Abstract
1. We have studied the effects of anoxia on the recovery of neural transmission between the perforant path and the dentate granule cells in the in vitro rat hippocampal slice. There is almost no recovery of the evoked population spike following 10 min of anoxia in slices from adult rats.
2. A 2 h exposure of slices to creatine markedly improves the recovery of the population spike (80% vs. 5%). The creatine pre-incubation builds up phosphocreatine levels in the slice and prevents the large fall in ATP during anoxia; ATP falls to 7·9 rather than 3·6 nM/mg protein. The intracellular pH of both groups falls to the same level during anoxia.
3. If calcium concentration in the medium is reduced to 0 while magnesium concentration is raised to 10 mM during anoxia the evoked response recovers to about 65%.
4. The data suggest that an attenuation of the fall in ATP or entry of calcium during anoxia protects the tissue against irreversible transmission damage. Thus both of these factors participate in the generation of this damage. It is not yet clear if they act independently or if one acts by altering the other.
5. In the post-anoxic recovery period the intracellular concentration of potassium is reduced by about 25%. However, it is still much higher than in slices that show only partial block of the evoked response when treated with ouabain. Therefore a fall in intracellular potassium 1 h after anoxia cannot explain the lack of recovery of the evoked response in adult tissue.
6. ATP levels in the post-anoxic recovery period are reduced from their pre-anoxic levels (9·7 vs. 13·9 nM/mg protein). However when azide or antimycin A are used to directly reduce ATP to the level found 1 h after anoxia the evoked response is reduced by only about 45%. Thus the reduced post-anoxic ATP levels are not sufficient to explain the loss of the evoked response in adult tissue.
7. The data show that the irreversible loss of transmission is not due to decreased cell ATP or to decreased cell K/Na levels 1 h after the anoxic period.
8. Since creatine pre-incubation protects against irreversible transmission loss this compound or one closely related to it may prove useful in attenuating irreversible brain damage in situ.
Full text
PDF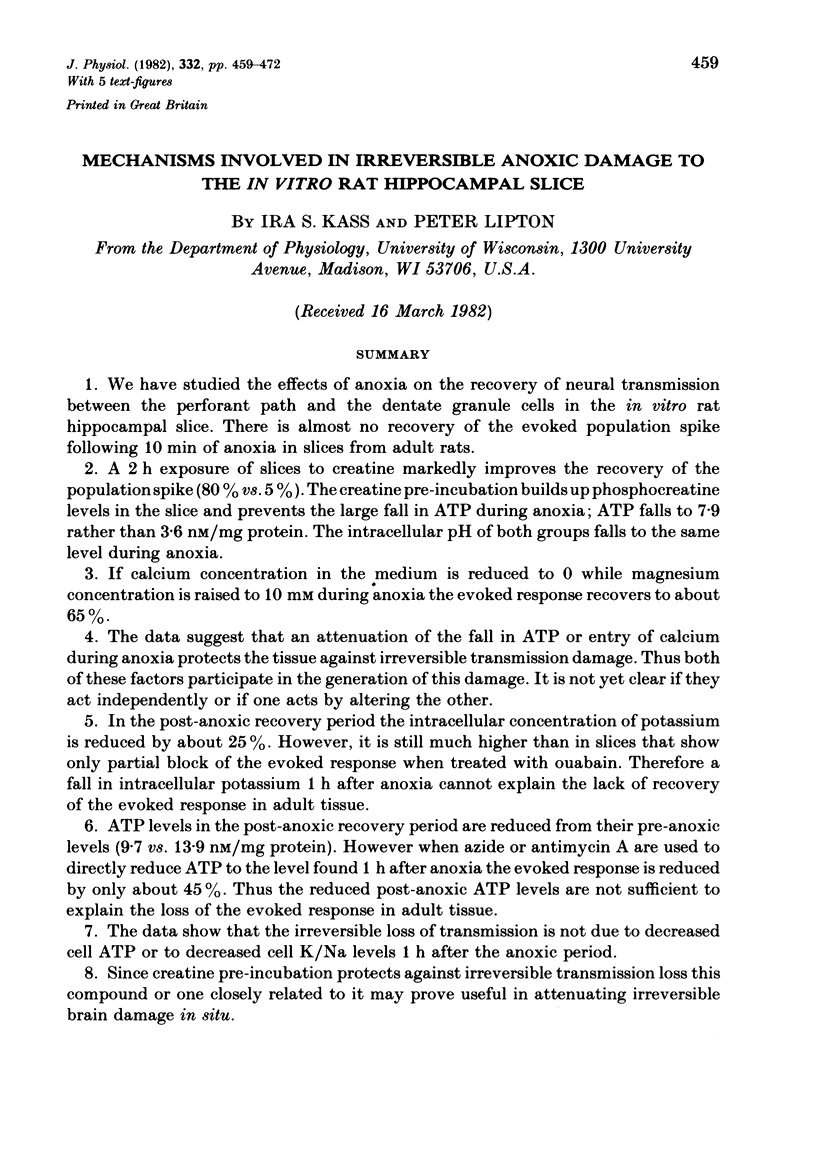
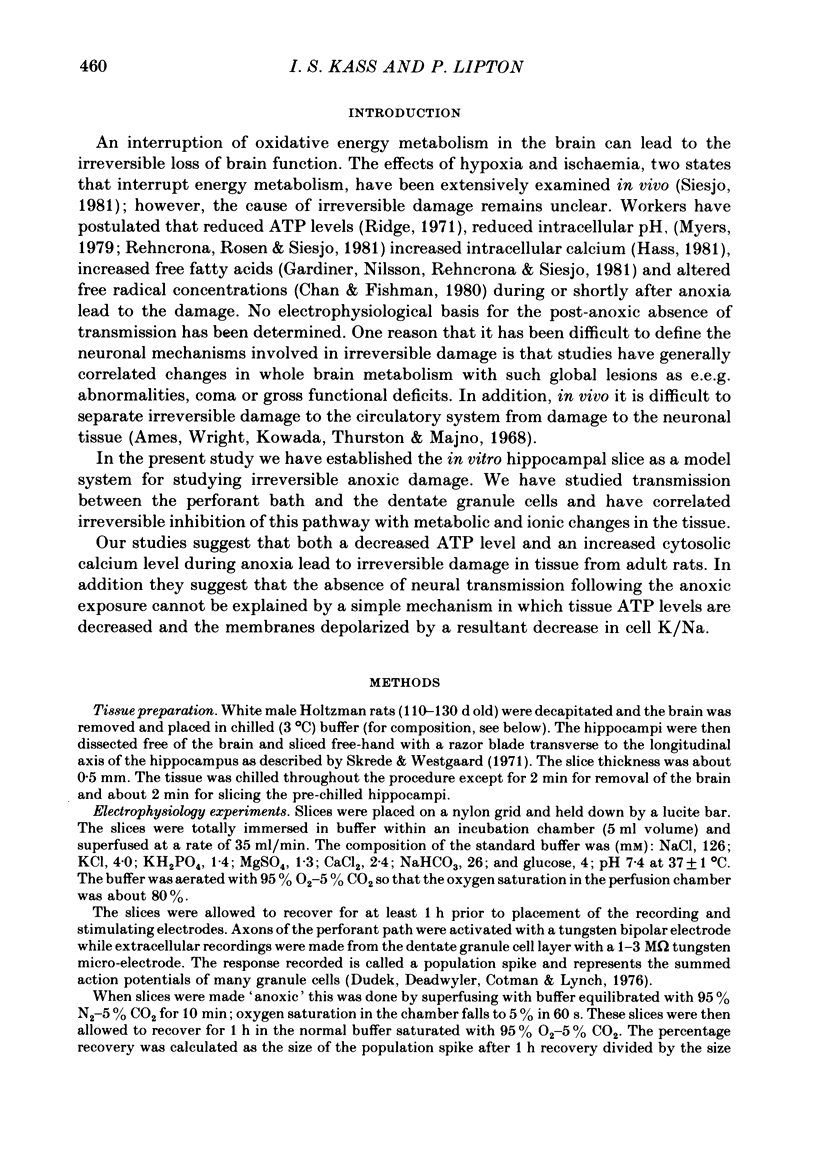
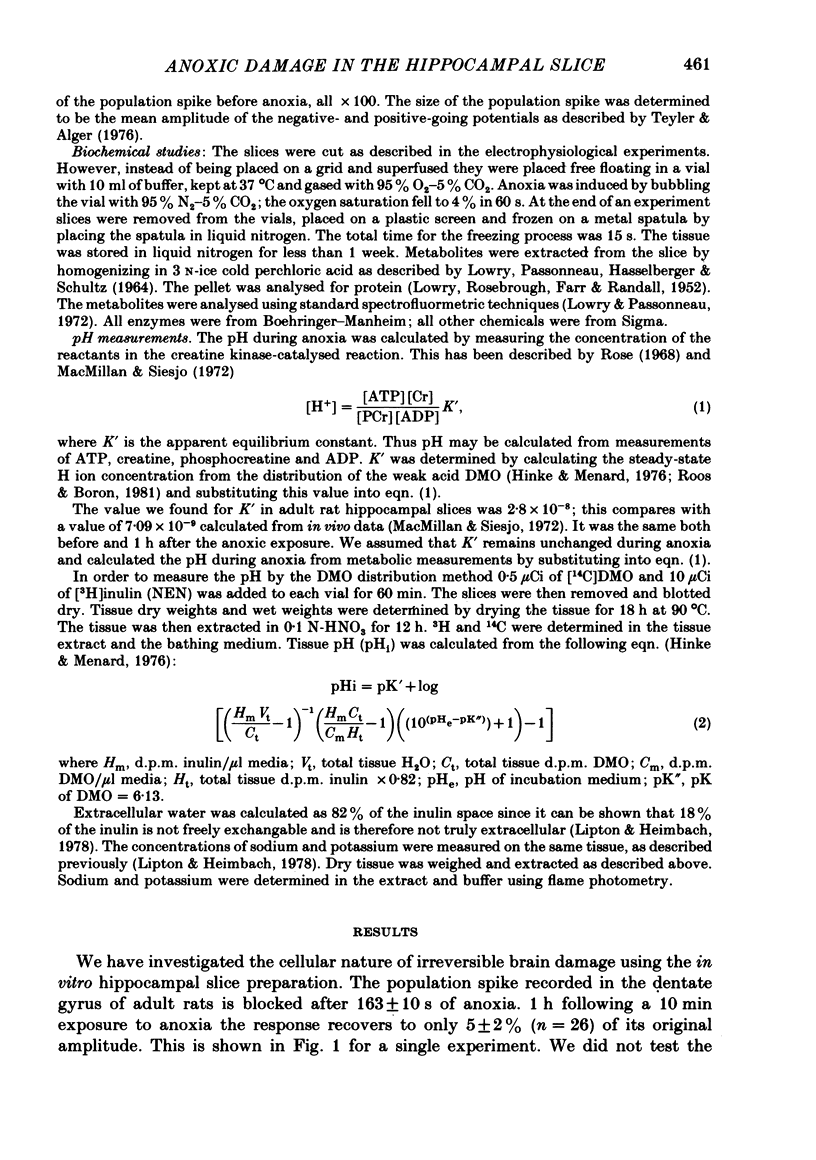
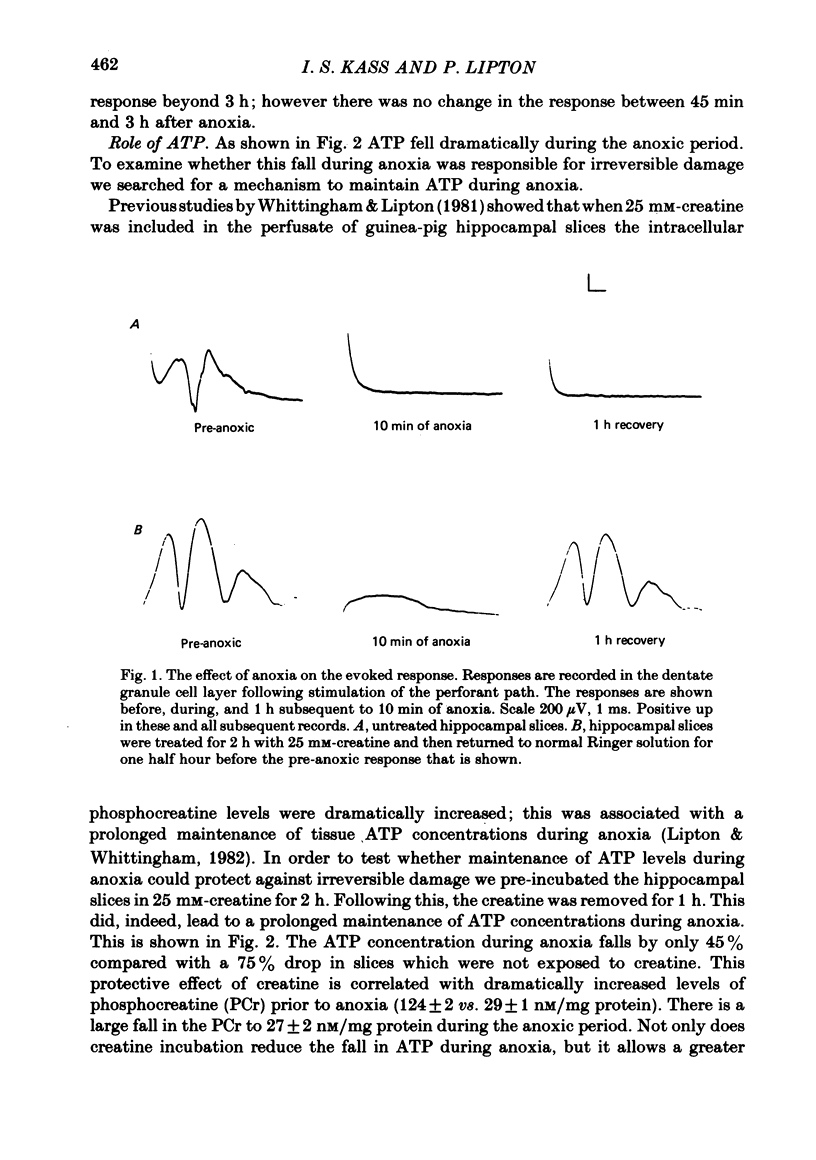
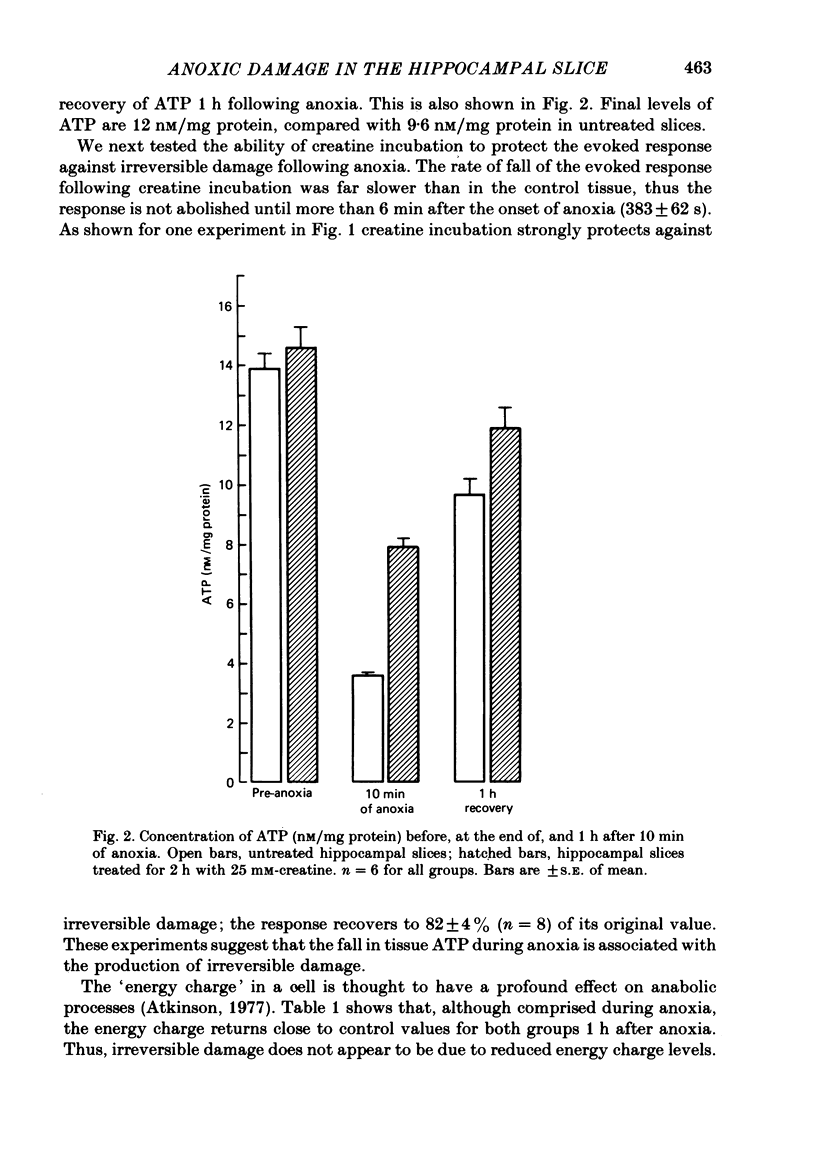
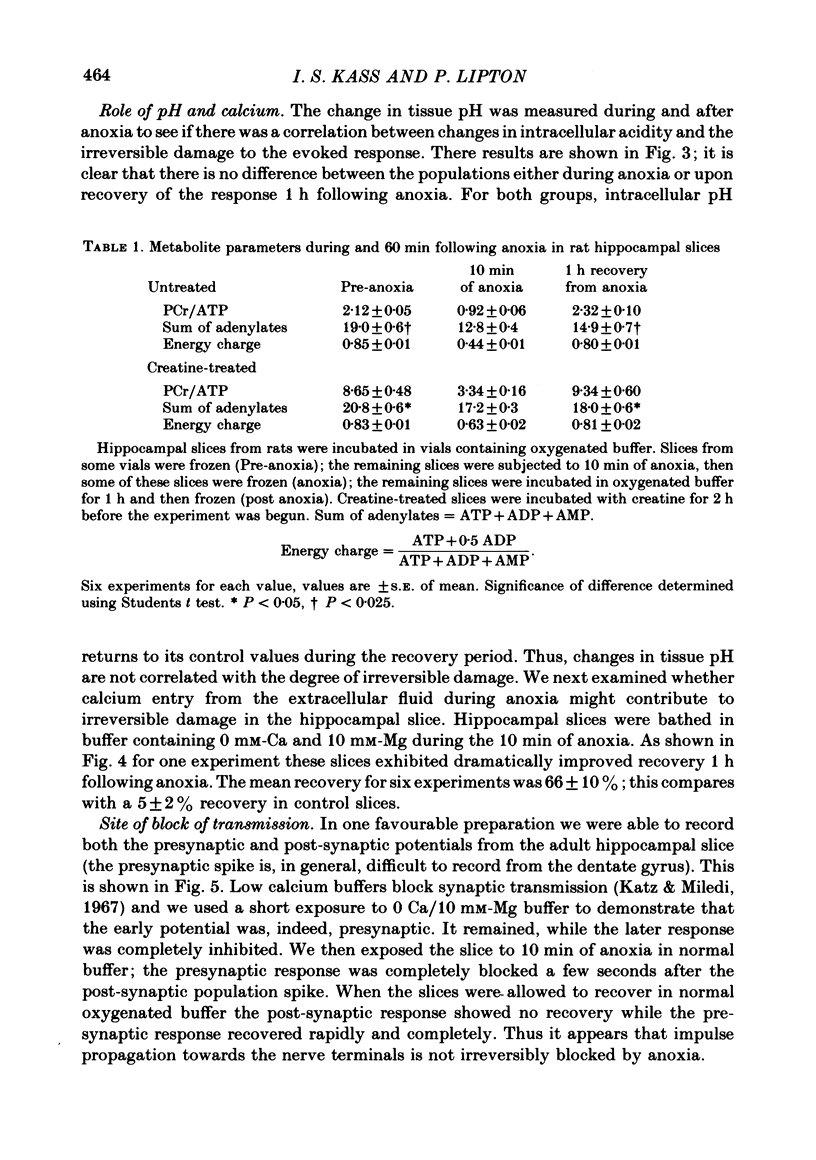
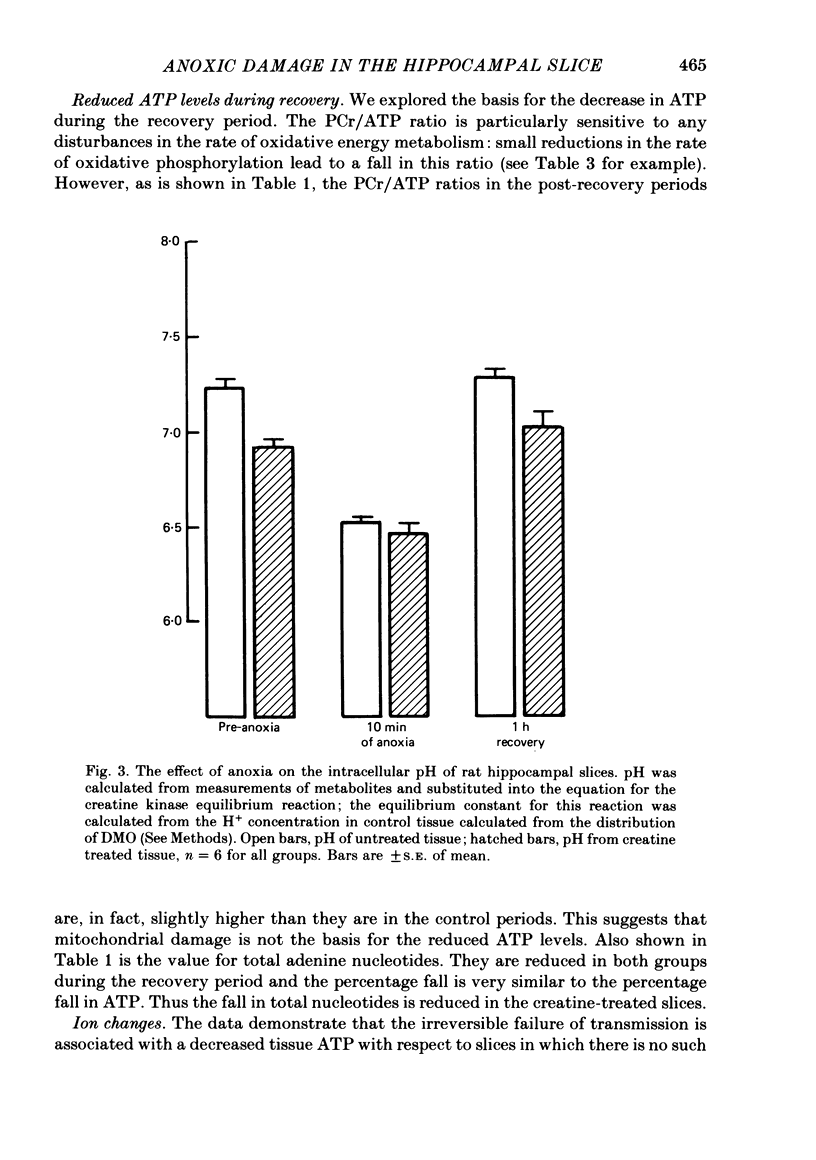
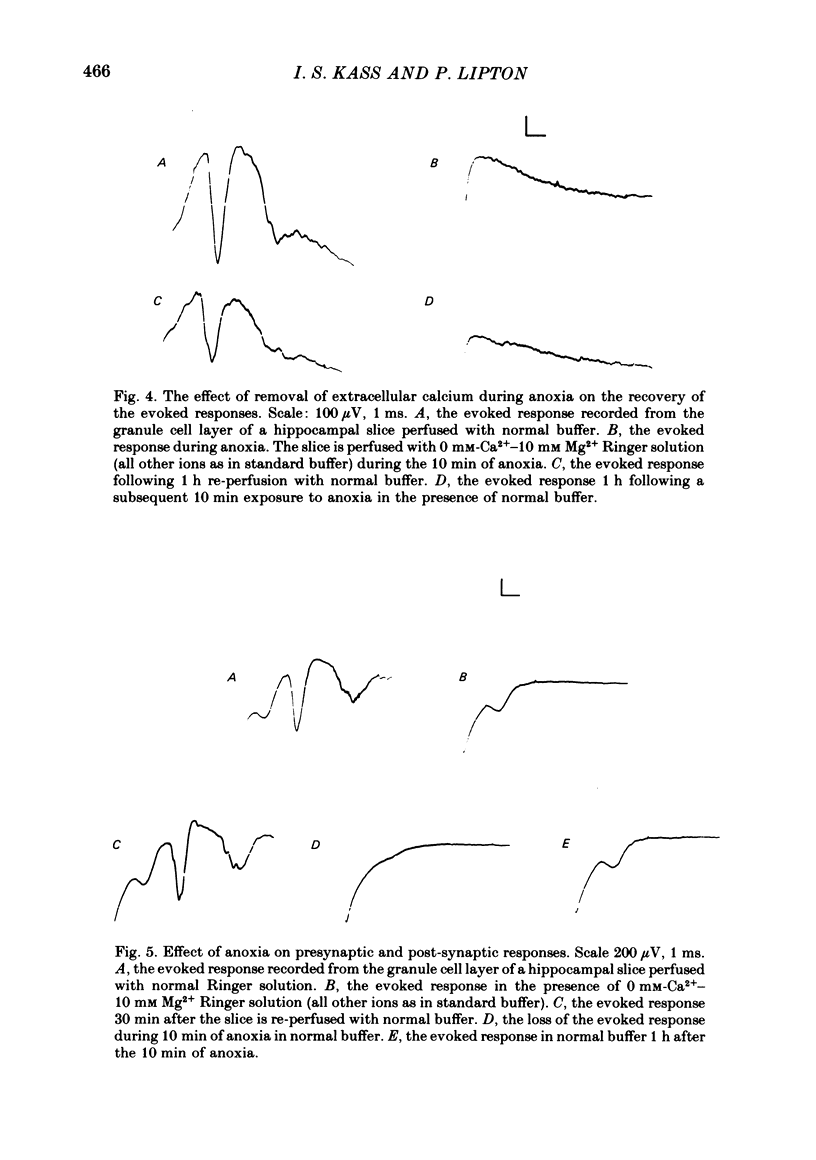
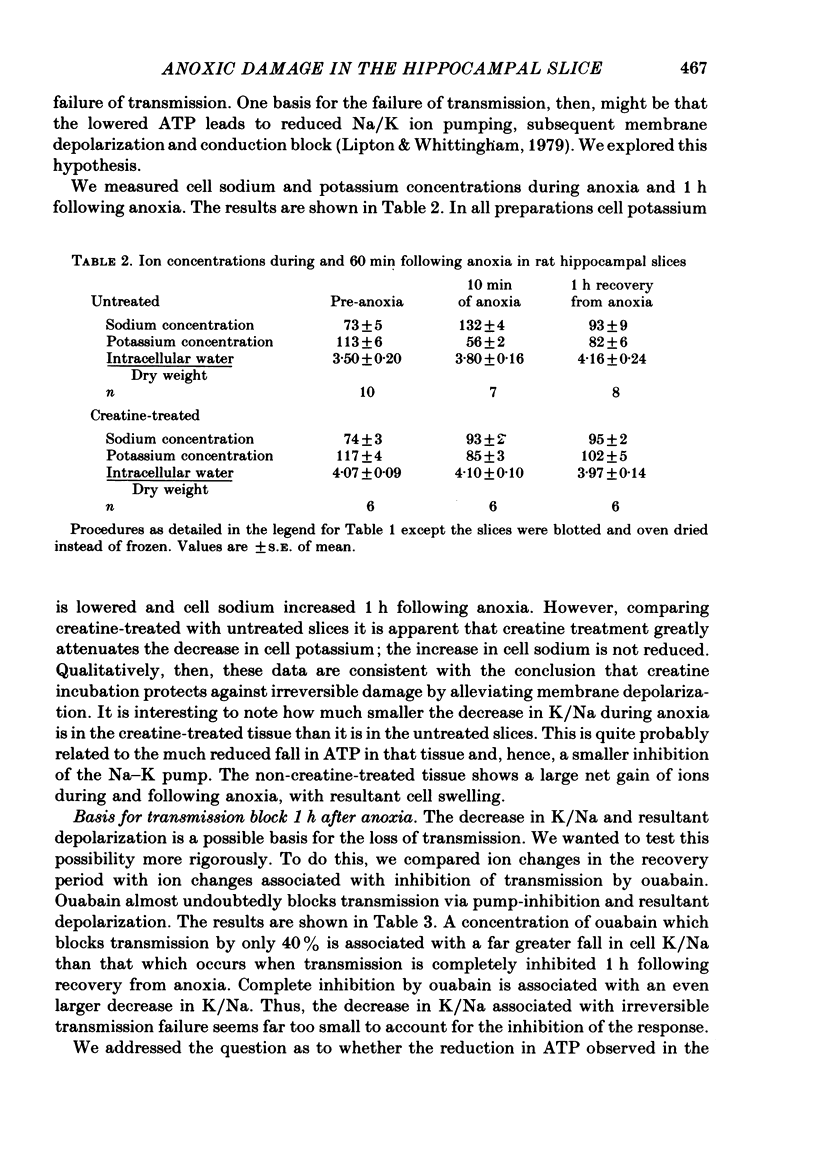
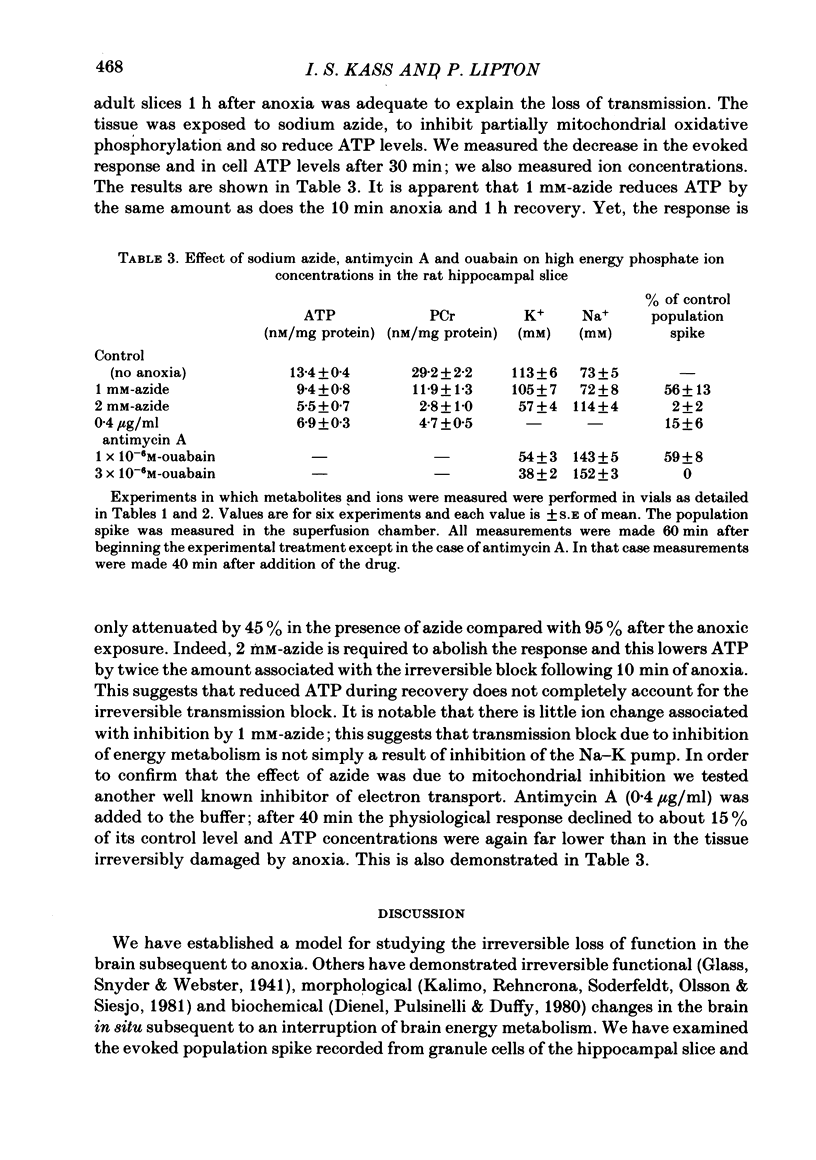
