Abstract
During prolonged periods of quiescence, the membrane potential of cells in the isolated canine coronary sinus, exposed to normal Tyrode solution containing 4 mM-K, declines to about -60 mV. The nature of the resting potential was investigated, in small strips of coronary sinus tissue mounted in a fast-flow system, by recording the membrane potential responses to sudden changes in the extracellular ionic environment. At extracellular K concentrations ([K]o) from 0 to 64 mM the resting potential was little affected by replacing all but 1 mM of external Cl ions with isethionate and methylsulphate ions. At [K]o levels from 4 to 150 mM the resting potential was reasonably well described by the Goldman-Hodgkin-Katz equation on the assumption that the intracellular K concentration ([K]i) was 155 mM and that the ratio of membrane permeability coefficients for Na and K, PNa/PK, was 0.07. In the presence of a high concentration of acetylcholine or carbachol (greater than or equal to 1 microM), the resting potentials at [K]o levels from 1 to 150 mM approximated K equilibrium potentials (EK) calculated on the assumption that [K]i was 155 mM. At [K]o levels less than or equal to 8 mM replacing most of the external Na with sucrose or Tris caused a substantial hyperpolarization, whereas application of 1-2 microM-tetrodotoxin caused only slight hyperpolarization. A transient hyperpolarization, due to enhanced electrogenic Na extrusion, was recorded on switching back to 4 mM-K following brief exposures to K-free solution; no transient hyperpolarization was recorded in the presence of 5 microM-acetylstrophanthidin. The acetylstrophanthidin itself caused a rapid depolarization of several millivolts. Preliminary conductance measurements made with two micro-electrodes in some smaller preparations indicate that the steady-state current-voltage relationship is N-shaped. We conclude that the low membrane potential of quiescent coronary sinus cells reflects not a low [K]i but rather a relatively high ratio PNa/PK, of about 0.07: the Na ions flow into the cells via predominantly TTX-insensitive pathways and are extruded by the electrogenic Na/K exchange pump, which thereby makes a substantial contribution to the resting potential.
