Abstract
Human peripheral T lymphocytes were studied at 20-24 degrees C using the gigaohm seal recording technique in whole-cell or outside-out patch conformations. The predominant ion channel present under the conditions employed was a voltage-gated K+ channel closely resembling delayed rectifier K+ channels of nerve and muscle. The maximum K+ conductance in ninety T lymphocytes ranged from 0.7 to 8.9 nS, with a mean of 4.2 nS. The estimated number of K+ channels per cell is 400, corresponding to a density of about three channels/micron2 apparent membrane area. The activation of K+ currents could be fitted by Hodgkin-Huxley type n4 kinetics. The K+ conductance in Ringer solution was half-maximal at -40 mV. The time constant of K+ current inactivation was practically independent of voltage except near the threshold for activating the K+ conductance. Recovery from inactivation was slow and followed complex kinetics. Steady-state inactivation was half-maximal at -70 mV, and was complete at positive potentials. Permeability ratios, relative to K+, determined from reversal potential measurements were: K+(1.0) greater than Rb+(0.77) greater than NH4+(0.10) greater than Cs+ (0.02) greater than Na+(less than 0.01). Currents through K+ channels display deviations from the independence principle. The limiting outward current increases when external K+ is increased, and Rb+ carries less inward current than expected from its relative permeability. Tail current kinetics were slowed about 2-fold by raising the external K+ concentration from 4.5 to 160 mM, and were 5 times slower in Rb+ Ringer solution than in K+ Ringer solution. Single K+ channel currents had two amplitudes corresponding to about 9 and 16 pS in Ringer solution. Replacing Ringer solution with isotonic K+ Ringer solution increased the unitary conductance and resulted in inward rectification of the unitary current-voltage relation. Comparable effects of external K+ were seen in the whole-cell conductance and instantaneous current-voltage relation. Several changes in the K+ conductance occurred during the first few minutes after achievement of the whole-cell conformation. Most are explainable by dissipation of a 10-20 mV junction potential between pipette solution and the cytoplasm, and by the use of a holding potential more negative than the resting potential. However, inactivation of K+ currents became faster and more complete, changes not accounted for by these mechanisms. K+ efflux through open K+ channels in intact lymphocytes, calculated from measured properties of K+ channels, can account for efflux values reported in resting lymphocytes, and for the increase in K+ efflux upon mitogenic stimulation.(ABSTRACT TRUNCATED AT 400 WORDS)
Full text
PDF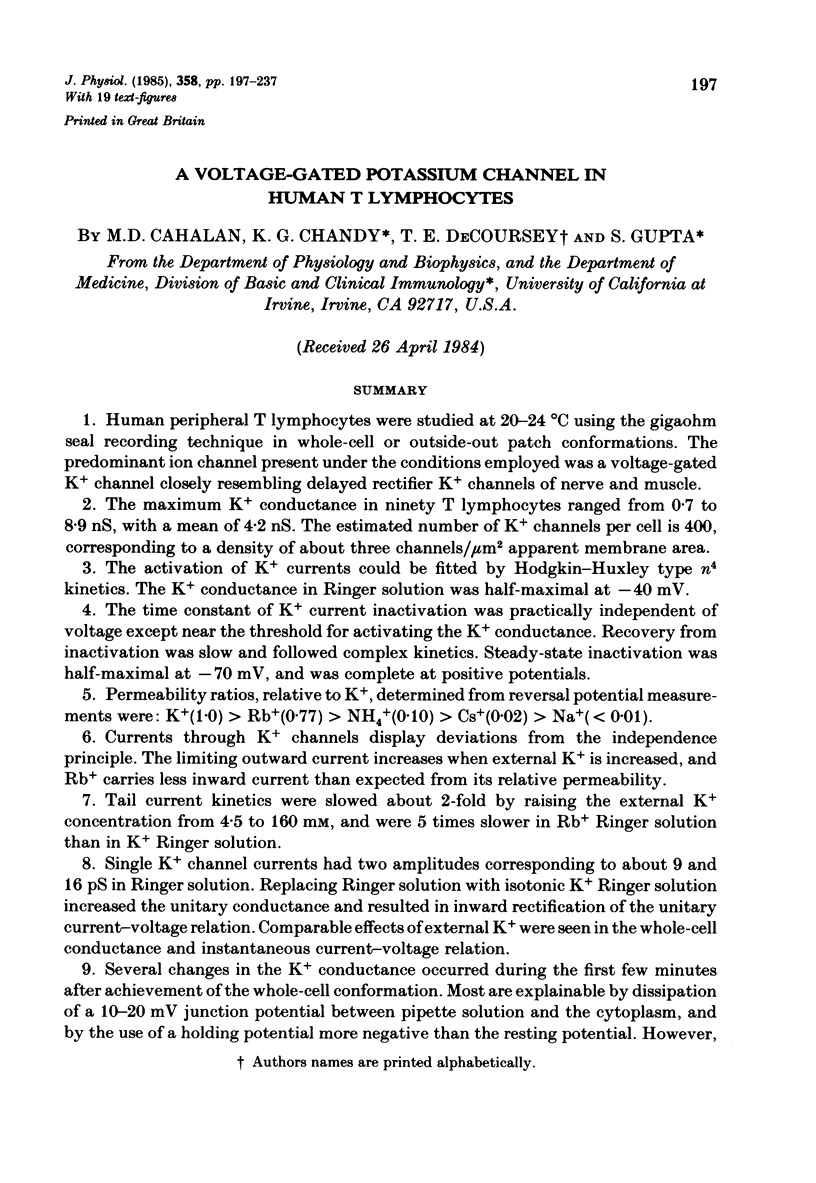
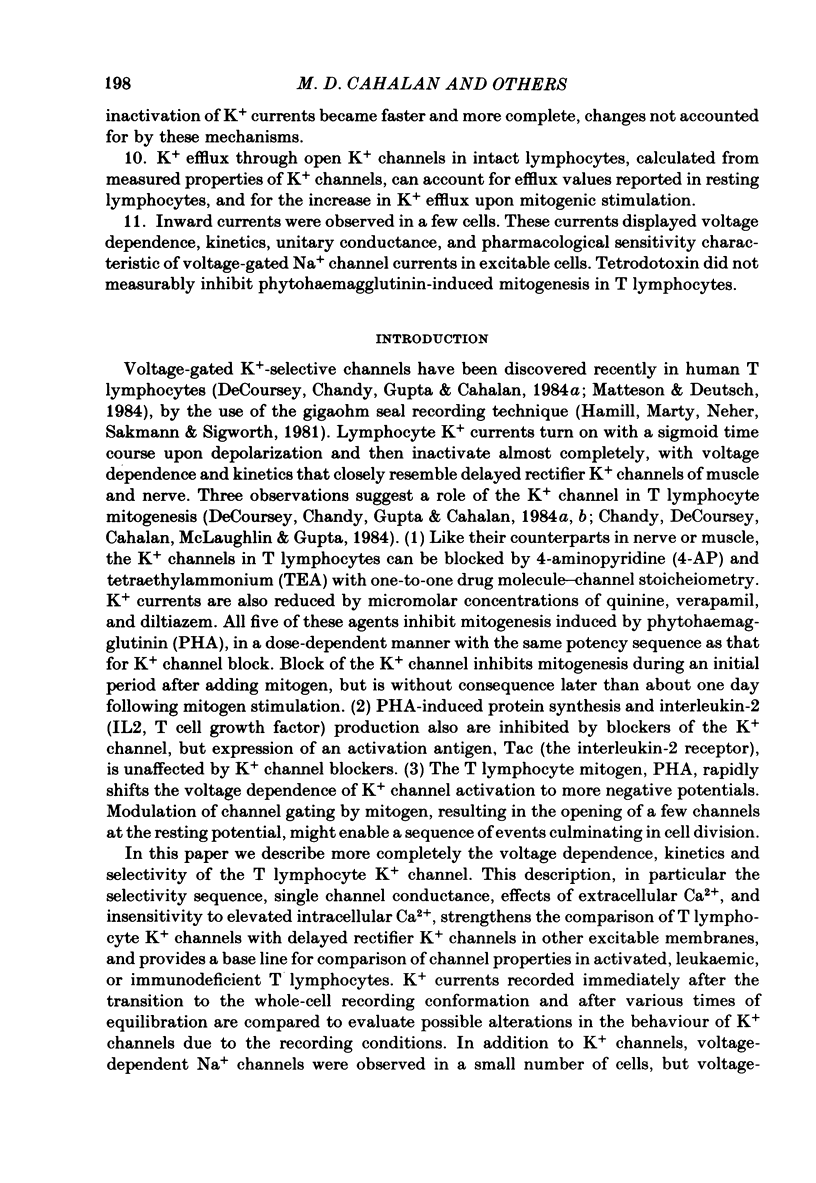
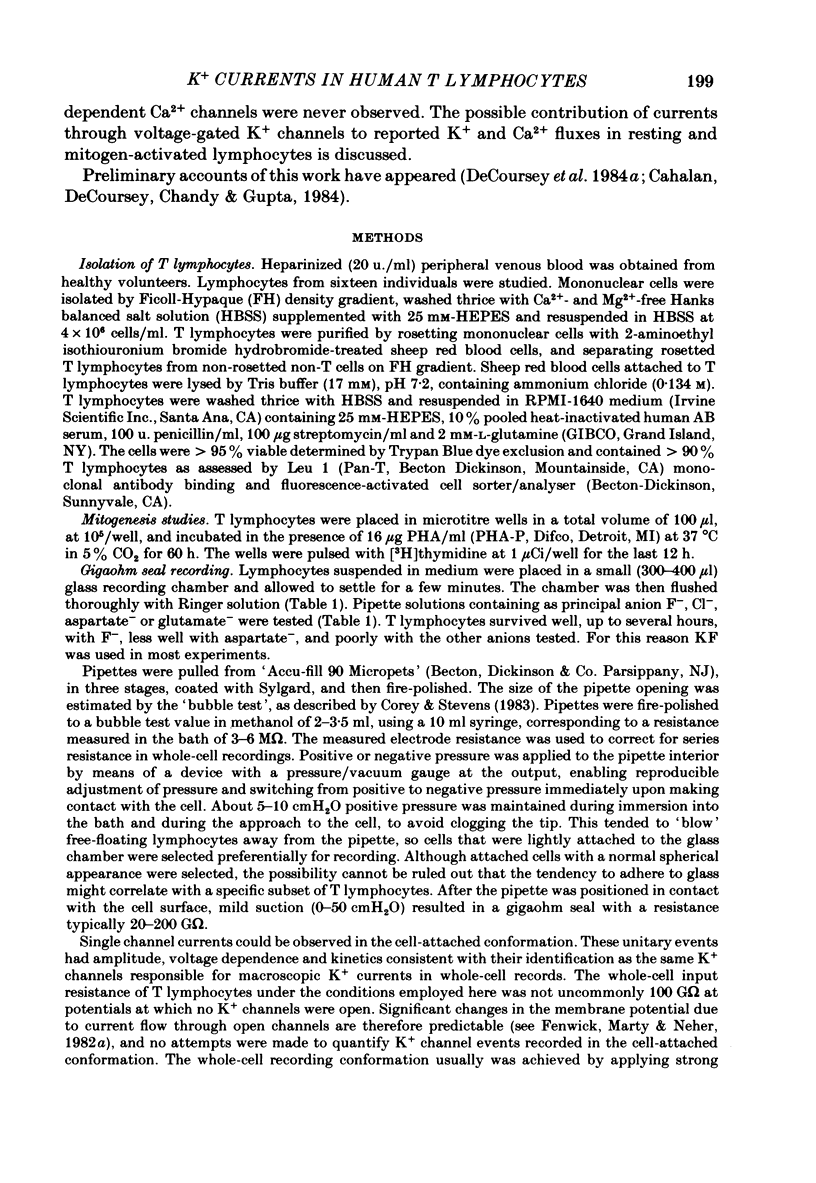
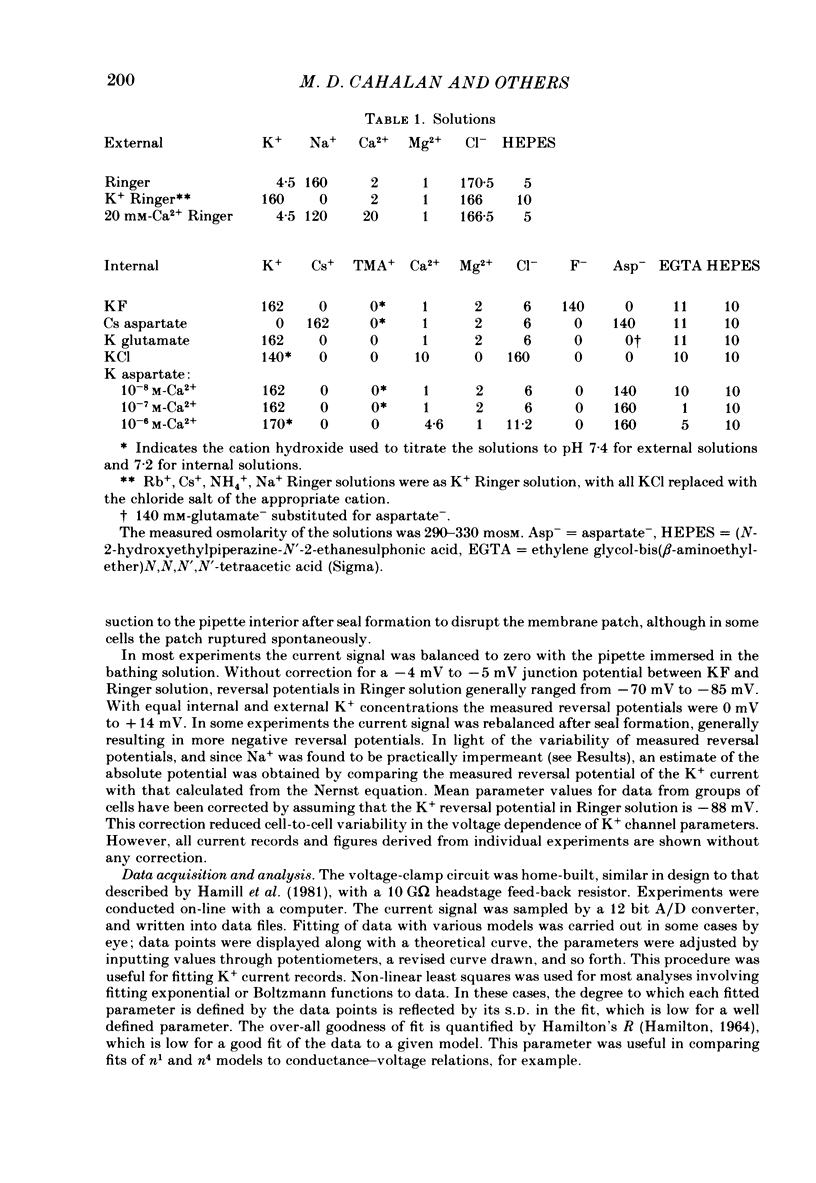
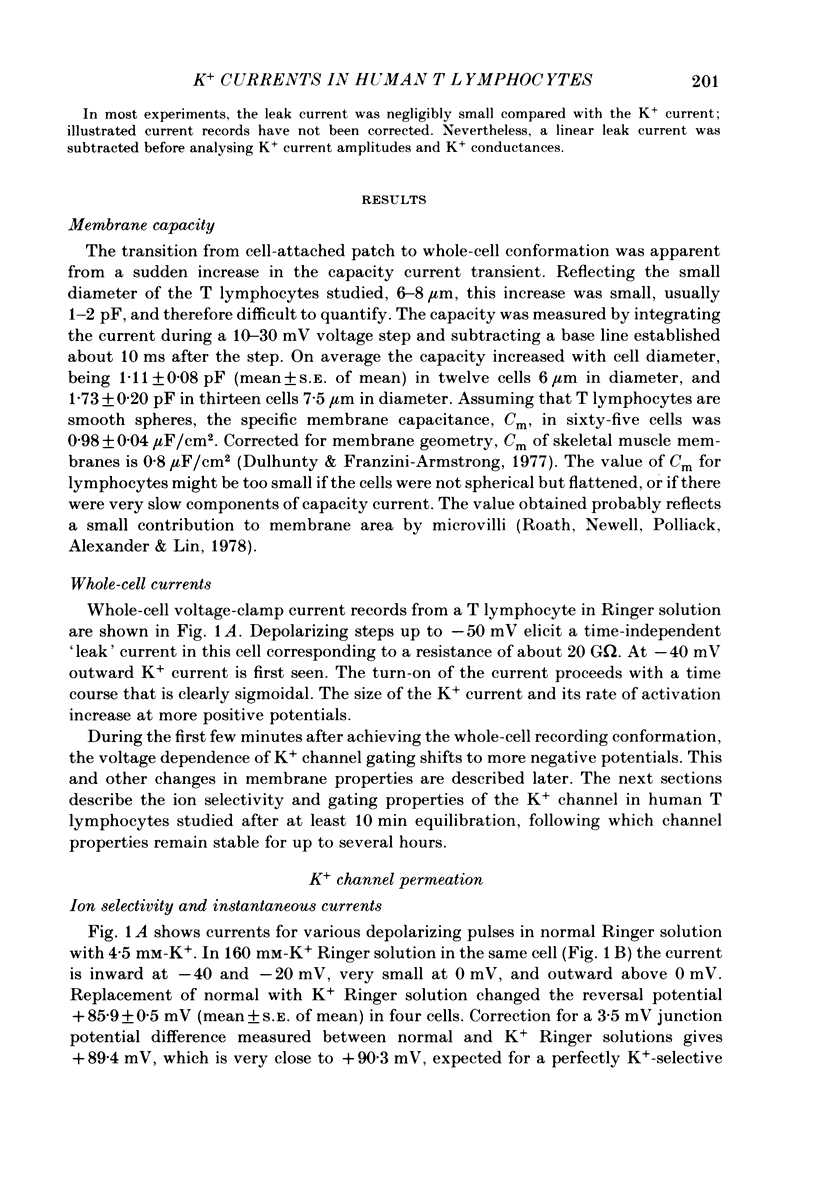
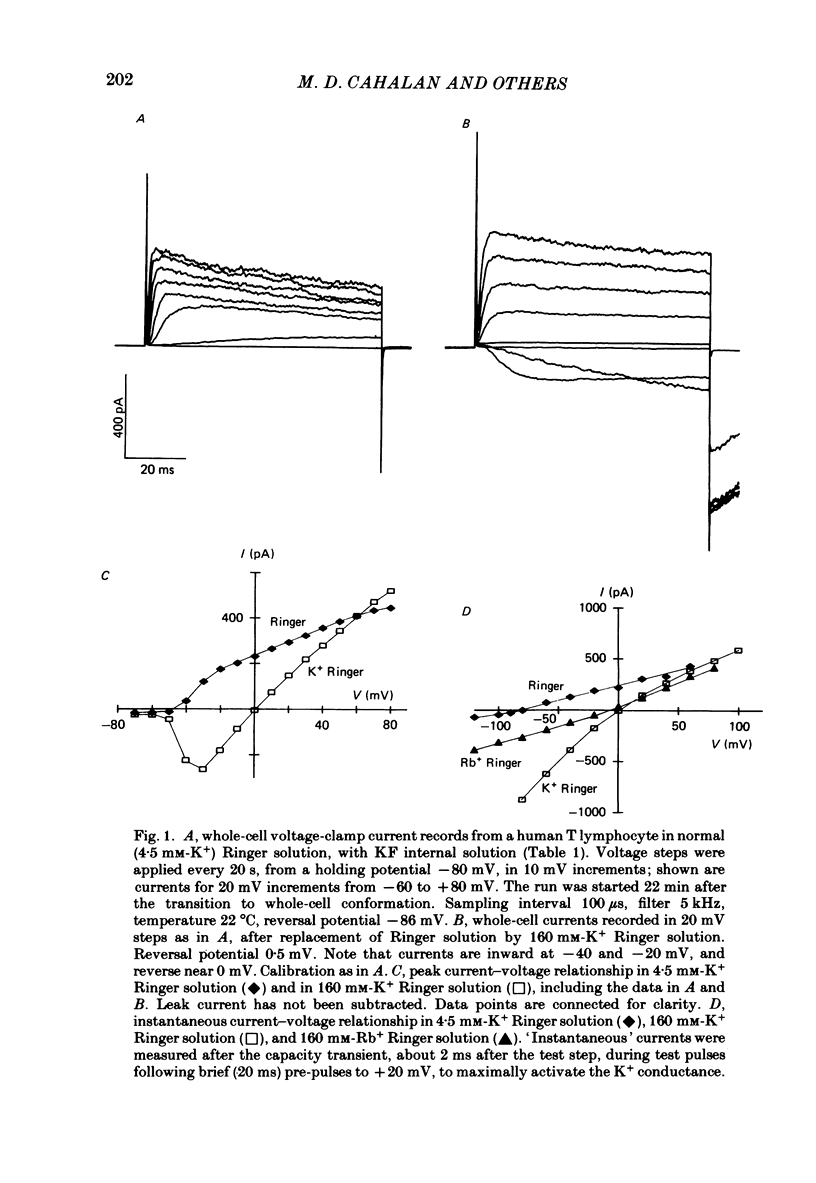
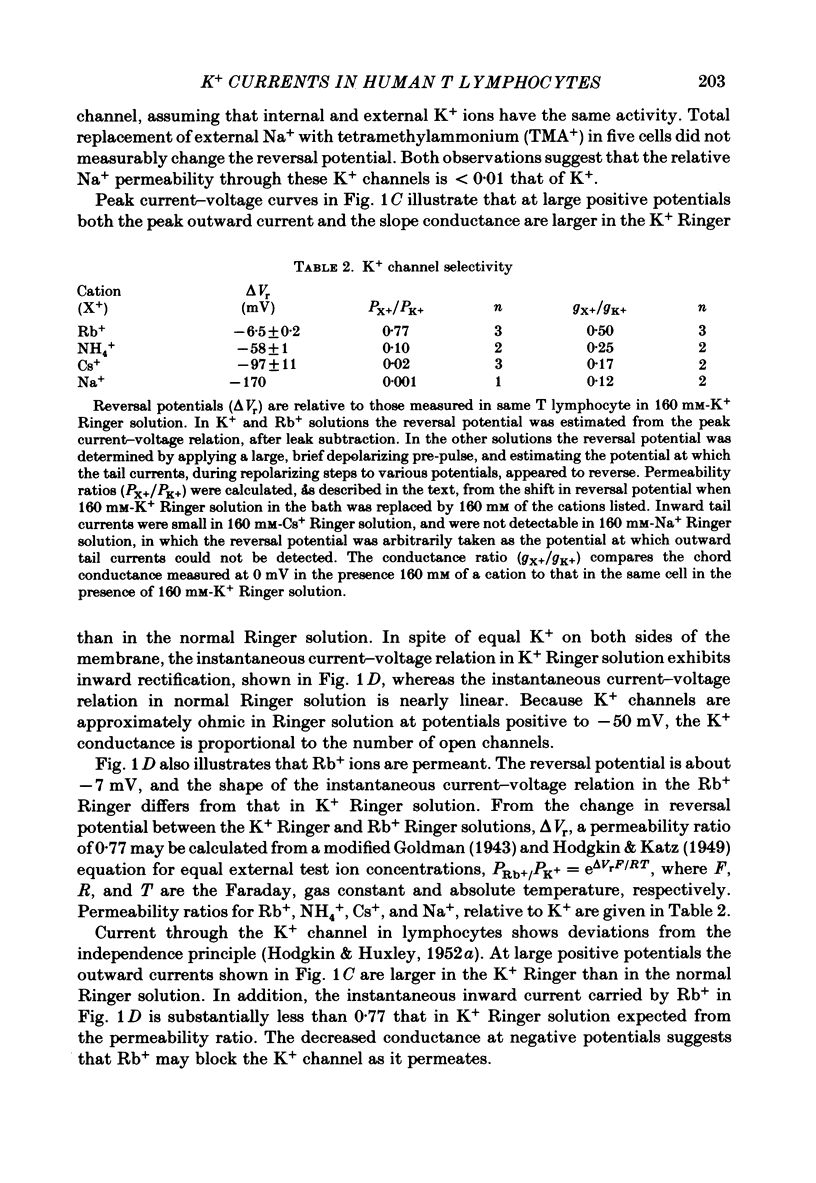
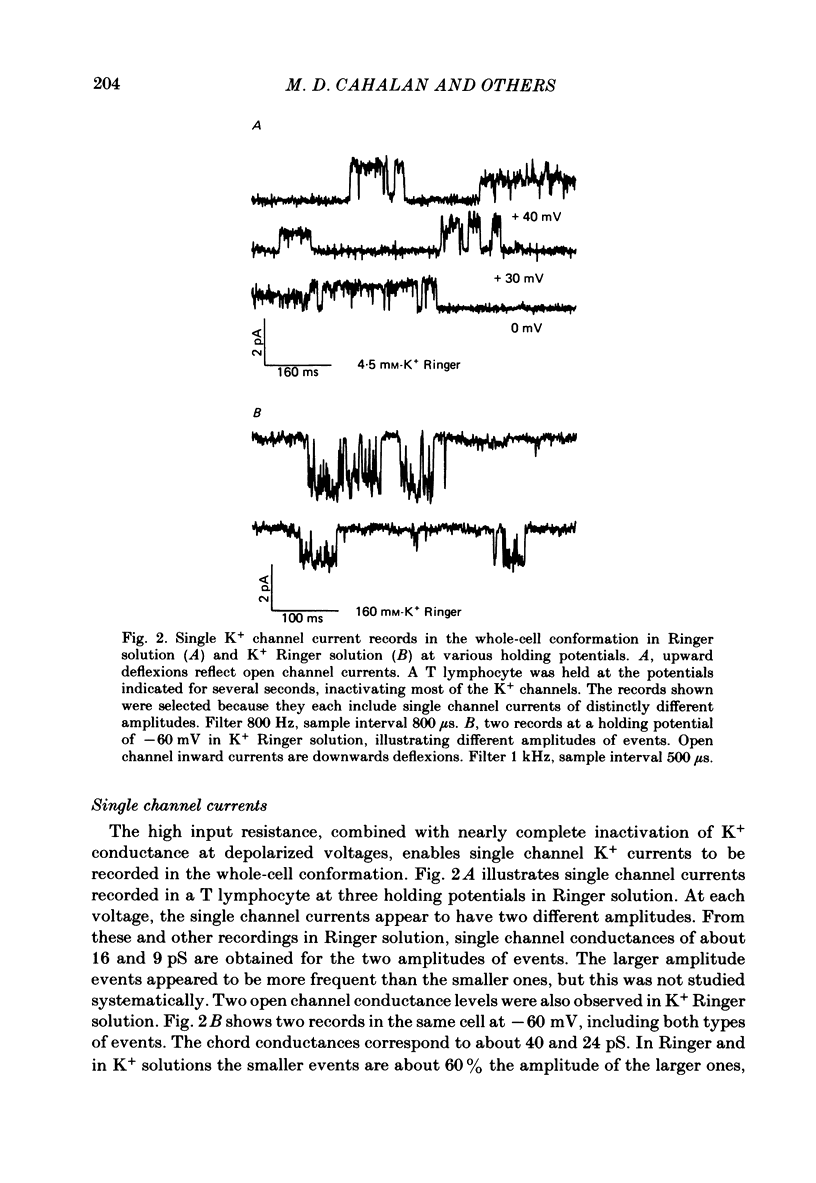
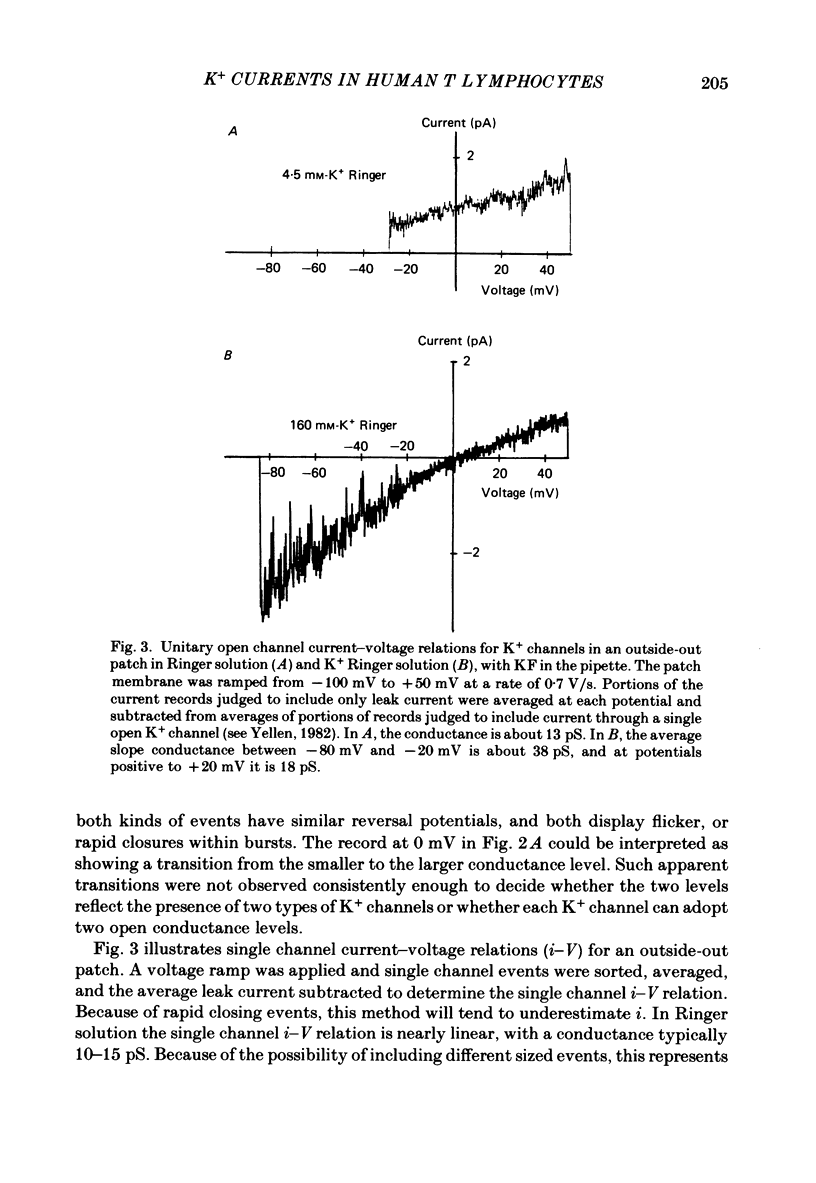
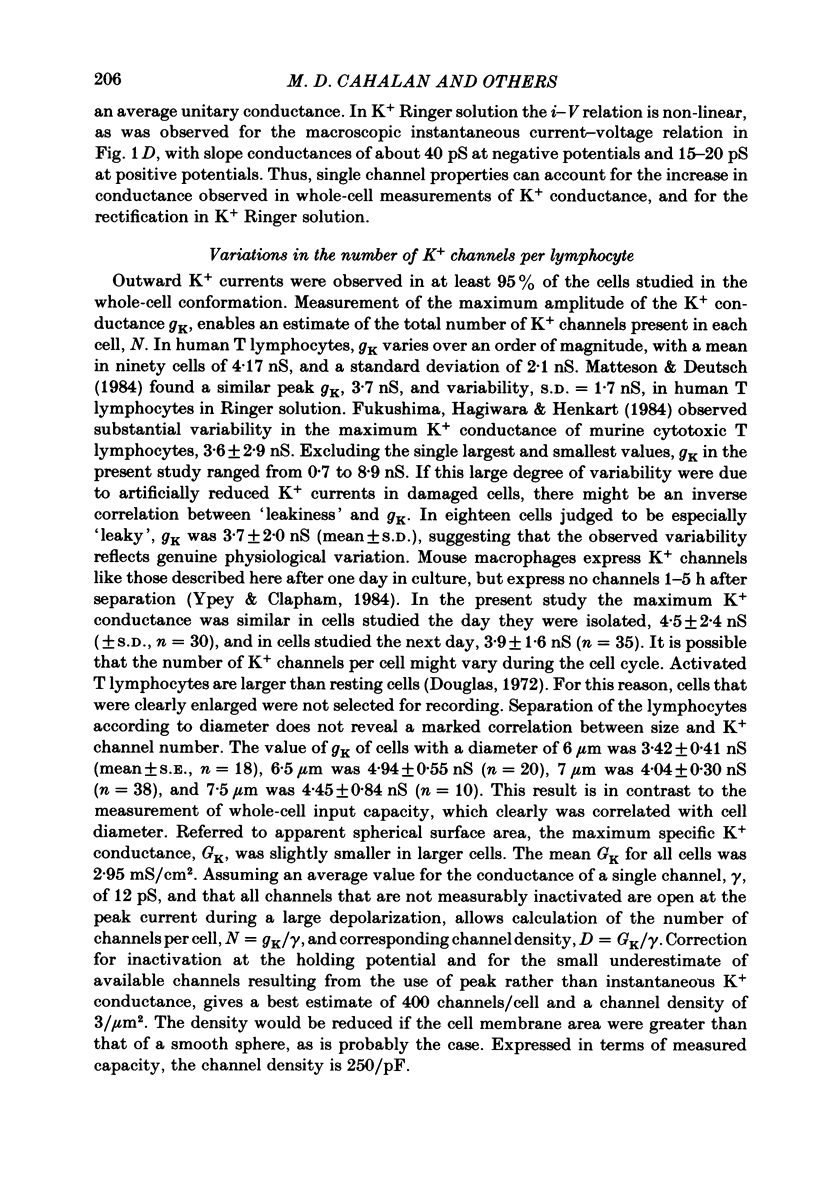
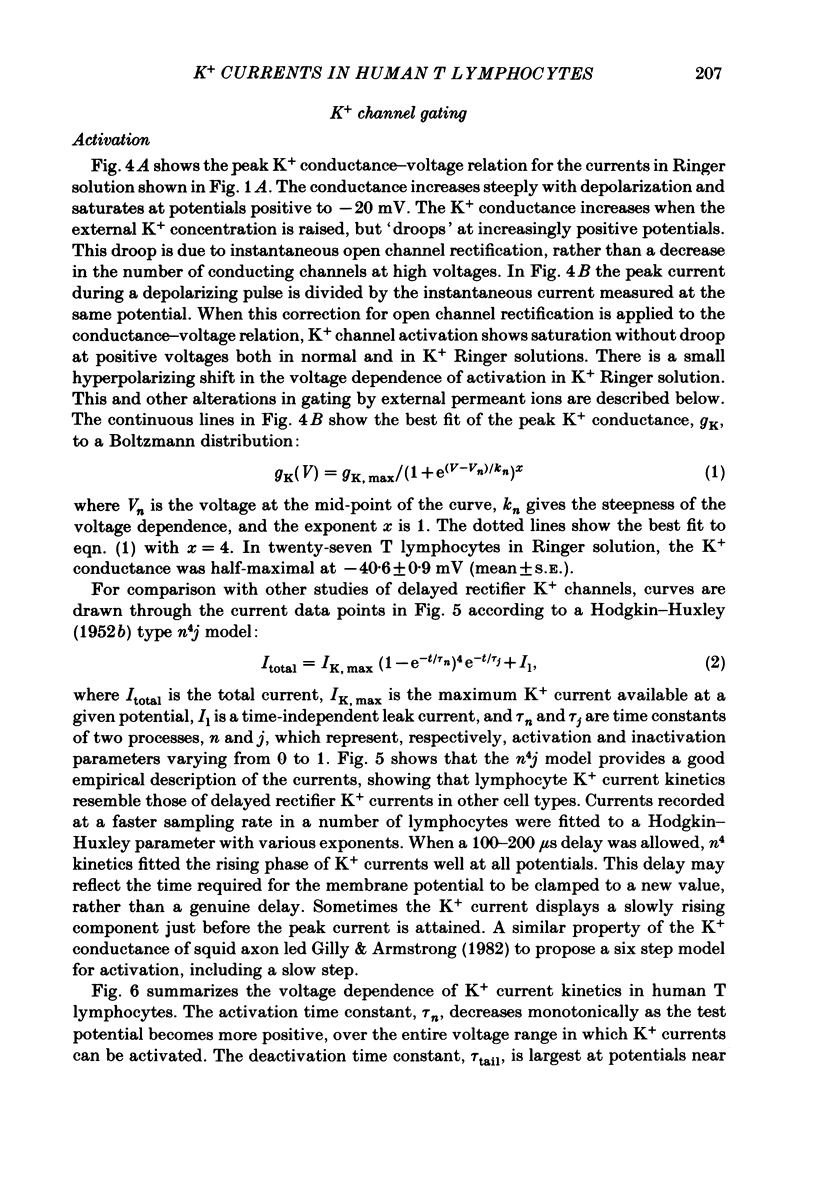
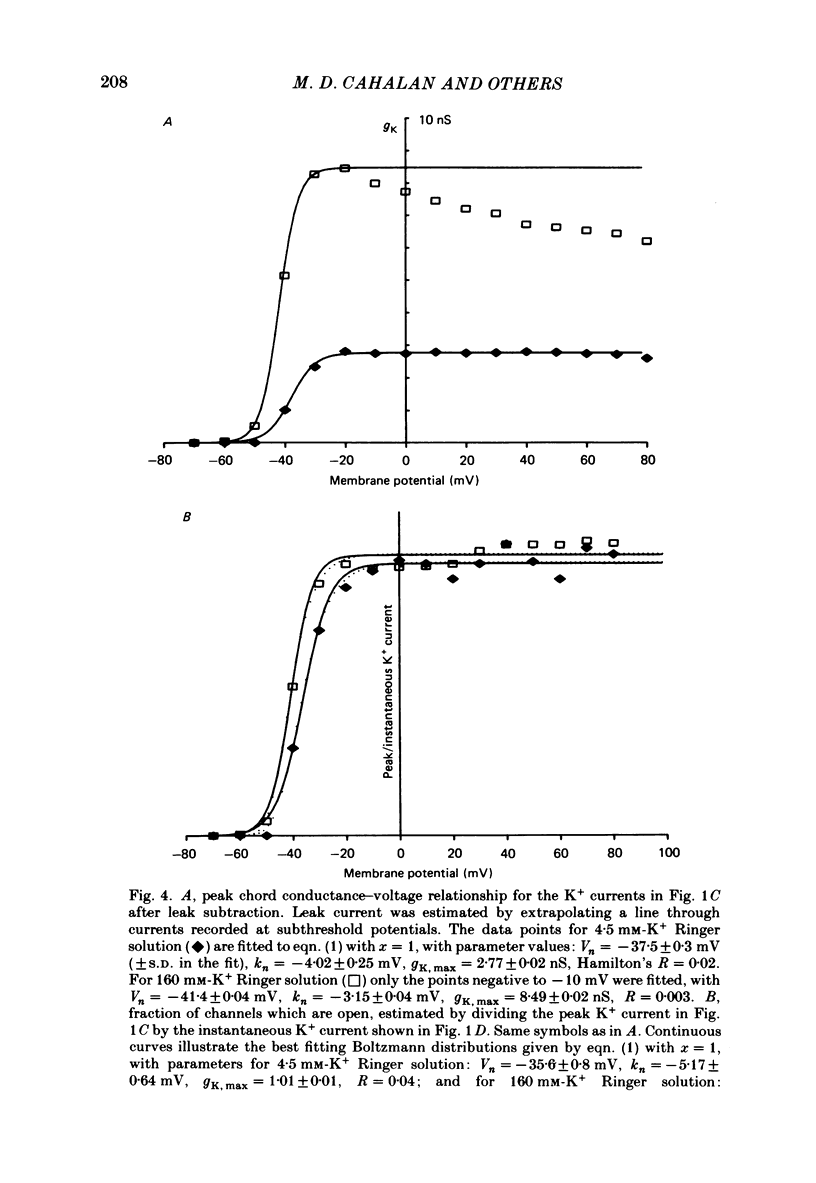
