Abstract
The inhibitory effect of cytoplasmic Ca on Na-pump-mediated Na-K exchange was investigated in intact red cells under conditions of constant cell volume, membrane potential and inorganic ion composition. The ionized cytoplasmic Ca concentration ( [Ca2+]i) was controlled using the ionophore A23187. In normal cells, ouabain-sensitive 24Na efflux was inhibited with an apparent affinity for [Ca2+]i which depended on the concentration of A23187; 50% inhibition required 20-40 microM and 160-300 microM-cytoplasmic Ca2+ with 10 microM and 0.63 microM-A23187 respectively. Cytoplasmic Ca also affected cell ATP content which fell rapidly on addition of A23187 and subsequently increased, steadied or continued to fall more slowly depending on the Ca and A23187 concentrations. Half-maximal fall required 5-15 microM and 110-170 microM-cytoplasmic Ca2+ at 10 microM and 0.63 microM-A23187 respectively. Removal of Ca from the cells failed to reverse either the Na pump inhibition or the fall in cell ATP. In ATP-enriched cells cytoplasmic Ca caused inhibition of ouabain-sensitive 24Na efflux in an A23187-dependent manner with apparent affinities for [Ca2+]i similar to those observed in the normal cells. Inhibition was complete at high [Ca2+]i. As in the normal cells, the ATP content of the cells fell in the presence of cytoplasmic Ca, but always remained above 1.2 m-mole/l. cells. This was higher than the ATP content of Ca-free normal intact cells. A23187 had no effect on the inhibition by Ca of ouabain-sensitive ATPase activity in isolated red cell membrane preparations. Both under conditions near optimal for Na-K-ATPase activity and under conditions resembling those in the cytoplasm, inhibition was half-maximal at about 25 microM-Ca2+ and in the latter case complete at below 400 microM-Ca2+. The apparent