Abstract
Incubation of rat hippocampal slices in solutions containing low Ca2+ and increased Mg2+ rapidly blocked synaptic responses and increased spontaneous firing of all the principal neurones. More remarkably, a rhythmic and synchronous bursting discharge developed, which was restricted to the CA1 population of pyramidal neurones. These 'field bursts' or 'spreading excitation' were rapidly abolished by restoring the Ca2+ to 2 mM, by increasing the Mg2+ to 6 mM or by decreasing K+ from 6 to 3 mM. The CA1 pyramidal cells depolarized after the change to the low-Ca2+ solution by about 10-20 mV. Individual field bursts were associated with a further depolarization of 10-12 mV surmounted by a burst of action potentials at about 20/s. This transient depolarization shift, recorded extracellularly as a negative field, could be attributed to the increase of [K+]o during the bursts, reaching 9-10 mM as measured by ion-sensitive electrodes. The bursts were followed by a hyperpolarization, seen extracellularly as a small soma-positive field, which was attributed to an electrogenic pump and/or a Ca2+-activated K+ conductance. Stimulation of the tightly packed pyramidal cell axons in the alveus elicited a train of population spikes, instead of the single spike normally seen, and could trigger a full field burst. Recordings of the alvear tract volley suggested that the repeated spikes arose within the pyramidal cells. Multiple recordings from CA1 revealed that field bursts usually, but by no means always, started near the caudal (subicular) end of the area. They spread through the cell layer at 0.04-0.12 m/s. The most rapid