Abstract
Dielectric spectra representing the frequency dependence of the complex permitivity at a range of depolarizations were obtained from voltage-clamped frog skeletal muscle membranes. This employed an analysis that derived the Fourier coefficients defining the capacitative transients to 10 mV steps as continuous functions of frequency, and so could examine closely the relevant frequencies at which non-linear components occurred. Non-linear capacitative components were identified through their appearance at lower frequencies than those of the linear components as obtained at the -85 mV control voltage, from spectra representing a logarithmic scale of frequencies. Permitivities from small depolarizing steps between about -75 and -50 mV gave single q beta dielectric loss peaks; the real permitivities declined monotonically with increasing frequency. Simple arc loci were obtained in the complex plane. With further depolarization, an additional q gamma loss peak at low frequencies and a resonant frequency in the real spectra occurred over a narrow voltage range around -45 mV. The complex loci then showed features implying an increased movement of charge not explicable through the simple effect of an electric field on a dielectric species. Spectra from small hyperpolarizing steps possessed only single dielectric loss peaks and real permitivities that declined monotonically with increasing frequency. However, in the complex plane, the loss tangents at the higher frequencies implied a population of two or more dielectric relaxations. The potential dependence of the frequency at maximum dielectric loss obtained from depolarizing steps showed a discontinuity at the onset of q gamma. In contrast, in hyperpolarizing responses, this dependence was smooth. The q beta relaxations obtained after q gamma was abolished by 1 mM-tetracaine gave dielectric spectra that were similar whether to depolarizing or hyperpolarizing potential steps. They gave single dielectric loss peaks and semicircular complex plane loci. The singularities in the dielectric spectra thus result from the q gamma charge movement component. They may reflect co-operative mechanisms that might also produce its steep voltage dependence and kinetics, and consequently those of the physiological processes it may control. These are discussed in terms of the mechanisms expected in allosteric proteins.
