Abstract
Ion-selective micro-electrodes have been used to measure K+ and Ca2+ activity changes in extracellular space beneath the surface of the neocortex and cerebellar cortex during current flow across the tissue surface in anaesthetized rats. Inward currents produced decreases of [K+]o and outward currents produced increases, with insignificant changes in [Ca2+]o. Changes of [K+]o were largest just under the surface of the tissue, but were detectable down to depths of ca. 1 mm. With appropriate sitting of electrodes in the cerebellar cortex, currents of 22 microA mm-2 for 400 sec produced changes averaging -42% for inward current and +66% for outward current. The [K+]o changes near the surface were most rapid immediately after the onset of current and more gradual after some tens of seconds. Deeper within the tissue the rate of change was more uniform and after the end of stimulation the return to base line was slower. The amplitude, depth dependence and time course of the [K+]o changes were in reasonable agreement with the results calculated for a model in which K+ moves partly through extracellular space but primarily through membranes and cytoplasm within the tissue. The [K+]o changes were not attributable to variations in neuronal activity, although unit activity could be modified by current, since alternating currents failed to produce [K+]o changes and neither 0.1 mM-tetrodotoxin nor 5 mM-Mn2+ abolished the changes. The [K+]o changes were not abolished by topically applied ouabain (4 X 10(-4) M), 2,4-dinitrophenol (20 mM) or iodoacetate (10 mM), or by asphyxiation. Consequently the [K+]o changes are not dependent on metabolism. The data