Abstract
1. We used external electrodes placed under precise visual control on motor endings of the mouse to record electrical activity promoted by nerve stimulation.
2. Three types of wave form have been observed in relation to well-defined electrode emplacements: (i) at the transition between myelinated and non-myelinated parts of the axon, the wave form consists of two negative deflexions preceded by a small positivity (preterminal response), (ii) at the main part of the terminal branches, we obtained a two component positive wave form (terminal response) and (iii) electrode positions in a narrow area between the former and the latter yielded triphasic (positive—negative—positive) wave forms (intermediate responses).
3. Since these responses could not be readily interpreted in terms of classical description of membrane currents associated with propagating action potentials, we used specific channel blocking agents to identify wave form components.
4. Bath application of tetraethylammonium or aminopyridines, or, better, a combination of both, suppressed delayed positive deflexions of terminal and intermediate responses and the late negative component of preterminal responses. Local inophoretic drug application showed that K channels are present only at the terminal part of the endings. K+ outflux promotes a local circuit whose sink is located at the preterminal part where it generates the late negative deflexion of the preterminal response.
5. Local application of tetrodotoxin suppressed the first negative component of preterminal responses but failed to affect electrical activity at the terminal part of the endings. This indicates that Na channels, and, therefore, action potential generation, are restricted to the preterminal part.
6. Suppression of K conductance revealed a slow inward current at the terminal part of the endings which could be identified as a Ca current. Ba2+ and Sr2+ could substitute for Ca2+ as inward current carriers.
7. Activation of spatially separated Na channels, on one side, and of K and Ca channels, on the other, generated ionic currents and separated local circuit currents which flow between preterminal and terminal parts (and vice versa). Thus, the