Abstract
1. The electrophysiological properties of dorsal horn neurones have been investigated in the immature rat in vitro spinal cord slice preparation. 2. Intracellular recordings from dorsal horn neurones show that direct or orthodromic stimulation generates action potentials followed by a brief after-hyperpolarization. Synaptic potentials were elicited by the activation of primary afferent fibres in the dorsal root. 3. Input resistance for dorsal horn neurones ranged from 48 to 267 M omega, and the membrane time constant was in the range of 4-19 ms. 4. In response to strong depolarizing currents dorsal horn neurones perfused with TTX and TEA frequently exhibit a slow regenerative depolarizing potential followed by a slow after-hyperpolarization. The depolarizing potential probably results from an influx of Ca. It is blocked by low concentration Ca, Co or Mn, and enhanced by high levels of extracellular Ca. 5. There is, in addition, a low-threshold Ca-dependent response which is activated at membrane potentials more negative than -65 mV and has a maximum rate of rise at the polarization level of about -80 mV. 6. The addition of Ba or TEA to the perfusing medium provided support for the Ca-dependence of the low- and high-threshold responses, and the lack of fast inactivation of the high-threshold Ca potential.
Full text
PDF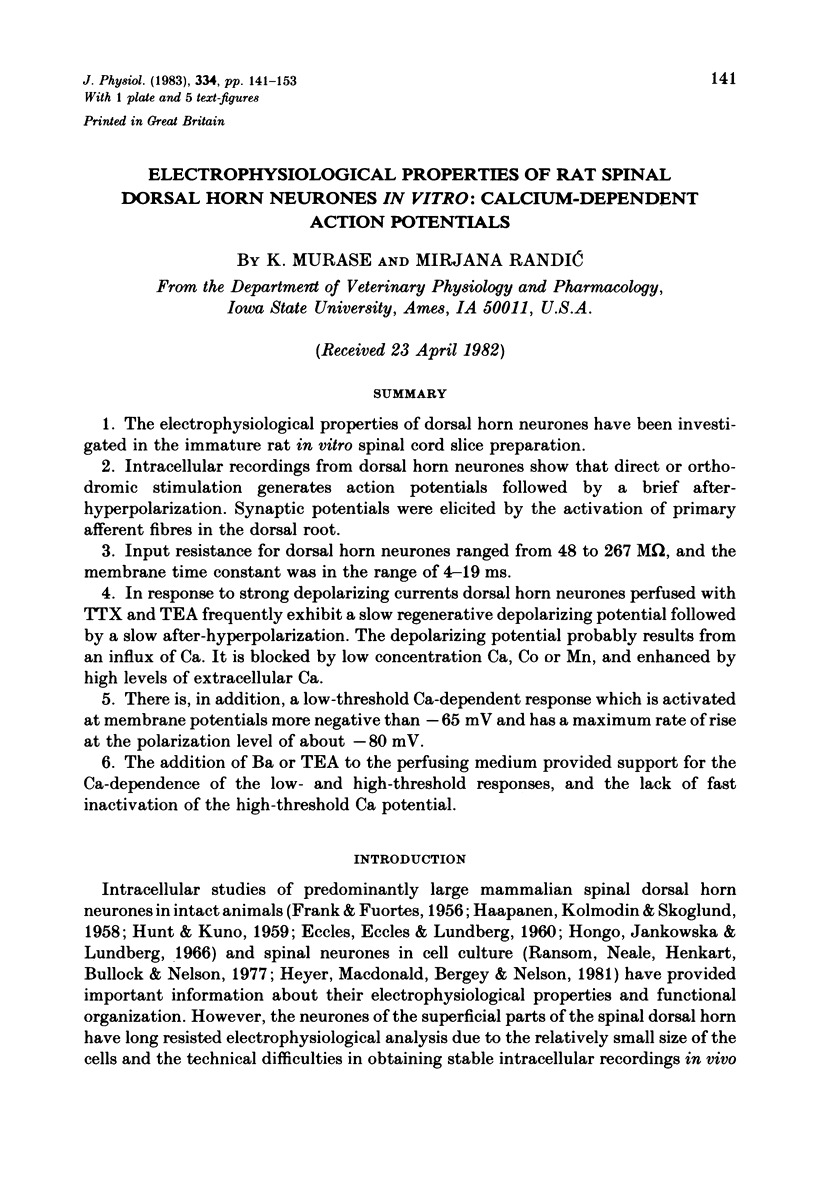
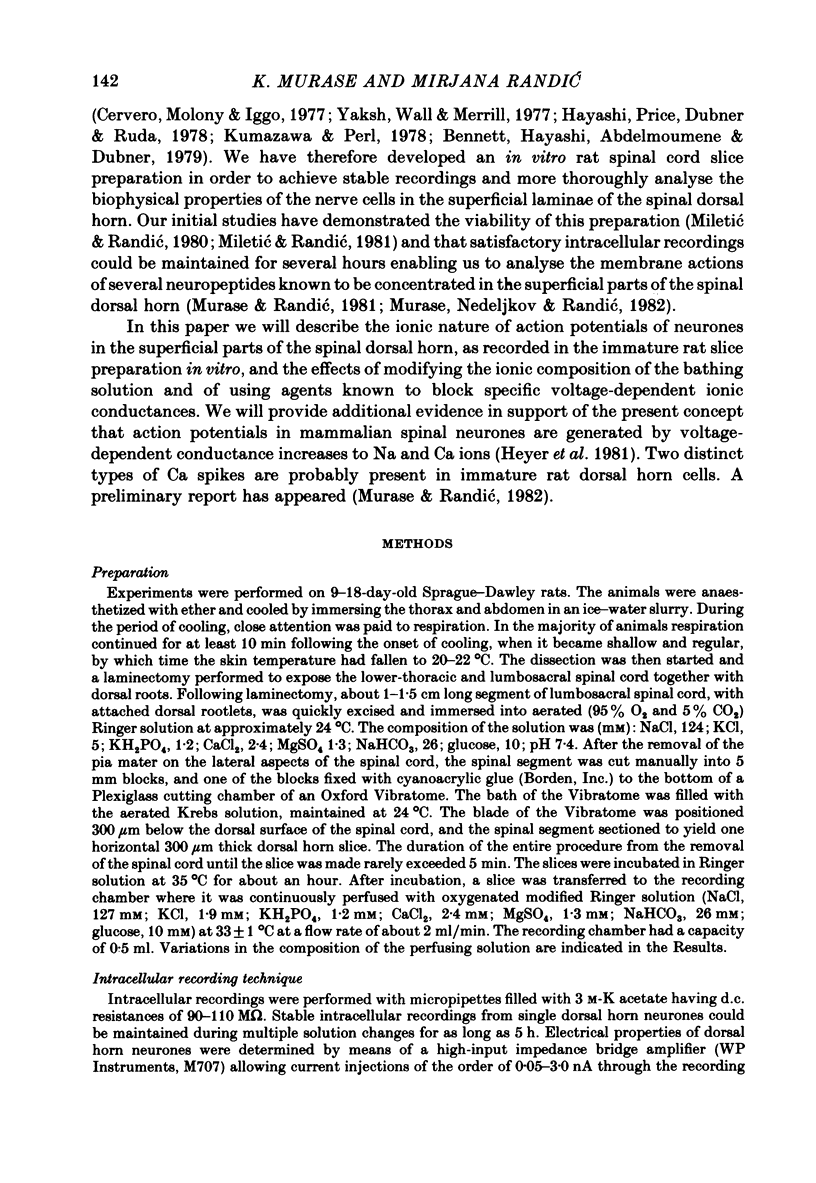
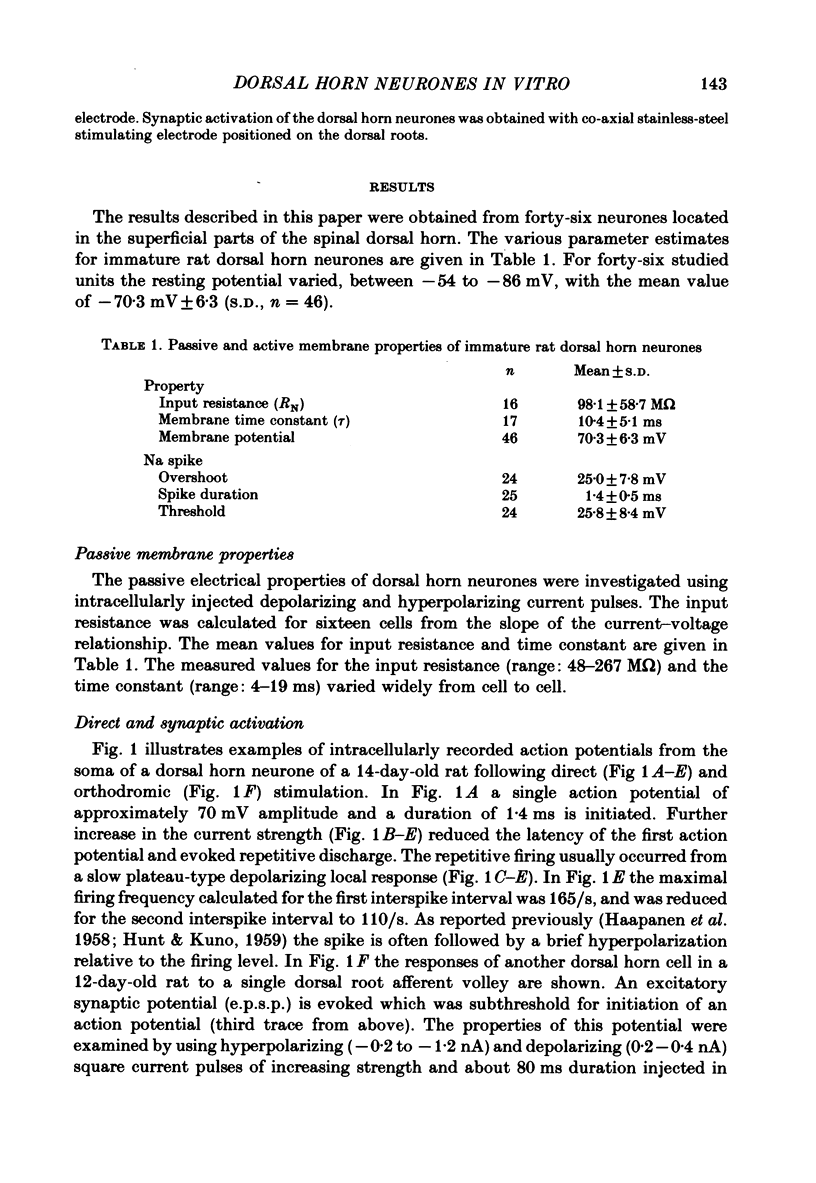
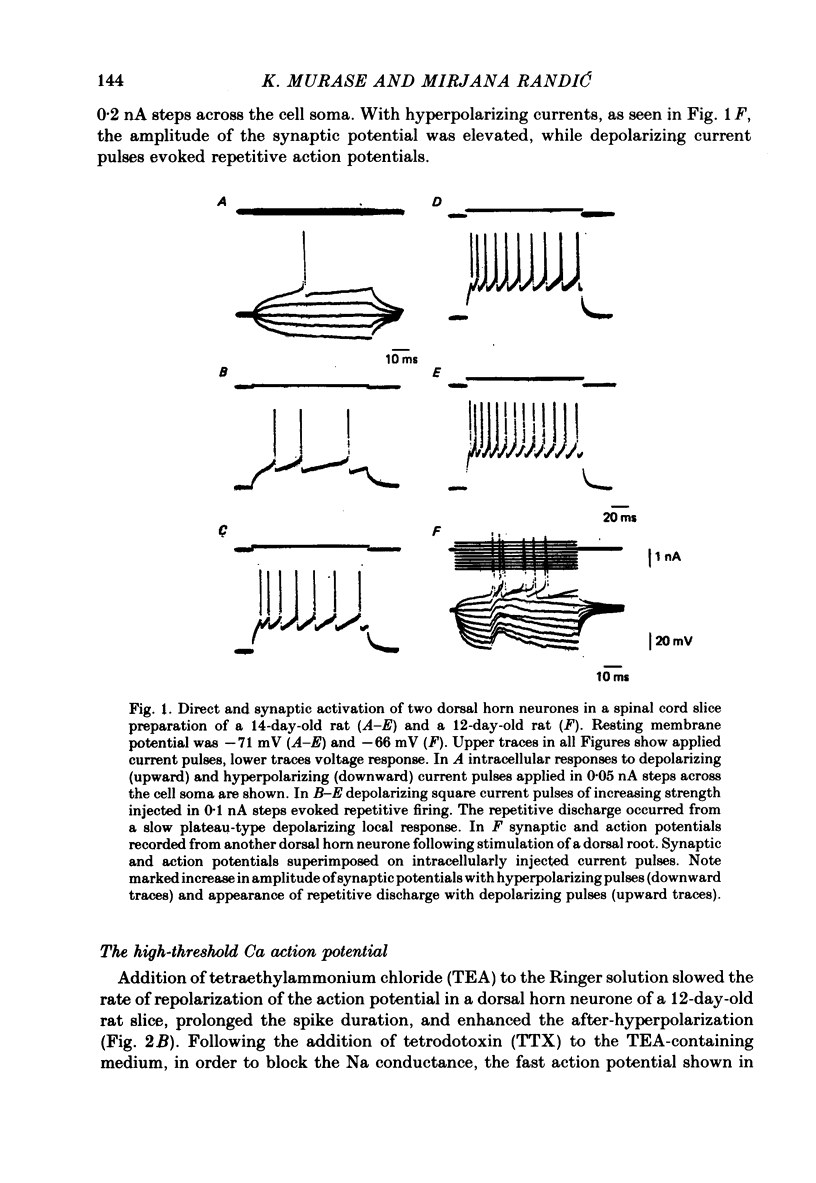
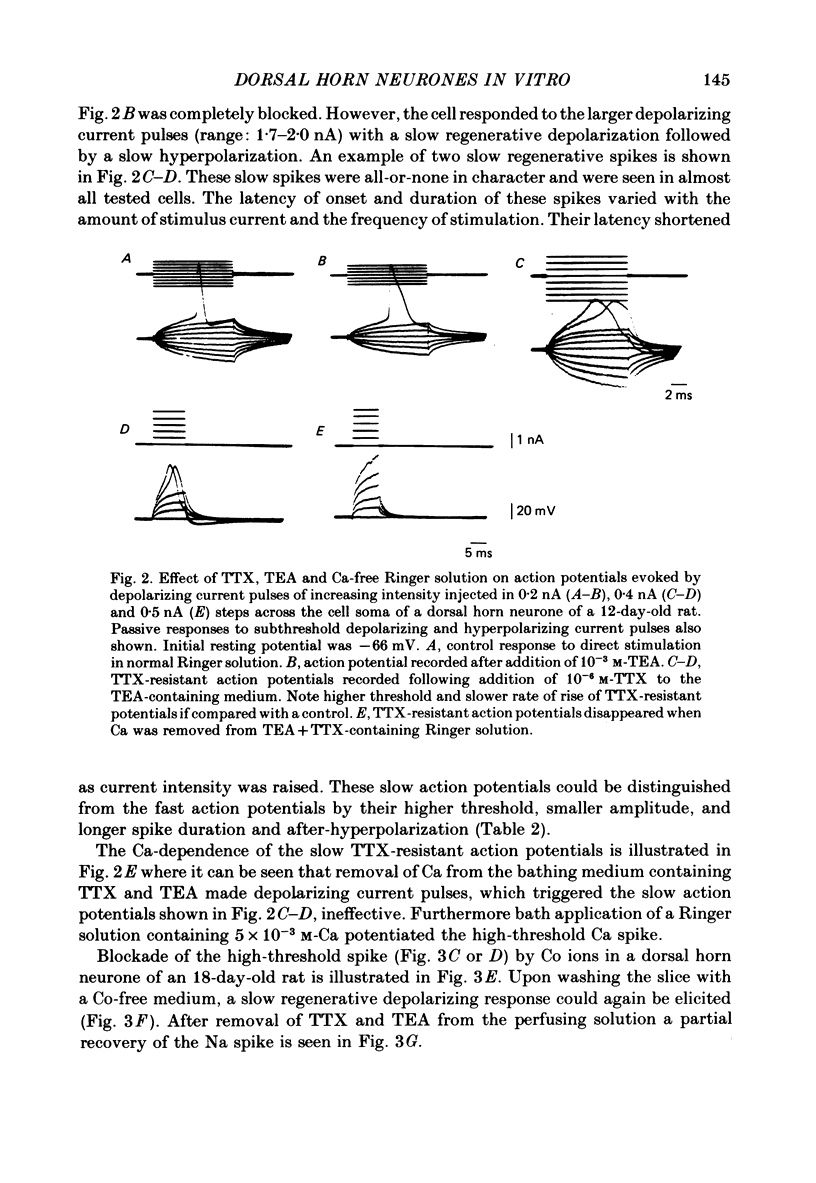
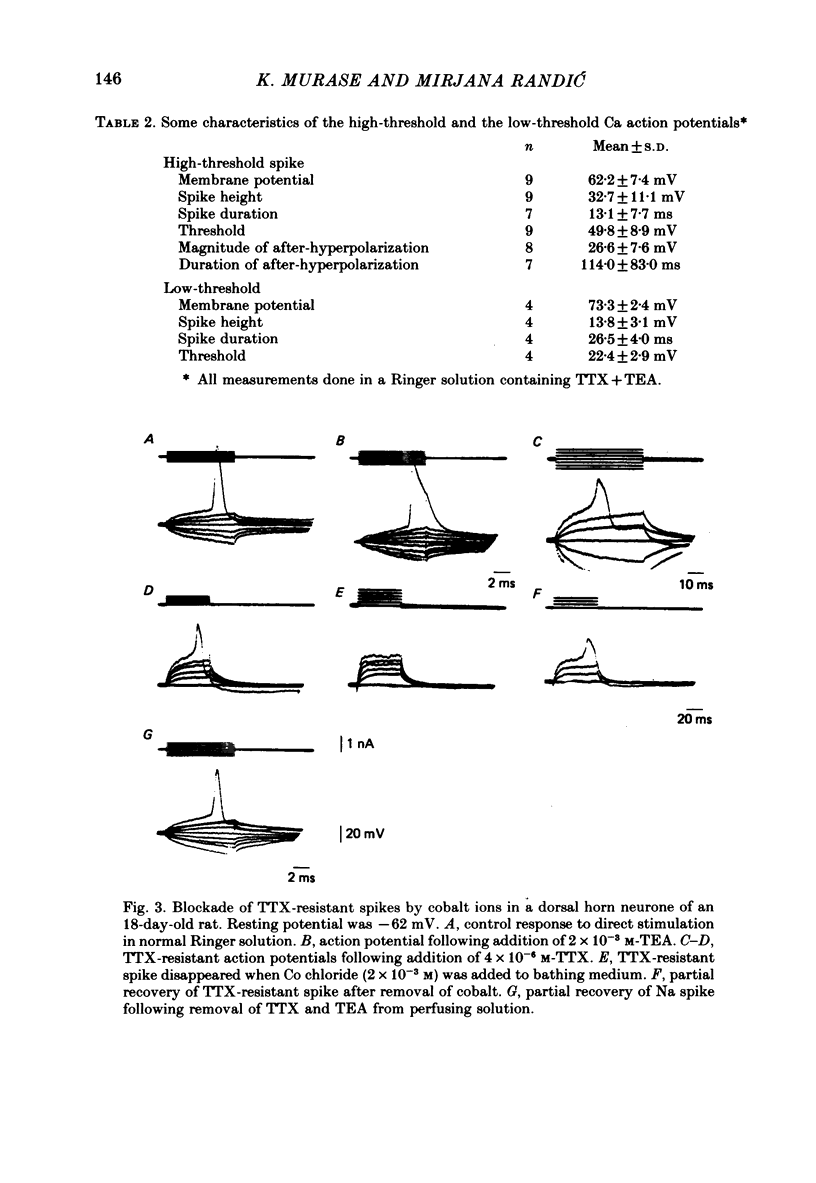
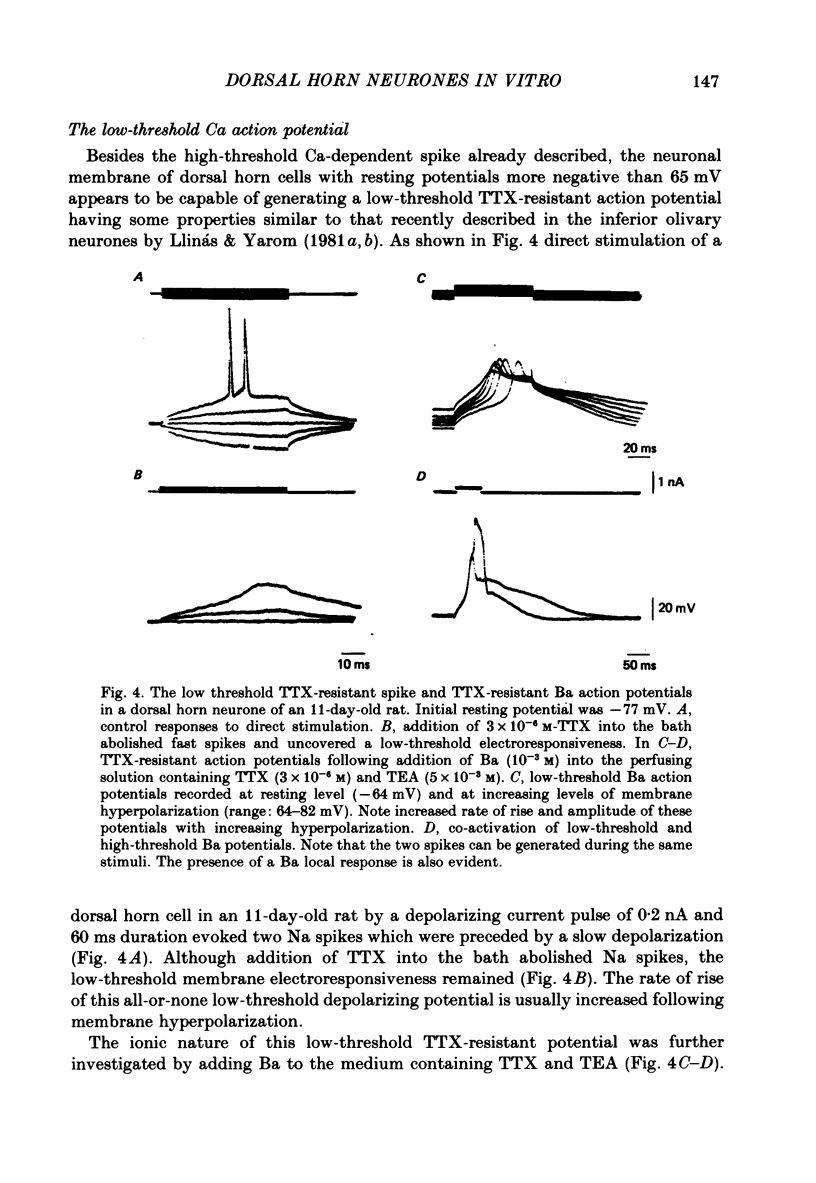
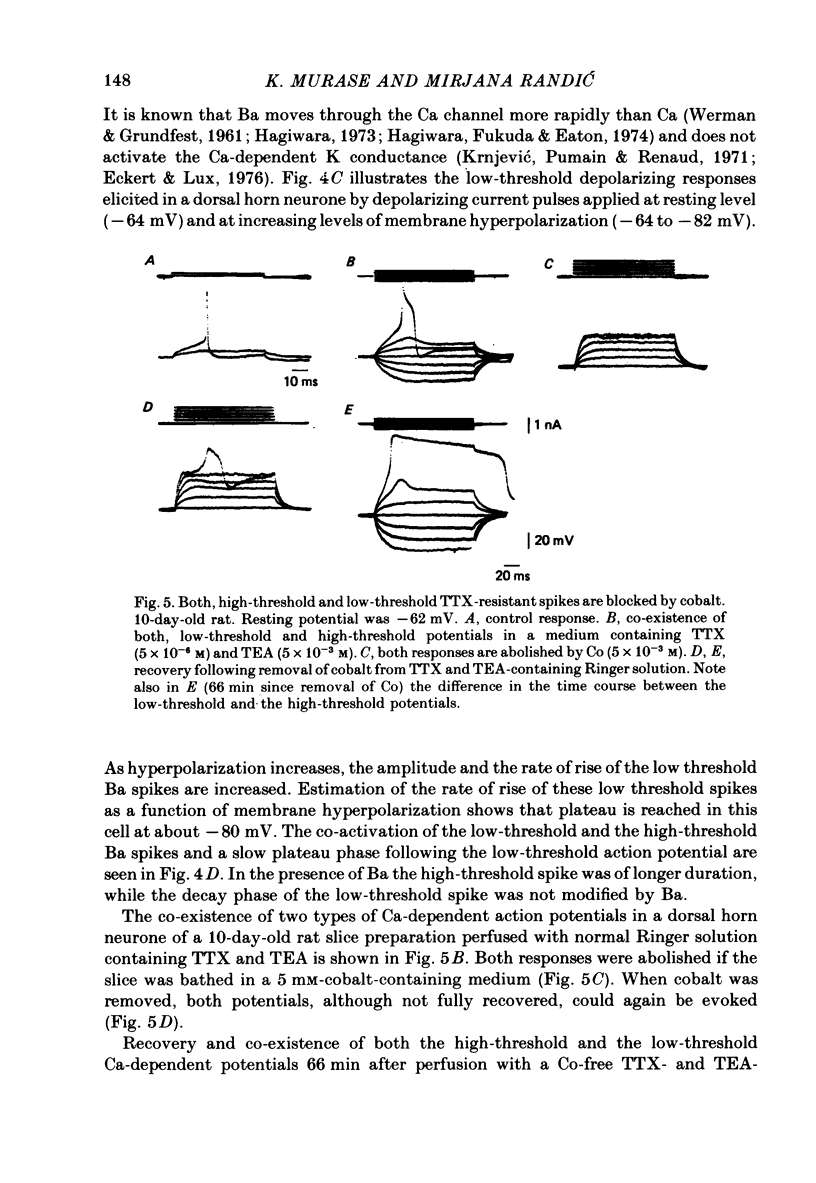
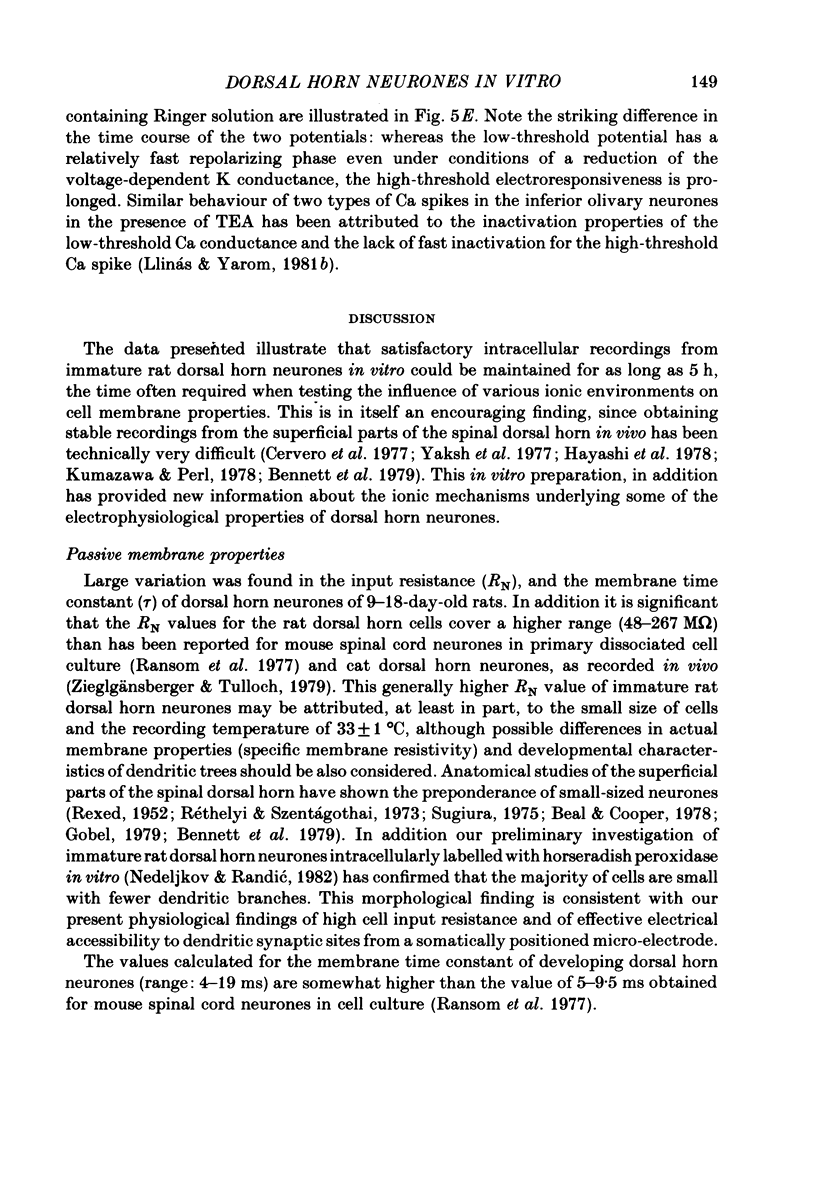
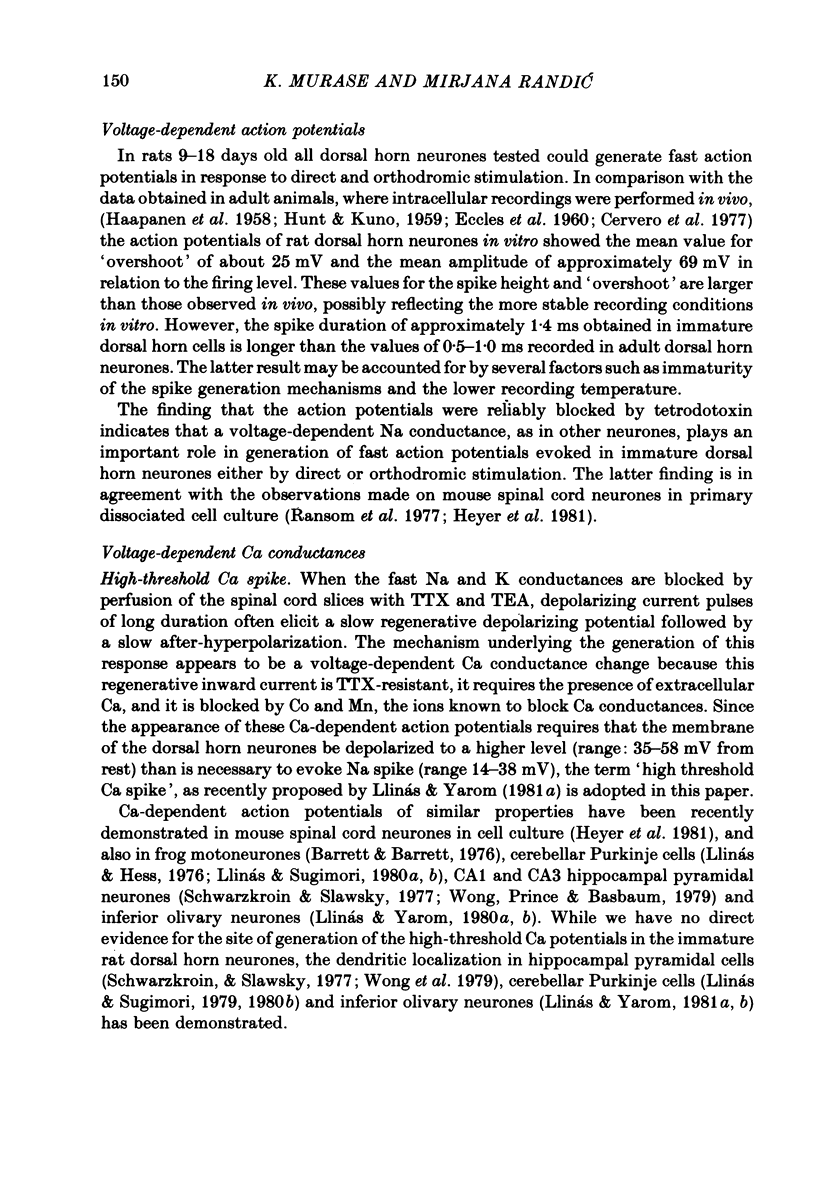
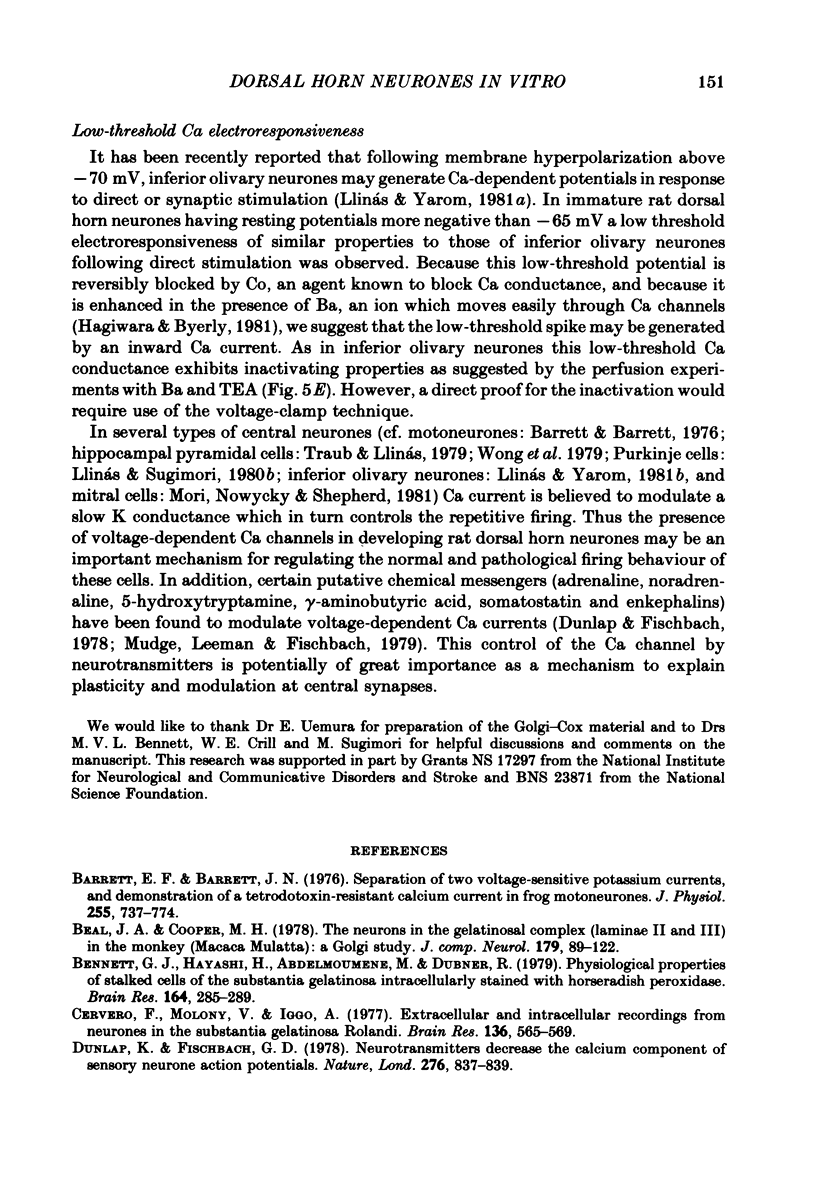
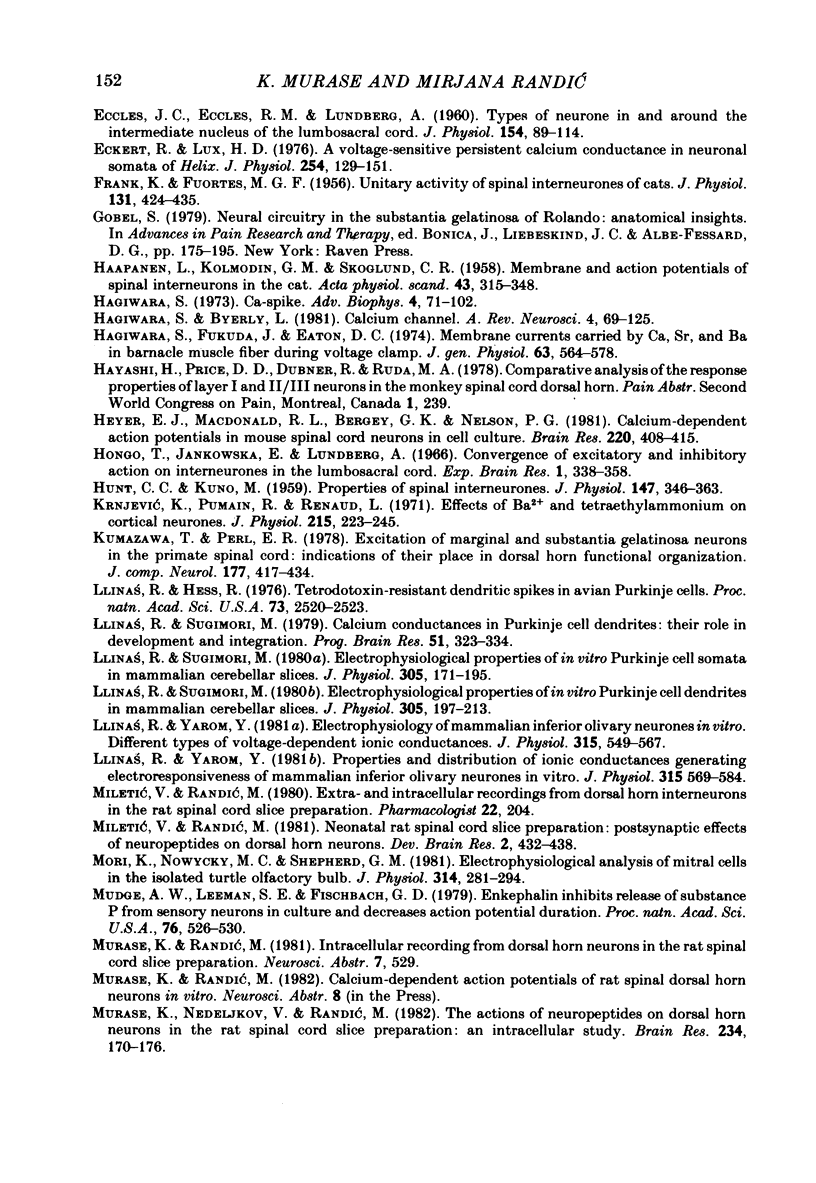
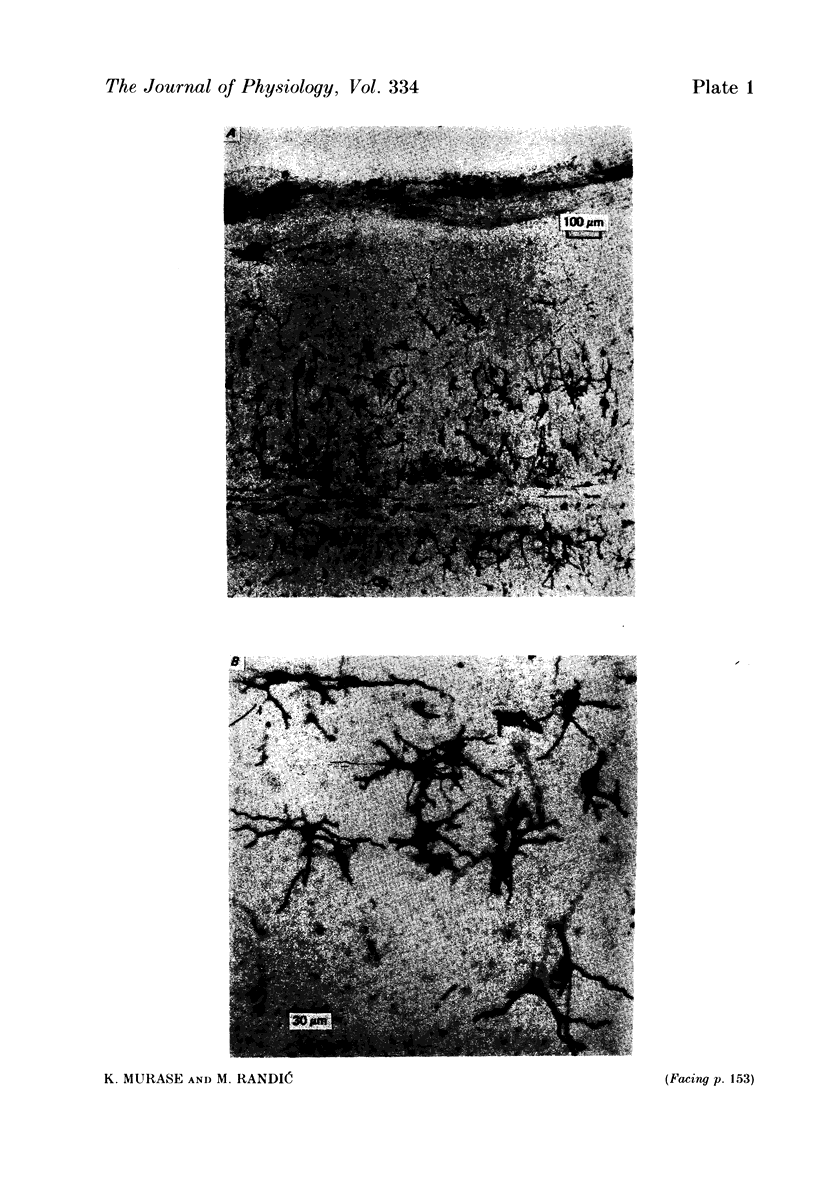
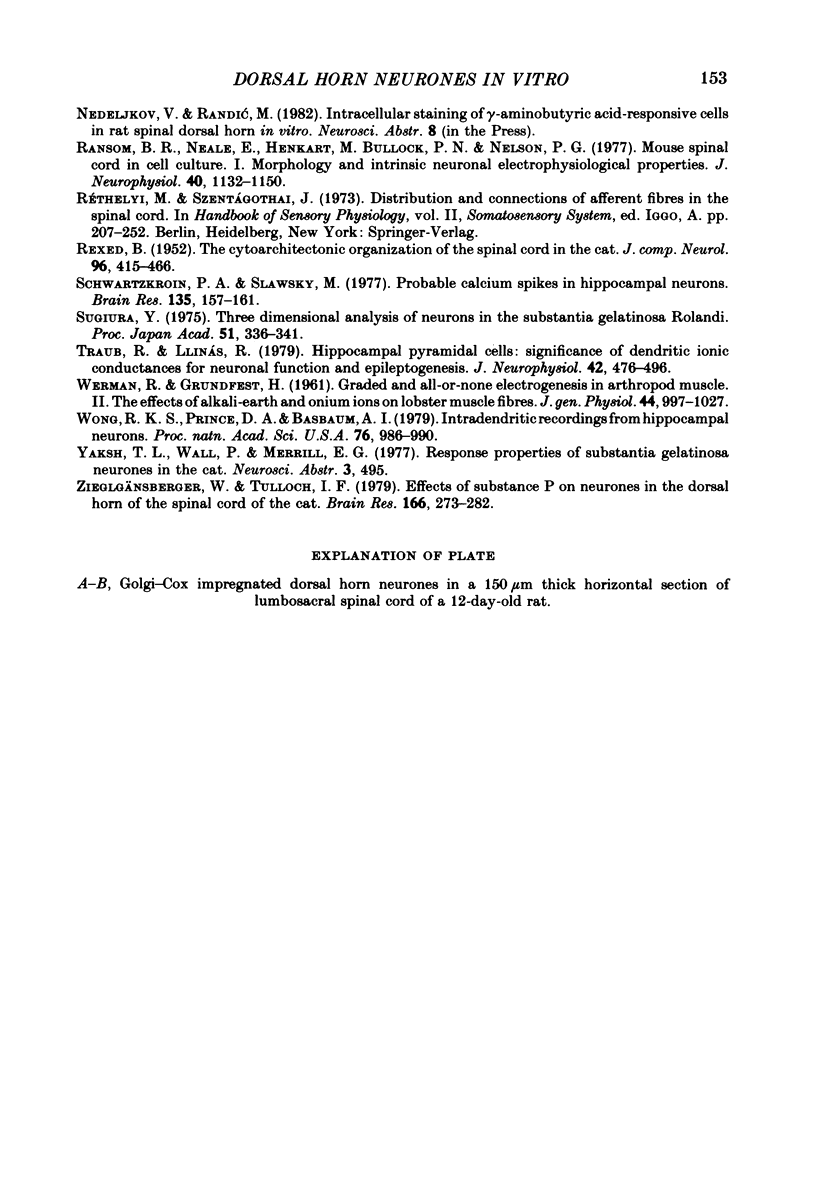
Images in this article
Selected References
These references are in PubMed. This may not be the complete list of references from this article.
- Barrett E. F., Barret J. N. Separation of two voltage-sensitive potassium currents, and demonstration of a tetrodotoxin-resistant calcium current in frog motoneurones. J Physiol. 1976 Mar;255(3):737–774. doi: 10.1113/jphysiol.1976.sp011306. [DOI] [PMC free article] [PubMed] [Google Scholar]
