Abstract
Voltage-clamp studies were carried out to compare currents through Ca2+ channels (ICa) with Na+ currents (Ins) through a non-selective cation conductance blocked by micromolar concentrations of external Ca2+. The gating of both currents was found to have similar time and voltage dependence. The amplitudes of ICa and Ins varied widely, but Ins was always large in fibres with large ICa, and small in fibres with small ICa. Both ICa and Ins were blocked by the specific Ca2+ channel blocker nifedipine, with half-blockage concentrations that were virtually identical (KD = 0.9 microM for ICa and 0.7 microM for Ins). ICa and Ins were also equally sensitive to block by diltiazem (KD = 80 microM). These parallels between Ins and ICa are most easily explained if Ins flows through Ca2+ channels. Apparently, Ca2+ channels bear high-affinity Ca2+-binding sites, and are highly permeable to monovalent cations when Ca2+ is absent. Ba2+ currents (IBa) and ICa were measured in external solutions containing mixtures of Ba2+ and Ca2+. IBa is blocked by Ca2+, as is Ins. Adding Ba2+ to Ca2+ produces only small or no increases in current, as if Ba2+ is only sparingly permeant when Ca2+ is present. Membrane currents in Ba2+/Ca2+ mixtures show anomalous mole-fraction behaviour, suggesting that Ca2+ channels are single-file, multi-ion pores. Complex current transients are observed