Abstract
Resting potential (r.p.) and muscarinic response mechanisms were studied in Xenopus laevis oocytes using the voltage-clamp technique. Insertion of micro-electrodes into the oocyte produced a 'shunt' membrane conductance which partially sealed after a few minutes. The oocyte resting potential (measured with a single intracellular electrode) ranged from -40 to -60 mV. Ouabain and low K+ solution depolarized both follicles and denuded oocytes. The electrogenic Na+-K+ pump was more active in the latter. In the presence of ouabain, the r.p. agreed with the constant field theory. alpha (PNa+/PK+) was 0.12 in follicles and 0.24 in denuded oocytes. beta (PCl-/PK+) was 0.4 in both. At [Na+]o lower than 70 mM, the r.p. deviated considerably from the constant field predictions. The relatively large value of alpha indicated the major role of Na+ in oocyte r.p. determination. The oocyte muscarinic response was separated into four distinct components: the fast depolarizing Cl- current, 'D1'; the slow depolarizing Cl- current, 'D2'; the slow hyperpolarizing K+ current, 'H'; and the large membrane Cl- current fluctuation, 'F'. The H response reversal potential showed a Nernst relationship to [K+] and was selectively blocked by intracellular injection of tetraethylammonium (TEA). The D1 and D2 reversal potential showed a Nernst relationship to [Cl-]. In Ca2+-deficient, EGTA-containing medium, D2 and F were abolished and D1 and H were reduced. Verapamil inhibited all responses. Increasing [Ca2+]o caused a significant increase in D1, D2 and F response amplitudes. Intracellular injection of 0.6-10 pmol guanosine 3',5'-cyclic monophosphate, induced a large outward K+ current, similar to the muscarinic H response.
Full text
PDF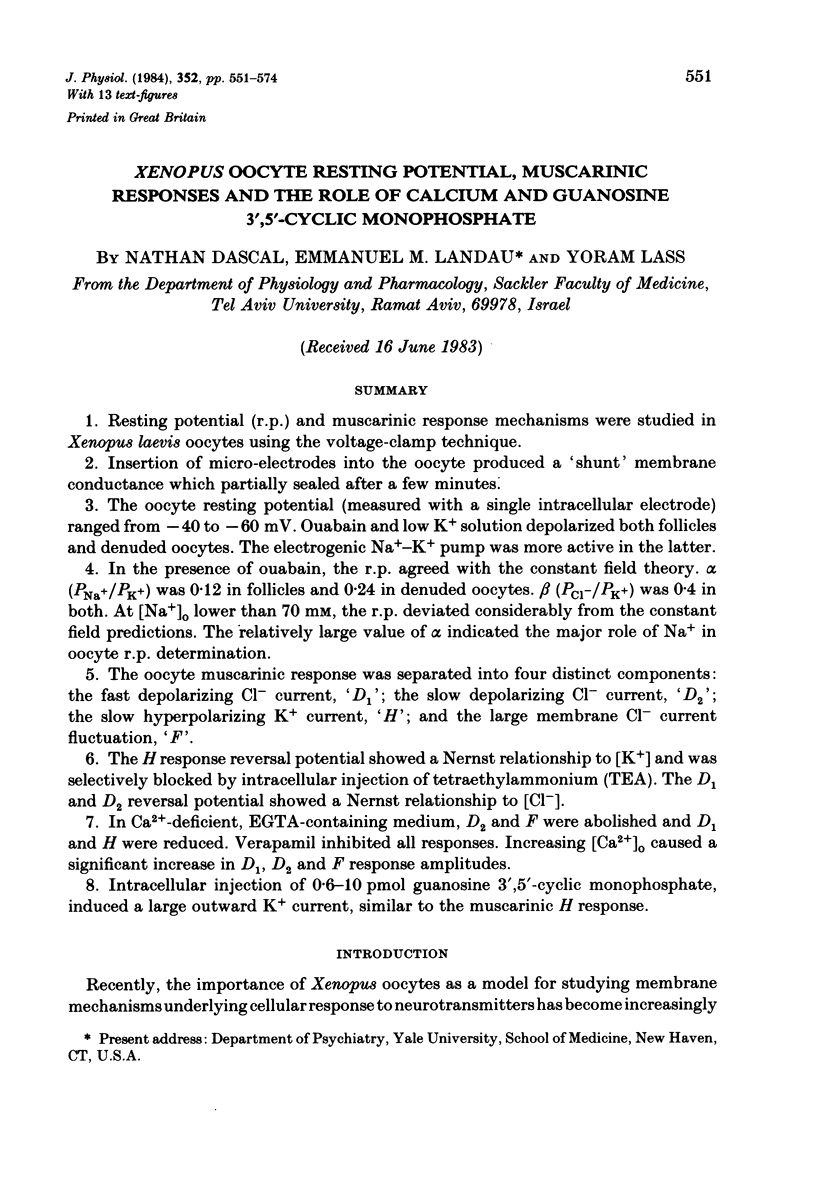
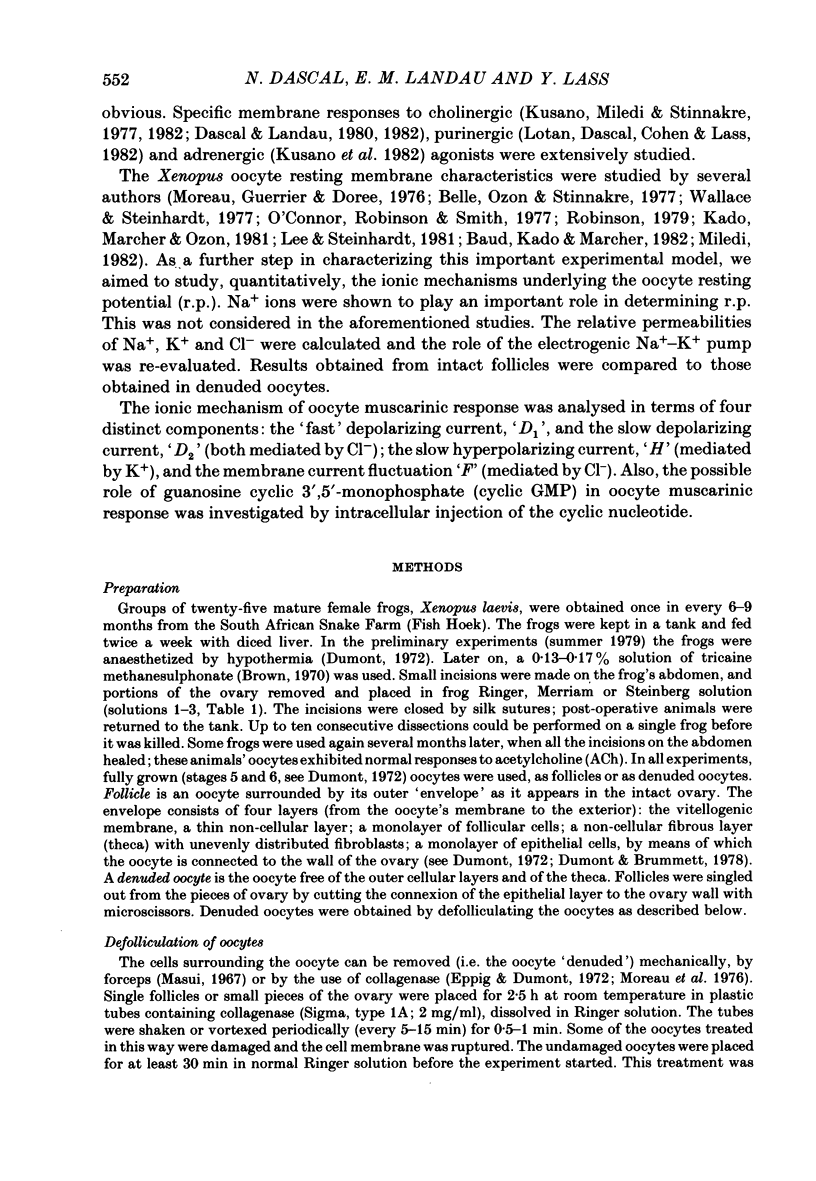
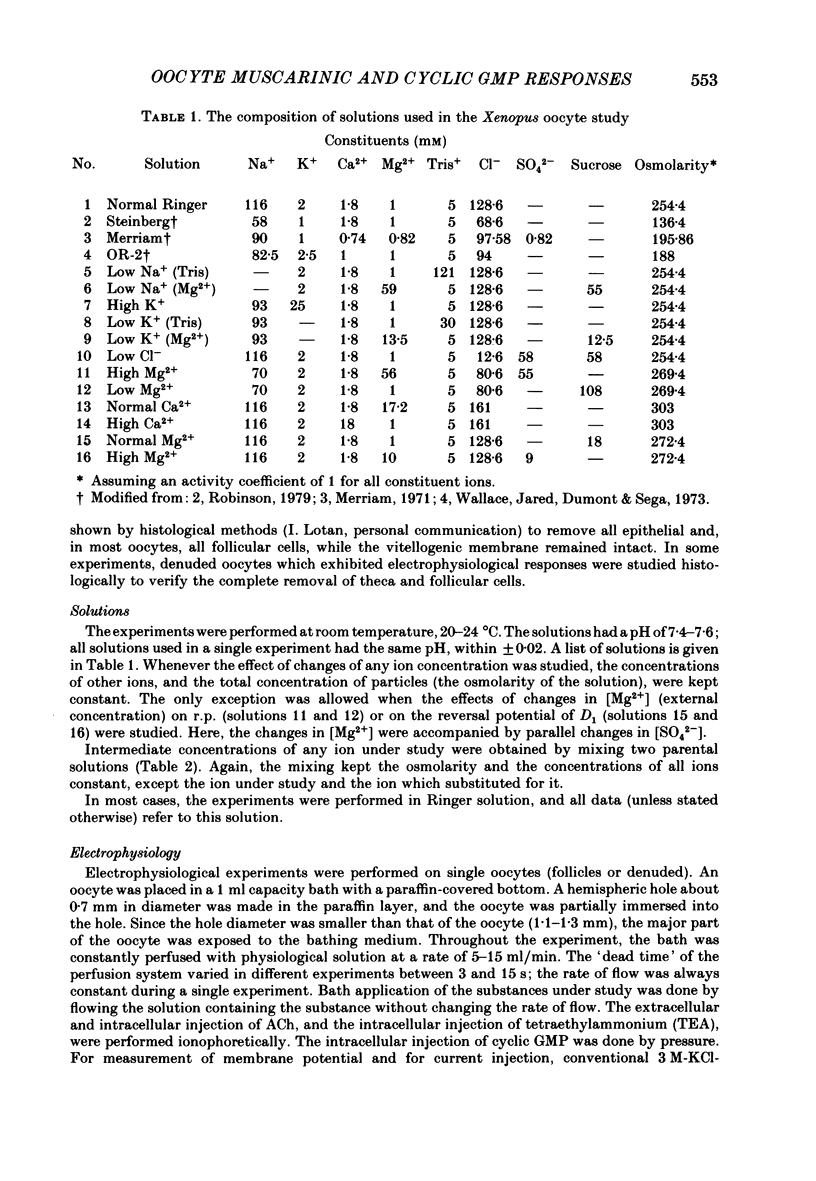
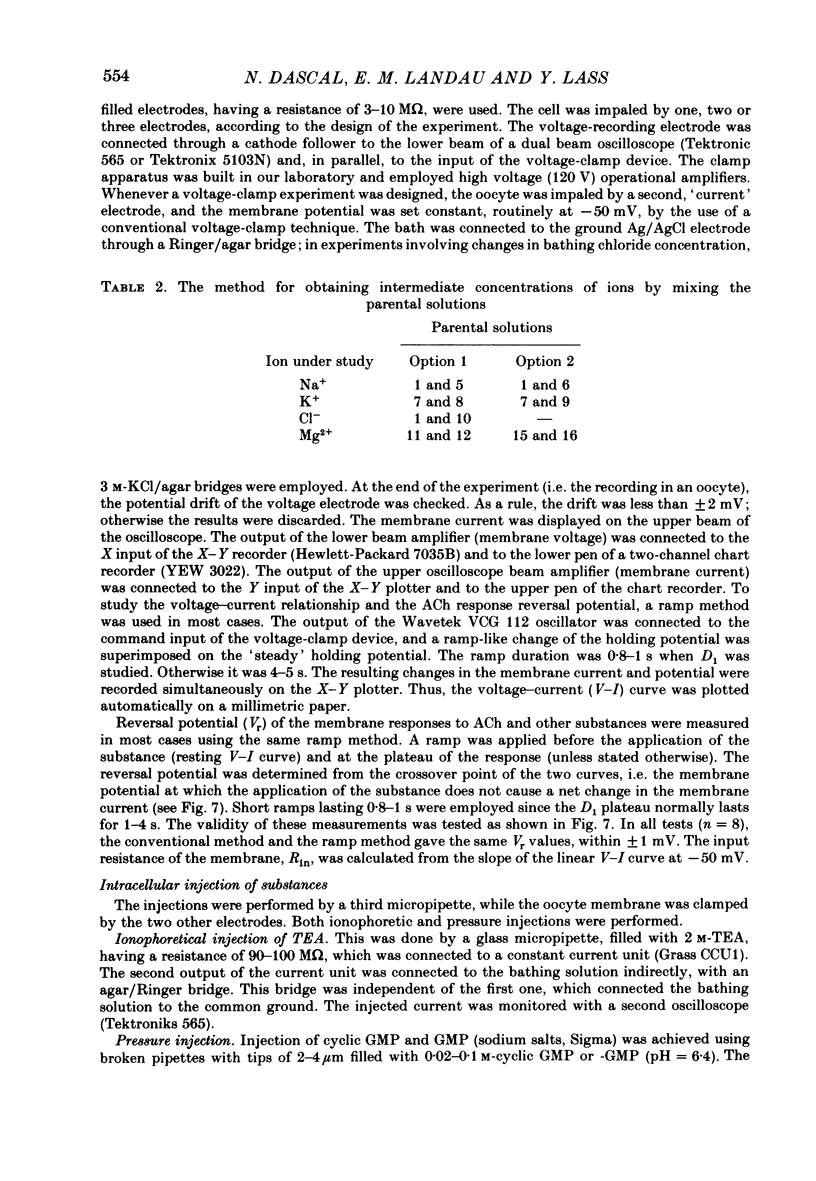
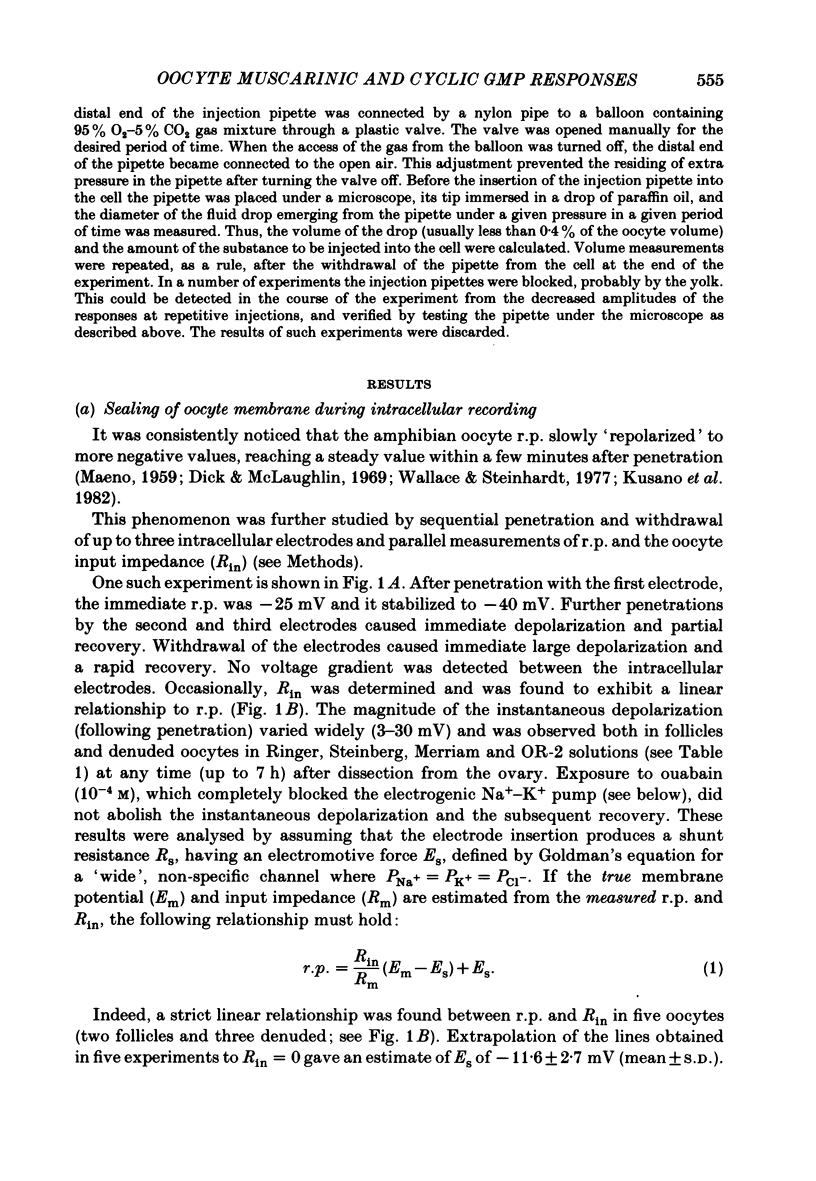
