Abstract
The excitability of developing rat optic nerves has been studied under conditions in which extracellular Cl- was replaced with other anions. In nerves younger than 3 days old, replacing Cl- with propionate or SO4(2-) usually led to spontaneous and repetitive cycling of extracellular K+ concentration ([K+]o). [K+]o reached peaks of 8-12 mM and then fell transiently below the base-line level of 5 mM before increasing again. This cycling behaviour continued, with a wave-length of 1-2 min, for as long as 2 h. Nerves older than 5 days either did not cycle or did so only transiently. Substitution of ten different anions for Cl- indicated that a minimum hydrated radius, between that of BrO3- and HCO3-, was necessary to induce cycling behaviour. Cycling behaviour was abolished by the Na+-channel blocker tetrodotoxin. Reduction of the bath [K+] to 2.5 mM slowed the frequency of spontaneous cycles; a bath [K+] of 1 mM abolished them. When the temperature was lowered, cycle frequency slowed. Substitution of large anions for Cl- enhanced axonal excitability. This was inferred from the prevalence of spontaneous action potentials during cycling behaviour, and from the generation of relatively large evoked increases of [K+]o. Cycling behaviour is hypothesized to result from a repetition of the following three processes: (i) spontaneous axonal firing elicits a gradual increase in [K+]o which increases axonal excitability and facilitates further K+ release, (ii) axonal firing and K+ release are eventually halted by a combination of depolarization