signals recorded at each point of motor endings correspond to the sum of ionic and passive currents entering (or leaving) the membrane at that point.
8. The present results represent a further example of heterogeneity of axonal membrane.
Full text
PDF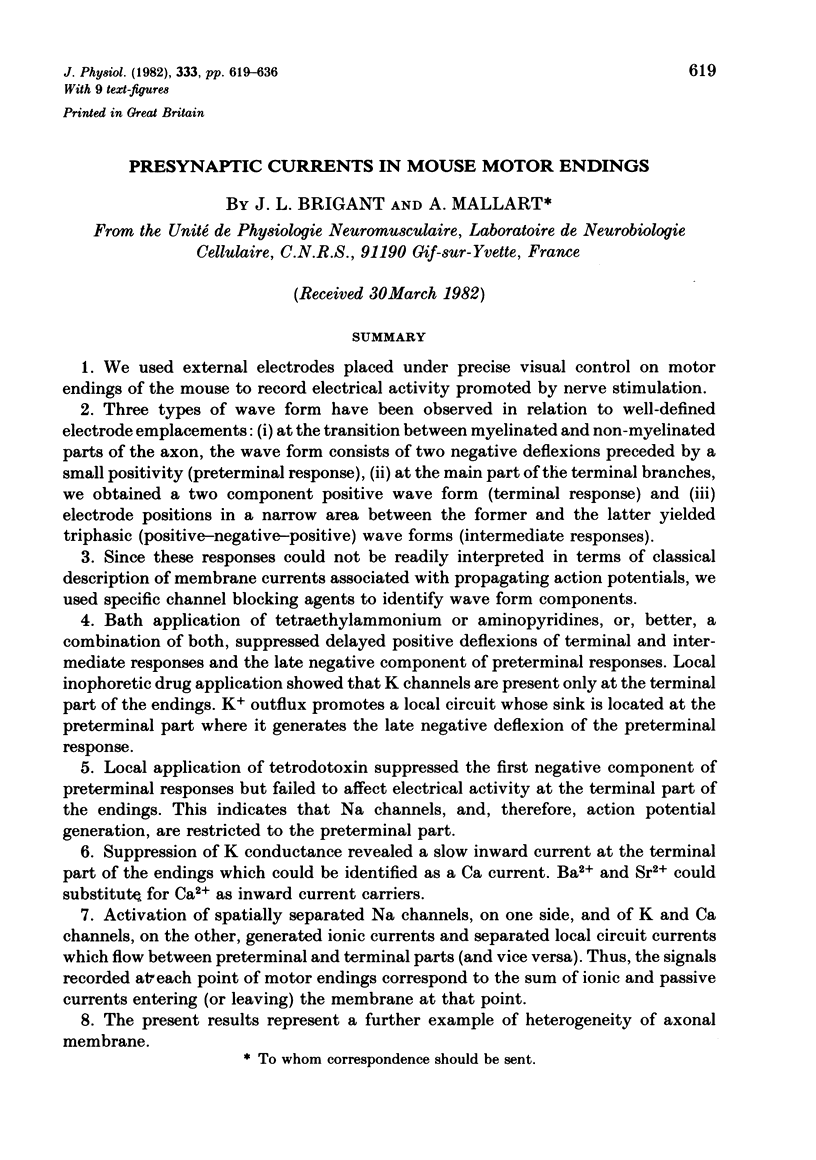
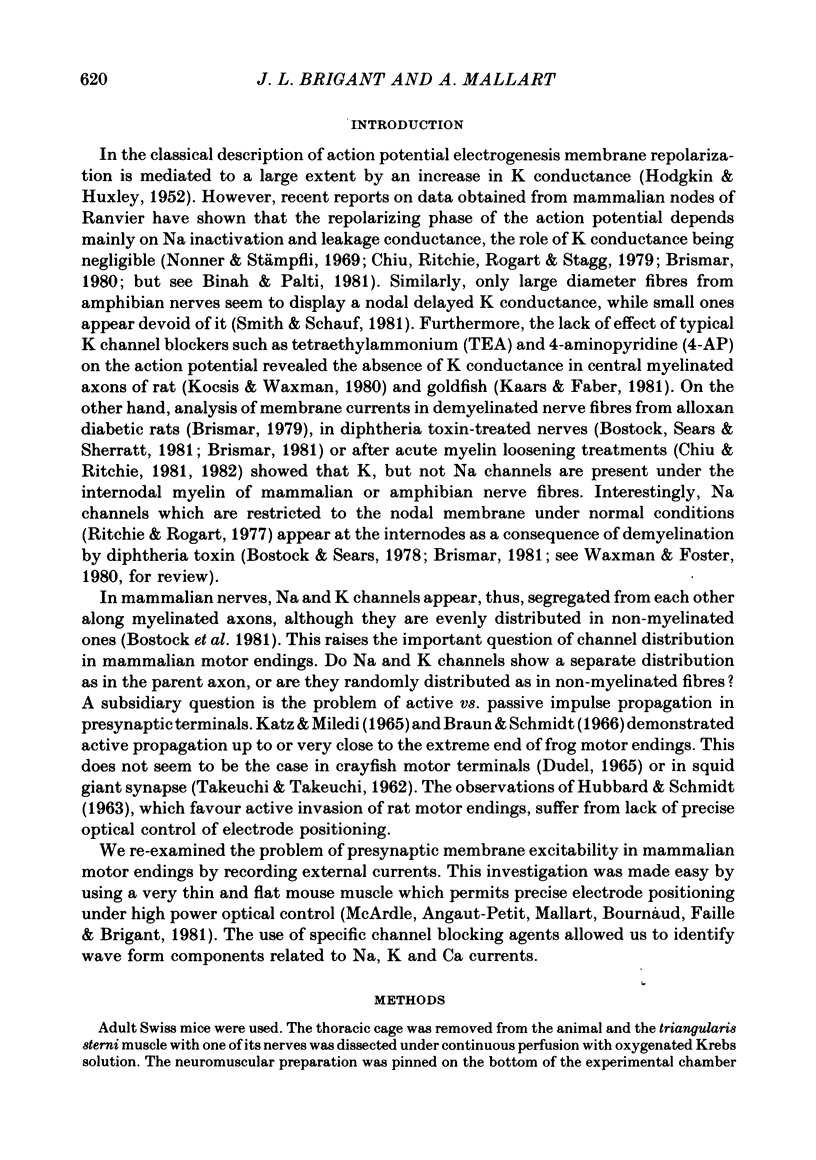
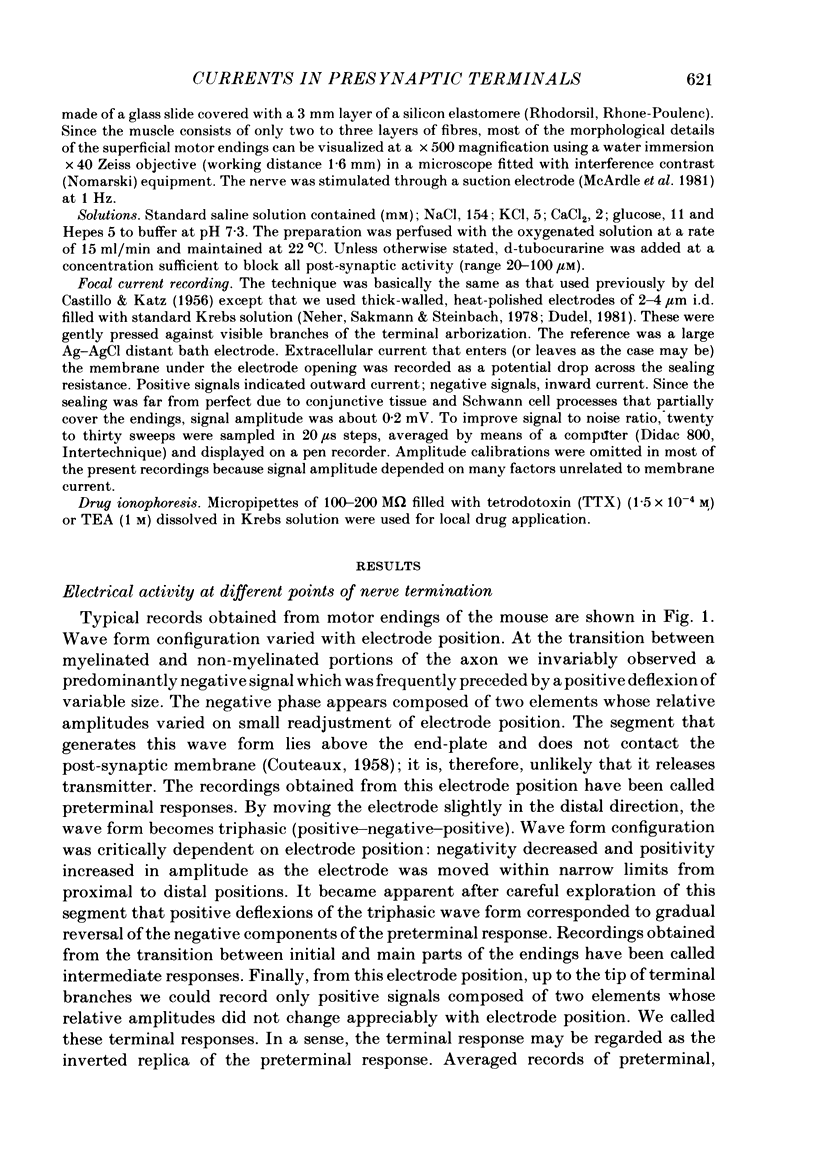
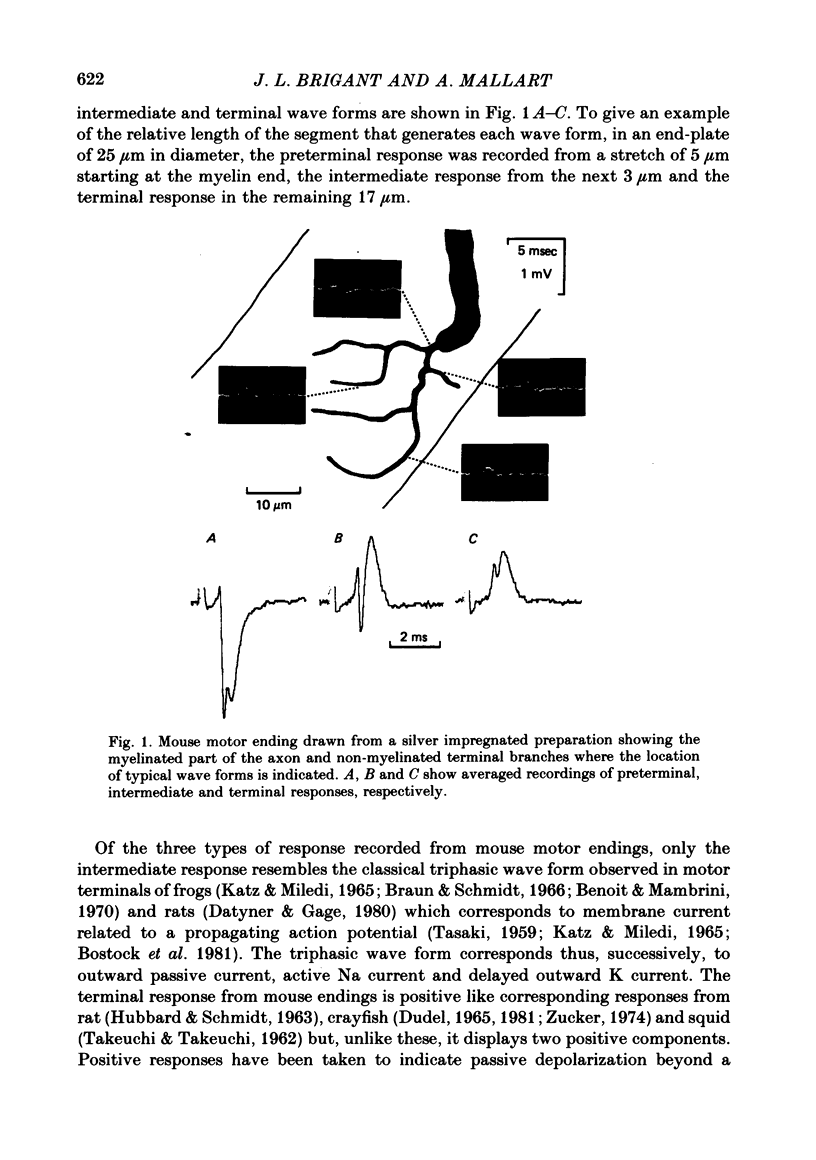
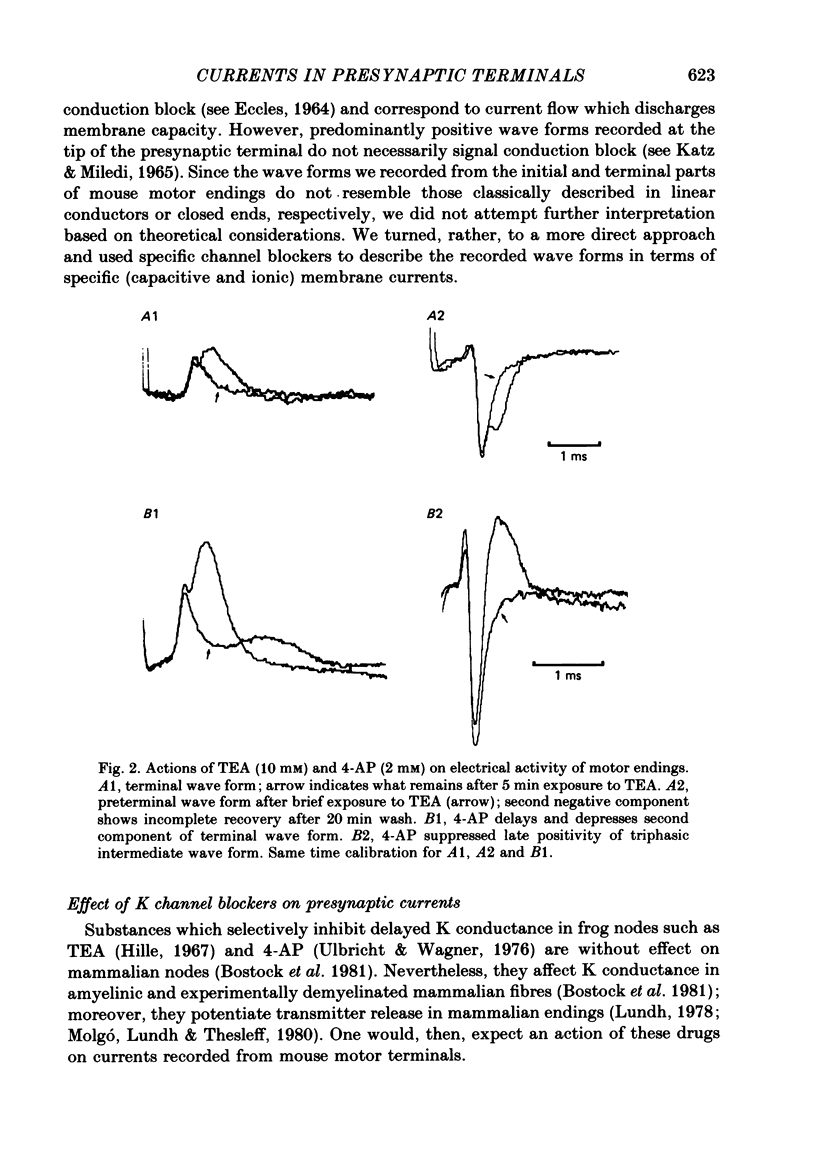
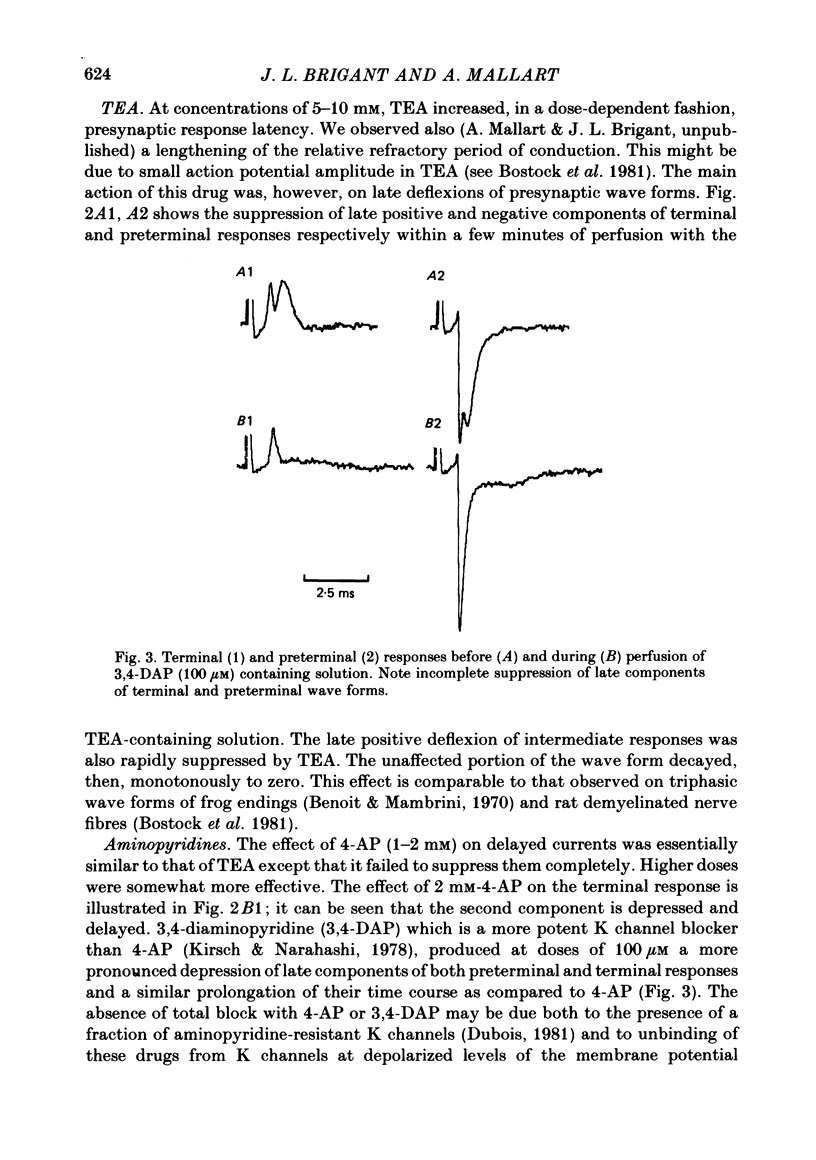
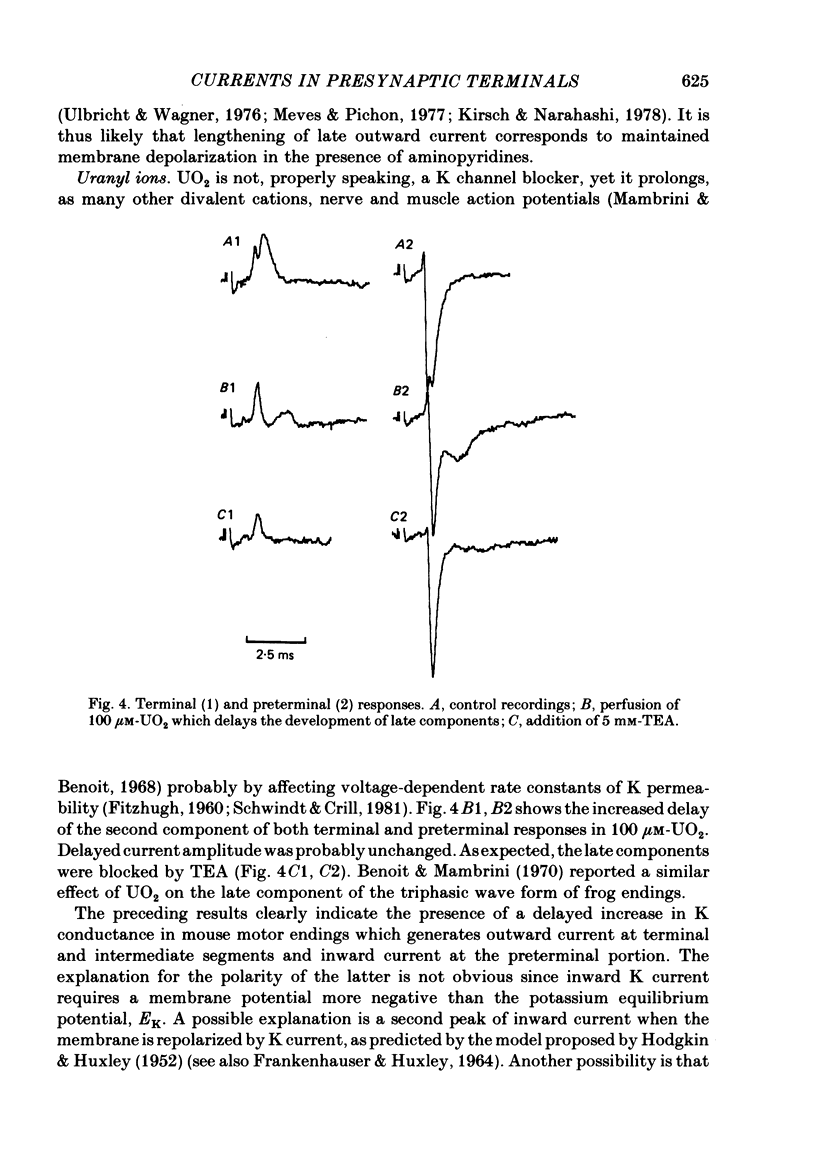
