Abstract
Mechanical and biochemical descriptions of the muscle cross-bridge cycle have been correlated. Skinned muscle fibres of rabbit psoas muscle in rigor were incubated in solutions containing approximately equal to 30 microM-Ca2+ ions and P3-1-(2-nitro)phenylethyladenosine-5'-triphosphate, 'caged ATP', an inert photolabile precursor of ATP. ATP was liberated from caged ATP within the fibres by pulses of 347 nm radiation from a frequency-doubled ruby laser. The mechanical responses of muscle fibres to the rapid increase of ATP concentration were monitored. Tension dropped briefly and then rose above the rigor value to the level characteristic of a steady active contraction. Liberation of ATP decreased in-phase stiffness (measured at 500 Hz) from the rigor level to a maintained value intermediate between rigor and relaxed values. Out-of-phase stiffness increased to a maintained level indicating a phase lead of tension with respect to imposed length oscillations. Rigor tension was varied prior to photolysis by slight alterations of fibre length. Tension traces starting at different rigor tensions converged to a common tension level at the same rate, whether or not Ca2+ was included in the medium. These data suggest that the rate of cross-bridge detachment by ATP from the rigor state is not influenced by Ca2+. Analysis of the tension records, in terms of sequential detachment and reattachment reactions, provided a measure of cross-bridge reattachment rate and an