Abstract
The relation between force and velocity was determined in sixteen trabeculae of rat right ventricle as a function of time during a twitch, of sarcomere length and of external Ca2+ concentration, [Ca2+]o. The trabeculae were studied in modified Krebs-Henseleit solution at 25 degrees C. Force was measured with a semiconductor strain gauge. Sarcomere length was measured with a laser diffraction system. A servomotor system was used in which control could be switched between sarcomere length, muscle length and force. Force-velocity relations were derived from load clamps and from contractions in which sarcomere length was initially held constant followed by a quick release and slower release of the sarcomeres at controlled velocity. Force-velocity relations were fitted by Hill's equation (Hill, 1938), (Po-P) b = (P+a) V, where P = force, V = velocity, Po = isometric force in mN/mm2 and a and b are constants. For [Ca2+]o = 2.5 mM, with both interventions the values (mean +/- S.D.) were: b = 1.00 +/- 0.45 micron/s; a = 9.52 +/- 5.60 mN/mm2; Vo measured = 13.6 +/- 3.0 micron/s; Vo calculated = 13.4 +/- 3.4 micron/s; Po measured = 96.5 +/- 25.0 mN/mm2; Po calculated = 119.3 +/- 34.5 mN/mm2. Vo rose with [Ca2+]o to a maximum at [Ca2+]o = 1.2 mM when Po was about 50% of maximum, while Po rose with [Ca2+]o to a maximum at above 2.5 mM. Vo rose with time during the twitch to a maximum at 25 ms following onset of contraction; Po was then about 50% of the maximum that was obtained at 120 ms. Vo increased with sarcomere length from zero at a sarcomere length