block, intracellular Na+ accumulation and hyperpolarization from electrogenic pumping, (iii) recovery of [K+]o to its minimal value depends on active K+ reuptake mediated by a highly stimulated axonal Na+-K+-ATPase. We conclude that a large proportion of the resting membrane conductance of optic nerve fibres is Cl- specific. A high Cl- conductance may stabilize fine central axons against the depolarizing effects of [K+]o increases.
Full text
PDF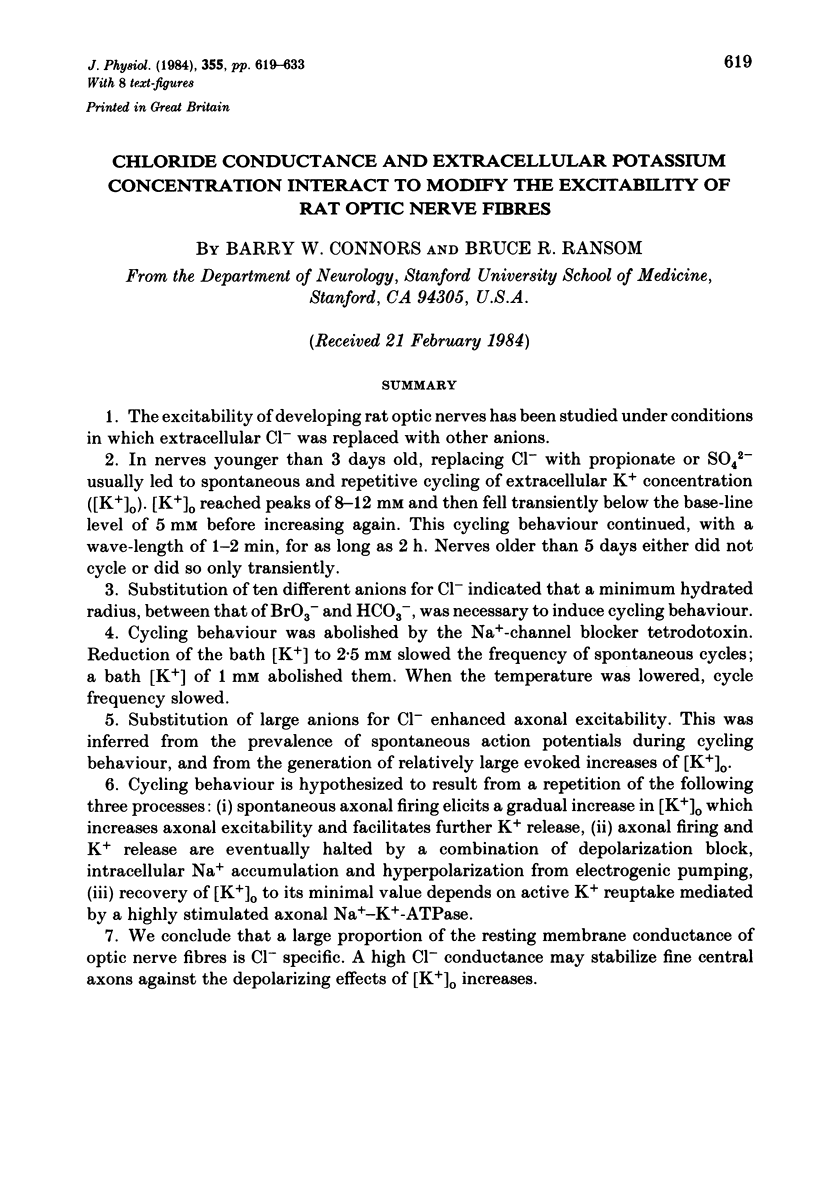
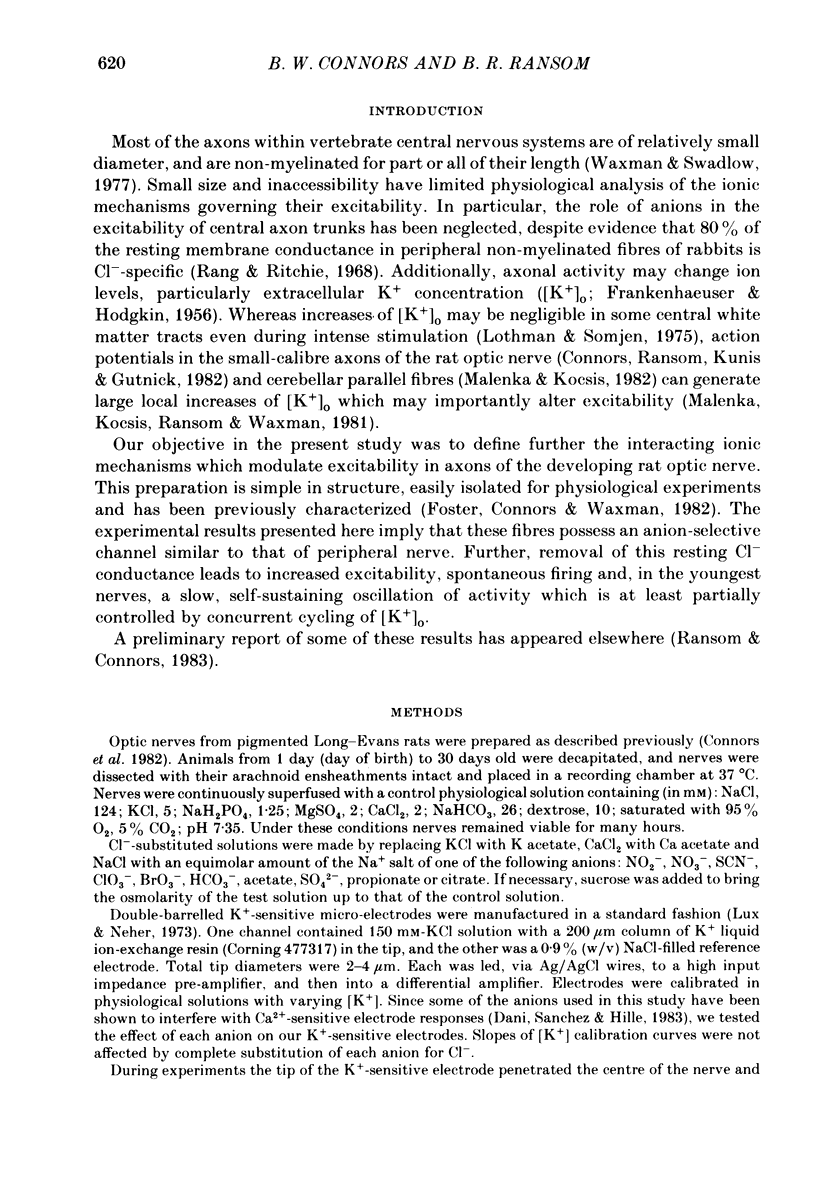
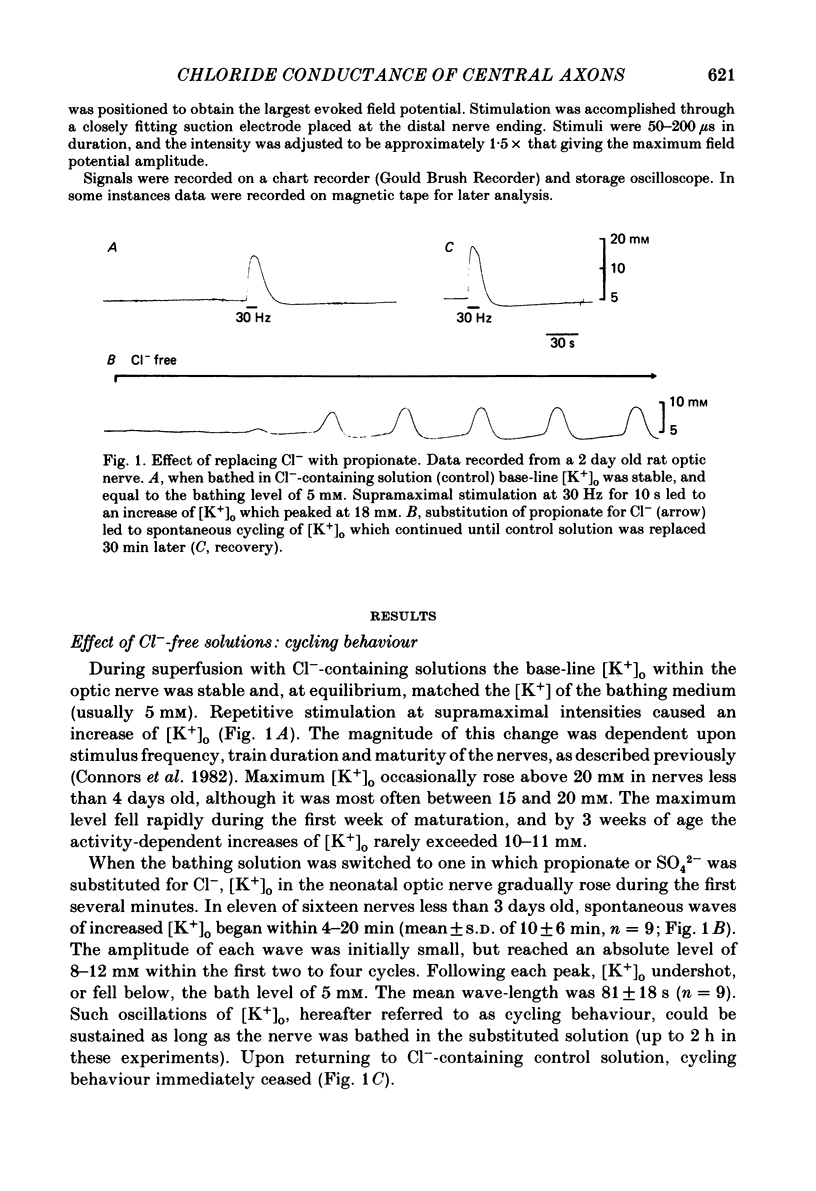
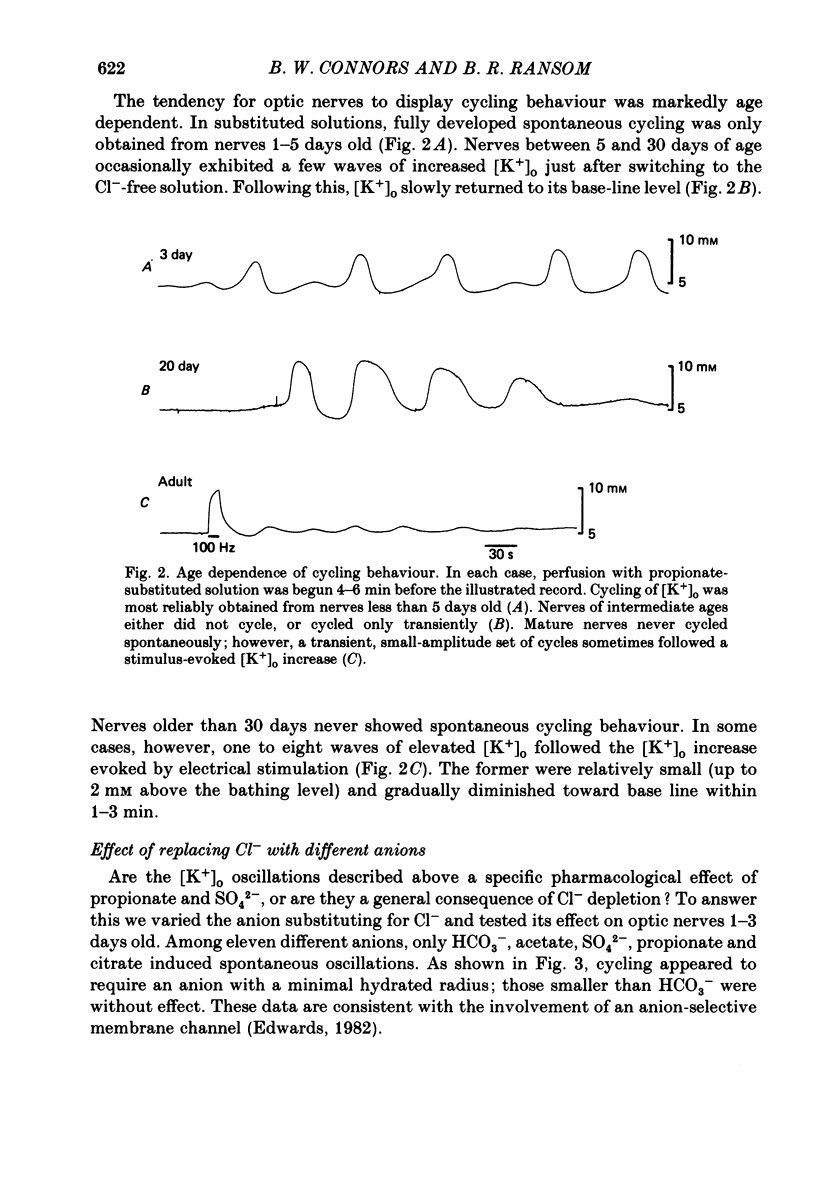
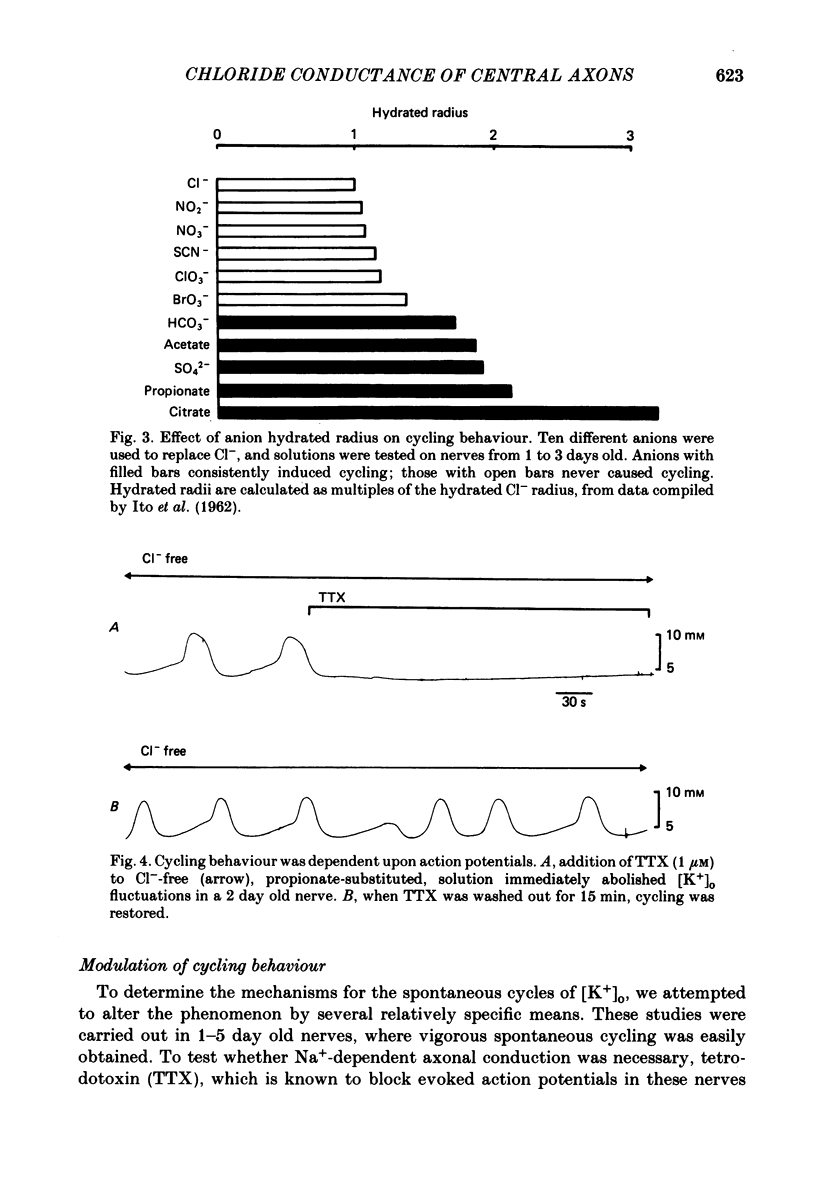
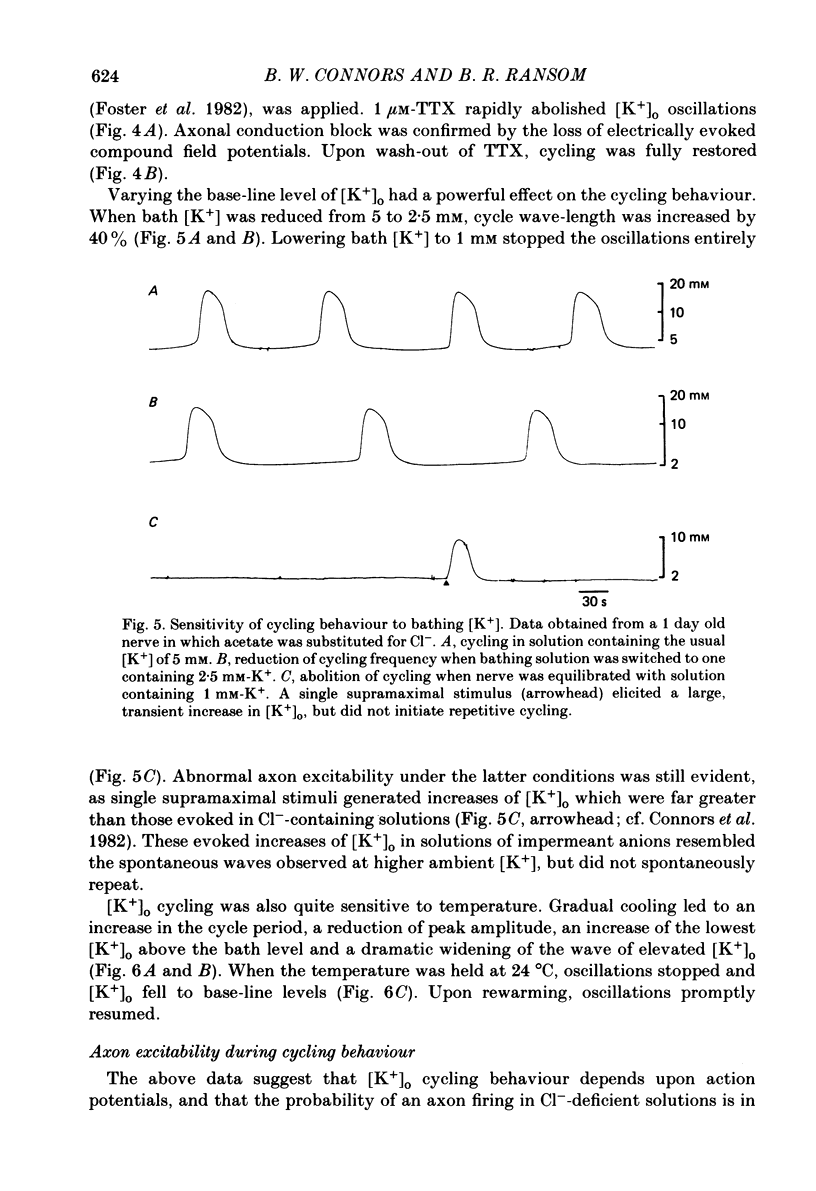
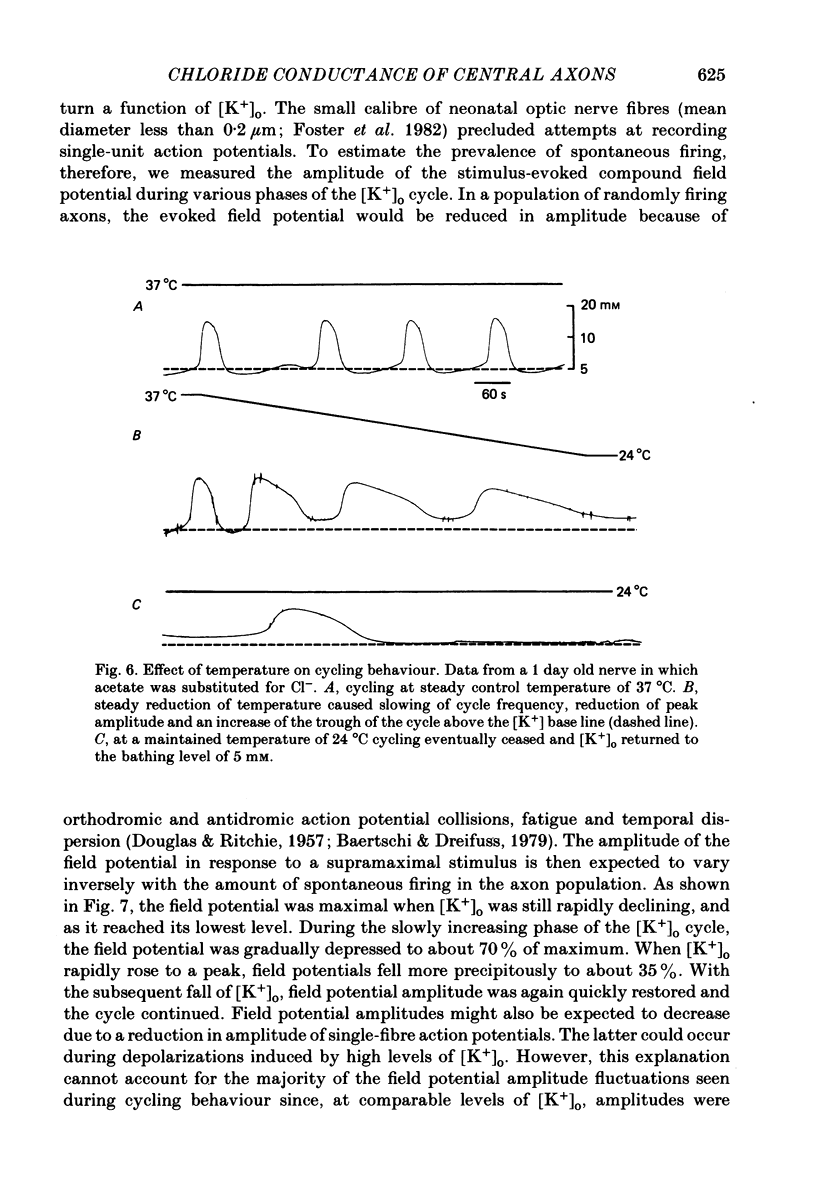
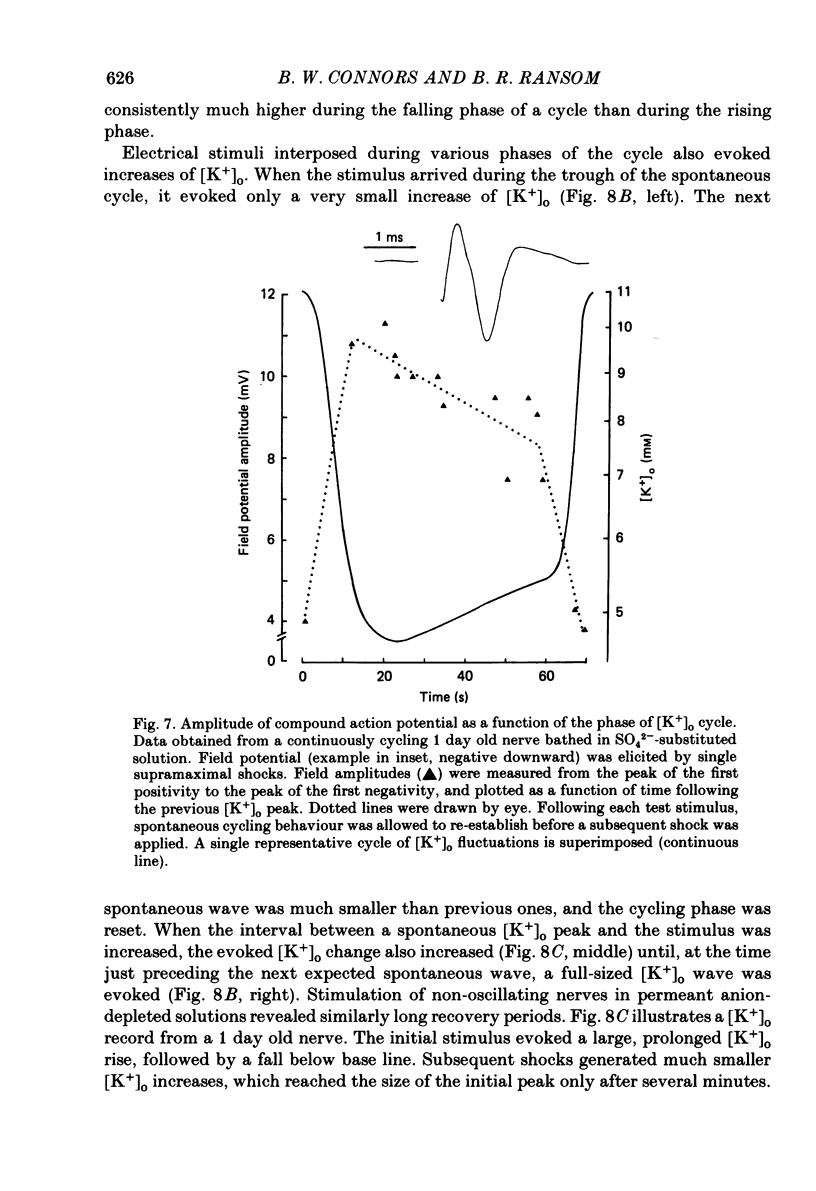
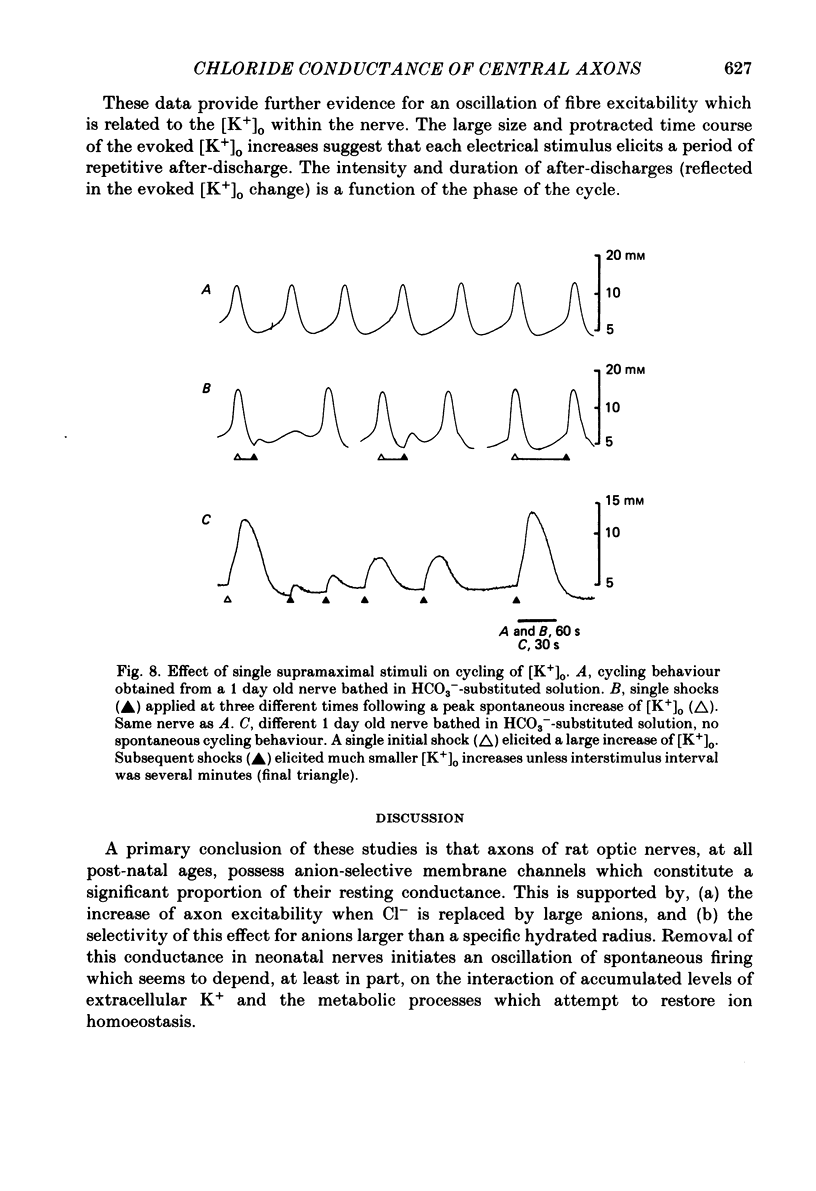
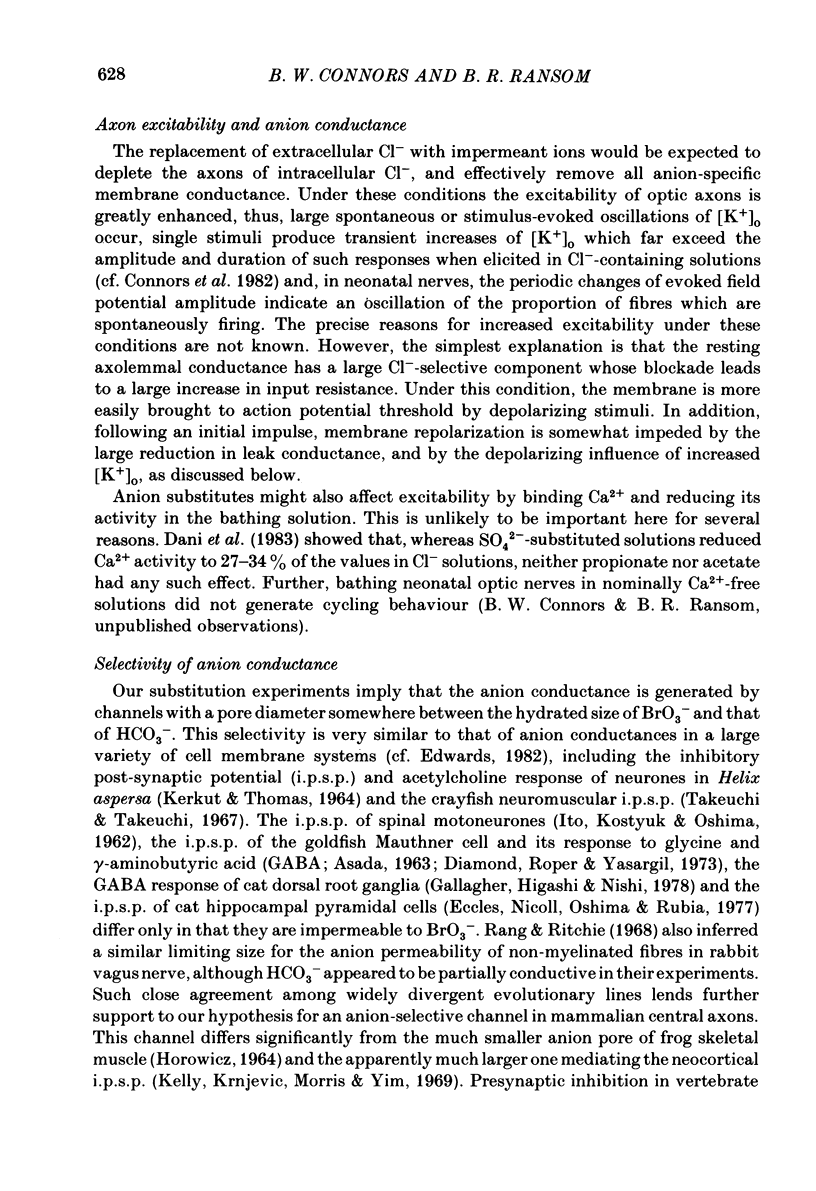
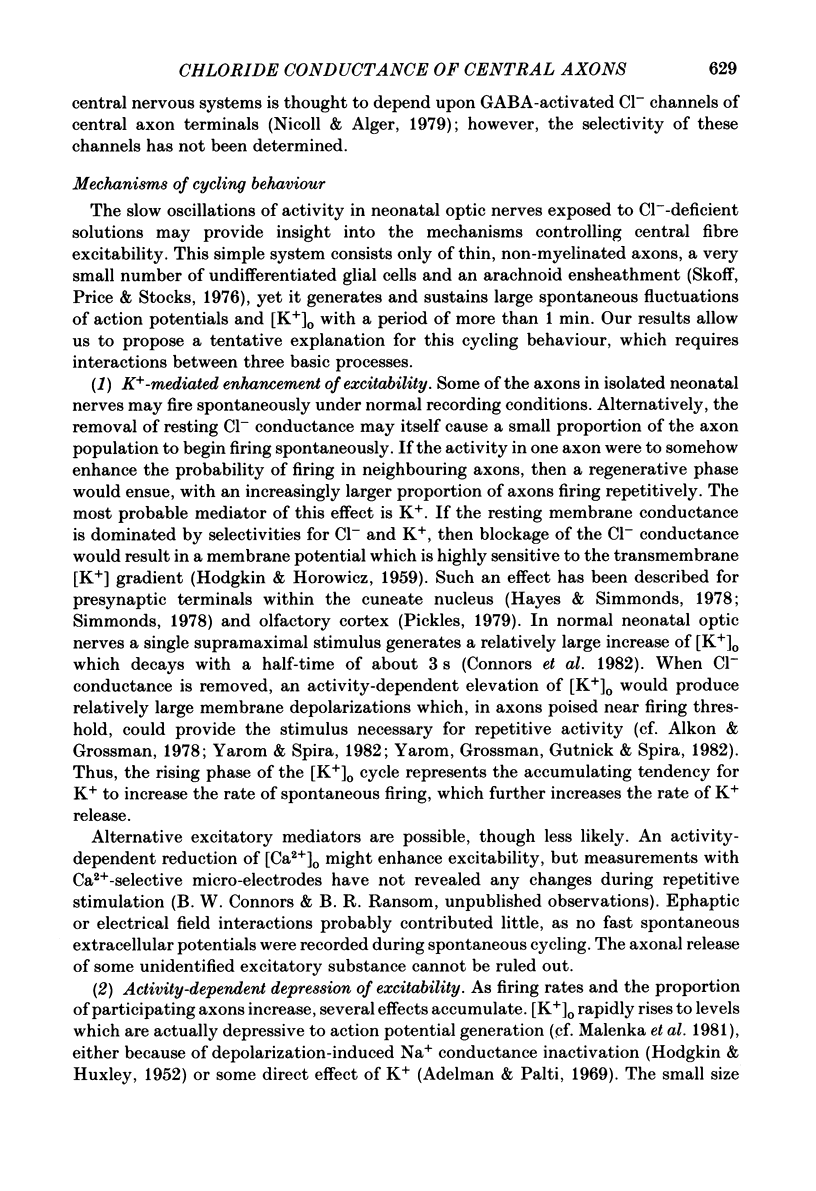
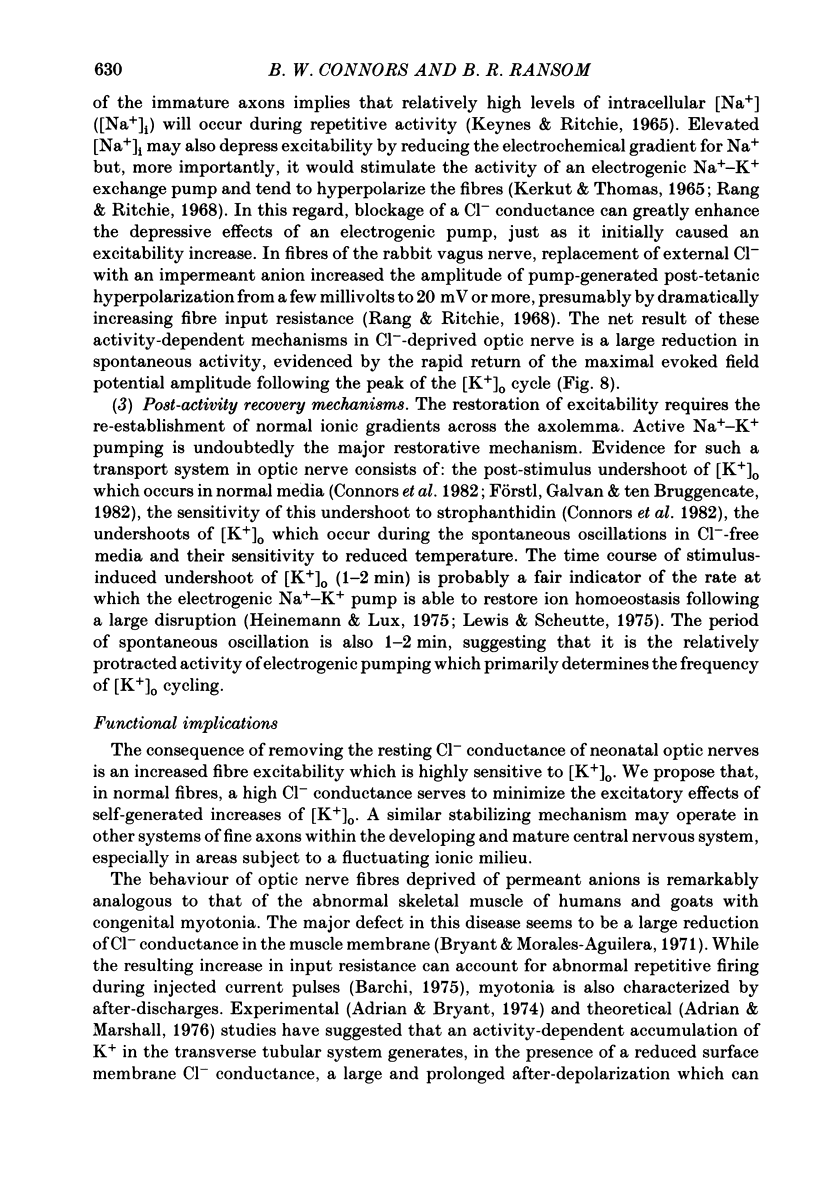
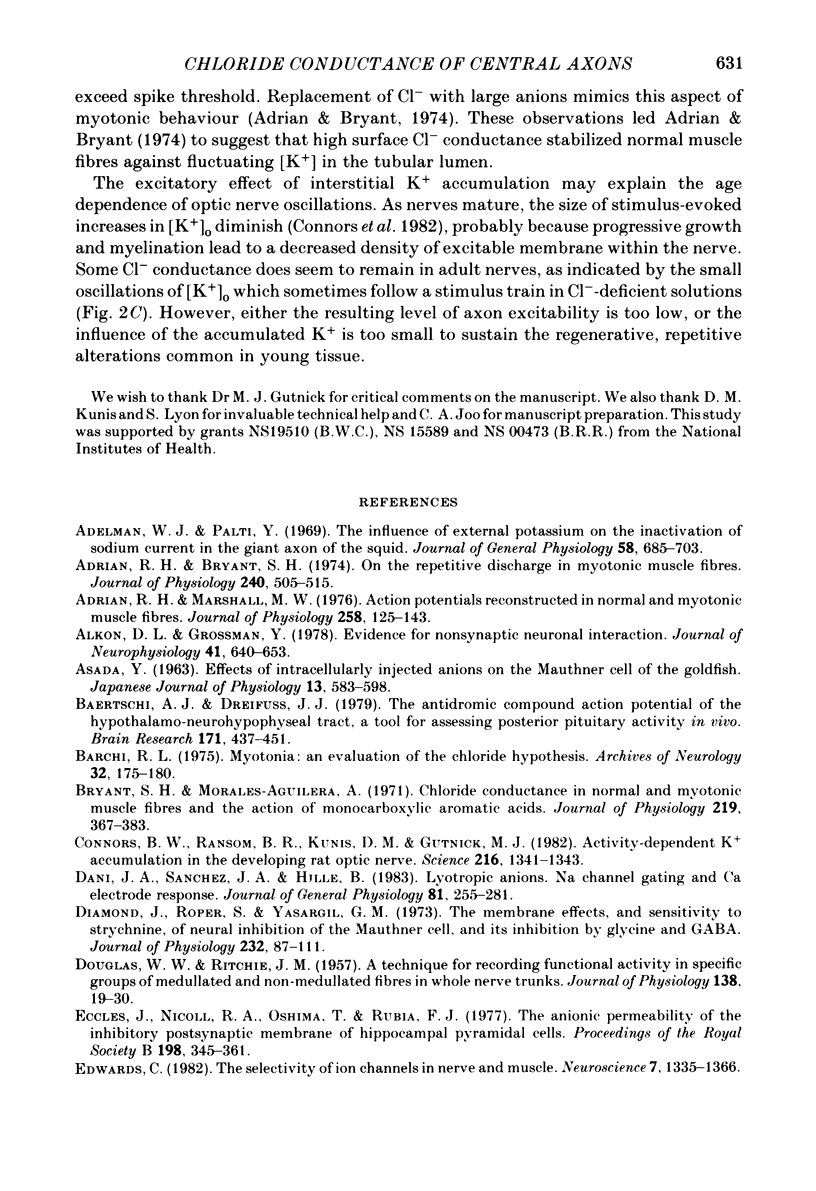
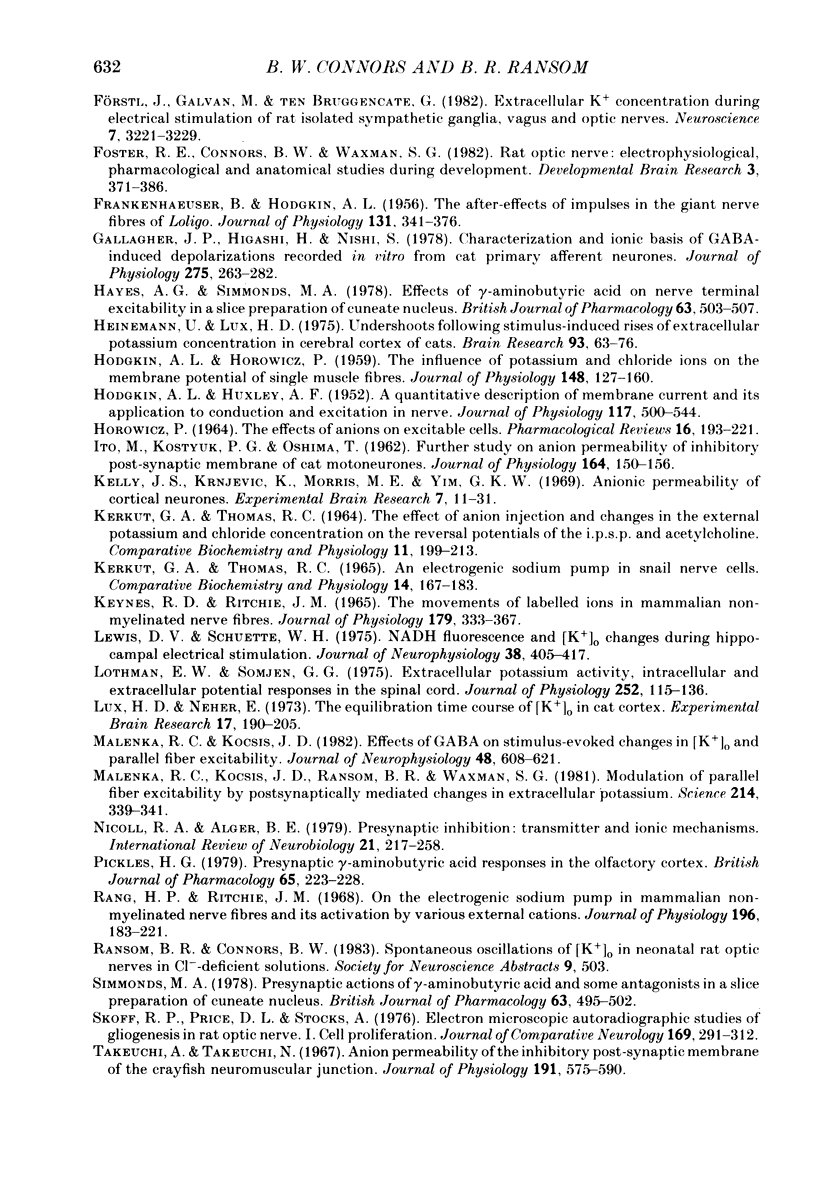
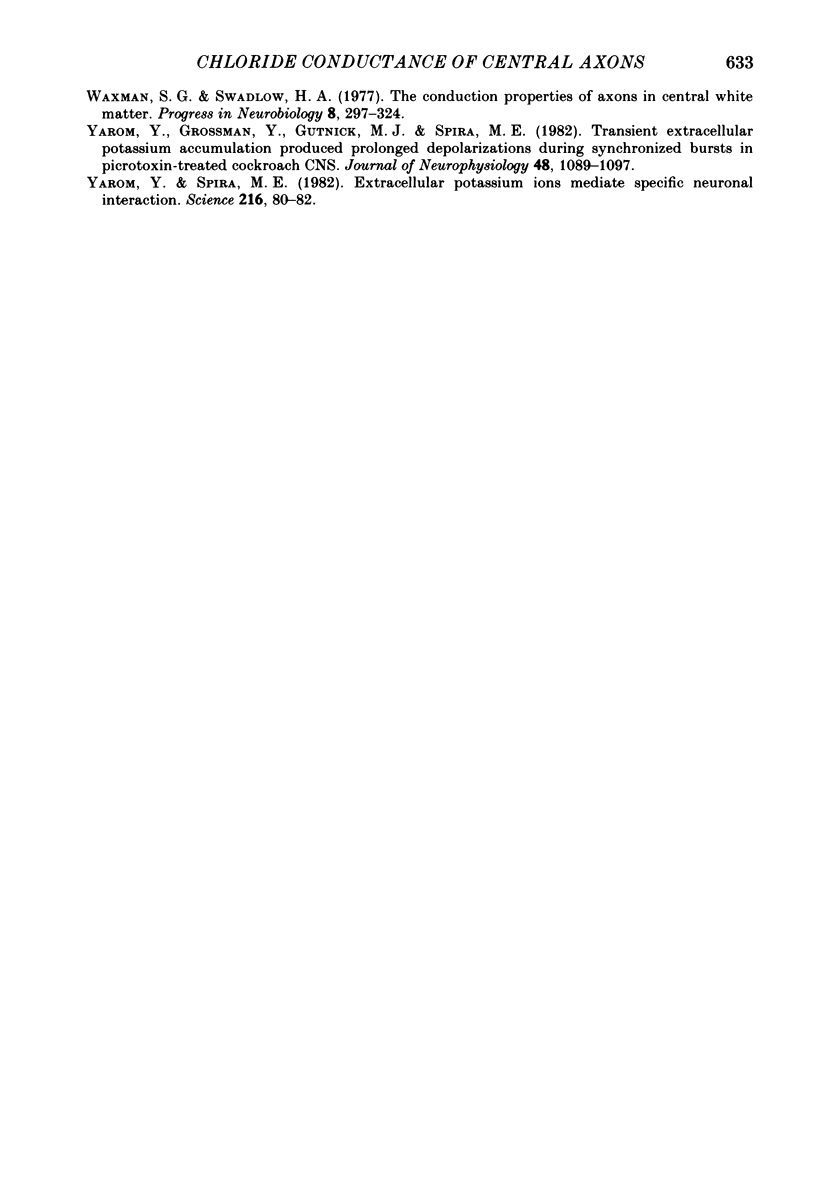
Selected References
These references are in PubMed. This may not be the complete list of references from this article.
- ASADA Y. EFFECTS OF INTRACELLULARLY INJECTED ANIONS ON THE MAUTHNER CELLS OF GOLDFISH. Jpn J Physiol. 1963 Dec 15;13:583–598. doi: 10.2170/jjphysiol.13.583. [DOI] [PubMed] [Google Scholar]
- Adelman W. J., Jr, Palti Y. The influence of external potassium on the inactivation of sodium currents in the giant axon of the squid, Loligo pealei. J Gen Physiol. 1969 Jun;53(6):685–703. doi: 10.1085/jgp.53.6.685. [DOI] [PMC free article] [PubMed] [Google Scholar]
