Abstract
1. The shapes of post-synaptic potentials (p.s.p.s) in cat motoneurones were compared with the time course of changes in firing probability during repetitive firing. Excitatory and inhibitory post-synaptic potentials (e.p.s.p.s and i.p.s.p.s) were evoked by electrical stimulation of peripheral nerve filaments. With the motoneurone quiescent, the shape of each p.s.p. was obtained by compiling post-stimulus averages of the membrane potential. Depolarizing current was then injected to evoke repetitive firing, and the post-stimulus time histogram of motoneurone spikes was obtained; this histogram reveals the primary features (peak and/or trough) of the cross-correlogram between stimulus and spike trains. The time course of the correlogram features produced by each p.s.p. was compared with the p.s.p. shape and its temporal derivative.
2. E.p.s.p.s of different sizes (0.15-3.1 mV, mean 0.75 mV) and shapes were investigated. The primary correlogram peak began, on the average, 0.48 msec after onset of the e.p.s.p., and reached a maximum 0.29 msec before the summit of the e.p.s.p; in many cases the correlogram peak was followed by a trough, in which firing rate fell below base-line rate. The height of the correlogram peak with respect to base-line firing rate increased in proportion to both the amplitude of the e.p.s.p.s and the magnitude of their rising slope (in these data, amplitude and rising slope also covaried).