Full text
PDF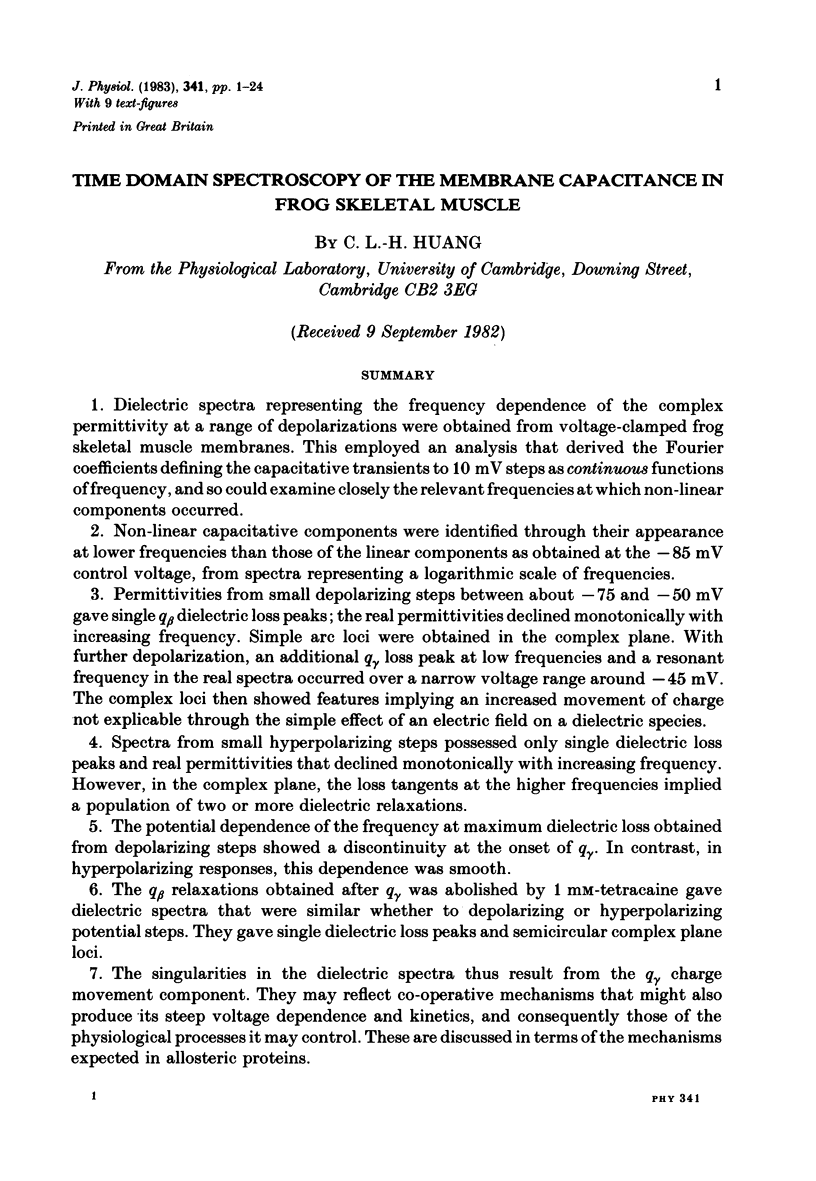
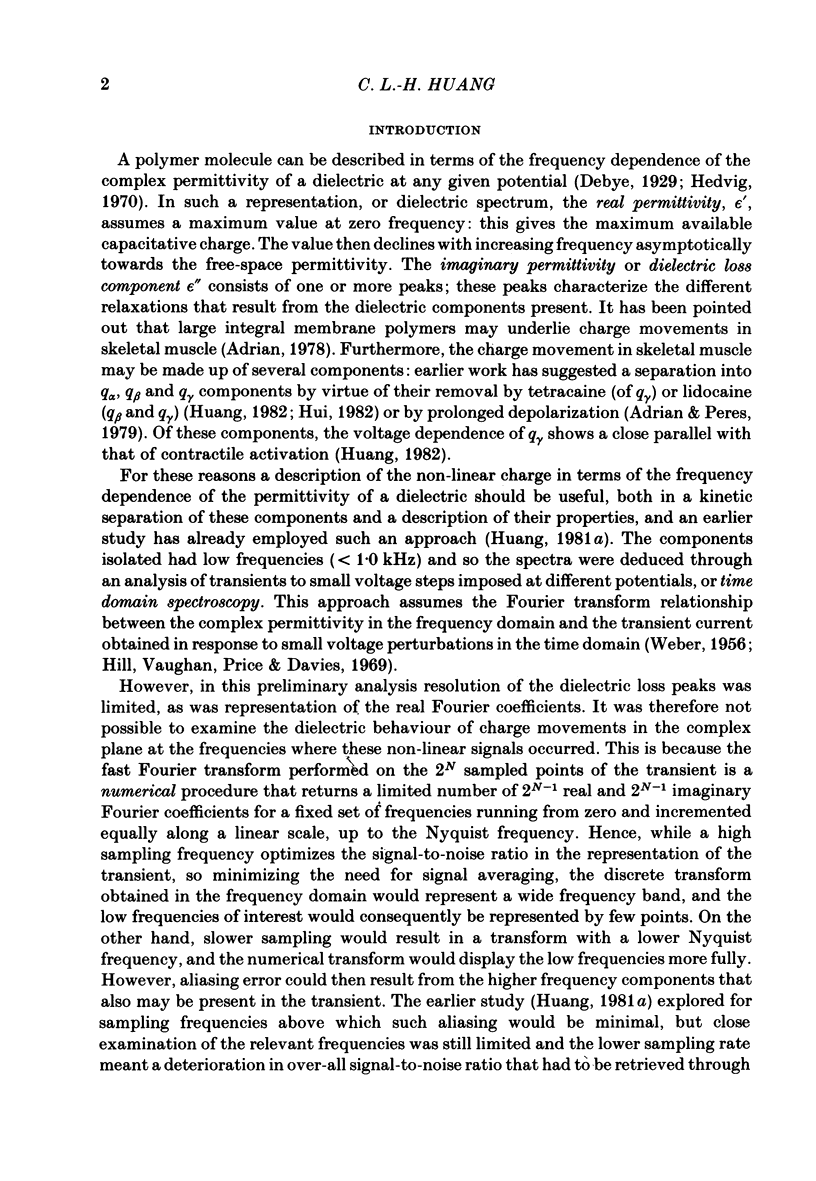
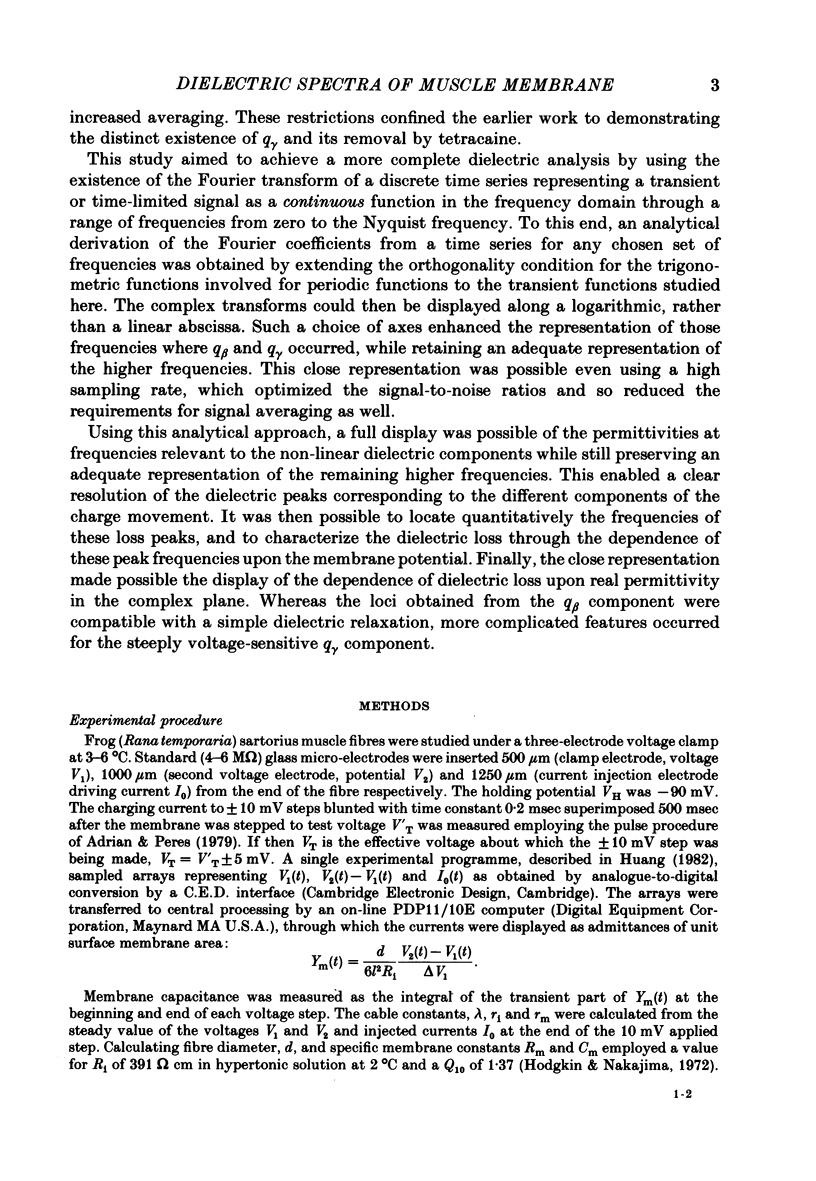
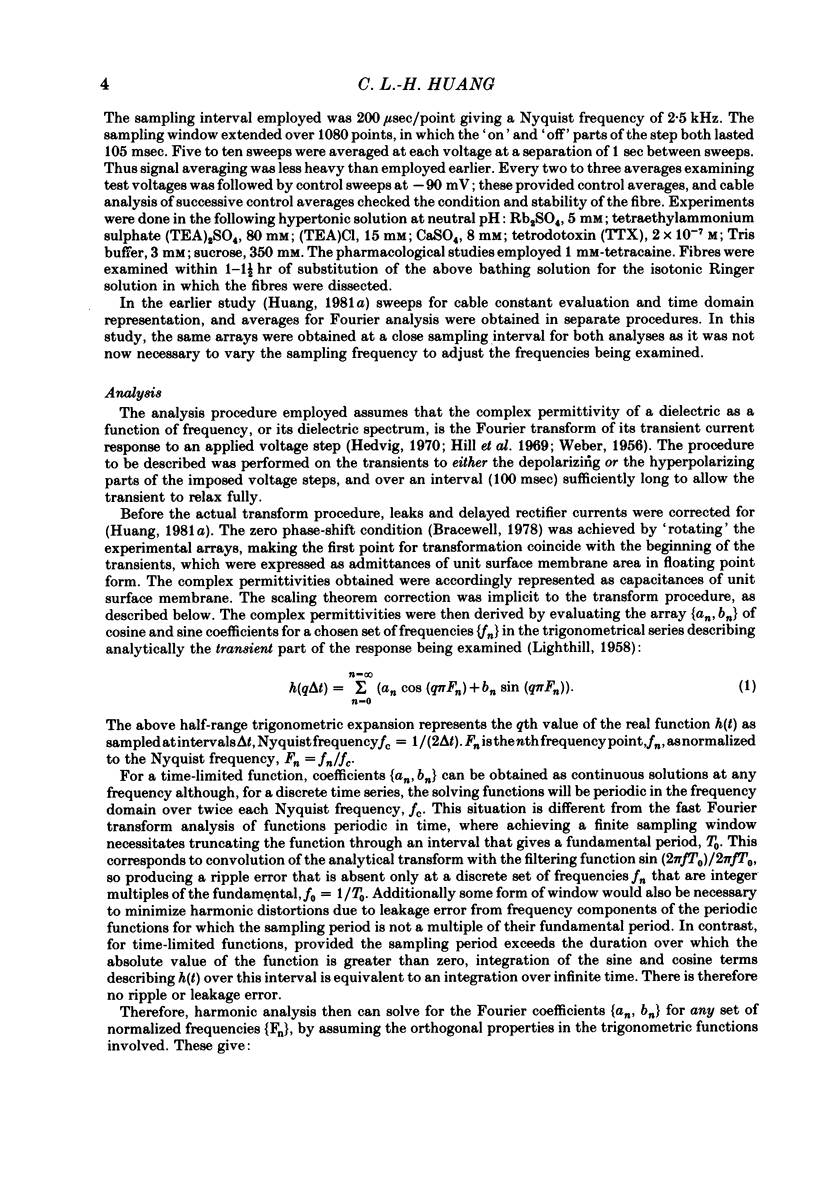
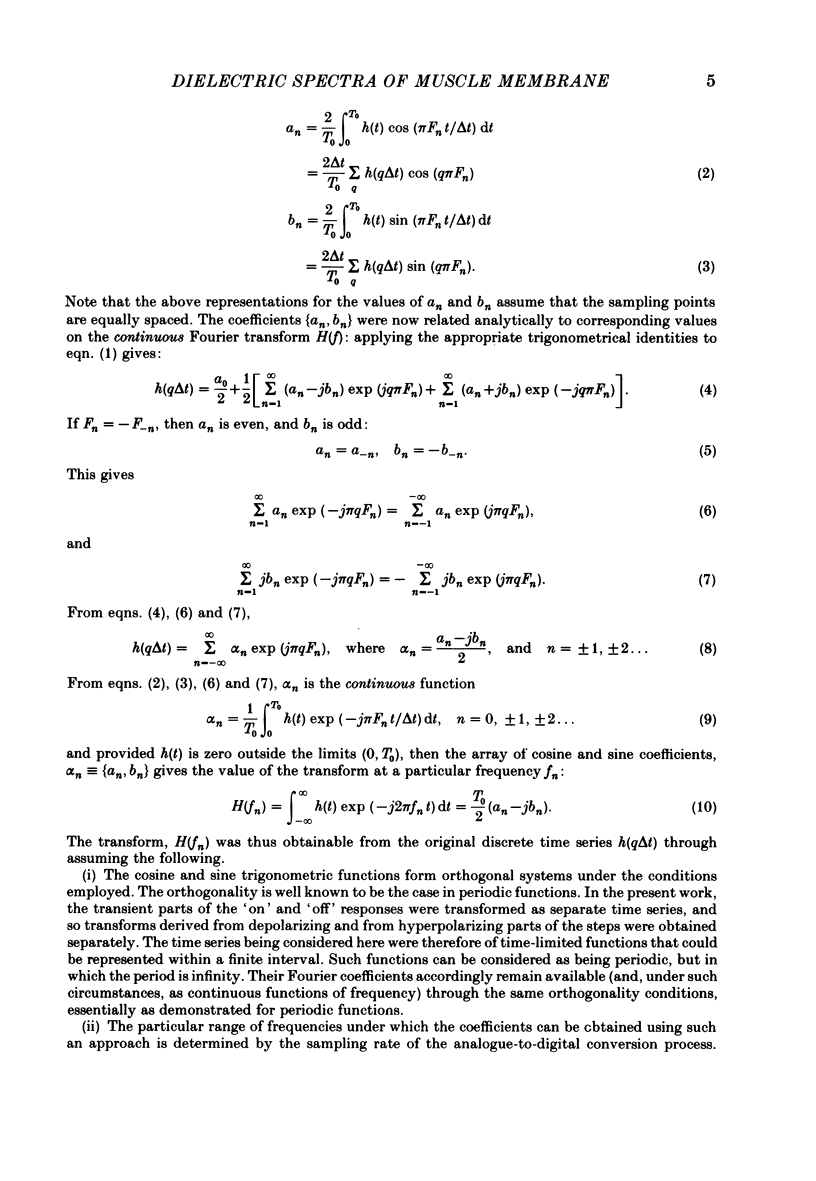
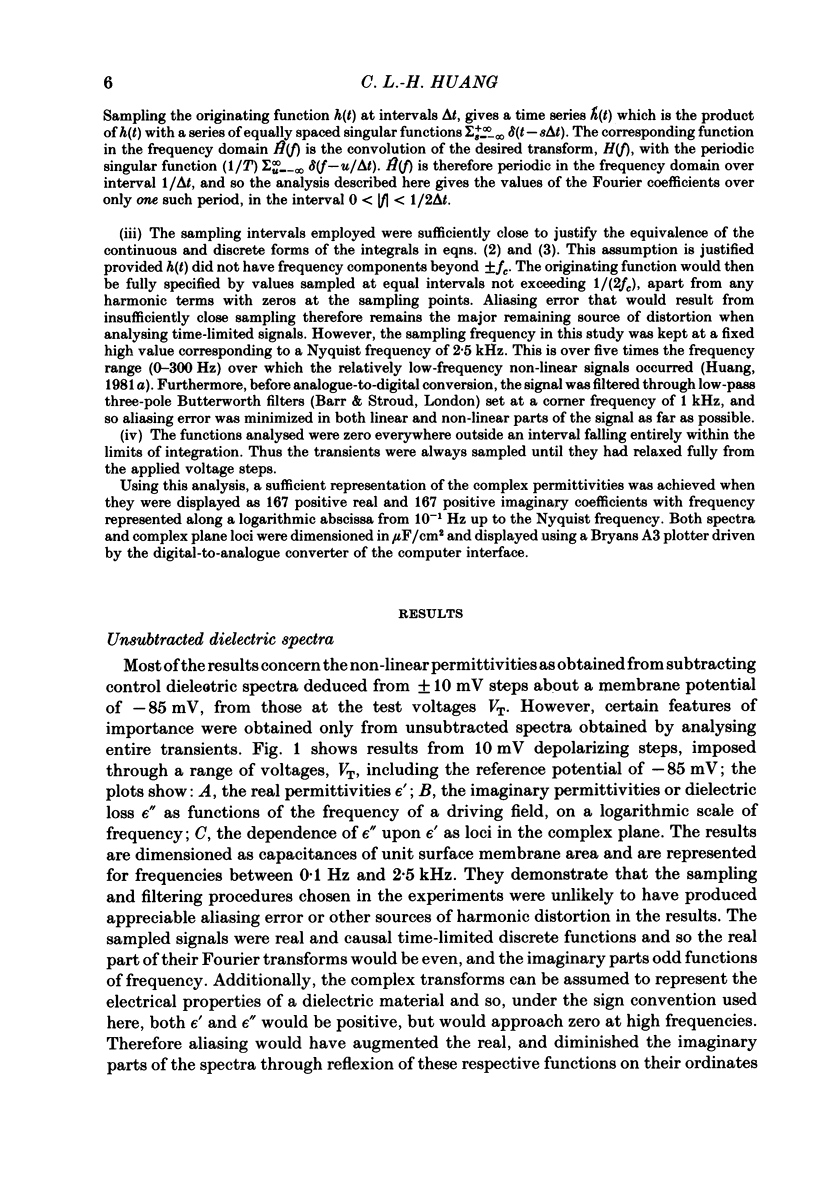
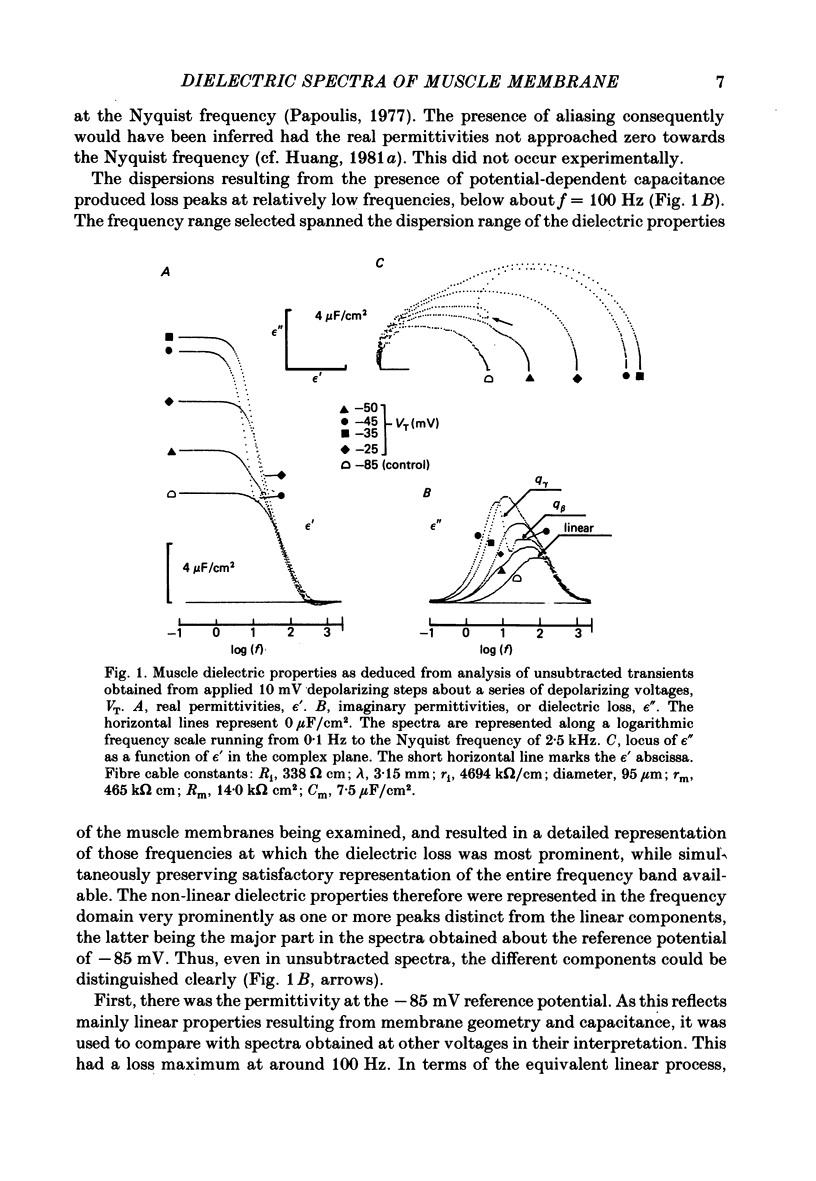
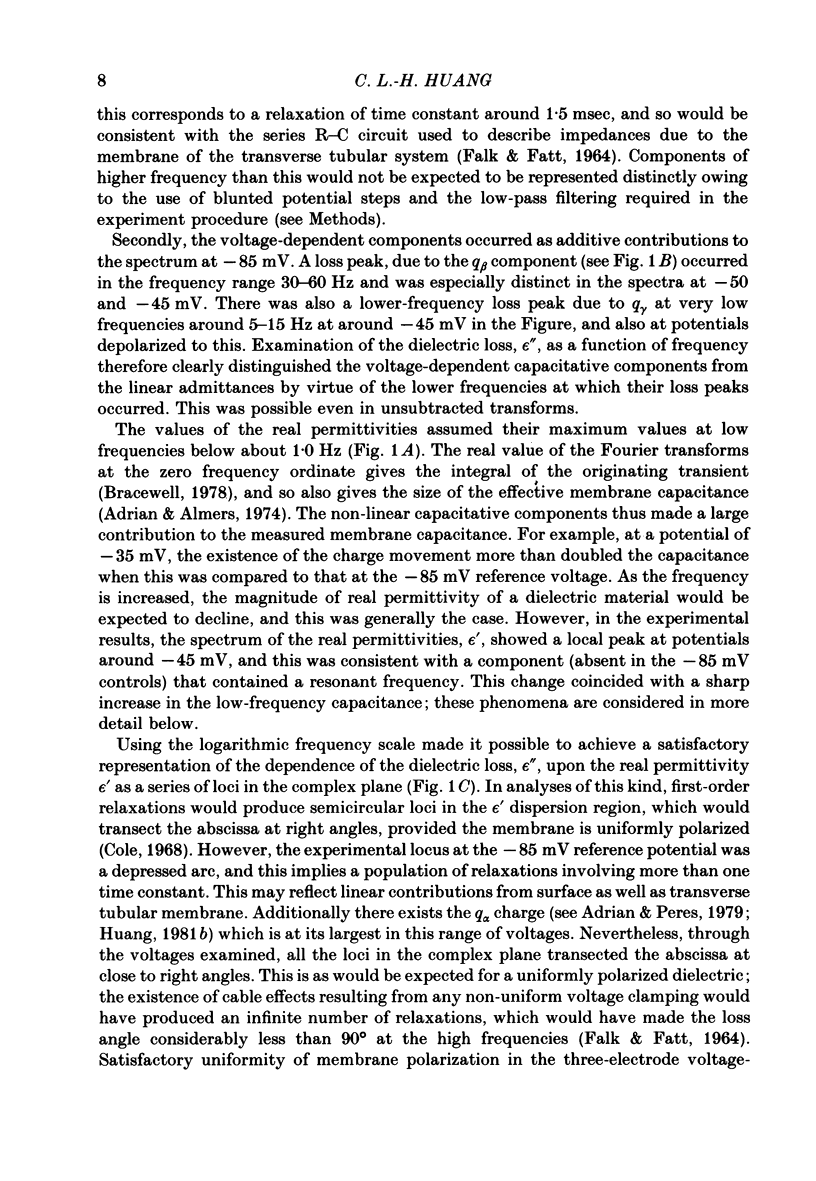
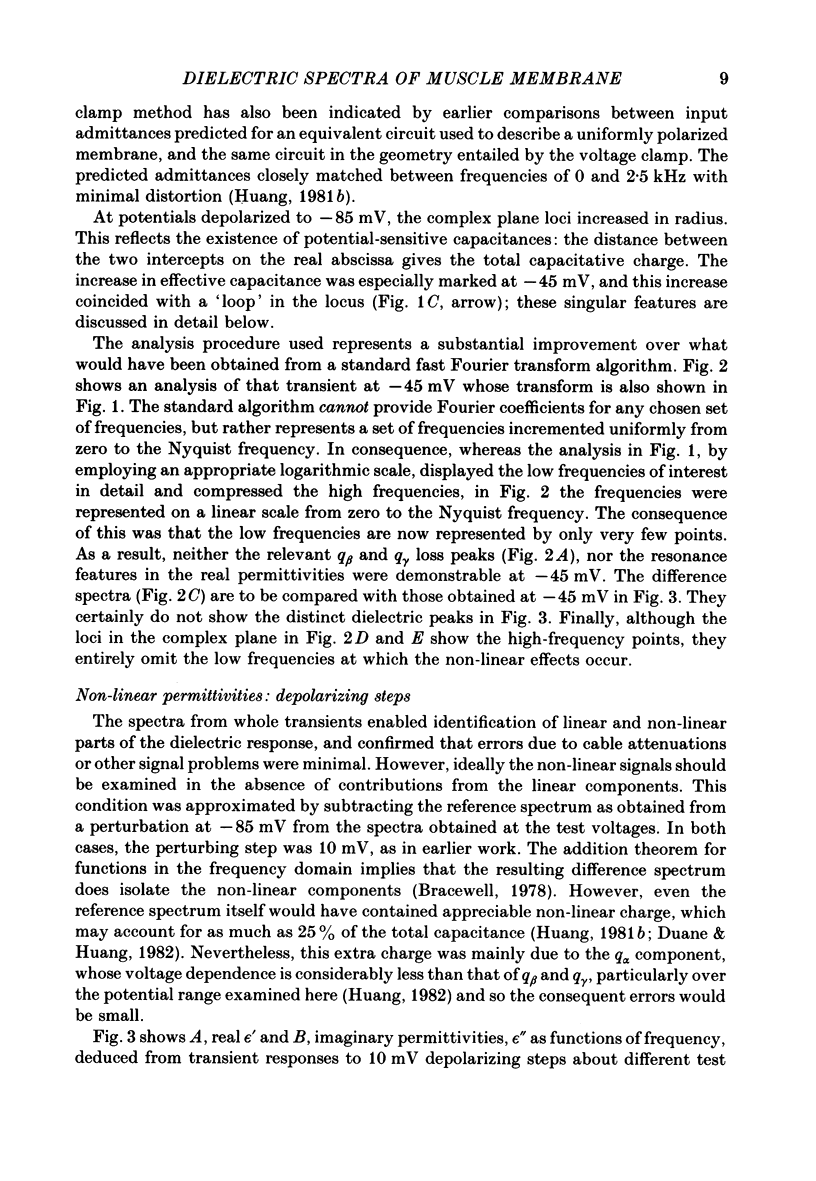
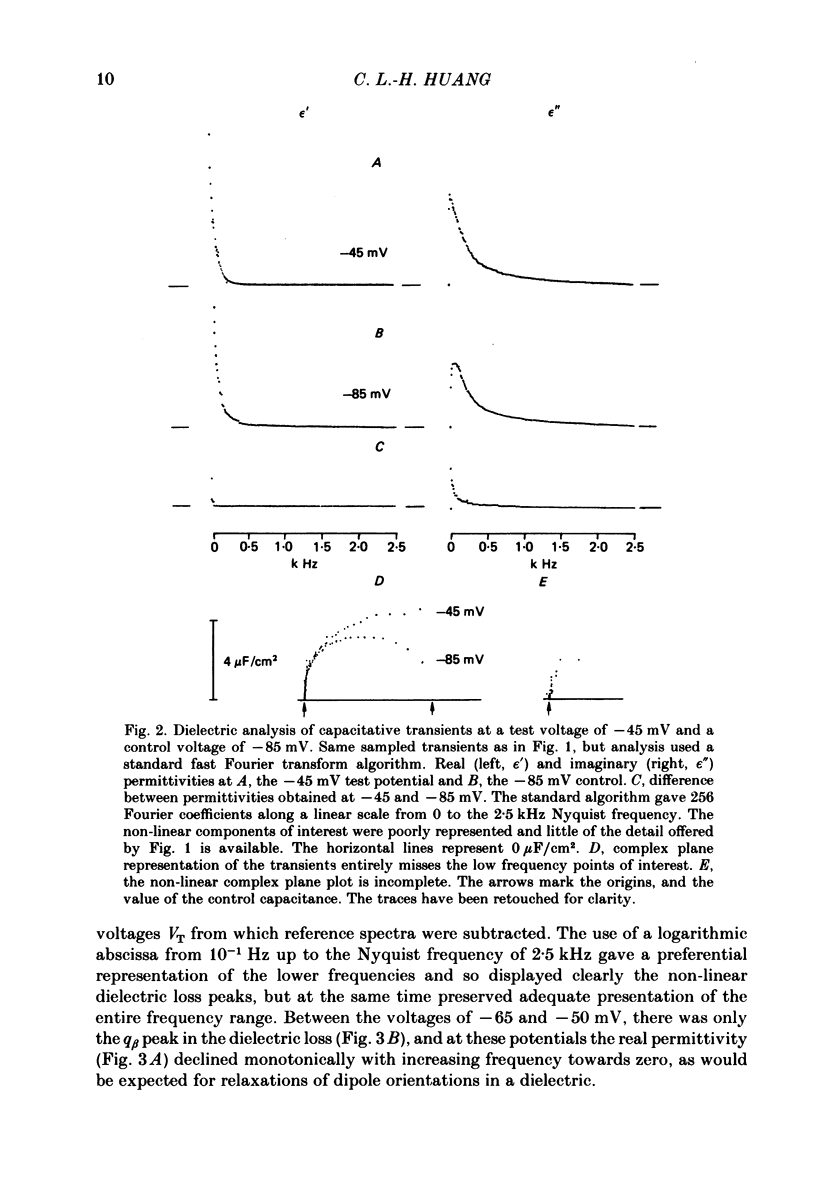
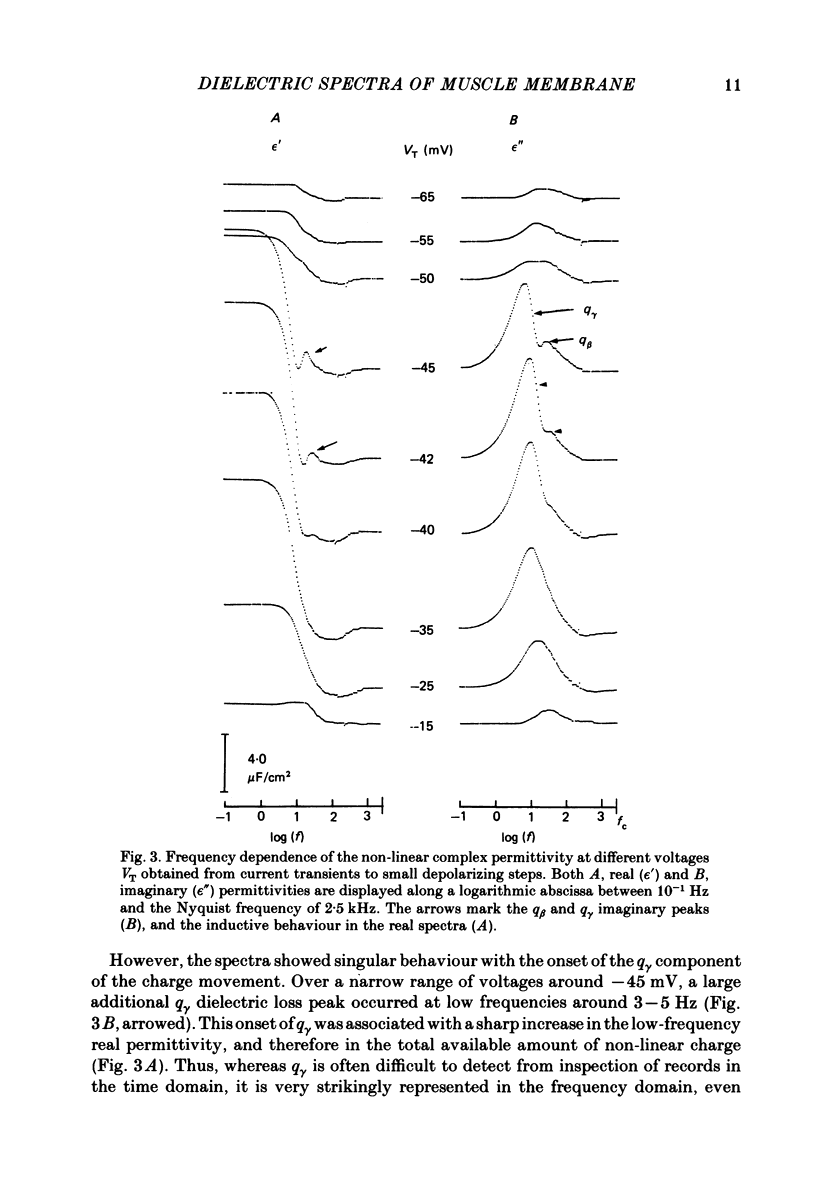
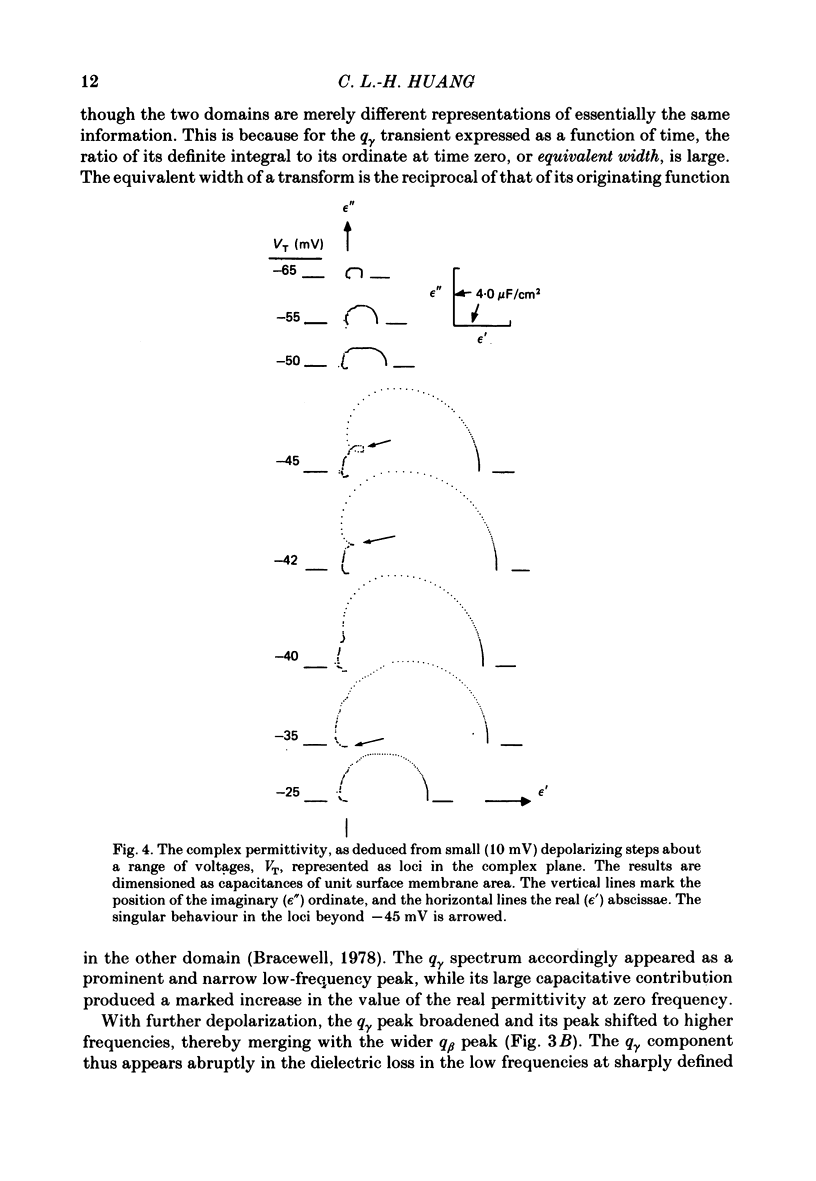
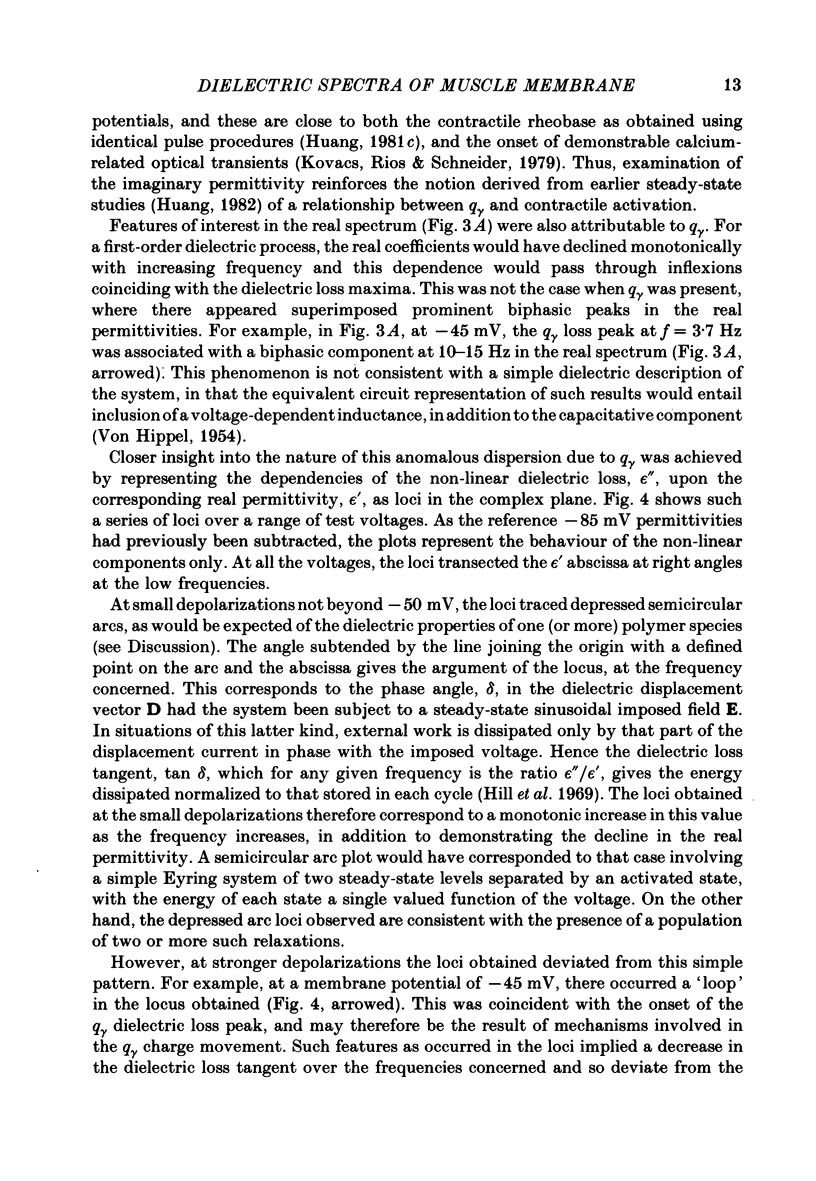
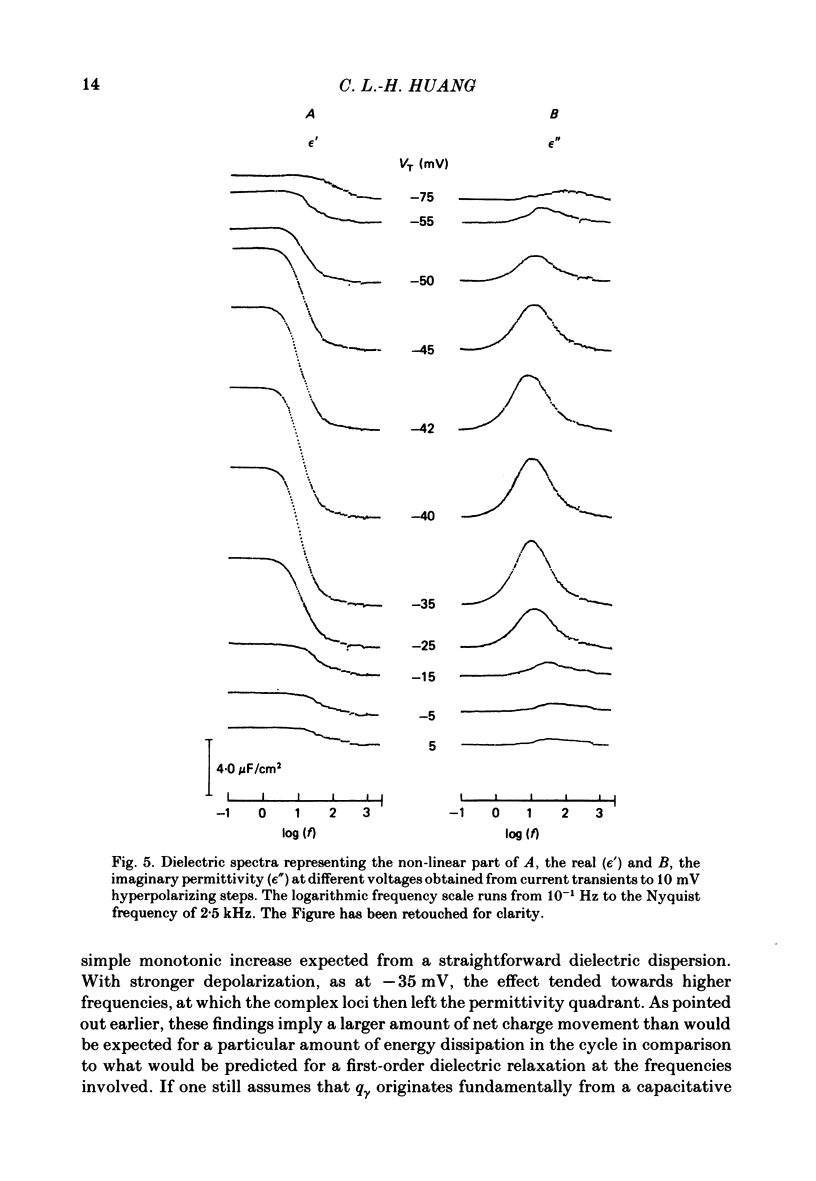
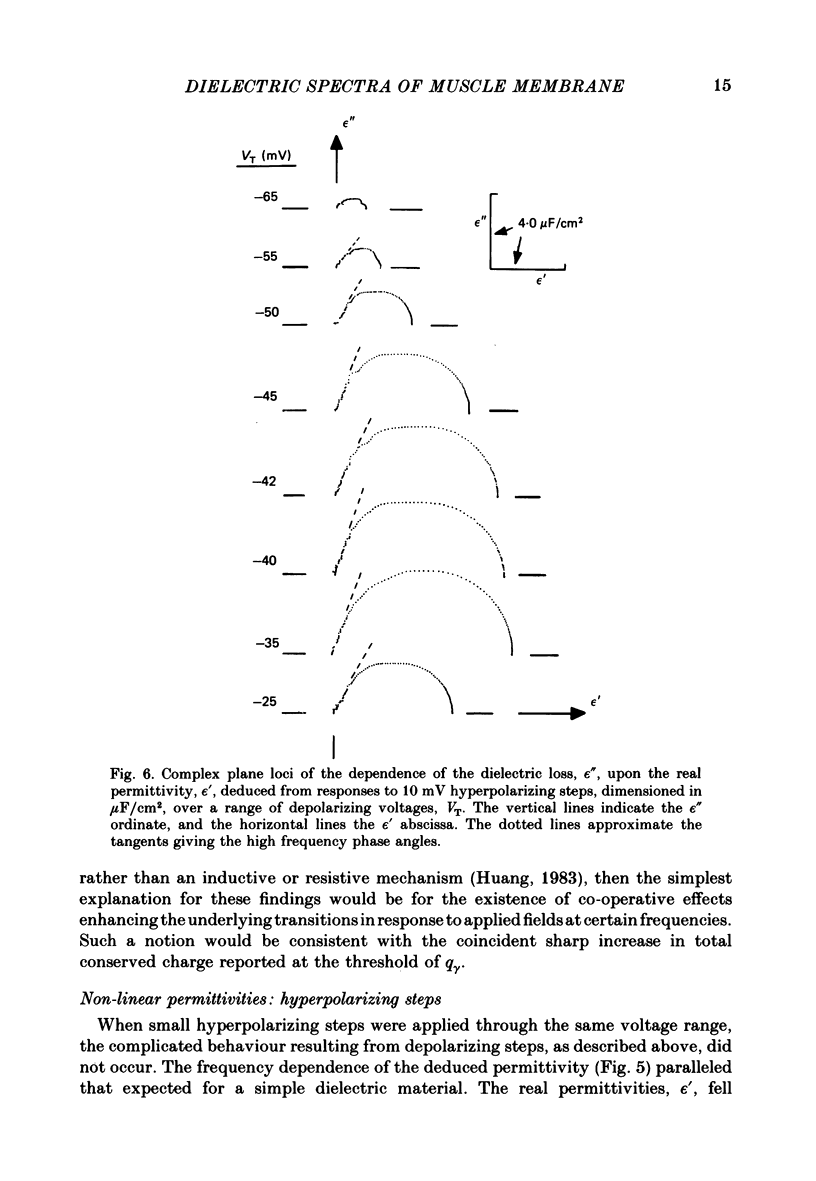
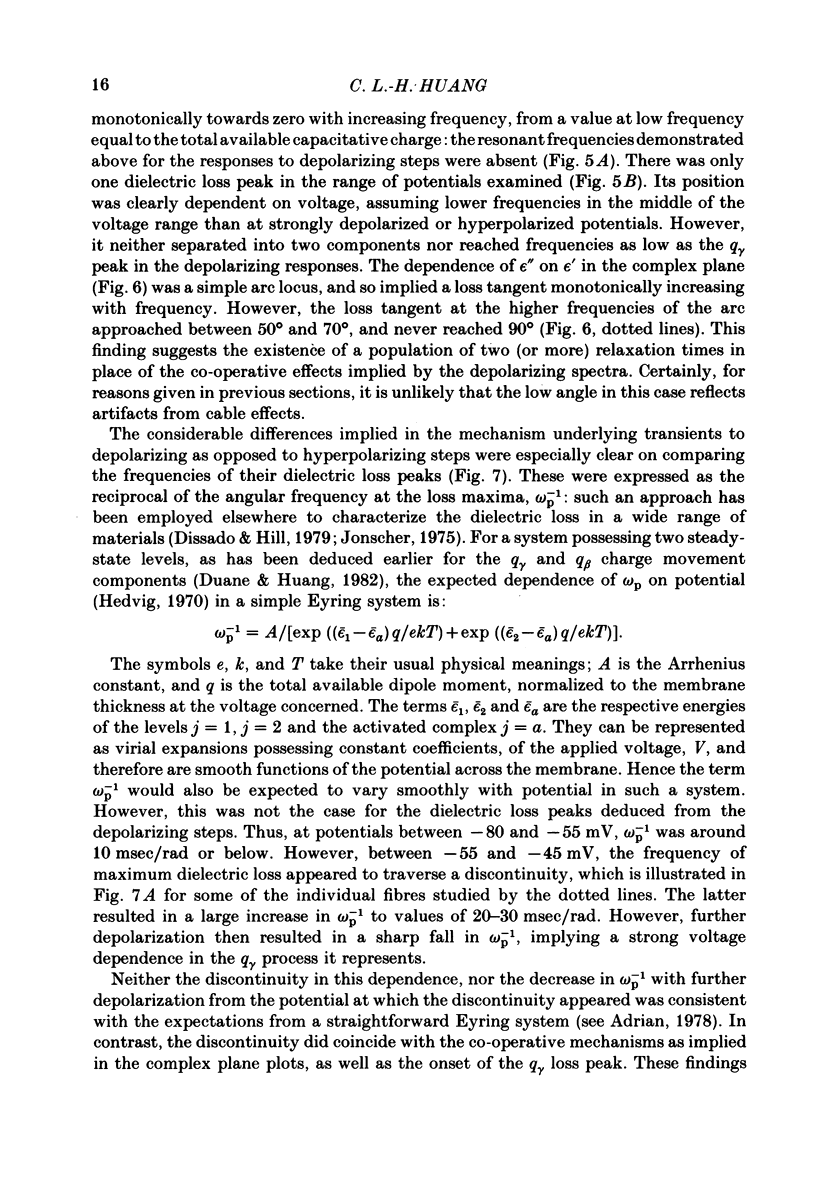
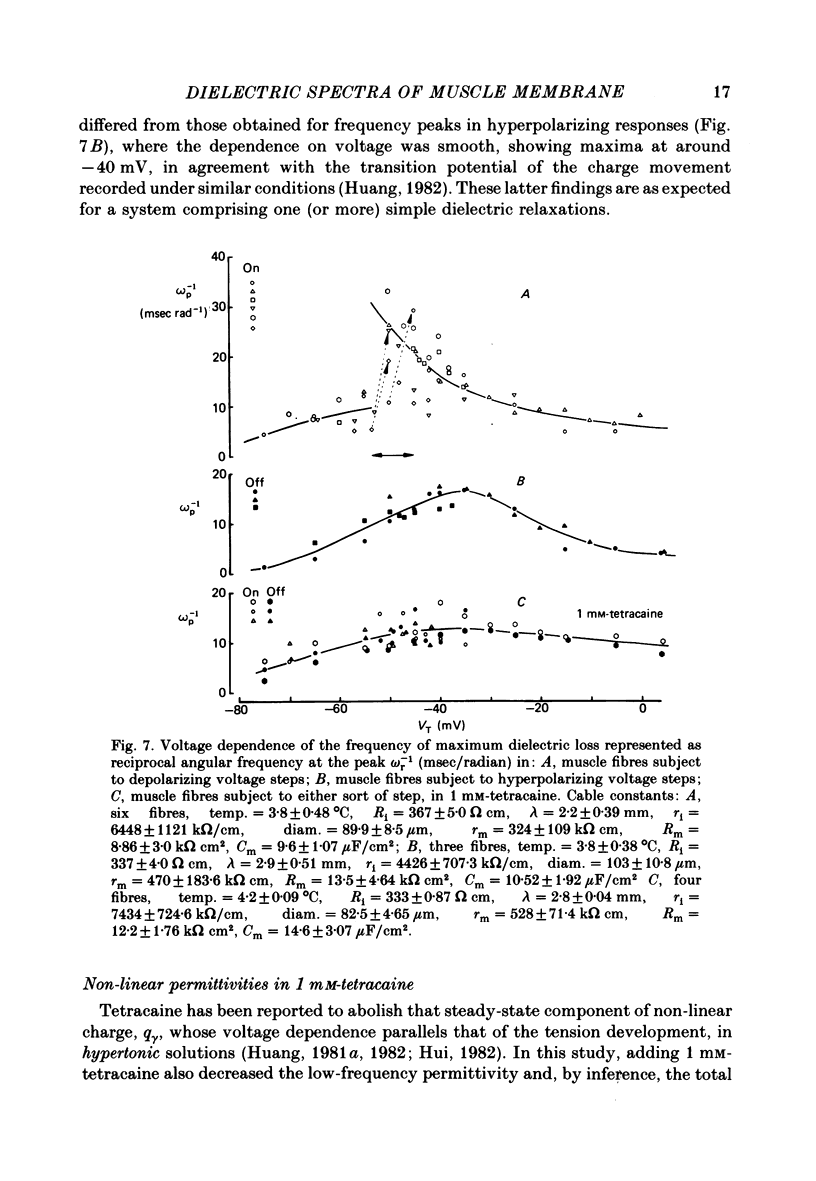
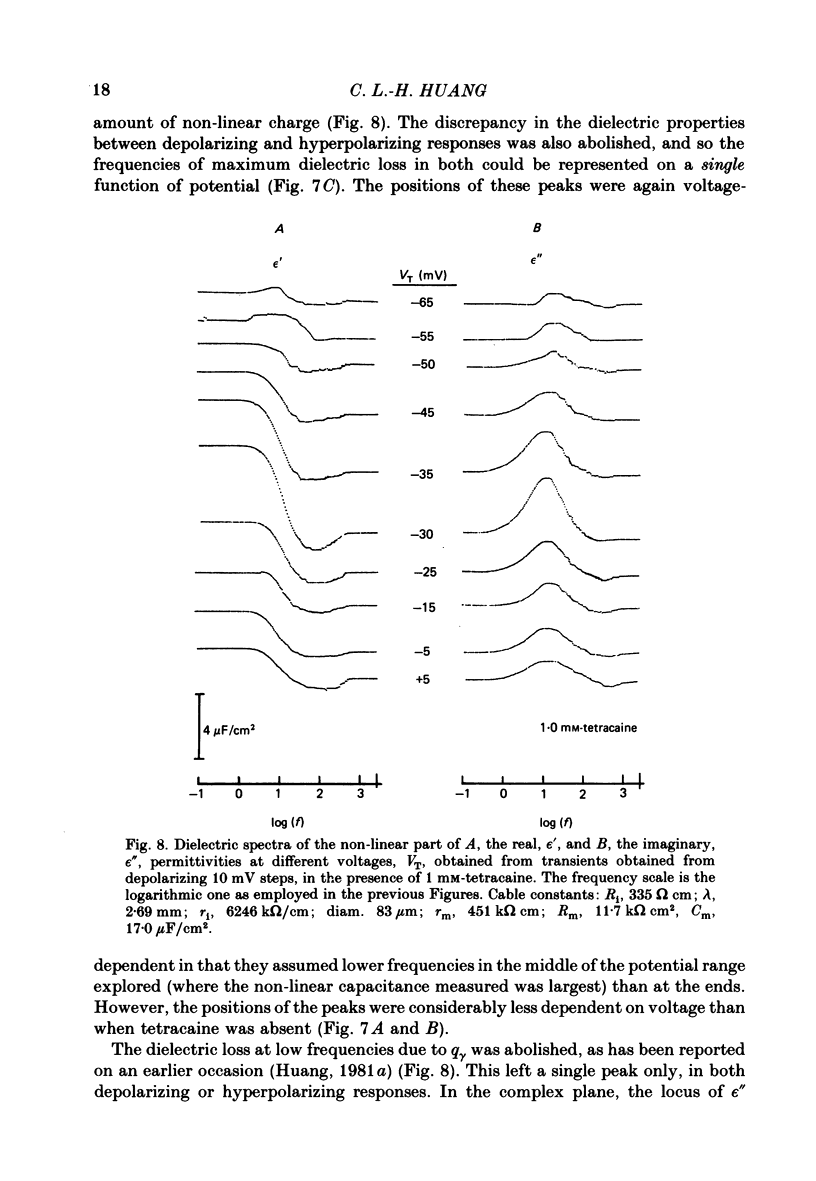
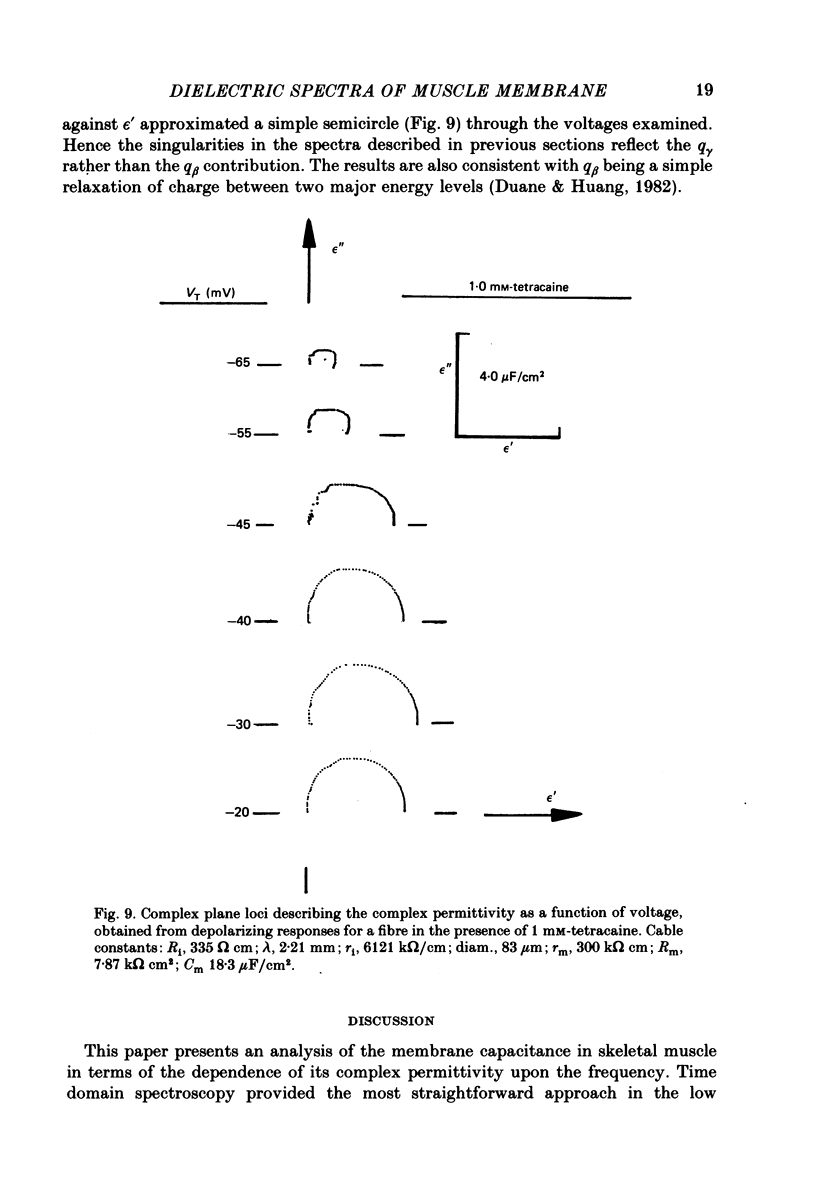
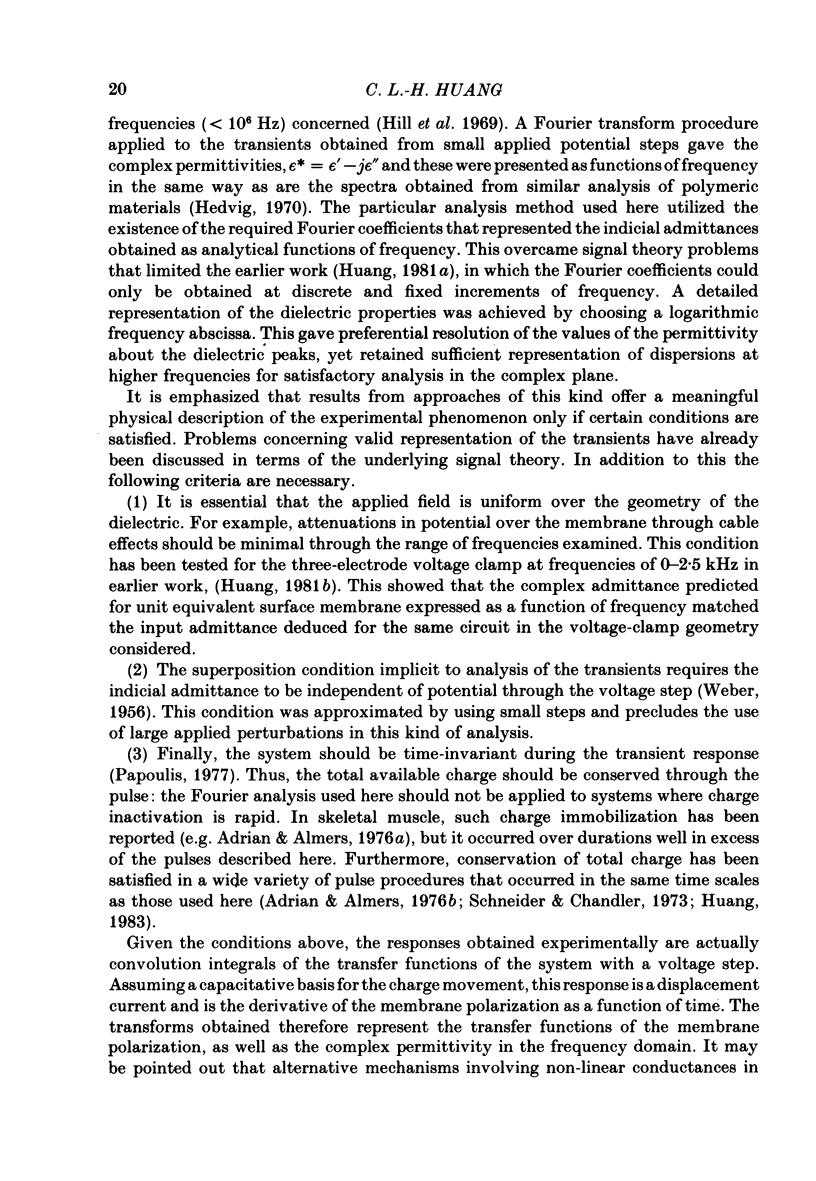
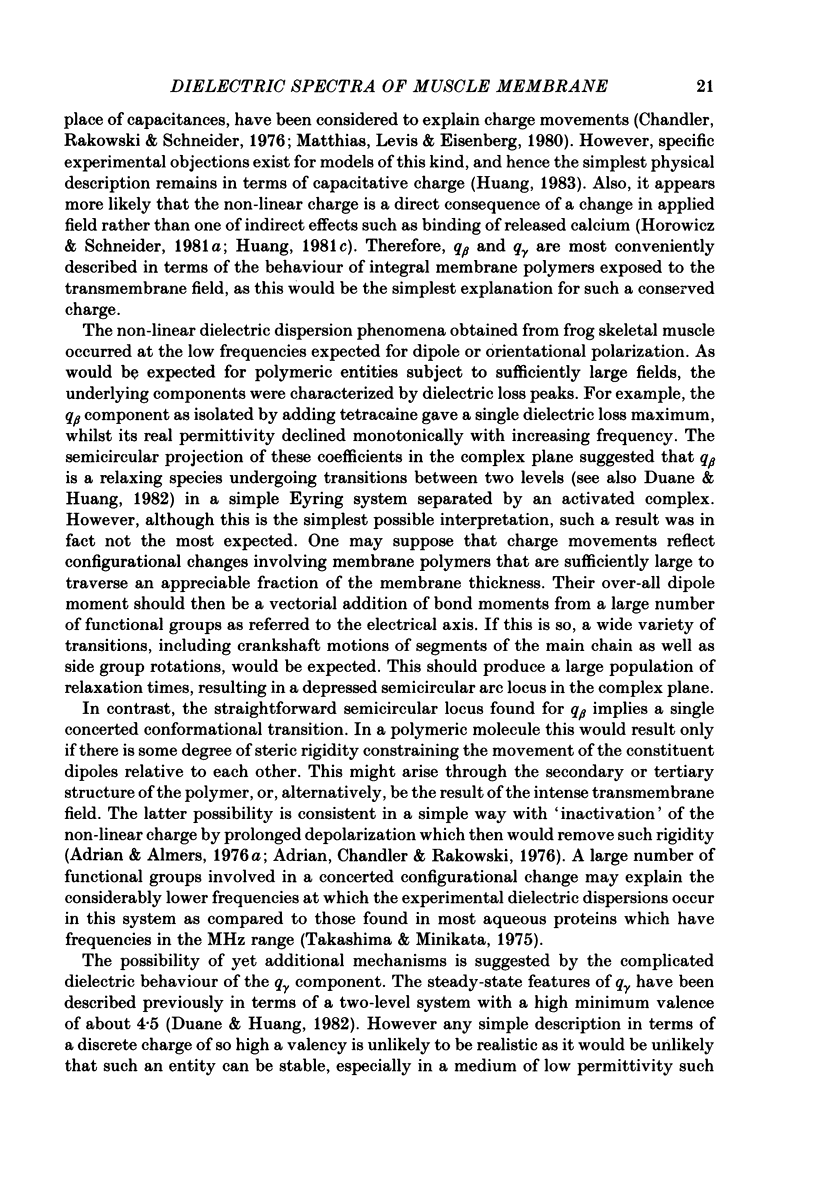
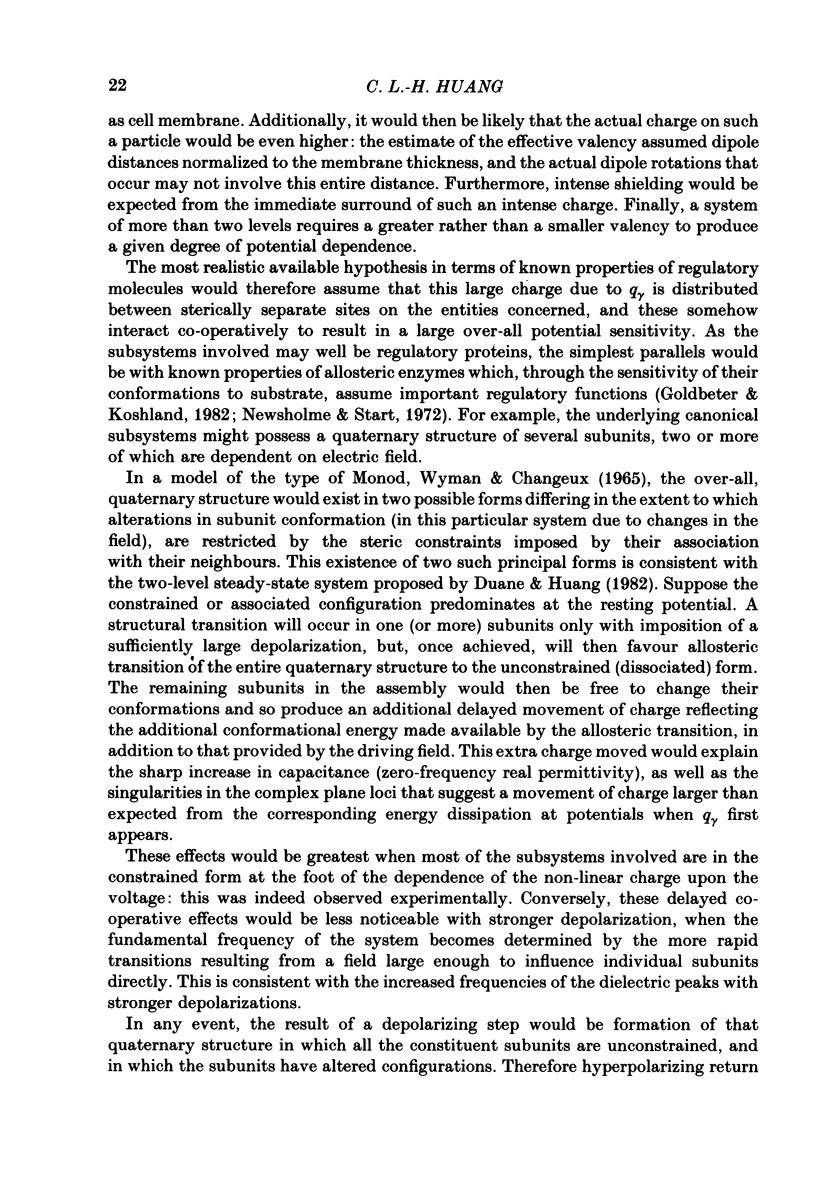
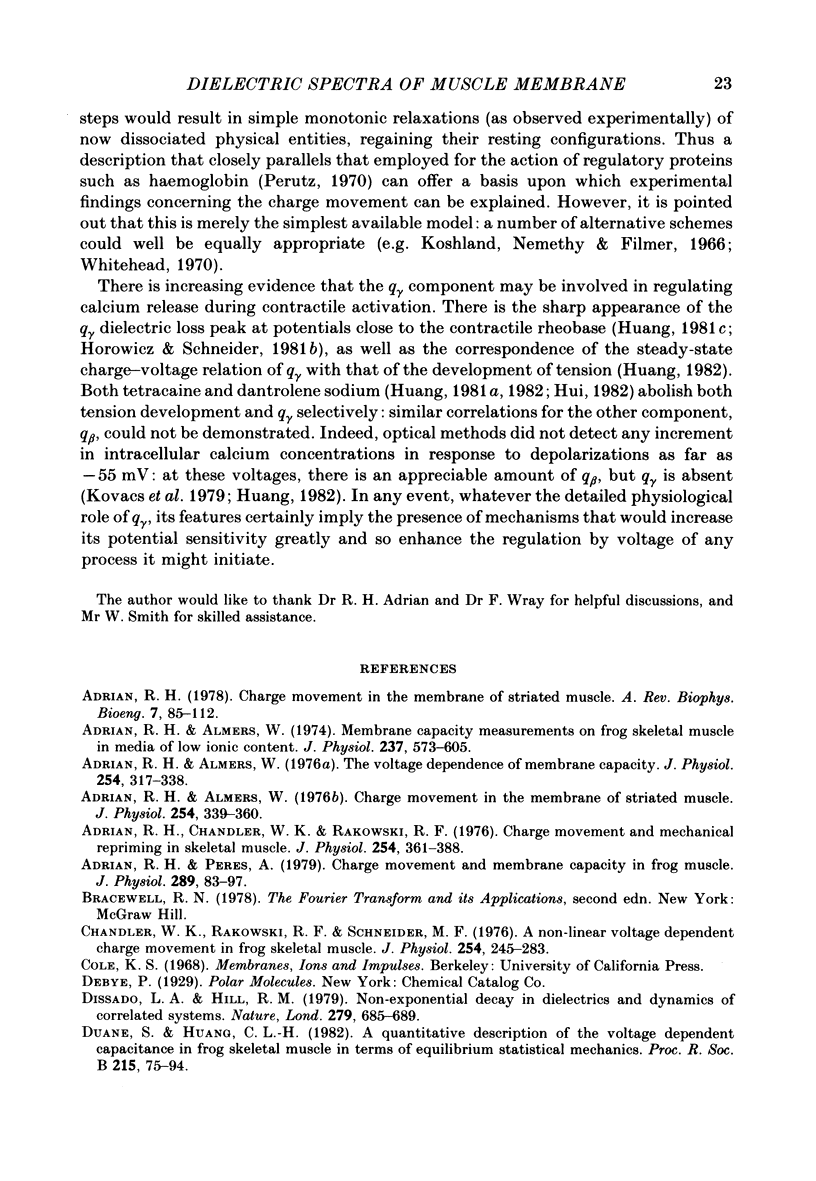
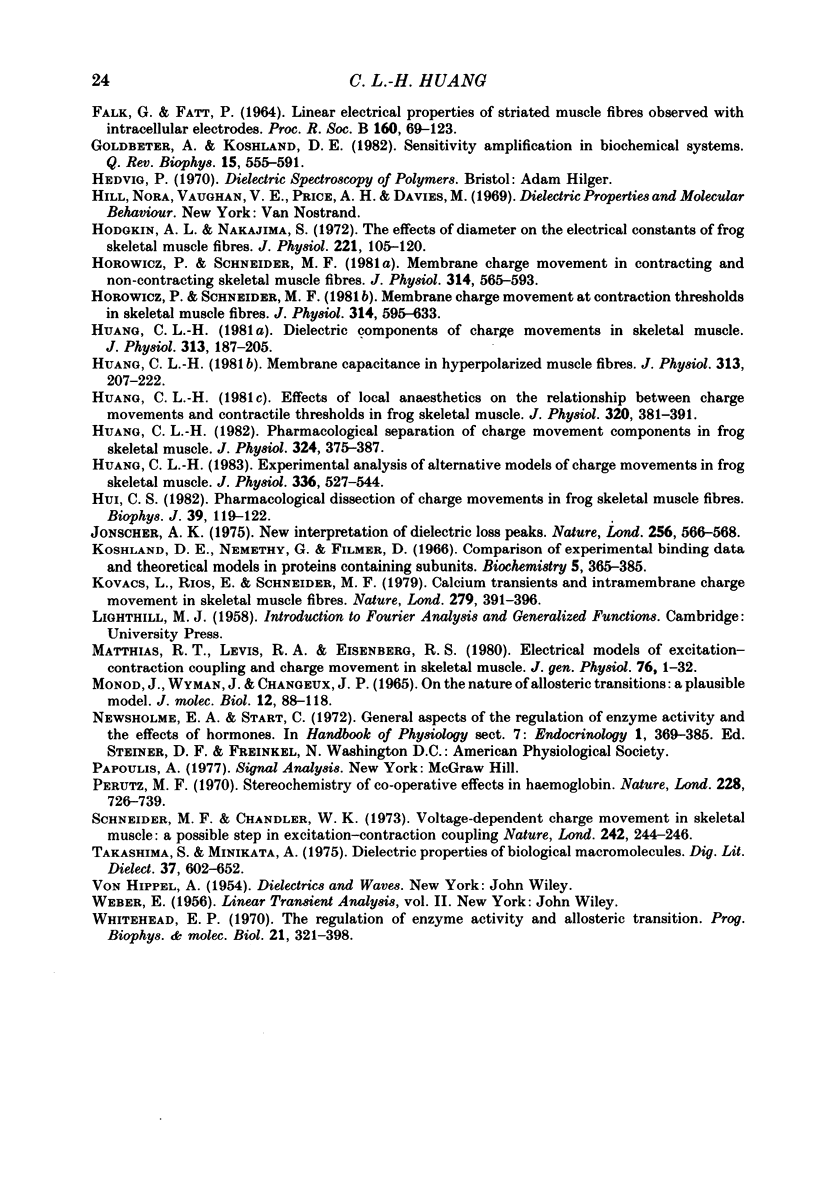
Selected References