under maintained depolarizations in Na+/Ca2+ and Ba2+/Ca2+ mixtures. They suggest that in ion mixtures, Ca2+ channels transport Ca2+ in preference to Na+ and Ba2+. Hence Ca2+ channels are selective for Ca2+, even though current amplitudes suggest that the Na+ or Ba2+ permeabilities in the absence of Ca2+ are as high as, or higher than, the Ca2+ permeability. We conclude that the selective permeability of Ca2+ channels depends on the presence of Ca2+. In model calculations, our observations are explained as a consequence of Ca2+ channels being single-file pores. It is proposed that Ca2+ channels derive much of their ion selectivity from high-affinity Ca2+ binding sites located in an otherwise unselective aqueous pore.
Full text
PDF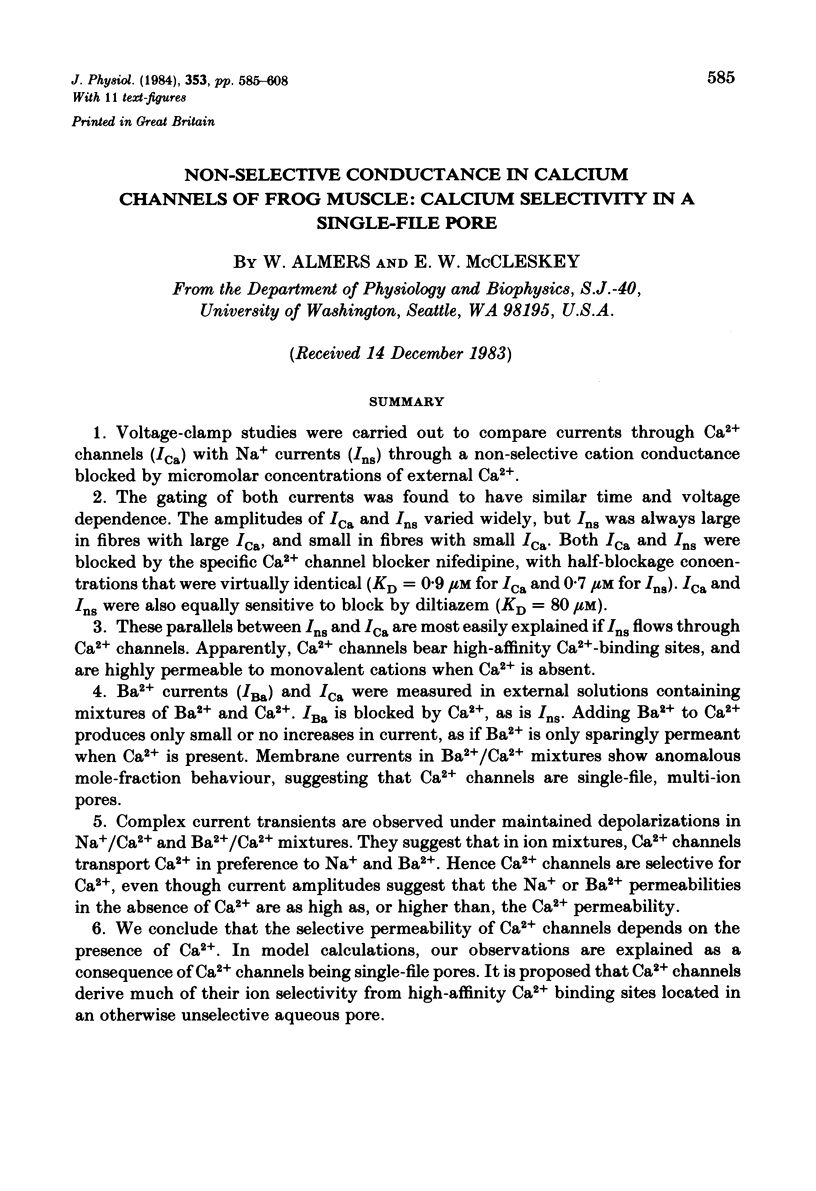
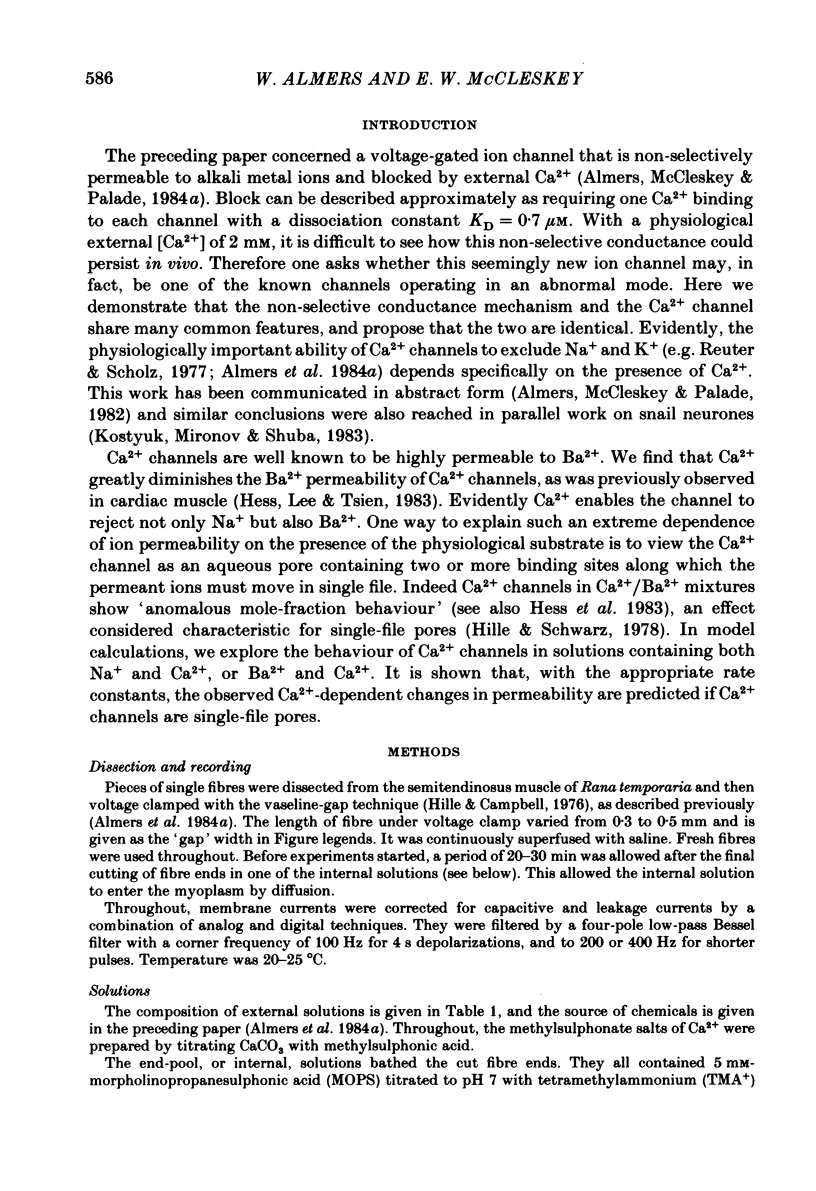
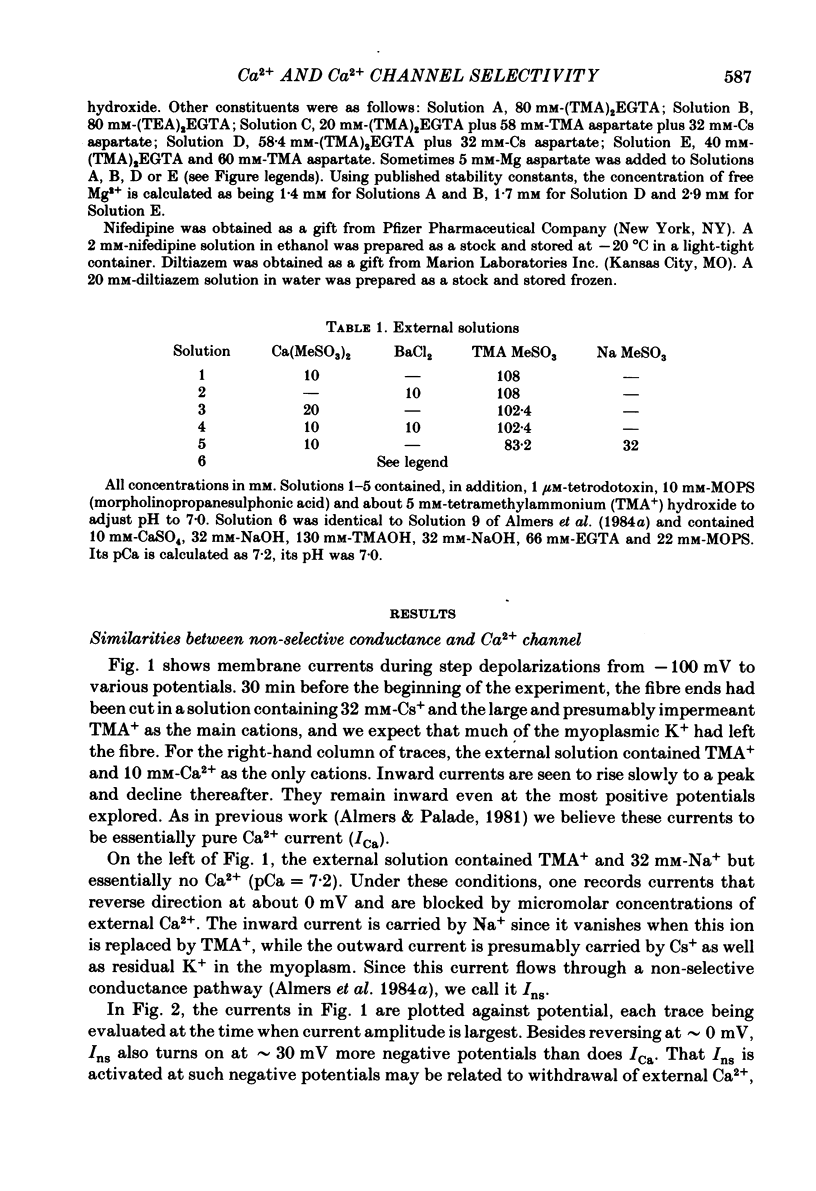
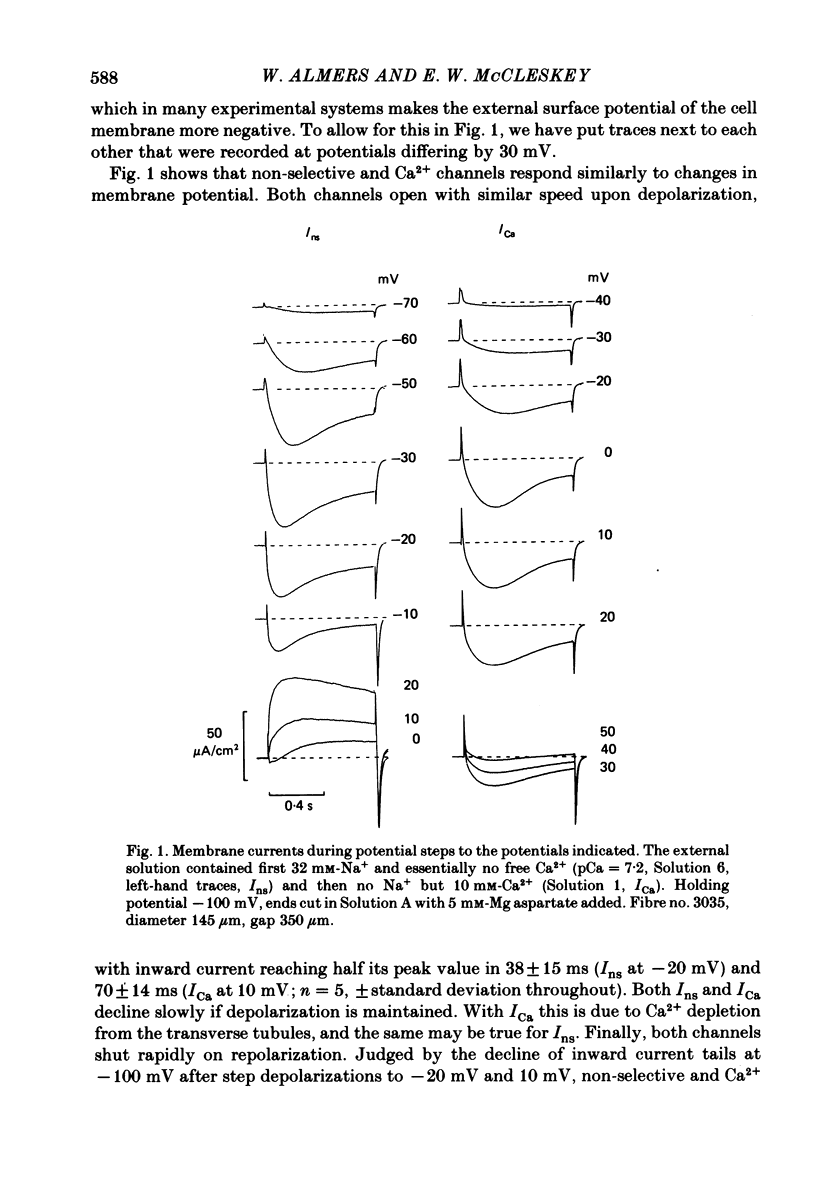