suggest that there is a selective mechanism for passive K+ transport in an electrochemical gradient within brain tissue that results in higher K+ fluxes than could be supported by ionic mobility in the extracellular fluid. This mechanism exists not only at the surface but within the brain parenchyma and may involve current flow through glial cells.
Full text
PDF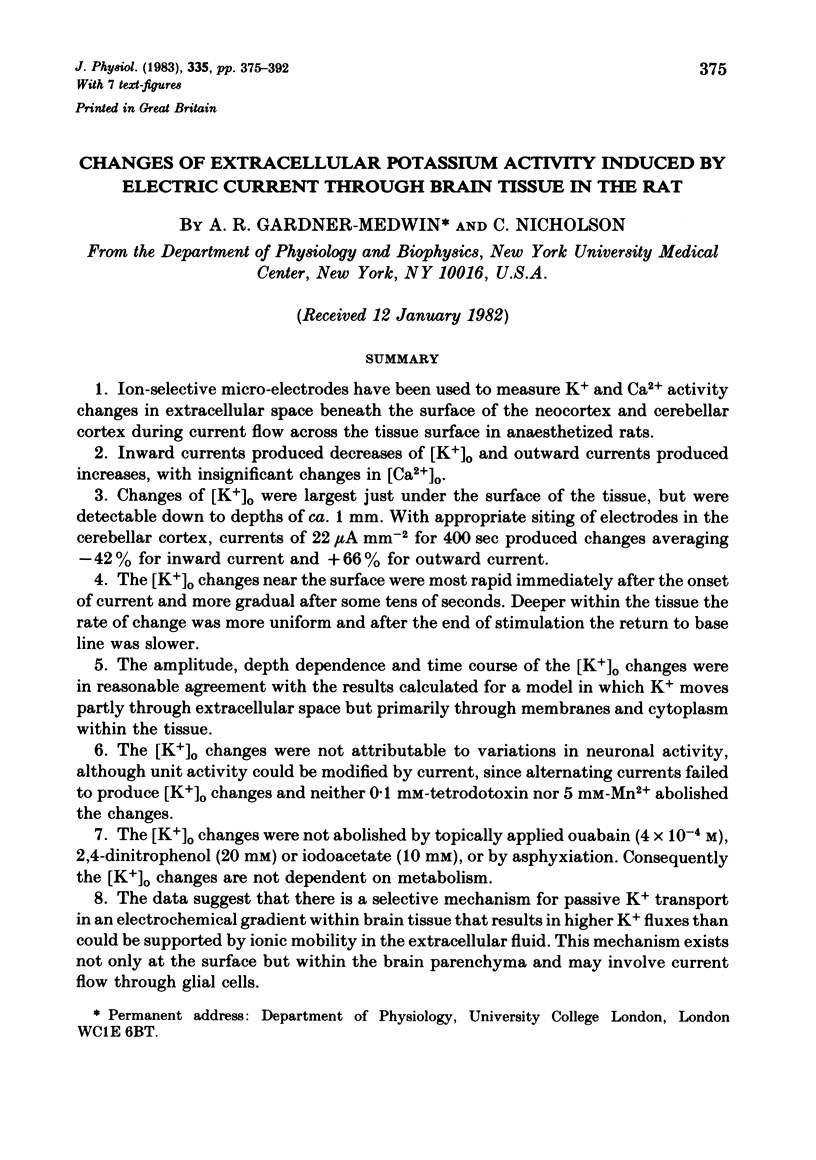
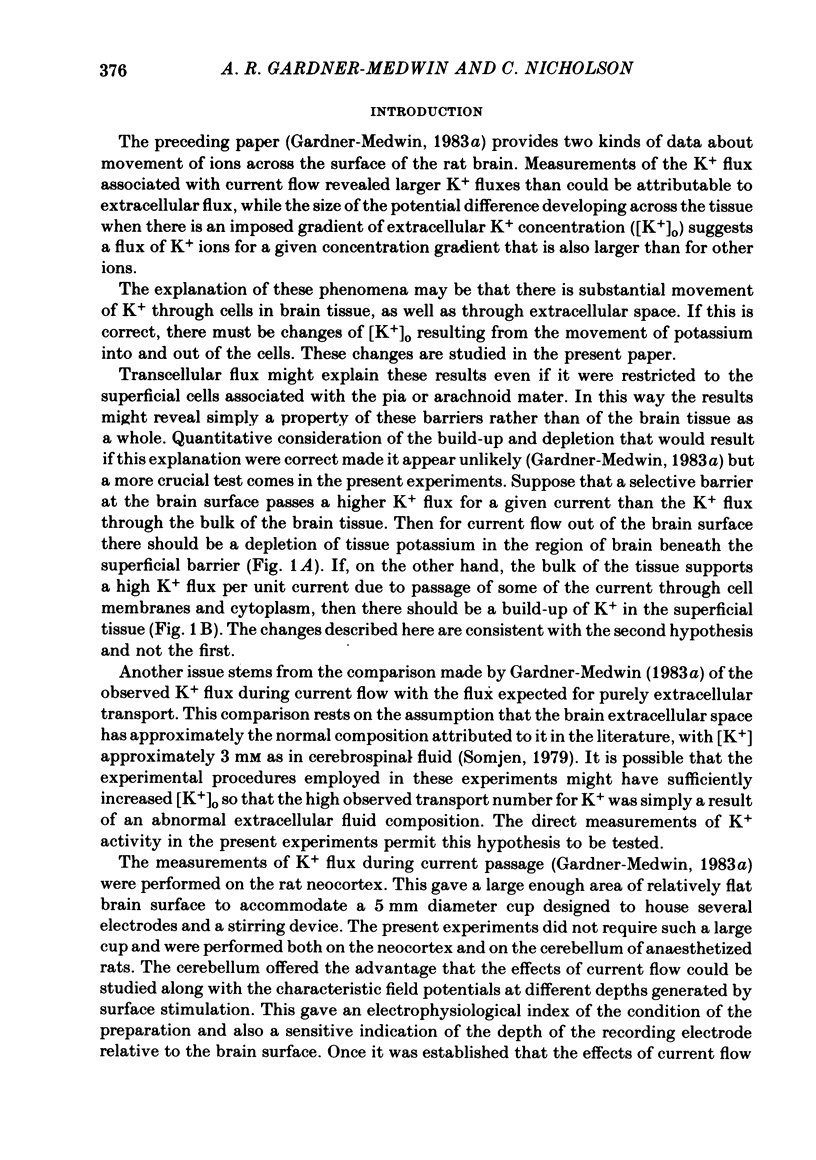
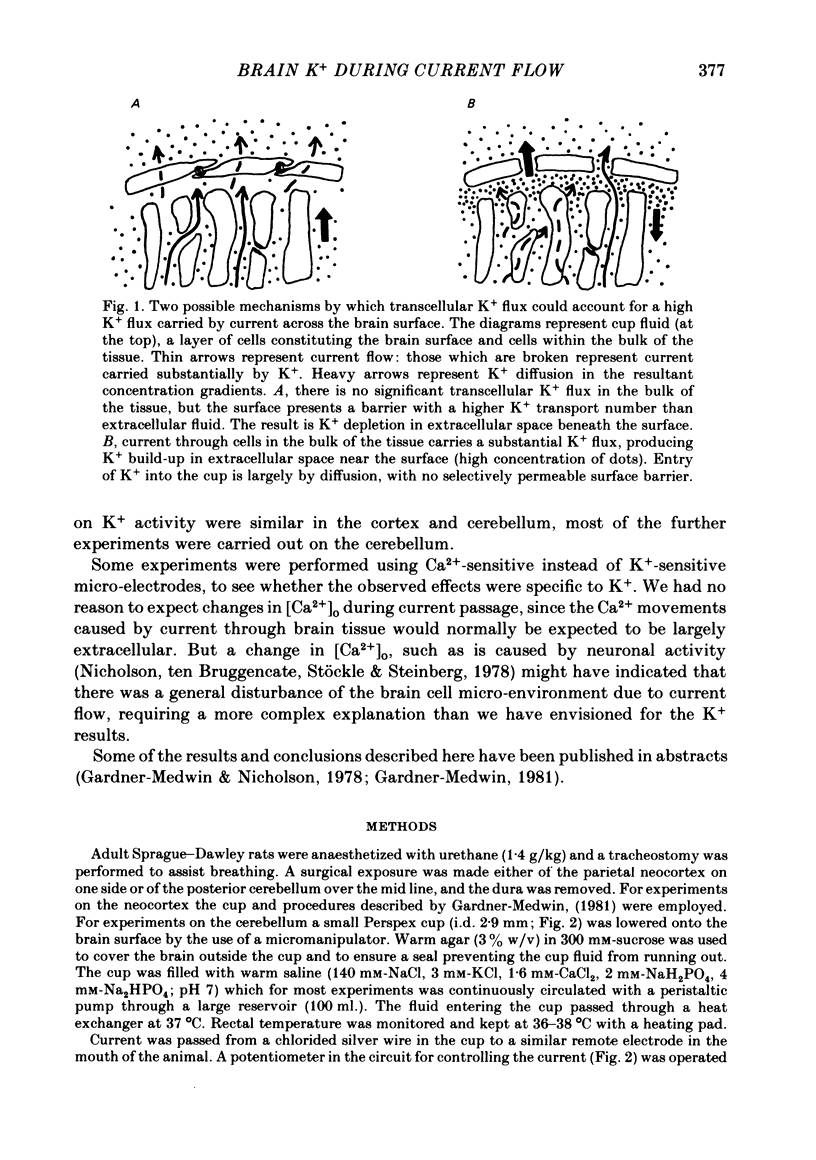
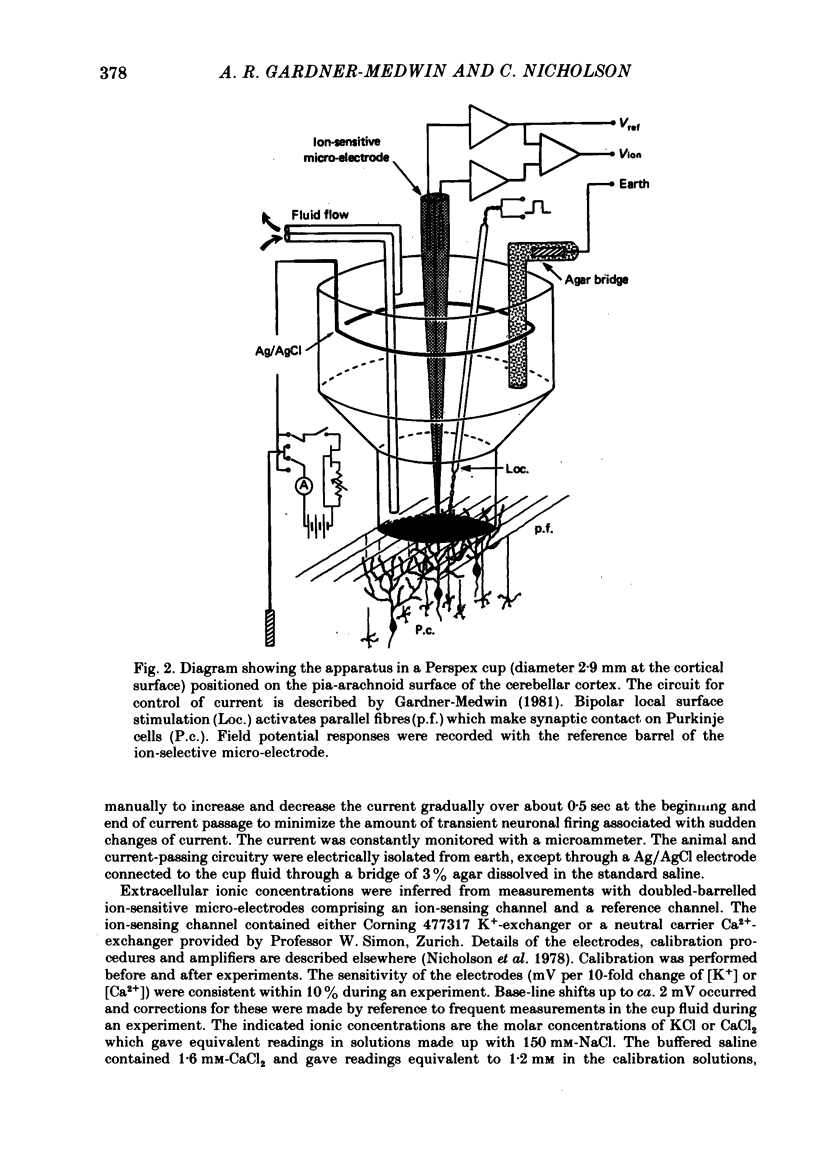
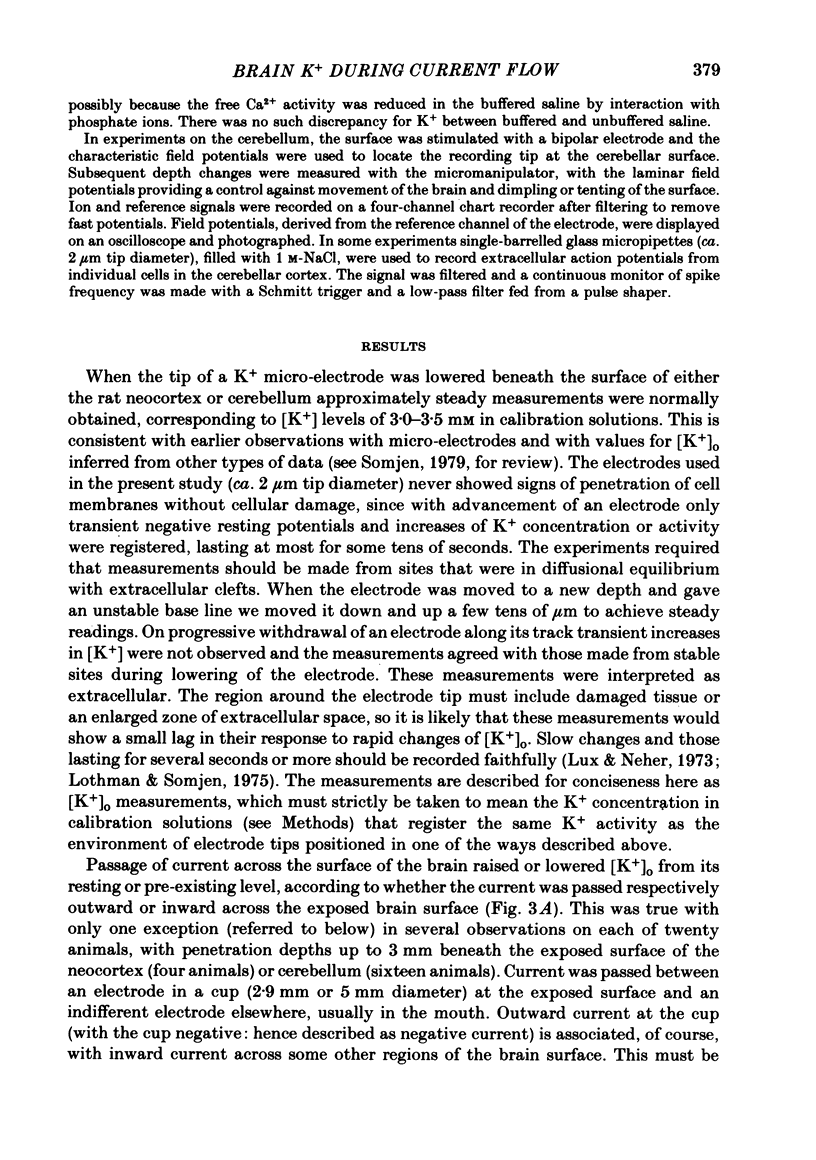
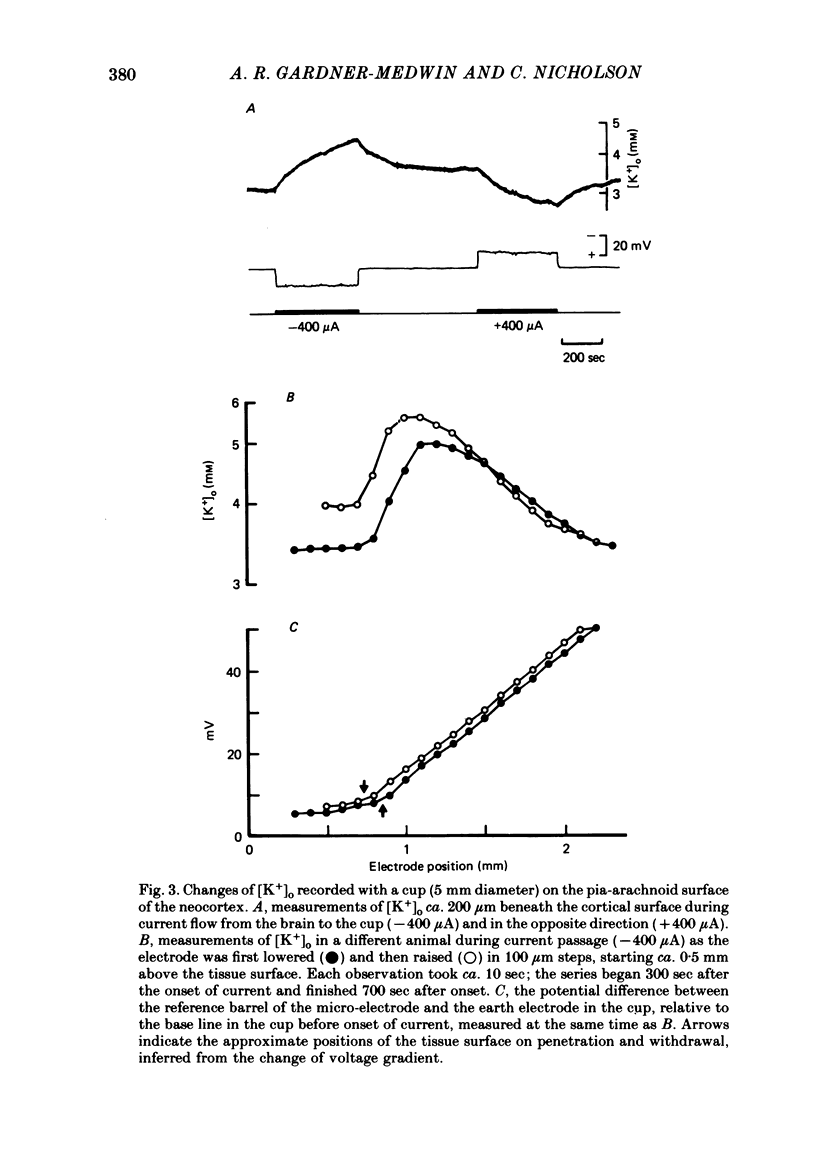
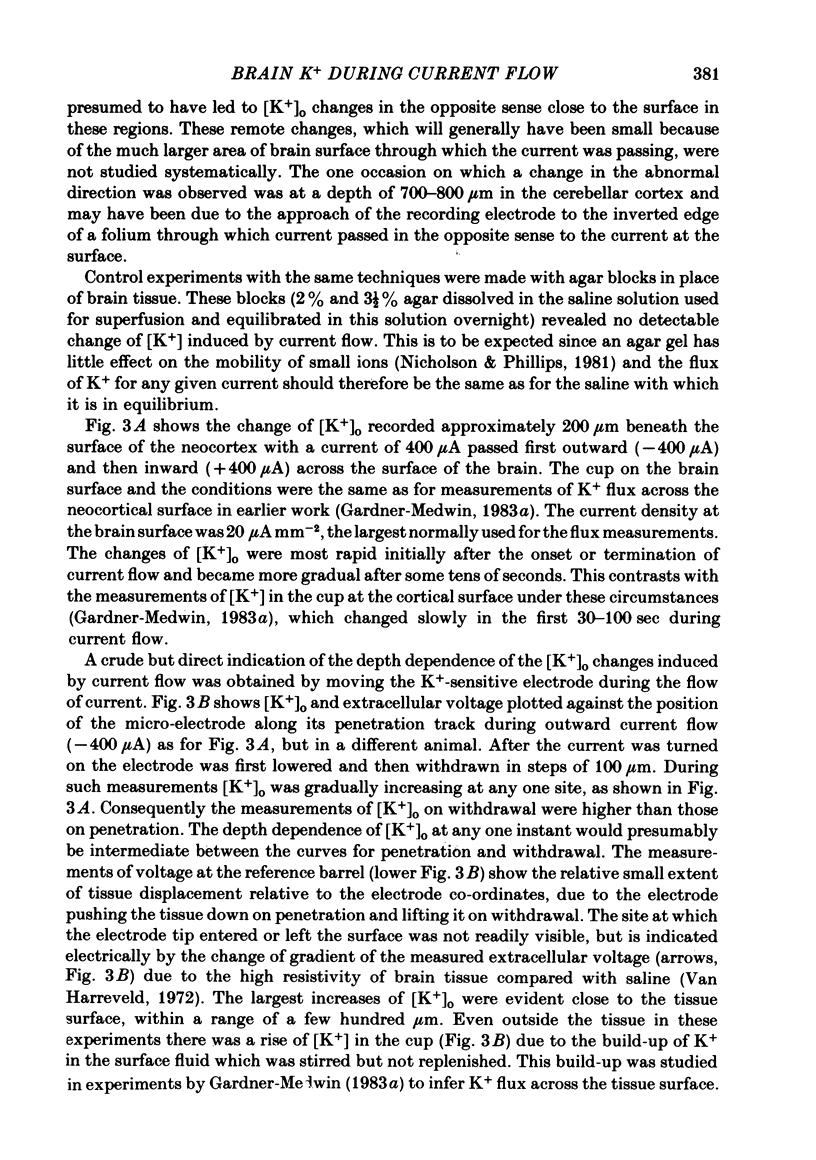
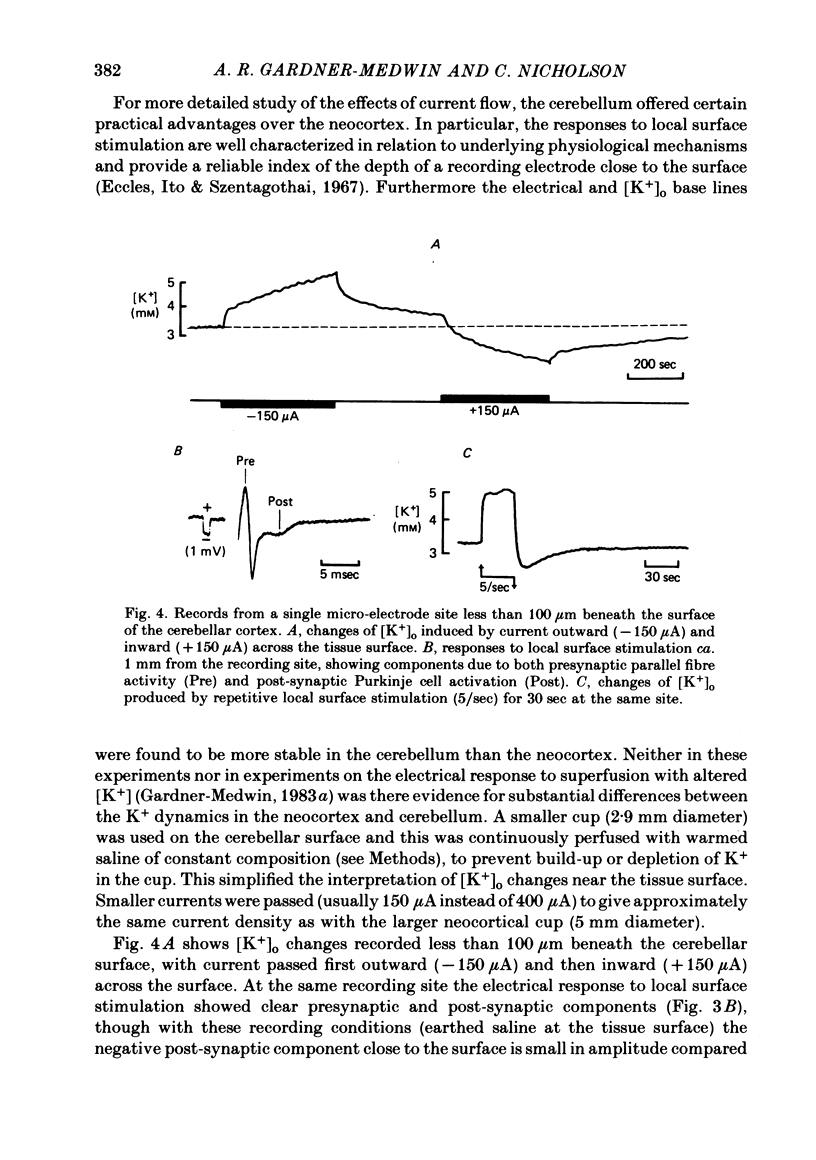
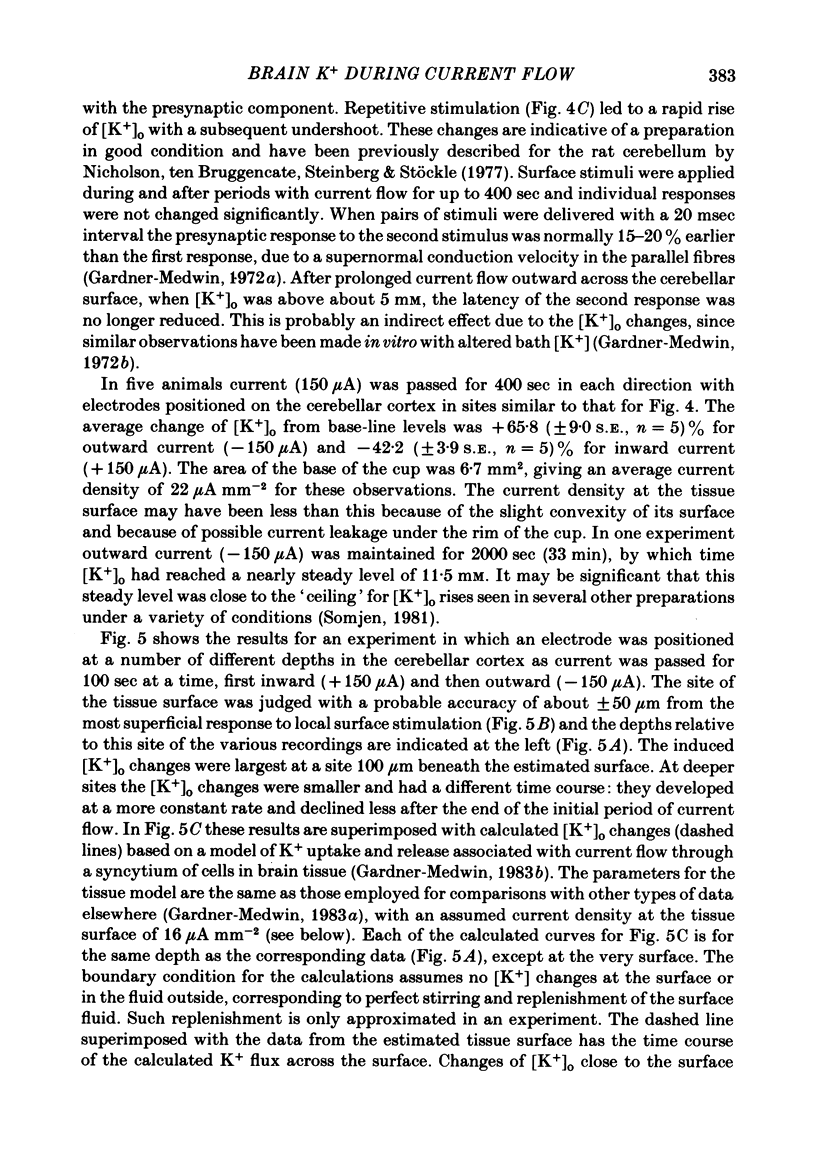
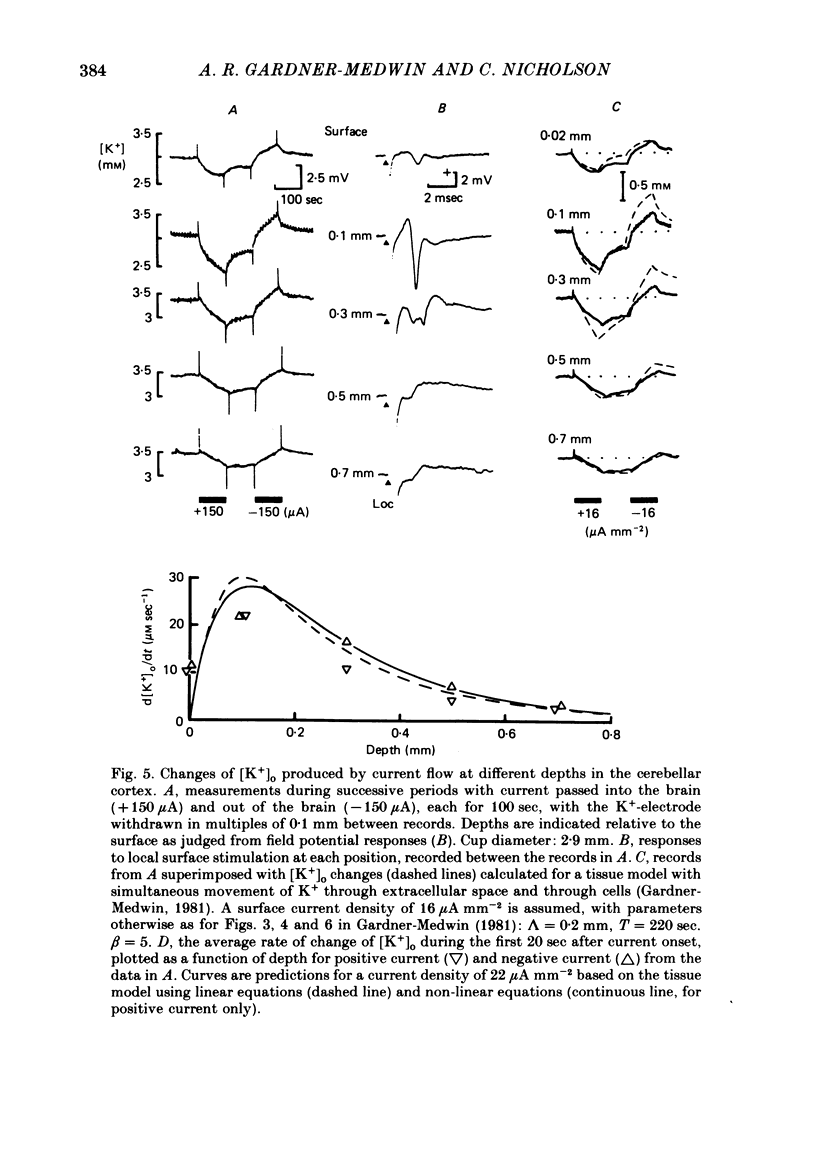
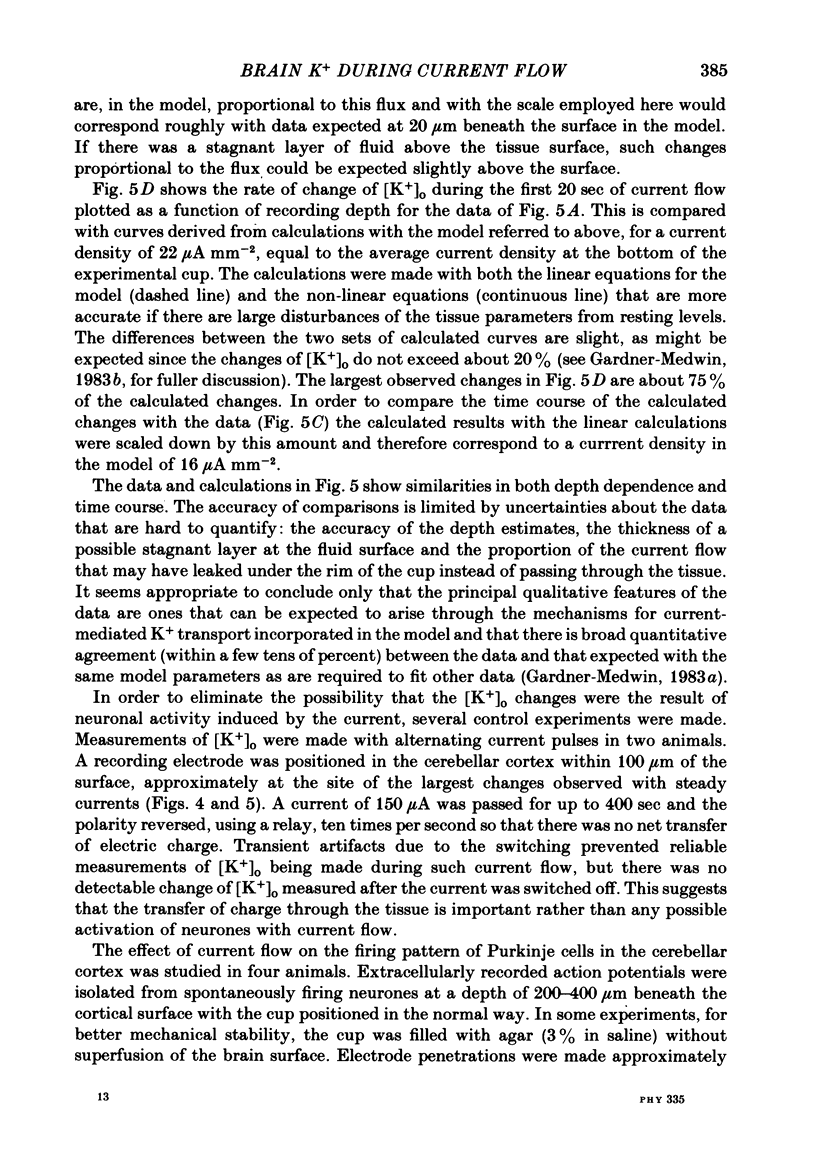

