Abstract
The flux of K+ produced by electric current across the pia-arachnoid surface of the neocortex of anaesthetized rats has been studied with K+-selective electrodes in a cup at the surface and with flame photometry. The potential differences developed across three regions of the rat brain (neocortex, cerebellum, hippocampus) have been measured as [K+] was altered in fluid at the surface. The experimental results have been related to those that would be expected (i) if K+ moved principally by diffusion in extracellular space and (ii) if current flow through cells makes a significant contribution to K+ transfer. K movement produced by current across the neocortical surface accounted for 0.06 of the transfer of electric charge with small currents in either direction (ca. 5 microA mm-2) and with larger currents out of the tissue. Large currents (ca. 20 microA mm-2) into the tissue produced less K+ movement, but still more than the fraction 0.012 expected for purely extracellular flux. Alternating current pulses (5 Hz) with zero net transfer of charge produced no flux of K+ across the surface, while alternation with unequal durations produced the same effects as the equivalent steady charge transfer. The K+ flux lagged behind the onset and cessation of current with a time constant ca. 45 sec, approximately as expected from calculations with a model of the tissue. A surface-negative potential shift averaging 2 mV was observed when [K+ ]at the brain surface was increased from 3 to 12 mM. The time for development of half of the full potential change was 20 sec, with the solution changes complete in less than 4 sec. These results are inconsistent with the hypothesis that K+ movement through brain tissue occurs principally through intercellular