Abstract
1. A spectrophotometric assay of the rates of penetration of oxaloacetate and l-malate into mitochondria is described. The assay is based on the measurement of the oxidation of intramitochondrial NADH by oxaloacetate and of the reduction of intramitochondrial NAD+ by malate. 2. The rate of entry of both oxaloacetate and l-malate into mitochondria is restricted, as shown by the fact that disruption of the mitochondrial structure can increase the rate of interaction between the dicarboxylic acids and intramitochondrial NAD+ and NADH by between 100- and 1000-fold. 3. The rates of entry of oxaloacetate and malate into liver, kidney and heart mitochondria increased by up to 50-fold on addition of a source of energy, either ascorbate plus NNN′N′-tetramethyl-p-phenylenediamine aerobically, or ATP anaerobically. 4. In the absence of a source of energy the changes in the concentrations of intramitochondrial NAD+ and NADH brought about by the addition of l-malate or oxaloacetate were followed by parallel changes in the concentrations of NADP+ and NADPH, indicating the presence in the mitochondria of an energy-independent transhydrogenase system. 5. The results are discussed in relation to the hypothesis that malate acts as a carrier of reducing equivalents between mitochondria and cytoplasm.
Full text
PDF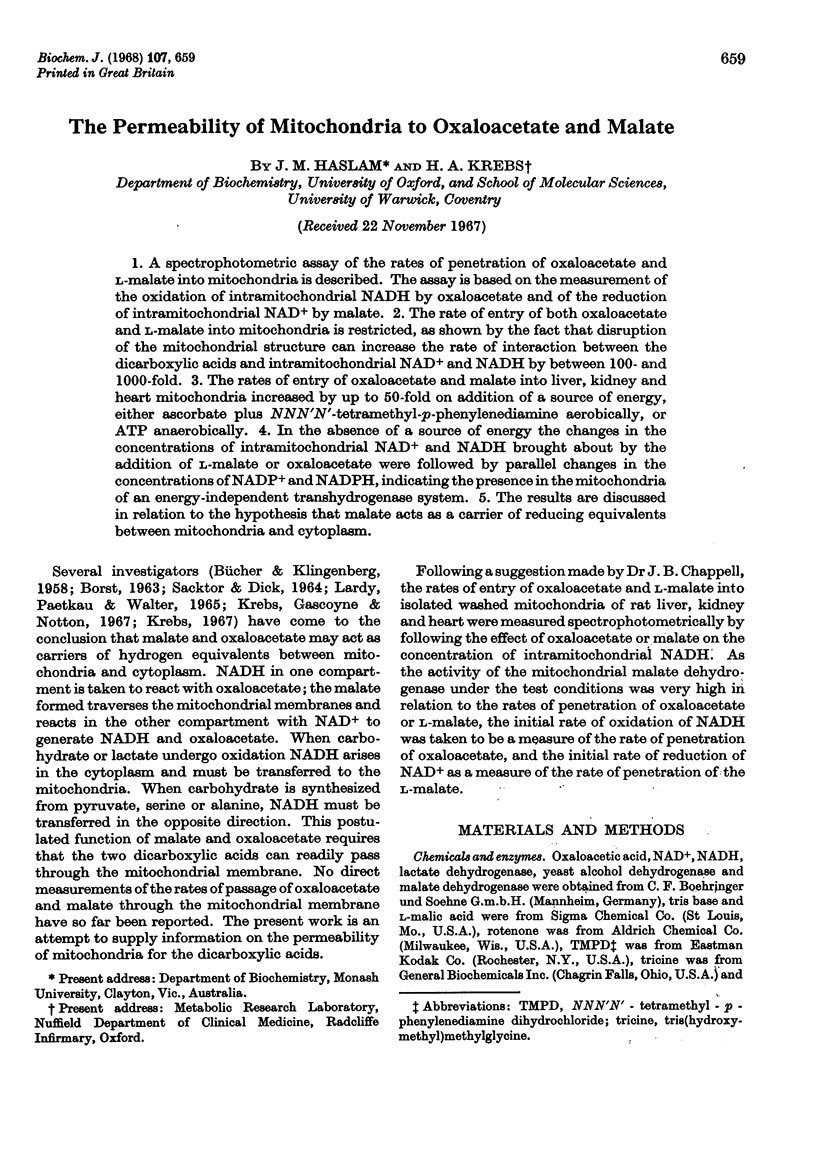
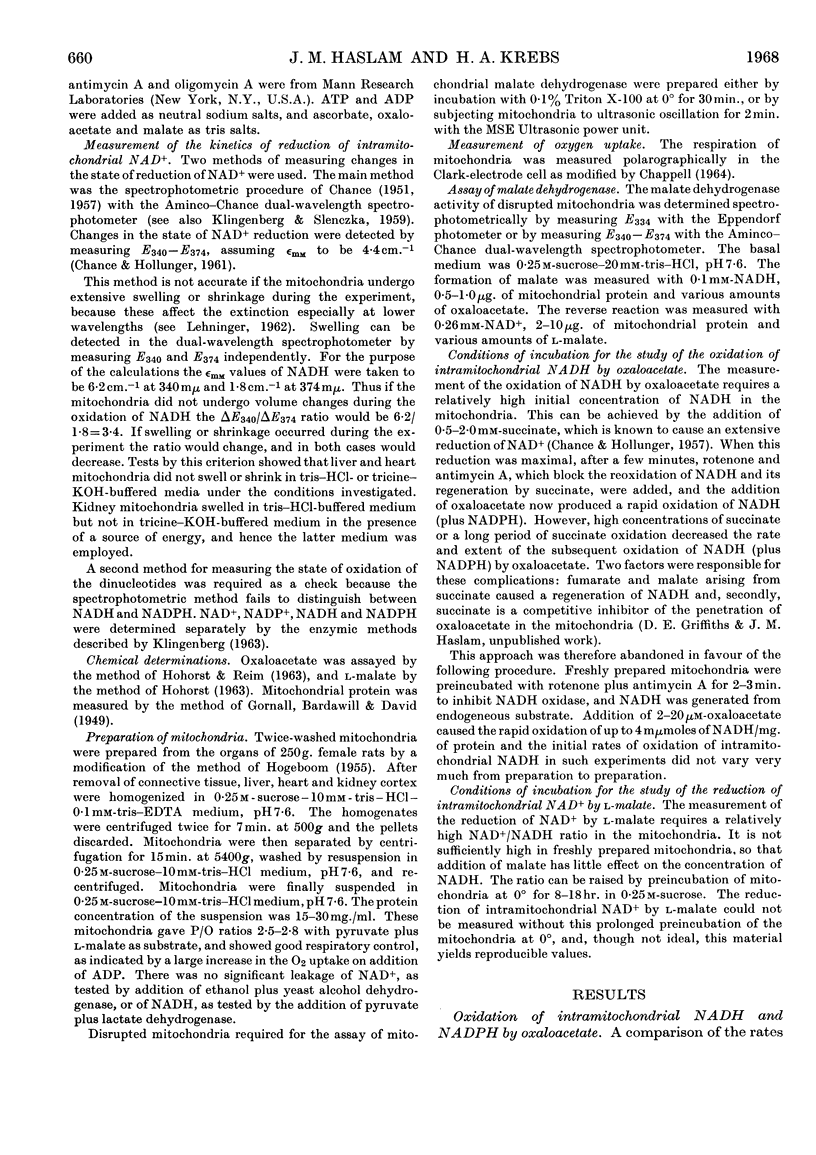
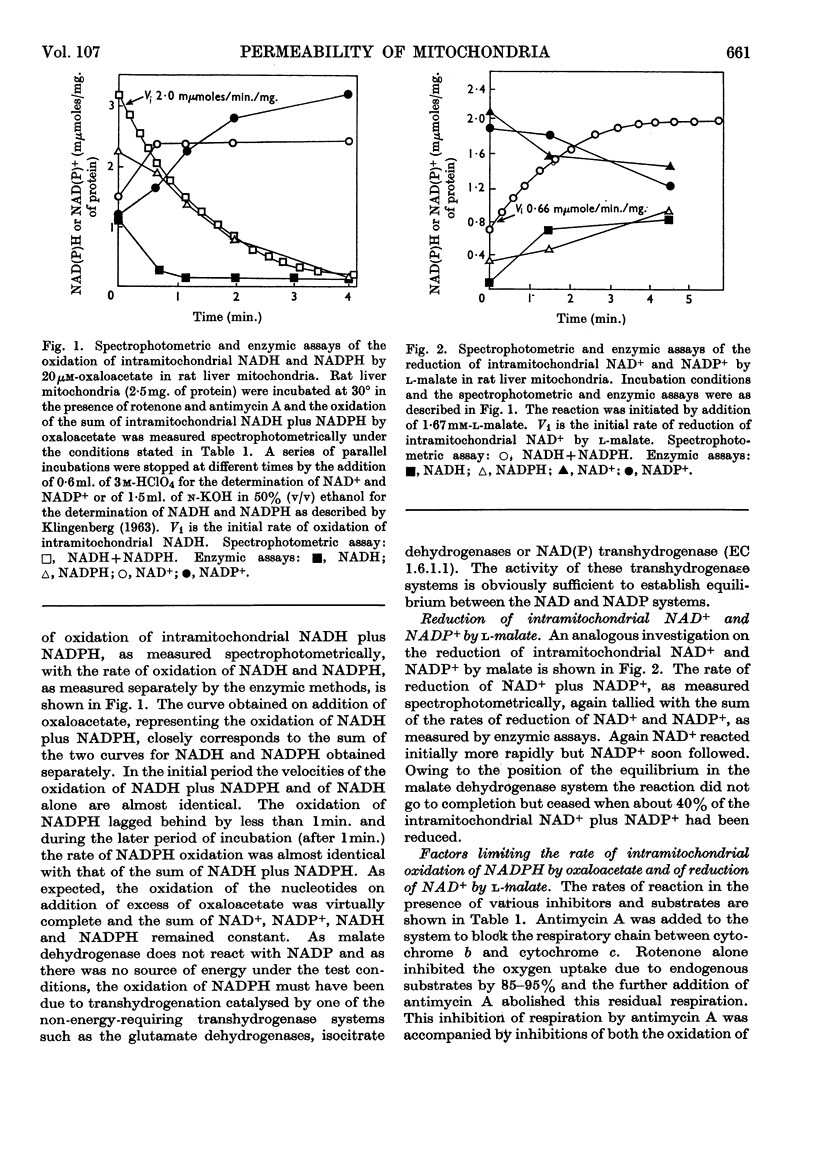
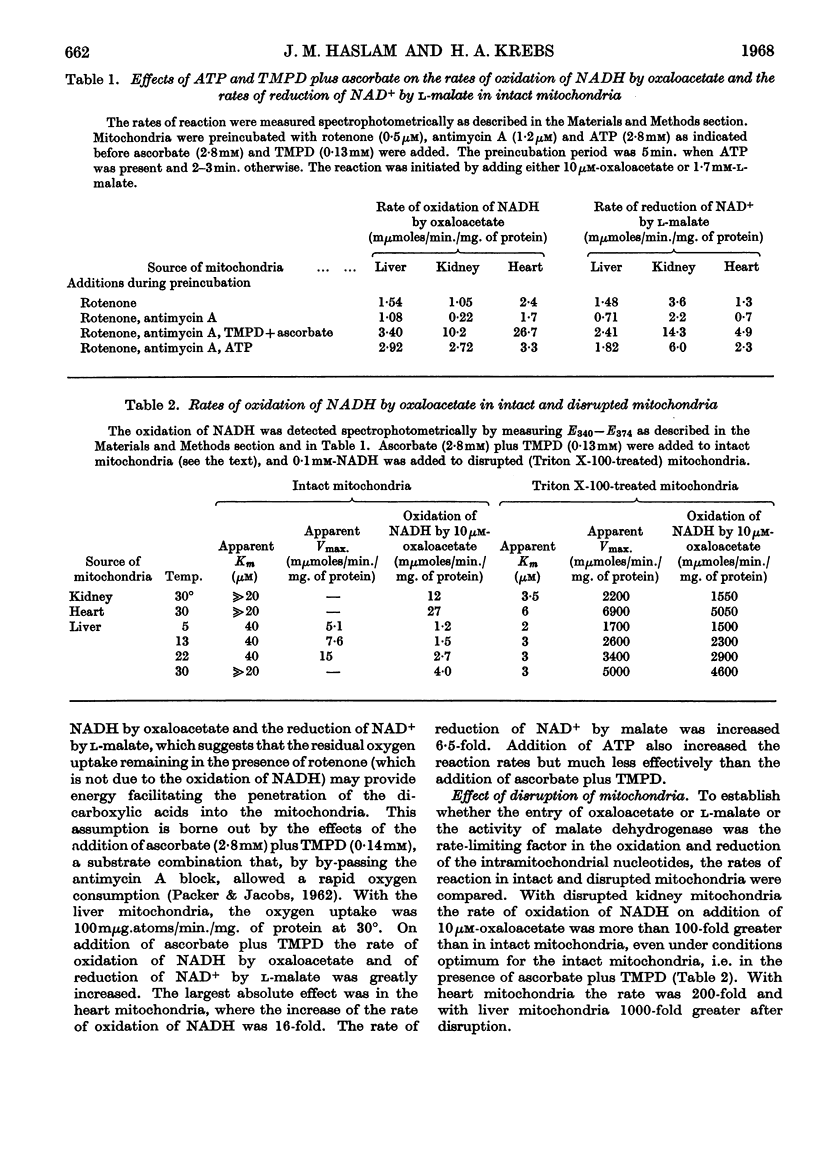
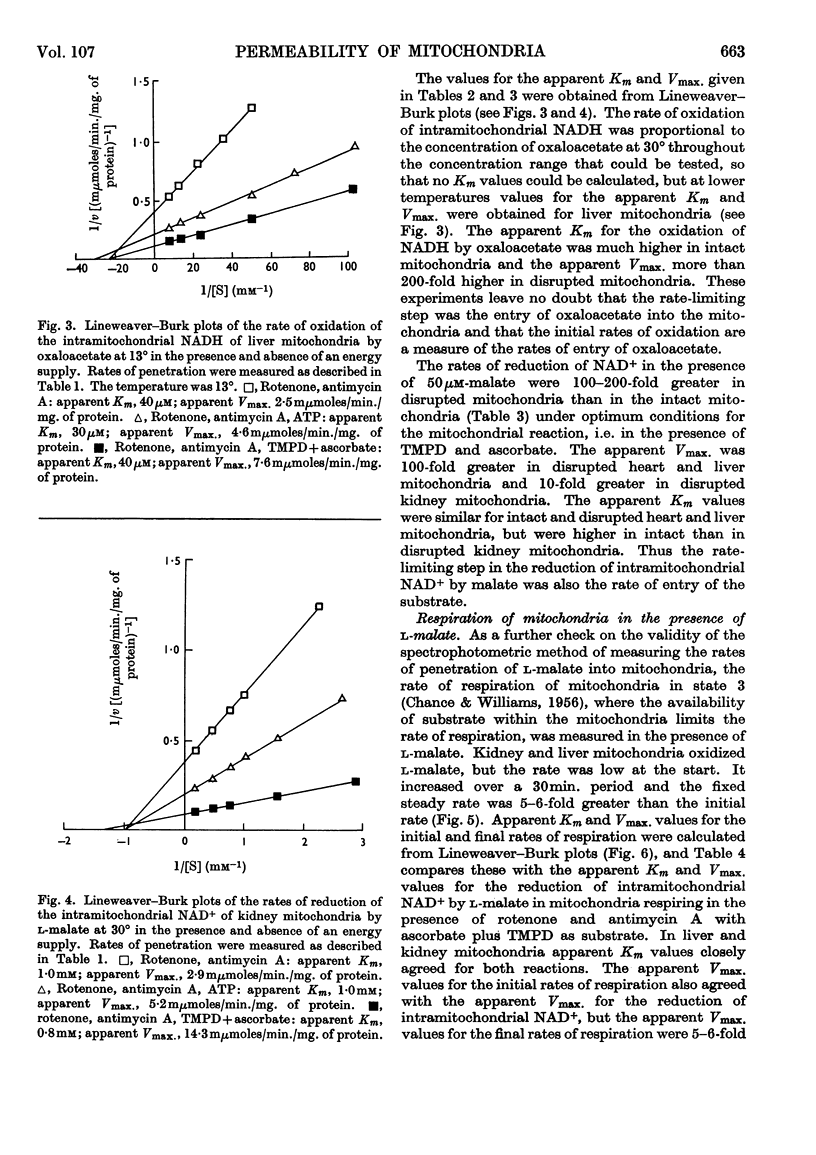
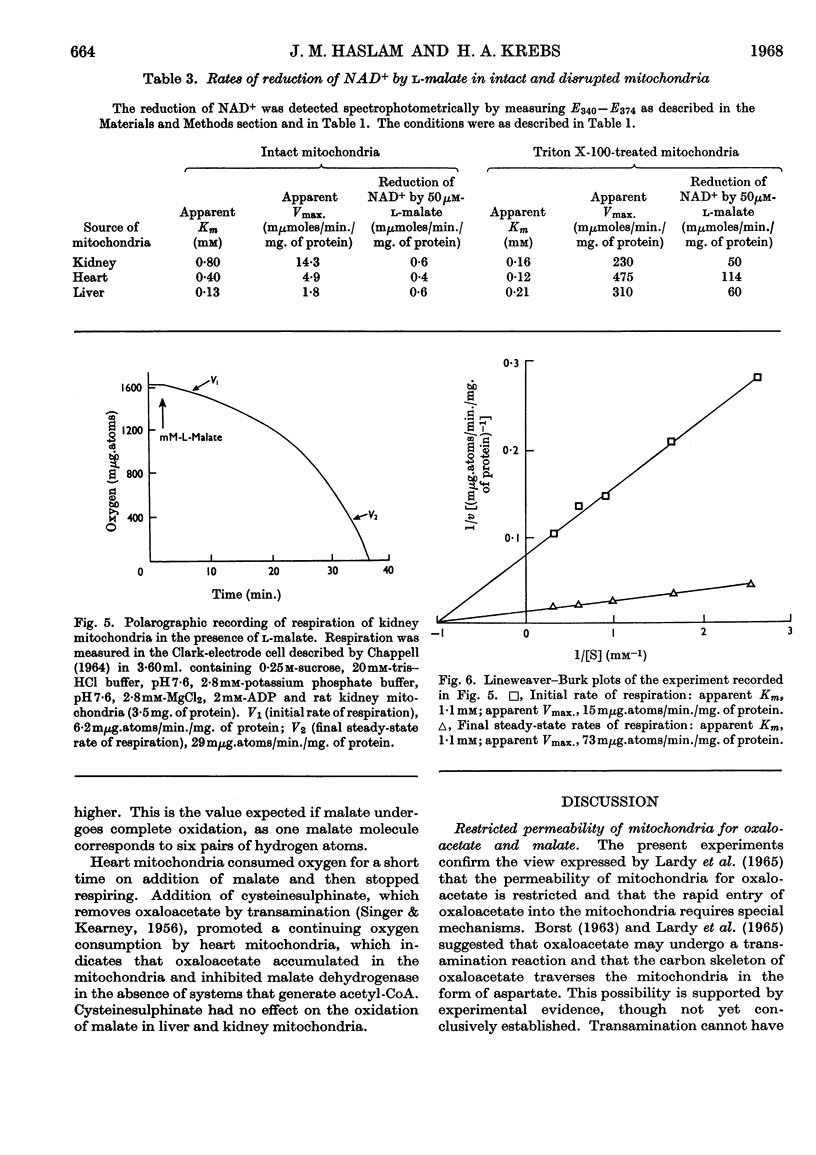
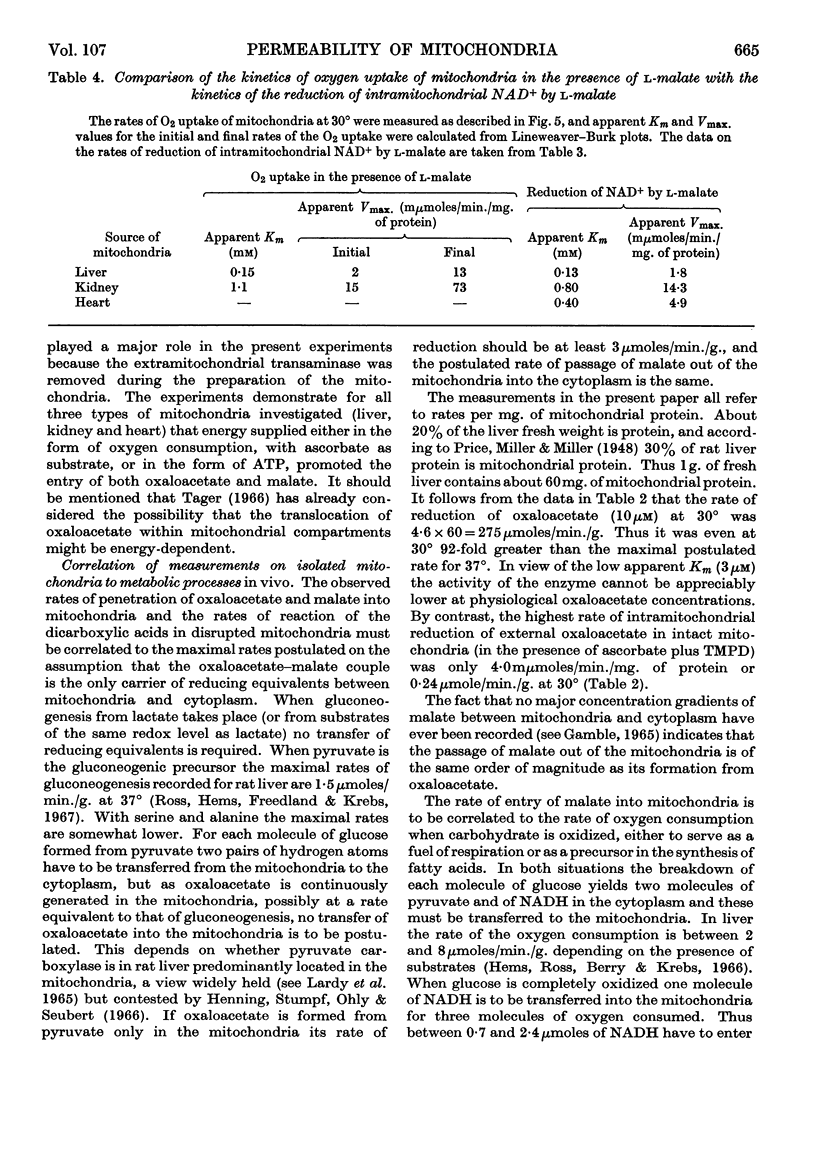