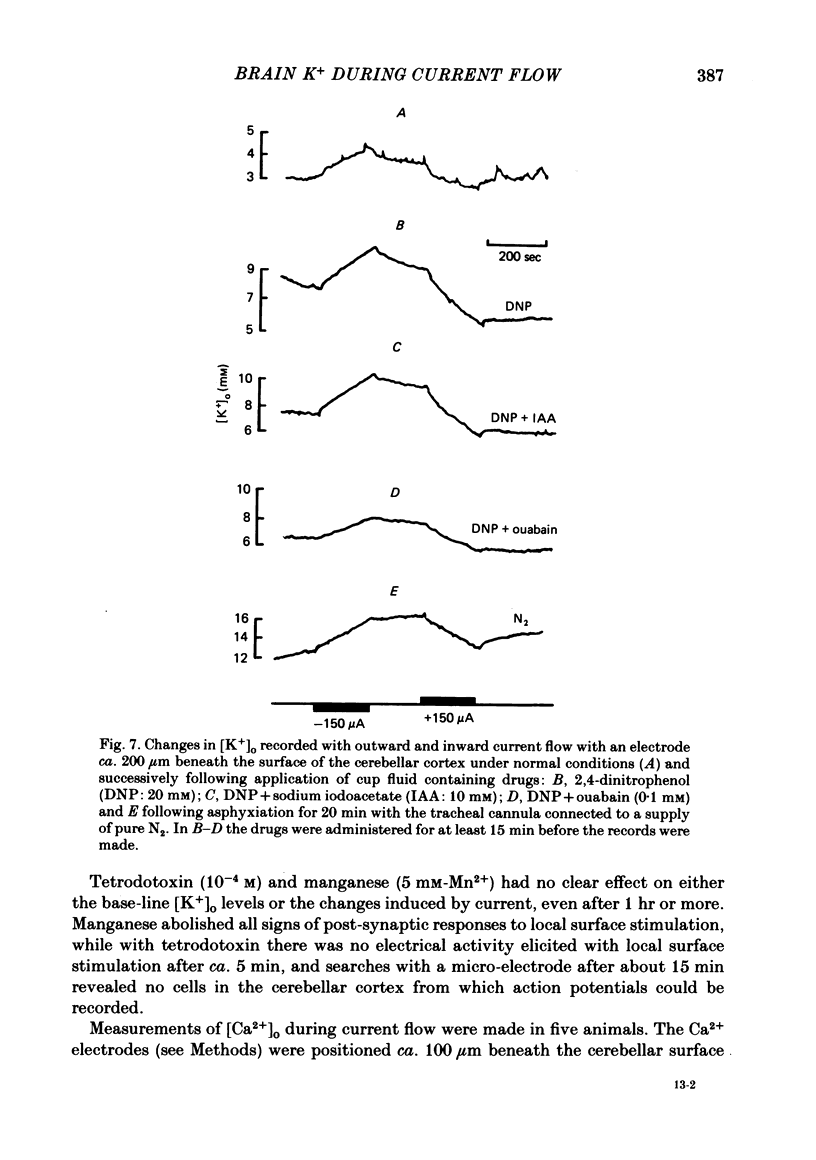
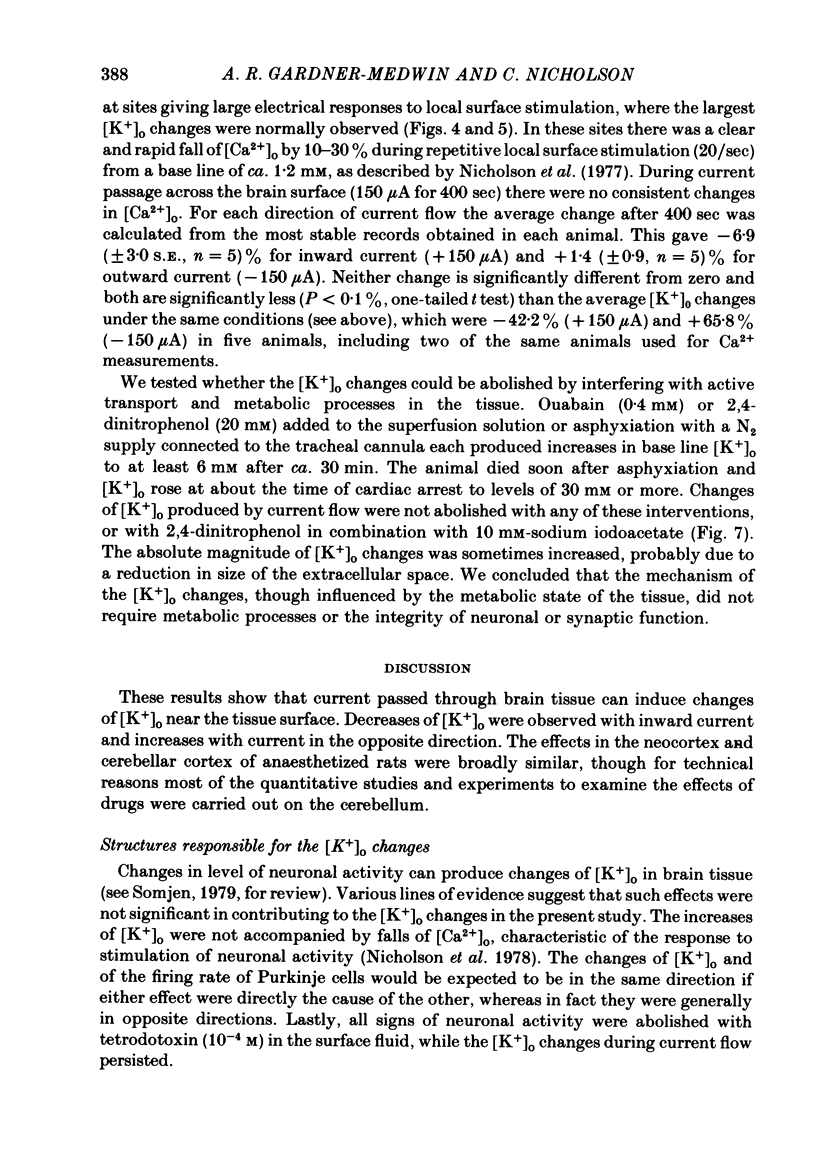
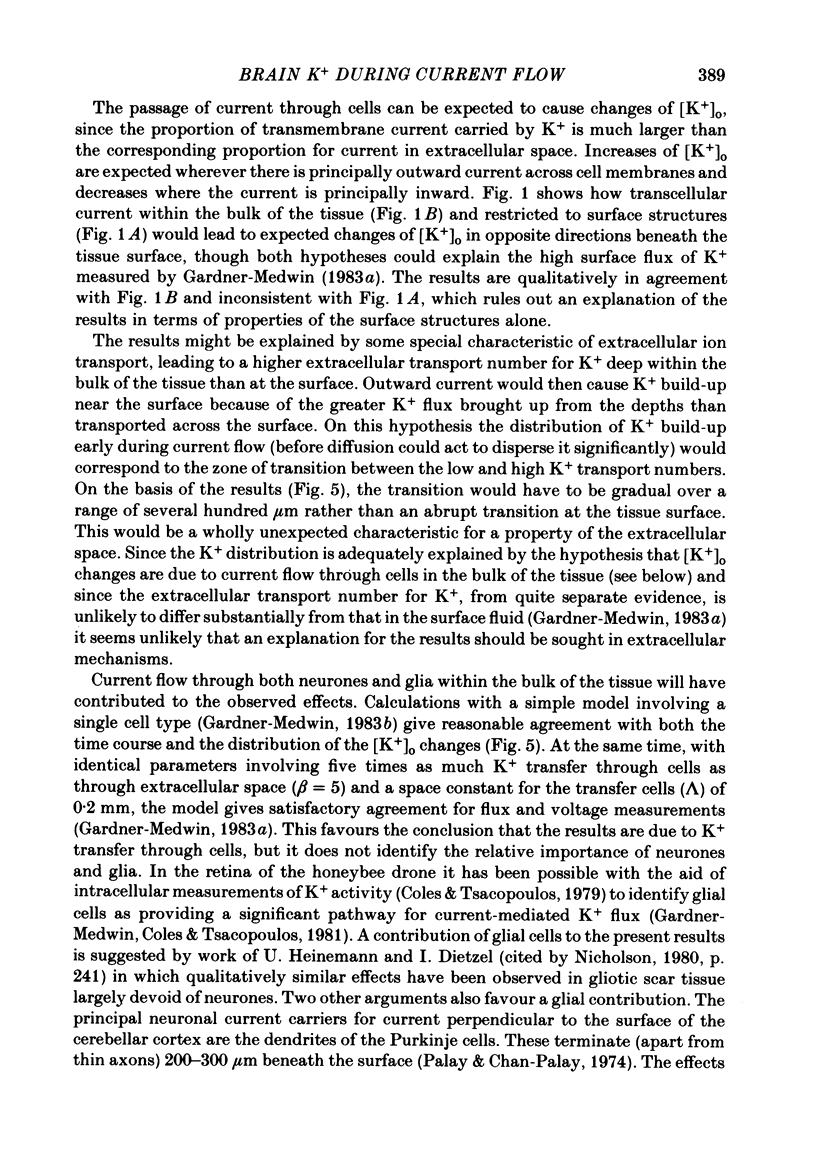
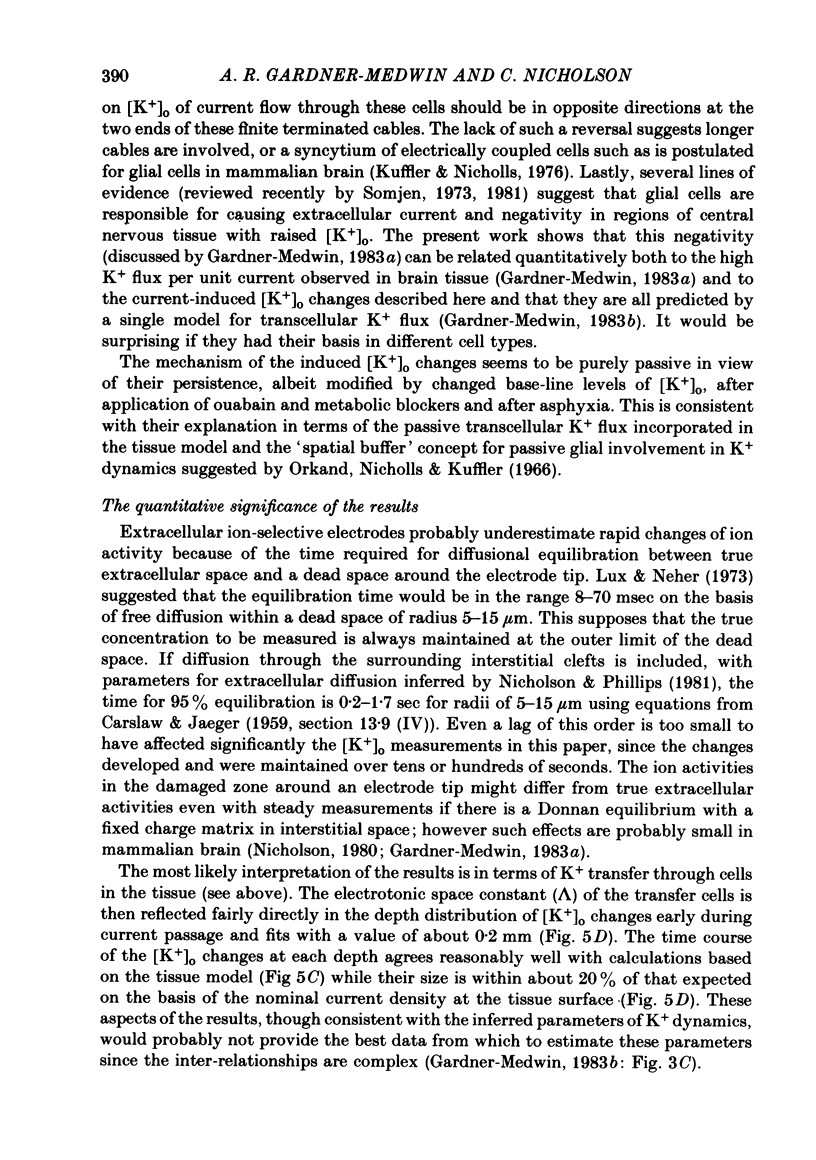
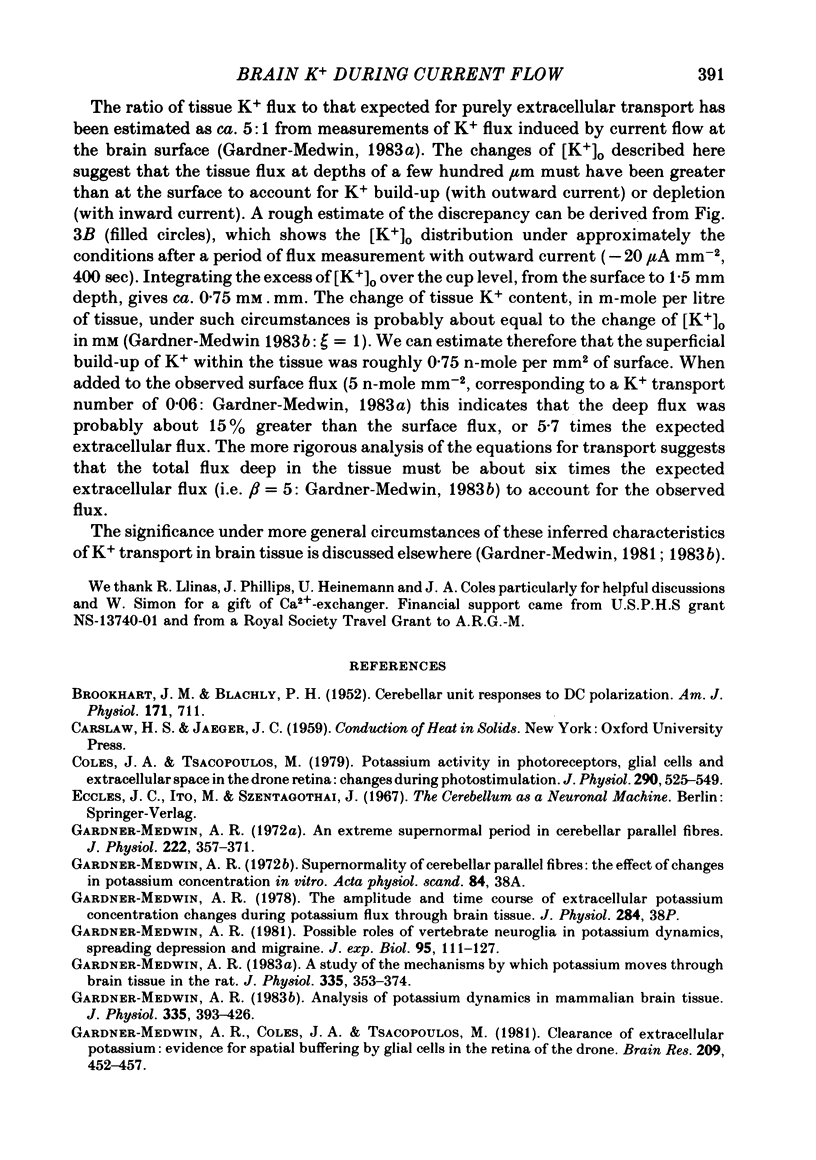
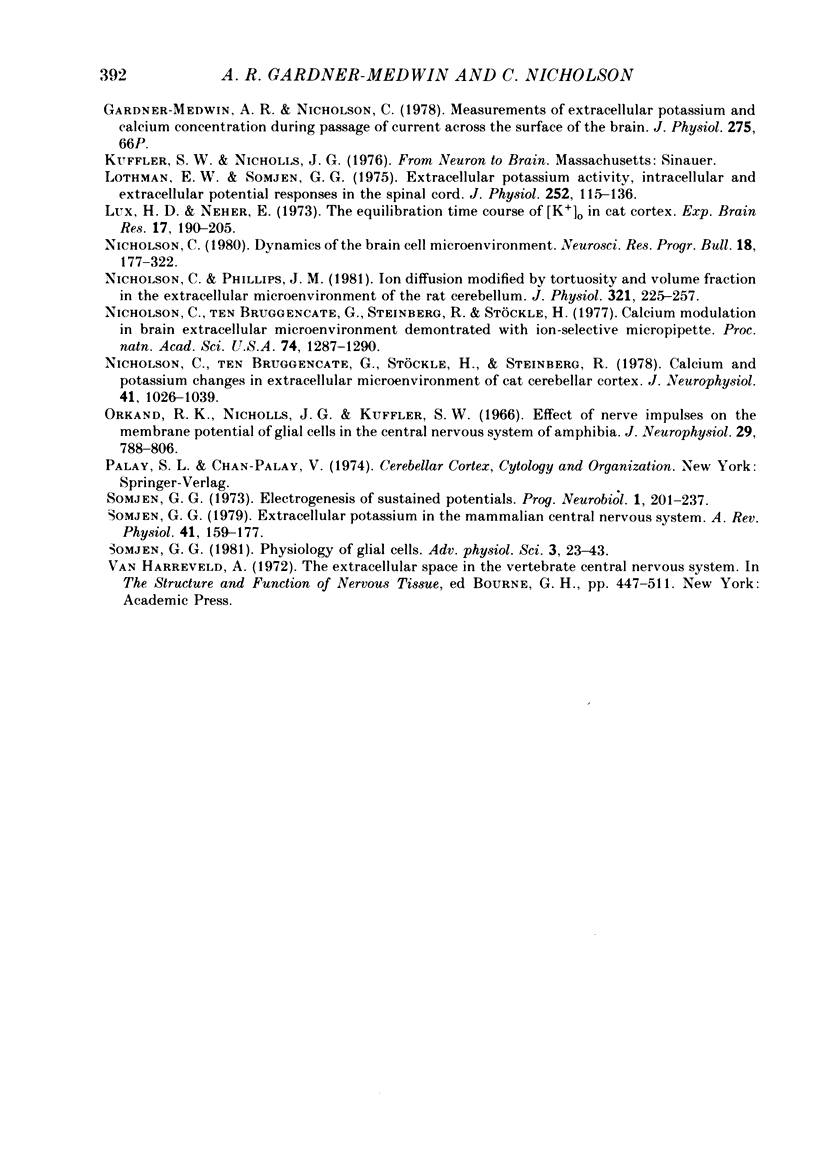
Selected References
These references are in PubMed. This may not be the complete list of references from this article.
- Coles J. A., Tsacopoulos M. Potassium activity in photoreceptors, glial cells and extracellular space in the drone retina: changes during photostimulation. J Physiol. 1979 May;290(2):525–549. doi: 10.1113/jphysiol.1979.sp012788. [DOI] [PMC free article] [PubMed] [Google Scholar]
- Gardner-Medwin A. R. A study of the mechanisms by which potassium moves through brain tissue in the rat. J Physiol. 1983 Feb;335:353–374. doi: 10.1113/jphysiol.1983.sp014539. [DOI] [PMC free article] [PubMed] [Google Scholar]
