Abstract
Trabeculae from guinea-pig ventricles were bathed in a physiological saline, were stimulated electrically and were stretched until isometric force production was maximal. They were then 'skinned' by superfusion for 30 min with a solution containing the non-ionic detergent, Triton X-100. The steady isometric force generated by these skinned trabeculae was measured in solutions of different pCa (-log10 [Ca2+]) and salt concentration to investigate the influence of salt concentration on (i) the maximum Ca2+-regulated force (at pCa approximately 3.8), (ii) the resting force (at pCa greater than 8) and (iii) the pCa required for 50% Ca2+ activation (pCa50). The apparent affinity constants of EGTA (ethyleneglycol-bis-(beta-aminoethylether)-N,N,N',N'-tetraacetic acid) for Ca2+ were measured in the solutions of different salt composition by a pH-metric method and these constants were used in the calculation of solution pCa. Increases in ionic strength (gamma/2) (and osmolarity) over the range 0.1-0.4 mol/l produced by the addition of various monovalent Cl or K salts resulted in an approximately linear decrease in the maximum Ca2+-regulated force. The inhibitory actions of the different salts were similar despite the differences in size and structure of their constituent ions. Increases in solution osmolarity alone, produced by the addition of sucrose up to 0.6 mol/l, did not significantly alter the maximum Ca2+-regulated force. It was concluded that the influence of monovalent salts on the maximum Ca2+-regulated force was due entirely to the changes in ionic