- Adrian R. H., Bryant S. H. On the repetitive discharge in myotonic muscle fibres. J Physiol. 1974 Jul;240(2):505–515. doi: 10.1113/jphysiol.1974.sp010620. [DOI] [PMC free article] [PubMed] [Google Scholar]
- Adrian R. H., Marshall M. W. Action potentials reconstructed in normal and myotonic muscle fibres. J Physiol. 1976 Jun;258(1):125–143. doi: 10.1113/jphysiol.1976.sp011410. [DOI] [PMC free article] [PubMed] [Google Scholar]
- Alkon D. L., Grossman Y. Evidence for nonsynaptic neuronal interaction. J Neurophysiol. 1978 May;41(3):640–653. doi: 10.1152/jn.1978.41.3.640. [DOI] [PubMed] [Google Scholar]
- Baertschi A. J., Dreifuss J. J. The antidromic compound action potential of the hypothalamo-neurohypophysial tract, a tool for assessing posterior pituitary activity in vivo. Brain Res. 1979 Aug 10;171(3):437–451. doi: 10.1016/0006-8993(79)91048-5. [DOI] [PubMed] [Google Scholar]
- Barchi R. L. Myotonia. An evaluation of the chloride hypothesis. Arch Neurol. 1975 Mar;32(3):175–180. doi: 10.1001/archneur.1975.00490450055007. [DOI] [PubMed] [Google Scholar]
- Bryant S. H., Morales-Aguilera A. Chloride conductance in normal and myotonic muscle fibres and the action of monocarboxylic aromatic acids. J Physiol. 1971 Dec;219(2):367–383. doi: 10.1113/jphysiol.1971.sp009667. [DOI] [PMC free article] [PubMed] [Google Scholar]
- Connors B. W., Ransom B. R., Kunis D. M., Gutnick M. J. Activity-dependent K+ accumulation in the developing rat optic nerve. Science. 1982 Jun 18;216(4552):1341–1343. doi: 10.1126/science.7079771. [DOI] [PubMed] [Google Scholar]
