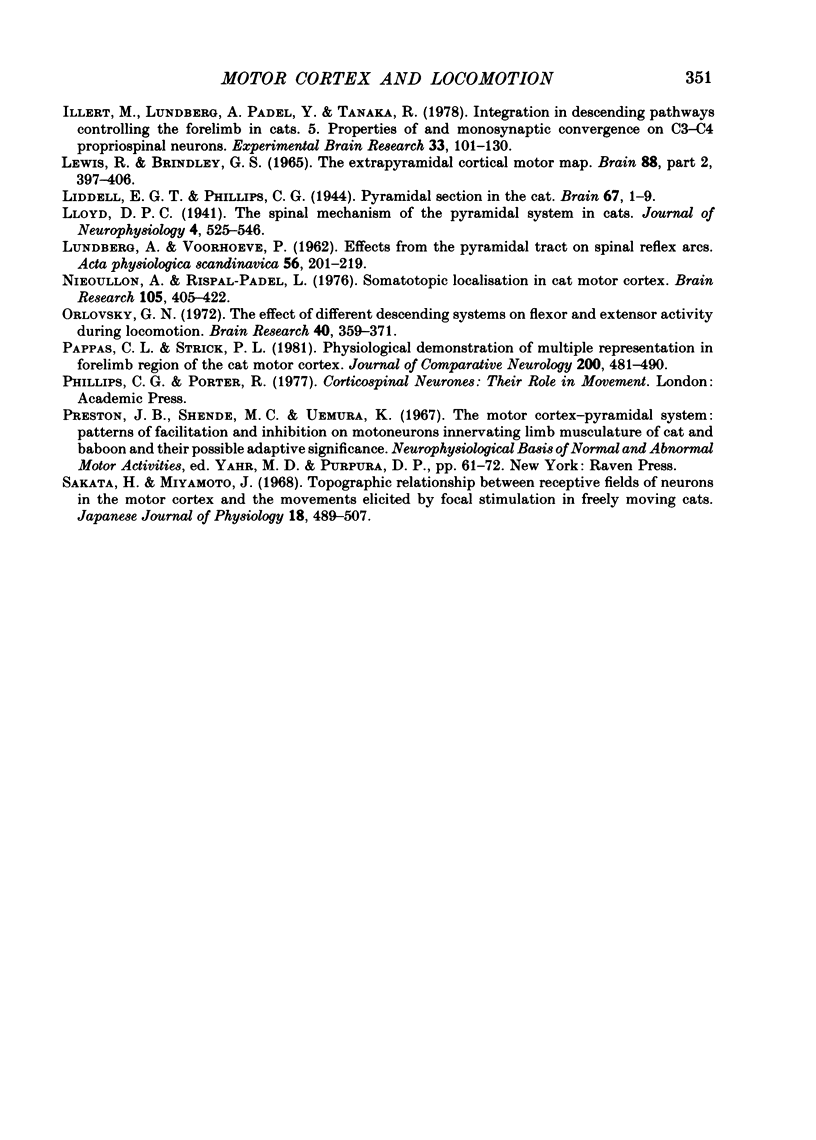
Selected References
These references are in PubMed. This may not be the complete list of references from this article.