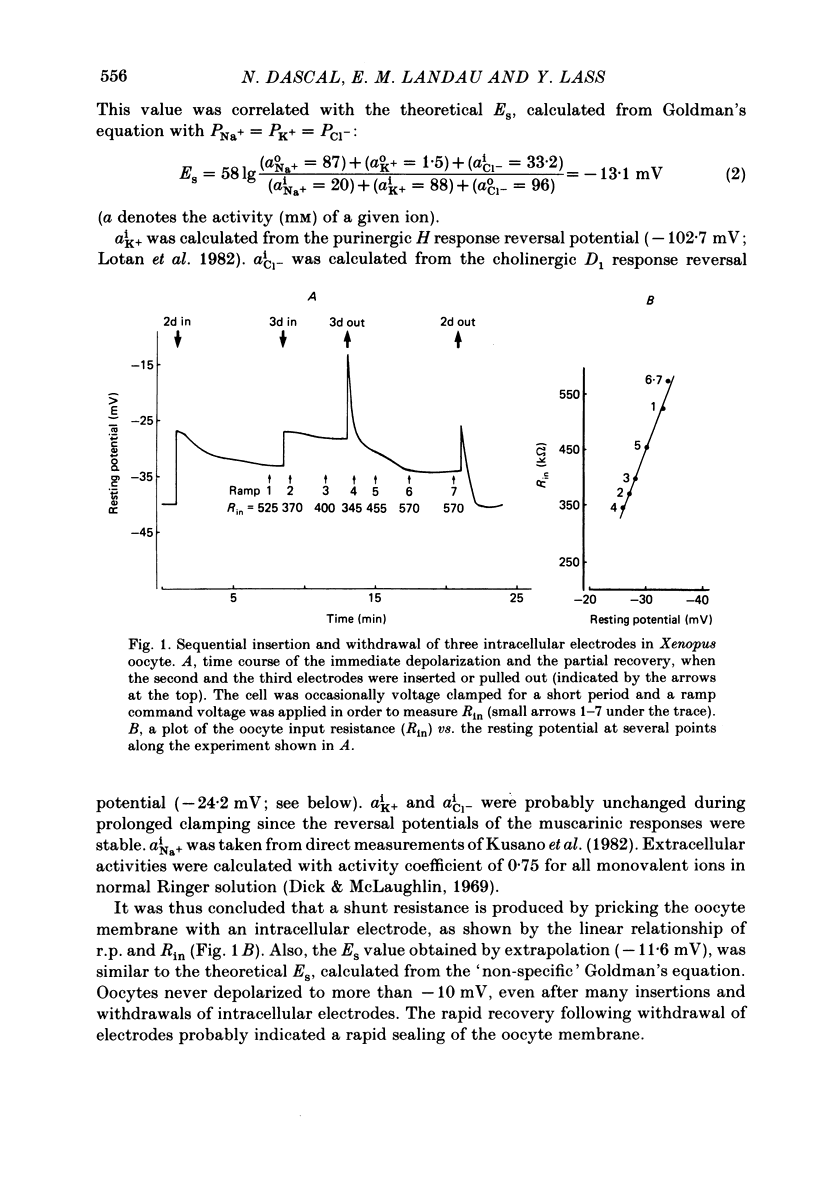
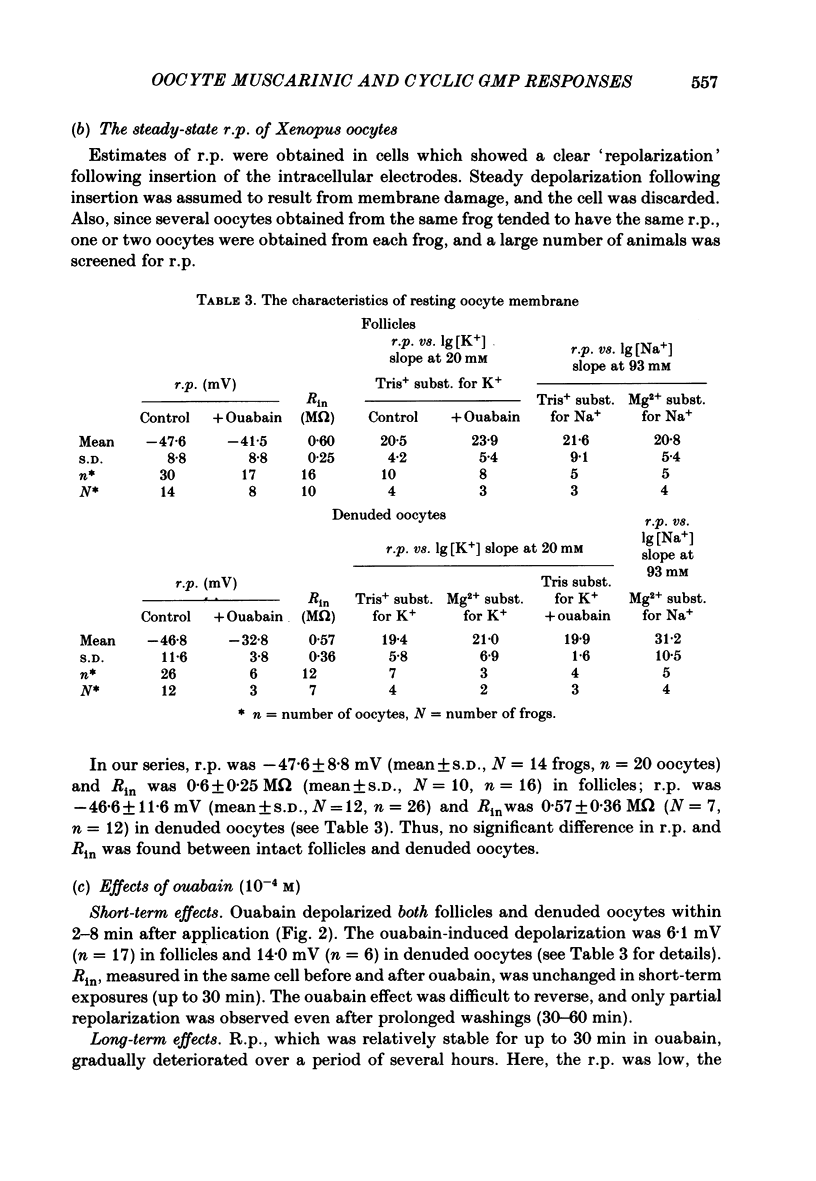
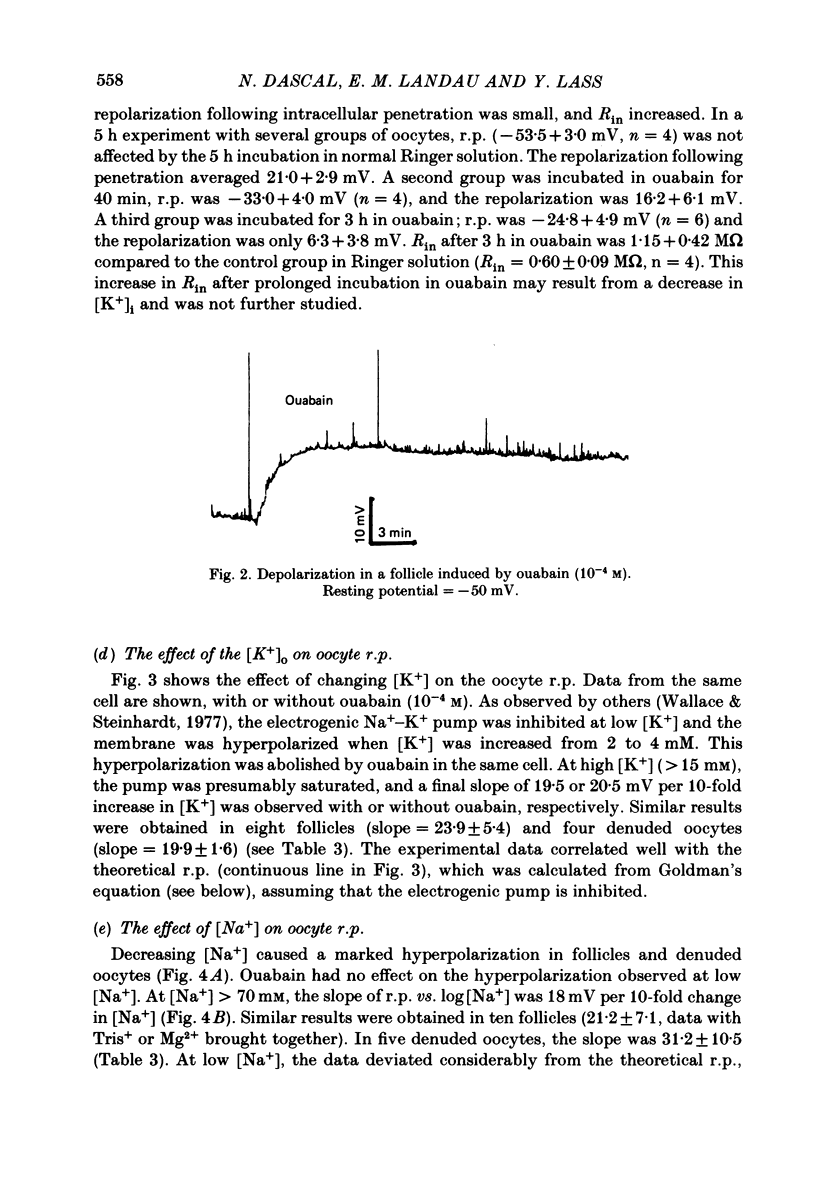
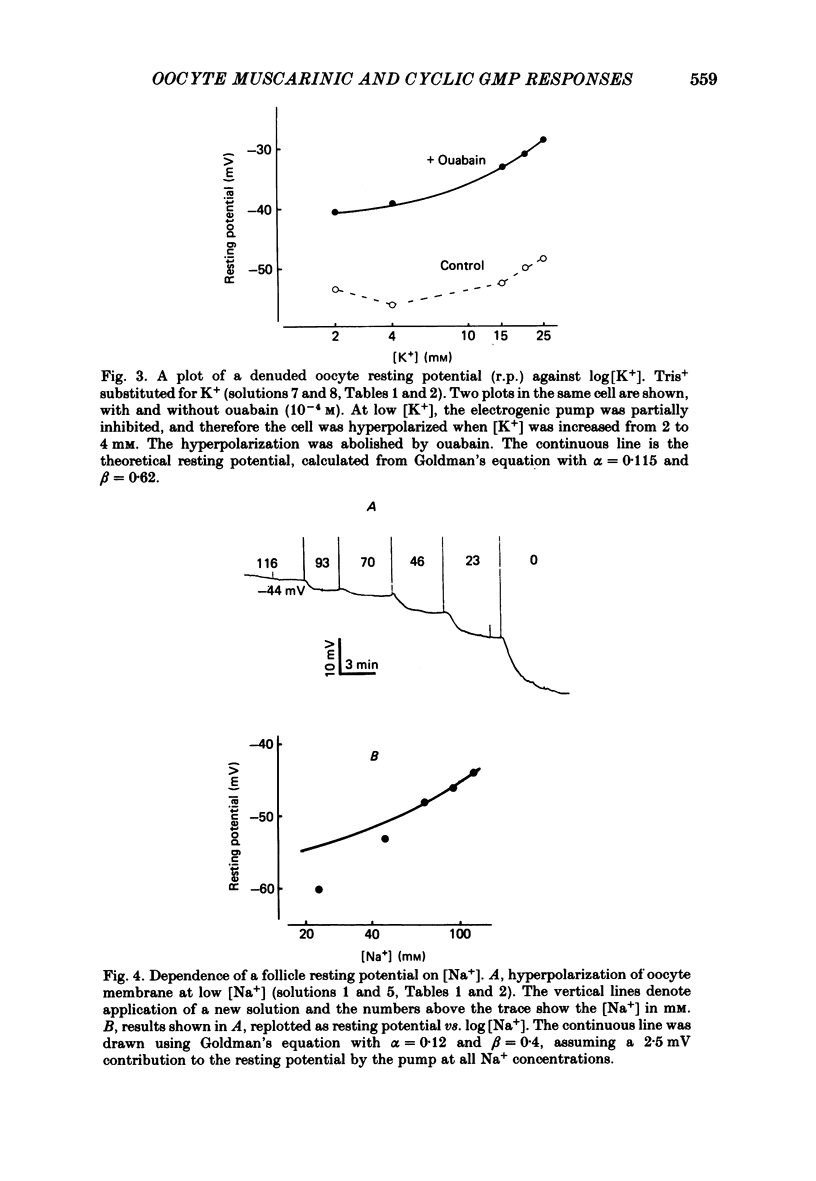
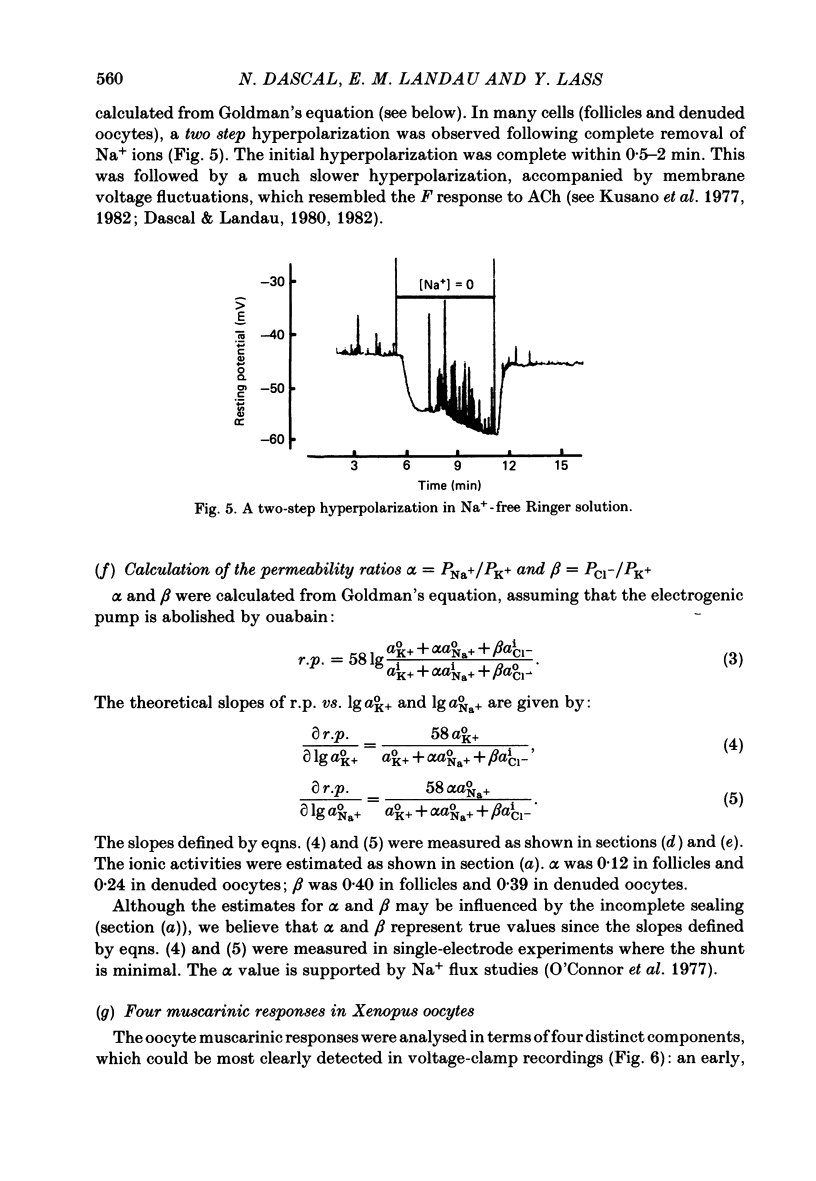
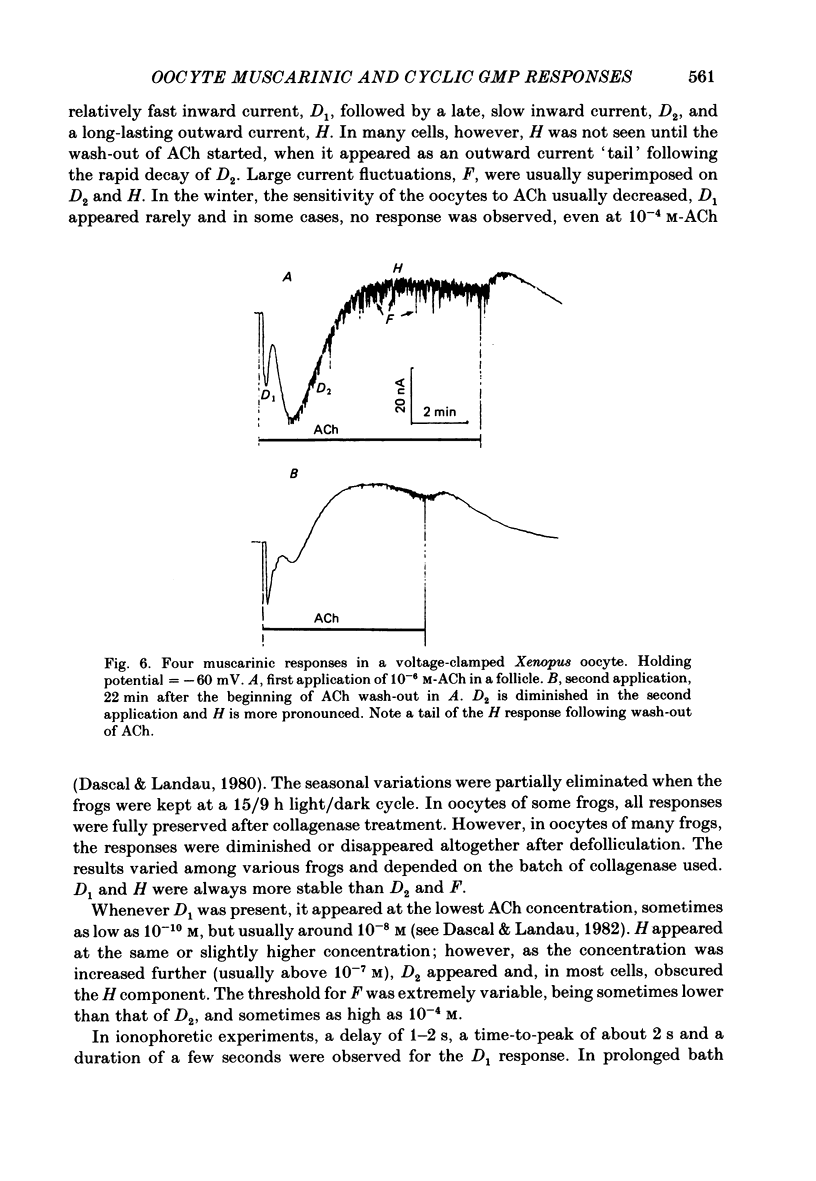
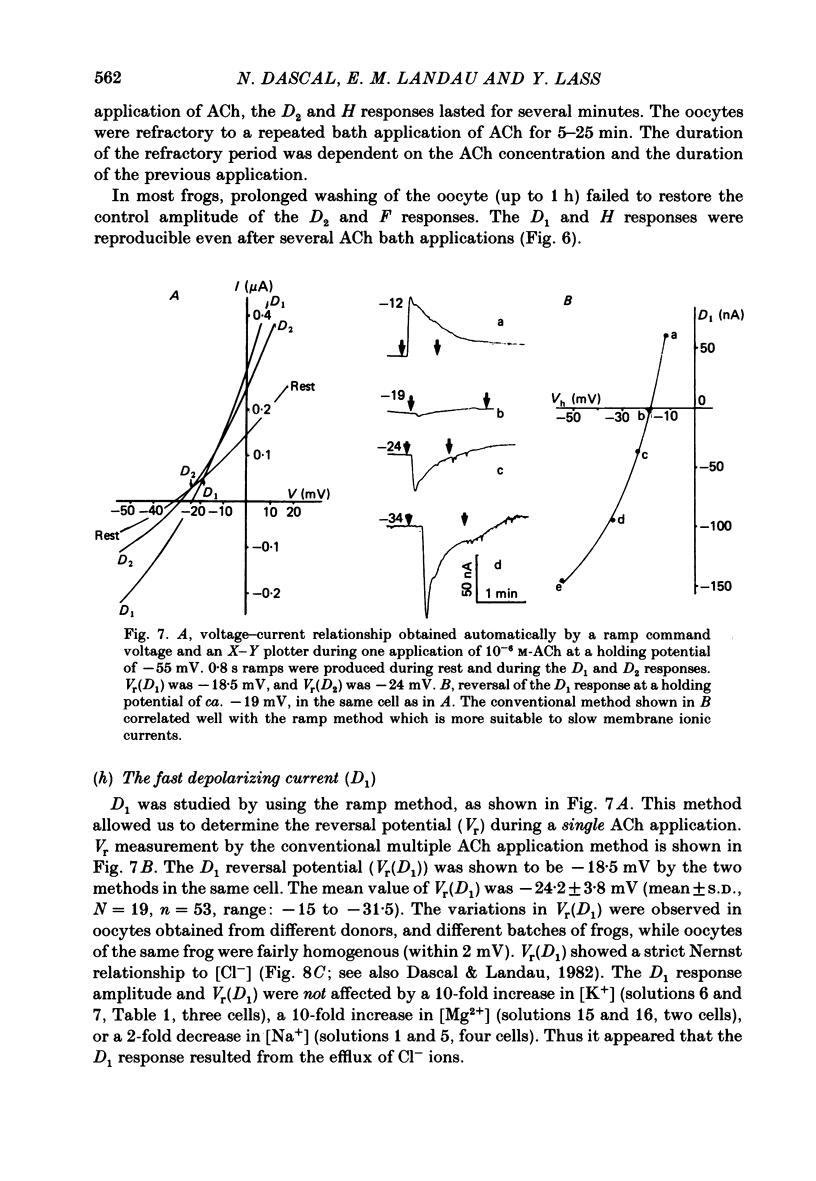
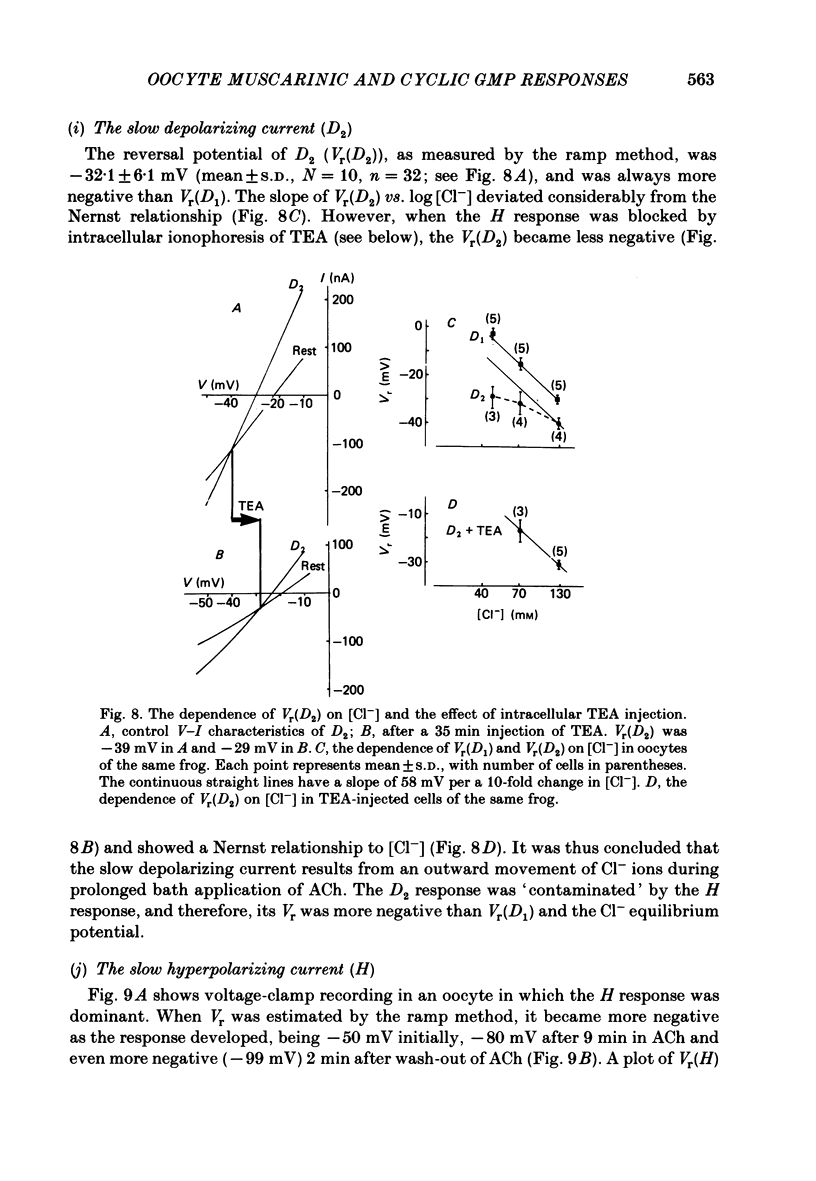
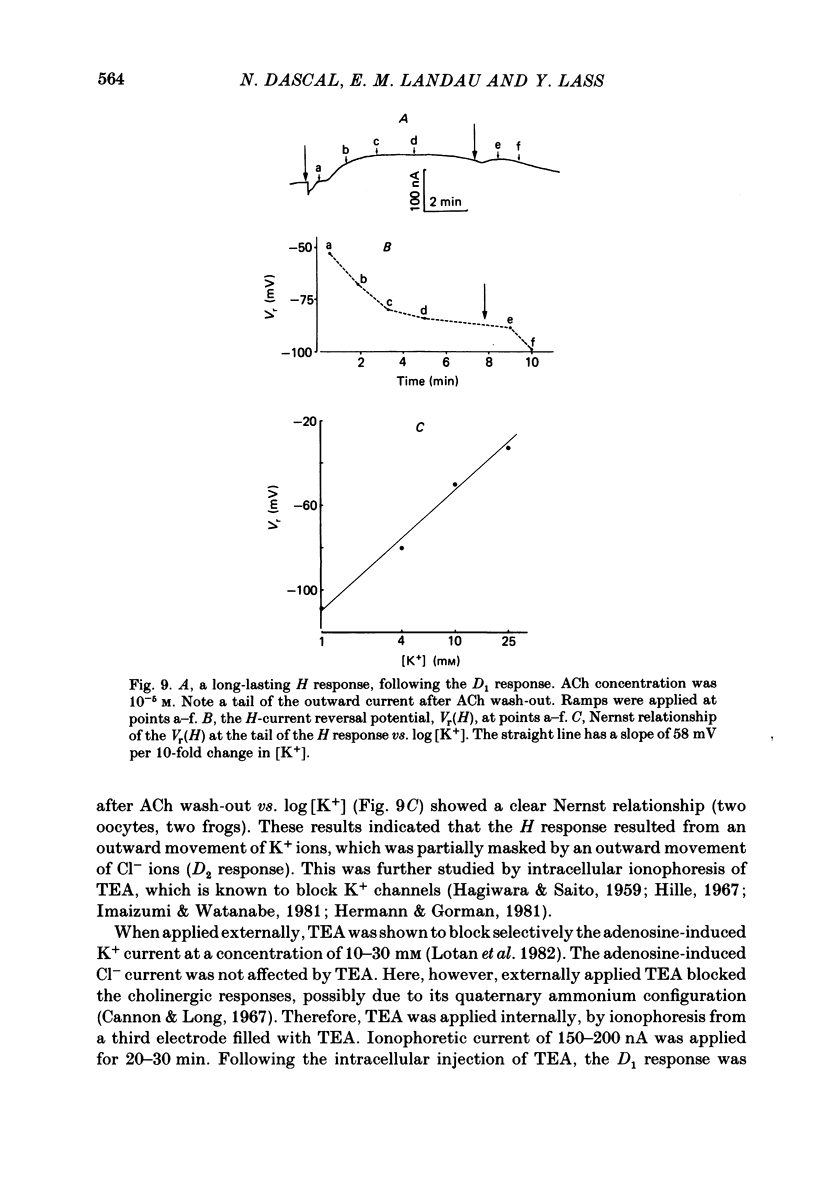
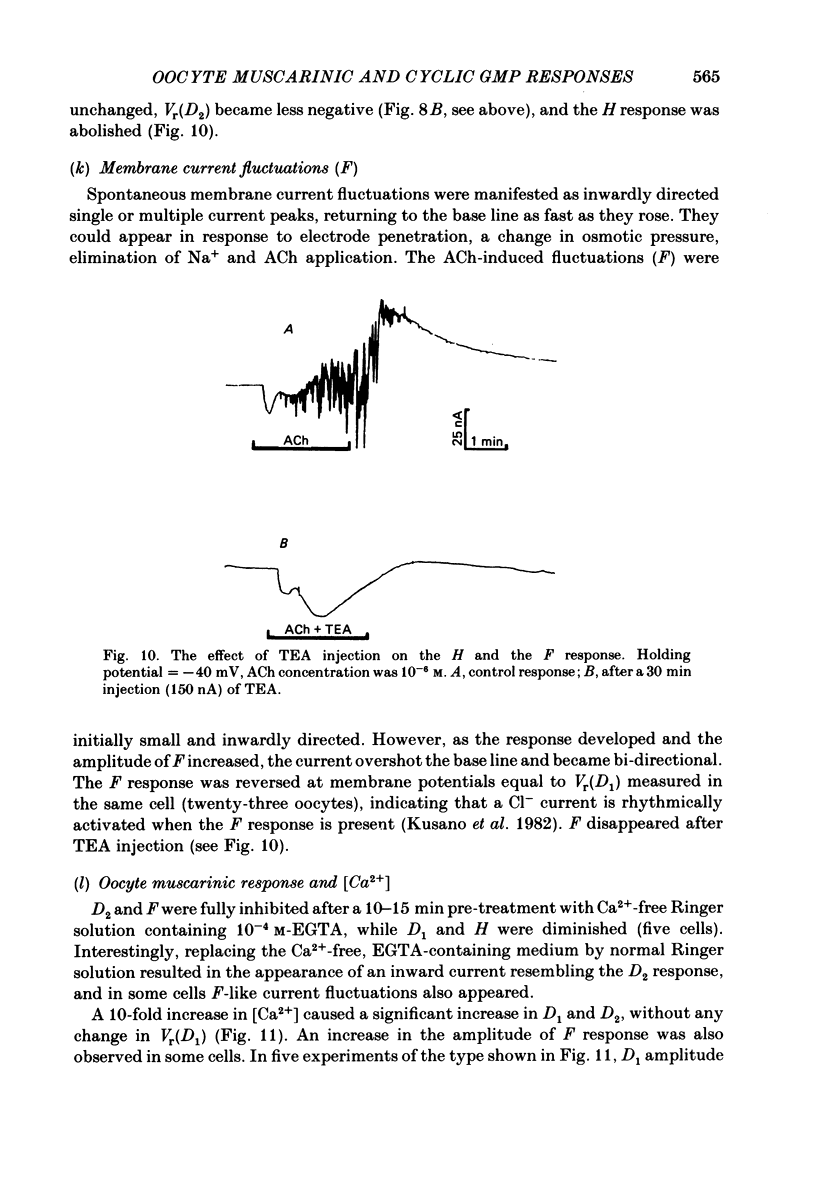
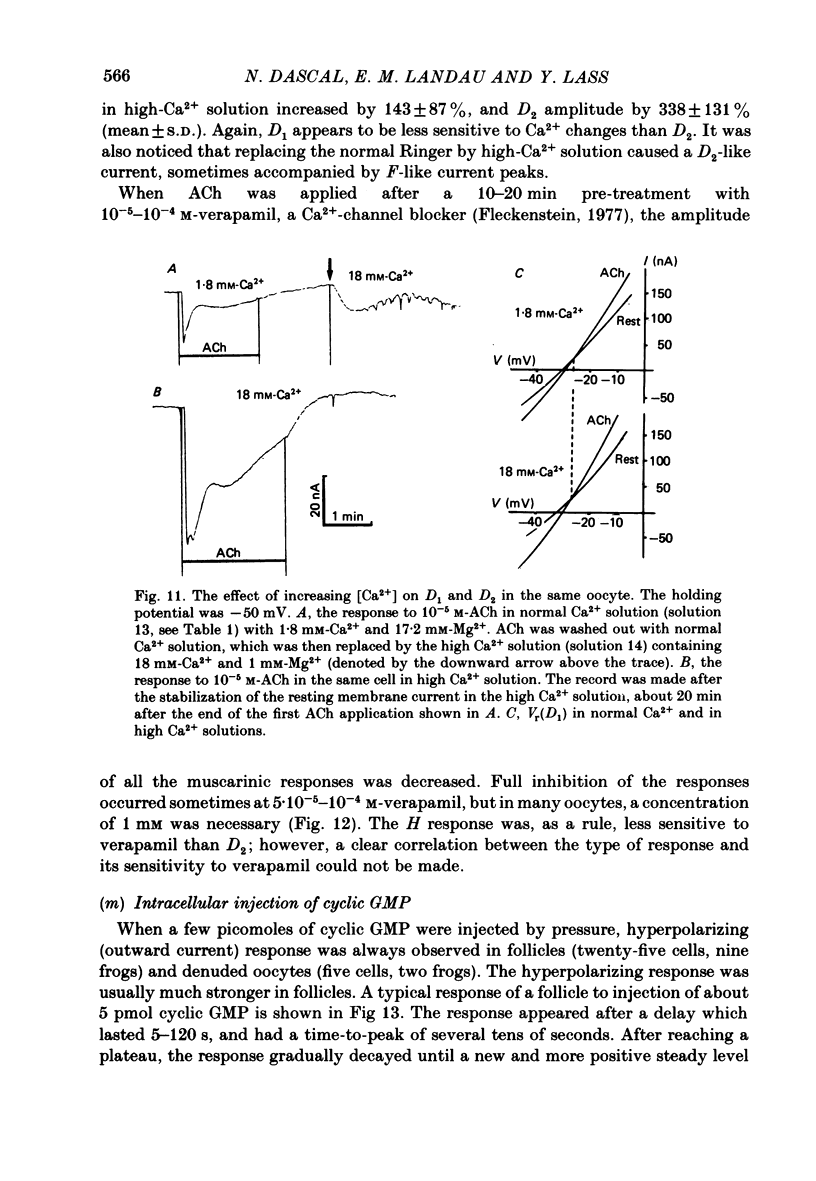
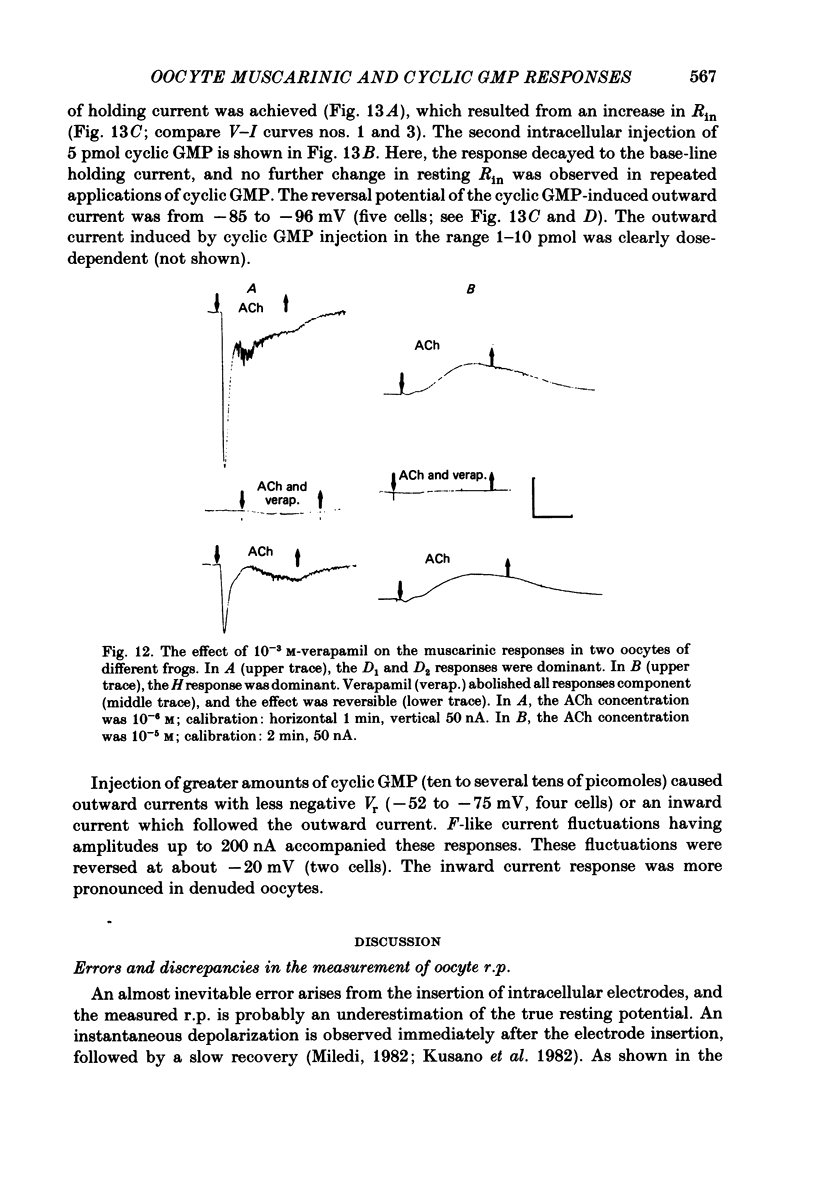
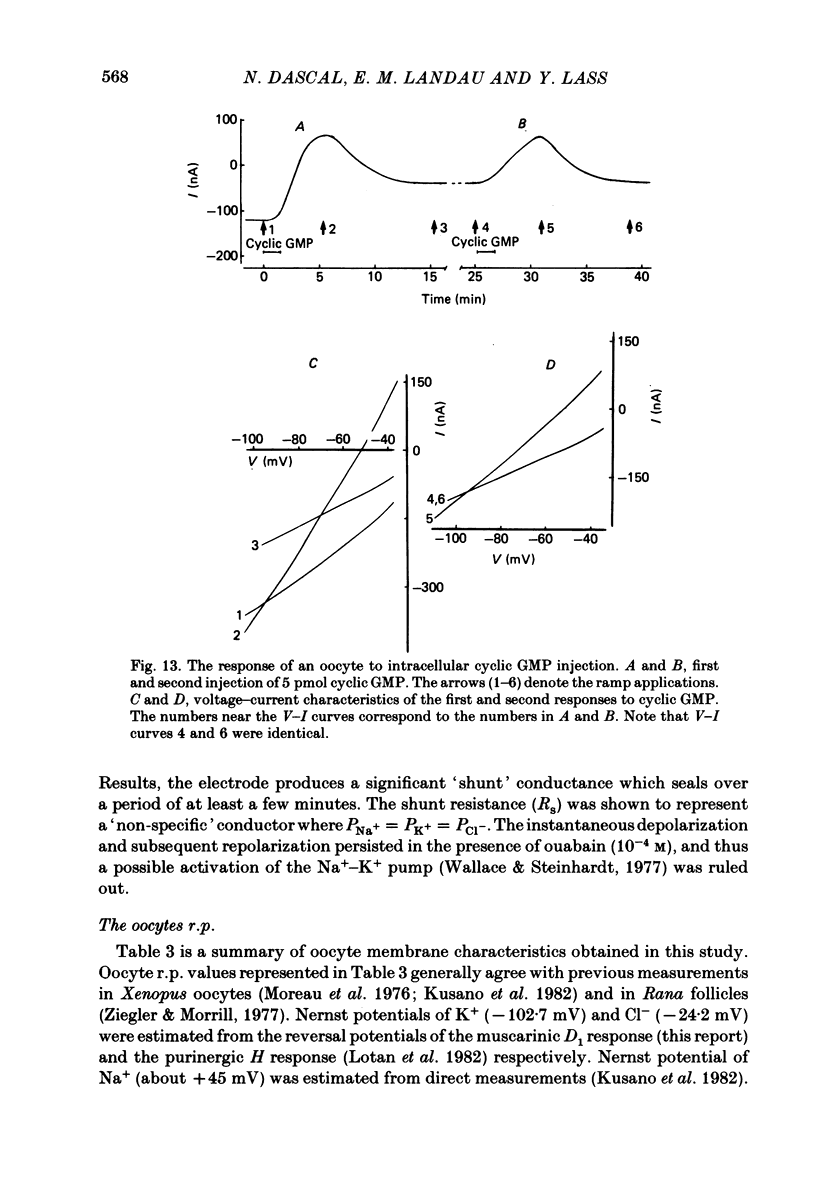
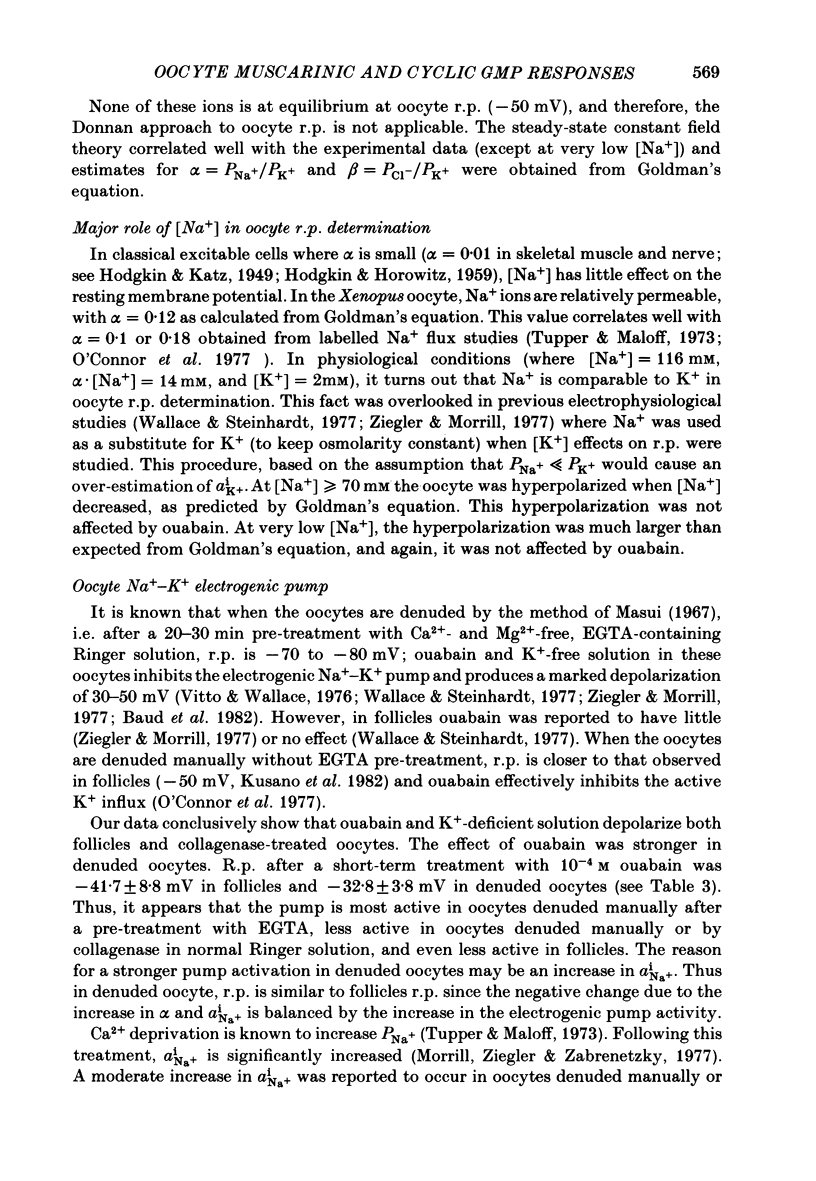
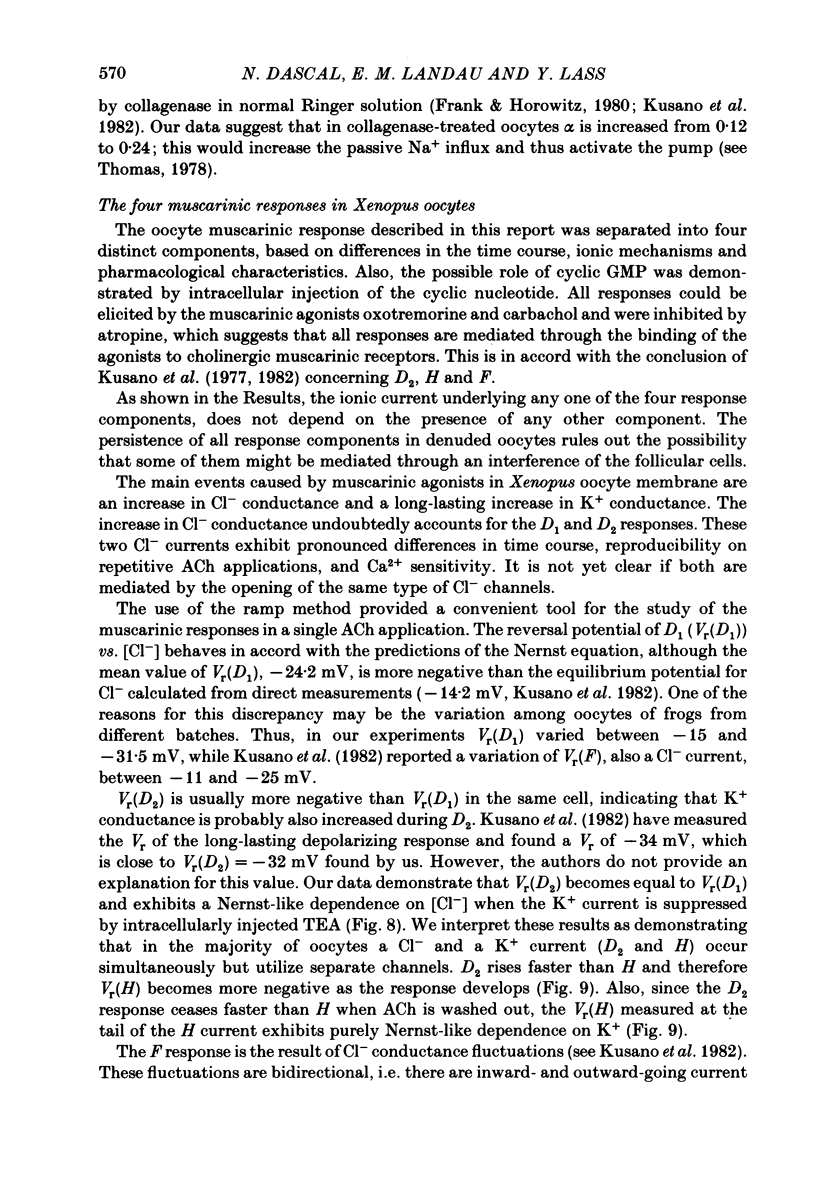
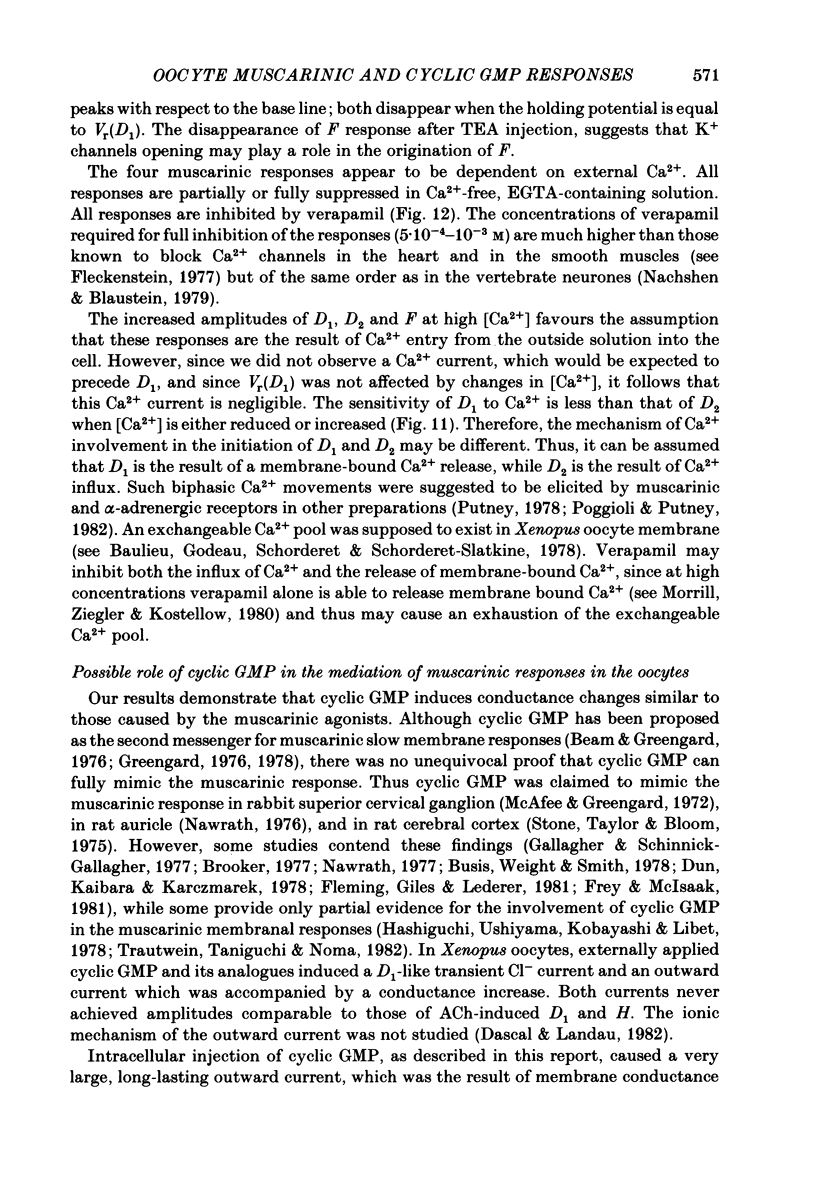
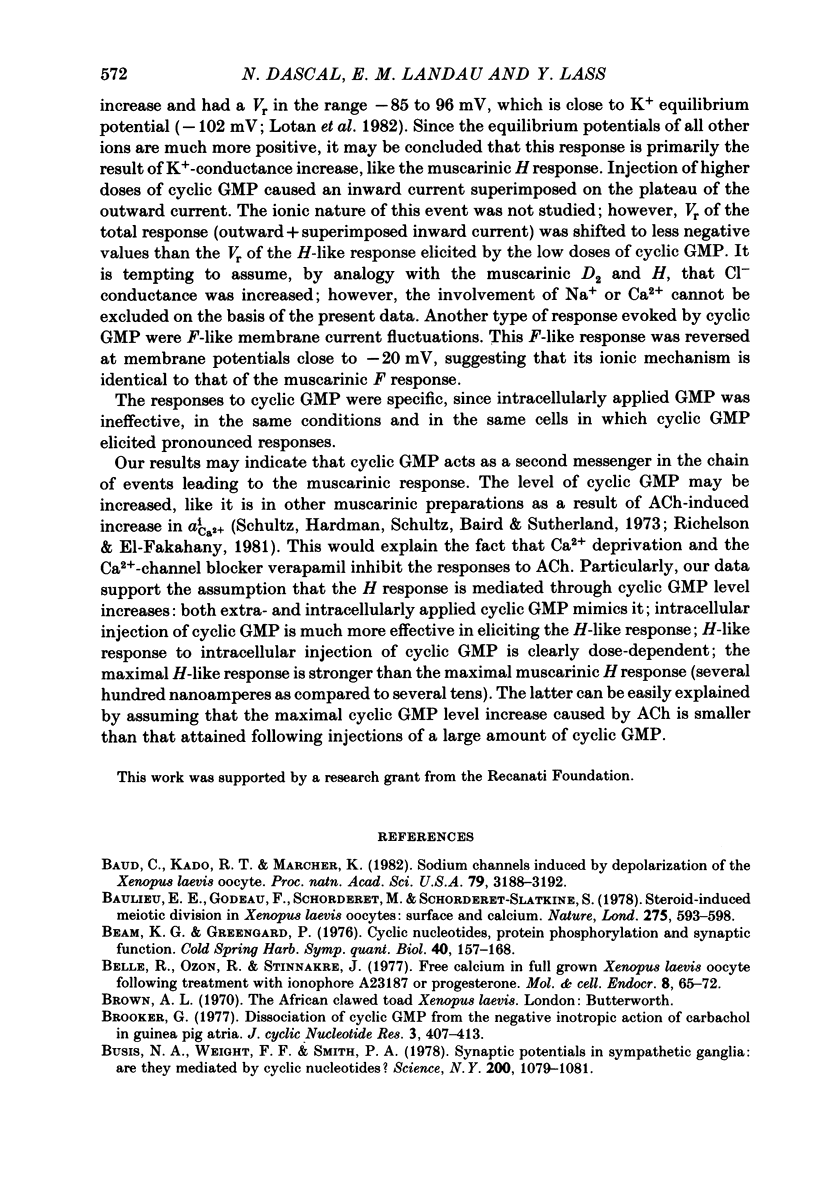
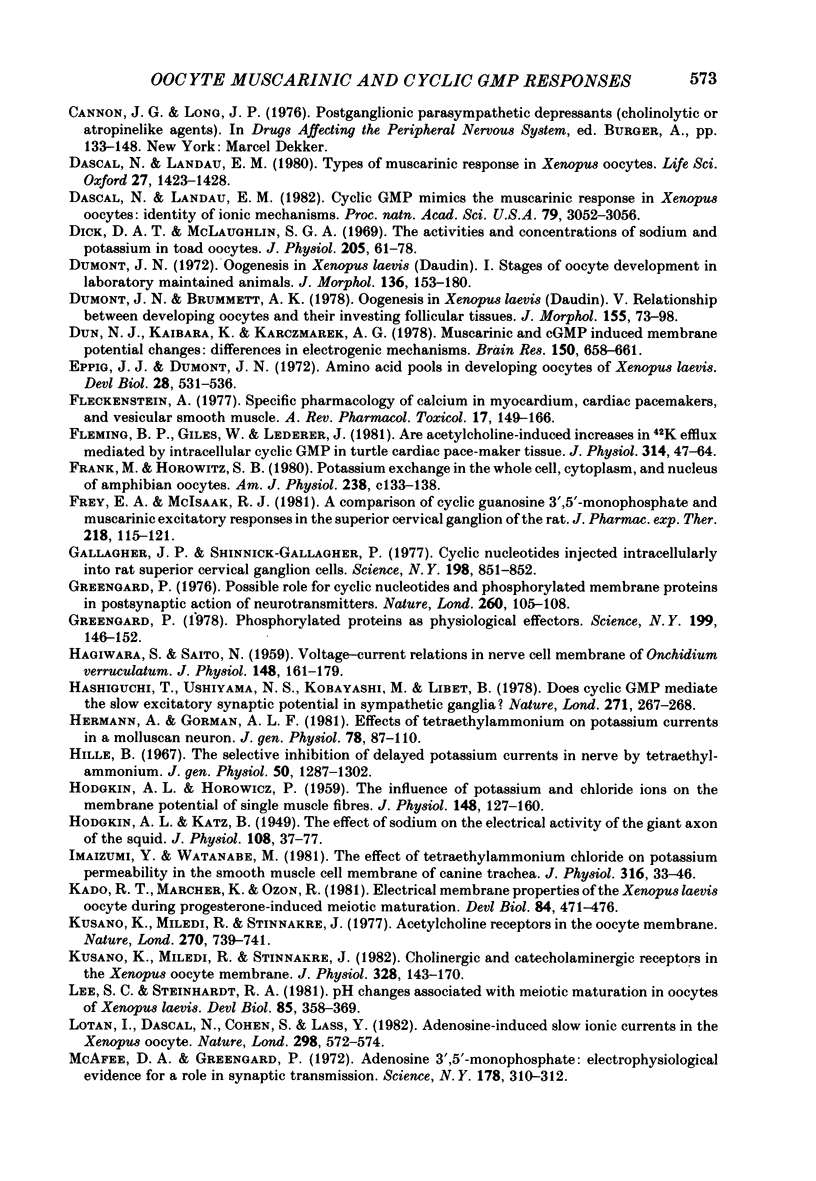
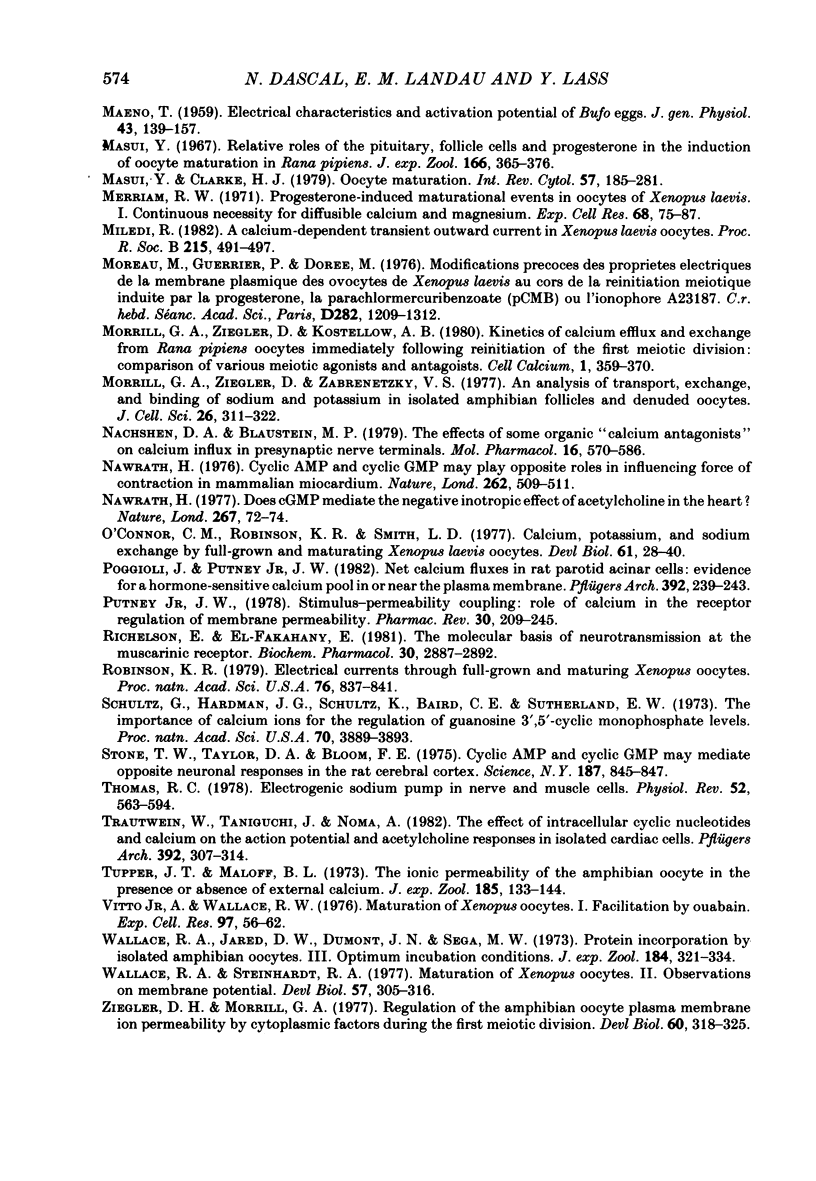
Selected References
These references are in PubMed. This may not be the complete list of references from this article.
- Baud C., Kado R. T., Marcher K. Sodium channels induced by depolarization of the Xenopus laevis oocyte. Proc Natl Acad Sci U S A. 1982 May;79(10):3188–3192. doi: 10.1073/pnas.79.10.3188. [DOI] [PMC free article] [PubMed] [Google Scholar]
- Baulieu E. E., Godeau F., Schorderet M., Schorderet-Slatkine S. Steroid-induced meiotic division in Xenopus laevis oocytes: surface and calcium. Nature. 1978 Oct 19;275(5681):593–598. doi: 10.1038/275593a0. [DOI] [PubMed] [Google Scholar]
- Beam K. G., Greengard P. Cyclic nucleotides, protein phosphorylation and synaptic function. Cold Spring Harb Symp Quant Biol. 1976;40:157–168. doi: 10.1101/sqb.1976.040.01.017. [DOI] [PubMed] [Google Scholar]
- Bellé R., Ozon R., Stinnakre J. Free calcium in full grown Xenopus laevis oocyte following treatment with ionophore A 23187 or progesterone. Mol Cell Endocrinol. 1977 Jul;8(1):65–72. doi: 10.1016/0303-7207(77)90018-1. [DOI] [PubMed] [Google Scholar]