3. The mean half-width of the correlogram peaks (0.65±0.28 msec (S.D.)) agreed better with the average half-width of the e.p.s.p. derivatives (0.55±0.33 msec) than with the half-width of the e.p.s.p.s (4.31±1.50 msec). The shape of the primary correlogram peak produced by simple e.p.s.p.s often resembled the temporal derivative of the e.p.s.p. rise. For larger e.p.s.p.s, the shape of the correlogram peak closely matched the e.p.s.p. derivative, while smaller e.p.s.p.s in appreciable synaptic noise often generated correlogram peaks somewhat wider than their derivatives. On the other hand, the match between the correlogram trough that followed the peak and the negative slope of the e.p.s.p. was better for the small e.p.s.p.s than for the large e.p.s.p.s; for large e.p.s.p.s the drop in firing rate during the trough was typically limited at zero. These relations were tested further by comparing the integral of the correlogram with the time course of the e.p.s.p. For large e.p.s.p.s, the correlogram integral matched the rising phase of the e.p.s.p. quite well, although it underestimated the rate of decline of the e.p.s.p.
4. Complex e.p.s.p.s with distinct components during their rising phase often produced correlogram peaks that did not accurately reflect the features in their temporal derivative. Temporal summation of large e.p.s.p.s and summation of their derivatives was linear, but the resulting correlogram peaks did not add linearly; the second correlogram peak was often smaller than the first. However, when small e.p.s.p.s were summed, the correlogram peaks more closely matched the e.p.s.p. derivatives.
5. Compound i.p.s.p.s produced primary correlogram troughs followed by a shallow compensatory peak. The width of the trough extended through the peak of the i.p.s.p., well into the falling phase of the i.p.s.p. During the trough the firing rate usually dropped to zero. Thus, the primary correlogram features produced by large i.p.s.p.s did not resemble any linear combination of the shape of the i.p.s.p. and/or its temporal derivative. Moreover, the integral of the correlogram did not resemble the i.p.s.p.
6. The major observations are consistent with a motoneurone model in which a membrane potential ramp approaches a voltage threshold for spike initiation. Near threshold, e.p.s.p.s superimposed on the ramp advance the occurrence of spikes to their rising phase, producing a correlogram peak resembling their temporal derivative. Synaptic noise would increase the probability of sampling the peak of the e.p.s.p., leading to wider correlogram peaks. I.p.s.p.s would delay the occurrence of spikes to their falling phase.
