Abstract
Calcium currents (ICa) were recorded in frog skeletal muscle fibres using the three-micro-electrode voltage-clamp technique. The sartorius muscle was bathed in TEA methanesulphonate saline with 350 mM-sucrose. 5 mM-3,4-diaminopyridine was added to the saline to minimize K+ currents. The I-V relationship for peak Ca2+ currents showed that ICa was detected at -40 mV and reached a maximum value at ca. -10 mV. No net inward current was recorded at potentials positive to ca. +40 mV. Remaining K+ currents (IK) were recorded by replacing 10 mM-Ca2+ with 5.5 mM-Co2+. They were not noticeably time-dependent up to +20 mV and would tend to diminish the amplitude of ICa without greatly affecting its time course. ICa tail currents could be separated from non-linear capacity currents. Tail currents were measured 5 msec after repolarization and extrapolated to the end of the pulse. ICa tail-current amplitudes at EK were measured with pulses of different durations. The envelope of tail-current amplitudes declined with a time course similar or identical to that of inward current during a maintained depolarization. Consequently, the decline of inward current cannot be explained by an increase of outward IK with time. ICa inactivated with 9 sec prepulses which did not elicit detectable ICa. The fitted h infinity curve had a mid point of -33.0 mV and a steepness of 6.3 mV. ICa between -30 mV and +20 