ATP-dependence of ouabain-sensitive Na efflux in the presence of cytoplasmic Ca was distinctly different in the normal and ATP-enriched cells but in both groups of cells it was similar for data obtained with high and low concentrations of A23187. The data for Na pump inhibition by cytoplasmic Ca in the intact cells were well fitted by several kinetic models involving either [Ca2+]i or CaATP as the inhibitory species and a low affinity dependence of pump activity on MgATP or total ATP. However, for any model, the apparent affinities for CaATP or for Ca2+ required to fit the ATPase data were 2.5-10 times higher than those required to fit the data for Na efflux.(ABSTRACT TRUNCATED AT 400 WORDS)
Full text
PDF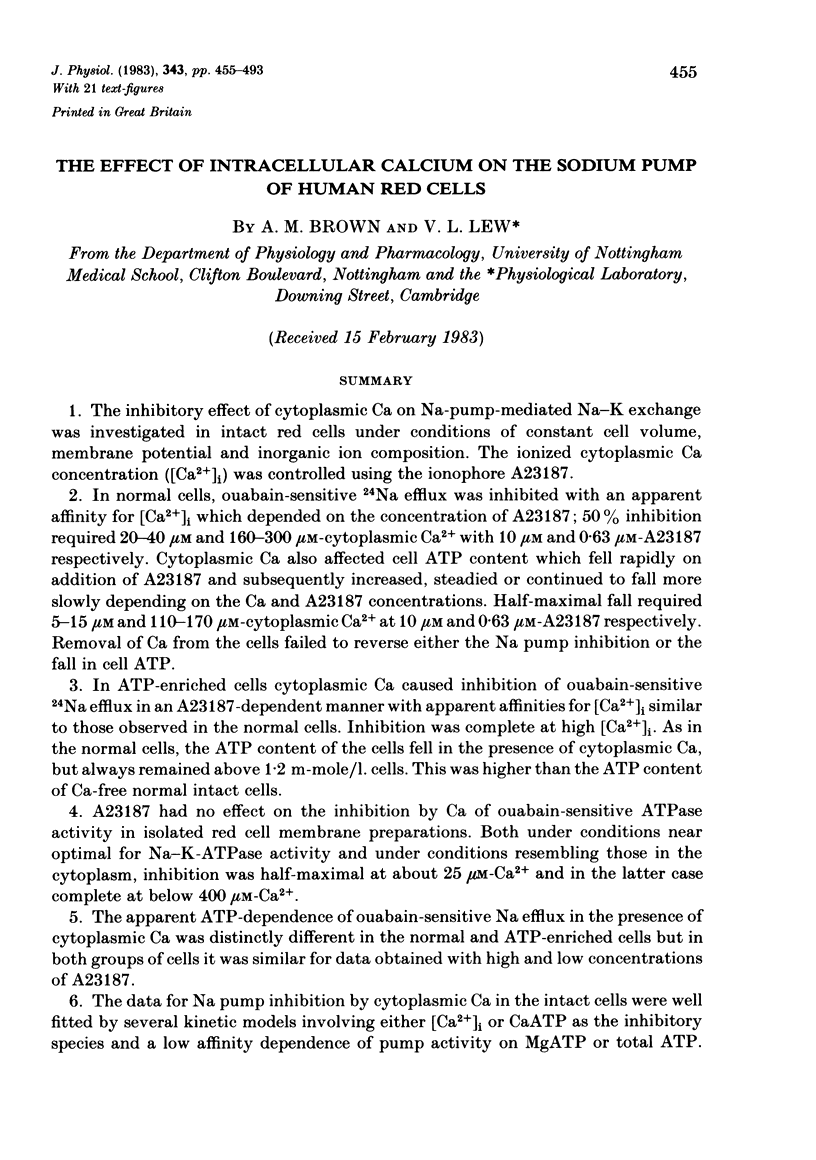
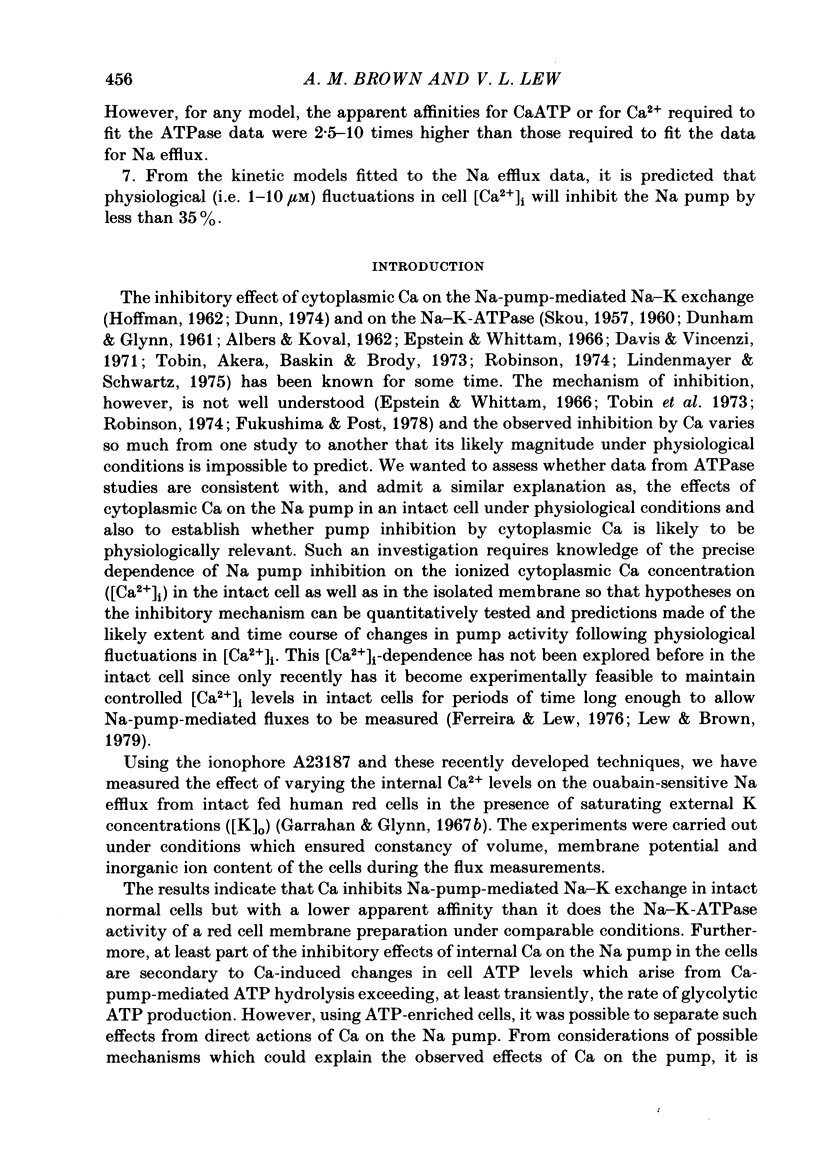
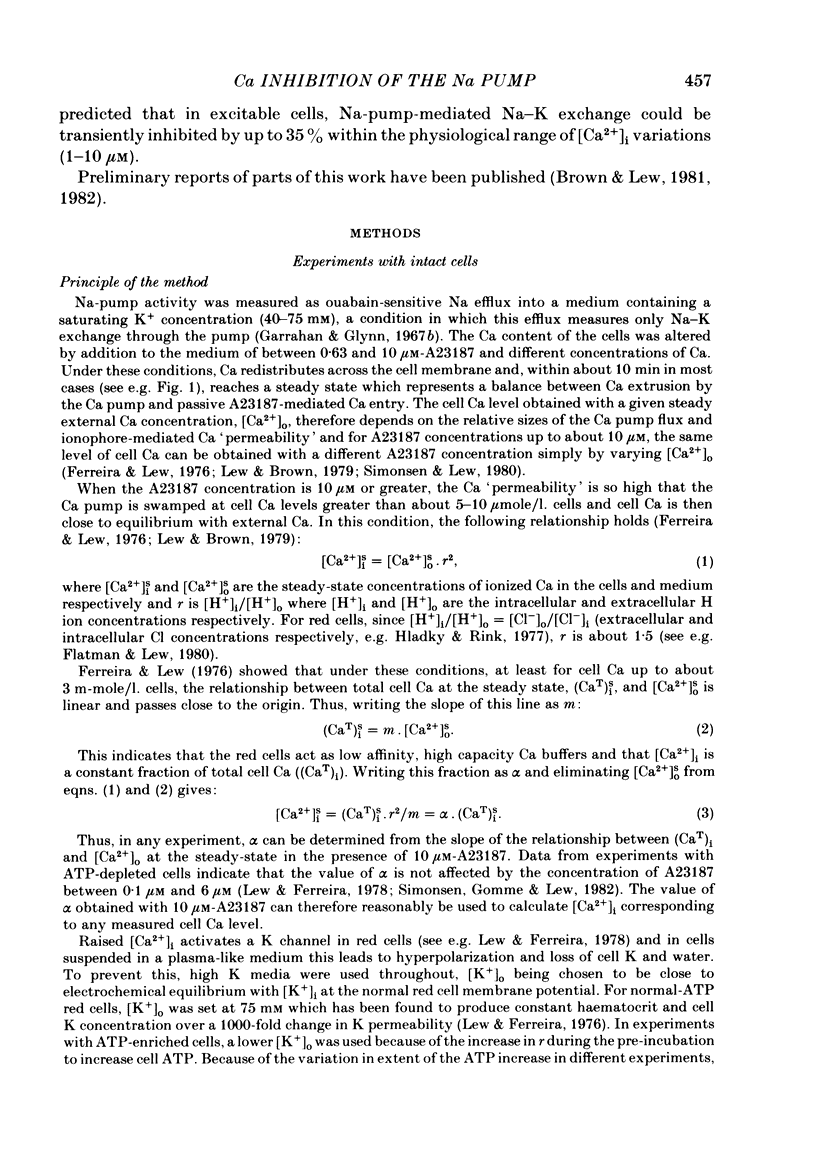
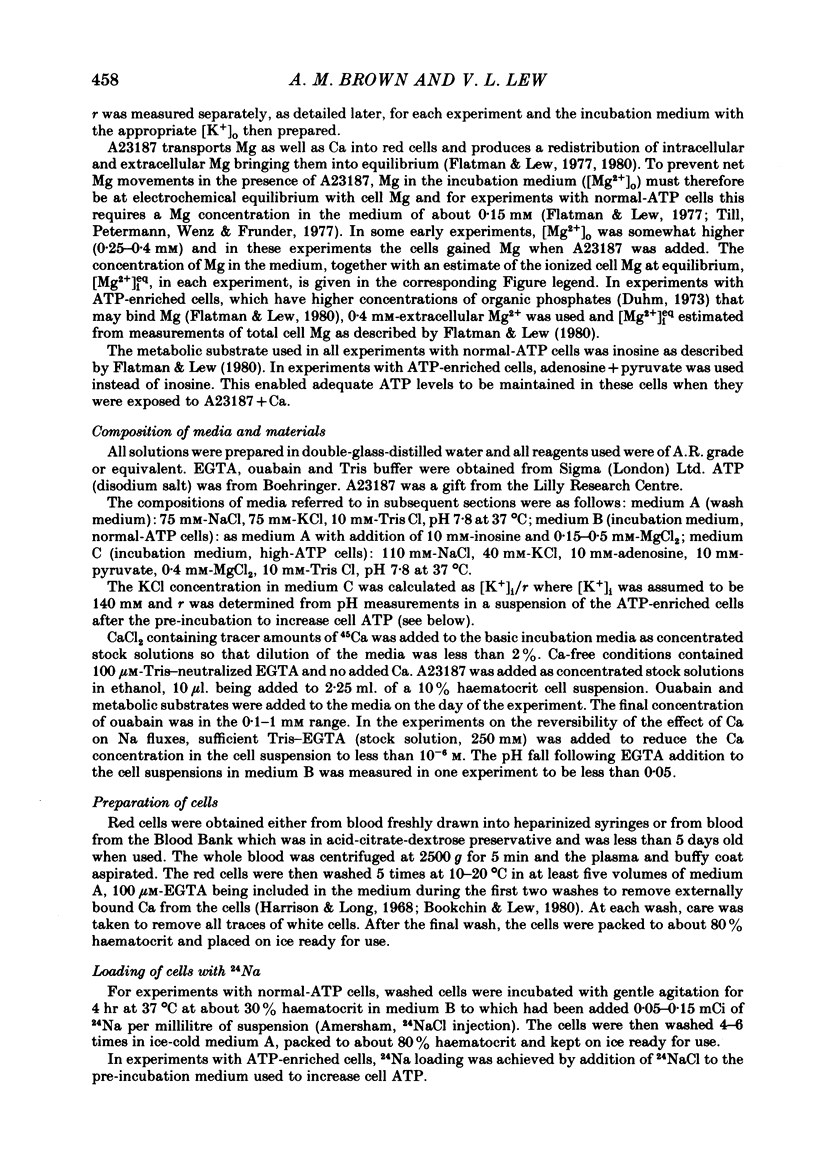
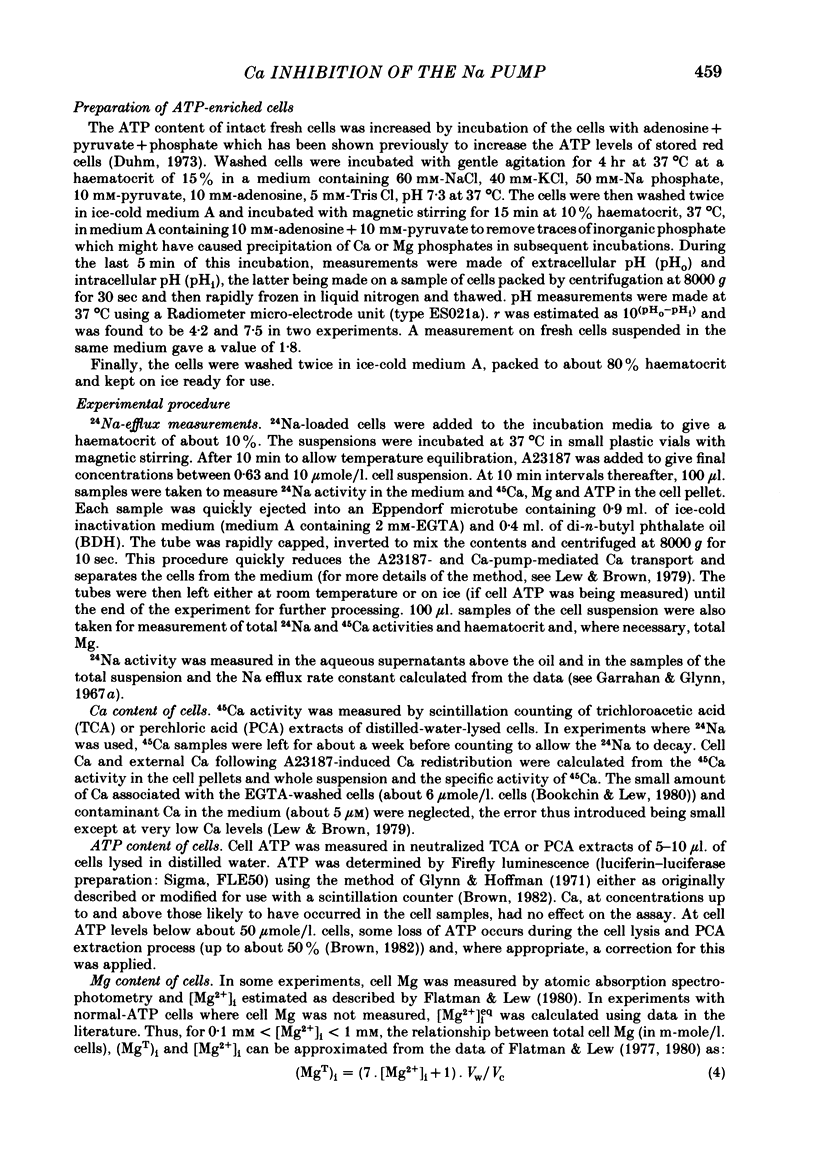
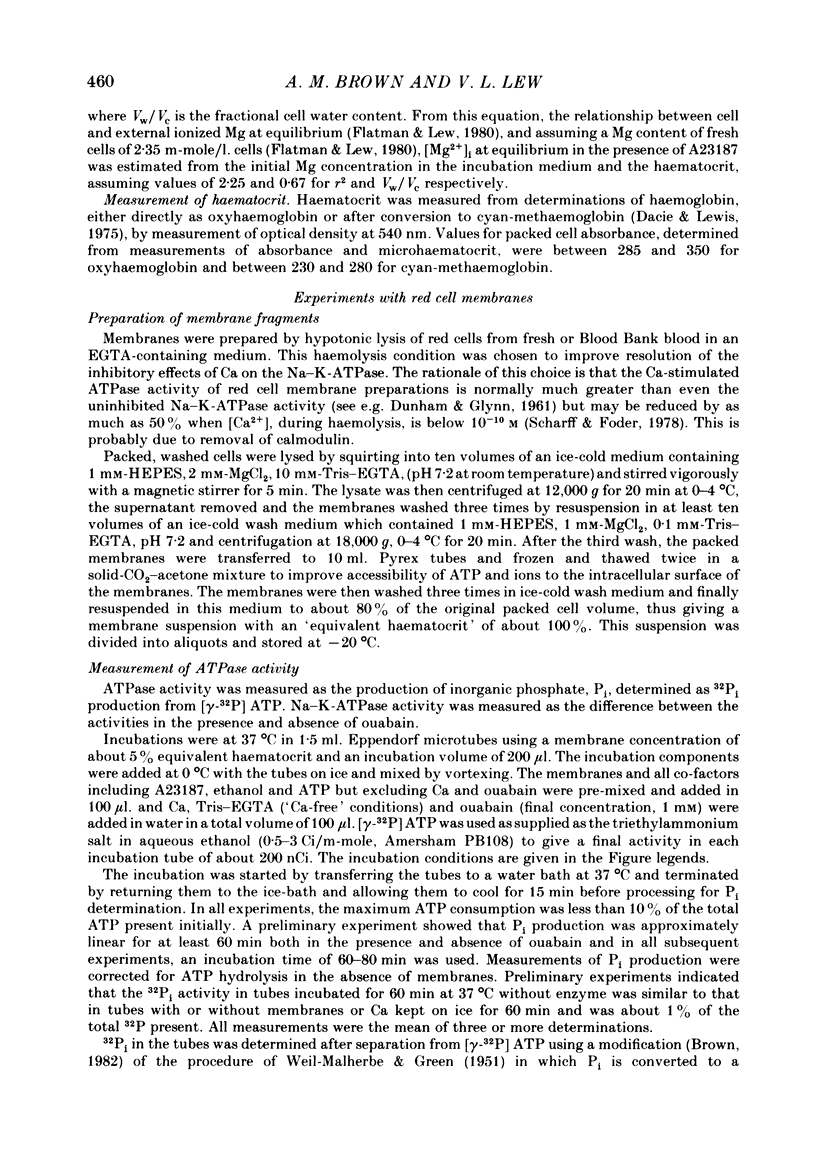
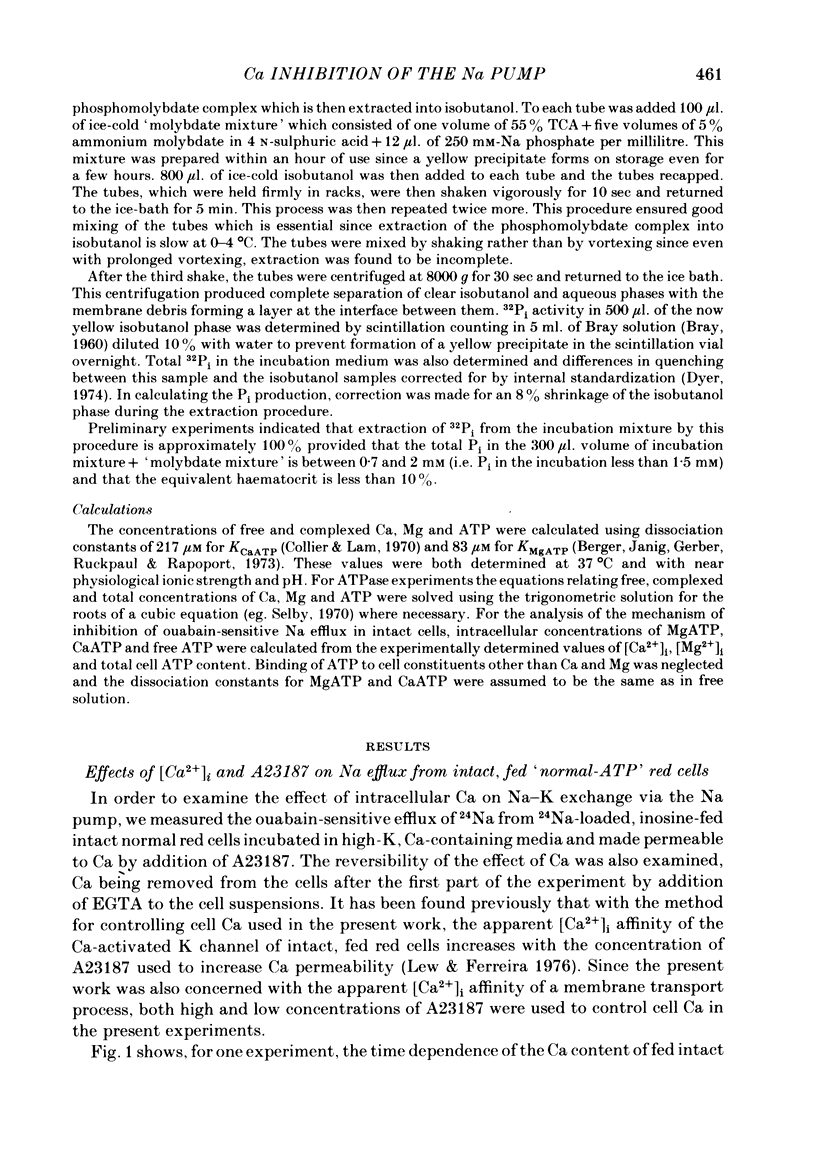
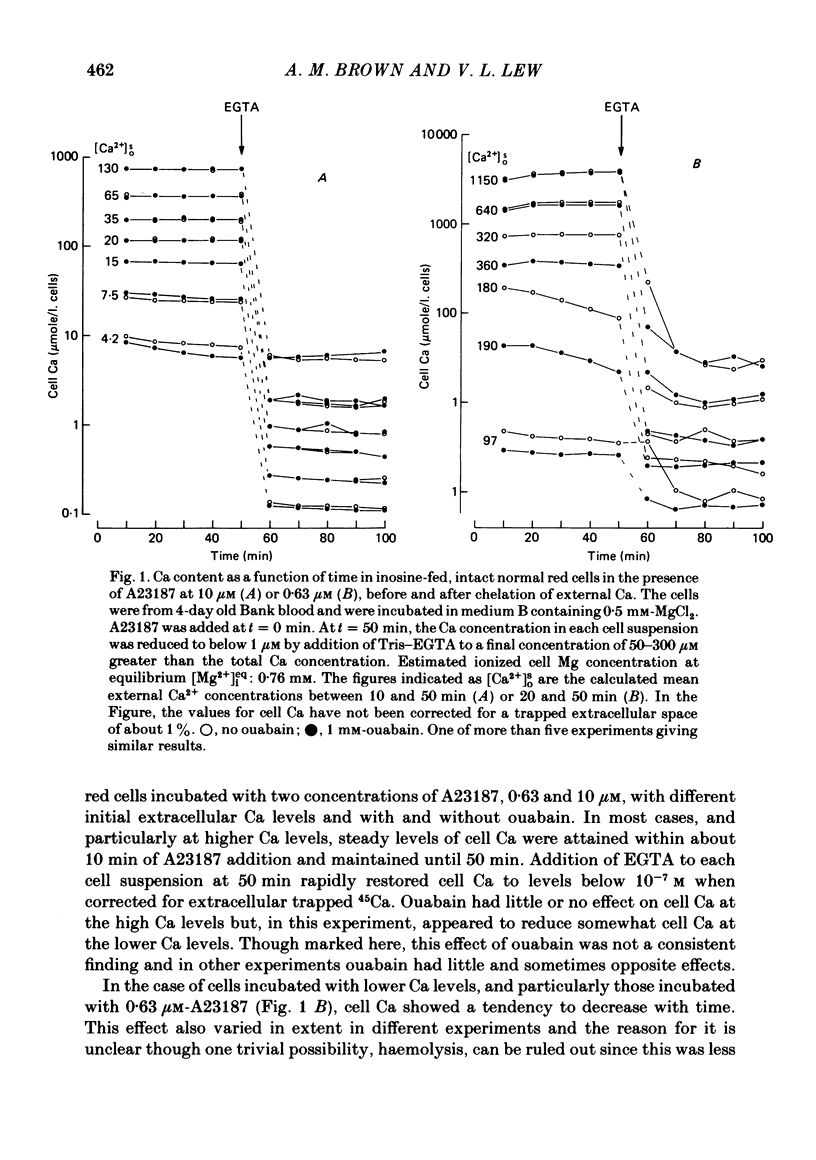
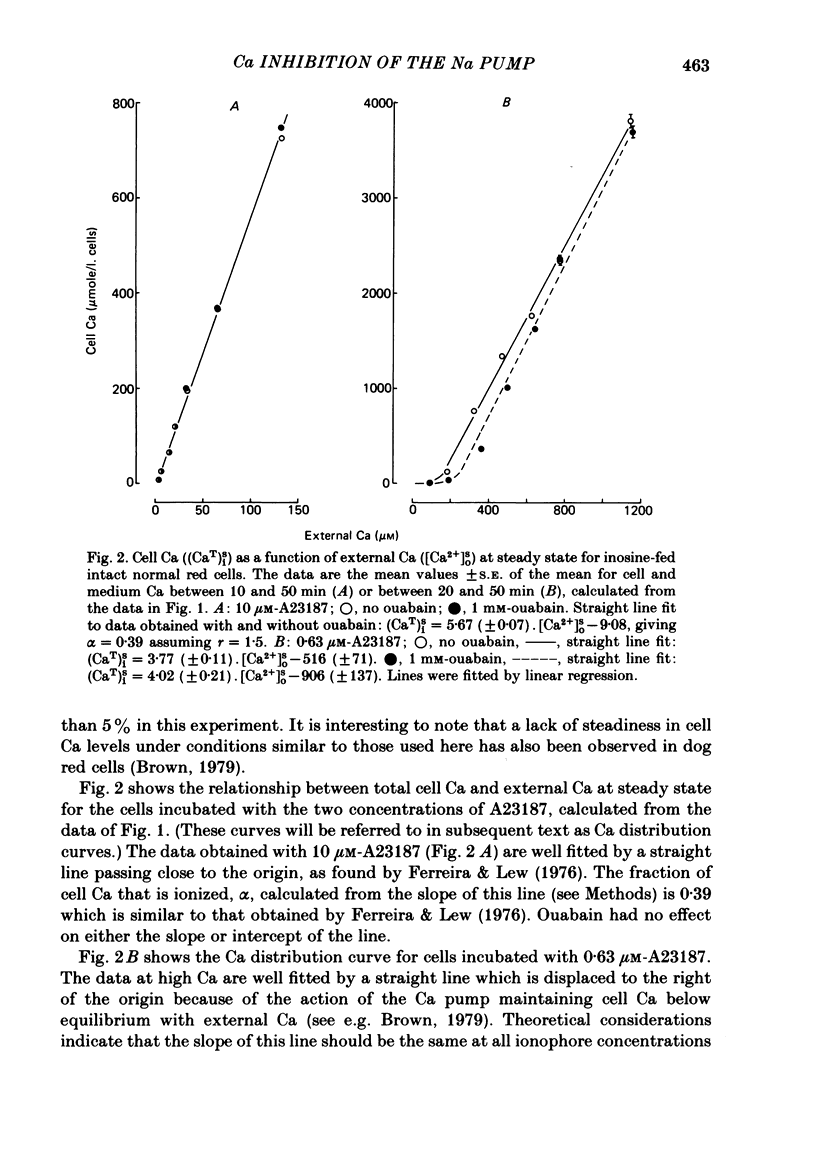
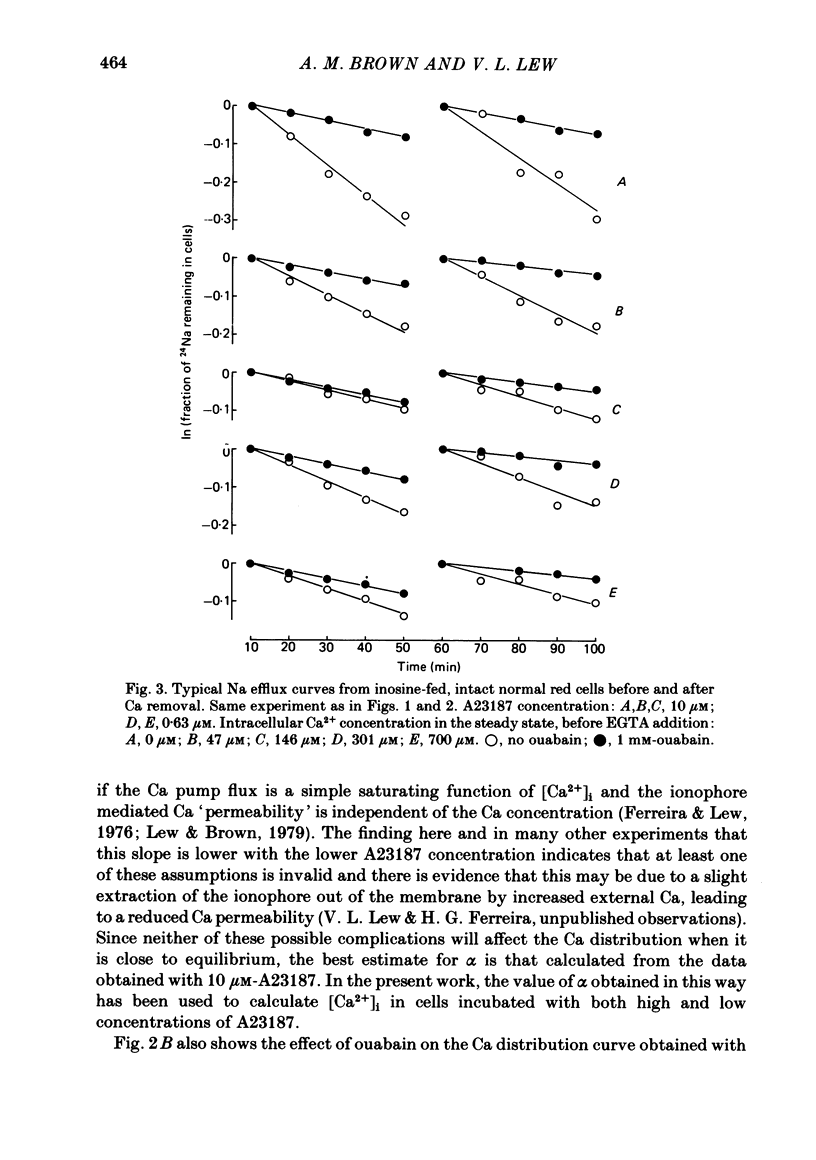
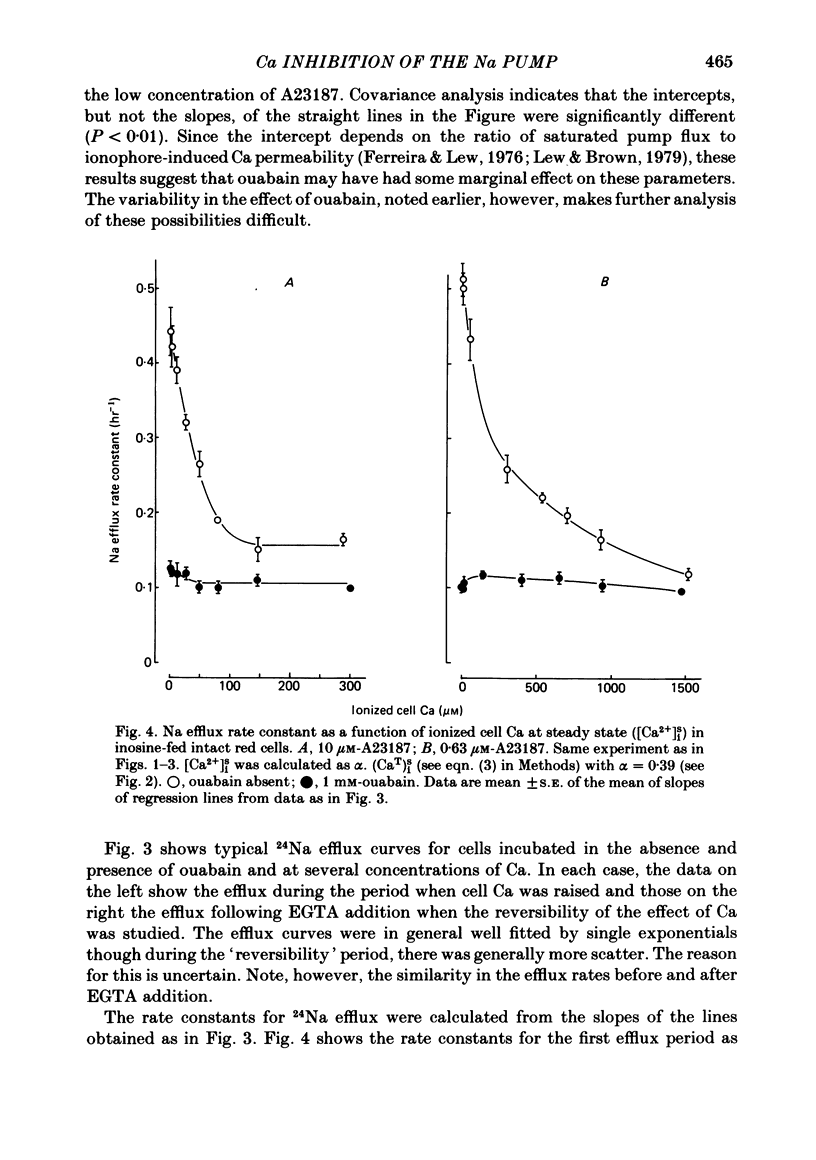
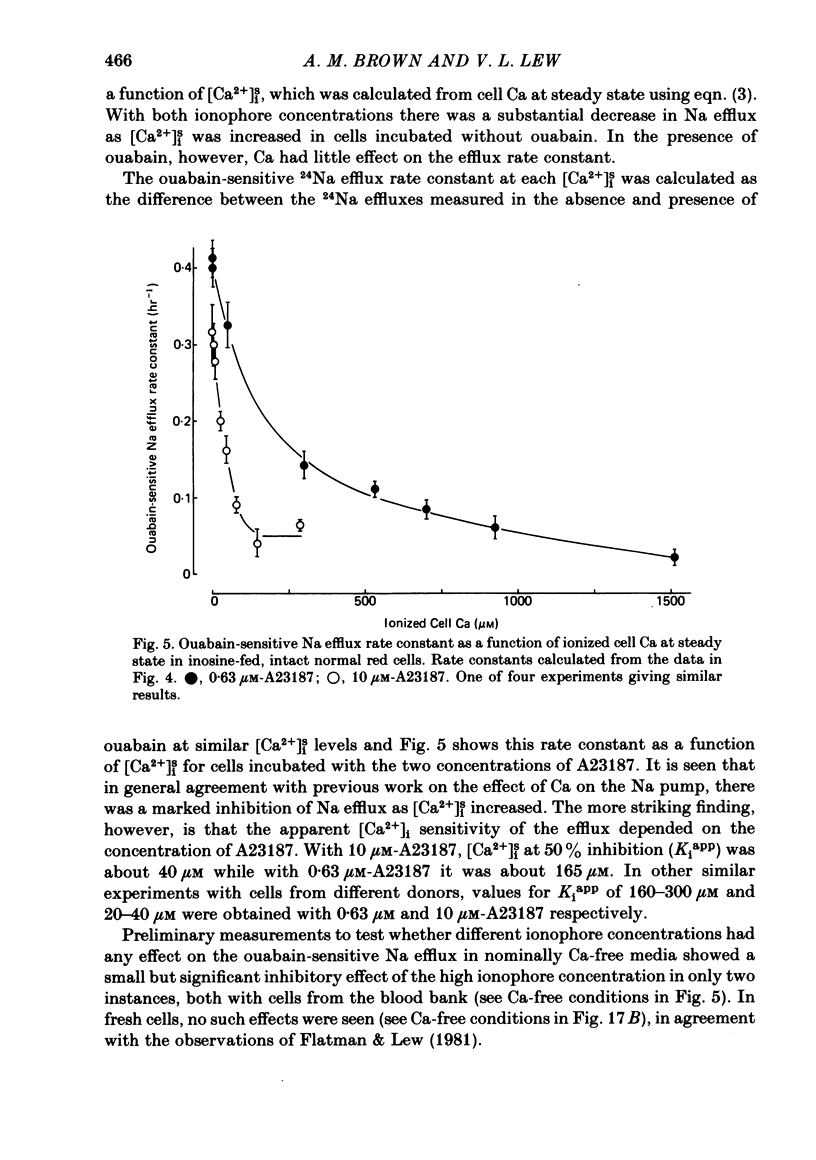