Full text
PDF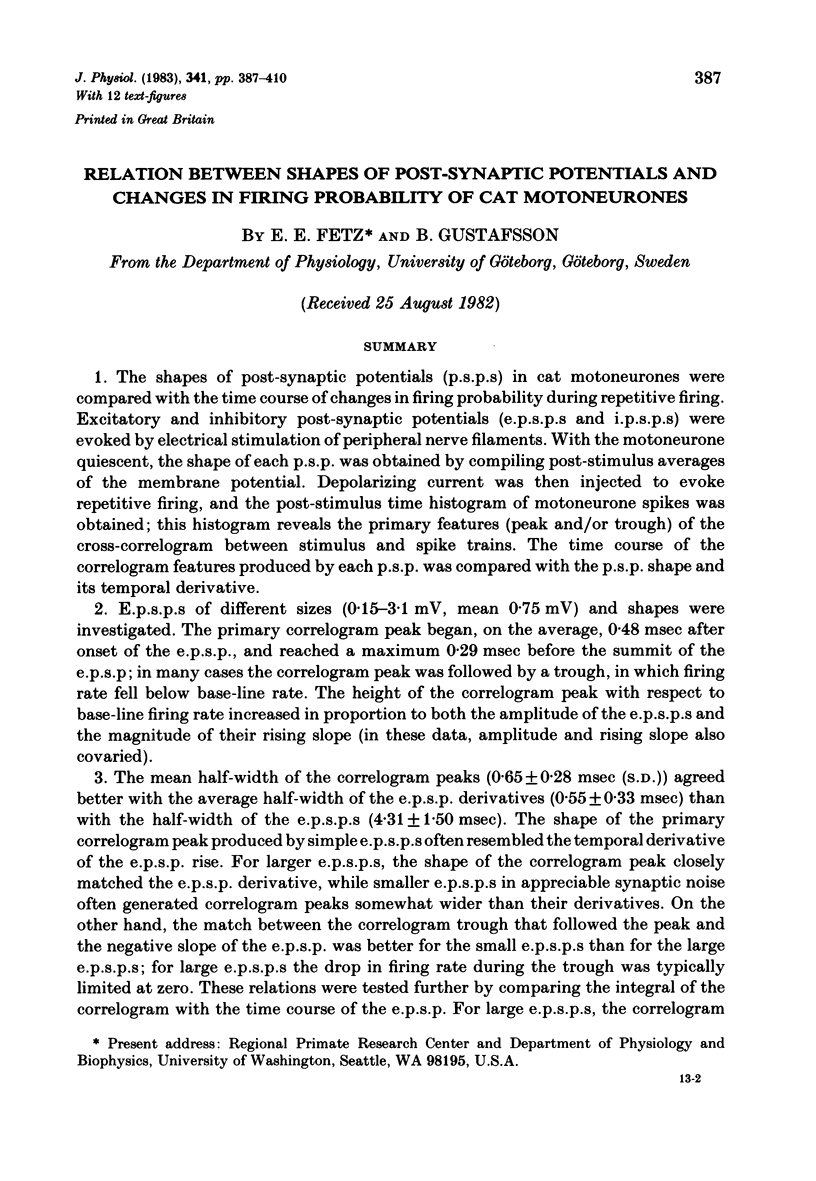
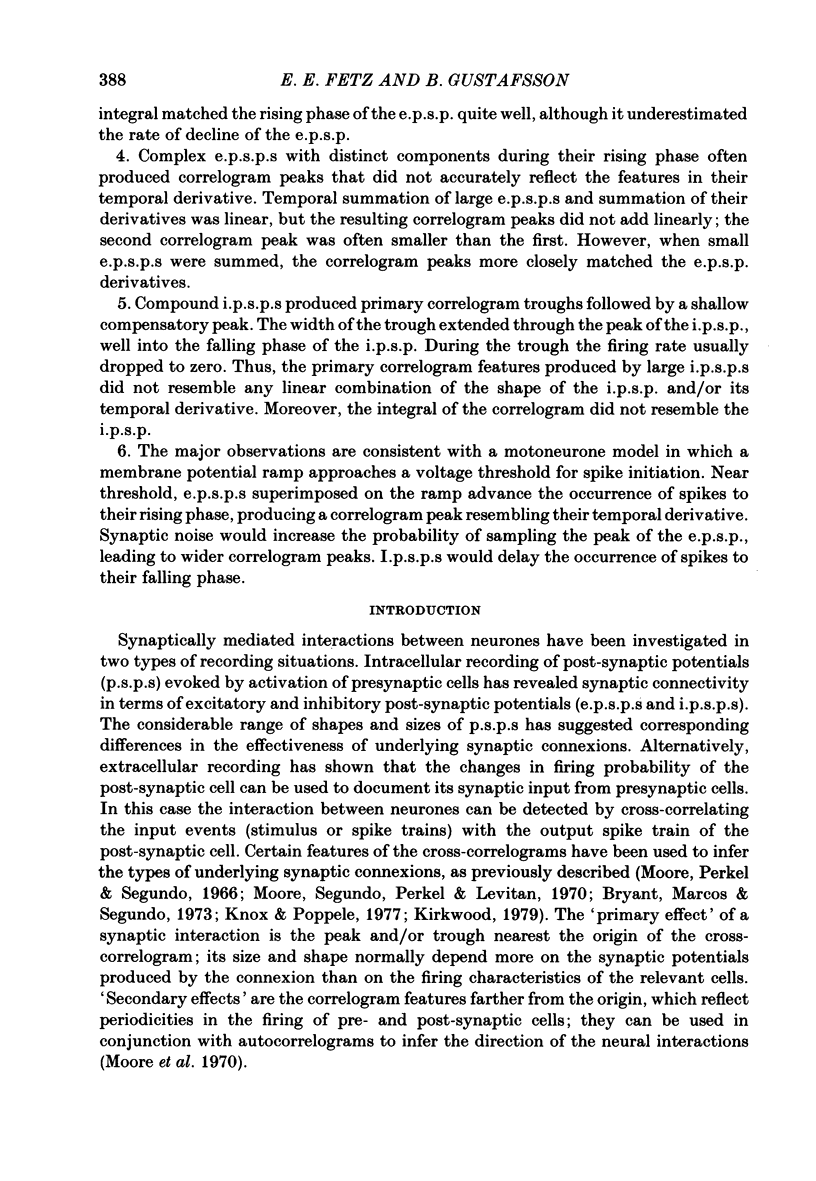
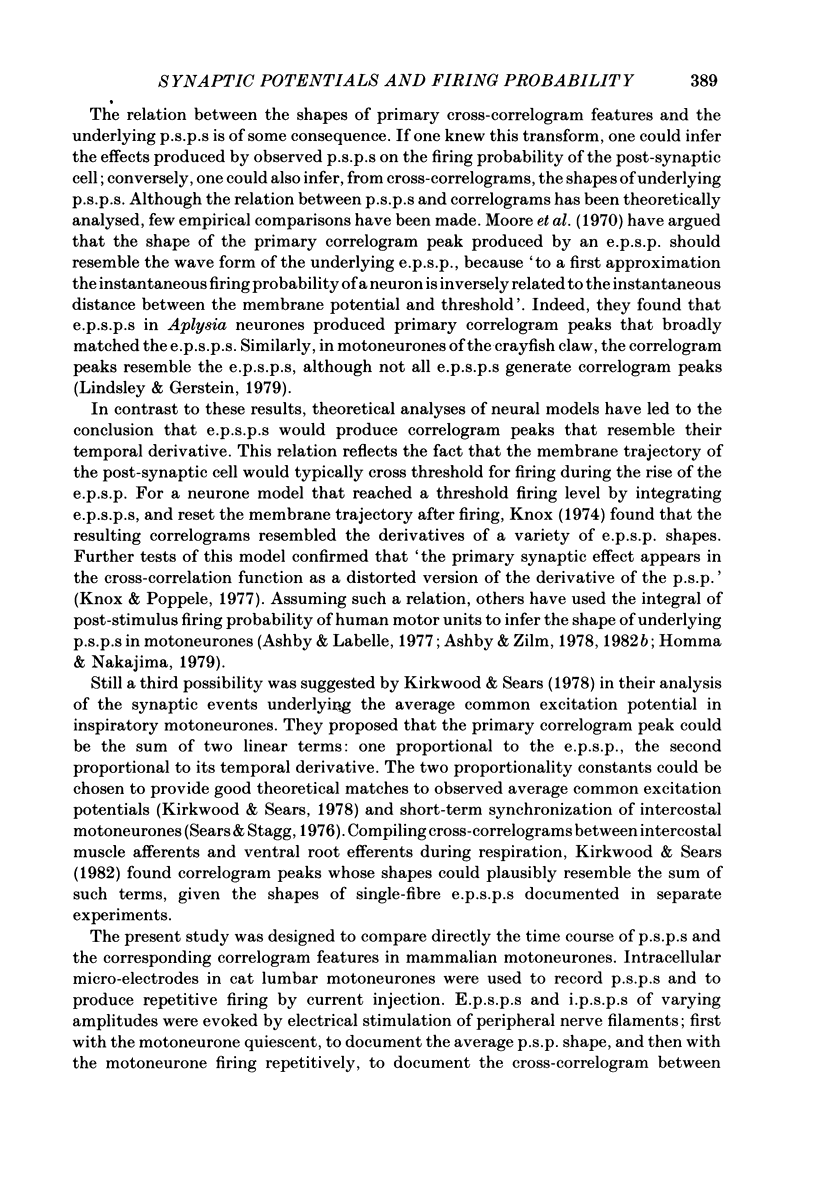
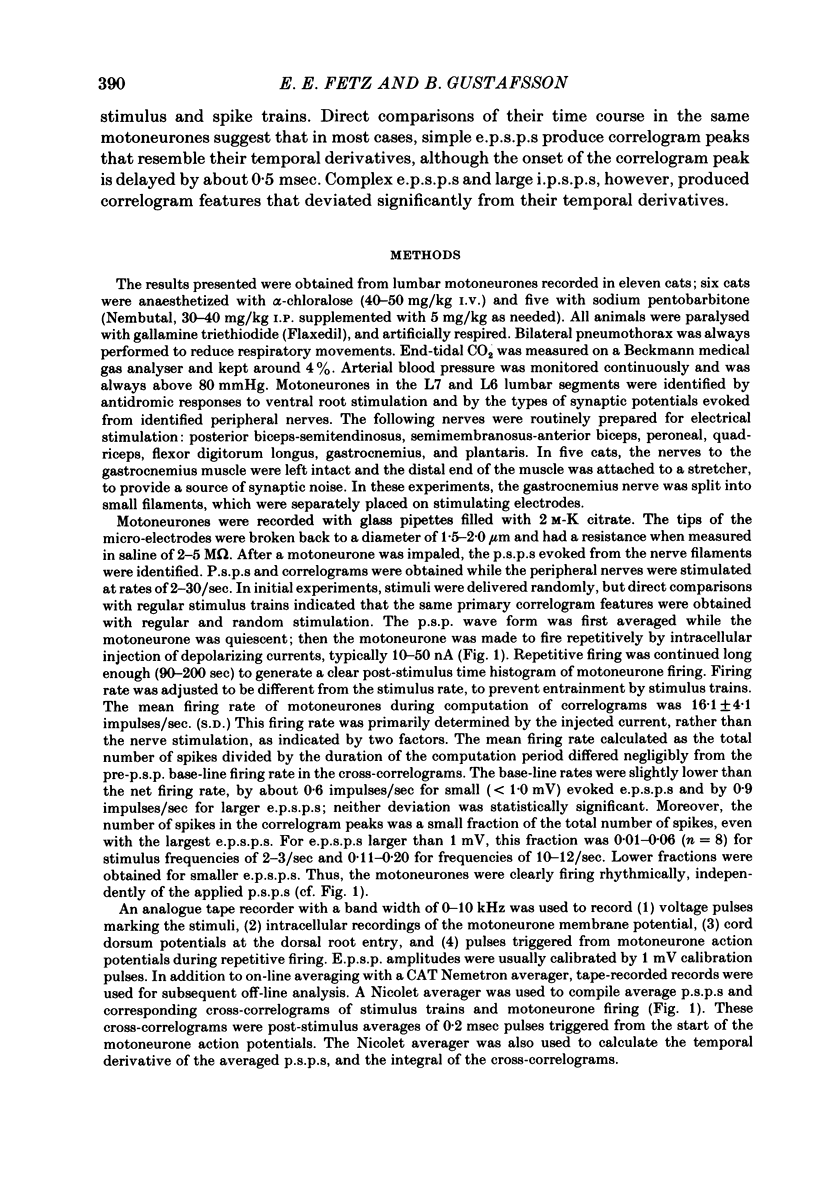
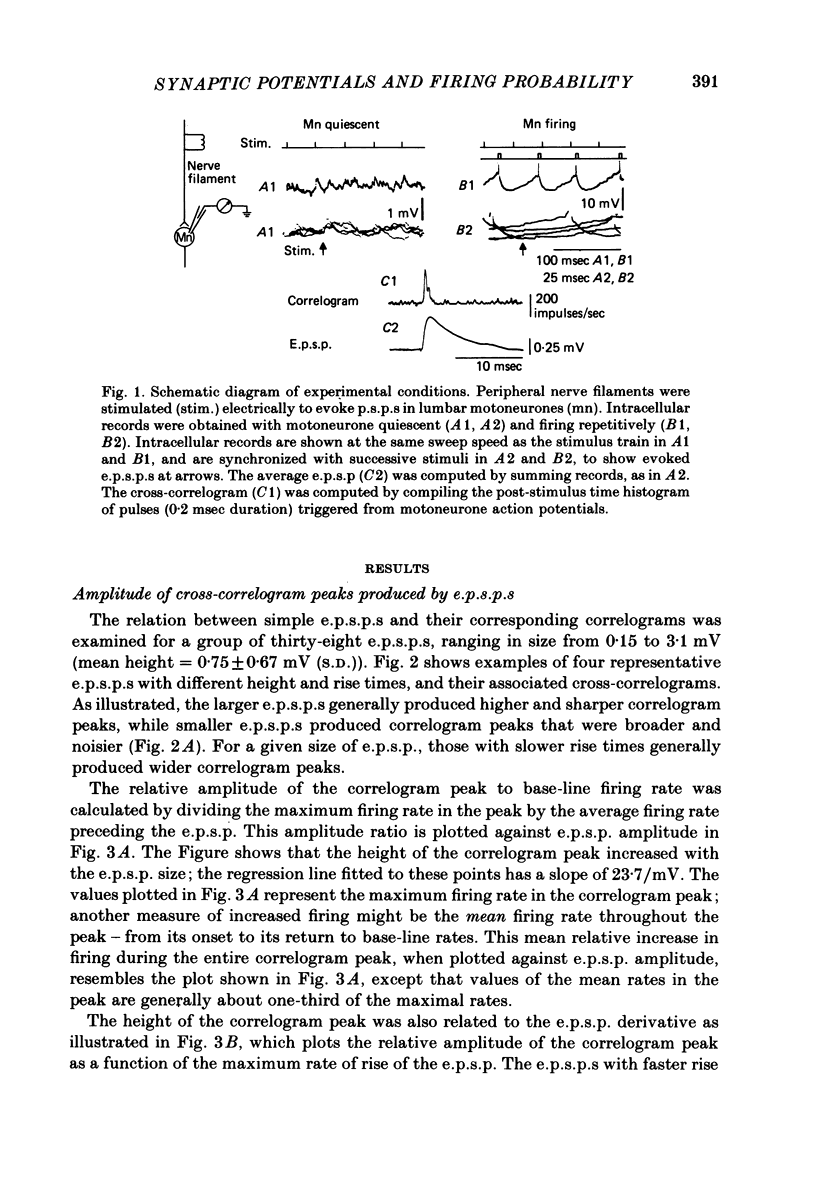
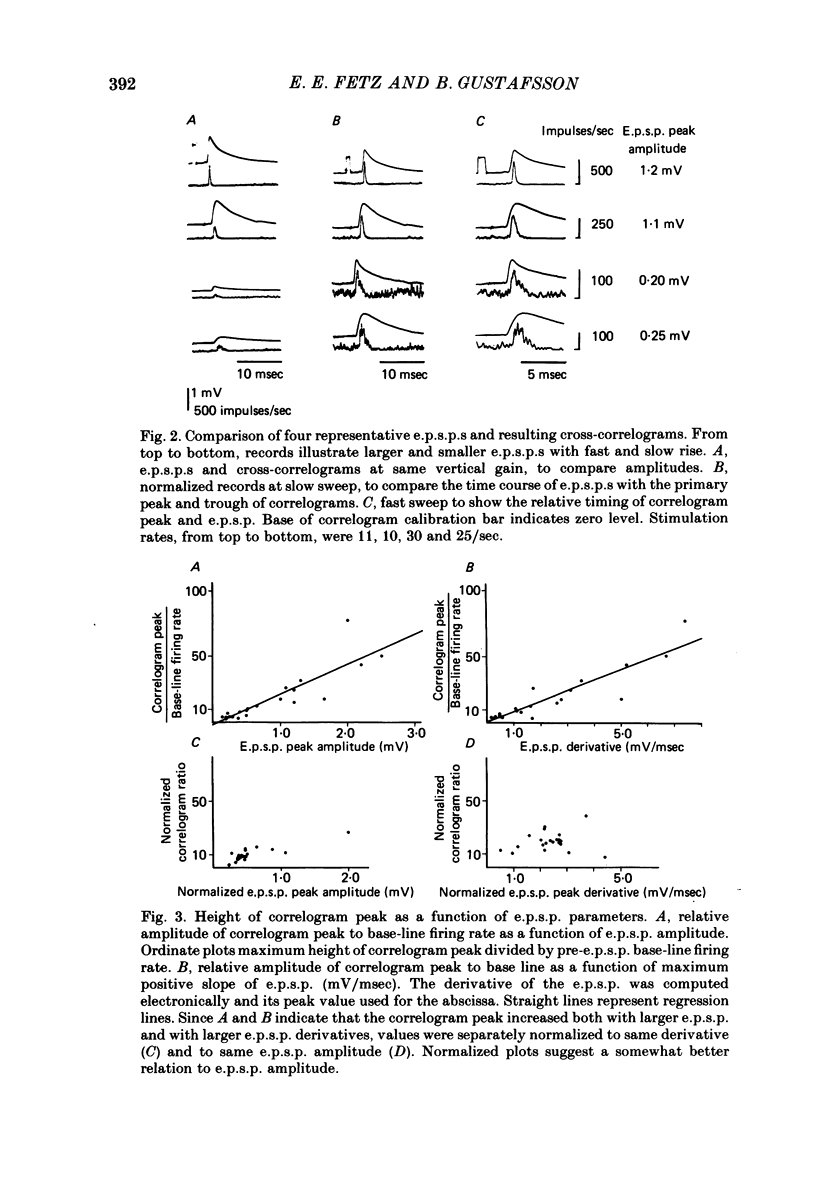
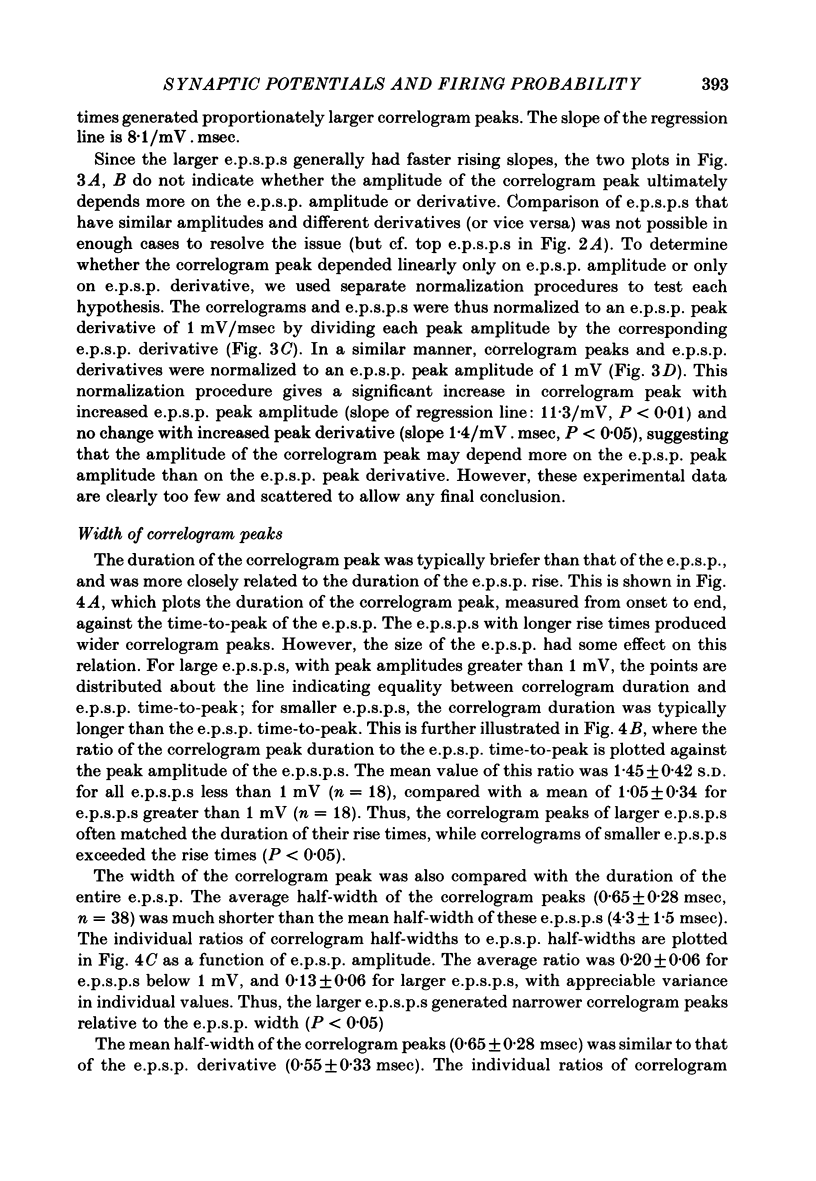
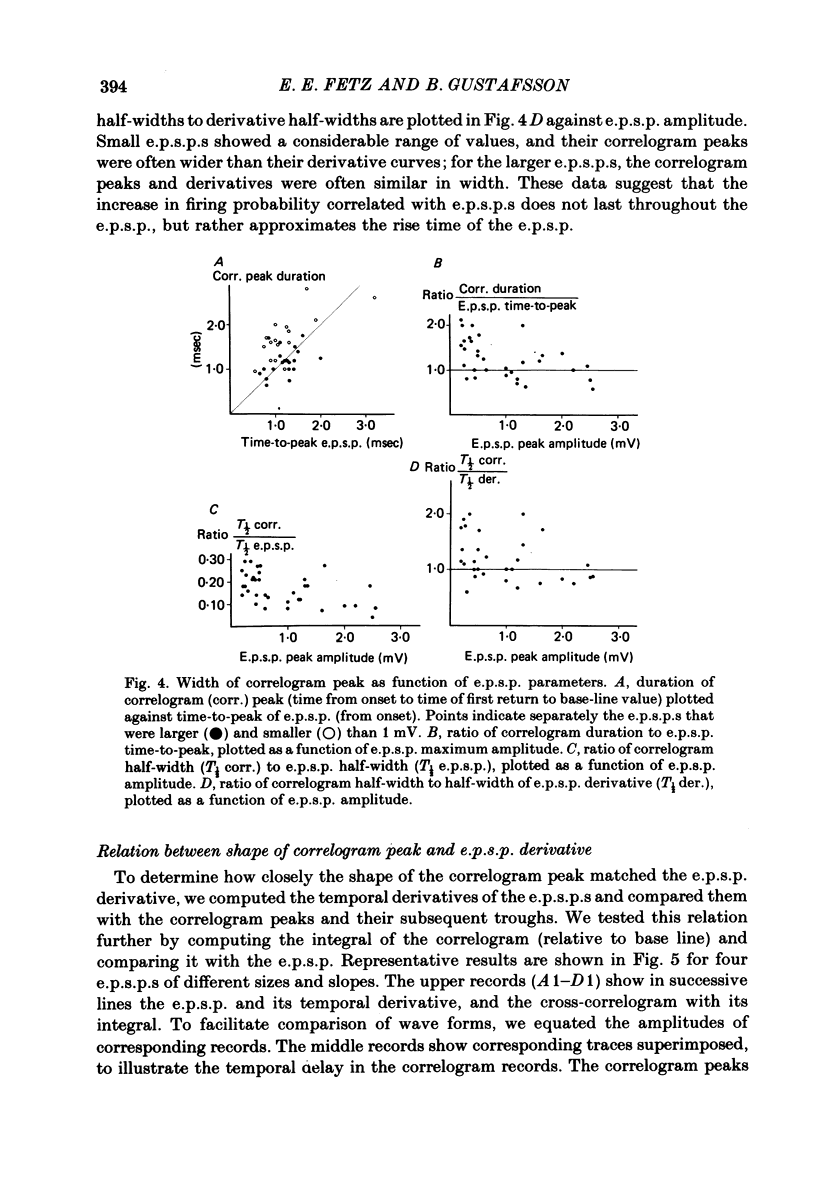
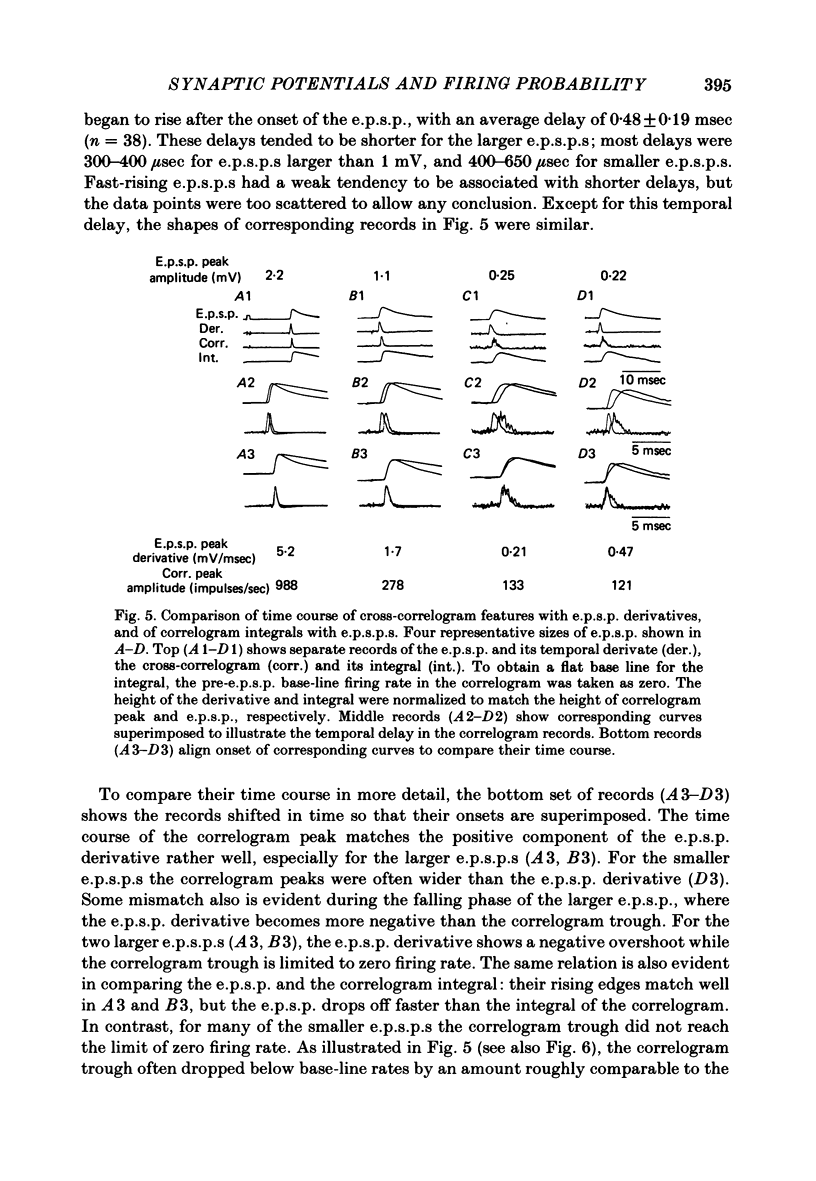
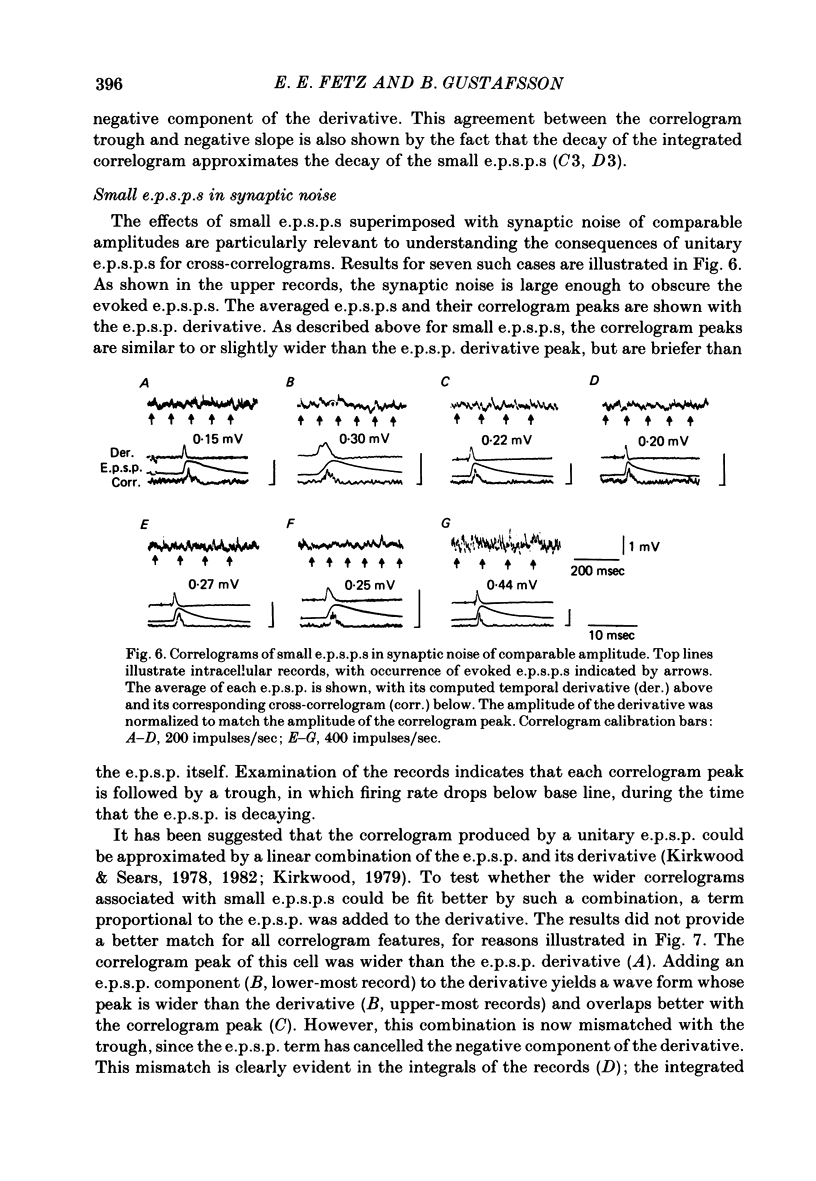
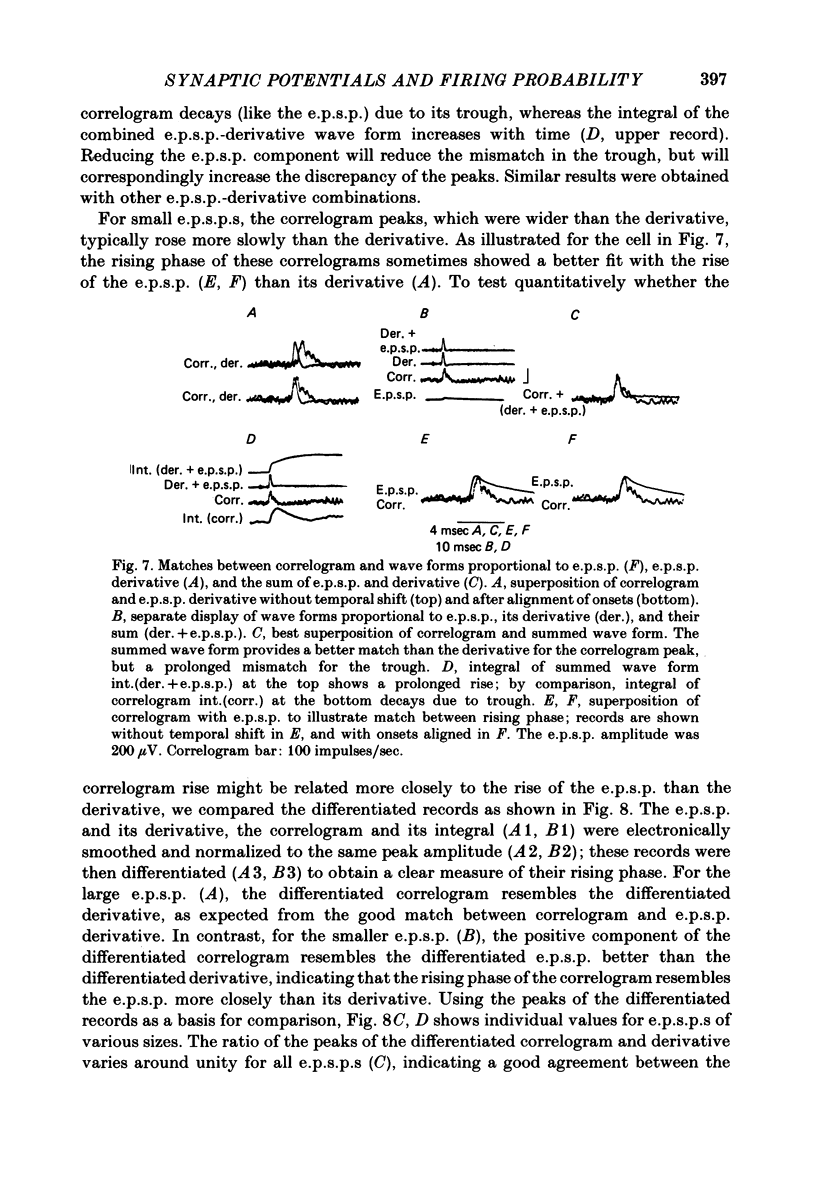
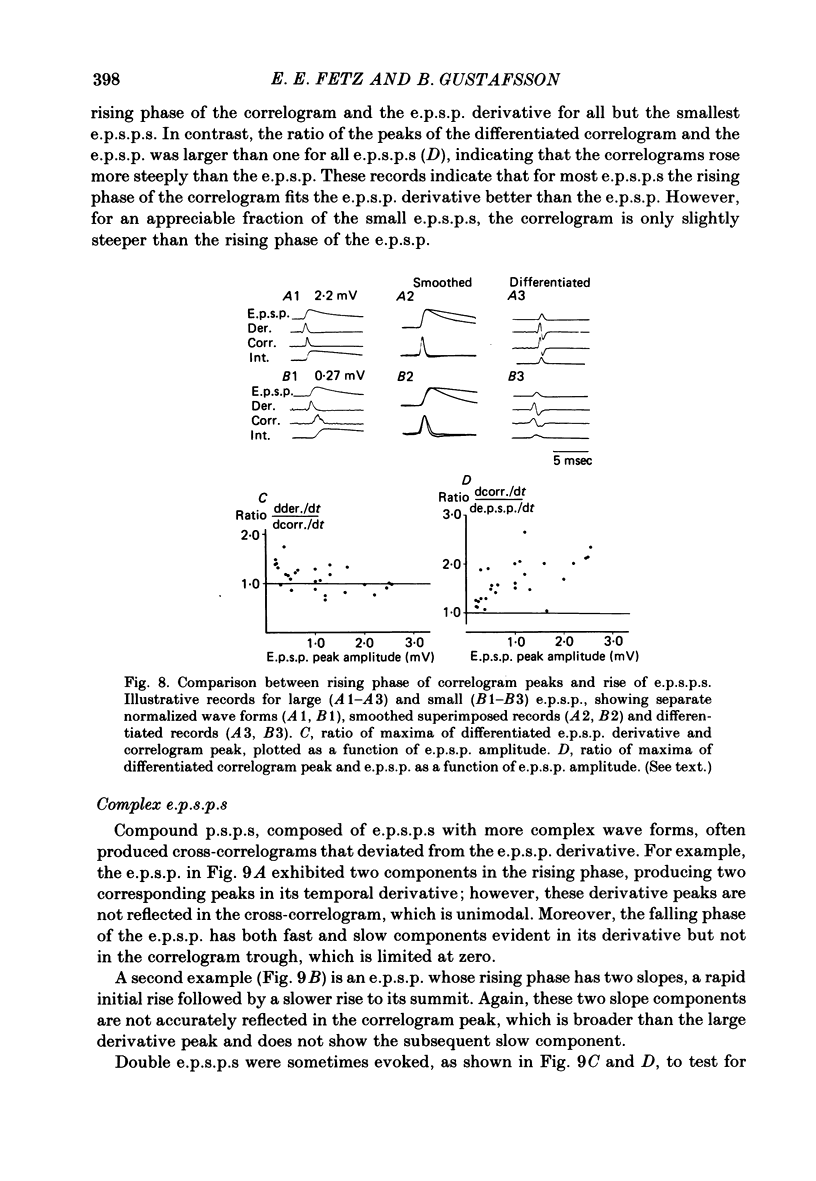
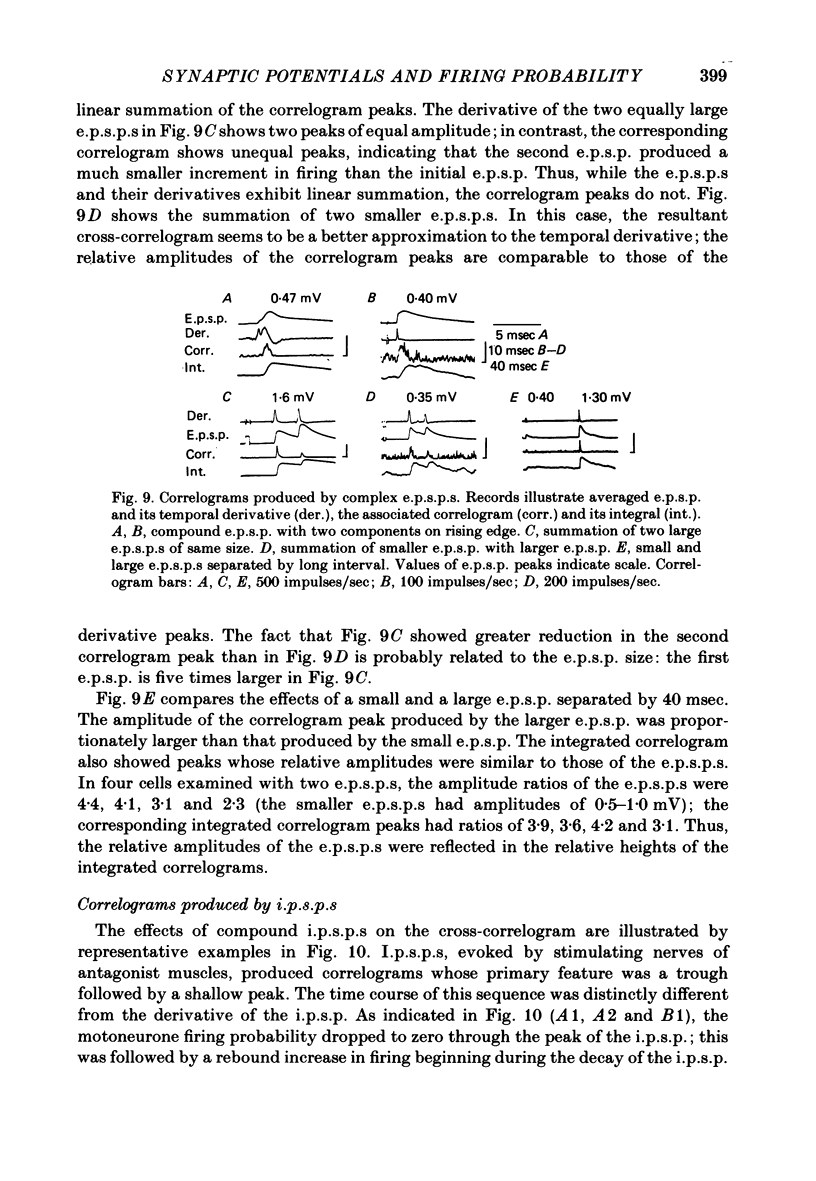
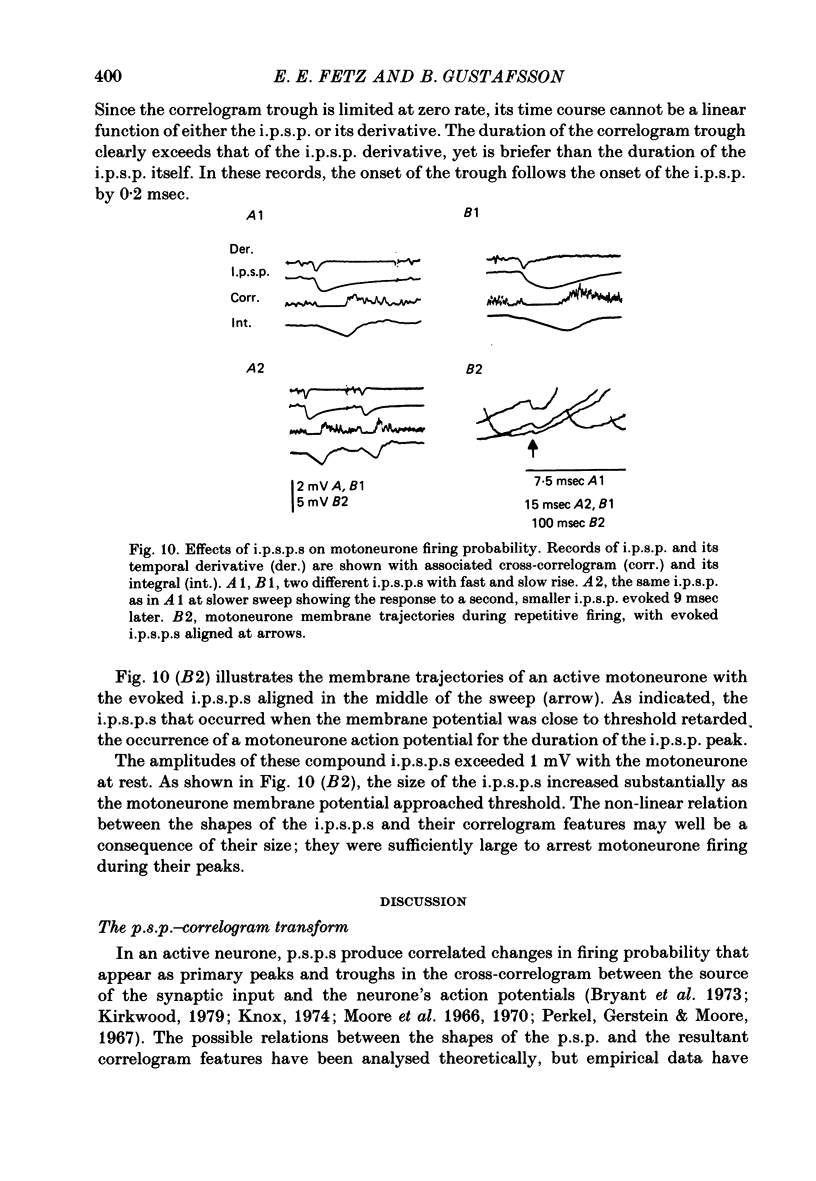
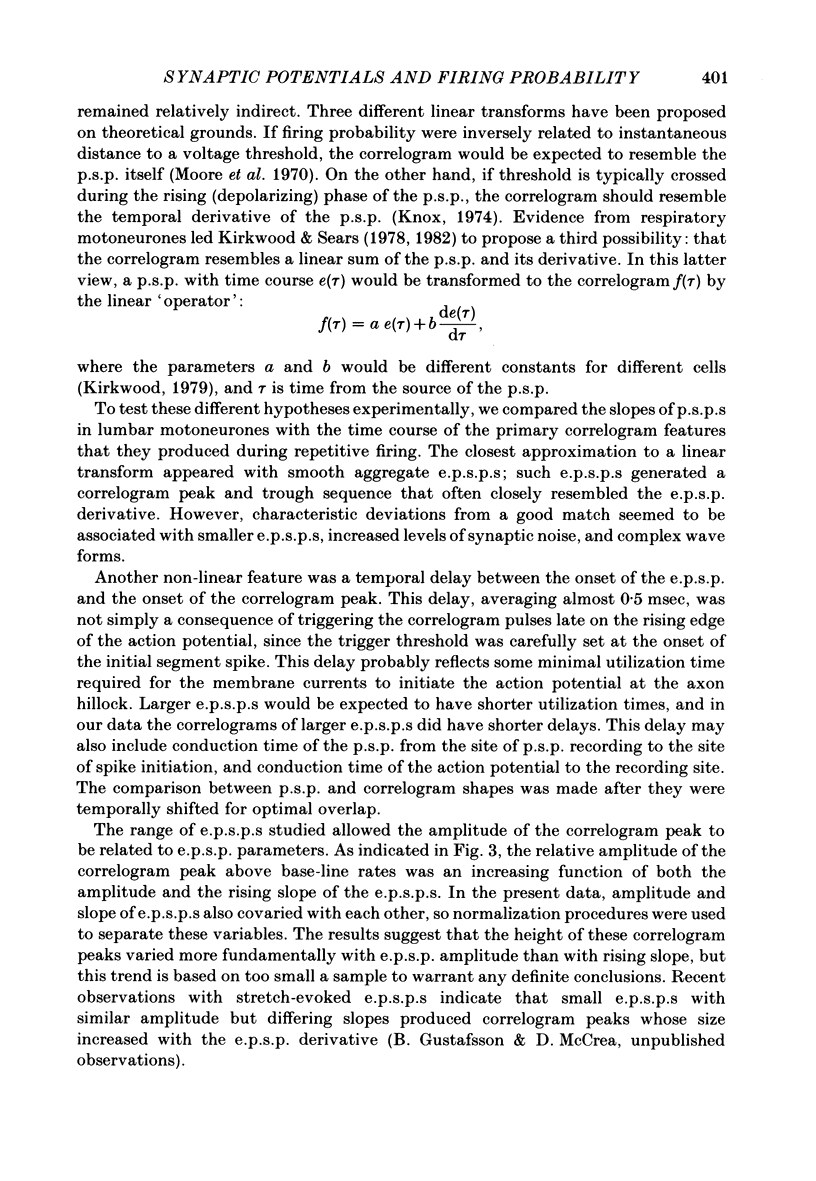
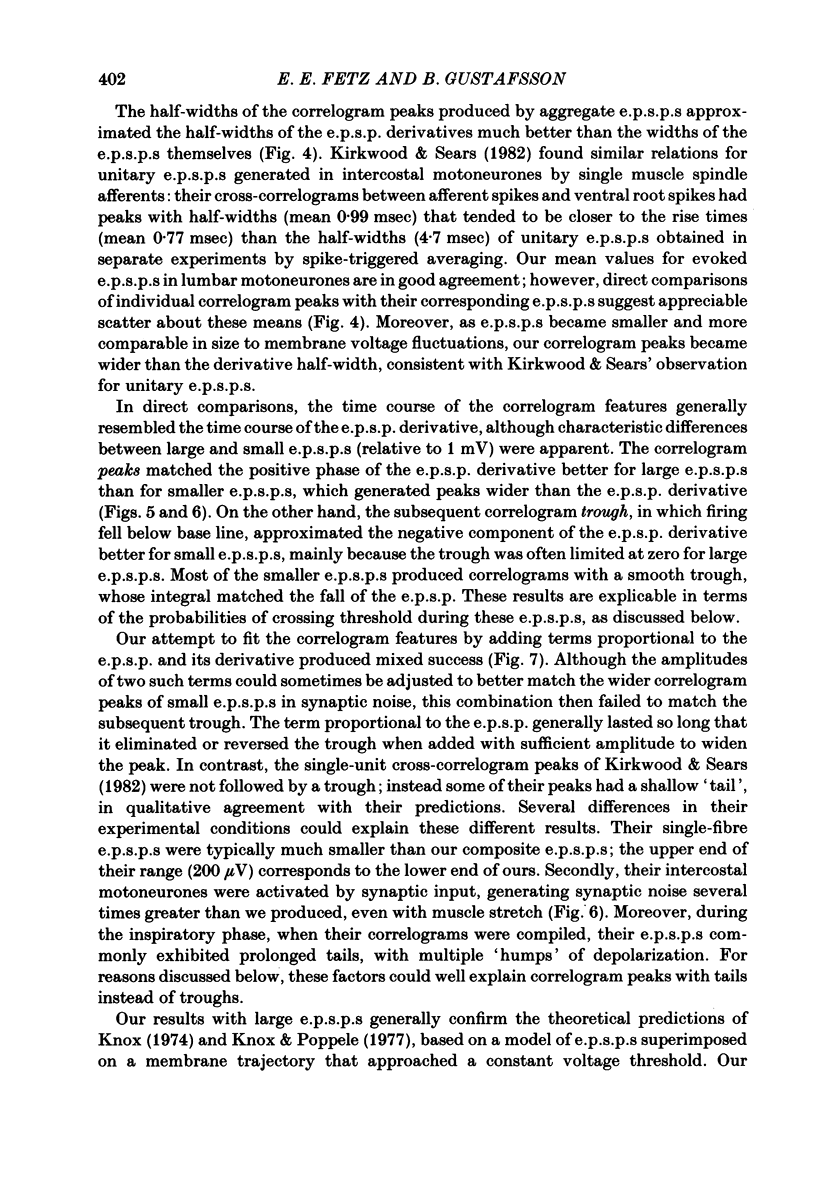
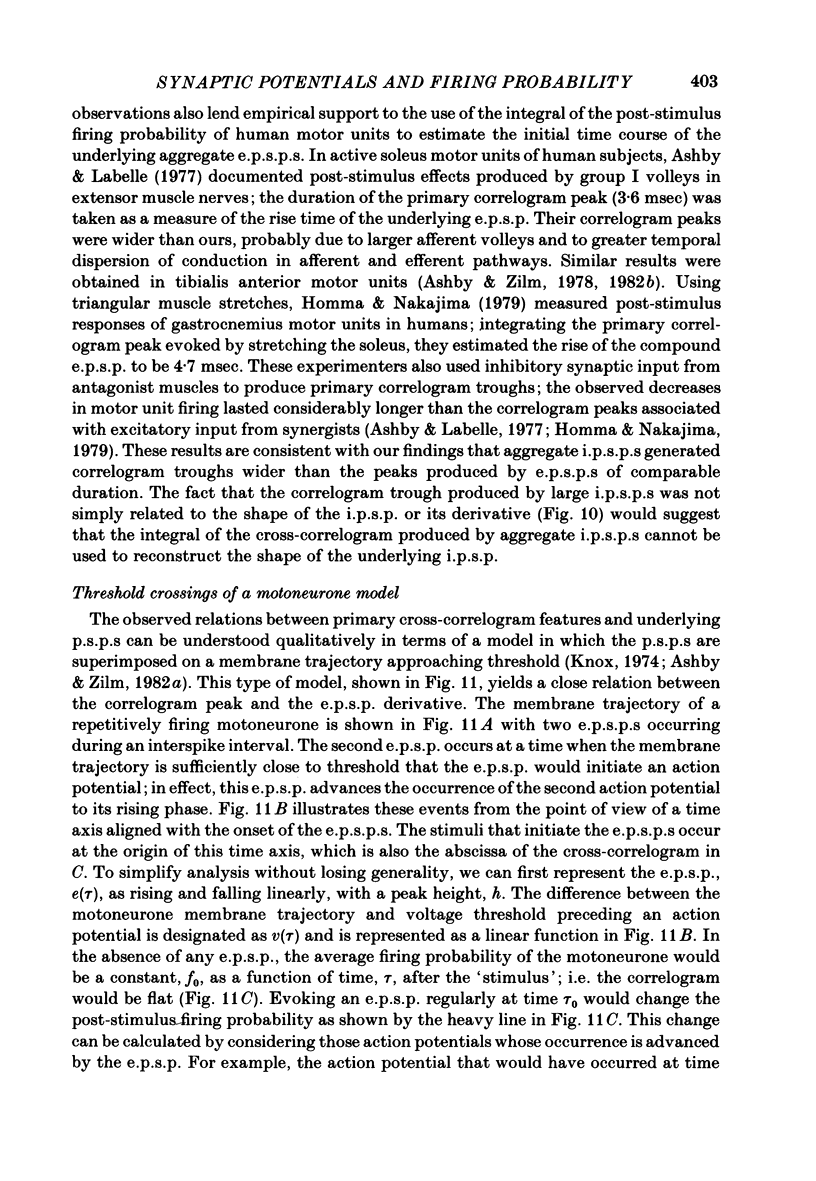
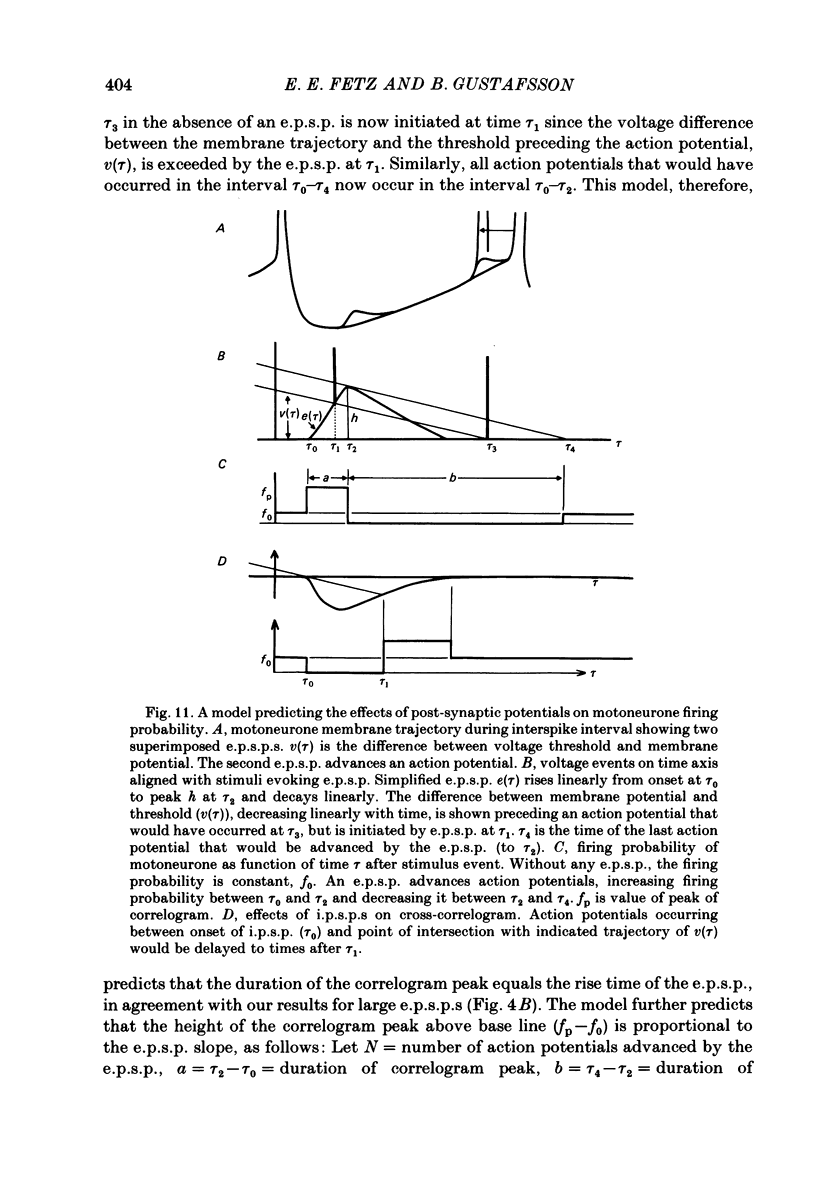
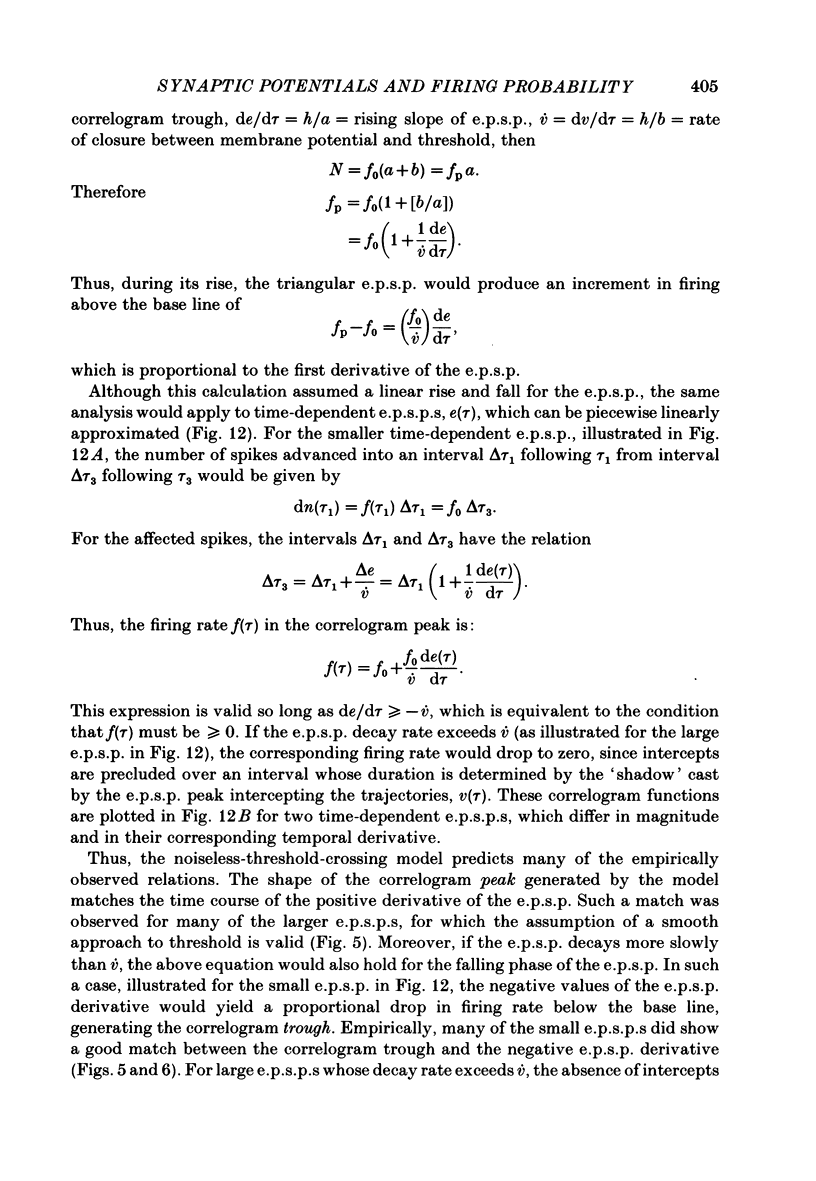
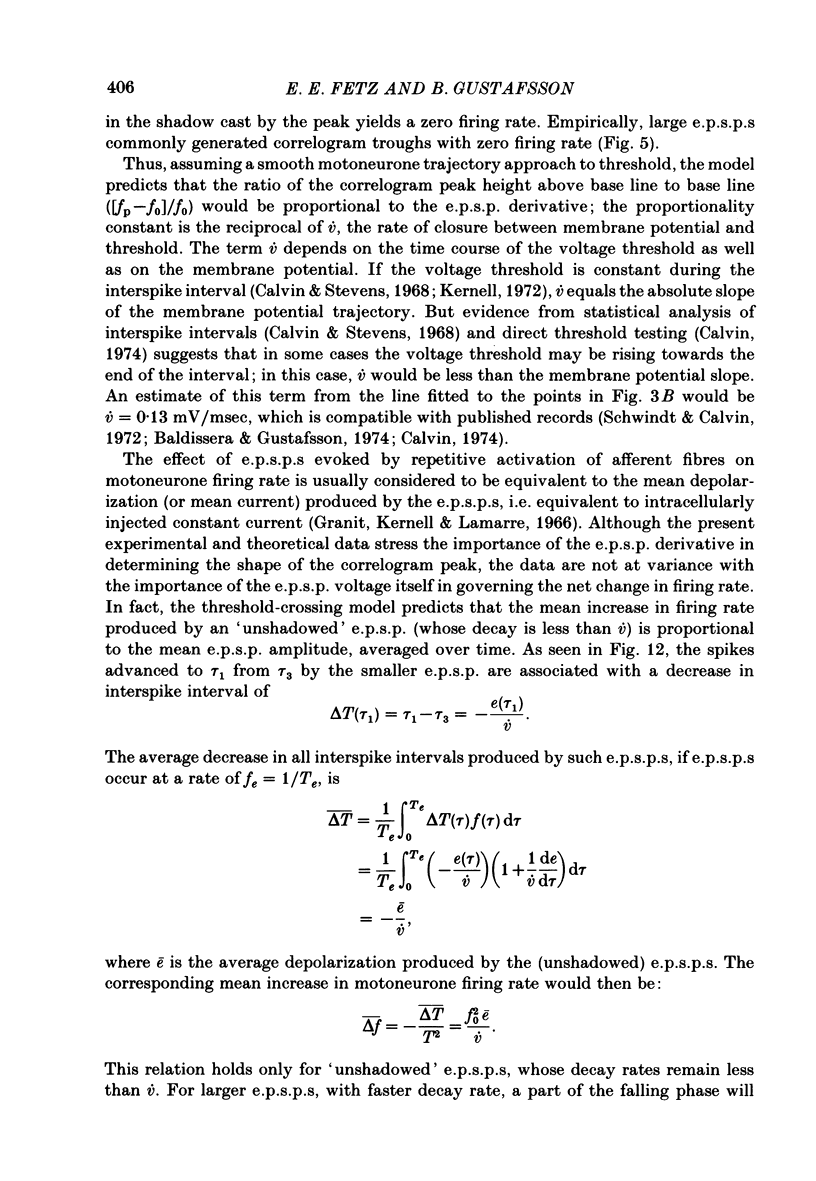
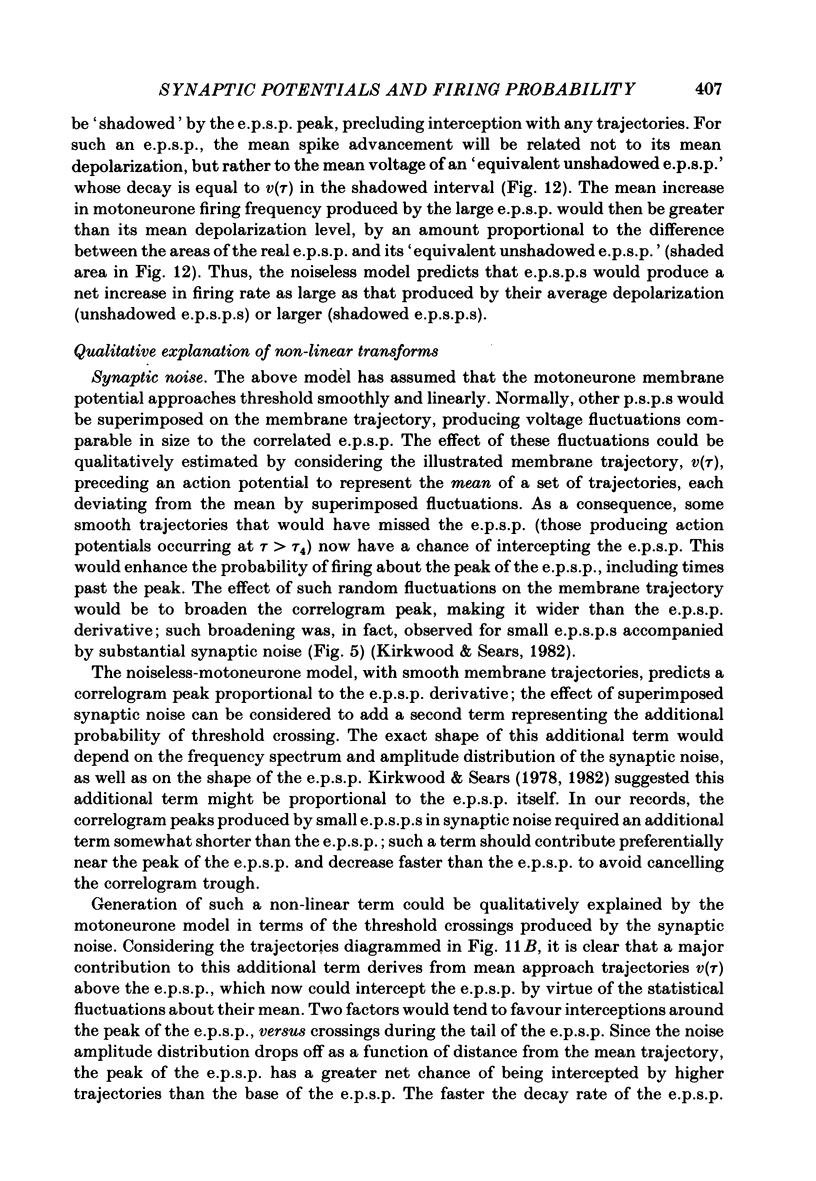
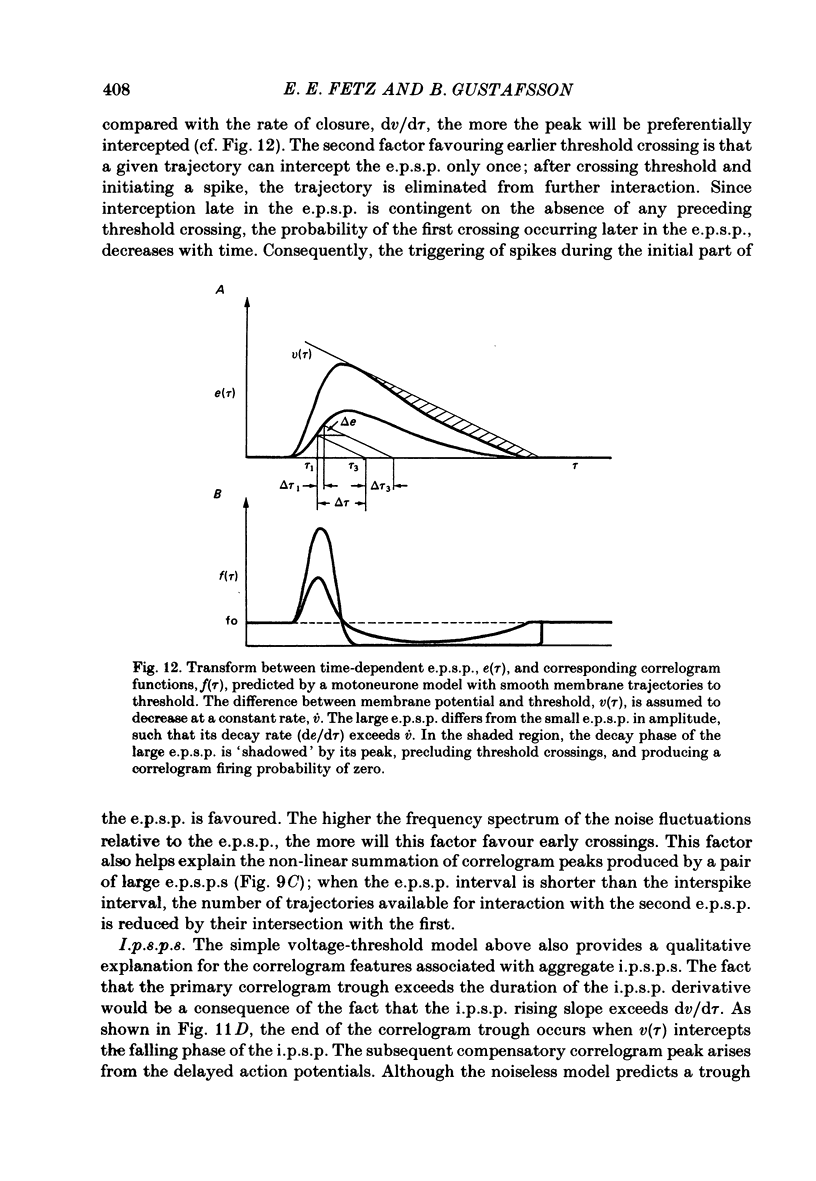
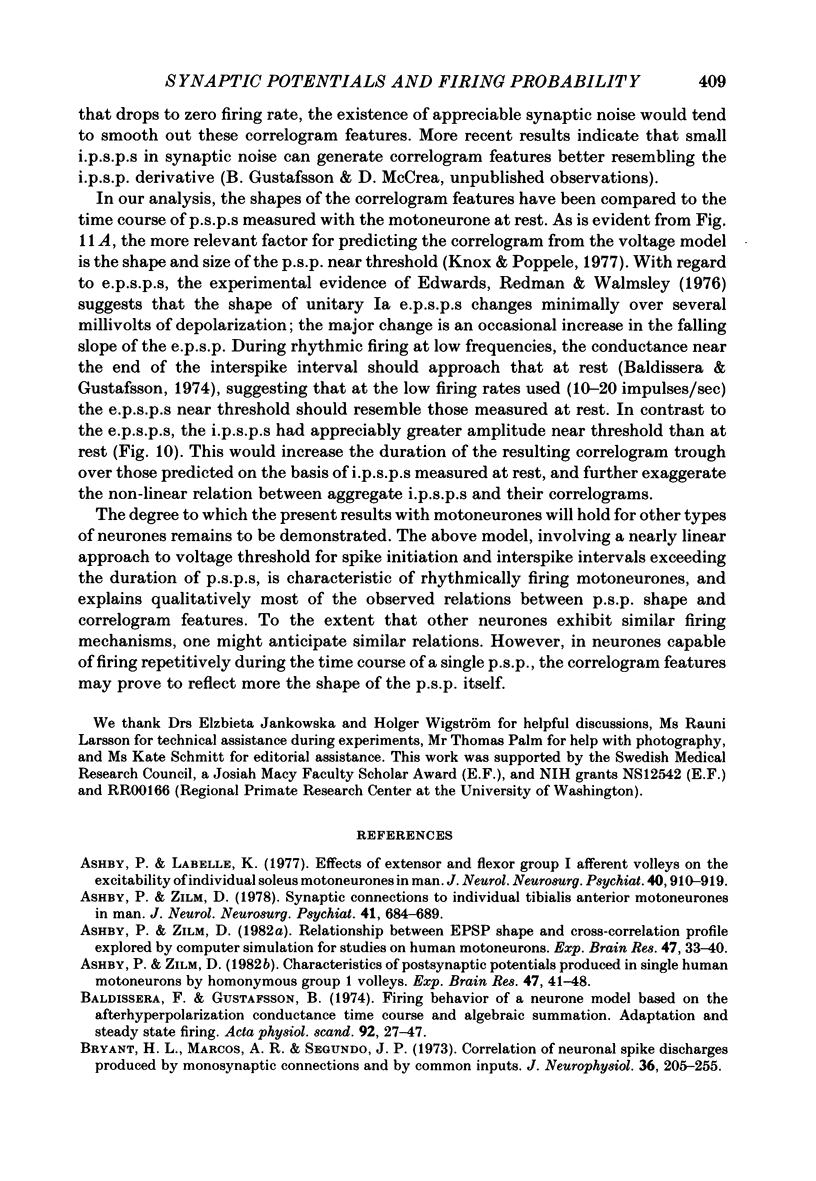
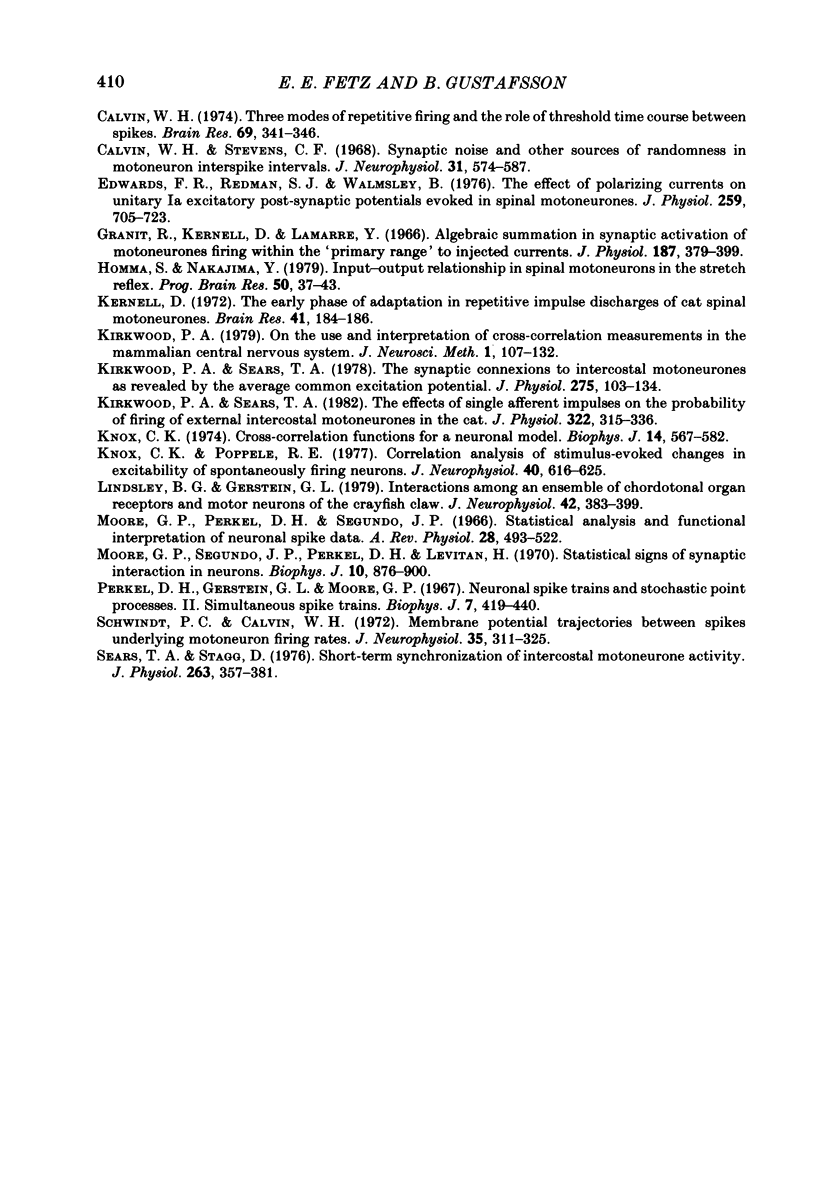
Selected References
These references are in PubMed. This may not be the complete list of references from this article.
