Abstract
1. Field potentials and extracellular potassium concentration, [K+]o, were recorded from the rat cerebellar cortex using ion-selective micro-electrodes, following micro-stimulation of the cerebellar surface. The compound action potential of the parallel fibres (p.f.s) showed changes indicative of a supernormal period (s.n.p) when conditioned by a previous p.f. volley, and was studied in relation to [K+]o. 2. Repetitive stimulation of the p.f.s (greater than 10 Hz) elicited an alternation in p.f. excitability from supernormality to subnormality simultaneous to a steady increase in [K+]o. 3. Superfusion with various levels of K+ led to changes in the p.f. conduction properties. Small increases in [K+]o above the resting 3.0 mM level led to an increase in p.f. conduction velocity while greater increases led to conduction slowing and eventually block. 4. Repetitive activation of a row of p.f.s elicited increases in [K+]o in the vicinity of neighbouring non-activated fibres. These fibres displayed an increase in excitability that was quantitatively related to [K+]o. 5. After introduction of 4-aminopyridine (4-AP; 100 microM) into the superfusate, a single stimulus would elicit relatively large (up to 15 mM) increases in [K+]o around neighbouring non-activated p.f.s. The excitability of the adjacent non-activated fibres was either increased or decreased, and was quantitatively related to [K+]o. 6. Strophanthidin application (15 microM) led to a slow and continuous increase in [K+]o. The excitability of the p.f.s initially increased as [K+]o increased, but subsequently decreased, eventually resulting in conduction block. 