- Gardner-Medwin A. R. An extreme supernormal period in cerebellar parallel fibres. J Physiol. 1972 Apr;222(2):357–371. doi: 10.1113/jphysiol.1972.sp009802. [DOI] [PMC free article] [PubMed] [Google Scholar]
- Gardner-Medwin A. R. Analysis of potassium dynamics in mammalian brain tissue. J Physiol. 1983 Feb;335:393–426. doi: 10.1113/jphysiol.1983.sp014541. [DOI] [PMC free article] [PubMed] [Google Scholar]
- Gardner-Medwin A. R., Coles J. A., Tsacopoulos M. Clearance of extracellular potassium: evidence for spatial buffering by glial cells in the retina of the drone. Brain Res. 1981 Mar 30;209(2):452–457. doi: 10.1016/0006-8993(81)90169-4. [DOI] [PubMed] [Google Scholar]
- Gardner-Medwin A. R., Nicholson C. Measurements of extracellular potassium and calcium concentration during passage of current across the surface of the brain [proceedings]. J Physiol. 1978 Feb;275:66P–67P. [PubMed] [Google Scholar]
- Gardner-Medwin A. R. Possible roles of vertebrate neuroglia in potassium dynamics, spreading depression and migraine. J Exp Biol. 1981 Dec;95:111–127. doi: 10.1242/jeb.95.1.111. [DOI] [PubMed] [Google Scholar]
- Gardner-Medwin A. R. The amplitude and time course of extracellular potassium concentration changes during potassium flux through brain tissue [proceedings]. J Physiol. 1978 Nov;284:38P–39P. [PMC free article] [PubMed] [Google Scholar]
- Lothman E. W., Somjen G. G. Extracellular potassium activity, intracellular and extracellular potential responses in the spinal cord. J Physiol. 1975 Oct;252(1):115–136. doi: 10.1113/jphysiol.1975.sp011137. [DOI] [PMC free article] [PubMed] [Google Scholar]