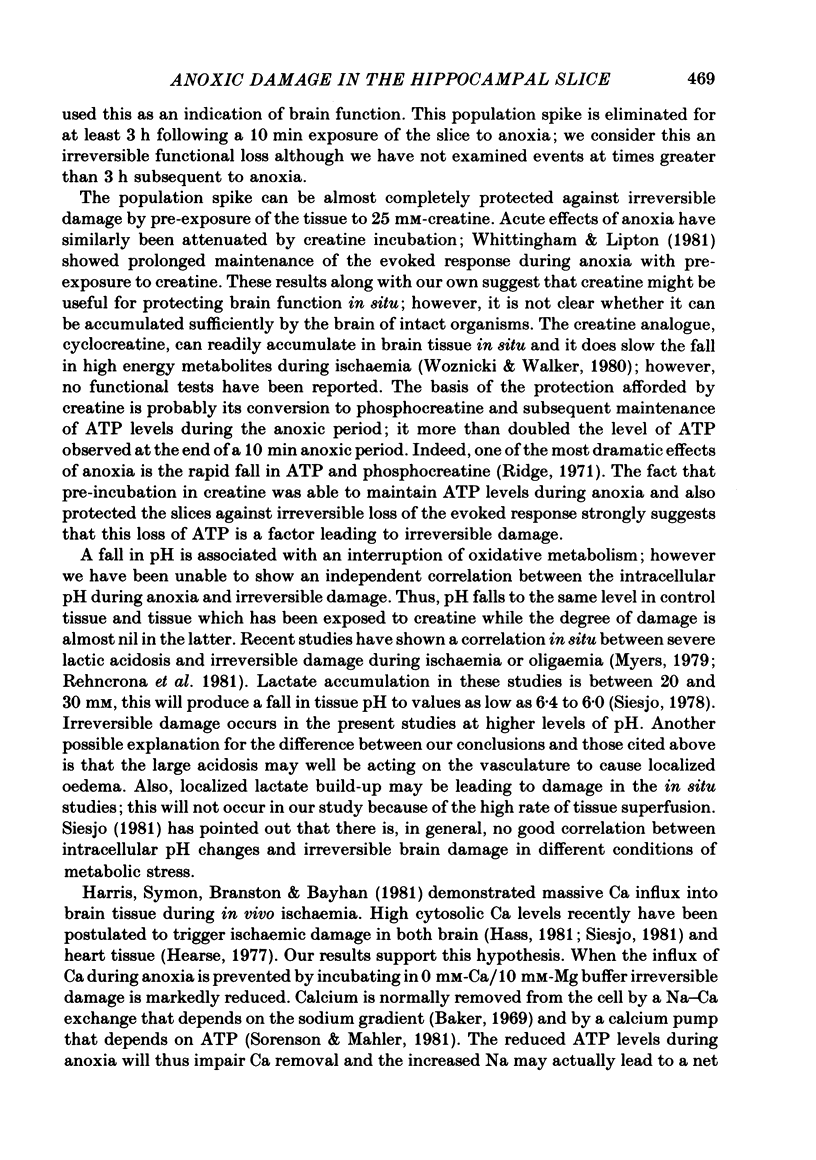
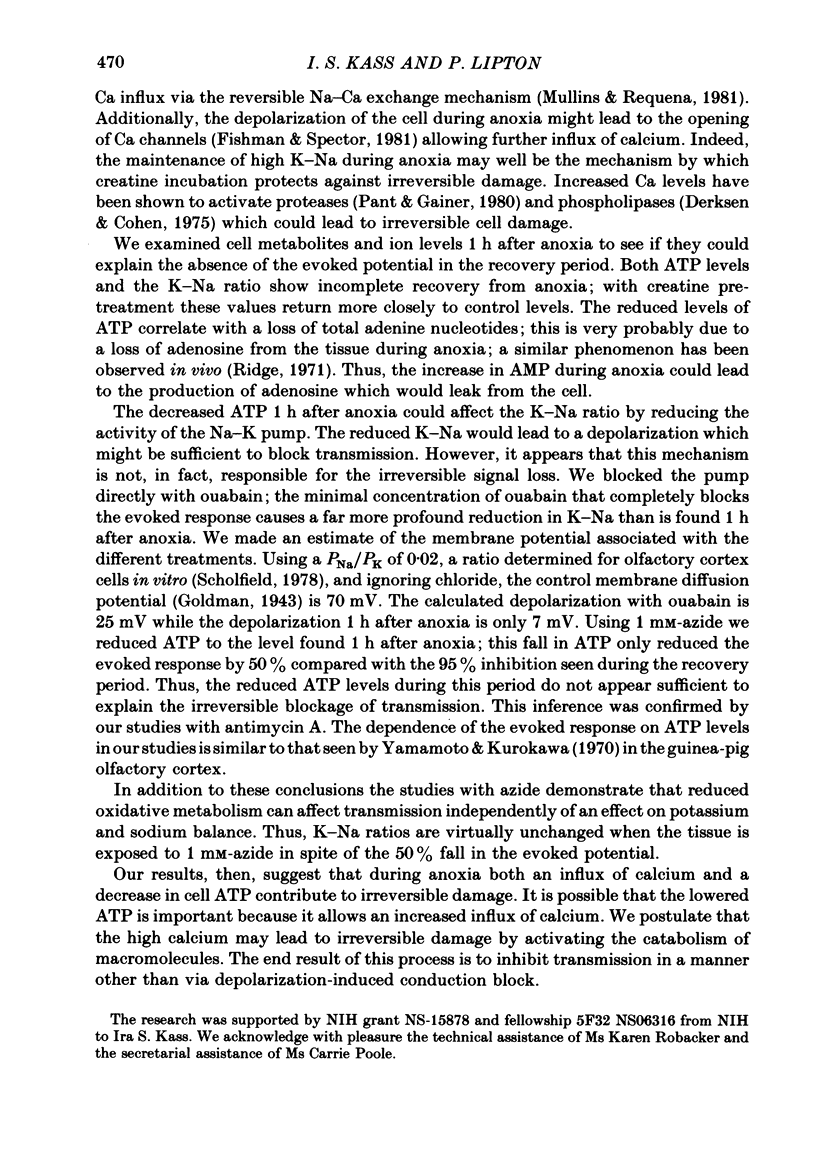
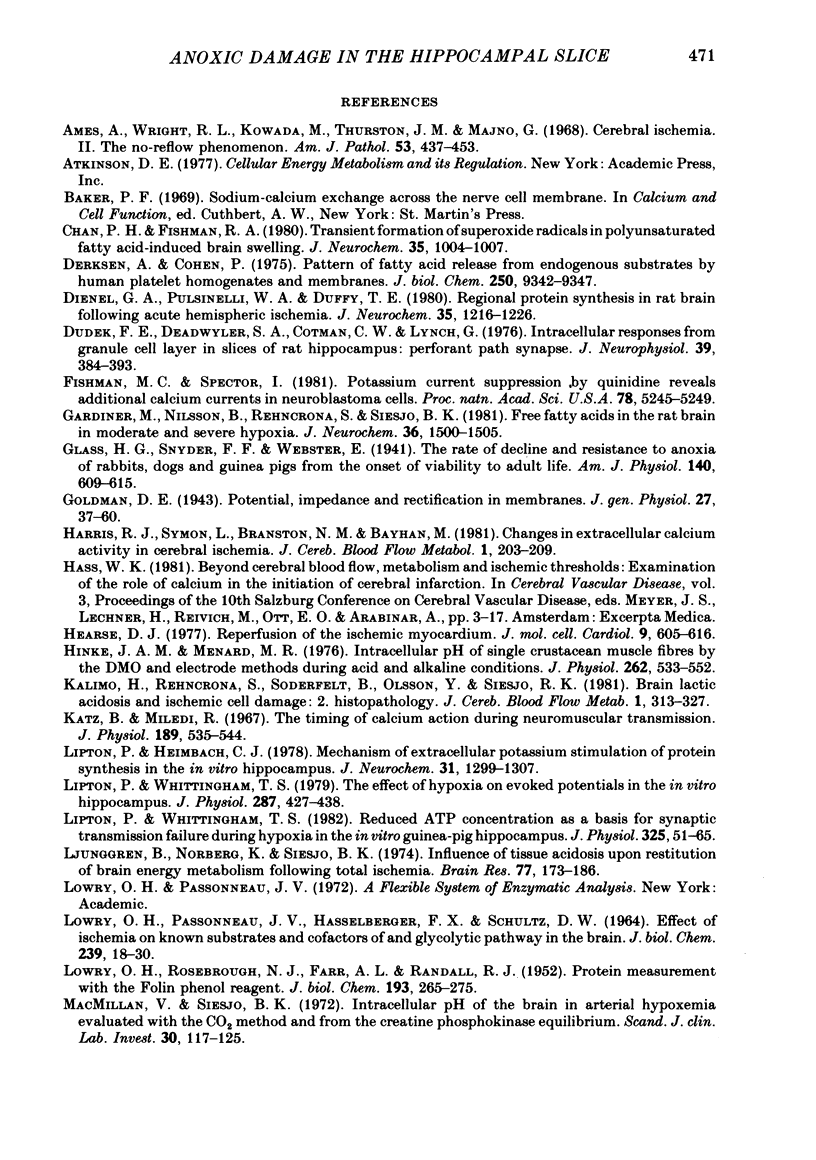
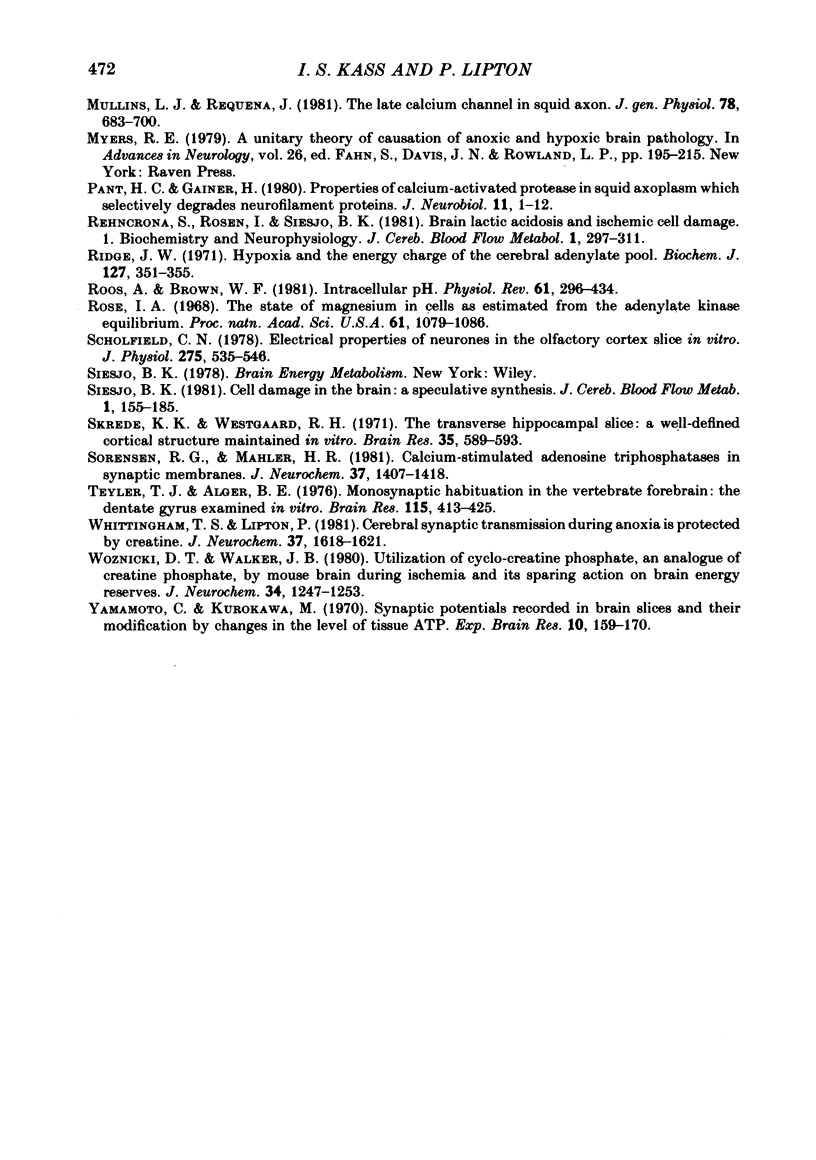
Selected References
These references are in PubMed. This may not be the complete list of references from this article.
- Ames A., 3rd, Wright R. L., Kowada M., Thurston J. M., Majno G. Cerebral ischemia. II. The no-reflow phenomenon. Am J Pathol. 1968 Feb;52(2):437–453. [PMC free article] [PubMed] [Google Scholar]
- Chan P. H., Fishman R. A. Transient formation of superoxide radicals in polyunsaturated fatty acid-induced brain swelling. J Neurochem. 1980 Oct;35(4):1004–1007. doi: 10.1111/j.1471-4159.1980.tb07100.x. [DOI] [PubMed] [Google Scholar]
- Derksen A., Cohen P. Patterns of fatty acid release from endogenous substrates by human platelet homogenates and membranes. J Biol Chem. 1975 Dec 25;250(24):9342–9347. [PubMed] [Google Scholar]
- Dienel G. A., Pulsinelli W. A., Duffy T. E. Regional protein synthesis in rat brain following acute hemispheric ischemia. J Neurochem. 1980 Nov;35(5):1216–1226. doi: 10.1111/j.1471-4159.1980.tb07878.x. [DOI] [PubMed] [Google Scholar]
- Dudek F. E., Deadwyler S. A., Cotman C. W., Lynch G. Intracellular responses from granule cell layer in slices of rat hippocampus: perforant path synapse. J Neurophysiol. 1976 Mar;39(2):384–393. doi: 10.1152/jn.1976.39.2.384. [DOI] [PubMed] [Google Scholar]