alternate measure of the detachment rate. Detachment from the rigor state was approximately proportional to the ATP concentration, with a second-order rate constant of at least 5 X 10(5) M-1 S-1. Reattachment with force generation had no detectable dependence on the concentration of ATP liberated by photolysis. A simple kinetic model of the cross-bridge cycle in terms of chemically defined intermediates was compatible with most of the experimental data. The ATP dependence of cross-bridge detachment, the kinetics of maintained cross-bridge reattachment in the presence of Ca2+, and transient reattachment and final relaxation in the absence of Ca2+ were explained. In this model, reversibility of cross-bridge attachment and the steps leading to force production allow the relatively high observed detachment rate to be accommodated with other data relating to active contraction. These data include the steady ATPase rate of active muscle fibres and the fewer attached cross-bridges in active contractions compared to rigor.
Full text
PDF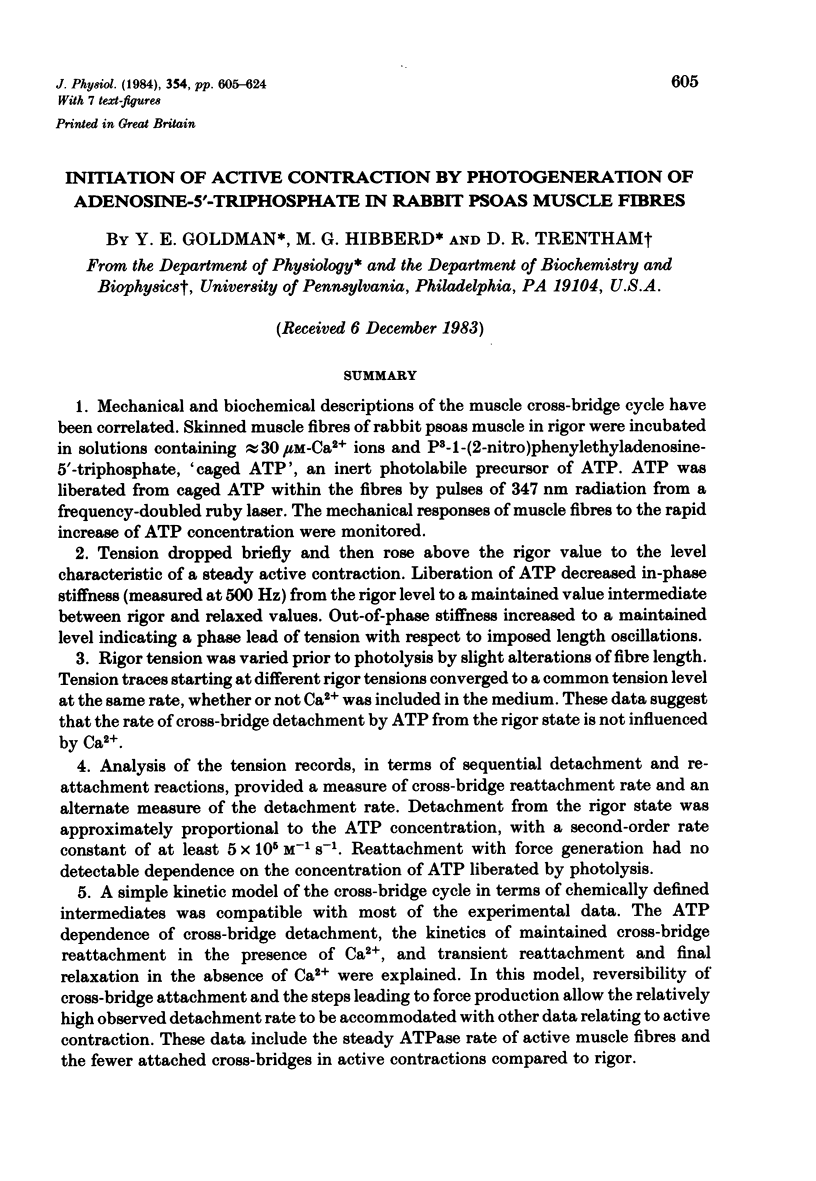
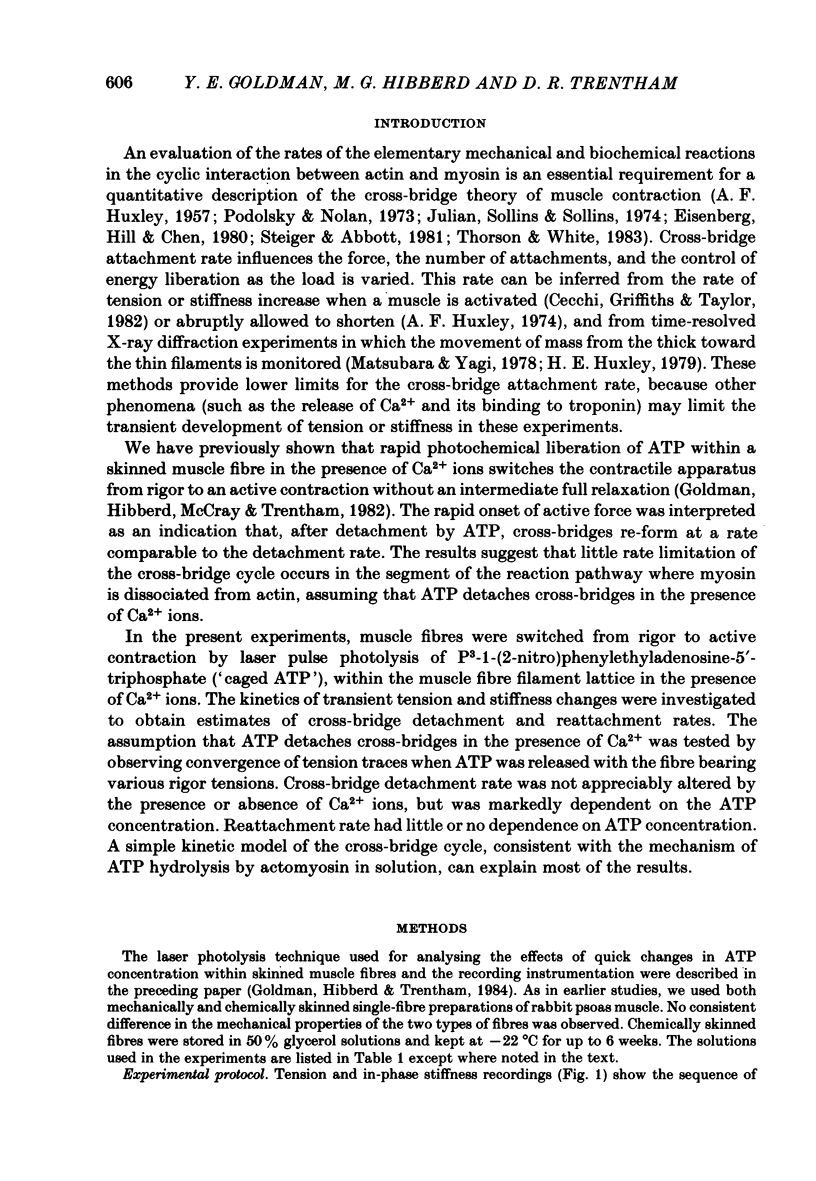
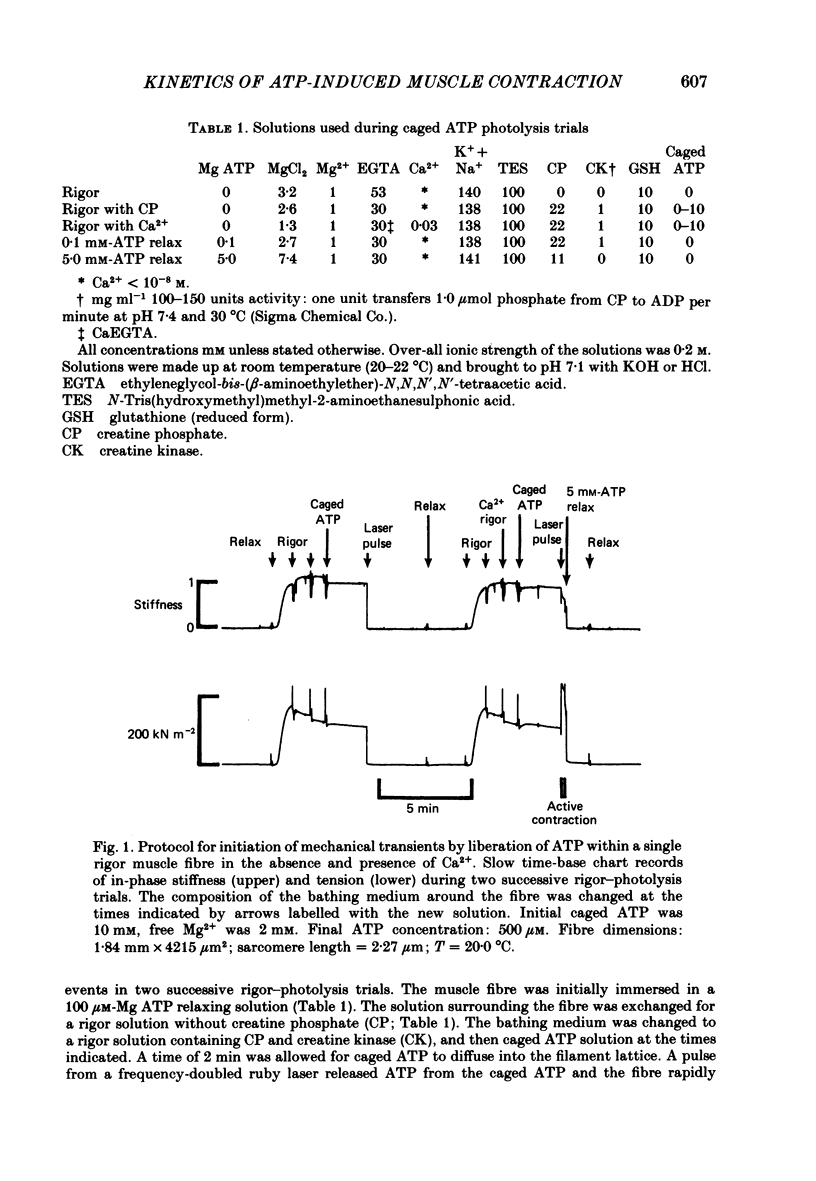
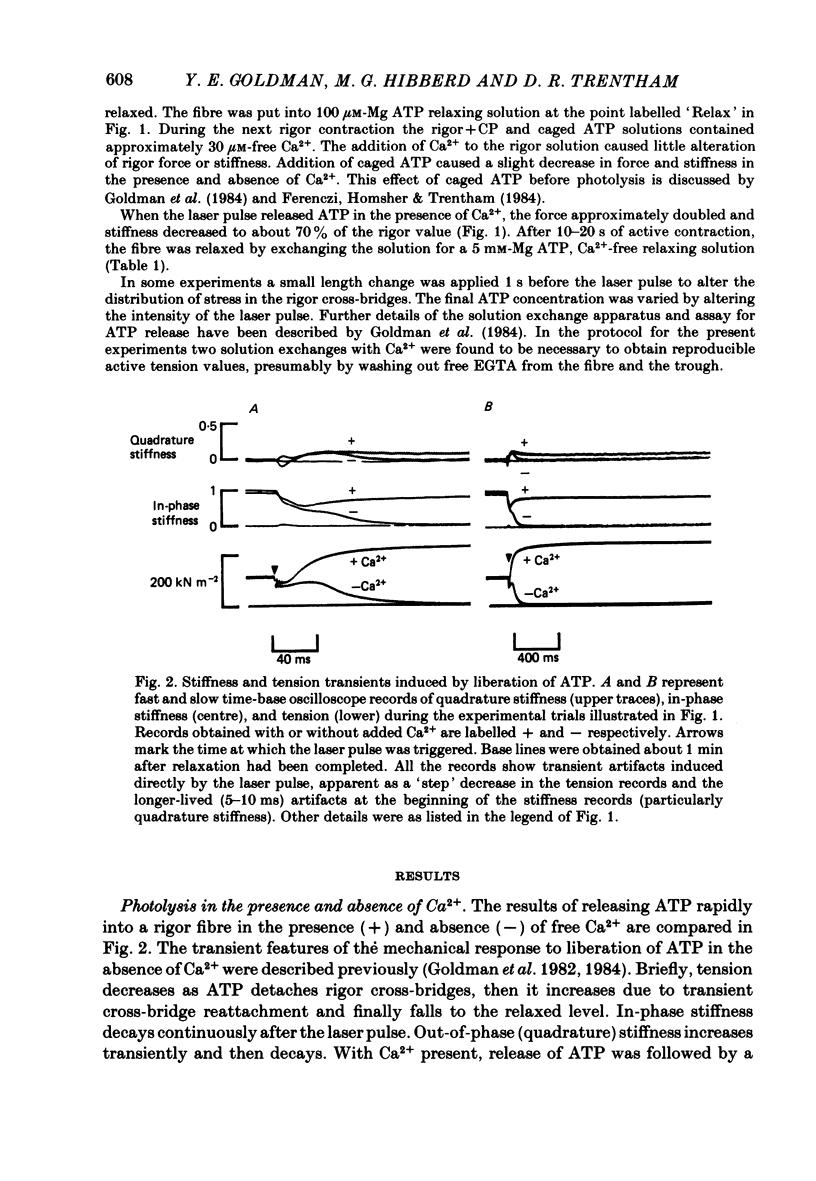
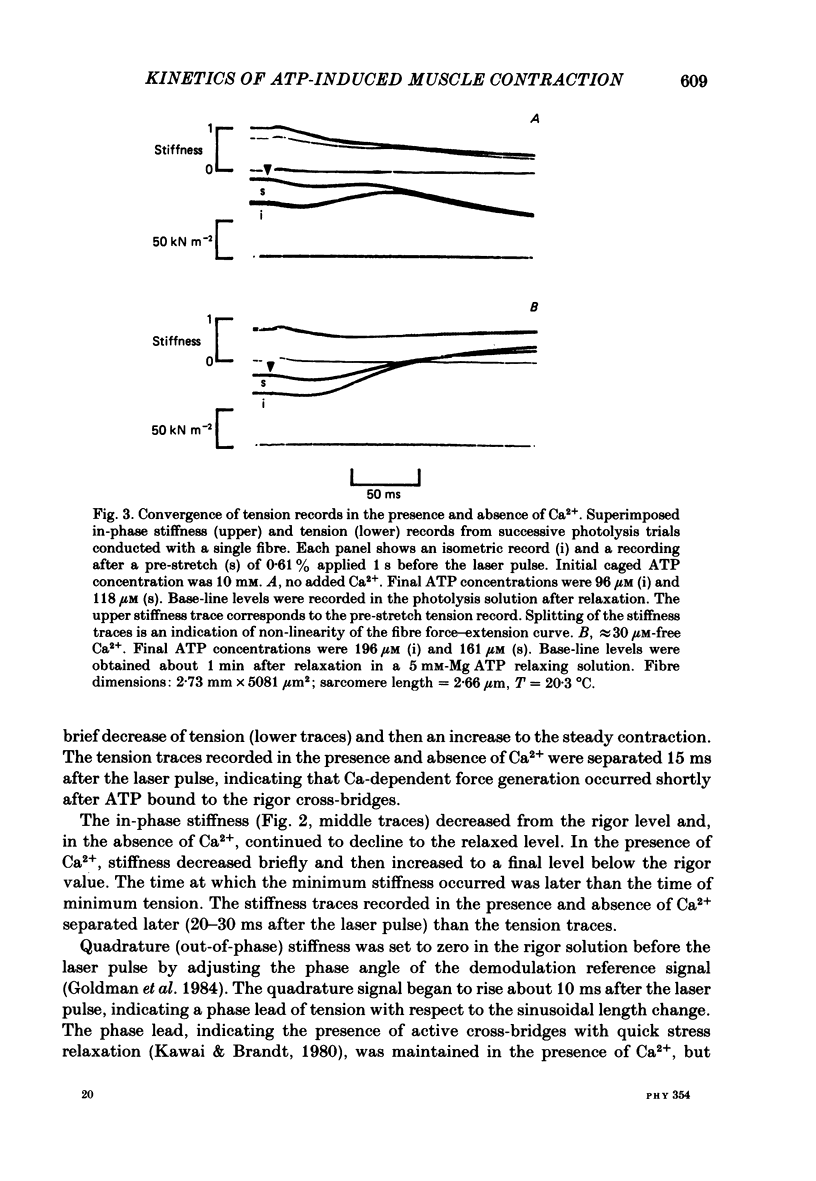
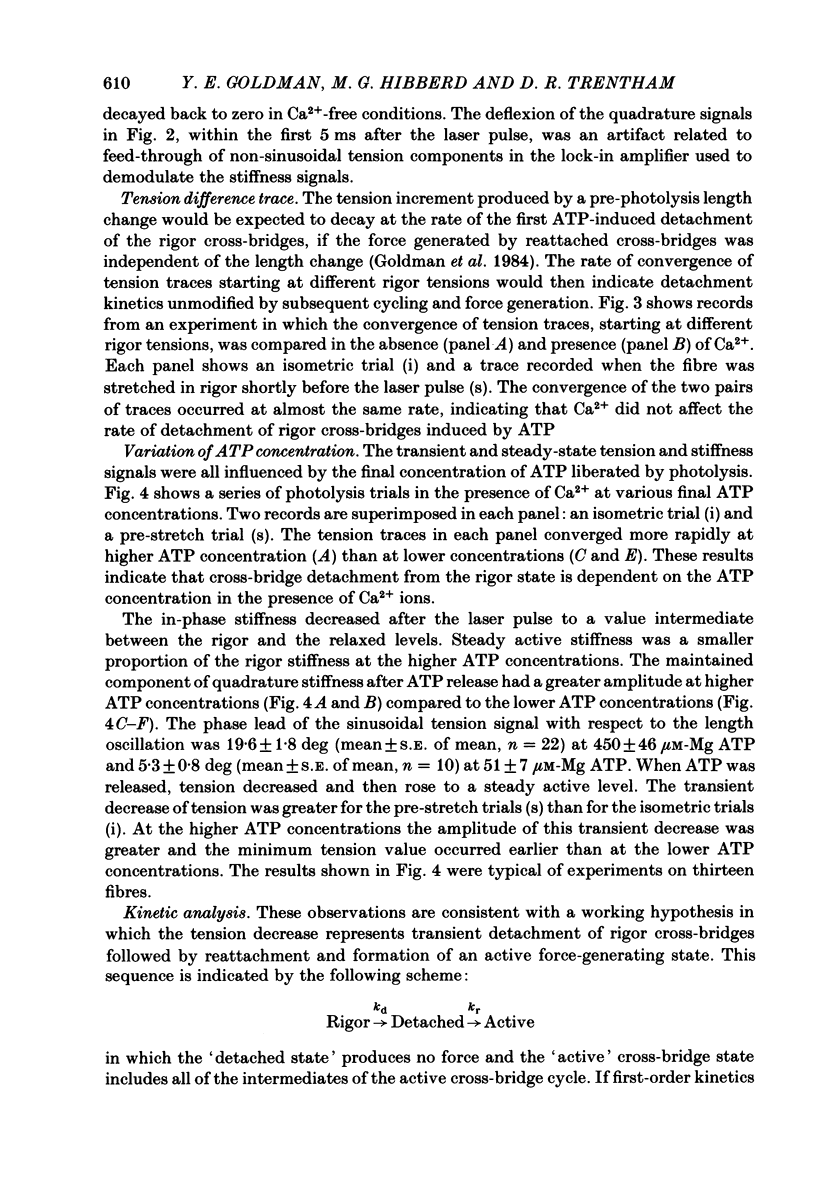
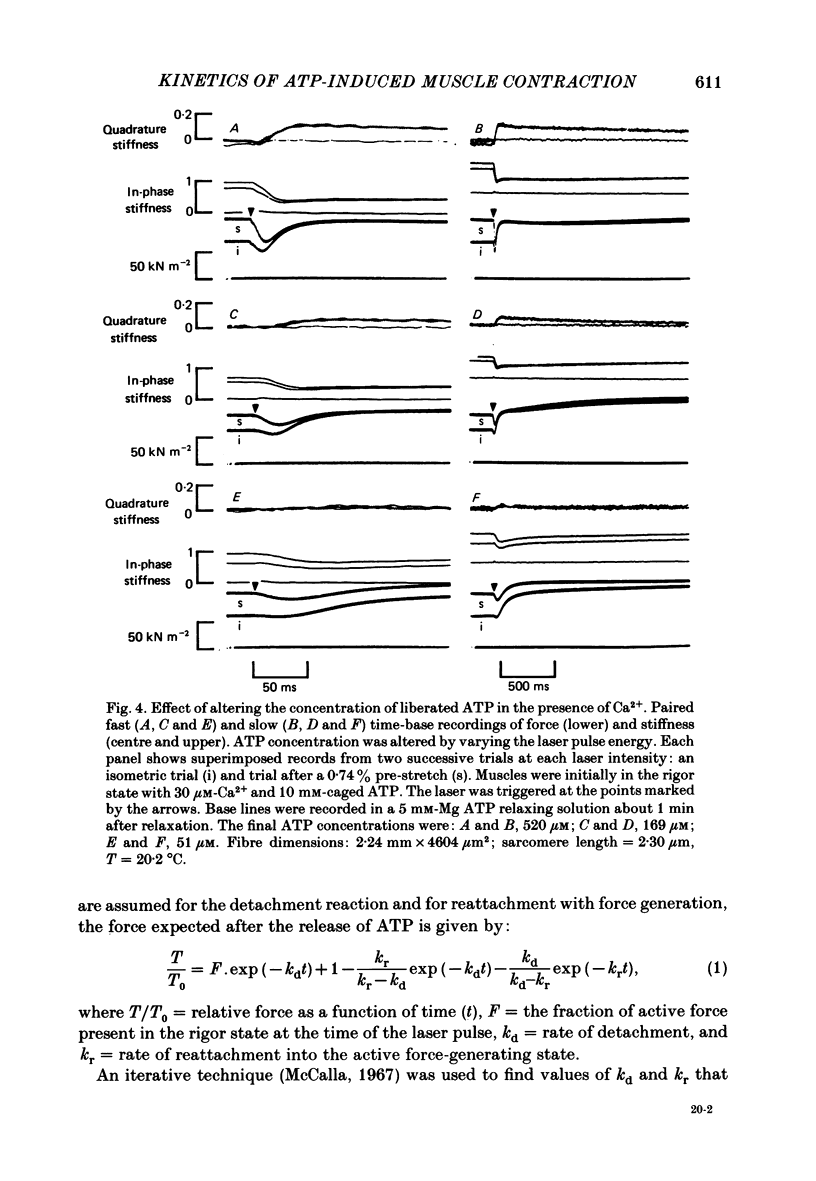
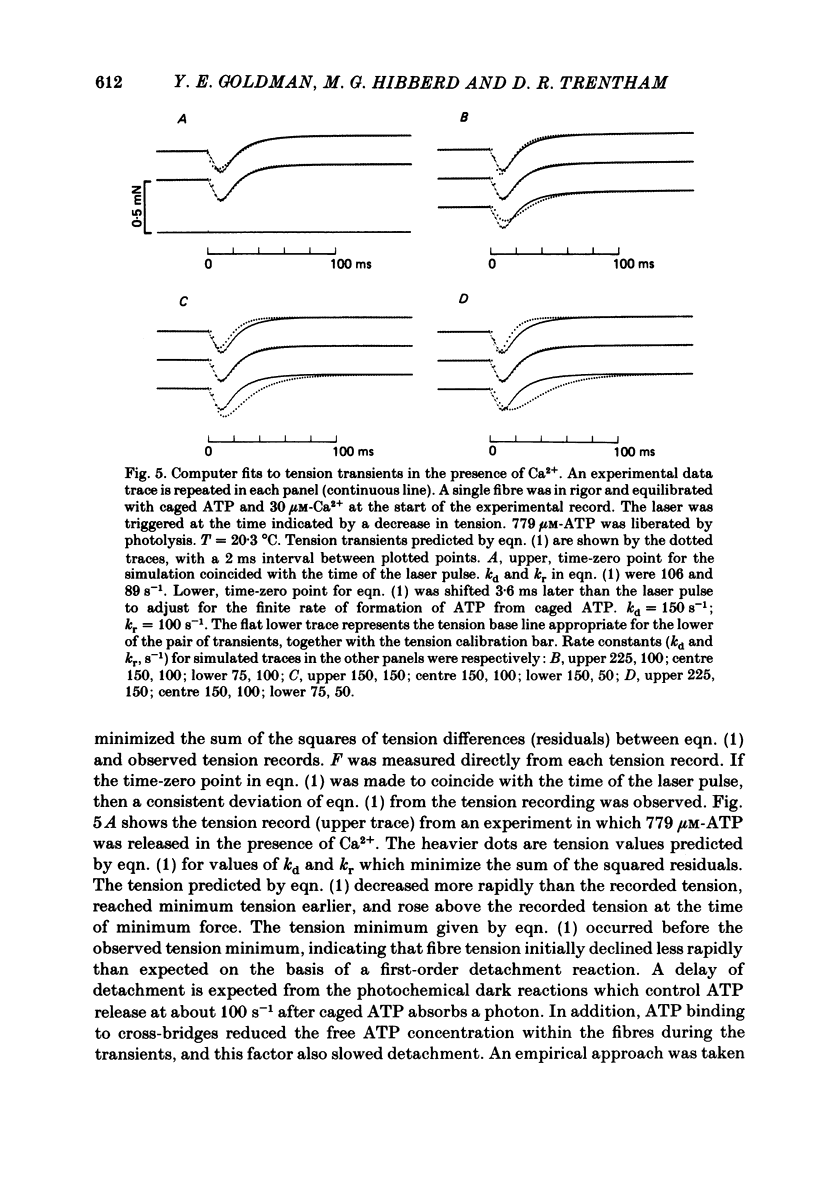
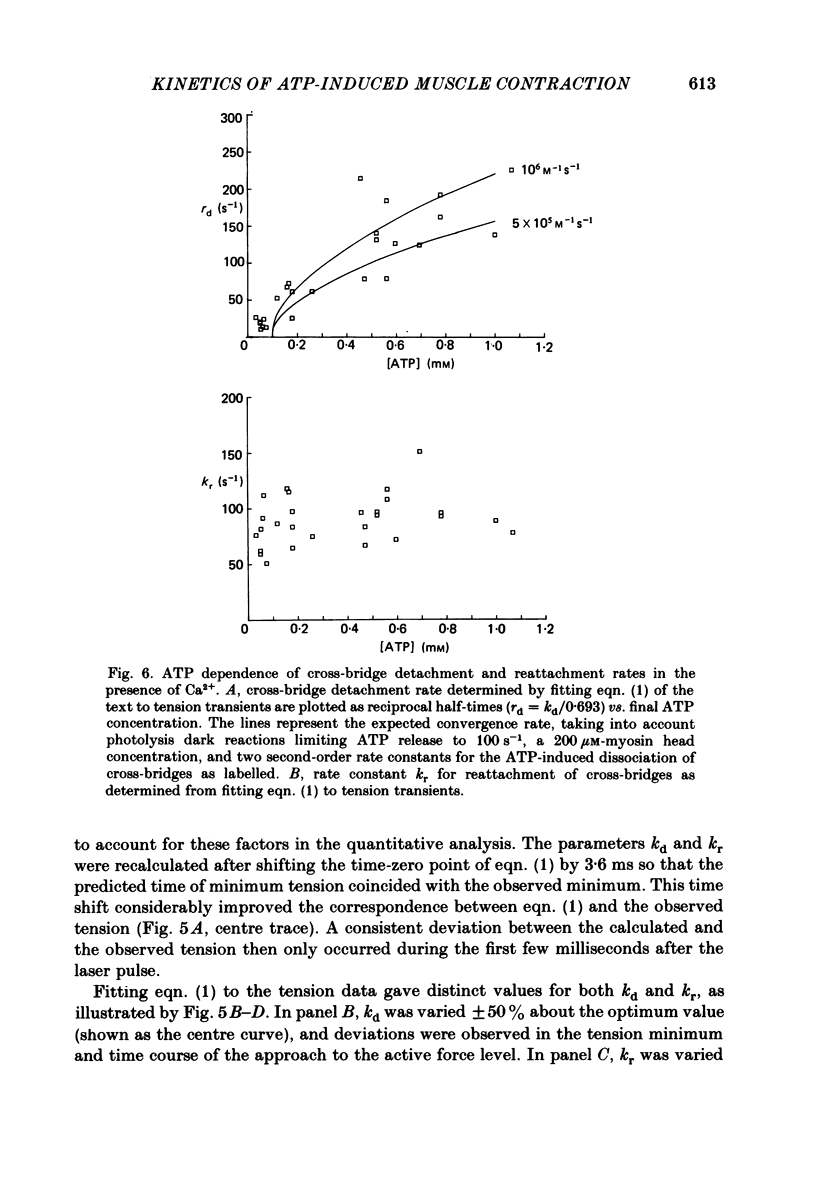
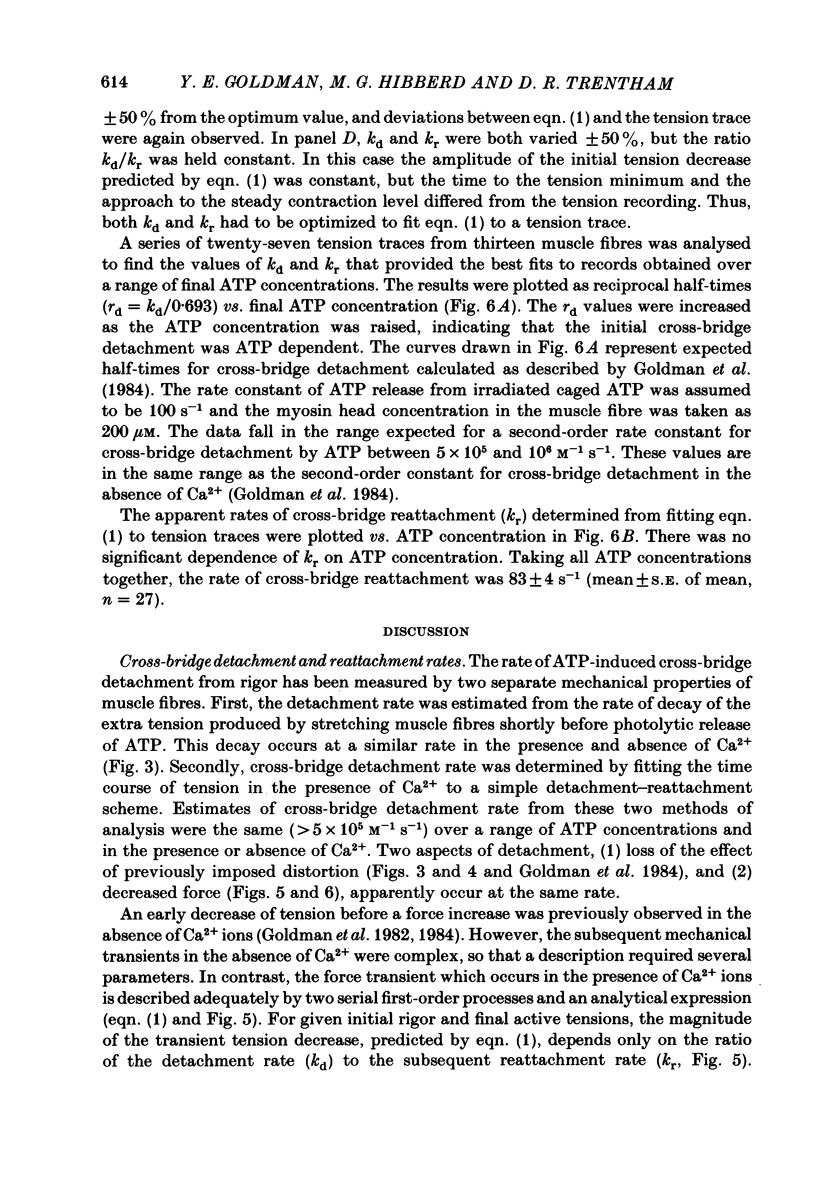
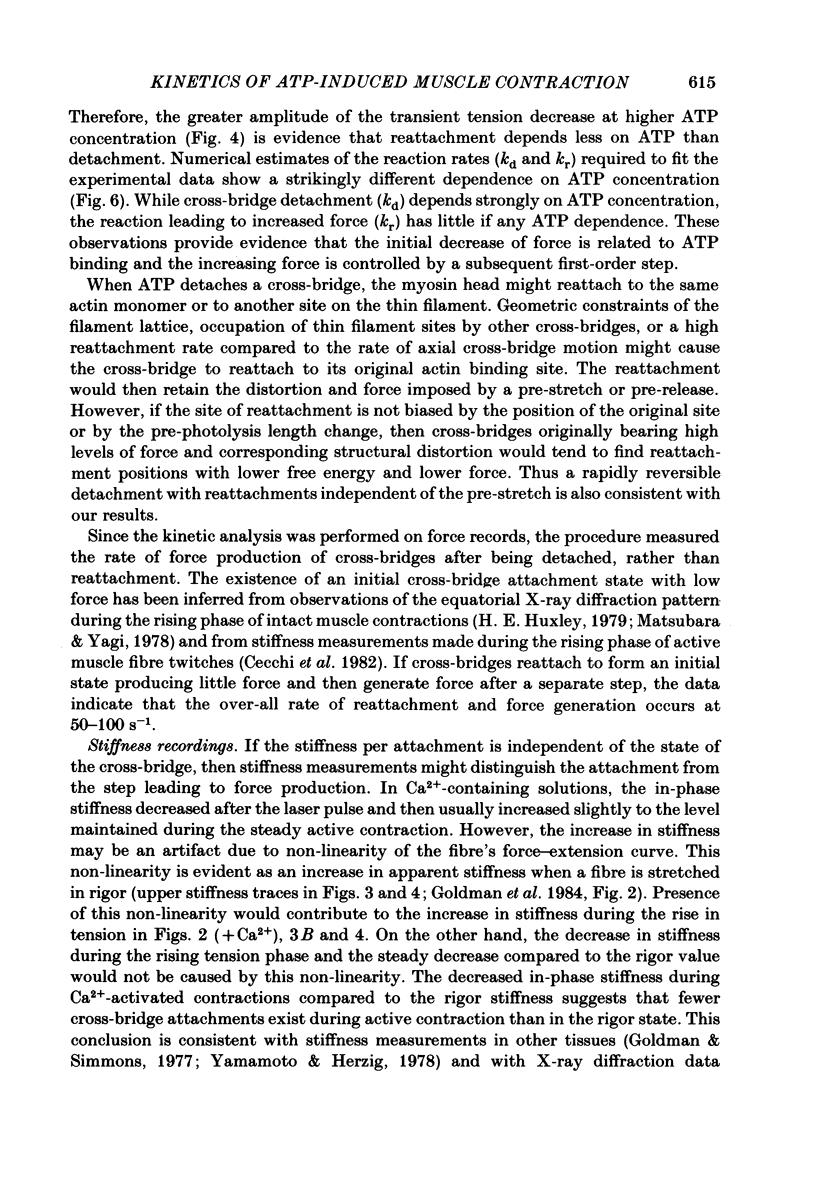