- Beal J. A., Cooper M. H. The neurons in the gelatinosal complex (Laminae II and III) of the monkey (Macaca mulatta): a Golgi study. J Comp Neurol. 1978 May 1;179(1):89–121. doi: 10.1002/cne.901790107. [DOI] [PubMed] [Google Scholar]
- Bennett G. J., Hayashi H., Abdelmoumene M., Dubner R. Physiological properties of stalked cells of the substantia gelatinosa intracellularly stained with horseradish peroxidase. Brain Res. 1979 Mar 23;164:285–289. doi: 10.1016/0006-8993(79)90022-2. [DOI] [PubMed] [Google Scholar]
- Cervero F., Molony V., Iggo A. Extracellular and intracellular recordings from neurones in the substantia gelatinosa Rolandi. Brain Res. 1977 Nov 18;136(3):565–569. doi: 10.1016/0006-8993(77)90082-8. [DOI] [PubMed] [Google Scholar]
- Dunlap K., Fischbach G. D. Neurotransmitters decrease the calcium ocmponent of sensory neurone action potentials. Nature. 1978 Dec 21;276(5690):837–839. doi: 10.1038/276837a0. [DOI] [PubMed] [Google Scholar]
- ECCLES J. C., ECCLES R. M., LUNDBERG A. Types of neurone in and around the intermediate nucleus of the lumbosacral cord. J Physiol. 1960 Nov;154:89–114. doi: 10.1113/jphysiol.1960.sp006566. [DOI] [PMC free article] [PubMed] [Google Scholar]