clefts, except where these movements involve very localized gradients. They are consistent with the conclusion that ca. 5 times as much K+ flux passes through cells (probably largely glial cells) as through extracellular space, with fluxes driven by either extracellular voltage or concentration gradients.
Full text
PDF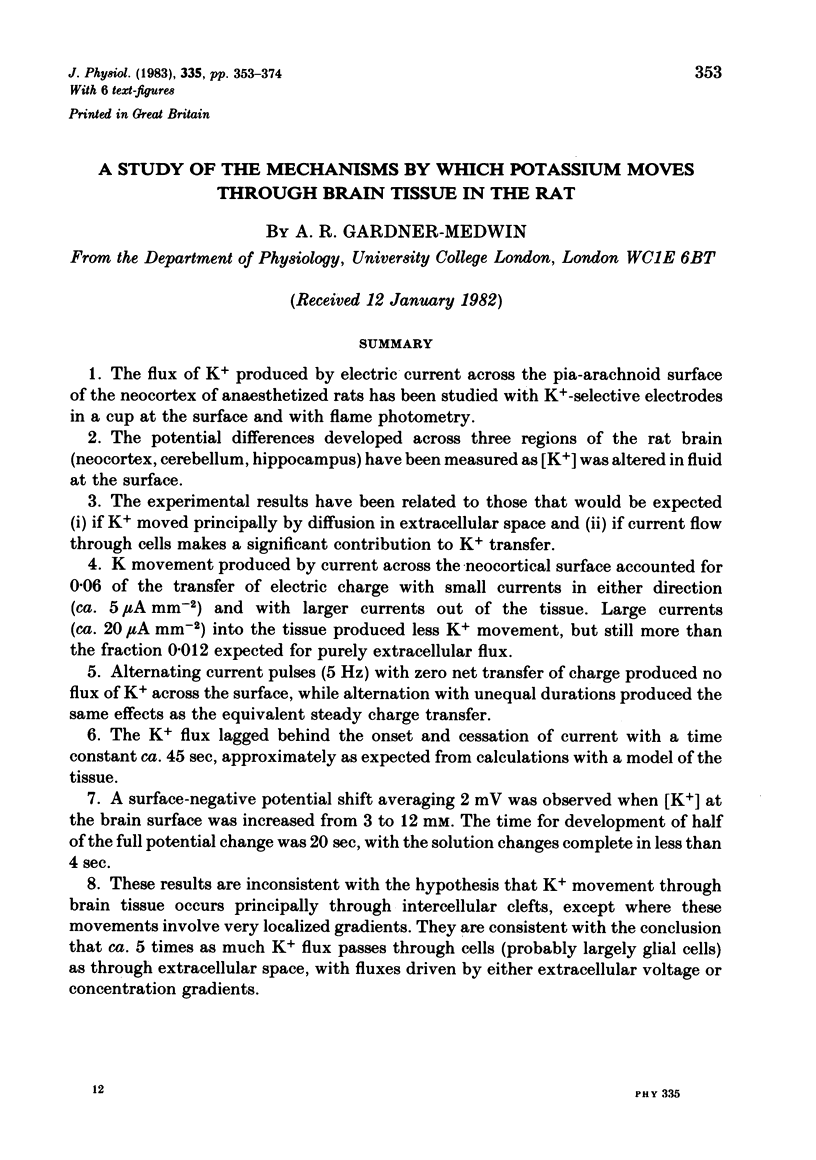
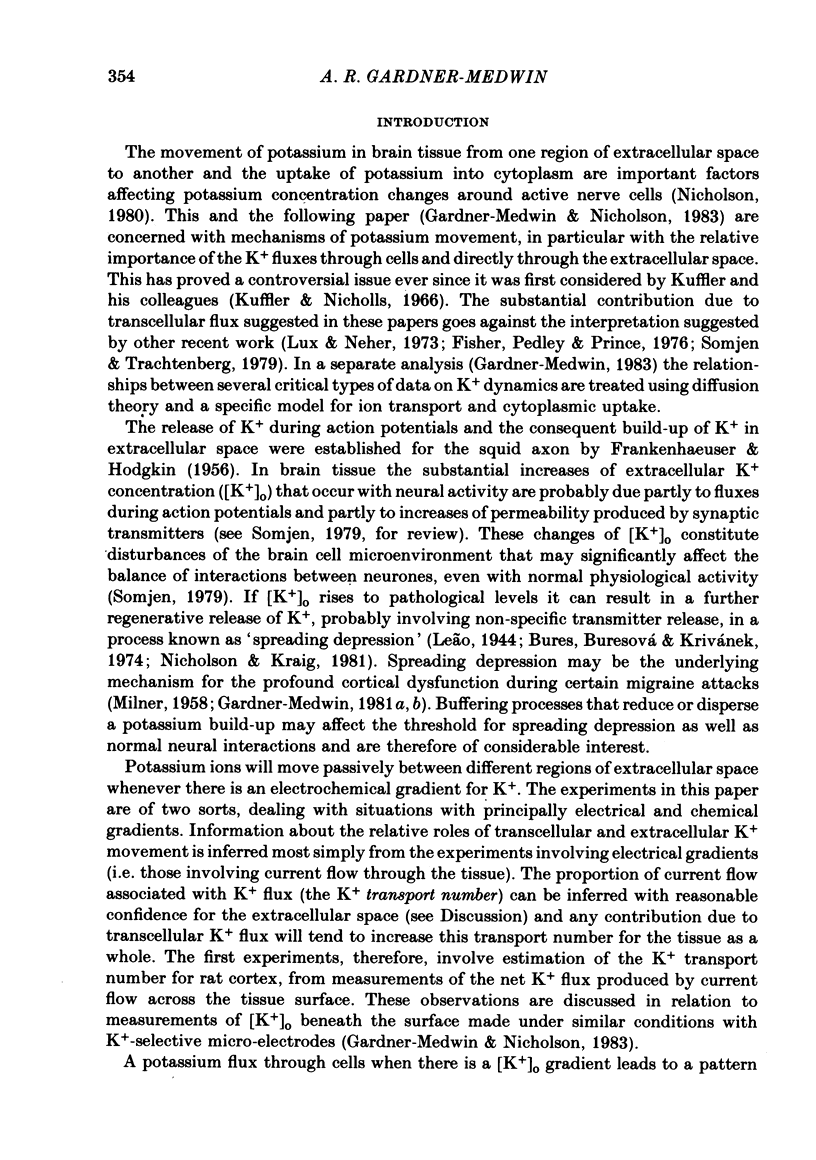
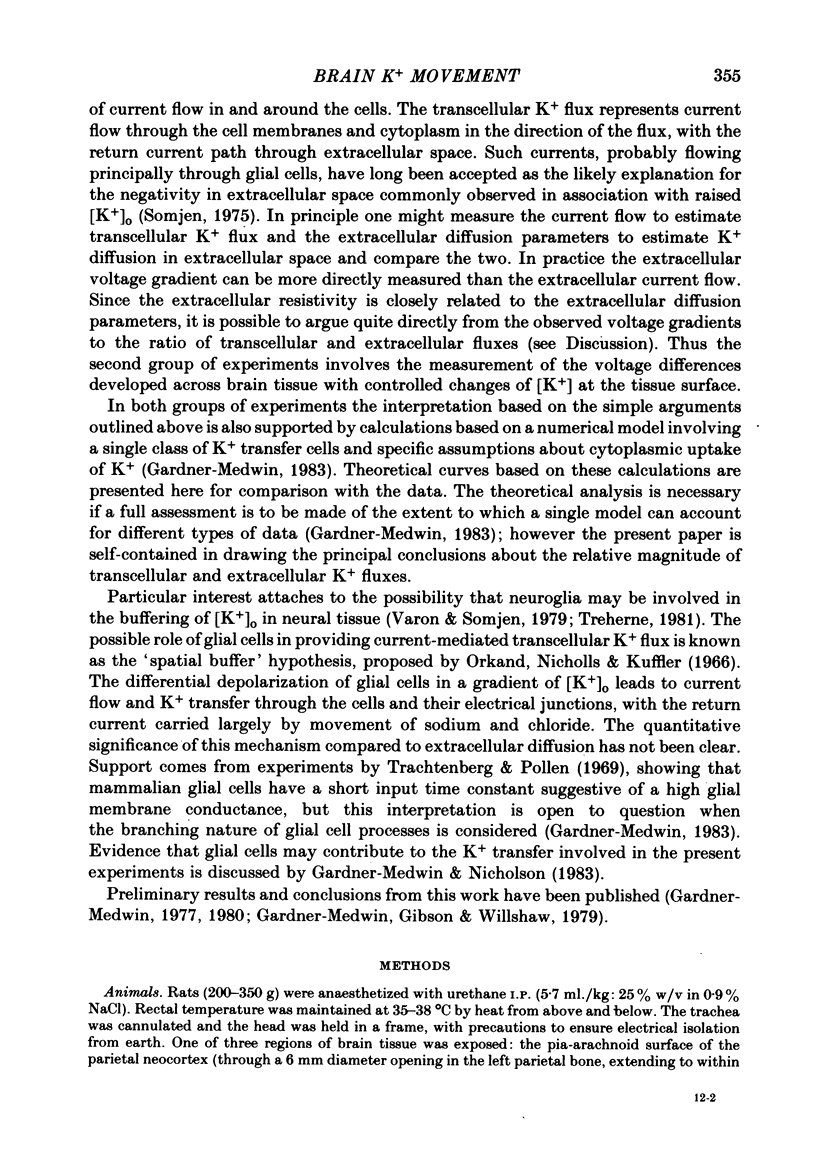