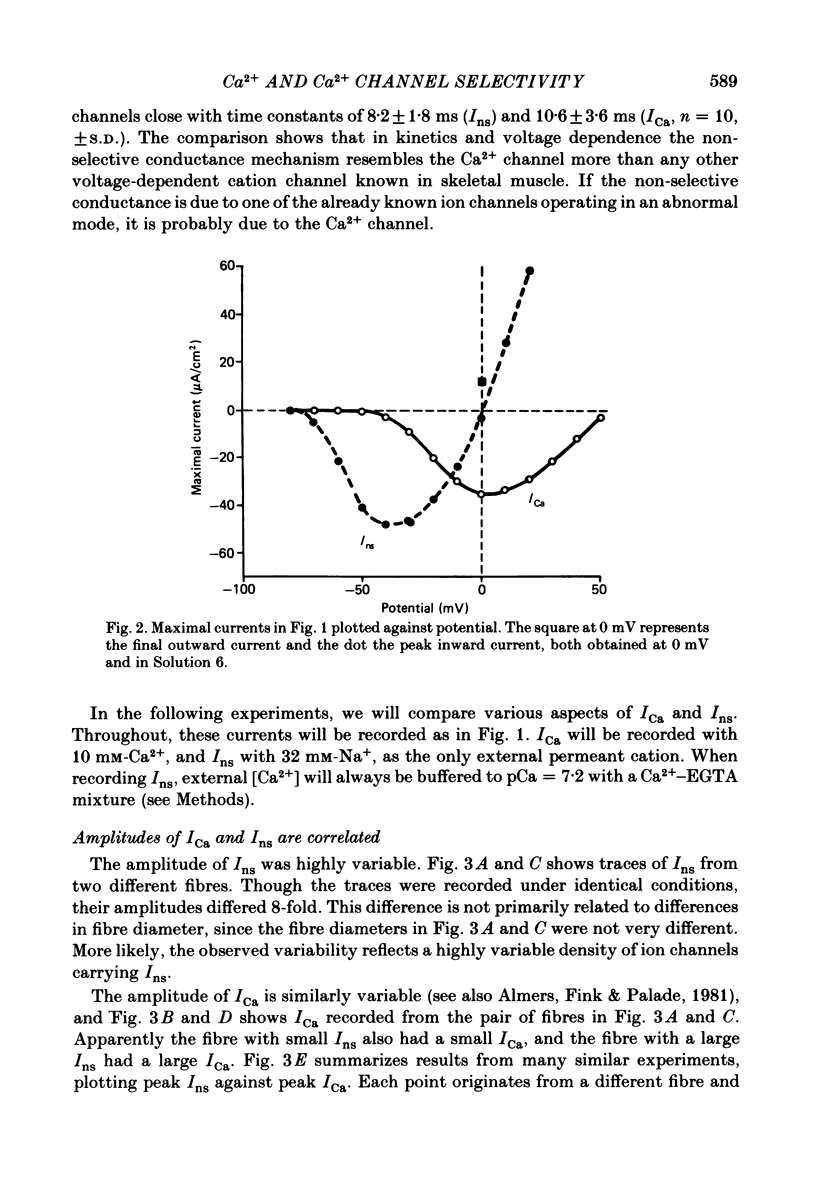
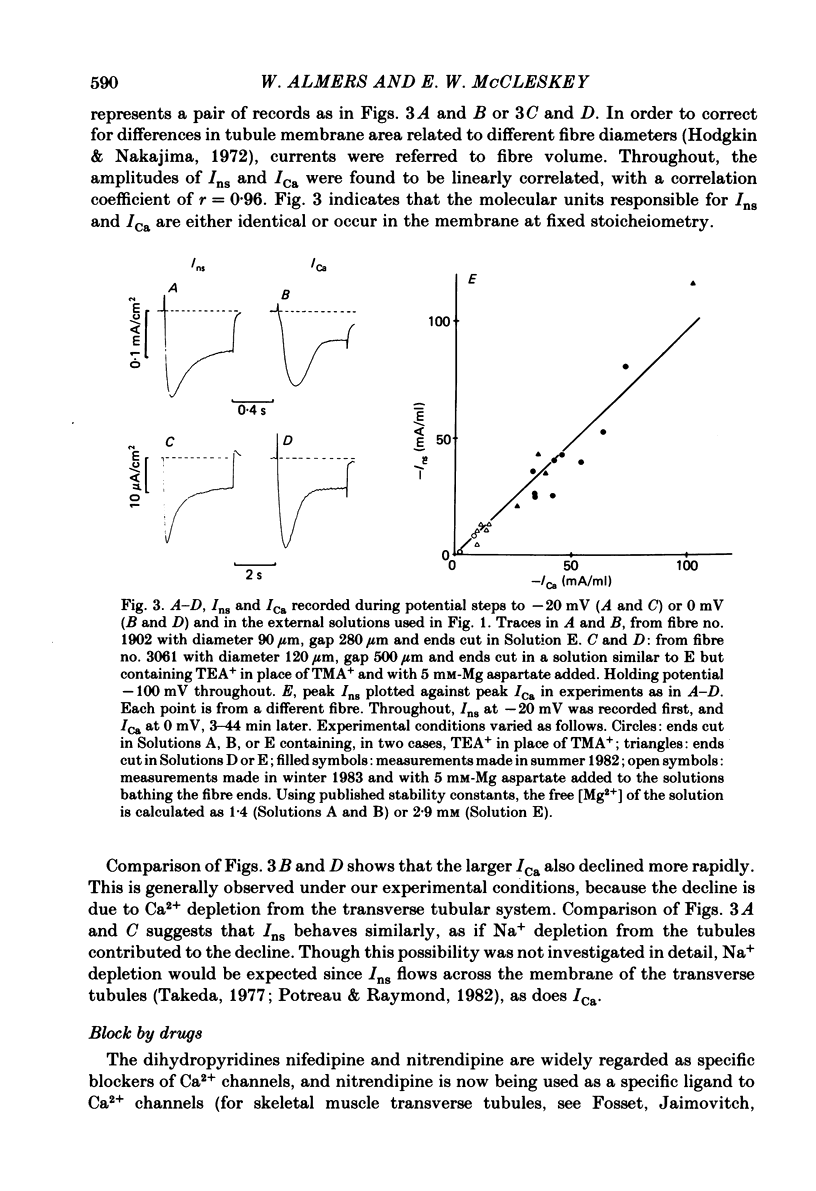
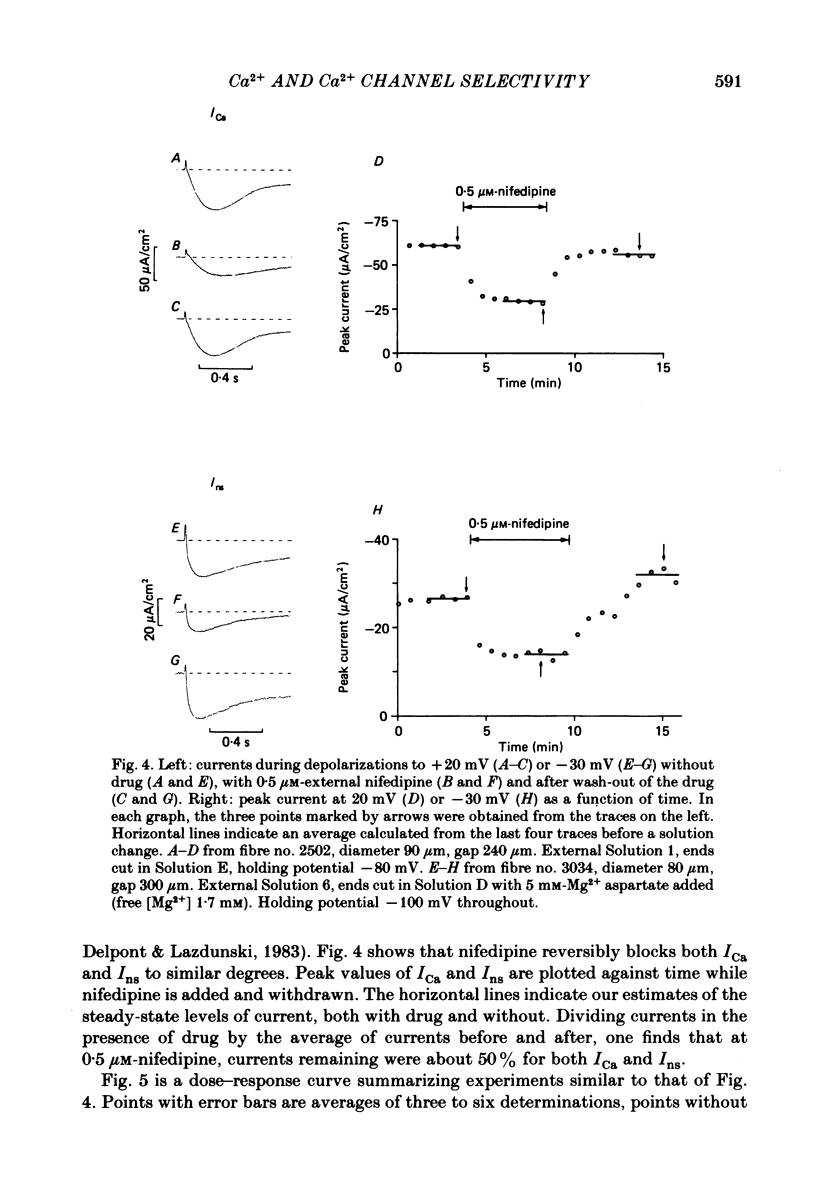
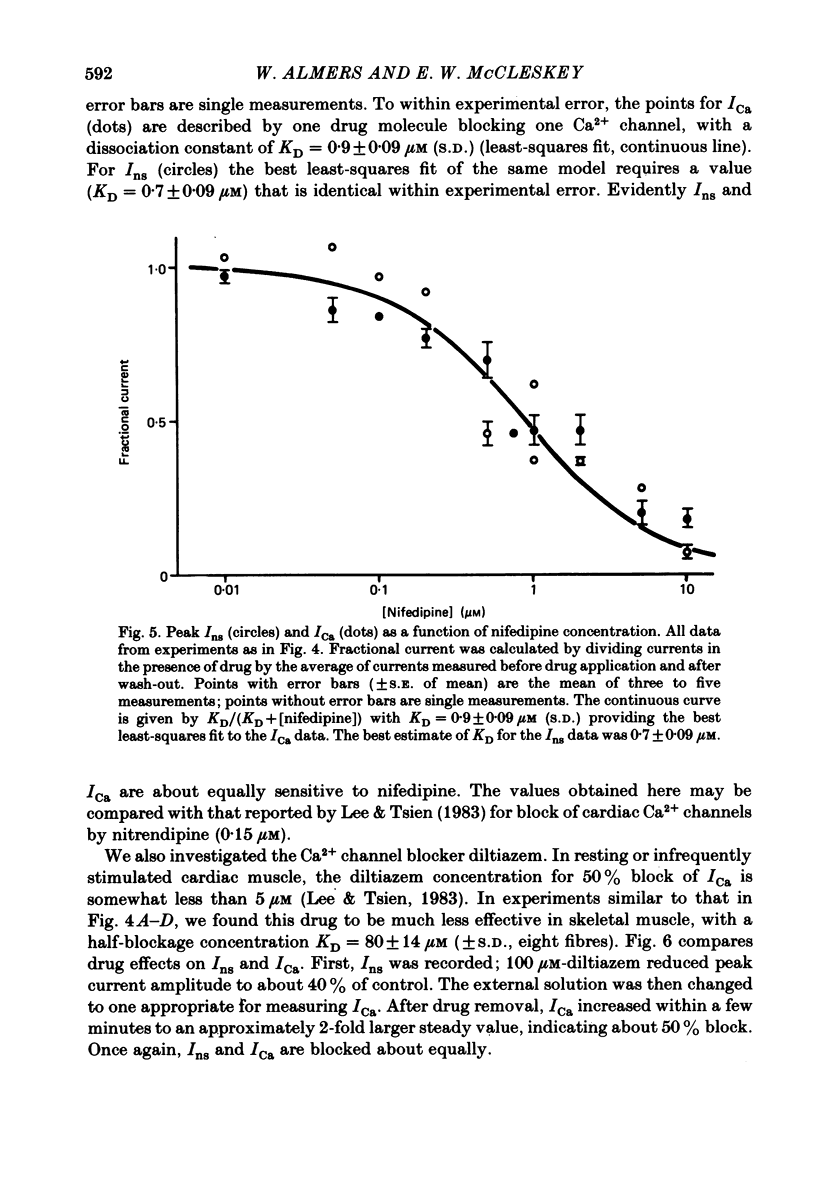
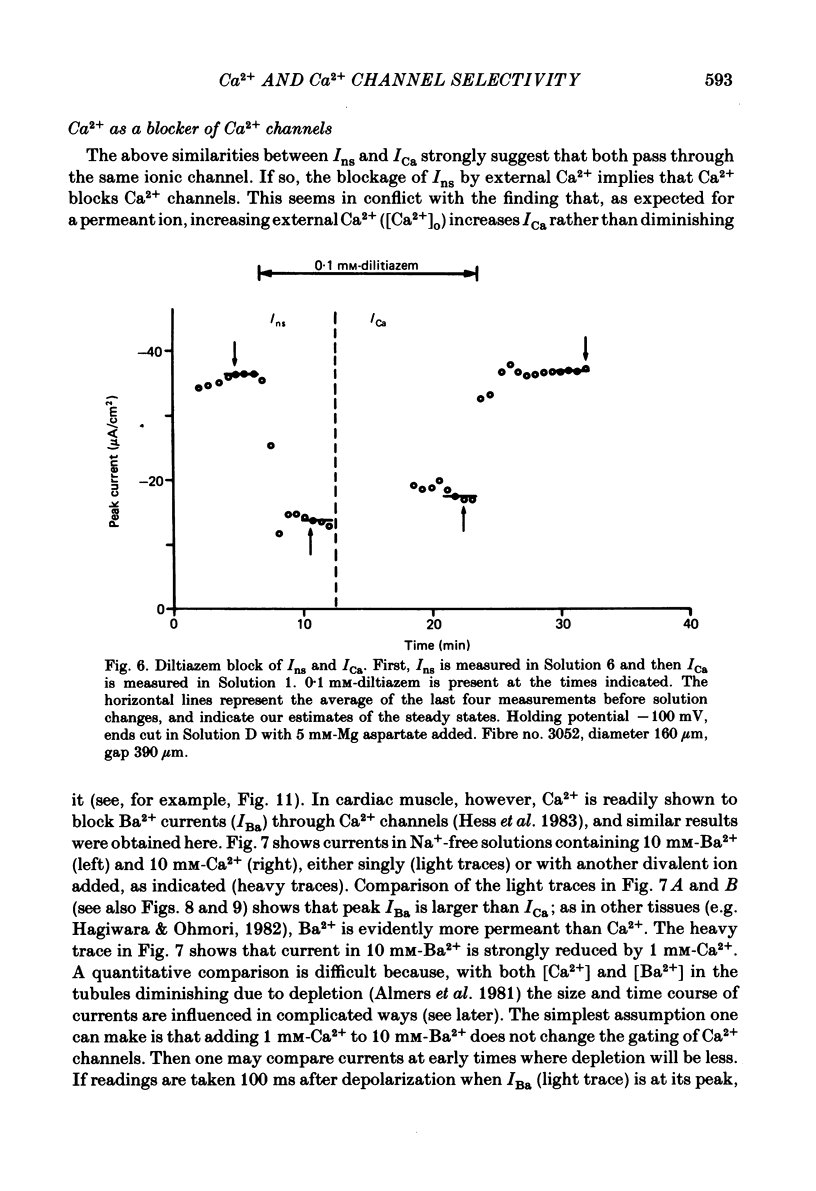
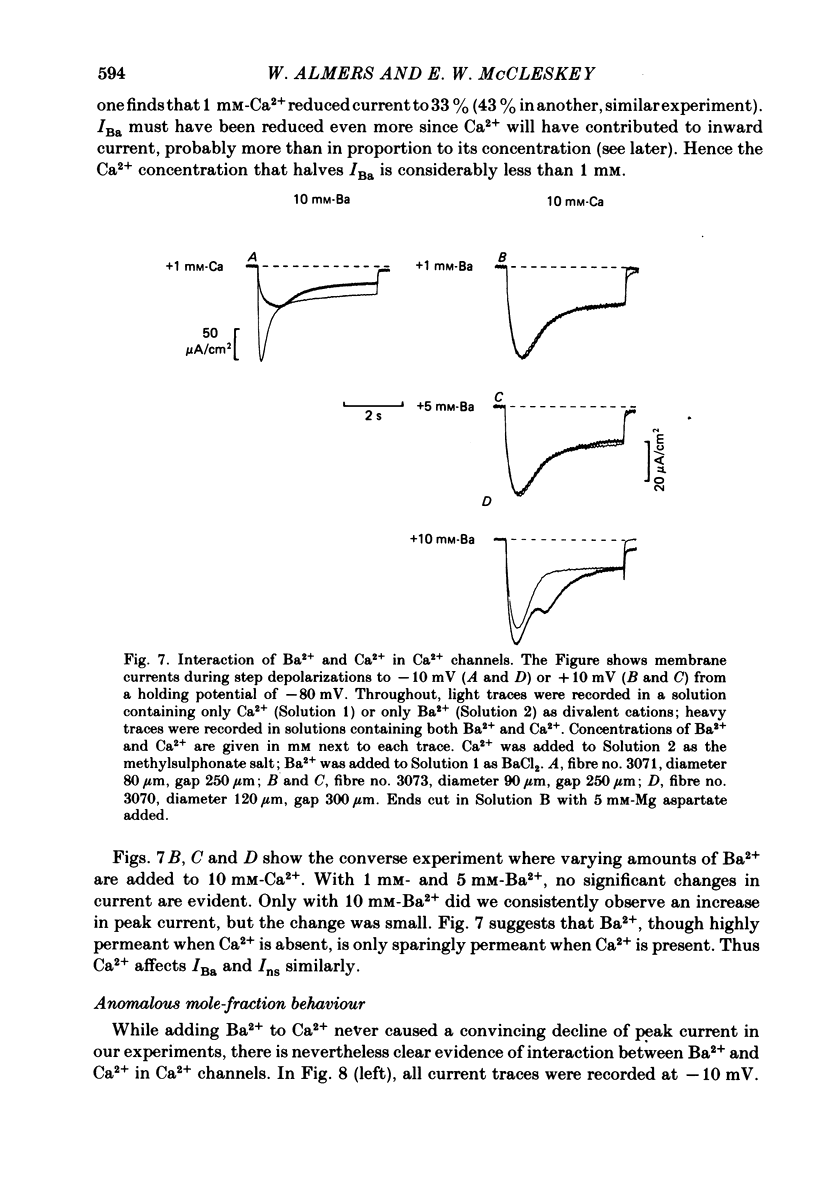
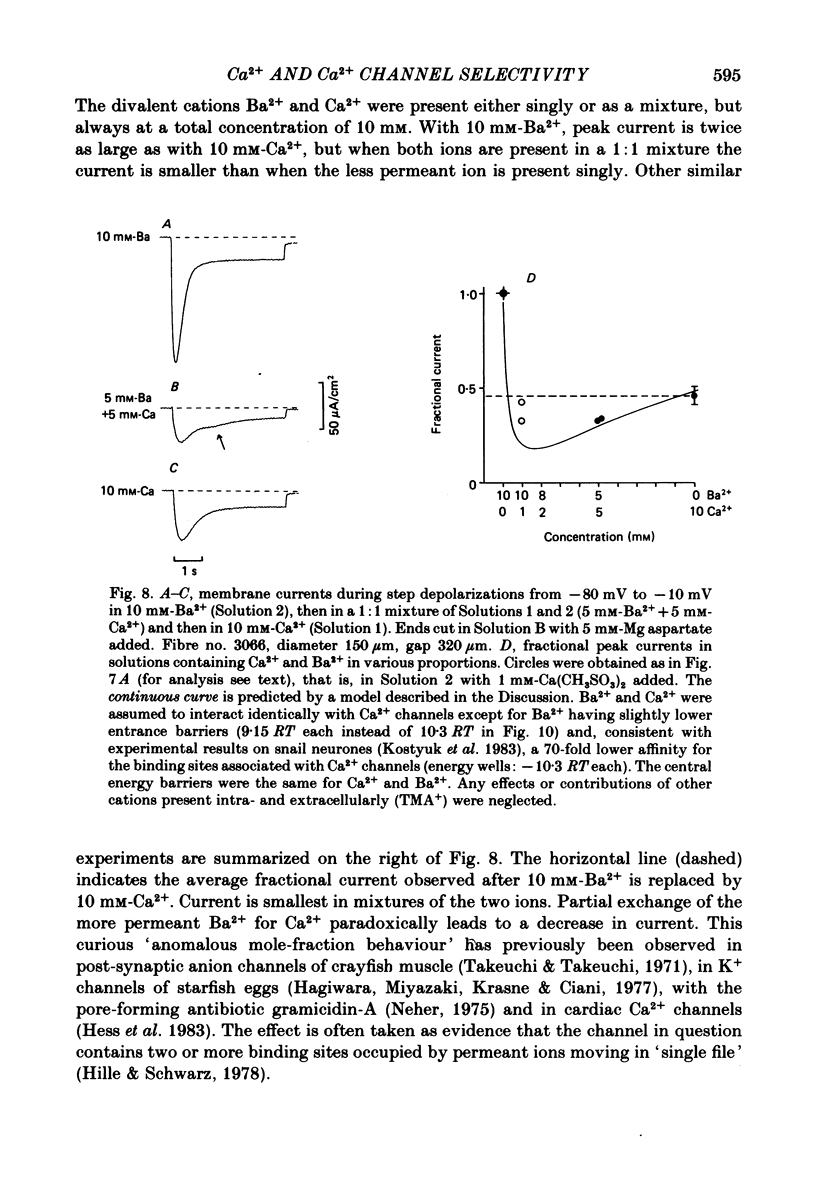
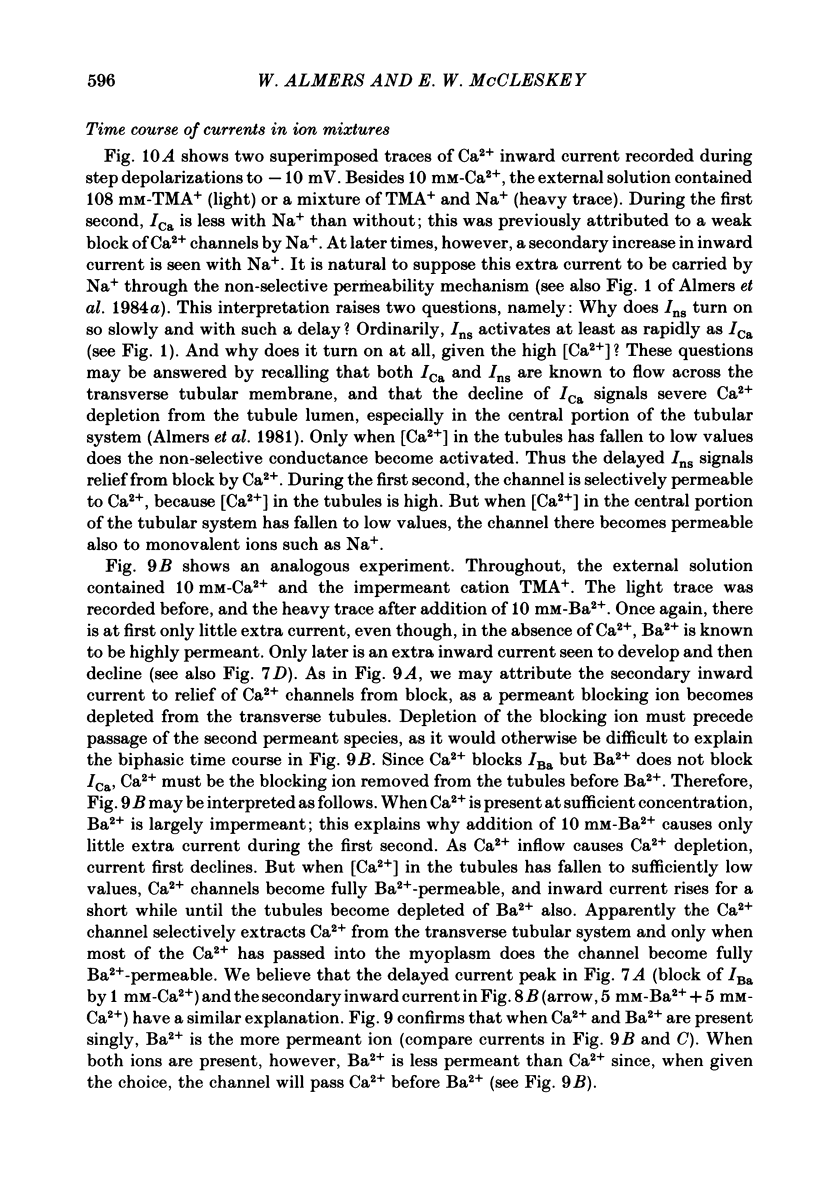
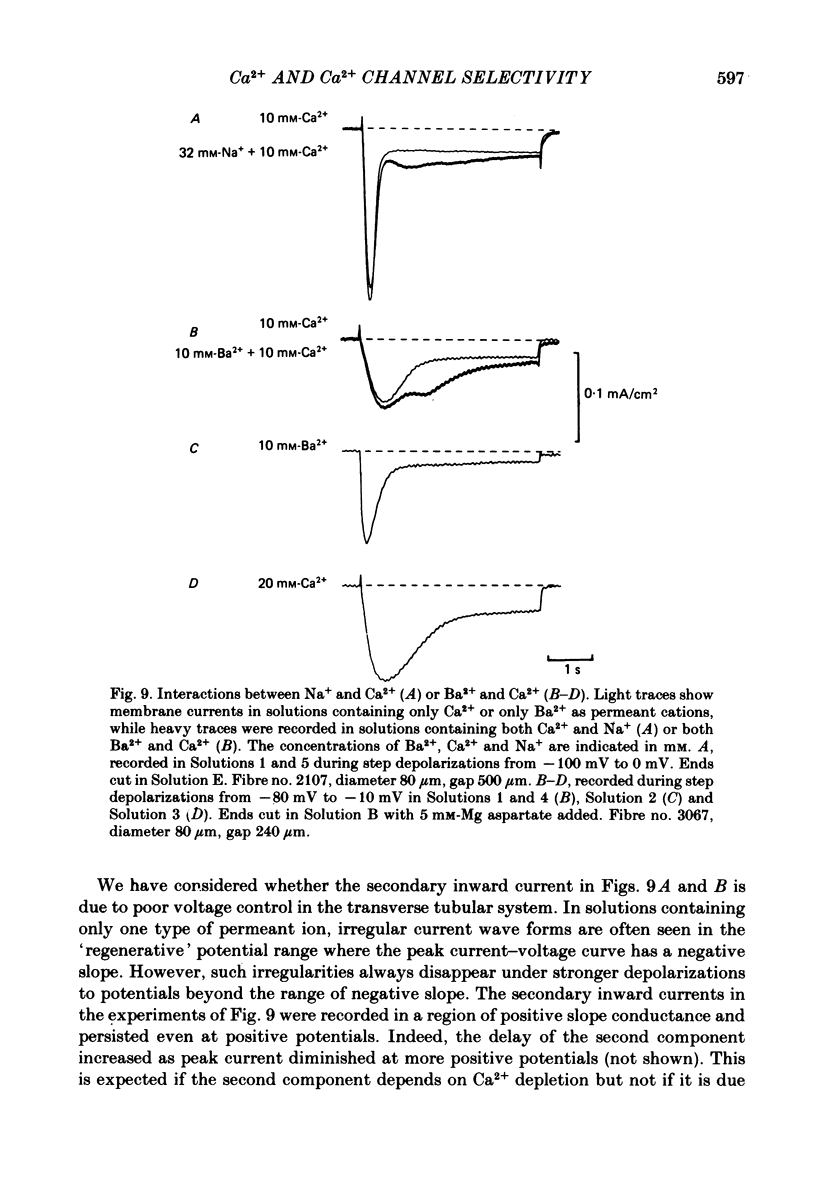
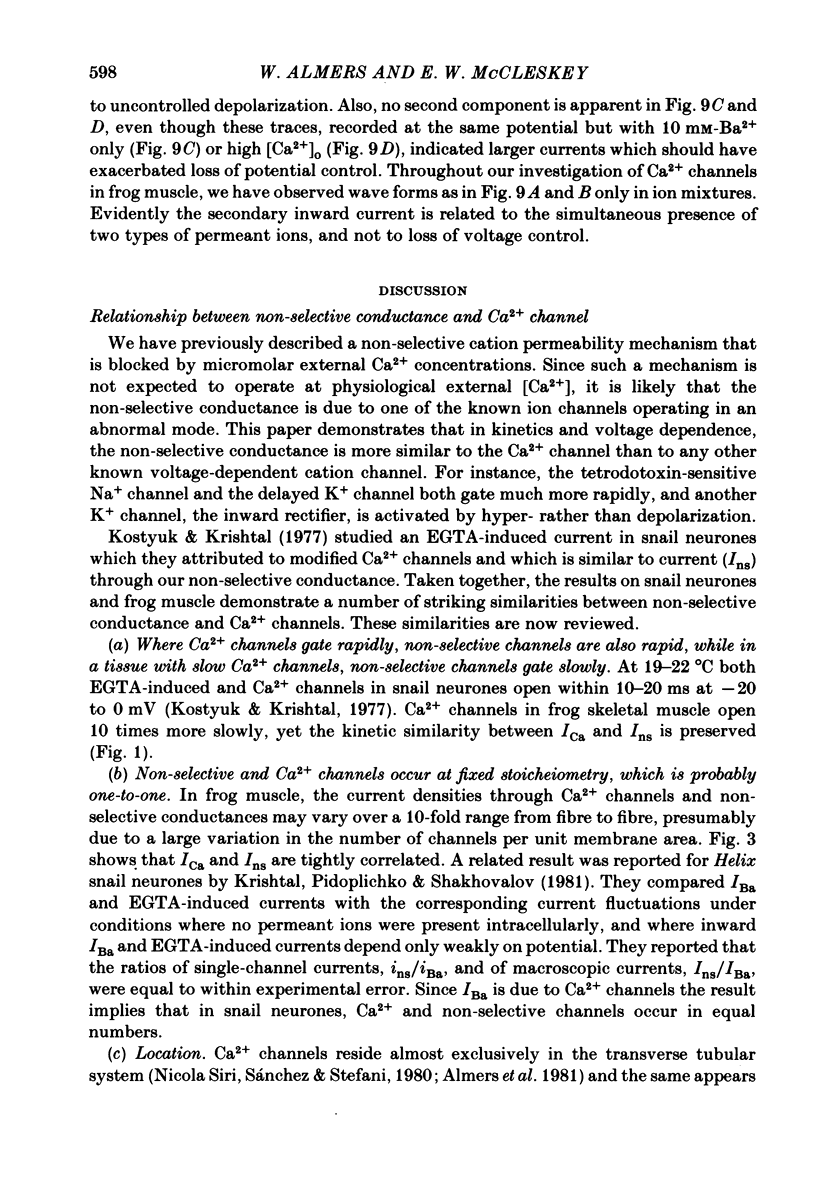
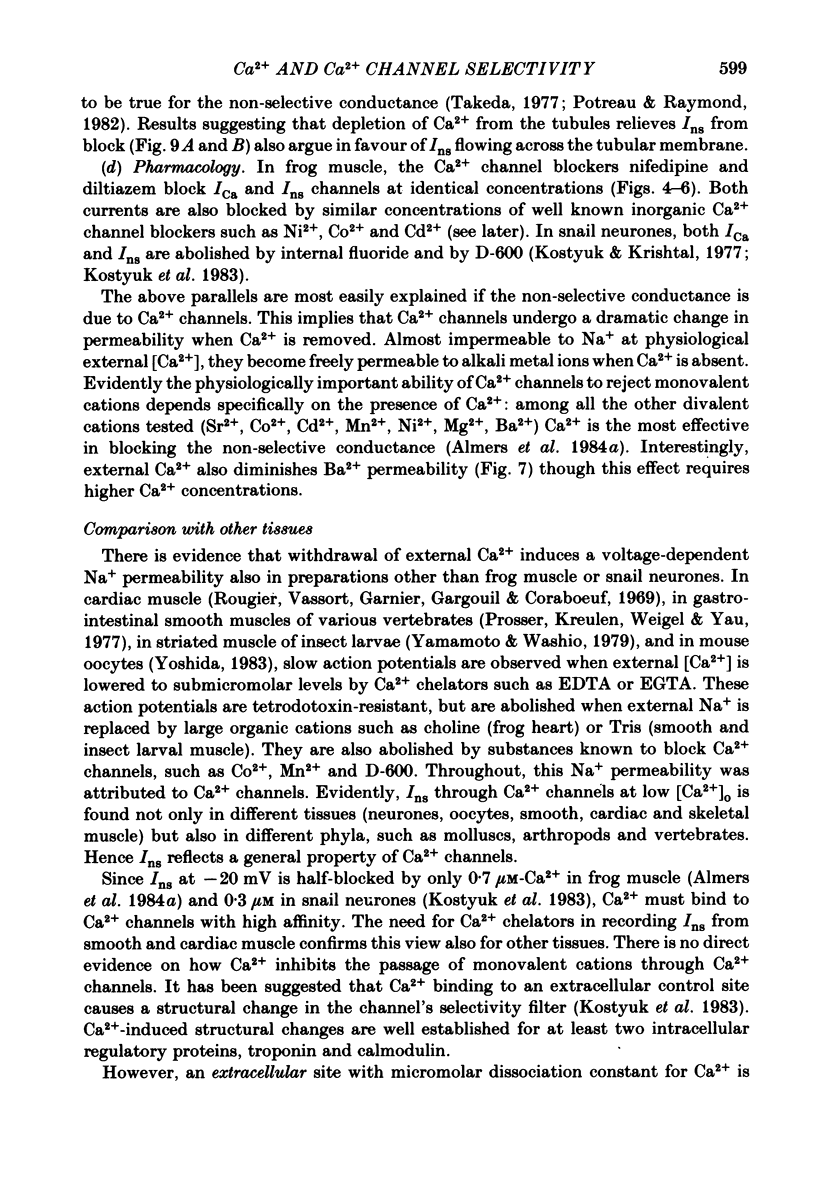
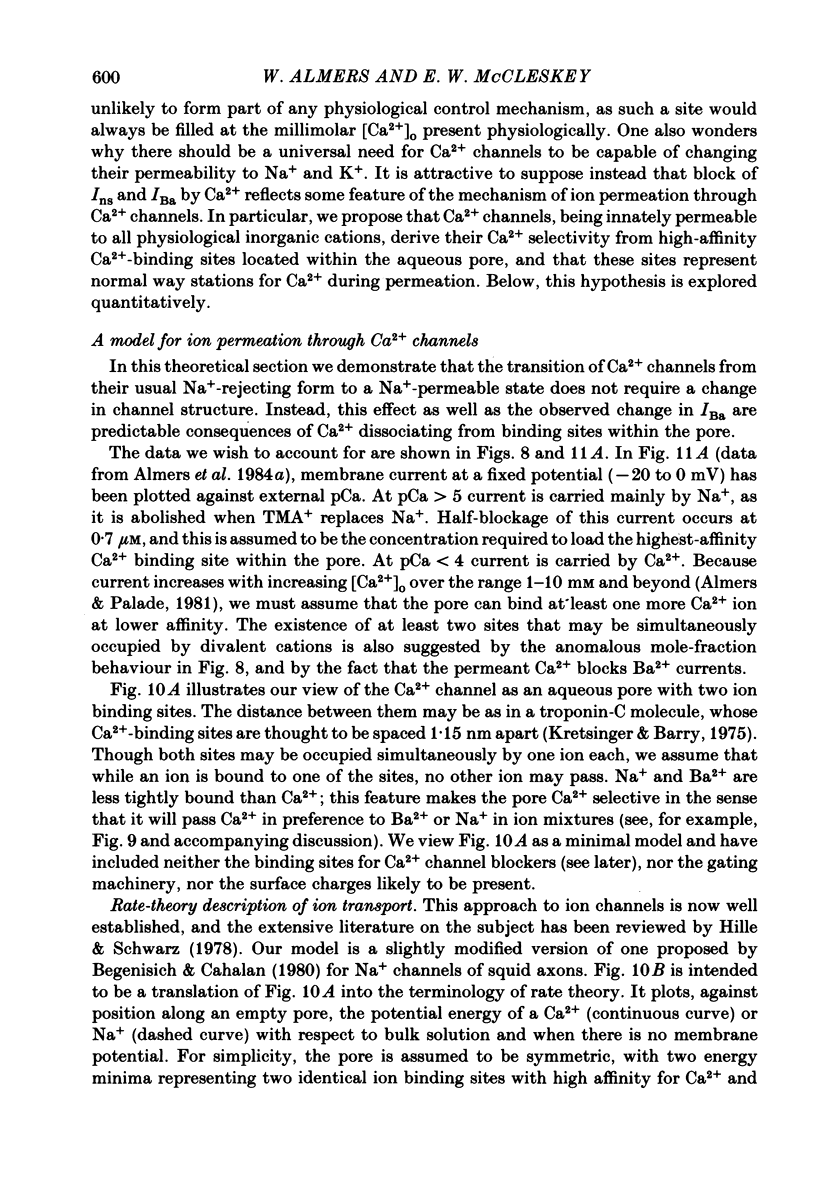