7. These experiments are consistent with the hypothesis that small increases in [K+]o may elicit an increase in p.f. excitability while greater increases lead to a decrease in p.f. excitability.
Full text
PDF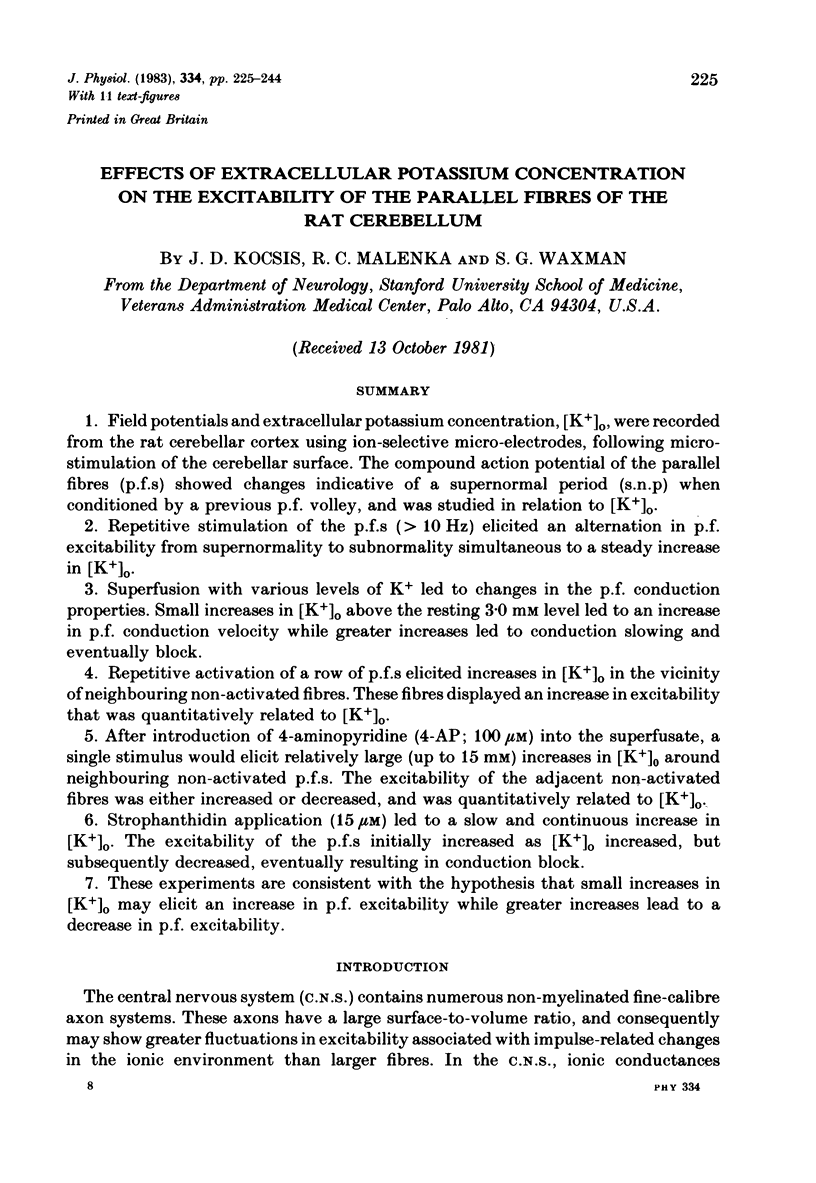
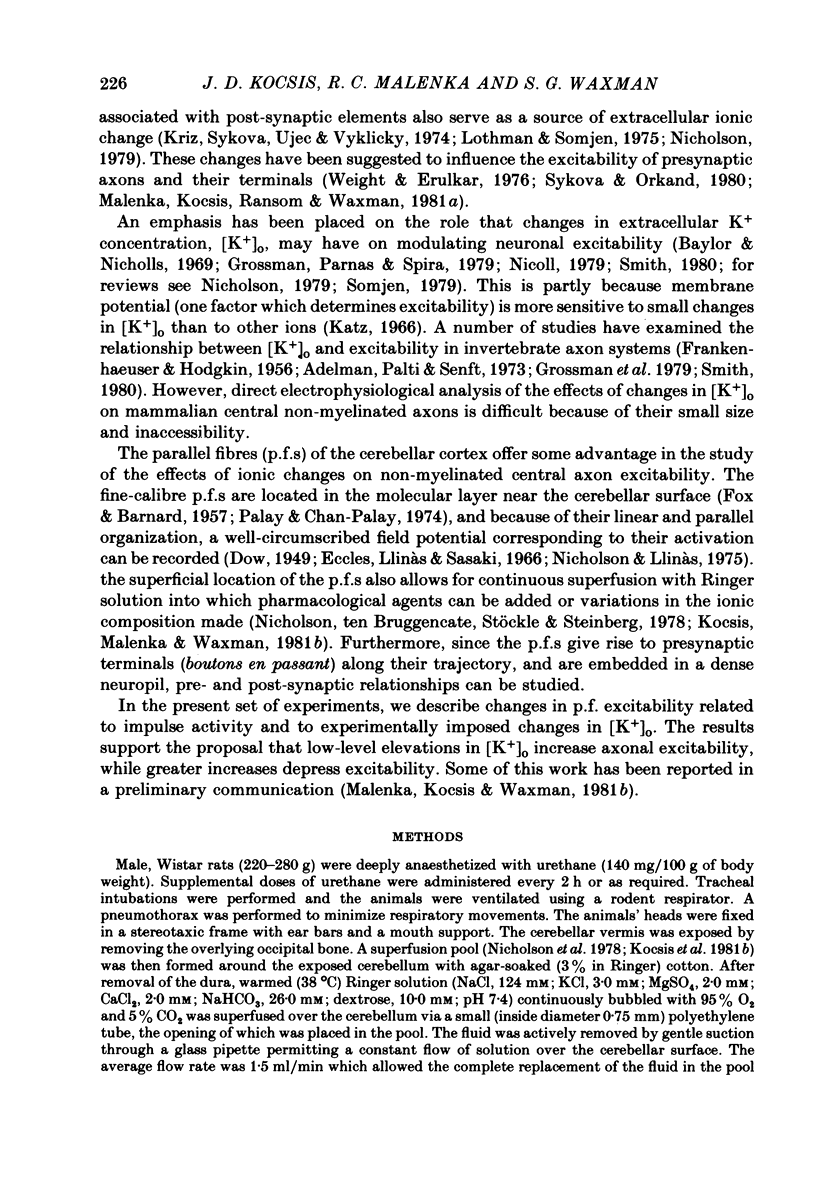
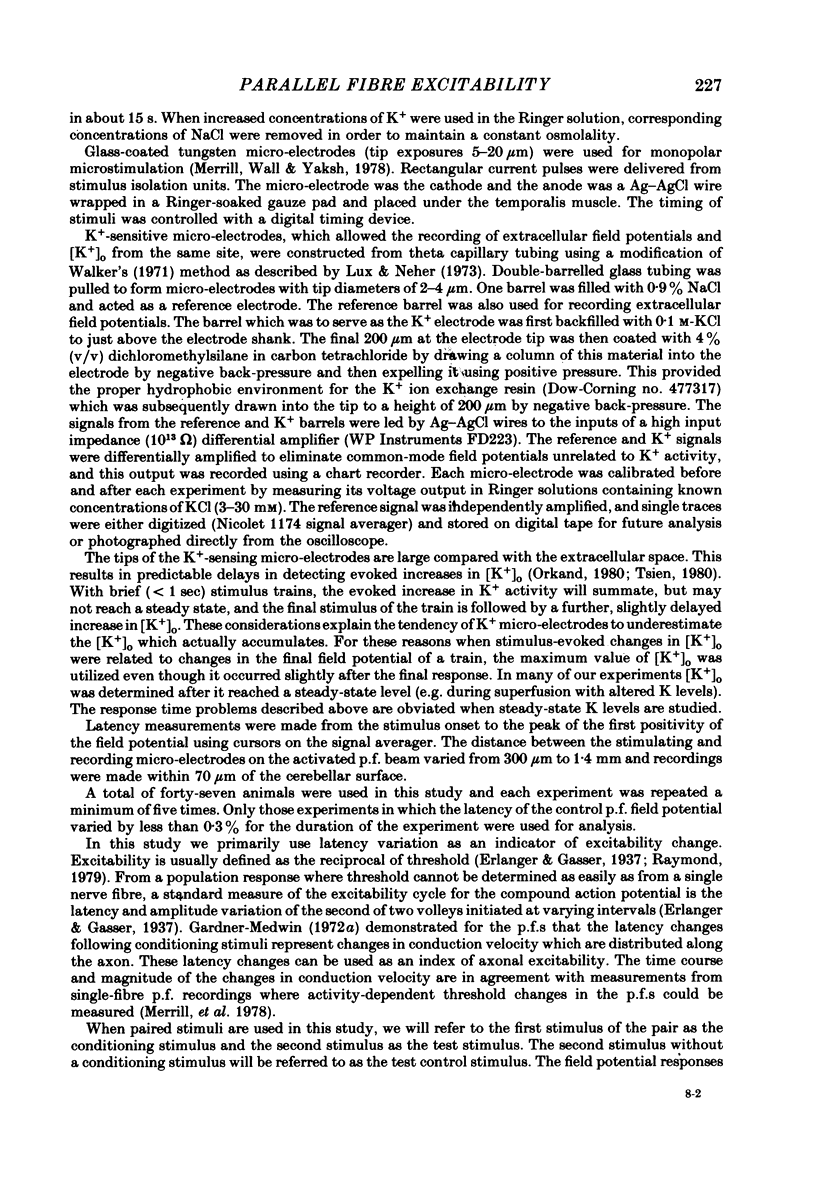