- Fishman M. C., Spector I. Potassium current suppression by quinidine reveals additional calcium currents in neuroblastoma cells. Proc Natl Acad Sci U S A. 1981 Aug;78(8):5245–5249. doi: 10.1073/pnas.78.8.5245. [DOI] [PMC free article] [PubMed] [Google Scholar]
- Gardiner M., Nilsson B., Rehncrona S., Siesjö B. K. Free fatty acids in the rat brain in moderate and severe hypoxia. J Neurochem. 1981 Apr;36(4):1500–1505. doi: 10.1111/j.1471-4159.1981.tb00592.x. [DOI] [PubMed] [Google Scholar]
- Goldman D. E. POTENTIAL, IMPEDANCE, AND RECTIFICATION IN MEMBRANES. J Gen Physiol. 1943 Sep 20;27(1):37–60. doi: 10.1085/jgp.27.1.37. [DOI] [PMC free article] [PubMed] [Google Scholar]
- Harris R. J., Symon L., Branston N. M., Bayhan M. Changes in extracellular calcium activity in cerebral ischaemia. J Cereb Blood Flow Metab. 1981;1(2):203–209. doi: 10.1038/jcbfm.1981.21. [DOI] [PubMed] [Google Scholar]
- Hearse D. J. Reperfusion of the ischemic myocardium. J Mol Cell Cardiol. 1977 Aug;9(8):605–616. doi: 10.1016/s0022-2828(77)80357-x. [DOI] [PubMed] [Google Scholar]
- Hinke J. A., Menard M. R. Intracellular pH of single crustacean muscle fibres by the DMO and electrode methods during acid and alkaline conditions. J Physiol. 1976 Nov;262(3):533–552. doi: 10.1113/jphysiol.1976.sp011609. [DOI] [PMC free article] [PubMed] [Google Scholar]