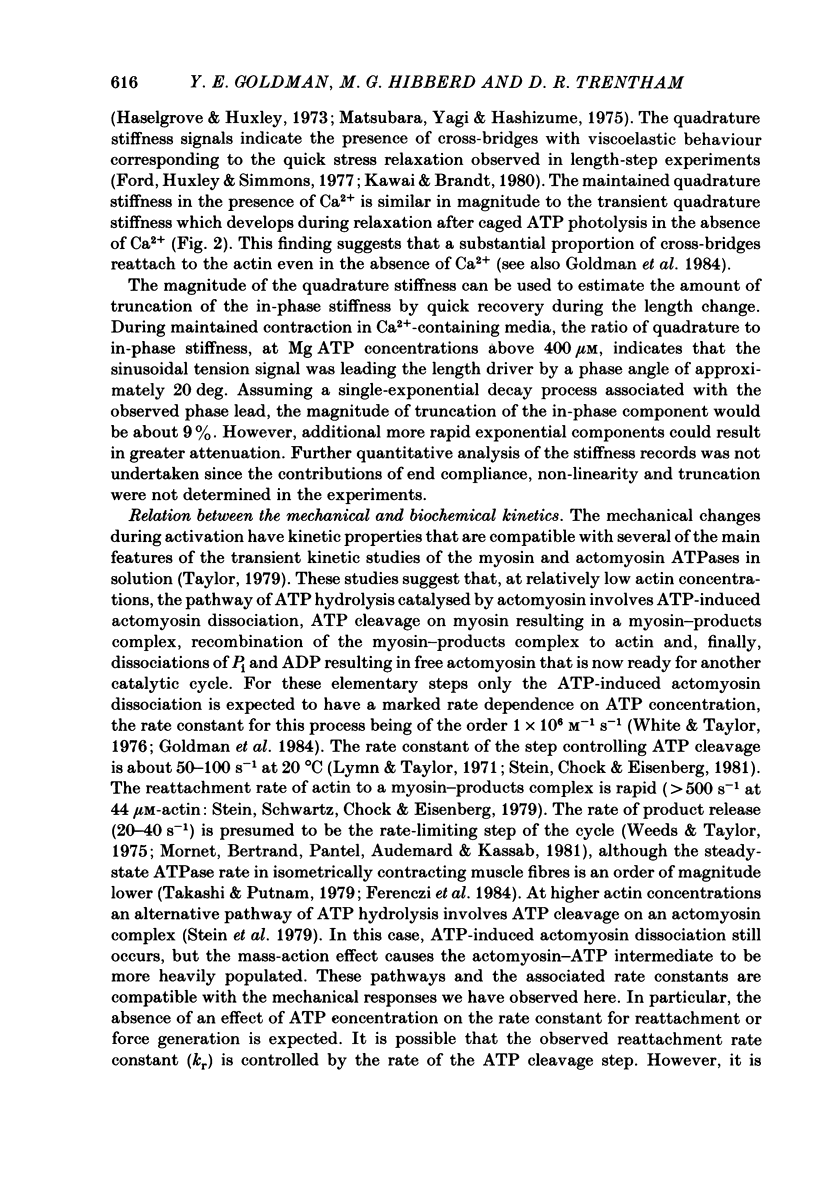
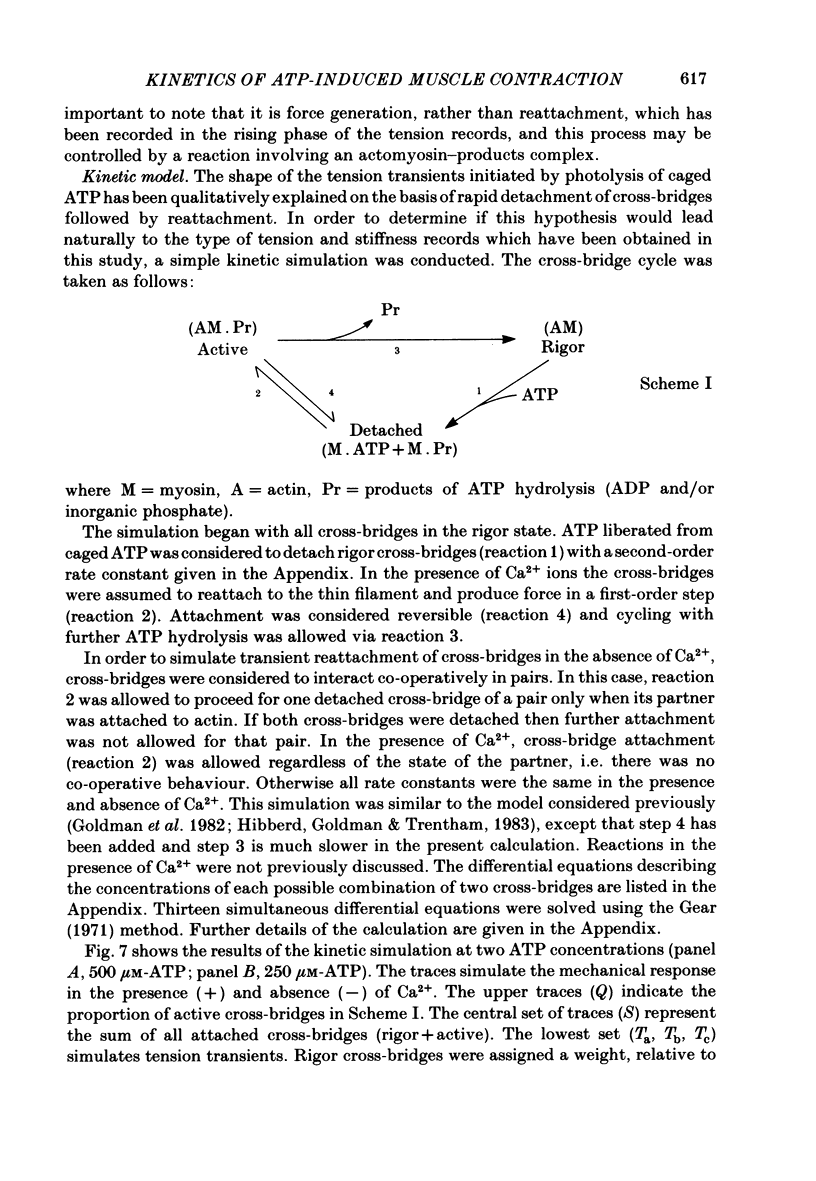
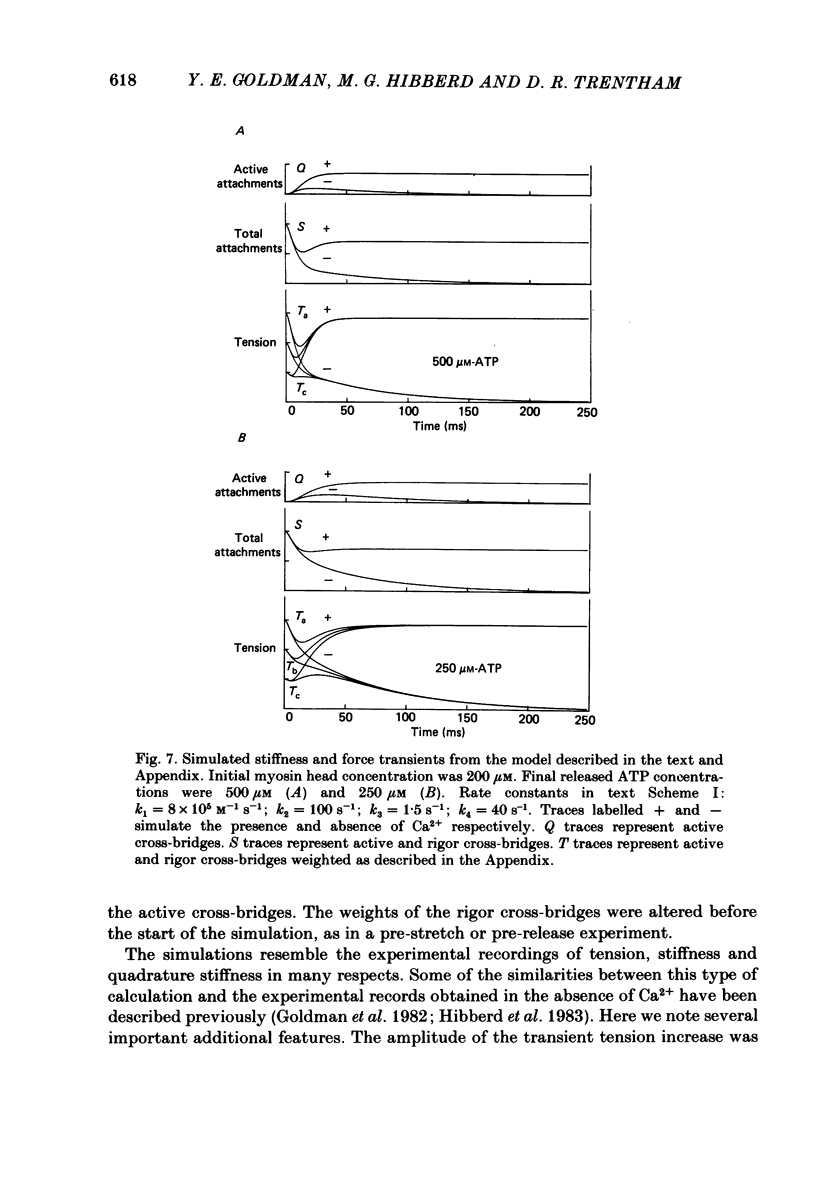
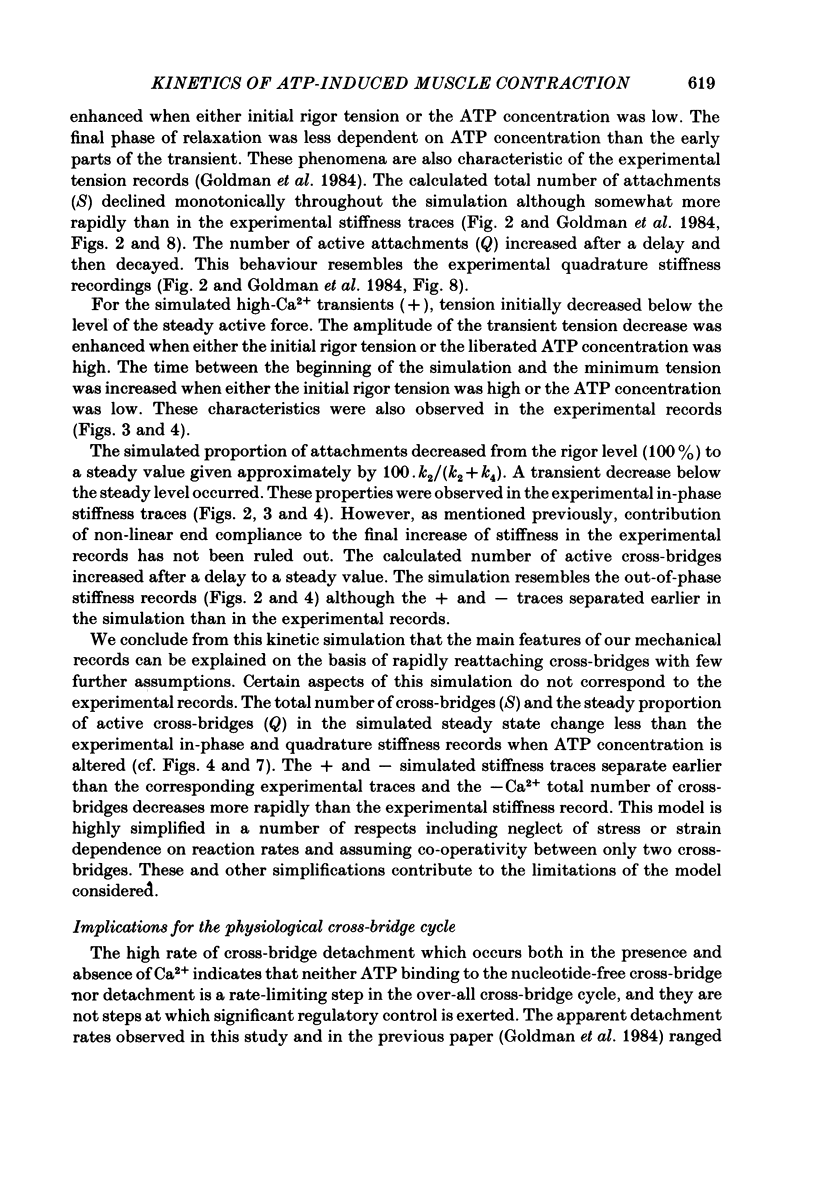
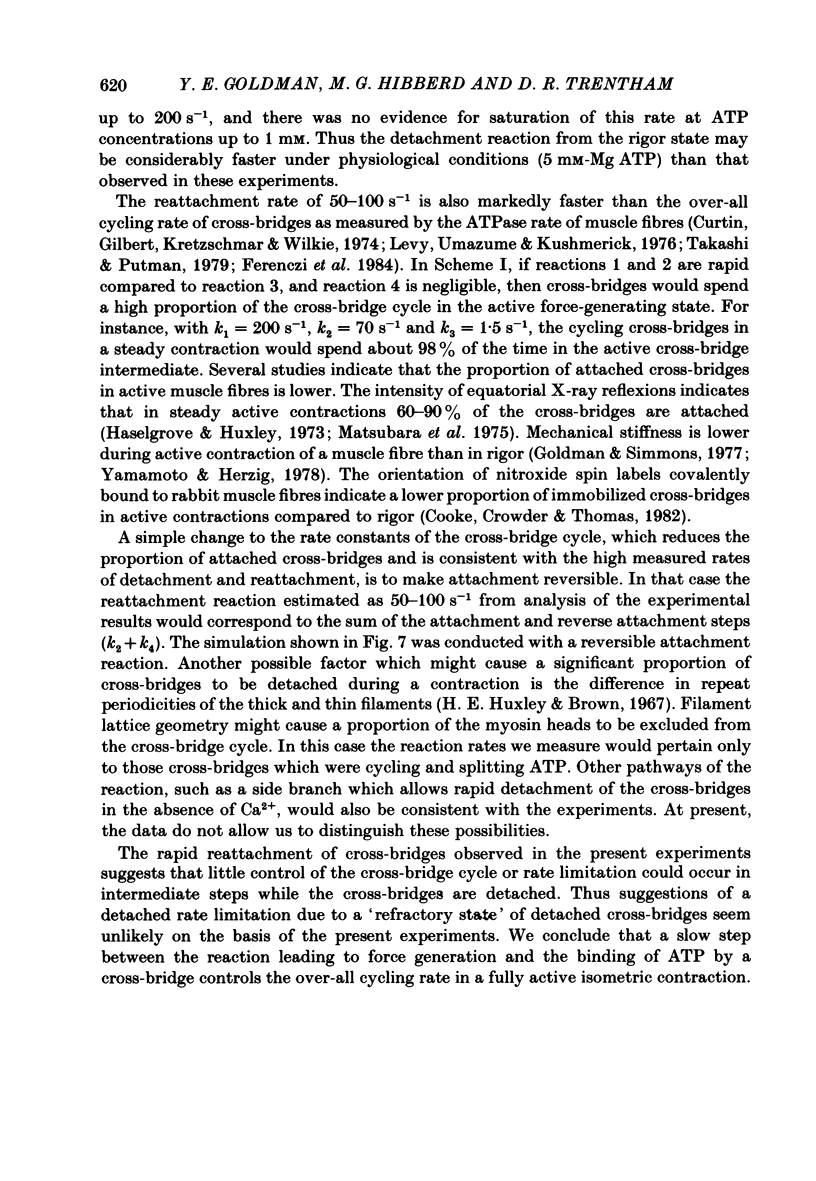
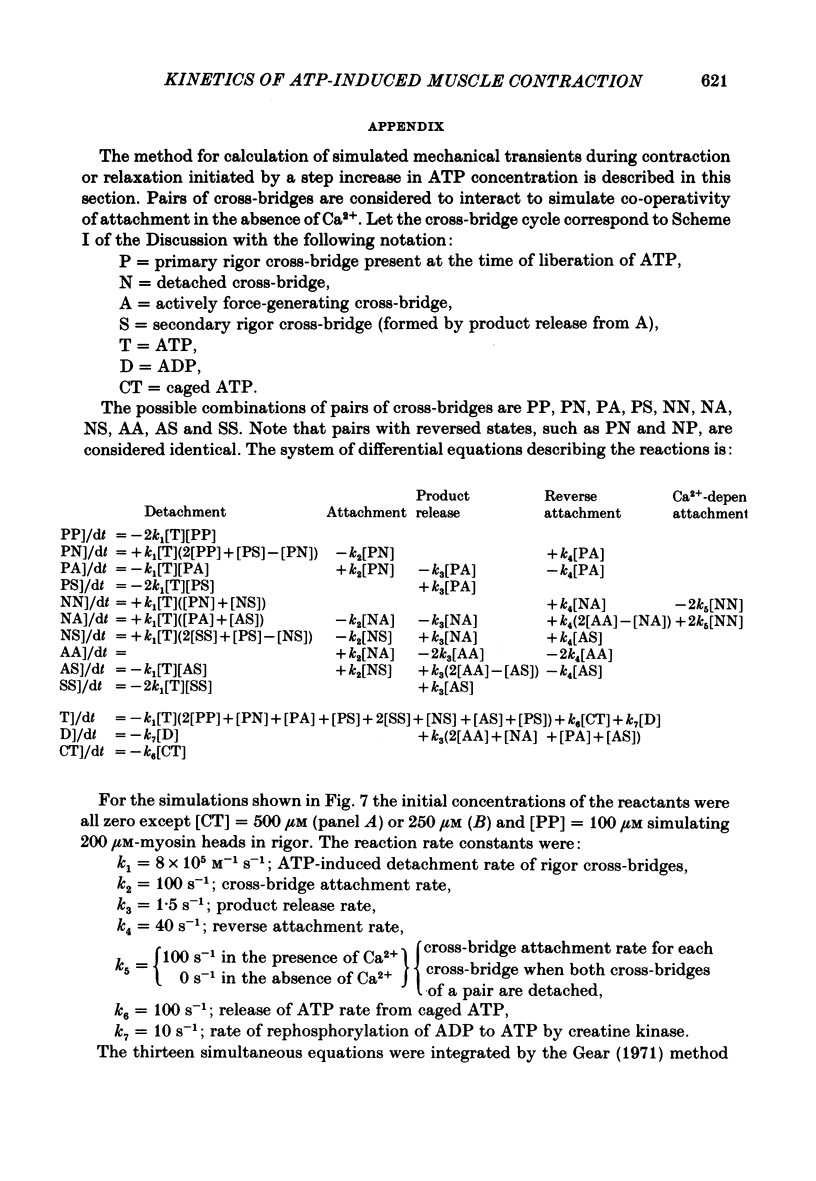
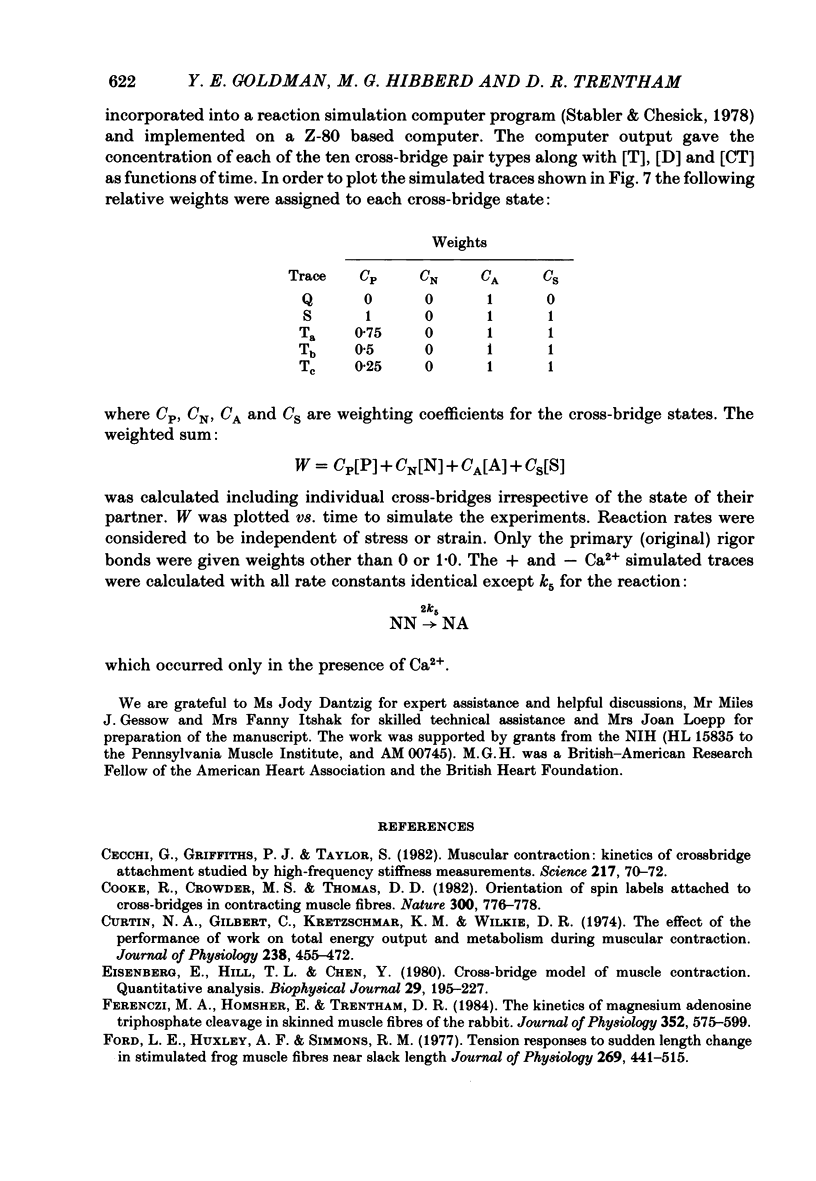
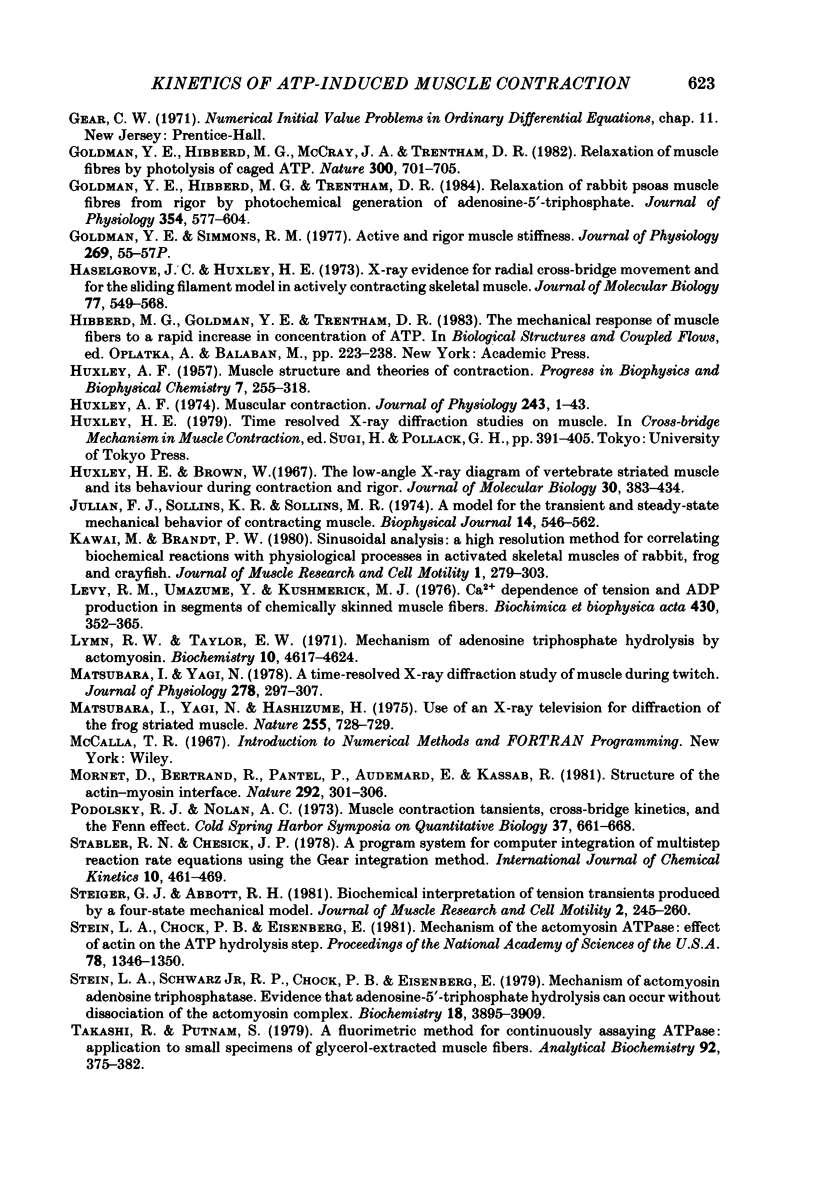
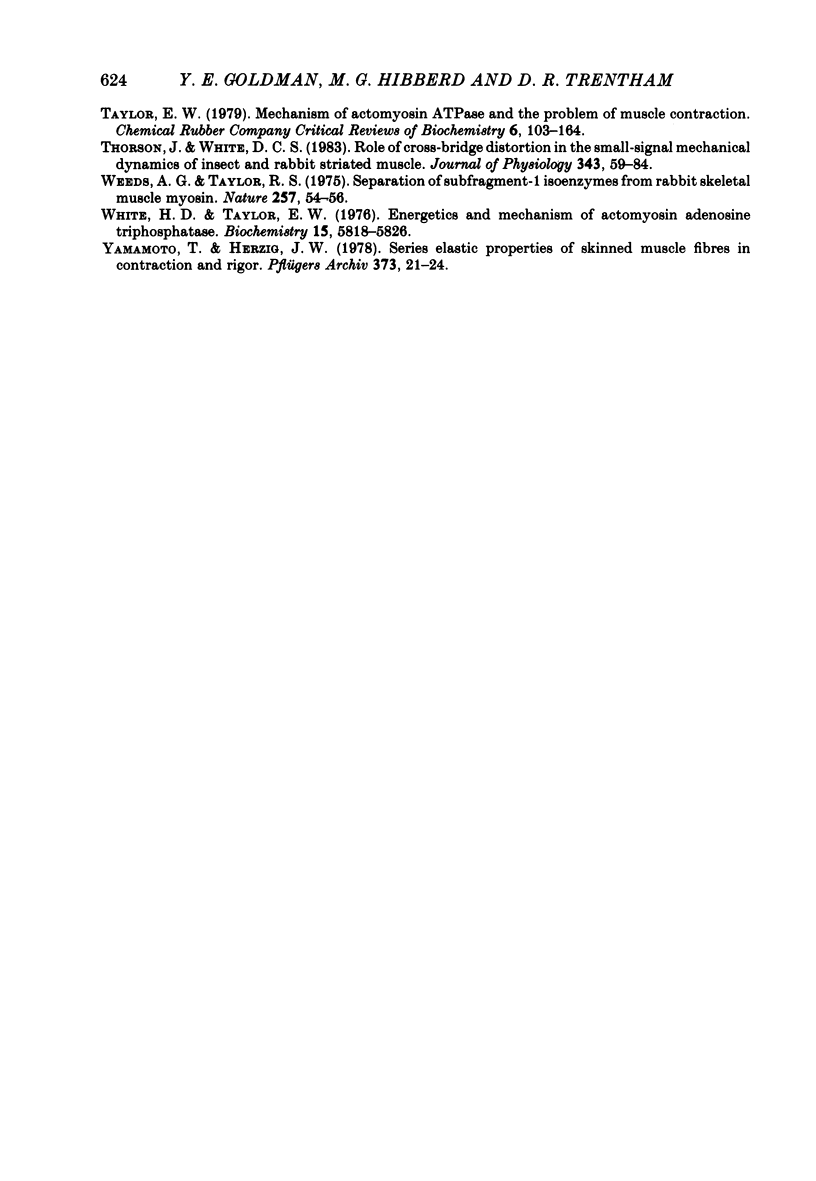
Selected References
These references are in PubMed. This may not be the complete list of references from this article.