mV could be described adequately using the Hodgkin-Huxley m3h relationship. The fitted m infinity curve had a mid point of -35.2 mV and a steepness of 9.9 mV. The limiting Ca2+ permeability PCa was 1.4 +/- 0.4 X 10(-4) cm/sec.
Full text
PDF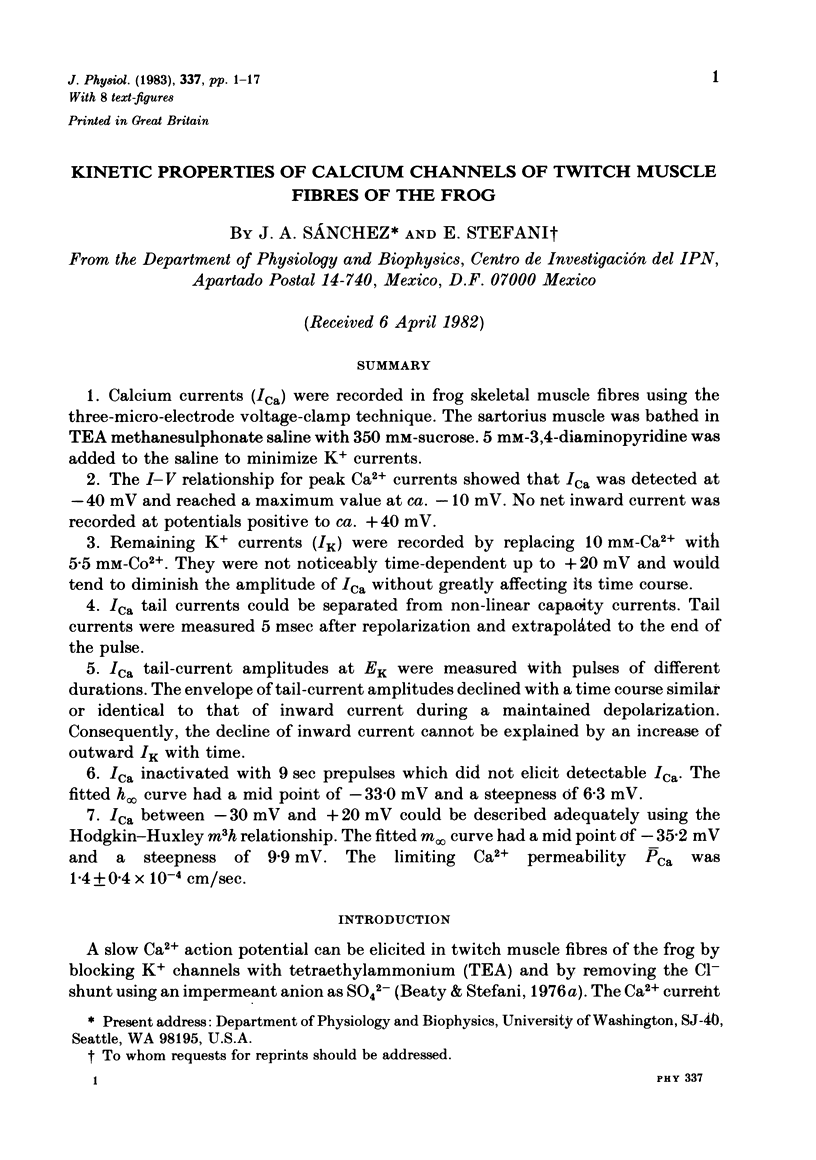
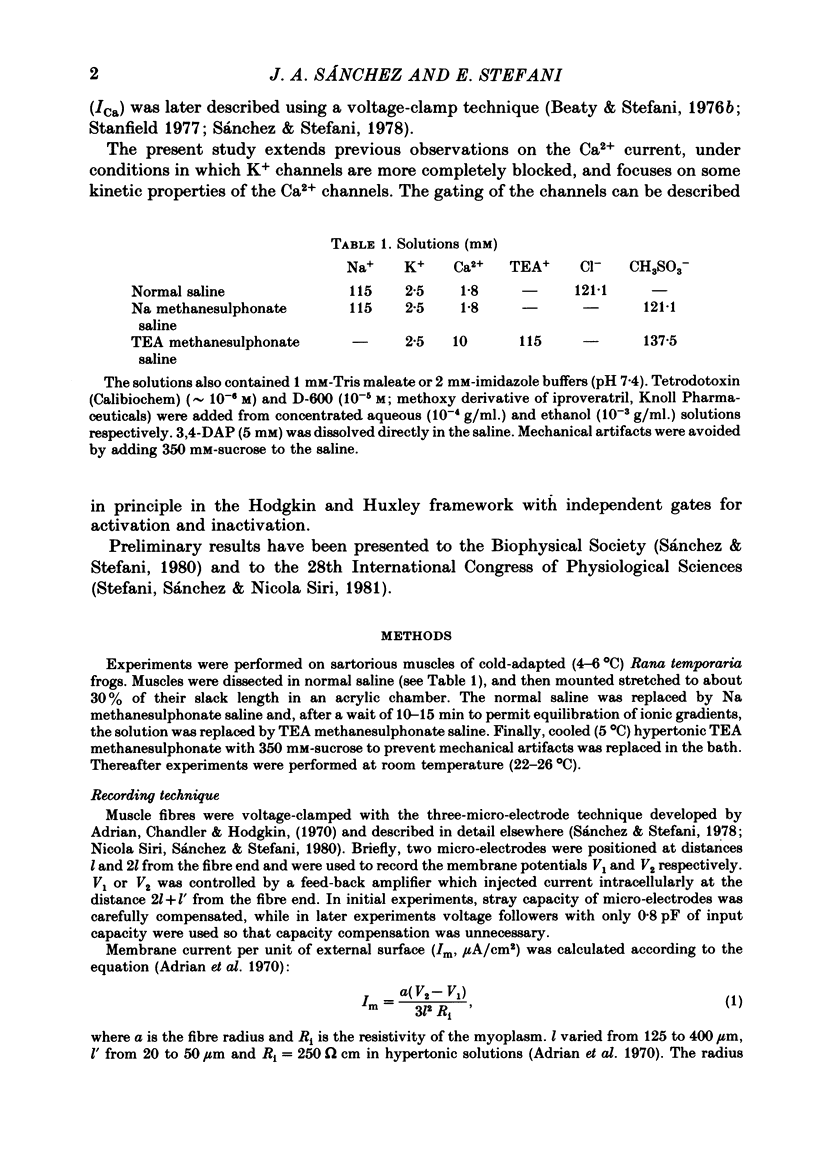
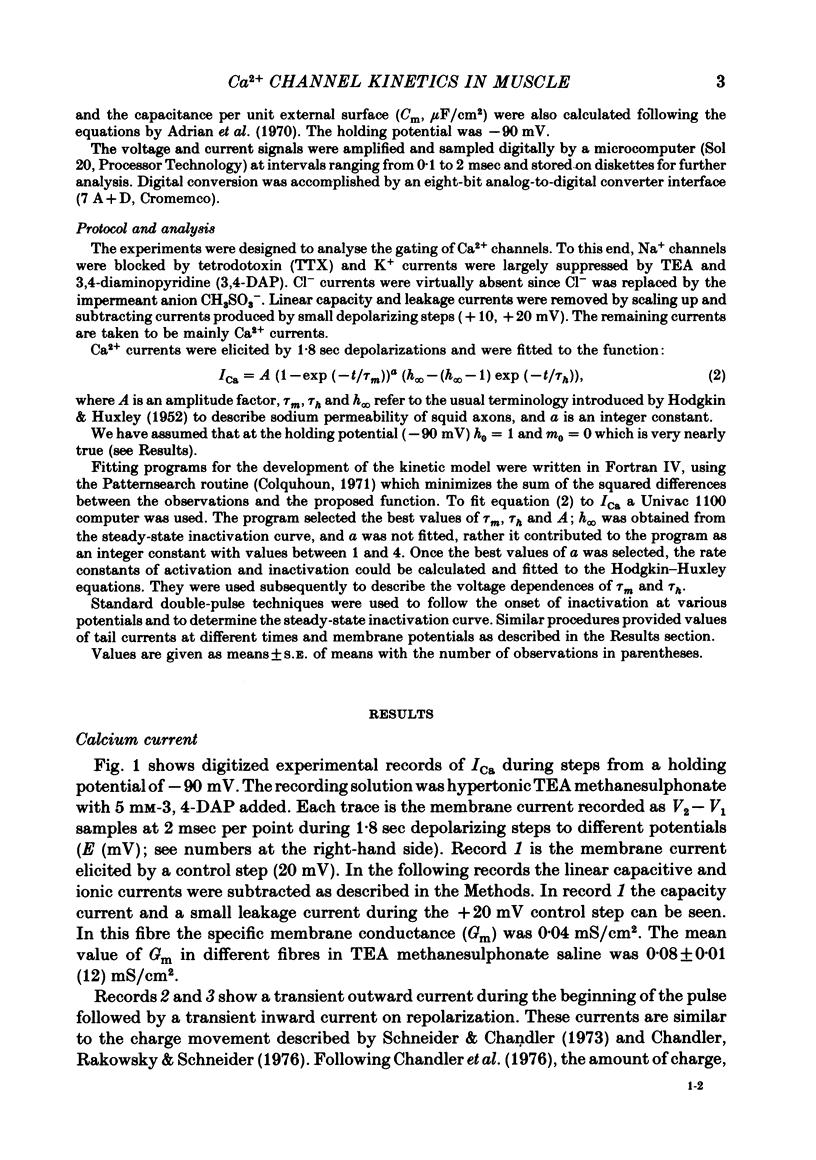