propagation was seen when the bursts had an abrupt onset; slower propagation (1-10 mm/s) occurred when the bursts started gradually, which generally was the case near the sites of burst initiation and termination. Usually the action potentials within each burst were synchronized into population spikes which spread across CA1 at 0.04-0.15 m/s. The site of initiation and the extent of the spread of these population spikes varied during each burst, as did their amplitude. The degree of spike synchronization was enhanced by various treatments expected to increase neuronal excitability. Measurements of transmembrane potential during the burst confirmed the role in the generation of population spikes of ephaptic or field interactions between the pyramidal cells. It is proposed that the increased firing of all neurones is due to the block of tonic inhibition, depression of after-hyperpolarization and to increased membrane excitability.(ABSTRACT TRUNCATED AT 400 WORDS)
Full text
PDF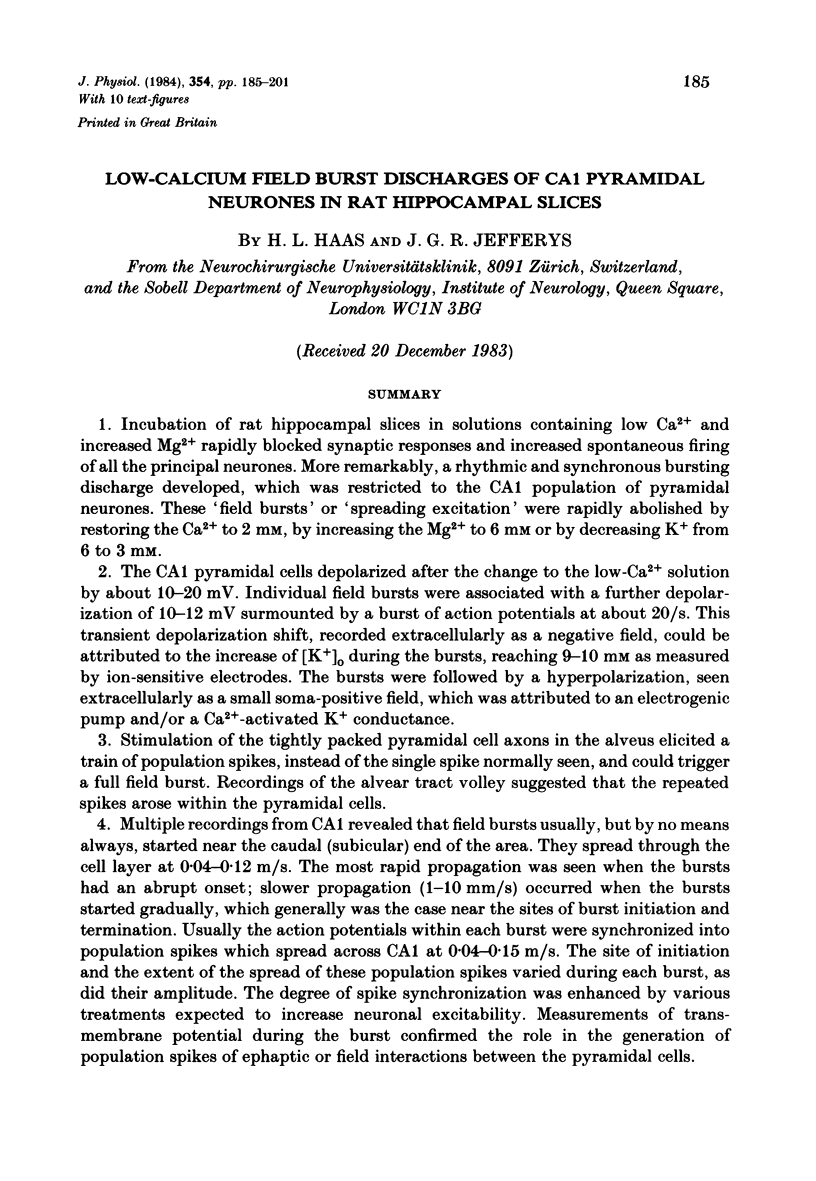
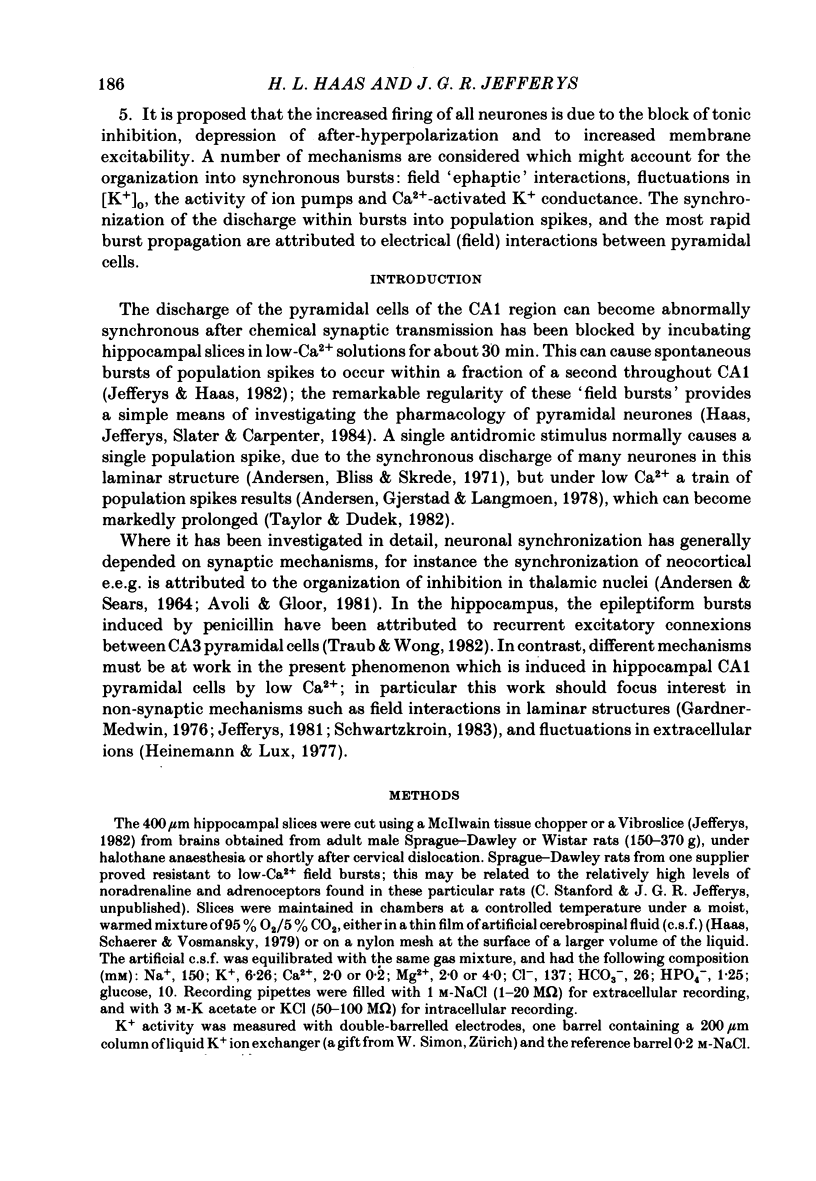
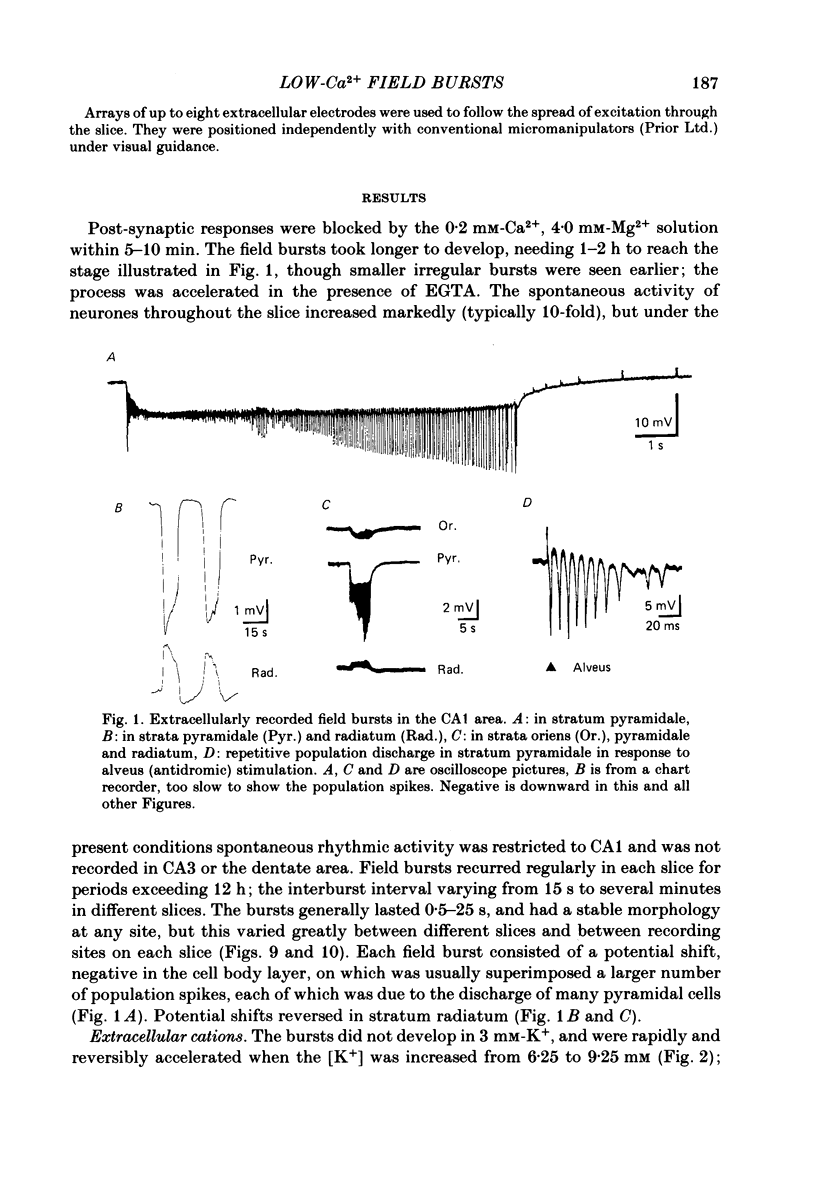
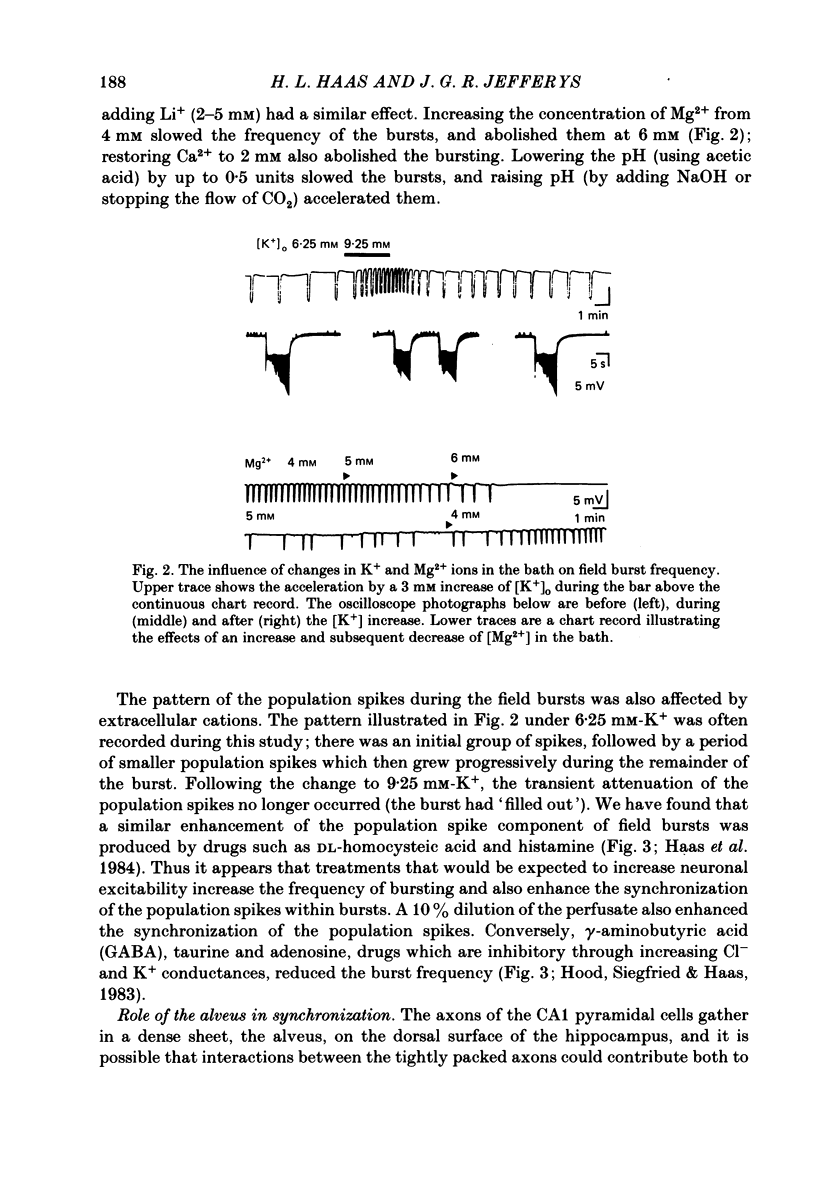
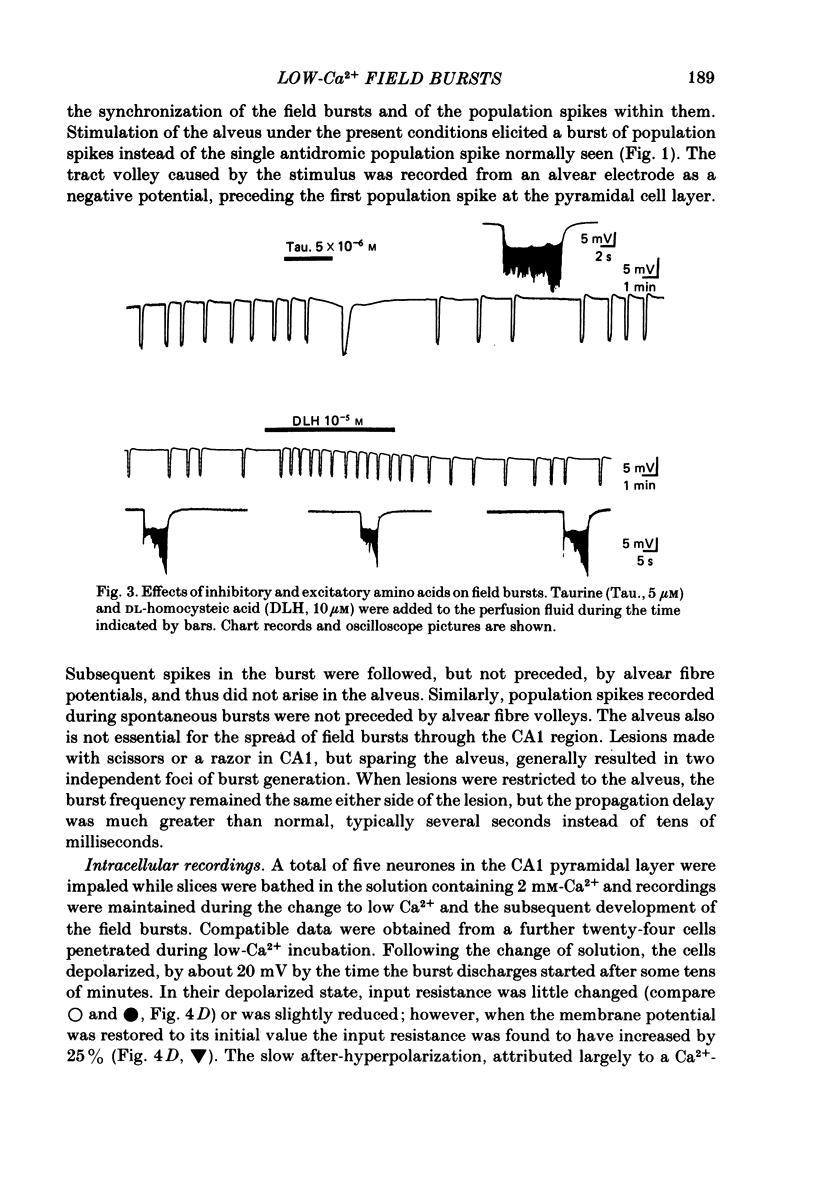
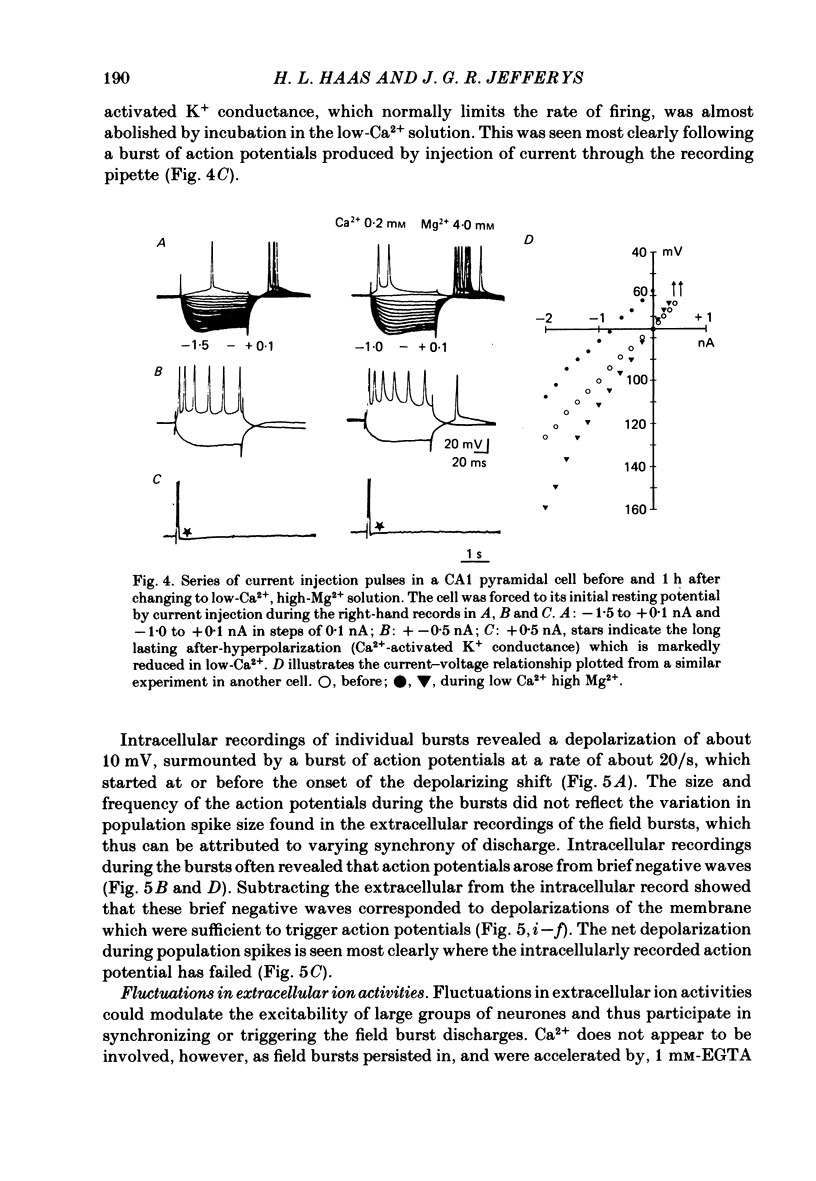
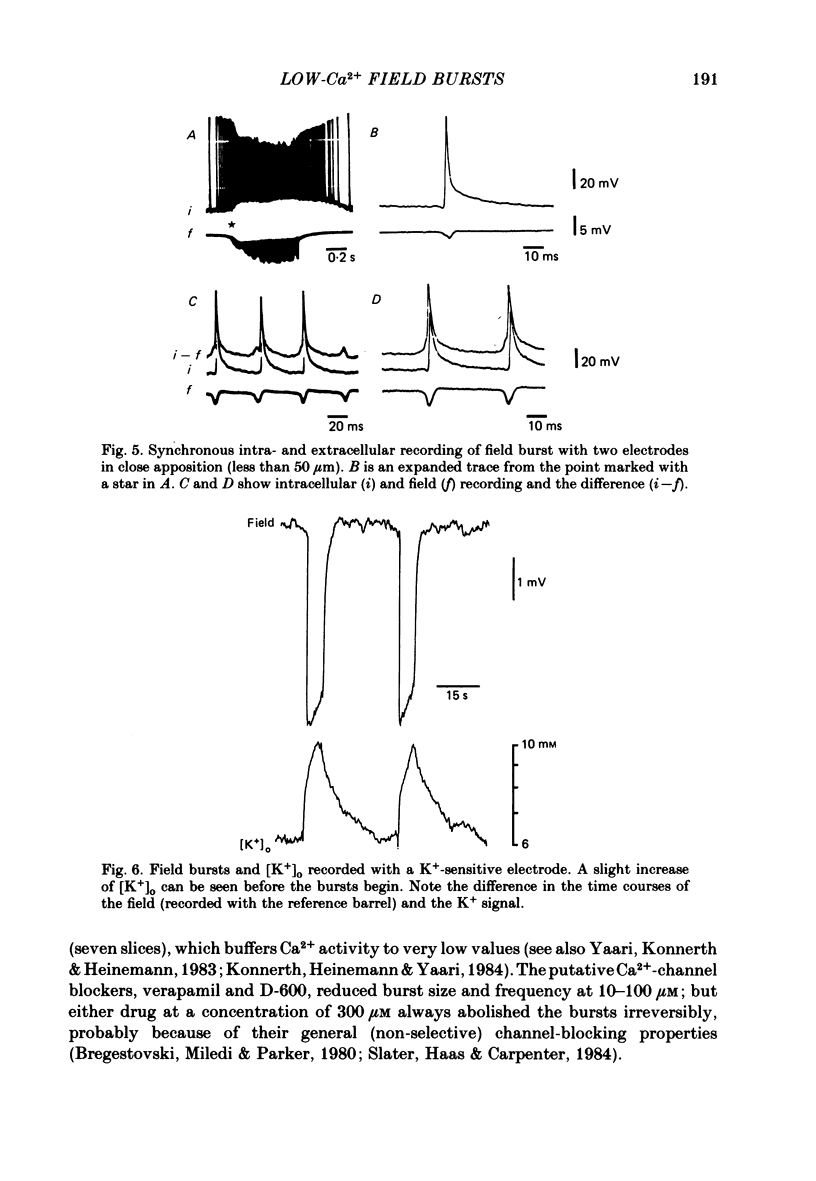
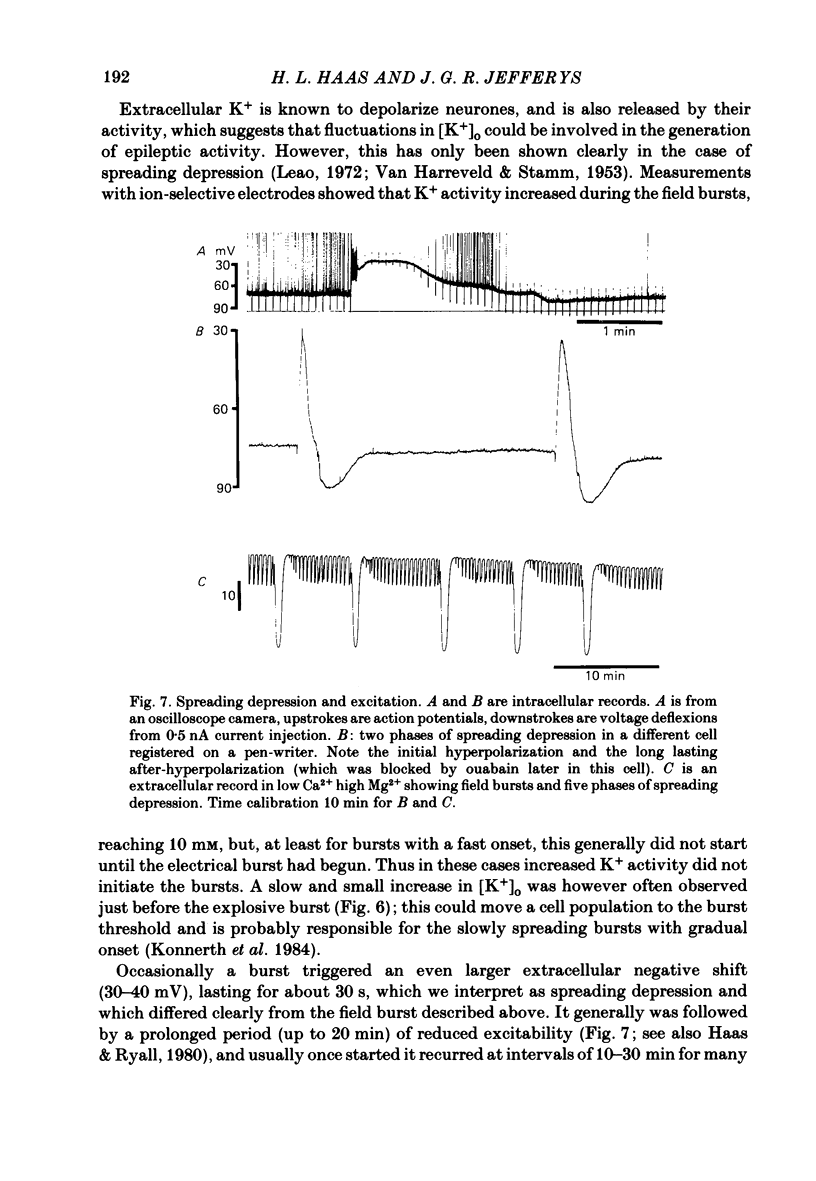
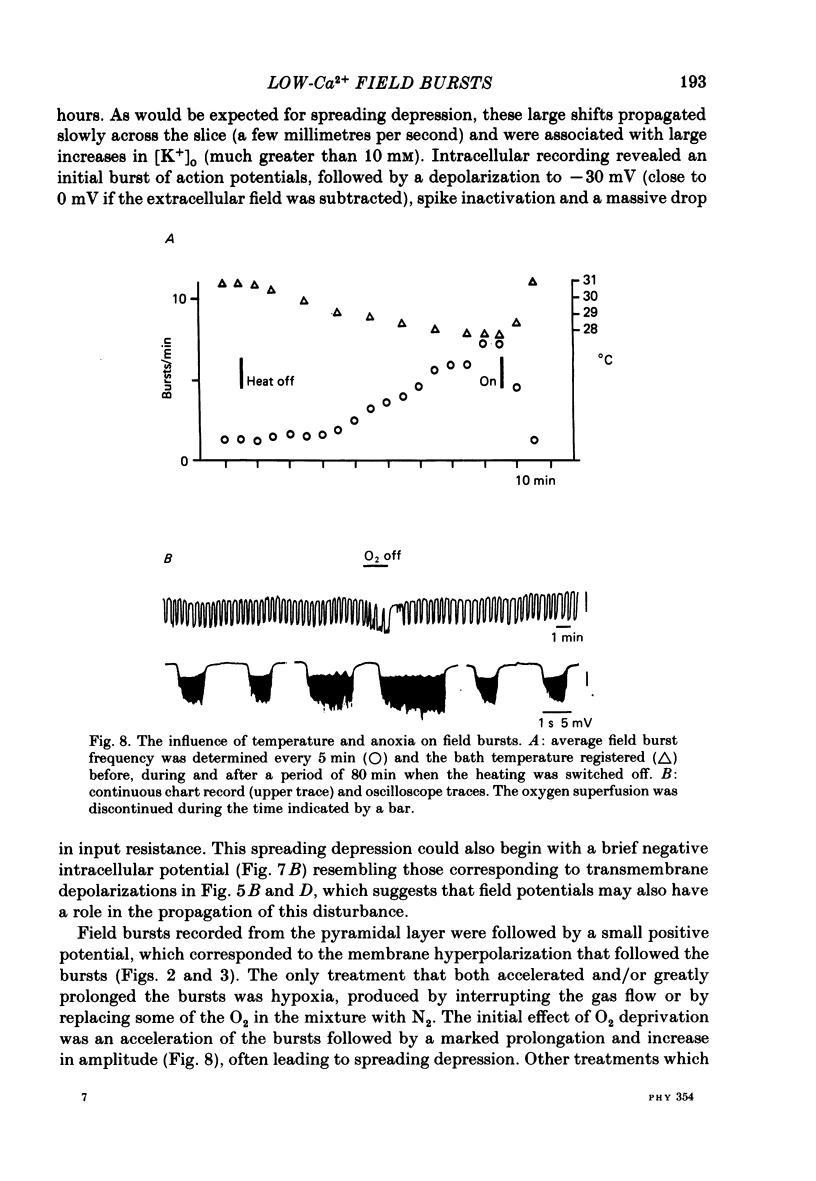
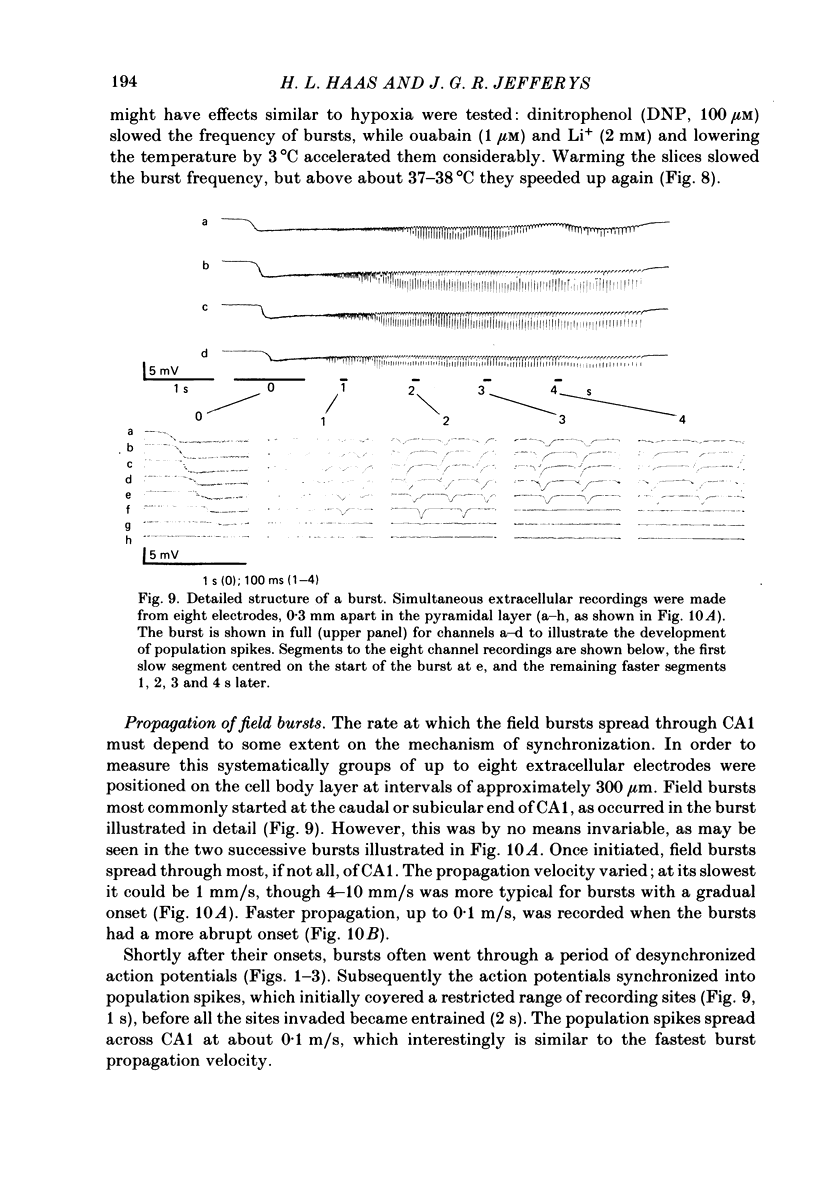
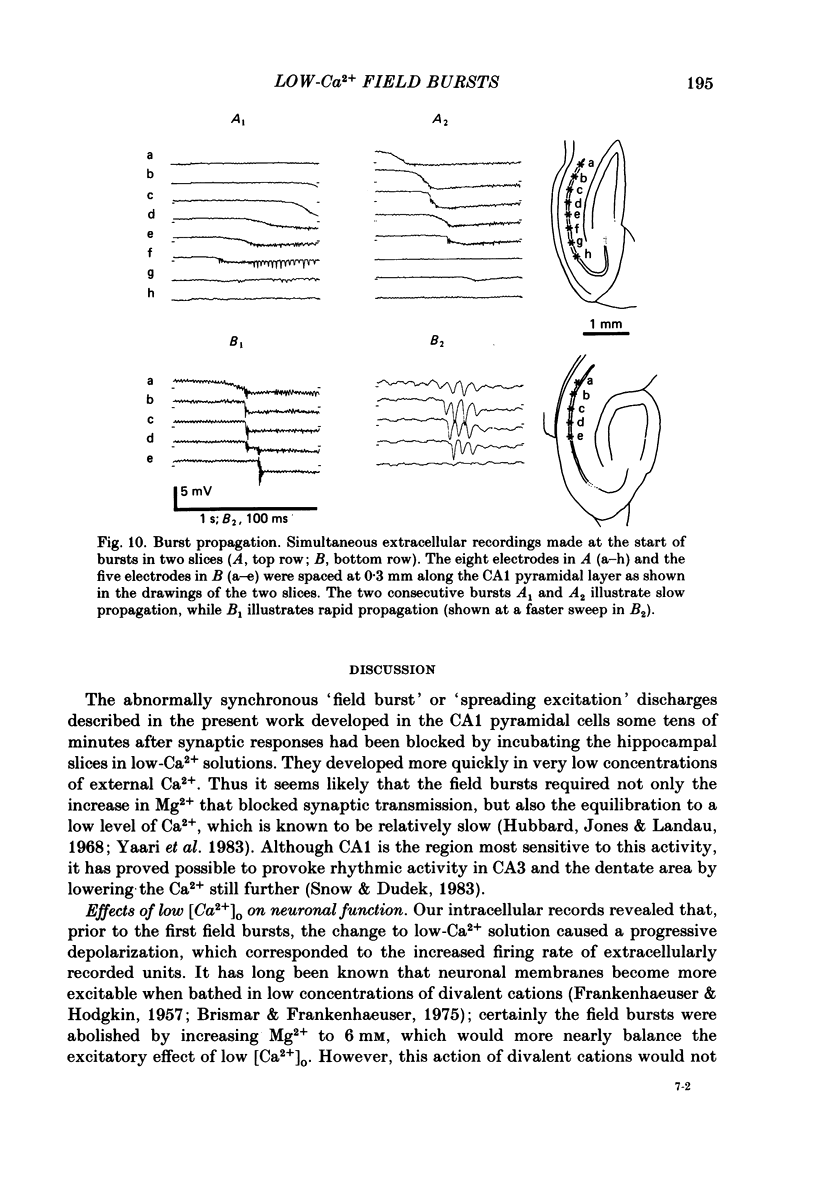
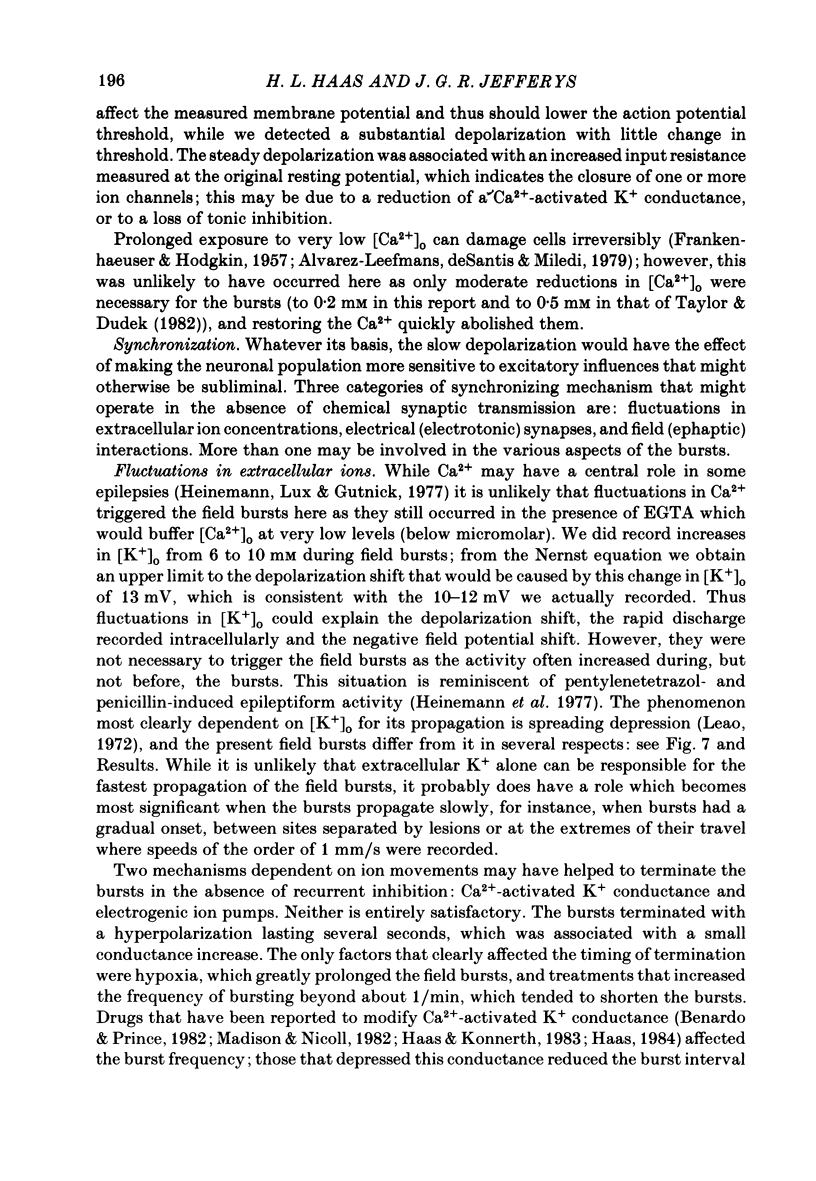
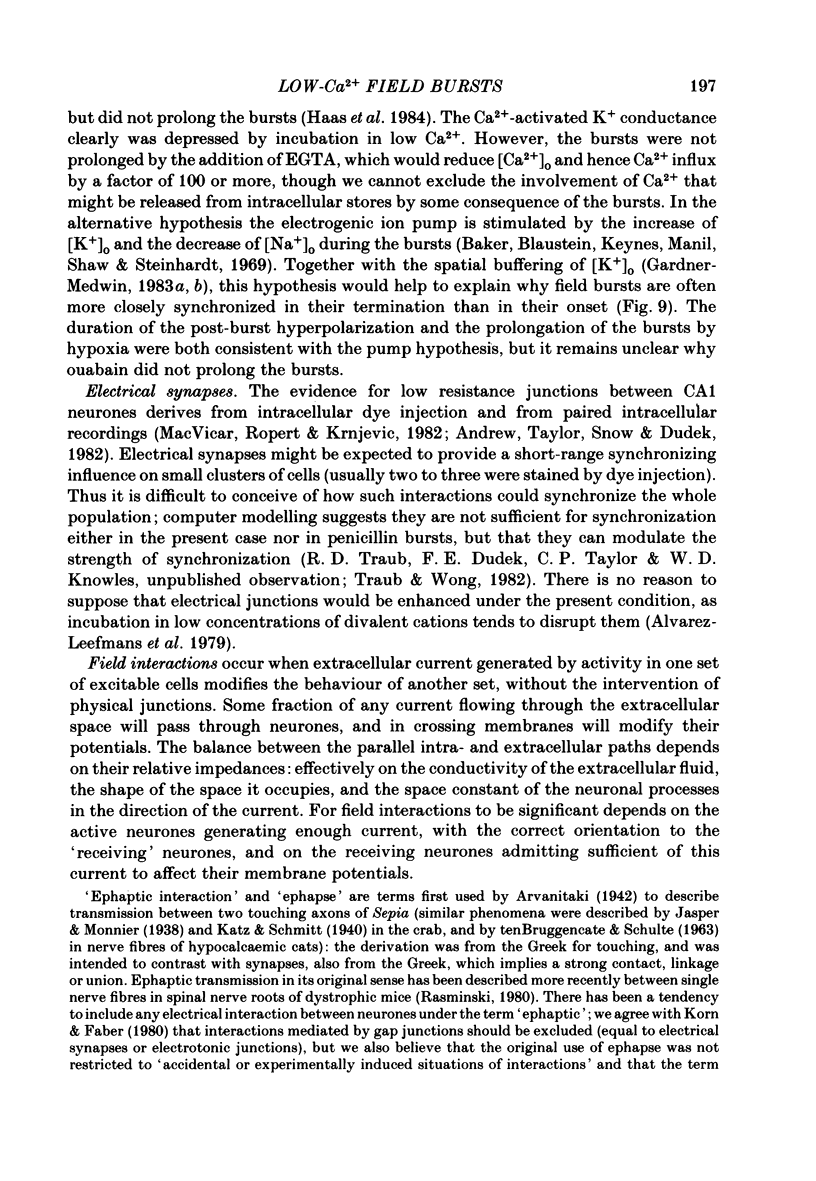
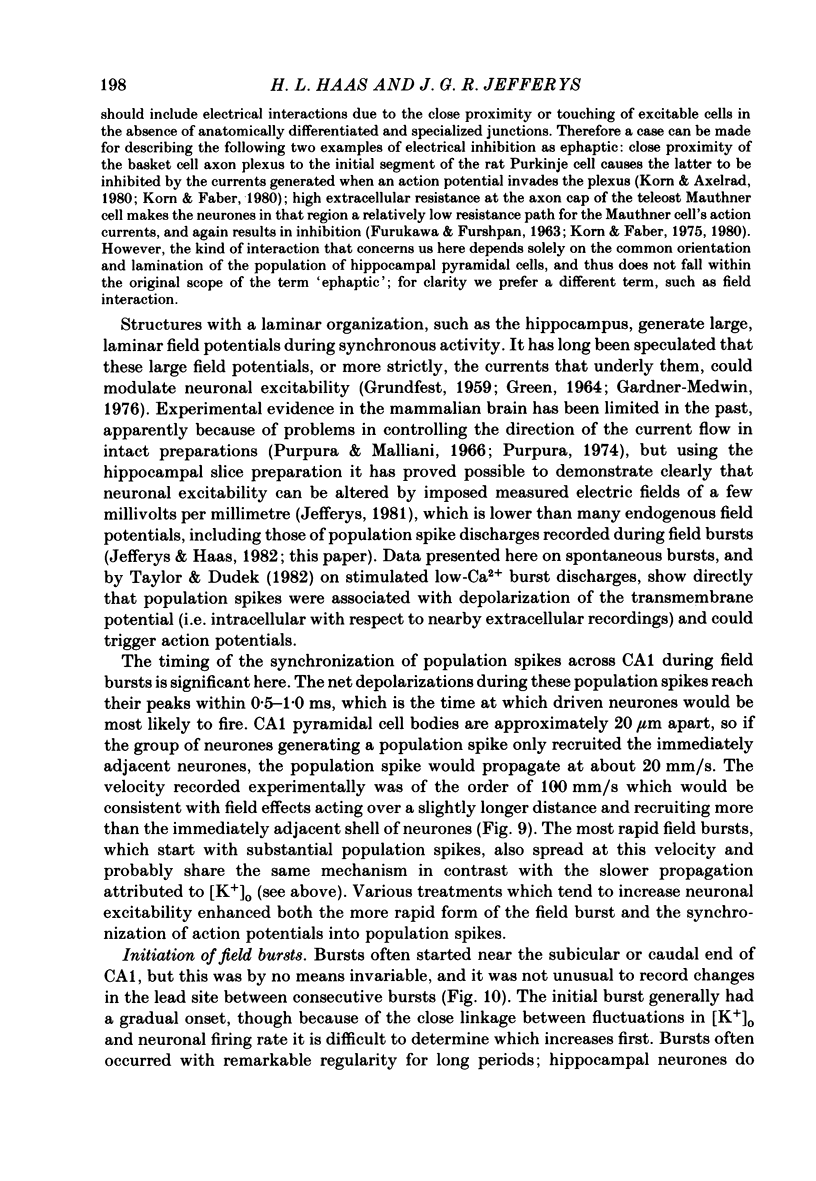
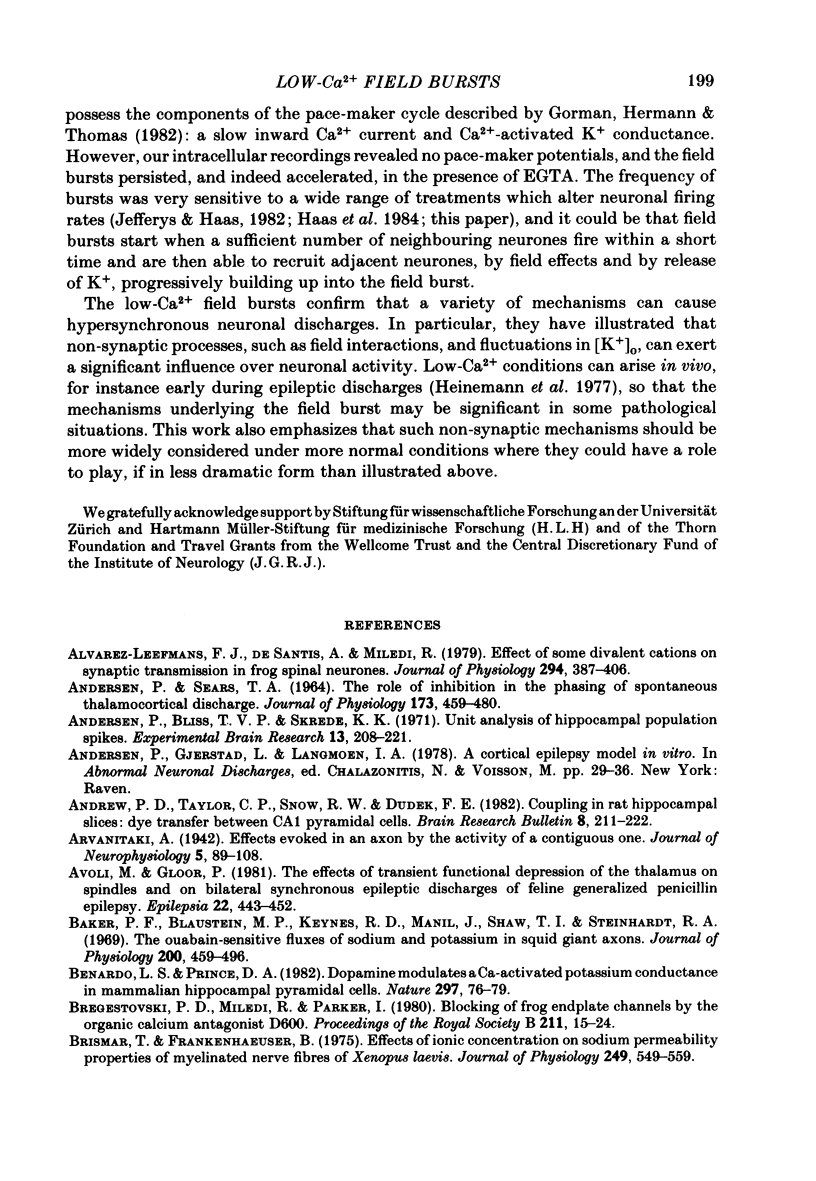
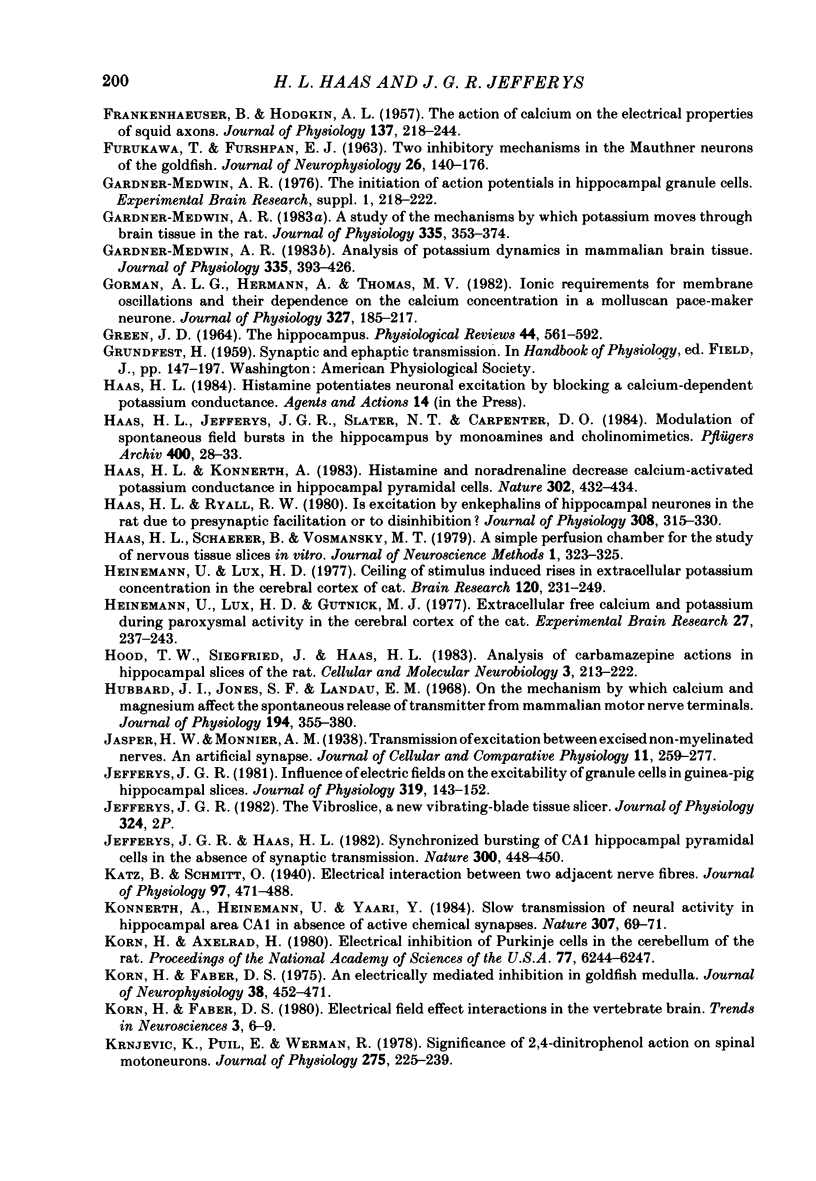