- Brooker G. Dissociation of cyclic GMP from the negative inotropic action of carbachol in guinea pig atria. J Cyclic Nucleotide Res. 1977 Dec;3(6):407–413. [PubMed] [Google Scholar]
- Busis N. A., Weight F. F., Smith P. A. Synaptic potentials in sympathetic ganglia: are they mediated by cyclic nucleotides? Science. 1978 Jun 2;200(4345):1079–1081. doi: 10.1126/science.206964. [DOI] [PubMed] [Google Scholar]
- Dascal N., Landau E. M. Cyclic GMP mimics the muscarinic response in Xenopus oocytes: identity of ionic mechanisms. Proc Natl Acad Sci U S A. 1982 May;79(9):3052–3056. doi: 10.1073/pnas.79.9.3052. [DOI] [PMC free article] [PubMed] [Google Scholar]
- Dascal N., Landau E. M. Types of muscarinic response in Xenopus oocytes. Life Sci. 1980 Oct 13;27(15):1423–1428. doi: 10.1016/0024-3205(80)90407-5. [DOI] [PubMed] [Google Scholar]
- Dick D. A., McLaughlin S. G. The activities and concentrations of sodium and potassium in toad oocytes. J Physiol. 1969 Nov;205(1):61–78. doi: 10.1113/jphysiol.1969.sp008951. [DOI] [PMC free article] [PubMed] [Google Scholar]
- Dumont J. N., Brummett A. R. Oogenesis in Xenopus laevis (Daudin). V. Relationships between developing oocytes and their investing follicular tissues. J Morphol. 1978 Jan;155(1):73–97. doi: 10.1002/jmor.1051550106. [DOI] [PubMed] [Google Scholar]
- Dumont J. N. Oogenesis in Xenopus laevis (Daudin). I. Stages of oocyte development in laboratory maintained animals. J Morphol. 1972 Feb;136(2):153–179. doi: 10.1002/jmor.1051360203. [DOI] [PubMed] [Google Scholar]
- Dun N. J., Kaibara K., Karczmar A. G. Muscarinic and cGMP induced membrane potential changes: differences in electrogenic mechanisms. Brain Res. 1978 Jul 21;150(3):658–661. doi: 10.1016/0006-8993(78)90833-8. [DOI] [PubMed] [Google Scholar]
- Eppig J. J., Jr, Dumont J. N. Amino acid pools in developing oocytes of Xenopus laevis. Dev Biol. 1972 Jul;28(3):531–536. doi: 10.1016/0012-1606(72)90036-x. [DOI] [PubMed] [Google Scholar]