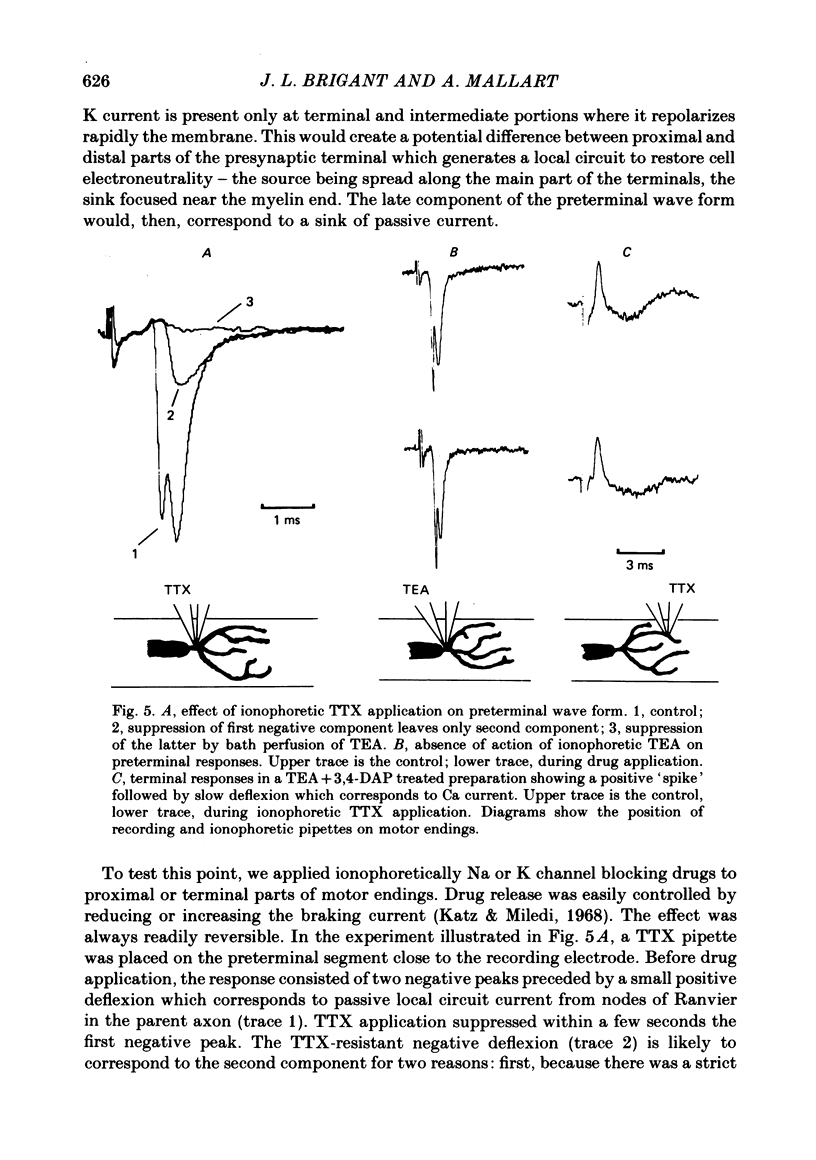
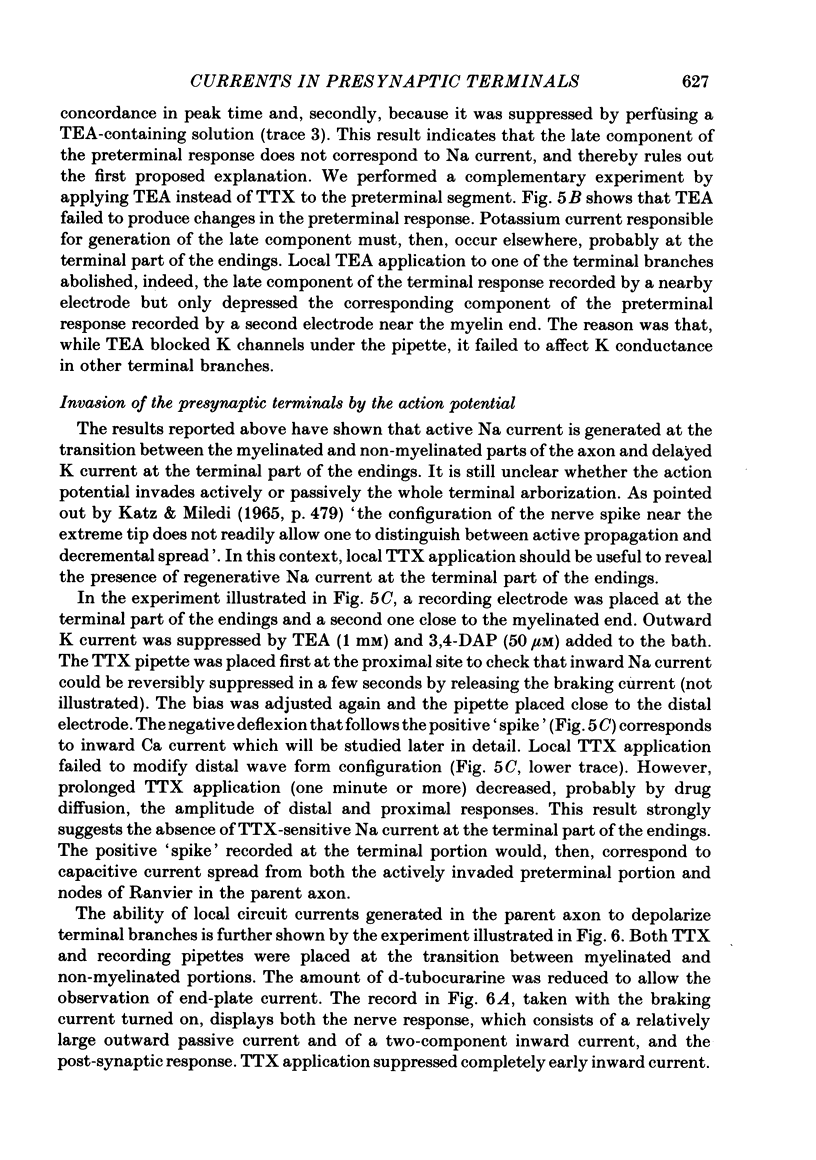
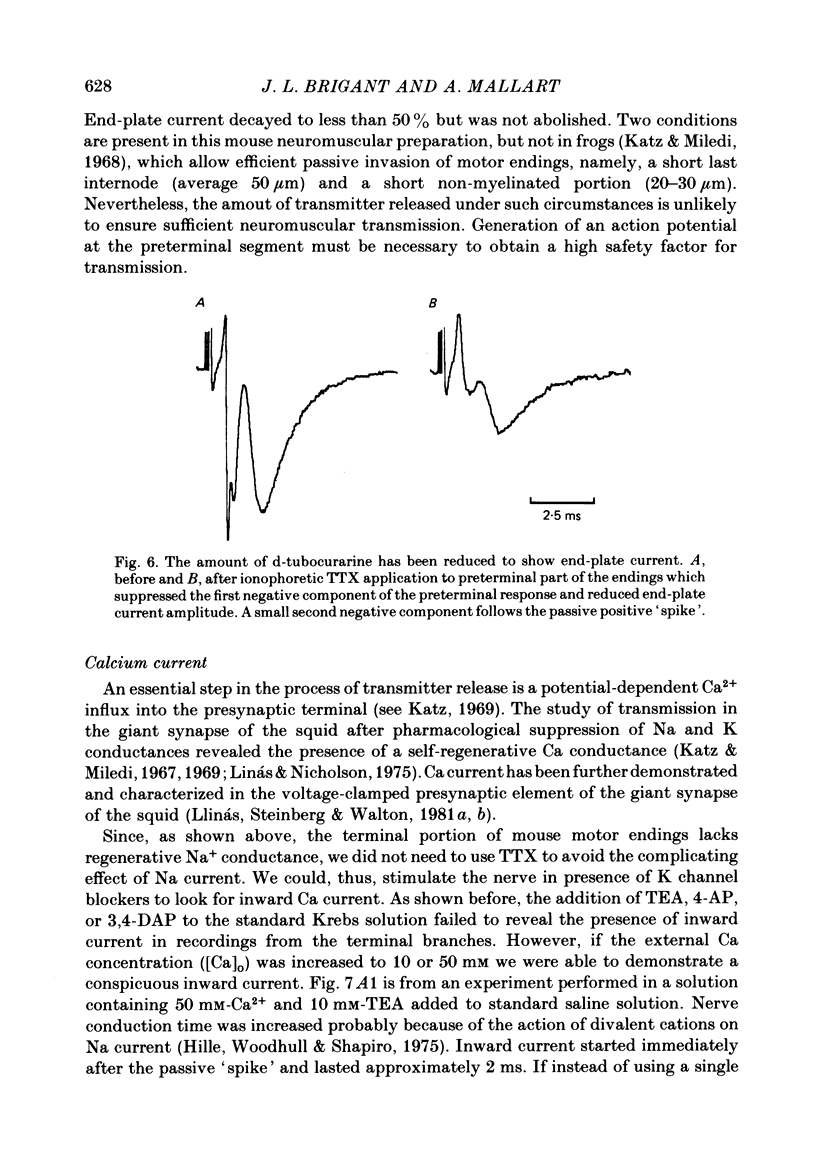
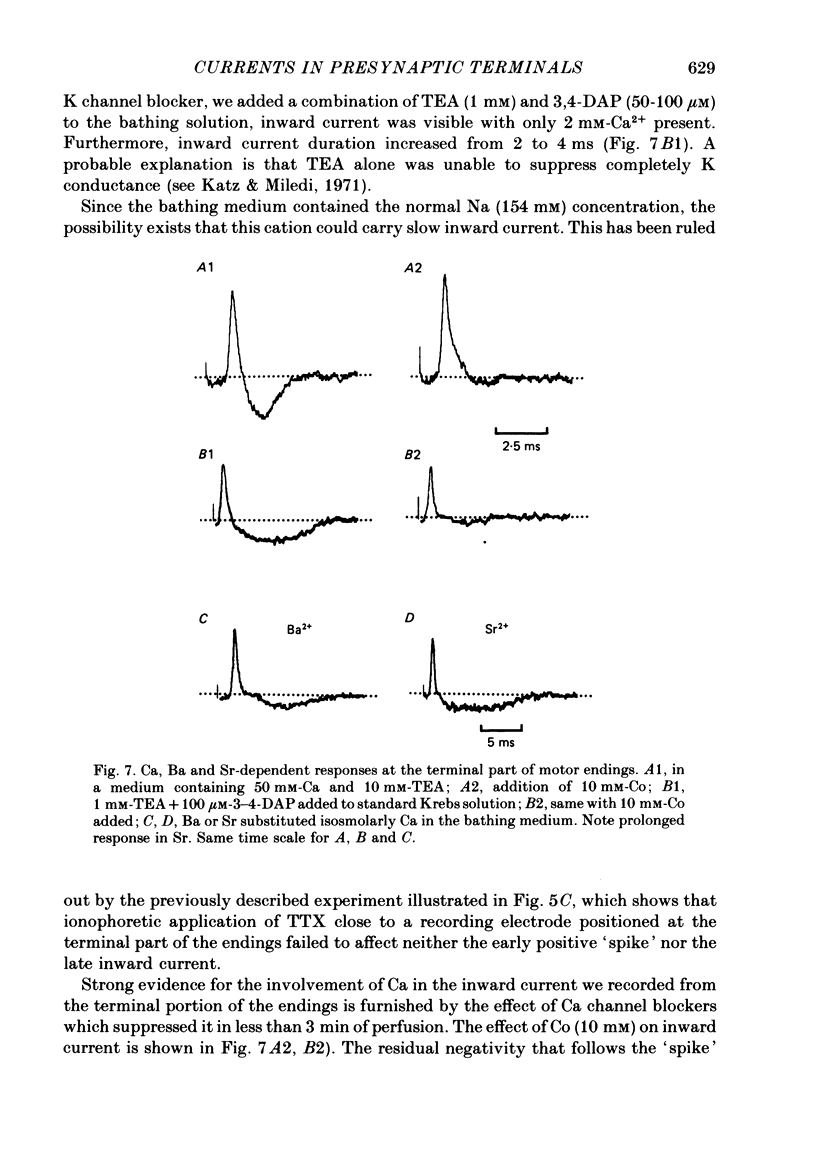
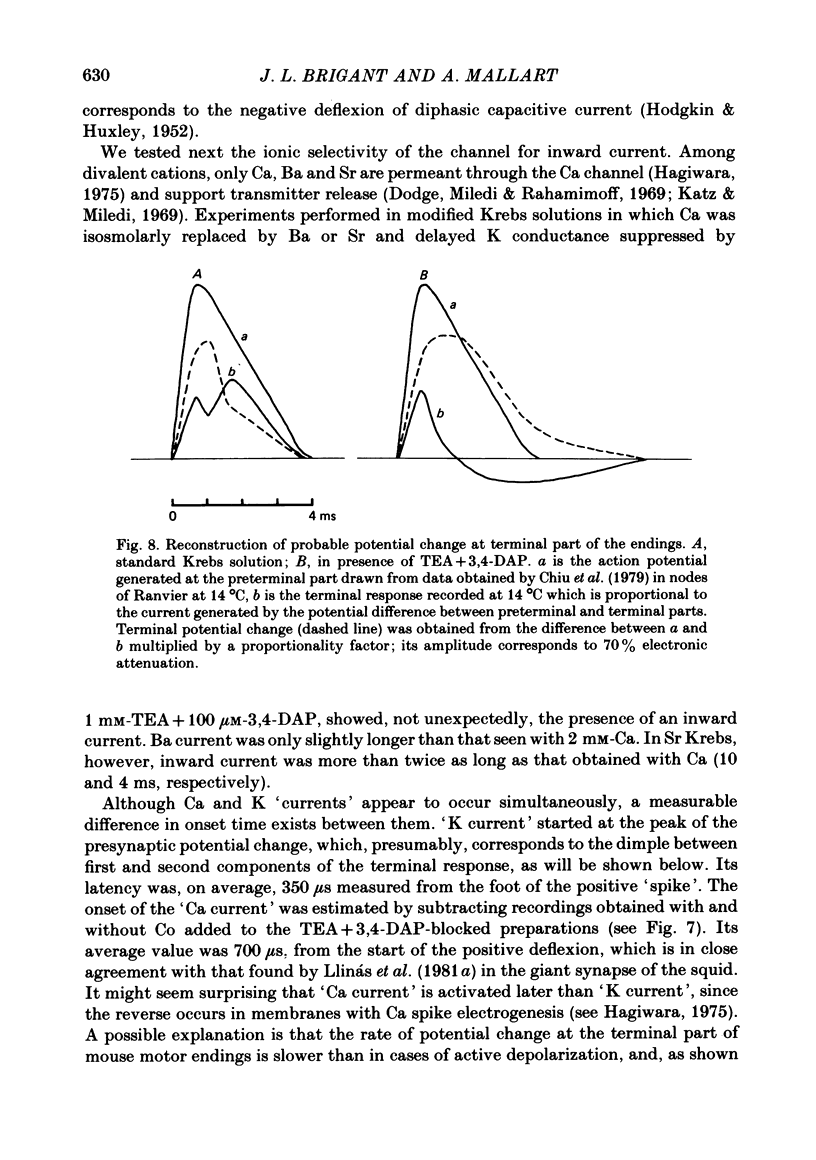
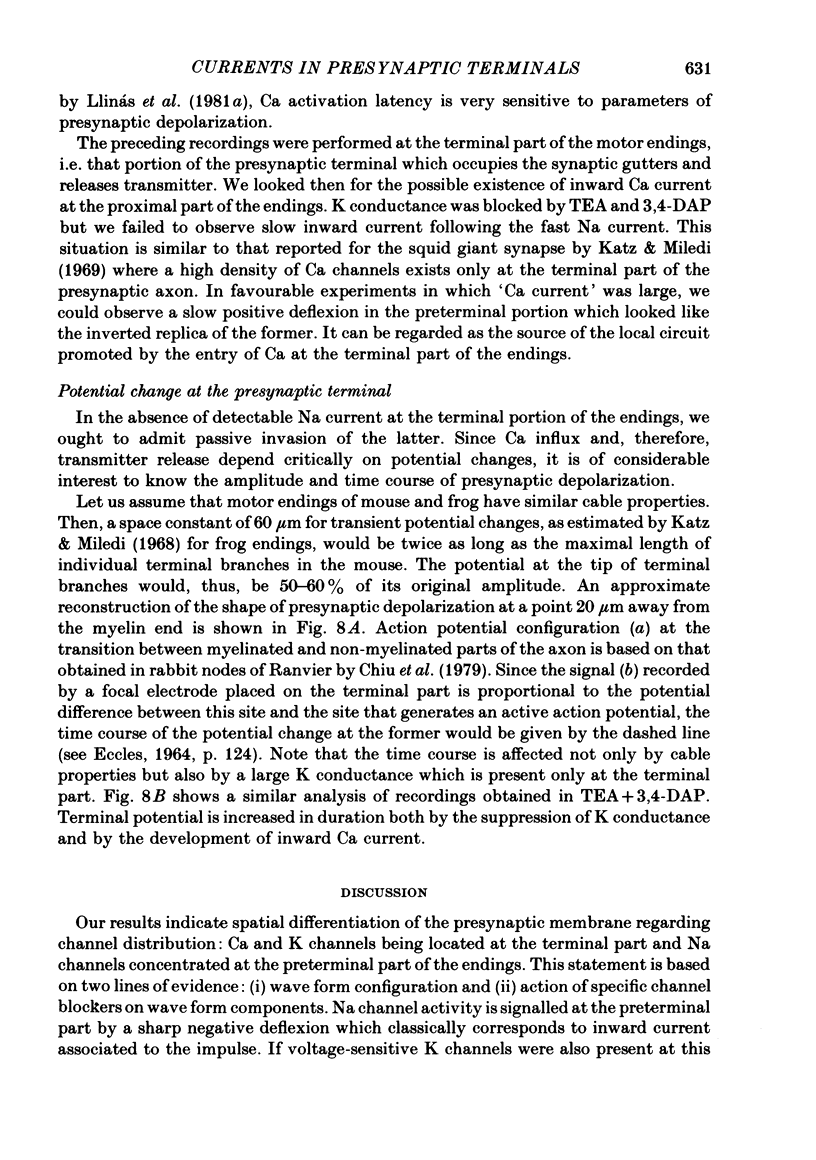
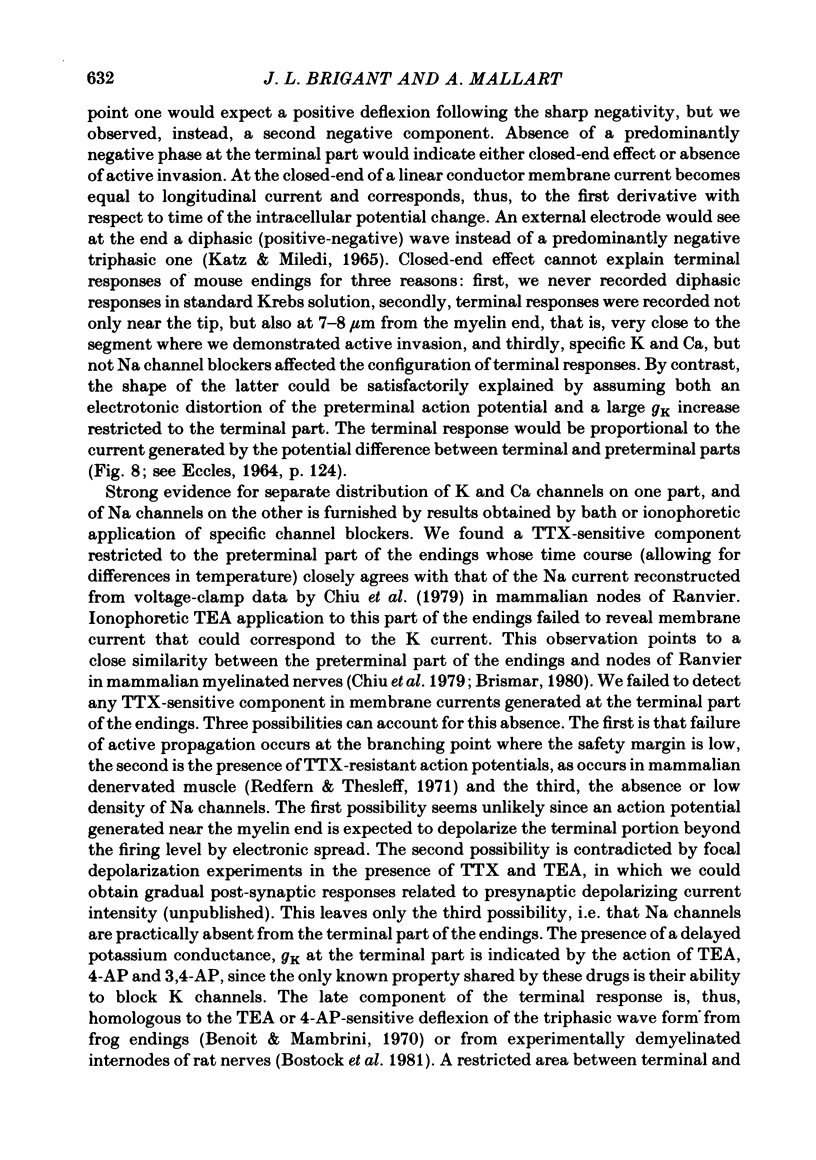
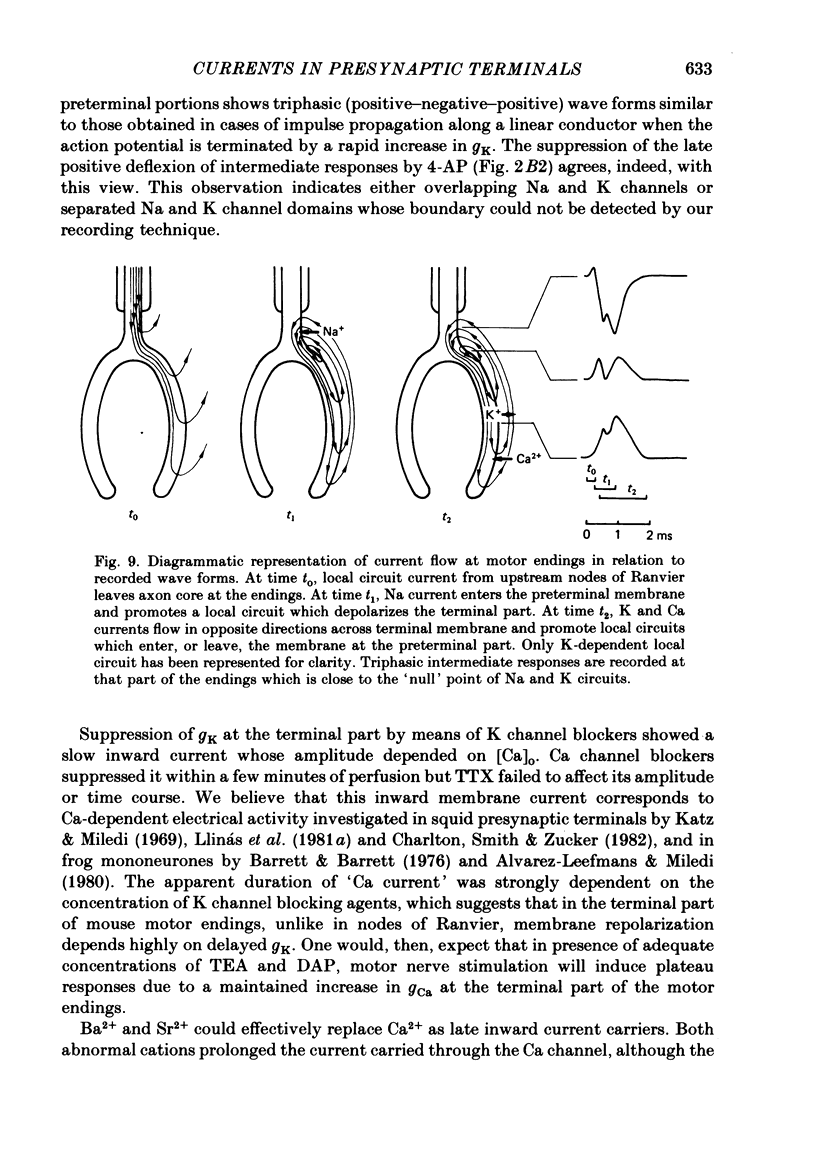
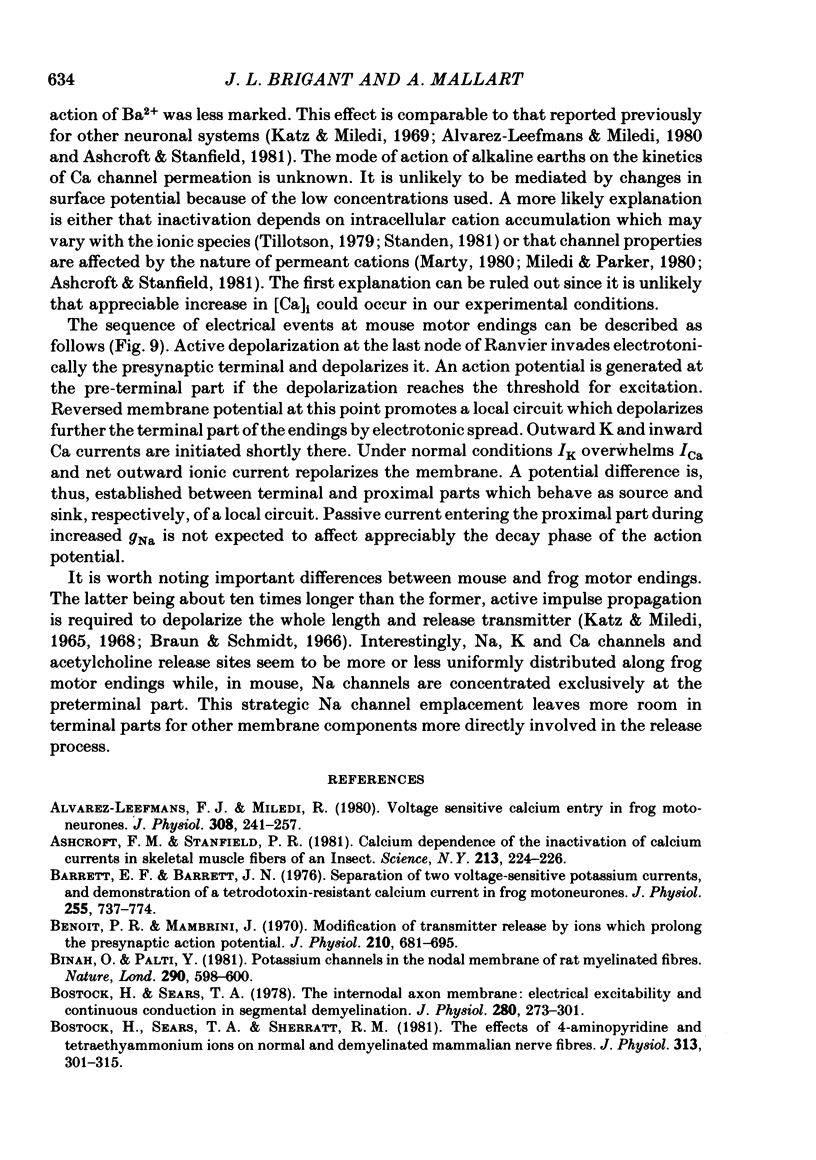
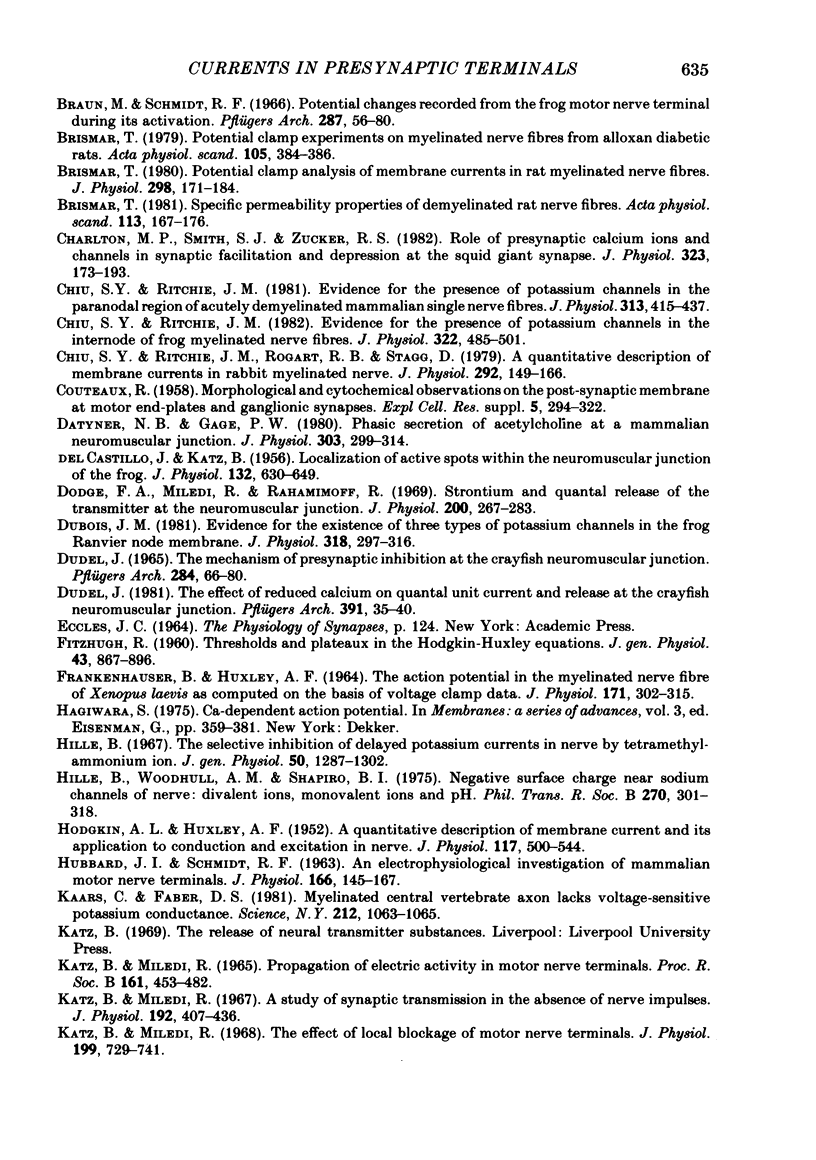
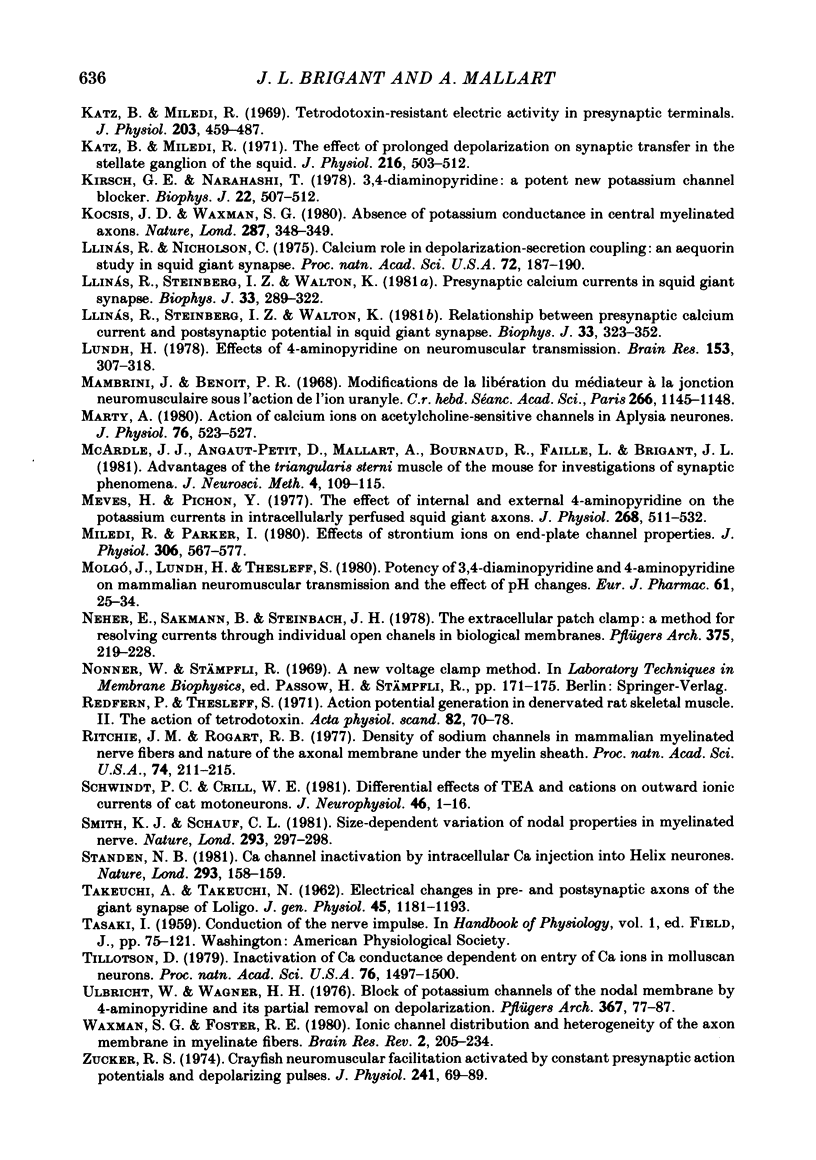
Selected References
These references are in PubMed. This may not be the complete list of references from this article.