- Lux H. D., Neher E. The equilibration time course of (K + ) 0 in cat cortex. Exp Brain Res. 1973 Apr 30;17(2):190–205. doi: 10.1007/BF00235028. [DOI] [PubMed] [Google Scholar]
- Nicholson C., Bruggencate G. T., Steinberg R., Stöckle H. Calcium modulation in brain extracellular microenvironment demonstrated with ion-selective micropipette. Proc Natl Acad Sci U S A. 1977 Mar;74(3):1287–1290. doi: 10.1073/pnas.74.3.1287. [DOI] [PMC free article] [PubMed] [Google Scholar]
- Nicholson C. Dynamics of the brain cell microenvironment. Neurosci Res Program Bull. 1980 Apr;18(2):175–322. [PubMed] [Google Scholar]
- Nicholson C., Phillips J. M. Ion diffusion modified by tortuosity and volume fraction in the extracellular microenvironment of the rat cerebellum. J Physiol. 1981 Dec;321:225–257. doi: 10.1113/jphysiol.1981.sp013981. [DOI] [PMC free article] [PubMed] [Google Scholar]
- Nicholson C., ten Bruggencate G., Stöckle H., Steinberg R. Calcium and potassium changes in extracellular microenvironment of cat cerebellar cortex. J Neurophysiol. 1978 Jul;41(4):1026–1039. doi: 10.1152/jn.1978.41.4.1026. [DOI] [PubMed] [Google Scholar]
- Orkand R. K., Nicholls J. G., Kuffler S. W. Effect of nerve impulses on the membrane potential of glial cells in the central nervous system of amphibia. J Neurophysiol. 1966 Jul;29(4):788–806. doi: 10.1152/jn.1966.29.4.788. [DOI] [PubMed] [Google Scholar]
- Somjen G. G. Electrogenesis of sustained potentials. Prog Neurobiol. 1973;1(3):201–237. [PubMed] [Google Scholar]
- Somjen G. G. Extracellular potassium in the mammalian central nervous system. Annu Rev Physiol. 1979;41:159–177. doi: 10.1146/annurev.ph.41.030179.001111. [DOI] [PubMed] [Google Scholar]