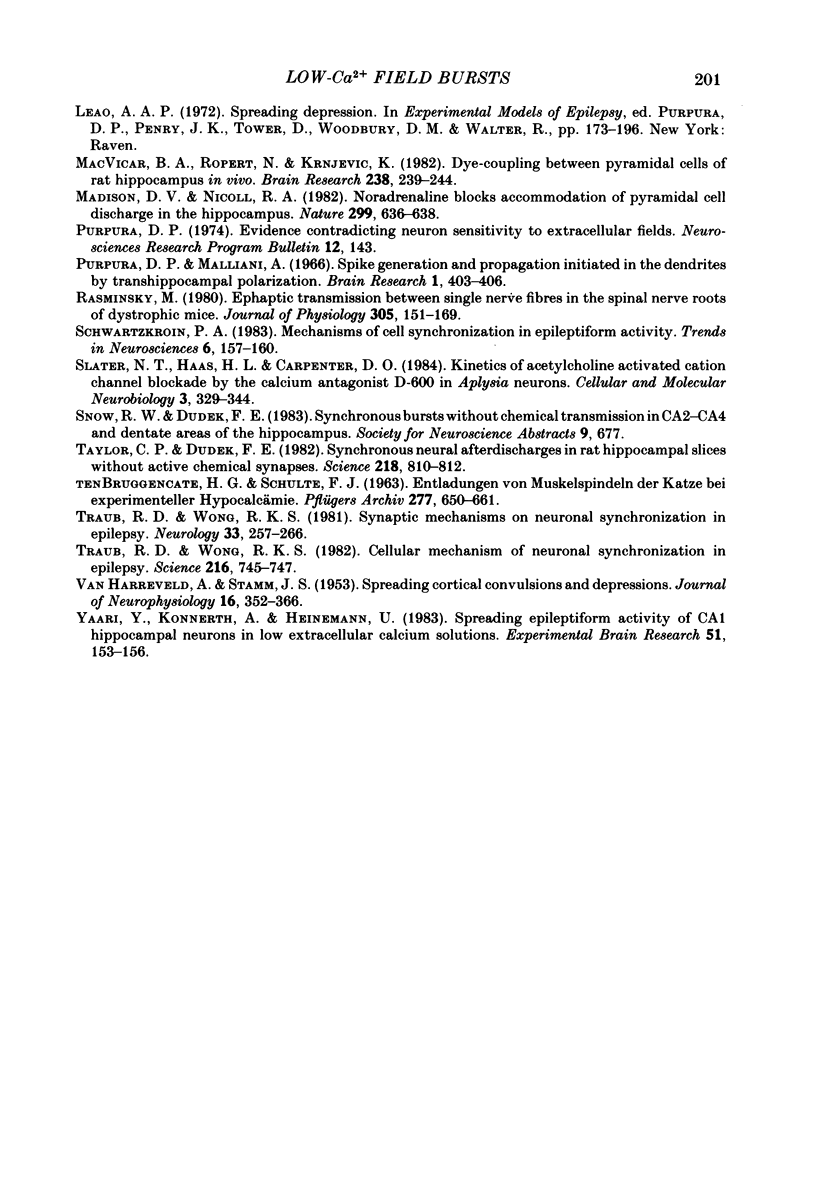
Selected References
These references are in PubMed. This may not be the complete list of references from this article.
- ANDERSEN P., SEARS T. A. THE ROLE OF INHIBITION IN THE PHASING OF SPONTANEOUS THALAMO-CORTICAL DISCHARGE. J Physiol. 1964 Oct;173:459–480. doi: 10.1113/jphysiol.1964.sp007468. [DOI] [PMC free article] [PubMed] [Google Scholar]
- Alvarez-Leefmans F. J., De Santis A., Miledi R. Effects of some divalent cations on synaptic transmission in frog spinal neurones. J Physiol. 1979 Sep;294:387–406. doi: 10.1113/jphysiol.1979.sp012936. [DOI] [PMC free article] [PubMed] [Google Scholar]
- Andersen P., Bliss T. V., Skrede K. K. Unit analysis of hippocampal polulation spikes. Exp Brain Res. 1971;13(2):208–221. doi: 10.1007/BF00234086. [DOI] [PubMed] [Google Scholar]
- Andrew R. D., Taylor C. P., Snow R. W., Dudek F. E. Coupling in rat hippocampal slices: dye transfer between CA1 pyramidal cells. Brain Res Bull. 1982 Feb;8(2):211–222. doi: 10.1016/0361-9230(82)90048-x. [DOI] [PubMed] [Google Scholar]
- Avoli M., Gloor P. The effects of transient functional depression of the thalamus on spindles and on bilateral synchronous epileptic discharges of feline generalized penicillin epilepsy. Epilepsia. 1981 Aug;22(4):443–452. doi: 10.1111/j.1528-1157.1981.tb06155.x. [DOI] [PubMed] [Google Scholar]
- Baker P. F., Blaustein M. P., Keynes R. D., Manil J., Shaw T. I., Steinhardt R. A. The ouabain-sensitive fluxes of sodium and potassium in squid giant axons. J Physiol. 1969 Feb;200(2):459–496. doi: 10.1113/jphysiol.1969.sp008703. [DOI] [PMC free article] [PubMed] [Google Scholar]