- Ashby P., Labelle K. Effects of extensor and flexor group I afferent volleys on the excitability of individual soleus motoneurones in man. J Neurol Neurosurg Psychiatry. 1977 Sep;40(9):910–919. doi: 10.1136/jnnp.40.9.910. [DOI] [PMC free article] [PubMed] [Google Scholar]
- Ashby P., Zilm D. Characteristics of postsynaptic potentials produced in single human motoneurons by homonymous group 1 volleys. Exp Brain Res. 1982;47(1):41–48. doi: 10.1007/BF00235884. [DOI] [PubMed] [Google Scholar]
- Ashby P., Zilm D. Relationship between EPSP shape and cross-correlation profile explored by computer simulation for studies on human motoneurons. Exp Brain Res. 1982;47(1):33–40. doi: 10.1007/BF00235883. [DOI] [PubMed] [Google Scholar]
- Ashby P., Zilm D. Synaptic connections to individual tibialis anterior motoneurones in man. J Neurol Neurosurg Psychiatry. 1978 Aug;41(8):684–689. doi: 10.1136/jnnp.41.8.684. [DOI] [PMC free article] [PubMed] [Google Scholar]
- Baldissera F., Gustafsson B. Firing behaviour of a neurone model based on the afterhyperpolarization conductance time course and algebraical summation. Adaptation and steady state firing. Acta Physiol Scand. 1974 Sep;92(1):27–47. doi: 10.1111/j.1748-1716.1974.tb05720.x. [DOI] [PubMed] [Google Scholar]
- Bryant H. L., Jr, Marcos A. R., Segundo J. P. Correlations of neuronal spike discharges produced by monosynaptic connections and by common inputs. J Neurophysiol. 1973 Mar;36(2):205–225. doi: 10.1152/jn.1973.36.2.205. [DOI] [PubMed] [Google Scholar]