- DOUGLAS W. W., RITCHIE J. M. A technique for recording functional activity in specific groups of medullated and non-medullated fibres in whole nerve trunks. J Physiol. 1957 Aug 29;138(1):19–30. doi: 10.1113/jphysiol.1957.sp005835. [DOI] [PMC free article] [PubMed] [Google Scholar]
- Dani J. A., Sanchez J. A., Hille B. Lyotropic anions. Na channel gating and Ca electrode response. J Gen Physiol. 1983 Feb;81(2):255–281. doi: 10.1085/jgp.81.2.255. [DOI] [PMC free article] [PubMed] [Google Scholar]
- Diamond J., Roper S., Yasargil G. M. The membrane effects, and sensitivity to strychnine, of neural inhibition of the Mauthner cell, and its inhibition by glycine and GABA. J Physiol. 1973 Jul;232(1):87–111. doi: 10.1113/jphysiol.1973.sp010258. [DOI] [PMC free article] [PubMed] [Google Scholar]
- Eccles J., Nicoll R. A., Oshima T., Rubia F. J. The anionic permeability of the inhibitory postsynaptic membrane of hippocampal pyramidal cells. Proc R Soc Lond B Biol Sci. 1977 Sep 19;198(1133):345–361. doi: 10.1098/rspb.1977.0102. [DOI] [PubMed] [Google Scholar]
- Edwards C. The selectivity of ion channels in nerve and muscle. Neuroscience. 1982 Jun;7(6):1335–1366. doi: 10.1016/0306-4522(82)90249-4. [DOI] [PubMed] [Google Scholar]
- FRANKENHAEUSER B., HODGKIN A. L. The after-effects of impulses in the giant nerve fibres of Loligo. J Physiol. 1956 Feb 28;131(2):341–376. doi: 10.1113/jphysiol.1956.sp005467. [DOI] [PMC free article] [PubMed] [Google Scholar]