- Fleckenstein A. Specific pharmacology of calcium in myocardium, cardiac pacemakers, and vascular smooth muscle. Annu Rev Pharmacol Toxicol. 1977;17:149–166. doi: 10.1146/annurev.pa.17.040177.001053. [DOI] [PubMed] [Google Scholar]
- Fleming B. P., Giles W., Lederer J. Are acetylcholine-induced increases in 42K efflux mediated by intracellular cyclic GMP in turtle cardiac pace-maker tissue? J Physiol. 1981 May;314:47–64. doi: 10.1113/jphysiol.1981.sp013689. [DOI] [PMC free article] [PubMed] [Google Scholar]
- Frank M., Horowitz S. B. Potassium exchange in the whole cell, cytoplasm, and nucleus of amphibian oocytes. Am J Physiol. 1980 Mar;238(3):C133–C138. doi: 10.1152/ajpcell.1980.238.3.C133. [DOI] [PubMed] [Google Scholar]
- Frey E. A., McIsaac R. J. A comparison of cyclic guanosine 3':5'-monophosphate and muscarinic excitatory responses in the superior cervical ganglion of the rat. J Pharmacol Exp Ther. 1981 Jul;218(1):115–121. [PubMed] [Google Scholar]
- Gallagher J. P., Shinnick-Gallagher P. Cyclic nucleotides injected intracellularly into rat superior cervical ganglion cells. Science. 1977 Nov 25;198(4319):851–852. doi: 10.1126/science.199943. [DOI] [PubMed] [Google Scholar]
- Greengard P. Phosphorylated proteins as physiological effectors. Science. 1978 Jan 13;199(4325):146–152. doi: 10.1126/science.22932. [DOI] [PubMed] [Google Scholar]
- Greengard P. Possible role for cyclic nucleotides and phosphorylated membrane proteins in postsynaptic actions of neurotransmitters. Nature. 1976 Mar 11;260(5547):101–108. doi: 10.1038/260101a0. [DOI] [PubMed] [Google Scholar]
- HAGIWARA S., SAITO N. Voltage-current relations in nerve cell membrane of Onchidium verruculatum. J Physiol. 1959 Oct;148:161–179. doi: 10.1113/jphysiol.1959.sp006279. [DOI] [PMC free article] [PubMed] [Google Scholar]
- HODGKIN A. L., HOROWICZ P. The influence of potassium and chloride ions on the membrane potential of single muscle fibres. J Physiol. 1959 Oct;148:127–160. doi: 10.1113/jphysiol.1959.sp006278. [DOI] [PMC free article] [PubMed] [Google Scholar]