These references are in PubMed. This may not be the complete list of references from this article.
- Adrian R. H., Almers W. Charge movement in the membrane of striated muscle. J Physiol. 1976 Jan;254(2):339–360. doi: 10.1113/jphysiol.1976.sp011235. [DOI] [PMC free article] [PubMed] [Google Scholar]
- Adrian R. H., Almers W. Membrane capacity measurements on frog skeletal muscle in media of low ion content. J Physiol. 1974 Mar;237(3):573–605. doi: 10.1113/jphysiol.1974.sp010499. [DOI] [PMC free article] [PubMed] [Google Scholar]
- Adrian R. H., Almers W. The voltage dependence of membrane capacity. J Physiol. 1976 Jan;254(2):317–338. doi: 10.1113/jphysiol.1976.sp011234. [DOI] [PMC free article] [PubMed] [Google Scholar]
- Adrian R. H., Chandler W. K., Rakowski R. F. Charge movement and mechanical repriming in skeletal muscle. J Physiol. 1976 Jan;254(2):361–388. doi: 10.1113/jphysiol.1976.sp011236. [DOI] [PMC free article] [PubMed] [Google Scholar]
- Adrian R. H. Charge movement in the membrane of striated muscle. Annu Rev Biophys Bioeng. 1978;7:85–112. doi: 10.1146/annurev.bb.07.060178.000505. [DOI] [PubMed] [Google Scholar]