- Benardo L. S., Prince D. A. Dopamine modulates a Ca2+-activated potassium conductance in mammalian hippocampal pyramidal cells. Nature. 1982 May 6;297(5861):76–79. doi: 10.1038/297076a0. [DOI] [PubMed] [Google Scholar]
- Bregestovski P. D., Miledi R., Parker I. Blocking of frog endplate channels by the organic calcium antagonist D600. Proc R Soc Lond B Biol Sci. 1980 Dec 31;211(1182):15–24. doi: 10.1098/rspb.1980.0155. [DOI] [PubMed] [Google Scholar]
- Brismar T., Frankenhaeuser B. Effects of ionic concentration on sodium permeability properties of myelinated nerve fibres of Xenopus laevis. J Physiol. 1975 Aug;249(3):549–559. doi: 10.1113/jphysiol.1975.sp011029. [DOI] [PMC free article] [PubMed] [Google Scholar]
- Dynamic patterns of brain cell assemblies. IV. Mixed systems. Influence of exogenous low-level currents. Evidence contradicting neuron sensitivity to extracellular fields. Neurosci Res Program Bull. 1974 Mar;12(1):143–144. [PubMed] [Google Scholar]
- FRANKENHAEUSER B., HODGKIN A. L. The action of calcium on the electrical properties of squid axons. J Physiol. 1957 Jul 11;137(2):218–244. doi: 10.1113/jphysiol.1957.sp005808. [DOI] [PMC free article] [PubMed] [Google Scholar]
- FURUKAWA T., FURSHPAN E. J. Two inhibitory mechanisms in the Mauthner neurons of goldfish. J Neurophysiol. 1963 Jan;26:140–176. doi: 10.1152/jn.1963.26.1.140. [DOI] [PubMed] [Google Scholar]
- GREEN J. D. THE HIPPOCAMPUS. Physiol Rev. 1964 Oct;44:561–608. doi: 10.1152/physrev.1964.44.4.561. [DOI] [PubMed] [Google Scholar]