- Foster R. E., Connors B. W., Waxman S. G. Rat optic nerve: electrophysiological, pharmacological and anatomical studies during development. Brain Res. 1982 Mar;255(3):371–386. doi: 10.1016/0165-3806(82)90005-0. [DOI] [PubMed] [Google Scholar]
- Förstl J., Galvan M., ten Bruggencate G. Extracellular K+ concentration during electrical stimulation of rat isolated sympathetic ganglia, vagus and optic nerves. Neuroscience. 1982;7(12):3221–3229. doi: 10.1016/0306-4522(82)90244-5. [DOI] [PubMed] [Google Scholar]
- Gallagher J. P., Higashi H., Nishi S. Characterization and ionic basis of GABA-induced depolarizations recorded in vitro from cat primary afferent neurones. J Physiol. 1978 Feb;275:263–282. doi: 10.1113/jphysiol.1978.sp012189. [DOI] [PMC free article] [PubMed] [Google Scholar]
- HODGKIN A. L., HOROWICZ P. The influence of potassium and chloride ions on the membrane potential of single muscle fibres. J Physiol. 1959 Oct;148:127–160. doi: 10.1113/jphysiol.1959.sp006278. [DOI] [PMC free article] [PubMed] [Google Scholar]
- HODGKIN A. L., HUXLEY A. F. A quantitative description of membrane current and its application to conduction and excitation in nerve. J Physiol. 1952 Aug;117(4):500–544. doi: 10.1113/jphysiol.1952.sp004764. [DOI] [PMC free article] [PubMed] [Google Scholar]
- HOROWICZ P. THE EFFECTS OF ANIONS ON EXCITABLE CELLS. Pharmacol Rev. 1964 Jun;16:193–221. [PubMed] [Google Scholar]
- Hayes A. G., Simmonds M. A. Effects of gamma-aminobutyric acid on nerve terminal excitability in a slice preparation of cuneate nucleus. Br J Pharmacol. 1978 Jul;63(3):503–507. doi: 10.1111/j.1476-5381.1978.tb07804.x. [DOI] [PMC free article] [PubMed] [Google Scholar]