- HODGKIN A. L., KATZ B. The effect of sodium ions on the electrical activity of giant axon of the squid. J Physiol. 1949 Mar 1;108(1):37–77. doi: 10.1113/jphysiol.1949.sp004310. [DOI] [PMC free article] [PubMed] [Google Scholar]
- Hashiguchi T., Ushiyama N. S., Kobayashi H., Libet B. Does cyclic GMP mediate the slow excitatory synaptic potential in sympathetic ganglia? Nature. 1978 Jan 19;271(5642):267–268. doi: 10.1038/271267a0. [DOI] [PubMed] [Google Scholar]
- Hermann A., Gorman A. L. Effects of tetraethylammonium on potassium currents in a molluscan neurons. J Gen Physiol. 1981 Jul;78(1):87–110. doi: 10.1085/jgp.78.1.87. [DOI] [PMC free article] [PubMed] [Google Scholar]
- Hille B. The selective inhibition of delayed potassium currents in nerve by tetraethylammonium ion. J Gen Physiol. 1967 May;50(5):1287–1302. doi: 10.1085/jgp.50.5.1287. [DOI] [PMC free article] [PubMed] [Google Scholar]
- Imaizumi Y., Watanabe M. The effect of tetraethylammonium chloride on potassium permeability in the smooth muscle cell membrane of canine trachea. J Physiol. 1981 Jul;316:33–46. doi: 10.1113/jphysiol.1981.sp013770. [DOI] [PMC free article] [PubMed] [Google Scholar]
- Kusano K., Miledi R., Stinnakre J. Acetylcholine receptors in the oocyte membrane. Nature. 1977 Dec 22;270(5639):739–741. doi: 10.1038/270739a0. [DOI] [PubMed] [Google Scholar]
- Kusano K., Miledi R., Stinnakre J. Cholinergic and catecholaminergic receptors in the Xenopus oocyte membrane. J Physiol. 1982 Jul;328:143–170. doi: 10.1113/jphysiol.1982.sp014257. [DOI] [PMC free article] [PubMed] [Google Scholar]
- Lee S. C., Steinhardt R. A. pH changes associated with meiotic maturation in oocytes of Xenopus laevis. Dev Biol. 1981 Jul 30;85(2):358–369. doi: 10.1016/0012-1606(81)90267-0. [DOI] [PubMed] [Google Scholar]