Full text
PDF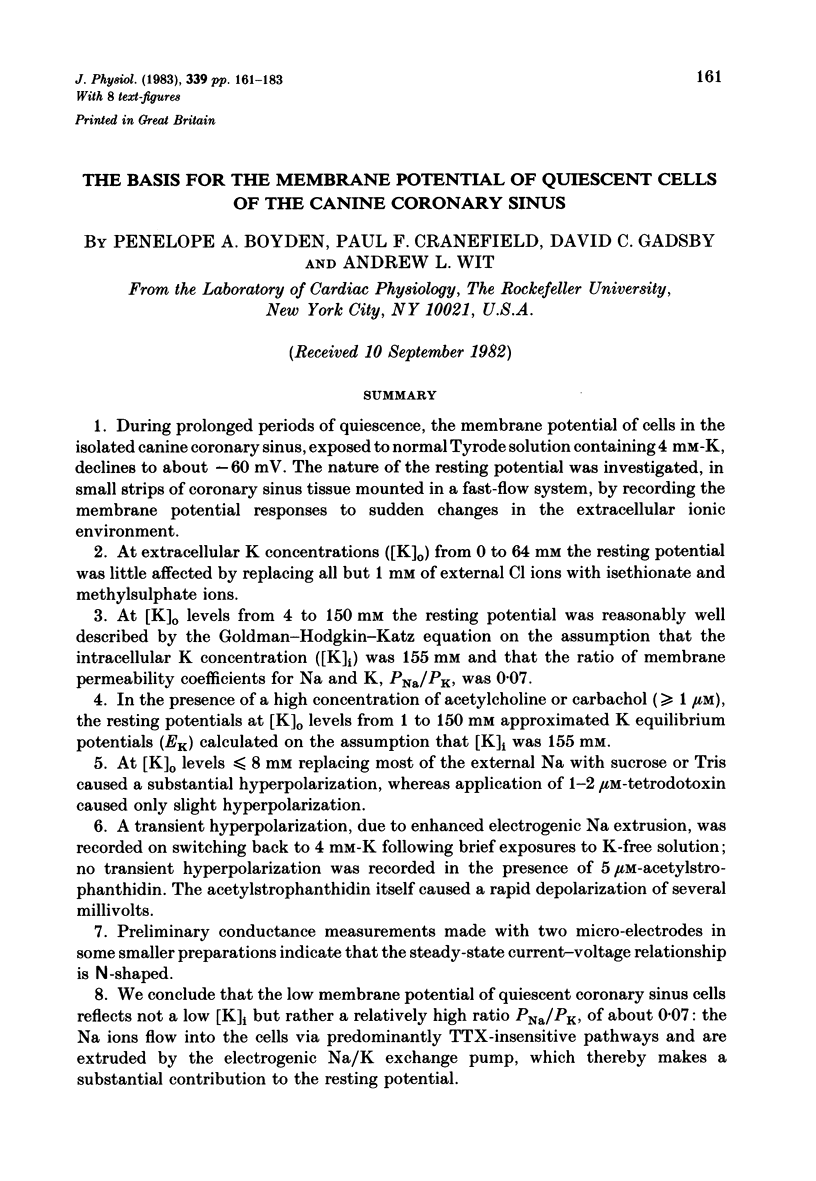
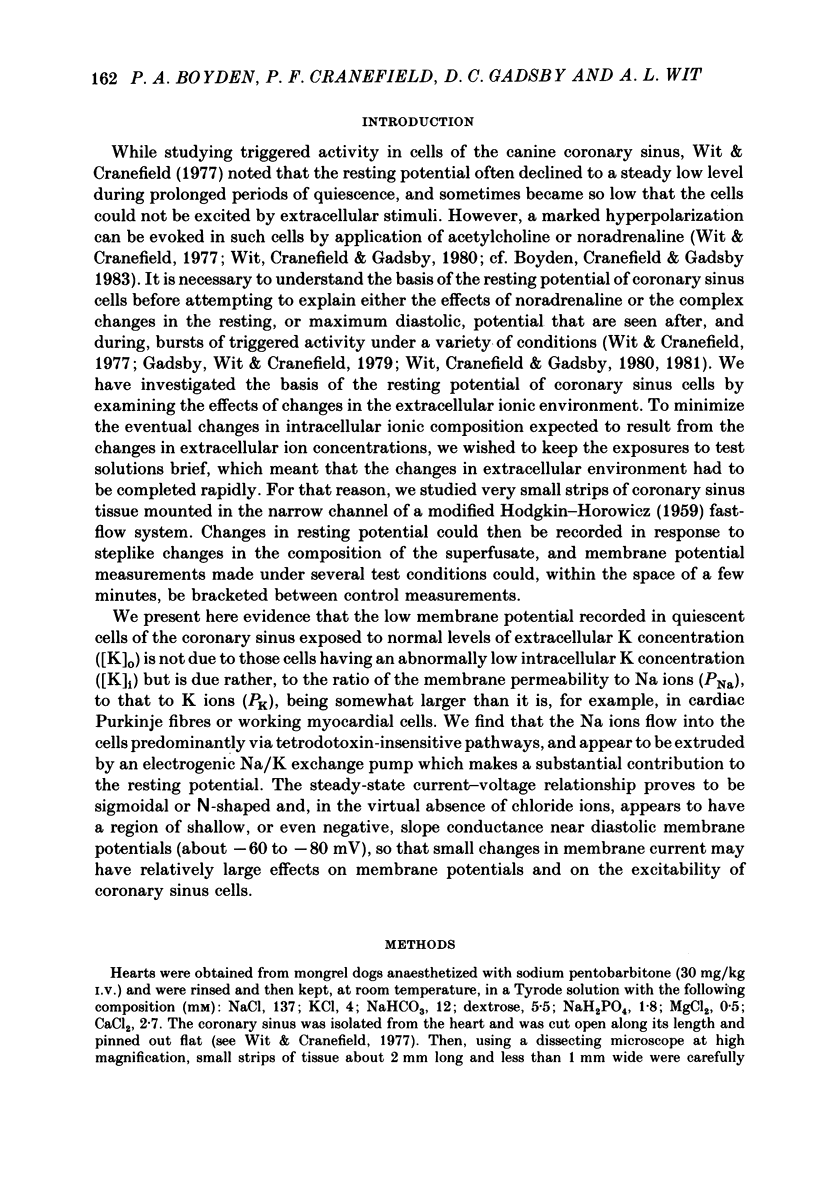
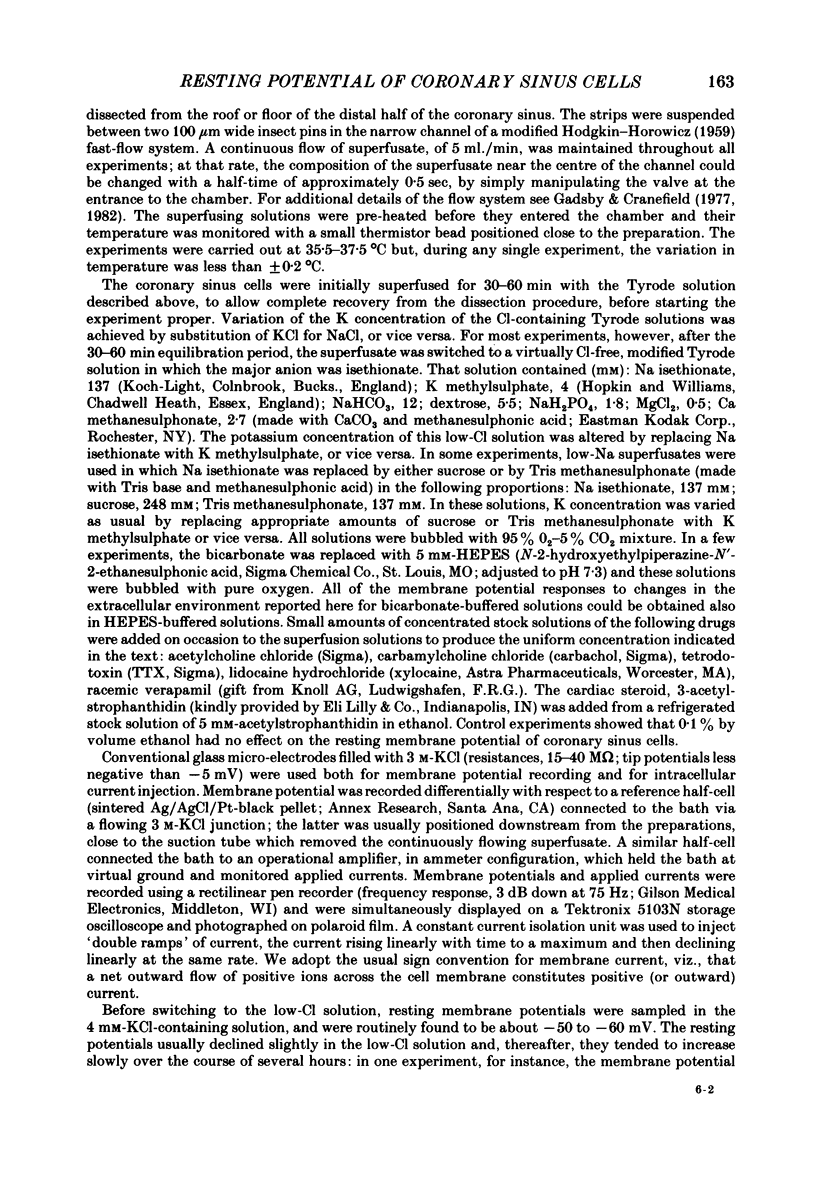
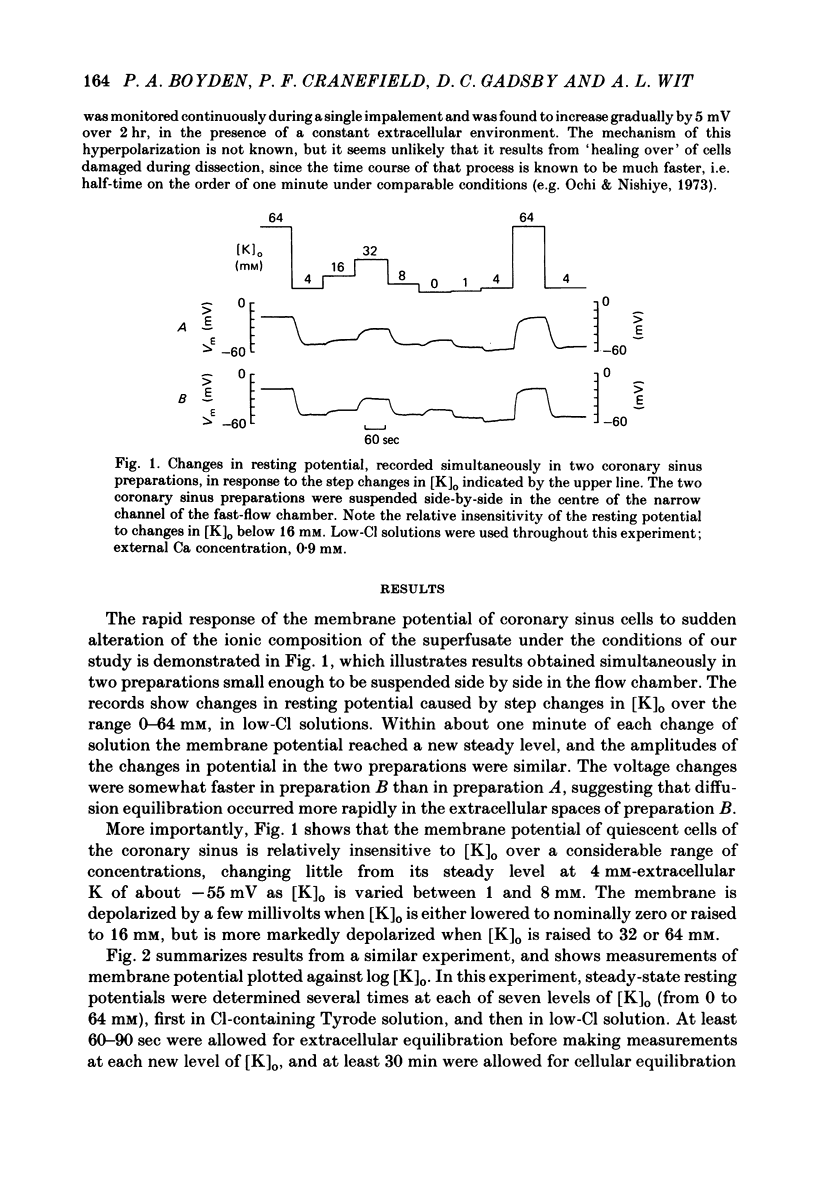
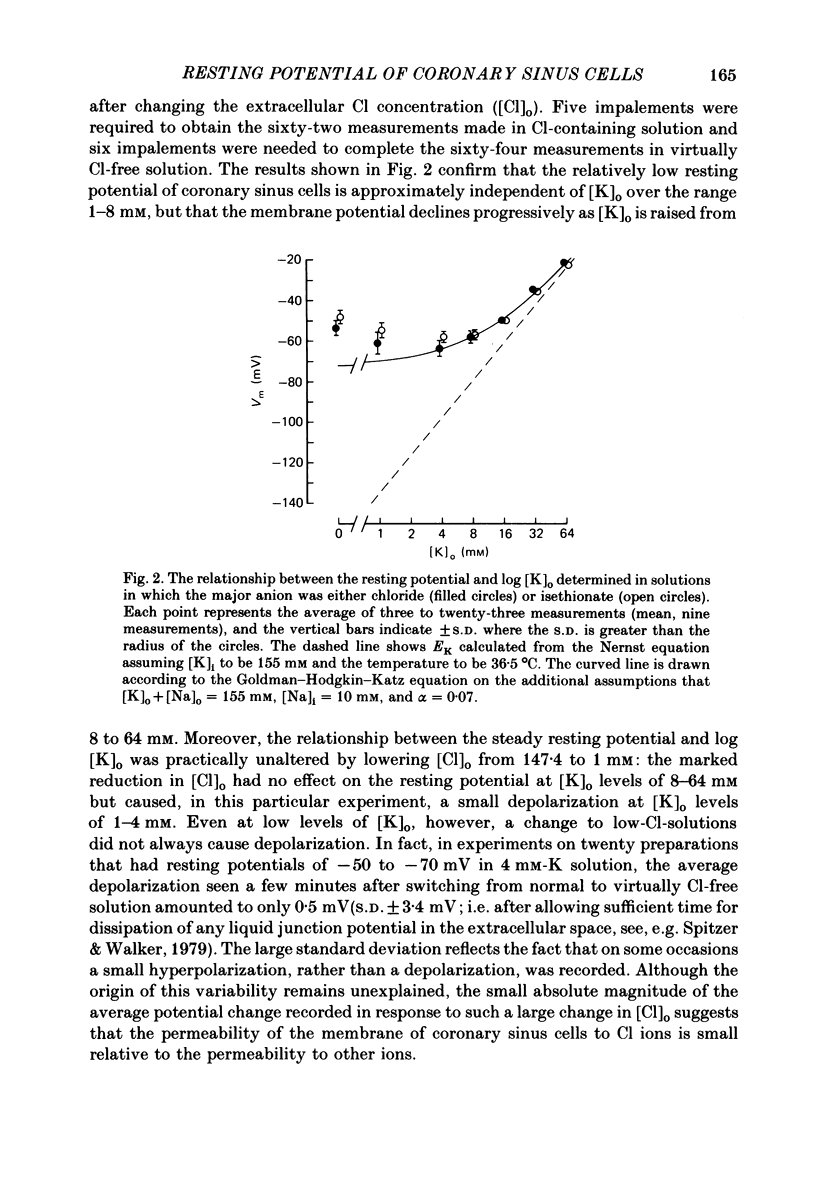
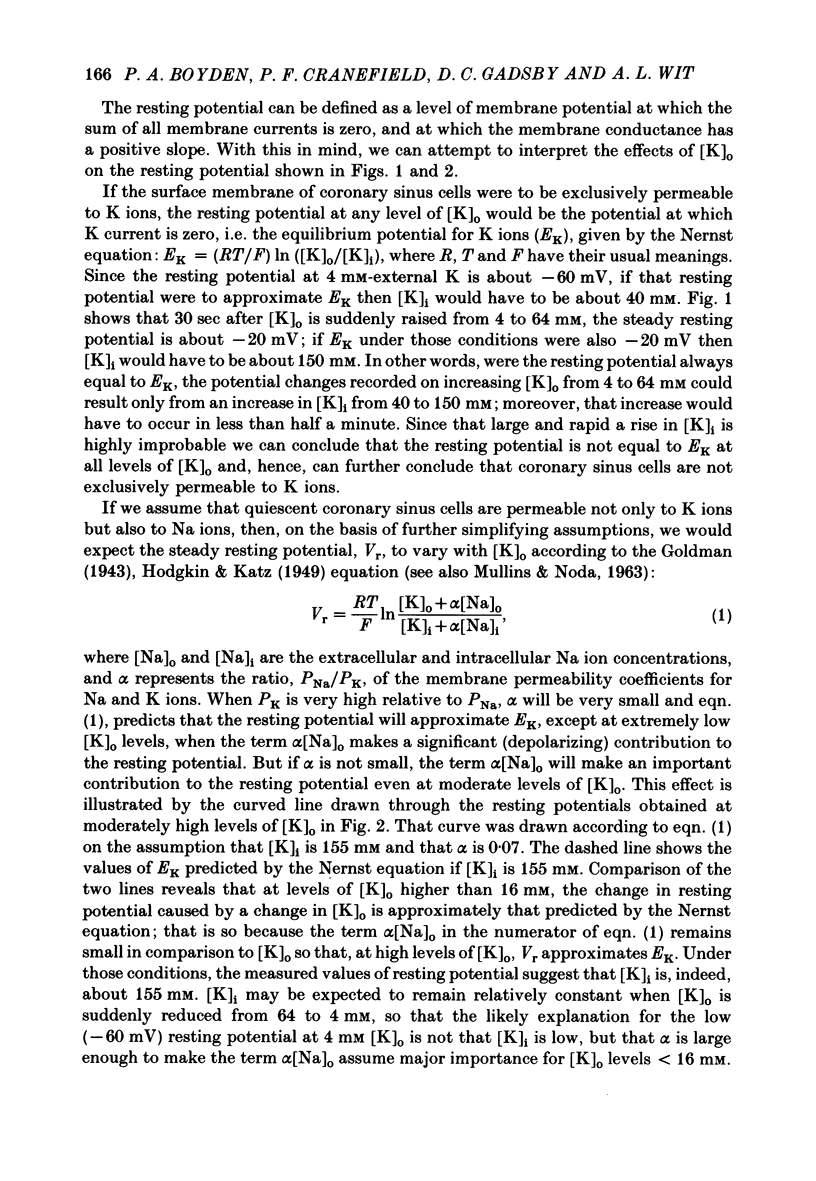
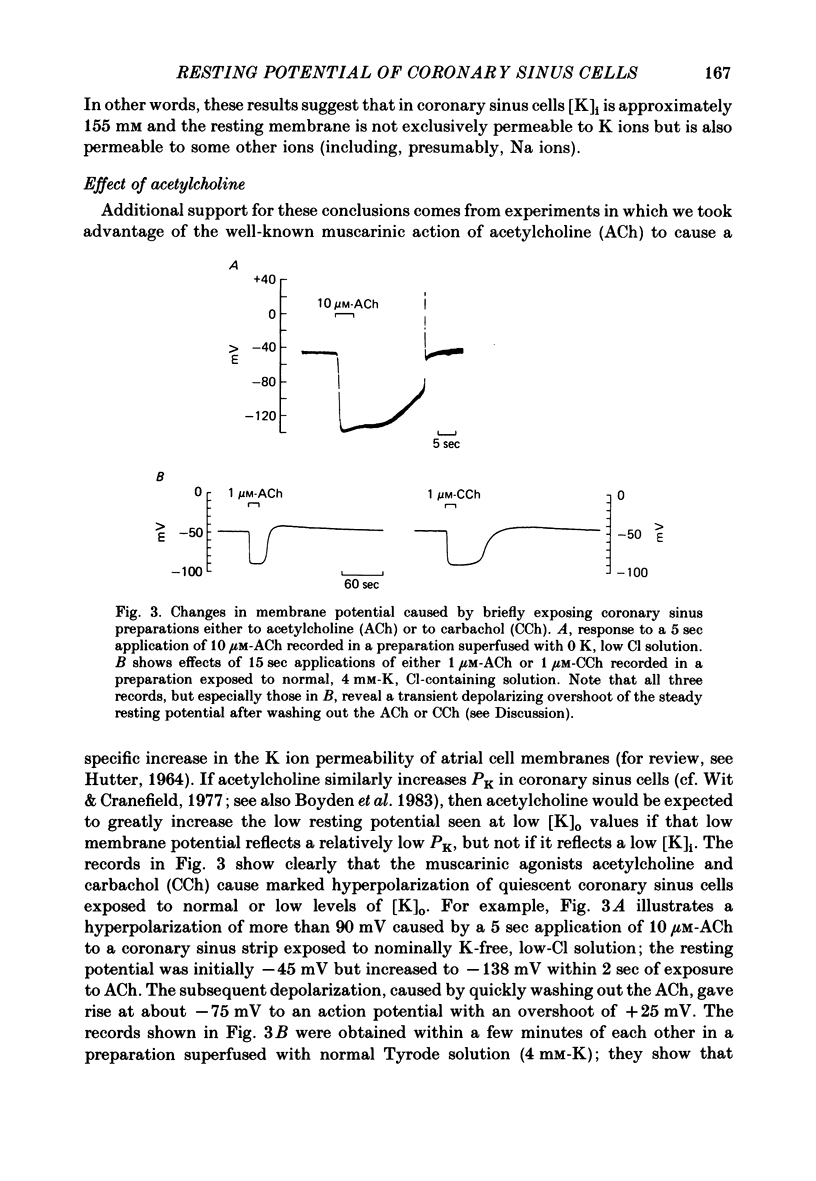
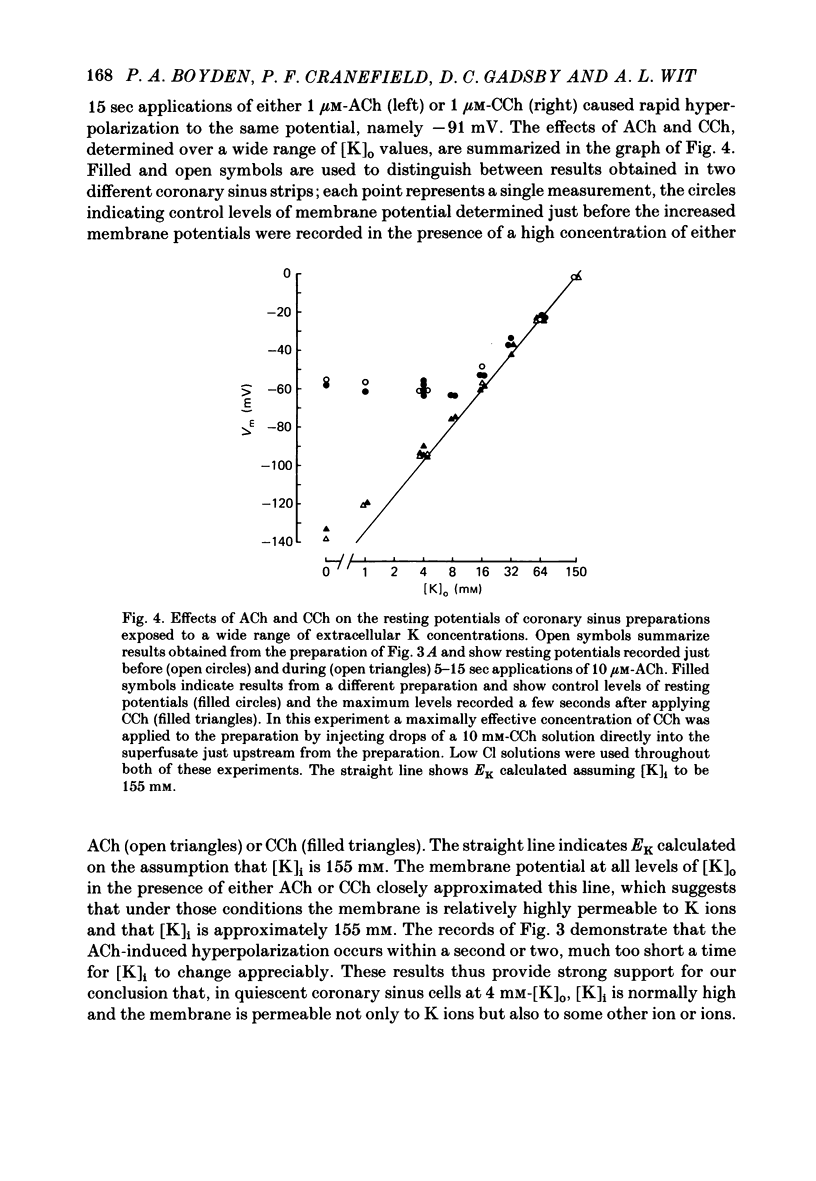
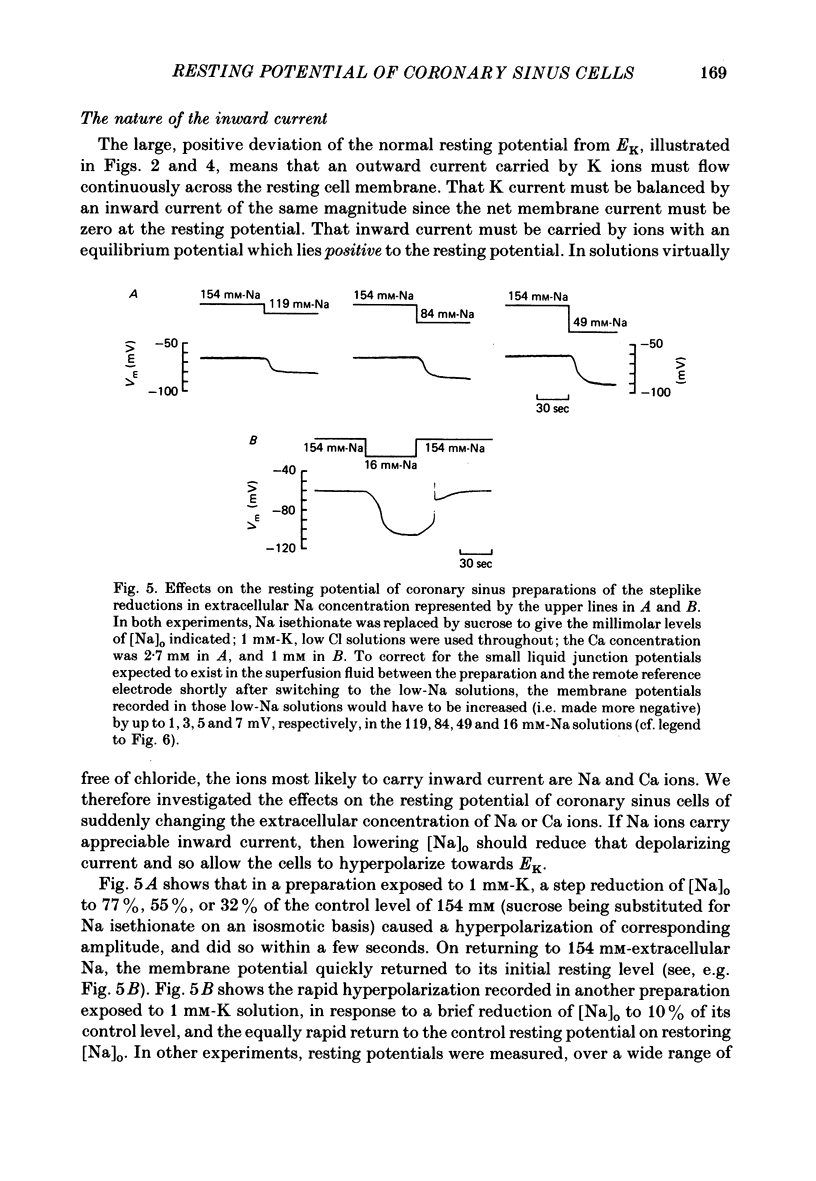
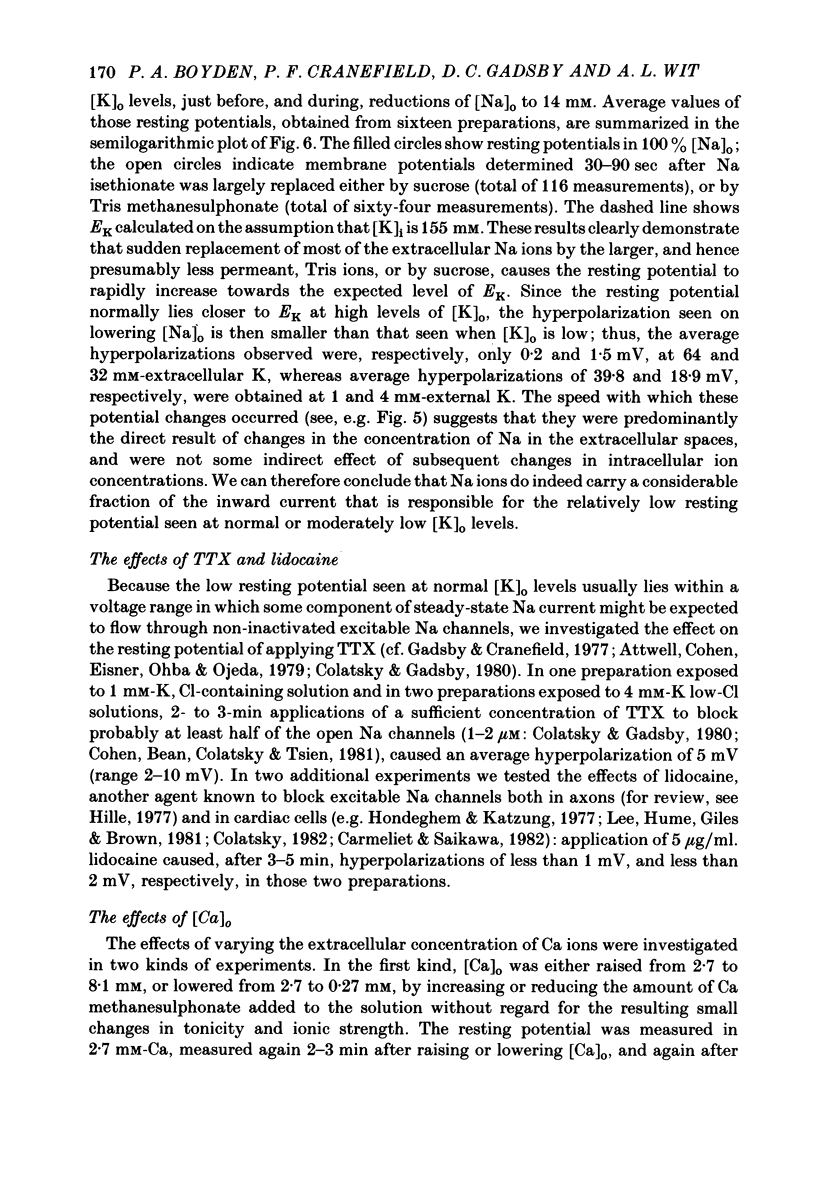
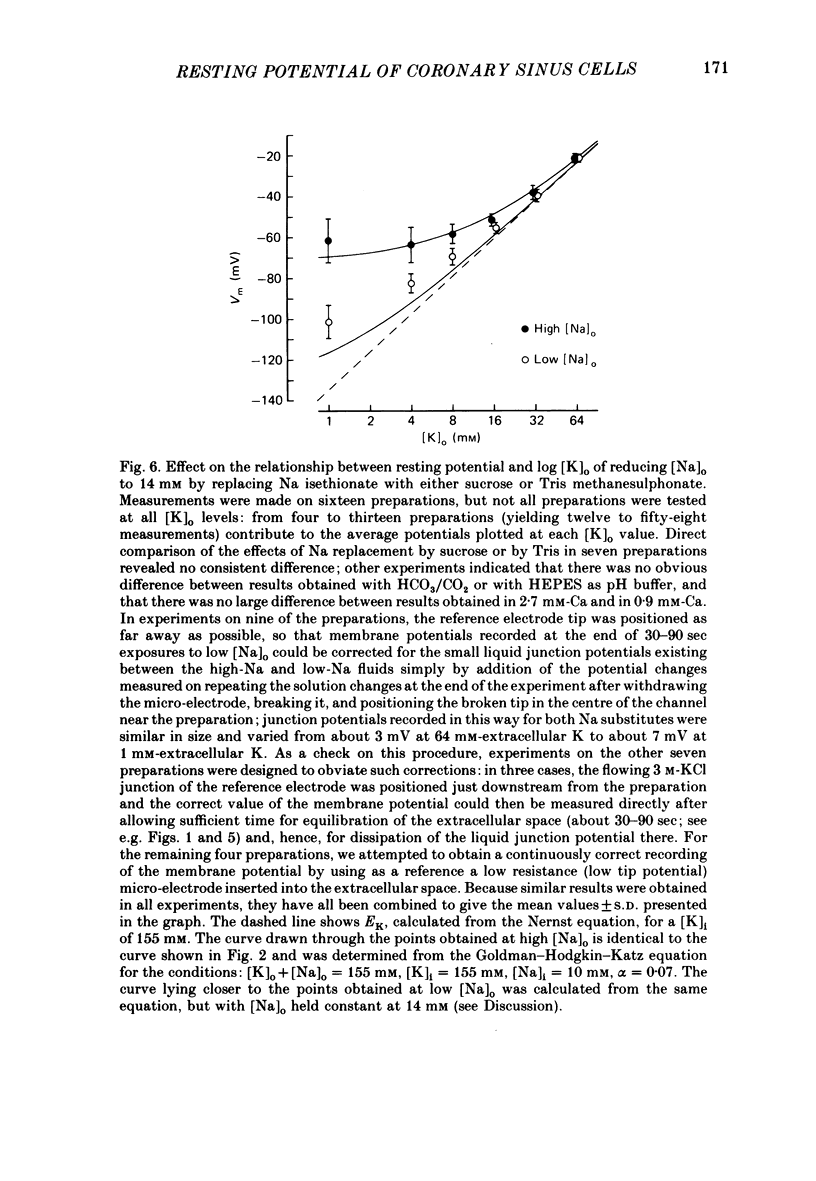
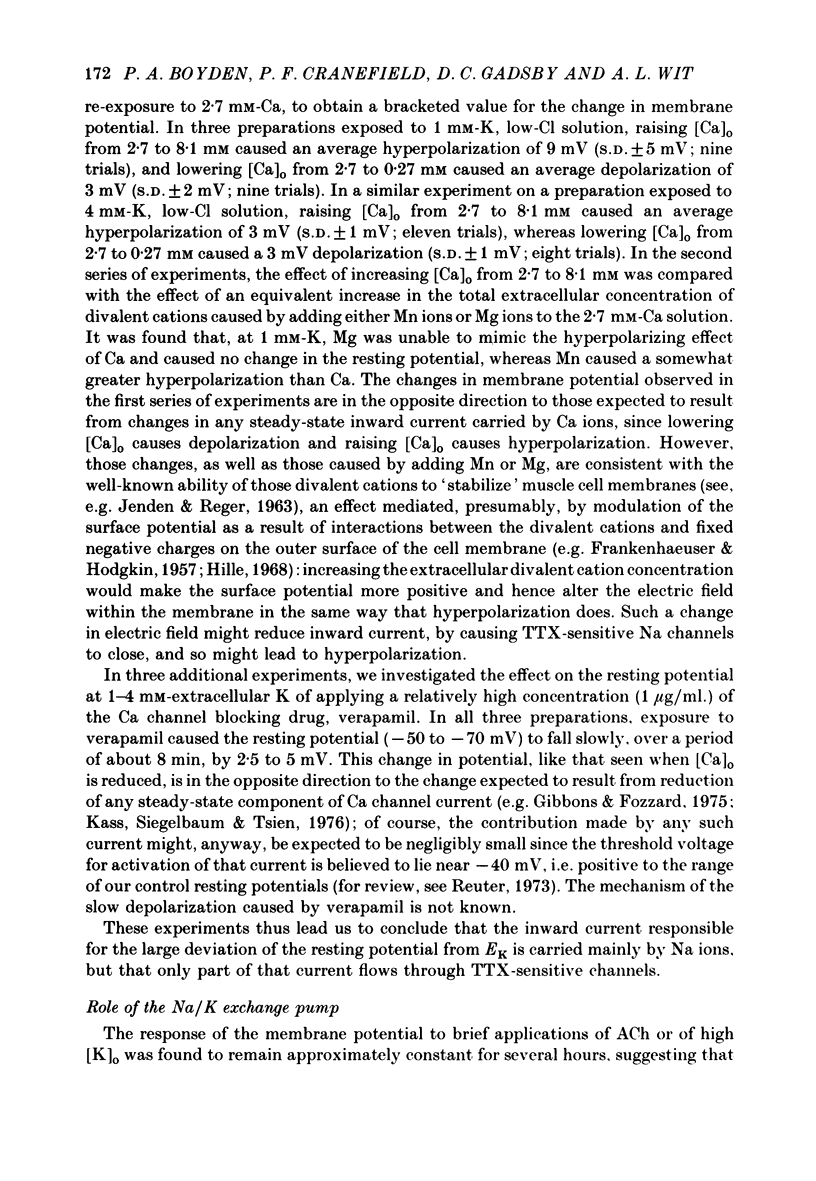
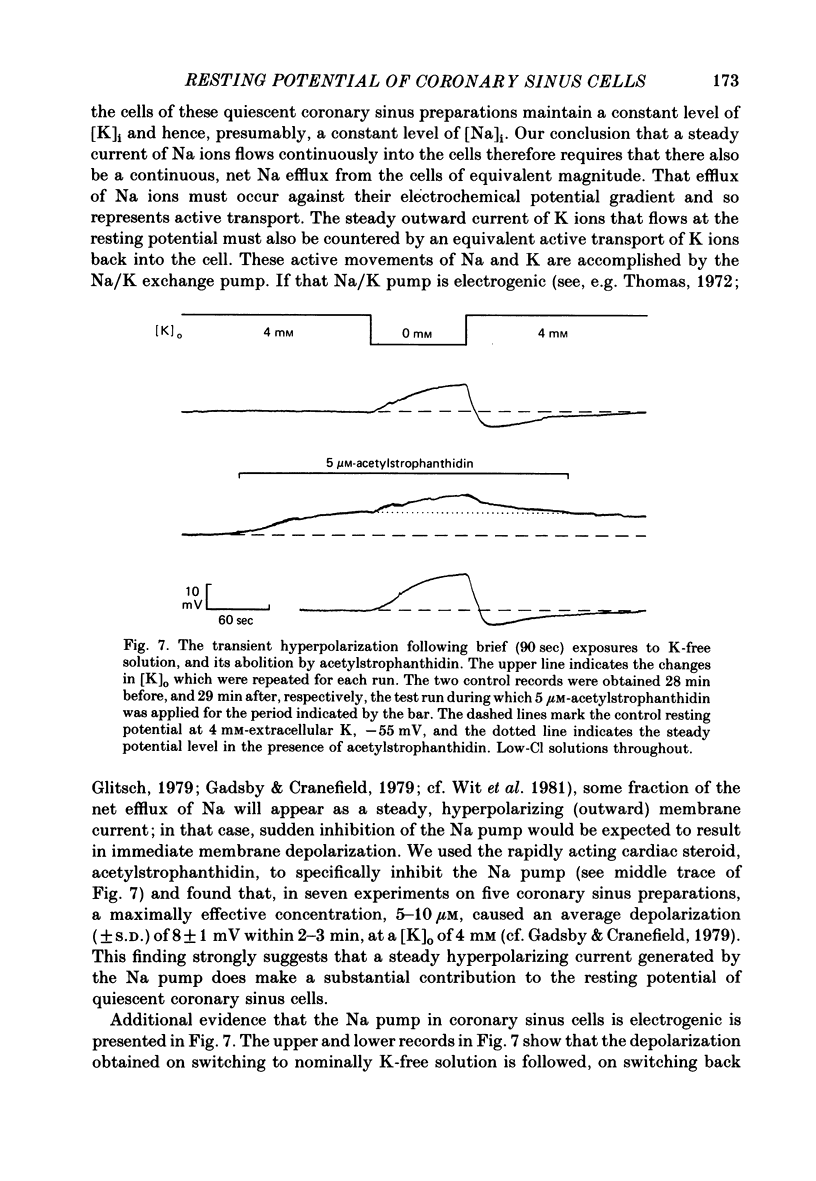
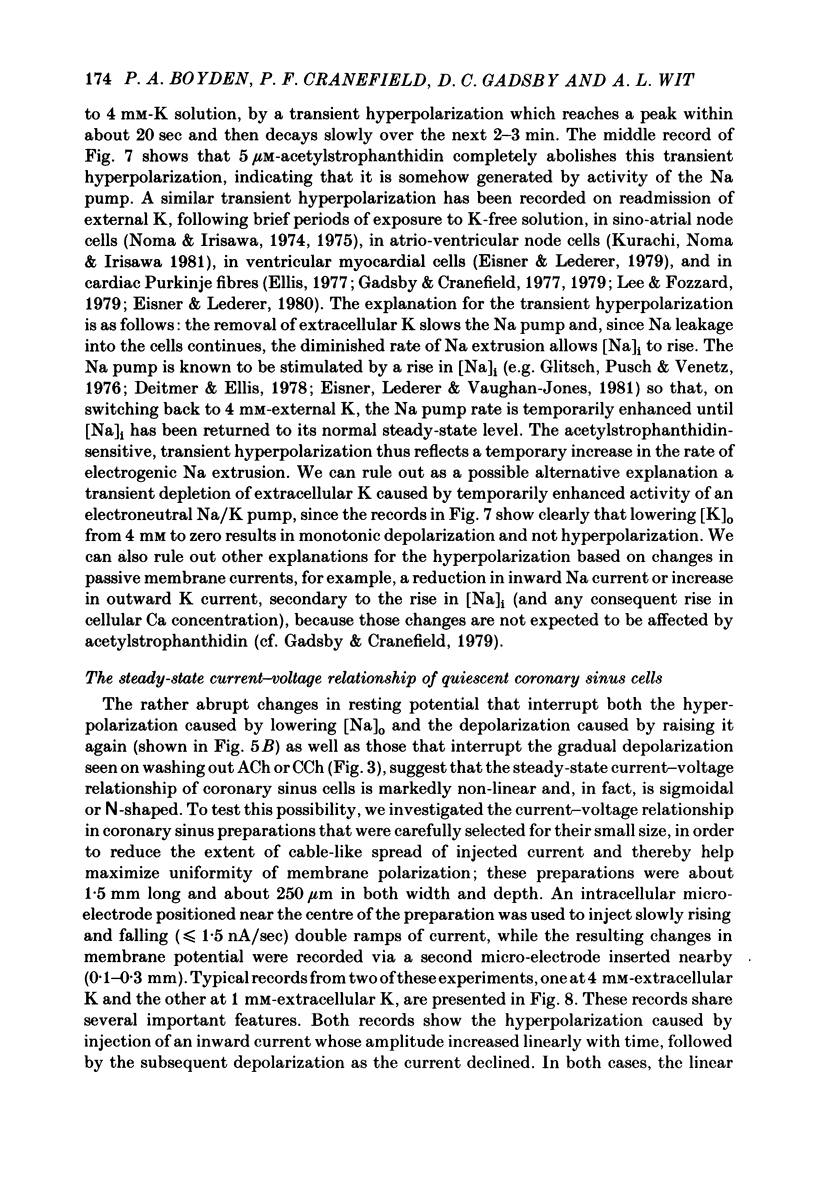
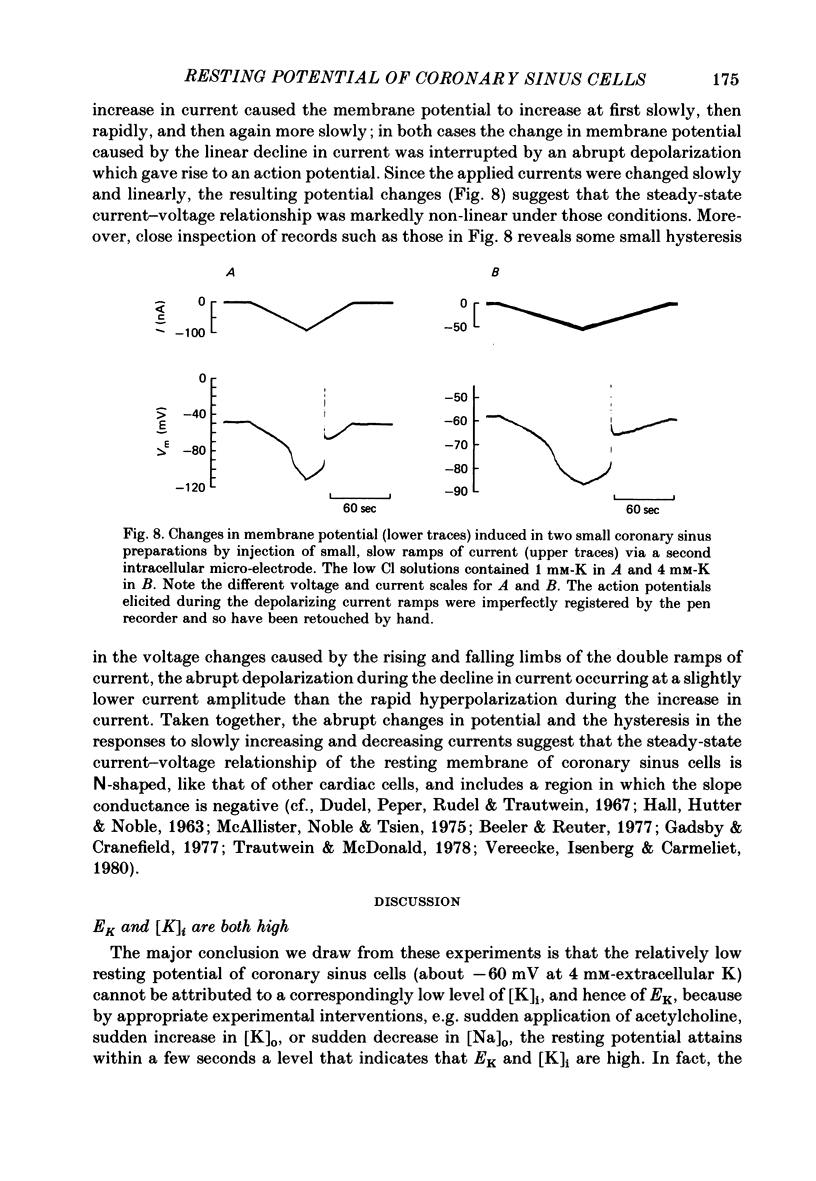
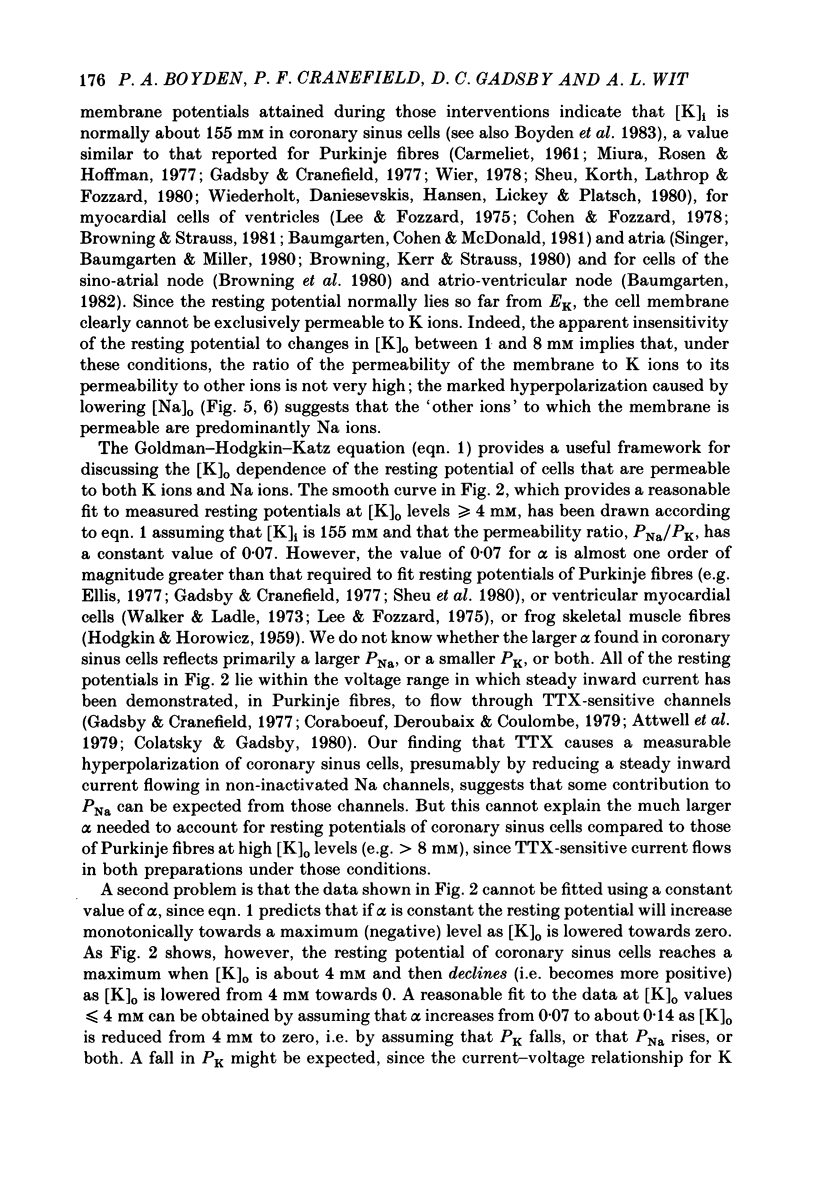
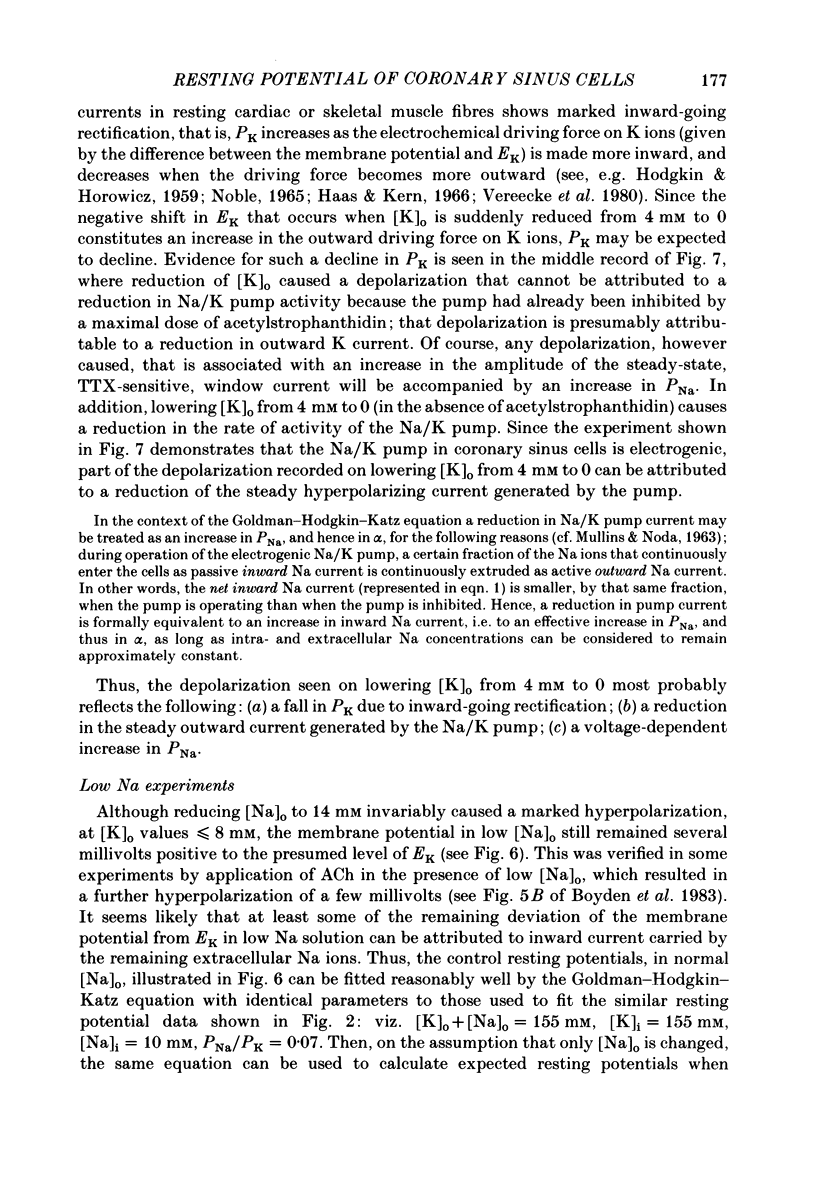
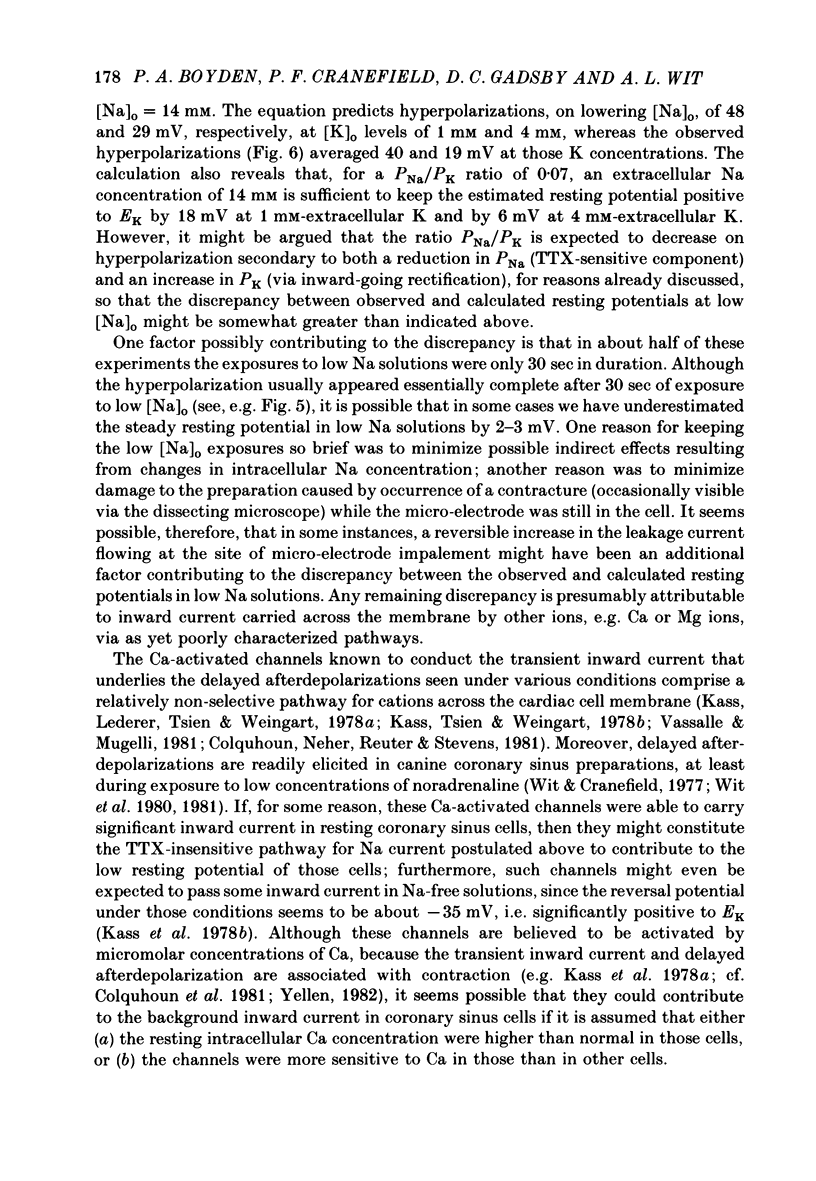
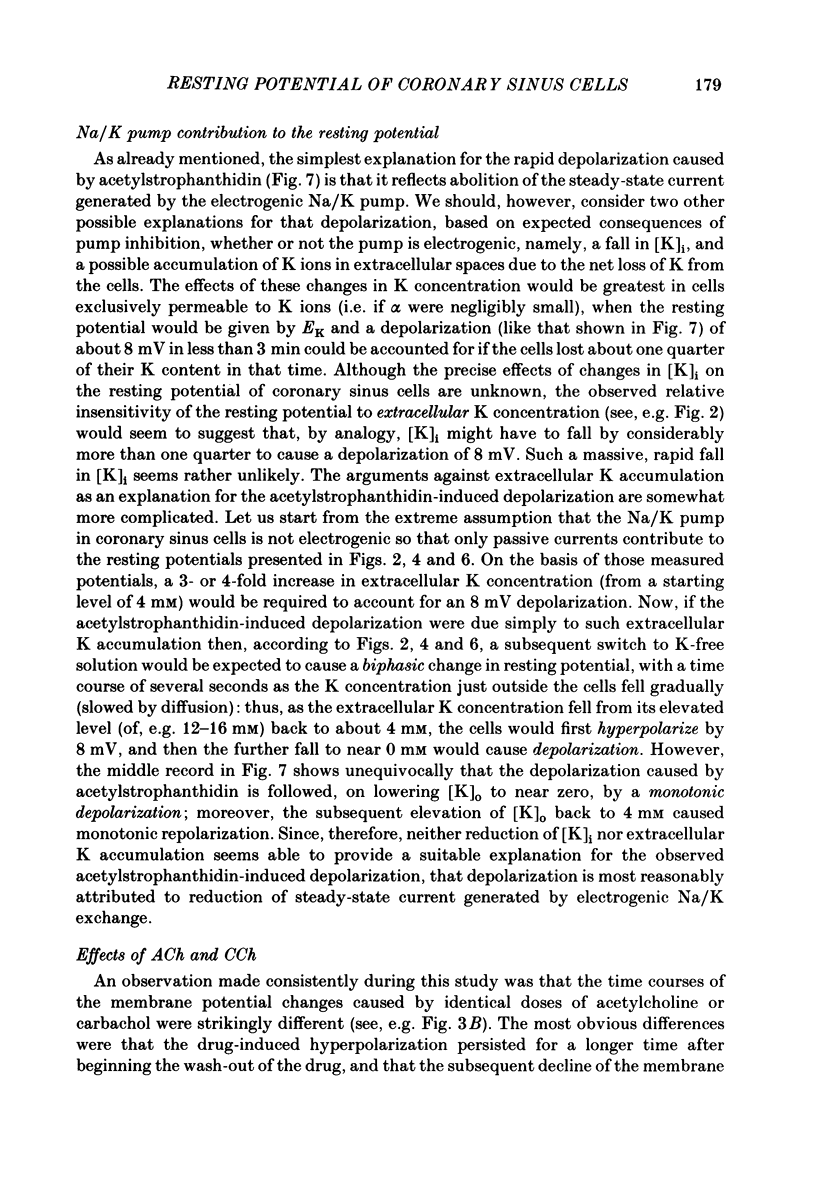
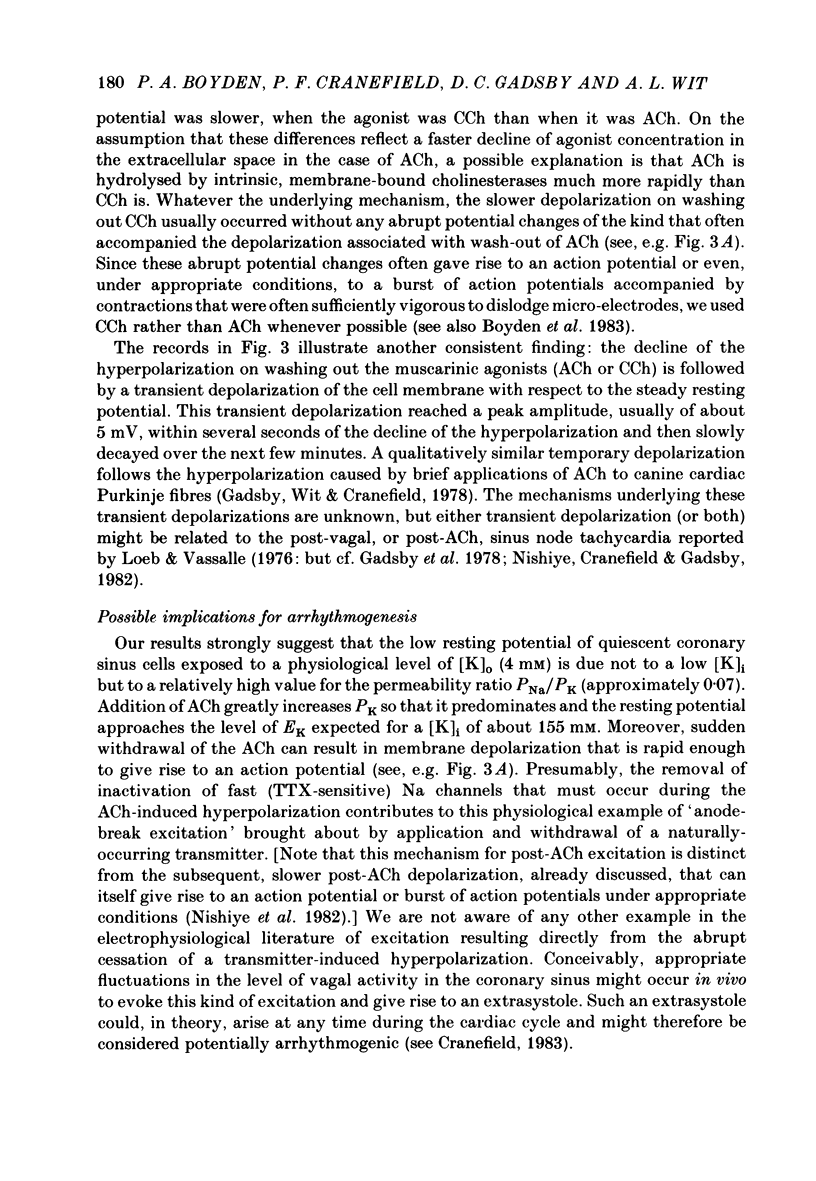
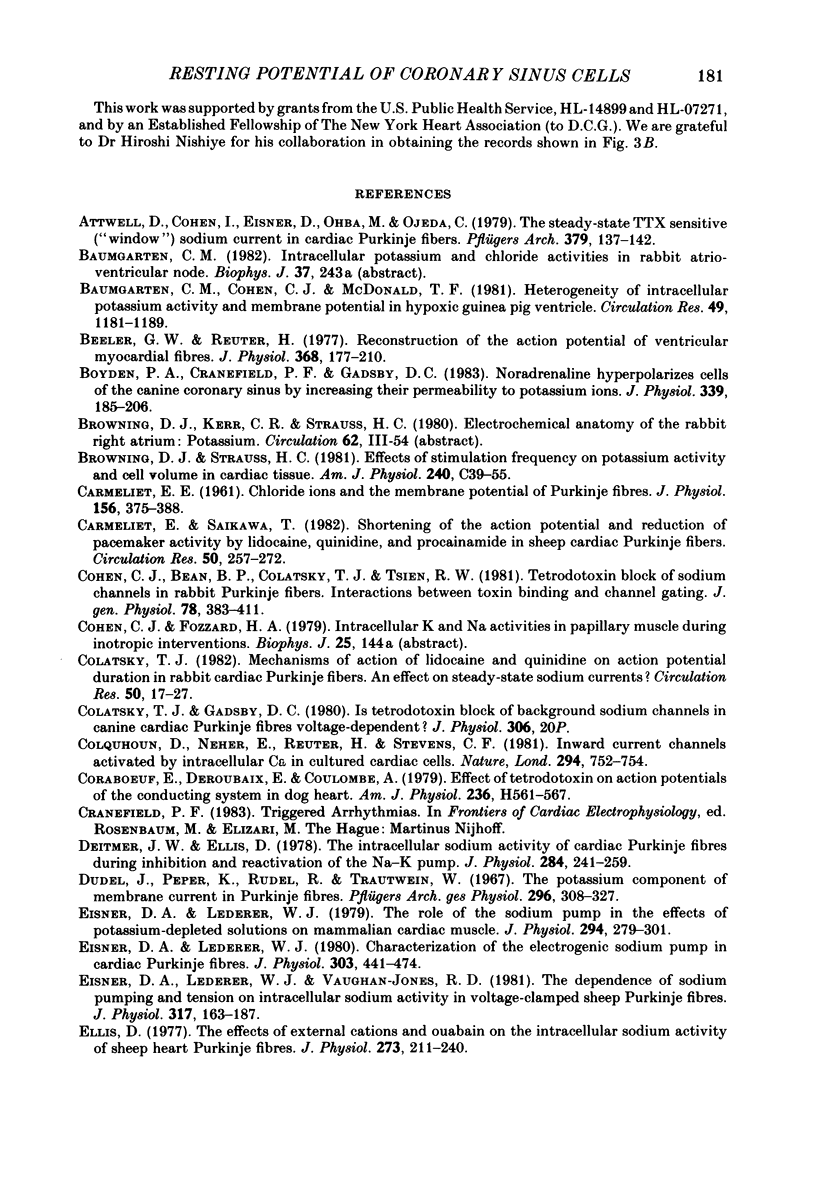
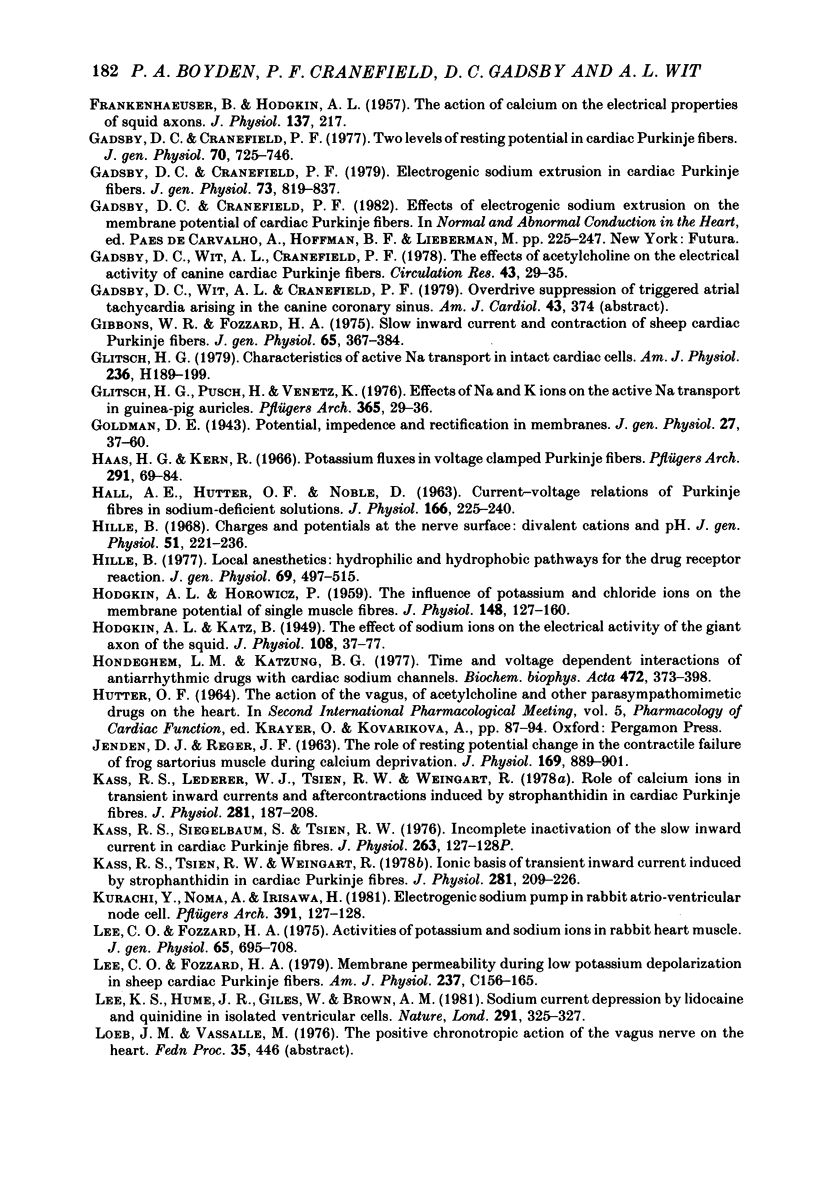
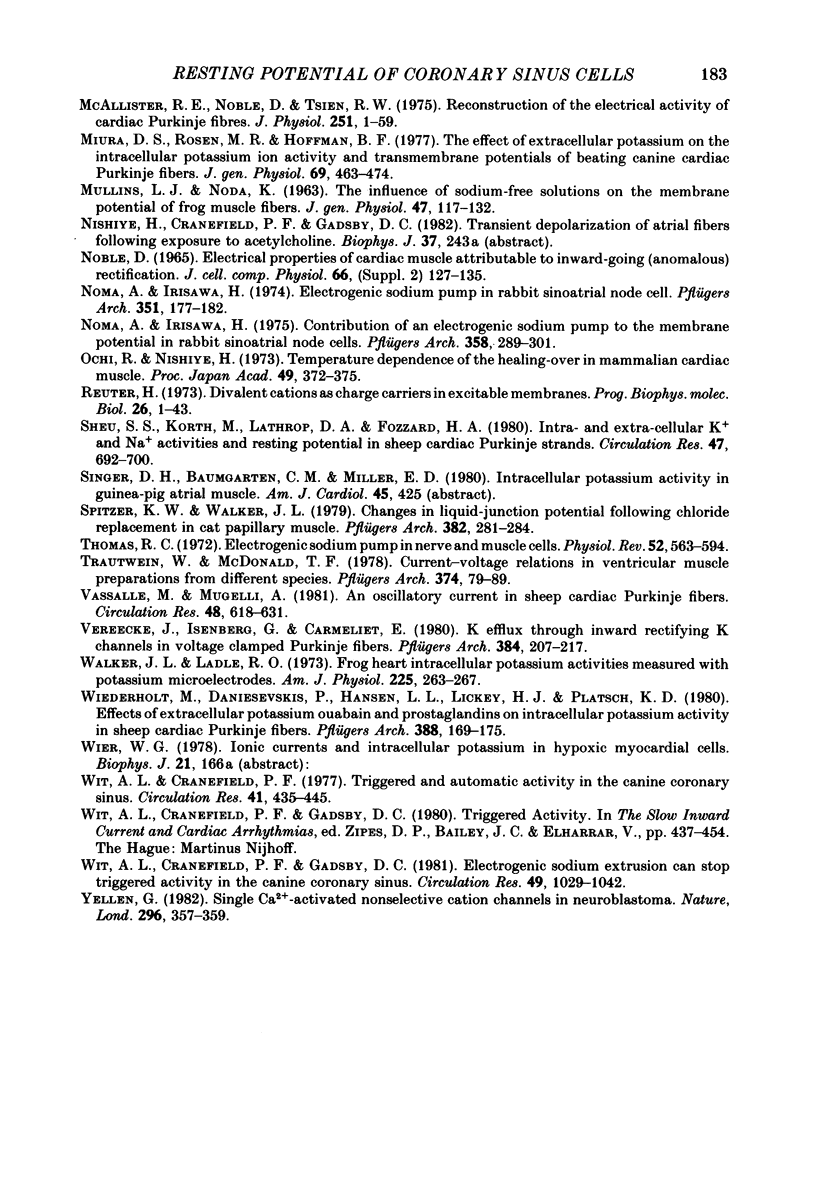
Selected References
These references are in PubMed. This may not be the complete list of references from this article.
- Attwell D., Cohen I., Eisner D., Ohba M., Ojeda C. The steady state TTX-sensitive ("window") sodium current in cardiac Purkinje fibres. Pflugers Arch. 1979 Mar 16;379(2):137–142. doi: 10.1007/BF00586939. [DOI] [PubMed] [Google Scholar]
- Baumgarten C. M., Cohen C. J., McDonald T. F. Heterogeneity of intracellular potassium activity and membrane potential in hypoxic guinea pig ventricle. Circ Res. 1981 Nov;49(5):1181–1189. doi: 10.1161/01.res.49.5.1181. [DOI] [PubMed] [Google Scholar]
- Beeler G. W., Reuter H. Reconstruction of the action potential of ventricular myocardial fibres. J Physiol. 1977 Jun;268(1):177–210. doi: 10.1113/jphysiol.1977.sp011853. [DOI] [PMC free article] [PubMed] [Google Scholar]