- Kalimo H., Rehncrona S., Söderfeldt B., Olsson Y., Siesjö B. K. Brain lactic acidosis and ischemic cell damage: 2. Histopathology. J Cereb Blood Flow Metab. 1981;1(3):313–327. doi: 10.1038/jcbfm.1981.35. [DOI] [PubMed] [Google Scholar]
- Katz B., Miledi R. The timing of calcium action during neuromuscular transmission. J Physiol. 1967 Apr;189(3):535–544. doi: 10.1113/jphysiol.1967.sp008183. [DOI] [PMC free article] [PubMed] [Google Scholar]
- LOWRY O. H., PASSONNEAU J. V., HASSELBERGER F. X., SCHULZ D. W. EFFECT OF ISCHEMIA ON KNOWN SUBSTRATES AND COFACTORS OF THE GLYCOLYTIC PATHWAY IN BRAIN. J Biol Chem. 1964 Jan;239:18–30. [PubMed] [Google Scholar]
- LOWRY O. H., ROSEBROUGH N. J., FARR A. L., RANDALL R. J. Protein measurement with the Folin phenol reagent. J Biol Chem. 1951 Nov;193(1):265–275. [PubMed] [Google Scholar]
- Lipton P., Heimbach C. J. Mechanism of extracellular potassium stimulation of protein synthesis in the in vitro hippocampus. J Neurochem. 1978 Nov;31(5):1299–1307. doi: 10.1111/j.1471-4159.1978.tb06255.x. [DOI] [PubMed] [Google Scholar]
- Lipton P., Whittingham T. S. Reduced ATP concentration as a basis for synaptic transmission failure during hypoxia in the in vitro guinea-pig hippocampus. J Physiol. 1982 Apr;325:51–65. doi: 10.1113/jphysiol.1982.sp014135. [DOI] [PMC free article] [PubMed] [Google Scholar]