- Adrian R. H., Peres A. Charge movement and membrane capacity in frog muscle. J Physiol. 1979 Apr;289:83–97. doi: 10.1113/jphysiol.1979.sp012726. [DOI] [PMC free article] [PubMed] [Google Scholar]
- Chandler W. K., Rakowski R. F., Schneider M. F. A non-linear voltage dependent charge movement in frog skeletal muscle. J Physiol. 1976 Jan;254(2):245–283. doi: 10.1113/jphysiol.1976.sp011232. [DOI] [PMC free article] [PubMed] [Google Scholar]
- Duane S., Huang C. L. A quantitative description of the voltage-dependent capacitance in frog skeletal muscle in terms of equilibrium statistical mechanics. Proc R Soc Lond B Biol Sci. 1982 Apr 22;215(1198):75–94. doi: 10.1098/rspb.1982.0029. [DOI] [PubMed] [Google Scholar]
- FALK G., FATT P. LINEAR ELECTRICAL PROPERTIES OF STRIATED MUSCLE FIBRES OBSERVED WITH INTRACELLULAR ELECTRODES. Proc R Soc Lond B Biol Sci. 1964 Apr 14;160:69–123. doi: 10.1098/rspb.1964.0030. [DOI] [PubMed] [Google Scholar]
- Goldbeter A., Koshland D. E., Jr Sensitivity amplification in biochemical systems. Q Rev Biophys. 1982 Aug;15(3):555–591. doi: 10.1017/s0033583500003449. [DOI] [PubMed] [Google Scholar]
