Abstract
Isolated cells from rat lacrimal glands were studied with patch-clamp techniques. Whole-cell and cell-attached recordings were obtained while the cells were stimulated by application of carbamylcholine or of the Ca ionophore, A23187. The results were compared with recordings of Ca-dependent channels obtained in isolated patches. Whole-cell recordings revealed two types of carbamylcholine-induced current. At low levels of stimulation, a specific class of Ca-dependent K channels was selectively activated ('BK channels'). With more intense stimulation an inward current, Ii, was obtained at the cell resting potential. Ii rose rather abruptly after a long delay. In several cells, Ii currents presented spontaneous oscillations. Both K and Ii current responses to carbamylcholine were due to activation of muscarinic receptors. Both responses were elicited by a rise of the intracellular Ca concentration. The immediate source of Ca was intracellular. Replacement of intracellular K with either Na or Cs blocked BK channels entirely, thus allowing the study of Ii currents free from K currents. Ii responses to carbamylcholine were, however, less frequently obtained in Na- or Cs-dialysed cells than in K-dialysed cells. In symmetrical NaCl solutions, Ii inverted at 0 mV. When replacing part of the intracellular or extracellular Cl with glutamate the reversal potential, Ei, was found to vary in the same direction as the equilibrium potential for Cl ions, ECl. In some experiments, Ei was close to ECl but in others Ei deviated strongly from ECl. These experiments suggested that Ii was mainly due to a Cl-selective conductance, and that another conductance type was contributing to