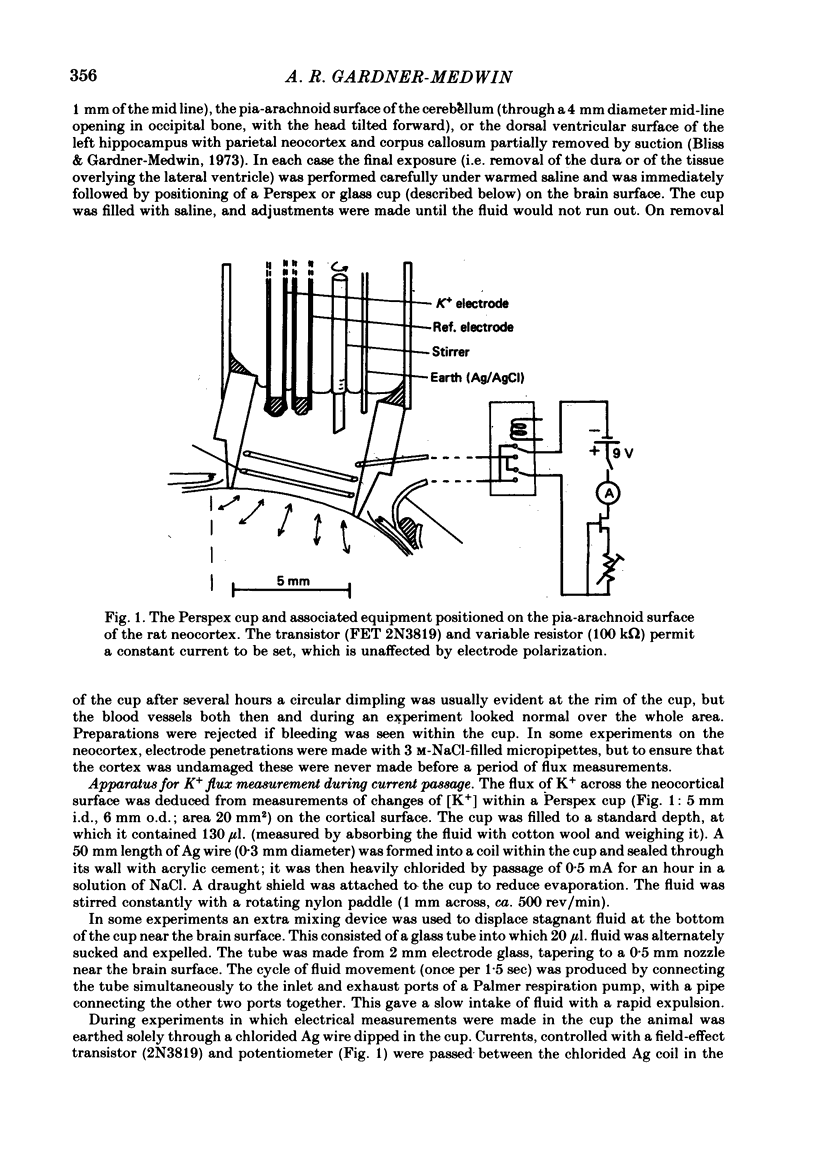
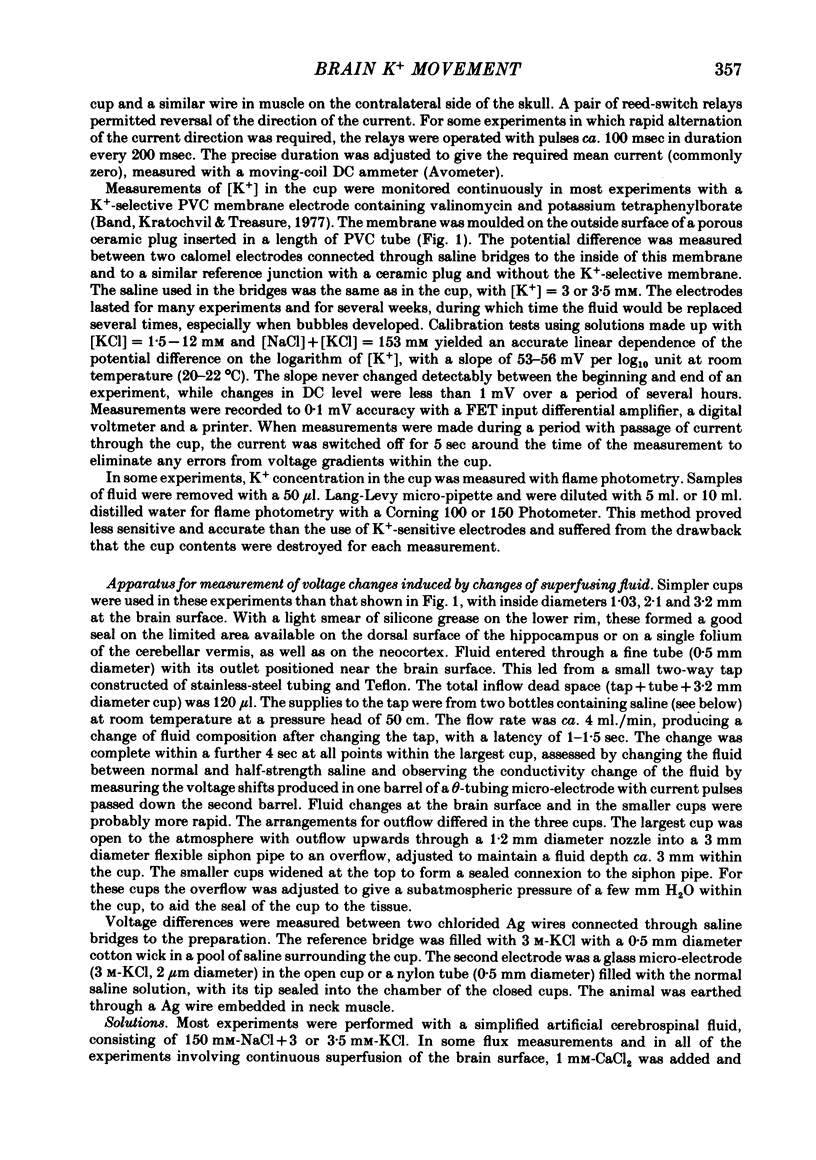
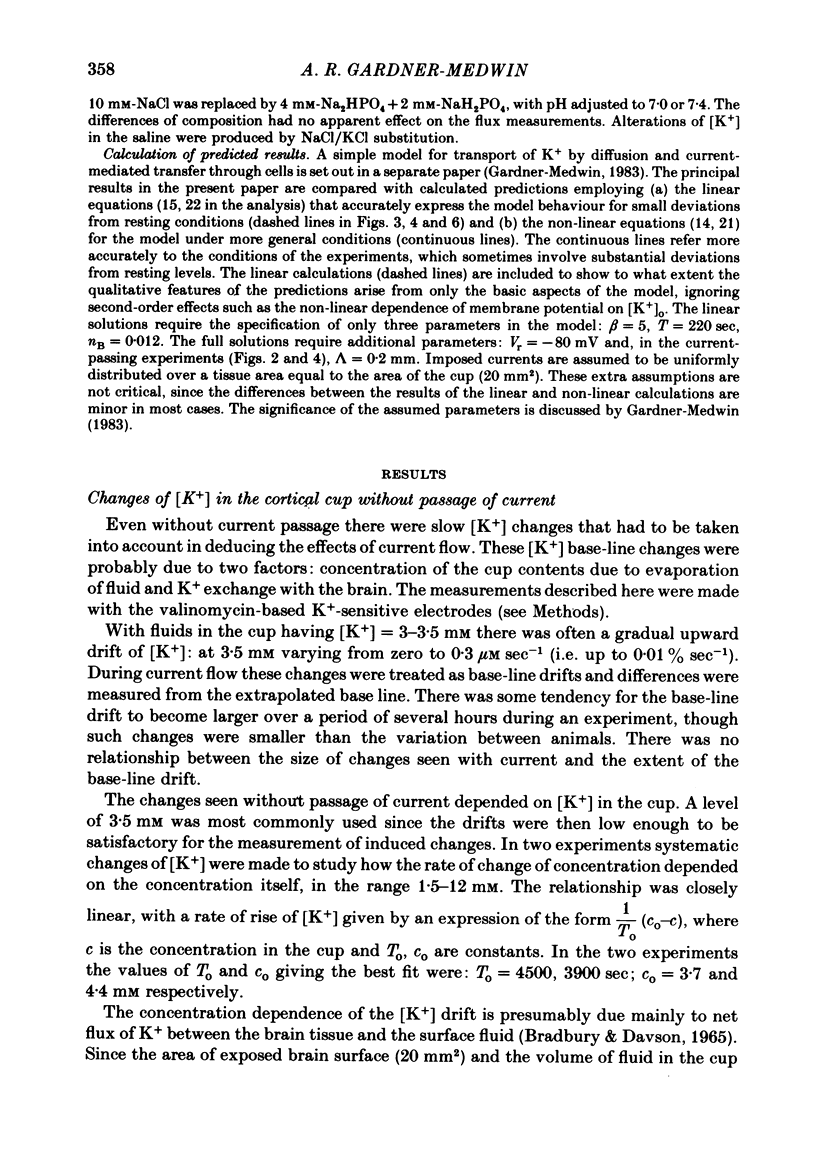
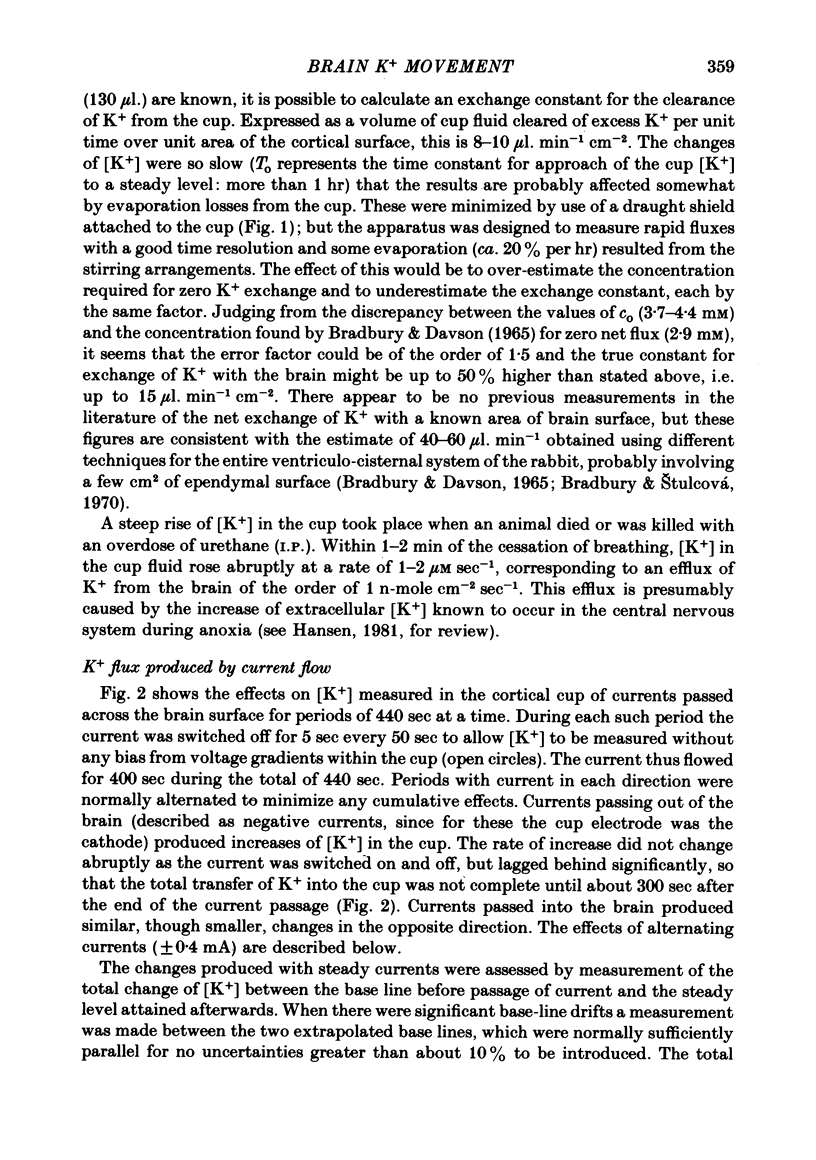
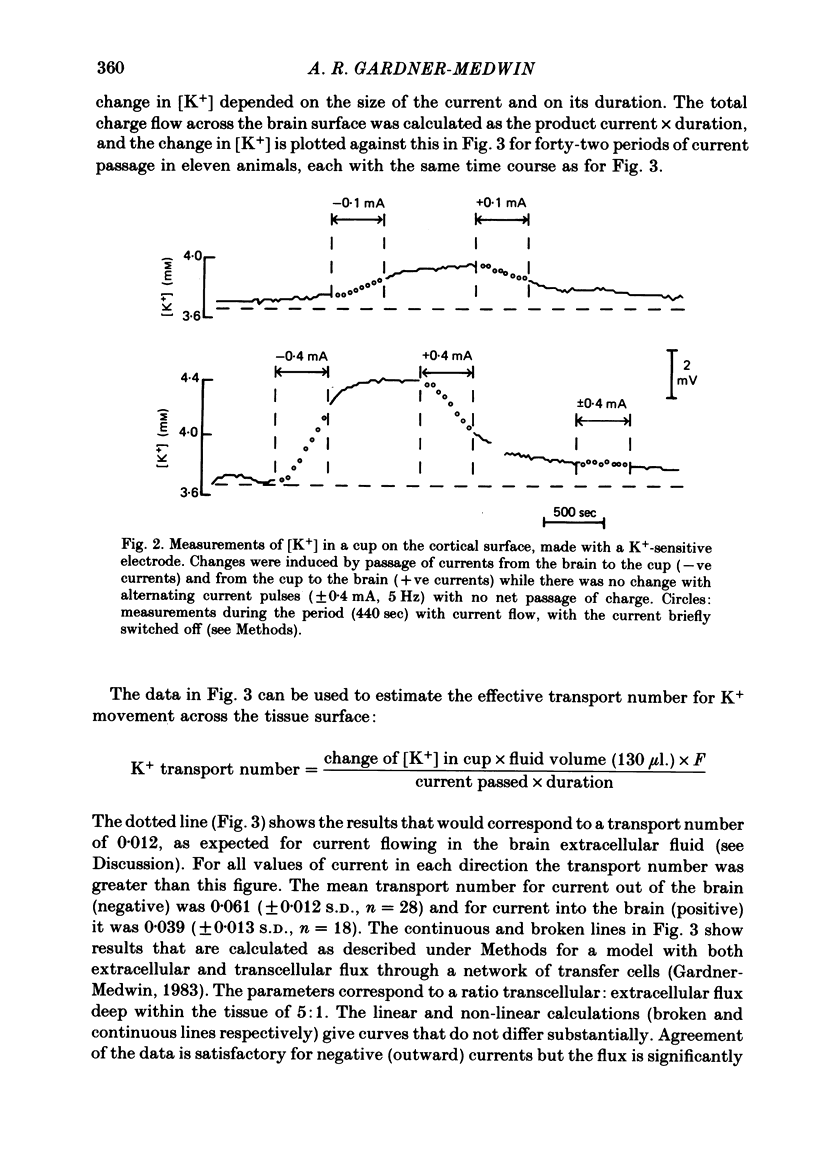
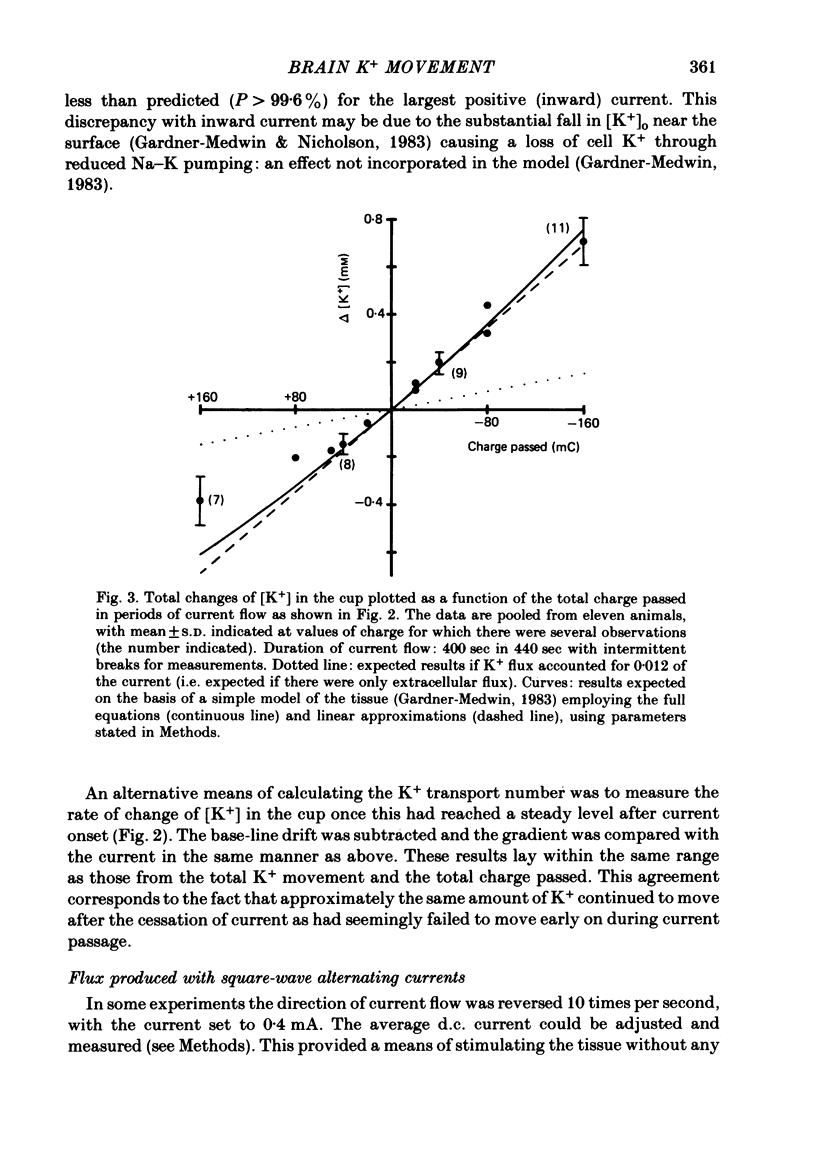
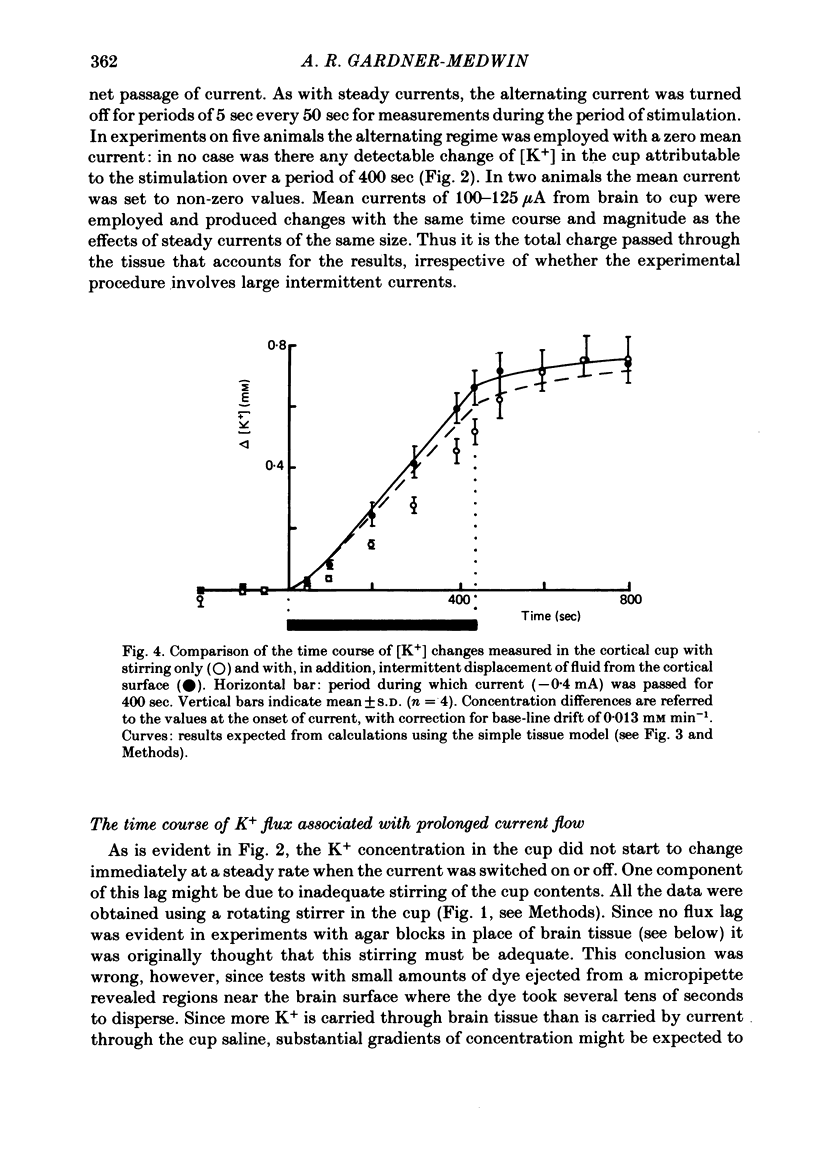
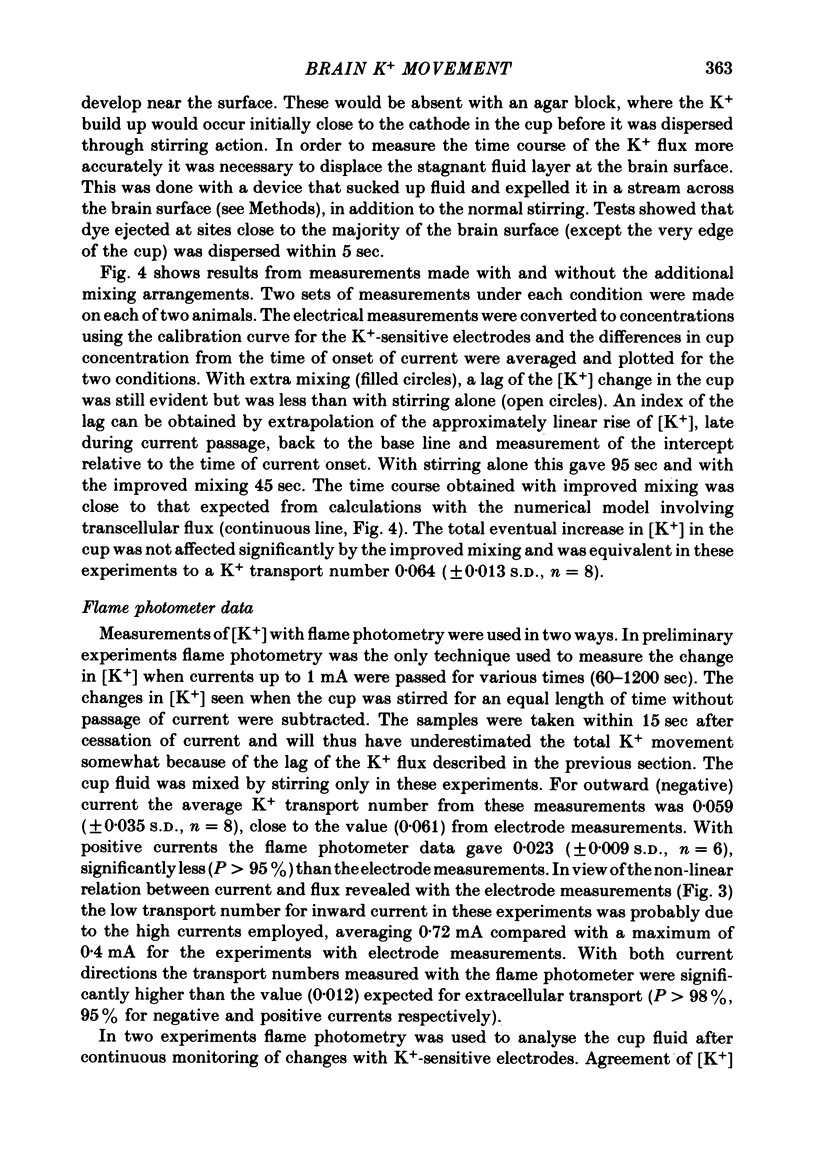
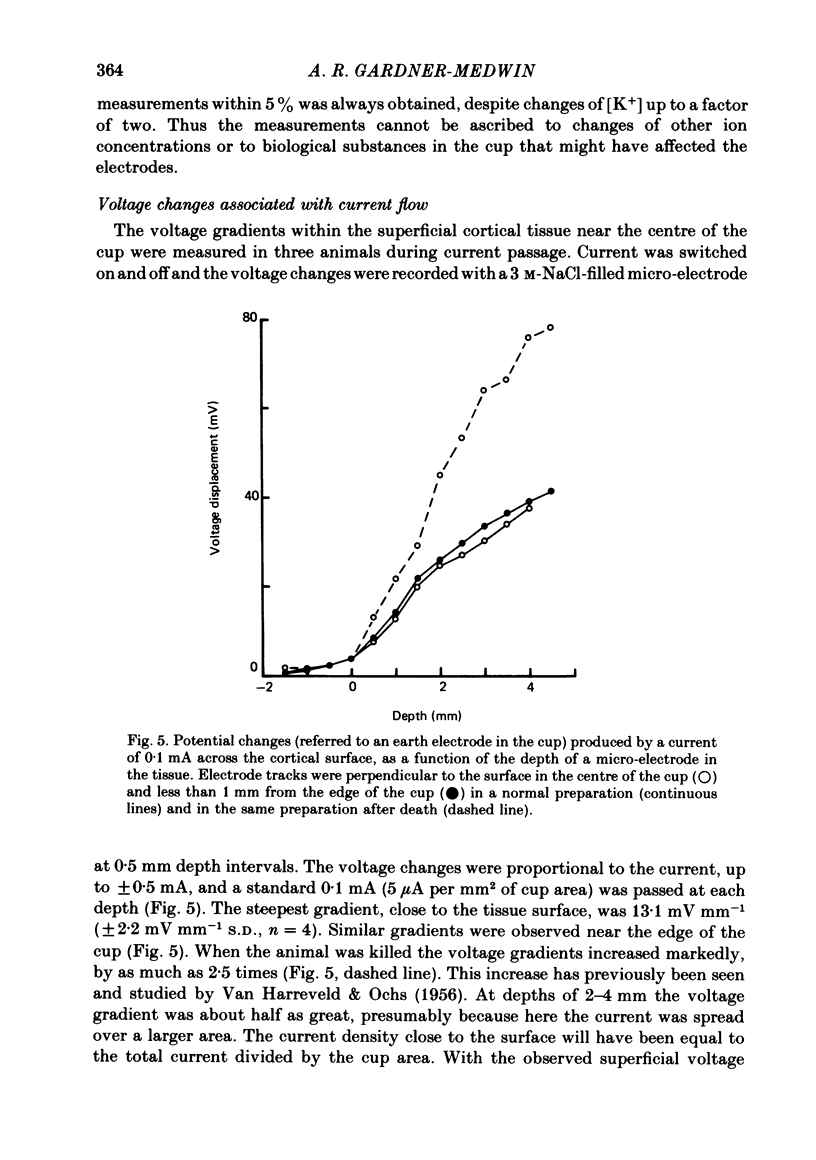
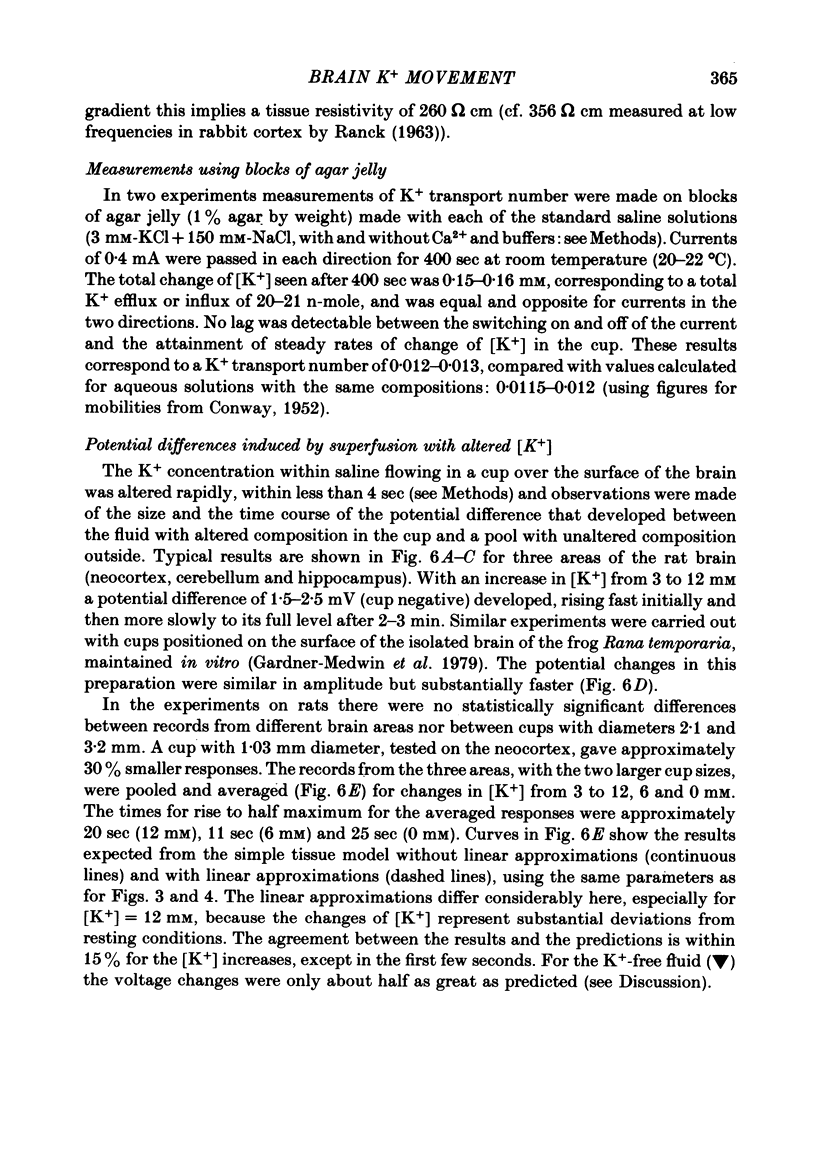
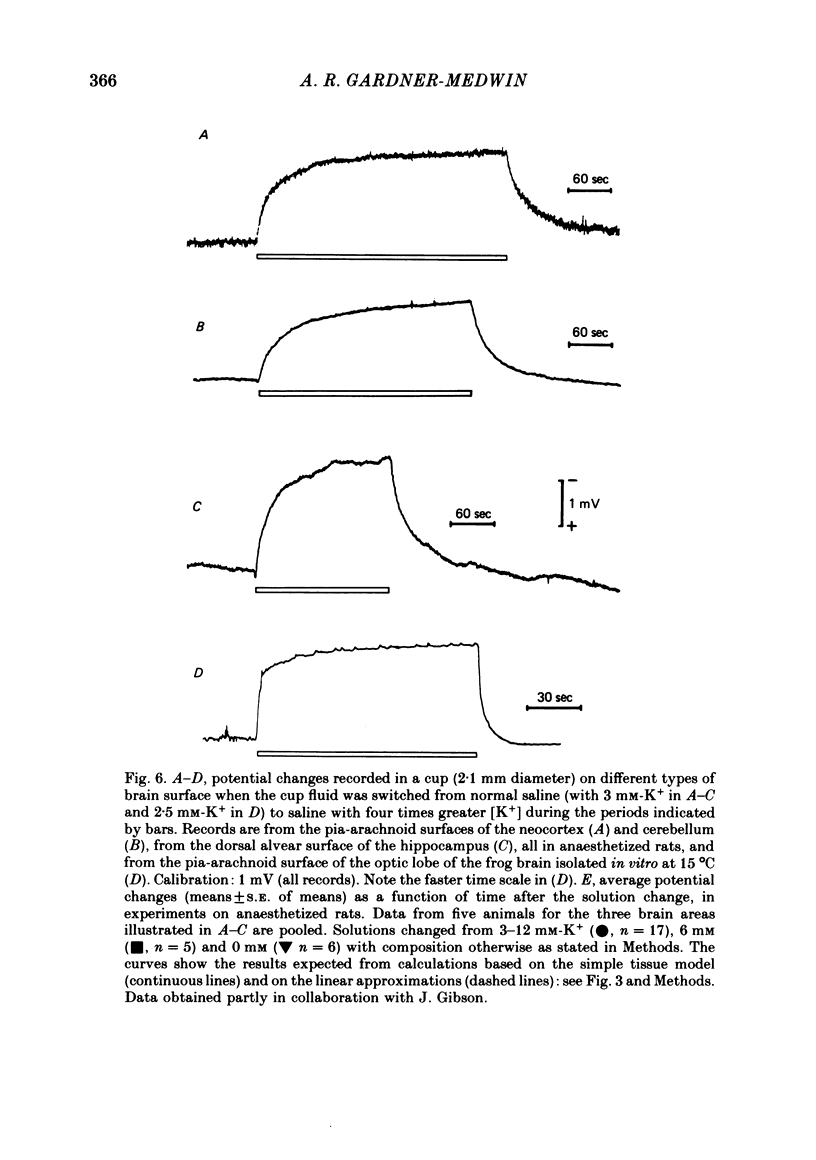