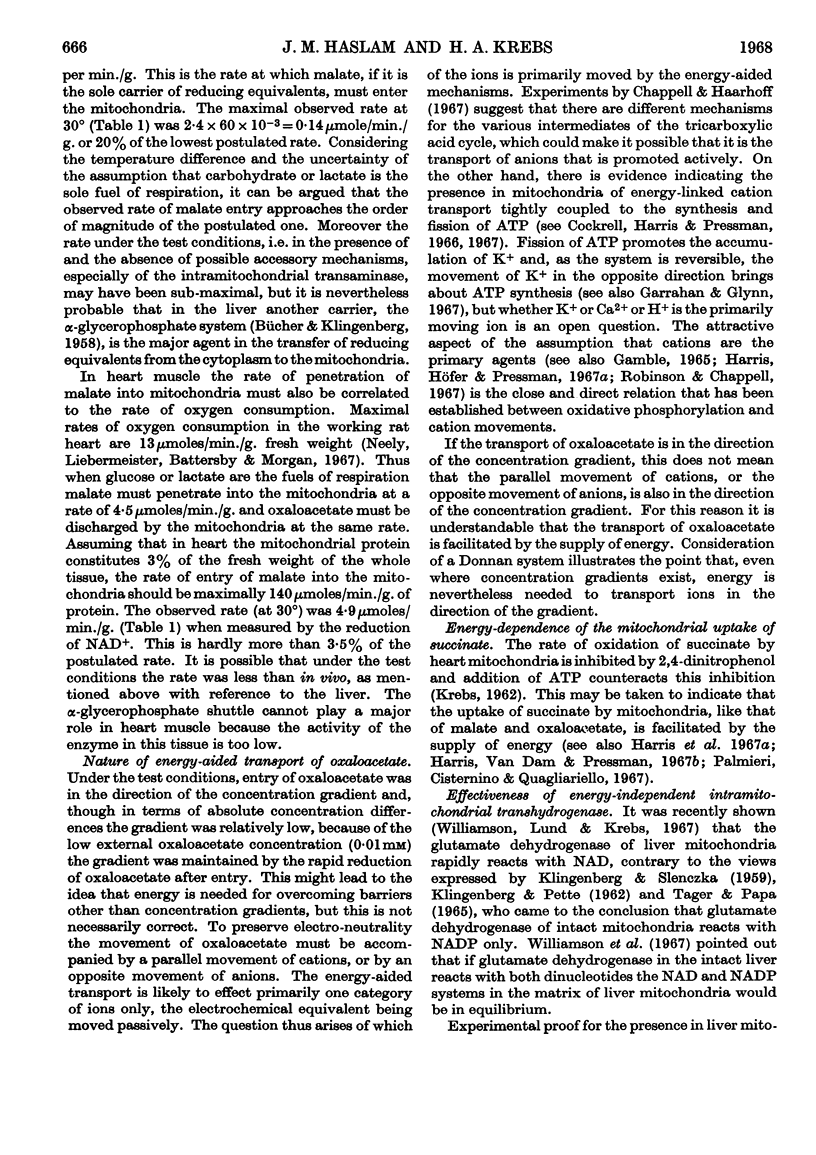
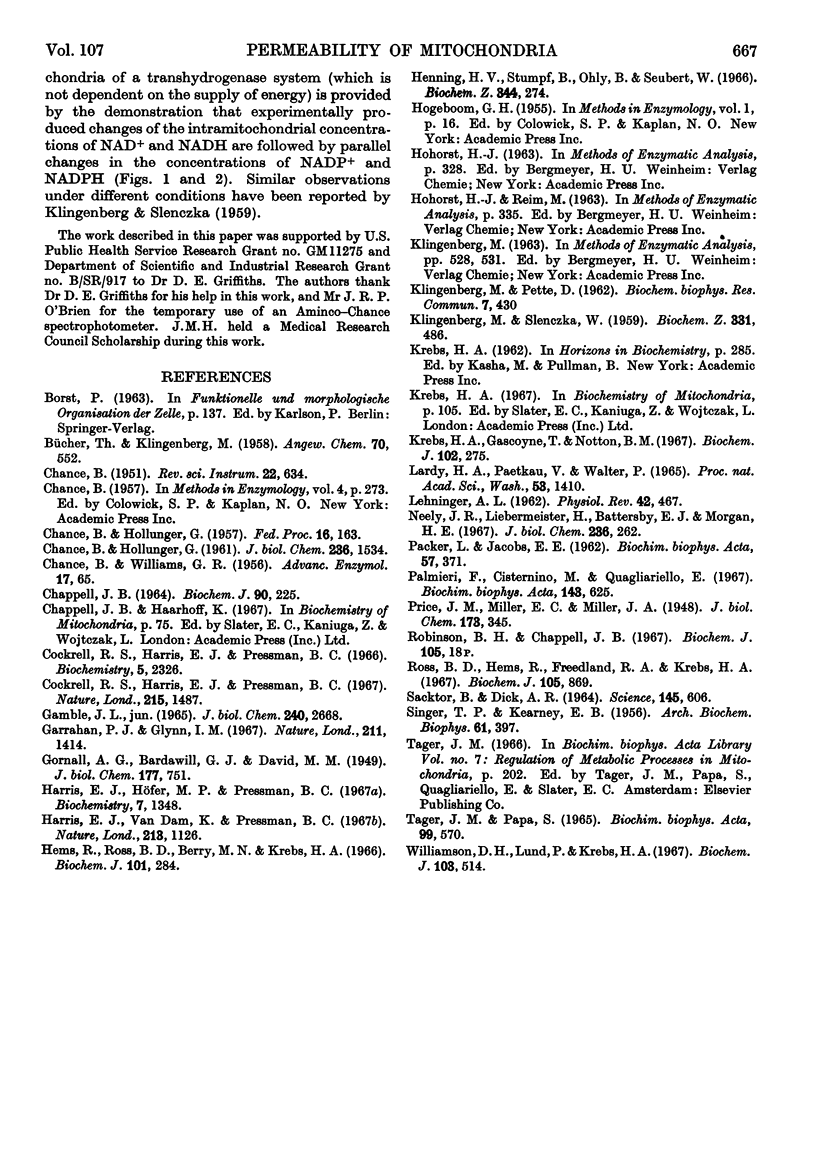
Selected References
These references are in PubMed. This may not be the complete list of references from this article.
- CHANCE B., HOLLUNGER G. The interaction of energy and electron transfer reactions in mitochondria. I. General properties and nature of the products of succinate-linked reduction of pyridine nucleotide. J Biol Chem. 1961 May;236:1534–1543. [PubMed] [Google Scholar]
- CHANCE B., WILLIAMS G. R. The respiratory chain and oxidative phosphorylation. Adv Enzymol Relat Subj Biochem. 1956;17:65–134. doi: 10.1002/9780470122624.ch2. [DOI] [PubMed] [Google Scholar]
- Chappell J. B. The oxidation of citrate, isocitrate and cis-aconitate by isolated mitochondria. Biochem J. 1964 Feb;90(2):225–237. doi: 10.1042/bj0900225. [DOI] [PMC free article] [PubMed] [Google Scholar]