strength and was not related to changes in solution osmolarity or to specific ion effects. Resting force was little affected by changes in salt concentration at ionic strengths of 0.2 mol/l and above. At lower ionic strengths the muscles exhibited an elevated resting force and an inability to relax completely after a Ca2+ -regulated contracture. A decrease in ionic strength from 0.2 to 0.1 mol/l produced by a reduction in KCl concentration raised the pCa50 from 5.56 to 5.85, i.e. the Ca2+ sensitivity was increased. However, the same increase in pCa50 was observed when the [K+] was lowered at constant ionic strength, osmolarity and [Cl-] by the replacement of KCl with tetramethylammonium Cl or choline Cl. Therefore, the change in pCa50 induced by an alteration in KCl concentration was due entirely to a specific inhibitory action of K+ on the Ca2+ sensitivity of cardiac myofibrils and was unrelated to changes in ionic strength, osmolarity and [Cl-]. Substitution of KCl 0.1 mol/l in the solutions by NaCl did not significantly alter the pCa50.(ABSTRACT TRUNCATED AT 400 WORDS)
Full text
PDF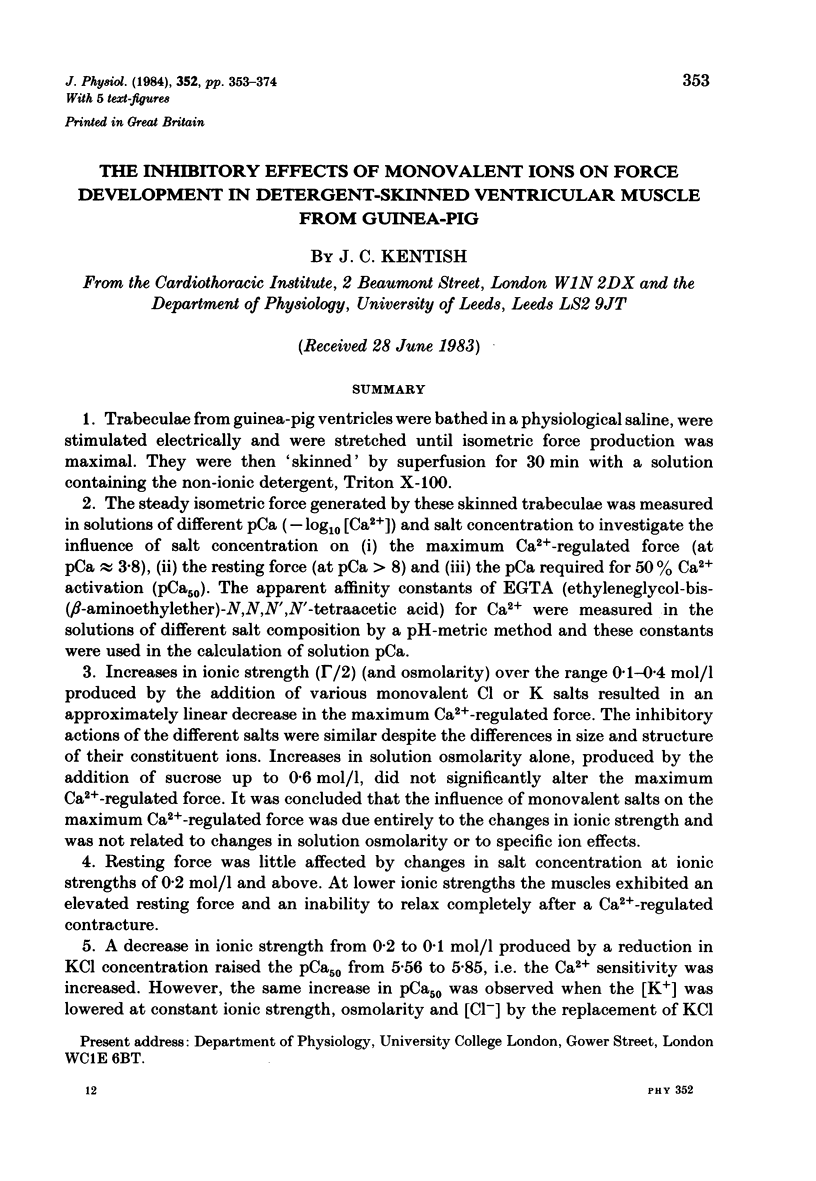
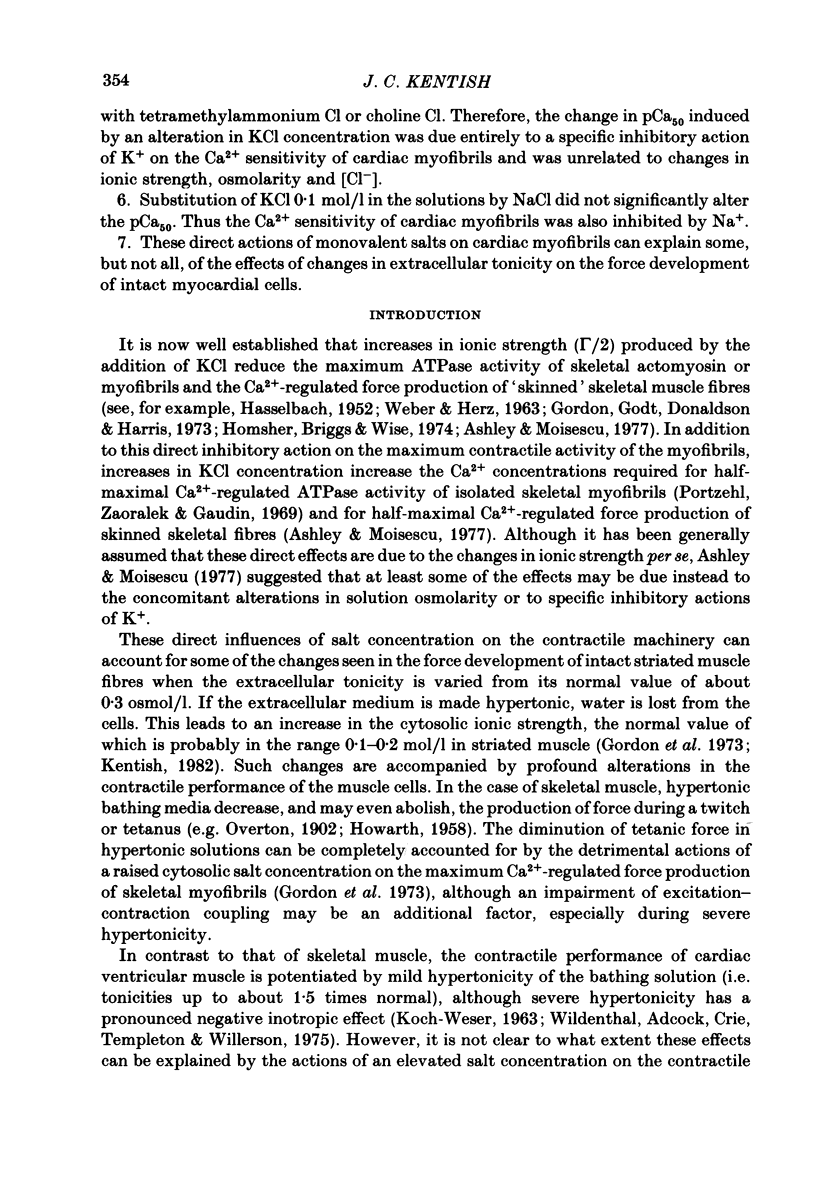
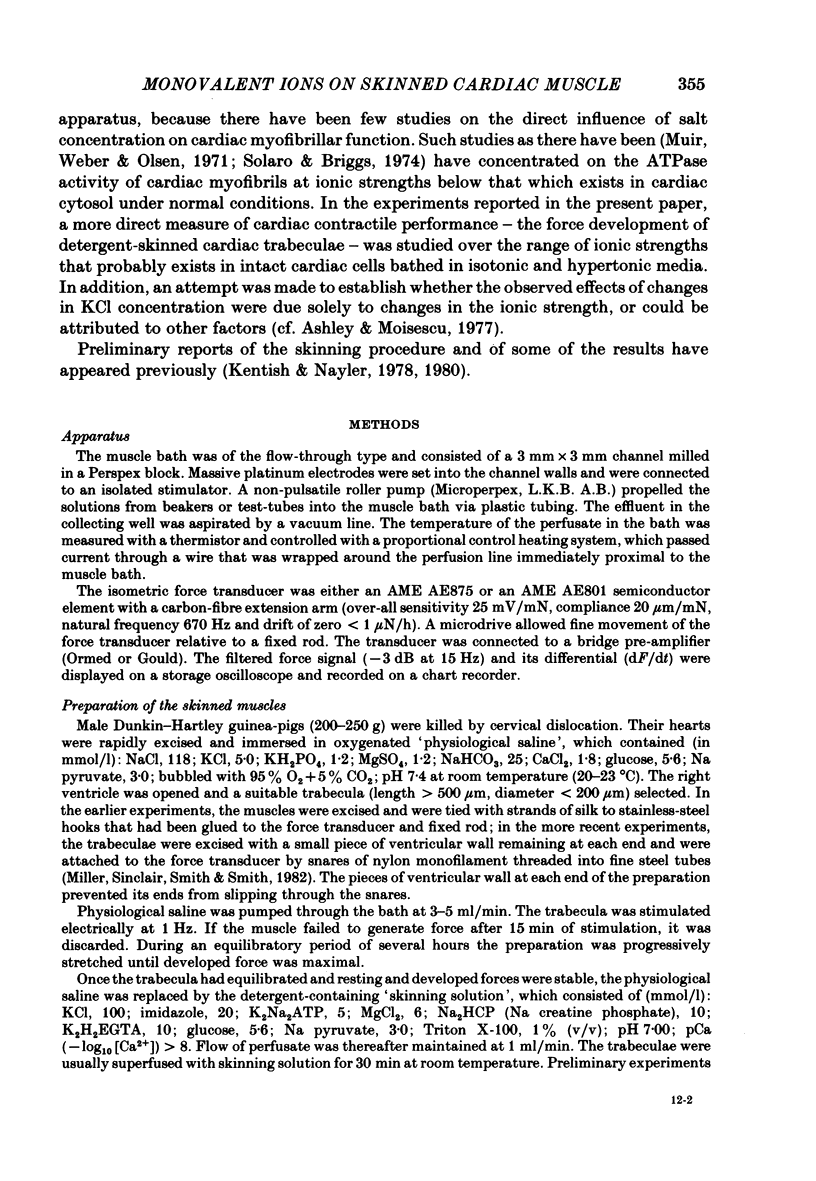
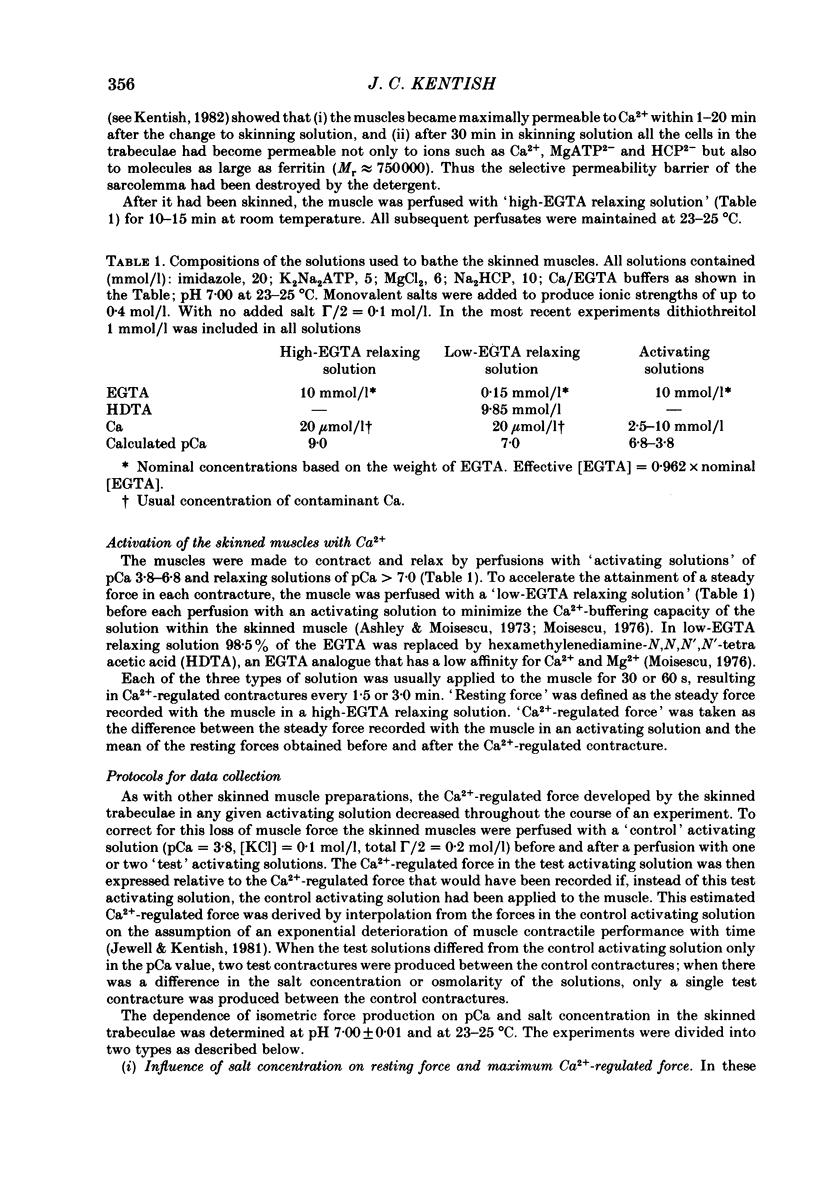
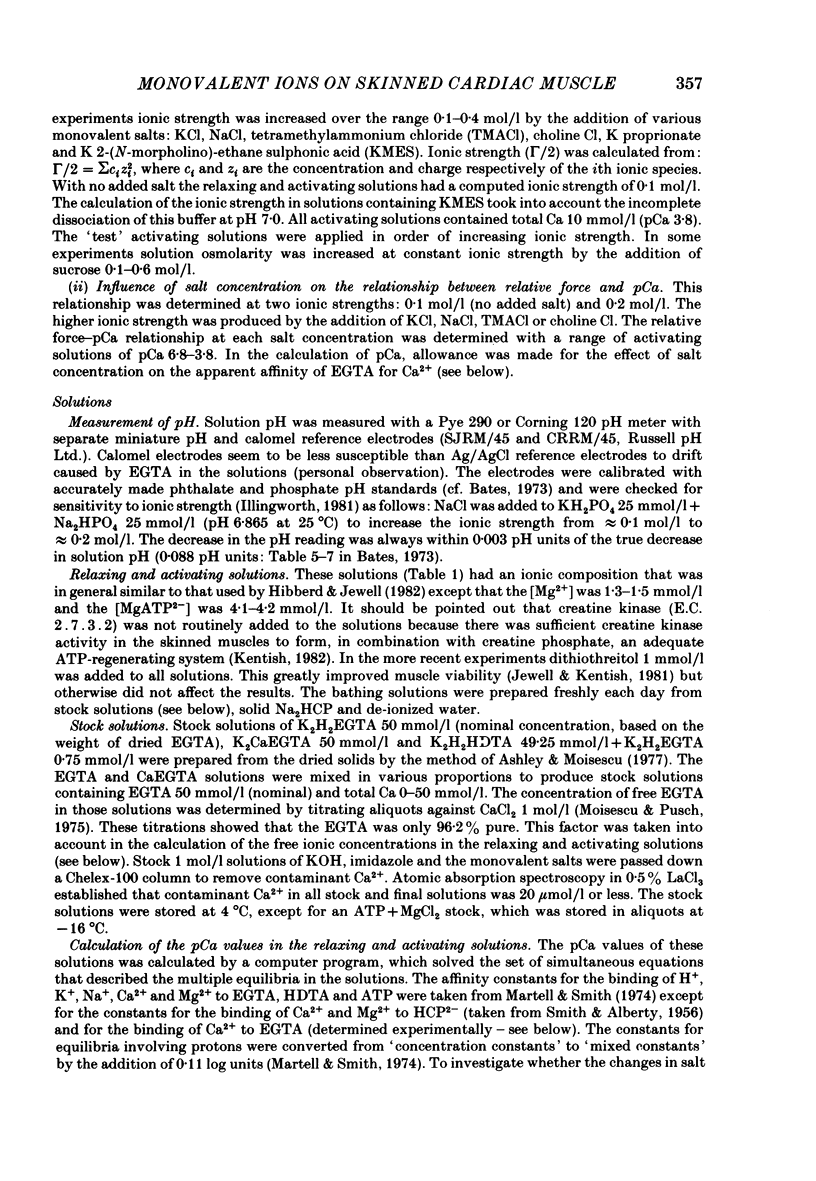
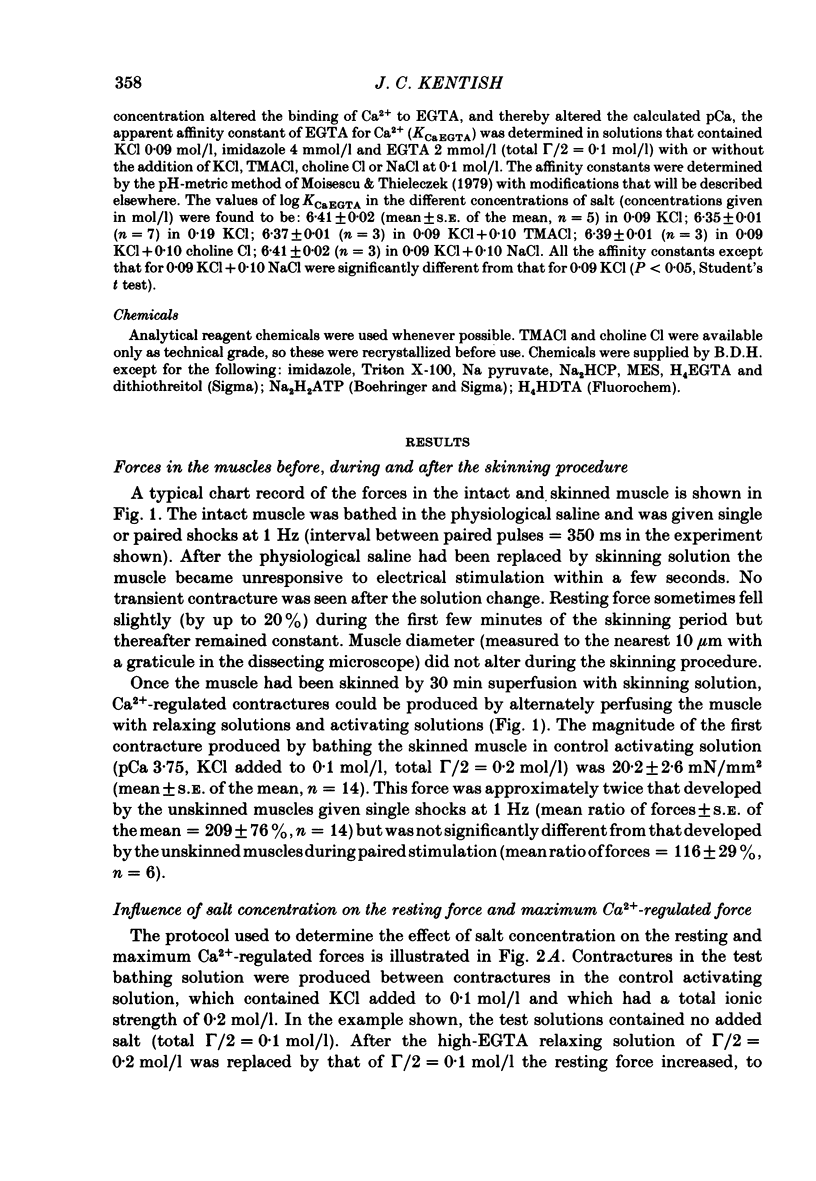
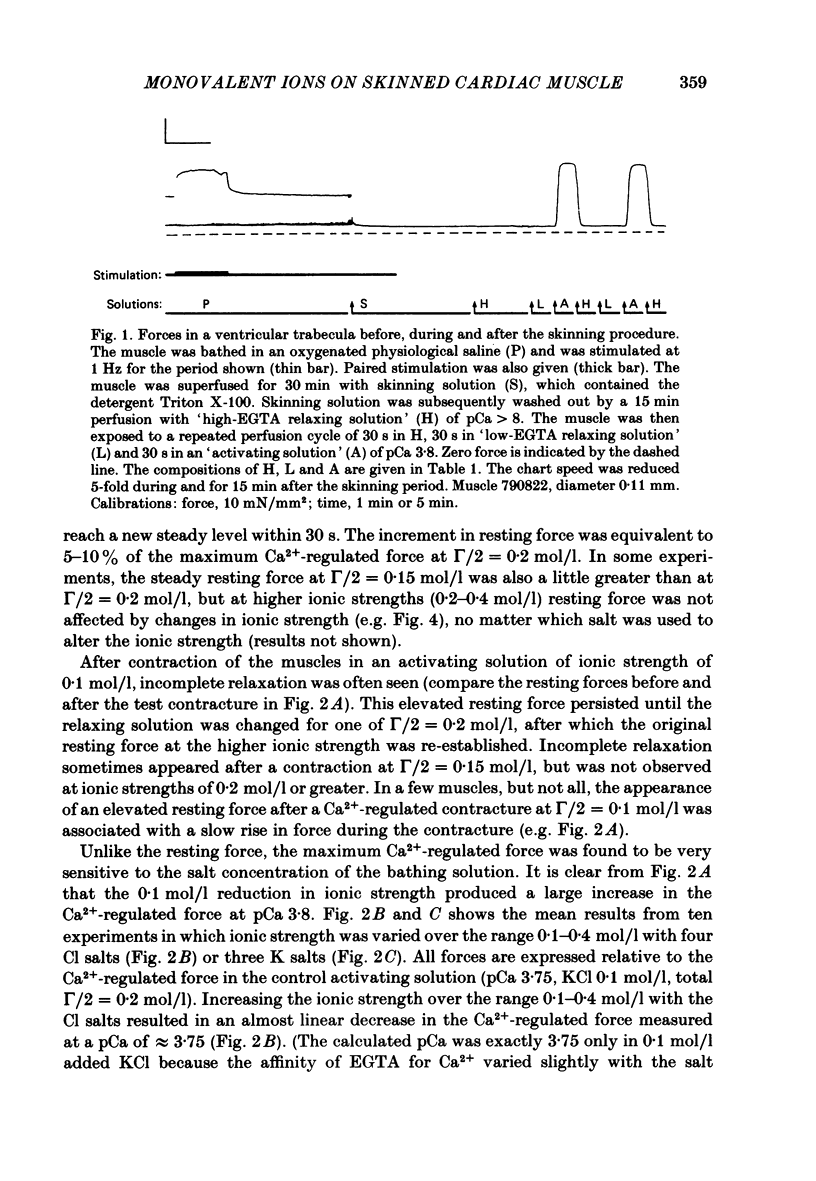
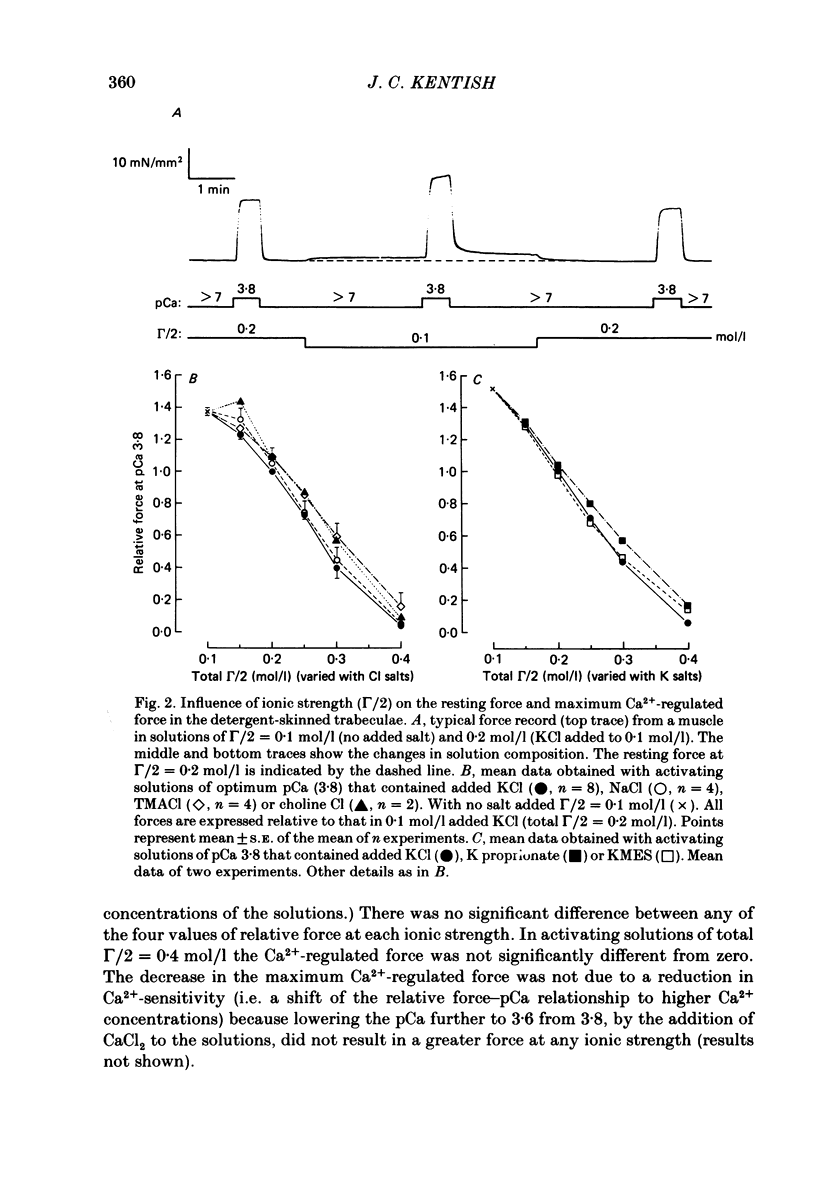
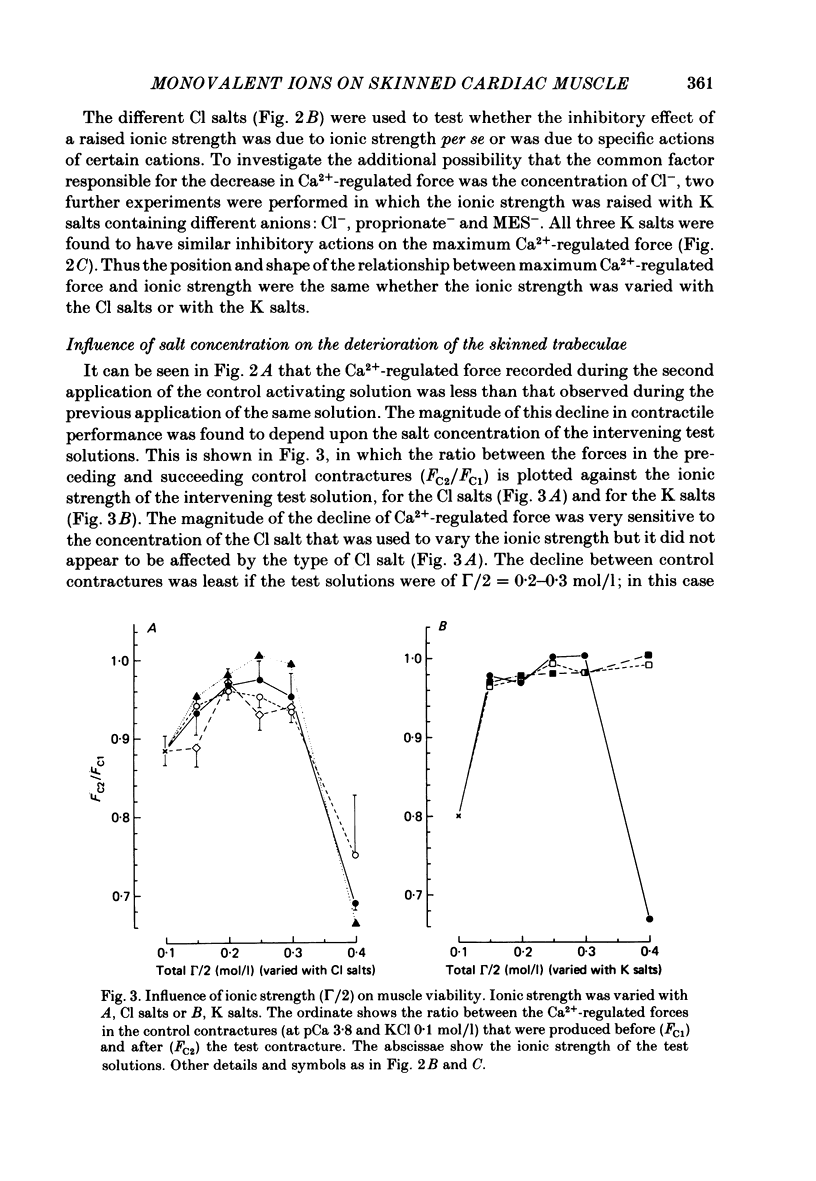
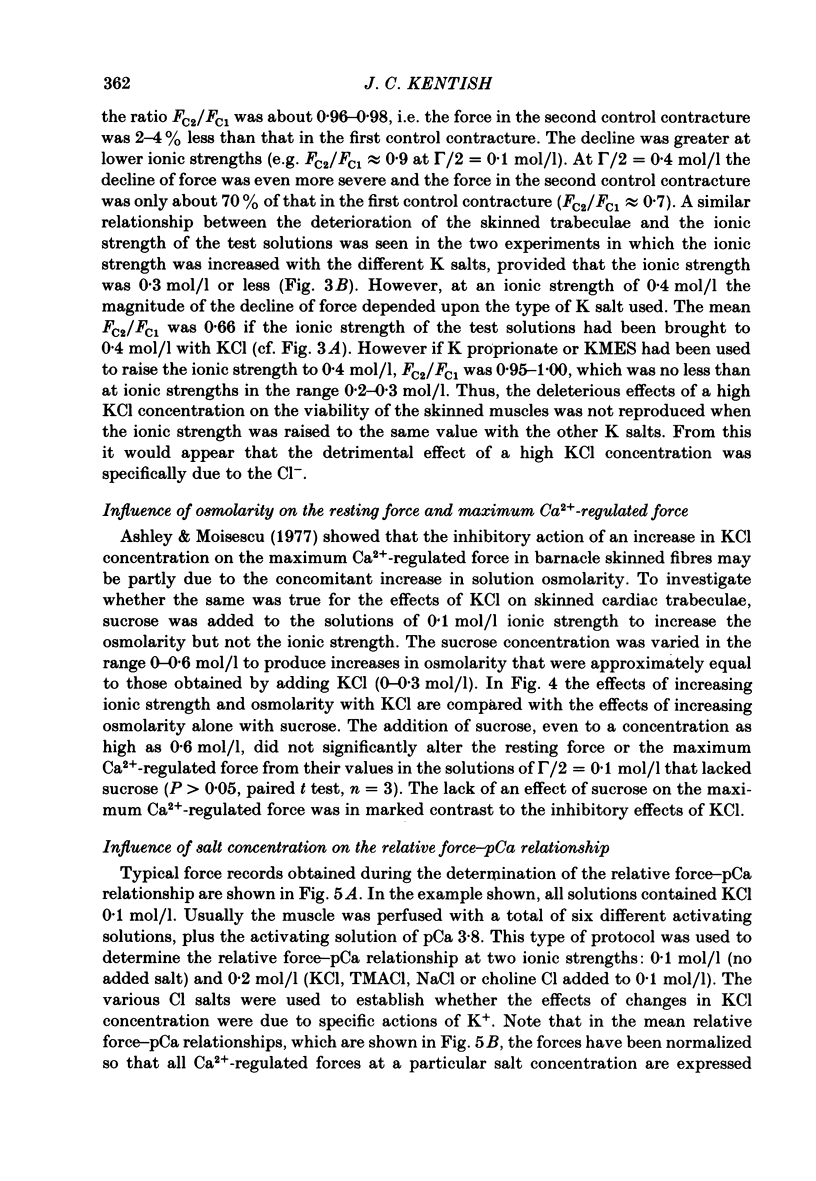
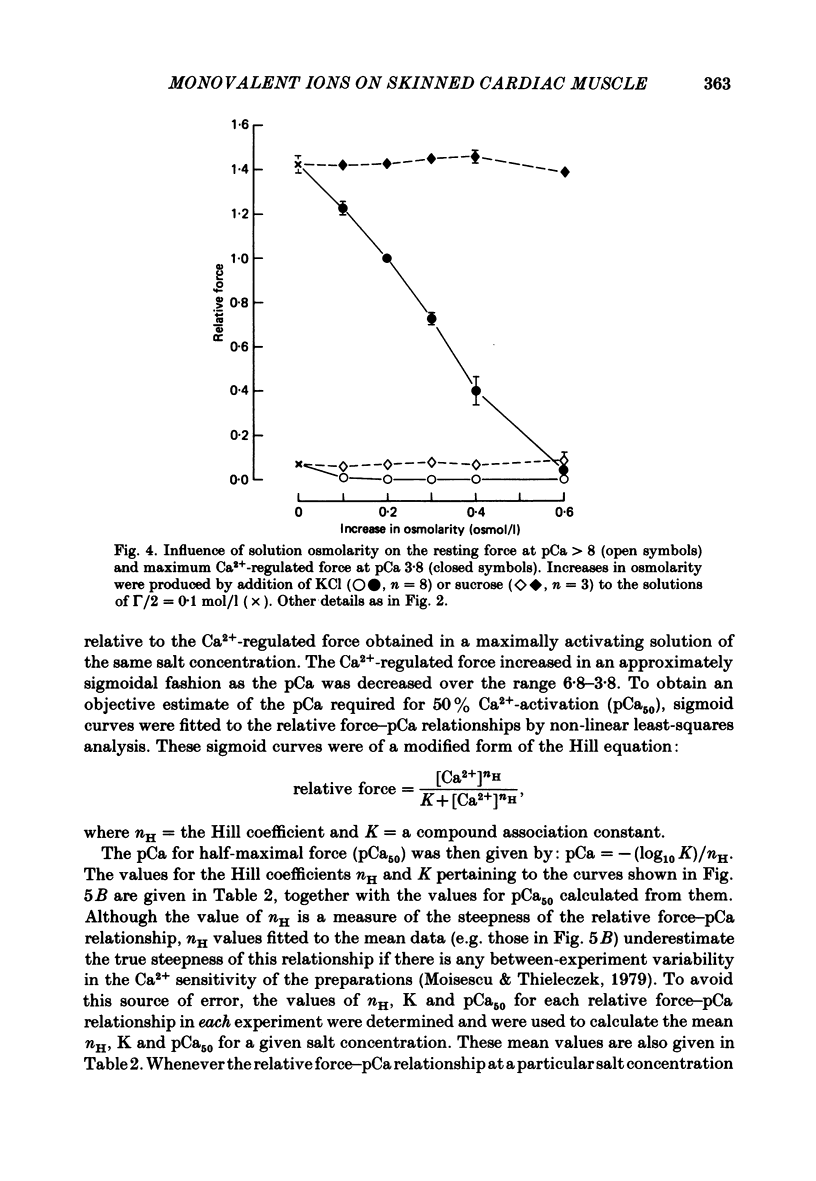
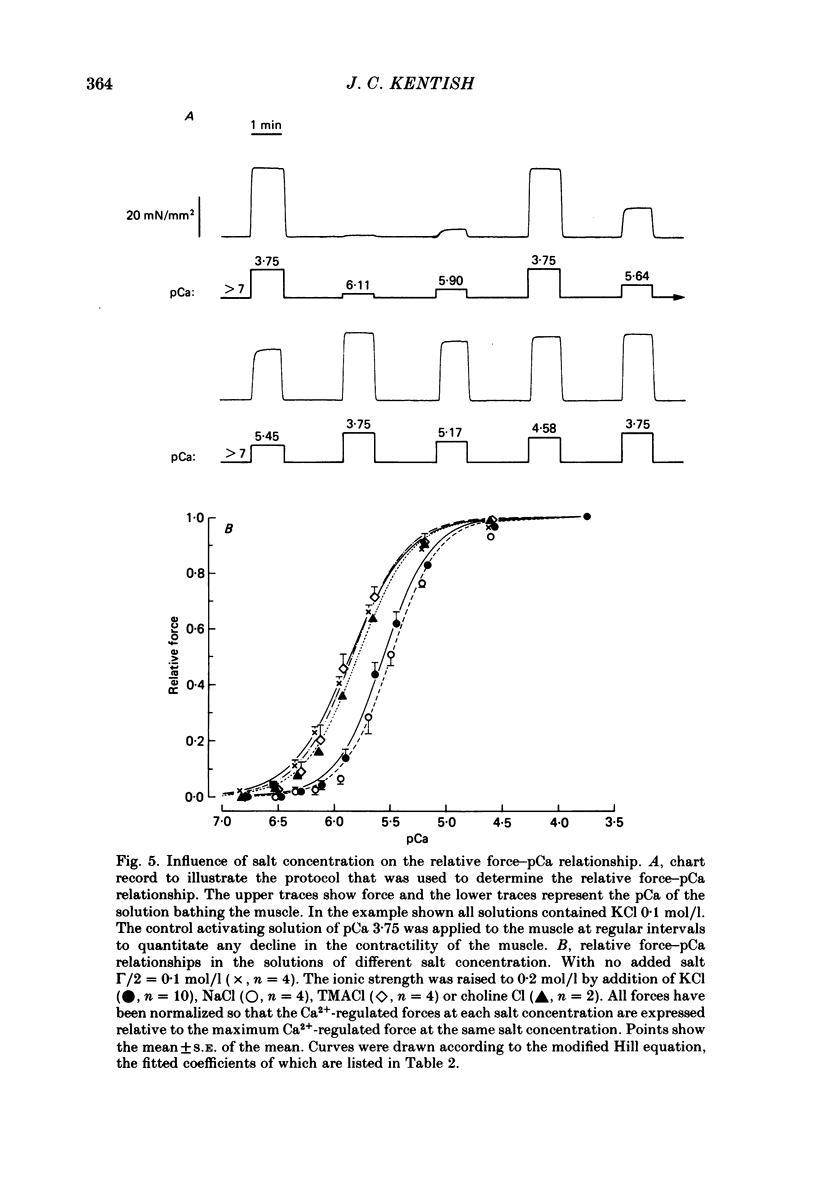