- Heinemann U., Lux H. D. Undershoots following stimulus-induced rises of extracellular potassium concentration in cerebral cortex of cat. Brain Res. 1975 Jul 25;93(1):63–76. doi: 10.1016/0006-8993(75)90286-3. [DOI] [PubMed] [Google Scholar]
- ITO M., KOSTYUK P. G., OSHIMA T. Further study on anion permeability of inhibitory post-synaptic membrane of cat motoneurones. J Physiol. 1962 Oct;164:150–156. doi: 10.1113/jphysiol.1962.sp007009. [DOI] [PMC free article] [PubMed] [Google Scholar]
- KERKUT G. A., THOMAS R. C. AN ELECTROGENIC SODIUM PUMP IN SNAIL NERVE CELLS. Comp Biochem Physiol. 1965 Jan;14:167–183. doi: 10.1016/0010-406x(65)90017-4. [DOI] [PubMed] [Google Scholar]
- KERKUT G. A., THOMAS R. C. THE EFFECT OF ANION INJECTION AND CHANGES IN THE EXTERNAL POTASSIUM AND CHLORIDE CONCENTRATION ON THE REVERSAL POTENTIALS OF THE IPSP AND ACETYLCHOLINE. Comp Biochem Physiol. 1964 Feb;11:199–213. doi: 10.1016/0010-406x(64)90163-x. [DOI] [PubMed] [Google Scholar]
- Kelly J. S., Krnjević K., Morris M. E., Yim G. K. Anionic permeability of cortical neurones. Exp Brain Res. 1969;7(1):11–31. doi: 10.1007/BF00236105. [DOI] [PubMed] [Google Scholar]
- Keynes R. D., Ritchie J. M. The movements of labelled ions in mammalian non-myelinated nerve fibres. J Physiol. 1965 Jul;179(2):333–367. doi: 10.1113/jphysiol.1965.sp007666. [DOI] [PMC free article] [PubMed] [Google Scholar]
- Lewis D. V., Schuette W. H. NADH fluorescence and [K+]o changes during hippocampal electrical stimulation. J Neurophysiol. 1975 Mar;38(2):405–417. doi: 10.1152/jn.1975.38.2.405. [DOI] [PubMed] [Google Scholar]