- Gardner-Medwin A. R. A study of the mechanisms by which potassium moves through brain tissue in the rat. J Physiol. 1983 Feb;335:353–374. doi: 10.1113/jphysiol.1983.sp014539. [DOI] [PMC free article] [PubMed] [Google Scholar]
- Gardner-Medwin A. R. Analysis of potassium dynamics in mammalian brain tissue. J Physiol. 1983 Feb;335:393–426. doi: 10.1113/jphysiol.1983.sp014541. [DOI] [PMC free article] [PubMed] [Google Scholar]
- Gorman A. L., Hermann A., Thomas M. V. Ionic requirements for membrane oscillations and their dependence on the calcium concentration in a molluscan pace-maker neurone. J Physiol. 1982 Jun;327:185–217. doi: 10.1113/jphysiol.1982.sp014227. [DOI] [PMC free article] [PubMed] [Google Scholar]
- Haas H. L., Jefferys J. G., Slater N. T., Carpenter D. O. Modulation of low calcium induced field bursts in the hippocampus by monoamines and cholinomimetics. Pflugers Arch. 1984 Jan;400(1):28–33. doi: 10.1007/BF00670532. [DOI] [PubMed] [Google Scholar]
- Haas H. L., Konnerth A. Histamine and noradrenaline decrease calcium-activated potassium conductance in hippocampal pyramidal cells. 1983 Mar 31-Apr 6Nature. 302(5907):432–434. doi: 10.1038/302432a0. [DOI] [PubMed] [Google Scholar]
- Haas H. L., Ryall R. W. Is excitation by enkephalins of hippocampal neurones in the rat due to presynaptic facilitation or to disinhibition? J Physiol. 1980 Nov;308:315–330. doi: 10.1113/jphysiol.1980.sp013473. [DOI] [PMC free article] [PubMed] [Google Scholar]
- Haas H. L., Schaerer B., Vosmansky M. A simple perfusion chamber for the study of nervous tissue slices in vitro. J Neurosci Methods. 1979 Dec;1(4):323–325. doi: 10.1016/0165-0270(79)90021-9. [DOI] [PubMed] [Google Scholar]