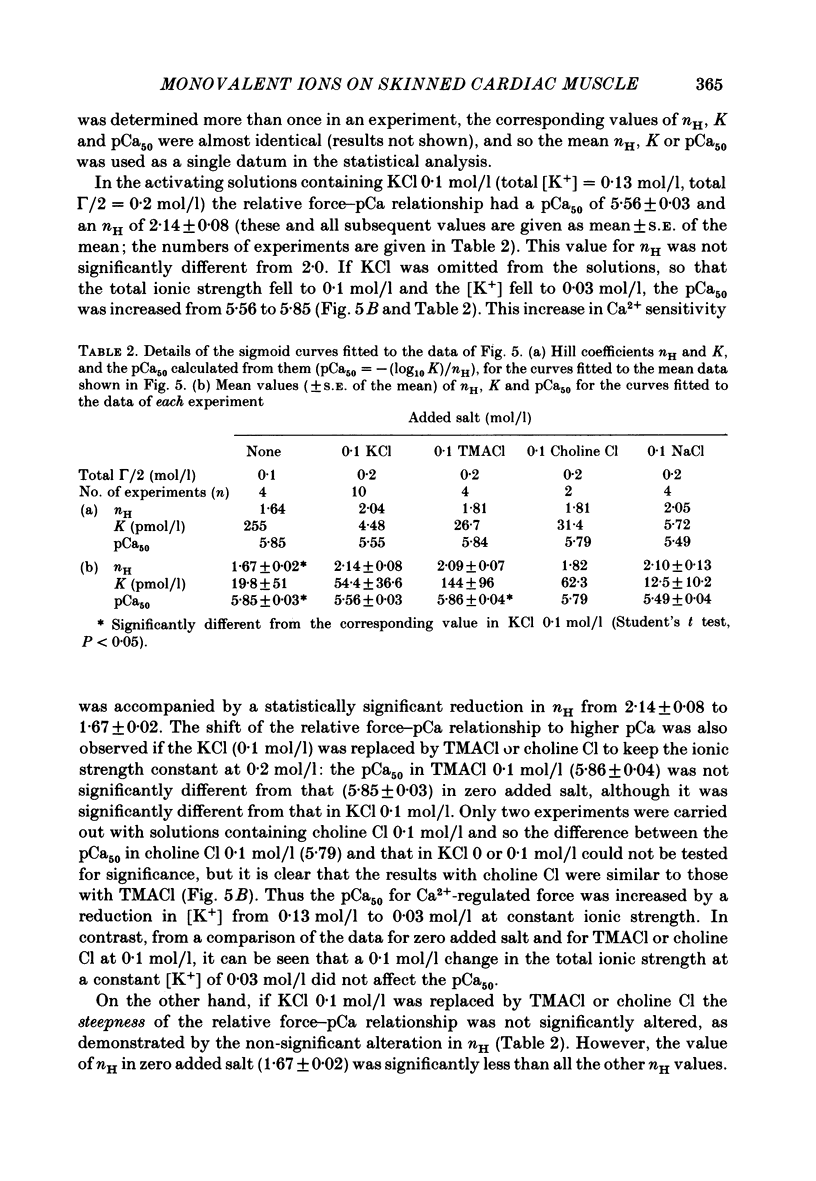
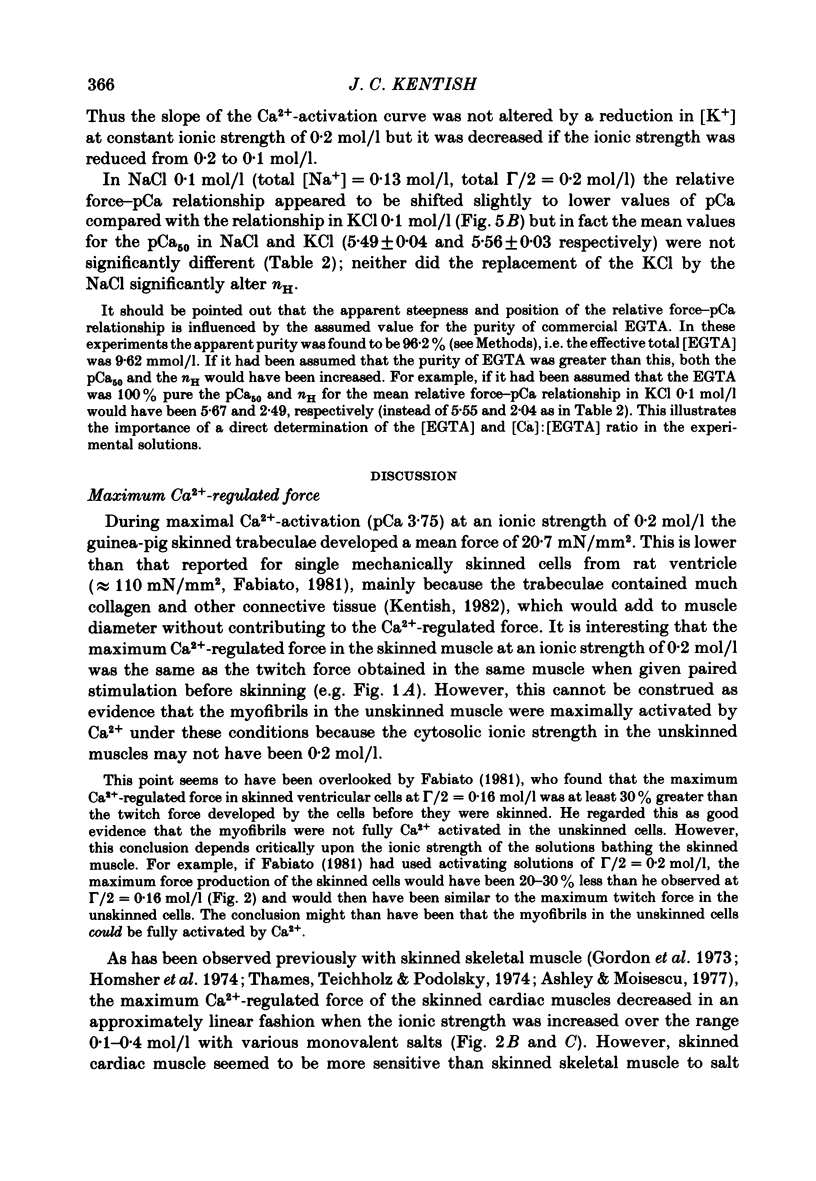
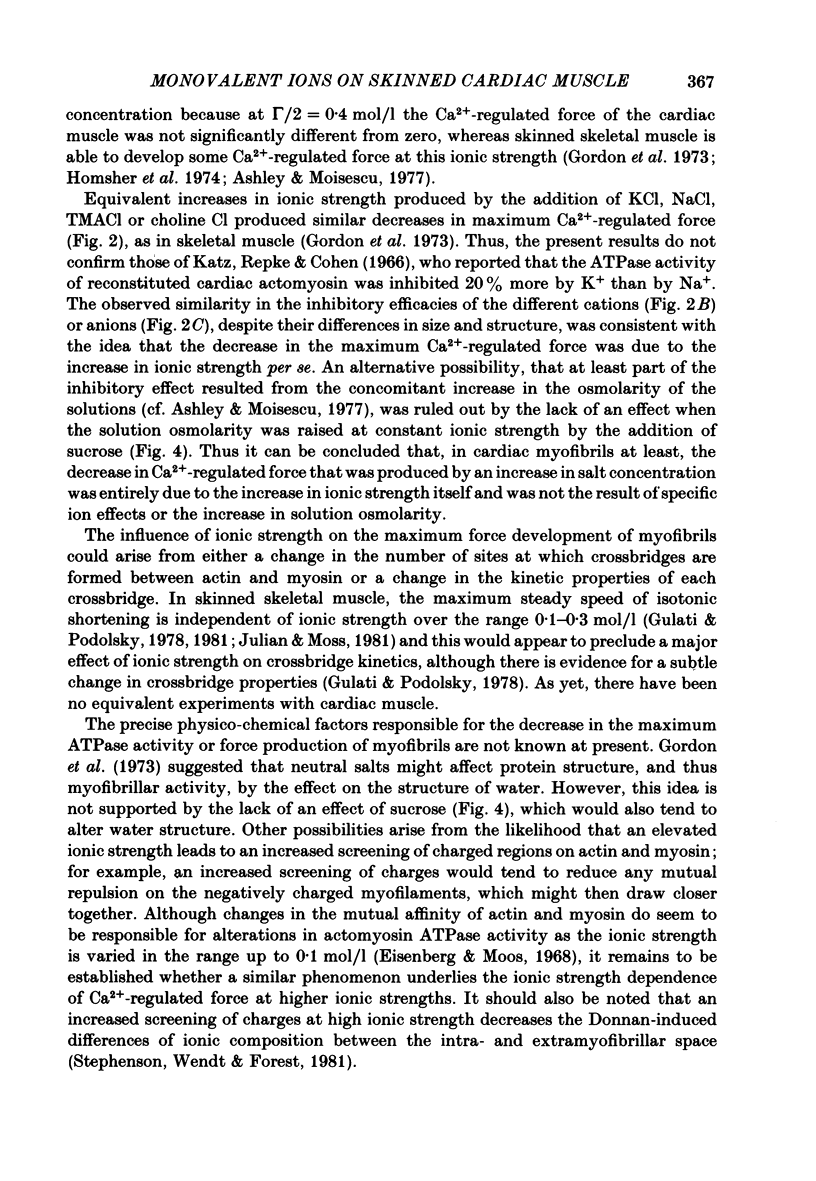
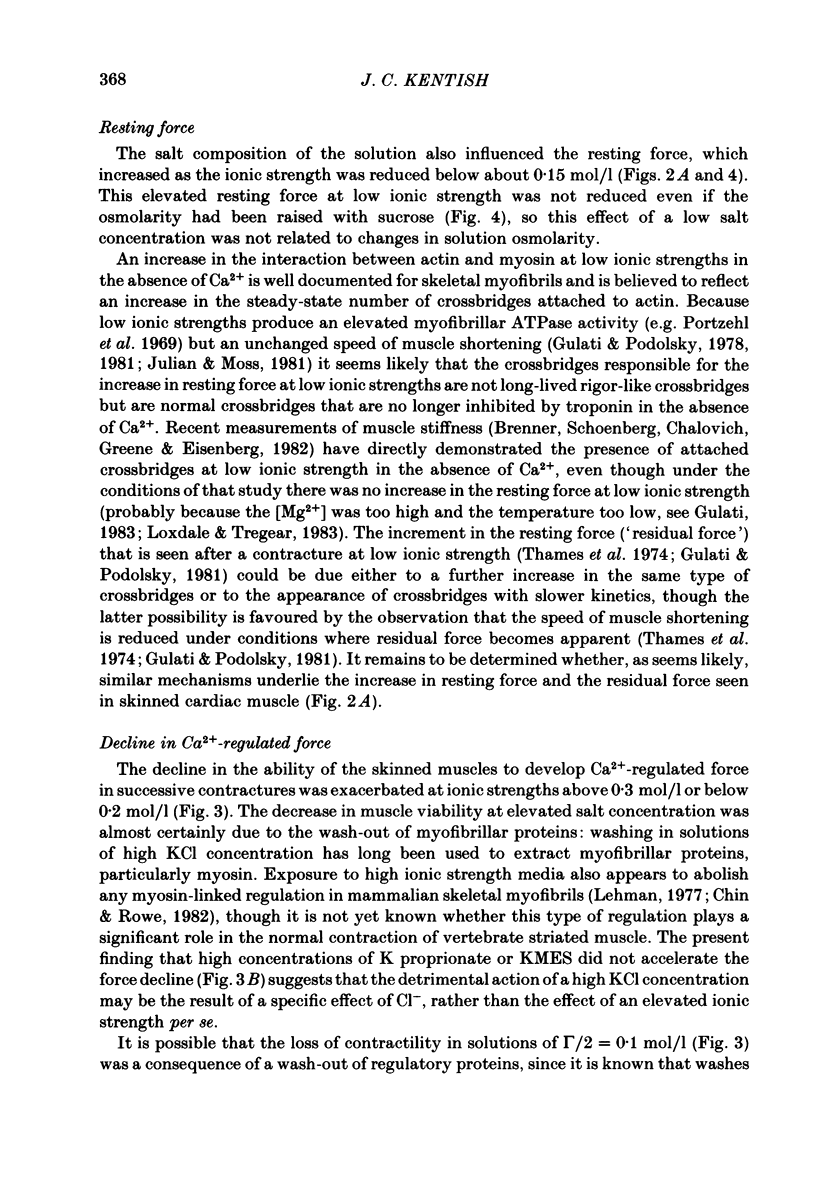
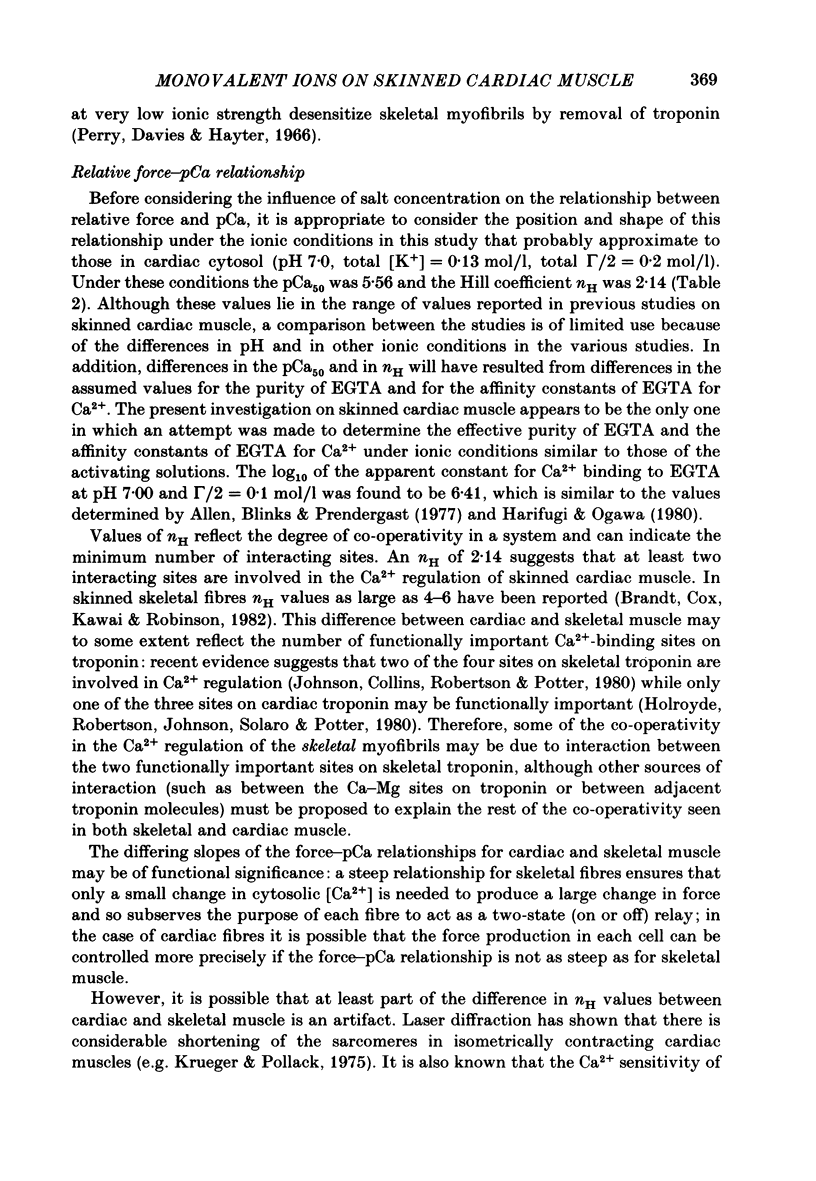
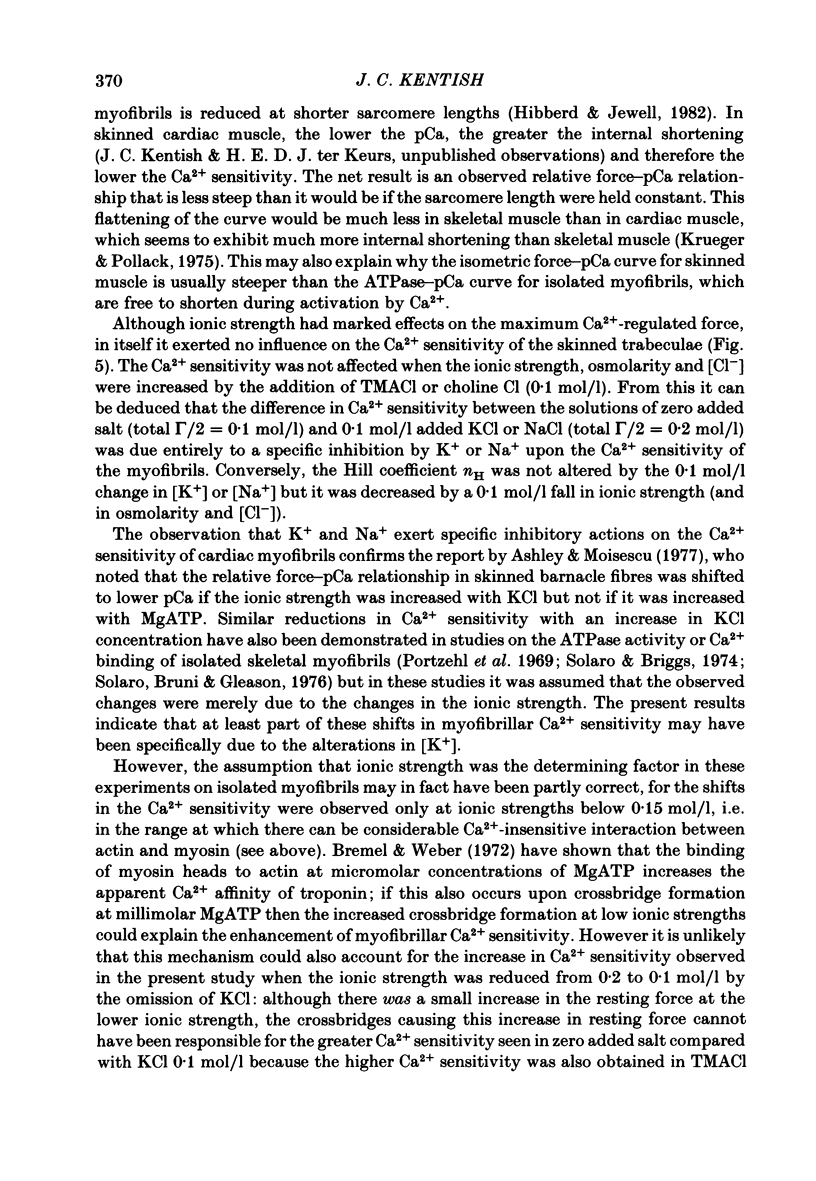
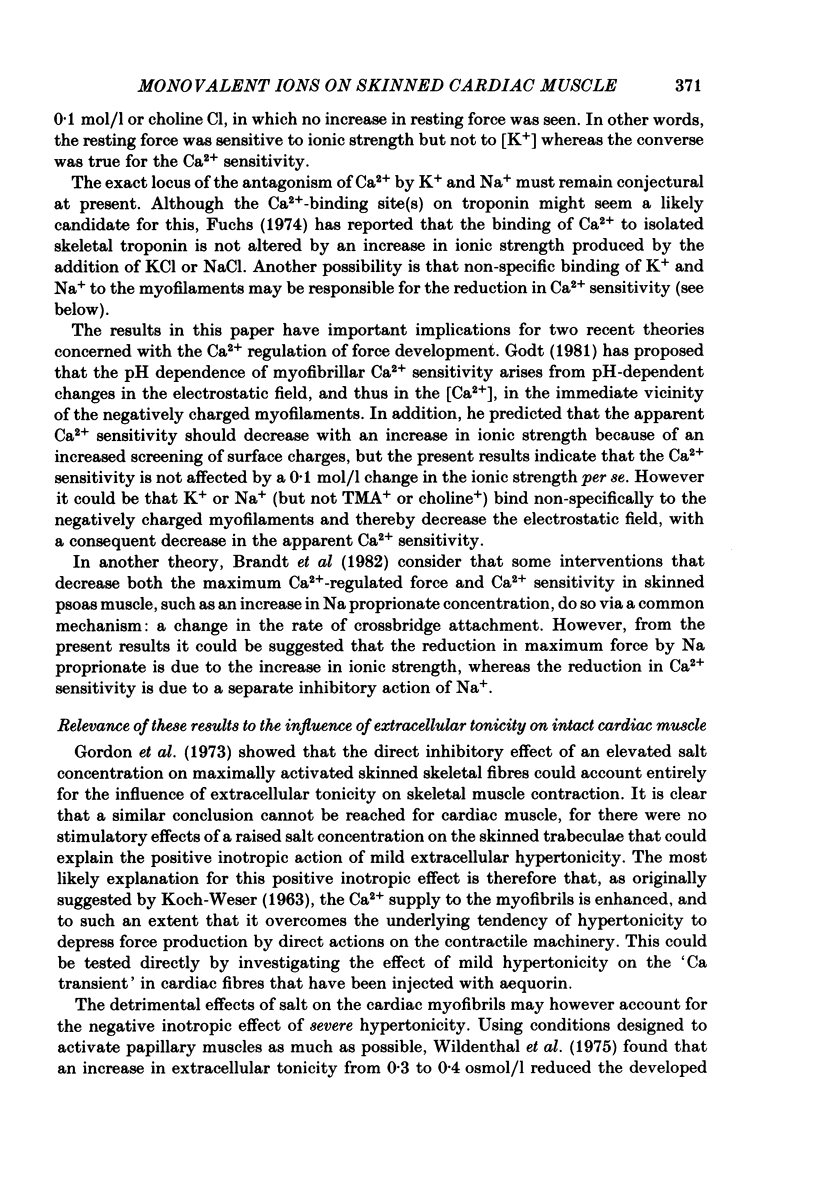
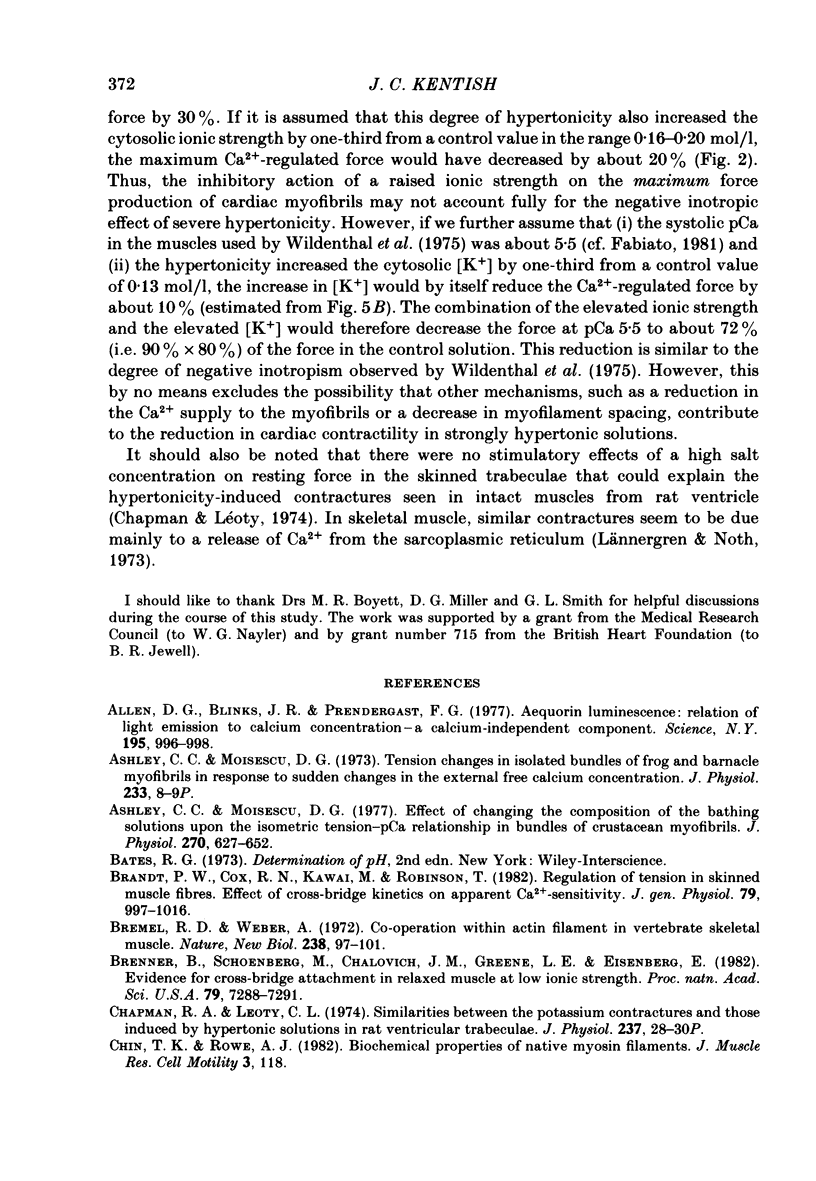
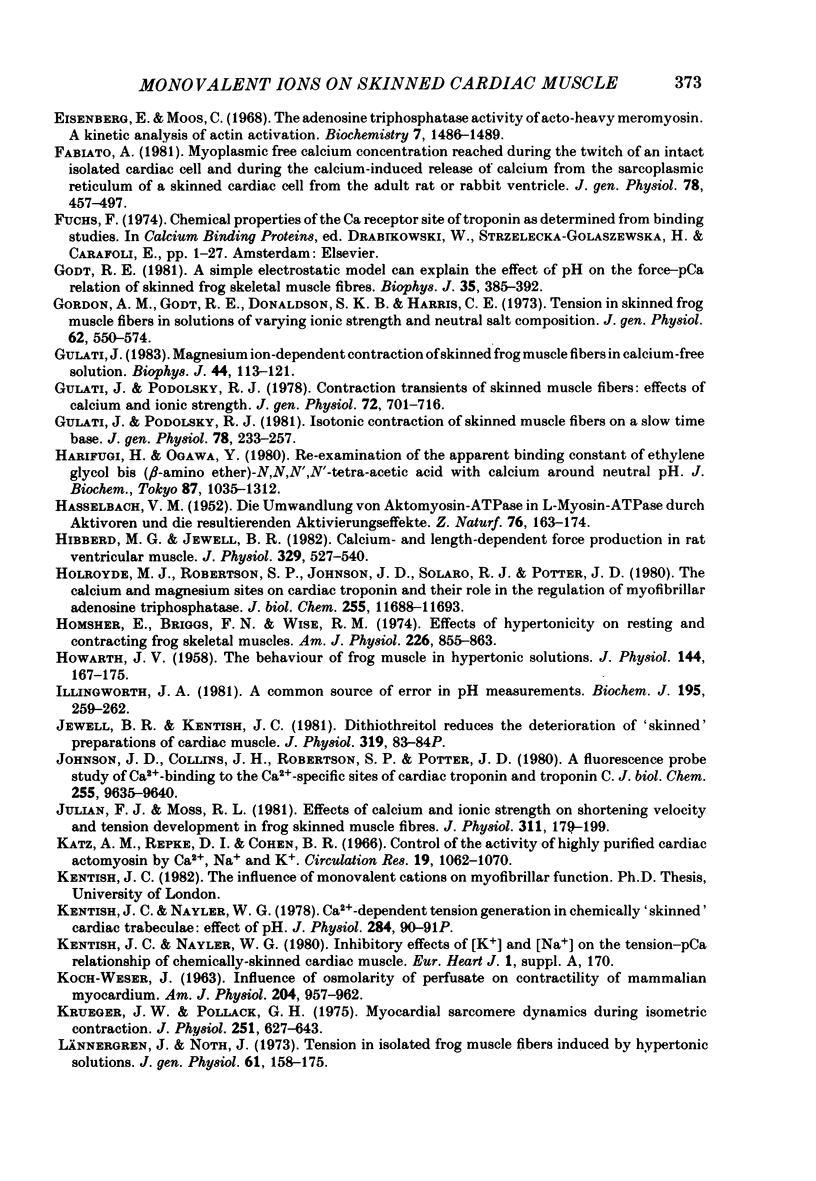
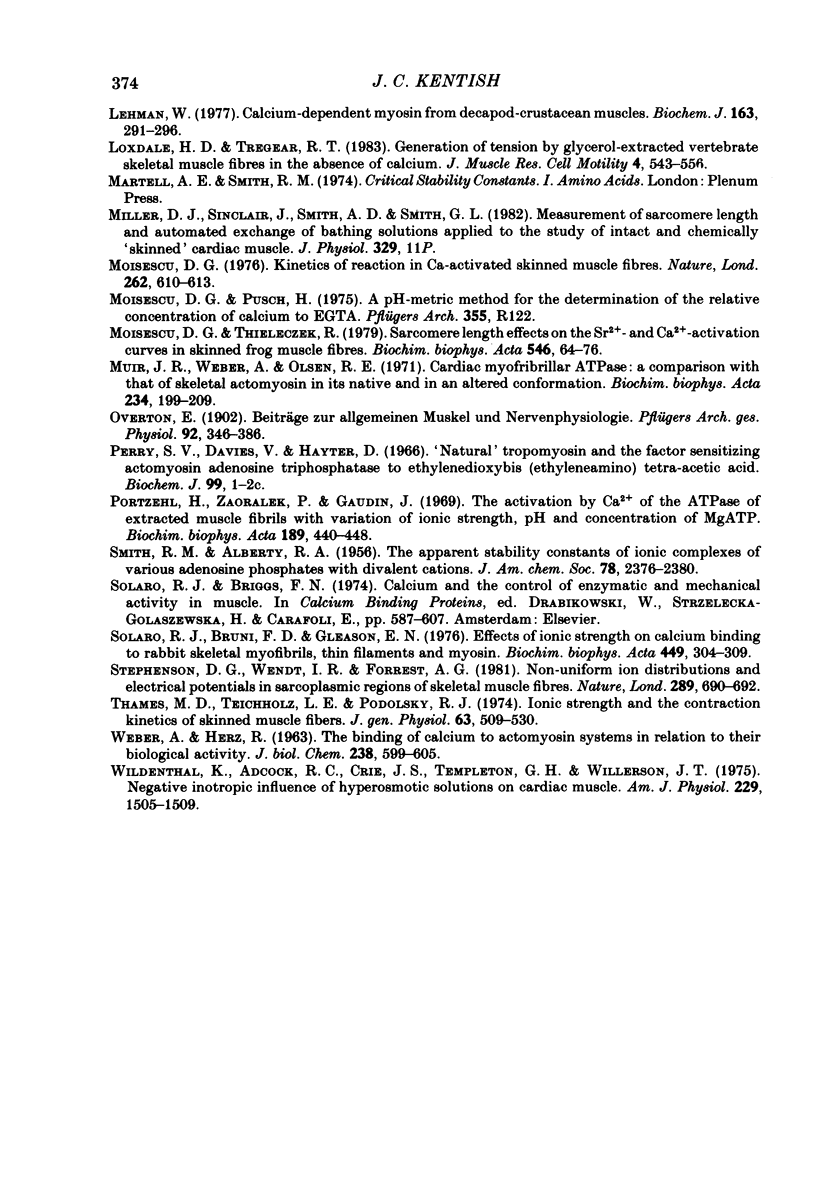
Selected References
These references are in PubMed. This may not be the complete list of references from this article.