Ii in variable proportions. It was found that, in K-free solutions, Ii had a reversal potential very close to ECl. Noise analysis showed that the Cl channels involved in Ii current had a unit conductance of about 1-2 pS in symmetrical NaCl solutions. At -60 mV, the mean channel open time derived from noise power spectra was about 200 ms. The activation of the Ca-dependent Cl channels was increased by depolarization. Voltage jumps elicited slow exponential relaxations. At -60 mV, the time constants of the relaxations were in the range 100-250 ms. Cell-attached recordings suggested that internal Ca activated three types of channel, depending on the Ca concentration: BK channels, 2-4 pS channels and 25 pS channels. Inside-out and outside-out patch conditions allowed a rough estimate to be made of the Ca concentration needed to activate each class of channel.(ABSTRACT TRUNCATED AT 400 WORDS)
Full text
PDF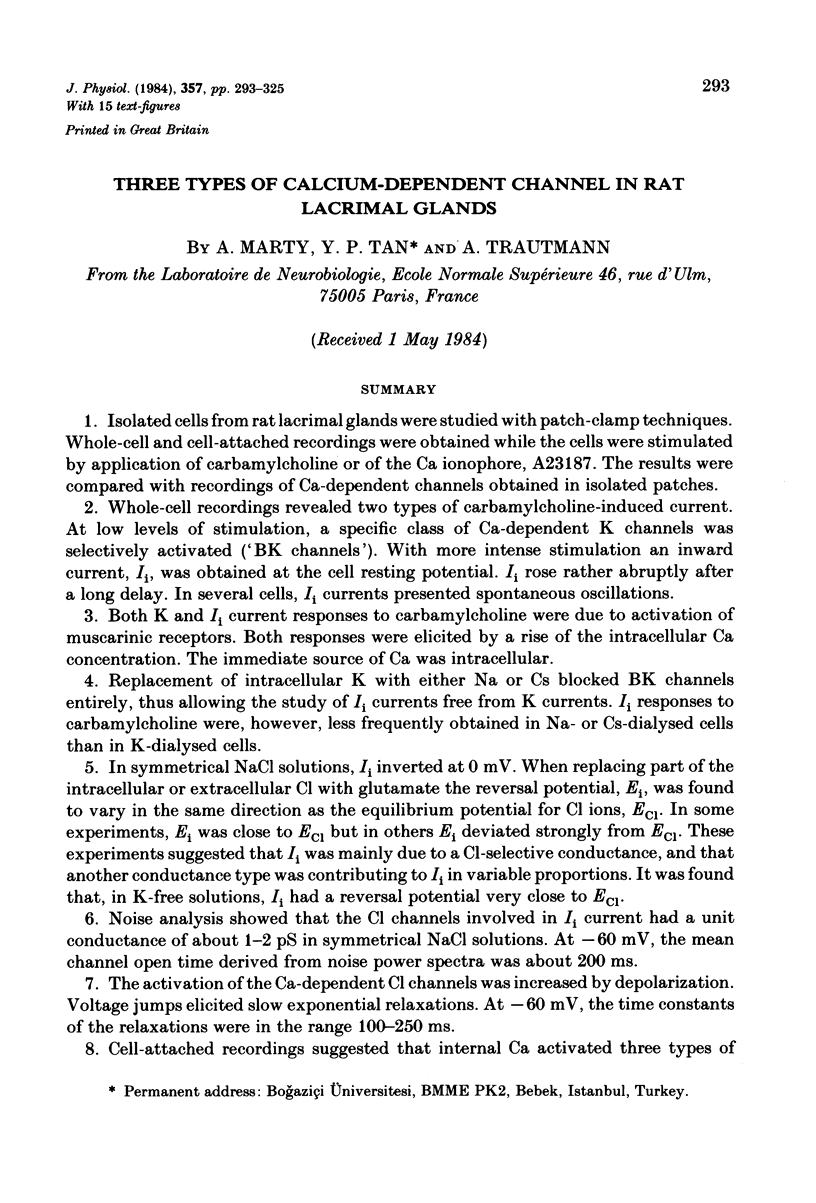
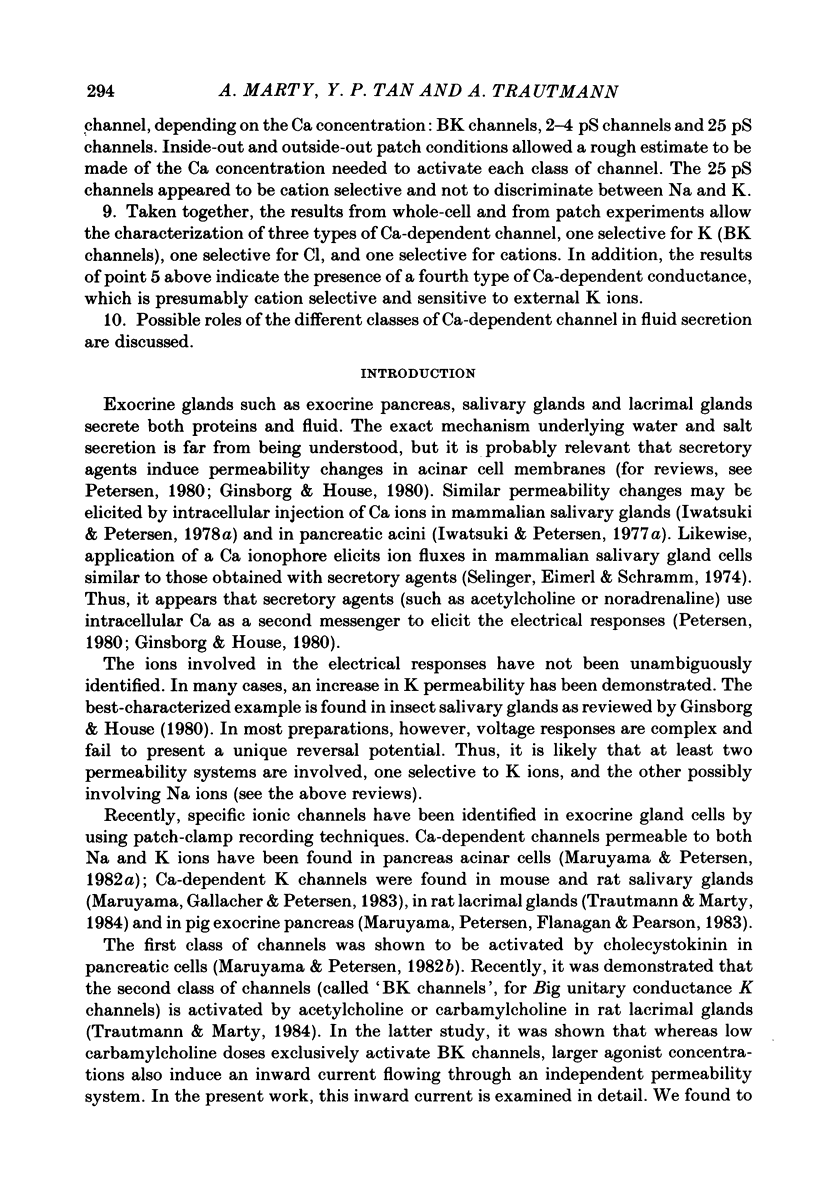
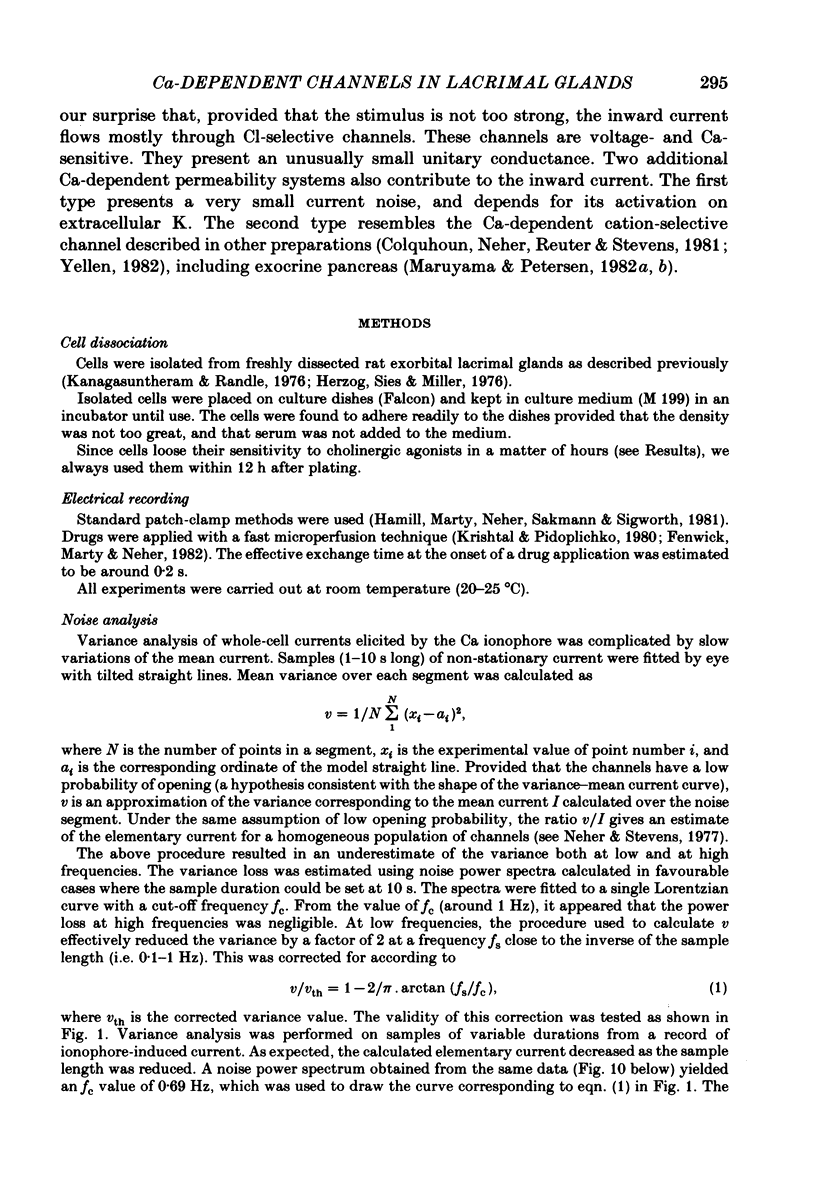
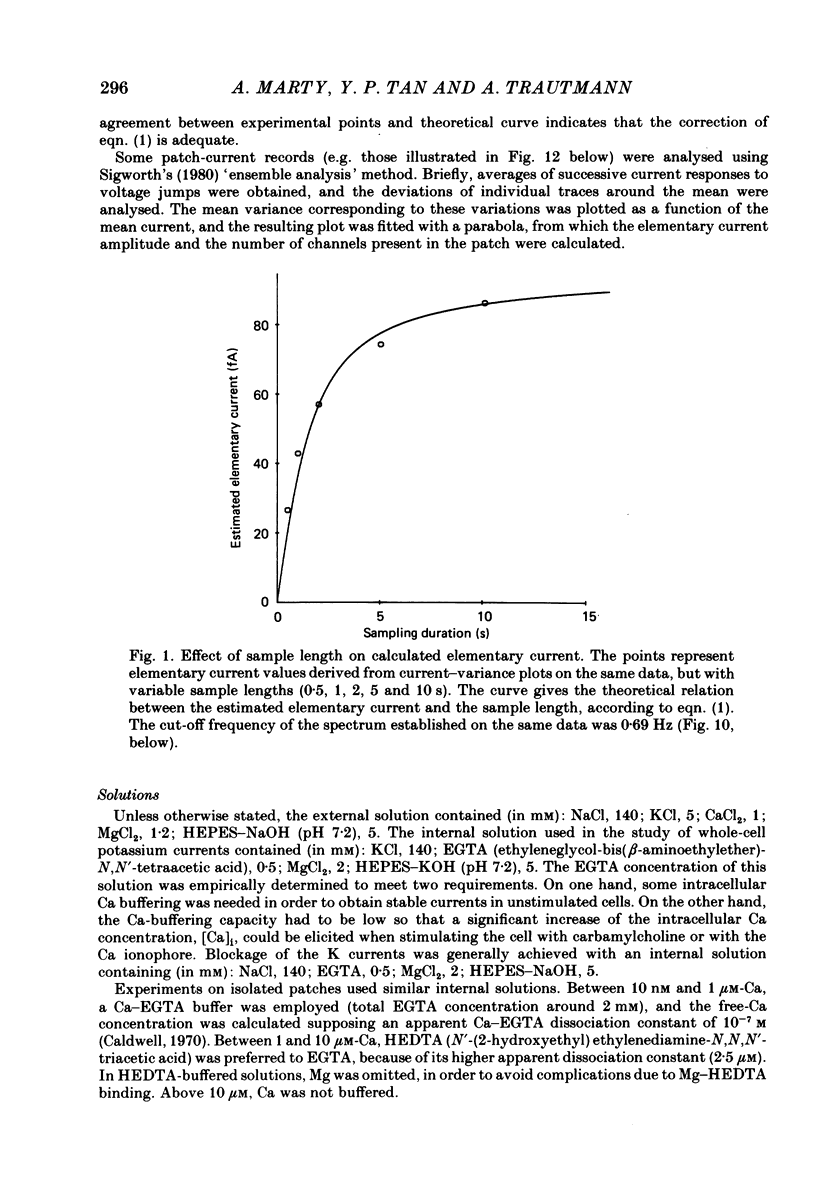
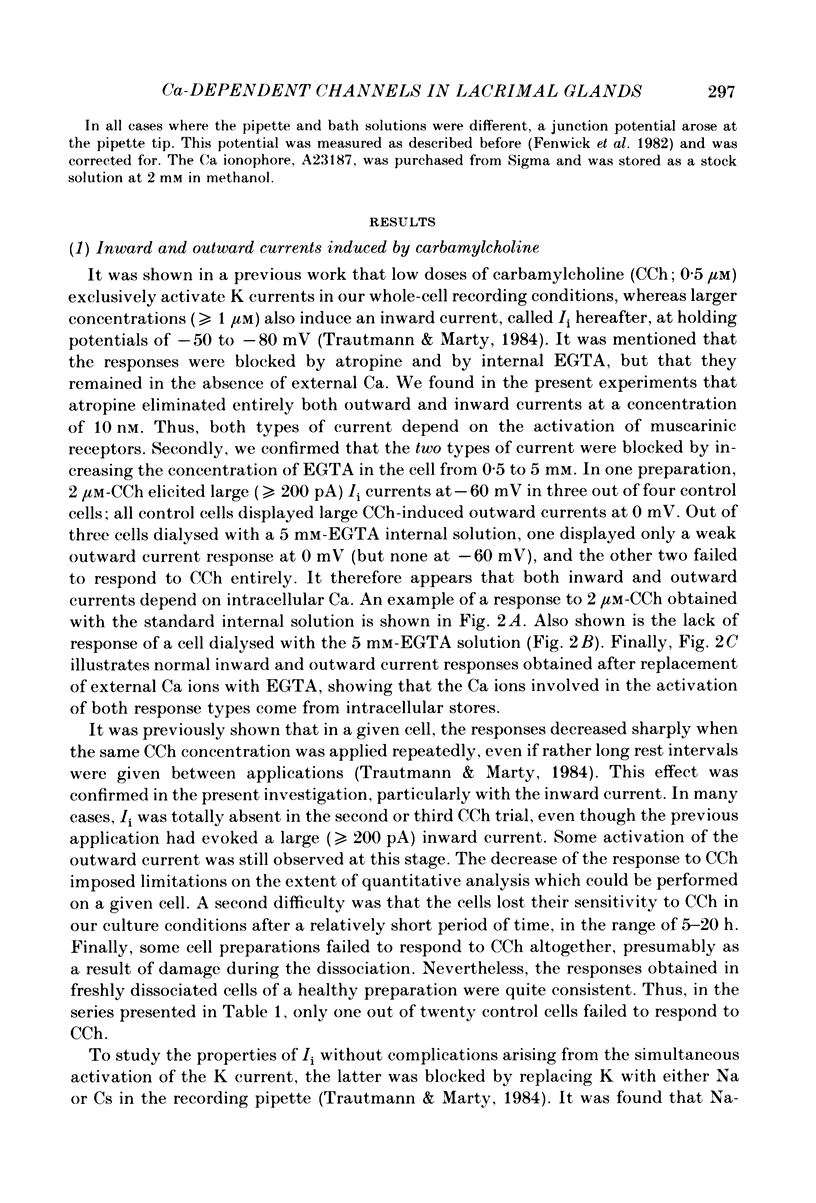