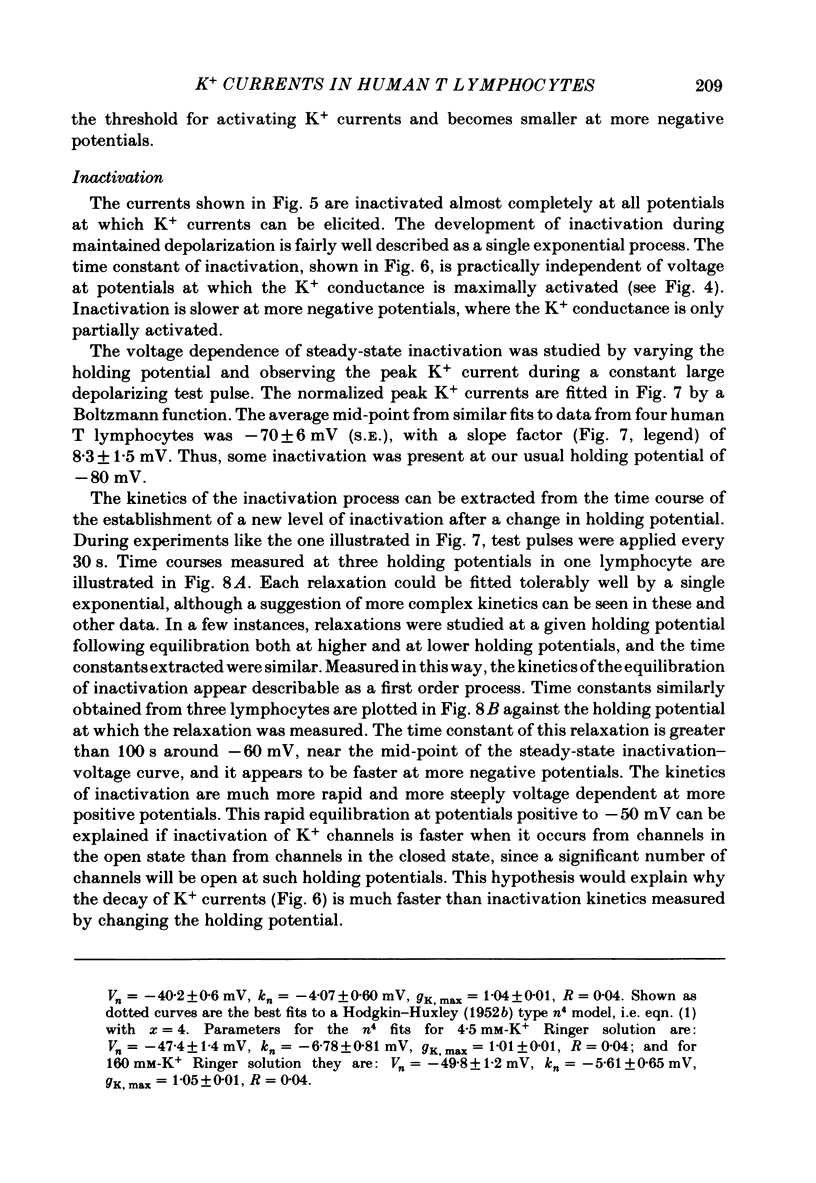
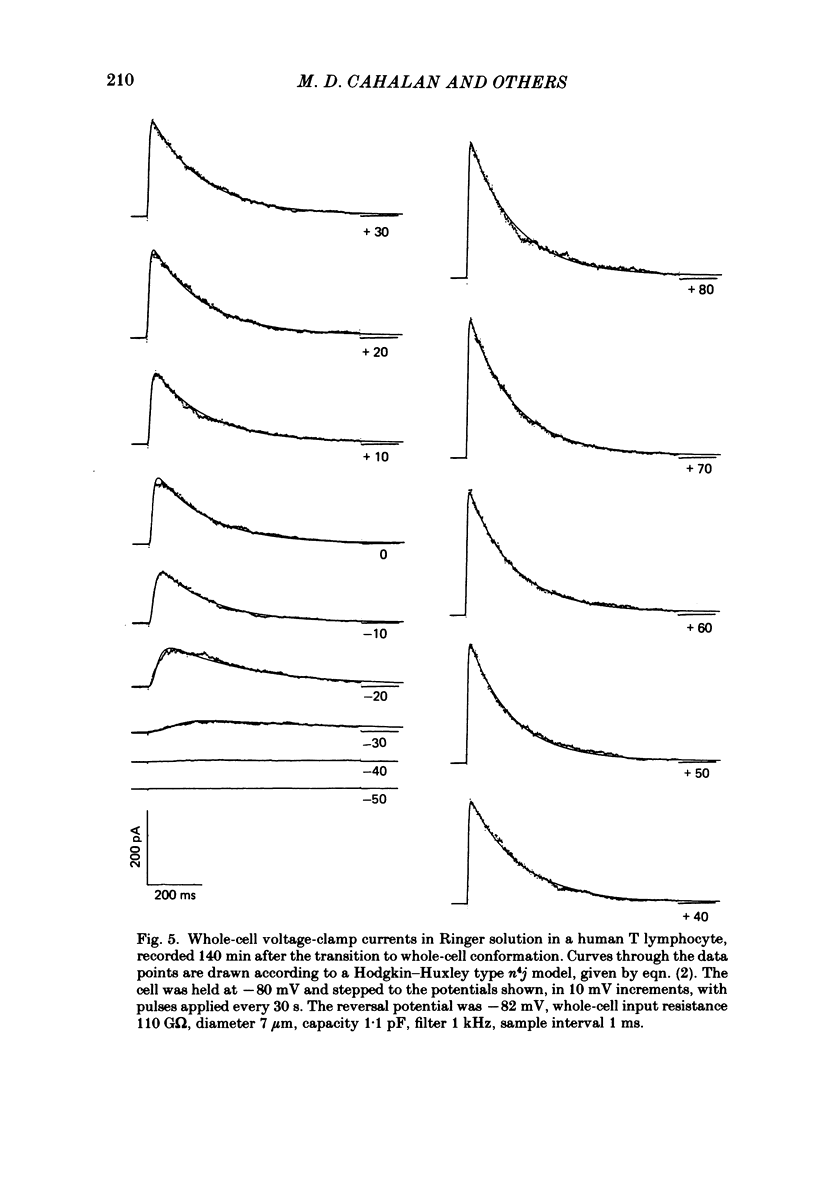
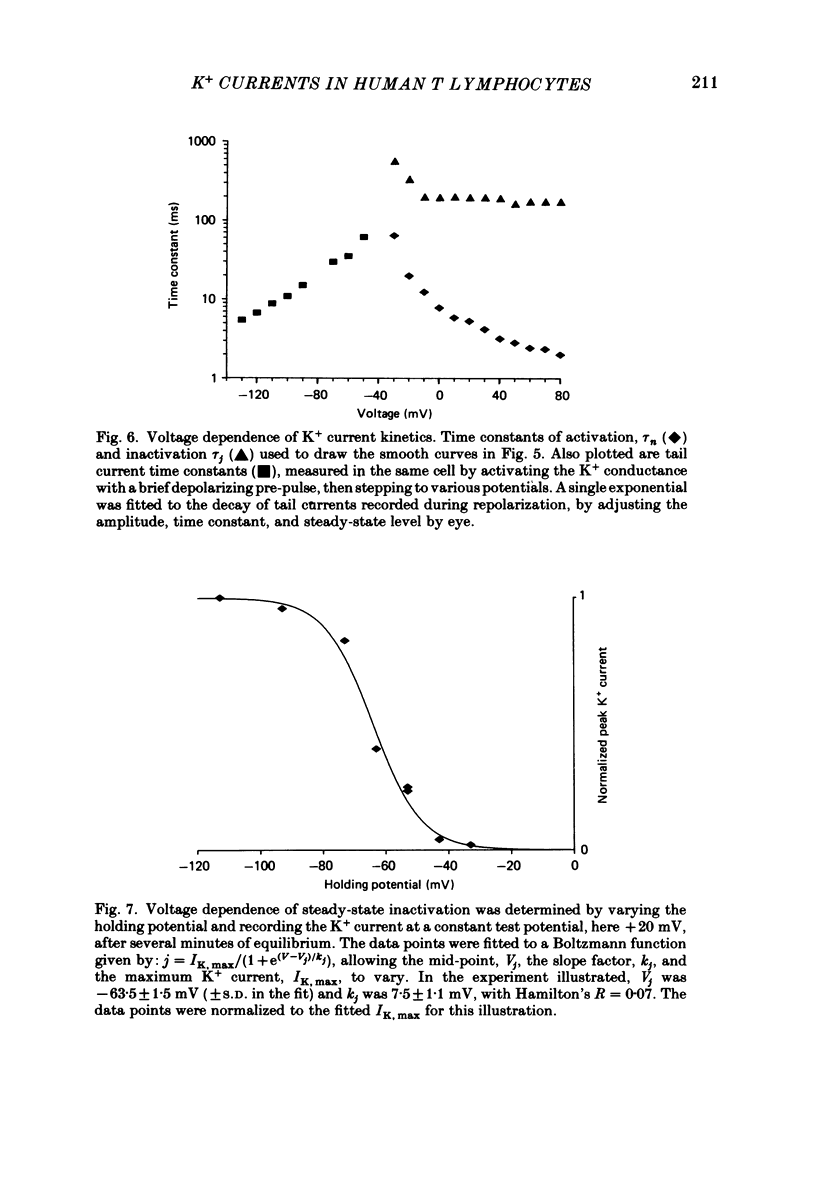
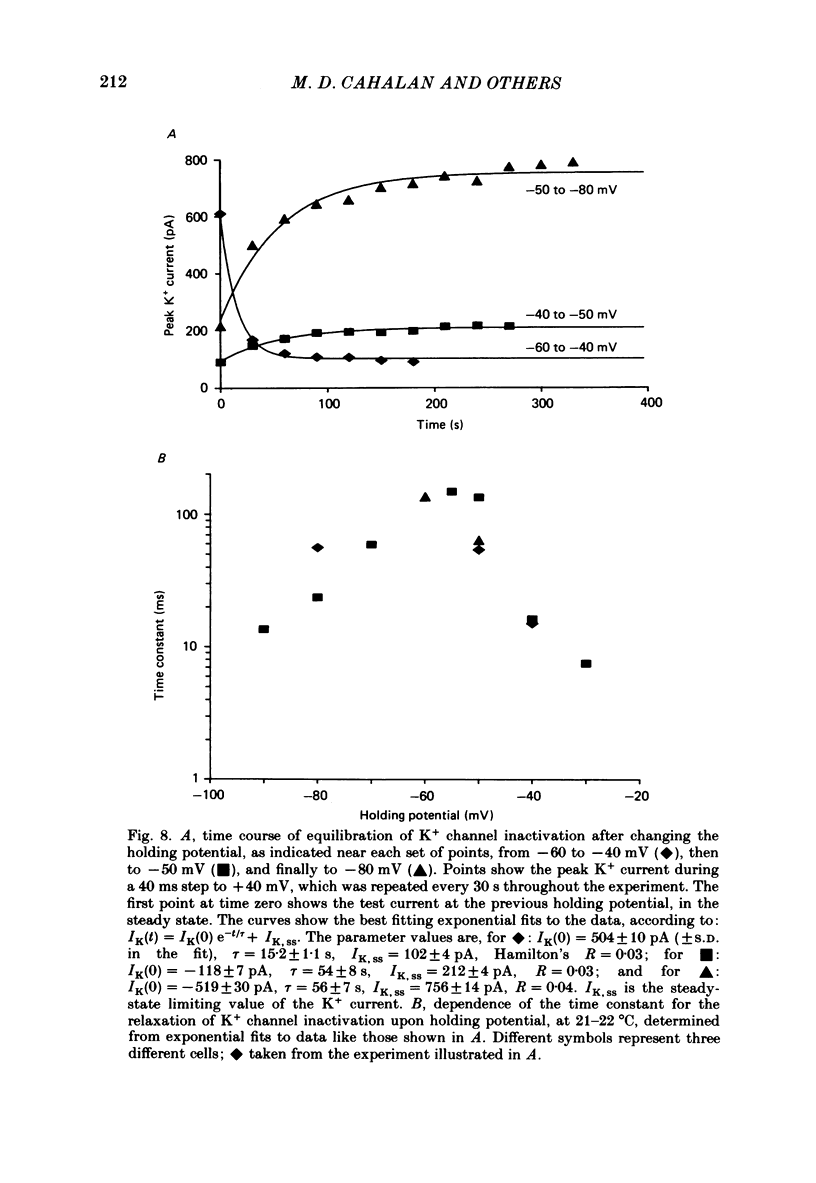
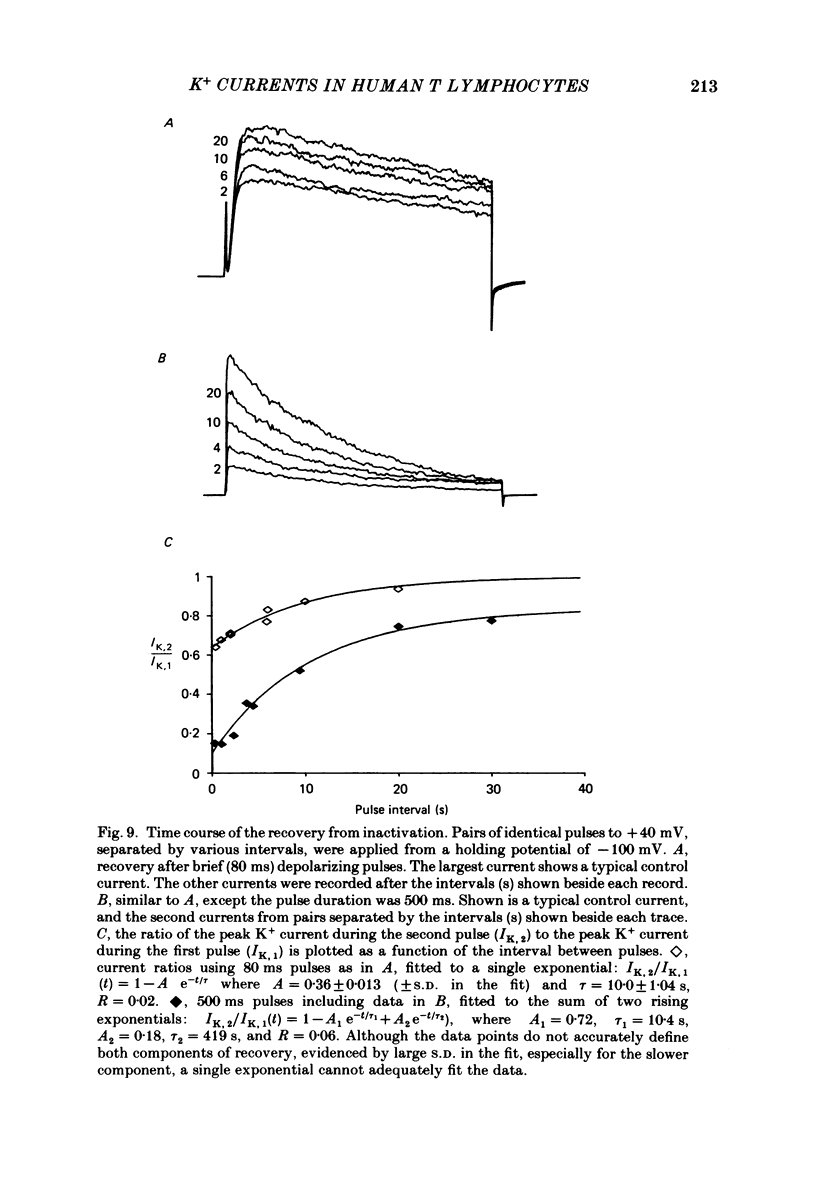
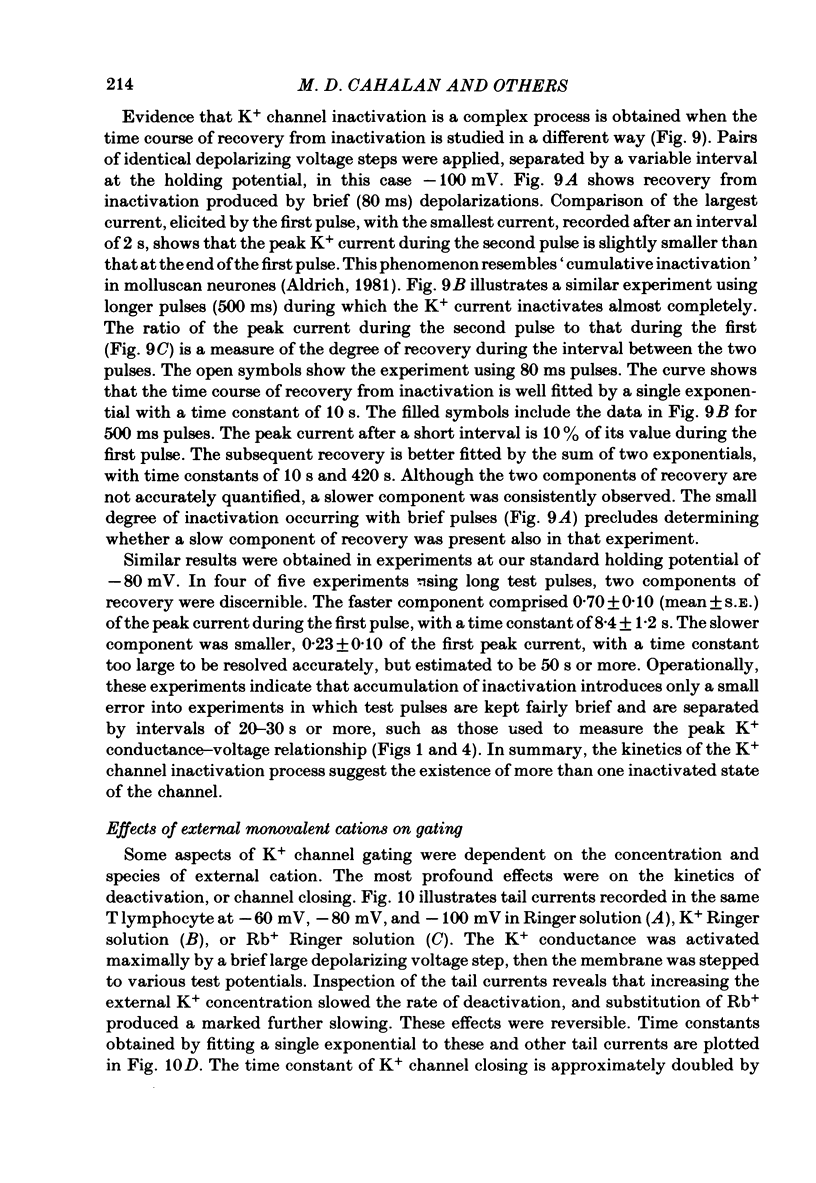
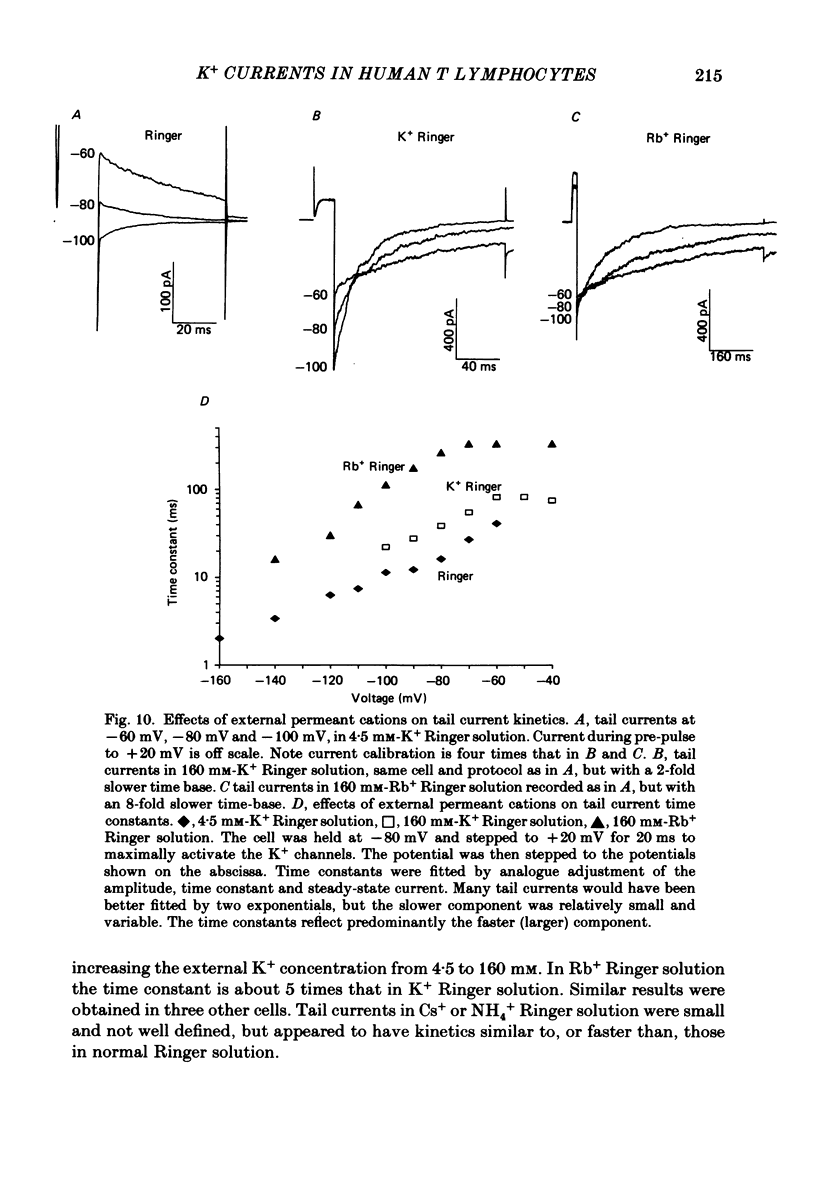
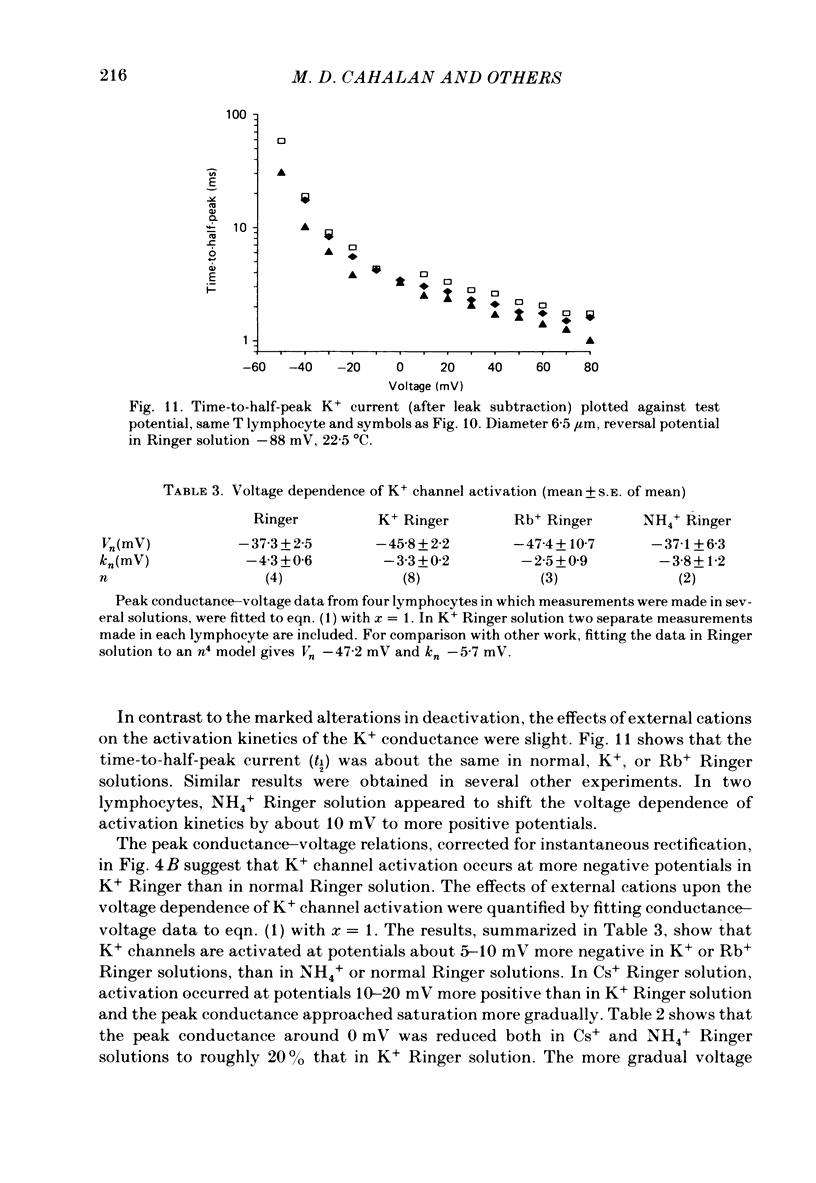
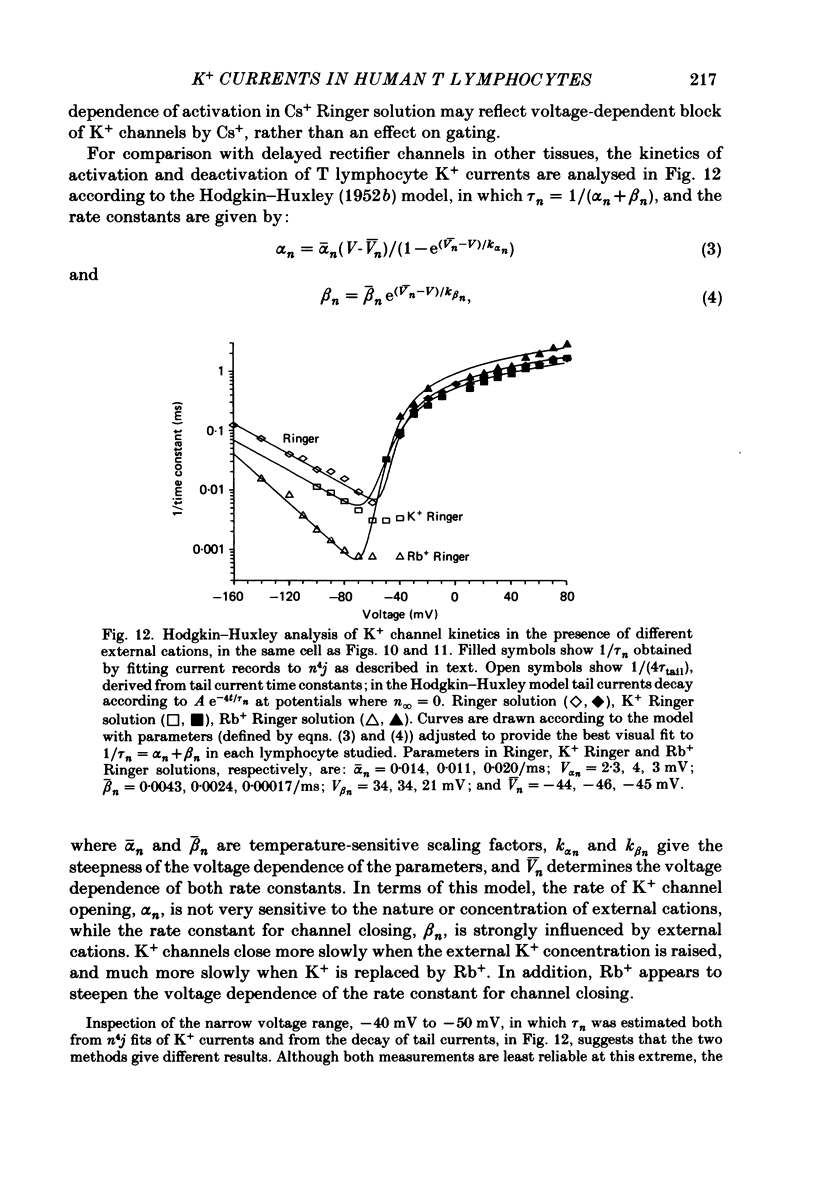
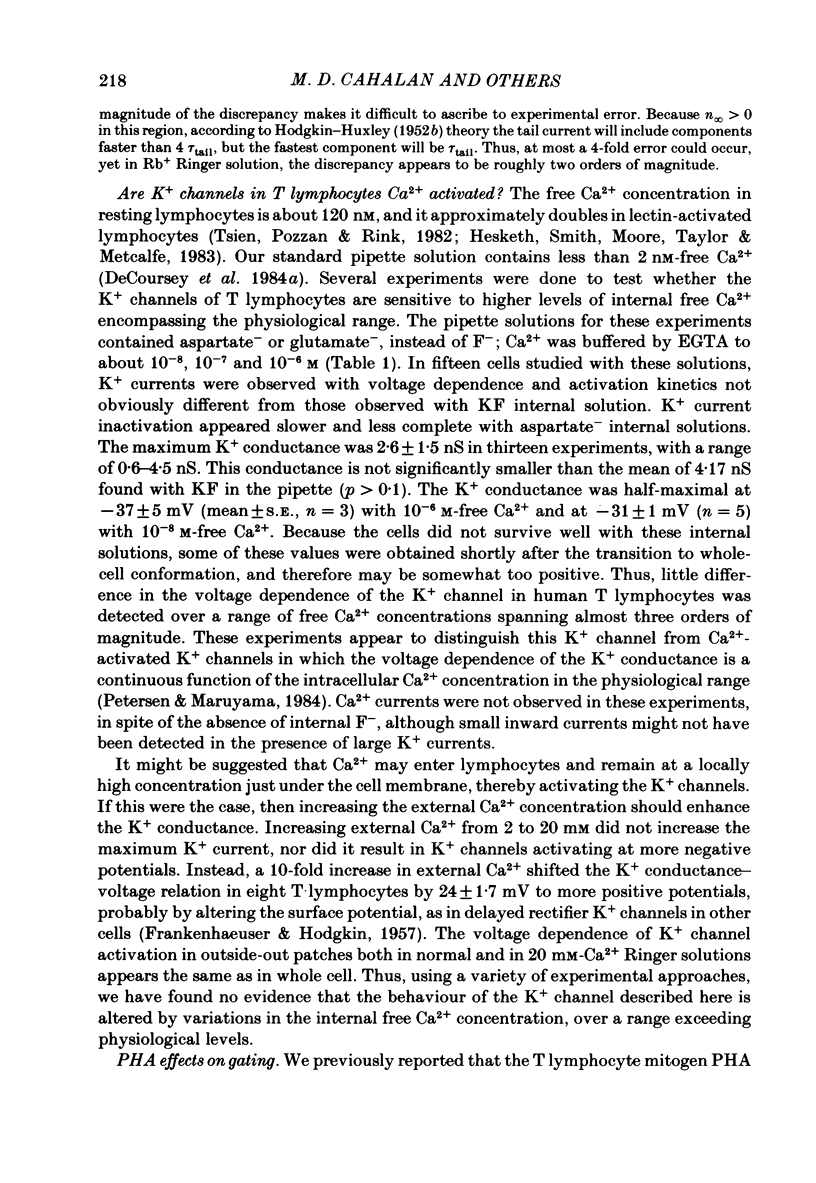
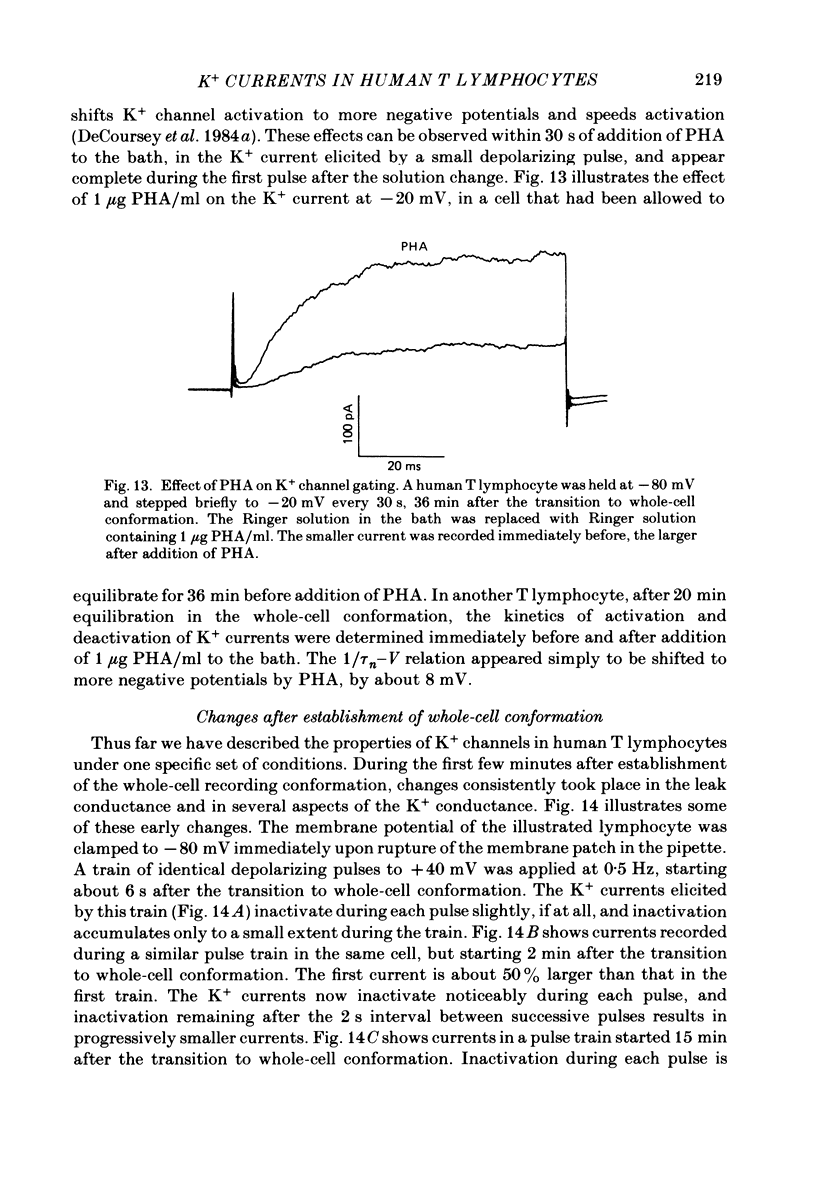
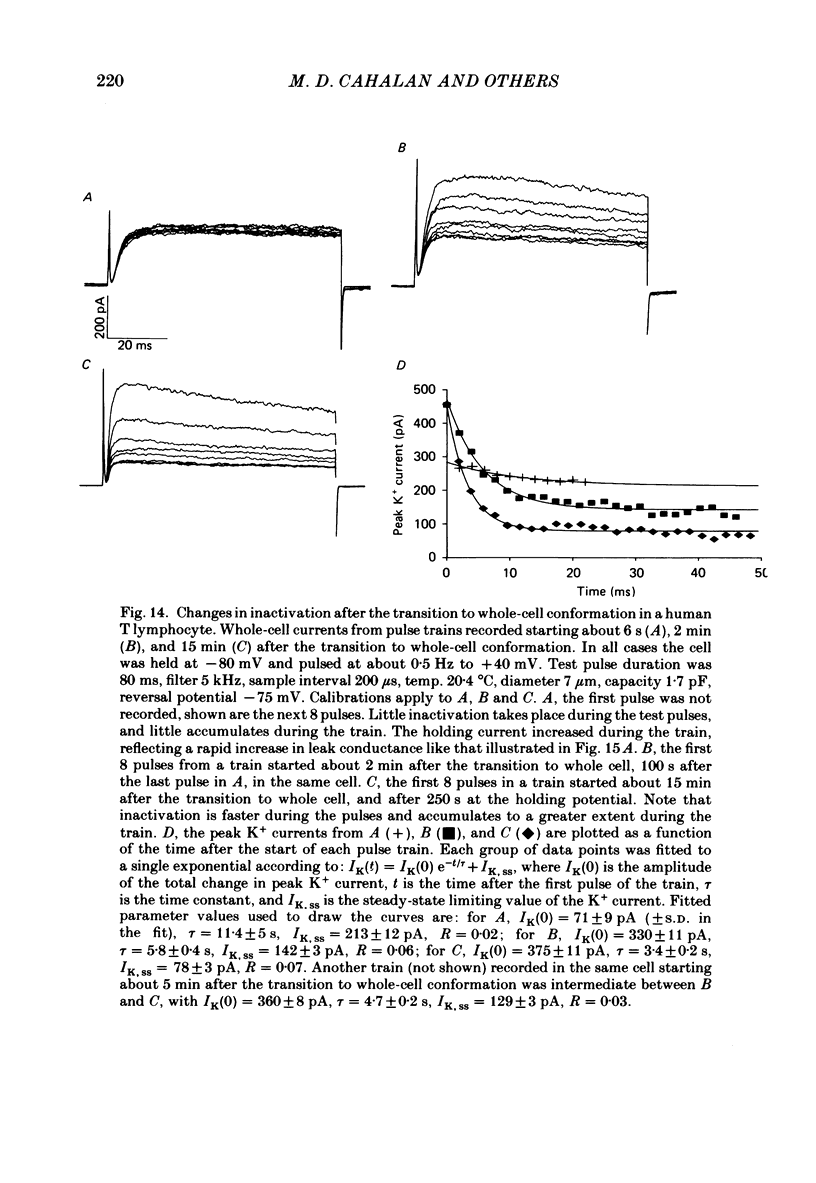
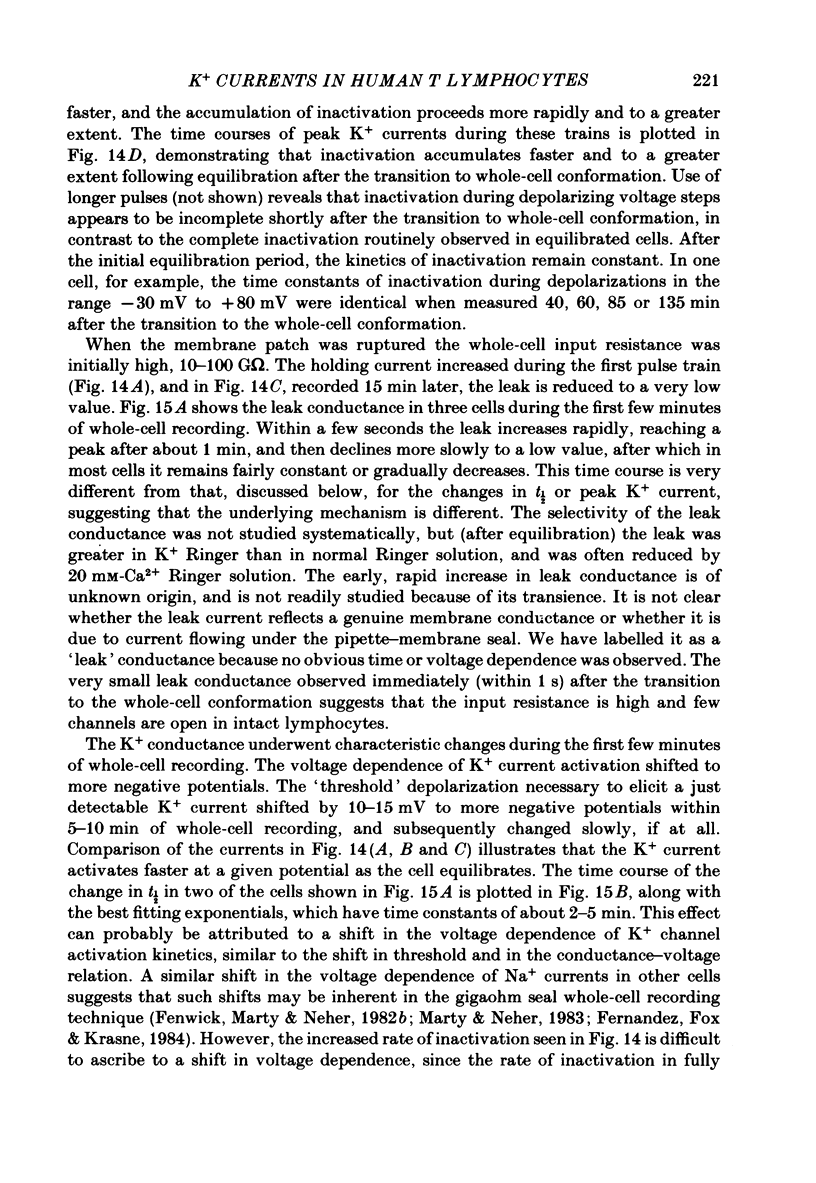
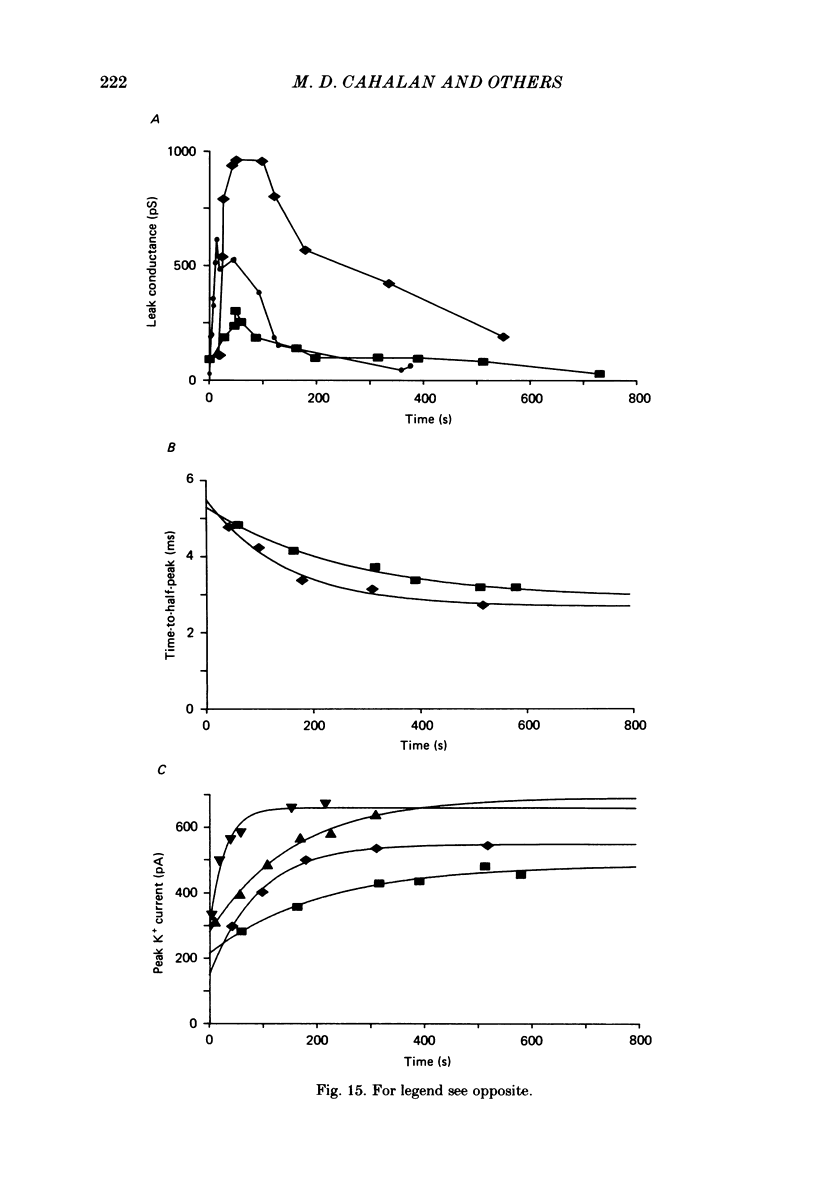
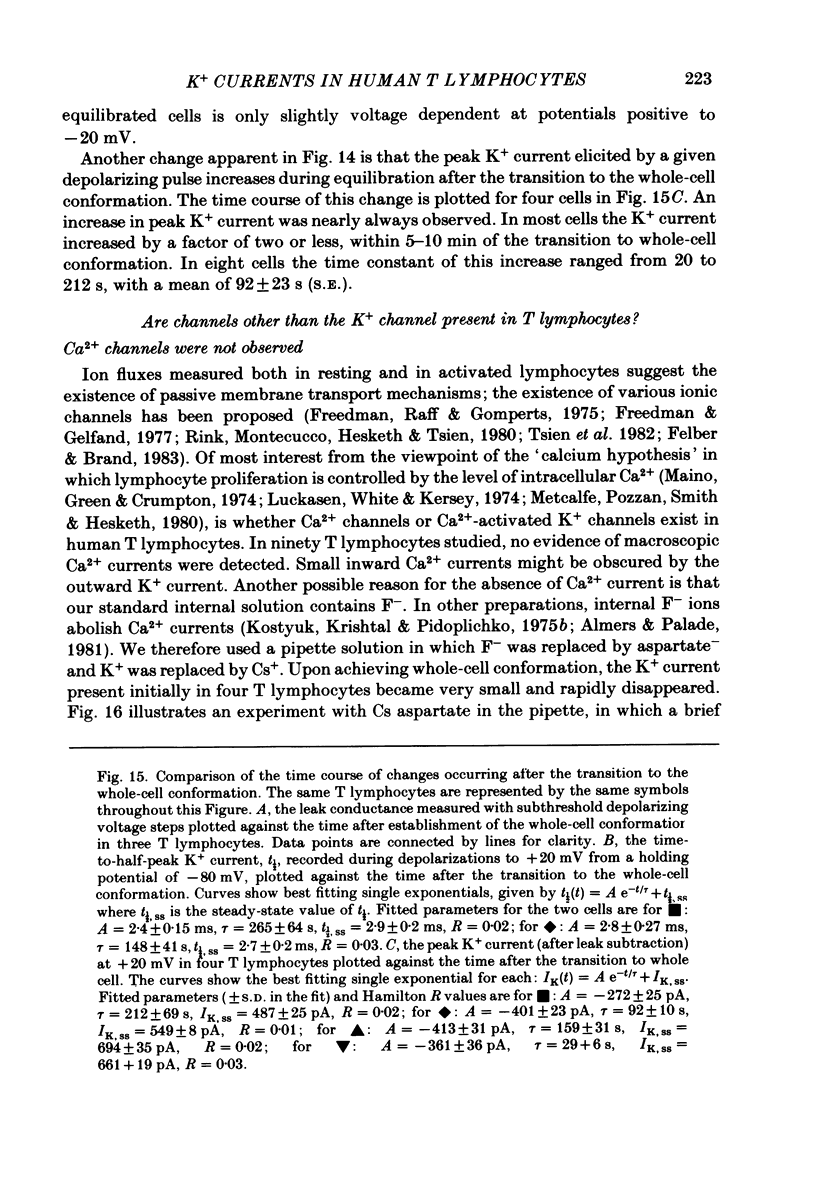
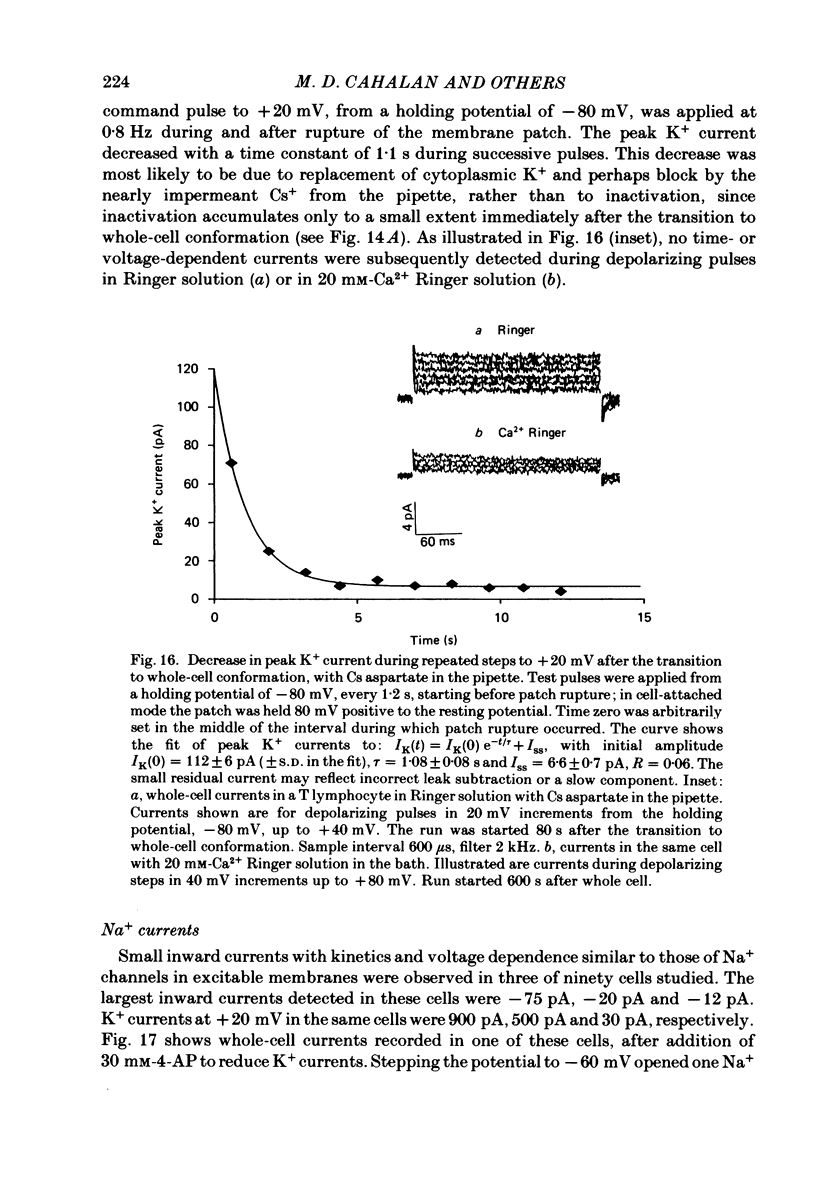
