Abstract
A method for mounting and rapidly perfusing small ventricular trabeculae (diameter around 250 micron) from either ferret or guinea-pig is described. Tension, membrane potential (Em) and intracellular Na activity (aiNa) were measured. aiNa was measured using Na-sensitive micro-electrodes. At room temperature (22-26 degrees C), [Na]o 155 mmol/l and [Ca]o 5.4 mmol/l, aiNa was 10.9 mmol/l +/- S.D. 4.2 mmol/l (n = 148). When [Na]o was reduced from 155 to 1.5 mmol/l contractures developed. These were about twitch height in guinea-pig but less than the twitch height in ferret. Associated with the development of the contracture there was a decrease in aiNa. The aiNa halved within 30 s. The decrease in aiNa was not influenced by changing pHo from 7.4 to 9.5, K-free solution or strophanthidin 50 mumol/l and was not passive since, even when the [Na]o was 1.5 mmol/l, the driving force for Na ions remained inward. The aiNa decreased if [Ca]o was increased and [Na]o decreased or vice versa. On the basis of these findings it is concluded that the decrease in aiNa is mainly due to Na/Ca exchange. Despite the large decrease in aiNa the [Ca]i, as monitored by tension changes, hardly increased. Since Ca uptake does occur in Na-free conditions in heart muscle it is proposed that the mitochondria take up Ca ions and so prevent an excessive rise in cytoplasmic Ca. Strophanthidin increased both aiNa and the withdrawal contracture, but collected results from a number of experiments showed no clear correlation between the initial aiNa and contracture amplitude. Strophanthidin