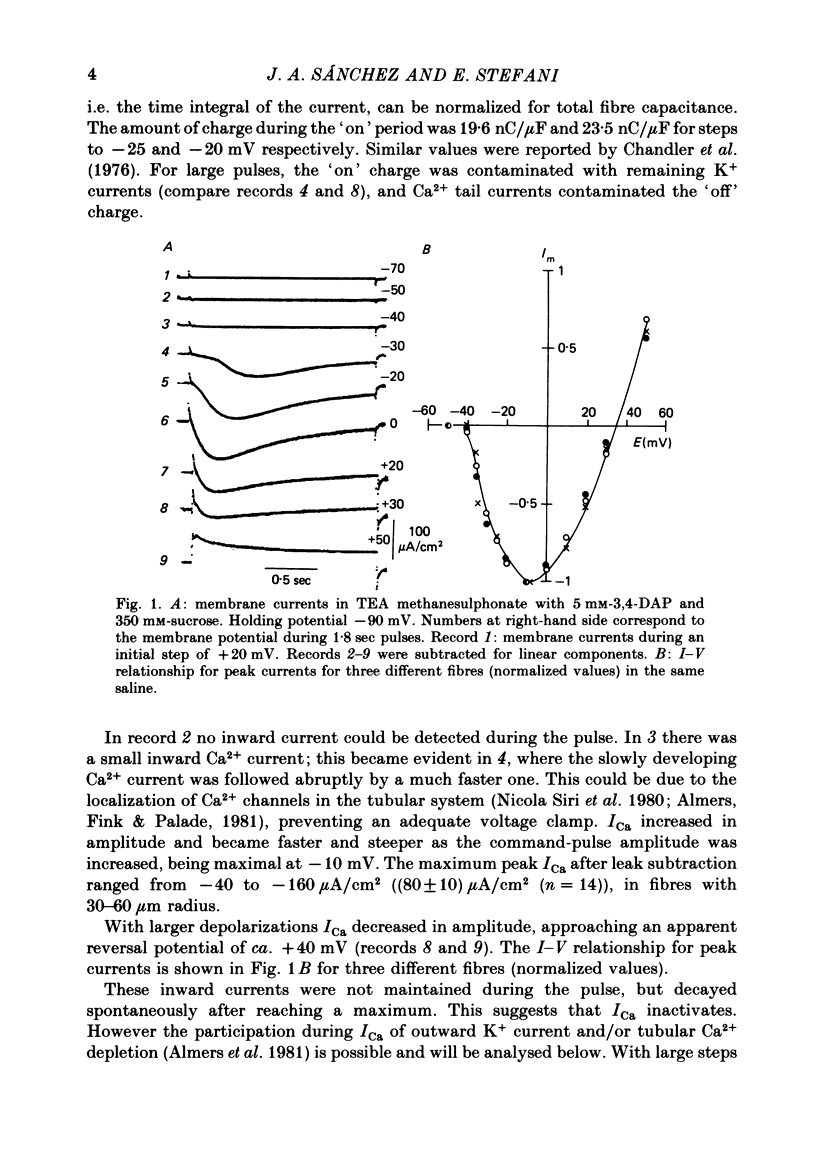
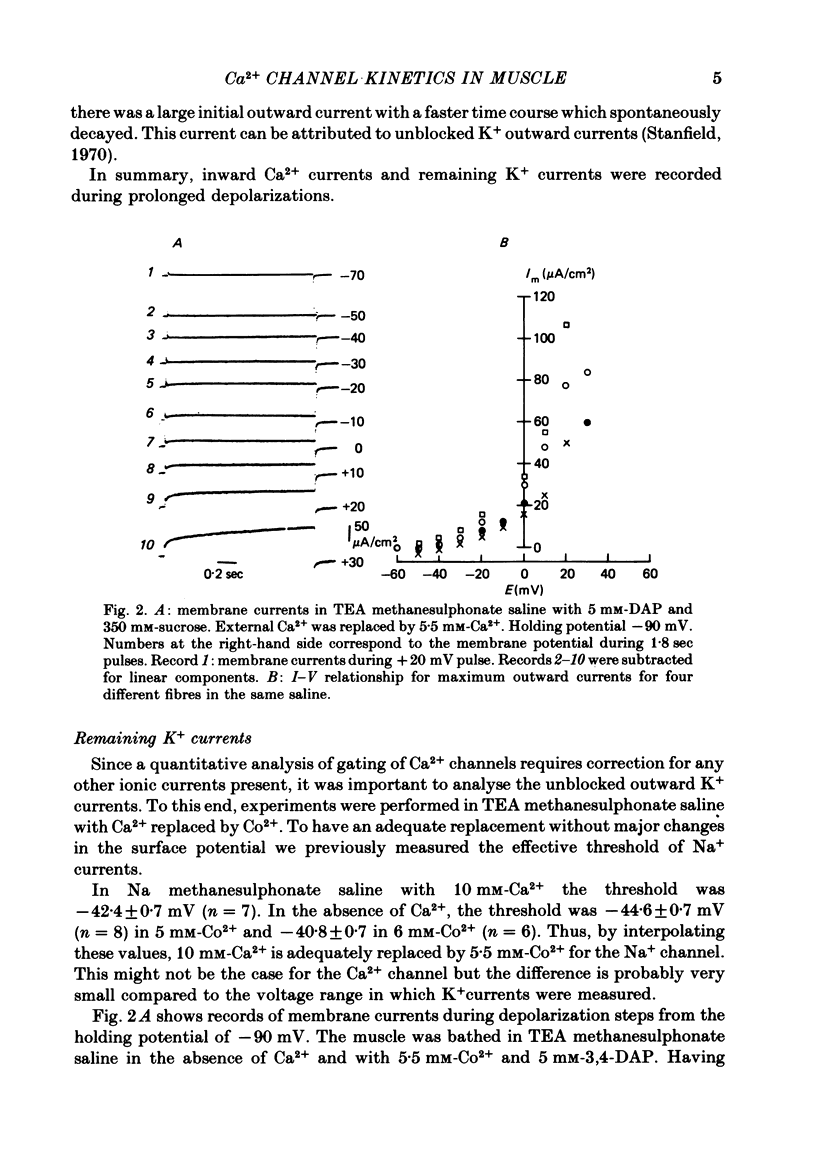
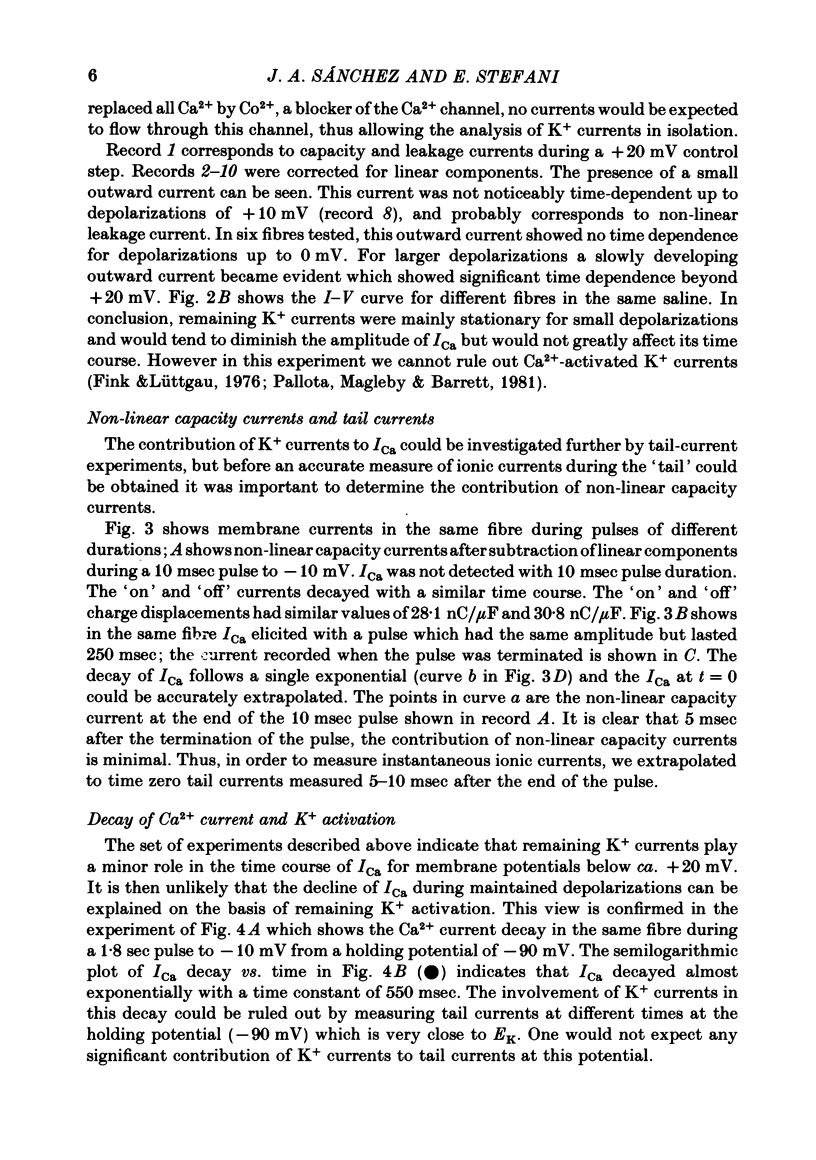
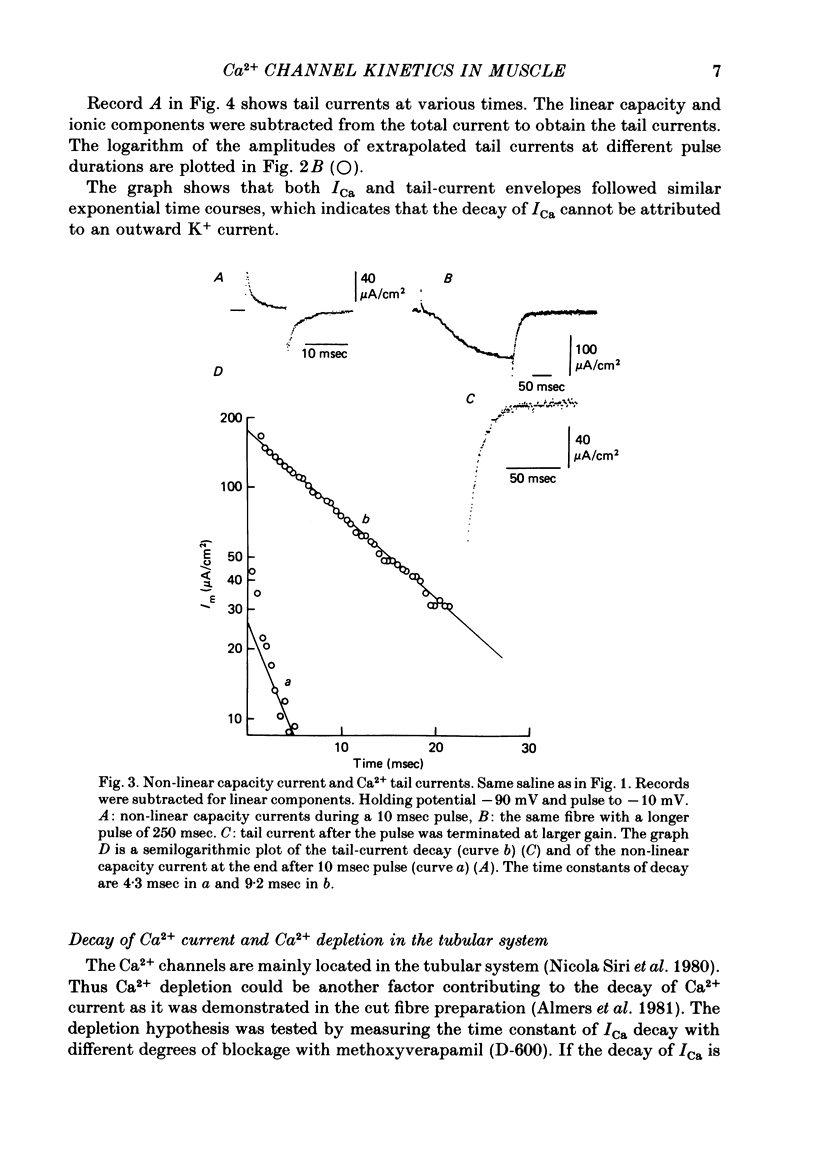
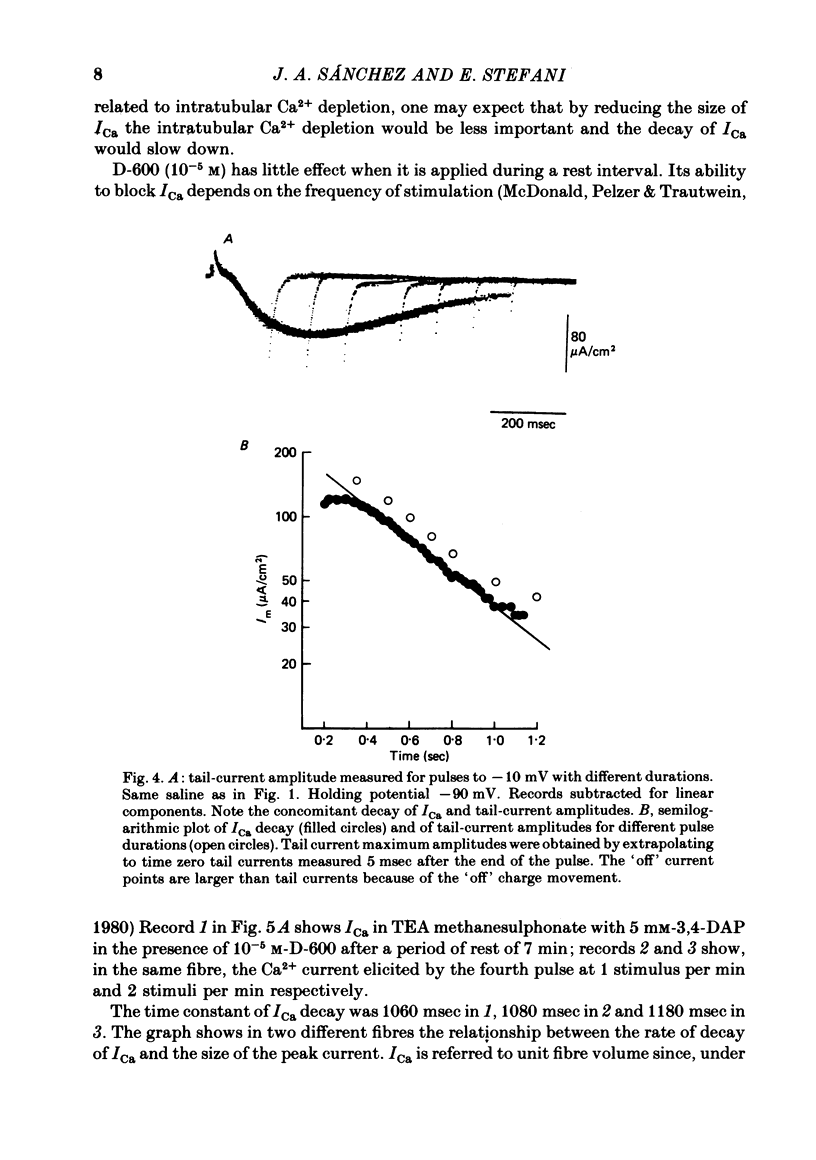
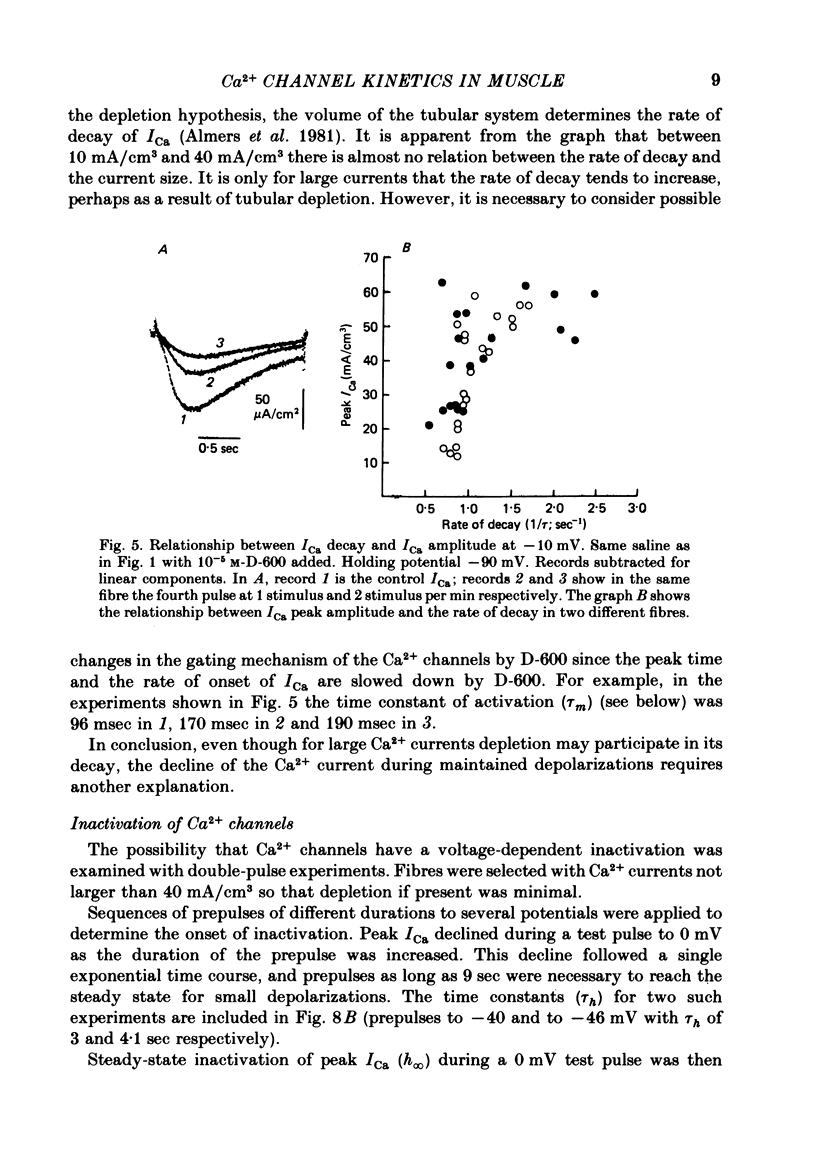
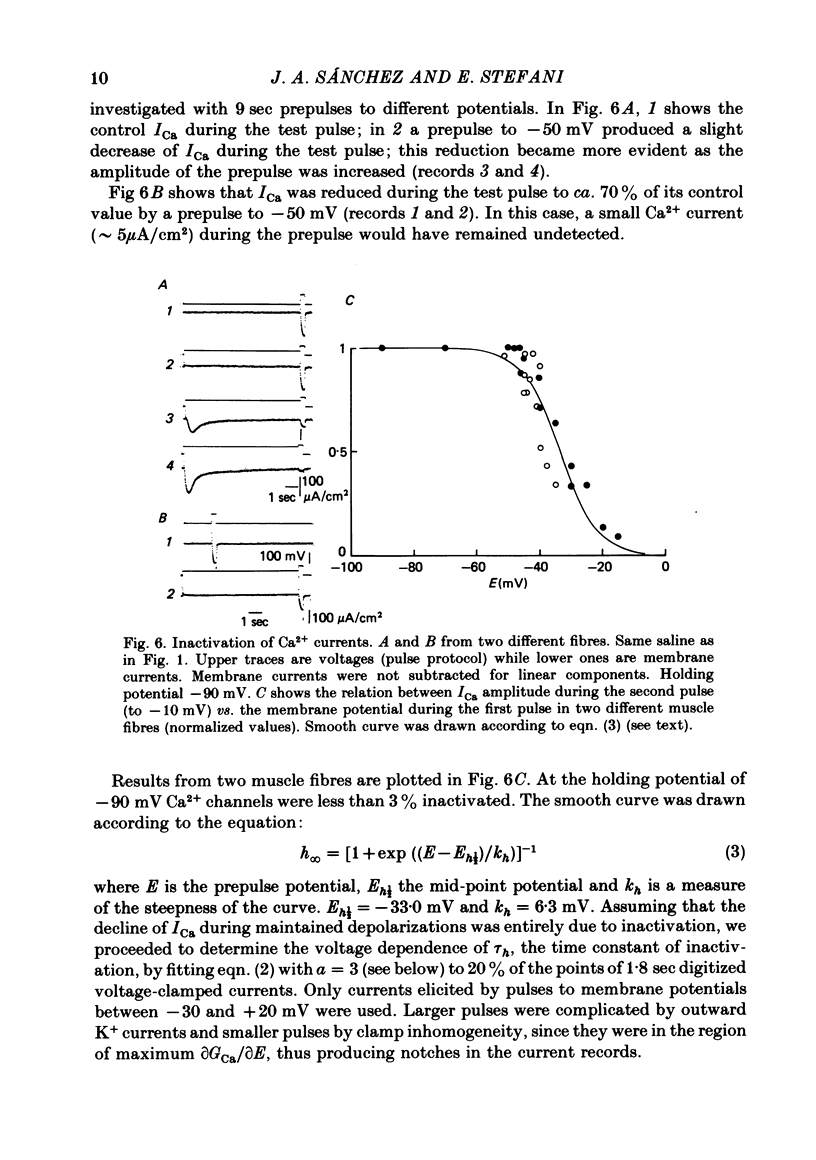
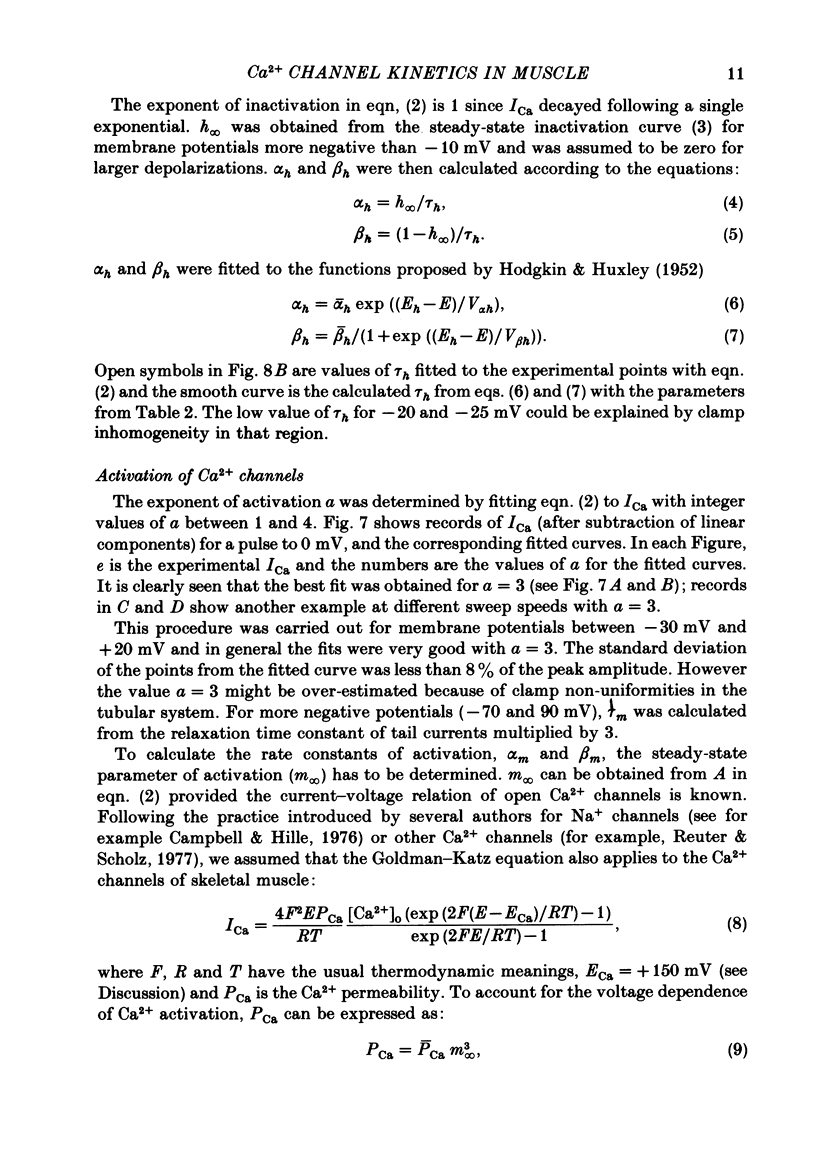