- Lipton P., Whittingham T. S. The effect of hypoxia on evoked potentials in the in vitro hippocampus. J Physiol. 1979 Feb;287:427–438. doi: 10.1113/jphysiol.1979.sp012668. [DOI] [PMC free article] [PubMed] [Google Scholar]
- Ljunggren B., Norberg K., Siesjö B. K. Influence of tissue acidosis upon restitution of brain energy metabolism following total ischemia. Brain Res. 1974 Sep 6;77(2):173–186. doi: 10.1016/0006-8993(74)90782-3. [DOI] [PubMed] [Google Scholar]
- MacMillan V., Siesjö B. K. Intracellular pH of the brain in arterial hypoxemia, evaluated with the CO 2 method and from the creatine phosphokinase equilibrium. Scand J Clin Lab Invest. 1972 Oct;30(2):117–125. doi: 10.3109/00365517209081100. [DOI] [PubMed] [Google Scholar]
- Mullins L. J., Requena J. The "late" Ca channel in squid axons. J Gen Physiol. 1981 Dec;78(6):683–700. doi: 10.1085/jgp.78.6.683. [DOI] [PMC free article] [PubMed] [Google Scholar]
- Myers R. E. A unitary theory of causation of anoxic and hypoxic brain pathology. Adv Neurol. 1979;26:195–213. [PubMed] [Google Scholar]
- Pant H. C., Gainer H. Properties of a calcium-activated protease in squid axoplasm which selectively degrades neurofilament proteins. J Neurobiol. 1980;11(1):1–12. doi: 10.1002/neu.480110102. [DOI] [PubMed] [Google Scholar]