- Lothman E. W., Somjen G. G. Extracellular potassium activity, intracellular and extracellular potential responses in the spinal cord. J Physiol. 1975 Oct;252(1):115–136. doi: 10.1113/jphysiol.1975.sp011137. [DOI] [PMC free article] [PubMed] [Google Scholar]
- Lux H. D., Neher E. The equilibration time course of (K + ) 0 in cat cortex. Exp Brain Res. 1973 Apr 30;17(2):190–205. doi: 10.1007/BF00235028. [DOI] [PubMed] [Google Scholar]
- Malenka R. C., Kocsis J. D. Effects of GABA on stimulus-evoked changes in [K+]o and parallel fiber excitability. J Neurophysiol. 1982 Sep;48(3):608–621. doi: 10.1152/jn.1982.48.3.608. [DOI] [PubMed] [Google Scholar]
- Malenka R. C., Kocsis J. D., Ransom B. R., Waxman S. G. Modulation of parallel fiber excitability by postsynaptically mediated changes in extracellular potassium. Science. 1981 Oct 16;214(4518):339–341. doi: 10.1126/science.7280695. [DOI] [PubMed] [Google Scholar]
- Nicoll R. A., Alger B. E. Presynaptic inhibition: transmitter and ionic mechanisms. Int Rev Neurobiol. 1979;21:217–258. doi: 10.1016/s0074-7742(08)60639-x. [DOI] [PubMed] [Google Scholar]
- Pickles H. G. Presynaptic gamma-aminobutyric acid responses in the olfactory cortex. Br J Pharmacol. 1979 Feb;65(2):223–228. doi: 10.1111/j.1476-5381.1979.tb07822.x. [DOI] [PMC free article] [PubMed] [Google Scholar]
- Rang H. P., Ritchie J. M. On the electrogenic sodium pump in mammalian non-myelinated nerve fibres and its activation by various external cations. J Physiol. 1968 May;196(1):183–221. doi: 10.1113/jphysiol.1968.sp008502. [DOI] [PMC free article] [PubMed] [Google Scholar]