- Boyden P. A., Cranefield P. F., Gadsby D. C. Noradrenaline hyperpolarizes cells of the canine coronary sinus by increasing their permeability to potassium ions. J Physiol. 1983 Jun;339:185–206. doi: 10.1113/jphysiol.1983.sp014711. [DOI] [PMC free article] [PubMed] [Google Scholar]
- Browning D. J., Strauss H. C. Effects of stimulation frequency on potassium activity and cell volume in cardiac tissue. Am J Physiol. 1981 Jan;240(1):C39–C55. doi: 10.1152/ajpcell.1981.240.1.C39. [DOI] [PubMed] [Google Scholar]
- CARMELIET E. E. Chloride ions and the membrane potential of Purkinje fibres. J Physiol. 1961 Apr;156:375–388. doi: 10.1113/jphysiol.1961.sp006682. [DOI] [PMC free article] [PubMed] [Google Scholar]
- Carmeliet E., Saikawa T. Shortening of the action potential and reduction of pacemaker activity by lidocaine, quinidine, and procainamide in sheep cardiac purkinje fibers. An effect on Na or K currents? Circ Res. 1982 Feb;50(2):257–272. doi: 10.1161/01.res.50.2.257. [DOI] [PubMed] [Google Scholar]
- Cohen C. J., Bean B. P., Colatsky T. J., Tsien R. W. Tetrodotoxin block of sodium channels in rabbit Purkinje fibers. Interactions between toxin binding and channel gating. J Gen Physiol. 1981 Oct;78(4):383–411. doi: 10.1085/jgp.78.4.383. [DOI] [PMC free article] [PubMed] [Google Scholar]
- Colatsky T. J. Mechanisms of action of lidocaine and quinidine on action potential duration in rabbit cardiac Purkinje fibers. An effect on steady state sodium currents? Circ Res. 1982 Jan;50(1):17–27. doi: 10.1161/01.res.50.1.17. [DOI] [PubMed] [Google Scholar]
- Colquhoun D., Neher E., Reuter H., Stevens C. F. Inward current channels activated by intracellular Ca in cultured cardiac cells. Nature. 1981 Dec 24;294(5843):752–754. doi: 10.1038/294752a0. [DOI] [PubMed] [Google Scholar]