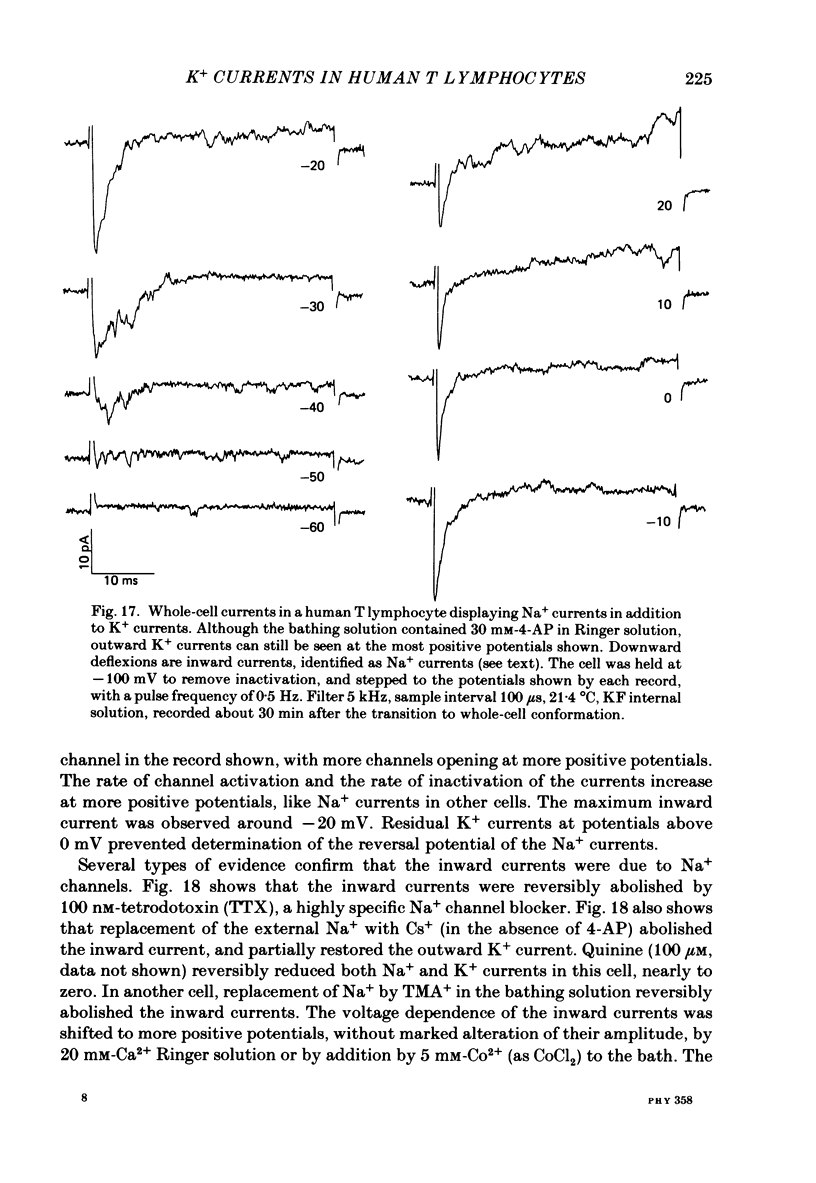
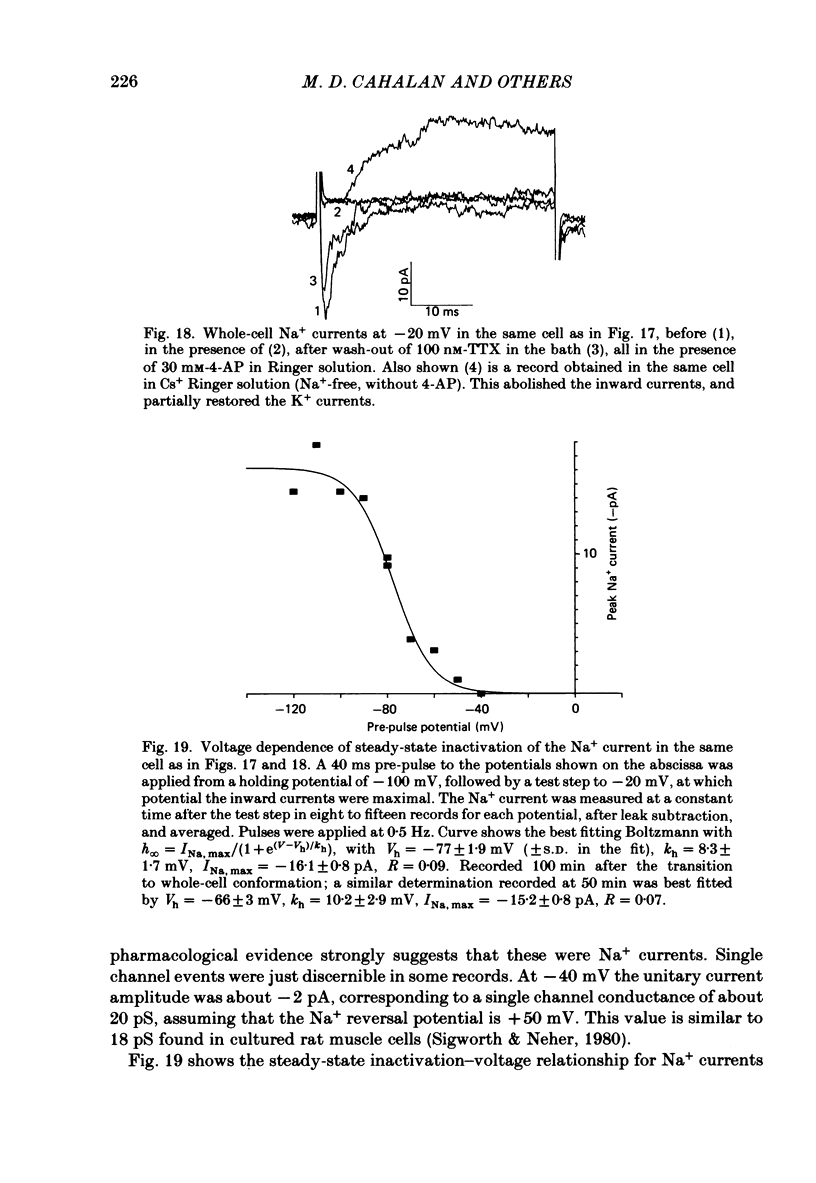
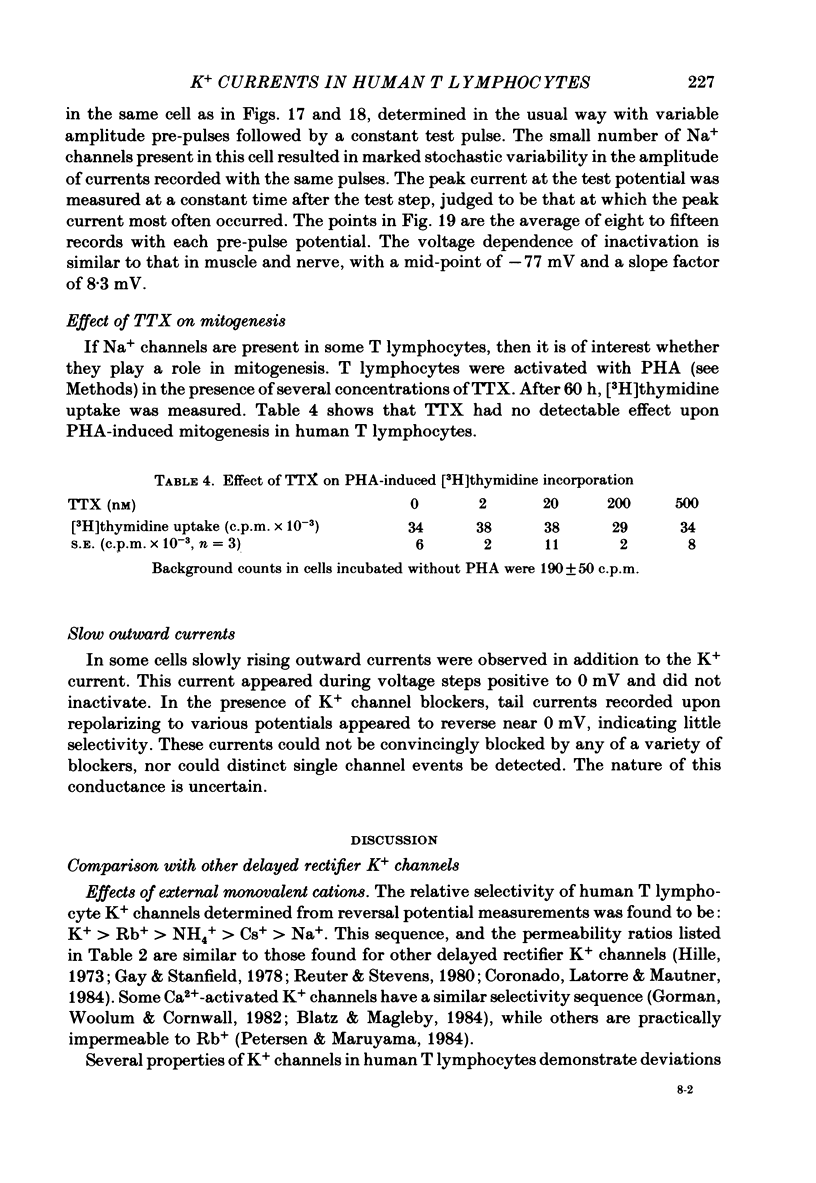
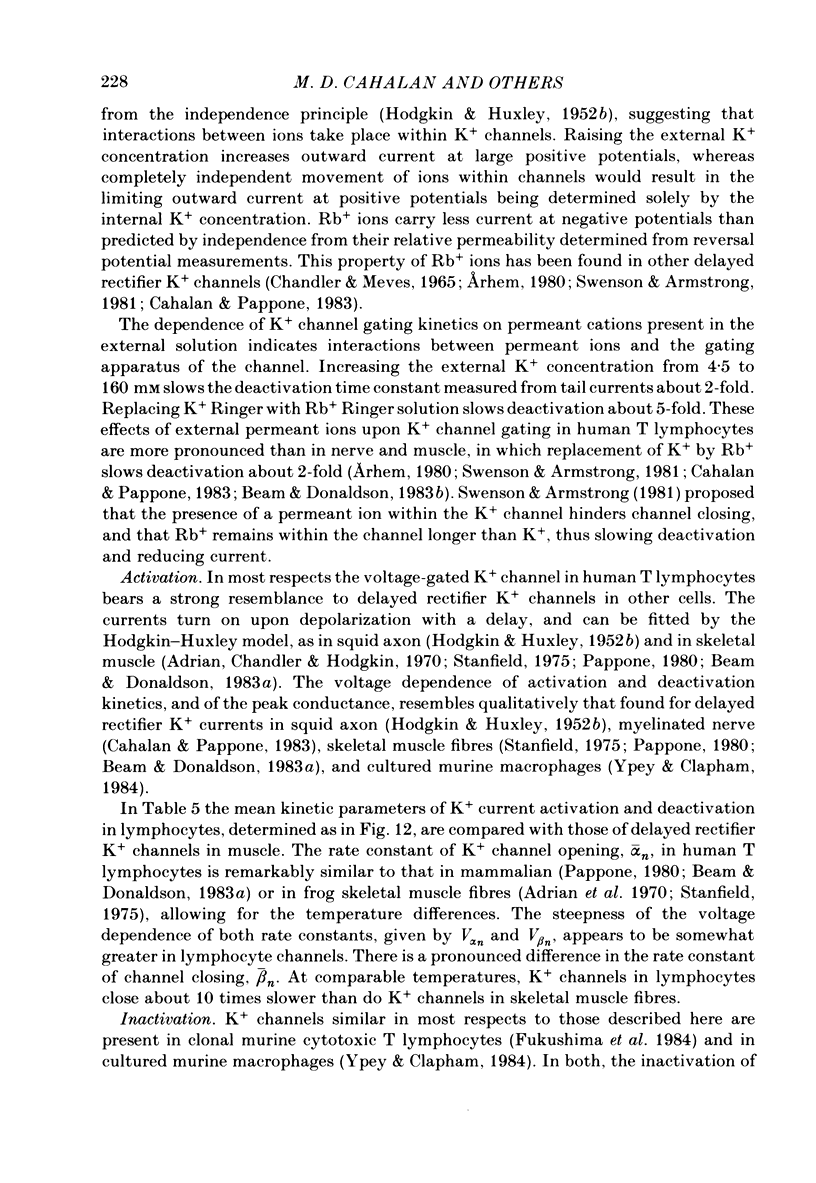
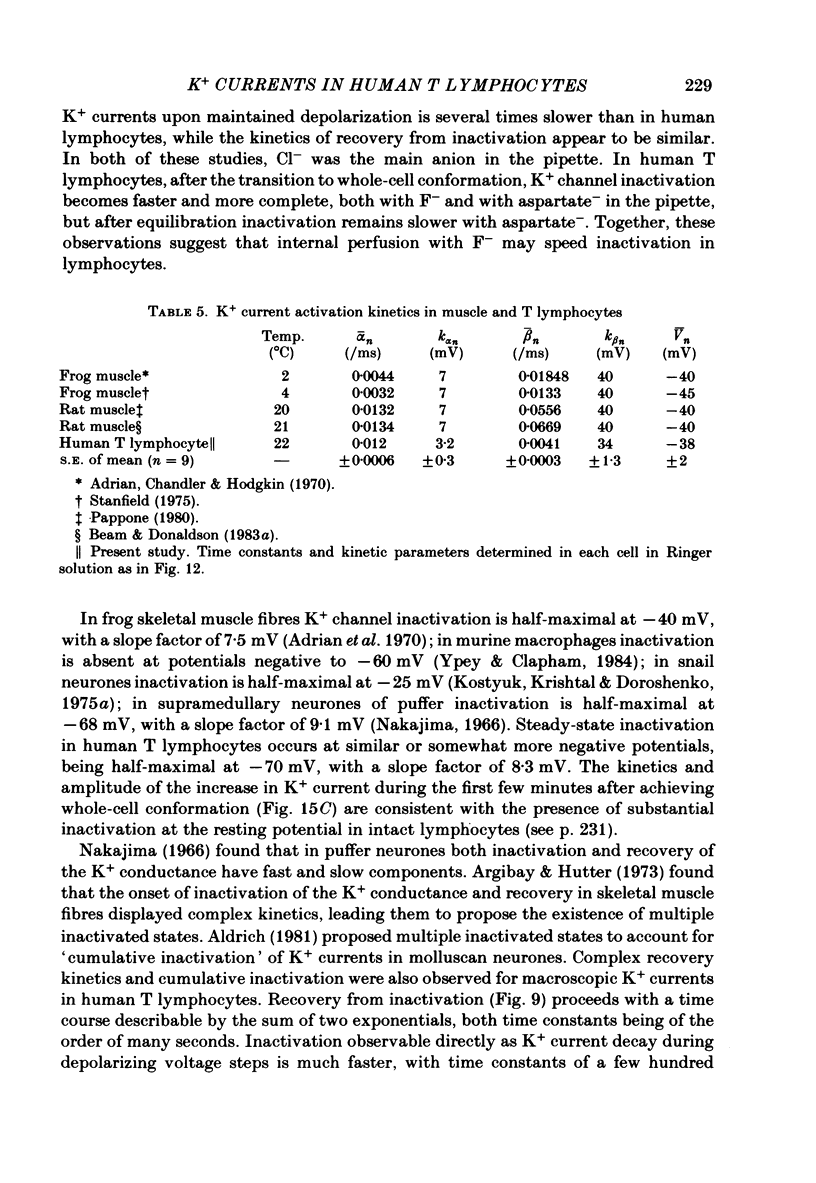
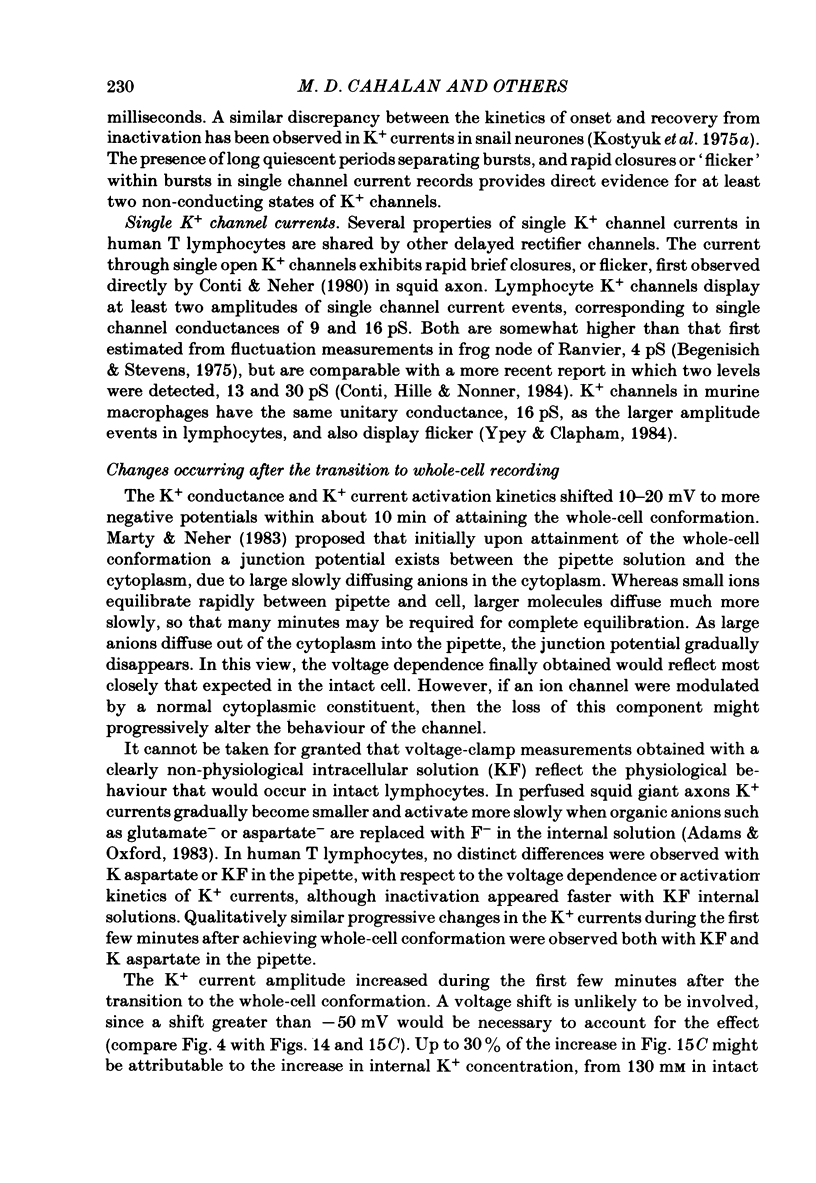
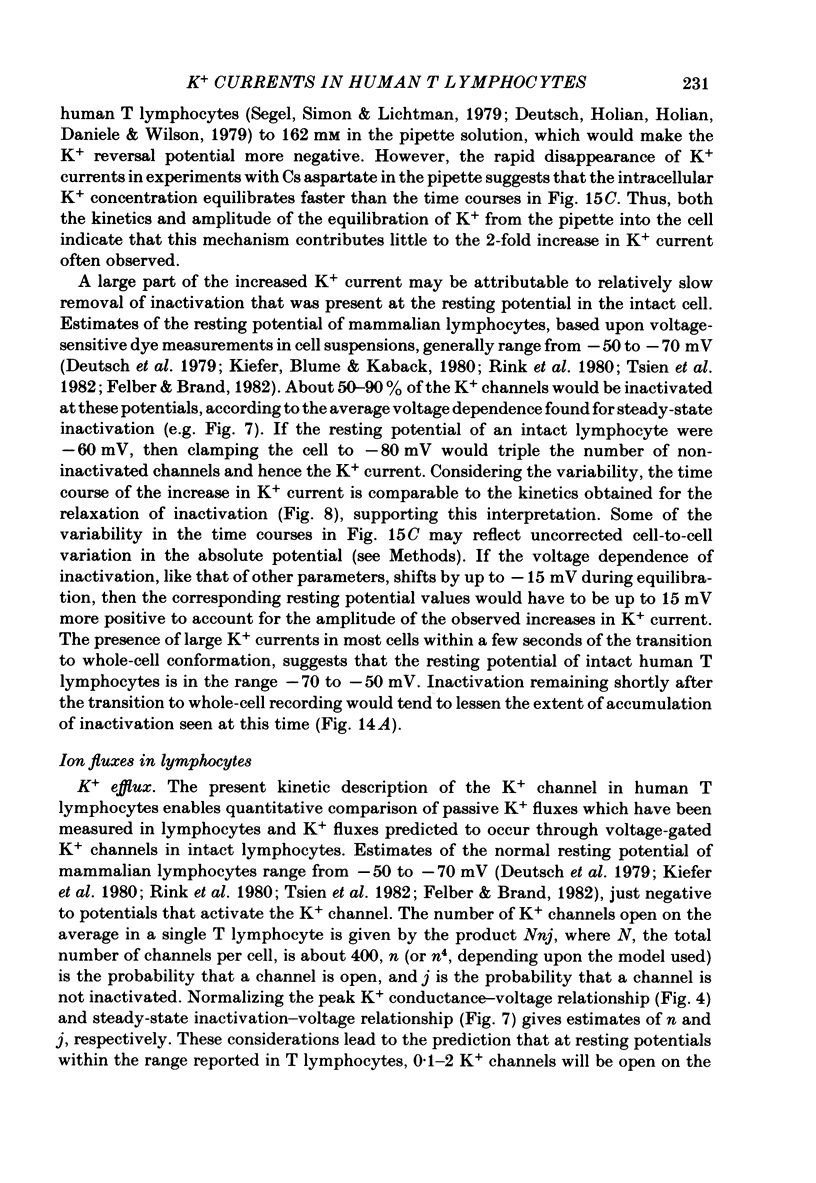
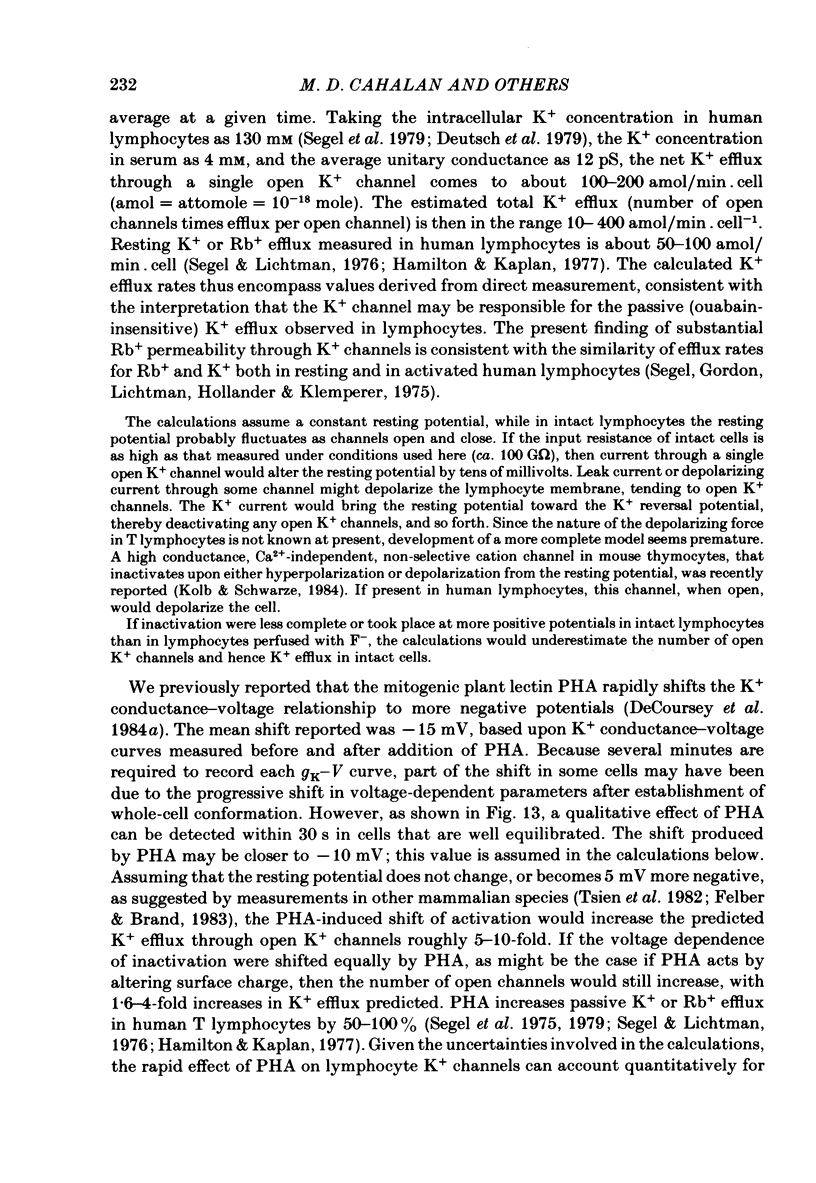
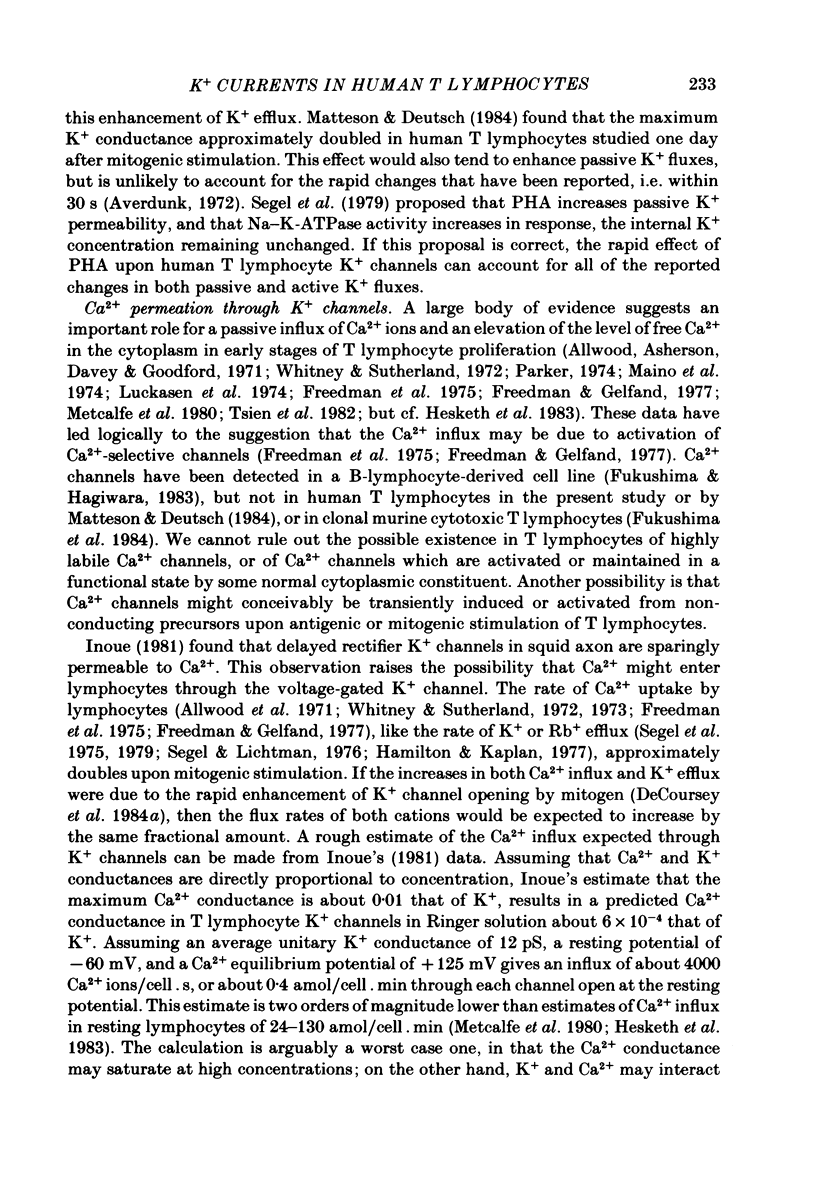
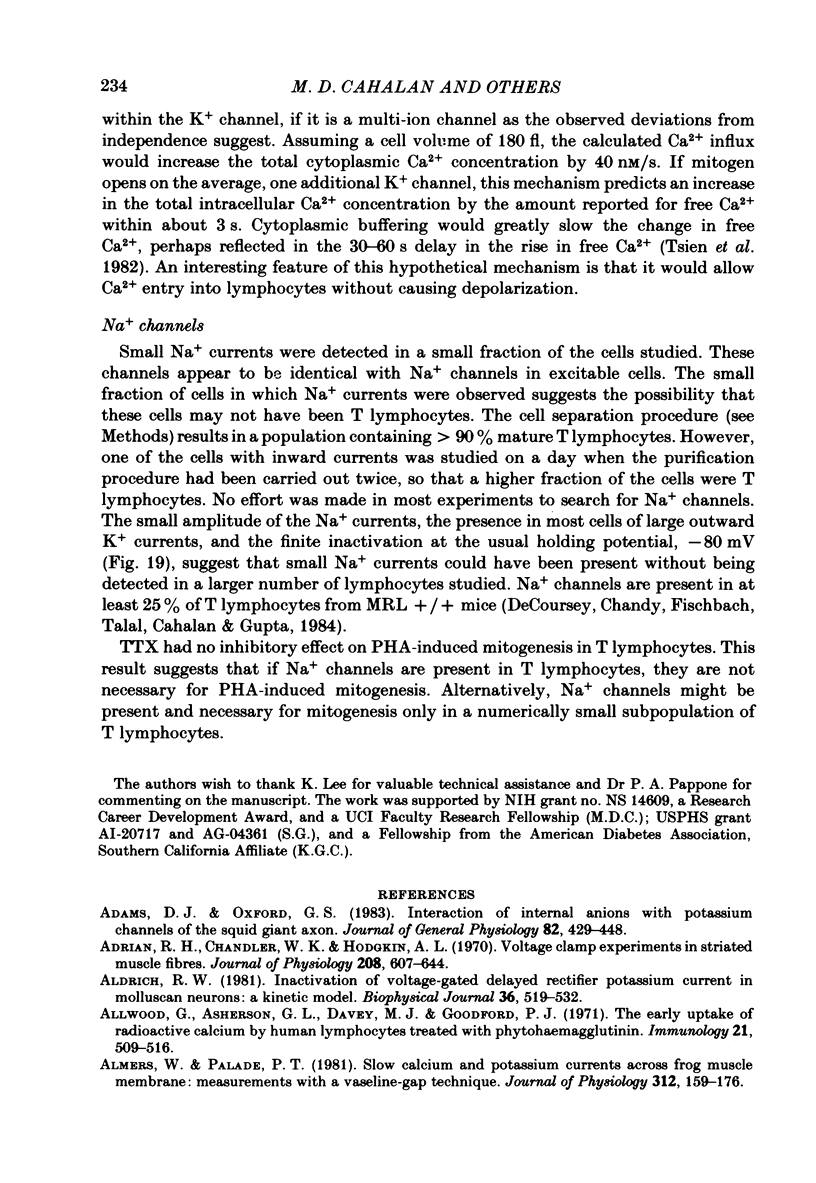
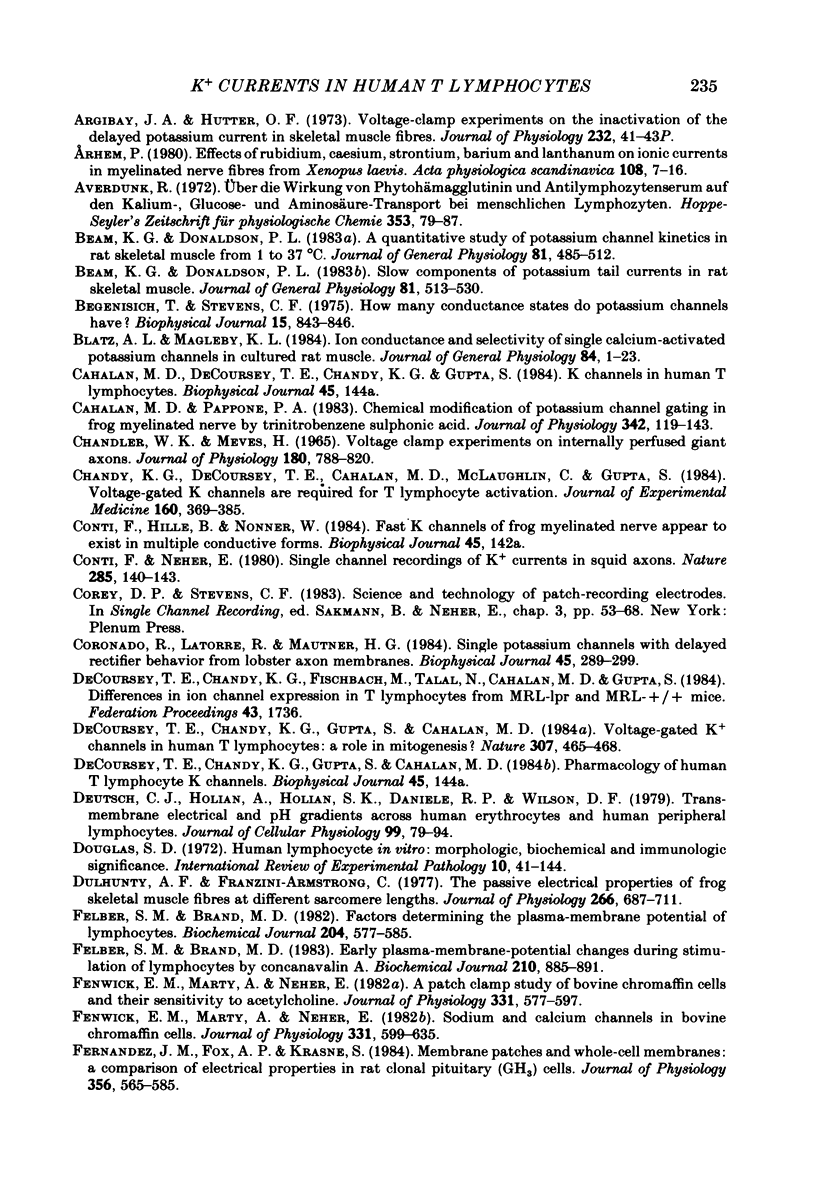
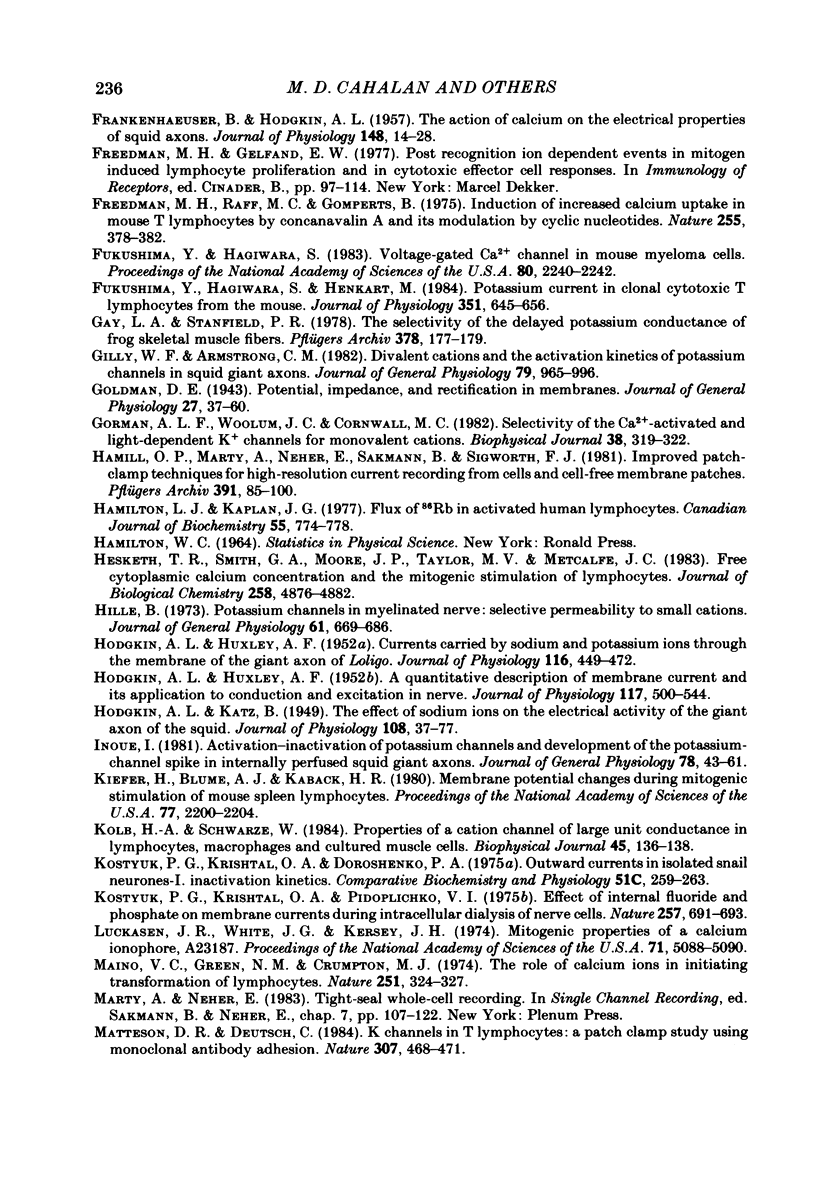
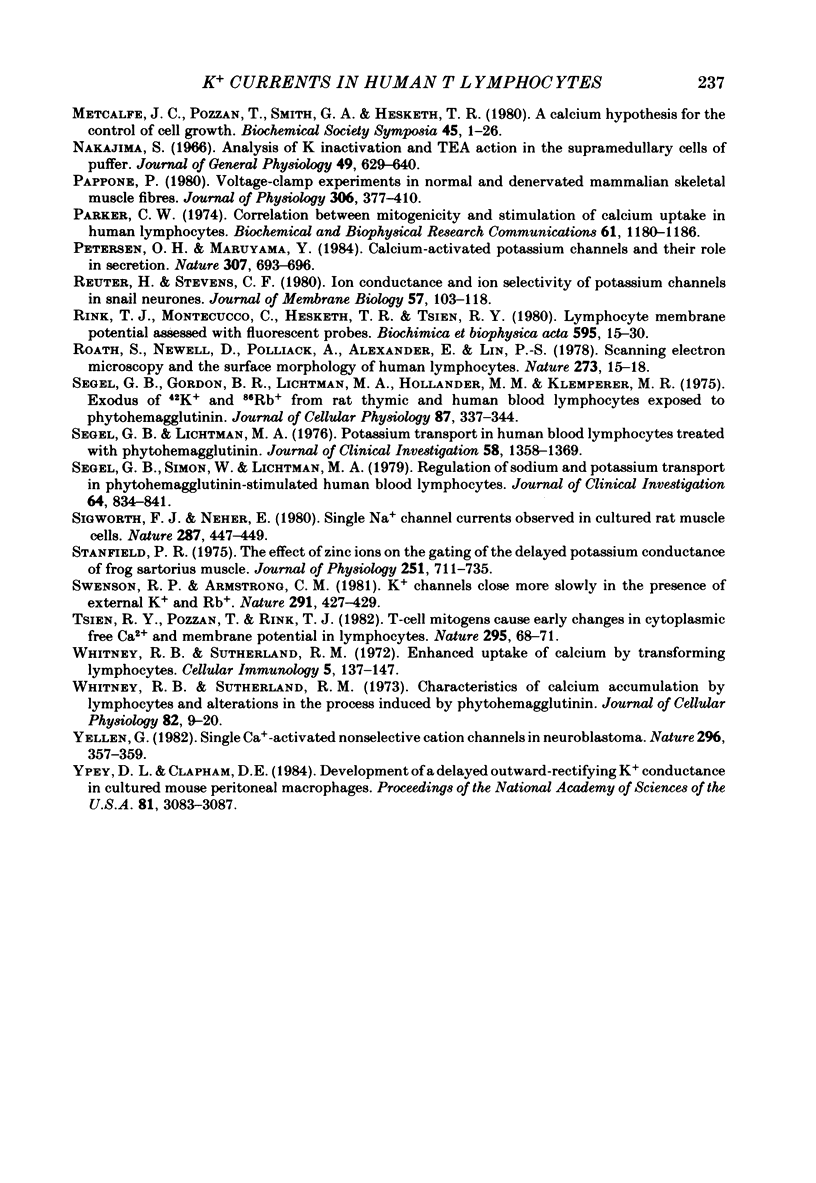
Selected References
These references are in PubMed. This may not be the complete list of references from this article.