- Hodgkin A. L., Nakajima S. The effect of diameter on the electrical constants of frog skeletal muscle fibres. J Physiol. 1972 Feb;221(1):105–120. doi: 10.1113/jphysiol.1972.sp009742. [DOI] [PMC free article] [PubMed] [Google Scholar]
- Horowicz P., Schneider M. F. Membrane charge moved at contraction thresholds in skeletal muscle fibres. J Physiol. 1981 May;314:595–633. doi: 10.1113/jphysiol.1981.sp013726. [DOI] [PMC free article] [PubMed] [Google Scholar]
- Horowicz P., Schneider M. F. Membrane charge movement in contracting and non-contracting skeletal muscle fibres. J Physiol. 1981 May;314:565–593. doi: 10.1113/jphysiol.1981.sp013725. [DOI] [PMC free article] [PubMed] [Google Scholar]
- Huang C. L. Dielectric components of charge movements in skeletal muscle. J Physiol. 1981;313:187–205. doi: 10.1113/jphysiol.1981.sp013658. [DOI] [PMC free article] [PubMed] [Google Scholar]
- Huang C. L. Effects of local anaesthetics on the relationship between charge movements and contractile thresholds in frog skeletal muscle. J Physiol. 1981 Nov;320:381–391. doi: 10.1113/jphysiol.1981.sp013956. [DOI] [PMC free article] [PubMed] [Google Scholar]