of 1.6 micron to a maximum at 1.85 micron. Between 1.85 micron and 2.3 micron, Vo was constant. At 1.85 micron, Po was about 60% of maximum Po. These results are compatible with the hypothesis that Vo is more sensitive than Po to the amount of Ca2+ bound to the contractile proteins, and that Vo reaches a maximal value with an amount of Ca2+ bound to the contractile proteins at which Po has obtained only about 50% of its maximal value.
Full text
PDF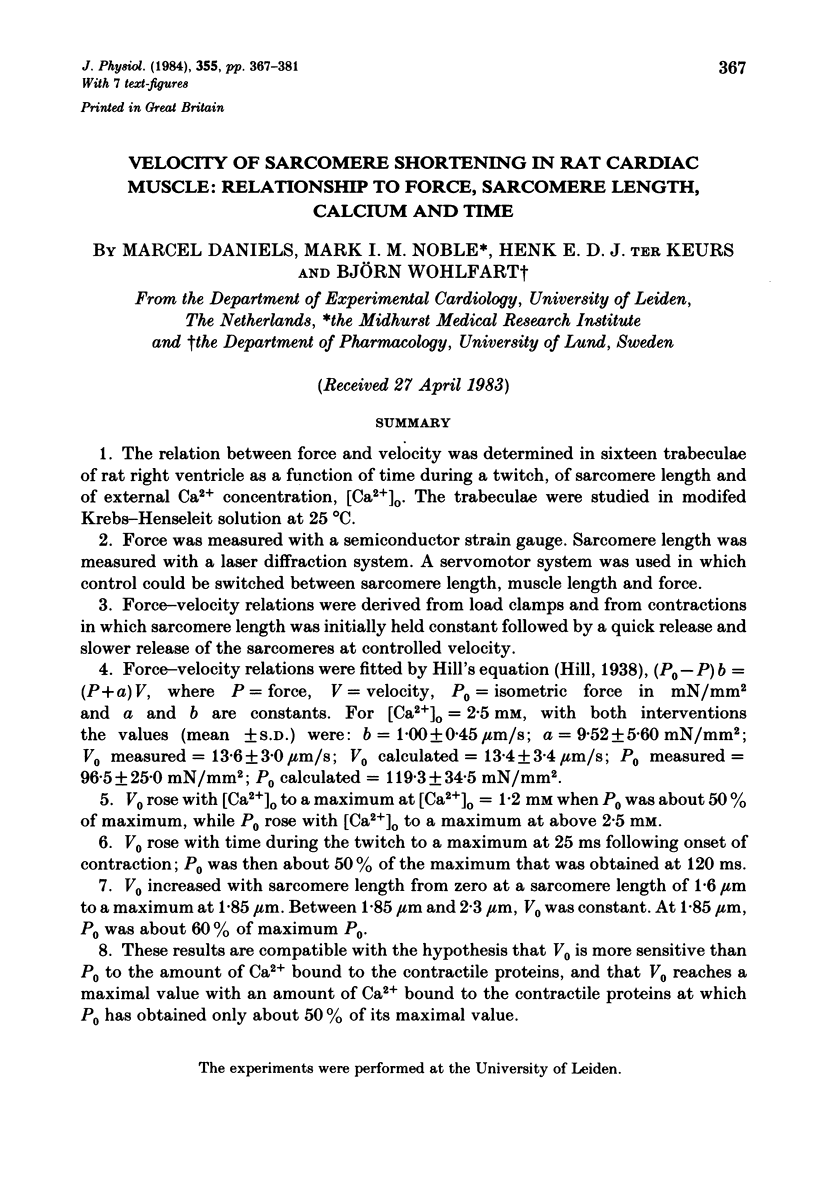
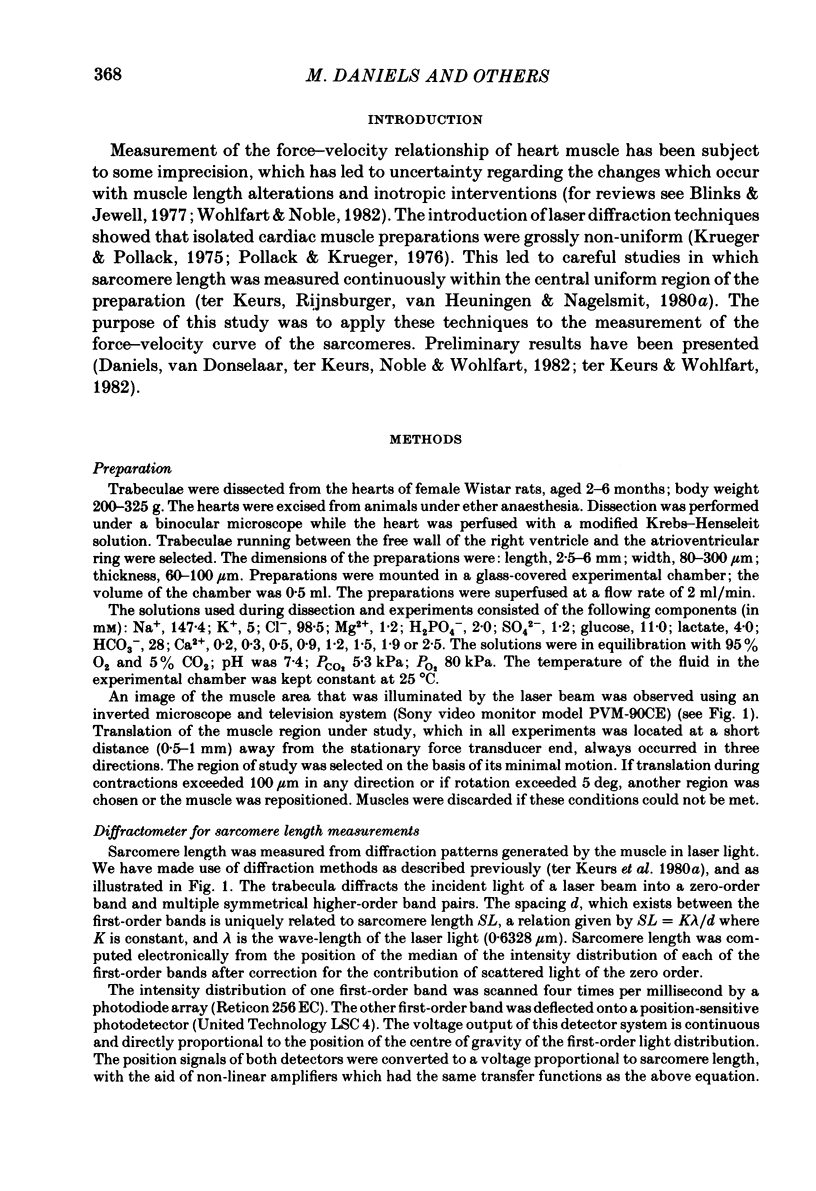
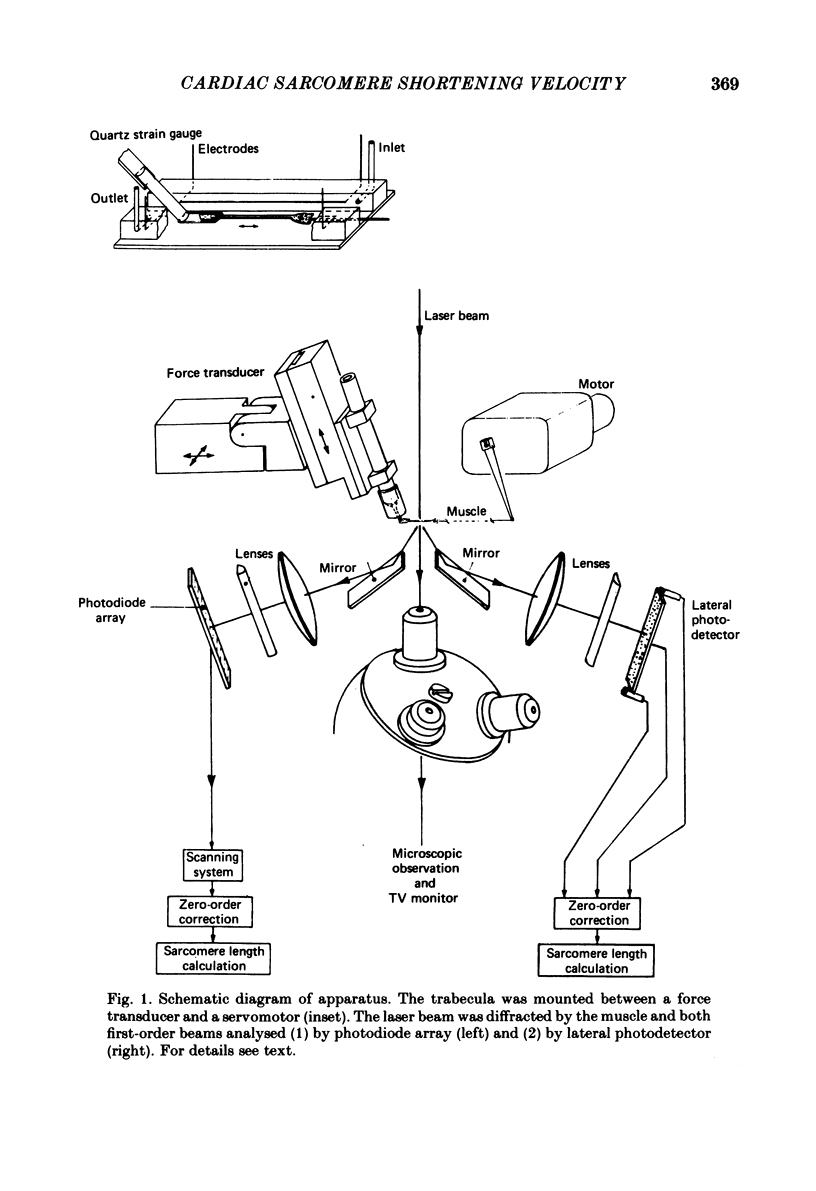
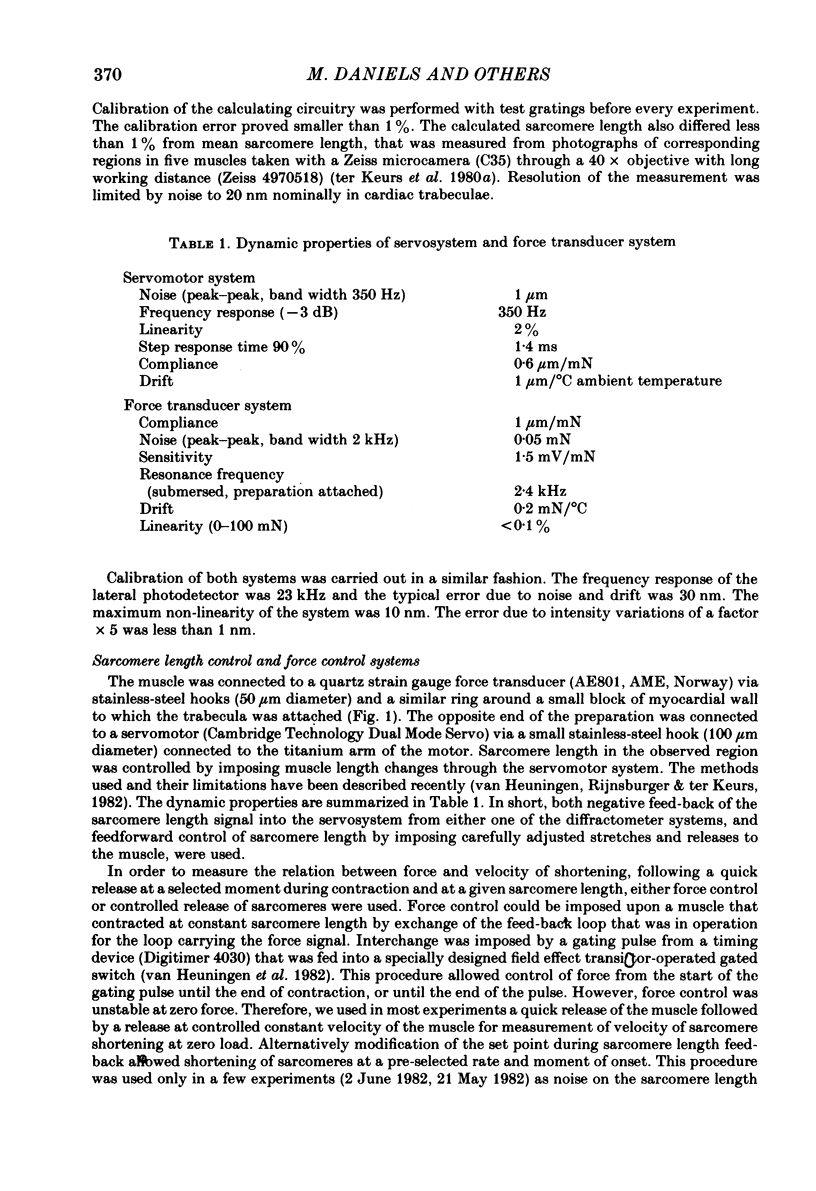
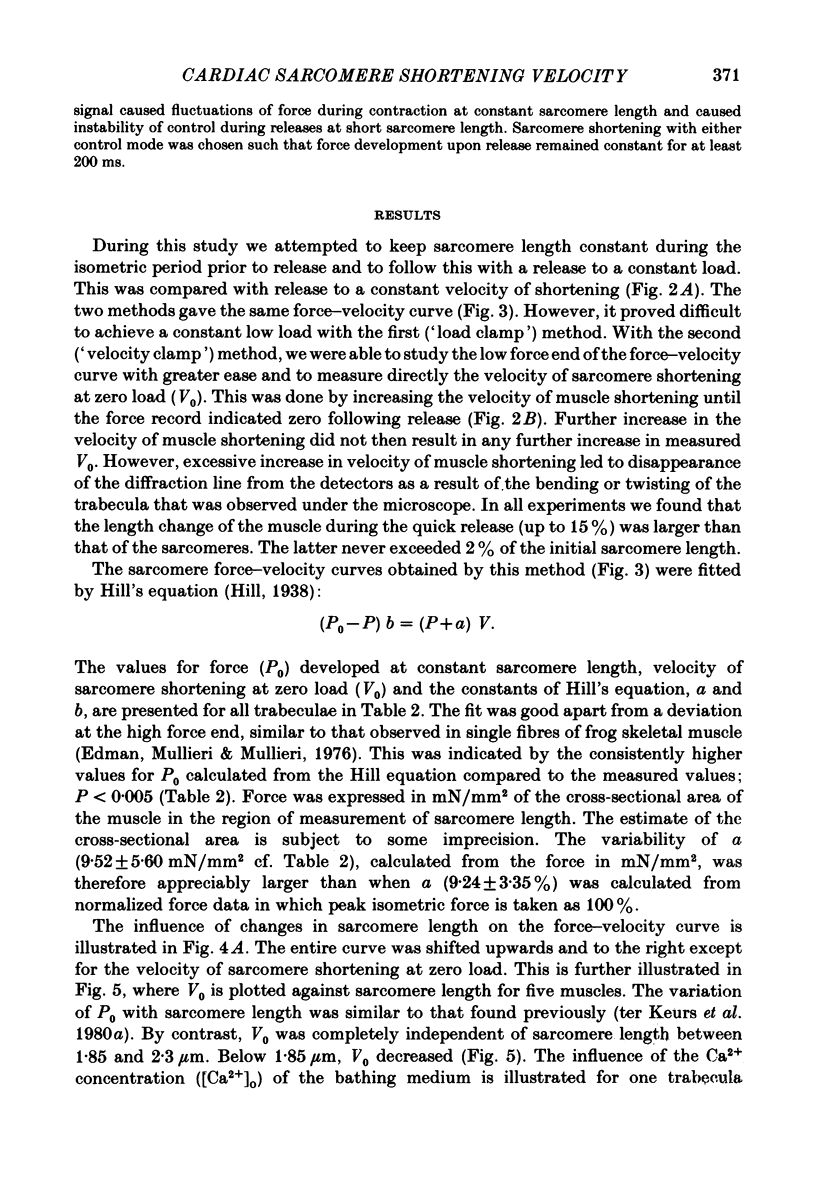
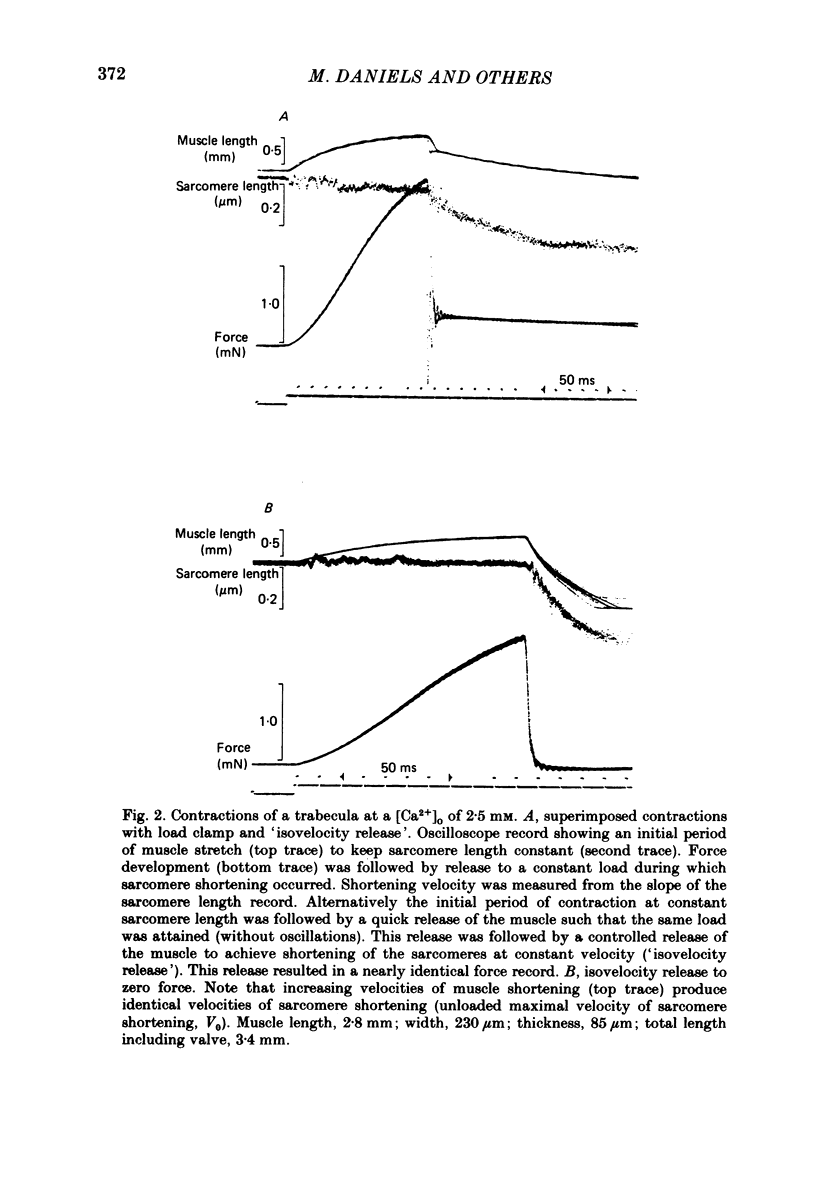
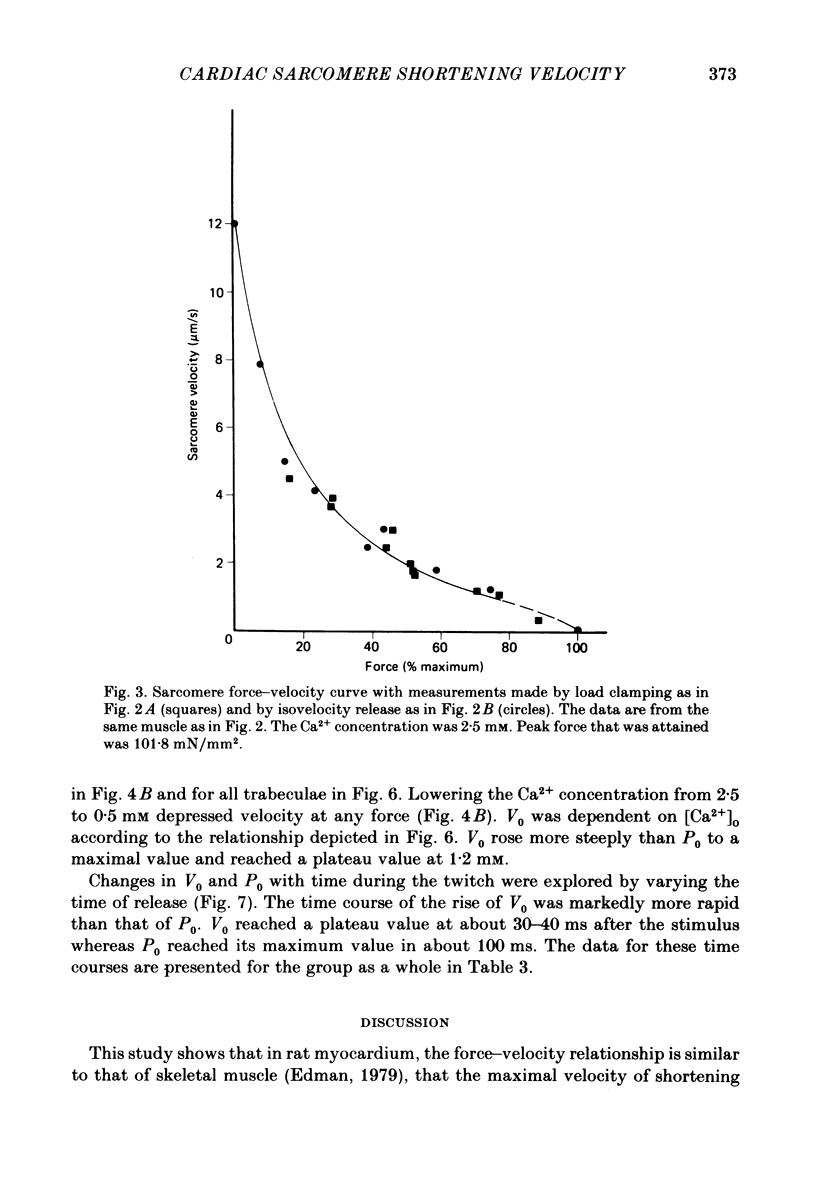
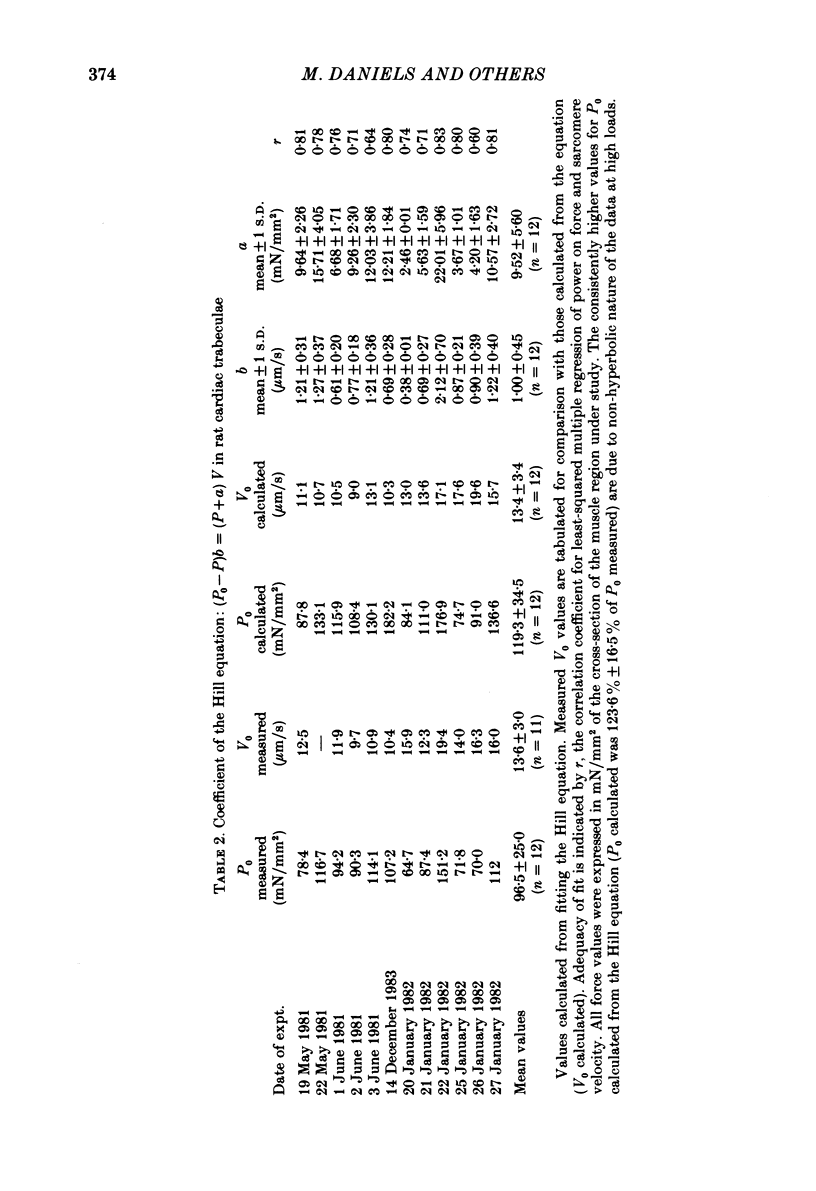