- Coraboeuf E., Deroubaix E., Coulombe A. Effect of tetrodotoxin on action potentials of the conducting system in the dog heart. Am J Physiol. 1979 Apr;236(4):H561–H567. doi: 10.1152/ajpheart.1979.236.4.H561. [DOI] [PubMed] [Google Scholar]
- Deitmer J. W., Ellis D. The intracellular sodium activity of cardiac Purkinje fibres during inhibition and re-activation of the Na-K pump. J Physiol. 1978 Nov;284:241–259. doi: 10.1113/jphysiol.1978.sp012539. [DOI] [PMC free article] [PubMed] [Google Scholar]
- Dudel J., Peper K., Rüdel R., Trautwein W. The potassium component of membrane current in Purkinje fibers. Pflugers Arch Gesamte Physiol Menschen Tiere. 1967;296(4):308–327. doi: 10.1007/BF00362531. [DOI] [PubMed] [Google Scholar]
- Eisner D. A., Lederer W. J. Characterization of the electrogenic sodium pump in cardiac Purkinje fibres. J Physiol. 1980 Jun;303:441–474. doi: 10.1113/jphysiol.1980.sp013298. [DOI] [PMC free article] [PubMed] [Google Scholar]
- Eisner D. A., Lederer W. J. The role of the sodium pump in the effects of potassium-depleted solutions on mammalian cardiac muscle. J Physiol. 1979 Sep;294:279–301. doi: 10.1113/jphysiol.1979.sp012930. [DOI] [PMC free article] [PubMed] [Google Scholar]
- Eisner D. A., Lederer W. J., Vaughan-Jones R. D. The dependence of sodium pumping and tension on intracellular sodium activity in voltage-clamped sheep Purkinje fibres. J Physiol. 1981 Aug;317:163–187. doi: 10.1113/jphysiol.1981.sp013819. [DOI] [PMC free article] [PubMed] [Google Scholar]
- Ellis D. The effects of external cations and ouabain on the intracellular sodium activity of sheep heart Purkinje fibres. J Physiol. 1977 Dec;273(1):211–240. doi: 10.1113/jphysiol.1977.sp012090. [DOI] [PMC free article] [PubMed] [Google Scholar]
- Gadsby D. C., Cranefield P. F. Electrogenic sodium extrusion in cardiac Purkinje fibers. J Gen Physiol. 1979 Jun;73(6):819–837. doi: 10.1085/jgp.73.6.819. [DOI] [PMC free article] [PubMed] [Google Scholar]
- Gadsby D. C., Cranefield P. F. Two levels of resting potential in cardiac Purkinje fibers. J Gen Physiol. 1977 Dec;70(6):725–746. doi: 10.1085/jgp.70.6.725. [DOI] [PMC free article] [PubMed] [Google Scholar]