- Cecchi G., Griffiths P. J., Taylor S. Muscular contraction: kinetics of crossbridge attachment studied by high-frequency stiffness measurements. Science. 1982 Jul 2;217(4554):70–72. doi: 10.1126/science.6979780. [DOI] [PubMed] [Google Scholar]
- Cooke R., Crowder M. S., Thomas D. D. Orientation of spin labels attached to cross-bridges in contracting muscle fibres. Nature. 1982 Dec 23;300(5894):776–778. doi: 10.1038/300776a0. [DOI] [PubMed] [Google Scholar]
- Curtin N. A., Gilbert C., Kretzschmar K. M., Wilkie D. R. The effect of the performance of work on total energy output and metabolism during muscular contraction. J Physiol. 1974 May;238(3):455–472. doi: 10.1113/jphysiol.1974.sp010537. [DOI] [PMC free article] [PubMed] [Google Scholar]
- Eisenberg E., Hill T. L., Chen Y. Cross-bridge model of muscle contraction. Quantitative analysis. Biophys J. 1980 Feb;29(2):195–227. doi: 10.1016/S0006-3495(80)85126-5. [DOI] [PMC free article] [PubMed] [Google Scholar]
- Ferenczi M. A., Homsher E., Trentham D. R. The kinetics of magnesium adenosine triphosphate cleavage in skinned muscle fibres of the rabbit. J Physiol. 1984 Jul;352:575–599. doi: 10.1113/jphysiol.1984.sp015311. [DOI] [PMC free article] [PubMed] [Google Scholar]
- Ford L. E., Huxley A. F., Simmons R. M. Tension responses to sudden length change in stimulated frog muscle fibres near slack length. J Physiol. 1977 Jul;269(2):441–515. doi: 10.1113/jphysiol.1977.sp011911. [DOI] [PMC free article] [PubMed] [Google Scholar]
- Goldman Y. E., Hibberd M. G., McCray J. A., Trentham D. R. Relaxation of muscle fibres by photolysis of caged ATP. Nature. 1982 Dec 23;300(5894):701–705. doi: 10.1038/300701a0. [DOI] [PubMed] [Google Scholar]
- Goldman Y. E., Hibberd M. G., Trentham D. R. Relaxation of rabbit psoas muscle fibres from rigor by photochemical generation of adenosine-5'-triphosphate. J Physiol. 1984 Sep;354:577–604. doi: 10.1113/jphysiol.1984.sp015394. [DOI] [PMC free article] [PubMed] [Google Scholar]
- Goldman Y. E., Simmons R. M. Active and rigor muscle stiffness [proceedings]. J Physiol. 1977 Jul;269(1):55P–57P. [PubMed] [Google Scholar]
- HUXLEY A. F. Muscle structure and theories of contraction. Prog Biophys Biophys Chem. 1957;7:255–318. [PubMed] [Google Scholar]
- Haselgrove J. C., Huxley H. E. X-ray evidence for radial cross-bridge movement and for the sliding filament model in actively contracting skeletal muscle. J Mol Biol. 1973 Jul 15;77(4):549–568. doi: 10.1016/0022-2836(73)90222-2. [DOI] [PubMed] [Google Scholar]
- Huxley A. F. Muscular contraction. J Physiol. 1974 Nov;243(1):1–43. [PMC free article] [PubMed] [Google Scholar]
- Huxley H. E., Brown W. The low-angle x-ray diagram of vertebrate striated muscle and its behaviour during contraction and rigor. J Mol Biol. 1967 Dec 14;30(2):383–434. doi: 10.1016/s0022-2836(67)80046-9. [DOI] [PubMed] [Google Scholar]
- Julian F. J., Sollins K. R., Sollins M. R. A model for the transient and steady-state mechanical behavior of contracting muscle. Biophys J. 1974 Jul;14(7):546–562. doi: 10.1016/S0006-3495(74)85934-5. [DOI] [PMC free article] [PubMed] [Google Scholar]
- Kawai M., Brandt P. W. Sinusoidal analysis: a high resolution method for correlating biochemical reactions with physiological processes in activated skeletal muscles of rabbit, frog and crayfish. J Muscle Res Cell Motil. 1980 Sep;1(3):279–303. doi: 10.1007/BF00711932. [DOI] [PubMed] [Google Scholar]
- Levy R. M., Umazume Y., Kushmerick M. J. Ca2+ dependence of tension and ADP production in segments of chemically skinned muscle fibers. Biochim Biophys Acta. 1976 May 14;430(2):352–365. doi: 10.1016/0005-2728(76)90091-8. [DOI] [PubMed] [Google Scholar]
- Lymn R. W., Taylor E. W. Mechanism of adenosine triphosphate hydrolysis by actomyosin. Biochemistry. 1971 Dec 7;10(25):4617–4624. doi: 10.1021/bi00801a004. [DOI] [PubMed] [Google Scholar]
- Matsubara I., Yagi N. A time-resolved X-ray diffraction study of muscle during twitch. J Physiol. 1978 May;278:297–307. doi: 10.1113/jphysiol.1978.sp012305. [DOI] [PMC free article] [PubMed] [Google Scholar]
- Matsubara I., Yagi N., Hashizume H. Use of an X-ray television for diffraction of the frog striated muscle. Nature. 1975 Jun 26;255(5511):728–729. doi: 10.1038/255728a0. [DOI] [PubMed] [Google Scholar]
- Mornet D., Bertrand R., Pantel P., Audemard E., Kassab R. Structure of the actin-myosin interface. Nature. 1981 Jul 23;292(5821):301–306. doi: 10.1038/292301a0. [DOI] [PubMed] [Google Scholar]
- Steiger J. G., Abbott R. H. Biochemical interpretation of tension transients produced by a four-state mechanical model. J Muscle Res Cell Motil. 1981 Sep;2(3):245–260. doi: 10.1007/BF00713264. [DOI] [PubMed] [Google Scholar]
- Stein L. A., Chock P. B., Eisenberg E. Mechanism of the actomyosin ATPase: effect of actin on the ATP hydrolysis step. Proc Natl Acad Sci U S A. 1981 Mar;78(3):1346–1350. doi: 10.1073/pnas.78.3.1346. [DOI] [PMC free article] [PubMed] [Google Scholar]
- Stein L. A., Schwarz R. P., Jr, Chock P. B., Eisenberg E. Mechanism of actomyosin adenosine triphosphatase. Evidence that adenosine 5'-triphosphate hydrolysis can occur without dissociation of the actomyosin complex. Biochemistry. 1979 Sep 4;18(18):3895–3909. doi: 10.1021/bi00585a009. [DOI] [PubMed] [Google Scholar]
- Takashi R., Putnam S. A fluorimetric method for continuously assaying ATPase: application to small specimens of glycerol-extracted muscle fibers. Anal Biochem. 1979 Jan 15;92(2):375–382. doi: 10.1016/0003-2697(79)90674-2. [DOI] [PubMed] [Google Scholar]
- Taylor E. W. Mechanism of actomyosin ATPase and the problem of muscle contraction. CRC Crit Rev Biochem. 1979;6(2):103–164. doi: 10.3109/10409237909102562. [DOI] [PubMed] [Google Scholar]
- Thorson J., White D. C. Role of cross-bridge distortion in the small-signal mechanical dynamics of insect and rabbit striated muscle. J Physiol. 1983 Oct;343:59–84. doi: 10.1113/jphysiol.1983.sp014881. [DOI] [PMC free article] [PubMed] [Google Scholar]
- Weeds A. G., Taylor R. S. Separation of subfragment-1 isoenzymes from rabbit skeletal muscle myosin. Nature. 1975 Sep 4;257(5521):54–56. doi: 10.1038/257054a0. [DOI] [PubMed] [Google Scholar]
- White H. D., Taylor E. W. Energetics and mechanism of actomyosin adenosine triphosphatase. Biochemistry. 1976 Dec 28;15(26):5818–5826. doi: 10.1021/bi00671a020. [DOI] [PubMed] [Google Scholar]
- Yamamoto T., Herzig J. W. Series elastic properties of skinned muscle fibres in contraction and rigor. Pflugers Arch. 1978 Jan 31;373(1):21–24. doi: 10.1007/BF00581144. [DOI] [PubMed] [Google Scholar]