may, therefore, have actions additional to increasing aiNa.
Full text
PDF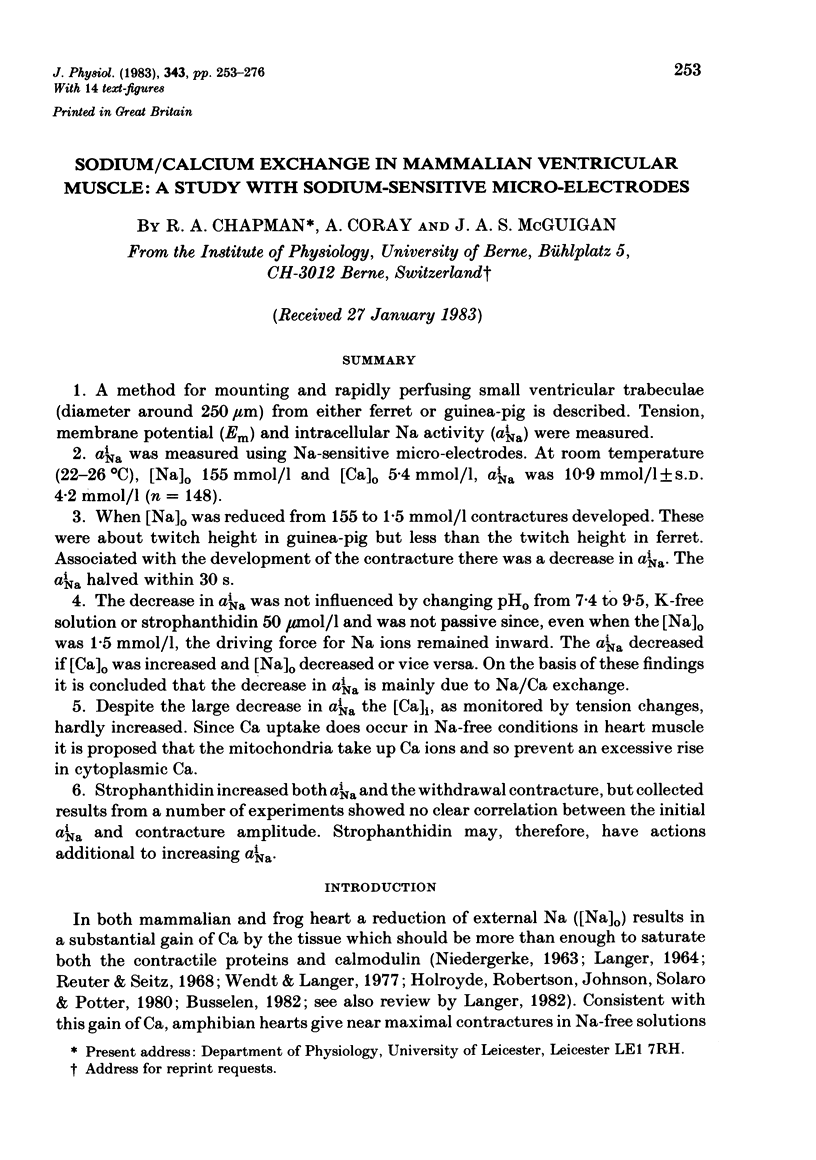
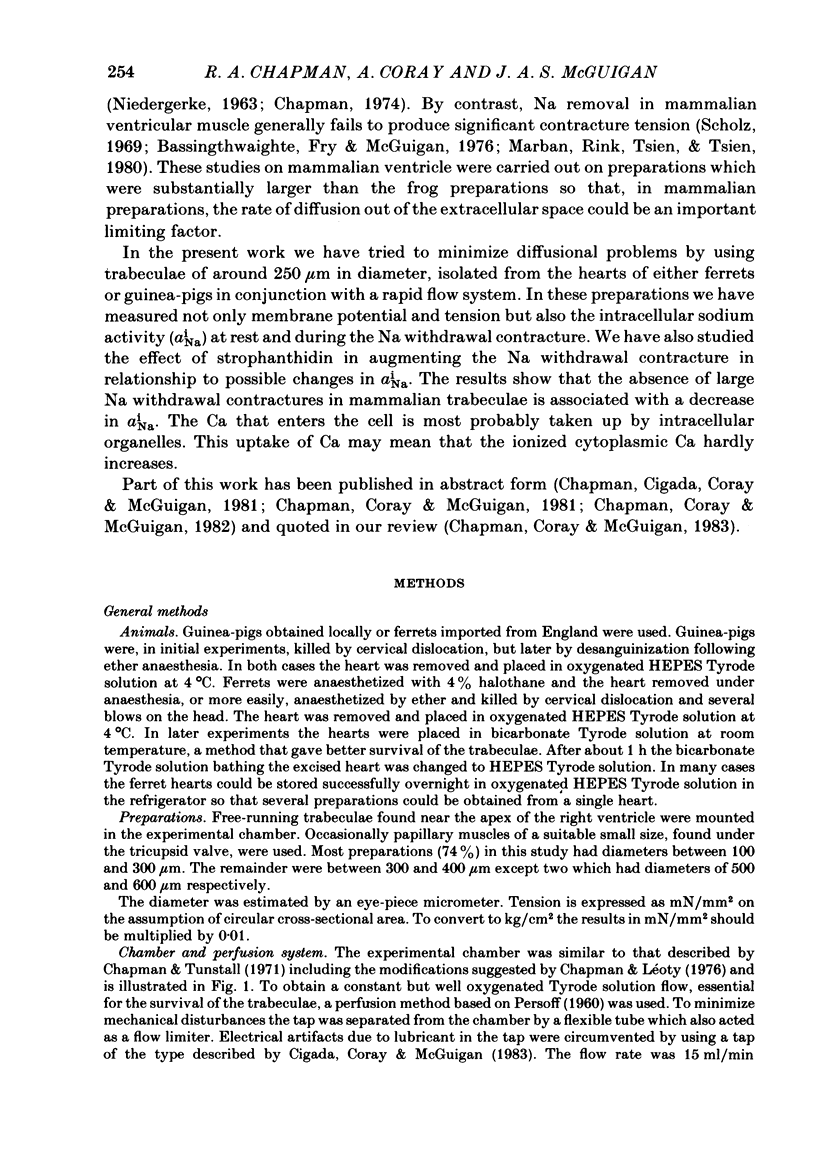
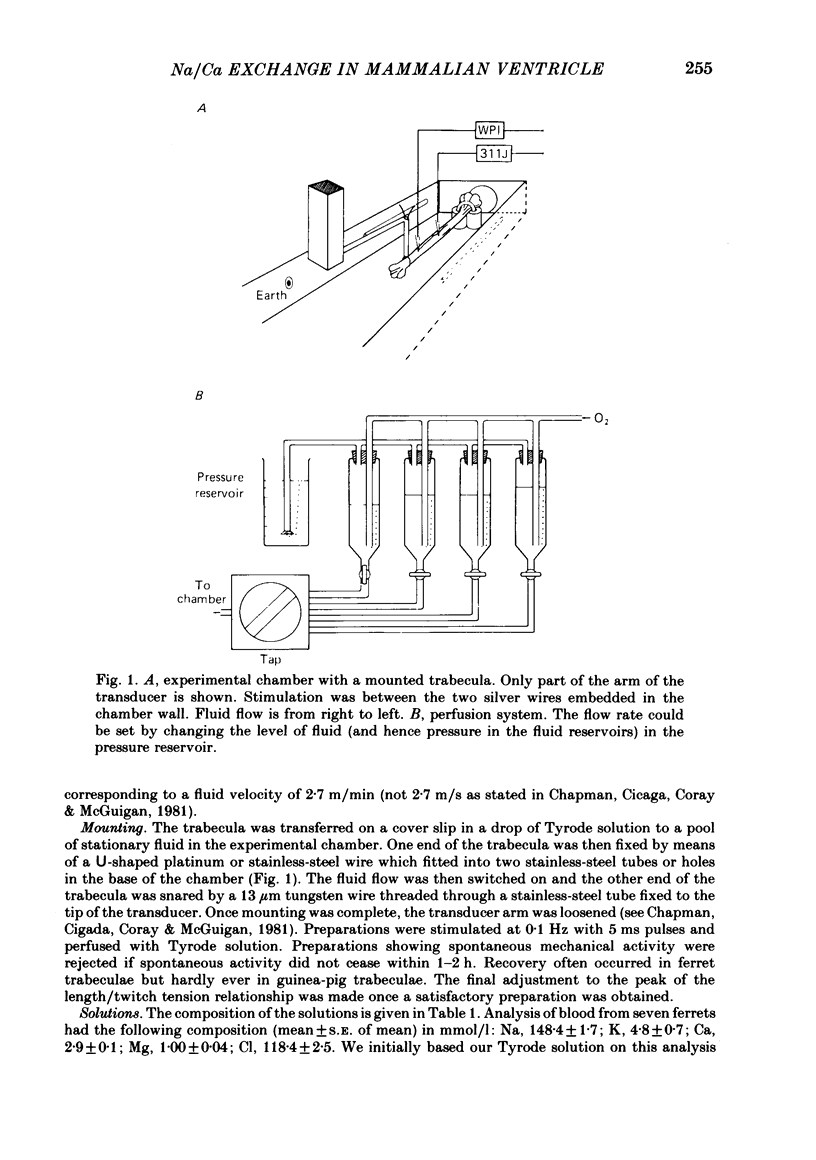
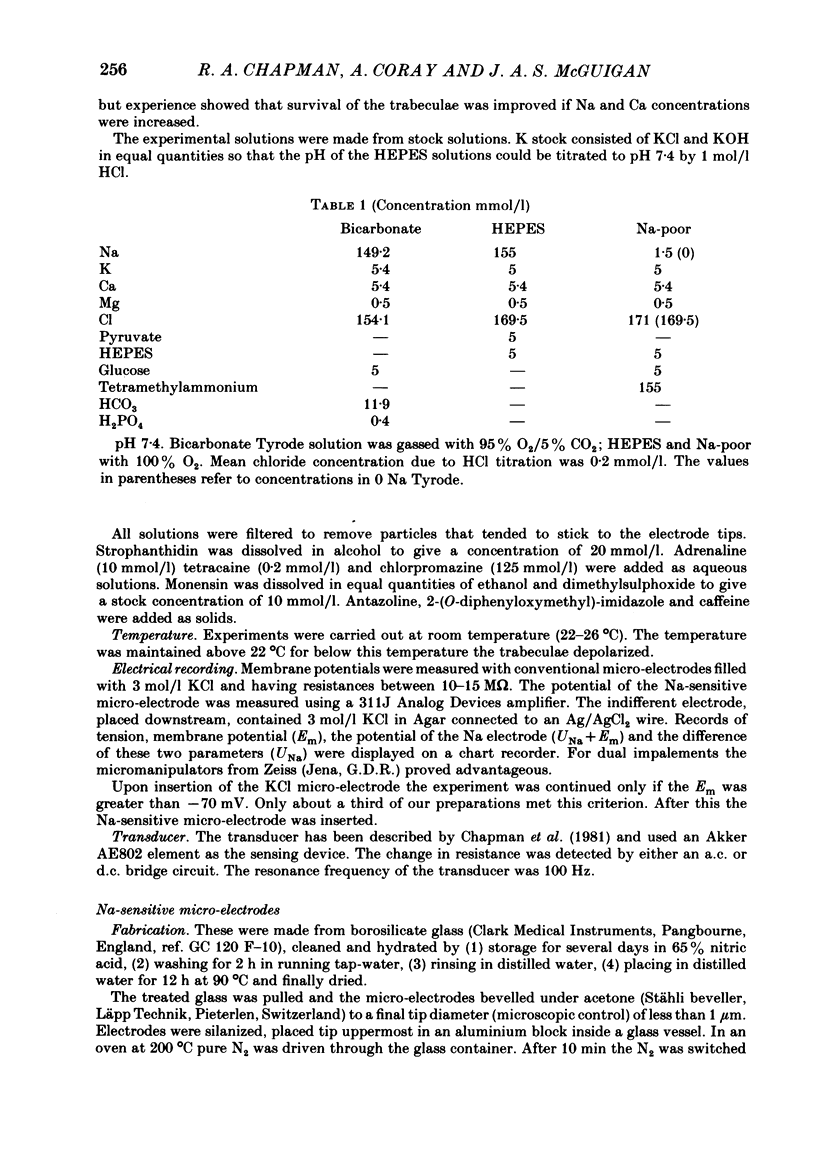
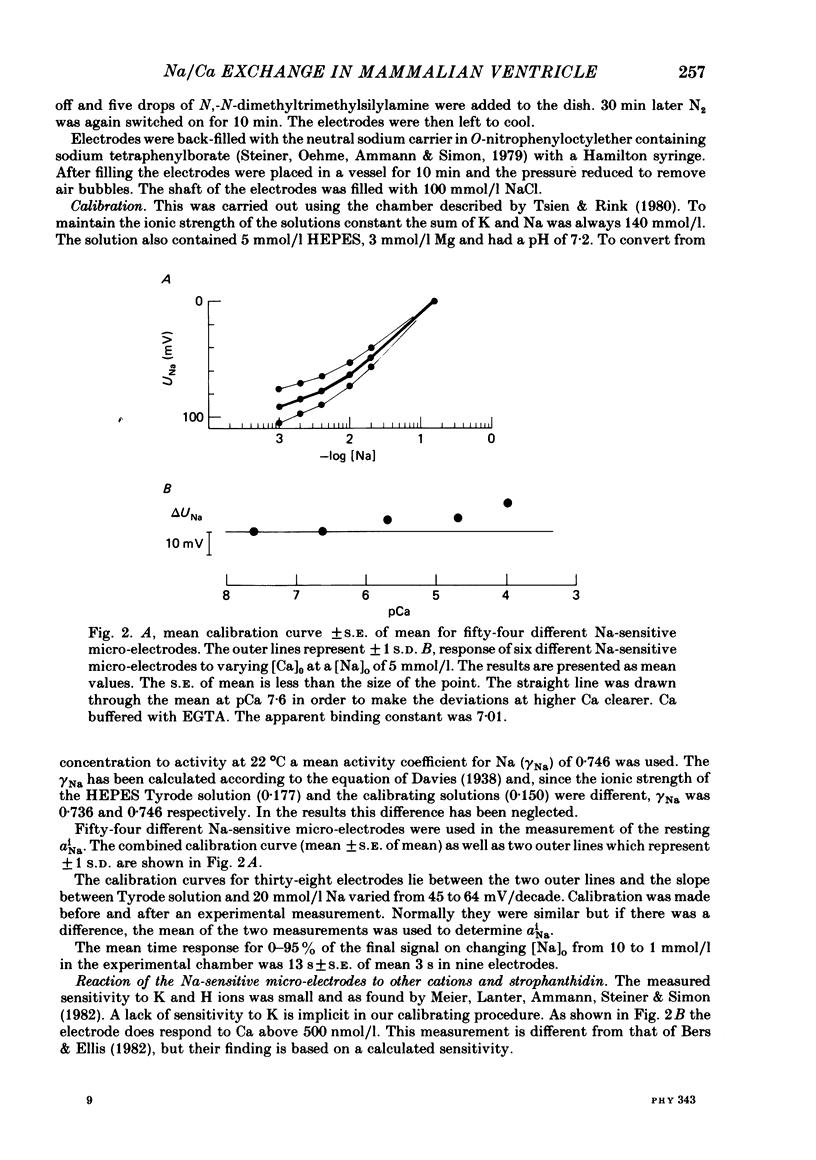
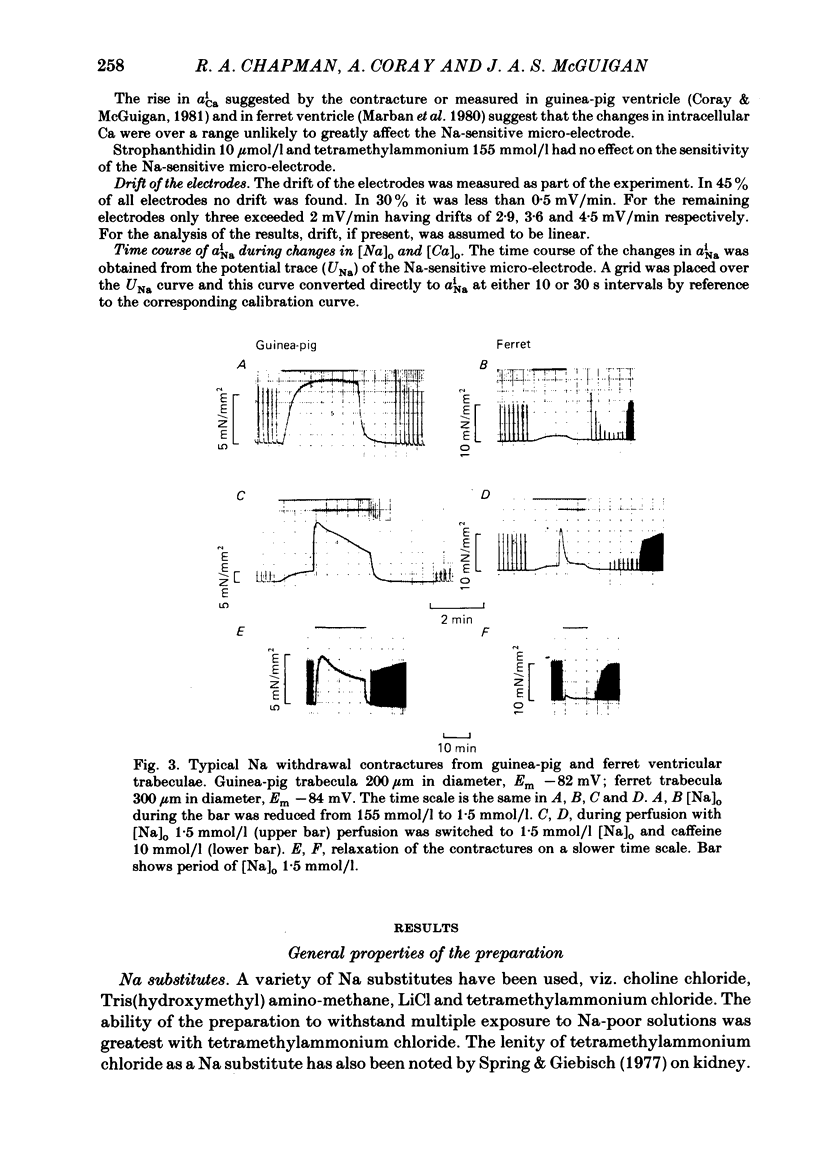
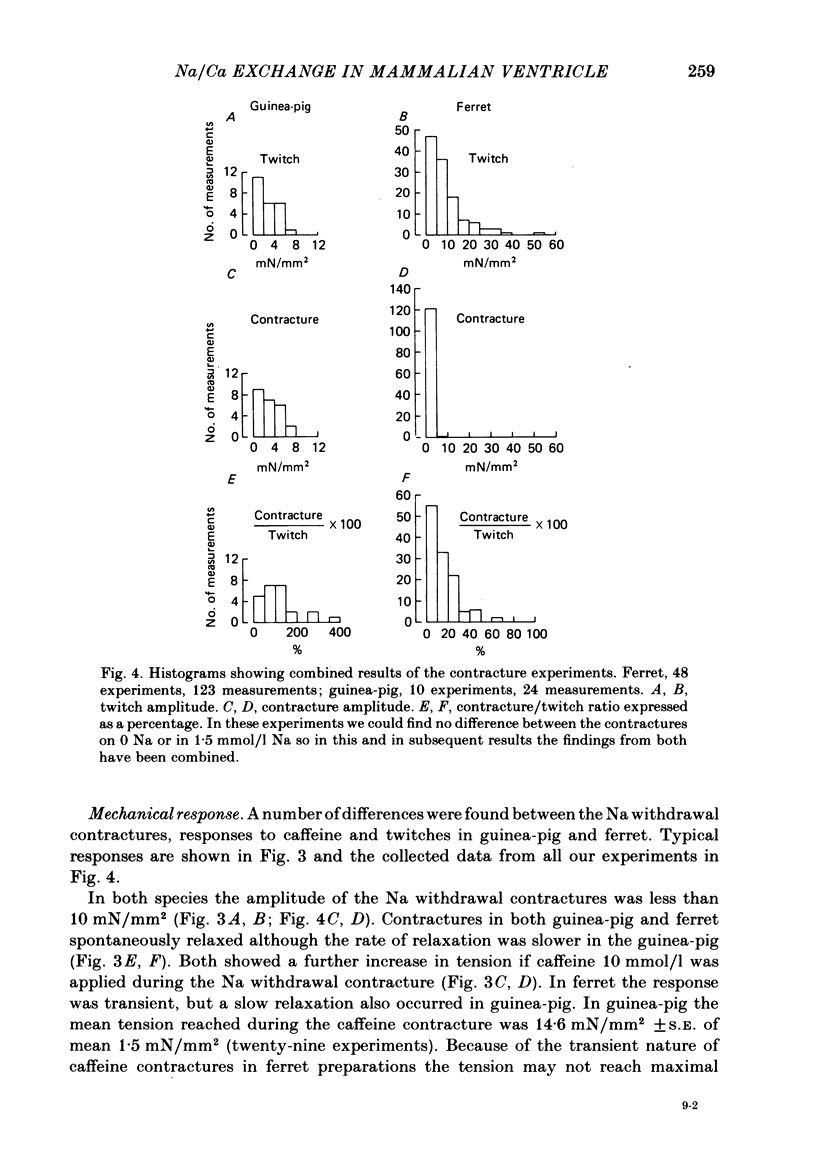
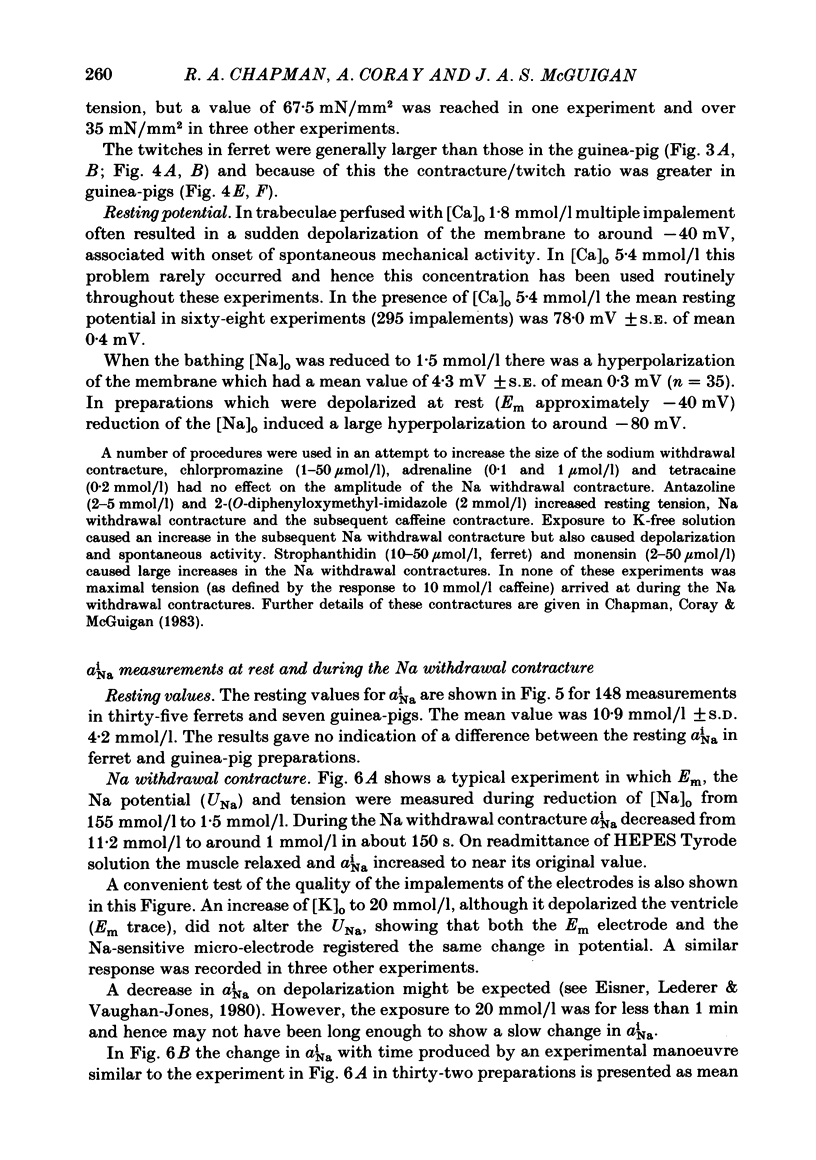