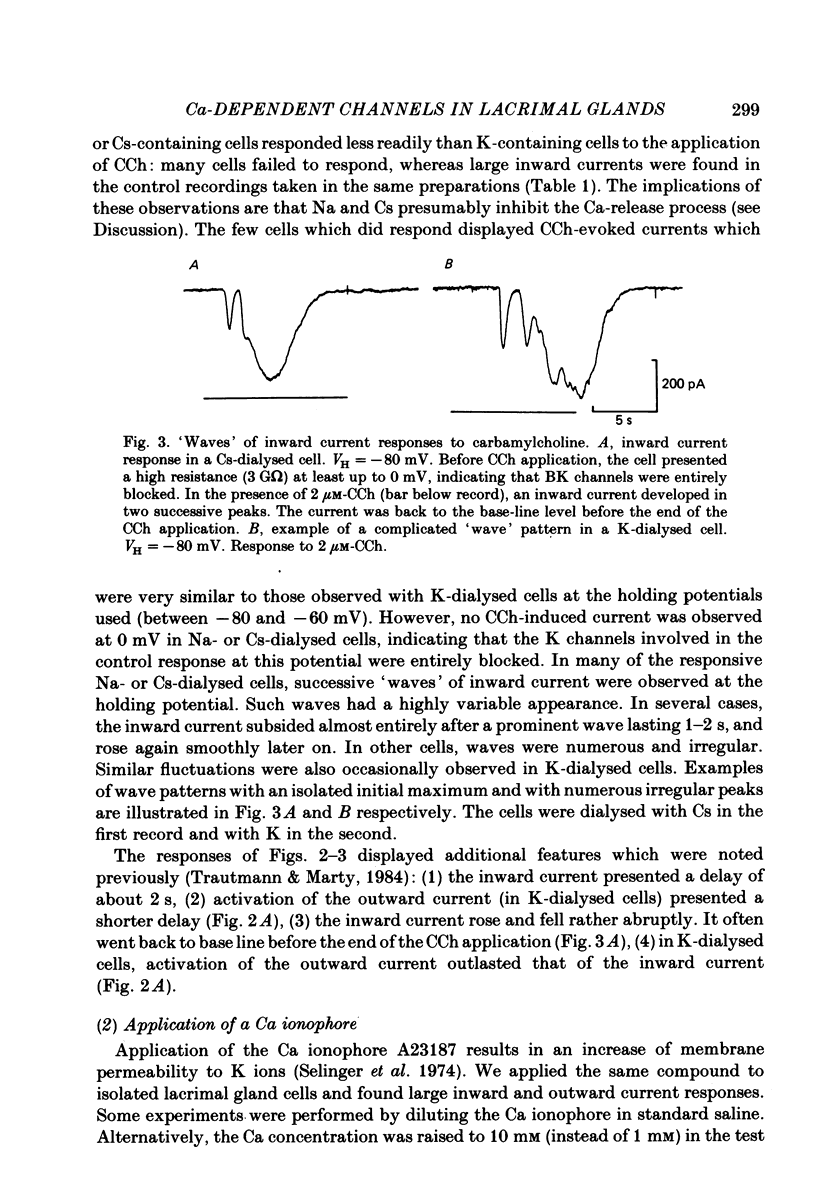
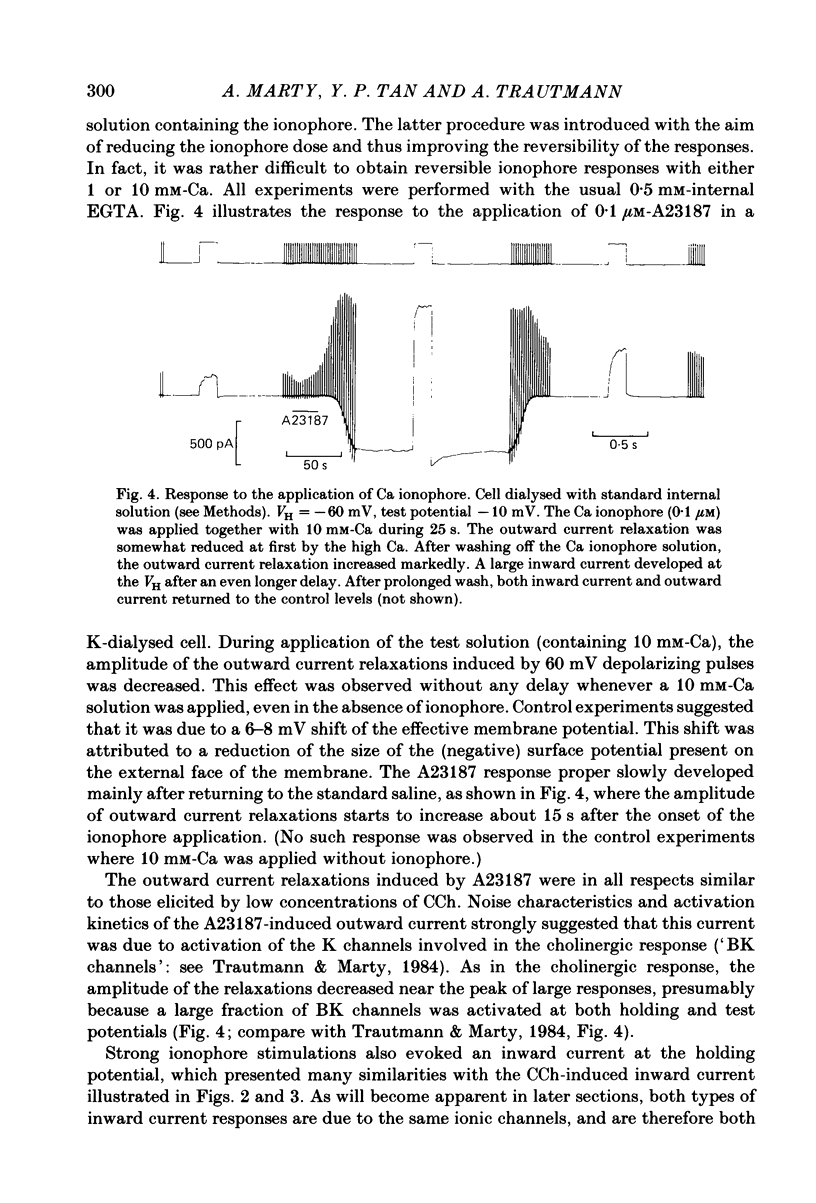
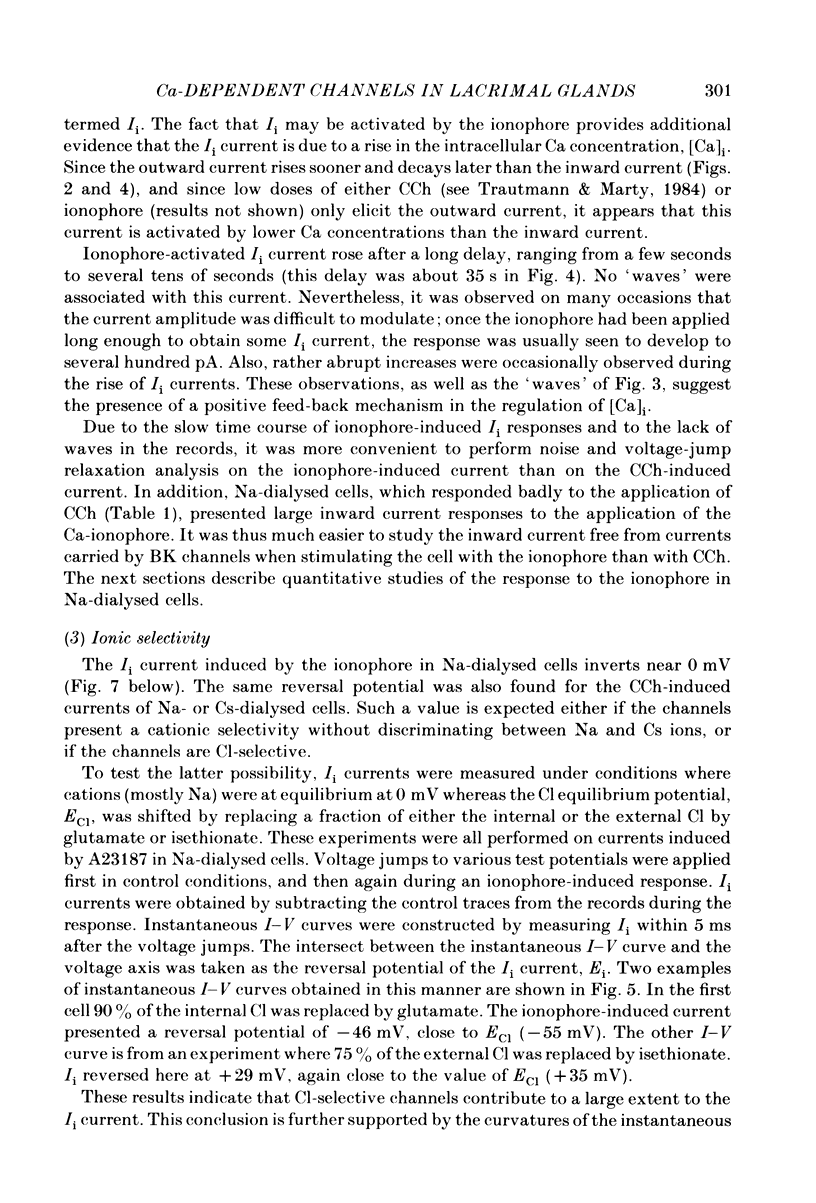
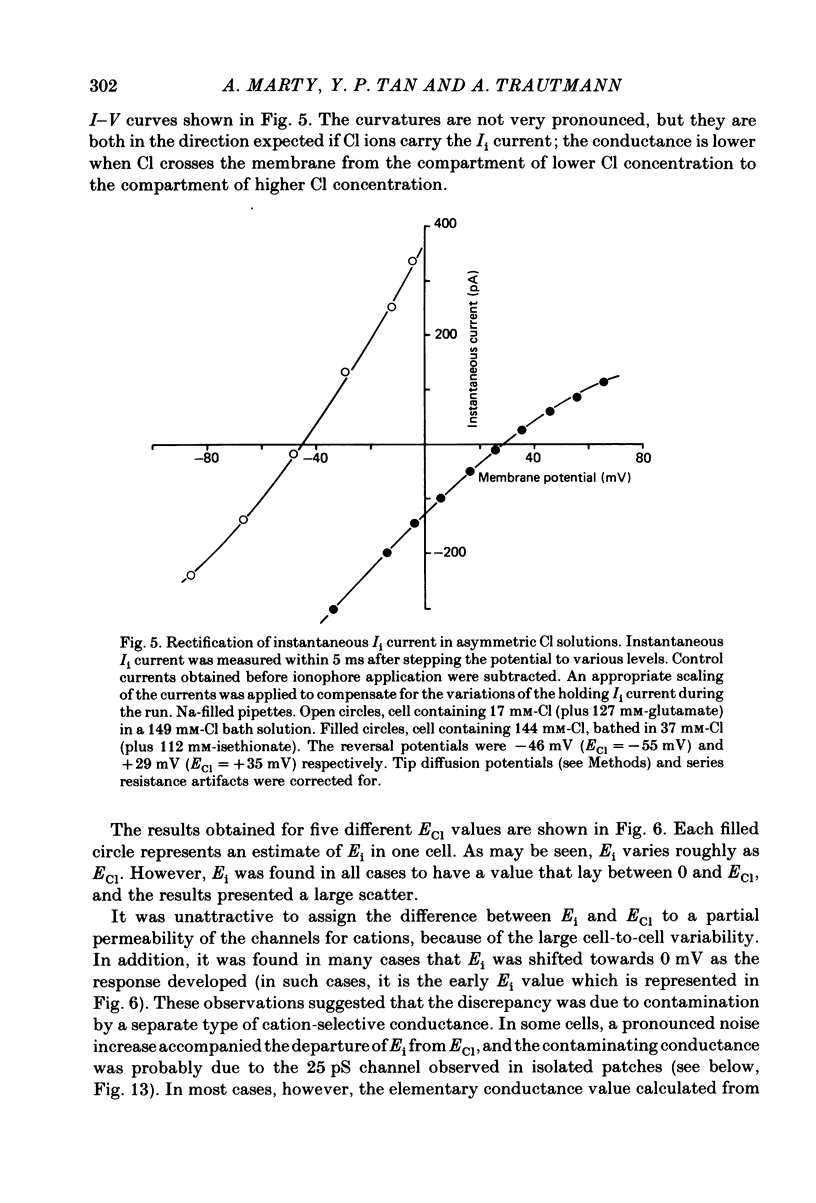
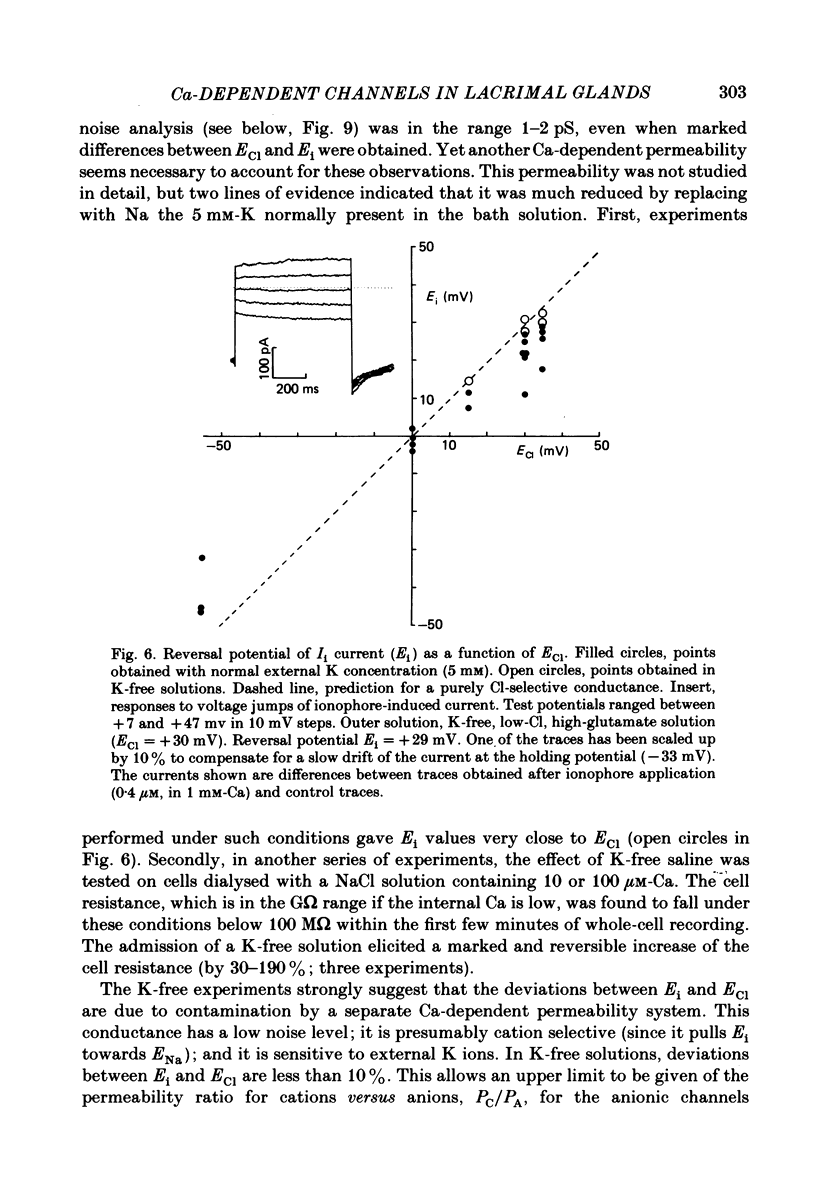
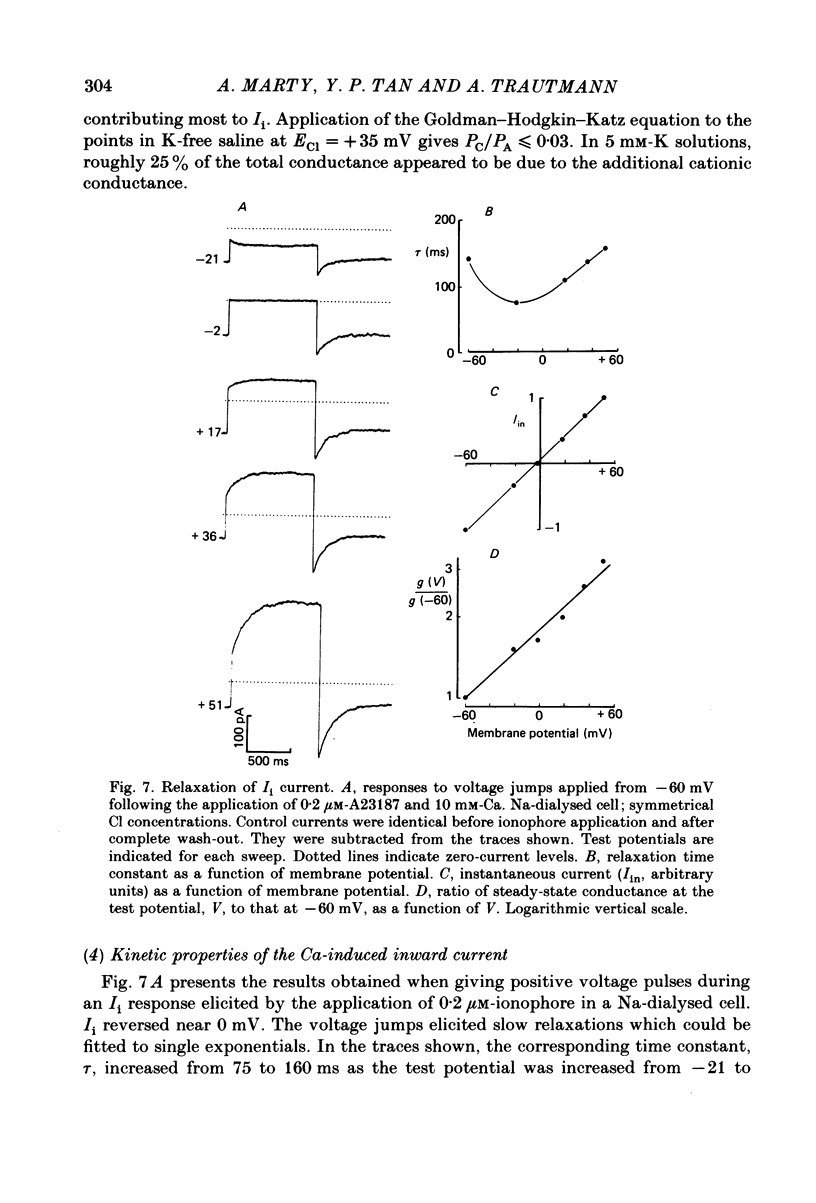
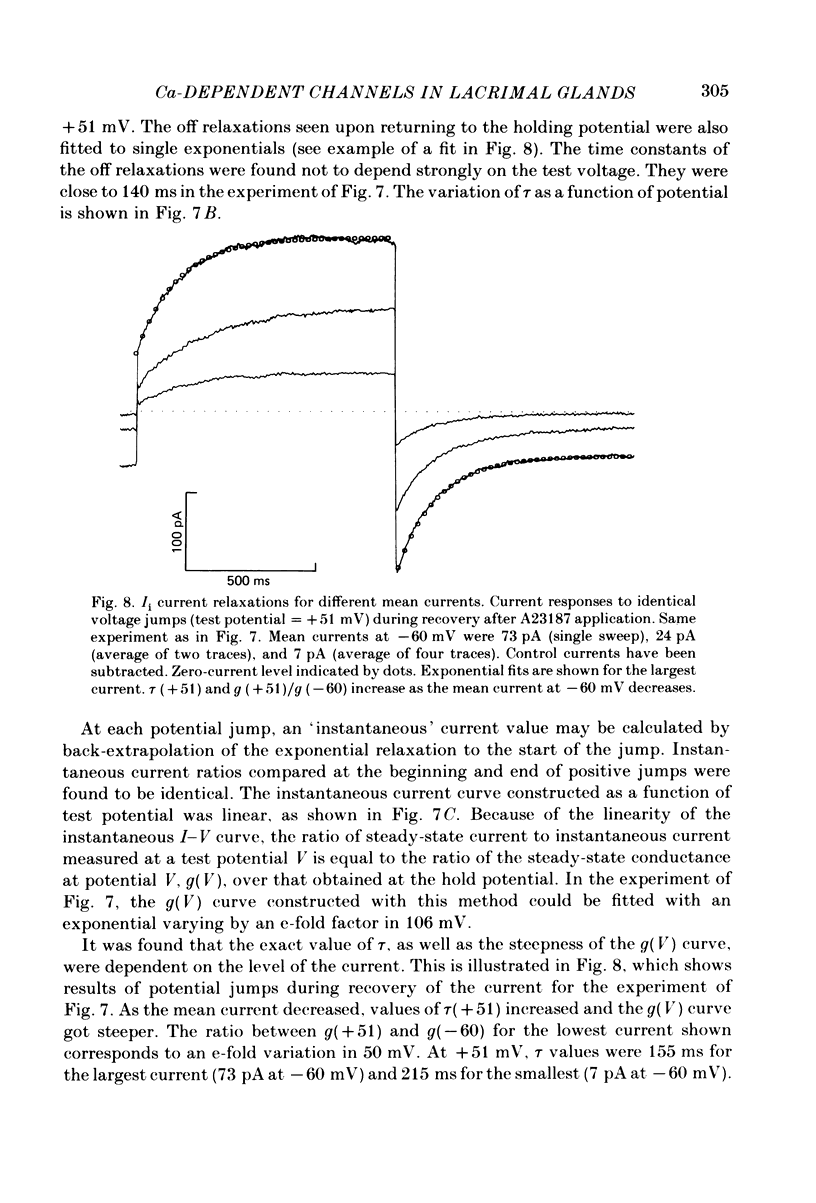
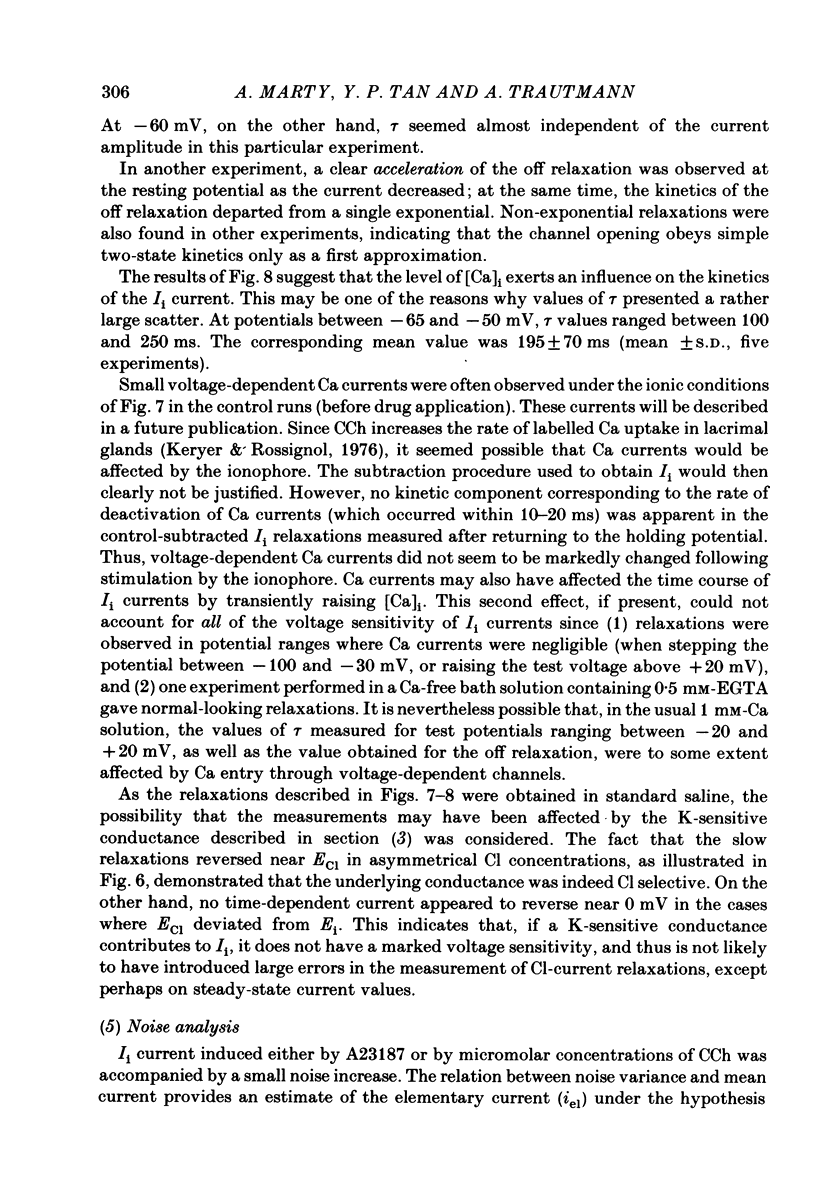

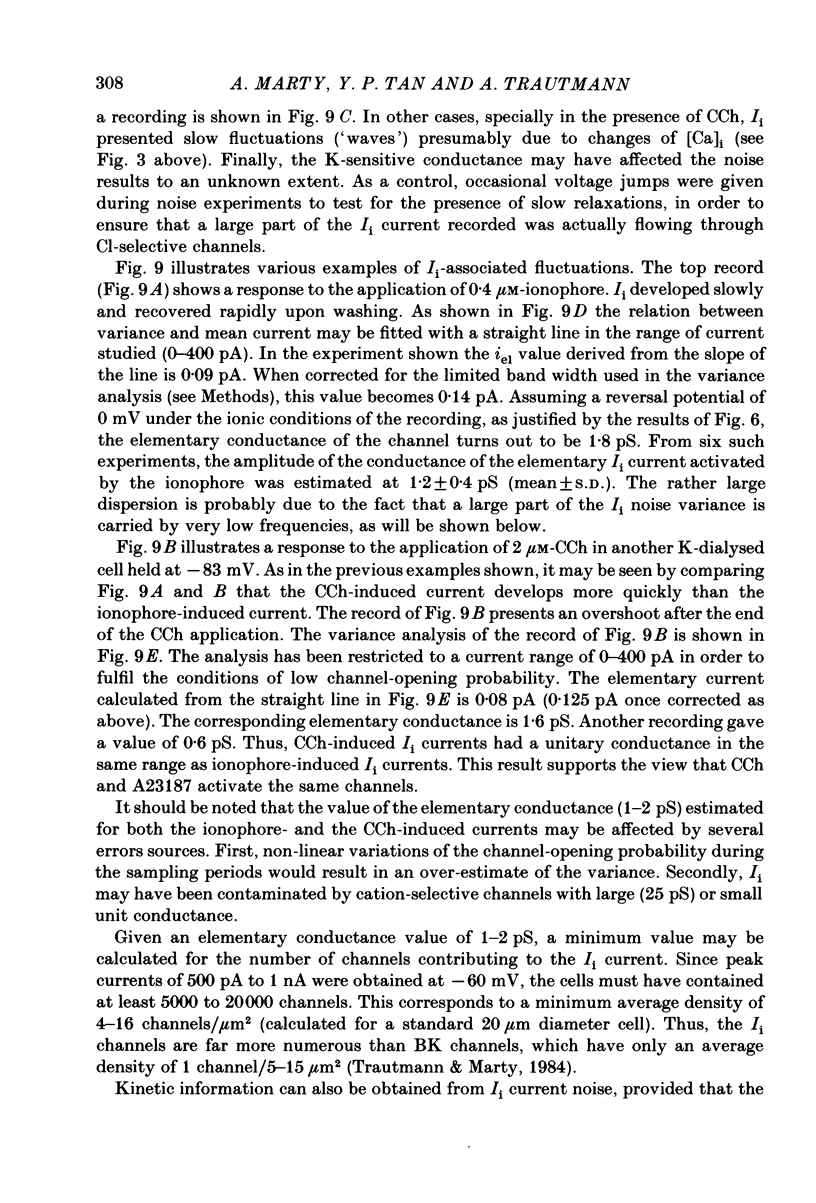
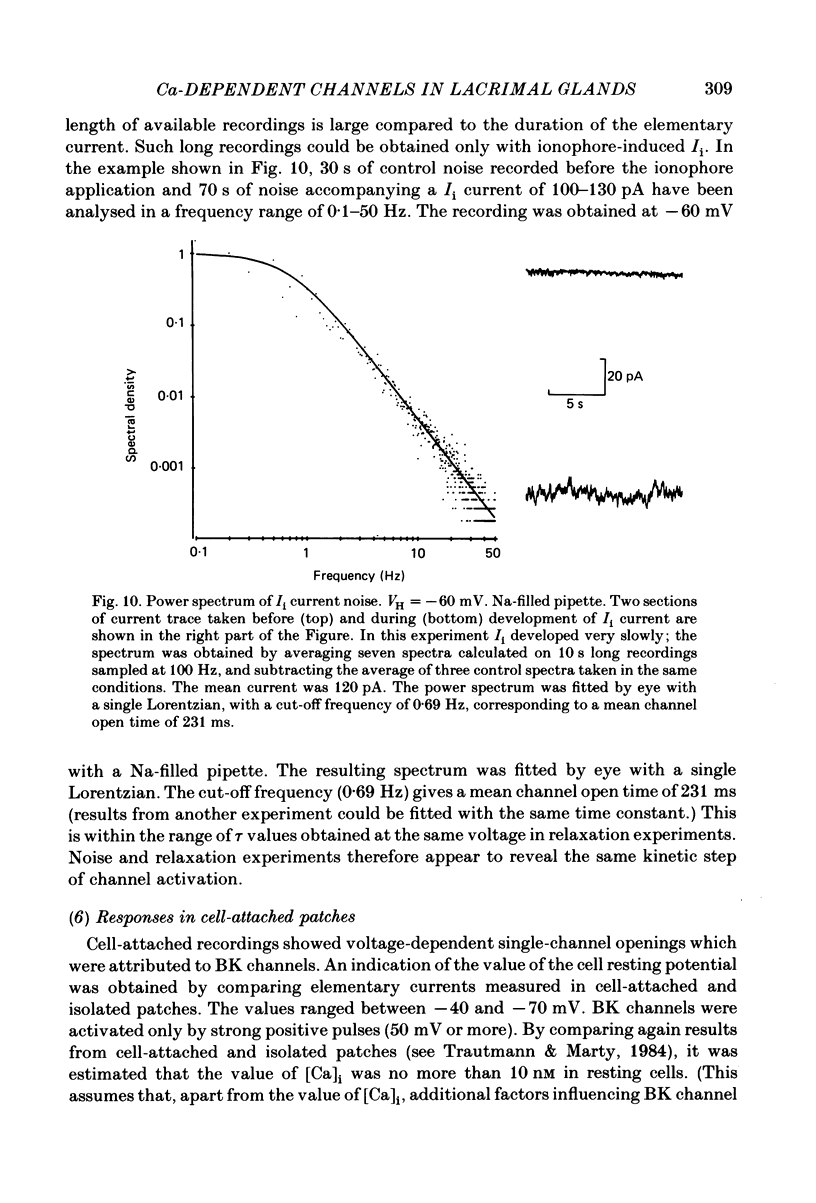
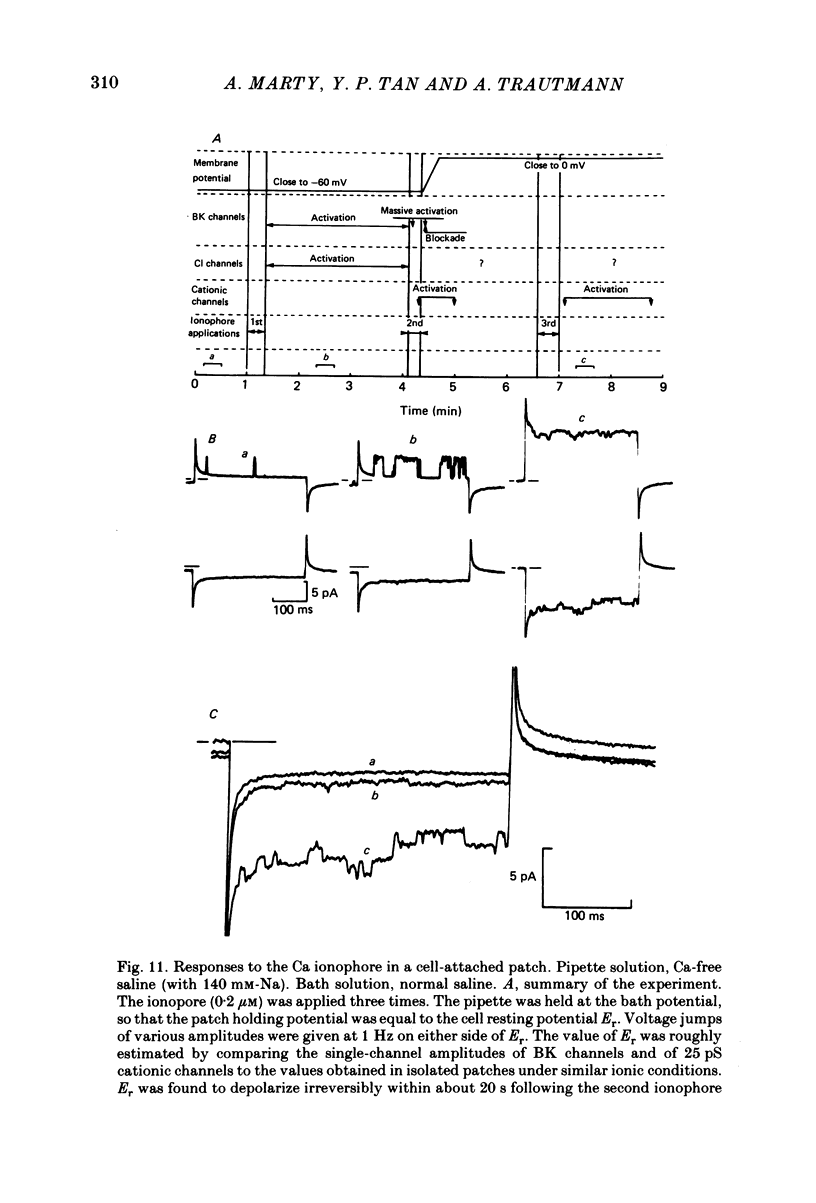
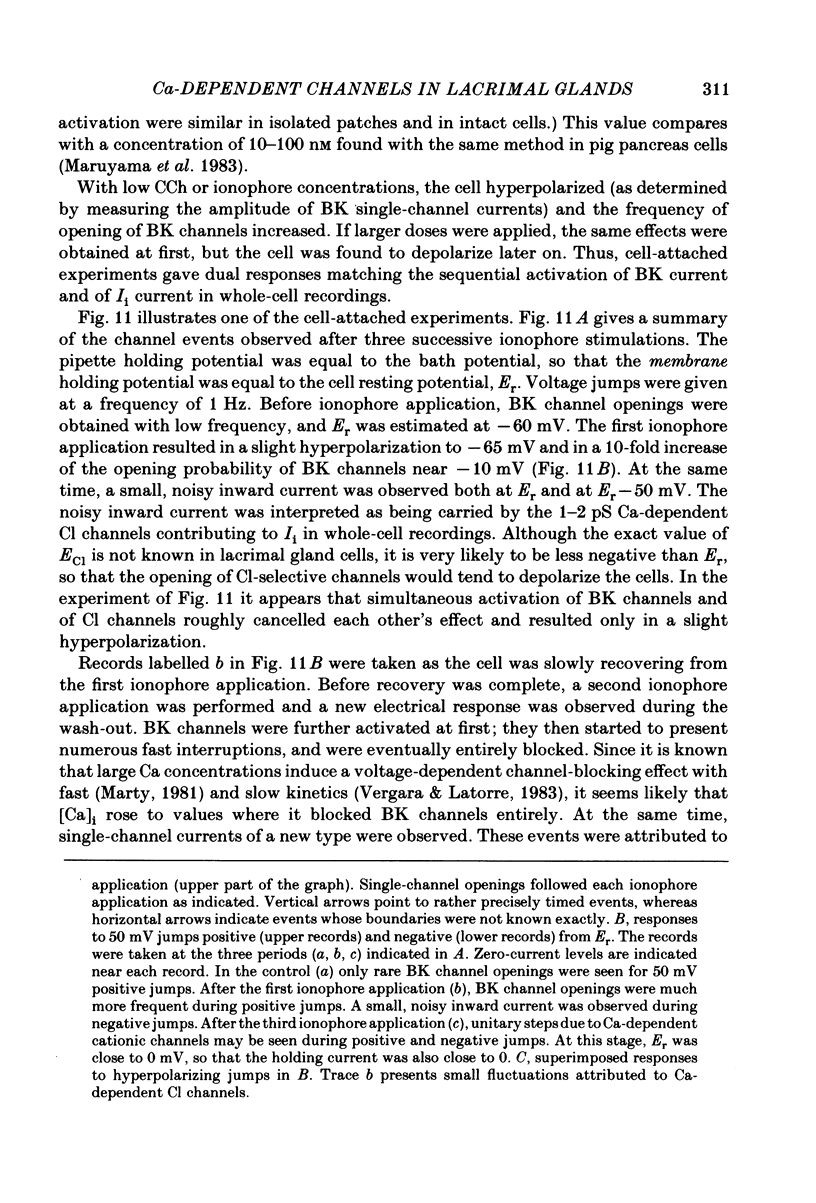
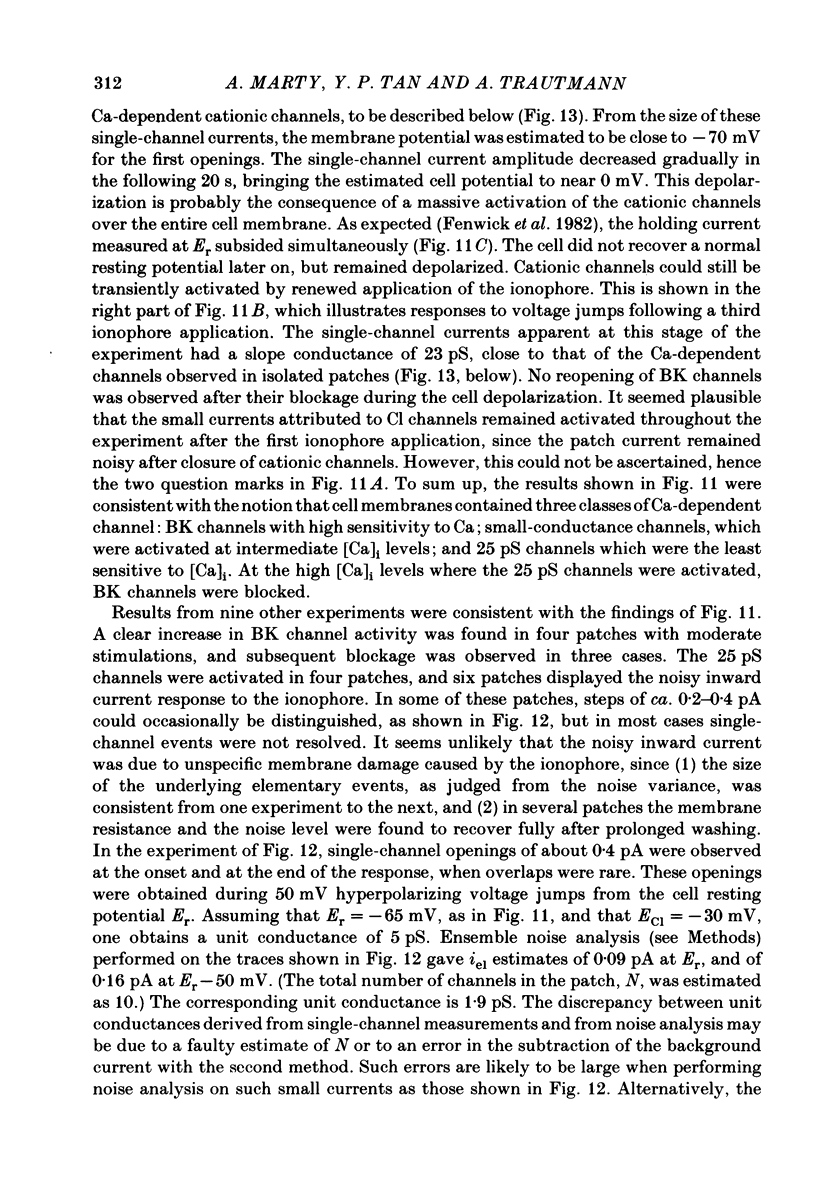

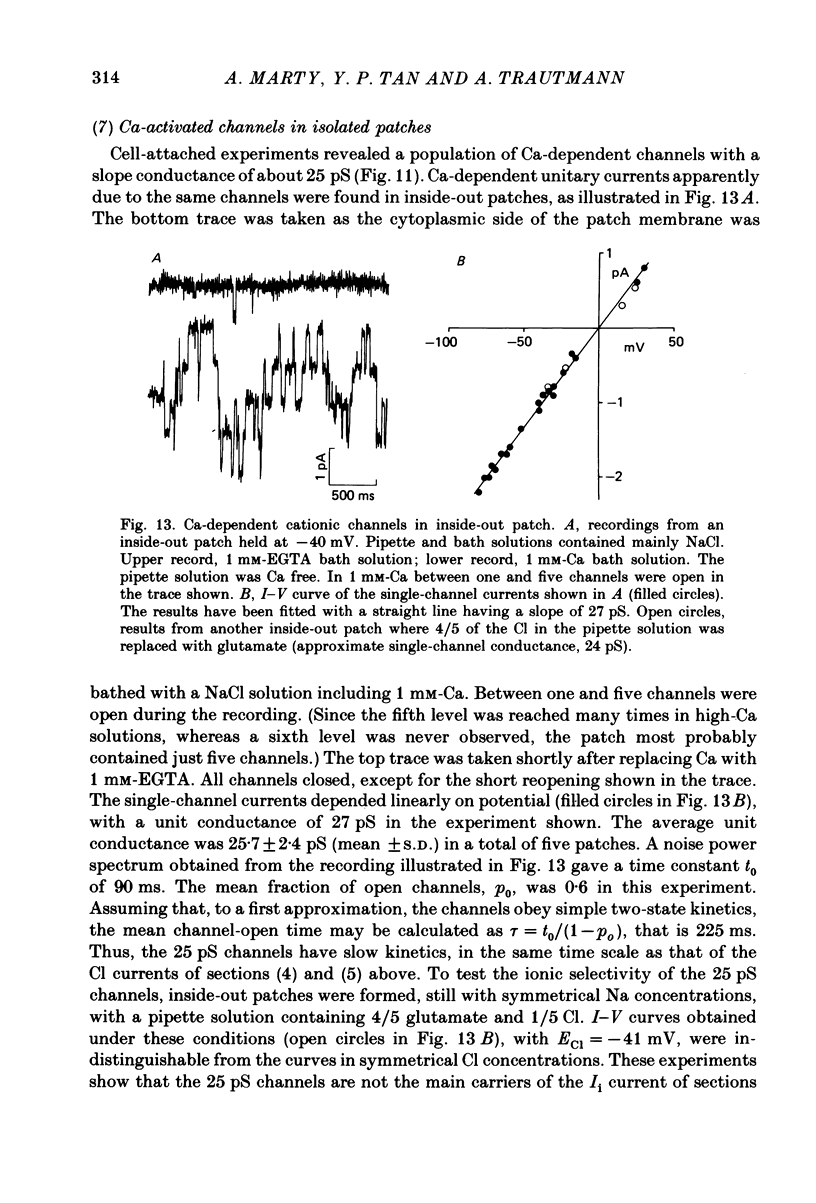