- Gadsby D. C., Wit A. L., Cranefield P. F. The effects of acetylcholine on the electrical activity of canine cardiac Purkinje fibers. Circ Res. 1978 Jul;43(1):29–35. doi: 10.1161/01.res.43.1.29. [DOI] [PubMed] [Google Scholar]
- Gibbons W. R., Fozzard H. A. Slow inward current and contraction of sheep cardiac Purkinje fibers. J Gen Physiol. 1975 Mar;65(3):367–384. doi: 10.1085/jgp.65.3.367. [DOI] [PMC free article] [PubMed] [Google Scholar]
- Glitsch H. G. Characteristics of active Na transport in intact cardiac cells. Am J Physiol. 1979 Feb;236(2):H189–H199. doi: 10.1152/ajpheart.1979.236.2.H189. [DOI] [PubMed] [Google Scholar]
- Goldman D. E. POTENTIAL, IMPEDANCE, AND RECTIFICATION IN MEMBRANES. J Gen Physiol. 1943 Sep 20;27(1):37–60. doi: 10.1085/jgp.27.1.37. [DOI] [PMC free article] [PubMed] [Google Scholar]
- HALL A. E., HUTTER O. F., NOBLE D. Current-voltage relations of Purkinje fibres in sodium-deficient solutions. J Physiol. 1963 Apr;166:225–240. doi: 10.1113/jphysiol.1963.sp007102. [DOI] [PMC free article] [PubMed] [Google Scholar]
- HODGKIN A. L., HOROWICZ P. The influence of potassium and chloride ions on the membrane potential of single muscle fibres. J Physiol. 1959 Oct;148:127–160. doi: 10.1113/jphysiol.1959.sp006278. [DOI] [PMC free article] [PubMed] [Google Scholar]
- HODGKIN A. L., KATZ B. The effect of sodium ions on the electrical activity of giant axon of the squid. J Physiol. 1949 Mar 1;108(1):37–77. doi: 10.1113/jphysiol.1949.sp004310. [DOI] [PMC free article] [PubMed] [Google Scholar]
- Haas H. G., Kern R. Potassium fluxes in voltage clamped Purkinje fibres. Pflugers Arch Gesamte Physiol Menschen Tiere. 1966;291(1):69–84. doi: 10.1007/BF00362653. [DOI] [PubMed] [Google Scholar]
- Hille B. Charges and potentials at the nerve surface. Divalent ions and pH. J Gen Physiol. 1968 Feb;51(2):221–236. doi: 10.1085/jgp.51.2.221. [DOI] [PMC free article] [PubMed] [Google Scholar]