- Eckert R., Lux H. D. A voltage-sensitive persistent calcium conductance in neuronal somata of Helix. J Physiol. 1976 Jan;254(1):129–151. doi: 10.1113/jphysiol.1976.sp011225. [DOI] [PMC free article] [PubMed] [Google Scholar]
- FRANK K., FUORTES M. G. Unitary activity of spinal interneurones of cats. J Physiol. 1956 Feb 28;131(2):424–435. doi: 10.1113/jphysiol.1956.sp005472. [DOI] [PMC free article] [PubMed] [Google Scholar]
- HAAPANEN L., KOLMODIN G. M., SKOGLUND C. R. Membrane and action potentials of spinal interneurons in the cat. Acta Physiol Scand. 1958 Oct 8;43(3-4):315–348. doi: 10.1111/j.1748-1716.1958.tb01598.x. [DOI] [PubMed] [Google Scholar]
- HUNT C. C., KUNO M. Properties of spinal interneurones. J Physiol. 1959 Sep 2;147:346–363. doi: 10.1113/jphysiol.1959.sp006248. [DOI] [PMC free article] [PubMed] [Google Scholar]
- Hagiwara S., Byerly L. Calcium channel. Annu Rev Neurosci. 1981;4:69–125. doi: 10.1146/annurev.ne.04.030181.000441. [DOI] [PubMed] [Google Scholar]
- Hagiwara S. Ca spike. Adv Biophys. 1973;4:71–102. [PubMed] [Google Scholar]
- Hagiwara S., Fukuda J., Eaton D. C. Membrane currents carried by Ca, Sr, and Ba in barnacle muscle fiber during voltage clamp. J Gen Physiol. 1974 May;63(5):564–578. doi: 10.1085/jgp.63.5.564. [DOI] [PMC free article] [PubMed] [Google Scholar]