- Heinemann U., Lux H. D. Ceiling of stimulus induced rises in extracellular potassium concentration in the cerebral cortex of cat. Brain Res. 1977 Jan 21;120(2):231–249. doi: 10.1016/0006-8993(77)90903-9. [DOI] [PubMed] [Google Scholar]
- Heinemann U., Lux H. D., Gutnick M. J. Extracellular free calcium and potassium during paroxsmal activity in the cerebral cortex of the cat. Exp Brain Res. 1977 Mar 30;27(3-4):237–243. doi: 10.1007/BF00235500. [DOI] [PubMed] [Google Scholar]
- Hood T. W., Siegfried J., Haas H. L. Analysis of carbamazepine actions in hippocampal slices of the rat. Cell Mol Neurobiol. 1983 Sep;3(3):213–222. doi: 10.1007/BF00710948. [DOI] [PMC free article] [PubMed] [Google Scholar]
- Hubbard J. I., Jones S. F., Landau E. M. On the mechanism by which calcium and magnesium affect the spontaneous release of transmitter from mammalian motor nerve terminals. J Physiol. 1968 Feb;194(2):355–380. doi: 10.1113/jphysiol.1968.sp008413. [DOI] [PMC free article] [PubMed] [Google Scholar]
- Jefferys J. G., Haas H. L. Synchronized bursting of CA1 hippocampal pyramidal cells in the absence of synaptic transmission. Nature. 1982 Dec 2;300(5891):448–450. doi: 10.1038/300448a0. [DOI] [PubMed] [Google Scholar]
- Jefferys J. G. Influence of electric fields on the excitability of granule cells in guinea-pig hippocampal slices. J Physiol. 1981;319:143–152. doi: 10.1113/jphysiol.1981.sp013897. [DOI] [PMC free article] [PubMed] [Google Scholar]
- Katz B., Schmitt O. H. Electric interaction between two adjacent nerve fibres. J Physiol. 1940 Feb 14;97(4):471–488. doi: 10.1113/jphysiol.1940.sp003823. [DOI] [PMC free article] [PubMed] [Google Scholar]