- Cockrell R. S., Harris E. J., Pressman B. C. Energetics of potassium transport in mitochondria induced by valinomycin. Biochemistry. 1966 Jul;5(7):2326–2335. doi: 10.1021/bi00871a022. [DOI] [PubMed] [Google Scholar]
- Cockrell R. S., Harris E. J., Pressman B. C. Synthesis of ATP driven by a potassium gradient in mitochondria. Nature. 1967 Sep 30;215(5109):1487–1488. doi: 10.1038/2151487a0. [DOI] [PubMed] [Google Scholar]
- GAMBLE J. L., Jr ACCUMULATION OF CITRATE AND MALATE BY MITOCHONDRIA. J Biol Chem. 1965 Jun;240:2668–2672. [PubMed] [Google Scholar]
- Garrahan P. J., Glynn I. M. Driving the sodium pump backwards to form adenosine triphosphate. Nature. 1966 Sep 24;211(5056):1414–1415. doi: 10.1038/2111414a0. [DOI] [PubMed] [Google Scholar]
- Harris E. J., Höfer M. P., Pressman B. C. Stimulation of mitochondrial respiration and phosphorylation by transport-inducing antibiotics. Biochemistry. 1967 May;6(5):1348–1360. doi: 10.1021/bi00857a018. [DOI] [PubMed] [Google Scholar]
- Harris E. J., van Dam K., Pressman B. C. Dependence of uptake of succinate by mitochondria on energy and its relation to potassium retention. Nature. 1967 Mar 18;213(5081):1126–1127. doi: 10.1038/2131126a0. [DOI] [PubMed] [Google Scholar]
- Hems R., Ross B. D., Berry M. N., Krebs H. A. Gluconeogenesis in the perfused rat liver. Biochem J. 1966 Nov;101(2):284–292. doi: 10.1042/bj1010284. [DOI] [PMC free article] [PubMed] [Google Scholar]
- KLINGENBERG M., PETTE D. Proportions of mitochondrial enzymes and pyridine nucleotides. Biochem Biophys Res Commun. 1962 Jun 4;7:430–432. doi: 10.1016/0006-291x(62)90329-7. [DOI] [PubMed] [Google Scholar]