- Lotan I., Dascal N., Cohen S., Lass Y. Adenosine-induced slow ionic currents in the Xenopus oocyte. Nature. 1982 Aug 5;298(5874):572–574. doi: 10.1038/298572a0. [DOI] [PubMed] [Google Scholar]
- MAENO T. Electrical characteristics and activation potential of Bufo eggs. J Gen Physiol. 1959 Sep;43:139–157. doi: 10.1085/jgp.43.1.139. [DOI] [PMC free article] [PubMed] [Google Scholar]
- Masui Y., Clarke H. J. Oocyte maturation. Int Rev Cytol. 1979;57:185–282. doi: 10.1016/s0074-7696(08)61464-3. [DOI] [PubMed] [Google Scholar]
- Masui Y. Relative roles of the pituitary, follicle cells, and progesterone in the induction of oocyte maturation in Rana pipiens. J Exp Zool. 1967 Dec;166(3):365–375. doi: 10.1002/jez.1401660309. [DOI] [PubMed] [Google Scholar]
- McAfee D. A., Greengard P. Adenosine 3',5'-monophosphate: electrophysiological evidence for a role in synaptic transmission. Science. 1972 Oct;178(58):310–312. doi: 10.1126/science.178.4058.310. [DOI] [PubMed] [Google Scholar]
- Merriam R. W. Progesterone-induced maturational events in oocytes of Xenopus laevis. I. Continuous necessity for diffusible calcium and magnesium. Exp Cell Res. 1971 Sep;68(1):75–80. doi: 10.1016/0014-4827(71)90588-x. [DOI] [PubMed] [Google Scholar]
- Miledi R. A calcium-dependent transient outward current in Xenopus laevis oocytes. Proc R Soc Lond B Biol Sci. 1982 Jul 22;215(1201):491–497. doi: 10.1098/rspb.1982.0056. [DOI] [PubMed] [Google Scholar]
- Moreau M., Guerrier P., Dorée M. Modifications précoces des propriétés électriques de la membrane plasmique des ovocytes de Xenopus laevis au cours de la réinitation méotique induite par la progestérone, le parachloromercuribenzoate (pCMB) ou l'ionophore A 23 187. C R Acad Sci Hebd Seances Acad Sci D. 1976 Mar 29;282(13):1309–1312. [PubMed] [Google Scholar]
- Morrill G. A., Ziegler D., Zabrenetzky V. S. An analysis of transport, exchange, and binding of sodium and potassium in isolated amphibian follicles and denuded oocytes. J Cell Sci. 1977 Aug;26:311–322. doi: 10.1242/jcs.26.1.311. [DOI] [PubMed] [Google Scholar]