- Konnerth A., Heinemann U., Yaari Y. Slow transmission of neural activity in hippocampal area CA1 in absence of active chemical synapses. Nature. 1984 Jan 5;307(5946):69–71. doi: 10.1038/307069a0. [DOI] [PubMed] [Google Scholar]
- Korn H., Axelrad H. Electrical inhibition of Purkinje cells in the cerebellum of the rat. Proc Natl Acad Sci U S A. 1980 Oct;77(10):6244–6247. doi: 10.1073/pnas.77.10.6244. [DOI] [PMC free article] [PubMed] [Google Scholar]
- Korn H., Faber D. S. An electrically mediated inhibition in goldfish medulla. J Neurophysiol. 1975 Mar;38(2):452–471. doi: 10.1152/jn.1975.38.2.452. [DOI] [PubMed] [Google Scholar]
- Krnjević K., Puil E., Werman R. Significance of 2,4-dinitrophenol action on spinal motoneurones. J Physiol. 1978 Feb;275:225–239. doi: 10.1113/jphysiol.1978.sp012187. [DOI] [PMC free article] [PubMed] [Google Scholar]
- MacVicar B. A., Ropert N., Krnjevic K. Dye-coupling between pyramidal cells of rat hippocampus in vivo. Brain Res. 1982 Apr 22;238(1):239–244. doi: 10.1016/0006-8993(82)90790-9. [DOI] [PubMed] [Google Scholar]
- Madison D. V., Nicoll R. A. Noradrenaline blocks accommodation of pyramidal cell discharge in the hippocampus. Nature. 1982 Oct 14;299(5884):636–638. doi: 10.1038/299636a0. [DOI] [PubMed] [Google Scholar]
- Purpura D. P., Malliani A. Spike generation and propagation initiated in dendrites by transhippocampal polarization. Brain Res. 1966 May-Jun;1(4):403–406. doi: 10.1016/0006-8993(66)90132-6. [DOI] [PubMed] [Google Scholar]
- Rasminsky M. Ephaptic transmission between single nerve fibres in the spinal nerve roots of dystrophic mice. J Physiol. 1980 Aug;305:151–169. doi: 10.1113/jphysiol.1980.sp013356. [DOI] [PMC free article] [PubMed] [Google Scholar]