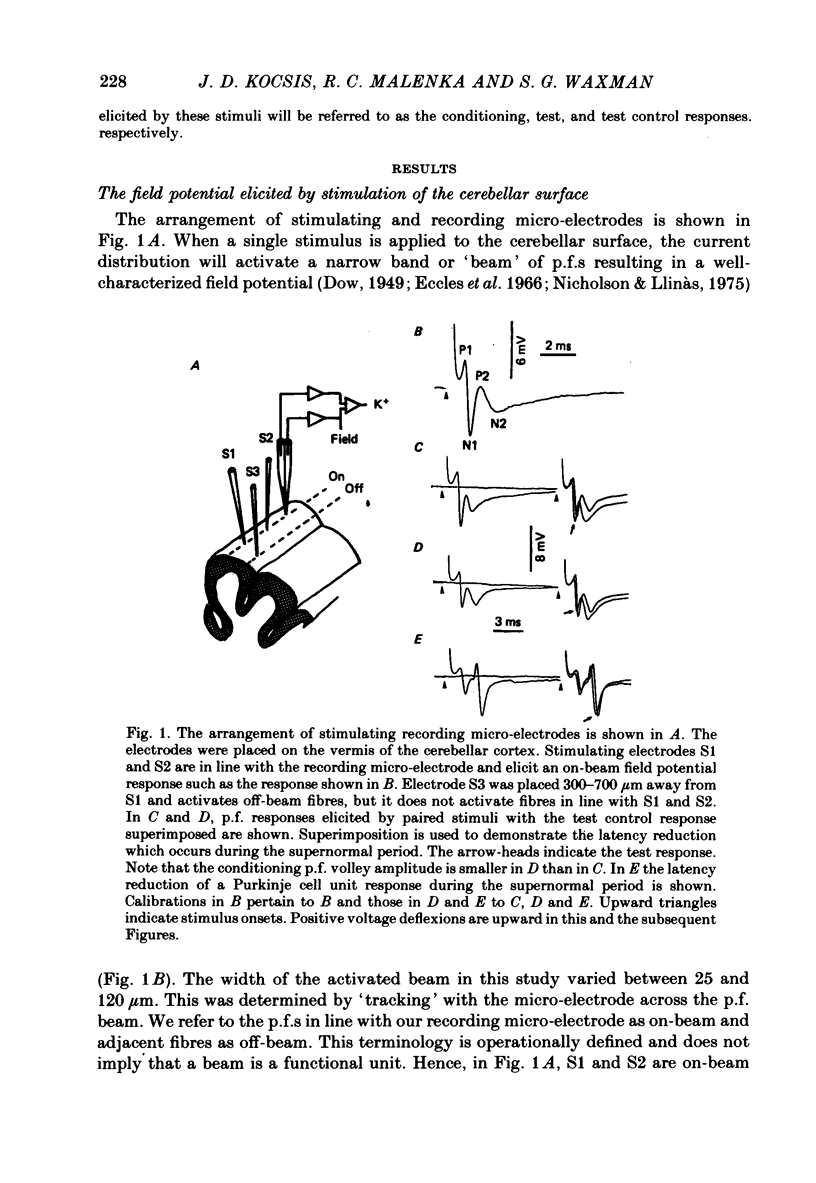
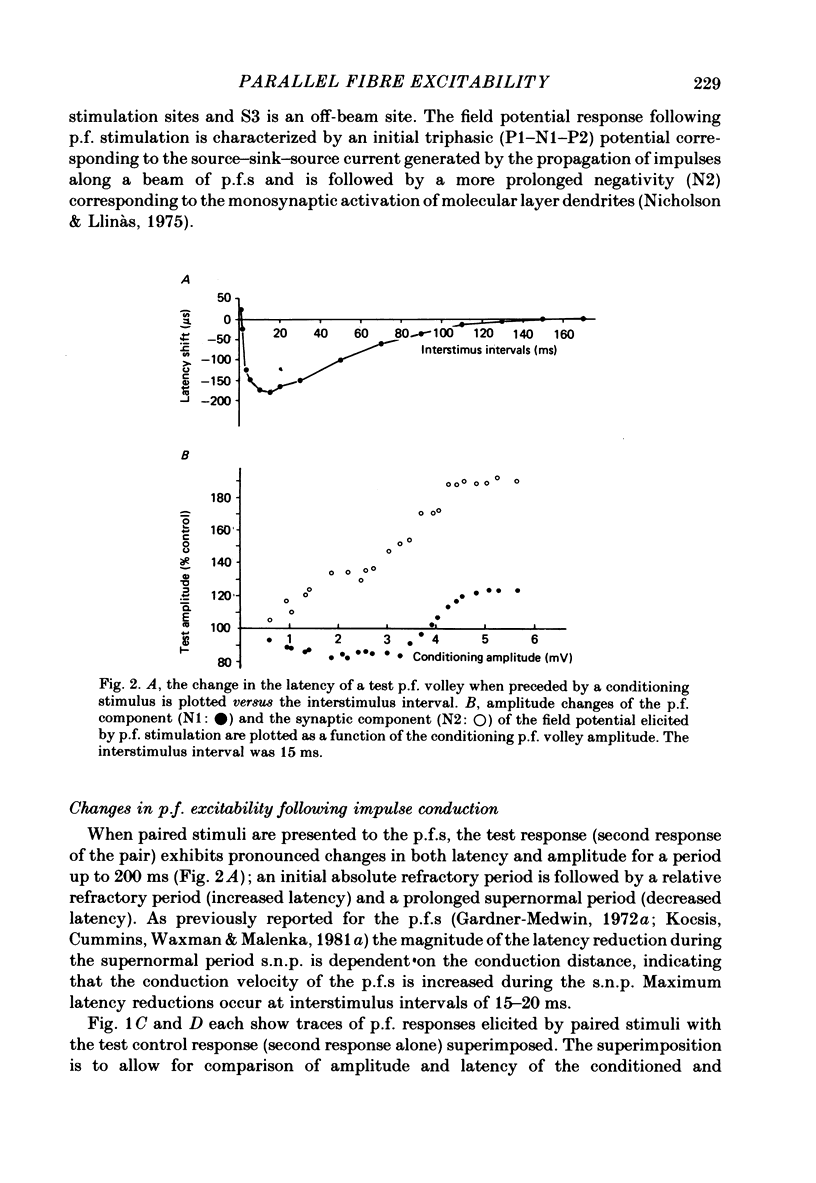
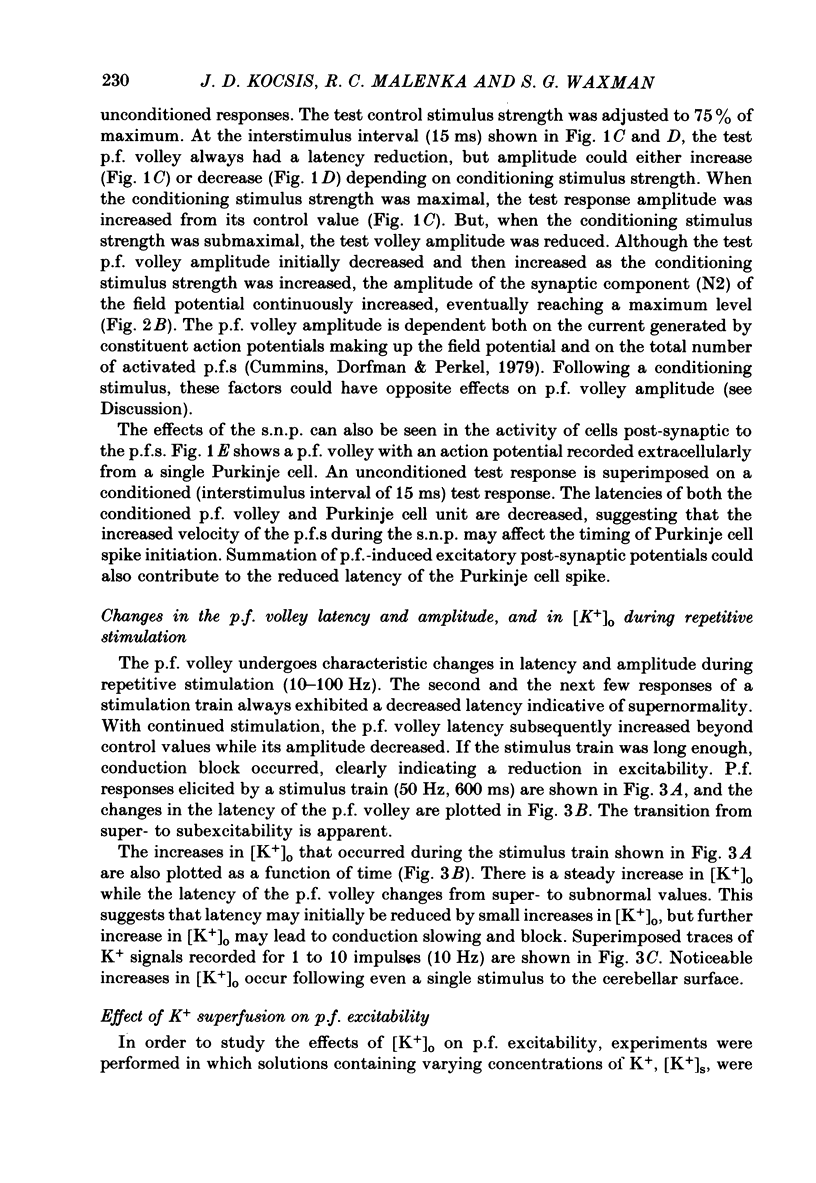
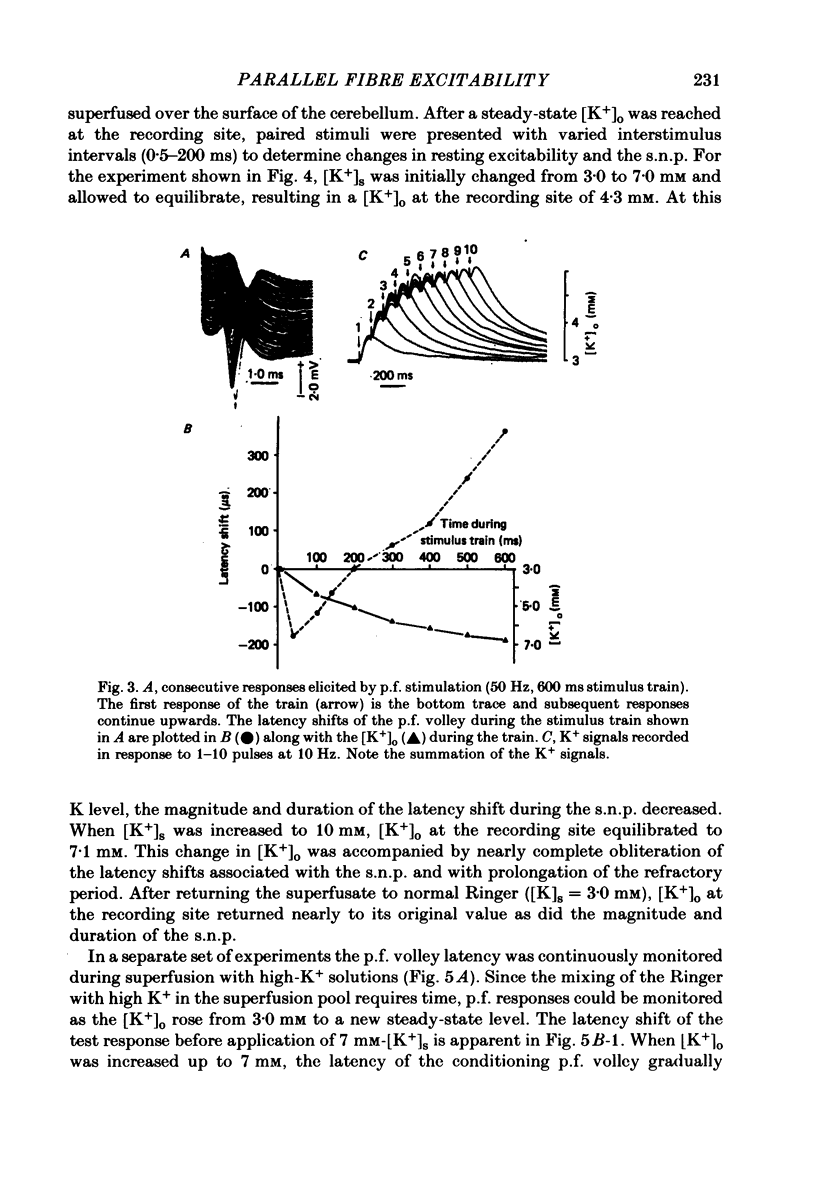
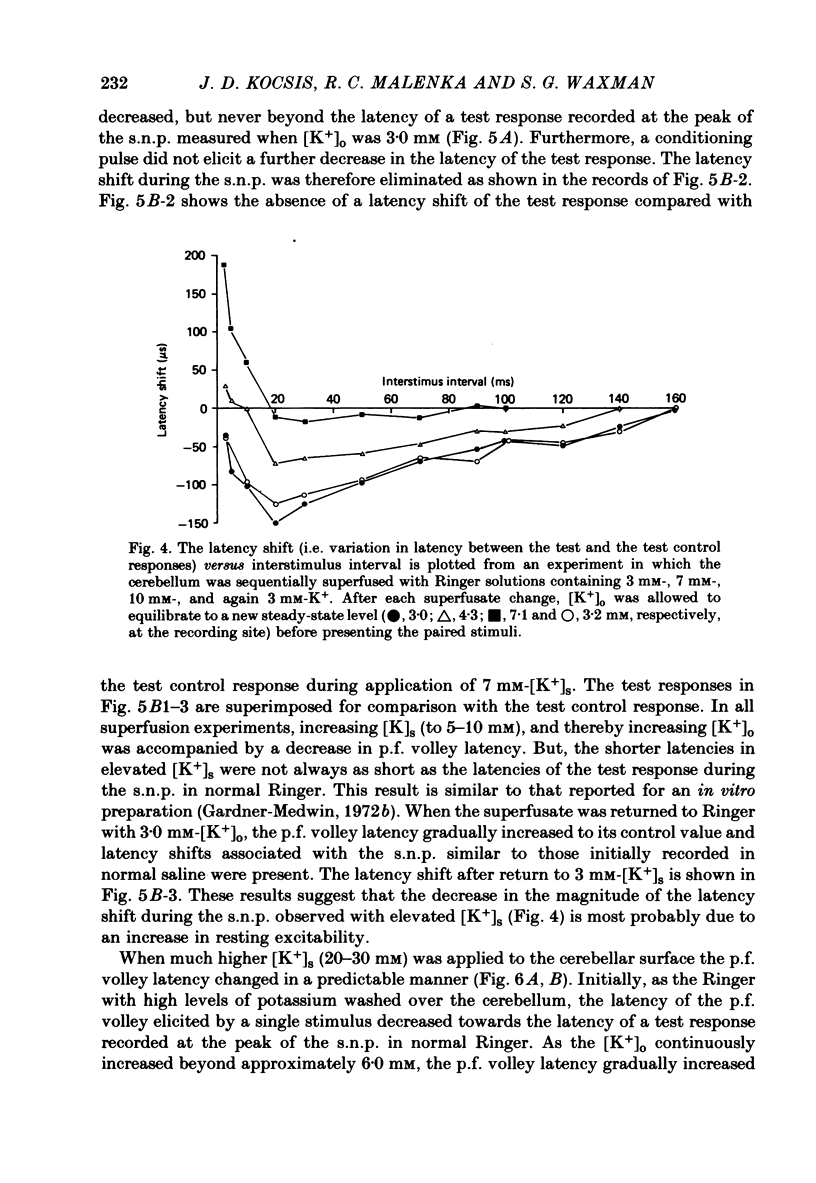
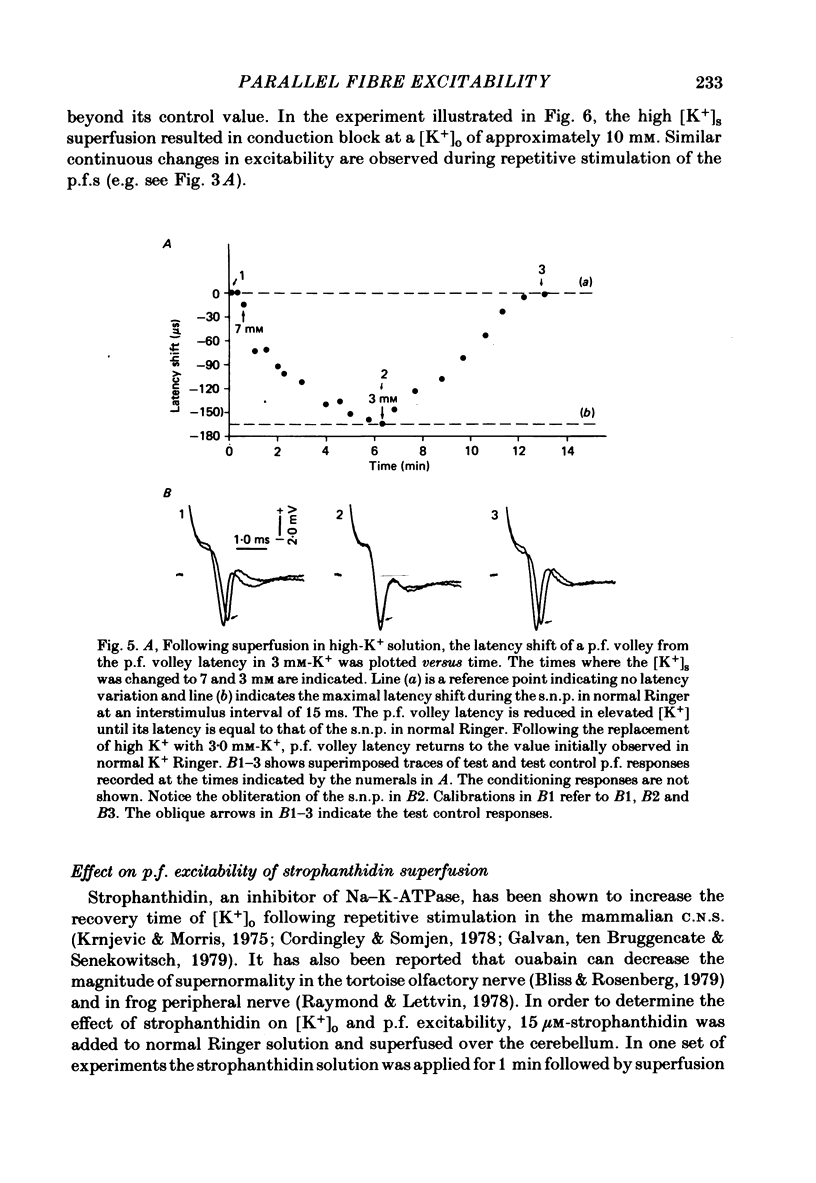
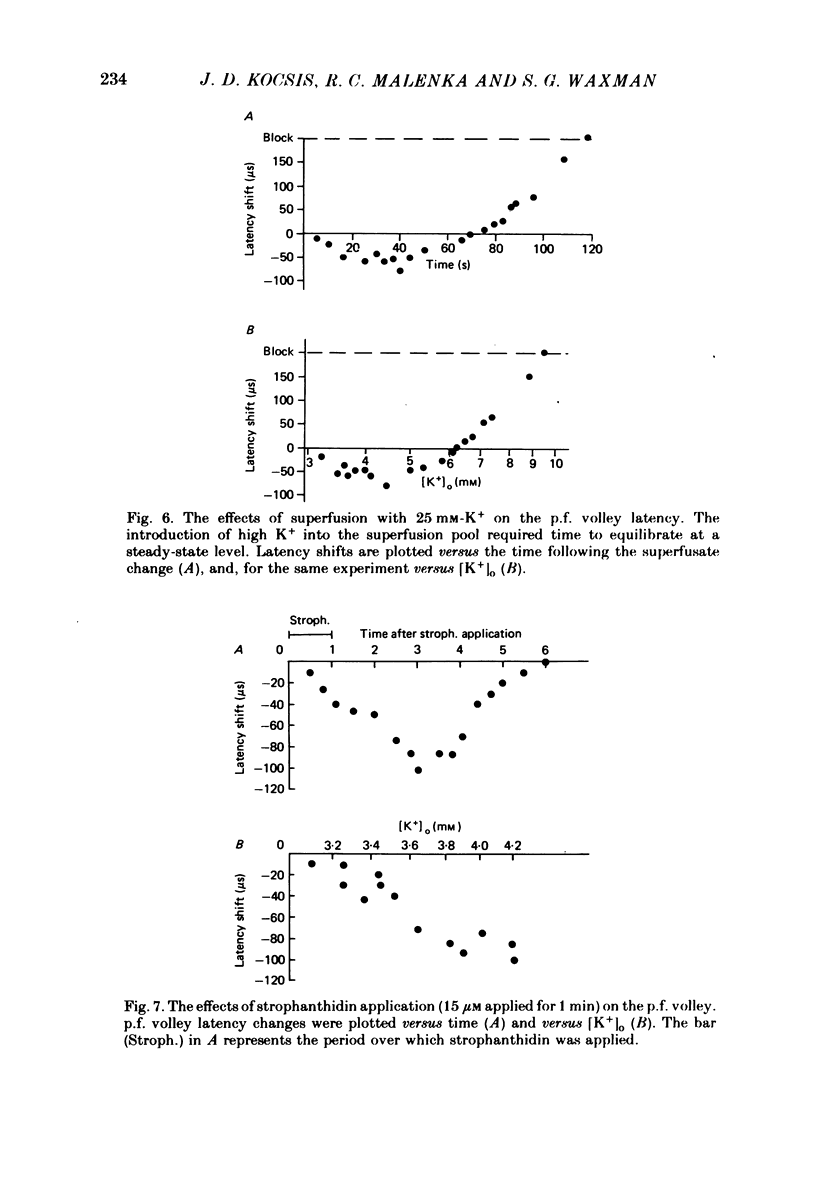
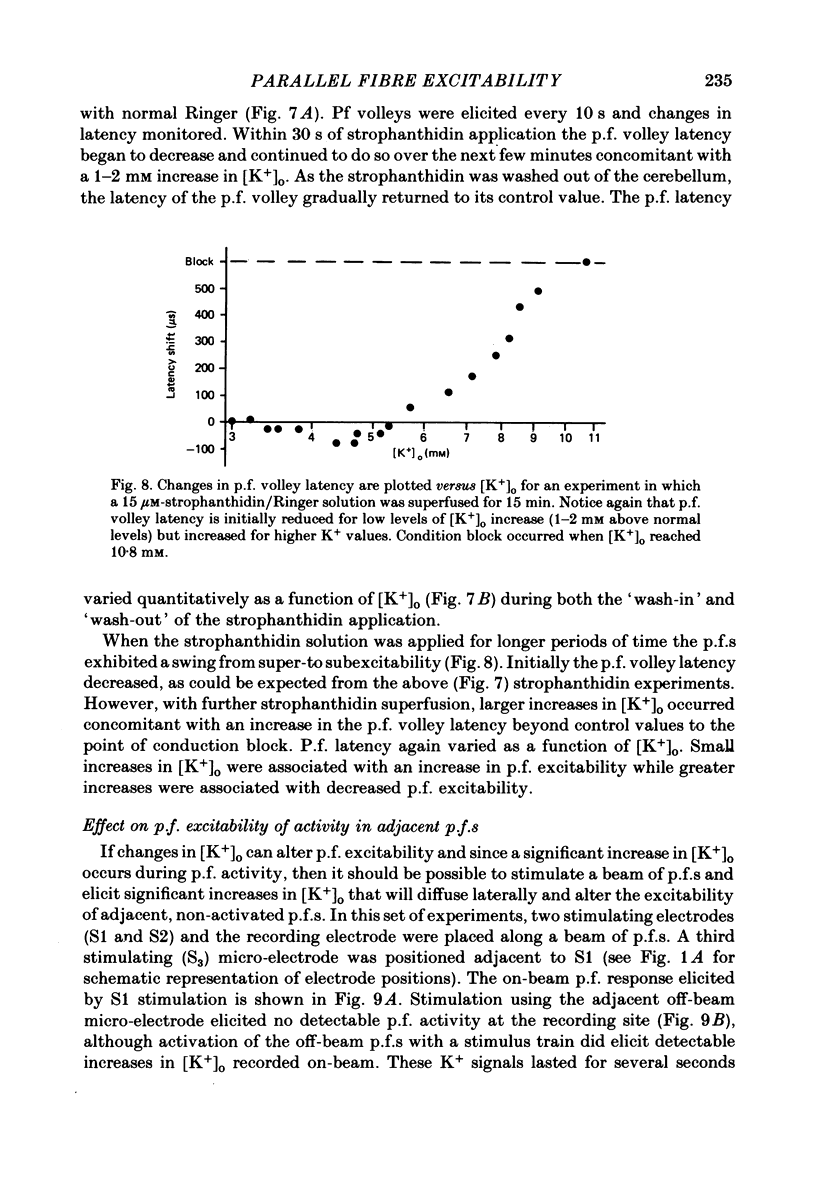
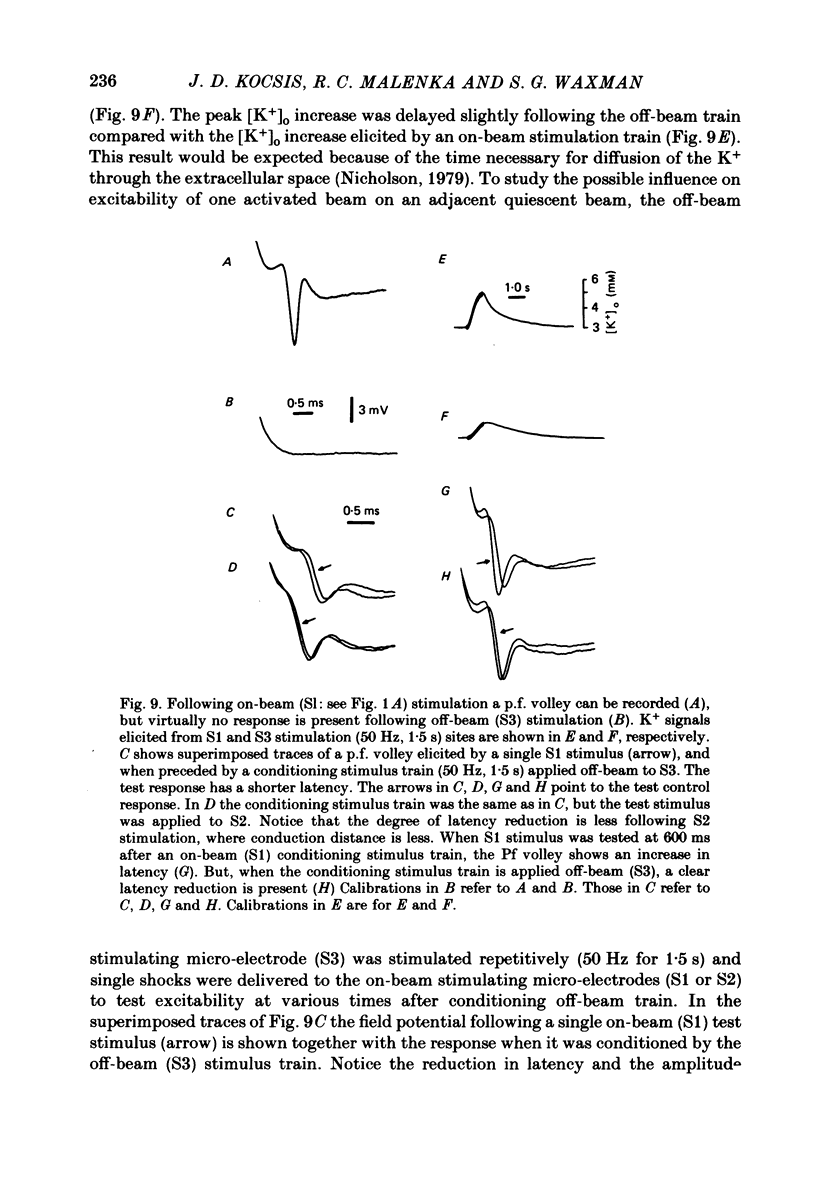
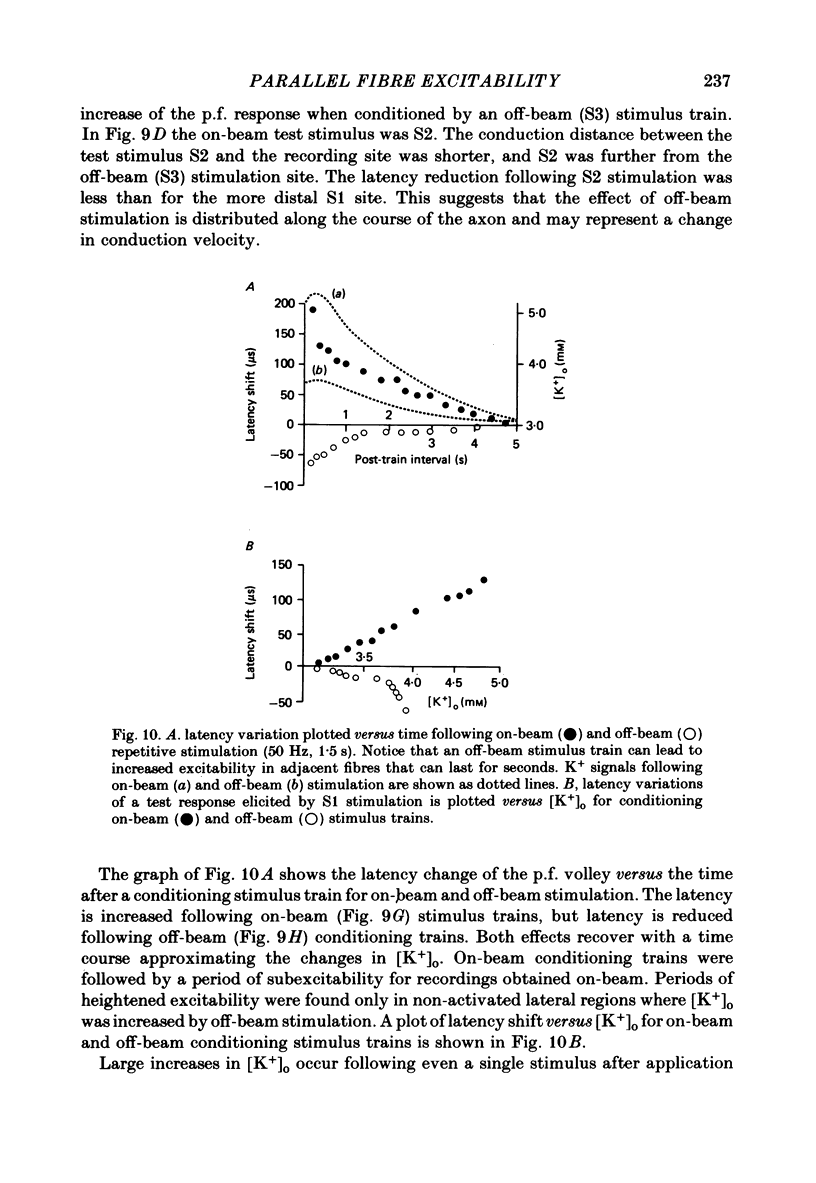