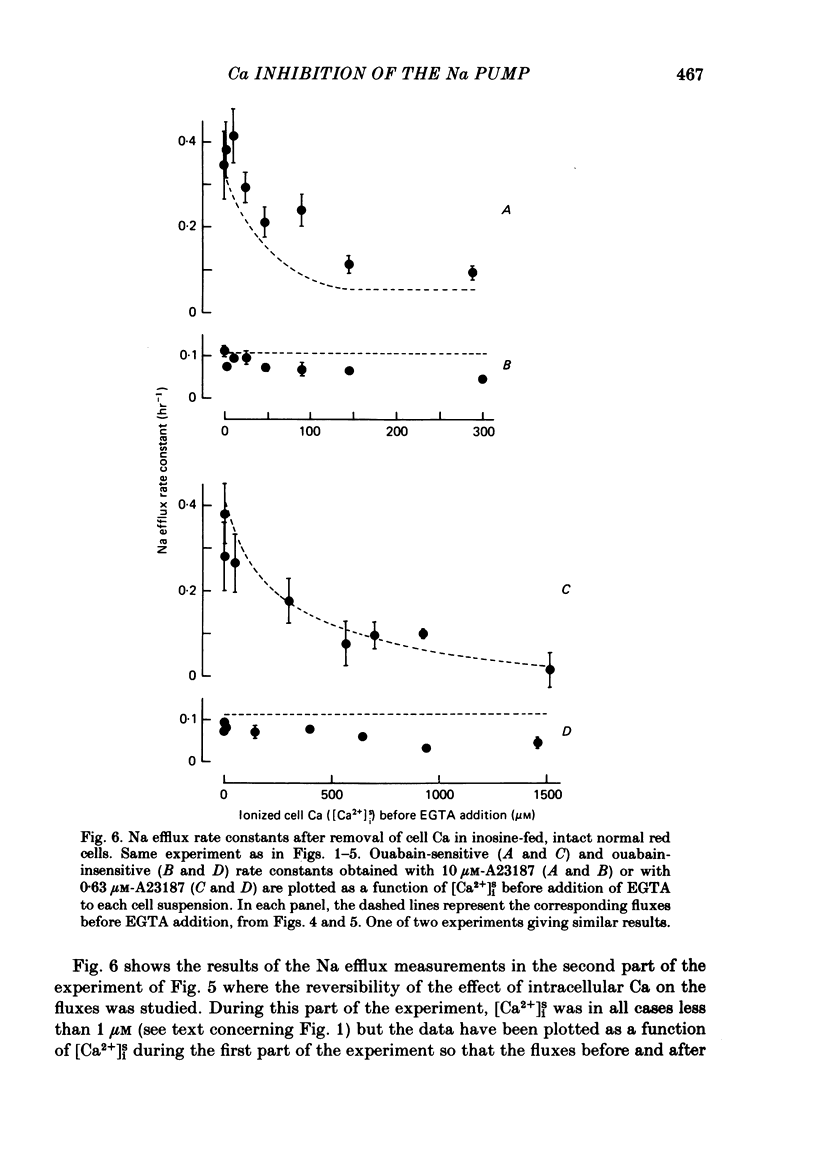
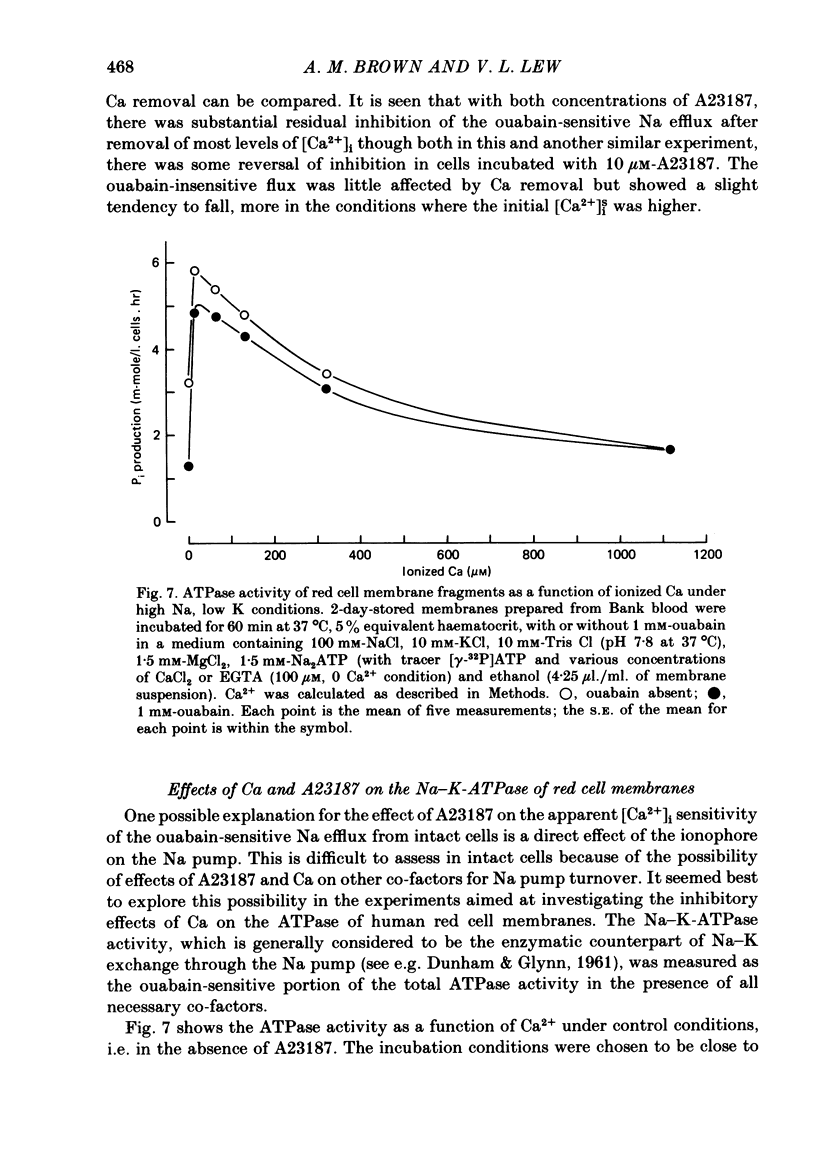
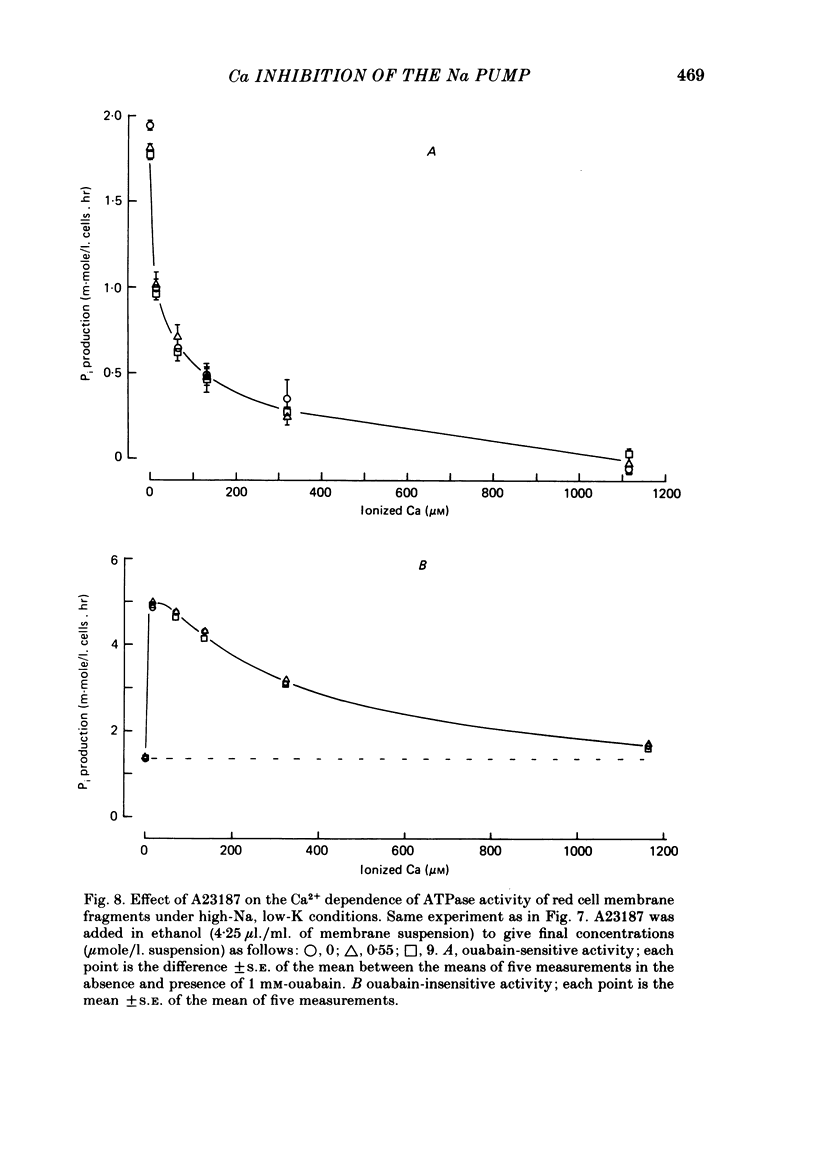
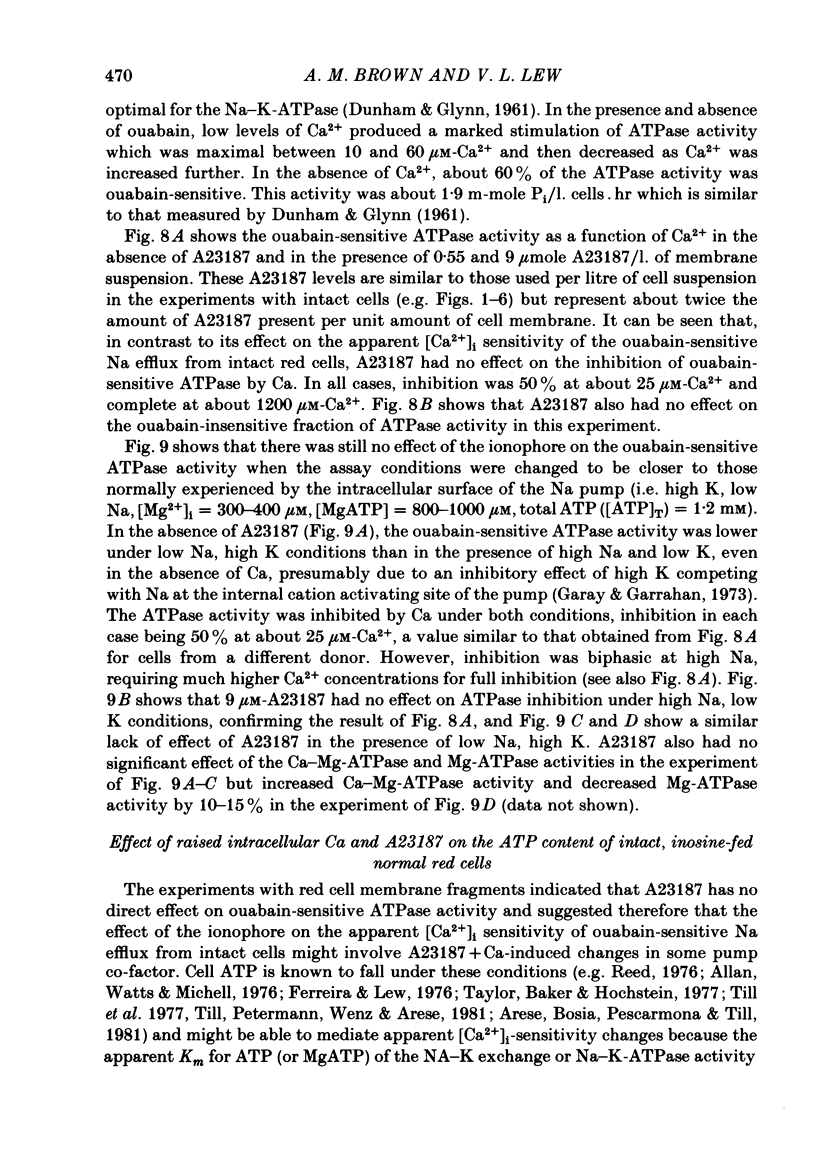
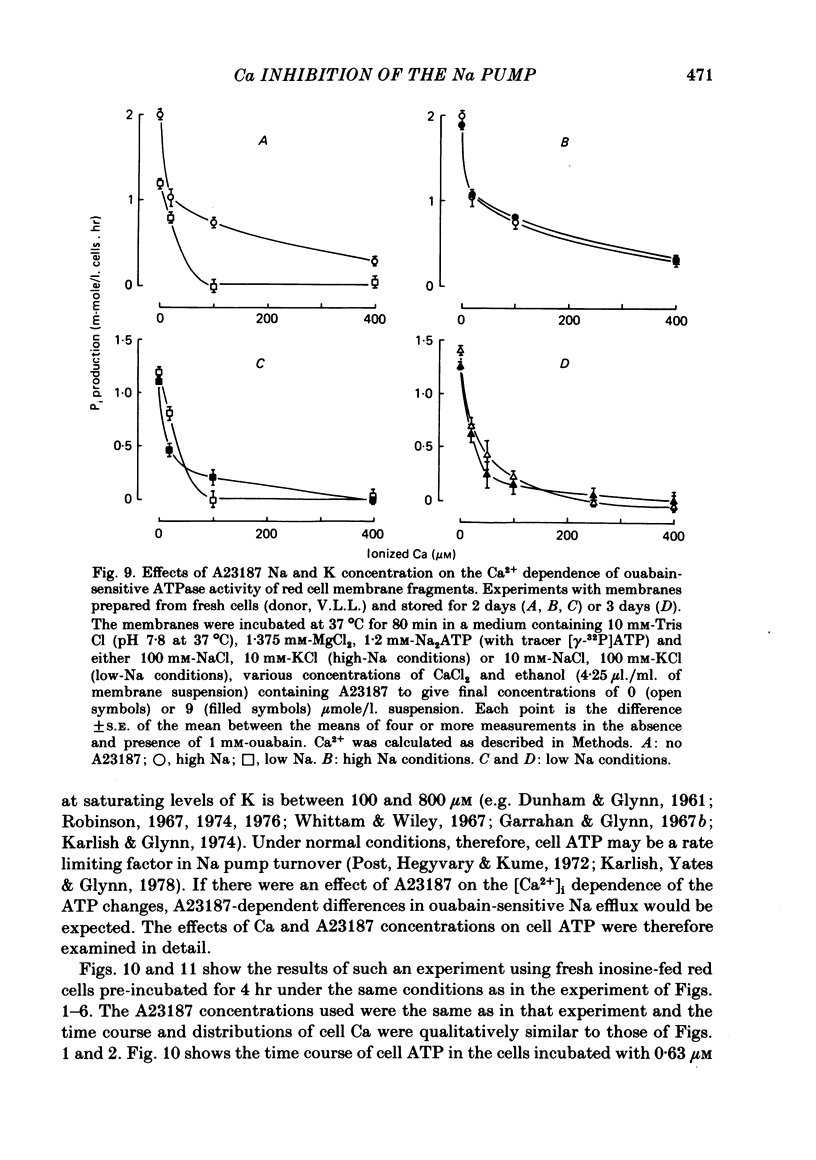
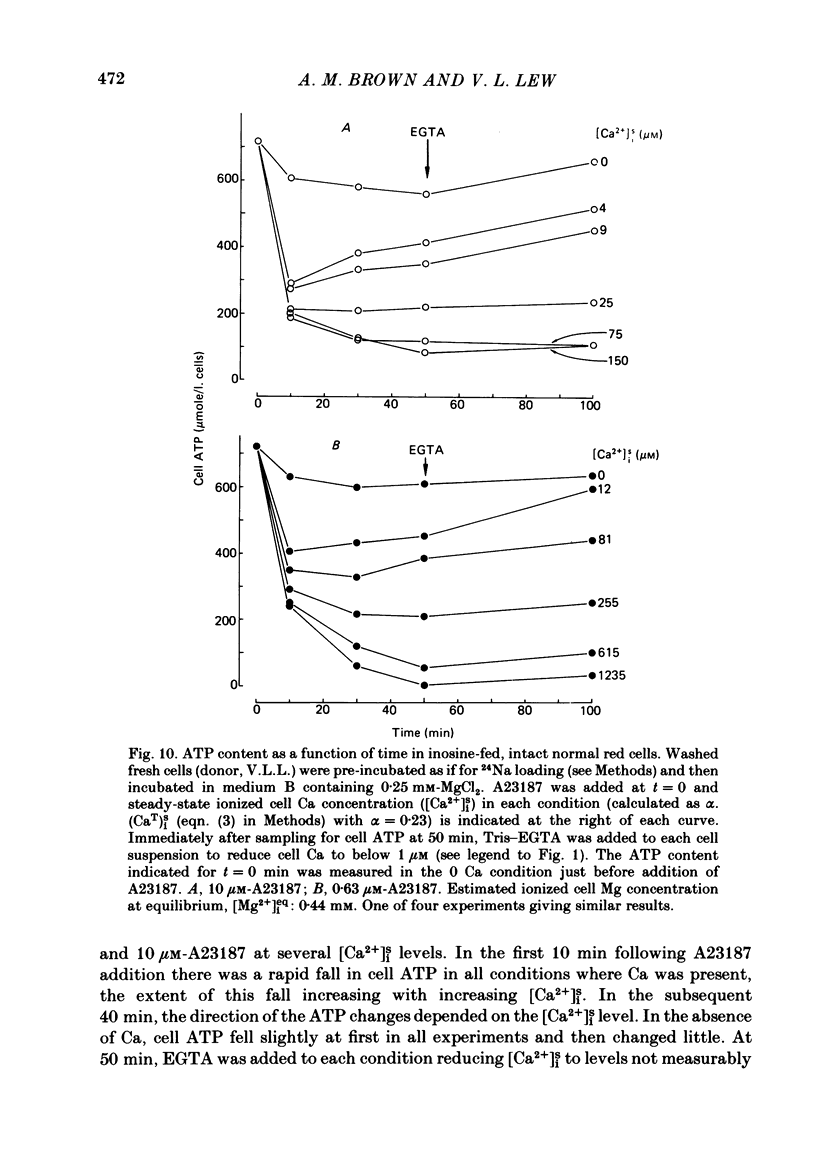
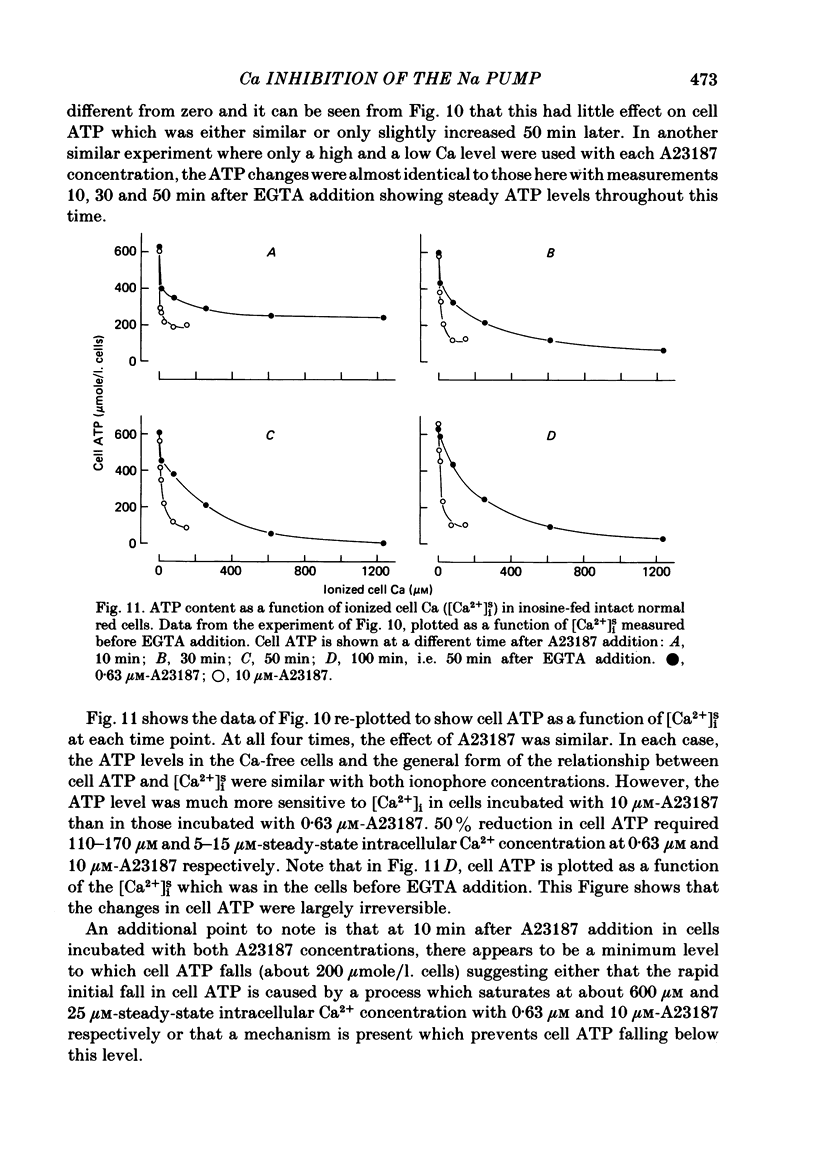
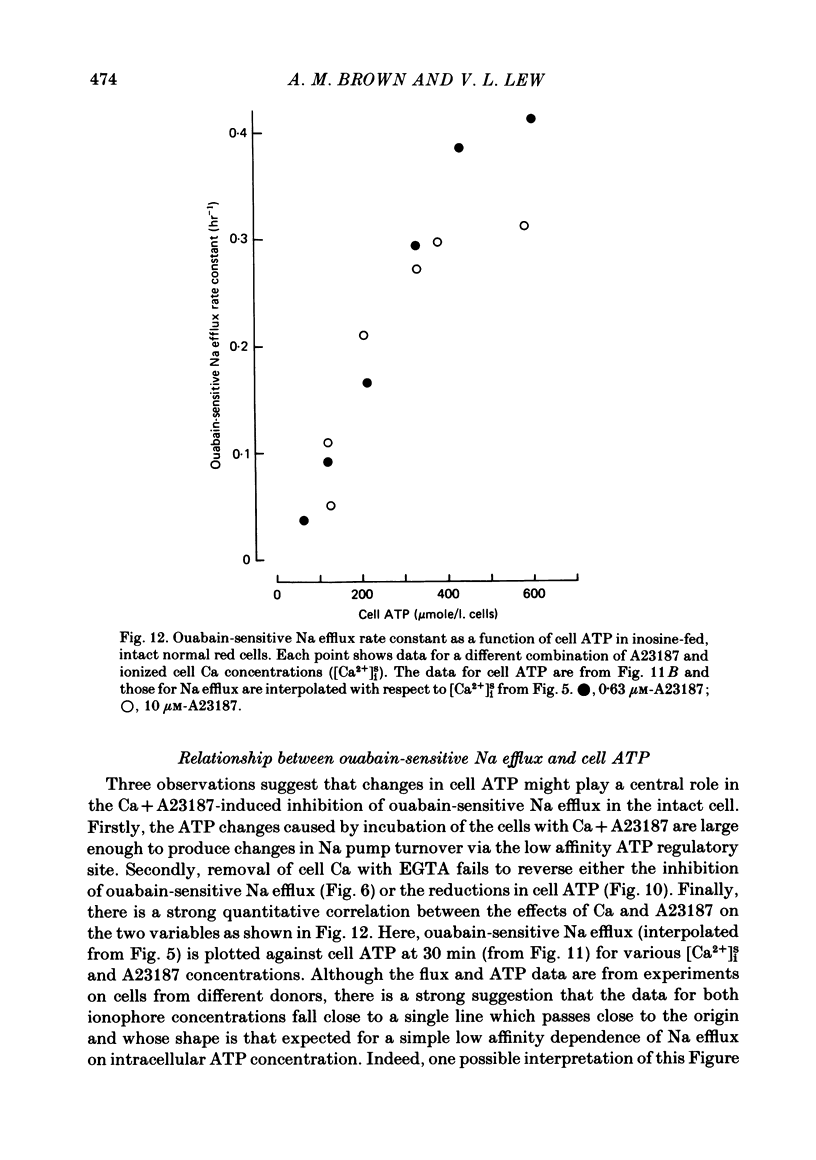
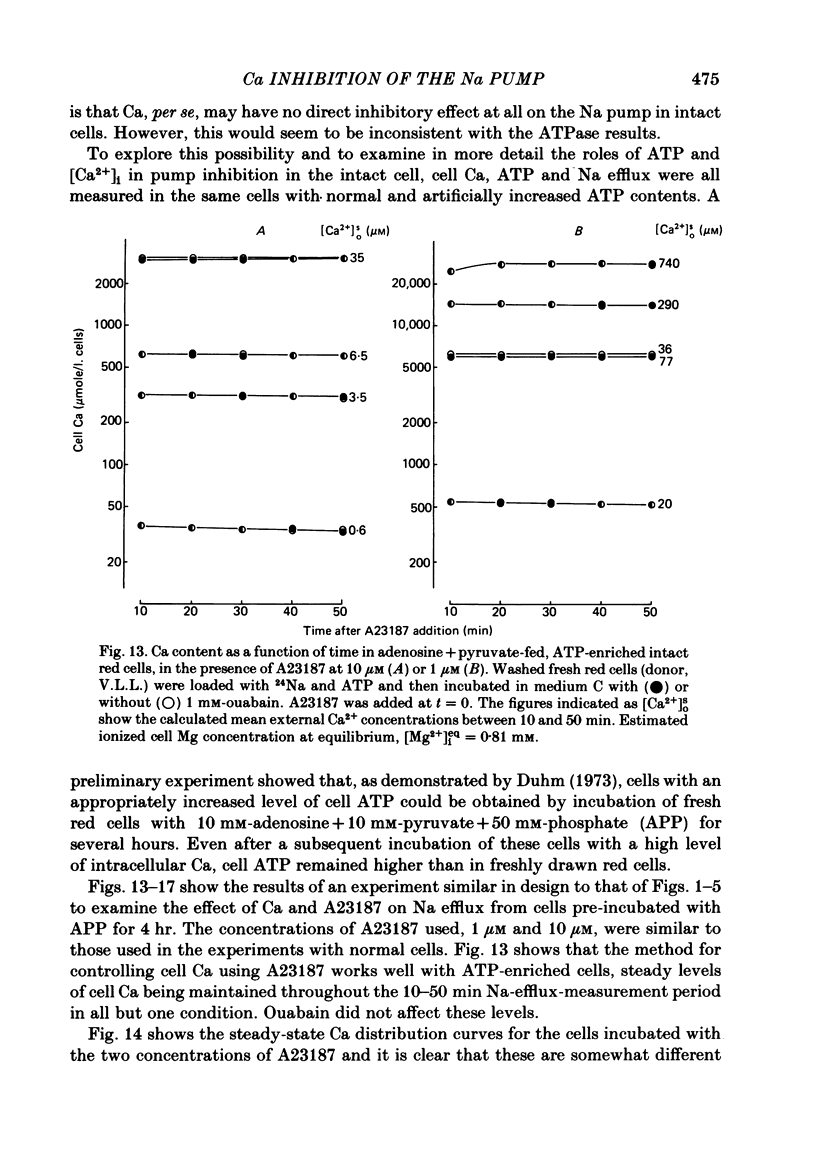
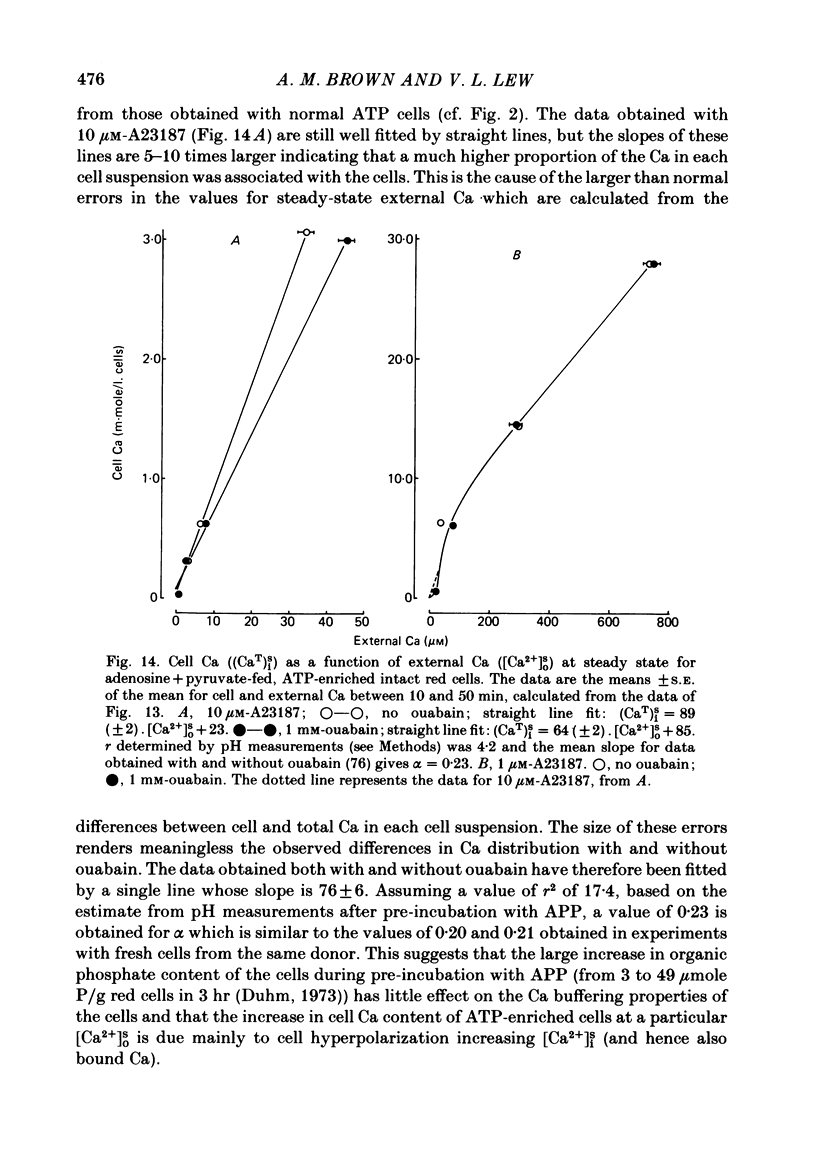
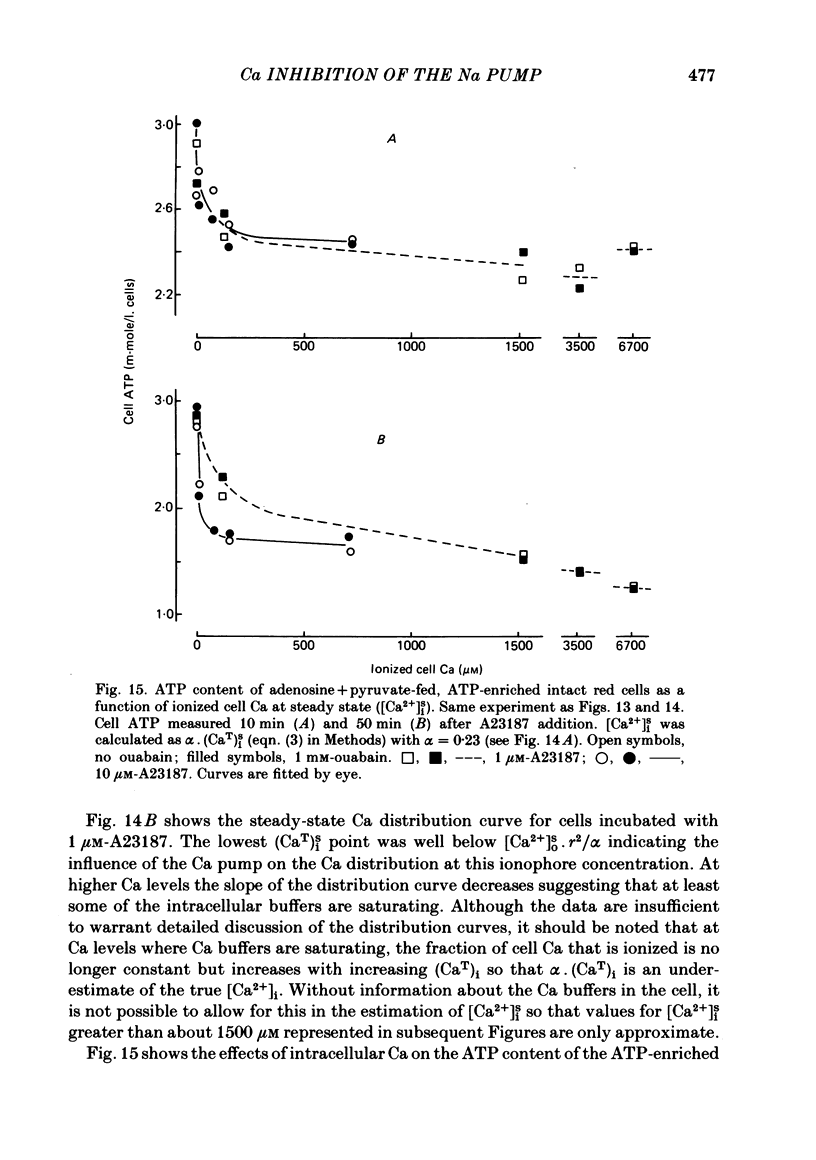
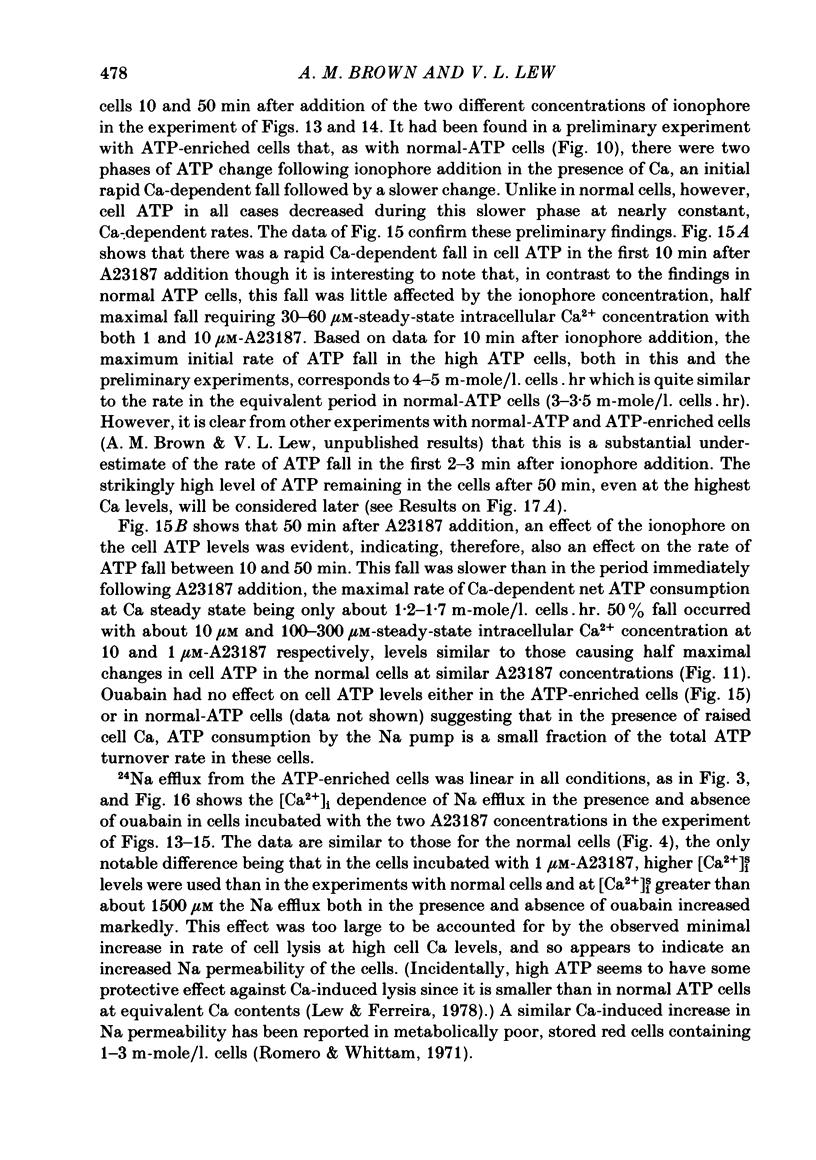
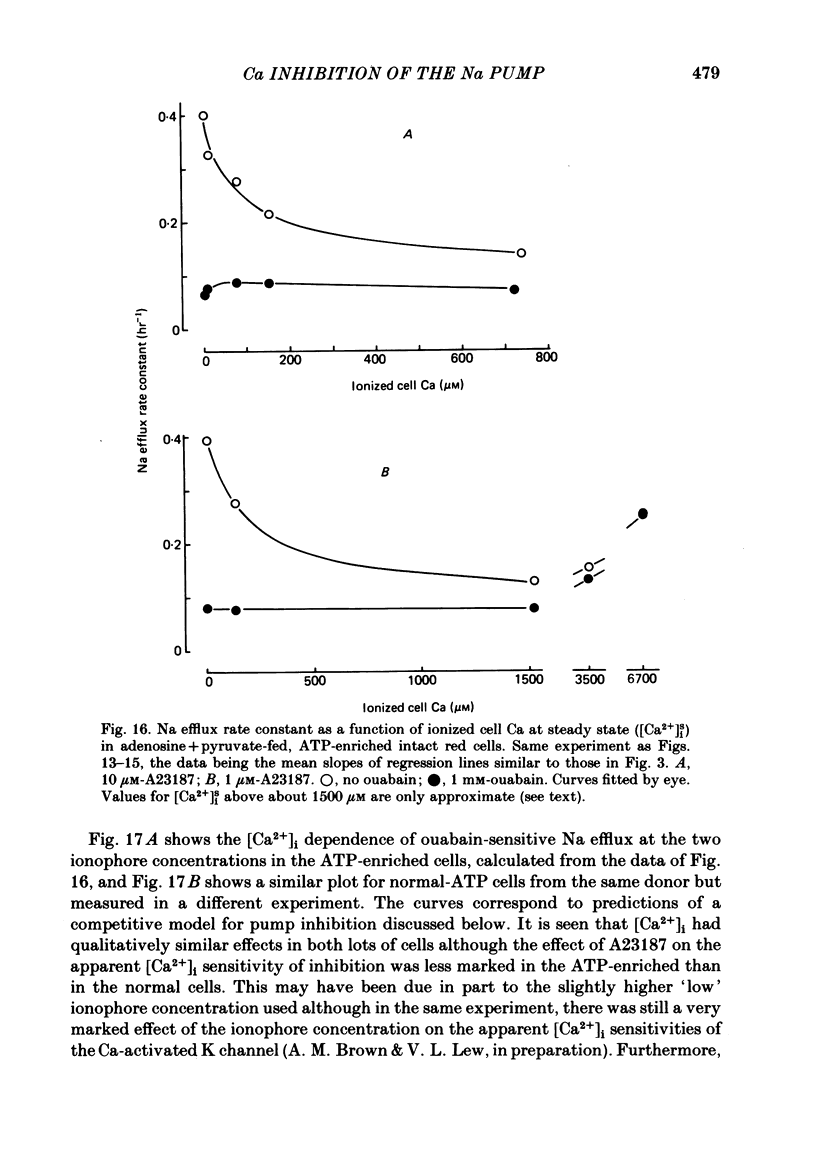
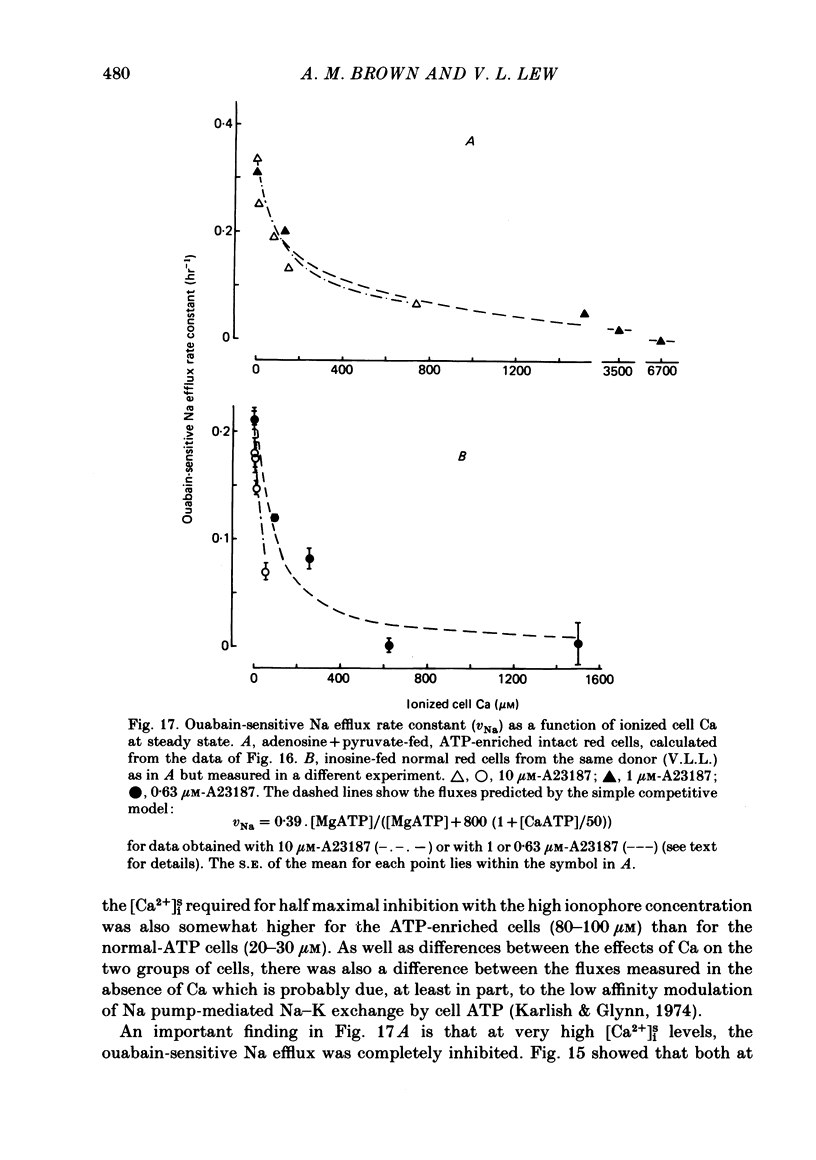
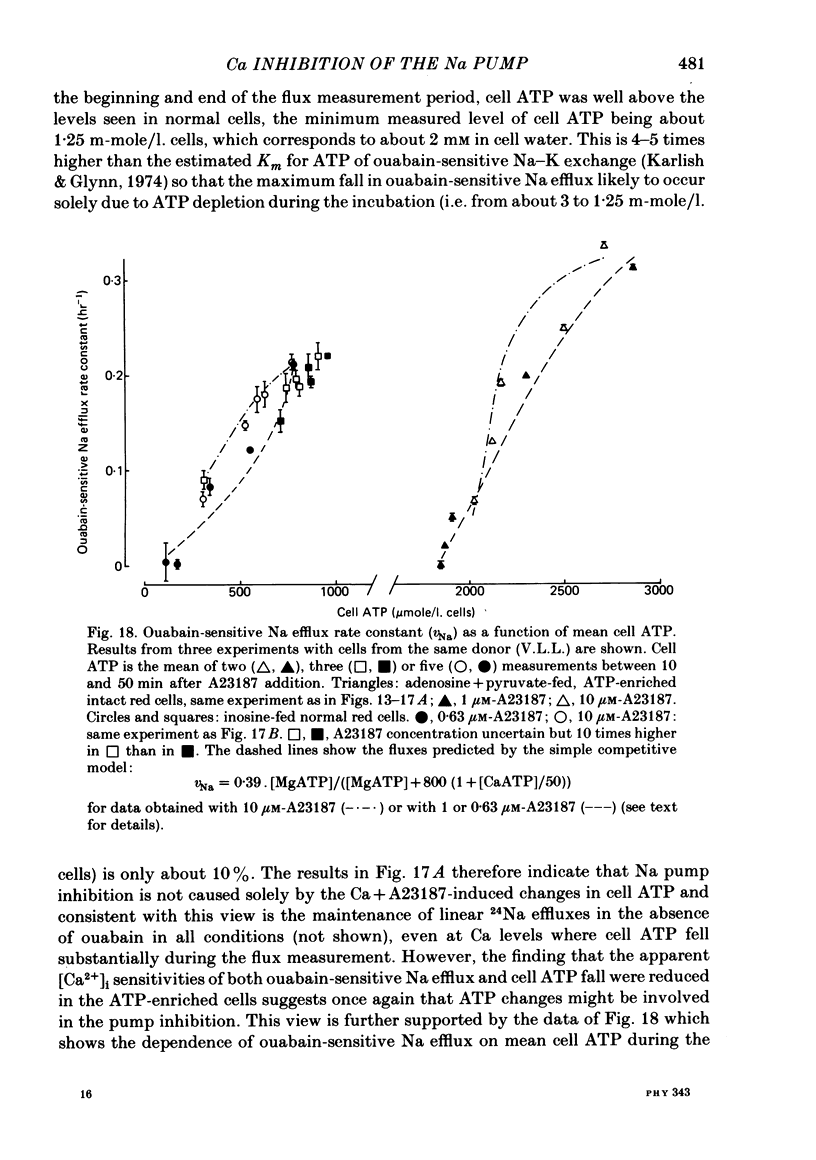