- Rehncrona S., Rosén I., Siesjö B. K. Brain lactic acidosis and ischemic cell damage: 1. Biochemistry and neurophysiology. J Cereb Blood Flow Metab. 1981;1(3):297–311. doi: 10.1038/jcbfm.1981.34. [DOI] [PubMed] [Google Scholar]
- Ridge J. W. Hypoxia and the energy charge of the cerebral adenylate pool. Biochem J. 1972 Apr;127(2):351–355. doi: 10.1042/bj1270351. [DOI] [PMC free article] [PubMed] [Google Scholar]
- Roos A., Boron W. F. Intracellular pH. Physiol Rev. 1981 Apr;61(2):296–434. doi: 10.1152/physrev.1981.61.2.296. [DOI] [PubMed] [Google Scholar]
- Rose I. A. The state of magnesium in cells as estimated from the adenylate kinase equilibrium. Proc Natl Acad Sci U S A. 1968 Nov;61(3):1079–1086. doi: 10.1073/pnas.61.3.1079. [DOI] [PMC free article] [PubMed] [Google Scholar]
- Scholfield C. N. Electrical properties of neurones in the olfactory cortex slice in vitro. J Physiol. 1978 Feb;275:535–546. doi: 10.1113/jphysiol.1978.sp012206. [DOI] [PMC free article] [PubMed] [Google Scholar]
- Siesjö B. K. Cell damage in the brain: a speculative synthesis. J Cereb Blood Flow Metab. 1981;1(2):155–185. doi: 10.1038/jcbfm.1981.18. [DOI] [PubMed] [Google Scholar]