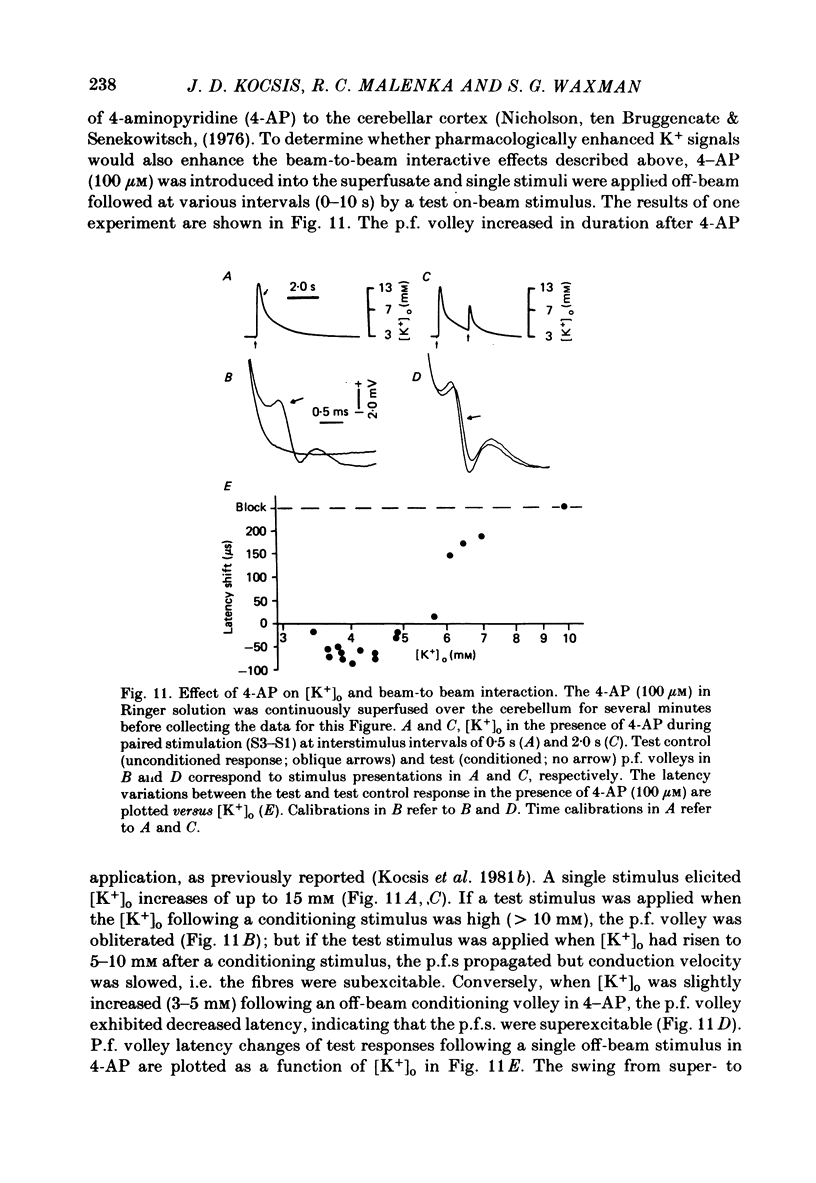
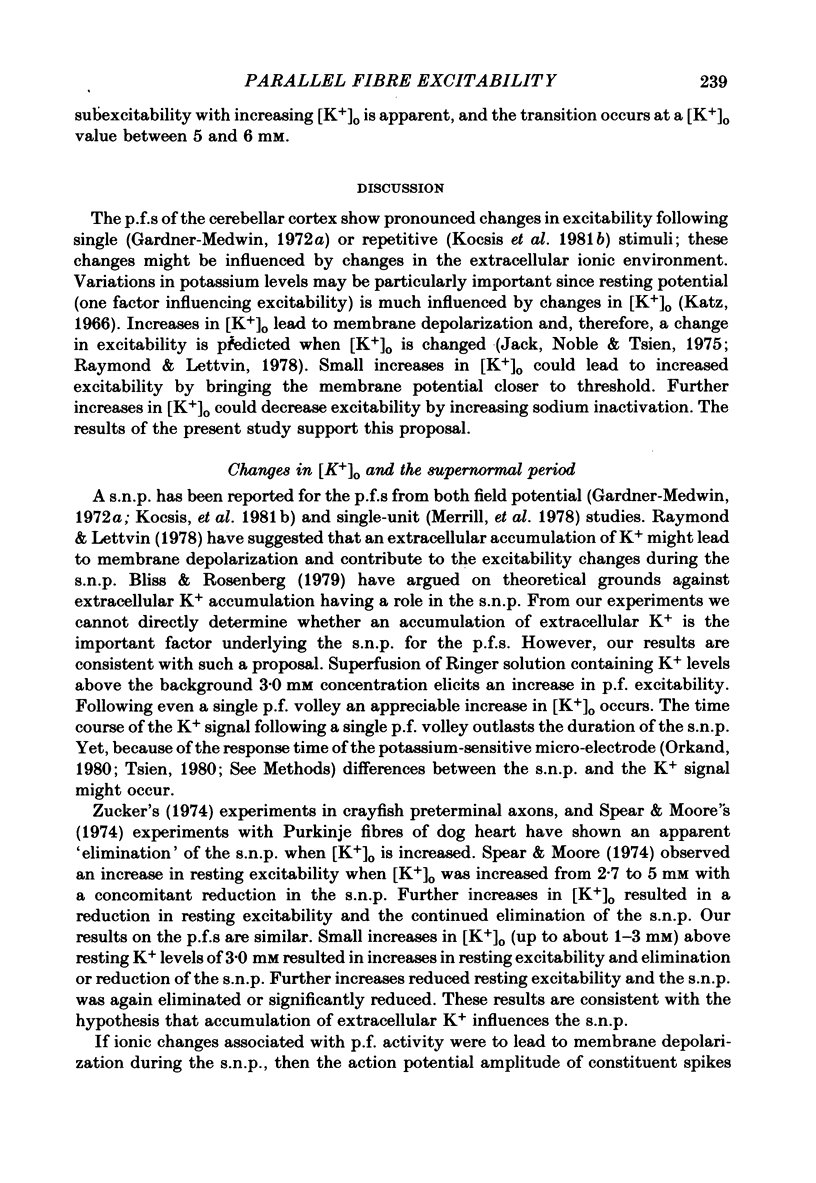
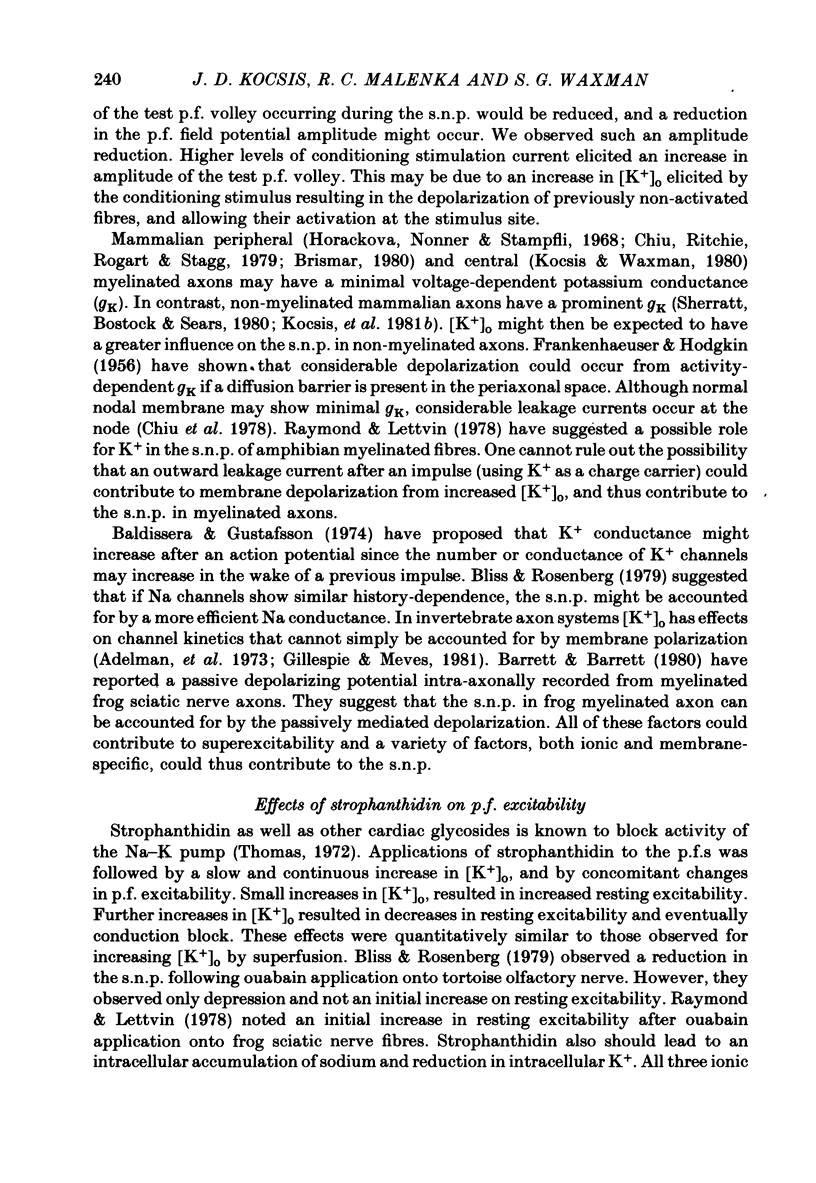
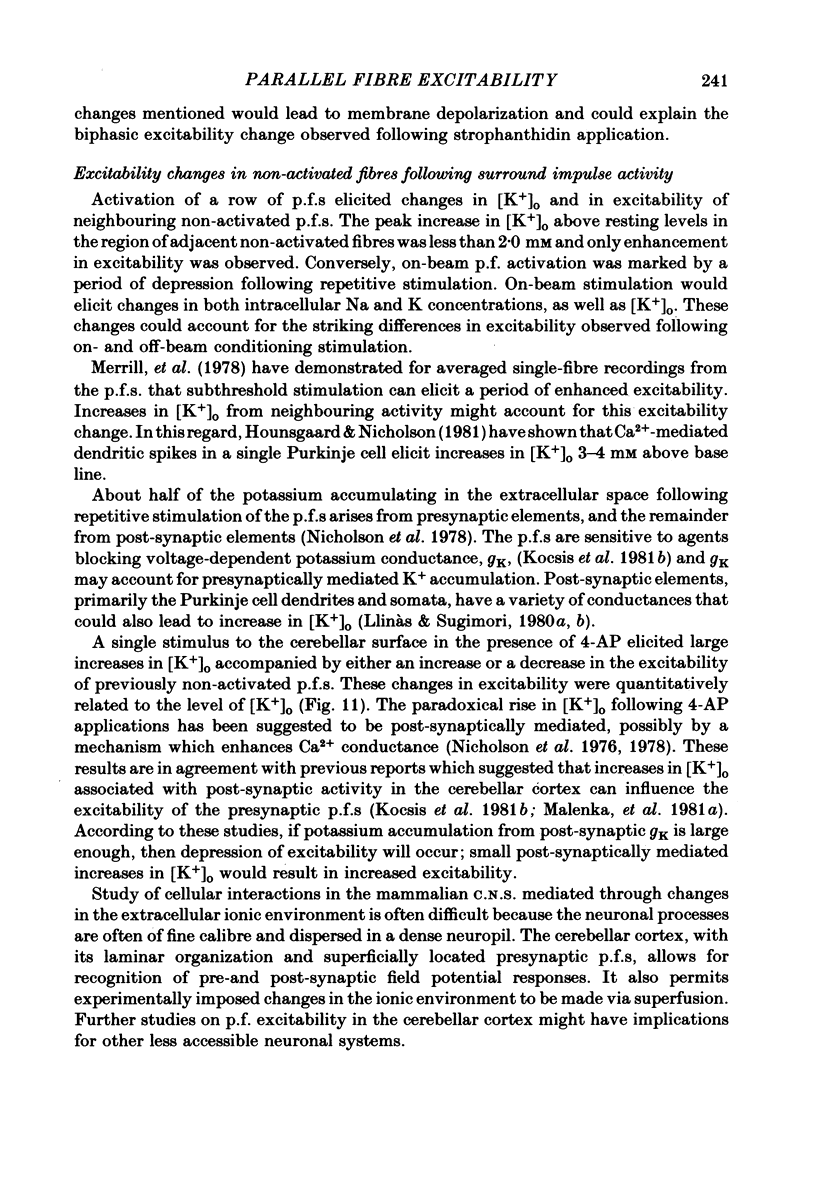
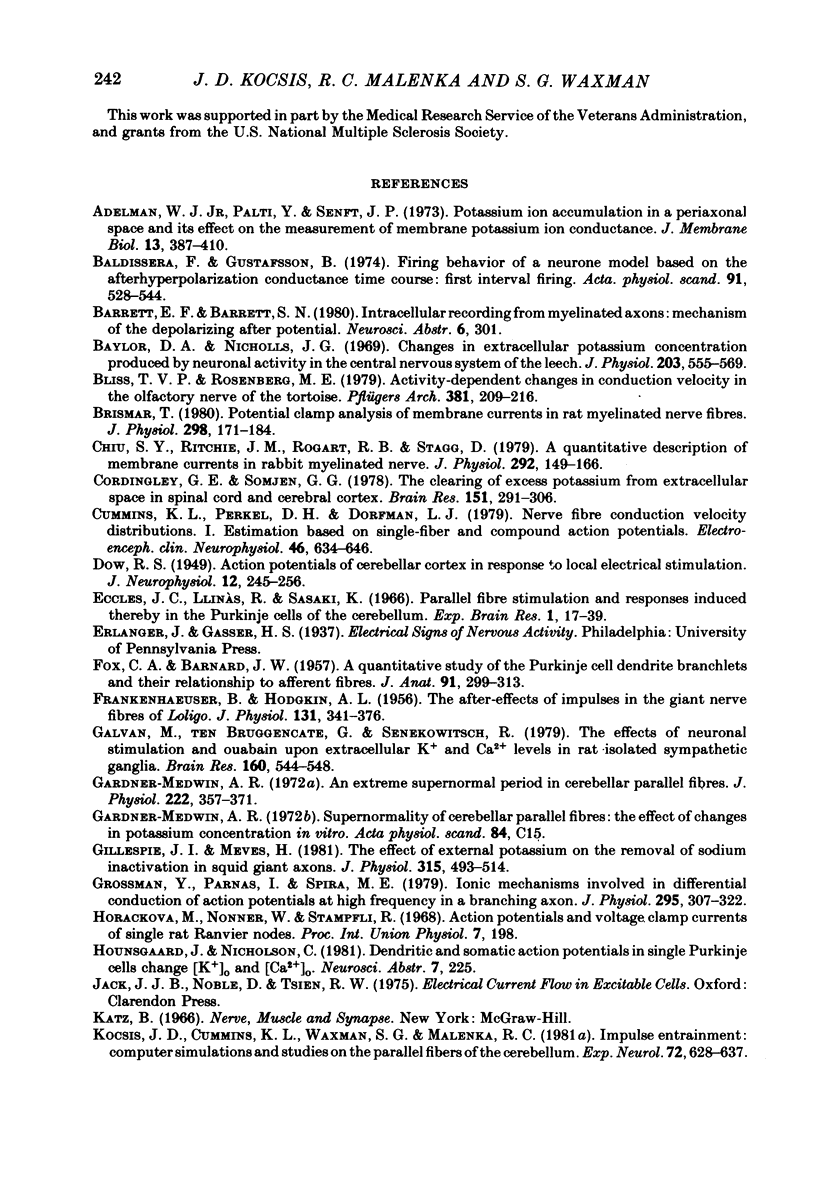
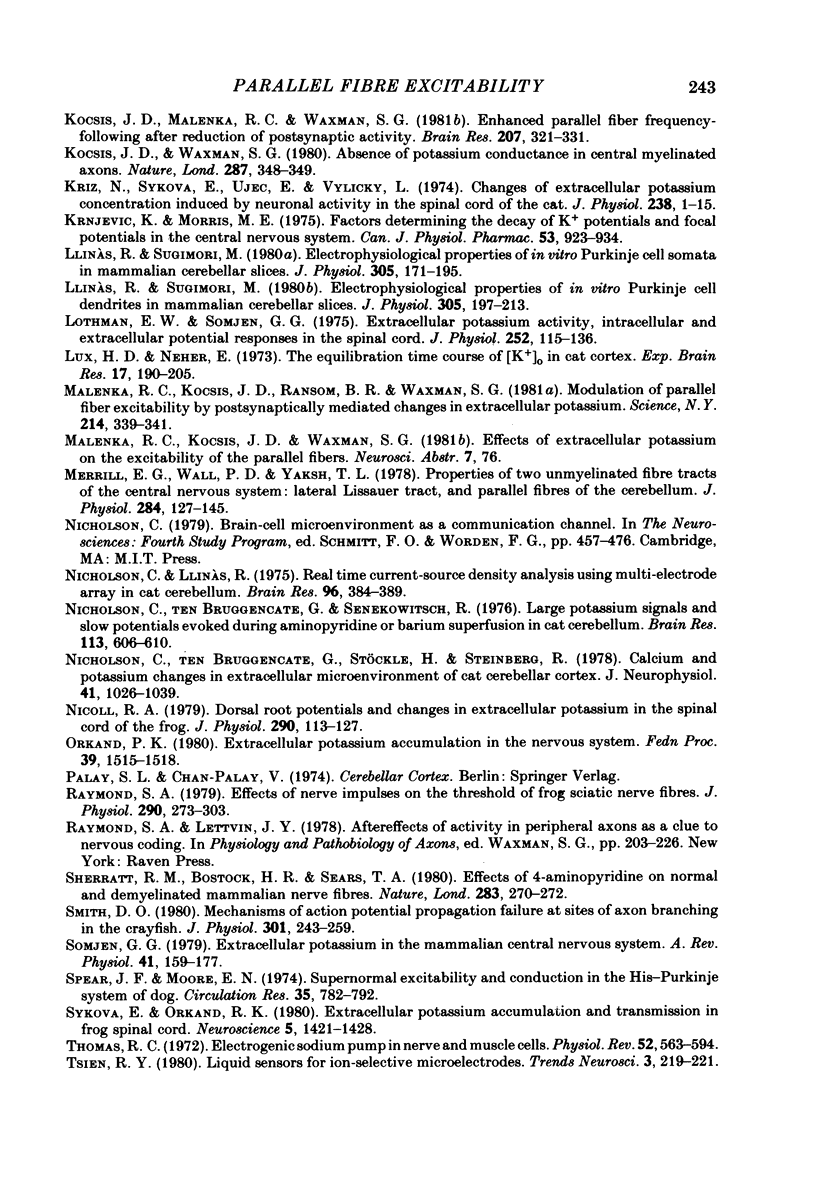
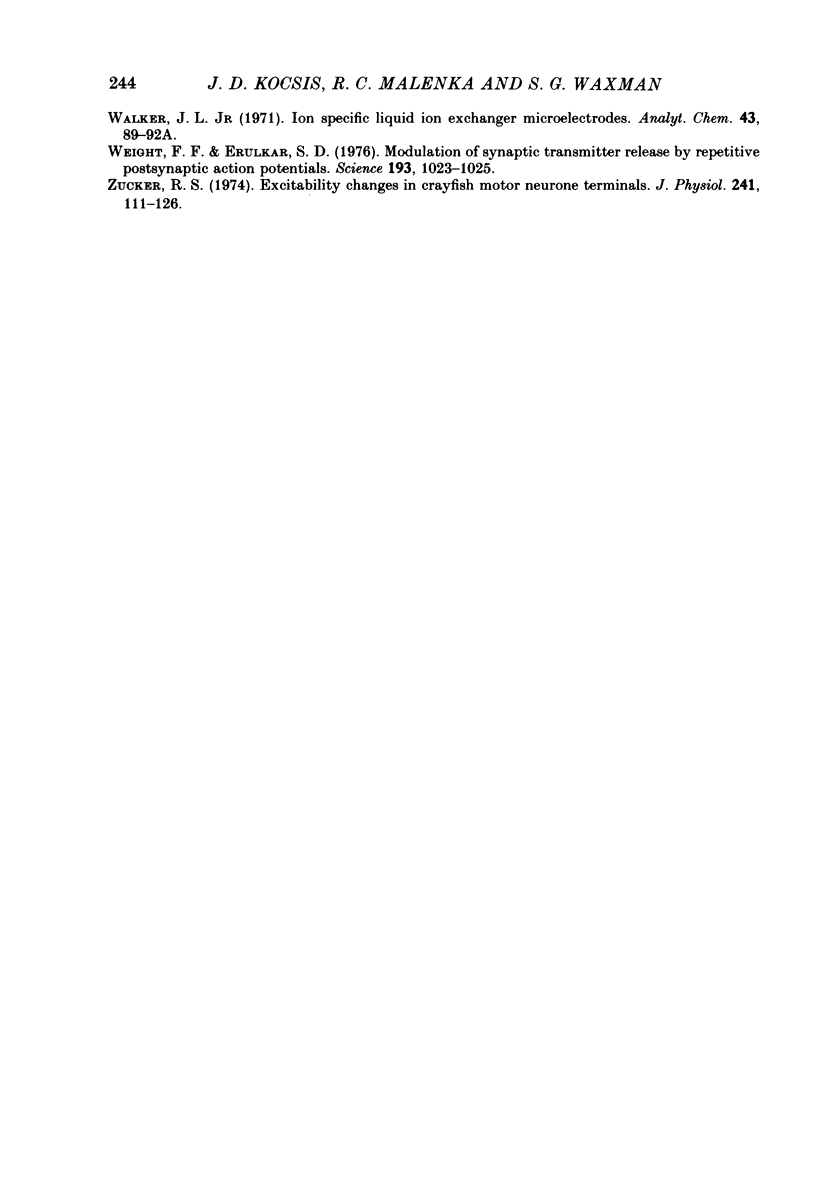
Selected References
These references are in PubMed. This may not be the complete list of references from this article.