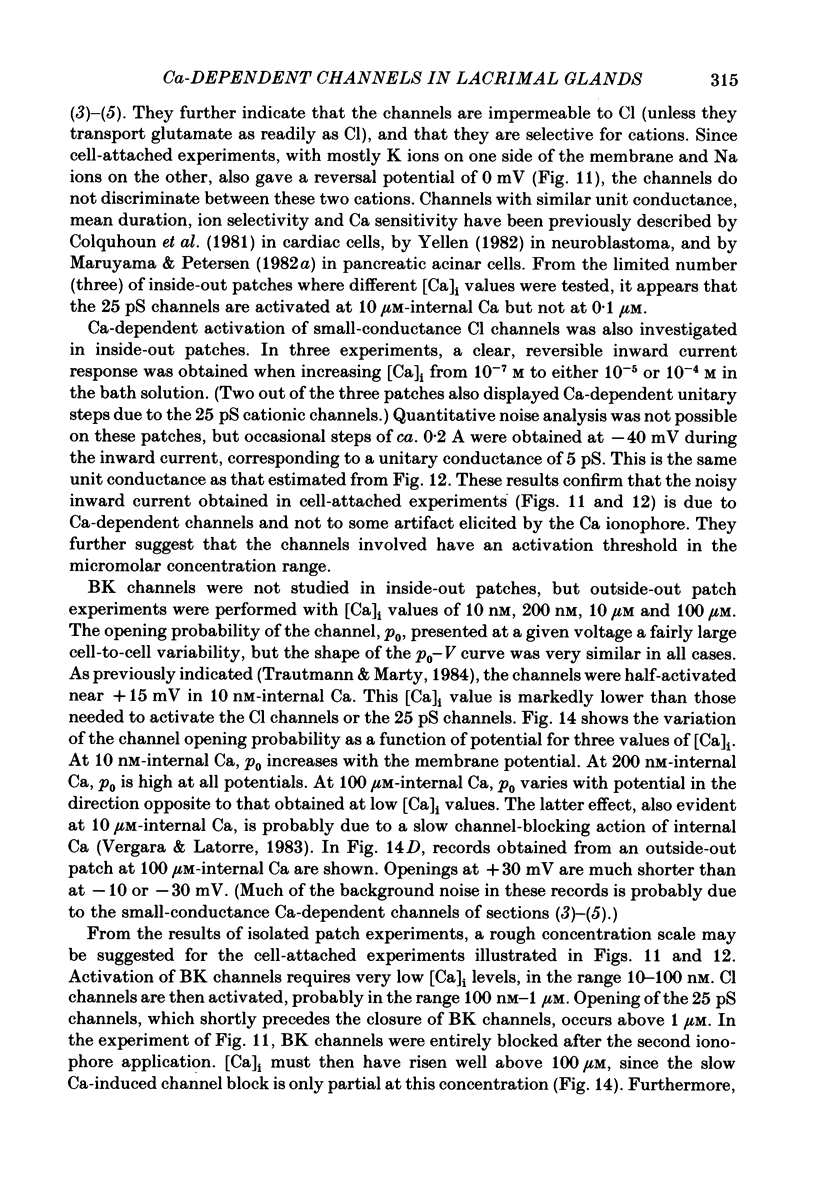
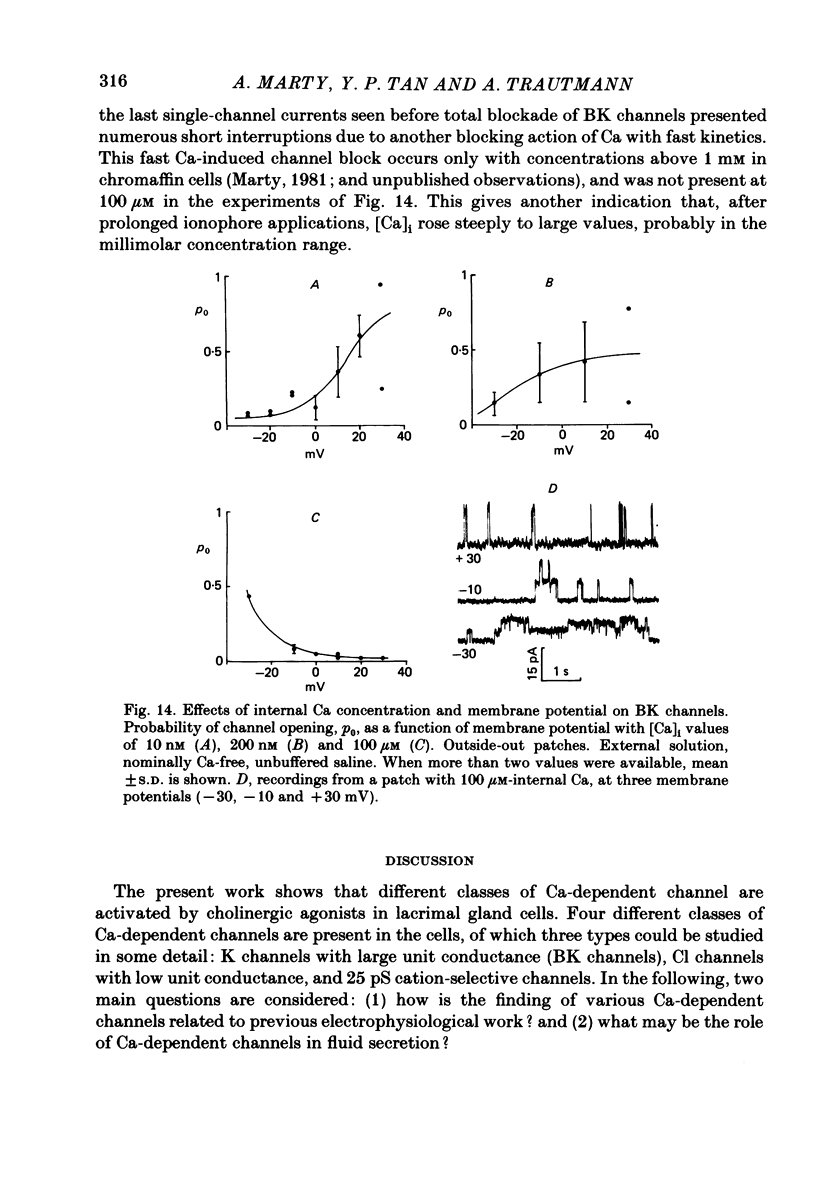
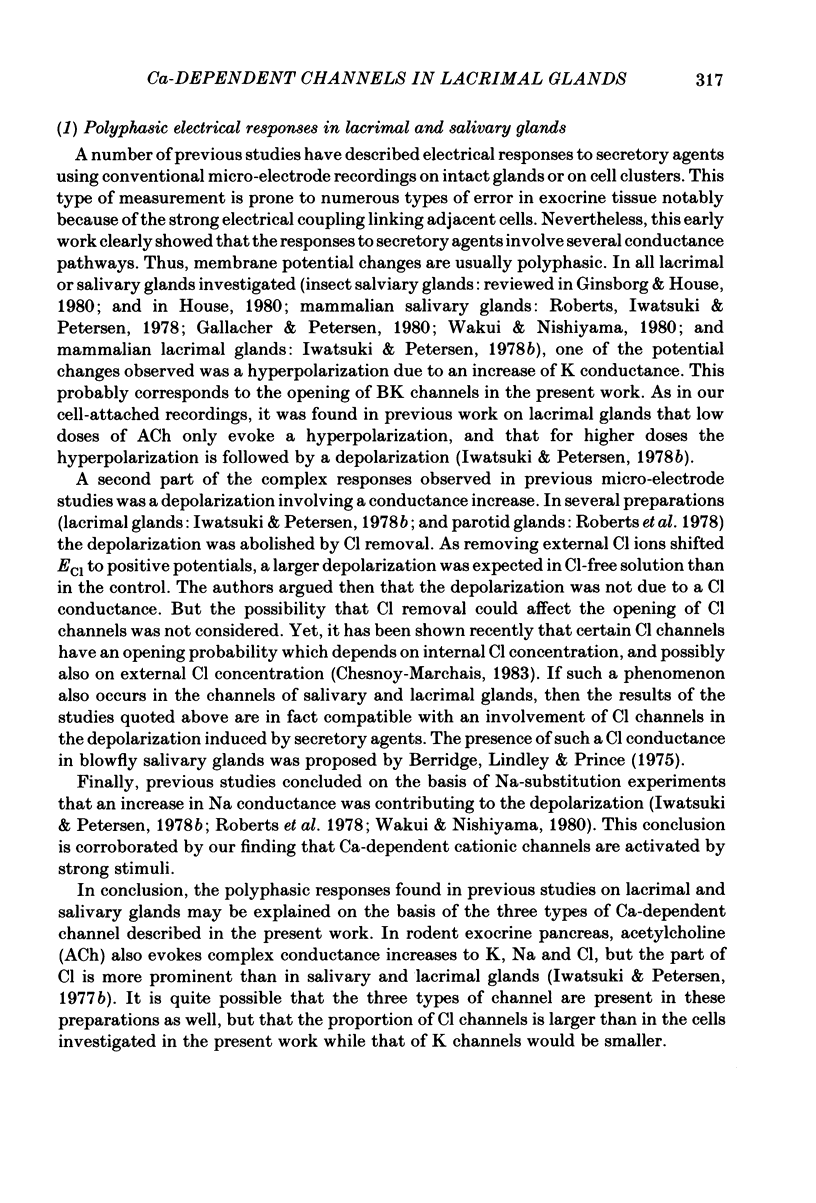
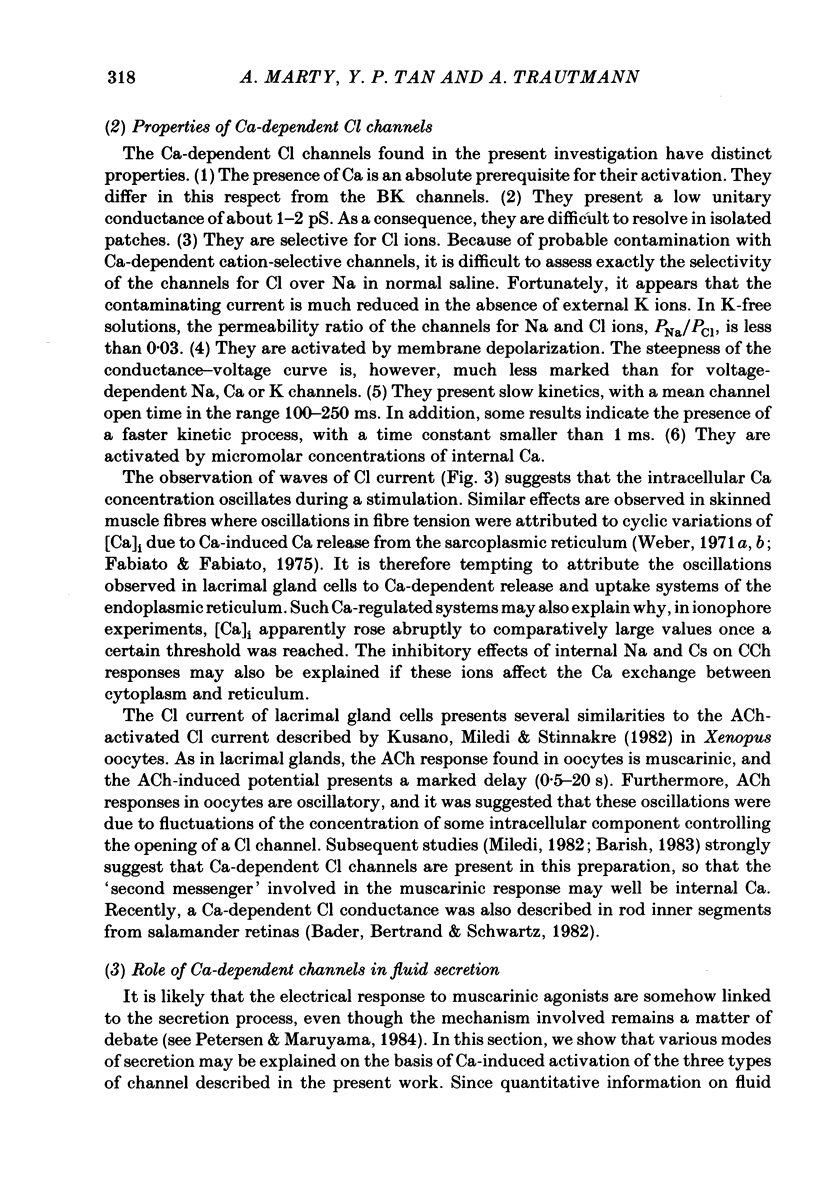
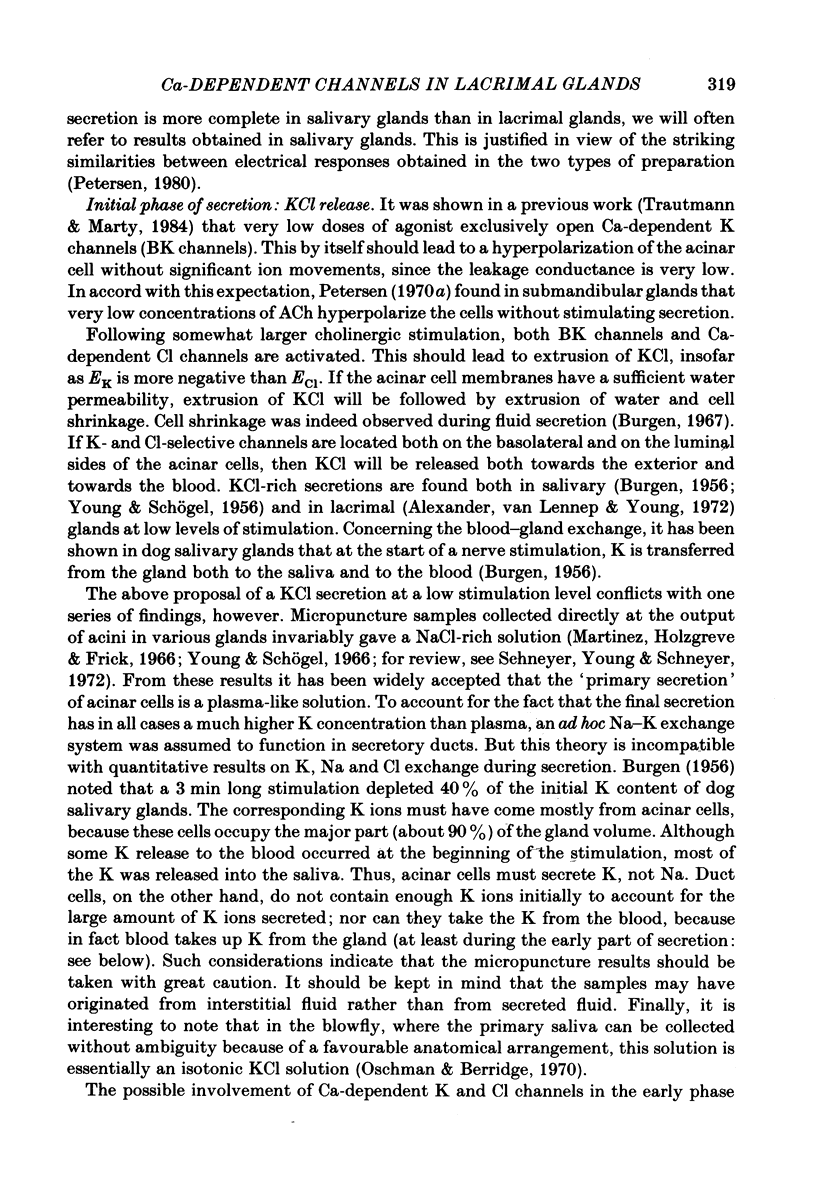
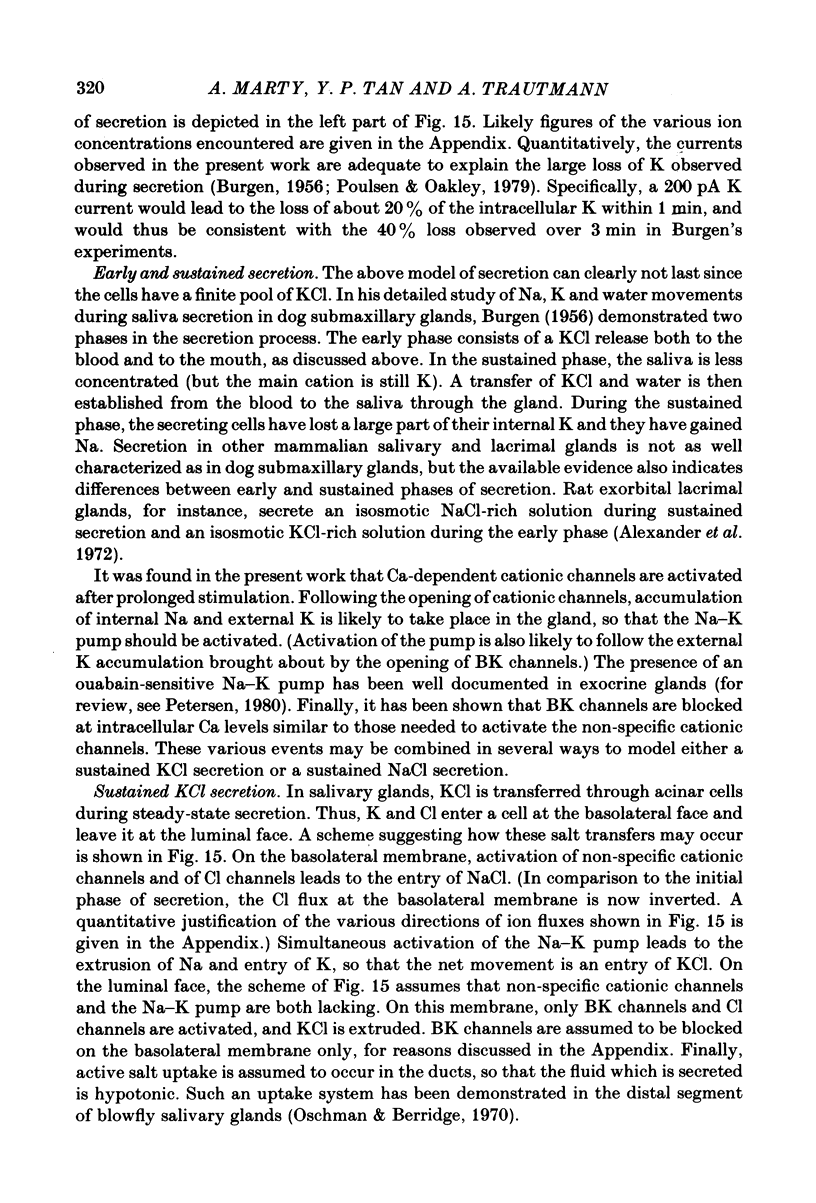
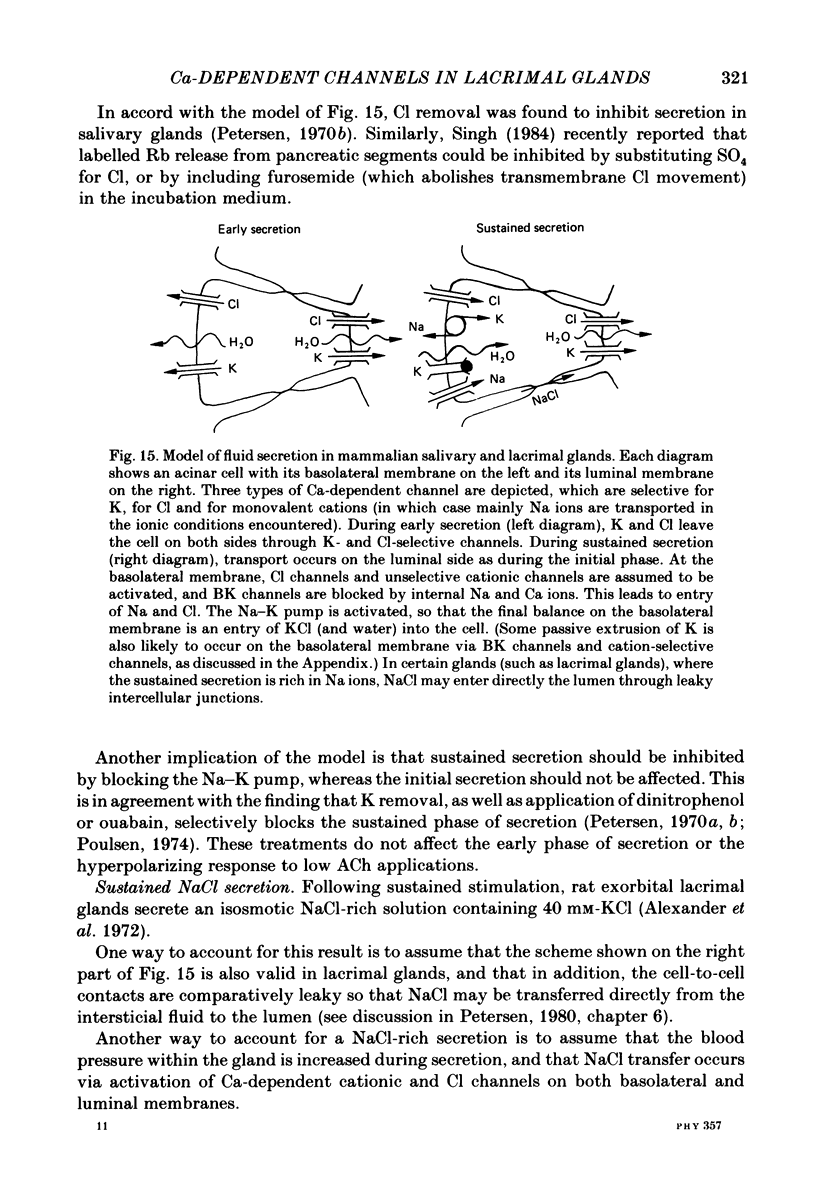
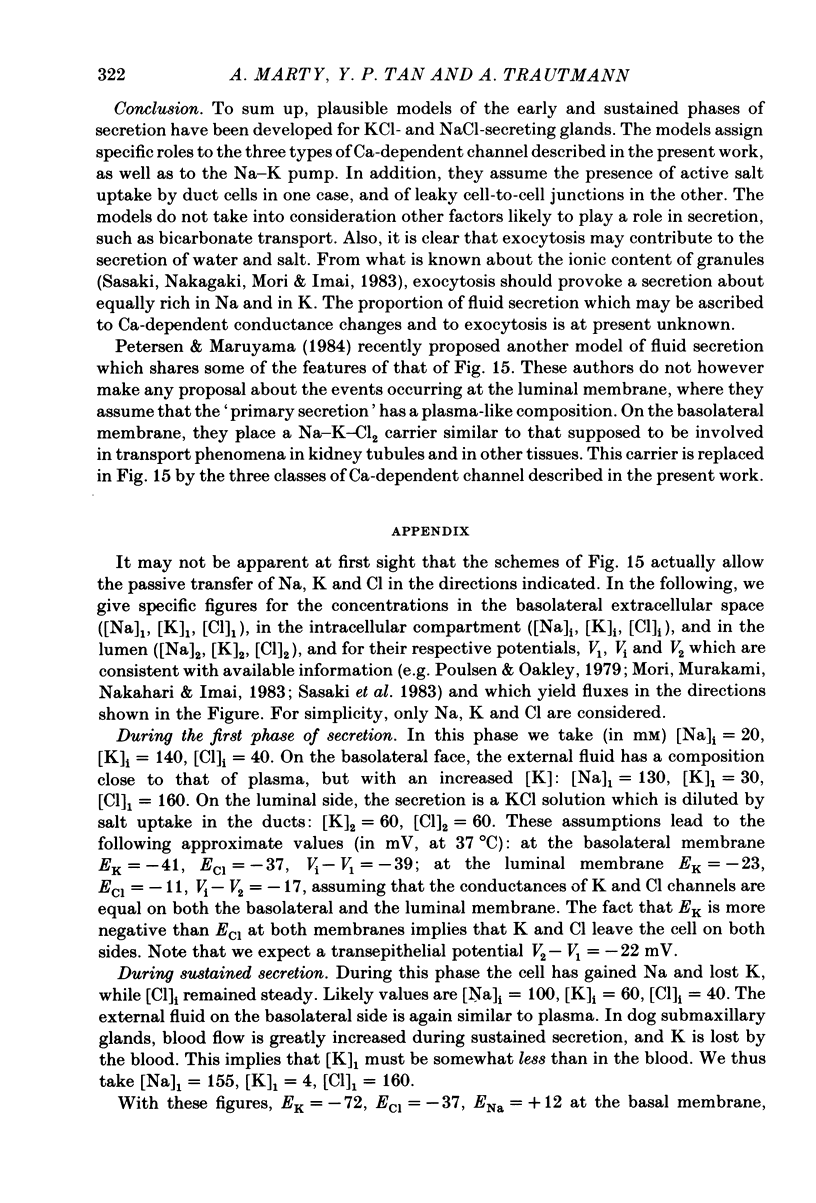
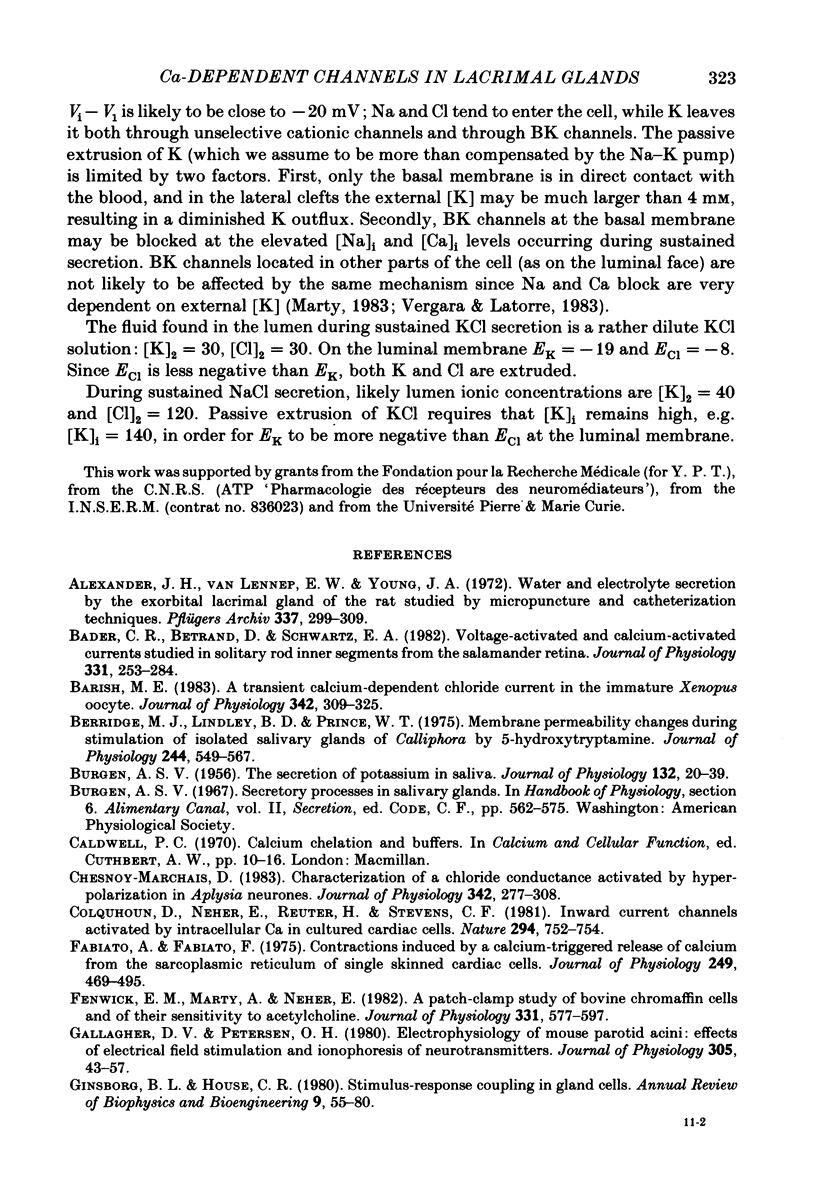
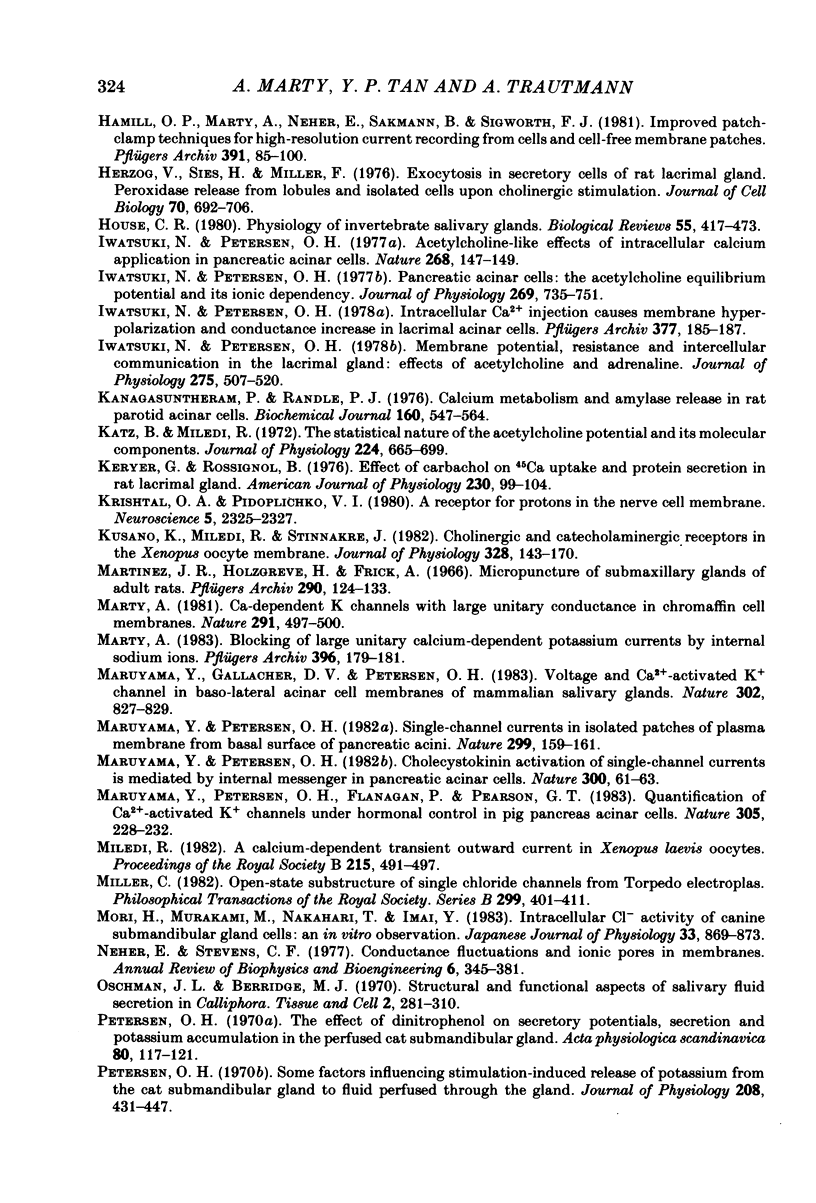
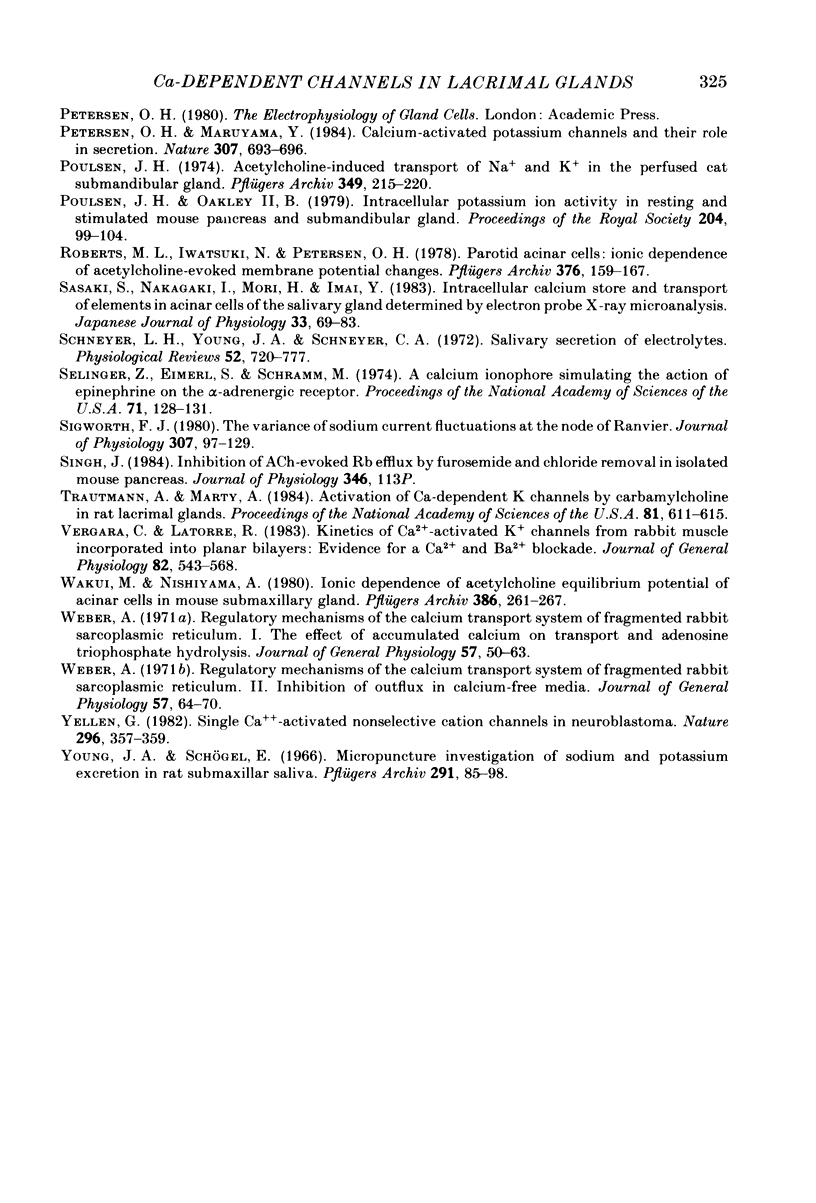
Selected References
These references are in PubMed. This may not be the complete list of references from this article.