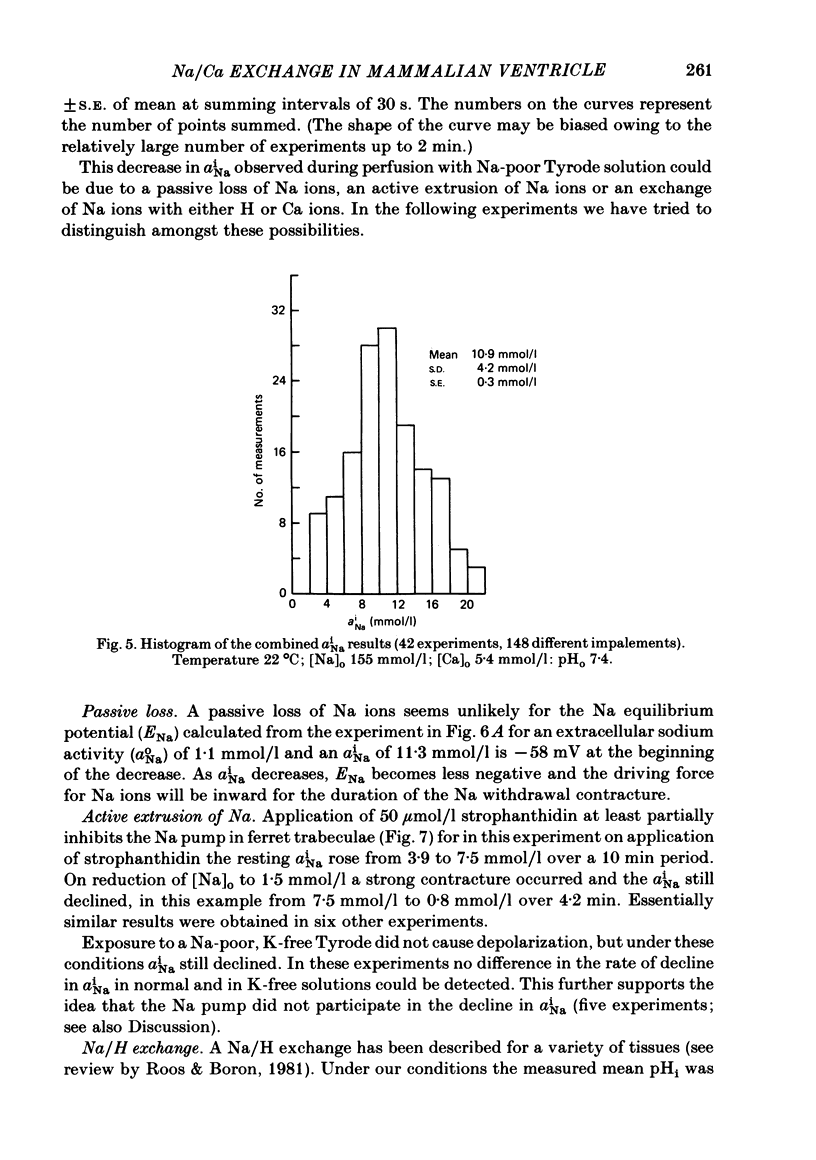
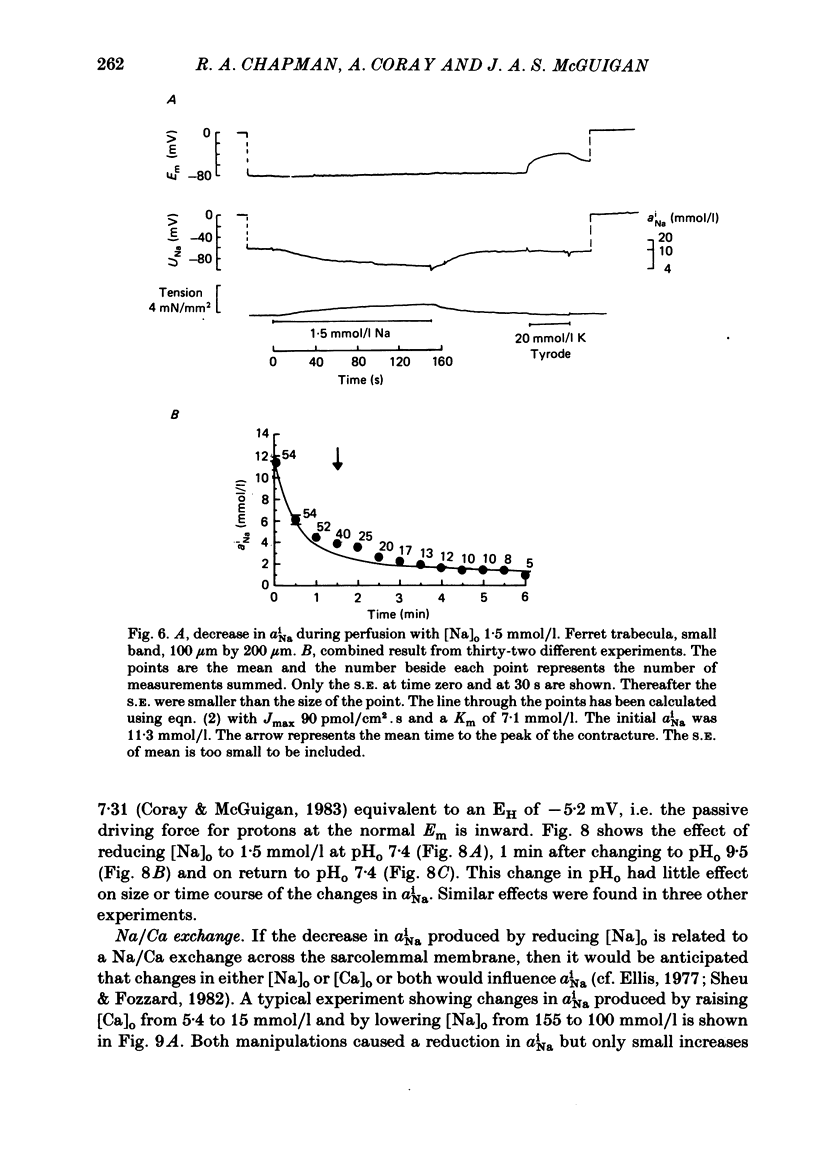
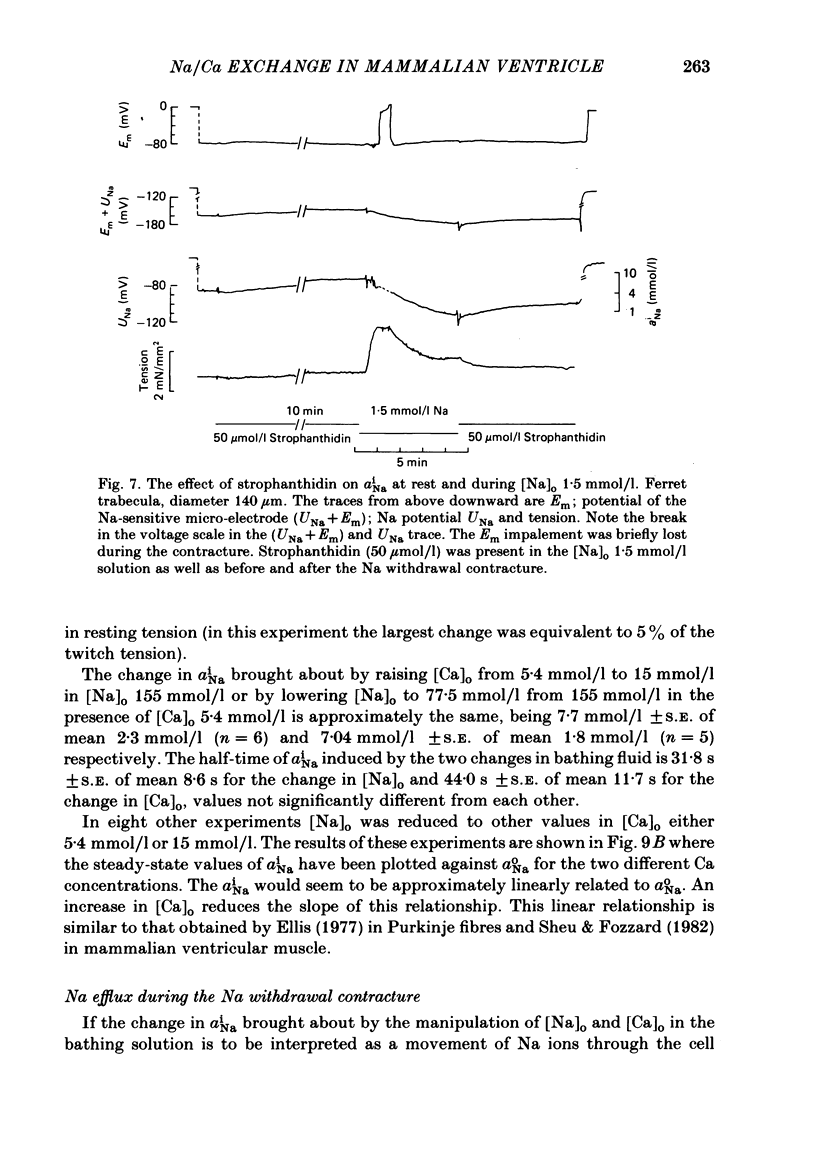
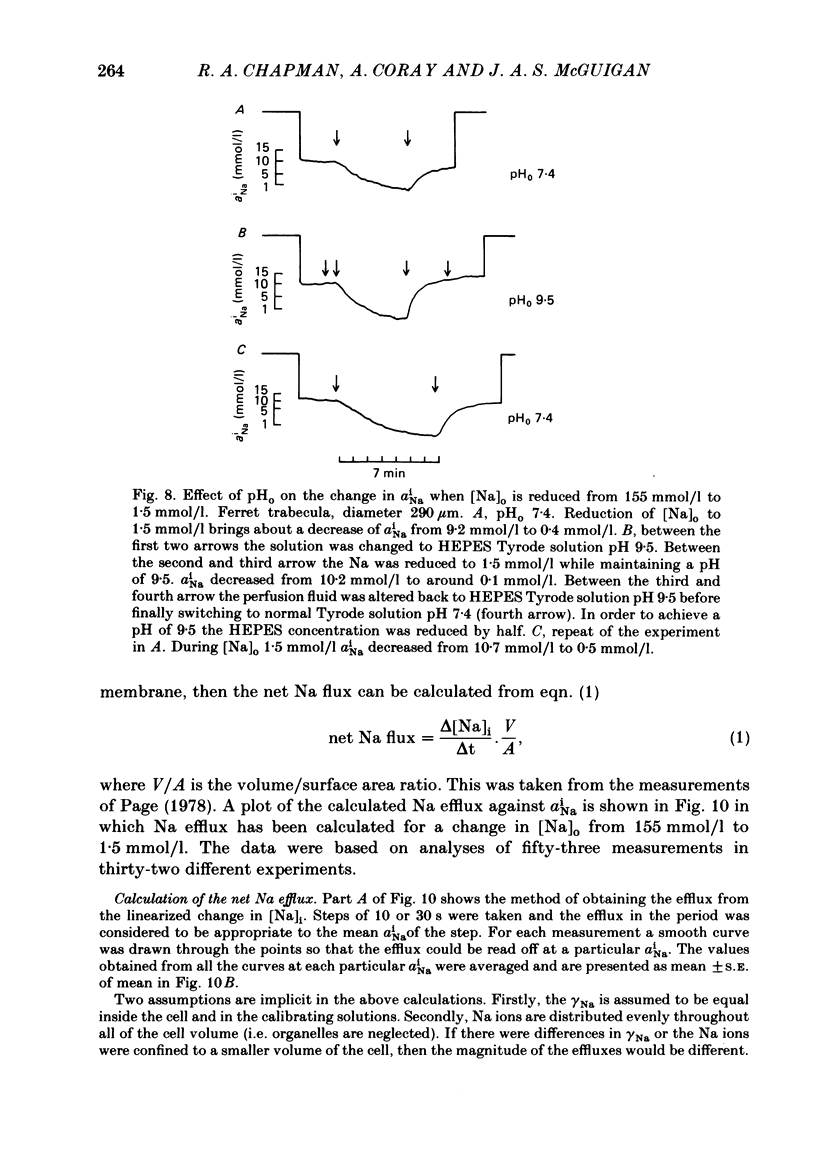
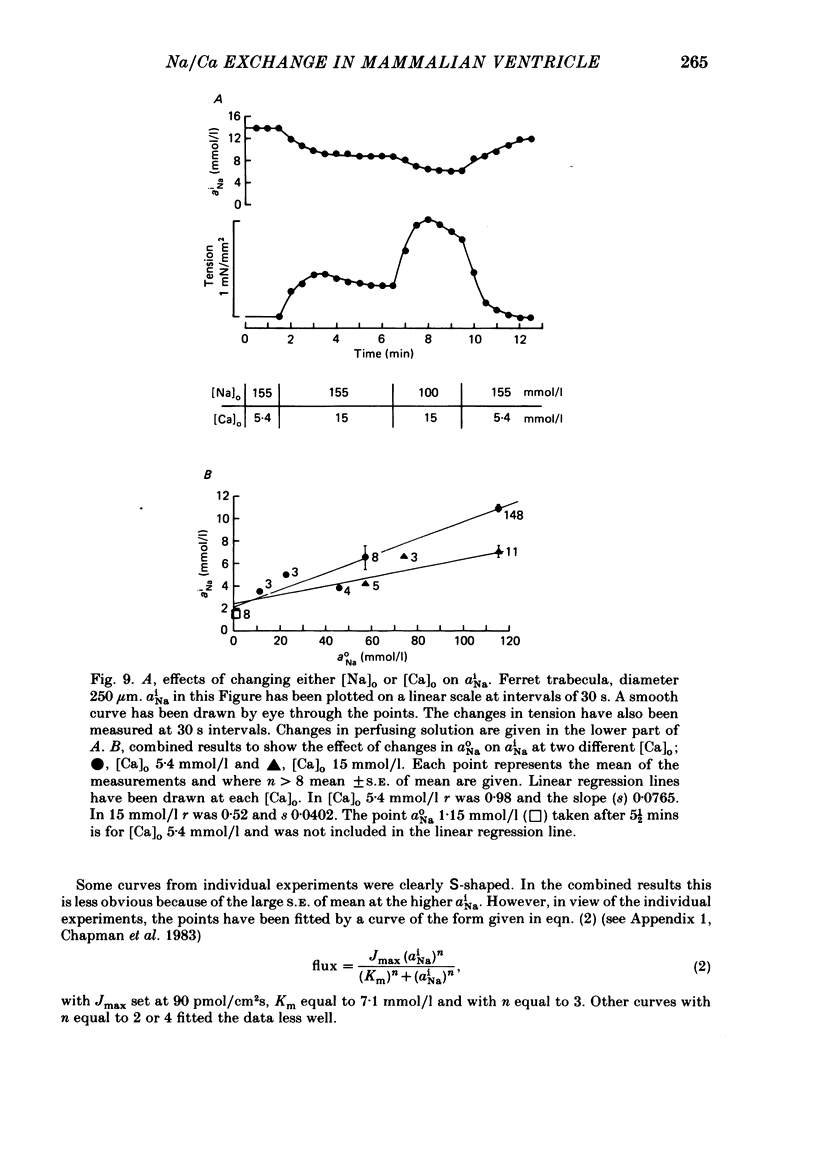
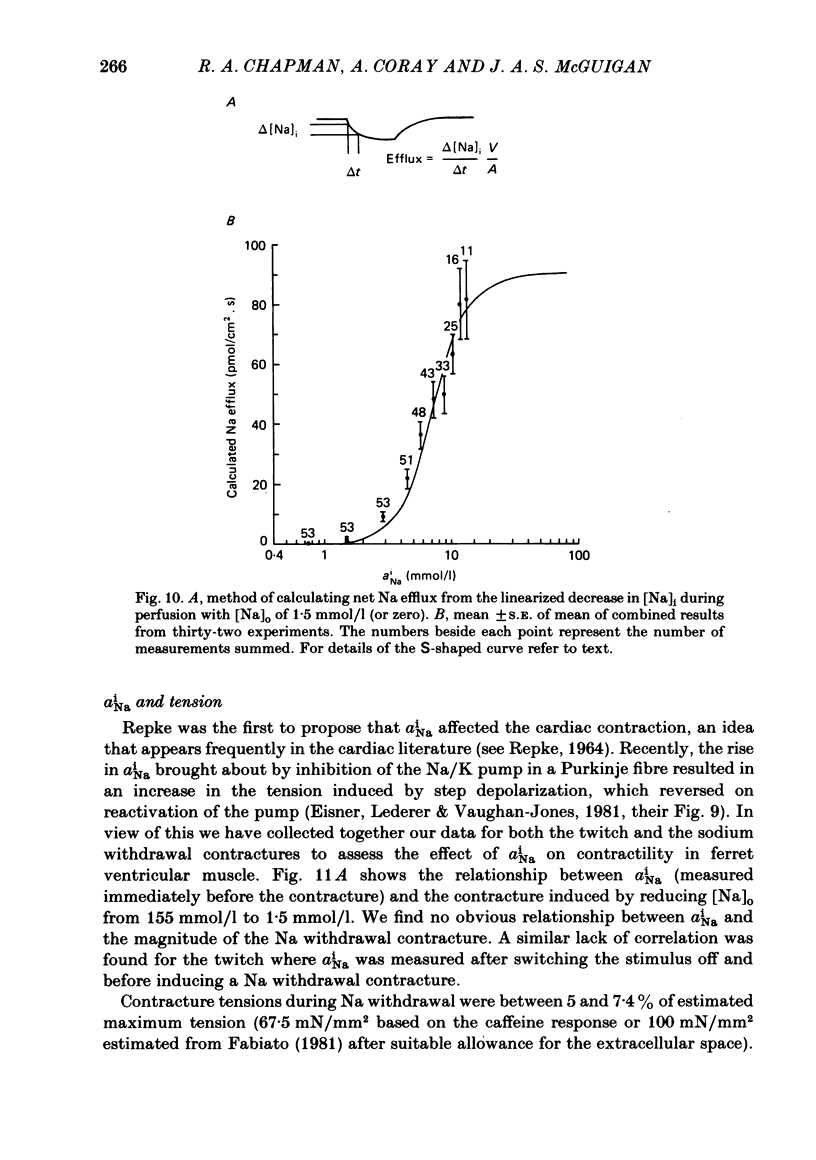
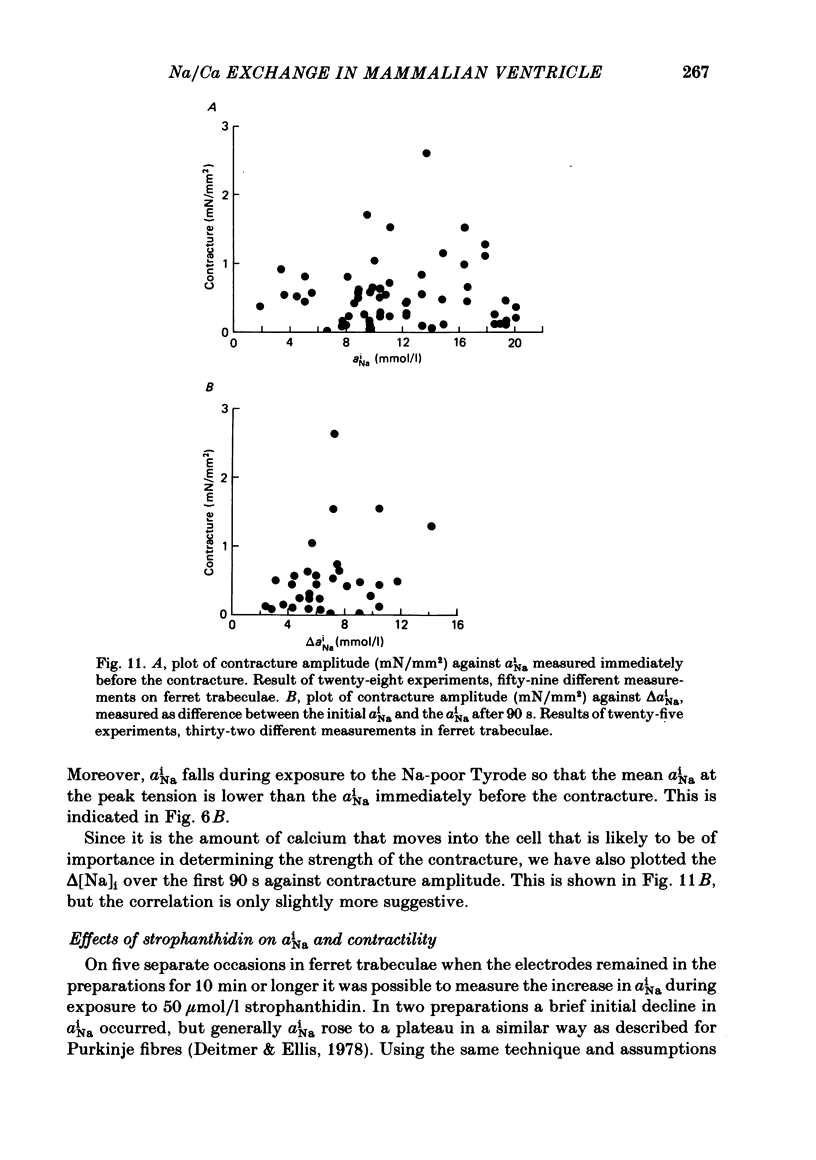
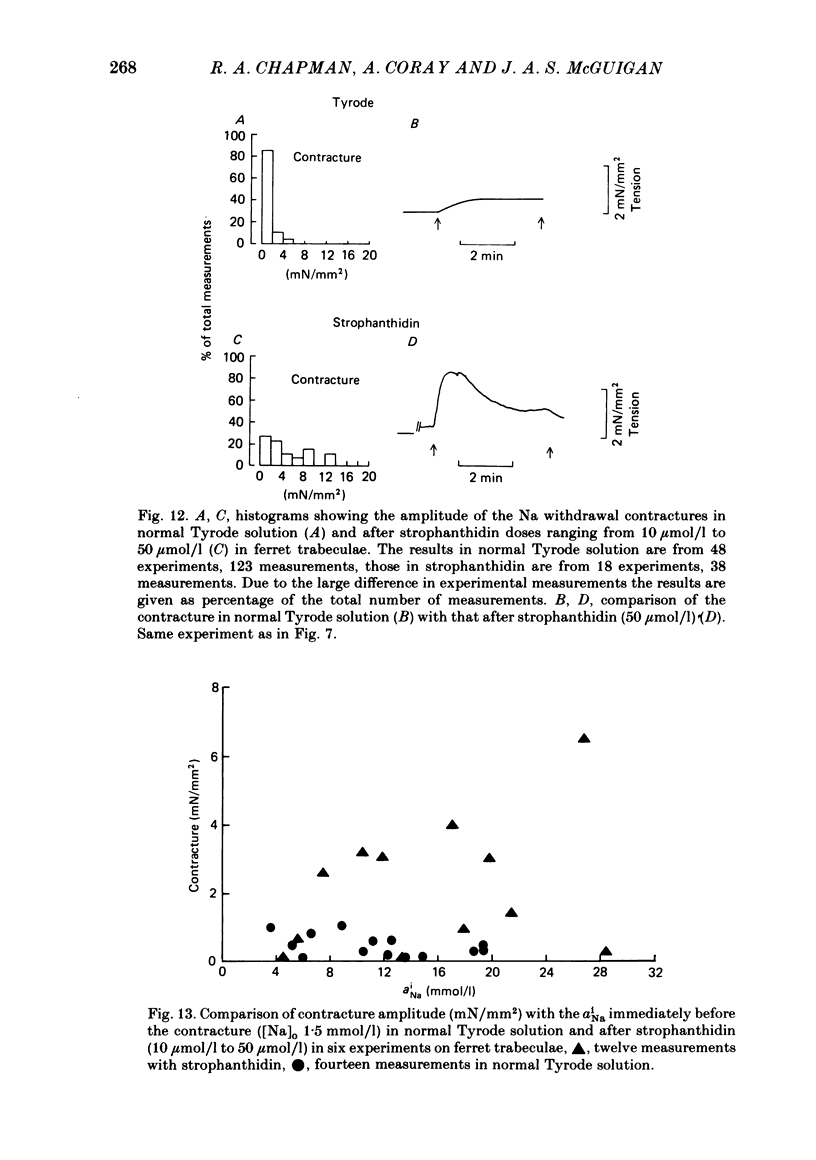
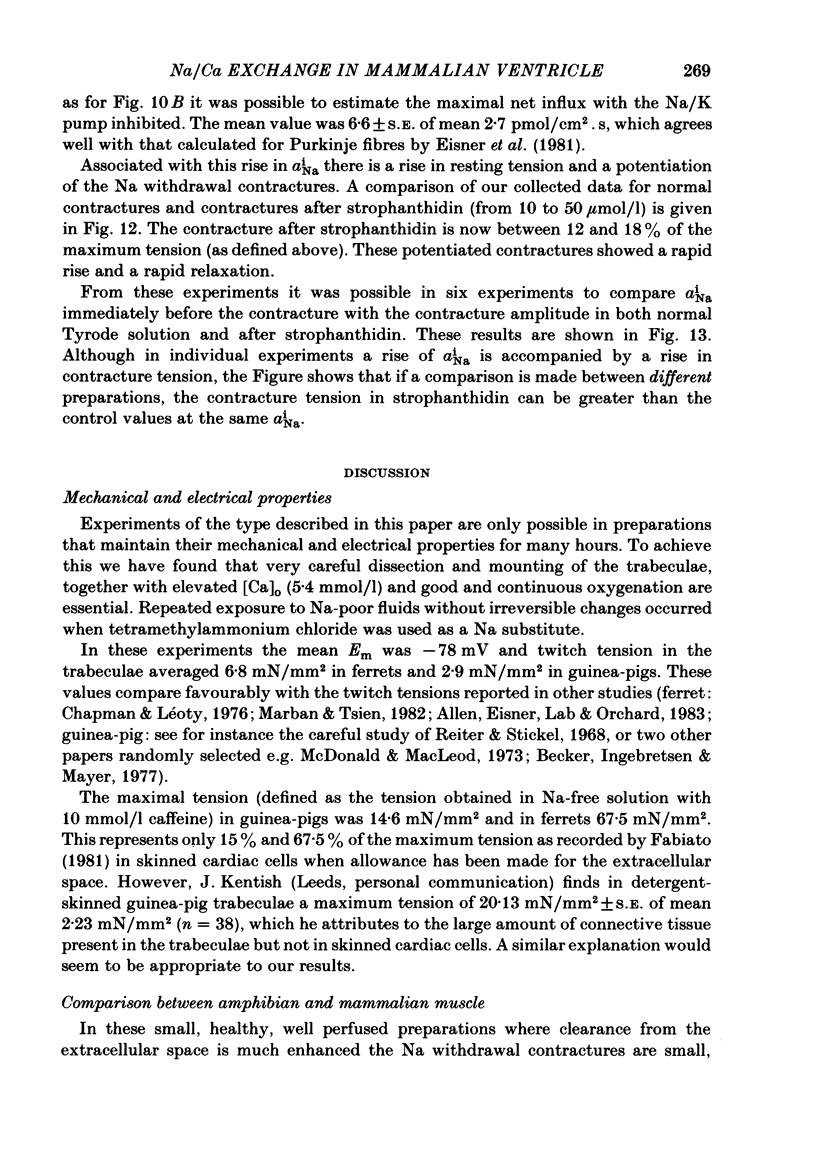