- Skrede K. K., Westgaard R. H. The transverse hippocampal slice: a well-defined cortical structure maintained in vitro. Brain Res. 1971 Dec 24;35(2):589–593. doi: 10.1016/0006-8993(71)90508-7. [DOI] [PubMed] [Google Scholar]
- Sorensen R. G., Mahler H. R. Calcium-stimulated adenosine triphosphatases in synaptic membranes. J Neurochem. 1981 Dec;37(6):1407–1418. doi: 10.1111/j.1471-4159.1981.tb06309.x. [DOI] [PubMed] [Google Scholar]
- Teyler T. J., Alger B. E. Monosynaptic habituation in the vertebrate forebrain: the dentate gyrus examined in vitro. Brain Res. 1976 Oct 22;115(3):413–425. doi: 10.1016/0006-8993(76)90358-9. [DOI] [PubMed] [Google Scholar]
- Whittingham T. S., Lipton P. Cerebral synaptic transmission during anoxia is protected by creatine. J Neurochem. 1981 Dec;37(6):1618–1621. doi: 10.1111/j.1471-4159.1981.tb06337.x. [DOI] [PubMed] [Google Scholar]
- Woznicki D. T., Walker J. B. Utilization of cyclocreatine phosphate, and analogue of creatine phosphate, by mouse brain during ischemia and its sparing action on brain energy reserves. J Neurochem. 1980 May;34(5):1247–1253. doi: 10.1111/j.1471-4159.1980.tb09966.x. [DOI] [PubMed] [Google Scholar]
- Yamamoto C., Kurokawa M. Synaptic potentials recorded in brain slices and their modification by changes in the level of tissue ATP. Exp Brain Res. 1970;10(2):159–170. doi: 10.1007/BF00234729. [DOI] [PubMed] [Google Scholar]