- Calvin W. H., Stevens C. F. Synaptic noise and other sources of randomness in motoneuron interspike intervals. J Neurophysiol. 1968 Jul;31(4):574–587. doi: 10.1152/jn.1968.31.4.574. [DOI] [PubMed] [Google Scholar]
- Calvin W. H. Three modes of repetitive firing and the role of threshold time course between spikes. Brain Res. 1974 Apr 5;69(2):341–346. doi: 10.1016/0006-8993(74)90012-2. [DOI] [PubMed] [Google Scholar]
- Edwards F. R., Redman S. J., Walmsley B. The effect of polarizing currents on unitary Ia excitatory post-synaptic potentials evoked in spinal motoneurones. J Physiol. 1976 Aug;259(3):705–723. doi: 10.1113/jphysiol.1976.sp011490. [DOI] [PMC free article] [PubMed] [Google Scholar]
- Granit R., Kernell D., Lamarre Y. Algebraical summation in synaptic activation of motoneurones firing within the 'primary range' to injected currents. J Physiol. 1966 Nov;187(2):379–399. doi: 10.1113/jphysiol.1966.sp008097. [DOI] [PMC free article] [PubMed] [Google Scholar]
- Homma S., Nakajima Y. Input-output relationship in spinal motoneurons in the stretch reflex. Prog Brain Res. 1979;50:37–43. doi: 10.1016/S0079-6123(08)60805-5. [DOI] [PubMed] [Google Scholar]