- Heyer E. J., MacDonald R. L., Bergey G. K., Nelson P. G. Calcium-dependent action potentials in mouse spinal cord neurons in cell culture. Brain Res. 1981 Sep 14;220(2):408–415. doi: 10.1016/0006-8993(81)91234-8. [DOI] [PubMed] [Google Scholar]
- Hongo T., Jankowska E., Lundberg A. Convergence of excitatory and inhibitory action on interneurones in the lumbosacral cord. Exp Brain Res. 1966;1(4):338–358. doi: 10.1007/BF00237706. [DOI] [PubMed] [Google Scholar]
- Krnjević K., Pumain R., Renaud L. Effects of Ba2+ and tetraethylammonium on cortical neurones. J Physiol. 1971 May;215(1):223–245. doi: 10.1113/jphysiol.1971.sp009466. [DOI] [PMC free article] [PubMed] [Google Scholar]
- Kumazawa T., Perl E. R. Excitation of marginal and substantia gelatinosa neurons in the primate spinal cord: indications of their place in dorsal horn functional organization. J Comp Neurol. 1978 Feb 1;177(3):417–434. doi: 10.1002/cne.901770305. [DOI] [PubMed] [Google Scholar]
- Llinás R., Hess R. Tetrodotoxin-resistant dendritic spikes in avian Purkinje cells. Proc Natl Acad Sci U S A. 1976 Jul;73(7):2520–2523. doi: 10.1073/pnas.73.7.2520. [DOI] [PMC free article] [PubMed] [Google Scholar]