- Allen D. G., Blinks J. R., Prendergast F. G. Aequorin luminescence: relation of light emission to calcium concentration--a calcium-independent component. Science. 1977 Mar 11;195(4282):996–998. doi: 10.1126/science.841325. [DOI] [PubMed] [Google Scholar]
- Ashley C. C., Moisescu D. G. Effect of changing the composition of the bathing solutions upon the isometric tension-pCa relationship in bundles of crustacean myofibrils. J Physiol. 1977 Sep;270(3):627–652. doi: 10.1113/jphysiol.1977.sp011972. [DOI] [PMC free article] [PubMed] [Google Scholar]
- Ashley C. C., Moisescu D. G. Tension changes in isolated bundles of frog and barnacle myofibrils in response to sudden changes in the external free calcium concentration. J Physiol. 1973 Aug;233(1):8P–9P. [PubMed] [Google Scholar]
- Brandt P. W., Cox R. N., Kawai M., Robinson T. Effect of cross-bridge kinetics on apparent Ca2+ sensitivity. J Gen Physiol. 1982 Jun;79(6):997–1016. doi: 10.1085/jgp.79.6.997. [DOI] [PMC free article] [PubMed] [Google Scholar]
- Bremel R. D., Weber A. Cooperation within actin filament in vertebrate skeletal muscle. Nat New Biol. 1972 Jul 26;238(82):97–101. doi: 10.1038/newbio238097a0. [DOI] [PubMed] [Google Scholar]
- Brenner B., Schoenberg M., Chalovich J. M., Greene L. E., Eisenberg E. Evidence for cross-bridge attachment in relaxed muscle at low ionic strength. Proc Natl Acad Sci U S A. 1982 Dec;79(23):7288–7291. doi: 10.1073/pnas.79.23.7288. [DOI] [PMC free article] [PubMed] [Google Scholar]
- Chapman R. A., Léoty C. Similarities between the potassium contractures and those induced by hypertonic solutions in rat ventricular trabeculae. J Physiol. 1974 Mar;237(2):28P–30P. [PubMed] [Google Scholar]
- Edman K. A., Flitney F. W. Laser diffraction studies of sarcomere dynamics during 'isometric' relaxation in isolated muscle fibres of the frog. J Physiol. 1982 Aug;329:1–20. doi: 10.1113/jphysiol.1982.sp014287. [DOI] [PMC free article] [PubMed] [Google Scholar]
- Eisenberg E., Moos C. The adenosine triphosphatase activity of acto-heavy meromyosin. A kinetic analysis of actin activation. Biochemistry. 1968 Apr;7(4):1486–1489. doi: 10.1021/bi00844a035. [DOI] [PubMed] [Google Scholar]
- Fabiato A. Myoplasmic free calcium concentration reached during the twitch of an intact isolated cardiac cell and during calcium-induced release of calcium from the sarcoplasmic reticulum of a skinned cardiac cell from the adult rat or rabbit ventricle. J Gen Physiol. 1981 Nov;78(5):457–497. doi: 10.1085/jgp.78.5.457. [DOI] [PMC free article] [PubMed] [Google Scholar]
- Godt R. E. A simple electrostatic model can explain the effect of pH upon the force-pCa relation of skinned frog skeletal muscle fibers. Biophys J. 1981 Aug;35(2):385–392. doi: 10.1016/S0006-3495(81)84797-2. [DOI] [PMC free article] [PubMed] [Google Scholar]
- Gordon A. M., Godt R. E., Donaldson S. K., Harris C. E. Tension in skinned frog muscle fibers in solutions of varying ionic strength and neutral salt composition. J Gen Physiol. 1973 Nov;62(5):550–574. doi: 10.1085/jgp.62.5.550. [DOI] [PMC free article] [PubMed] [Google Scholar]
- Gulati J. Magnesium ion-dependent contraction of skinned frog muscle fibers in calcium-free solution. Biophys J. 1983 Oct;44(1):113–121. doi: 10.1016/S0006-3495(83)84283-0. [DOI] [PMC free article] [PubMed] [Google Scholar]
- Gulati J., Podolsky R. J. Contraction transients of skinned muscle fibers: effects of calcium and ionic strength. J Gen Physiol. 1978 Nov;72(5):701–715. doi: 10.1085/jgp.72.5.701. [DOI] [PMC free article] [PubMed] [Google Scholar]
- Gulati J., Podolsky R. J. Isotonic contraction of skinned muscle fibers on a slow time base: effects of ionic strength and calcium. J Gen Physiol. 1981 Sep;78(3):233–257. doi: 10.1085/jgp.78.3.233. [DOI] [PMC free article] [PubMed] [Google Scholar]
- HOWARTH J. V. The behaviour of frog muscle in hypertonic solutions. J Physiol. 1958 Nov 10;144(1):167–175. doi: 10.1113/jphysiol.1958.sp006093. [DOI] [PMC free article] [PubMed] [Google Scholar]
- Harafuji H., Ogawa Y. Re-examination of the apparent binding constant of ethylene glycol bis(beta-aminoethyl ether)-N,N,N',N'-tetraacetic acid with calcium around neutral pH. J Biochem. 1980 May;87(5):1305–1312. doi: 10.1093/oxfordjournals.jbchem.a132868. [DOI] [PubMed] [Google Scholar]
- Hibberd M. G., Jewell B. R. Calcium- and length-dependent force production in rat ventricular muscle. J Physiol. 1982 Aug;329:527–540. doi: 10.1113/jphysiol.1982.sp014317. [DOI] [PMC free article] [PubMed] [Google Scholar]
- Holroyde M. J., Robertson S. P., Johnson J. D., Solaro R. J., Potter J. D. The calcium and magnesium binding sites on cardiac troponin and their role in the regulation of myofibrillar adenosine triphosphatase. J Biol Chem. 1980 Dec 25;255(24):11688–11693. [PubMed] [Google Scholar]
- Homsher E., Briggs F. N., Wise R. M. Effects of hypertonicity on resting and contracting frog skeletal muscles. Am J Physiol. 1974 Apr;226(4):855–863. doi: 10.1152/ajplegacy.1974.226.4.855. [DOI] [PubMed] [Google Scholar]
- Illingworth J. A. A common source of error in pH measurements. Biochem J. 1981 Apr 1;195(1):259–262. doi: 10.1042/bj1950259. [DOI] [PMC free article] [PubMed] [Google Scholar]
- Johnson J. D., Collins J. H., Robertson S. P., Potter J. D. A fluorescent probe study of Ca2+ binding to the Ca2+-specific sites of cardiac troponin and troponin C. J Biol Chem. 1980 Oct 25;255(20):9635–9640. [PubMed] [Google Scholar]
- Julian F. J., Moss R. L. Effects of calcium and ionic strength on shortening velocity and tension development in frog skinned muscle fibres. J Physiol. 1981 Feb;311:179–199. doi: 10.1113/jphysiol.1981.sp013580. [DOI] [PMC free article] [PubMed] [Google Scholar]
- KOCH-WESER J. Influence of osmolarity of perfusate on contractility of mammalian myocardium. Am J Physiol. 1963 Jun;204:957–962. doi: 10.1152/ajplegacy.1963.204.6.957. [DOI] [PubMed] [Google Scholar]
- Katz A. M., Repke D. I., Cohen B. R. Control of the activity of highly purified cardiac actomyosin by Ca2+, Na+ and K+. Circ Res. 1966 Dec;19(6):1062–1070. doi: 10.1161/01.res.19.6.1062. [DOI] [PubMed] [Google Scholar]
- Kentish J. C., Nayler W. G. Ca2+-dependent tension generation in chemically 'skinned' cardiac trabeculae: effect of pH [proceedings]. J Physiol. 1978 Nov;284:90P–91P. [PubMed] [Google Scholar]
- Krueger J. W., Pollack G. H. Myocardial sarcomere dynamics during isometric contraction. J Physiol. 1975 Oct;251(3):627–643. doi: 10.1113/jphysiol.1975.sp011112. [DOI] [PMC free article] [PubMed] [Google Scholar]
- Lehman W. Calcium ion-dependent myosin from decapod-crustacean muscles. Biochem J. 1977 May 1;163(2):291–296. doi: 10.1042/bj1630291. [DOI] [PMC free article] [PubMed] [Google Scholar]
- Loxdale H. D., Tregear R. T. Generation of tension by glycerol-extracted vertebrate skeletal muscle fibres in the absence of calcium. J Muscle Res Cell Motil. 1983 Oct;4(5):543–556. doi: 10.1007/BF00712114. [DOI] [PubMed] [Google Scholar]
- Lännergren J., Noth J. Tension in isolated frog muscle fibers induced by hypertonic solutions. J Gen Physiol. 1973 Feb;61(2):158–175. doi: 10.1085/jgp.61.2.158. [DOI] [PMC free article] [PubMed] [Google Scholar]
- Moisescu D. G. Kinetics of reaction in calcium-activated skinned muscle fibres. Nature. 1976 Aug 12;262(5569):610–613. doi: 10.1038/262610a0. [DOI] [PubMed] [Google Scholar]
- Moisescu D. G., Thieleczek R. Sarcomere length effects on the Sr2+- and Ca2+-activation curves in skinned frog muscle fibres. Biochim Biophys Acta. 1979 Apr 11;546(1):64–76. doi: 10.1016/0005-2728(79)90170-1. [DOI] [PubMed] [Google Scholar]
- Muir J. R., Weber A., Olson R. E. Cardiac myofibrillar ATPase: a comparison with that of fast skeletal actomyosin in its native and in an altered conformation. Biochim Biophys Acta. 1971 May 11;234(2):199–209. doi: 10.1016/0005-2728(71)90075-2. [DOI] [PubMed] [Google Scholar]
- Portzehl H., Zaoralek P., Gaudin J. The activation by Ca2+ of the ATPase of extracted muscle fibrilsith variation of ionic strength, pH and concentration of MgATP. Biochim Biophys Acta. 1969;189(3):440–448. doi: 10.1016/0005-2728(69)90175-3. [DOI] [PubMed] [Google Scholar]
- Solaro R. J., Bruni F. D., Gleason E. N. Effects of ionic strength on calcium binding to rabbit skeletal myofibrils, thin filaments and myosin. Biochim Biophys Acta. 1976 Nov 9;449(2):304–309. doi: 10.1016/0005-2728(76)90142-0. [DOI] [PubMed] [Google Scholar]
- Stephenson D. G., Wendt I. R., Forrest Q. G. Non-uniform ion distributions and electrical potentials in sarcoplasmic regions of skeletal muscle fibres. Nature. 1981 Feb 19;289(5799):690–692. doi: 10.1038/289690a0. [DOI] [PubMed] [Google Scholar]
- Thames M. D., Teichholz L. E., Podolsky R. J. Ionic strength and the contraction kinetics of skinned muscle fibers. J Gen Physiol. 1974 Apr;63(4):509–530. doi: 10.1085/jgp.63.4.509. [DOI] [PMC free article] [PubMed] [Google Scholar]
- WEBER A., HERZ R. The binding of calcium to actomyosin systems in relation to their biological activity. J Biol Chem. 1963 Feb;238:599–605. [PubMed] [Google Scholar]
- Wildenthal K., Adcock R. C., Crie J. S., Templeton G. H., Willerson J. T. Negative inotropic influence of hyperosmotic solutions on cardiac muscle. Am J Physiol. 1975 Dec;229(6):1505–1509. doi: 10.1152/ajplegacy.1975.229.6.1505. [DOI] [PubMed] [Google Scholar]