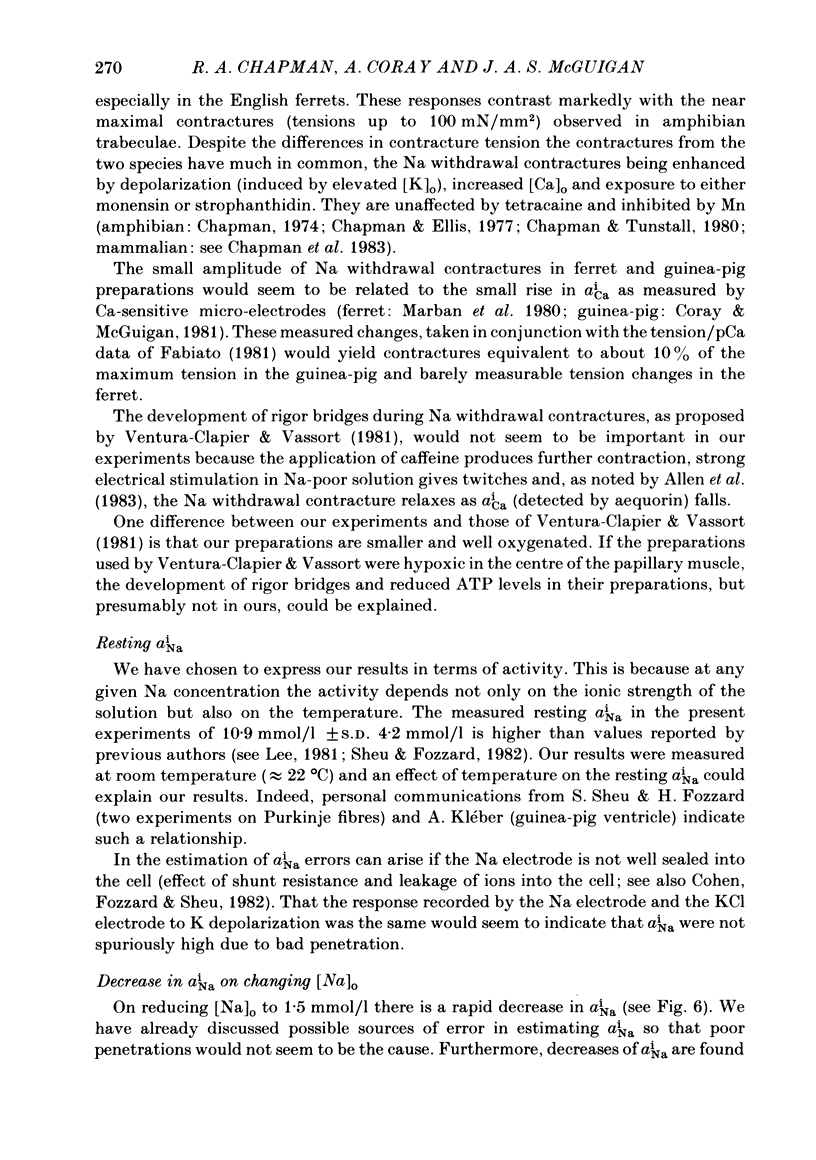
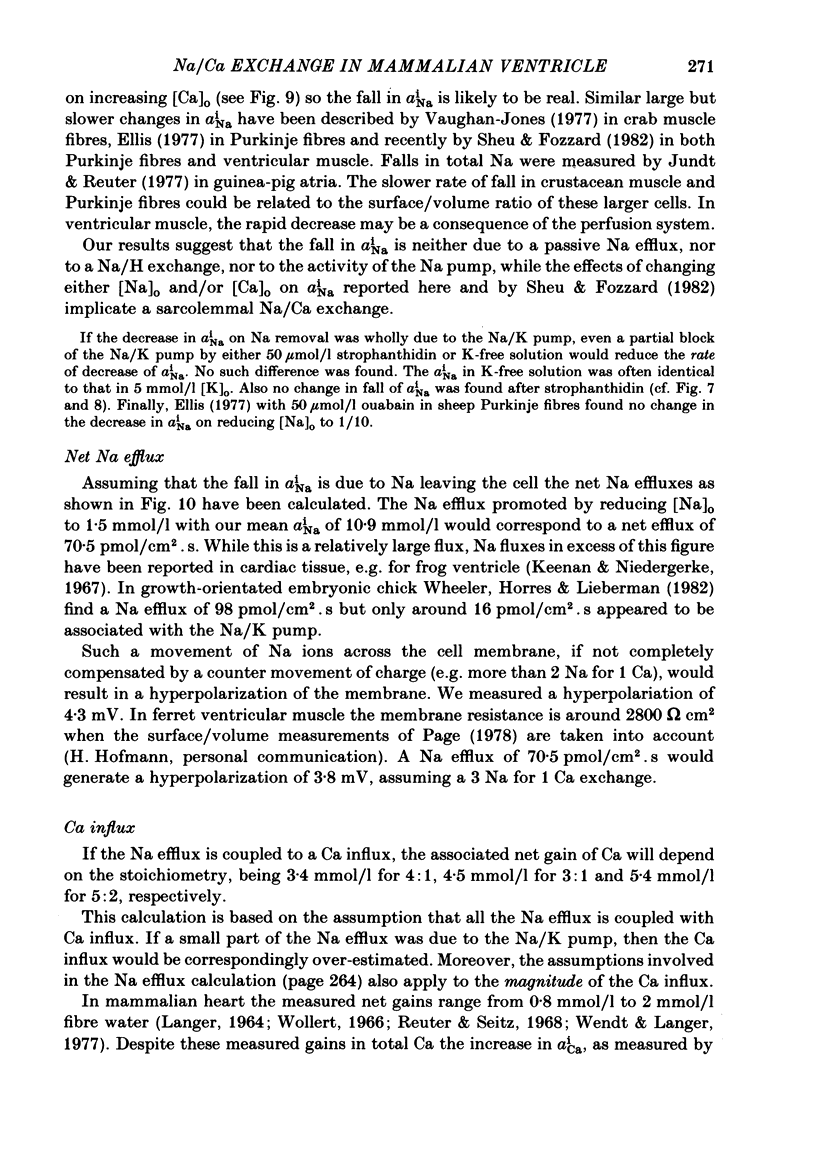
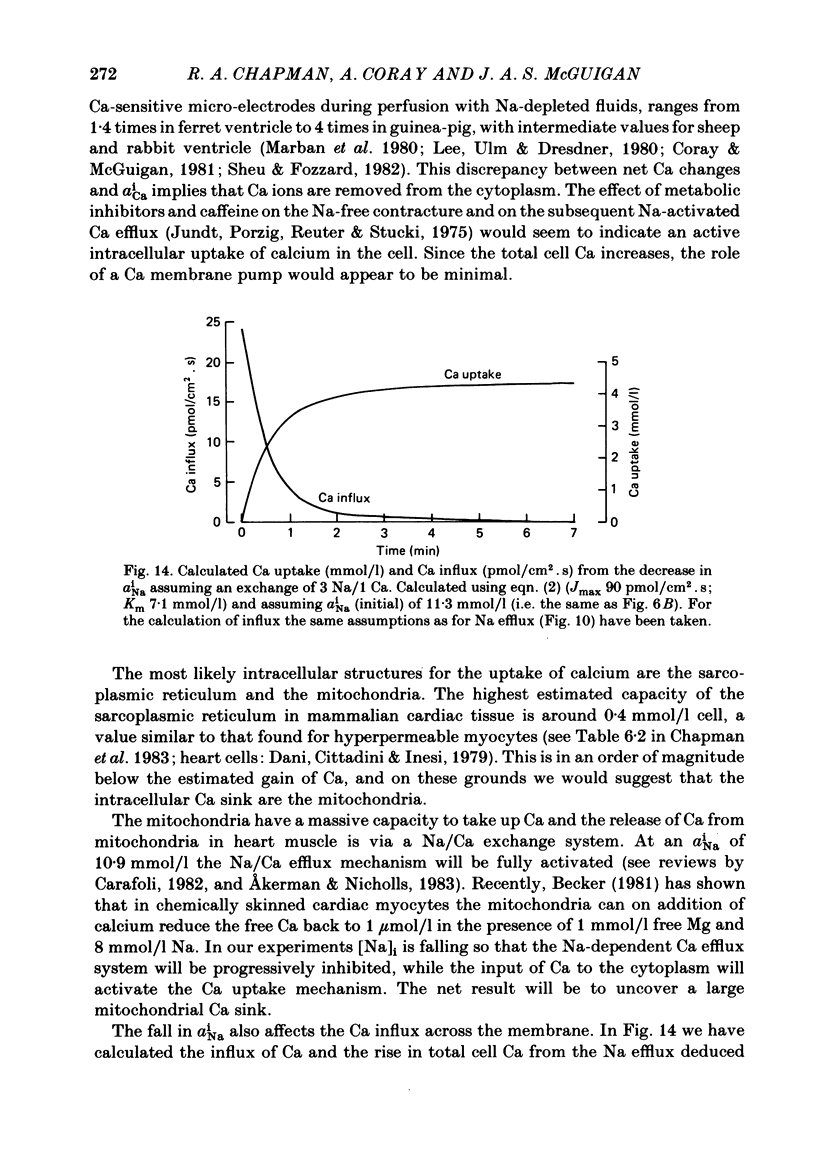
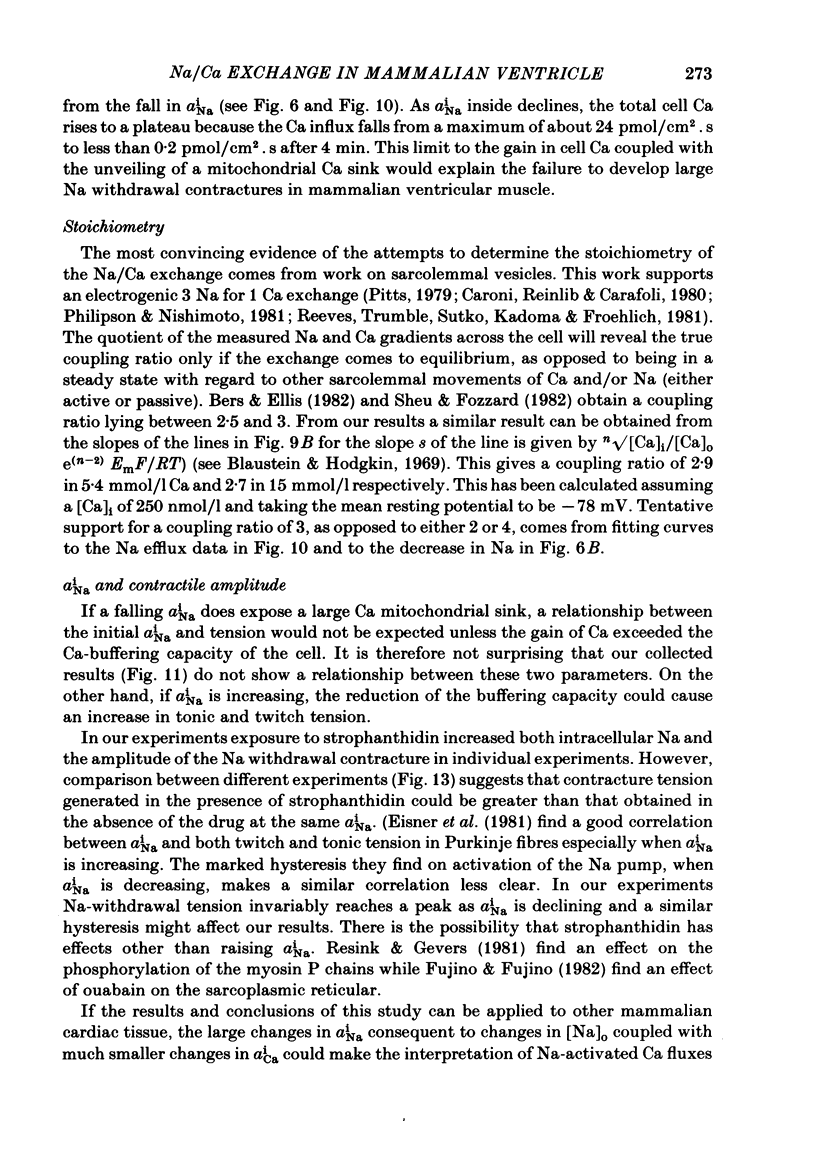
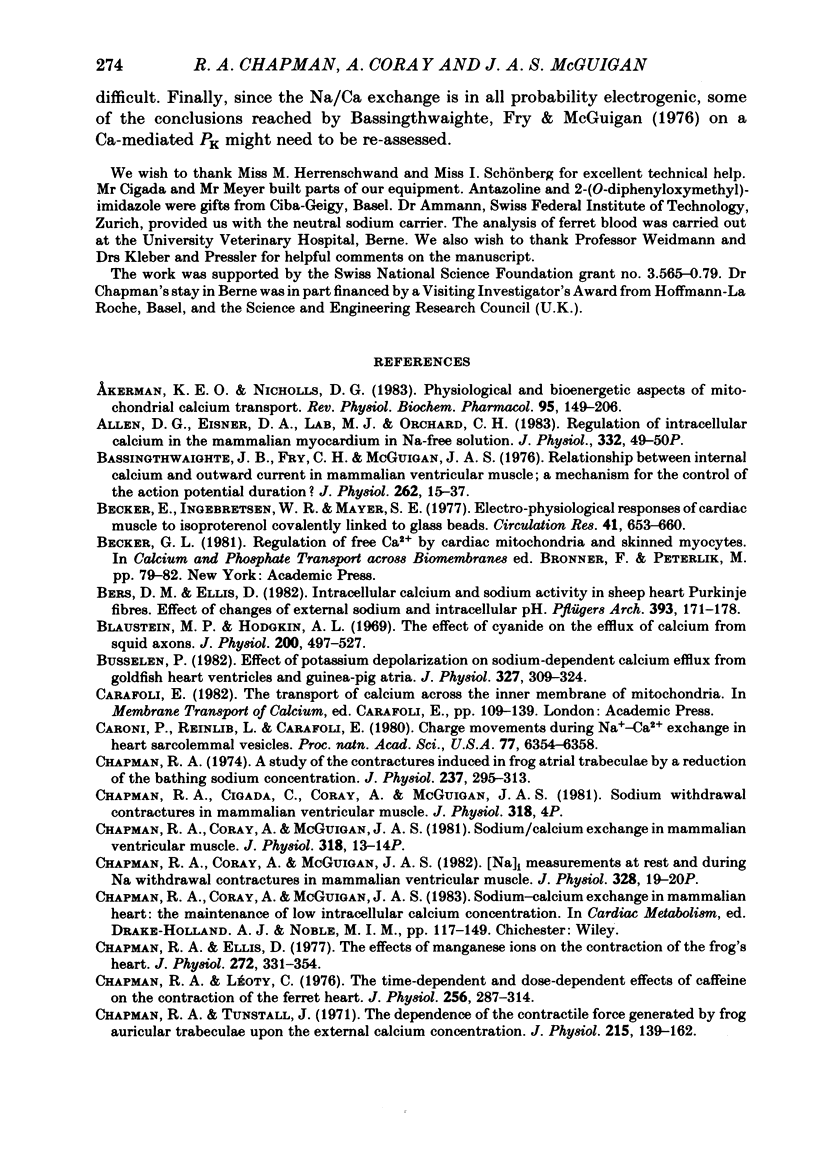
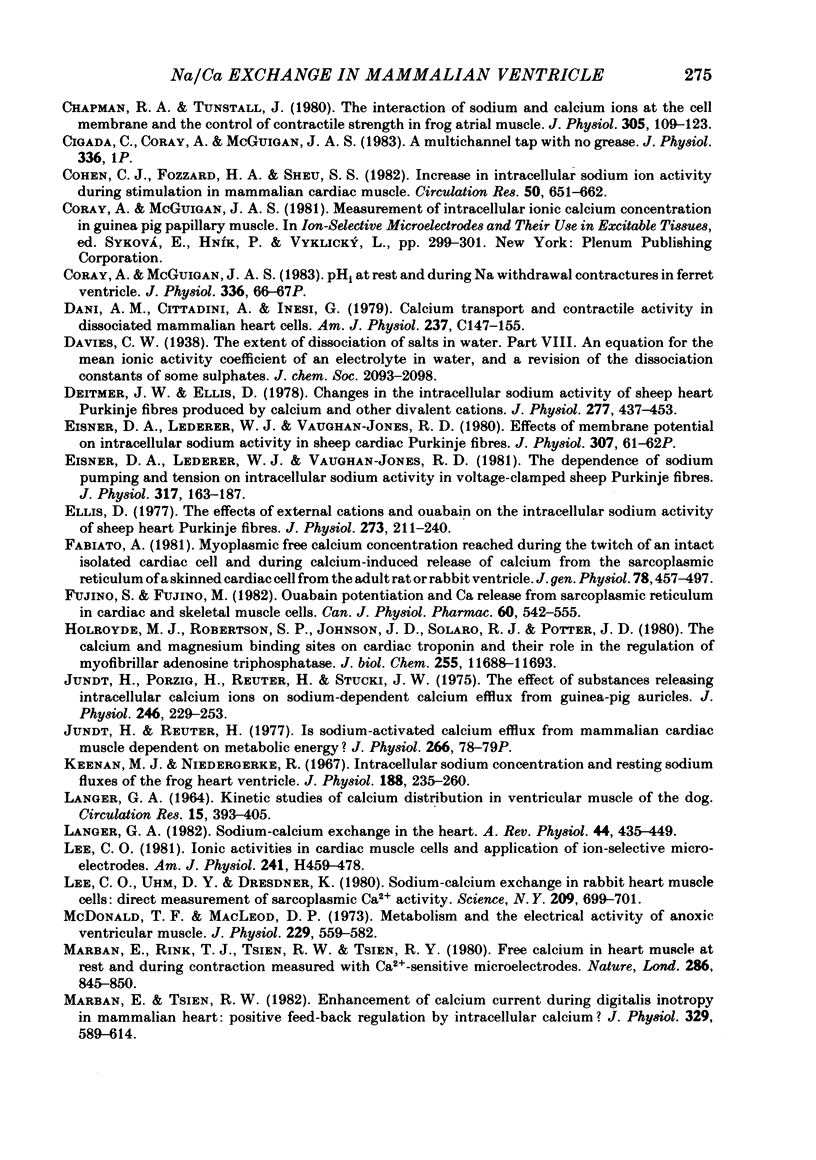
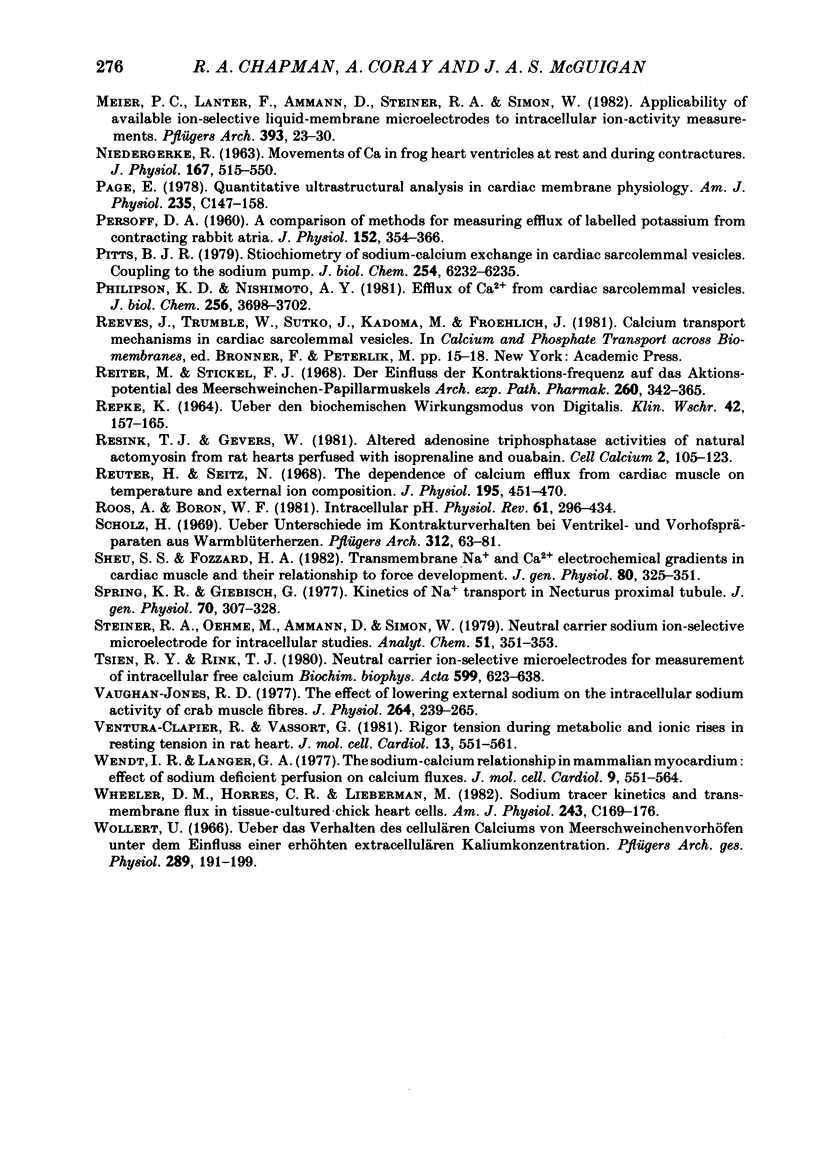
Selected References
These references are in PubMed. This may not be the complete list of references from this article.