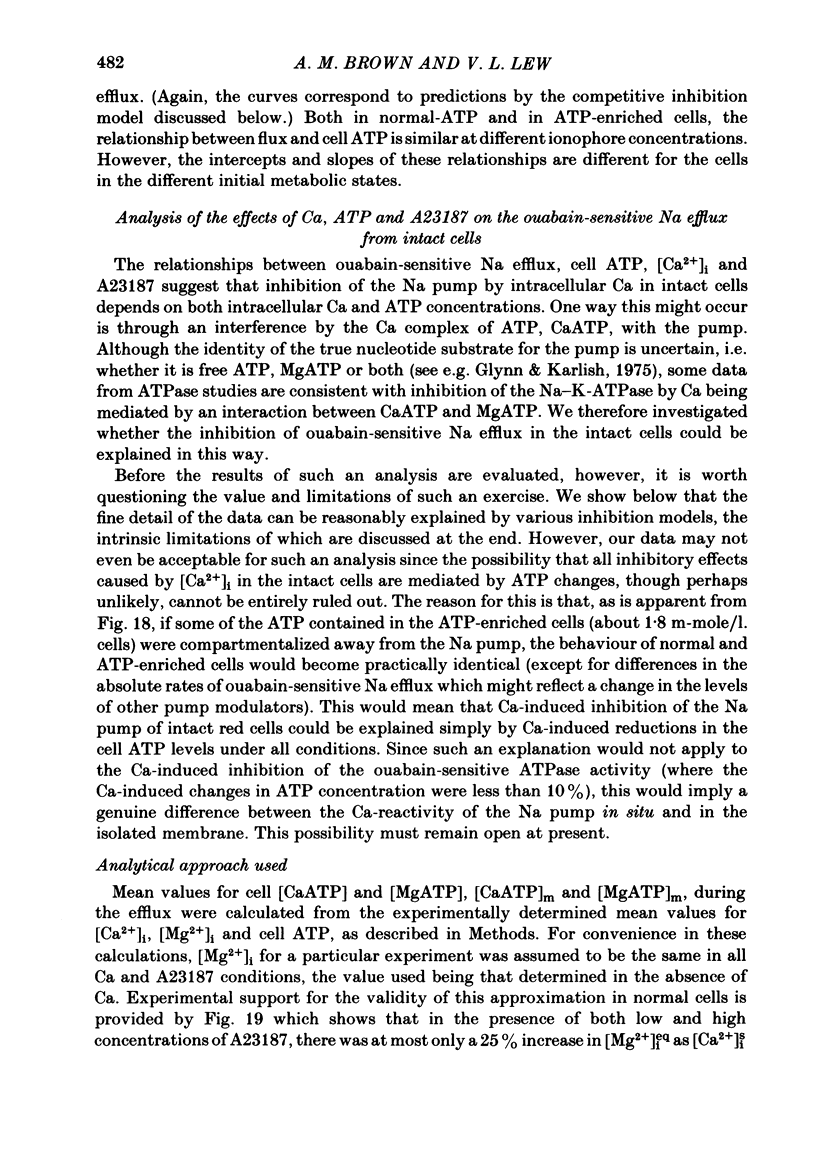
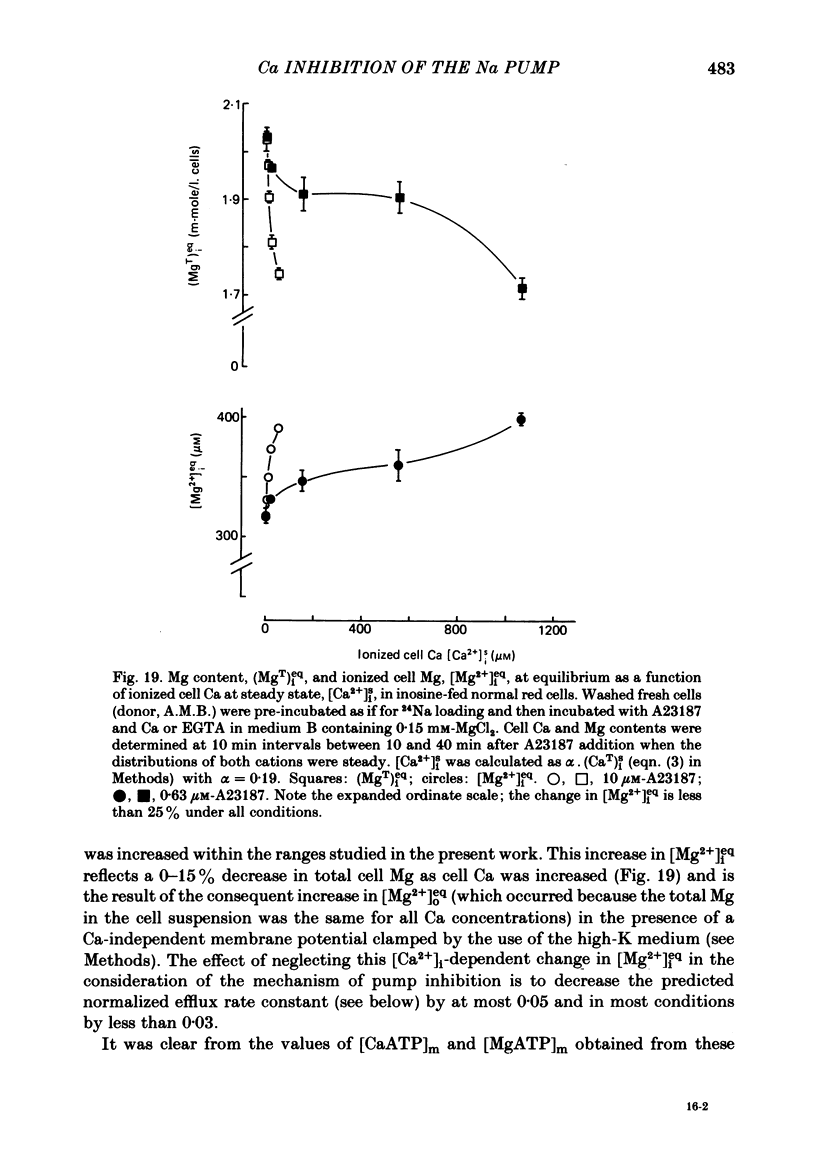
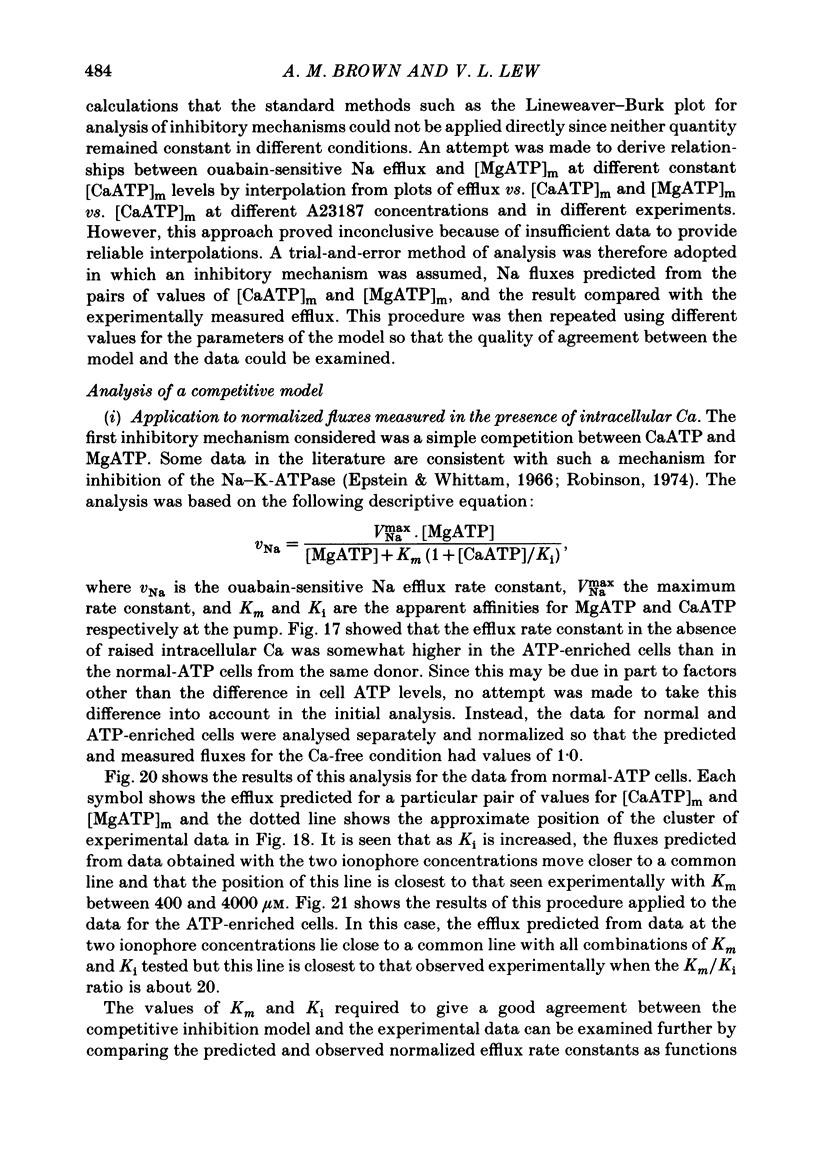
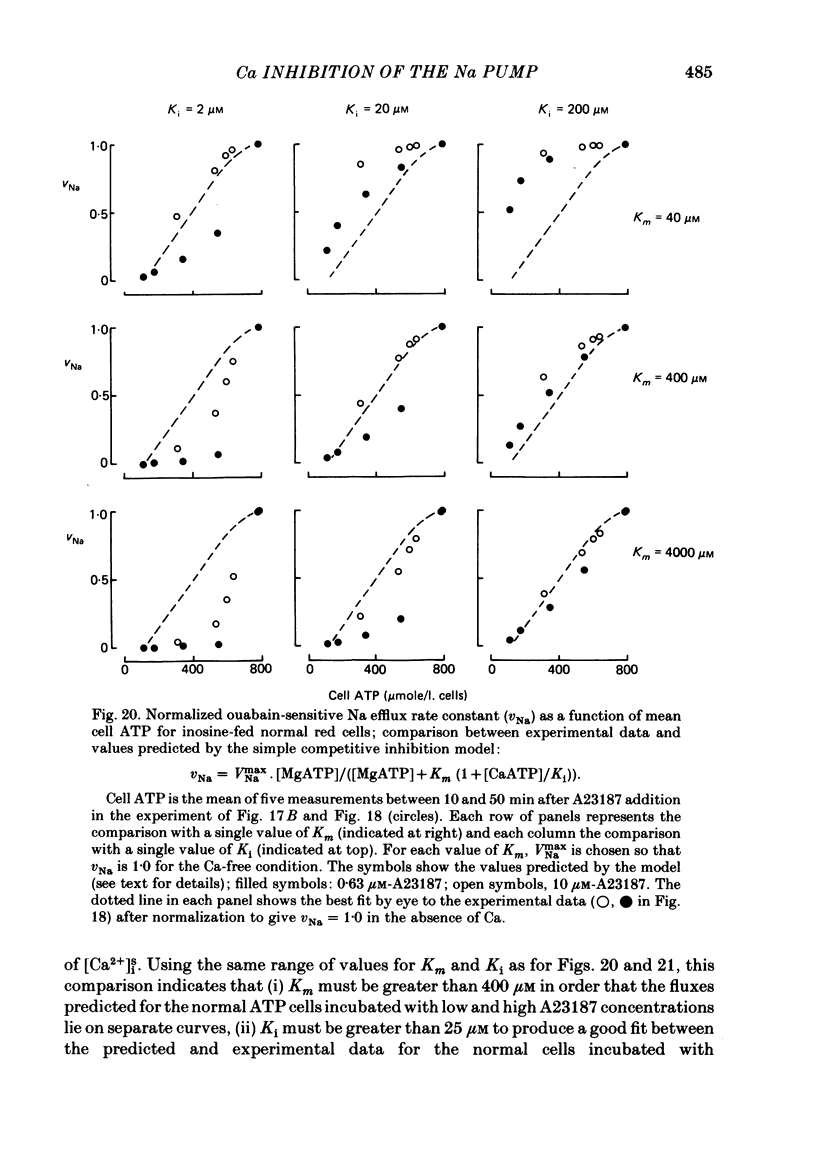
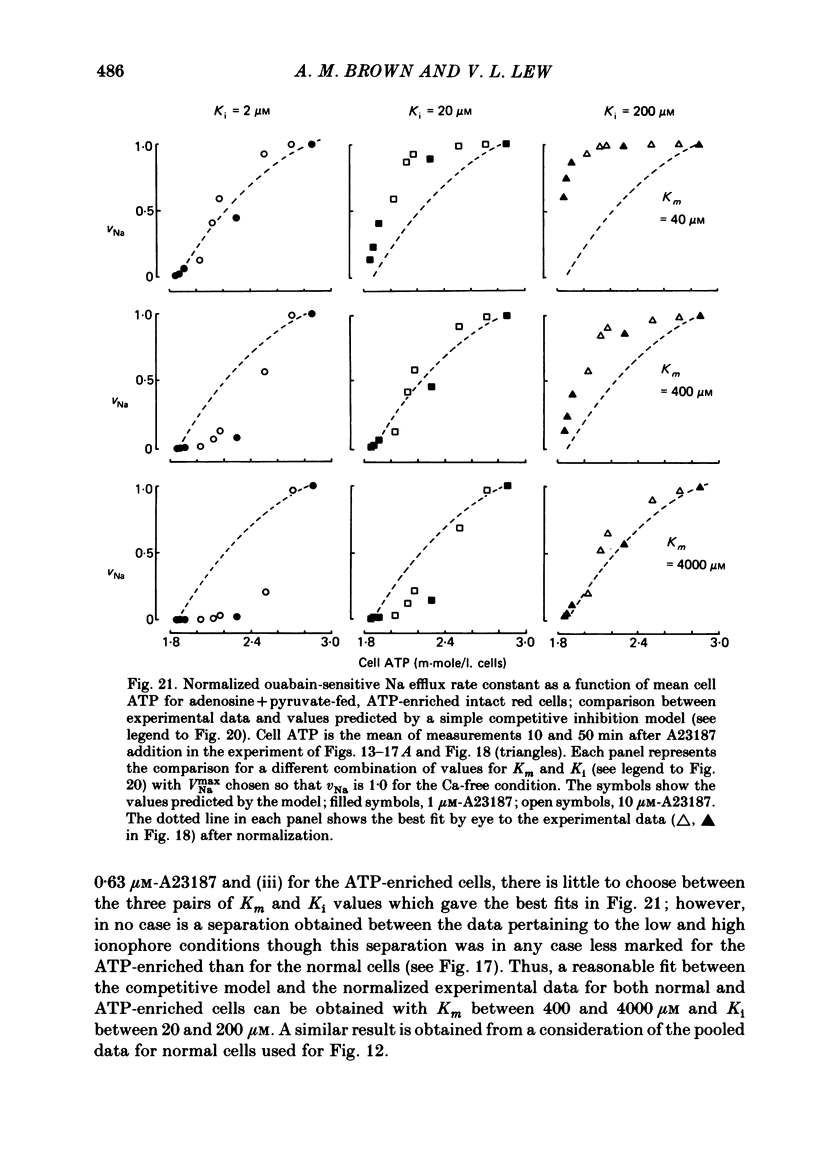
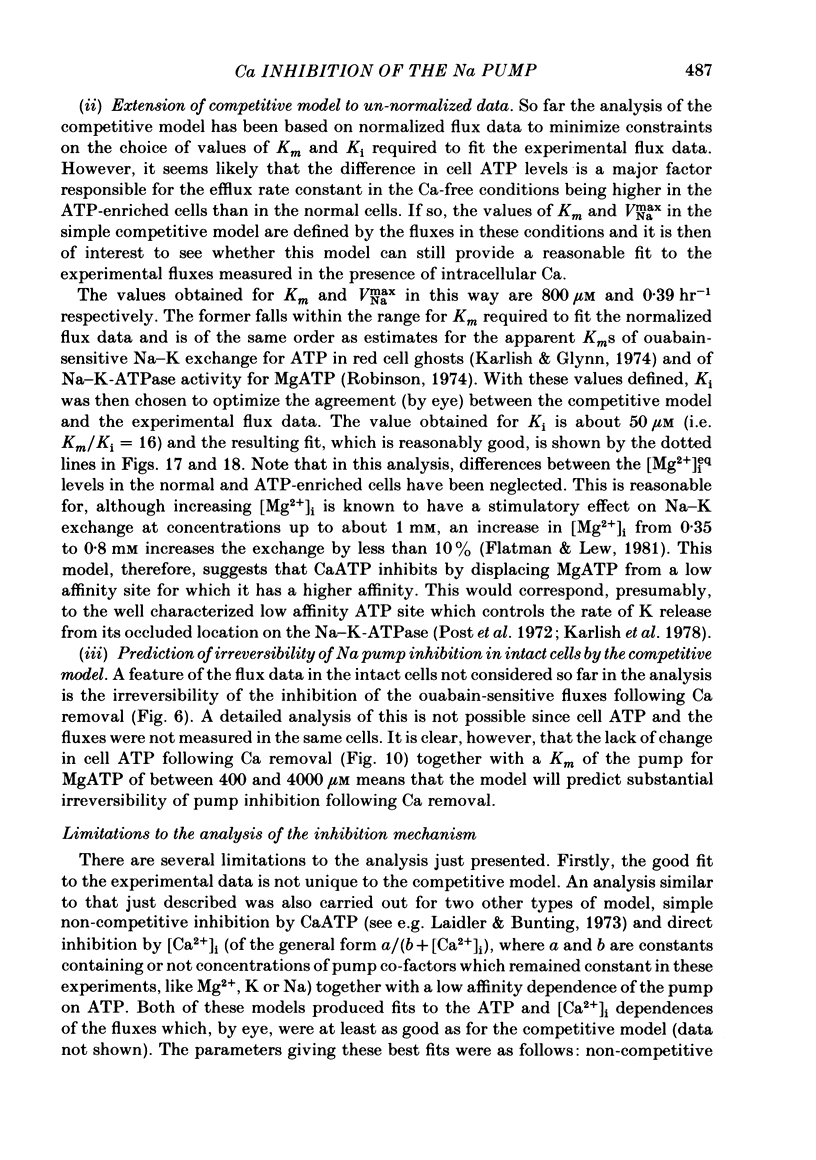
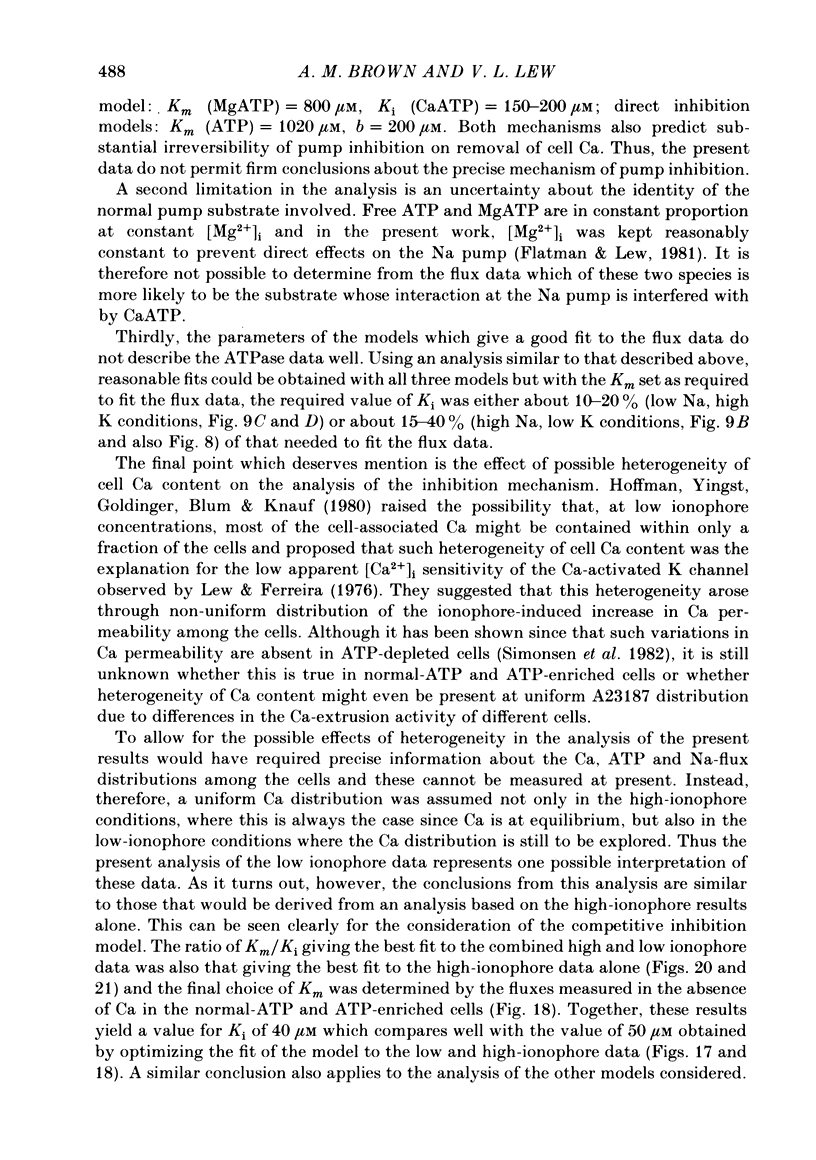
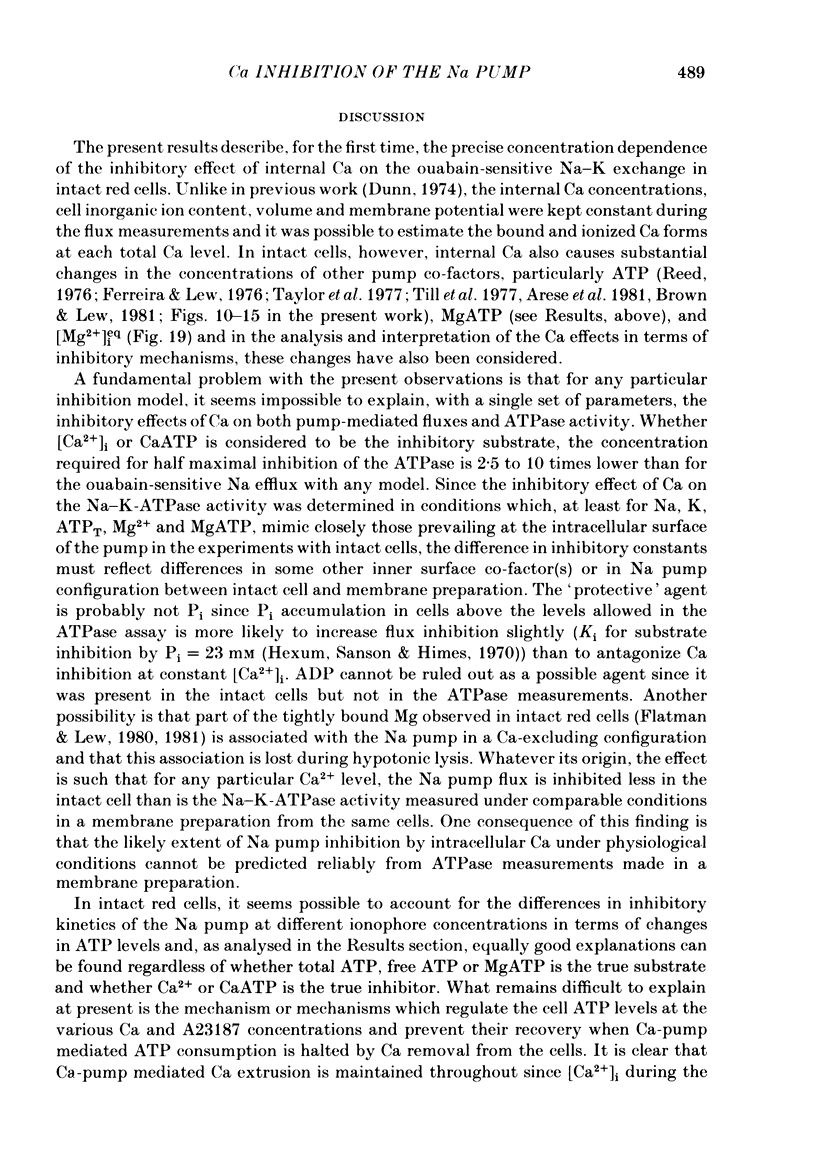
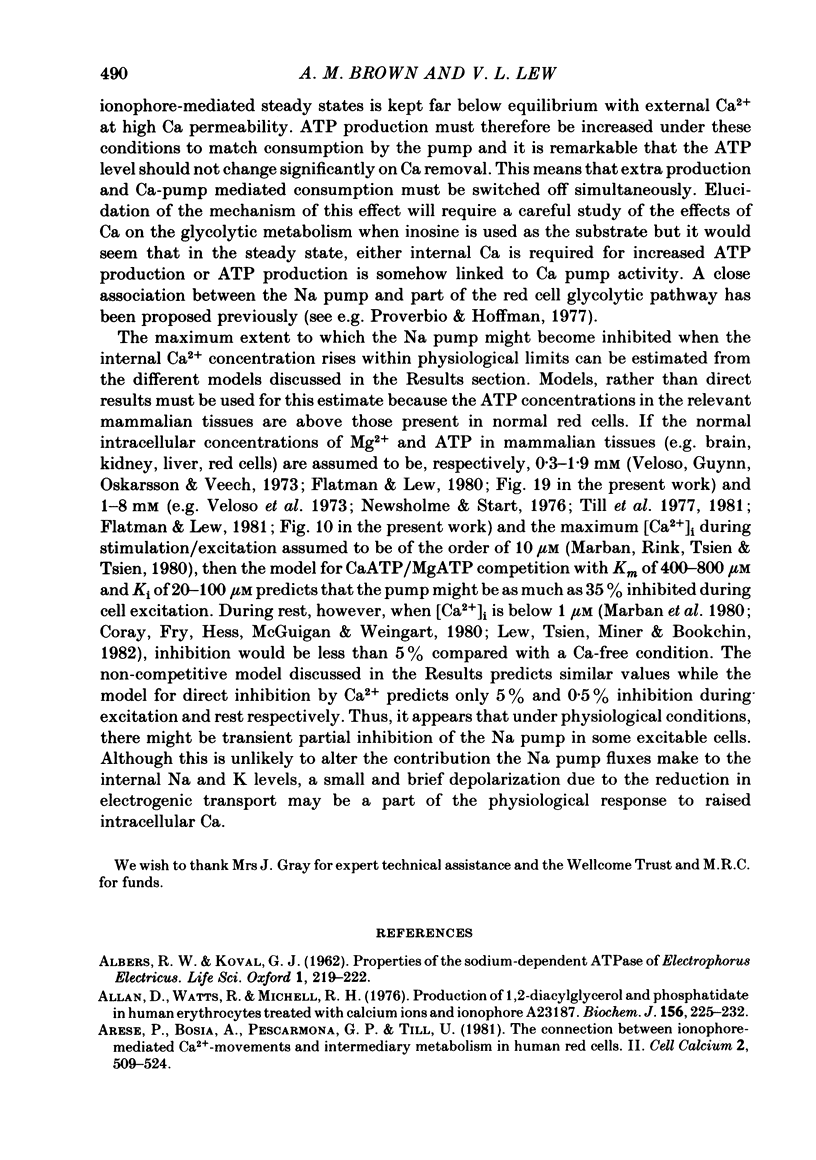
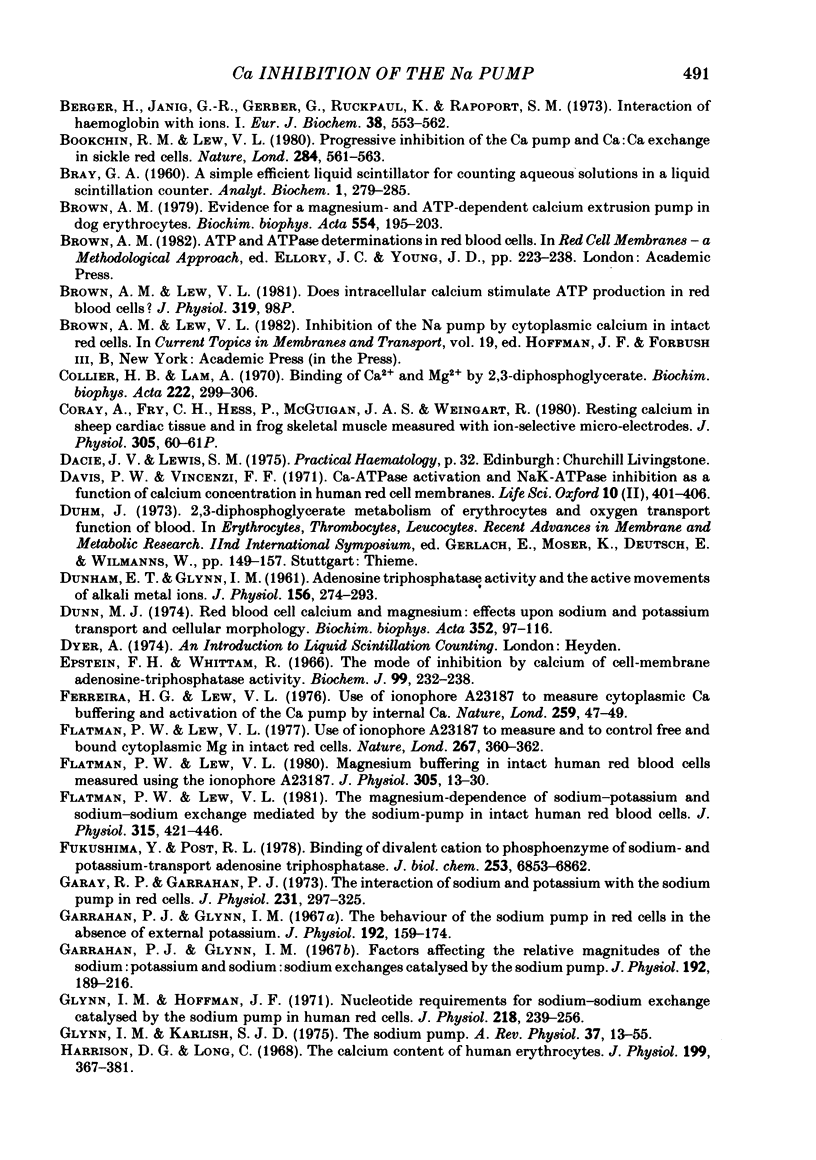
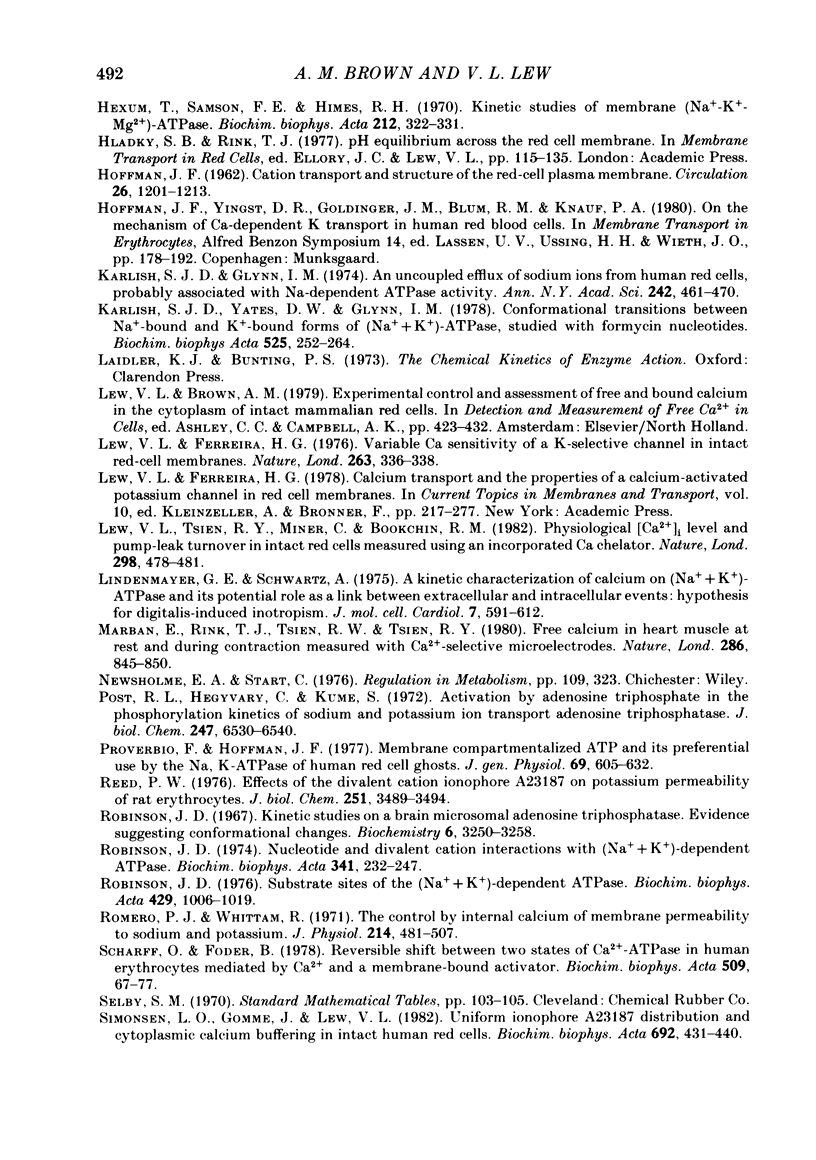
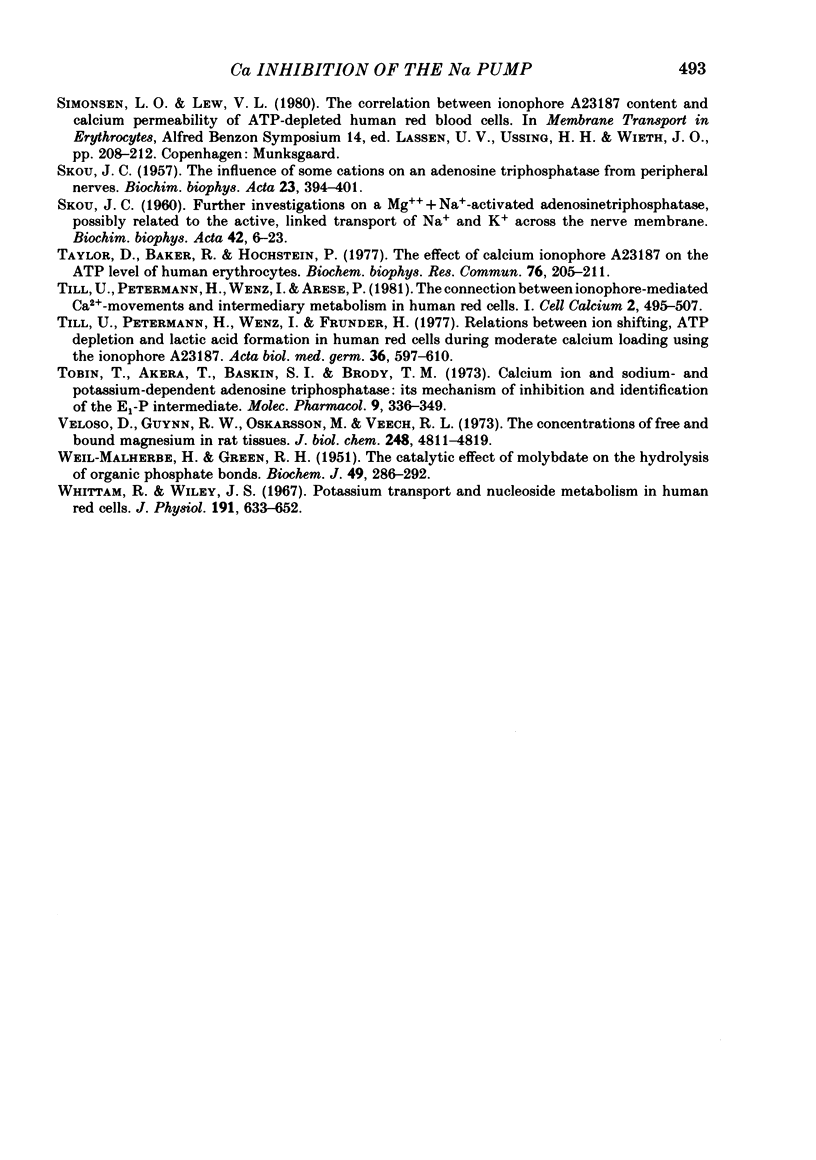
Selected References
These references are in PubMed. This may not be the complete list of references from this article.