- Nachshen D. A., Blaustein M. P. The effects of some organic "calcium antagonists" on calcium influx in presynaptic nerve terminals. Mol Pharmacol. 1979 Sep;16(2):576–586. [PubMed] [Google Scholar]
- Nawrath H. Cyclic AMP and cyclic GMP may play opposing roles in influencing force of contraction in mammalian myocardium. Nature. 1976 Aug 5;262(5568):509–511. doi: 10.1038/262509b0. [DOI] [PubMed] [Google Scholar]
- Nawrath H. Does cyclic GMP mediate the negative inotropic effect of acetylcholine in the heart? Nature. 1977 May 5;267(5606):72–74. doi: 10.1038/267072a0. [DOI] [PubMed] [Google Scholar]
- O'Connor C. M., Robinson K. R., Smith L. D. Calcium, potassium, and sodium exchange by full-grown and maturing Xenopus laevis oocytes. Dev Biol. 1977 Nov;61(1):28–40. doi: 10.1016/0012-1606(77)90339-6. [DOI] [PubMed] [Google Scholar]
- Poggioli J., Putney J. W., Jr Net calcium fluxes in rat parotid acinar cells: evidence for a hormone-sensitive calcium pool in or near the plasma membrane. Pflugers Arch. 1982 Jan;392(3):239–243. doi: 10.1007/BF00584303. [DOI] [PubMed] [Google Scholar]
- Putney J. W., Jr Stimulus-permeability coupling: role of calcium in the receptor regulation of membrane permeability. Pharmacol Rev. 1978 Jun;30(2):209–245. [PubMed] [Google Scholar]
- Richelson E., El-Fakahany E. The molecular basis of neurotransmission at the muscarinic receptor. Biochem Pharmacol. 1981 Nov 1;30(21):2887–2891. doi: 10.1016/0006-2952(81)90248-3. [DOI] [PubMed] [Google Scholar]
- Robinson K. R. Electrical currents through full-grown and maturing Xenopus oocytes. Proc Natl Acad Sci U S A. 1979 Feb;76(2):837–841. doi: 10.1073/pnas.76.2.837. [DOI] [PMC free article] [PubMed] [Google Scholar]
- Schultz G., Hardman J. G., Schultz K., Baird C. E., Sutherland E. W. The importance of calcium ions for the regulation of guanosine 3':5'-cyclic monophosphage levels. Proc Natl Acad Sci U S A. 1973 Dec;70(12):3889–3893. doi: 10.1073/pnas.70.12.3889. [DOI] [PMC free article] [PubMed] [Google Scholar]