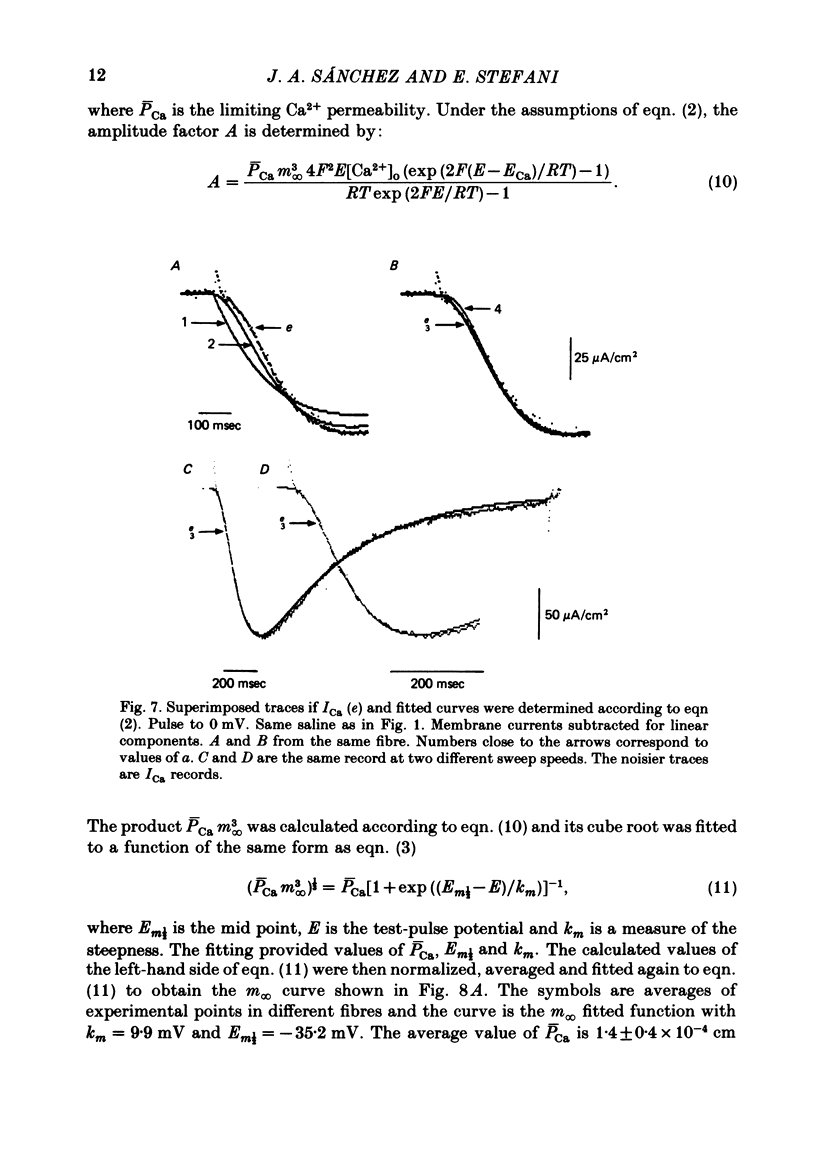
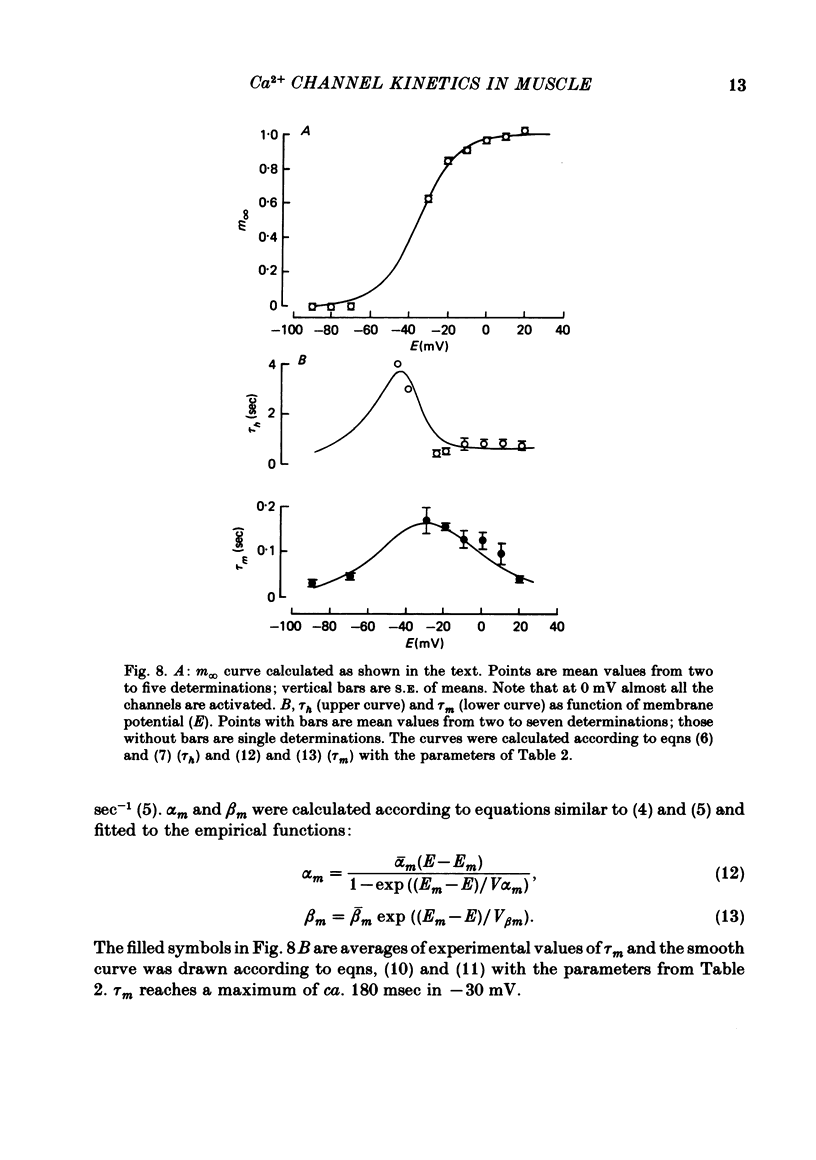
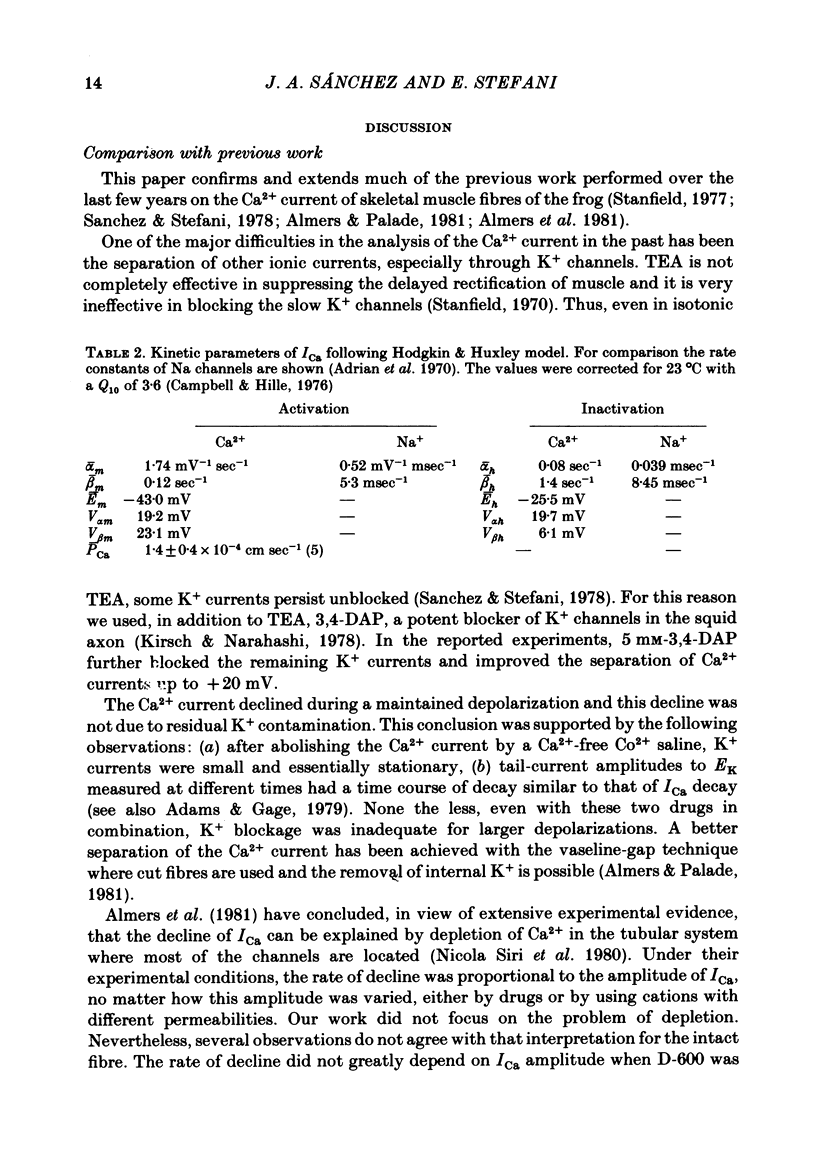
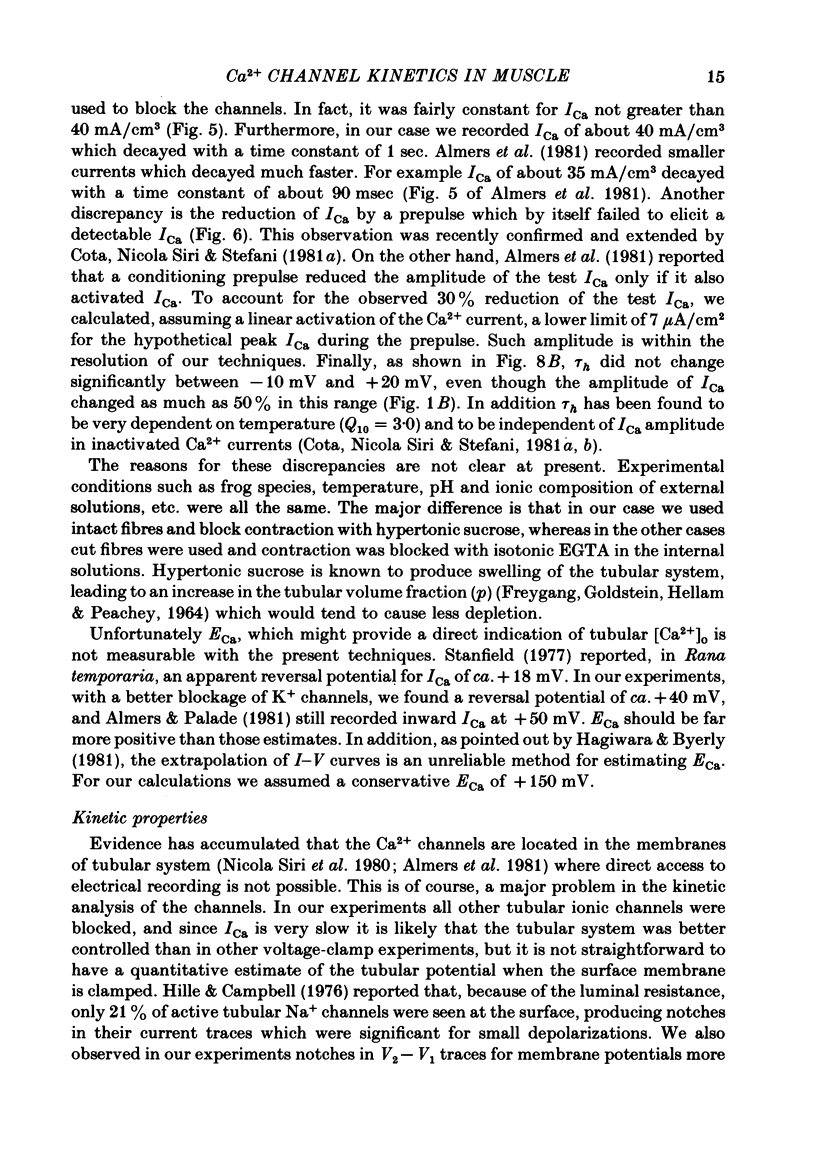
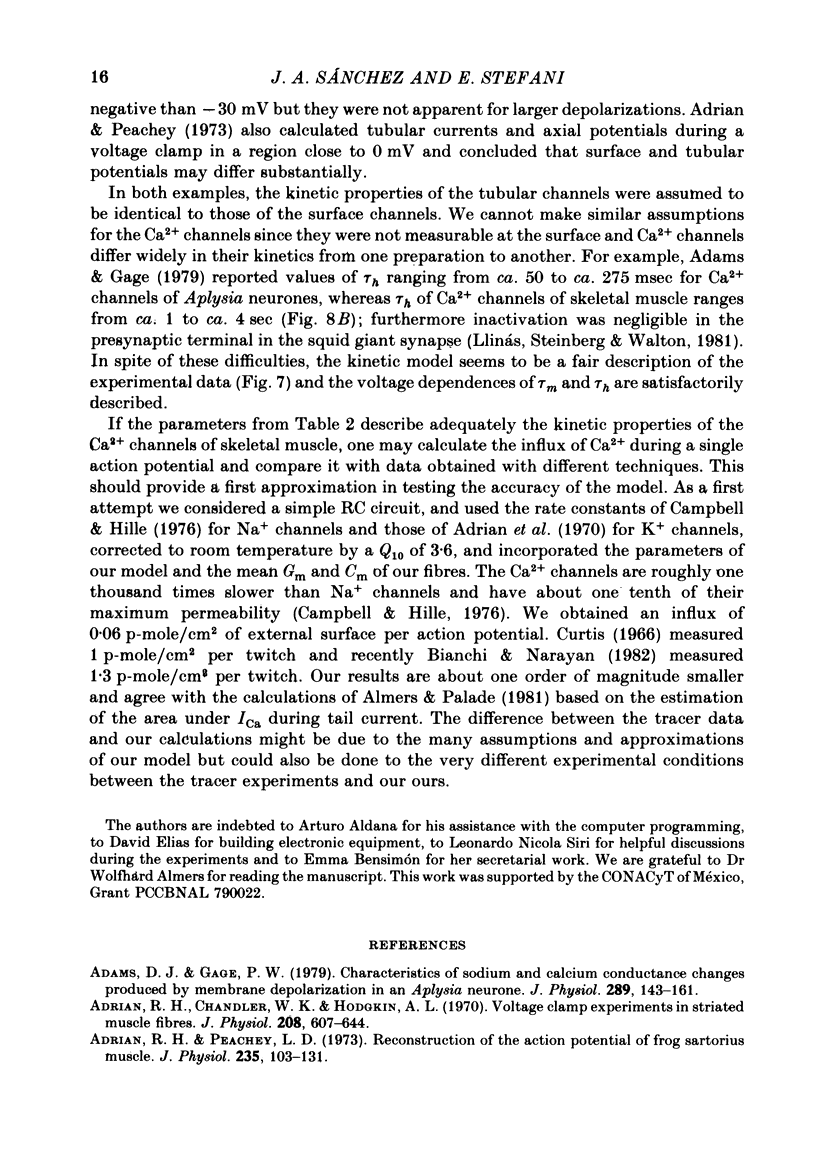
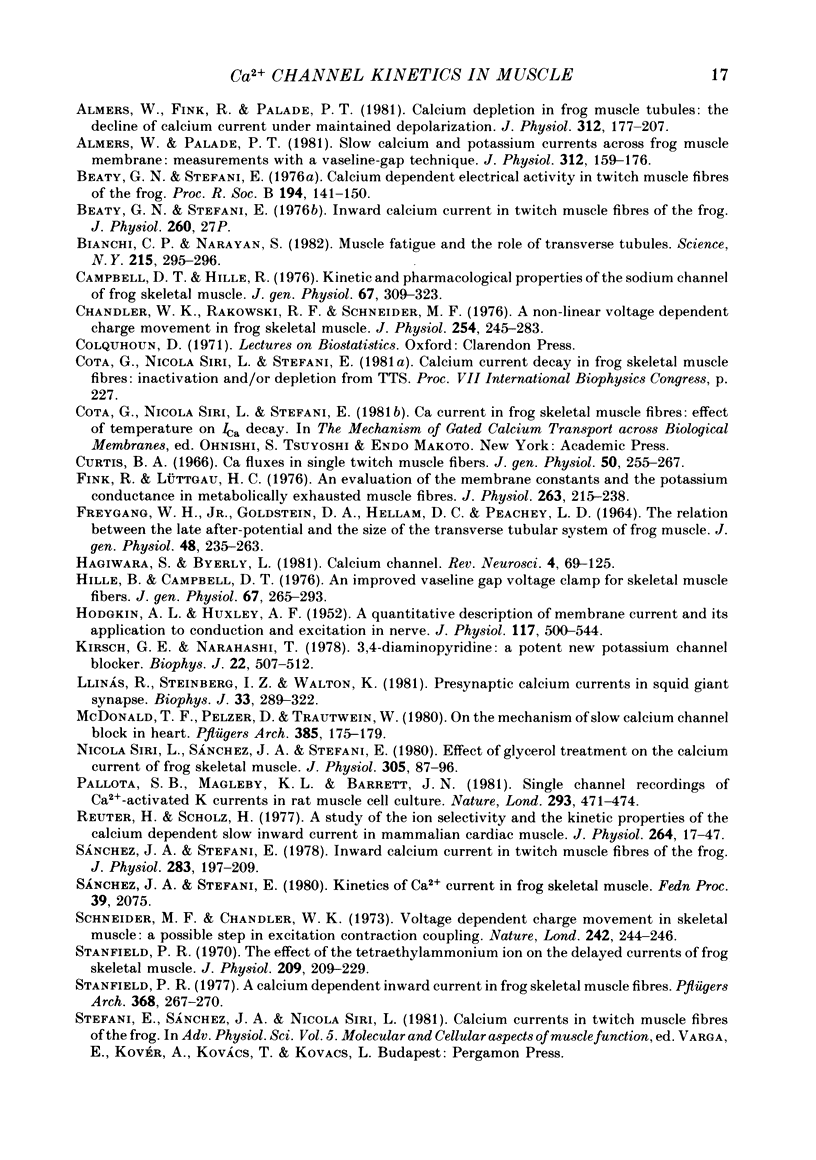
Selected References
These references are in PubMed. This may not be the complete list of references from this article.