- Kernell D. The early phase of adaptation in repetitive impulse discharges of cat spinal motoneurones. Brain Res. 1972 Jun 8;41(1):184–186. doi: 10.1016/0006-8993(72)90626-9. [DOI] [PubMed] [Google Scholar]
- Kirkwood P. A. On the use and interpretation of cross-correlations measurements in the mammalian central nervous system. J Neurosci Methods. 1979 Aug;1(2):107–132. doi: 10.1016/0165-0270(79)90009-8. [DOI] [PubMed] [Google Scholar]
- Kirkwood P. A., Sears T. A. The effects of single afferent impulses on the probability of firing of external intercostal motoneurones in the cat. J Physiol. 1982 Jan;322:315–336. doi: 10.1113/jphysiol.1982.sp014039. [DOI] [PMC free article] [PubMed] [Google Scholar]
- Kirkwood P. A., Sears T. A. The synaptic connexions to intercostal motoneurones as revealed by the average common excitation potential. J Physiol. 1978 Feb;275:103–134. doi: 10.1113/jphysiol.1978.sp012180. [DOI] [PMC free article] [PubMed] [Google Scholar]
- Knox C. K. Cross-correlation functions for a neuronal model. Biophys J. 1974 Aug;14(8):567–582. doi: 10.1016/S0006-3495(74)85936-9. [DOI] [PMC free article] [PubMed] [Google Scholar]
- Knox C. K., Poppele R. E. Correlation analysis of stimulus-evoked changes in excitability of spontaneously firing neurons. J Neurophysiol. 1977 May;40(3):616–625. doi: 10.1152/jn.1977.40.3.616. [DOI] [PubMed] [Google Scholar]