- Hille B. Local anesthetics: hydrophilic and hydrophobic pathways for the drug-receptor reaction. J Gen Physiol. 1977 Apr;69(4):497–515. doi: 10.1085/jgp.69.4.497. [DOI] [PMC free article] [PubMed] [Google Scholar]
- Hondeghem L. M., Katzung B. G. Time- and voltage-dependent interactions of antiarrhythmic drugs with cardiac sodium channels. Biochim Biophys Acta. 1977 Nov 14;472(3-4):373–398. doi: 10.1016/0304-4157(77)90003-x. [DOI] [PubMed] [Google Scholar]
- JENDEN D. J., REGER J. F. THE ROLE OF RESTING POTENTIAL CHANGES IN THE CONTRACTILE FAILURE OF FROG SARTORIUS MUSCLES DURING CALCIUM DEPRIVATION. J Physiol. 1963 Dec;169:889–901. doi: 10.1113/jphysiol.1963.sp007302. [DOI] [PMC free article] [PubMed] [Google Scholar]
- Kass R. S., Lederer W. J., Tsien R. W., Weingart R. Role of calcium ions in transient inward currents and aftercontractions induced by strophanthidin in cardiac Purkinje fibres. J Physiol. 1978 Aug;281:187–208. doi: 10.1113/jphysiol.1978.sp012416. [DOI] [PMC free article] [PubMed] [Google Scholar]
- Kass R. S., Siegelbaum S., Tsien R. W. Incomplete inactivation of the slow inward current in cardiac Purkinje fibres [proceedings]. J Physiol. 1976 Dec;263(1):127P–128P. [PubMed] [Google Scholar]
- Kass R. S., Tsien R. W., Weingart R. Ionic basis of transient inward current induced by strophanthidin in cardiac Purkinje fibres. J Physiol. 1978 Aug;281:209–226. doi: 10.1113/jphysiol.1978.sp012417. [DOI] [PMC free article] [PubMed] [Google Scholar]
- Lee C. O., Fozzard H. A. Activities of potassium and sodium ions in rabbit heart muscle. J Gen Physiol. 1975 Jun;65(6):695–708. doi: 10.1085/jgp.65.6.695. [DOI] [PMC free article] [PubMed] [Google Scholar]
- Lee C. O., Fozzard H. A. Membrane permeability during low potassium depolarization in sheep cardiac Purkinje fibers. Am J Physiol. 1979 Sep;237(3):C156–C165. doi: 10.1152/ajpcell.1979.237.3.C156. [DOI] [PubMed] [Google Scholar]