- Adkins R. J., Cegnar M. R., Rafuse D. D. Differential effects of lesions of the anterior and posterior sigmoid gyri in cats. Brain Res. 1971 Jul 23;30(2):411–414. doi: 10.1016/0006-8993(71)90092-8. [DOI] [PubMed] [Google Scholar]
- Armstrong D. M., Drew T. Discharges of pyramidal tract and other motor cortical neurones during locomotion in the cat. J Physiol. 1984 Jan;346:471–495. doi: 10.1113/jphysiol.1984.sp015036. [DOI] [PMC free article] [PubMed] [Google Scholar]
- Armstrong D. M., Drew T. Electromyographic responses evoked in muscles of the forelimb by intracortical stimulation in the cat. J Physiol. 1985 Oct;367:309–326. doi: 10.1113/jphysiol.1985.sp015826. [DOI] [PMC free article] [PubMed] [Google Scholar]
- Armstrong D. M., Drew T. Locomotor-related neuronal discharges in cat motor cortex compared with peripheral receptive fields and evoked movements. J Physiol. 1984 Jan;346:497–517. doi: 10.1113/jphysiol.1984.sp015037. [DOI] [PMC free article] [PubMed] [Google Scholar]
- Armstrong D. M., Drew T. Topographical localization in the motor cortex of the cat for somatic afferent responses and evoked movements. J Physiol. 1984 May;350:33–54. doi: 10.1113/jphysiol.1984.sp015187. [DOI] [PMC free article] [PubMed] [Google Scholar]
- Asanuma H., Arnold A., Zarzecki P. Further study on the excitation of pyramidal tract cells by intracortical microstimulation. Exp Brain Res. 1976 Dec 22;26(5):443–461. doi: 10.1007/BF00238820. [DOI] [PubMed] [Google Scholar]
- Asanuma H. Recent developments in the study of the columnar arrangement of neurons within the motor cortex. Physiol Rev. 1975 Apr;55(2):143–156. doi: 10.1152/physrev.1975.55.2.143. [DOI] [PubMed] [Google Scholar]
- Asanuma H., Stoney S. D., Jr, Abzug C. Relationship between afferent input and motor outflow in cat motorsensory cortex. J Neurophysiol. 1968 Sep;31(5):670–681. doi: 10.1152/jn.1968.31.5.670. [DOI] [PubMed] [Google Scholar]
- Drew T., Rossignol S. Phase-dependent responses evoked in limb muscles by stimulation of medullary reticular formation during locomotion in thalamic cats. J Neurophysiol. 1984 Oct;52(4):653–675. doi: 10.1152/jn.1984.52.4.653. [DOI] [PubMed] [Google Scholar]
- Eidelberg E., Yu J. Effects of corticospinal lesions upon treadmill locomotion by cats. Exp Brain Res. 1981;43(1):101–103. doi: 10.1007/BF00238815. [DOI] [PubMed] [Google Scholar]
- Illert M., Lundberg A., Padel Y., Tanaka R. Integration in descending motor pathways controlling the forelimb in the cat. 5. Properties of and monosynaptic excitatory convergence on C3--C4 propriospinal neurones. Exp Brain Res. 1978 Sep 15;33(1):101–130. doi: 10.1007/BF00238798. [DOI] [PubMed] [Google Scholar]
- Illert M., Lundberg A., Tanaka R. Integration in descending motor pathways controlling the forelimb in the cat. 1. Pyramidal effects on motoneurones. Exp Brain Res. 1976 Dec 22;26(5):509–519. doi: 10.1007/BF00238824. [DOI] [PubMed] [Google Scholar]
- Illert M., Lundberg A., Tanaka R. Integration in descending motor pathways controlling the forelimb in the cat. 3. Convergence on propriospinal neurones transmitting disynaptic excitation from the corticospinal tract and other descending tracts. Exp Brain Res. 1977 Sep 28;29(3-4):323–346. doi: 10.1007/BF00236174. [DOI] [PubMed] [Google Scholar]
- LUNDBERG A., VOORHOEVE P. Effects from the pyramidal tract on spinal reflex arcs. Acta Physiol Scand. 1962 Nov-Dec;56:201–219. doi: 10.1111/j.1748-1716.1962.tb02498.x. [DOI] [PubMed] [Google Scholar]
- Lewis R., Brindley G. S. The extrapyramidal cortical motor map. Brain. 1965 Jun;88(2):397–406. doi: 10.1093/brain/88.2.397. [DOI] [PubMed] [Google Scholar]
- Nieoullon A., Rispal-Padel L. Somatotopic localization in cat motor cortex. Brain Res. 1976 Apr 9;105(3):405–422. doi: 10.1016/0006-8993(76)90590-4. [DOI] [PubMed] [Google Scholar]
- Orlovsky G. N. The effect of different descending systems on flexor and extensor activity during locomotion. Brain Res. 1972 May 26;40(2):359–371. doi: 10.1016/0006-8993(72)90139-4. [DOI] [PubMed] [Google Scholar]
- Pappas C. L., Strick P. L. Physiological demonstration of multiple representation in the forelimb region of the cat motor cortex. J Comp Neurol. 1981 Aug 20;200(4):481–490. doi: 10.1002/cne.902000403. [DOI] [PubMed] [Google Scholar]
- Sakata H., Miyamoto J. Topographic relationship between the receptive fields of neurons in the motor cortex and the movements elicited by focal stimulation in freely moving cats. Jpn J Physiol. 1968 Aug 15;18(4):489–507. doi: 10.2170/jjphysiol.18.489. [DOI] [PubMed] [Google Scholar]