- Alvarez-Leefmans F. J., Miledi R. Voltage sensitive calcium entry in frog motoneurones. J Physiol. 1980 Nov;308:241–257. doi: 10.1113/jphysiol.1980.sp013470. [DOI] [PMC free article] [PubMed] [Google Scholar]
- Ashcroft F. M., Stanfield P. R. Calcium dependence of the inactivation of calcium currents in skeletal muscle fibers of an insect. Science. 1981 Jul 10;213(4504):224–226. doi: 10.1126/science.213.4504.224. [DOI] [PubMed] [Google Scholar]
- Barrett E. F., Barret J. N. Separation of two voltage-sensitive potassium currents, and demonstration of a tetrodotoxin-resistant calcium current in frog motoneurones. J Physiol. 1976 Mar;255(3):737–774. doi: 10.1113/jphysiol.1976.sp011306. [DOI] [PMC free article] [PubMed] [Google Scholar]
- Benoit P. R., Mambrini J. Modification of transmitter release by ions which prolong the presynaptic action potential. J Physiol. 1970 Oct;210(3):681–695. doi: 10.1113/jphysiol.1970.sp009235. [DOI] [PMC free article] [PubMed] [Google Scholar]
- Binah O., Palti Y. Potassium channels in the nodal membrane of rat myelinated fibres. Nature. 1981 Apr 16;290(5807):598–600. doi: 10.1038/290598a0. [DOI] [PubMed] [Google Scholar]
- Bostock H., Sears T. A., Sherratt R. M. The effects of 4-aminopyridine and tetraethylammonium ions on normal and demyelinated mammalian nerve fibres. J Physiol. 1981;313:301–315. doi: 10.1113/jphysiol.1981.sp013666. [DOI] [PMC free article] [PubMed] [Google Scholar]
- Bostock H., Sears T. A. The internodal axon membrane: electrical excitability and continuous conduction in segmental demyelination. J Physiol. 1978 Jul;280:273–301. doi: 10.1113/jphysiol.1978.sp012384. [DOI] [PMC free article] [PubMed] [Google Scholar]
- Braun M., Schmidt R. F. Potential changes recorded from the frog motor nerve terminal during its activation. Pflugers Arch Gesamte Physiol Menschen Tiere. 1966;287(1):56–80. doi: 10.1007/BF00362454. [DOI] [PubMed] [Google Scholar]
- Brismar T. Potential clamp analysis of membrane currents in rat myelinated nerve fibres. J Physiol. 1980 Jan;298:171–184. doi: 10.1113/jphysiol.1980.sp013074. [DOI] [PMC free article] [PubMed] [Google Scholar]
- Brismar T. Potential clamp experiments on myelinated nerve fibres from alloxan diabetic rats. Acta Physiol Scand. 1979 Mar;105(3):384–386. doi: 10.1111/j.1748-1716.1979.tb06356.x. [DOI] [PubMed] [Google Scholar]
- Brismar T. Specific permeability properties of demyelinated rat nerve fibres. Acta Physiol Scand. 1981 Oct;113(2):167–176. doi: 10.1111/j.1748-1716.1981.tb06878.x. [DOI] [PubMed] [Google Scholar]
- COUTEAUX R. Morphological and cytochemical observations on the post-synaptic membrane at motor end-plates and ganglionic synapses. Exp Cell Res. 1958;14(Suppl 5):294–322. [PubMed] [Google Scholar]
- Charlton M. P., Smith S. J., Zucker R. S. Role of presynaptic calcium ions and channels in synaptic facilitation and depression at the squid giant synapse. J Physiol. 1982 Feb;323:173–193. doi: 10.1113/jphysiol.1982.sp014067. [DOI] [PMC free article] [PubMed] [Google Scholar]
- Chiu S. Y., Ritchie J. M. Evidence for the presence of potassium channels in the internode of frog myelinated nerve fibres. J Physiol. 1982 Jan;322:485–501. doi: 10.1113/jphysiol.1982.sp014051. [DOI] [PMC free article] [PubMed] [Google Scholar]
- Chiu S. Y., Ritchie J. M. Evidence for the presence of potassium channels in the paranodal region of acutely demyelinated mammalian single nerve fibres. J Physiol. 1981;313:415–437. doi: 10.1113/jphysiol.1981.sp013674. [DOI] [PMC free article] [PubMed] [Google Scholar]
- Chiu S. Y., Ritchie J. M., Rogart R. B., Stagg D. A quantitative description of membrane currents in rabbit myelinated nerve. J Physiol. 1979 Jul;292:149–166. doi: 10.1113/jphysiol.1979.sp012843. [DOI] [PMC free article] [PubMed] [Google Scholar]
- DEL CASTILLO J., KATZ B. Localization of active spots within the neuromuscular junction of the frog. J Physiol. 1956 Jun 28;132(3):630–649. doi: 10.1113/jphysiol.1956.sp005554. [DOI] [PMC free article] [PubMed] [Google Scholar]
- DUDEL J. THE MECHANISM OF PRESYNAPTIC INHIBITION AT THE CRAYFISH NEUROMUSCULAR JUNCTION. Pflugers Arch Gesamte Physiol Menschen Tiere. 1965 May 10;284:66–80. doi: 10.1007/BF00412368. [DOI] [PubMed] [Google Scholar]
- Datyner N. B., Gage P. W. Phasic secretion of acetylcholine at a mammalian neuromuscular junction. J Physiol. 1980 Jun;303:299–314. doi: 10.1113/jphysiol.1980.sp013286. [DOI] [PMC free article] [PubMed] [Google Scholar]
- Dodge F. A., Jr, Miledi R., Rahamimoff R. Strontium and quantal release of transmitter at the neuromuscular junction. J Physiol. 1969 Jan;200(1):267–283. doi: 10.1113/jphysiol.1969.sp008692. [DOI] [PMC free article] [PubMed] [Google Scholar]
- Dubois J. M. Evidence for the existence of three types of potassium channels in the frog Ranvier node membrane. J Physiol. 1981 Sep;318:297–316. doi: 10.1113/jphysiol.1981.sp013865. [DOI] [PMC free article] [PubMed] [Google Scholar]
- Dudel J. The effect of reduced calcium on quantal unit current and release at the crayfish neuromuscular junction. Pflugers Arch. 1981 Jul;391(1):35–40. doi: 10.1007/BF00580691. [DOI] [PubMed] [Google Scholar]
- FITZHUGH R. Thresholds and plateaus in the Hodgkin-Huxley nerve equations. J Gen Physiol. 1960 May;43:867–896. doi: 10.1085/jgp.43.5.867. [DOI] [PMC free article] [PubMed] [Google Scholar]
- FRANKENHAEUSER B., HUXLEY A. F. THE ACTION POTENTIAL IN THE MYELINATED NERVE FIBER OF XENOPUS LAEVIS AS COMPUTED ON THE BASIS OF VOLTAGE CLAMP DATA. J Physiol. 1964 Jun;171:302–315. doi: 10.1113/jphysiol.1964.sp007378. [DOI] [PMC free article] [PubMed] [Google Scholar]
- HODGKIN A. L., HUXLEY A. F. A quantitative description of membrane current and its application to conduction and excitation in nerve. J Physiol. 1952 Aug;117(4):500–544. doi: 10.1113/jphysiol.1952.sp004764. [DOI] [PMC free article] [PubMed] [Google Scholar]
- HUBBARD J. I., SCHMIDT R. F. An electrophysiological investigation of mammalian motor nerve terminals. J Physiol. 1963 Apr;166:145–167. doi: 10.1113/jphysiol.1963.sp007096. [DOI] [PMC free article] [PubMed] [Google Scholar]
- Hagiwara S. Ca-dependent action potential. Membranes. 1975;3:359–381. [PubMed] [Google Scholar]
- Hille B. The selective inhibition of delayed potassium currents in nerve by tetraethylammonium ion. J Gen Physiol. 1967 May;50(5):1287–1302. doi: 10.1085/jgp.50.5.1287. [DOI] [PMC free article] [PubMed] [Google Scholar]
- Hille B., Woodhull A. M., Shapiro B. I. Negative surface charge near sodium channels of nerve: divalent ions, monovalent ions, and pH. Philos Trans R Soc Lond B Biol Sci. 1975 Jun 10;270(908):301–318. doi: 10.1098/rstb.1975.0011. [DOI] [PubMed] [Google Scholar]