- Lee K. S., Hume J. R., Giles W., Brown A. M. Sodium current depression by lidocaine and quinidine in isolated ventricular cells. Nature. 1981 May 28;291(5813):325–327. doi: 10.1038/291325a0. [DOI] [PubMed] [Google Scholar]
- MULLINS L. J., NODA K. THE INFLUENCE OF SODIUM-FREE SOLUTIONS ON THE MEMBRANE POTENTIAL OF FROG MUSCLE FIBERS. J Gen Physiol. 1963 Sep;47:117–132. doi: 10.1085/jgp.47.1.117. [DOI] [PMC free article] [PubMed] [Google Scholar]
- McAllister R. E., Noble D., Tsien R. W. Reconstruction of the electrical activity of cardiac Purkinje fibres. J Physiol. 1975 Sep;251(1):1–59. doi: 10.1113/jphysiol.1975.sp011080. [DOI] [PMC free article] [PubMed] [Google Scholar]
- Miura D. S., Hoffman B. F., Rosen M. R. The effect of extracellular potassium on the intracellular potassium ion activity and transmembrane potentials of beating canine cardiac Purkinje fibers. J Gen Physiol. 1977 Apr;69(4):463–474. doi: 10.1085/jgp.69.4.463. [DOI] [PMC free article] [PubMed] [Google Scholar]
- Noma A., Irisawa H. Contribution of an electrogenic sodium pump to the membrane potential in rabbit sinoatrial node cells. Pflugers Arch. 1975 Aug 12;358(4):289–301. doi: 10.1007/BF00580527. [DOI] [PubMed] [Google Scholar]
- Noma A., Irisawa H. Electrogenic sodium pump in rabbit sinoatrial node cell. Pflugers Arch. 1974;351(2):177–182. doi: 10.1007/BF00587436. [DOI] [PubMed] [Google Scholar]
- Reuter H. Divalent cations as charge carriers in excitable membranes. Prog Biophys Mol Biol. 1973;26:1–43. doi: 10.1016/0079-6107(73)90016-3. [DOI] [PubMed] [Google Scholar]
- Sheu S. S., Korth M., Lathrop D. A., Fozzard H. A. Intra- and extracellular K+ and Na+ activities and resting membrane potential in sheep cardiac purkinje strands. Circ Res. 1980 Nov;47(5):692–700. doi: 10.1161/01.res.47.5.692. [DOI] [PubMed] [Google Scholar]
- Spitzer K. W., Walker J. L. Changes in liquid-junction potential following chloride replacement in cat papillary muscle. Pflugers Arch. 1979 Nov;382(3):281–284. doi: 10.1007/BF00583715. [DOI] [PubMed] [Google Scholar]