- Huang C. L. Experimental analysis of alternative models of charge movement in frog skeletal muscle. J Physiol. 1983 Mar;336:527–543. doi: 10.1113/jphysiol.1983.sp014596. [DOI] [PMC free article] [PubMed] [Google Scholar]
- Huang C. L. Membrane capacitance in hyperpolarized muscle fibres. J Physiol. 1981;313:207–222. doi: 10.1113/jphysiol.1981.sp013659. [DOI] [PMC free article] [PubMed] [Google Scholar]
- Huang C. L. Pharmacological separation of charge movement components in frog skeletal muscle. J Physiol. 1982 Mar;324:375–387. doi: 10.1113/jphysiol.1982.sp014118. [DOI] [PMC free article] [PubMed] [Google Scholar]
- Hui C. S. Pharmacological dissection of charge movement in frog skeletal muscle fibers. Biophys J. 1982 Jul;39(1):119–122. doi: 10.1016/S0006-3495(82)84498-6. [DOI] [PMC free article] [PubMed] [Google Scholar]
- Koshland D. E., Jr, Némethy G., Filmer D. Comparison of experimental binding data and theoretical models in proteins containing subunits. Biochemistry. 1966 Jan;5(1):365–385. doi: 10.1021/bi00865a047. [DOI] [PubMed] [Google Scholar]