- Simmonds M. A. Presynaptic actions of gamma-aminobutyric acid and some antagonists in a slice preparation of cuneate nucleus. Br J Pharmacol. 1978 Jul;63(3):495–502. doi: 10.1111/j.1476-5381.1978.tb07803.x. [DOI] [PMC free article] [PubMed] [Google Scholar]
- Skoff R. P., Price D. L., Stocks A. Electron microscopic autoradiographic studies of gliogenesis in rat optic nerve. I. Cell proliferation. J Comp Neurol. 1976 Oct 1;169(3):291–312. doi: 10.1002/cne.901690303. [DOI] [PubMed] [Google Scholar]
- Takeuchi A., Takeuchi N. Anion permeability of the inhibitory post-synaptic membrane of the crayfish neuromuscular junction. J Physiol. 1967 Aug;191(3):575–590. doi: 10.1113/jphysiol.1967.sp008269. [DOI] [PMC free article] [PubMed] [Google Scholar]
- Waxman S. G., Swadlow H. A. The conduction properties of axons in central white matter. Prog Neurobiol. 1977;8(4):297–324. doi: 10.1016/0301-0082(77)90009-0. [DOI] [PubMed] [Google Scholar]
- Yarom Y., Grossman Y., Gutnick M. J., Spira M. E. Transient extracellular potassium accumulation produced prolonged depolarizations during synchronized bursts in picrotoxin-treated cockroach CNS. J Neurophysiol. 1982 Nov;48(5):1089–1097. doi: 10.1152/jn.1982.48.5.1089. [DOI] [PubMed] [Google Scholar]
- Yarom Y., Spira M. E. Extracellular potassium ions mediate specific neuronal interaction. Science. 1982 Apr 2;216(4541):80–82. doi: 10.1126/science.6278595. [DOI] [PubMed] [Google Scholar]