- Krebs H. A., Gascoyne T., Notton B. M. Generation of extramitochondrial reducing power in gluconeogenesis. Biochem J. 1967 Jan;102(1):275–282. doi: 10.1042/bj1020275. [DOI] [PMC free article] [PubMed] [Google Scholar]
- LEHNINGER A. L. Water uptake and extrusion by mitochondria in relation to oxidative phosphorylation. Physiol Rev. 1962 Jul;42:467–517. doi: 10.1152/physrev.1962.42.3.467. [DOI] [PubMed] [Google Scholar]
- Lardy H. A., Paetkau V., Walter P. Paths of carbon in gluconeogenesis and lipogenesis: the role of mitochondria in supplying precursors of phosphoenolpyruvate. Proc Natl Acad Sci U S A. 1965 Jun;53(6):1410–1415. doi: 10.1073/pnas.53.6.1410. [DOI] [PMC free article] [PubMed] [Google Scholar]
- PACKER L., JACOBS E. E. Coupling of phosphorylation to terminal segments of the mitochondrial respiratory chain. Biochim Biophys Acta. 1962 Feb 26;57:371–373. doi: 10.1016/0006-3002(62)91132-0. [DOI] [PubMed] [Google Scholar]
- Palmieri F., Cisternino M., Quagliariello E. Inhibition of uptake and oxidation of succinate in rat-liver mitochondria. Biochim Biophys Acta. 1967;143(3):625–627. doi: 10.1016/0005-2728(67)90068-0. [DOI] [PubMed] [Google Scholar]
- Ross B. D., Hems R., Freedland R. A., Krebs H. A. Carbohydrate metabolism of the perfused rat liver. Biochem J. 1967 Nov;105(2):869–875. doi: 10.1042/bj1050869. [DOI] [PMC free article] [PubMed] [Google Scholar]
- SACKTOR B., DICK A. R. OXIDATION OF EXTRAMITOCHONDRIAL DIPHOSPHOPYRIDINE NUCLEOTIDE BY VARIOUS TISSUES OF THE MOUSE. Science. 1964 Aug 7;145(3632):606–607. doi: 10.1126/science.145.3632.606. [DOI] [PubMed] [Google Scholar]
- SINGER T. P., KEARNEY E. B. Intermediary metabolism of L-cysteinesulfinic acid in animal tissues. Arch Biochem Biophys. 1956 Apr;61(2):397–409. doi: 10.1016/0003-9861(56)90363-0. [DOI] [PubMed] [Google Scholar]
- Tager T. M., Papa S. On the nicotinamide nucleotide specificity of glutamate dehydrogenase in rat-liver mitochondria. Biochim Biophys Acta. 1965 Jun 22;99(3):570–572. doi: 10.1016/s0926-6593(65)80217-x. [DOI] [PubMed] [Google Scholar]
- Williamson D. H., Lund P., Krebs H. A. The redox state of free nicotinamide-adenine dinucleotide in the cytoplasm and mitochondria of rat liver. Biochem J. 1967 May;103(2):514–527. doi: 10.1042/bj1030514. [DOI] [PMC free article] [PubMed] [Google Scholar]