- Slater N. T., Haas H. L., Carpenter D. O. Kinetics of acetylcholine-activated cation channel blockade by the calcium antagonist D-600 in Aplysia neurons. Cell Mol Neurobiol. 1983 Dec;3(4):329–344. doi: 10.1007/BF00734714. [DOI] [PMC free article] [PubMed] [Google Scholar]
- TEN BRUGGENCATEH, SCHULTE F. J. ENTLADUNGEN VON MUSKELSPINDELN DER KATZE BEI EXPERIMENTELLER HYPOCALCAEMIE. Pflugers Arch Gesamte Physiol Menschen Tiere. 1963;277:650–661. doi: 10.1007/BF00363283. [DOI] [PubMed] [Google Scholar]
- Taylor C. P., Dudek F. E. Synchronous neural afterdischarges in rat hippocampal slices without active chemical synapses. Science. 1982 Nov 19;218(4574):810–812. doi: 10.1126/science.7134978. [DOI] [PubMed] [Google Scholar]
- Traub R. D., Wong R. K. Cellular mechanism of neuronal synchronization in epilepsy. Science. 1982 May 14;216(4547):745–747. doi: 10.1126/science.7079735. [DOI] [PubMed] [Google Scholar]
- Traub R. D., Wong R. K. Synaptic mechanisms underlying interictal spike initiation in a hippocampal network. Neurology. 1983 Mar;33(3):257–266. doi: 10.1212/wnl.33.3.257. [DOI] [PubMed] [Google Scholar]
- VAN HARREVELD A., STAMM J. S. Spreading cortical convulsions and depressions. J Neurophysiol. 1953 Jul;16(4):352–366. doi: 10.1152/jn.1953.16.4.352. [DOI] [PubMed] [Google Scholar]
- Yaari Y., Konnerth A., Heinemann U. Spontaneous epileptiform activity of CA1 hippocampal neurons in low extracellular calcium solutions. Exp Brain Res. 1983;51(1):153–156. doi: 10.1007/BF00236813. [DOI] [PubMed] [Google Scholar]