- Lindsey B. G., Gerstein G. L. Interactions among an ensemble of chordotonal organ receptors and motor neurons of the crayfish claw. J Neurophysiol. 1979 Mar;42(2):383–399. doi: 10.1152/jn.1979.42.2.383. [DOI] [PubMed] [Google Scholar]
- Moore G. P., Perkel D. H., Segundo J. P. Statistical analysis and functional interpretation of neuronal spike data. Annu Rev Physiol. 1966;28:493–522. doi: 10.1146/annurev.ph.28.030166.002425. [DOI] [PubMed] [Google Scholar]
- Moore G. P., Segundo J. P., Perkel D. H., Levitan H. Statistical signs of synaptic interaction in neurons. Biophys J. 1970 Sep;10(9):876–900. doi: 10.1016/S0006-3495(70)86341-X. [DOI] [PMC free article] [PubMed] [Google Scholar]
- Perkel D. H., Gerstein G. L., Moore G. P. Neuronal spike trains and stochastic point processes. II. Simultaneous spike trains. Biophys J. 1967 Jul;7(4):419–440. doi: 10.1016/S0006-3495(67)86597-4. [DOI] [PMC free article] [PubMed] [Google Scholar]
- Schwindt P. C., Calvin W. H. Membrane-potential trajectories between spikes underlying motoneuron firing rates. J Neurophysiol. 1972 May;35(3):311–325. doi: 10.1152/jn.1972.35.3.311. [DOI] [PubMed] [Google Scholar]
- Sears T. A., Stagg D. Short-term synchronization of intercostal motoneurone activity. J Physiol. 1976 Dec;263(3):357–381. doi: 10.1113/jphysiol.1976.sp011635. [DOI] [PMC free article] [PubMed] [Google Scholar]