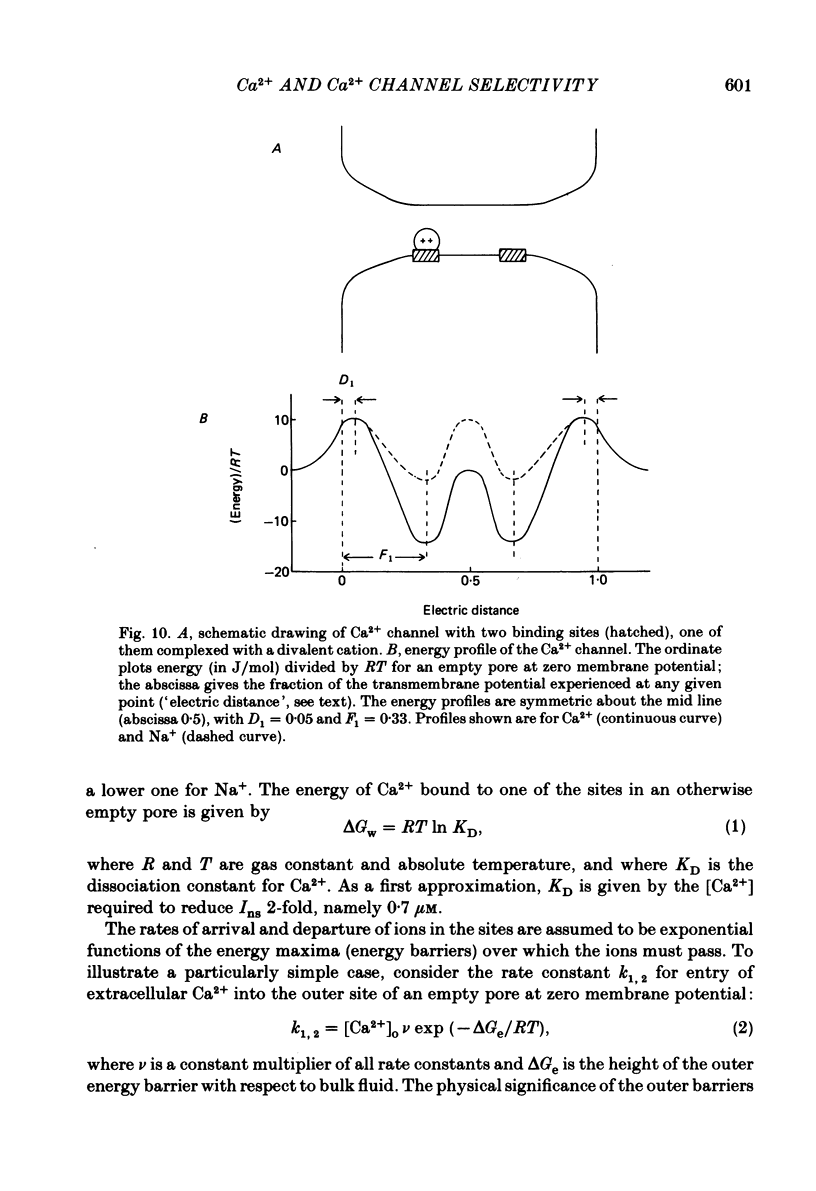
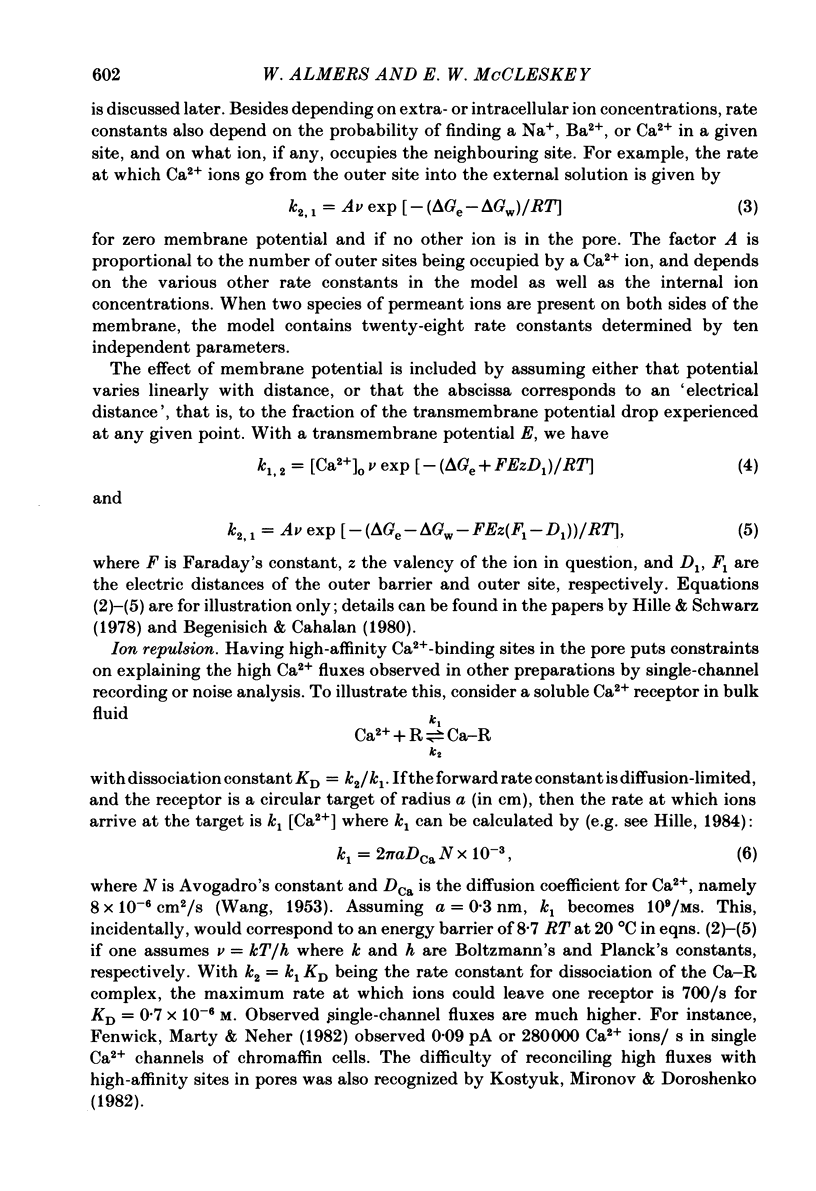
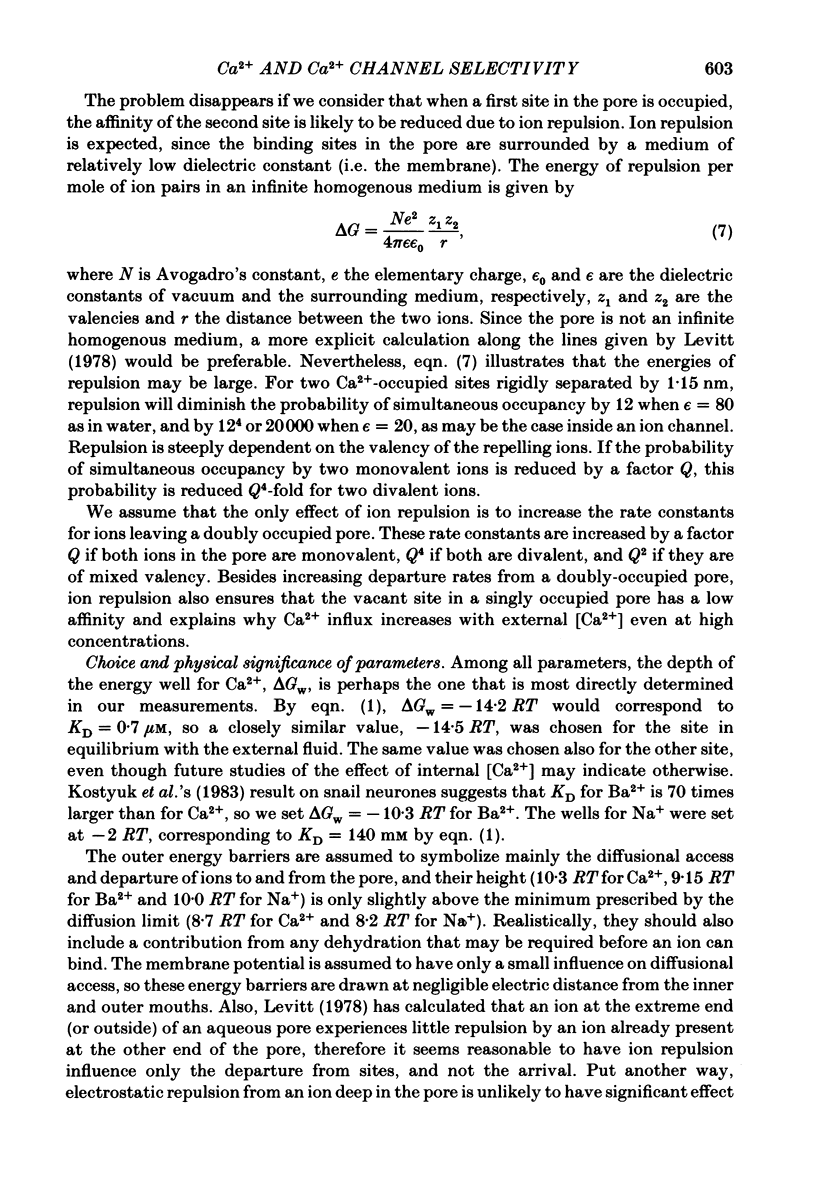
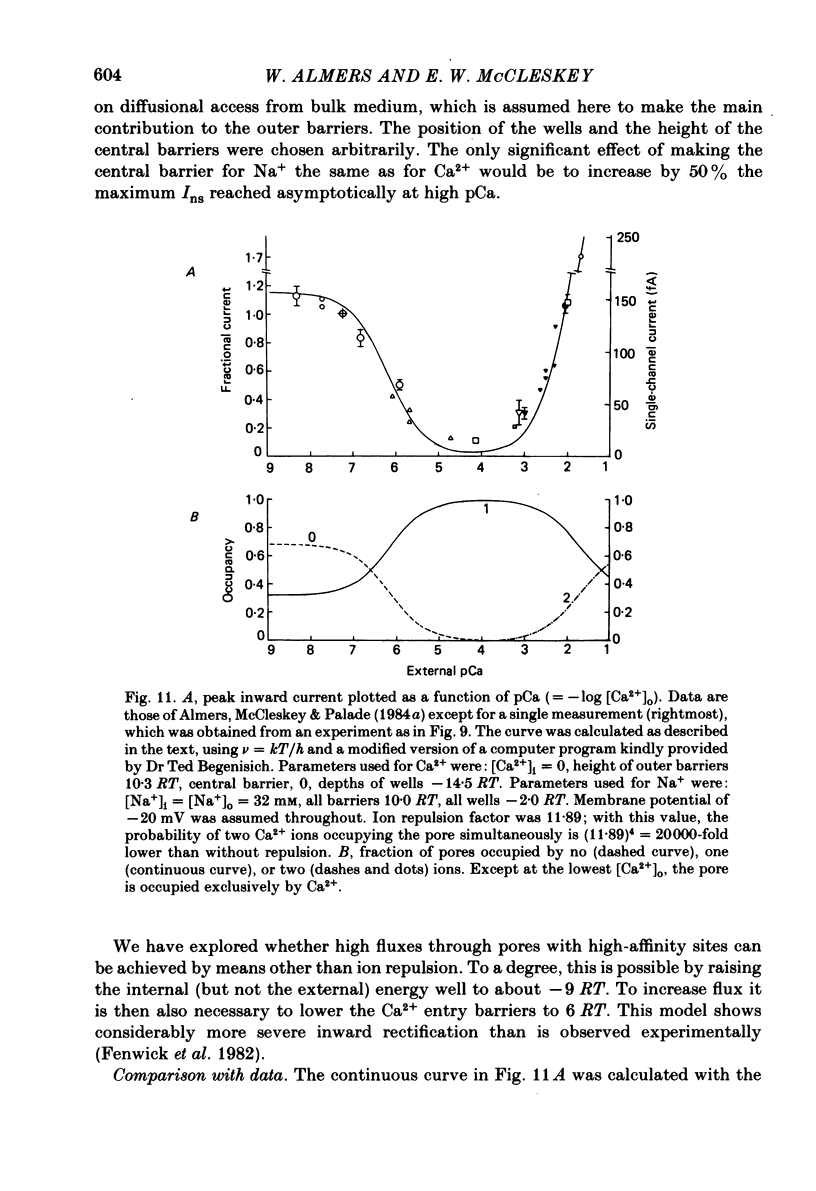
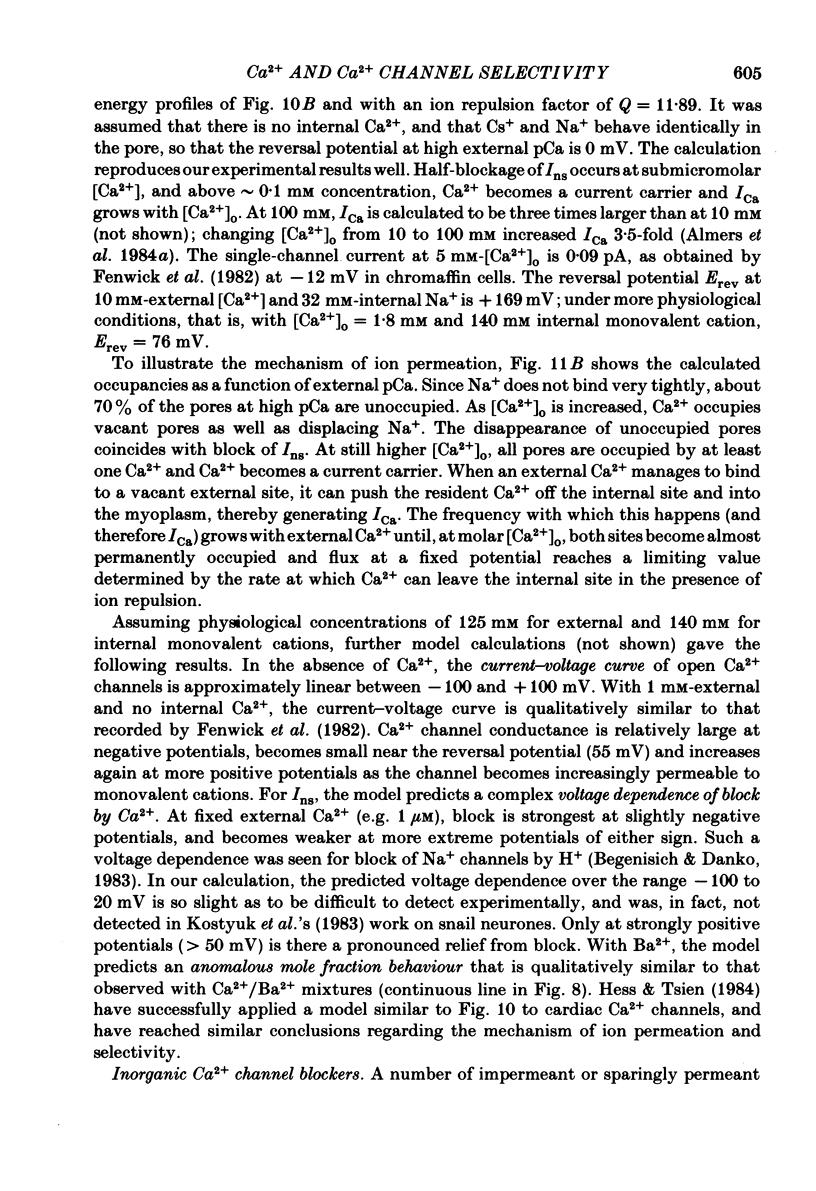
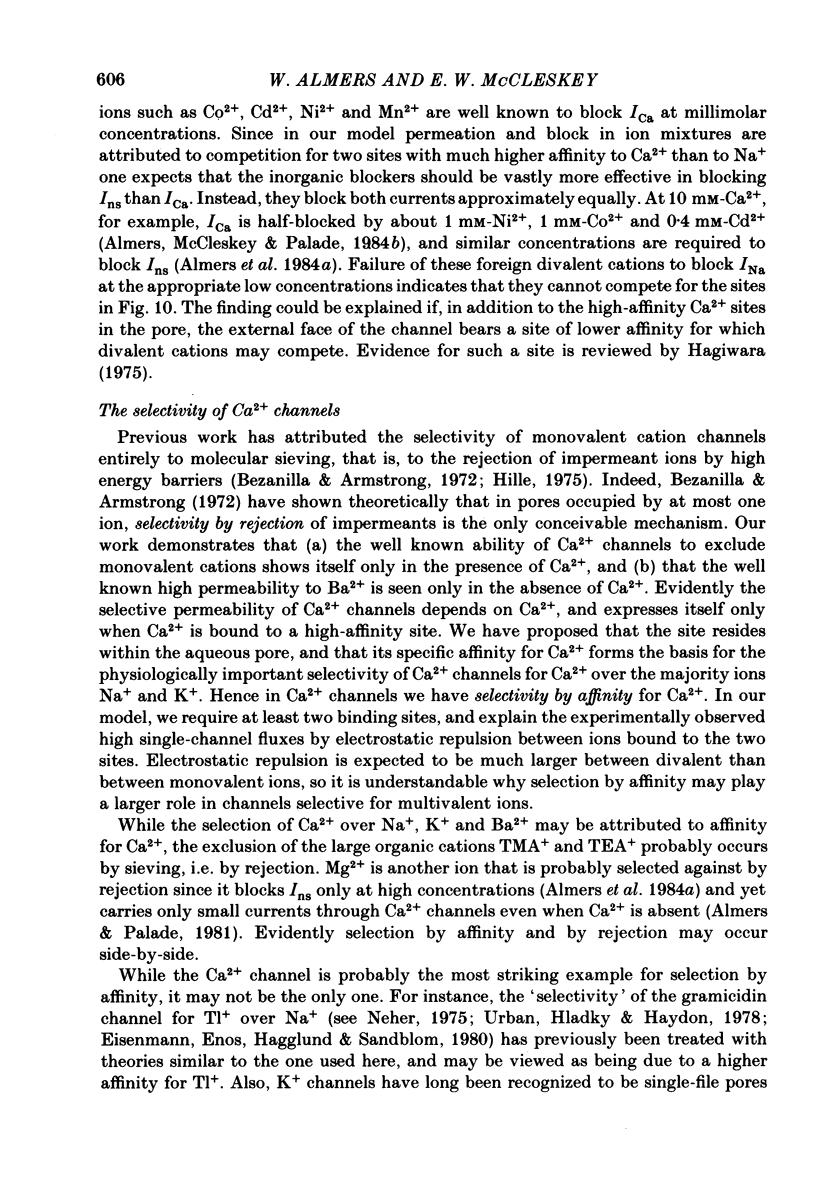
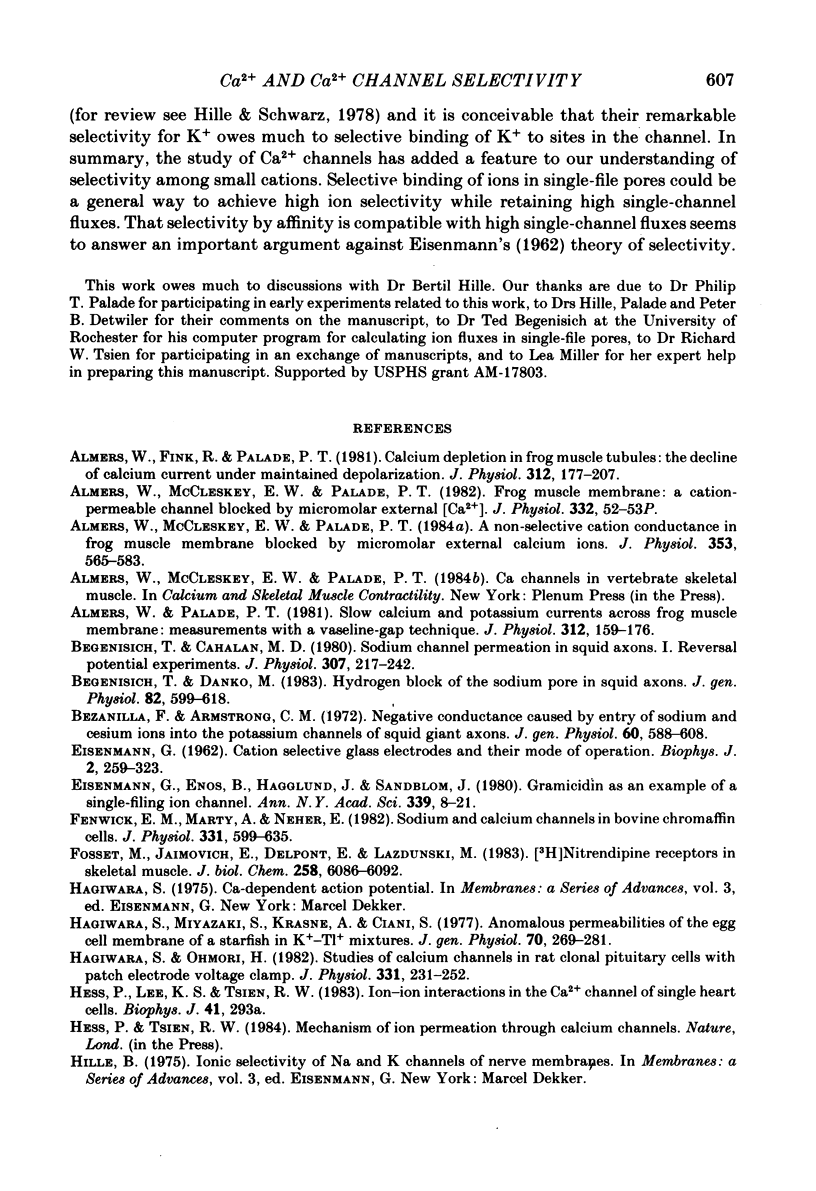
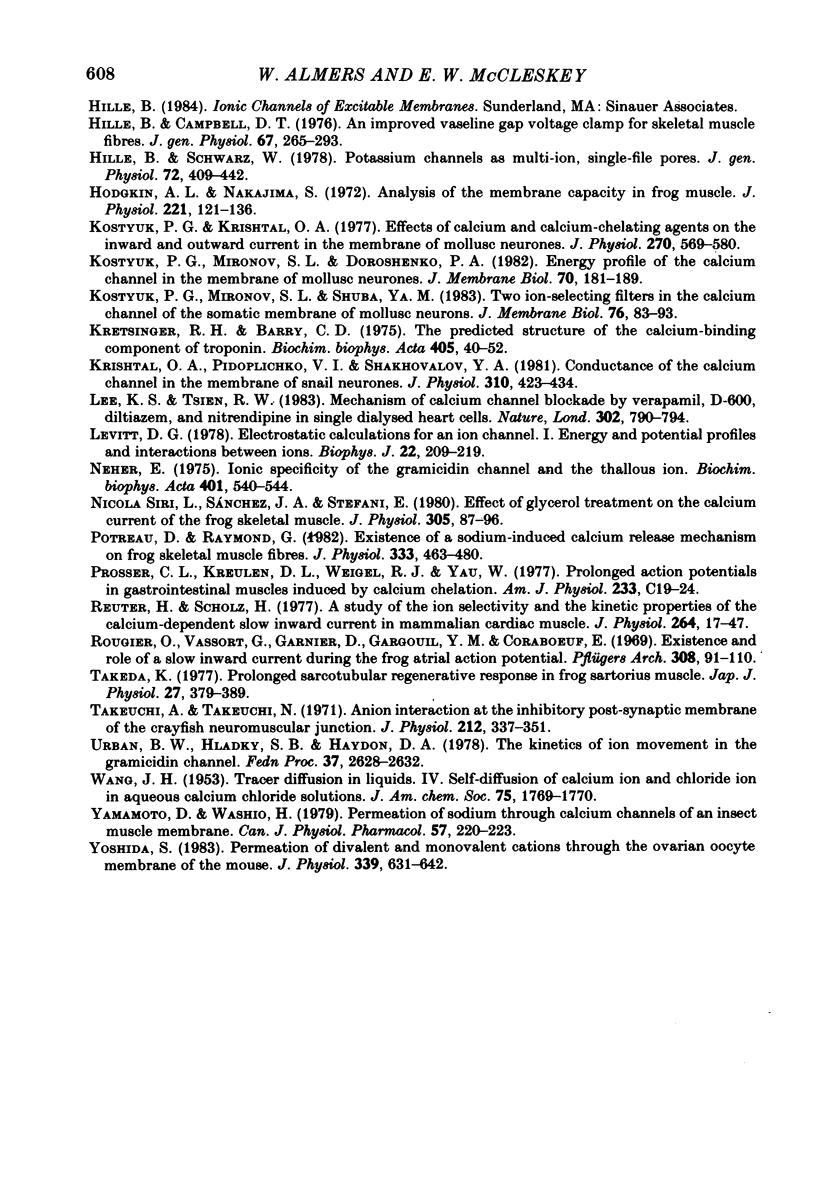
Selected References
These references are in PubMed. This may not be the complete list of references from this article.