- Bassingthwaighte J. B., Fry C. H., McGuigan J. A. Relationship between internal calcium and outward current in mammalian ventricular muscle; a mechanism for the control of the action potential duration? J Physiol. 1976 Oct;262(1):15–37. doi: 10.1113/jphysiol.1976.sp011583. [DOI] [PMC free article] [PubMed] [Google Scholar]
- Becker E., Ingebretsen W. R., Jr, Mayer S. E. Electrophysiological responses of cardiac muscle to isoproterenol covalently linked to glass beads. Circ Res. 1977 Nov;41(5):653–660. doi: 10.1161/01.res.41.5.653. [DOI] [PubMed] [Google Scholar]
- Bers D. M., Ellis D. Intracellular calcium and sodium activity in sheep heart Purkinje fibres. Effect of changes of external sodium and intracellular pH. Pflugers Arch. 1982 Apr;393(2):171–178. doi: 10.1007/BF00582941. [DOI] [PubMed] [Google Scholar]
- Blaustein M. P., Hodgkin A. L. The effect of cyanide on the efflux of calcium from squid axons. J Physiol. 1969 Feb;200(2):497–527. doi: 10.1113/jphysiol.1969.sp008704. [DOI] [PMC free article] [PubMed] [Google Scholar]
- Busselen P. Effect of potassium depolarization on sodium-dependent calcium efflux from goldfish heart ventricles and guinea-pig atria. J Physiol. 1982 Jun;327:309–324. doi: 10.1113/jphysiol.1982.sp014233. [DOI] [PMC free article] [PubMed] [Google Scholar]
- Caroni P., Reinlib L., Carafoli E. Charge movements during the Na+-Ca2+ exchange in heart sarcolemmal vesicles. Proc Natl Acad Sci U S A. 1980 Nov;77(11):6354–6358. doi: 10.1073/pnas.77.11.6354. [DOI] [PMC free article] [PubMed] [Google Scholar]
- Chapman R. A. A study of the contractures induced in frog atrial trabeculae by a reduction of the bathing sodium concentration. J Physiol. 1974 Mar;237(2):295–313. doi: 10.1113/jphysiol.1974.sp010483. [DOI] [PMC free article] [PubMed] [Google Scholar]
- Chapman R. A., Ellis D. The effects of manganese ions on the contraction of the frog's heart. J Physiol. 1977 Nov;272(2):331–354. doi: 10.1113/jphysiol.1977.sp012047. [DOI] [PMC free article] [PubMed] [Google Scholar]
- Chapman R. A., Léoty C. The time-dependent and dose-dependent effects of caffeine on the contraction of the ferret heart. J Physiol. 1976 Apr;256(2):287–314. doi: 10.1113/jphysiol.1976.sp011326. [DOI] [PMC free article] [PubMed] [Google Scholar]
- Chapman R. A., Tunstall J. The dependence of the contractile force generated by frog auricular trabeculae upon the external calcium concentration. J Physiol. 1971 May;215(1):139–162. doi: 10.1113/jphysiol.1971.sp009462. [DOI] [PMC free article] [PubMed] [Google Scholar]
- Chapman R. A., Tunstall J. The interaction of sodium and calcium ions at the cell membrane and the control of contractile strength in frog atrial muscle. J Physiol. 1980 Aug;305:109–123. doi: 10.1113/jphysiol.1980.sp013353. [DOI] [PMC free article] [PubMed] [Google Scholar]
- Cohen C. J., Fozzard H. A., Sheu S. S. Increase in intracellular sodium ion activity during stimulation in mammalian cardiac muscle. Circ Res. 1982 May;50(5):651–662. doi: 10.1161/01.res.50.5.651. [DOI] [PubMed] [Google Scholar]
- Dani A. M., Cittadini A., Inesi G. Calcium transport and contractile activity in dissociated mammalian heart cells. Am J Physiol. 1979 Sep;237(3):C147–C155. doi: 10.1152/ajpcell.1979.237.3.C147. [DOI] [PubMed] [Google Scholar]
- Deitmer J. W., Ellis D. Changes in the intracellular sodium activity of sheep heart Purkinje fibres produced by calcium and other divalent cations. J Physiol. 1978 Apr;277:437–453. doi: 10.1113/jphysiol.1978.sp012283. [DOI] [PMC free article] [PubMed] [Google Scholar]
- Eisner D. A., Lederer W. J., Vaughan-Jones R. D. The dependence of sodium pumping and tension on intracellular sodium activity in voltage-clamped sheep Purkinje fibres. J Physiol. 1981 Aug;317:163–187. doi: 10.1113/jphysiol.1981.sp013819. [DOI] [PMC free article] [PubMed] [Google Scholar]
- Ellis D. The effects of external cations and ouabain on the intracellular sodium activity of sheep heart Purkinje fibres. J Physiol. 1977 Dec;273(1):211–240. doi: 10.1113/jphysiol.1977.sp012090. [DOI] [PMC free article] [PubMed] [Google Scholar]
- Fabiato A. Myoplasmic free calcium concentration reached during the twitch of an intact isolated cardiac cell and during calcium-induced release of calcium from the sarcoplasmic reticulum of a skinned cardiac cell from the adult rat or rabbit ventricle. J Gen Physiol. 1981 Nov;78(5):457–497. doi: 10.1085/jgp.78.5.457. [DOI] [PMC free article] [PubMed] [Google Scholar]
- Fujino S., Fujino M. Ouabain potentiation and Ca release from sarcoplasmic reticulum in cardiac and skeletal muscle cells. Can J Physiol Pharmacol. 1982 Apr;60(4):542–555. doi: 10.1139/y82-074. [DOI] [PubMed] [Google Scholar]
- Holroyde M. J., Robertson S. P., Johnson J. D., Solaro R. J., Potter J. D. The calcium and magnesium binding sites on cardiac troponin and their role in the regulation of myofibrillar adenosine triphosphatase. J Biol Chem. 1980 Dec 25;255(24):11688–11693. [PubMed] [Google Scholar]
- Jundt H., Porzig H., Reuter H., Stucki J. W. The effect of substances releasing intracellular calcium ions on sodium-dependent calcium efflux from guinea-pig auricles. J Physiol. 1975 Mar;246(1):229–253. doi: 10.1113/jphysiol.1975.sp010888. [DOI] [PMC free article] [PubMed] [Google Scholar]
- Jundt H., Reuter H. Is sodium-activated calcium efflux from mammalian cardiac muscle dependent on metabolic energy [proceedings]? J Physiol. 1977 Mar;266(1):78P–79P. [PubMed] [Google Scholar]
- Keenan M. J., Niedergerke R. Intracellular sodium concentration and resting sodium fluxes of the frog heart ventricle. J Physiol. 1967 Jan;188(2):235–260. doi: 10.1113/jphysiol.1967.sp008136. [DOI] [PMC free article] [PubMed] [Google Scholar]
- LANGER G. A. KINETIC STUDIES OF CALCIUM DISTRIBUTION IN VENTRICULAR MUSCLE OF THE DOG. Circ Res. 1964 Nov;15:393–405. doi: 10.1161/01.res.15.5.393. [DOI] [PubMed] [Google Scholar]
- Langer G. A. Sodium-calcium exchange in the heart. Annu Rev Physiol. 1982;44:435–449. doi: 10.1146/annurev.ph.44.030182.002251. [DOI] [PubMed] [Google Scholar]
- Lee C. O. Ionic activities in cardiac muscle cells and application of ion-selective microelectrodes. Am J Physiol. 1981 Oct;241(4):H459–H478. doi: 10.1152/ajpheart.1981.241.4.H459. [DOI] [PubMed] [Google Scholar]
- Lee C. O., Uhm D. Y., Dresdner K. Sodium-calcium exchange in rabbit heart muscle cells: direct measurement of sarcoplasmic Ca2+ activity. Science. 1980 Aug 8;209(4457):699–701. doi: 10.1126/science.7394527. [DOI] [PubMed] [Google Scholar]
- Marban E., Rink T. J., Tsien R. W., Tsien R. Y. Free calcium in heart muscle at rest and during contraction measured with Ca2+ -sensitive microelectrodes. Nature. 1980 Aug 28;286(5776):845–850. doi: 10.1038/286845a0. [DOI] [PubMed] [Google Scholar]
- Marban E., Tsien R. W. Enhancement of calcium current during digitalis inotropy in mammalian heart: positive feed-back regulation by intracellular calcium? J Physiol. 1982 Aug;329:589–614. doi: 10.1113/jphysiol.1982.sp014321. [DOI] [PMC free article] [PubMed] [Google Scholar]
- McDonald T. F., MacLeod D. P. Metabolism and the electrical activity of anoxic ventricular muscle. J Physiol. 1973 Mar;229(3):559–582. doi: 10.1113/jphysiol.1973.sp010154. [DOI] [PMC free article] [PubMed] [Google Scholar]
- Meier P. C., Lanter F., Ammann D., Steiner R. A., Simon W. Applicability of available ion-selective liquid-membrane microelectrodes to intracellular ion-activity measurements. Pflugers Arch. 1982 Mar;393(1):23–30. doi: 10.1007/BF00582386. [DOI] [PubMed] [Google Scholar]
- NIEDERGERKE R. MOVEMENTS OF CA IN FROG HEART VENTRICLES AT REST AND DURING CONTRACTURES. J Physiol. 1963 Jul;167:515–550. doi: 10.1113/jphysiol.1963.sp007166. [DOI] [PMC free article] [PubMed] [Google Scholar]
- PERSOFF D. A. A comparison of methods for measuring efflux of labelled potassium from contracting rabbit atria. J Physiol. 1960 Jul;152:354–366. doi: 10.1113/jphysiol.1960.sp006492. [DOI] [PMC free article] [PubMed] [Google Scholar]
- Page E. Quantitative ultrastructural analysis in cardiac membrane physiology. Am J Physiol. 1978 Nov;235(5):C147–C158. doi: 10.1152/ajpcell.1978.235.5.C147. [DOI] [PubMed] [Google Scholar]
- Pappenheimer J. R. Bayliss-Starling Memorial Lecture (1982). Induction of sleep by muramyl peptides. J Physiol. 1983 Mar;336:1–11. doi: 10.1113/jphysiol.1983.sp014561. [DOI] [PMC free article] [PubMed] [Google Scholar]
- Philipson K. D., Nishimoto A. Y. Efflux of Ca2+ from cardiac sarcolemmal vesicles. Influence of external Ca2+ and Na+. J Biol Chem. 1981 Apr 25;256(8):3698–3702. [PubMed] [Google Scholar]
- Pitts B. J. Stoichiometry of sodium-calcium exchange in cardiac sarcolemmal vesicles. Coupling to the sodium pump. J Biol Chem. 1979 Jul 25;254(14):6232–6235. [PubMed] [Google Scholar]
- REPKE K. UBER DEN BIOCHEMISCHEN WIRKUNGSMODUS VON DIGITALIS. Klin Wochenschr. 1964 Feb 15;42:157–165. doi: 10.1007/BF01482616. [DOI] [PubMed] [Google Scholar]
- Reiter M., Stickel F. J. Der Einfluss der Kontraktionsfrequenz auf das Aktionspotential des Meerschweinchem-Papillarmuskels. Naunyn Schmiedebergs Arch Exp Pathol Pharmakol. 1968;260(4):342–365. [PubMed] [Google Scholar]
- Reuter H., Seitz N. The dependence of calcium efflux from cardiac muscle on temperature and external ion composition. J Physiol. 1968 Mar;195(2):451–470. doi: 10.1113/jphysiol.1968.sp008467. [DOI] [PMC free article] [PubMed] [Google Scholar]
- Roos A., Boron W. F. Intracellular pH. Physiol Rev. 1981 Apr;61(2):296–434. doi: 10.1152/physrev.1981.61.2.296. [DOI] [PubMed] [Google Scholar]
- Scholz H. Uber Unterschiede im Kontrakturverhalten bei Ventrikel- und Vorhofspräparaten aus Warmblüterherzen. Pflugers Arch. 1969;312(3):63–81. doi: 10.1007/BF00588532. [DOI] [PubMed] [Google Scholar]
- Sheu S. S., Fozzard H. A. Transmembrane Na+ and Ca2+ electrochemical gradients in cardiac muscle and their relationship to force development. J Gen Physiol. 1982 Sep;80(3):325–351. doi: 10.1085/jgp.80.3.325. [DOI] [PMC free article] [PubMed] [Google Scholar]
- Spring K. R., Giebisch G. Kinetics of Na+ transport in Necturus proximal tubule. J Gen Physiol. 1977 Sep;70(3):307–328. doi: 10.1085/jgp.70.3.307. [DOI] [PMC free article] [PubMed] [Google Scholar]
- Tsien R. Y., Rink T. J. Neutral carrier ion-selective microelectrodes for measurement of intracellular free calcium. Biochim Biophys Acta. 1980 Jul;599(2):623–638. doi: 10.1016/0005-2736(80)90205-9. [DOI] [PubMed] [Google Scholar]
- Vaughan-Jones R. D. The effect of lowering external sodium on the intracellular sodium activity of crab muscle fibres. J Physiol. 1977 Jan;264(1):239–265. doi: 10.1113/jphysiol.1977.sp011666. [DOI] [PMC free article] [PubMed] [Google Scholar]
- Ventura-Clapier R., Vassort G. Rigor tension during metabolic and ionic rises in resting tension in rat heart. J Mol Cell Cardiol. 1981 Jun;13(6):551–561. doi: 10.1016/0022-2828(81)90326-6. [DOI] [PubMed] [Google Scholar]
- Wendt I. R., Langer G. A. The sodium-calcium relationship in mammalian myocardium: effect of sodium deficient perfusion on calcium fluxes. J Mol Cell Cardiol. 1977 Jul;9(7):551–564. doi: 10.1016/s0022-2828(77)80370-2. [DOI] [PubMed] [Google Scholar]
- Wheeler D. M., Horres C. R., Lieberman M. Sodium tracer kinetics and transmembrane flux in tissue-cultured chick heart cells. Am J Physiol. 1982 Sep;243(3):C169–C176. doi: 10.1152/ajpcell.1982.243.3.C169. [DOI] [PubMed] [Google Scholar]