- Adams D. J., Gage P. W. Characteristics of sodium and calcium conductance changes produced by membrane depolarization in an Aplysia neurone. J Physiol. 1979 Apr;289:143–161. doi: 10.1113/jphysiol.1979.sp012729. [DOI] [PMC free article] [PubMed] [Google Scholar]
- Adrian R. H., Chandler W. K., Hodgkin A. L. Voltage clamp experiments in striated muscle fibres. J Physiol. 1970 Jul;208(3):607–644. doi: 10.1113/jphysiol.1970.sp009139. [DOI] [PMC free article] [PubMed] [Google Scholar]
- Adrian R. H., Peachey L. D. Reconstruction of the action potential of frog sartorius muscle. J Physiol. 1973 Nov;235(1):103–131. doi: 10.1113/jphysiol.1973.sp010380. [DOI] [PMC free article] [PubMed] [Google Scholar]
- Almers W., Fink R., Palade P. T. Calcium depletion in frog muscle tubules: the decline of calcium current under maintained depolarization. J Physiol. 1981 Mar;312:177–207. doi: 10.1113/jphysiol.1981.sp013623. [DOI] [PMC free article] [PubMed] [Google Scholar]
- Almers W., Palade P. T. Slow calcium and potassium currents across frog muscle membrane: measurements with a vaseline-gap technique. J Physiol. 1981 Mar;312:159–176. doi: 10.1113/jphysiol.1981.sp013622. [DOI] [PMC free article] [PubMed] [Google Scholar]
- Beaty G. N., Stefani E. Calcium dependent electrical activity in twitch muscle fibres of the frog. Proc R Soc Lond B Biol Sci. 1976 Aug 27;194(1114):141–150. doi: 10.1098/rspb.1976.0070. [DOI] [PubMed] [Google Scholar]
- Beaty G. N., Stefani E. Inward calcium current in twitch muscle fibres of the frog [proceedings]. J Physiol. 1976 Sep;260(2):27P–27P. [PubMed] [Google Scholar]
- Bianchi C. P., Narayan S. Muscle fatigue and the role of transverse tubules. Science. 1982 Jan 15;215(4530):295–296. doi: 10.1126/science.7053577. [DOI] [PubMed] [Google Scholar]
- Campbell D. T., Hille B. Kinetic and pharmacological properties of the sodium channel of frog skeletal muscle. J Gen Physiol. 1976 Mar;67(3):309–323. doi: 10.1085/jgp.67.3.309. [DOI] [PMC free article] [PubMed] [Google Scholar]
- Chandler W. K., Rakowski R. F., Schneider M. F. A non-linear voltage dependent charge movement in frog skeletal muscle. J Physiol. 1976 Jan;254(2):245–283. doi: 10.1113/jphysiol.1976.sp011232. [DOI] [PMC free article] [PubMed] [Google Scholar]
- Curtis B. A. Ca fluxes in single twitch muscle fibers. J Gen Physiol. 1966 Nov;50(2):255–267. doi: 10.1085/jgp.50.2.255. [DOI] [PMC free article] [PubMed] [Google Scholar]
- FREYGANG W. H., Jr, GOLDSTEIN D. A., HELLAM D. C., PEACHEY L. D. THE RELATION BETWEEN THE LATE AFTER-POTENTIAL AND THE SIZE OF THE TRANSVERSE TUBULAR SYSTEM OF FROG MUSCLE. J Gen Physiol. 1964 Nov;48:235–263. doi: 10.1085/jgp.48.2.235. [DOI] [PMC free article] [PubMed] [Google Scholar]
- Fink R., Lüttgau H. C. An evaluation of the membrane constants and the potassium conductance in metabolically exhausted muscle fibres. J Physiol. 1976 Dec;263(2):215–238. doi: 10.1113/jphysiol.1976.sp011629. [DOI] [PMC free article] [PubMed] [Google Scholar]
- HODGKIN A. L., HUXLEY A. F. A quantitative description of membrane current and its application to conduction and excitation in nerve. J Physiol. 1952 Aug;117(4):500–544. doi: 10.1113/jphysiol.1952.sp004764. [DOI] [PMC free article] [PubMed] [Google Scholar]
- Hagiwara S., Byerly L. Calcium channel. Annu Rev Neurosci. 1981;4:69–125. doi: 10.1146/annurev.ne.04.030181.000441. [DOI] [PubMed] [Google Scholar]
- Hille B., Campbell D. T. An improved vaseline gap voltage clamp for skeletal muscle fibers. J Gen Physiol. 1976 Mar;67(3):265–293. doi: 10.1085/jgp.67.3.265. [DOI] [PMC free article] [PubMed] [Google Scholar]
- Kirsch G. E., Narahashi T. 3,4-diaminopyridine. A potent new potassium channel blocker. Biophys J. 1978 Jun;22(3):507–512. doi: 10.1016/S0006-3495(78)85503-9. [DOI] [PMC free article] [PubMed] [Google Scholar]
- Llinás R., Steinberg I. Z., Walton K. Presynaptic calcium currents in squid giant synapse. Biophys J. 1981 Mar;33(3):289–321. doi: 10.1016/S0006-3495(81)84898-9. [DOI] [PMC free article] [PubMed] [Google Scholar]
- McDonald T. F., Pelzer D., Trautwein W. On the mechanism of slow calcium channel block in heart. Pflugers Arch. 1980 May;385(2):175–179. doi: 10.1007/BF00588699. [DOI] [PubMed] [Google Scholar]
- Pallotta B. S., Magleby K. L., Barrett J. N. Single channel recordings of Ca2+-activated K+ currents in rat muscle cell culture. Nature. 1981 Oct 8;293(5832):471–474. doi: 10.1038/293471a0. [DOI] [PubMed] [Google Scholar]
- Reuter H., Scholz H. A study of the ion selectivity and the kinetic properties of the calcium dependent slow inward current in mammalian cardiac muscle. J Physiol. 1977 Jan;264(1):17–47. doi: 10.1113/jphysiol.1977.sp011656. [DOI] [PMC free article] [PubMed] [Google Scholar]
- Sanchez J. A., Stefani E. Inward calcium current in twitch muscle fibres of the frog. J Physiol. 1978 Oct;283:197–209. doi: 10.1113/jphysiol.1978.sp012496. [DOI] [PMC free article] [PubMed] [Google Scholar]
- Schneider M. F., Chandler W. K. Voltage dependent charge movement of skeletal muscle: a possible step in excitation-contraction coupling. Nature. 1973 Mar 23;242(5395):244–246. doi: 10.1038/242244a0. [DOI] [PubMed] [Google Scholar]
- Siri L. N., Sánchez J. A., Stefani E. Effect of glycerol treatment on the calcium current of frog skeletal muscle. J Physiol. 1980 Aug;305:87–96. doi: 10.1113/jphysiol.1980.sp013351. [DOI] [PMC free article] [PubMed] [Google Scholar]
- Stanfield P. R. A calcium dependent inward current in frog skeletal muscle fibres. Pflugers Arch. 1977 Apr 25;368(3):267–270. doi: 10.1007/BF00585206. [DOI] [PubMed] [Google Scholar]
- Stanfield P. R. The effect of the tetraethylammonium ion on the delayed currents of frog skeletal muscle. J Physiol. 1970 Jul;209(1):209–229. doi: 10.1113/jphysiol.1970.sp009163. [DOI] [PMC free article] [PubMed] [Google Scholar]