- Adelman W. J., Jr, Palti Y., Senft J. P. Potassium ion accumulation in a periaxonal space and its effect on the measurement of membrane potassium ion conductance. J Membr Biol. 1973 Nov 8;13(4):387–410. doi: 10.1007/BF01868237. [DOI] [PubMed] [Google Scholar]
- Baldissera F., Gustafsson B. Firing behaviour of a neurone model based on the afterhyperpolarization conductance time course. First interval firing. Acta Physiol Scand. 1974 Aug;91(4):528–544. doi: 10.1111/j.1748-1716.1974.tb05708.x. [DOI] [PubMed] [Google Scholar]
- Baylor D. A., Nicholls J. G. Changes in extracellular potassium concentration produced by neuronal activity in the central nervous system of the leech. J Physiol. 1969 Aug;203(3):555–569. doi: 10.1113/jphysiol.1969.sp008879. [DOI] [PMC free article] [PubMed] [Google Scholar]
- Bliss T. V., Rosenberg M. E. Activity-dependent changes in conduction velocity in the olfactory nerve of the tortoise. Pflugers Arch. 1979 Sep;381(3):209–216. doi: 10.1007/BF00583251. [DOI] [PubMed] [Google Scholar]
- Brismar T. Potential clamp analysis of membrane currents in rat myelinated nerve fibres. J Physiol. 1980 Jan;298:171–184. doi: 10.1113/jphysiol.1980.sp013074. [DOI] [PMC free article] [PubMed] [Google Scholar]
- Chiu S. Y., Ritchie J. M., Rogart R. B., Stagg D. A quantitative description of membrane currents in rabbit myelinated nerve. J Physiol. 1979 Jul;292:149–166. doi: 10.1113/jphysiol.1979.sp012843. [DOI] [PMC free article] [PubMed] [Google Scholar]
- Cordingley G. E., Somjen G. G. The clearing of excess potassium from extracellular space in spinal cord and cerebral cortex. Brain Res. 1978 Aug 4;151(2):291–306. doi: 10.1016/0006-8993(78)90886-7. [DOI] [PubMed] [Google Scholar]
- Cummins K. L., Perkel D. H., Dorfman L. J. Nerve fiber conduction-velocity distributions. I. Estimation based on the single-fiber and compound action potentials. Electroencephalogr Clin Neurophysiol. 1979 Jun;46(6):634–646. doi: 10.1016/0013-4694(79)90101-9. [DOI] [PubMed] [Google Scholar]
- Eccles J. C., Llinás R., Sasaki K. Parallel fibre stimulation and the responses induced thereby in the Purkinje cells of the cerebellum. Exp Brain Res. 1966;1(1):17–39. doi: 10.1007/BF00235207. [DOI] [PubMed] [Google Scholar]
- FOX C. A., BARNARD J. W. A quantitative study of the Purkinje cell dendritic branchlets and their relationship to afferent fibres. J Anat. 1957 Jul;91(3):299–313. [PMC free article] [PubMed] [Google Scholar]
- FRANKENHAEUSER B., HODGKIN A. L. The after-effects of impulses in the giant nerve fibres of Loligo. J Physiol. 1956 Feb 28;131(2):341–376. doi: 10.1113/jphysiol.1956.sp005467. [DOI] [PMC free article] [PubMed] [Google Scholar]
- Galvan M., Bruggencate G. T., Senekowitsch R. The effects of neuronal stimulation and ouabain upon extracellular K+ and Ca2+ levels in rat isolated sympathetic ganglia. Brain Res. 1979 Jan 19;160(3):544–548. doi: 10.1016/0006-8993(79)91084-9. [DOI] [PubMed] [Google Scholar]
- Gardner-Medwin A. R. An extreme supernormal period in cerebellar parallel fibres. J Physiol. 1972 Apr;222(2):357–371. doi: 10.1113/jphysiol.1972.sp009802. [DOI] [PMC free article] [PubMed] [Google Scholar]
- Gillespie J. I., Meves H. The effect of external potassium on the removal of sodium inactivation in squid giant axons. J Physiol. 1981 Jun;315:493–514. doi: 10.1113/jphysiol.1981.sp013760. [DOI] [PMC free article] [PubMed] [Google Scholar]
- Grossman Y., Parnas I., Spira M. E. Mechanisms involved in differential conduction of potentials at high frequency in a branching axon. J Physiol. 1979 Oct;295:307–322. doi: 10.1113/jphysiol.1979.sp012970. [DOI] [PMC free article] [PubMed] [Google Scholar]
- Kocsis J. D., Cummins K. L., Waxman S. G., Malenka R. C. Impulse entrainment: computer simulations and studies on the parallel fibers of the cerebellum. Exp Neurol. 1981 Jun;72(3):628–637. doi: 10.1016/0014-4886(81)90011-x. [DOI] [PubMed] [Google Scholar]
- Kocsis J. D., Malenka R. C., Waxman S. G. Enhanced parallel fiber frequency-following after reduction of postsynaptic activity. Brain Res. 1981 Mar 2;207(2):321–331. doi: 10.1016/0006-8993(81)90367-x. [DOI] [PubMed] [Google Scholar]
- Kocsis J. D., Waxman S. G. Absence of potassium conductance in central myelinated axons. Nature. 1980 Sep 25;287(5780):348–349. doi: 10.1038/287348a0. [DOI] [PubMed] [Google Scholar]
- Krnjević K., Morris M. E. Factors determining the decay of K+ potentials and focal potentials in the central nervous system. Can J Physiol Pharmacol. 1975 Oct;53(5):923–934. doi: 10.1139/y75-126. [DOI] [PubMed] [Google Scholar]
- Kríz N., Syková E., Ujec E., Vyklický L. Changes of extracellular potassium concentration induced by neuronal activity in the sinal cord of the cat. J Physiol. 1974 Apr;238(1):1–15. doi: 10.1113/jphysiol.1974.sp010507. [DOI] [PMC free article] [PubMed] [Google Scholar]
- Llinás R., Sugimori M. Electrophysiological properties of in vitro Purkinje cell dendrites in mammalian cerebellar slices. J Physiol. 1980 Aug;305:197–213. doi: 10.1113/jphysiol.1980.sp013358. [DOI] [PMC free article] [PubMed] [Google Scholar]
- Llinás R., Sugimori M. Electrophysiological properties of in vitro Purkinje cell somata in mammalian cerebellar slices. J Physiol. 1980 Aug;305:171–195. doi: 10.1113/jphysiol.1980.sp013357. [DOI] [PMC free article] [PubMed] [Google Scholar]
- Lothman E. W., Somjen G. G. Extracellular potassium activity, intracellular and extracellular potential responses in the spinal cord. J Physiol. 1975 Oct;252(1):115–136. doi: 10.1113/jphysiol.1975.sp011137. [DOI] [PMC free article] [PubMed] [Google Scholar]
- Lux H. D., Neher E. The equilibration time course of (K + ) 0 in cat cortex. Exp Brain Res. 1973 Apr 30;17(2):190–205. doi: 10.1007/BF00235028. [DOI] [PubMed] [Google Scholar]
- Malenka R. C., Kocsis J. D., Ransom B. R., Waxman S. G. Modulation of parallel fiber excitability by postsynaptically mediated changes in extracellular potassium. Science. 1981 Oct 16;214(4518):339–341. doi: 10.1126/science.7280695. [DOI] [PubMed] [Google Scholar]
- Merrill E. G., Wall P. D., Yaksh T. L. Properties of two unmyelinated fibre tracts of the central nervous system: lateral Lissauer tract, and parallel fibres of the cerebellum. J Physiol. 1978 Nov;284:127–145. doi: 10.1113/jphysiol.1978.sp012531. [DOI] [PMC free article] [PubMed] [Google Scholar]
- Nicholson C., Bruggencate G. T., Senekowitsch R. Large potassium signals and slow potentials evoked during aminopyridine or barium superfusion in cat cerebellum. Brain Res. 1976 Sep 3;113(3):606–610. doi: 10.1016/0006-8993(76)90063-9. [DOI] [PubMed] [Google Scholar]
- Nicholson C., Kraig R. P. Chloride and potassium changes measured during spreading depression in catfish cerebellum. Brain Res. 1975 Oct 17;96(2):384–389. doi: 10.1016/0006-8993(75)90752-0. [DOI] [PubMed] [Google Scholar]
- Nicholson C., ten Bruggencate G., Stöckle H., Steinberg R. Calcium and potassium changes in extracellular microenvironment of cat cerebellar cortex. J Neurophysiol. 1978 Jul;41(4):1026–1039. doi: 10.1152/jn.1978.41.4.1026. [DOI] [PubMed] [Google Scholar]
- Nicoll R. A. Dorsal root potentials and changes in extracellular potassium in the spinal cord of the frog. J Physiol. 1979 May;290(2):113–127. doi: 10.1113/jphysiol.1979.sp012763. [DOI] [PMC free article] [PubMed] [Google Scholar]
- Orkand R. K. Extracellular potassium accumulation in the nervous system. Fed Proc. 1980 Apr;39(5):1515–1518. [PubMed] [Google Scholar]
- Raymond S. A. Effects of nerve impulses on threshold of frog sciatic nerve fibres. J Physiol. 1979 May;290(2):273–303. doi: 10.1113/jphysiol.1979.sp012771. [DOI] [PMC free article] [PubMed] [Google Scholar]
- Smith D. O. Mechanisms of action potential propagation failure at sites of axon branching in the crayfish. J Physiol. 1980 Apr;301:243–259. doi: 10.1113/jphysiol.1980.sp013202. [DOI] [PMC free article] [PubMed] [Google Scholar]
- Somjen G. G. Extracellular potassium in the mammalian central nervous system. Annu Rev Physiol. 1979;41:159–177. doi: 10.1146/annurev.ph.41.030179.001111. [DOI] [PubMed] [Google Scholar]
- Spear J. F., Moore E. N. Supernormal excitability and conduction in the His-Purkinje system of the dog. Circ Res. 1974 Nov;35(5):782–792. doi: 10.1161/01.res.35.5.782. [DOI] [PubMed] [Google Scholar]
- Syková E., Orkand R. K. Extracellular potassium accumulation and transmission in frog spinal cord. Neuroscience. 1980;5(8):1421–1428. doi: 10.1016/0306-4522(80)90003-2. [DOI] [PubMed] [Google Scholar]
- Thomas R. C. Electrogenic sodium pump in nerve and muscle cells. Physiol Rev. 1972 Jul;52(3):563–594. doi: 10.1152/physrev.1972.52.3.563. [DOI] [PubMed] [Google Scholar]
- Weight F. F., Erulkar S. D. Modulation of synaptic transmitter release by repetitive postsynaptic action potentials. Science. 1976 Sep 10;193(4257):1023–1025. doi: 10.1126/science.7839. [DOI] [PubMed] [Google Scholar]
- Zucker R. S. Excitability changes in crayfish motor neurone terminals. J Physiol. 1974 Aug;241(1):111–126. doi: 10.1113/jphysiol.1974.sp010643. [DOI] [PMC free article] [PubMed] [Google Scholar]