- Kovács L., Ríos E., Schneider M. F. Calcium transients and intramembrane charge movement in skeletal muscle fibres. Nature. 1979 May 31;279(5712):391–396. doi: 10.1038/279391a0. [DOI] [PubMed] [Google Scholar]
- MONOD J., WYMAN J., CHANGEUX J. P. ON THE NATURE OF ALLOSTERIC TRANSITIONS: A PLAUSIBLE MODEL. J Mol Biol. 1965 May;12:88–118. doi: 10.1016/s0022-2836(65)80285-6. [DOI] [PubMed] [Google Scholar]
- Mathias R. T., Levis R. A., Eisenberg R. S. Electrical models of excitation-contraction coupling and charge movement in skeletal muscle. J Gen Physiol. 1980 Jul;76(1):1–31. doi: 10.1085/jgp.76.1.1. [DOI] [PMC free article] [PubMed] [Google Scholar]
- Perutz M. F. Stereochemistry of cooperative effects in haemoglobin. Nature. 1970 Nov 21;228(5273):726–739. doi: 10.1038/228726a0. [DOI] [PubMed] [Google Scholar]
- Schneider M. F., Chandler W. K. Voltage dependent charge movement of skeletal muscle: a possible step in excitation-contraction coupling. Nature. 1973 Mar 23;242(5395):244–246. doi: 10.1038/242244a0. [DOI] [PubMed] [Google Scholar]
- Whitehead E. The regulation of enzyme activity and allosteric transition. Prog Biophys Mol Biol. 1970;21:321–397. doi: 10.1016/0079-6107(70)90028-3. [DOI] [PubMed] [Google Scholar]