- ALBERS R. W., KOVAL G. J. Properties of the sodium-dependent ATPase of Electrophorus electricus. Life Sci. 1962 May;1:219–222. doi: 10.1016/0024-3205(62)90022-x. [DOI] [PubMed] [Google Scholar]
- Allan D., Watts R., Michell R. H. Production of 1,2-diacylglycerol and phosphatidate in human erythrocytes treated with calcium ions and ionophore A23187. Biochem J. 1976 May 15;156(2):225–232. doi: 10.1042/bj1560225. [DOI] [PMC free article] [PubMed] [Google Scholar]
- Berger H., Jänig G. R., Gerber G., Ruckpaul K., Rapoport S. M. Interaction of haemoglobin with ions. Interactions among magnesium, adenosine 5'-triphosphate, 2,3-bisphosphoglycerate, and oxygenated and deoxygenated human haemoglobin under simulated intracellular conditions. Eur J Biochem. 1973 Oct 18;38(3):553–562. doi: 10.1111/j.1432-1033.1973.tb03090.x. [DOI] [PubMed] [Google Scholar]
- Bookchin R. M., Lew V. L. Progressive inhibition of the Ca pump and Ca:Ca exchange in sickle red cells. Nature. 1980 Apr 10;284(5756):561–563. doi: 10.1038/284561a0. [DOI] [PubMed] [Google Scholar]
- Brown A. M. Evidence for a magnesium- and ATP-dependent calcium extrusion pump in dog erythrocytes. Biochim Biophys Acta. 1979 Jun 13;554(1):195–203. doi: 10.1016/0005-2736(79)90018-x. [DOI] [PubMed] [Google Scholar]
- Collier H. B., Lam A. Binding of Ca2+ and Mg2+ by 2,3-diphosphoglycerate. Biochim Biophys Acta. 1970 Nov 24;222(2):299–306. doi: 10.1016/0304-4165(70)90117-0. [DOI] [PubMed] [Google Scholar]
- DUNHAM E. T., GLYNN I. M. Adenosinetriphosphatase activity and the active movements of alkali metal ions. J Physiol. 1961 Apr;156:274–293. doi: 10.1113/jphysiol.1961.sp006675. [DOI] [PMC free article] [PubMed] [Google Scholar]
- Davis P. W., Vincenzi F. F. Ca-ATPase activation and NaK-ATPase inhibition as a function of calcium concentration in human red cell membranes. Life Sci II. 1971 Apr 8;10(7):401–406. doi: 10.1016/0024-3205(71)90051-8. [DOI] [PubMed] [Google Scholar]
- Dunn M. J. Red blood cell calcium and magnesium: effects upon sodium and potassium transport and cellular morphology. Biochim Biophys Acta. 1974 May 30;352(1):97–116. doi: 10.1016/0005-2736(74)90182-5. [DOI] [PubMed] [Google Scholar]
- Epstein F. H., Whittam R. The mode of inhibition by calcium of cell-membrane adenosine-triphosphatase activity. Biochem J. 1966 Apr;99(1):232–238. doi: 10.1042/bj0990232. [DOI] [PMC free article] [PubMed] [Google Scholar]
- Ferreira H. G., Lew V. L. Use of ionophore A23187 to measure cytoplasmic Ca buffering and activation of the Ca pump by internal Ca. Nature. 1976 Jan 1;259(5538):47–49. doi: 10.1038/259047a0. [DOI] [PubMed] [Google Scholar]
- Flatman P. W., Lew V. L. Magnesium buffering in intact human red blood cells measured using the ionophore A23187. J Physiol. 1980 Aug;305:13–30. doi: 10.1113/jphysiol.1980.sp013346. [DOI] [PMC free article] [PubMed] [Google Scholar]
- Flatman P. W., Lew V. L. The magnesium dependence of sodium-pump-mediated sodium-potassium and sodium-sodium exchange in intact human red cells. J Physiol. 1981 Jun;315:421–446. doi: 10.1113/jphysiol.1981.sp013756. [DOI] [PMC free article] [PubMed] [Google Scholar]
- Flatman P., Lew V. L. Use of ionophore A23187 to measure and to control free and bound cytoplasmic Mg in intact red cells. Nature. 1977 May 26;267(5609):360–362. doi: 10.1038/267360a0. [DOI] [PubMed] [Google Scholar]
- Fukushima Y., Post R. L. Binding of divalent cation to phosphoenzyme of sodium- and potassium-transport adenosine triphosphatase. J Biol Chem. 1978 Oct 10;253(19):6853–6862. [PubMed] [Google Scholar]
- Garay R. P., Garrahan P. J. The interaction of sodium and potassium with the sodium pump in red cells. J Physiol. 1973 Jun;231(2):297–325. doi: 10.1113/jphysiol.1973.sp010234. [DOI] [PMC free article] [PubMed] [Google Scholar]
- Garrahan P. J., Glynn I. M. Facftors affecting the relative magnitudes of the sodium:potassium and sodium:sodium exchanges catalysed by the sodium pump. J Physiol. 1967 Sep;192(1):189–216. doi: 10.1113/jphysiol.1967.sp008296. [DOI] [PMC free article] [PubMed] [Google Scholar]
- Garrahan P. J., Glynn I. M. The behaviour of the sodium pump in red cells in the absence of external potassium. J Physiol. 1967 Sep;192(1):159–174. doi: 10.1113/jphysiol.1967.sp008294. [DOI] [PMC free article] [PubMed] [Google Scholar]
- Glynn I. M., Hoffman J. F. Nucleotide requirements for sodium-sodium exchange catalysed by the sodium pump in human red cells. J Physiol. 1971 Oct;218(1):239–256. doi: 10.1113/jphysiol.1971.sp009612. [DOI] [PMC free article] [PubMed] [Google Scholar]
- Glynn I. M., Karlish S. J. The sodium pump. Annu Rev Physiol. 1975;37:13–55. doi: 10.1146/annurev.ph.37.030175.000305. [DOI] [PubMed] [Google Scholar]
- HOFFMAN J. F. Cation transport and structure of the red-cell plasma membrane. Circulation. 1962 Nov;26:1202–1213. doi: 10.1161/01.cir.26.5.1201. [DOI] [PubMed] [Google Scholar]
- Harrison D. G., Long C. The calcium content of human erythrocytes. J Physiol. 1968 Dec;199(2):367–381. doi: 10.1113/jphysiol.1968.sp008658. [DOI] [PMC free article] [PubMed] [Google Scholar]
- Hexum T., Samson F. E., Jr, Himes R. H. Kinetic studies of membrane (Na+-K+-Mg2+)-ATPase. Biochim Biophys Acta. 1970 Aug 15;212(2):322–331. doi: 10.1016/0005-2744(70)90213-5. [DOI] [PubMed] [Google Scholar]
- Karlish S. J., Glynn I. M. An uncoupled efflux of sodium ions from human red cells, probably associated with Na-dependent ATPase activity. Ann N Y Acad Sci. 1974;242(0):461–470. doi: 10.1111/j.1749-6632.1974.tb19110.x. [DOI] [PubMed] [Google Scholar]
- Karlish S. J., Yates D. W., Glynn I. M. Conformational transitions between Na+-bound and K+-bound forms of (Na+ + K+)-ATPase, studied with formycin nucleotides. Biochim Biophys Acta. 1978 Jul 7;525(1):252–264. doi: 10.1016/0005-2744(78)90219-x. [DOI] [PubMed] [Google Scholar]
- Lew V. L., Ferreira H. G. Variable Ca sensitivity of a K-selective channel in intact red-cell membranes. Nature. 1976 Sep 23;263(5575):336–338. doi: 10.1038/263336a0. [DOI] [PubMed] [Google Scholar]
- Lew V. L., Tsien R. Y., Miner C., Bookchin R. M. Physiological [Ca2+]i level and pump-leak turnover in intact red cells measured using an incorporated Ca chelator. Nature. 1982 Jul 29;298(5873):478–481. doi: 10.1038/298478a0. [DOI] [PubMed] [Google Scholar]
- Lindenmayer G. E., Schwartz A. A kinetic characterization of calcium on (Na+ + K+)-ATPase and its potential role as a link between extracellular and intracellular events: hypothesis for digitalis-induced inotropism. J Mol Cell Cardiol. 1975 Aug;7(8):591–612. doi: 10.1016/0022-2828(75)90117-0. [DOI] [PubMed] [Google Scholar]
- Marban E., Rink T. J., Tsien R. W., Tsien R. Y. Free calcium in heart muscle at rest and during contraction measured with Ca2+ -sensitive microelectrodes. Nature. 1980 Aug 28;286(5776):845–850. doi: 10.1038/286845a0. [DOI] [PubMed] [Google Scholar]
- Post R. L., Hegyvary C., Kume S. Activation by adenosine triphosphate in the phosphorylation kinetics of sodium and potassium ion transport adenosine triphosphatase. J Biol Chem. 1972 Oct 25;247(20):6530–6540. [PubMed] [Google Scholar]
- Proverbio F., Hoffman J. F. Membrane compartmentalized ATP and its preferential use by the Na,K-ATPase of human red cell ghosts. J Gen Physiol. 1977 May;69(5):605–632. doi: 10.1085/jgp.69.5.605. [DOI] [PMC free article] [PubMed] [Google Scholar]
- Reed P. W. Effects of divalent cation ionophore A23187 on potassium permeability of rat erythrocytes. J Biol Chem. 1976 Jun 10;251(11):3489–3494. [PubMed] [Google Scholar]
- Robinson J. D. Kinetic studies on a brain microsomal adenosine triphosphatase. Evidence suggesting conformational changes. Biochemistry. 1967 Oct;6(10):3250–3258. doi: 10.1021/bi00862a034. [DOI] [PubMed] [Google Scholar]
- Robinson J. D. Nucleotide and divalent cation interactions with the (Na+ plus K+)-dependent ATPase. Biochim Biophys Acta. 1974 Mar 21;341(1):232–247. doi: 10.1016/0005-2744(74)90084-9. [DOI] [PubMed] [Google Scholar]
- Robinson J. D. Substrate sites for the (Na+ + K+)-dependent ATPase. Biochim Biophys Acta. 1976 May 13;429(3):1006–1019. doi: 10.1016/0005-2744(76)90345-4. [DOI] [PubMed] [Google Scholar]
- Romero P. J., Whittam R. The control by internal calcium of membrane permeability to sodium and potassium. J Physiol. 1971 May;214(3):481–507. doi: 10.1113/jphysiol.1971.sp009445. [DOI] [PMC free article] [PubMed] [Google Scholar]
- SKOU J. C. The influence of some cations on an adenosine triphosphatase from peripheral nerves. Biochim Biophys Acta. 1957 Feb;23(2):394–401. doi: 10.1016/0006-3002(57)90343-8. [DOI] [PubMed] [Google Scholar]
- Scharff O., Foder B. Reversible shift between two states of Ca2+-ATPase in human erythrocytes mediated by Ca2+ and a membrane-bound activator. Biochim Biophys Acta. 1978 May 4;509(1):67–77. doi: 10.1016/0005-2736(78)90008-1. [DOI] [PubMed] [Google Scholar]
- Simonsen L. O., Gomme J., Lew V. L. Uniform ionophore A23187 distribution and cytoplasmic calcium buffering in intact human red cells. Biochim Biophys Acta. 1982 Nov 22;692(3):431–440. doi: 10.1016/0005-2736(82)90394-7. [DOI] [PubMed] [Google Scholar]
- Taylor D., Baker R., Hochstein P. The effect of calcium ionophore A23187 on the ATP level of human erythrocytes. Biochem Biophys Res Commun. 1976 May 23;76(2):205–211. doi: 10.1016/0006-291x(77)90712-4. [DOI] [PubMed] [Google Scholar]
- Till U., Petermann H., Wenz I., Frunder H. Relations between ion shifting, ATP depletion and lactic acid formation in human red cells during moderate calcium loading using the ionophore A 23187. Acta Biol Med Ger. 1977;36(3-4):597–610. [PubMed] [Google Scholar]
- Tobin T., Akera T., Baskin S. I., Brody T. M. Calcium ion and sodium- and potassium-dependent adenosine triphosphatase: its mechanism of inhibition and identification of the E 1 -P intermediate. Mol Pharmacol. 1973 May;9(3):336–349. [PubMed] [Google Scholar]
- Veloso D., Guynn R. W., Oskarsson M., Veech R. L. The concentrations of free and bound magnesium in rat tissues. Relative constancy of free Mg 2+ concentrations. J Biol Chem. 1973 Jul 10;248(13):4811–4819. [PubMed] [Google Scholar]
- WEIL-MALHERBE H., GREEN R. H. The catalytic effect of molybdate on the hydrolysis of organic phosphate bonds. Biochem J. 1951 Aug;49(3):286–292. [PMC free article] [PubMed] [Google Scholar]
- Whittam R., Wiley J. S. Potassium transport and nucleoside metabolism in human red cells. J Physiol. 1967 Aug;191(3):633–652. doi: 10.1113/jphysiol.1967.sp008272. [DOI] [PMC free article] [PubMed] [Google Scholar]