- Alexander J. H., van Lennep E. W., Young J. A. Water and electrolyte secretion by the exorbital lacrimal gland of the rat studied by micropuncture and catheterization techniques. Pflugers Arch. 1972;337(4):299–309. doi: 10.1007/BF00586647. [DOI] [PubMed] [Google Scholar]
- BURGEN A. S. The secretion of potassium in saliva. J Physiol. 1956 Apr 27;132(1):20–39. doi: 10.1113/jphysiol.1956.sp005500. [DOI] [PMC free article] [PubMed] [Google Scholar]
- Bader C. R., Bertrand D., Schwartz E. A. Voltage-activated and calcium-activated currents studied in solitary rod inner segments from the salamander retina. J Physiol. 1982 Oct;331:253–284. doi: 10.1113/jphysiol.1982.sp014372. [DOI] [PMC free article] [PubMed] [Google Scholar]
- Barish M. E. A transient calcium-dependent chloride current in the immature Xenopus oocyte. J Physiol. 1983 Sep;342:309–325. doi: 10.1113/jphysiol.1983.sp014852. [DOI] [PMC free article] [PubMed] [Google Scholar]
- Berridge M. J., Lindley B. D., Prince W. T. Membrane permeability changes during stimulation of isolated salivary glands of Calliphora by 5-hydroxytryptamine. J Physiol. 1975 Jan;244(3):549–567. doi: 10.1113/jphysiol.1975.sp010812. [DOI] [PMC free article] [PubMed] [Google Scholar]
- Chesnoy-Marchais D. Characterization of a chloride conductance activated by hyperpolarization in Aplysia neurones. J Physiol. 1983 Sep;342:277–308. doi: 10.1113/jphysiol.1983.sp014851. [DOI] [PMC free article] [PubMed] [Google Scholar]
- Colquhoun D., Neher E., Reuter H., Stevens C. F. Inward current channels activated by intracellular Ca in cultured cardiac cells. Nature. 1981 Dec 24;294(5843):752–754. doi: 10.1038/294752a0. [DOI] [PubMed] [Google Scholar]
- Fabiato A., Fabiato F. Contractions induced by a calcium-triggered release of calcium from the sarcoplasmic reticulum of single skinned cardiac cells. J Physiol. 1975 Aug;249(3):469–495. doi: 10.1113/jphysiol.1975.sp011026. [DOI] [PMC free article] [PubMed] [Google Scholar]
- Fenwick E. M., Marty A., Neher E. A patch-clamp study of bovine chromaffin cells and of their sensitivity to acetylcholine. J Physiol. 1982 Oct;331:577–597. doi: 10.1113/jphysiol.1982.sp014393. [DOI] [PMC free article] [PubMed] [Google Scholar]
- Gallacher D. V., Petersen O. H. Electrophysiology of mouse parotid acini: effects of electrical field stimulation and ionophoresis of neurotransmitters. J Physiol. 1980 Aug;305:43–57. doi: 10.1113/jphysiol.1980.sp013348. [DOI] [PMC free article] [PubMed] [Google Scholar]
- Ginsborg B. L., House C. R. Stimulus-response coupling in gland cells. Annu Rev Biophys Bioeng. 1980;9:55–80. doi: 10.1146/annurev.bb.09.060180.000415. [DOI] [PubMed] [Google Scholar]
- Hamill O. P., Marty A., Neher E., Sakmann B., Sigworth F. J. Improved patch-clamp techniques for high-resolution current recording from cells and cell-free membrane patches. Pflugers Arch. 1981 Aug;391(2):85–100. doi: 10.1007/BF00656997. [DOI] [PubMed] [Google Scholar]
- Herzog V., Sies H., Miller F. Exocytosis in secretory cells of rat lacrimal gland. Peroxidase release from lobules and isolated cells upon cholinergic stimulation. J Cell Biol. 1976 Sep;70(3):692–706. doi: 10.1083/jcb.70.3.692. [DOI] [PMC free article] [PubMed] [Google Scholar]
- Iwatsuki N., Petersen O. H. Acetylcholine-like effects of intracellular calcium application in pancreatic acinar cells. Nature. 1977 Jul 14;268(5616):147–149. doi: 10.1038/268147a0. [DOI] [PubMed] [Google Scholar]
- Iwatsuki N., Petersen O. H. Intracellular Ca2+ injection causes membrane hyperpolarization and conductance increase in lacrimal acinar cells. Pflugers Arch. 1978 Nov 14;377(2):185–187. [PubMed] [Google Scholar]
- Iwatsuki N., Petersen O. H. Membrane potential, resistance, and intercellular communication in the lacrimal gland: effects of acetylcholine and adrenaline. J Physiol. 1978 Feb;275:507–520. doi: 10.1113/jphysiol.1978.sp012204. [DOI] [PMC free article] [PubMed] [Google Scholar]
- Iwatsuki N., Petersen O. H. Pancreatic acinar cells: the acetylcholine equilibrium potential and its ionic dependency. J Physiol. 1977 Aug;269(3):735–751. doi: 10.1113/jphysiol.1977.sp011926. [DOI] [PMC free article] [PubMed] [Google Scholar]
- Kanagasuntheram P., Randle P. J. Calcium metabolism and amylase release in rat parotid acinar cells. Biochem J. 1976 Dec 15;160(3):547–564. doi: 10.1042/bj1600547. [DOI] [PMC free article] [PubMed] [Google Scholar]
- Katz B., Miledi R. The statistical nature of the acetycholine potential and its molecular components. J Physiol. 1972 Aug;224(3):665–699. doi: 10.1113/jphysiol.1972.sp009918. [DOI] [PMC free article] [PubMed] [Google Scholar]
- Keryer G., Rossignol B. Effect of carbachol on 45Ca uptake and protein secretion in rat lacrimal gland. Am J Physiol. 1976 Jan;230(1):99–104. doi: 10.1152/ajplegacy.1976.230.1.99. [DOI] [PubMed] [Google Scholar]
- Krishtal O. A., Pidoplichko V. I. A receptor for protons in the nerve cell membrane. Neuroscience. 1980;5(12):2325–2327. doi: 10.1016/0306-4522(80)90149-9. [DOI] [PubMed] [Google Scholar]
- Kusano K., Miledi R., Stinnakre J. Cholinergic and catecholaminergic receptors in the Xenopus oocyte membrane. J Physiol. 1982 Jul;328:143–170. doi: 10.1113/jphysiol.1982.sp014257. [DOI] [PMC free article] [PubMed] [Google Scholar]
- Martinez J. R., Holzgreve H., Frick A. Micropuncture study of submaxillary glands of adult rats. Pflugers Arch Gesamte Physiol Menschen Tiere. 1966;290(2):124–133. doi: 10.1007/BF00363690. [DOI] [PubMed] [Google Scholar]
- Marty A. Blocking of large unitary calcium-dependent potassium currents by internal sodium ions. Pflugers Arch. 1983 Feb;396(2):179–181. doi: 10.1007/BF00615524. [DOI] [PubMed] [Google Scholar]
- Marty A. Ca-dependent K channels with large unitary conductance in chromaffin cell membranes. Nature. 1981 Jun 11;291(5815):497–500. doi: 10.1038/291497a0. [DOI] [PubMed] [Google Scholar]
- Maruyama Y., Gallacher D. V., Petersen O. H. Voltage and Ca2+-activated K+ channel in baso-lateral acinar cell membranes of mammalian salivary glands. Nature. 1983 Apr 28;302(5911):827–829. doi: 10.1038/302827a0. [DOI] [PubMed] [Google Scholar]
- Maruyama Y., Petersen O. H. Cholecystokinin activation of single-channel currents is mediated by internal messenger in pancreatic acinar cells. Nature. 1982 Nov 4;300(5887):61–63. doi: 10.1038/300061a0. [DOI] [PubMed] [Google Scholar]
- Maruyama Y., Petersen O. H., Flanagan P., Pearson G. T. Quantification of Ca2+-activated K+ channels under hormonal control in pig pancreas acinar cells. Nature. 1983 Sep 15;305(5931):228–232. doi: 10.1038/305228a0. [DOI] [PubMed] [Google Scholar]
- Maruyama Y., Peterson O. H. Single-channel currents in isolated patches of plasma membrane from basal surface of pancreatic acini. Nature. 1982 Sep 9;299(5879):159–161. doi: 10.1038/299159a0. [DOI] [PubMed] [Google Scholar]
- Miledi R. A calcium-dependent transient outward current in Xenopus laevis oocytes. Proc R Soc Lond B Biol Sci. 1982 Jul 22;215(1201):491–497. doi: 10.1098/rspb.1982.0056. [DOI] [PubMed] [Google Scholar]
- Miller C. Open-state substructure of single chloride channels from Torpedo electroplax. Philos Trans R Soc Lond B Biol Sci. 1982 Dec 1;299(1097):401–411. doi: 10.1098/rstb.1982.0140. [DOI] [PubMed] [Google Scholar]
- Mori H., Murakami M., Nakahari T., Imai Y. Intracellular Cl- activity of canine submandibular gland cells: an in vitro observation. Jpn J Physiol. 1983;33(5):869–873. doi: 10.2170/jjphysiol.33.869. [DOI] [PubMed] [Google Scholar]
- Neher E., Stevens C. F. Conductance fluctuations and ionic pores in membranes. Annu Rev Biophys Bioeng. 1977;6:345–381. doi: 10.1146/annurev.bb.06.060177.002021. [DOI] [PubMed] [Google Scholar]
- Oschman J. L., Berridge M. J. Structural and functional aspects of salivary fluid section in Calliphora. Tissue Cell. 1970;2(2):281–310. doi: 10.1016/s0040-8166(70)80021-0. [DOI] [PubMed] [Google Scholar]
- Petersen O. H., Maruyama Y. Calcium-activated potassium channels and their role in secretion. Nature. 1984 Feb 23;307(5953):693–696. doi: 10.1038/307693a0. [DOI] [PubMed] [Google Scholar]
- Petersen O. H. Some factors influencing stimulation-induced release of potassium from the cat submandibular gland to fluid perfused through the gland. J Physiol. 1970 Jun;208(2):431–447. doi: 10.1113/jphysiol.1970.sp009129. [DOI] [PMC free article] [PubMed] [Google Scholar]
- Petersen O. H. The effect of dinitrophenol on secretory potentials, secretion and potassium accumulation in the perfused cat submandibular gland. Acta Physiol Scand. 1970 Sep;80(1):117–121. doi: 10.1111/j.1748-1716.1970.tb04775.x. [DOI] [PubMed] [Google Scholar]
- Poulsen J. H. Acetylcholine-induced transport of Na+ and K+ in the perfused cat submandibular gland. Pflugers Arch. 1974 Jul 9;349(3):215–220. doi: 10.1007/BF00592449. [DOI] [PubMed] [Google Scholar]
- Poulsen J. H., Oakley B., 2nd Intracellular potassium ion activity in resting and stimulated mouse pancreas and submandibular gland. Proc R Soc Lond B Biol Sci. 1979 Mar 26;204(1154):99–104. doi: 10.1098/rspb.1979.0015. [DOI] [PubMed] [Google Scholar]
- Roberts M. L., Iwatsuki N., Petersen O. H. Parotid acinar cells: ionic dependence of acetylcholine-evoked membrane potential changes. Pflugers Arch. 1978 Sep 6;376(2):159–167. doi: 10.1007/BF00581579. [DOI] [PubMed] [Google Scholar]
- Sasaki S., Nakagaki I., Mori H., Imai Y. Intracellular calcium store and transport of elements in acinar cells of the salivary gland determined by electron probe X-ray microanalysis. Jpn J Physiol. 1983;33(1):69–83. doi: 10.2170/jjphysiol.33.69. [DOI] [PubMed] [Google Scholar]
- Schneyer L. H., Young J. A., Schneyer C. A. Salivary secretion of electrolytes. Physiol Rev. 1972 Jul;52(3):720–777. doi: 10.1152/physrev.1972.52.3.720. [DOI] [PubMed] [Google Scholar]
- Selinger Z., Eimerl S., Schramm M. A calcium ionophore simulating the action of epinephrine on the alpha-adrenergic receptor. Proc Natl Acad Sci U S A. 1974 Jan;71(1):128–131. doi: 10.1073/pnas.71.1.128. [DOI] [PMC free article] [PubMed] [Google Scholar]
- Sigworth F. J. The variance of sodium current fluctuations at the node of Ranvier. J Physiol. 1980 Oct;307:97–129. doi: 10.1113/jphysiol.1980.sp013426. [DOI] [PMC free article] [PubMed] [Google Scholar]
- Trautmann A., Marty A. Activation of Ca-dependent K channels by carbamoylcholine in rat lacrimal glands. Proc Natl Acad Sci U S A. 1984 Jan;81(2):611–615. doi: 10.1073/pnas.81.2.611. [DOI] [PMC free article] [PubMed] [Google Scholar]
- Vergara C., Latorre R. Kinetics of Ca2+-activated K+ channels from rabbit muscle incorporated into planar bilayers. Evidence for a Ca2+ and Ba2+ blockade. J Gen Physiol. 1983 Oct;82(4):543–568. doi: 10.1085/jgp.82.4.543. [DOI] [PMC free article] [PubMed] [Google Scholar]
- Wakui M., Nishiyama A. Ionic dependence of acetylcholine equilibrium potential of acinar cells in mouse submaxillary gland. Pflugers Arch. 1980 Aug;386(3):261–267. doi: 10.1007/BF00587477. [DOI] [PubMed] [Google Scholar]
- Weber A. Regulatory mechanisms of the calcium transport system of fragmented rabbit sarcoplasmic rticulum. I. The effect of accumulated calcium on transport and adenosine triphosphate hydrolysis. J Gen Physiol. 1971 Jan;57(1):50–63. doi: 10.1085/jgp.57.1.50. [DOI] [PMC free article] [PubMed] [Google Scholar]
- Weber A. Regulatory mechanisms ofthe calcium transport system of ramented rabbit sarcoplasmic rticulum. II. Inhibition of outflux in calcium-free media. J Gen Physiol. 1971 Jan;57(1):64–70. doi: 10.1085/jgp.57.1.64. [DOI] [PMC free article] [PubMed] [Google Scholar]
- Yellen G. Single Ca2+-activated nonselective cation channels in neuroblastoma. Nature. 1982 Mar 25;296(5855):357–359. doi: 10.1038/296357a0. [DOI] [PubMed] [Google Scholar]
- Young J. A., Schögel E. Micropuncture investigation of sodium and potassium excretion in rat submaxillary saliva. Pflugers Arch Gesamte Physiol Menschen Tiere. 1966;291(1):85–98. doi: 10.1007/BF00362654. [DOI] [PubMed] [Google Scholar]