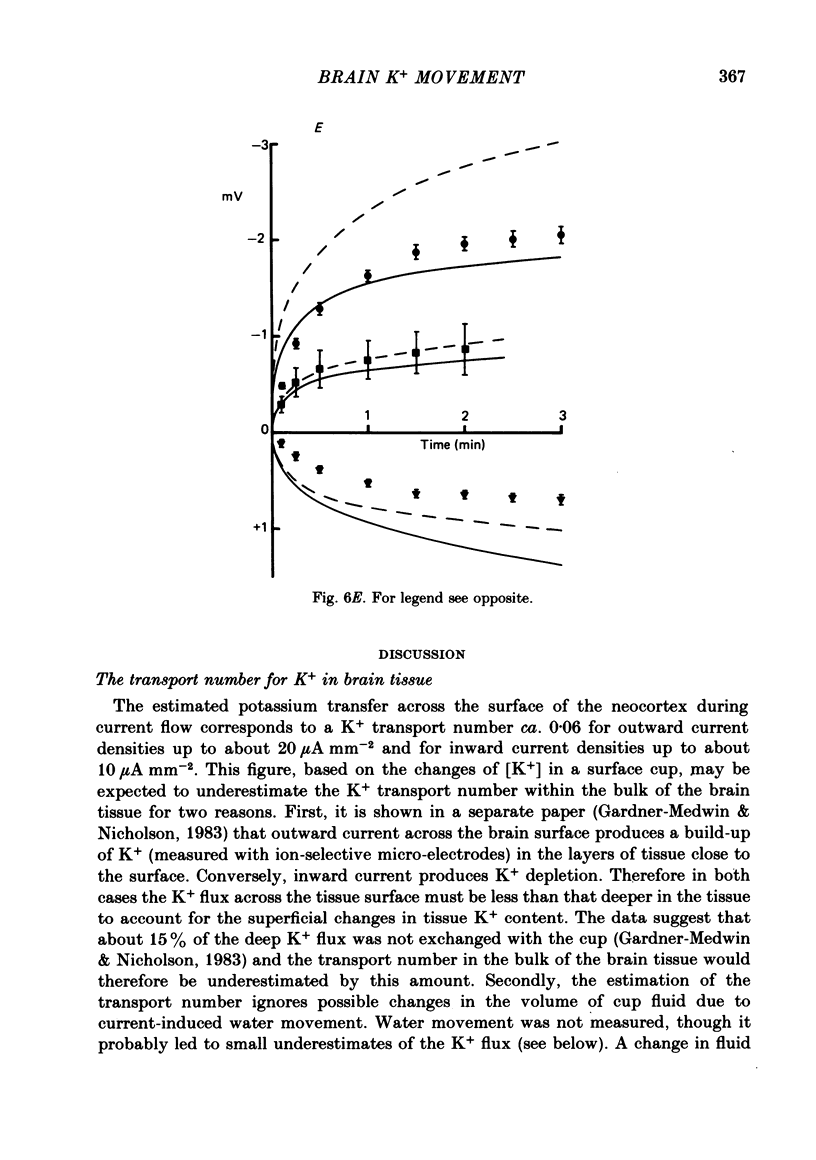
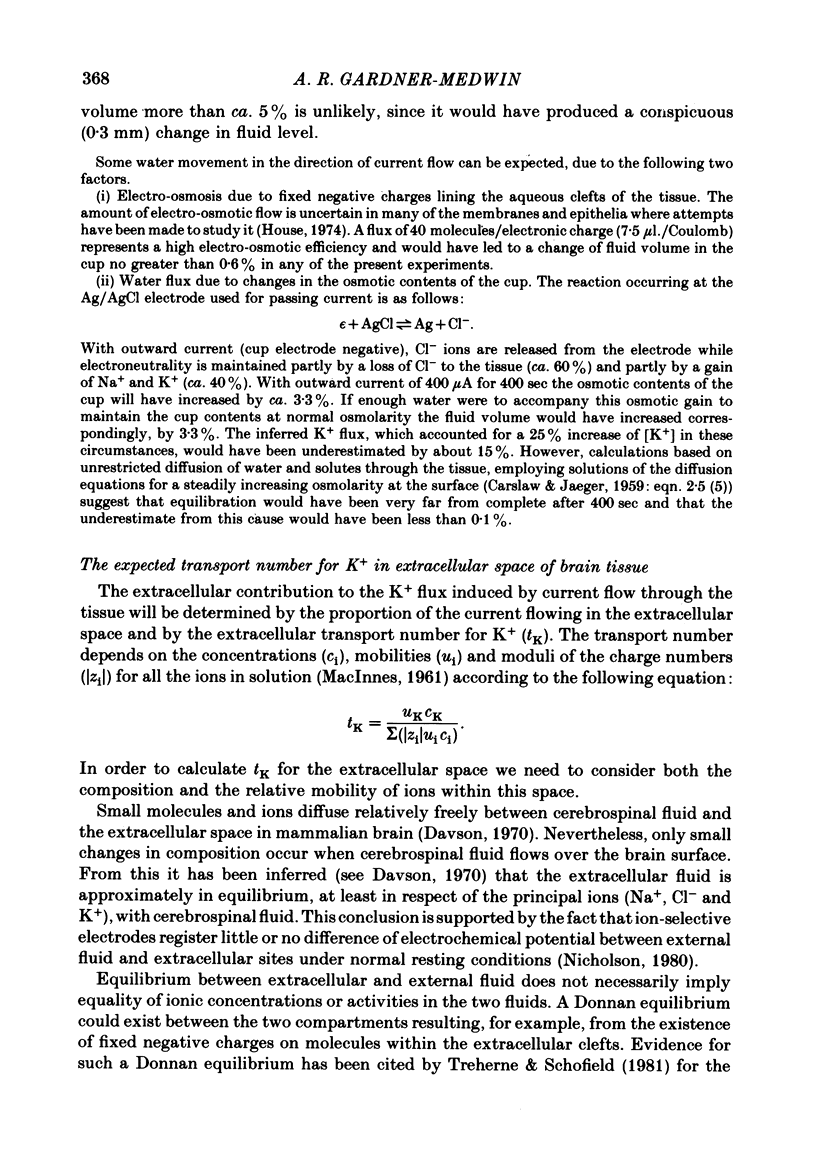
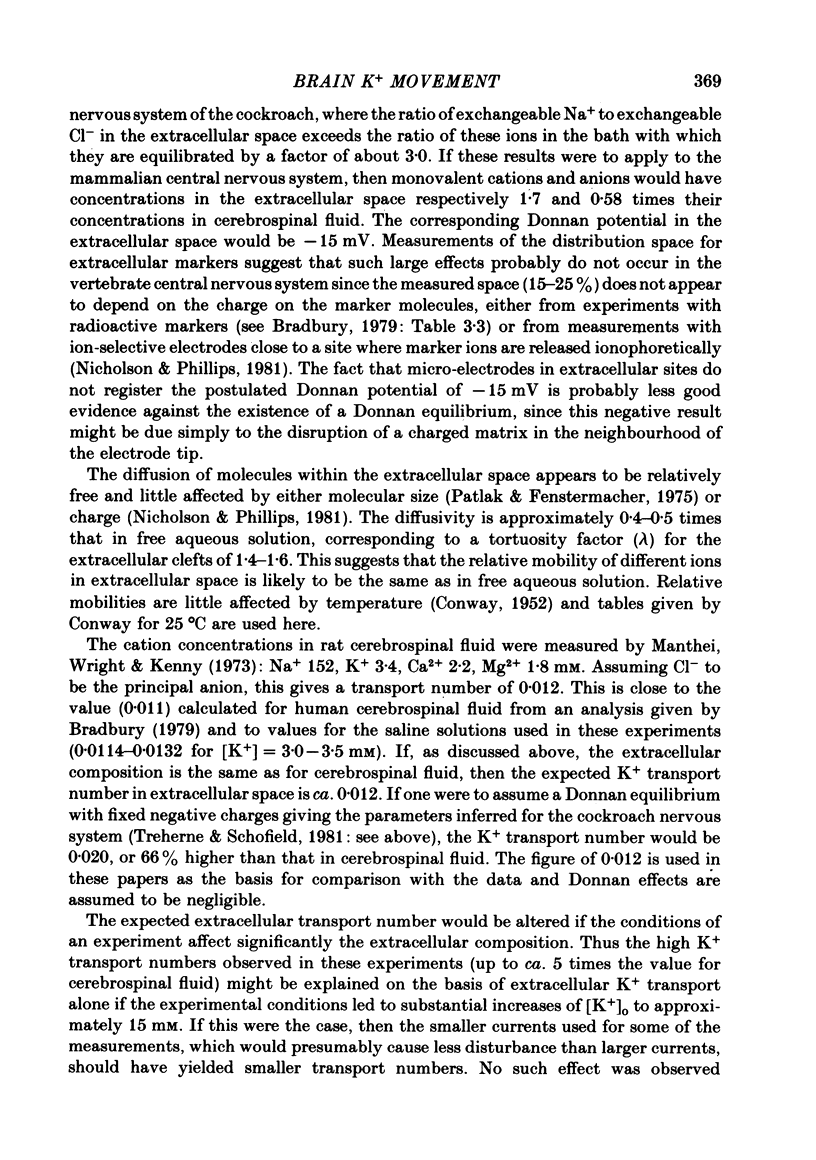
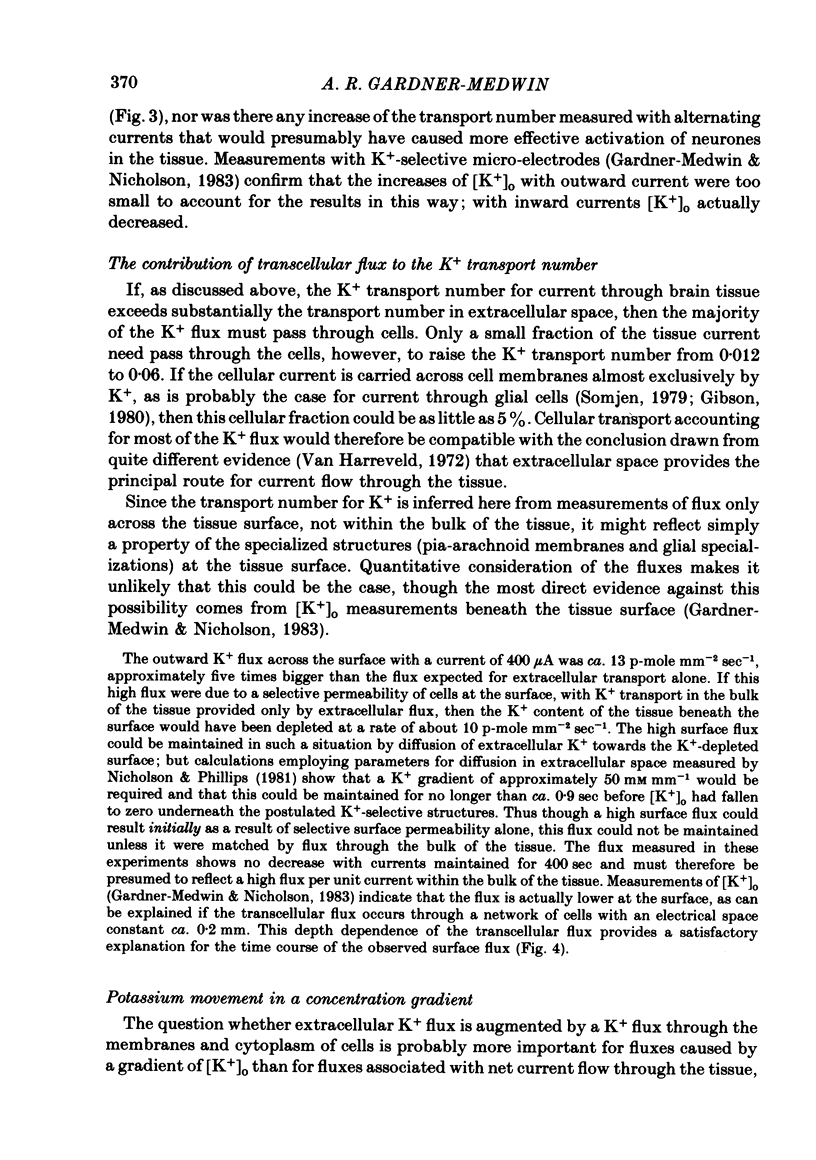
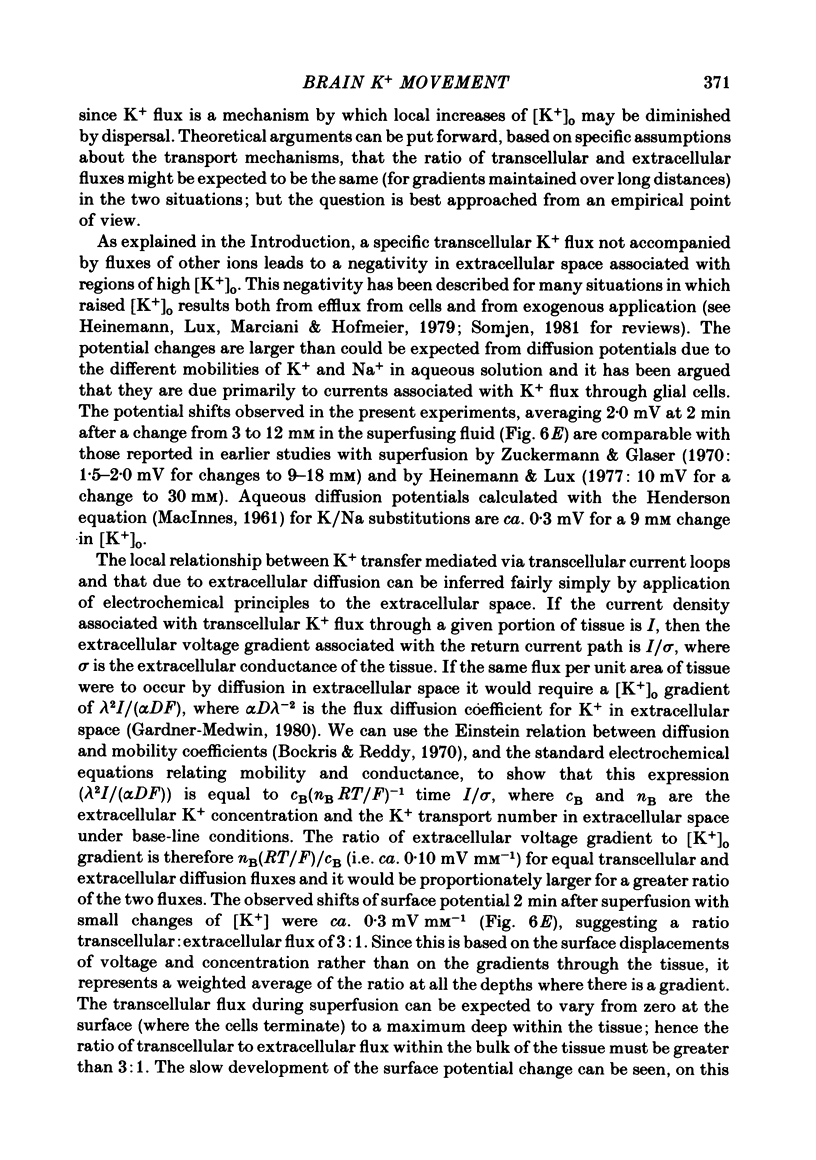
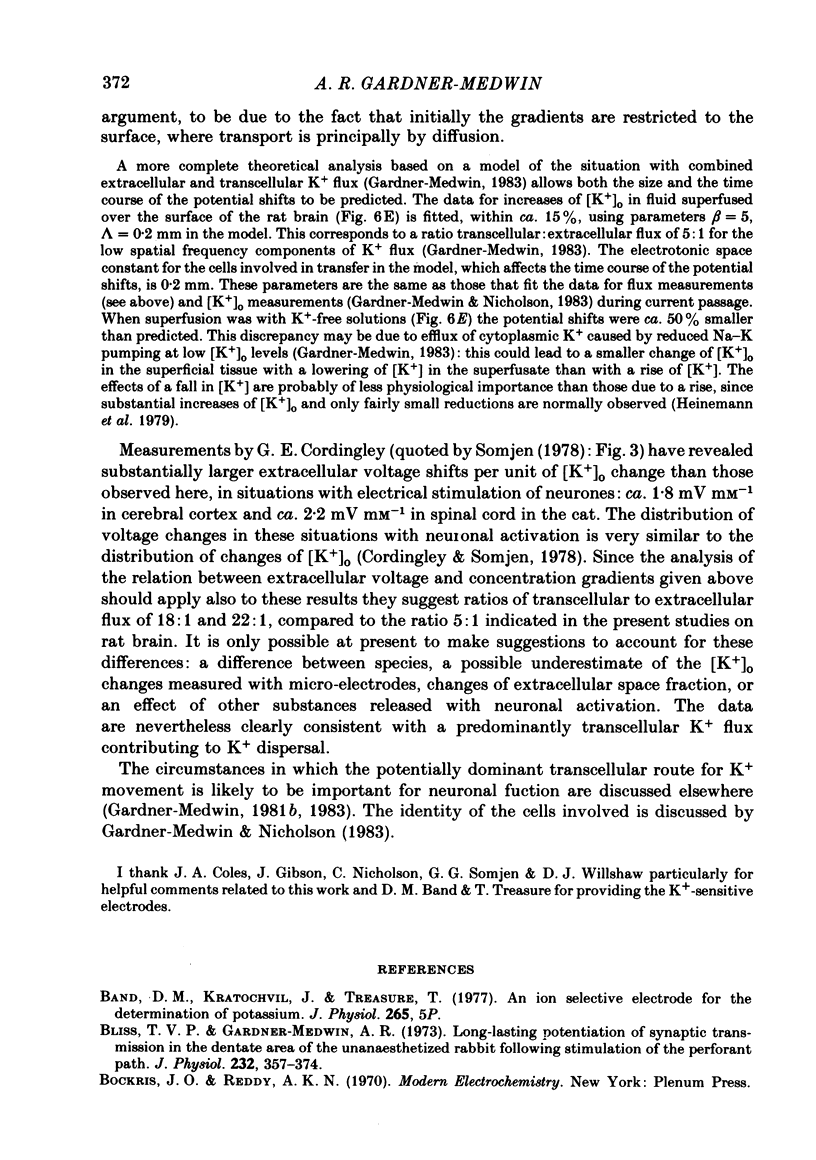
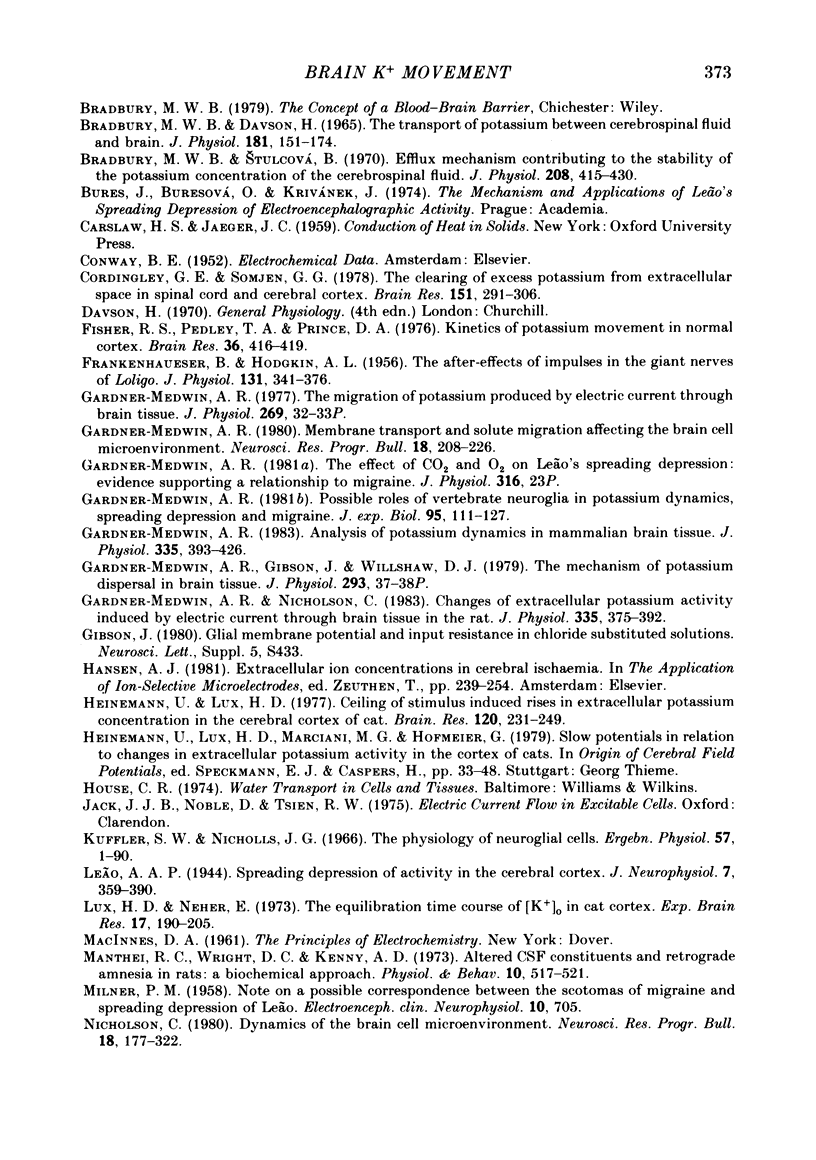
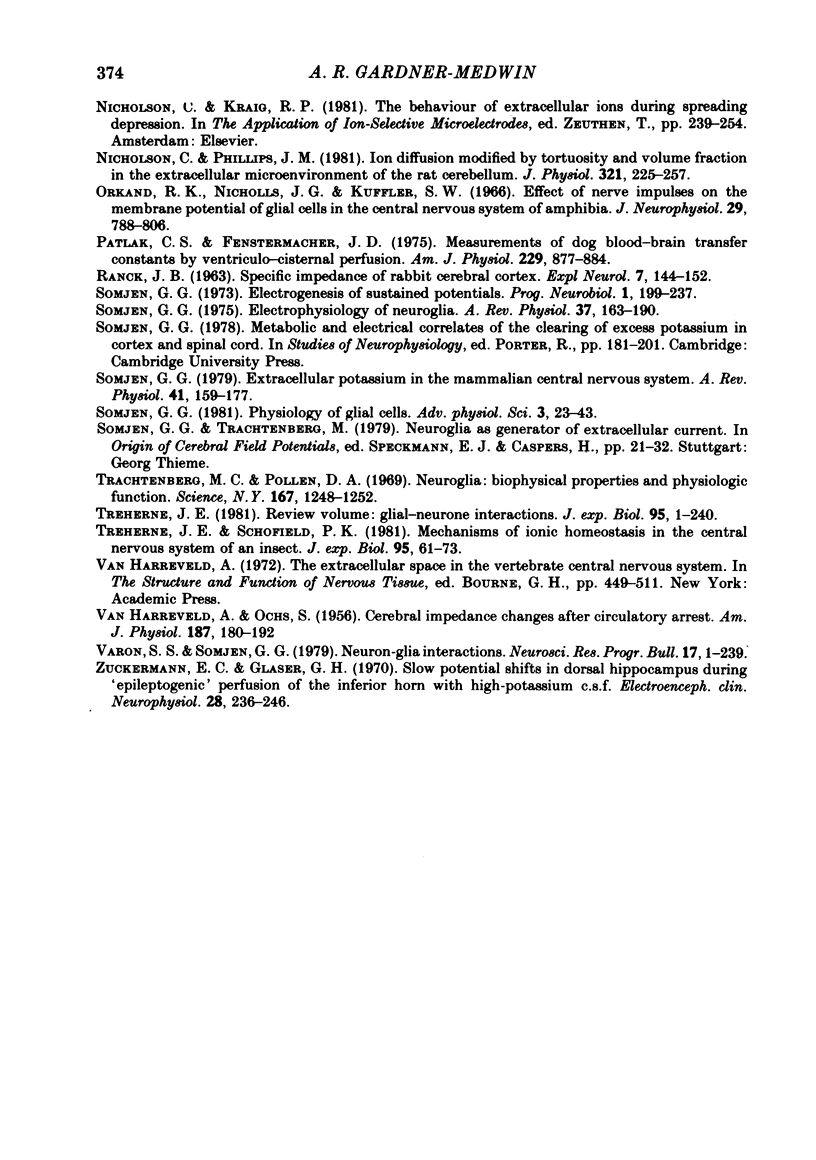
Selected References
These references are in PubMed. This may not be the complete list of references from this article.