- Thomas R. C. Electrogenic sodium pump in nerve and muscle cells. Physiol Rev. 1972 Jul;52(3):563–594. doi: 10.1152/physrev.1972.52.3.563. [DOI] [PubMed] [Google Scholar]
- Trautwein W., McDonald T. F. Current-voltage relations in ventricular muscle preparations from different species. Pflugers Arch. 1978 Apr 25;374(1):79–89. doi: 10.1007/BF00585700. [DOI] [PubMed] [Google Scholar]
- Vassalle M., Mugelli A. An oscillatory current in sheep cardiac Purkinje fibers. Circ Res. 1981 May;48(5):618–631. doi: 10.1161/01.res.48.5.618. [DOI] [PubMed] [Google Scholar]
- Vereecke J., Isenberg G., Carmeliet E. K efflux through inward rectifying K channels in voltage clamped Purkinje fibers. Pflugers Arch. 1980 Apr;384(3):207–217. doi: 10.1007/BF00584555. [DOI] [PubMed] [Google Scholar]
- Walker J. L., Ladle R. O. Frog heart intracellular potassium activities measured with potassium microelectrodes. Am J Physiol. 1973 Jul;225(1):263–267. doi: 10.1152/ajplegacy.1973.225.1.263. [DOI] [PubMed] [Google Scholar]
- Wiederholt M., Danisevskis P., Hansen L. L., Lichey H. J., Platsch K. D. The effects of extracellular potassium, ouabain, and prostaglandins on intracellular potassium activity in sheep cardica Purkinje fibers. Pflugers Arch. 1980 Nov;388(2):169–175. doi: 10.1007/BF00584124. [DOI] [PubMed] [Google Scholar]
- Wit A. L., Cranefield P. F., Gadsby D. C. Electrogenic sodium extrusion can stop triggered activity in the canine coronary sinus. Circ Res. 1981 Oct;49(4):1029–1042. doi: 10.1161/01.res.49.4.1029. [DOI] [PubMed] [Google Scholar]
- Wit A. L., Cranefield P. F. Triggered and automatic activity in the canine coronary sinus. Circ Res. 1977 Oct;41(4):434–445. doi: 10.1161/01.res.41.4.434. [DOI] [PubMed] [Google Scholar]
- Yellen G. Single Ca2+-activated nonselective cation channels in neuroblastoma. Nature. 1982 Mar 25;296(5855):357–359. doi: 10.1038/296357a0. [DOI] [PubMed] [Google Scholar]