- Llinás R., Sugimori M. Calcium conductances in Purkinje cell dendrites: their role in development and integration. Prog Brain Res. 1979;51:323–334. doi: 10.1016/S0079-6123(08)61312-6. [DOI] [PubMed] [Google Scholar]
- Llinás R., Sugimori M. Electrophysiological properties of in vitro Purkinje cell dendrites in mammalian cerebellar slices. J Physiol. 1980 Aug;305:197–213. doi: 10.1113/jphysiol.1980.sp013358. [DOI] [PMC free article] [PubMed] [Google Scholar]
- Llinás R., Sugimori M. Electrophysiological properties of in vitro Purkinje cell somata in mammalian cerebellar slices. J Physiol. 1980 Aug;305:171–195. doi: 10.1113/jphysiol.1980.sp013357. [DOI] [PMC free article] [PubMed] [Google Scholar]
- Llinás R., Yarom Y. Electrophysiology of mammalian inferior olivary neurones in vitro. Different types of voltage-dependent ionic conductances. J Physiol. 1981 Jun;315:549–567. doi: 10.1113/jphysiol.1981.sp013763. [DOI] [PMC free article] [PubMed] [Google Scholar]
- Llinás R., Yarom Y. Properties and distribution of ionic conductances generating electroresponsiveness of mammalian inferior olivary neurones in vitro. J Physiol. 1981 Jun;315:569–584. doi: 10.1113/jphysiol.1981.sp013764. [DOI] [PMC free article] [PubMed] [Google Scholar]
- Mori K., Nowycky M. C., Shepherd G. M. Electrophysiological analysis of mitral cells in the isolated turtle olfactory bulb. J Physiol. 1981 May;314:281–294. doi: 10.1113/jphysiol.1981.sp013707. [DOI] [PMC free article] [PubMed] [Google Scholar]
- Mudge A. W., Leeman S. E., Fischbach G. D. Enkephalin inhibits release of substance P from sensory neurons in culture and decreases action potential duration. Proc Natl Acad Sci U S A. 1979 Jan;76(1):526–530. doi: 10.1073/pnas.76.1.526. [DOI] [PMC free article] [PubMed] [Google Scholar]
- Murase K., Nedeljkov V., Randić M. The actions of neuropeptides on dorsal horn neurons in the rat spinal cord slice preparation: an intracellular study. Brain Res. 1982 Feb 18;234(1):170–176. doi: 10.1016/0006-8993(82)90483-8. [DOI] [PubMed] [Google Scholar]
- Ransom B. R., Neale E., Henkart M., Bullock P. N., Nelson P. G. Mouse spinal cord in cell culture. I. Morphology and intrinsic neuronal electrophysiologic properties. J Neurophysiol. 1977 Sep;40(5):1132–1150. doi: 10.1152/jn.1977.40.5.1132. [DOI] [PubMed] [Google Scholar]