- Band D. M., Kratochvil J., Treasure T. An ion selective electrode for the determination of potassium [proceedings]. J Physiol. 1977 Feb;265(1):5P–6P. [PubMed] [Google Scholar]
- Bliss T. V., Gardner-Medwin A. R. Long-lasting potentiation of synaptic transmission in the dentate area of the unanaestetized rabbit following stimulation of the perforant path. J Physiol. 1973 Jul;232(2):357–374. doi: 10.1113/jphysiol.1973.sp010274. [DOI] [PMC free article] [PubMed] [Google Scholar]
- Bradbury M. W., Davson H. The transport of potassium between blood, cerebrospinal fluid and brain. J Physiol. 1965 Nov;181(1):151–174. doi: 10.1113/jphysiol.1965.sp007752. [DOI] [PMC free article] [PubMed] [Google Scholar]
- Bradbury M. W., Stulcová B. Efflux mechanism contributing to the stability of the potassium concentration in cerebrospinal fluid. J Physiol. 1970 Jun;208(2):415–430. doi: 10.1113/jphysiol.1970.sp009128. [DOI] [PMC free article] [PubMed] [Google Scholar]
- Cordingley G. E., Somjen G. G. The clearing of excess potassium from extracellular space in spinal cord and cerebral cortex. Brain Res. 1978 Aug 4;151(2):291–306. doi: 10.1016/0006-8993(78)90886-7. [DOI] [PubMed] [Google Scholar]
- FRANKENHAEUSER B., HODGKIN A. L. The after-effects of impulses in the giant nerve fibres of Loligo. J Physiol. 1956 Feb 28;131(2):341–376. doi: 10.1113/jphysiol.1956.sp005467. [DOI] [PMC free article] [PubMed] [Google Scholar]
- Gardner-Medwin A. R. Analysis of potassium dynamics in mammalian brain tissue. J Physiol. 1983 Feb;335:393–426. doi: 10.1113/jphysiol.1983.sp014541. [DOI] [PMC free article] [PubMed] [Google Scholar]
- Gardner-Medwin A. R., Gibson J. L., Willshaw D. J. The mechanism of potassium dispersal in brain tissue [proceedings]. J Physiol. 1979 Aug;293:37P–38P. [PubMed] [Google Scholar]
- Gardner-Medwin A. R., Nicholson C. Changes of extracellular potassium activity induced by electric current through brain tissue in the rat. J Physiol. 1983 Feb;335:375–392. doi: 10.1113/jphysiol.1983.sp014540. [DOI] [PMC free article] [PubMed] [Google Scholar]
- Gardner-Medwin A. R. Possible roles of vertebrate neuroglia in potassium dynamics, spreading depression and migraine. J Exp Biol. 1981 Dec;95:111–127. doi: 10.1242/jeb.95.1.111. [DOI] [PubMed] [Google Scholar]
- Gardner-Medwin A. R. The migration of potassium produced by electric current through brain tissue [proceedings]. J Physiol. 1977 Jul;269(1):32P–33P. [PubMed] [Google Scholar]
- Glial-neurone interactions. J Exp Biol. 1981 Dec;95:1–240. doi: 10.1242/jeb.95.1.1. [DOI] [PubMed] [Google Scholar]
- Heinemann U., Lux H. D. Ceiling of stimulus induced rises in extracellular potassium concentration in the cerebral cortex of cat. Brain Res. 1977 Jan 21;120(2):231–249. doi: 10.1016/0006-8993(77)90903-9. [DOI] [PubMed] [Google Scholar]
- Kuffler S. W., Nicholls J. G. The physiology of neuroglial cells. Ergeb Physiol. 1966;57:1–90. [PubMed] [Google Scholar]
- Lux H. D., Neher E. The equilibration time course of (K + ) 0 in cat cortex. Exp Brain Res. 1973 Apr 30;17(2):190–205. doi: 10.1007/BF00235028. [DOI] [PubMed] [Google Scholar]
- MILNER P. M. Note on a possible correspondence between the scotomas of migraine and spreading depression of Leão. Electroencephalogr Clin Neurophysiol. 1958 Nov;10(4):705–705. doi: 10.1016/0013-4694(58)90073-7. [DOI] [PubMed] [Google Scholar]
- Manthei R. C., Wricht D. C., Kenny A. D. Altered CSF constituents and retrograde amnesia in rats: a biochemical approach. Physiol Behav. 1973 Mar;10(3):517–521. doi: 10.1016/0031-9384(73)90214-x. [DOI] [PubMed] [Google Scholar]
- Nicholson C. Dynamics of the brain cell microenvironment. Neurosci Res Program Bull. 1980 Apr;18(2):175–322. [PubMed] [Google Scholar]
- Nicholson C., Phillips J. M. Ion diffusion modified by tortuosity and volume fraction in the extracellular microenvironment of the rat cerebellum. J Physiol. 1981 Dec;321:225–257. doi: 10.1113/jphysiol.1981.sp013981. [DOI] [PMC free article] [PubMed] [Google Scholar]
- OCHS S., VAN HARREVELD A. Cerebral impedance changes after circulatory arrest. Am J Physiol. 1956 Sep;187(1):180–192. doi: 10.1152/ajplegacy.1956.187.1.180. [DOI] [PubMed] [Google Scholar]
- Orkand R. K., Nicholls J. G., Kuffler S. W. Effect of nerve impulses on the membrane potential of glial cells in the central nervous system of amphibia. J Neurophysiol. 1966 Jul;29(4):788–806. doi: 10.1152/jn.1966.29.4.788. [DOI] [PubMed] [Google Scholar]
- Patlak C. S., Fenstermacher J. D. Measurements of dog blood-brain transfer constants by ventriculocisternal perfusion. Am J Physiol. 1975 Oct;229(4):877–884. doi: 10.1152/ajplegacy.1975.229.4.877. [DOI] [PubMed] [Google Scholar]
- RANCK J. B., Jr Specific impedance of rabbit cerebral cortex. Exp Neurol. 1963 Feb;7:144–152. doi: 10.1016/s0014-4886(63)80005-9. [DOI] [PubMed] [Google Scholar]
- Somjen G. G. Electrogenesis of sustained potentials. Prog Neurobiol. 1973;1(3):201–237. [PubMed] [Google Scholar]
- Somjen G. G. Electrophysiology of neuroglia. Annu Rev Physiol. 1975;37:163–190. doi: 10.1146/annurev.ph.37.030175.001115. [DOI] [PubMed] [Google Scholar]
- Somjen G. G. Extracellular potassium in the mammalian central nervous system. Annu Rev Physiol. 1979;41:159–177. doi: 10.1146/annurev.ph.41.030179.001111. [DOI] [PubMed] [Google Scholar]
- Trachtenberg M. C., Pollen D. A. Neuroglia: biophysical properties and physiologic function. Science. 1970 Feb 27;167(3922):1248–1252. doi: 10.1126/science.167.3922.1248. [DOI] [PubMed] [Google Scholar]
- Treherne J. E., Schofield P. K. Mechanisms of ionic homeostasis in the central nervous system of an insect. J Exp Biol. 1981 Dec;95:61–73. doi: 10.1242/jeb.95.1.61. [DOI] [PubMed] [Google Scholar]
- Varon S. S., Somjen G. G. Neuron-glia interactions. Neurosci Res Program Bull. 1979 Feb;17(1):1–239. [PubMed] [Google Scholar]
- Zuckermann E. C., Glaser G. H. Slow potential shifts in dorsal hippocampus during "epileptogenic" perfusion of the inferior horn with high-potassium CSF. Electroencephalogr Clin Neurophysiol. 1970 Mar;28(3):236–246. doi: 10.1016/0013-4694(70)90159-8. [DOI] [PubMed] [Google Scholar]