- Adams D. J., Oxford G. S. Interaction of internal anions with potassium channels of the squid giant axon. J Gen Physiol. 1983 Oct;82(4):429–448. doi: 10.1085/jgp.82.4.429. [DOI] [PMC free article] [PubMed] [Google Scholar]
- Adrian R. H., Chandler W. K., Hodgkin A. L. Voltage clamp experiments in striated muscle fibres. J Physiol. 1970 Jul;208(3):607–644. doi: 10.1113/jphysiol.1970.sp009139. [DOI] [PMC free article] [PubMed] [Google Scholar]
- Aldrich R. W. Inactivation of voltage-gated delayed potassium current in molluscan neurons. A kinetic model. Biophys J. 1981 Dec;36(3):519–532. doi: 10.1016/S0006-3495(81)84750-9. [DOI] [PMC free article] [PubMed] [Google Scholar]
- Allwood G., Asherson G. L., Davey M. J., Goodford P. J. The early uptake of radioactive calcium by human lymphocytes treated with phytohaemagglutinin. Immunology. 1971 Sep;21(3):509–516. [PMC free article] [PubMed] [Google Scholar]
- Almers W., Palade P. T. Slow calcium and potassium currents across frog muscle membrane: measurements with a vaseline-gap technique. J Physiol. 1981 Mar;312:159–176. doi: 10.1113/jphysiol.1981.sp013622. [DOI] [PMC free article] [PubMed] [Google Scholar]
- Argibay J. A., Hutter O. F. Voltage-clamp experiments on the inactivation of the delayed potassium current in skeletal muscle fibres. J Physiol. 1973 Jul;232(1):41P–43P. [PubMed] [Google Scholar]
- Arhem P. Effects of rubidium, caesium, strontium, barium and lanthanum on ionic currents in myelinated nerve fibres from Xenopus laevis. Acta Physiol Scand. 1980 Jan;108(1):7–16. doi: 10.1111/j.1748-1716.1980.tb06494.x. [DOI] [PubMed] [Google Scholar]
- Averdunk R. Uber die Wirkung von Phytohänagglutinin und Antilymphozytenserum auf den Kalium-, Glucose- und Aminosäure-Transport bei menschlichen Lymphozyten. Hoppe Seylers Z Physiol Chem. 1972 Jan;353(1):79–87. doi: 10.1515/bchm2.1972.353.1.79. [DOI] [PubMed] [Google Scholar]
- Beam K. G., Donaldson P. L. A quantitative study of potassium channel kinetics in rat skeletal muscle from 1 to 37 degrees C. J Gen Physiol. 1983 Apr;81(4):485–512. doi: 10.1085/jgp.81.4.485. [DOI] [PMC free article] [PubMed] [Google Scholar]
- Beam K. G., Donaldson P. L. Slow components of potassium tail currents in rat skeletal muscle. J Gen Physiol. 1983 Apr;81(4):513–530. doi: 10.1085/jgp.81.4.513. [DOI] [PMC free article] [PubMed] [Google Scholar]
- Begenisich T., Stevens C. F. How many conductance states do potassium channels have? Biophys J. 1975 Aug;15(8):843–846. doi: 10.1016/S0006-3495(75)85858-9. [DOI] [PMC free article] [PubMed] [Google Scholar]
- Blatz A. L., Magleby K. L. Ion conductance and selectivity of single calcium-activated potassium channels in cultured rat muscle. J Gen Physiol. 1984 Jul;84(1):1–23. doi: 10.1085/jgp.84.1.1. [DOI] [PMC free article] [PubMed] [Google Scholar]
- Cahalan M. D., Pappone P. A. Chemical modification of potassium channel gating in frog myelinated nerve by trinitrobenzene sulphonic acid. J Physiol. 1983 Sep;342:119–143. doi: 10.1113/jphysiol.1983.sp014843. [DOI] [PMC free article] [PubMed] [Google Scholar]
- Chandler W. K., Meves H. Voltage clamp experiments on internally perfused giant axons. J Physiol. 1965 Oct;180(4):788–820. doi: 10.1113/jphysiol.1965.sp007732. [DOI] [PMC free article] [PubMed] [Google Scholar]
- Chandy K. G., DeCoursey T. E., Cahalan M. D., McLaughlin C., Gupta S. Voltage-gated potassium channels are required for human T lymphocyte activation. J Exp Med. 1984 Aug 1;160(2):369–385. doi: 10.1084/jem.160.2.369. [DOI] [PMC free article] [PubMed] [Google Scholar]
- Conti F., Neher E. Single channel recordings of K+ currents in squid axons. Nature. 1980 May 15;285(5761):140–143. doi: 10.1038/285140a0. [DOI] [PubMed] [Google Scholar]
- Coronado R., Latorre R., Mautner H. G. Single potassium channels with delayed rectifier behavior from lobster axon membranes. Biophys J. 1984 Jan;45(1):289–299. doi: 10.1016/S0006-3495(84)84155-7. [DOI] [PMC free article] [PubMed] [Google Scholar]
- DeCoursey T. E., Chandy K. G., Gupta S., Cahalan M. D. Voltage-gated K+ channels in human T lymphocytes: a role in mitogenesis? Nature. 1984 Feb 2;307(5950):465–468. doi: 10.1038/307465a0. [DOI] [PubMed] [Google Scholar]
- Deutsch C. J., Holian A., Holian S. K., Daniele R. P., Wilson D. F. Transmembrane electrical and pH gradients across human erythrocytes and human peripheral lymphocytes. J Cell Physiol. 1979 Apr;99(1):79–93. doi: 10.1002/jcp.1040990110. [DOI] [PubMed] [Google Scholar]
- Douglas S. D. Human lymphocyte growth in vitro: morphologic, biochemical, and immunologic significance. Int Rev Exp Pathol. 1971;10:41–114. [PubMed] [Google Scholar]
- Dulhunty A. F., Franzini-Armstrong C. The passive electrical properties of frog skeletal muscle fibres at different sarcomere lengths. J Physiol. 1977 Apr;266(3):687–711. doi: 10.1113/jphysiol.1977.sp011788. [DOI] [PMC free article] [PubMed] [Google Scholar]
- Felber S. M., Brand M. D. Early plasma-membrane-potential changes during stimulation of lymphocytes by concanavalin A. Biochem J. 1983 Mar 15;210(3):885–891. doi: 10.1042/bj2100885. [DOI] [PMC free article] [PubMed] [Google Scholar]
- Felber S. M., Brand M. D. Factors determining the plasma-membrane potential of lymphocytes. Biochem J. 1982 May 15;204(2):577–585. doi: 10.1042/bj2040577. [DOI] [PMC free article] [PubMed] [Google Scholar]
- Fenwick E. M., Marty A., Neher E. A patch-clamp study of bovine chromaffin cells and of their sensitivity to acetylcholine. J Physiol. 1982 Oct;331:577–597. doi: 10.1113/jphysiol.1982.sp014393. [DOI] [PMC free article] [PubMed] [Google Scholar]
- Fenwick E. M., Marty A., Neher E. Sodium and calcium channels in bovine chromaffin cells. J Physiol. 1982 Oct;331:599–635. doi: 10.1113/jphysiol.1982.sp014394. [DOI] [PMC free article] [PubMed] [Google Scholar]
- Fernandez J. M., Fox A. P., Krasne S. Membrane patches and whole-cell membranes: a comparison of electrical properties in rat clonal pituitary (GH3) cells. J Physiol. 1984 Nov;356:565–585. doi: 10.1113/jphysiol.1984.sp015483. [DOI] [PMC free article] [PubMed] [Google Scholar]
- Freedman M. H., Raff M. C. Induction of increased calcium uptake in mouse T lymphocytes by concanavalin A and its modulation by cyclic nucleotides. Nature. 1975 May 29;255(5507):378–382. doi: 10.1038/255378a0. [DOI] [PubMed] [Google Scholar]
- Fukushima Y., Hagiwara S., Henkart M. Potassium current in clonal cytotoxic T lymphocytes from the mouse. J Physiol. 1984 Jun;351:645–656. doi: 10.1113/jphysiol.1984.sp015268. [DOI] [PMC free article] [PubMed] [Google Scholar]
- Fukushima Y., Hagiwara S. Voltage-gated Ca2+ channel in mouse myeloma cells. Proc Natl Acad Sci U S A. 1983 Apr;80(8):2240–2242. doi: 10.1073/pnas.80.8.2240. [DOI] [PMC free article] [PubMed] [Google Scholar]
- Gay L. A., Stanfield P. R. The selectivity of the delayed potassium conductance of frog skeletal muscle fibers. Pflugers Arch. 1978 Dec 28;378(2):177–179. doi: 10.1007/BF00584453. [DOI] [PubMed] [Google Scholar]
- Gilly W. F., Armstrong C. M. Divalent cations and the activation kinetics of potassium channels in squid giant axons. J Gen Physiol. 1982 Jun;79(6):965–996. doi: 10.1085/jgp.79.6.965. [DOI] [PMC free article] [PubMed] [Google Scholar]
- Goldman D. E. POTENTIAL, IMPEDANCE, AND RECTIFICATION IN MEMBRANES. J Gen Physiol. 1943 Sep 20;27(1):37–60. doi: 10.1085/jgp.27.1.37. [DOI] [PMC free article] [PubMed] [Google Scholar]
- Gorman A. L., Woolum J. C., Cornwall M. C. Selectivity of the Ca2+-activated and light-dependent K+ channels for monovalent cations. Biophys J. 1982 Jun;38(3):319–322. doi: 10.1016/S0006-3495(82)84565-7. [DOI] [PMC free article] [PubMed] [Google Scholar]
- HODGKIN A. L., HUXLEY A. F. A quantitative description of membrane current and its application to conduction and excitation in nerve. J Physiol. 1952 Aug;117(4):500–544. doi: 10.1113/jphysiol.1952.sp004764. [DOI] [PMC free article] [PubMed] [Google Scholar]
- HODGKIN A. L., HUXLEY A. F. Currents carried by sodium and potassium ions through the membrane of the giant axon of Loligo. J Physiol. 1952 Apr;116(4):449–472. doi: 10.1113/jphysiol.1952.sp004717. [DOI] [PMC free article] [PubMed] [Google Scholar]
- HODGKIN A. L., KATZ B. The effect of sodium ions on the electrical activity of giant axon of the squid. J Physiol. 1949 Mar 1;108(1):37–77. doi: 10.1113/jphysiol.1949.sp004310. [DOI] [PMC free article] [PubMed] [Google Scholar]
- Hamill O. P., Marty A., Neher E., Sakmann B., Sigworth F. J. Improved patch-clamp techniques for high-resolution current recording from cells and cell-free membrane patches. Pflugers Arch. 1981 Aug;391(2):85–100. doi: 10.1007/BF00656997. [DOI] [PubMed] [Google Scholar]
- Hamilton L. J., Kaplan J. G. Flux of 86Rb in activated human lymphocytes. Can J Biochem. 1977 Jul;55(7):774–778. doi: 10.1139/o77-113. [DOI] [PubMed] [Google Scholar]
- Hesketh T. R., Smith G. A., Moore J. P., Taylor M. V., Metcalfe J. C. Free cytoplasmic calcium concentration and the mitogenic stimulation of lymphocytes. J Biol Chem. 1983 Apr 25;258(8):4876–4882. [PubMed] [Google Scholar]
- Hille B. Potassium channels in myelinated nerve. Selective permeability to small cations. J Gen Physiol. 1973 Jun;61(6):669–686. doi: 10.1085/jgp.61.6.669. [DOI] [PMC free article] [PubMed] [Google Scholar]
- Inoue I. Activation-inactivation of potassium channels and development of the potassium-channel spike in internally perfused squid giant axons. J Gen Physiol. 1981 Jul;78(1):43–61. doi: 10.1085/jgp.78.1.43. [DOI] [PMC free article] [PubMed] [Google Scholar]
- Kiefer H., Blume A. J., Kaback H. R. Membrane potential changes during mitogenic stimulation of mouse spleen lymphocytes. Proc Natl Acad Sci U S A. 1980 Apr;77(4):2200–2204. doi: 10.1073/pnas.77.4.2200. [DOI] [PMC free article] [PubMed] [Google Scholar]
- Kolb H. A., Schwarze W. Properties of a cation channel of large unit conductance in lymphocytes, macrophages and cultured muscle cells. Biophys J. 1984 Jan;45(1):136–138. doi: 10.1016/S0006-3495(84)84139-9. [DOI] [PMC free article] [PubMed] [Google Scholar]
- Kostyuk P. G., Krishtal O. A., Pidoplichko V. I. Effect of internal fluoride and phosphate on membrane currents during intracellular dialysis of nerve cells. Nature. 1975 Oct 23;257(5528):691–693. doi: 10.1038/257691a0. [DOI] [PubMed] [Google Scholar]
- Luckasen J. R., White J. G., Kersey J. H. Mitogenic properties of a calcium ionophore, A23187. Proc Natl Acad Sci U S A. 1974 Dec;71(12):5088–5090. doi: 10.1073/pnas.71.12.5088. [DOI] [PMC free article] [PubMed] [Google Scholar]
- Maino V. C., Green N. M., Crumpton M. J. The role of calcium ions in initiating transformation of lymphocytes. Nature. 1974 Sep 27;251(5473):324–327. doi: 10.1038/251324b0. [DOI] [PubMed] [Google Scholar]
- Matteson D. R., Deutsch C. K channels in T lymphocytes: a patch clamp study using monoclonal antibody adhesion. Nature. 1984 Feb 2;307(5950):468–471. doi: 10.1038/307468a0. [DOI] [PubMed] [Google Scholar]
- Metcalfe J. C., Pozzan T., Smith G. A., Hesketh T. R. A calcium hypothesis for the control of cell growth. Biochem Soc Symp. 1980;45:1–26. [PubMed] [Google Scholar]
- Nakajima S. Analysis of K inactivation and TEA action in the supramedullary cells of puffer. J Gen Physiol. 1966 Mar;49(4):629–640. doi: 10.1085/jgp.49.4.629. [DOI] [PMC free article] [PubMed] [Google Scholar]
- Pappone P. A. Voltage-clamp experiments in normal and denervated mammalian skeletal muscle fibres. J Physiol. 1980 Sep;306:377–410. doi: 10.1113/jphysiol.1980.sp013403. [DOI] [PMC free article] [PubMed] [Google Scholar]
- Parker C. W. Correlation between mitogenicity and stimulation of calcium uptake in human lymphocytes. Biochem Biophys Res Commun. 1974 Dec 23;61(4):1180–1186. doi: 10.1016/s0006-291x(74)80408-0. [DOI] [PubMed] [Google Scholar]
- Petersen O. H., Maruyama Y. Calcium-activated potassium channels and their role in secretion. Nature. 1984 Feb 23;307(5953):693–696. doi: 10.1038/307693a0. [DOI] [PubMed] [Google Scholar]
- Reuter H., Stevens C. F. Ion conductance and ion selectivity of potassium channels in snail neurones. J Membr Biol. 1980 Dec 15;57(2):103–118. doi: 10.1007/BF01868997. [DOI] [PubMed] [Google Scholar]
- Rink T. J., Montecucco C., Hesketh T. R., Tsien R. Y. Lymphocyte membrane potential assessed with fluorescent probes. Biochim Biophys Acta. 1980;595(1):15–30. doi: 10.1016/0005-2736(80)90243-6. [DOI] [PubMed] [Google Scholar]
- Roath S., Newell D., Polliack A., Alexander E., Lin P. S. Scanning electron microscopy and the surface morphology of human lymphocytes. Nature. 1978 May 4;273(5657):15–18. doi: 10.1038/273015a0. [DOI] [PubMed] [Google Scholar]
- Segel G. B., Gordon B. R., Lichtman M. A., Hollander M. M., Klemperer M. R. Exodus of 42K+ and 86Rb+ from rat thymic and human blood lymphocytes exposed to phytohemagglutinin. J Cell Physiol. 1976 Mar;87(3):337–343. doi: 10.1002/jcp.1040870309. [DOI] [PubMed] [Google Scholar]
- Segel G. B., Lichtman M. A. Potasssium transport in human blood lymphocytes treated with phytohemagglutinin. J Clin Invest. 1976 Dec;58(6):1358–1369. doi: 10.1172/JCI108591. [DOI] [PMC free article] [PubMed] [Google Scholar]
- Segel G. B., Simon W., Lichtman M. A. Regulation of sodium and potassium transport in phytohemagglutinin-stimulated human blood lymphocytes. J Clin Invest. 1979 Sep;64(3):834–841. doi: 10.1172/JCI109531. [DOI] [PMC free article] [PubMed] [Google Scholar]
- Sigworth F. J., Neher E. Single Na+ channel currents observed in cultured rat muscle cells. Nature. 1980 Oct 2;287(5781):447–449. doi: 10.1038/287447a0. [DOI] [PubMed] [Google Scholar]
- Stanfield P. R. The effect of zinc ions on the gating of the delayed potassium conductance of frog sartorius muscle. J Physiol. 1975 Oct;251(3):711–735. doi: 10.1113/jphysiol.1975.sp011118. [DOI] [PMC free article] [PubMed] [Google Scholar]
- Swenson R. P., Jr, Armstrong C. M. K+ channels close more slowly in the presence of external K+ and Rb+. Nature. 1981 Jun 4;291(5814):427–429. doi: 10.1038/291427a0. [DOI] [PubMed] [Google Scholar]
- Tsien R. Y., Pozzan T., Rink T. J. T-cell mitogens cause early changes in cytoplasmic free Ca2+ and membrane potential in lymphocytes. Nature. 1982 Jan 7;295(5844):68–71. doi: 10.1038/295068a0. [DOI] [PubMed] [Google Scholar]
- Whitney R. B., Sutherland R. M. Characteristics of calcium accumulation by lymphocytes and alterations in the process induced by phytohemagglutinin. J Cell Physiol. 1973 Aug;82(1):9–20. doi: 10.1002/jcp.1040820103. [DOI] [PubMed] [Google Scholar]
- Whitney R. B., Sutherland R. M. Enhanced uptake of calcium by transforming lymphocytes. Cell Immunol. 1972 Sep;5(1):137–147. doi: 10.1016/0008-8749(72)90091-3. [DOI] [PubMed] [Google Scholar]
- Yellen G. Single Ca2+-activated nonselective cation channels in neuroblastoma. Nature. 1982 Mar 25;296(5855):357–359. doi: 10.1038/296357a0. [DOI] [PubMed] [Google Scholar]
- Ypey D. L., Clapham D. E. Development of a delayed outward-rectifying K+ conductance in cultured mouse peritoneal macrophages. Proc Natl Acad Sci U S A. 1984 May;81(10):3083–3087. doi: 10.1073/pnas.81.10.3083. [DOI] [PMC free article] [PubMed] [Google Scholar]