- Stone T. W., Taylor D. A., Bloom F. E. Cyclic AMP and cyclic GMP may mediate opposite neuronal responses in the rat cerebral cortex. Science. 1975 Mar 7;187(4179):845–847. doi: 10.1126/science.163488. [DOI] [PubMed] [Google Scholar]
- Thomas R. C. Electrogenic sodium pump in nerve and muscle cells. Physiol Rev. 1972 Jul;52(3):563–594. doi: 10.1152/physrev.1972.52.3.563. [DOI] [PubMed] [Google Scholar]
- Trautwein W., Taniguchi J., Noma A. The effect of intracellular cyclic nucleotides and calcium on the action potential and acetylcholine response of isolated cardiac cells. Pflugers Arch. 1982 Feb;392(4):307–314. doi: 10.1007/BF00581624. [DOI] [PubMed] [Google Scholar]
- Tupper J. T., Maloff B. L. The ionic permeability of the amphibian oocyte in the presence or absence of external calcium. J Exp Zool. 1973 Jul;185(1):133–144. doi: 10.1002/jez.1401850113. [DOI] [PubMed] [Google Scholar]
- Vitto A., Jr, wallace R. A. Maturation of Xenopus oocytes. I. Facilitation by ouabain. Exp Cell Res. 1976 Jan;97:56–62. doi: 10.1016/0014-4827(76)90654-6. [DOI] [PubMed] [Google Scholar]
- Wallace R. A., Jared D. W., Dumont J. N., Sega M. W. Protein incorporation by isolated amphibian oocytes. 3. Optimum incubation conditions. J Exp Zool. 1973 Jun;184(3):321–333. doi: 10.1002/jez.1401840305. [DOI] [PubMed] [Google Scholar]
- Wallace R. A., Steinhardt R. A. Maturation of Xenopus oocytes. II. Observations on membrane potential. Dev Biol. 1977 Jun;57(2):305–316. doi: 10.1016/0012-1606(77)90217-2. [DOI] [PubMed] [Google Scholar]
- Ziegler D., Morrill G. A. Regulation of the amphibian oocyte plasma membrane ion permeability by cytoplasmic factors during the first meiotic division. Dev Biol. 1977 Oct 1;60(1):318–325. doi: 10.1016/0012-1606(77)90129-4. [DOI] [PubMed] [Google Scholar]