- Almers W., Fink R., Palade P. T. Calcium depletion in frog muscle tubules: the decline of calcium current under maintained depolarization. J Physiol. 1981 Mar;312:177–207. doi: 10.1113/jphysiol.1981.sp013623. [DOI] [PMC free article] [PubMed] [Google Scholar]
- Almers W., McCleskey E. W., Palade P. T. A non-selective cation conductance in frog muscle membrane blocked by micromolar external calcium ions. J Physiol. 1984 Aug;353:565–583. doi: 10.1113/jphysiol.1984.sp015351. [DOI] [PMC free article] [PubMed] [Google Scholar]
- Almers W., Palade P. T. Slow calcium and potassium currents across frog muscle membrane: measurements with a vaseline-gap technique. J Physiol. 1981 Mar;312:159–176. doi: 10.1113/jphysiol.1981.sp013622. [DOI] [PMC free article] [PubMed] [Google Scholar]
- Begenisich T. B., Cahalan M. D. Sodium channel permeation in squid axons. I: Reversal potential experiments. J Physiol. 1980 Oct;307:217–242. doi: 10.1113/jphysiol.1980.sp013432. [DOI] [PMC free article] [PubMed] [Google Scholar]
- Begenisich T., Danko M. Hydrogen ion block of the sodium pore in squid giant axons. J Gen Physiol. 1983 Nov;82(5):599–618. doi: 10.1085/jgp.82.5.599. [DOI] [PMC free article] [PubMed] [Google Scholar]
- Bezanilla F., Armstrong C. M. Negative conductance caused by entry of sodium and cesium ions into the potassium channels of squid axons. J Gen Physiol. 1972 Nov;60(5):588–608. doi: 10.1085/jgp.60.5.588. [DOI] [PMC free article] [PubMed] [Google Scholar]
- EISENMAN G. Cation selective glass electrodes and their mode of operation. Biophys J. 1962 Mar;2(2 Pt 2):259–323. doi: 10.1016/s0006-3495(62)86959-8. [DOI] [PMC free article] [PubMed] [Google Scholar]
- Eisenman G., Enos B., Hägglund J., Sandblom J. Gramicidin as an example of a single-filing ionic channel. Ann N Y Acad Sci. 1980;339:8–20. doi: 10.1111/j.1749-6632.1980.tb15964.x. [DOI] [PubMed] [Google Scholar]
- Fenwick E. M., Marty A., Neher E. Sodium and calcium channels in bovine chromaffin cells. J Physiol. 1982 Oct;331:599–635. doi: 10.1113/jphysiol.1982.sp014394. [DOI] [PMC free article] [PubMed] [Google Scholar]
- Fosset M., Jaimovich E., Delpont E., Lazdunski M. [3H]nitrendipine receptors in skeletal muscle. J Biol Chem. 1983 May 25;258(10):6086–6092. [PubMed] [Google Scholar]
- Hagiwara S., Miyazaki S., Krasne S., Ciani S. Anomalous permeabilities of the egg cell membrane of a starfish in K+-Tl+ mixtures. J Gen Physiol. 1977 Sep;70(3):269–281. doi: 10.1085/jgp.70.3.269. [DOI] [PMC free article] [PubMed] [Google Scholar]
- Hagiwara S., Ohmori H. Studies of calcium channels in rat clonal pituitary cells with patch electrode voltage clamp. J Physiol. 1982 Oct;331:231–252. doi: 10.1113/jphysiol.1982.sp014371. [DOI] [PMC free article] [PubMed] [Google Scholar]
- Hille B., Campbell D. T. An improved vaseline gap voltage clamp for skeletal muscle fibers. J Gen Physiol. 1976 Mar;67(3):265–293. doi: 10.1085/jgp.67.3.265. [DOI] [PMC free article] [PubMed] [Google Scholar]
- Hille B., Schwarz W. Potassium channels as multi-ion single-file pores. J Gen Physiol. 1978 Oct;72(4):409–442. doi: 10.1085/jgp.72.4.409. [DOI] [PMC free article] [PubMed] [Google Scholar]
- Hodgkin A. L., Nakajima S. Analysis of the membrane capacity in frog muscle. J Physiol. 1972 Feb;221(1):121–136. doi: 10.1113/jphysiol.1972.sp009743. [DOI] [PMC free article] [PubMed] [Google Scholar]
- Kostyuk P. G., Krishtal O. A. Effects of calcium and calcium-chelating agents on the inward and outward current in the membrane of mollusc neurones. J Physiol. 1977 Sep;270(3):569–580. doi: 10.1113/jphysiol.1977.sp011969. [DOI] [PMC free article] [PubMed] [Google Scholar]
- Kretsinger R. H., Barry C. D. The predicted structure of the calcium-binding component of troponin. Biochim Biophys Acta. 1975 Sep 9;405(1):40–52. doi: 10.1016/0005-2795(75)90312-8. [DOI] [PubMed] [Google Scholar]
- Krishtal O. A., Pidoplichko V. I., Shakhovalov Y. A. Conductance of the calcium channel in the membrane of snail neurones. J Physiol. 1981 Jan;310:423–434. doi: 10.1113/jphysiol.1981.sp013558. [DOI] [PMC free article] [PubMed] [Google Scholar]
- Lee K. S., Tsien R. W. Mechanism of calcium channel blockade by verapamil, D600, diltiazem and nitrendipine in single dialysed heart cells. Nature. 1983 Apr 28;302(5911):790–794. doi: 10.1038/302790a0. [DOI] [PubMed] [Google Scholar]
- Levitt D. G. Electrostatic calculations for an ion channel. I. Energy and potential profiles and interactions between ions. Biophys J. 1978 May;22(2):209–219. doi: 10.1016/S0006-3495(78)85485-X. [DOI] [PMC free article] [PubMed] [Google Scholar]
- Prosser C. L., Kreulen D. L., Weigel R. J., Yau W. Prolonged potentials in gastrointestinal muscles induced by calcium chelation. Am J Physiol. 1977 Jul;233(1):C19–C24. doi: 10.1152/ajpcell.1977.233.1.C19. [DOI] [PubMed] [Google Scholar]
- Reuter H., Scholz H. A study of the ion selectivity and the kinetic properties of the calcium dependent slow inward current in mammalian cardiac muscle. J Physiol. 1977 Jan;264(1):17–47. doi: 10.1113/jphysiol.1977.sp011656. [DOI] [PMC free article] [PubMed] [Google Scholar]
- Rougier O., Vassort G., Garnier D., Gargouil Y. M., Coraboeuf E. Existence and role of a slow inward current during the frog atrial action potential. Pflugers Arch. 1969;308(2):91–110. doi: 10.1007/BF00587018. [DOI] [PubMed] [Google Scholar]
- Siri L. N., Sánchez J. A., Stefani E. Effect of glycerol treatment on the calcium current of frog skeletal muscle. J Physiol. 1980 Aug;305:87–96. doi: 10.1113/jphysiol.1980.sp013351. [DOI] [PMC free article] [PubMed] [Google Scholar]
- Takeda K. Prolonged sarcotubular regenerative response in frog sartorius muscle. Jpn J Physiol. 1977;27(3):379–389. doi: 10.2170/jjphysiol.27.379. [DOI] [PubMed] [Google Scholar]
- Takeuchi A., Takeuchi N. Anion interaction at the inhibitory post-synaptic membrane of the crayfish neuromuscular junction. J Physiol. 1971 Jan;212(2):337–351. doi: 10.1113/jphysiol.1971.sp009328. [DOI] [PMC free article] [PubMed] [Google Scholar]
- Urban B. W., Hladky S. B., Haydon D. A. The kinetics of ion movements in the gramicidin channel. Fed Proc. 1978 Oct;37(12):2628–2632. [PubMed] [Google Scholar]
- Yamamoto D., Washio H. Permeation of sodium through calcium channels of an insect muscle membrane. Can J Physiol Pharmacol. 1979 Feb;57(2):220–222. doi: 10.1139/y79-033. [DOI] [PubMed] [Google Scholar]
- Yoshida S. Permeation of divalent and monovalent cations through the ovarian oocyte membrane of the mouse. J Physiol. 1983 Jun;339:631–642. doi: 10.1113/jphysiol.1983.sp014739. [DOI] [PMC free article] [PubMed] [Google Scholar]