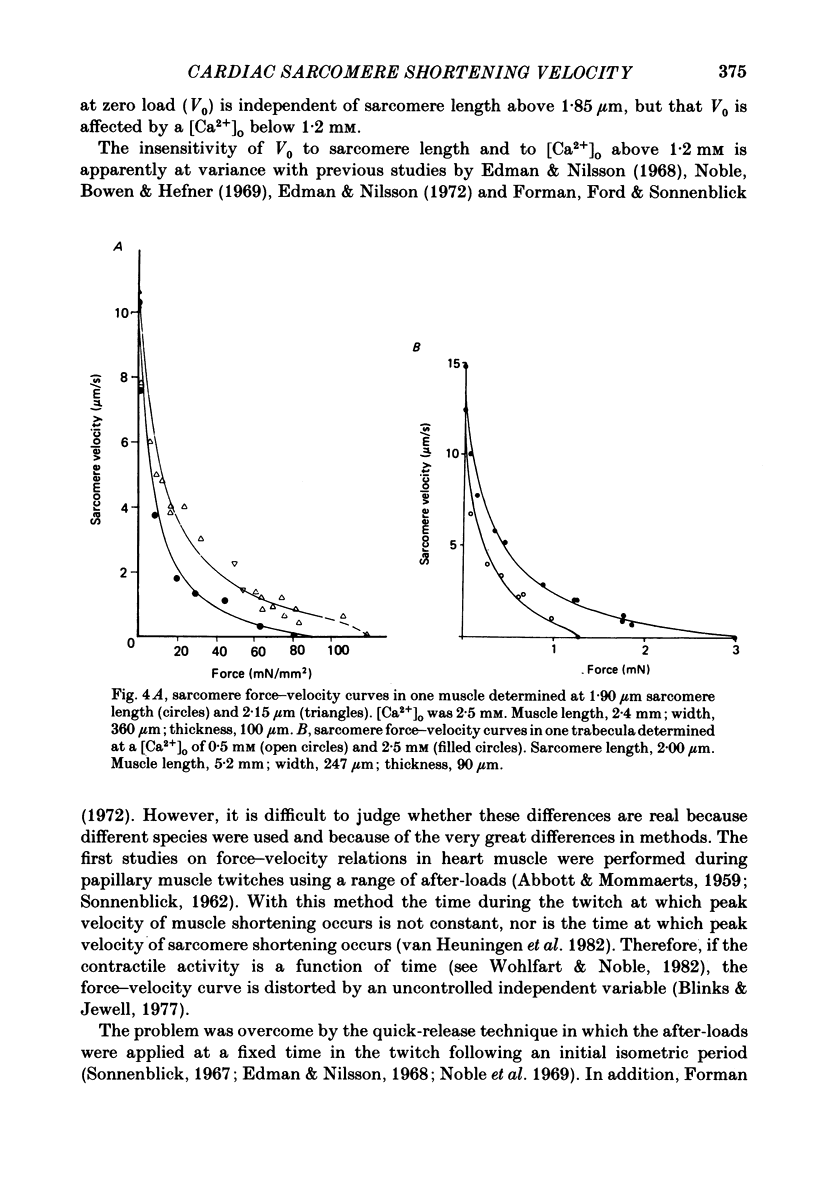
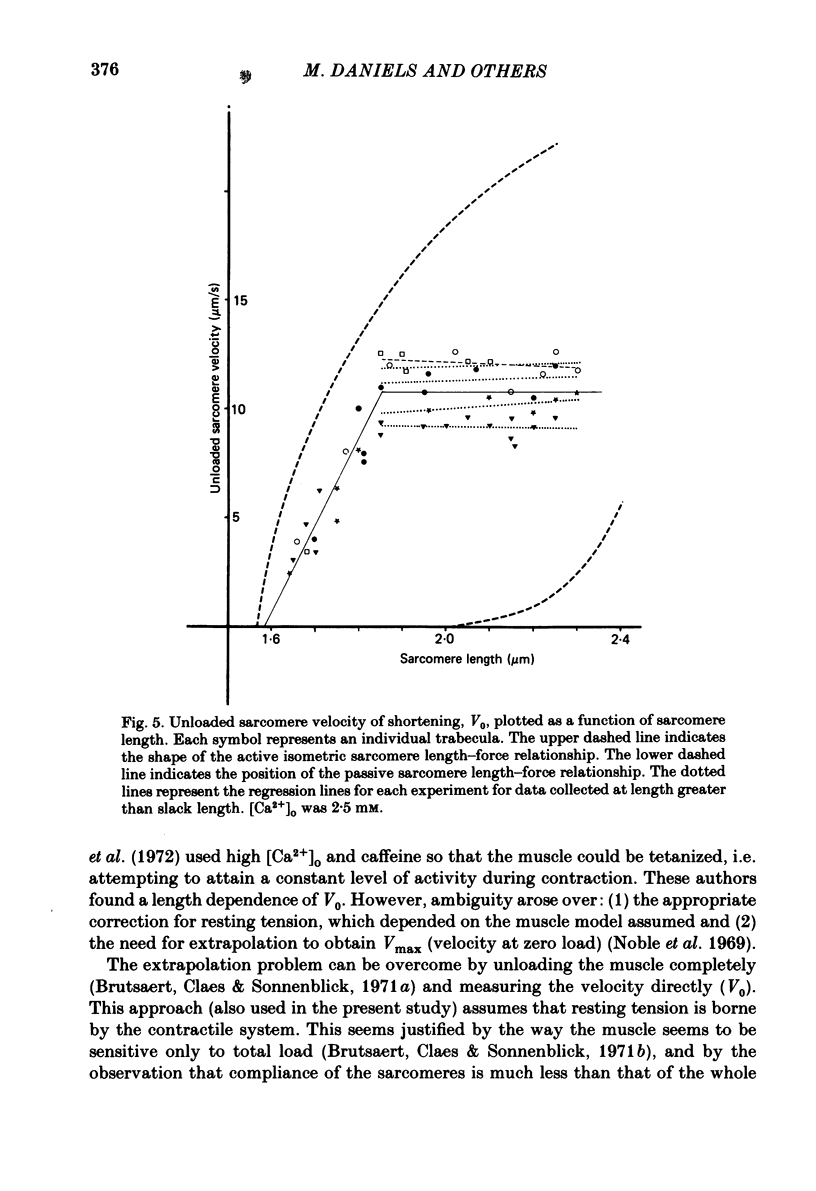
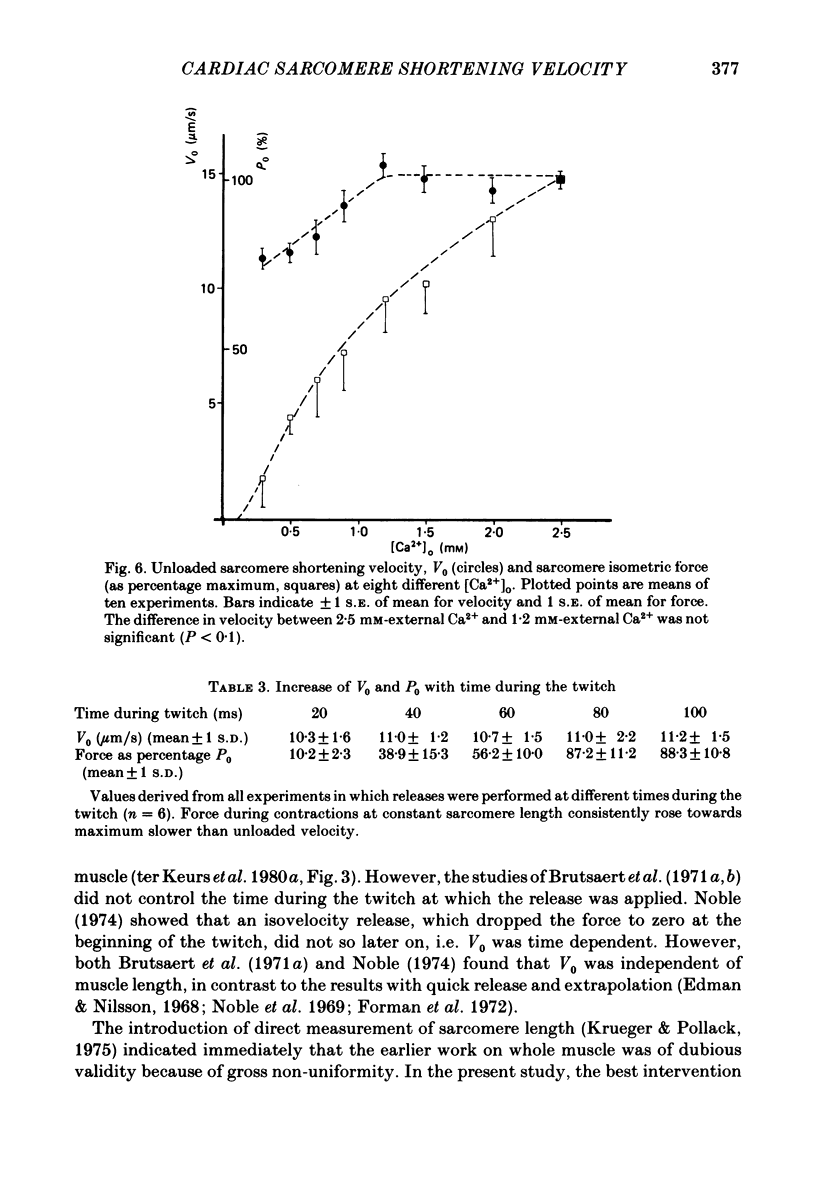
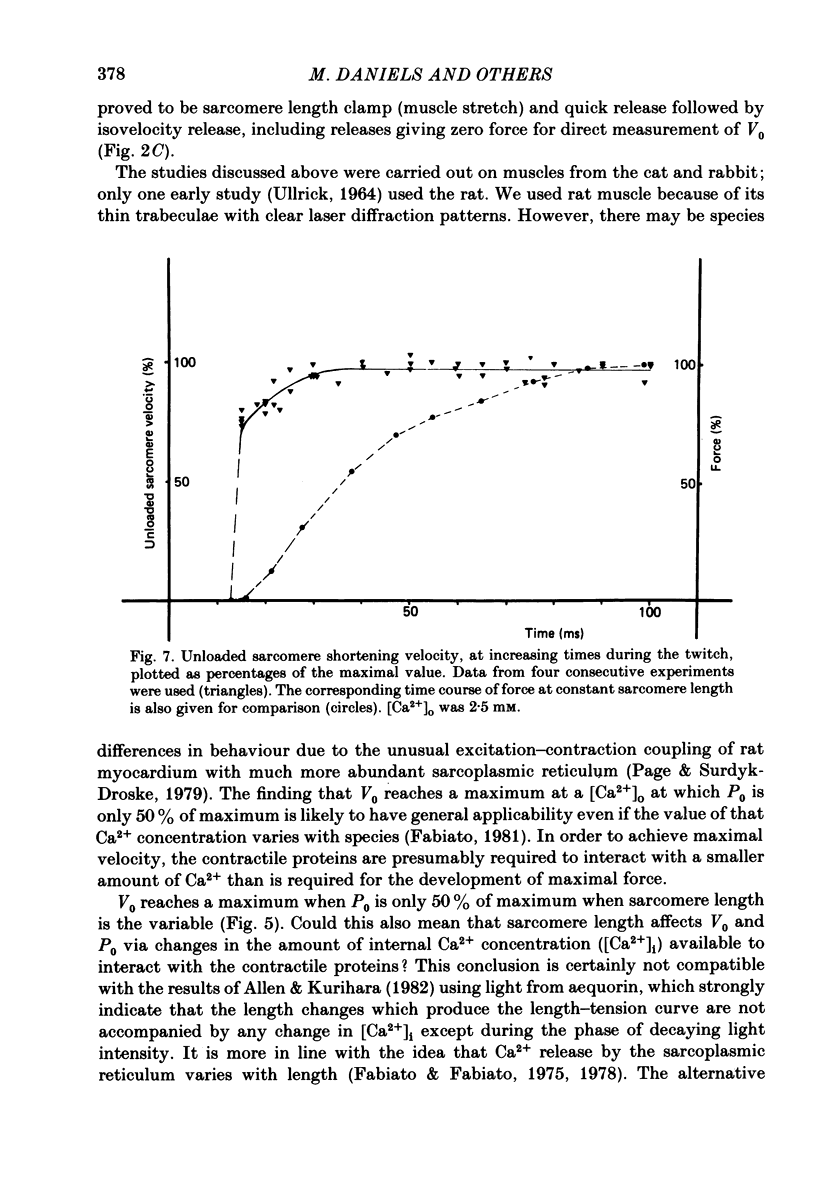
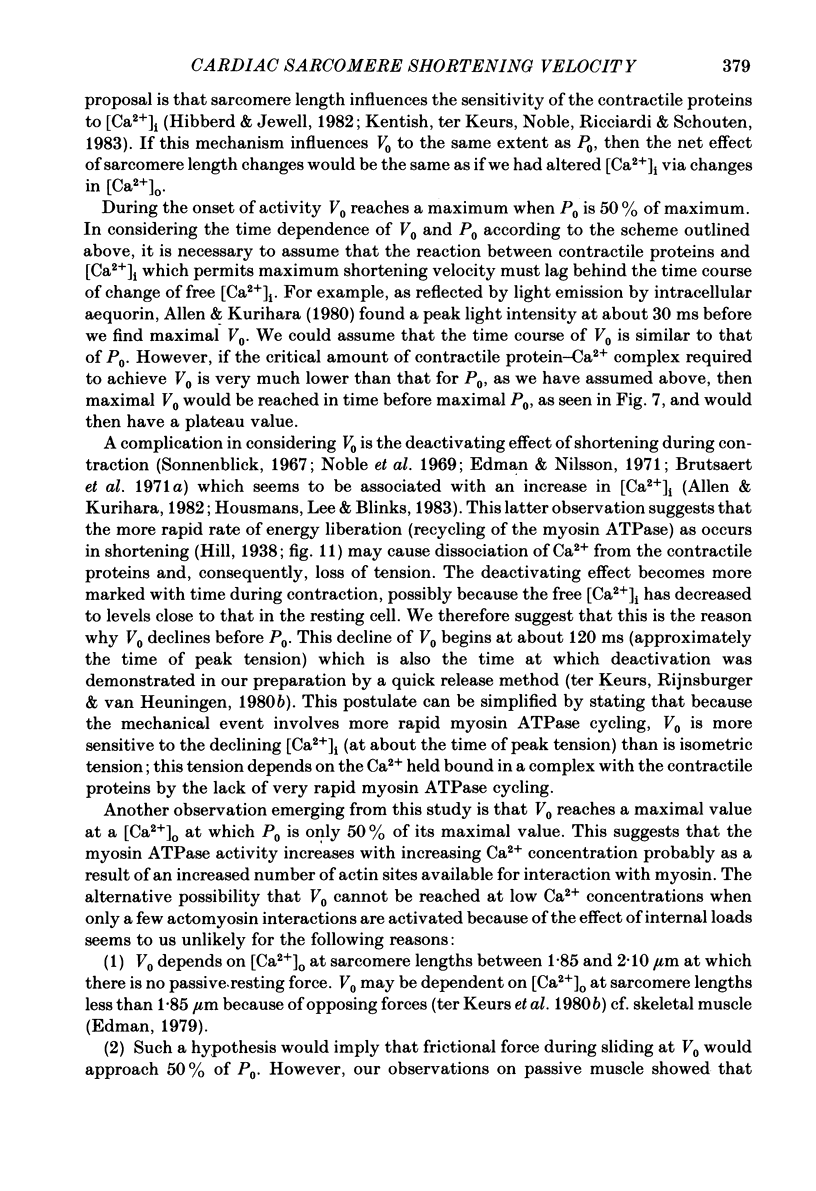
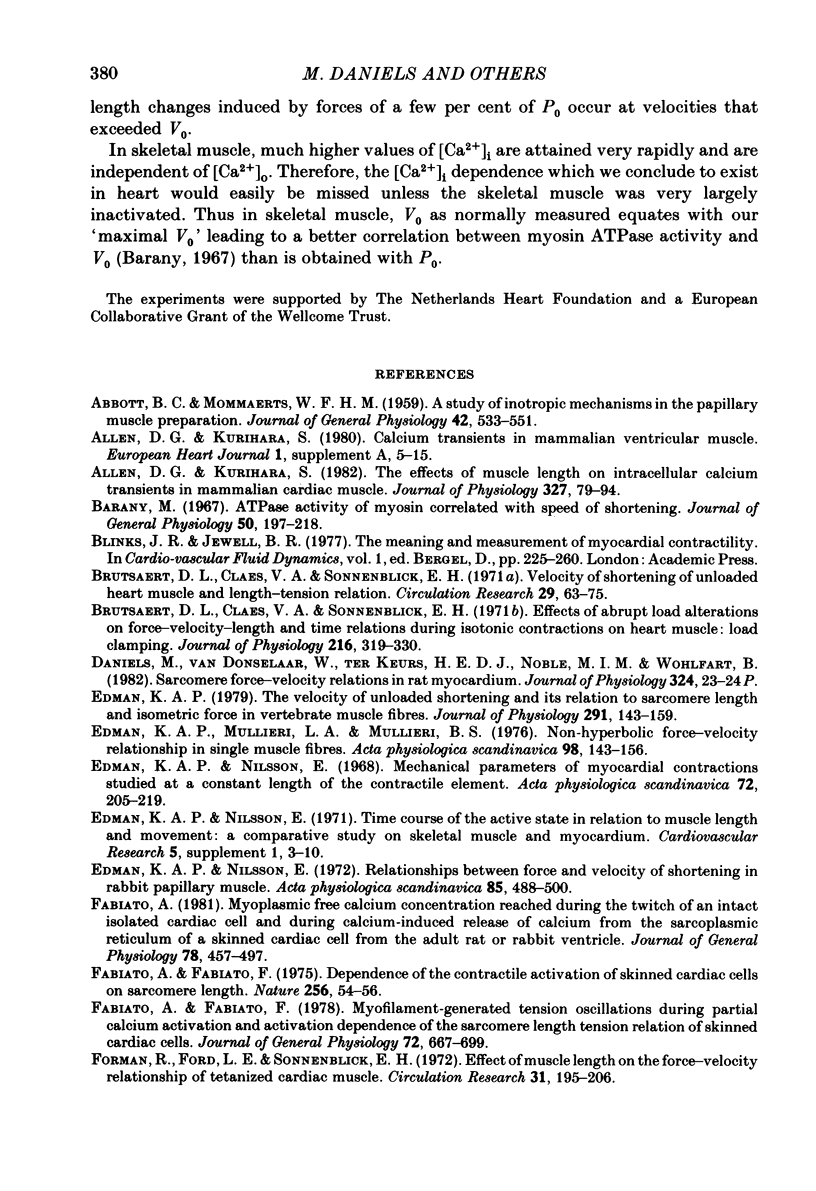
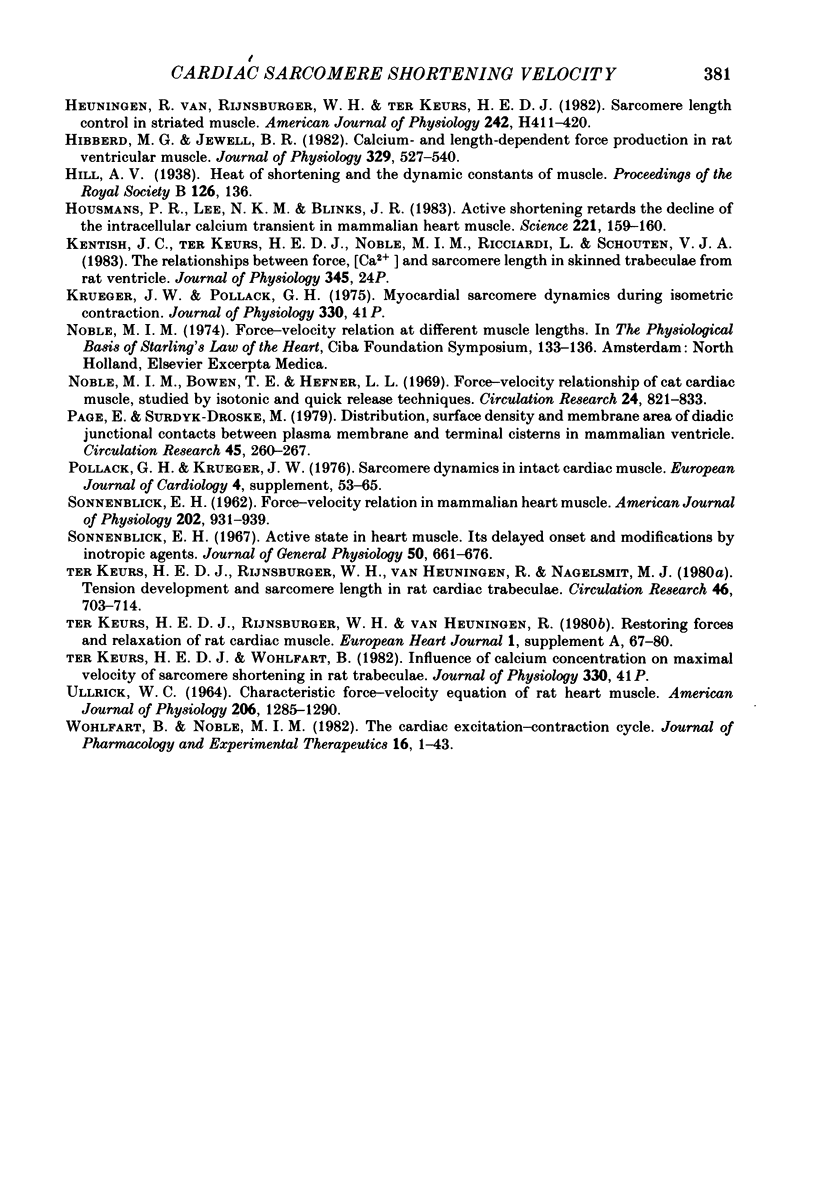
Selected References
These references are in PubMed. This may not be the complete list of references from this article.