- Schwartzkroin P. A., Slawsky M. Probable calcium spikes in hippocampal neurons. Brain Res. 1977 Oct 21;135(1):157–161. doi: 10.1016/0006-8993(77)91060-5. [DOI] [PubMed] [Google Scholar]
- Traub R. D., Llinás R. Hippocampal pyramidal cells: significance of dendritic ionic conductances for neuronal function and epileptogenesis. J Neurophysiol. 1979 Mar;42(2):476–496. doi: 10.1152/jn.1979.42.2.476. [DOI] [PubMed] [Google Scholar]
- WERMAN R., GRUNDFEST H. Graded and all-or-none electrogenesis in arthropod muscle. II. The effects of alkali-earth and onium ions on lobster muscle fibers. J Gen Physiol. 1961 May;44:997–1027. doi: 10.1085/jgp.44.5.997. [DOI] [PMC free article] [PubMed] [Google Scholar]
- Wong R. K., Prince D. A., Basbaum A. I. Intradendritic recordings from hippocampal neurons. Proc Natl Acad Sci U S A. 1979 Feb;76(2):986–990. doi: 10.1073/pnas.76.2.986. [DOI] [PMC free article] [PubMed] [Google Scholar]
- Zieglgänsberger W., Tulloch I. F. Effects of substance P on neurones in the dorsal horn of the spinal cord of the cat. Brain Res. 1979 Apr 27;166(2):273–282. doi: 10.1016/0006-8993(79)90213-0. [DOI] [PubMed] [Google Scholar]