- KATZ B., MILEDI R. PROPAGATION OF ELECTRIC ACTIVITY IN MOTOR NERVE TERMINALS. Proc R Soc Lond B Biol Sci. 1965 Feb 16;161:453–482. doi: 10.1098/rspb.1965.0015. [DOI] [PubMed] [Google Scholar]
- Kaars C., Faber D. S. Myelinated central vertebrate axon lacks voltage-sensitive potassium conductance. Science. 1981 May 29;212(4498):1063–1065. doi: 10.1126/science.7233201. [DOI] [PubMed] [Google Scholar]
- Katz B., Miledi R. A study of synaptic transmission in the absence of nerve impulses. J Physiol. 1967 Sep;192(2):407–436. doi: 10.1113/jphysiol.1967.sp008307. [DOI] [PMC free article] [PubMed] [Google Scholar]
- Katz B., Miledi R. Tetrodotoxin-resistant electric activity in presynaptic terminals. J Physiol. 1969 Aug;203(2):459–487. doi: 10.1113/jphysiol.1969.sp008875. [DOI] [PMC free article] [PubMed] [Google Scholar]
- Katz B., Miledi R. The effect of local blockage of motor nerve terminals. J Physiol. 1968 Dec;199(3):729–741. doi: 10.1113/jphysiol.1968.sp008675. [DOI] [PMC free article] [PubMed] [Google Scholar]
- Katz B., Miledi R. The effect of prolonged depolarization on synaptic transfer in the stellate ganglion of the squid. J Physiol. 1971 Jul;216(2):503–512. doi: 10.1113/jphysiol.1971.sp009537. [DOI] [PMC free article] [PubMed] [Google Scholar]
- Kirsch G. E., Narahashi T. 3,4-diaminopyridine. A potent new potassium channel blocker. Biophys J. 1978 Jun;22(3):507–512. doi: 10.1016/S0006-3495(78)85503-9. [DOI] [PMC free article] [PubMed] [Google Scholar]
- Kocsis J. D., Waxman S. G. Absence of potassium conductance in central myelinated axons. Nature. 1980 Sep 25;287(5780):348–349. doi: 10.1038/287348a0. [DOI] [PubMed] [Google Scholar]
- Llinás R., Nicholson C. Calcium role in depolarization-secretion coupling: an aequorin study in squid giant synapse. Proc Natl Acad Sci U S A. 1975 Jan;72(1):187–190. doi: 10.1073/pnas.72.1.187. [DOI] [PMC free article] [PubMed] [Google Scholar]
- Llinás R., Steinberg I. Z., Walton K. Presynaptic calcium currents in squid giant synapse. Biophys J. 1981 Mar;33(3):289–321. doi: 10.1016/S0006-3495(81)84898-9. [DOI] [PMC free article] [PubMed] [Google Scholar]
- Llinás R., Steinberg I. Z., Walton K. Relationship between presynaptic calcium current and postsynaptic potential in squid giant synapse. Biophys J. 1981 Mar;33(3):323–351. doi: 10.1016/S0006-3495(81)84899-0. [DOI] [PMC free article] [PubMed] [Google Scholar]
- Lundh H. Effects of 4-aminopyridine on neuromuscular transmission. Brain Res. 1978 Sep 22;153(2):307–318. doi: 10.1016/0006-8993(78)90409-2. [DOI] [PubMed] [Google Scholar]
- Mambrini J., Benoit P. R. Modifications de la libération du médiateur à la jonction neuromusculaire sous l'action de l'ion uranyle. C R Acad Sci Hebd Seances Acad Sci D. 1968 Mar 11;266(11):1145–1148. [PubMed] [Google Scholar]
- Marty A. Action of calcium ions on acetylcholine-sensitive channels in Aplysia neurones. J Physiol (Paris) 1980 Sep;76(5):523–527. [PubMed] [Google Scholar]
- McArdle J. J., Angaut-Petit D., Mallart A., Bournaud R., Faille L., Brigant J. L. Advantages of the triangularis sterni muscle of the mouse for investigations of synaptic phenomena. J Neurosci Methods. 1981 Aug;4(2):109–115. doi: 10.1016/0165-0270(81)90044-3. [DOI] [PubMed] [Google Scholar]
- Meves H., Pichon Y. The effect of internal and external 4-aminopyridine on the potassium currents in intracellularly perfused squid giant axons. J Physiol. 1977 Jun;268(2):511–532. doi: 10.1113/jphysiol.1977.sp011869. [DOI] [PMC free article] [PubMed] [Google Scholar]
- Miledi R., Parker I. Effects of strontium ions on end-plate channel properties. J Physiol. 1980 Sep;306:567–577. doi: 10.1113/jphysiol.1980.sp013415. [DOI] [PMC free article] [PubMed] [Google Scholar]
- Molgó J., Lundh H., Thesleff S. Potency of 3,4-diaminopyridine and 4-aminopyridine on mammalian neuromuscular transmission and the effect of pH changes. Eur J Pharmacol. 1980 Jan 11;61(1):25–34. doi: 10.1016/0014-2999(80)90378-7. [DOI] [PubMed] [Google Scholar]
- Neher E., Sakmann B., Steinbach J. H. The extracellular patch clamp: a method for resolving currents through individual open channels in biological membranes. Pflugers Arch. 1978 Jul 18;375(2):219–228. doi: 10.1007/BF00584247. [DOI] [PubMed] [Google Scholar]
- Redfern P., Thesleff S. Action potential generation in denervated rat skeletal muscle. II. The action of tetrodotoxin. Acta Physiol Scand. 1971 May;82(1):70–78. doi: 10.1111/j.1748-1716.1971.tb04943.x. [DOI] [PubMed] [Google Scholar]
- Ritchie J. M., Rogart R. B. Density of sodium channels in mammalian myelinated nerve fibers and nature of the axonal membrane under the myelin sheath. Proc Natl Acad Sci U S A. 1977 Jan;74(1):211–215. doi: 10.1073/pnas.74.1.211. [DOI] [PMC free article] [PubMed] [Google Scholar]
- Schwindt P. C., Crill W. E. Differential effects of TEA and cations on outward ionic currents of cat motoneurons. J Neurophysiol. 1981 Jul;46(1):1–16. doi: 10.1152/jn.1981.46.1.1. [DOI] [PubMed] [Google Scholar]
- Smith K. J., Schauf C. L. Size-dependent variation of nodal properties in myelinated nerve. Nature. 1981 Sep 24;293(5830):297–299. doi: 10.1038/293297a0. [DOI] [PubMed] [Google Scholar]
- Standen N. B. Ca channel inactivation by intracellular Ca injection into Helix neurones. Nature. 1981 Sep 10;293(5828):158–159. doi: 10.1038/293158a0. [DOI] [PubMed] [Google Scholar]
- TAKEUCHI A., TAKEUCHI N. Electrical changes in pre- and postsynaptic axons of the giant synapse of Loligo. J Gen Physiol. 1962 Jul;45:1181–1193. doi: 10.1085/jgp.45.6.1181. [DOI] [PMC free article] [PubMed] [Google Scholar]
- Tillotson D. Inactivation of Ca conductance dependent on entry of Ca ions in molluscan neurons. Proc Natl Acad Sci U S A. 1979 Mar;76(3):1497–1500. doi: 10.1073/pnas.76.3.1497. [DOI] [PMC free article] [PubMed] [Google Scholar]
- Ulbricht W., Wagner H. H. Block of potassium channels of the nodal membrane by 4-aminopyridine and its partial removal on depolarization. Pflugers Arch. 1976 Nov 30;367(1):77–87. doi: 10.1007/BF00583659. [DOI] [PubMed] [Google Scholar]
- Waxman S. G., Foster R. E. Ionic channel distribution and heterogeneity of the axon membrane in myelinated fibers. Brain Res. 1980 Oct;203(2):205–234. doi: 10.1016/0165-0173(80)90008-9. [DOI] [PubMed] [Google Scholar]
- Zucker R. S. Crayfish neuromuscular facilitation activated by constant presynaptic action potentials and depolarizing pulses. J Physiol. 1974 Aug;241(1):69–89. doi: 10.1113/jphysiol.1974.sp010641. [DOI] [PMC free article] [PubMed] [Google Scholar]