- ABBOTT B. C., MOMMAERTS W. F. A study of inotropic mechanisms in the papillary muscle preparation. J Gen Physiol. 1959 Jan 20;42(3):533–551. doi: 10.1085/jgp.42.3.533. [DOI] [PMC free article] [PubMed] [Google Scholar]
- Allen D. G., Kurihara S. Calcium transients in mammalian ventricular muscle. Eur Heart J. 1980;Suppl A:5–15. doi: 10.1093/eurheartj/1.suppl_1.5. [DOI] [PubMed] [Google Scholar]
- Allen D. G., Kurihara S. The effects of muscle length on intracellular calcium transients in mammalian cardiac muscle. J Physiol. 1982 Jun;327:79–94. doi: 10.1113/jphysiol.1982.sp014221. [DOI] [PMC free article] [PubMed] [Google Scholar]
- Brutsaert D. L., Claes V. A., Sonnenblick E. H. Effects of abrupt load alterations on force-velocity-length and time relations during isotonic contractions of heart muscle: load clamping. J Physiol. 1971 Jul;216(2):319–330. doi: 10.1113/jphysiol.1971.sp009527. [DOI] [PMC free article] [PubMed] [Google Scholar]
- Brutsaert D. L., Claes V. A., Sonnenblick E. H. Velocity of shortening of unloaded heart muscle and the length-tension relation. Circ Res. 1971 Jul;29(1):63–75. doi: 10.1161/01.res.29.1.63. [DOI] [PubMed] [Google Scholar]
- Bárány M. ATPase activity of myosin correlated with speed of muscle shortening. J Gen Physiol. 1967 Jul;50(6 Suppl):197–218. doi: 10.1085/jgp.50.6.197. [DOI] [PMC free article] [PubMed] [Google Scholar]
- Edman K. A., Mulieri L. A., Scubon-Mulieri B. Non-hyperbolic force-velocity relationship in single muscle fibres. Acta Physiol Scand. 1976 Oct;98(2):143–156. doi: 10.1111/j.1748-1716.1976.tb00234.x. [DOI] [PubMed] [Google Scholar]
- Edman K. A., Nilsson E. Relationships between force and velocity of shortening in rabbit papillary muscle. Acta Physiol Scand. 1972 Aug;85(4):488–500. doi: 10.1111/j.1748-1716.1971.tb05286.x. [DOI] [PubMed] [Google Scholar]
- Edman K. A., Nilsson E. The mechanical parameters of myocardial contraction studied at a constant length of the contractile element. Acta Physiol Scand. 1968 Jan-Feb;72(1):205–219. doi: 10.1111/j.1748-1716.1968.tb03843.x. [DOI] [PubMed] [Google Scholar]
- Edman K. A. The velocity of unloaded shortening and its relation to sarcomere length and isometric force in vertebrate muscle fibres. J Physiol. 1979 Jun;291:143–159. doi: 10.1113/jphysiol.1979.sp012804. [DOI] [PMC free article] [PubMed] [Google Scholar]
- Fabiato A., Fabiato F. Dependence of the contractile activation of skinned cardiac cells on the sarcomere length. Nature. 1975 Jul 3;256(5512):54–56. doi: 10.1038/256054a0. [DOI] [PubMed] [Google Scholar]
- Fabiato A., Fabiato F. Myofilament-generated tension oscillations during partial calcium activation and activation dependence of the sarcomere length-tension relation of skinned cardiac cells. J Gen Physiol. 1978 Nov;72(5):667–699. doi: 10.1085/jgp.72.5.667. [DOI] [PMC free article] [PubMed] [Google Scholar]
- Fabiato A. Myoplasmic free calcium concentration reached during the twitch of an intact isolated cardiac cell and during calcium-induced release of calcium from the sarcoplasmic reticulum of a skinned cardiac cell from the adult rat or rabbit ventricle. J Gen Physiol. 1981 Nov;78(5):457–497. doi: 10.1085/jgp.78.5.457. [DOI] [PMC free article] [PubMed] [Google Scholar]
- Forman R., Ford L. E., Sonnenblick E. H. Effect of muscle length on the force-velocity relationship of tetanized cardiac muscle. Circ Res. 1972 Aug;31(2):195–206. doi: 10.1161/01.res.31.2.195. [DOI] [PubMed] [Google Scholar]
- Hibberd M. G., Jewell B. R. Calcium- and length-dependent force production in rat ventricular muscle. J Physiol. 1982 Aug;329:527–540. doi: 10.1113/jphysiol.1982.sp014317. [DOI] [PMC free article] [PubMed] [Google Scholar]
- Housmans P. R., Lee N. K., Blinks J. R. Active shortening retards the decline of the intracellular calcium transient in mammalian heart muscle. Science. 1983 Jul 8;221(4606):159–161. doi: 10.1126/science.6857274. [DOI] [PubMed] [Google Scholar]
- Noble M. I., Bowen T. E., Hefner L. L. Force-velocity relationship of cat cardiac muscle, studied by isotonic and quick-release techniques. Circ Res. 1969 Jun;24(6):821–833. doi: 10.1161/01.res.24.6.821. [DOI] [PubMed] [Google Scholar]
- Page E., Surdyk-Droske M. Distribution, surface density, and membrane area of diadic junctional contacts between plasma membrane and terminal cisterns in mammalian ventricle. Circ Res. 1979 Aug;45(2):260–267. doi: 10.1161/01.res.45.2.260. [DOI] [PubMed] [Google Scholar]
- Pollack G. H., Krueger J. W. Sarcomere dynamics in intact cardiac muscle. Eur J Cardiol. 1976 May;4 (Suppl):53–65. [PubMed] [Google Scholar]
- SONNENBLICK E. H. Force-velocity relations in mammalian heart muscle. Am J Physiol. 1962 May;202:931–939. doi: 10.1152/ajplegacy.1962.202.5.931. [DOI] [PubMed] [Google Scholar]
- Sonnenblick E. H. Active state in heart muscle. Its delayed onset and modification by inotropic agents. J Gen Physiol. 1967 Jan;50(3):661–676. doi: 10.1085/jgp.50.3.661. [DOI] [PMC free article] [PubMed] [Google Scholar]
- ULLRICK W. C. CHARACTERISTIC FORCE-VELOCITY EQUATION OF RAT HEART MUSCLE. Am J Physiol. 1964 Jun;206:1285–1298. doi: 10.1152/ajplegacy.1964.206.6.1285. [DOI] [PubMed] [Google Scholar]
- Wohlfart B., Noble M. I. The cardiac excitation-contraction cycle. Pharmacol Ther. 1982;16(1):1–43. doi: 10.1016/0163-7258(82)90030-4. [DOI] [PubMed] [Google Scholar]
- ter Keurs H. E., Rijnsburger W. H., van Heuningen R., Nagelsmit M. J. Tension development and sarcomere length in rat cardiac trabeculae. Evidence of length-dependent activation. Circ Res. 1980 May;46(5):703–714. doi: 10.1161/01.res.46.5.703. [DOI] [PubMed] [Google Scholar]
- van Heuningen R., Rijnsburger W. H., ter Keurs H. E. Sarcomere length control in striated muscle. Am J Physiol. 1982 Mar;242(3):H411–H420. doi: 10.1152/ajpheart.1982.242.3.H411. [DOI] [PubMed] [Google Scholar]